- 1Department of Microbiology, Faculty of Pharmacy, University of Granada, Granada, Spain
- 2Biomedical Research Centre (CIBM), Institute of Biotechnology, University of Granada, Granada, Spain
A polyphasic taxonomic approach was conducted to characterize the bacterial strain B22T isolated from the rhizospheric soil of the halophyte Salicornia hispanica. This strain is aerobic, Gram-negative, rod-shaped, catalase and oxidase positive, motile, reduces nitrates and chemoheterotrophic. It is halotolerant, exhibiting optimal growth at 28°C and pH 7.0 in the presence of 0.5-2.5% (w/v) of NaCl. The B22T genome size is 5.7 Mbp, with a G+C content of 60.5 mol%. This strain has the capacity to promote tomato growth by producing siderophores, indole-3-acetic acid and enzymes such as phytase and acid phosphatase. Additionally, strain B22T produces a quorum quenching (QQ) enzyme capable of degrading synthetic N-acylhomoserine lactones (AHLs) as well as those produced by phytopathogens. The interference of plant pathogen communication reduced virulence in tomato fruits and plants. Phylogenetic analysis revealed that the closest relatives of strain B22T was Pseudomonas tehranensis SWRI 196T. The average nucleotide identity values between strain B22T and P. tehranensis SWRI 196T was 95.1% while digital DNA-DNA hybridization values was 64.5% The main cellular fatty acids of strain B22T were C16:0, summed feature 3 (C16:1 ω7c/C16:1 ω6c) and summed feature 8 (C18:1 ω7c/C18:1 ω6c). The major polar lipids identified were diphosphatidylglycerol and phosphatidylethanolamine, while the predominant respiratory quinone was ubiquinone (Q-9). Based on genomic, phylogenetic and chemotaxonomic data, strain B22T (=CECT 31209; =LMG33902) represents a novel species within the genus Pseudomonas. The name Pseudomonas halotolerans sp. nov. is proposed. Additionally, this study highlights the potential of P. halotolerans as a sustainable biocontrol agent due to its plant growth-promoting activity in tomato plants and its ability to reduce phytopathogen virulence factors, mitigating damage to fruits and plants.
1 Introduction
Global agriculture faces a complex array of challenges as it strives to feed a growing population, projected to reach nearly 10 billion by 2050 (FAO, 2018). Intensive farming practices, combined with the widespread use of chemical fertilizers and pesticides, have led to significant environmental degradation, including pollution, loss of beneficial soil microbes, and reduced biodiversity (Hartmann et al., 2015; Kopittke et al., 2019; Tripathi et al., 2020). One of the most serious threats to crop productivity and food security worldwide is the spread of pest and plant diseases. The misuse and overuse of agrochemicals have significantly contributed to the development of resistant phytopathogens, further complicating efforts to manage plant diseases (FAO, 2023). Addressing these challenges is a priority in European agricultural policies, which emphasize the need for sustainable alternatives to traditional, chemical-based practices. These approaches aim to protect ecosystems while supporting food security (Schneider et al., 2023; Finger and Möhring, 2024; FAO, 2023).
Beneficial plant associated microorganisms are well-documented as an eco-friendly strategy to reduce the use of agrochemical compounds due to their capability of promoting plant growth (plant growth-promoting bacteria, PGPB) and/or protecting against phytopathogens (biological control agents, BCAs) (Berg et al., 2017; Singh, 2018; Jiao et al., 2021).
Regarding to Pseudomonas genus, several plant-associated species have been recognized for their beneficial interaction with plants and their role in the biocontrol of phytopathogens (Weller, 2007; Navarro-Monsterrat and Taylor, 2023). Such is the case of P. chlororaphis (Arrebola et al., 2019; Raio and Puopolo, 2021), P. fluorescens (Al-Karablieh et al., 2022), P. protegens (Bakaeva et al., 2022; Ramette et al., 2011), P. putida (Weimer et al., 2020; Costa-Gutiérrez et al., 2022), and P. segetis (Rodríguez et al., 2020). These species exhibit a range of beneficial traits, including nutrient solubilization, phytohormone production, nitrogen fixation and induction of systemic resistance (ISR). Their biocontrol capabilities are attributed to diverse mechanisms, such as the production of bioactive metabolites like phenazines, pyoverdines, cyclic lipopeptides and volatile compounds with antimicrobial activities (Raio and Puopolo, 2021; Al-Karablieh et al., 2022; Balthazar et al., 2022; Ajijah et al., 2023; Navarro-Monsterrat and Taylor, 2023). Additionally, the remarkable adaptability of Pseudomonas strains to diverse environmental conditions enables effective root colonization and competitive exclusion of pathogens. Together, these traits contribute to improve plant health and resilience (Garrido-Sanz et al., 2023).
Among the mechanisms for biocontrol, the disruption of quorum sensing (QS) systems in plant pathogens has emerged as a promising, eco-friendly alternative for managing plant diseases, reducing reliance on agrochemicals (Defoirdt, 2018; Verma et al., 2021; Sharma et al., 2022; Zhu et al., 2023). QS is a cell-density-dependent communication system mediated by the diffusion of signaling molecules, such as N-acylhomoserine lactones (AHLs) in Gram-negative bacteria, and coordinates behaviors critical to pathogenicity (Baltenneck et al., 2021; Von Bodman et al., 2003). These QS-regulated traits include the production of hydrolytic enzymes, biofilm formation, toxin production, and host invasion, as observed in phytopathogens such as Dickeya solani (Crépin et al., 2012; Potrykus et al., 2018), Erwinia amylovora (Venturi et al., 2004; Piqué et al., 2015), Pantoea agglomerans (Cruz et al., 2024), Pectobacterium atrosepticum (Smadja et al., 2004), P. carotovorum (Moleleki et al., 2017; Pollumaa et al., 2012) and Pseudomonas syringae (Quinones et al., 2005; Cheng et al., 2016).
One extensively studied mechanisms to interfere QS is quorum quenching (QQ), which involves the enzymatic degradation or modification of AHLs via lactonases, acylases, or oxidoreductases (Grandclément et al., 2016). Studies have demonstrated that heterologous expression of QQ enzymes in bacterial phytopathogens or co-cultivation with AHL-degrading bacteria reduces virulence, often resulting in decreased infection severity (Zhou et al., 2022; Zhu et al., 2023). In the case of Pseudomonas species, the strains P. segetis P6, P. nitroreducens W-7 and P. multirresinivorans QL-9a have reported to attenuate bacterial phytopathogen virulence through QQ mechanisms (Rodríguez et al., 2020; Liu et al., 2023).
This study aimed to achieve several objectives. The primary aim was to taxonomically identify the strain B22, which was isolated from the rhizospheric soil of a halophyte plant in the El Saladar de El Margen, Granada, southern Spain. Furthermore, this study analyzed its PGP and QQ activities and investigated its potential application as biocontrol agent. These properties underscore its suitability for integration into sustainable agricultural practices.
2 Material and methods
2.1 Bacterial strains, media, compounds and culture conditions
Strain B22T was isolated from the rhizospheric soil of Salicornia hipanica in El Saladar del Margen, Cúllar, Granada, Spain (37° 38’ 50.6’’N, 2° 37’ 22.2’’ W). Pseudomonas tehranensis SWRI 196T (LMG 32044), P. alvandae SWRI 17T (LMG 32056) and P. canavaninivorans HB 002T (LMG 32336) were used for taxonomic identification. The AHL-producing plant pathogenic strains used in this work were Dickeya solani LMG 25993T, Pectobacterium atrosepticum CECT 314T, P. carotovorum subsp. carotovorum CECT 225T, Pseudomonas syringae pv. tomato DC3000 and Pantoea agglomerans CFBP 11141. All of them were routinely grown in Luria Bertani (LB) media at 28°C.
The AHL biosensor strains Chromobacterium subtsugae CV026 (Harrison and Soby, 2020) and C. violaceum VIR07 (Morohoshi et al., 2008) were grown in LB medium while Agrobacterium tumefaciens NTL4 (pZLR4) (Shaw et al., 1997) was cultured in LB medium supplemented with 2.5 mmol L-1 CaCl2 x 2H20 and 2.5 mmol L-1 MgSO4 x 7H20 (LB/MC) or Agrobacterium broth (AB) medium (Chilton et al., 1974). The antibiotics kanamycin (Km) and gentamicin (Gm) were used in final concentrations of 50 μg mL−1 for the growth of the biosensor strains. All strains were grown at 28°C and at 120 rpm in an orbital shaker.
2.2 Phylogenetic 16S rRNA gene analysis
Genomic DNA of strain B22T was extracted using the X-DNA Purification Kit (Xtrem Biotech S.L., Granada, Spain). The 16S rRNA gene was then amplified using universal bacterial primers 16F27 and 16R1488. The resulting PCR product was purified and cloned into the pGEM®-T vector (Promega).
Direct sequencing of the PCR-amplified DNA was performed, and the DNA sequence was compared with reference 16S rRNA gene sequences from GenBank and EMBL databases via the NCBI Genome Database using EzBioCloud server (Yoon et al., 2017).
Phylogenetic analysis was conducted using the Molecular Evolutionary Genetics Analysis (MEGA) software version X (Kumar et al., 2018), incorporating multiple sequence alignments with CLUSTAL OMEGA (Sievers et al., 2011). Evolutionary distances and clustering were determined using the neighbor-joining and maximum-likelihood methods. Cluster stability was assessed through bootstrap analysis with 1,000 replications.
2.3 Multilocus sequence analysis
To construct a more robust phylogenetic tree, a multigene approach was used. MLSA was conducted by concatenating the sequences of four housekeeping genes (16S rRNA, gyrB, rpoD, and rpoB). Housekeeping gene sequences from other Pseudomonas species were retrieved from the genomes deposited in NCBI GenBank database. Multiple sequence alignment of the concatenated gene sequences was performed using the MUSCLE algorithm embedded in MEGA X, followed by manual verification to identify and correct alignment errors.
A phylogenetic tree was generated using the neighbor-joining (NJ) algorithm, with 1000 bootstrap replicates to assess the robustness of the branching.
2.4 Genome sequencing and assembly
Genomic DNA of strain B22T, which was extracted as described above, was sequenced by the Illumina MiSeq methodology at the STAB VIDA facility (Caparica, Portugal) with 2 x 150-bp paired-end reads. The reads were trimmed using software tools implemented in the BBMap project (https://sourceforge.net/project/bbmap/) (Bushnell, 2016) to remove the adapters and low-quality bases and de novo assembled using SPADES v3.11.1 (Bankevich et al., 2012). CheckM v1.0.18 (Parks et al., 2015) and Quast v5.0.2 (Gurevich et al., 2013) were used for assembly quality checked. The genome of strain B22T was annotated using RASTtk v1.073 (Aziz et al., 2008; Overbeek et al., 2014; Brettin et al., 2015) and deposited in GenBank/EMBL/DDBJ under the accession number JBCNTJ010000000.
2.5 In silico ANI and DDH
Average nucleotide identity (ANI) was determined by using OrthoANI software (Lee et al., 2016). For digital DNA–DNA hybridization (dDDH), values were calculated using the BLAST+ algorithm via the DSMZ Genome-to-Genome Distance Calculator (GGDC 3.0) web service at http://ggdc.dsmz.de/ (Meier-Kolthoff et al., 2013, 2022). The results presented in this study are based on the recommended Formula 2 (identities/HSP length), which is independent of genome length, ensuring robustness even when using incomplete draft genomes. The results of the strain described in this study were additionally validated using the Type (Strain) Genome Server [TYGS (Meier-Kolthoff et al., 2022)] in January 2025.
2.6 Analysis of the core orthologous genes
A core genome analysis of strain B22T and 14 closest related bacteria based on their 16S rRNA percentage of similarity for which their genome was available, downloaded from the NCBI RefSeq database, was also performed using Bacterial Pan Genome Analysis (BPGA) software (Chaudhari et al., 2016) with the default parameters. After obtaining the core of these 15 bacterial genomes, all protein orthologs belonging to the core genome were concatenated and aligned by MAFFT (Katoh and Standley, 2013). A phylogenomic tree of the core genes of the species was then constructed using MEGA X software according to the maximum-likelihood method, incorporating 1,000 bootstrap replicates to evaluate the robustness of the branching.
2.7 Phenotypic and chemotaxonomic characterization
The colonies were observed on LB agar after 48 h of incubation at 28°C. The oxidase and catalase were determined. Salinity tolerance and optimal growth conditions of strain B22T were determined at 28°C on LB agar plates supplemented with 0; 0.5; 2.5; 5; 7.5; 12 and 25% (w/v) of NaCl adjusting the pH 7. The pH growth range and optimum pH were analyzed on LB agar plates, testing from 4 to 10 pH unit intervals. The temperature range for growth and the optimum temperature were determined on LB plates at 4; 10; 15; 20; 25; 30; 37 and 40°C. In both cases 1% NaCl w/v was selected.
Phenotypic characteristics of strain B22T related with the in vitro plant-growth-promoting (PGP) activities and rhizosphere competence were evaluated: amylase (Barrow and Feltham, 1993), caseinase (Barrow and Feltham, 1993), cellulase (Tasse et al., 2010), acid phosphatase (Baird-Parker, 1963), alkaline phosphatase (Pikovskaya, 1948), phytase (Hosseinkhani and Hosseinkhani, 2009), gelatinase (Tindall et al., 2007), lecithinase (Larpent and Larpent-Gourgand, 1957), quitinase, hydrolysis of Tween 20 and Tween 80 (Sierra, 1957), production of indole-3-acetic (IAA) (Naik et al., 2008) and siderophores (Alexander and Zuberer, 1991).
Other biochemical tests were performed using inoculum of B22T grown in optimal conditions in API 20NE, API 50CH, API ZYM and BIOLOG GEN III according to the manufacturer’s instructions. In addition, DNAase (Jeffris et al., 1957) and hemolysin (Columbia blood agar plates, Difco) were detected.
The cellular fatty acids were analyzed at the Spanish Type Culture Collection (CECT) in Valencia, Spain, following the instruction of the Microbial Identification System Operating Manual (MIDI, 2008). For this, the cell mass of the B22T strain was obtained after growing for 24 h in LB at 28°C and 1% NaCl w/v.
