- Department of Pediatrics, Baylor College of Medicine, USDA-ARS Children’s Nutrition Research Center, Houston, TX, United States
Introduction: Spinach produces an array of unique flavonoids not commonly found in other fruits and vegetables. These molecules likely serve as defense agents against biotic and abiotic stress and may have health beneficial properties for humans. Current methods to analyze spinach flavonoids are incomplete and only capture a portion of this uncharacterized pathway. A comprehensive analysis method is needed to determine how genetics, environmental conditions, and other factors influence spinach flavonoid biosynthesis.
Methods: We developed and validated a high-throughput extraction and ultra high-performance liquid chromatography tandem mass spectrometry (UHPLC-MS/MS) method to separate and quantify 39 spinach flavonoid species in 11.5 minutes. Spinach flavonoids without authentic standards were putatively identified using MS/MS fragmentation experiments, precursor scans, and matches to high-resolution MS literature reports.
Results: Our extraction method enables up to 48 samples to be extracted in 60 minutes with recovery estimates between 100.5 – 107.8%. To assess the suitability of our method and generate benchmark estimates for 39 spinach flavonoids, we grew a panel of 30 genetically diverse spinach accessions and compared quantification data generated with a traditional or our high-throughput approach. Data generated by either approach were comparable, estimating total flavonoid averages of 75.1 – 170.1 or 93.1 – 187.26 mg/100 g fresh weight for the high-throughput and traditional method, respectively.
Discussion: Many estimates generated by our analysis method represent the first quantitative literature reports of these compounds. These experiments indicate that our extraction and analysis method is efficient, robust, and an important tool needed to study the biosynthesis and biological role of spinach flavonoids.
Introduction
Flavonoids are secondary metabolites contributing to the pigmentation of flowers (aiding in pollination), quenching UV light in leaves, and protection against environmental biotic and abiotic stress (Takahashi and Ohnishi, 2004; Griesbach, 2005). Spinach (Spinacia oleracea) produces a remarkable concentration of flavonoids with varying reports between 82 – 224 mg/100 g fresh weight (Gil et al., 1999; Pandjaitan et al., 2005). Spinach-derived flavonoids, with reported antioxidant and anti-inflammatory properties, have been associated with a reduced risk of developing certain cancers, neurodegenerative, and cardiovascular diseases (Lomnitski et al., 2000; Edenharder et al., 2001; Nyska et al., 2001; Takahashi and Ohnishi, 2004; Grosso et al., 2017; Singh et al., 2018b; Hamsalakshmi et al., 2022). As research surrounding spinach flavonoids and their effects on human health continues to garner interest, lack of a comprehensive extraction and analysis methods represents a significant gap in knowledge. Moreover, the concentration range of spinach flavonoids remains unclear as many studies only focus on a few varieties of spinach and a few common flavonoids.
Spinach flavonoids are typically extracted by first homogenizing fresh or frozen spinach in a blender or mortar and pestle, followed by the addition of varying amounts of polar solvents including acetone, ethanol, water, and methanol, with the latter two being used most frequently (Edenharder et al., 2001; Koh et al., 2012; Singh et al., 2017). Samples are then centrifuged and flavonoids can be quantified from filtered supernatants (Cho et al., 2008; Koh et al., 2012; Singh et al., 2017, 2018a, 2018b). Current extraction methods tend to be time-consuming, and more efficient methods are needed to profile larger populations. Quantification of spinach flavonoids is generally done by reverse phase liquid chromatography tandem mass spectrometry (LC-MS/MS), although other less sensitive approaches have also been implemented such as photodiode array detector (Howard et al., 2002; Pandjaitan et al., 2005; Singh et al., 2018b). Through 1H and 13C NMR, as well as mass spectrometry, over 40 flavonoid species have been identified in spinach (Ferreres et al., 1997; Singh et al., 2018b). However, most published methods only consider 5-10 of these flavonoids with the most comprehensive method quantifying up to 17 (Koh et al., 2012). Despite a lack of authentic standards for most of these compounds, common product ions have been determined (e.g. 330 m/z) for many of these flavonoids using accurate mass collision-induced dissociation (CID) experiments (Singh et al., 2019). These data make it possible to create a comprehensive analysis method that simultaneously quantifies most of the known flavonoids in spinach. Utilizing an efficient extraction technique with a comprehensive quantification method would enable scientists to determine the diversity of flavonoids in spinach frequently utilized by consumers. These data could better inform investigators seeking to determine the role of spinach flavonoids in human health.
To gain additional insight into the diversity of spinach flavonoids, we developed a high-throughput extraction (48 samples/hour) and comprehensive quantification method (39 flavonoids/11.5 minutes) using high-performance liquid chromatography tandem mass spectrometry (UHPLC-MS/MS). We performed MS/MS fragmentation experiments on each analyte and report both common and unique molecular signatures of spinach flavonoids. In addition to traditional validation experiments, we profiled a panel of 30 genetically diverse spinach accessions using both a validated and high-throughput extraction method to determine how flavonoid estimates and population structures differed due to methodology. Here, we summarize this series of experiments that validated our methods and provide benchmark concentrations for spinach flavonoids in a genetically diverse population.
Materials and methods
Chemical and reagents
Methanol (MeOH; LC-MS grade), water (LC-MS grade), formic acid (FA; LC-MS grade), and taxifolin (99.99%) were purchased from Thermo Fisher Scientific (Pittsburgh, PA, United States). Naringin (≥95% purity), naringenin (≥95%), and quercetin-3-glucoside (≥95%) were purchased from Sigma-Aldrich (St. Louis, MO, United States). Spinacetin (>95%) and jaceidin (>95%) were purchased from Key Organics (Camelford, United Kingdom). Patuletin (≥98%) was purchased from Extrasynthese (Genay, France). Acetonitrile (LC-MS grade) was purchased from VWR (Radnor, PA, United States).
Standard curve and internal standard preparation
Unless stated otherwise, all solutions were prepared in 1:1 MeOH:H2O + 0.1% FA to maximize analyte solubility and chromatographic resolution. Stock solutions of each authentic standard were made by dissolving weighed powders in MeOH + 0.1% FA and diluting 1:1 with H2O + 0.1% FA to final concentrations of 0.1 mg/mL. Thirty μL of each stock solution was combined into a 4 mL glass vial and 36 μL of 20 μM taxifolin was added (4% of final volume). This concentration of taxifolin was selected as it generates a signal intensity that is intermediate to the range of intensities seen for all analytes quantified by our method. An additional 714 μL of 1:1 MeOH:H2O + 0.1% FA was added to bring the final volume of the stock solution to 900 μL. Eight additional 4 mL vials with 576 μL of 1:1 MeOH:H2O + 0.1% FA and 24 μL of 20 μM taxifolin were created. A 300 μL aliquot of the stock solution was added into the following vial and the dilution series was propagated seven more times (final vial was 6561x diluted). Depending on the analyte, concentrations ranged from 9.03 nmol/vial to 0.63 pmol/vial. Due to the unavailability of glycosylated spinach flavonoid standards, these analytes were quantified relative to a ratio of quercetin-3-glucoside to taxifolin (Singh et al., 2017; Grace et al., 2022).
Solutions of 200 nmol naringin and naringenin were also prepared for spike recovery experiments and used to routinely monitor extraction efficiency for each sample. These molecules are not detectable in spinach but have similar chromatographic characteristics as glycosylated and aglycone spinach flavonoids, respectively.
