- 1Corn Insects and Crop Genetics Research Unit, USDA-ARS, Ames, IA, United States
- 2Department of Tropical Plant and Soil Sciences, University of Hawaii at Manoa, Honolulu, HI, United States
- 3Molecular, Cellular and Developmental Biology Program, Iowa State University, Ames, IA, United States
The ga1 locus of maize confers unilateral cross incompatibility, preventing cross pollination between females carrying the incompatible Ga1-s allele and males not carrying a corresponding compatible allele. To characterize this system at the molecular level, we carried out a transcript profiling experiment in which silks from near isogenic lines carrying the Ga1-s and ga1 alleles were compared. While several differentially expressed genes were identified, only one mapped to the known location of ga1. This gene is a pectin methylesterase (PME), which we designated as ZmPme3, and is present and expressed only in Ga1-s genotypes. While a functional ZmPME3 is not present in the ga1 genotypes examined, a pectin methylesterase gene cluster is found in ga1 genotypes. The gene cluster in W22 contains 58 tandem full-length or partial PME pseudo genes. These data combined with a wealth of previously published data on the involvement of PMEs in pollen tube growth suggest a role for cell wall modification enzymes in the pollen exclusion component of Ga1-s gametophytic incompatibility. Consistent with this role, a third allele which lacks the female function of Ga1-s, Ga1-m, has a mutationally inactivated version of ZmPme3.
Introduction
Maize is a wind-pollinated plant, where pollen grains released from anthers on the tassel are dispersed to the female silks on the ear. Unlike many other plants, maize male and female flowers are separate, resulting in high rates of cross-pollination. Several cross incompatibility systems have been identified in maize. These systems may have provided a reproductive barrier between maize and teosinte (Evans and Kermicle, 2001; Kermicle and Evans, 2005) in the evolution of modern maize. Cross incompatibility systems also have practical application in limiting undesired pollen transmission between different market classes of corn, such as dent and popcorn (Nelson, 1953).
One of the first genetic studies in maize following the rediscovery of Mendel's laws was a study of cross incompatibility between maize and popcorn (Correns, 1901). The genetics of this and other cross incompatibility systems in maize have been studied intensely since then and have been reviewed (Nelson, 1994). One of these systems was designated Gametophyte factor (Mangelsdorf and Jones, 1926) or Ga (now called Ga1) because of the involvement of the gamete in cross incompatibility. In the Ga1 system, cross incompatibility is conferred by a factor in silks of plants carrying the Ga1-strong (Ga1-s) allele, unless the male gamete also carries the Ga1-s allele. Cross incompatibility is therefore unilateral, providing a reproductive barrier between ga1 pollen and silks containing Ga1-s, but not between Ga1-s pollen and ga1/ga1 silks. The Ga1-s allele is common in popcorn and annual teosinte (Kermicle et al., 2006), while most other maize varieties carry the ga1 allele and are therefore susceptible to the fertilization barrier imposed by Ga1-s. While most dent corn can freely cross pollinate, crosses between dent corn and popcorn must usually be carried out with the popcorn as the male parent.
The Ga1-s allele performs two functions, 1. Excluding pollen not carrying the Ga1-s allele and 2. Pollinating Ga1-s silks. We refer to these functions as the female and male functions, respectively. Cross compatibility is thus conferred by the lack of an incompatibility system in both parents as in the case of ga1 or by a compatible interaction between the male and female factors of the Ga1-s allele (Jones, 1924). Characterization of the Ga1-m allele containing only the male function (Jimenez and Nelson, 1965) validated the idea that the ga1 locus conferred two functions and further demonstrated that these functions are genetically separable. Ga1-m/Ga1-m plants can overcome the Ga1-s crossing barrier, but their silks are receptive to pollination by all ga1 alleles; Ga1-s, Ga1-m and ga1. Thus, Ga1-m performs the male function but not the female function of the locus.
Kermicle and Evans (2005) used disomic pollen grains derived from a tertiary trisomic plant carrying both Ga1-s and ga1 to demonstrate that presence of Ga1-s in pollen is sufficient to overcome the pollination barrier. This is consistent with a model requiring congruity of the pollen and silk alleles, as opposed to the alternative hypothesis of active rejection of ga1 by the Ga1-s allele in silk tissue. One possibility raised by this observation is that the ga1 allele is actually a null allele. If ga1 is null due to the absence of an active gene that is present only in Ga1-s genotypes, identification of the gene of interest will be difficult because all currently sequenced and assembled genomes carry only the ga1 allele.
Maize pollen tubes have been shown to have an altered growth rate (Zhang et al., 2012) and morphology when grown on incompatible silks (Lu et al., 2014). In the Ga1 system, this morphology consists of an erratic growth pattern, with the pollen tube often outside of the transmitting tract of the pistil. Data suggest this behavior may be a result of altered pollen tube cell wall growth (Bosch and Hepler, 2006). Several studies illustrate the importance of pectin methylesterase and pectin methylesterase inhibitors in regulating pollen tube growth by altering the balance between strength and plasticity of the apical cell wall of the pollen tube (reviewed in Krichevsky et al., 2007; Zonia and Munnik, 2009).
The Ga1-s female function has been genetically mapped (Bloom and Holland, 2011), as has the male function (Zhang et al., 2012). In spite of mapping different functions, the region identified in these studies overlaps substantially. The most precise mapping data suggests that the male function is contained on a 100 kb region of maize chromosome 4 based on B73 RefGen_v2 (Liu et al., 2014). Despite the presence of only a few candidate genes in that region, the causative Ga1-s gene has yet to be identified. However if Ga1-s is not present in ga1 backgrounds, identification through a candidate gene approach based on ga1 genomes such as B73 may not be effective.
The objective of this study is to gain insight into the molecular mechanism of Ga1 function. We conducted a transcriptomic study of unpollinated silks from Ga1-s (W22) and ga1 (W22) which identified ZmPme3 as a candidate gene for the female function of Ga1-s. Proteomics, genetic mapping and genomic sequences of ga1 alleles support this hypothesis. The results of this study, together with data in the literature suggest a role for cell wall enzymes in the molecular genetic function of the Ga1 system. This discovery allows for new avenues for testing hypotheses about the molecular mechanism of gametophytic incompatibility systems.
