- 1Hubei Collaborative Innovation Centre for Grain Industry/Engineering Research Centre of Ecology and Agricultural Use of Wetland, Ministry of Education, Yangtze University, Jingzhou, China
- 2Guangdong Provincial Key Laboratory of Biotechnology for Plant Development, School of Life Sciences, South China Normal University, Guangzhou, China
- 3Tasmanian Institute for Agriculture, University of Tasmania, Hobart, TAS, Australia
Arabidopsis is used as a model species in numerous physiological and genetic studies. Most of them employ parafilm-wrapped sterile culture. Here we demonstrate that this method is prone to potential artifacts and can lead to erroneous conclusions. We compared the effect of different sealing methods including air-permeable paper tape and traditional parafilm on Arabidopsis seedling growth, root development and gene expression network. Although seedlings grown in Petri dishes after 1 week sealed with paper tape showed a similar growth phenotype to that of parafilm-sealed seedlings, more than 700 differentially expressed genes (DEG) were found, including stress and nutrition-responsive genes. In addition, more H2O2 was accumulated in the tissues of parafilm-sealed plants. After 14 days of growth, paper tape-sealed plants grew much better than parafilm-sealed ones and accumulated higher chlorophyll content, with 490 DEGs found. After 3 weeks of growth, paper tape-sealed plants had higher chlorophyll and better growth compared to parafilm-sealed ones; and only 10 DEGs were found at this stage. Thus, the obvious phenotype observed at the latter stage was a result of differential gene expression at earlier time points, mostly of defense, abiotic stress, nutrition, and phytohormone-responsive genes. More O2 content was detected inside paper tape-sealed Petri dishes at early growth stage (7 days), and distinct difference in the CO2 content was observed between parafilm-sealed and paper tape-sealed Petri dishes. Furthermore, the carbon source also influenced seedlings growth with different sealing methods. In conclusion, conventional sealing using parafilm was not the optimal choice, most likely because of the limited gas exchange and a consequent stress caused to plants.
Introduction
Arabidopsis thaliana belongs to the Cruciferae and is known as a “plant fly” due to its broad use in genetic studies and the fast growth cycle, simple morphological characteristics, as well as strong vitality. The genome sequence of Arabidopsis thaliana was the first amongst plants to be completely sequenced and published at the end of 2000. Arabidopsis also has a large mutant library and has long been used as a model organism in biological studies. The results of Arabidopsis thaliana research in botany can help deepen the understanding of other plants and improve the application of Arabidopsis to important crops (Meinke et al., 1998; Eckardt, 2001).
Plant tissue culture is a tool for basic research and with potential applications in agriculture, horticulture, and forestry industry of important plant bioactive compounds. Plant tissue culture has wide application, encompassing vector and vector-free genetic transformation of cells, embryo rescue, somatic embryogenesis, and commercial clonal propagation. Much plant biotechnology requires at least one stage where plant tissue culture is obligatory. Because of the short cycle and sensitivity to various stimuli, many researchers choose sterile culture to study seed germination rates and response to phytohormones or other chemical substances (Thorpe, 2006), as well as use Petri dish-grown plants to study mechanisms of tolerance to a broad range of abiotic stresses such as salinity or heavy metal toxicity.
The requirement for both sterility and the avoidance of dehydration in plant tissue cultures can impose sealing requirements that severely limit the rate of gas exchange in and out of the culture vessel. Sealing with parafilm to protect tissue cultures from infection and desiccation is the most used method. However, the use of the parafilm may potentially interfere with the free exchange of gasses between the cultured material and the outside atmosphere. This shortcoming can have unwelcome consequences for culture performance because of the strong physiological impact of the gasses involved, notably, O2, CO2, and C2H4 (Jackson et al., 1994). Oxygen and CO2 are principal substrates or products of aerobic respiration and photosynthesis and thus intrinsic to the most basic life-sustaining metabolic pathways of plant cells.
Despite the self-evident importance, researchers often neglect the gaseous environment for tissue cultures and used parafilm-sealed Petri dishes, for convenience purpose. Here we show that this approach is flawed, as it may result in an altered phenotype, gas composition, and gene expression pattern, hence, make a major impact on data interpretation.
