- 1Key Laboratory of Agricultural Water Resources, Hebei Laboratory of Agricultural Water-Saving, Center for Agricultural Resources Research, Institute of Genetics and Developmental Biology, Chinese Academy of Sciences, Shijiazhuang, China
- 2University of Chinese Academy of Sciences, Beijing, China
Drought is a major environmental stress that severely restricts plant growth and crop productivity. A previous study showed that TaWRKY2 from wheat (Triticum aestivum) plays an important role in drought stress tolerance. In the present study, we isolated the promoter of TaWRKY2 and identified multiple regulatory cis-elements in the promoter region. The activity of the TaWRKY2 promoter was induced by drought, salt, heat, and abscisic acid (ABA). We also generated TaWRKY2-overexpressing transgenic wheat, and found that the transgenic seedlings exhibited significantly enhanced tolerance to drought stress, as evidenced by a higher survival rate and lower water loss rate of detached leaves compared with wild type (WT) plants. In addition, the transgenic lines had higher contents of free proline, soluble sugar, and chlorophyll. During a prolonged period of drought stress before the heading stage, the growth of WT plants was inhibited, whereas the TaWRKY2-overexpressing lines progressed to the heading stage. The increased grain yield of the transgenic wheat lines reflected the cumulative effects of longer panicle length, more kernels per spike, and greater aboveground biomass. Our findings show that TaWRKY2 can enhance drought tolerance and increase grain yield in wheat, thus providing a promising candidate target for improving the drought tolerance of wheat cultivars through genetic engineering.
Introduction
Wheat (Triticum aestivum) is one of the most important staple crops worldwide, and its production in many regions is severely impacted by drought; thus, improving the drought tolerance of wheat through the breeding of cultivars is an important step toward ensuring food security.
Reflecting their sessile nature, plants have developed a fairly complex stress response system to deal with drought via changes at the physiological, morphological, and molecular levels (Todaka et al., 2012; Lata et al., 2015; Tiwari et al., 2017). Transcription factors (TFs) can synchronously regulate the expression of many downstream drought-regulated genes and thus mediate the response to drought in plants (Singh and Laxmi, 2015; Gahlaut et al., 2016). The overexpression of some stress-responsive TFs can enhance abiotic stress tolerance in transgenic plants (Saad et al., 2013). For instance, the Arabidopsis homeodomain-leucine zipper TF gene EDT1/HDG11 not only conferred drought tolerance, but also increased grain yield in transgenic rice (Yu et al., 2013). The Oryza sativa TF gene OsMYB2 was found to be induced by salt and cold, and transgenic rice overexpressing OsMYB2 showed increased tolerance to various abiotic stresses by regulating the relative expression levels of diverse genes involved in abiotic stress responses (Yang et al., 2012).
With the rapid development of genetic engineering to improve abiotic stress tolerance in plants, TFs may be a practical and an effective class of targets for improving stress tolerance in wheat. Several examples from previous studies using transgenic wheat are described below. TaERF3-overexpressing transgenic wheat seedlings exhibited significantly enhanced tolerance to both salt and drought stresses compared with untransformed wheat (Rong et al., 2014). TaPIE1 overexpression significantly enhanced resistance to freezing stress (Zhu et al., 2014). TaHsfC2a-overexpressing transgenic wheat showed improved thermal tolerance (Hu et al., 2017). GmDREB1, a soybean TF gene, was also shown to improve salt stress tolerance in transgenic wheat (Jiang Q. et al., 2014).
The WRKY TFs family not only imparts tolerance against abiotic stresses, but also regulates plant growth and development (Jiang et al., 2012; Zhu et al., 2013; Tripathi et al., 2014; Xu et al., 2014). In recent years, many WRKY genes have been identified in a variety of plants (Fan et al., 2015; He et al., 2016; Xu et al., 2016; Wei et al., 2017). Recently, most studies of WRKY TFs have based on functional analyses of responses to abiotic stress, such as drought and salt, with functional validation conducted only in the model plants (Wang et al., 2013; Xu et al., 2014; Qin et al., 2015). However, functional validation of the vast majority of WRKYs has yet to be confirmed in non-model plants, particularly wheat, in which WRKY-mediated drought resistance mechanisms have not been studied. We previously reported that TaWRKY2 was induced in response to drought stress. Additionally, overexpression of TaWRKY2 conferred tolerance to drought stress in transgenic Arabidopsis (Niu et al., 2012). However, to the best of our knowledge, the roles of TaWRKY2 in the drought stress response of wheat and its functional mechanisms in wheat have not been reported.
To investigate how TaWRKY2 induces drought stress responses, we cloned the promoter of the TaWRKY2 gene and identified the regulatory cis-elements in the promoter region in the present study. Transgenic wheat generated by conducting Agrobacterium-mediated transformation with TaWRKY2-overexpressing vectors were used to confirm the function of TaWRKY2, and the physiological mechanisms of drought resistance were analyzed. In addition, the effects of TaWRKY2 overexpression on wheat yield were studied. Taken together, the present findings indicate that TaWRKY2 contributes to drought tolerance and increases yield.
Materials and Methods
Plant Materials
The promoter of TaWRKY2 (NCBI ID: EU665425.1) was cloned from Xifeng20, a common drought-tolerant wheat variety. Fielder, a spring wheat variety, was used as the recipient for the TaWRKY2-overexpressing transformation. T1–T3 transgenic wheat plants. Nicotiana benthamiana were used for β-glucuronidase (GUS) histochemical analysis.
Cloning of the TaWRKY2 Promoter and GUS Histochemical Analysis
The TaWRKY2 promoter was cloned from Xifeng20 using specific primers (PW2-F and PW2-R). PCR was performed in a 50-μl reaction volume containing 200 ng genomic DNA, 0.4 μM dNTPs, 0.3 μM each primer and 1 U KOD FX (Toyobo, Shanghai, China). The PCR program used was 94°C for 2 min; 40 cycles of 98°C for 10 s, 55°C for 30 s, and 68°C for 2.5 min; final extension at 72°C for 10 min; and storage at 4°C. The cloned sequence was inserted into pCAMBIA1391 to obtain PTaWRKY 2::GUS for GUS histochemical analysis. The PTaWRKY 2::GUS construct was introduced into Agrobacterium tumefaciens strain GV3101 and transformed into N. benthamiana leaves. Two days after being cultured under 14 h light/10 h dark at 25°C, the injected N. benthamiana was treated with different abiotic stresses. The GUS assay was performed as previously described (Zhao et al., 2011). The histochemical staining was conducted by incubating the materials in prepared buffer containing 5-bromo-4-chloro-3-indolyl-β-D-glucuronic acid for 12 h at 37°C in the dark followed by clearing with 75% ethanol.
