- 1Department of Plant Sciences, Centre de recherche et d’innovation sur les végétaux, Université Laval, Québec, QC, Canada
- 2Department of Forest Ecology and Management, Swedish University of Agricultural Sciences, Umeå, Sweden
- 3Agriculture and Agri-Food Canada, Quebec Research and Development Centre, Québec, QC, Canada
- 4Department of Soil and Agri-Food Engineering, Centre de recherche et d’innovation sur les végétaux, Université Laval, Québec, QC, Canada
Organic nitrogen is now considered a significant source of N for plants. Although organic management practices increase soil organic C and N content, the importance of organic N as a source of crop N under organic farming management systems is still poorly understood. While dual-labeled (13C and 15N) molecule methods have been developed to study amino acid uptake by plants, multiple biases may arise from pre-uptake mineralization by microorganisms or post-uptake metabolism by the plant. We propose the combination of different isotopic analysis methods with molecule isotopologues as a novel approach to improve the accuracy of measured amino acid uptake rates in the total N budget of cucumber seedlings and provide a better characterization of post-uptake metabolism. Cucumber seedlings were exposed to solutions containing L-Ala-1-13C,15N or U-L-Ala-13C3,15N, in combination with ammonium nitrate, at total N concentrations ranging from 0 to 15 mM N and at inorganic/organic N ratios from 10:1 to 500:1. Roots and shoots were then subjected to bulk stable isotope analysis (BSIA) by Isotope Ratio Mass Spectrometry (IRMS), and to compound-specific stable isotope analysis (CSIA) of the free amino acids by Gas Chromatography – Combustion – Isotope Ratio Mass Spectrometry (GC-C-IRMS). Plants exposed to a lower inorganic:organic N ratio acquired up to 6.84% of their N from alanine, compared with 0.94% at higher ratio. No 13C from L-Ala-1-13C,15N was found in shoot tissues suggesting that post-uptake metabolism of Ala leads to the loss of the carboxyl-C as CO2. CSIA of the free amino acids in roots confirmed that intact Ala is indeed taken up by the roots, but that it is rapidly metabolized. C atoms other than from the carboxyl group and amino-N from Ala are assimilated in other amino acids, predominantly Glu, Gln, Asp, and Asn. Uptake rates reported by CSIA of the free amino acids are nevertheless much lower (16–64 times) than those reported by BSIA. Combining the use of isotopologues of amino acids with compound-specific isotope analysis helps reduce the bias in the assessment of organic N uptake and improves the understanding of organic N assimilation especially in the context of organic horticulture.
Introduction
Nitrogen is usually considered as being more readily available for plant uptake in its inorganic forms, NO3- and NH4+ (Nacry et al., 2013). However, it is now well established that plants can also take up N directly as organic molecules (Näsholm et al., 2009) such as amino acids and peptides, as most of the transporters and related genes are known, at least for Arabidopsis thaliana (Rentsch et al., 2007; Tegeder and Rentsch, 2010). The capacity to use organic N allows plants to access a wide array of N sources (Paungfoo-Lonhienne et al., 2012; Warren, 2014), and thus it is important to consider the availability of organic molecules in fertilization planning in agriculture.
The uptake of organic N (ON) was, at first, considered a secondary source of N, mostly useful in environments with low inorganic N (IN) availability such as arctic or boreal ecosystems (Näsholm et al., 1998; Henry and Jefferies, 2003; Schimel and Bennett, 2004; Zhou et al., 2013; Hu et al., 2017). Conversely, more recent studies on agricultural (Näsholm et al., 2000; Okamoto and Okada, 2004; Jämtgård et al., 2008; Ge et al., 2009; Gioseffi et al., 2012; Brackin et al., 2015; Moran-Zuloaga et al., 2015) and sub-tropical plants (Wei et al., 2015) demonstrated that ON uptake accounts for a significant portion of the N budget of the plant, although IN is still often the preferred N source. In addition, organic molecules have been found to be the preferred N source for some tree species, even when IN is abundant (e.g., beech, see Li et al., 2015). Organic N molecules have the advantage of providing reduced N along with a C source, which could improve the C budget and N use efficiency of the plant by minimizing the energy required for N reduction and assimilation (Franklin et al., 2017; Ganeteg et al., 2017). It has also been established that plants can take up ON even in the presence of IN (Gioseffi et al., 2012; Gruffman et al., 2014; Czaban et al., 2016), sometimes with no effect on ON uptake rate (e.g., in wheat, Gioseffi et al., 2012). Nonetheless, knowledge of the effect of varying availability of IN relative to ON (IN:ON ratio) on the uptake of ON, at varying total N concentrations, is still missing and could help determine the nutritional contexts in which ON uptake is most relevant.
Organic agriculture is increasingly considered as a realistic and more sustainable alternative to conventional intensive agriculture for meeting the global challenges in food safety and security (Reganold and Wachter, 2016). In order to meet the requirements for organic certification, N must be provided to the crops entirely or predominantly as organic residues. Specifically, greenhouse crops require very high levels of fertilization (Cuijpers et al., 2008; Wang et al., 2017; Dorais and Schwarz, 2018). The synchronization of N supply by organic fertilizers with N demand by the crop was identified as one of the most important challenges in the next years for improving yields, while reducing environmental impacts of greenhouse organic horticulture (Reeve et al., 2016; Tittarelli et al., 2016). Organic management of soils generally increases the total organic C (Ge et al., 2011) and the concentration of free amino acids in the soil solution (Ge et al., 2012). Paradoxically, the uptake of organic N molecules has rarely been studied in open field organic horticulture (Song et al., 2010), and never in greenhouse organic horticulture where applications of organic amendments are frequent. Better knowledge of the significance of the uptake of organic N molecules in the global N budget of organic horticultural plants, especially in greenhouses, could help improving the planning of organic fertilization.
The use of dual-labeled (13C and 15N) organic molecules combined with bulk stable isotope analysis (BSIA) is one of the key methods used for ON root uptake experiments. Monitoring the 13C intake along with 15N, following exposure of the roots to a dual-labeled amino acid, increases the certainty that the isotopic enrichment is due to intact amino acid uptake, rather than uptake of IN following mineralization of the labeled amino acid. Universally labeled molecules (UL), in which all the C and N atoms are of the heavy isotope type, are typically used for ON root uptake experiments (e.g., Näsholm et al., 1998; Ge et al., 2009; Czaban et al., 2016; Franklin et al., 2017). They are available at a reasonable cost and have the advantage of providing a strong isotopic signal in the plant. The monitoring of ON uptake with BSIA can be biased by pre-uptake metabolism of the intact molecule by soil microbes or by post-uptake metabolism (Sauheitl et al., 2009; Rasmussen et al., 2010) and 13CO2 respiration inside the plant (Ganeteg et al., 2017). Compound specific isotope analysis (CSIA) by gas chromatography – combustion – isotope-ratio mass spectrometry (GC-C-IRMS) has recently been used in the study of amino acid uptake to reduce the bias associated with the use of BSIA, by monitoring the isotopic signature specifically in amino acids of interest (Sauheitl et al., 2009). Analysis of the intact universally labeled amino acid using liquid chromatography – mass spectrometry (LC-MS) is another CSIA method for detection of the intact amino acid molecule taken up by the plant (Gaudin et al., 2014; Czaban et al., 2016; Ganeteg et al., 2017). Due to the rapid metabolism of amino acids following their uptake, these methods are not free from biases in the evaluation of intact ON uptake and for these reasons underestimate uptake (Ganeteg et al., 2017). It is nevertheless a tool with great potential for determining uptake rates and for studying the post-uptake metabolism of ON and its related C inside the plant. Using all of these tools, the duration of the exposure of the plants to the labeled solution and their subsequent processing must be planned with care due to the fast processes of degradation in the soil (or nutrient solution) and plant metabolism after uptake. Moran-Zuloaga et al. (2015) compared the use of UL and position-specific labeled (PSL) amino acids and reported that UL amino acids overestimate the uptake of intact molecules measured by BSIA, relative to PSL amino acids. They suggest using amino acids with only the carboxyl-C atom as 13C, because this C is usually the first to be removed from the molecule by decarboxylation from the action of soil microorganisms or plant metabolism. Thus, the use of PSL amino acids may provide more accurate estimates of uptake rates of intact amino acids compared to UL amino acids. More specifically, using CSIA in an experiment combining the use of UL and PSL molecules would provide information on the fate of specific C atoms from the labeled amino acid taken up by the plant.
The objective of the present study was to characterize the significance of organic N acquisition as alanine in the total N budget of cucumber seedlings grown under different N forms and concentrations, and IN:ON ratios relevant to greenhouse organic horticulture. The underlying hypothesis was that cucumber plants rely mostly on inorganic N as N source but, when exposed to low IN:ON ratios or low total N availability, organic N source could become significant. To discriminate between the N sources utilized by the plants, we used dual labeled Alanine (13C and 15N) as source of organic N. We also wanted to test the combined approach of using CSIA with PSL amino acids to better understand the fate of amino acids taken up within the cucumber plant following exposure to labeled Alanine.
Materials and Methods
Experimental Setup and Labeled Solutions
The experiment was set up as five complete randomized blocks, with 19 plants per block. Each plant was exposed to one of 19 different labeled solutions for a period of 2 h. The labeled solutions were a mixture of dual-labeled L-Alanine (either L-Ala-1-13C,15N with 13C at the carboxyl-C, or universally labeled L-Ala-13C3,15N; purchased from Sigma-Aldrich) as ON source, and unlabeled ammonium nitrate (NH4NO3) as IN source.
The contents of labeled solutions are detailed in Table 1. The maximum N concentration (15 mM) is the suggested N concentration in fertigation for conventional greenhouse horticulture of cucumber in Ontario, Canada (OMAFRA, 2010). Solutions S1–S9 consist of a combination of three IN:ON ratios, at three different total N concentrations, using Ala-1-13C,15N as the ON source, to test how varying N concentration and IN:ON ratio affect Ala uptake. Solutions S7–S18 aimed at comparing the use of UL and PSL Ala, in presence or absence of IN. Solutions S13–S18 contain U-Ala-13C3,15N as the ON source. Solutions S13–S15 are the same as S7–S9 (IN:ON ratio of 10:1, at three different total N concentrations), but with the different types of labeled Ala. Solutions S10–S12 and S16–S18 contain only amino acids, without ammonium nitrate, at the same Ala concentrations as, respectively, solutions S7–S9 and S13–S15. CSIA was performed on free amino acids in roots and shoots from plants exposed to solutions S8, S11, S14, S17, and S0.
