- State Key Laboratory for Agrobiotechnology, Key Laboratory of Crop Heterosis and Utilization (MOE), Beijing Key Laboratory of Crop Genetic Improvement, China Agricultural University, Beijing, China
Wheat (Triticum aestivum L.) yield and quality are adversely affected by heat, drought, or the combination of these two stresses in many regions of the world. A phosphoenolpyruvate carboxylase kinase-related kinase gene, TaPEPKR2, was identified from our previous heat stress-responsive transcriptome analysis of heat susceptible and tolerant wheat cultivars. Based on the wheat cultivar Chinese Spring genome sequence, TaPEPKR2 was mapped to chromosome 5B. Expression analysis revealed that TaPEPKR2 was induced by heat and polyethylene glycol treatment. To analyze the function of TaPEPKR2 in wheat, we transformed it into the wheat cultivar Liaochun10, and observed that the transgenic lines exhibited enhanced heat and dehydration stress tolerance. To examine whether TaPEPKR2 exhibits the same function in dicotyledonous plants, we transformed it into Arabidopsis, and found that its overexpression functionally enhanced tolerance to heat and dehydration stresses. Our results imply that TaPEPKR2 plays an important role in both heat and dehydration stress tolerance, and could be utilized as a candidate gene in transgenic breeding.
Introduction
Heat and drought stress and their combination during the growing season are major environmental factors affecting the production and quality of wheat worldwide. A 5.5% decrease in global wheat yields was caused by heat stress between 1980–2008 (Lobell et al., 2011), while drought stress caused considerable yield loss and high economic costs in more than 50% of wheat cultivation areas (Ashraf, 2010). Therefore, research into the genetic mechanism of heat and drought stress tolerance is getting increasingly important.
Plants have developed a range of response mechanisms to adjust to abiotic stress, especially molecular responses to maintain normal activities (Shinozaki and Dennis, 2003; Ashraf, 2010; Mittler et al., 2012; Qu et al., 2013). Genes responding to abiotic stress are essential for enhancing abiotic stress tolerance, and an understanding of these is crucial to developing abiotic stress-tolerant crops.
Protein kinases regulate key aspects of cellular function, including responses to external signals. In Arabidopsis, around 4% of predicted genes encode typical protein kinases (Hrabak et al., 2003). Phosphoenolpyruvate carboxylase kinase (PPCK)-related kinases (PEPKRs) are unique to plants and belong to the CDPK-SnRK superfamily (Hrabak et al., 2003). PPCKs are calcium-independent protein kinases that function in crassulacean acid metabolism and C4 plants (Vidal and Chollet, 1997; Nimmo et al., 2001; Agetsuma et al., 2004). Predicted PEPKR proteins in Arabidopsis contain both N- and C-terminal extensions with no similarity to the non-catalytic domains of other kinases in this superfamily (Hrabak et al., 2003). Thus far, their functions remain unknown.
In this study, we isolated the coding and promoter region of TaPEPKR2, and analyzed the expression pattern of TaPEPKR2 under heat and dehydration stress conditions. Finally, a transgenic approach was used to investigate the function of TaPEPKR2 in both wheat and Arabidopsis under heat and dehydration stress conditions.
Materials and Methods
Plant Materials, Growth Conditions, and Stress Treatments
The common wheat variety “TAM107,” which has a heat-tolerant phenotype and was released by Texas A&M University in 1984, was used for gene cloning and expression analysis. Growth conditions and stress treatments of wheat were as previously described (Zang et al., 2017a,b). Briefly, the sprouted seeds were grown on moistened filter paper at 22°C/18°C (day/night), 12 h/12 h (light/dark), and 60% humidity in a growth chamber. For high-temperature treatments, seedlings were transferred to another growth chamber maintained at 40°C. For dehydration stress treatments, water was replaced by PEG-6000 (20%). Leaves were collected from the seedlings at different time points, frozen immediately in liquid nitrogen and stored at -80°C for RNA isolation.
Common wheat cultivar Liaochun10 (LC10) and Arabidopsis thaliana ecotype Col-0 was used for genetic transformation.
Cloning and Sequence Analysis
Total RNA was extracted from 7-day-old seedlings using TRIzol reagent (Invitrogen), and purified RNA was treated with DNase I. Subsequently, 2 μg of total RNA was reverse transcribed by M-MLV reverse transcriptase according to the instruction (Promega). Based on the probe sequence (Ta.10701.1.S1_at), a pair of gene-specific primers TaPEPKR2-L/R was used to amplify TaPEPKR2. Primer sequences are listed in Supplementary Table S1 (1, 2).
Database searches of nucleotide and deduced amino acid sequences of the TaPEPKR2 homologs were analyzed by NCBI/GenBank/Blast. Sequence alignment and similarity comparisons were performed by DNAMAN software. The functional domains of TaPEPKR2 were identified by SMART programs1.
Expression Pattern Analysis of TaPEPKR2 in Wheat
Quantitative real-time PCR (RT-qPCR) was performed to determine the relative expression level of TaPEPKR2 with specific primers. The 2-ΔΔCT method (Livak and Schmittgen, 2001) was used to quantify the relative expression of TaPEPKR2, and the wheat β-actin gene was used as a reference. Each experiment was repeated three times independently. Supplementary Table S1 (3, 4, 7, 8) lists the RT-qPCR primers.
Transgenic Constructs of TaPEPKR2 and Genetic Transformation in Wheat
The coding DNA sequence (CDS) of TaPEPKR2 (Supplementary File S1) driven by the maize ubiquitin promoter was inserted into the binary vector pBract806. The resulting expression constructs were utilized for genetic transformation. LC10 immature embryos were utilized for wheat transformation via the particle bombardment method. The presence of a TaPEPKR2 transgene was verified by PCR using primers listed in Supplementary Table S1 (5, 6). In total, five transgenic events (T1–T5) were produced and three lines (T3–T5) with higher expression levels were selected for further analysis.
Thermotolerance Assay in Wheat
The thermotolerance assay in wheat was performed as previously described (Zang et al., 2017a). Seeds of LC10 and TaPEPKR2 transgenic lines were grown in pots containing potting soil under the above-mentioned conditions. 5-day-old seedlings were transferred to a growth chamber at 45°C for 18 h, typically beginning at 09:00 h, and were then shifted to 22°C for recovery. The phenotypes were photographed 5 days after the treatment.
