- 1Yinshanbeilu Grassland Eco-hydrology National Observation and Research Station, China Institute of Water Resources and Hydropower Research, Beijing, China
- 2Institute of Water Resources for Pastoral Area, Ministry of Water Resources, Hohhot, China
- 3Wuhai City Water Bureau, Inner Mongolia Autonomous Region, Wuhai Soil and Water Conservation Workstation, Wuhai, Inner Mongolia, China
Understanding water sources and utilization strategies is essential for the water use patterns of vegetation restoration species and achieving sustainable vegetation restoration. The water use strategies of Corethrodendron scoparium and Calligonum mongolicum were studied in the afforestation area on the northeastern edge of the Tengger Desert, to provide scientific guidance for regional vegetation restoration and stand structure adjustment. We utilize hydrogen and oxygen isotope techniques and the MixSIAR model to calculate the contribution rates of these two plant species to various potential water sources from June to October. By calculating the PS index, we determined the competitive relationship of C. scoparium and C. mongolicum towards different water sources. The results showed that the soil moisture content in the 0-80 cm soil layer changed significantly due to rainfall and evaporation, but stabilized with increasing depth. Shallow soil water shows enriched stable isotope composition, while the isotope of groundwater is relatively stable, and the isotope of precipitation is more enriched than that of groundwater. The main water source of C. scoparium and C. mongolicum was soil moisture. The utilization rate of 0-40 cm soil layer was 27% (C.scoparium) and 33% (C.mongolicum), and the utilization rate of 40-80 cm soil layer was 32% (C.scoparium) and 25% (C.mongolicum). The average proportional similarity index (PS index) between the two species was 95.67%, indicating a competitive relationship with water resources. When the surface layer (0-40) soil moisture is high (July, August), both species preferentially absorb water from this layer, and the water competition is reduced. The average PS index is 89%. When the surface layer (0-40) was deficient in soil moisture (June, September and October), the water competition increased, and the PS index was 97.83%. This study emphasized the adaptation strategies of these shrubs to arid environments and found that it provided key insights for optimizing vegetation density and species composition in desert aerial seeding areas and ensuring sustainable ecological restoration in the study area.
1 Introduction
Water is the key factor limiting the growth of artificial vegetation in arid and semi-arid regions (Chen et al., 2014). The availability and utilization patterns of water by plants reflect the ecosystem’s response to environmental water conditions, with eco-hydrological processes playing a decisive role in the function and evolutionary trajectory of the soil-vegetation system (Beyer et al., 2018). Therefore, studying plant water use is crucial for understanding the survival adaptability of species in aerial-seeding afforestation areas and for selecting sand-stabilizing vegetation. Since aerial seeding alters the original water balance and the spatial distribution of soil moisture in sandy areas, determining the mechanisms of water use by plants in arid and semi-arid regions is essential for the construction of artificial vegetation in these areas (Wang et al., 2017).
Stable isotope techniques are an important method for accurately determining the sources and proportions of water used by plants (Ehleringer and Dawson, 1992; Renée Brooks et al., 2009; Guillaume et al., 2014; David et al., 2017). By comparing the hydrogen and oxygen stable isotope values in the xylem water of plant stems with those of different water sources, these techniques provide insights into the water-use relationships of plants. In studies of plant water utilization, plants of different life forms generally utilize water from different sources, which is related to their root system types. Typically, trees and deep-rooted shrubs can access deep soil water or groundwater (Wei et al., 2013), while shallow-rooted shrubs and perennial herbaceous plants rely on shallow or intermediate soil water (Liu et al., 2011). In areas with higher precipitation, plants tend to use shallow soil water (Chen et al., 2016); in drier climates, they are more inclined to use deeper soil water and groundwater (Zhao et al., 2008). De Wispelaere et al. (Lien et al., 2017) studied seasonal variations in the water sources of plants in arid and semi-arid regions, finding that plants mainly use deep soil water and groundwater during the dry season, while relying on rainfall during the wet season. Dai et al (Dai et al., 2015). analyzed the water use patterns of Haloxylon ammodendron and Haloxylon persicum naturally distributed on dune tops and interdune lowlands in the Gurbantunggut Desert, revealing that these plants primarily utilize deep soil water and groundwater. In summary, the current research on plant water sources mainly focuses on hilly woodlands, forests, deserts, and riparian forests. However, there are few reports on the differences in water sources of artificial plants in arid and semi-arid areas, especially in the aerial seeding afforestation area on the northeastern edge of the Tengger Desert.
The Tengger Desert is located in the arid and semi-arid area of the Inner Mongolia Autonomous Region in northern China. In the 1980 s, China began large-scale aerial seeding afforestation in the Tengger Desert. After more than 30 years of development, the vegetation coverage of the Tengger Desert has been effectively improved. It has greatly promoted the ecological restoration of the Tengger Desert and realized the transformation from the past ‘ sand into human retreat ‘ to the present ‘ human into sand retreat ‘. In 1992, the vegetation coverage of the aerial seeding afforestation area in the northeastern margin of the Tengger Desert increased from less than 5% to more than 20% on average after more than 30 years of restoration, mainly due to the formation of plant communities dominated by C.scoparium and C.mongolicum in the aerial seeding area. The regional landform has developed from the original mobile dunes to fixed or semi-fixed dunes. However, with the large-scale sand-fixing afforestation, the contradiction between supply and demand of soil moisture is increasing, and vegetation in some high-density areas is even degraded. In addition, with the warming of the climate, the seasonal drought in the Tengger Desert occurs frequently, and the drought and water shortage seriously threaten the ecological restoration and sustainable vegetation construction in the desert area. However, water is the main limiting factor for vegetation restoration in the northeastern margin of the Tengger Desert. Some sand control plants with unreasonable planting density increase water consumption. What is the change of soil moisture in the plant community formed on this basis? After aerial seeding afforestation, what are the water sources used by the main artificial plants in the sandy area during the growing season, and are there any differences in their water sources? What kind of water use relationship does the plant have and what kind of water use strategy is reflected? Is there a competitive relationship between the mixed mode of C.scoparium and C.mongolicum in the utilization of soil moisture in this area? These issues remain to be studied.
Therefore, in this study, from June to October 2023, in the 1992 aerial seeding area in the northeastern margin of the Tengger Desert in Alxa Left Banner, Alxa League, the meteorological elements such as precipitation and temperature were monitored by small weather stations, and the hydrogen and oxygen stable isotope composition of atmospheric precipitation, plant xylem water, groundwater and soil water were measured. The MixSIAR model was used to study the water source characteristics and water use relationship of C.scoparium and C.mongolicum in different months, so as to clarify the water use strategy of C.scoparium and C.mongolicum, analyze the competitive relationship between C.scoparium and C.mongolicum on soil water use, and master the water use strategy of aerial seeding plants in detail. It provides reference for vegetation construction and regional desertification control in Tengger Desert.
2 Materials and methods
2.1 Overview of the study area
The study area is located in the 1992 aerial-seeding afforestation zone on the northeastern edge of the Tengger Desert, Alxa Left Banner, Alxa League, as shown in Figure 1. The climate in the region is characterized by a mid-temperate continental climate. Although the area receives little rainfall, most of it is concentrated in July and August, with an annual average precipitation of only 123.33 mm. The total rainfall in June-October 2023 is 36.8 mm as shown in Table 1. The annual average temperature is 7.8°C, with extreme temperatures reaching up to 39°C. The average annual evaporation is 2258.8 mm, with a total of 3181 hours of sunshine per year, an average wind speed of about 4 m/s, and approximately 45 days of strong winds annually. The region is primarily dominated by the aerial seeding shrubs C.scoparium and C.mongolicum, along with the native shrub Artemisia ordosica. Herbaceous plants in the area include Allium mongolicum, Stipa capillata, Grubovia dasyphylla, and Agriophyllum squarrosum. The soil in the study area exhibits zonal distribution, including types such as gray desert soil, gray-calcic soil, and brown-calcic soil.
