- 1State Key Laboratory of Urban and Regional Ecology, Research Center for Eco-Environmental Sciences, Chinese Academy of Sciences, Beijing, China
- 2University of Chinese Academy of Sciences, Beijing, China
- 3Global Ecology Unit, Facultat de Biociencies, CREAF-CSIS-UAB, Barcelona, Spain
- 4Laboratory of Geospatial Technology for the Middle and Lower Yellow River Regions, College of Environment and Planning, Henan University, Kaifeng, China
- 5Key Laboratory of Vegetation Restoration and Management of Degraded Ecosystems, South China Botanical Garden, Chinese Academy of Sciences, Guangzhou, China
- 6School of Earth Sciences and Resources, China University of Geosciences, Beijing, China
Nitrogen (N) deposition and precipitation could profoundly influence the structure and function of forest ecosystems. However, conventional studies with understory additions of nitrogen and water largely ignored canopy-associated ecological processes and may have not accurately reflected the natural situations. Additionally, most studies only made sampling at one time point, overlooked temporal dynamics of ecosystem response to environmental changes. Here we carried out a field trial in a mixed deciduous forest of China with canopy addition of N and water for 4 years to investigate the effects of increased N deposition and precipitation on the diversity and community composition of arbuscular mycorrhizal (AM) fungi, the ubiquitous symbiotic fungi for the majority of terrestrial plants. We found that (1) in the 1st year, N addition, water addition and their interactions all exhibited significant influences on AM fungal community composition; (2) in the 2nd year, only water addition significantly reduced AM fungal alpha-diversity (richness and Shannon index); (3) in the next 2 years, both N addition and water addition showed no significant effect on AM fungal community composition or alpha-diversity, with an exception that water addition significantly changed AM fungal community composition in the 4th year; (4) the increment of N or water tended to decrease the abundance and richness of the dominant genus Glomus and favored other AM fungi. (5) soil pH was marginally positively related with AM fungal community composition dissimilarity, soil NH4+-N and N/P showed significant/marginal positive correlation with AM fungal alpha-diversity. We concluded that the effect of increased N deposition and precipitation on AM fungal community composition was time-dependent, mediated by soil factors, and possibly related to the sensitivity and resilience of forest ecosystem to environmental changes.
Introduction
Arbuscular mycorrhizal (AM) fungi can form mutualistic symbioses with the majority of terrestrial plants (Smith and Read, 2008) and provide vital ecological services such as improving plant mineral nutrition (Li et al., 2006; Subramanian et al., 2006), enhancing plant tolerance to biotic (Elsen et al., 2008; Affokpon et al., 2011) and abiotic stresses (e.g., flooding, high temperature) (Fougnies et al., 2007; Li et al., 2009; Zhu et al., 2011; Camprubi et al., 2012), altering the composition and diversity of plant communities and influencing the productivity, structure and stability of ecosystems (van Der Heijden et al., 1998, 2008; Jansa et al., 2008), and intensifying the resilience of ecosystem to global climate change (Martínez-García et al., 2017). In view of their ecological significance, investigation on AM fungal diversity and community assemblage has become hot topics in soil ecology in recent years. AM fungal community assembly could be predicted by both niche theory which assumes that the competition among species for limited resources and the differentiation of niche space across species allow species coexistence, emphasizing the importance of determined processes in structuring community assembly (Leibold and McPeek, 2006), and neutral theory which presumes that all species are ecologically equivalent, emphasizing the significance of stochastic processes and dispersal limitation depending on spatial scales (Hubbell, 2001). At global and regional scales, neutral theory weighs more than the ecological niche theory, and AM fungal distribution pattern is mainly shaped by geographical distance and climate factors. However, the ecological niche theory dominates at local scale and small scale, and the effects of host plants and the soil properties on AM fungal community become more important than geographical distance restriction (Chen et al., 2018).
In recent decades, global climate change driven by anthropogenic disturbance has been intensified and impacted the structure and function of multiple aquatic and terrestrial ecosystems (Marino et al., 2017). As an important component of global climate change, nitrogen (N) deposition and its ecological consequences have attracted serious concerns. At global scale, it is estimated that the N deposition rate has increased nearly 34 Tg N yr-1 in 1860–100 Tg N yr-1 in 1995 and may increase up to 200 Tg N yr-1 in 2050 (Galloway et al., 2004). Increased N deposition could cause negative effects on terrestrial ecosystems, such as biodiversity loss, soil acidification, productivity decline, nutrient imbalance and forest degradation (Vitousek et al., 1997; Magill et al., 2004; Hogberg et al., 2006; Bobbink et al., 2010; Lu et al., 2014). Most previous studies on N deposition are carried out in Europe and North America, while studies on N deposition and its ecological consequences in China are rather limited. As a fact, with the rapid development, China is also experiencing increasing N deposition, especially in its central and southeastern areas (Jia et al., 2014). The mean wet N deposition over China has increased nearly 25% from 1990s to 2000s (Jia et al., 2014), and the N deposition rate in China is predicted to continually increase in the coming decades (Liu et al., 2013).
Besides increased N deposition, changes in precipitation patterns is also an important component of global change. According to IPCC report, heavy precipitation events including the frequency and intensity of heavy precipitation over land regions increased markedly in recent years. In many mid-latitude regions, mean precipitation will gradually increase in the 21st century (Integrated Professional Competency Course [IPCC], 2013). Increased precipitation could change species richness and alter the plant community structure and aboveground net primary productivity (ANPP) in arid and semi-arid (water-limited) steppe ecosystems (Yang et al., 2011; Zeppel et al., 2014; Ren et al., 2015). Intensified precipitation can also elevate the risk of soil nutrient leaching (e.g., N, P) (Martínez-García et al., 2017). Moreover, under natural conditions, multiple global changes may occur simultaneously and interact with each other (Harpole et al., 2007). The impacts of N deposition on the ecosystem structure and function would be substantially altered by precipitation (Harpole et al., 2007; Yang et al., 2011; Araya et al., 2013). Previous studies showed that precipitation increment could alleviate the negative effects of increased N deposition by increasing the mobility and leaching of soil inorganic N (Li et al., 2016; Sun et al., 2017). More extensive studies on the interactive effects of N deposition and precipitation on ecosystems are still expected.
Up to date, very limited information is available as for the responses of belowground ecosystem especially for soil microbial communities, to climate changes (Li et al., 2016). Definitely more attention should be paid to the soil microorganisms which play important roles in nutrient cycling, organic matter decomposition, primary production, regulation of greenhouse emissions and other ecosystem functions (Philippot et al., 2013; Wagg et al., 2014; Delgado-Baquerizo et al., 2017). As an important functional group of soil microbes, AM fungi directly bridge up plant and soil, and are selected as model organism for studying belowground-aboveground interactions. It has been well documented that increased N deposition decreased the abundance (van Diepen et al., 2007, 2010; Camenzind et al., 2014, 2016), richness (Camenzind et al., 2014; Liu et al., 2014; Chen et al., 2017) and diversity (Chen et al., 2017), and altered the community composition (van Diepen et al., 2011; Chen et al., 2014; Zheng et al., 2014; Kim et al., 2015) of AM fungi. Precipitation increment can also decrease the abundance and alpha-diversity (Chen et al., 2017), and alter community composition (Gao et al., 2016; Chen et al., 2017) of AM fungi in semiarid (water-limited) steppe ecosystem. However, as far as we know, there were only limited reports on the interaction of N deposition and precipitation on AM fungal communities, and they all were carried out in water-limited steppe ecosystems (Li et al., 2015; Chen et al., 2017). The interaction could be different in humid forest ecosystem from arid/semi-arid steppe ecosystems. In addition, most previous studies on the ecological impacts of precipitation increment or N deposition on forest ecosystems largely ignored many canopy-associated ecological processes by understory addition of N or water. The canopy-associated processes may include N uptake by leaves, epiphytes and microbes; immobilization in decaying leaves or other dead organic matters; volatilization as water evaporates; and transformation of inorganic N to organic N (Zhang et al., 2015). Undoubtedly, canopy processes are more important in forest than in grassland and should not be neglected. As seen in report, the percentage of retained N from N deposition by forest canopy could vary in different studies from 1∼5% to 10∼25%, depending on forest type and N deposition intensity (Zhang et al., 2015).
Furthermore, AM fungal community structure exhibits seasonal dynamics and interannual variability (Husband et al., 2002; Hazard et al., 2014). Husband et al. (2002) investigated the diversity and distribution of AM fungi colonizing tree seedling roots for 2 years in the tropical forest on Barro Colorado Island, Republic of Panama. They found that dominant AM fungal types in the first year were nearly entirely replaced by previously rare types in the following year; Hazard et al. (2014) investigated the effects of biosolids on AM fungal communities in grassland and arable agroecosystems and found that the effect of seasonality exceeded that of biosolids application. The AM fungal community compositions (using T-RFLP method) associated with Lolium perenne shifted with seasonality and year, some dominant AM fungi (e.g., T-RFs associated with Rhizophagus irregularis) were present in roots throughout and between years, others were only present seasonally (e.g., HinfI-HEX 422), and rarer species fluctuated in presence and frequency. However, many studies only made one sampling and overlooked the temporal dynamics of AM fungal community in response to environmental changes.
As a result, we conducted a field trial in a mixed forest in China’s climate transition zone from subtropical to warm temperate climate to investigate the impacts of N deposition and precipitation increment on AM fungal community with canopy N and water addition. We carried out field investigation and collected soil samples every year since the experiment establishment in 2013, and analyzed AM fungal diversity and community structure by using the high throughput sequencing technology. We hypothesized that (1) canopy N or water addition would significantly decrease AM fungal richness, Shannon diversity index and change community composition; (2) N and water addition interactively shape AM fungal community; (3) the effects of N and water addition on AM fungal community would be time-dependent. To the best of our knowledge, this study for the first time investigated the interactive effects of increased N deposition and precipitation on AM fungal diversity and community composition in a forest ecosystem and is expected to allow better understanding of the impacts of climate changes on the forest ecosystems.