Generation of TaPEPKR2 Transgenic Arabidopsis Plants
The CDS of TaPEPKR2 was amplified and cloned into the binary vector pB2GW7 using the gateway method. Agrobacterium tumefaciens strain GV3101 containing this binary construct was used to transform Arabidopsis plants by the floral dip method. Transformants were selected on 1/2 MS medium containing Basta (125 μL/L), followed by PCR amplification of positive clones. In total, seven transgenic lines (L1–L7) were produced and three lines (L1, L3, and L5) were selected for further analysis.
Thermotolerance Assay in Arabidopsis
The thermotolerance assay in Arabidopsis was performed as previously described (Zang et al., 2017a,b). Surface-sterilized seeds of WT and TaPEPKR2 transgenic lines were sown on MS solid medium. The plated 7-day-old seedlings were exposed to 45°C for 120 min in an illuminated growth chamber, then shifted to 22°C to the previous day/night cycle for 5–7 days recovery. Phenotypes before and after heat treatment were photographically documented.
Ion Leakage Assay
Electrolyte leakage was measured as previously described (Camejo et al., 2005; Zang et al., 2017a,b). Leaf segments of uniform maturity were cut into disks and washed three times with de-ionized water to eliminate external residues. Six disks were placed in test tube flasks with 20 mL of de-ionized water and incubated at 42°C for 1 h. After incubation at room temperature for 24 h, the conductivity of the solutions was determined with a Horiba Twin Cond B-173 conductivity meter (HORIBA Ltd., Kyoto, Japan) and noted as T1. Next, the samples were boiled for 15 min to kill the tissues, followed by incubation at room temperature for 24 h. The conductivities of the solutions were then recorded as T2. Ion permeability was calculated as T1/T2. The experiment was repeated three times independently. Student’s t-test in Microsoft excel was used to determine the presence of significant differences (∗P < 0.05, ∗∗P < 0.01).
Dehydration Tolerance Assay
In Arabidopsis, 10-day-old seedlings of WT and TaPEPKR2 transgenic lines germinated on MS medium solidified with 0.8% agar were planted in identical pots, which contained 30 g mixed soil (vermiculite: humus = 1:1) and were added the same volume of water. Then, they were cultured in the greenhouse under optimum growth conditions (16-h-light/8-h-dark cycle, 150 μmol m-2 s-1, 22°C/18°C, 60% humidity). For dehydration tolerance assay, seedlings were subjected to water deprivation for 25 days, and then re-watered for about ∼1 week. In wheat, seeds of LC10 and TaPEPKR2 transgenic lines were planted in water. 5-day-old seedlings of WT and TaPEPKR2 transgenic lines were placed in water and 20% PEG conditions for 5 days. Student’s t-test in Microsoft excel was used to determine the presence of significant differences (∗P < 0.05).
Results
Cloning of a TaPEPKR2 Gene From TAM107
Microarray analysis with the Affymetrix GeneChip® Wheat Genome Array previously indicated that probe “Ta.10701.1.S1_at” was induced 22.73-fold after 40°C treatment for 1 h (Qin et al., 2008). Based on this probe sequence, we cloned the open reading frame (ORF) of TaPEPKR2 from wheat cultivar “TAM107” (previously named TaSTK, GenBank Accession No. GU213488.1). The complete ORF of TaPEPKR2 is 1347 bp and encodes a polypeptide of 448 amino acid residues. Based on the wheat cultivar Chinese Spring genome sequence, TaPEPKR2 was mapped to chromosome 5B. The protein sequence showed homology with PEPKR2 family members from other plant species, including Zea mays (ZmPEPKR2, 81.46%), Oryza sativa (OsPEPKR2, 81.98%), and A. thaliana (AtPEPKR2, 53.18%) (Figure 1). SMART analysis indicated that the amino acid sequence of TaPEPKR2 possessed a S_TKc motif, with both N- and C-terminal extensions (Figure 1 and Supplementary Figure S1).
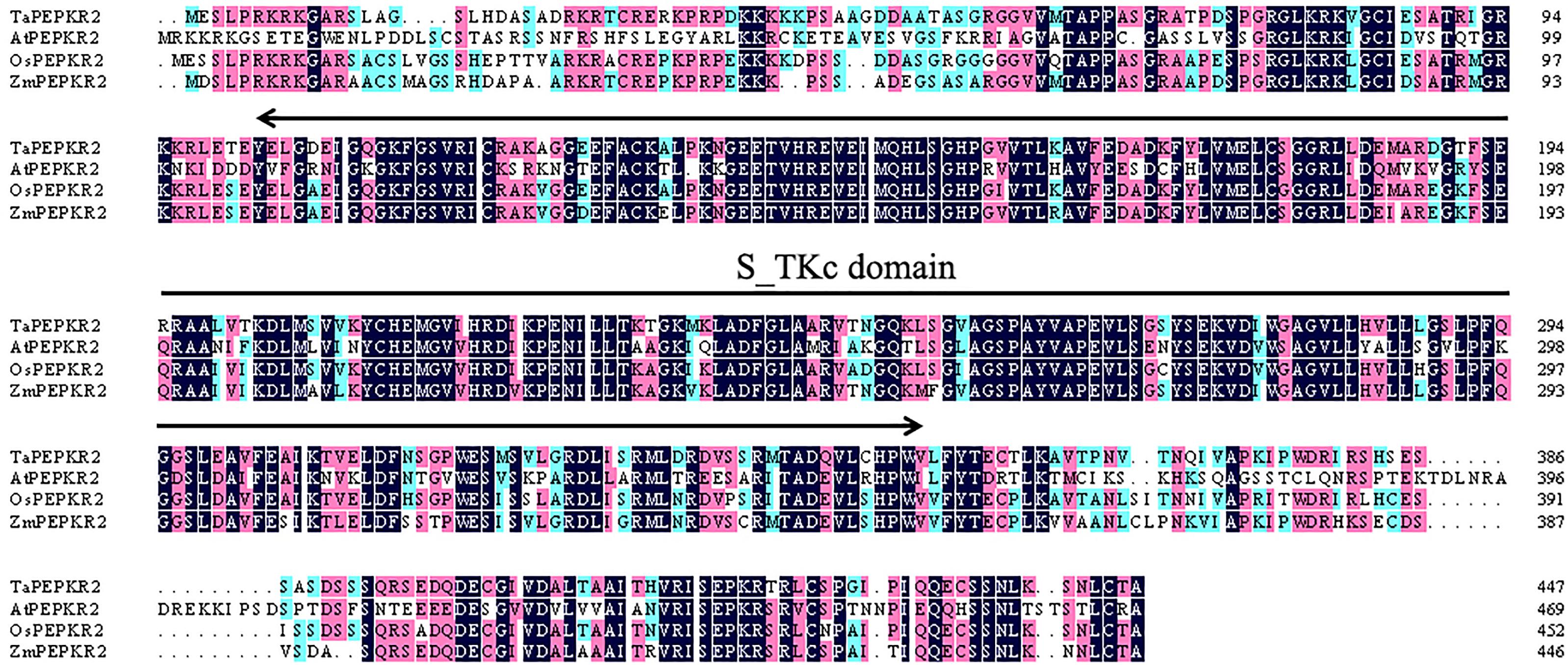
FIGURE 1. Sequence alignment of TaPEPKR2 and orthologous genes from Arabidopsis, rice, and maize. Regions corresponding to the S_TKc domain are indicated by the SMART program.