2.2 Research methods
2.2.1 Plot setup and investigation
The study area is located in the northeast edge of Tengger Desert of Alxa Left Banner in 1992. The study area was initially unvegetated. After more than 30 years of aerial seeding afforestation vegetation restoration, a vegetation pattern dominated by mixed plant communities of C.scoparium and C.mongolicum has been formed. From June to October 2023, a mixed plot of C.scoparium and C.mongolicum was selected in the study area. Firstly, the current vegetation status in the study area was investigated. The basic situation of different plant community samples is shown in Table 2. The survey indicators include plant height, crown width, base diameter, shrub coverage, and herbaceous plants. Based on the vegetation survey data, the standard growth parameters of representative plants were determined. Using these parameters as the standard, three healthy and representative strains of C.scoparium and C.mongolicum were selected for experimental sampling.
2.2.2 Sample collection
1. Plant Sample Collection: The experiment was conducted in June 2023. In different plots within the study area, healthy and mature C.scoparium and C.mongolicum plants were selected. Non-green lignified twigs (diameter 0.1-0.3 cm, length 3-5 cm) were clipped, peeled, and quickly placed into screw-top glass vials, which were then sealed with sealing film. The vials were immediately stored in portable ice boxes and transported back to the laboratory for freezing (below -20°C) for hydrogen and oxygen isotope analysis.
2. Soil Sample Collection: Soil samples were collected at half the canopy radius from where the plant samples were taken, at depths of 0-20 cm, 20-40 cm, 40-60 cm, 60-80 cm, 80-100 cm, 100-120 cm, 120-140 cm, 140-160 cm, 160-180 cm, and 180-200 cm. Three replicates were taken for each layer, with sampling times matching those of the plant samples. Each soil layer sample was divided into two parts: one part was quickly placed in sample bottles, sealed with sealing film, and stored in portable insulated boxes for transport to the laboratory for hydrogen and oxygen isotope value analysis. The other part was placed in aluminum boxes and brought back to the laboratory for soil moisture content measurement using the drying method.
3. Groundwater Sample Collection: Groundwater samples were taken from the nearest well in the study area.
4. The precipitation samples were taken from the standard rainfall buckets placed in the study area. The precipitation samples were collected immediately after each precipitation. The samples were sealed in a 10 ml glass bottle and brought back to the laboratory for stable isotope determination. Due to the scarcity of rainfall in the study area, only rainfall from 4 effective rainfall events can be collected.
2.2.3 Water extraction and isotope determination
The processing and measurements in this study were conducted in the laboratory of the Institute of Water Resources for Pastoral Areas, Ministry of Water Resources. Plant and soil samples were processed using a low-temperature vacuum distillation system to extract water. The extracted water was then sealed and refrigerated for later analysis. The δ18O values of groundwater, precipitation, and the extracted water from plant xylem and soil were measured using a liquid water isotope analyzer from LGR. The δ value is widely used worldwide as a standard method to measure the number of hydrogen and oxygen stable isotopes, that is, the deviation of the isotope ratio in the sample from its reference (Vienna Standard Mean Sea Water) by one thousandth (Fry, 2015). The formula is (1):
In the formula, δX is δD or δ18O (‰), R is the isotope ratio, Rsample is the ratio of heavy and light isotope abundance of elements in the sample (18O/16O, D/H); Rstandard is the ratio of stable isotope abundance of international common standard (18O/16O, D/H stable isotope using VSMOW).
2.2.4 Calculation of soil water content and the proportion of potential water sources used by plants
The soil water content was determined by drying method. The soil samples brought back to the laboratory were dried in a 105°C blast oven for 12 h, and the dry weight was weighed. The soil water content was calculated by the formula (Cao et al., 2018).
In the formula, W is the mass water content (%), W0 is the weight of aluminum box, W1 is the weight of aluminum box + wet soil, and W2 is the weight of aluminum box and dried soil.
The MixSIAR model is a Bayesian mixing model that runs in R and can be used to determine the proportion of different potential water sources utilized by plants. It has the capability to handle any number of isotope values simultaneously, analyze multiple potential sources at once, operate quickly, and account for differences between isotope values. The Bayesian isotope mixing model MixSIAR estimates the proportion of potential water sources utilized by plants. It incorporates numerous factors related to multiple sources and their uncertainties, which enhances the accuracy and rigor of the model (Stock and Semmens, 2017). During model execution, the isotope values of the woody tissues of C.scoparium and C.mongolicum were input as mixing data into the MixSIAR model. The average values and standard deviations of isotope values from different soil depths (0-20, 20-40, 40-60, 60-80, 80-100, 100-120, 120-140, 140-160, 160-180, 180-200 cm) and groundwater were input as source data into the MixSIAR model. Since no isotope fractionation occurs during plant water uptake (Renée Brooks et al., 2009), the average values and standard deviations of potential sources were set to 0 as difference data for the MixSIAR model. The Monte Carlo Markov Chain run length was set to “short,” and the error term choice was set to “residual,” after which the model was executed (Wang et al., 2017).
2.2.5 Proprtional similarity index
The proprtional similarity index (PS) is an indicator of niche overlap between organisms. The larger the PS index, the more the niche overlap between the two species, and the greater the competition for the same water source; the smaller the PS index, indicating that the niche overlap between the two species is not obvious, and the competition for the same resource is smaller (Stefan et al., 2016). In this study, PS index was used to evaluate the water use of C.scoparium and C.mongolicum. The specific calculation formula is shown in Formula 3:
In the formula: P1i and P2i represent the utilization ratio of the two plants to the i water source, respectively.
2.2.6 Data analysis
This study used stable oxygen isotopes for analysis. Data were analyzed using SPSS 19.0, with one-way ANOVA employed to compare the significance of differences in soil moisture content across different soil depths and the δ18O values of woody tissue water. The MixSIAR model was used to analyze the contribution ratios of potential water sources to the plants. Graphs were created using Origin 8.0 software.
3 Results and analysis
3.1 Characteristics of soil water content
NOTE: Each graph represents the following sample plots for: (a) C.scoparium; (b) C.mongolicum; Different lowercase letters indicated that there were significant differences between different soil layers in the same plot (P< 0.05).