Materials and Methods
All wild-type (ecotype Col-0) were surface sterilized with 20% bleach containing 0.1% Tween 20 for 15 min and then washed approximately five times with sterilized water. Seeds were sown on Murashige and Skoog medium, followed by cold treatment in the dark for 2 days. For sucrose deficiency treatment, all the preparations for the MS medium were the same except no addition of sucrose. MS medium composition was as follows: potassium nitrate, 1.9 g; ammonium nitrate, 1.65 g; calcium chloride, 0.33 g; potassium phosphate monobasic, 0.17 g; magnesium sulfate, 0.18 g; boric acid, 0.0062 g; manganese sulfate monohydrate, 0.0169 g; molybdic acid (sodium salt), 0.00025 g; zinc sulfate heptahydrate, 0.0086 g; potassium iodide, 0.00083 g; copper sulfate pentahydrate: 0.000025 g; ferrous sulfate heptahydrate, 0.0152 g; myo-inositol, 0.1 g; glycine, 0.002 g; thiamine hydrochloride, 0.0001 g; pyridoxine HCl, 0.005 g; niacin, 0.0005 g; ethylene diamine tetraacetic acid, 0.03726 g. MS powder was purchased from Solarbio (Beijing, Cat NO. M8521). For 1 L MS medium, 4.42 g powder was used and 8 g agarose and 20 g sucrose were added. Media pH was adjusted to 6.5–6.6 with 1N NaOH/HCl (not buffered). Parafilm was purchased from Parafilm M Laboratory Film, and the paper tape was from 3M Yuwell company (China). For the parafilm we used 10.2 cm parafilm in length, and we wrapped the Petri dish around the circumference twice. The same was done for the paper tape. The single-use plastic Petri dishes were used. Light intensity was 150 umol s-1 m-2. Around thirty uniform seedlings were grown on each Petri dishes. Seedlings were grown in a plant growth room under a 16-h light/8-h dark cycle at 22°C.
Gas Content, Water Content, and pH Value Measurement
Gas detector tubes (GASTEC, Japan) were used to measure the gas content in the Petri dish with seedlings after growing for 1, 2, and 3 weeks. The detecting layer of the tubes contained reaction particles and chemical reactions happen when they meet certain type of gas. For oxygen, measuring range was 6–24%, the color of detecting layer changed from black to white and the reaction principle is O2+4TiCl3+6H2O→4TiO2+12HCl.
For CO2, the measuring range is 100–2000 ppm and the color of detecting layer changed from pale red to orange, as a result of the following chemical reaction: CO2+2KOH→K2CO3+H2O. For ethylene, the measuring range was 0.2–50 ppm and the color of detecting layer changed from pale yellow to blue following CH2:CH2+PbSO4+(NH4)2MoO4→Molybdenum blue reaction. The Gastec sampling pump together with Gastec detector tubes were used. A fresh detector tube was inserted into the pump inlet. Two minutes later, the concentration level at the interface where the stained reagent meets the unstained reagent was read. Before conducting experiments with plants, we have conducted a series of methodological experiments checking kinetics of O2 and CO2 concentration changes in sealed Petri dishes with no plants in them. After 2 days, the measured values were not different from those for respective atmospheric concentrations (20.9–21.0% and 420–425 ppm, respectively) and in a full agreement with reported literature data. This data was accepted as a “time zero” point. Four replicates were used for every treatment.
Diaminobenzidine Staining
Diaminobenzidine (DAB) staining was performed as described previously (Chen et al., 2015). In brief, young seedlings were soaked in 1 mg/ml DAB solution (pH = 5.5) for 1 h and then immersed in 75% ethanol until the leaves turned white. The H2O2 signal (indicated by the pink or brown color) was observed under a stereoscope (Leica M205C). Eight seedlings were chosen for staining for every treatment and this experiment was repeated twice with similar results.
RNA-Seq Analysis
To construct RNA libraries with a VAHTS mRNA-seq V3 Library Prep Kit for Illumina, 1 μg of total RNA was used. The procedure included polyA-selected RNA extraction, RNA fragmentation, random hexamer-primed reverse transcription, and 150 nt paired-end sequencing by Illumina HiSeq X-ten. Libraries were quantified using qPCR according to the qPCR Quantification Protocol Guide. To estimate expression levels, the RNA-seq reads were mapped to the Arabidopsis reference genome using TopHat, which is capable of reporting split-read alignments across splice junctions. Transcript counts were calculated, and the relative transcript abundances were measured in FPKM (Fragments Per Kilobase of exon per Million fragments mapped) using Cufflinks. We excluded transcripts with zeroed FPKM values of more than one for total samples. We added 1 to FPKM values of filtered transcripts to facilitate log2 transformation. Filtered data were transformed logarithmically and normalized using a quantile normalization method. For each transcript, we calculated fold change between case and control. Differentially expressed transcripts were determined by adjusting fold change ≥ 2 of more than at least one of total comparisons.
