- 1Department of Life Sciences, University of Modena and Reggio Emilia, Reggio Emilia, Italy
- 2Department of Agricultural and Food Sciences (DISTAL), Alma Mater Studiorum - University of Bologna, Bologna, Italy
- 3Institute of Agri−Food, Animal and Environmental Sciences (ICA3), Universidad de O’Higgins, San Fernando, Chile
- 4Center Agriculture Food Environment (C3A), University of Trento, San Michele all’Adige, Italy
Genera Pseudomonas and Xanthomonas include bacterial species that are etiological agents of several diseases of major vegetable crops, such as tomato, pepper, bean, cabbage and cauliflower. The bacterial pathogens of those genera may cause severe crop damage, leading to symptoms like leaf spots, wilting, blights, and rotting. These plant pathogens can affect propagation materials and spread rapidly through plant tissues, contaminated soils, or water sources, making them challenging to control using conventional chemical products alone. Biopesticides, such as essential oils (EOs), are nowadays studied, tested and formulated by employing nano- and micro-technologies as innovative biological control strategies to obtain more sustainable products using less heavy metal ions. Moreover, there is a growing interest in exploring new biological control agents (BCAs), such as antagonistic bacterial and fungal species or bacteriophages and understanding their ecology and biological mechanisms to control bacterial phytopathogens. These include direct competition for nutrients, production of antimicrobial compounds, quorum quenching and indirect induction of systemic resistance. Optimisation of the biocontrol potential goes through the development of nanoparticle-based formulations and new methods for field application, from foliar sprays to seed coatings and root inoculation, aimed to improve microbial stability, shelf life, controlled release and field performance. Overall, the use of biological control in horticultural crops is an area of research that continues to advance and shows promising potential. This review aims to provide an in-depth exploration of commercially accessible biocontrol solutions and innovative biocontrol strategies, with a specific focus on the management of bacterial diseases in vegetable crops caused by Pseudomonas and Xanthomonas species. In this article, we highlighted the advancements in the development and use of EOs and other BCAs, emphasizing their potential or shortcomings for sustainable disease management. Indeed, despite the reduced dependence on synthetic pesticides and enhanced crop productivity, variable regulatory frameworks, compatibility among different BCAs, and consistent performance under field conditions are among the current challenges to their commercialization and use. The review seeks to contribute valuable insights into the evolving landscape of biocontrol in vegetable crops and to provide guidance for more effective and eco-friendly solutions against plant bacterial diseases.
1 Introduction
The world’s population will grow by 39% over the next two decades, reaching 9.1 billion by 2050 (www.fao.org). Thus, it will be necessary to increase world food production by 60% as there will be more people to feed, and agriculture has to become more productive. Vegetables are essential sources of the micronutrients needed for healthier diets and enable consumers to tap the nutritional power of vegetables (Schreinemachers et al., 2018). Many economically important vegetable crops, including tomato (Solanum lycopersici L.), pepper (Capsicum annuum L.), beans (Phaseolus vulgaris L.), cabbage (Brassica oleracea var. capitata L.) and cauliflower (Brassica oleracea var. botrytis L.) suffer from bacterial infections, which are thought to account for yield losses of 5–10% (Holtappels et al., 2021). Gram-negative bacterial species belonging to Pseudomonas and Xanthomonas genera represent the most relevant and destructive plant pathogenic bacteria (Mansfield et al., 2012).
Bactericides, copper-based products, and/or antibiotics (e.g., streptomycin, kasugamycin, tetracyclines) have a long history and are still the main strategies readily available and used for bacterial disease management. However, chemical control of bacterial pathogens still results in a problematic and even ineffective control due to their frequent polycyclic nature and the lack of systemic antibacterial substances (Oerke, 2006), therefore not providing a solution to plant infection or disease eradication (Dewdney and Graham, 2017). The use of chemical pesticides is also associated with a loss in their efficacy because of the co-selection of antimicrobial resistance (AMR) to specific active substances, such as copper compounds and antibiotics (Miller et al., 2022), reported mainly as prevalent in Pseudomonas spp. and Xanthomonas spp (Cooksey, 1994; Islam et al., 2024). Moreover, chemical pesticide use can result in potentially detrimental consequences, impacting the human population, environmental health, and ecology of the phytobiome. Thus, there is a need for alternative solutions that are durable, sustainable, accessible to farmers, specific in their target, and environmentally friendly, mainly where conventional approaches are limited or compromised.
One possible alternative is represented by biopesticides, defined as “pesticides derived from natural materials that can manage pests by specific biological effects or actions” (Koul, 2023). This definition allows to include under the “biopesticide umbrella” the three major classes listed by the US Environmental Protection Agency (EPA): 1) biochemicals (i.e., pheromones and botanicals), 2) microbes (i.e., bacteria, fungi, viruses, and protozoans), and 3) plant-incorporated-protectants (PIPs, i.e., genetically engineered crops) (https://www.epa.gov/ingredients-used-pesticide-products/what-are-biopesticides#advantages).
Botanicals (e.g., plant extracts, EOs) have a broad antibacterial spectrum that inhibits the growth of phytopathogens through the interaction of their hydrophobic components with the lipids present in the cell membrane of microorganisms, resulting in metabolic damages and bacterial cell death (Da Silva et al., 2021).
The hydrophobic nature of EOs is conferred by several molecules such as terpenes and terpenoids, but also other compounds as alcohols, aldehydes, aliphatic hydrocarbons, acyclic esters or lactones; this nature allows the interaction against the cytoplasmic membrane by inducing alterations of it, by increasing cell permeability, that leads to leakage of the cell contents, or by causing alterations in cell structure and functionality (Nazzaro et al., 2013).
In particular, EOs are a mixture of volatile oils produced as a secondary metabolite in different aromatic plant species (Bakkali et al., 2008), showing the potential as safe, biodegradable alternatives to conventional toxic chemical bactericides in agriculture (Assadpour et al., 2024). Recent studies have proven the antimicrobial effectiveness of EOs against antibiotic-resistant bacteria (ARB), candidating them as a potential sustainable solution to the management of ARB phytopathogens (Di Vito et al., 2019). Moreover, EOs may play important roles in the plant immune system, acting either as antimicrobials or as signal molecules for the activation of the plant defenses (Choudhary et al., 2021).
Besides, the effectiveness of microbiological control agents (mBCAs) in controlling plant diseases is characterized by multiple modes of action: (a) competition for resources (e.g., oxygen, carbon, nitrogen, and other essential resources); (b) antibiosis via effects of toxic secondary metabolites; (c) hyperparasitism, where the antagonist acts as a predator and exploits the pathogen as a prey; (d) induced systemic resistance (ISR) in planta against invading plant pathogens; (e) stimulation by better nutrient absorption and/or by affecting plant hormone pathways (e.g., rhizosphere bacteria and fungi).
Although bacteriophages (phageBCAs) are viruses that specifically infect and replicate in bacteria as antimicrobial agents, they should not be considered conventional mBCAs together with other true microorganisms (i.e., bacteria and fungi) (Stefani et al., 2021). phageBCAs can replicate and spread through lytic life cycles, leading to the degradation of bacterial hosts and exhibiting a high degree of specificity and persistence/proliferation in the environment (Buttimer et al., 2017; Rabiey et al., 2020). These are the most important determinants that confer control characteristics to phageBCAs as a promising multifaceted tool for the management of bacterial disease (Farooq et al., 2022).
It is widely accepted that the use of these biopesticides does not guarantee the level of crop protection achieved using a single formulated antibiotic compound. However, growers could employ several compounds of different origins or modes of action to achieve such a control level by using only biocontrol strategies during the spread of the epidemic. Moreover, it is worth mentioning that the real long-term advantage of the use of more sustainable bioproducts is their multifaceted nature. Indeed, their application could significantly reduce the possibility of upcoming bacterial resistance due to their several modes of action.
However, there are other various limitations to the use of biopesticides, compared to the use of synthetic pesticides in agriculture, such as: lower efficacy, that is highly dependent on environmental field conditions (e.g., heat, UV light, desiccation), and a slower rate in the control of plant diseases, by limiting pathogen population through multiple modes of action but not a complete control (Ayilara et al., 2023). Besides, the complexity of the regulatory framework for registration, the decreased availability in the market, and the still wide skepticism of these products by farmers (Stefani et al., 2021).
2 Tomato and pepper, Fabaceae and Brassicaceae (cabbage and cauliflower): Pseudomonas and Xanthomonas bacterial diseases and their management
2.1 Tomato and pepper
Tomato and pepper are among the most cultivated vegetables crops worldwide, with over seven million hectares of cultivated area and more than 186 and 37 million metric tons of tomato and pepper produced, respectively (FAO - Food and Agriculture Organization of the United Nations, 2024). Among the most challenging bacterial diseases of pepper and tomato, bacterial spot and bacterial speck can cause significant reductions in field crop yields, especially if the infection appears early in the vegetative season (Ji et al., 2006); additionally, their causal agents are seed-borne and seed-transmitted, thus posing specific threat to the seed industry. Bacterial spot caused by Xanthomonas vesicatoria (Xv), Xanthomonas euvesicatoria pv. euvesicatoria (Xee), Xanthomonas euvesicatoria pv. perforans (Xep) and Xanthomonas hortorum pv. gardneri (Xhg) is a worldwide disease causing yield losses of up to 50% on tomatoes under warm and rainy weather (Buttimer et al., 2017). Xanthomonads enter tomato plants primarily through stomata, lenticels, and wounds, causing necrotic lesions on leaves with a polygonal shape. On fruits, symptoms are scab-like, raised, and whitish lesions, leading to decreased market value (EPPO, 2023). These causal agents are included in the A2 list of quarantine pathogens of the European and Mediterranean Plant Protection Organization (EPPO A1 and A2 Lists of Pests Recommended for Regulation as Quarantine Pests PM 1/2(32); https://gd.eppo.int/download/standard/2/pm1-002-32-en_A1A2_2023.pdf).
Pseudomonas syringae pv. tomato (Pst), the causal agent of bacterial speck, is categorized as a quarantine pest in Mexico, Egypt, Jordan, and China (https://gd.eppo.int/taxon/PSDMTM/categorization). Bacterial speck bacterial speck is more severe under cool (i.e., between 18 and 25°C) and humid conditions, in which typical symptoms show small and dark leaf lesions, usually surrounded by a chlorotic halo that is provoked by the coronatine toxin and necrotic spots along stems and on fruits. Pst cells can evade the plant as exudates from necrotic lesions and spread around (Xhemali et al., 2024).
Pst, Xv, Xee, Xep and Xhg are widely distributed in different geographical regions, probably as the result of trading infected seeds or transplants. Thus, the management strategy should include using pathogen‐free, certified seed or disease‐free transplants (Xhemali et al., 2024). In addition, the removal of potential inoculum sources, such as volunteer plants and infected host plants, should be carried out promptly. Field isolation from infected host plants in close proximity, accompanied by sanitation, physical removal and disposal of diseased crop material, and crop rotation with non‐hosts, should be followed as Integrated Pest Management (IPM). However, the most common disease control strategies for both bacterial diseases are based on preventive application of copper-based products, alone or in combination with dithiocarbamate fungicides and antibiotics (where allowed), which poses a severe threat to human health, environment and the development of copper and/or antibiotic-resistant strains (Lamichhane et al., 2018; Šević et al., 2019). An additional control method of Pst race 0 is represented by the use of commercial tomato cultivars carrying the resistance gene Pto, which interacts with the avirulence gene of the plant pathogen (e.g., avrptoB in Pst DC3000) by limiting its spread during an epidemic occurrence (Martin et al., 1993). Recently, a study concerning the characterization of the hrpZ gene, present in the hrc/hrp pathogenicity domain, was carried out by analyzing Pst strains isolated in different regions in Egypt performed pathogenicity investigations to study the level of virulence with specific RFLP-PCR, along with molecular marker system, and correlated to the development of the symptoms (El-Fatah et al., 2024).
On the contrary, commercial pepper and tomato varieties exhibiting complete resistance to bacterial spot are not available (Adhikari et al., 2020). In addition to qualitative resistance available against Pst race 0 strains mentioned above, quantitative resistance against Pst race 1 strains have been identified in wild tomato Solanum habrochaites accessions LA1777 and LA2109, and Solanum lycopersicoides accession LA295 (Mazo-Molina et al., 2020; Thapa et al., 2015). For bacterial spot, quantitative trait loci (QTL) associated with resistance were also identified in wild Solanum pimpenellifolium accession LA2533 or cultivated tomato relatives Solanum lycopersicum var. cerasiformae PI114490, tomato lines Hawaii 7998 and Hawaii 7981 (Hassan et al., 2024; Sharma and Bhattarai, 2019). More recently, the breeding between two tomato varieties, the susceptible line Ohio 88119 and the resistant line Ohio 9834 carrying the resistance locus (Rx3), was used to locate the locus responsible for the bacterial spot resistance against the race T1 in Xee (Meng et al., 2022). However, complete resistance has yet to be obtained because it is impeded by the emergence of Pst race 1 or by as many as four Xanthomonad species on tomato. Moreover, overcoming the identified resistant germplasm, multi-genic control of the resistance, linkage drag, non-additive components of the resistance, and the negative correlation between fruit quality and disease resistance have made the introgression of resistance even more challenging (Adhikari et al., 2020).
