- 1Key Laboratory of Saline–alkali Soil Improvement and Utilization (Saline–alkali Land in Arid and Semiarid Regions), Ministry of Agriculture and Rural Affairs, Institute of Agricultural Resources and Environment, Xinjiang Academy of Agricultural Sciences, Urumchi, China
- 2Institute of Plant Protection, Chinese Academy of Agricultural Sciences, Beijing, China
- 3Jia Sixie College of Agriculture, Weifang University of Science and Technology, Weifang, Shandong, China
- 4Farmland Irrigation Research Institute, Chinese Academy of Agricultural Sciences, Xinxiang, Henan, China
Cold stress (CS) is one of the main factors that limits the crop or plant growth and development in many regions of the world. Many researchers have been endeavoring to break the natural temperature barrier to grow plants in extremely cold areas or to alleviate erratic cold devastation on crops in temperate or subtropical regions for years. Numerous studies and research papers published recently for the last two decades have proven that exogenous substances (ESs) are effective and practical in helping plants tolerate CS. Here, we systematically summarize and characterize all 72 ESs that have been tried against CS, analyze research hotspots in the Web of Science database from 2000 to 2024 using VOSviewer with the keywords “cold stress” and “exogenous substances”, and grouped them accordingly. Based on their underlying mechanisms, five categories of ESs are clearly defined, described and discussed: 1) enhancement of cell osmotic adjustment, 2) improvement of antioxidant pathways, 3) involvement in phytohormone regulation, 4) promotion of photosynthesis; 5) enrichment of nutritional status. After clarifying these five categories, a detailed plant responses and their possible interactions through a signal cross-talk are proposed and followed by discussions on future perspectives on using ESs to fortify plants against CS. The accumulative knowledge and information provided here will be ultimately used to increase plant productivity and agricultural sustainability under CS through chemical and microbial approaches.
1 Introduction
Plants live in an environment with a constantly changing temperature and are greatly affected by its downshift that seriously impacts their normal growth, development and productivity, and limits their geographical habitat (Wang et al., 2017). Approximately, 64% of our earth’s land has the lowest average temperature below 0°C (Rihan et al., 2017), which should include the plants under cold (0 - 15°C) and freezing stress (<0°C). Due to their adaptability through the chronological evolution, plants in tropical and subtropical regions usually do not survive sporadic freezing, while those living in temperate regions are tolerant to cold stress (CS) or resilient against freezing by acclimation (Jeon and Kim, 2013). While being under CS, plants and crops reduce their growth productivity, posing a serious threat to food security (Zhang et al., 2022b). With an intensified global warming and unexpected temperature fluctuations due to climate changes, extreme weather conditions occur more frequently and plants/crops are suffering from increasingly severe colds and freezing calamities, causing a devastating yield loss or prominent crop loss (Liu et al., 2022a). Therefore, it has become of great significance and is imperative to understand how CS undermines plant health and impacts crop production and how plants respond to CS and the underlying mechanisms involved in alleviation of CS through application of exogenous substances (ESs).
With recent advances and achievements in molecular biology and CS researches, the complexity and diversity of plant responses to CS and their corresponding mechanisms have been realized and recognized more clearly for the last two decades, which includes but is not limited to various physiological, biochemical, and molecular changes. First and foremost, plants under CS seem to accumulate excessive reactive oxygen species (ROS) resulting in damage of cell membranes, leakage of cell fluids, impairment of normal physiological functions, increment of malondialdehyde content, peroxidation of plasma membranes, and excessive oxidative activities (Chen et al., 2021). Secondly, the chlorophyll synthesis in plants is hindered, various photosynthetic enzymes are deactivated, photosynthesis is inhibited, and photosynthetic rate decreased (Ding et al., 2019). Thirdly, plants respond to CS with noticeable changes in the content of osmoregulatory substances such as soluble sugars, soluble proteins, and proline as well as the water content of plant cells, resulting in osmotic adjustments (Fàbregas et al., 2020). Fourthly, plants under CS tend to enhance the anaerobic respiration, causing the protein denaturation, abnormal hormone levels, and changes in root activity (Ji et al., 2010) that all impairs water absorption, desiccation of aboveground plant tissues (Aroca and Ruiz Lozano, 2012; Hussain et al., 2018), and consequently reduction of root growth and development (Nezhadahmadi et al., 2013; Hassan et al., 2017). Finally, plants are able to sense coming cold and activate expressions of CS-related genes through signal cross-talks to regulate all responses mentioned above in neutralizing the CS impact (Qari et al., 2022) and alleviating its damages. However, this type of self-regulated responses and tolerance in plants is rather limited and species-dependent.
In order to improve the stress resistance in plants, researchers have made countless attempts in finding promising ESs for that purpose and some of them have proven to be effective and applicable (Feng et al., 2023b). Thus, inducing or enhancing positive responses in plants to reverse CS by ESs has become an important research area aiming at revealing functions of ESs and providing their theoretical underlying mechanisms. Although ESs are not magic cures against plant freezing stress and there is a large amount of research literature on them that needs to be carefully analyzed and summarized, we have mainly focused on analyzing the basic mechanisms of ESs that help plants alleviate CS. So far, 72 ESs have been reported to mitigate plant CS in the literatures published during the last two decades around the world and their relevant mechanisms summarized and detailed (Table 1). According to their chemical composition and source (Cheng et al., 2020), ESs are divided into five categories: inorganic salts, organic compounds, plant hormones, plant extracts, and multi-element complexes. While the classification based on chemical composition and source (Cheng et al., 2020) provides one way to categorize ESs, we also adopt another approach considering other aspects. We divide ESs into the chemical or microbial group first and then separate chemical substances into the subgroup of organic (polyamines, polyphenols, peptides, polyols, sugars, esters, hormones, vitamins, amino acids, organic acids, plant growth regulators) and inorganic compounds.
Due to extensive research advances achieved for the last 25 years and accumulative findings available in recent literatures, we have thoroughly reviewed the most recent and outstanding studies that were conducted in using different ESs to alleviate plant CS, summarized their mechanisms involved in responses against different levels of CS through structural, physiological, biochemical, and molecular changes. The review we present here is to shed some light on the future research directions, provide some assistance in finding an appropriate ESs for alleviation of plant CS to some degree, and ultimately solve the problems associated with plants under CS.
2 Research development and advance of ESs in alleviating CS in plants
2.1 Overview
The research on ESs to alleviate plant CS started in the 1940s and has been an international focus in plant biology and physiology. This review uses the keywords “cold stress”, “exogenous substances”, “cold resistance” and “cold tolerance” to search for research papers published between 2000 and 2024 in the Web of Science database. A total of 49956 articles are found and included in the whole collection of the database. From a comparison between countries, Chinese scholars have published a total of 15027 papers (30.1%), American scholars have published 9649 articles (19.3%), Canadian scholars have published 2400 articles (4.8%), German scholars have published 2346 articles (4.7%), Indian scholars have published 2186 articles (4.4%), Japanese scholars have published 2062 articles (4.1%), and the scholars from other countries have published 16286 articles (32.6%). Based on this, a run chart of the number of documents published on cold stress by different countries during 2000 - 2024 has been formed (Figure 1). Figure 1 indicates that more attentions and efforts have been given to the CS studies, such as in China where the number of papers published has increased by more than 100 times in the last 25 years. The global trend of CS research interest also reflects indirectly the frequent occurrence of extreme weather conditions and the increasingly prevalent issues pertaining to the global food availability and demand. So, it has become obvious that the world is paying more attention to plant CS and its possible solution through ESs application.
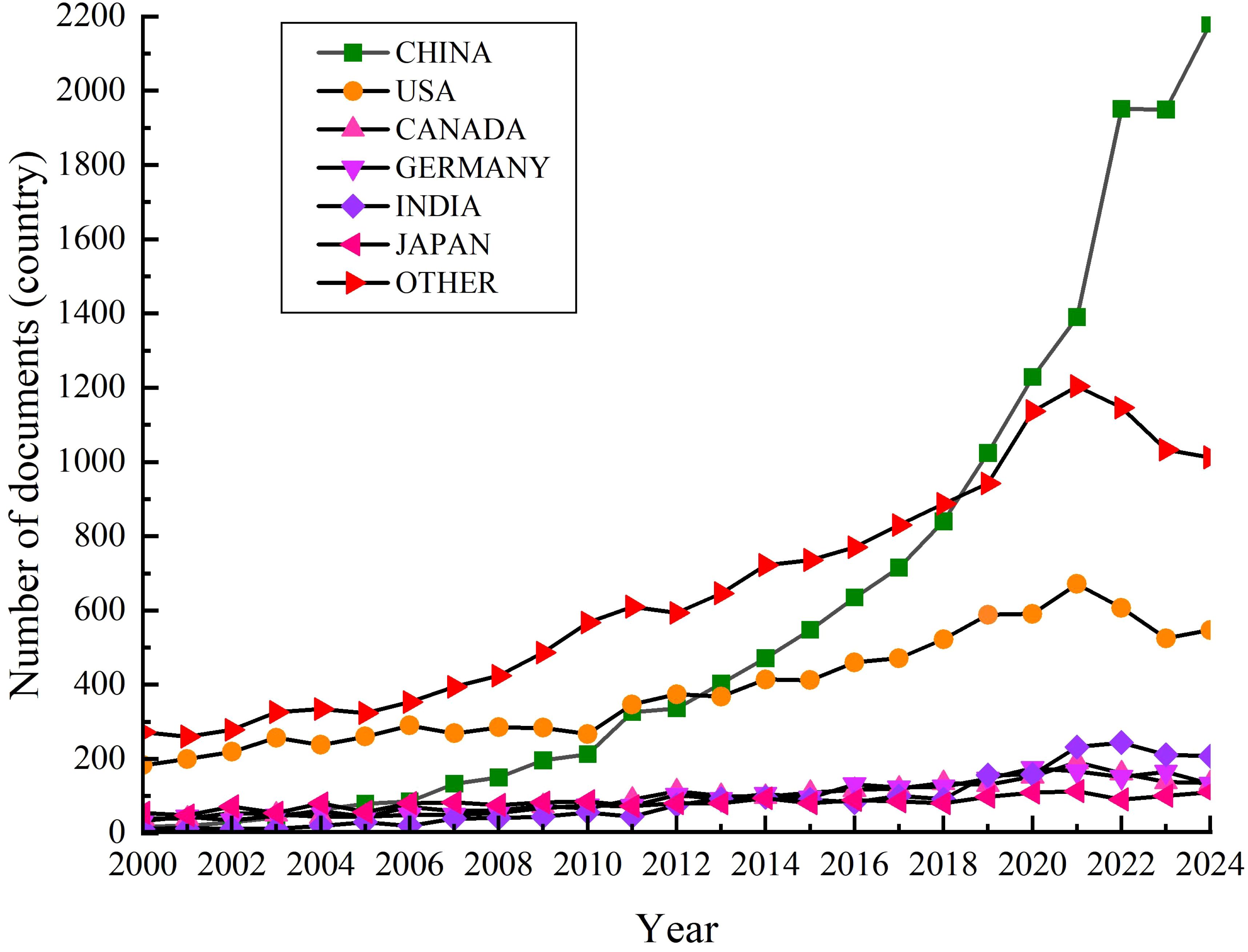
Figure 1. Trends in the number of articles published on cold stress with different countries from 2000 to 2024.
