- 1Faculty of Life and Environmental Science, Shimane University, Matsue, Japan
- 2Genomics Division, National Institute of Agricultural Sciences, Rural Development Administration, Jeonju, South Korea
- 3Iwate Biotechnology Research Center, Kitakami, Japan
Floral shape in higher plants typically requires genetic regulation through MADS transcription factors. In Japan, hundreds of azalea cultivars including flower shape mutations have been selected from the diversity of endogenous species and natural hybrids since the early 17th century, the Edo era (1603–1867). The long-lasting trait, known as “Misome-shō” in Japanese, has been identified in several species and cultivar groups of evergreen azaleas (Rhododendron L.) from three hundred years ago in Japan. However, the natural mutation conferring the long-lasting trait in azalea remains unknown. Here, we showed MADS-box gene mutations in long-lasting flowers, R. kaempferi ‘Nikkō-misome,’ R. macrosepalum ‘Kochō-zoroi,’ R. indicum ‘Chōjyu-hō,’ and R. × hannoense ‘Amagi-beni-chōjyu.’ All of the long-lasting flowers exhibited small-sized corollas with stomata during long blooming. In the long-lasting flowers, transcript of the APETALA3 (AP3)/DEFICIENS (DEF) homolog was reduced, and an LTR-retrotransposon was independently inserted into exons 1, 2, and 7 or an unknown sequence in exon 1 in gDNA of each cultivar. This insertion apparently abolished the normal mRNA sequence of the AP3/DEF homolog in long-lasting flowers. Also, long-lasting flowers were shown from F2 hybrids that had homozygous ap3/def alleles. Therefore, we concluded that the loss of function of the AP3/DEF homolog through a transposable element insertion may confer a stable long-lasting mutation in evergreen azaleas.
Introduction
Species of the genus Rhododendron (Ericaceae), subgenus Tsutsusi, section Tsutsusi are important genetic resources for evergreen azalea cultivars used as ornamental shrubs or potted azaleas in many regions worldwide, including Asia, Europe, and America. Japan has many wild evergreen azalea species, and hundreds of azalea cultivars have been selected from natural populations of endogenous azalea species and hybrids since the Edo era (1603–1867) (Yamazaki, 1996). A monograph on azaleas, “Kinshū-makura” (Figure 1A), edited in 1692, described more than 300 azalea cultivars, and several of the cultivars described in this monograph still exist and have unique flower and leaf characteristics (Ito, 1984). This monograph provided the first description of ‘Misome-guruma’ (Figure 1B), a cultivar possessing small light-red flowers, long stamens, and a long blooming season, with the flowers turning greenish in June (Ito, 1984). The ‘Misome-guruma’ has been defined as “Misome-shō,” a long-lasting trait in Japanese, and “Misome-shō” species have been identified in several azalea cultivars. The long-lasting flower mechanisms remain largely unknown; however, the trait is characterized by a temporal color change and a long-lasting corolla (Figures 1C–E) that is smaller than other evergreen azaleas and may be derived from the sepaloid corolla (Kobayashi et al., 2010; Gobara et al., 2017). In genetic analysis by crossing of azaleas, normal flowers were shown from all F1 progenies between normal and long-lasting flowers, and long-lasting flowers were detected from 1/3 F2 progenies. Therefore, long-lasting flower was shown as recessive trait to normal flower and was controlled by single gene (Gobara et al., 2017).
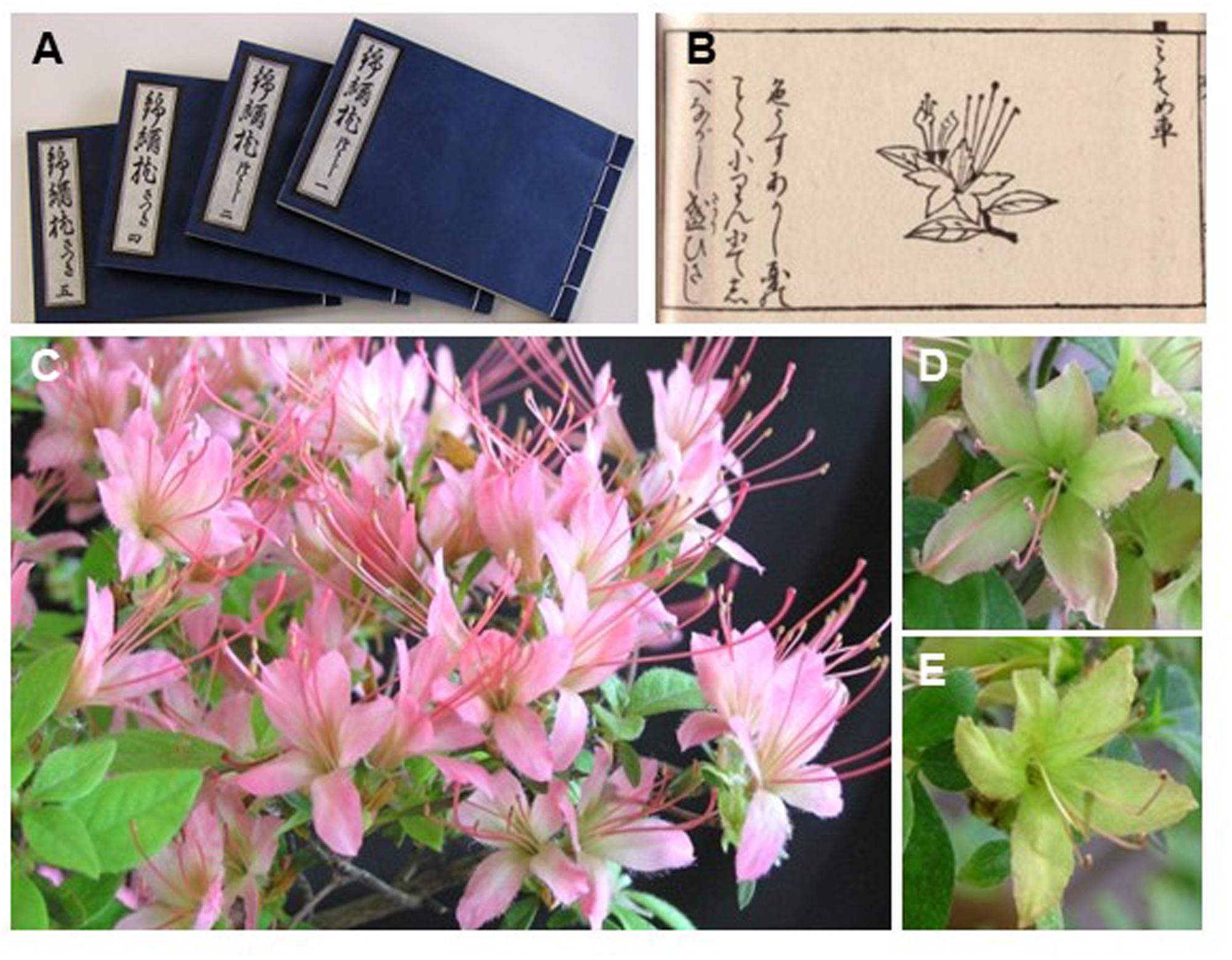
FIGURE 1. Information of long-lasting trait in Japanese azalea. (A,B) Photograph of “Kinshū-makura” (A) and ‘Misome-guruma’ (B), edited in 1692. (C–E) Flower photos of Rhododendron kaempferi ‘Nikkō-misome’ after just flowering (C), approximately 50 days (D), and approximately 100 days (E).
The corolla mutant of long-lasting flowers is likely associated with the ABC model. Flower development has been studied using Arabidopsis and Antirrhinum as model plants (Coen and Meyerowitz, 1991). In these model plants, floral homeotic mutant have been described converting to other organs. Most of these mutants have been found from different mutant alleles including one or more MADS-box gene encoding putative transcription factors (Weigel and Meyerowitz, 1994). The floral homeotic MADS-box genes have been classified into three classes by different function according to ABC model (Coen and Meyerowitz, 1991; Weigel and Meyerowitz, 1994). In summary, for Arabidopsis, the A-class gene APETALA1 (AP1) controls sepal formation in whorl 1; AP1 and two B-class genes [APETALA3 (AP3) and PISTILLATA (PI)] together control petal formation in whorl 2; the B-class genes and the C-class gene AGAMOUS (AG) together control stamen formation in whorl 3; and AG alone control carpel formation in whorl 4. The B-class PI and AP3 proteins are functional partners and form a heterodimer for petal formation (Yang et al., 2003). A loss-of-function mutation in either PI or AP3 produces sepals in two outer whorls of flowers (Jack et al., 1992). Also, in case of Antirrhinum majus, similar mutant phenotypes are described for mutation of the gene GLOBOSA (GLO) and/or DEFICIENS (DEF), homologs to PI and AP3, respectively (Sommer et al., 1990; Tröbner et al., 1992). In B-class gene mutants, B-class loss-of-function mutants have sepal or sepaloid petal instead of petals in the second whorl in model and horticultural plants (Sommer et al., 1990; Tröbner et al., 1992; Weigel and Meyerowitz, 1994; De Byzova et al., 2004; Vandenbussche et al., 2004; Drea et al., 2007; Hirai et al., 2010; Gong et al., 2017). In B-class genes, the natural corolla mutation of long-lasting azaleas might be associated with the AP3/DEF gene, as the sepaloid petals or sepals in whorl 2 were produced from artificial ap3/def and/or the pi/glo mutant (Vandenbussche et al., 2004), whereas the stamens in whorl 3 were not observed in artificial and natural pi/glo mutants in horticulture plants (Yao et al., 2001; Vandenbussche et al., 2004). Thus, we postulated that the long-lasting flower is due to mutation homologs of AP3/DEF in the azalea.