2.2 Fabaceae
The Fabaceae family is one of the most important vegetables that are consumed by people from every part of the world, among which beans are the third legume species with the highest economic relevance with an annual global yield of over 27 million metric tons (Fernandes Gomes et al., 2020; FAO - Food and Agriculture Organization of the United Nations, 2024).
Among the bacterial plant pathogens, Pseudomonas savastanoi pv. phaseolicola (Psph), Xanthomonas axonopodis pv. phaseoli (Xaph) and Xanthomonas citri pv. fuscans (Xcf) are the causal agents of halo blight, common blight and fuscous blight, respectively. These are the most important pathogens concerning direct crop damage and their further dissemination at long distances through bean seeds (Agarwal and Sinclair, 1997). Psph is a serious seed-borne pathogen that needs a low optimal temperature (less than 25°C) to survive and start the pathogenic process. Due to its rapid spread, even low levels of Psph primary infection can result in severe epidemics at optimal weather conditions (Schaad et al., 1995). The symptoms start from water-soaked lesions/spots on leaves, pods, and stems that develop in yellowish haloes on leaves (Arnold et al., 2011). Seeds are often asymptomatic, but they might carry primary inoculum sources (latent infections). Looking at Xanthomonads, Xaph and Xcf are seed-borne severe pathogens favored, in direct crop damage, by higher temperature (28-30°C). The symptomatology affects all the aerial parts of the bean plant, such as leaves, pods, and stems. These plant pathogenic bacteria provoke water-soaked spots that become necrotic, surrounded in the leaves by a yellow halo; even seeds may be symptomatic, showing lesions distributed all over the coat or close to the hilum area. The latent infections on seeds are the most dangerous because of the bacterial spread at long distances, as it happens for Psph (EFSA Panel on Plant Health (PLH), 2014).
In the field, in case of an epidemic spread, the control of these pathogens is mainly achieved through the application of copper-based products or antibiotics, where they are allowed. Still, the diagnostic analyses of bean seeds represent the most effective method to avoid infections that might start from contaminated/infected seeds (EFSA Panel on Plant Health (PLH), 2014) to have pathogen-free propagation material. In addition, as concerns the control of Psph, breeding for resistance could be another effective method to control that pathogen: based on gene-for-gene interactions, Psph is divided into nine races depending on the presence of different avr genes that interact with R genes (NLR resistance genes) of the host plant. Indeed, not all bean cultivars are susceptible to Psph (Arnold et al., 2011). The gene-for-gene interaction regarding Xaph is still being studied, and it involves the proposed dominant gene xap-1 (Zapata et al., 2011). Thus, to date, there is no complete data on resistant bean cultivars.
2.3 Brassicaceae (cabbage and cauliflower)
The Brassicaceae family, which includes approximately 3.700 species, produces ornamental flowers, edible vegetables, and oilseed plants. It represents one of the ten most economically important vegetables in world agricultural and food markets, where cabbages and cauliflowers are among the most produced brassica with an annual global yield of ca. 73 and 26 million metric tons, respectively (FAO - Food and Agriculture Organization of the United Nations, 2024).
Black rot, caused by Xanthomonas campestris pv. campestris (Xcc) is considered one of the most important and destructive diseases affecting the quality and yield of Brassicaceae, in particular for the Brassica family, where it can reduce cabbage yield from 50 to 60% annually (Kong et al., 2021). Xcc is primarily a seed-borne disease, but this pathogenic bacterium can also be transmitted by wounding, water splash irrigation, or wind-driven rain. Here, a critical epidemiological aspect of Xcc is played by the possible rapid spread in transplants with the sprinkling irrigation systems used by plant growers. Moreover, Xcc can survive in the soil associated with plant debris for up to two years in the case of harsh stem residues and cold temperatures (Gazdik et al., 2021), persisting therefore between brassica crop rotations and acting as a source of primary inoculum. Under conducive environmental conditions (i.e., high humidity and temperatures of 25–30°C), Xcc inoculum can enter the host plant vascular system through hydathodes or wounds along the leaf margin, causing V-shaped necrotic lesions (Köhl and van der Wolf, 2005). In most cases, Xcc moves systemically throughout the plant, causing leaf wilting, rot and, in case of severe infections, plant death (Greer et al., 2023). Several preventive agricultural practices and cultural methods (e.g., certified and treated seeds; Brassica cultivation in 3-year field rotations; cleaning and sterilization of field equipment; crop residue management; elimination of other host plants of the pathogen), similar to those used for Xanthomonads and Pst, have been reported for controlling Xcc (Vicente and Holub, 2013).
On the contrary, the use of chemical pesticides in disease management has been shown to be often ineffective because they are applied when symptoms are visible and the disease is already established (Liu et al., 2022). Resistant cultivars represent one of the most effective approaches to control black rot, reducing the overall cost and chemical pollution. Nevertheless, the complex differentiation of Xcc in 11 physiological races, where races 1 and 4 are the most virulent and widespread, hampers the development of Xcc-resistant breeding lines. This is because only a few germplasms have clear race-specific resistance, and for cabbage, for example, the resources with high resistance are rare (Kong et al., 2021). Concerning the host range, in 2014, several Xcc strains were isolated from winter oilseed rape (Brassica napus L.); such strains from winter oilseed rape exhibited greater genetic diversity and had higher specificity towards this host isolates from the other brassicas (Jelušić et al., 2021).
3 Current status and legislation of biopesticides
During the last decades, evidence of significant advances in biopesticides and their applications is highlighted by the constant growth of the global biopesticides market. It reached a value of US$ 6.7 billion in 2023, which is expected to touch a value of US$ 13.9 billion by 2028, with a compound annual growth rate (CAGR) of 15.9% during 2023 - 2028 (https://www.marketsandmarkets.com/Market-Reports/biopesticides-267.html). However, it still represents about 10% of the global chemical pesticide market, estimated at around $79.3 billion in 2023.
Currently, there are hundreds of registered biopesticides worldwide, with more than 200 in the USA, 60 in the EU, 300 in China, and 400 in India (Chakraborty et al., 2023). Marrone (2024) reported that 396 out of 567 registered biopesticides in Brazil are used by conventional growers (among which 60.4% are microbials and 9% are botanicals). These data highlight that biopesticide development becomes an essential component of the integrated pest management (IPM) concept. However, their registration framework continues to be very challenging within the biopesticide industry, often using the same process as conventional chemical pesticides. Laws and policies regulating pesticide development, registration and use vary from country to country (e.g., USA, UE, Cina, India, Brazil), with a non-uniform model that can simplify their registration process and promote the use of biopesticides (Arora et al., 2016). Different global agencies, such as the International Organization for Biological Control (IOBC), the European and Mediterranean Plant Protection Organization (EPPO) and the Organization for Economic and Co-operative Development (OECD), made efforts to provide some flexibility to biopesticide regulation. However, progress towards harmonization and work sharing still required progress through the development of guidance and working documents.
The USA has a simpler pesticide regulatory process than many other countries, such as Europe, with a distinct procedure for biopesticides and chemicals. In the United States, biopesticides are registered under the Pesticide Registration Improvement Act (PRIA) by involving the Environmental Protection Agency (EPA) and the Food and Drug Administration (FDA). The process has a lower cost and faster timeline than chemicals (~ 1–2 years to approve a new active biopesticide ingredient), a lower submission fee for small businesses, allowing continued innovation and more products on the market (Marrone, 2023). On the other hand, EU legislation for placing on the market biopesticides is not treated as a specific category (European Commission, 2009), but two categories of plant protection products (PPPs) are recognized: chemical (including biochemical and botanicals) and microbial pesticides. (10) The Reg. EC 1107/2009 defines the two-step regulatory process of a biopesticide that includes: (i) evaluation and approval of the active substances (a.s.) at the EU level, followed by the (ii) evaluation of PPPs in zonal level (3 administrative EU zones: northern, central, southern) and authorization by the concerned Member States. The regulatory process steps of evaluation are made based on the following requirements: a) sufficiently effective to control a target disease/pest on the specific crop(s); b) impact on human and animal health; c) Fate and behavior in the environment (e.g., persistence, bioaccumulation, potential for long-range environmental transport, ecotoxicology, residue definition, impact on non-target species, impact on biodiversity and the ecosystem).
As regards Asian Countries, particularly China, the Institute for the Control of Agrochemicals of the Agriculture Ministry is responsible for the registration and regulation of biopesticides. They are broadly categorized into six categories: botanical, microbial, biochemical, biological, genetically modified organisms (GMOs), and agro-antibiotics based on Chinese data requirements for pesticide registration (Wang et al., 2022). In India, the regulatory centers are represented by the Central Integrated Pest Management Centre (CIPMC) at Faridabad and the National Centre for IPM (NCPM) under the Indian Agricultural Research Council; on the other hand, the Directorate of Biological Control and the marketing of biopesticides to farmers is under the responsibility of the Ministry of Agriculture and Farmers Welfare and the Department of Biotechnology (DBT) (Chakraborty et al., 2023). Meanwhile, in Africa, several nations use a variety of guidelines to create systems for the registration and control of biopesticide regulation aimed at the management of diseases. A regional inventory of the regulatory environments was conducted by six African countries as West African regions (Kenya, Uganda, Ethiopia, Tanzania, Nigeria) and Ghana, as part of the Commercial Products (COMPRO II) project, which is overseen by the International Institute of Tropical Agriculture (IITA) (Arora et al., 2016).
Moreover, different authorities (i.e., the EU Commission, European Food Safety Authority, Competent Evaluation and Authorization Authorities of the 27 EU Member States) are involved in the biopesticides evaluation and authorization processes. This results in a laborious and more prolonged process (approval procedure of a.s. ~ 2–4 years, followed by those of new PPPs ~ 1–2 years) compared with the United States, South America, and Asia (Karamaouna et al., 2023). Moreover, when the previous evaluation system of the EU was based on regulation 91/414/EEC and then followed by directive 1107/2009, just 26% of registered Plant Protection Products (PPP) and active substances were annulled in agriculture (Nawaz et al., 2023). Arora et al. (2016) highlighted another complex issue surrounding the regulation of biopesticides having multiple modes of action (i.e., biofertilizer/phytostimulators and biocontrol agents), as in the case of fluorescent Pseudomonads. They can be sold based on their plant growth-promoting properties rather than as plant protection products, escaping scrutiny from regulators regarding their efficacy and safety because there are no particular regulatory mechanisms to check agronomic efficacy and the risks associated with human, animal or plant health or to the environment before biofertilizer commercialization. Indeed, while the European Union and countries like Brazil, India, and China have made progress in this area, the USA market lacks clear regulation guidelines for biofertilizer production and sale, highlighting the lack of a globally coordinated uniform regulatory policy (Santos et al., 2024).
4 Commercial biopesticides
During the last few years, a limited number of new chemical formulations have been marketed by the industrial sector as a consequence of the low perceived market value of conventional bactericides and the uncertainty of acquiring registration for plant disease management (Sharma et al., 2020). Moreover, the cost and time associated with the development process of new chemical bactericides have been a significant barrier to commercialization: it can exceed $250 million and take over a decade to bring the drugs to the field (Ma et al., 2023). In contrast, biopesticide development cost ranges from $5–25 million, with a time to market of 5–7 years (https://croplife.org/wp-content/uploads/2024/02/Time-and-Cost-To-Market-CP-2024.pdf, accessed on 21 September 2024). In this regard, biopesticides require less intensive regulatory scrutiny and leverage existing fermentation and formulation technologies, resulting in lower overall costs that make biopesticides a more attractive option for industrial companies and growers (Samada and Tambunan, 2020). Although biopesticides are eco-friendly, reducing harmful residues and promoting biodiversity, regionally variable regulatory frameworks, efficient formulations and application technologies remain significant challenges to their commercialization and use (Butu et al., 2022).
Regarding the formulation of commercial biopesticides, it poses important challenges to guarantee the development of high-quality preparations with stable shelf life and proven efficacy products that can be implemented in the field for potential marketability. Indeed, factors such as temperature, moisture, UV radiation and certain plant-produced chemicals can negatively impact the viability and efficacy of biopesticides, leading to an increase in the application frequency with an increase in costs (Buttimer et al., 2017). Other fundamental aspects to be taken into consideration for the most efficient formulations are the ecology and biology of biopesticides, the pathosystem, the environment and the application niche, the inoculation techniques (e.g., foliar spray, soil spray, soil drench, soil irrigation, seed coating) and types of irrigation systems (e.g., sprinkler irrigation, drip irrigation) involved in the cropping system (Bejarano and Puopolo, 2020).