Sample material
To maximize genetic variation, 30 spinach accessions previously phenotyped and genotyped were selected and grown (Qin et al., 2017; Hayes et al., 2020). Selections were made based on reported differences in carotenoid and mineral concentrations as well as genetic features that segregated accessions into unique subgroups. Metadata about each accession can be found in Supplementary Table 1. Before sowing, seeds were osmoprimed in tap water and stored in a 4 °C refrigerator for five days to enhance germination rates. Osmoprimed seeds were sown into one-gallon pots filled with HFC/20 Growing mix (Jolly Gardener Products; Oviedo, FL) and thinned to six plants per pot. Plants were grown in a PGW36 walk-in growth chamber (Conviron; Winnipeg, Canada) maintained at 20 ± 0.5°C/15 ± 0.5°C day and night temperatures, respectively, with a 12 hour photoperiod (300 μmol/m2/s of photosynthetically active radiation) and pot positions were randomized on a daily basis. Relative humidity was maintained at 50 ± 10%. Plants were irrigated with deionized water until germination and then sub-irrigated with a nutrient solution containing: 1.2 mM KNO3, 0.8 mM Ca(NO3)2, 0.8 mM NH4NO3, 0.2 mM MgSO4, 0.3 mM KH2PO4, 25 μM CaCl2, 25 μM H3BO3, 2 μm MnSO4, 2 μM ZnSO4, 0.5 μM CuSO4, 0.5 μM H2MoO4, 0.1 μM NiSO4, and 10 μM Fe (III)-N, N′-ethylenebis[2-(2-hydroxyphenyl)-glycine] (Sprint 138; Becker-Underwood Inc.; Ames, IA) according to Qin and colleagues (Qin et al., 2017). Plants were harvested when each plant within a pot had six to eight fully developed leaves (approximately 35 days after sowing). Tissue was frozen at -80 °C. Frozen samples were homogenized in a ratio of 1:1 spinach:MilliQ H2O with a VWR Model 250 polytron at 20,000 RPM for ~one minute. Homogenate was aliquoted and immediately stored at -80°C until extraction and analysis.
Quality control material and pseudo-standard preparation
A quality control (QC) composite sample was created by blending equal parts of the 30 accessions referenced above into a single homogenate. This homogenate was aliquoted for spike recovery experiments, intra/interday variation experiments, and used for MS/MS validation studies described below.
A methanolic extract of the QC material was diluted 1:1 with H2O + 0.1% FA and semi-purified using a solid phase extraction (SPE; StrataX 33 μM, 30 mg, 3 mL tube; Phenomenex, Torrance, CA, United States) method adapted from Redan and others (Redan et al., 2017). Spinach flavonoids were eluted from cartridges using 500 μL of MeOH + 0.1% FA and the eluent was diluted 1:1 with H2O + 0.1% FA. These samples were used for multiple reaction monitoring (MRM) optimization experiments.
Flavonoid extraction
Traditional method
Spinach samples (500 mg ± 10 mg) were extracted using a validated extraction protocol (Mohamedshah et al., 2022). Samples were spiked (200 μL) with a 1:1 solution of 200 nmol naringin and 200 nmol naringenin in 1:1 MeOH:H2O + 0.1% FA. Samples were extracted with 5 mL of 98:2 MeOH: FA, vortexed for 1 minute, sonicated for 20 minutes, and centrifuged for 5 minutes at 3700 RPM at 4°C in a VWR Mega Star 4.0R. The supernatant was decanted into 15 mL borosilicate glass tubes. This extraction process was repeated twice more but sonicated for only 10 minutes.
High throughput method
A high-throughput extraction using a Tecan Freedom EVO 150 (Tecan; Mannedorf, Switzerland) was adapted from previous methodology validated for carotenoids and modified for water soluble flavonoids (Dzakovich et al., 2023). Samples (100 ± 5 mg) were spiked with 100 μL of 1:1 200 nmol naringin to 200 nmol naringenin internal standard solution. Two stainless steel balls (Grainger #4RJH5, ⅛ inch diameter) were then inserted into each sample tube. After several minutes to allow internal standards to equilibrate with sample matrix, 1 mL of 98:2 MeOH: FA was added to each sample. Samples were then extracted in a Spex SamplePrep 2010 Geno/Grinder for 45 seconds at 1400 RPM and centrifuged for 3 minutes at 13,000 RPM. The supernatant was collected into 15 mL borosilicate glass tubes and the extraction process was repeated twice more.
Drying, resolubilizing, and filtering
For both extraction methods, samples were loaded into a Labconco RapidVap (Kansas City, MO, United States) with lid heater for drying. While maintaining a temperature of 35 °C and 35% speed, the vacuum was gradually decreased in 20 mbar increments from a starting pressure of 280 mbar to 10 mbar. After drying, samples were capped and stored at -80°C until quantification. For samples extracted using the traditional method, 5 mL of 1:1 MeOH:H2O + 0.1% FA was added to each tube and sonicated (~15 seconds) with agitation to resuspend dried residue on tube walls. Samples were vortexed and a 1 mL aliquot was removed and diluted in 4 mL of 1:1 MeOH:H2O + 0.1% FA with internal standard (3800 μL of 1:1 MeOH:H2O + 0.1% FA + 200 μL of 20 μM taxifolin in the same carrier solvent) in a separate tube. This dilution step ensured analyte concentrations were within linear detection limits of the instrument. For the high-throughput extraction, 200 μL of 20 μM taxifolin solution was spiked into each tube prior to adding 4800 μL of 1:1 MeOH:H2O + 0.1% FA. Samples were then sonicated as described above. For both extraction methods, redissolved samples were vortexed and filtered through a 0.2 μm PTFE syringe filter into HPLC vials and run immediately.
Flavonoid quantification
Spinach flavonoids were separated on a Waters C18 Acquity bridged ethylene hybrid (BEH) 2.1x100 mm, 1.7 mm particle size column installed in a ThermoFisher Vanquish Horizon UHPLC with a column chamber maintained at 40 °C interfaced with a ThermoFisher TSQ Altis triple quadrupole mass spectrometer. The autosampler was maintained at 12 °C to minimize sample oxidation. A 0.5 mL/min gradient was developed consisting of mobile phase A (LC-MS grade water + 0.1% FA) and mobile phase B (LC-MS grade acetonitrile + 0.1% FA) were adjusted as follows: 0% B to 6.0% B over 0.5 minutes, 6.0% B to 9.0% B for 1.5 minutes, 9% B to 13% B over 1.0 minute, 13% B to 35% B over 1.5 minutes, 35% B to 75% B over 2.91 minutes, 75% B to 90% B over 0.09 minutes, 90% B held for 2.0 minutes, 90% B to 0% B over 0.5 minutes, hold at 0% B for an additional 1.5 minutes to equilibrate the column. Each run was a total of 11.5 minutes and the needle was washed twice for 5 seconds using MeOH + 0.1% FA followed by water. To minimize matrix buildup on the mass spectrometer sampling cone and optics, column eluent was routed to waste for the first 1.9 minutes and the last 4.0 minutes of each run utilizing a post-column diverter valve. Mass spectrometer source and MRM parameters can be found in Table 1. Dwell times were calculated for each analyte to ensure 12-15 points per peak. Since glycosylated spinach flavonoids are not commercially available, quantification was relative to quercetin-3-glucoside using the linear portion of a 9-point standard curve. The aglycones jaceidin, patuletin, and spinacetin were quantified against commercially available authentic standards. Peak areas were normalized to taxifolin spiked into samples and standards to correct for within- and between-day instrument variability.
Limit of detection and quantification
Six replicates of the lowest concentration calibrant from standard curves for quercetin-3-glucoside, patuletin, spinacetin, and jaceidin were used to calculate limit of detection (LOD; 3:1 signal to noise) and limit of quantification (LOQ; 10:1 signal to noise) (Vial and Jardy, 1999). A weighting factor for the signal to noise for each analyte’s lowest standard curve calibrant was calculated to estimate moles on column at 10:1 or 3:1 signal to noise.
Spike addition experiments
Quality control samples (100 mg ± 5 mg) were randomly divided into two different groups of six tubes, 1) tubes only containing spinach homogenate; 2) tubes containing spinach homogenate spiked with standards. Additionally, another 6 tubes only spiked with standards were also utilized. 20 μL spikes consisted of: 200 nmol naringin, 200 nmol naringenin, and 50 nmol jaceidin. Samples underwent a traditional extraction and were analyzed using the LC-MS/MS method described above. Spike recovery was calculated using the following formula:
Intra/interday variability experiments
The intra/interday variability experiments compared the quantification values from six replicates from the same quality control material over three days. Each day, six randomly selected samples (100 mg ± 5 mg) in 2 mL tubes were spiked with 100 μL of a 1:1 mixture of 200 nM naringin and 200 nM naringenin, accompanied with three internal standard-only tubes. The samples then underwent a high-throughput extraction. Intra and interday variability was calculated by determining the coefficient of variation for each analyte within a day or between days, respectively.
Random effects modeling and data visualization
Random effects modeling and data visualization were conducted using R v4.4.1 (R Development Core Team, 2018). Random effects models were run to partition variance associated with genetic background, extraction type, and replicate using the package lme4 (Bates et al., 2015). The following model was used:
In the above model, Ŷijk represents an analyte estimate within the ith genotype, jth extraction method, and kth replicate; μ represents the population mean of an analyte; Gi represents the contribution from genetic factors for the ith genotype of the population; Ej represents the contribution from extraction for the jth extraction method; Rk(EJ) represents the contribution of the kth replicate within extraction method and can be interpreted as within-method variation; and ϵijk represents the residual error.
