- 1School of Agricultural, Forest, Food and Environmental Sciences, University of Basilicata, Potenza, Italy
- 2Pyrenean Institute of Ecology (IPE-CSIC), Zaragoza, Spain
- 3Department of Crop and Forest Sciences, Agrotecnio Center, University of Lleida, Lleida, Spain
- 4Department of Forest Mycology and Plant Pathology, Swedish University of Agricultural Sciences, Uppsala, Sweden
Forest decline induced by climate change is a global phenomenon that affects many tree species, mainly in drought-prone areas as the Mediterranean region. In southern Italy, several oak species have shown decline symptoms and elevated mortality since the 2000s due to drought stress. However, it remains to be answered whether decline occurred alone or whether a pathogen was also involved. To this aim, we compared two coexisting oak species in a forest located in southern Italy which are assumed to be less (Quercus cerris) and more tolerant to drought (Quercus pubescens). We sampled fifteen couples of neighboring declining (D) and non-declining (ND) trees of both species. Wood cores were taken from all trees to perform dendrochronological analyses to detect the decline onset and link it to potential climatic drivers. Carbon isotope ratios (d13C) were analyzed in wood of the two vigor classes to compare their water-use efficiency. Phytophthora presence was also assessed in soil samples from ten D-ND couples of trees per species. The oak species most affected by drought-induced decline in terms of leaf shedding and mortality was Q. cerris, i.e., the least tolerant to drought. In both species, the D trees showed a reduced growth rate compared with ND trees from 2000 onward when drought and warming intensified. Q. pubescens showed higher growth sensitivity to precipitation, temperature and drought than Q. cerris. This sensitivity to climate was magnified in D trees whose growth decreased in response to warm and dry conditions during the prior winter and the late summer. The Q. pubescens D trees were more efficient in their water use than ND trees before the growth divergence between D and ND trees amplified. In the studied area, Phytophthora quercina was isolated from 40% of the sampled trees, and tended to be more frequent amongst ND than amongst D trees. Our data suggests that droughts and warm summer conditions triggered oak decline. The high prevalence of P. quercina in the studied area warrants further study as a potential predisposing factor.
Introduction
Forest decline is a complex phenomenon characterized by a loss in tree vigor and increased mortality, and it is usually triggered by extreme climate events (e.g., droughts) but also involves pathogens and pests (Manion, 1991; Sangüesa-Barreda et al., 2015).
In oaks, climate warming and altered precipitation regimes may negatively affect the carbon and water use of trees and indirectly benefit root pathogens (e.g., Phytophthora spp.) causing the dead of fine roots and making trees more susceptible to water shortage (Wargo, 1996; Führer, 1998; Thomas et al., 2002; Haavik et al., 2015). However, it remains to be answered to what degree severe climatic stressors (drought or heat waves) trigger forest decline making trees more vulnerable to biotic stressors (root pathogens).
Drought and high evapotranspiration rates linked to warmer conditions have been found to reduce radial growth, increase stress and trigger forest decline in many oak species worldwide (Tainter et al., 1990; Jenkins and Pallardy, 1995; Pedersen, 1998; Andersson et al., 2011; Corcuera et al., 2004a,b; Sohar et al., 2014). Comparisons of tree-ring width series through time has revealed that declining trees showed lower radial-growth rates than non-declining trees about 5–25 years prior to the decline onset or before tree death (Haavik et al., 2011; Levaniè et al., 2011; Helama et al., 2016; Cailleret et al., 2017; Gentilesca et al., 2017; Colangelo et al., 2017a, 2018). Declining trees are usually characterized by recent low growth rates but also by leaf shedding and architectural anomalies such as abundant epicormic and dead shoots (Drobyshev et al., 2007).
The most likely mechanism causing drought-induced decline in anisohydric species as many ring-porous oak species is hydraulic failure (McDowell et al., 2008). Such prolific use of water during drought leads to a reduction of stem water potential and an elevated loss in hydraulic conductivity of declining individuals showing intense crown desiccation and leaf shedding (Nardini et al., 2013). If unusually severe and sustained droughts modify C supply (photosynthesis, leaf area) and demand (growth, root production) in oaks, and alter aboveground and belowground C reserves and translocation, trees could become more susceptible to necrotrophic root-rot pathogens (Oliva et al., 2014) such as several Phytophthora species (Brasier, 1996; Jung et al., 1999, 2000). Drought and soil pathogens can synergistically amplify stress and cause the decline and death of oaks (Haavik et al., 2015).
In Europe, Phytophthora quercina has been commonly associated with oak root rot and decline predominantly in wet sites (Jung et al., 1999, 2000; Vettraino et al., 2002; Balci and Halmschlager, 2003; Jönsson, 2004). However, it remains unclear whether Phytophthora is the main trigger for oak decline episodes, because the relationships between environmental conditions, root damage caused by the pathogen and the loss in tree vigor are poorly understood (Jönsson et al., 2005; Jönsson, 2006). Although associations between oak crown transparency and the presence of Phytophthora species in the rhizosphere have been reported (Jung et al., 2000; Vettraino et al., 2002), information on the growth rates of coexisting non-declining and declining trees which show disease development is still missing (Jönsson et al., 2005).
Drought stress has been considered among the main triggering factors of oak decline, but it remains to be answered if both drought and pathogens synergistically contribute to the long-term loss in growth and tree vigor. Furthermore, to our best knowledge there are not experiments which investigated the effect of both stressors under controlled conditions on our studied species, except for other Quercus species (see for instance Sànchez et al., 2002 on Q. ilex where P. cinnamomi was investigated).
Here we use tree-ring width data to reconstruct growth and pinpoint the onset of decline in two affected oak species coexisting in the same forest located in southern Italy (Quercus pubescens, Quercus cerris). This comparison of the two species allows testing if the species more abundant in xeric sites (Q. pubescens) was less affected by drought-induced decline than the species from mesic sites which is assumed to be less tolerant to water shortage (Q. cerris). Both Q. pubescens and Q. cerris seem to be susceptible to P. quercina, because incidence of the pathogen has been previously reported in stands of these oak species (Vettraino et al., 2002; Balci and Halmschlager, 2003). Although differences in drought tolerance among oak species are widely reported in literature, there are only few comparative studies on Mediterranean oaks (e.g., Dreyer et al., 1992; Valentini et al., 1992; Tognetti et al., 1999). Based on the distribution of the main oak species in southern Italy, the following ranking in drought tolerance can be suggested: Q. robur (least tolerant) < Q. cerris < Q. frainetto < Q. pubescens < Q. ilex < Q. suber (most tolerant).
We hypothesize that the severe 2000s droughts triggered oak decline, and that Phytophthora quercina was involved in the decline syndrome either as predisposing or as a contributing factor. To test this idea, we reconstructed the tree-ring growth, isotopic signature of declining oaks and we assessed the association between the presence of symptoms of decline and Phytophthora infestation.
Materials and Methods
Study Area, Tree Species and Forest Decline
The study site (Gorgoglione forest, 40° 21′ 51″ N, 16° 10′ 34″ E, 800–850 m a.s.l.) is located in the mountainous Basilicata region, southern Italy. It has a mean slope of 25%. The study area is a mixed high forest with a mean density of 600 stems ha-1 dominated by Quercus cerris L. (71%) followed by Quercus pubescens L. (25%) and by other broadleaf species (4%). No recent disturbance has been reported for the study sites (e.g., insect outbreaks or fires) and no silvicultural treatment has been applied in the last five decades. In the past, these oak forests were traditionally managed as coppices for firewood and timber production in combination with livestock grazing. Soils are a mixture of sand, silt, and clay textures.
Climate in the study area is Mediterranean (cool dry-summer climate according to the Köppen classification), characterized by dry and warm summers (total precipitation from June to August is 93 mm) and wet and mild winters (total precipitation from December to February is 230 mm) with mean annual temperature of 11.6°C and annual precipitation of 722 mm (data from Gorgoglione station, 40° 24′ N, 16° 09′ E, 796 m). The warmest and coldest months are August (mean temperature of 20.5°C) and January (mean temperature of 3.7°C), respectively, whereas the driest and wettest months are July (22 mm) and November (96 mm). Drought occurs from June to August.
The two studied tree species showed recent drought-induced decline symptoms since the early 2000s (shoot dieback, leaf loss and withering, growth decline, and high mortality). Regarding the incidence of the decline syndrome according to local reports, annual oak mortality in the study area affected ca. 450 ha and shifted from 5 to 10% from 2002 to 2004. In the most affected stands, more than 50% of mature specimens showed decline symptoms and 15% were dead, while the remaining individuals (35%) were non-declining trees.
Long-Term Climate and Drought Data
Due to the shortness and heterogeneity of local climate data we used gridded (0.25° resolution) climate data from the E-OBS dataset version 13.0 (Haylock et al., 2008) to quantify climate trends and climate-growth associations for the period 1950–2016. Climate was extracted from the 0.25° grid with coordinates 40.00–40.25° N, 16.00–16.25° E. To evaluate drought-growth associations we downloaded the Standardized Precipitation Evapotranspiration Index (SPEI) for the 0.5° grid where the study sites are located and considering 1–24 months long scales using the Global SPEI database webpage1. The SPEI is a multiscalar drought index, which considers the effects of temperature and evapotranspiration on drought severity and indicates wet (positive SPEI values) and dry (negative SPEI values) conditions (Vicente-Serrano et al., 2010).