We used ammonium nitrate as IN source to avoid the possible interaction between N uptake and the presence of another cation. According to Roosta and Schjoerring (2007), cucumber growth is not hindered by ammonium toxicity when ammonium is provided in equimolar concentration with nitrate. The choice of Alanine (Ala) as organic N source was based on the results of a previous greenhouse experiment on cucumber in which organic N amendments were provided by a mixture of blood meal and feather meal in a peat moss-based organic substrate (Dion et al., 2017). The analysis of cucumber xylem sap samples and soil water extracts showed that Ala, which was the most abundant amino acid in the substrate, was also present in the xylem sap showing its ability to be taken up by cucumber. The 10:1, 100:1, and 500:1 IN:ON ratios are approximations of the ratios of inorganic-N to, respectively, soluble organic-N, amino acid-N and Ala-N in the soil that were observed in the same experiment.
Plant Growth and Sampling
A total of 116 seeds of cucumber (Cucumis sativus cv. Verdun) were placed between two layers of wet brown paper in 100 × 20 mm petri dishes (two seeds per dish) and let at room temperature for germination. The paper was kept wet daily by the addition of deionized water. After 4 days, when the cotyledons started to unfold, the seedlings, still in their brown paper to support the roots, were transferred in 50 mL tubes (one seedling per tube), with a few mL of deionized water to keep the paper moist. The tubes were placed in a growth chamber for 1 h the first day, 2 h the second day, 10 h the third day, and the entire day henceforth, to acclimate the plants to growth chamber conditions. The growth chamber provided the following conditions: 25°C temperature, relative humidity of 75% and 750 μmol ⋅ m-2 ⋅s-1 PPFD at leaf height, with a photoperiod of 18 h. Four days after their transfer into 50 mL tubes, when the cotyledons were fully expanded and the first leaf started to unfold, damaged seedlings were discarded and 19 healthy seedlings per complete randomized block, for a total of 95 plants, were transferred in 250 mL Mason jars (one plant per jar) filled with the following nutritive solution: 0.85 g ⋅ L-1 calcium nitrate, 1.15 g ⋅ L-1 of 6-11-31 (N-P2O5-K2O) hydroponic fertilizer (Plant-Prod, Brampton, ON, Canada) and 0.013 g ⋅ L-1 L-Alanine. The resulting mineral content of the growth solution was (mg ⋅ L-1): 203 N (IN:ON ratio 100:1), 55.2 P, 296 K, 34.5 Mg, 208 Ca, 40 S, 3.45 Fe, 0.69 Mn, 0.23 Zn, 0.0046 Cu, and 0.31 B.
In order to limit size difference among plants at the time of sampling, the seeds were separated in two groups: the first one for blocks 1–3, and the second one for blocks 4 and 5. The seeds of blocks 4 and 5 were humidified 3 days after those of blocks 1–3, and all following manipulations were also delayed 3 days relative to blocks 1–3.
Exposure to the labeled solution was performed when the plants in the first block had two true leaves fully unfolded. One complete block was sampled each day, for 5 consecutive days. The plants were sampled in sequence from the lowest to the highest 13C and 15N concentrations in order to limit contamination. The roots of each plant were submerged once in a 5 mM CaCl2 solution and twice in deionized water (the solution and the deionized water were replaced after each plant), then placed in a new 250 mL Mason jar containing 195 mL of the labeled solution. The plants were exposed 2 h to the solution, then sampled. The roots and the shoot were separated. The roots were again submerged once in CaCl2 5 mM and twice in deionized water. The shoot was also washed with deionized water in order to remove potential fertilizer residues. The volume of the remaining (not taken up by the plant) labeled solution was measured in a graduated cylinder. Fifteen milliliter of this solution was sampled and frozen for subsequent NO3- and NH4+ analysis by ion chromatography (ICS-1100 for NH4+ and ICS-2100 for NO3-; Dionex Corporation, Sunnyvale, CA, United States) for calculating the IN uptake. The clean roots and shoots were placed separately in pre-weighted Al envelopes and immediately frozen in liquid N. They were then kept at -80°C for a few days before being freeze-dried, weighed and ground to powder in a mortar with pestle.
Isotopic Analyses
Dried and ground samples were enclosed in tin capsules and sent to the Stable Isotope Facility at the University of California, Davis, CA, United States, for BSIA of the 13C and 15N enrichment along with total C and N content (PDZ Europa ANCA-GLS elemental analyzer interfaced to a PDZ Europa 20-20 isotope ratio mass spectrometer; Sercon Ltd., Crewe, Cheshire, United Kingdom).
Based on the method described by Walsh et al. (2014), the remaining of samples exposed to solutions S0, S8, S11, S14, and S17 were used for CSIA of 13C and 15N in free amino acids by gas chromatography – combustion – isotope ratio mass spectrometry (GC-C-IRMS), in a Trace GC Ultra gas chromatograph, coupled to a Thermo Delta V Plus isotope ratio mass spectrometer through a GC IsoLink (Thermo Scientific, Waltham, MA, United States) at the Stable Isotope Facility at the University of California. Free amino acids were extracted in ethanol 80% for 30 min. Analyzed amino acids were Ala, Asx, Glx, Gly, Ile, Leu, Lys, Met, Phe, Pro, Thr, Tyr, and Val. Gln and Glu are grouped as “Glx” and Asn and Asp are grouped as “Asx,” because the columns used did not allow complete separation of the corresponding peaks. Lys, Met, Tyr, and Thr were below the limit of quantification in all samples.
Quantification of Free Amino Acids
Plant samples exposed to solutions S0, S8, S11, S14, and S17 yielded just enough dry material for CSIA. Since GC-C-IRMS does not allow quantification of the amino acids enriched in 13C or 15N, free amino acids were quantified in other samples and extrapolated to the plants submitted to CSIA. Free amino acids from the freeze-dried and ground samples exposed to solutions S1, S5, S7, S9, S10, and S12 were extracted by suspending 0.2 g leaves and 0.1 g roots in, respectively, 7 and 4 mL 80% ethanol (Carpena-Ruiz et al., 1989). The ethanol extracts were evaporated, then the residue containing the amino acids was re-solubilized in water. The amino acids were derivatized using the AccQ⋅TagTM Ultra method, before quantification in a UPLC (Waters Corporation, Milford, MA, United States). The concentrations of free amino acids in samples that were quantified are presented in Supplementary Figure 1. The correspondence between samples in which free amino acids were quantified (S1, S5, S7, S9, S10, and S12) and samples submitted to CSIA (S0, S8, S11, S14, and S17) is detailed in Supplementary Table 1.
Uptake Calculations
The 13C and 15N excess in root and shoot samples were calculated using the difference in atom% between samples exposed to the labeled and unlabeled (S0 in Table 1) solutions and converted to μmol (15N or eq-13C) ⋅ g-1 dw ⋅ h-1 using the dry weight and total C or N content of the samples. In order to allow a comparison of the assessment of 13C enrichment by both types of labeled Ala (Ala-1-13C,15N or U-Ala-13C3,15N), the excess in μmol equivalent 13C (“eq-13C”) was estimated by dividing by 3 the 13C excess in samples exposed to U-Ala-13C3,15N. The assimilation of 13C and 15N in free amino acids was expressed in nmol (15N or eq-13C) ⋅ g-1 dw ⋅ h-1, by multiplying the free amino acid 13C or 15N enrichment (in nmol 15N or eq-13C ⋅ mol-1 AA ⋅ h-1) by the free amino acid concentration (mol AA ⋅ g-1 dw).
The IN uptake was calculated using the NO3- and NH4+ depletion in the labeled solution and the volume of solution absorbed by the roots. This allowed to express the Ala uptake as mmol eq-13C or 15N ⋅ mol-1 total N, in order to study the proportion of total N uptake due to Ala uptake.
Statistical Analyses
Statistical analyses were performed using R 3.4.3 (R Core Team, 2018) with packages aod 1.3 (Lesnoff and Lancelot, 2012; for Wald test), lme4 1.1–15 (Bates et al., 2015), lmerTest 2.0–36 (Kuznetsova et al., 2017), and multcomp 1.4–8 (Hothorn et al., 2008).
An ANOVA followed by a Dunnett test (α = 0.05) was first performed comparing the bulk 13C and 15N enrichments of shoots and roots of the plants exposed to all labeled solutions (S1–S18) with those exposed to the non-labeled control solution (S0).
A non-linear regression following the Michaelis-Menten kinetics was performed on the eq-13C and the 15N rate of enrichment (v) against the Ala-N concentration [Ala] of the solution to which the plant was exposed, in order to estimate the maximum uptake rate, Km, and the affinity constant, Vmax, parameters.
In all curves, the eq-13C or 15N enrichment of plants exposed to S0 was used for 0 mM Ala. The Wald test was used to perform pairwise comparisons of the parameters among treatments. The P-values were adjusted according to the Benjamini–Hocheberg procedure with Q = 0.05.
Given the high number of different treatment solutions, they were separated in two sub-experiments in regard to the statistical analyses. Km and Vmax parameters were compared among the three curves given by the different IN:ON ratios with Ala-1-13C,15N (solutions S1–S9 in Table 1) to test how varying N concentration and IN:ON ratio affect Ala uptake. They were also compared among the four curves resulting from the use of the two types of labeled Ala, with and without IN (solutions S7–S18), to compare the use of UL and PSL Ala, in presence or absence of IN.
As a complementary data analysis, a two-way and a three-way ANOVAs (α = 0.05) with mixed-effect models were performed to test the effects of total N concentration, the IN:ON ratio and their interaction (solutions S1–S9) and the effects of the type of isotopic labeling, the Ala concentration, the presence/absence of NH4NO3 and their interactions (solutions S7–S18) on the eq-13C or 15N enrichment in roots and shoots. Blocks were considered to be a random effect. The results are presented in Supplementary Tables 3, 4.
