- 1School of Life Sciences, Chongqing University, Chongqing, China
- 2School of Basic Medical Sciences, North Sichuan Medical College, Nanchong, China
- 3Department of Cellular and Structural Biology, University of Texas Health Science Center at San Antonio, San Antonio, TX, United States
- 4College of Agronomy and Biotechnology, Southwest University, Chongqing, China
- 5Plant Biotechnology Institute, National Research Council of Canada, Saskatoon, SK, Canada
Phytophthora infestans (P. infestans) is the causal agent of potato late blight, which caused the devastating Irish Potato Famine during 1845-1852. Until now, potato late blight is still the most serious threat to potato growth and has caused significant economic losses worldwide. Melatonin can induce plant innate immunity against pathogen infection, but the direct effects of melatonin on plant pathogens are poorly understood. In this study, we investigated the direct effects of melatonin on P. infestans. Exogenous melatonin significantly attenuated the potato late blight by inhibiting mycelial growth, changing cell ultrastructure, and reducing stress tolerance of P. infestans. Notably, synergistic anti-fungal effects of melatonin with fungicides on P. infestans suggest that melatonin could reduce the dose levels and enhance the efficacy of fungicide against potato late blight. A transcriptome analysis was carried out to mine downstream genes whose expression levels were affected by melatonin. The analysis of the transcriptome suggests that 66 differentially expressed genes involved in amino acid metabolic processes were significantly affected by melatonin. Moreover, the differentially expressed genes associated with stress tolerance, fungicide resistance, and virulence were also affected. These findings contribute to a new understanding of the direct functions of the melatonin on P. infestans and provide a potential ecofriendly biocontrol approach using a melatonin-based paradigm and application to prevent potato late blight.
Introduction
Phytophthora infestans is a notorious oomycete pathogen, as the causal agent of potato late blight, which triggered the Great Irish Famine in the mid-nineteenth century (Pearson, 2011). Potato late blight is the most serious threat to potato plants and causes significant economic losses worldwide (Haverkort et al., 2008). In recent decades, several chemical fungicides have been developed and applied for controlling potato late blight by targeting the typical mechanisms of impairing the respiratory chain, disrupting metabolic homeostasis and inhibiting RNA polymerase of P. infestans (Matheron and Porchas, 2000; Mitani et al., 2001; Gullino et al., 2010). However, the highly complex and huge genome of P. infestans confers this pathogen the capacity of rapid evolution to overcome these chemical fungicides by altering a single gene (Haas et al., 2009). Moreover, it is important that the environmental, health and safety concerns associated with the chemical fungicides be taken into consideration. Thus, the need to develop alternatives to fungicide-based approaches that offers less toxicity, more target-specific and environmental-sustainability is desired and preferred.
Melatonin, a natural product also referred as N-acetyl-5-methoxytryptamine, is widely present in animals, plants, and microbes (Arendt, 2005; Pandi-Perumal et al., 2006; Reiter et al., 2014). Initially, its function is known to regulate circadian rhythms. Subsequently, other functions including the modulation of mood, sleep, metabolism, and antioxidant are also established in diverse organisms (Reiter et al., 2009, 2015, 2016; Arnao and Hernandezruiz, 2015; Manchester et al., 2015). Specifically, melatonin has been reported to be effective in inhibiting the cell growth in some human pathogens by impairing their mitochondrial functions, inhibiting biofilm formation, and reducing intracellular substrates (Tekbas et al., 2008; Elmahallawy et al., 2014; Yang et al., 2014). For example, melatonin inhibits Leishmania via impairing the mitochondrial functions (Elmahallawy et al., 2014). Besides, melatonin mediated inhibition of the growth of a variety of cancer cells, such as hepatoma via the interference of fatty acid metabolism (Blask et al., 1999a,b; Sauer et al., 2001), colorectal cancer via the decrease of MT1 (melatonin receptor 1) (Farriol et al., 2000; Nemeth et al., 2011), pituitary tumor via the disturbance of nuclear receptor (Karasek et al., 2003). Importantly, a number of clinical trials have also confirmed that the use of melatonin is an effective measure to control a variety of disease, including the infectious diseases caused by pathogenic bacteria or virus, such as sepsis, Herpes (Sanchezbarcelo et al., 2010).
In plants, melatonin increases the resistance against pathogens via activating the expression of defense genes, elevating NO production, and thickening the cell wall (Yin et al., 2013; Lee et al., 2014, 2015; Qian et al., 2015; Shi et al., 2015, 2016; Zhao et al., 2015; Lee and Back, 2016; Wei et al., 2017). For example, various pathogenesis-related (PR) and defense genes that are activated by salicylic acid (SA) and ethylene (ET), have also been shown to be induced by melatonin in the Arabidopsis and tobacco (Lee et al., 2014, 2015). Melatonin also induces NO production, which plays an important role in plant innate immunity response against bacterial pathogen attacks (Shi et al., 2015). It is also observed that melatonin is effective for thickening cell wall by inducing the accumulation of cellulose, galactose, xylose, and callose in plants to prevent pathogen infection (Qian et al., 2015; Zhao et al., 2015). Recently, studies on melatonin against plant pathogens indirectly by triggering the plant immunity have been explored. However, little is known about the direct interaction between melatonin and plant pathogens (Arnao and Hernandezruiz, 2015).
In this study, we investigated the direct effects of melatonin on P. infestans. The results and observations showed that the mycelial growth, cell ultrastructure, stress tolerance, and fungicide susceptibility of P. infestans could be significantly altered in the presence of melatonin. Because of the lack of the potato varieties resistant to late blight, the control of this notorious disease largely depends on the high dosage and frequency of fungicide application. Interestingly, the dosage of fungicide can be significantly reduced when it was combined with melatonin, which would be important for human health and the environment. Majority of the previous transcriptome studies focused on the host-P. infestans interaction (Gao et al., 2013; Ali et al., 2014; Frades et al., 2015; Ah-Fong et al., 2017), but our analysis of transcriptome were focused to reveal the underlying mechanism of melatonin against P. infestans. The key findings of this study will be useful to explore the melatonin-based alternate approaches for the late blight control in potato.
Materials and Methods
P. infestans Strain, Media, and Culture Conditions
T30-4 (A1 mating type) is the sequenced P. infestans isolate that is commonly used in laboratory research worldwide (Haas et al., 2009). It was provided by Dr. Suomeng Dong, Nanjing Agriculture University, China. The strain was cultured on Rye A agar at 18°C in the dark as described in previous report (Avrova et al., 2003).
Effects of Melatonin on Late Blight of Potato Infected by P. infestans T30-4
P. infestans strain T30-4 was cultured on Rye A agar at 18°C in the dark for 14 days (Avrova et al., 2003). Then, the potato leaves and the tuber slices (2 cm × 3 cm × 3 mm in size) were sprayed with melatonin solution in varying concentrations (0, 1, 3, 6, 8, 10 mM) dissolved in DMSO, and the same volumes of DMSO as the control, and the water also as the control (CK) (excluding the influence on leaves and tubers by melatonin or DMSO). Then, the 7-mm-diameter T30-4 mycelial disks were put on the potato tuber slices and leaves (the melatonin team and DMSO team), then incubated at 18°C with a 12-h/12-h light/dark cycle for 5 days. The size of each lesion was measured then analyzed with the Student's t-test. Three biological repeats were performed for each experiment.
Measuring the Effects of Melatonin on Mycelial Growth of P. infestans
The 7-mm-diameter T30-4 mycelial disks were cultured on Rye A agar plates supplemented with varying concentrations of melatonin solution (0, 1, 1.5, 2, 3, and 5 mM), and the same volumes of DMSO as the control. The diameters of the colony were measured at the 9th day and 14th day. The inhibition rates were calculated using the diameter of control colony (D), drug-treated colony (M) as follows: [(D – M)/(D – 0.7)] × 100%. All experiments were repeated three times.
Measurements of the Effects of Melatonin on Cell Viability of P. infestans
MTT, 3-(4, 5-dimethyl-2-thiazolyl)-2, 5-diphenyl-2H-tetrazolium bromide can be converted to formazan by the mitochondrial reductase of living cells. The 7-mm-diameter T30-4 mycelial disks were cultured on 96-well plates supplemented with DMSO (as control), melatonin (0, 3, 6, 10 mM), Infinito (0, 0.01, 0.05, 0.1 ml/L, ml/L stands for the volume of fungicide in 1 L water), and various combinations of melatonin + Infinito (3 + 0.01, 6 + 0.05, 10 mM + 0.1 ml/L), and incubated at 18°C for 24 h. After 24 h, 10 μL MTT solution was added and incubated at 18°C for 4 h. Then, the samples were measured in an automatic microplate reader working at a wavelength of 490 nm. The cell viability (%) = [A (drug) – A (mock)]/[A (control) – A (mock)].
Electron Microscopy
The T30-4 was cultured in liquid Rye A agar medium containing 6 mM melatonin and the same volumes of DMSO were set as the control, then incubated at 18°C in the dark for 9 days. Next, the mycelia were collected, fixed, observed for scanning electron microscope (SEM) and transmission electron microscope (TEM) as described in the literatures (Cao et al., 2014; Chen et al., 2017).
Stress Tolerance Assay
(1). The 7-mm-diameter T30-4 mycelial disks were cultured on Rye A agar plates with different supplements: DMSO (as a control), melatonin (3 mM), NaCl (0.1 M)/H2O2 (0.1 mM), and a combination of melatonin + NaCl/H2O2. Then the disks were incubated at 18°C in the dark for 14 days.
(2). The 7-mm-diameter T30-4 mycelial disks were cultured on Rye A agar plates with different treatments: DMSO (as a control), melatonin (3 mM), UV (1,350 Mw/mm2) for 30 mins/37°C for 2 h/4°C for 24 h, and a combination of melatonin + UV/37°C/4°C. Then, the disks were incubated at 18°C in the dark for 14 days.
(3). After the 14 days, we measured the diameter of the colony and calculated the inhibition rates. All experiments were repeated three times.
Analysis of Synergism/Antagonism Effect of the Combination of Melatonin and Fungicide on P. infestans
Infinito is a famous fungicide, manufactured by Bayer (Germany), mainly composed of Fluopicolide and Propamocab hydrochloride. The 7-mm-diameter T30-4 mycelial disks were cultured on Rye A agar plates supplemented with DMSO (as control), melatonin solution in DMSO (0, 1, 2, 3 mM), fungicide (0, 0.001, 0.005, 0.01 ml/L, ml/L stands for the volume of fungicide in 1 L water), and various combinations of melatonin + Infinito (1 + 0.001, 1 + 0.005, 1 + 0.01, 2 + 0.001, 2 + 0.005, 2 + 0.01, 3 + 0.001, 3 + 0.005, 3 mM + 0.01 ml/L), and incubated at 18°C for 14 days. Then, the diameters of the colony were measured and the inhibition rates were analyzed by using the Student's t-test. Each datum was from the average of three independent biological replicates. The interaction between melatonin and fungicide (Infinito) was quantitatively measured using Combination index (CI) value (Xiong et al., 2017). The levels of reagent interaction were defined following the previous report (Chou, 2006), synergism (CI < 1), additive effect (CI = 1), or antagonism (CI > 1). The percentage of growth value was calculated using the diameter of 14-day-old DMSO-treated control colony (D), and drug-treated colony (T) as follows: [(T-0.7)/(D-0.7)] × 100. IC50 (The half maximal inhibitory concentration) and CI values were calculated by using the CompuSyn software (Chou and Talalay, 1984). The affected value (Fa) indicated the growth inhibition of colony by drug was calculated using the program's instructions as follows: (100 – % growth value)/100.