Materials and Methods
Study Site
The study site was located in Jigongshan (JGS) National Nature Reserve (31°46′-31°52′ N, 114°01′-114°06′ E) representing a transitional zone from subtropical to warm temperate climate in China (Zhang et al., 2015). Dominant tree species in the forest are Quercus acutissima Carruth., Quercus variabilis Bl. and Liquidambar formosana Hance. Both oaks form ectomycorrhiza, while Liquidambar formosana Hance can form arbuscular mycorrhiza. In addition, there are some understory shrubs, such as Lindera glauca (Sieb.et Zucc.) Bl and Rubus lambertianus Ser., and herbs such as Ophiopogon japonicas (Linn. f.) Ker-Gawl. and Phaenosperma globosa Munro ex Benth. which also can form arbuscular mycorrhiza. The soil type is Ferri-Udic Argosols (yellow brown soils) and the pH value is 5.0-6.0.
According to the meteorological data from 1951 to 2011, the local mean annual temperature (MAT) is 15.2°C and the mean annual precipitation (MAP) is 1119 mm. 80% of the precipitation occurs in April- October. The average annual air humidity is 79%. The background N deposition rate in rainfall is 19.6 kg N ha-1yr-1, in which the NH4+/NO3- ratio is close to 1 (Zhang et al., 2015; Zhang et al., 2018). More information of JGS Reserve were described by Zhang et al. (2015).
Experimental Design
This experiment was set up as a fully randomized two-factor block design with four blocks, each block included four plots, and each plot was 17 m in radius. Within each block, each plot was randomly assigned with one of the four treatments: control (CK, ambient environment), canopy addition of N (CN), canopy addition of water (CW), and canopy additions of both N and water (CNW). In order to prevent the interference among treatments, a 20 m buffer zone was left between any two plots and a PVC isolation board with depth of 1 m was installed in the middle of the buffer zone.
The N application rate was 50 kg ha-1 yr-1, which is predicted to occur in the near future in this region by Liu et al. (2013). The amount of water addition was 30% of the MAP (336 mm), and the magnitude was within the range of model predictions for future precipitation increment induced by warming in Northern Hemisphere by Yang et al. (2015). Nitrogen was added as NH4NO3 solution (7.7 mmol/L), both the solvent and water are from the local lake. Nitrogen and water were added during the growing season from April to October, and N was applied once a month (totally seven times per year), while water was added once a week (12 mm per week) to prevent surface runoff (Shi et al., 2018). The treatment dates were determined according to the phenology of the forest, i.e., the first time was conducted 1 week before all buds began to open (mid-April), and the last was conducted as leaf litter began to fall (mid-October) (Shi et al., 2016).
All the treatments except CK were realized with a forest canopy spraying system built in the center of the plots. This system can pump N solution or water to a height of 35 m (almost 5 m above the forest canopy) through PVC pipes (10 cm in diameter) which were fixed on the supporting tower. The N solution or water was evenly sprayed onto the forest canopy of the plot by four sprinklers with different spraying range and could be rotated 360° freely (Shi et al., 2018). The working rules and efficiency of the system were described in details by Zhang et al. (2015) and Shi et al. (2016, 2018).
Soil Sampling and Laboratory Analysis
We made sampling after the seventh treatment every year (mid or late November or December depending on the weather condition). To minimize the impact of sampling position as far as possible, 5 dominant trees which are evenly distributed in the 20 × 20 m2 core area of each plot were selected as target trees. Two soil cores (3 cm diameter, 10 cm depth) within the range of 2 m from each target tree, 10 soil cores in total per plot were taken and mixed into one soil sample. The fresh soil samples were transported to laboratory on ice bag, passed through a 2-mm sieve, and divided into 2 subsamples. One was freeze-dried for the DNA extraction, the other was air dried for analysis of soil physicochemical properties.
Soil moisture content was measured gravimetrically by oven-drying the fresh soil samples to constant weight at 105°C. Soil NH4+-N and NO3--N were extracted with 2 mol L-1 KCl (a soil to water ratio of 1: 5) and measured using a continuous flow analyzer (SAN++, Skakar, Breda, Holland). Soil available N (AN) was the sum of NH4+-N and NO3--N. Soil pH was determined in a soil/water suspension [1: 2.5 (w/v)] by PB-10 pH-meter (Sartorius, Göttingen, Germany). Soil organic carbon (SOC) was measured according to Walkley and Black (1934). Total N (TN) was determined on an element analyzer (Vario EL Ш[scale=0.5]img001, Elementar, Germany). Soil C/N ratio (C/N) was calculated based on SOC and TN. Soil available phosphorus (AP) was extracted with 0.5 M NaHCO3 and measured using a colorimetric method (Murphy and Riley, 1962). Soil N/P ratio (N/P) was calculated based on AN and AP.
Soil DNA was extracted from 0.25 g freeze-dried soil sample by using the Power-Soil® DNA Isolation Kit (MO BIO Laboratories, San Diego, CA, United States) according to the manufacturer’s instructions. Each soil DNA sample was diluted (1:5) with sterilized Milli-Q water. We conducted a nested PCR with primer pairs AML1/AML2 (Lee et al., 2008) and AMV4.5NF/AMDGR (Sato et al., 2005). The first PCR was conducted with a total volume of 25 μl, which contained 2.5 μl 10 × Ex Taq Buffer (Mg2++plus), 2.0 μl dNTP mixture, 0.25 μl Ex Taq (5U/μl)(TaKaRa, Dalian, China), 1.0 μl (10 mg/ml) BSA (TaKaRa, Dalian, China), 0.5 μl (10 μM) of each primer, 17.25 μl sterilized water and 1.0 μl DNA template. The PCR program of amplification are as follows: 94°C for 3 min; 35 cycles at 94°C for 45 s, 51°C for 40 s, 72°C for 1 min; followed by 72°C for 10 min and 16°C for 2 min. The first PCR products were diluted (1:10) with sterilized Milli-Q water, and then used as a template for the second PCR amplification (25 μl) under the following conditions: 94°C for 3 min; 35 cycles at 94°C for 40 s, 58°C for 1 min and 72°C for 1 min, followed by 72°C for 10 min and 4°C for 2 min. The volume of the second PCR was the same as the first. An Eppendorf Mastercycler pro-thermocycler (Eppendorf, Hamburg, Germany) was used for PCR amplification. The PCR products were separated through a 1.5% agarose gel in 1×TAE, bands were excised, and purified with the 0.8×Agencourt AMPure XP Beads (Beckman Coulter Inc., Boulevard Brea, CA, United States). The amount of DNA in the purified PCR products was measured using a Qubit 2.0 Fluorometer (Thermo Fisher Scientific Inc., Hudson, NH, United States). The final products were mixed at equimolar concentrations and then subjected to sequencing on the Illumina MiSeq platform with MiSeq Reagent Kit v3 at Shanghai Hanyu Biotech Co., Ltd.
Bioinformatics
The initial quality filtering and assembling of paired-end reads were performed by Shanghai Hanyu Biotech Co., Ltd. Raw sequence data were processed in Trimmomatic v 0.32 (Bolger et al., 2014). Main flows are as follows: (1) Remove the reads with N bases; (2) Remove the low-quality bases (Q value < 20); (3) Remove the reads itself and its pairing reads whose length is less than 50 bp. Paired-end reads were assembled by Mothur v.1.32.1 (Schloss et al., 2009) permitting 1 bp mismatches of primer bases. Sequences with maxhomop > 8, or shorter than 200 bp were removed. Chimeras were checked in Chimera.uchime v.4.2 (Edgar et al., 2011). OTUs clustering was achieved in Usearch v.9.0.2132_i86linux32 (Edgar, 2010) with a 97% identity threshold. Taxonomic assignment was performed by blasting the representative sequence of each OTU against NCBI GenBank and MaarjAM database (Öpik et al., 2010), a conservative approach was followed for the species identification: considering only identifications with > 97% similarity, >90% coverage and >200 BLAST score value (Supplementary Table S1). In order to further identify the taxonomic information for all AM fungal OTUs, we constructed a neighbor joining phylogenic tree (Supplementary Figure S1) in MEGA v5 (Tamura et al., 2011) with default parameters except that bootstrap replication was set at 1,000 with the Kimura 2 – parameter model. Representative sequences from each encountered AM fungal OTU have been deposited in GenBank (accession numbers MH205770 - MH205915).
Statistical Analysis
All statistical analyses were conducted in R (R Development Core Team). OTU tables are subsampled to the median according to de Ca’rcer et al. (2011) as the dataset of AM fungal community composition, and then used to calculate the richness and Shannon diversity index (H’). Shannon diversity index (H’) was calculated using the function ‘diversity’ in R package ‘vegan’ (Oksanen et al., 2013). To analyze the effects of canopy N and water addition and their interactions on AM fungal richness, Shannon diversity index (H’) and soil properties, two-way analysis of variance (ANOVA) was conducted, followed by Duncan’s multiple range test. The significant difference was accepted at P < 0.05. Before evaluating the effects of canopy N and water additions and their interactions on AM fungal community composition, the data of AM fungal community composition was sqrt transformed, and then a two-way permutational multivariate analysis of variance (PERMANOVA) (Anderson, 2001) was performed using the function ‘adonis2’ in R package ‘vegan’ (Oksanen et al., 2013) with 9999 permutations. In order to further confirm the differences in the composition of AM fungal communities among treatments, we used the functions ‘mrpp’, ‘adonis’ and ‘anosim’ in R package ‘vegan’ (Oksanen et al., 2013) with 9999 permutations. To analyze the influence of soil properties (AP, pH, SOC, TN, C/N, moisture, NH4+-N, NO3--N, AN, N/P) on the AM fungal richness and Shannon diversity index (H’), we performed Pearson correlation analysis. To explore the relationship between AM fungal community composition dissimilarity and soil properties, Mantel and partial Mantel test were carried out using functions ‘mantel’ and ‘mantel.partial’ in R package ‘vegan’ (Oksanen et al., 2013) with 9999 permutations. In all the analyses involved in AM fungal richness, Shannon diversity and soil properties, in order to discover outliers Dixon’s Q test was used at 95% confidence level (Dean and Dixon, 1951) and to satisfy the assumption of normality, some soil properties were log or sqrt transformed: in the 1st year, C/N and N/P were log transformed; in the 2nd year, NH4+-N was sqrt transformed; in the 3rd year, SOC and N/P were log transformed, TN was sqrt transformed; in the 4th year, AP was sqrt transformed, SOC, C/N and AN were log transformed.