An ANOVA of the effects of the kind of labeled molecule (I), the presence or absence of IN (N) and their interaction (I × N) on the eq-13C and 15N enrichment of the free amino acids was performed (α = 0.05) for each amino acid, among the four treatments concerned (S8, 11, 14, and 17). Blocks were included as a random effect.
Results
Both the shoots and roots of plants were significantly enriched in 15N in all treatments involving Ala-1-13C,15N (Figures 1B,D; solutions S1–S12). Furthermore, some 13C did enter the roots in most of those treatments (Figure 1C) but did not reach the shoots (Figure 1A). In response to plant exposure to solutions containing U-Ala-13C3,15N, we observed that 13C and 15N reached cucumber shoots and roots at all concentrations (Figure 1; solutions S13–S18). The linear regressions of 13C enrichment against 15N enrichment (following method of uptake calculation from Näsholm et al., 1998) within each treatment are presented in Supplementary Figure 2 and Supplementary Table 2.
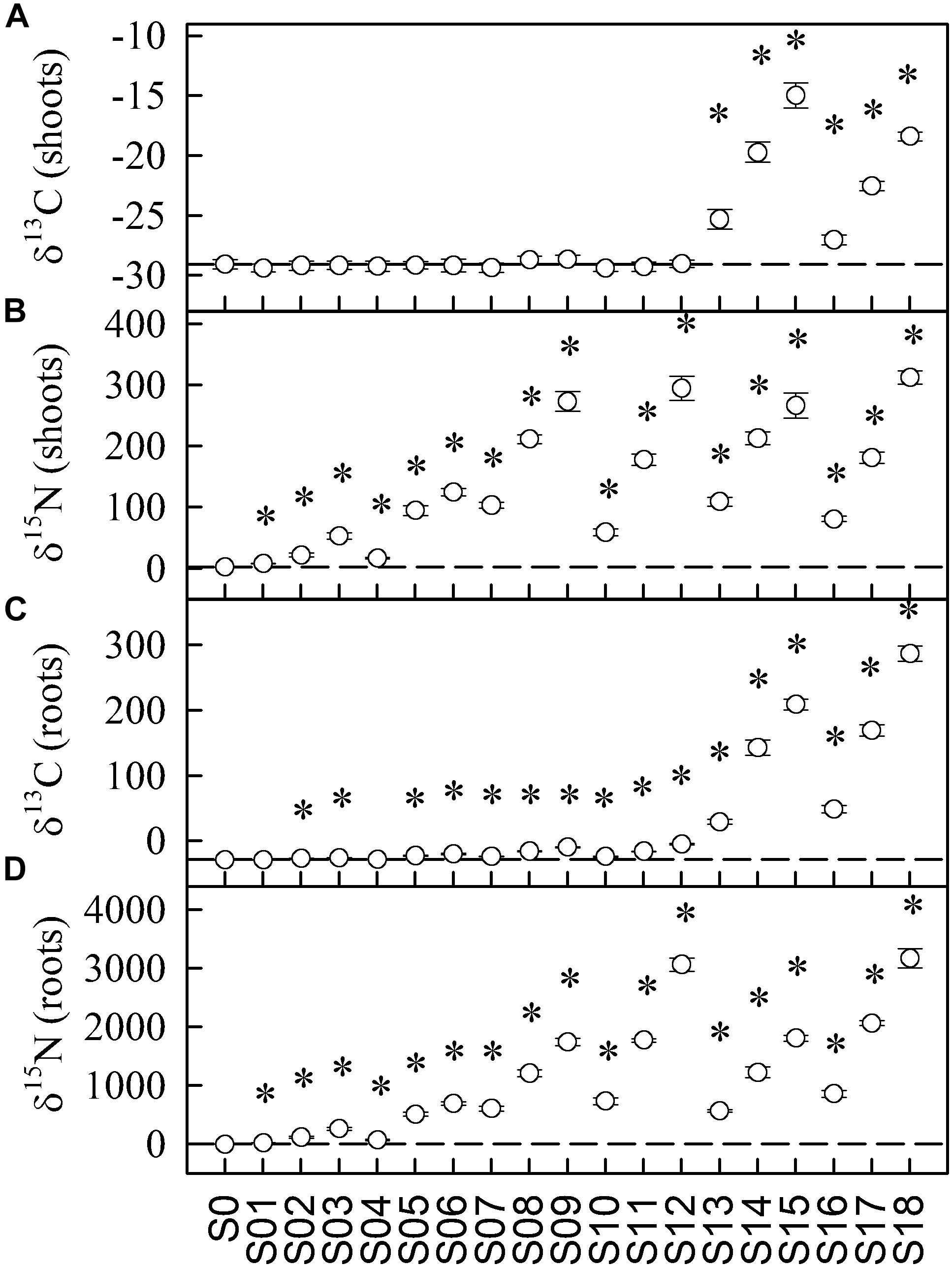
FIGURE 1. 13C (A,C) and 15N (B,D) content (expressed as δ values obtained by BSIA) of shoots (A,B) and roots (C,D) of cucumber seedlings exposed to 19 different labeled solutions. See Table 1 for the detailed content of the solutions. Stars indicate that the shoots or roots of the plants exposed to these solutions were significantly enriched in 13C or 15N relative to the unlabeled control solution (SO), according to the Dunnett test (α = 0.05). Values are means ± SE. Horizontal dashed lines are the δ13C and δ15N values of plants exposed to the unlabeled control solution. For all treatments, n = 5, except S1, 2, 3, 4, 5, 6, 15, and 18, for which n = 4.
The uptake of Ala followed Michaelis-Menten kinetics correspondingly to the concentration of Ala to which the roots were exposed. In a solution with a lower IN:ON ratio, the cucumber plants had an affinity constant (Km) and a maximum uptake rate (Vmax) significantly higher than when exposed to a higher IN:ON ratio (Table 2A and Figures 2A,B). IN uptake increased by 25 and 37% when raising the IN:ON ratio from 10:1 to 100:1, but decreased five and nine-fold at a IN:ON ratio of 500:1, when exposed to a total N concentration of, respectively, 5 and 15 mM (Figure 2C).
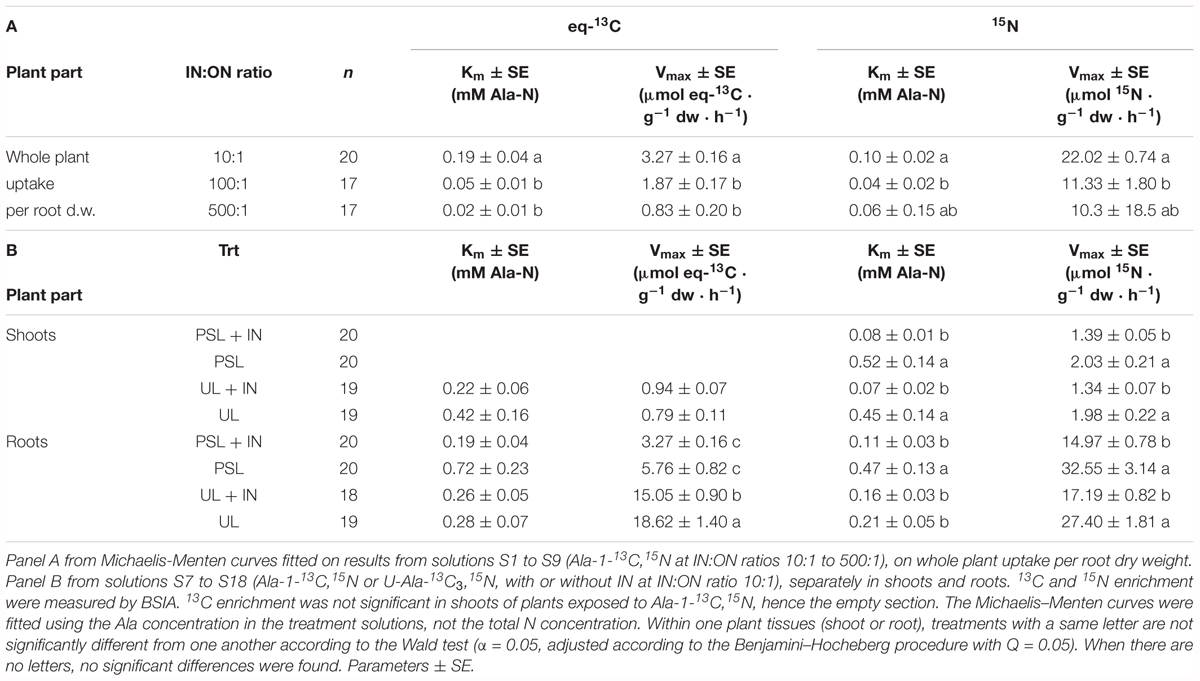
TABLE 2. Km and Vmax parameters of eq-13C and 15N uptake from labeled Alanine in cucumber seedlings.