Coordinates for 2-D scores plots were generated using the packages FactoMineR and Factoextra, scaling variable means to zero and standard deviation one, as input data for principal components analysis (PCA) (Lê et al., 2008). ggplot2 and MoMA color palette were used for visualization (Wickham, 2016; Mills, 2024).
Results and discussion
Development and validation of UHPLC-MS/MS method
Precursor ion discovery
To maximize the detection, separation, and quantification of flavonoids that are unique to spinach, a combined literature search and precursor ion scan approach was utilized. Previous mass spectrometry based approaches revealed over 20 species of flavonoids present in spinach and provide valuable fragmentation information that can be used to putatively identify molecules in the absence of authentic standards (Cho et al., 2008). For example, most of the flavonoids identified by Cho and colleagues generated either a 333 or 347 m/z ion in positive mode. High-resolution mass spectrometry enabled the discovery of glucuronidated flavonoids in spinach as well as several other glycosylated molecules (Singh et al., 2017, 2018a, 2019). Merging the findings of the last 20 years of method development yields a list of 39 flavonoid molecules discovered in spinach with varying degrees of information available about them (Supplementary Tables 2, 5). We utilized this information to determine if each of these compounds could be detected in methanolic spinach extracts.
We created a methanolic extract of the quality control material described above and injected it into a ThermoFisher Vanquish UHPLC system in tandem with a TSQ Altis triple quadrupole mass spectrometer operated in negative mode. Using a gradient developed for anthocyanins (Mengist et al., 2020), analytes were separated on a Waters Acquity BEH column (2.1 x 100 mm; 1.7 μm particle size). We developed selected ion recordings (SIRs) for each candidate flavonoid. Additionally, we ran precursor ion scans by leveraging fragmentation information available about common core structures found in most spinach flavonoids (Figure 1) to eliminate isobaric peaks that are not flavonoids. A variety of collision energies were tested to find precursor ions that produced product ions consistent with patuletin (e.g. 316 m/z), spinacetin (e.g. 330 m/z), and jaceidin (e.g. 344 m/z) as well as other product ions produced by carbon monoxide/dioxide loses, M-122 losses, demethylation, and retro-Diels-Alder reactions (Fabre et al., 2001; McNab et al., 2009; Dimkić et al., 2020). Unoptimized MRM scans helped further reduce extraneous peaks using previously validated product ions (Singh et al., 2018a, 2019; Grace et al., 2022). Product ion scans of the remaining chemical features, their relative retention order, and matches to database/literature reference helped putatively identify the remaining chemical features where commercial standards are not available. A summary of this information can be found in Supplementary Tables 2, 5. Spectral information generated by our study could likely aid molecular fingerprinting and identification efforts aiming to discover novel flavonoids and their metabolites in plants, animal models, and humans (Ivey et al., 2017; Demarque et al., 2020; Chen et al., 2021; Mannochio-Russo et al., 2022).
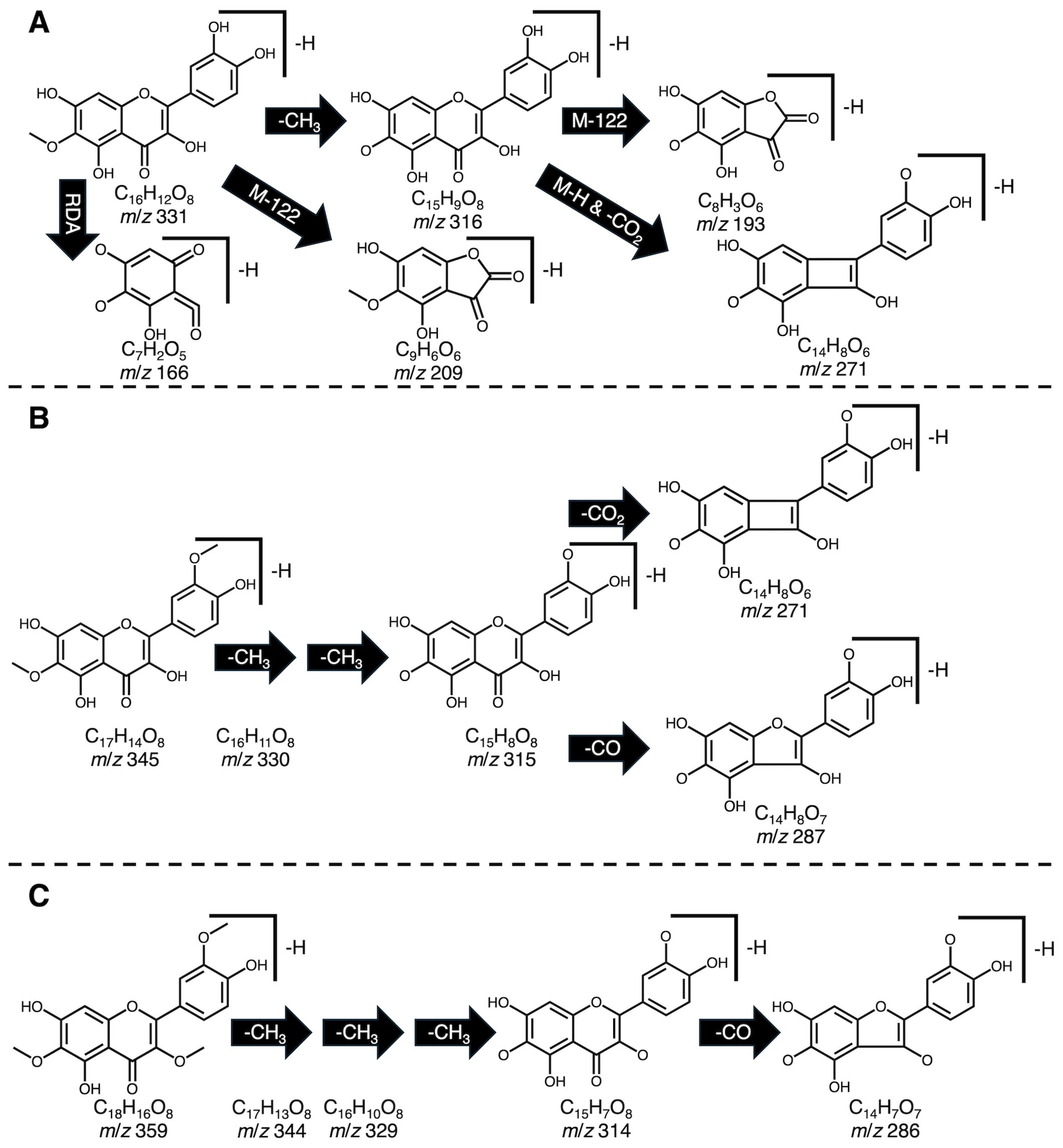
Figure 1. Example fragmentation patterns reported for patuletin (A), spinacetin (B), and jaceidin (C) including proton loss (M-H), retro Diels-Alder (RDA), demethylation (-CH3), carbon monoxide loss (-CO), carbon dioxide loss (-CO2). Fragmentation patterns were used to guide identification efforts to screen for potential precursor molecules containing these motifs.
Internal standard selection
We explored five different internal standard candidates commonly used in flavonoid analysis including naringenin, naringin, ethyl gallate, phlorizin, and taxifolin (Mengist et al., 2020; Grace et al., 2022; Mohamedshah et al., 2022). Ethyl gallate and phlorizin were eliminated due to their structural dissimilarity to spinach flavonoids, stability, and ionization efficiency. Spikes of 200 μM naringin/naringenin (200 or 100 μL depending on extraction method) generated peak areas consistent with those seen in spinach flavonoids quantified by our method, eluted near analytes, and are not naturally present in spinach in detectable amounts.
Optimization of MS parameters
Source temperature parameters (ion transfer tube and vaporizer temperatures) were optimized by successively injecting a mixture of quercetin-3-glucoside, naringin, naringenin, spinacetin, jaceidin, and patuletin into the TSQ Altis at temperatures ranging from 275 to 375 °C at 25 °C intervals. At a flow rate of 0.5 mL/min, 350 °C for both source parameters resulted in the highest average signal intensity for these analytes.