2.2 Analysis of hotspots in international research reports
VOSviewer is used to analyze literature collected from the Web of Science database under keywords of “cold stress”, “exogenous substances”, “cold resistance” and “cold tolerance” during last 25 years (2000-2024) for a research hotspot map (Figure 2). Six hot topics have emerged from the analysis: crop response, crop varieties, growth stages, climate change, types of ESs, and underlying mechanisms, while Melatonin (MT), Nitric oxide (NO), Abscisic acid (ABA), Salicylic acid (SA), Calcium chloride (CaCl2) and ethylene are the most studied ESs to alleviate CS. In terms of the mechanisms associated with the effect of ESs on mitigating CS, “improving antioxidant system and maintaining membrane structure stability”, “hormone regulation”, “enhancing cell osmotic regulation ability”, “improving photosynthetic system” and “improving the nutritional status” are the hot topics derived from the literature analysis. Therefore, we believe that the plant tolerant mechanisms pertaining to effect of ESs on CS alleviation should be separated into 5 groups accordingly: 1) enhancement of cell osmotic adjustment, 2) improvement of antioxidant pathways, 3) involvement in phytohormone regulation, 4) promotion of photosynthesis; 5) enrichment of nutritional status.
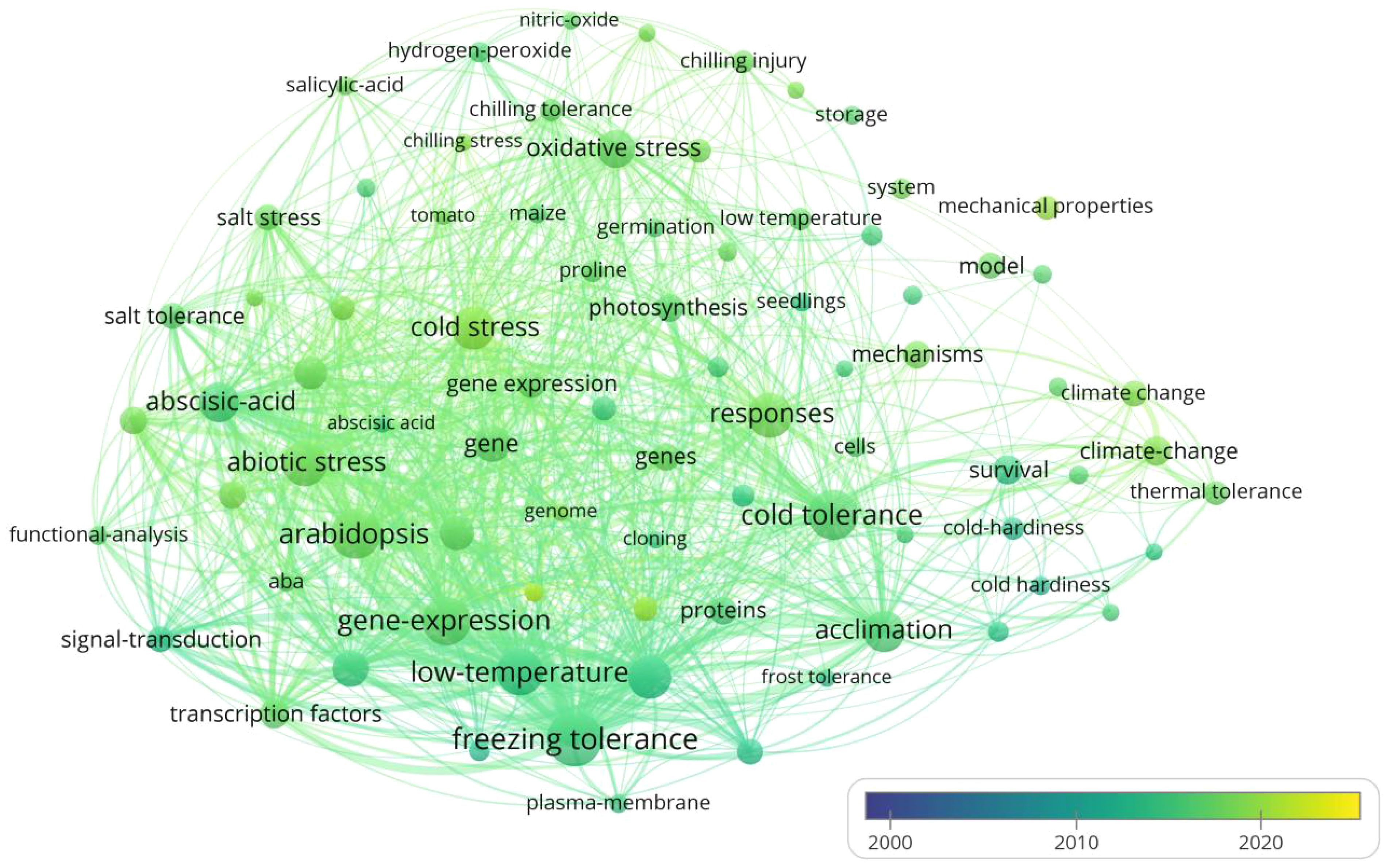
Figure 2. Hotspot analysis of English literatures resulted from searching keywords of “cold stress”, “exogenous substances”, “cold resistance” and “cold tolerance” during last 25 years. The size of each dot represents the focal length of the keywords in the literature, and the line between two points represents closeness of their coupling relationship.
3 The mechanism of ESs underlying plant CS
3.1 Enhancement of cell osmotic adjustment
While being under CS, plants manage to increase their cell fluid, maintain the osmotic pressure in cells, and protect membrane components through accumulating various osmotic adjusting metabolites (Khan et al., 2017) as the first defensive line of cold resistance against an osmotic imbalance likely caused by the exosmosis of cell fluid (Ren et al., 2013). Some ESs have been identified to induce synthesis of osmoregulation substances. For example, spraying propyl gallate (PG) on cucumber plants under CS could increase the soluble sugar content, reduce the cell osmotic potential, and maintain the integrity, structure and function of cucumber proteins in cucumber leaves (Gao et al., 2022). Qian et al. (2021) used 1 μL L-1 1-methylcyclopropene (1-MCP) to fumigate peach fruit for 12 hours, resulting in an increased content of proline (Pro) and phenylpeptide amino acid (PA) for enhanced cold tolerance in fruits through regulating cell osmotic pressure. Also, exogenous proline could be used on pepper callus as a signal molecule to stimulate defense pathways in endogenous proline biosynthesis and improve the accumulation of endogenous proline and antifreeze proteins against CS (Koç, 2013). Spraying 5 mM ferulic acid (FA) on tomatoes under cold stress induced the gene expression of C-repeat binding factors (CBF) in transcription pathway, increased the expression of SlMAPK3, SlCBF1, and SlICE1, and promoted the accumulation of proline and soluble proteins (Shu et al., 2022). Similar effect of ESs on priming chickpea (Cicer arietinum L.) seeds for cold tolerance was observed through soaking seeds with 5 µM GAs under CS to maintain a high relative water content and low electrolyte leakage, maintain water balance, and promote the damaged cells to divide and elongate (Aziz and Pekşen, 2020).
Some beneficial microbes have proven to participate in osmotic adjustment through inoculating tomato seeds with Trichoderma harzianum AK20G strain to reduce the lipid peroxidation rate and electrolyte leakage and increase the leaf water content and proline accumulation (Ghorbanpour et al., 2018). Arbuscular mycorrhizal fungi (AMF) was also used to colonize plant roots to enhance cold tolerance in rice and to form hyphae vesicles that facilitate rice roots to absorb nutrients, promote proline accumulation through enhancing glutamate (Glu) and ornithine (Orn) synthesis (Liu et al., 2022b).
External application of PLD helps the signaling pathway cope with short-term CS in barley seedlings by regulating the balance between proline and ROS levels. In contrast, the decrease in PLD activity in response to long-term cold stress does not affect proline levels. The lipid signaling triggered by PLD plays a key role in both short-term and long-term cold stress in barley (Peppino Margutti et al., 2017).
So, it can be seen that this CS mitigating mechanism mainly works through improving the cell osmoregulation which is likely related to the antioxidant metabolism and membrane structure. Interestingly, this type of osmotic damages caused by cold may not be as severe as those caused by drought or salt stress, so there are not enough studies on using ESs for osmoregulation. Perhaps that’s why there are relatively fewer studies on using ESs to further enhance osmoregulation substances. However, priming the osmotic regulation ability of seed or seedling cells may be still meaningful for plants to develop a sustainable cold tolerance in the field and needs more attention and effort.
