- 1Department of Grassland Science, College of Animal Science and Technology, Northeast Agricultural University, Harbin, China
- 2Grassland Station of Heilongjiang, Harbin, China
- 3Institute of Forage and Grassland Science, Heilong Academy of Agricultural Science, Harbin, China
Introduction: Drought stress severely impacts plant productivity, particularly in non-cultivated species such as Allium senescens.L. However, the role of rhizosphere and endophytic bacterial communities in enhancing drought tolerance remains underexplored.
Methods: We used 16S rRNA amplicon sequencing to investigate microbial communities in the rhizosphere, roots, bulbs, and leaves of A. senescens under simulated drought conditions using PEG-6000 (CK, 5%, 15%, 25%) and post-rehydration recovery. Alpha and beta diversity, phylogenetic relationships, and functional predictions were analyzed.
Results: Drought stress reduced rhizosphere bacterial diversity by 42% but increased leaf diversity by 52%. The 15% PEG treatment marked a key threshold for community shifts. Streptomyces and Ralstonia were significantly enriched under drought, and functional predictions indicated their involvement in osmotic regulation and phytohormone synthesis. Post-rehydration partially restored microbial composition in aerial tissues but not in the rhizosphere.
Discussion: These findings suggest that drought induces niche-specific microbial adaptations and that bacterial community structure plays a critical role in drought resilience. This study provides insights into plant-microbe interactions and offers a basis for developing microbial strategies to improve drought tolerance in Allium species.
1 Introduction
Drought has the potential to disrupt the normal growth and development of plants (Li et al., 2020; Kvgk and Ramarao, 2019). More specifically, drought affects plant germination, growth, physiology, leaf water content, photosynthesis, stomatal function, chlorophyll content, flowering and maturity, plant height, and biomass (Todorova et al., 2022; Onyemaobi et al., 2021; Javed et al., 2022; Wan et al., 2022; Pour-Aboughadareh et al., 2020). Drought can lead to oxidative damage and membrane peroxidation in plants by disrupting osmotic regulation and signal transduction processes, resulting in toxic effects and triggering a cascade of issues, including a reduction in plant yield (Duke et al., 2017; Kumar et al., 2020). Studies have shown that drought affects the growth and development of Allium plants. Drought stress led to a substantial decline in both the absolute and relative water content of Allium schoenoprasum L. leaves. Additionally, it elevates the osmotic pressure within the leaf sap and intensifies leaf transpiration while concurrently suppressing the production of reactive oxygen species (Egert and Tevini, 2002). Investigations into the aldehyde dehydrogenase (ALDH) superfamily of garlic (Allium sativum L.) and colleagues revealed that drought conditions lead to the down-regulation of the majority of AsALDH genes (Twaij et al., 2023). AsTCP17 protein, as a positive regulator, was involved in drought resistance of A. senescens (Fu et al., 2023).
Endophytes are defined as bacteria or fungi that inhabit the intercellular spaces or compartments of various tissues and organs in healthy plants, either at a specific stage or throughout their entire life cycle (Hallmann et al., 1997). These endophytes have co-evolved over an extended period with their host plants, establishing a stable and mutually advantageous symbiotic relationship (Hardoim et al., 2015). Bacterial endophytes commonly inhabit intercellular spaces in plants (Hardoim et al., 2015). Endophytes are known to colonize intercellular spaces within various plant components such as roots, leaves, stems, flowers, and seeds (Kandel et al., 2015; Mitter et al., 2017; Glassner et al., 2017). The extent of colonization can range from being confined to specific tissues to encompass the entire plant. However, the question remains whether endophytes must migrate to particular organs or tissues to optimally fulfill the roles of the identified endophytes (Hardoim et al., 2015).
The plant rhizosphere microbial community is the first microbial layer of plant defense, followed by endophytes, as has been widely reported (Dini-Andreote, 2020; Zhang and Mason, 2022). The presence of many beneficial bacteria in the rhizosphere and roots of plants confers various benefits to plants, including increased plant biomass, improved plant nutrient uptake and utilization, improved plant stress tolerance, and better adaptation to the environment (Cao et al., 2023; Papik et al., 2020). Endophytes facilitate plant growth by fixing nitrogen, producing plant hormones, acquiring nutrients, and enhancing tolerance to abiotic and biotic stresses (Kandel et al., 2017).
Drought substantially modifies the biomass, diversity, and structure of the endosphere and rhizosphere microbial communities, often leading to changes or disturbances in ecosystem processes and plant community dynamics (Seaton et al., 2022; Naylor and Coleman-Derr, 2018; Naylor et al., 2017). In cases in which drought conditions do not result in irreversible harm to crops, prompt regulatory intervention can promote normal growth and development (De Vries et al., 2020; Mega et al., 2019). It is believed that endophytic bacteria in host plants improve their capacity to adapt to biotic and abiotic stress conditions. Drought selectively recruits more drought-tolerant plants depending on the bacteria, which benefits the host (Liu et al., 2021; Ullah et al., 2019). Beneficial microbes can produce permeable material through biofilm formation and undergo morphological changes to enhance the drought resistance capacity, increase the availability of soil nutrients, promote organic matter decomposition, and secrete plant hormones to enhance plant drought resistance (Marasco et al., 2021; Santos-Medellín et al., 2021).
Currently, limited research has been conducted on the drought resistance mechanisms of the Allium species. Allium senescens L. (A. senescens), one member of the Allium genus, exhibits robust drought tolerance coupled with a high degree of adaptability and diverse practical applications (Munim et al., 2023). Specifically, the A. senescens is used for its ornamental value (Zhang et al., 2014), but more importantly, it has a genetic link to the cultivated onion Allium cepa and can be used as a source of trait genes in breeding strategies (Mallor et al., 2014). However, the underlying mechanism of drought tolerance in A. senescens remains elusive, potentially involving alterations in the composition of the rhizosphere and the endophytic bacterial community. The specific dynamics of rhizosphere and endophytic bacterial community diversity respond to drought stress in this plant species have not been fully elucidated. Therefore, we investigated the impact of drought stress on the rhizosphere and endophytic bacterial communities of A. senescens using microbiome sequencing techniques to elucidate the mechanisms by which the bacteria enhance the drought tolerance of plant species. Meanwhile, this study may not only contribute to the mechanism of bacteria enhancing plant drought tolerance and enriches the significance of endophytic bacteria resources in Allium plants, but also has potential application value for agricultural production practice. We hypothesized that 1) the diversity of the rhizosphere bacterial community was higher than that of the endophytic bacterial community, 2) the drought resistance of A. senescens is related to rhizosphere and endophytic bacteria, and 3) drought stress will affect the rhizosphere and endophytic bacterial community of A. senescens.
2 Material and methods
2.1 Plant sampling and drought stress
High-quality seeds of A. senescens, sourced from the Key Laboratory of Forage Breeding at Northeast Agricultural University, underwent a two-step sterilization protocol to ensure uniformity and minimize contamination: immersion in 75% ethanol (v/v) for 5 minutes followed by 10% sodium hypochlorite solution (v/v) for 10 minutes. After thorough rinsing with distilled water and air-drying, the seeds were sown in sterile Petri dishes (50 seeds per dish) lined with filter paper and cultivated under controlled conditions (25°C, constant hydration) until germination. Seedlings reaching 10 cm in height were transplanted into pots filled with soil (8-10 seedlings per pot), with a total of 25 pots arranged in a lighted culture chamber maintained at 22°C, 3200 K light intensity, and controlled humidity, with weekly watering to sustain optimal growth. To simulate drought stress, 20 cm-tall seedlings were subjected to a gradient of polyethylene glycol (PEG-6000) concentrations: a control group (CK) irrigated with distilled water, three drought stress groups (D2: 5%, D3: 15%, D4: 25% PEG-6000), and four corresponding rehydration groups (R1-R4) that resumed regular watering for one week after a two-week drought period.
The experimental nutrient soil was procured from a Harbin City, Heilongjiang Province flower market. It consisted of peat soil, leaf mold, and vermiculite mixed in a ratio of 2:1:1.
Each treatment was replicated five times, with 500 mL of PEG-6000 solution or distilled water applied every six days. Drought treatments lasted for two weeks, followed by the rehydration phase for applicable groups. Treatment details are summarized in Table 1:
After the treatments, samples of leaves, bulbs, roots, and rhizosphere soil were collected from each group. Each sample type was collected in triplicate to ensure biological replicability. Samples were immediately flash-frozen in liquid nitrogen and stored at -80°C for subsequent analyses.
2.2 Experimental procedure
2.2.1 DNA extraction and sample quality control
Rhizosphere soil genomic DNA was extracted using a TianGen magnetic bead soil genomic DNA extraction kit (TianGen, BeiJing, China). Genomic DNA was isolated from roots, bulbs, and leaves using the Hexadecyl trimethyl ammonium bromide (CTAB) method. The purity and concentration of DNA were determined using 1% agarose gel electrophoresis. To achieve a suitable concentration for subsequent analyses, an aliquot of the DNA sample was placed in a centrifuge tube and diluted with sterile water to a final concentration of 1 ng/μL.
2.2.2 Amplicon generation
The V57 region of the 16S rRNA gene was amplified by polymerase chain reaction (PCR). The primer sequences used were 5’-AACMGGATTAGATACCCKG-3’ and 5’-ACGTCATCCCCCCACCTTCC-3.’ Each PCR mixture was prepared by adding 15 μL of Phusion High-Fidelity PCR Master Mix (New England Biolabs, Ipswich, USA) along with 0.2 μM of each primer and a DNA template of 10 ng. The thermal cycling protocol began with a denaturation step at 98°C for 1 min, followed by 30 amplification cycles, including denaturation at 98°C for 10 s, annealing at 50°C for 30 s, and extension at 72°C for 30 s, and a final extension at 72°C for an additional 5 min.
2.2.3 PCR products quantification and qualification
PCR products were analyzed using 2% agarose gel electrophoresis. Subsequently, the qualified products were purified with magnetic beads and their concentrations were determined by enzyme labeling. Equal amounts of these samples were combined based on PCR product concentrations. Thereafter, the mixed PCR products were analyzed by 2% agarose gel electrophoresis. To recover the target band, a universal DNA purification recovery kit (TianGen, BeiJing, China) was used.
