- 1ICAR-Directorate of Onion and Garlic Research, Pune, India
- 2ICAR-National Research Centre for Grapes, Pune, India
- 3N.M. College of Agriculture, Navsari Agricultural University, Navsari, India
Allium tuberosum, commonly known as garlic chives, is an underutilized Allium species despite its significant culinary value for its mild garlic flavor and therapeutic potential due to the presence of sulphur-containing compounds with antimicrobial, anti-inflammatory, and antioxidant properties. This study assessed the cultivation potential of A. tuberosum in the non-traditional agro-climatic region of the Western Ghats, focusing on the effects of cultivars and seasonal variations on growth, yield, and quality in two-year field trials. Among the accessions tested, A. tuberosum Kazakhstan CGN-1587 demonstrated the highest yield, producing 157.01 tons of green foliage per hectare. Bright sunshine seasons positively influenced both yield and quality, while the monsoon season induced morphological changes such as increased stem length, reduced leaf width, and decreased stem girth, traits generally considered inferior for market quality. The monsoon also led to increased waste generation, highlighting the need for careful management during this period. Nutritional analysis revealed high concentrations of potassium (5355 mg/kg), phosphorus (691 mg/kg), and sulphur (2484 mg/kg), while biochemical profiling identified bioactive compounds such as flavonoids (3.19 mg/g) and organosulfur compounds, including Allyl Methyl Thiosulfinate (269.00 mg/kg), which contribute to the plant’s notable health benefits. These findings support the suitability of A. tuberosum for year-round cultivation in the Western Ghats and its potential for commercialization, especially in regions with similar climatic conditions.
1 Introduction
The genus Allium characterized by its distinctive scented underground bulb is one of the largest plant genera comprising nearly 1000 species with Allium cepa (onion) and Allium sativum (garlic) being commercially important crops (Ochar and Kim, 2023). The genus includes some underutilized and semi-domesticated species which are equally potential for commercialization. These species have significant culinary uses, primarily adding flavor and savor to food. The flavor arises from the chemical transformation of S-alk(en)yl cysteine sulfoxides into volatile compounds, including S-methyl cysteine sulfoxide, S-allyl cysteine sulfoxide, and S-trans-prop-1-enyl cysteine sulfoxide, among others (Jones et al., 2004). Despite multifaceted uses, many species within the genus remain largely underutilized, which is a missed opportunity considering their importance for food and medicinal properties as alliums are rich in secondary metabolites like organosulfur compounds, flavonoids, phenols, saponins, alkaloids, and polysaccharides. By virtue of this, they offer a range of nutritional, biological, and health benefits, such as antimicrobial, antioxidant, antitumor, immunomodulatory, antidiabetic, and anti-inflammatory properties (Riaz et al., 2020; Yan et al., 2022; Kim et al., 2023). Processed products in the form of dried bulbs, leaves, buds and flowers from wild Allium species like A. tuberosum, A. angulsorum, A. auriculatum, A. carolinianum, A. griffithianum, A. hummile, A. roylei, and A. wallichii are in demand for their culinary applications and extended shelf life (Negi et al., 2006).
A.tuberosum commonly known as garlic chives, Chinese chives, or Oriental garlic, remains an underutilized species despite its substantial culinary and medicinal applications. Native to Southeast Asia and often cultivated in home gardens in northeast India (Pandey et al., 2014), this perennial species thrives in moderate climates and is particularly valued for its mild garlic flavor due to the presence of methiin, alliin other sulfur compounds (Xia et al., 2022). It is especially useful in dishes where raw garlic would be too overpowering, such as salads, egg dishes, soups, stews, stir-fries etc. The flowers are also edible and can be used as a garnish. In several countries including China, Philippines, Korea, and Thailand, garlic chives are a well-known vegetable (Saito, 2020; Alam et al., 2023.
Past research on A. tuberosum has revealed a rich profile of essential micronutrients and vitamins vital for various bodily functions, including immune response, bone health, and red blood cell formation (Bastaki et al., 2021). Medicinally, A. tuberosum seed extract is valued for its aphrodisiac properties (Guohua et al., 2009). The presence of organosulfur compounds, similar to those found in garlic, enhances its therapeutic potential by providing antimicrobial (Khalid et al., 2014), anti-inflammatory, anti-cancer (Park et al., 2013), antidiabetic benefits (Tang et al., 2017) etc.
Field surveys and research have highlighted the edible uses of A. tuberosum in the Himalayas, particularly in the Garhwal and Kumaon regions (Joseph and Pradheep, 2019). A. tuberosum consumed as raw or cooked vegetables, spices, and seasonings. Beyond these primary regions, it is gaining importance in the plains and other non-traditional areas, serving as an alternative to leaf-purpose onion cultivation, which faces challenges like susceptibility to biotic and abiotic stresses and seasonal availability cycles (Huang et al., 2016; Khandagale et al., 2022). Pandey et al. (2014) stated that A. tuberosum has the potential for commercial cultivation in different onion and garlic growing part of the India. The ICAR-DOGR in Pune has been working on primary studies for A. tuberosum in this new geographical setup to cater for a year-round supply and developed a value chain model (Kale et al., 2024). Recent agronomic research has focused on improving the cultivation practices of A. tuberosum to enhance its productivity and quality. Studies have found that the use of slow-release fertilizers and mulching increased the growth, nutrient use efficiency, weed control, yield and biological value of A. tuberosum (Wang et al., 2020; Adamczewska-Sowinska and Turczuk, 2016; Kim, 2010).
The economic importance of A. tuberosum extends beyond its culinary and medicinal uses. The plant has significant market value due to its increasing popularity in gourmet cuisine and traditional medicine (Kalasee and Mittraparp-arthorn, 2023; Alam et al., 2023). The demand for fresh and processed products example dried leaves and flower buds, is rising globally, driven by consumer preferences for natural and health-promoting foods (Imahori, 2022; Liu et al., 2021; Kale et al., 2024b). Its unique flavor due to the presence of sulfur-containing volatiles such as Diallyl disulfides (Xia et al., 2022) and health benefits like antidiabetic, hepatoprotective, and antitumor nature (Tang et al., 2017) make it a sought-after ingredient in high-end restaurants and health food stores. Additionally, the development of value-added products including powder and feed capsules, is opening new avenues for commercial explorations (Annual report ICAR-DOGR, 2021). Western Ghats, characterized by its climatic variability and ecological diversity, offer a promising environment for agricultural diversification, particularly in response to the limitations faced by traditional crops such as onions and garlic, which are prone to biotic and abiotic stresses (Huang et al., 2016). This study aims to assess the adaptability of Allium tuberosum in this ecologically unique region, focusing on developing sustainable cultivation practices that can enhance agricultural diversity and resilience. Given that the Western Ghats present a warmer climate compared to the native habitat of A. tuberosum, this research seeks to explore the potential of year-round cultivation, overcoming the seasonal constraints imposed by harsh winters.
In light of significant agronomic variations, such as sowing methods (either from seed or bulb), planting layouts, and intercultural operations, this study will establish foundational guidelines for optimizing these practices in the Western Ghats. The primary objective is to evaluate the cultivation viability and nutritional profile of A. tuberosum in non-traditional geographical regions, with an emphasis on the Western Ghats. By analyzing its adaptability to novel environmental conditions, this research aims to provide a comprehensive framework for popularizing A. tuberosum, contributing to agricultural diversification and nutritional security. Furthermore, the study seeks to identify commercial opportunities for A. tuberosum within the expanding health food market, offering farmers and agribusinesses access to a profitable and emerging agricultural niche.
2 Materials and methods
The Allium germplasm in the collection was cultivated in normal conditions of western ghats to assess their production performance in the region. Three accessions viz. A. tuberosum Kazakhstan CGN-1587, A. tuberosum CGN-116418 & A. tuberosum CGN-16373 referred to as AT1, AT2 & AT3 respectively for convenience, were used in the present study for production performance and assessment of nutraceutical properties. The nutritional and biochemical profile of the best-suited A. tuberosum line was done to assist the commercialization by advocating its health and related benefits.
2.1 Location, soil and general weather conditions
The study was conducted at the ICAR-Directorate of Onion and Garlic Research (ICAR-DOGR), Rajgurunagar, India. The region falls under the Western Maharashtra Plain Zone agroclimatic zone as per the classification by ICAR (Ahmad et al., 2017). This is a region of the regur (black) soil. Wheat, gram, millets, cotton, pulses, groundnut, and oilseeds are the main crops in the rain-fed areas, while in the irrigated areas, sugarcane, onion and wheat, are cultivated. The experimental site is situated at 18° 50’ 33.5112’’ N and 73°53’5.7732’’ E with an elevation of 553.8 m above MSL. The average annual temperature ranges from 5.5°C to 42.0°C, while the average annual rainfall is 669 mm. The predominant soil type at the site is black vertisol with a pH of 7.27, an electrical conductivity of 0.26 dS/m, and a soil organic carbon (SOC) content of 6.98 g/kg. The available nutrient status of the soil is favorable for crop growth, with nitrogen at 219.82 kg/ha, phosphorus at 52.82 kg/ha, potassium at 824.0 kg/ha and sulphur at 28.54 kg/ha. The general weather conditions prevailing during the experiment period are depicted in Figure 1.
