- 1Department of Environmental and Biological Chemistry, Chungbuk National University, Cheongju, South Korea
- 2Indigenous and Frontier Technology Research Centre, Chennai, India
Soil salinization is one of the most serious abiotic stress factors affecting plant productivity through reduction of soil water potential, decreasing the absorptive capacity of the roots for water and nutrients. A weighted meta-analysis was conducted to study the effects of arbuscular mycorrhizal fungi (AMF) inoculation in alleviating salt stress in C3 and C4 plants. We analyzed the salt stress influence on seven independent variables such as chlorophyll, leaf area, photosynthetic rate (Amax), stomatal conductance (Gs), transpiration rate (E), relative water content (RWC), and water use efficiency (WUE) on AMF inoculated plants. Responses were compared between C3 and C4 plants, AMF species, plant functional groups, level of salinity, and environmental conditions. Our results showed that AMF inoculated plants had a positive impact on gas exchange and water status under salt stress. The total chlorophyll contents of C3 plants were higher than C4 plants. However, C3 plants responses regarding Gs, Amax, and E were more positive compared to C4 plants. The increase in Gs mainly maintained E and it explains the increase in Amax and increase in E. When the two major AMF species (Rhizophagus intraradices and Funnelliformis mosseae) were considered, the effect sizes of RWC and WUE in R. intraradices were lower than those in F. mosseae indicating that F. mosseae inoculated plants performed better under salt stress. In terms of C3 and C4 plant photosynthetic pathways, the effect size of C4 was lower than C3 plants indicating that AMF inoculation more effectively alleviated salt stress in C3 compared to C4 plants.
Introduction
Salinity, especially in the very dry areas of the world, limits crop production seriously. It negatively impacts plant water potential and ionic balance through compounding effects of osmotic stress and/or Na+ and Cl− cytotoxicity resulting in significant reduction of plant growth and crop production (Zhu, 2001; Chinnusamy et al., 2005; Teakle et al., 2006; Munns and Tester, 2008). These changes affect plant growth by impairing metabolic processes and decrease photosynthetic efficiency (Munns and Tester, 2008). Tolerance and sensitivity to salt stress greatly vary among plant species but many studies have indicated that the decrease in growth of plants under saline conditions is linked to the decline in photosynthesis and related metabolic processes (Stepien and Klobus, 2006) thereby affecting the other important biological activities such as cell growth (Munns et al., 2006; Geissler et al., 2009). One of the most immediate plant responses to soil salinity is the reduction of the stomatal aperture. This, in turn, leads to reduced stomatal conductance (Gs), leaf transpiration rate (E), and photosynthetic rate (light-saturated photosynthetic rate under ambient conditions) (Amax) (Bethke and Drew, 1992; Koyro, 2006; Lu et al., 2009). This could be one of the probable reasons for the difference in resistance to stress in plants.
Photosynthesis has changed the biochemical processes on Earth by utilizing the energy from the sun in the course of carbon fixation. In the C4 pathway, the Calvin cycle is optimized by a more efficient concentration of CO2 reacting to RuBisCO. This minimizes photorespiration and enhances the plant's utilization of water and nitrogen. There were at least 60 occasions of independent evolution leading to several thousand plant species diverging from their C3 lineage developing C4 photosynthetic pathway (Reyna-Llorens and Hibberd, 2017). C4 photosynthesis greatly minimizes photorespiration and allowing stomatal function while producing sugar in a more efficient way to that of C3 plants. As a consequence, C4 plants grow faster and have greater biomass and plant productivity relative to plants with C3 photosynthesis. A comparative study performed on C3 and C4 systems indicated that increase in mass by C4 plants is linked to their tolerance to abiotic stresses (Ali et al., 2002). Nevertheless, the responses of particular plant species belonging to C3 and C4 photosynthetic type showing high-stress tolerance, may not be true for all species from the same family (Chapin, 1991; Ali et al., 2002; Niu et al., 2006). The increased production of biomass along with more efficient use of water leads to the notion that C4 plants are more tolerant to salt stress and are better adapted to conditions in semi-arid and arid regions. However, they are able to reach the same photosynthetic rate as C3 plants but with much smaller stomatal aperture and therefore much less water loss (Way et al., 2014).
Responses of mycorrhizal plants to salt stress in relation to the exchange of O2-CO2 and water use efficiency are complex. These depend on the level of salinity, metabolic CO2 assimilation, and biological form. Mutualistic interaction of arbuscular mycorrhiza fungi (AMF) residing on the root endosphere of many terrestrial plants is capable of mitigating salinity stress and promoting continued growth (Al-Karaki, 2000; Porcel et al., 2012). There are numerous studies showing positive AMF-inoculated plant responses on the alleviation of salt stress, but the magnitudes of effect greatly differ among various studies (Evelin et al., 2009; Porcel et al., 2012). These differences could be attributed to the compounding effects of salinity, different types of mycorrhizal plant used and the complicated interactions between these factors. There is also a paucity of information on the relative importance and magnitude of AMF symbiotic features such as the type of AMF species, AMF richness, plant species, and root morphology on the mitigation of the damaging effects of salinity in both C3 and C4 photosynthetic groups. While a lot of literature available on the comparative responses such as elevated metabolic processes of C3 and C4 photosynthetic types, transport involving plastids, CO2 concentration and water availability, shoot and root biomass, nitrogen availability and competition, stomatal conductance and transpiration rate (Wand et al., 1999; Derner et al., 2003; Niu et al., 2006; Caird et al., 2007; Tang et al., 2009; Weber and Caemmerer, 2010), only limited information is available on their gas exchange and their water status under salt stress. Meta-analysis is a numerical way of analyzing potential experimental factors that causes variations among studies when individual and independent data from different studies are collected and collated (Rosenberg et al., 2000; Treseder, 2004; Lehmann et al., 2014; Yang et al., 2014, 2015; Pellegrino et al., 2015).
Up to date and to our knowledge, no comprehensive studies have yet been conducted to assess how gas exchange and water status are related in C3 and C4 photosynthetic plants and their response to AMF inoculation under saline condition. In this study, a meta-analysis was conducted within over published studies spanning the period 1987–2017 dealing with plant gas exchange (Amax, Gs, and E) and water status (relative water content-RWC) and water use efficiency (WUE) responses toward AMF inoculation under salt stress. Specifically, we hypothesized different responses between C3 and C4 photosynthetic groups of plants to AMF inoculation under the influence of salinity stress.
Materials and Methods
Literature Search and Data Collection
The database was prepared by searching and retrieving cited references in the Web of Knowledge™. Keywords related to AMF were used particularly: AM fungal, AM fungi, fungal, fungi, mycorrhiza, mycorrhizal, mycorrhizae, arbuscular, and AMF. Keywords related to plant responses and salt stress include gas exchange, photosynthetic efficiency, stomatal conductance, transpiration, water use efficiency and water status, under the saline condition, salinity stress, and salt stress. Screening and selection were done on the searches in order to include studies containing quantitatively measured C3 and C4 plant responses after mycorrhizal inoculation under salt stress especially parameters on gas exchange and water status. Initial screening brought about 657 research publications meticulously reassessed to meet criteria for inclusion in the study: (i) studies with response variables on photosynthesis, stomatal conductance, transpiration, chlorophyll, water status (ii) studies with a treatment containing one or more AMF species (iii) studies with non-inoculated control (iv) experiments performed under salt stress. Based on our inclusion criteria 587 publications were excluded, and the list was refined to 69 publications (from 1987 until 2017) (Datasheet, Appendix S1). From the 69 publications, 540 trials were identified for the comparative analysis of gas exchange and water status response to AMF inoculation under salt stress (Supplementary Information Dataset), all of which hypothetically passed the criteria of selection for inclusion in the study.