Materials and Methods
Aniline Blue Staining of Pollen Tubes
Pollen tubes shown in Figure 1 were visualized following silk fixation using a combination of methods performed by Lausser et al. (2010) and Zhang et al. (2012). Briefly, pollen tubes were allowed to germinate for a predetermined time before silk tissue was harvested and incubated in FAA (10:85:5 v/v/v formaldehyde: 95% ethanol: acetic acid) for 24 h at 4°C. Silks were then slowly rehydrated by washing in 75, 50, 25% ethanol for 3 min each. Samples were washed in 0.1 M potassium phosphate buffer (pH 8.0) before a 2 h incubation in 8 M NaOH. Silk tissue was stained in 0.1% aniline blue (Fisher Scientific, Pittsburgh, PA) dissolved in 0.1 potassium phosphate overnight at 4°C. Silks were placed on glass slides and pollen tubes were visualized at near UV excitation using a fluorescent microscope (Nikon Diaphot 300). At least 10 silks per plant were measured and three biological replicates were completed for each experiment. In transmitting tracts with more than one pollen tube germinated, measurements were recorded from the pollen grain closest to the ovule.
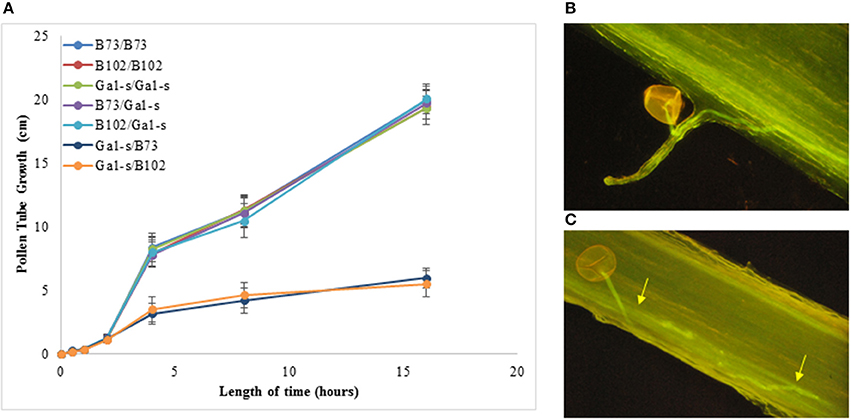
Figure 1. Pollen tube growth in compatible vs. incompatible pollinations. Pollen tubes from compatible and incompatible pollinations were fixed and stained with aniline blue at fixed times after pollination (A) Length of pollen tubes over time in compatible vs. incompatible pollinations. Error bars represent the standard deviation for three independent experiments. (B) Aniline blue staining in vivo of pollen tubes in a compatible pollination. Pollen tubes grow straight once inside the transmitting tract (40X magnification). (C) Aniline blue staining in vivo of pollen tubes in an incompatible pollination. Incompatible pollen tubes do not grow straight and are sometimes seen exiting and reentering the transmitting tract (arrows).
RNA Isolation for RNA-Seq and qRT-PCR
W22 (ga1) and Ga1-s (W22) (Maize Genetic Stock Center number 401D) plants were grown in the greenhouse at Iowa State University. Three biological replicates of unpollinated, emerged silks from each genotype were collected for RNA isolation. Following isolation, RNA was sent to the DNA facility at Iowa State University for single-end RNA sequencing on Illumina MiSeq with a read length of 50 bp.
For qRT-PCR analysis, W22 (ga1), Ga1-s (W22) and Hp301 were grown in the USDA greenhouse at Iowa State University. Five to seven biological replicates of unpollinated, emerged silks from each genotype were collected for RNA isolation by Qiagen RNeasy Plant kit.
RNA-Seq and Bioinformatic Analysis
Sequencing was performed at the Iowa State University on a MiSeq. Libraries were sequenced for 51 cycles. TopHat (version 2.0.3, Trapnell et al., 2009) was used to align reads to the B73 reference genome (version 3 ref). The program samtools (Li et al., 2009) was used to remove unreliably mapped reads. The resulting mapping files (bam) were imported into the statistical program R (R Core Team, 2016) using the Bioconductor package Rsamtools (Morgan et al., 2010). Mapped reads were submitted to NCBI SRA under BioProject PRJNA382364.
The R graphics program ggplot2 (Wickham, 2009) was used to compare sample replicates for technical reproducibility (data not shown). Samples of low quality were not used in further analyses. The Bioconductor package edgeR (Robinson and Smyth, 2007, 2008; Robinson et al., 2010; McCarthy et al., 2012) was used for single factor, pairwise comparisons to calculate normalization factors, estimate tagwise dispersion and determine differential expression (DEG) based in comparison to the reference genome B73_v3. 1452 DEG were identified at a cutoff of p > 0.05. (Table S3).
RNA-Seq reads were also assembled de novo through the Trinity package. RSEM was used to estimate abundance after mapping reads back to assembled transcripts using bowtie2. The raw counts for transcripts were then normalized (upper quartile) and differential gene expression (DEG) analysis was performed (three independent methods were used: edgeR, voom, DESeq2). EdgeR results are presented in this paper. ORFs were predicted from these transcripts and predicted peptides were generated. Annotation of the assembled transcripts was performed using Trinnotate which uses a curated dataset from swissprot to predict function as well as the pfam database, tmhmm, signalp, and gene ontology for additional annotation. Some sequences that were reported as differentially expressed most likely resulted from a sequence polymorphism between the two genotypes sequenced because a corresponding transcript with minor sequence differences and approximately the same read count was also found on this list. These transcripts were manually removed from the gene list shown in Table 1, but are still present in Table S1.
qRT-PCR of ZmPme3
Equal amounts (0.5 ng) of total RNA were used in a one step RT/qPCR reaction with SuperScript® III Platinum® SYBR® Green One-Step qRT-PCR kit (Invitrogen #11736-059). 18S was used as an internal control and ZmPme3 primers (Table S4) were used to detect transcripts. ZmPme3 ΔCt levels were normalized to 18S RNA levels and ZmPme3 transcript levels in Ga1-s and Hp301 are expressed as relative to those of W22.
