- 1Department of Botany and Microbiology, Faculty of Science, King Saud University, Riyadh, Saudi Arabia
- 2Department of Botany, Sri Pratap College, Srinagar, India
- 3Botany Department, Faculty of Science, South Valley University, Qena, Egypt
- 4Biology Department, College of Applied Medical Sciences, Taif University, Taif, Saudi Arabia
- 5Department of Plant Production, Faculty of Food and Agricultural Sciences, King Saud University, Riyadh, Saudi Arabia
- 6Mycology and Plant Disease Survey Department, Agriculture Research Center, Plant Pathology Research Institute, Giza, Egypt
- 7Pharmaceutical Biotechnology, Amity Institute of Pharmacy, Amity University, Uttar Pradesh, India
- 8Department of Chemistry, Centre for Environmental and Marine Studies, University of Aveiro, Aveiro, Portugal
- 9Centre for Environmental Research, Near East University, Lefkosa, Cyprus
This work examined the role of exogenously applied calcium (Ca; 50 mM) and potassium (K; 10 mM) (alone and in combination) in alleviating the negative effects of cadmium (Cd; 200 μM) on growth, biochemical attributes, secondary metabolites and yield of chickpea (Cicer arietinum L.). Cd stress significantly decreased the length and weight (fresh and dry) of shoot and root and yield attributes in terms of number of pods and seed yield (vs. control). Exhibition of decreases in chlorophyll (Chl) a, Chl b, and total Chl was also observed with Cd-exposure when compared to control. However, Cd-exposure led to an increase in the content of carotenoids. In contrast, the exogenous application of Ca and K individually as well as in combination minimized the extent of Cd-impact on previous traits. C. arietinum seedlings subjected to Cd treatment exhibited increased contents of organic solute (proline, Pro) and total protein; whereas, Ca and K-supplementation further enhanced the Pro and total protein content. Additionally, compared to control, Cd-exposure also caused elevation in the contents of oxidative stress markers (hydrogen peroxidase, H2O2; malondialdehyde, MDA) and in the activity of antioxidant defense enzymes (superoxide dismutase, SOD; catalase, CAT; ascorbate peroxidase, APX; glutathione reductase, GR). Ca, K, and Ca + K supplementation caused further enhancements in the activity of these enzymes but significantly decreased contents of H2O2 and MDA, also that of Cd accumulation in shoot and root. The contents of total phenol, flavonoid and mineral elements (S, Mn, Mg, Ca and K) that were also suppressed in Cd stressed plants in both shoot and root were restored to appreciable levels with Ca- and K-supplementation. However, the combination of Ca + K supplementation was more effective in bringing the positive response as compared to individual effect of Ca and K on Cd-exposed C. arietinum. Overall, this investigation suggests that application of Ca and/or K can efficiently minimize Cd-toxicity and eventually improve health and yield in C. arietinum by the cumulative outcome of the enhanced contents of organic solute, secondary metabolites, mineral elements, and activity of antioxidant defense enzymes.
Introduction
Cadmium (Cd), a naturally occurring element having no known beneficial role is a highly toxic environmental pollutant (Mobin and Khan, 2007; Shamsi et al., 2008; Asgher et al., 2015). Cd can enter the environment via multiple pathways including atmospheric deposition, wastewater irrigation, phosphatic fertilizers, usage of metal-containing pesticides and many industrial processes (Amirjani, 2012; Abdel Latef, 2013). Plants can easily uptake Cd by roots and transport it to shoots, which eventually cause toxicity there (Talukdar, 2012; Abdel Latef, 2013). Accumulation of Cd brings complex changes in plants at physiological, biochemical and genetic levels (El-Beltagi and Mohamed, 2013). The most obvious symptoms are: (i) inhibition of seed germination and suppression of plant growth (Siddique et al., 2012; Abdel Latef, 2013), (ii) degeneration of chlorophyll (Chl) synthesis and disturbance in Calvin cycle enzymes leading to decrease in photosynthesis (Mobin and Khan, 2007; Shamsi et al., 2008), and (iii) induction of stomatal closure due to its effect on the water balance of the plant (Perfus-Barbeoch et al., 2002). Additionally, tissue/organ-Cd-burden can alter carbohydrates, proline (Pro) and protein contents (Siddique et al., 2012; Abdel Latef, 2013; El-Beltagi and Mohamed, 2013; Mondal, 2013), perturb the absorption of nutrients such as N, P, K, Ca, Mg, Mn, Cu, Zn, Fe, and Ni (Sandalio et al., 2001; Siddique et al., 2012; Abdel Latef, 2013), and can also elevate the reactive oxygen species (ROS) levels (Ahmad et al., 2010, 2015). The excessive or non-metabolized ROS can lead to oxidation of organic molecules like lipids (Ahmad et al., 2010, 2015). The lipid peroxidation is generally reflected by increased concentration of malondialdehyde (MDA) content (Abdel Latef, 2013; Ahmad et al., 2015; Anjum et al., 2015).
Plant can sustain cellular ROS-attack through important endogenous protective strategies consisting of enzymatic and non-enzymatic systems (Ahmad et al., 2008, 2010, 2015, 2016a,b; Hasanuzzaman and Fujita, 2011, 2013). Antioxidant defense enzymes like superoxide dismutase (SOD), catalase (CAT), ascorbate peroxidase (APX), and glutathione reductase (GR) have been reported to reduce the concentrations of superoxide () and hydrogen peroxide (H2O2) content in plants (Ahmad et al., 2015). Accumulation of protective solutes like Pro and protein in the leaf is a unique plant response to heavy metal stress (El-Beltagi and Mohamed, 2013). Total phenols and flavonoids got induced under low concentrations of metal stress in plants; however, their levels can decline at higher metal concentrations (Cetin et al., 2014). During the last decade, efforts were made to control the level and improve the efficiency of above mentioned defense system components by exogenously supplying mineral elements (Anjum et al., 2012; Nazar et al., 2012) and phytohormones (Khan et al., 2012; Asgher et al., 2015) to the stressed plants.
Calcium (Ca) is a divalent cation and an essential element that plays important role in the structure and permeability of cell membranes, plant cell division and elongation, carbohydrate translocation and N-metabolism (White, 2000; El-Beltagi and Mohamed, 2013). Ca also plays regulatory role in plant cell metabolism, signal transduction and in the absorption of nutrients across cell membranes (Talukdar, 2012; El-Beltagi and Mohamed, 2013). Recent studies showed a major role of Ca in alleviating the inhibitory effects of Cd on growth and physiological processes of plants (Suzuki, 2005; Siddique et al., 2012; El-Beltagi and Mohamed, 2013; Ahmad et al., 2015). Potassium (K) is also an essential macronutrient and the most abundant cation in plant tissues. It consists of up to 10% on the basis of dry weight (Zhao et al., 2003; Siddique et al., 2012). K plays multifarious roles in plant growth and development as it stimulates cell elongation and preserves osmoregulation, and is also involved in stomatal movement, photosynthesis, decrease uptake of Cd, synthesis of soluble carbohydrates, protein, and soluble nitrogen containing compounds (Zhao et al., 2003; Siddique et al., 2012; Zorb et al., 2014). It also acts as a cofactor for many enzymes and activates several enzymes by changing their conformation through the stabilization of pH between 7.0 and 8.0 (Mengel, 2007; Siddique et al., 2012).
Legumes are more sensitive to Cd toxicity than cereals and grasses and thus they can lead to severe suppression of biomass and yield production even under minute quantities of Cd (Shamsi et al., 2008). Chickpea (Cicer arietinum L.) is an essential legume crop grown worldwide because it serves as a prime source of proteins for the growing population and it is also used as green manure and fodder for animals. Apart from the proteins, the seeds are also rich in fats and carbohydrates (Rasool et al., 2013, 2015). Insights into C. arietinum responses to Cd-exposure, and potential strategies for minimization of Cd-impacts are meager in literature. Additionally, the efficacy of Ca and K in alleviating Cd stress has not been tested in legumes such as C. arietinum. So, this work was undertaken to check whether exogenous application of Ca and K can protect C. arietinum health and productivity against Cd exposure. Notably, important physio-biochemical parameters, enzymatic activities and the status of organic osmolyte (Pro) and secondary metabolites were assessed to understand potential mechanisms underlying of Cd-tolerance in C. arietinum.
Materials and Methods
Plant Culture and Exposure Conditions
Seeds of chickpea (Cicer arietinum L.) were sown in pots containing peat, perlite and sand (1:1:1, v/v/v) under glasshouse conditions. Four-days old germinated C. arietinum seedlings were shifted to pots (one plant per pot) supplemented with nutrient solution (200 ml pot−1). Seedlings were allowed to grow for one more week at average day/night temperature of 24°C/15°C. The 11-day-old-plants were treated with different concentrations of Cd (CdSO4.8H2O) dissolved in nutrient solution with or without spray of Ca (CaCl2) and K (KCl2). Treatments consisted of: C- Nutrient solution alone (control); C + Ca: 0 μM Cd + 50 mM Ca + 0 mM K; C + K: 0 μM Cd + 0 mM Ca + 10 mM K; C + Ca + K: 0 μM Cd + 50 mM Ca + 10 mM K; Cd stress alone: 200 μM Cd + 0 mM Ca + 0 mM K; Cd + Ca: 200 μM Cd + 50 mM Ca + 0 mM K; Cd + K: 200 μM Cd + 0 mM Ca +10 mM K; Cd + Ca + K: 200 μM Cd + 50 mM Ca + 10 mM K.