Data Acquisition
The number of replication or sample size (n), the mean and the standard deviation (SD) of the control as well as the treatment (AMF inoculation) under salinity stress are necessary for meta-analysis for independent studies. Dexter (GAVO data center, http://dc.zah.uni-heidelberg.de/sdexter/) was used to estimate means and errors from published figures.
Categorical Independent/Moderator Variables
Fixed factors related to the responses of C3 and C4 plant gas exchange and water status after AMF inoculation under salt stress conditions were categorically analyzed. Specific fixed factors were as follow:
Photosynthetic types were grouped into two levels: AMF-inoculated C3 and C4 plant groups were tested for significant differences in their photosynthetic state and water status during salinity stress.
Chlorophyll content was divided into three parameters: chlorophyll a, chlorophyll b and total chlorophyll. This allowed the testing for significant tissue differences and chlorophyll content mediated by inoculation of AMF.
The gas exchange includes three parameters: Photosynthetic rate, stomatal conductance, and leaf transpiration rate.
Water status had two parameters: relative water content (measured from photosynthetically active tissues) and water use efficiency (the ratio of net photosynthetic rate per transpiration rate).
AMF richness is divided into single and mixed levels.
The use of AMF belonging to only one species was categorized as “single species inoculum” and was dominated by members of Glomeraceae. Most of the studies were conducted on those species compared to those of other species. Moreover, we did not get enough studies for meta-analysis for other species. Co-inoculation with more than one AMF species was categorized as mixed species inoculum.
Plant species and plant family: There were 40 plant host species included for analysis spanning members of different families particularly Anacardiaceae, Asteraceae, Caryophyllaceae, Chenopodiaceae, Euphorbiaceae, Fabaceae, Lamiaceae, Liliaceae, Malvaceae, Moraceae, Poaceae, Rutaceae, Solanaceae, and Verbenaceae. The plants were classified by using the PLANTS database of the USDA, Natural Resources Conservation Service (http://plants.usda.gov/java/).
Plant group comprised of two levels: monocot and dicot. Classification of the plants was done according to the PLANTS database of the USDA, Natural Resources Conservation Service (http://plants.usda.gov/java/).
Life cycle: Plants were categorized as annual or perennial for the plant life cycle.
Plant growth habit: This categorical variable was described as herbaceous, grass, shrub, and woody form of plants.
Soil textural type was grouped into five: clay loamy, loamy, sandy, sandy loamy, and silty soil. Soil textural classification was done following the soil database of the USDA, Natural Resources Conservation Service (https://www.nrcs.usda.gov/wps/portal/nrcs/detail/soils/research/guide/).
Soil salinity was defined in three categories: low, moderate and high. Categorization of the level of imposed soil salinity was done following USDA Natural Resources Conservation Service. Low soil salinity has an EC ≤ 4 dS m−1; moderate soil salinity ranged from 4 to 8 dS m−1, and; higher than 8 dS m−1 was high salinity.
The experimental condition comprised of two levels: greenhouse included all experiments were done under protected and controlled set-up (i.e., pot trails); and field, containing all outdoor studies (i.e., soil trails).
Statistics
The metric for the AMF inoculation response under salt stress was computed as the natural log of the response ratio (ln R) which showed the effect size of the AMF inoculation on gas exchange and water status. The ln R is a measure of outcome in an experimental group to that of the control group. The ln R calculations and statistical analysis were conducted using the MetaWin v2.1 software (Rosenberg et al., 2000).
In this calculation, R represents the response ratio, ln R represents natural log of the response ratio, V lnR denotes variance of ln R, XC denotes the mean of the control (plants under salt stress having no AMF), XE is the mean of the treatment (plants under salt stress were inoculated with AMF), SC represents the standard deviation of the control, SE denotes the standard deviation of the treatment, NC is the control replication number and NE is the treatment replication number (Rosenberg et al., 2000). A positive value of ln R indicates a beneficial AMF mediated effect while negative values represent a detrimental effect. A permutation procedure containing 3,999 iterations was run for the computation of P-values since the effect sizes violated the assumption of normality. To calculate the confidence intervals (CIs), a bootstrapping approach was done with implemented bias correction (Adams et al., 1997). Two univariate random effects meta-analysis were conducted corresponding to the two effect sizes as well as related datasets. These assessed the whole effect of AMF inoculation on the plants' gas exchange and water status. Heterogeneity in the effect sizes was calculated using Q statistics (Lehmann and Rillig, 2015), and was compared against a chi-squared distribution with n-1 degrees of freedom (Lehmann and Rillig, 2015). A dataset indicates more heterogeneity than expected due to errors in sampling if the calculated Q is significant (Cooper, 1998). Furthermore, categorical independent variables were analyzed to find the observed variability in the datasets. Therefore, the significance level of the random value between-level difference of categorical moderators was investigated and a significant level at <0.05 was considered statistically significant.
Results
Overall AMF Inoculation Effects on Gas Exchange and Water Status
Irrespective of photosynthetic type, an overall positive effect of AMF inoculation on gas exchange and water status under salt stress was observed in both C3 and C4 plants (Figure 1; Table 1). The results showed positive effect sizes of Amax, Gs, and E across studies (Figure 1A; Table 1). Also, total chlorophyll, chlorophyll a and chlorophyll b contents of AMF-inoculated plants under salt stress had increased effect size, compared to un-inoculated plants. Moreover, leaf area, water use efficiency, and relative water content also had significantly increased effect sizes (Table 1). In terms of the C3 and C4 photosynthetic pathways, the effect size of C4 plants were lower than those in C3 plants (Figure 1B). Though the total chlorophyll content was high in both C3 and C4 plant, the C3 plants had higher chlorophyll content compared to C4 plants. Under the same saline conditions, the effect sizes of Amax, leaf area, and E in C3 plants were higher than C4 plants. Whereas, the effect sizes of stomatal conductance and relative water content were higher in C4 plants than C3 plants. Among AMF species, R. intraradices inoculated C3 plants had more positive effect size values for Gs and E than C4 plants (Figure 2). For moderator variables plant functional groups, growth habits, and types, categorical analyses showed significant influence on effect sizes (Figure 3). The effect sizes of Amax, Gs, and E under high salinity were higher in C3 plants than in C4 plants (Figure 4).