Field Sampling and Tree-Ring Data
In the field, first we randomly located seven circular plots (radius of 15 m) to estimate the density of trees with different vigor. Then, we sampled dominant trees of the two oak species. We selected pairs of neighboring (located at less than 20 m apart) declining or symptomatic (D trees) and non-declining or asymptomatic (ND trees). Declining oaks (hereafter D trees) were considered those with crown transparency higher than 50%, whereas non-declining oaks (hereafter ND trees) were considered those with transparency lower than 50%. Crown transparency was estimated by a visual assessment performed by two independent observations on the same tree using binoculars (Colangelo et al., 2017a). Using other crown-transparency thresholds (40 and 60%) the main results presented here did not change (cf. Colangelo et al., 2017a). Note, however, that recently dead trees could have been logged by people from nearby villages so our data may underestimate the actual density of D and recently dead trees.
The diameter at breast height (dbh), i.e., at 1.3 m, and height of each tree were measured using tapes and a laser rangefinder, respectively. To study radial growth we extracted one increment core per tree at breast height (1.3 m) using 5-mm Pressler increment borers. We avoided taking two cores per tree due to restrictions imposed by forest managers for preserving the study stands.
To quantify radial-growth changes in trees of contrasting vigor we used dendrochronology. In total we sampled 42 D and 32 ND Quercus cerris trees and 17 D and 21 ND Quercus pubescens trees. Within each ND-D couple, trees were located 10–15 m apart at maximum. Wood samples were air–dried and the surface of the cores was cut using a sledge core microtome (Gärtner and Nievergelt, 2010). Tree rings were visually cross-dated and measured with precision of 0.01 mm using a binocular microscope coupled to a computer with the LINTAB package (RINNTECH, Heidelberg, Germany). The COFECHA program (Holmes, 1983) was used to evaluate the visual cross-dating of tree-ring series. To estimate age at 1.3 m we counted the number of annual rings. In cores without pith, the missing distance was estimated by fitting a template of concentric circles with known radii to the curve of the innermost rings, which allowed the estimation of the missing radius length and transforming it into the number of missing rings (Camarero et al., 2015).
To quantify climate- and drought-growth relationships, first we removed the long–term trends of tree-ring width series by detrending them through the Friedman super smoother, which preserves high-frequency (annual) variability in the resulting ring-width indices. In addition, an autoregressive model was applied to each detrended series to remove most of the first-order autocorrelation related to the previous-year of growth. We obtained series at the tree level of dimensionless ring-width indices. Lastly, a biweight robust mean was used to obtain mean chronologies of ND and D trees at each site. Chronology development and standardization were carried out using the ARSTAN program (Cook and Krusic, 2005).
Climate-growth associations were calculated for the common and best-replicated period 1950–2016, considering monthly climatic variables (mean maximum and minimum temperatures, precipitation). The window of analyses included form the previous September to current September based on previous studies (Tessier et al., 1994). Drought-growth associations were calculated for the same time period using 1- to 24-month long SPEI values obtained from January to December.
Finally, we calculated dendrochronological statistics to compare the ring-width series of ND and D trees. Specifically, we calculated the first-order autocorrelation of ring-width data (AR1) and the mean sensitivity of ring-width indices (MS) which assesses the relative variability in width between consecutive rings (Briffa and Jones, 1990).
Carbon Isotopes in Wood
We compared carbon isotope ratios (13C/12C, δ13C) in wood between non-declining (n = 5 trees) and declining (n = 5 trees) individuals of each species. We considered three different 5-year periods including different phased of the decline event (2002–2006, 2007–2011, and 2012–2016). The wood samples were dried in the oven at 70°C for 48 h and ground to a fine powder. Wood aliquots (0.001 g) were weighed on a microbalance (AX205 Mettler Toledo, OH, United States) into tin foil capsules and combusted to CO2 using a Flash EA-1112 elemental analyzer interfaced with a Finnigan MAT Delta C isotope ratio mass spectrometer (Thermo Fisher Scientific Inc., MA, United States). Isotope analyses were conducted at the Isotope Lab of the “Istituto di Geoscienze e Georisorse” (CNR; Udine, Italy). Stable isotope ratios were expressed as per mil deviations using the δ notation relative to Vienna Pee Dee Belemnite (VPDB). The standard deviation for repeated analyses was better than 0.1‰
To account for the δ13C depletion of atmospheric CO2 (δ13Ca) due to the combustion of fossil fuels, we calculated C isotope discrimination in wood (Δ13C) from δ13Ca and plant δ13Cp following Farquhar et al. (1989):
δ13Ca was obtained from Graven et al. (2017).
Soil Characteristics
Soils were sampled at ten points separated by 10 m and located within the sampled stands. Soils were taken at a depth of 15–30 cm. Soils were characterized them by measuring the following variables: texture, C and N concentrations, pH and electrical conductivity. Soil texture was determined with laser diffraction method in Coulter Mastersizer (2000) and clay content was corrected (Taubner et al., 2009). Soil C and N concentrations and the C/N ratio were determined with an elemental analyzer (Elementar Vario MAX N/CM, Hanau, Germany).
Detection of Phytophthora Presence
We focused on the detection of Phytophthora since this pathogen has been often associated with decline and mortality of oak stands across Europe (Jung et al., 1999, 2000). To evaluate whether dieback was associated with soilborne Phytophthora infection we sampled the rhizosphere of twenty trees (five defoliated and five healthy trees ×2 tree species). Approximately 400 gr of shallow soil were collected after removing the organic layer from three points situated below the crown of each oak taken at a mean 25 cm depth in the A horizon. Samples were pooled for each tree. Soil baiting was carried out following Jung’s (2009) using fresh leaves of Valencian oak (Quercus faginea) as baits. Baits were inspected regularly during 10 days. Necrotic spots were plated on CMA-PARPBH medium (Jeffers and Martin, 1986) and then incubated at 20°C in darkness. Within 2–4 days, Phytophthora-like colonies were transferred onto V8 agar media (Erwin and Ribeiro, 1996), and stored at 20°C. DNA from 14 Phytophthora-like isolates was extracted following the NaOH extraction procedure described by Wang et al. (1993). The ITS region was amplified using the ITS6f and ITS4 primers (White et al., 1990; Cooke and Duncan, 1997), following the PCR settings specified in Samils et al. (2011). PCR product was sequenced by Macrogen (South Korea), and a BLAST search was later performed on the Phytophthora ID curated database2, establishing a minimum identity threshold for species identification of 98%.
Statistical Analyses
Tree characteristics of the two tree vigor classes were compared between species and between vigor classes using t or Mann-Whitney U tests in the case of variables that followed or did not follow normal distributions, respectively. To assess trends in monthly climate data (mean maximum and minimum temperatures, precipitation) we used the non-parametric tau statistic (τ) considering climate data for the 1950–2016 period. We used the Wilcoxon rank-sum test to check if mean tree-ring width differed between ND and D trees because this non-parametric statistic is robust against deviations from normal distributions and the presence of temporal autocorrelation (Gibbons and Chakraborti, 2011).
Results
Climate Trends and Drought Variability
Significant (P < 0.05) and positive trends in mean maximum temperatures of January (τ = 0.26), July (τ = 0.17), October (τ = 0.20), and November (τ = 0.21) were found for the period 1950–2016 (Figure 1). All months excepting February showed significant and positive trends in mean minimum temperatures, being particularly strong in July–August (τ = 0.40). Only December showed a significant negative trend in precipitation (τ = -0.18), followed by May (τ = -0.14). Lastly, the May SPEI calculated at 11-month long scales showed dry conditions (SPEI < -1.75) in 1957, 1995, 2000, 2002, and 2013, whereas wet conditions (SPEI > 1.75) occurred in 1980, 2006, and 2009.