3.2 Improvement of antioxidant pathways
Under CS, a large amount of ROS accumulates in plant cells. ROS can act as a signaling substance in cells to induce gene expressions and protein syntheses for cold tolerance in plants (Su et al., 2019), but it can also interfere with normal oxidative activities (Heidarvand and Maali Amiri, 2013), disrupt the stability of proteins or protein complexes, reduce the activity of ROS scavenging enzymes, and lead to photoinhibition of photosynthesis and damaged cell membranes (Siddiqui and Cavicchioli, 2006). Introducing ESs can enhance antioxidant capacity of plants to remove superoxide anions, hydrogen peroxide (H2O2), malondialdehyde and other peroxides through activating the enzymes involved in the antioxidant pathway and promoting the production of non-enzymatic antioxidant substances (Feng et al., 2023a). Non enzymatic antioxidant substances refer to small molecule compounds with antioxidant properties, including the human metabolite uric acid and intracellular synthesized glutathione, as well as vitamin E, vitamin C, carotenoids (such as astaxanthin, lutein, zeaxanthin, etc.), selenium, copper and other nutrients, and phytochemicals such as tea polyphenols. The effect of ESs on stabilizing the oxidative system does not only help plants avoid potential damages caused by oxidative stress but also effectively protect plant cells from abnormal changes in the membrane spatial configuration, permeability, or leakage. Moreover, some ESs have been found to act as signaling molecules to promote plant cold regulatory and defensive activities against cold (Song et al., 2015). Moreover, some TF families encoding the cold tolerance include DREB, WRKY, NAC, MYB, AP2, ERF, and bHLH (Yang et al., 2018), among which CBF1, CBF2, CBF3, CBF4, ICE1, ICE2, CAMTA3, MYB15, ZAT12, COR15a, and COR15b can regulate the cold signaling and cold stress. With the application of ESs, TFs balanced the ROS content in plants through the indirect action, alleviated the oxidative damage of cell membranes, maintained the normal metabolism of plants, and improved cold tolerance (Ritonga and Chen, 2020). Exogenous SA was reported to induce an accumulation or biosynthesis of some extracellular proteins in barley, regulate the activities of cytoplasmic extracellular antioxidant enzymes and ice nucleation, and diversify extracellular proteins for enhanced cold tolerance against CS (Mutlu et al., 2013; Turkyilmaz Unal et al., 2015). Lei et al. (2017) found that γ-Polyglutamic acid (γ-PGA) secreted by bacteria could increase the H2O2 content in root cells of rapeseed seedlings and transmit H2O2 to leaves through the Ca2+ signaling channel to activate antioxidant enzymes for removal of H2O2 and various peroxide metabolites in enhancing cold tolerance. The postharvest γ-Aminobutyric acid (GABA) treatment of cucumber fruit was reported to increase antioxidant enzyme activity, reduce ROS accumulation (Malekzadeh et al., 2017), protect cell membranes from impairment due to CS, which is consistent with the findings concluded from the study on exogenous GABA treatment of tomato seedlings under CS (Abd Elbar et al., 2021). Zhang et al. (2021) injected Flavobacterium succinate (a plant growth promoting microbe) into the roots of ryegrass seedlings to enhance cold tolerance through the secreted IAA, dissolved phosphorus, and enriched iron carriers to increase the biomass, antioxidant enzyme activity, soluble sugars, and proline content, reduce the relative conductivity and malondialdehyde content, and keep the redox balance in check (Zhang et al., 2021). More examples of the effect of ESs on mitigating CS have shown by using exogenous boron (B) to enhance plant antioxidant enzyme activity and reduce the negative impact of ROS (Ghassemi et al., 2021) and applying exogenous CO2 to increase the activity of antioxidant enzymes and the concentration of osmoregulation substances in wheat leaves for an adjustment of cold tolerance in wheat offspring through the sucrose metabolism (Li et al., 2020). It has come to a consensus that the negative effect caused by excessive accumulation of ROS can be alleviated through various ESs applications (Table 2), but the relationship between ROS and major metabolites such as proline is still unclear.
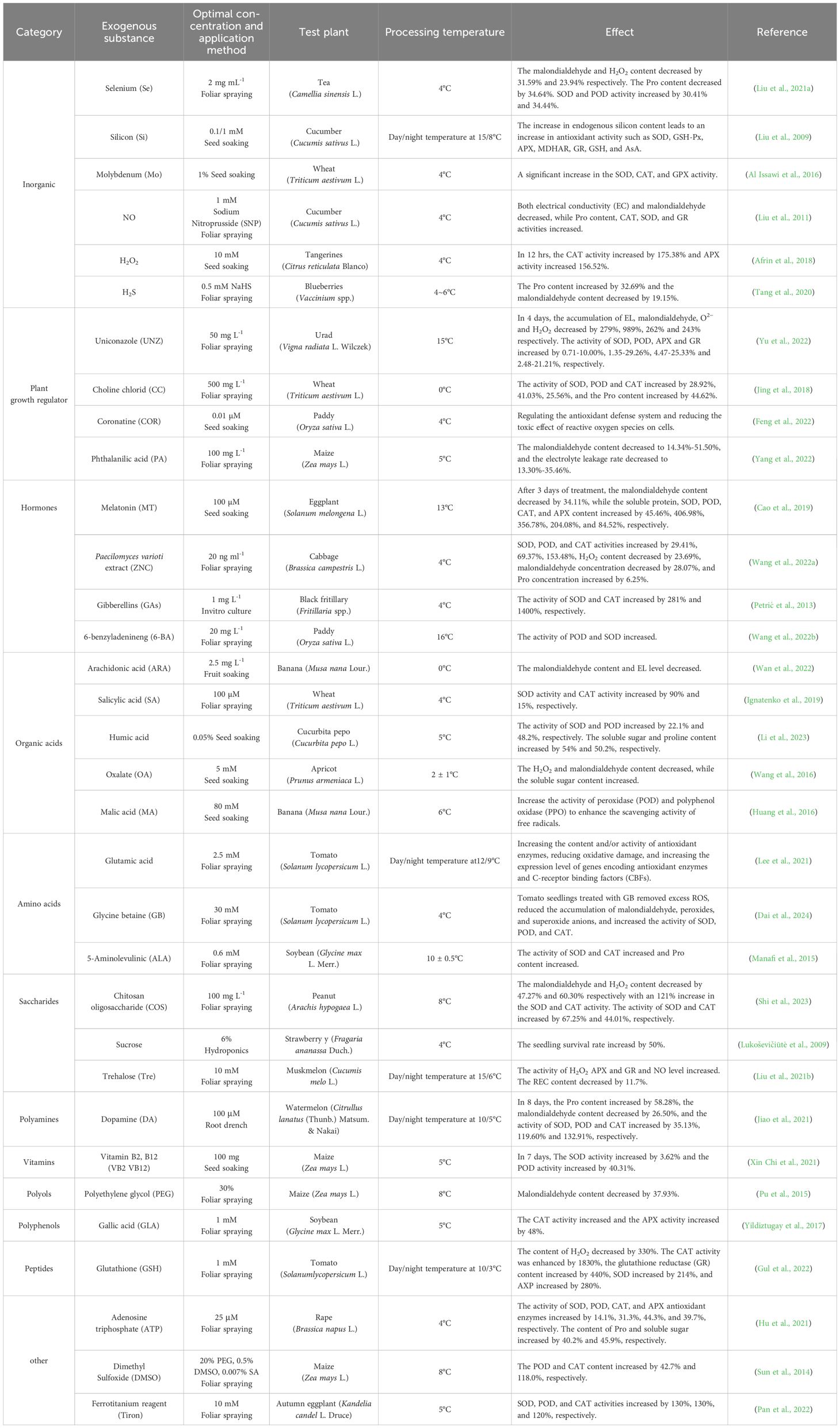
Table 2. Optimal concentration and application method of exogenous substances used to improve antioxidant system.
3.3 Phytohormone regulation
Plant hormones are essential in regulating plant growth, development and reproduction serving also as signaling molecules that respond to stress and widely participating in crop physiological processes as cross-talk messengers (Li et al., 2017). It has been noticed that plant cold resistance is induced and primed through a disruption of the hormone balance to begin with (Huang et al., 2021) and any ES that interfere with the phytohormone balance has the potential to be used for adjustment of plant cold tolerance. Under CS, plant growth hormones (such as auxin, gibberellin, cytokinin, etc.) usually sense changes such as reduced metabolism, obstructed transportation and disturbed signal transduction, leading to an inhibition of plant growth. However, low temperature always reduces the water availability in plant cells, causing osmotic stress and stimulating the ABA synthesis (Raza et al., 2023). ABA proves to play an important role in many cellular processes. Endogenous ABA is transported from roots to leaves and accumulates in guard cells to regulate stomatal closure and reduce the transpiration rate and cell growth (Raza et al., 2023). A foliar application of exogenous ABA helps activate the antioxidant defense system mediated by nitric oxide (NO), prevent excessive accumulation of ROS, and alleviate oxidative damage caused by cold stress (Dong et al., 2017). Therefore, some ESs used to adjust the phytohormone balance in plants under CS can improve plant cold tolerance. Exogenous MT was recognized to activate the endogenous hormone metabolic pathways, promote the amount of endogenous hormones SA, JA, Auxin (IAA), and reduce the levels of ABA, ethylene (ETH), and GAs, which is conducive to the growth and development of red bean seedlings under CS (Chen et al., 2021). Exogenous Methyl Jasmonate (MeJA) proves to promote the expression of genes that modulate the jasmonic acid biosynthesis in plants under CS, leading to an increase in endogenous jasmonic acid content, thereby enhancing the expression of three jasmonic acid responsive transcription factors and improving artemisinin biosynthesis (Liu et al., 2017). Zuo et al. (2022) used 15 mM Pro to soak corn seeds and found that Pro alleviated the decrease in IAA, zeatin nucleoside, and GA content in corn embryos, reduced the increase in the ABA level, which alleviated the inhibitory effect of cold on seed germination. The application of Zinc enhanced the synthesis of cytokinin (CTK) and IAA in rice tillering buds under CS, promoted the transportation of IAA from tillering buds to other parts of rice plants, and improved the resistance of rice against CS (Liu et al., 2022d). Exogenous 2, 4 epibrassinolide (EBR) was found to increase the content of GAs and IAA in tomato seedlings, accelerated the tomato growth rate, and alleviated the damage caused by CS (Heidari et al., 2021). Spermidine (Spd) promoted wheat seed germination by regulating the content of abscisic acid and gibberellin, as well as starch degradation (Gu et al., 2019).
Mechanisms of exogenous substances involved in enhancing plant cold tolerance are shown in Figure 3. At present, research on cold tolerance through regulating endogenous hormone levels in plants through ESs should have drawn more attention and investment and the interactive pathways between endogenous and exogenous hormones should be further explored.