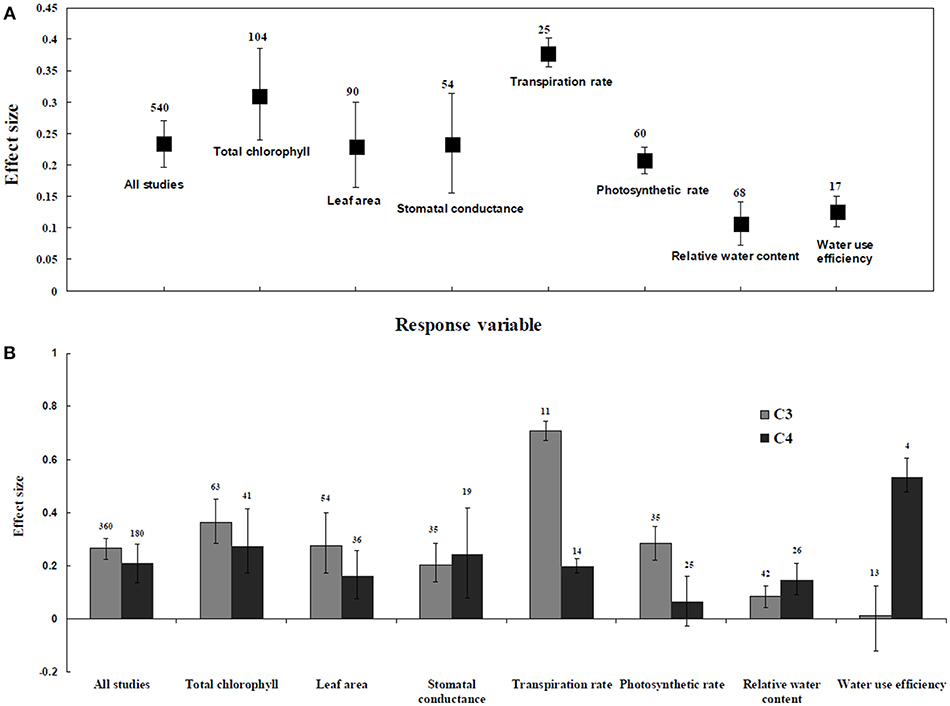
Figure 1. Comparative gas exchange and water status responses of arbuscular mycorrhizal fungi (AMF) inoculated plants under salt stress. (A) Overall analysis (B) C3 and C4 plants response. Error bars are means ± BS CIs. Where the CIs do not overlap each other, the effect size for a parameter is significant at P < 0.05. The number of trials included in the meta-analysis is denoted above the bar.
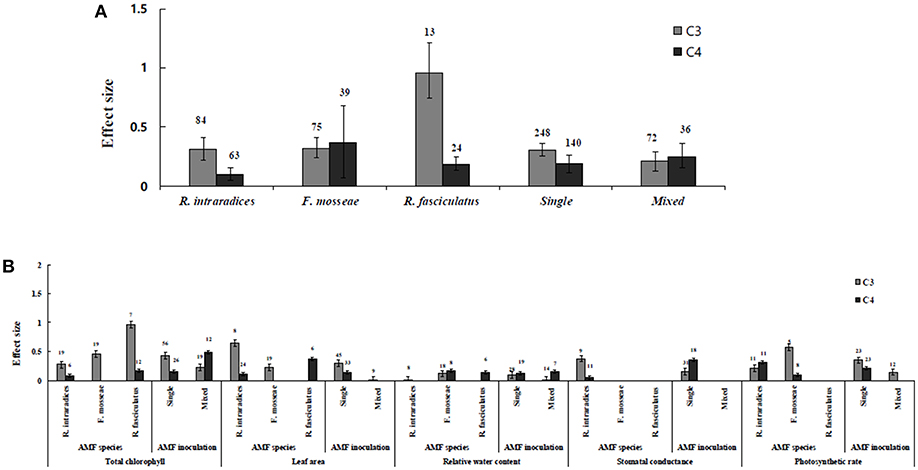
Figure 2. AMF species-categorical analysis. (A) Overall AMF species and AMF inoculation response to gas exchange and water status. (B) Response variables reaction to AMF species and AMF inoculation. Error bars are means ± BS CIs. Where the CIs do not overlap each other, the effect size for a parameter is significant at P < 0.05. The number of trials included in the meta-analysis is denoted above the bar.
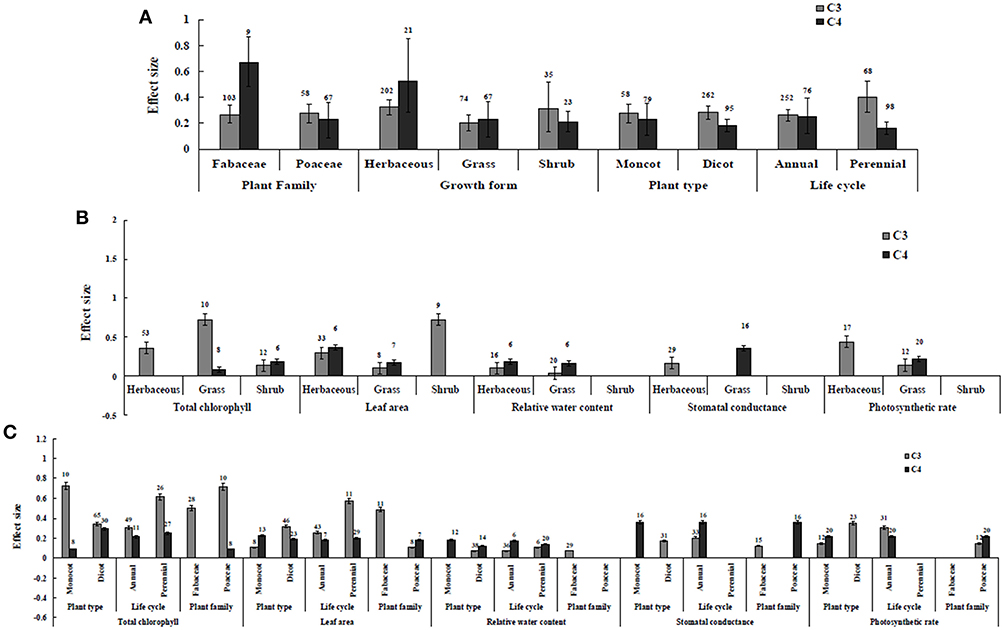
Figure 3. Plant functional groups-categorical analysis. (A) Overall plant functional groups response to gas exchange and water status. (B) Individual response variables reaction to plant growth form. (C) Individual response variables response to plant type, life cycle, and plant family. Error bars are means ± BS CIs. Where the CIs do not overlap each other, the effect size for a parameter is significant at P < 0.05. The number of trials included in the meta-analysis is denoted above the bar.
