- 1Key Laboratory of Adaptation and Evolution of Plateau Biota, Northwest Institute of Plateau Biology, Chinese Academy of Sciences, Xining, China
- 2University of Chinese Academy of Sciences, Beijing, China
- 3School of Ecology and Environmental Resources, Qinghai University for Nationalities, Xining, China
- 4School of Life Sciences, Southwest University, Chongqing, China
In vascular plants, R2R3-MYB transcription factors are important regulators of secondary cell wall formation. Although 192 annotated R2R3 MYB genes were identified in the poplar genome, only a few members were characterized to participate in the regulation of the secondary cell wall biosynthesis. In this paper, we identify an R2R3-MYB transcription factor, PtoMYB74, which is predicted to be an ortholog of Arabidopsis AtMYB61, a transcription activator that regulates the secondary cell wall formation, lignin biosynthesis, stomatal aperture, and the mucilage of seed coat. PtoMYB74 is mainly expressed in the stems, especially in the xylem tissues and organs. PtoMYB74 as a transcriptional activator is localized to the nucleus. The overexpression of PtoMYB74 increased the secondary cell wall thickness of vessels in transgenic poplar and changed the secondary cell wall compositions. The expression levels of lignin and cellulose biosynthetic genes were elevated in the transgenic poplar overexpressing PtoMYB74 compared to the wild type, while there was no change in the xylan biosynthetic genes. Transcriptional activation assays demonstrated that PtoMYB74 could activate the promoters of structural genes in the lignin and cellulose biosynthetic pathways. Taken together, our data show that PtoMYB74 positively regulates the secondary cell wall biosynthesis in poplar.
Introduction
Wood, which is mainly composed of a secondary cell wall, is utilized for a wide range of applications, such as wood pulping, paper making, house construction, biofuels, and bio-functional coating (Pilate et al., 2004). The secondary cell wall, which develops from beneath the primary cell wall, is mainly made of xylan, lignin, and cellulose (Douglas, 1996; Boudet, 2000). Owing to the massive economic values of wood, understanding the molecular mechanism of the secondary wall formation will be helpful for the improvement of wood quality through genetic engineering (Kubo et al., 2005).
In Arabidopsis thaliana, genetic and molecular analyses have identified a number of NAC and MYB transcription factors involved in the secondary wall formation, and recently an NAC-MYB-mediated transcriptional network regulating the secondary cell wall formation has been constructed (Zhong and Ye, 2014). In this complicated network, a couple of secondary-wall-related NAC transcription factors, including SND1, NST1/2/3, and VND1/2/3/4/5/6/7 have been characterized as master switches that control many secondary wall-associated genes (Zhong et al., 2006; Mitsuda et al., 2007; Ohashi-Ito et al., 2010; Yamaguchi et al., 2011; Zhong and Ye, 2015). These NAC genes can directly or indirectly promote the expression of a great number of MYB genes (Zhong and Ye, 2015). Among these downstream target genes, AtMYB58/63/85 have been demonstrated as lignin-specific transcription factors (Zhou et al., 2009; Nakano et al., 2010), while AtMYB26/32/41/44/46/61/83/103 are shown to participate in the biosynthesis of secondary cell wall (Jin et al., 2000; Preston et al., 2004; Yang et al., 2007; Ko et al., 2009; McCarthy et al., 2009; Romano et al., 2012; Öhman et al., 2013; Kosma et al., 2014). In addition, Arabidopsis MYB26 is also identified to up-regulate the expression of NST1 and NST2 genes (Yang et al., 2007; Ko et al., 2014).
In the past decade, it has been well-demonstrated that the regulation of the secondary wall biosynthesis by the NAC master switches is a conserved mechanism in vascular plants, and similar transcriptional regulatory networks have been established in woody plants, including Eucalyptus gunnii, spruce (Picea glauca), and poplar (Ohtani et al., 2011; Li et al., 2012). In poplar, six pairs of SND, VND, and NST homologs (PtrWNDs/PtrVNSs), such as PtrWND2B/6B and PtrVNS12/16, were identified to activate the expression of the secondary wall biosynthetic genes and induce ectopic deposition of lignin, xylan, and cellulose (Zhong et al., 2010, 2011; Ohtani et al., 2011; Li et al., 2012). These NAC proteins directly control the expression of several downstream genes, such as PtrMYB2/3/20/21, which are considered as the second-level master switches (Bomal et al., 2008). Dominant repressed expression of the MYB master switch genes led to a decrease in the secondary wall thickness in the woody tissues of transgenic plants (Patzlaff et al., 2003; Goicoechea et al., 2005; Bomal et al., 2008; McCarthy et al., 2010; Zhong et al., 2010, 2011), indicating their regulatory roles in secondary wall formation. In addition to the MYB genes mentioned above, a number of other MYB transcription factors have been shown to promote the up-regulation of the expression of some biosynthetic genes for xylan, lignin, and cellulose, thereby suggesting the complexity of the transcriptional regulatory network of wood formation (Zhong et al., 2011; Zhong and Ye, 2015). For example, the ortholog of AtMYB58/63, PtrMYB28, could activate the promoter activities of the lignin biosynthesis genes (Zhong and Ye, 2009). Overexpression of PtrMYB152, an ortholog of AtMYB43, improved lignin biosynthesis in transgenic Arabidopsis (Li et al., 2014). In our previous studies, PtoMYB92 has been demonstrated to be a transcriptional activator of secondary wall biosynthetic pathway in Populus tomentosa (Li et al., 2015). The ectopic expression of PtoMYB216 resulted in an increase of lignin content and changes in the xylan and cellulose components (Tian et al., 2013).
AtMYB61, an R2R3-MYB gene, is identified as a regulatory factor of plant growth and development (Romano et al., 2012). For example, AtMYB61 controlled the stomatal aperture in response to light signals and diurnal cycle (Liang et al., 2005), and positively regulated the mucilage in its seed coat (Penfield et al., 2001). Recently, other studies also revealed that AtMYB61 is involved in the regulation of xylem formation and lateral root development (Newman et al., 2004). Previous studies have shown that PtMYB8, the Pinus taeda ortholog of Arabidopsis AtMYB61, participates in the secondary wall formation as a positive regulator (Bomal et al., 2008). In this paper, we have characterized the function of PtoMYB74, an ortholog of AtMYB61, in the secondary cell wall biosynthesis in poplar. PtoMYB74 is preferentially expressed in the stems, especially the xylem tissues. Overexpression of PtoMYB74 in poplar and Arabidopsis resulted in a change of secondary cell wall thicknesses in vessels, and significantly increased the contents of lignin and cellulose. Furthermore, we demonstrated that PtoMYB74 could activate the expression of some poplar key enzyme genes (C3H3, C4H2, CCR2, CCOAOMT1, F5H2, and CesA2B). These results suggest that PtoMYB74 should play an important role in the positive regulation of secondary wall biosynthesis in poplar.
Materials and Methods
Plant Materials and Growth Conditions
The seeds of Arabidopsis thaliana (ecotype Columbia) were germinated and cultured on glass culture dishes containing MS medium (containing 30 g/L sucrose and 8.5 g/L Agar) for 2 weeks (Murashige and Skoog, 1962). The seedlings were transferred into nutrient soil for further growth in an illumination incubator with a culture condition of 20–23°C, 16/8 h light/dark cycle, 555 μmol m-2 s-1 supplemental light, and 80% humidity. The nutrient soil is a mixture of peat (Klasmann-Deilmann, Germany), vermiculite (Sanbao, China), and perlite (Longshan, China) in the proportion 6:3:1.
Poplar plants (Populus tomentosa Carr. clone 741) were transplanted from the culture dishes to customized pots and grown in the greenhouse. The conditions for seedlings culture in the greenhouse were 23–25°C, 16/8 h light/dark cycle, 278 μmol m-2 s-1 supplemental light, and 60% humidity (Li et al., 2015).
Cloning of Full-Length PtoMYB74 cDNA From P. tomentosa
Total RNA was extracted from the poplar leaves and cDNA was synthesized by reverse transcript polymerase chain reaction (RT-PCR). According to the sequence of PtrMYB74 (XM_002321591.2/Potri.015G082700.1), the cDNA fragment (1116 bp) of PtoMYB74 was amplified with gene-specific primers (Supplementary Table S1) and inserted into the cloning vector pMD19-T (TaKaRa) with the DNA Ligation Kit Solution I (TaKaRa). After sequencing, the fragment was subcloned into the plant binary vector pCXSN (Chen et al., 2009; Supplementary Figure S1). The resulting construct, pCXSN-PtoMYB74, was transferred into Agrobacterium tumefaciens stain EHA105 via the freeze-thaw method.
Phylogenetic Analysis of PtoMYB74
For phylogenetic analysis, the amino acid sequences of R2R3 MYB genes, known to participate in the secondary wall formation, were acquired by the basic local alignment search tool (BLAST1). The multiple sequence alignment was then carried out with the DNAMAN8 (Altschul et al., 1990) software. The phylogenetic tree was constructed with the MEGA 6.06 software using the neighbor-joining (NJ) method with 2500 bootstrap replications under the p-distance model.
RNA Extraction and RT-qPCR Analysis
Total RNA (TRIzol-extracted) was separately extracted from the Arabidopsis stems and various poplar tissues (including the young leaf, old leaf, root, stem, phloem, xylem, and petiole), and then genomic DNA was removed from the extracted RNA by DNAse treatment. The bark was separated from the poplar stem after girdling, and the phloem was obtained after scraping off all the epidermis and periderm. Meanwhile, the xylem was obtained after cutting away the pith from the rest of the stem. The first strand of cDNA was then synthesized by RT-PCR according to the manufacturer’s instructions (PrimeScriptTM RT Master Mix, TaKaRa). The RT-qPCR assay was performed as described previously (Nolan et al., 2006), and the 2-ΔΔCt method was used to analyze the relative changes in gene expression (Livak and Schmittgen, 2001). RT-qPCR was performed using the Novostar-SYBR Supermix (Novoprotein, Shanghai, China) in a 96-well plate with the Thermal Cycler Dice Real Time System TP800 (TaKaRa, Japan) machine, and the Thermal Cycler Dice Real Time System Software Ver.5.00 was used for data collection and analysis. In each reaction (25 μl PCR reaction system), 12.5 μl Novostar-SYBR Supermix, 0.5 μM per primer pair, 15 ng of cDNA, and some amount of ultra-pure water were used. The initial denaturing conditions was 95°C for 30 s, followed by 40 cycles at 95°C for 5 s, and 60°C for 30 s. A melting curve was run after the PCR cycles, and the time was 95°C for 15 s, 60°C for 30 s, and 95°C for 15 s. The poplar 18S rRNA was used as a control to calculate the relative expression level in WT and transgenic poplar (Figures 2A, 5A, 7 and Supplementary Figure S4), while the Arabidopsis UBC gene was used as a reference gene in WT and transgenic Arabidopsis (Figure 4B and Supplementary Figure S3). The expression level of Pto18S/AtUBC was determined and set to 1.0. Three biological replicates were used for each sample and each RT-PCR reaction had three technical replicates. The tool Primer-BLAST2 was used to test the specificity of each primer pair, and the melting curve of each primer pair showed one single peak and a specific single product. The standard curve was used to test the amplification efficiency of each primer pair, and each primer pair had an appropriate PCR amplification efficiency (90.7–97.3%). The description of the RT-qPCR data followed all the MIQE-guidelines (Bustin et al., 2009).
Semi-Quantitative RT-PCR Assays
The reverse transcribed cDNA samples (from Trizol-extracted Arabidopsis RNA) were used for semi-quantitative RT-PCR, and the AtUBC gene was used as an internal control. The conditions for semi-quantitative RT-PCR included an initial denaturation step at 94°C for 5 min, 28 cycles of 94°C for 45 s, 56°C for 45 s, and 72°C for 90 s, and an extension step at 72°C for 10 min. The amplification products were resolved by 1% (w/v) agarose gel electrophoresis and visualized with EB (ethidium bromide) under UV light.
Subcellular Localization of PtoMYB74
The coding sequence (CDS) of PtoMYB74 was amplified with gene-specific primers (Supplementary Table S1) from the full-length cDNA, and ligated to the binary vector pCX-DG (Chen et al., 2009) which contains a GFP reporter gene. The resulting construct containing the GFP and the full-length PtoMYB74 CDS under the control of CaMV 35S promoter was infiltrated into the epidermal cells of tobacco leaves as described previously (Sparkes et al., 2006) and cultured in dark for 24 h. After 3 days, the GFP signal in the infected tobacco leaves was detected using an Olympus FV2000 confocal microscope at 488 nm laser.