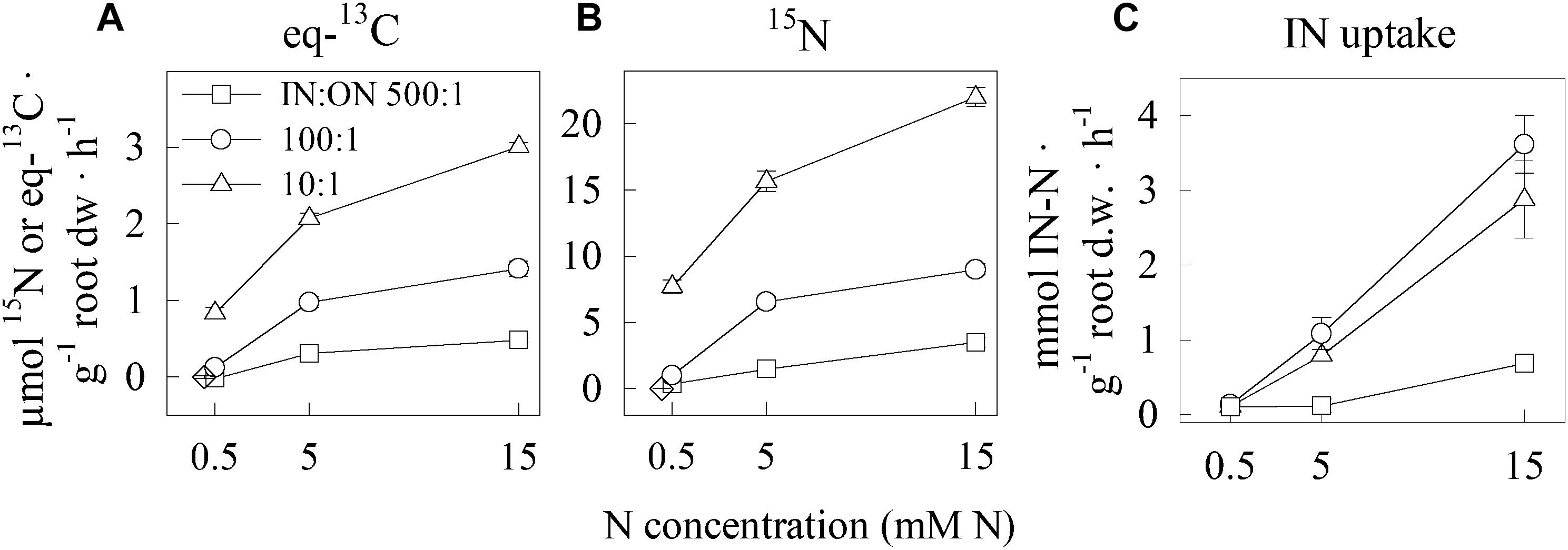
FIGURE 2. Influence of total N concentration and IN:ON ratio on eq-13C (A) and 15N (B) enrichment in whole plants of cucumber seedlings per root dry weight based on BSIA, and on the IN uptake by the plant (C) based on IN depletion in the solution. Plants were exposed to nine different solutions (S1–S9 in Table 1) of three total N concentrations × three different IN:ON ratios (IN = NH4NO3-N and ON = Alanine-1-13C,15N-N): 500:1 (□), 100:1 (∘) and 10:1 (∆). Diamonds (◇) are enrichment at 0 mM N. Values are mean ± SE. n = 4 for each ratio in plants exposed to 0.5 and 5 mM N; n = 5 for the others.
When reducing the total N concentration from 15 to 0.5 mM N, the fraction of total N taken up from Ala increased 2.9- and 6.6-fold (on an eq-13C basis) for ratios 10:1 and 100:1, respectively (Figure 3A). At ratio 10:1, for which this increase was the steepest, the fraction increased from 8.35‰ in the 15 mM N solution to 68.38‰ in the 0.5 mM N solution on a 15N enrichment basis (Figure 3B).
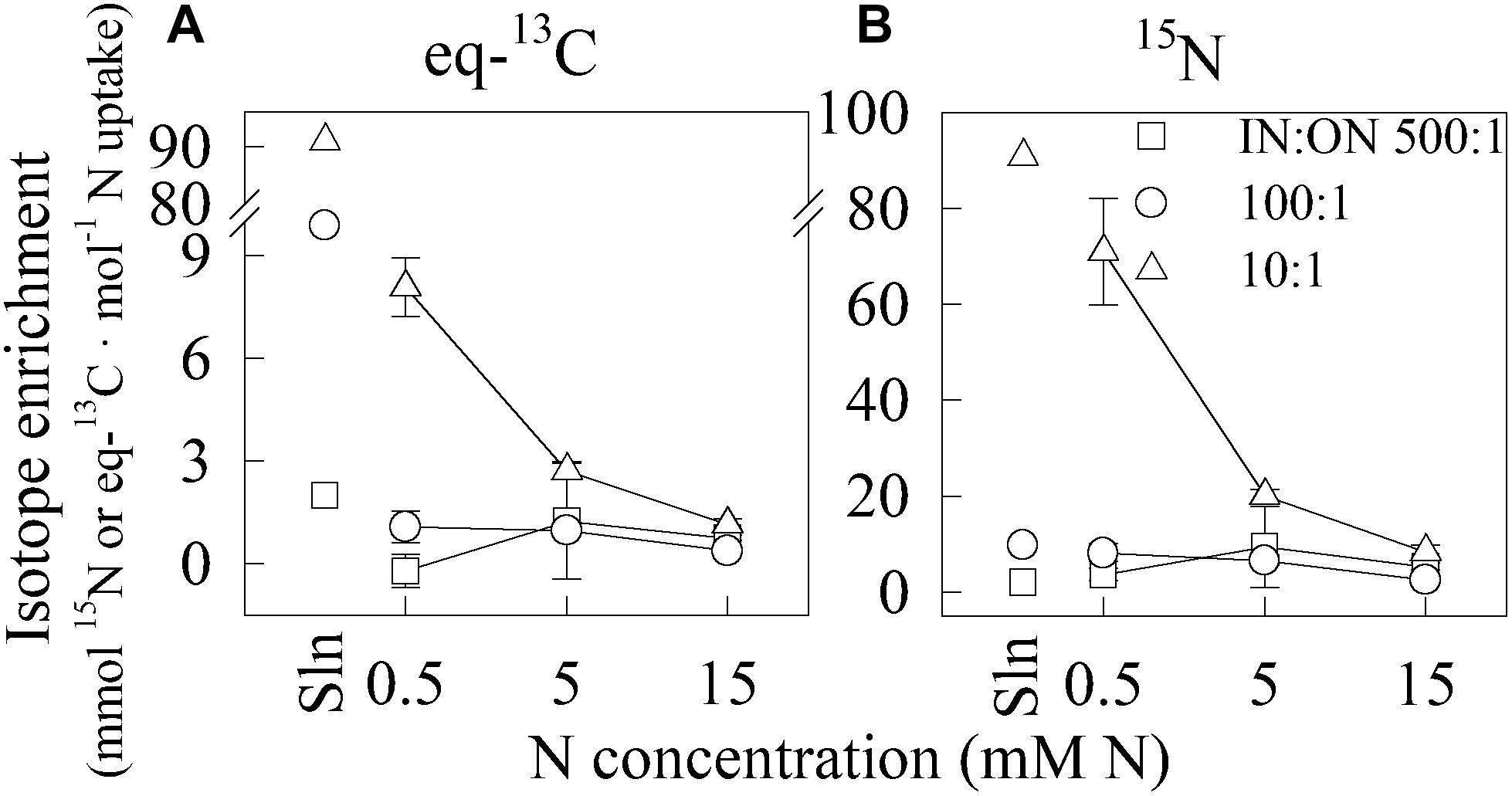
FIGURE 3. Effect of total N concentration and IN:ON ratio on eq-13C (A) and 15N (B) enrichment per total N uptake in cucumber seedlings, based on BSIA. Plants were exposed to three different concentrations of total N at three different IN:ON ratios (IN = NH4NO3-N and ON = Alanine-1-13C,15N-N): 500:1 (□), 100:1 (∘) and 10:1 (∆). Values are means ± SE. Points on the left (“Sln”) indicate the IN:ON ratio in the solution (mmol Ala ⋅ mol-1 total N). n = 4 for plants exposed to 0.5 and 5 mM N; n = 5 for the others.
The maximum eq-13C uptake rate (Vmax) was ca. 4 times higher in plants exposed to solutions containing U-Ala-13C3,15N than to Ala-1-13C,15N (Figure 4C and Table 2B). This difference was not significant in shoots and roots when calculated from 15N enrichment. In absence of NH4NO3, the Km and Vmax for 15N enrichment were ca. twice as high as in presence of NH4NO3 in plants exposed to both types of labeled Ala. This difference was, however, not significant in plants exposed to Ala-1-13C,15N, when calculated from 13C enrichment.
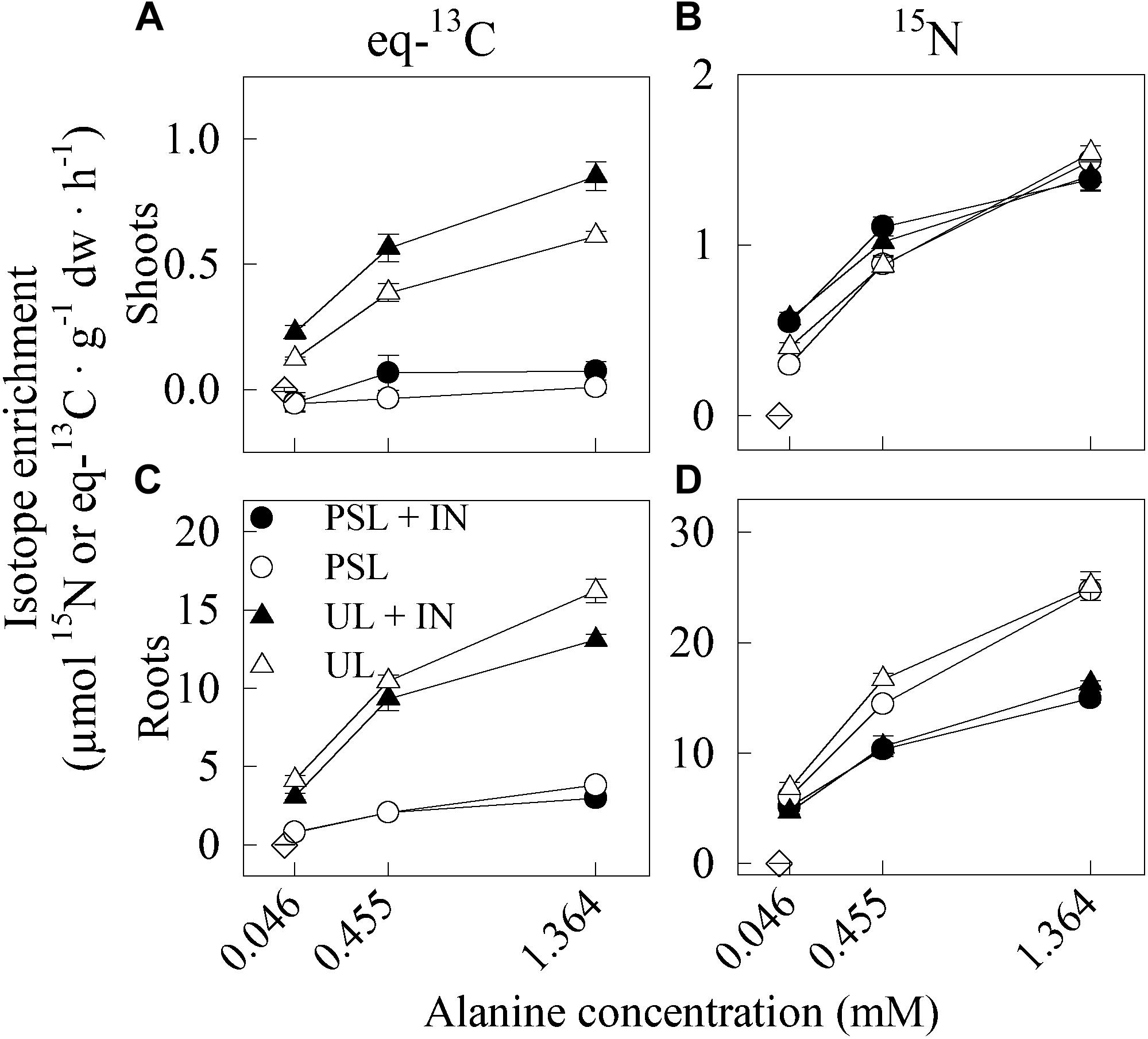
FIGURE 4. Influence of the presence or absence of IN (at a IN:ON ratio of 10:1) and of the different labeling of Ala on 13C-eq (A,C) and 15N (B,D) enrichment in shoots (A,B) and roots (C,D) of cucumber seedlings, based on BSIA. Plants were exposed to three different concentrations of Ala-1-13C,15N (∘) or U-Ala-13C3,15N (∆), with (black) or without (white) NH4NO3 (see solutions S7–S18 in Table 1). Values are means ± SE. Diamonds (◇) indicate the enrichment at 0 mM N. Treatments exposed to 0.455 mM Ala were also analyzed by CSIA (see Figure 5). n = 5 for all treatments, except for solutions containing 1.364 mM U-Ala-13C3,15N (S15 and 18), for which n = 4.