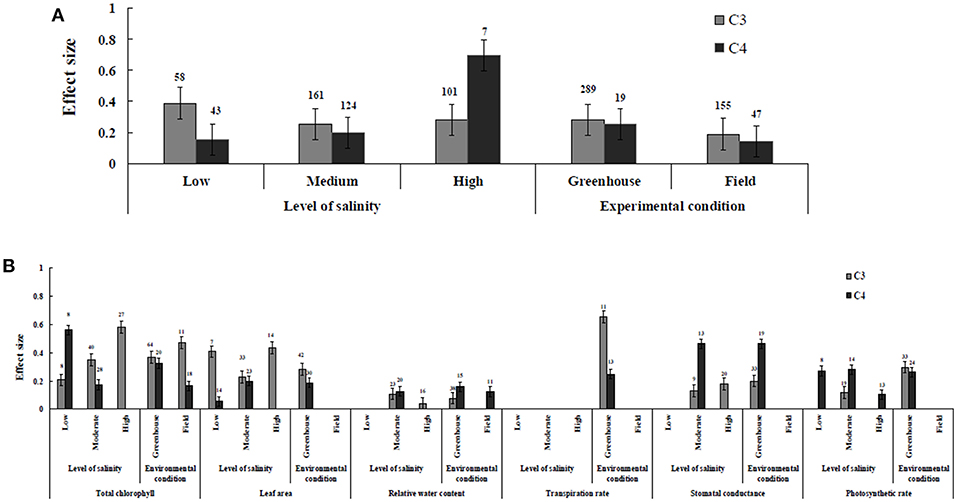
Figure 4. The level of salinity and environmental conditions—categorical analysis. (A) Overall level of salinity and environmental conditions response to gas exchange and water status. (B) Individual response variables reaction to level of salinity and environmental conditions. Error bars are means ± BS CIs. Where the CIs do not overlap each other, the effect size for a parameter is significant at P < 0.05. The number of trials included in the meta-analysis is denoted above the bar.
C3 and C4 Plants Response to Water Status and Water Content
RWC and WUE were the main indicators reflecting the water status of plants suffering from salinity. The RWC and WUE had positive effect sizes (P < 0.0001) (Figure 1). However, RWC and WUE effect sizes differed according to the level of salinity under low, moderate and high salinity. Plant growth forms differed in effect sizes in terms of RWC and WUE where highest WUE was exhibited in grasses while highest RCW though lower WUE was observed in herbaceous plants. When the two major AMF species (R. intraradices and F. mosseae) were considered, the effect sizes of RWC and WUE in R. intraradices were lower than those in F. mosseae indicating that F. mosseae inoculated plants performed well under salt stress. The effect sizes of RWC and WUE in C4 plants were higher than C3 plants under the same saline conditions. Mycorrhizal C3 and C4 plants under salt stress performed differently for relative water content. While both respond favorably, C4 plants showed higher effect size values than C3 plants. Among the AMF fungi, F. mosseae shows relatively increased relative water content than other species in both C3 and C4 plants under salt stress (Figure 2B). Moreover, single inoculation showed a significant increase in relative water content than mixed inoculation in C3 plants whereas C4 plant results were exactly opposite. However, these results should be treated with caution due to low sample size. There were no significant differences for other experimental conditions and functional groups in terms of relative water content under soil salinity stress.
C3 and C4 Plants Response to Chlorophyll
Categorical analyses indicated significant differences between AMF species, plant species, plant family, plant type and growth form on both C3 and C4 plants. Annual and perennial plants had a significant different effect within C3 plants. Whereas, experimental conditions showed no significant differences under salt stress in C3 plants. For the moderators like AMF richness, soil type, and level of salinity no significant effects were detectable. Among AMF species, the highest and the lowest effect size values for total chlorophyll were observed in C3 plants inoculated with R. intraradices and Glomus sp. and R. fasciculatus. While Glomus sp., R. fasciculatus, and R. intraradices had the lowest effect size in C4 plants, F. mosseae demonstrated highest effect size (treat the results with caution due to low sample size) (Figure 2B). Categorical analysis of growth forms showed herbaceous plants with the lowest and grass with the highest effect size values in C3 plants. Whereas, in C4 plants, grasses and shrubs had the lowest and herbaceous plants had the highest effect size values (Figure 3B). However, these results are to be considered with caution due to low sample size. Among the families investigated, Fabaceae recorded the lowest while Poaceae had the highest effect size values in C3 plants (Figure 3C). There were no significant differences exhibited by other plant functional groups under salt stress. Other plant functional groups showed no significant differences under salt stress.
C3 and C4 Plants Response to Leaf Area
AMF inoculated plants varied in their impact on leaf area. In contrast to relative water content and stomatal conductance, AMF inoculated C3 plants showed significantly higher effect size values than C4 plants. While the categorical analyses showed significant differences for all the moderators studied in C3 plants, C4 plants showed significant variation only for plant species, plant family, lifestyle, life cycle, and growth form. Among family, Fabaceae showed the highest effect size (Figure 3C). Annual and perennial C3 plants had the highest effect size values when plant life cycle was tested on leaf area. In addition, under the plant group, dicot plants had the highest effect size in both C3 and C4 plants. Among plant functional groups, grass, and herbaceous plants in C3 plants and herbaceous and grass plants in C4 plants had the lowest effect size values with shrubs in C3 plants and herbaceous forms in C4 plants displaying the highest effect size values. As expected, the level of salinity significantly influenced the leaf area of C3 plants (Figure 4B). Consequently, the moderate and low level of salinity had the lowest and high level of salinity the highest effect size values.
C3 and C4 Plants Response to Stomatal Conductance and Photosynthetic Rate
Highest stomatal conductance was observed in AMF inoculated C4 plants. Among AMF species, R. intraradices showed a significant increase in stomatal conductance of C3 plants (Figure 2B). While the significant difference was observed in AMF richness of C3 plants, mixed inocula showed highest effect size than those of single inocula though the results of which are to be treated with caution due to low sample size. The C3 plants also had a significant positive effect on plant species, plant family, plant group, growth habit, soil type, the level of salinity and experimental condition (P = 0.006). Whereas, C4 plants had a significant positive effect only on soil type. Sandy soil had a high effect size value than those of loamy soil. AMF had a positive overall effect on photosynthetic rate under saline conditions. While no significant (P > 0.05) effect was observed in C3 plants, AMF had a significant positive effect on C4 plants under saline condition. Among AMF species, R. intraradices showed a significant increase in effect size in C4 plants. Hence, C4 plants were tested additionally for a possible compounding effect of categorical variables and found no significant difference between studies. However, in the case of photosynthetic rate, an opposite trend was observed with C3 plants showing significant variations and C4 plants (P > 0.05) showing no significant positive effect. Also, the level of salinity significantly influenced the photosynthetic rate of C4 plants. Among level salinity, moderate level of salinity in C4 plants had the highest effect size values compared to those of C3 plants (Figure 4B).