3.4 Promotion of photosynthesis
As a key physiological process in plants, photosynthesis is highly sensitive to and regulated by temperature fluctuations (Zhu et al., 2018) due to the involvement of many catalytic enzymes. Cold impacts on the photosynthetic system including the photosynthetic pigments, photosynthetic parameters and chlorophyll fluorescence responses, likely affecting all components involved such as the structure and function of chloroplast, stomata, chlorophylls, transpiration rate (Tr), intercellular CO2 concentration, apparent quantum efficiency, dark respiration rate, photochemical efficiency, non-photochemical quenching coefficient, chlorophyll fluorescence parameters enzyme activity, photosynthetic electron transfer, carbon assimilation process, and photochemical reactions (Li and Cui, 2018; Lambers et al., 2008). Researches have proven that suitable ESs are useful to alleviate CS impacts on the photosynthetic system. Applying SA during the winter wheat seedling stage significantly improved the photosynthesis and photochemical efficiency of the photosystem II, effectively alleviated CS impacts on the net photosynthetic rate, plant height, biomass, and yield, and significantly prolonged the wheat growth window (Wang et al., 2021a). Exogenous Tre also proved to be effective on salvage impairments of wheat grain due to CS at booting stage. The alleviation obviously occurred through enhancing nitrogen assimilation, increasing the content of endogenous Spd, ascorbic acid (GSH) and AsA, promoting the GSH/AsA cycle, and alleviating an inhibition of wheat floret development (Liang et al., 2021). Moreover, additional exogenous phosphorus (P) fertilizer helped slow down the senescence of wheat flag leaves, increase green leaf area and photosynthetic capacity, improve assimilation accumulation, promote the transportation of assimilates to grains, thereby reduce wheat yield loss due to CS (Xu et al., 2022). Overall, the chlorophyll content is the basis of photosynthesis and reflects the photosynthetic efficiency of a plant, therefore, there is a need for systematic researches on such chlorophylls as indicators of photosynthetic effects regulated by ESs, and their corresponding changes of photosynthetic characteristics. The ESs related to improving the photosynthetic system and their optimal application concentrations are detailed in Table 3.
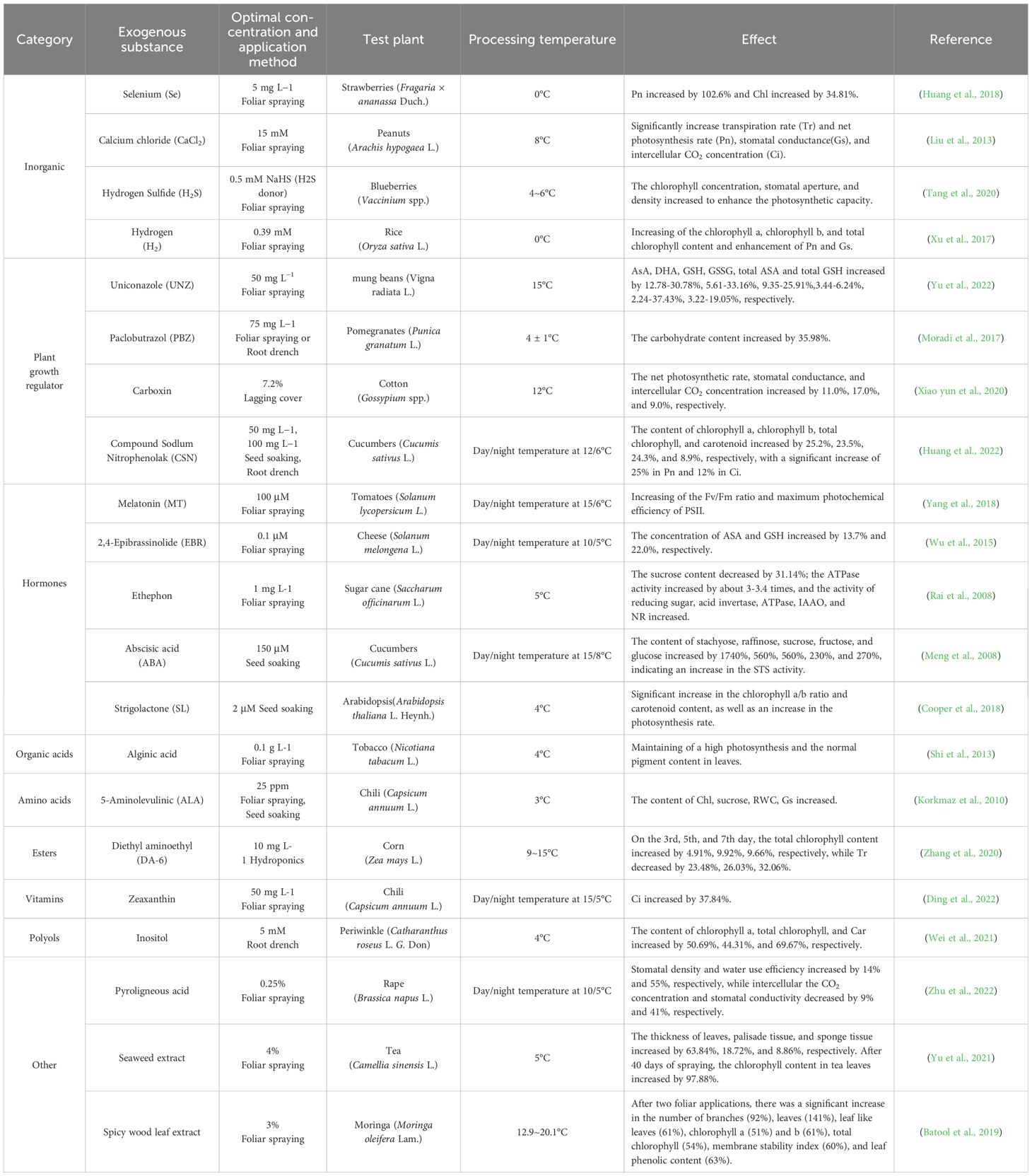
Table 3. The optimal concentration of exogenous substances for partial improvement of photosynthesis.
3.5 Enrichment of nutritional status
Under CS conditions, plant growths and metabolisms are significantly affected due to a reduced or limited absorption and utilization of minerals and nutrients. In plants, coldness hinders physiological activities and slows down all metabolisms, especially in roots. It also decreases the permeability of cell membranes throughout the whole plants (Zhang and Sonnewald, 2017). To help plants fight against CS, some ESs have demonstrated their property in enriching the nutritional status in plants so as to alleviate CS. They can be used as nutrients themselves alone or as to promote the nutrient absorption and transport, redistribution and metabolic activities. In that regard, some beneficial microbes have been found to help plants grow and develop normally under CS such as plant growth promoting bacteria (PGPB) that were considered to stimulate nitrogen fixation, nutrient dissolution, phytohormone synthesis and to regulate iron carrier production, chitinase activity, antibiotic and cyanide production in plants (Selvakumar et al., 2010). Supplementing Put in plants under CS activated the arginine polyamine pathway in red palm, induced the synthesis of endogenous arginine (Arg), improved the synthesis and conversion rate of endogenous arginine, and reduced the decomposition rate of endogenous arginine (Sun et al., 2021). Rai et al. (2008) found that the application of potassium (K), ethephon and zinc (Zn) to sugarcane at 5°C increased the germination rate by 80%, 50% and 40%, respectively, promoted the activities of sugar - reducing enzymes, acid invertase, ATPase, indole acetic acid oxidase (IAAO) and nitrite reductase (NR), and enhanced the oxidative irritability and nitrogen content to stimulate the growth of buds. Pepper seeds treated with chitosan demonstrated an enhanced activity of chitinase and glucanase that thereby increased the germination rate and better protected seedlings from fungal diseases under CS (Samarah et al., 2020). As a beneficial bacterium in soil, Bacillus subtilis was able to colonize, grow and help absorb nutrients through participating in the nitrogen fixation, secretion of antibiotics, activation of proteases, and dissolution of inorganic and organic phosphorus in roots of maize seedlings (Zhang et al., 2022a). Similarly, Pseudomonas strains that were introduced to roots proved to synthesize IAA in vegetables under CS by stimulating the seed and tuber germination, triggering the plant cell division and proliferation, and controlling the vegetative growth to alleviate CS damages (Tsavkelova et al., 2006; Spaepen and Vanderleyden, 2011). Overall, most studies on enrichment of nutritional status with ESs have focused on the absorption and utilization of nutrients, and further researches should be aimed to improve nutrient redistribution so that more nutrients to be available and utilized for growth and development for plant cold tolerance.
4 Summary
With a global climate change and frequent occurrence of extreme weather, the plant CS is inevitable. Research has shown that ESs play a positive role in enhancing plant tolerance/resistance against CS and therefore has drawn many researchers’ attention to determine on mechanisms involved in both plant cold tolerance against CS and application of ESs in alleviating adversary effects of CS. Up to date, about 72 ESs have been identified and evaluated for their possible use in plants to tolerate CS during the past 25 years and ESs research efforts have expanded from a few chemicals for structural, morphological and preliminary physiological adaptations at the beginning to the current status involving in various ESs on biochemical, molecular, and microbial functions and their interactions and crosstalks through signal transmission throughout the entire regulatory system. This review of over a hundred research papers here has grouped mechanisms of ESs in alleviating plant CS into 5 categories with a summarized elaboration (Figure 4). However, due to the differences and uniqueness of each plant species, the whole picture of a plant’s adaptation to cold through using ESs to adjust its morphological traits, internal physiological structures, gene regulation and biochemistry is not fully drawn, therefore, the undertakings of the researches on ESs will continue, especially when extreme temperature downshifts become more frequent.