Pathogenicity Test of P. infestans by Using the Combination of Melatonin and Fungicide
P. infestans strain T30-4 was cultured on Rye A agar at 18°C in the dark for 14 days (Avrova et al., 2003). Then, potato leaves and tuber slices (2 cm × 3 cm × 3 mm in size) were sprayed with DMSO (as control), melatonin solution (3, 6, 8 mM), Infinito (0.0025, 0.025, 0.25 ml/L), and melatonin + Infinito (3 + 0.0025, 3 + 0.025, 3 + 0.25, 6 + 0.0025, 6 + 0.025, 6 + 0.25, 8 + 0.0025, 8 + 0.025, 8 mM + 0.25 ml/L). The 7-mm-diameter T30-4 mycelial disks were put on the potato tuber slices and leaves, and incubated at 18°C with a 12-h/12-h light/dark cycle for 5 days. Then, the size of each lesion was measured and analyzed with the Student's t-test. Three biological repeats were performed for each experiment.
Transcriptome Assay and the Dataset Analysis
The T30-4 was chosen for the RNA-Seq (Haas et al., 2009). The T30-4 was cultured for 14 days in liquid Rye A agar medium at 18°C in the dark. Then the mycelia were transplanted to liquid Rye A agar medium containing with 3 mM melatonin (IC50: half maximal inhibitory concentration) and the same volumes of DMSO as the control, where mycelia were cultured at 18°C in the dark for 24 h. The mycelia were collected and frozen in liquid nitrogen for transcriptome studies. The total RNA was extracted from the samples using the RNAprep Pure Plant Kit (TianGen Biotech, Beijing, China). Three biological replicates were employed in the transcriptome experiments. The sequencing libraries were generated using the NEBNext® Ultra™ RNA Library Prep Kit for Illumina® (NEB, USA) following the manufacturer's recommendations. These libraries were sequenced on an Illumina Hiseq platform and 125 bp/150 bp paired-end reads were generated. Reference genome and gene model annotation files were downloaded from the genome website directly. Paired-end clean reads were aligned to the reference genome using TopHat v2.0.12. HTSeq v0.6.1 was used to count the numbers of reads mapped to each gene. Then, FPKM of each gene was calculated based on the length of the gene and read counts mapped to this gene. Differential expression analysis was performed using the DESeq R package (1.18.0). Genes with an adjusted p < 0.05 found by DESeq were regarded as the differentially expressed ones. Gene Ontology (GO) enrichment analysis of differentially expressed genes was implemented by the GO seq R package. GO terms with corrected p < 0.05 were considered as significant ones. The KOBAS software was used to test the statistical enrichment of differential expression genes in KEGG pathway. The transcriptome datasets were submitted to NCBI and the accession number—PRJNA415528.
Real-Time Quantitative RT-PCR (qRT-PCR) Assay
The mycelia were cultured and collected as described in RNA-seq. The total RNA was extracted from the mycelia by using the RNAprep Pure Plant Kit (TianGen Biotech, Beijing, China). The total RNA (1 μg) was used for reverse transcription reaction by using the PrimeScript RT Kit (TAKARA Biotech). After that, the TransStart TopGreen qPCR Super Mix (TransGen Biotech) was used for the qRT-PCR assays by a Bio-Rad CFX96 System. Primer sequences for qRT-PCR were listed in Table S1. Each datum was averaged from three independent biological replicates.
Results and Discussion
Potato Late Blight Can Be Significantly Attenuated by Melatonin in Vivo
It has been proven that melatonin displays multiple effects on the cell growth and development of plants, animals and humans (Pandi-Perumal et al., 2006; Reiter et al., 2014), but the impact of melatonin on P. infestans has not been explored. For this aim, the T30-4 strain, was inoculated on the potato leaves and tuber slices which were pretreated with DMSO (mock) and various concentrations of melatonin solution. The in vivo assay showed that the lesion sizes of leaves and tuber slices infected by P. infestans were gradually reduced by increasing melatonin concentrations, and no toxic effects to leaves or tuber even at the highest concentration of melatonin were observed (Figures 1A–D). The occurrence of potato late blight can be prevented on leaves and tuber slices when the concentration of melatonin reached 10 mM (Figures 1A–D). Thus, these in vivo observations showed that the potato late blight can be significantly attenuated by using melatonin.
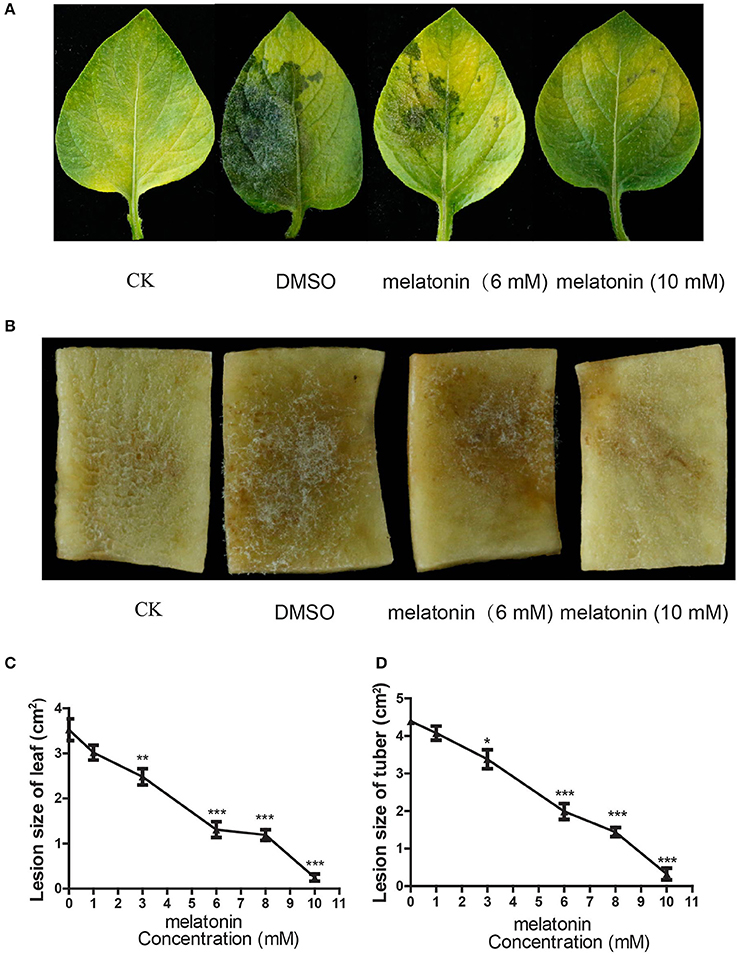
Figure 1. Melatonin suppress potato late blight in a dose dependent manner. (A,B) Disease symptoms of potato late blight on leaves (A) and fresh tuber slices (B) were captured after inoculation of T30-4 strain for 5 days. The leaves and slices were pretreated with water (CK, without inoculation with P. infestans), DMSO, and different concentrations of melatonin. (C,D) The dose-effect curves of melatonin against P. infestans on potato leaves (C) and tuber slices (D). (*P < 0.05, **P < 0.01, ***P < 0.001). Each data point was average of three independent biological replicates.
Suppressing the Mycelial Growth of P. infestans by Melatonin in Vitro
The previous studies have shown that melatonin can act as an important signal to induce innate immunity in plants (Yin et al., 2013; Lee et al., 2014, 2015; Qian et al., 2015; Shi et al., 2015, 2016; Zhao et al., 2015; Lee and Back, 2016; Wei et al., 2017). To exclude the effects of plant immunity induced by melatonin on the occurrence of potato late blight, we next investigated whether melatonin can directly suppress the cell growth of P. infestans. Toward this objective, the strain T30-4 was cultured on the medium supplemented with different concentrations of melatonin. When higher concentrations of melatonin were used, slower growth of mycelia was observed (Figures 2A,B). The IC50 of melatonin appeared at 3 mM for T30-4 (Figures 2A,B). The mycelial growth was completely inhibited at a melatonin concentration of 5 mM (Figures 2A,B). Furthermore, after being incubated for 24 h with different concentrations of melatonin, the mycelial cell viability was tested by the MTT reduction assay. This assay showed that the cell viability was decreased with increases in melatonin concentration (Figure 2C). These in vitro observations suggested that melatonin could directly suppress the cell growth of P. infestans to attenuate the potato late blight, which was consistent with the previous studies that exogenous melatonin directly resists the human pathogens in vitro, including Leishmania infantum, Candida parapsilosis, Staphylococcus aureus (Tekbas et al., 2008; Elmahallawy et al., 2014; Yang et al., 2014).
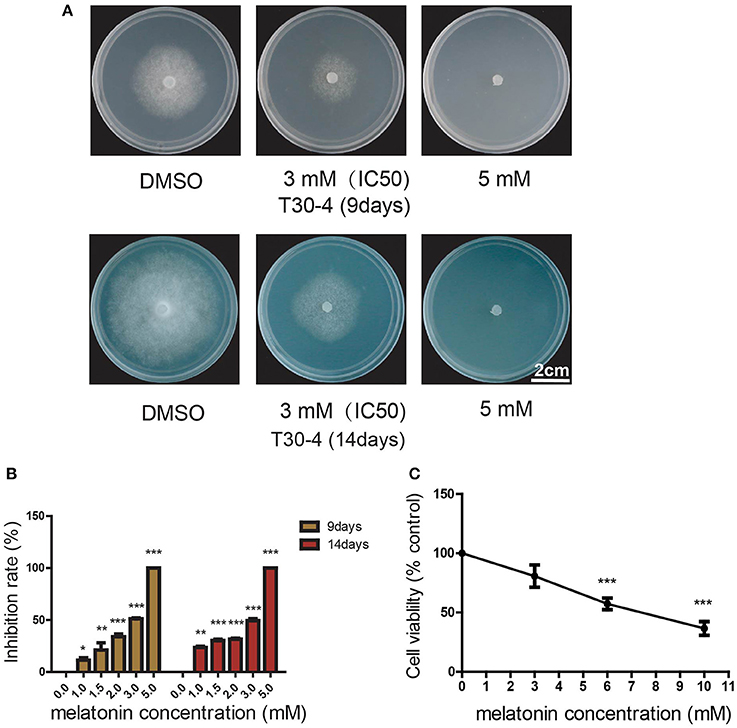
Figure 2. Melatonin can suppress the growth of P. infestans in vitro. (A) Mycelial phenotypes of P. infestans grown on the medium supplemented with melatonin for 9 days (upper panel) and 14 days (below panel). The strain T30-4 was cultured for on Rye A agar amended with 3 or 5 mM melatonin. (B) Dose-dependent effect of melatonin on the mycelial inhibition rate of T30-4 at 9 and 14 days. The strain T30-4 was treated with DMSO (control) and melatonin at 1, 1.5, 2, 3, and 5 mM, respectively (*P < 0.05, **P < 0.01, ***P < 0.001). Each data point was averaged of three independent biological replicates. (C) MTT reduction assay showed that cell viability of P. infestans T30-4 under melatonin treatment for 24 h. The strain T30-4 was treated with DMSO (control) and melatonin at 3, 6, 10 mM (*P < 0.05, **P < 0.01, ***P < 0.001).