The analysis of polar lipids and respiratory quinones in strain B22T was performed by the Identification Service at DSMZ in Braunschweig, Germany.
2.8 In vivo Plant-growth-promoting and colonization assays
To test the PGP activity of B22T, an in vivo assay was performed by inoculating tomato plants (Solanum lycopersicum L. var. Roma) with this bacterium. After the sterilization of the seeds, they were planted in seedbeds (20 x 7 cm) using sterile vermiculite as a substrate. Sterile water was added until the field capacity was reached, and it was covered with plastic film to maintain humidity. The seedlings were maintained in a greenhouse throughout the duration of the experiment, with a relative humidity (RH) of 60%, temperatures of 25°C during the day and 20°C at night, and a long-day photoperiod (light:dark, 16:8 hours). During a month, the plant seedlings were irrigated weekly with 250 μL of a bacterial suspension (108 CFU mL-1), while the control group was irrigated with sterile distilled water. The experiment was carried out with 12 replicates. Once the treatment had concluded, the plants were harvested and the length, dry weight and thickness of the stem were determined (Sánchez et al., 2023).
Another assay to investigate the colonization properties of B22T was also performed using the model plant Arabidopsis thaliana (Lee et al., 2020; Furci et al., 2021). Five sterile seeds were placed 2 cm apart in a square Petri dish (10 x 10 cm) containing Murashige & Skoog medium at 0.8% (w/v) agar and a final sucrose concentration of 2.5 g L-1. In vitro cultures were maintained under controlled conditions in a phytotron (Hederahelix Ing.) with a long day photoperiod (light:dark, 16:8 h), at 25°C day and 20°C night, relative humidity 60-80% and a luminosity of 250 microsiemens/cm2/sg. After one week of growth, the seedlings were inoculated with 1 μL of the bacterial suspension (108 CFU mL−1) at 1 cm from the base of the plant stem. The same volume of sterile distilled water was inoculated in the control and three replicates were made per treatment. The plates were maintained under controlled conditions as mentioned above for another 7 days. After this period, root colonization was observed by differential interference contrast microscopy between slide and coverslip, using 70% (v/v) glycerol as mounting medium in a LEICA DM5500B microscope.
2.9 Quorum-quenching activity: range of AHLs, identification and localization of the enzyme
The QQ activity of the B22T strain against synthetic AHLs was assessed through a well-diffusion agar-plate assay (Torres et al., 2013). In brief, each AHL was added to an overnight culture in LB medium of the B22T strain, at a final concentration of 10μM, followed by a 24-hour incubation at 28°C. A sterile LB medium supplemented with AHLs served as negative control. The remaining AHLs were identified in the supernatant of each sample, which was then placed in wells on LB agar plates covered with C. subtsugae CV026 or C. violaceum VIR07, or on AB agar plates added with 80 μg mL−1 of 5-bromo-4-chloro-3-indolyl-ß-D-galactopyranoside (Xgal) and overlaid with A. tumefaciens NTL4 (pZLR4). The plates were then incubated at 28°C for 24 hours to observe the appearance of purple or blue coloration around each well. This assay was conducted three times for validation.
The synthetic AHLs (Sigma-Aldrich, Saint Louis, USA) used were C6-HSL (N-hexanoyl-DL-homoserine lactone), C8-HSL (N-octanoyl-DL-homoserine lactone), 3-O-C8-HSL (N-3-oxo-octanoyl-DL-homoserine lactone), C10-HSL (N-decanoyl-DL-homoserine lactone), 3-OH-C10-HSL (N-3-hydroxydecanoyl-DL-homoserine lactone), C12-HSL (N-dodecanoyl-DL-homoserine lactone) and 3-O-C12-HSL (N-3-oxo-dodecanoyl-DL-homoserine lactone).
To determine whether the QQ enzyme of B22T was a lactonase type, an acidification assay was carried out (Yates et al., 2002). Briefly, C12-HSL was added to a 24-hour incubation at 28°C. A sterile LB medium supplemented with C12-HSL served as the negative control. Then, the culture and the control were centrifuged, and the pH of the supernatants was adjusted to 2.0 with HCl 1N. Acidified supernatants were then incubated for 24 h at 28°C. The remaining amount of C12-HSL was extracted twice with 1 volume of dichloromethane. Dried extracts were resuspended in acetonitrile and analyzed by HPLC/MS (Torres et al., 2013) as well as by well-diffusion agar-plate assay using A. tumefaciens NTL4 (pZLR4) as biosensor strain.
The cellular localization of QQ activity was identified in the supernatant and crude cellular extract (CCE) fractions from a 24 h culture of strain B22T following the methodology previously described. Supernatant and CCE were obtained as described previously and filtered through a 0.22 μm-pore membrane filter (Romero et al., 2014).
To identify genes encoding for QQ activity in strain B22T, the whole genome annotated using RAST was used, and potential genes were tested. A protein encoding a predicted acylhomoserine lactone acylase was selected WP_343468083.1 (782 aa) and used to design the specific primers B22-acilgen-Fw: 5’atgtccgggcagttatcgagg-3’ and B22-acilgen-Rev: 5’-ttagtgcgcggccaccctgc-3’. A PCR fragment was amplified and cloned into pGEM-T cloning vector (Promega) following the manufacturer’s instructions. Then, the plasmid construction was transferred into Escherichia coli DH5α and the AHL-degradation activity was tested in LB media supplemented with IPTG and AHLs (C10-HSL and C12-HSL) as described above.
2.10 Interference of QS systems of plant pathogenic bacteria by in vitro co-culture assays
Previous to determine the QQ activity, we determine if the strain B22T interferes the growth of the five plant pathogenic bacteria in an antagonist assay based in a well diffusion method (Frikha-Gargouri et al., 2017). Briefly, 24 h culture of each pathogen was used to prepare an overlay on LB agar plates and 100 μL of aliquots of the filtered supernatant from a 5-day culture of strain B22T were placed on previously prepared wells. After 48 h incubation at 28°C, plates were examined for the appearance of growth inhibition halos surrounding the wells.
QQ activity of strain B22T upon phytopathogenic bacteria was determined by co-culture assays (Torres et al., 2016; Rodríguez et al., 2020). Briefly, 24 h cultures of each pathogen (109 CFU mL-1) were co-cultured with 24 h culture of strain B22T (109 CFU mL-1) at a ratio of 1:100 in LB medium and incubated for 24 h. As controls, each bacterium was cultured in similar conditions. Then, AHLs from each co-cultures y monocultures were detected using the well-diffusion agar-plate assay as described above, and C. subtsugae CV026 and A. tumefaciens NTL4 (pZLR4) as bioindicator strains. The abundance of strain B22T and each pathogen in the co-cultures were quantified by serial dilutions and plate counts, using LB medium and LB medium supplemented with 5% (w/v) NaCl and King B agar.
The interference of strain B22 with virulence-associated cellular functions controlled by QS in the pathogens was evaluated by spotting 10 μL of co-cultures and monocultures on different media. Particularly, the following phenotypic characteristics were evaluated: production of acid and alkaline phosphatase (Pikovskaya, 1948; Baird-Parker, 1963); siderophore production (Alexander and Zuberer, 1991); production of hydrolytic enzymes such as amylase and caseinase (Barrow and Feltham, 1993), DNAase (Jeffris et al., 1957), lipases (hydrolysis of Tween 20 and Tween 80) and gelatinase (Tindall et al., 2007).
2.11 Virulence assays in potato and carrot slices
The interference of strain B22T with the virulence of D. solani LMG 25993T and P. atrosepticum CECT 314T was evaluated on potato slices (Solanum tuberosum). Infection of P. carotovorum subsp. carotovorum CECT 225T was tested on potato and carrot (Daucus carota) slices while P. agglomerans CFBP 11141 was evaluated in cherry tomatoes (S. lycopersicum L. var. cerasiforme) (Garge and Nerurkar, 2016; Rodríguez et al., 2020). Briefly, vegetables were surface sterilized. Then, potatoes and carrots were cut into slices and placed in Petri dishes while cherry tomatoes were placed entirely with wet filter paper to maintain moisture. The following treatments were conducted for each pathogen: sterilized distillated water, monoculture of pathogen, monoculture of strain B22T, pathogen: B22T co-culture (1:100). Each bacterial culture (109 CFU mL-1) was centrifuged, resuspended in sterilized distillated water and 15 μL of each condition was inoculated into the tomatoes, carrot and potato slices. Nine replicates of each treatment were conducted, and the assay was repeated three times. After 2–5 days of incubation (depending on each pathogen) at 28°C, maceration areas were calculated by image analysis using ImageJ software (Schneider et al., 2012).
2.12 Virulence assays in tomato plants
The impact of AHL degradation of P. syringae pv. tomato DC3000 by strain B22T was evaluated in tomato plants (S. lycopersicum L. var. Roma) following the technique described elsewhere (Yan et al., 2008). Briefly, tomato seeds were surface-sterilized and sown in pots as described above (section 2.7.). Three pots containing 50 seeds per pot were used for each treatment: sterile distilled water (negative control), strain B22T, the pathogen (positive control) and the pathogen: B22T co-culture. The pots were kept in an indoor greenhouse during a long-day photoperiod (16:8h, light:dark) at 25°C and watered with 50 mL sterile distilled water twice a week. After 3 weeks, the pots were exposed to 100% humidity for 16 h to induces stomatal opening and then sprayed with a 5 mL washed cell suspension of P. syringae pv. tomato DC3000 (109 CFU mL-1), co-culture of pathogen with strain B22T (ratio 1:100), strain B22T (109 CFU mL-1) and sterile distilled water. Relative humidity was maintained at 100% for a further 24h to facilitate pathogen infection. Finally, after 4 weeks post-inoculation, affected (necrotic and chlorotic leaves, dead leaves) and unaffected shoot (healthy leaves) were counted and followed by plant harvesting (López-Escudero et al., 2004). The unaffected and affected shoot and roots length total dry weight of 20 plants per treatment were determined.
The chlorophyll fluorescence (Fv/Fm ratio) in tomato leaves was determined using a fluorometer (Handy PEA Hansatech Instruments Ltd.) by measuring the fluorescence on the adaxial side of the leaf for a time of 30 minutes. The parameters of basal fluorescence (F0), maximum fluorescence (Fm), variable fluorescence (Fv), (Fv= Fm-F0), and the ratio (Fv/Fm), an indicator of the functionality of the photochemical conversion of light energy in photosystem II, were obtained (Passari et al., 2019).
2.13 Statistical analysis
The data analysis for this study was conducted using GraphPad Prism 9, Statistical Package for the Social Sciences (SPSS) software. The normality of the data was evaluated through either the Shapiro-Wilk test or the D’Agostino and Pearson test. A one-way ANOVA followed by Tukey’s post hoc test was employed for comparing group means. A 95% confidence interval was applied in all analyses.
3 Results
3.1 Phylogenetic and MLSA analyses of strain B22T
Strain B22T was isolated from the rhizospheric soil of the halophyte Salicornia hispanica. The almost complete 16 rRNA gene sequence ( ̴1,500 bp) of strain B22T was obtained by PCR, which was identical to that extracted from its genomic sequence. Strain B22T showed the highest sequence identity to Pseudomonas tehranensis SWRI 196T (99.86%), P. alvandae SWRI 17T (99,66%) and P. canavaninivorans HB 002T (99,59%), while identities below 97% were obtained with other species from Pseudomonas genus. Phylogenetic analysis of its 16S rRNA gene sequences and other related strains through a phylogenetic tree reconstruction using the maximum-likelihood algorithm showed that this strain is a member of the genus Pseudomonas and forms a cluster with the P. tehranensis species, which showed the highest sequence similarity (Figure 1). A similar phylogenetic distribution was obtained when the neighbor-joining and maximum parsimony algorithms were used (Supplementary Figure S1).
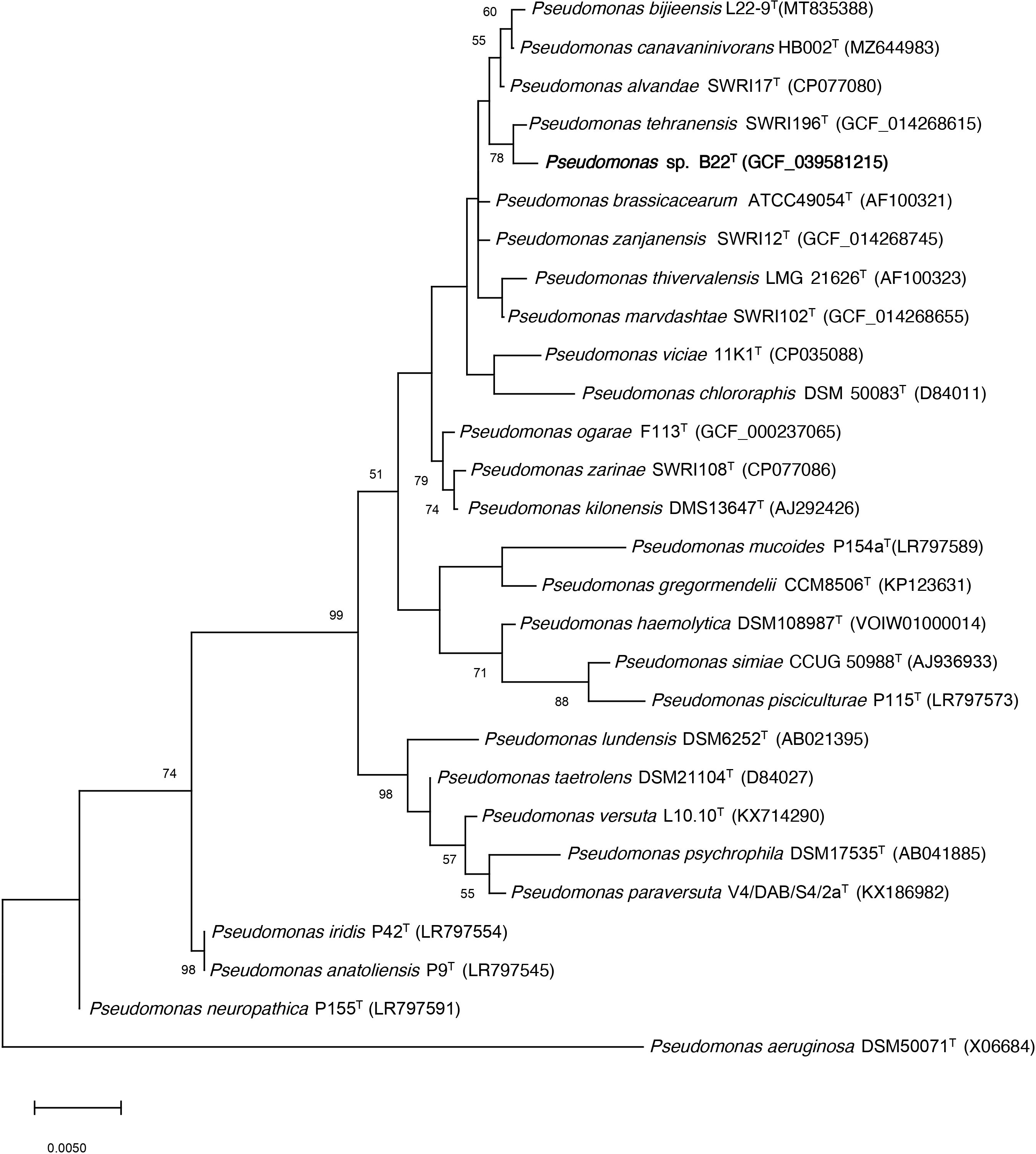
Figure 1. Phylogenetic position of the strain B22T 16S rRNA gene sequence (bold) and its relationship with other related species by using the maximum-likelihood algorithm. The GenBank/EMBL/DDBJ accession number of each sequence is shown in parenthesis. Bootstrap values are expressed as percentages of 1,000 replications, and those >50% are shown at branch points. Bar shows sequence divergence. Bar−0.005 substitutions per nucleotide position. P. aeruginosa DSM50071T sequence was used as an outgroup.