Cd was applied to pots every week from the first day of treatment (i.e., 11-day-old plants) up to day 30 (41-day-old plants) excluding the control which was supplied with nutrient solution only. CaCl2 (50 mM) and KCl2 (10 mM) were mixed with Tween-20, and sprayed to plants with a manual sprayer (10 ml plant−1) every alternate day, from the 7th day of the treatment-initiation. The Ca and K concentrations were selected based on our preliminary experiments. Each treatment was replicated five times in a randomized block design and each replicate included 5 plants.
Estimations and Bioassays
Growth and Crop Yield
At the end of the experiment the plants were taken out of the pots and washed well with tap water. Shoot and root length was measured with a scale. Fresh weight (FW) of shoot and root was measured directly by weighing the fresh samples. For the dry weight (DW), the samples were oven dried for 24 h at 80°C and then weighed. Yield parameters (pods and seeds) were also measured manually.
Cadmium Accumulation
The Cd accumulation in the shoot and root tissues was determined using a Perkin-Elmer (Analyst Model 300) atomic absorption spectrophotometer. The heavy metal content was expressed as μg g−1.
Photosynthetic Pigments
The photosynthetic pigments were determined by the method of Hiscox and Israelstam (1979). The absorbances were read at 480, 510, 645, 663 nm by spectrophotometer (Beckman 640 D, USA) with DMSO as blank.
Proline and Protein
The Pro content in leaves was estimated by the method of Bates et al. (1973). The absorbance was measured spectrophotometerically (Beckman 640 D, USA) at 520 nm and toluene was used as blank.
The method of Bradford (1976) was employed for the estimation of protein from leaves. The absorbance was measured at 595 nm by spectrophotometer (Beckman 640 D, USA) with bovine serum albumin as blank.
Total Phenols and Flavonoids
The leaf sample was grounded and extracted with methanol at room temperature. The extract containing phenolic content was reduced by Folin-Ciocalteu reagent using the method of Chun et al. (2003). The mg gallic acid equivalent (GAE) g−1 of extract (mg g−1) expresses the total phenolic content. The flavonoids content was estimated as per colorimetric method described by Zhishen et al. (1999). Catechin was used as standard for the calibration curve. The absorbance was read at 510 nm by spectrophotometer (Beckman 640 D, USA) and flavonoids content was expressed as mg catechin equivalents g−1 of extract (mg g−1).
H2O2 and MDA
H2O2 in fresh leaves was estimated by the method of Velikova et al. (2000). The absorbance was read at 390 nm by spectrophotometer (Beckman 640 D, USA). For the estimation of MDA content in fresh leaves, the method of Heath and Packer (1968) was employed. The absorbances were recorded at 532 and 600 nm spectrophotometerically (Beckman 640 D, USA) for the calculation of MDA equivalents. Thiobarbituric acid (1%) in 20% trichloroacetic acid was used as control.
Enzyme Extractions and Assays
Fresh leaves (0.5 g/sample) were homogenized in presence of 100 mM Tris-HCl (5.0 ml, pH 7.5), 5.0 mM DTT (Dithiothreitol), 10 mM MgCl2, 1.0 mM EDTA (Ethylenediaminetetraacetic acid), 5.0 mM magnesium acetate and 1.5% Polyvinylpyrrolidone (PVP)-40. The reaction mixture was supplemented with serine and cysteine proteinase inhibitors [1.0 mM Phenylmethanesulfonyl fluoride (PMSF) + 1.0 μg/ml aproptinin]. The homogenate was centrifuged at 10,000 × g for 15 min (4°C) after the filtration through cheesecloth. The supernatants collected served as sources for determination of SOD (EC 1.15.1.1), CAT (EC 1.11.1.6), and GR (EC 1.6.4.2) activities. For the determination of APX activity, leaf sample was separately grounded in a homogenizing medium containing 2.0 mM ascorbic acid (AsA).
SOD activity was determined by the method of Van Rossum et al. (1997) after the photoreduction of nitrobluetetrazolium (NBT). The absorbance was recorded spectrophotometerically (Beckman 640 D, USA) at 560 nm. One unit of SOD is the quantity of protein that hampers 50% photoreduction of NBT and the activity was expressed as enzyme unit (EU) mg−1 protein.
The method of Luck (1974) was employed for the assay of CAT activity. The absorbance was recorded at 240 nm by spectrophotometer (Beckman 640 D, USA) and EU mg−1 protein expresses the CAT activity. The method of Nakano and Asada (1981) was used for the assay of APX activity (EC 1.11.1.11). The absorbance was measured spectrophotometerically (Beckman 640 D, USA) at 290 nm and EU mg−1 protein expresses the APX activity. One unit of APX is the quantity of protein used to break down 1.0 μmol of substrate per min at 25°C.
The activity of other important enzyme GR was estimated by the method of Carlberg and Mannervik (1985). The absorbance was read at 340 nm by spectrophotometer (Beckman 640 D, USA). GR activity was expressed as μmol NADPH oxidized min−1 (EU mg−1 protein).
Nutritional Elements
Dried shoot and root samples (0.1 g) were powdered and digested in H2SO4/HNO3 mixture (1/5, v/v) for 24 h, then were treated with HNO3/HClO4 mixture (5/1, v/v). Atomic absorption spectrophotometer (Analyst 300, Perkin-Elmer, Germany) was used for the estimation of elemental content (S, Mn, Mg, Ca, and K) in both shoot and root.
Statistical Analysis
Statistical analysis was executed utilizing one-way analysis of variance (ANOVA) followed by Duncan's Multiple Range Test (DMRT). The values are mean ± standard error (SE) of five replicates in each group. P ≤ 0.05 were considered as significant.
Results
Growth, Biomass Yield, and Yield Attributes
The length and weight (fresh and dry) of shoot and root and yield attributes in terms of number of pods and seed yield in C. arietinum subjected to Ca and/or K under Cd stress and normal conditions are presented in Table 1. Cd stress reduced the shoot length, root length, shoot FW, shoot DW, root FW, root DW, number of pods and seed yield by 58.25, 62.50, 41.26, 21.80, 48.17, 34.84, 49.60, and 52.62%, respectively in comparison with control. Application of Ca and K enhanced the shoot length by 66.76, 39.05, and 95.54% and root length by 34.79, 54.09, and 107.60% with Cd + Ca, Cd + K and Cd + Ca + K, respectively compared to Cd treated plants alone (Table 1). The shoot FW and DW were also elevated by the supplementation of Ca and K and the maximum increase in shoot FW and DW was 30.59 and 20.60%, respectively with Cd + Ca + K treatment compared to plants treated with Cd alone (Table 1). The root FW and DW also increased with addition of Ca and K and the highest increase in root FW (30.98%) and root DW (24.41%) was observed at Cd + Ca + K treatment over the plants treated with Cd alone (Table 1). The supplementation of Ca and K improved the pods by 29.42, 25.05, and 68.51% with Cd + Ca, Cd + K and Cd + Ca + K treatments, respectively relative to plants treated with Cd alone. The seed yield also increased by 52.45% with Cd + Ca, 47.13% with Cd + K and 92.21% with Cd + Ca + K treatments over the Cd treated plants alone (Table 1). Control plants treated with Ca and K showed significant elevation in above parameters compared to control plants alone and the more effective treatment was the combination of Ca + K compared to their individual effect (Table 1).
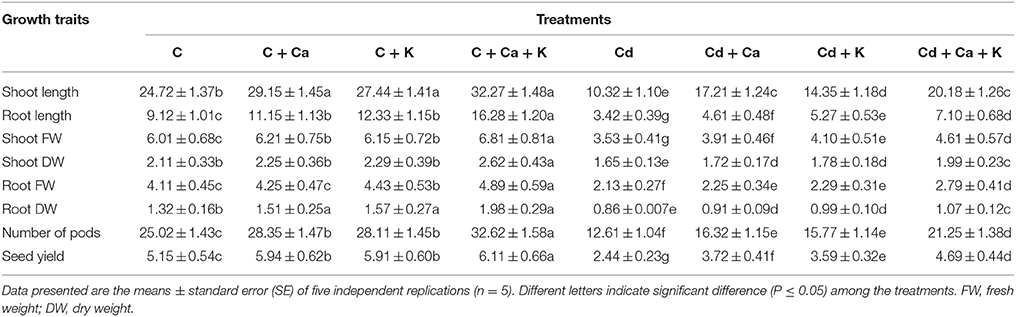
Table 1. Effect of calcium (Ca) and potassium (K) alone and in combination on shoot and root length (cm plant−1), shoot FW and DW (g plant−1), root FW and DW (g plant−1), number of pods (pod plant−1) and seed yield (g plant−1) in chickpea (Cicer arietinum L.) plants under normal and cadmium (Cd) stress conditions.
Cadmium Accumulation
The accumulation of Cd was about 2.40-fold in root as compared to shoot. Cd stressed plants treated with Ca and K recorded the suppression of Cd accumulation by 69.04, 61.72, and 78.29% with Cd + Ca, Cd + K and Cd + Ca + K, respectively in shoot compared to shoot in Cd treated plants alone. The root Cd accumulation was also reduced by 82.39% with Cd + Ca, 69.76% with Cd + K and 85.87% with Cd + Ca + K treatments relative to plants treated with Cd alone (Table 2).

Table 2. Effect of calcium (Ca) and potassium (K) alone and in combination on cadmium (Cd) content in shoot and root (μmol g−1 DW) in chickpea (Cicer arietinum L.) seedlings under normal and cadmium (Cd) stress conditions.