The 15N and eq-13C assimilations in free amino acids are presented in Figure 5, along with the ANOVAs of the effects of isotopic labeling types, the presence of IN and their interaction (P-values presented in Supplementary Table 5). Glx (i.e., the sum of Gln and Glu) accounted for ca. 30 times the 13C transfer and 2.5 times the 15N transfer to the shoots relative to all other amino acids combined. In general, the type of labeled molecule (“I” effect in Figure 5) impacted the 13C assimilation in amino acids, whereas the presence or absence of IN (“N” effect in Figure 5) affected the 15N assimilation. Specifically, in roots, 15N assimilation in amino acids was 12% (Glx) to 274% (Asx, i.e., the sum of Asn and Asp) higher in plants exposed to Ala alone than in plants which were exposed to Ala with IN (Figure 5D). In shoots, all amino acids were enriched in 15N, with no difference between the two types of labeled Ala (Figure 5C). However, plants exposed to IN along with ON assimilated in their shoots up to 25, 23, and 50% more 15N as, respectively, free Ala, Asx, and Glx than plants exposed to ON only. The eq-13C assimilation was much higher for all amino acids in plants exposed to U-Ala-13C3,15N than to Ala-1-13C,15N, except for glycine in the shoots; this difference, although significant, was only 5.7% for Ala in the roots (Figures 5A,B). Glu and Gln (Glx) were the amino acids in which 13C was most assimilated in the roots exposed to U-Ala-13C3,15N, accounting for 85 and 76% (respectively with and without IN) of the 13C in all free amino acids. In roots exposed to Ala-1-13C,15N, Ala accounted for 50 and 59% (respectively with and without IN) of the 13C present in all free amino acids. Shoots of plants exposed to Ala-1-13C,15N were significantly depleted in 13C in Leu (Figure 5A; Ile was not significantly depleted according to Dunnett test, data not shown).
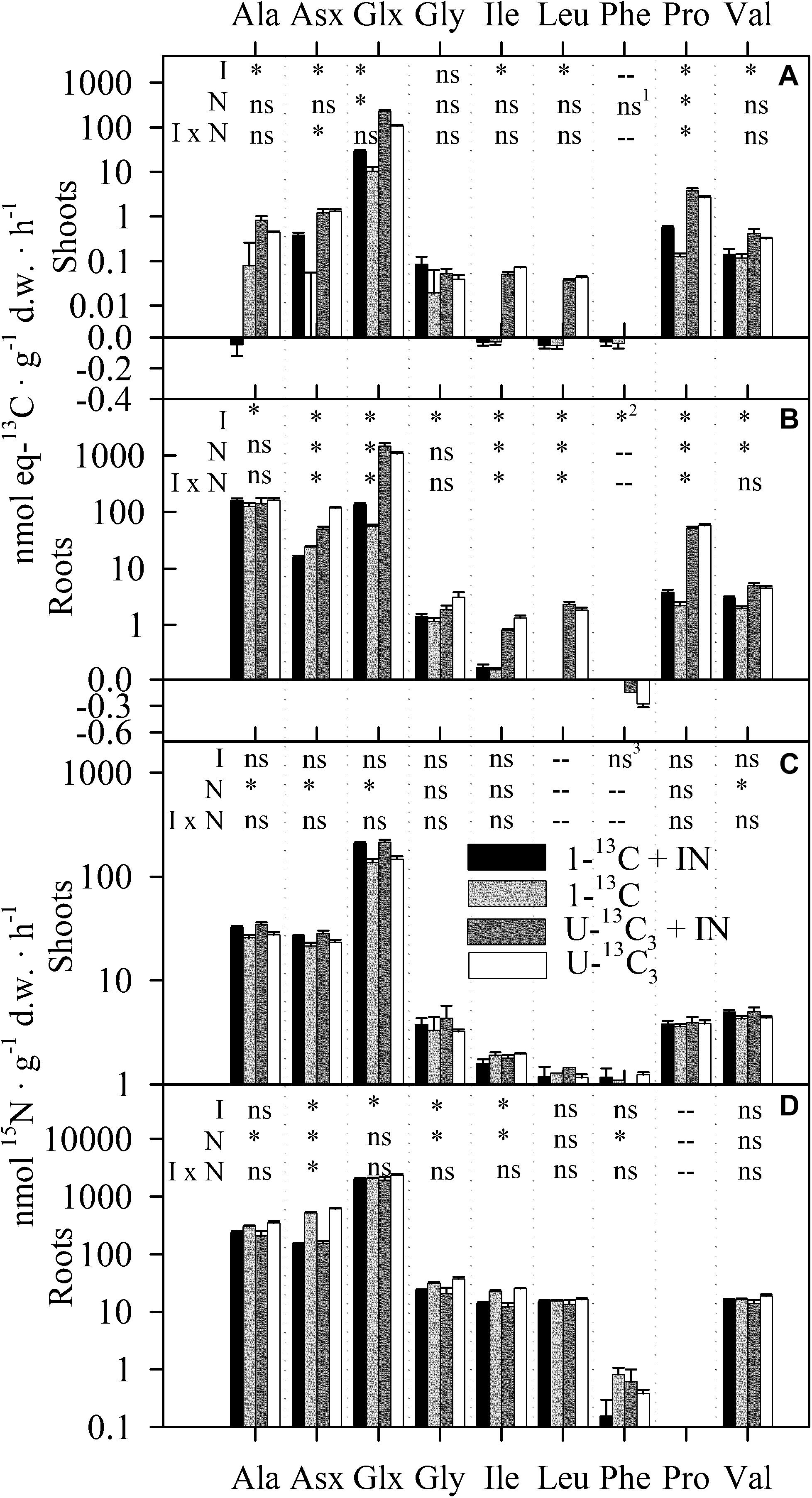
FIGURE 5. Compound specific isotope analysis (CSIA) by GC-C-IRMS of 13C (A,B) and 15N (C,D) assimilation in free amino acids in roots and shoots of cucumber seedlings exposed to 0.455 mM Ala-1-13C,15N or U-Ala-13C3,15N, with or without NH4NO3 at an IN:ON ratio of 10:1. The scale of the vertical axis is logarithmic over zero and linear below zero. The results of the ANOVA of the effects of the type of isotopologue (I), the presence of IN (N) and their interaction (I × N) are presented: ∗ indicates significance (α = 0.05) and ns means nonsignificant. – indicates that the effect could not be analyzed because of data below the limit of quantification: 1ANOVA comparing S8 (1-13C + IN) and S11 (1-13C) only; 2S11and S17 (U-13C3) only; 3only one data point for S11, thus one-way ANOVA comparing S8, S14 (U-13C3 + IN) and S17. See Table 1 for details on the content of the labeled solutions. n = 5 within each treatment.
A comparison of the eq-13C and 15N uptake values reported by CSIA or BSIA, after exposure to Ala-1-13C,15N or U-Ala-13C3,15N alone (solutions S11 and S17 in Table 1), is presented in Table 3. Exposing plants to Ala-1-13C,15N instead of U-Ala-13C3,15N resulted in a fivefold decrease in the eq-13C uptake monitored by BSIA in the roots, and the 13C enrichment in the shoots was below detection limits. Calculating the eq-13C and 15N assimilation in free Ala by CSIA resulted in uptake rates 16–64 times lower than when monitored by BSIA. When summing up the eq-13C or 15N assimilation in all free amino acids, the total enrichment accounted for 10–29% of the enrichment monitored by BSIA, suggesting that 10–29% of the eq-13C and 15N taken up as intact Ala is present as free amino acids in roots and shoots of the plant, 2 h after the beginning of exposure to the solution.
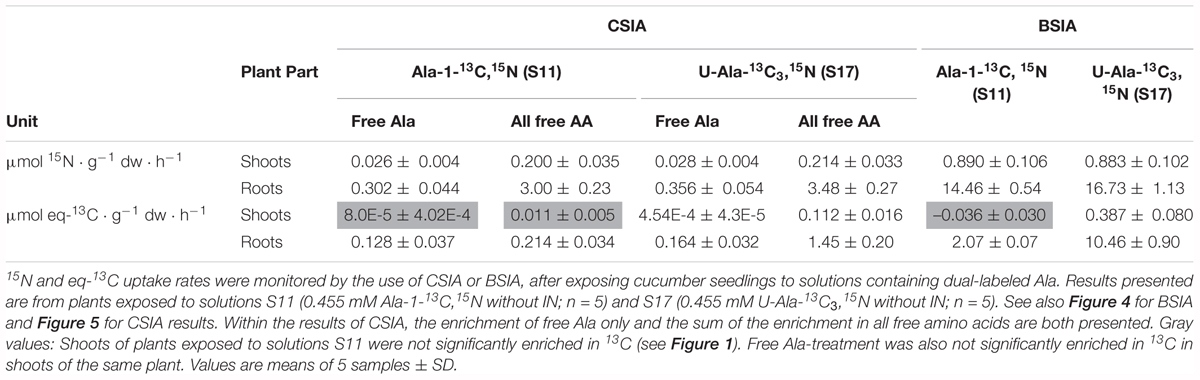
TABLE 3. Comparison of results from six different analytical methods for estimation of intact Ala uptake.