The MLSA resulting Neighbor-joining tree, obtained by concatenating the sequences of four housekeeping genes (16S rRNA, gyrB, rpoD, and rpoB) also indicated that B22T and Pseudomonas tehranensis SWRI196T forms a cluster with a bootstrap value of 100% (Supplementary Figure S2).
3.2 Whole-genome sequencing and assembly
The draft genome of strain B22T was manually curated, resulting in a genome size of over 5.7 Mbp across 32 contigs. The assembly quality was evaluated using the Quality Assessment Tool for Genome Assemblies (QUAST), yielding an N50 value of 237,4 kb, an L50 of 9, and approximately 300X coverage, indicating high-quality sequencing. Annotation of the draft genome using PGAP (Tatusova et al., 2016) identified 5014 protein-coding genes (PCGs). This genome sequence was deposited in the GenBank/EMBL/DDBJ database under accession number JBCNTJ010000000 and was used for further analysis.
3.3 In silico G+C content, ANI and DDH calculations
The in silico analysis of the G+C content in the draft genome of strain B22T revealed a value of 60.5 mol%. Average nucleotide identity (ANI) values, calculated using OrthoANI, yielded a similarity between strain B22T and P. tehranensis SWRI 196T of 95.61%, within the limit of the species delimitation threshold of 95–96% (Chun et al., 2018). The values for strain B22T and other related species were below this threshold (Supplementary Table S1).
Digital DNA-DNA hybridization (dDDH) analysis of the whole-genome sequences of strain B22T and closely related species also produced values below the species delineation threshold (70%) (Supplementary Table S1).
3.4 Phylogenetic analysis of core orthologous proteins
The concatenated alignment of 3093 core orthologous proteins from strain B22T and related species of the genus Pseudomonas, was used to construct a maximum-likelihood phylogenetic tree. The resulting tree is shown in Figure 2.
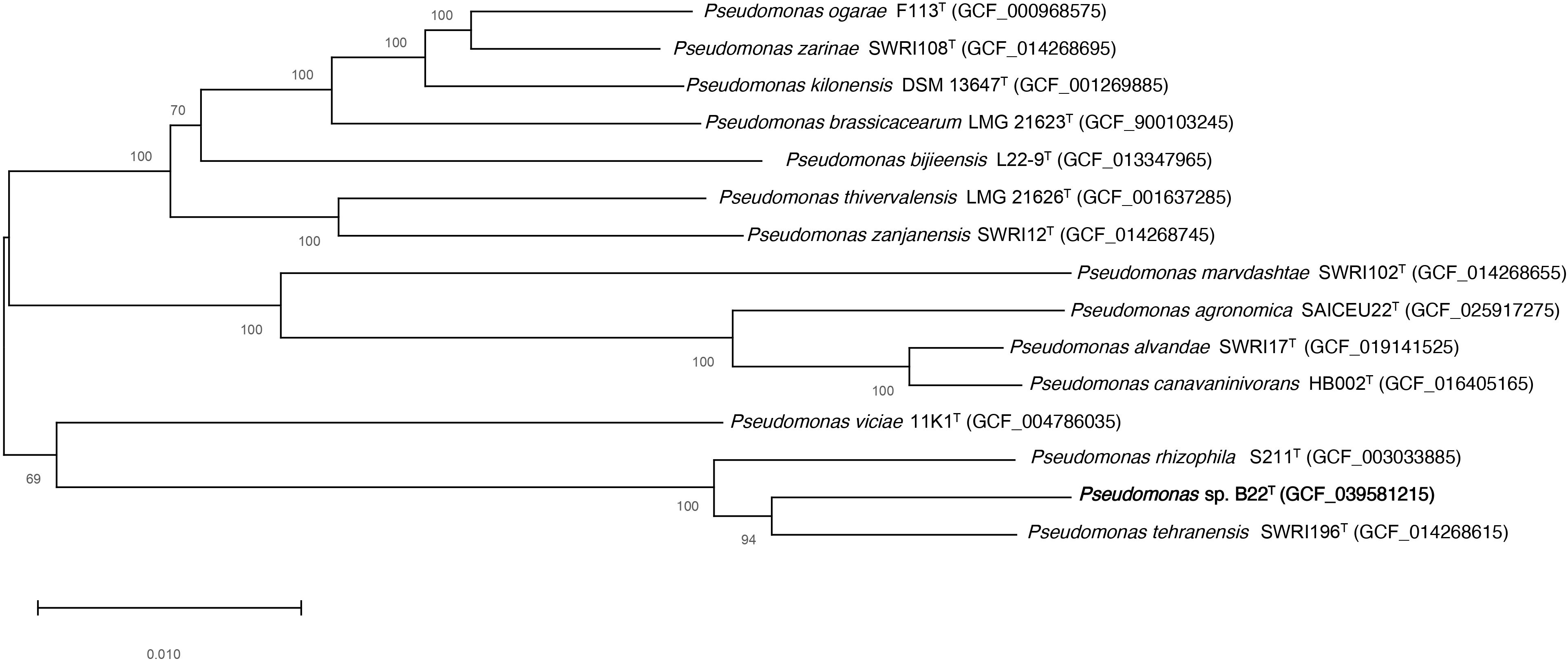
Figure 2. Tree constructed according to the maximum-likelihood method based on 3093 core orthologous proteins of the strain B22T (bold) and the available genomes of Pseudomonas related species. Bootstrap values are expressed as percentages of 1,000 replications, and those over 50% are shown at branch points. Bar−0.010 substitutions per nucleotide position.
3.5 Chemotaxonomic characteristics
The predominant cellular fatty acids of the strain B22T were summed feature 3 (contains C16:1 ω7c and/or C16:1 ω6c) (41.07%), C16:0 (28.87%), and summed feature 8 (contains C18:1 ω7c and C18:1 ω6c) (12.04%). The major polar lipids of strain B22T were diphosphatidylglycerol and phosphatidylethanolamine. Other polar lipids present were phosphatidylglycerol, an unidentified glycolipid, an unidentified phospholipid and an unidentified lipid (Supplementary Figure S3). The predominant respiratory quinone of strain B22T was ubiquinone-9 (Q-9).
3.6 Morphological, physiological and biochemical characteristics
Cells of strain B22T were Gram-negative, rod-shape and motile (Supplementary Figure S4). It forms creamy small colonies after 48 h of incubation in LB agar plates. Growth was positive on Simmons citrate agar and fluorescent colonies were detected on King B agar. Strain B22T did not produce endospores. It was catalase and oxidase positive. It was aerobe, with growth temperatures ranging from 4 to 40°C and with an optimum temperature at 28°C. It grew in pH range from 5 to 10 with pH 7 as optimum. Strain B22T resulted to be halotolerant as it grew from 0 to 5% (w/v) of NaCl, with 0.5-2.5% (w/v) being the optimum concentration. The differential characteristics of this strain with respect to the most closely related species, P. tehranensis SWRI 196T, P. alvandae SWRI 17T and P. canavaninivorans HB 002T were listed in Table 1. For example, strain B22T doesn’t assimilate D-trehalose, D-serine, D-sorbitol, ß-hydroxy-phenylacetic acid while P. tehranensis SWRI 196T does. B22T is sensitive to fusidic acid while P. tehranensis SWRI 196T is resistant. More phenotypical characteristics of strain B22T were detailed in the species description and in Supplementary Tables S2, S3.
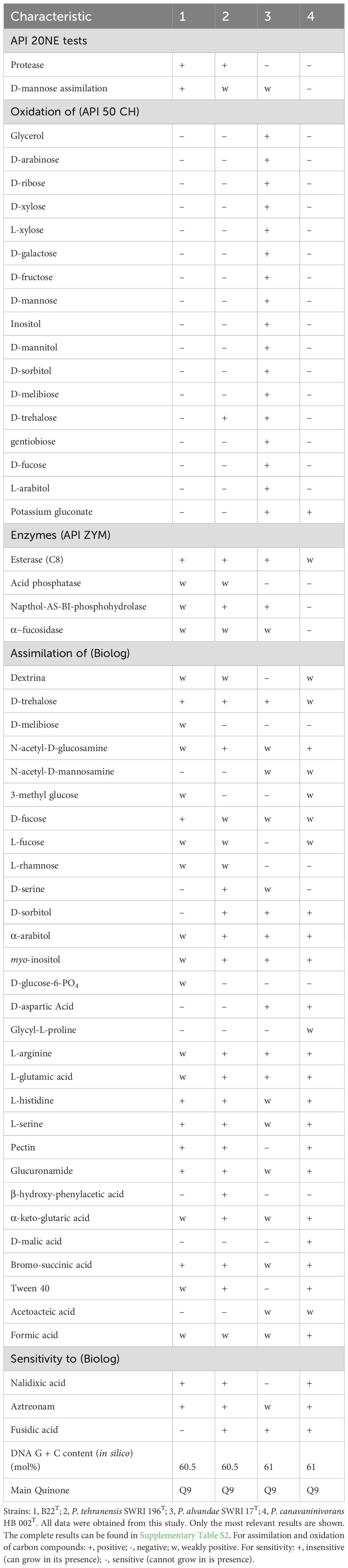
Table 1. Differential characteristic between strain B22T with respect to its closest species of the Pseudomonas genus.
Concerning the PGP activity, strain B22T resulted positive for the hydrolysis of Tween 20 and Tween 80 as well as for phytase and acid phosphatase. It also produced IAA and siderophores. Nevertheless, it did not produce amylase, cellulase, caseinase, lecithinase, alkaline phosphatase and chitinase (Supplementary Table S3).
3.7 Growth promotion and colonization assays
To assess the PGP activity of the halotolerant bacteria in tomato plants 28 days post-inoculation, the plants’ morphological characteristics were evaluated. Plant dry weight and length were significantly higher compared to non-inoculated plants 114.73 ± 62.07 mg vs 19.34 ± 2.83 cm and 27.6 ± 3.51 cm vs 19.34 ± 2.83 cm, respectively (Figures 3A, B). A similar pattern was observed for stem diameter, with plants inoculated with the B22T strain showing the largest stem diameters compared to the non-inoculated plants 0.9 ± 0.35 mm vs 0.31 ± 0.1 mm, respectively.
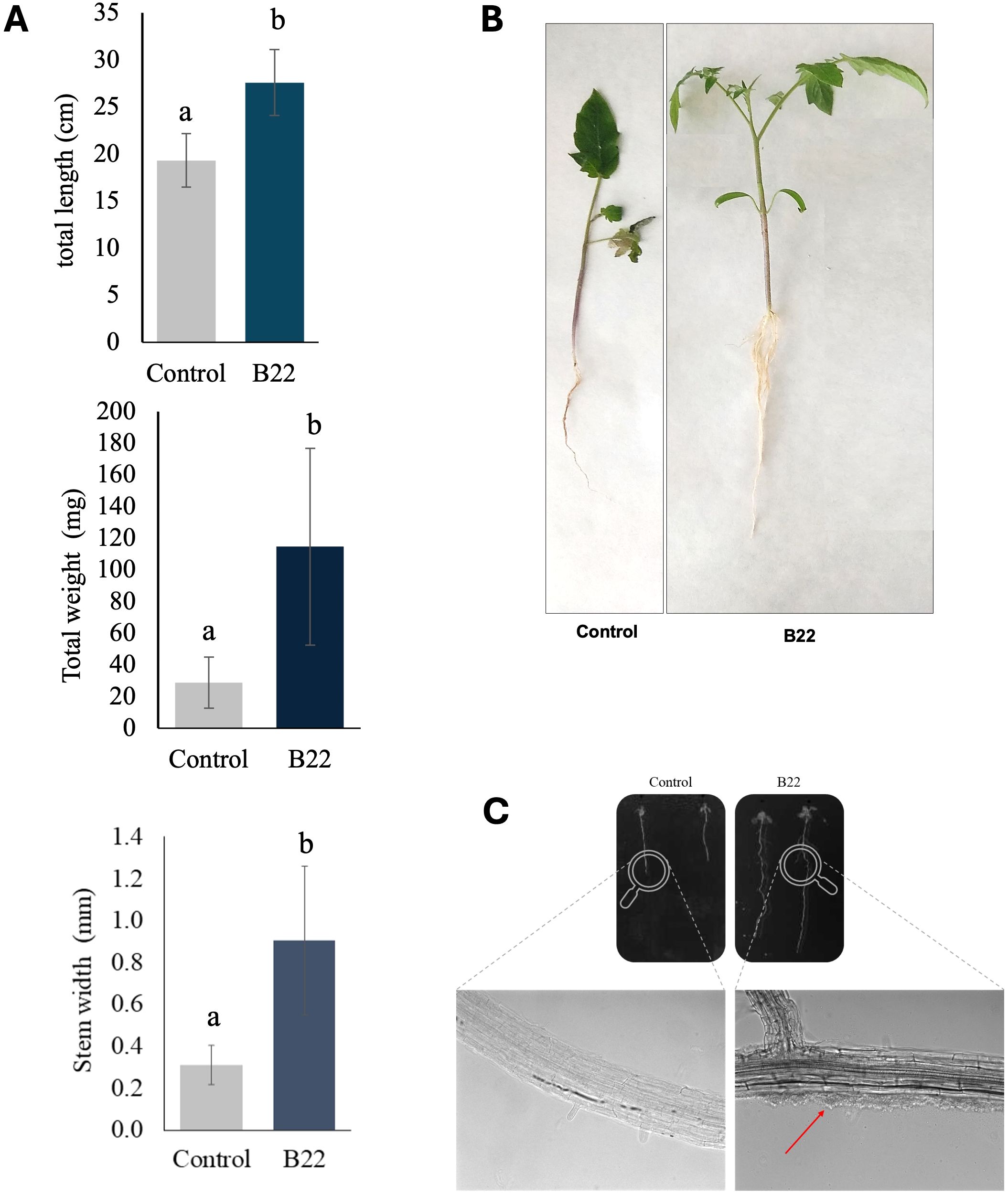
Figure 3. Plant growth-promoting assay of B22T. (A) Effects on plant height, dry weight and stem diameter of tomato plants. Values are expressed as mean ± standard deviation. Data presented are means ± SE (n = 12). Different letters indicate significant differences according to Tukey’s test (p < 0.05). (B) Photographs of the tomato plants. (C) Microscopy observation of the colonization of A. thaliana roots by strain B22T. Differential interference contrast (DIC) micrographs taken at 200X.