Photosynthetic Pigment Content
Chl a, Chl b and total Chl decreased by 48.02, 46.51, and 47.69%, respectively in Cd treated plants relative to control (Table 3). On the other hand, Cd stress significantly increased carotenoids content by 35.89% over the control. However, application of Ca or K alone as well as in combination improved photosynthetic pigments in Cd stressed plants. An increase by 44.30, 69.56, 50.00, and 45.28% in Chl a, Chl b, total Chl and carotenoids, respectively was observed with treatment Cd + Ca + K compared to the plants treated with Cd alone. Control plants supplemented with Ca and K alone as well as in combination also showed increase in pigment content (Table 3).
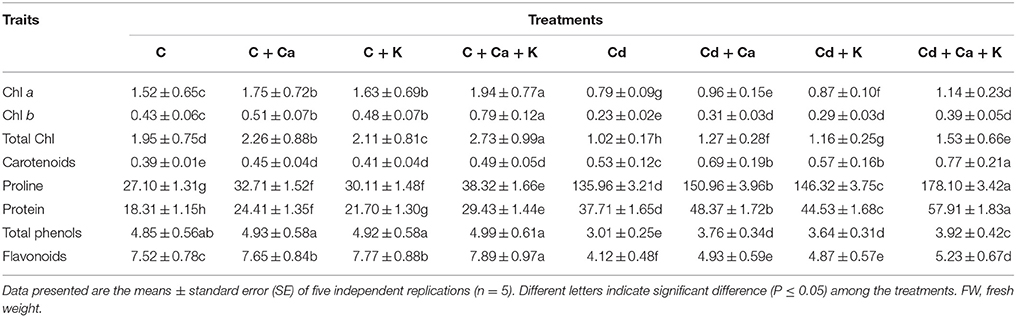
Table 3. Effect of calcium (Ca) and potassium (K) alone and in combination on the contents of chlorophyll (Chl) (mg g−1 FW), proline (μg g−1 FW), protein (mg g−1 FW), total phenols (mg gallic acid equivalent (GAE) g−1extract) and flavonoids content (mg Catechin g−1extract) in chickpea (Cicer arietinum L.) leaves under normal and cadmium (Cd) stress conditions.
Proline and Protein Contents
The results related to the effect of Ca and K on Pro and protein content in Cd stressed chickpea plants are presented in Table 3. The Pro and protein contents increased by 5- and 2-fold, respectively in Cd stressed plants relative to control. Supplementation of Ca and K showed further accumulation in Pro and protein content and the maximum increase in Pro (30.99%) and protein (53.57%) was recorded in plants treated with Cd + Ca + K treatment over the plants treated with Cd alone. Application of Ca and K in combination was more effective in control as well as in treated plants (Table 3).
Total Phenols and Flavonoids
The total phenols and flavonoids contents were declined by 37.93 and 45.21% in Cd stressed plants comparison to control plants (Table 3). However, supplementation of Ca and K increased the total phenols by 24.91, 20.93, and 30.23% with Cd + Ca, Cd + K and Cd +Ca + K treatments, respectively as compared to plants treated with Cd alone. Insignificant increase in total phenols was observed by the application of Ca and K individually as well as in combination to control plants (Table 3). Flavonoids content also enhanced by 19.66% with Cd + Ca, 18.20% with Cd + K and 26.94% with Cd + Ca + K treatments relative to Cd treated plants alone (Table 3). Ca and K in combination were proved to me more beneficial as compared to their individual effect in both control and Cd treated plants. Significant increase in flavonoids content was observed in plants supplied with Ca and K individually as well as in combination (Table 3).
H2O2 and MDA
Cd treatment led to a sharp accumulation of H2O2 (191.24%) and MDA (49.05%) over the control plants. Addition of Ca and K to Cd treated plants reduced the H2O2 accumulation by 27.68% with Cd+Ca, 21.99% with Cd + K and 49.20% with Cd + Ca + K treatments compared to plants treated with Cd alone. The MDA content also decreased by 16.56, 8.06, and 27.39% with Cd + Ca, Cd + K and Cd + Ca + K, respectively relative to Cd treated plants alone (Figures 1A,B).
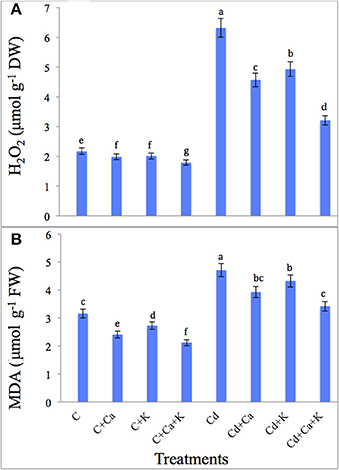
Figure 1. Effect of calcium (Ca) and potassium (K) alone and in combination on H2O2 (A), and lipid peroxidation (MDA) (B) in chickpea seedlings under cadmium (Cd) stress. Data presented are the means ± SE (n = 5). Different letters indicate significant difference (P ≤ 0.05) among the treatments.
Activities of Antioxidant Enzymes
Cd stress boosted the SOD, CAT, APX, and GR activity by 67.99, 83.90, 183.67, and 215.81%, respectively as compared to control plants. Cd treated plants supplemented with Ca and K further enhanced the activities of SOD by 21.08%, CAT by 14.03%, APX by 70.43% and GR by 53.52% with Cd + Ca + K treatments over the plants treated with Cd alone (Figures 2A–D). Control plants supplemented with Ca and K individually as well as in combination also boosted antioxidant activity in untreated plants (Figures 2A–D).
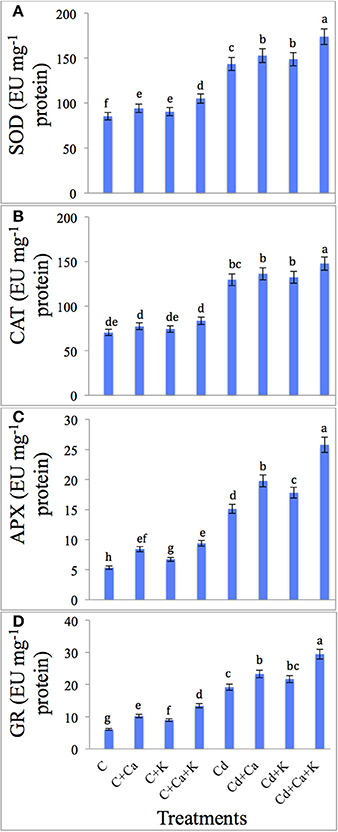
Figure 2. Effect of calcium (Ca) and potassium (K) alone and in combination on the activity of superoxide dismutase (SOD) (A), catalase (CAT) (B), ascorbate peroxidase (APX) (C), and glutathione reductase (GR) (D) in chickpea seedlings under cadmium (Cd) stress. Data presented are the means ± SE (n = 5). Different letters indicate significant difference (P ≤ 0.05) among the treatments.
Mineral Elements
Cd stress significantly affected the pools of mineral nutrients in C. arietinum shoot and root. S, Mn, Mg, Ca and K contents in shoot were declined by 36.74, 64.04, 47.14, 33.84, and 49.20%, respectively with Cd treatment under the control plants. The root mineral elements also showed decline by 48.21, 16.52, 18.36, 14.15, and 37.22% in S, Mn, Mg, Ca, and K, respectively with Cd treatment relative to control plants. The application of Ca and K individually as well as in combination improved the mineral elements in both shoot and root under Cd toxicity. The combined effect of Ca + K proved to be more beneficial in comparison to individual effects of Ca and K in restoring the mineral nutrients in shoot and root in control as well as in Cd treated plants (Table 4).
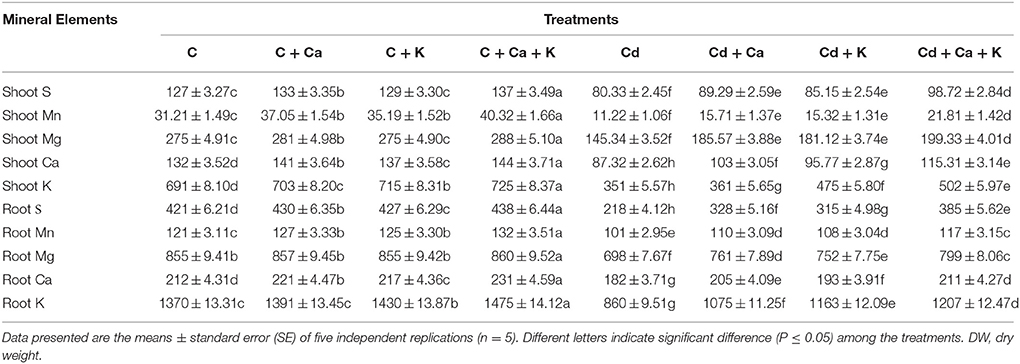
Table 4. Effect of calcium (Ca) and potassium (K) alone and in combination on mineral elements content in shoot and root (μg g−1 DW) of chickpea (Cicer arietinum L.) plants under normal and cadmium (Cd) stress conditions.