Yeast Activation Assay
To examine the PtoMYB74 transcription activity, the PtoMYB74 CDS was cloned into the vector pGBKT7. The resulting construct pGBKT7-PtoMYB74 was transformed into the yeast strain Saccharomyces cerevisiae Gold2 through the PEG-LiAc method (Zaragoza et al., 2004), while the pGBKT7 empty vector was used as a negative control. The transformants were grown on a synthetic dropout (SD) medium lacking tryptophan (Trp). The positive clones were then transferred into the SD medium lacking Trp, histidine (His), and adenine (Ade) for transcriptional activation activity analysis by staining with X-α-gal.
Overexpression of PtoMYB74 in Arabidopsis and P. tomentosa
The pCXSN-PtoMYB74 construct was transformed into 30-day-old Arabidopsis plants by the floral dip method (Zhang et al., 2006). Transgenic plants were selected on ½ MS medium containing 50 mg/L hygromycin (Hyg) and 400 mg/L cefotaxime (Cef).
For poplar transformation, the pCXSN-PtoMYB74 vector was transformed into P. tomentosa by the leaf disk method, as described previously (Jia et al., 2010). The transgenic plants were cultured on the woody plant medium (WPM; McCown and Lloyd, 1981) with 9 mg/L Hyg, 400 mg/L Cef, 30 g/L sucrose, and 8.5 g/L Agar. The survived seedlings were characterized by PCR and RT-qPCR. The positive transgenic plants were transferred into the soil and grown in the greenhouse.
Isolation and Analysis of PtoMYB74 Promoter
According to the genomic sequence of PtrMYB74 (Potri. 015G082700.13), the upstream flanking sequence (1500 bp) was used to design the gene-specific primers for PtoMYB74 Promoter. The promoter fragment (1562 bp) of PtoMYB74 was isolated from the P. tomentosa genomic DNA with the gene-specific primers listed in Supplementary Table S1. The first single-base of 5′UTR sequence (307 bp) was set as the transcriptional start site. The fragment was ligated to the binary vector pCX-GUS-P (Chen et al., 2009) containing a GUS reporter gene. The resulting vector was transformed into A. tumefaciens EHA105 strain for poplar transformation. The transgenic seedlings were used for GUS staining performed as instructed by Jefferson (1987).
Transcriptional Activation Assay
The pCXSN-PtoMYB74 vector was used as an effector construct. The promoter fragments of the CCOAOMT1, CCR2, C3H3, C4H2, F5H2, GT8D, GT43B, and CesA2B genes were amplified by the gene-specific primers listed in Supplementary Table S1, respectively, and then inserted into the reporter vectors to drive the GUS reporter gene. The co-transfection of reporter and effector constructs was performed in the leaves of Nicotiana benthamiana for transient expression by the agroinfiltration method (Sparkes et al., 2006). The GUS activity was measured using the 4-MUG (4-methylumbelliferyl-β-D-glucuronide) assay (Chen et al., 2005). After 3 days of infiltration, the tobacco leaves (100 mg each sample) were dealt with the freeze grinding technology and extracted with 1 ml GUS extraction buffer (50 mM sodium phosphate buffer, pH 7.0, 10 mM EDTA, 0.1% SDS, 0.1% β-mercaptoethanol, 0.1% triton-X 100) and then centrifuged (12000 rpm, 5 min, 4°C). After that, 100 μl of the supernatant was mixed with 900 μl MUG assay buffer (GUS extraction buffer containing 10 mM MUG) and incubated at 37°C for 60 min. The reaction was terminated by mixing 200 μl of aliquots with 800 μl 200 mM Na2CO3. The fluorescence intensity was measured and recorded in a Hitachi F-7000 fluorospectrophotometer with excitation at 365 nm and emission at 455 nm. The GUS activity was calculated as p mol MU mg min-1 protein-1.
Scanning Electron Microscopy (SEM)
Transverse stem sections were hand-cut from different internodes of poplar plants with a razor blade. A Phenom Pure FEI SEM was used to examine the hand-cut sections, and the Revolution 1.6.1 software was employed to measure the radial width of xylem (μm) and the vessel cell wall thickness (μm).
Histochemical Staining
Histochemical staining and observation of the stem cross-sections of Arabidopsis and poplar plants for lignin detection was performed as previously described (Chai et al., 2014; Li et al., 2015). In Phloroglucinol-HCl staining, after treatment with 37% HCl for 30 s, the sections were stained with 1.0% (w/v) phloroglucinol for 30 s (Li et al., 2015). In Toluidine Blue O (TBO) staining, the sections were stained with 1.0% (w/v) TBO for 30 s immediately (Chai et al., 2014). The stem cross-sections were then observed under a Zeiss Axio microscope (Zeiss, Oberkochen, Germany). Meanwhile, the stem cross-sections were dissected transversely with razor blades and directly observed under a scanning electron microscope (Phenom Pure FEI, United States) following the manual’s recommendations.
Chemical Analysis of Secondary Cell Wall Components
The components of the secondary wall (lignin, cellulose, and xylan) were measured as previously described (Li et al., 2015). Briefly, the Klason and acetyl bromide (AcBr) methods were used to measure the lignin content (Iiyama and Wallis, 1988; Dence, 1992), while the Van Soest method was used to determine the xylan and cellulose contents (Van Soest and Wine, 1967).
Statistical Analysis
The statistical analysis of all the experimental data is performed with Student’s t-test program4. Significant differences among data were evaluated via one-way ANOVA. ∗p < 0.05; ∗∗p < 0.01.
GenBank Accession Numbers
The accession numbers of genes in the paper are listed in Supplementary Table S2.
Results
Isolation and Characterization of PtoMYB74 From P. tomentosa
To investigate the function of poplar MYB74 in poplar, the 1116 bp open reading frame sequence of PtoMYB74, encoding a protein with 371 amino acid residues, was isolated from P. tomentosa by RT-PCR. The predicted molecular weight of PtoMYB74 was approximately 41.91 kDa, and its calculated isoelectric point (pI) was 6.44. The PtoMYB74 protein consists of two conserved MYB domains at the N-terminal region, and each domain contains a Helix-Turn-Helix (HTH) motif (Figure 1A). The sequence analysis of PtoMYB74 with other R2R3 MYB proteins showed that PtoMYB74 and PtoMYB216 (Tian et al., 2013) are a paralogous pair of genes with 89.74% identities in their R2R3 domain regions (Figure 1A). PtoMYB74 also shares high amino acid identities with other R2R3 MYB transcription factors, such as AtMYB61 (83.76%) in A. thaliana, PtMYB8 (82.05%) in P. taeda, and HvMYB3 (75.63%) in Hordeum vulgare L. (Figure 1A). The phylogenetic analysis revealed that PtoMYB74 had close relationships with PtMYB4, PtMYB8, HvMYB3, PtoMYB170, PtoMYB216, and AtMYB61 (Figure 1B), which are known to play important roles in the secondary cell wall formation (Wissenbach et al., 1993; Patzlaff et al., 2003; Bomal et al., 2008; Romano et al., 2012; Tian et al., 2013; Xu et al., 2017). Interestingly, we also found that PtoMYB74 had close relationships with some lower plant species (Selaginella moellendorffii and Spirodela polyrhiza) (Figure 1B). The results suggest that the R2R3 MYB transcription factors are very conservative in the protein evolution of Plants.
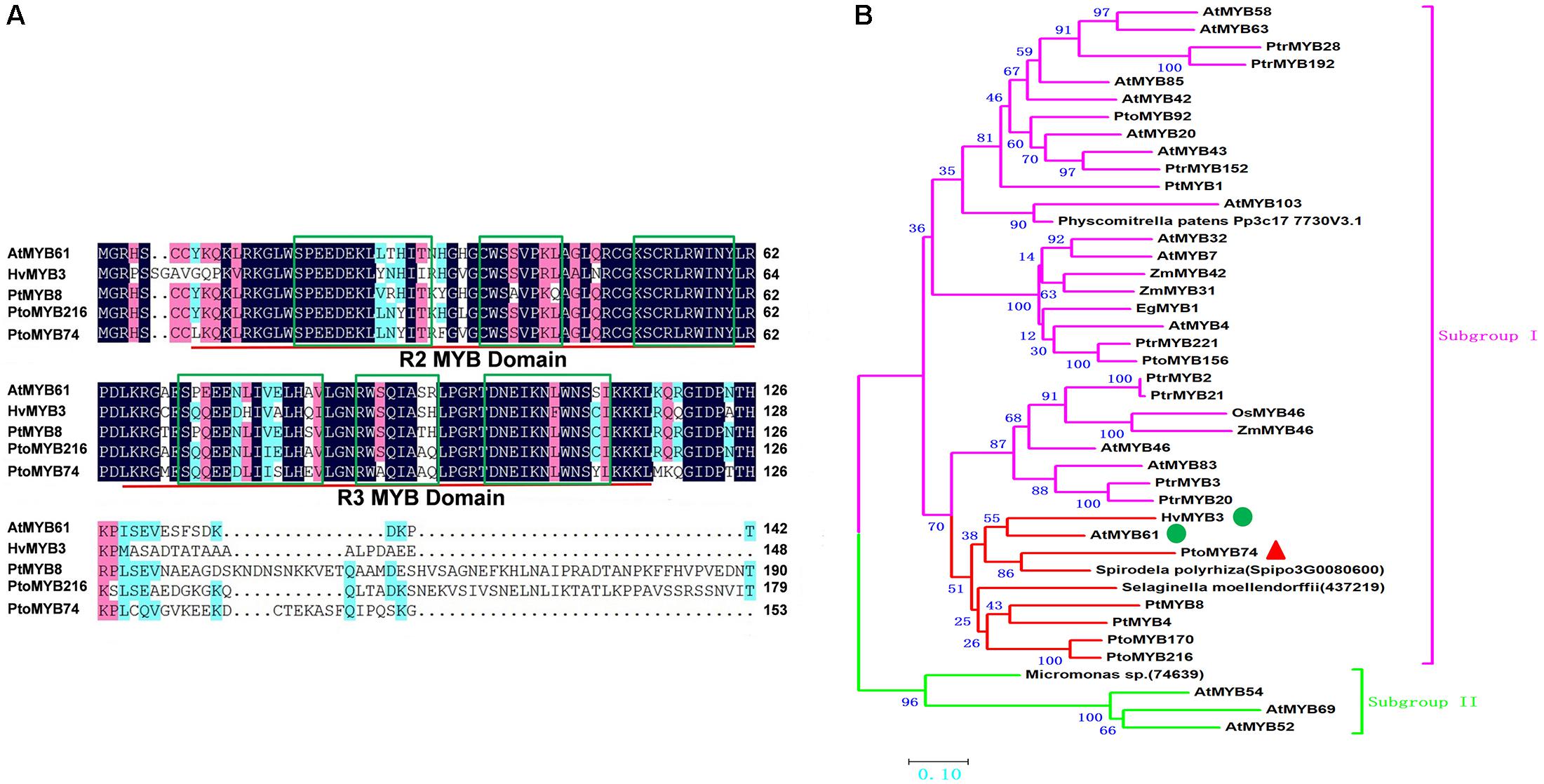
FIGURE 1. PtoMYB74 is highly conserved with many R2R3-MYB transcription factors. (A) Amino acid sequence alignment of the N-terminal region of PtoMYB74 with AtMYB61 (A. thaliana), HvMYB3 (H. vulgare), PtMYB8 (P. taeda), and PtoMYB216 (P. tomentosa). Sequences were aligned with the software of DNAMAN 8. Numbers on the left side refer to its sequence positions. The R2 and R3 repeats are indicated with red bars, and the Helix-Turn-Helix motifs are marked with green boxes. Blue and pink shades are used to show the identification and similarities of the amino acid residues, respectively. (B) Phylogenetic analysis of PtoMYB74 with selected R2R3-MYB transcription factors which are involved in secondary cell wall formation. Full-length of amino acid sequences were aligned with Clustal W, and the phylogenetic tree was constructed using MEGA 6.0 using the neighbor-joining method (2500 bootstrap replicates) and p-distance model. Bar = 0.1 substitutions per site. The Subgroup I is indicated with fuchsine bars, and the Subgroup II is marked with mint green bars. In the Subgroup I, PtoMYB74 and its homologous genes are prominently displayed in the red bars. GenBank accession numbers of MYB transcription factors sequences are given in the Supplementary Table S2.