Influencing the Ultrastructure of P. infestans by Melatonin
To understand the growth inhibition phenotypes of P. infestans caused by melatonin treatment, the cell ultrastructure of the melatonin-treated P. infestans was investigated using scanning electron microscopy (SEM) and transmission electron microscopy (TEM). As shown in Figures 3A,B, the disorganized hyphal growth pattern, and aberrant and distorted hyphae were observed in melatonin-treated P. infestans in comparison with the untreated controls; this could result in the inhibition of hyphal growth of P. infestans. In melatonin-treated P. infestans, the branched hyphae and blunt hyphal tip (Figures 3B,D), possibly was a result of suppressing the apical dominance of the hyphal tip that could lead to the inhibition of hyphal elongation (Semighini and Harris, 2008; Zhao et al., 2011). Furthermore, the TEM analysis showed that more vacancies and fewer lipid droplets appeared in the cells of P. infestans with melatonin treatment compared to the control (Figures 3E,F), indicating that the melatonin treatment resulted in the decrease of lipid droplets in P. infestans. It is well-known that lipid droplets play an important role in various biological processes, such as facilitation of cell growth, propagation as well as infection of fungus, viral and bacterium (Bago et al., 2002; Stehr et al., 2012; Radulovic et al., 2013; Kobae et al., 2014; Bi et al., 2016). Thus, the reduction of lipid droplets could result in the inhibition of growth of P. infestans.
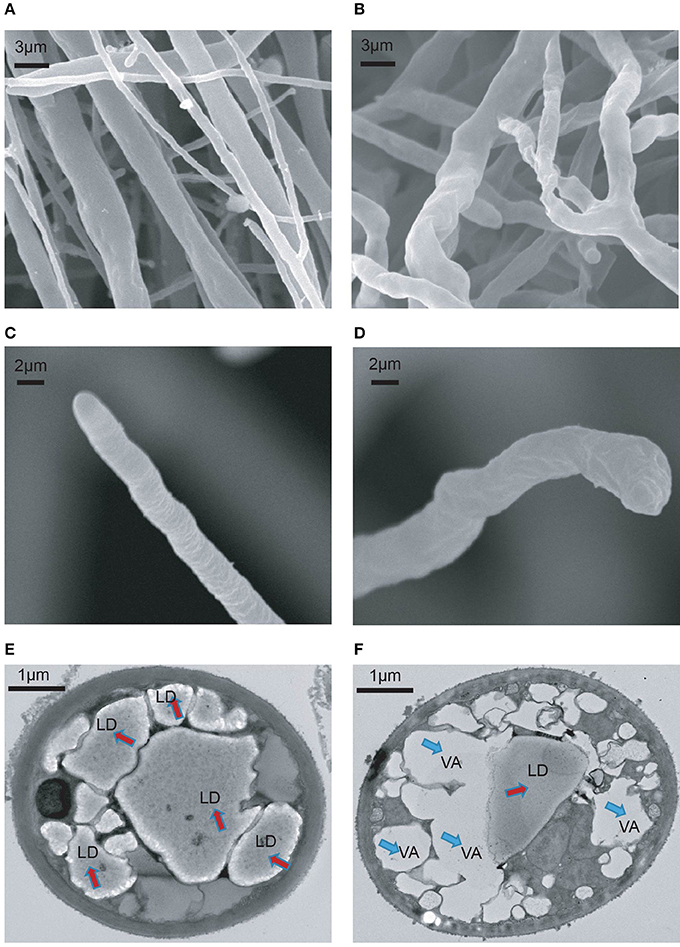
Figure 3. SEM and TEM analysis of melatonin treated P. infestans. Ultrastructure of (A) hyphal surface untreated by melatonin documented by SEM, (B) hyphal surface treated by melatonin documented by SEM, (C) hyphal tip untreated by melatonin documented by SEM, (D) hyphal tip treated by melatonin documented by SEM, (E) hypha untreated by melatonin documented by TEM. LP, lipid droplet, (F) hypha treated by melatonin documented by TEM. VA, vacancy.
Enhanced Susceptibility of P. infestans to Various Environmental Stresses in the Presence of Melatonin
The tolerance of P. infestans to environmental stresses derived from salt, heat, cold and Ultra Violate (UV) radiation can promote the successful occurrence and epidemics of potato late blight (Ortizurquiza and Keyhani, 2014). We therefore explored the tolerance of P. infestans to these stresses and the combination of these stresses with melatonin. The mycelial growth of P. infestans was inhibited when using salt, UV, heat, H2O2 and cold, respectively (Figures 4A–C,E,G,I,K,M), indicating that P. infestans was sensitive to these types of environmental stresses. Interestingly, the mycelial growth of P. infestans was more severely inhibited by the pairwise combination of melatonin and these stresses (UV/salt/heat) than that of melatonin or any of these stresses alone (Figures 4A–F,I,J,M). These results indicated that melatonin reduced the stress tolerance of P. infestans under challenging environmental conditions.
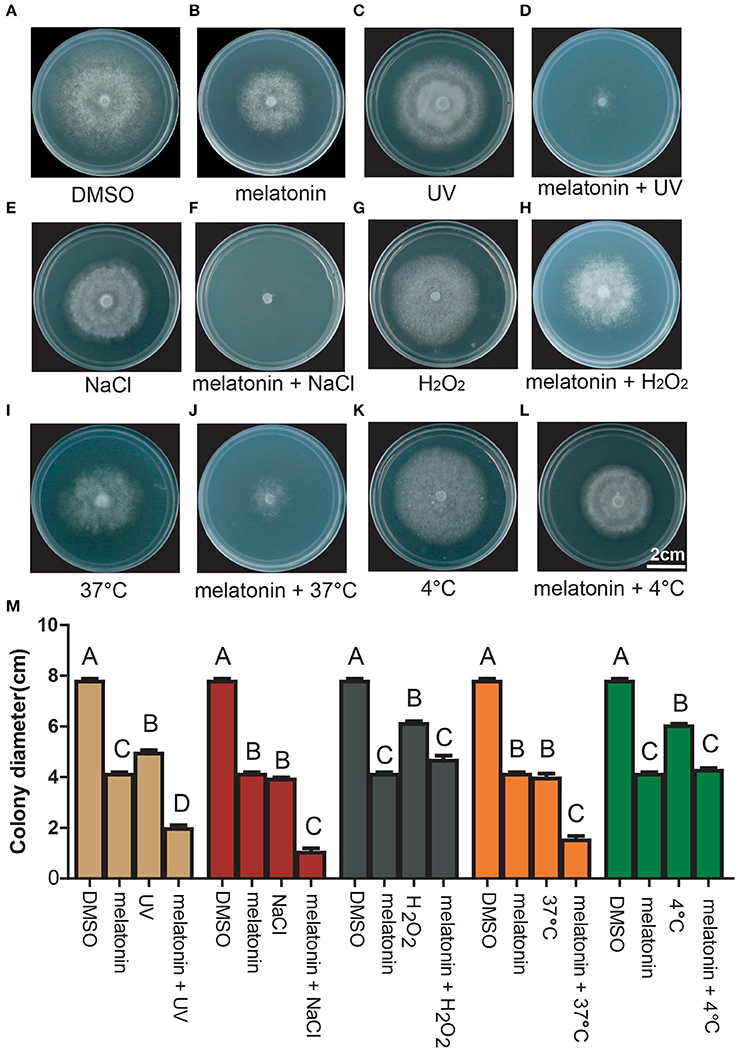
Figure 4. Growth pattern of P. infestans in response to the treatment of DMSO, melatonin, stress, and melatonin + stress. Colony morphology of P. infestans grown on Rye A agar medium treated by (A–L) DMSO, 3 mM melatonin, UV, 3 mM melatonin + UV, 0.1 M NaCl, 3 mM melatonin + 0.1 M NaCl, 0.1 mM H2O2, 3 mM melatonin + 0.1 mM H2O2, 37°C, 3 mM melatonin + 37°C, 4°C, 3 mM melatonin + 4°C. (M) Colony diameter of P. infestans grown on Rye A agar medium treated by DMSO, 3 mM melatonin, UV/NaCl/H2O2/37°C/4°C, 3 mM melatonin + UV/NaCl/H2O2/37°C/4°C. Capitalized letters indicate significant difference, p < 0.01.
Melatonin and Chemical Fungicide Synergistically Inhibit the Growth of P. infestans in Vitro
Currently, the control of potato late blight mainly depends on high dosage and frequency of fungicide applications. These practices result in many health and environmental issues. Reduced fungicide doses are important for a sustainable environment. It has been reported that melatonin could be used as a synergist to reduce the use of some drugs and enhance the drug effect, for example, vincristine, atorvastatin, vitamin C and E (Lissoni et al., 1999; Gitto et al., 2001; Casadozapico et al., 2010; Dayoub et al., 2011). We therefore examined whether the synergistic effects exist in combined application of melatonin and fungicide against P. infestans. Infinito is one of the most effective, expensive and extensively used chemical fungicides for potato late blight control. The results showed that the inhibitory effects of T30-4 treated with Infinito + melatonin was more significantly enhanced than that of Infinito or melatonin alone (Figures 5A,B); this suggested that melatonin can promote P. infestans to become more susceptible to Infinito. The IC50s of melatonin, Infinito, and melatonin + Infinito were also examined, and the values were 3 mM, 0.005 ml/L, 2 mM + 0.0005 ml/L, respectively. A significant reduction of Infinito dose (10-fold reduction) was generated by co-applying melatonin (Figure 5A), which indicated that the chemical fungicide dose was decreased when combined with melatonin for potato late blight control. Moreover, CI < 1 was observed, confirming that the synergistic effects exist in the melatonin + Infinito (Figure 5C). The MTT reduction assay also confirmed that the cell viability of T30-4 treated with Infinito + melatonin was more significantly decreased than that of Infinito or melatonin alone (Figure 5D). Thus, these observations provide new insights into the synergistic anti-fungal effects of Infinito with melatonin on P. infestans, and expand the understanding of the synergistic effect of melatonin (Lissoni et al., 1999; Casadozapico et al., 2010; Dayoub et al., 2011).