As shown in Figure 2, in general, the soil water content of C.scoparium community in the study area decreased first and then increased with the increase of month, and the soil water content of C.mongolicum community increased first, then decreased and then increased with the increase of month (Equation 1). In June, the change of soil water content was consistent with the change of soil depth. With the increase of soil depth, the water content increased first and then decreased. The soil water content of C.scoparium plants in 0-20 cm was relatively low, which was 0.29%, and the soil water content in 40-60 cm was higher, which was 0.97%. The soil water content of C.mongolicum plants in 0-20 cm was relatively low, which was 0.23%, and the soil water content in 100-120 cm was higher, which was 0.67%. In July, the soil water content of C.scoparium and C.mongolicum plants reached the maximum at 180-200cm and 20-40cm, respectively, 0.63% and 1.13%. In August, the soil water content of C.scoparium and C.mongolicum plants reached the maximum at 60-80cm and 180-200cm, respectively, 0.74% and 0.78%. In September, the soil water content of C.scoparium and C.mongolicum reached the maximum at 20-40cm and 140-160cm, respectively, 0.80% and 0.93%. In October, the soil water content of C.scoparium and C.mongolicum reached the maximum at 60-80cm and 160-180cm, respectively, 0.89% and 0.9%. The soil water content in different layers showed significant variations between the two species (C.scoparium and C.mongolicum) across different months (P< 0.05). For C.scoparium, the soil water content in the 0-20 cm layer was significantly lower than that in the 40-60 cm layer in June (P< 0.05). Similarly, for C.mongolicum, the soil water content in the 0-20 cm layer was significantly lower than that in the 100-120 cm layer in June (P< 0.05). In July, the soil water content in the 180-200 cm layer for C.scoparium and the 20-40 cm layer for C.mongolicum were significantly higher than other layers (P< 0.05). These results indicate that the two species exhibit distinct patterns of soil water utilization, with C.scoparium relying more on deeper soil layers during certain periods, while C.mongolicum shows a preference for shallower layers.
3.2 Hydrogen and oxygen stable isotope characteristics of different water sources
Based on the precipitation collected during the experimental period, the Local Meteoric Water Line (LMWL) for the study area was determined from the hydrogen and oxygen stable isotope values of the precipitation: δD = 7.47δ18O + 7.11 (R² = 0.79) (Figure 3). Its slope is lower than the Global Meteoric Water Line (GMWL): δD = 8δ18O + 10 (Craig, 1961), indicating that the precipitation in the study area experienced the effect of dry air during and produced secondary evaporation. The isotope variation range of the precipitation was: δ18O from -3.23‰ to 2.17‰ and δD from -22.82‰ to 25.88‰, with significant fluctuations. The isotope variation range of the groundwater was: δ18O from -8.28‰ to -7.38‰ and δD from -65.18‰ to -63.62‰, with smaller fluctuations. The isotope variation range of the soil water was: δ18O from -8.13‰ to 4.39‰ and δD from -61.36‰ to -31.68‰ (Equation 2). The Soil Water Line (SWL) equation was δD = 2.06δ18O - 42.13 (R² = 0.60), with both the slope and intercept lower than that of the LMWL, suggesting that the soil water was influenced by secondary evaporation.
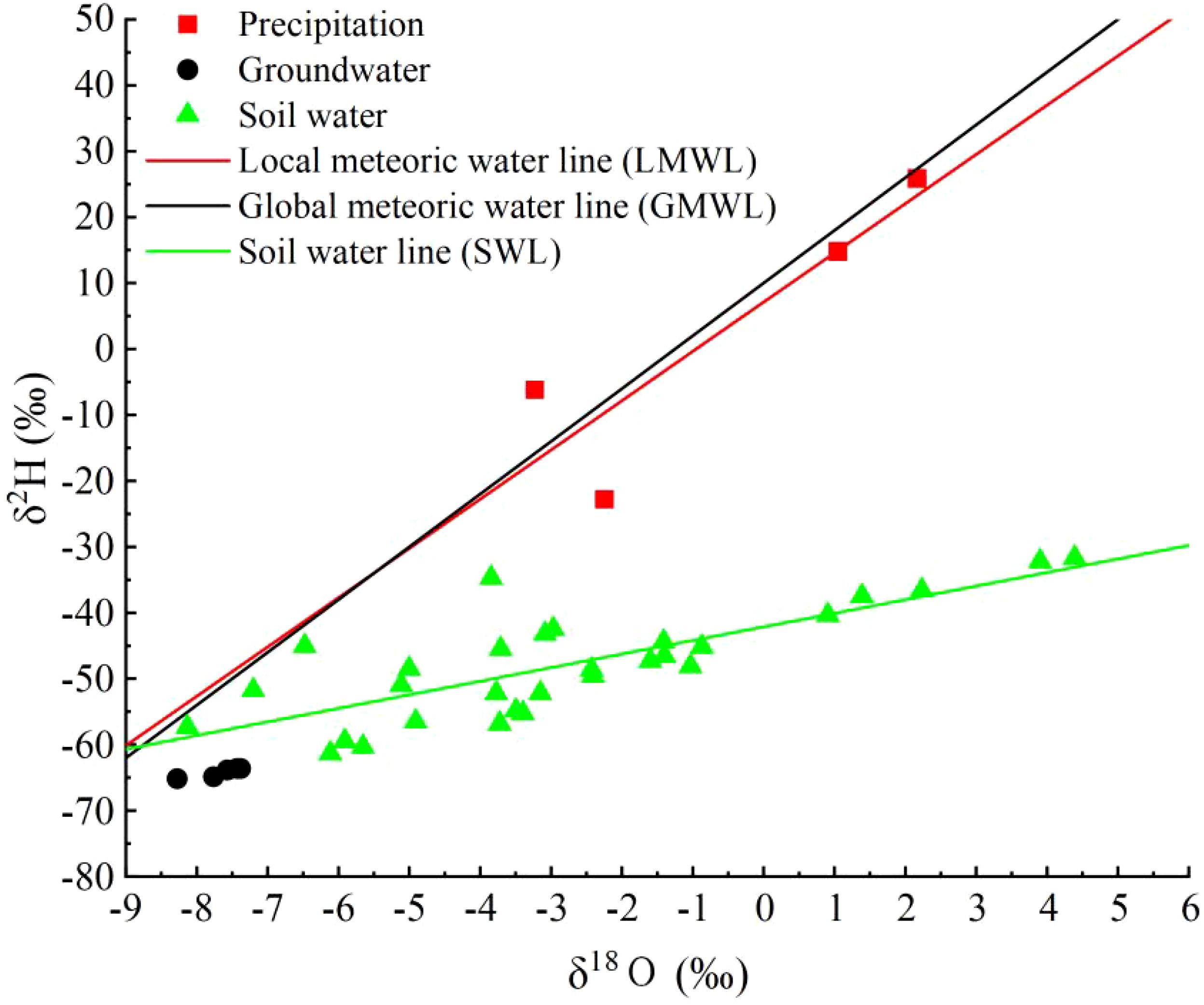
Figure 3. The distribution characteristics of atmospheric precipitation line, soil water line, precipitation and soil water δD and δ18O in the study area.
The isotopic distribution characteristics of plant xylem water are shown in Table 3. For C.scoparium, the xylem water isotope range was: δ18O from 2.89‰ to 12.92‰ and δD from -45.11‰ to -32.21‰; for C.mongolicum, the xylem water isotope range was: δ18O from -0.09‰ to 8.62‰ and δD from -47.02‰ to -9.67‰. The monthly variations in xylem water isotopes for the two plants were as follows: for C.scoparium, the highest mean values of δD and δ18O were -32.21‰ and 12.92‰, respectively, both occurring in August, while the lowest values were -45.11‰ and 2.89‰, both in October; for C.mongolicum, the highest δD and δ18O values were -9.67‰ and 8.62‰, both in September, while the lowest were -47.02‰ and -0.09‰, also in September. For C.scoparium, the δD and δ18O values showed significant differences between June and August (P< 0.05), with the highest values observed in August. For C.mongolicum, the δD and δ18O values exhibited significant differences between June and September (P< 0.05), with the highest values observed in September. The study found that the δD and δ18O values of groundwater samples collected in different months were relatively consistent, indicating that the hydrogen and oxygen stable isotope composition of groundwater in the study area is relatively stable. However, a comparison of hydrogen and oxygen isotopes between groundwater and precipitation showed significant differences, with precipitation isotope values being more enriched than groundwater, indicating that precipitation in the study area almost does not contribute to groundwater recharge.