In a previous study, we isolated two full-length sequences of PI/GLO from R. obtusum (Cheon et al., 2016) and a partial length of the AP3/DEF homolog from R. pulchrum (Cheon et al., 2011) and reported that PI/GLO and AP3/DEF homologs participated in corolla development in the evergreen azaleas (Tasaki et al., 2012a; Cheon et al., 2016). In this study, to further characterize long-lasting flowers in evergreen azaleas, including R. kaempferi ‘Nikkō-misome,’ R. macrosepalum ‘Kochō-zoroi,’ R. indicum ‘Chōjyu-hō,’ and R. × hannoense ‘Amagi-beni-chōjyu,’ and to understand how mutant genes result in long-lasting flowers, we investigated the floral morphologies and B-class genes using the cultivars and progenies from a cross between normal and long-lasting flowers.
Materials and Methods
Plant Materials
The normal-flower azaleas R. kaempferi (Figure 2A), R. macrosepalum (Figure 2B), and R. indicum ‘Ōsakazuki’ (Figure 2C), Kurume hybrid ‘Wakakaede,’ and R. oldhamii, the long-lasting flower azaleas R. kaempferi ‘Nikkō-misome’ (Figures 1C, 2D), R. macrosepalum ‘Kochō-zoroi’ (Figure 2E), R. indicum ‘Chōjyu-hō’ (Figure 2F), and R. × hannoense ‘Amagi-beni-chōjyu’ (Figure 2G), and several progenies between normal and long-lasting flowers used in this study were obtained from the azalea resources collection of the Plant Breeding Laboratory of the Faculty of Life and Environmental Sciences of Shimane University (Figure 1). For the extraction of genomic DNA (gDNA) and total RNA, three leaves, ten floral buds, and five flowers were collected from plant materials. These samples were immediately frozen in liquid nitrogen and stored at -80°C until the extraction of gDNAs and total RNAs.
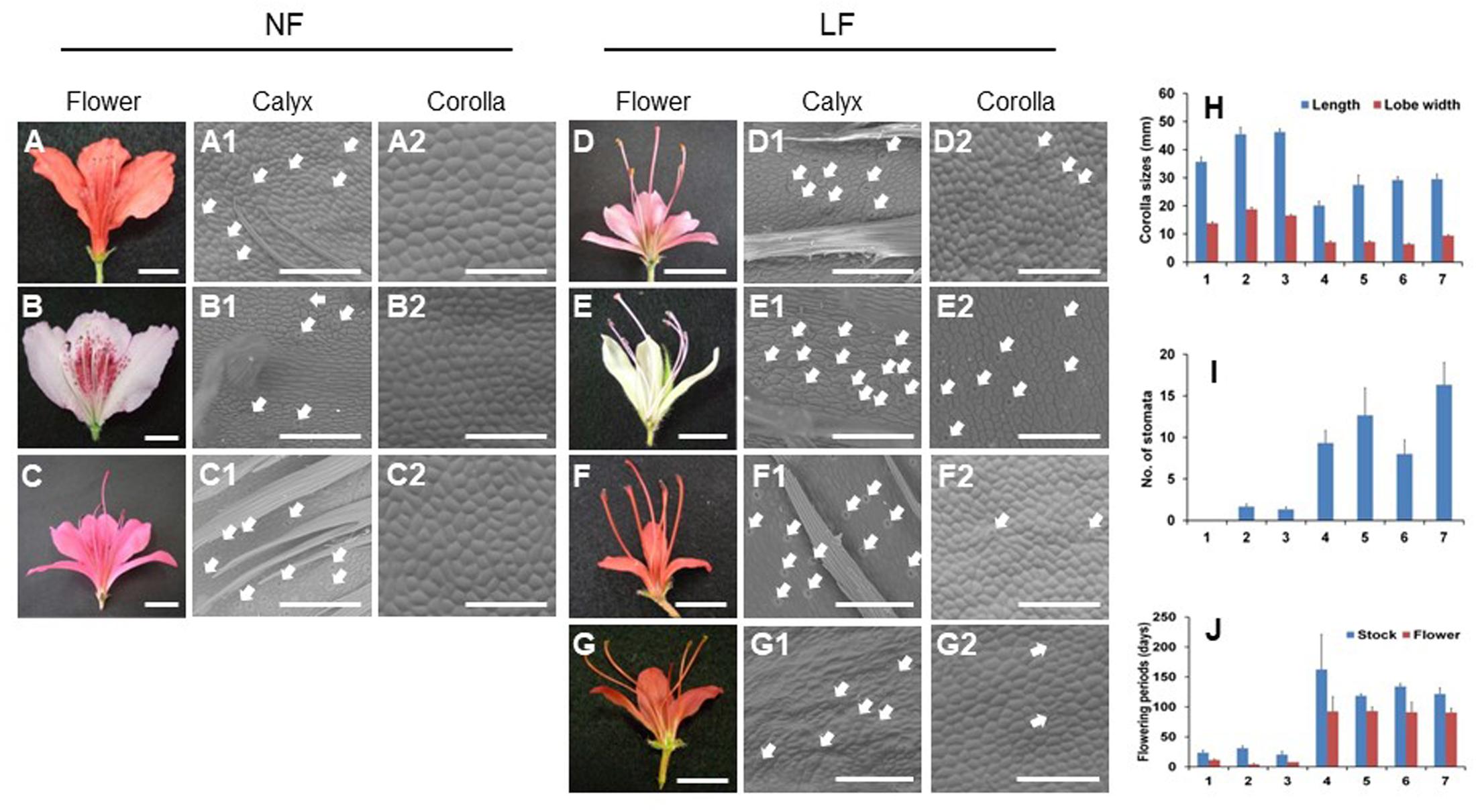
FIGURE 2. Floral morphological analysis of normal (NF) and long-lasting flowers (LF). (A–G2) Photos of flowers (A–G) and abaxial epidermal cells of calyx (A1–G1) and corollas (A2–G2) in normal (A–C) and long-lasting flowers (D–G). (H–J) Analyses of floral phenotypes, such as corolla sizes (mm) (H), number of stomata (I), and flowering periods (days) (J). The bars for flowers and abaxial epidermal cells indicate 1 cm and 250 μm, respectively. Closed arrows indicate stomata. The error bars indicate the mean ± SE of three biological replicates. The following numbers indicate the azaleas cultivars; 1, R. kaempferi (A–A2); 2, R. macrospalum (B–B2); 3, R. indicum ‘Ōsakazuki’ (C–C2); 4, R. kaempferi ‘Nikkō-misome’ (D–D2); 5, R. macrosepalum ‘Kochō-zoroi’ (E–E2); 6, R. indicum ‘Chōjyu-hō’ (F–F2); 7, R. × hannoense ‘Amagi-beni-chōjyu’ (G–G2).