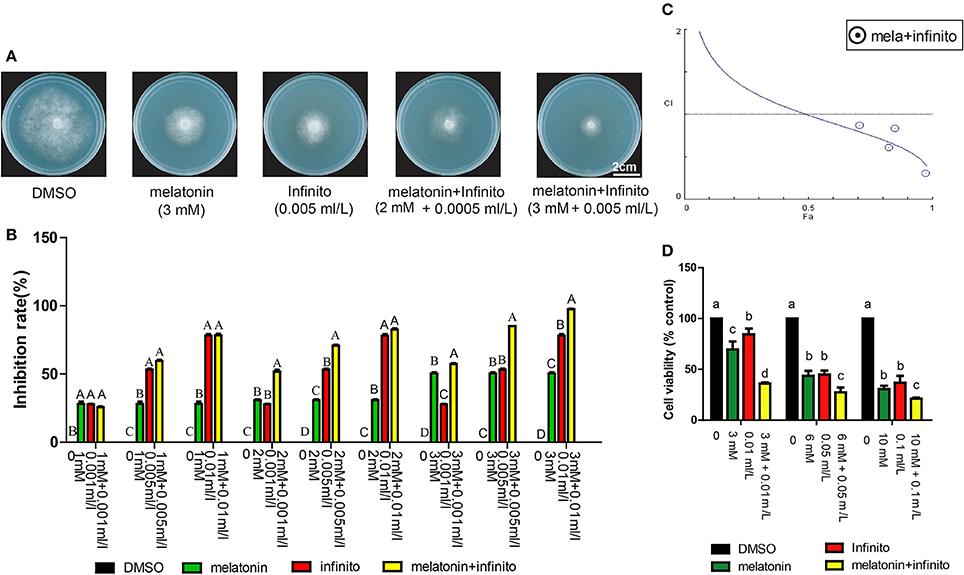
Figure 5. Synergistic growth inhibition of P. infestans induced by co-application of the melatonin and Infinito. (A) Colony morphology on Rye A agar medium that contains DMSO, 3 mM melatonin (IC50), 0.005 ml/L Infinito (IC50), 2 mM melatonin + 0.0005 ml/L Infinito (IC50), 3 mM melatonin + 0.005 ml/L Infinito. (B) Mycelial inhibition rate of P. infestans T30-4 under DMSO, melatonin, Infinito, and melatonin + Infinito treatment for 14 days. Each data point was averaged from three independent biological replicates. Capitalized letters indicate significant difference, p < 0.01. (C) Fa-CI curve showed synergism (CI < 1) generated by melatonin + Infinito treatment. (D) MTT reduction assay showed that cell viability of P. infestans T30-4 under DMSO, melatonin, Infinito, and melatonin + Infinito treatment for 24 h. Lower case letters indicate significant difference, p < 0.05.
Melatonin Reduced the Fungicide Dose for Potato Late Blight Control in Vivo
Next, the in vivo assay of synergistic inhibition was conducted. When treated with melatonin + Infinito, the lesion sizes infected by P. infestans on the potato leaves and tuber slices were much smaller than that of melatonin or Infinito alone (Figures 6A–D, and Figures S1A,B). The 6 mM melatonin + 0.025 ml/L Infinito was the optimal concentration among these combinations (the lowest concentration of Infinito for reaching the same small lesion size, while the concentration of melatonin was modest) (Figures S1A,B). A significant reduction of Infinito dose (10-fold reduction) was achieved by the combination with melatonin in vivo (Figures 6E,F). This result revealed an eco-friendly potential for the reduced use of fungicides by co-application of melatonin in potato late blight control.
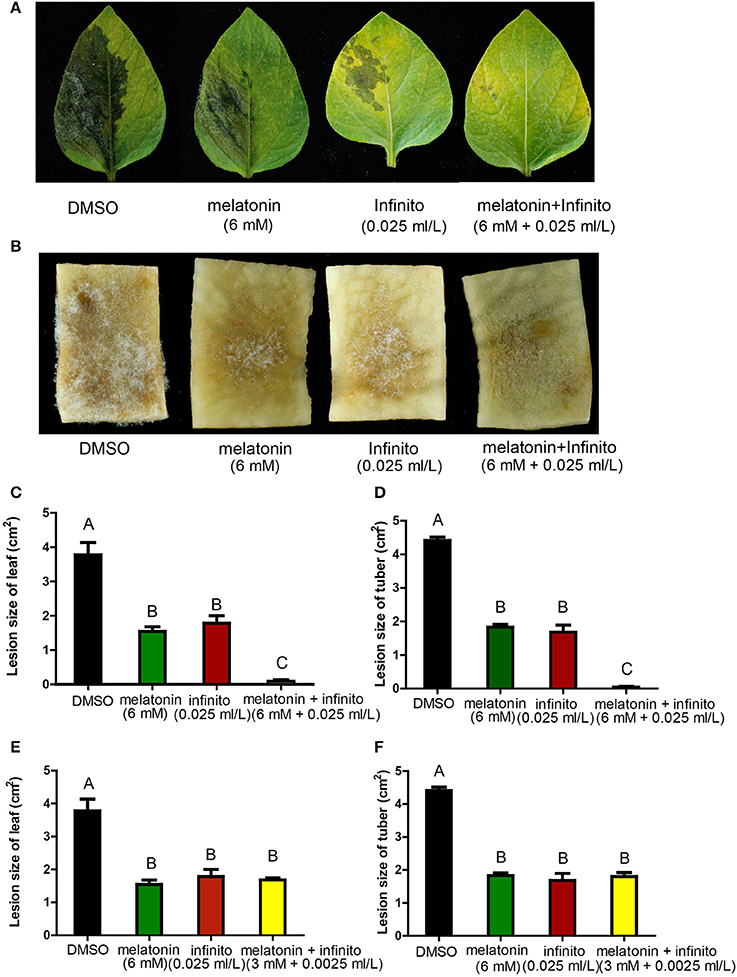
Figure 6. Disease symptoms of potato late blight on leaves and tubers slices infected by P. infestans for 5 days in the presence of melatonin and Infinito. (A) Disease symptoms of potato leaves with the treatment of DMSO, 6 mM melatonin, 0.025 ml/L Infinito, 6 mM melatonin + 0.025 ml/L Infinito, respectively, after 5 days. (B) Disease symptoms of potato tuber slices with the treatment of DMSO, 6 mM melatonin, 0.025 ml/L Infinito, 6 mM melatonin + 0.025 ml/L Infinito, respectively, after 5 days. (C) Lesion size of leaves with the treatment of 6 mM melatonin, 0.025 ml/L Infinito, 6 mM melatonin + 0.025 ml/L Infinito, respectively, after 5 days. (D) Lesion size of tuber slices with the treatment of 6 mM melatonin, 0.025 ml/L Infinito, 6 mM melatonin + 0.025 ml/L Infinito, respectively, after 5 days. (E) The lesion size of leaves infected by P. infestans treated with 6 mM melatonin, 0.025 ml/L Infinito, 3 mM melatonin + 0.0025 ml/L Infinito, respectively, after 5 days. (F) The lesion size of tuber slices under the treatment of 6 mM melatonin, 0.025 ml/L Infinito, 3 mM melatonin + 0.0025 ml/L Infinito, respectively, after 5 days. Each data point was averaged of three independent biological replicates. Capitalized letters indicate significant difference, p < 0.01
Analysis of Melatonin Effects on P. infestans by the Transcriptome
To further investigate the underlying molecular mechanism about the roles of melatonin in the growth and development of P. infestans, we performed transcriptome analysis to assess the global transcriptional profile revealing differentially expressed genes (DEGs) induced by melatonin. The T30-4 strain was treated with DMSO (mock) and 3 mM melatonin for 24 h and the samples were collected for transcriptome assay. After trimming for quality and adapter sequences, 45.79 and 47.89 million transcriptome reads were obtained with the treatments of 3 mM melatonin and DMSO as the control, respectively (Figure 7A), of which 85.73, 5.88, and 79.85% reads could be mapped to the annotated genome, multiple genes, and unique genes of P. infestans, respectively (Figure 7B). 1,279 DEGs out of 10,844 detected genes were identified in the melatonin- treated samples in contrast to DMSO-treated one (Table S2). Among these DEGs, 809 were significantly up-regulated and 470 significantly down-regulated (Figure 7C). The heat-map also showed that melatonin treatment was tend to increase the gene expression level (FPKM) of the DEGs (Figure 7D and Figure S2, Table S3).
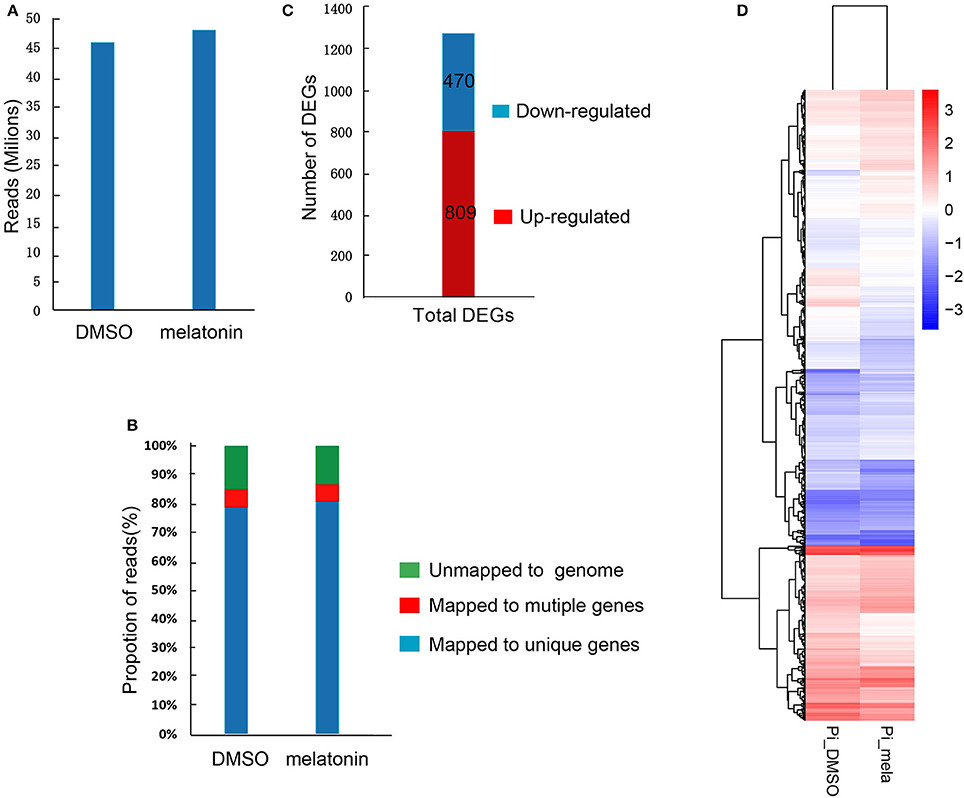
Figure 7. Summary of the basic information for the transcriptome data. (A) High-quality clean reads from high-throughput sequencing. (B) Proportions of high-quality clean reads of unmapped to genes, mapped to unique genes and multiple genes. (C) The number of differentially expressed genes (DEGs). (D) The heat-map of DEGs induced by melatonin.