Discussion
In many regions of the world, high soil salinity affects plants, compounding the effects of pedospheric and atmospheric water deficits recurrently faced by plants throughout their life cycle (Chaves et al., 2009). Salt stress adversely affect plant growth by disturbing the physiological mechanisms including reduction of cell water potential, stomatal conductance, photosynthetic rate, gas exchange, and disruption of membrane integrity among others (Abdel Latef and Miransari, 2014) through ionic toxicity and osmotic stress (Zhang and Shi, 2013; Pan et al., 2016). Interaction of AMF with plants could alleviate salt stress-induced reduction in plant health, productivity, leaf area, and biomass together with improved root to shoot dry mass ratio (Sheng et al., 2008; Hajiboland et al., 2010; Yang et al., 2016; Elhindi et al., 2017). The beneficial symbiosis arose partly due to modification of the fungi's environment and development of extensive mycelial extensions modulating water absorption and retention, soil volume and AMF-host water relations (Harris-Valle et al., 2018). Additionally, these effects are metabolically connected to Na+ exclusion, facilitating down-regulation of toxic Na+ build-up concomitant to selective absorption of K+ due to AMF-plant symbiosis (Jahromi et al., 2008; Sheng et al., 2008; Evelin et al., 2009; Porcel et al., 2012; Chandrasekaran et al., 2016). In our previous meta-analysis study (Chandrasekaran et al., 2014), we established that the improved growth of mycorrhiza inoculated plants in saline environments was partly related to mycorrhizal-mediated nutrient uptake and growth enhancement of host plants. Recently, with the quantitative analytical evidence, we showed a positive influence of AMF inoculation in both C3 and C4 photosynthetic groups grown under salt stress condition in terms of nutrient absorption and growth. A more competitive K+ ion absorption was exhibited by C4 compared to C3 plants (Chandrasekaran et al., 2016).
In this study, results showed that AMF has an overall positive effect on C3 and C4 plants gas exchange and water status under salt stress. The AMF mediated effect on total chlorophyll, chlorophyll a, chlorophyll b, leaf area, photosynthetic rate, and transpiration rate of C3 plants were higher than C4 plants. Whereas, C4 plants were found to have a higher effect size on stomatal conductance, RWC, and WUE. The AMF inoculated plants were able to display higher photosynthetic capacity under salt stress, showing the capacity of AMF to mitigate salt stress (Zuccarini, 2007; Evelin et al., 2009; Abdel Latef and Chaoxing, 2014). Symbiotic association of plants to AMF resulted in upregulated chloroplast gene expression, RppsbA, and RppsbD during different levels of salt stress and only at 100 mM NaCl, respectively. These, in turn, endow the plant with higher PSII efficiency then enhanced photosynthetic capacity during salt stress conditions (Chen et al., 2017). Previous studies also showed that greater chlorophyll represents higher rates of photosynthesis and carbon fixation, sustaining AMF-plant symbiosis (Wright et al., 1998; Elhindi et al., 2017). This study also confirmed that the response of AMF plants to total chlorophyll response of plants is greater than Amax, Gs, leaf area, and RWC. This is consonance with the earlier observations (Hajiboland et al., 2010; Wu and Zou, 2010; Abdel Latef and Chaoxing, 2011, 2014) where AMF plants have higher photosynthetic activity due to higher chlorophyll content. Moreover, the meta-analysis data also showed that the total chlorophyll content of C3 plants was greater than C4 plants. This could be the reason for decreased Amax in C4 plants compared to C3 plants. Exclusion of toxic Na+ due to inhibited Na+ transport caused by AMF colonization increased chlorophyll content and continued photosynthetic machinery. Increased absorption of Mg2+ has also been reported to increase the chlorophyll content in mycorrhiza inoculated plants (Zhu et al., 2010) where the AMF maintained absorption of Mg2+ under salinized soils in spite of the antagonistic effects of increased Na+ concentration (Giri et al., 2003; Talaat and Shawky, 2014).
Regression analysis based on the effect sizes of all plants showed that Gs had a close relationship to E (P < 0.05, R2 = 0.19), as previous work reported, the role of stomata in the control of transpiration can be defined as the relative change in E for a given relative change in Gs (Jones, 1998; Yan et al., 2016). Therefore, across all studies, we found that an increase in Gs could explain the variation in E, which is higher than that of Amax, indicating that maintaining plant water status may the most important function under salt stress in AMF inoculated plants. We also found a significant relationship (P < 0.05, R2 = 0.12) between C4 plants leaf area and total chlorophyll under salt stress. Moreover, we found a significant positive relationship (P < 0.05, R2 = 0.25) between Gs and Amax in AMF inoculated C4 plants under salt stress. In C4 plants, the increase in Gs indicates the increase in Amax, suggesting that the increase in Gs played a more important role in the increase in Amax under salt stress in the C4 plants. We did not find a significant relationship in AMF inoculated C3 plants under salt stress. Therefore, the regulation of Gs is related to species and genotype, making it difficult to define a pattern of photosynthetic responses to salt stress.
Plant-AMF symbiosis improves water status which also facilitates plant growth and photosynthesis with a positive effect on relative water content due to AMF colonization (Chen et al., 2017). The extensive hyphal extensions of mycorrhiza allow higher hydraulic conductivity even when water potential is low. This is added to the effect of higher stomatal conductance and transpiration which also improve water status (Kapoor et al., 2008; Sheng et al., 2008). Overall, the studies show a decrease in C3 mycorrhizal plants RWC and stomatal conductance as compared to C4 mycorrhizal plants under saline condition. In the present study, C4 plants like Zea mays and Allium sativum showed significantly increased effect sizes in RWC. This effect was more noticeable in Z. mays, which could be related to high stomatal conductance. F. mosseae also significantly increased the RWC content and chlorophyll level of plants under salt stress (Al-Khaliel, 2010). This resulted in a more enhanced gas exchange capacity in plants inoculated with mycorrhiza. Previous studies also show the role of AMF on the absorption of much-needed nutrients in the soil along with improved stomatal conductance with a subsequent increase in transpiration (Sheng et al., 2008; Hoeksema et al., 2010).
The increasing concentration of plant cellular Na+ and Cl− ions under salt stress causes a reduction in the cellular osmotic potential as free water is bound causing a state of physiological drought (Fuzy et al., 2008). On the other hand, AMF generally alters root architecture along with the formation of elaborate hyphal extensions permitting enhanced root conductance (Kothari et al., 1990; Yang et al., 2015) eventually leading to improved water content when compared to plants without symbiotic mycorrhizal fungi (Sheng et al., 2008). AMF associated with plant roots mediates improved hydraulic conductivity which could result in the better relative water content of plants even under environmental stress such as soil salinity (Kapoor et al., 2008). It was related to the ability of mycorrhizal plants to accumulate solutes enabling the AMF inoculated plants to adjust their osmotic potential than in non-mycorrhizal plants (Al-Garni, 2006). The increase in stomatal conductance of AMF plants, observed in this study, also has been reported to increase the transpiration under salt stress (Sheng et al., 2008). All these parameters combine to enhance WUE in mycorrhizal plant leading to improvement in the gas exchange capacity in the AMF plants (Elhindi et al., 2017). AMF symbiosis to plant hosts results to formation of an extensive hyphal network that enables AMF plants to absorb water and nutrients facilitating better photosynthetic rate and water osmotic potential (Hoeksema et al., 2010; Veresoglou et al., 2012; Yang et al., 2016). The current study is in agreement with earlier work (Augé et al., 2008) where Amax, E, and Gs parameters improved in relation to the degree of mycorrhizal inoculation over uninoculated plants experiencing damaging effects of salt stress. AMF plants show an increased transpiration rate in the leaves linked to increased stomatal conductance essential for photosynthesis and transport of carbon to the mycorrhiza (Auge', 2001; Maggio et al., 2004; Choe et al., 2006). For instance, the water content in the leaves of Jatropha curcas improved under salt stress due to mycorrhiza (Kumar et al., 2010) because of the continued water absorbance in the roots coupled with better stomatal conductance and transpiration (Jahromi et al., 2008).