Results
Effects of Canopy N and Water Addition on Soil Properties
In the 1st year, N addition significantly decreased soil pH. Significant interactive effect of N and water addition on soil C/N was observed: without water addition, N addition had negative effect on soil C/N; with water addition, the effect became positive. In the 2nd year, N addition significantly increased soil NH4+-N; water addition significantly increased soil AP, but decreased soil NH4+-N, AN and N/P. To the 3rd and 4th year, there were no significant effect of N or water addition on any soil properties, except that water addition significantly increased AN in the 3rd year (Table 1 and Supplementary Table S2).
Overall Miseq-Sequencing Information and OTU Delineation
After filtering out chimeras, 624,153 sequences were kept and clustered into 567 OTUs. The sequences of AM fungi accounted for 74.90% (467,479/624,153) of the total sequences and the AM fungal OTUs accounted for 25.75% (146/567) of the total OTUs. Moreover, the majority of AM fungal sequences (62.65%, 292,883/467,479) and OTUs (64.38%, 94/146) belonged to genus Glomus, followed by Acaulospora sequences (32.58 %, 152,330/ 467,479) and OTUs (22.60 %, 33/146), while the others covered 7 genera from 6 families, 4 orders (Figure 1).
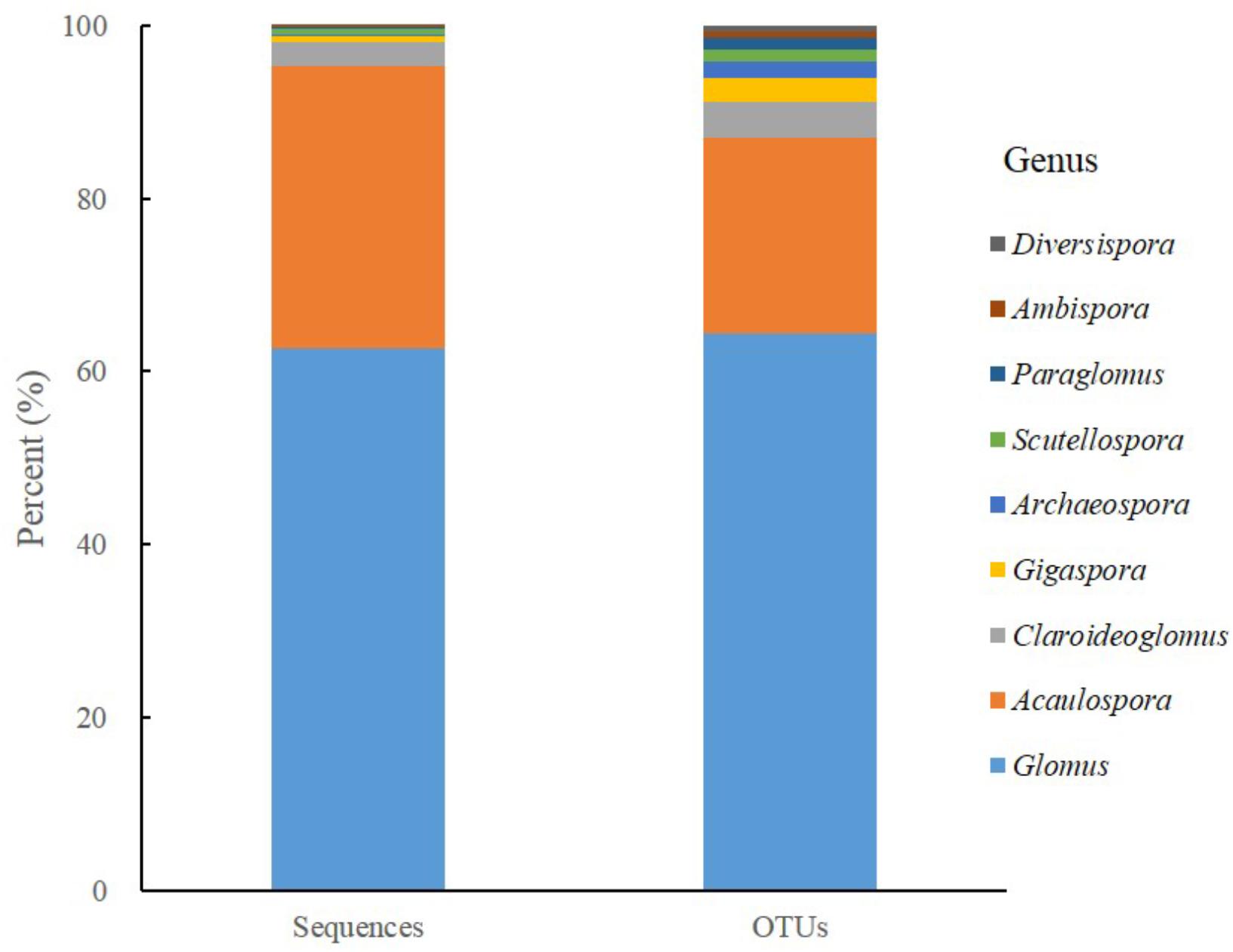
Figure 1. The proportional distributions of sequences and derived AM fungal OTUs detected in all soil samples.
Effects of Canopy N and Water Addition on AM Fungal Alpha-Diversity and Community Composition
In the 1st year, both N and water addition significantly changed AM fungal community composition. There was also significant interactive effect of N and water addition on AM fungal community composition (Tables 2, 3). However, no significant treatment effects were detected on AM fungal alpha-diversity (Figure 2). Moreover, N addition marginally decreased the relative abundance of Glomus (Figure 3A) but marginally increased Acaulospora relative abundance (Figure 3B). Simultaneously, water addition also marginally increased the relative abundance of Acaulospora, but showed no significant effect on Glomus. No significant interactions were found of N and water additions on the relative abundance of these two dominant genera.
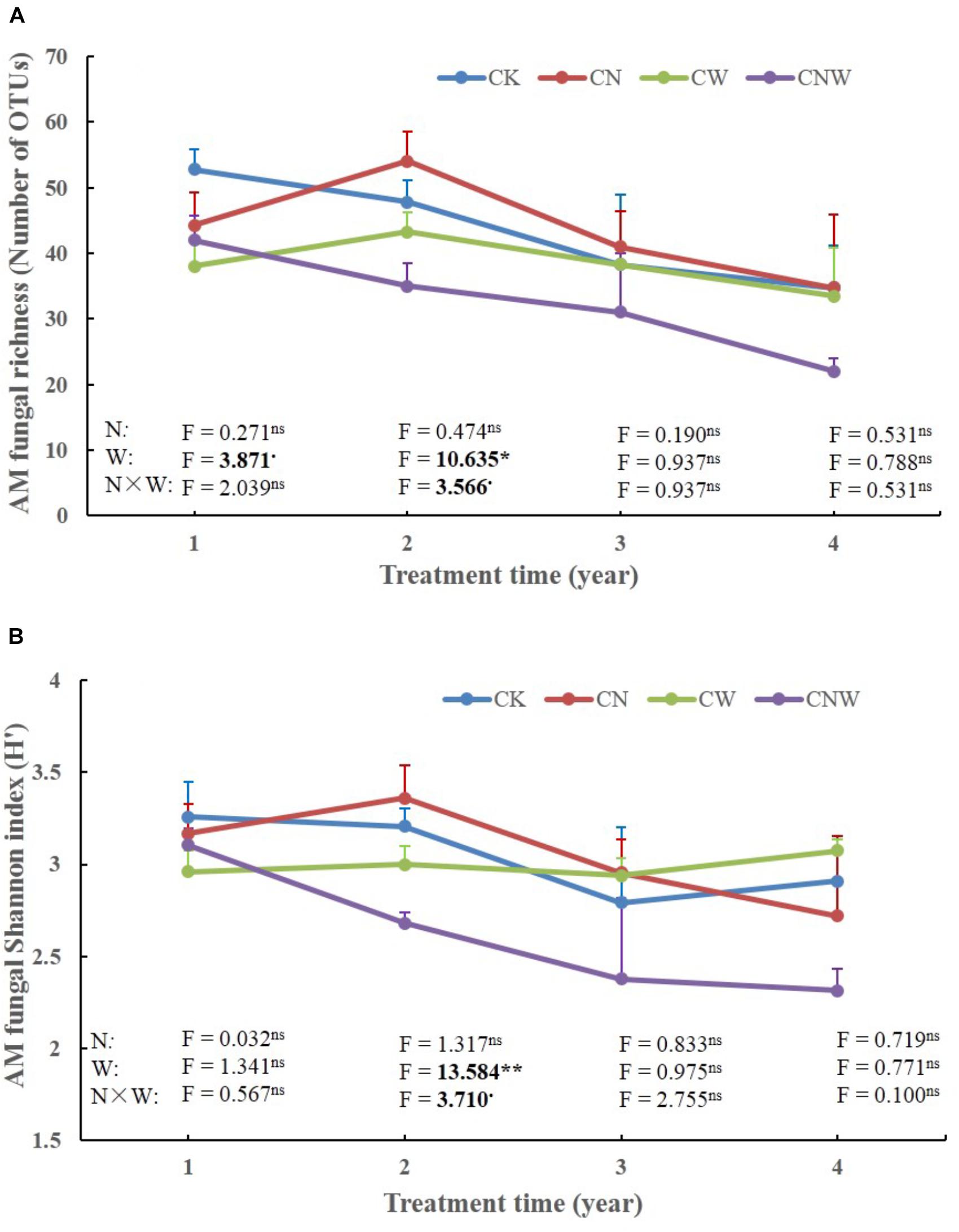
Figure 2. Effects of canopy additions of N and water on AM fungal richness (A) and Shannon diversity index (H’) (B) over time (4 years). CK, control; CN, canopy addition of N; CW, canopy addition of water; CNW, canopy additions of N and water. N, N addition; W, water addition; N × W, the results were the interaction. Significance of treatment effect was determined by two-way ANOVA. ∗∗p < 0.01; ∗p < 0.05; ⋅p < 0.1; ns, not significant.