TaPEPKR2 Is Induced by Heat and Dehydration Stress Treatment
Relative expression levels of TaPEPKR2 in wheat under heat and 20% PEG stress conditions were determined by RT-qPCR using gene-specific primers (Figure 2). The relative expression of TaPEPKR2 increased and peaked at 1 h after heat treatment at 40°C and then decreased, but increased mRNA abundance was maintained (Figure 2A). Following treatment with 20% PEG, TaPEPKR2 expression increased gradually and peaked at 12 h (Figure 2B). These results indicate that TaPEPKR2 can be induced by heat and 20% PEG treatment.
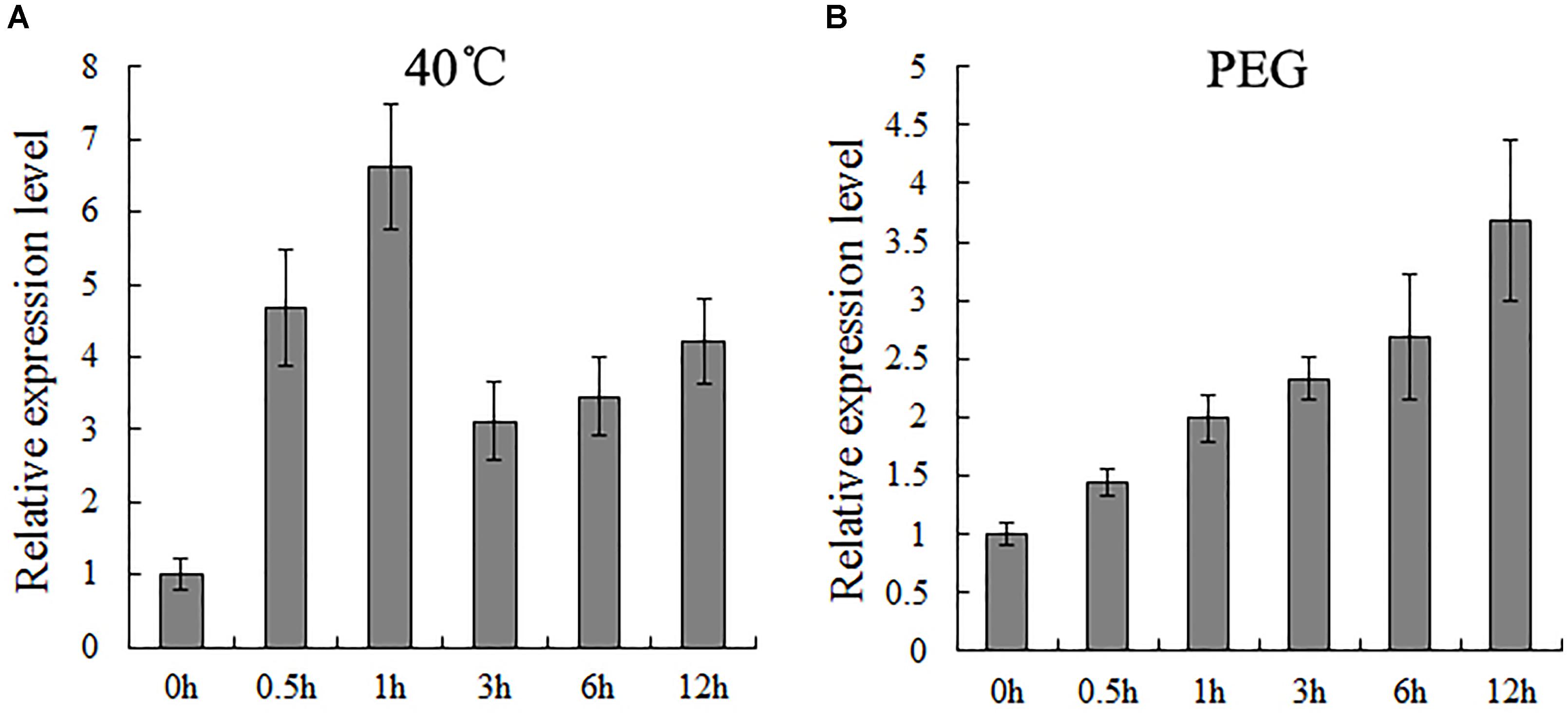
FIGURE 2. Relative expression of TaPEPKR2 under heat (A) and dehydration (B) stress conditions, as determined by RT-qPCR. Data represent the mean of three replicates ± SD.
Overexpression of TaPEPKR2 in Wheat Conferred Heat Tolerance at the Seedling Stage
To understand the function of TaPEPKR2, we transformed it into wheat cultivar LC10 under the control of the maize ubiquitin promoter by particle bombardment. A total of five transgenic lines were analyzed over T1 and T2 generations by PCR analysis with specific corresponding primers. Three lines (L3, L4, and L5) that exhibited TaPEPKR2 up-regulation in shoots at the seedling stage (Supplementary Figure S2) were selected for further analysis.
To examine the applicability of TaPEPKR2 for thermotolerance transgenic breeding, we characterized the phenotypes of TaPEPKR2 transgenic wheat at various developmental stages. Under optimum growth conditions, no visible difference was found between transgenic and WT plants (Figure 3A). However, after heat treatment and the recovery stage, WT plants wilted slightly more rapidly than TaPEPKR2 transgenic lines (Figure 3A). Electrolyte leakage is an indicator to reflect heat stress-induced membrane injury. Thus, we evaluated electrolyte leakage with detached leaves after heat treatment. The results showed that transgenic lines exhibited significantly reduced electrolyte leakage compared with LC10 (Figure 3B).