The colonization of plant roots was studied in Arabidopsis thaliana through microscopy. The micrographs obtained by differential interference contrast showed small bacterial masses at the base of the root hairs of plants treated with the strain B22T strain, and the absence of bacteria in the control roots (Figure 3C).
3.8 Characterization of the AHL degradation activity of strain B22T
The ability of AHL degradation of strain B22T was tested against a wide range of synthetic AHLs through a well-diffusion agar-plate assay. Strain B22T was able to completely degrade the following AHLs: C6-HSL, C10-HSL, 3-OH-C10-HSL, C12-HSL and 3-oxo-C12-HSL as the corresponding biosensor strains (C. subtsugae CV026, C. violaceum VIR07 and A. tumefaciens NTL4 pZLR4) were not activated. Nevertheless, C8-HSL and 3-oxo-C8-HSL were not degraded (Supplementary Figure S5).
To determine whether the enzymatic activity of strain B22T was due to a AHL lactonase type, an acidification assay to test the lactone ring closure was conducted using C12-HSL, an AHL totally degraded by this strain. The remaining AHLs in the acidified cell-free supernatant (pH 2) as well as in the control (cell-free supernatant at pH 7) were detected by a well-diffusion agar-plate assay using A. tumefaciens NTL4 (pLR4) as biosensor strain. The AHL concentration was not restored in the acid conditions. This result was confirmed by HPLC-MRM, suggesting that QQ activity in this strain was not caused by an AHL lactonase (Supplementary Figure S6A).
The cellular localization of the enzyme was assessed by testing C10-HSL and C12-HSL degrading activity of strain B22T in cell-free supernatant (SN) and crude cellular extract (CCE). Well-diffusion agar-plate assay with C. violaceum VIR07 and A. tumefaciens NTL4 (pZLR4) showed that QQ activity was detected in SN, whilst no AHL degradation was seen in CCE, suggesting that the enzyme was secreted (Supplementary Figure S6B).
To identify the gene responsible for AHL-degradation activity in strain B22T, the automatic annotation of the whole genome was used to select a gene that encodes potential acylase protein WP_343468083.1 (782 aa). A DNA fragment of ~2349 bp was amplified by PCR, using designed specific primers, purified and cloned in the pGEM-T vector and then, expressed in E. coli DH5α. The QQ activity of the plasmid construction pGEMT-B22 against C10-HSL and C12-HSL was confirmed by the well-diffusion agar-plate assay (Supplementary Figure S6C). In addition, BLASTp analysis revealed that the predicted protein encoded by the identified gene WP_343468083.1 (782 aa) shared 95.27% and 93.48% identity with the acylase sequences from P. fluorescens and P. tehranensis, respectively, both of which are annotated as members of the penicillin acylase family.
3.9 Interference of bacterial phytopathogen QS systems and impact on associated phenotypes by strain B22T
The QQ activity of strain B22T was tested against the AHLs produced by D. solani LMG 25993T, P. atrosepticum CECT 314T, P. carotovorum subsp. carotovorum CECT 225T, P. agglomerans CFBP 11141 and P. syringae pv. tomato DC3000 to evaluate its potential use for biocontrol. In these pathogens, AHLs have been characterized and correlated to the expression of virulence factors. Firstly, an antagonism assay was conducted to evaluate whether strain B22T interferes with the growth of each pathogen and no inhibitory effect was detected (data not shown). The five plant pathogens were co-cultured with strain B22T in LB medium at a ratio of 1:100 for 24 h. Monocultures of each bacterium were used in each case as controls. The concentration of each bacterium was determined by counting plate using selective media and they remained stable through the experiment. Then, AHLs were detected by well-diffusion agar-plate assay with A. tumefaciens NTL4 (pLRZ4) and C. subtsugae CV026 as bioindicator strains. The results indicated that under our assay conditions, strain B22T degraded almost completely the AHLs produced by D. solani LMG 25993T and P. agglomerans CFBP 11141 as no activation of the biosensors were visualized in their respectively co-cultures. In respect of co-cultures of strain B22T and the pathogens P. atrosepticum CECT 314T, P. carotovorum subsp. carotovorum CECT 225T and P. syringae pv. tomato DC3000, a partial AHL degradation was observed (Supplementary Figure S7A).
Based on the capacity of strain B22T to degrade AHLs produced by the tested phytopathogens, similar co-cultures were conducted and used to evaluate the effect on the production of cellular functions regulated by QS in each pathogen. Since strain B22T produced numerous PGP traits, the possible reduction of some phenotypic characteristics in the pathogens could not detected (Supplementary Table S3). As results, some phenotypes of pathogens were inhibited when co-cultured with strain B22T. The production of alkaline phosphatase and lecithinase in D. solani; the production of Tween 20 in P. atrosepticum and the production of amylase in P. agglomerans was inhibited in the presence of strain B22T while a reduction in DNAase production was observed in P. carotovorum. Regarding P. syringae pv. tomato, no traits were found to be affected in our phenotypic assays (Supplementary Figure 7B).
3.10 Bioassay of strain B22T in controlling phytopathogens on vegetables and fruits and tomato plants
To evaluate whether the AHL degradation produced by strain B22T in co-culture with the plant pathogens has an impact on their in vivo virulence, different assays on fruits and plants were conducted. Co-cultures of strain B22T with the pathogens were prepared as described above. Additionally, sterile water and monoculture of each strain were used as controls. Mono and co-cultures of D. solani, P. atrosepticum and P. carotovorum were inoculated on the surface of potato slices. In the case of P. carotovorum, cultures were also inoculated on the surface of carrot slices. Mono and co-cultures of B22T with P. agglomerans were inoculated in cherry tomatoes. As it is shown in Figure 4 and Supplementary Figure S8, under our assay conditions, strain B22T drastically reduced the virulence capacity of D. solani and P. atrosepticum to produce soft rot due to maceration area of 5.46 ± 1.79% and 5.22 ± 0.52% respectively, while a maceration area of 65.38 ± 3.21% and 14.96 ± 1.25% was originated by each pathogen in monoculture respectively. Regarding P. carotovorum, strain B22T significantly diminished the capacity of the pathogen to induce soft rot in slice carrots (7.06 ± 0.58%) and potatoes (18.19 ± 0.06%) as compared to the 46.17 ± 3.12% and 34.44 ± 8.40% observed by the pathogen in monoculture respectively. In the case of P. agglomerans, cherry tomatoes inoculated with the pathogen exhibited tissue damage (25 ± 0.01%) while a significant reduction of the infection symptoms were observed in the presence of strain B22T (2.5 ± 0.01%). In all of assays conducted with the different types of vegetables, the inoculation of strain B22T did not originate any infection symptoms.
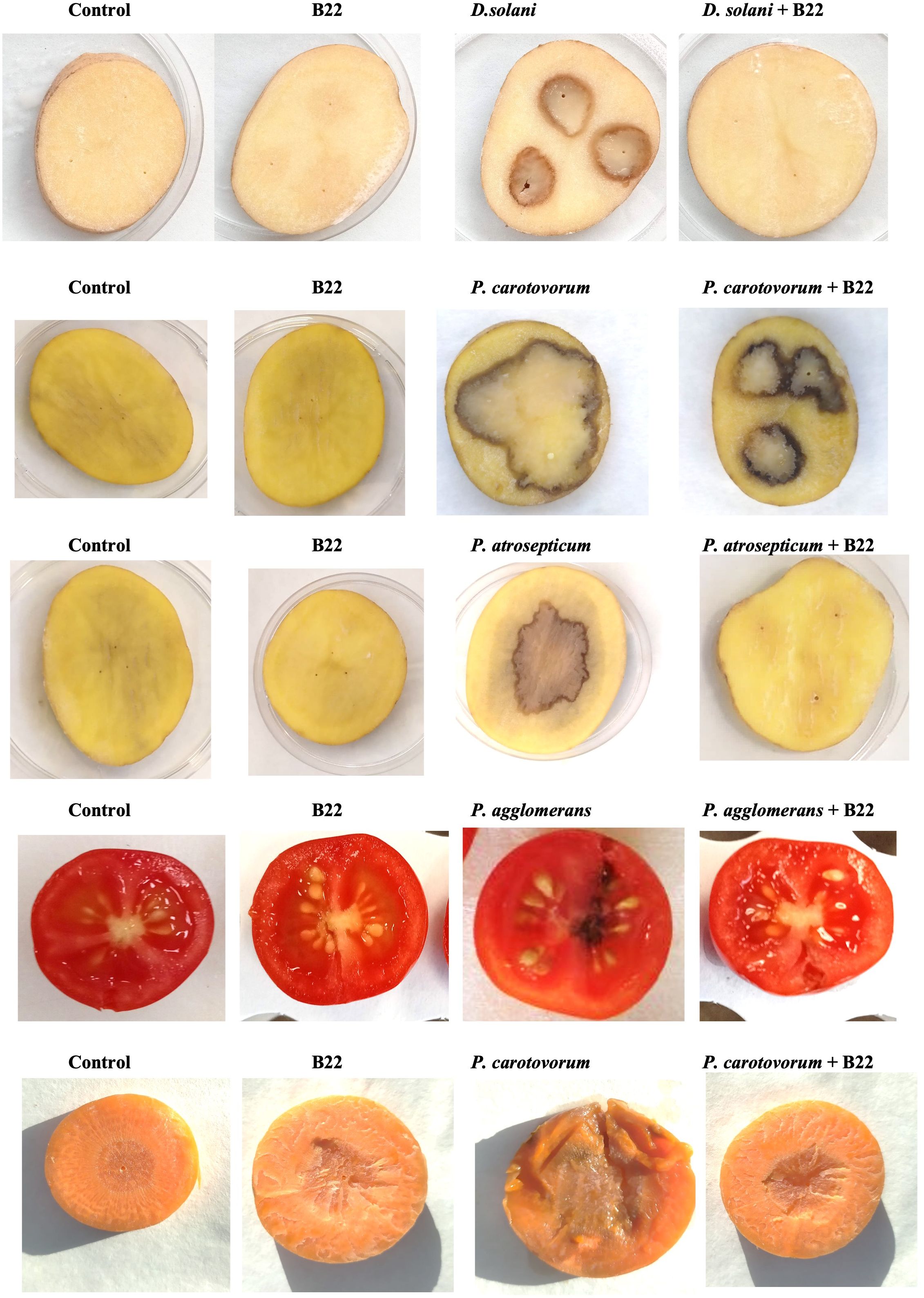
Figure 4. Virulence assay in potato tuber, carrot slices and cherry tomatoes. Assessment of virulence and maceration of cultures and co-cultures of strain B22T and different pathogens inoculated on the surface of potato and carrot slices after 2 days of incubation and cherry tomatoes after 5 days of incubation. Sterile water was used as negative control.
The use of strain B22T as biocontrol agent was also evaluate by conducting in vivo assays of tomato plants infected with P. syringae pv. tomato DC3000. As it is shown in Figure 5, tomato plants treated with the pathogen:strain B22T co-culture showed less infection symptoms than those infected with the pathogen alone. Indeed, tomato plants treated with the co-culture showed a significative increase of 31.49% in healthy leaves as compared to plants inoculated with the pathogen. Moreover, the number of necrotic/chlorotic leaves in plants treated with the co-culture was 0 while the value was 37.5% in those plants treated with the pathogen alone. Negative control and strain B22T-treated plants showed some damaged leaves associated with natural senescence.