2.2.4 Library preparation and sequencing
Sequencing libraries were prepared using the NEB Next® Ultra™ II FS DNA PCR-free Library Prep Kit (New England Biolabs, Ipswich, USA), following the manufacturer’s instructions, and incorporating index sequences. Library quality was assessed using a Qubit Fluorometer and validated via real-time PCR for quantification, as well as by electrophoresis on an Agilent Bioanalyzer (Novogene, BeiJing, China). Subsequently, the quantified libraries were pooled and sequenced on Illumina platforms (Novogene, BeiJing, China), with the procedure tailored to meet the desired library concentration and required data output volume.
2.2.5 Amplicon sequence variants denoise and species annotation
Denoising was performed with DADA2 or the Deblur module in the Qiime 2 software to obtain initial Amplicon Sequence Variants (ASVs). Species annotation was performed using the Qiime 2 software. The Silva Database was used as the annotation database. Multiple sequence alignment was performed using the Qiime 2 software. The absolute abundance of ASVs was normalized using a standard sequence number corresponding to the sample with the lowest number of sequences. Subsequent analyses of alpha and beta diversity were performed based on the output-normalized data.
2.3 Statistical analysis
This study employed Python 3.6.13, R 4.0.3(Lucent, USA), and Perl 5.26.2 were used for data analysis and mapping throughout the analysis process. The distribution histogram of the relative abundance in Perl was drawn using the SVG function. A heatmap was generated in R using the pheatmap function. Flower diagrams were produced perl with SVG function. One hundred genera with the highest abundance in the samples were selected and performed sequence alignment to draw the phylogenetic tree in perl with SVG function. To analyze the alpha diversity, richness, and uniformity of the communities in the sample, alpha and beta diversity were calculated using Qiime 2. The UPGMA map was drawn using the upgma.tre function in Qiime 2, and a clustering tree was constructed on the UPGMA based on the weighted UniFrac distance matrix. Principal coordinates analysis (PCoA) and non-metric multidimensional scaling (NMDS) analyses were performed using the ade4 and ggplot2 packages in R 4.0.3. Linear discriminant analysis effect size (LEfSe) analysis was used an exclusive package called Lefse.
3 Results
3.1 Species composition of bacterial community in A. senescens
High-throughput sequencing identified 45 phyla, 126 classes, and 944 genera across rhizosphere soil, roots, bulbs, and leaves of A. senescens. Cyanobacteriota were dominant in non-stressed leaves (98%) and bulbs (96%), whereas Pseudomonadota prevailed in roots (48%) and rhizosphere soil (50%) (Figures 1a, 1b). Drought stress induced significant taxonomic shifts: Cyanobacteriota abundance declined across all tissues, particularly in leaves (98% to 82% under 25% PEG), while Actinobacteria increased in leaves (CK: 0.5% vs. D3: 8.2%) and Bacillota in bulbs (CK: 1.1% vs. D3: 4.7%) (Figure 1a). At the genus level, Chloroplast (51.5%) and Streptomyces (3.6%) were dominant in control plants (Figures 2a, 2b). The term “Chloroplast” refers to sequences associated with photosynthetic bacteria, such as Cyanobacteriota, likely derived from chloroplast DNA detected in the samples. Under 15% PEG treatment, Streptomyces surged to 8.4% in roots (P < 0.001), while Ralstonia increased 2.3-fold in leaves (Figure 2a). The enrichment of Streptomyces suggests its potential role in drought adaptation through osmolyte production and phytohormone regulation.
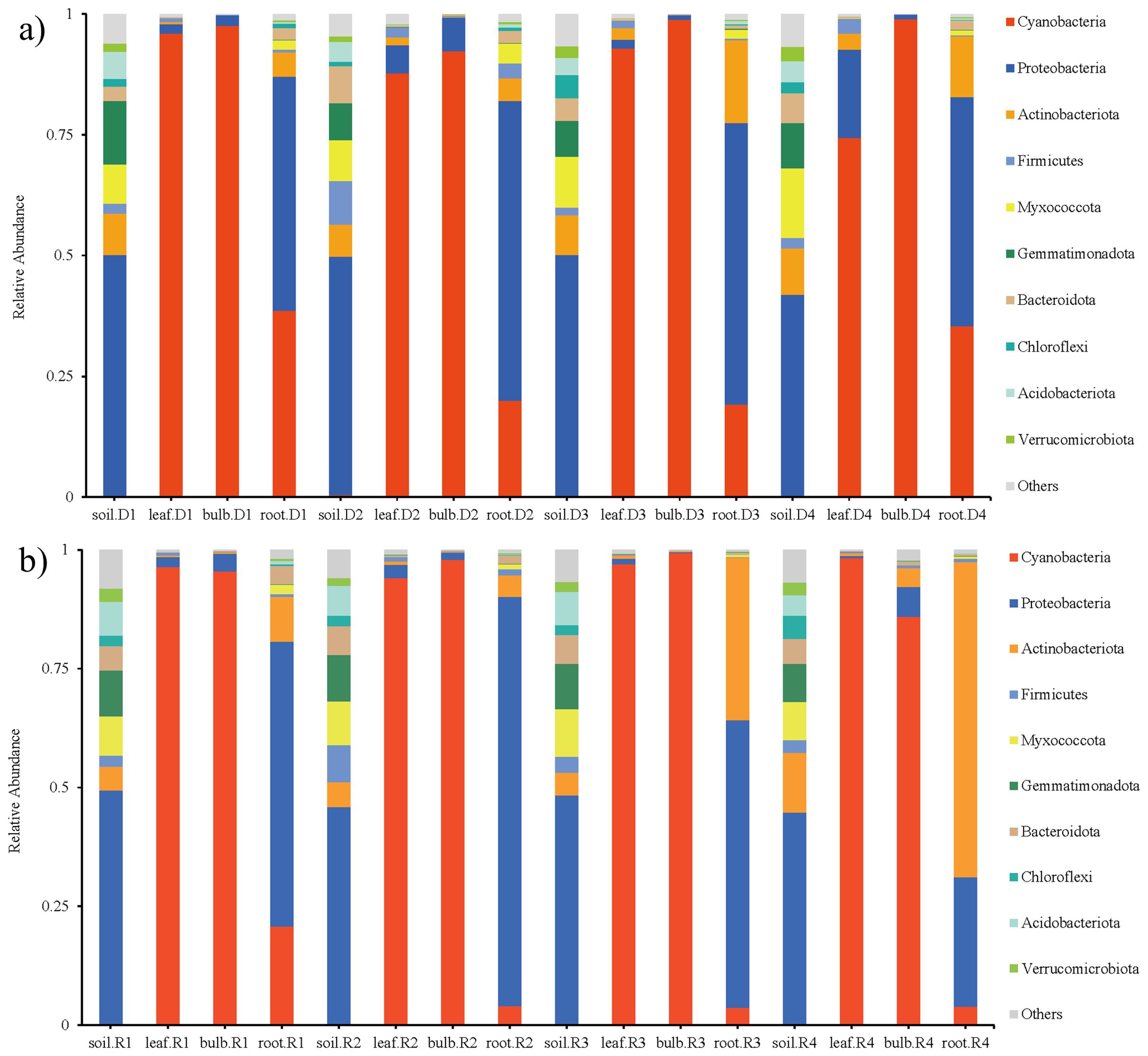
Figure 1. Species abundance of endophytic bacteria in A. senescens after drought stress (a) and re-watering (b) at the phylum level.
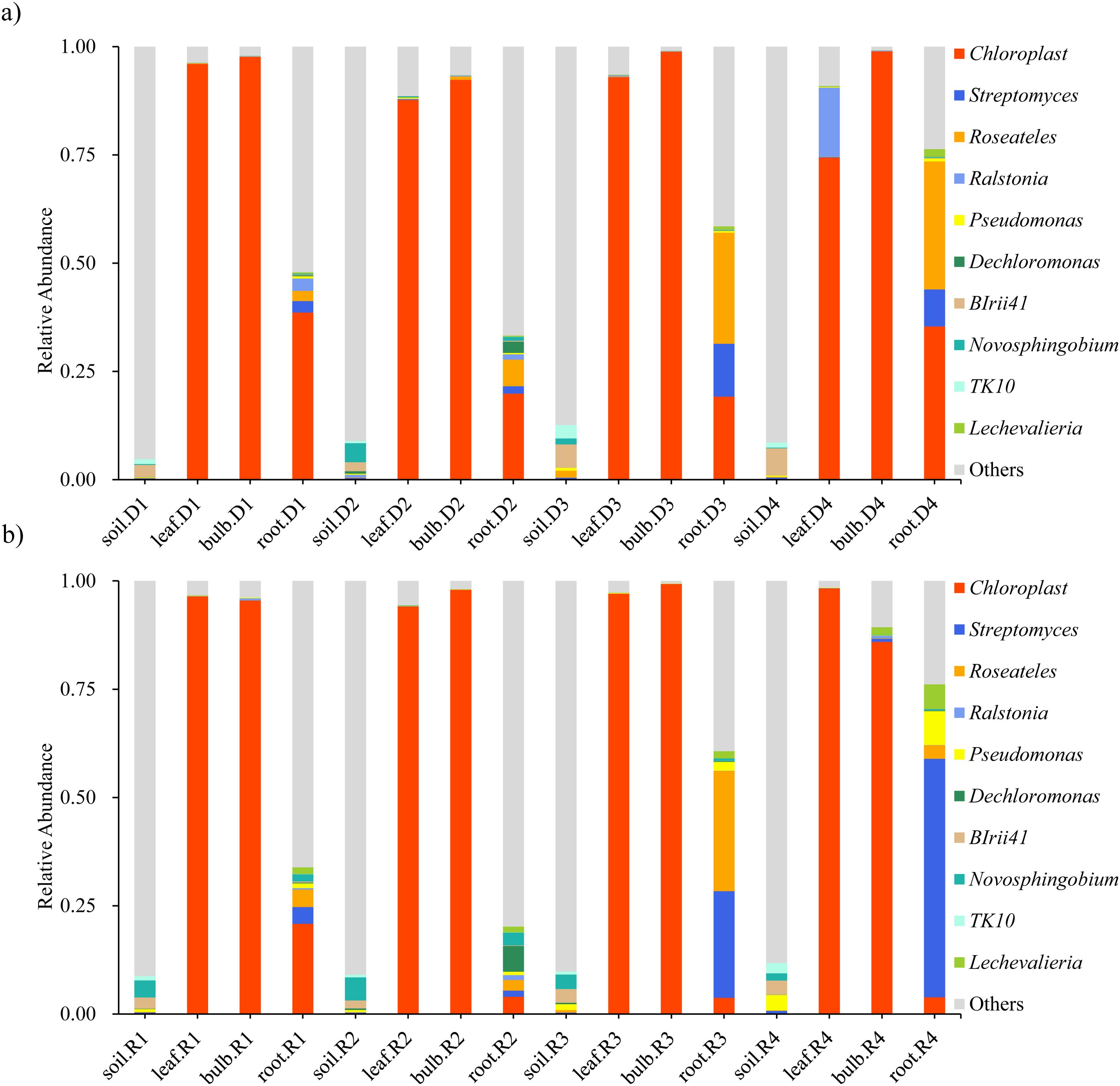
Figure 2. Species abundance of endophytic bacteria in A. senescens after drought stress (a) and re-watering (b) at the genus level.