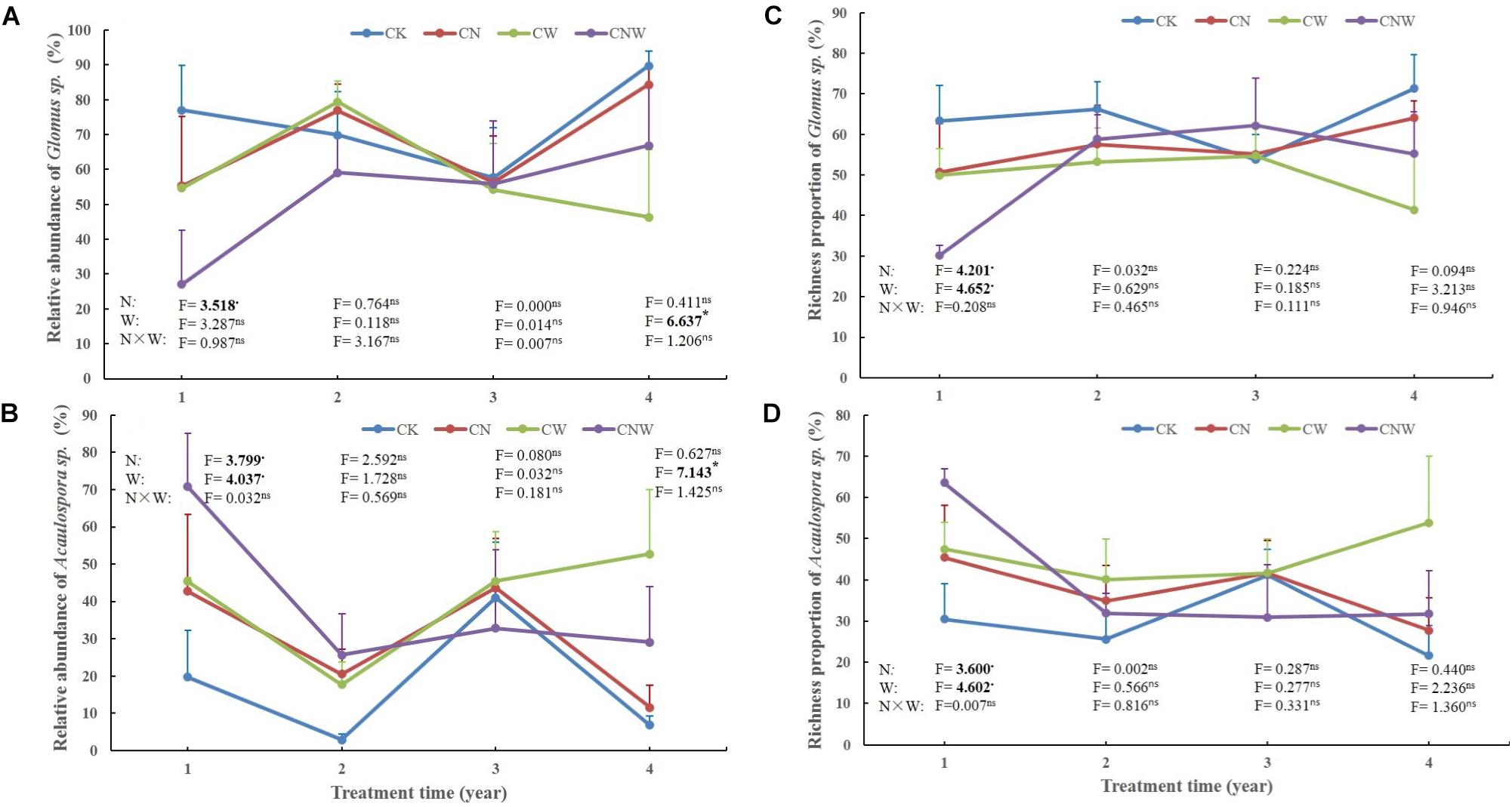
Figure 3. Effects of canopy additions of N and water on the abundance and richness of Glomus sp. (A,C) and Acaulospora sp. (B,D) over time (4 years). CK, control; CN, canopy addition of N; CW, canopy addition of water; CNW, canopy additions of N and water. N, N addition; W, water addition; N × W, the interaction. Significance of treatment effect was determined by two-way ANOVA. ∗∗p < 0.01; ∗p < 0.05; ⋅p < 0.1; ns, not significant.
In the 2nd year, water addition significantly decreased AM fungal richness and Shannon index (Figure 2), but did not change AM fungal community composition (Tables 2, 3). By contrast, N addition showed no significant effect on AM fungal alpha-diversity or community composition (Figure 2 and Tables 2, 3). Also no significant interactions between N and water addition were observed on AM fungal alpha-diversity or community composition (Figure 2 and Table 2). In addition, both N and water additions showed marginally positive effects to the richness of Acaulospora, in contrast, the marginally negative effects were found on Glomus richness (Figures 3C,D).
In the next 2 years, both N and water additions did not show significant effects on AM fungal alpha-diversity or community composition, with an exception that water addition significantly changed AM fungal community composition, accompanied by significant raise in Acaulospora abundance and significant decline in that of Glomus (Figures 2, 3A,B and Table 2).
Correlation Between AM Fungal Richness, Shannon Index, Community Composition Dissimilarity and Soil Properties
Only soil NH4+-N and N/P were marginally positively correlated with AM fungal richness (Table 4), and significantly positively correlated with AM fungal Shannon index (Table 4). No other significant correlations were observed between AM fungal biodiversity and soil properties.
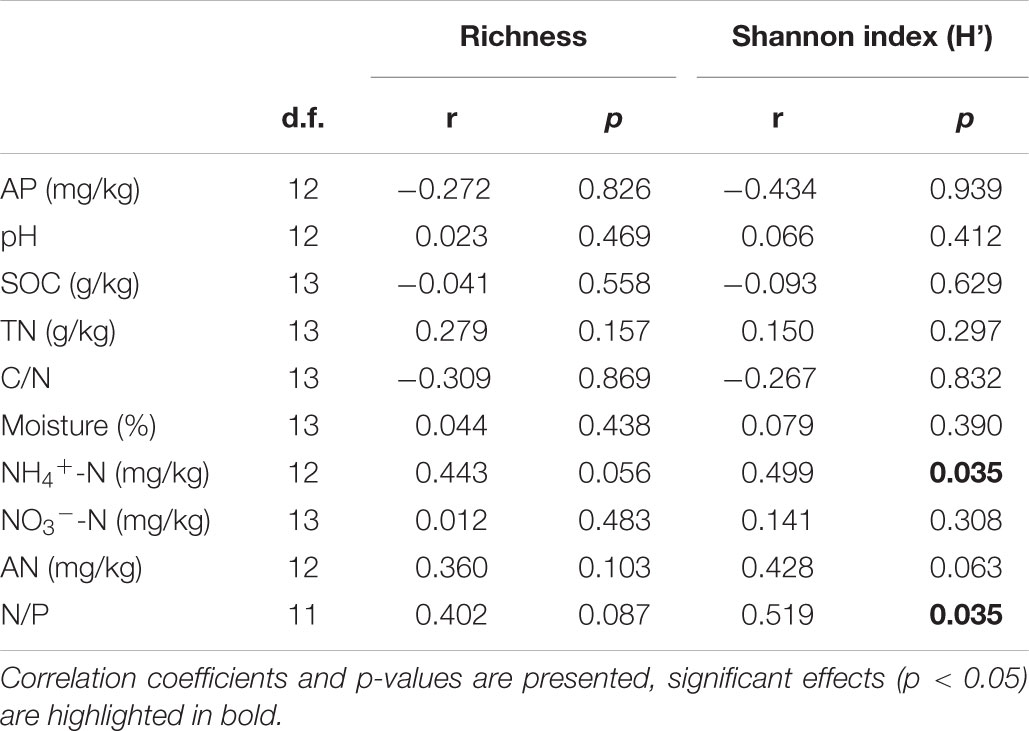
Table 4. Pearson correlation analysis between AM fungal richness, Shannon index (H’) and soil properties in the 2nd year.
Mantel and partial Mantel test indicated that only soil pH showed marginally positive correlation with the dissimilarity among AM fungal community composition among different treatments (Table 5).
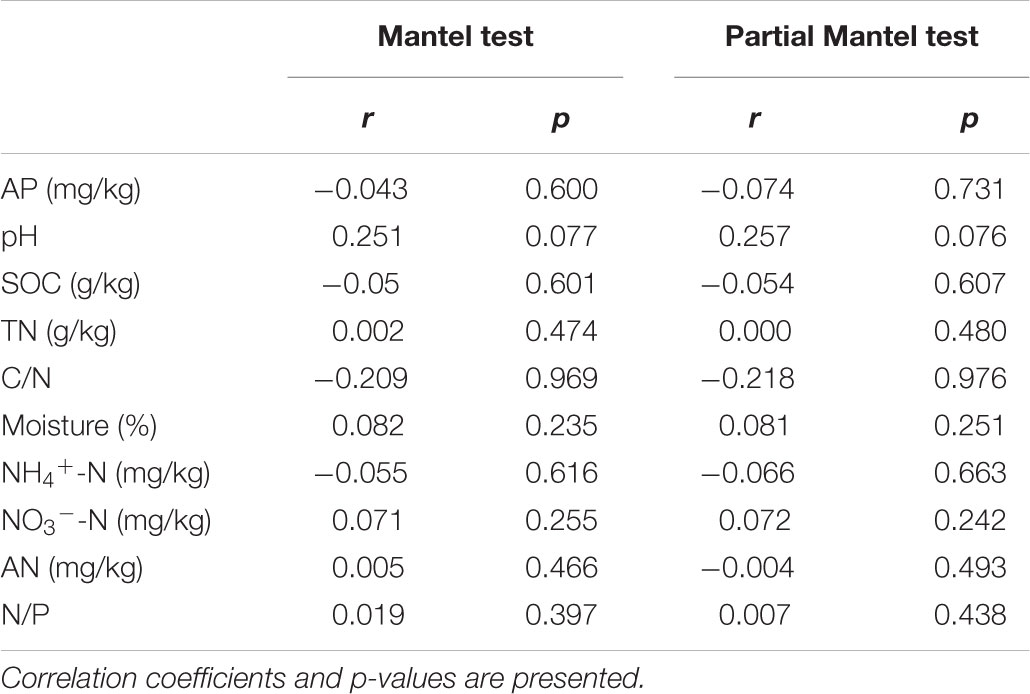
Table 5. Mantel and partial Mantel test of AM fungal community composition with the matrices of soil properties in the 1st year.