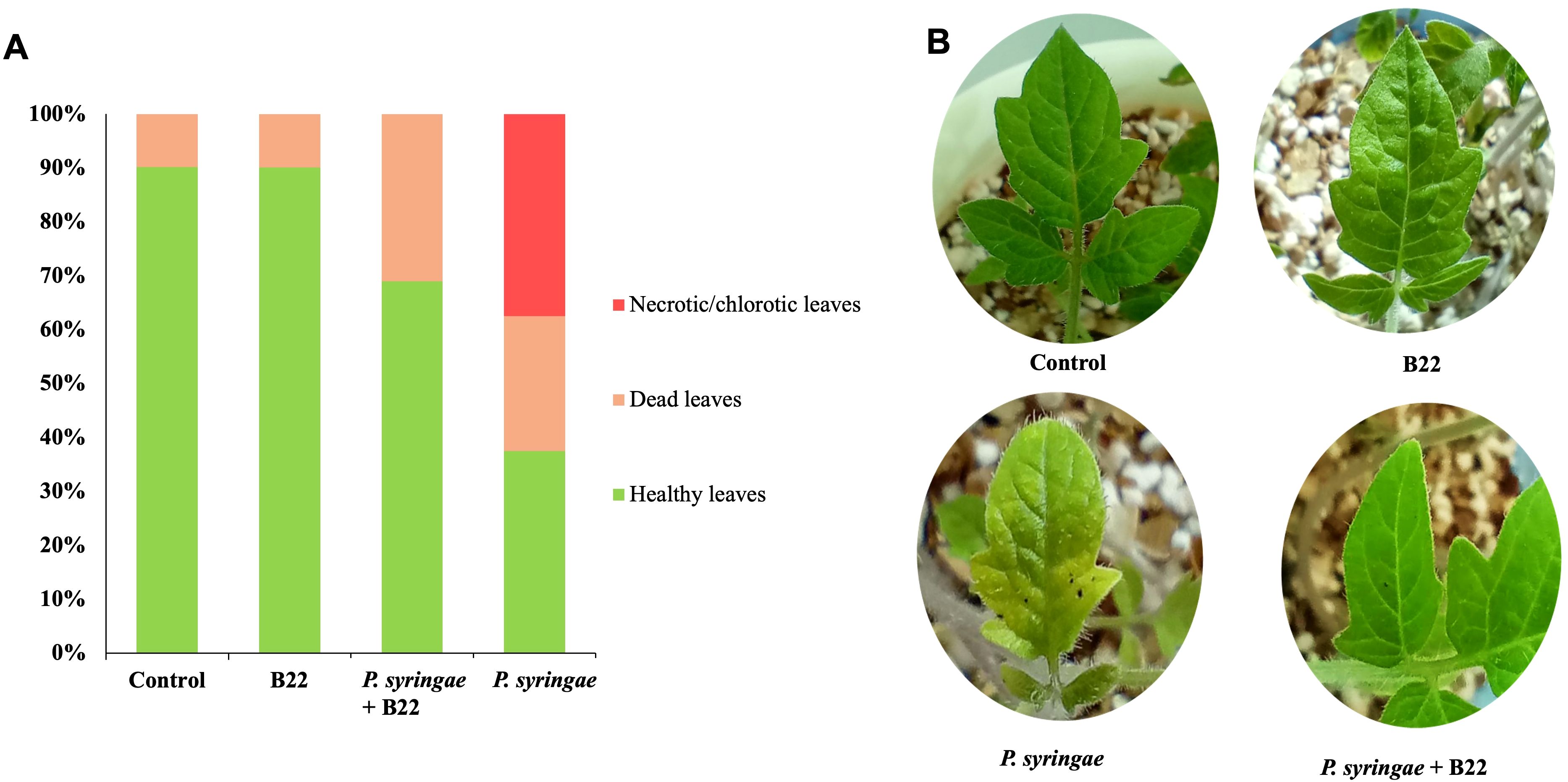
Figure 5. Infection assay in tomato plants treated with sterile water (control), strain B22T and Pseudomonas syringae pv. tomato DC3000 mono-cultures and phytopathogen- B22T co-culture. (A) Total percentage of healthy, necrotic/chlorotic and dead leaves after each treatment. (B). Photograps showing the necrotic symtoms on leaves after treatment.
Regarding fluorescence parameters, plants exposed to the monoculture of B22T and the pathogen:strain B22T co-culture showed an increase of the optimum quantum yield of PSII, represented by the Fv/Fm ratio, compared with plants inoculated with the pathogen (7 and 15% respectively) (data not shown). These results indicated the benefits of the strain B22T that clearly attenuated the virulence of the pathogen.
4 Discussion
Bacterial plant diseases represent a major threat to agricultural productivity, causing severe economic losses and compromising the quality and safety of crops. To address this challenge, sustainable strategies are being developed to replace or minimize the use of chemical treatments, which often contribute to the emergence of resistant bacterial strains. One promising approach involves the use of microorganisms as biostimulants and biocontrol agents (Sharma et al., 2022; Chaudhary et al., 2024). In this context, among biocontrol mechanisms, as QS regulates numerous cellular functions, including virulence factors, the enzymatic degradation of AHL signal molecules in phytopathogenic bacteria has emerged as a promising and sustainable strategy to combat bacterial infection (Grandclément et al., 2016; Hartmann et al., 2021; Zhu et al., 2023).
In this study, we taxonomically identified the strain B22T, which was isolated from the rhizospheric soil of Salicornia hispanica and evaluated its PGP and QQ properties to control bacterial plant infections.
In respect of the genomic analyses, the in silico analysis of the G+C content revealed a value of 60.5 mol%, which falls within the reported range average for the Pseudomonas genus (61.2 mol%) (Hesse et al., 2018). The OrthoANI similarity value between strain B22T and P. tehranensis SWRI 196T was 95.61%, placing it within the species delimitation threshold of 95–96% (Richter and Rosselló-Móra, 2009; Chun et al., 2018). The digital DNA-DNA hybridization values between strain B22T and its closely relatives were clearly bellow of the 70% species delineation threshold (Stackebrandt and Goebel, 1994), reinforcing its classification as a novel species. Phylogenetic analysis based on the concatenated alignment of 3,093 core orthologous proteins from strain B22T and related Pseudomonas species further supports the propose taxonomic placement.
Regarding to the cellular fatty acids profile and the predominant respiratory quinone of strain B22T were similar to those of its closest related species within the Pseudomonas genus. Among phenotypic tests performed, slight differences were observed between strain B22T and the closest relatives as expected since they belong to the P. corrugata subgroup (Poli et al., 2024).
Taken together, genomic, phylogenetic and chemotaxonomic analyses strongly indicate that strain B22T represents a novel species, for which the name Pseudomonas halotolerans is proposed.
Strain B22T belongs to a genus in which certain species such as P. fluorescens, P. chlororaphis, P. protegens, P. putida and P. segetis have demonstrated considerable potential as PGP and biocontrol agents against plant diseases. They can efficiently colonize the rhizosphere and produce beneficial effects through various mechanisms, including the production of bioactive compounds such as hormones, enzymes, antibiotics and siderophores, as well as the induction of systemic resistance in plants (Vejan et al., 2016; Gouda et al., 2018; Arrebola et al., 2019; Rodríguez et al., 2020; Raio and Puopolo, 2021; Al-Karablieh et al., 2022; Bakaeva et al., 2022; Ajijah et al., 2023).
We assessed the PGP properties of strain B22T through an in vivo experiment with tomato plants under sterile conditions. Our findings revealed that strain B22T significantly enhanced all measured growth parameters in tomato plants compared to the control group (increase of 30%, 74.8% and 65.5% in total length, weight and stem width). Notably, plants treated with strain B22T exhibited a remarkable increase in root length and weight, highlighting its strong root growth stimulation activity. These results could be due to several traits linked to its ability to promote plant growth identified in strain B22T, including phosphate solubilization (through acid and phytase activities), and the production of IAA, siderophores, and enzymes. These biochemical activities enhance plant nutrition as reported in other bacteria (Lavakush et al., 2014; Prasad et al., 2015). In addition, as strain B22T was isolated from the rhizosphere, its ability to colonize plant roots was confirmed using the model organism A. thaliana, reinforcing its potential as an effective root-associated growth-promoting agent.
The plant-growth promotion on tomato plants has been evaluated in several Pseudomonas species by other authors. However, no studies have been conducted on the most phylogenetically related species needed for comparison with our data. It has been reported that the inoculation with P. geniculata in tomato plants increased aerial and root biomass by 7 and 9%, respectively (Gopalakrishnan et al., 2015), while P. fluorescens enhanced aerial dry weight by 4.7% (Siddiqui et al., 2001). Notably, inoculation with P. segetis led to significant increases in aerial and root dry weight (19.28% and 21.54%, respectively), although no substantial differences were observed in shoot and root length of tomato plants (Rodríguez et al., 2020).
The QQ activity of strain B22T exhibited versatility for a wide range of AHLs (C6-C12-HSL), with or without different chemical substitutions, except for C8-HSL and 3-oxo-C8-HSL. The enzymatic mechanism responsible for this QQ activity was not attributed to an AHL lactonase but was more likely mediated by another type of enzyme, such as an acylase or oxidoreductase. The heterologous expression of a putative QQ enzyme-encoding gene identified in the annotated genome of strain B22T, combined with BLASTp analysis of the predicted protein, strongly support the hypothesis that the QQ enzyme from strain B22T belong to the acylase family.
AHL acylases has been identified more frequently than lactonases in other Pseudomonas species such as PvdQ (Bokhove et al., 2010) and QuiP (Huang et al., 2003) from P. aeruginosa; HacA and HacB from P. syringae (Shepherd and Lindow, 2009); acylase from P. segetis P6 (Rodríguez et al., 2020), P. multiresinivorans QL-9a (Liu et al., 2023) and Pseudomonas sp. HS-18 (Wang et al., 2022). Studies have shown that AHL acylases exhibit greater substrate specificity than lactonases and degrade long-chain AHLs more efficiently than those with short side chains (Yu et al., 2018). However, strain B22T has demonstrated to possess a wide AHL degradation profile.
AHL-degrading enzymes have been widely studied due to their ability to degrade AHL molecules through catalytic processes without the need to penetrate bacterial cells. This characteristic makes them less invasive than QSIs while potentially exhibiting bactericidal effects (Bzdrenga et al., 2017). Additionally, it has been suggested that plant growth-promoting bacteria may enhance their competitiveness in root colonization and contribute to the biocontrol of AHL-dependent plant pathogens through their AHL-degrading activity (Hartmann et al., 2021).
Since strain B22T exhibited significant QQ activity against a wide range of synthetic AHLs, we evaluated its ability to degrade AHLs produced by plant bacterial pathogen in co-cultures to assess its potential as biocontrol agent in agriculture. Our results showed that strain B22T completely degraded AHLs produced by D. solani and P. agglomerans, while partial degradation was observed in the co-cultures with P. atrosepticum, P. carotovorum subsp. carotovorum and P. syringae pv. tomato DC3000. In addition to AHL degradation, the suppression of QS-regulated phenotypes further supports the QQ activity of strain B22T. In our co-culture experiments, the impact of AHL degradation on the production of some enzymatic activities in plant pathogens could not be tested, as strain B22T itself produces several hydrolytic enzymes. However, we observed a significant reduction in the production of alkaline phosphatase and lecithinase in D. solani, reduction of Tween 20 hydrolysis in P. atrosepticum and the suppression of amylase production in P. agglomerans was significantly reduced in the presence of strain B22T. The observed impact of these phenotypes is in concordance with previous studies demonstrating the role of QS in controlling virulence factors, including the production of hydrolytic enzymes in plant pathogens (Faure and Dessaux, 2007; Helman and Chernin, 2015; Garge and Nerurkar, 2016; Reina et al., 2019).
Moreover, to support the potential of strain B22T as a biocontrol agent, vegetable infection assays demonstrated its ability to attenuate the virulence of multiple plant pathogens. The addition of strain B22T significantly reduced soft rot development caused by D. solani (91.64%) and P. atrosepticum (65%). Similarly, the virulence of P. carotovorum was markedly reduced in both potato (45.6%) and carrot (85.5%) slices. Notably, results obtained with P. agglomerans (60%) in cherry tomatoes indicate that strain B22T can mitigate tissue damage beyond typical soft rot pathogens, suggesting broader biocontrol potential. These results are according to previous studies reporting the use of AHL-degrading bacteria to attenuate phytopathogen virulence (Vega et al., 2020; Rodríguez et al., 2020; Liu et al., 2023; Roca et al., 2024). It has been shown that the production of cell-wall degrading enzymes (PCWDE) promotes the destruction of plant tissue and is in many bacteria regulated by AHLs (Garge and Nerurkar, 2016).
In vivo assays on tomato plants infected with P. syringae pv. tomato DC3000 provides further evidence of strain B22T’s protective role. Plants treated with the co-culture containing strain B22T exhibited a significant increase in healthy leaves and a complete absence of necrotic or chlorotic symptoms compared with those exposed to the pathogen alone, highlight its effectiveness in reducing disease severity. This finding aligns with previous studies demonstrating the use of QQ bacteria to mitigate foliar pathogen virulence (Vega et al., 2020). Photosynthesis is one of the most important processes affected by the infection with bacterial pathogens and photosystem II (PSII) may play a significant role in the plant’s defense (Zou et al., 2005). Previous studies observed the decrease of the maximum quantum efficiency of PSII (Fv/Fm) in plants treated with P. syringae compared to uninfected control plants (Bonfig et al., 2006; Rodríguez-Moreno et al., 2008). In this study the treatment of tomato plants with the monoculture of B22T and the pathogen:strain B22T co-culture increased significantly the ratio Fv/Fm compared to the plants treated with the phytopathogen. Similar results were found previously in tomato plants inoculated with the actinobacteria Streptomyces thermocarboxydus as a biocontrol agent to control Fusarium wilt disease (Passari et al., 2019). These results suggest that strain B22T not only promotes tomato plants growth but may also enhances plant defense responses, further limiting pathogen impact.
This significant reduction in virulence observed in our assays suggests that strain B22T may interfere with the pathogenicity mechanisms of these bacteria, potentially through AHL degradation and other mechanisms that need to be elucidated. Notably, strain B22T did not inhibit pathogen growth, as bacterial concentrations remained stable throughout the experiment. However, the biocontrol mechanisms in certain Pseudomonas spp., have been linked to the production of antibiotics, siderophores and volatile organic compounds (Arseneault et al., 2016; Raio and Puopolo, 2021).
Previous studies have shown that plant-associated bacteria can attenuate pathogen induced QS-dependent plant infections by degrading AHL molecules (Vega et al., 2020; Rodríguez et al., 2020; Liu et al, 2023; Roca et al., 2024). Notably, the heterologous expression of QQ enzymes in bacterial pathogens, such as P. carotovorum subsp. carotovorum (Crépin et al., 2012; Torres et al., 2017; Dong et al., 2000), D. chrysanthemi (Hosseinzadeh et al., 2017) and Burkholderia cenocepacia (Wang et al., 2022), has been found to drastically reduce AHL accumulation and virulence.