Nowadays, conventional biopesticide formulations are usually based on very few variants: powder, granulated or liquid forms, with a wide range of carrier materials, protectants, and optimized delivery systems, which can facilitate their integration into comprehensive disease management programs for vegetable growers (Bonaterra et al., 2022). Commercially accessible biocontrol solutions that control bacterial plant disease are still few and in the beginning phases (Lahlali et al., 2022). However, for these commercial biopesticides, the several technological challenges listed above were surmounted. A list of some commercially available biocontrol products against Pseudomonas spp. and Xanthomonas spp. bacterial diseases of vegetable crops are documented in Table 1, together with trade names, crops, target pests, territories marketed and web links. The majority of commercial EOs are characterized by their direct antibacterial action, such as cell wall destruction, telomerase inhibition, cell membrane damage leading to loss of cytoplasmic content and ergosterol depletion against the bacterial pathogens affecting tomato, pepper, bean, cabbage and cauliflower. For EOs, common application strategies resulting in efficient control of bacterial diseases are based on ground sprays, aerial foliar sprays and to soil or crops through irrigation systems (i.e., chemigation).
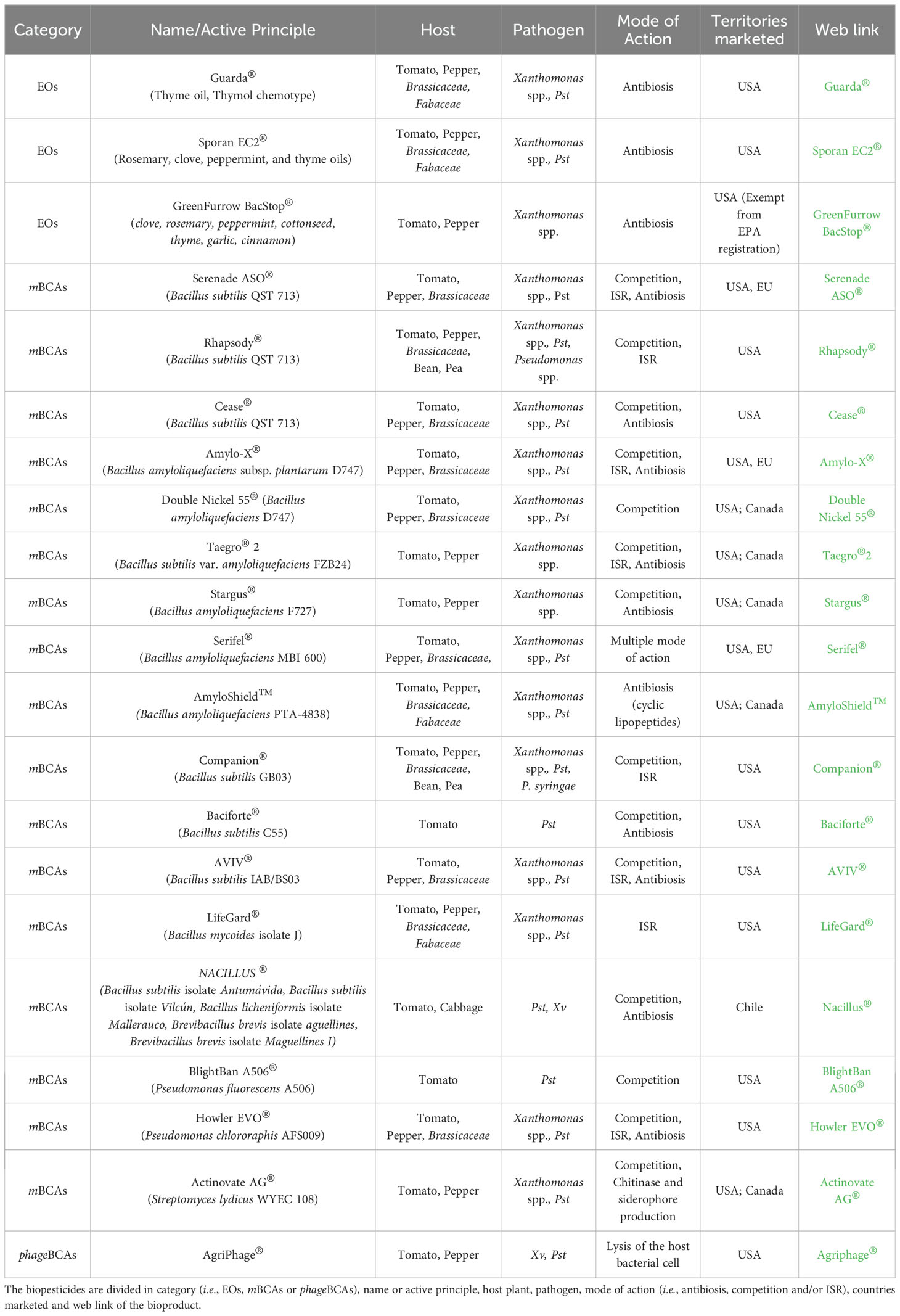
Table 1. A broad description of some commercial biopesticides applied in the control of Pseudomonas spp. and Xanthomonas spp. bacterial diseases on tomato and pepper, Fabaceae and Brassicaceae.
Among commercial mBCAs for the control of bacterial diseases, twelve bacteria are registered in the EU as active ingredients: Bacillus amyloliquefaciens strains QST 713 (formerly B. subtilis), AH2, MBI 600, FZB24 and IT 45, Bacillus amyloliquefaciens subsp. plantarum strain D747, Bacillus subtilis strain IAB/BS03, Pseudomonas spp. strain DSMZ 13134, Pseudomonas chlororaphis strain MA 342, Streptomyces K61 and Streptomyces lydicus strain WYEC 108 (https://food.ec.europa.eu/plants/pesticides/eu-pesticides-database_en, accessed on 10 October 2024). Meanwhile, eight mBCAs are marketed exclusively in USA, Chile, Brazil or Canada: Pseudomonas fluorescens strain A506, Bacillus subtilis strains C55, GB03, N5, Brevibacillus parabrevis strain N4, Bacillus cereus strains N6, N7, Bacillus amyloliquefaciens strains F727 and PTA-4838, Bacillus mycoides strain J and finally the Pseudomonas chlororaphis strain AFS009 (https://www.epa.gov/ingredients-used-pesticide-products/biopesticide-active-ingredients, accessed on 11 October 2024) (Table 1). These biopesticides are available in several formulations (e.g., liquid, powder, wettable powder, water dispensable granule) and characterized by different modes of actions, such as: (i) competition, antibiosis and ISR, provided by Serenade ASO®, Amylo-X®, Taegro®2, Serifel®, AVIV® and Howler EVO®; (ii) competition and ISR, provided by Rhapsody® and Companion®; (iii) competition and antibiosis, provided by Cease®, Stargus®, Baciforte®, Nacillus®; (iv) competition, provided by Actinovate AG®, Double Nickel 55® and BlightBan A506®; (v) ISR, provided by LifeGard® and finally through (vi) antibiosis by AmyloShield™.
Different application methods (i.e., seed treatments, soil drench, in-furrow, ground spray, aerial spray, chemigation) during the entire cropping season of tomato, pepper, bean, cabbage and cauliflower are possible. Conversely, the commercial availability of phageBCAs is very limited. Among the Pseudomonas spp. and Xanthomonas spp. considered in this review, only AgriPhage® (OmniLytics, Inc., Sant Lake City, UT, USA) has specific applications for controlling both bacterial spot and speck of tomato and pepper. Its liquid formulation relies on ground and aerial spray applications as preventive treatments when conditions are conducive to heavy disease pressure or when the first disease symptoms are visible. As argued above, the variety of possible formulations has also increased, thanks to the increased technologies in such fields; this made biopesticides more functional to the grower needs and for large-scale employment, provided by supposed lower costs of production. The liquid or dry formulations have been improved in conservation characteristics, and they may have longer life-shelf than that in the past, at different temperature conditions; that partly compensates the high costs of the biopesticides, which in some cases may be triple compared to some conventional copper compounds (Tazzari, 2019).
5 Recent publications on novel biopesticide candidates
Systematic literature research from 2019-2024 years survey) was carried out to summarize the most recent progress and the current research trends on identifying and testing BCAs based on EOs, mBCAs and phageBCAs. The recent studies listed in Table 2 reported biopesticide candidates for sustainable control of diseases caused by Pseudomonas spp. and Xanthomonas spp. on vegetable crops, together with their mode of action, experimental assay levels (i.e., in vitro, growth chamber, greenhouse or field conditions) and references.
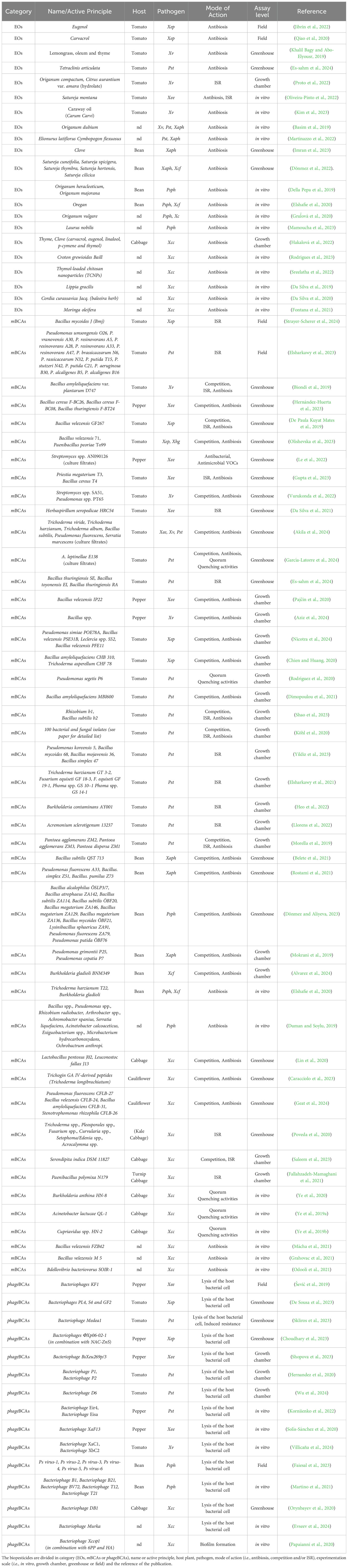
Table 2. Recent (2019-2024 years survey) BCAs tested for Pseudomonas syringae pv. tomato, Pseudomonas savastanoi pv. phaseolicola and Xanthomonas spp. control on tomato and pepper, bean, cabbage and cauliflower.
EOs are basically obtained through hydro- or steam distillation of plant tissues and by cold pressing of Citrus spp. fruit peel; a co-product of the distillation is the hydrolate, which stands for aromatic water containing approx. 0.1% EOs mixture (Di Vito et al., 2019). To date, the hydrolates are basically studied for their activities against human bacterial pathogens, since Proto et al. (2022) evaluated their direct in vitro activity against Xv. Nowadays, the majority of studies on EOs concern the evaluation of the antimicrobial activity in vitro against plant bacterial pathogens. In several works it was highlighted the ability of EOs extracted from plant species such as Thymus spp., Origanum spp., Eucalyptus spp., or Mentha spp. among others, in inhibiting the in vitro growth through diffusion or dilution methods against Pst, Xv, Xee on tomato, Xcf and Psph on bean, and Xcc on cabbage, or in affecting the capability to produce biofilms by observations with scanning electron microscopy (SEM) of Xv (Proto et al., 2022), Pst and Psph (Della Pepa et al., 2019; Jamshidi et al., 2023). Other studies took into account, again in vitro, the antimicrobial activity of carvacrol and thymol, or other essential oils extracted by Moringa oleifera against Xcc and by observing the impact of the EOs on Xcc vitality, motility, and biofilm’s formation on cabbage seeds (Hakalová et al., 2022). Concerning the activity against Xaph on beans, few studies have evaluated the efficacy of EOs obtained by Satureja spp. and clove in significantly inhibiting the pathogen growth under greenhouse experiments (Dönmez et al., 2022; Imran et al., 2023). Likewise, different EOs have highlighted their antibacterial capability to control pathogen infections in planta for Xep, Xv, Pst on tomato (Es-sahm et al., 2024; Jibrin et al., 2022; Khalil Bagy and Abo-Elyousr, 2019; Qiao et al., 2020), and for Xcc on cabbage (Hakalová et al., 2022) and on radish (Raphanus sativus L.) (Fontana et al., 2021). Besides the in vivo antibacterial activity of EOs described above, Proto et al. (2022) demonstrated the potential of Origanum compactum and a hydrolate obtained from Citrus aurantium by acting through the plant host and triggering the ISR: root treatments of tomato plants significantly reduced the bacterial leaf spot severity caused by experimental inoculation of Xv with respect to untreated control plants. On beans, Imran et al. (2023) showed the production of eugenol in plants treated with clove oil, which acts against Xaph through both direct pathogen inhibition and enhancing the plant immune system by triggering the expression level of PR proteins.