Overexpression Vector Construction and Transformation
A 1,407-bp fragment corresponding to the TaWRKY2 coding sequence (CDS) was amplified from the vector pBIN121-TaWRKY2 that was introduced into Arabidopsis thaliana (Columbia) (Niu et al., 2012) with specific primers (Ta2-F and Ta2-R). The CDS was subsequently cloned into the SpeI and BamhI sites of pMWB122, a binary expression vector with an ubiquitin promoter. The recombinant vector pMWB122-TaWRKY2 was transformed into a spring wheat variety, Fielder, using Agrobacterium-mediated transformation. The transformation was performed by Xingguo Ye’s group from the Chinese Academy of Agricultural Sciences.
PCR and Quantitative Reverse Transcription-PCR Analyses
Genomic DNA was isolated from leaves of wheat using the cetyl-trimethyl-ammonium bromide (CTAB) method. A 381-bp fragment was amplified by PCR using specific primers (TaW2-F and TaW2-R). PCR reactions were performed in a 20-μl reaction volume, containing 50 ng wheat genomic DNA, 0.4 μM of each primer, and 1 U Taq polymerase (TransGen Biotech, Beijing, China). The PCR program used was 94°C for 2 min; followed by 32 cycles of 94°C for 30 s, 56°C for 30 s, and 72°C for 40 s; final extension at 72°C for 10 min; and storage at 4°C. The amplified fragments were separated on a 1.0% agarose gel.
Total RNA was extracted from wheat leaves using TRIZOL reagent (TransGEN, Beijing, China). cDNA was synthesized using the TansSript kit (TransGEN, Beijing, China). qRT-PCR was used to analyze transcript levels of TaWRKY2 (QT-Ta2-F and QT-Ta2-R) and eight drought-related genes following the methods described in Niu et al. (2012). Wheat Actin was used as an internal reference to normalize all data (Taactin F and Taactin R). All primers were listed in Supplementary Table S1.
Southern Blot
The transgenic TaWRKY2 gene copy number was determined by Southern blot. Genomic DNA was extracted from the leaves of 3-week-old transgenic wheat plants by using the CTAB method (Sambrook et al., 1989). Each sample was digested with HindIII (TaKaRa, Dalian, China) overnight, and the digested DNA was electrophoresed on 0.8% agarose gels and then transferred onto a nylon Hybond-N+ membrane (Roche). The DNA fragments of the ubiquitin promoter were amplified with specific primers (UbiF and UbiR; Supplementary Table S1) and were labeled with digoxigenin (DIG) and used as probes to hybridize with the digested DNA on the membrane. The hybridization and detection steps were performed following the instructions for the DIG High Prime DNA Labeling and Detection Starter Kit II (Roche).
Drought Treatment and Measurement of Drought-Related Indicators
For the drought tolerance assays at the seedling stage, the wheat plants were cultured in plastic containers (depth: 8 cm, diameter: 8 cm) containing mixed soil (1:1 nutritional soil:vermiculite), with nine plants per pot. All plants were grown in a culture room under standard growth conditions (14 h light at 24°C/10 h dark at 18°C). After the full development of the three leaves of each plant, the wheat seedlings were subjected to drought treatment by withholding water for 8–11 days before re-watering. Meanwhile, the soil relative water content (SRWC) and leaf relative water content (LRWC) were calculated as formulas in Supplementary Table S3. The water loss rate of detached leaves was calculated as previously described (Niu et al., 2012). Free proline, chlorophyll, soluble sugar, and H2O2 contents in leaves were measured following the kit introductions (Comin, Suzhou, China). Each data point corresponds to three replicates.
For the analysis of TaWRKY2 effects on the heading stage, wheat plants were cultured in the plastic containers (depth: 30 cm, diameter: 25 cm) in a greenhouse. Approximately 20–25 days before the heading stage, the following treatments were applied: (1) wild type (WT) and transgenic wheat plants were grown under normal condition until harvest, and (2) the same plants were subjected to 20–25 days of water-withholding (drought treatment), followed by re-watering until harvest. The entire experiment was carried out at room temperature.
Agronomic traits data were collected from the harvested transgenic wheat and WT plants under normal and drought conditions mentioned above for statistical analysis.
Results
Analysis of TaWRKY2 Promoter
It has been found that the expression of TaWRKY2 was induced by drought (Niu et al., 2012). To further understand the mechanism underlying this phenomena, we cloned the 2,166-bp DNA fragment upstream of the ATG start codon of TaWRKY2 from the wheat variety Xifeng20 (Figure 1A). Additionally, we analyzed the promoter region to identify regulatory cis-elements using PlantCARE1. As shown in Figure 1B and Supplementary Table S2, numerous cis-elements were identified in the TaWRKY2 promoter region. Consistent with the hypothesized function in stress tolerance, one abscisic acid (ABA)-responsive element (ABRE) cis-element involved in ABA response and two MBS cis-elements (V-myb avian myeloblastosis viral oncogene homolog (MYB) binding site) involved in drought response were found in the TaWRKY2 promoter. Unexpectedly, the TaWRKY2 promoter also contained cis-elements involved in plant hormone signaling with functions in plant growth regulation and stress response, including elements associated with gibberellic acid (GA), methyl jasmonate acid (MeJA), and salicylic acid (SA). These findings suggest that TaWRKY2 plays an important role in plant responses to drought and that it may also modulate plant development through hormone regulation under stress conditions. GUS histochemical analysis showed that the activity of the TaWRKY2 promoter was up-regulated by drought, salt, heat, and ABA (Figure 1C).