2.2 Selection of tubers, planting and general operations
Three A. tuberosum accessions were evaluated employing a factorial random block design. The accessions of A. tuberosum, constituted one factor, while eight age windows each comprising a three-month unit, formed the second factor. The selected planting material i.e. rhizomes from the three-year-old mother batch were carefully extracted and separated from the clump. No treatment was applied to the rhizomes, which were then planted at 25×25cm spacing on a raised bed of 1.2 m width and 15 cm height. Five replicates of each A. tuberosum line were planted, with each replicate consisting of 35 hills arranged in seven rows of five hills each. Two drip laterals with emitters spaced 30 cm apart were installed over the bed for irrigation. Weeding was the primary interculture operation in the research field. According to the age windows, the third, fourth, sixth, and eighth windows exhibited higher weed infestation rates, necessitating frequent weeding. Irrigation scheduling was managed based on field moisture conditions, with the second, third, fifth, and sixth age windows requiring less frequent irrigation compared to other dry and hot seasonal windows.
2.3 Observation schedule and data analyses
The establishment of planting was followed by observations commencing in the first month. Morphological parameters were recorded before cutting, while green foliage yield, waste generated and dry matter content were recorded after each monthly cutting. Plant height (cm), number of tillers, number of leaves, leaf length (cm), leaf width (mm), stem length (cm), stem width (mm), and crop spread (cm) were measured to determine the growth and morphology of selected A. tuberosum accessions. Crop spread was calculated as the average diameter of the clump spread over the ground. Five plants were randomly selected and tagged in each replication. The number of leaves per plant was calculated by counting the total number of leaves from each tagged plant. The data was analyzed using basic statistics and a two-factorial randomized block design analysis of variance. The quality of green foliage cuttings was further evaluated in terms of waste-to-yield ratio and stem length-to-plant height ratio.
2.4 The analysis of the nutritional and biochemical profile of A. tuberosum
2.4.1 Estimation of proximate composition
The proximate composition of the A. tuberosum leaves was analyzed following the standard food analysis methods described in the Association of Official Analytical Chemists. The moisture was determined using a hot air oven as per AOAC 952.08, 2016 (Cunniff and Washington, 1997), ash content was estimated by heating plant sample in a muffle furnace for about 5-6 h at 500°C (Official Methods of Analysis of AOAC, 2016; Cunniff and Washington, 1997) whereas the acid insoluble ash content was determined by dissolving ash in dilute hydrochloric acid (10% w/w) (Official Methods of Analysis of AOAC, 2016; (Cunniff and Washington, 1997), the liquid was filtered through an ashless filter paper and thoroughly washed with hot water. The filter paper was then ignited in the original dish, cooled and weighed. The crude lipid was extracted from a moisture-free sample with petroleum ether (60-80°C) in a Soxhlet apparatus for about 6-8 h. Estimation of crude fiber content in the plant materials was carried out by treating the fat and moisture-free materials with 1.25% dilute acid and 1.25% alkali followed by washing with water and ignition of the residue. The crude protein was determined using the micro Kjeldahl method (AOAC 992.23, 2016; Sarkar et al., 2020). Total fat was determined according to the acid hydrolysis method (AOAC 948.15, 2016; Cunniff and Washington, 1997), using a Soxhlet extractor at 60°C until constant weight. Dietary fiber was determined according to the enzymatic gravimetric method (AOAC 985.29, 2016; Cunniff and Washington, 1997), digested leaf samples with heat using alpha-amylase, protease, and amyloglucosidase at 60°C, respectively, then added ethanol to samples to precipitate fiber (McCleary, 2023; Prosky et al., 1985).
The total carbohydrate content was estimated as described in the method of Hedge et al. (1962) by calculation having estimated all the other fractions by proximate analysis i.e. % Available carbohydrates = 100 - [% Moisture + % Ash + % Fat +% Protein + % Fiber]. The % organic matter was calculated as per James (2013). The energy content was determined by multiplying the values obtained for protein, fat and available carbohydrate by 4.00, 9.00 and 4.00, respectively and adding up the values. Total sugar, reducing sugar, and non-reducing sugar were calculated as per the Lane and Eynon method (James, 2013) which measures sugar as reducing sugar and total sugar as invert sugar. Non-reducing sugar is determined by subtracting the total reducing sugar from the total sugar and multiplying the remainder with a 0.95 factor.
2.4.2 Estimation of minerals
The analysis of the mineral composition of A. tuberosum leaves was done using a spectrometer as per AOAC 990.08:2023 (Szydłowska and Szłyk, 2003; Piatkowska et al., 2015; Isaac and Johnson, 1985). Plant material was taken in a pre-cleaned and constantly weighed silica crucible and heated in a muffle furnace at 400°C till there was no evolution of smoke. The crucible was cooled at room temperature in a desiccator and carbon-free ash was moistened with concentrated sulphuric acid and heated on a heating mantle till fumes of sulphuric acid ceased to evolve. The crucible with sulphated ash was then heated in a muffle furnace at 600°C till the weight of the content was constant. One gram of sulphated ash obtained above was dissolved in 100 ml of 5% hydrochloric acid (HCl) to obtain the solution ready for determination of mineral elements through atomic absorption spectroscopy (AAS) (AA 800, Perkin-Elmer, Germany). A standard solution for each element was prepared and calibration curves were drawn for each element using AAS (Indrayan et al., 2005). Total nitrogen was estimated using the modified Kjeldahl method as per IS 7219 (Bremner and Mulvaney, 1982; Sáez-Plaza et al., 2013; Sarkar et al., 2014). The total Sulphur content was estimated by inductively coupled plasma-optical emission spectrometry (ICP-OES) (iCAP PRO-X-Duo, Thermo-Scientific, MA, USA) after sample preparation with tetramethylammonium hydroxide (TMAH) (Tavares et al., 2020; Hansen et al., 2013).
2.5 Estimation of phenolic acids and flavonoids
2.5.1 Total phenol content
The total phenolic content of the samples was quantitatively analyzed using the Folin-Ciocalteu reagent, following the procedure (Blainski et al., 2013). Gallic acid served as the standard for this assay. The absorbance of the reaction mixture was measured at a wavelength of 750 nm using a UV-Vis spectrophotometer (Model no. UV1800, Shimadzu Corporation, Japan). The phenolic content in the samples was determined by comparing the absorbance values with a standard curve prepared using known concentrations of gallic acid (1, 2, 5, 7, 10, 20 and 50 ppm). The results were expressed as milligrams of Gallic Acid Equivalent per gram of dry weight (mg GAE/g DW).
2.5.2 Total flavonoid content
The total flavonoid content was determined using a modified version of the aluminium chloride colorimetric method, with quercetin as the reference standard (Sulastri et al., 2018). In this assay, the sample extracts were reacted with aluminium chloride, and the resulting complex’s absorbance was measured at 510 nm. The flavonoid content was quantified by comparing the absorbance readings against a calibration curve generated with quercetin standards by a UV-Vis spectrophotometer (Model no. UV1800, Shimadzu Corporation, Japan). The results were articulated in terms of milligrams of Quercetin Equivalents per gram of fresh weight (mg QE/g FW).