Expression Patterns of PtoMYB74
To determine the PtoMYB74 expression pattern in poplar, the total RNA was extracted from different poplar tissues. The RT-qPCR analysis showed that the transcripts of PtoMYB74 could be detected in all the organs and tissues, with higher expression levels in the stems, especially in the xylem tissues (Figure 2A).
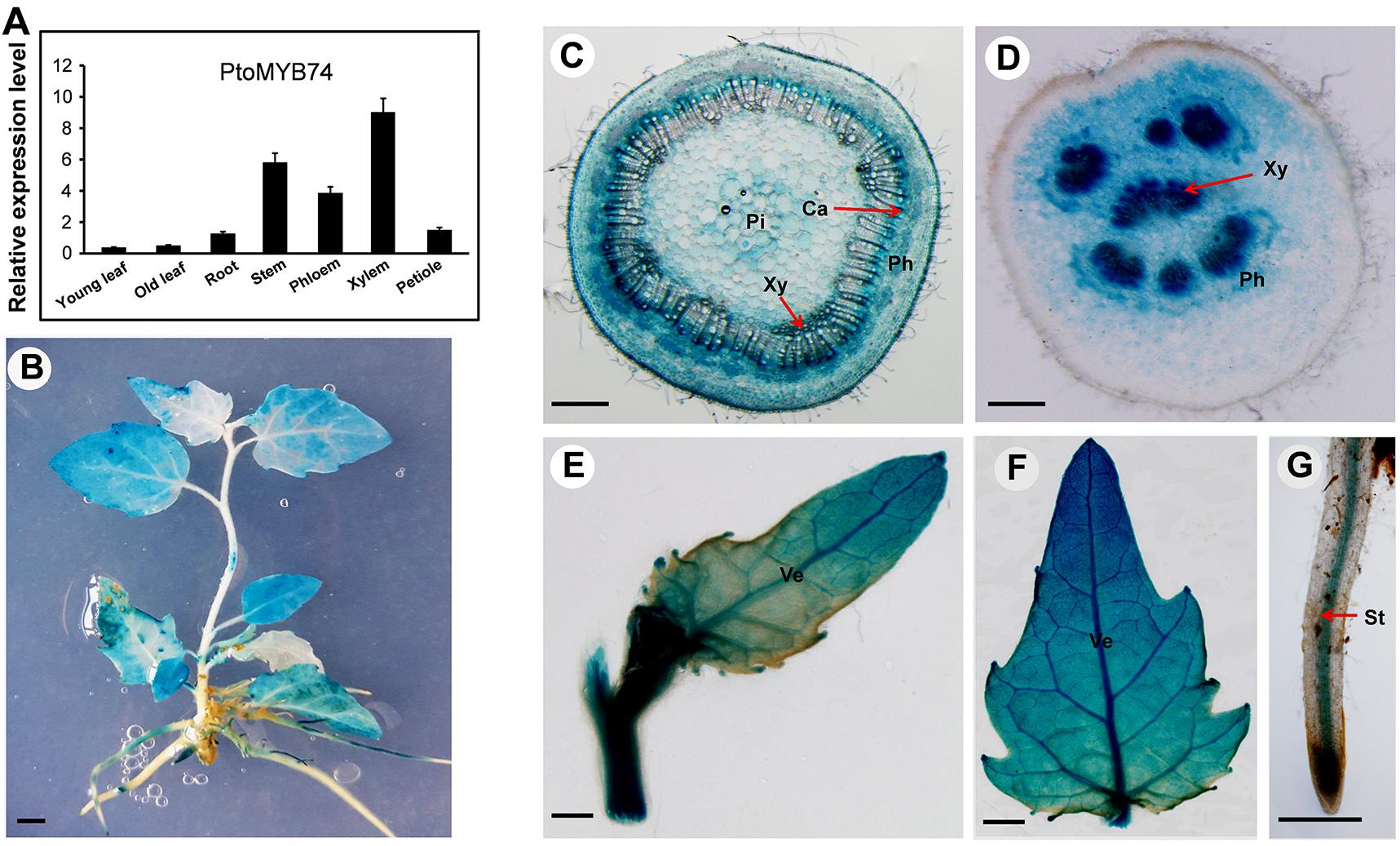
FIGURE 2. Express patterns of PtoMYB74 in vascular blade, tissues, and organs of poplar. (A) RT-qPCR analysis of the relative expression levels of PtoMYB74 in seven different tissues of wild-type poplar. The poplar 18S rRNA was used as an internal reference. Error bars represent ± SD from three biological repeats. (B–F) The PtoMYB74 gene promoter-driven GUS expression vector was introduced into P. tomentosa Carr. The GUS expression was detected in seedlings (B) and various tissues of PtoMYB74:GUS plants, including the stem (C), petiole (D), young leaf (E), old leaf (F), and root (G). Ca, cambium; Ph, phloem; Pi, pith; St, stele; Ve, vein; Xy, xylem. The red arrow indicates the different tissues of poplar. Bars: B = 5 mm; C,D = 500 mm; E,F = 2 mm; G = 100 mm.
To further investigate its spatial and temporal expression profiles, the 5′-flanking sequence of PtoMYB74 was isolated and fused with a GUS reporter gene. After transformation, the histochemical staining results revealed that the GUS activity was detected in all organs and tissues of the transgenic proPtoMYB74:GUS poplar plants (Figure 2B). PtoMYB74 was predominantly expressed in vessels of different tissues (Figures 2C–G). In the stem, the cambium and xylem had strong GUS activities, while the pith and phloem exhibited relatively weak staining (Figure 2C). In the petiole, the GUS expression was observed in both xylem and phloem, but the GUS activity was relatively higher in the xylem (Figure 2D). In the young leaf, the GUS gene was predominantly expressed in the veins (Figure 2E), while the mature leaf had stronger GUS staining (Figure 2F). In the root, the GUS activity was mainly detected in the vascular cylinder (Figure 2G). Similar results were also obtained in transgenic proPtoMYB74:GUS Arabidopsis (Supplementary Figure S2). These results are consistent with the RT-qPCR data above (Figure 2A), suggesting that PtoMYB74 may participate in the secondary cell wall biosynthesis of poplar.
Subcellular Localization of PtoMYB74
To examine its subcellular localization, the CDS of PtoMYB74 was fused to the downstream of a GFP reporter gene driven by the CaMV 35S promoter. The resulting construct was introduced into the tobacco leaves by a transient expression system (Sparkes et al., 2006). As shown in Figure 3A, the GFP fluorescence was detected exclusively in the nuclei, which was co-localized by DAPI staining, thus indicating that PtoMYB74 is a nucleus-localized protein.
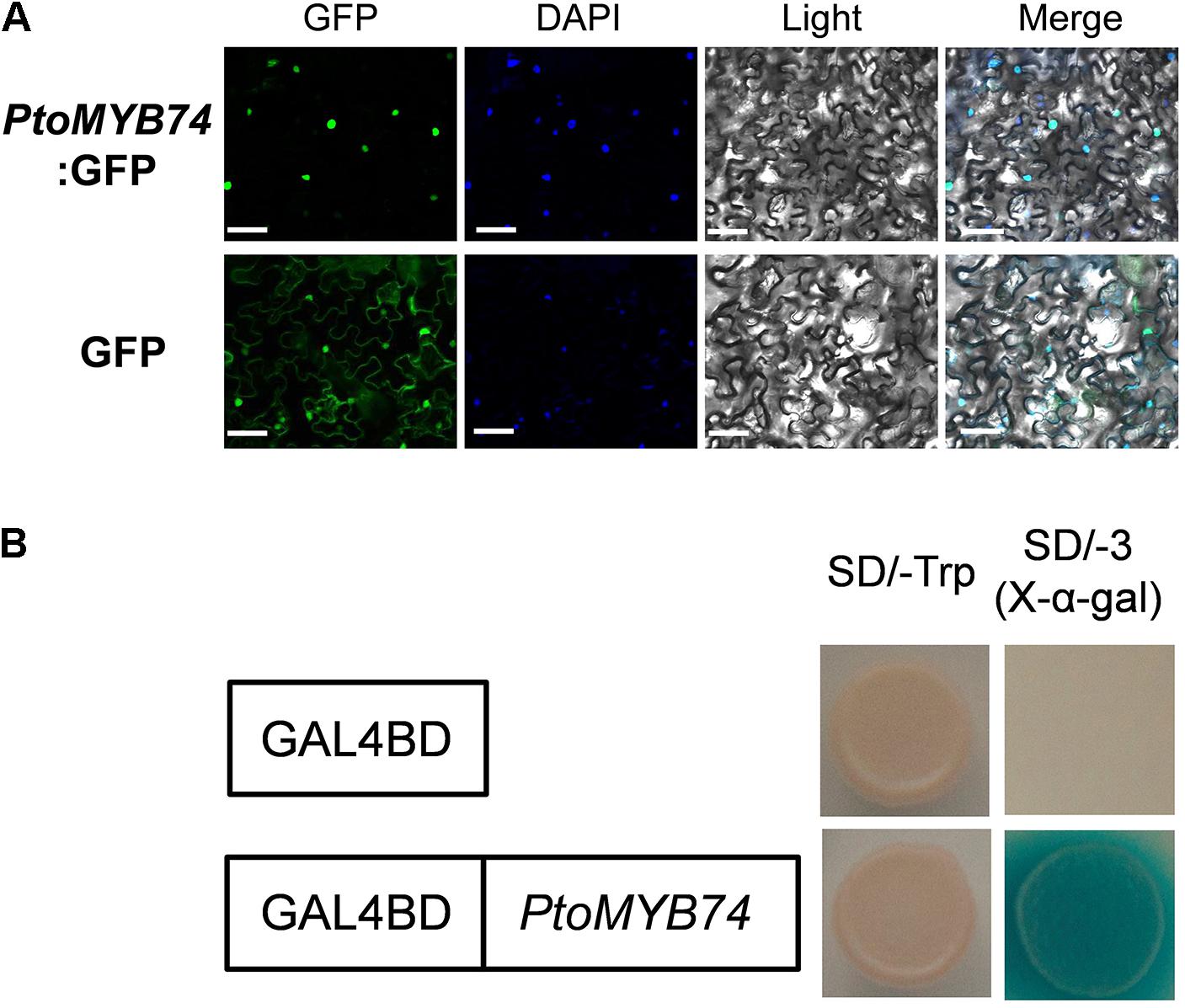
FIGURE 3. Subcellular localization and transcriptional activity analysis of PtoMYB74. (A) Confocal images of localization of GFP-PtoMYB74 in tobacco leaf epidermal cells. Bright field and fluorescent micrographs show the nuclear localization of GFP-PtoMYB74 and free GFP expressed both in nuclear and cytomembrane localization in tobacco (Nicotiana benthamiana) leaf epidermal cells. DAPI (4′,6-Diamidino-2-phenylindole dihydrochloride), a nuclear staining dye. Scale bars = 10 μm. (B) The transcriptional activity assays in yeast cells. The yeast carrying GAL4BD-PtoMYB74 grew on a medium without Trp, His, and Ade (SD/–AHT) and induced the expression activity of X-α-Gal, while the negative control was not.
PtoMYB74 Is a Transcriptional Activator
To investigate the transcriptional activity of PtoMYB74, we fused it with the GAL4 DNA binding domain (GAL4BD). The yeast recombinant transformants expressing the GAL4BD:PtoMYB74 fusion protein grew well on the SD medium lacking Trp, His, and Ade, and was able to metabolize X-α-Gal to produce blue colonies (Figure 3B), indicating that PtoMYB74 acts as a transcriptional activator in poplar.