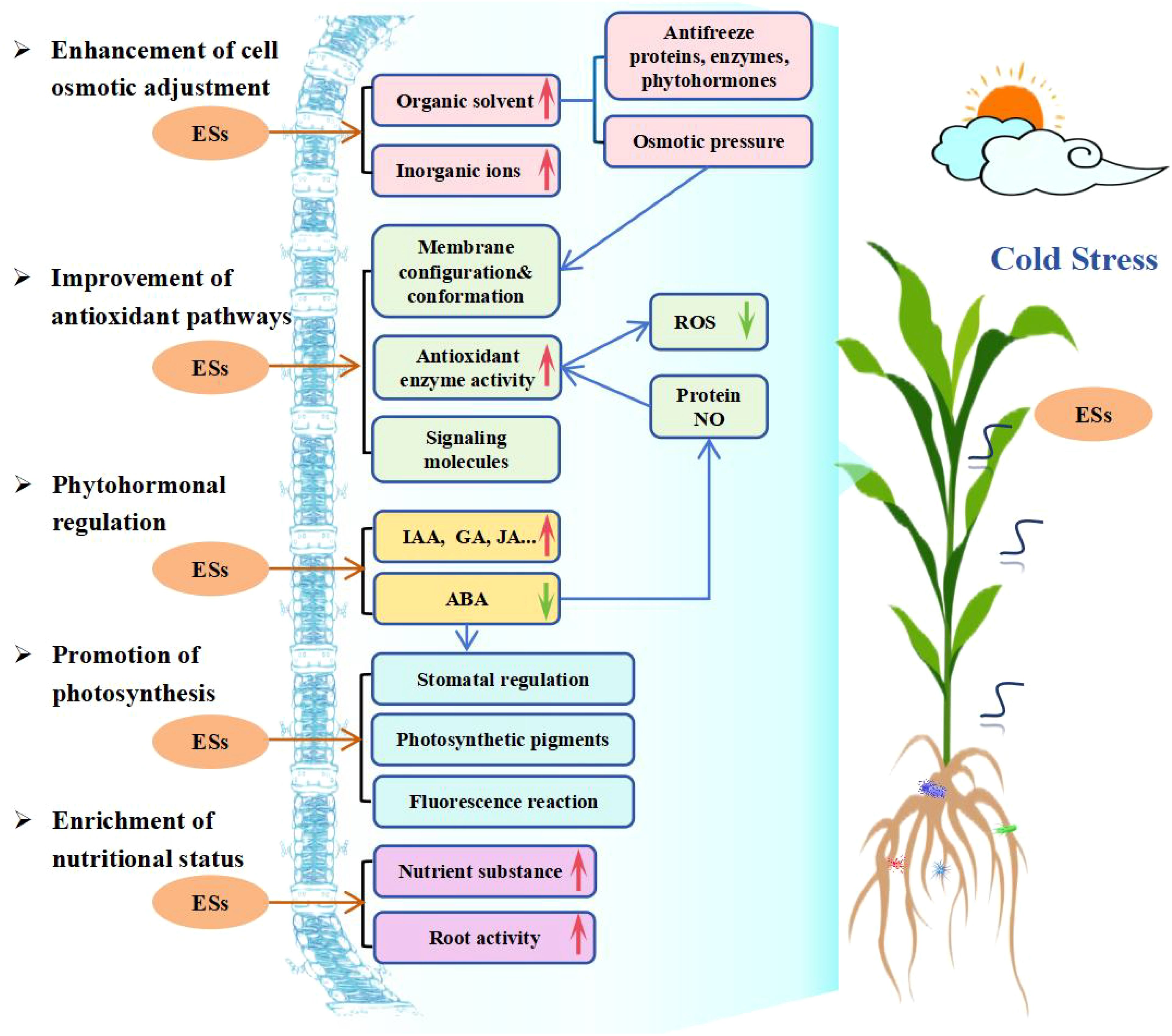
Figure 4. Mechanisms of exogenous substances (ESs) involved in enhancing plant cold tolerance. Red and green arrows indicate promotion/increase or inhibition/decrease, respectively.
5 Outlook
For ESs to achieve their expected efficacy in CS alleviation, the application method can be critically important. So far, the foliar spraying, seed soaking, root irrigation, hydroponics, and inoculation are five application methods that have been used. Among those, leaf spraying seems to be the most commonly used application, especially on seedlings due to the fact that leaves provide the largest area to receive sprayed ESs and can respond to them instantly. However, ESs should be applied according to their chemical properties, the action sites of ESs, type of crops, and growth stage of plants since the function, mechanism and efficacy of ESs may vary under certain circumstances. At present, there is no unified standard for the amount and duration of use of ESs in most studies, resulting in a lack of reference basis for the use of ESs and a bias in understanding the effects of ESs. So, this review has provided a detailed list of the optimal application method, concentration, plant growth stage that are derived from literatures and can be used as a reference provided any of those ESs are intended to be used for further studies. In addition, in case there are situations where multiple stresses occur simultaneously, use of couple or multiple ESs can be attempted to induce more resilience in plants against abiotic stresses, and for that purpose, this review can be used to find relevant information of each individual ESs. In addition, most studies on using ESs to alleviate CS in plants are conducted on seedlings in a laboratory or greenhouse settings and a field trial should be more informative and practical to verify the efficacy or “priming” effect of ESs on field crops.
Cold stress drives plants to respond with the structural and physiological adjustments through sensing the temperature downshift, transmitting a stress signal to interior of cells, and ultimately inducing gene expressions in response to CS. Certain ESs demonstrate their efficacy in inducing the expressions of certain genes encoding for cold tolerance and antifreeze proteins including their precursors (Rayirath et al., 2009). However, all these plant responses to CS relating to gene expressions are presumably initiated by the cold receptors that sense the low temperature and turn on the “molecular switch”. CBF seems to be one of those protein receptors activated under CS and subsequently to trigger a series of signal responsive pathways and interactions among various defensive mechanisms, activate the expression of multiple downstream CS responsive genes, enhance cold resistance, and enable plants to adapt and survive under CS. Therefore, the CBF signaling pathway can be a reinforcement of plant tolerance against CS other than the preexisted mechanisms such as structural adaptation, adjustment of membrane lipids, production of antifreeze proteins, etc. Moreover, any ES that affects gene expressions, such as CBF should be explored further to induce a possible and temporary “dormancy” in plant seedlings prior to the incoming low temperature with reduced metabolisms and a minimized growth rate for their tolerance against temporary CS.
This review has elaborated in great detail on positive effects of ESs on alleviation of plant CS, but we strongly believe that they are not sufficient and effective to mitigate the damages brought upon by extreme cold/freezing conditions beyond plant can endure. Finally, we suggested that some research areas be further focused and endeavored to reveal more fundamental responses and their underlying mechanism in plants against CS: 1) the relationship between ROS and major metabolites such as proline; 2) the interaction and constraint pathways between endogenous hormones that are regulated by ESs; 3) the changes pertaining to photosynthetic processes that are regulated by ESs; 4) the post transcriptional process and post translational modification of plant cold tolerance genes; and 5) the low-temperature cold signal regulatory network and cold signal transduction/crosstalks in other crops.
Overall, this review theoretically summarizes the underlying mechanisms of ESs involved in enhancing cold tolerance and alleviating CS in plants and provides comprehensive perspectives for the future research with paramount knowledge and various directions on chemistry, microbiology, plant physiology, and molecular biology. With information collectively provided in the review, further research can be focused on developing actual work plans and novel ES-based strategies to CS related issues and possible impact on plant growth, crop production, yield loss and food security.
Author contributions
DF: Conceptualization, Funding acquisition, Writing – review & editing, Writing – original draft. MZ: Investigation, Software, Writing – original draft. JX: Formal Analysis, Writing – original draft. WX: Funding acquisition, Writing – review & editing. QG: Methodology, Software, Writing – original draft. JL: Writing – review & editing. CL: Writing – original draft, Writing – review & editing. XS: Writing – original draft, Writing – review & editing.
Funding
The author(s) declare that financial support was received for the research and/or publication of this article. This study was supported by Major Science and Technology Special Projects of Xinjiang Uygur Autonomous Region 2024A03008-3, by “Tianshan Elite Scholars” Program for Innovative Leading Talents in Science and Technology (No. 2022TSYCLJ0039), and by the Project of Fund for Stable Support to Agricultural Sci-Tech Renovation (No. xjnkywdzc-2024001-04).
Conflict of interest
The authors declare that the research was conducted in the absence of any commercial or financial relationships that could be construed as a potential conflict of interest.
Publisher’s note
All claims expressed in this article are solely those of the authors and do not necessarily represent those of their affiliated organizations, or those of the publisher, the editors and the reviewers. Any product that may be evaluated in this article, or claim that may be made by its manufacturer, is not guaranteed or endorsed by the publisher.
References
Abd Elbar, O. H., Elkelish, A., Niedbała, G., Farag, R., Wojciechowski, T., Mukherjee, S., et al. (2021). Protective effect of γ-aminobutyric acid against chilling stress during reproductive stage in tomato plants through modulation of sugar metabolism, chloroplast integrity, and antioxidative defense systems. Front. Plant Sci. 12, 663750. doi: 10.3389/fpls.2021.663750
Afrin, S., Tahjib Ul Arif, M., Sakil, M. A., Sohag, A. A. M., Polash, M. A. S., Hossain, M. A. (2018). Hydrogen peroxide priming alleviates chilling stress in rice (Oryza sativa L.) by enhancing oxidant scavenging capacity. Fundam Appl. Agric. 4, 713–722. doi: 10.5455/faa.13554
Al Issawi, M., Rihan, H. Z., Al Shmgani, H., Fuller, M. P. (2016). Molybdenum application enhances antioxidant enzyme activity and COR15a protein expression under cold stress in wheat. J. Plant Interact. 11, 5–10. doi: 10.1080/17429145.2015.1129074
Aroca, R., Ruiz Lozano, J. M. (2012). “Regulation of root water uptake under drought stress conditions,” in Plant Responses to Drought Stress: From Morphological to Molecular Features. Ed. Aroca, R. (Springer Berlin Heidelberg, Berlin, Heidelberg), 113–127.