Discussion
Cd is a non-essential element and its accumulation hampers the growth and development of the plant (Abdel Latef, 2013; Ahmad et al., 2015; Asgher et al., 2015). The suppression in growth under Cd toxicity may be attributed to restricted water and nutritional uptake by plant roots (Dinakar et al., 2009), disturbance in photosynthesis (Dong et al., 2005), reduction of cell wall components and changes in carbohydrates metabolism (Abdel Latef, 2013; Hussain et al., 2013). Our results are in harmony with Mondal (2013) who reported that the reduction of root growth may be due to direct interference of Cd with some hydrolytic enzymes, which plays a pivotal role in transporting the food to the primary root and shoot. He also reported that the reduction in the shoot length may be due to the direct inhibition of cell elongation or cell division, retarded root growth and low nutrients/water transport to the upper parts of shoot (Mondal, 2013). Foliar application of Ca and K alone as well as in combination mitigated the negative impact of Cd toxicity and enhanced the plant growth and yield attributes of chickpea plants (Figure 3), indicating the direct or indirect role of both elements in improving plant growth and biomass yield even under heavy metal stress. Ca may favor cell elongation and cell expansion, finally enhancing the plant growth and was also reported by Hernandez and Almansa (2002) in pea and Abdel Latef (2011a) in canola. This finding is in consistence with Ismail (2008) who reported that supplementation of Ca mitigated the growth inhibition induced by Cd toxicity in common bean. Our previous work on mustard also confirmed that Ca alleviated the Cd induced suppression in growth and biomass yield (Ahmad et al., 2015). Eller and Brix (2016) also proved that shoot and root biomass was enhanced by addition of Ca to Cd stressed Brassica juncea plants. K plays a vital role in nullifying the harmful effects of stress on plants as it serves as a catalyst for various enzymatic processes that are essential for plant growth and development (Cakmak, 2005). Deficiency of K in plants has been reported to decrease the rate of net photosynthesis and ribulose-1, 5-bisphosphate carboxylase activity (Cakmak, 2005; Weng et al., 2007). Song et al. (2015) have reported that application of K restricted the fresh weight of peach (Prunus persica) seedlings under Zn-toxicity. According to the present study Ca and K in combination proved to be more effective than individual Ca and K and the results are in conformity with the finding of Siddique et al. (2012) in Vicia faba under Cd toxicity.
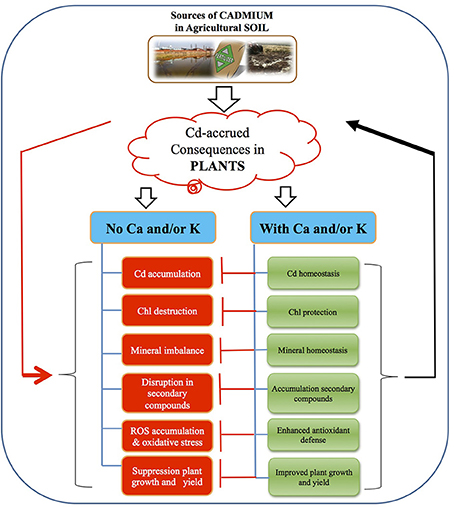
Figure 3. A possible model showing the strategy of calcium (Ca) and/or potassium (K) induced cadmium tolerance in chickpea. Exposure of C. arietinum to Cd caused an increased in uptake and accumulation of Cd in plant cells. Elevated cellular-Cd induced chlorophyll (Chl)-destruction, ionic disorder, disruption in secondary metabolites, generation of reactive oxygen species (ROS), leading to suppression of plant growth and yield. On the other side, supplementation of Ca and or K resulted into mineral nutrient homeostasis, increased Chl content, accumulation of secondary compounds and higher antioxidant capacity all of which contributed to the mitigation of Cd-induced damage, leading to improved plant growth and yield.
Cd decreased the number of pods and seed yield which coincides with the findings of Siddhu and Khan (2012) in Phaseolus mungo. Fatoba et al. (2012) also reported the decrease in yield parameters with increasing concentration of Cd in Arachis hypogaea and Glycine max. The negative effects of Cd stress on yield attributes have also been reported by Kumar and Dhingra (2005) in mungbean and Ali Khan and Siddhu (2006) in Vigna mungo. Application of Ca and K enhanced the yield parameters under Cd stress in the current study that may be attributed to (i) increased mineral and water uptake, (ii) restoration of photosynthetic pigments and rehabilitation of other metabolic processes affected by Cd stress.
The accumulation of Cd was more in root compared to shoot and the results coincided with previous reports on different plant species (Amirjani, 2012; Mondal, 2013). The root is first entry point for immobilization of Cd by means of the cell wall and extracellular carbohydrates (Amirjani, 2012). The lower accumulation of Cd in shoot may be due to the retention of Cd ions in the root and preventing the transport of excess Cd to the aerial parts of C. arietinum. The application of Ca and K individually or in combination reduced accumulation of Cd in both root and shoot in the present study (Figure 3). The protection provided by Ca for alleviating Cd toxicity can be due to various mechanisms like (i) the displacement of cell-surface toxic cations by Ca reduced the negativity of plasma membrane and mitigation the deleterious effect of cationic toxicants (Kinraide, 1998) (ii) elimination of anxious Cd by the formation and exudation of Cd/Ca containing crystals from the trichome head cells (Choi et al., 2001) (iii) Cd uptake via Ca channels blockers, diltiazem, verapmil, nifedipine and nitrendipine (Hinkle et al., 1987) (iv) competition for metal ion influx (Suzuki, 2005), and (v) complexing Cd with phytochelatins such as metalothionin and cysteine-rich proteins (Suzuki et al., 2002; Song et al., 2004). The impact of K fertilizers on plant accumulation of Cd has rarely been studied in detail earlier. Availability of K minimizes the uptake of Cd as both ions (Cd and K) are in competition for the same transmembrane carrier (Clarkson and Luttge, 1989; Rivetta et al., 1997). Noraho and Gaur (1995) also reported that K application resulted in less Cd uptake in Lemna polyrrhiza. The molecular mechanism how K decreases uptake of Cd needs further elucidation.
Our data regarding the photosynthetic pigments (Chl a, b, and total Chl) in C. arietinum showed decline with Cd stress. This decline may be due to (i) inhibition of photosynthetic electron transport chain (Siddique et al., 2012), (ii) inhibition of the sulfhydryl requiring enzymes of Chl biosynthesis (Siddique et al., 2012), (iii) damage of PS II reaction center in the leaf (Mondal, 2013), (iv) replacement the central Mg2+ of Chl in plants to prevent light harvesting (Abdel Latef, 2013), (v) reduction the uptake of P which contributes to pigment biosynthesis (Abdel Latef, 2013) (vi) induction of lipid peroxidation, which causes degradation of photosynthetic pigments (Abdel Latef, 2013; Ahmad et al., 2015). Carotenoids are categorized in lipophilic antioxidant group and have the ability to scavenge various forms of ROS such as singlet oxygen (1O2) (Mourato et al., 2012). Carotenoids act as precursors to signaling molecules that have a positive impact on plant growth and development and responses under biotic and abiotic stress (El-Beltagi and Mohamed, 2013). Interestingly, in our study, the synthesis of carotenoids decreased the oxidative damage induced by Cd toxicity and is also reported by El-Beltagi and Mohamed (2013) in Pisum sativum and Abdel Latef and Abu Alhmad (2013) in broad bean with copper stress. Supplementing the medium with Ca enhanced photosynthetic pigments in Vicia faba (Siddique et al., 2012) and Brassica juncea (Ahmad et al., 2015) under Cd toxicity. Ca served as secondary messenger for cytokinin action in improving synthesis of Chl (Lechowski and Bialczyk, 1993). K also plays a remarkable role in development of photosynthetic pigments by preventing degradation of newly formed Chl and aminolevulinic acid (ALA) (Siddique et al., 2012). Song et al. (2015) also reported that application of K enhanced the photosynthetic efficiency by increasing chlorophyll content in zinc stressed P. persica. Thus, the improvements in photosynthetic pigments are due to the combined effect of Ca and K that provides tolerance to C. arietinum plants against Cd stress (Figure 3).
Pro accumulation under Cd exposure appears to be prevalent among plants (Zhao, 2011; Siddique et al., 2012; El-Beltagi and Mohamed, 2013). Pro plays a pivotal role in protection of plants from abiotic and biotic stresses (Ahmad et al., 2010, 2011, 2012a,b, 2014, 2015; Siddique et al., 2012; Abdel Latef and Tran, 2016). The possible roles of Pro in heavy metals toxicity can be due to (i) its ability to balance the plant water potential (Fariduddin et al., 2009), (ii) its ability to save enzymes from inhibition (Sharma and Dietz, 2006), (iii) its function as metal chelator (Sharma et al., 1998), and (v) its antioxidant property (Zhao, 2011). The accumulation of protein during heavy metal stress may furnish a reserve form of N that could be re-utilized later and helps in osmoregulation. The greater increase in protein content under Cd stress was followed by a significant decline in growth. Thus, it may be suggested that under these conditions, C. arietinum transfer most of the synthesized protein from a state of growth to a state of osmoregulation (survival). Thus, C. arietinum under Cd stress synthesized the protein for survival rather than for growth. However, application of Ca and K together was found to be efficient for augmenting further accumulation of Pro and protein under Cd stress. Ca signaling is involved in Probiosynthesis (Verslues and Sharma, 2010). Calmodulin, a Ca signaling unit has been reported to activate MYB (myeloblastosis) transcription factor which further activates different downstream genes including Δ1- pyrroline-5-carboxlyate synthetase 1 (P5CS1) (Yoo et al., 2005). Phospholipase “C” is another calcium signaling component which upregulated the expression of P5CS1 gene in Arabidopsis under salt stress (Parre et al., 2007). The Pro biosynthesis occurs through the activation of the precursors like glutemic acid, arginine and ornithine (Stewart and Bogess, 1977) and N-acetyl gutamic acid (Morris et al., 1969). K has the ability to interact with arginine in synergistic way enhancing the Pro content (Nageswararao et al., 1981). The accumulation of protein by application of Ca in the present study is in accordance with Abdel Latef (2011a) in canola plants under seawater stress. Recently, Yousuf et al. (2015) also reported that, application of K and Ca fertilizers regained the protein content in salt-stressed Indian mustard. K supplementation also enhanced the protein content in salt stressed wheat plants (Zheng et al., 2008). K has a leading role in plant protein synthesis (Blevins, 1985). The role of Ca and K in enhancing protein content may also be attributed to enhanced protein synthesis, decreased proteolysis, lowering of enzyme denaturation that were disturbed during abiotic stress (Levitt, 1980).