2.5.3 Antioxidant activity (DPPH assay)
The antioxidant activity of the sample extracts was evaluated using the DPPH (2,2-diphenyl-1-picrylhydrazyl) radical scavenging assay (Scherer and Godoy, 2009). The procedure involved adding 50 µL of the sample extract to 1.950 mL of a DPPH solution, which was prepared in 95% methanol to achieve an initial absorbance of 0.8 to 0.9 at 515 nm. The mixture was incubated at room temperature in the dark for 90 minutes to allow the reaction to occur. Post incubation, the decrease in absorbance was measured at 515 nm using a spectrophotometer (Model no. UV1800, Shimadzu Corporation, Japan). The antioxidant activity was quantified as the percentage of DPPH radical inhibition, calculated using the following formula:
This percentage indicates the capacity of the sample to scavenge DPPH radicals, thereby reflecting its antioxidant activity
2.6 Estimation of vitamins
The estimation of vitamins was outsourced to an ISO/IEC 17025:2017 accredited laboratory (Food Hygiene & Health Laboratory (FHHL), Pune, India). The stock standard solutions of vitamins B1, B2, B3, B5 and B6, B7 & B9 were prepared by dissolving 25 mg of each standard in 1 ml 0.1M hydrochloric acid in a 25 ml standard volumetric flask. For the preparation of standard stock solutions of vitamin B9 and B2, 25 mg of each standard were dissolved in one ml 0.1 M sodium hydroxide in a 25 ml standard volumetric flask. The standard solution was stored in amber-glass bottles in the refrigerator at 4°C. The working standards were prepared by diluting with phosphate buffer (1 M, pH 5.5). For the preparation of the sample solution, plant materials were washed with distilled water. The washed plant materials were cut into very small pieces, frozen in liquid nitrogen and kept at 20°C until analysis. One gram of each of the freeze-dried samples was soaked in 10 ml water and extracted with 1 ml 0.1 M NaOH and 10 ml phosphate buffer (1 M, pH 5.5) was added to it and kept in the dark for 24 hours. The solution was first filtered through a Whatman No. 1 filter paper and the resulting filtrate was taken in a 25 ml volumetric flask and the solution was topped up to the mark with HPLC grade water. The sample solution was filtered through a 0.45 µm membrane filter before injection into the LC system. The stock solutions of the sample were kept in a refrigerator for further use. The chromatographic analysis was carried out following the method described by Seal et al. (2017) with minor modifications on an LC-MS/MS system (API 5500-MS system connected to Exion LC, Sciex, Foster City, CA, USA). The mobile phase contains acetonitrile (Solvent A) and aqueous trifluoro acetic acid (TFA, 0.01% v/v) (Solvent B), the column was thermostatically controlled at 220°C and the injection volume was kept at 20 ml. A gradient elution was performed by varying the proportion of solvent A to solvent B. The total analysis time per sample was 35 minutes. Estimation of vitamin B4 was done using LC-MS (API 5500-MS system connected to Exion LC, Sciex, Foster City, CA, USA) as per AOAC official method 2012.18 (Martin et al., 2013).
To quantify fat-soluble vitamins A, E, and K in A. tuberosum leaves the AOAC official method 2014.02 was followed (Giménez and Martin, 2018). Freshly harvested leaves were immediately submerged in liquid nitrogen to halt enzymatic degradation and stored at -80°C. For analysis, the frozen leaves were pulverized under liquid nitrogen and a weighed portion was extracted using a solvent system optimized for all three vitamins (methanol and buffered aqueous solution). The extract was then analyzed by UHPLC (LC 2030C-3D-Plus, Shimadzu Corporation, Japan) with a PDA detector for vitamin A and fluorescence detector for vitamins E and K. Separation was achieved using a suitable chromatographic column and optimized mobile phase conditions as outlined in the AOAC method.
For estimation of Vitamin C (Ascorbic acid), two grams (2 g) of the sample was dissolved in 50 ml of a 20% metaphosphoric acid-acetic acid mixture. After filtration, 10 mL of the filtrate was titrated with a solution of 2,6-dichlorophenolindophenol (DCPIP) at 0.5 g/L until a persistent pink coloration was observed. The final content of vitamin C was calculated using the following formula:
Where:
Vitamin C: Vitamin C content (mg/100 g),
∁DCPIP: Concentration of DCPIP solution (0.5 g/L),
Veq: Volume (mL) of DCPIP used for titration,
W: Weight (g) of A. tuberosum sample.
2.7 Estimation of other biochemical & physiochemical properties and compounds
The major Sulphur active compounds viz. Allicin, AMThs (Allyl methyl thiosulphinate) and ATPThs (Allyl trans-1-propenyl thiosulphinate) were estimated by Liquid Chromatography Tandem Mass Spectrometry (LC-MS/MS) (Thangasamy et al., 2018; Singh et al., 2020). commercially available certified reference standards alliin (S-alliin-L-cysteine sulphoxide) having purity >90% and MS grade solvents were used. Alliin stock solution was prepared at a specific concentration and stored at -20°C. Allicin standard solution was generated from the alliin stock using a commercially available or purified alliinase enzyme, following a conversion process with centrifugation and storage at -80°C. Approximately 100 g of leaf sample was chopped, and crushed and a specific weight was extracted with water following a modified version of the method by Khar et al. (2011). The final extract was filtered and injected into the LC-MS/MS system (API 5500 Q-trap LC-MS/MS, AB Sciex, Canada) for analysis with preoptimized instrument parameters (Singh et al., 2020). The analytical method was validated according to a specific guideline, assessing linearity, limit of detection, limit of quantification, and precision.
The total soluble solids were measured using a refractometer as per the AOAC 932.12.2023 and the results were reported in °B. The pH was estimated using a pH meter at 10% solution as per ISO 11289:1993. Titrable acidity was measured by titration with 0.1N NaOH using phenolphthalein indicator as per Appendix A of IS 13242:2018.
2.8 Experimental design and statistical analysis
The study employed a factorial randomized block design to evaluate the effects of age windows (eight windows each of three months’ unit) and A. tuberosum accessions (three) on production performance. Descriptive statistics were used to summarize the collected data (e.g., mean, standard deviation). A two-factor analysis of variance (ANOVA) was then conducted to determine the statistical significance of the main effects (age windows and accessions) and their interaction effect on the measured parameters. This analysis helps identify if there are significant differences in the responses (e.g., plant height, yield) between the different age windows and accessions, and if these differences depend on each other. The nutritional and biochemical profile of the superior Allium tuberosum line, i.e. A. tuberosum CGN Kaz was represented with a simple mean value along with standard error.
3 Results
The performance of underutilized alliums was studied over two years, analyzing production data to assess the effects of age, season, and inherent characteristics on growth, yield, and quality.
3.1 Crop growth, productivity and produce quality
3.1.1 Morphology
The morphological traits of Allium tuberosum accessions exhibited significant differences across various age windows, particularly in plant height, tiller number, crop spread, leaf width, and stem length (Table 1). The plant height ranged from 21.53 cm to 34.61 cm, depending on the accession and age window, with AT1 showing the greatest height at 270 DAT (34.61 cm). Seasonal factors had a more profound impact on plant height than the age window alone, as indicated by higher values recorded during periods of bright sunshine hours i.e. April to June (age window 90 DAT to 180 DAT and 450 DAT to 540 DAT). The tiller counts also increased significantly with age, starting from approximately 2 tillers at 90 DAT and reaching over 43 tillers per plant at 720 DAT, with AT1 consistently producing the highest number of tillers. In terms of crop spread, AT1 exhibited the widest spread of 18.52 cm at 720 DAT, followed by AT3 and AT2.
The variations in leaf width and stem length were similarly pronounced across different age windows. AT1 had the widest leaves at 720 DAT (8.28 cm), while the longest stem length was also observed at 720 DAT, with AT1 and AT3 recording lengths of 7.81 cm and 7.9 cm, respectively. The leaf count showed moderate differences across accessions, with AT1 consistently producing a higher number of leaves, peaking at 5.17 leaves per plant at 540 DAT. These results suggest that both genetic makeup and the age window strongly influenced the morphology of the accessions, with seasonal effects playing an equally important role in shaping plant structure, especially during windows of bright sunshine.
Tables 2, 3 show that statistical significance was evident for most morphological traits. The least-square difference (LSD) values from the tables confirmed that the differences in plant height, tiller count, crop spread, and other morphological parameters were indeed significant across accessions and age windows, further reinforcing the impact of age and genetic variation on plant structure. This significance emphasizes the necessity of considering both genetic factors and environmental conditions when evaluating the morphology of A. tuberosum.