Discussion
This study investigated the effects of canopy additions of N and water on AM fungal diversity and community composition for consecutive 4 years and the results indicated that canopy N addition significantly changed AM fungal community composition, however, this effect was time-dependent, only occurred in the 1st year. While, the effect of water addition overwhelmed that of N addition, which not only changed the community composition, but also decreased the alpha-diversity of AM fungi, and these consequences were also time-dependent and only occurred in the earlier stages (1st/2nd year). In addition, the increment of N or water tended to decrease the abundance and richness of the most dominant genus Glomus and favored other AM fungi. The effects of N/water addition on AM fungal community composition were potentially mediated by soil properties, such as pH, NH4+-N and N/P.
Effects of Canopy N and Water Addition on Soil Properties
Nitrogen deposition usually leads to soil acidification (Lu et al., 2014; Tian and Niu, 2015; Chen et al., 2017), our study was not an exception (N addition significantly decreased soil pH in the 1st year), although we differently practiced canopy N addition. Possible reasons for soil acidification resulting from N deposition include: (1) NH4+ ions are absorbed by plant roots, while H+ will be released into soil, causing soil acidification (Smith and Read, 2008); (2) NH4+ ions are converted into nitrites and further converted into nitrates, producing H+ leading to soil acidification (Azevedo et al., 2013); (3) NH4+ ions displacing base cations (Ca2+, Mg2+, K+, Na+) and the increasing loss of metal cations could reduce soil buffering capacity against acidification (Tian and Niu, 2015; Lucas et al., 2016). Following soil acidification, soil microbial community composition and activity could be changed (Wei et al., 2013). Soil acidification can also result in the loss of plant species across multiple ecosystems (Azevedo et al., 2013) and the suppression of plant growth and carbon (C) sequestration (Schulte-Uebbing and de Vries, 2018).
In the 2nd year, water addition significantly decreased soil NH4+-N and AN, which may attributed to significant leaching (Martínez-García et al., 2017) and runoff. The loss of N can cause negative impacts to the environment and human, such as eutrophication of water body and decline of crop productivity, which will likely be aggravated by intensive heavy precipitation events (Martínez-García et al., 2017). On the other hand, N addition significantly increased soil NH4+-N, consistent with many previous studies (Chen et al., 2014, 2017; Zhang et al., 2014). The increase of soil NH4+-N can increase the productivity of N-limited ecosystems such as grassland and forest in temperate zone (Aber et al., 1998; Bai et al., 2010); However, excessive N supply can also lead to the accumulation of reactive nitrogen in soil to a toxic level for plant (Wei et al., 2013) and other soil organisms, such as nematodes and fungi (Eno et al., 1955). In the study of Wei et al. (2013), NH4+ concentration showed negative relationships with plant composition; in Eno et al. (1955), the fungi and nematode numbers were decreased under all N addition levels. Compared to control, only 0.6% of the nematodes and 4.9% of the fungi survived under N addition level of 608 mg kg-1.
Effects of Canopy N and Water Addition on AM Fungal Alpha-Diversity
In our study, N addition did not significantly decrease AM fungal richness and Shannon index, which failed to support our first hypothesis, also inconsistent with previous studies in forest (Camenzind et al., 2014), agriculture (Liu et al., 2014) and alpine meadow ecosystems (Zheng et al., 2014). In the study of Camenzind et al. (2014) and Liu et al. (2014), N addition significantly decreased AM fungal richness, while in the study of Zheng et al. (2014), N addition had significant positive effect on AM fungal alpha-diversity. How AM fungi respond to N addition is probably influenced by local environmental conditions, plant communities, intensity and frequency of N addition, experimental duration and other unknown factors (Porras-Alfaro et al., 2007; Wang et al., 2018). In this study, the ecosystem type is forest, which has higher species diversity and stability (strong resistance) than meadow, and agriculture ecosystems. More importantly, the mode of N application in our study was canopy spraying, different from Camenzind et al. (2014), in which N was directly added to the soil. As known, many canopy processes could substantially affect the consequences of N addition, however, the extent of the impact has not yet been clarified. In addition, although the total amount of N applied in Camenzind et al. (2014) was the same as this study, but the frequency of N application was different (7 times a year in this study, versus twice a year in Camenzind’s). Low frequency with high rate could very likely over-estimate the effect of N deposition, as Zhang et al. (2014) confirmed the overestimation of plant species loss of N addition at high rates and low frequency in a temperate steppe.
In the present study, water addition significantly decreased AM fungal richness and Shannon diversity index in the 2nd year, in support of our first hypothesis, also consistent with Gao et al. (2016) and Chen et al. (2017) in steppe ecosystem. By Pearson correlation analysis, we found that NH4+-N and N/P were marginally positively correlated with AM fungal richness and significantly positively correlated with AM fungal Shannon diversity index. Meanwhile, NH4+-N and N/P were indeed significantly decreased after water addition, possibly due to run-off and leaching of N from soil (Martínez-García et al., 2017). The decrease of NH4+-N may have intensified the competition among species leading to the loss of AM fungal niche, while lost niche could finally lead to decrease of AM fungal diversity (Dickie, 2007; Gao and Guo, 2013). Moreover, water addition could affect soil nutrient balance including N/P ratio, which can largely affect AM fungal community composition (Verbruggen et al., 2015).
Effects of Canopy N and Water Addition on AM Fungal Composition
In the 1st year, N addition significantly changed AM fungal community composition, in support of our first hypothesis, and consistent with van Diepen et al. (2011) and Camenzind et al. (2014), although the mode of N addition were different. The underlying mechanisms for the N effects on AM fungal community composition could be: (1) N addition increased the availability of soil N and reduced the cost in uptake of N by plant, so the plant dependence on mycorrhizal fungi decreased, and the amount of C allocated to mycorrhiza also decreased, which finally strengthened the competition among AM fungal species, led to changes in AM fungal community composition (Huang et al., 2014). (2) N addition led to soil acidification, which can directly affect spore germination and mycelial development (Rousk et al., 2010). More importantly, different AM fungi prefer different optimum pH, so changes in soil pH may lead to changes in community composition of AM fungi (An et al., 2008). Soil acidification caused by N addition may have stronger direct influence on soil microbial community composition than indirectly through the changes in plant community (Wei et al., 2013). At the same time, AM fungal community composition was also significantly altered by water addition, supported our first hypothesis, and in agreement with Gao et al. (2016) and Chen et al. (2017) although their studies were carried out in water-limited ecosystems. Precipitation increment may directly change soil water status and affect the physiological activity of AM fungi. Furthermore, increased precipitation can indirectly affect AM fungi via influencing the soil characteristics and plant communities. For example, in the study of Chen et al. (2017), changes in soil pH and plant species richness could shift AM fungal community composition. Gao et al. (2016) found that increased precipitation could alter fungal community composition through influencing soil moisture, NO3--N and root turnover.
The significant interactions between N and water addition on AM fungal community composition confirmed our second hypothesis, but inconsistent with Li et al. (2015) who observed no significant interactive effect of N and water increment in a semiarid grassland ecosystem after 8 years of experimental treatment. Chen et al. (2017) found that although there was no significant interactive effect of N and precipitation increment on AM fungal diversity, but significant interactive effect was observed on the relative abundance of some AM fungal OTUs. One possible explanation was experimental duration, as in our study the interaction was only observed after 1 year of treatment.
Moreover, we also confirmed that the effect of N and water addition on AM fungal community was time-dependent, in support of our third hypothesis. The results of Yang et al. (2016) demonstrated that under field conditions, AM fungal richness increased and community composition shifted after 15 days waterlogging. However, the time resolution in our study is year-based, therefore, more sampling at finer time scales is expected to test how quickly AM fungi respond to environmental changes. In addition, in a Mediterranean grassland, increased precipitation during rainy seasons significantly altered plant community and soil fungal community structure (Suttle et al., 2007; Hawkes et al., 2011), but in the dry season, fungal community did not respond to water addition in a different Mediterranean grassland (Barnard et al., 2013). Koyama et al. (2018) suggested that besides water amounts, timing of water manipulations can also be an important influencing factor, however, the present study did not involve the timing of water addition, which could be addressed in future research. In the meta-analysis by Wang et al. (2018), they found that N addition didn’t change fungal richness significantly when the experimental duration was within 5 years or longer than 10 years, but had significant influence when the treatment duration was 5 ∼10 years. This study only lasted for 4 years, next we will continue to sample and study the long-term ecological effects of increased N deposition and precipitation.
Changes in the composition and structure of plant community may affect the amount and quality of C input to belowground thus affecting soil microbial biomass, activity, and community structure (Meier and Bowman, 2008; Treseder, 2008; Liu et al., 2016). For instance, in the meta-analysis of Liu et al. (2016), plant lignin, plant protein and soil lignin were significantly increased by 7.13, 25.94, 7.30%, respectively following N addition. On the one hand, the increase of litter quality could promote microbial growth and biomass accumulation; on the other hand, the increase of recalcitrant C compounds (e.g., lignin) could result in the decrease of C availability to soil microbes, inhibiting microbial growth and activity (Treseder, 2008). Moreover, community composition of some specific microbial groups can change under N additions, for example, the diversity of ectomycorrhizal fungi and the richness of fungal decomposers decreased after N fertilization or deposition (Treseder, 2008). It should be further noted that, as symbiotic fungi, AM fungi have host preference (Sanders, 2003; Croll et al., 2008), and their community composition and structure are closely linked to plant community characteristics (Öpik et al., 2010; Kivlin et al., 2011; Xu et al., 2016). At the regional scale, a significant relationship between AM fungal community composition and plant was observed by Xu et al. (2016). The results of Li et al. (2015) indicated that the AM fungal abundance and OTU richness were significantly correlated with the 7-year averaged ANPP and aboveground biomass of plant functional groups after 8-years N and water additions. In the study of Chen et al. (2017), significant correlation between plant species richness and AM fungal taxonomic composition was also recorded. Therefore, further research incorporating plant community data is still needed.