Discussion
Alanine Uptake
Our results showed that cucumber seedlings exposed to solutions containing IN and Alanine N can take up part of their N as intact Ala at concentrations typically used in organic greenhouse horticulture. This is in agreement with previous studies on plant uptake of amino acids in presence of inorganic N (Ge et al., 2009; Gioseffi et al., 2012; Gruffman et al., 2014; Czaban et al., 2016).
In soil, IN and ON occur at different ratios, e.g., during the season or after application of fertilizer. What is unique in the present study is the investigation of this aspect, specifically of different ratios of IN and ON and different concentrations relevant to organic greenhouse horticulture of cucumber. The plants were able to increase their total ON uptake when exposed to a higher total N concentration and Ala concentration within each IN:ON ratio in presence of IN (Figure 2). In accordance with kinetic studies of amino acid uptake (Soldal and Nissen, 1978; Gruffman et al., 2014), the increase in uptake was not linear but followed Michaelis-Menten kinetics (Figure 2 and Table 2). The uptake of Ala was highest at the smallest ratio (10:1) which correlates to the smallest proportion of IN to ON but also to the largest proportions of Ala (Figure 2). This confirms the capacity of plants to benefit from increased ON availability. Plants possess both high and low affinity amino acid transporters, with Km values, respectively, <50 μM and >500 μM (Henry and Jefferies, 2003; Rentsch et al., 2007; Tegeder and Rentsch, 2010). Low-affinity transporters (high Km) usually provide passive, high rate transport, but only manage to take up amino acids at a high concentration. High-affinity transporters (low Km) rely on active, low rate transport, but achieve uptake at lower substrate concentration. The Km and Vmax values reported here are based on the net uptake, spanning the action of multiple types of amino acid transporters. A higher Km suggests that a higher proportion of the uptake is the result of the action of low affinity transporters. Accordingly, the Km and Vmax values observed at a IN:ON ratio of 10:1 were higher than at ratio 100:1 (not significantly different from the 500:1 ratio, which had higher standard errors). This indicates that the low-affinity amino acid transporters can take advantage of the higher presence of ON relative to IN.
In absence of NH4NO3, the roots exposed to Ala took up more N as Ala than in presence of both NH4NO3 and Ala. Removing altogether the IN from the solution affected Ala uptake in the same direction as decreasing the IN:ON ratio; the ensuing increase in Ala-N uptake can thus be attributable to the need for the plant to find alternative N sources. This hypothesis was also confirmed in white clover (Czaban et al., 2016). Moreover, some species adapted to low IN environments (Gruffman et al., 2014), or even agricultural plants such as wheat (Gioseffi et al., 2012) can have their ON uptake unaffected by the presence or absence of nitrate and rely on organic N sources for a significant part of their N budget. In our experiment, the uptake of Ala measured in roots was in agreement with this hypothesis, though higher quantities of C and N were transferred from labeled Ala to the shoots in presence of NH4NO3 than when exposed to Ala alone (Figure 4; ANOVA in Supplementary Table 4). There were, however, no significant differences in Km and Vmax values. This is in contradiction with the results for white clover reported by Czaban et al. (2016), who found a decrease in Arg transfer to the shoot in presence of IN. The recent proposition by Franklin et al. (2017) that the C assimilated from organic N sources increases N use efficiency (NUE) could help explaining our results: the C cost associated to the additional uptake of N from inorganic source may further stimulate the transfer of C from the roots to the shoots in order to contribute to N assimilation at the shoot level. Since the only C source available for uptake by the roots in our experiment was Ala, the 15N included in the molecule could have come along and stimulated a higher IN uptake. Besides, an increase in NUE due to a greater C availability can explain the much higher IN uptake observed at IN:ON ratios of 10:1 and 100:1 than at 500:1 (Figure 2C). Gruffman et al. (2014) also observed a slightly higher nitrate uptake in Scots pine seedlings pre-exposed to a high concentration of Arg and IN. Gioseffi et al. (2012), however, reported a decrease in nitrate uptake when exposing wheat plants to Gly along with nitrate, relative to nitrate alone; so did Thornton and Robinson (2005) after exposing ryegrass to a mixed nitrate – ammonium – glycine solution, relative to a nitrate-only solution, and Stoelken et al. (2010) after exposing European beech to a mixed nitrate – ammonium – Arg or Gln solution, relative to nitrate and ammonium only. In the four cases, however, the N concentration of the treatment solution was much lower (respectively 0.5, 3, 0.66, and 3.5 mM IN) than in our experiment (5 and 15 mM total N). The increased presence of C as Ala not only was beneficial, but a certain amount of it appeared essential to attain a high IN uptake rate. This effect encourages fertilization of crops with an organic source of N to complement highly concentrated mineral N fertilization (>5 mM N), such as encountered in conventional greenhouse fertigation.
In all studied solutions, the ratio of Ala-N uptake to total N uptake was always lower than the ratio of available Ala-N to total N in the solution (Figure 3). This finding supports the well-accepted assumption that roots have a preference for IN over amino acids in agricultural contexts (Okamoto and Okada, 2004; Schimel and Bennett, 2004; Nacry et al., 2013), even at ON concentrations representative of greenhouse organic horticulture. Indeed, at total N concentration of 15 mM N, only 0.4–0.9% of the total N that was taken up by seedlings came from Ala (Figure 3B). The highest proportion of Ala, 6.8%, was taken up at a IN:ON ratio of 10:1 and a total N concentration of 0.5 mM, which is, however, too low for horticulture application in greenhouse context.
Using a dual-labeled amino acid with only the carboxyl C as 13C for assessing its intact uptake rate had the intended purpose of reducing the biases occurring when relying on universally labeled amino acids, due to their pre- and post-uptake metabolism. The enrichment in eq-13C observed using U-Ala-13C3,15N was much higher than that found with Ala-1-13C,15N, even when accounting for the presence of three 13C atoms in the former compared to only one in the later (hence the use of eq-13C instead of 13C). The bias associated with universally labeled amino acids in the determination of intact amino acid uptake can result from many sources. First, mineralization of the labeled molecules can occur outside the roots in the rhizosphere, mainly by microorganisms (Schimel and Bennett, 2004), but also to a lesser extent by exoenzymes secreted in root exudates (Czaban et al., 2016). Second, post-uptake metabolism of the amino acid such as decarboxylation (Moran-Zuloaga et al., 2015) can reduce the isotopic enrichment. Moreover, no eq-13C enrichment was detected in shoots of plants exposed to Ala-1-13C,15N based on BSIA, whereas there was eq-13C transferred to the shoots of plants exposed to U-Ala-13C3,15N (Figure 1A). This further supports the occurrence of a rapid post-uptake metabolism of amino acids, mainly affecting the carboxyl-C (Moran-Zuloaga et al., 2015). It must, however, be considered that the natural abundance of 13C (1.07 at.% 13C in shoots of plants exposed to the control solution S0) was higher than for 15N (0.37 at.% 15N in S0), and that the concentration of C (39.47% C in S0) was higher than the concentration of N (4.06% in S0) in plants. This resulted in a larger dilution of 13C from labeled Ala in samples compared to 15N, making detection of 13C excess by BSIA harder than for 15N. Indeed, a small but significant eq-13C enrichment was monitored for free Asx, Glx, Pro, and Val in shoots of plants exposed to Ala-1-13C,15N (Figure 5A). In any case, albeit the various biases encountered in the calculation of the intact uptake rate, exposing the plant to UL alanine is still informative on the total uptake and assimilation of N and C provided by Ala.
Alanine Assimilation
Plants possess an array of catabolic pathways for amino acids, which allow the reuse of amino acids and recycling of N (Hildebrandt et al., 2015; Yoneyama et al., 2016). The isotopic enrichment of each amino acid, obtained by CSIA, in combination with both position-specific and universally labeled Ala, provides insightful information on the fate of 13C and 15N along those pathways. It thus allows for a clear demonstration that, in addition to being taken up intact, amino acids can be assimilated into the plant’s metabolism.
Carbon and N from free Ala can be incorporated in the amino acid metabolism through pyruvate (C) and Glu (N), from the action of alanine aminotransferase (Hildebrandt et al., 2015). This same enzyme has previously been studied for the positive effect of its reverse reaction on N use efficiency (Ala production from pyruvate and Glu), in a context of mineral fertilization only (Good et al., 2007; Shrawat et al., 2008). Pyruvate is then integrated to acetyl-CoA, with the loss, as CO2, of the carboxyl-C (13C in our experiment) of the amino acid. Acetyl-CoA is integrated in the citrate cycle, and both C atoms originally from Ala can be integrated in Glu and Asp (Gaufichon et al., 2016), thus explaining the high eq-13C enrichment in those two amino acids after exposure to U-Ala-13C3,15N. Since the carboxyl-C of Ala is lost as CO2, it is not surprising that a very low eq-13C enrichment was observed in amino acids other than Ala in the plants exposed to Ala-1-13C,15N, in which only the carboxyl-C was 13C-labeled. The 13C assimilation in free amino acids other than Ala that was found even after exposure to Ala-1-13C,15N could be explained at least in part by the uptake of carbonate resulting from the decarboxylation of Ala outside the roots (Vuorinen et al., 1989; Sauheitl et al., 2009; Rasmussen et al., 2010): bicarbonate can be used in the carboxylation of phosphoenolpyruvate, resulting in the production of malate that can be integrated into the citrate cycle. Yet, in roots, free Ala had a similar eq-13C enrichment after exposure to both types of labeled Ala; it is a strong indicator that the 13C enrichment observed in free Ala came from intact uptake, both when exposing the plant to Ala-1-13C,15N and U-Ala-13C3,15N.