Positive and negative ionization modes were explored since existing mass spectrometry approaches for spinach flavonoids have reported both (Cho et al., 2008; Singh et al., 2017, 2018a). We consistently found that while our analytes ionized in both modes, negative mode tended to result in higher signal to noise ratios compared to positive mode. Moreover, there is a greater abundance of fragmentation information for spinach flavonoids in negative mode allowing for more confident identification (Singh et al., 2017, 2018a, 2019).
To optimize MRMs, semi-purified flavonoid extracts described earlier were infused into the TSQ Altis source. The flow rate of the UHPLC was maintained at 0.5 mL/min and eluent composition was adjusted to match the ratio of mobile phases where each analyte was determined to elute. The TSQ Altis systematically tested spray voltages (1500 – 5000 V), sheath gas (0 – 60 arbitrary units), auxiliary gas (0 – 25 arbitrary units), sweep gas (0 – 20 arbitrary units), RF lens voltage (30 – 250 V), and collision energy (5 – 55 V) seeking five product ions per analyte. For all parameters except RF lens voltage and collision energy, average values were determined to provide the most parsimonious setpoint for all analytes. The top two MRMs with the highest signal to noise ratio were retained for quantification and other pertinent MS information can be found in Table 1. Additional qualifying product ions for each analyte as well as structures and spectra can be found in the Supplementary Information (Supplementary Tables 2, 5). Windows of 15 seconds on each side of a retention time were added to maximize the instrument’s duty cycle and allow for 12-15 points across a peak. Analytes available as authentic standards were optimized as outlined above but without SPE.
Chromatographic gradient
We initially used a chromatographic gradient developed for separating anthocyanins using both Waters BEH and HSS T3 2.1 x 100 mm (1.7 μm particle size) C18 columns (Mengist et al., 2020). Generally, the BEH column exhibited more favorable separation of relatively polar spinach flavonoids compared to the HSS T3. The original gradient used 1% FA as a modifier for their mobile phase A to aid in pH maintenance, chromatography, and ionization nuances important for anthocyanins. We found that switching to 0.1% FA for both mobile phases slightly improved signal of most analytes, ostensibly from a reduction in formate adducts, and lowers the overall cost of mobile phase preparation. To shorten the overall runtime of our method to 11.5 minutes, we initiated a jump to 90% B (acetonitrile + 0.1% FA) shortly after our final analyte eluted followed by an equilibration period allowing for the column to be stabilized by the following injection cycle. A two-phase wash cycle of MeOH + 0.1% FA followed by H2O + 0.1% FA ensured minimal carryover. Multiple analytes in our method exhibited isomers that have been previously reported in the literature (Cho et al., 2008; Singh et al., 2017). Each of these isomers were chromatographically resolved from their respective counterparts allowing for separate quantification. All 39 spinach flavonoids analyzed by our method are separated in under 3 minutes with the remaining time devoted to column cleaning and equilibration.
LOD/LOQ
UV-Vis spectral information for many spinach flavonoids have been reported (Cho et al., 2008; Singh et al., 2018a; Grace et al., 2022), but overwhelmingly similar spectral characteristics make it impossible to distinguish many spinach flavonoids from one another. Mass spectrometry allows for the development of highly selective and sensitive methods while overcoming chromatography issues such as co-elution. Limits of detection and quantification do not appear to be published for previous methods developed for these compounds, so we sought to calculate these values here. Our method was able to detect jaceidin, patuletin, spinacetin, and quercetin-3-glucoside at sub-femtomole-on-column quantities (Table 2). Assuming the same extraction, redissolve, and injection conditions, we estimate that flavonoids at 23.6 pmol/gram spinach tissue or higher could be quantified. Sensitivity at this level enables high-throughput, micro-extraction methods where little sample (< 100 mg) is required.
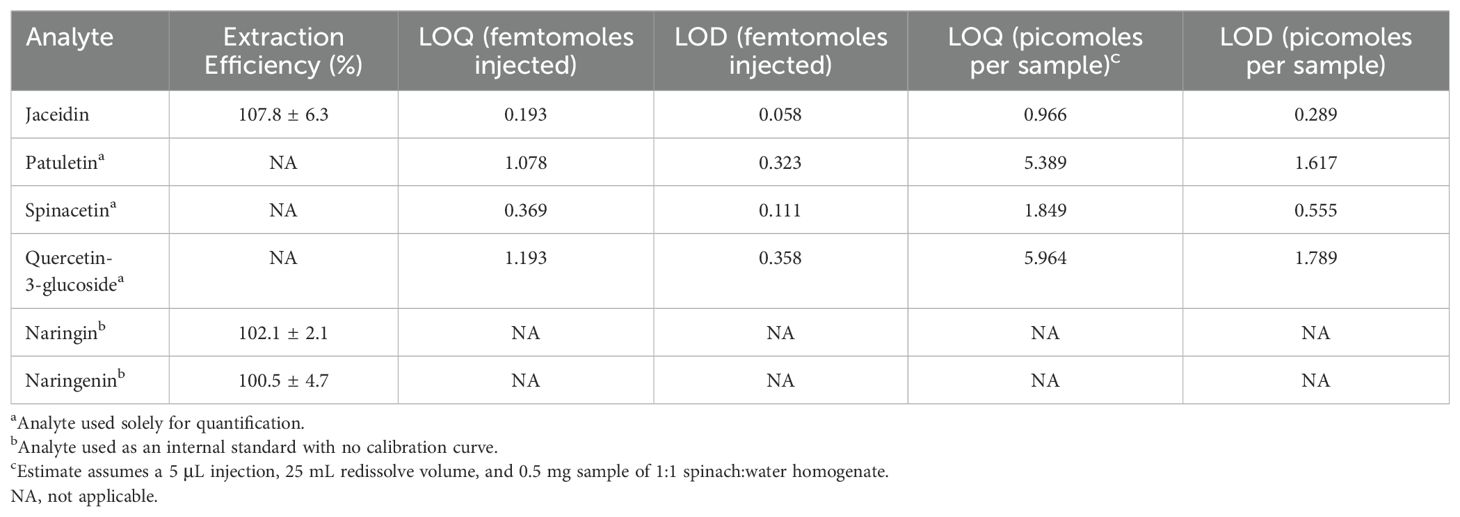
Table 2. Extraction efficiency of commercially available spinach flavonoids and structurally similar internal standards.
Validation of extraction
Given the speed of our chromatographic gradient, the possibility to merge our analysis method with a high-throughput extraction method poses an exciting opportunity to quantitatively profile large populations of spinach in a relatively short period of time. Miniaturized extractions are quickly gaining popularity as they reduce solvent usage, less labor-intensive, and are often amenable to automation compared to traditional methods. We developed a high-throughput protocol based on previously validated approaches for vegetables and cereals (Dzakovich et al., 2020, 2023) making modifications for spinach. We conducted spike recovery and intra/interday variability experiments to determine the suitability of our high-throughput approach. Additionally, we compared both the traditional extraction method with our high-throughput approach by profiling a genetically diverse population of spinach. Published spinach flavonoid values are sparse and infrequently use multiple genotypes (Howard et al., 2002; Pandjaitan et al., 2005; Cho et al., 2008; Schlering et al., 2020).
Spike recovery
A spike addition experiment allowed us to generate quantitative recovery estimates from our high-throughput method. We excluded spinacetin and patuletin from this experiment because these aglycones are rarely present in detectable levels in spinach. Naringin (102.1 ± 2.1%) and naringenin (100.5 ± 4.7%) had recovery estimates similar to jaceidin (107.8 ± 6.3%), which is naturally present in our sample matrix (Table 2). These findings support that our high-throughput extraction works efficiently with analytes varying in polarity. Our recovery estimates mirror or exceed estimates for similar molecules (Furrer et al., 2017; Mengist et al., 2020; Mohamedshah et al., 2022).
Intra/interday variability
Inter and intraday variability experiments allowed us to quantify the combined variation of our high-throughput extraction method as well as our analysis. A single individual extracted six randomly selected samples and analyzed them using our UHPLC-MS/MS method. This experiment was repeated twice more by the same individual to determine interday variation. Our findings indicate that our high-throughput extraction can reliably estimate analyte concentrations both within and between days (Table 3). The use of taxifolin as an internal standard helped compensate for day-day variation that is common in mass spectrometers.