To investigate the detailed functions of melatonin's effects on P. infestans, the GO terms and KEGG pathways were analyzed. In the transcriptome, among the top 30 GO terms and KEGG pathways (sorted by their p-values in the descending order; Tables S4A,B, S5A,B), there were 19 GO terms and 26 KEGG pathways associated with the metabolism of carbohydrates, lipids, nucleic acid, amino acid, cofactors and vitamins (Tables 1, 2). Notably, among 19 GO terms and 26 KEGG pathways, the down-regulated genes were predominated in 14 GO terms and 14 KEGG pathways (Table 3), suggesting that the various metabolic processes in P. infestans are dramatically suppressed after melatonin treatment.
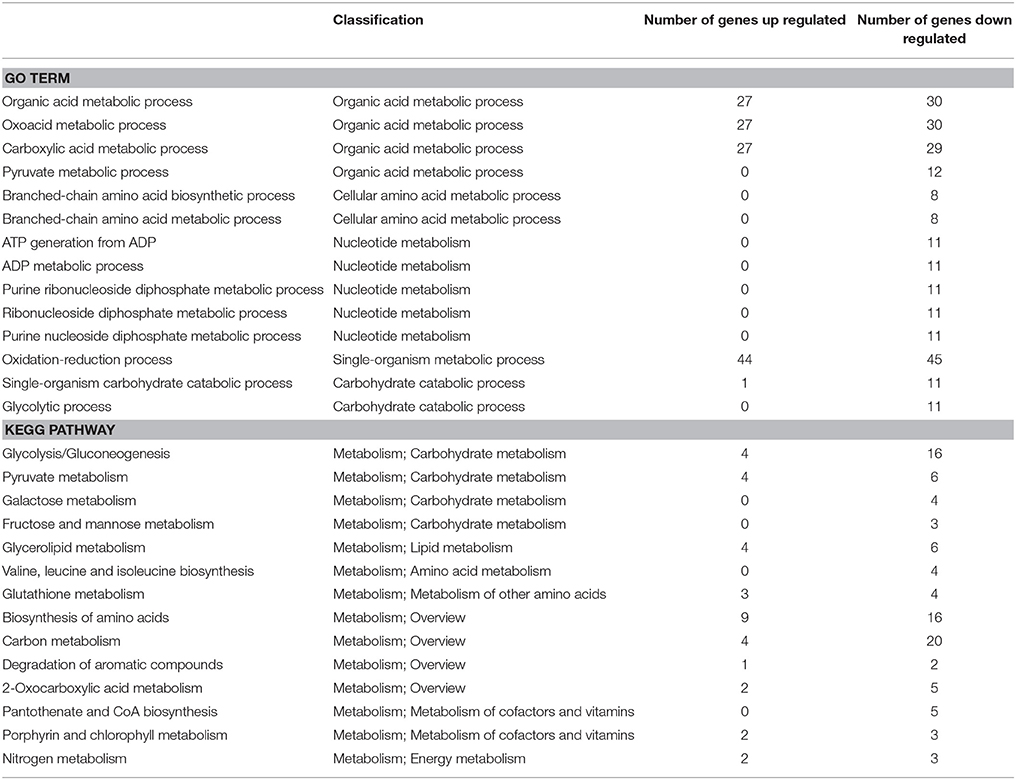
Table 3. The prominent down-regulated genes in 14 GO terms and 14 KEGG pathways related to metabolism in transcriptome.
Differentially Expressed Genes Related to Amino Acid Metabolism of P. infestans Were Significantly Affected by Melatonin
Among these altered metabolic processes in transcriptome (top 30 GO terms and KEGG pathways), the number of GO terms and KEGG pathways related to amino acid metabolism was the largest (three GO terms and ten KEGG pathways) (Table 4, Table S6A). This result indicated that the amino acid metabolism was the most significantly targeted metabolic process by melatonin. Among these GO terms and KEGG pathways associated with amino acid metabolism (Tables S6A,B), the biosynthesis of amino acids was the overview pathway in which the down-regulated genes were predominant (Table 3). Particularly, the genes encoding rate-limiting enzymes: pyruvate kinase, fructose-bisphosphate aldolase, isocitrate dehydrogenase [NADP] were down-regulated as well (Table 5, Tables S6A,B). The genetic mutation of these rate-limiting enzymes can reduce amino acid metabolism levels (Heneka et al., 2001; Malay et al., 2005; Gupta and Bamezai, 2010; Grace et al., 2015; Lv et al., 2017), indicating that the amino acid metabolism was suppressed after melatonin treatment. Collectively, the results and observations from the transcriptome suggest that amino acid metabolism of P. infestans is likely the main target of melatonin.
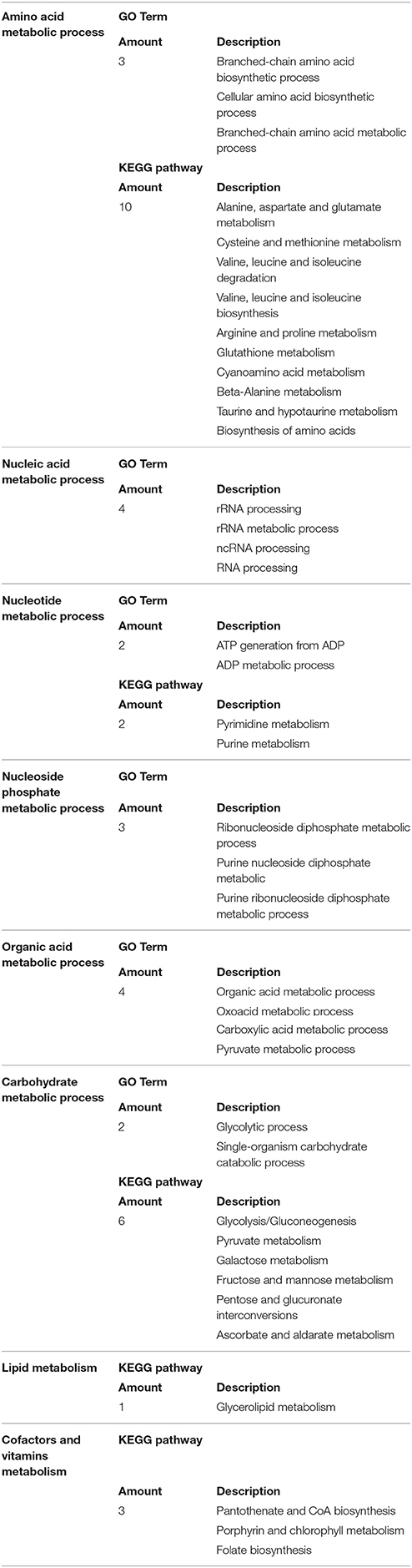
Table 4. The GO term or KEGG pathway related to metabolism of amino acid, carbohydrate, nucleic acid, lipid.
Carbohydrate metabolism and lipid metabolism were also altered in transcriptome (Tables 1, 2, 4 and Tables S6C,D). For example, the down-regulation of the rate-limiting enzyme 6-phosphofructokinase (PFK) in the transcriptome (Table S6C) would reduce the carbohydrate metabolism levels of P. infestans by melatonin (Raben et al., 1995; Wegener and Krause, 2002). The down-regulation of another rate-limiting enzyme fatty acid synthase (FASN) in transcriptome (Table S6D) documented that the suppression of lipid metabolism of P. infestans by melatonin (Smith et al., 2003), which is possibly the reason for the decrease in lipid droplets observed in TEM analysis of melatonin-treated samples.
In summary, the transcriptome analysis showed that multiple metabolic processes were affected by melatonin in P. infestans, especially amino acid metabolism. The perturbation of amino acid metabolism leads to cell death (Mizushima, 2005; Green et al., 2014), indicating that the disruption of amino acid metabolism homeostasis by melatonin may significantly contribute to the inhibition of growth in P. infestans. The previous study also showed that amino acid metabolism of P. infestans may be an important metabolic target for fungicides (Grenvillebriggs et al., 2005). Additionally, the amino acid metabolism has been a target to control the plant pathgens by some fungicides, such as pyrimethanil (Milling and Richardson, 1995). Thus, the results from this study imply that the target of amino acid metabolism in P. infestans by melatonin could be a novel alternative for the biocontrol of potato late blight.
Differentially Expressed Genes Related to Stress Tolerance of P. infestans Were Affected by Melatonin
Though metabolic processes in P. infestans were significantly suppressed after melatonin treatment, the expression of some DEGs related to stress tolerance was also affected by melatonin. The transcriptome data showed that 15 DEGs were related to stress tolerance (Table 6). Among them, 9 DEGs were down-regulated and 6 DEGs were up-regulated. Especially, two DEGs encoding the heat shock 70 kDa protein (HSP70) were significantly down-regulated. The HSP70 is one of the most prominent stress proteins and can be induced by a variety of stresses, such as UV radiation, heat shock, hypertonicity and low temperature (Tavaria et al., 1996; Alfieri et al., 2002; Fulgentini et al., 2015). This result showed that the deregulation of genes associated with the stress tolerance and especially the down-regulation of the genes encoding the HSP70, probably causing the suppression of stress tolerance of P. infestans under the melatonin treatment.
Differentially Expressed Genes Related to Drug Resistance of P. infestans Were Affected by Melatonin
The transcriptome data also showed that the expression of some DEGs related to drug resistance was also affected by melatonin. The down-regulation of drug resistance genes by melatonin treatment was possibly the reason for the synergistic effects with Infinito. For example, among the 25 most down-regulated differentially expressed genes, there were two genes encoding major facilitator superfamilies (MFS) (Figure S3). MFS is regarded as a major mechanism of drug resistance by transporting the drug from the cytoplasm to extracellular space (Kumar et al., 2013). The overexpression of MFS can confer the cell with resistant to many toxic compounds, while the mutant of MFS cannot (Morschhauser, 2010; Kumar et al., 2013). These results suggested that the down-regulation of MFS gene expression could lead to the reduction of the drug resistance of P. infestans. Thus, the down-regulated MFS in transcriptome could weaken the drug resistance of P. infestans, leading to the synergic effects of melatonin combination with Infinito, such as reducing the use and increasing cytotoxicity of Infinito. Furthermore, consistent with the previous study, melatonin is able to overcome the clofarabine resistance in leukemic cell lines: combination of melatonin and clofarabine significantly increase cytotoxicity in resistant leukemic cell lines (Yamanishi et al., 2015).