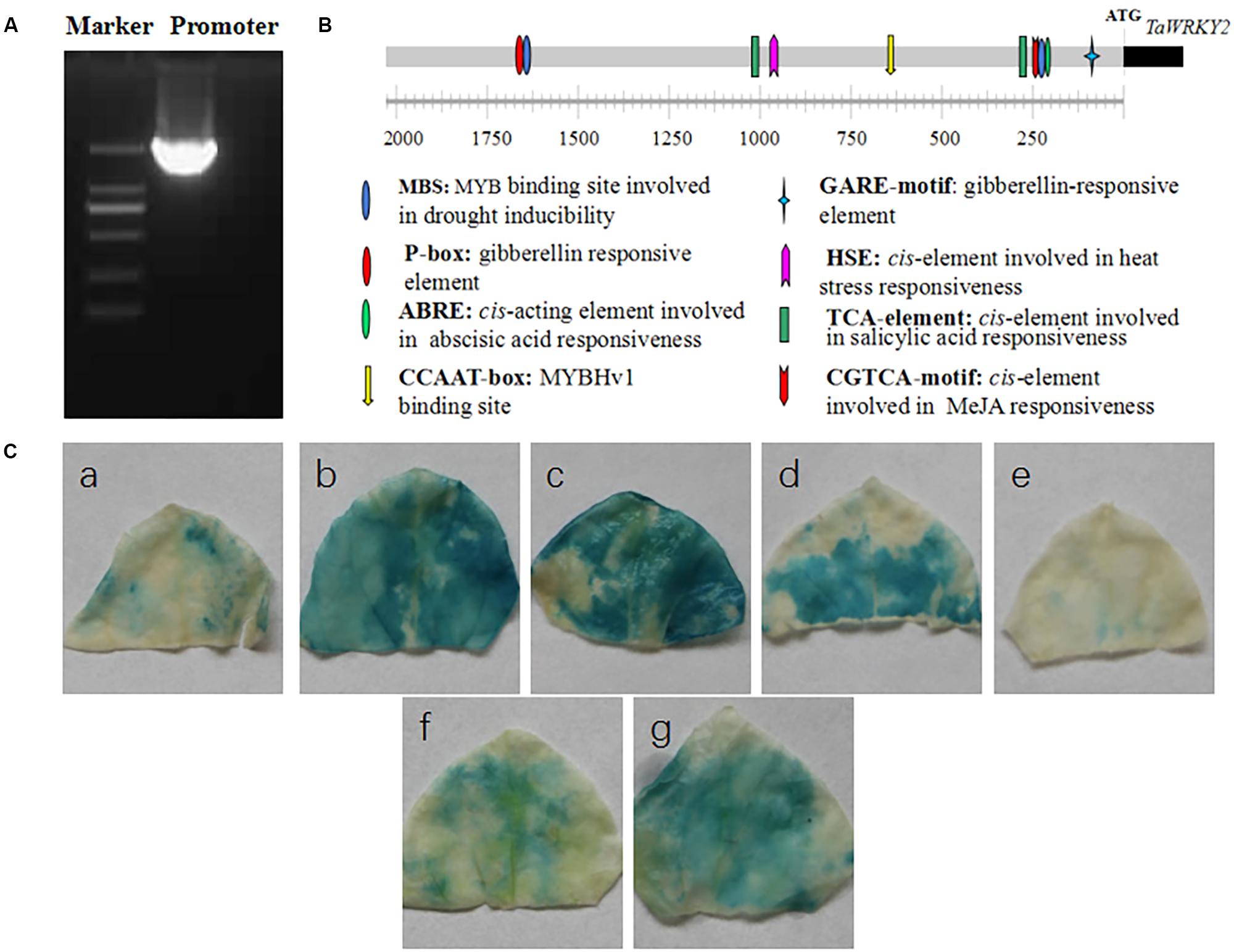
FIGURE 1. Promoter cloning and analysis of TaWRKY2. (A) The 2,166-bp promoter upstream of the ATG start codon of TaWRKY2. (B) Analysis of the cis-regulatory promoter elements. (C) Responsiveness of the promoter to the following abiotic stresses: (a) control, (b) drought (20% polyethylene glycol for 24 h), (c) salt (300 mM NaCl for 24 h), (d) heat (37°C for 12 h), (e) cold (4°C for 12 h), (f) sprayed with H2O for 24 h, and (g) sprayed with ABA (200 mM for 24 h).
Molecular Characterization of Transgenic Wheat Plants
To better understand the function of TaWRKY2 in wheat, we generated transgenic wheat lines expressing TaWRKY2 driven by an Ubi promoter. To identify the positive transgenic wheat lines, exogenous TaWRKY2 was detected in the T1, T2, and T3 generations and confirmed by PCR using specific primers to amplify a 381-bp DNA segment spanning UBI promoter and TaWRKY2 (Figure 2A). We isolated three independent transgenic lines (L2, L13, and L20) in which the exogenous TaWRKY2 was authenticated from the T1 to T3 generations, whereas no amplification was detected in WT plants (Figure 2B). qRT-PCR analysis confirmed that the relative expression levels of TaWRKY2 in the three transgenic lines mentioned above were significantly higher than the levels in WT plants (Figure 2C). As shown in Figure 2D, Southern blot analyses confirmed that the transformed TaWRKY2 gene was indeed integrated into the genomes of lines L2, L13, and L20, with one copy present in each transgenic line (the unclear result for L13 in Figure 2D, supplemented with the blot shown in Supplementary Figure S1). These results confirmed that TaWRKY2 was integrated into the wheat genome and expressed.
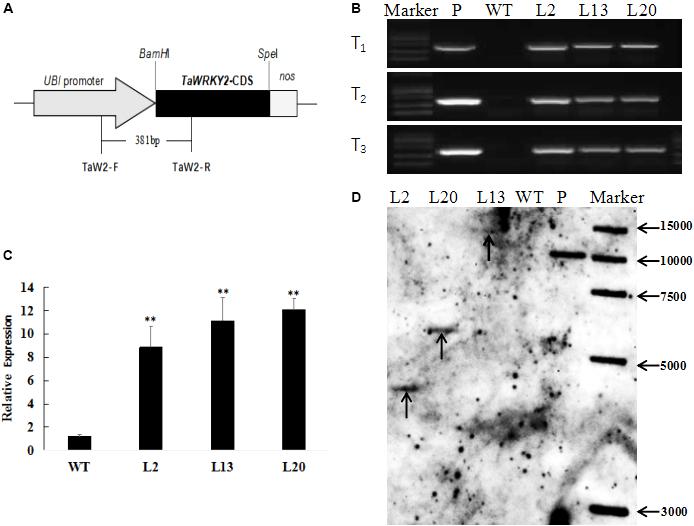
FIGURE 2. Molecular characterization of transgenic wheat. (A) Schematic diagram of the transformation vector pMWB122-TaWRKY2. (B) PCR analysis of the three transgenic lines from T1 to T3. (C) Relative expression of TaWRKY2 in transgenic wheat. (D) Southern blot analysis of HindIII digested genomic DNAs from untransformed and T3 transgenic plants. WT, wild type; P, plasmid. Asterisks represent statistically significant difference compared WT (Student’s t-test, ∗P < 0.05, ∗∗P < 0.01).
TaWRKY2 Enhances the Drought Tolerance of Transgenic Wheat at the Seedling Stage
To investigate the contribution of TaWRKY2 to drought tolerance, 2-week-old seedlings of three independent T3 transgenic wheat lines (L2, L13, and L20) and WT wheat were subjected to water withholding (the LRWCs at each time point are shown in Supplementary Table S3). Before the drought treatment (drought treatment day 0, SRWC: 78.3%), there was no discernible difference between the WT and transgenic lines (Figure 3A). Eight days after drought treatment (SRWC: 31.5%), most leaves of all the transgenic lines remained upright, whereas those in WT plants started wilting (Figure 3A). After 11 days (SRWC: 12.4%), the transgenic wheat showed less wilting compared with WT (Figure 3A). After re-watering for 6 days (SRWC: 62.0%), almost all of the plants from the three transgenic lines recovered, with survival rates of 93–100%. In contrast, the WT wheat had a significantly low survival rate of 70% (Figure 3B). The water loss rate of detached leaves from the three transgenic wheat lines was significantly lower than that of the WT leaves at nine time points over a 12-h time course (Figure 3C). These results show that TaWRKY2 significantly improved drought tolerance in the transgenic wheat seedlings.