Amplification and Sequencing of ZmPme3 in Ga1-s Lines
PCR primers were designed using the de novo assembled transcript sequence to amplify the corresponding genomic sequence in four pieces (Table S4). Using genomic DNA from the maize genetic stock center stock 401D which carries the genotype Ga1-s/Ga1-s in a W22 background, Hp301 which is a popcorn line known to be Ga1-s/Ga1-s(obtained from the North Central Region Plant Introduction Station), W22 (ga1/ga1)(obtained from the North Central Region Plant Introduction Station) and NC390/NC394(Ga1-m/Ga1-m)(provided by Dr. M. Goodman, NCSU), products were amplified and sequenced in both directions at the Iowa State University DNA Facility. NAM B73 × Hp301 seeds were obtained from the Maize Genetics Stock Center (maizecoop.cropsci.uiuc.edu/).
Genetic Mapping of ZmPme3 in the Hp301xB73 NAM Population
The NAM lines used to map the ga1 locus with recombination breakpoints in the region of Ga1-s (Bloom and Holland, 2011) were screened for the presence of the active PME gene sequence by PCR (Table 2). NAM B73 × Hp301 seeds were obtained from the Maize Genetics Stock Center (maizecoop.cropsci.uiuc.edu/). Two primer pairs (PME marker and PME_A, Table S4) which distinguish the active ZmPme3 from the inactive genes in W22, were used. The PCR results were compared to the published pollen exclusion phenotypes of these lines (Bloom and Holland, 2011).
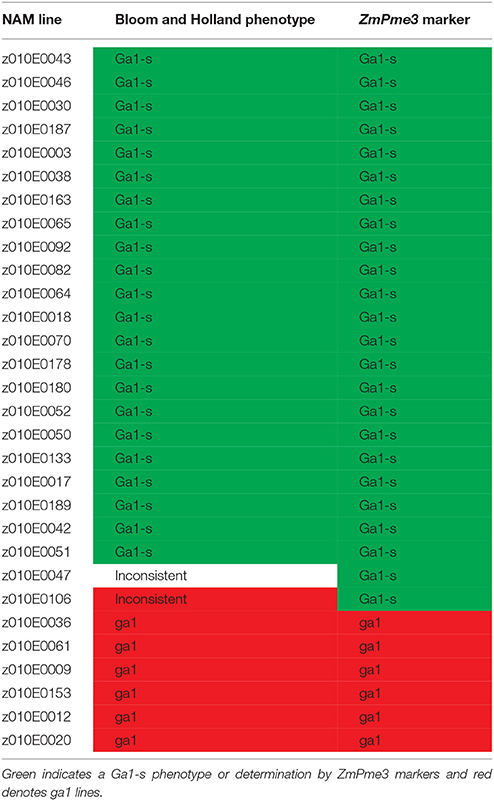
Table 2. Mapping ZmPme3 to chromosome 4 using the NAM Hp301 × B73 lines that were used to determine the ga1 locus (Bloom and Holland, 2011).
Dot Plot and Alignments of PME Pseudo Gene Cluster Members from W22
To visualize the structure of the W22 PME repeat region, the 3 Mb portion of the Chromosome 4 genomic DNA sequence containing regions of PME homology was analyzed using YASS (Noé and Kucherov, 2005) by plotting the sequence against itself (Figure 3). Genomic sequences of each PME pseudo gene were extracted from the genomic sequence using the genome coordinates produced by YASS. The resulting PME pseudogene sequences were aligned using BioEdit and Clustal W. The genome coordinates of the aligned sequences was used to produce Figure 4.
Proteomic Analysis of Ga1-s Tissues
Pollen protein extract was produced from 20 mg of mature pollen grains by mortar and pestle (Zhu et al., 2011). Pistil (pre- and post-pollination) protein extract was produced by grinding tissue in liquid nitrogen using a mortar and pestle into a fine powder that was extracted with trichloroacetic acid (Méchin et al., 2007). Pollen and pistil protein extracts were analyzed by the Protein Facility at Iowa State University as a fee-for-service. The isotopic label-free relative quantification method used was as follows; 100 μg of each protein extract was digested in solution using a trypsin/Lys-C protease mix. Samples were dried down and spiked with 250 fmol of peptide retention time calibration (PRTC) standards during reconstitution. PRTC standards contained an equimolar mixture of 15 known peptides to allow for the quantification of unknown peptides. Newly digested peptides were then separated by liquid chromatography before analysis by MS/MS using a Q Exactive Hybrid Quadrupole-Orbitrap Mass Spectrometer (Fisher Scientific, Waltham, MA). The resulting spectral data was compared to theoretical fragmentation patterns using the MASCOT search engine (Matrix Science, London, UK) to identify the most likely protein accessions by considering the highest-scoring peptide sequence for each input fragment and alignment of 2 or more high-scoring peptide sequences to a single accession. A trinity-predicted peptide database from silk transcript de novo assembly was used to make protein identifications.
Results
Pollen Tubes Show Aberrant Growth Patterns in Incompatible Pollinations of Maize Silks
To confirm pollen tube growth arrest (Lu et al., 2014) and slow growth rate (Zhang et al., 2012) characteristic of ga1 pollen on Ga1-s silks (Lu et al., 2014) in our genetic materials, pollen tubes from compatible and incompatible pollinations were visualized. In compatible pollinations, pollen tubes were straight and grew within the transmitting tract of the silk to the ovule. In incompatible pollinations of ga1 (W22) pollen on Ga1-s (W22) silks, the pollen tubes had a slower rate of growth (Zhang et al., 2012), were not straight and often left the transmitting tract while growing (Figure 1C).