Aziz, T., Pekşen, E. (2020). Seed priming with gibberellic acid rescues chickpea (Cicer arietinum L.) from chilling stress. Acta Physiol. Plant 42, 139. doi: 10.1007/s11738-020-03124-x
Batool, S., Khan, S., Basra, S. M. A. (2019). Foliar application of moringa leaf extract improves the growth of moringa seedlings in winter. South Afr. J. Bot. 129, 347–353. doi: 10.1016/j.sajb.2019.08.040
Cao, Q., Li, G., Cui, Z., Yang, F., Jiang, X., Diallo, L., et al. (2019). Seed Priming with Melatonin Improves the Seed Germination of Waxy Maize under Chilling Stress via Promoting the Antioxidant System and Starch Metabolism. Sci. Rep. 9, 15044. doi: 10.1038/s41598-019-51122-y
Chen, Z., Jin, X., Li, H., Zhou, W., Qiang, B., Liu, J., et al. (2021). Effects of exogenous melatonin on growth, photosynthetic fluorescence characteristics and yield components of red bean. Crop J. 37, 88–94. doi: 10.27122/d.cnki.ghlnu.2021.000086
Cheng, L., An, F., Xie, G., Wang, L., Zhang, X., Wang, J. (2020). Research progress of plant cold resistance regulator substances. Trop. Agric. Sci. 40, 65–75. doi: 10.12008/j.issn.1009-2196.2020.05.011
Cooper, J. W., Hu, Y., Beyyoudh, L., Yildiz Dasgan, H., Kunert, K., Beveridge, C. A., et al. (2018). Strigolactones positively regulate chilling tolerance in pea and in Arabidopsis. Plant Cell Environ. 41, 1298–1310. doi: 10.1111/pce.13147
Dai, T., Ban, S., Han, L., Li, L., Zhang, Y., Zhang, Y., et al. (2024). Effects of exogenous glycine betaine on growth and development of tomato seedlings under cold stress. Front. Plant Sci. 15, 1332583. doi: 10.3389/fpls.2024.1332583
Ding, D., Li, J., Xie, J., Li, N., Bakpa, E. P., Han, K., et al. (2022). Exogenous zeaxanthin alleviates low temperature combined with low light induced photosynthesis inhibition and oxidative stress in pepper (Capsicum annuum L.) plants. Curr. Issues Mol. Biol. 44, 2453–2471. doi: 10.3390/cimb44060168
Ding, H., Wang, M., Xie, J., Xiong, X., Hu, X., Qin, Y. (2019). Plant response to low temperature stress and research progress. Jiangsu Agric. Sci. 47, 31–36. doi: 10.15889/j.issn.1002-1302.2019.14.007
Dong, N., Qi, J., Li, Y., Chen, Y., Hao, Y. (2017). Effects of abscisic acid and nitric oxide on chilling resistance and activation of the antioxidant system in walnut shoots in vitro. J. Amer Soc. Hort Sci. 142, 376–384. doi: 10.21273/JASHS04197-17
Fàbregas, N., Yoshida, T., Fernie, A. R. (2020). Role of Raf-like kinases in SnRK2 activation and osmotic stress response in plants. Nat. Commun. 11, 6184. doi: 10.1038/s41467-020-19977-2
Feng, D., Gao, Q., Liu, J., Tang, J., Hua, Z., Sun, X. (2023a). Categories of exogenous substances and their effect on alleviation of plant salt stress. Eur. J. Agron. 142, 126656. doi: 10.1016/j.eja.2022.126656
Feng, D., Wang, X., Gao, J., Zhang, C., Liu, H., Liu, P., et al. (2023b). Exogenous calcium: Its mechanisms and research advances involved in plant stress tolerance. Front. Plant Sci. 14. doi: 10.3389/fpls.2023.1143963
Feng, T., Zhang, Y., He, M., Zhang, M., Li, Z., Zhou, Y., et al. (2022). Coronatine alleviates cold stress by improving growth and modulating antioxidative defense system in rice (Oryza sativa L.) seedlings. Plant Growth Regul. 96, 283–291. doi: 10.1007/s10725-021-00775-9
Gao, W., Li, P., Che, H., Zhao, M. (2022). Propyl gallate affects physiological and biochemical characteristics of cucumber seedlings under low temperature stress. Chin. Cucurbits Veg 35, 70–74. doi: 10.16861/j.cnki.zggc.20220808.001
Ghassemi, S., Delangiz, N., Asgari Lajayer, B., Saghafi, D., Maggi, F. (2021). Review and future prospects on the mechanisms related to cold stress resistance and tolerance in medicinal plants. Acta Ecol. Sin. 41, 120–129. doi: 10.1016/j.chnaes.2020.09.006
Ghorbanpour, A., Salimi, A., Ghanbary, M. A. T., Pirdashti, H., Dehestani, A. (2018). The effect of Trichoderma harzianum in mitigating low temperature stress in tomato (Solanum lycopersicum L.) plants. Sci. Hortic. 230, 134–141. doi: 10.1016/j.scienta.2017.11.028
Gu, X., Wei, B., Liao, Y., Wen, X., Liu, Y. (2019). Spermidine promotes starch degradation to relieve the inhibitory effect of chilling on wheat seed germination. Acta Physiol. Plant 41, 32. doi: 10.1007/s11738-019-2821-1
Gul, N., Ahmad, P., Wani, T. A., Tyagi, A., Aslam, S. (2022). Glutathione improves low temperature stress tolerance in pusa sheetal cultivar of Solanum lycopersicum. Sci. Rep. 12, 12548. doi: 10.1038/s41598-022-16440-8
Hassan, M. U., Aamer, M., Chattha, M. U., Ullah, M. A., Sulaman, S., Nawaz, M., et al. (2017). The role of potassium in plants under drought stress: mini. J. Basic Appl. Sci. 13, 268–271. doi: 10.6000/1927-5129.2017.13.44
Heidari, P., Entazari, M., Ebrahimi, A., Ahmadizadeh, M., Vannozzi, A., Palumbo, F., et al. (2021). Exogenous EBR ameliorates endogenous hormone contents in tomato species under low-temperature stress. Horticulturae 7, 84. doi: 10.3390/horticulturae7040084
Heidarvand, L., Maali Amiri, R. (2013). Physio-biochemical and proteome analysis of chickpea in early phases of cold stress. J. Plant Physiol. 170, 459–469. doi: 10.1016/j.jplph.2012.11.021
Hu, X., Li, Y., Zhang, X., Liang, J., Zhang, T. (2021). Effects of exogenous ATP on cold tolerance of rapeseed seedlings. Plant Res. 41, 302–311. doi: 10.7525/j.issn.1673-5102.2021.02.018
Huang, H., Jian, Q., Jiang, Y., Duan, X., Qu, H. (2016). Enhanced chilling tolerance of banana fruit treated with Malic acid prior to low-temperature storage. Postharvest Biol. Technol. 111, 209–213. doi: 10.1016/j.postharvbio.2015.09.008
Huang, B., Li, W., Sun, M., Yan, Y., Wang, J., He, C., et al. (2022). Effect of compound sodium nitrophenolate on seed germination and seedling cold tolerance of cucumber under low temperature. J. Nucl. Agric. Sci. 36, 845–855. doi: 10.11869/j.issn.100-8551.2022.04.0845
Huang, C., Qin, N., Sun, L., Yu, M., Hu, W., Qi, Z. (2018). Selenium improves physiological parameters and alleviates oxidative stress in strawberry seedlings under low-temperature stress. Int. J. Mol. Sci. 19, 1913. doi: 10.3390/ijms19071913
Huang, C., Tian, Y., Zhang, B., Hassan, M. J., Li, Z., Zhu, Y. (2021). Chitosan (CTS) alleviates heat-induced leaf senescence in creeping bentgrass by regulating chlorophyll metabolism, antioxidant defense, and the heat shock pathway. Molecules 26, 5337. doi: 10.3390/molecules26175337
Hussain, H. A., Hussain, S., Khaliq, A., Ashraf, U., Anjum, S. A., Men, S., et al. (2018). Chilling and drought stresses in crop plants: implications, cross talk, and potential management opportunities. Front. Plant Sci. 9. doi: 10.3389/fpls.2018.00393
Ignatenko, A., Talanova, V., Repkina, N., Titov, A. (2019). Exogenous salicylic acid treatment induces cold tolerance in wheat through promotion of antioxidant enzyme activity and proline accumulation. Acta Physiol. Plant 41, 80. doi: 10.1007/s11738-019-2872-3
Jeon, J., Kim, J. (2013). Cold stress signaling networks in Arabidopsis. J. Plant Biol. 56, 69–76. doi: 10.1007/s12374-013-0903-y
Ji, S., Dai, S., Liu, W. (2010). Research progress on the mechanism of plant response to low temperature stress. Life Sci. 22 (10), 1013–1019. doi: 10.13376/j.cbls/2010.10.006
Jiao, C., Lan, G., Sun, Y., Wang, G., Sun, Y. (2021). Dopamine alleviates chilling stress in watermelon seedlings via modulation of proline content, antioxidant enzyme activity, and polyamine metabolism. J. Plant Growth Regul. 40, 277–292. doi: 10.1007/s00344-020-10096-2
Jing, E., Gao, X., Li, Z., Zhang, L., Yu, X., Ren, Y., et al. (2018). Physiological regulatory effects of choline chloride on the low temperature tolerance of wheat seedlings. J. Wheat Crops 38, 748–755. doi: 10.7606/j.issn.1009-1041.2018.06.16
Khan, T. A., Fariduddin, Q., Yusuf, M. (2017). Low-temperature stress: is phytohormones application a remedy? Environ. Sci. pollut. Res. 24, 21574–21590. doi: 10.1007/s11356-017-9948-7
Koç, E. (2013). The effect of exogenous proline and salicylic acid application on proline and apoplastic protein in cold tolerance of pepper callus cultures. Int. J. Agric. Biol. 15 (2), 382–385.