Overexpression of PtoMYB74 Promotes Secondary Cell Wall Formation in Stems of Transgenic Plants
To determine the role of PtoMYB74 in the secondary cell wall biosynthesis, PtoMYB74 was expressed in Arabidopsis under the control of the CaMV 35S promoter. More than 30 PtoMYB74-overexpression transgenic lines were generated and 3 homozygous lines (L1, -6, and -11) harboring a single copy of the transgene was selected for further experiments (Figure 4A and Supplementary Figure S3). The relative expression levels of PtoMYB74 in transgenic plants were examined by semi-quantitative RT-PCR (Figure 4B) and RT-qPCR (Figure 4C). The PtoMYB74 expression level was the highest in Line 1 (Figures 4B,C), and the Line 6 and Line 11 also had higher expression levels compared to the wild type Arabidopsis (Figures 4B,C). There was no significant difference in the phenotypes among the wild-type and PtoMYB74-overexpression transgenic plants (Figure 4A). Anatomical cross-sections of 40-day-old Arabidopsis plants were used for histochemical staining. The results showed that the overexpression of PtoMYB74 caused stronger lignification in the xylem of transgenic plants, compared to the wild-type plants (Figure 4D). In PtoMYB74-overexpression transgenic Arabidopsis plants, the lignin were also ectopically deposited in the walls of pith parenchyma cells (Figure 4D), which normally is not secondary walled and not lignified. Similar results were also obtained from lignin autofluorescence under UV light (Figure 4D). To count the xylem variations in the stems of PtoMYB74-overexpression transgenic Arabidopsis plants, we measured the xylem radial width and the vessel cell wall thickness (Supplementary Table S3). The xylem width of PtoMYB74-overexpression transgenic Arabidopsis plants had significantly increased by 50.83–108.33% compared to the wild-type plants, while the vessel cell wall thickness of PtoMYB74-overexpression transgenic Arabidopsis plants had significantly increased by 24.14–62.65% compared to the wild-type plants (Supplementary Table S3). The results indicate that PtoMYB74 is involved in the regulation of secondary wall deposition in the xylem tissues of transgenic Arabidopsis.
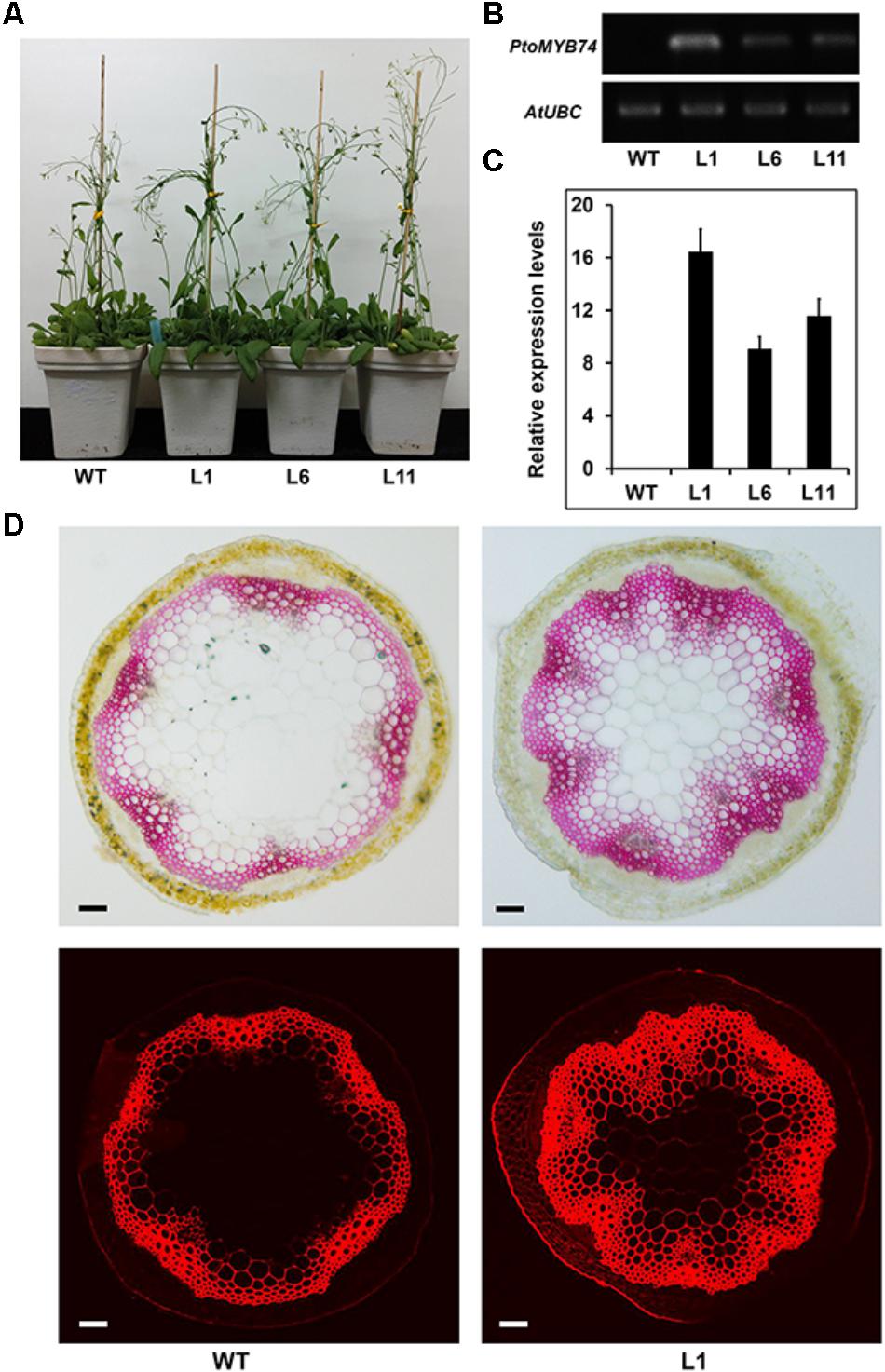
FIGURE 4. Phenotypes of transgenic Arabidopsis overexpressing PtoMYB74. (A) Representative 30-day-old wild-type and transgenic lines (L1, L6, and L11). (B,C) The relative expression levels of PtoMYB74 in different lines (L1, L6, and L11). Semi-quantitative RT-PCR analyses of PtoMYB74 in transgenic Arabidopsis plants (B); Quantitative RT-PCR analyses of PtoMYB74 in transgenic Arabidopsis plants (C). (D) The phloroglucinol-HCl staining and lignin autofluorescence in wild-type and PtoMYB74 overexpression line (L1). Bars: C = 500 μm.
The 35S:PtoMYB74 construct was further introduced into the Chinese white poplar (P. tomentosa Carr.). Over 25 putative transgenic plants were obtained and the integration of the transgene in the transgenic plants was confirmed by PCR with gene-specific primers of the hygromycin phosphotransferase (HPT) gene (Supplementary Table S1 and Supplementary Figure S4A). Three independent transgenic lines (L1, -5, and -8) with high expression levels of PtoMYB74 were used for further experiments (Figure 5A and Supplementary Figure S4B). The stem diameters of the transgenic plants overexpressing PtoMYB74 displayed a significant increase compared to the wild-type plants (Figures 5B,C and Supplementary Figure S5). Phloroglucinol staining revealed a stronger lignification in xylem of transgenic 35S:PtoMYB74 lines (Figures 6C,D), compared to the wild type (Figures 6A,B). Meanwhile, more fiber cells in the sixth internode were observed in PtoMYB74-overexpression plants (17–23 layers) (Figure 6D), while only 9–13 layers of fiber cells were found in the wild-type plants (Figure 6B). Similar results were also obtained in other internodes of the transgenic and wild-type plants (Supplementary Figure S6). More lignin autofluorescence under UV light was detected in the transverse sections of transgenic 35S:PtoMYB74 lines (Figures 6I–K) as compared to that in the wild-type plants (Figures 6E–G). Interestingly, we also observed the ectopic deposition of lignin in pith cells of transgenic 35S:PtoMYB74 lines (Figure 6I). It is worth mentioning that we also observed the cambium in stems of wild type and PtoMYB74-overexpression poplar (Supplementary Figure S7). More cambium cell layers in the sixth internode was observed in PtoMYB74-overexpression plants (6–12 layers) (Supplementary Figures S7A,C), while only 3–7 layers of cambium cells were found in the wild-type plants (Supplementary Figures S7B,D). Furthermore, the xylem radial width and the vessel cell walls of transgenic lines overexpressing PtoMYB74 were significantly thicker than that in the wild type (Figures 6H,L and Table 1). The xylem width of poplar transgenic 35S:PtoMYB74 lines had significantly increased by 12.90–33.47% compared to the wild-type plants, while the vessel cell wall thickness of poplar transgenic 35S:PtoMYB74 lines had significantly increased by 29.59–61.22% compared to the wild-type plants (Table 1). The phenotype analysis and statistical data in poplar are in line with that in Arabidopsis. These results showed that PtoMYB74 positively regulated the secondary cell wall formation in poplar, especially the ectopic deposition of lignin.
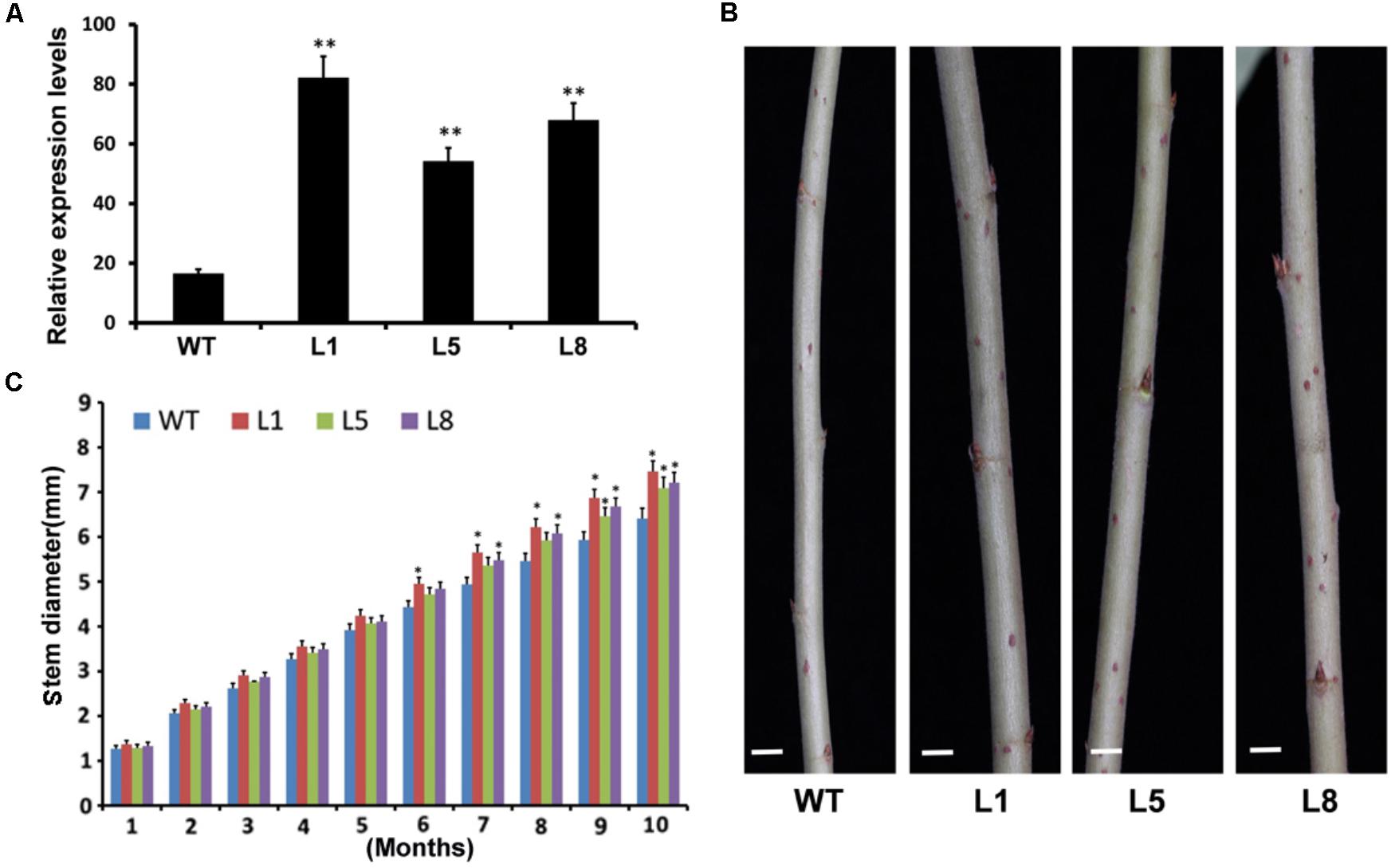
FIGURE 5. Phenotypes of transgenic poplar overexpressing PtoMYB74. (A) The expression levels of PtoMYB74 in wild-type and three independent transgenic lines by RT-qPCR (after MIQE-guidelines). The poplar 18S rRNA was used as an internal reference. (B) Stem microphotographs of the 10-month-old wild-type and PtoMYB74 overexpression lines (L1, L5, and L8). (C) Stem diameters of wild-type and PtoMYB74 overexpression lines (L1, L5, and L8). Error bars represent ± SD from three biological repeats. Student’s t-test: ∗P < 0.05; ∗∗P < 0.01. Bars: C = 5 mm.