A Pectin Methylesterase Is Expressed in Ga1-s and Not in ga1 and Is Homologous to Sequences at the ga1 Locus
To identify candidate genes for the Ga1-s allele, we sequenced transcripts from the silks of two near isogenic lines differing at the ga1 locus (Ga1-s/Ga1-s vs ga1/ga1) in the inbred background W22. The sequencing reads generated by this study were deposited in the National Center for Biotechnology Short Read Archive (NCBI SRA BioProject accession PRJNA382364). There were 65,780,803 reads from the ga1/ga1 sample and 65,261,178 reads from the Ga1-s/Ga1-s sample. Since genetic experiments suggest that ga1 may be a null allele, it could be missing from ga1 genotypes such as W22 and B73 and mapping reads to these genotypes would not be expected to identify transcripts from the Ga1-s gene. We therefore processed the transcript data by de novo assembly of the pooled RNA reads. There were 69,160 Trinity assembled transcripts and 61,891 “genes” identified. We then identified differentially expressed genes (DEGs) using edgeR with a FDR of < 0.001. At that cutoff, there are 1958 significant DEG, with 1005 of them upregulated in Ga1-s silks (Table 1, Table S1). We used Trinnotate and BLAST to identify the assembled transcripts where 706 transcripts had significant BLAST hits, leaving 1252 without identification.
Keeping with the hypothesis that the Ga1-s transcripts are missing from ga1 genotypes, we focused on genes that were upregulated in Ga1-s. The most significantly differentially expressed (based on FDR) of the genes upregulated in Ga1-s is a putative pectinesterase/pectinesterase inhibitor 38. Among the de novo assembled transcripts, there is only one Pme38-like transcript and the reads used to assemble it came overwhelmingly from Ga1-s samples (Supplemental File). A BLAST search was performed against the B73 genome (RefGen3) with the 40 most significant DEG that were up regulated in Ga1-s to determine potential genome localizations. Only the pectinesterase gene hit within the ga1 locus on chromosome 4. A hevein-like preproprotein resides just outside the locus.
Two genes identified in the B73 genome that lie within the 100 kb region of interest on chromosome 4 such as GRMZM2G027021 (GTP-binding protein hflX) and GRMZM2G039983 (Wave-dampened 2) were detected in the de novo assembly (Trinity_13706 and Trinity_17477). Both genes appear to be expressed in silks but not differentially. There do not appear to be genotype differences in transcript sequence for Trinity_17477 (Wave-dampened 2) despite SNPs for GRMZM2G039983 genomic sequence between Ga1-s, Ga1-m and ga1 (data not shown).
Ga1-s Genotypes Have a PME Gene That Is Lacking in ga1 Genotypes
In order to confirm that the de novo assembled PME transcript is encoded by a gene present in Ga1-s plants and is not a bioinformatic artifact, we used PCR to amplify genomic DNA from Ga1-s(W22) and Hp301 (a popcorn line known to be Ga1-s/Ga1-s). We amplified a genomic DNA sequence consistent with the computationally derived transcript (Figure 2A). The same PCR reactions were done in ga1(W22) and while amplification products were produced, they were not identical in sequence to Ga1-s and Hp301 and did not encode functional PMEs. Thus, the hits from the BLAST search with the PME transcript against the B73 genome were likely to be sequences with homology to the PME within the chromosome 4 region of interest. The PME coding sequence from Ga1-s(W22) and Hp301 is 1578 bases long with a 99-bp intron and is predicted to encode transcripts identical to the sequence from the de novo assembly. We designated the gene encoding this transcript ZmPme3. According to the literature, the coding sequence of PME genes range from 1.2 to 3.0 kb long (Wang et al., 2013). We used Hmmer to search for Pfam families within the predicted protein sequence and found only the PME domain (PF01095) with a signal peptide.
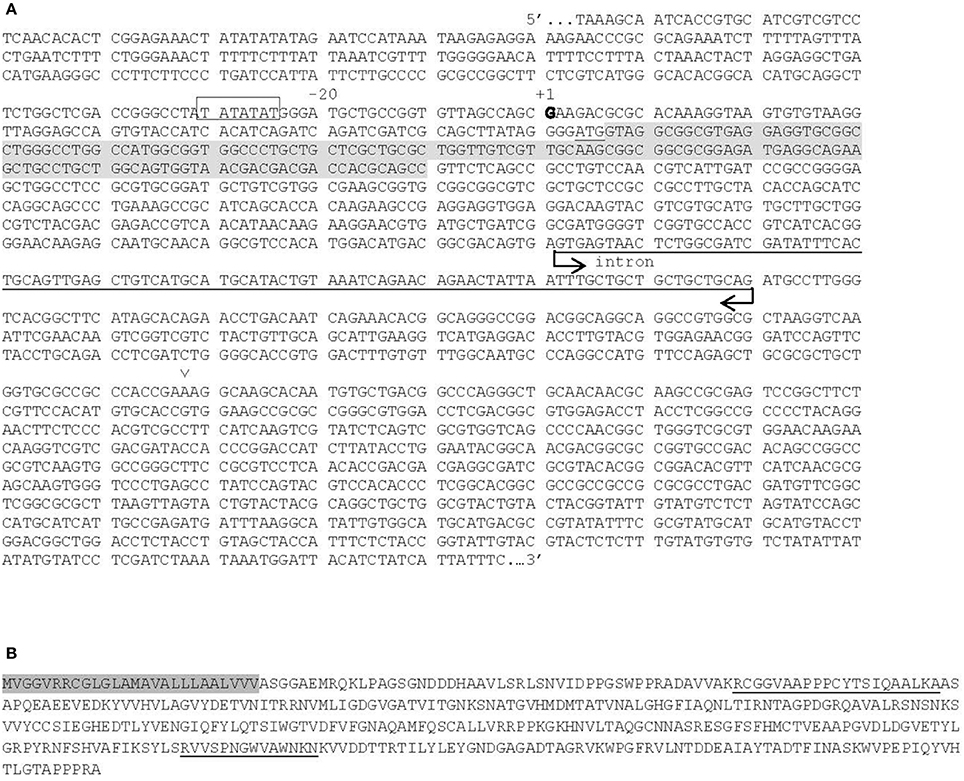
Figure 2. Genomic and predicted protein sequences of ZmPme3. (A) Genomic sequence of ZmPme3, with predicted TATA sequence in a box, +1 base is in bold, ATG start and TGA stop codons are underlined, predicted signal sequence in gray, and intron indicated by arrows. The position of the “GA” insertion in the Gal-m hybrid NC390/NC394 is indicated by arrows. (B) Predicted protein sequence of ZmPme3 with peptides detected my LC-MS underlined and predicted signal peptide highlighted.