An important observation from this study is that strain B22T did not cause any visible infection symptoms in any tested vegetables and plants, highlighting its safety as a potential biocontrol agent. This aspect is crucial for agricultural applications, ensuring that introducing strain B22T does not pose risks of pathogenicity to crops. To date, although QQ activity has been identified in certain Pseudomonas strains (Huang et al., 2003; Shepherd and Lindow, 2009; Bokhove et al., 2010; Wang et al., 2022; Liu et al., 2023) but relative few studies have described PGP strains of Pseudomonas capable of degrading AHL signal molecules produced by bacterial plant pathogens (Rodríguez et al., 2020).
Overall, our results demonstrate that strain B22T exhibits a broad-spectrum QQ activity, mediated by a secreted enzyme distinct from AHL lactonases. The potential biocontrol application of strain B22T is further supported by its ability to degrade AHLs without negatively affecting pathogen growth. This characteristic makes it a promising candidate for sustainable plant protection strategies that target QS without exerting selective pressure on bacterial populations, which could otherwise lead to resistance development.
4.1 Description of Pseudomonas halotolerans sp. nov.
Pseudomonas halotolerans (ha.lo.to′le.rans. Gr. n. hals salt; L. pres. part. tolerans tolerating; N.L. part. adj. halotolerans referring to the ability of the organism to tolerate high salt concentrations).
Cells of this species are aerobic, Gram-negative, non-sporeforming, motile and rod-shaped. In LB agar plates, cells can grow forming creamy and small colonies. Growth occurs in 0-5% (w/v) NaCl (optimum 0.5-2.5%), at pH varying from pH 5 to 10 (optimum pH 7), and within the temperature range of 4-40° C (optimum 28° C). Catalase and oxidase tests are both positive. Results obtained with Biolog GEN III microplates indicate that cells can use the following substrates as carbon and energy sources: D-trehalose, sucrose, α-D-glucose, D-mannose, D-fructose, D-galactose, D-fucose, inosine, D-mannitol, glycerol, D-fructose 6-PO4, L-alanine, L-aspartic acid, L-histidine, L-pyroglutamic acid, L-serine, pectin, D-galacturonic acid, L-galacturonic acid lactone, D-gluconic acid, D-glucuronic acid, glucuronamide, mucic acid, quinic acid, D-saccharic acid, methyl pyruvate, L-lactic acid, citric acid, L-malic acid, bromo-succinic acid, gamma-amino-butyric acid β-hydroxyl-D L-butyric acid, propionic acid and acetic acid. All other substrates in the Biolog GEN III panel did not or only weakly support cell growth. Based on Biolog Gen III sensitivity assays, cells of this species can grow at 1% w/v sodium lactate, and are resistant to troleandomycin, rifamycin SV, lincomycin, guanidine HCl, niaproof 4, vancomycin, tetrazolium violet, tetrazolium blue, lithium chloride, potassium tellurite, nalidixic acid, aztreonam and sodium butyrate. Cell growth was inhibited by all other compounds tested within the Biolog GEN III sensitivity assays.
Results obtained with API 50 CH and API 20NE strips indicate that cells of this species oxidize or assimilate of the following substrates: L-arabinose, D-glucose, aesculin, sucrose, D-mannose, D-mannitol, potassium gluconate, trisodium citrate, capric acid and malic acid. Cells of this species exhibited the following enzymatic activities detected by API 20NE and API ZYM: reduction of nitrates to nitrites, gelatine hydrolysis, leucine arylamidase, esterase (C8) and weakly positive for esterase (C4), lipase (C14), valine arylamide, trypsin and naphtol-AS-BI-phosphohydrolase, acid phosphatase and α-fucosidase. Acids from glucose were negative. Strain B22T resulted positive for the hydrolysis of Tween 20 and Tween 80 as well as for phytase and acid phosphatase. It also produced IAA and siderophores. It did not produce amylase, cellulase, caseinase, lecithinase, alkaline phosphatase and chitinase.
The main cellular fatty acids of strain B22T were C16:0, summed feature 3 (C16:1 ω7c/C16:1 ω6c) and summed feature 8 (C18:1 ω7c/C18:1 ω6c). The major polar lipids identified were diphosphatidylglycerol and phosphatidylethanolamine, while the predominant respiratory quinone was ubiquinone (Q-9).
The genome of P. halotolerans B22T consists of a circular chromosome of 5.7 Mbp, G+C value of 60.5 mol% and 5014 protein-coding genes. The whole genome sequence of P. halotolerans B22T has been deposited al NCBI and is publicly available at NCBI GeneBank accs. no. JBCNTJ010000000.
The type strain is B22T (= CECT31209 = LMG33902), isolated from the rhizospheric soil of the halophyte plant Salicornia hispanica.
5 Conclusions
In this study, we characterized a novel Pseudomonas species isolated from the rizospheric soil of the halophite plant Salicornia hispanica. Using a polyphasic taxonomy approach, we demonstrate that strain B22T represents a novel species within the Pseudomonas corrugata subgroup. Furthermore, this study underscores the potential of P. halotolerans as a sustainable biocontrol agent, given its PGP activity in tomato plants and its ability to reduce phytopathogen virulence factors, thereby mitigating damage to fruits and plants. These attributes suggest that P. halotolerans could play a crucial role in enhancing plant health and reducing disease-related losses in agriculture.
Data availability statement
The datasets presented in this study can be found in online repositories. The names of the repository/repositories and accession number(s) can be found in the article/Supplementary Material.
Author contributions
PS: Formal analysis, Investigation, Writing – review & editing. IC: Formal analysis, Investigation, Writing – review & editing. FM: Conceptualization, Methodology, Resources, Writing – original draft. IS: Conceptualization, Formal analysis, Funding acquisition, Investigation, Methodology, Project administration, Supervision, Visualization, Writing – review & editing. IL: Conceptualization, Funding acquisition, Investigation, Methodology, Project administration, Supervision, Visualization, Writing – original draft, Writing – review & editing.
Funding
The author(s) declare that financial support was received for the research and/or publication of this article. This research was supported by the following grants: PDI2023-1501540B-I00 funded by MICIU/AEI/10.13039/501100011033 and by ERDF/EU; PID2019-106704RB-I00 funded by MCIN/AEI/10.13039/501100011033 and B-AGR-222-UGR20 funded by Consejería de Universidad, Investigación e Innovación de la Junta de Andalucía and ERDF A way of making Europe. Patricia Sánchez is supported by the grant B-AGR-222-UGR20 funded by Consejería de Universidad, Investigación e Innovación de la Junta de Andalucía and ERDF A way of making Europe.
Conflict of interest
The authors declare that the research was conducted in the absence of any commercial or financial relationships that could be construed as a potential conflict of interest.
Generative AI statement
The author(s) declare that no Generative AI was used in the creation of this manuscript.
Publisher’s note
All claims expressed in this article are solely those of the authors and do not necessarily represent those of their affiliated organizations, or those of the publisher, the editors and the reviewers. Any product that may be evaluated in this article, or claim that may be made by its manufacturer, is not guaranteed or endorsed by the publisher.
Supplementary material
The Supplementary Material for this article can be found online at: https://www.frontiersin.org/articles/10.3389/fpls.2025.1605131/full#supplementary-material
References
Ajijah, N., Fiodor, A., Dziurzynski, M., Stasiuk, R., Pawlowska, J., Dziewit, L., et al. (2023). Biocontrol potential of Pseudomonas protegens ML15 against Botrytis cinerea causing gray mold on postharvest tomato (Solanum lycopersicum var. cerasiforme). Front. Plant Sci. 14. doi: 10.3389/fpls.2023.1288408
Alexander, D. and Zuberer, D. (1991). Use of chrome azurol S reagents to evaluate siderophore production by rhizosphere bacteria. Biol. Fert. Soils 12, 39–45. doi: 10.1007/BF00369386
Al-Karablieh, N., Al-Shomali, I., Al-Elaumi, L., and Hasan, K. (2022). Pseudomonas fluorescens NK4 siderophore promotes plant growth and biocontrol in cucumber. J. Appl. Microbiol. 133, 1414–1421. doi: 10.1111/jam.15645
Arrebola, E., Tienda, S., Vida, C., De Vicente, A., and Cazorla, F. M. (2019). Fitness features involved in the biocontrol interaction of Pseudomonas chlororaphis with host plants: the case study of PcPCL1606. Front. Microbiol. 10. doi: 10.3389/fmicb.2019.00719
Arseneault, T., Goyer, C., and Filion, M. (2016). Biocontrol of potato common scab is associated with high Pseudomonas fluorescens LBUM223 populations and phenazine-1-carboxylic acid biosynthetic transcript accumulation in the potato geocaulosphere. Phytopathology 106, 963–970. doi: 10.1094/PHYTO-01-16-0019-R
Aziz, R. K., Bartels, D., Best, A. A., DeJongh, M., Disz, T., Edwards, R. A., et al. (2008). The RAST server: rapid annotations using subsystems technology. BMC Genomics 9, 75. doi: 10.1186/1471-2164-9-75
Baird-Parker, A. C. (1963). A classification of micrococci and staphylococci based on physiological and biochemical tests. J. Gen. Microbiol. 30, 409–427. doi: 10.1099/00221287-30-3-409
Bakaeva, M., Chetverikov, S., Timergalin, M., Feoktistova, A., Rameev, T., Chetverikova, D., et al. (2022). PGP-bacterium Pseudomonas protegens improves bread wheat growth and mitigates herbicide and drought stress. Plants (Basel). 11, 3289. doi: 10.3390/plants11233289
Baltenneck, J., Reverchon, S., and Hommais, F. (2021). Quorum sensing regulation in phytopathogenic bacteria. Microorganisms 9, 239. doi: 10.3390/microorganisms9020239
Balthazar, C., Joly, D. L., and Filion, M. (2022). Exploiting beneficial Pseudomonas spp. for cannabis production. Front. Microbiol. 12. doi: 10.3389/fmicb.2021.833172
Bankevich, A., Nurk, S., Antipov, D., Gurevich, A. A., Dvorkin, M., Kulikov, A. S., et al. (2012). SPAdes: a new genome assembly algorithm and its applications to single-cell sequencing. J. Comput. Biol. 19, 455–477. doi: 10.1089/cmb.2012.0021
Barrow, G. I. and Feltham, R. K. A. (1993). Cowan and Steel’s manual for the identification of medical bacteria, 3rd edition (Cambridge: Cambridge University Press).
Berg, G., Köberl, M., Rybakova, D., Müller, H., Grosch, R., and Smalla, K. (2017). Plant microbial diversity is suggested as the key to future biocontrol and health trends. FEMS Microbiol. Ecol. 93, 5. doi: 10.1093/femsec/fix050
Bokhove, M., Jimenez, P. N., Quax, W. J., and Dijkstra, B. W. (2010). The quorum-quenching N-acyl homoserine lactone acylase PvdQ is an Ntn-hydrolase with an unusual substrate-binding pocket. Proc. Natl. Acad. Sci. U.S.A. 107, 686–691. doi: 10.1073/pnas.0911839107
Bonfig, K. B., Schreiber, U., Gabler, A., Roitsch, T., and Berger, S. (2006). Infection with virulent and avirulent P. syringae strains differentially affects photosynthesis and sink metabolism in Arabidopsis leaves. Planta 225, 1–12. doi: 10.1007/s00425-006-0303-3
Brettin, T., Davis, J. J., Disz, T., Edwards, R. A., Gerdes, S., Olsen, G. J., et al. (2015). RASTtk: A modular and extensible implementation of the RAST algorithm for building custom annotation pipelines and annotating batches of genomes. Sci. Rep. 5, 8365. doi: 10.1038/srep08365
Bushnell, B. (2016).BBMap short read aligner. Available online at: http://sourceforge.net/projects/bbmap (Accessed January 14, 2025).
Bzdrenga, J., Daudé, D., Rémy, B., Jacquet, P., Plener, L., Elias, M., et al. (2017). Biotechnological applications of quorum quenching enzymes. Chemico-Biological Interact. 267, 104–115. doi: 10.1016/j.cbi.2016.05.028
Chaudhari, N. M., Gupta, V. K., and Dutta, C. (2016). BPGA- an ultra-fast pan-genome analysis pipeline. Sci. Rep. 6, 24373. doi: 10.1038/srep24373
Chaudhary, R., Nawaz, A., Kattak, Z., Butt, M. A., Fouillaud, M., Dufossé, L., et al. (2024). Microbial bio-control agents: a comprehensive analysis on sustainable pest management in agriculture. J. Agr. Food. Res. 18, 101421. doi: 10.1016/j.jafr.2024.101421
Cheng, F., Ma, A., Zhuang, X., He, X., and Zhuang, G. (2016). N-(3-oxo-hexanoyl)-homoserine lactone has a critical contribution to the quorum-sensing-dependent regulation in phytopathogen Pseudomonas syringae pv. tabaci 11528. FEMS Microbiol. Let. 363, fnw265. doi: 10.1093/femsle/fnw265
Chilton, M. D., Currier, T. C., Farrand, S. K., Bendich, A. J., Gordon, M. P., and Nester, E. W. (1974). Agrobacterium tumefaciens DNA and PS8 bacteriophage DNA not detected in crown gall tumors. Proc. Natl. Acad. Sci. U.S.A. 71, 3672–3676. doi: 10.1073/pnas.71.9.3672
Chun, J., Oren, A., Ventosa, A., Christensen, H., Arahal, D. R., da Costa, M. S., et al. (2018). Proposed minimal standards for the use of genoma data for the taxonomy of prokaryotes. Int. J. Syst. Evol. Microbiol. 68, 461–466. doi: 10.1099/ijsem.0.002516
Costa-Gutiérrez, S. B., Adler, C., Espinosa-Urgel, M., and de Cristóbal, R. E. (2022). Pseudomonas putida and its close relatives: mixing and mastering the perfect tune for plants. Appl. Microbiol. Biotechnol. 106, 3351–3367. doi: 10.1007/s00253-022-11881-7
Crépin, A., Beury-Cirou, A., Barbey, C., Farmer, C., Hélias, V., Burini, J.-F., et al. (2012). N-acyl homoserine lactones in diverse Pectobacterium and Dickeya plant pathogens: diversity, abundance, and involvement in virulence. Sensors 12, 3484–3497. doi: 10.3390/s120303484
Cruz, A. A., Cabeo, M., Durán-Viseras, A., Sampedro, I., and Llamas, I. (2024). Interference of AHL signal production in the phytophatogen Pantoea agglomerans as a sustainable biological strategy to reduce its virulence. Microbiol. Res. 285, 127781. doi: 10.1016/j.micres.2024.127781
Defoirdt, T. (2018). Quorum-sensing systems as targets for antivirulence therapy. Trends Microbiol. 26, 313–328. doi: 10.1016/j.tim.2017.10.005
Dong, Y. H., Xu, J. L., Li, X. Z., and Zhang, L. H. (2000). AiiA, an enzyme that inactivates the acylhomoserine lactone quorum-sensing signal and attenuates the virulence of Erwinia carotovora. Proc. Natl. Acad. Sci. U.S.A. 97, 3526–3531. doi: 10.1073/pnas.97.7.3526
FAO (2018). The Future of Food and Agriculture-Alternative Pathways to 2050 (Rome, Italy: FAO), 60, ISBN: ISBN 9789251309896. Summary Version.