Conclusion
Increased N deposition and precipitation have significant interactive effect on AM fungal diversity and community composition, while precipitation increment have stronger effect on AM fungal community structure than increased N deposition in the forest ecosystem. The effect of N deposition and precipitation on AM fungal community composition was time-dependent, mediated by soil factors, and possibly related to the sensitivity and resilience of forest ecosystem to global changes. In the future, we will consider finer and broader time scales and take into account the plant data to achieve comprehensive understanding of AM fungal ecology in the forest ecosystem.
Author Contributions
AZ conducted the experiments, analyzed the data, and drafted the manuscript. LL conceived the study, received financial support, and conducted parts of the experiments. BC, TX, and WX performed the data analysis. LS provided some basic data. WZ was responsible for the operation of this experimental platform. SF and HF designed and established this experimental platform. BC revised the manuscript.
Conflict of Interest Statement
The authors declare that the research was conducted in the absence of any commercial or financial relationships that could be construed as a potential conflict of interest.
Acknowledgments
This study was supported by the National Natural Science Foundation of China (41877050, 31300446, and 41401286).
Supplementary Material
The Supplementary Material for this article can be found online at: https://www.frontiersin.org/articles/10.3389/fpls.2018.01842/full#supplementary-material
References
Aber, J., Mcdowell, W., Nadelhoffer, K., Magill, A., Berntson, G., Kamakea, M., et al. (1998). Nitrogen saturation in temperate forest ecosystems. Bioscience 48, 921–934. doi: 10.2307/1313296
Affokpon, A., Coyne, D. L., Lawouin, L., Tossou, C., Agbèdè, R. D., and Coosemans, J. (2011). Effectiveness of native West African arbuscular mycorrhizal fungi in protecting vegetable crops against root-knot nematodes. Biol. Fertil. Soils 47, 207–217. doi: 10.1007/s00374-010-0525-1
An, G. H., Miyakawa, S., Kawahara, A., Osaki, M., and Ezawa, T. (2008). Community structure of arbuscular mycorrhizal fungi associated with pioneer grass species Miscanthus sinensis in acid sulfate soils: habitat segregation along pH gradients. Soil Sci. Plant Nutr. 54, 517–528. doi: 10.1111/j.1747-0765.2008.00267.x
Anderson, M. J. (2001). A new method for non-parametric multivariate analysis of variance. Austral Ecol. 26, 32–46. doi: 10.1111/j.1442-9993.2001.01070.x
Araya, Y. N., Gowing, D. J., and Dise, N. (2013). Does soil nitrogen availability mediate the response of grassland composition to water regime? J. Veg. Sci. 24, 506–517. doi: 10.1111/j.1654-1103.2012.01481.x
Azevedo, L. B., van Zelm, R., Jan Hendriks, A., Bobbink, R., and Huijbregts, M. A. J. (2013). Global assessment of the effects of terrestrial acidification on plant species richness. Environ. Pollut. 174, 10–15. doi: 10.1016/j.envpol.2012.11.001
Bai, Y. F., Wu, J. G., Clark, C. M., Naeem, S., Pan, Q., Huang, J. H., et al. (2010). Tradeoffs and thresholds in the effects of nitrogen addition on biodiversity and ecosystem functioning: evidence from inner Mongolia grasslands. Glob. Change Biol. 16, 358–372. doi: 10.1111/j.1365-2486.2009.01950.x
Barnard, R. L., Osborne, C. A., and Firestone, M. K. (2013). Responses of soil bacterial and fungal communities to extreme desiccation and rewetting. ISME J. 7, 2229–2241. doi: 10.1038/ismej.2013.104
Bobbink, R., Hicks, K., Galloway, J., Spranger, T., Alkemade, R., Ashmore, M., et al. (2010). Global assessment of nitrogen deposition effects on terrestrial plant diversity: a synthesis. Ecol. Appl. 20, 30–59. doi: 10.1890/08-1140.1
Bolger, A. M., Lohse, M., and Usadel, B. (2014). Trimmomatic: a flexible trimmer for Illumina sequence data. Bioinformatics 30, 2114–2120. doi: 10.1093/bioinformatics/btu170
Camenzind, T., Hempel, S., Homeier, J., Horn, S., Velescu, A., Wilcke, W., et al. (2014). Nitrogen and phosphorus additions impact arbuscular mycorrhizal abundance and molecular diversity in a tropical montane forest. Glob. Change Boil. 20, 3646–3659. doi: 10.1111/gcb.12618
Camenzind, T., Homeier, J., Dietrich, K., Hempel, S., Hertel, D., Krohn, A., et al. (2016). Opposing effects of nitrogen versus phosphorus additions on mycorrhizal fungal abundance along an elevational gradient in tropical montane forests. Soil Biol. Biochem. 94, 37–47. doi: 10.1016/j.soilbio.2015.11.011
Camprubi, A., Abril, M., Estaun, V., and Calvet, C. (2012). Contribution of arbuscular mycorrhizal symbiosis to the survival of psammophilic plants after sea water flooding. Plant Soil 351, 97–105. doi: 10.1007/s11104-011-0933-5
Chen, B. D., Li, X. J., Xu, T. L., and Xie, W. (2018). Research progress in the biogeography of arbuscular mycorrhizal fungi. Acta Ecol. Sin. 38, 1167–1175.
Chen, Y. L., Xu, Z. W., Xu, T. L., Veresoglou, S. D., Yang, G. W., and Chen, B. D. (2017). Nitrogen deposition and precipitation induced phylogenetic clustering of arbuscular mycorrhizal fungal communities. Soil Biol. Biochem. 115, 233–242. doi: 10.1016/j.soilbio.2017.08.024
Chen, Y. L., Zhang, X., Ye, J. S., Han, H. Y., Wan, S. Q., and Chen, B. D. (2014). Six-year fertilization modifies the biodiversity of arbuscular mycorrhizal fungi in a temperate steppe in Inner Mongolia. Soil Biol. Biochem. 69, 371–381. doi: 10.1016/j.soilbio.2013.11.020
Croll, D., Wille, L., Gamper, H. A., Mathimaran, N., Lammers, P. J., Corradi, N., et al. (2008). Genetic diversity and host plant preferences revealed by simple sequence repeat and mitochondrial markers in a population of the arbuscular mycorrhizal fungus Glomus intraradices. New Phytol. 178, 672–687. doi: 10.1111/j.1469-8137.2008.02381.x
de Ca’rcer, D. A., Denman, S. E., McSweeney, C., and Mark Morrison, M. (2011). Evaluation of subsampling-based normalization strategies for tagged high throughput sequencing data sets from gut microbiomes. Appl. Environ. Microbiol. 77, 8795–8798. doi: 10.1128/AEM.05491-11
Dean, R. B., and Dixon, W. J. (1951). Simplified statistics for small numbers of observations. Anal. Chem. 23, 636–638. doi: 10.1021/ac60052a025
Delgado-Baquerizo, M., Eldridge, D. J., Ochoa, V., Gozalo, B., Singh, B. K., and Maestre, F. T. (2017). Soil microbial communities drive the resistance of ecosystem multifunctionality to global change in drylands across the globe. Ecol. Lett. 20, 1295–1305. doi: 10.1111/ele.12826
Dickie, I. A. (2007). Host preference, niches and fungal diversity. New. Phytol. 174, 230–233. doi: 10.1111/j.1469-8137.2007.02055.x
Edgar, R. C. (2010). Search and clustering orders of magnitude faster than blast. Bioinformatics 26, 2460–2461. doi: 10.1093/bioinformatics/btq461
Edgar, R. C., Haas, B. J., Clemente, J. C., Quince, C., and Knight, R. (2011). Uchime improves sensitivity and speed of chimera detection. Bioinformatics 27, 2194–2200. doi: 10.1093/bioinformatics/btr381
Elsen, A., Gervacio, D., Swennen, R., and De Waele, D. (2008). AMF-induced biocontrol against plant parasitic nematodes in Musa sp.: a systemic effect. Mycorrhiza 18, 251–256. doi: 10.1007/s00572-008-0173-6
Eno, C. F., Blue, W. G., and Good, J. M. (1955). The effect of anhydrous ammonia on nematodes, fungi, bacteria, and nitrification in some florida soils1. Soil Sci. Soc. Am. J. 19, 55–58. doi: 10.2136/sssaj1955.03615995001900010013x
Fougnies, L., Renciot, S., Muller, F., Plenchette, C., Prin, Y., Faria, D., et al. (2007). Arbuscular mycorrhizal colonization and nodulation improve flooding tolerance in Pterocarpus officinalis Jacq. seedlings. Mycorrhiza 17, 159–166. doi: 10.1007/s00572-006-0085-2
Galloway, J. N., Dentener, F. J., Capone, D. G., Boyer, E. W., Howarth, R. W., Seitzinger, S. P., et al. (2004). Nitrogen cycles: past, present, and future. Biogeochemistry 70, 153–226. doi: 10.1007/s10533-004-0370-0
Gao, C., and Guo, L. D. (2013). Distribution pattern and maintenance of ectomycorrhizal fungus diversity. Biodivers. Sci. 21, 488–498. doi: 10.3724/SP.J.1003.2013.11055
Gao, C., Kim, Y. C., Zheng, Y., Yang, W., Chen, M. L., Ji, N. N., et al. (2016). Increased precipitation, rather than warming exerts strong influence on arbuscular mycorrhizal fungal community in a semiarid steppe ecosystem. Botany 94, 1–11. doi: 10.1139/cjb-2015-0210
Harpole, W. S., Potts, D. L., and Suding, K. N. (2007). Ecosystem responses to water and nitrogen amendment in a California grassland. Glob. Change Biol. 13, 2341–2348. doi: 10.1111/j.1365-2486.2007.01447.x
Hawkes, C. V., Kivlin, S. N., Rocca, J. D., Huguet, V., Thomsen, M. A., and Suttle, K. B. (2011). Fungal community responses to precipitation. Glob. Change Biol. 17, 1637–1645. doi: 10.1111/j.1365-2486.2010.02327.x
Hazard, C., Boots, B., Keith, A. M., Mitchell, D. T., Schmidt, O., Doohan, F. M., et al. (2014). Temporal variation outweighs effects of biosolids applications in shaping arbuscular mycorrhizal fungi communities on plants grown in pasture and arable soils. Appl. Soil Ecol. 82, 52–60. doi: 10.1016/j.apsoil.2014.05.007
Hogberg, P., Fan, H., Quist, M., Binkley, D. A. N., and Tamm, C. O. (2006). Tree growth and soil acidification in response to 30 years of experimental nitrogen loading on boreal forest. Glob. Change Biol. 12, 489–499. doi: 10.1111/j.1365-2486.2006.01102.x
Huang, Y. Y., Olbrecht, L., Yang, X. X., and He, J. S. (2014). Effects of nutrient additions on the arbuscular mycorrhizal fungal colonization in the alpine meadow on the Tibetan plateau. Acta Sci. Nat. Univ. Pekinensis 50, 911–918. doi: 10.13209/j.0479-8023.2014.074
Hubbell, S. P. (2001). The Unified Neutral Theory of Biodiversity and Biogeography. Monographs in Population Biology. Princeton, NJ: Princeton University Press.