We further confirmed the expression data by qRT-PCR. RNA was isolated from unpollinated, exposed silks from W22, Ga1-s(W22) and Hp301. Using two primer pairs for ZmPme3, relative expression levels were 17- to 97-fold higher in Ga1-s genotypes than W22 (Figure S1). ΔCt values for ZmPme3 from W22 samples were near no template control levels as would be expected if this genotype lacks the ZmPMme3 gene (Figure S1).
The Gene Encoding ZmPME3 Maps to the ga1 Locus in an Hp301 × B73 Mapping Population
While we identified sequences homologous to ZmPme3 in the genomic region known to contain ga1 using BLAST, the genome position of ZmPme3 was not yet clear. To determine whether ZmPme3 maps to the ga1 locus, we mapped ZmPme3 in the Hp301 × B73 RIL population developed for the NAM project (McMullen et al., 2009). Based on previously published information about this population (Bloom and Holland, 2011) we screened the lines used to map the ga1 locus with recombination breakpoints in the region of Ga1-s for the presence of the active PME gene sequence by PCR. ZmPme3 was detected in the NAM lines that excluded pollen and was not detected in lines that did not exclude pollen (Bloom and Holland, 2011; Table 2). These data confirmed that ZmPme3 from Ga1-s is in the ga1 interval that Bloom and Holland identified.
A protein Encoded by the ZmPme3 Gene Is Detectable in Ga1-s Silks, But Not in ga1 Silks
To determine if the ZmPME3 protein showed an expression pattern similar to the ZmPme3 transcript, protein extracts from mature pollen and silk tissue pre- and post-pollination were subjected to mass spectrometry analysis. Six samples were analyzed, all in the W22 background; Ga1-s and ga1 mature pollen, Ga1-s and ga1 silks, ga1 silks pollinated with Ga1-s pollen (compatible) and Ga1-s silks pollinated with ga1 pollen (incompatible). Identification of the peptides generated was performed by matching resulting peptide masses to the predicted proteins from the de novo assembled transcripts of ZmPme3. Peptides from ZmPME3 (Trinity_DN41247) were detected in both Ga1-s unpollinated and pollinated silks at a coverage of 7.8% (Figure 2B). Peptides from ZmPME3 were detected in neither of the pollen samples nor the ga1 unpollinated and pollinated silks. We conclude that the ZmPME3 protein expression is consistent with the expression of the ZmPme3 transcript.
Plants Containing the Ga1-m Allele Contain a Non-functional ZmPme3 Gene
The Ga1-m allele lacks the female function of the ga1 locus, so the sequence of ZmPme3 in this genotype was of great interest. If ZmPme3 is responsible for the female function of Ga1-s, we would expect it to be non-functional in Ga1-m genotypes. Through PCR and sequencing of ZmPme3 in the Ga1-m/Ga1-m containing hybrid NC390/NC394, we found a 2 base insertion that causes a frameshift resulting in a truncated and likely inactive protein (Figure 2A). This observation is consistent with the hypothesis that ZmPme3 is involved in the female function of Ga1-s.
Structure of the PME Repeat Region of Chromosome 4 (W22/B73)
The transcript profiling with de novo assembly and proteomics experiments presented here show that the Ga1-s genotypes examined have a single active differentially expressed PME gene, however we observed BLAST hits to PME sequences in the ga1 genotypes as well. Our initial observation of PME-like sequences in a ga1 genotype was when RNA reads were mapped to B73_RefGen v3 (Schnable et al., 2009). The top 40 most significant (lowest FDR) upregulated genes are all annotated in AGPv3 as transposable elements, with 34 out of 40 on chromosome 4 and within a 2 Mb region containing the ga1 locus but outside of the smallest 100 kb mapped region identified by Liu et al. (2014). To characterize these gene models further, homology was examined by BLAST. We examined the 34 genes from the ga1 locus on chromosome 4 and all but one have pectinesterase/pectinesterase inhibitor 38, 17, or 46 as their top BLAST hit with the one remaining sequence having pectin methylesterase (PME) 63 as its top BLAST hit. The remaining six genes fall on other chromosomes and either have no significant BLAST hits, are related to kinesin (GRMZM2G327923) or nucleobase-ascorbate transporter 12 (GRMZM2G031728).
We compared the de novo assembled transcript to the B73 genomic sequence and the co-linear W22 sequence in the genetically mapped region of interest. In doing this, we found that this region contains a cluster of 58 PME-like sequences. In a 1.1 MB region of W22 there are 58 full length and partial PME genes (Figure 4; Table S2). All but three have pectinesterase/pectinesterase inhibitor 38/17 as their top BLAST hit. The remaining three have Pme63 as their top hit, but appear to be partial genes. By aligning the 58 PME sequences from W22 and the assembled transcript, there does appear to be an intron in the W22 sequences. Although similar to the differentially expressed transcript, none of the B73 or W22 sequences matched it exactly. Using the ExPASy.org translate tool, we found that none of these genes have a full-length open reading frame, even accounting for an intron, while the de novo assembled transcript does. In several cases, a simple one base difference introduces a stop codon in the W22 sequence. All PME-like sequences in the gene cluster are oriented in the same direction. The 1.1 MB region containing the gene cluster has a complex organization of nested repeats (Figure 3), however the PME-like sequences are dispersed throughout the region with no obvious association with a larger repeat unit. Alignment of the PME-like sequences according to their genome position (Figure 4) shows that partial PME sequences are interspersed with complete sequences. In some cases the sequence organization suggests that partial sequences are derived from insertions into complete PME sequences because partial sequences adjacent to and on either side of some PME-like sequences are contiguous.