Differentially Expressed Genes Related to Virulence of P. infestans Were Affected by Melatonin
The transcriptome data also showed that the expression of some DEGs related to virulence was also affected by melatonin. P. infestans, like other pathogens, can employ proteins related to virulence to facilitate colonization and infection, such as the apoplastic effectors which are secreted into the plant extracellular, cytoplasmic effectors which are translocated into the plant cell, Cytochrome P450, (Haas et al., 2009; Tian et al., 2009; Dong et al., 2014). Elicitins are apoplastic effectors for helping pathogen invasion into plants in a negative manner (Schornack et al., 2009). The reduced expression of elicitins help the pathogen to evade the host innate immunity, and the mutations of elicitin INF1 of P. infestans could cause the disease lesions (Kamoun et al., 1998; Schornack et al., 2009). Our transcriptome data showed that six genes encoding elicitin proteins were up-regulated but two were down-regulated in response to melatonin treatment (Table 7), suggesting that the virulence of P. infestans was reduced by melatonin. Most of these virulence-related proteins likely help pathogen to more access and entry into plants and in causing serious disease, by higher expression, such as: CBEL (apoplastic effector), Crinkler protein (CRN) (more than 200) (cytoplasmic effectors), and many Cytochrome P450 (Beyer et al., 2001; Gaulin et al., 2002; Haas et al., 2009). For example, the CRN protein mutant reduced the virulence of pathogen (Amaro et al., 2017). The down-regulated genes encoding CRN, CBEL, and Cytochrome P450 were major in the transcriptome data (Table 7), indicating that the virulence of P. infestans was impaired by melatonin. Thus, deregulation of genes by melatonin associated with the virulence might reduce the virulence of the pathogen invading the host, which could be one of the reasons for the attenuation of potato late blight in our observations.
Differentially Expressed Genes Related to Circadian Rhythms of P. infestans Treated by Melatonin Were Not Observed
The function of melatonin is well known to regulate circadian rhythms. The circadian rhythms also may regulate the physiological function of fungus, including virulence and mycelia (Liu and Bellpedersen, 2006; Brody et al., 2010; Hevia et al., 2015). Therefore, we investigated whether melatonin altered circadian rhythms-related genes of P. infestans. However, the core circadian rhythms genes of fungus, such as FRQ, WC-1,WC-2 (Liu and Bellpedersen, 2006; Salichos and Rokas, 2010), were not observed in the transcriptome data; the GO terms or KEGG pathway involved in circadian rhythms were also not detected in transcriptome data (Tables S4, S5). This observation likely caused by the continuous darkness growth condition of P. infestans in this study.
Validation of Gene Expression Profiles Using RT-qPCR
To confirm these transcriptome-based observations, nine DEGs encoding rate-limiting enzymes in metabolism, and four DEGs involved in pathogenesis were selected for the real-time PCR (RT-qPCR) based confirmation studies. The results were highly consistent with these in the in transcriptome dataset (Figure S4), suggesting that the datasets derived from the transcriptome assay are reliable and valid.
Conclusion
In this study, exogenous application of melatonin significantly attenuated the potato late blight by inhibiting mycelial growth, changing cell ultrastructure, reducing the virulence and impairing stress tolerance of P. infestans. Importantly, melatonin reduced the doses and enhanced the effects of fungicide for potato late blight control, because of the synergistic inhibitory effects generated by co-treating with melatonin and fungicide. The analysis of the transcriptome suggests that the inhibitory effects of melatonin on P. infestans is most likely due to altering the homeostasis of amino acid metabolism. Furthermore, melatonin also altered the expression of important factors associated to stress tolerance, fungicide resistance and virulence. These observations also revealed the direct inhibitory effects of melatonin on the growth of P. infestans for the first time and provided new insights into the mechanisms of the direct interaction between melatonin and plant pathogens. Melatonin treatment showed some advantages in for the control of potato late blight in comparison with traditional chemical fungicides and potential application valuable in the cultivation of resistant variety. Firstly, the commonly used chemical fungicides usually display toxicity to humans and animals, whereas melatonin shows less toxicity even under high concentrations. Secondly, as a potential synergist, melatonin has great potential to reduce the use of chemical fungicides. It not only maximizes the efficiency of chemical fungicides and minimizes the use of chemical fungicides, but also reduces fungicide pollution. Additionally, there is potential to develop novel transgenic potato plants which may not only produce more melatonin but also generate the small RNA targeting the key regulators of amino acid metabolism in P. infestans, by manipulating the key genes controlling the biogenesis of melatonin in potato and amino acid metabolism in P. infestans, respectively.
Author Contributions
MR and SZ designed the research; SZ, XZ, SF, SL, LJ, and YW performed research; MR, SZ, RR, ZL, and RD analyzed data; MR, SZ, RR wrote the paper.
Conflict of Interest Statement
The authors declare that the research was conducted in the absence of any commercial or financial relationships that could be construed as a potential conflict of interest.
Acknowledgments
This financial support from the Grants: the National Natural Science Foundation of China (No. 31672206); National Key R&D Program for Crop Breeding (No. 2016YFD0100306); Chongqing Social Undertakings and People's Livelihood Science and technology innovation Project: Tuber Crop Special New Varieties Creating and Clean & Safe Processing (No. CSTC2015shms-ztzx80003); Fundamental Research Funds for the Central Universities (No. 106112017CDJQJ29883) are acknowledged.
Supplementary Material
The Supplementary Material for this article can be found online at: https://www.frontiersin.org/articles/10.3389/fpls.2017.01993/full#supplementary-material
Figure S1. (A) The lesion size of leaves infected by P. infestans treated with DMSO, melatonin, Infinito, melatonin + Infinito, respectively, after 5 days. (B) The lesion size of tuber slices treated with DMSO, melatonin, Infinito, melatonin + Infinito, respectively, after 5 days.
Figure S2. The detail heat-map of total DEGs induced by melatonin.
Figure S3. The top 25 down-regulated genes. 1. Putative uncharacterized protein; 2. Hypothetical protein O9G_005006; 3. Unknown protein; 4.Crinkler (CRN) family protein; 5. Unknown protein; 6. Unknown protein; 7. 12-oxophytodienoate reductase, putative; 8. Putative uncharacterized protein; 9. Putative uncharacterized protein; 10. Unknown protein; 11. Alcohol dehydrogenase, putative; 12. Major Facilitator Superfamily (MFS); 13. Putative uncharacterized protein; 14. Unknown protein; 15. Alcohol dehydrogenase, putative; 16. Secreted RxLR effector peptide protein, putative; 17. Putative uncharacterized protein; 18. Putative uncharacterized protein; 19. Putative uncharacterized protein; 20. Secreted RxLR effector peptide protein, putative; 21. Aquaporin, putative; 22. Major Facilitator Superfamily (MFS); 23. Putative uncharacterized protein; 24. Putative uncharacterized protein; 25. Putative uncharacterized protein.
Figure S4. Expression levels of 9 genes encoding rate-limiting enzymes and 4 genes related to pathogenesis in the 3 mM melatonin treatment for 24 h. 1. 6-phosphofructokinase, putative; 2. Pyruvate kinase; 3. Fructose-bisphosphate aldolase; 4. Glycerol-3-phosphate O-acyltransferase, putative; 5. Isocitrate dehydrogenase [NADP]; 6. Aconitate hydratase, putative; 7. Acetyl-CoA carboxylase, putative; 8. Glucokinase, putative; 9. Glucokinase, putative; 10. Crinkler (CRN) family protein, putative; 11. Cytochrome P450, putative; 12. Cellulose binding elicitor lectin (CBEL), putative; 13. Elicitin INF2A-like protein.
References
Ah-Fong, A. M., Kim, K. S., and Judelson, H. S. (2017). RNA-seq of life stages of the oomycete Phytophthora infestans reveals dynamic changes in metabolic, signal transduction, and pathogenesis genes and a major role for calcium signaling in development. BMC Genomics 18:198. doi: 10.1186/s12864-017-3585-x
Alfieri, R. R., Bonelli, M. A., Petronini, P. G., and Borghetti, A. F. (2002). Stabilization of hsp70 mRNA on prolonged cell exposure to hypertonicity. Biochim. Biophys. Acta 1592, 135–140. doi: 10.1016/S0167-4889(02)00291-4
Ali, A., Alexandersson, E., Sandin, M., Resjo, S., Lenman, M., Hedley, P. E., et al. (2014). Quantitative proteomics and transcriptomics of potato in response to Phytophthora infestans in compatible and incompatible interactions. BMC Genomics 15:497. doi: 10.1186/1471-2164-15-497
Amaro, T. M., Thilliez, G. J., Motion, G. B., and Huitema, E. (2017). A perspective on CRN proteins in the genomics age: evolution, classification, delivery and function revisited. Front. Plant Sci. 8:99. doi: 10.3389/fpls.2017.00099
Arendt, J. (2005). Melatonin: characteristics, concerns, and prospects. J. Biol. Rhythms 20, 291–303. doi: 10.1177/0748730405277492
Arnao, M. B., and Hernandezruiz, J. (2015). Functions of melatonin in plants: a review. J. Pineal Res. 59, 133–150. doi: 10.1111/jpi.12253
Avrova, A. O., Venter, E., Birch, P. R., and Whisson, S. C. (2003). Profiling and quantifying differential gene transcription in Phytophthora infestans prior to and during the early stages of potato infection. Fungal Genet. Biol. 40, 4–14. doi: 10.1016/S1087-1845(03)00063-X
Bago, B., Zipfel, W., Williams, R. M., Jun, J., Arreola, R., Lammers, P. J., et al. (2002). Translocation and utilization of fungal storage lipid in the arbuscular mycorrhizal symbiosis. Plant Physiol. 128, 108–124. doi: 10.1104/pp.010466
Beyer, K., Binder, A., Boller, T., and Collinge, M. (2001). Identification of potato genes induced during colonization by Phytophthora infestans. Mol. Plant Pathol. 2, 125–134. doi: 10.1046/j.1364-3703.2001.00059.x
Bi, K., He, Z., Gao, Z., Zhao, Y., Fu, Y., Cheng, J., et al. (2016). Integrated omics study of lipid droplets from Plasmodiophora brassicae. Sci. Rep. 6:36965. doi: 10.1038/srep36965
Blask, D. E., Sauer, L. A., Dauchy, R. T., Holowachuk, E. W., and Ruhoff, M. S. (1999a). New actions of melatonin on tumor metabolism and growth. Neurosignals 8, 49–55. doi: 10.1159/000014568
Blask, D. E., Sauer, L. A., Dauchy, R. T., Holowachuk, E. W., Ruhoff, M. S., and Kopff, H. S. (1999b). Melatonin inhibition of cancer growth in vivo involves suppression of tumor fatty acid metabolism via melatonin receptor-mediated signal transduction events. Cancer Res. 59, 4693–4701.