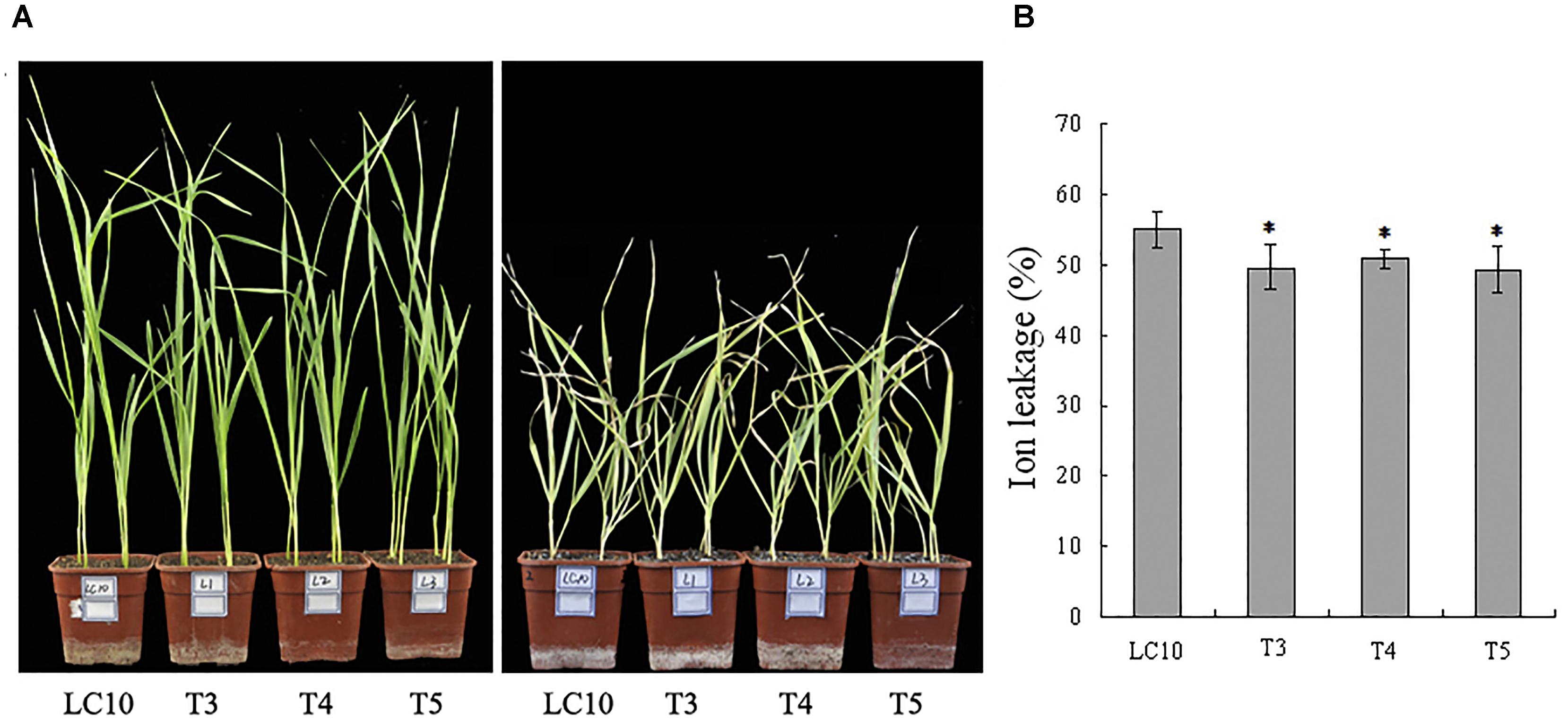
FIGURE 3. Heat tolerance assay of TaPEPKR2 transgenic wheat seedlings. (A) Phenotypes of 10-day-old LC10 and three TaPEPKR2 transgenic lines before heat treatment (left). 5-day-old LC10 and three TaPEPKR2 transgenic wheat lines were treated at 45°C for 18 h, then recovered at 22°C for 5 days. Images were taken post-recovery (right). (B) Ion leakage assay of the transgenic seedlings in panel (A) after heat treatment. The symbol “∗” indicates significance at P < 0.05.
TaPEPKR2 Overexpression in Wheat Enhanced Tolerance to Dehydration Stress
Because TaPEPKR2 was induced by 20% PEG treatment, this suggested that TaPEPKR2 may be involved in dehydration stress tolerance. As expected, TaPEPKR2 transgenic lines showed significantly higher total root lengths in the presence of 10% PEG than WT (Figure 4). These results indicate that the overexpression of TaPEPKR2 in wheat conferred dehydration stress tolerance.
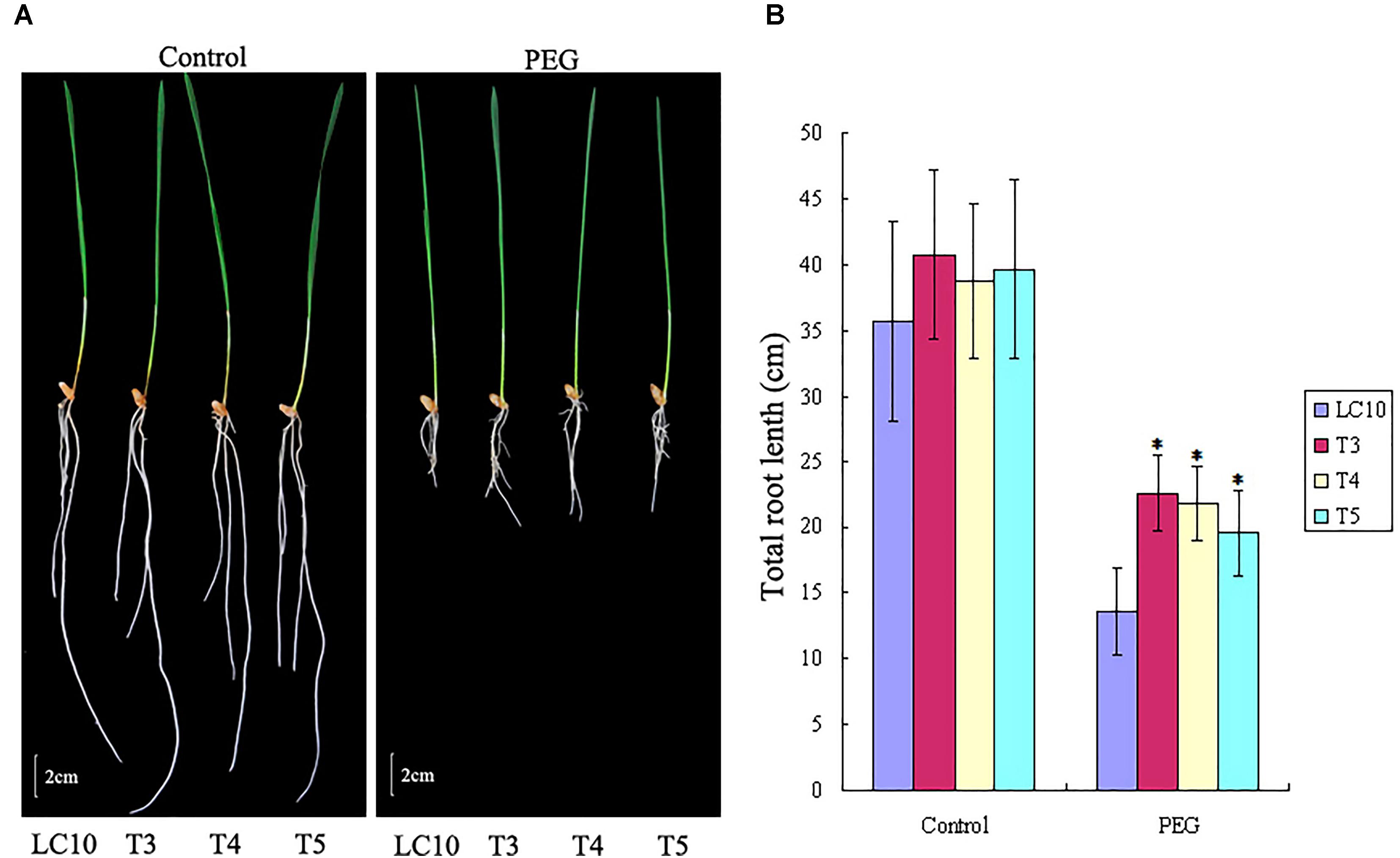
FIGURE 4. Dehydration stress tolerance assay of TaPEPKR2 transgenic wheat seedlings. (A) Phenotypes of 10-day-old LC10 and transgenic lines overexpressing TaPEPKR2 under control and 20% PEG conditions. (B) Total root length of 10-day-old LC10 and transgenic seedlings under stress conditions. The symbol “∗” indicates significance at P < 0.05.
TaPEPKR2 Overexpression in Dicotyledonous Arabidopsis Also Enhanced Tolerance to Heat and Dehydration Stresses