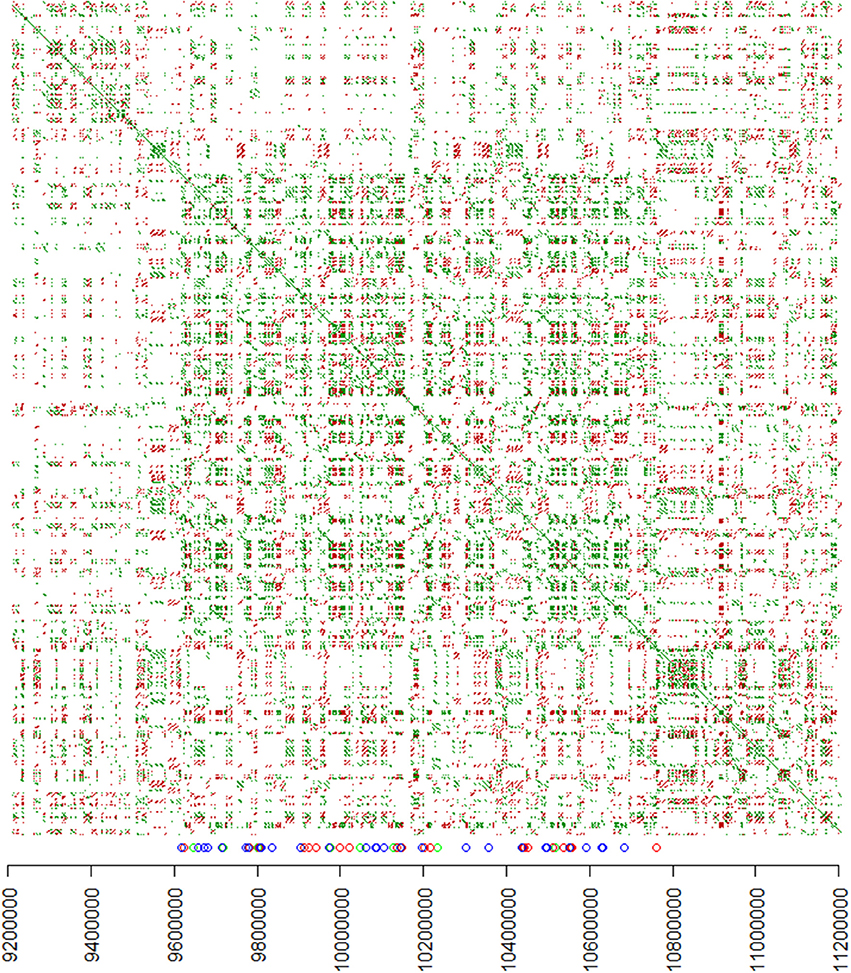
Figure 3. Structure of the PME repeat region in W22. A dot plot was constructed by plotting sequence matches with the indicated coordinates from the W22 chromosome 4 genomic sequence on both axes. Green dots indicate matching sequences on the forward strand and red dots indicate matching sequences on the reverse strand. On the X-axis, blue, green, and red circles indicate the positions of full-length, medium length, and short PME-like sequences, respectively.
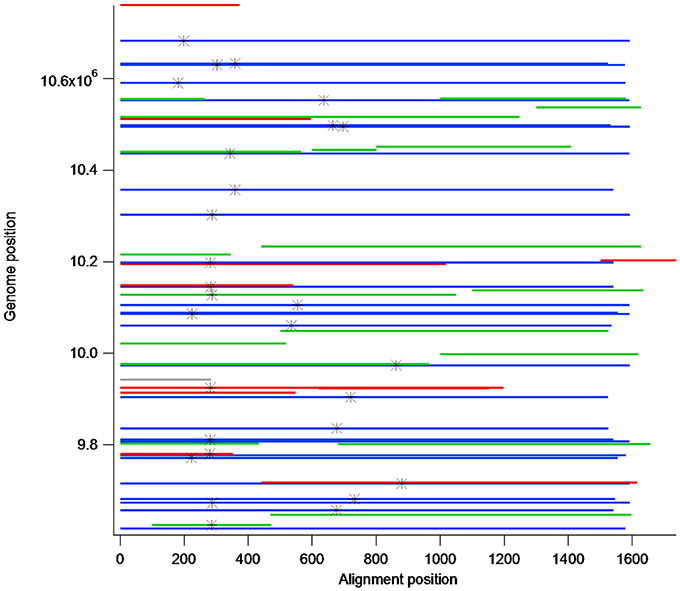
Figure 4. Alignment of 58 W22 PME-like sequences and their relative chromosome 4 genomic positions. Blue bars are full length PME-like sequences, green and red bars are middle and short length sequences, respectively. The positions of the first in-frame stop codon are indicated by an asterisk.
Discussion
We report the discovery and characterization of a new maize pectinesterase gene and protein that is expressed in silks of Ga1-s but not ga1 plants and maps to the ga1 locus. We have named this gene ZmPme3. The genetic map position, ZmPme3 allele sequences, RNA expression and protein expression are consistent with the hypothesis that ZmPME3 is involved in Ga1 function. Additional work is needed to establish the role of ZmPME3 in the process of Ga1 gametophytic incompatibility. Genetic disruption of ZmPme3 and overexpression in transgenic plants, as well as heterologous expression and activity studies, could all be used to provide evidence of ZmPME3 function. Until these studies are complete, ZmPME3 should be considered a candidate gene for Ga1 function. The observations that Ga1 has different functions in different tissues suggest that the locus is functionally complex and presence of the Ga1-m allele suggests that it is genetically complex as well. Any molecular explanation of Ga1 function will need to address these complexities.
PMEs have been shown to alter pollen tube growth in pollen tube cell walls in Arabidopsis (Jiang et al., 2005; Tian et al., 2006) and tobacco (Bosch et al., 2005; Bosch and Hepler, 2006). The likely mechanism for this activity is deesterification of homogalacturanan in the growing tip of pollen tubes. This alters the rigidity of the pollen tube cell wall, impairing its ability to grow directly toward the ovule. The growth behavior of pollen tubes with altered PME activity in these studies is consistent with the observations of incompatible pollen tube growth in Ga1-s silks presented here and elsewhere (Lu et al., 2014). An active version of ZmPme3 is not found in either Ga1-m or ga1 genotypes surveyed, which lack the Ga1-s female function. Genetic evidence suggests that the lack of the female function in ga1 may be because ga1 is a null allele of the ga1 locus. Plants with the Ga1-m allele are able to pollinate Ga1-s but can also be pollinated by ga1. They therefore have the male function but lack the female function of the ga1 locus. Our observation that Ga1-m contains a mutationally inactivated allele of ZmPme3 would predict that inactivation of ZmPme3 would result in the loss of the female function while the male function of Ga1 is preserved. The male function is genetically separable from the female function in Ga1-m, suggesting that it is encoded by a separate but tightly linked gene from ZmPme3.