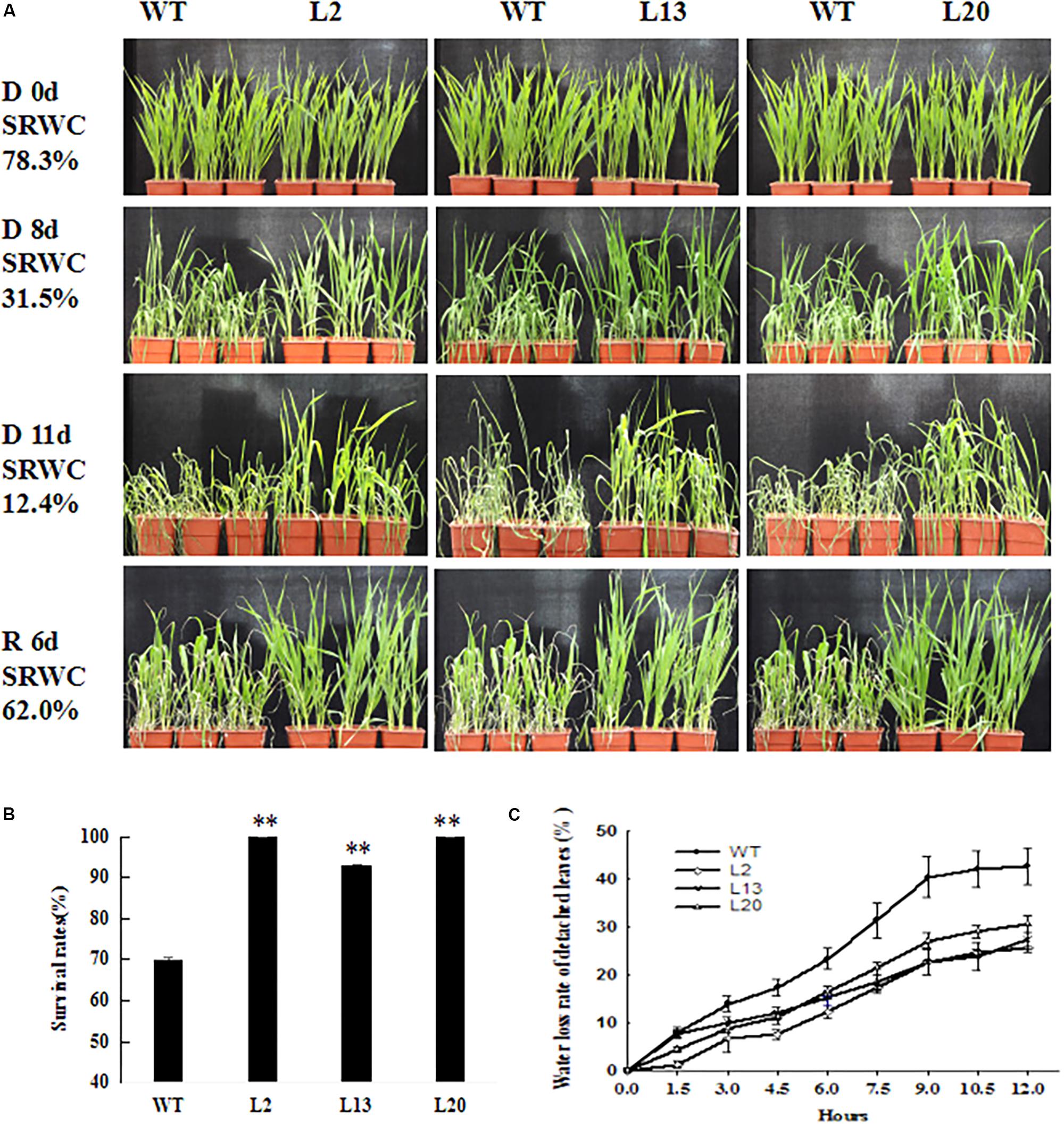
FIGURE 3. (A) Drought-resistant phenotypes. (B) Survival rates of WT and T3 TaWRKY2 overexpressing lines. (C) Water loss rate of detached leaves. D 0d, drought for 0 days; D 8d, drought for 8 days; D 11d, drought for 11 days; R 6d, re-watering for 6 days; WT, wild type. Asterisks represent statistically significant difference compared WT (Student’s t-test, ∗P < 0.05, ∗∗P < 0.01).
Drought Resistance Indexes in the Transgenic Wheat
The levels of osmolytes (free proline and soluble sugar), the accumulation of H2O2, and the chlorophyll content in leaves, have been widely used as important indicators of drought resistance (Szabados and Savoure, 2010). For all four parameters, the transgenic lines did not differ significantly from WT under normal growth condition (Figure 4). However, after drought treatment for 5 days, H2O2 accumulation in the leaves of transgenic plants was lower compared with WT (Figure 4A). The soluble sugar and free proline content were significantly enhanced in the transgenic wheat lines compared with WT, suggesting that TaWRKY2 overexpression can help improve the accumulation of soluble sugar and proline in leaves under drought stress (Figures 4B,C). The chlorophyll content in the transgenic wheat lines was significantly higher than that in WT plants (Figure 4D). Thus, TaWRKY2 overexpression led to physiological changes that may have contributed to the enhanced drought tolerance in the transgenic wheat lines.