The decrease in total phenols and flavonoids content subjected to Cd stress is corroborated with the findings of Kapoor et al. (2014). Lachman et al. (2005) also reported the decrease in flavonoids content with increasing concentration of Cd in barley. Bai et al. (2004) reported that flavonoids play a great role in metal chelation. The increase in Cd content decreased the free flavonoids content in the present study. Low concentration of Cd stress increases the flavonoids content in many plants; however, at higher concentrations a decrease was observed (Cetin et al., 2014) as also observed in present study. Phenolic compounds played a key role in protecting the plants against toxic effects induced by different stresses. Total phenols and flavonoids have the antioxidant property because they are electron-donating agents (Michalak, 2006), thus scavenge ROS. Phenolic compounds are synthesized quickly after stress exposure through the signaling processes (Bais et al., 2002; Li et al., 2005). Application of Ca and K increased the total phenols and flavonoids content under Cd stress (Figure 3). The mechanism underlying previous process is still unclear. However, Ca and K-mediated increase the total phenols and flavonoids can be possible since: (i) Ca and K hamper the uptake of Cd as described above, (ii) Ca has a role in signaling and helps in upregulation of respective genes for polyphenols biosynthesis (Xu et al., 2014), and (iii) the reduced Cd in the cells has the ability to stimulate the phenylalanine ammonia lyase (PAL) enzyme activity, an important enzyme in phenylpropanoid biosynthesis (Kuthanova et al., 2004).
H2O2 production is very harmful for many biochemical reactions; however, it also plays a signaling role in plant growth and development (Mazid et al., 2011). H2O2 also interferes with the Calvin cycle that finally leads to decreased rate of photosynthesis (Hussain et al., 2013) and is usually proclaimed stress criterion in plants (Mourato et al., 2012). Cd stress causes lipid peroxidation and is a result of oxidative stress (Abdel Latef, 2013). MDA is the end product of peroxidation of lipid membranes and its concentration is ordinarily considered as a general criterion of lipid peroxidation as well as the stress level (Shamsi et al., 2008; Abdel Latef, 2011a, 2013; Ahmad et al., 2015; Anjum et al., 2015). Cd stress induced the production of ROS resulting in malfunctioning of biomolecules (Ahmad et al., 2015, 2016a). Cd-induced loss of membrane permeability, coupled with increased MDA production, has also been observed by Abdel Latef (2013) in pepper (Capsicum annuum) and Hussain et al. (2013) in Zea mays seedlings. Our results are also in agreement with findings of Li et al. (2016) who reported Cd-induced oxidative stress via an enhanced production of H2O2 and MDA contents in Arabidopsis. However, the Cd stressed plants that were subjected to Ca and K alone as well as in combination, showed less accumulation of H2O2 and MDA content (Figure 3). This might be due to the activity of antioxidant enzymes and Pro accumulation which have an adaptive significance, because of their role in detoxification of free radicals thus reducing oxidative damage to membranes under Cd stress (Siddique et al., 2012; Li et al., 2016). Talukdar (2012) reported that mitigation of Cd-induced stress by Ca application was due to decline in the levels of H2O2 accumulation and consequently protects the membranes from oxidative damage. Li et al. (2016) reported that Ca protection against Cd-induced oxidative injuries is achieved through avoidance of H2O2 generation as well as the reduction of Cd uptake. Ca binds to the membrane phospholoipids thus stabilizing the lipid bilayer and providing the structural integrity (Hirschi, 2004; Yousuf et al., 2015) and is exhibited by the reduced MDA content in the plants treated with Ca (Siddique et al., 2012; Talukdar, 2012; Li et al., 2016). K plays an active role in reducing ROS production by decreasing the activity of NAD(P)H oxidases and maintaining photosynthetic electron transport (Cakmak, 2005; Siddique et al., 2012).
Plants synthesize numerous antioxidant enzymes as defense mechanism to cope with the oxidative stress (Foyer and Shigeoka, 2011). The antioxidant enzymes such as SOD, CAT, APX and GR are important components for preventing the oxidative stress in plants (Li et al., 2016). SOD is the first line of defense that catalyzes the dismutation of radicals to H2O2 and O2 (Alscher et al., 2002; Mourato et al., 2012). H2O2 is also toxic to plant cells and CAT carries out its decomposition to water and oxygen (Ahmad et al., 2015). APX showed much attraction toward H2O2 as compared to CAT, advocating that they have different roles in ROS detoxification. CAT is accountable for the elimination of surplus H2O2 and APX is labile for maintaining lower concentrations of H2O2 (Mittler, 2002; Mourato et al., 2012). GR is important in maintaining the pool of reduced glutathione by H2O2 removal and by activating the ascorbate-glutathione cycle (Asada, 1999; Abdel Latef, 2011b). In this work, antioxidant enzymes (SOD, CAT, APX, and GR) increased markedly under Cd stress. The increase in antioxidant enzyme activity might be due to the overproduction of H2O2, which would produce oxidative damages in Cd stressed plants (Li et al., 2016). The elevation in the activities of these enzymes showed that C. arietinum had the ability to acclimate Cd stress by developing an antioxidant defense system. In our work, GR was stimulated more than other antioxidant enzymes under Cd stress suggesting that GR is a major player in H2O2-scavenging in Cd stressed plants. An elevated activities of antioxidant enzymes and lower hydrogen peroxide and lipid peroxidation with Ca application suggested that Ca prevented the structural and functional deterioration of cell membranes (Abdel Latef, 2011a; Ahmad et al., 2015; Figure 3). Recent studies reported that application of Ca was found to be effective in enhancing the tolerance of plant to Cd stress by enhancing antioxidant systems (Siddique et al., 2012; Talukdar, 2012; El-Beltagi and Mohamed, 2013). The enhanced activity of antioxidant enzymes may also be due to the active role of K, which activates more than 60 enzyme systems which aid in photosynthesis, regulate stomatal opening and nutrient-translocation, and enhancement in starch synthesis (Shamsi et al., 2008). Under stress, K also plays great role in protein synthesis, e.g., thioredoxin, glutaredoxin, cyclophilin which facilitate regeneration of the reduced form of peroxiredoxins participate in reducing the ROS formation in plants under biotic and abiotic stress (Tripathi et al., 2009; Siddique et al., 2012). Increases in the activities of all four-enzymes led to decrease in the level of both H2O2 and MDA content in response to application of Ca and K. Consequently, this ensured recovery of plant growth by mitigating the oxidative damage induced by Cd treatment in chickpea plants (Figure 3).
Increased Cd concentration also decreased the uptake of mineral elements as reported by Gonçalves et al. (2009). The plant nutrients and Cd ions can compete for the same transporters; therefore, presence of Cd can result in mineral nutrients deficiency (Nazar et al., 2012; Figure 3). Rivelli et al. (2014) also reported that increasing concentration of Cd decreased the essential mineral uptake in sunflower. Exogenous application of Ca and K alleviated the negative effect of Cd on these nutrients in the present study (Figures 2A–D). Application of Ca and K might have decreased the Cd-competition for the same transporter. Ca also blocks the entry of Cd in root through Ca channels, and controls the translocation of root-Cd to leaves. Thus, applied Ca to Cd-exposed plants can restore their transpiration and photosynthesis (Hayakawa et al., 2011).
Conclusions
It is inferred from the results of present work that exogenous application of Ca and/or K mitigated the negative effects of Cd on C. arietinum through osmoregulation, by adjusting the membrane stability, photosynthetic pigments and lowering H2O2 and MDA contents. Further, protection under Cd stress was achieved via improved activities of antioxidant enzymes such as SOD, CAT, APX, and GR. Supplementation of Ca and K improved the secondary metabolites that were suppressed by Cd-exposure. The study also confirmed Cd-accrued decreases in the uptake of essential mineral elements and the crop yield. Contrarily, exogenously applied Ca and K improved the crop yield through the enhancing uptake of mineral elements and the biosynthesis of photosynthetic pigments. Thus, application of mineral nutrients could be a strategy to improve the growth, development and economic yield in plants/crops growing in toxic metal-polluted soils.
Author Contributions
PA, AA, EA, and AH designed and performed the experimental work. PA, AA, EA, and AH have written the manuscript. MS, NAA and SG contributed in discussion part. MS and SG have done the statistical analysis, and formatting of the paper.
Conflict of Interest Statement
The authors declare that the research was conducted in the absence of any commercial or financial relationships that could be construed as a potential conflict of interest.
Acknowledgments
The authors would like to extend their sincere appreciation to their institutions and acknowledges Deanship of Scientific Research at King Saud University for Funding the Research Group Project no RGP-271.