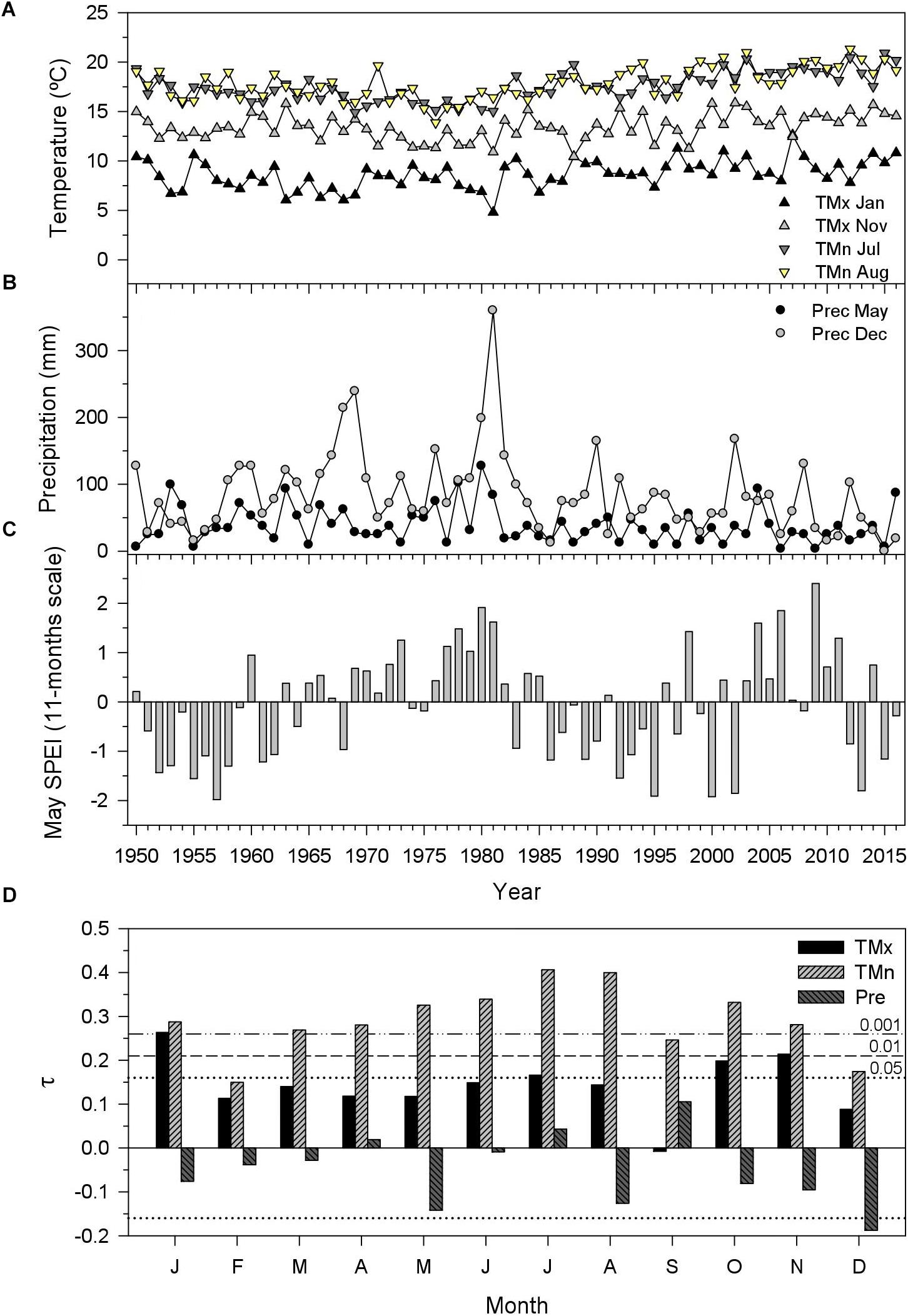
FIGURE 1. Climate trends and drought patterns in the study area. The selected climate variables correspond to monthly mean maximum (TMx) and minimum (TMn) temperatures (A) and precipitation [(B) Prec] showing the most pronounced trends for the 1950–2016 period as assessed through the tau (τ) statistic (D). The drought severity was assessed using the May Standardized Precipitation Evapotranspiration Index (SPEI) for 11-months long scales (C), which showed high associations to tree growth (see Figure 4).
Density and Size of Declining and Non-declining Trees
The densities of D trees were 262 trees ha-1 (64% of trees) and 43 trees ha-1 (30% of trees) in Q. cerris and Q. pubescens, respectively (Table 1). ND trees had thicker stems (greater dbh) and were taller than D trees in both species, but age did not differ between the two vigor classes (Table 1).

TABLE 1. Main structural characteristics of the non-declining (ND) and declining (D) oak trees sampled in Gorgoglione forest.
Growth Patterns and Comparisons of the Two Vigor Classes
Radial growth increased in the 1901s, from the 1930s to 1940s, in the late 1950s and 1960s, in the and early 1970s, and in the 2000s and 2010s; but it decreased in the 1920s, early 1950s and early 1960s, 1980s, and 1990s (Figure 2A). Tree-ring width was higher in ND than in D trees for the periods 2014–2016 and 2010–2016 in Q. pubescens and Q. cerris, respectively (Figure 2A). Considering the common period (1950–2016) ND trees had wider rings than D trees (Q. pubescens, 0.94 vs. 0.73 mm; Q. cerris, 1.22 vs. 1.07 mm), whilst the rest of dendrochronological statistics did not differ between the two vigor classes (Table 2). It is also remarkable the higher correlations of the individual series with the mean series of each vigor class observed in Q. cerris as compared with Q. pubescens, indicating a higher coherence and a higher responsiveness to climate of the former species as compared with the latter.
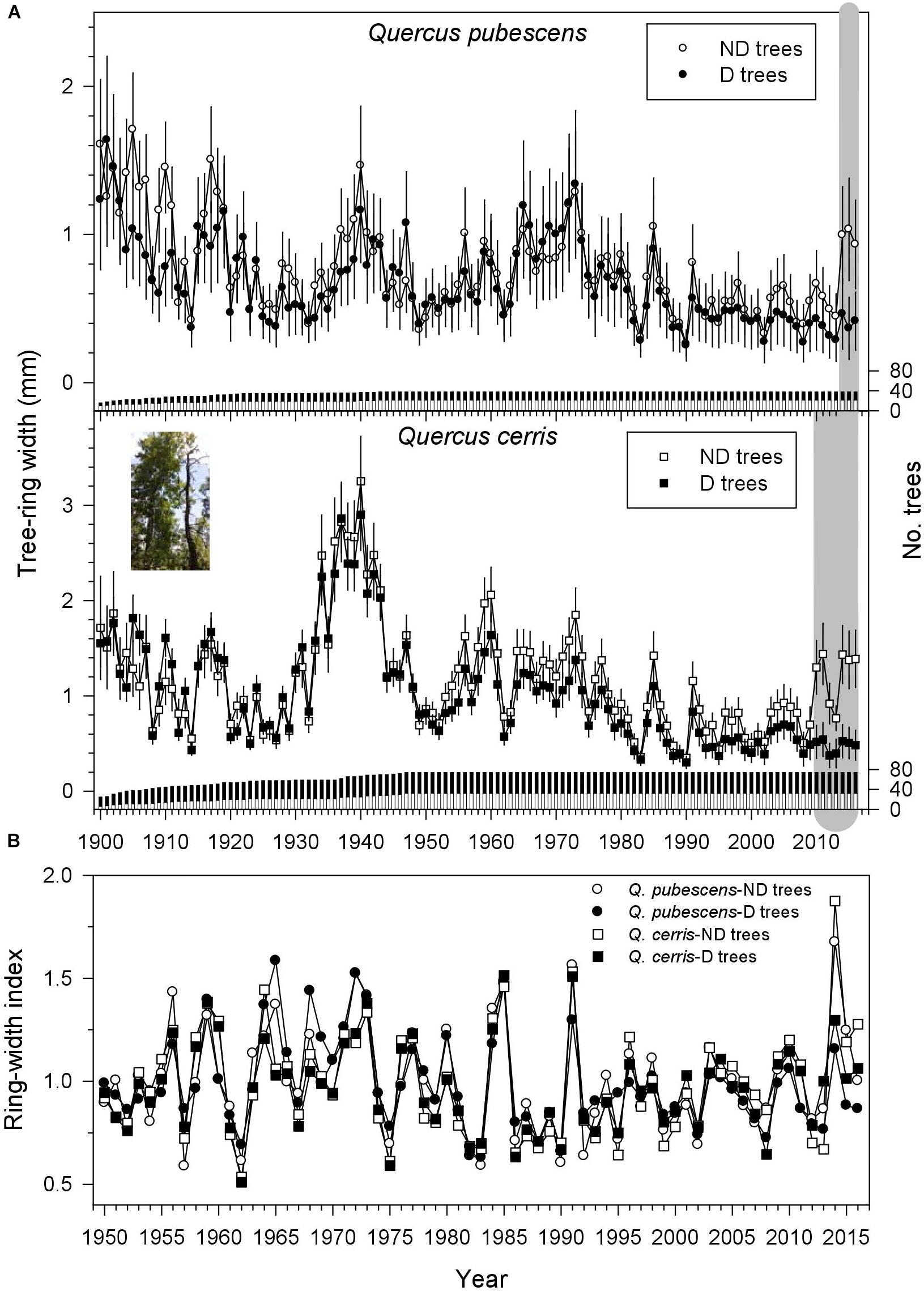
FIGURE 2. Radial-growth patterns from 1900 to 2016 of non-declining (ND) and declining (D) trees in (A) Q. pubescens and Q. cerris and (B) residual ring-width chronologies for the best-replicated period (1950–2016). The right y axes show the sample depth. In the plot (A) the gray areas indicate periods when tree-ring width of ND trees was significantly (P < 0.05) higher than in D trees according to Wilcoxon rank-sum tests. Values are mean tree-ring widths ± SE. The inset shows one of the sampled couples of ND and D Q. cerris trees.