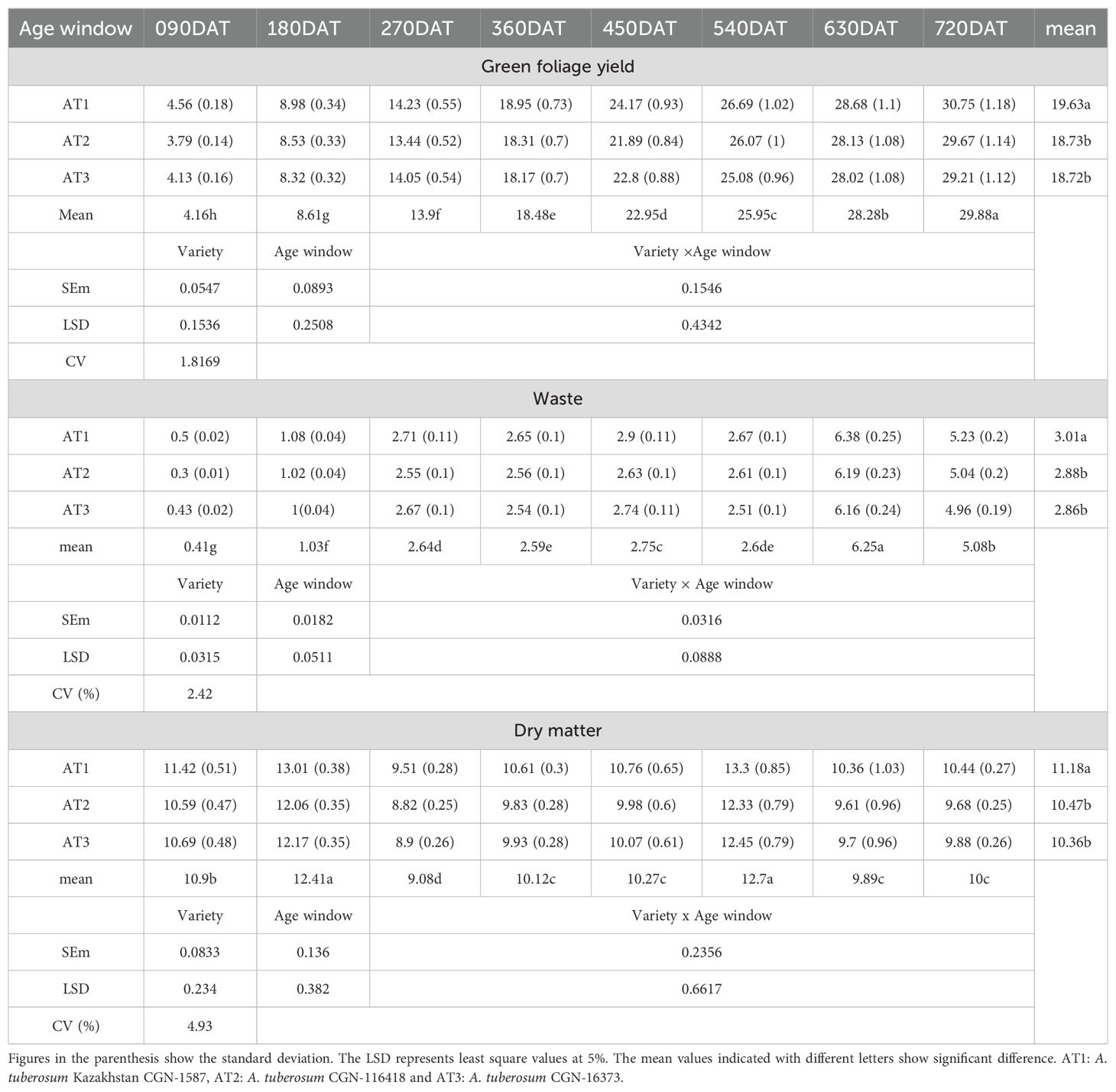
Table 2. Green foliage yield and related parameters of A. tuberosum accessions at Western Ghat condition.
3.1.2 Crop growth
The crop growth patterns over the study period, specifically regarding tillering, crop spread, and dry matter content, showed steady increases with age across all accessions. Tillering, which began with an average of around 2.5 tillers at 90 DAT, grew progressively to over 43 tillers by 720 DAT. AT1 consistently led in tiller production across all age windows, although the other accessions (AT2 and AT3) followed closely behind. Similarly, crop spread increased with age, starting at 3.75 cm at 90 DAT and reaching a maximum of 18.52 cm at 720 DAT for AT1, while AT3 and AT2 showed slightly smaller, yet significant, values. Dry matter content was influenced by both crop age and seasonal variations. The highest dry matter yields were recorded during the 90–180 DAT and 450–540 DAT windows, coinciding with periods of bright sunshine and elevated temperatures. AT1 maintained the highest average dry matter content (11.18%), while AT2 and AT3 trailed behind slightly. This seasonal trend demonstrates the strong interplay between environmental conditions and crop physiology, with the brightest sunshine hours contributing to higher dry matter accumulation.
The significant differences between accessions, as shown by the LSD values, underscore the importance of considering both genetic traits and environmental conditions when evaluating the crop growth of A. tuberosum. The growth patterns observed suggest that tillering and dry matter content are not only dependent on age but also heavily influenced by external factors such as sunshine hours and temperature, further validating the role of environmental variables in crop development.
3.1.3 Yield and quality of harvest
The quality of the harvest was assessed through a range of parameters, including green foliage yield, waste generated, the waste-to-yield ratio, and the stem-to-leaf ratio. Fresh foliage yield increased significantly with crop age across all accessions, with AT1 producing the highest overall yield. In the 630-720 DAT window, AT1 yielded 30.75 tons/ha, whereas AT2 and AT3 produced slightly lower yields of 29.67 tons/ha and 29.21 tons/ha, respectively. Over the two years, AT1 accumulated a total yield of 157.01 tons/ha, significantly higher than the other accessions, illustrating its superior productivity. However, the higher productivity also led to a proportional increase in waste generation, with AT1 producing 6.38 tons/ha of waste in the 540-630 DAT window, while AT2 and AT3 followed closely behind. The waste-to-yield ratio was highest during the 180-270 and 540-630 DAT windows, coinciding with the monsoon season, which negatively impacted foliage quality.
The stem-to-leaf ratio, which served as a critical measure of harvest quality, indicated that a higher ratio corresponded to poorer quality, as the crop is primarily cultivated for its leaves. Moreover, the results suggest that seasonal variations, particularly during monsoon windows, had a profound effect on yield quality. The stem-to-leaf and waste-to-yield ratios were notably higher during these periods, confirming that environmental conditions played a critical role in determining harvest quality. These findings, combined with the significant LSD values, further validate the hypothesis that age, genetics, and seasonality collectively influence both yield and its quality (Figures 2, 3).
3.2 The association of different growth and yield parameters
The correlations between different morphological and yield parameters of A. tuberosum as given in Figure 4 reveal intriguing results. The green foliage yield was found positively correlated with the tillering. As these tillers are added with the age of the plant, it widens the crop spread which is supported by a highly positive correlation between crop spread with yield (r = 0.89) and the tillers (r = 0.98). However, this crop spread increment was also associated with inferior quality traits i.e. the waste (r = 0.89) and stem-to-leaf ratio (r = 0.58). This hints at a possible trade-off i.e. increased vegetative growth might come at the cost of generating more stem and inferior quality harvest. This connection is further strengthened by the strong positive correlation between waste and waste-to-yield ratio (waste-waste to yield ratio: r = 0.813, green foliage yield-waste to yield ratio: r = 0.836). Interestingly, the table reveals some counterpoints. A negative correlation of both leaf width and stem girth with the number of tillers (leaf width-tillers: -0.237, stem girth-tillers: r = -0.523) was observed.
In addition to the aforementioned correlations, other interesting patterns emerged from the correlogram. The leaf length showed a positive yet moderate correlation with green foliage yield (r = 0.55), implying that longer leaves contribute somewhat to the overall yield, though not as significantly as tillering. On the contrary, the stem-to-leaf ratio was negatively correlated with yield quality parameters like leaf width (r = -0.42), indicating that a higher stem-to-leaf ratio might reduce leaf quality, potentially due to a thicker, less desirable stem structure. Moreover, the waste-to-yield ratio was inversely related to both leaf length (r = -0.31) and leaf width (r = -0.36), reinforcing that plants with thinner or shorter leaves might generate more waste relative to the total yield, pointing to an indirect relationship between morphological attributes and overall yield efficiency. These additional correlations highlight the complexity of the trade-offs between vegetative growth and yield quality in Allium tuberosum.
3.3 The principal component analysis
Figure 5 shows that the flat portion of the curve begins at component 4, indicating we should use the first three principal components. The first three principal components have eigenvalues greater than 1 (Table 3). The eigen analysis also indicated that PC1, PC2 and PC3 accounted for 50.9%, 19.8% and 14.1% of the variance, respectively. These principal components accounted for 84.8% of the total variability.
The coefficient of the principal component (Eigenvectors) (Table 2) revealed that the first principal component had large positive associations with waste, stem-to-leaf ratio, green foliage yield, crop spread, waste-to-yield ratio, stem length and the number of tillers, so this component primarily measures continuing stability at 630DAT. The second component had large negative associations with dry matter %, green foliage, crop spread, number of tillers and leaf width, so this component primarily measures yield at 720DAT. The third component had positive associations with the number of leaves, stem girth, plant height, and dry matter %, so this component mostly measures steady variability at 90DAT and 180DAT. The principal component analysis - biplot (PC1 vs PC2) visually shown in Figure 6.
3.4 The Nutritional and biochemical profile of A. tuberosum Kazakhstan CGN-1587
3.4.1 The proximate analysis & mineral composition
The proximate analysis of A. tuberosum Kazakhstan CGN-1587 (AT1) revealed a high moisture content of 88.94% in the fresh leaf cuttings (Table 4). This translates to a low caloric density of 36.13 Kcal per 100g. Interestingly, this moisture content is slightly higher than reported by Pooja (2021), suggesting latent variations based on cultivation practices. Rest, the ash content of 1.47 g per 100g, with a minimal acid-insoluble fraction (0.04 g), indicates the presence of dietary minerals. The low-fat content (0.85 g per 100g) and the lower cholesterol (<0.50 g per 100g) of AT1 are promising, especially considering the commendable ratio of unsaturated to saturated fats. The presence of a notable amount of dietary fiber (2.56 g per 100g) and protein (3.10 g per 100g) in AT1 is important. Both, the protein and dietary fiber content are quite higher than the A. cepa bulbs (1.1 g and 1.7g per 100g respectively) (Bhattacharjee et al., 2013; Mahmood et al., 2021).