In summary, our meta-analysis synthesizes AMF plant responses under salt stress in terms of water status and gas exchange and the interaction of these factors. Based on our data sets and the analysis, we conclude that the AMF inoculation not only increases the gas exchange performances but also ameliorate the plant water status in plants under salt stress. Mycorrhizal C3 plants more positively responded in terms of gas exchange compared to C4 plants. The increase in Gs primarily increased E under saline conditions. This increase in Gs could explain the increase in A and of the increase in E under salt stress and its role in the increase of A in C3 plants. Hence, it could be concluded that choosing appropriate AMF for a specific host plant could help alleviate salinity stress and will help use saline soils for the cultivation of crop plants. From the results of this study, it is obvious that F. mosseae is a preferred mycorrhizal partner invoking a positive response in C3 plants only to be followed by C4 plants.
Author Contributions
MuC performed most of the experimental work and wrote the manuscript. MaC, SS, KK, and TS helped with the analysis of the meta-analysis data, and with the discussion of the statistics. MuC performed the meta-analysis. MuC, TS, MaC, and KK contributed to the design of the experiments. MuC, TS, and SS edited the final version of the manuscript.
Funding
This study was supported by Basic Science Research Program through the National Research Foundation (NRF) funded by the Ministry of Education, Science and Technology (2015R1A2A1A05001885), Republic of Korea.
Conflict of Interest Statement
The authors declare that the research was conducted in the absence of any commercial or financial relationships that could be construed as a potential conflict of interest.
Acknowledgments
The authors would also like to thank Dr. Denver Walitang for his suggestions while revising the manuscript.
Supplementary Material
The Supplementary Material for this article can be found online at: https://www.frontiersin.org/articles/10.3389/fpls.2019.00457/full#supplementary-material
References
Abdel Latef, A. A., and Chaoxing, H. (2011). Effect of arbuscular mycorrhizal fungi on growth, mineral nutrition, antioxidant enzymes activity and fruit yield of tomato grown under salinity stress. Sci. Hortic. 127, 228–233. doi: 10.1016/j.scienta.2010.09.020
Abdel Latef, A. A., and Chaoxing, H. (2014). Does the inoculation with Glomus mosseae improve salt tolerance in pepper plants? J. Plant Growth Regul. 33, 644–653. doi: 10.1007/s00344-014-9414-4
Abdel Latef, A. A., and Miransari, M. (2014). “The role of arbuscular mycorrhizal fungi in alleviation of salt stress,” in Use of Microbes for the Alleviation of Soil Stresses Volume 2: Alleviation of Soil Stress by PGPR and Mycorrhizal Fungi. ed M. Miransari (New York, NY: Springer), 23–38.
Adams, D. C., Gurevitch, J., and Rosenberg, M. S. (1997). Resampling tests for meta-analysis of ecological data. Ecology 78, 1277–1283. doi: 10.1890/0012-9658(1997)078[1277:RTFMAO]2.0.CO;2
Al-Garni, S. M. S. (2006). Increasing NaCl-salt tolerance of a halophytic plant Phragmites australis by mycorrhizal symbiosis. Am. Eur. J. Agric. Environ. Sci. 1, 119–126.
Ali, N. A., Bernal, M. P., and Ater, M. (2002). Tolerance and bioaccumulation of copper in Phragmites australis and Zea mays. Plant Soil. 239, 103–111. doi: 10.1023/A:1014995321560
Al-Karaki, G. N. (2000). Growth and mineral acquisition by mycorrhizal tomato grown under salt stress. Mycorrhiza 10, 51–4. doi: 10.1007/s005720000055
Al-Khaliel, A. S. (2010). Effect of salinity stress on mycorrhizal association and growth response of peanut infected by Glomus mosseae. Plant Soil Environ. 56, 318–24. doi: 10.17221/204/2009-PSE
Auge', R. M. (2001). Water relations, drought and vesicular-arbuscular mycorrhizal symbiosis. Mycorrhiza 11, 3–42. doi: 10.1007/s005720100097
Augé, R. M., Toler, H. D., Sams, C. E., and Nasim, G. (2008). Hydraulic conductance and water potential gradients in squash leaves showing mycorrhiza-induced increases in stomatal conductance. Mycorrhiza 18, 115–121. doi: 10.1007/s00572-008-0162-9
Bethke, P. C., and Drew, M. C. (1992). Stomatal and non-stomatal components to inhibition of photosynthesis in leaves of Capsicum annum during progressive exposure to NaCl salinity. Plant Physiol. 99, 219–226. doi: 10.1104/pp.99.1.219
Caird, M. A., Richards, J. H., and Donovan, L. A. (2007). Nighttime stomatal conductance and transpiration in C3 and C4 plants. Plant Physiol. 143, 4–10. doi: 10.1104/pp.106.092940
Chandrasekaran, M., Kim, K., Krishnamoorthy, R., Walitang, D., Sundaram, S., Joe, M., et al. (2016). Mycorrhizal symbiotic efficiency on C3 and C4 plants under salinity stress – a meta-analysis. Front. Microbiol. 7:1246. doi: 10.3389/fmicb.2016.01246
Chandrasekaran, M., Sonia, B., Hu, S., Oh, S. H., and Sa, T. (2014): A meta-analysis of arbuscular mycorrhizal effects on plants grown under salt stress. Mycorrhiza 24, 611–625. doi: 10.1007/s00572-014-0582-7
Chapin, F. S. (1991). “Effects of multiple environmental stresses on nutrient availability and use,” in Response of Plant to Multiple Stresses, eds H. A. Mooney, W. Winner, E. J. Pell (San Diego, CA: Physiological Ecology Series Academic Press), 67–88.
Chaves, M. M., Flexas, J., and Pinheiro, C. (2009). Photosynthesis under drought and salt stress: regulation mechanisms from whole plant to cell. Ann. Bot. 103, 551–560. doi: 10.1093/aob/mcn125
Chen, J., Zhang, H., Zhang, X., and Tang, M. (2017). Arbuscular mycorrhizal symbiosis alleviates salt stress in black locust through improved photosynthesis, water status, and K+/Na+ homeostasis. Front. Plant Sci. 8:1739. doi: 10.3389/fpls.2017.01739
Chinnusamy, V., Jagendorf, A., and Zhu, J. K. (2005). Understanding and improving salt tolerance in plants. Crop Sci. 45, 437–448. doi: 10.2135/cropsci2005.0437
Choe, K., Toler, H., Lee, J., Ownley, B., Stutz, J., Moore, J., et al. (2006). Mycorrhizal symbiosis and responses of sorghum plants to combined drought and salinity stresses. J. Plant Physiol. 163, 517–528. doi: 10.1016/j.jplph.2005.05.003
Cooper, H. (1998). Synthesizing Research: A Guide for Literature Reviews. 3rd edn. Thousand Oaks, CA: Sage Publications, 216.