3.3 Sources of soil moisture in different soil layers
As a stable isotope, δ 18O is very stable and is not prone to decay. In addition, δ 18O is able to record the movement process of water well, because the δ 18O value is used to study the soil water source. As shown in Figure 4, the δ18O values of soil water exhibit a decreasing trend with increasing soil depth. The potential water sources for the plants in the study area include shallow soil water (0-40 cm), upper soil water (40-80 cm), middle soil water (80-120 cm), lower soil water (120-160 cm), and deep soil water (160-200 cm). The soil layers that intersect with or have δ18O values closest to the δ18O values of the xylem water of C.scoparium and C.mongolicum represent the primary water uptake layers for these plants. Consequently, in June, C.scoparium primarily utilized shallow soil water, C.mongolicum primarily utilized upper and middle soil water. In July, C.scoparium primarily utilized upper soil water, C.mongolicum primarily utilized shallow soil water. In August, C.scoparium primarily utilized shallow and upper soil water, C.mongolicum primarily utilized upper and middle soil water. In September and October, C.scoparium primarily utilized shallow soil water, C.mongolicum primarily utilized shallow and upper soil water.
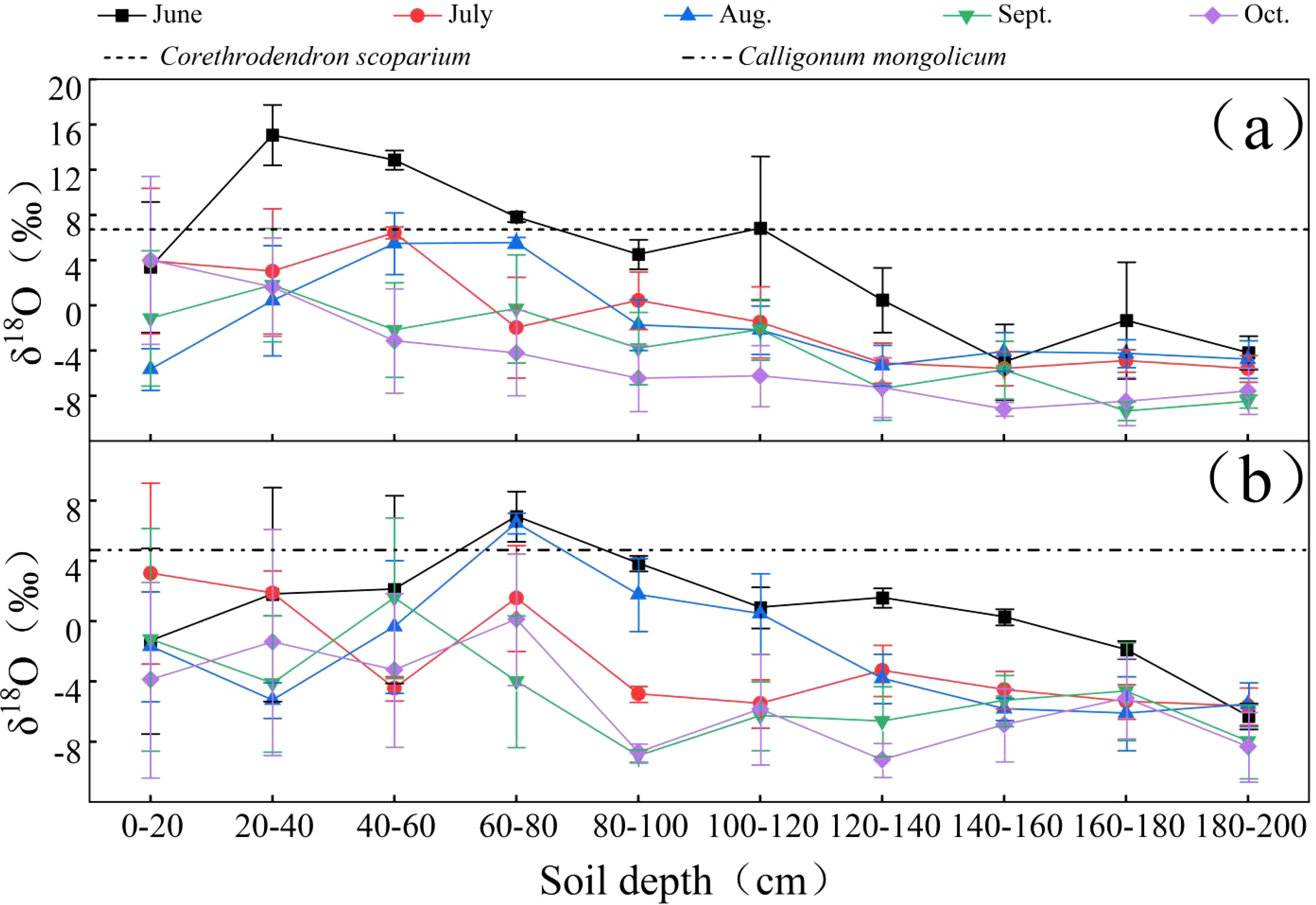
Figure 4. Comparison of δ18O values of soil water and plant xylem in different plots NOTE: Each graph represents the following sample plots for: (a) C.scoparium; (b) C.mongolicum.
3.4 Analysis of water use sources of plants
As shown in Figure 5, throughout the entire growing season, C.scoparium primarily utilized upper soil water, with a utilization rate of 33.2%. C.mongolicum mainly relied on shallow soil water, with utilization rates of 32.4%. The two species had the lowest utilization rates of deep soil water, at 11.3% and 12.5% respectively. However, the contribution rates of soil water from different layers to C.scoparium and C.mongolicum varied across different months. In June, which is outside the main growing season, 64.2% of the water absorbed by C.scoparium came from soil layers above 120 cm, mainly relying on shallow and upper soil water. C.mongolicum absorbed 71.4% of its water from soil layers below 80 cm, primarily depending on lower soil water. In the mid-growing season (July-August), C.scoparium absorbed 63.1%-73.5% of its water from soil layers above 80 cm, primarily relying on shallow soil water. C.mongolicum absorbed 47.8%-73% of its water from soil layers above 80 cm, mainly relying on shallow and upper soil water. In the late growing season (September-October), C.scoparium absorbed 58.8%-63.8% of its water from soil layers above 80 cm, mainly relying on shallow soil water. C.mongolicum absorbed 61.9%-75% of its water from soil layers above 80 cm, mainly relying on shallow soil water.