References
Abdel Latef, A. A. (2011a). Ameliorative effect of calcium chloride on growth, antioxidant enzymes, protein patterns and some metabolic activities of canola (Brassica napus L.) under seawater stress. J. Plant Nutr. 34, 1303–1320. doi: 10.1080/01904167.2011.580817
Abdel Latef, A. A. (2011b). Influence of arbuscular mycorrhizal fungi and copper on growth, accumulation of osmolyte, mineral nutrition and antioxidant enzyme activity of pepper (Capsicum annuum L.). Mycorrhiza 21, 495–503. doi: 10.1007/s00572-010-0360-0
Abdel Latef, A. A. (2013). Growth and some physiological activities of pepper (Capsicum annuum L.) in response to cadmium stress and mycorrhizal symbiosis. J. Agri. Sci. Tech. 15, 1437–1448.
Abdel Latef, A. A., and Abu Alhmad, M. F. (2013). Strategies of copper tolerance in root and shoot of broad bean (Vicia faba L.). Pak. J. Agri. Sci. 50, 323–328.
Abdel Latef, A. A., and Tran, L.-S. P. (2016). Impacts of priming with silicon on the growth and tolerance of maize plants to alkaline stress. Front. Plant Sci. 7:243. doi: 10.3389/fpls.2016.00243
Ahmad, P., Abd_Allah, E. F., Hashem, A., Sarwat, M., and Gucel, S. (2016a). Exogenous application of selenium mitigates cadmium toxicity in Brassica juncea L. (Czern & Cross) by up-regulating antioxidative system and secondary metabolites. J. Plant Growth Regul. (in press). doi: 10.1007/s00344-016-9592-3
Ahmad, P., Abdel Latef, A. A., Hashem, A., Abd_Allah, E. F., Gucel, S., and Tran, L. S. P. (2016b). Nitric oxide mitigates salt stress by regulating levels of osmolytes and antioxidant enzymes in chickpea. Front. Plant Sci. 7:347. doi: 10.3389/fpls.2016.00347
Ahmad, P., Hakeem, K. R., Kumar, A., Ashraf, M., and Akram, N. A. (2012a). Salt-induced changes in photosynthetic activity and oxidative defense system of three cultivars of mustard (Brassica juncea L.). Afr. J. Biotechnol. 11, 2694–2703. doi: 10.5897/AJB11.3203
Ahmad, P., Jaleel, C. A., and Sharma, S. (2010). Antioxidative defence system, lipid peroxidation, proline metabolizing enzymes and biochemical activity in two genotypes of Morus alba L. subjected to NaCl stress. Russ. J. Plant Physiol. 57, 509–517. doi: 10.1134/S1021443710040084
Ahmad, P., Nabi, G., and Ashraf, M. (2011). Cadmium-induced oxidative damage in mustard [Brassica juncea (L.) Czern. & Coss.] plants can be alleviated by salicylic acid. South Afr. J. Bot. 77, 36–44. doi: 10.1016/j.sajb.2010.05.003
Ahmad, P., Ozturk, M., and Gucel, S. (2012b). Oxidative damage and antioxidants induced by heavy metal stress in two cultivars of mustard (L) plants. Fres. Environ. Bull. 21, 2953–2961.
Ahmad, P., Ozturk, M., Sharma, S., and Gucel, S. (2014). Effect of sodium carbonate-induced salinity-alkalinity on some key osmoprotectants, protein profile, antioxidant enzymes, and lipid peroxidation in two mulberry (Morus alba L.) cultivars. J. Plant Interact. 9, 460–467. doi: 10.1080/17429145.2013.855271
Ahmad, P., Sarwat, M., Bhat, N. A., Wani, M. R., Kazi, A. G., and Tran, L. S. (2015). Alleviation of cadmium toxicity in Brassica juncea L. (Czern. & Coss.) by calcium application involves various physiological and biochemical strategies. PLoS ONE 10:e0114571. doi: 10.1371/journal.pone.0114571
Ahmad, P., Sarwat, M., and Sharma, S. (2008). Reactive oxygen species, antioxidants and signaling in plants. J. Plant Biol. 51, 167–173. doi: 10.1007/BF03030694
Ali Khan, M. A., and Siddhu, G. (2006). Phytotoxic effect of cadmium (Cd) on physiology of Urd bean [Vigna mungo (L.) Heeper]. Adv. Plant Sci. 19, 439–444.
Alscher, R. G., Erturk, N., and Heath, L. S. (2002). Role of superoxide dismutases (SODs) in controlling oxidative stress in plants. J. Exp. Bot. 53, 1331–1341. doi: 10.1093/jexbot/53.372.1331
Amirjani, M. R. (2012). Effects of cadmium on wheat growth and some physiological factors. Int. J. Soil. Eros. 2, 50–58.
Anjum, N. A., Gill, S. S., Umar, S., Ahmad, I., Duarte, A. C., and Pereira, E. (2012). Improving growth and productivity of oleiferous Brassicas under changing environment: significance of nitrogen and sulphur nutrition, and underlying mechanisms. Sci. World J. 2012:657808. doi: 10.1100/2012/657808
Anjum, N. A., Sofo, A., Scopa, A., Roychoudhury, A., Gill, S. S., Iqbal, M., et al. (2015). Lipids and proteins-major targets of oxidative modifications in abiotic stressed plants. Environ. Sci. Pollut. Res. 22, 4099–4121. doi: 10.1007/s11356-014-3917-1
Asada, K. (1999). The water-water cycle in chloroplasts: scavenging of active oxygens and dissipation of excess photons. Annu. Rev. Plant Physiol. Plant Mol. Biol. 50, 601–639. doi: 10.1146/annurev.arplant.50.1.601
Asgher, M., Khan, M. I. R., Anjum, N. A., and Khan, N. A. (2015). Minimizing toxicity of cadmium in plants - role of plant growth regulators. Protoplasma 252, 399–413. doi: 10.1007/s00709-014-0710-4
Bai, Y., Song, F., Chen, M., Xing, J., Liu, Z., and Liu, S. (2004). Characterization of the rutin-metal complex by electrospray ionisation tandem mass spectrometry. Analytical Sci. 20, 1147–1151. doi: 10.2116/analsci.20.1147
Bais, H. P., Walker, T. S., Schweizer, H. P., and Vivanco, J. M. (2002). Root specific elicitation and antimicrobial activity of rosmarinic acid in hairy root cultures of Ocimum basilicum. Plant Physiol. Biochem. 40, 983–995. doi: 10.1016/S0981-9428(02)01460-2
Bates, L., Waldren, P. P., and Teare, J. D. (1973). Rapid determination of free proline of water stress studies. Plant Soil 39, 205–207. doi: 10.1007/BF00018060
Blevins, D. G. (1985). Role of potassium in protein metabolism in plants in Potassium in Agriculture, ed R. D. Munson (Madison, WI: ASA-CSSA-SSSA), 413–424.
Bradford, M. M. (1976). A rapid and sensitive method for the quantization of microgram quantities of pro- tein using the principle of protein-dye binding. Anal. Biochem. 72, 248–259. doi: 10.1016/0003-2697(76)90527-3
Cakmak, I. (2005). The role of potassium in alleviating detrimental effects of abiotic stresses in plants. J. Plant Nutr. Soil Sci. 168, 521–530. doi: 10.1002/jpln.200420485
Carlberg, I., and Mannervik, B. (1985). Glutathione reductase. Methods Enzymol. 113, 484–490. doi: 10.1016/S0076-6879(85)13062-4
Cetin, E. S., Babalik, Z., Hallac-Turk, F., and Gokturk-Baydar, N. (2014). The effects of cadmium chloride on secondary metabolite production in Vitis vinifera cv. cell suspension cultures. Biol. Res. 47:47. doi: 10.1186/0717-6287-47-47
Choi, Y. E., Harada, E., Wada, M., Tsuboi, H., Mortia, Y., Kusano, T., et al. (2001). Detoxification of cadmium in tobacco plant: formation and active excretion of crystals containing cadmium and calcium through trichomes. Planta 213, 45–50. doi: 10.1007/s004250000487
Chun, O. K., Kim, D. O., and Lee, C. Y. (2003). Superoxide radical scavenging activity of the major polyphenols in fresh plums. J. Agricul. Food Chem. 51, 8067–8072. doi: 10.1021/jf034740d
Clarkson, D. T., and Luttge, U. (1989). Mineral nutrition. Divalent cations, transport and compartmentalization. Prog. Bot. 51, 93–112. doi: 10.1007/978-3-642-75154-7_7
Dinakar, N., Nagajyothi, P. C., Suresh, S., Damodharam, T., and Suresh, C. (2009). Cadmium induced changes on proline, antioxidant enzymes, nitrate and nitrite reductases in Arachis hypogaea L. J. Environ. Biol. 30, 289–294.
Dong, J., Wu, F., and Zhang, G. (2005). Effect of cadmium on growth and photosynthesis of tomato seedlings. Zhejiang Univ. Sci. B. 6, 974–980. doi: 10.1631/jzus.2005.B0974
El-Beltagi, H. S., and Mohamed, H. I. (2013). Alleviation of cadmium toxicity in Pisum sativum L. seedlings by calcium chloride. Not. Bot. Horti. Agrobo. 41, 157–168.
Eller, F., and Brix, H. (2016). Influence of low calcium avaliability on cadmium uptake and translocation in a fast-growing shrub and a metal accumulating herb. AOB Plant. 8:plv143.