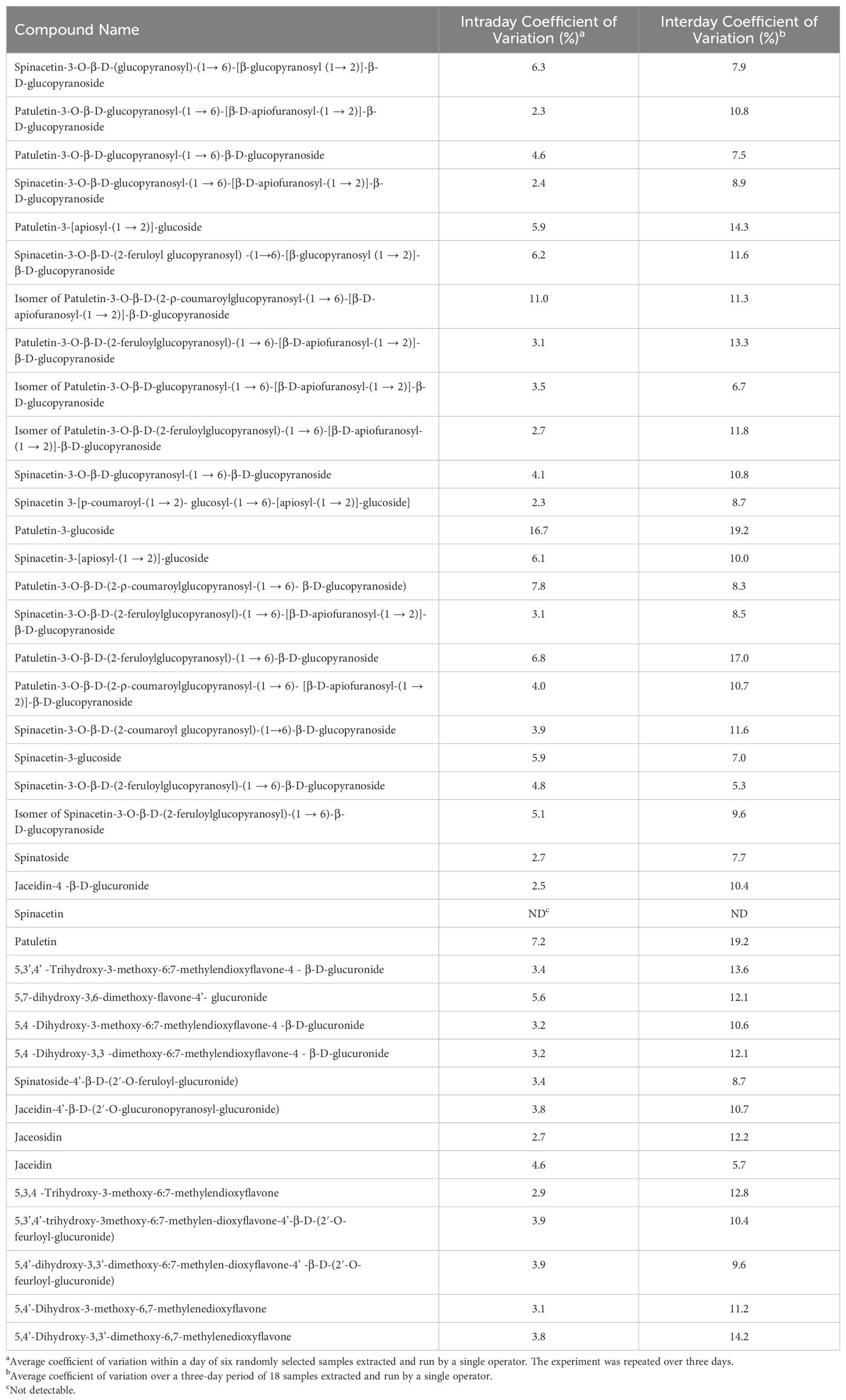
Table 3. Intraday and interday coefficient of variation values for analytes separated and quantified by our UHPLC-MS/MS method extracted using our high-throughput method.
Spinach germplasm survey
To further test our high-throughput extraction and simultaneously address the lack of comprehensive, quantitative information on spinach flavonoids, we profiled a population of 30 spinach accessions. Accessions include both commonly grown/consumed spinach cultivars that are commercially available as well as wild-collected accessions curated by the USDA Germplasm Resources Information Network (USDA-GRIN). Details about the individual accessions as well as analyte concentrations generated by both traditional and high-throughput extractions can be found in the Supplementary Information (Supplementary Tables 1, 3). Total flavonoid concentrations ranged from 75.1 – 170.1 mg/100 g fresh weight and approximately 70% of total flavonoids was represented by 519.08, 801.21, 521.09, 533.09, 787.19, and 977.26 m/z (Supplementary Tables 3, 4). Using the traditional method, data ranged from 93.1 – 187.26 mg/100 g fresh weight. Both datasets align well with previous reports of flavonoid concentrations in spinach (Cho et al., 2008; Grace et al., 2022). Critically, the proportion of each flavonoid compared to total flavonoids in each method were nearly identical (Supplementary Table 4). This finding indicates that differences between extraction methods are largely due to differences in extraction efficiency. Given that we used the same solvent systems in both extraction types, sonication used in the traditional extraction method (40 minutes total) likely allowed for more complete solvent penetration and destruction of spinach tissue compared to homogenization. Our data additionally indicate that spinach produces substantially greater amounts of flavonoids compared to many other fruits and vegetables (Harnly et al., 2006; Slimestad et al., 2008). Given the limited environmental variability in our study, the range of concentrations seen in our data primarily reflect genetic differences. Environmental aspects such as light and temperature could drastically alter the concentrations of spinach flavonoids (Howard et al., 2002; Cho et al., 2008; Schlering et al., 2020). While overall trends in data were similar between extraction methods (Supplementary Tables 3, 4), some differences exist.
Principal components analysis visualized the population structure as a function of extraction and illustrates that differences within and among accessions was slightly less pronounced in the high-throughput data (Figure 2). Notably, replicates of the same accession were further apart, indicating less similarity. A larger portion of variance explained by principal component 1 allowed for a clearer delineation among different accessions in the traditionally extracted samples. In practice, population structures were nearly identical and an end-user such as a plant breeder would be able to generalize trends using either method.
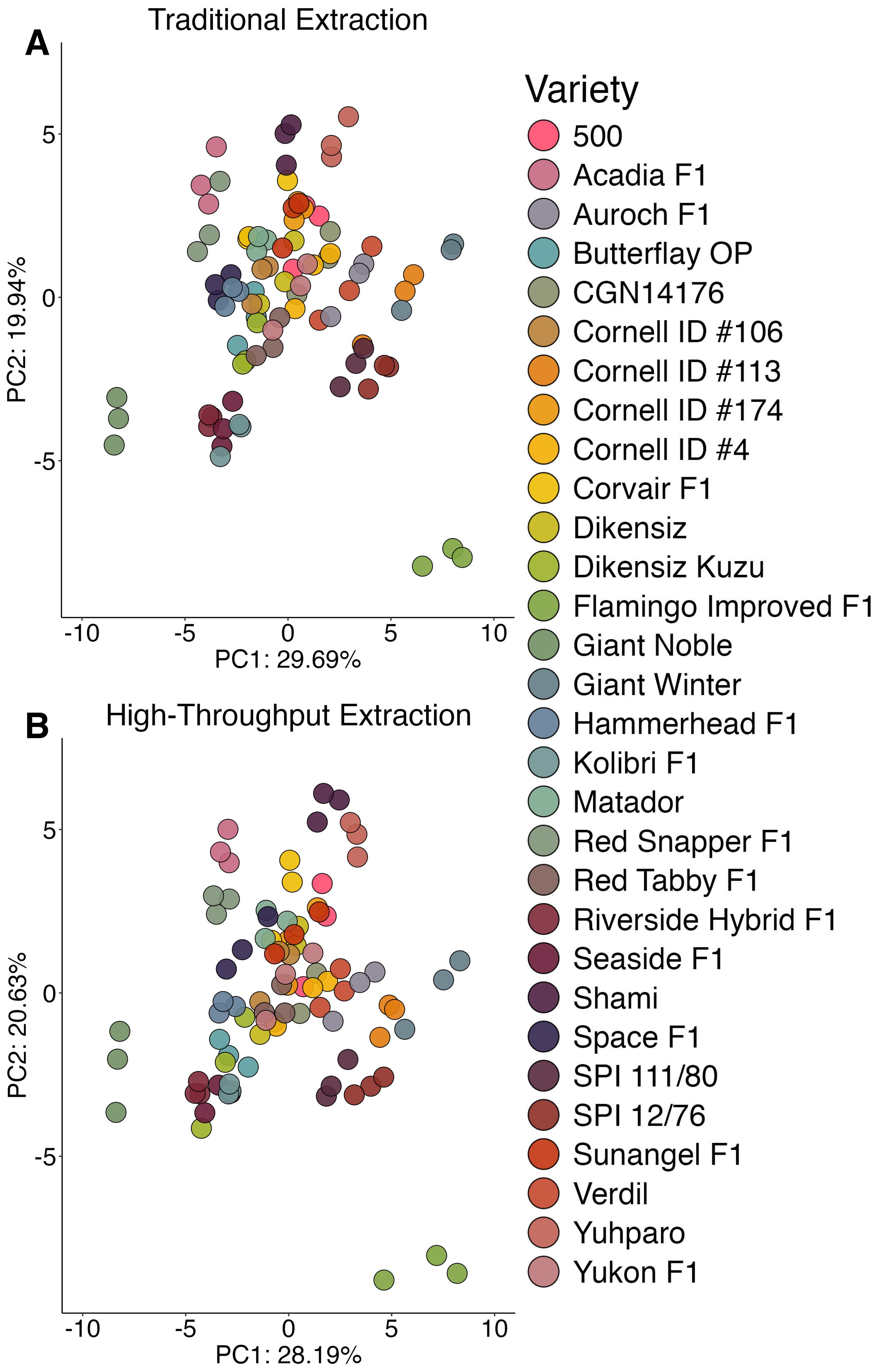
Figure 2. Principal components analysis 2-D scores plot displaying the population structure generated by the traditional extraction (A) or high-throughput extraction (B). Variable means were scaled to zero with a standard deviation of one.