In total, eight steps were included: RNA extraction (Trizol method), rRNA and fragment removal, obtaining first-strand cDNA, obtaining second-strand cDNA, A-tailing addition, adapter ligation, purification and size selection and PCR amplification. Raw data were calculated as the FPKM of each transcript in each sample using Cufflinks software.
Gene ontology (GO) enrichment analysis was performed using the EasyGO gene ontology enrichment analysis tool1. The GO term enrichment was calculated using a hypergeometric distribution with a P value cut off of 0.01. P values obtained by Fisher’s exact test were adjusted with FDR for multiple comparisons to detect overrepresented GO terms (Xin and Zhen, 2007).
Three biological repeats were prepared for RNA-seq analysis and the data was generated in the same experiment.
Results
Effects of Different Sealing Methods on the Growth of Arabidopsis thaliana
The growth rate, fresh weight, chlorophyll content, and root length were similar between 7-day-old parafilm-sealed and paper tape-sealed seedlings. However, after 2 weeks of growth, the paper tape-sealed seedlings had more vigorous growth, higher biomass, and their shoots possessed more large green leaves (higher chlorophyll content, Figure 1C), compared with parafilm-sealed seedlings. The difference became even more prominent after 3 weeks of growth between seedlings using the two different sealing methods (Figure 1). Similar trends were observed for the root growth (Table 1). No difference in the root length/appearance