Interestingly, PMEIs are typically structurally similar to PMEs, but they contain an additional inhibitor domain on the N terminus of the protein (reviewed in Pelloux et al., 2007). This raises the possibility that the silk specific PME identified here is actually a PMEI and the transcript we identified is incomplete. Several observations suggest this is not the case. First, the intron/exon structure is more similar to a PME than a PMEI (Duan et al., 2016). Second, the gene encoding the differentially expressed silk PME contains predicted promoter elements including TATA and CAAT boxes. This suggests that the gene contains the transcriptional start site and we are not missing an exon containing a PMEI domain. Finally, the differentially expressed silk PME is predicted to contain a signal sequence. Such a sequence would target the PME to the extracellular space. This is consistent with ZmPME3 having a role in pollen exclusion which would require the PME to be present in the extracellular space to interact with pollen tubes. Since signal sequences are removed during translation, it is likely that the start codon identified here is correct.
Conclusions
We have identified a pectin methylesterase gene designated as ZmPme3 that is present in the silks of Ga1-s plants and maps to the ga1 locus. This gene is lacking in ga1 genotypes but present and mutationally inactive in Ga1-m lines which lack the female function of Ga1-s. We also identify a novel cluster of PME pseudogenes that map at the ga1 locus in ga1 genotypes. We propose a role for PME in the molecular mechanism of pollen exclusion that provides a framework for hypothesis testing and new potential targets for discovery in other maize gametophytic incompatibility systems.
Accession Numbers
The W22 genome sequence has been released under the Toronto Agreement, GenBank Bioproject PRJNA311133. RNA-Seq data is deposited in the NCBI Short Read Archive accession number SRP111127.
Author Contributions
AM analyzed and interpreted data, designed and performed the experiments, wrote and revised the manuscript. MM conceived of the work and generated the RNA-Seq data. RH acquired and interpreted data. MS conceived of the work, analyzed data, wrote and revised the manuscript. All authors contributed to the discussion and approved the final manuscript.
Funding
This work was supported in part by a grant from USDA-NIFA-OREI (2014-05340) as well as USDA-ARS CRIS (3050-21000-063-00D) project funds.
Conflict of Interest Statement
The authors declare that the research was conducted in the absence of any commercial or financial relationships that could be construed as a potential conflict of interest.
Acknowledgments
The authors wish to thank Abby Petefish for technical support, the Maize Genetics Stock Center, Dr. Major Goodman and Dr. Jim Holland for the genetic stocks used in this work, the ISU Bioinformatics Facility for assistance with processing RNA-Seq data, Dr. Matt Evans for helpful discussions and Dr. Erik Vollbrecht sharing for the W22 genome sequence prior to publication. Mention of trade names or commercial products in this publication is solely for the purpose of providing specific information and does not imply recommendation or endorsement by the U.S. Department of Agriculture.
Supplementary Material
The Supplementary Material for this article can be found online at: https://www.frontiersin.org/articles/10.3389/fpls.2017.01926/full#supplementary-material
References
Bloom, J. C., and Holland, J. B. (2011). Genomic localization of the maize cross-incompatibility gene, Gametophyte factor 1 (ga1). Maydica 56, 379–387.
Bosch, M., and Hepler, P. K. (2006). Silencing of the tobacco pollen pectin methylesterase NtPPME1 results in retarded in vivo pollen tube growth. Planta 223, 736–745. doi: 10.1007/s00425-005-0131-x
Bosch, M., Cheung, A. Y., and Hepler, P. K. (2005). Pectin methylesterase, a regulator of pollen tube growth. Plant Physiol. 138, 1334–1346. doi: 10.1104/pp.105.059865
Correns, C. (1901). Bastarde zwischen Maisrassem, mit besonderer Berucksichtung der Xenien. Biblio. Bot. 53, 1–161.
Duan, W., Huang, Z., Song, X., Liu, T., Liu, H., Hou, X., et al. (2016). Comprehensive analysis of the polygalacturonase and pectin methylesterase genes in Brassica rapa shed light on their different evolutionary patterns. Sci. Rep. 6:25107. doi: 10.1038/srep25107
Evans, M. M. S., and Kermicle, J. L. (2001). Teosinte crossing barrier1, a locus governing hybridization of teosinte with maize. Theor. Appl. Genet. 103, 259–265. doi: 10.1007/s001220100549
Jiang, L., Yang, S. L., Xie, L. F., Puah, C. S., Zhang, X. Q., Yang, W. C., et al. (2005). VANGUARD1 encodes a pectin methylesterase that enhances pollen tube growth in the Arabidopsis style and transmitting tract. Plant Cell 17, 584–596. doi: 10.1105/tpc.104.027631
Jimenez, T., and Nelson, O. E. (1965). A new fourth chromosome gametophyte locus in maize. J. Hered. 56, 259–263. doi: 10.1093/oxfordjournals.jhered.a107433
Jones, D. F. (1924). Selective fertilization among the gametes from the same individuals. Proc. Natl. Acad. Sci.U.S.A. 10, 218–221. doi: 10.1073/pnas.10.6.218
Kermicle, J. L., and Evans, M. M. S. (2005). Pollen-pistil barriers to crossing in maize and teosinte result from incongruity rather than active rejection. Sex. Plant Reprod. 18, 187–194. doi: 10.1007/s00497-005-0012-2
Kermicle, J. L., Taba, S., and Evans, M. M. S. (2006). The Gametophyte-1 locus and reproductive isolation among Zea mays subspecies. Maydica 51, 219–225.
Krichevsky, A., Kozlovsky, S. V., Tian, G. W., Chen, M. H., Zaltsman, A., and Citovsky, V. (2007). How pollen tubes grow. Dev. Biol. 303, 405–420. doi: 10.1016/j.ydbio.2006.12.003
Lausser, A., Kliwer, I., Srilunchang, K. O., and Dresselhaus, T. (2010). Sporophytic control of pollen tube growth and guidance in maize. J. Exp. Bot. 61, 673–682. doi: 10.1093/jxb/erp330
Liu, X., Sun, H., Wu, P., Tian, Y., Cui, D., Xu, C., et al. (2014). Fine mapping of the maize cross- Incompatibility locus gametophytic factor 1 (ga1) using a homogeneous population. Crop Sci. 54, 873–881. doi: 10.2135/cropsci2013.09.0598
Li, H., Handsaker, B., Wysoker, A., Fennell, T., Ruan, J., Homer, N., et al. (2009). The sequence alignment/map format and SAMtools. Bioinformatics 25, 2078–2079. doi: 10.1093/bioinformatics/btp352
Lu, Y., Kermicle, J. L., and Evans, M. M. S. (2014). Genetic and cellular analysis of cross-incompatibility in Zea mays. Plant Reprod. 27, 19–29. doi: 10.1007/s00497-013-0236-5
Mangelsdorf, P. C., and Jones, D. F. (1926). The expression of mendelian factors in the gametophyte of maize. Genetics 11, 423–455.