3.2 Bacterial community structure and composition of A. senescens
Heatmap analysis highlighted distinct microbial niches, with Gemmatimonas and Haliangium uniquely enriched in the rhizosphere, potentially involved in soil nutrient cycling (Figure 3). Bulbs and leaves shared 68% of ASVs, including Ensifer, also known as Sinorhizobium, suggesting potential nitrogen-fixing roles in aerial tissues. Drought stress amplified niche-specific enrichment: Ensifer (leaves), Uliginosibacterium (bulbs), and Bacteroides (rhizosphere) were significantly enriched under 5% PEG (Figure 3). Post-rehydration, these taxa declined, but Anaeromyxobacter and Pseudomonas persisted in roots, indicating their potential involvement in stress resilience (Figure 3).
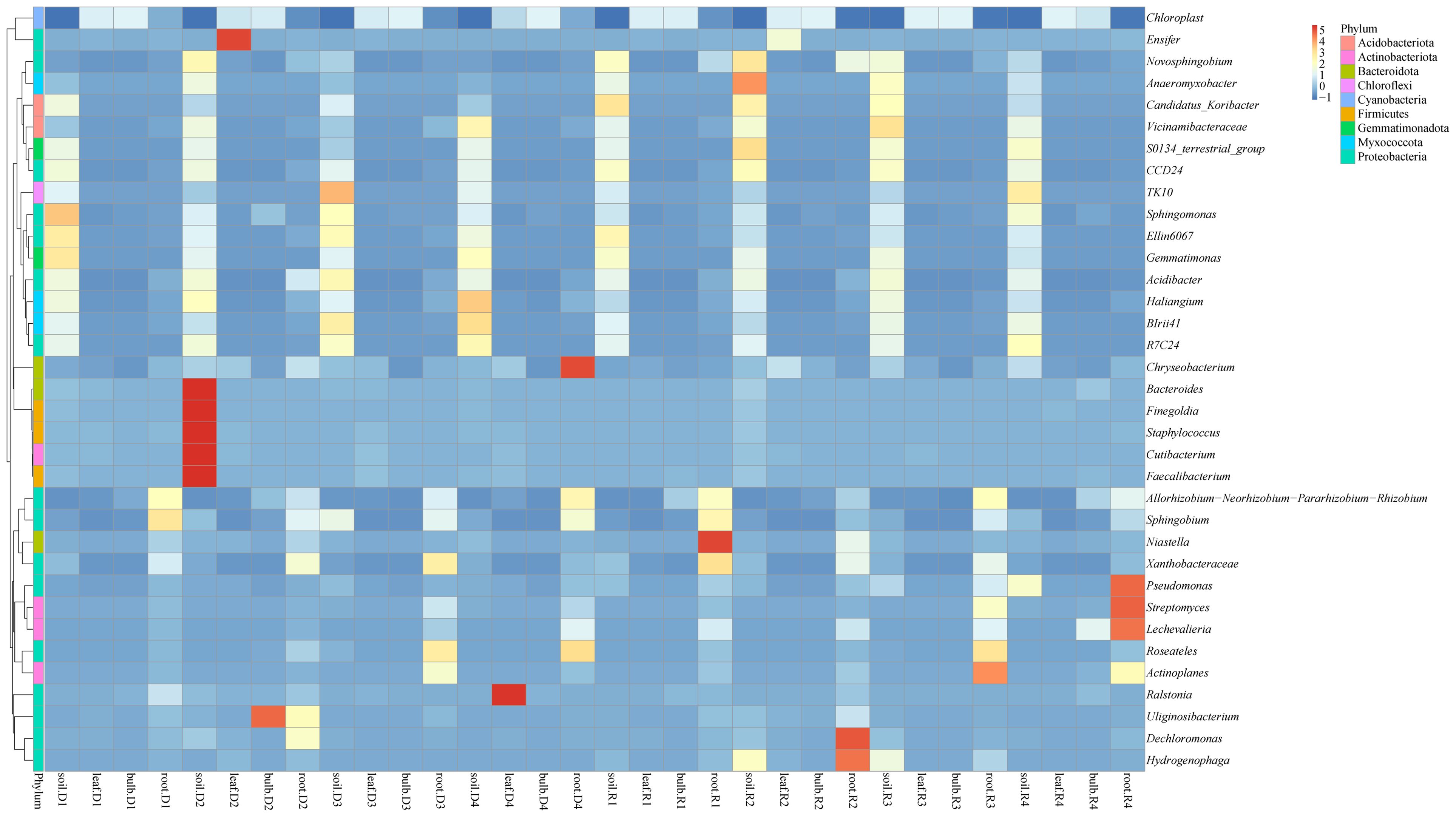
Figure 3. Cluster heat map of species abundance at the genus level of A. senescens after drought stress and re-watering.
The composition of the endophytic bacterial communities within different parts of A. senescens exhibited a higher degree of similarity (Figure 4). The leaves of A. senescens contained 68 ASVs, and drought stress induced the development of distinct ASVs, particularly in the 15% and 25% drought treatment groups, where rehydration correspondingly reduced the ASVs count (Figure 4a). The bulbs of A. senescens harbored 37 shared ASVs, with drought stress having a minimal impact on this community (Figure 4b). Application of drought stress at 5% and 15% resulted in increased bulb-associated ASVs, which conversely decreased after rewatering. At 25% drought stress, bulb ASVs decreased under drought conditions, but increased after rewatering. In the roots, 223 ASVs were identified as being shared (Figure 4c). Drought stress exceeding 15% led to a reduction in root-associated ASVs, which was temporarily alleviated by re-watering. The rhizosphere soil of A. senescens harbored 770 ASVs (Figure 4d). Drought stress significantly depleted the ASVs count in the rhizosphere soil, a condition that was not rectified following re-watering. Notably, the highest number of unique ASVs was identified in rhizosphere soil, followed by root, leaf, and bulb tissues.
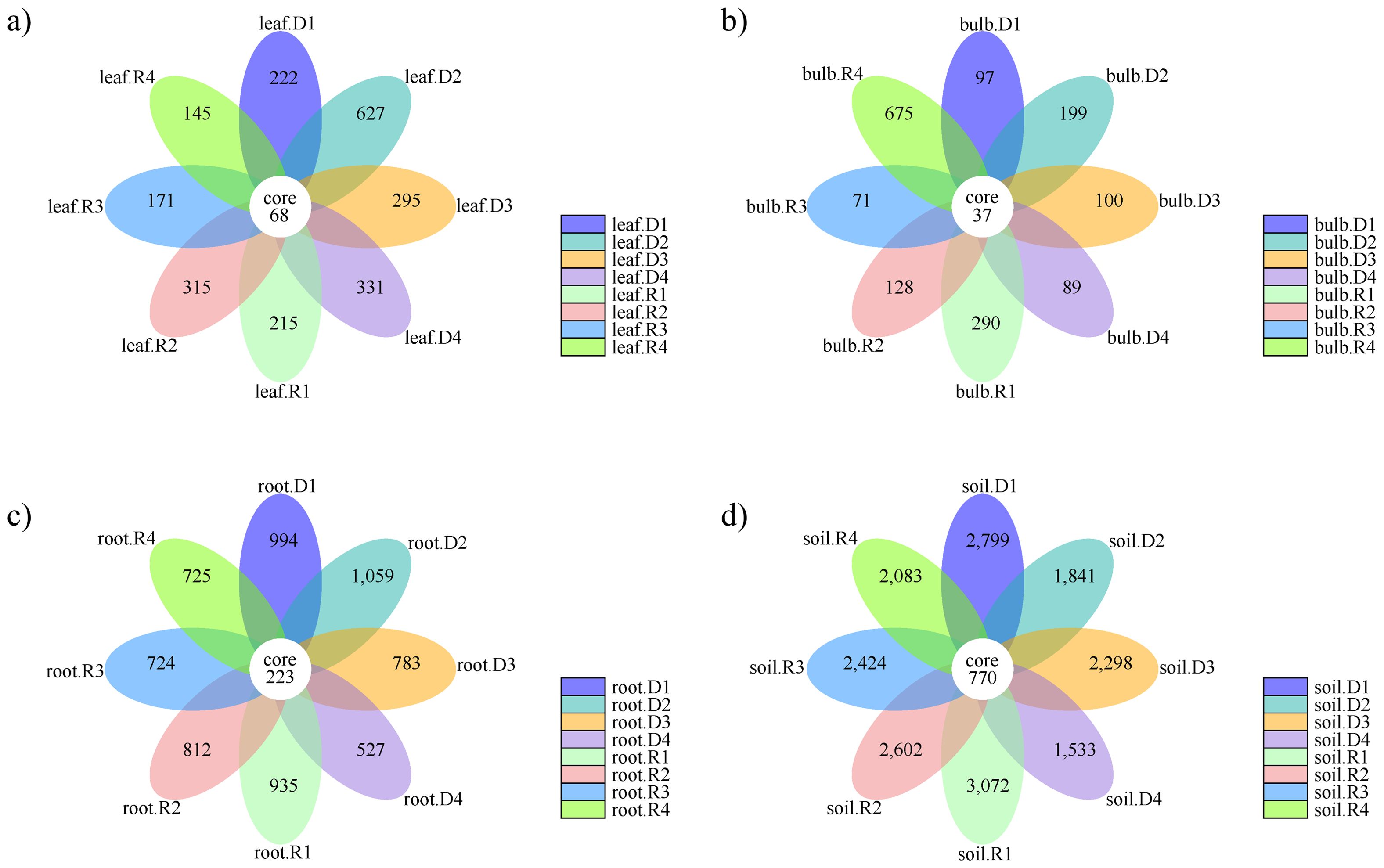
Figure 4. Number of ASVs in the bacterial community of A. senescens after drought stress and re-watering. (a) Flower plot of leaf of A. senescens, (b) Flower plot of bulb of A. senescens, (c) Flower plot of root of A. senescens, (d) Flower plot of rhizosphere soil of A. senescens.