Derner, J. D., Johnson, H. B., Kimball, B. A., Pinter, P. J., Polley, H. W., Tischler, C. R., et al. (2003). Above and below-ground responses of C3 –C4 species mixtures to elevated CO2 and water availability. Global Change Biol. 9, 452–460. doi: 10.1046/j.1365-2486.2003.00579.x
Elhindi, K. M., El-Din, A. S., and Elgorban, A. M. (2017). The impact of arbuscular mycorrhizal fungi in mitigating salt-induced adverse effects in sweet basil (Ocimum basilicum L.), Saudi J. Biol. Sci. 24, 170–179. doi: 10.1016/j.sjbs.2016.02.010
Evelin, H., Kapoor, R., and Giri, B. (2009). Arbuscular mycorrhizal fungi in alleviation of salt stress: a review. Ann. Bot. 104, 1263–1280. doi: 10.1093/aob/mcp251
Fuzy, A., Biro, B., Toth, T., Hildebrandt, U., and Bothe, H. (2008). Drought, but not salinity, determines the apparent effectiveness of halophytes colonized by arbuscular mycorrhizal fungi. J. Plant Physiol. 165, 1181–1192. doi: 10.1016/j.jplph.2007.08.010
Geissler, N., Hussin, S., and Koyro, H. W. (2009). Interactive effects of NaCl salinity, elevated atmospheric CO2 concentration on growth, photosynthesis, water relations and chemical composition of the potential cash crop halophyte Aster tripolium L. Environ. Exp. Bot. 65, 220–231 doi: 10.1016/j.envexpbot.2008.11.001
Giri, B., Kapoor, R., and Mukerji, K. G. (2003). Influence of arbuscular mycorrhizal fungi and salinity on growth, biomass, and mineral nutrition of Acacia auriculiformis. Biol. Fertil. Soils 38, 170–175. doi: 10.1007/s00374-003-0636-z
Hajiboland, R., Aliasgharzadeh, N., Laiegh, S. F., and Poschenreider, C. (2010). Colonization with arbuscular mycorrhizal fungi improves salinity tolerance of tomato (Solanum lycopersicum L.) plants. Plant Soil 331, 313–327 doi: 10.1007/s11104-009-0255-z
Harris-Valle, C., Esqueda, M., Gutiérrez, A., Castellanos, A. E., Gardea, A. A., and Berbara, R. (2018). Physiological response of Cucurbita pepo var. pepo mycorrhized by Sonoran Desert native arbuscular fungi to drought and salinity stresses. Braz. J. Microbiol. 49, 45–53. doi: 10.1016/j.bjm.2017.04.005
Hoeksema, J. D., Chaudhary, V. B., Gehring, C. A., Johnson, N. C., Karst, J., Koide, R. T., et al. (2010): A meta-analysis of context-dependency in plant response to inoculation with mycorrhizal fungi. Ecol. Lett. 13, 394–407. doi: 10.1111/j.1461-0248.2009.01430.x.
Jahromi, F., Aroca, R., Porcel, R., and Ruiz-Lozano, J. M. (2008). Influence of salinity on the in vitro development of Glomus intraradices and on the in vivo physiological and molecular responses of mycorrhizal lettuce plants. Microbial. Ecol. 55, 45–53. doi: 10.1007/s00248-007-9249-7
Jones, H. G. (1998). Stomatal control of photosynthesis and transpiration. J. Exp. Bot. 49, 387–398. doi: 10.1093/jxb/49.Special_Issue.387
Kapoor, R., Sharma, D., and Bhatnagar, A. K. (2008). Arbuscular mycorrhizae in micropropagation systems and their potential applications. Sci. Horticult. 116, 227–239. doi: 10.1016/j.scienta.2008.02.002
Kothari, S. K., Marschner, H., and George, E. (1990). Effect of VA mycorrhizal fungi and rhizosphere microorganism on root and shoot morphology, growth and water relations of maize. New Phytol. 116, 303–311. doi: 10.1111/j.1469-8137.1990.tb04718.x
Koyro, H. W. (2006): Effect of salinity on growth, photosynthesis, water relations and solute composition of the potential cash crop halophyte Plantago coronopus (L.). Environ. Exp. Bot. 56, 136–146. doi: 10.1016/j.envexpbot.2005.02.001.
Kumar, A., Sharma, S., and Mishra, S (2010). Influence of arbuscular mycorrhizal (AM) fungi and salinity on seedling growth, solute accumulation and mycorrhizal dependency of Jatropha curcas L. J. Plant Growth Regul. 29, 297–306. doi: 10.1007/s00344-009-9136-1
Lehmann, A., and Rillig, M. C. (2015), Arbuscular mycorrhizal contribution to copper, manganese iron nutrient concentrations in crops: a meta-analysis. Soil Biol. Biochem. 81, 147–158. doi: 10.1016/j.soilbio.2014.11.013
Lehmann, A., Veresoglou, S. D., Leifheit, E. F., and Rillig, M. C. (2014). Arbuscular mycorrhizal influence on zinc nutrition in crop plants: a meta-analysis. Soil Biol. Biochem. 69, 123–131. doi: 10.1016/j.soilbio.2013.11.001
Lu, K. X., Cao, B. H., Feng, X. P., He, Y., and Jiang, D. A. (2009). Photosynthetic response of salt-tolerant and sensitive soybean varieties. Photosynthetica 47, 381–387. doi: 10.1007/s11099-009-0059-7
Maggio, A., Pascale, S. D., Angelino, G., Ruggiero, C., and Barbieri, G. (2004). Physiological response of tomato to saline irrigation in longterm salinized soils. Eur. J. Agron. 21, 149–159. doi: 10.1016/S1161-0301(03)00092-3
Munns, R., James, R. A., and Lauchli, A. (2006). Approaches to increasing the salt tolerance of wheat and other cereals. J. Exp. Bot. 57, 1025–1043. doi: 10.1093/jxb/erj100
Munns, R., and Tester, M. (2008). Mechanisms of salinity tolerance. Annu. Rev. Plant Biol. 59, 651–681. doi: 10.1146/annurev.arplant.59.032607.092911
Niu, S., Zhang, Y., Yuan, Z., Liu, W., Huang, J., and Wan, S. (2006). Effects of interspecific competition and nitrogen seasonally on the photosynthetic characteristics of C3 and C4 grasses. Environ. Exp. Bot. 57, 270–277. doi: 10.1016/j.envexpbot.2005.06.004
Pan, Y. Q., Guo, H., Wang, S. M., Zhao, B., Zhang, J. L., Ma, Q., et al. (2016). The photosynthesis, Na+/K+ homeostasis and osmotic adjustment of Atriplex canescens in Response to Salinity, Front. Plant Sci. 7:848. doi: 10.3389/fpls.2016.00848
Pellegrino, E., Opik, M., Bonari, E., and Ercoli, L. (2015). Responses of wheat to arbuscular mycorrhizal fungi: a meta-analysis of field studies from 1975 to 2013. Soil Biol. Biochem. 84, 210–217. doi: 10.1016/j.soilbio.2015.02.020
Porcel, R., Aroca, R., and Ruiz-Lozano, J. M. (2012). Salinity stress alleviation using arbuscular mycorrhizal fungi: a review. Agron. Sustain. Dev. 32, 181–200. doi: 10.1007/s13593-011-0029-x
Reyna-Llorens, I., and Hibberd, J. M. (2017). Recruitment of pre-existing networks during the evolution of C4 photosynthesis. Phil. Trans. R. Soc. B 372:20160386. doi: 10.1098/rstb.2016.0386
Rosenberg, N. J., Adams, D. C., and Gurevitch, J. (2000). MetaWin: Statistical Software for Meta-Analysis Version 2.0. Sunderland, MA: Sinauer.