To further explore small differences in population structures between extraction methods, we utilized random effects modeling to partition variance associated with genetic background, extraction method, replication, and within-method variability (Figure 3). In general, genetic background explained most variation (40.0% – 97.5%) compared to extraction method (0% – 49.0%). One notable exception is spinacetin (345 m/z) which was only detectable in our traditional extraction method. This finding suggests that spinacetin detected in the traditionally extracted samples was possibly from the hydrolysis of glycosylated flavonoids due to low pH and long sonication times. Another important trend is that the contribution to total variance caused by the extraction method tended to increase inversely to analyte polarity. Analytes in Figure 3 are in order of retention time from top to bottom which to a large degree is controlled by polarity. Analyte estimates were universally lower in the high-throughput extracted samples indicating that these later-eluting molecules were not extracted as effectively with a homogenization-based approach (Supplementary Tables 3, 4). Later eluting analytes in our method tend to be lower molecular weight. Given our observation about Spinacetin, another possibility is that some molecules that are higher in the traditional extraction are hydrolysis products of larger, more glycosylated flavonoids. For example, 519.08 m/z could feasibly be derived from 695.12 m/z if the ferulic acid residue was hydrolyzed during extraction. However, there is no indication that pools of 695.12 m/z are being exhausted in the traditional extraction given that its estimated concentration is higher than that of the high-throughput method (Supplementary Tables 3, 4). Thus, differences between extraction efficiency of these more nonpolar molecules is the most likely explanation. Sonication used in the traditional extraction method helped improve extraction of all flavonoids by effective solvent penetration and matrix breakdown. While previous work has shown that methanolic solvent systems work well for most spinach flavonoids, some of the more nonpolar species benefit from other solvents such as acetone (Singh et al., 2018a).Extraction efficiency could be further improved by the use of different solvents (e.g. acetone), solvent mixtures, and/or including sonication at each extraction step. These modifications may more broadly capture spinach flavonoids at a wider range of polarities.
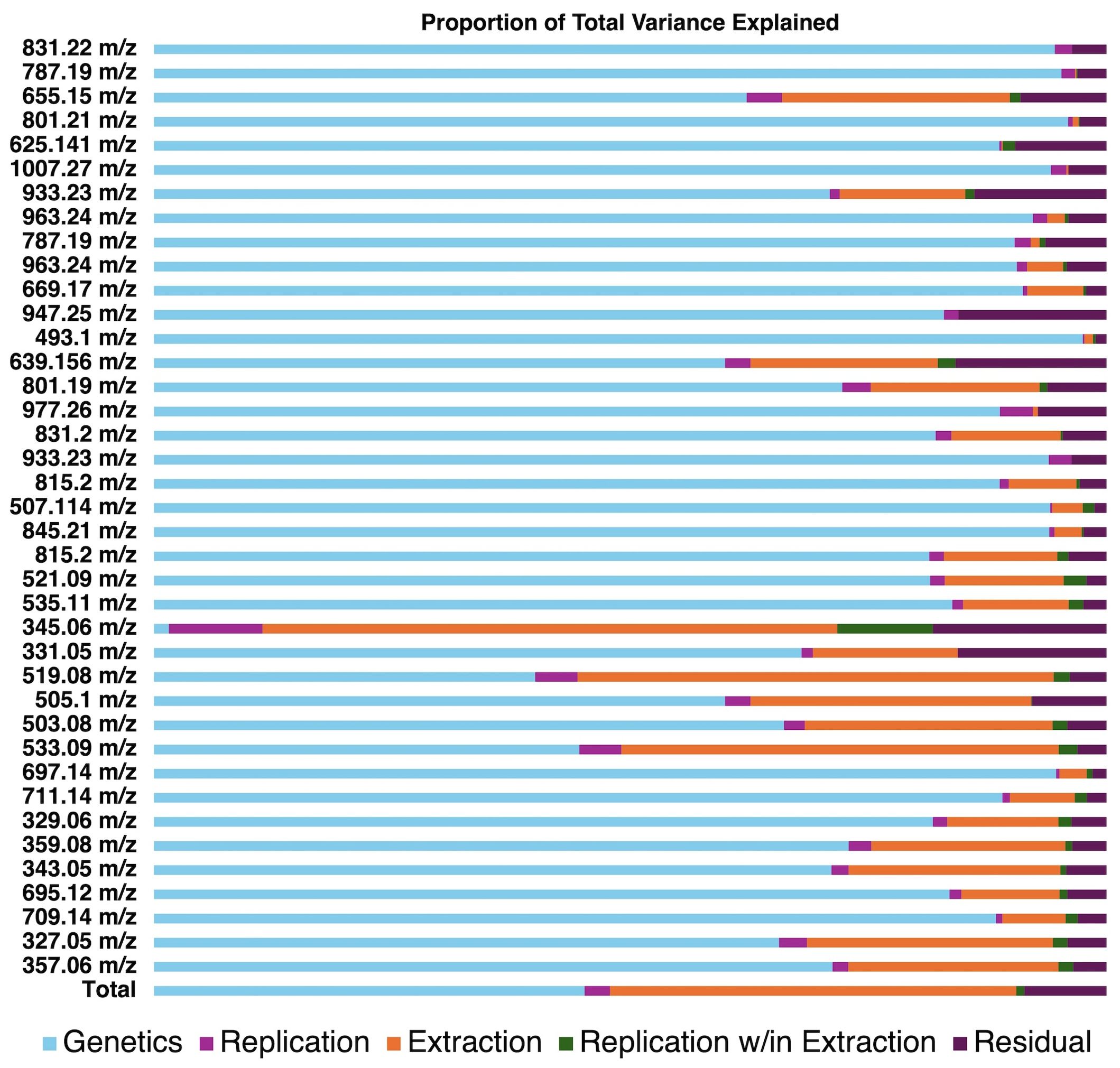
Figure 3. Visualization of variance estimates for each analyte profiled by our UHPLC-MS/MS method. Estimates were generated through random effects modeling separating the proportion of variance explained by genetics, replication, extraction method, replication within method, and residual error.
A key advantage of the high-throughput method is that 48 samples can be simultaneously extracted in roughly 60 minutes while using significantly less solvent (3 mL/sample compared to 15 mL/sample). The high-throughput extraction approach could be utilized by researchers interested in profiling large populations of spinach, and the traditional approach could be later utilized on a subset of samples that require additional accuracy and precision.