To investigate the phylogenetic relationships among genus-level species, we retrieved representative sequences from the top 100 genera using multiple sequence alignment (Figure 5). The majority of the bacteria within A. senescens were classified as Pseudomonadota, with a considerable number of strains also belonging to Actinobacteria, Bacteroidetes, and Bacillota.
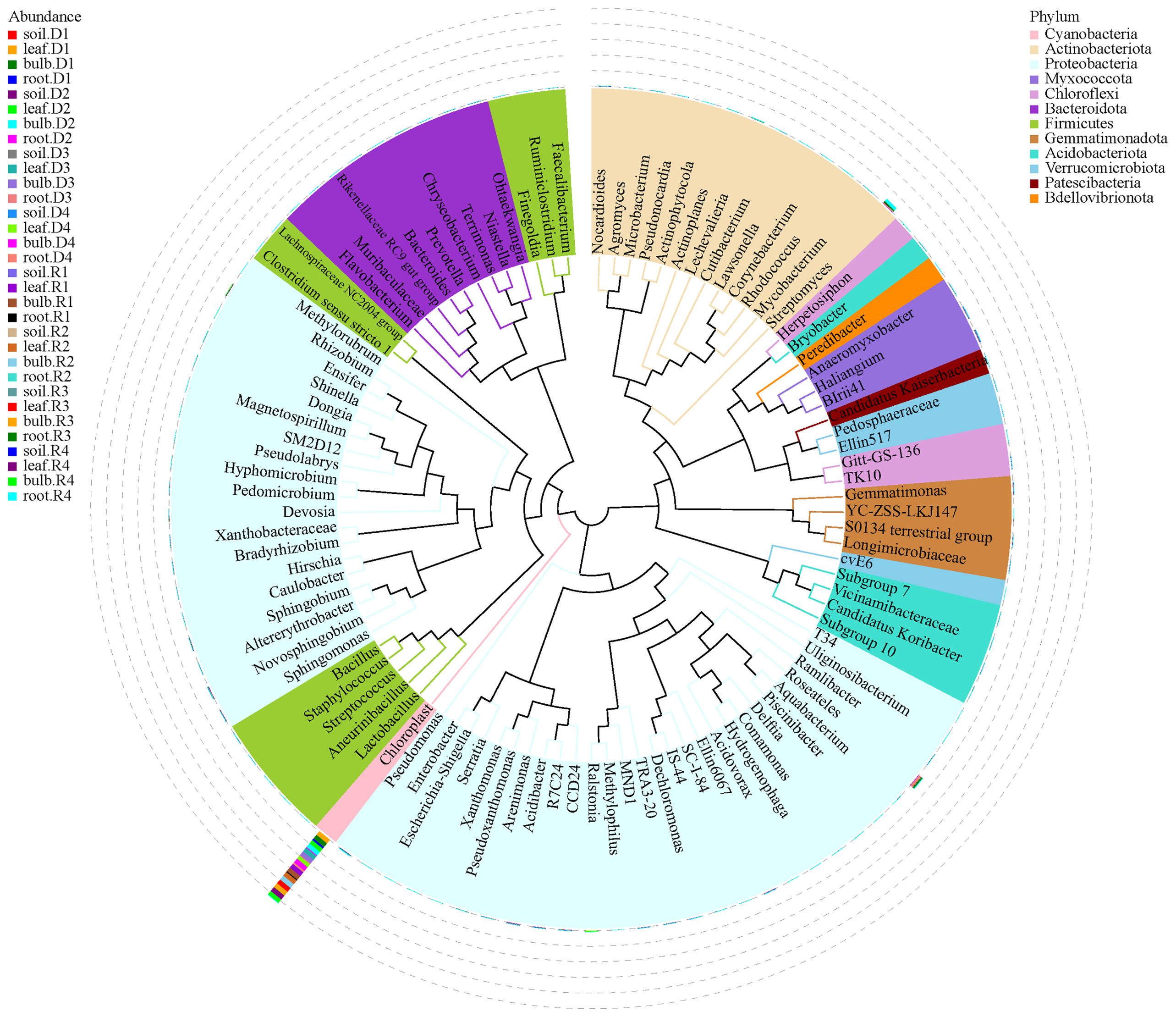
Figure 5. Phylogenetic tree of endophytic bacteria in A. senescens under drought stress and re-watering. The phylogenetic tree was constructed using the representative sequence of the genus-level species; the color of the branch and the fan represents its corresponding door, and the stacking histogram outside the fan ring represents the abundance distribution information of the genus in different samples. (The left legend is the sample information, and the right legend is the classification information at the phylum level corresponding to the genus level species).
3.3 The α diversity of bacteria in A. senescens
Alpha diversity indices exhibited tissue- and treatment-specific trends (Table 2). Rhizosphere soil displayed the highest species richness (Chao1: 850 ± 32), which declined by 42% under 15% PEG (493 ± 28; P < 0.01). Conversely, bulb diversity transiently increased at 5% PEG (Chao1: 162 ± 12 vs. CK: 120 ± 15; P < 0.05) but dropped at 25% PEG (89 ± 10; P < 0.01). Leaves showed elevated Shannon diversity under drought (CK: 2.1 ± 0.2 vs. D3: 3.2 ± 0.3; P < 0.01), suggesting stress-induced microbial niche expansion. These trends indicate that microbial adaptation under drought varies across plant tissues, with rhizosphere soil experiencing the most pronounced diversity loss.
3.4 The β diversity of endophytic bacteria in A. senescens
PCoA based on unweighted UniFrac distances revealed significant microbial differentiation across tissues (PERMANOVA, R² = 0.67, P = 0.001), with the first two axes explaining 83.9% of variance (Figure 6a). Drought stress amplified divergence, particularly in roots (Figure 6b). NMDS analysis (Stress value = 0.06) confirmed tighter clustering of bulb and leaf microbiomes, contrasting with rhizosphere heterogeneity (Figure 6b). UPGMA clustering further highlighted tissue-specific patterns, with roots and rhizosphere forming distinct clades (Figure 7). These findings suggest that drought disrupts microbial stability in belowground compartments while aerial tissues maintain greater compositional consistency.
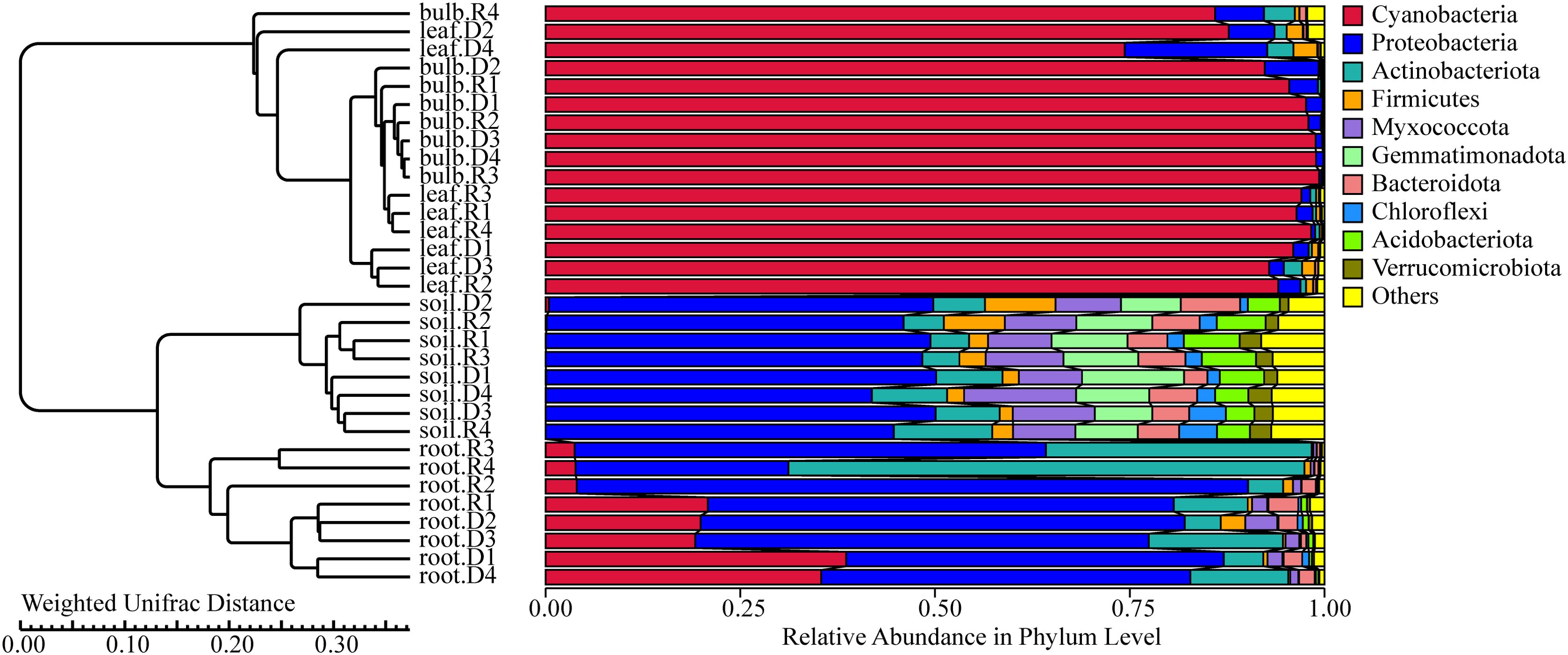
Figure 6. UPGMA dendrogram of A. senescens bacteria after drought stress and re-watering based on Weighted Unifrac distance.