To characterize the biological functions of TaPEPKR2, we overexpressed it in Arabidopsis. RT-qPCR results indicated that all seven transgenic lines showed high expression levels of TaPEPKR2, with highest expression detected in transgenic line L7 (Figure 5A). To further validate the function of TaPEPKR2 in plant tolerance to heat and dehydration stresses, we observed the phenotypes of TaPEPKR2 transgenic Arabidopsis at various developmental stages but found no morphological differences between transgenic lines and WT (data not shown).
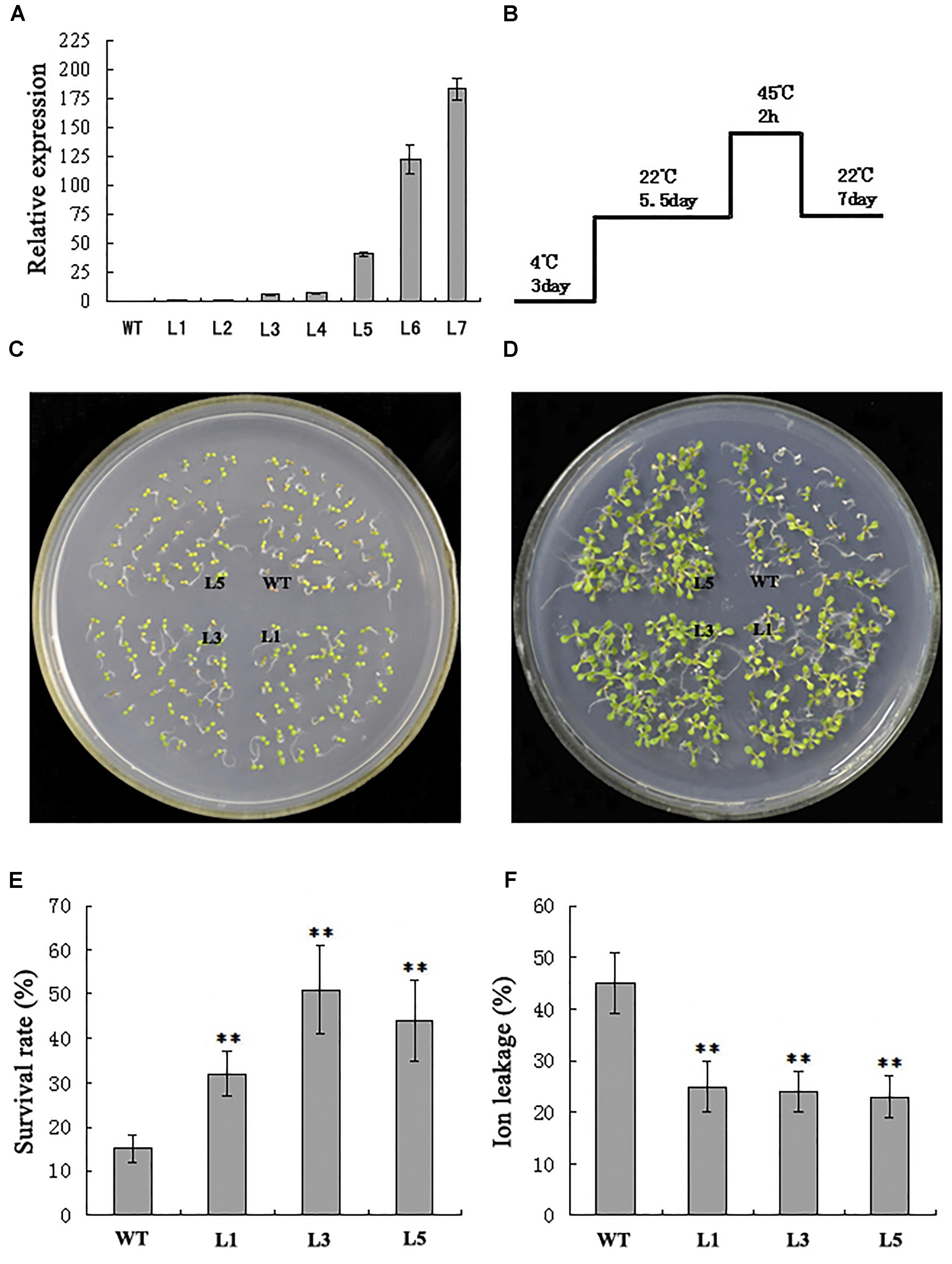
FIGURE 5. Effect of TaPEPKR2 overexpression on heat tolerance in transgenic Arabidopsis. (A) Real-time PCR of transgenic Arabidopsis lines overexpressing TaPEPKR2. L1–L7 are seven individual TaPEPKR2 transgenic lines. Relative TaPEPKR2 expression in transgenic line L1 was set as the control. Data are the means of three replicates ± SD. (B) The heat treatment regime. (C) Seedlings grown for 5.5 days at 22°C were used as the control. (D) Phenotypes of WT, L1, L3, and L5 after heat treatment and recovery at 22°C for 7 days. (E) Survival rates of three TaPEPKR2 transgenic lines (L1, L3, and L5) and WT after heat treatment. Data are the mean of three replicates ± SD (n = 60 for each experiment). (F) Ion leakage assay of the seedlings in panel (D) at 42°C for 1 h. Data are the means ± SD of three replicates; ∗∗P < 0.01 (Student’s t-test).