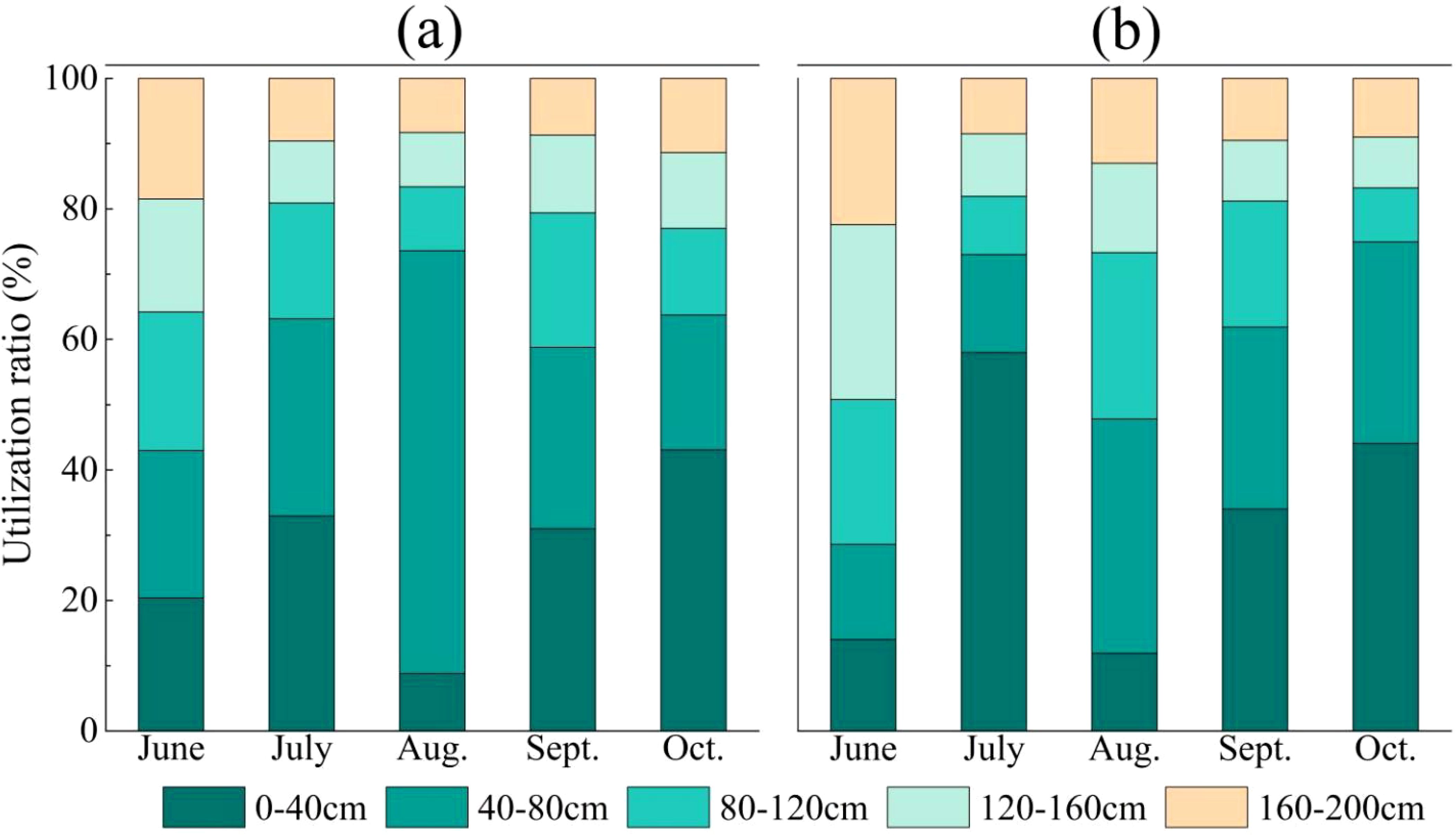
Figure 5. The utilization rate of potential water sources by two plants in different months NOTE: Each graph represents the following sample plots for: (a) C.scoparium; (b) C.mongolicum.
3.5 Competition relationship of plant water source
In arid and semi-arid regions, plant competition for water resources is a common phenomenon. As shown in Figure 6, the PS index between the two plants is relatively high, which shows that C.scoparium and C.mongolicum have more use of the same water source (Equation 3). During the whole study period, the average PS index of C.scoparium and C.mongolicum was 95.67%, indicating that there was a competitive relationship between the water use of C.scoparium and C.mongolicum, but there were differences in the water use relationship between C.scoparium and C.mongolicum in different months. In September, the PS index of soil moisture in 0-200cm of C.scoparium and C.mongolicum was the highest, which was 99.29%, indicating that the water sources of C.scoparium and C.mongolicum were the same in September, the water use patterns were basically the same, and the water competition relationship was the strongest. The soil moisture PS index of 0-200 cm in August was the lowest, which was 91.82%, indicating that the main water sources of C.scoparium and C.mongolicum were different, the water use patterns were different, and the water competition relationship was relatively weak. In June, July and October, the PS index was smaller, indicating that the water use patterns of C.scoparium and C.mongolicum were different, and the water competition relationship was weak. At different depths, the PS indexes of 0-200 cm C.scoparium and C.mongolicum in June, September and October were all greater than 95%, indicating that the water competition relationship between C.scoparium and C.mongolicum was the strongest. In July 120-160 cm and August 0-40 cm, 160-200 cm C.scoparium and C.mongolicum, the PS indexes were 87.45%, 85.60% and 85.60%, respectively, which were lower than those in other soil layers, indicating that the water competition relationship between C.scoparium and C.mongolicum was the weakest.
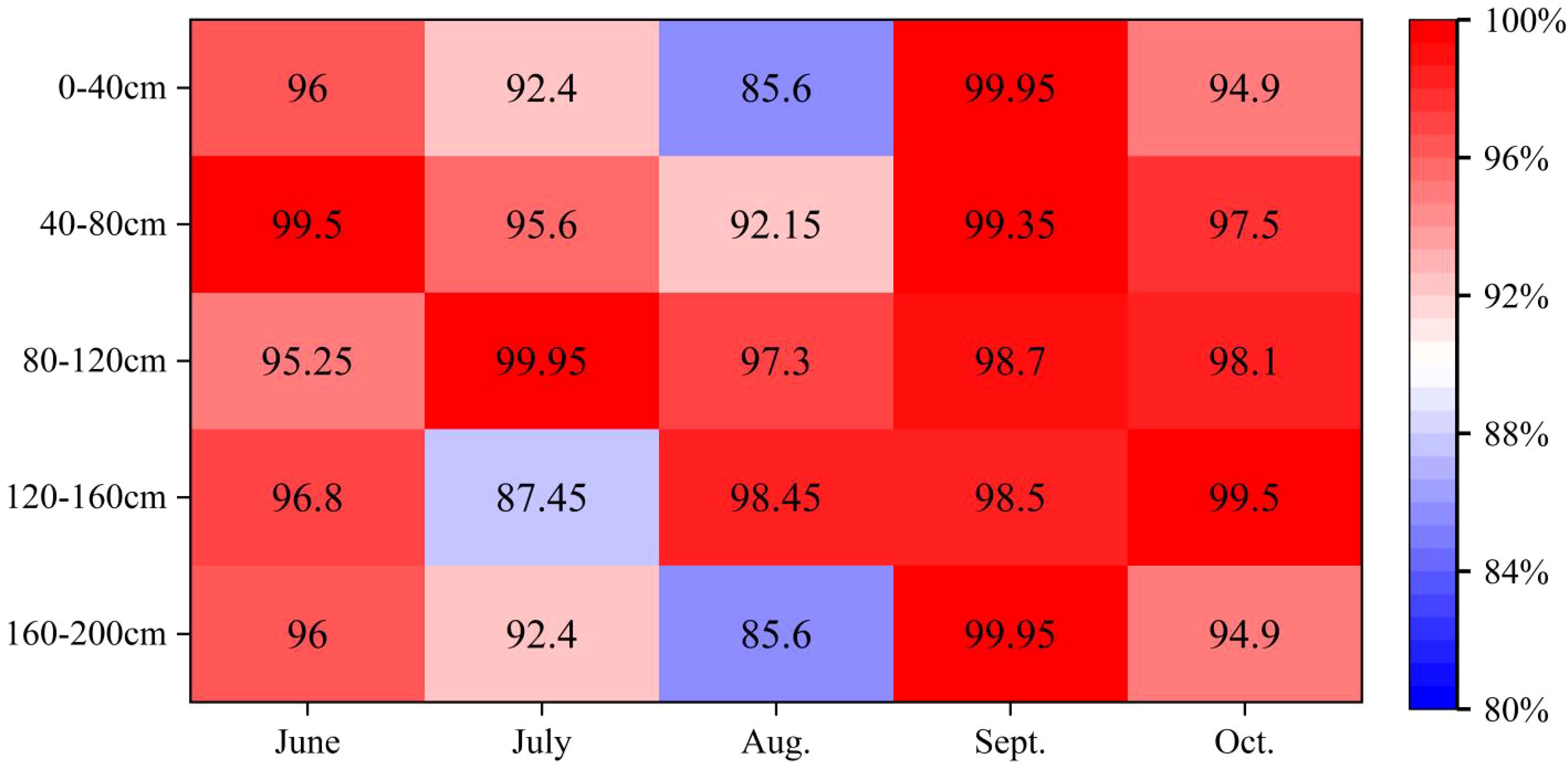
Figure 6. Comparison of PS index of two aerial seeding plants NOTE: PS: Proportional similarity index; PS = 0 indicates that there is no competition between the two plants. The PS index was between 0-100%, indicating that there was a competitive relationship between the two plants. And the larger the PS index, the stronger the competitive relationship; PS = 100% indicated that the competition between the two species reached the maximum.