FAO (2023).Antimicrobial resistance. Available online at: https://www.fao.org/antimicrobial-resistance/key-sectors/plant-production/en/ (Accessed February 7, 2025).
Faure, D. and Dessaux, Y. (2007). Quorum sensing as a target for developing control strategies for the plant pathogen. Pectobacterium. Eur. J. Plant Pathol. 119, 353–365. doi: 10.1007/s10658-007-9149-1
Finger, R. and Möhring, N. (2024). The emergence of pesticide-free crop production systems in Europe. Nat. Plants 10, 360–366. doi: 10.1038/s41477-024-01650-x
Frikha-Gargouri, O., Ben Abdallah, D., Bhar, I., and Tounsi, S. (2017). Antibiosis and bmyB gene presence as prevalent traits for the selection of efficient Bacillus biocontrol agents against crown gall disease. Front. Plant Sci. 8. doi: 10.3389/fpls.2017.01363
Furci, L., Pascual-Pardo, D., and Ton, J. (2021). A rapid and non-destructive method for spatial–temporal quantification of colonization by Pseudomonas syringae pv. tomato DC3000 in Arabidopsis and tomato. Plant Methods 17, 126. doi: 10.1186/s13007-021-00826-2
Garge, S. S. and Nerurkar, A. S. (2016). Attenuation of quorum sensing regulated virulence of Pectobacterium carotovorum subsp. carotovorum through an AHL lactonase produced by Lysinibacillus sp. Gs50. PloS One 11, 1–23. doi: 10.1371/journal.pone.0167344
Garrido-Sanz, D., Čaušević, S., Vacheron, J., Heiman, C. M., Sentchilo, V., van der Meer, J. R., et al. (2023). Changes in structure and assembly of a species-rich soil natural community with contrasting nutrient availability upon establishment of a plant-beneficial Pseudomonas in the wheat rhizosphere. Microbiome 11, 214. doi: 10.1186/s40168-023-01660-5
Gopalakrishnan, S., Srinivas, V., Prakash, B., Sathya, A., and Vijayabharathi, R. (2015). Plant growth-promoting traits of Pseudomonas geniculata isolated from chickpea nodules. Biotech 5, 653–661. doi: 10.1007/s13205-014-0263-4
Gouda, S., Kerry, R. G., Das, G., Paramithiotis, S., Shin, H. S., and Patra, J. K. (2018). Revitalization of plant growth promoting rhizobacteria for sustainable development in agriculture. Microb. Res. 206, 131–140. doi: 10.1016/j.micres.2017.08.016
Grandclément, C., Tannières, M., Moréra, S., Dessaux, Y., and Faure, D. (2016). Quorum quenching: role in nature and applied developments. FEMS Microbiol. Rev. 40, 86–116. doi: 10.1093/femsre/fuv038
Gurevich, A., Saveliev, V., Vyahhi, N., and Tesler, G. (2013). QUAST: quality assessment tool for genome assemblies. Bioinformatics 29, 1072–1075. doi: 10.1093/bioinformatics/btt086
Harrison, A. M. and Soby, S. D. (2020). Reclassification of Chromobacterium violaceum ATCC 31532 and its quorum biosensor mutant CV026 to Chromobacterium subtsugae. AMB Express 10, 202. doi: 10.1186/s13568-020-01140-1
Hartmann, M., Frey, B., Mayer, J., Mäder, P., and Widmer, F. (2015). Distinct soil microbial diversity under long-term organic and conventional farming. ISME J. 9, 1177–1194. doi: 10.1038/ismej.2014.210
Hartmann, A., Klink, S., and Rothballer, M. (2021). Importance of N-Acyl-homoserine lactone-based quorum sensing and quorum quenching in pathogen control and plant growth promotion. Pathogens 10, 1561. doi: 10.3390/pathogens1012156
Helman, Y. and Chernin, L. (2015). Silencing the mob: disrupting quorum sensing as a means to fight plant disease. Mol. Plant Pathol. 16, 316–329. doi: 10.1111/mpp.12180
Hesse, C., Schulz, F., Bull, C. T., Shaffer, B. T., Yan, Q., Shapiro, N., et al. (2018). Genome-based evolutionary history of Pseudomonas spp. Environ. Microbiol. 20, 2142–2159. doi: 10.1111/1462-2920.14130
Hosseinkhani, B. and Hosseinkhani, G. (2009). Analysis of phytase producing bacteria (Pseudomonas sp.) from poultry faeces and optimization of this enzyme production. Afr. J. Biotechnol. 8, 4229–4232.
Hosseinzadeh, S., Shams-Bakhsh, M., and Sadeghizadeh, M. (2017). Attenuation and quantitation of virulence gene expression in quorum-quenched Dickeya chrysanthemi. Arch. Microbiol. 199, 51–61. doi: 10.1007/s00203-016-1276-7
Huang, J. J., Han, J., Zhang, L., and Leadbetter, J. R. (2003). Utilization of acyl-homoserine lactone quorum signals for growth by a soil pseudomonad and Pseudomonas aeruginosa PAO1. Appl. Environ. Microbiol. 69, 5941–5949. doi: 10.1128/AEM.69.10.5941-5949.2003
Jeffris, C. D., Holtman, D. F., and Guse, D. G. (1957). Rapid method for determining the activity of microorganisms on nucleic acids. J. Bacteriol. 73, 590–591. doi: 10.1128/jb.73.4.590-591.1957
Jiao, X., Takishita, Y., Zhou, G., and Smith, D. L. (2021). Plant associated rhizobacteria for biocontrol and plant growth enhancement. Front. Plant Sci. 12. doi: 10.3389/fpls.2021.634796
Katoh, K. and Standley, D. M. (2013). MAFFT multiple sequence alignment software version 7: improvements in performance and usability. MBE 30, 772–780. doi: 10.1093/molbev/mst010
Kopittke, P. M., Menzies, N. W., Wang, P., McKenna, B. A., and Lombi, E. (2019). Soil and the intensification of agriculture for global food security. Environ. Int. 132, 105078. doi: 10.1016/j.envint.2019.105078
Kumar, S., Stecher, G., Li, M., Knyaz, C., and Tamura, K. (2018). MEGA X: molecular evolutionary genetics analysis across computing platforms. Mol. Biol. Evol. 35, 1547–1549. doi: 10.1093/molbev/msy096
Larpent, J. P. and Larpent-Gourgand, M. (1957). M´emento technique de microbiologie (Paris: Technique et Documentation Lavoisier).
Lavakush, J. Y., Verma, J. P., Jaiswal, D. K., and Kumar, A. (2014). Evaluation of PGPR and different concentration of phosphorus level on plant growth, yield and nutrient content of rice (Oryza sativa). Ecol. Eng. 62, 123–128. doi: 10.1016/j.ecoleng.2013.10.013
Lee, I., Kim, Y. O., Park, S.-C., and Chun, J. (2016). OrthoANI: an improved algorithm and software for calculating average nucleotide identity. Int. J. Syst. Evol. Microbiol. 66, 1100–1103. doi: 10.1099/ijsem.0.000760
Lee, S., Trịnh, C. S., Lee, W. J., Jeong, C. Y., Truong, H. A., Chung, N., et al. (2020). Bacillus subtilis strain L1 promotes nitrate reductase activity in Arabidopsis and elicits enhanced growth performance in Arabidopsis, lettuce, and wheat. J. Plant Res. 133, 231–244. doi: 10.1007/s10265-019-01160-4
Liu, S., Zhu, X., Yan, Z., Liu, H., Zhang, L., Chen, W., et al. (2023). The isolate Pseudomonas multiresinivorans QL-9a quenches the quorum sensing signal and suppresses plant soft rot disease. Plants 12, 3037. doi: 10.3390/plants12173037
López-Escudero, F. J., Del Río, C., Caballero, J. M., and Blanco-López, M. A. (2004). Evaluation of olive cultivars for resistance to Verticillium dahliae. Eur. J. Plant Pathol. 110, 79–85. doi: 10.1023/B:EJPP.0000010150.08098.2d
Meier-Kolthoff, J. P., Auch, A. F., Klenk, H.-P., and Göker, M. (2013). Genome sequence-based species delimitation with confidence intervals and improved distance functions. BMC Bioinform. 14, 60. doi: 10.1186/1471-2105-14-60
Meier-Kolthoff, J. P., Carbasse, J. S., Peinado-Olarte, R. L., and Göker, M. (2022). TYGS and LPSN: a database tandem for fast and reliable genome based classification and nomenclature of prokaryotes. Nucleic Acids Res. 50, D801–D807. doi: 10.1093/nar/gkab902
MIDI. (2008). Sherlock Microbial Identification System Operating Manual, version 6.1 (Newark, DE: MIDI Inc.).
Moleleki, L. N., Pretorius, R. G., Tanui, C. K., Mosina, G., and Theron, J. (2017). A quorum sensing-defective mutant of Pectobacterium carotovorum ssp. brasiliense 1692 is attenuated in virulence and unable to occlude xylem tissue of susceptible potato plant stems. Mol. Plant Pathol. 18, 32–44. doi: 10.1111/mpp.12372
Morohoshi, T., Kato, M., Fukamachi, K., Kato, N., and Ikeda, T. (2008). N-Acylhomoserine lactone regulates violacein production in Chromobacterium violaceum type strain ATCC 12472. FEMS Microbiol. Lett. 279, 124–130. doi: 10.1111/j.1574-6968.2007.01016.x
Naik, P., Sahoo, N., Goswami, D., Ayyadurai, N., and Sakthivel, N. (2008). Genetic and functional diversity among fluorescent pseudomonads isolated from the rhizosphere of banana. Microb. Ecol. 56, 492–504. doi: 10.1007/s00248-008-9368-9
Navarro-Monsterrat, E. D. and Taylor, C. G. (2023). T6SS: A key to Pseudomonas’s success in biocontrol. Microorganisms 11, 2718. doi: 10.3390/microorganisms11112718
Overbeek, R., Olson, R., Pusch, G. D., Olsen, G. J., Davis, J. J., Disz, T., et al. (2014). The SEED and the Rapid Annotation of microbial genomes using Subsystems Technology (RAST). Nucleic Acids Res. 42, D206–D214. doi: 10.1093/nar/gkt1226
Parks, D. H., Imelfort, M., Skennerton, C. T., Hugenholtz, P., and Tyson, G. W. (2015). CheckM: assessing the quality of microbial genomes recovered from isolates, single cells, and metagenomes. Genome Res. 25, 1043–1055. doi: 10.1101/gr.186072.114
Passari, A. K., Upadhyaya, K., Singh, G., Abdel- Azeem, A. M., Thankappan, S., Uthandi, S., et al. (2019). Enhancement of disease resistance, growth potential, and photosynthesis in tomato (Solanum lycopersicum) by inoculation with an endophytic actinobacterium, Streptomyces thermocarboxydus strain BPSAC147. PloS One 14, e0219014. doi: 10.1371/journal.pone.0219014
Pikovskaya, R. I. (1948). Mobilization of phosphorus in soil in connection with the vital activity of some microbial species. Microbiology 17, 362–370.
Piqué, N., Minana-Galbis, D., Merino, S., and Tomás, J. M. (2015). Virulence factors of Erwinia amylovora: a review. Int. J. Mol. Sci. 16, 12836–12854. doi: 10.3390/ijms160612836
Poli, N., Keel, C. J., and Garrido-Sanz, D. (2024). Expanding the Pseudomonas diversity of the wheat rhizosphere: four novel species antagonizing fungal phytopathogens and with plant-beneficial properties. Front. Microbiol. 15, 1440341. doi: 10.3389/fmicb.2024.1440341
Pollumaa, L., Alamae, T., and Mae, A. (2012). Quorum sensing and expression of virulence in pectobacteria. Sensors 12, 3327–3349. doi: 10.3390/s120303327
Potrykus, M., Hugouvieux-Cotte-Pattat, N., and Lojkowska, E. (2018). Interplay of classic Exp and specific Vfm quorum sensing systems on the phenotypic features of Dickeya solani strains exhibiting different virulence levels. Mol. Plant Pathol. 19, 1238–1251. doi: 10.1111/mpp.12614
Prasad, R., Kumar, M., and Varma, A. (2015). Role of PGPR in soil fertility and plant health in Plant-growth-promoting rhizobacteria (PGPR) and medicinal plants. Eds. Egamberdieva, D., Shrivastava, S., and Varma, A. (Cham: Springer), 247–260.