Bacterial pathogens penetrate the plant through natural openings (e.g., stomata, hydathodes) or through wounds, where organs of the plant are equally susceptible to the plant pathogen survival and the subsequent penetration into the host (Akhavan et al., 2013). Therefore, EOs are studied to prevent the penetration of plant bacterial pathogens and to avoid the following infection of the host (DuPont et al., 2023). Unfortunately, they have shown problems with their stability and persistence on the plant organ’s surfaces, primarily due to their rapid evaporation and their unequal coverage of the treatment. Besides, the increase in the EO’s concentration may provoke phytotoxic effects on the organs (Borges et al., 2018; Proto et al., 2022). This is the reason why EOs need co-formulants able to improve the distribution and reduce the evaporation of the active principle (Proto et al., 2022). In fact, various solid and liquid formulations of EOs have been assayed to enhance their handling, stability, and controlled release. Innovative “green” solid formulations for encapsulation, such as microencapsulation and nanoparticles, offer prolonged and sustained release of active compounds compared to their liquid counterparts (Dunan et al., 2023). In two recent studies, EOs have been employed against Xcc in combination with other antimicrobial agents, such as chitosan nanoparticles, highlighting the potential of these carriers for the delivery of EO biopesticides for effective plant disease management (Sreelatha et al., 2022). Likewise, liquid nanoemulsions have demonstrated a superior ability to enhance the stability, water solubility, and biological activity of EOs, making them more effective than their direct application (López et al., 2021).
In Table 2, Bacillus spp. and Pseudomonas spp. have been the most widely studied microorganisms in controlling diverse bacterial phytopathogens through their multiple modes of action, including resource competition, antibiosis and ISR. Solely two studies have been conducted under field conditions, both of them on tomato, by testing the ISR potential of Bacillus mycoides isolate J (the active ingredient of the biofungicide LifeGard® WG; Marin et al., 2019; Strayer-Scherer et al., 2024; Tariq et al., 2020) and Pseudomonas spp (Elsharkawy et al., 2023). The biopesticide Bacillus mycoides J resulted not effective in controlling Xep by foliar treatments, nor in combination with Kocide® 3000 (copper hydroxide); meanwhile, Pseudomonas resinovorans A47, Pseudomonas brassicacearum N32, and Pseudomonas putida T15 showed a considerable decrease in bacterial speck severity in comparison to Pst control, by triggering the SA immune response pathway and increasing the peroxidase and polyphenol oxidase activities. In a greenhouse study, root treatments with Bacillus amyloliquefaciens spp. plantarum strain D747 (active principle of Amylo-X® and Double Nickel 55®) upregulated the SA signaling pathway in tomato plants. There, the phytopathometric assessment showed a significant reduction of the bacterial spot Xv pathogen severity at levels similar to those of streptomycin sulfate and acibenzolar-S-methyl (ASM) treated plants (Biondi et al., 2019). Streptomyces spp. and Bacillus spp. were also investigated for their capability to produce volatile organic compounds (VOCs), such as dimethyl sulfide and trimethyl sulfide, and cyclic lipopeptides in the culture filtrates, which were able to significantly inhibit the growth of Xee compared to streptomycin sulfate and untreated controls in in planta assays, respectively (Le et al., 2022; Pajčin et al., 2020).
Besides, Trichoderma spp. were the most tested fungal mBCAs, mainly investigated against tomato and cabbage bacterial diseases under growth chamber and greenhouse conditions. These experiments evaluated the production and excretion of fungal secondary metabolites and antimicrobial peptides present in the culture filtrates against Xee, Xv, Pst and Xcc (Akila et al., 2024; Caracciolo et al., 2023). Additionally, different Trichoderma spp. have proved their ability to elicit ISR on tomato and cabbage plants and reduce Pst and Xcc severity compared to untreated control plants, respectively (Elsharkawy et al., 2021; Poveda et al., 2020). Other fungal mBCAs were promising in controlling bacterial diseases by up-regulating defense pathways dependent on SA and/or JA signaling pathways, such as Fusarium equiseti and Phoma spp. against Pst, and Serendipita indica against Xcc (Elsharkawy et al., 2021; Saleem et al., 2023).
Notably, a promising strategy has been studied for controlling diseases by quorum quenching (QQ) mode of action. QQ involves the enzymatic degradation of N-acyl homoserine lactones (AHLs) signal molecules attenuating virulence and reducing the infection of bacterial pathogens (Uroz et al., 2009). Under greenhouse conditions, Rodríguez et al. (2020) showed the QQ potential of Pseudomonas segetis strain P6 on tomato plants infected by Pst. In in vitro assays, QQ biocontrol mechanisms were reported for the bacterial isolates Acinetobacter lactucae QL-1 (Ye et al., 2019a), Cupriavidus spp.HN-2 (Ye et al., 2019b), Burkholderia anthina HN-8 (Ye et al., 2020) against Xcc and the fungal isolate Alternaria leptinellae E138 against Pst (García-Latorre et al., 2024).
Among phageBCAs, their efficacy has been reported in different papers, underscoring satisfactory results on the control of bacterial spot and speck, halo blight, and black rot. They described results obtained in vitro or in growth chamber experiments on phageBCAs isolation, characterization and testing for the lysis ability against Xv (Villicaña et al., 2024), Xee (Shopova et al., 2023; Solís-Sánchez et al., 2020), Pst (Hernandez et al., 2020; Wu et al., 2024), Psph (Martino et al., 2021) and Xcc (Evseev et al., 2024). In vitro, the bacteriophage Xccφ1, alone or formulated in combination with 6-pentyl-α-pyrone (6PP) and hydroxyapatite (HA) nanocrystals, proved the capability to interfere with the gene pathways involved in the formation of Xcc biofilm. 6PP, produced by Trichoderma atroviride, was tested for its antibiotic and plant metabolome interference properties; instead, HA nanocrystals were assessed for bacteriophage delivery and as an enhancer of its biological activities and stability (Papaianni et al., 2020).
In greenhouse experiments, spray application of phageBCAs resulted in disease reduction of pepper bacterial spot (i.e., Xep) at levels higher than those achieved by the use of copper hydroxide (De Sousa et al., 2023). For Pst, Skliros et al. (2023) reported the Pseudomonas phage Medea1 biocontrol potential through its (i) lytic nature and ability (ii) and by upregulating SA and abscisic acid (ABA) defense pathways by foliar spray and root drenching applications, respectively. During a four-year study, phageBCAs were assessed in combination with copper hydroxide and acibenzolar-S-methyl (ASM) as a part of integrated disease management practices in pepper fields, improving the efficacy of single phageBCAs and providing a consistent bacterial spot control compared to plants sprayed with water only (Šević et al., 2019).
PhageBCAs offer promising biocontrol potential in agriculture, as highlighted by the above-described studies. However, the most significant limiting environmental factor is the phage’s vulnerability to UV light, which can drastically reduce their viability. Nonetheless, recent studies highlighted the effectiveness of phageBCA formulations with skimmed milk and corn flour, or riboflavin, to control Psph and Xcc compared to plants sprayed with copper or water, respectively, under greenhouse and open field conditions (Faiesal et al., 2023; Orynbayev et al., 2020). All these examples of studies on EOs and mBCAs highlight the increased need for biopesticides and, at the same time, the increased variability of available BCAs, which implicitly might prevent treatments based only on one or two strains or naturals. It is clear that the complete protection of the crop from bacterial diseases is challenging; applying integrated control methods, from the seed diagnostic analyses to the chemical compounds treatment to the biopesticides employment and to optimal agronomical measures, will not be possible. The increase in biopesticide employment, in any case, avoids the accumulation of toxic compounds at the soil level, and the increased variety may enrich the soil microflora, being environmentally advantageous. Furthermore, nano-formulations of N-acetyl cysteine zinc sulfide (NAC-ZnS) has shown to significantly improve both UV stability and the antimicrobial efficacy of bacteriophages against the tomato bacterial spot pathogen Xep, making them more effective at sunlight exposure (Choudhary et al., 2023).
6 Perspectives and future challenges
The gap between studies on BCAs and their translation into commercial products in the EU, for instance, might be mainly determined by the inadequacy of BCAs, characterized at the laboratory level, to all the requirements of the registration process, such as eco-toxicological risks. Another possible answer might be found in the inefficacy of BCAs applied in the field or in the greenhouse under real-scale conditions, which may be due to the poor attention that the scientific community gives to the research focused on their production, formulation and delivery (Bejarano and Puopolo, 2020). Furthermore, other main limitations comparing the full adoption of biopesticides are the high cost of the commercial products compared to the available conventional agrochemical products, the inability to meet the global market demand and the extreme variability of the methods used for the bio-formulations (Fenibo et al., 2021).
Bio-formulation is, indeed, one of the critical points for the gap between research studies and real field conditions, as discussed above for EOs. In fact, the evaporation of the EO active principle strongly limits the stability and persistence of the treatment that aims to directly inhibit pathogen survival at the epiphytic phase and its penetration into the host. To overcome the persistence limit, some new commercially available formulations of EO mixtures are composed of a recently patented micro-clay (Patent N° EP 3071039-28.09.2016), amended with a low percentage of heavy metal as copper or zinc to enhance the effectiveness of the EOs. Other attempts to employ nano-technology were carried out against Xcc in in vitro assays by evaluating the effect of Thymol-Loaded Chitosan Nanoparticles against the pathogen (Sreelatha et al., 2022). Against other bacterial pathogens, such as Xanthomonas fragariae on strawberry, these combined formulations have demonstrated their efficacy, either in vitro and in planta experiments, under controlled and field conditions (Biondi et al., 2022).
To strengthen the efficacy of mBCA applications, innovative formulation approaches are being developed to improve microbial stability, shelf life, and field performance, ensuring their viability and effectiveness under field conditions (Saberi Riseh et al., 2022). Research and companies involved in biopesticide development are exploring formulations made by means of emulsions, encapsulations, hydrogels, and nanoproducts, each of which offers distinct advantages for microbial stability and controlled release in agricultural applications. Nanoformulations and microencapsulation technologies, in particular, have been shown to improve the residual action of biopesticide, potentially expanding their practical field use by increasing persistence in agriculture conditions (Damalas and Koutroubas, 2018; Hernandez-Tenorio et al., 2022). Alginate-based microcapsules have demonstrated potential for encapsulating plant biocontrol bacteria due to their biocompatibility, biodegradability, and capacity to support long-term microbial survival. However, more studies are needed to verify their efficacy in disease management (Saberi Riseh et al., 2021). Biopolymer-based formulations, such as those combining polydopamine particles with whey protein isolates, have been shown to significantly improve both UV stability and the antimicrobial efficacy of phageBCAs, making them more effective in agricultural environments (Huang and Nitin, 2020). For mBCAs, what really lacks are studies on their survival in the host organs over a long period, as up to one month, in order to well define the persistence of the active alive principle.
Besides the formulation challenges, the compatibility among BCAs represents an additional criticism in the framework of integrated and non-integrated pathogen management. In particular, the compatibility between EOs and other BCAs has not yet been studied, neither in vitro: the risk, in this case, could be the inhibition of mBCA by EOs treatment (e.g., negative effect of EOs toward the bacterial biofilm formation). This important aspect can also directly affect the colonization, survival and persistence of the mBCA in the cropping systems (Sreelatha et al., 2022). Considering the compatibility among multiple mBCA strains or species in vitro, different rapid methods are available to assess their possible coexistence and synergy (Vanneste et al., 1992). Under greenhouse or field conditions, on the contrary, the time of the experiments is significantly longer, and several times, there were no positive correlations between the in vitro and in planta results. Thus, the first screening rounds using in vitro tests on a large number of isolates followed by in planta testing of a selected group of candidates may not exploit the entire potential of antagonists. For instance, microbial antagonists combining various modes of action may be excluded by in vitro screening with a bias on a specific mode of action (Köhl et al., 2020).
7 Conclusions
The diseases caused by the genera Pseudomonas and Xanthomonas on tomato, pepper, bean, cabbage and cauliflower include several bacterial species affecting the crops yearly and causing significant economic losses in various countries. Chemical control of these plant pathogenic bacteria still results in problems and ineffectiveness, not providing a solution to plant infection or disease eradication. Moreover, the adverse effects on human health and the environment stressed the importance of ecological alternatives for managing plant pathogenic Pseudomonas syringae pv. tomato, Pseudomonas savastanoi pv. phaseolicola and Xanthomonas spp. Nowadays, the cost and time for developing new chemical bactericides have been a significant barrier to commercialization compared to developing biopesticides. Nevertheless, commercially accessible BCAs for managing bacterial diseases are few in the beginning phases. Among commercial mBCAs, only a few bacteria are registered in the EU as active ingredients, and others are marketed exclusively in the American continent. For EOs, three products are available for the market in the USA, significantly limiting their availability worldwide. So far, for the antibacterial products based on phageBCAs, the commercially available product is one in the USA, against bacterial speck and spot of tomato and pepper. Studies on EOs, mBCAs, and phageBCAs in the last five years have shown promising but variable results, with only a few trials conducted in field conditions. The lack of a uniform regulatory model for pesticide development, registration, and use is a significant barrier to the widespread use of biopesticides. This non-uniformity underscores the urgent need for policy changes that can simplify the registration process and promote the use of biopesticides. Such changes could facilitate the translation of academic research into practical agricultural solutions, potentially revolutionizing pest management in agriculture.