was found between paper and parafilm-sealed seedlings after 7 days of growth, however, the root surface area, average diameter, root volume, and root tip number of paper-sealed Arabidopsis thaliana were greater than those of parafilm-sealed seedlings after 14 days of growth. After 21 days of growth, the root length, average diameter, root volume, and root tip numbers of paper-sealed seedlings were significantly greater than those of Arabidopsis thaliana with parafilm sealing (Table 1). These results indicated that plants grown with the use of the paper tape maintained a more advanced rooting system, and vigor as well as a higher proliferation capacity of Arabidopsis and the “excellent water vapor permeability” of parafilm may not be as good as the manufacturer mentioned in the description of the product.
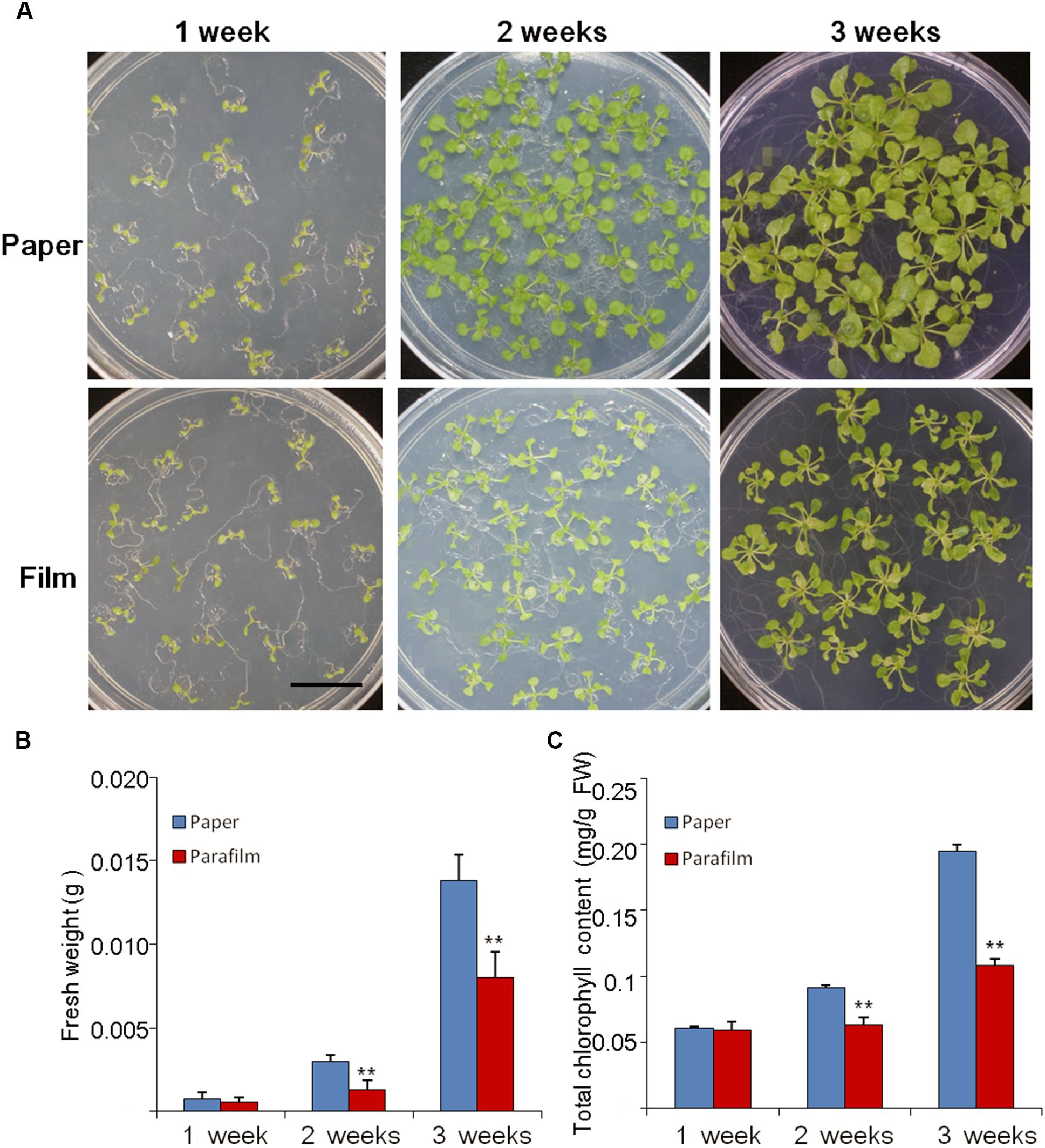
Figure 1. Phenotype (A), biomass (B), and chlorophyll content (C) of Col seedlings grown on MS medium for 1, 2, and 3 weeks with the different sealing methods. One (of six) representative plates are shown. For (B), entire seedlings were used. Data are mean ± SD (n = 6). Data labeled with asterisks are significant at p < 0.05(Student’s t-test). For each treatment, seedlings in six plates were used for measurement and experiments repeated for three times with similar results.
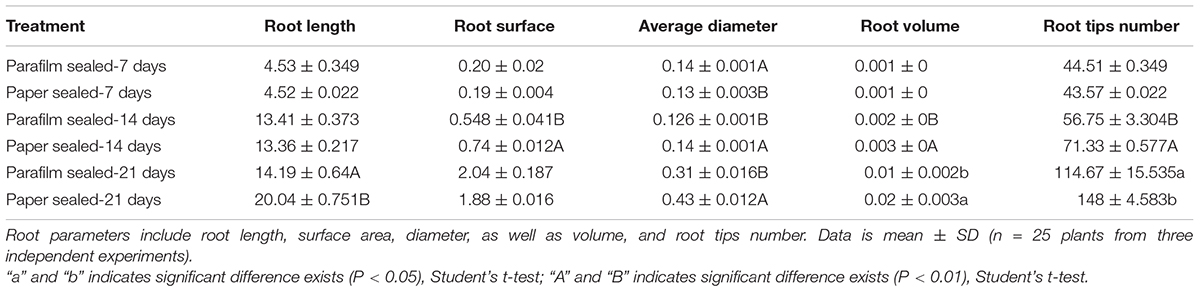
Table 1. Effect of parafilm and paper sealing methods on parameters of the root systems of Arabidopsis seedlings after 1, 2, and 3 weeks in culture.