Sheng, M., Tang, M., Chan, H., Yang, B., Zhang, F., and Huang, Y. (2008). Influence of arbuscular mycorrhizae on photosynthesis and water status of maize plants under salt stress. Mycorrhiza 18, 287–296. doi: 10.1007/s00572-008-0180-7
Stepien, P., and Klobus, G. (2006). Water relations and photosynthesis in Cucumis sativus L. leaves under salt stress. Biol. Plantarum 50, 610–6. doi: 10.1007/s10535-006-0096-z
Talaat, N. B., and Shawky, B. T. (2014). Protective effects of arbuscular mycorrhizal fungi on wheat (Triticum aestivum L.) Plants exposed to salinity. Environ. Exp. Bot. 98, 20–31. doi: 10.1016/j.envexpbot.2013.10.005
Tang, J., Xu, L., Chen, X., and Hu, S. (2009). Interaction between C4 barnyard grass and C3 upland rice under elevated CO2: Impact of mycorrhizae. Acta Oecol. 35, 227–235. doi: 10.1016/j.actao.2008.10.005
Teakle, N. L., Real, D., and Colmer, T. D. (2006). Growth and ion relations in response to combined salinity and waterlogging in the perennial forage legumes Lotus corniculatus and Lotus tenuis. Plant Soil. 289, 369–383. doi: 10.1007/s11104-006-9146-8
Treseder, K. K. (2004). The extent of mycorrhizal colonization of roots and its influence on plant growth and phosphorus content. Plant Soil. 371, 1–13. doi: 10.1007/s11104-013-1681-5
Veresoglou, S. D., Menexes, G., and Rillig, M. C. (2012). Do arbuscular mycorrhizal fungi affect the allometric partition of host plant biomass to shoots and roots? A meta-analysis of studies from 1990 to 2010. Mycorrhiza 22, 227–35. doi: 10.1007/s00572-011-0398-7
Wand, S. J., Midgley, G. F., Jones, M. H., and Curtis, P. S. (1999). Response of wild C4 and C3 (Poaceae) species to elevated atmospheric CO2 concentration: a meta analytic test of current theories and perceptions. Global Change Biol. 5, 723–41. doi: 10.1046/j.1365-2486.1999.00265.x
Way, D. A., Katul, G. G., Manzoni, S., and Vico, G. (2014). Increasing water use efficiency along the C3 to C4 evolutionary pathway: a stomatal optimization perspective. J. Exp. Bot. 65, 3683–3693. doi: 10.1093/jxb/eru205
Weber, A. P. M., and Caemmerer, S. V. (2010). Plastid transport and metabolism of C3 and C4 plants comparative analysis and possible biotechnological exploration. Curr. Opin. Plant Biol. 13, 257–265. doi: 10.1016/j.pbi.2010.01.007
Wright, D. P., Read, D. J., and Scholes, J. D. (1998). Mycorrhizal sink strength influences whole plant carbon balance of Trifolium repens L. Plant Cell Environ. 21, 881–91. doi: 10.1046/j.1365-3040.1998.00351.x
Wu, Q. S., and Zou, Y. N. (2010). Beneficial roles of arbuscular mycorrhizas in citrus seedlings at temperature stress. Sci. Hortic. 125, 289–293. doi: 10.1016/j.scienta.2010.04.001
Yan, W., Zhong, Y., and Shangguan, Z. (2016). A meta-analysis of leaf gas exchange and water status responses to drought. Sci. Rep. 6:20917. doi: 10.1038/srep20917
Yang, H., Zhang, Q., Dai, Y., Liu, Q., Tang, J., Bian, X., et al. (2015). Effects of arbuscular mycorrhizal fungi on plant growth depend on root system: a meta-analysis. Plant Soil. 389, 361–374. doi: 10.1007/s11104-014-2370-8
Yang, H. S., Dai, Y. J., Wang, X. H., Zhang, Q., Zhu, L. Q., and Bian, X. M. (2014). Meta-analysis of interactions between arbuscular mycorrhizal fungi and biotic stressors. Sci. World J. 2014:746507. doi: 10.1155/2014/746506
Yang, H. S., Xu, J., Guo, Y., Koide, R. T., Dai, Y., Xu, M., et al. (2016). Predicting plant response to arbuscular mycorrhizas: the role of host functional traits, Fungal Ecol. 20, 79–83. doi: 10.1016/j.funeco.2015.12.001
Zhang, J. L., and Shi, H. (2013). Physiological and molecular mechanisms of plant salt tolerance. Photosynth. Res. 115, 1–22. doi: 10.1007/s11120-013-9813-6
Zhu, J. K. (2001). Plant salt tolerance. Trends Plant Sci. 6, 66–71. doi: 10.1016/S1360-1385(00)01838-0
Zhu, X. C., Song, F. B., and Xu, H. W (2010). Arbuscular mycorrhizae improve low temp stress in maize via alterations in host water status and photosynthesis. Plant Soil. 331, 129–137. doi: 10.1007/s11104-009-0239-z
Keywords: arbuscular mycorrhizal fungi, plants, meta-analyis, salt stress, gas exchange, water status
Citation: Chandrasekaran M, Chanratana M, Kim K, Seshadri S and Sa T (2019) Impact of Arbuscular Mycorrhizal Fungi on Photosynthesis, Water Status, and Gas Exchange of Plants Under Salt Stress–A Meta-Analysis. Front. Plant Sci. 10:457. doi: 10.3389/fpls.2019.00457
Received: 26 November 2018; Accepted: 26 March 2019;
Published: 16 April 2019.
Edited by:
Ricardo Aroca, Estación Experimental del Zaidín (EEZ), SpainReviewed by:
Jan Graefe, Leibniz-Institut für Gemüse- und Zierpflanzenbau (IGZ), GermanyWalter Chitarra, Council for Agricultural and Economics Research, Italy
Copyright © 2019 Chandrasekaran, Chanratana, Kim, Seshadri and Sa. This is an open-access article distributed under the terms of the Creative Commons Attribution License (CC BY). The use, distribution or reproduction in other forums is permitted, provided the original author(s) and the copyright owner(s) are credited and that the original publication in this journal is cited, in accordance with accepted academic practice. No use, distribution or reproduction is permitted which does not comply with these terms.
*Correspondence: Tongmin Sa, dG9tc2FAY2h1bmdidWsuYWMua3I=
†Present Address: Murugesan Chandrasekaran, Department of Food Science and Biotechnology, Sejong University, Seoul, South Korea
Mak Chanratana, Cambodian Agricultural Research and Development Institute (CARDI), Phnom Penh, Cambodia