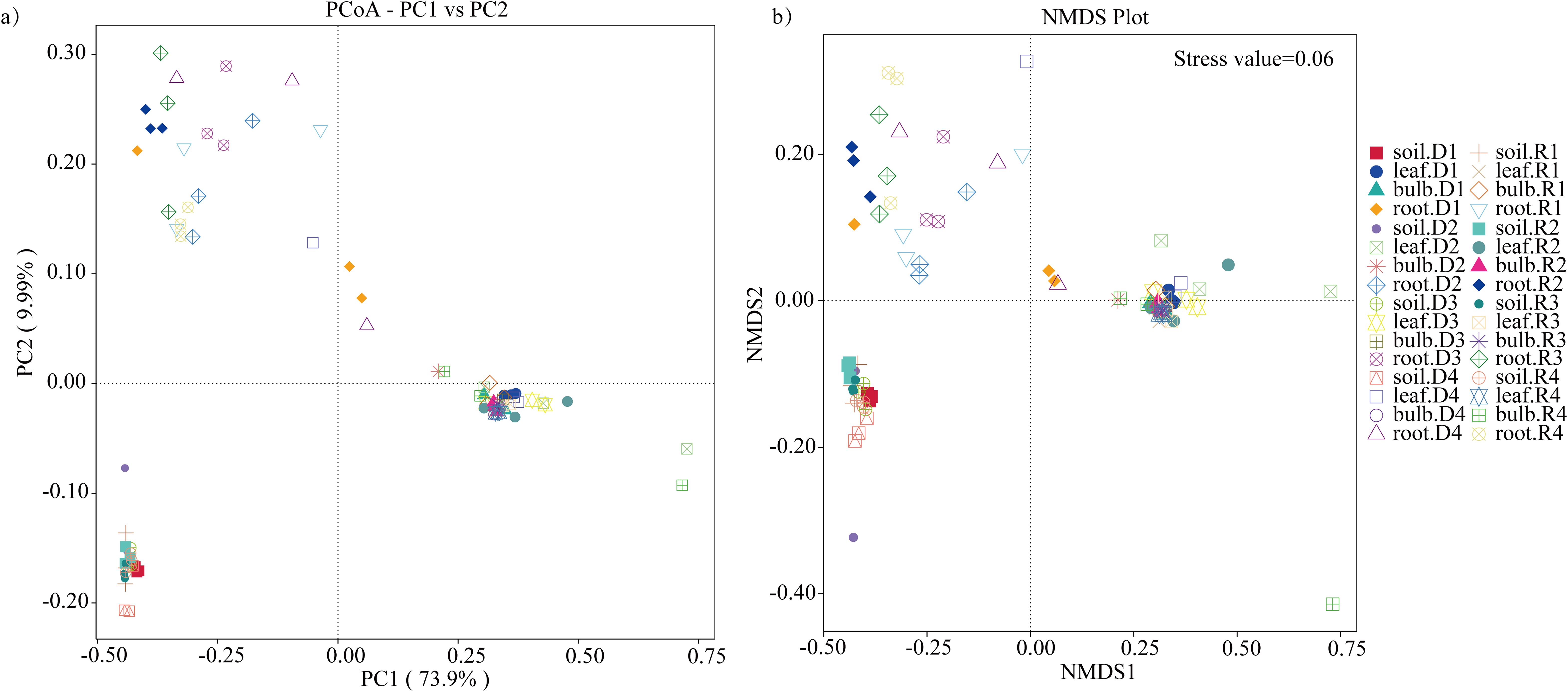
Figure 7. β-diversity of bacteria in A. senescens after drought stress and re-watering. (a) PCoA analysis of bacterial community in A. senescens, (b) NMDS analysis of bacterial community in A. senescens.
3.5 Statistical test analysis of endophytic bacteria in A. senescens
LEfSe identified 32 drought-responsive taxa (LDA > 4.0). In rhizosphere soil, Myxococcota (class: Deltaproteobacteria) and Bacillaceae were enriched under 15% PEG (Figure 8a). Roots exhibited Sandaracinaceae dominance at 5% PEG, while leaves under 25% PEG showed Bacilli and Ralstonia enrichment (Figures 8b, c). Post-rehydration, Actinoplanes and Hydrogenophaga became signature taxa in roots (Figure 8b). The persistence of Actinoplanes suggests its role in post-drought recovery, potentially through secondary metabolite production.
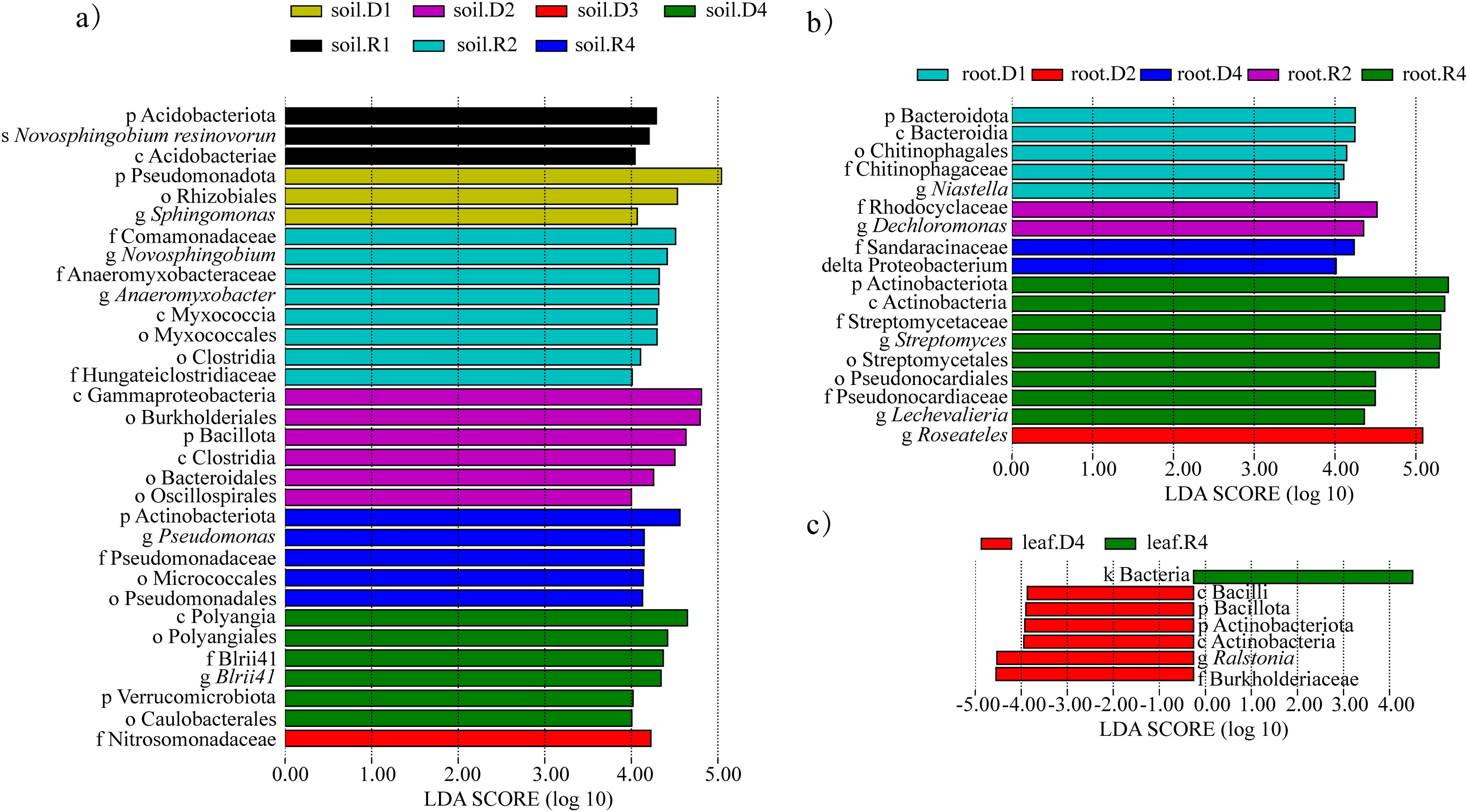
Figure 8. Significantly different bacteria in A. senescens after drought stress and re-watering. (a) LEfSe analysis of bacterial community in rhizosphere soil, (b) LEfSe analysis of bacterial community in root, (c) LEfSe analysis of bacterial community in leaf.
3.6 Bacterial network analysis of A. senescens
Network analysis revealed tissue-specific interaction patterns. In leaves, Niastella correlated positively with Hydrogenophaga (R = 1.0, P < 0.01), while Bacillus negatively linked to Chloroplast (R = -0.94, P < 0.05) (Figure 9a). In bulbs (Figure 9b), Cutibacterium and Hirschia showed a positive correlation (R = 1.0, P < 0.01), and Rhizobium and Chloroplast showed the maximum negative correlation (R = -0.87, P < 0.05). In roots, Prevotella and Methylorubrum showed the largest positive correlation (R = 1.0, P < 0.01), and Niastella and Roseateles showed the largest negative correlation (R = -0.87, P < 0.05) (Figure 10a). Rhizosphere soil displayed strong antagonism between Finegoldia and BIrii41 (R = -0.96, P < 0.01) (Figure 10b).