Flower Morphological Investigation
The length of the corolla and the width of the corolla lobe were measured in 3–10 fully expanded flowers per individual plant (Hang et al., 2010; Gobara et al., 2017). The observation of abaxial epidermal cells was performed using a Scanning Electron Microscope (HITACHI TM3000) (Cheon et al., 2016). The stomatal density of abaxial surface in the corolla was determined using Suzuki’s Universal Microprinting Method (SUMP; SUMP Laboratory) and a light microscope (Nikon) (Tasaki et al., 2012b). The date of flower opening and abscission was investigated in both the stock and the flower (Gobara et al., 2017).
mRNA Analysis of AP3/DEF Homologs
Ten floral buds from each plant and five flowers from wild type and cultivar of R. kaempferi were collected and used for RNA extraction, AP3/DEF homolog isolation, semi-quantitative reverse transcription-PCR (semi-qRT-PCR), and RT-qPCR. Total RNA was extracted by RNeasy plant mini kit (Qiagen) and treated with DNase I (Promega) as previously described (Cheon et al., 2011). These RNA samples were subjected to semi-qRT-PCR and RT-qPCR using ReverTra-Ace (TOYOBO) according to the manufacturer’s instructions. The R. kaempferi total RNA sample and the 5′-Full RACE Core Set (TaKaRa) was used for 5′ RACE PCR with the partial sequence of the AP3/DEF homolog (Cheon et al., 2011). The primers of full-length AP3/DEF homolog, from 5′ untranslated region (UTR) to 3′ UTR, were P1-F (TCTCTTCCTCAACCGAGATCTG) and P1-R (GGTTCTACAACATCACAAGTGC) (Supplementary Figure S1A). PCR analysis was used to identify the full-length AP3/DEF homolog in the cDNA of R. kaempferi. Each 10 μl reaction mixture contained 1 × Ex Taq buffer, 200 μM dNTPs, 0.2 μM of each primer, 1.25 U Ex Taq (Takara), and 25 ng cDNA template. The reaction conditions were as follows: preheating at 94°C for 1 min; 35 cycles of denaturation at 94°C for 30 s, annealing at 60°C for 30 s, and extension at 72°C for 30 s; and final extension at 72°C for 1 min. The fragments were cloned into the pGEM-T easy vector (Promega), transformed into DH5α (Nippon gene) and subsequently sequenced using the BigDye Terminator version 3.1 Cycle Sequencing Kit (Applied Biosystems) and an ABI 3130 genetic analyser (Applied Biosystems). The deduced amino acid sequences were aligned by the NCBI BLASTp function and Genetyx ver.10 (Software Development Co.).
For expression analysis, semi-qRT-PCR was performed for B-class genes. For AP3/DEF homolog, each 10 μl reaction mixture contained 1 × Ex Taq buffer, 200 μM dNTPs, 0.2 μM of each primer, 1.25 U Ex-Taq (Takara), and 2.5 ng cDNA template. The primer sets were P1-F and P1-R for set 1, P1-F and P2-R for set 2, P3-F and P3-R for set 3, P4-F and P4-R for set 4, and P5-F and P1-R for set 5 (Supplementary Figure S1A). The reaction conditions were as follows: preheating at 94°C for 1 min; 35 cycles of denaturation at 94°C for 10 s/30 s, annealing at 61°C or 63°C for 10 s/30 s, and extension at 72°C for 10 s/30 s; and final extension at 72°C for 1 min. Also, expression analysis of AP3/DEF and PI/GLO homologs in each floral organ was performed according to previous study (Tasaki et al., 2012a). RT-qPCR with one biological and three technical replicates was performed using the Thermal Cycler Dice Real-Time System, SYBR Premix Ex Taq II (TaKaRa) and primers (Supplementary Table S1). The housekeeping gene ACTIN was used to normalize the RT-qPCR output (Cheon et al., 2011).
Genomic DNA Analysis of the AP3/DEF Homolog
gDNAs were extracted from the young leaves of plants using the modified CTAB method (Kobayashi et al., 1998). P-F1 and P-R1 primers were used for AP3/DEF homolog isolation in gDNA of R. kaempferi. However, because the homolog was not amplified by these primers in each long-lasting cultivar, inverse PCR (Ochman et al., 1988) was performed after digesting R. kaempferi gDNA with HindIII. Finally, the full-sized AP3/DEF homolog, including the 5′ upstream region, was isolated from the gDNAs of R. kaempferi and long-lasting flowers. Each 10 μl reaction mixture contained 1 × PrimeSTAR GXL buffer, 200 μM dNTPs, 0.2 μM of each primer, 1.25 U PrimeSTAR GXL DNA polymerase, and 25 ng gDNA in R. kaempferi or each 25 μl reaction mixture contained 50 ng gDNA template of long-lasting flowers with 5P-F1 (TTCCGACCCAACTCACATAC) and/or 5P-F2 (CGCAAGTCCCAACTCACATA), and P1-R primers. The conditions for R. kaempferi and long-lasting flowers were as follows: preheating at 98°C for 10 min; 30–35 cycles of denaturation at 98°C for 10 s, annealing at 60°C for 15 s, and extension at 68°C for 3–12 min; and final extension at 68°C for 15 min. The PCR products were amplified with PrimeSTAR GXL DNA polymerase (TaKaRa) and acquired from overhanging dA at the 3′-ends using an A-overhang mixture from the Mighty TA-cloning Reagent Set for PrimeSTAR, followed by sequencing as described above. For analysis of mutant region in azalea AP3/DEF sequences, R. kaempferi mRNA and gDNA sequences of AP3/DEF homologs were used as reference. After exon and intron positions of AP3/DEF homologs were analyzed using mRNA and gDNA sequences of R. kaempferi, the isolated AP3/DEF sequences from gDNAs of R. kaempferi, R. kaempferi ‘Nikkō-misome,’ R. macrosepalum ‘Kochō-zoroi,’ R. indicum ‘Chōjyu-hō,’ and R. × hannoense ‘Amagi-beni-chōjyu’ were aligned by Genetyx ver.10 (Software Development Co.). PCR analysis for mutant allele was performed using Ex-taq (TaKaRa) and primers (Supplementary Table S1).
Results
Corolla Mutations Develop to Long-lasting Flowers
To analyze the floral phenotype of long-lasting flowers, we investigated several corolla morphologies (Figure 2). Each floral organ of long-lasting flowers existed in each whorl as normal flowers (Figures 2A–G). The abaxial epidermal cells of corollas in long-lasting flowers were smaller compared with normal flowers and were similar to the abaxial epidermal cells of calyx (Figures 2A1–G2). However, the length and lobe width of the corollas in long-lasting flowers were also smaller compared with normal flowers (Figure 2H), and a higher number of stomata were observed on the abaxial surface of the corollas in long-lasting flowers compared with normal flowers (Figures 2A2–G2,I). The flowering periods, which facilitate corolla maintenance after flowering, were longer in long-lasting flowers than in normal flowers (Figure 2J). Thus, based on these observations of floral morphologies, we confirmed that long-lasting flowers have small-sized corollas with stomata during long blooming period and that the corollas of long-lasting flowers show the conversion of normal corollas to sepaloid corollas, whereas the stamens remain unaffected.
Isolation of AP3/DEF Homolog from R. kaempferi
We cloned the azalea AP3/DEF homolog using a PCR approach. Based on a partial RpAP3 sequence, the region from MADS domain to 3′ UTRs, in previously study (Cheon et al., 2011), the 5′ UTR were further amplified by using two nested PCR primers (Supplementary Table S1). Ten clones containing the 5′ UTR fragments were sequenced. Subsequently, when we cloned the azalea AP3/DEF homolog by using a PCR approach with a P1 primer set, two clones were detected from R. kaempferi (Supplementary Figure S1A). These cloned mRNA sequences involved a MADS-domain, a K-box, and the C-terminal region (Sommer et al., 1990); and showed 76% amino sequence identity with the DEF genes of A. majus (Supplementary Figure S1B). The putative azalea AP3/DEF homologs were named RkAP3a (approximately 824 bp, DDBJ accession number AB853117) and RkAP3b (approximately 826 bp, DDBJ Acc. No. AB853118), respectively.
Abnormal Sequences of AP3/DEF Homologs Affected to Reduce Expression in Long-lasting Flowers
PCR analyses were carried out to investigate the mRNA structure and expression level of the azalea AP3/DEF and PI/GLO homolog in floral buds and organs (Figure 3). In PCR products of primer set 1, P1-F and P1-R (Figure 3A and Supplementary Figure S1A), the PCR product predicted for the full-size sequence (approximately 800-bp) was detected from all normal flowers (Figure 3B). However, the normal sized band was not detected from long-lasting flowers and multi-bands were detected from R. indicum ‘Chōjyu-hō’ in especially (Figure 3B). When we analyzed the sequences of multi bands, insertion and deletion of sequences were detected from five clones, which have 1340-, 874-, 742-, 642-, and 168-bp sizes, of AP3/DEF homolog in R. indicum ‘Chōjyu-hō’ (Figure 4). Moreover, PCR analysis was carried out to investigate why the full-sized AP3/DEF homolog was not amplified (Figure 3C). The amplified PCR product (approximately 250 bp) of primer set 2, P1-F and P2-R, was not detected from R. macrosepalum ‘Kochō-zoroi’ or R. × hannoense ‘Amagi-beni-chōjyu,’ and the PCR product (approximately 135 bp) expression of primer set 3, P3-F and P3-R, in long-lasting flowers were lower than in normal flowers. The PCR product (approximately 260 bp) of primer set 4, P4-F and P4-R, was detected from the cDNA of floral buds in all plant materials. The PCR product (approximately 284 bp) of primer set 5, P5-F and P1-R, was not detected from R. kaempferi ‘Nikkō-misome.’ In floral organs, PI/GLO homolog was mainly expressed from corolla and stamen in both normal and long-lasting flowers, whereas AP3/DEF homolog was not expressed from all floral organs in long-lasting flowers (Figure 3D). In addition to semi-qRT-PCR, the results of RT-qPCR showed that the expression of the AP3/DEF homolog in floral buds of long-lasting cultivars was lower than that in floral buds of normal cultivars (Figure 3E).