4 Discussion
4.1 Soil moisture research
Soil moisture is primarily influenced by external factors such as evapotranspiration and atmospheric precipitation, with the impact of these external conditions gradually weakening as soil depth increases (Miriam et al., 2016; Shan et al., 2020). The soil water content in 0 ~ 60cm of C.scoparium and C.mongolicum plots was large and varied greatly. In the 100 ~ 200cm soil layer, the soil water content tends to be stable. This situation may be that the density of vegetation root system in deep soil is relatively small, and the root system absorbs less water from the soil. Because root absorption is one of the main ways of soil water consumption, the low root density leads to the relative reduction of deep soil water consumption, which helps to maintain the stability of water content. Compared with the surface soil, the deep soil is less affected by direct sunlight and air flow, so the evaporation is weaker. This further reduces the loss of deep soil moisture and helps to maintain a stable state of water content. Additionally, local climate variations, different vegetation types, and the combination of different soils directly affect the distribution of soil moisture. Differences in soil physical and chemical properties lead to heterogeneity in soil moisture (Miriam et al., 2016; Nicole et al., 2016).
4.2 Differences in plant water sources and water use relationship
Analysis of the relationship between atmospheric precipitation, plant water, soil water, and groundwater hydrogen and oxygen isotopes in the study area reveals that both the intercept and slope of the atmospheric precipitation equation are greater than those of the soil water equation. This indicates that precipitation primarily recharges soil water in the study area, while soil water is significantly affected by non-equilibrium evaporation. The minimal variation in groundwater hydrogen and oxygen isotopes suggests a stable isotopic composition, likely due to the deep burial of groundwater layers, which are less influenced by environmental factors compared to soil water. Consequently, the hydrogen and oxygen isotopes in the groundwater of the aerial seeding forestation area on the northeastern edge of the Tengger Desert are almost unaffected by evaporative fractionation. Due to their drought tolerance, resistance to sand burial, and rapid growth, C.scoparium and C.mongolicum have become important plants for sand control in the region in recent years. Moreover, plants are sensitive to changes in soil moisture and can adjust their water use strategies in time according to changes in soil moisture (Bush et al., 2010). The research findings indicate that during the growing season in the aerial seeding forestation area on the northeastern edge of the Tengger Desert, these plants primarily utilize soil water, with precipitation and groundwater contributing less than soil water. C.scoparium and C.mongolicum mainly rely on soil water in 0-40 cm and 40-80 cm soil layers, with a total contribution rate of more than 50%. The variation in soil water usage across different months is primarily influenced by rainfall; when rainfall is abundant, soil water is replenished, leading to changes in the plants’ soil water utilization. In August, when rainfall is higher, C.scoparium and C.mongolicum utilize soil water from the 40-80 cm layer, likely because rainfall recharges the soil water, allowing water to infiltrate and become available to plants at that depth. Wang et al (Wang et al., 2017, Wang et al., 2019). found that in Vitex negundo, water is mainly obtained from the surface soil layer (0-40 cm), and then shifts to the deep soil layer (120-300 cm) as the season progresses. Then the seasonal water use characteristics of Spiraea pubescens and Hippophae rhamnoides were investigated. The results showed that during the growing season, about 80% of the water source of H.rhamnoides and S.pubescens came from the 0-120 cm soil layer. Zhao et al (Zhao et al., 2021). studied the water use of Salix psammophila and Caragana korshinskii and found that the use of 0-30 cm soil water was shifted in July and August, with an average contribution rate of 61% and 51%, respectively. Ding et al (Ding et al., 2023). found that evergreen and deciduous tree species mainly use 0-50 cm soil water in summer. The above studies are similar to the results of this study. At the same time, we also found that plants tend to use it preferentially when the shallow soil moisture is sufficient (Hasselquist and Allen, 2009). It also shows that C.scoparium and C.mongolicum respond to different environments through spatial differences in water use.
4.3 Effects of plant roots on soil water use
Plant roots are one of the primary means by which plants absorb water, and they play a crucial role in determining the sources of water that plants utilize (Liu et al., 2011; Davi et al., 2012). We studied the root system. As shown in Table 4, the main roots of the two plants are distributed between 0-3m, while the lateral roots of the two plants are developed, mainly horizontally distributed between 0-1.5m, and the water absorption of the plants is mainly carried out by the lateral roots. Therefore, the utilization of water by plants is closely related to the distribution of roots. When the water conditions are different, the absorption of water by plant roots will also be different. In this study, C.scoparium mainly used 0-80 cm soil water from June to October, but the contribution rate of surface soil water was slightly higher. And C.scoparium mainly uses 0-40 cm soil water. The reason is that the lateral root system of C.scoparium is developed, and the lateral root grows horizontally (Ma et al., 2012). It will preferentially use 0-40 cm soil moisture, and the contribution rate to deep 120-200 cm soil moisture in June is higher than that in other months, indicating that in the period of drought or less rainfall, the developed main roots of C.scoparium will choose to use deeper soil moisture. The root system of C.mongolicum is developed, and the main root will grow to 2-3m, while the horizontal root system is mainly distributed in the surface and shallow layers. Therefore, C.mongolicum mainly uses 0-80cm soil water, and when the rainfall is scarce in June, it will also increase the use of soil water in other soil layers. This reflects the adaptive strategies of C.scoparium and C.mongolicum on water use. In plant-water systems in arid regions, plants have the ability to redistribute water absorbed by roots at different depths, so they can selectively absorb and utilize various types of water sources to meet their growth needs (Zeng and Ma, 2013).
Nippert et al. (Jesse and Alan, 2007) found that when shallow soil water is insufficient to sustain normal plant growth, the root system of plants shifts from a shallow water uptake mode to a deeper water uptake mode. Consistent with our research findings, C.mongolicum utilizes soil water from the 120-160 cm and deep 160-200 cm layers during June when water availability is poor. However, in July and August, when rainfall is more abundant, the well-developed lateral roots of C.mongolicum prioritize the use of soil water from the 0-40 cm and 40-80 cm layers, reducing reliance on deeper water sources. This indicates that C.mongolicum is well-adapted to the soil moisture conditions in this region. The differing water uptake sources for the root systems of C.scoparium and C.mongolicum reflect their adaptation to the varying moisture conditions of the study area, showcasing the co-evolution of these two plants in this environment (Hao and Li, 2021). Therefore, the horizontal and vertical distribution of plant roots in the soil is one of the reasons for the differences in water uptake depth and sources among different plants.