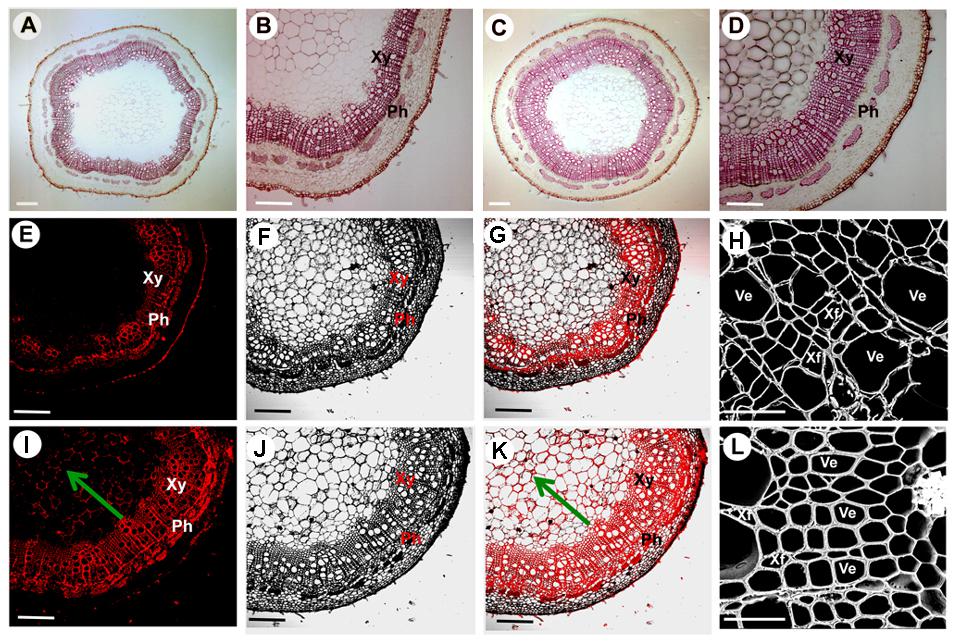
FIGURE 6. Effects of PtoMYB74 overexpression on secondary cell wall thickening of poplar stems. (A–D) Stem sections from the sixth internode of 10-month-old wild-type and transgenic plants were stained with phloroglucinol-HCl. (E–K) Lignin auto-fluorescence of stem sections at the sixth internode in PtoMYB74-overexpressing transgenic and wild-type poplar under UV lights were detected by confocal fluorescence microscopy system. (H,L) Scanning electron micrographs of stem cross-sections at the sixth internode in PtoMYB74-overexpressing transgenic and wild-type poplars. Stem cross-sections used in these images were from the wild type (A,B,E,F,G,H) and transgenic poplars (C,D,I,J,K,L). Ph, phloem; Xy, xylem; Ve, vessel; Xf, xylary fiber. The green arrow indicates the pith of stems. Scale bars: A,C = 500 μm; B,D,E,F,G,I,J,K = 200 μm; H,L = 10 μm.
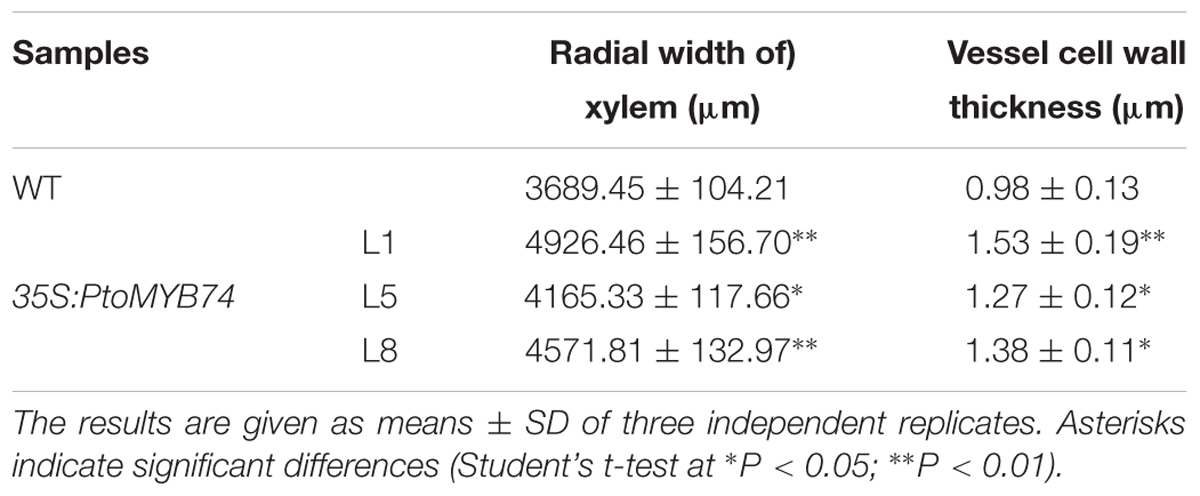
TABLE 1. Radial width of xylem and cell wall thickness of vessels in stems of WT and PtoMYB74 transgenic poplar plants.
To quantify the changes of secondary wall components, the lignin, cellulose, and xylan contents were determined in the stems of transgenic 35S:PtoMYB74 lines via both Klason and acetyl bromide (AcBr) methods. The results revealed that the Klason lignin contents in the stems of transgenic 35S:PtoMYB74 lines were significantly increased by 13.39–21.53% compared to the wild-type plants (Table 2). Similar results were also obtained from the AcBr method (Table 2). Moreover, overexpression of PtoMYB74 appeared to have no significant effect on the xylan biosynthesis, while higher cellulose levels (10.13–12.46%) were detected in transgenic 35S:PtoMYB74 lines (Table 2).
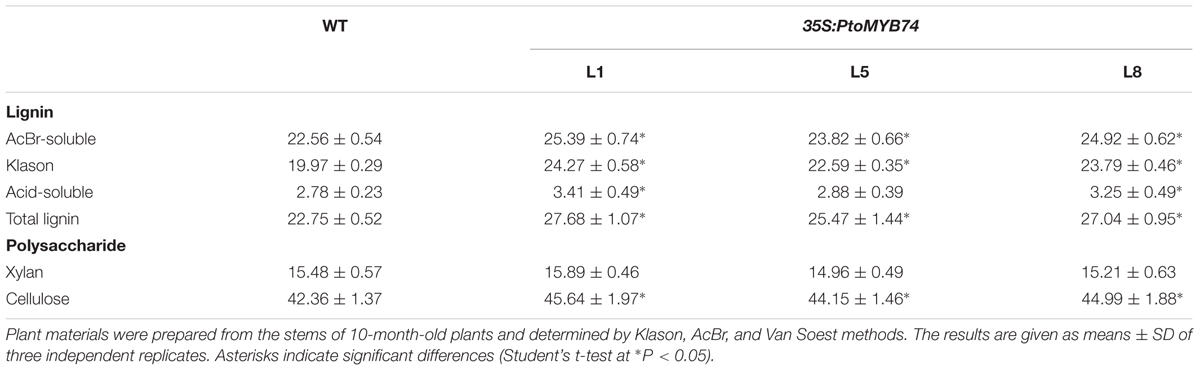
TABLE 2. Secondary cell wall composition analysis of the stems in wild-type and PtoMYB74 overexpression plants.
Overexpression of PtoMYB74 Improves the Expression of Secondary Wall Biosynthetic Genes in Transgenic Poplar
Given that the constitutive expression of PtoMYB74 led to the ectopic deposition of lignin and secondary wall thickening in the stems of transgenic poplar, we further investigated whether the key enzyme genes involved in the secondary wall biosynthetic pathway were activated by PtoMYB74. The RT-qPCR analysis revealed that the relative expression levels of all the lignin synthetic genes (CAD1, C3H3, COMT2, C4H2, CCOAOMT1, CSE1, HCT1, F5H2, CCR2, PAL1, and 4CL5) (Shi et al., 2010; Vanholme et al., 2013) and two cellulose synthetic genes (CesA2B and CesA3A) (Joshi et al., 2004; Djerbi et al., 2005) were elevated in the PtoMYB74 overexpressing lines (Figure 7A), consistent with an increase of lignin and cellulose contents in the transgenic plants (Table 2). However, the expression of three xylan synthetic genes (GT8D, GT43B, and GT43D) (Lee et al., 2011) was not activated in the transgenic plants (Figure 7A). In addition, all of the secondary wall-associated NAC and MYB transcription factors, except PtrNAC156 and PtrMYB128, were not induced in the transgenic lines compared to the wild-type plants (Figure 7B). These results suggested that PtoMYB74 may positively regulate the secondary wall biosynthesis by activating the lignin and cellulose biosynthetic genes in poplar.
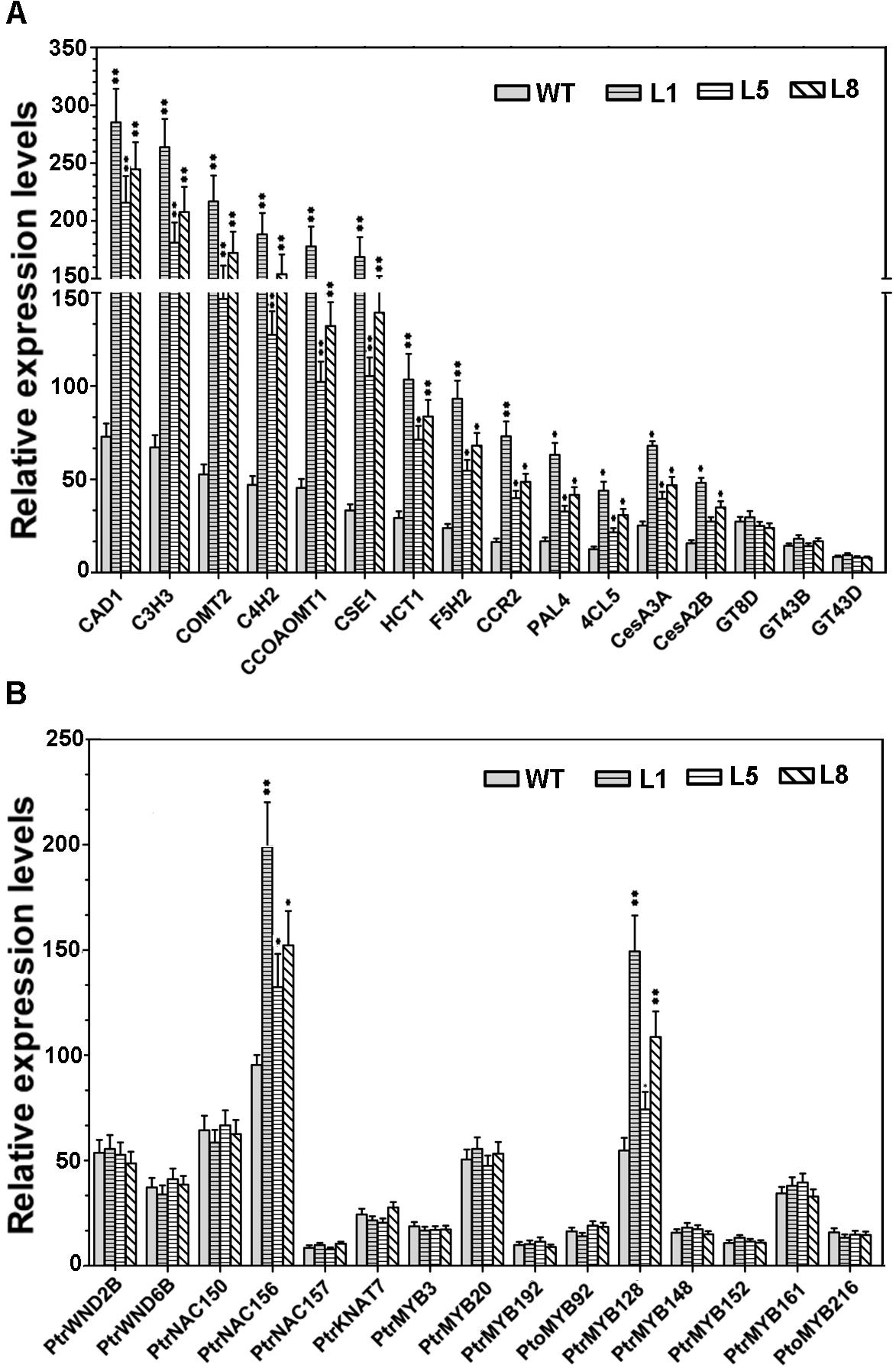
FIGURE 7. The expression of several secondary wall biosynthetic genes and secondary wall-associated transcription factors in the stems of wild-type and PtoMYB74 overexpression poplar plants by RT-qPCR (after MIQE-guidelines). (A) Key enzyme genes in secondary cell wall biosynthetic pathway. (B) Transcription factors involved in the regulation of secondary cell wall biosynthesis. The poplar 18S rRNA was used as an internal reference. Error bars represent ± SD from three biological repeats. Student’s t-test: ∗P < 0.05; ∗∗P < 0.01.