Fariduddin, Q., Khanam, S., Hasan, S. A., Ali, B., Hayat, S., and Ahmad, A. (2009). Effect of 28-homobrassinolide on the drought stress-induced changes in photosynthesis and antioxidant system of Brassica juncea L. Acta Physiol. Plant. 31, 889–897. doi: 10.1007/s11738-009-0302-7
Fatoba, P. O., Ogunkunle, C. O., and Salihu, B. Z. (2012). Toxic effects of cadmium (Cd) and Lead (Pb) on growth and productivity of Arachis hypogaea (L) and Glycine max (L). J. Asian Sci. Res. 2, 254–259.
Foyer, C. H., and Shigeoka, S. (2011). Understanding oxidative stress and antioxidant functions to enhance photosynthesis. Plant Physiol. 155, 93–100. doi: 10.1104/pp.110.166181
Gonçalves, J. F., Antes, F. G., Maldaner, J., Pereira, L. B., Tabaldi, L. A., Rauber, R., et al. (2009). Cadmium and mineral nutrient accumulation in potato plantlets grown under cadmium stress in two different experimental culture conditions. Plant Physiol. Biochem. 47, 814–821. doi: 10.1016/j.plaphy.2009.04.002
Hasanuzzaman, M., and Fujita, M. (2011). Selenium pretreatment upregulates the antioxidant defense and methylglyoxal detoxification system and confers enhanced tolerance to drought stress in rapeseed seedlings. Biol. Trace Elem. Res. 143, 1758–1776. doi: 10.1007/s12011-011-8998-9
Hasanuzzaman, M., and Fujita, M. (2013). Exogenous sodium nitroprusside alleviates arsenic-induced oxidative stress in wheat (Triticum aestivum L.) seedlings by enhancing antioxidant defense and glyoxalase system. Ecotoxicol. 22, 584–596. doi: 10.1007/s10646-013-1050-4
Hayakawa, N., Tomioka, R., and Takenaka, C. (2011). Effects of calcium on cadmium uptake and transport in the tree species Gamblea innovans. Soil Sci. Plant Nutr. 57, 691–695. doi: 10.1080/00380768.2011.608196
Heath, R. L., and Packer, L. (1968). Photoperoxidation in isolated chloroplasts. I. Kinetics and stoichiometry of fatty acid peroxidation. Arch. Biochem. Biophy. 125, 189–198. doi: 10.1016/0003-9861(68)90654-1
Hernandez, J. A., and Almansa, M. S. (2002). Short term effects of salt stress on antioxidant systems and leaf water relations of pea leaves. Physiol Plant. 115, 251–257. doi: 10.1034/j.1399-3054.2002.1150211.x
Hinkle, P. M., Kinsella, P. A., and Osterhoudt, K. C. (1987). Cadmium uptake and toxicity via voltage-sensitive calcium channels. J. Biol. Chem. 262, 16333–16337.
Hirschi, K. D. (2004). The calcium conundrum. both versatile nutrient and specific signal. Plant Physiol. 136, 2438–2442. doi: 10.1104/pp.104.046490
Hiscox, J. D., and Israelstam, G. F. (1979). A method for the extraction of chlorophyll from leaf tissue without maceration. Can. J. Bot. 57, 1332–1334. doi: 10.1139/b79-163
Hussain, I., Akhtar, S., Ashraf, M. A., Rasheed, R., Siddiqi, E. H., and Ibrahim, M. (2013). Response of maize seedlings to cadmium application after different time intervals. Agronomy 2013:169610. doi: 10.1155/2013/169610
Ismail, M. A. (2008). Involvement of Ca2+ in alleviation of Cd2+ toxicity in common bean (Phaseolus vulgaris L.) plants. Res. J. Agric. Biol. Sci. 4, 203–209.
Kapoor, D., Kaur, S., and Bhardwaj, R. (2014). Physiological and biochemical changes in Brassica juncea Plants under Cd-induced stress. Biomed Res. Int. 2014:726070. doi: 10.1155/2014/726070
Khan, N. A., Nazar, R., Iqbal, N., and Anjum, N. A. (2012). Phytohormones and Abiotic stress Tolerance in Plants. Berlin; Heidelberg: Springer.
Kinraide, T. B. (1998). Three mechanisms for the calcium alleviation of mineral toxicities. Plant Physiol. 118, 513–520. doi: 10.1104/pp.118.2.513
Kumar, S., and Dhingra, H. R. (2005). Sexual reproduction and cadmium partitioning in two mungbean genotypes raised in soils contaminated with cadmium. Ind. J. Plant Physiol. 10, 151–157.
Kuthanova, A., Gemperlova, L., Zelenkova, S., Eder, J., Machackovai Opatrny, Z., and Cvikrova, M. (2004). Cytological changes and alterations in polyamine contents induced by cadmium in tobacco BY-2 cells. Plant Physiol. Biochem. 42, 149–156. doi: 10.1016/j.plaphy.2003.11.003
Lachman, J., Dudjak, J., Miholová, D., Kolihová, D., and Pivec, V. (2005). Effect of cadmium on flavonoid content in young barley (Hordeum sativum L.) plants. Plant Soil Environ. 51, 513–516.
Lechowski, Z., and Bialczyk, J. (1993). Calcium mediated cytokinin action on chlorophyll synthesis in isolated embryo of Scots pine. Biol. Plant. 35, 53–62. doi: 10.1007/BF02921119
Levitt, J. (1980). Responses of Plant to Environmental Stresses. Water, Radiation, Salt and Other Stresses. New York, NY: Academic Press.
Li, W., Koike, K., Asada, Y., Yoshikawa, T., and Nikaido, T. (2005). Rosmarinic acid production by Coleus forskohlii hairy root cultures. Plant Cell Tiss. Org. 80, 151–155. doi: 10.1007/s11240-004-9541-x
Li, P., Zhao, C., Zhang, Y., Wang, X., Wang, X., Wang, J., et al. (2016). Calcium alleviates cadmium-induced inhibition on root growth by maintaining auxin homeostasis in Arabidopsis seedlings. Protoplasma 253, 185–200. doi: 10.1007/s00709-015-0810-9
Luck, H. (1974). Catalase in Methods of Enzymatic Analysis, Vol. II, eds J. Bergmeyer and M. Grabi (New York, NY: Academic Press), 885–890.
Mazid, M., Khan, T. A., and Mohammad, F. (2011). Potential of NO and H2O2 as signaling molecules in tolerance to abiotic stress in plants. J. Indus. Res. Technol. 1, 56–68.
Mengel, K. (2007). “Potassium in Handbook of Plant Nutrition, eds A. V. Barker and D. J. Pilbeam (Boca Raton, FL: CRC Press), 91–120.
Michalak, A. (2006). Phenolic compounds and their antioxidant activity in plants growing under heavy metal stress. Polish J. Environ. Stud. 15, 523–530.
Mittler, R. (2002). Oxidative stress, antioxidants and stress tolerance. Trend. Plant Sci. 7, 405–410. doi: 10.1016/S1360-1385(02)02312-9
Mobin, M., and Khan, N. A. (2007). Photosynthetic activity, pigment composition and antioxidative response of two mustard (Brassica juncea) cultivars differing in photosynthetic capacity subjected to cadmium stress. J. Plant Physiol. 164, 601–610. doi: 10.1016/j.jplph.2006.03.003
Mondal, N. K. (2013). Effect of varying cadmium stress on chickpea (Cicer arietinum L.) seedlings: an ultrastructural study. Ann. Environ. Sci. 7, 59–70.
Morris, C. J., Thompson, J. F., and Johnson, C. M. (1969). Metabolism of glutamic acid and N-acetylglutamic acid in leaf discs and cell-free extracts of higher plants. Plant Physiol. 44, 1023–1026. doi: 10.1104/pp.44.7.1023
Mourato, M., Reis, R., and Martins, L. L. (2012). “Characterization of plant antioxidative system in response to abiotic stresses in A Focus on Heavy Metal Toxicity, Advances in Selected Plant Physiology Aspects, ed G. Montanaro (Rijeka: InTech), 23–44.
Nageswararao, R. C., Krishnasastry, K. S., and Udayakumar, M. (1981). Role of potassium in proline metabolism. i. conversion of precursors into proline under stress conditions in K-sufficient and K-deficient plants. Plant Sci. Lett. 23, 327–334. doi: 10.1016/0304-4211(81)90044-4
Nakano, Y., and Asada, K. (1981). Hydrogen peroxide is scavenged by ascorbate-specific peroxidase in spinach chloroplasts. Plant Cell Physiol. 22, 867–880.