4.4 Competition relationship of plant water source
The utilization of the same resources and the interspecific relationship between the two tree species under the same habitat conditions can be revealed by the niche overlap index. There are two possibilities for niche overlap between tree species: First, there is a competitive relationship between tree species sharing resources; second, there is a niche separation relationship between resource utilization among tree species, which includes temporal and spatial differences (Han et al., 2023; Li et al., 2024). In this study, PS index was selected as an index to characterize the niche overlap between plants. The study found that the PS index of the two plants was basically higher, indicating that there was a strong water competition between C.scoparium and C.mongolicum in most periods. This conclusion is similar to the results of (Tang et al., 2018). During the study period, the PS index of C.scoparium and C.mongolicum was higher. Combined with water use, both plants would use soil moisture in 0-80 cm soil layer. When the water competition between the two plants increased, C.scoparium would reduce the use of soil moisture in 0-80 cm soil layer, and C.mongolicum would increase the use of soil moisture in 0-80 cm soil layer. The variation characteristics of soil water uptake at different depths of the two species have similar responses to the competition of water sources between plants, which can be explained by niche separation. In September, the PS index of the two plants was higher than that in other months, indicating that the competition for soil moisture was the largest in September. The reason is that we speculate that under the condition of relatively high soil moisture content in September, due to the relative concentration of water resources and the strong demand for water by plants, coupled with niche overlap, root distribution conflict, soil texture and structural differences, the competition for water between plants will be fierce and complex. The degree of competition between the two plants for water sources is different in different periods, suggesting that this is an adaptation process of plants to changes in soil water conditions. At the same time, it shows that the two plants can achieve the purpose of coexistence through niche separation under continuous water resources competition.
5 Conclusions
The study investigated the water use strategies and competitive relationships of C. scoparium and C. mongolicum in the aerial afforestation area at the northeastern margin of the Tengger Desert.
1. Groundwater shows relatively stable hydrogen and oxygen stable isotopes, while precipitation isotopes are more enriched. Affected by precipitation and evaporation, stable isotopes of shallow soil moisture are enriched.
2. The main water source of C. scoparium and C. mongolicum was soil moisture, and the utilization rate was 45% and 41.1%, respectively. The water contribution rate of soil moisture in 0-80 cm soil layer to plant growth was the highest, reaching 60% and 57%, respectively. C. scoparium is more dependent on 40-80 cm soil moisture, and C. mongolicum is more dependent on 0-40 cm soil moisture.
3. The average proportional similarity index (PS index) between the two species was 95.67%, indicating a competitive relationship with water resources. When the surface layer (0-40) soil moisture is high (July, August), both species preferentially absorb water from this layer, and the water competition is reduced. The average PS index is 89%. When the surface layer (0-40) was deficient in soil moisture (June, September and October), the water competition increased, and the PS index was 97.83%.
Data availability statement
The original contributions presented in the study are included in the article/supplementary material. Further inquiries can be directed to the corresponding author.
Author contributions
ZZ: Software, Writing – original draft. GT: Investigation, Methodology, Writing – review & editing. JL: Data curation, Investigation, Methodology, Writing – review & editing. WM: Data curation, Formal Analysis, Writing – review & editing. ZL: Conceptualization, Supervision, Writing – review & editing.
Funding
The author(s) declare that financial support was received for the research and/or publication of this article. This research was funded by The Inner Mongolia Autonomous Region Science and Technology Plan Project ‘ Research on New Format Technology for Efficient Utilization of Water Resources in Aerial Seeding Afforestation Area of Tengger Desert in Yellow River Basin’ (2022YFHH0096); IWHR Research&Development Support Program (MK0145B022021) and the Project of Creating Ordos National Sustainable Development Agenda Innovation Demonstration Zone (2022EEDSKJXM005).
Acknowledgments
The author is very grateful to you for your participation in the research platform provided by the Institute of Water Resources for Pastoral Area, Ministry of Water Resources, for the support provided by all the projects, and for the joint efforts of all the authors to complete this article. The author thanks the reviewers for their help and advice, which helps us improve the quality of the manuscript.
Conflict of interest
The authors declare that the research was conducted in the absence of any commercial or financial relationships that could be construed as a potential conflict of interest.
The reviewer ZD declared a past co-authorship with the author(s) JL to the handling editor.
Generative AI statement
The author(s) declare that no Generative AI was used in the creation of this manuscript.
Publisher’s note
All claims expressed in this article are solely those of the authors and do not necessarily represent those of their affiliated organizations, or those of the publisher, the editors and the reviewers. Any product that may be evaluated in this article, or claim that may be made by its manufacturer, is not guaranteed or endorsed by the publisher.
References
Beyer, M., Hamutoko, J. T., Wanke, H., Gaj, M., Koeniger, P. (2018). Examination of deep root water uptake using anomalies of soil water stable isotopes, depth-controlled isotopic labeling and mixing models. J. Hydrol (Amst). 566, 122–136. doi: 10.1016/j.jhydrol.2018.08.060
Bush, S. E., Hultine, K. R., Sperry, J. S., Ehleringer, J. R. (2010). Calibration of thermal dissipation sap flow probes for ring- and diffuse-porous trees. Tree Physiol. 30, 1545. doi: 10.1093/treephys/tpq096
Cao, Z. M., Lorenzo, R., Cheyenne, S., Zhang, W. L., Leonardo, L., Ma, X. M. (2018). The impact of cerium oxide nanoparticles on the physiology of soybean (Glycine max (L.) Merr.) under different soil moisture conditions. Environ. Sci. Pollut. Res. 25, 930–939. doi: 10.1007/s11356-017-0501-5
Chen, J., Xu, Q., Gao, D. Q., Song, A. Y., Hao, Y. G., Ma, Y. B. (2016). Differential water use strategies among selected rare and endangered species in West Ordos Desert of China. Chin. J. Plant Ecol. 10, 660–669. doi: 10.1093/jpe/rtw059
Chen, L. X., Zhang, Z. Q., Melanie, Z., Liu, C. F., Guo, J. T., Zhu, J. Z., et al. (2014). Response of transpiration to rain pulses for two tree species in a semiarid plantation. Int. J. Biometeorol. 58, 1569–1581. doi: 10.1007/s00484-013-0761-9
Craig, H. (1961). Isotopic variations in meteoric waters. Science. 133, 1702–1703. doi: 10.1126/science.133.3465.1702