Korkmaz, A., Korkmaz, Y., Demirkıran, A. R. (2010). Enhancing chilling stress tolerance of pepper seedlings by exogenous application of 5-aminolevulinic acid. Environ. Exp. Bot. 67, 495–501. doi: 10.1016/j.envexpbot.2009.07.009
Lee, H. J., Lee, J. H., Wi, S., Jang, Y., An, S., Choi, C. K., et al. (2021). Exogenously applied glutamic acid confers improved yield through increased photosynthesis efficiency and antioxidant defense system under chilling stress condition in Solanum lycopersicum L. cv. Dotaerang Dia. Sci. Hortic. 277, 109817. doi: 10.1016/j.scienta.2020.109817
Lei, P., Pang, X., Feng, X., Li, S., Chi, B., Wang, R., et al. (2017). The microbe-secreted isopeptide poly-γ-glutamic acid induces stress tolerance in Brassica napus L. seedlings by activating crosstalk between H2O2 and Ca2+. Sci. Rep. 7, 1–15. doi: 10.1038/srep41618
Li, X., Cui, H. (2018). Research progress on plant photosynthetic physiology under low temperature stress. Shandong For. Sci. Technol. 48, 90–94. doi: 10.3969/j.issn.1002-2724.2018.06.027
Li, H., Kong, F., Tang, T., Luo, Y., Gao, H., Xu, J., et al. (2023). Physiological and transcriptomic analyses revealed that humic acids improve low-temperature stress tolerance in zucchini (Cucurbita pepo L.) seedlings. Plants 12 (3), 548. doi: 10.3390/plants12030548
Li, H., Liu, S., Guo, J., Liu, F., Song, F., Li, X. (2020). Effect of the transgenerational exposure to elevated CO2 on low temperature tolerance of winter wheat: Chloroplast ultrastructure and carbohydrate metabolism. J. Agron. Crop Sci. 206, 773–783. doi: 10.1111/jac.v206.6
Li, X., Yang, Y., Zhang, L., Zuo, S., Li, L., Jiao, J., et al. (2017). Regulation on contents of endogenous hormones and Asr1 gene expression of maize seedling by exogenous ABA under low-temperature stress. Acta Agron. Sin. 43, 141–148. doi: 10.3724/SP.J.1006.2017.00141
Liang, Z., Luo, J., Wei, B., Liao, Y., Liu, Y. (2021). Trehalose can alleviate decreases in grain number per spike caused by low-temperature stress at the booting stage by promoting floret fertility in wheat. J. Agron. Crop Sci. 207, 717–732. doi: 10.1111/jac.v207.4
Liu, Z., Bi, S., Meng, J., Liu, T., Li, P., Yu, C., et al. (2022b). Arbuscular mycorrhizal fungi enhanced rice proline metabolism 3under low temperature with nitric oxide involvement. J. Front. Plant Sci. 13, 962460. doi: 10.3389/fpls.2022.962460
Liu, Y. F., Han, X. R., Zhan, X. M., Yang, J. F., Wang, Y. Z., Song, Q. B., et al. (2013). Regulation of calcium on peanut photosynthesis under low night temperature stress. J. Integr. Agric. 12, 2172–2178. doi: 10.1016/S2095-3119(13)60411-6
Liu, K., Li, S., Han, J., Zeng, X., Ling, M., Mao, J., et al. (2021a). Effect of selenium on tea (Camellia sinensis) under low temperature: Changes in physiological and biochemical responses and quality. Environ. Exp. Bot. 188, 104475. doi: 10.1016/j.envexpbot.2021.104475
Liu, J. J., Lin, S. H., Xu, P. L., Wang, X. J., Bai, J. G. (2009). Effects of exogenous silicon on the activities of antioxidant enzymes and lipid peroxidation in chilling-stressed cucumber leaves. Agric. Sci. China 8, 1075–1086. doi: 10.1016/S1671-2927(08)60315-6
Liu, G., Liu, F., Wang, Y., Liu, X. (2022a). A novel long noncoding RNA CIL1 enhances cold stress tolerance in Arabidopsis. Plant Sci. 323, 111370. doi: 10.1016/j.plantsci.2022.111370
Liu, Z., Meng, J., Sun, Z., Su, J., Luo, X., Song, J., et al. (2022d). Zinc application after low temperature stress promoted rice tillers recovery: Aspects of nutrient absorption and plant hormone regulation. Plant Sci. 314, 111104. doi: 10.1016/j.plantsci.2021.111104
Liu, T., Shi, J., Li, M., Ye, X., Qi, H. (2021b). Trehalose triggers hydrogen peroxide and nitric oxide to participate in melon seedlings oxidative stress tolerance under cold stress. Environ. Exp. Bot. 184, 104379. doi: 10.1016/j.envexpbot.2021.104379
Liu, W., Wang, H., Chen, Y., Zhu, S., Chen, M., Lan, X., et al. (2017). Cold stress improves the production of artemisinin depending on the increase in endogenous jasmonate. Biotechnol. Appl. Biochem. 64, 305–314. doi: 10.1002/bab.2017.64.issue-3
Liu, X., Wang, L., Liu, L., Guo, Y., Ren, H. (2011). Alleviating effect of exogenous nitric oxide in cucumber seedling against chilling stress. Afr. J. Biotechnol. 10, 4380–4386. doi: 10.5897/AJB10.812
Lukoševičiūtė, V., Rugienius, R., Sasnauskas, A., Stanys, V., Bobinas, Č. (2009). Impact of exogenous sucrose, raffinose and proline on cold acclimation of strawberry in vitro. Acta Hortic. 2 (839), 203–208. doi: 10.17660/ActaHortic.2009.839.24
Malekzadeh, P., Khosravi Nejad, F., Hatamnia, A. A., Sheikhakbari Mehr, R. (2017). Impact of postharvest exogenous γ-aminobutyric acid treatment on cucumber fruit in response to chilling tolerance. Physiol. Mol. Biol. Plants 23, 827–836. doi: 10.1007/s12298-017-0475-2
Manafi, E., Modarres Sanavy, S. A. M., Aghaalikhani, M., Dolatabadian, A. (2015). Exogenous 5-Aminolevulenic acid promotes antioxidative defence system, photosynthesis and growth in soybean against cold stress. Not Sci. Biol. 7, 486–494. doi: 10.15835/nsb749654
Meng, F. Z., Hu, L. P., Wang, S. H., Sui, X. L., Wei, L., Wei, Y. X., et al. (2008). Effects of exogenous abscisic acid (ABA) on cucumber seedling leaf carbohydrate metabolism under low temperature. Plant Growth Regul. 56, 233–244. doi: 10.1007/s10725-008-9303-6
Moradi, S., Baninasab, B., Gholami, M., Ghobadi, C. (2017). Paclobutrazol application enhances antioxidant enzyme activities in pomegranate plants affected by cold stress. J. Hortic. Sci. Biotechnol. 92, 65–71. doi: 10.1080/14620316.2016.1224605
Mutlu, S., Karadağoğlu, Ö, Atici, Ö, Nalbantoğlu, B. (2013). Protective role of salicylic acid applied before cold stress on antioxidative system and protein patterns in barley apoplast. Biol. Plant 57, 507–513. doi: 10.1007/s10535-013-0322-4
Nezhadahmadi, A., Prodhan, Z. H., Faruq, G. (2013). Drought tolerance in wheat. Sci. World J. 2013, 610721. doi: 10.1155/tswj.v2013.1
Pan, X., Zheng, C., Liu, W., Chen, J., Ding, W. (2022). Effects of titanium and iron reagents on photosynthetic physiological characteristics of candela seedlings under low temperature stress and recovery. Anhui Agric. Sci. 50, 120–123 + 140. doi: 10.3969/j.issn.0517-6611.2022.12.030
Peppino Margutti, M., Reyna, M., Meringer, M. V., Racagni, G. E., Villasuso, A. L. (2017). Lipid signalling mediated by PLD/PA modulates proline and H2O2 levels in barley seedlings exposed to short- and long-term chilling stress. Plant Physiol. Biochem. 113, 149–160. doi: 10.1016/j.plaphy.2017.02.008
Petrić, M., Jevremović, S., Trifunović, M., Tadić, V., Milošević, S., Dragićević, M., et al. (2013). The effect of low temperature and GA3 treatments on dormancy breaking and activity of antioxidant enzymes in Fritillaria meleagris bulblets cultured in vitro. Acta Physiol. Plant 35, 3223–3236. doi: 10.1007/s11738-013-1357-z
Pu, Y., Jiang, H., Yu, Y., Liu, R., Yang, Y., Wu, Y. (2015). Effects of polyethylene glycol on physiological and biochemical indexes of maize seedlings under low temperature stress. Anhui Agric. Sci. 43, 5–6. doi: 10.13989/j.cnki.0517-6611.2015.14.002
Qari, S. H., Hassan, M. U., Chattha, M. U., Mahmood, A., Naqve, M., Nawaz, M., et al. (2022). Melatonin induced cold tolerance in plants: physiological and molecular responses. Front. Plant Sci. 13, 843071. doi: 10.3389/fpls.2022.843071
Qian, C., Ji, Z., Zhu, Q., Qi, X., Li, Q., Yin, J., et al. (2021). Effects of 1-MCP on proline, polyamine, and nitric oxide metabolism in postharvest peach fruit under chilling stress. Hortic. Plant J. 7, 188–196. doi: 10.1016/j.hpj.2020.12.007
Rai, R. K., Singh, P., Shrivastava, A. K., Suman, A. (2008). Modulation of low-temperature-induced biochemical changes in bud and root band zones of sugar cane sets by potassium, zinc, and ethrel for improving sprouting. J. Agric. Food Chem. 56, 11976–11982. doi: 10.1021/jf802696h
Rayirath, P., Benkel, B., Mark Hodges, D., Allan-Wojtas, P., MacKinnon, S., Critchley, A. T., et al. (2009). Lipophilic components of the brown seaweed, ascophyllum nodosum, enhance freezing tolerance in arabidopsis thaliana. Planta 230, 135–147.
Raza, A., Charagh, S., Najafi-Kakavand, S., Abbas, A., Shoaib, Y., Anwar, S., et al. (2023). Role of phytohormones in regulating cold stress tolerance: physiological and molecular approaches for developing cold-smart crop plants. Plant Stress 8, 100152. doi: 10.1016/j.stress.2023.100152
Ren, J., Huang, Z., Zeng, L., Shi, Z. (2013). A review of physiological reaction mechanism of pla nts exposed to low temperature stress. World For Res. 26, 15–20. doi: 10.13348/j.cnki.sjlyyj.2013.06.011
Rihan, H. Z., Al-Issawi, M., Fuller, M. P. (2017). Advances in physiological and molecular aspects of plant cold tolerance. J. Plant Interact. 12 (1), 143–157.