Glutamate and Glutamine are at the heart of IN assimilation through the GS/GOGAT pathway. Nitrate taken up by the roots is reduced to NO2- by Nitrate reductase, to NH4+ by Nitrite reductase, included in Glutamine by Glutamine synthetase, then transaminated to Glutamate by Glutamate synthase (Xu et al., 2012). After that, N can be transferred to Ala also by the action of alanine aminotransferase (Good et al., 2007). The 14N from ammonium nitrate can thus dilute the 15N pool of Alanine (thus decreasing its δ15N), with little effect on its δ13C. This can explain how Ala in roots exposed to ON + IN was depleted in 15N relative to roots exposed to ON alone, while there was no effect of the presence of IN on δ13C (Figures 5B,D). Moreover, Glu and Gln (Glx) were by far the main free amino acids responsible for the transport to the shoots of the 13C and 15N taken up by the roots, suggesting rapid metabolism of Ala after its uptake. Glu and Gln being at the center of the N assimilation pathways, it is to be expected that a significant portion of the assimilated C and N would become part of them. Proline, a direct product of Glu, was also strongly enriched in 13C.
One unexpected result is the 13C depletion observed in Leu (also present, but not significant in Phe and Ile according to Dunnett test; data not shown) in shoots exposed to Ala-1-13C,15N. Leu is a down-product from pyruvate (Galili et al., 2016). Downstream in the metabolic pathway leading to Leu, the carboxyl-C from pyruvate is lost as CO2, and two C from Acetyl-CoA are included. The non-carboxyl C atoms from Ala-1-13C,15N (thus also in pyruvate produced from the catabolism of Ala), and the two C from Acetyl-CoA (that can also be coming from the two non-carboxyl C in pyruvate) could be depleted in 13C depending on the source material used for their production. This could explain the 13C depletion of Leu, although confirming this is outside the scope of this study.
Comparison of BSIA With CSIA
Unsurprisingly, the rates of 13C and 15N assimilation in free amino acids determined by BSIA were much higher (between 16 and 64 times) than those observed using CSIA. This is in accordance with the results of Sauheitl et al. (2009), who found a 1.6–8 times higher uptake rate when using BSIA compared with CSIA after exposing plants to dual-labeled amino acids. The larger difference that we observed between BSIA and CSIA as compared to Sauheitl et al. (2009) could be due to the fact that we performed CSIA on free amino acids compared to their CSIA analysis of samples containing amino acids from proteins, or to our shorter exposure to labeled solution (2 h compared to 24 h for Sauheitl et al., 2009). Our analysis targeting only free amino acids and our short incubation time likely decreased the proportion of amino acids incorporated in proteins and could give a better snapshot of the rapid post-uptake metabolism of amino acids. However, considering the rapid post-uptake metabolism unraveled by CSIA, BSIA of plants exposed to a PSL amino acid appears more accurate in the estimation of intact Ala uptake rate.
Conclusion
The use of CSIA and BSIA, in combination with PSL and universally labeled Alanine, provided unique information on the uptake and assimilation of labeled Ala in free amino acids in cucumber seedlings. Our results contribute to the increasing awareness that uptake of ON in the form of intact amino acids is possible and significant for the plant N budget. In the context of field organic horticulture, understanding the post-uptake metabolism of different amino acids will help predict and model the nutritive value of different organic N fertilizers for the plant. However, at high N concentration such as that used for greenhouse crops, amino acid uptake appears to have only a marginal role in the total crop N budget. Future research on ON uptake should be widened to different crops and agricultural practices, such as organic farming, since the preferred source of N varies from one species to another and, for a given species, among different nutritional contexts. A substantial achievement would be to quantify the global ON uptake in field conditions and adjust recommendations for N fertilization accordingly.
Author Contributions
P-PD designed and performed the experiment, analyzed the data and wrote the first version of the article. SP and MD obtained the funding for the project. SP, MD, and SJ participated in the planning of the experiment. AB contributed to the analysis of amino acids. SP, MD, SJ, and AB helped in the interpretation of the results and revised the manuscript.
Funding
This project was funded by the Ministère de l’Agriculture, des Pêcheries et de l’Alimentation du Québec (MAPAQ) through the Agri-Food Innov’Action program (grant number IA115292) and by the Fonds de recherche du Québec - Nature et Technologies (FRQNT).
Conflict of Interest Statement
The authors declare that the research was conducted in the absence of any commercial or financial relationships that could be construed as a potential conflict of interest.
Acknowledgments
We thank B. Dion-Morin and J. Bourassa for assistance in the lab. M. Thériault contributed to the initial planning of the experiment.
Supplementary Material
The Supplementary Material for this article can be found online at: https://www.frontiersin.org/articles/10.3389/fpls.2018.01596/full#supplementary-material
Abbreviations
BSIA, Bulk stable isotope analysis; CSIA, Compound specific isotope analysis; IN, Inorganic nitrogen; ON, Organic nitrogen; PSL, Position-specific labeling; UL, Universally labeled.
References
Bates, D., Maechler, M., Bolker, B. M., and Walker, S. C. (2015). Fitting linear mixed-effects models using lme4. J. Stat. Softw. 67, 1–48.
Brackin, R., Nasholm, T., Robinson, N., Guillou, S., Vinall, K., Lakshmanan, P., et al. (2015). Nitrogen fluxes at the root-soil interface show a mismatch of nitrogen fertilizer supply and sugarcane root uptake capacity. Sci. Rep. 5:15727. doi: 10.1038/srep15727
Carpena-Ruiz, R., Sopeña, A., and Ramon, A. (1989). Extraction of free amino acids from tomato leaves. Plant Soil 119, 251–254. doi: 10.1007/BF02370416
Cuijpers, W., Van der Burgt, G., and Voogt, W. (2008). “Nitrogen balances in Dutch organic greenhouse production,” in Proceedings from the 16th IFOAM Organic World Congress, Modena.
Czaban, W., Jämtgård, S., Näsholm, T., Rasmussen, J., Nicolaisen, M., and Fomsgaard, I. S. (2016). Direct acquisition of organic N by white clover even in the presence of inorganic N. Plant Soil 407, 1–17. doi: 10.1007/s11104-016-2896-z
Dion, P. P., Jämtgård, S., Thériault, M., Bertrand, A., Pepin, S., and Dorais, M. (2017). “Xylem sap organic N content is linked to soil N composition in an organic greenhouse cucumber crop,” in Proceedings Book of the XVIII International Plant Nutrition Colloquium with Boron and Manganese Satellite Meetings, eds A. Carstensen, K. Laursen, and J. Schoerring (Denmark: University of Copenhagen), 498–499.
Dorais, M., and Schwarz, D. (2018). “Chapter 11: Organic tomato,” in Tomato: Crop Production Science in Horticulture Series, 2nd Edn, ed. E. Heuvelink (Netherlands: Wageningen University).
Franklin, O., Cambui, C. A., Gruffman, L., Palmroth, S., Oren, R., and Näsholm, T. (2017). The carbon bonus of organic nitrogen enhances nitrogen use efficiency of plants. Plant Cell Environ. 40, 25–35. doi: 10.1111/pce.12772
Galili, G., Amir, R., and Fernie, A. R. (2016). “The regulation of essential amino acid synthesis and accumulation in plants,” in Annual Review of Plant Biology, Vol. 67, ed. S. S. Merchant (Palo Alto: Annual Reviews), 153.
Ganeteg, U., Ahmad, I., Jämtgård, S., Aguetoni-Cambui, C., Inselsbacher, E., Svennerstam, H., et al. (2017). Amino acid transporter mutants of Arabidopsis provides evidence that a non-mycorrhizal plant acquires organic nitrogen from agricultural soil. Plant Cell Environ. 40, 413–423.
Gaudin, Z., Cerveau, D., Marnet, N., Bouchereau, A., Delavault, P., Simier, P., et al. (2014). Robust method for investigating nitrogen metabolism of 15N labeled amino acids using AccQ⋅Tag Ultra Performance liquid chromatography-photodiode array-electrospray ionization-mass spectrometry: Application to a parasitic plant-plant interaction. Anal. Chem. 86, 1138–1145. doi: 10.1021/ac403067w
Gaufichon, L., Rothstein, S. J., and Suzuki, A. (2016). Asparagine metabolic pathways in Arabidopsis. Plant Cell Physiol. 57, 675–689. doi: 10.1093/pcp/pcv184
Ge, T., Nie, S., Wu, J., Shen, J., Xiao, H., Tong, C., et al. (2011). Chemical properties, microbial biomass, and activity differ between soils of organic and conventional horticultural systems under greenhouse and open field management: a case study. J. Soils Sediments 11, 25–36. doi: 10.1007/s11368-010-0293-4
Ge, T., Yuan, H., Roberts, P., Jones, D. L., Qin, H., Tong, C., et al. (2012). Amino acid and peptide dynamics in horticultural soils under conventional and organic management strategies. J. Soils Sediments 12, 323–333. doi: 10.1007/s11368-011-0457-x
Ge, T., Song, S., Roberts, P., Jones, D. L., Huang, D., and Iwasaki, K. (2009). Amino acids as a nitrogen source for tomato seedlings: the use of dual-labeled (13C, 15N) glycine to test for direct uptake by tomato seedlings. Environ. Exp. Bot. 66, 357–361. doi: 10.1016/j.envexpbot.2009.05.004
Gioseffi, E., de Neergaard, A., and Schjoerring, J. K. (2012). Interactions between uptake of amino acids and inorganic nitrogen in wheat plants. Biogeosciences 9, 1509–1518. doi: 10.5194/bg-9-1509-2012
Good, A. G., Johnson, S. J., De Pauw, M., Carroll, R. T., and Savidov, N. (2007). Engineering nitrogen use efficiency with alanine aminotransferase. Can. J. Bot. 85, 252–262. doi: 10.1139/B07-019
Gruffman, L., Jämtgård, S., and Näsholm, T. (2014). Plant nitrogen status and co-occurrence of organic and inorganic nitrogen sources influence root uptake by Scots pine seedlings. Tree Physiol. 34, 205–213. doi: 10.1093/treephys/tpt121
Henry, H. A. L., and Jefferies, R. L. (2003). Interactions in the uptake of amino acids, ammonium and nitrate ions in the Arctic salt-marsh grass, Puccinellia phryganodes. Plant Cell Environ. 26, 419–428. doi: 10.1046/j.1365-3040.2003.00973.x
Hildebrandt, T. M., Nesi, A. N., Araujo, W. L., and Braun, H.-P. (2015). Amino acid catabolism in plants. Mol. Plant 8, 1563–1579. doi: 10.1016/j.molp.2015.09.005
Hothorn, T., Bretz, F., and Westfall, P. (2008). Simultaneous inference in general parametric models. Biom. J. 50, 346–363. doi: 10.1002/bimj.200810425
Hu, B., Zhou, M., Bilela, S., Simon, J., Dannenmann, M., Liu, X., et al. (2017). Nitrogen nutrition of native and introduced forest tree species in N-limited ecosystems of the Qinling Mountains, China. Trees Struct. Funct. 31, 1189–1202. doi: 10.1007/s00468-017-1537-3
Jämtgård, S., Näsholm, T., and Huss-Danell, K. (2008). Characteristics of amino acid uptake in barley. Plant Soil 302, 221–231.