Carbon Source Is an Important Factor That Influences Plant Growth
Young seedlings grown in a Petri dish are frequently used for nutrition tests, because nutrient concentration in the media can be easily controlled. We tested the response of seedlings of the different sealing methods under sugar deficient conditions. We found seedlings in paper-sealed Petri dishes grew much better than those in parafilm-sealed ones after 2 weeks, the difference became even more pronounced after 3 weeks of growth (Figure 2). The most logical explanation for this fact would be a possible difference in the gas composition and its influence on the most important reactions in plants: photosynthesis and respiration. If this is the case, then these findings suggest that the starvation (carbon source cutdown) response may be closely linked with the gas environment. Possessing the impeded transpiration under sealed conditions, seedlings appear to have higher demands for nutrients and carbon. Therefore, false results may be obtained if this factor is not taken into account.
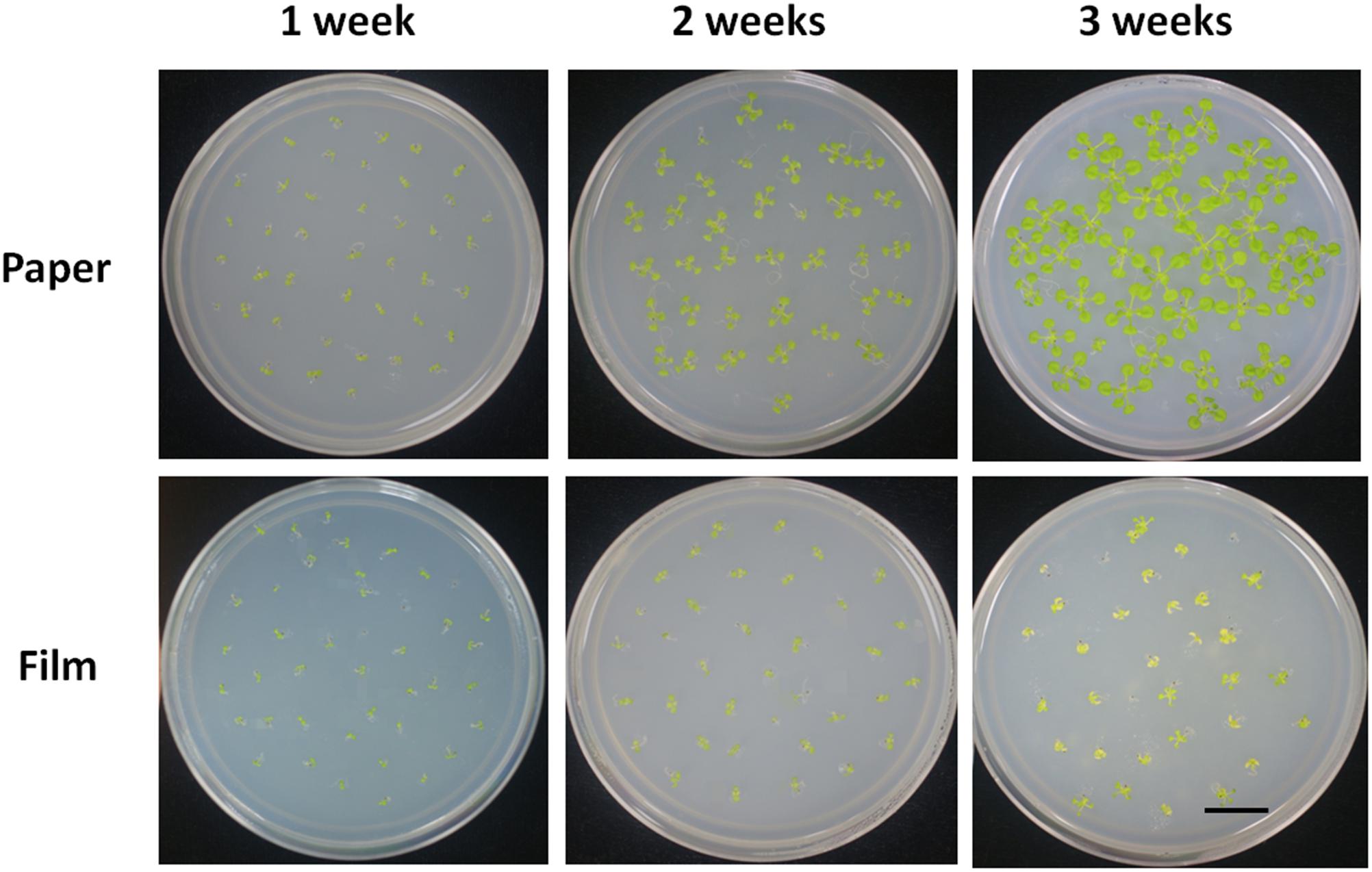
Figure 2. Phenotype of Col seedlings grown on MS medium without sucrose for 1, 2, and 3 weeks with the different sealing methods. For each treatment, four replicates were used.