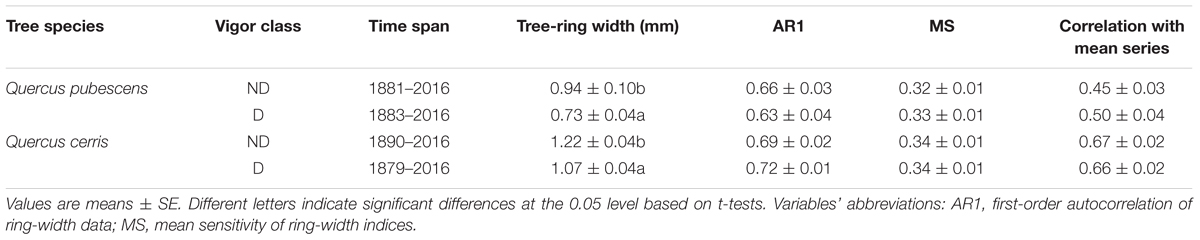
TABLE 2. Tree-ring width statistics of the non-declining (ND) and declining (D) oak trees sampled in Gorgoglione forest and calculated for the common and best-replicated period 1950–2016.
During the common period 1950–2016, sharp growth reductions in both species and vigor classes (e.g., 1957, 1962, 1983, 1986, 2002, and 2012–2013) usually corresponded to dry conditions, whereas growth increases corresponded to wet conditions (1960, 1972–1973, 1977, 1980, 1985, 1998, 2004, and 2009; compare Figures 1C, 2B). An abrupt increase of the ring-width index was observed in ND trees of both species in 2014. The four ring-width chronologies were highly and significantly correlated during 1950–2016 suggesting a high coherence and similar responses to climate (Figure 2B), but the highest and lowest correlations were observed for Q. cerris ND and D trees (r = 0.90, P < 0.001) and for Q. cerris ND and Q. pubescens D trees (r = 0.68, P < 0.001), respectively.
Growth Responses to Climate and Drought
Both Q. pubescens and Q. cerris growth responded negatively to warm previous September conditions, regardless the tree vigor (Figure 3). However, the growth of Q. pubescens D trees was sensitive to high maximum and minimum temperatures in January, April and from current July to September. In Q. cerris, D trees were more sensitive to warm April conditions, whilst warm conditions in current September were negatively associated to growth of both vigor classes. In Q. cerris, high minimum temperatures in the prior late autumn and early winter (November and December) enhanced growth of ND trees. Wet January conditions benefitted growth of both species, particularly of D trees, whereas wet current September conditions (and also in the prior September for Q. pubescens) improved growth of ND trees.
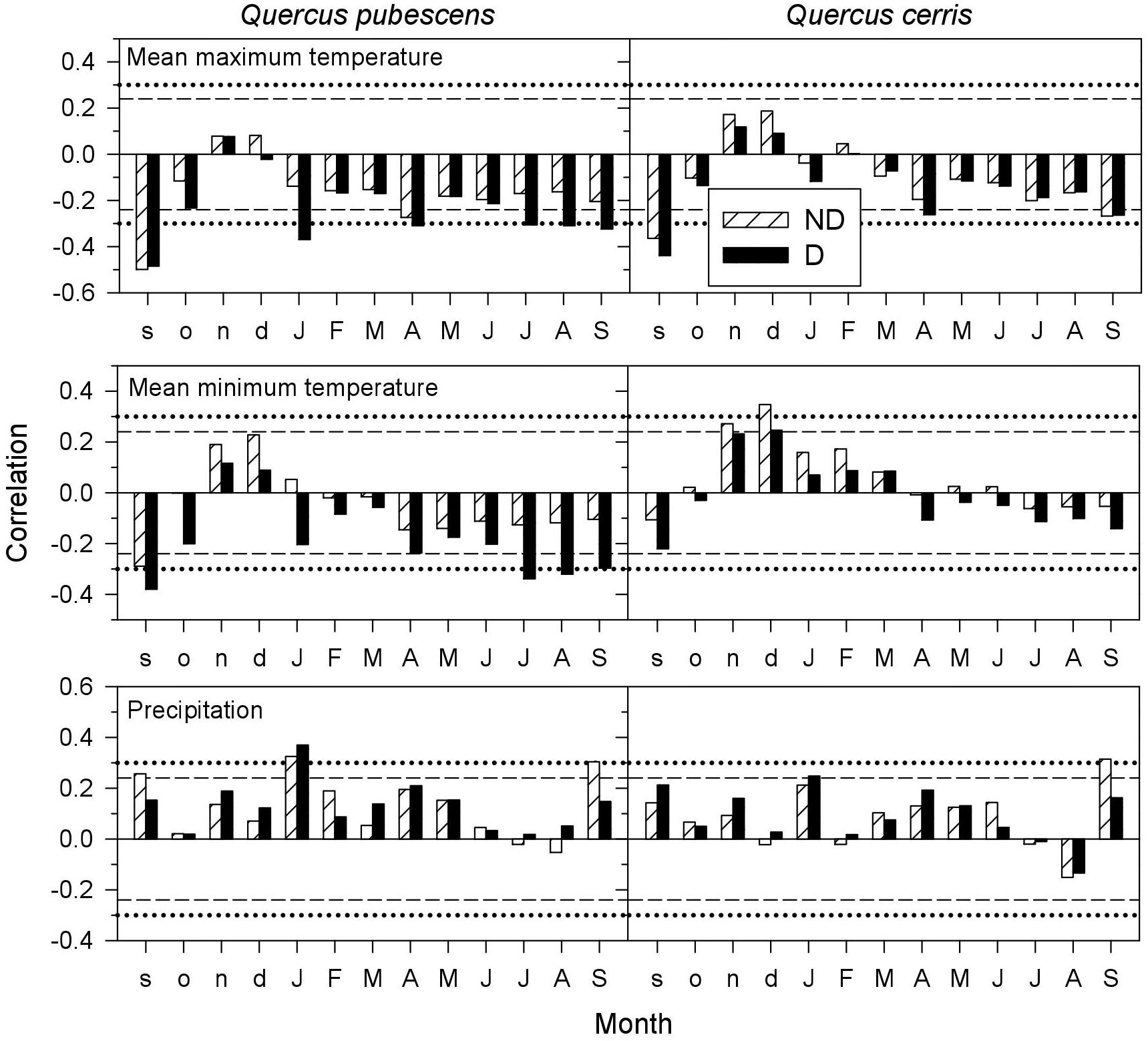
FIGURE 3. Climate-growth relationships (Pearson correlations) in declining (D, filled bars) and non-declining (ND, hatched bars) trees of Quercus pubescens and Quercus cerris. The dashed and dotted horizontal lines indicate the 0.05 and 0.01 significance levels.
In all species and vigor classes, the maximum positive correlations between ring-width indices and SPEI (r = 0.37–0.42) were found for May and considering 11-months long scales, i.e., from the previous July up to May (Figure 4) The highest correlations with May SPEI calculated at 11-months scales were found for Q. pubescens D trees (r = 0.42). In Q. cerris ND trees, very high correlations (r = 0.38) were also observed with January SPEI and considering a 2-month scale.
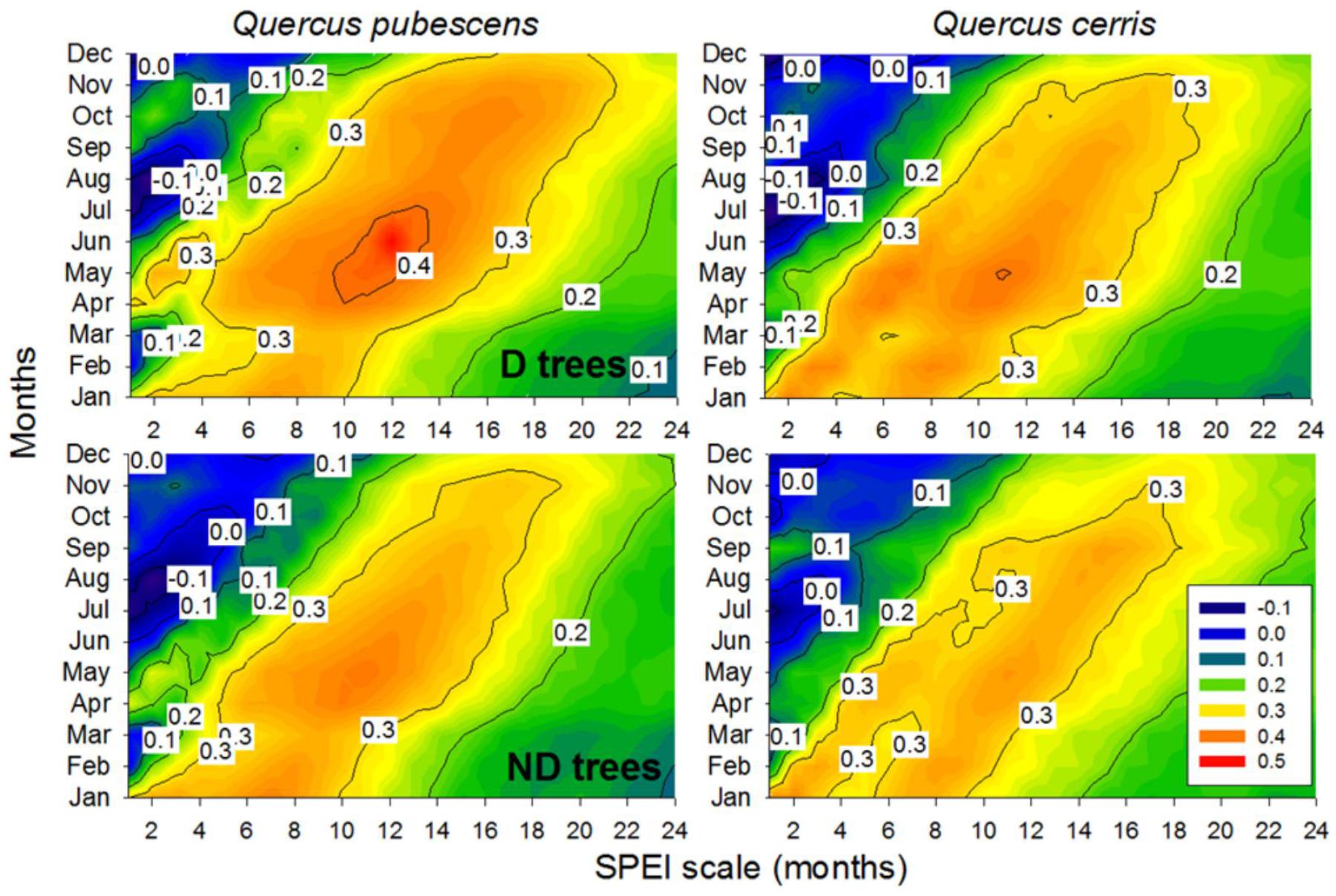
FIGURE 4. Drought-growth relationships (Pearson correlations) in declining (D) and non-declining (ND) trees of Quercus pubescens and Quercus cerris. The color scale shows the correlations between ring-width indices and the Standardized Precipitation Evapotranspiration Index (SPEI) calculated at 1–24 months long scales (x axes) from January to December (y axes). Significance levels are as in Figure 2.