Kuznetsova, A., Brockhoff, P. B., and Christensen, R. H. B. (2017). lmertest package: tests in linear mixed effects models. J. Stat. Softw. 82:26.
Lesnoff, M., and Lancelot, R. (2012). aod: Analysis of Overdispersed Data. Available at: http://cran.r-project.org/package=aod
Li, X., Rennenbergl, H., and Simon, J. (2015). Competition for nitrogen between Fagus sylvatica and Acer pseudoplatanus seedlings depends on soil nitrogen availability. Front. Plant Sci. 6:302. doi: 10.3389/fpls.2015.00302
Moran-Zuloaga, D., Dippold, M., Glaser, B., and Kuzyakov, Y. (2015). Organic nitrogen uptake by plants: reevaluation by position-specific labeling of amino acids. Biogeochemistry 125, 359–374. doi: 10.1007/s10533-015-0130-3
Nacry, P., Bouguyon, E., and Gojon, A. (2013). Nitrogen acquisition by roots: physiological and developmental mechanisms ensuring plant adaptation to a fluctuating resource. Plant Soil 370, 1–29. doi: 10.1007/s11104-013-1645-9
Näsholm, T., Ekblad, A., Nordin, A., Giesler, R., Hogberg, M., and Hogberg, P. (1998). Boreal forest plants take up organic nitrogen. Nature 392, 914–916. doi: 10.1038/31921
Näsholm, T., Huss-Danell, K., and Hogberg, P. (2000). Uptake of organic nitrogen in the field by four agriculturally important plant species. Ecology 81, 1155–1161.
Näsholm, T., Kielland, K., and Ganeteg, U. (2009). Uptake of organic nitrogen by plants. New Phytol. 182, 31–48. doi: 10.1111/j.1469-8137.2008.02751.x
Okamoto, M., and Okada, K. (2004). Differential responses of growth and nitrogen uptake to organic nitrogen in four gramineous crops. J. Exp. Bot. 55, 1577–1585. doi: 10.1093/jxb/erh167
OMAFRA. (2010). Growing Greenhoue Vegetables in Ontario. Toronto, ON: Ministry of Agriculture Food and Rural Affairs.
Paungfoo-Lonhienne, C., Visser, J., Lonhienne, T. G. A., and Schmidt, S. (2012). Past, present and future of organic nutrients. Plant Soil 359, 1–18. doi: 10.1007/s11104-012-1357-6
R Core Team. (2018). R: A Language and Environment for Statistical Computing. Vienna: R Foundation for Statistical Computing.
Rasmussen, J., Sauheitl, L., Eriksen, J., and Kuzyakov, Y. (2010). Plant uptake of dual-labeled organic N biased by inorganic C uptake: results of a triple labeling study. Soil Biol. Biochem. 42, 524–527. doi: 10.1016/j.soilbio.2009.11.032
Reeve, J. R., Hoagland, L. A., Villalba, J. J., Carr, P. M., Atucha, A., Cambardella, C., et al. (2016). “Chapter Six - Organic farming, soil health, and food quality: considering possible links,” in Advances in Agronomy, ed. D. L. Sparks (Cambridge, MA: Academic Press), 319–367. doi: 10.1016/bs.agron.2015.12.003
Reganold, J. P., and Wachter, J. M. (2016). Organic agriculture in the twenty-first century. Nat. Plants 2, 15221–15221.
Rentsch, D., Schmidt, S., and Tegeder, M. (2007). Transporters for uptake and allocation of organic nitrogen compounds in plants. FEBS Lett. 581, 2281–2289. doi: 10.1016/j.febslet.2007.04.013
Roosta, H. R., and Schjoerring, J. K. (2007). Effects of ammonium toxicity on nitrogen metabolism and elemental profile of cucumber plants. J. Plant Nutr. 30, 1933–1951. doi: 10.1080/01904160701629211
Sauheitl, L., Glaser, B., and Weigelt, A. (2009). Advantages of compound-specific stable isotope measurements over bulk measurements in studies on plant uptake of intact amino acids. Rapid Commun. Mass Spectrom. 23, 3333–3342. doi: 10.1002/rcm.4255
Schimel, J. P., and Bennett, J. (2004). Nitrogen mineralization: challenges of a changing paradigm. Ecology 85, 591–602. doi: 10.1890/03-8002
Shrawat, A. K., Carroll, R. T., DePauw, M., Taylor, G. J., and Good, A. G. (2008). Genetic engineering of improved nitrogen use efficiency in rice by the tissue-specific expression of alanine aminotransferase. Plant Biotechnol. J. 6, 722–732. doi: 10.1111/j.1467-7652.2008.00351.x
Soldal, T., and Nissen, P. (1978). Multiphasic uptake of amino-acids by barley roots. Physiol. Plant. 43, 181–188. doi: 10.1111/j.1399-3054.1978.tb02561.x
Song, S. W., Lehne, P., Le, J. G., Ge, T. D., and Huang, D. F. (2010). Yield, fruit quality and nitrogen uptake of organically and conventionally grown muskmelon with different inputs of nitrogen, phosphorus, and potassium. J. Plant Nutr. 33, 130–141. doi: 10.1080/01904160903394622
Stoelken, G., Simon, J., Ehlting, B., and Rennenberg, H. (2010). The presence of amino acids affects inorganic N uptake in non-mycorrhizal seedlings of European beech (Fagus sylvatica). Tree Physiol. 30, 1118–1128.
Tegeder, M., and Rentsch, D. (2010). Uptake and partitioning of amino acids and peptides. Mol. Plant 3, 997–1011. doi: 10.1093/mp/ssq047
Thornton, B., and Robinson, D. (2005). Uptake and assimilation of nitrogen from solutions containing multiple N sources. Plant Cell Environ. 28, 813–821.
Tittarelli, F., Båth, B., Ceglie, F. G., Garcia, M. C., Möller, K., Reents, H. J., et al. (2016). Soil Fertility Management in Organic Greenhouses in Europe. BioGreenhouse COST Action FA 1105. Available at: www.biogreenhouse.org
Vuorinen, A., Vapaavuori, E., and Lapinjoki, S. (1989). Time-course of uptake of dissolved inorganic carbon through willow roots. Physiol. Plant. 77, 33–38. doi: 10.1111/j.1399-3054.1989.tb05974.x
Walsh, R. G., He, S., and Yarnes, C. T. (2014). Compound-specific δ13C and δ15N analysis of amino acids: a rapid, chloroformate-based method for ecological studies. Rapid Commun. Mass Spectrom. 28, 96–108. doi: 10.1002/rcm.6761
Wang, X.-X., Zhao, F., Zhang, G., Zhang, Y., and Yang, L. (2017). Vermicompost improves tomato yield and quality and the biochemical properties of soils with different tomato planting history in a greenhouse study. Front. Plant Sci. 8:1978. doi: 10.3389/fpls.2017.01978
Warren, C. R. (2014). Organic N molecules in the soil solution: what is known, what is unknown and the path forwards. Plant Soil 375, 1–19. doi: 10.1007/s11104-013-1939-y
Wei, L. L., Chen, C. R., and Yu, S. (2015). Uptake of organic nitrogen and preference for inorganic nitrogen by two Australian native Araucariaceae species. Plant Ecol. Divers. 8, 259–264. doi: 10.1080/17550874.2013.871656
Xu, G. H., Fan, X. R., and Miller, A. J. (2012). “Plant nitrogen assimilation and use efficiency,” in Annual Review Plant Biology, ed. S. S. Merchant (Palo Alto: Annual Reviews), 153–182.
Yoneyama, T., Tanno, F., Tatsumi, J., and Mae, T. (2016). Whole-plant dynamic system of nitrogen use for vegetative growth and grain filling in rice plants (Oryza sativa L.) as revealed through the production of 350 Grains from a germinated seed over 150 days: a review and synthesis. Front. Plant Sci. 7:1151. doi: 10.3389/fpls.2016.01151
Keywords: amino acid, assimilation, compound-specific stable isotope analysis, cucumber, nitrogen, organic horticulture, position-specific labeling, uptake
Citation: Dion P-P, Jämtgård S, Bertrand A, Pepin S and Dorais M (2018) Organic Nitrogen Uptake and Assimilation in Cucumis sativus Using Position-Specific Labeling and Compound-Specific Isotope Analysis. Front. Plant Sci. 9:1596. doi: 10.3389/fpls.2018.01596
Received: 29 August 2018; Accepted: 15 October 2018;
Published: 06 November 2018.
Edited by:
Victoria Fernandez, Universidad Politécnica de Madrid (UPM), SpainReviewed by:
Daniel Moran, Max-Planck-Institut für Chemie, GermanyJudy Simon, Universität Konstanz, Germany
Copyright © 2018 Dion, Jämtgård, Bertrand, Pepin and Dorais. This is an open-access article distributed under the terms of the Creative Commons Attribution License (CC BY). The use, distribution or reproduction in other forums is permitted, provided the original author(s) and the copyright owner(s) are credited and that the original publication in this journal is cited, in accordance with accepted academic practice. No use, distribution or reproduction is permitted which does not comply with these terms.
*Correspondence: Pierre-Paul Dion, pierre-paul.dion.1@ulaval.ca Martine Dorais, martine.dorais.1@ulaval.ca