Seven-day-old TaPEPKR2-OE and WT seedlings grown at 22°C were subjected to heat treatment at 45°C for 2 h (Figure 5B). Before the treatment, there was no evident difference between transgenic and WT lines (Figure 5C), whereas after the treatment, the survival rate of transgenic lines L3 and L5 was much higher than that of WT seedlings (Figures 5D,E). Electrolyte leakage of detached leaves was evaluated under heat stress conditions. The results showed that detached leaves of WT plants had released more electrolytes than transgenic leaves (Figure 5F). These results indicate that TaPEPKR2 overexpression in Arabidopsis also enhances thermotolerance.
To further investigate the performance of transgenic TaPEPKR2Arabidopsis plants, lines L1, L3, and L5 were selected for dehydration tolerance tests before bolting (Figure 6). After dehydration stress (1 week after watering), around 95% of WT seedlings died, whereas 35–70% of TaPEPKR2 transgenic seedlings survived (Figures 6A,B). We further validated relative water loss rates of detached leaves (Figures 6C,D). Compared with WT plants, lines L1, L3, and L5 displayed lower relative water loss rates, and the final relative water content of detached rosette leaves from lines L3 and L5 was significantly higher than that of controls. These results indicated that the overexpression of TaPEPKR2 resulted in dehydration tolerance in Arabidopsis.
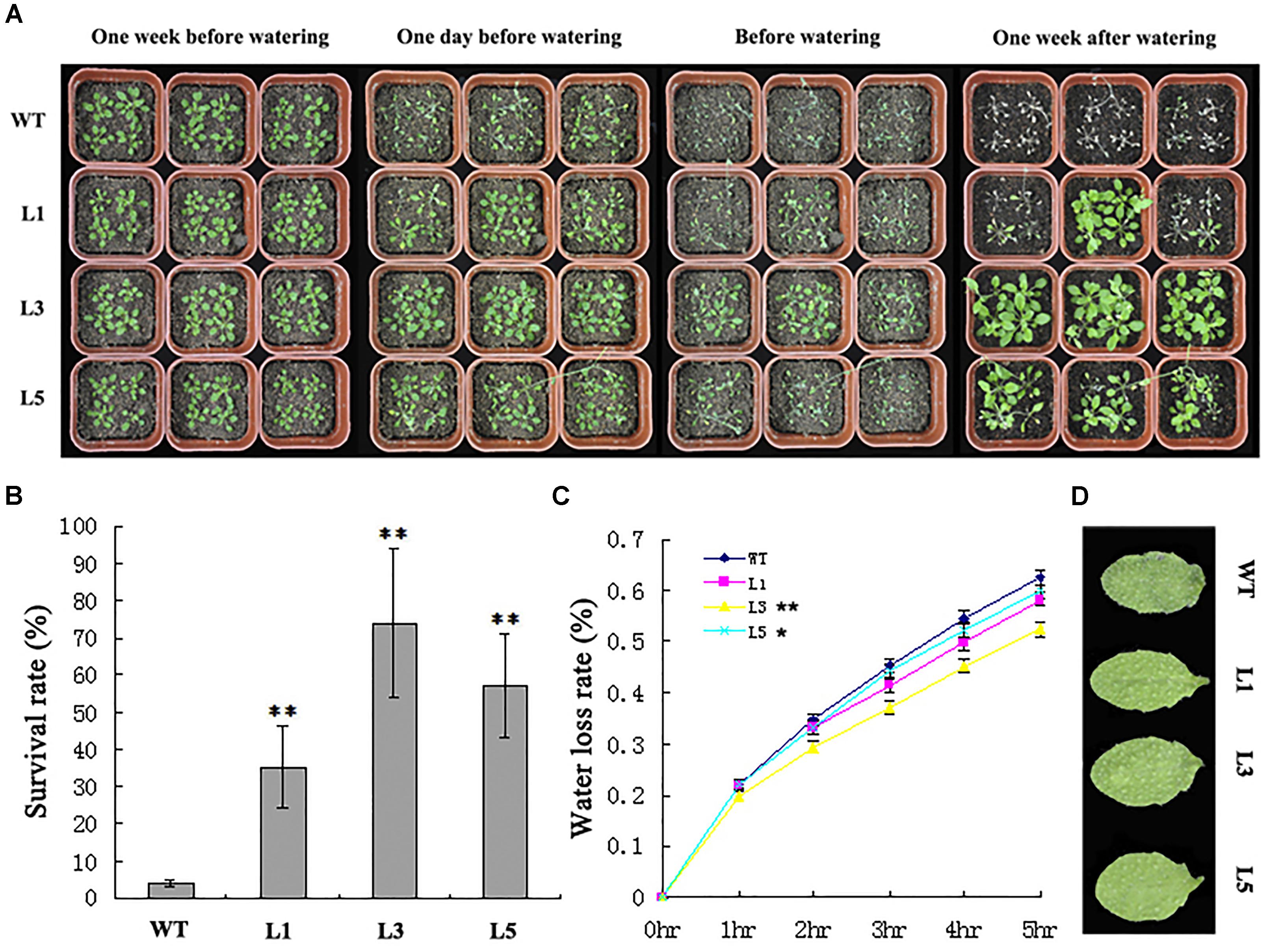
FIGURE 6. TaPEPKR2 transgenic plants show improved dehydration tolerance. (A) WT and TaPEPKR2 transgenic seedlings grown in soil were subjected to water deprivation for 25 days, then re-watered for ∼1 week. (B) Survival rates of WT and TaPEPKR2 transgenic plants after dehydration treatment. Data are the mean ± SD of three replicates (n = 30 for each experiment); ∗∗P < 0.01 (Student’s t-test). (C) Relative water loss rate of WT and TaPEPKR2 transgenic plants. Data are the mean ± SD of three replicates; ∗P < 0.05, ∗∗P < 0.01 (Student’s t-test). (D) Leaf wilting of detached leaves from WT and transgenic plants after 5 h.