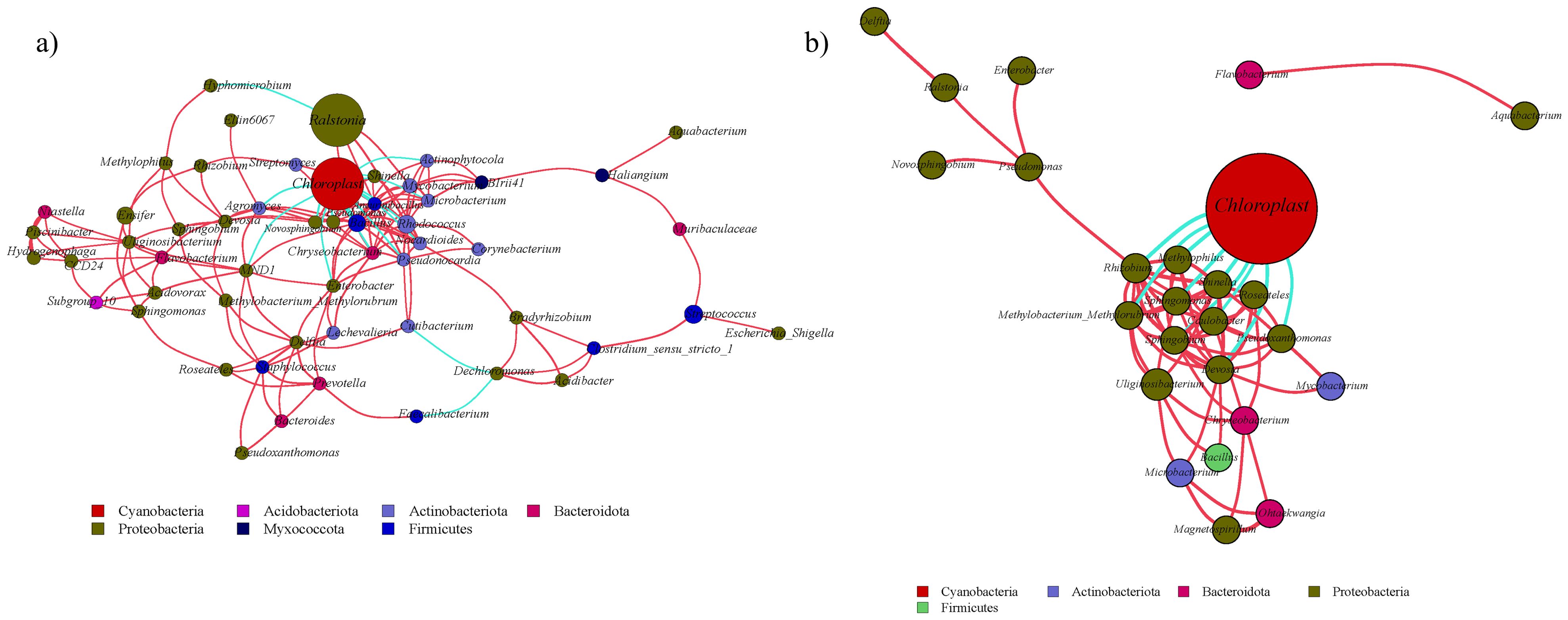
Figure 9. Analysis of endophytic bacterial network in the aboveground part of A. senescens after drought stress and re-watering. (a) Correlation network analysis of bacterial community in leaf of A. senescens, (b) Correlation network analysis of bacterial community in bulb of A. senescens.
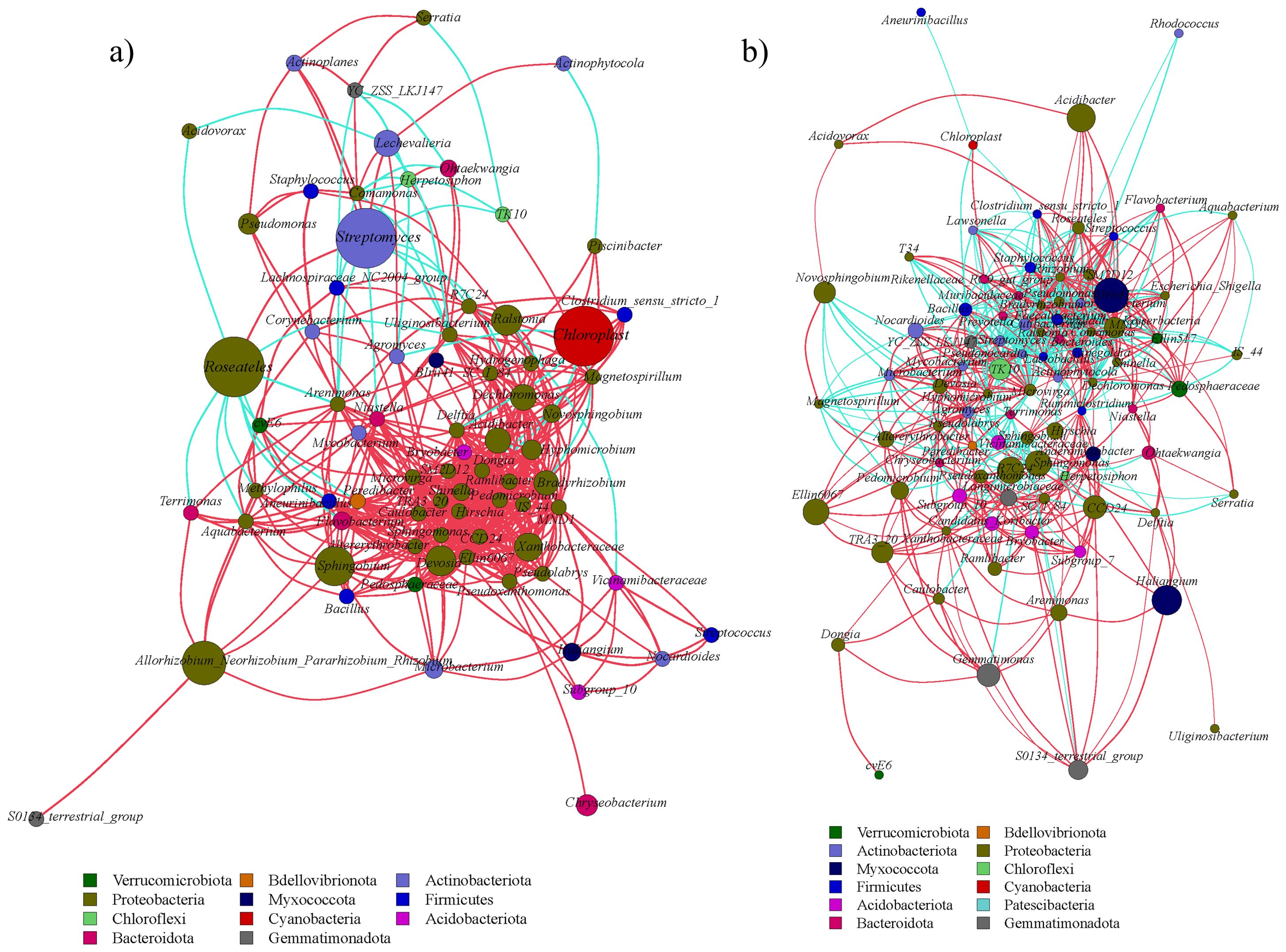
Figure 10. Analysis of bacterial network in the underground part of A. senescens after drought stress and re-watering. (a) Correlation network analysis of bacterial community in root of A. senescens, (b) Correlation network analysis of bacterial community in rhizosphere soil of A. senescens.
3.7 Function prediction of endophytic bacteria in A. senescens
PICRUSt functional predictions indicated significant metabolic shifts under drought. Pathways associated with transporters (5.71% of predicted functions), DNA repair (2.32%), and photosynthetic proteins (1.63%) were enriched, suggesting enhanced microbial adaptation mechanisms (Figure 11). Notably, Streptomyces-associated phenylalanine biosynthesis pathways were significantly upregulated under drought conditions, aligning with its role in stress adaptation.
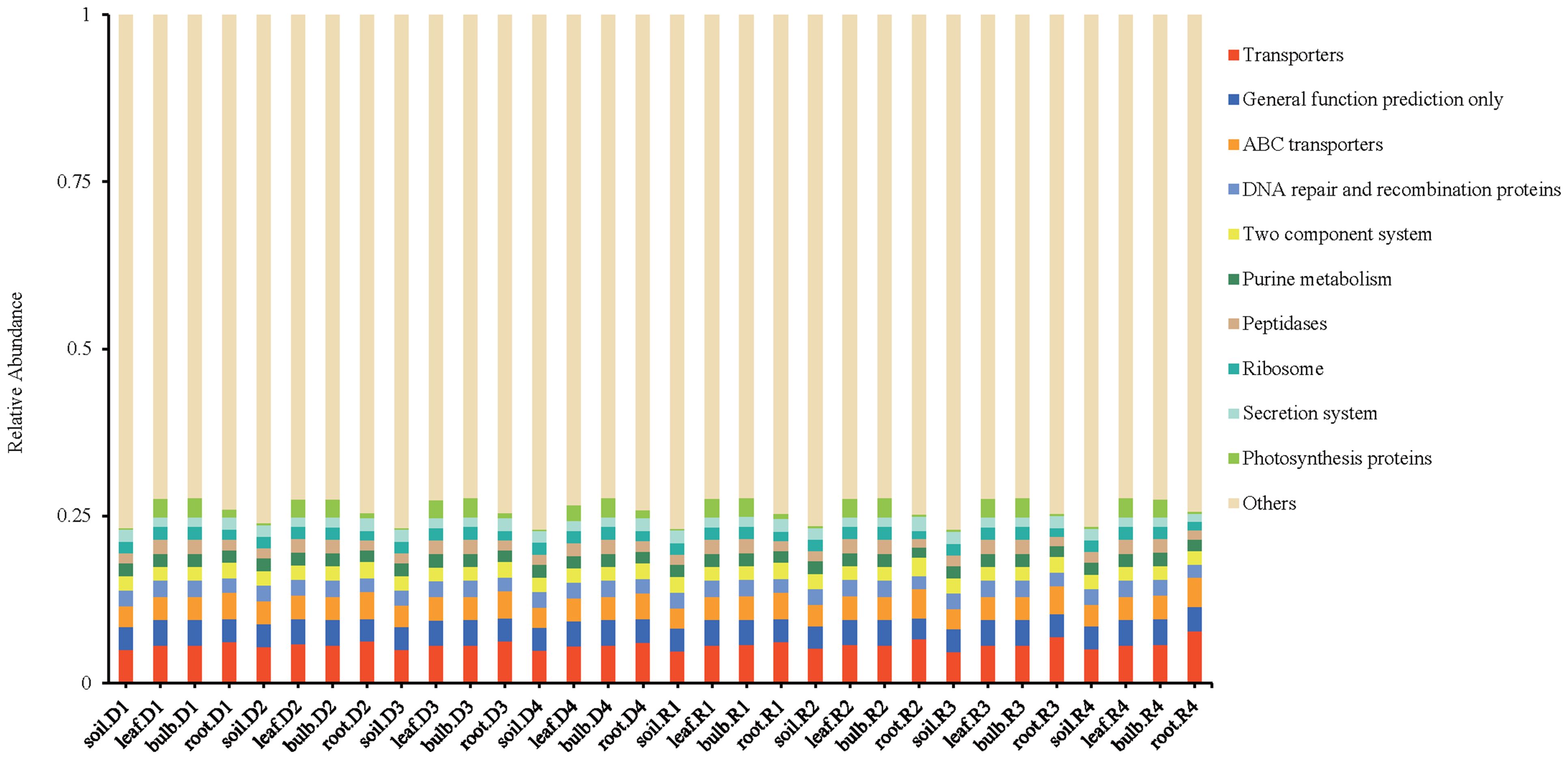
Figure 11. PICRUSt function prediction of bacteria in A. senescens after drought stress and re-watering.