Ritonga, F. N., Chen, S. (2020). Physiological and molecular mechanism involved in cold stress tolerance in plants. Plants 9, 560. doi: 10.3390/plants9050560
Samarah, N. H., Al Quraan, N. A., Massad, R. S., Welbaum, G. E. (2020). Treatment of bell pepper (Capsicum annuum L.) seeds with chitosan increases chitinase and glucanase activities and enhances emergence in a standard cold test. Sci. Hortic. 269, 109393. doi: 10.1016/j.scienta.2020.109393
Selvakumar, G., Kundu, S., Joshi, P., Nazim, S., Gupta, A. D., Gupta, H. S. (2010). Growth promotion of wheat seedlings by Exiguobacterium acetylicum 1P (MTCC 8707) a cold tolerant bacterial strain from the Uttarakhand Himalayas. Indian J. Microbiol. 50, 50–56. doi: 10.1007/s12088-009-0024-y
Shi, X., Sun, Z., Xue, X., Xu, H., Wu, Y., Zhang, Y., et al. (2023). Amelioration of hypothermia-induced damage on peanut by exogenous application of chitooligosaccharide. Agriculture 13, 217. doi: 10.3390/agriculture13010217
Shi, Y., Yang, Y., Fan, D., Liu, W. (2013). Physiological analysis of foliar spraying alginic acid and KH_2PO_4 on alleviating low temperature stress in tobacco. J. Henan Agric. Univ 47, 667–670. doi: 10.16445/j.cnki.1000-2340.2013.06.017
Shu, P., Li, Y., Li, Z., Xiang, L., Sheng, J., Shen, L. (2022). Ferulic acid enhances chilling tolerance in tomato fruit by up-regulating the gene expression of CBF transcriptional pathway in MAPK3-dependent manner. Postharvest Biol. Technol. 185, 111775. doi: 10.1016/j.postharvbio.2021.111775
Siddiqui, K. S., Cavicchioli, R. (2006). Cold-adapted enzymes. Annu. Rev. Biochem. 75, 403–433. doi: 10.1146/annurev.biochem.75.103004.142723
Song, Y., Diao, Q., Qi, H. (2015). Polyamine metabolism and biosynthetic genes expression in tomato (Lycopersicon esculentum Mill.) seedlings during cold acclimation. Plant Growth Regul. 75, 21–32. doi: 10.1007/s10725-014-9928-6
Spaepen, S., Vanderleyden, J. (2011). Auxin and plant-microbe interactions. Cold Spring Harb. Perspect. Biol. 3, a001438. doi: 10.1101/cshperspect.a001438
Su, Z., Burchfield, J. G., Yang, P., Humphrey, S. J., Yang, G., Francis, D., et al. (2019). Global redox proteome and phosphoproteome analysis reveals redox switch in Akt. Nat. Commun. 10, 5486. doi: 10.1038/s41467-019-13114-4
Sun, G., Cao, M., Zhang, H., Yu, H., Zhao, X. (2014). Effects of different concentrations of PEG, dimethyl sulfoxide and salicylic acid on the cold resistance of maize seeds. Maize Sci. 22, 86–90. doi: 10.13597/j.cnki.maize.science.2014.03.019
Sun, X., Yuan, Z., Wang, B., Zheng, L., Tan, J. (2021). Exogenous putrescine activates the arginine-polyamine pathway and inhibits the decomposition of endogenous polyamine in Anthurium andraeanum under chilling stress. Sci. Hortic. 282, 110047. doi: 10.1016/j.scienta.2021.110047
Tang, X., An, B., Cao, D., Xu, R., Wang, S., Zhang, Z., et al. (2020). Improving photosynthetic capacity, alleviating photosynthetic inhibition and oxidative stress under low temperature stress with exogenous hydrogen sulfide in blueberry seedlings. Front. Plant Sci. 11, 108. doi: 10.3389/fpls.2020.00108
Tsavkelova, E. A., Klimova, S. Y., Cherdyntseva, T. A., Netrusov, A. I. (2006). Microbial producers of plant growth stimulators and their practical use: A review. Appl. Biochem. Microbiol. 42, 117–126. doi: 10.1134/S0003683806020013
Turkyilmaz Unal, B., Mentis, O., Akyol, E. (2015). Effects of exogenous salicylic acid on antioxidant activity and proline accumulation in apple (Malus domestica L.). Hortic. Environ. Biotechnol. 56, 606–611. doi: 10.1007/s13580-015-0049-6
Wan, C., Tan, P., Zeng, C., Liu, Z. (2022). Arachidonic acid treatment combined with low temperature conditioning mitigates chilling injury in cold-stored banana fruit. Int. J. Food Sci. Technol. 57, 210–223. doi: 10.1111/ijfs.15403
Wang, Z., Cao, J., Jiang, W. (2016). Changes in sugar metabolism caused by exogenous oxalic acid related to chilling tolerance of apricot fruit. Postharvest Biol. Technol. 114, 10–16. doi: 10.1016/j.postharvbio.2015.11.015
Wang, Q., Liu, Z., Peng, C. E., Meng, H., Zhao, H., Wang, H., et al. (2022a). Mechanism of paecilomyces variotii extract in inducing pakchoi resistance to low-temperature stress. J. Nucl. Agric. Sci. 36, 473–480. doi: 10.11869/j.issn.100-8551.2022.02.0473
Wang, W., Wang, X., Huang, M., Cai, J., Zhou, Q., Dai, T., et al. (2021a). Alleviation of field low-temperature stress in winter wheat by exogenous application of salicylic acid. J. Plant Growth Regul. 40, 811–823. doi: 10.1007/s00344-020-10144-x
Wang, S., Yang, S., He, D., Yi, Y., Fu, Y., Yin, D., et al. (2022b). Exogenous 6-benzyladenine treatment alleviates cold stress in early japonica rice at booting in Northeast China. Agron. J. 114, 2905–2919. doi: 10.1002/agj2.v114.5
Wang, Y., Zhu, M., Yang, Y., Alam, I., Cheng, X., Qin, T., et al. (2017). Exogenous application of abscisic acid (ABA) enhances chilling tolerance in seedlings of napier grass (Pennisetum purpureum Schum.). Agric. Sci. Technol. 18, 417–423. doi: 10.16175/j.cnki.1009-4229.2017.03.008
Wei, X., Gao, C., Chang, C., Tang, Z. (2021). Metabolic regulation characteristics of exogenous inositol in relieving low temperature injury of periwinkle. Acta Physiol. Plant 57, 2247–2257. doi: 10.13592/j.cnki.ppj.2021.0256
Wu, X. X., Ding, H. D., Chen, J. L., Zhu, Z. W., Zha, D. S. (2015). Exogenous spray application of 24-epibrassinolide induced changes in photosynthesis and anti-oxidant defences against chilling stress in eggplant (Solanum melongena L.) seedlings. J. Hortic. Sci. Biotechnol. 90, 217–225. doi: 10.1080/14620316.2015.11513175
Xiao yun, Z., Meng li, L. I. U., Jin, L. I., Jun gao, Z., Jing, L., Peng cheng, D. U., et al. (2020). Effects of the carboxin from seed coating formulation on the cotton seed germination characteristics under low temperature stress. Chin. J. Agrometeorol 41, 495. doi: 10.3969/j.issn.1000-6362.2020.08.003
Xin Chi, Y., Yang, L., Jiang Zhao, C., Muhammad, I., Bo Zhou, X., De Zhu, H. (2021). Effects of soaking seeds in exogenous vitamins on active oxygen metabolism and seedling growth under low-temperature stress. Saudi J. Biol. Sci. 28, 3254–3261. doi: 10.1016/j.sjbs.2021.02.065
Xu, S., Jiang, Y., Cui, W., Jin, Q., Zhang, Y., Bu, D., et al. (2017). Hydrogen enhances adaptation of rice seedlings to cold stress via the reestablishment of redox homeostasis mediated by miRNA expression. Plant Soil 414, 53–67. doi: 10.1007/s11104-016-3106-8
Xu, H., Wu, Z., Xu, B., Sun, D., Hassan, M. A., Cai, H., et al. (2022). Optimized phosphorus application alleviated adverse effects of short-term low-temperature stress in winter wheat by enhancing photosynthesis and improved accumulation and partitioning of dry matter. Agronomy 12 (7), 1700. doi: 10.3390/agronomy12071700
Yang, D., Wang, Q., He, L., Wang, Y., Sun, H., Ren, J., et al. (2022). Effects of N-phenyl-phthalamic acid on antioxidant system and nitrogen metabolism in maize seedlings under low temperature stress. J. Maize Sci. 3, 63–71. doi: 10.13597/j.cnki.maize.science.20220309
Yang, X. L., Xu, H., Li, D., Gao, X., Li, T. L., Wang, R. (2018). Effect of melatonin priming on photosynthetic capacity of tomato leaves under low-temperature stress. Photosynthetica 56, 884–892. doi: 10.1007/s11099-017-0748-6
Yildiztugay, E., Ozfidan Konakci, C., Kucukoduk, M. (2017). Improvement of cold stress resistance via free radical scavenging ability and promoted water status and photosynthetic capacity of gallic acid in soybean leaves. J. Soil Sci. Plant Nutr. 17, 366–384. doi: 10.4067/S0718-95162017005000027
Yu, M., Fan, B., Yuan, Q., Hou, J., Yu, S., Han, X., et al. (2021). Effect of seaweed extract on cold resistance of tea plant. J. Tea Communication 48 (04), 652–662.
Yu, M., Huang, L., Feng, N., Zheng, D., Zhao, J. (2022). Exogenous uniconazole enhances tolerance to chilling stress in mung beans (Vigna radiata L.) through cross talk among photosynthesis, antioxidant system, sucrose metabolism, and hormones. J. Plant Physiol. 276, 153772. doi: 10.1016/j.jplph.2022.153772
Zhang, J., Li, S., Cai, Q., Wang, Z., Cao, J., Yu, T., et al. (2020). Exogenous diethyl aminoethyl hexanoate ameliorates low temperature stress by improving nitrogen metabolism in maize seedlings. PLoS One 15, e0232294. doi: 10.1371/journal.pone.0232294
Zhang, X., Ma, T., Wang, Y., Yang, K. (2022a). Mitigation effect of bacillus on maize germination low temperature stress. Heilongjiang Agric. Sci. 6, 11–17. doi: 10.11942/j.issn.1002-2767.2022.06.001
Zhang, X., She, M., Li, H., Jing, Song, Jiang, H., et al. (2021). The growth-promoting mechanism of Flavobacterium succinate and its physiological regulation on the growth and stress resistance of perennial ryegrass. Acta Grassl 29, 1704. doi: 10.11733/j.issn.1007-0435.2021.08013
Zhang, H., Sonnewald, U. (2017). Differences and commonalities of plant responses to single and combined stresses. Plant J. 90, 839–855. doi: 10.1111/tpj.2017.90.issue-5
Zhang, Z., Yuan, L., Ma, Y., Kang, Z., Zhou, F., Gao, Y., et al. (2022b). Exogenous 5-aminolevulinic acid alleviates low-temperature damage by modulating the xanthophyll cycle and nutrient uptake in tomato seedlings. Plant Physiol. Biochem. 189, 83–93. doi: 10.1016/j.plaphy.2022.08.013
Zhu, K., Liu, J., Luo, T., Zhang, K., Khan, Z., Zhou, Y., et al. (2022). Wood vinegar impact on the growth and low-temperature tolerance of rapeseed seedlings. Agronomy 12 (10), 2453. doi: 10.3390/agronomy12102453
Zhu, X., Liu, S., Sun, L., Song, F., Liu, F., Li, X. (2018). Cold tolerance of photosynthetic electron transport system is enhanced in wheat plants grown under elevated CO2. Front. Plant Sci. 9. doi: 10.3389/fpls.2018.00933
Keywords: cold stress, exogenous substances, cold tolerance, chemical regulation, microbial regulation
Citation: Feng D, Zhang M, Xu J, Gao Q, Liu J, Li C, Sun X and Xu W (2025) Revelation of mechanisms associated with strengthening plant cold tolerance through using exogenous substances. Front. Plant Sci. 16:1478692. doi: 10.3389/fpls.2025.1478692
Received: 10 August 2024; Accepted: 10 March 2025;
Published: 07 April 2025.
Edited by:
Shifeng Cao, Zhejiang Wanli University, ChinaReviewed by:
Klára Kosová, Crop Research Institute (CRI), CzechiaGyanendra Kumar Rai, Sher-e-Kashmir University of Agricultural Sciences and Technology, India
Mohammad Shah Jahan, Sher-e-Bangla Agricultural University, Bangladesh
Copyright © 2025 Feng, Zhang, Xu, Gao, Liu, Li, Sun and Xu. This is an open-access article distributed under the terms of the Creative Commons Attribution License (CC BY). The use, distribution or reproduction in other forums is permitted, provided the original author(s) and the copyright owner(s) are credited and that the original publication in this journal is cited, in accordance with accepted academic practice. No use, distribution or reproduction is permitted which does not comply with these terms.
*Correspondence: Xiaoan Sun, c2Vhbm55eEBvdXRsb29rLmNvbQ==; Wanli Xu, d2x4dTIwMDVAMTYzLmNvbQ==
†These authors have contributed equally to this work