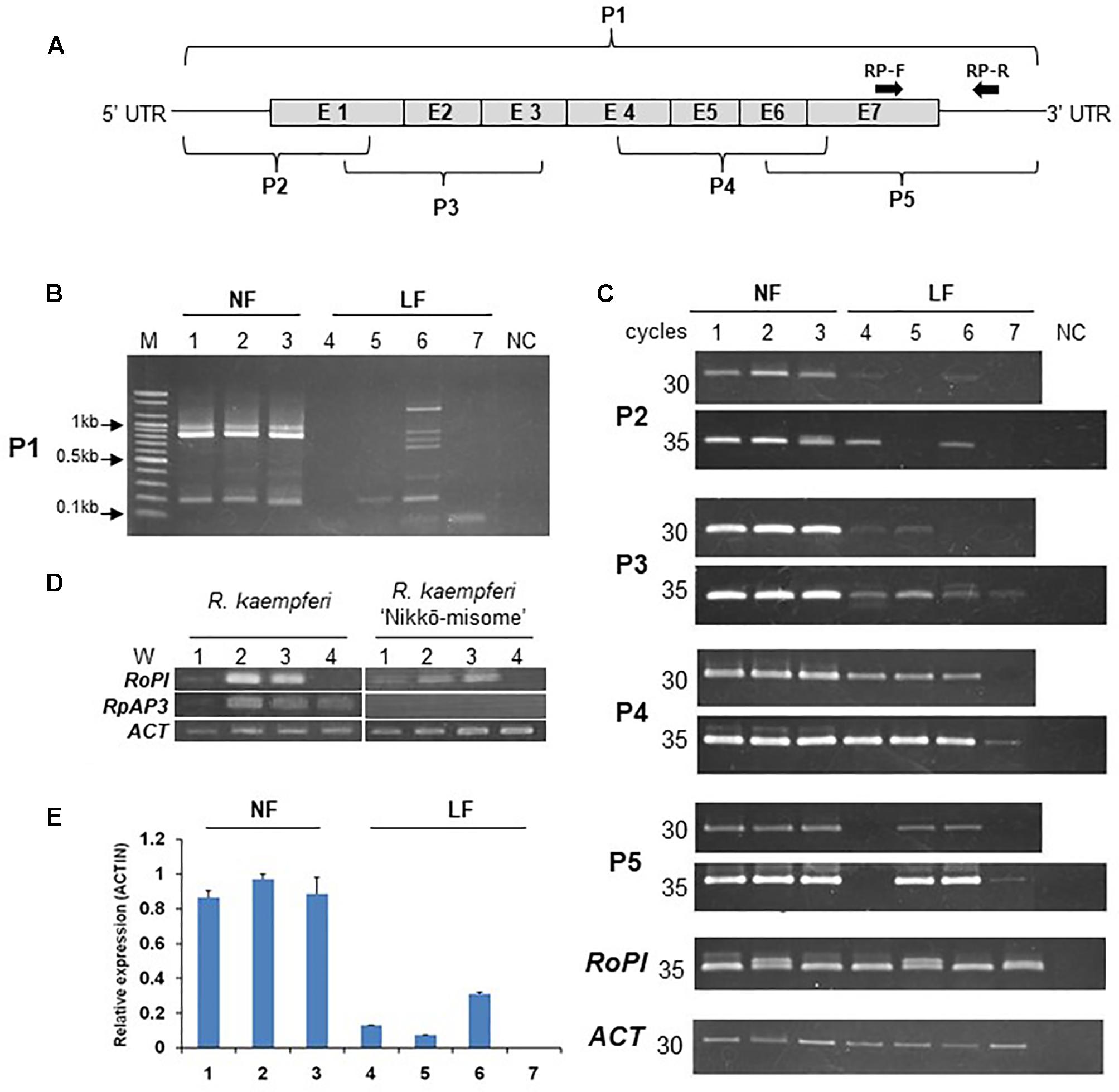
FIGURE 3. Expression and structure analysis of the AP3/DEF homolog in the cDNA of floral buds and organs in normal (NF) and long-lasting flowers (LF). (A) Schematic diagram of the AP3/DEF homolog sequences according to Supplementary Figure S1A. P1–5 and RP-F and -R indicate primer set in this study. (B,C) Analysis of PCR amplification using semi-qRT-PCR; analysis of AP3/DEF homolog using P1-F and -R primer set (B) and other primers (C). M and NC indicate 100 bp DNA ladder (Bioneer) and negative control, respectively. (D) Expression analysis of RoPI and RkAP3 genes using semi-qRT-PCR in floral organs of R. kaempferi and its cultivar ‘Nikkō-misome.’ W1–4 indicate calyx, corolla, stamens, and carpels, respectively. (E) Expression analysis of the AP3/DEF homolog using RT-qPCR. The error bars indicate the mean ± SE of three technical replicates. The numbers correspond to the plant materials; normal flowers R. kaempferi (1), R. macrospalum (2), and R. indicum ‘Ōsakazuki’ (3) and long-lasting flowers R. kaempferi ‘Nikkō-misome’ (4), R. macrosepalum ‘Kochō-zoroi’ (5), R. indicum ‘Chōjyu-hō’ (6), and R. × hannoense ‘Amagi-beni-chōjyu’ (7).
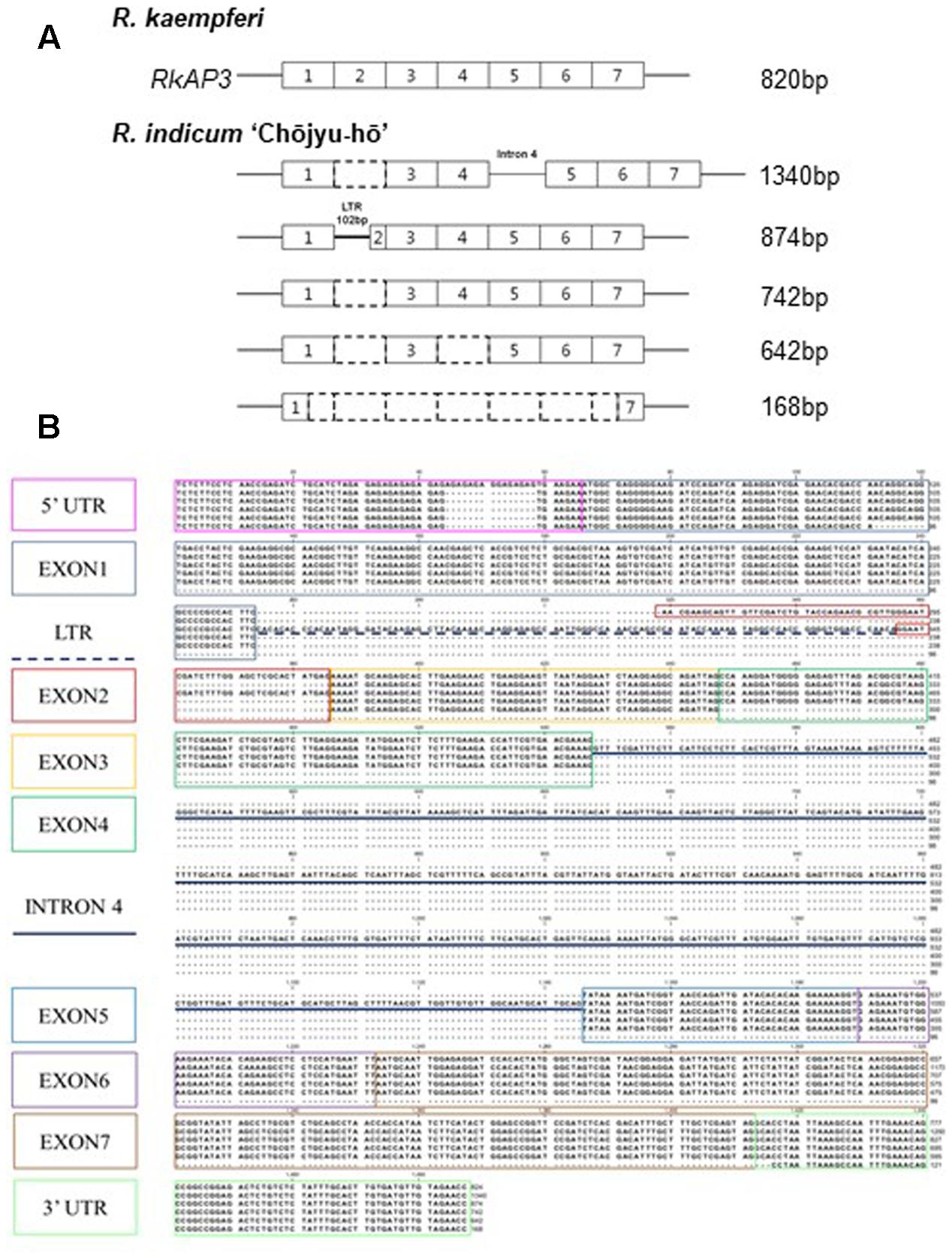
FIGURE 4. Sequence analysis of the AP3/DEF homolog transcript variants in R. indicum ‘Chōjyu-hō’ (Figure 3B). (A) Schematic diagrams of AP3/DEF homolog sequences in R. indicum ‘Chōjyu-hō.’ (B) Sequences of transcript variants. The colored boxes indicate the region of mRNA sequences corresponding to the same color lines.