The analysis of AT1 reveals a rich mineral profile dominated by essential macro-elements. Potassium (5355.30 mg/kg), followed closely by phosphorous (691.68 mg/kg), calcium (1126.99 mg/kg) and magnesium (569.39 mg/kg). In the macro-elements, a noteworthy aspect is the substantial amount of Sulphur (2484.97 ± 104 mg/kg) present in AT1. This aligns with the characteristic pungent aroma of Alliums, attributed to various volatile Sulphur compounds (Abe et al., 2020). Similarly, as the assimilation of selenium is carried out through the metabolic absorption route of sulfur (Barak and Goldman, 1997) the concentration of Se was found 42.17 mg/kg which is in line with the previous findings (Pilon-Smits et al., 2009). The presence of essential micronutrients like manganese (5.62 mg/kg), zinc (5.34 mg/kg), copper (1.62 mg/kg), and iron (39.74 mg/kg) further strengthen the nutritional value of AT. Additionally, the low sodium content (14.72 mg/kg) was also reported. The B, Cu and Zn content was comparatively lower in concentration than the A. cepa leaves as reported by Ferreira et al. (2022).
3.4.2 The vitamin profile
The vitamin profile of A tuberosum (AT1) reveals a captivating interplay of water-soluble and fat-soluble vitamins, impacting human health (Table 4). AT stands out for its particularly high content of vitamin B4, also known as choline (264.45 mg/kg) which is approximately four times more than in A. cepa bulb as reported by Pareek et al. (2017). While the content of these B vitamins is not exceptionally high, their combined presence contributes to the overall nutritional value of AT1. Interestingly, pyridoxine (vitamin B6) is present in very low quantities (0.053 ± 0.0045 mg/kg), suggesting that AT may not be a significant dietary source for this particular vitamin. The presence of vitamin C (18.12 mg/100g) highlights AT1’s contribution to immune function and antioxidant activity (Carr and Maggini, 2017). This vitamin C quantity is quite less than previous reports where Żurawik and Jadczak (2008) reported 43.0 - 56.1 mg/100g of L-ascorbic acid in the leaves A. tuberosum. The presence of vitamin E (5.38 mg/100g) further strengthens the antioxidant properties of AT1 (Traber and Atkinson, 2007). Vitamin E helps to protect cells from free radical damage, which has been linked to various chronic diseases (Traber and Atkinson, 2007). While vitamin K content is relatively low (85.00 mcg/100g), it can contribute modestly to blood clotting and bone health (Shearer and Newman, 2008). The limited detection of folates (vitamin B9) and biotin (vitamin B7) suggests that AT1 may not be a primary source of these essential nutrients.
3.4.3 The biochemical properties
The reported biochemical properties of AT1 shed light on some interesting aspects that can influence its sensory characteristics, preservation, and culinary applications (Table 4). A slightly acidic pH of 6.7 in a 10% AT solution indicated its weakly acidic nature (Sánchez-Rodríguez et al., 2019). Further investigation revealed the presence of organic acids (0.36 g/100g) within AT1, evident from the titrable acidity value. The total soluble solids (TSS) measured at 7.93°Brix signifies the concentration of soluble solids present in AT1 which is lower than some previous reports i.e. 8.6°Brix as reported by Pooja (2021). This TSS content is at par with that of Welsh onion leaves (7.51 ± 0.31°Brix) and lower than A. cepa leaves (5.87 ± 0.18) as reported by Masahiro Yuasa et al. (2022).
The biochemical profile of A. tuberosum, revealed a promising composition with various health-promoting compounds (Table 4). The presence of a significant amount of total phenolics (1.187 mg GAE/g fresh weight basis) suggests strong antioxidant property (Bindon et al., 2010). This is further supported by the high free radical scavenging activity (64.45% RSA DPPH). These findings align with observations in other Allium species for their antioxidant properties (Rahman, 2007). Interestingly, A. tuberosum displayed a unique profile of organosulfur compounds. While allicin, the characteristic pungent compound of garlic (A. sativum), was present at relatively low levels (16.60 mg kg-1), the dominant organosulfur compound was allyl methyl thiosulfinate (AMThs) at a considerably higher concentration (269.00 mg kg-1). This suggests A. tuberosum might possess a distinct flavor and potentially different biological activities compared to garlic (Lanzotti, 2006). The presence of flavonoids (3.19 mg/g) and ascorbic acid (18.12 mg/g) adds further value to the nutritional profile of AT1.
4 Discussion
The two-year production study highlight the suitability of A. tuberosum cultivation at Western Ghats as the yield scenarios of the green leaf cuttings were comparable with the traditional growing belt. The A. tuberosum yielded 157.01 tons/ha in two years by monthly cuttings, which was three times higher than the reported by Wang et al. (2020). Where the A. tuberosum chives production in main growing regions (i.e China, Uzbekisthan, Kazakisthan, Eastern Europe and Korea) is limited by the winter low temperatures, the Western ghat conditions provide a year-round growth season with monthly cutting intervals. More importantly, the leaf purpose market of Allium species is majorly dominated by A. cepa cultivars. It faces a seasonal slag due to monsoon and high summer heat months. Comparably the yield variations in case of A. tuberosum are less and can be projected as alternative to later as also supported by consumer studies by Kale et al. (2024b). The yield related studies carried out for A. tuberosum (Rathore et al., 2020; Pooja 2021) were mostly short durational, thus this study is the first insight at Indian planes for the comparable multi-year production.
The temporal and varietal variations in A. tuberosum growth dynamics present compelling insights into optimizing cultivation strategies, especially when considering the intricate interaction between environmental factors and plant morphology. Seasonal fluctuations, particularly the heightened sensitivity of AT1 to photoperiod and temperature, reaffirm the species’ dependence on these external variables for growth regulation (Rathore et al., 2020). Notably, the monsoon season’s influence, leading to an exaggerated stem elongation, supports the hypothesis of light-seeking behavior in low-sunlight conditions (Golan et al., 2024). However, this adaptive elongation, while advantageous for light capture, results in a proportional reduction in stem girth and leaf width. These findings invite deeper exploration into the physiological trade-offs plants make to cope with fluctuating environmental constraints. The distinctive patterns observed in foliage yield, especially the progressive increase in tillering, reveal a double-edged sword. Although an increase in tillers is correlated with higher yields, it simultaneously escalates waste production, a phenomenon corroborated by Żurawik and Jadczak (2008) and Uddin et al. (2019). This apparent yield-waste paradox challenges conventional notions of productivity, suggesting that beyond a certain threshold, the drive for higher foliage production may compromise overall harvest quality. Producers must thus navigate this trade-off, optimizing tillering while controlling waste accumulation to balance economic returns with quality.
A critical reflection on the crop’s morphological adjustments under different seasonal windows reveals fascinating behavioral responses. For instance, the reduction in leaf width during the monsoon, despite lower light availability, contradicts the general expectation of increased leaf area in low-light environments. This anomaly suggests a possible compensatory mechanism where the plant may prioritize structural integrity over expansive leaf growth to maintain balance, a notion in line with Brand (1997) and Sladek et al. (2009) but contrast with the findings of Marenco and Reis (1998) and Sladek et al. (2009). This observation invites further investigation into the physiological underpinnings of such adaptive strategies, particularly in light-limited environments.
The results of this study establish A. tuberosum (AT1) as a nutritionally rich crop, exhibiting significant concentrations of potassium, phosphorus, and sulphur, which are vital for cardiovascular health and metabolic regulation (Schwalfenberg and Genuis, 2017; Humphries and Dart, 2015). The high sulfur content enhances its culinary appeal and suggests therapeutic applications (Tang et al., 2017). Furthermore, the presence of organosulfur compounds, particularly allyl propyl disulfide, comparable with Singh et al. (2020) other Allium species as reported by Rathore et al. (2020). positions AT1 as a possible mitigator of oxidative stress associated with chronic diseases (Rahman, 2007). The favorable fat profile and low caloric content of AT1 further support its role in dietary management for individuals with metabolic disorders and low calory diets (Campos, 2018; Thompkinsons et al., 2014).