Husband, R., Herre, E. A., Turner, S. L., Gallery, R., and Young, J. P. W. (2002). Molecular diversity of arbuscular mycorrhizal fungi and patterns of host association over time and space in a tropical forest. Mol. Ecol. 11, 2669–2678. doi: 10.1046/j.1365-294X.2002.01647.x
Integrated Professional Competency Course [IPCC] (2013). “Climate change 2013: the physical science basis,” in Working Group I Contribution to the IPCC Fifth Assessment Report, eds T. F. Stocker, D. Qin, G.-K. Plattner, M. Tignor, S. K. Allen, J. Boschung, et al. (Cambridge: Cambridge University Press).
Jansa, J., Smith, F. A., and Smith, S. E. (2008). Are there benefits of simultaneous root colonization by different arbuscular mycorrhizal fungi? New Phytol. 177, 779–789. doi: 10.1111/j.1469-8137.2007.02294.x
Jia, Y., Yu, G., He, N., Zhan, X., Fang, H., Sheng, W., et al. (2014). Spatial and decadal variations in inorganic nitrogen wet deposition in China induced by human activity. Sci. Rep. 4:3763. doi: 10.1038/srep03763
Kim, Y. C., Gao, C., Zheng, Y., He, X. H., Yang, W., Chen, L., et al. (2015). Arbuscular mycorrhizal fungal community response to warming and nitrogen addition in a semiarid steppe ecosystem. Mycorrhiza 25, 267–276. doi: 10.1007/s00572-014-0608-1
Kivlin, S. N., Hawkes, C. V., and Treseder, K. K. (2011). Global diversity and distribution of arbuscular mycorrhizal fungi. Soil Biol. Biochem. 43, 2294–2303. doi: 10.1016/j.soilbio.2011.07.012
Koyama, A., Steinweg, J. M., Haddix, M. L., Dukes, J. S., and Wallenstein, M. D. (2018). Soil bacterial community response to altered precipitation and temperature regimes in an old field grassland are mediated by plants. FEMS Microbiol. Ecol. 94:fix156. doi: 10.1093/femsec/fix156
Lee, J., Lee, S., and Young, J. P. W. (2008). Improved PCR primers for the detection and identification of arbuscular mycorrhizal fungi. FEMS Microbiol. Ecol. 65, 339–349. doi: 10.1111/j.1574-6941.2008.00531.x
Leibold, M. A., and McPeek, M. A. (2006). Coexistence of the niche and neutral perspectives in community ecology. Ecology 87, 1399–1410. doi: 10.1890/0012-9658 (2006)87[1399:COTNAN]2.0.CO;2
Li, H., Smith, S. E., Holloway, R. E., Zhu, Y., and Smith, F. A. (2006). Arbuscular mycorrhizal fungi contribute to phosphorus uptake by wheat grown in a phosphorus-fixing soil even in the absence of positive growth responses. New Phytol. 172, 536–543. doi: 10.1111/j.1469-8137.2006.01846.x
Li, H., Xu, Z. W., Yang, S., Li, X. B., Top, E. M., Wang, R., et al. (2016). Responses of soil bacterial communities to nitrogen deposition and precipitation increment are closely linked with aboveground community variation. Microb. Ecol. 71, 974–989. doi: 10.1007/s00248-016-0730-z
Li, S. L., Zhang, Y. L., Chen, D. M., Ma, J., and Guo, S. X. (2009). Effect of arbuscular mycorrhizal fungi on physiology and biochemistry of tree peony under high temperature stress. Chin. Agric. Sci. Bull. 25, 154–157.
Li, X. L., Zhu, T. Y., Peng, F., Chen, Q., Lin, S., Christie, P., et al. (2015). Inner Mongolian steppe arbuscular mycorrhizal fungal communities respond more strongly to water availability than to nitrogen fertilization. Environ. Microbiol. 17, 3051–3068. doi: 10.1111/1462-2920.12931
Liu, J., Wu, N. N., Wang, H., Sun, J. F., Peng, B., Jiang, P., et al. (2016). Nitrogen addition affects chemical compositions of plant tissues, litter and soil organic matter. Ecology 97, 1796–1806. doi: 10.1890/15-1683.1
Liu, W., Jiang, S., Zhang, Y., Yue, S., Christie, P., and Murray, P. J. (2014). Spatiotemporal changes in arbuscular mycorrhizal fungal communities under different nitrogen inputs over a 5-year period in intensive agricultural ecosystems on the north China plain. FEMS Microbial. Ecol. 90, 436–453. doi: 10.1111/1574-6941.12405
Liu, X., Zhang, Y., Han, W., Tang, A., Shen, J., and Cui, Z. (2013). Enhanced nitrogen deposition over China. Nature 494, 459–462. doi: 10.1038/nature11917
Lu, X. K., Mao, Q. G., Gilliam, F. S., Luo, Y. Q., and Mo, J. M. (2014). Nitrogen deposition contributes to soil acidification in tropical ecosystems. Glob. Change Biol. 20, 3790–3801. doi: 10.1111/gcb.12665
Lucas, R. W., Klaminder, J., Futter, M. N., Bishop, K. H., Egnell, G., Laudon, H., et al. (2016). A meta-analysis of the effects of nitrogen additions on base cations: implications for plants, soils, and streams. For. Ecol. Manag. 262, 95–104. doi: 10.1016/j.foreco.2011.03.018
Magill, A. H., Aber, J. D., Currie, W. S., Nadelhoffer, K. J., Martin, M. E., and McDowell, W. H. (2004). Ecosystem response to 15 years of chronic nitrogen additions at the Harvard Forest LTER, Massachusetts, USA. For. Ecol. Manag. 196, 7–28. doi: 10.1016/j.foreco.2004.03.033
Marino, N. A. C., Srivastava, D. S., Macdonald, A. A. M., Leal, J. S., Campos, A. B. A., and Farjalla, V. F. (2017). Rainfall and hydrological stability alter the impact of top predators on food web structure and function. Glob. Change Biol. 23, 673–685. doi: 10.1111/gcb.13399
Martínez-García, L. B., De Deyn, G. B., Pugnaire, F. I., Kothamasi, D., and Mga, V. D. H. (2017). Symbiotic soil fungi enhance ecosystem resilience to climate change. Glob. Change Biol. 23, 5228–5236. doi: 10.1111/gcb.13785
Meier, C. L., and Bowman, W. D. (2008). Links between plant litter chemistry, species diversity, and below-ground ecosystem function. Proc. Natl. Acad. Sci. U.S.A. 105, 19780–19785. doi: 10.1073/pnas.0805600105
Murphy, J., and Riley, J. P. (1962). A modified single solution method for determination of phosphate in natural waters. Anal. Chim. Acta 27, 31–36. doi: 10.1016/S0003-2670(00)88444-5
Oksanen, J., Blanchet, F. G., Kindt, R., Legendre, P., Minchin, P. R., O’hara, R. B., et al. (2013). Vegan: Community Ecology Package. Available at: http://CRAN.R-project.org/package=vegan [accessed July 25, 2013].