Conclusion
Here, we present the first comprehensive UHPLC-MS/MS method for spinach flavonoids as well as an accompanying high-throughput extraction method. Our method can separate and quantify 39 flavonoids uniquely produced by spinach in 11.5 minutes. Spectral information generated by our study may aid in the development of biomarkers for spinach consumption and/or the discovery of additional flavonoids. Our UHPLC-MS/MS method features excellent sensitivity; low femtomole-on-column quantification limits enabling miniaturized extractions to be used in tandem. A limitation of our method is that the absence of authentic standards for spinach flavonoids make it impossible to know the true ionization efficiency of each molecule. Future studies are needed to establish ionization efficiency ratios between quercetin-3-glucoside and authentic spinach standards to more accurately estimate their concentrations. We also developed and tested a high-throughput extraction method (48 samples/hour; 3 mL solvent/sample), which can save researchers time and reduce solvent usage. The high-throughput extraction approach could be utilized by a researcher interested in profiling a large population of spinach, and the traditional approach could be later utilized on a subset of samples that require additional accuracy and precision for the more nonpolar flavonoids. Given sporadic reports in the literature, the data presented here represents benchmark values and ranges for many spinach flavonoids. Our tools enable these understudied analytes to be examined in a variety of contexts and open new research avenues in plant biology, food science, and human health research.
Data availability statement
The original contributions presented in the study are included in the article/Supplementary Material. Further inquiries can be directed to the corresponding author.
Author contributions
MD: Conceptualization, Data curation, Formal analysis, Funding acquisition, Investigation, Methodology, Project administration, Resources, Software, Supervision, Validation, Visualization, Writing – original draft, Writing – review & editing. EL: Data curation, Formal analysis, Methodology, Writing – review & editing. AT: Data curation, Formal analysis, Methodology, Writing – review & editing. SC: Data curation, Formal analysis, Methodology, Resources, Validation, Writing – review & editing.
Funding
The author(s) declare that financial support was received for the research and/or publication of this article. Financial support for these studies was provided by USDA-ARS CRIS project 3092-51000-061-000 as well as the Texas Children’s Hospital Pediatric Pilot Award 69513-I.
Acknowledgments
We thank David Brenner and the USDA-ARS GRIN for providing some of the germplasm used in this study as well as Massimo Iorizzo of North Carolina State University for advice on curating this population. We also thank Brian Chlouber for assistance with plant growth and harvest. We appreciate the advice of Bhimanagouda Patil of Texas A&M University for suggestions regarding internal standards and extraction methodology.
Conflict of interest
The authors declare that the research was conducted in the absence of any commercial or financial relationships that could be construed as a potential conflict of interest.
Publisher’s note
All claims expressed in this article are solely those of the authors and do not necessarily represent those of their affiliated organizations, or those of the publisher, the editors and the reviewers. Any product that may be evaluated in this article, or claim that may be made by its manufacturer, is not guaranteed or endorsed by the publisher.
Author disclaimer
The findings and conclusions in this publication are those of the authors and should not be construed to represent any official USDA or U.S. Government determination or policy. Mention of trade names or commercial products in this publication is solely for the purpose of providing specific information and does not imply recommendation or endorsement by the U.S. Department of Agriculture. The USDA is an equal opportunity provider and employer.
Supplementary material
The Supplementary Material for this article can be found online at: https://www.frontiersin.org/articles/10.3389/fpls.2025.1496200/full#supplementary-material
References
Bates, D., Mächler, M., Bolker, B., Walker, S. (2015). Fitting linear mixed-effects models using lme4. J. Stat. Software 67, 1–48. doi: 10.18637/jss.v067.i01
Chen, L., Lu, W., Wang, L., Xing, X., Chen, Z., Teng, X., et al. (2021). Metabolite discovery through global annotation of untargeted metabolomics data. Nat. Methods 18, 1377–1385. doi: 10.1038/s41592-021-01303-3
Cho, M. J., Howard, L. R., Prior, R. L., Morelock, T. (2008). Flavonoid content and antioxidant capacity of spinach genotypes determined by high-performance liquid chromatography/mass spectrometry. J. Sci. Food Agric. 88, 1099–1106. doi: 10.1002/jsfa.3206
Demarque, D. P., Dusi, R. G., de Sousa, F. D. M., Grossi, S. M., Silvério, M. R. S., Lopes, N. P., et al. (2020). Mass spectrometry-based metabolomics approach in the isolation of bioactive natural products. Sci. Rep. 10, 1051. doi: 10.1038/s41598-020-58046-y
Dimkić, I., Petrović, M., Gavrilović, M., Gašić, U., Ristivojević, P., Stanković, S., et al. (2020). New perspectives of purple starthistle (Centaurea calcitrapa) leaf extracts: phytochemical analysis, cytotoxicity and antimicrobial activity. AMB Expr 10, 183. doi: 10.1186/s13568-020-01120-5
Dzakovich, M. P., Debelo, H., Albertsen, M. C., Che, P., Jones, T. J., Simon, M. K., et al. (2023). Trait stacking simultaneously enhances provitamin A carotenoid and mineral bioaccessibility in biofortified Sorghum bicolor. Food Funct. 14, 7053–7065. doi: 10.1039/D2FO03606A
Dzakovich, M. P., Hartman, J. L., Cooperstone, J. L. (2020). ). A high-throughput extraction and analysis method for steroidal glycoalkaloids in tomato. Front. Plant Sci. 11. doi: 10.3389/fpls.2020.00767
Edenharder, R., Keller, G., Platt, K. L., Unger, K. K. (2001). Isolation and characterization of structurally novel antimutagenic flavonoids from spinach (Spinacia oleracea). J. Agric. Food Chem. 49, 2767–2773. doi: 10.1021/jf0013712
Fabre, N., Rustan, I., de Hoffmann, E., Quetin-Leclercq, J. (2001). Determination of flavone, flavonol, and flavanone aglycones by negative ion liquid chromatography electrospray ion trap mass spectrometry. J. Am. Soc. Mass Spectrometry 12, 707–715. doi: 10.1016/S1044-0305(01)00226-4
Ferreres, F., Castañer, M., Tomás-Barberán, F. A. (1997). Acylated flavonol glycosides from spinach leaves (Spinacia oleracea). Phytochemistry 45, 1701–1705. doi: 10.1016/S0031-9422(97)00244-6
Furrer, A., Cladis, D. P., Kurilich, A., Manoharan, R., Ferruzzi, M. G. (2017). Changes in phenolic content of commercial potato varieties through industrial processing and fresh preparation. Food Chem. 218, 47–55. doi: 10.1016/j.foodchem.2016.08.126
Gil, M. I., Ferreres, F., Tomás-Barberán, F. A. (1999). Effect of postharvest storage and processing on the antioxidant constituents (Flavonoids and vitamin C) of fresh-cut spinach. J. Agric. Food Chem. 47, 2213–2217. doi: 10.1021/jf981200l
Grace, M. H., Hoskin, R. T., Hayes, M., Iorizzo, M., Kay, C., Ferruzzi, M. G., et al. (2022). Spray-dried and freeze-dried protein-spinach particles; effect of drying technique and protein type on the bioaccessibility of carotenoids, chlorophylls, and phenolics. Food Chem. 388, 133017. doi: 10.1016/j.foodchem.2022.133017
Griesbach, R. J. (2005). “Biochemistry and Genetics of Flower Color,” in Plant Breeding Reviews (Oxford, UK: John Wiley & Sons, Ltd), 89–114. doi: 10.1002/9780470650301.ch4