McCarthy, D. J., Chen, Y., and Smyth, G. K. (2012). Differential expression analysis of multifactor RNA-Seq experiments with respect to biological variation. Nucleic. Acids Res. 40, 4288–4297. doi: 10.1093/nar/gks042
McMullen, M. D., Kresovich, S., Villeda, H. S., Bradbury, P., Li, H., Sun, Q., et al. (2009). Genetic properties of the maize nested association mapping population. Science 325, 737–40. doi: 10.1126/science.1174320
Méchin, V., Damerval, C., and Zivy, M. (2007). Total Protein Extraction with TCA-Acetone. Methods Mol. Biol. 355, 1–8.
Morgan, M., Pages, H., Obenchain, V., and Hayden, N. (2010). Rsamtools: Binary Alignment (BAM), FASTA, Variant Call (BCF), and Tabix File Import. R package version 1.18. 2.
Nelson, O. E. (1994). “The gametophyte factors of maize,” in The Maize Handbook, eds V. Freeling and M. Walbot (New York, NY: Springer), 496–503.
Nelson, O. E. (1953). A genic substitute for isolation in hybrid corn seed production. Econ. Bot. 7, 382–384. doi: 10.1007/BF02930812
Noé, L., and Kucherov, G. (2005). YASS: enhancing the sensitivity of DNA similarity search. Nucleic. Acids Res. 33, W540–W543. doi: 10.1093/nar/gki478
Pelloux, J., Rustérucci, C., and Mellerowicz, E. J. (2007). New insights into pectin methylesterase structure and function. Trends Plant Sci. 12, 267–277. doi: 10.1016/j.tplants.2007.04.001
R Core Team (2016). R: A Language and Environment For Statistical Computing. Vienna: R Foundation for Statistical Computing. Available online at: http://www.R-project.org/
Robinson, M. D., McCarthy, D. J., and Smyth, G. (2010). edgeR: a Bioconductor package for differential expression analysis of digital gene expression data. Bioinformatics 26, 139–140. doi: 10.1093/bioinformatics/btp616
Robinson, M. D., and Smyth, G. K. (2007). Moderated statistical tests for assessing differences in tag abundance. Bioinformatics 23, 2881–2887. doi: 10.1093/bioinformatics/btm453
Robinson, M. D., and Smyth, G. K. (2008). Small-sample estimation of negative binomial dispersion, with applications to SAGE data. Biostatistics 9, 321–332. doi: 10.1093/biostatistics/kxm030
Schnable, P., Ware, D., Fulton, R., and Stein, J. (2009). The B73 maize genome: complexity, diversity, dynamics. Science 326, 1112–1115. doi: 10.1126/science.1178534
Tian, G. W., Chen, M. H., Zaltsman, A., and Citovsky, V. (2006). Pollen-specific pectin methylesterase involved in pollen tube growth. Dev. Biol. 294, 83–91. doi: 10.1016/j.ydbio.2006.02.026
Trapnell, C., Pachter, L., and Salzberg, S. L. (2009). TopHat: discovering splice junctions with RNA-Seq. Bioinformatics 25, 1105–1111. doi: 10.1093/bioinformatics/btp120
Wang, M., Yuan, D., Gao, W., Li, Y., Tan, J., and Zhang, X. (2013). A comparative genome analysis of PME and PMEI families reveals the evolution of pectin metabolism in plant cell walls. PLoS ONE 8:e72082. doi: 10.1371/journal.pone.0072082
Wickham, H. (2009). ggplot2 Elegant Graphics for Data Analysis. Available online at: http://had.co.nz/ggplot2/
Zhang, H., Liu, X., Zhang, Y., Jiang, C., Cui, D., Liu, H., et al. (2012). Genetic analysis and fine mapping of the Ga1-S gene region conferring cross-incompatibility in maize. Theor. Appl. Genet., 124, 459–465. doi: 10.1007/s00122-011-1720-7
Zhu, Y., Zhao, P., Wu, X., Wang, W., Scali, M., and Cresti, M. (2011). Proteomic identification of differentially expressed proteins in mature and germinated maize pollen. Acta Physiol. Plant. 33, 1467–1474. doi: 10.1007/s11738-010-0683-7
Keywords: gametophytic incompatibility, pollen, pistil, Zea mays, pectin methylesterase
Citation: Moran Lauter AN, Muszynski MG, Huffman RD and Scott MP (2017) A Pectin Methylesterase ZmPme3 Is Expressed in Gametophyte factor1-s (Ga1-s) Silks and Maps to that Locus in Maize (Zea mays L.). Front. Plant Sci. 8:1926. doi: 10.3389/fpls.2017.01926
Received: 22 September 2017; Accepted: 24 October 2017;
Published: 07 November 2017.
Edited by:
Maurice Bosch, Aberystwyth University, United KingdomReviewed by:
Huabang Chen, Institute of Genetics and Developmental Biology (CAS), ChinaThomas C. Hoegemeyer, University of Nebraska Lincoln, United States
Copyright © 2017 Moran Lauter, Muszynski, Huffman and Scott. This is an open-access article distributed under the terms of the Creative Commons Attribution License (CC BY). The use, distribution or reproduction in other forums is permitted, provided the original author(s) or licensor are credited and that the original publication in this journal is cited, in accordance with accepted academic practice. No use, distribution or reproduction is permitted which does not comply with these terms.
*Correspondence: Adrienne N. Moran Lauter, YWRyaWVubmUubW9yYW5sYXV0ZXJAYXJzLnVzZGEuZ292