4 Discussion
Our study provides a comprehensive analysis of how drought stress and subsequent rehydration reshape the rhizosphere and endophytic bacterial communities in A. senescens, a species renowned for its drought resilience. By employing high-throughput sequencing, we revealed tissue-specific microbial dynamics: drought reduced bacterial diversity in rhizosphere soil and roots but increased abundance in bulbs and leaves. These findings align with recent reports on Allium sativum (Munim et al., 2023), suggesting a conserved strategy among Allium species to reallocate microbial resources under stress. Notably, 15% PEG-6000 emerged as a critical threshold, inducing the most pronounced shifts in community structure (Figure 4). This parallels observations in crops like sugarcane (Liu et al., 2021), where moderate drought intensity triggers microbial functional remodeling. Compared with the non-drought stress group, under 15% -PEG stress treatment, the abundance of Streptomyces, Roseateles, Pseudomonas and TK10 increased at the genus level, which was an important reason for the drought resistance of A. senescens. Streptomyces and Pseudomonas are generally accepted as drought-tolerant bacterial genera that form close associations with plants to provide host resilience to drought stress (Liu et al., 2024; Khan et al., 2025). It was found that Roseateles had greater resilience under osmotic stress (Lengrand et al., 2024). In addition, TK10 also can resist abiotic stress (Wang et al., 2024). At the same time, another important reason for the drought resistance of A. senescens is the change of rhizosphere and endophytic bacterial community diversity. Alpha analysis (Table 2) showed that under 15% -PEG stress treatment, the Shannon index of rhizosphere soil, root and leaf bacterial communities of A. senescens became larger, and the stability of the community was enhanced, thus improving the ability of A. senescens to resist drought stress (Eisenhauer et al., 2012).
However, unlike Allium tuberosum (Huang, 2019), A. senescens bulbs and leaves were overwhelmingly dominated by Cyanobacteriota (> 90%), potentially leveraging their nitrogen-fixing capacity (Papik et al., 2020) to offset drought-induced nutrient limitations. Conversely, Pseudomonadota dominated the rhizosphere (50%), likely facilitating nutrient acquisition through metabolic versatility, as evidenced by enriched pathways such as ABC transporters and two-component systems (Figure 11). These results highlight both conserved and species-specific adaptations within the Allium genus, underscoring the need for tailored studies on plant-microbe interactions in undercharacterized species. The latest research shows that AsTCP17 can upregulate the AtSVP expression, a key transcription factor gene in the ABA pathway, to enhance the drought-tolerance of the A. senescens (Fu et al., 2023). Further studies have found that CPMMV virus small interfering RNA (vsiRNA) enhances drought tolerance by targeting the host gene PvTCP2, reducing its transcriptional repressor, promoting autophagy and stomatal opening (Wu et al., 2024). The mechanism of TCP transcription factors family interacting with microorganisms to improve plant drought tolerance needs further study.
Central to our findings is the drought-induced enrichment of Streptomyces, a genus whose relative abundance surged 3.2-fold in rhizosphere soil and 2.5-fold in leaves under 15% PEG treatment (Figures 8a, c). This aligns with its documented role in enhancing drought tolerance in crops like sugarcane (Pang et al., 2024) and rice (Liu et al., 2024). Functional predictions (Figure 11) suggest Streptomyces may bolster host resilience through osmoprotectant synthesis (e.g., proline via proB), phytohormone modulation (e.g., IAA-driven root elongation), and pathogen suppression via antibiotic production. Equally notable was the incomplete recovery of certain taxa post-rehydration, such as rhizosphere BIrii41 and leaf Roseateles (Figure 4). This hysteresis effect, previously observed in maize (Santos-Medellín et al., 2021), implies that drought may impose lasting impacts on microbial network stability, potentially affecting plant recovery trajectories. Such insights challenge the assumption of full microbial resilience after stress alleviation and emphasize the need to integrate temporal dynamics into drought adaptation models.
While our study advances understanding of A. senescens-microbe interactions, several limitations merit consideration. First, focusing solely on bacterial communities overlooks the potential contributions of fungi, particularly arbuscular mycorrhizal fungi known to enhance drought tolerance (Dini-Andreote, 2020). Future work should adopt a multi-kingdom approach, combining ITS and 16S sequencing. Second, although PICRUSt predictions offer functional insights, empirical validation through strain isolation and in planta assays is critical. For instance, targeted cultivation of Streptomyces sp. AS15 could clarify its role in phenylalanine biosynthesis—a pathway linked to drought resistance (Pang et al., 2024). Finally, controlled greenhouse conditions may oversimplify field realities; validating these findings in natural arid ecosystems, where soil heterogeneity and microbial competition prevail, remains essential.
Translating these discoveries into practice holds promise for sustainable agriculture. The drought-responsive taxa identified here, particularly Streptomyces and Roseateles, represent prime candidates for bioinoculant development. Field trials could assess their efficacy in enhancing yield stability in Allium crops under water-limited conditions. Moreover, the partial post-drought recovery of rhizosphere communities (Figure 4d) suggests that microbial management—such as targeted amendments during early recovery phases—could accelerate ecosystem rehabilitation. Integrating these microbial strategies with breeding programs, perhaps via CRISPR editing to optimize plant-microbe synergy, may further bolster drought resilience.
In conclusion, our work elucidates the dynamic interplay between A. senescens and its microbiome under drought stress, revealing both universal and novel adaptation mechanisms. By bridging ecological observations with functional predictions, we provide a framework for harnessing microbial resources to mitigate climate-driven agricultural challenges. Future studies integrating multi-omics approaches and field validation will be pivotal in unlocking the full potential of plant-microbe partnerships in arid agroecosystems.
5 Conclusion
In this study, we investigated the effects of drought stress and re-watering on the diversity and composition of bacterial communities in the leaves, bulbs, roots, and rhizosphere soil of A. senescens. The dominant bacteria in each niche were different, and the diversity of rhizosphere bacteria was much higher than that of the endophytic bacteria. The composition and diversity of the bacterial rhizosphere and endophytic bacteria were affected by drought and re-watering, and 15% PEG-6000 treatment had the greatest effect on the diversity of the bacterial rhizosphere and endophytic bacteria. Roseateles, Streptomyces, and other bacteria are important regulators of drought resistance in A. senescens. This study enhances our understanding of the rhizosphere and endophytic bacterial communities in A. senescens.
Data availability statement
The datasets generated for this study can be found in the NCBI Sequence Reads Archive (SRA) with the accession numbers SAMN47255586-SAMN47255681 for 16S rRNA, under the BioProject, PRJNA1230695.
Author contributions
XSo: Data curation, Formal analysis, Investigation, Methodology, Resources, Software, Writing – original draft, Writing – review & editing. HL: Conceptualization, Data curation, Formal analysis, Writing – review & editing. CF: Supervision, Validation, Writing – review & editing. JhL: Investigation, Writing – review & editing. JX: Writing – review & editing, Data curation. XSu: Writing – review & editing, Validation. JlL: Validation, Writing – review & editing, Supervision. LQ: Writing – review & editing, Funding acquisition, Project administration.
Funding
The author(s) declare that financial support was received for the research and/or publication of this article. This study was supported by the National Natural Science Foundation of China (grant No. 32271770) and National Natural Science Foundation of Heilongjiang Province (grant No. YQ2023C013).
Conflict of interest
The authors declare that the research was conducted in the absence of any commercial or financial relationships that could be construed as a potential conflict of interest.
Generative AI statement
The author(s) declare that no Generative AI was used in the creation of this manuscript.
Publisher’s note
All claims expressed in this article are solely those of the authors and do not necessarily represent those of their affiliated organizations, or those of the publisher, the editors and the reviewers. Any product that may be evaluated in this article, or claim that may be made by its manufacturer, is not guaranteed or endorsed by the publisher.
References
Cao, Y., Du, P., Yin, B., Zhou, S., Li, Z., Zhang, X., et al. (2023). Melatonin and dopamine enhance waterlogging tolerance by modulating ROS scavenging, nitrogen uptake, and the rhizosphere microbial community in Malus hupehensis. Plant Soil 483, 475–493. doi: 10.1007/s11104-022-05759-w
De Vries, F., Griffiths, R., Knight, C., Nicolitch, O., Williams, A. (2020). Harnessing rhizosphere microbiomes for drought-resilient crop production. Sci. Rep. 368, 270–274. doi: 10.1126/science.aaz5192
Dini-Andreote, F. (2020). Endophytes: the second layer of plant defense. Trends Plant Sci. 25, 319–322. doi: 10.1016/j.tplants.2020.01.007
Duke, N. C., Kovacs, J. M., Griffiths, A. D., Preece, L., Hill, D. J. E., Van Oosterzee, P., et al. (2017). Large-scale dieback of mangroves in Australia’s Gulf of Carpentaria: a severe ecosystem response, coincidental with an unusually extreme weather event. Mar. Freshw. Res. 68, 1816–1829. doi: 10.1071/mf16322
Egert, M., Tevini, M. (2002). Influence of drought on some physiological parameters symptomatic for oxidative stress in leaves of chives (Allium schoenoprasum). Environ. Exp. Bot. 48, 43–49. doi: 10.1016/S0098-8472(02)00008-4
Eisenhauer, N., Scheu, S., Jousset, A. (2012). Bacterial diversity stabilizes community productivity. PLoS One 7, e34517. doi: 10.1371/journal.pone.0034517
Fu, X. H., Zhao, J., Cao, D. D., He, C. X., Wang, Z. Y., Jiang, Y. B., et al. (2023). Characteristics and expression of the TCP transcription factors family in Allium senescens reveal its potential roles in drought stress responses. Biocell 47, 905–917. doi: 10.32604/biocell.2023.026930
Glassner, H., Zchori-Fein, E., Yaron, S., Sessitsch, A., Sauer, U., Compant, S. (2017). Bacterial niches inside seeds of Cucumis melo L. Plant Soil. 422, 101–113. doi: 10.1007/s11104-017-3175-3
Hallmann, J., Quadt-Hallmann, A., Mahaffee, W., Kloepper, J. (1997). Bacterial endophytes in agricultural crops. Can. J. Microbiol. 43, 895–914. doi: 10.1139/m97-131
Hardoim, P. R., Van Overbeek, L. S., Berg, G., Pirttilä, A. M., Compant, S., Campisano, A., et al. (2015). The hidden world within plants: Ecological and evolutionary considerations for defining functioning of microbial endophytes. Microbiol. Mol. Biol. Rev. 79, 293–320. doi: 10.1128/mmbr.00050-14
Huang, Y. (2019). Illumina-based Analysis of Endophytic Bacterial Diversity of four Allium species. Sci. Rep. 9, 15271. doi: 10.1038/s41598-019-51707-7
Javed, A., Ahmad, N., Ahmed, J., Hameed, A., Ashraf, A., Zafar, S., et al. (2022). Grain yield, chlorophyll and protein contents of elite wheat genotypes under drought stress. J. King Saud Univ. - Sci. 34, 102279. doi: 10.1016/j.jksus.2022.102279
Kandel, S. L., Herschberger, N., Kim, S. H., Doty, S. L. (2015). Diazotrophic endophytes of poplar and willow for growth promotion of rice plants in nitrogen-limited conditions. Crop Sci. 55, 1765–1772. doi: 10.2135/cropsci2014.08.0570
Kandel, S. L., Joubert, P. M., Doty, S. L. (2017). Bacterial endophyte colonization and distribution within plants. Microorganisms 5, 77. doi: 10.3390/microorganisms5040077
Khan, V., Princi, Mubashshir, M., Umar, S., Iqbal, N. (2025). Methyl jasmonate and Pseudomonas fluorescens synergistically boost antioxidative defense, secondary metabolites, and osmolyte production to enhance drought resilience in mustard. J. Plant Growth Regul. 44, 198–216. doi: 10.1007/s00344-024-11310-1
Kumar, V. V. S., Verma, R. K., Yadav, S. K., Yadav, P., Watts, A., Rao, M. V., et al. (2020). CRISPR-Cas9 mediated genome editing of drought and salt tolerance (OsDST) gene in indica mega rice cultivar MTU1010. Physiol. Mol. Biol. Plants 26, 1099–1110. doi: 10.1007/s12298-020-00819-w
Kvgk, V. P., Ramarao, G. V. (2019). Various plant’s responses and strategies to cope with the water deficit: A review. J. Pharmacognosy Phytochem. 8, 159–168.