Soil Features and Phytophthora Presence
Soils were of silt loam texture with mean percentages of 55, 32, and 13% for silt, sand and clay. The mean (±SE) soil C and N concentrations were 3.21 ± 0.24 and 0.17 ± 0.01%, respectively (mean C/N ratio is 20.5 ± 4.0). The soil pH was 7.5 ± 0.1 and the soil electrical conductivity was 184.0 ± 8.7 ms cm-1 indicating slight salinity.
We isolated Phytophthora quercina in 40% of the sampled trees. Phytophthora tended (p = 0.068, Chi-square test without Yates correction) to be more frequent on ND than on D trees (same proportion was observed for Q. pubescens and Q. cerris). The low number of infected trees per defoliation class did not allow the comparison of tree ring width between D and ND trees. Nevertheless, comparison of tree-ring width data between Phytophthora-infected and -uninfected trees (regardless of the defoliation class) within each tree species for the periods 2014–2016 and 2010–2016 did not reveal significant differences (Q. pubescens, Mann-Whitney U = 81–149, P = 0.41–0.62; Q. cerris, Mann-Whitney U = 271–319, P = 0.43–0.69; see Supporting Information, Supplementary Figure S1).
Wood C Isotopes
Regardless the vigor class, Q. pubescens showed higher d13C values (mean ± SE = -27.33 ± 0.22‰) than Q. cerris (-27.45 ± 0.19‰), but differences between the two species were not significant (t = 0.42, P = 0.67). Considering the two vigor classes, D trees (-26.85 ± 0.27‰) showed significantly higher d13C values than ND trees (-27.79 ± 0.29‰) in Q. pubescens (t = 2.35, P = 0.026), but differences were not significant in the case of Q. cerris (D trees, -27.39 ± 0.30‰; ND trees, -27.53 ± 0.25‰; t = 0.36, P = 0.72; Table 3). Considering different 5-year periods, d13C and Δ13C were higher in D than in ND trees of Q. pubescens for the period 2007–2011.
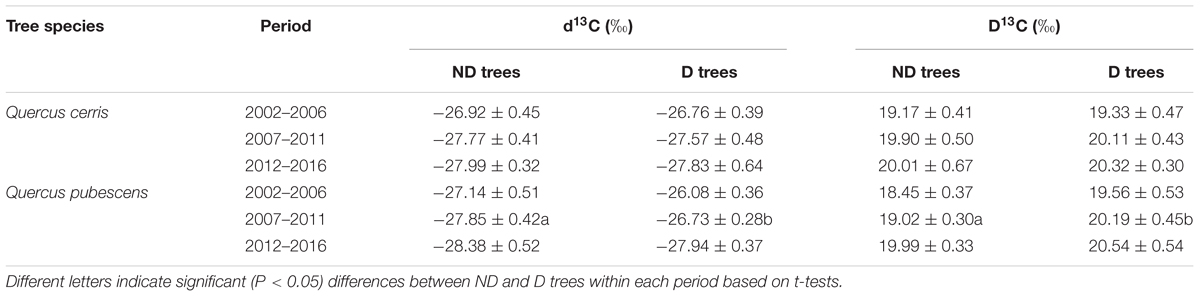
TABLE 3. Wood C isotope radios (d13C) and discrimination (Δ13C) in non-declining (ND) and declining (D) Q. cerris and Q. pubescens individuals considering three 5-year periods.
Discussion
The study area has experienced a rise in minimum temperatures since 1950 and this warming trend coincided with the occurrence of severe droughts in the early 2000s and early 2010s. As expected, these warm and dry conditions probably triggered the oak decline phenomena affecting more Q. cerris (less tolerant to drought) than Q. pubescens (more tolerant to drought). Similarly, Gentilesca et al. (2017), in a recent review, reported drought as the main driver triggering oak decline within the Mediterranean area, although other causes (i.e., increasing temperature, low temperature, tree nutritional status, overaging, excessive stand density or pathogens attack) could have also exacerbated decline.
In this study, the oak decline was characterized by conspicuous leaf shedding and high mortality rates which were preceded by recent divergences in radial growth between ND and D trees (2010–2016 in Q. cerris, 2014–2016 in Q. pubescens).
Our findings agree with previous studies in the region which found that ND trees were larger (thicker diameter, longer stems) than D trees (Colangelo et al., 2017a,b). However, they do not support global reviews suggesting that the tallest trees are more vulnerable to drought-induced hydraulic failure (Bennett et al., 2015). This discrepancy may be explained by several factors making smaller, but dominant, trees more vulnerable to drought damage. First, it is possible that oaks forming short stems develop more shallow roots or do not have access to deep soil-water pools, but this aspect remains understudied. Second, tree height is related to the size of the stem sapwood water reserves and may determine hydraulic capacitance and vulnerability to drought (Zhang et al., 2009).
Considering the period 1950–2016, ND trees presented slightly higher growth rates than D trees in both oak species (29 and 14% higher in Q. pubescens and Q. cerris, respectively). The divergence in growth between both vigor classes was recent, thus indicating that the growth reduction started about 3–7 years in Q. pubescens and Q. cerris, respectively, before the decline intensified and trees started to die. This pattern indicates that slow-growing trees were prone to show decline and does not concur with other studies showing that trees growing vigorously produce vessels of wider lumen and are more prone to xylem embolism and decline (Levaniè et al., 2011; Voltas et al., 2013). In Quercus rubra currently D trees were growing at slower rates than ND trees but D trees grew more in the past than ND trees suggesting that rapid growth early in development led to later decline and mortality (Haavik et al., 2011). However, we did not observe this pattern characterized by D oaks showing rapid growth early in life. The growth increase in the 1930s, which was more evident in Q. cerris than in Q. pubescens, suggests past management in the study forest, probably logging of trees for timber or firewood. This past legacy effects could interact with recent climate warming if formerly logged trees were growing better and were also less vulnerable to drought damage (Camarero et al., 2011), but this potential interaction merits further research.
As previously observed, we also found that radial growth of Mediterranean deciduous oaks is enhanced by mild and wet spring conditions and that the decline was triggered by severe droughts and warm conditions during the growing season (Tessier et al., 1994; Corona et al., 1995; Amorini et al., 1996; Haavik et al., 2011; Alla and Camarero, 2012). Climate-growth associations were usually stronger in non-declining than in declining trees, particularly in the sensitive Q. pubescens, which agrees with Romagnoli et al. (2018). In oaks growth responses to drought are often lagged 1 year and they are characterized by leaf shedding, shoot dieback and the lack of latewood production leading to altered patterns of hydraulic conductivity (Corcuera et al., 2004a,b; Di Filippo et al., 2010). We also observed those patterns but working with mature and dominant individuals in a high forest, whereas other studies on the decline of Mediterranean oak species using dendroecology were developed on coppice stands (e.g., Amorini et al., 1996; Di Filippo et al., 2010). Spring drought was the main triggering factor of dieback in these studies where declining trees often showed lower growth rates than non-declining trees prior (about 20 years before in Q. cerris) to the onset of the decline episode (Amorini et al., 1996). In recently dead Q. robur trees, growth of dying trees was also lower than in surviving trees 1–25 years prior to death (Andersson et al., 2011). By contrast, here the ND-D divergence in growth was recent and lasted from 3 (Q. pubescens) to 7 (Q. cerris) years. These different periods indicate distinct periods of stress and complex interactions between climate stress (drought) and perhaps opportunistic pathogens causing dieback and tree death (Marcais and Bréda, 2006).