Öpik, M., Vanatoa, A., Vanatoa, E., Moora, M., Davison, J., Kalwij, J. M., et al. (2010). The online database MaarjAM reveals global and ecosystemic distribution patterns in arbuscular mycorrhizal fungi (Glomeromycota). New Phytol. 188, 223–241. doi: 10.1111/j.1469-8137.2010.03334.x
Philippot, L., Spor, A., Hénault, C., Bru, D., Bizouard, F., Jones, C. M., et al. (2013). Loss in microbial diversity affects nitrogen cycling in soil. ISME J. 7, 1609–1619. doi: 10.1038/ismej.2013.34
Porras-Alfaro, A., Herrera, J., Natvig, D. O., and Sinsabaugh, R. L. (2007). Effect of long-term nitrogen fertilization on mycorrhizal fungi associated with a dominant grass in a semiarid grassland. Plant Soil 296, 65–75. doi: 10.1007/s11104-007-9290-9
Ren, H., Xu, Z., Huang, J., Lü, X., Zeng, D. H., Yuan, Z., et al. (2015). Increased precipitation induces a positive plant-soil feedback in a semi-arid grassland. Plant Soil 389, 211–223. doi: 10.1007/s11104-014-2349-5
Rousk, J., Brookes, P. C., and Bååth, E. (2010). The microbial plfa composition as affected by ph in an arable soil. Soil Biol. Biochem. 42, 516–520. doi: 10.1016/j.soilbio.2009.11.026
Sanders, I. R. (2003). Preference, specificity and cheating in the arbuscular mycorrhizal symbiosis. Trends Plant Sci. 8, 143–145. doi: 10.1016/S1360-1385(03)00012-8
Sato, K., Suyama, Y., Saito, M., and Sugawara, K. (2005). A new primer for discrimination of arbuscular mycorrhizal fungi with polymerase chain reaction-denature gradient gel electrophoresis. Grassl. Sci. 51, 179–181. doi: 10.1111/j.1744-697X.2005.00023.x
Schloss, P. D., Westcott, S. L., Ryabin, T., Hall, J. R., Hartmann, M., Hollister, E. B., et al. (2009). Introducing mothur: open-source, platform-independent, community-supported software for describing and comparing microbial communities. Appl. Environ. Microbiol. 75, 7537–7541. doi: 10.1128/AEM.01541-09
Schulte-Uebbing, L., and de Vries, W. (2018). Global-scale impacts of nitrogen deposition on tree carbon sequestration in tropical, temperate, and boreal forests: a meta-analysis. Glob. Change Biol. 24, e416–e431. doi: 10.1111/gcb.13862
Shi, L. L., Zhang, H. Z., Liu, T., Mao, P., Zhang, W. Y., Shao, Y. H., et al. (2018). An increase in precipitation exacerbates negative effects of nitrogen deposition on soil cations and soil microbial communities in a temperate forest. Environ. Pollut. 235, 293–301. doi: 10.1016/j.envpol.2017.12.083
Shi, L. L., Zhang, H. Z., Liu, T., Zhang, W., Shao, Y. H., Ha, D. L., et al. (2016). Consistent effects of canopy vs. understory nitrogen addition on the soil exchangeable cations and microbial community in two contrasting forests. Sci. Total Environ. 553, 349–357. doi: 10.1016/j.scitotenv.2016.02.100
Subramanian, K. S., Santhanakrishnan, P., and Balasubramanian, P. (2006). Responses of field grown tomato plants to arbuscular mycorrhizal fungal colonization under varying intensities of drought stress. Sci. Hortic. 107, 245–253. doi: 10.1016/j.scienta.2005.07.006
Sun, S., Zhao, H., Xing, F., Bai, Z., Gao, Y., Dong, Y., et al. (2017). Response of soil microbial community structure to increased precipitation and nitrogen addition in a semiarid meadow steppe. Eur. J. Soil Sci. 68, 524–536. doi: 10.1111/ejss.12441
Suttle, K. B., Thomsen, M. A., and Power, M. E. (2007). Species interactions reverse grassland responses to changing climate. Science 315, 640–642. doi: 10.1126/science.1136401
Tamura, K., Peterson, D., Peterson, N., Stecher, G., Nei, M., and Kumar, S. (2011). MEGA5: molecular evolutionary genetics analysis using maximum likelihood, evolutionary distance, and maximum parsimony. Mol. Biol. Evol. 28, 2731–2739. doi: 10.1093/molbev/msr121
Tian, D. S., and Niu, S. L. (2015). A global analysis of soil acidification caused by nitrogen addition. Environ. Res. Lett. 10:024019. doi: 10.1088/1748-9326/10/2/024019
Treseder, K. K. (2008). Nitrogen additions and microbial biomass: a meta-analysis of ecosystem studies. Ecol. Lett. 11, 1111–1120. doi: 10.1111/j.1461-0248.2008.01230.x
van Der Heijden, M. G., Bardgett, R. D., and Van Straalen, N. M. (2008). The unseen majority: soil microbes as drivers of plant diversity and productivity in terrestrial ecosystems. Ecol. Lett. 11, 296–310. doi: 10.1111/j.1461-0248.2007.01139.x
van Der Heijden, M. G., Klironomos, J. N., Ursic, M., Moutoglis, P., Streitwolf-Engel, R., Boller, T., et al. (1998). Mycorrhizal fungal diversity determines plant biodiversity, ecosystem variability and productivity. Nature 396, 69–72. doi: 10.1038/23932
van Diepen, L. T., Lilleskov, E. A., and Pregitzer, K. S. (2011). Simulated nitrogen deposition affects community structure of arbuscular mycorrhizal fungi in northern hardwood forests. Mol. Ecol. 20, 799–811. doi: 10.1111/j.1365-294X.2010.04969.x
van Diepen, L. T., Lilleskov, E. A., Pregitzer, K. S., and Miller, R. M. (2007). Decline of arbuscular mycorrhizal fungi in northern hardwood forests exposed to chronic nitrogen additions. New Phytol. 176, 175–183. doi: 10.1111/j.1469-8137.2007.02150.x
van Diepen, L. T., Lilleskov, E. A., Pregitzer, K. S., and Miller, R. M. (2010). Simulated nitrogen deposition causes a decline of intra-and extraradical abundance of arbuscular mycorrhizal fungi and changes in microbial community structure in northern hardwood forests. Ecosystems 13, 683–695. doi: 10.1007/s10021-010-9347-0
Verbruggen, E., Xiang, D., Chen, B. D., Xu, T. L., and Rillig, M. C. (2015). Mycorrhizal fungi associated with high soil N:P ratios are more likely to be lost upon conversion from grasslands to arable agriculture. Soil Biol. Biochem. 86, 1–4. doi: 10.1016/j.soilbio.2015.03.008
Vitousek, P. M., Aber, J. D., Howarth, R. W., Likens, G. E., Matson, P. A., Schindler, D. W., et al. (1997). Human alteration of the global nitrogen cycle: source and consequences. Ecol. Appl. 7, 737–750. doi: 10.1890/1051-0761 (1997)007[0737:HAOTGN]2.0.CO;2
Wagg, C., Bender, S. F., Widmer, F., and Mg, V. D. H. (2014). Soil biodiversity and soil community composition determine ecosystem multifunctionality. Proc. Natl. Acad. Sci. U.S.A. 111, 5266–5270. doi: 10.1073/pnas.1320054111
Walkley, A., and Black, I. A. (1934). An examination of the Degtjareff method for determining organic carbon in soils: effect of variations in digestion conditions and of inorganic soil constituents. Soil Sci. 63, 251–263. doi: 10.1097/00010694-194704000-00001
Wang, C., Liu, D. W., and Bai, E. (2018). Decreasing soil microbial diversity is associated with decreasing microbial biomass under nitrogen addition. Soil Biol. Biochem. 120, 126–133. doi: 10.1016/j.soilbio.2018.02.003
Wei, C., Yu, Q., Bai, E., Lü, X., Li, Q., Xia, J., et al. (2013). Nitrogen deposition weakens plant-microbe interactions in grassland ecosystems. Glob. Chang Biol. 19, 3688–3697. doi: 10.1111/gcb.12348
Xu, T. L., Veresoglou, S. D., Chen, Y. L., Rillig, M. C., Xiang, D., Daniel, O., et al. (2016). Plant community, geographic distance and abiotic factors play different roles in predicting AMF biogeography at the regional scale in northern china. Environ. Microbiol. Rep. 8, 1048–1057. doi: 10.1111/1758-2229.12485
Yang, H., Yang, L., Wu, M., Zhang, Z., Li, L., and Wan, S. Q. (2011). Plant community responses to nitrogen addition and increased precipitation: the importance of water availability and species traits. Glob. Change Biol. 17, 2936–2944. doi: 10.1111/j.1365-2486.2011.02423.x
Yang, H. S., Koide, R. T., and Zhang, Q. (2016). Short-term waterlogging increases arbuscular mycorrhizal fungal species richness and shifts community composition. Plant Soil 404, 373–384. doi: 10.1007/s11104-016-2850-0
Yang, S. L., Ding, Z. L., Li, Y. Y., Wang, X., Jiang, W. Y., and Huang, X. F. (2015). Warming-induced northwestward migration of the East Asian monsoon rain belt from the Last Glacial Maximum to the mid-Holocene. Proc. Natl. Acad. Sci. U.S.A. 112, 13178–13183. doi: 10.1073/pnas.1504688112
Zeppel, M. J. B., Wilks, J. V., and Lewis, J. D. (2014). Impacts of extreme precipitation and seasonal changes in precipitation on plants. Biogeosciences 11, 3083–3093. doi: 10.5194/bg-11-3083-2014
Zhang, S. K., Rossi, S., Huang, J. G., Jiang, S. W., Yu, B. Y., Zhang, W., et al. (2018). Intra-annual dynamics of xylem formation in Liquidambar formosana subjected to canopy and understory N addition. Front. Plant Sci. 9:79. doi: 10.3389/fpls.2018.00079
Zhang, W., Shen, W., Zhu, S., Wan, S., Luo, Y., Yan, J., et al. (2015). Can canopy addition of nitrogen better illustrate the effect of atmospheric nitrogen deposition on forest ecosystem? Sci. Rep. 5:11245. doi: 10.1038/srep11245
Zhang, Y. H., Lü, X. T., Isbell, F., Stevens, C., Han, X., He, N. P., et al. (2014). Rapid plant species loss at high rates and at low frequency of N addition in temperate steppe. Glob. Change Biol. 20, 3520–3529. doi: 10.1111/gcb.12611
Zheng, Y., Kim, Y. C., Tian, X. F., Chen, L., Yang, W., Gao, C., et al. (2014). Differential responses of arbuscular mycorrhizal fungi to nitrogen addition in a near pristine Tibetan alpine meadow. FEMS Microbiol. Ecol. 89, 594–605. doi: 10.1111/1574-6941.12361
Keywords: nitrogen deposition, precipitation, AM fungi, forest ecosystem, community composition, climate change, canopy
Citation: Zhao A, Liu L, Xu T, Shi L, Xie W, Zhang W, Fu S, Feng H and Chen B (2018) Influences of Canopy Nitrogen and Water Addition on AM Fungal Biodiversity and Community Composition in a Mixed Deciduous Forest of China. Front. Plant Sci. 9:1842. doi: 10.3389/fpls.2018.01842
Received: 25 June 2018; Accepted: 27 November 2018;
Published: 11 December 2018.
Edited by:
Ying Ma, University of Coimbra, PortugalReviewed by:
Raffaella Balestrini, Istituto per la Protezione Sostenibile delle Piante (IPSP), ItalyVikki L. Rodgers, Babson College, United States
Aleš Látr, Symbiom, Czechia
Copyright © 2018 Zhao, Liu, Xu, Shi, Xie, Zhang, Fu, Feng and Chen. This is an open-access article distributed under the terms of the Creative Commons Attribution License (CC BY). The use, distribution or reproduction in other forums is permitted, provided the original author(s) and the copyright owner(s) are credited and that the original publication in this journal is cited, in accordance with accepted academic practice. No use, distribution or reproduction is permitted which does not comply with these terms.
*Correspondence: Baodong Chen, YmRjaGVuQHJjZWVzLmFjLmNu
†These authors have contributed equally to this work