Insertion Mutation Detected in AP3/DEF Homolog of Long-lasting Flowers
To analyze evidence of abnormal mRNA sequences, AP3/DEF homologs were isolated from gDNA samples of R. kaempferi and all long-lasting flowers (Figure 5). For isolation of AP3/DEF homolog gDNA sequences, PCR amplifications were performed using 5P-F1, 5P-F2, and P1-R primers, which designed on 5′ upstream region in R. kaempferi. Approximately 3.5-, 4-, and 5-kb sized bands were detected from normal flowers, R. macrosepalum ‘Kochō-zoroi,’ and R. indicum ‘Chōjyu-hō,’ respectively, and over 10-kb sized bands were detected from R. kaempferi ‘Nikkō-misome’ and R. × hannoense ‘Amagi-beni-chōjyu’ (Figure 5A). PCR products of 5P-F1 and 5P-F2 primers were used for cloning of AP3/DEF homolog in R. kaempferi. However, PCR products of either 5P-F1 and/or 5P-F2 primers were used to analyze AP3/DEF gDNA sequences in long-lasting flowers, because too large-sized bands were detected from gDNAs of long-lasting flowers. The length of the RkAP3a and RkAP3b sequences, including the 5′ upstream region, seven exons, and six introns, was 3,772 (DDBJ Acc. No. AB861603) and 3,804 bp (DDBJ Acc. No. AB861604), respectively (Supplementary Figure S1C). The sequence analysis in long-lasting flowers predicted full-length sequences of the AP3/DEF homolog region from R. kaempferi ‘Nikkō-misome,’ R. macrosepalum ‘Kochō-zoroi,’ R. indicum ‘Chōjyu-hō,’ and R. × hannoense ‘Amagi-beni-chōjyu’ as approximately 17,102 bp (RkAP3NM, DDBJ Acc. No. AB861605), 4,507 bp (RmAP3KZ, DDBJ Acc. No. AB861606), 6,887 bp (RiAP3CH, DDBJ Acc. No. AB861607), and 17,070 bp (RhAP3AC, DDBJ Acc. No. AB861608), respectively (Figure 5B).
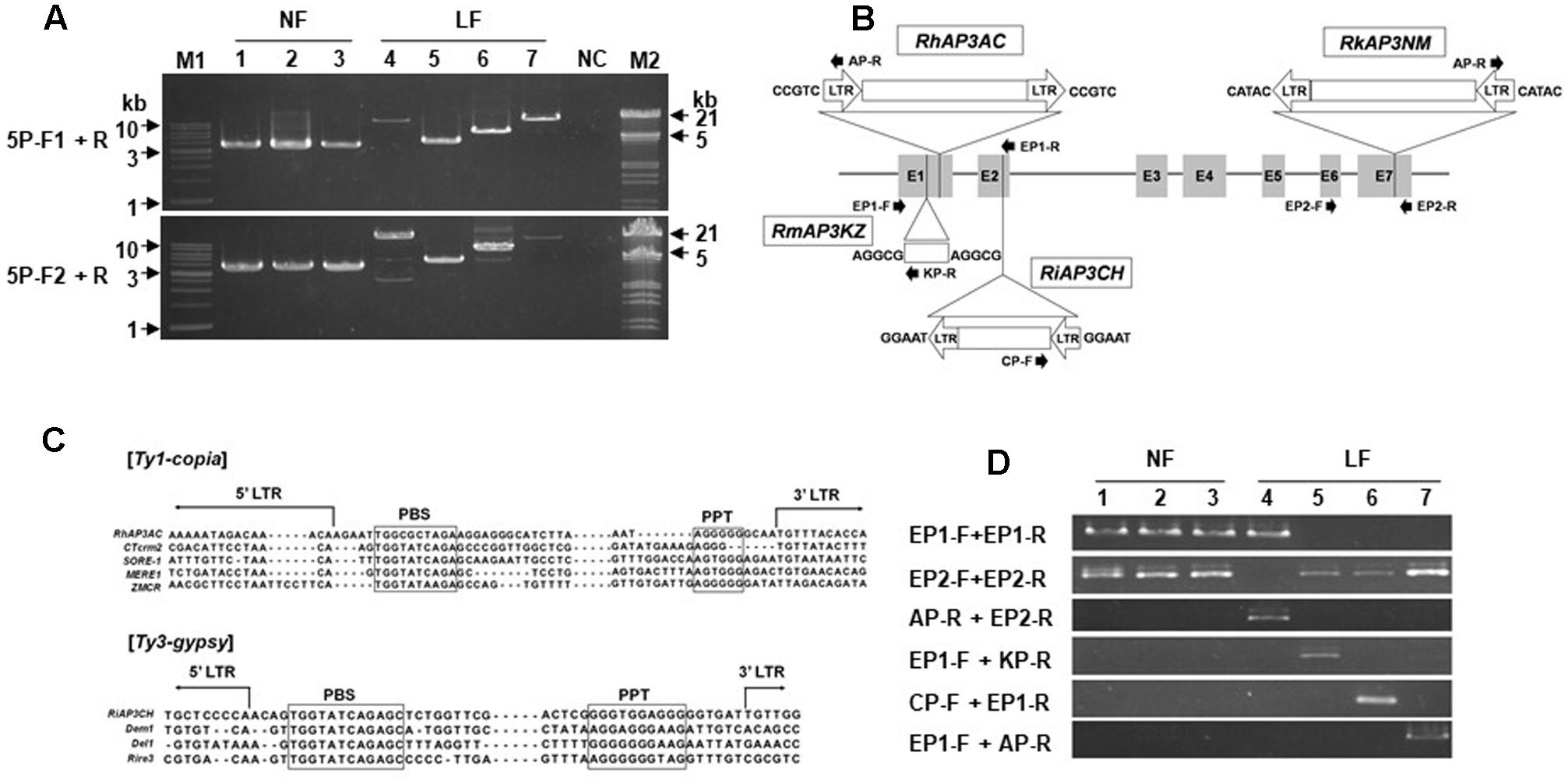
FIGURE 5. The AP3/DEF homolog analysis in gDNA from normal (NF) and long-lasting flowers (LF). (A) gDNA fragments were amplified from normal and long-lasting flowers by using primers 5P-F1, 5P-F2, and P1-R. M1, M2, and NC indicate hyperladder1 kb (Bioline) and lambda DNA/EcoRI+HindIII conventional DNA digest marker (Promega), and negative control, respectively. (B) Schematic diagram of the structures of the AP3/DEF homolog in long-lasting flowers. E1–7 and lines indicate each exon and each intron, respectively. The open arrows and boxes indicate insertions of TEs. The five nucleotides indicate TSD sequences. The closed arrows indicate primers for TE analysis. (C) Comparison among the sequences of LTR-retrotransposons. The boxes indicate the sequences of the PBS and PPT regions. The names of LTR-retrotransposons included RhAP3AC, Rhododendron × hannoense ‘Amagi-beni-chōjyu’; CTerm2, Malus × domestica; SORE-1, Glycine max; MERE1, Medicago truncatula; ZMCR, Zea mays; RiAP3CH, R. indicum ‘Chōjyu-hō’; Dem1, Malus × domestica; Del1, Lilium henryi; and Rire3, Oryza sativa. (D) PCR analysis for TE confirmation. Normal flowers R. kaempferi (1), R. macrospalum (2), and R. indicum ‘Ōsakazuki’ (3) and long-lasting flowers R. kaempferi ‘Nikkō-misome’ (4), R. macrosepalum ‘Kochō-zoroi’ (5), R. indicum ‘Chōjyu-hō’ (6), and R. × hannoense ‘Amagi-beni-chōjyu’ (7).