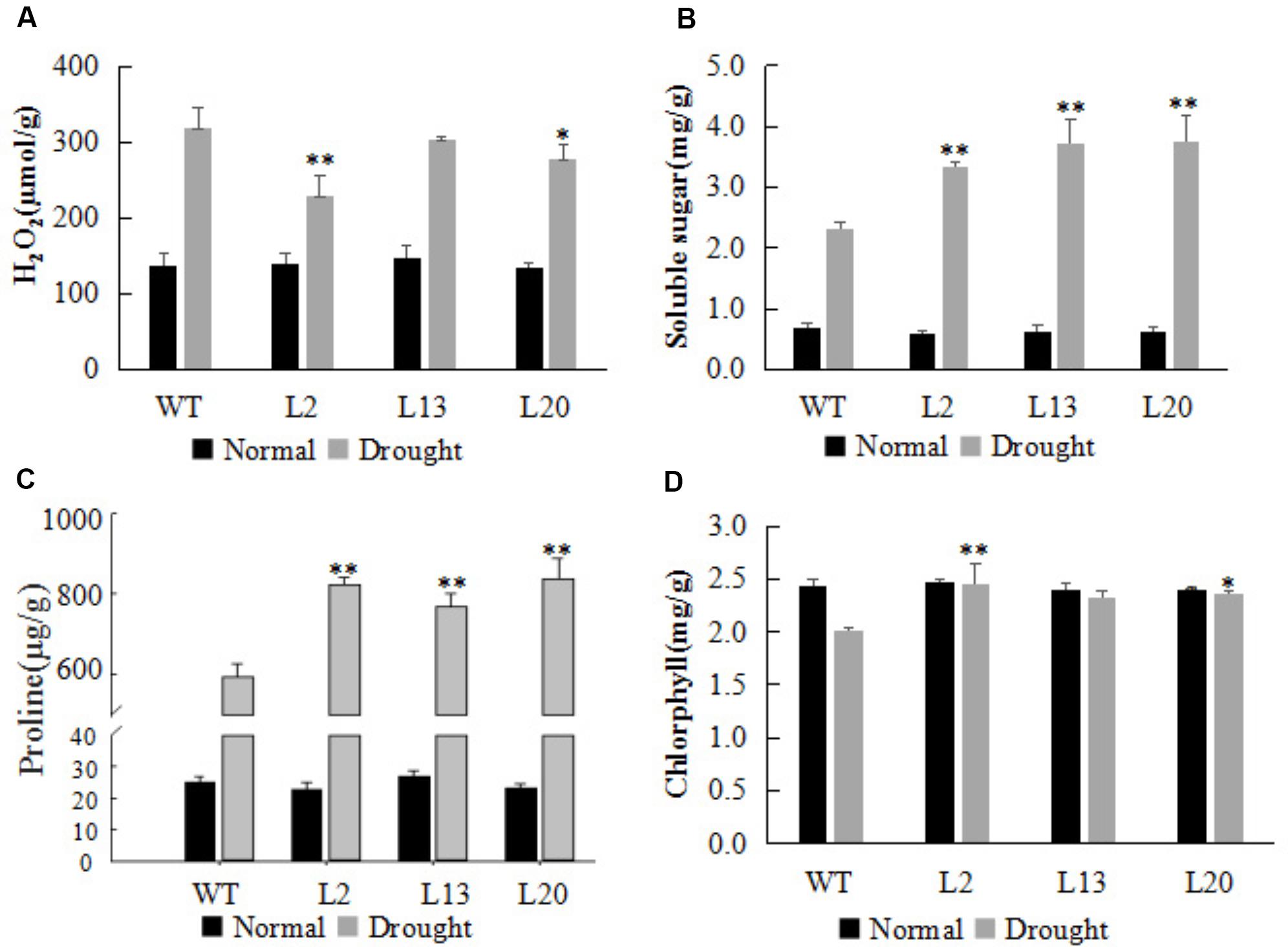
FIGURE 4. Changes in resistance indices in TaWRKY2 overexpressing transgenic wheat (A) H2O2 content. (B) Soluble sugar content. (C) Proline content. (D) Chlorophyll content. Asterisks represent statistically significant difference compared WT (Student’s t-test, ∗P < 0.05, ∗∗P < 0.01).
TaWRKY2 Effects on Wheat Heading Under Drought Stress
To test drought tolerance of transgenic wheat during heading stage, we applied drought treatment approximately 20–25 days prior to the wheat heading stage. The heading date of the transgenic wheat lines (L13 and L20) and WT plants were synchronous, and no significant differences were observed under normal conditions (Figure 5A). Although all of the plants had germinated at the same time, the transgenic plants progressed to the heading stage after drought treatment for 20–25 days, while WT growth was inhibited before heading (Figure 5B). After re-watering, the inhibitory effect of drought stress on the WT plants was removed. These results indicate that TaWRKY2 overexpression could promote wheat heading under drought stress, which is beneficial in terms of life cycle completion under drought stress.
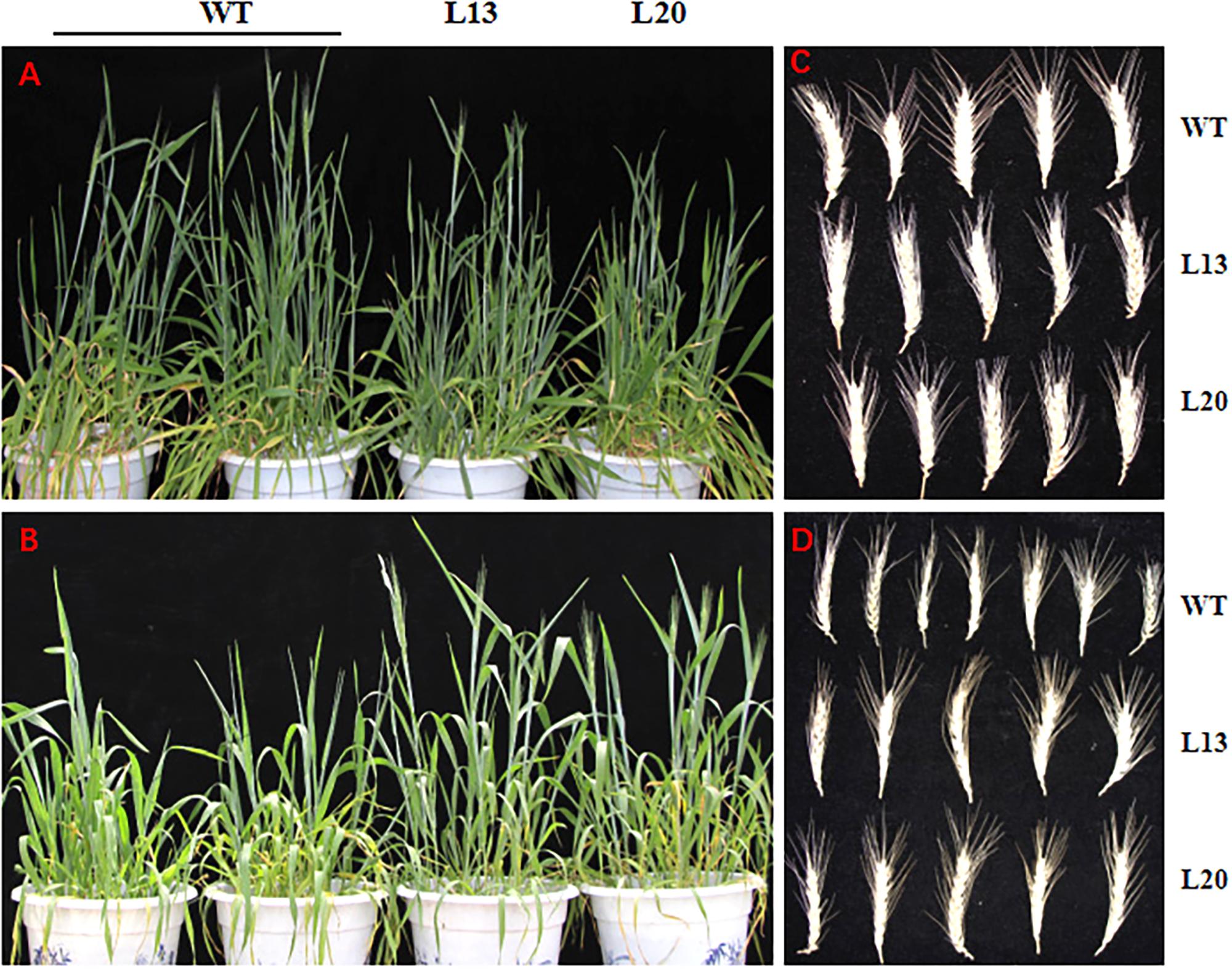
FIGURE 5. (A) Normal growth condition. (B) Drought stress for 20–25 days. (C) Wheat spikes under normal condition. (D) Wheat spikes under drought stress.