Discussion
In the present study, we identified a wheat PEP carboxylase kinase-related kinase gene, TaPEPKR2, that we showed to be involved in both heat and dehydration stress tolerance. SMART analysis revealed that the TaPEPKR2 protein sequence possessed a S_TKc motif (Supplementary Figure S1). These results suggested that TaPEPKR2 is a typical serine–threonine protein kinase gene. Sequence alignment indicated that TaPEPKR2 shares 81.98% similarity with OsPEPKR2, 81.46% similarity with ZmPEPKR2, and 53.18% similarity with AtPEPKR2, suggesting that it is highly conserved. The S_TKc motif of PEPKRs showed the highest homology with PPCKs, however, PEPKR proteins contain both N- and C-terminal extensions without the PPCK superfamily (Hrabak et al., 2003). These results indicated that PEPKR and PPCK functions have commonalities but also exhibit differences.
The upstream sequence of TaPEPKR2 was analyzed for the presence of cis-acting elements by using the PlantCARE database (Supplementary Table S2). We found the promoter region of TaPEPKR2 contained the putative CGTCA-motif/TGACG-motif, ABRE, Skn-1-motif/GCN4, TC-rich repeats, GARE-motif/TATC-box, TCA-element, MBS and WUN-motif, indicating the expression of TaPEPKR2 could be regulated by several types of stresses, including abiotic and biotic stresses. The expression pattern is a direct indication of gene involvement in developmental events. In this study, TaPEPKR2 expression was induced during heat and dehydration stress (Figure 2); however, there was no report of TaPEPKR2 homologs from other species previously. The expression pattern of TaPEPKR2 homologous genes was analyzed in the Arabidopsis Weigelworld Database2 and Plant Expression Database3. In Arabidopsis, AtPEPKR2 was induced by heat and drought stress within a small range. In durum wheat cv. Cappelli, neither heat stress nor drought stress alone could induce TdPEPKR2 expression, however, this was achieved by a combination of the two. In durum wheat cv. Ofanto, TdPEPKR2 was induced by heat stress within a small range, but not by drought stress. However, their combination significantly induced TdPEPKR2 expression. In common, wheat drought sensitive cv. A24-39, TaPEPKR2 was weakly induced by drought stress, while in common wheat drought tolerant cv. Y12-3, TaPEPKR2 was significantly induced by drought stress (Krugman et al., 2010). In barley caryopsis, the orthologous gene of TaPEPKR2 (probe Barley1_05454) was induced by 0.5, 3, and 6 h heat stress treatment (Mangelsen et al., 2010). The expression pattern of TaPEPKR2 and its orthologous genes induced by high temperature and dehydration hinted the function of PEPKR2 in heat and drought tolerance.
Our results revealed that overexpression of TaPEPKR2 in wheat imparted tolerance to heat and dehydration stresses compared to wild type plants. It was fist report about function of PEPKRs in plant. PEPKRs belonged to the CDPK-SnRK superfamily (Hrabak et al., 2003). Not surprisingly, many of these kinases have been implicated in response to both heat and/or dehydration stresses. PEPKRs also called PPCK related kinases and shared catalytic domain with PPCK. Previous studies have concluded that PPCK activity in rice positively regulates PEPC activity during exposure to osmotic stress (Feria et al., 2016; Liu et al., 2017). Furthermore, PPCK gene expression and activity are regulated by signaling molecules such as Ca2+ and H2O2 (Liu et al., 2017). Are there PEPRKs regulatory proteins which play important role in calcium and ROS homeostasis under abiotic stress? What potential specific substrates they have? Further studies are needed to determine the molecular mechanisms involved in enhancing heat and dehydration stresses tolerance in TaPEPKR2-OE plants. Relative expression levels of the genes functioning in ABA biosynthesis (ABA1, AT5G67030), signaling (ABI3, AT3G24650), heat shock protein 70 (HSP70, AT3G12580), and 17.6A (HSP17.6A, AT5G12030) were investigated in TaPEPKR2 transgenic Arabidopsis plants (Supplementary Figure S3). The expressions of ABA1 and HSP70 were found to be unchanged, however, ABI3 and HSP17.6A were elevated constitutively. These results present some clues of TaPEPKR2 regulating ABA signaling and heat shock proteins in heat and dehydration stresses.
Author Contributions
QS, HP, and XZ designed the research. XZ, XG, KH, FW, and XT performed the research. XZ, XG, HP, ZN, YY, ZH, and MX analyzed the data. XZ and HP wrote the paper. All authors read and approved the final manuscript.
Funding
This work was supported by the National Natural Science Foundation of China (31571747) and National Key Project for Research on Transgenic (2016ZX08002-002).
Conflict of Interest Statement
The authors declare that the research was conducted in the absence of any commercial or financial relationships that could be construed as a potential conflict of interest.
Supplementary Material
The Supplementary Material for this article can be found online at: https://www.frontiersin.org/articles/10.3389/fpls.2018.01710/full#supplementary-material
FIGURE S1 | The functional domain of the TaPEPKR2 protein sequence identified by the SMART program.
FIGURE S2 | PCR analysis of TaPEPKR2 transgenic wheat plants. (A) Confirmation of TaPEPKR2 insertion in LC10 by PCR analysis of H2O, LC10, PC, L3, L4, and L5 transgenic plants. PC: Ubi::TaPEPKR2 vector was used as the positive control. (B) Relative expression of TaPEPKR2 as shown by RT-qPCR. β-actin was used as the internal control. Data are the mean ± SD of three independent biological replicates.
FIGURE S3 | Relative expression level of ABA1 (A), ABI3 (B), HSP70 (C), and HSP17.6A (D) in 10-day-old TaPEPKR2 transgenic Arabidopsis plants, as determined by RT-qPCR. Data represent the mean of three replicates ± SD.
TABLE S1 | Primer sequences used in this study.
TABLE S2 | Cis-elements present in the promoters of TaPEPKR2.
FILE S1 | Coding sequence of TaPEPKR2.
Footnotes
- ^http://smart.embl-heidelberg.de/
- ^http://jsp.weigelworld.org/expviz/expviz.jsp
- ^http://www.plexdb.org/
References
Agetsuma, M., Tuchida, Y., Imanari, E., Furumoto, T., and Izui, K. (2004). Regulatory phosphorylation of PEP carboxylase (PEPC) in C-4 photosynthesis: redox regulation of a cytosolic protein kinase (PK) activity in vivo. Plant Cell Physiol. 45, S154–S154.