Notably, the plant’s micronutrient profile includes selenium, zinc, copper, and iron, which are essential for metabolic and immune functions (Elmadfa and Meyer, 2019). The Selenium’s countable presence justifies their common assimilation through the metabolic absorption route of sulphur as reported by González-Morales et al. (2017) and Jiang et al. (2021). The vitamins present, particularly B vitamins, vitamin C, and vitamin E bolster AT1’s antioxidant and immune-supportive properties (Mehedint and Zeisel, 2013; Doseděl et al., 2021). Overall, AT1 emerges as a significant player in dietary strategies aimed at combatting chronic diseases. To optimize the benefits of AT1, further research is necessary to validate them which is crucial for translating its nutritional advantages into effective dietary interventions.
5 Conclusion and implication
This study offers a comprehensive analysis of the agronomic performance, yield potential, quality traits, and nutritional profile of A. tuberosum under the specific environmental conditions of the Western Ghats. The A. tuberosum Kazakhstan CGN-1587 line demonstrated the highest foliage yield, making it ideal for large-scale cultivation. The outcomes of the study give a deeper understanding of the complex interactions between agronomic traits and overall crop performance. The key implications of the study are as follows:
● The study guides farmers in tailoring harvesting schedules and spatial arrangements to specific seasonal conditions for a stable and reliable yields.
● The A. tuberosum Kazakhstan CGN-1587 line’s superior yield and quality traits suggest significant potential for vegetable supply chains and the production of value-added products.
● High bioactive compound content stages A. tuberosum Kazakhstan CGN-1587 as an attractive ingredient for health and wellness products, contributing to nutritional security in regions with prevalent deficiencies.
● Resilience to seasonal variability, with minimal yield fluctuations, highlight A. tuberosum’s high adaptability to diverse environmental conditions in the region.
Developing guidelines and best practices based on these insights will help farmers adopt optimal cultivation techniques. These findings promotes sustainable and economically viable farming practices, by expanding the cultivation in unconventional zones. The findings provide valuable insights for optimizing and commercializing A. tuberosum, benefiting farmers, entrepreneurs, processing industries, and supply chain developers.
Data availability statement
The original contributions presented in the study are included in the article/supplementary material. Further inquiries can be directed to the corresponding authors.
Author contributions
AG: Data curation, Formal analysis, Investigation, Methodology, Software, Writing – original draft, Writing – review & editing. RK: Conceptualization, Project administration, Resources, Supervision, Writing – review & editing. YK: Writing – review & editing, Conceptualization, Resources. HB: Writing – review & editing. SG: Formal analysis, Investigation, Visualization, Writing – original draft. SS: Investigation, Writing – original draft. AS: Formal analysis, Investigation, Resources, Writing – original draft. YG: Visualization, Writing – original draft, Formal analysis. KK: Writing – review & editing, Visualization, Writing – original draft. VM: Funding acquisition, Project administration, Resources, Writing – review & editing.
Funding
The author(s) declare financial support was received for the research and/or publication of this article. This work was financially supported by the ICAR-Directorate of Onion and Garlic Research, Pune, India.
Conflict of interest
The authors declare that the research was conducted in the absence of any commercial or financial relationships that could be construed as a potential conflict of interest.
Publisher’s note
All claims expressed in this article are solely those of the authors and do not necessarily represent those of their affiliated organizations, or those of the publisher, the editors and the reviewers. Any product that may be evaluated in this article, or claim that may be made by its manufacturer, is not guaranteed or endorsed by the publisher.
References
Abe, K., Hori, Y., Myoda, T. (2020). Volatile compounds of fresh and processed garlic. Exp. Ther. Med 19, 1585–1593. doi: 10.3892/etm.2019.8394
Adamczewska-Sowinska, K., Turczuk, J. (2016). Yielding and biological value of garlic chives (Allium tuberosum Rottl. ex Spreng.) depending to the type of mulch. J. Elementology 21, 7–19. doi: 10.5601/jelem.2015.20.2.910
Ahmad, L., Habib Kanth, R., Parvaze, S., Sheraz Mahdi, S., Ahmad, L., Habib Kanth, R., et al. (2017). Agro-climatic and agro-ecological zones of India.
Alam, A., Al Arif Jahan, A., Bari, M. S., Khandokar, L., Mahmud, M. H., Junaid, M., et al. (2023). Allium vegetables: Traditional uses, phytoconstituents, and beneficial effects in inflammation and cancer. Crit. Rev. Food Sci. Nutr 63, 6580–6614. doi: 10.1080/10408398.2022.2036094
Annual Report ICAR-DOGR. (2021). Available online at: https://dogr.icar.gov.in/images/AnnualReport/ar2021_c.pdf (Accessed May 25, 2024).
Barak, P., Goldman, I. L. (1997). Antagonistic relationship between selenate and sulfate uptake in onion (Allium cepa): implications for the production of organosulfur and organoselenium compounds in plants. J. Agric. Food Chem 45, 1290–1294. doi: 10.1021/jf960729k
Bastaki, S. M., Ojha, S., Kalasz, H., Adeghate, E. (2021). Chemical constituents and medicinal properties of Allium species. Mol. Cell. Biochem 476, 4301–4321. doi: 10.1007/s11010-021-04213-2
Bhattacharjee, S., Sultana, A., Sazzad, M. H., Islam, M. A., Ahtashom, M., Asaduzzaman, M. (2013). Analysis of the proximate composition and energy values of two varieties of onion (Allium cepa L.) bulbs of different origin: A comparative study. Int. J. Nutr. Food Sci 2, 246–253.
Bindon, K. A., Smith, P. A., Kennedy, J. A. (2010). Interaction between grape-derived proanthocyanidins and cell wall material. 1. Effect on proanthocyanidin composition and molecular mass. J. Agric. Food Chem 58, 2520–2528. doi: 10.1021/jf1022274
Blainski, A., Lopes, G. C., De Mello, J. C. P. (2013). Application and analysis of the folin ciocalteu method for the determination of the total phenolic content from Limonium brasiliense L. Molecules 18, 6852–6865. doi: 10.3390/molecules18066852
Brand, M. H. (1997). Shade influences plant growth, leaf color, and chlorophyll content of Kalmia latifolia L. cultivars. Hortic. Sci 32, 206–208. doi: 10.21273/HORTSCI.32.2.206
Bremner, J. M., Mulvaney, C. S. (1982). Nitrogen—total. Methods Soil analysis: Part 2 Chem. microbiological properties 9, 595–624.
Campos, C. (2018). Weight management for patients with type 2 diabetes: impact of newer antidiabetic therapies on body weight. J. Family Pract 67, S61–S61.
Carr, A. C., Maggini, S. (2017). Vitamin C and immune function. Nutrients 9, p.1211. doi: 10.3390/nu9111211
Cunniff, P., Washington, D. (1997). Official methods of analysis of AOAC International. J. AOAC Int 80, 127A.
Doseděl, M., Jirkovský, E., Macáková, K., Krčmová, L. K., Javorská, L., Pourová, J., et al. (2021). Vitamin C—sources, physiological role, kinetics, deficiency, use, toxicity, and determination. Nutrients 13, 615. doi: 10.3390/nu13020615
Elmadfa, I., Meyer, A. L. (2019). The role of the status of selected micronutrients in shaping the immune function. Endocrine Metab. Immune Disorders-Drug Targets (Formerly Curr. Drug Targets-Immune Endocrine Metab. Disorders) 19, 1100–1115. doi: 10.2174/1871530319666190529101816
Ferreira Bertino, N. M., Grangeiro, L. C., CecílioFilho, A. B., Nogueira, H. C., de Oliveira, A. K. S., Alves, A. A., et al. (2022). Quality and agronomic biofortification of onion as a function of fertilization with micronutrients. J. Plant Nutr 45, 2251–2262. doi: 10.1080/01904167.2022.2027971
Giménez, E. C., Martin, F. (2018). Vitamin B12 (cyanocobalamin) in infant formula adult/pediatric nutritional formula by liquid chromatography with ultraviolet detection: collaborative study, final action 2014.02. J. AOAC Int 101, 1112–1118. doi: 10.5740/jaoacint.17-0452
Golan, G., Weiner, J., Zhao, Y., Schnurbusch, T. (2024). Agroecological genetics of biomass allocation in wheat uncovers genotype interactions with canopy shade and plant size. New Phytologist. 242, 107–120. doi: 10.1111/nph.v242.1
González-Morales, S., Pérez-Labrada, F., García-Enciso, E. L., Leija-Martínez, P., Medrano-Macías, J., Dávila-Rangel, I. E., et al. (2017). Selenium and sulfur to produce Allium functional crops. Molecules 22, p.558. doi: 10.3390/molecules22040558
Guohua, H., Yanhua, L., Rengang, M., Dongzhi, W., Zhengzhi, M., Hua, Z. (2009). Aphrodisiac properties of Allium tuberosum seeds extract. J. ethnopharmacology 122, 579–582. doi: 10.1016/j.jep.2009.01.018
Hansen, T. H., De Bang, T. C., Laursen, K. H., Pedas, P., Husted, S., Schjoerring, J. K. (2013). Multielement plant tissue analysis using ICP spectrometry. Plant Mineral Nutrients: Methods Protoc 953, 121–141. doi: 10.1007/978-1-62703-152-3_8
Hedge, J. E., Hofreiter, B. T., Whistler, R. L. (1962). Carbohydrate chemistry. Acad. Press New York 17, 371–380.