Insertion Sequences in Azalea AP3/DEF Homolog Are LTR-Retrotransposon or Unknown Sequence
Inserted sequences and regions were revealed by sequence comparison among isolated sequences (Figure 5B). The inserted RkAP3NM sequence in was approximately 13,488 bp and located in exon 7. This sequence contained both 688-bp long-terminal repeats (LTRs) in the same orientation. The inset in RhAP3AC was approximately 13,420 bp and located in exon 1. This sequence contained both 690-bp LTRs in the same orientation. The LTR-retrotransposon in both RkAP3NM and RhAP3AC had similar sequences, but the inserted sequence in RkAP3NM was oriented in the opposite direction from RhAP3AC. In addition, the insert in RiAP3CH was 3,206 bp and located in exon 2. This insert contained the same 359-bp LTRs on both the left and right sides. The other insert in RmAP3KZ was 815 bp and located in exon 1. Although this inserted sequence did not correspond to the LTRs of RkAP3NM and RiAP3CH, the inserted sequence in RmAP3KZ was predicted as an LTR, reflecting the presence of TA and CA sequences, which are start and stop sequences (Kumar and Bennetzen, 1999), respectively, in other LTR-retrotransposons. Compared with part of the primer binding (PBS) site and polypurine tract (PPT) among other transposable elements (TEs) (Smyth et al., 1989; Kumekawa et al., 1999), we proposed that the TE in both RkAP3NM and RhAP3AC were Ty1-copia type and the TE in RiAP3CH was a Ty3-gypsy type (Figure 5C).
Moreover, to confirm the mutant gene status in long-lasting flowers, we searched the gDNA of long-lasting flowers using multiplex-PCR with three primers (Figure 5B and Supplementary Table S1) designed on exon and putative TE sequences. The PCR product obtained using the EP1 primer set was amplified in normal and RkAP3NM samples, and the PCR product obtained using the EP2 primer set was amplified from all of the samples, except RkAP3NM. In addition, the PCR products of the other primer sets were amplified from each region containing a TE insertion (Figure 5D). The long-lasting flower contained the mutant gene, but not the normal gene, suggesting that the TE insertion likely leads to long-lasting flowers and that the long-lasting flowers likely carry homozygous mutant genes.
Long-lasting Mutant Was Caused by ap3/def Alleles
To determine whether the mutant allele is essential for long-lasting flowers, we investigated floral phenotypes and genotypes using cultivars (n = 4) and individual progenies (n = 12) through multiplex-PCR with three primers (Supplementary Table S1) that were used to distinguish each ap3/def mutant allele in evergreen azaleas based on differences in the TE insertion regions (Figure 6). All of the F1 progenies resulting from the cross between normal and long-lasting flowers showed normal flowers, whereas F2 progenies showed normal and long-lasting flowers (Figures 6A–C). Consistent with the observed phenotypes, normal genes were detected in all of the F1 progenies and one F2 progeny, and only mutant alleles, both RiAP3CH and RmAP3KZ, were detected from the two F2 progenies with long-lasting flowers, whereas normal and mutant genes, RiAP3CH or RmAP3KZ, were detected from the F2 progenies with normal flowers (Figure 6D). As heterozygous alleles, combination of normal and mutant flowers, in F1 and F2 leads to normal flower development and the homozygous mutant alleles permit long-lasting flower development in F2, we concluded that long-lasting flowers are conferred through homozygous ap3/def mutant alleles.
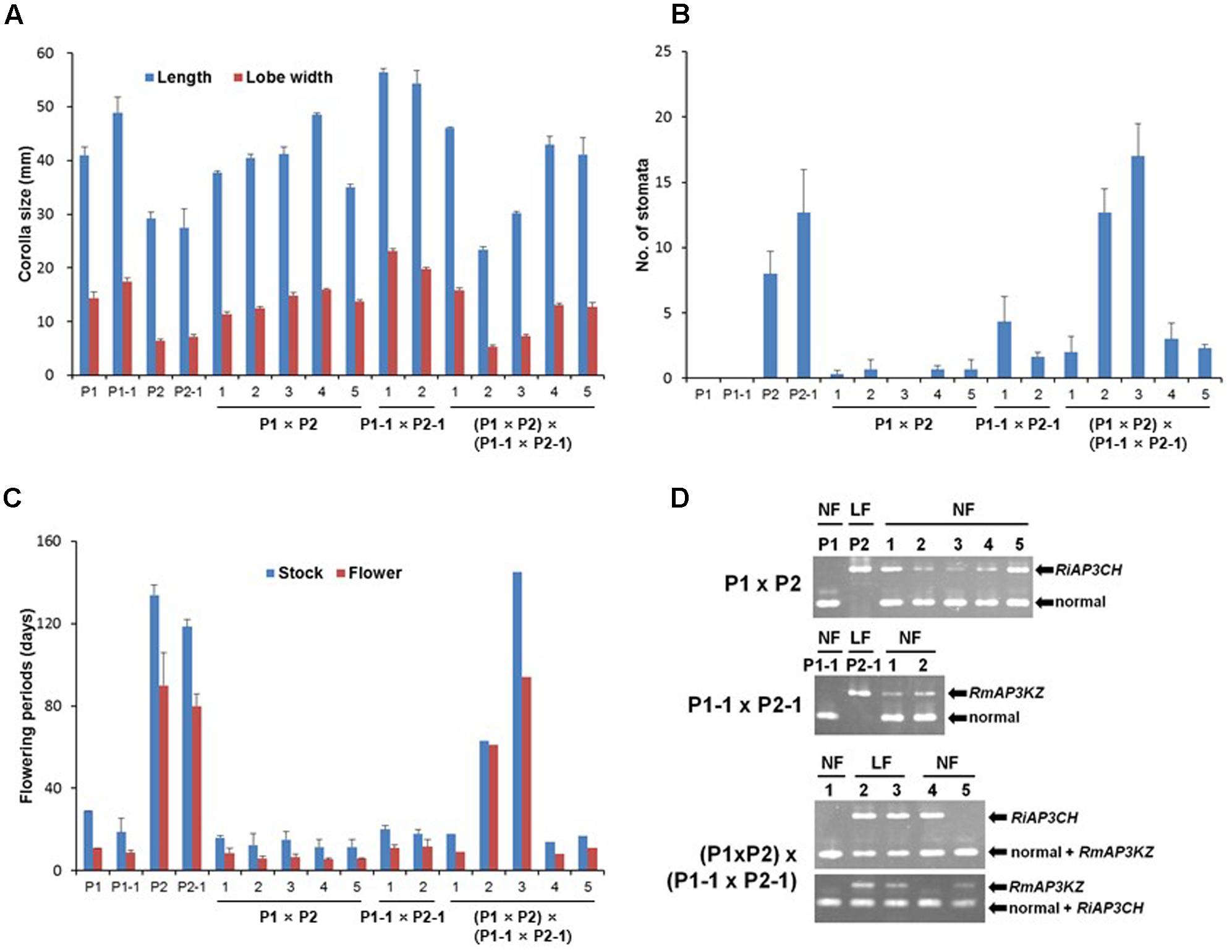
FIGURE 6. Investigation of floral morphology and mutant gene in progenies resulting from a cross between normal (NF) and long-lasting flowers (LF). (A–C) Analysis of floral morphology, such as corolla sizes (mm) (A), number of stomata (B), and flowering periods (days) (C). (D) Mutant allele analysis using PCR and mutant gene-specific primers (Supplementary Table S1). P1, Kurume hybrid ‘Wakakaede’; P1-1, R. oldhamii; P2, R. indicum ‘Chōjyu-hō’; P2-1, R. macroseplaum ‘Kochō-zoroi’; F1, P1 × P2; F1-1, P1-1 × P2-1; F2, (P1 × P2) × (P1-1 × P2-1).
Discussion
Our investigation of floral morphology and molecular characters in long-lasting flowers showed that the characteristics of evergreen azaleas with flowers, including long-lasting, small-sized, and sepaloid corollas, are unique to these species and are rarely found in other horticultural plants.