TaWRKY2 Transgenic Plants Had Increased Yield Under Drought Stress
Grain yield is an integrated parameter, and yield improvement is the ultimate goal of genetic engineering or conventional crossbreeding effects. After harvesting under both normal and drought conditions, some yield-related agronomic traits of TaWRKY2 transgenic lines (L13 and L20) and WT were quantified. Under normal conditions, no significant differences in agronomic traits were found between the transgenic lines and WT plants, except for aboveground biomass between WT and L20 plants (Table 1 and Figure 5C). Under drought stress, the aboveground biomass of transgenic line L13 increased slightly but not significantly compared with that of WT plants. However, the aboveground biomass of L20 was significantly greater than that of WT. Both transgenic lines had a longer panicle length (Figure 5D), more kernels per spike, and greater yield per plant compared WT. There was no significant difference in plant height between transgenic and WT plants under normal and drought stress conditions, respectively.
TaWRKY2 Activates the Expression of Drought Stress-Responsive Genes
To investigate whether TaWRKY2 overexpression affected the expression of stress-responsive genes, the expression of eight previously reported drought-responsive genes were examined in transgenic plants and WT wheat grown under normal condition using qRT-PCR (Figure 6).
Eight genes investigated encode two DREBs (DREB1 and DREB3), a glutathione S-transferase (GST6), an ERF (ERF5a), a tonoplast intrinsic protein (TIP2), a WRKY (WRKY19), a lipid transfer protein (LTP1), an aquaporin (AQP7). Compared with WT, the DREB1, DREB3, GST6, ERF5a, TaWRKY19, and TIP2 gene expressions were significantly enhanced in TaWRKY2-transgenic plants whereas LTP1 and AQP7 expressions were not significantly changed. These results indicated that TaWRKY2 overexpression regulated the expression of the drought-related genes.
Discussion
Technological advances have raised the possibility of improving drought tolerance in plants, potentially through the manipulation of TFs associated with stress responses. These TFs including ABRE-binding proteins, zinc finger proteins, NAM/ATAF/CUC, (NAC), V-myb avian myeloblastosis viral oncogene homolog (MYB), and APETALA2/ethylene responsive factor (AP2/ERF) can regulate a large number of downstream genes. Notably, a few of these TFs have been analyzed in studies using genetic engineering to improve tolerance to abiotic and biotic stresses (Agarwal et al., 2006). Previous research has firmly established that WRKY TFs play crucial roles in regulating plant abiotic stress responses (?; Jiang Y. et al., 2014; Kayum et al., 2014; Wang et al., 2014; Yan et al., 2014). Understanding the roles and mechanisms of wheat WRKYs in regulating stress responses is vital for understanding plant adaptations to environmental stress. Although a previous study showed that TaWRKY2 expression was induced by multiple abiotic stresses and that TaWRKY2 overexpression conferred tolerance to drought stress in transgenic Arabidopsis (Niu et al., 2012), no evidence was provided for the role of WRKY2 in regulating drought response in wheat.
In the present study, the TaWRKY2 promoter was analyzed for putative plant regulatory cis-elements using the Plant CARE database, and several regulatory cis-elements related to abiotic stress responses were identified. Histochemical GUS analysis demonstrated that the activity of the TaWRKY2 promoter could be up-regulated by drought, salt, heat, and ABA but not cold, which is consistent with the previous finding that TaWRKY2 expression was not induced by cold (Niu et al., 2012).
To explore the role of TaWRKY2 in drought stress adaptations in wheat, we generated TaWRKY2-overexpressing wheat lines via transformation. The results of molecular characterization (PCR, qRT-PCR, and Southern blot) indicated that exogenous TaWRKY2 was successfully transformed into acceptor wheat and that high TaWRKY2 expression levels were stably inherited in the three transgenic lines from the T1 to the T3 generations. The transgenic wheat lines may therefore be used for further functional validating of TaWRKY2 in wheat in future work.
Our drought tolerance assays showed that TaWRKY2 overexpression significantly enhanced drought tolerance at the seedling stage in transgenic wheat, as evidenced by the higher survival rate and lower water loss rate of detached leaves compared with WT plants. Meanwhile, changes in physiological parameters, including H2O2 content, soluble sugars, proline content, and chlorophyll content after stress treatment, were better protected from oxidative and osmotic damages. These results suggest that TaWRKY2 may serve as an important positive regulator for adaptations of wheat to drought stress at the seedling stage. During a prolonged exposure to drought stress before the heading stage, the growth of WT plants was inhibited, whereas the TaWRKY2-overexpressing lines were able to progress to the heading stage. Growth recovery was observed in WT plants only after re-watering. These results show that TaWRKY2 overexpression can promote wheat heading to facilitate life cycle completion under drought stress.
Crop production is greatly impacted by complex environmental factors. Some studies have shown that overexpression of stress-related TFs may increase crop yield (Oh et al., 2009; Yu et al., 2013; Zhao et al., 2014; Shavrukov et al., 2016). However, little is presently known about the effects of WRKY TFs on wheat yield. Notably, the present findings demonstrate that TaWRKY2 overexpression in wheat increased aboveground biomass and grain yield under drought stress. The transgenic wheat also had longer panicle length and more kernels per spike than WT plants under drought stress, indicating that these agronomic traits contributed to the increased yield.
The expression levels of several previously reported drought-responsive genes such as DREBs, GST6, ERF5a, TIP2, and WRKY19 were up-regulated in the TaWRKY2-transgenic plants. The DREB3 overexpression in transgenic wheat has been found to increase drought stress tolerance (Shavrukov et al., 2016). GST is ROS-Scavenging enzyme and is known to be involved in redox homeostasis of cells under various stress conditions (Huang et al., 2013). ERF5a and TIP2 are involved in plant response to drought and salt stress (Wang et al., 2011; Eini et al., 2013). WRKY19 positively contributes to plant tolerance to drought, salt, and cold stresses in transgenic Arabidopsis (Niu et al., 2012). These findings indicated that TaWRKY2 contributes to drought stress tolerance in wheat possibly through the activation of a range of drought-related genes.
Overall, TaWRKY2 promoted drought stress tolerance and increased the yield of wheat. This study provides a foundation for investigating wheat responses to drought stress and identifies a candidate target gene, TaWRKY2, for improving drought stress tolerance in wheat and other cereals.