The higher responsiveness to year-to-year climate variability of Q. pubescens compared to Q. cerris corresponds to the highest vulnerability to drought damage of Q. cerris. These results agree with previous dendroecological research (Garfi, 2000; Cherubini et al., 2003). It is noticeable that Q. pubescens growth mainly responded to January precipitation whereas Q cerris growth responded more to warm November-December mean minimum temperatures suggesting a higher sensitivity of Q. cerris to warm winter conditions. This could explain the vulnerability to drought of Q. cerris since we detected the most marked decrease of precipitation and increase in maximum temperatures during winter. Interestingly, the growth responsiveness to climate was more marked in D than in ND trees of Q. pubescens trees and the growth loss in response to warm night conditions from July to August observed in D confirms their highest sensitivity to summer drought as confirmed the SPEI-growth analyses.
Q. pubescens shows higher and more variable radial-growth rates than Q. cerris and also a better post-drought resilience or recovery capacity (Tognetti et al., 2007). According to these authors both oak species are able tolerate some drought stress but Q. pubescens better tolerates water shortage than Q. cerris by uptaking deep soil water and using it more efficiently. Overall, this suggests that Q. pubescens can better tolerate drought stress than Q. cerris in terms of wood production and associated processes (carbon uptake and storage, hydraulic conductivity, fine root production, etc.).
We isolated Phytophthora quercina from the rhizosphere of 40% of the trees that we sampled. Whether the observed prevalence may pose a threat for these stands is unknown, but the fact that our isolation frequency of P. quercina was four times higher than the one reported in the north of Italy (Vettraino et al., 2002), may suggest that further monitoring could be carried out. The fact that soil sampling was carried out in winter, when the pathogen is less active, may have underestimated its true incidence, and could indicate that P. quercina is even more widespread than reported. P. quercina is a primary and aggressive pathogen, which causes root necrosis and dieback of fine roots leading to tree decline and death (Jung et al., 1999). In this area, P. quercina was observed more frequently in healthy than in declining trees, indicating that the pathogen did not seem to play a crucial role in the observed decline as a contributing factor. However, the tendency to isolate the pathogen from healthy trees suggests that P. quercina could instead have played a role in the onset of decline, as a predisposing factor (sensu Manion), although the lower number of samples collected in this survey requires caution about the possible role played by the pathogen. In other ecosystems in which Phytophthora spp. also act as primary pathogens, a similar pattern has been observed. For instance, P. alni was more frequently isolated from cankers in relatively symptomless alder trees rather than from highly symptomatic trees (Redondo et al., 2015). Further studies comparing the frequency of decline in areas with and without P. quercina should be carried out in order to confirm the role of P. quercina as predisposing factor. Considering not only the presence, but other more quantitative measures of inoculum could also help elucidating the role of this pathogen in the decline of this area. In parallel, managers could improve forest resilience against Phytophthora attacks by improving soil drainage and restricting further spread or by selecting genotypes resistant to this soil-borne pathogen (Sena et al., 2018).
The carbon isotope discrimination (Δ13C) was used as surrogate of intrinsic water-use efficiency (WUEi), i.e., the ratio between the photosynthesis (A) and the stomatal conductance (g) rates (cf. Ripullone et al., 2004). The D trees of Q. pubescens present a higher WUEi than conspecific ND trees for the period 2007–2011 when the growth divergence between D and ND trees increased. This could be explained because those D trees presented a persistent growth reduction in response to warm and dry conditions during the growing season but at the same time reduced g by forcing stomatal closure, and probably showed a reduction in hydraulic conductivity as is common in anisohydric oak species (Granda et al., 2017). It is unclear why the difference in WUEi between D and ND Q. pubescens trees diminished in the most recent period but it could be a common response to severe dry conditions regardless the vigor class. By contrast, D trees showed lower WUEi than ND trees after the dieback onset in Quercus frainetto, which was associated to higher g and water loss through transpiration (Colangelo et al., 2017a). In Q. robur, dying trees had also lower WUEi than surviving trees (Levaniè et al., 2011), which was interpreted as lower A or to higher g (Farquhar et al., 1989). The difference in WUEi between ND and D trees was found in Q. pubescens but not in Q. cerris which can be attributed to their different ecophysiological behaviors. When these species coexist, Q. pubescens shows a more conservative water-use strategy than Q. cerris, since Q. pubescens presents higher WUEi, and lower photosynthesis rates, stomatal conductance rates and negative midday water potential than Q. cerris (Tognetti et al., 2007). These complex responses related to WUEi indicate the need to increase the samples collection and more studies considering different decline episodes, using several functioning (growth, wood anatomy) and ecophysiological proxies (C and O isotope discrimination) and involving tree species with diverse susceptibility to drought and pathogen damage (Gessler et al., 2018).
Conclusion
Drought and warmer minimum temperatures triggered oak decline in the 2000s and 2010s. Such drier conditions affected more negatively Q. cerris than Q. pubescens. The more tolerant Q. pubescens was also the most responsive to climate and drought in terms of wood production, particularly declining trees. The sensitive Q. pubescens declining trees showed higher water-use efficiency than non-declining trees of the same species before the growth divergence between these two vigor classes started in 2014. Phytophthora quercina was isolated from both oak species, and its incidence tended to be higher in non-declining than in declining trees, suggesting a possible role as predisposing factor. However, considering the reduced number of individual surveyed in this field experiment further studies are needed to support this hypothesis. These findings illustrate complex responses to drought and soil-borne pathogens of coexisting oak species suggesting different tolerances to the combined effects of climate and biotic stressors under the forecasted warmer and drier conditions.
Author Contributions
MC, JC, MB, TG, and FR conceived the idea and contributed to writing the manuscript. MC, TG, and FR carried out the field work. The dataset was analyzed by JC, MC, and JO. Phytophthora analyses were carried out by JO and M-AR. All authors contributed to the interpretation of the results and the revision, discussion, and approval of the final draft.
Funding
This research was supported by the project “Alarm of Forest Mortality in Southern Italy” (Gorgoglione Administration, Basilicata Region, Italy) and by the CGL2015-69186-C2-1-R project (Spanish Ministry of Science).
Conflict of Interest Statement
The authors declare that the research was conducted in the absence of any commercial or financial relationships that could be construed as a potential conflict of interest.
Acknowledgments
MC was supported by the Ph.D. program in Agricultural, Forest and Food Science (University of Basilicata, Italy). We thank the framework of the COST network STReESS for facilitating collaborative work. We acknowledge the E-OBS dataset from the EU-FP6 project ENSEMBLES (http://ensembles-eu.metoffice.com) and the data providers in the ECA&D project (http://www.ecad.eu).
Supplementary Material
The Supplementary Material for this article can be found online at: https://www.frontiersin.org/articles/10.3389/fpls.2018.01595/full#supplementary-material
Footnotes
References
Alla, A. Q., and Camarero, J. J. (2012). Contrasting responses of radial growth and wood anatomy to climate in a Mediterranean ring-porous oak: implications for its future persistence or why the variance matters more than the mean. Eur. J. For. Res. 131, 1537–1550. doi: 10.1007/s10342-012-0621-x
Amorini, E., Biocca, M., Manetti, M. C., and Motta, E. (1996). A dendroecological study in a declining oak coppice stand. Ann. Sci. For. 53, 731–742. doi: 10.1051/forest:19960249
Andersson, M., Milberg, P., and Bergman, K.-O. (2011). Low pre-death growth rates of oak (Quercus robur L.)–Is oak death a long-term process induced by dry years? Ann. For. Sci. 68, 159–168. doi: 10.1007/s13595-011-0017-y
Balci, Y., and Halmschlager, E. (2003). Incidence of Phytophthora species in oak forests in Austria and their possible involvement in oak decline. For. Pathol. 33, 157–174. doi: 10.1046/j.1439-0329.2003.00318.x
Bennett, A. C., McDowell, N. G., Allen, C. D., and Anderson-Teixeira, K. J. (2015). Larger trees suffer most during drought in forests worldwide. Nat. Plants 1:15139. doi: 10.1038/nplants.2015.139
Brasier, C. M. (1996). Phytophthora cinnamomi and oak decline in southern Europe. Environmental constraints including climate change. Ann. Sci. For. 53, 347–358. doi: 10.1051/forest:19960217
Briffa, K. R., and Jones, P. D. (1990). Basic Chronology Statistics and Assessment. In: Methods of Dendrochronology: Applications in the Environmental Sciences, eds E. R. Cook, and L. A. Kairiukstis (Dordrecht: Kluwer Academic Publishers), 137–152.