We have shown that azalea AP3/DEF homolog has functions similar to those of Antirrhinum DEF gene in regulation of long-lasting flower development (Schwarz-Sommer et al., 1992). The Antirrhinum def mutants have several phenotypes as chlorantha and nicotianoides flowers that are smaller and their petals show green color indicating sepaloid features. In addition, gloifera petals are morphologically indistinguishable from sepals, except that these sepaloid whorl 2 organs are larger than sepals in the whorl 1 and that their position in the mature mutant flower resembles the position of wild type petals of the upper and lower lobes. In analysis of Antirrhinum GLO and DEF expressions, DEF gene is lowly expressed than GLO gene expression in chlorantha and nicotianoides flowers, both genes were not detected from gloifera flower buds (Sommer et al., 1990; Schwarz-Sommer et al., 1992; Tröbner et al., 1992). In other plants, an investigation of the relationship between the observed phenotype and PhDEF gene expression in the Petunia hybrid artificial phdef mutants showed that the corolla was converted to sepals in the whorl 2, where PhDEF gene expression was found to be significantly reduced (Vandenbussche et al., 2004). When AP3 homolog expression is repressed in oilseed rape (Brassica napus) and poppies (Papaver somniferum) flower mutants, petals of the transformed plants are converted to sepaloid petals, which are smaller than normal petals; furthermore, in these mutant plants, stomata grow from the sepaloid petal in the same manner as the sepal (De Byzova et al., 2004; Drea et al., 2007). Also, Hirai et al. (2010) suggested that the reduced expressions of TGDEFA and TGDEFB are associated in the floral development of the viridiflora tulip (‘White Dream’ and ‘Spring Green’), which is partially composed of sepaloid tepals in the first and second whorl. As case of herbaceous peony (Paeonia lactiflora), spontaneous corolla mutant in natural condition was closely associated with selective expression alterations of duplicated AP3 and PI genes, because down-regulation of duplicated B-class genes might result in reduction of AP3-PI heterodimer in sepaloid corolla (Gong et al., 2017). Although phenotype is different, Malus × domestica PI (MdPI) mutant, which inserted retrotransposon in natural condition, was weakly expressed in mutant plant; some apples produce parthenocarpic fruit, resulting in LTR-retrotransposon insertion in the MdPI MADS transcription factor; Intron 4 of MdPI has insertions in ‘Rae Ime,’ and intron 6 has insertions in ‘Spencer Seedless’ and ‘Wellington Bloomless.’ In these mutant cultivars, flowers have no petals or stamens but two whorls of five sepals and an increased number of styles and carpels (Yao et al., 2001), due to insertion into the common MADS transcription factor. In this study, long-lasting flowers have functional stamens (Figure 6) and azalea AP3/DEF homolog was expressed in floral buds of plant materials, except R. × hannoense ‘Amagi-beni-chojyu’ in RT-qPCR, but results of semi-qRT-PCR and sequence analysis indicated that the abnormal mRNA sequence of azalea AP3/DEF exist in floral buds of all long-lasting cultivars (Figures 3, 4). Moreover, TE or unknown sequences were detected from gDNA sequences in AP3/DEF homologs of long-lasting flowers (Figure 5). Thus, the loss-of-function of the AP3/DEF homolog in long-lasting flowers were caused by the insertion of a retrotransposon or putative LTR sequence, and these inserted sequences might be affected by mRNA splicing of the AP3/DEF homolog in long-lasting flowers, because gene expression or the structure encoded proteins can be occurred by mutations due to TE insertions in or near genes (Kumar and Bennetzen, 1999; Lisch, 2013).
The original species of four long-lasting flowers were distributed at different site and the original species have been recorded since 17th century in Japan (Ito, 1984; Yamazaki, 1996). The geographic origin of the azalea species indicates the possibility of independent events of mutation, because azalea cultivars were selected from natural hybrid population and the selected cultivars have been propagated by cutting (Kobayashi, 2013). We have analyzed azalea AP3/DEF gDNA sequences of four flower mutants and found insertions in all four cultivars; ‘Nikkō-misome’ and ‘Amagi-beni-chōjyu’ have the nearly same insertion sequence in exon 7 and exon 1, respectively, but ‘Kochō-zoroi’ and ‘Chōjyu-hō’ have different insertion sequence in exon 1 and exon 2, respectively (Figure 5B). The different TE insertion regions at same gene are good evidence that ap3/def mutant is included in the development of long-lasting flower in these azaleas. In addition, when we analyzed normal and mutant alleles from individual azalea cultivars and its progenies, the non-functional AP3/DEF mutant gene was detected from long-lasting flowers but normal allele was not detected (Figures 5D, 6). The results corresponded to petal loss plant in apetalous mutant of Nigella; the mutant was recessive trait that indeed caused by the TE insertion in AP3-3 gene, and the non-functional AP3-3 gene was existed as homozygous for petal loss (Zhang et al., 2013). In previous study of azalea, long-lasting flower was shown as recessive trait to normal corollas and was controlled by single gene (Gobara et al., 2017). From this, the results of mutant allele investigation in cultivars and progenies suggest that whereas all long-lasting flowers are homozygous for the ap3/def mutant alleles, normal flowers are either heterozygous (normal allele/mutant allele) or homozygous (normal alleles). Despite the phenotypic similarity of Antirrhinum def mutants (Sommer et al., 1990), it is still difficult to conclude that azalea AP3/DEF homolog was participated to long-lasting flower development. A difference between azalea ap/def homolog and Antirrhinum DEF mutants is that the azalea long-lasting flowers produce corollas, which have long maintenance period. Phenotypic and molecular evidence show that corollas in long-lasting flowers are related to mutations in azalea mutant allele. The corolla persisting period of long-lasting flower after flowering was long, approximately 100 days (Figures 1C–E, 2J,6C). When we analyzed normal and mutant allele from individual azalea cultivars and its progenies, the non-functional azalea ap3/def mutant allele was detected from long-lasting flowers but normal allele was not detected (Figures 5D, 6D). The sepaloid corolla of long-lasting flowers may affect flower longevity, because the period of sepal persisting is longer than the period of petal persisting after flowering in evergreen azaleas. Although the maintenance of PeMADS6, which might have the function of GLO/PI-like genes in Phalaenopsis in orchids, transcripts in flower through flowering to senescence corresponds to the long-lasting flower longevity in orchids; transgenic plants overexpressing PeMADS6 or PI, respectively, had a 3.4- or 2.1-fold higher flower longevity than wild-type Arabidopsis plants (Tsai et al., 2005), the relationship of AP3/DEF-like gene and flower longevity has not been reported. Thus, the results in this study showed that the long-lasting flower would be derived from the sepaloid corolla in long-lasting flowers and would be caused by a mutation of the AP3/DEF homolog, although more experiments are needed to clarify mechanism of long flower longevity.
The flower quality of the current long-lasting flowers is a desirable trait (Kobayashi et al., 2010; Kobayashi, 2013). To breed long-lasting trait into commercial azalea cultivars, azalea breeding have attempted. However, the breeding process is slow when traditional crossing methods are used in wood plants because of the long juvenile period required. With the identification of the AP3/DEF homolog sequence, genetic transformation could be used to generate high-quality, long-lasting flowers by the down-regulation of AP3/DEF homolog expression using RNAi techniques together with transgenic systems developed for azalea cultivars. This study firstly revealed LTR-retrotransposons in azalea cultivars and the TEs into AP3/DEF homolog may also be used as a gene base marker for the selection of the long-lasting trait at the juvenile period in evergreen azalea breeding. Collectively, our results increase the current understanding of long-lasting flowers. With regard to the nature of the mutation at azalea AP3/DEF homolog, this naturally occurring TE-induced phenotypic change may be especially important for variety flower in horticultural plants, as it affects an essential trait for adaptive plant evolution. From more than 300 years ago, unique mutant flowers have been collected from natural population, reflecting the natural selection and maintenance of TE-induced mutant flowers, and Japanese people have been enjoyed mutant flowers as ornamental plant.
Author Contributions
Experimental design: NK, AN, and K-SC. Experiments: K-SC and KT. Data analysis: K-SC, AN, and KT. Manuscript preparation: K-SC, KT, AN, and NK. Supervision, funding, and reagents: NK and AN.
Funding
This study was financially supported through a Grant-in-Aid for Scientific Research (KAKENHI Nos. 23241076 and 26292017) from the Japan Society for the Promotion of Science (JSPS).
Conflict of Interest Statement
The authors declare that the research was conducted in the absence of any commercial or financial relationships that could be construed as a potential conflict of interest.
Supplementary Material
The Supplementary Material for this article can be found online at: https://www.frontiersin.org/articles/10.3389/fpls.2017.02239/full#supplementary-material
FIGURE S1 | Sequence and structure information for RkAP3a and RkAP3b. (A) The cDNA sequences of RkAP3a and RkAP3b isolated from R. kaempferi are shown in the top and bottom lines, respectively. The dots and boxes indicate sequence identity and different sequences between RkAP3a and RkAP3b mRNA sequences, respectively. The M and asterisk indicate the start and stop codons, respectively. Primers and directions are indicated with horizontal arrows. The intron positions are indicated with vertical arrows. (B) Comparison of the amino acids among AP3, DEF, RkAP3a, and RkAP3b. The box indicates the amino acid sequence of the euAP3 motif. (C) The gDNA structure of RkAP3a and RkAP3b. Dotted lines, bold lines, thin lines, and gray boxes indicate the 5′ upstream region, 5′ and 3′ UTR, introns, and exons, respectively.
TABLE S1 | Primers used for the sequence and mutant gene analysis in normal and long-lasting flower types.