Dai, Y., Zheng, X. J., Tang, L. S., Li, Y. (2015). Stable oxygen isotopes reveal distinct water use patterns of two Haloxylon species in the Gurbantonggut Desert. Plant Soil. 389, 73–87. doi: 10.1007/s11104-014-2342-z
Davi, R. R., Lucas de, C. R. S., Randoll, V., Leonel da, S. L. S., Augusto, C. F. (2012). Depth of water uptake in woody plants relates to groundwater level and vegetation structure along a topographic gradient in a neotropical savanna. Environ. Exp. Botany. 77, 259–266. doi: 10.1016/j.envexpbot.2011.11.025
David, R. B., Emily, S. S., Steven, J. H. (2017). Revisiting streamside trees that do not use stream water: can the two water worlds hypothesis and snowpack isotopic effects explain a missing water source? Ecohydrol. 10, e1771. doi: 10.1002/eco.1771
Ding, F. J., Yuan, C. J., Zhou, T., Cheng, J., Wu, P., Ye, Y. Y. (2023). Water-use strategies and habitat adaptation of four tree species in karstic climax forest in maolan. Water. 15, 203. doi: 10.3390/w15010203
Ehleringer, J. R., Dawson, T. E. (1992). Water uptake by plants: perspectives from stable isotope composition. Plant Cell Environ. 15, 1073–1082. doi: 10.1111/j.1365-3040.1992.tb01657.x
Guillaume, B., Jean, M., Nico, G., Jessica, M., Véronique, L., Hélène, C., et al. (2014). Determination of spatiotemporal variability of tree water uptake using stable isotopes (δ18O, δ2H) in an alluvial system supplied by a high-altitude watershed, Pfyn forest, Switzerland. Ecohydrol. 7, 319–333. doi: 10.1002/eco.1347
Han, L., Liu, L. L., Peng, L., Wang, N. N., Zhou, P. (2023). Mixing of tree species with the same water use strategy might lead to deep soil water deficit. For. Ecol. Manage. 534, 120876. doi: 10.1016/j.foreco.2023.120876
Hao, S., Li, F. D. (2021). Water sources of the typical desert vegetation in Ebinur Lake basin. Acta Geogr. Sin. 76, 1649–1661. doi: 10.11821/dlxb202107006
Hasselquist, N. J., Allen, M. F. (2009). Increasing demands on limited water resources: Consequences for two endangered plants in Amargosa Valley, USA. Am. J. Botany. 96, 620–626. doi: 10.3732/ajb.0800181
Jesse, B. N., Alan, K. K. (2007). Soil water partitioning contributes to species coexistence in tallgrass prairie. Oikos. 116, 1017–1029. doi: 10.1111/j.0030-1299.2007.15630.x
Li, J. T., Mu, J., Shen, K. P., Guo, Y., Bai, X. J., Zang, L. P., et al. (2024). Niche and interspecific association of dominant woody plants in Camellia luteoflora community. Acta Ecol. Sin. 44, 283–294. doi: 10.20103/j.stxb.202212273676
Lien, D. W., Samuel, B., Pedro, H., Andreas, H., Dirk, V., Pascal, B. (2017). Plant water resource partitioning and isotopic fractionation during transpiration in a seasonally dry tropical climate. Biogeosciences. 14, 73–88. doi: 10.5194/bg-14-73-2017
Liu, Y. H., Xu, Z., Rodney, D., Chen, W. L., An, S. Q., Liu, S. R., et al. (2011). Analyzing relationships among water uptake patterns, rootlet biomass distribution and soil water content profile in a subalpine shrubland using water isotopes. Eur. J. Soil Biol. 47, 380–386. doi: 10.1016/j.ejsobi.2011.07.012
Ma, H. T., Wang, R., Sun, Q. (2012). Study on root distribution and soil moisture difference of two sand-fixing shrubs. For. Sci. Technol. 10, 6–8. doi: 10.13456/j.cnki.lykt.2012.10.008
Miriam, P., José, M., María, P., Nilda, S., Mercè, V., Adriano, C. (2016). Multi-temporal evaluation of soil moisture and land surface temperature dynamics using in situ and satellite observations. Remote Sens (Basel). 8, 587. doi: 10.3390/rs8070587
Nicole, A. L. A., Wilfred, O., Sonja, S., Glyn Bengough, A., Nadeem, S., Mike, B. (2016). Rainfall infiltration and soil hydrological characteristics below ancient forest, planted forest and grassland in a temperate northern climate. Ecohydrol. 9, 585–600. doi: 10.1002/eco.1658
Renée Brooks, J., Holly, R. B., Rob, C., Jeffrey, J. M. (2009). Ecohydrologic separation of water between trees and streams in a Mediterranean climate. Nat. Geoscience. 3, 100–104. doi: 10.1038/ngeo722
Shan, Y. L., Xie, J. C., Lei, N., Dong, Q. G. (2020). Soil moisture characteristics of a typical slope in the watershed of the loess plateau for gully land consolidation project. E3S Web Conf. 199, 00006. doi: 10.1051/E3SCONF/202019900006
Stefan, T., Yann, S., He, J. S., Andy, H., Michael, S. L. (2016). Spatio-temporal water uptake patterns of tree saplings are not altered by interspecific interaction in the early stage of a subtropical forest. For. Ecol. Manage. 367, 52–61. doi: 10.1016/j.foreco.2016.02.018
Stock, B. C., Semmens, B. X. (2017). MixSIAR GUI user manual. Version3.1.2.[2017-07-08] Available online at: https://github.com/brianstock/MixSIAR (Accessed September 18, 2024).
Tang, Y.K, Wu, X., Chen, Y. M., Wen, J., Xie, Y. L., Lu, S. B. (2018). Water use strategies for two dominant tree species in pure and mixed plantations of the semiarid Chinese Loess Plateau. Ecohydrol. 11, e1943. doi: 10.1002/eco.1943
Wang, J., Fu, B. J., Lu, N., Wang, S., Zhang, L. L. (2019). Water use characteristics of native and exotic shrub species in the semi-arid Loess Plateau using an isotope technique. Agriculture Ecosystems Environment. 276, 55–63. doi: 10.1016/j.agee.2019.02.015
Wang, J., Fu, B. J., Lu, N., Zhang, L. (2017). Seasonal variation in water uptake patterns of three plant species based on stable isotopes in the semi-arid Loess Plateau. Sci. Total Environ. 609, 27–37. doi: 10.1016/j.scitotenv.2017.07.133
Wei, Y. F., Fang, J., Liu, S., Zhao, X. Y., Li, S. G. (2013). Stable isotopic observation of water use sources of Pinus sylvestris var. mongolica in Horqin Sandy Land, China. Trees. 27, 1249–1260. doi: 10.1007/s00468-013-0873-1
Zeng, Q., Ma, J. Y. (2013). Plant water sources of different habitats and its environmental indication in heihe river basin. J. Glaciol. Geocryol. 35, 148–155. doi: 10.7522/j.issn.1000-0240.2013.0017
Zhao, L. J., Xiao, H. L., Cheng, G. D., Song, Y. X., Zhao, L., Li, C. Z., et al. (2008). A preliminary study of water sources of riparian plants in the lower reaches of the heihe basin. Acta Geosci. Sin. 29, 709–718. doi: 10.3321/j.issn:1006-3021.2008.06.008
Keywords: Tengger Desert, aerial seeding, dominant shrubs, hydrogen and oxygen isotopes, water source
Citation: Zhao Z, Tang G, Li J, Wu M and Zhang L (2025) Water source of artificial plants in the northeastern margin of Tengger Desert based on hydrogen and oxygen stable isotopes. Front. Plant Sci. 16:1523085. doi: 10.3389/fpls.2025.1523085
Received: 05 November 2024; Accepted: 01 April 2025;
Published: 05 May 2025.
Edited by:
Yalin Hu, Fujian Agriculture and Forestry University, ChinaReviewed by:
Zhi Dong, Shandong Agricultural University, ChinaXi Qi, Fujian Agriculture and Forestry University, China
Copyright © 2025 Zhao, Tang, Li, Wu and Zhang. This is an open-access article distributed under the terms of the Creative Commons Attribution License (CC BY). The use, distribution or reproduction in other forums is permitted, provided the original author(s) and the copyright owner(s) are credited and that the original publication in this journal is cited, in accordance with accepted academic practice. No use, distribution or reproduction is permitted which does not comply with these terms.
*Correspondence: Guodong Tang, MTgyNDcxNTg2OTBAMTYzLmNvbQ==
†These authors share first authorship