Nazar, R., Iqbal, N., Masood, A., Khan, M. I. R., Syeed, S., and Khan, N. A. (2012). Cadmium toxicity in plants and role of mineral nutrients in its alleviation. Am. J. Plant Sci. 3, 1476–1489. doi: 10.4236/ajps.2012.310178
Noraho, N., and Gaur, J. P. (1995). Effect of cations, including heavy metals, on cadmium uptake by Lemna polyrhiza L. Biometal 8, 95–98. doi: 10.1007/BF00142006
Parre, E., Ghars, M. A., Leprince, A. S., Thiery, L., Lefebvre, D., Bor- denave, M., et al. (2007). Calcium signaling via phospholipase C is essential for proline accumulation upon ionic but not nonionic hyperosmotic stresses in Ara- bidopsis. Plant Physiol. 144, 503–512. doi: 10.1104/pp.106.095281
Perfus-Barbeoch, L., Leonhardt, N., Vavasseur, A., and Forestier, C. (2002). Heavy metal toxicity: cadmium permeates through calcium channels and disturbs the plant water status. Plant J. 32, 539–548. doi: 10.1046/j.1365-313X.2002.01442.x
Rasool, S., Ahmad, A., Siddiqi, T. O., and Ahmad, P. (2013). Changes in growth, lipid peroxidation and some key antioxidant enzymes in chickpea genotypes under salt stress. Acta Physiol. Plant. 35, 1039–1050. doi: 10.1007/s11738-012-1142-4
Rasool, S., Ahmad, P., Rehman, M., Arif, A., and Anjum, N. A. (2015). Achieving crop stress tolerance and improvement—an overview of genomic techniques. Appl. Biochem. Biotechnol. 177, 1395–1408. doi: 10.1007/s12010-015-1830-9
Rivelli, A. R., Puschenreiter, M., and De Maria, S. (2014). Assessment of cadmium uptake and nutrient content in sunflower plants grown under Cd stress. Plant Soil Environ. 60, 80–86.
Rivetta, A., Negrini, N., and Cocucci, M. (1997). Involvement of Ca2+-calmodulin in Cd2+ toxicity during the early phases of radish (Raphanus sativus L.) seed germination. Plant Cell Environ. 20, 600–608. doi: 10.1111/j.1365-3040.1997.00072.x
Sandalio, L. M., Dalurzo, H. C., Gómez, M., Romero-Puertas, M. C., and del Rio, L. A. (2001). Cadmium-induced changes in the growth and oxidative metabolism of pea plants. J. Exp. Bot. 52, 2115–2126.
Shamsi, I. H., Jilani, G., Zhang, G. P., and Kang, W. (2008). Cadmium stress tolerance through potassium nutrition in soybean. Asian J. Chem. 20, 1099–1108.
Sharma, S. S., and Dietz, K. J. (2006). The significance of amino acids and amino acid-derived molecules in plant responses and adaptation to heavy metal stress. J. Exp. Bot. 57, 711–726. doi: 10.1093/jxb/erj073
Sharma, S. S., Schat, H., and Vooijs, R. (1998). In vitro alleviation of heavy metal induced enzyme inhibition by proline. Phytochem. 46, 1531–1535. doi: 10.1016/S0031-9422(98)00282-9
Siddhu, G., and Khan, M. A. A. (2012). Effects of cadmium on growth and metabolism of Phaseolus mungo. J. Environ. Biol. 3, 173–179.
Siddique, M. H., Al-Whaibi, M. H., Sakran, A. H., Basalah, M. O., and Ali, H. M. (2012). Effect of calcium and potassium on antioxidant system of Vicia faba L. under cadmium stress. Int. J. Mol. Sci. 13, 6604–6619. doi: 10.3390/ijms13066604
Song, W. Y., Martinoia, E., Lee, J., Kim, D., Kim, D. Y., Vogt, E., et al. (2004). A novel family of cys-rich membrane proteins mediates cadmium resistance in Arabidopsis. Plant Physiol. 135, 1027–1039. doi: 10.1104/pp.103.037739
Song, Z. Z., Duan, C. L., Guo, S. L., Yang, Y., Feng, Y. F., Ma, R. J., et al. (2015). Potassium contributes to zinc stress tolerance in peach (Prunus persica) seedlings by enhancing photosynthesis and the antioxidant defense system. Genet. Mol. Res. 14, 8338–8835. doi: 10.4238/2015.July.27.22
Stewart, C. R., and Bogess, S. F. (1977). The effect of wilting on the conversion of arginine, ornithine, and glutamate to proline in bean leaves. Plant Sci. Lett. 8, 147–153. doi: 10.1016/0304-4211(77)90025-6
Suzuki, N. (2005). Alleviation by calcium of cadmium-induced root growth inhibition in Arabidopsis seedlings. Plant Biotechnol. 22, 19–25. doi: 10.5511/plantbiotechnology.22.19
Suzuki, N., Yamaguchi, Y., Koizumi, N., and Sano, H. (2002). Functional characterization of a heavy metal binding protein Cdl19 from Arabidopsis. Plant J. 32, 165–173. doi: 10.1046/j.1365-313X.2002.01412.x
Talukdar, D. (2012). Exogenous calcium alleviates the impact of cadmium-induced oxidative stress in Lens culinaris Medic. seedlings through modulation of antioxidant enzyme activities. J. Crop Sci. Biotechnol. 15, 325–334. doi: 10.1007/s12892-012-0065-3
Tripathi, B. N., Bhatt, I., and Dietz, K. J. (2009). Peroxiredoxins: a less studied component of hydrogen peroxide detoxification in photosynthetic organisms. Protoplasma 235, 3–15. doi: 10.1007/s00709-009-0032-0
Van Rossum, M. W. P. C., Alberda, M., and Van der Plas, L. H. W. (1997). Role of oxidative damage in tulip bulb scale micropropagation. Plant Sci. 130, 207–216. doi: 10.1016/S0168-9452(97)00215-X
Velikova, V., Yordanov, I., and Edreva, A. (2000). Oxidative stress and some antioxidant systems in acid rain-treated bean plants. Protective role of exogenous polyamines. Plant Sci. 151, 59–66. doi: 10.1016/S0168-9452(99)00197-1
Verslues, P. E., and Sharma, S. (2010). Proline metabolism and its implications for plant-environment interaction. Arabidopsis Book. 8:e0140. doi: 10.1199/tab.0140
Weng, X. Y., Zheng, C. J., Xu, H. X., and Sun, J. Y. (2007). Characteristics of photosynthesis and functions of the water-water cycle in rice (Oryza sativa) leaves in response to potassium deficiency. Physiol. Plant. 131, 614–621. doi: 10.1111/j.1399-3054.2007.00978.x
White, P. J. (2000). Calcium channels in higher plants. Biochem. Biophys. Acta 1465, 171–189. doi: 10.1016/S0005-2736(00)00137-1
Xu, W., Peng, H., Yang, T., Whitaker, B., Huang, L., Sun, J., et al. (2014). Effect of calcium on strawberry fruit flavonoid pathway gene expression and anthocyanin accumulation. Plant Physiol. Biochem. 82, 289–298. doi: 10.1016/j.plaphy.2014.06.015
Yoo, R. H., Park, C. Y., Kim, J. C., Heo, W. D., Cheong, M. S., Park, H. C., et al. (2005). Direct interaction of a divergent CaM isoform and the transcription factor, MYB2, enhances salt tolerance in Arabidopsis. J. Biol. Chem. 280, 3697–3706. doi: 10.1074/jbc.M408237200
Yousuf, P. Y., Ahmad, A., Hemant Ganie, A. H., Aref, I. M., and Iqbal, M. (2015). Potassium and calcium application ameliorates growth and oxidative homeostasis in salt-stressed indian mustard (Brassica juncea) Plants. Pak. J. Bot. 47, 1629–1639.
Zhao, Y. (2011). Cadmium accumulation and antioxidative defenses in leaves of Triticum aestivum L. and Zea mays L. Afr. J. Biotechnol. 10, 2936–2943.
Zhao, Z. Q., Zhu, Y. G., Li, H. Y., Smith, S. E., and Smith, F. A. (2003). Effects of forms and rates of potassium fertilizers on cadmium uptake by two cultivars of spring wheat (Triticum aestivum L.). Environ. Intern. 29, 973–978. doi: 10.1016/S0160-4120(03)00081-3
Zheng, Y., Jia, A., Ning, T., Xu, J., Li, Z., and Jiang, G. (2008). Potassium nitrate application alleviates sodium chloride stress in winter wheat cultivars differing in salt tolerance. J. Plant Physiol. 165, 1455–1465. doi: 10.1016/j.jplph.2008.01.001
Zhishen, J., Mengcheng, T., and Jianming, W. (1999). The determination of flavonoid contents in mulberry and their scavenging effects on superoxide radicals. Food Chem. 64, 555–559. doi: 10.1016/S0308-8146(98)00102-2
Keywords: antioxidant enzymes, cadmium toxicity, chickpea, oxidative stress, organic solutes, secondary metabolites, yield attributes
Citation: Ahmad P, Abdel Latef AA, Abd_Allah EF, Hashem A, Sarwat M, Anjum NA and Gucel S (2016) Calcium and Potassium Supplementation Enhanced Growth, Osmolyte Secondary Metabolite Production, and Enzymatic Antioxidant Machinery in Cadmium-Exposed Chickpea (Cicer arietinum L.). Front. Plant Sci. 7:513. doi: 10.3389/fpls.2016.00513
Received: 24 January 2016; Accepted: 01 April 2016;
Published: 27 April 2016.
Edited by:
Shabir Hussain Wani, SKUAST-Kashmir, IndiaReviewed by:
Adriano Sofo, Università degli Studi della Basilicata, ItalyBu-Jun Shi, University of Adelaide, Australia
Copyright © 2016 Ahmad, Abdel Latef, Abd_Allah, Hashem, Sarwat, Anjum and Gucel. This is an open-access article distributed under the terms of the Creative Commons Attribution License (CC BY). The use, distribution or reproduction in other forums is permitted, provided the original author(s) or licensor are credited and that the original publication in this journal is cited, in accordance with accepted academic practice. No use, distribution or reproduction is permitted which does not comply with these terms.
*Correspondence: Parvaiz Ahmad, cGFydmFpemJvdEB5YWhvby5jb20=