Ashraf, M. (2010). Inducing drought tolerance in plants: recent advances. Biotechnol. Adv. 28, 169–183. doi: 10.1016/j.biotechadv.2009.11.005
Camejo, D., Rodriguez, P., Morales, A., Dell’amico, J. M., Torrecillas, A., and Alarcón, J. J. (2005). High temperature effects on photosynthetic activity of two tomato cultivars with different heat susceptibility. J. Plant Physiol. 162, 281–289. doi: 10.1016/j.jplph.2004.07.014
Feria, A. B., Bosch, N., Sanchez, A., Nieto-Ingelmo, A. I., De La Osa, C., Echevarría, C., et al. (2016). Phosphoenolpyruvate carboxylase (PEPC) and PEPC-kinase (PEPC-k) isoenzymes in Arabidopsis thaliana: role in control and abiotic stress conditions. Planta 244, 901–913. doi: 10.1007/s00425-016-2556-9
Hrabak, E. M., Chan, C. W., Gribskov, M., Harper, J. F., Choi, J. H., Halford, N., et al. (2003). The Arabidopsis CDPK-SnRK superfamily of protein kinases. Plant Physiol. 132, 666–680. doi: 10.1104/pp.102.011999
Krugman, T., Chague, V., Peleg, Z., Balzergue, S., Just, J., Korol, A. B., et al. (2010). Multilevel regulation and signalling processes associated with adaptation to terminal drought in wild emmer wheat. Funct. Integr. Genomics 10, 167–186. doi: 10.1007/s10142-010-0166-3
Liu, X., Li, X., Zhang, C., Dai, C., Zhou, J., Ren, C., et al. (2017). Phosphoenolpyruvate carboxylase regulation in C4-PEPC-expressing transgenic rice during early responses to drought stress. Physiol. Plant. 159, 178–200. doi: 10.1111/ppl.12506
Livak, K. J., and Schmittgen, T. D. (2001). Analysis of relative gene expression data using real-time quantitative PCR and the 2(T)(-Delta Delta C) method. Methods 25, 402–408. doi: 10.1006/meth.2001.1262
Lobell, D. B., Schlenker, W., and Costa-Roberts, J. (2011). Climate trends and global crop production since 1980. Science 333, 616–620. doi: 10.1126/science.1204531
Mangelsen, E., Wanke, D., Kilian, J., Sundberg, E., Harter, K., Jansson, C., et al. (2010). Significance of light, sugar, and amino acid supply for diurnal gene regulation in developing barley caryopses. Plant Physiol. 153, 14–33. doi: 10.1104/pp.110.154856
Mittler, R., Finka, A., and Goloubinoff, P. (2012). How do plants feel the heat? Trends Biochem. Sci. 37, 118–125. doi: 10.1016/j.tibs.2011.11.007
Nimmo, H. G., Fontaine, V., Hartwell, J., Jenkins, G. I., Nimmo, G. A., and Wilkins, M. B. (2001). PEP carboxylase kinase is a novel protein kinase controlled at the level of expression. New Phytol. 151, 91–97. doi: 10.1046/j.1469-8137.2001.00155.x
Qin, D., Wu, H., Peng, H., Yao, Y., Ni, Z., Li, Z., et al. (2008). Heat stress-responsive transcriptome analysis in heat susceptible and tolerant wheat (Triticum aestivum L.) by using wheat genome array. BMC Genomics 9:432. doi: 10.1186/1471-2164-9-432
Qu, A. L., Ding, Y. F., Jiang, Q., and Zhu, C. (2013). Molecular mechanisms of the plant heat stress response. Biochem. Biophys. Res. Commun. 432, 203–207. doi: 10.1016/j.bbrc.2013.01.104
Shinozaki, K., and Dennis, E. S. (2003). Cell signalling and gene regulation - global analyses of signal transduction and gene expression profiles - editorial overview. Curr. Opin. Plant Biol. 6, 405–409. doi: 10.1016/S1369-5266(03)00093-1
Vidal, J., and Chollet, R. (1997). Regulatory phosphorylation of C-4 PEP carboxylase. Trends Plant Sci. 2, 230–237. doi: 10.1016/S1360-1385(97)01046-7
Zang, X., Geng, X., Wang, F., Liu, Z., Zhang, L., Zhao, Y., et al. (2017a). Overexpression of wheat ferritin gene TaFER-5B enhances tolerance to heat stress and other abiotic stresses associated with the ROS scavenging. BMC Plant Biol. 17:14. doi: 10.1186/s12870-016-0958-2
Zang, X. S., Geng, X. L., Liu, K. L., Wang, F., Liu, Z. S., Zhang, L., et al. (2017b). Ectopic expression of TaOEP16-2-5B, a wheat plastid outer envelope protein gene, enhances heat and drought stress tolerance in transgenic Arabidopsis plants. Plant Sci. 258, 1–11. doi: 10.1016/j.plantsci.2017.01.011
Keywords: TaPEPKR2, heat stress, dehydration stress, PEP carboxylase kinase-related kinase, wheat, Arabidopsis
Citation: Zang X, Geng X, He K, Wang F, Tian X, Xin M, Yao Y, Hu Z, Ni Z, Sun Q and Peng H (2018) Overexpression of the Wheat (Triticum aestivum L.) TaPEPKR2 Gene Enhances Heat and Dehydration Tolerance in Both Wheat and Arabidopsis. Front. Plant Sci. 9:1710. doi: 10.3389/fpls.2018.01710
Received: 21 May 2018; Accepted: 02 November 2018;
Published: 23 November 2018.
Edited by:
Dae-Jin Yun, Konkuk University, South KoreaReviewed by:
Guangxiao Yang, Huazhong University of Science and Technology, ChinaKlára Kosová, Crop Research Institute (CRI), Czechia
Copyright © 2018 Zang, Geng, He, Wang, Tian, Xin, Yao, Hu, Ni, Sun and Peng. This is an open-access article distributed under the terms of the Creative Commons Attribution License (CC BY). The use, distribution or reproduction in other forums is permitted, provided the original author(s) and the copyright owner(s) are credited and that the original publication in this journal is cited, in accordance with accepted academic practice. No use, distribution or reproduction is permitted which does not comply with these terms.
*Correspondence: Huiru Peng, cGVuZ2h1aXJ1QGNhdS5lZHUuY24=; cGVuZ2h1aXJ1MTQ1MkAxNjMuY29t