Grosso, G., Micek, A., Godos, J., Pajak, A., Sciacca, S., Galvano, F., et al. (2017). Dietary flavonoid and lignan intake and mortality in prospective cohort studies: systematic review and dose-response meta-analysis. Am. J. Epidemiol. 185, 1304–1316. doi: 10.1093/aje/kww207
Hamsalakshmi, Alex, A. M., Arehally Marappa, M., Joghee, S., Chidambaram, S. B. (2022). Therapeutic benefits of flavonoids against neuroinflammation: a systematic review. Inflammopharmacol 30, 111–136. doi: 10.1007/s10787-021-00895-8
Harnly, J. M., Doherty, R. F., Beecher, G. R., Holden, J. M., Haytowitz, D. B., Bhagwat, S., et al. (2006). Flavonoid content of U.S. Fruits, vegetables, and nuts. J. Agric. Food Chem. 54, 9966–9977. doi: 10.1021/jf061478a
Hayes, M., Pottorff, M., Kay, C., Van Deynze, A., Osorio-Marin, J., Lila, M. A., et al. (2020). In vitro bioaccessibility of carotenoids and chlorophylls in a diverse collection of spinach accessions and commercial cultivars. J. Agric. Food Chem. 68, 3495–3505. doi: 10.1021/acs.jafc.0c00158
Howard, L. R., Pandjaitan, N., Morelock, T., Gil, M. I. (2002). Antioxidant capacity and phenolic content of spinach as affected by genetics and growing season. J. Agric. Food Chem. 50, 5891–5896. doi: 10.1021/jf020507o
Ivey, K. L., Rimm, E. B., Kraft, P., Clish, C. B., Cassidy, A., Hodgson, J., et al. (2017). Identifying the metabolomic fingerprint of high and low flavonoid consumers. J. Nutr. Sci. 6, e34. doi: 10.1017/jns.2017.27
Koh, E., Charoenprasert, S., Mitchell, A. E. (2012). Effect of organic and conventional cropping systems on ascorbic acid, vitamin C, flavonoids, nitrate, and oxalate in 27 varieties of spinach (Spinacia oleracea L.). J. Agric. Food Chem. 60, 3144–3150. doi: 10.1021/jf300051f
Lê, S., Josse, J., Husson, F. (2008). FactoMineR: an R package for multivariate analysis. J. Stat. Software 25, 1–18. doi: 10.18637/jss.v025.i01
Lomnitski, L., Foley, J. F., Grossman, S., Shaul, V. B., Maronpot, R. R., Moomaw, C. R., et al. (2000). Effects of apocynin and natural antioxidant from spinach on inducible nitric oxide synthase and cyclooxygenase-2 induction in lipopolysaccharide-induced hepatic injury in rat. Pharmacol. Toxicol. 87, 18–25. doi: 10.1111/j.0901-9928.2000.870104.x
Mannochio-Russo, H., de Almeida, R. F., Nunes, W. D. G., Bueno, P. C. P., Caraballo-Rodríguez, A. M., Bauermeister, A., et al. (2022). Untargeted metabolomics sheds light on the diversity of major classes of secondary metabolites in the malpighiaceae botanical family. Front. Plant Sci. 13. doi: 10.3389/fpls.2022.854842
McNab, H., Ferreira, E. S. B., Hulme, A. N., Quye, A. (2009). Negative ion ESI–MS analysis of natural yellow dye flavonoids—An isotopic labelling study. Int. J. Mass Spectrometry 284, 57–65. doi: 10.1016/j.ijms.2008.05.039
Mengist, M. F., Burtch, H., Debelo, H., Pottorff, M., Bostan, H., Nunn, C., et al. (2020). Development of a genetic framework to improve the efficiency of bioactive delivery from blueberry. Sci. Rep. 10, 17311. doi: 10.1038/s41598-020-74280-w
Mohamedshah, Z., Hayes, M., Chadwick-Corbin, S., Neilson, A. P., Ferruzzi, M. G. (2022). Bioaccessibility, gut microbial metabolism and intestinal transport of phenolics from 100% Concord grape juice and whole grapes are similar in a simulated digestion and fecal fermentation model. Food Funct. 13, 4315–4330. doi: 10.1039/D1FO04226B
Mills, B. R. (2024). _MoMAColors: Color Palettes Inspired by Artwork at the Museum of Modern Art in New York City_. R package version 0.0.0.9000, commit 6f5d75decc3f0063b199d5f0127d63c950528b8a, vailable at: https://github.com/BlakeRMills/MoMAColors.
Nyska, A., Lomnitski, L., Spalding, J., Dunson, D. B., Goldsworthy, T. L., Grossman, S., et al. (2001). Topical and oral administration of the natural water-soluble antioxidant from spinach reduces the multiplicity of papillomas in the Tg.AC mouse model. Toxicol. Lett. 122, 33–44. doi: 10.1016/S0378-4274(01)00345-9
Pandjaitan, N., Howard, L. R., Morelock, T., Gil, M. I. (2005). Antioxidant capacity and phenolic content of spinach as affected by genetics and maturation. J. Agric. Food Chem. 53, 8618–8623. doi: 10.1021/jf052077i
Qin, J., Shi, A., Mou, B., Grusak, M. A., Weng, Y., Ravelombola, W., et al. (2017). Genetic diversity and association mapping of mineral element concentrations in spinach leaves. BMC Genomics 18, 941. doi: 10.1186/s12864-017-4297-y
Redan, B. W., Albaugh, G. P., Charron, C. S., Novotny, J. A., Ferruzzi, M. G. (2017). Adaptation in caco-2 human intestinal cell differentiation and phenolic transport with chronic exposure to blackberry (Rubus sp.) extract. J. Agric. Food Chem. 65, 2694–2701. doi: 10.1021/acs.jafc.7b00027
Schlering, C., Zinkernagel, J., Dietrich, H., Frisch, M., Schweiggert, R. (2020). Alterations in the chemical composition of spinach (Spinacia oleracea L.) as provoked by season and moderately limited water supply in open field cultivation. Horticulturae 6, 25. doi: 10.3390/horticulturae6020025
Singh, J., Jayaprakasha, G. K., Patil, B. S. (2017). Rapid ultra-high-performance liquid chromatography/quadrupole time-of-flight tandem mass spectrometry and selected reaction monitoring strategy for the identification and quantification of minor spinacetin derivatives in spinach. Rapid Commun. Mass Spectrometry 31, 1803–1812. doi: 10.1002/rcm.7967
Singh, J., Jayaprakasha, G. K., Patil, B. S. (2018a). An optimized solvent extraction and characterization of unidentified flavonoid glucuronide derivatives from spinach by UHPLC-HR-QTOF-MS. Talanta 188, 763–771. doi: 10.1016/j.talanta.2018.06.025
Singh, J., Jayaprakasha, G. K., Patil, B. S. (2018b).Extraction, Identification, and Potential Health Benefits of Spinach Flavonoids: A Review. In: Advances in Plant Phenolics: From Chemistry to Human Health (American Chemical Society). Available online at: https://pubs.acs.org/doi/pdf/10.1021/bk-2018-1286.ch006 (Accessed January 27, 2025).
Singh, A., Singh, P., Kumar, B., Kumar, S., Dev, K., Maurya, R. (2019). Detection of flavonoids from Spinacia oleracea leaves using HPLC-ESI-QTOF-MS/MS and UPLC-QqQLIT-MS/MS techniques. Natural Product Res. 33, 2253–2256. doi: 10.1080/14786419.2018.1489395
Slimestad, R., Fossen, T., Verheul, M. J. (2008). The flavonoids of tomatoes. J. Agric. Food Chem. 56, 2436–2441. doi: 10.1021/jf073434n
Takahashi, A., Ohnishi, T. (2004). The significance of the study about the biological effects of solar ultraviolet radiation using the Exposed Facility on the International Space Station. Biol. Sci. Space 18, 255–260. doi: 10.2187/bss.18.255
Vial, J., Jardy, A. (1999). Experimental comparison of the different approaches to estimate LOD and LOQ of an HPLC method. Anal. Chem. 71, 2672–2677. doi: 10.1021/ac981179n
Keywords: phytochemicals, mass spectrometry, genetic diversity, high-throughput phenotyping, quantitative analysis
Citation: Dzakovich MP, Le EA, Tak AL and Chacko SK (2025) A comprehensive UHPLC-MS/MS and extraction method reveals flavonoid profile and concentration are diverse in spinach (Spinacia oleracea L.). Front. Plant Sci. 16:1496200. doi: 10.3389/fpls.2025.1496200
Received: 13 September 2024; Accepted: 31 March 2025;
Published: 28 April 2025.
Edited by:
Christelle M. Andre, The New Zealand Institute for Plant and Food Research Ltd, New ZealandReviewed by:
Wanpeng Xi, Southwest University, ChinaAzazahemad A. Kureshi, Pharmanza Herbal Private Limited, India
Copyright © 2025 Dzakovich, Le, Tak and Chacko. This is an open-access article distributed under the terms of the Creative Commons Attribution License (CC BY). The use, distribution or reproduction in other forums is permitted, provided the original author(s) and the copyright owner(s) are credited and that the original publication in this journal is cited, in accordance with accepted academic practice. No use, distribution or reproduction is permitted which does not comply with these terms.
*Correspondence: Michael P. Dzakovich, bWljaGFlbC5kemFrb3ZpY2hAdXNkYS5nb3Y=