References
Cheon, K.-S., Nakatsuka, A., and Kobayashi, N. (2011). Isolation and expression pattern of genes related to flower initiation in the evergreen azalea, Rhododendron × pulchrum ‘Oomurasaki’. Sci. Hortic. 130, 906–912. doi: 10.1016/j.scienta.2011.09.001
Cheon, K.-S., Nakatsuka, A., and Kobayashi, N. (2016). Mutant PI/GLO homolog confers the hose-in-hose flower phenotype in Kurume azaleas. Hort. J. 85, 380–387. doi: 10.2503/hortj.MI-138
Coen, E. S., and Meyerowitz, E. M. (1991). The war of the whorls: genetic interactions controlling flower development. Nature 353, 31–37. doi: 10.1038/353031a0
De Byzova, M., Verduyn, C., De Brouwer, D., and De Block, M. (2004). Transforming petals into sepaloid organs in Arabidopsis and oilseed rape: implementation of the hairpin RNA-mediated gene silencing technology in an organ-specific manner. Planta 218, 379–387. doi: 10.1007/s00425-003-1117-1
Drea, S., Hileman, L. C., De Martino, G., and Irish, V. F. (2007). Functional analyses of genetic pathways controlling petal specification in poppy. Development 134, 4157–4166. doi: 10.1242/dev.013136
Gobara, Y., Nakatska, A., Cheon, K.-S., and Kobayashi, N. (2017). Floral morphology and inheritance of the long-lasting flower trait (misome-sho) in Japanese evergreen azalea cultivars. Hort. Res. 16, 383–390. doi: 10.2503/hrj.16.383
Gong, P., Ao, X., Liu, G., Cheng, F., and He, C. (2017). Duplication and whorl-specific down-regulation of the obligate AP3–PI heterodimer genes explain the origin of Paeonia lactiflora plants with spontaneous corolla mutation. Plant Cell Physiol. 58, 411–425. doi: 10.1093/pcp/pcw204
Hang, N. T. T., Miyajima, I., Ureshino, K., Masuda, J.-I., and Okubo, H. (2010). Comparison of morphological characteristics of Rhododendron simsii planch. Distributed in Vietnam and Japan. J. Fac. Agric. Kyushu Univ. 55, 233–237.
Hirai, M., Ochiai, T., and Kanno, A. (2010). The expression of two DEFICIENS-like genes was reduced in the sepaloid tepals of viridiflora tulips. Breed. Sci. 60, 110–120. doi: 10.1270/jsbbs.60.110
Jack, T., Brockman, L. L., and Meyerowitz, E. M. (1992). The homeotic gene APETALA3 of Arabidopsis thaliana encodes a MADS box and is expressed in petals and stamens. Cell 68, 683–697. doi: 10.1016/0092-8674(92)90144-2
Kobayashi, N. (2013). Evaluation and application of evergreen azalea resources of Japan. Acta Hortic. 990, 213–219. doi: 10.17660/ActaHortic.2013.990.24
Kobayashi, N., Horikoshi, T., Katsuyama, H., Handa, T., and Takayanagi, K. (1998). A simple and efficient DNA extraction method for plants, especially woody plants. Plant Tissue Cult. Biotechnol. 4, 76–80.
Kobayashi, N., Ishihara, M., Ohtani, M., Nakatsuka, A., Cheon, K. S., Mizuta, D., et al. (2010). Evaluation and application of the long-lasting flower trait (Misome-sho) of azalea cultivars. Acta Hortic. 855, 165–168. doi: 10.17660/ActaHortic.2010.855.23
Kumar, A., and Bennetzen, J. L. (1999). Plant retrotransposons. Annu. Rev. Genet. 33, 479–532. doi: 10.1146/annurev.genet.33.1.479
Kumekawa, N., Ohtsubo, H., Horiuchi, T., and Ohtsubo, E. (1999). Identification and characterization of novel retrotransposons of the gypsy type in rice. Mol. Gen. Genet. 260, 593–602. doi: 10.1007/s004380050933
Lisch, D. (2013). How important are transposons for plant evolution? Nat. Rev. Genet. 14, 49–61. doi: 10.1038/nrg3374
Ochman, H., Gerber, A. S., and Hart, D. L. (1988). Genetic applications of an inverse polymerase chain reaction. Genetics 120, 621–623.
Schwarz-Sommer, Z., Hue, I., Huijser, P., Flor, P. J., Hansen, R., Tetens, F., et al. (1992). Characterization of the Antirrhinum floral homeotic MADS-box gene deficiens: evidence for DNA binding and autoregulation of its persistent expression throughout flower development. EMBO J. 11, 251–263.
Smyth, D. R., Kalitsis, P., Joseph, J. L., and Sentry, J. W. (1989). Plant retrotransposon from Lilium henryi is related to Ty3 of yeast and the gypsy group of Drosophila. Proc. Natl. Acad. Sci. U.S.A. 86, 5015–5019. doi: 10.1073/pnas.86.13.5015
Sommer, H., Beltrán, J. P., Huijser, P., Pape, H., Lönnig, W. E., Saedler, H., et al. (1990). Deficiens, a homeotic gene involved in the control of flower morphogenesis in Antirrhinum majus: the protein shows homology to transcription factors. EMBO J. 9, 605–613.
Tasaki, K., Nakatsuka, A., Cheon, K.-S., Koga, M., and Kobayashi, N. (2012a). Morphological and expression analyses of MADS genes in Japanese traditional narrow- and/or staminoid-petaled cultivars of Rhododendron kaempferi planch. Sci. Hortic. 134, 191–199. doi: 10.1016/j.scienta.2011.11.013
Tasaki, K., Nakatsuka, A., and Kobayashi, N. (2012b). Morphological analysis of narrow-petaled cultivars of Rhododendron macrosepalum maxim. J. Japan. Soc. Hortic. Sci. 81, 72–79. doi: 10.2503/jjshs1.81.72
Tröbner, W., Ramirez, L., Motte, P., Hue, I., Huijser, P., Lönnig, W. E., et al. (1992). GLOBOSA: a homeotic gene which interacts with DEFICIENS in the control of Antirrhinum floral organogenesis. EMBO J. 11, 4693–4704.
Tsai, W. C., Lee, P. F., Chen, H. I., Hsiao, Y. Y., Wei, W. J., Pan, Z. J., et al. (2005). PeMADS6, a GLOBOSA/PISTILLATA-like gene in Phalaenopsis equestris involved in petaloid formation, and correlated with flower longevity and ovary development. Plant Cell Physiol. 46, 1125–1139. doi: 10.1093/pcp/pci125
Vandenbussche, M., Zethof, J., Royaert, S., Weterings, K., and Gerats, T. (2004). The duplicated B-class heterodimer model: whorl-specific effects and complex genetic interactions in Petunia hybrida flower development. Plant Cell 16, 741–754. doi: 10.1105/tpc.019166
Weigel, D., and Meyerowitz, E. M. (1994). The ABCs of floral homeotic genes. Cell 78, 203–209. doi: 10.1016/0092-8674(94)90291-7
Yamazaki, T. (1996). A Revision of the Genus Rhododendron in Japan, Taiwan, Korea, and Sakhalin. Tokyo: Tsumura Laboratory.
Yang, Y., Fanning, L., and Jack, T. (2003). The K domain mediates heterodimerization of the Arabidopsis floral organ identity proteins, APETALA3 and PISTILLATA. Plant J. 33, 47–59. doi: 10.1046/j.0960-7412.2003.01473.x
Yao, J.-L., Dong, Y.-H., and Morris, B. A. M. (2001). Parthenocarpic apple fruit production conferred by transposon insertion mutations in a MADS-box transcription factor. Proc. Natl. Acad. Sci. U.S.A. 98, 1306–1311. doi: 10.1073/pnas.98.3.1306
Keywords: long-lasting flower, corolla mutant, AP3/DEF homolog, transcriptional factors, retrotransposon, evergreen azalea
Citation: Cheon K-S, Nakatsuka A, Tasaki K and Kobayashi N (2018) Long-lasting Corolla Cultivars in Japanese Azaleas: A Mutant AP3/DEF Homolog Identified in Traditional Azalea Cultivars from More Than 300 Years Ago. Front. Plant Sci. 8:2239. doi: 10.3389/fpls.2017.02239
Received: 06 November 2017; Accepted: 20 December 2017;
Published: 09 January 2018.
Edited by:
Akira Kanno, Tohoku University, JapanReviewed by:
Chaoying He, State Key Laboratory of Systematic and Evolutionary Botany, Institute of Botany, Chinese Academy of Sciences, ChinaMasaru Nakano, Niigata University, Japan
Copyright © 2018 Cheon, Nakatsuka, Tasaki and Kobayashi. This is an open-access article distributed under the terms of the Creative Commons Attribution License (CC BY). The use, distribution or reproduction in other forums is permitted, provided the original author(s) or licensor are credited and that the original publication in this journal is cited, in accordance with accepted academic practice. No use, distribution or reproduction is permitted which does not comply with these terms.
*Correspondence: Nobuo Kobayashi, bmtvYmF5YXNoaUBsaWZlLnNoaW1hbmUtdS5hYy5qcA==