Huang, Y. H., Mao, Z. C., Xie, B. Y. (2016). Chinese leek (Allium tuberosum Rottler ex Sprengel) reduced disease symptom caused by root-knot nematode. J. Integr. Agric 15, 364–372. doi: 10.1016/S2095-3119(15)61032-2
Humphries, E. S., Dart, C. (2015). Neuronal and cardiovascular potassium channels as therapeutic drug targets: promise and pitfalls. J. Biomol Screen 20, 1055–1073. doi: 10.1177/1087057115601677
Imahori, Y. (2022). Crop development–chinese chive. In Edible Alliums: Botany Production Uses Ed. Rabinowitch, H., Thomas, B. (CABI), 169–181.
Indrayan, A. K., Sharma, S., Durgapal, D., Kumar, N., Kumar, M. (2005). Determination of nutritive value and analysis of mineral elements for some medicinally valued plants from Uttaranchal. Curr. Sci 89, 1252–1255.
Isaac, R. A., Johnson, W. C. (1985). Elemental analysis of plant tissue by plasma emission spectroscopy: Collaborative study. J. Assoc. Off. Analytical Chem 68, 499–505. doi: 10.1093/jaoac/68.3.499
James, C. S. (2013). Analytical chemistry of foods (United States: Springer Science & Business Media).
Jiang, H., Lin, W., Jiao, H., Liu, J., Chan, L., Liu, X., et al. (2021). Uptake, transport, and metabolism of selenium and its protective effects against toxic metals in plants: A review. Metallomics 13, p.mfab040. doi: 10.1093/mtomcs/mfab040
Jones, M. G., Hughes, J., Tregova, A., Milne, J., Tomsett, A. B., Collin, H. A. (2004). Biosynthesis of the flavour precursors of onion and garlic. J. Exp. Bot 55, 1903–1918. doi: 10.1093/jxb/erh138
Joseph John, K., Pradheep, K. (2019). Wild relatives of horticultural crops: PGR management in Indian context. 451–480.
Kalasee, B., Mittraparp-arthorn, P. (2023). Phytochemicals, antioxidant, and antibacterial activities of fresh and dried Chinese chive (Allium tuberosum Rottler) leaf extracts. ASEAN J. Sci. Technological Rep 26, 1–10. doi: 10.55164/ajstr.v26i4.249336
Kale, R. B., Gavhane, A. D., Gadge, S. S., Gaikwad, S. Y., Singh, S., Khandagale, K., et al. (2024b). From Field to Fork: revitalizing underutilized Alliums through value chain development. Front. Sustain. Food Syst 8, 1427167. doi: 10.3389/fsufs.2024.1427167
Khalid, N., Ahmed, I., Latif, M. S. Z., Rafique, T., Fawad, S. A. (2014). Comparison of antimicrobial activity, phytochemical profile and minerals composition of garlic Allium sativum and Allium tuberosum. J. Korean Soc. Appl. Biol. Chem 57, 311–317. doi: 10.1007/s13765-014-4021-4
Khandagale, K., Roylawar, P., Randive, P., Karuppaiah, V., Soumia, P. S., Shirsat, D., et al. (2022). Isolation and Expression Profiling of Insecticidal Lectins from Wild Alliums Against Onion Thrips (Thrips tabaci Lindeman). Proc Natl Acad Sci India Sect B Biol Sci. 92, 451–459.
Khar, A., Banerjee, K., Jadhav, M. R., Lawande, K. E. (2011). Evaluation of garlic ecotypes for allicin and other allyl thiosulphinates. Food Chem 128, 988–996. doi: 10.1016/j.foodchem.2011.04.004
Kim, J. H. (2010). Effects of mulching materials on growth of allium tuberosum rottler and weed control. Korean J. Plant Resour 23, 242–247.
Kim, S.-H., Yoon, J. B., Han, J., Seo, Y. Am, Kang, B.-H., Lee, J., et al. (2023). Green onion (Allium fistulosum): an aromatic vegetable crop esteemed for food, nutritional and therapeutic significance. Foods 12, 4503. doi: 10.3390/foods12244503
Lanzotti, V. (2006). The analysis of onion and garlic. J. Chromatogr. A 1112, 3–pp22. doi: 10.1016/j.chroma.2005.12.016
Liu, N., Tong, J., Hu, M., Ji, Y., Wang, B., Liang, H., et al. (2021). Transcriptome landscapes of multiple tissues highlight the genes involved in the flavor metabolic pathway in Chinese chive (Allium tuberosum). Genomics 113, 2145–2157. doi: 10.1016/j.ygeno.2021.05.005
Mahmood, N., Muazzam, M. A., Ahmad, M., Hussain, S., Javed, W. (2021). Phytochemistry of Allium cepa L.(Onion): An overview of its nutritional and pharmacological importance. Sci. Inq. Rev 5, 41–59. doi: 10.32350/sir/53.04
Marenco, R. A., Reis, A. C. S. (1998). Shading as an environmental factor affecting the growth of Ischaemum rugosum, Vol. 10. 107–112.
Martin, F., Gimenez, C., Fontannaz, P., Kilinc, T., Campos-Giménez, E., Dowell, D. (2013). Choline in infant formula and adult/pediatric nutritional Formula by ultra-high-performance liquid chromatography/ Tandem mass spectrometry: AOAC First Action 2012.18. J. AOAC Int 96, 1396–1399. doi: 10.5740/jaoacint.13-195
McCleary, B. V. (2023). Measurement of dietary fiber: Which AOAC Official Method of Analysis SM to use. J. AOAC Int 106, 917–930. doi: 10.1093/jaoacint/qsad051
Mehedint, M. G., Zeisel, S. H. (2013). Choline's role in maintaining liver function: new evidence for epigenetic mechanisms. Curr. Opin. Clin. Nutr. Metab. Care 16, 339–345. doi: 10.1097/MCO.0b013e3283600d46
Negi, K. S., Rathore, S. S., Pant, V. K., Muneem, K. C. (2006). Addition to the rare and economically useful plant germplasm Allium clarkei Hook f. from Uttarakhand. Indian J. Plant Genet. Resour 19, 128–129.
Ochar, K., Kim, S.-H. (2023). Conservation and global distribution of onion (Allium cepa L.) germplasm for agricultural sustainability. Plants 12, 3294. doi: 10.3390/plants12183294
Official Methods of Analysis of AOAC INTERNATIONAL. (2016). 20th edn. (Rockville, MD, USA: AOAC International).
Pandey, A., Pradheep, K., Gupta, R. (2014). Chinese chives (Allium tuberosum Rottler ex Sprengel): a home garden species or a commercial crop in India. Genet. Resour. Crop Evol 61, 1433–1440. doi: 10.1007/s10722-014-0144-z
Pareek, S., Sagar, N. A., Sharma, S., Kumar, V. (2017). “Onion (allium cepa L.),” in Fruit and vegetable phytochemicals: chemistry and human health, 2nd, 1145–1162.
Park, H. S., Choi, E. J., Lee, J. H., Kim, G. H. (2013). Evaluation of Allium vegetables for anti-Adipogenic, anti-Cancer, and anti-inflammatory activities InVitro. J. Life Sci 5, 127–132. doi: 10.1080/09751270.2013.11885219
Piatkowska, E., Kopec, A., Leszczynska, T. (2015). Basic chemical composition, content of micro-and macroelements and antioxidant activity of different varieties of garlic's leaves Polish origin. Żywność Nauka Technologia Jakość 1, 181–192. doi: 10.15193/zntj/2015/98/014
Pilon-Smits, E. A., Quinn, C. F., Tapken, W., Malagoli, A. (2009a). Physiological functions of beneficial elements. Curr. Opin. Plant Biol 12, 267–274. doi: 10.1016/j.pbi.2009.04.009
Pooja, A. E. (2021). Performance evaluation of underutilized edible alliums (Doctoral dissertation, Department of Plantation crops and spices, College of Agriculture, Vellanikkara), Thrissur, Kerala.