Lengrand, S., Dubois, B., Pesenti, L., Debode, F., Legrève, A. (2024). Humic substances increase tomato tolerance to osmotic stress while modulating vertically transmitted endophytic bacterial communities. Front. Plant Sci. 15. doi: 10.3389/fpls.2024.1488671
Li, S., Wan, L., Nie, Z., Li, X. (2020). Fractal and Topological Analyses and Antioxidant Defense Systems of Alfalfa (Medicago sativa L.) Root System under Drought and Rehydration Regimes. Agronomy 10, 805. doi: 10.3390/agronomy10060805
Liu, H., Li, J., Singh, B. K. (2024). Harnessing co-evolutionary interactions between plants and Streptomyces to combat drought stress. Nat. Plants 10, 1159–1171. doi: 10.1038/s41477-024-01749-1
Liu, Q., Zhao, X., Liu, Y., Xie, S., Xing, Y., Dao, J., et al. (2021). Response of sugarcane rhizosphere bacterial community to drought stress. Front. Microbiol. 12. doi: 10.3389/fmicb.2021.716196
Mallor, C., Arnedo-Andrés, M. S., Garcés-Claver, A. (2014). Assessing the genetic diversity of Spanish Allium cepa landraces for onion breeding using microsatellite markers. Scientia Hortic. 170, 24–31. doi: 10.1016/j.scienta.2014.02.040
Marasco, R., Fusi, M., Rolli, E., Ettoumi, B., Tambone, F., Borin, S., et al. (2021). Aridity modulates belowground bacterial community dynamics in olive tree. Environ. Microbiol. 23, 6275–6291. doi: 10.1111/1462-2920.15764
Mega, R., Abe, F., Kim, J.-S., Tsuboi, Y., Tanaka, K., Kobayashi, H., et al. (2019). Tuning water-use efficiency and drought tolerance in wheat using abscisic acid receptors. Nat. Plants 5, 153–159. doi: 10.1038/s41477-023-01416-x
Mitter, B., Pfaffenbichler, N., Flavell, R., Compant, S., Antonielli, L., Petric, A., et al. (2017). A new approach to modify plant microbiomes and traits by introducing beneficial bacteria at flowering into progeny seeds. Front. Microbiol. 8. doi: 10.3389/fmicb.2017.00011
Munim, T. B., Jameel, I. L., Al-Shammari, R., Mahmudul, H., Roksana, A. K., Sunzid, A. M. P., et al. (2023). Identification and characterization of aldehyde dehydrogenase (ALDH) gene superfamily in garlic and expression profiling in response to drought, salinity, and ABA. Gene 15, 147215. doi: 10.1016/j.gene.2023.147215
Naylor, D., Coleman-Derr, D. (2018). Drought stress and root-associated bacterial communities. Front. Plant Sci. 8. doi: 10.3389/fpls.2017.02223
Naylor, D., Degraaf, S., Purdom, E., Coleman-Derr, D. (2017). Drought and host selection influence bacterial community dynamics in the grass root microbiome. ISME J. 11, 2691–2704. doi: 10.1038/ismej.2017.118
Onyemaobi, O., Sangma, H., Garg, G., Wallace, X., Kleven, S., Suwanchaikasem, P., et al. (2021). Reproductive stage drought tolerance in wheat: Importance of stomatal conductance and plant growth regulators. Genes 12, 1742. doi: 10.3390/genes12111742
Pang, F., Solanki, M. K., Xing, Y. X., Dong, D. F., Wang, Z. (2024). Streptomyces improves sugarcane drought tolerance by enhancing phenylalanine biosynthesis and optimizing the rhizosphere environment. Plant Physiol. Biochem. 217, 109236. doi: 10.1016/j.plaphy.2024.109236
Papik, J., Folkmanova, M., Polivkova-Majorova, M., Suman, J., Uhlik, O. (2020). The invisible life inside plants: Deciphering the riddles of endophytic bacterial diversity. Biotechnol. Adv. 44, 107614. doi: 10.1016/j.bioteChadv.2020.107614
Pour-Aboughadareh, A., Mohammadi, R., Etminan, A., Shooshtari, L., Maleki-Tabrizi, N., Poczai, P. (2020). Effects of drought stress on some agronomic and morpho-physiological traits in durum wheat genotypes. Sustainability 12, 5610. doi: 10.3390/su12145610
Santos-Medellín, C., Liechty, Z., Edwards, J., Nguyen, B., Huang, B., Weimer, B. C., et al. (2021). Prolonged drought imparts lasting compositional changes to the rice root microbiome. Nat. Plants 7, 1065–1077. doi: 10.1038/s41477-021-00967-1
Seaton, F. M., Reinsch, S., Goodall, T., White, N., Jones, D. L., Griffiths, R. I., et al. (2022). Long-term drought and warming alter soil bacterial and fungal communities in an upland heathland. Ecosystems 25, 1279–1294. doi: 10.1007/s10021-021-00715-8
Todorova, D., Aleksandrov, V., Anev, S., Sergiev, I. (2022). Photosynthesis alterations in wheat plants induced by herbicide, soil drought or flooding. Agronomy 12, 390. doi: 10.3390/agronomy12020390
Twaij, B. M., Ibraheem, L. J., Al-Shammari, R. H. H., Hasan, M., Khoko, R. A., Ahomed, M. S., et al. (2023). Identification and characterization of aldehyde dehydrogenase (ALDH) gene superfamily in garlic and expression profiling in response to drought, salinity, and ABA. Gene 860, 147215. doi: 10.1016/j.gene.2023.147215
Ullah, A., Nisar, M., Ali, H., Hazrat, A., Hayat, K., Keerio, A. A., et al. (2019). Drought tolerance improvement in plants: an endophytic bacterial approach. Appl. Microbiol. Biotechnol. 103, 7385–7397. doi: 10.1007/s00253-019-10045-4
Wan, C., Dang, P., Gao, L., Wang, J., Tao, J., Qin, X., et al. (2022). How does the environment affect wheat yield and protein content response to drought? A meta-analysis. Front. Plant Sci. 13. doi: 10.3389/fpls.2022.896985
Wang, M., Chen, X. X., Hamid, Y., Yang, X. E. (2024). Evaluating the response of the soil bacterial community and lettuce growth in a fluorine and cadmium co-contaminated yellow soil. Toxics 12, 459. doi: 10.3390/toxics12070459
Wu, X. Y., Chen, S. T., Zhang, Z. X., Zhou, W. X., Sun, T., Ning, K., et al. (2024). A viral small interfering RNA-host plant mRNA pathway modulates virus-induced drought tolerance by enhancing autophagy. Plant Cell 36, 3219–3236. doi: 10.1093/plcell/koae158
Zhang, H., Lu, S. S., Wu, J., Jiang, Y., Lu, Y. M., Zhao, H. E. (2014). Effect of substrate depth on 18 non-succulent herbaceous perennials for extensive green roofs in a region with a dry spring. Ecol. Eng. 71, 490–500. doi: 10.1016/j.ecoleng.2014.07.033
Keywords: Allium senescens L., drought stress, endophytic bacteria community, rhizosphere bacteria community, Streptomyces
Citation: Song X, Li H, Fu C, Li J, Xiang J, Sun X, Liu J and Qin L (2025) Diversity changes of rhizosphere and endophytic bacteria in Allium senescens L. under drought stress and rewatering. Front. Plant Sci. 16:1571736. doi: 10.3389/fpls.2025.1571736
Received: 06 February 2025; Accepted: 25 March 2025;
Published: 07 May 2025.
Edited by:
Wang Junfeng, Northeast Normal University, ChinaReviewed by:
Photini V. Mylona, Hellenic Agricultural Organisation (HAO), GreeceLi li Nan, Gansu Agricultural University, China
Copyright © 2025 Song, Li, Fu, Li, Xiang, Sun, Liu and Qin. This is an open-access article distributed under the terms of the Creative Commons Attribution License (CC BY). The use, distribution or reproduction in other forums is permitted, provided the original author(s) and the copyright owner(s) are credited and that the original publication in this journal is cited, in accordance with accepted academic practice. No use, distribution or reproduction is permitted which does not comply with these terms.
*Correspondence: Ligang Qin, cWlubGlnYW5nQG5lYXUuZWR1LmNu
†These authors have contributed equally to this work