Brody, S., Oelhafen, K., Schneider, K., Perrino, S., Goetz, A., Wang, C., et al. (2010). Circadian rhythms in neurospora crassa: downstream effectors. Fungal Genet. Biol. 47, 159–168. doi: 10.1016/j.fgb.2009.09.006
Cao, Y., Du, M., Luo, S., and Xia, Y. (2014). Calcineurin modulates growth, stress tolerance, and virulence in Metarhizium acridum and its regulatory network. Appl. Microbiol. Biotechnol. 98, 8253–8265. doi: 10.1007/s00253-014-5876-3
Casadozapico, S., Rodriguezblanco, J., Garciasantos, G., Martin, V., Sanchezsanchez, A. M., Antolin, I., et al. (2010). Synergistic antitumor effect of melatonin with several chemotherapeutic drugs on human Ewing sarcoma cancer cells: potentiation of the extrinsic apoptotic pathway. J. Pineal Res. 48, 72–80. doi: 10.1111/j.1600-079X.2009.00727.x
Chen, X., Liu, Y., Keyhani, N. O., Xia, Y., and Cao, Y. (2017). The regulatory role of the transcription factor Crz1 in stress tolerance, pathogenicity, and its target gene expression in Metarhizium acridum. Appl. Microbiol. Biotechnol. 101, 5033–5043. doi: 10.1007/s00253-017-8290-9
Chou, T. C. (2006). Theoretical basis, experimental design, and computerized simulation of synergism and antagonism in drug combination studies. Pharmacol. Rev. 58, 621–681. doi: 10.1124/pr.58.3.10
Chou, T. C., and Talalay, P. (1984). Quantitative analysis of dose-effect relationships: the combined effects of multiple drugs or enzyme inhibitors. Adv. Enzyme Regul. 22, 27–55. doi: 10.1016/0065-2571(84)90007-4
Dayoub, J. C., Ortiz, F., Lopez, L. C., Venegas, C., Pinozumaquero, A. D., Roda, O., et al. (2011). Synergism between melatonin and atorvastatin against endothelial cell damage induced by lipopolysaccharide. J. Pineal Res. 51, 324–330. doi: 10.1111/j.1600-079X.2011.00892.x
Dong, S., Stam, R., Cano, L. M., Song, J., Sklenar, J., Yoshida, K., et al. (2014). Effector specialization in a lineage of the Irish potato famine pathogen. Science 343, 552–555. doi: 10.1126/science.1246300
Elmahallawy, E. K., Jimenez-Aranda, A., Martinez, A. S., Rodriguez-Granger, J., Navarro-Alarcon, M., Gutierrez-Fernandez, J., et al. (2014). Activity of melatonin against Leishmania infantum promastigotes by mitochondrial dependent pathway. Chem. Biol. Interact. 220, 84–93. doi: 10.1016/j.cbi.2014.06.016
Farriol, M., Venereo, Y., Orta, X., Castellanos, J. M., and Segoviasilvestre, T. (2000). In vitro effects of melatonin on cell proliferation in a colon adenocarcinoma line. J. Appl. Toxicol. 20, 21–24. doi: 10.1002/(SICI)1099-1263(200001/02)20:13.0.CO;2-M
Frades, I., Abreha, K. B., Prouxwera, E., Lankinen, A., Andreasson, E., and Alexandersson, E. (2015). A novel workflow correlating RNA-seq data to Phythophthora infestans resistance levels in wild Solanum species and potato clones. Front. Plant Sci. 6:718. doi: 10.3389/fpls.2015.00718
Fulgentini, L., Passini, V., Colombetti, G., Miceli, C., La Terza, A., and Marangoni, R. (2015). UV radiation and visible light induce hsp70 gene expression in the antarctic psychrophilic ciliate euplotes focardii. Microb. Ecol. 70, 372–379. doi: 10.1007/s00248-015-0566-y
Gao, L., Tu, Z. J., Millett, B. P., and Bradeen, J. M. (2013). Insights into organ-specific pathogen defense responses in plants: RNA-seq analysis of potato tuber-Phytophthora infestans interactions. BMC Genomics 14:340. doi: 10.1186/1471-2164-14-340
Gaulin, E., Jauneau, A., Villalba, F., Rickauer, M., Esquerretugaye, M., and Bottin, A. (2002). The CBEL glycoprotein of Phytophthora parasitica var-nicotianae is involved in cell wall deposition and adhesion to cellulosic substrates. J. Cell Sci. 115, 4565–4575. doi: 10.1242/jcs.00138
Gitto, E., Tan, D., Reiter, R. J., Karbownik, M., Manchester, L. C., Cuzzocrea, S., et al. (2001). Individual and synergistic antioxidative actions of melatonin: studies with vitamin E, vitamin C, glutathione and desferrrioxamine (desferoxamine) in rat liver homogenates. J. Pharm. Pharmacol. 53, 1393–1401. doi: 10.1211/0022357011777747
Grace, R. F., Zanella, A., Neufeld, E. J., Morton, D. H., Eber, S., Yaish, H., et al. (2015). Erythrocyte pyruvate kinase deficiency: 2015 status report. Am. J. Hematol. 90, 825–830. doi: 10.1002/ajh.24088
Green, D. R., Galluzzi, L., and Kroemer, G. (2014). Metabolic control of cell death. Science 345:1250256. doi: 10.1126/science.1250256
Grenvillebriggs, L. J., Avrova, A. O., Bruce, C. R., Williams, A., Whisson, S. C., Birch, P. R. J., et al. (2005). Elevated amino acid biosynthesis in Phytophthora infestans during appressorium formation and potato infection. Fungal Genet. Biol. 42, 244–256. doi: 10.1016/j.fgb.2004.11.009
Gullino, M. L., Tinivella, F., Garibaldi, A., Kemmitt, G. M., Bacci, L., and Sheppard, B. L. (2010). Mancozeb: past, present, and future. Plant Dis. 94, 1076–1086. doi: 10.1094/PDIS-94-9-1076
Gupta, V., and Bamezai, R. N. K. (2010). Human pyruvate kinase M2: a multifunctional protein. Protein Sci. 19, 2031–2044. doi: 10.1002/pro.505
Haas, B. J., Kamoun, S., Zody, M. C., Jiang, R. H., Handsaker, R. E., Cano, L. M., et al. (2009). Genome sequence and analysis of the Irish potato famine pathogen Phytophthora infestans. Nature 461, 393–398. doi: 10.1038/nature08358
Haverkort, A. J., Boonekamp, P. M., Hutten, R. C. B., Jacobsen, E., Lotz, L. A. P., Kessel, G. J. T., et al. (2008). Societal costs of late blight in potato and prospects of durable resistance through cisgenic modification. Potato Res. 51, 47–57. doi: 10.1007/s11540-008-9089-y
Heneka, M. T., Wiesinger, H., Dumitrescu-Ozimek, L., Riederer, P., Feinstein, D. L., and Klockgether, T. (2001). Neuronal and glial coexpression of argininosuccinate synthetase and inducible nitric oxide synthase in Alzheimer disease. J. Neuropathol. Exp. Neurol. 60, 906–916. doi: 10.1093/jnen/60.9.906
Hevia, M. A., Canessa, P., Mulleresparza, H., and Larrondo, L. F. (2015). A circadian oscillator in the fungus Botrytis cinerea regulates virulence when infecting Arabidopsis thaliana. Proc. Natl. Acad. Sci. U.S.A. 112, 8744–8749. doi: 10.1073/pnas.1508432112
Kamoun, S., Van West, P., Vleeshouwers, V. G. A. A., De Groot, K. E., and Govers, F. (1998). Resistance of nicotiana benthamiana to phytophthora infestans is mediated by the recognition of the elicitor protein INF1. Plant Cell 10, 1413–1425. doi: 10.1105/tpc.10.9.1413
Karasek, M., Gruszka, A., Lawnicka, H., Kunertradek, J., and Pawlikowski, M. (2003). Melatonin inhibits growth of diethylstilbestrol-induced prolactin-secreting pituitary tumor in vitro: possible involvement of nuclear RZR/ROR receptors. J. Pineal Res. 34, 294–296. doi: 10.1034/j.1600-079X.2003.00046.x
Kobae, Y., Gutjahr, C., Paszkowski, U., Kojima, T., Fujiwara, T., and Hata, S. (2014). Lipid droplets of arbuscular mycorrhizal fungi emerge in concert with arbuscule collapse. Plant Cell Physiol. 55, 1945–1953. doi: 10.1093/pcp/pcu123
Kumar, S., Mukherjee, M. M., and Varela, M. F. (2013). Modulation of bacterial multidrug resistance efflux pumps of the major facilitator superfamily. Int. J. Bacteriol. 2013:204141. doi: 10.1155/2013/204141
Lee, H. Y., and Back, K. (2016). Mitogen-activated protein kinase pathways are required for melatonin-mediated defense responses in plants. J. Pineal Res. 60, 327–335. doi: 10.1111/jpi.12314
Lee, H. Y., Byeon, Y., and Back, K. (2014). Melatonin as a signal molecule triggering defense responses against pathogen attack in Arabidopsis and tobacco. J. Pineal Res. 57, 262–268. doi: 10.1111/jpi.12165
Lee, H. Y., Byeon, Y., Tan, D., Reiter, R. J., and Back, K. (2015). Arabidopsis serotonin N-acetyltransferase knockout mutant plants exhibit decreased melatonin and salicylic acid levels resulting in susceptibility to an avirulent pathogen. J. Pineal Res. 58, 291–299. doi: 10.1111/jpi.12214
Lissoni, P., Barni, S., Mandalà, M., Ardizzoia, A., Paolorossi, F., Vaghi, M., et al. (1999). Decreased toxicity and increased efficacy of cancer chemotherapy using the pineal hormone melatonin in metastatic solid tumour patients with poor clinical status. Eur. J. Cancer 35, 1688–1692. doi: 10.1016/S0959-8049(99)00159-8
Liu, Y., and Bellpedersen, D. (2006). circadian rhythms in neurospora crassa and other filamentous fungi. Eukaryotic Cell 5, 1184–1193. doi: 10.1128/EC.00133-06
Lv, P., Tang, W., Wang, P., Cao, Z., and Zhu, G. (2017). Enzymatic characterization and functional implication of two structurally different isocitrate dehydrogenases from Xylella fastidiosa. Biotechnol. Appl. Biochem. doi: 10.1002/bab.1560. [Epub ahead of print].