Prosky, L., Asp, N. G., Furda, I., Devries, J. W., Schweizer, T. F., Harland, B. F. (1985). Determination of total dietary fiber in foods and food products: collaborative study. J. Assoc. Off. Analytical Chem 68, 677–679. doi: 10.1093/jaoac/68.4.677
Rahman, M. S. (2007). Allicin and other functional active components in garlic: Health benefits and bioavailability. Int. J. Food Properties 10, 245–268. doi: 10.1080/10942910601113327
Rathore, N., Gayen, R., Mahajan, V., Thangasamy, A., Ghodke, P. (2020). Studies on leaf yield and association analysis in underutilized and cultivated Allium species. Int. J. Chem. Stud 8, 57–61. doi: 10.22271/chemi.2020.v8.i3a.9467
Riaz, A., Lagnika, C., Luo, H., Dai, Z., Nie, M., Hashim, M. M., et al. (2020). Chitosan-based biodegradable active food packaging film containing Chinese chive (Allium tuberosum) root extract for food application. Int. J. Biol. Macromolecules 150, 595–604. doi: 10.1016/j.ijbiomac.2020.02.078
Sáez-Plaza, P., Navas, M. J., Wybraniec, S., Michałowski, T., Asuero, A. G. (2013). An overview of the Kjeldahl method of nitrogen determination. Part II. Sample preparation, working scale, instrumental finish, and quality control. Crit. Rev. Analytical Chem 43, 224–272. doi: 10.1080/10408347.2012.751787
Saito, S. (2020). “Chinese chives allium tuberosum rottl,” in In Onions and allied crops (Boca Raton: CRC Press), 219–230.
Sánchez-Rodríguez, L., Ali, N. S., Cano-Lamadrid, M., Noguera-Artiaga, L., Lipan, L., Carbonell-BarraChina, Á.A., et al. (2019). “Flavors and aromas,” in Postharvest physiology and biochemistry of fruits and vegetables (Cambridge: Woodhead Publishing), 385–404.
Sarkar, S., Mondal, M., Ghosh, P., Saha, M., Chatterjee, S. (2020). Quantification of total protein content from some traditionally used edible plant leaves: a comparative study. J. Medicinal Plant Stud 8, 166–170. doi: 10.22271/plants.2020.v8.i4c.1164
Sarkar, D., Sheikh, A. A., Batabyal, K., Mandal, B. (2014). Boron estimation in soil, plant, and water samples using spectrophotometric methods. Commun. Soil Sci. Plant Anal 45, 1538–1550. doi: 10.1080/00103624.2014.904336
Scherer, R., Godoy, H. T. (2009). Antioxidant activity index (AAI) by the 2, 2-diphenyl-1-picrylhydrazyl method. Food Chem 112, 654–658. doi: 10.1016/j.foodchem.2008.06.026
Schwalfenberg, G. K., Genuis, S. J. (2017). The importance of magnesium in clinical healthcare. Scientifica (Cairo) 2017, 4179326. doi: 10.1155/2017/4179326
Seal, T., Chaudhuri, K., Pillai, B. (2017). Water soluble vitamin estimation in five wild edible fruits consumed by the tribal people of north-eastern region in India by high performance liquid chromatography. Int. J. Chem. Stud 5, 1576–1584.
Shearer, M. J., Newman, P. (2008). Metabolism and cell biology of vitamin K. Thromb. haemostasis 100, 530–547.
Singh, P., Mahajan, V., TP, A. S., Banerjee, K., Jadhav, M. R., Kumar, P., et al. (2020). Comparative evaluation of different Allium accessions for allicin and other allyl thiosulphinates. Ind. Crops Products 147, p.112215. doi: 10.1016/j.indcrop.2020.112215
Sladek, B. S., Henry, G. M., Auld, D. L. (2009). Evaluation of zoysia grass genotypes for shade tolerance. Hortic. Sci 44, 1447–1451.
Sulastri, E., Zubair, M. S., Anas, N. I., Abidin, S., Hardani, R., Yulianti, R. (2018). Total phenolic, total flavonoid, quercetin content and antioxidant activity of standardized extract of Moringa oleifera leaf from regions with different elevation. Pharmacognosy J. 10, 104–108. doi: 10.5530/pj.2018.6s.20
Szydłowska-Czerniak, A., Szłyk, E. (2003). Spectrophotometric determination of total phosphorus in rape seeds and oils at various stages of technological process: calculation of phospholipids and non-hydratable phospholipids contents in rapeseed oil. Food Chem 81, 613–619. doi: 10.1016/S0308-8146(02)00562-9
Tang, X., Olatunji, O. J., Zhou, Y., Hou, X. (2017). Allium tuberosum: Antidiabetic and hepatoprotective activities. Food Res. Int 102, 681–689. doi: 10.1016/j.foodres.2017.08.034
Tavares, K. D. N., Lucena, I. O. D., Toaldo, I. M., Haas, I. C. D. S., Luna, A. S., de Gois, J. S. (2020). Optimized sample preparation for sulfur determination in animal feed by inductively coupled plasma–optical emission spectrometry (ICP-OES) with correlation to the total protein content. Analytical Lett 53, 2252–2265. doi: 10.1080/00032719.2020.1736090
Thangasamy, A., Gorrepati, K. G. K., Ahammed, T. P. S., Savalekar, R. K., Banerjee, K. B. K., Sankar, V., et al. (2018). Comparison of organic and conventional farming for onion yield, biochemical quality, soil organic carbon, and microbial population. Arch. Agron. Soil Sci 64, 219–230. doi: 10.1080/03650340.2017.1341045
Thompkinson, D. K., Bhavana, V., Kanika, P. (2014). Dietary approaches for management of cardio-vascular health-a review. J. Food Sci. Technol 51, 2318–2330. doi: 10.1007/s13197-012-0661-8
Traber, M. G., Atkinson, J. (2007). Vitamin E, antioxidant and nothing more. Free Radical Biol. Med 43, 4–pp15. doi: 10.1016/j.freeradbiomed.2007.03.024
Uddin, A. J., Alam, M. M., Husna, M. A., Raisa, I., Rakibuzzaman, M. (2019). Thiourea application frequency on growth and yield of Garlic Chives (Allium tuberosum). Int. J. Bus. Soc Sci. Res 7, 115–118.
Wang, C., Lv, J., Coulter, J. A., Xie, J., Yu, J., Li, J., et al. (2020). Slow-release fertilizer improves the growth, quality, and nutrient utilization of wintering Chinese chives (Allium tuberosum Rottler ex Spreng.). Agronomy 10, p.381. doi: 10.3390/agronomy10030381
Xia, S.-W., Hang, L.-F., Ali, S., Xu, X.-Y., Liu, Y.-J., Yan, Q.-Q., et al. (2022). Biosynthesis and Metabolism of Garlic Odor Compounds in Cultivated Chinese Chives (Allium tuberosum) and Wild Chinese Chives (Allium hookeri). Int. J. Mol. Sci 23, 7013. doi: 10.3390/ijms23137013
Yan, J. K., Zhu, J., Liu, Y., Chen, X., Wang, W., Zhang, H., et al. (2022). Recent advances in research on Allium plants: functional ingredients, physiological activities, and applications in agricultural and food sciences. Crit. Rev. Food Sci. Nutr 63, 8107–8135. doi: 10.1080/10408398.2022.2056132
Yuasa, M., Ueno, M., Kawabeta, K., Morikawa, M., Uemura, M., Matsuzawa, T., et al. (2022). Taste characteristics, volatile components, sensory properties, and antioxidant activity of fresh onion (Allium cepa L.) leaves. Bull. Natl. Res. Centre 46, p.270. doi: 10.1186/s42269-022-00958-y
Keywords: underutilized Allium, non-traditional cultivation, yield optimization, seasonal effects, Western Ghats agro-climatic region
Citation: Gavhane AD, Kale RB, Khade Y, Bhandari HR, Gaikwad SY, Singh S, Shabeer T. P. A, Garde YA, Khandagale K and Mahajan V (2024) Cultivation viability of Allium tuberosum L. in the Western Ghats: insights into crop dynamics, yield and quality. Front. Plant Sci. 15:1480510. doi: 10.3389/fpls.2024.1480510
Received: 14 August 2024; Accepted: 25 October 2024;
Published: 18 November 2024.
Edited by:
Giuseppe Mannino, University of Turin, ItalyReviewed by:
Beppe Benedetto Consentino, University of Palermo, ItalyMiroslawa Chwil, University of Life Sciences of Lublin, Poland
Copyright © 2024 Gavhane, Kale, Khade, Bhandari, Gaikwad, Singh, Shabeer T. P., Garde, Khandagale and Mahajan. This is an open-access article distributed under the terms of the Creative Commons Attribution License (CC BY). The use, distribution or reproduction in other forums is permitted, provided the original author(s) and the copyright owner(s) are credited and that the original publication in this journal is cited, in accordance with accepted academic practice. No use, distribution or reproduction is permitted which does not comply with these terms.
*Correspondence: Rajiv B. Kale, cmFqaXYua2FsZUBpY2FyLmdvdi5pbg==; Vijay Mahajan, dmlqYXkubWFoYWphbkBpY2FyLmdvdi5pbg==; Yogesh Khade, eW9nZXNoLmtoYWRlQGljYXIuZ292Lmlu; Kiran Khandagale, a2lyYW5raGFuZGFnYWxlMjUzQGdtYWlsLmNvbQ==