Transactivation assays were used to determine whether PtoMYB74 is capable of activating the promoters of some key enzyme genes in the secondary wall biosynthetic pathway. Transient expression experiments in tobacco leaves showed that PtoMYB74 could activate the promoters of biosynthetic genes for lignin (CCOAOMT1, CCR2, C3H3, C4H2, and F5H2) and cellulose (CesA2B) (Figure 8). In addition, the promoters of GT8D and GT43B were not activated by PtoMYB74. These results indicate that PtoMYB74 functions as a transcription activator of secondary cell wall biosynthesis, and preferentially regulates lignin and cellulose biosynthesis during wood formation in poplar.
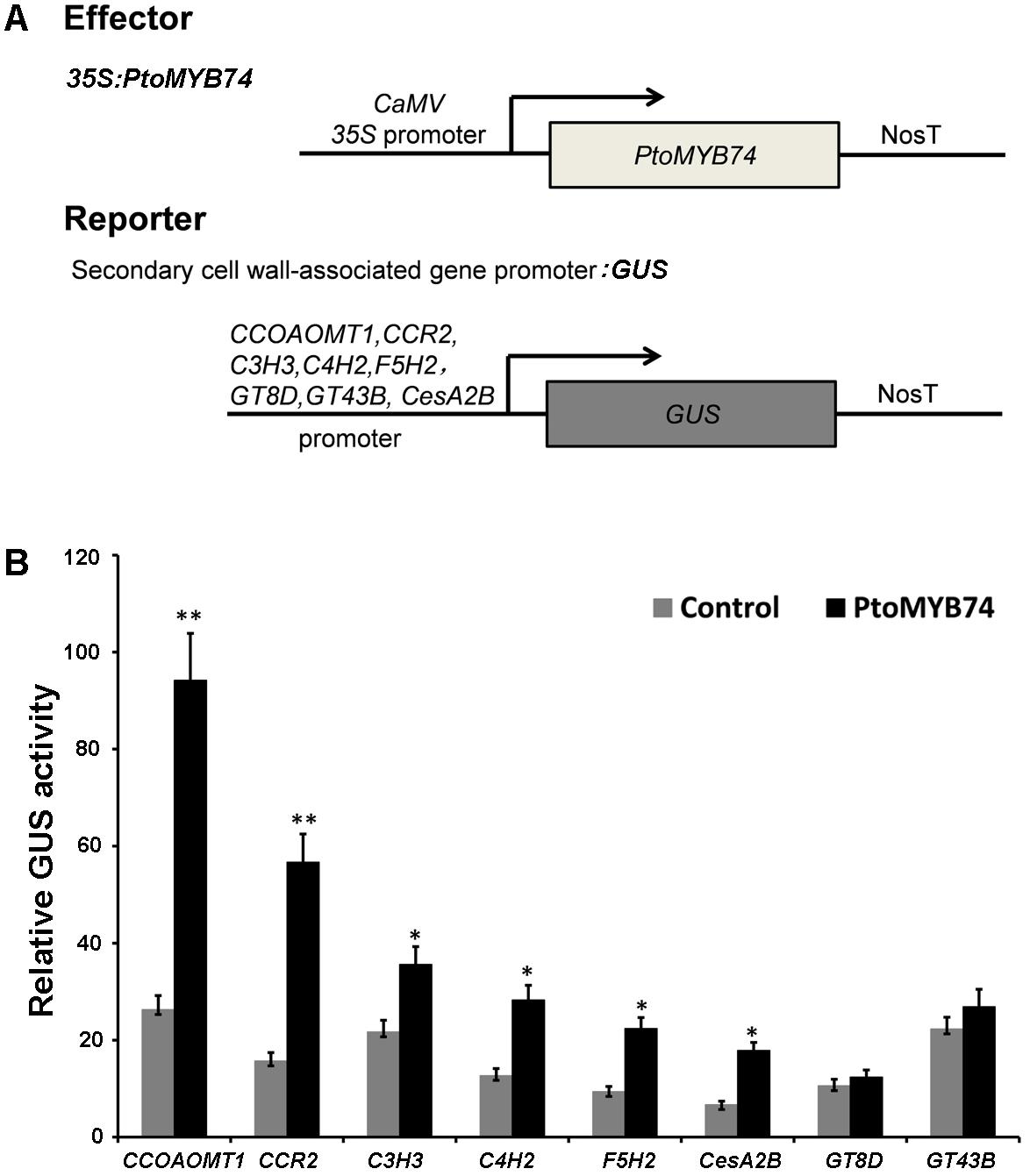
FIGURE 8. Activation of the promoters of some secondary cell wall biosynthetic genes by PtoMYB74. (A) Diagrams of the effector and reporter constructs used for transactivation analysis. The effector construct is the PtoMYB74 cDNA driven by the 35S promoter. The reporter constructs contain the GUS reporter genes driven by the promoters of some secondary cell wall biosynthetic genes, including CCOAOMT1, CCR2, C3H3, C4H2, F5H2, GT8D, GT43B, and CesA2B. (B) Transactivation analysis shows that PtoMYB74 activated individually the CCOAOMT1/CCR2/C3H3/C4H2/F5H2/CesA2B promoter-driven expression of the GUS reporter gene. GUS activity in leaves transfected with the reporter gene alone was used as a control. Error bars represent ± SD of three biological replicates. Student’s t-test: ∗P < 0.05; ∗∗P < 0.01.
Discussion
Wood formation is a very complex process that requires the coordinated and regulated expression of secondary cell wall biosynthesis in higher plants. A subset of the secondary wall biosynthetic genes, including CAD, C3H, COMT, C4H, CCOAOMT, CSE, HCT, F5H, CCR, PAL, 4CL, CesA, CSLC, and GT, have been isolated and characterized in various plant species (Djerbi et al., 2005; Betancur et al., 2010; Shi et al., 2010; Yin et al., 2010; Carroll et al., 2012; Rennie et al., 2012). The expression of these key enzyme genes was controlled by the secondary cell wall transcriptional regulatory network (Zhong et al., 2010; Zhong and Ye, 2014). In annual model plants, such as A. thaliana and tobacco, many NAC and MYB transcription factors have been identified to be involved in the regulation of the secondary cell wall formation through comprehensive molecular and genetic studies (Zhong and Ye, 2014). The transcriptional regulation mechanism of the secondary cell wall formation is more complicated and sophisticated in woody plants. For example, a number of R2R3-MYB transcription factors responsible for the secondary cell wall formation have been functionally characterized in poplar, such as PtrMYB3, PtrMYB20, PtrMYB152, PtoMYB92, PtoMYB216, and PdMYB221 (McCarthy et al., 2010; Tian et al., 2013; Wang et al., 2014; Tang et al., 2015).
In the present study, we have isolated an R2R3 MYB transcription factor PtoMYB74, a homologous gene of Arabidopsis MYB61, and identified that it is involved in the transcriptional regulation of secondary cell wall biosynthesis. As shown in Figure 1A, PtoMYB74 contains a highly conserved R2R3-MYB region, and shares high similarities with AtMYB61 (Romano et al., 2012), HvMYB3 (Wissenbach et al., 1993), PtoMYB216 (Tian et al., 2013), and PtMYB8 (Bomal et al., 2008). Phylogenetic analysis displayed that PtoMYB74 also had a high homology with the MYB transcription factors of Selaginella moellendorffii and Spirodela polyrhiza (Figure 1A). The results indicated that the MYB transcription factors were very conservative in the cell wall formation of vascular plants. Expression pattern analysis showed that PtoMYB74 is predominantly expressed in the vascular tissues (Figure 2), which are mainly made up of the xylem and phloem, thereby suggesting its potential roles in the transcriptional regulation of secondary cell wall biosynthesis. Overexpression of PtoMYB74 not only significantly increased the cell wall thickness of the xylem vessels in the stems of Arabidopsis and poplar but also significantly increased the radial width of the xylem compared to that in the wild-type plants (Figures 4–6, Table 1, and Supplementary Table S3). Consistent with the morphological and anatomical observations, the contents of lignin and cellulose were elevated in the stems of the PtoMYB74 overexpressing transgenic poplar, compared to the wild-type plants (Table 2). The lignin deposition in the parenchyma cells (Figure 4D) and piths (Figures 4D, 6) was also observed, and the phenotypes of the PtoMYB74 overexpressing transgenic Arabidopsis were very similar to the mutants of atelp-1 (Zhong et al., 2000) and atwrky12-1 (Wang et al., 2010). PtoMYB74 may also participate in the plants lignification process in response to wounding or infection (Zhong et al., 2000; Wang et al., 2010). However, the mechanism of poplar MYB transcription factors’ function in the parenchyma cells and pith cell wall formation is still unclear. Meanwhile, we also found that the xylem tissue of transgenic 35S:PtoMYB74 lines had more cell layers (Figure 6). Interestingly, the PtoMYB74-overexpression transgenic plants also had more layers in the cambium cells (Supplementary Figure S7). The results indicated that PtoMYB74 can affect the cell division of cambial cells and differentiation into xylem vessels. Genome-wide expression profiling of xylem and phloem-cambium isolated from the root-hypocotyl of Arabidopsis showed that the class III HD-ZIP and KANADI transcription factors could function as regulators of radial patterning during secondary growth, and the G2-like, NAC, AP2, MADS, and MYB transcription factors may play important roles as regulators of xylem or phloem cell differentiation and activity (Zhao et al., 2005). However, further work about the roles of the NAC/MYB transcription factors in the xylem or phloem cell differentiation and activity has not been carried out. Similarly, the links between the NAC/MYB transcription factors (including PtoMYB74) and cambial cells differentiation into xylem vessels are still unclear. The research about cell division of cambial cells and differentiation into xylem vessels has been concentrated in the model of peptide signaling (Chen et al., 2014; Nieminen et al., 2015). It is worthy to take on the effects of the NAC/MYB transcription factors on the cell division of cambial cells and differentiation into xylem vessels during further research. Taken together, a subset of experimental results suggests that the poplar PtoMYB74 may function as a positive genetic regulator to take part in the secondary cell wall biosynthesis during wood formation.