Author Contributions
HG contributed to experimental implementation, data analysis, and manuscript preparation. YW and PX contributed to experimental implementation. ZZ conceived and designed the study.
Funding
This work was supported by The National Key Research and Development Program of China (2016YFD0100605 and 2016YFD0100102-11).
Conflict of Interest Statement
The authors declare that the research was conducted in the absence of any commercial or financial relationships that could be construed as a potential conflict of interest.
Acknowledgments
We thank the Group of Xingguo Ye (Institute of Crop Sciences, Chinese Academy of Agricultural Sciences) for performing the transgenic wheat transformation and instructing the Southern blot experiment, and Professors Xigang Liu and Dongping Lu for reviewing this manuscript.
Supplementary Material
The Supplementary Material for this article can be found online at: https://www.frontiersin.org/articles/10.3389/fpls.2018.00997/full#supplementary-material
FIGURE S1 | Southern blot analysis of HindIII digested genomic DNAs from WT and T3 transgenic plants.
TABLE S1 | Primer sequences used for PCR, qRT-PCR, and probes in this study.
TABLE S2 | Distribution and function of cis-acting regulatory elements in TaWRKY2 promoter.
TABLE S3 | Leaf relative water content in WT and transgenic lines.
Footnotes
References
Agarwal, P. K., Agarwal, P., Reddy, M. K., and Sopory, S. K. (2006). Role of DREB transcripcion factors in abiotic and biotic stress tolerance in plants. Plant Cell Rep. 25, 1263–1274. doi: 10.1007/s00299-006-0204-8
Eini, O., Yang, N., Pyvovarenko, T., Pillman, K., Bazanova, N., Tikhomirov, N., et al. (2013). Complex regulation by apetala2 domain-containing transcription factors revealed through analysis of the stress-responsive TdCor410b promoter from durum wheat. PLoS One 8:e58713. doi: 10.1371/journal.pone.0058713
Fan, X. Q., Guo, Q., Xu, P., Gong, Y. Y., Shu, H. M., Yang, Y., et al. (2015). Transcriptome wide identification of salt-responsive members of the WRKY gene family in Gossypium aridum. PLoS One 10:e126148. doi: 10.1371/journal.pone.0126148
Gahlaut, V., Jaiswal, V., Kumar, A., and Gupta, P. K. (2016). Transcription factors involved in drought tolerance and their possible role in developing drought tolerant cultivars with emphasis on wheat (Triticum aestivum L.). Theor. Appl. Genet. 129, 2019–2042. doi: 10.1007/s00122-016-2794-z
He, G. H., Xu, J. Y., Wang, Y. X., Liu, J. M., Li, P. S., Chen, M., et al. (2016). Drought-responsive WRKY transcription factor genes TaWRKY1 and TaWRKY33 from wheat confer drought and/or heat resistance in Arabidopsis. BMC Plant Biol. 16:116. doi: 10.1186/s12870-016-0806-4
Huang, X. S., Wang, W., Zhang, Q., and Liu, J. H. (2013). A basic helix-loop-helix transcription factor, PtrbHLH, of Poncirus trifoliata confers cold tolerance and modulates peroxidase-mediated scavenging of hydrogen peroxide. Plant Physiol. 162, 1178–1194. doi: 10.1104/pp.112.210740
Hu, X., Chen, D., Lynne Mclntyre, C., Fernanda Dreccer, M., Zhang, Z. B., Drenth, J. et al. (2017). Heat shock factor C2a serves as a proactive mechanism for heat protection in developing grains in wheat via an ABA-mediated regulatory pathway. Plant Cell Environ. 41, 79–98. doi: 10.1111/pce.12957
Jiang, Q., Zheng, H., Zhang, H., and Ma, Y. (2014). Overexpression of GmDREB1 improves salt tolerance in transgenic wheat and leaf protein response to high salinity. Crop J. 2, 120–131. doi: 10.1016/j.cj.2014.02.003
Jiang, Y., Zeng, B., Zhao, H. N., Zhang, M., Xie, S. J., and Lai, J. S. (2012). Genome-wide transcription factor gene prediction and their expressional tissue-specificities in maize. J. Integr. Plant Biol. 54, 616–630. doi: 10.1111/j.1744-7909.2012.01149.x
Jiang, Y., Duan, Y., Yin, J., Ye, S., Zhu, J., Zhang, F., et al. (2014). Genome-wide identification and characterization of the Populus WRKY transcription factor family and analysis of their expression in response to biotic and abiotic stresses. J. Exp. Bot. 65, 6629–6644. doi: 10.1093/jxb/eru381
Kayum, M. A., Jung, H. J., Park, J. I., Ahmed, N. U., Saha, G., and Yang, T. J. (2014). Identification and expression analysis of WRKY family genes under biotic and abiotic stresses in Brassica rapa. Mol. Genet. Genomics 290, 79–95. doi: 10.1007/s00438-014-0898-1
Lata, C., Muthamilarasan, M., and Prasad, M. (2015). Drought Stress Responses and Signal Transduction in Plants[M]//Elucidation of Abiotic Stress Signaling in Plants. New York, NY: Springer, 195–225.
Niu, C. F., Wei, W., Zhou, Q. Y., Tian, A. G., Hao, Y. J., Zhang, W. K., et al. (2012). Wheat WRKY genes TaWRKY2 and TaWRKY19 regulate abiotic stress tolerance in transgenic Arabidopsis plants. Plant Cell Environ. 35, 1156–1170. doi: 10.1111/j.1365-3040.2012.02480.x
Oh, S. J., Kim, Y. S., Kwon, C. W.,Park, H. K., Jeong, J. S., and Kim, J. K. (2009). Overexpression of the transcription factor AP37 in rice improves grain yield under drought conditions. Plant Physiol. 150, 1368–1379. doi: 10.1104/pp.109.137554
Qin, Y. X., Tian, Y. C., Han, L., and Yang, X. C. (2013). Constitutive expression of a salinity-induced wheat WRKY transcription factor enhances salinity and ionic stress tolerance in transgenic Arabidopsis thaliana. Biochem. Biophys. Res. Commun. 441, 476–481. doi: 10.1016/j.bbrc.2013.10.088
Qin, Y. X., Tian, Y. C., and Liu, X. Z. (2015). A wheat salinity-induced WRKY transcription factor TaWRKY93 confers multiple abiotic stress tolerance in Arabidopsis thaliana. Biochem. Biophys. Res. Commun. 464, 428–433. doi: 10.1016/j.bbrc.2015.06.128
Rong, W., Qi, L., Wang, A. Y., Ye, X. G., Du, L. P., Liang, H. X., et al. (2014). The ERF transcription factor TaERF3 promotes tolerance to salt and drought stresses in wheat. Plant Biotechnol. J. 12, 468–479. doi: 10.1111/pbi.12153
Saad, A. S., Li, X., Li, H. P., Huang, T., Gao, C. S., Guo, M. W., et al. (2013). A rice stress-responsive NAC gene enhances tolerance of transgenic wheat to drought and salt stresses. Plant Sci. 203–204, 33–40. doi: 10.1016/j.plantsci.2012.12.016
Sambrook, J., Fritsch, E. F., and Maniatis, T. (1989). Molecular Cloning: A Laboratory Manual, 2nd Edn. New York, NY: Cold Spring Harbor Laboratory.