Cailleret, M., Jansen, S., Robert, E. M. R., Desoto, L., Aakala, T., Antos, J. A., et al. (2017). A synthesis of radial growth patterns preceding tree mortality. Glob. Change Biol. 23, 1675–1690. doi: 10.1111/gcb.13535
Camarero, J. J., Bigler, C., Linares, J. C., and Gil-Pelegrín, E. (2011). Synergistic effects of past historical logging and drought on the decline of Pyrenean silver fir forests. For. Ecol. Manage. 262, 759–769. doi: 10.1016/j.foreco.2011.05.009
Camarero, J. J., Gazol, A., Galván, J. D., Sangüesa-Barreda, G., and Gutiérrez, E. (2015). Disparate effects of global-change drivers on mountain conifer forests: warming-induced growth enhancement in young trees vs. CO2 fertilization in old trees from wet sites. Glob. Change Biol. 21, 738–749. doi: 10.1111/gcb.12787
Cherubini, P., Gartner, B. L., Tognetti, R., Bräker, O. U., Schoch, W., and Innes, J. L. (2003). Identification, measurement and interpretation of tree rings in woody species from Mediterranean climates. Biol. Rev. Camb. Philos. Soc. 78, 119–148. doi: 10.1017/S1464793102006000
Colangelo, M., Camarero, J. J., Battipaglia, G., Borghetti, M., De Micco, V., Gentilesca, T., et al. (2017a). A multi-proxy assessment of dieback causes in a Mediterranean oak species. Tree Physiol. 37, 617–631. doi: 10.1093/treephys/tpx002
Colangelo, M., Camarero, J. J., Borghetti, M., Gazol, A., Gentilesca, T., and Ripullone, F. (2017b). Size matters a lot: drought-affected Italian oaks are smaller and show lower growth prior to tree death. Front. Plant Sci. 8:135. doi: 10.3389/fpls.2017.00135
Colangelo, M., Camarero, J. J., Ripullone, F., Gazol, A., Sánchez-Salguero, R., Oliva, J., et al. (2018). Drought decreases growth and increases mortality of coexisting native and introduced tree species in a temperate floodplain forest. Forests 9:205. doi: 10.3390/f9040205
Cook, E. R., and Krusic, P. J. (2005). ARSTAN Software, version 4.4h3. Palisades, NY: Lamont Doherty Earth Observatory.
Cooke, D. E. L., and Duncan, J. M. (1997). Phylogenetic analysis of Phytophthora species based on ITS1 and ITS2 sequences of the ribosomal RNA gene repeat. Mycol. Res. 101, 667–677. doi: 10.1017/S0953756296003218
Corcuera, L., Camarero, J. J., and Gil-Pelegrín, E. (2004a). Effects of a severe drought on growth and wood-anatomical properties of Quercus faginea. IAWA J. 25, 185–204. doi: 10.1163/22941932-90000360
Corcuera, L., Camarero, J. J., and Gil-Pelegrín, E. (2004b). Effects of a severe drought on Quercus ilex radial growth and xylem anatomy. Trees 18, 83–92. doi: 10.1007/s00468-003-0284-9
Corona, P., Romagnoli, M., and Torrini, L. (1995). Stem annual increments as ecobiological indicators in Turkey oak (Quercus cerris L.). Trees 10, 13–19. doi: 10.1007/BF00197774
Di Filippo, A., Alessandrini, A., Biondi, F., Blasi, S., Portoghesi, L., and Piovesan, G. (2010). Climate change and oak growth decline: dendroecology and stand productivity of a Turkey oak (Quercus cerris L.) old stored coppice in Central Italy. Ann. For. Sci. 67:706. doi: 10.1051/forest/2010031
Dreyer, E., Granier, A., Breda, N., Cochard, H., Epron, D., and Aussenac, G. (1992). “Oak trees under drought constraints: ecophysiological aspects,” in Proceedings of the International Congress: Recent Advances in Studies on Oak Decline, (Brindisi: Selva di Fasano), 293–322.
Drobyshev, I., Linderson, H., and Sonesson, K. (2007). Relationship between crown condition and tree diameter growth in southern Swedish oaks. Env. Monit. Assessm. 128, 61–73. doi: 10.1007/s10661-006-9415-2
Erwin, D. C., and Ribeiro, O. K. (1996). Phytophthora Diseases Worldwide. St. Paul, MN: The American Phytopathological Society.
Farquhar, G. D., Ehleringer, J. R., and Hubick, K. T. (1989). Carbon isotope discrimination and photosynthesis. Annu. Rev. Plant Physiol. 40, 503–537. doi: 10.1146/annurev.pp.40.060189.002443
Führer, E. (1998). “Oak decline in central Europe: a synopsis of hypotheses,” in Proceedings: Population Dynamics, Impacts, and Integrated Management of Forest Defoliating Insects, eds M. L. McManus, A. M. Liebhiold (Banska Stiavnica: U.S. Department of Agriculture Forest Service), 7–24.
Garfi, G. (2000). Climatic signal in tree–rings of Quercus pubescens s.l. and Celtis australis L. in South–eastern Sicily. Dendrochronologia 18, 41–51.
Gärtner, H., and Nievergelt, D. (2010). The core-microtome: a new tool for surface preparation on cores and time series analysis of varying cell parameters. Dendrochronologia 28, 85–92. doi: 10.1016/j.dendro.2009.09.002
Gentilesca, T., Camarero, J. J., Colangelo, M., Nolè, A., and Ripullone, F. (2017). Drought-induced oak decline in the western Mediterranean region: an overview on current evidences, mechanisms and management options to improve forest resilience. iFor. Biogeosci. For 10, 796–806. doi: 10.3832/ifor2317-010
Gessler, A., Cailleret, M., Joseph, J., Schönbeck, L., Schaub, M., Lehmann, M., et al. (2018). Drought induced tree mortality –a tree-ring isotope based conceptual model to assess mechanisms and predispositions. New Phytol. 219, 485–490. doi: 10.1111/nph.15154
Gibbons, J. D., and Chakraborti, S. (2011). Nonparametric Statistical Inference. New York, NY: CRC Press. doi: 10.1007/978-3-642-04898-2_420
Granda, E., Alla, A. Q., Laskurain, N. A., Loidi, J., Sánchez-Lorenzo, A., and Camarero, J. J. (2017). Coexisting oak species, including rear-edge populations, buffer climate stress through xylem adjustments. Tree Physiol. 38, 159–172. doi: 10.1093/treephys/tpx157
Graven, H., Allison, C. E., Etheridge, D. M., Hammer, S., Keeling, R. F., Levin, I., et al. (2017). Compiled records of carbon isotopes in atmospheric CO2 for historical simulations in CMIP6. Geosci. Model Dev. 10, 4405–4417. doi: 10.5194/gmd-10-4405-2017
Haavik, L. J., Billings, S. A., Guldin, J. M., and Stephen, F. M. (2015). Emergent insects, pathogens and drought shape changing patterns in oak decline in North America and Europe. For. Ecol. Manage. 354, 190–205. doi: 10.1016/j.foreco.2015.06.019
Haavik, L. J., Stahle, D. W., and Stephen, F. M. (2011). Temporal aspects of Quercus rubra L. decline and relationship to climate in the Ozark and Ouachita Mountains, Arkansas. Can. J. For. Res. 41, 773–781. doi: 10.1139/x11-018
Haylock, M. R., Hofstra, N., Klein Tank, A. M. G., Klok, E. J., Jones, P. D., and New, M. (2008). A European daily high-resolution gridded data set of surface temperature and precipitation for 1950–2006. J. Geophys. Res. 113:D20119.