Author contributions
DG: Conceptualization, Writing – original draft, Writing – review & editing. EB: Conceptualization, Writing – original draft, Writing – review & editing. NB: Writing – original draft. NQ: Writing – original draft. FM: Writing – original draft. GP: Writing – original draft, Conceptualization, Writing – review & editing. SP: Conceptualization, Writing – original draft, Writing – review & editing.
Funding
The author(s) declare that no financial support was received for the research and/or publication of this article.
Acknowledgments
The authors would like to thank Prof. E. Stefani (Department of Life Sciences, University of Modena and Reggio Emilia, Italy), for the critical comments on the manuscript.
Conflict of interest
The authors declare that the research was conducted in the absence of any commercial or financial relationships that could be construed as a potential conflict of interest.
The author(s) declared that they were an editorial board member of Frontiers, at the time of submission. This had no impact on the peer review process and the final decision.
Generative AI statement
The author(s) declare that no Generative AI was used in the creation of this manuscript.
Publisher’s note
All claims expressed in this article are solely those of the authors and do not necessarily represent those of their affiliated organizations, or those of the publisher, the editors and the reviewers. Any product that may be evaluated in this article, or claim that may be made by its manufacturer, is not guaranteed or endorsed by the publisher.
References
Adhikari, P., Adhikari, T. B., Louws, F. J., Panthee, D. R. (2020). Advances and challenges in bacterial spot resistance breeding in tomato (Solanum lycopersicum L.). Int. J. Mol. Sci. 21, 1734. doi: 10.3390/ijms21051734
Agarwal, V., Sinclair, J. (1997). Principles of seed pathology, Second Edition (Boca Ratón: CRC Press). doi: 10.1201/9781482275650
Akhavan, A., Bahar, M., Askarian, H., Lak, M. R., Nazemi, A., Zamani, Z. (2013). Bean common bacterial blight: Pathogen epiphytic life and effect of irrigation practices. SpringerPlus 2, 41. doi: 10.1186/2193-1801-2-41
Akila, A. H., Ali, M. A. S., Khairy, A. M., Elnahal, A. S. M., Alfassam, H. E., Rudayni, H. A., et al. (2024). Biological control of tomato bacterial leaf spots and its impact on some antioxidant enzymes, phenolic compounds, and pigment content. Biology 13, 369. doi: 10.3390/biology13060369
Alvarez, F., Grispi, J. A., Montecchia, M. S., Draghi, W. O., Cabrera, G. M., Romero, A. M., et al. (2024). Burkholderia gladioli BNM349 as a promising source of bacterial metabolites for biocontrol of common bacterial blight of bean. BioControl 70, 131–144. doi: 10.1007/s10526-024-10284-z
Arnold, D. L., Lovell, H. C., Jackson, R. W., Mansfield, J. W. (2011). Pseudomonas syringae pv. phaseolicola: From ‘has bean’ to supermodel. Mol. Plant Pathol. 12, 617–627. doi: 10.1111/j.1364-3703.2010.00697.x
Arora, N. K., Verma, M., Prakash, J., Mishra, J. (2016). “Regulation of biopesticides: Global concerns and policies,” in Bioformulations: For Sustainable Agriculture. Eds. Arora, N. K., Mehnaz, S., Balestrini, R. (Springer, India), 283–299. doi: 10.1007/978-81-322-2779-3_16
Assadpour, E., Can Karaça, A., Fasamanesh, M., Mahdavi, S. A., Shariat-Alavi, M., Feng, J., et al. (2024). Application of essential oils as natural biopesticides; recent advances. Crit. Rev. Food Sci. Nutr. 64, 6477–6497. doi: 10.1080/10408398.2023.2170317
Ayilara, M. S., Adeleke, B. S., Akinola, S. A., Fayose, C. A., Adeyemi, U. T., Gbadegesin, L. A., et al. (2023). Biopesticides as a promising alternative to synthetic pesticides: A case for microbial pesticides, phytopesticides, and nanobiopesticides. Front. Microbiol. 14. doi: 10.3389/fmicb.2023.1040901
Aziz, S., Jamshed, S. A., Mukhtar, T., Irshad, G., Ijaz, S. S., Raja, M. U. (2024). Evaluation of Bacillus spp. As biocontrol agents against chili leaf spot caused by Xanthomonas vesicatoria. J. Plant Dis. Prot. 131, 987–997. doi: 10.1007/s41348-024-00866-5
Bakkali, F., Averbeck, S., Averbeck, D., Idaomar, M. (2008). Biological effects of essential oils – A review. Food Chem. Toxicol. 46, 446–475. doi: 10.1016/j.fct.2007.09.106
Basim, H., Turgut, K., Kaplan, B., Basim, E., Turgut, A. (2019). The potential application of Origanum dubium Boiss. Essential oil as a seed protectant against bean and tomato seed-borne bacterial pathogens. Acta Scientiarum Polonorum Hortorum Cultus 18 (3), 79–86. doi: 10.24326/asphc.2019.3.8
Bejarano, A., Puopolo, G. (2020). “Bioformulation of microbial biocontrol agents for a sustainable agriculture,” in How Research Can Stimulate the Development of Commercial Biological Control Against Plant Diseases, vol. 21 . Eds. Cal, A., Melgarejo, P., Magan, N. (Cham, Switzerland: Springer International Publishing), 275–293. doi: 10.1007/978-3-030-53238-3_16
Belete, T., Bastas, K. K., Francesconi, S., Balestra, G. M. (2021). Biological effectiveness of Bacillus subtilis on common bean bacterial blight. J. Plant Pathol. 103, 249–258. doi: 10.1007/s42161-020-00727-8
Biondi, E., Biondo, N., Lucchi, P., Vibio, M., Tazzari, L., Cardoni, M., et al. (2022). Strawberry angular leafspot: Efficacy of biocontrol agents and acibenzolar-S-methyl towards Xanthomonas fragariae. J. Plant Pathol. 104, 1207–1280. doi: 10.1007/s42161-022-01234-8
Biondi, E., Perez, S., Proto, M. R., Pagliarani, P., Bertaccini, A. (2019). Bacillus amyloliquefaciens var. Plantarum strain D747: Directly active and resistance inducer towards bacterial leaf spot of tomato. J. Plant Pathol. 101, 811–848. doi: 10.1007/s42161-019-00394-4
Bonaterra, A., Badosa, E., Daranas, N., Francés, J., Roselló, G., Montesinos, E. (2022). Bacteria as biological control agents of plant diseases. Microorganisms 10, 1759. doi: 10.3390/microorganisms10091759
Borges, D. F., Lopes, E. A., Fialho Moraes, A. R., Soares, M. S., Visôtto, L. E., Oliveira, C. R., et al. (2018). Formulation of botanicals for the control of plant-pathogens: A review. Crop Prot. 110, 135–140. doi: 10.1016/j.cropro.2018.04.003
Buttimer, C., McAuliffe, O., Ross, R. P., Hill, C., O’Mahony, J., Coffey, A. (2017). Bacteriophages and bacterial plant diseases. Front. Microbiol. 8. doi: 10.3389/fmicb.2017.00034
Butu, M., Rodino, S., Butu, A. (2022). Chapter 3 - Biopesticide formulations -current challenges and future perspectives. Biopesticides. Advances in Bio-inoculant Science, 19–29. doi: 10.1016/B978-0-12-823355-9.00010-9
Caracciolo, R., Sella, L., De Zotti, M., Bolzonello, A., Armellin, M., Trainotti, L., et al. (2023). Efficacy of Trichoderma longibrachiatum Trichogin GA IV Peptaibol analogs against the black rot pathogen Xanthomonas campestris pv. campestris and other phytopathogenic bacteria. Microorganisms 11, 480. doi: 10.3390/microorganisms11020480
Chakraborty, N., Mitra, R., Pal, S., Ganguly, R., Acharya, K., Minkina, T., et al. (2023). Biopesticide consumption in India: Insights into the current trends. Agriculture 13, 557. doi: 10.3390/agriculture13030557
Chien, Y.-C., Huang, C.-H. (2020). Biocontrol of bacterial spot on tomato by foliar spray and growth medium application of Bacillus amyloliquefaciens and Trichoderma asperellum. Eur. J. Plant Pathol. 156, 995–1003. doi: 10.1007/s10658-020-01947-5
Choudhary, M., Pereira, J., Davidson, E. B., Colee, J., Santra, S., Jones, J. B., et al. (2023). Improved persistence of bacteriophage formulation with nano N -acetylcysteine–zinc sulfide and tomato bacterial spot disease control. Plant Dis. 107, 3933–3942. doi: 10.1094/PDIS-02-23-0255-RE
Choudhary, S., Zehra, A., Mukarram, M., Wani, K. I., Naeem, M., Khan, M. M. A., et al. (2021). Salicylic acid-mediated alleviation of soil boron toxicity in Mentha arvensis and Cymbopogon flexuosus: Growth, antioxidant responses, essential oil contents and components. Chemosphere 276, 130153. doi: 10.1016/j.chemosphere.2021.130153
Cooksey, D. A. (1994). Molecular mechanisms of copper resistance and accumulation in bacteria. FEMS Microbiol. Rev. 14, 381–386. doi: 10.1111/j.1574-6976.1994.tb00112.x
Damalas, C., Koutroubas, S. (2018). Current status and recent developments in biopesticide use. Agriculture 8, 13. doi: 10.3390/agriculture8010013
Da Silva, B. D., Bernardes, P. C., Pinheiro, P. F., Fantuzzi, E., Roberto, C. D. (2021). Chemical composition, extraction sources and action mechanisms of essential oils: Natural preservative and limitations of use in meat products. Meat Sci. 176, 108463. doi: 10.1016/j.meatsci.2021.108463
Da Silva, R. S., De Oliveira, M. M. G., De Melo, J. O., Blank, A. F., Corrêa, C. B., Scher, R., et al. (2019). Antimicrobial activity of Lippia gracilis essential oils on the plant pathogen Xanthomonas campestris pv. campestris and their effect on membrane integrity. Pesticide Biochem. Physiol. 160, 40–48. doi: 10.1016/j.pestbp.2019.06.014
Da Silva, R. S., De Oliveira, M. M. G., Silva, K. P., Da Silva Vasconcelos Rodrigues, I., Dos Santos Pinto, V., Blank, A. F., et al. (2020). Synergistic effect of Cordia curassavica Jacq. Essential oils association against the phytopathogen Xanthomonas campestris pv. campestris. Environ. Sci. Pollution Res. 27, 4376–4389. doi: 10.1007/s11356-019-06631-8
Della Pepa, T., Elshafie, H. S., Capasso, R., De Feo, V., Camele, I., Nazzaro, F., et al. (2019). Antimicrobial and phytotoxic activity of Origanum heracleoticum and O. majorana essential oils growing in Cilento (southern Italy). Molecules 24, 2576. doi: 10.3390/molecules24142576
De Paula Kuyat Mates, A., De Carvalho Pontes, N., De Almeida Halfeld-Vieira, B. (2019). Bacillus velezensis GF267 as a multi-site antagonist for the control of tomato bacterial spot. Biol. Control 137, 104013. doi: 10.1016/j.biocontrol.2019.104013
De Sousa, D. M., Janssen, L., Rosa, R. B., Belmok, A., Yamada, J. K., Corrêa, R. F. T., et al. (2023). Isolation, characterization, and evaluation of putative new bacteriophages for controlling bacterial spot on tomato in Brazil. Arch. Virol. 168, 222. doi: 10.1007/s00705-023-05846-y
Dewdney, M. M., Graham, J. H. (2017). 2017–2018 Florida citrus production guide. Citrus canker. 107–110. Eds Institute Food Agric. Sci., University of Florida.