Shavrukov, Y., Baho, M., Lopato, S., and Langridge, P. (2016). The TaDREB3 transgene transferred by conventional crossings to different genetic backgrounds of bread wheat improves drought tolerance. Plant Biotechnol. J. 14, 313–322. doi: 10.1111/pbi.12385
Singh, D., and Laxmi, A. (2015). Transcriptional regulation of drought response: a tortuous network of transcriptional factors. Front. Plant Sci. 6:895. doi: 10.3389/fpls.2015.00895
Szabados, L., and Savoure, A. (2010). Proline: a multifunctional amino acid. Trends Plant Sci. 15, 89–97. doi: 10.1016/j.tplants.2009.11.009
Tiwari, S., Lata, C., Chauhan, P. S., Prasad, V., and Prasad, M. (2017). A functional genomic perspective on drought signalling and its crosstalk with phytohormone-mediated signalling pathways in plants. Curr. Genomics 18, 469–482. doi: 10.2174/1389202918666170605083319
Todaka, D., Nakashima, K., Shinozaki, K., and Yamaguchi-Shinozaki, K. (2012). Toward understanding transcriptional regulatory networks in abiotic stress responses and tolerance in rice. Rice 5:6. doi: 10.1186/1939-8433-5-6
Tripathi, P., Rabara, R. C., and Rushton, P. J. (2014). A systems biology perspective on the role of WRKY transcription factors in drought responses in plants. Planta 239, 255–266. doi: 10.1007/s00425-013-1985-y
Wang, C., Deng, P., Chen, L., Wang, X., Ma, H., Hu, W., et al. (2013). A wheat WRKY transcription factor TaWRKY10 confers tolerance to multiple abiotic stresses in transgenic tobacco. PLoS One 8:e65120. doi: 10.1371/journal.pone.0065120
Wang, S. J., Wang, J. Y., Yao, W. J., Zhou, B., Li, R., and Jiang, T. (2014). Expression patterns of WRKYgenes in di-haploid Populus simonii × P. nigra in response to salinity stress revealed by quantitative real-time PCR and RNA sequencing. Plant Cell Rep. 33, 1687–1696. doi: 10.1007/s00299-014-1647-y
Wang, X., Li, Y., Ji, W., Bai, X., Cai, H., Zhu, D., et al. (2011). A novel Glycine soja tonoplast intrinsic protein gene respond to abiotic stress and depressed salt and dehydration tolerance in transgenic Arabidopsis thaliana. J. Plant Physiol. 168, 1241–1248. doi: 10.1016/j.jplph.2011.01.016
Wei, S. G., Ma, X. J., Pan, L. M., Miao, J. H., Fu, J., Bai, L. H., et al. (2017). Transcriptome analysis of Taxillusi chinensis (DC.) danser seeds in response to water loss. PLoS One 12:e0169177. doi: 10.1371/journal.pone.0169177
Xu, H., Watanabe, K. A., Zhang, L., and Shen, Q. J. (2016). WRKY transcription factor genes in wild rice Oryza nivara. DNA Res. 23, 311–323. doi: 10.1093/dnares/dsw025
Xu, Q., Feng, W. J., Peng, H. R., Ni, Z. F., and Sun, Q. X. (2014). TaWRKY71, a WRKY transcription factor from wheat, enhances tolerance to abiotic stress in transgenic Arabidopsis thaliana. Cereal Res. Commun. 42, 47–57. doi: 10.1556/CRC.2013.0051
Yang, A., Dai, X., and Zhang, W. H. (2012). A R2R3-type MYB gene, OsMYB2, is involved in salt, cold, and dehydration tolerance in rice. J. Exp. Bot. 63, 2541–2556. doi: 10.1093/jxb/err431
Yan, H., Jia, H., Chen, X., Hao, L., An, H., and Guo, X. (2014). The cotton WRKY transcription factor GhWRKY17 functions in drought and salt stress in transgenic Nicotiana benthamiana through ABA signalling and the modulation of reactive oxygen species production. Plant Cell Physiol. 55, 2060–2076. doi: 10.1093/pcp/pcu133
Yu, L. H., Chen, X., Wang, Z., Wang, S. M., Wang, Y. P., Zhu, Q. S., et al. (2013). Arabidopsis enhanced drought tolerance1/HOMEODOMAINGLABROUS11 confers drought tolerance in transgenic rice without yield penalty. Plant Physiol. 162, 1378–1391. doi: 10.1104/pp.113.217596
Zhao, D., Derkx, A. P., Liu, D. C., Buchner, P., and Hawkesford, M. J. (2014). Overexpression of a NAC transcription factor delays leaf senescence and increases grain nitrogen concentration in wheat. Plant Biol. 17, 904–913. doi: 10.1111/plb.12296
Zhao, Y., Wang, T., Zhang, W., and Li, X. (2011). SOS3 mediates lateral root development under low salt stress through regulation of auxin redistribution and maxima in Arabidopsis. New Phytol. 189, 1122–1134. doi: 10.1111/j.1469-8137.2010.03545.x
Zhu, X., Liu, S., Meng, C., Qin, L., Kong, L., and Xia, G. (2013). WRKY transcription factors in wheat and their induction by biotic and abiotic stress. Plant Mol. Biol. Rep. 31, 1053–1067. doi: 10.1007/s11105-013-0565-4
Keywords: TaWRKY2, transgenic wheat, transcription factors, yield, drought
Citation: Gao H, Wang Y, Xu P and Zhang Z (2018) Overexpression of a WRKY Transcription Factor TaWRKY2 Enhances Drought Stress Tolerance in Transgenic Wheat. Front. Plant Sci. 9:997. doi: 10.3389/fpls.2018.00997
Received: 28 November 2017; Accepted: 19 June 2018;
Published: 07 August 2018.
Edited by:
Viswanathan Chinnusamy, Indian Agricultural Research Institute (ICAR), IndiaReviewed by:
Charu Lata, National Botanical Research Institute (CSIR), IndiaLingaraj Sahoo, Indian Institute of Technology Guwahati, India
Copyright © 2018 Gao, Wang, Xu and Zhang. This is an open-access article distributed under the terms of the Creative Commons Attribution License (CC BY). The use, distribution or reproduction in other forums is permitted, provided the original author(s) and the copyright owner(s) are credited and that the original publication in this journal is cited, in accordance with accepted academic practice. No use, distribution or reproduction is permitted which does not comply with these terms.
*Correspondence: Zhengbin Zhang, enpiQHNqemlhbS5hYy5jbg==