Malay, A. D., Allen, K. N., and Tolan, D. R. (2005). Structure of the thermolabile mutant aldolase B, A149P: molecular basis of hereditary fructose intolerance. J. Mol. Biol. 347, 135–144. doi: 10.1016/j.jmb.2005.01.008
Manchester, L. C., Cotomontes, A., Boga, J. A., Andersen, L. P., Zhou, Z., Galano, A., et al. (2015). Melatonin: an ancient molecule that makes oxygen metabolically tolerable. J. Pineal Res. 59, 403–419. doi: 10.1111/jpi.12267
Matheron, M., and Porchas, M. (2000). Impact of azoxystrobin, dimethomorph, fluazinam, fosetyl-Al, and metalaxyl on growth, sporulation, and zoospore cyst germination of three Phytophthora spp. Plant Dis. 84, 454–458. doi: 10.1094/PDIS.2000.84.4.454
Milling, R. J., and Richardson, C. J. (1995). Mode of action of the anilino-pyrimidine fungicide pyrimethanil. 2. Effects on enzyme secretion in Botrytis cinerea. Pesticide Sci. 45, 43–48. doi: 10.1002/ps.2780450107
Mitani, S., Araki, S., Yamaguchi, T., Takii, Y., Ohshima, T., and Matsuo, N. (2001). Antifungal activity of the novel fungicide cyazofamid against Phytophthora infestans and other plant pathogenic fungi in vitro. Pestic. Biochem. Physiol. 70, 92–99. doi: 10.1006/pest.2001.2541
Mizushima, N. (2005). The pleiotropic role of autophagy: from protein metabolism to bactericide. Cell Death Diff. 12, 1535–1541. doi: 10.1038/sj.cdd.4401728
Morschhauser, J. (2010). Regulation of multidrug resistance in pathogenic fungi. Fungal Genet. Biol. 47, 94–106. doi: 10.1016/j.fgb.2009.08.002
Nemeth, C., Humpeler, S., Kallay, E., Mesteri, I., Svoboda, M., Rogelsperger, O., et al. (2011). Decreased expression of the melatonin receptor 1 in human colorectal adenocarcinomas. J. Biol. Regul. Homeost. Agents 25, 531–542.
Ortizurquiza, A., and Keyhani, N. O. (2014). Stress response signaling and virulence: insights from entomopathogenic fungi. Curr. Genet. 61, 239–249. doi: 10.1007/s00294-014-0439-9
Pandi-Perumal, S. R., Srinivasan, V., Maestroni, G. J., Cardinali, D. P., Poeggeler, B., and Hardeland, R. (2006). Melatonin: nature's most versatile biological signal? FEBS J. 273, 2813–2838. doi: 10.1111/j.1742-4658.2006.05322.x
Pearson, K. (2011). Potato: a history of the propitious esculent (review). J. World Hist. 22, 372–374. doi: 10.1353/jwh.2011.0044
Qian, Y., Tan, D., Reiter, R. J., and Shi, H. (2015). Comparative metabolomic analysis highlights the involvement of sugars and glycerol in melatonin-mediated innate immunity against bacterial pathogen in Arabidopsis. Sci. Rep. 5:15815. doi: 10.1038/srep15815
Raben, N., Exelbert, R., Spiegel, R., Sherman, J. B., Nakajima, H., Plotz, P., et al. (1995). Functional expression of human mutant phosphofructokinase in yeast: genetic defects in French Canadian and Swiss patients with phosphofructokinase deficiency. Am. J. Hum. Genet. 56, 131–141.
Radulovic, M., Knittelfelder, O., Cristobal-Sarramian, A., Kolb, D., Wolinski, H., and Kohlwein, S. D. (2013). The emergence of lipid droplets in yeast: current status and experimental approaches. Curr. Genet. 59, 231–242. doi: 10.1007/s00294-013-0407-9
Reiter, R. J., Mayo, J. C., Tan, D., Sainz, R. M., Alatorrejimenez, M. A., and Qin, L. (2016). Melatonin as an antioxidant: under promises but over delivers. J. Pineal Res. 61, 253–278. doi: 10.1111/jpi.12360
Reiter, R. J., Paredes, S. D., Manchester, L. C., and Tan, D. (2009). Reducing oxidative/nitrosative stress: a newly-discovered genre for melatonin. Crit. Rev. Biochem. Mol. Biol. 44, 175–200. doi: 10.1080/10409230903044914
Reiter, R. J., Tan, D. X., and Galano, A. (2014). Melatonin: exceeding expectations. Physiology 29, 325–333. doi: 10.1152/physiol.00011.2014
Reiter, R. J., Tan, D., Zhou, Z., Cruz, M. H. C., Fuentesbroto, L., and Galano, A. (2015). Phytomelatonin: assisting plants to survive and thrive. Molecules 20, 7396–7437. doi: 10.3390/molecules20047396
Salichos, L., and Rokas, A. (2010). The diversity and evolution of circadian clock proteins in fungi. Mycologia 102, 269–278. doi: 10.3852/09-073
Sanchezbarcelo, E. J., Mediavilla, M. D., Tan, D., and Reiter, R. J. (2010). Clinical uses of melatonin: evaluation of human trials. Curr. Med. Chem. 17, 2070–2095. doi: 10.2174/092986710791233689
Sauer, L. A., Dauchy, R. T., and Blask, D. E. (2001). Polyunsaturated fatty acids, melatonin, and cancer prevention. Biochem. Pharmacol. 61, 1455–1462. doi: 10.1016/S0006-2952(01)00634-7
Schornack, S., Huitema, E., Cano, L. M., Bozkurt, T. O., Oliva, R., Van Damme, M., et al. (2009). Ten things to know about oomycete effectors. Mol. Plant Pathol. 10, 795–803. doi: 10.1111/j.1364-3703.2009.00593.x
Semighini, C. P., and Harris, S. D. (2008). Regulation of apical dominance in aspergillus nidulans hyphae by reactive oxygen species. Genetics 179, 1919–1932. doi: 10.1534/genetics.108.089318
Shi, H., Chen, Y., Tan, D. X., Reiter, R. J., Chan, Z., and He, C. (2015). Melatonin induces nitric oxide and the potential mechanisms relate to innate immunity against bacterial pathogen infection in Arabidopsis. J. Pineal Res. 59:102. doi: 10.1111/jpi.12244
Shi, H., Wei, Y., and He, C. (2016). Melatonin-induced CBF/DREB1s are essential for diurnal change of disease resistance and CCA1 expression in Arabidopsis. Plant Physiol. Biochem. 100, 150–155. doi: 10.1016/j.plaphy.2016.01.018
Smith, S., Witkowski, A., and Joshi, A. K. (2003). Structural and functional organization of the animal fatty acid synthase. Prog. Lipid Res. 42, 289–317. doi: 10.1016/S0163-7827(02)00067-X
Stehr, M., Elamin, A. A., and Singh, M. (2012). Cytosolic lipid inclusions formed during infection by viral and bacterial pathogens. Microbes Infecti. 14, 1227–1237. doi: 10.1016/j.micinf.2012.08.001
Tavaria, M., Gabriele, T., Kola, I., and Anderson, R. L. (1996). A hitchhiker's guide to the human Hsp70 family. Cell Stress Chaperones 1, 23–28. doi: 10.1379/1466-1268(1996)001<0023:AHSGTT>2.3.CO;2
Tekbas, O. F., Ogur, R., Korkmaz, A., Kilic, A., and Reiter, R. J. (2008). Melatonin as an antibiotic: new insights into the actions of this ubiquitous molecule. J. Pineal Res. 44, 222–226. doi: 10.1111/j.1600-079X.2007.00516.x
Tian, M., Chaudhry, F., Ruzicka, D. R., Meagher, R. B., Staiger, C. J., and Day, B. (2009). Arabidopsis actin-depolymerizing factor AtADF4 mediates defense signal transduction triggered by the Pseudomonas syringae effector AvrPphB. Plant Physiol. 150, 815–824. doi: 10.1104/pp.109.137604
Wegener, G., and Krause, U. (2002). Different modes of activating phosphofructokinase, a key regulatory enzyme of glycolysis, in working vertebrate muscle. Biochem. Soc. Trans. 30, 264–270.
Wei, Y., Hu, W., Wang, Q., Zeng, H., Li, X., Yan, Y., et al. (2017). Identification, transcriptional and functional analysis of heat-shock protein 90s in banana (Musa acuminata L.) highlight their novel role in melatonin-mediated plant response to Fusarium wilt. J. Pineal Res. 62:e12347. doi: 10.1111/jpi.12367
Xiong, F., Zhang, R., Meng, Z., Deng, K., Que, Y., Zhuo, F., et al. (2017). Brassinosteriod Insensitive 2 (BIN2) acts as a downstream effector of the Target of Rapamycin (TOR) signaling pathway to regulate photoautotrophic growth in Arabidopsis. New Phytol. 213, 233–249. doi: 10.1111/nph.14118
Yamanishi, M., Narazaki, H., and Asano, T. (2015). Melatonin overcomes resistance to clofarabine in two leukemic cell lines by increased expression of deoxycytidine kinase. Exp. Hematol. 43, 207–214. doi: 10.1016/j.exphem.2014.11.001
Yang, H. P., Tsang, P. C., and Tsang, P. W. (2014). Melatonin inhibits biofilm formation in Candida parapsilosis. J. Mycol. Med. 24, 360–361. doi: 10.1016/j.mycmed.2014.05.003
Yin, L., Wang, P., Li, M., Ke, X., Li, C., Liang, D., et al. (2013). Exogenous melatonin improves Malus resistance to Marssonina apple blotch. J. Pineal Res. 54, 426–434. doi: 10.1111/jpi.12038
Zhao, C., Waalwijk, C., De Wit, P. J. G. M., Der Lee, T. V., and Tang, D. (2011). EBR1, a novel Zn 2 Cys 6 transcription factor, affects virulence and apical dominance of the hyphal tip in fusarium graminearum. Mol. Plant Microbe Interact. 24, 1407–1418. doi: 10.1094/MPMI-06-11-0158
Keywords: Phytophthora infestans, melatonin, potato late blight, stress tolerance, fungicide susceptibility, transcriptome
Citation: Zhang S, Zheng X, Reiter RJ, Feng S, Wang Y, Liu S, Jin L, Li Z, Datla R and Ren M (2017) Melatonin Attenuates Potato Late Blight by Disrupting Cell Growth, Stress Tolerance, Fungicide Susceptibility and Homeostasis of Gene Expression in Phytophthora infestans. Front. Plant Sci. 8:1993. doi: 10.3389/fpls.2017.01993
Received: 26 August 2017; Accepted: 06 November 2017;
Published: 21 November 2017.
Edited by:
Jens Staal, Ghent University, BelgiumReviewed by:
Susan Breen, Commonwealth Scientific and Industrial Research Organisation (CSIRO), AustraliaChenglong Liu, Texas A&M University, United States
Copyright © 2017 Zhang, Zheng, Reiter, Feng, Wang, Liu, Jin, Li, Datla and Ren. This is an open-access article distributed under the terms of the Creative Commons Attribution License (CC BY). The use, distribution or reproduction in other forums is permitted, provided the original author(s) or licensor are credited and that the original publication in this journal is cited, in accordance with accepted academic practice. No use, distribution or reproduction is permitted which does not comply with these terms.
*Correspondence: Liang Jin, amlubGlhbmc0MDAyQDEyNi5jb20=
Maozhi Ren, cmVubWFvemhpQGNxdS5lZHUuY24=
†These authors have contributed equally to this work.