Dimopoulou, A., Theologidis, I., Benaki, D., Koukounia, M., Zervakou, A., Tzima, A., et al. (2021). Direct antibiotic activity of bacillibactin broadens the biocontrol range of Bacillus amyloliquefaciens MBI600. mSphere 6, e00376–e00321. doi: 10.1128/mSphere.00376-21
Di Vito, M., Bellardi, M. G., Mondello, F., Modesto, M., Michelozzi, M., Bugli, F., et al. (2019). Monarda citriodora hydrolate vs essential oil comparison in several anti-microbial applications. Industrial Crops Products 128, 206–212. doi: 10.1016/j.indcrop.2018.11.007
Dönmez, M. F., Aliyeva, Z. (2023). Biological control of bean halo blight disease (Pseudomonas savastanoi pv. Phaseolicola) with antagonist bacterial strains. Gesunde Pflanzen 75, 815–824. doi: 10.1007/s10343-022-00746-8
Dönmez, M. F., Uysal ŞahiN, B., Usanmaz Bozhüyük, A. (2022). Antibacterial activity of plant essential oils obtained from Satureja species against Xanthomonas phaseoli pv. phaseoli and Xanthomonas citri subspp. Fuscans. J. Institute Sci. Technol. 12, 91–103. doi: 10.21597/jist.867758
Duman, K., Soylu, S. (2019). Characterization of plant growth-promoting traits and antagonistic potentials of endophytic bacteria from bean plants against Pseudomonas syringae pv. Phaseolicola. Plant Prot. Bull. 59, 59–69. doi: 10.16955/bitkorb.597214
Dunan, L., Malanga, T., Benhamou, S., Papaiconomou, N., Desneux, N., Lavoir, A.-V., et al. (2023). Effects of essential oil-based formulation on biopesticide activity. Industrial Crops Products 202, 117006. doi: 10.1016/j.indcrop.2023.117006
DuPont, S. T., Cox, K., Johnson, K., Peter, K., Smith, T., Munir, M., et al. (2023). Evaluation of biopesticides for the control of Erwinia amylovora in apple and pear. J. Plant Pathol. 106, 889–901. doi: 10.1007/s42161-023-01372-7
EFSA Panel on Plant Health (PLH) (2014). Scientific opinion on the pest categorisation of Xanthomonas axonopodis pv. phaseoli and Xanthomonas fuscans subspp. fuscans. EFSA J. 12, 3856. doi: 10.2903/j.efsa.2014.3856
El-Fatah, B., Imran, M., Abo-Elyousr, K. A. M., Mahmoud, A. F. (2024). Isolation of Pseudomonas syringae pv. tomato strains causing bacterial speck disease of tomato and marker-based monitoring for their virulence. Mol. Biol. Rep. 50, 4917–4930. doi: 10.1007/s11033-023-08302-x
Elshafie, H. S., Nuzzaci, M., Logozzo, G., Gioia, T., Camele, I. (2020). Biological investigations on the role of hydrogel formulations containing bioactive natural agents against some common phytopathogens of Phaseolus vulgaris L. and seed germination. J. Biol. Res. - Bollettino Della Società Italiana Di Biol. Sperimentale 93 (2), 114–122. doi: 10.4081/jbr.2020.9219
Elsharkawy, M. M., Khedr, A. A., Mehiar, F., El-Kady, E. M., Alwutayd, K. M., Behiry, S. I. (2023). Rhizobacterial colonization and management of bacterial speck pathogen in tomato by Pseudomonas spp. Microorganisms 11, 1103. doi: 10.3390/microorganisms11051103
Elsharkawy, M. M., Khedr, A. A., Mehiar, F., El-Kady, E. M., Baazeem, A., Shimizu, M. (2021). Suppression of Pseudomonas syringae pv. Tomato infection by rhizosphere fungi. Pest Manage. Sci. 77, 4350–4356. doi: 10.1002/ps.6468
EPPO (2023). PM 7/110 (2) Xanthomonas spp. (Xanthomonas euvesicatoria pv. Euvesicatoria, Xanthomonas hortorum pv. gardneri, Xanthomonas euvesicatoria pv. perforans, Xanthomonas vesicatoria) causing bacterial spot of tomato and sweet pepper. EPPO Bull. - Standard Diagn. 53, 558–579. doi: 10.1111/epp.12960
Es-sahm, I., Esserti, S., Dich, J., Smaili, A., Rifai, L. A., Faize, L., et al. (2024). Common Bacillus mitigate tomato verticillium wilt and bacterial specks when combined with an essential oil extract. Rhizosphere 29, 100865. doi: 10.1016/j.rhisph.2024.100865
European Commission (2009). Regulation (EC) No 1107/2009 of the European Parliament and of the Council of 21 October 2009 concerning the placing of plant protection products on the market and repealing Council Directives 79/117/EEC and 91/414/EEC. Off. J. Eur. Union 52. Available at: https://eur-lex.europa.eu/eli/reg/2009/1107/oj (Accessed November 24, 2024).
Evseev, P. V., Tarakanov, R. I., Vo, H. T. N., Suzina, N. E., Vasilyeva, A. A., Ignatov, A. N., et al. (2024). Characterisation of new Foxunavirus phage Murka with the potential of Xanthomonas campestris pv. campestris control. Viruses 16, 198. doi: 10.3390/v16020198
Faiesal, A., Bondok, A., El Dougdoug, N. (2023). Controlling Pseudomonas syringae causing halo blight on bean plants via biocontrol-formulated bacterial viruses. Egyptian Acad. J. Biol. Sciences G. Microbiol. 15, 151–167. doi: 10.21608/eajbsg.2023.303299
Fallahzadeh-Mamaghani, V., Golchin, S., Shirzad, A., Mohammadi, H., Mohamadivand, F. (2021). Characterization of Paenibacillus polymixa N179 as a robust and multifunctional biocontrol agent. Biol. Control 154, 104505. doi: 10.1016/j.biocontrol.2020.104505
FAO - Food and Agriculture Organization of the United Nations (2024). FAOSTAT. Available online at: https://www.fao.org/faostat/en/ (Accessed November 24, 2024).
Farooq, T., Hussain, M. D., Shakeel, M. T., Tariqjaveed, M., Aslam, M. N., Naqvi, S. A. H., et al. (2022). Deploying viruses against phytobacteria: Potential use of phage cocktails as a multifaceted approach to combat resistant bacterial plant pathogens. Viruses 14, 171. doi: 10.3390/v14020171
Fenibo, E. O., Ijoma, G. N., Matambo, T. (2021). Biopesticides in sustainable agriculture: A critical sustainable development driver governed by green chemistry principles. Front. Sustain. Food Syst. 5. doi: 10.3389/fsufs.2021.619058
Fernandes Gomes, A. P., Da Costa, A. C. C., Massae Yokoo, E., Matos Fonseca, V. D. (2020). Impact of bean consumption on nutritional outcomes amongst adolescents. Nutrients 12, 1083. doi: 10.3390/nu12041083
Fontana, R., Caproni, A., Buzzi, R., Sicurella, M., Buratto, M., Salvatori, F., et al. (2021). Effects of Moringa oleifera leaf extracts on Xanthomonas campestris pv. campestris. Microorganisms 9, 2244. doi: 10.3390/microorganisms9112244
García-Latorre, C., Rodrigo, S., Santamaria, O. (2024). Biological control of Pseudomonas syringae in tomato using filtrates and extracts produced by Alternaria leptinellae. Horticulturae 10, 334. doi: 10.3390/horticulturae10040334
Gazdik, F., Magnus, S., Roberts, S. J., Baranski, R., Cechova, J., Pokluda, R., et al. (2021). Persistence of Xanthomonas campestris pv. campestris in field soil in central Europe. Microorganisms 9, 591. doi: 10.3390/microorganisms9030591
Geat, N., Singh, D., Singh, D., Saha, P., Jatoth, R., Babu, P. L. (2024). Assessing the efficacy of phyllospheric growth-promoting and antagonistic bacteria for management of black rot disease of cauliflower incited by Xanthomonas campestris pv. campestris. Folia Microbiologica 69, 789–804. doi: 10.1007/s12223-023-01106-3
Grahovac, J., Pajcin, I., Vlajkov, V., Roncevic, Z., Dodic, J., Cvetkovic, D., et al. (2021). Xanthomonas campestris biocontrol agent: Selection, medium formulation and bioprocess kinetic analysis. Chem. Industry Chem. Eng. Q. 27, 131–142. doi: 10.2298/CICEQ200508032G
Greer, S. F., Surendran, A., Grant, M., Lillywhite, R. (2023). The current status, challenges, and future perspectives for managing diseases of brassicas. Front. Microbiol. 14. doi: 10.3389/fmicb.2023.1209258
Gruľová, D., Caputo, L., Elshafie, H. S., Baranová, B., De Martino, L., Sedlák, V., et al. (2020). Thymol chemotype Origanum vulgare L. essential oil as a potential selective bio-based herbicide on monocot plant species. Molecules 25, 595. doi: 10.3390/molecules25030595
Gupta, S., Pandey, S., Nandi, S. P., Singh, M. (2023). Modulation of ethylene and ROS-scavenging enzymes by multifarious plant growth-promoting endophytes in tomato (Solanum lycopersicum) plants to combat Xanthomonas-induced stress. Plant Physiol. Biochem. 202, 107982. doi: 10.1016/j.plaphy.2023.107982
Hakalová, E., Čechová, J., Tekielska, D. A., Eichmeier, A., Pothier, J. F. (2022). Combined effect of thyme and clove phenolic compounds on Xanthomonas campestris pv. campestris and biocontrol of black rot disease on cabbage seeds. Front. Microbiol. 13. doi: 10.3389/fmicb.2022.1007988
Hassan, J. A., Diplock, N., Chau-Ly, I. J., Calma, J., Boville, E., Yee, S., et al. (2024). Solanum pimpinellifolium exhibits complex genetic resistance to Pseudomonas syringae pv. tomato. Front. Plant Sci. 15. doi: 10.3389/fpls.2024.1416078
Heo, A. Y., Koo, Y. M., Choi, H. W. (2022). Biological control activity of plant growth promoting rhizobacteria Burkholderia contaminans AY001 against tomato Fusarium wilt and bacterial speck diseases. Biology 11, 619. doi: 10.3390/biology11040619
Hernandez, C. A., Salazar, A. J., Koskella, B. (2020). Bacteriophage-mediated reduction of bacterial speck on tomato seedlings. Phage 1, 205–212. doi: 10.1089/phage.2020.0027
Hernández-Huerta, J., Tamez-Guerra, P., Gomez-Flores, R., Delgado-Gardea, Ma. C.E., Robles-Hernández, L., Gonzalez-Franco, A. C., et al. (2023). Pepper growth promotion and biocontrol against Xanthomonas euvesicatoria by Bacillus cereus and Bacillus thuringiensis formulations. PeerJ 11, e14633. doi: 10.7717/peerj.14633
Hernandez-Tenorio, F., Miranda, A. M., Rodríguez, C. A., Giraldo-Estrada, C., Sáez, A. A. (2022). Potential strategies in the biopesticide formulations: A bibliometric analysis. Agronomy 12, 2665. doi: 10.3390/agronomy12112665
Holtappels, D., Fortuna, K., Lavigne, R., Wagemans, J. (2021). The future of phage biocontrol in integrated plant protection for sustainable crop production. Curr. Opin. Biotechnol. 68, 60–71. doi: 10.1016/j.copbio.2020.08.016
Huang, K., Nitin, N. (2020). Food-grade microscale dispersion enhances UV stability and antimicrobial activity of a model bacteriophage (T7) for reducing bacterial contamination (Escherichia coli) on the plant surface. J. Agric. Food Chem. 68, 10920–10927. doi: 10.1021/acs.jafc.0c02795
Imran, M., Abo-Elyousr, K. A. M., AL-Harbi, M. S., Ali, E. F., Sallam, N. M. A., Khalil Bagy, H. M. M. (2023). Antibacterial efficacy of clove essential oil against Xanthomonas phaseoli pv. phaseoli and its influence on pathogen responses in bean. Gesunde Pflanzen 75, 431–440. doi: 10.1007/s10343-022-00721-3
Islam, T., Haque, M. A., Barai, H. R., Istiaq, A., Kim, J.-J. (2024). Antibiotic resistance in plant pathogenic bacteria: Recent data and environmental impact of unchecked use and the potential of biocontrol agents as an eco-friendly alternative. Plants 13, 1135. doi: 10.3390/plants13081135
Jamshidi, N., Darvishnia, M., Bazgir, E., Mirzaei Najafgholi, H., Ghodoum Parizipour, M. H., Aeini, M. (2023). Antibacterial activity of some plant-derived essential oils against plant pathogenic bacteria. J. Plant Dis. Prot. 130, 853–865. doi: 10.1007/s41348-023-00765-1
Jelušić, A., Berić, T., Mitrović, P., Dimkić, I., Stanković, S., Marjanović-Jeromela, A., et al. (2021). New insights into the genetic diversity of Xanthomonas campestris pv. campestris isolates from winter oilseed rape in Serbia. Plant Pathol. 70, 35–49. doi: 10.1111/ppa.13273
Ji, P., Campbell, H. L., Kloepper, J. W., Jones, J. B., Suslow, T. V., Wilson, M. (2006). Integrated biological control of bacterial speck and spot of tomato under field conditions using foliar biological control agents and plant growth-promoting rhizobacteria. Biol. Control 36, 358–367. doi: 10.1016/j.biocontrol.2005.09.003
Jibrin, M. O., Liu, Q., Garrett, T. J., Jones, J. B., Zhang, S. (2022). Potential and metabolic pathways of eugenol in the management of Xanthomonas perforans, a pathogen of bacterial spot of tomato. Int. J. Mol. Sci. 23, 14648. doi: 10.3390/ijms232314648
Karamaouna, F., Economou, L. P., Lykogianni, M., Mantzoukas, S., Eliopoulos, P. A. (2023). “Biopesticides in the EU: state of play and perspectives after the green deal for agriculture,” in Development and Commercialization of Biopesticides (London, UK: Elsevier), 213–239. doi: 10.1016/B978-0-323-95290-3.00004-2
Khalil Bagy, H. M. M., Abo-Elyousr, K. A. M. (2019). Antibacterial activity of some essential oils on bacterial spot disease of tomato plant caused by Xanthomonas axonopodis pv. vesicatoria. Int. J. Phytopathol. 8, 53–61. doi: 10.33687/phytopath.008.02.2967
Kim, J. Y., Kim, J. Y., Park, J. Y., Kim, J.-S., Seo, M.-K., Shin, M.-K., et al. (2023). Synergistic bactericidal effects of carvone and β-lactams against Xanthomonas campestris pv. vesicatoria. Appl. Biol. Chem. 66, 44. doi: 10.1186/s13765-023-00803-4
Köhl, J., Medeiros, F. H. V., Lombaers-van Der Plas, C., Groenenboom-de Haas, L., Van Den Bosch, T. (2020). Efficacies of bacterial and fungal isolates in biocontrol of Botrytis cinerea and Pseudomonas syringae pv. tomato and growth promotion in tomato do not correlate. Biol. Control 150, 104375. doi: 10.1016/j.biocontrol.2020.104375
Köhl, J., van der Wolf, J. (2005). Alternaria brassicicola and Xanthomonas campestris pv. campestris in organic seed production of Brassicae: Epidemiology and seed infection (Wageningen: Plant Research International B.V.), 1–15. Available at: https://edepot.wur.nl/17130 (Accessed November 24, 2024).