In previous studies, many MYB transcription factors have been shown to act as transcription activators of secondary cell wall biosynthesis (McCarthy et al., 2010; Tian et al., 2013; Zhong and Ye, 2014; Tang et al., 2015). The transcriptional activity assays showed that PtoMYB74 encodes a nucleus-localized protein (Figure 3A) and activated the β-galactosidase reporter gene expression in the GAL4BD-based self-activation system (Figure 3B), indicating that it is localized to the nucleus and functions as a transcriptional activator. Overexpression of PtoMYB74 in poplar increased the expression of secondary wall-associated key enzyme genes (CAD1, C3H3, COMT2, C4H2, CCOAOMT1, CSE1, HCT1, F5H2, CCR2, PAL1, 4CL5 CesA2B, and CesA3A) and two wood-formation-related transcription factors (PtrNAC156 and PtrMYB128) (Figure 7). Moreover, the CCOAOMT1, CCR2, C3H3, C4H2, F5H2, and CesA2B promoters were activated by PtoMYB74 in the tobacco transient expression system (Figure 8), indicating that these genes are possible direct targets of PtoMYB74. Further studies are required to test this speculation by chromatin immunoprecipitation assays or electrophoretic mobility shift assays. In addition, a previous study has reported that AtMYB61 regulated the stomatal aperture and enhanced the drought tolerance in Arabidopsis (Romano et al., 2012). In our previous studies, the function of PtoMYB216 has been heavily focused on manipulation of lignin biosynthesis (Tian et al., 2013), while the PtoMYB170 positively regulates the lignin deposition during wood formation in poplar and confers drought tolerance in transgenic Arabidopsis (Xu et al., 2017). In this research, we have demonstrated that PtoMYB74 not only promoted the lignin deposition in poplar and Arabidopsis but also increased the accumulation of cellulose. As the functional orthologs of AtMYB61, the three R2R3 MYB family members (PtoMYB74, PtoMYB216, and PtoMYB170) have functional redundancy in lignin biosynthesis and functional differentiation in stress response and substance metabolism. From the phylogenetic analysis and amino acid sequence alignment (Figure 1; Tian et al., 2013; Xu et al., 2017), we can learn that they are highly conserved in the N-terminal region, especially the R2R3-MYB domain. On comparing with the expression patterns of the three MYB transcription factors (Figure 2; Tian et al., 2013; Xu et al., 2017), we have found that they all have high expression levels in lignin-rich tissues (xylem and phloem). These should supply partial evidence for their functional redundancy in the lignin biosynthesis. The amino acid sequence alignment also shows that they are diverse and complicated in the C-terminal region (Figure 1; Tian et al., 2013; Xu et al., 2017), and these variations may sustain functional divergences. Promoter analysis showed that they also have various elements to respond to diverse stresses, except for AC element (Figure 2 and Supplementary Figure S2; Tian et al., 2013; Zhong et al., 2013; Xu et al., 2017). Unlike PtoMYB74 and PtoMYB216, PtoMYB170 had higher expression levels in young leaves, and this may explain why PtoMYB170 positively confers the drought tolerance connecting with promoter analysis (Xu et al., 2017). Apart from PtoMYB216 and PtoMYB170, PtoMYB74 also promotes the cellulose accumulation (Table 2). Promoter analysis showed that PtoMYB74 has several elements to respond to cellulose synthesis, and the results of RT-qPCR and transcriptional activity analysis supply the data for this analysis (Figures 7, 8). AtMYB61 also positively regulates the mucilage in its seed coat (Penfield et al., 2001), and a similar research is difficult for poplar because of its growth cycle.
In summary, this study has identified PtoMYB74 as a poplar ortholog of AtMYB61 that is involved in the secondary wall biosynthesis via the activation of key synthetic enzyme genes in the biosynthetic pathways of lignin and cellulose. Constitutive expression of PtoMYB74 increased the secondary cell wall thickness of the xylem vessels in the stems of transgenic poplar and caused ectopic deposition of lignin and cellulose. Our results may provide some new information about the complex and sophisticated regulatory network of secondary wall biosynthesis in poplar, and help us better understand the wood formation.
Author Contributions
KL and CL designed this work. CL and XM performed the experiments, analyzed the data, and drafted the manuscript. HY and YF analyzed the data and drafted the manuscript. KL revised the manuscript. All authors approved the final revision of the manuscript for publication.
Funding
This work was supported by the National Natural Science Foundation of China (31370672, 31500216, 31500245, and 31500544) and the “One Hundred Talents Program” of the Chinese Academy of Sciences.
Conflict of Interest Statement
The authors declare that the research was conducted in the absence of any commercial or financial relationships that could be construed as a potential conflict of interest.
Supplementary Material
The Supplementary Material for this article can be found online at: https://www.frontiersin.org/articles/10.3389/fpls.2018.01262/full#supplementary-material
Footnotes
- ^ https://blast.ncbi.nlm.nih.gov/Blast.cgi
- ^ https://www.ncbi.nlm.nih.gov/tools/primer-blast/
- ^ https://phytozome.jgi.doe.gov/pz/portal.html
- ^ http://www.graphpad.com/quickcalcs/ttest1.cfm
References
Altschul, S. F., Gish, W., Miller, W., Myers, E. W., and Lipman, D. J. (1990). Basic local alignment search tool. J. Mol. Biol. 215, 403–410. doi: 10.1016/S0022-2836(05)80360-2
Betancur, L., Singh, B., Rapp, R. A., Wendel, J. F., Marks, M. D., Roberts, A. W., et al. (2010). Phylogenetically distinct cellulose synthase genes support secondary wall thickening in Arabidopsis shoot trichomes and cotton fiber. J. Integr. Plant Biol. 52, 205–220. doi: 10.1111/j.1744-7909.2010.00934.x
Bomal, C., Bedon, F., Caron, S., Mansfield, S. D., Levasseur, C., Cooke, J. E., et al. (2008). Involvement of Pinus taeda MYB1 and MYB8 in phenylpropanoid metabolism and secondary cell wall biogenesis: a comparative in planta analysis. J. Exp. Bot. 59, 3925–3939. doi: 10.1093/jxb/ern234
Boudet, A. M. (2000). Lignins and lignification: selected issues. Plant Physiol. Biochem. 38, 81–96. doi: 10.1016/S0981-9428(00)00166-2
Bustin, S. A., Benes, V., Garson, J. A., Hellemans, J., Huggett, J., Kubista, M., et al. (2009). The MIQE guidelines: minimum information for publication of quantitative real-time PCR experiments. Clin. Chem. 55, 611–622. doi: 10.1373/clinchem.2008.112797
Carroll, A., Mansoori, N., Li, S., Lei, L., Vernhettes, S., Visser, R. G., et al. (2012). Complexes with mixed primary and secondary cellulose synthases are functional in Arabidopsis plants. Plant Physiol. 160, 726–737. doi: 10.1104/pp.112.199208
Chai, G., Qi, G., Cao, Y., Wang, Z., Yu, L., Tang, X., et al. (2014). Poplar PdC3H17 and PdC3H18 are direct targets of PdMYB3 and PdMYB21, and positively regulate secondary wall formation in Arabidopsis and poplar. New Phytol. 203, 520–534. doi: 10.1111/nph.12825
Chen, J., Liu, F., Tang, Y., Yuan, Y., and Guo, Q. (2014). Transcriptome sequencing and profiling of expressed genes in phloem and xylem of ramie (Boehmeria nivea L. Gaud). PLoS one 9:e110623. doi: 10.1371/journal.pone.0110623
Chen, S., Helliwell, C. A., Wu, L. M., Dennis, E. S., Upadhyaya, N. M., Narayana, M., et al. (2005). A novel T-DNA vector design for selection of transgenic lines with simple transgene integration and stable transgene expression. Funct. Plant Biol. 32, 671–681. doi: 10.1071/FP05072
Chen, S., Songkumarn, P., Liu, J., and Wang, G. L. (2009). A versatile zero background T-vector system for gene cloning and functional genomics. Plant Physiol. 150, 1111–1121. doi: 10.1104/pp.109.137125
Dence, C. W. (1992). The Determination of Lignin. Methods in Lignin Chemistry. Berlin: Springer, 33–61. doi: 10.1007/978-3-642-74065-7_3
Djerbi, S., Lindskog, M., Arvestad, L., Sterky, F., and Teeri, T. T. (2005). The genome sequence of black cottonwood (Populus trichocarpa) reveals 18 conserved cellulose synthase (CesA) genes. Planta 221, 739–746. doi: 10.1007/s00425-005-1498-4
Douglas, C. J. (1996). Phenylpropanoid metabolism and lignin biosynthesis: from weeds to trees. Trends Plant Sci. 1, 171–178. doi: 10.1016/1360-1385(96)10019-4
Goicoechea, M., Lacombe, E., Legay, S., Mihaljevic, S., Rech, P., Jauneau, A., et al. (2005). EgMYB2, a new transcriptional activator from Eucalyptus xylem, regulates secondary cell wall formation and lignin biosynthesis. Plant J. 43, 553–567. doi: 10.1111/j.1365-313X.2005.02480.x
Iiyama, K., and Wallis, A. (1988). An improved acetyl bromide procedure for determining lignin in woods and wood pulps. Wood Sci. Technol. 22, 271–280. doi: 10.1007/BF00386022
Jefferson, R. A. (1987). Assaying chimeric genes in plants: the GUS gene fusion system. Plant Mol. Biol. Rep. 5, 387–405. doi: 10.1007/BF02667740
Jia, Z., Gou, J., Sun, Y., Yuan, L., Tang, Q., Yang, X., et al. (2010). Enhanced resistance to fungal pathogens in transgenic Populus tomentosa carr. by overexpression of an nsLTP-like antimicrobial protein gene from motherwort (Leonurus japonicus). Tree Physiol. 30, 1599–1605. doi: 10.1093/treephys/tpq093
Jin, H., Cominelli, E., Bailey, P., Parr, A., Mehrtens, F., Jones, J., et al. (2000). Transcriptional repression by AtMYB4 controls production of UV-protecting sunscreens in Arabidopsis. EMBO J. 19, 6150–6161. doi: 10.1093/emboj/19.22.6150
Joshi, C. P., Bhandari, S., Ranjan, P., Kalluri, U. C., Liang, X., Fujino, T., et al. (2004). Genomics of cellulose biosynthesis in poplars. New Phytol. 164, 53–61. doi: 10.1111/j.1469-8137.2004.01155.x
Ko, J. H., Jeon, H. W., Kim, W. C., Kim, J. Y., and Han, K. H. (2014). The MYB46/MYB83-mediated transcriptional regulatory programme is a gatekeeper of secondary wall biosynthesis. Ann. Bot. 114, 1099–1107. doi: 10.1093/aob/mcu126
Ko, J. H., Kim, W. C., and Han, K. H. (2009). Ectopic expression of MYB46 identifies transcriptional regulatory genes involved in secondary wall biosynthesis in Arabidopsis. Plant J. 60, 649–665. doi: 10.1111/j.1365-313X.2009.03989.x
Kosma, D. K., Murmu, J., Razeq, F. M., Santos, P., Bourgault, R., Molina, I., et al. (2014). AtMYB41 activates ectopic suberin synthesis and assembly in multiple plant species and cell types. Plant J. 80, 216–229. doi: 10.1111/tpj.12624
Kubo, M., Udagawa, M., Nishikubo, N., Horiguchi, G., Yamaguchi, M., Ito, J., et al. (2005). Transcription switches for protoxylem and metaxylem vessel formation. Gene Dev. 19, 1855–1860. doi: 10.1101/gad.1331305
Lee, C., Teng, Q., Zhong, R., and Ye, Z.-H. (2011). Molecular dissection of xylan biosynthesis during wood formation in poplar. Mol. Plant 4, 730–747. doi: 10.1093/mp/ssr035
Li, C., Wang, X., Lu, W., Liu, R., Tian, Q., Sun, Y., et al. (2014). A poplar R2R3-MYB transcription factor, PtrMYB152, is involved in regulation of lignin biosynthesis during secondary cell wall formation. Plant Cell Tissue Organ. Cult. 119, 553–563. doi: 10.1007/s11240-014-0555-8
Li, C., Wang, X., Ran, L., Tian, Q., Fan, D., and Luo, K. (2015). PtoMYB92 is a transcriptional activator of the lignin biosynthetic pathway during secondary cell wall formation in Populus tomentosa. Plant Cell Physiol. 56, 2436–2446. doi: 10.1093/pcp/pcv157
Li, Q., Lin, Y.-C., Sun, Y.-H., Song, J., Chen, H., Zhang, X.-H., et al. (2012). Splice variant of the SND1 transcription factor is a dominant negative of SND1 members and their regulation in Populus trichocarpa. Proc. Natl. Acad. Sci. U.S.A. 109, 14699–14704. doi: 10.1073/pnas.1212977109
Liang, Y.-K., Dubos, C., Dodd, I. C., Holroyd, G. H., Hetherington, A. M., and Campbell, M. M. (2005). AtMYB61, an R2R3-MYB transcription factor controlling stomatal aperture in Arabidopsis thaliana. Curr. Biol. 15, 1201–1206. doi: 10.1016/j.cub.2005.06.041
Livak, K. J., and Schmittgen, T. D. (2001). Analysis of relative gene expression data using real-time quantitative PCR and the 2-ΔΔCt method. Methods 25, 402–408. doi: 10.1006/meth.2001.1262
McCarthy, R. L., Zhong, R., Fowler, S., Lyskowski, D., Piyasena, H., Carleton, K., et al. (2010). The poplar MYB transcription factors, PtrMYB3 and PtrMYB20, are involved in the regulation of secondary wall biosynthesis. Plant Cell Physiol. 51, 1084–1090. doi: 10.1093/pcp/pcq064
McCarthy, R. L., Zhong, R., and Ye, Z.-H. (2009). MYB83 is a direct target of SND1 and acts redundantly with MYB46 in the regulation of secondary cell wall biosynthesis in Arabidopsis. Plant Cell Physiol. 50, 1950–1964. doi: 10.1093/pcp/pcp139
McCown, B. H., and Lloyd, G. (1981). Woody plant medium (WPM)-a mineral nutrient formulation for microculture of woody plant-species. Am. Soc. Hortic. Sci. 16, 453–453.