Helama, S., Sohar, K., Läänelaid, A., Mäkelä, H. M., and Raisio, J. (2016). Oak decline as illustrated through plant-climate interactions near the northern edge of species range. Bot. Rev. 82, 1–23. doi: 10.1007/s12229-016-9160-3
Holmes, R. L. (1983). Computer–assisted quality control in tree–ring dating and measurement. Tree Ring Res. 43, 69–78. doi: 10.1016/j.dib.2018.08.019
Jeffers, S. N., and Martin, S. B. (1986). Comparison of two media selective for Phytophthora and Pythium species. Plant Dis. 70, 1038–1043. doi: 10.1094/PD-70-1038
Jenkins, M. A., and Pallardy, S. G. (1995). The influence of drought on red oak group species growth and mortality in the Missouri Ozarks. Can. J. For. Res. 25, 1119–1127. doi: 10.1139/x95-124
Jönsson, U. (2004). Phytophthora species and oak decline – can a weak competitor cause significant root damage in a nonsterilized acidic forest soil? New Phytol. 162, 211–222. doi: 10.1111/j.1469-8137.2004.01016.x
Jönsson, U. (2006). A conceptual model for the development of Phytophthora disease in Quercus robur. New Phytologist 171, 55–68. doi: 10.1111/j.1469-8137.2006.01743.x
Jönsson, U., Jung, T., Sonesson, K., and Rosengren, U. (2005). Relationships between health of Quercus robur, occurrence of Phytophthora species and site conditions in southern Sweden. Plant Pathol. 54, 502–511. doi: 10.1111/j.1365-3059.2005.01228.x
Jung, T. (2009). Beech decline in Central Europe driven by the interaction between Phytophthora infections and climatic extremes. For. Pathol. 39, 73–94. doi: 10.1111/j.1439-0329.2008.00566.x
Jung, T., Blaschke, H., and Oßwald, W. (2000). Involvement of soilborne Phytophthora species in Central European oak decline and the effect of site factors on the disease. Plant Pathol. 49, 706–718. doi: 10.1046/j.1365-3059.2000.00521.x
Jung, T., Cooke, D. E. L., Blaschke, H., Duncan, J. M., and Oßwald, W. (1999). Phytophthora quercina sp. nov., causing root rot of European oaks. Mycol. Res. 103, 785–798. doi: 10.1017/S0953756298007734
Levanič, T., Čater, M., and McDowell, N. G. (2011). Associations between growth, wood anatomy, carbon isotope discrimination and mortality in a Quercus robur forest. Tree Physiol. 31, 298–308. doi: 10.1093/treephys/tpq111
Marcais, B., and Bréda, N. (2006). Role of an opportunistic pathogen in the decline of stressed oak trees. J. Ecol. 94, 1214–1223. doi: 10.1111/j.1365-2745.2006.01173.x
McDowell, N. G., Pockman, W. T., Allen, C. D., Breshears, D. D., Cobb, N., Kolb, T., et al. (2008). Mechanisms of plant survival and mortality under drought: why do some plants survive while others succumb to drought? New Phytol. 178, 719–739. doi: 10.1111/j.1469-8137.2008.02436.x
Nardini, A., Battistuzzo, M., and Savi, T. (2013). Shoot desiccation and hydraulic failure in temperate woody angiosperms during an extreme summer drought. New Phytol. 200, 322–329. doi: 10.1111/nph.12288
Oliva, J., Stenlid, J., and Martínez-Vilalta, J. (2014). The effect of fungal pathogens on the water and carbon economy of trees: implications for drought-induced mortality. New Phytol. 203, 1028–1035. doi: 10.1111/nph.12857
Pedersen, B. S. (1998). The role of stress in the mortality of Midwestern oaks as indicated by growth prior to death. Ecology 79, 79–93. doi: 10.1890/0012-9658(1998)079[0079:TROSIT]2.0.CO;2
Redondo, M. A., Boberg, J., Olsson, C. H. B., and Oliva, J. (2015). Winter conditions correlate with Phytophthora alni subspecies distribution in Southern Sweden. Phytopathology 105, 1191–1197. doi: 10.1094/PHYTO-01-15-0020-R
Ripullone, F., Lauteri, M., Grassi, G., Amato, M., and Borghetti, M. (2004). Variation in nitrogen supply changes water-use efficiency of Pseudotsuga menziesii and Populus x euroamericana: a comparison of three approaches to determine water-use efficiency. Tree Physiol. 24, 671–679. doi: 10.1093/treephys/24.6.671
Romagnoli, M., Moroni, S., Recanatesi, F., Salvati, F., and Mugnozza, G. S. (2018). Climate factors and oak decline based on tree-ring analysis. A case study of peri-urban forest in the Mediterranean area. Urban For. Urban Green. 34, 17–28. doi: 10.1016/j.ufug.2018.05.010
Samils, B., Ihrmark, K., Kaitera, J., Stenlid, J., and Barklund, P. (2011). New genetic markers for identifying Cronartium flaccidum and Peridermium pini and examining genetic variation within and between lesions of Scots pine blister rust in Sweden. Fungal Biol. 115, 1303–1311. doi: 10.1016/j.funbio.2011.09.009
Sànchez, M. E., Ferraz, P. C. J., and Trapero-Casas, A. (2002). Phytophthora disease of Quercus ilex in South-Western Spain. For. Pathol. 32, 5–18. doi: 10.1046/j.1439-0329.2002.00261.x
Sangüesa-Barreda, G., Camarero, J. J., Oliva, J., Montes, F., and Gazol, A. (2015). Past logging, drought and pathogens interact and contribute to forest dieback. Agric. For. Meteorol. 208, 85–94. doi: 10.1016/j.agrformet.2015.04.011
Sena, K., Crocker, E., Vincelli, P., and Barton, C. (2018). Phytophthora cinnamomi as a driver of forest change: Implications for conservation and management. For. Ecol. Manage. 409, 799–807. doi: 10.1016/j.foreco.2017.12.022
Sohar, K., Helama, S., Läänelaid, A., Raisio, J., and Tuomenvirta, H. (2014). Oak decline in a southern Finnish forest as affected by a drought sequence. Geochronometria 41, 92–103. doi: 10.2478/s13386-013-0137-2
Tainter, F. H., Retzlaff, W. A., Starkey, D. A., and Oak, S. W. (1990). Decline of radial growth in red oaks is associated with short-term changes in climate. Eur. J. For. Pathol. 20, 95–105. doi: 10.1111/j.1439-0329.1990.tb01277.x
Taubner, H., Roth, B., and Tippkotter, R. (2009). Determination of soil texture: comparison of the sedimentation method and the laser-diffraction analysis. J. Plant Nutr. Soil Sci. 172, 161–171. doi: 10.1002/jpln.200800085
Tessier, L., Nola, P., and Serre-Bachet, F. (1994). Deciduous Quercus in the Mediterranean region – tree-ring/climate relationships. New Phytol. 126, 355–367. doi: 10.1093/treephys/tpu037
Thomas, F. M., Blank, R., and Hartmann, G. (2002). Abiotic and biotic factors and their interactions as causes of oak decline in Central Europe. For. Pathol. 32, 277–307. doi: 10.1046/j.1439-0329.2002.00291.x
Tognetti, R., Cherubini, P., Marchi, S., and Raschi, A. (2007). Leaf traits and tree rings suggest different water-use and carbon assimilation strategies by two co-occurring Quercus species in a Mediterranean mixed-forest stand in Tuscany. Italy. Tree Physiol. 27, 1741–1751. doi: 10.1093/treephys/27.12.1741
Tognetti, R., Longobucco, A., Miglietta, F., and Raschi, A. (1999). Water relations, stomatal response and transpiration of Quercus pubescens trees during summer in a Mediterranean carbon dioxide spring. Tree Physiol. 19, 261–270. doi: 10.1093/treephys/19.4-5.261
Valentini, R., Scarascia-Mugnozza, G., Giordano, E., and Vannini, A. (1992). “Water relations of Mediterranean oaks. Possible influences on their dieback,” in Proceedings of the International Congress Recent Advances in Studies on Decline (Brindisi: Selva di Fasano), 439–446.
Vettraino, A. M., Barzanti, G. P., Bianco, M. C., Ragazzi, A., Capretti, P., Paoletti, E., et al. (2002). Occurrence of Phytophthora species in oak stands in Italy and their association with declining oak trees. For. Pathol. 32, 19–28. doi: 10.1046/j.1439-0329.2002.00264.x
Vicente-Serrano, S. M., Beguería, S., and López-Moreno, J. I. (2010). A multi-scalar drought index sensitive to global warming: the Standardized Precipitation Evapotranspiration Index -SPEI. J Clim. 23, 1696–1718. doi: 10.1175/2009JCLI2909.1
Voltas, J., Camarero, J. J., Carulla, D., Aguilera, M., Oriz, A., and Ferrio, J. P. (2013). A retrospective, dual-isotope approach reveals individual predispositions to winter-drought induced tree dieback in the southernmost distribution limit of Scots pine. Plant, Cell Environ. 36, 1435–1448. doi: 10.1111/pce.12072
Wang, H., Qi, M., and Cutler, A. J. (1993). A simple method of preparing plant samples for PCR. Nucleic Acids Res. 21, 4153–4154. doi: 10.1093/nar/21.17.4153
Wargo, P. M. (1996). Consequences of environmental stress on oak: predisposition to pathogens. Ann. For. Sci. 53, 359–368. doi: 10.1051/forest:19960218
White, T., Bruns, T., Lee, S., and Taylor, J. (1990). “Amplification and direct sequencing of fungal ribosomal RNA genes for phylogenetics,” in PCR Protocols: A Guide to Methods and Applications, eds M. Innis, D. Gelfand, J. Sninsky, and T. White (San Diego, CA: Academic Press), 315–322.
Zhang, Y. J., Meinzer, F. C., Hao, G. Y., Scholz, F. G., Bucci, S. J., Takahashi, F. S. C., et al. (2009). Size-dependent mortality in a Neotropical savanna tree: the role of height-related adjustments in hydraulic architecture and carbon allocation. Plant Cell Environ. 32, 1456–1466. doi: 10.1111/j.1365-3040.2009.02012.x
Keywords: carbon isotopes, dendroecology, drought stress, forest dieback, Phytophthora species, Quercus cerris, Quercus pubescens, water-use efficiency
Citation: Colangelo M, Camarero JJ, Borghetti M, Gentilesca T, Oliva J, Redondo M-A and Ripullone F (2018) Drought and Phytophthora Are Associated With the Decline of Oak Species in Southern Italy. Front. Plant Sci. 9:1595. doi: 10.3389/fpls.2018.01595
Received: 05 July 2018; Accepted: 15 October 2018;
Published: 05 November 2018.
Edited by:
Risto Kasanen, University of Helsinki, FinlandReviewed by:
Joaquín Duque-Lazo, Universidad de Córdoba, SpainJesus V. Jorrin Novo, Universidad de Córdoba, Spain
Copyright © 2018 Colangelo, Camarero, Borghetti, Gentilesca, Oliva, Redondo and Ripullone. This is an open-access article distributed under the terms of the Creative Commons Attribution License (CC BY). The use, distribution or reproduction in other forums is permitted, provided the original author(s) and the copyright owner(s) are credited and that the original publication in this journal is cited, in accordance with accepted academic practice. No use, distribution or reproduction is permitted which does not comply with these terms.
*Correspondence: Michele Colangelo, michelecolangelo3@gmail.com