Kong, C., Chen, G., Yang, L., Zhuang, M., Zhang, Y., Wang, Y., et al. (2021). Germplasm screening and inheritance analysis of resistance to cabbage black rot in a worldwide collection of cabbage (Brassica oleracea var. capitata) resources. Scientia Hortic. 288, 110234. doi: 10.1016/j.scienta.2021.110234
Korniienko, N., Kharina, A., Zrelovs, N., Jindřichová, B., Moravec, T., Budzanivska, I., et al. (2022). Isolation and characterization of two lytic phages efficient against phytopathogenic bacteria from Pseudomonas and Xanthomonas genera. Front. Microbiol. 13. doi: 10.3389/fmicb.2022.853593
Koul, O. (2023). “Biopesticides: Commercial opportunities and challenges,” in Development and Commercialization of Biopesticides (London, UK: Elsevier), 1–23. doi: 10.1016/B978-0-323-95290-3.00009-1
Lahlali, R., Ezrari, S., Radouane, N., Kenfaoui, J., Esmaeel, Q., El Hamss, H., et al. (2022). Biological control of plant pathogens: A global perspective. Microorganisms 10, 596. doi: 10.3390/microorganisms10030596
Lamichhane, J. R., Osdaghi, E., Behlau, F., Köhl, J., Jones, J. B., Aubertot, J.-N. (2018). Thirteen decades of antimicrobial copper compounds applied in agriculture. A review. Agron. Sustain. Dev. 38, 28. doi: 10.1007/s13593-018-0503-9
Le, K. D., Yu, N. H., Park, A. R., Park, D.-J., Kim, C.-J., Kim, J.-C. (2022). Streptomyces spp. AN090126 as a biocontrol agent against bacterial and fungal plant diseases. Microorganisms 10, 791. doi: 10.3390/microorganisms10040791
Lin, Y.-C., Chung, K.-R., Huang, J.-W. (2020). A synergistic effect of chitosan and lactic acid bacteria on the control of cruciferous vegetable diseases. Plant Pathol. J. 36, 157–169. doi: 10.5423/PPJ.OA.01.2020.0004
Liu, Z., Wang, H., Wang, J., Lv, J., Xie, B., Luo, S., et al. (2022). Physical, chemical, and biological control of black rot of Brassicaceae vegetables: A review. Front. Microbiol. 13. doi: 10.3389/fmicb.2022.1023826
Llorens, E., Scalschi, L., Sharon, O., Vicedo, B., Sharon, A., García-Agustín, P. (2022). Jasmonic acid pathway is required in the resistance induced by Acremonium sclerotigenum in tomato against Pseudomonas syringae. Plant Sci. 318, 111210. doi: 10.1016/j.plantsci.2022.111210
López, M. D., Cantó-Tejero, M., Pascual-Villalobos, M. J. (2021). New insights into biopesticides: Solid and liquid formulations of essential oils and derivatives. Front. Agron. 3. doi: 10.3389/fagro.2021.763530
Ma, Y., Wang, Y.-R., He, Y.-H., Ding, Y.-Y., An, J.-X., Zhang, Z.-J., et al. (2023). Drug repurposing strategy part 1: From approved drugs to agri-bactericides leads. J. Antibiotics 76, 27–51. doi: 10.1038/s41429-022-00574-y
Mácha, H., Marešová, H., Juříková, T., Švecová, M., Benada, O., Škríba, A., et al. (2021). Killing effect of Bacillus velezensis FZB42 on a Xanthomonas campestris pv. campestris (Xcc) strain newly isolated from cabbage Brassica oleracea convar. capitata (L.): A metabolomic study. Microorganisms 9, 1410. doi: 10.3390/microorganisms9071410
Mamoucha, S., Prombona, A., Galeou, A. (2023). Evaluation of the antibacterial activity of essential oil of Laurus nobilis against Pseudomonas syringae pv. phaseolicola and potential biocidal action. Hellenic Plant Prot. J. 16, 29–39. doi: 10.2478/hppj-2023-0005
Mansfield, J., Genin, S., Magori, S., Citovsky, V., Sriariyanum, M., Ronald, P., et al. (2012). Top 10 plant pathogenic bacteria in molecular plant pathology. Mol. Plant Pathol. 13, 614–629. doi: 10.1111/j.1364-3703.2012.00804.x
Marin, V. R., Ferrarezi, J. H., Vieira, G., Sass, D. C. (2019). Recent advances in the biocontrol of Xanthomonas spp. World J. Microbiol. Biotechnol. 35, 72. doi: 10.1007/s11274-019-2646-5
Marrone, P. G. (2023). “Biopesticide commercialization in North America,” in Development and Commercialization of Biopesticides (London, UK: Elsevier), 173–202. doi: 10.1016/B978-0-323-95290-3.00017-0
Marrone, P. G. (2024). Status of the biopesticide market and prospects for new bioherbicides. Pest Manage. Sci. 80, 81–86. doi: 10.1002/ps.7403
Martin, G. B., Brommonschenkel, S. H., Chunwongse, J., Frary, A., Ganal, M. W., Spivey, R., et al. (1993). Map-based cloning of a protein kinase gene conferring disease resistance in tomato. Science 262, 1432–1436. doi: 10.1126/science.7902614
Martinazzo, A. P., Braga, R. D. O., Teodoro, C. E. D. S. (2022). Alternative control of phytopathogenic bacteria with essential oils of Elionurus latiflorus and Cymbopogon flexuosus. Ciec. e Natura 44, e25. doi: 10.5902/2179460X67338
Martino, G., Holtappels, D., Vallino, M., Chiapello, M., Turina, M., Lavigne, R., et al. (2021). Molecular characterization and taxonomic assignment of three phage isolates from a collection infecting Pseudomonas syringae pv. Actinidiae and P. syringae pv. Phaseolicola from northern Italy. Viruses 13, 2083. doi: 10.3390/v13102083
Mazo-Molina, C., Mainiero, S., Haefner, B. J., Bednarek, R., Zhang, J., Feder, A., et al. (2020). Ptr1 evolved convergently with RPS2 and Mr5 to mediate recognition of AvrRpt2 in diverse solanaceous species. Plant J. 103, 1433–1445. doi: 10.1111/tpj.14810
Meng, G., Xiao, Y., Li, A., Qian, Z., Xie, Y., Yang, L., et al. (2022). Mapping and characterization of the Rx3 gene for resistance to Xanthomonas euvesicatoria pv. euvesicatoria race T1 in tomato. Theor. Appl. Genet. 135, 1637–1656. doi: 10.1007/s00122-022-04059-2
Miller, S. A., Ferreira, J. P., LeJeune, J. T. (2022). Antimicrobial use and resistance in plant agriculture: A One Health perspective. Agriculture 12, 289. doi: 10.3390/agriculture12020289
Mokrani, S., Rai, A., Belabid, L., Cherif, A., Cherif, H., Mahjoubi, M., et al. (2019). Pseudomonas diversity in western Algeria: Role in the stimulation of bean germination and common bean blight biocontrol. Eur. J. Plant Pathol. 153, 397–415. doi: 10.1007/s10658-018-1566-9
Morella, N. M., Zhang, X., Koskella, B. (2019). Tomato seed-associated bacteria confer protection of seedlings against foliar disease caused by Pseudomonas syringae. Phytobiomes J. 3, 177–190. doi: 10.1094/PBIOMES-01-19-0007-R
Nawaz, A., Zafar, S., Shahzadi, M., Bukhari, S. M. A. U. S., Khan, N., Shah, A. A., et al. (2023). Bacteriophages: An overview of the control strategies against phytopathogens. Egyptian J. Biol. Pest Control 33, 108. doi: 10.1186/s41938-023-00751-7
Nazzaro, F., Fratianni, F., De Martino, L., Coppola, R., De Feo, V. (2013). Effect of essential oils on pathogenic Bacteria. Pharmaceuticals 6, 1451–1474. doi: 10.3390/ph6121451
Nicotra, D., Ghadamgahi, F., Ghosh, S., Anzalone, A., Dimaria, G., Mosca, A., et al. (2024). Genomic insights and biocontrol potential of ten bacterial strains from the tomato core microbiome. Front. Plant Sci. 15. doi: 10.3389/fpls.2024.1437947
Odooli, S., Roghanian, R., Ghasemi, Y., Mohkam, M., Emtiazi, G. (2021). Predatory and biocontrol potency of Bdellovibrio bacteriovorus toward phytopathogenic strains of Pantoea spp. And Xanthomonas campestris in the presence of exo-biopolymers: In vitro and in vivo assessments. Int. Microbiol. 24, 399–413. doi: 10.1007/s10123-021-00177-x
Oerke, E.-C. (2006). Crop losses to pests. J. Agric. Sci. 144, 31–43. doi: 10.1017/S0021859605005708
Olishevska, S., Nickzad, A., Restieri, C., Dagher, F., Luo, Y., Zheng, J., et al. (2023). Bacillus velezensis and Paenibacillus peoriae strains effective as biocontrol agents against Xanthomonas bacterial spot. Appl. Microbiol. 3, 1101–1119. doi: 10.3390/applmicrobiol3030076
Oliveira-Pinto, P. R., Mariz-Ponte, N., Gil, R. L., Cunha, E., Amorim, C. G., Montenegro, M. C. B. S. M., et al. (2022). Montmorillonite nanoclay and formulation with Satureja montana essential oil as a tool to alleviate Xanthomonas euvesicatoria load on Solanum lycopersicum. Appl. Nano 3, 126–142. doi: 10.3390/applnano3030009
Orynbayev, A. T., Dzhalilov, F. S. U., Ignatov, A. N. (2020). Improved efficacy of formulated bacteriophage in control of black rot caused by Xanthomonas campestris pv. campestris on cabbage seedlings. Arch. Phytopathol. Plant Prot. 53, 379–394. doi: 10.1080/03235408.2020.1745487
Pajčin, I., Vlajkov, V., Frohme, M., Grebinyk, S., Grahovac, M., Mojićević, M., et al. (2020). Pepper bacterial spot control by Bacillus velezensis: Bioprocess solution. Microorganisms 8, 1463. doi: 10.3390/microorganisms8101463
Papaianni, M., Ricciardelli, A., Fulgione, A., d’Errico, G., Zoina, A., Lorito, M., et al. (2020). Antibiofilm activity of a Trichoderma metabolite against Xanthomonas campestris pv. campestris, alone and in association with a phage. Microorganisms 8, 620. doi: 10.3390/microorganisms8050620
Poveda, J., Zabalgogeazcoa, I., Soengas, P., Rodríguez, V. M., Cartea, M. E., Abilleira, R., et al. (2020). Brassica oleracea var. Acephala (kale) improvement by biological activity of root endophytic fungi. Sci. Rep. 10, 20224. doi: 10.1038/s41598-020-77215-7
Proto, M. R., Biondi, E., Baldo, D., Levoni, M., Filippini, G., Modesto, M., et al. (2022). Essential oils and hydrolates: Potential tools for defense against bacterial plant pathogens. Microorganisms 10, 702. doi: 10.3390/microorganisms10040702
Qiao, K., Liu, Q., Huang, Y., Xia, Y., Zhang, S. (2020). Management of bacterial spot of tomato caused by copper-resistant Xanthomonas perforans using a small molecule compound carvacrol. Crop Prot. 132, 105114. doi: 10.1016/j.cropro.2020.105114
Rabiey, M., Roy, S. R., Holtappels, D., Franceschetti, L., Quilty, B. J., Creeth, R., et al. (2020). Phage biocontrol to combat Pseudomonas syringae pathogens causing disease in cherry. Microbial Biotechnol. 13, 1428–1445. doi: 10.1111/1751-7915.13585
Rodrigues, T. C., Gois, I. B., Fernandes, R. P. M., Blank, A. F., Sandes, R. D. D., Leite Neta, M. T. S., et al. (2023). Chemical characterization and antimicrobial activity of essential oils from Croton grewioides Baill. Accessions on the phytopathogen Xanthomonas campestris pv. campestris. Pesticide Biochem. Physiol. 193, 105454. doi: 10.1016/j.pestbp.2023.105454