Mitsuda, N., Iwase, A., Yamamoto, H., Yoshida, M., Seki, M., Shinozaki, K., et al. (2007). NAC transcription factors, NST1 and NST3, are key regulators of the formation of secondary walls in woody tissues of Arabidopsis. Plant Cell 19, 270–280. doi: 10.1105/tpc.106.047043
Murashige, T., and Skoog, F. (1962). A revised medium for rapid growth and bio assays with tobacco tissue cultures. Physiol. Plant. 15, 473–497. doi: 10.1111/j.1399-3054.1962.tb08052.x
Nakano, Y., Nishikubo, N., Goué, N., Ohtani, M., Yamaguchi, M., Katayama, Y., et al. (2010). MYB transcription factors orchestrating the developmental program of xylem vessels in Arabidopsis roots. Plant Biotechnol. J. 27, 267–272. doi: 10.5511/plantbiotechnology.27.267
Newman, L. J., Perazza, D. E., Juda, L., and Campbell, M. M. (2004). Involvement of the R2R3-MYB, AtMYB61, in the ectopic lignification and dark-photomorphogenic components of the det3 mutant phenotype. Plant J. 37, 239–250. doi: 10.1046/j.1365-313X.2003.01953.x
Nieminen, K., Blomster, T., Helariutta, Y., and Mähönen, A. P. (2015). Vascular cambium development. Arabidopsis Book 13:e0177. doi: 10.1199/tab.0177
Nolan, T., Hands, R. E., and Bustin, S. A. (2006). Quantification of mRNA using real-time RT-PCR. Nat. Protoc. 1, 1559–1582. doi: 10.1038/nprot.2006.236
Ohashi-Ito, K., Oda, Y., and Fukuda, H. (2010). Arabidopsis VASCULAR-RELATED NAC-DOMAIN6 directly regulates the genes that govern programmed cell death and secondary wall formation during xylem differentiation. Plant Cell 22, 3461–3473. doi: 10.1105/tpc.110.075036
Öhman, D., Demedts, B., Kumar, M., Gerber, L., Gorzsás, A., Goeminne, G., et al. (2013). MYB103 is required for FERULATE-5-HYDROXYLASE expression and syringyl lignin biosynthesis in Arabidopsis stems. Plant J. 73, 63–76. doi: 10.1111/tpj.12018
Ohtani, M., Nishikubo, N., Xu, B., Yamaguchi, M., Mitsuda, N., Goué, N., et al. (2011). A NAC domain protein family contributing to the regulation of wood formation in poplar. Plant J. 67, 499–512. doi: 10.1111/j.1365-313X.2011.04614.x
Patzlaff, A., McInnis, S., Courtenay, A., Surman, C., Newman, L. J., Smith, C., et al. (2003). Characterisation of a pine MYB that regulates lignification. Plant J. 36, 743–754. doi: 10.1046/j.1365-313X.2003.01916.x
Penfield, S., Meissner, R. C., Shoue, D. A., Carpita, N. C., and Bevan, M. W. (2001). MYB61 is required for mucilage deposition and extrusion in the Arabidopsis seed coat. Plant Cell 13, 2777–2791. doi: 10.1105/tpc.13.12.2777
Pilate, G., Déjardin, A., Laurans, F., and Leplé, J. C. (2004). Tension wood as a model for functional genomics of wood formation. New Phytol. 164, 63–72. doi: 10.1111/tpj.13157
Preston, J., Wheeler, J., Heazlewood, J., Li, S. F., and Parish, R. W. (2004). AtMYB32 is required for normal pollen development in Arabidopsis thaliana. Plant J. 40, 979–995. doi: 10.1111/j.1365-313X.2004.02280.x
Rennie, E. A., Hansen, S. F., Baidoo, E. E., Hadi, M. Z., Keasling, J. D., and Scheller, H. V. (2012). Three members of the Arabidopsis glycosyltransferase family 8 are xylan glucuronosyltransferases. Plant Physiol. 159, 1408–1417. doi: 10.1104/pp.112.200964
Romano, J. M., Dubos, C., Prouse, M. B., Wilkins, O., Hong, H., Poole, M., et al. (2012). AtMYB61, an R2R3-MYB transcription factor, functions as a pleiotropic regulator via a small gene network. New Phytol. 195, 774–786. doi: 10.1111/j.1469-8137.2012.04201.x
Shi, R., Sun, Y.-H., Li, Q., Heber, S., Sederoff, R., and Chiang, V. L. (2010). Towards a systems approach for lignin biosynthesis in Populus trichocarpa: transcript abundance and specificity of the monolignol biosynthetic genes. Plant Cell Physiol. 51, 144–163. doi: 10.1093/pcp/pcp175
Sparkes, I. A., Runions, J., Kearns, A., and Hawes C. (2006). Rapid, transient expression of fluorescent fusion proteins in tobacco plants and generation of stably transformed plants. Nat. Protoc. 1, 2019–2025. doi: 10.1038/nprot.2006.286
Tang, X., Zhuang, Y., Qi, G., Wang, D., Liu, H., Wang, K., et al. (2015). Poplar PdMYB221 is involved in the direct and indirect regulation of secondary wall biosynthesis during wood formation. Sci. Rep. 5:12240. doi: 10.1038/srep12240
Tian, Q., Wang, X., Li, C., Lu, W., Yang, L., Jiang, Y., et al. (2013). Functional characterization of the poplar R2R3-MYB transcription factor PtoMYB216 involved in the regulation of lignin biosynthesis during wood formation. PLoS One 8:e76369. doi: 10.1371/journal.pone.0076369
Van Soest, P. J., and Wine, R. H. (1967). Use of detergents in the analysis of fibrous feeds. IV. Determination of plant cell-wall constituents. J. Assoc. Off. Anal. Chem. 50, 50–55.
Vanholme, R., Cesarino, I., Rataj, K., Xiao, Y., Sundin, L., Goeminne, G., et al. (2013). Caffeoyl shikimate esterase (CSE) is an enzyme in the lignin biosynthetic pathway in Arabidopsis. Science 341, 1103–1106. doi: 10.1126/science.1241602
Wang, H., Avci, U., Nakashima, J., Hahn, M. G., Chen, F., and Dixon, R. A. (2010). Mutation of WRKY transcription factors initiates pith secondary wall formation and increases stem biomass in dicotyledonous plants. Proc. Natl. Acad. Sci. U.S.A. 107, 22338–22343. doi: 10.1073/pnas.1016436107
Wang, S., Li, E., Porth, I., Chen, J.-G., Mansfield, S. D., and Douglas, C. J. (2014). Regulation of secondary cell wall biosynthesis by poplar R2R3 MYB transcription factor PtrMYB152 in Arabidopsis. Sci. Rep. 4:5054. doi: 10.1038/srep05054
Wissenbach, M., Überlacker, B., Vogt, F., Becker, D., Salamini, F., and Rohde, W. (1993). Myb genes from Hordeum vulgare: tissue-specific expression of chimeric Myb promoter/Gus genes in transgenic tobacco. Plant J. 4, 411–422. doi: 10.1046/j.1365-313X.1993.04030411.x
Xu, C., Fu, X., Liu, R., Guo, L., Ran, L., Li, C., et al. (2017). PtoMYB170 positively regulates lignin deposition during wood formation in poplar and confers drought tolerance in transgenic Arabidopsis. Tree physiol. 37, 1713–1726. doi: 10.1093/treephys/tpx093
Yamaguchi, M., Mitsuda, N., Ohtani, M., Ohme-Takagi, M., Kato, K., and Demura, T. (2011). VASCULAR-RELATED NAC-DOMAIN 7 directly regulates the expression of a broad range of genes for xylem vessel formation. Plant J. 66, 579–590. doi: 10.1111/j.1365-313X.2011.04514.x
Yang, C., Xu, Z., Song, J., Conner, K., Barrena, G. V., and Wilson, Z. A. (2007). Arabidopsis MYB26/MALE STERILE35 regulates secondary thickening in the endothecium and is essential for anther dehiscence. Plant Cell 19, 534–548. doi: 10.1105/tpc.106.046391
Yin, Y., Chen, H., Hahn, M. G., Mohnen, D., and Xu, Y. (2010). Evolution and function of the plant cell wall synthesis-related glycosyltransferase family 8. Plant Physiol. 154, 1729–1746. doi: 10.1104/pp.110.154229
Zaragoza, M. V., Lewis, L. E., Sun, G., Wang, E., Li, L., Said-Salman, I., et al. (2004). Identification of the TBX5 transactivating domain and the nuclear localization signal. Gene 330, 9–18. doi: 10.1016/j.gene.2004.01.017
Zhang, X., Henriques, R., Lin, S.-S., Niu, Q.-W., and Chua, N.-H. (2006). Agrobacterium-mediated transformation of Arabidopsis thaliana using the floral dip method. Nat. Protoc. 1, 641–646. doi: 10.1038/nprot.2006.97
Zhao, C., Craig, J. C., Petzold, H. E., Dickerman, A. W., and Beers, E. P. (2005). The xylem and phloem transcriptomes from secondary tissues of the Arabidopsis root-hypocotyl. Plant Physiol. 138, 803–818. doi: 10.1104/pp.105.060202
Zhong, R., Demura, T., and Ye, Z.-H. (2006). SND1, a NAC domain transcription factor, is a key regulator of secondary wall synthesis in fibers of Arabidopsis. Plant Cell 18, 3158–3170. doi: 10.1105/tpc.106.047399
Zhong, R., Lee, C., and Ye, Z.-H. (2010). Functional characterization of poplar wood-associated NAC domain transcription factors. Plant Physiol. 152, 1044–1055. doi: 10.1104/pp.109.148270
Zhong, R., McCarthy, R. L., Haghighat, M., and Ye, Z.-H. (2013). The poplar MYB master switches bind to the SMRE site and activate the secondary wall biosynthetic program during wood formation. PLoS One 8:e69219. doi: 10.1371/journal.pone.0069219
Zhong, R., McCarthy, R. L., Lee, C., and Ye, Z.-H. (2011). Dissection of the transcriptional program regulating secondary wall biosynthesis during wood formation in poplar. Plant Physiol. 157, 1452–1468. doi: 10.1104/pp.111.181354
Zhong, R., Ripperger, A., and Ye, Z.-H. (2000). Ectopic deposition of lignin in the pith of stems of two Arabidopsis mutants. Plant Physiol. 123, 59–70. doi: 10.1104/pp.123.1.59
Zhong, R., and Ye, Z.-H. (2009). Transcriptional regulation of lignin biosynthesis. Plant Signal. Behav. 4, 1028–1034. doi: 10.4161/psb.4.11.9875
Zhong, R., and Ye, Z.-H. (2014). Complexity of the transcriptional network controlling secondary wall biosynthesis. Plant Sci. 229, 193–207. doi: 10.1016/j.plantsci.2014.09.009
Zhong, R., and Ye, Z.-H. (2015). The Arabidopsis NAC transcription factor NST2 functions together with SND1 and NST1 to regulate secondary wall biosynthesis in fibers of inflorescence stems. Plant Signal. Behav. 10:e989746. doi: 10.4161/15592324.2014.989746
Keywords: Populus, R2R3-MYB, transcription factors, secondary cell wall, transgenic, genetic
Citation: Li C, Ma X, Yu H, Fu Y and Luo K (2018) Ectopic Expression of PtoMYB74 in Poplar and Arabidopsis Promotes Secondary Cell Wall Formation. Front. Plant Sci. 9:1262. doi: 10.3389/fpls.2018.01262
Received: 04 February 2018; Accepted: 10 August 2018;
Published: 09 October 2018.
Edited by:
Shucai Wang, Northeast Normal University, ChinaReviewed by:
Hua Cassan Wang, Université de Toulouse, FranceGuohua Chai, Qingdao Institute of Bioenergy and Bioprocess Technology (CAS), China
Copyright © 2018 Li, Ma, Yu, Fu and Luo. This is an open-access article distributed under the terms of the Creative Commons Attribution License (CC BY). The use, distribution or reproduction in other forums is permitted, provided the original author(s) and the copyright owner(s) are credited and that the original publication in this journal is cited, in accordance with accepted academic practice. No use, distribution or reproduction is permitted which does not comply with these terms.
*Correspondence: Keming Luo, a2VtaW5nbEBzd3UuZWR1LmNu; bHVva2VtaW5nQGhvdG1haWwuY29t