Quinones, B., Dulla, G., and Lindow, S. E. (2005). Quorum sensing regulates exopolysaccharide production, motility, and virulence in Pseudomonas syringae. Mol. Plant Microbe Inter. 18, 682–693. doi: 10.1094/mpmi-18-0682
Raio, A. and Puopolo, G. (2021). Pseudomonas chlororaphis metabolites as biocontrol promoters of plant health and improved crop yield. World J. Microbiol. Biotechnol. 37, 99. doi: 10.1007/s11274-021-03063-w
Ramette, A., Frapolli, M., Fischer-Le Saux, M., Gruffaz, C., Meyer, J.-M., Défago, G., et al. (2011). Pseudomonas protegens sp. nov., Widespread Plant-Protecting Bacteria Producing the Biocontrol Compounds 2,4-Diacetylphloroglucinol and Pyoluteorin. Syst. Appl. Microbiol. 34, 180–188. doi: 10.1016/j.syapm.2010.10.005
Reina, J. C., Torres, M., and Llamas, I. (2019). Stenotrophomonas maltophilia AHL-degrading strains isolated from marine invertebrate microbiota attenuate the virulence of Pectobacterium carotovorum and Vibrio corallilyticus. Mar. Biotechnol. 21, 276–290. doi: 10.1007/s10126-019-09879-w
Richter, M. and Rosselló-Móra, R. (2009). Shifting the genomic gold standard for the prokaryotic species definition. Proc. Natl. Acad. Sci. U.S.A. 106, 19126–19131. doi: 10.1073/pnas.0906412106
Roca, A., Cabeo, M., Enguindanos, C., Martínez-Checa, F., Sampedro, I., and Llamas, I. (2024). Potential of the quorum-quenching and plant-growth promoting halotolerant Bacillus toyonensis AA1EC1 as biocontrol agent. Microb. Biotechnol. 17, e14420. doi: 10.1111/1751-7915.14420
Rodríguez, M., Torres, M., Blanco, L., Béjar, V., Sampedro, I., and Llamas, I. (2020). Plant growth-promoting activity and quorum quenching-mediated biocontrol of bacterial phytopathogens by Pseudomonas segetis strain P6. Sci. Rep. 10, 4121. doi: 10.1038/s41598-020-61084-1
Rodríguez-Moreno, L., Pineda, M., Soukupová, J., Macho, A. P., Beuzón, C. R., Barón, M., et al. (2008). Early detection of bean infection by Pseudomonas syringae in asymptomatic leaf areas using chlorophyll fluorescence imaging. Photosynthesis Res. 96, 27–35. doi: 10.1007/s11120-007-9278-6
Romero, M., Muras, A., Mayer, C., Buján, N., Magariños, B., and Otero, A. (2014). In vitro quenching of fish pathogen Edwardsiella tarda AHL production using marine bacterium Tenacibaculum sp. strain 20J cell extracts. Dis. Aquat. Organ. 108, 217–225. doi: 10.3354/dao02697
Sánchez, P., Castro-Cegri, A., Sierra, S., Garrido, D., Llamas, I., Sampedro, I., et al. (2023). The synergy of halotolerant PGPB and mauran mitigates salt stress in tomato (Solanum lycopersicum) via osmoprotectants accumulation. Physiol. Plant 175, e14111. doi: 10.1111/ppl.14111
Schneider, K., Barreiro-Hurle, J., and Rodríguez-Cerezo, E. (2023). Pesticide reduction amidst food and feed security concerns in Europe. Nat. Food 4, 746–750. doi: 10.1038/s43016-023-00834-6
Schneider, C. A., Rasband, W. S., and Eliceiri, K. W. (2012). NIH image to imagej: 25 years of image analysis. Nat. Methods 9, 671–675. doi: 10.1038/nmeth.2089
Sharma, A., Abrahamian, P., Carvalho, R., Choudhary, M., Paret, M. L., Vallad, G. E., et al. (2022). Future of bacterial disease management in crop production. Annu. Rev. Phytopathol. 60, 259–282. doi: 10.1146/annurev-phyto-021621-121806
Shaw, P. D., Ping, G., Daly, S. L., Cha, C., Cronan, J. E., Rinehart, K. L., et al. (1997). Detecting and characterizing N-acyl-homoserine lactone signal molecules by thin-layer chromatography. Proc. Natl. Acad. Sci. U.S.A. 94, 6036–6041. doi: 10.1073/pnas.94.12.6036
Shepherd, R. W. and Lindow, S. E. (2009). Two dissimilar N-acyl-homoserine lactone acylases of Pseudomonas syringae influence colony and biofilm morphology. Appl. Environ. Microbiol. 75, 45–53. doi: 10.1128/AEM.01723-08
Siddiqui, Z. A., Iqbal, A., and Mahmood, I. (2001). Effects of Pseudomonas fluorescens and fertilizers on the reproduction of Meloidogyne incognita and growth of tomato. Appl. Soil Ecol. 16, 179–185. doi: 10.1016/S0929-1393(00)00083-4
Sierra, G. (1957). A simple method for the detection of lipolytic activity of micro-organisms and some observations on the influence of the contact between cells and fatty substrates. Antonie Van Leeuwenhoek 23, 15–22. doi: 10.1007/BF02545855
Sievers, F., Wilm, A., Dineen, D., Gibson, T. J., Karplus, K., Li, W., et al. (2011). Fast, scalable generation of high-quality protein multiple sequence alignments using Clustal Omega. Mol. Syst. Biol. 7, 539. doi: 10.1038/msb.2011.75
Singh, I. (2018). Plant growth promoting rhizobacteria (PGPR) and their various mechanisms for plant growth enhancement in stressful conditions-A review. Eur. J. Biol. Res. 8, 191–213. doi: 10.5281/zenodo.1455995
Smadja, B., Latour, X., Faure, D., Chevalier, S., Dessaux, Y., and Orange, N. (2004). Involvement of N-acylhomoserine lactones throughout plant infection by Erwinia carotovora subsp. atroseptica (Pectobacterium atrosepticum). Mol. Plant Microbe Interact. 17, 1269–1278. doi: 10.1094/mpmi.2004.17.11.1269
Stackebrandt, E. and Goebel, B. (1994). Taxonomic note: A place for DNA-DNA reassociation and 16S rRNA sequence analysis in the present species definition in bacteriology. Int. J. Syst. Bacteriol. 44, 846–849. doi: 10.1099/00207713-44-4-846
Tasse, L., Bercovici, J., Pizzut-Serin, S., Robe, P., Tap, J., Klopp, C., et al. (2010). Functional metagenomics to mine the human gut microbiome for dietary fiber catabolic enzymes. Genome Res. 20, 1605–1612. doi: 10.1101/gr.108332.110
Tatusova, T., DiCuccio, M., Badretdin, A., Chetvernin, V., Nawrocki, E. P., Zaslavsky, L., et al. (2016). NCBI prokaryotic genome annotation pipeline. Nucleic Acids Res. 44, 6614–6624. doi: 10.1093/nar/gkw569
Tindall, B. J., Sikorski, J., Smibert, R. M., and Krieg, N. R. (2007). Phenotypic characterization and the principles of comparative systematics. In Methods for General and Molecular Microbiology, 3rd ed. Reddy, C. A., Beveridge, T. J., Breznak, J. A., Marzluf, G., and Schmidt, T. M. (Washington, DC USA: Snyder ASM Press) pp. 330–393.
Torres, M., Romero, M., Prado, S., Dubert, J., Tahrioui, A., Otero, A., et al. (2013). N-acylhomoserine lactone-degrading bacteria isolated from hatchery bivalve larval cultures. Microbiol. Res. 168, 547–554. doi: 10.1016/j.micres.2013.04.011
Torres, M., Rubio-Portillo, E., Anton, J., Ramos-Espla, A. A., Quesada, E., and Llamas, I. (2016). Selection of the N-Acylhomoserine lactone-degrading bacterium Alteromonas stellipolaris PQQ-42 and of its potential for biocontrol in aquaculture. Front. Microbiol. 7. doi: 10.3389/fmicb.2016.00646
Torres, M., Uroz, S., Salto, R., Fauchery, L., Quesada, E., and Llamas, I. (2017). HqiA, a novel quorum-quenching enzyme which expands the AHL lactonase family. Sci. Rep. 7, 943. doi: 10.1038/s41598-017-01176-7
Tripathi, S., Srivastava, P., Devi, R., and Bhadouria, R. (2020). “Chapter 2-Influence of synthetic fertilizers and pesticides on soil health and soil microbiology,” in Agrochemicals detection, treatment and remediation. Eds. Narasimha, M. and Prasad, V. (Butterworth-Heinemann), 25–54. doi: 10.1016/B978-0-08-103017-2.00002-7
Vega, C., Rodríguez, M., Llamas, I., Béjar, V., and Sampedro, I. (2020). Silencing of phytopathogen communication by the halotolerant PGPR Staphylococcus equorum strain EN21. Microorganisms 8, 42. doi: 10.3390/microorganisms8010042
Vejan, P., Abdullah, R., Khadiran, T., Ismail, S., and Nasrulhaq, B. A. (2016). Role of plant growth promoting rhizobacteria in agricultural sustainability: a review. Molecules 21, 573. doi: 10.3390/molecules21050573
Venturi, V., Venuti, C., Devescovi, G., Lucchese, C., Friscina, A., Degrassi, G., et al. (2004). The plant pathogen Erwinia amylovora produces acyl-homoserine lactone signal molecules in vitro and in planta. FEMS Microbiol. Lett. 241, 179–183. doi: 10.1016/j.femsle.2004.10.015
Verma, R., Das, A., Sarmah, D. K., Narzary, P. R., Sharma, S., Kaman, P. K., et al. (2021). A review article: anti-quorum sensing agents as a potential replacement for antibiotics in Phytobacteriology. Pharm. Innov. J. 10, 121–125. doi: 10.22271/tpi.2021.v10.i11b.9200
Von Bodman, S. B., Bauer, W. D., and Coplin, D. L. (2003). Quorum sensing in plant-pathogenic bacteria. Annu. Rev. Phytopathol. 41, 455–482. doi: 10.1146/annurev.phyto.41.052002.095652
Wang, H., Lin, Q., Dong, L., Wu, W., Liang, Z., Dong, Z., et al. (2022). A bacterial isolate capable of quenching both diffusible signal factor- and N-acylhomoserine lactone-family quorum sensing signals shows much enhanced biocontrol potencies. J. Agric. Food. Chem. 70, 7716–7726. doi: 10.1021/acs.jafc.2c01299
Weimer, A., Kohlstedt, M., Volke, D. C., Nikel, P. I., and Wittmann, C. (2020). Industrial biotechnology of Pseudomonas putida: advances and prospects. Appl. Microbiol. Biotechnol. 104, 7745–7766. doi: 10.1007/s00253-020-10811-9
Weller, D. M. (2007). Pseudomonas biocontrol agents of soilborne pathogens: looking back over 30 years. Phytopathology 97, 250–256. doi: 10.1094/PHYTO-97-2-0250
Yan, S., Liu, H., Mohr, T. J., Jenrette, J., Chiodini, R., Zaccardelli, M., et al. (2008). Role of recombination in the evolution of the model plant pathogen Pseudomonas syringae pv. tomato DC3000, a very atypical tomato strain. Appl. Environ. Microbiol. 74, 3171–3181. doi: 10.1128/AEM.00180-08
Yates, E. A., Philipp, B., Buckley, C., Atkinson, S., Chhabra, S. R., Sockett, R. E., et al. (2002). N-Acylhomoserine lactones undergo lactonolysis in a pH-, temperature-, and acyl chain length-dependent manner during growth of Yersinia pseudotuberculosis and Pseudomonas aeruginosa. Infect. Immun. 70, 5635–5646. doi: 10.1128/IAI.70.10.5635-5646.2002
Yoon, S.-H., Ha, S.-M., Kwon, S., Lim, J., Kim, Y., Seo, H., et al. (2017). Introducing EzBioCloud: a taxonomically united database of 16S rRNA gene sequences and whole-genomeassemblies. Int. J. Syst. Evol. Microbiol. 67, 1613–1617. doi: 10.1099/ijsem.0.001755
Yu, M., Liu, N., Zhao, Y., and Zhang, X. (2018). Quorum quenching enzymes of marine bacteria and implication in aquaculture. J. Ocean Univ. China 48, 34–43. doi: 10.16441/j.cnki.hdxb.20170202
Zhou, Z., Wu, X., Li, J., Zhang, Y., Huang, Y., Zhang, W., et al. (2022). A novel quorum quencher, Rhodococcus pyridinivorans XN-36, is a powerful agent for the biocontrol of soft rot disease in various host plants. Biol. Control 169, 104889. doi: 10.1016/j.biocontrol.2022.104889
Zhu, X., Chen, W.-J., Bhatt, K., Zhou, Z., Huang, Y., Zhang, L.-H., et al. (2023). Innovative microbial disease biocontrol strategies mediated by quorum quenching and their multifaceted applications: a review. Front. Plant Sci. 13. doi: 10.3389/fpls.2022.1063393
Zou, J., Rodriguez-Zas, S., Aldea, M., Li, M., Zhu, J., Gonzalez, D. O., et al. (2005). Expression profiling soybean response to Pseudomonas syringae reveals new defense-related genes and rapid HR-specific downregulation of photosynthesis. Mol. Plant-Microbe interactions. 18, 1161–1174. doi: 10.1094/MPMI-18-1161
Keywords: Pseudomonas, plant-growth promoting bacteria, quorum quenching, phytopathogen, halotolerant bacteria
Citation: Sánchez P, Castillo I, Martínez-Checa F, Sampedro I and Llamas I (2025) Pseudomonas halotolerans sp. nov., a halotolerant biocontrol agent with plant-growth properties. Front. Plant Sci. 16:1605131. doi: 10.3389/fpls.2025.1605131
Received: 02 April 2025; Accepted: 30 April 2025;
Published: 21 May 2025.
Edited by:
Jean Luiz Simoes-Araujo, Embrapa Agrobiology, BrazilReviewed by:
Cesar Arriagada, University of La Frontera, ChileXinguo Li, Shandong Academy of Agricultural Sciences, China
Copyright © 2025 Sánchez, Castillo, Martínez-Checa, Sampedro and Llamas. This is an open-access article distributed under the terms of the Creative Commons Attribution License (CC BY). The use, distribution or reproduction in other forums is permitted, provided the original author(s) and the copyright owner(s) are credited and that the original publication in this journal is cited, in accordance with accepted academic practice. No use, distribution or reproduction is permitted which does not comply with these terms.
*Correspondence: Inmaculada Sampedro, aXNhbXBlZHJvQHVnci5lcw==