Rodríguez, M., Torres, M., Blanco, L., Béjar, V., Sampedro, I., Llamas, I. (2020). Plant growth-promoting activity and quorum quenching-mediated biocontrol of bacterial phytopathogens by Pseudomonas segetis strain P6. Sci. Rep. 10, 4121. doi: 10.1038/s41598-020-61084-1
Rostami, S., Hasanzadeh, N., Rajaei, S., Golnaraghi, A. R., Azizinezhad, R. (2021). A study on endophytic bacteria isolated from wild legumes against Xanthomonas phaseoli. Appl. Entomology Phytopathol. 89, 1–16. doi: 10.22092/jaep.2020.343449.1356
Saberi Riseh, R., Hassanisaadi, M., Vatankhah, M., Soroush, F., Varma, R. S. (2022). Nano/microencapsulation of plant biocontrol agents by chitosan, alginate, and other important biopolymers as a novel strategy for alleviating plant biotic stresses. Int. J. Biol. Macromolecules 222, 1589–1604. doi: 10.1016/j.ijbiomac.2022.09.278
Saberi Riseh, R., Skorik, Y. A., Thakur, V. K., Moradi Pour, M., Tamanadar, E., Noghabi, S. S. (2021). Encapsulation of plant biocontrol bacteria with alginate as a main polymer material. Int. J. Mol. Sci. 22, 11165. doi: 10.3390/ijms222011165
Saleem, S., Ragasova, L. N., Tekielska, D., Fidurski, M., Sekara, A., Pokluda, R. (2023). Serendipita indica as a plant growth promoter and biocontrol agent against black rot disease in cabbage grown in a phytotron. Agriculture 13, 2048. doi: 10.3390/agriculture13112048
Samada, L. H., Tambunan, U. S. F. (2020). Biopesticides as promising alternatives to chemical pesticides: A review of their current and future status. Online J. Biol. Sci. 20, 66–76. doi: 10.3844/ojbsci.2020.66.76
Santos, F., Melkani, S., Oliveira-Paiva, C., Bini, D., Pavuluri, K., Gatiboni, L., et al. (2024). Biofertilizer use in the United States: definition, regulation, and prospects. Appl. Microbiol. Biotechnol. 108, 511. doi: 10.1007/s00253-024-13347-4
Schaad, N., Cheong, S., Tamaki, S., Hatziloukas, E., Panopoulos, N. J. (1995). A combined biological and enzymatic amplification (BIO-PCR) Technique to Detect Pseudomonas syringae pv. phaseolicola in bean seed extracts. Phytopathology 85, 243. doi: 10.1094/Phyto-85-243
Schreinemachers, P., Simmons, E. B., Wopereis, M. C. S. (2018). Tapping the economic and nutritional power of vegetables. Global Food Secur. 16, 36–45. doi: 10.1016/j.gfs.2017.09.005
Šević, M., Gašić, K., Ignjatov, M., Mijatović, M., Prokić, A., Obradović, A. (2019). Integration of biological and conventional treatments in control of pepper bacterial spot. Crop Prot. 119, 46–51. doi: 10.1016/j.cropro.2019.01.006
Shao, Z., Schenk, P. M., Dart, P. (2023). Phyllosphere bacterial strains Rhizobium b1 and Bacillus subtilis b2 control tomato leaf diseases caused by Pseudomonas syringae pv. tomato and Alternaria solani. J. Appl. Microbiol. 134, lxad139. doi: 10.1093/jambio/lxad139
Sharma, S., Bhattarai, K. (2019). Progress in developing bacterial spot resistance in tomato. Agronomy 9, 26. doi: 10.3390/agronomy9010026
Sharma, A., Shukla, A., Attri, K., Kumar, M., Kumar, P., Suttee, A., Singh, G., et al. (2020). Global trends in pesticides: A looming threat and viable alternatives. Ecotoxicol. Environ. Saf. 201, 110812. doi: 10.1016/j.ecoenv.2020.110812
Shopova, E., Brankova, L., Ivanov, S., Urshev, Z., Dimitrova, L., Dimitrova, M., et al. (2023). Xanthomonas euvesicatoria-specific bacteriophage BsXeu269p/3 reduces the spread of bacterial spot disease in pepper plants. Plants 12, 3348. doi: 10.3390/plants12193348
Skliros, D., Papazoglou, P., Gkizi, D., Paraskevopoulou, E., Katharios, P., Goumas, D. E., et al. (2023). In planta interactions of a novel bacteriophage against Pseudomonas syringae pv. tomato. Appl. Microbiol. Biotechnol. 107, 3801–3815. doi: 10.1007/s00253-023-12493-5
Solís-Sánchez, G. A., Quiñones-Aguilar, E. E., Fraire-Velázquez, S., Vega-Arreguín, J., Rincón-Enríquez, G. (2020). Complete genome sequence of XaF13, a novel bacteriophage of Xanthomonas vesicatoria from Mexico. Microbiol. Resource Announcements 9 (5), 10.1128/mra.01371-19. doi: 10.1128/mra.01371-19
Sreelatha, S., Kumar, N., Yin, T. S., Rajani, S. (2022). Evaluating the antibacterial activity and mode of action of thymol-loaded chitosan nanoparticles against plant bacterial pathogen Xanthomonas campestris pv. campestris. Front. Microbiol. 12. doi: 10.3389/fmicb.2021.792737
Stefani, E., Obradović, A., Gašić, K., Altin, I., Nagy, I. K., Kovács, T. (2021). Bacteriophage-mediated control of phytopathogenic Xanthomonads: A promising green solution for the future. Microorganisms 9, 1056. doi: 10.3390/microorganisms9051056
Strayer-Scherer, A. L., Sharpe, S. R., Louws, F. J., Meadows, I. M. (2024). Evaluation of systemic acquired resistance inducers for the management of copper and streptomycin resistant strains of Xanthomonas perforans on tomato. Crop Prot. 175, 106430. doi: 10.1016/j.cropro.2023.106430
Tariq, M., Khan, A., Asif, M., Khan, F., Ansari, T., Shariq, M., et al. (2020). Biological control: a sustainable and practical approach for plant disease management. Acta Agriculturae Scandinavica, Section B —. Soil Plant Sci. 70, 507–524. doi: 10.1080/09064710.2020.1784262
Tazzari, L. (2019). Valutazione di bio-formulati nei confronti dell’agente della maculatura Angolare della fragola. University of Bologna, Bologna (IT.
Thapa, S. P., Miyao, E. M., Davis, R. M., Coaker, G. (2015). Identification of QTLs controlling resistance to Pseudomonas syringae pv. tomato race 1 strains from the wild tomato, Solanum habrochaites LA1777. Theor. Appl. Genet. 128, 681–692. doi: 10.1007/s00122-015-2463-7
Uroz, S., Dessaux, Y., Oger, P. (2009). Quorum Sensing and Quorum Quenching: The Yin and Yang of bacterial communication. ChemBioChem 10, 205–216. doi: 10.1002/cbic.200800521
Vanneste, J. L., Yu, J., Beer, S. V. (1992). Role of antibiotic production by Erwinia herbicola Eh252 in biological control of Erwinia amylovora. J. Bacteriology 174, 2785–2796. doi: 10.1128/jb.174.9.2785-2796.1992
Vicente, J. G., Holub, E. B. (2013). Xanthomonas campestris pv. campestris (cause of black rot of crucifers) in the genomic era is still a worldwide threat to brassica crops. Mol. Plant Pathol. 14, 2–18. doi: 10.1111/j.1364-3703.2012.00833.x
Villicaña, C., Rubí-Rangel, L. M., Amarillas, L., Lightbourn-Rojas, L. A., Carrillo-Fasio, J. A., León-Félix, J. (2024). Isolation and characterization of two novel genera of jumbo bacteriophages infecting Xanthomonas vesicatoria isolated from agricultural regions in Mexico. Antibiotics 13, 651. doi: 10.3390/antibiotics13070651
Vurukonda, S. S. K. P., Giovanardi, D., Stefani, E. (2022). Identification, evaluation and selection of a bacterial endophyte able to colonize tomato plants, enhance their growth and control Xanthomonas vesicatoria, the causal agent of the spot disease. Can. J. Plant Pathol. 44, 219–234. doi: 10.1080/07060661.2021.1980822
Wang, X., Chi, Y., Li, F. (2022). Exploring China stepping into the dawn of chemical pesticide-free agriculture in 2050. Front. Plant Sci. 13. doi: 10.3389/fpls.2022.942117
Wu, Q., An, N., Fang, Z., Li, S., Xiang, L., Liu, Q., et al. (2024). Characteristics and whole-genome analysis of a novel Pseudomonas syringae pv. tomato bacteriophage D6 isolated from a karst cave. Virus Genes 60, 295–308. doi: 10.1007/s11262-024-02064-9
Xhemali, B., Giovanardi, D., Biondi, E., Stefani, E. (2024). Tomato and pepper seeds as pathways for the dissemination of phytopathogenic bacteria: A constant challenge for the seed industry and the sustainability of crop production. Sustainability 16, 1808. doi: 10.3390/su16051808
Ye, T., Zhang, W., Feng, Z., Fan, X., Xu, X., Mishra, S., et al. (2020). Characterization of a novel Quorum-Quenching bacterial strain, Burkholderia anthina HN-8, and its biocontrol potential against black rot disease caused by Xanthomonas campestris pv. Campestris. Microorganisms 8, 1485. doi: 10.3390/microorganisms8101485
Ye, T., Zhou, T., Fan, X., Bhatt, P., Zhang, L., Chen, S. (2019a). Acinetobacter lactucae strain QL-1, a novel Quorum Quenching candidate against bacterial pathogen Xanthomonas campestris pv. Campestris. Front. Microbiol. 10. doi: 10.3389/fmicb.2019.02867
Ye, T., Zhou, T., Li, Q., Xu, X., Fan, X., Zhang, L., et al. (2019b). Cupriavidus spp. HN-2, a novel Quorum Quenching bacterial isolate, is a potent biocontrol agent against Xanthomonas campestris pv. campestris. Microorganisms 8, 45. doi: 10.3390/microorganisms8010045
Yildiz, H. N., Altinok, H. H., Dikilitas, M., Günacti, H., Ay, T. (2023). Suppression of tomato bacterial speck disease (Pseudomonas syringae pv. Tomato (Okabe) Young, Dye, & Wilkie) via induced systemic resistance by Pseudomonas and Bacillus strains. Botany 101, 391–399. doi: 10.1139/cjb-2022-0066
Keywords: bacterial plant diseases, vegetables, essential oils, microbiological control agents, bacteriophages
Citation: Giovanardi D, Biondi E, Biondo N, Quiroga N, Modica F, Puopolo G and Pérez Fuentealba S (2025) Sustainable and innovative biological control strategies against Pseudomonas syringae pv. tomato, Pseudomonas savastanoi pv. phaseolicola and Xanthomonas spp. affecting vegetable crops: a review. Front. Plant Sci. 16:1536152. doi: 10.3389/fpls.2025.1536152
Received: 28 November 2024; Accepted: 07 April 2025;
Published: 30 April 2025.
Edited by:
Roberta Marra, University of Naples Federico II, ItalyReviewed by:
Guillermo A. Galvan, Universidad de la República, UruguayRaghavendra Aminedi, Indian Council of Agricultural Research (ICAR), India
Aisha Lawan Idris, Henan Agricultural University, China
Copyright © 2025 Giovanardi, Biondi, Biondo, Quiroga, Modica, Puopolo and Pérez Fuentealba. This is an open-access article distributed under the terms of the Creative Commons Attribution License (CC BY). The use, distribution or reproduction in other forums is permitted, provided the original author(s) and the copyright owner(s) are credited and that the original publication in this journal is cited, in accordance with accepted academic practice. No use, distribution or reproduction is permitted which does not comply with these terms.
*Correspondence: Set Pérez Fuentealba, c2V0LnBlcmV6QHVvaC5jbA==