Gas Content in Petri Dishes With Paper Tape and Parafilm Sealing
To test the above hypothesis that impeded gas exchange was a major factor differentiating between plant performance in parafilm- and paper-sealed Petri dishes, we measured the content of O2, CO2, and ethylene in the Petri dishes with seedlings growing for 7, 14, and 21 days (Table 2). For O2 content, paper-sealed Petri dish consist more O2 compared to parafilm-sealed ones after 7-day growth. This trend reversed after 14 days growth and O2 concentration was the same at other time points. Ethylene cannot be detected during the whole growing process, despite the detection limit of the gas tube was as little as 0.05 ppm. CO2 content was significantly higher in parafilm sealed Petri dishes after 7-day (4.2% higher), and the difference became even higher at 21-day (33.9%) while it showed a trend reversal after 14 days growth (16.2% lower).
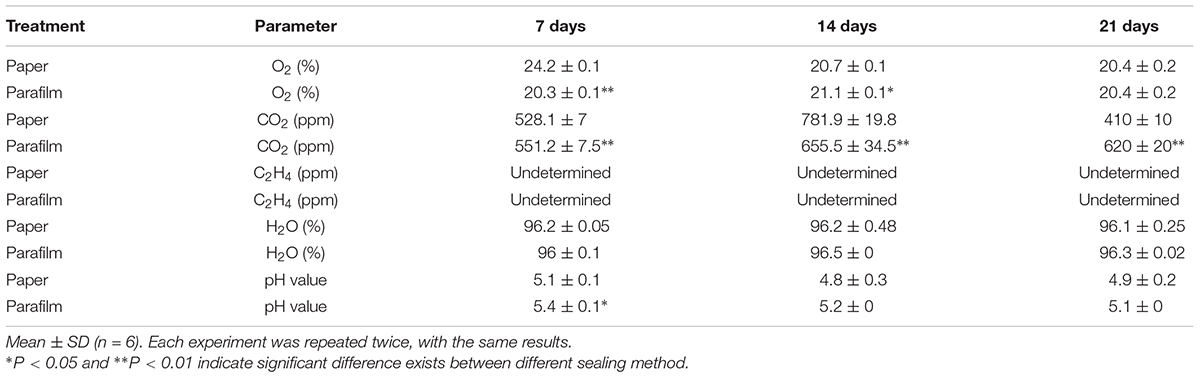
Table 2. Concentration of oxygen, carbon dioxide, ethylene in the Petri dishes, and moisture content in the media with seedlings growing for 7, 14, and 21 days.
The water content ranged from 96 to 97.2% and no difference was between different sealing methods. Msogoya et al. (2008) reported that autoclaving cause a significant drop of pH (from 6.8 to 5.8) value; different gelling agaents and autoclaving conditions resulted in different drop extent of pH value (Owen et al., 1991). In our experiment, pH value of media in parafilm sealed Petri dishes (7-day growth) was higher than paper tape sealed ones and no significant difference was found in other time points. Media pH level may influence nutrient uptake (Ramage and Williams, 2002), rooting and cellular growth (Leifert et al., 1992; Klerk et al., 2008), and plant gene expression (Lager et al., 2010).
Effect of Different Sealing Methods on H2O2 Burst
Altered oxygen availability may potentially result in differential production of reactive oxygen species (ROS) by plant cells (Mehdy, 1994; Baker and Orlandi, 1995; Low and Merida, 2010). Thus, we tested H2O2 levels in parafilm and paper tape-sealed plants by DAB staining after 1 and 2 weeks of growth. As shown in Figure 3, after 7 days of growth, the production of H2O2, indicated by brown coloration in seedlings, was much higher in the leaf vain, central growth point and root of paper-sealed seedlings than that in those tissues of parafilm-sealed seedlings. In contrast, the H2O2 signal intensity was almost the same in 2-week-old seedlings using the two sealing methods (Figure 3).
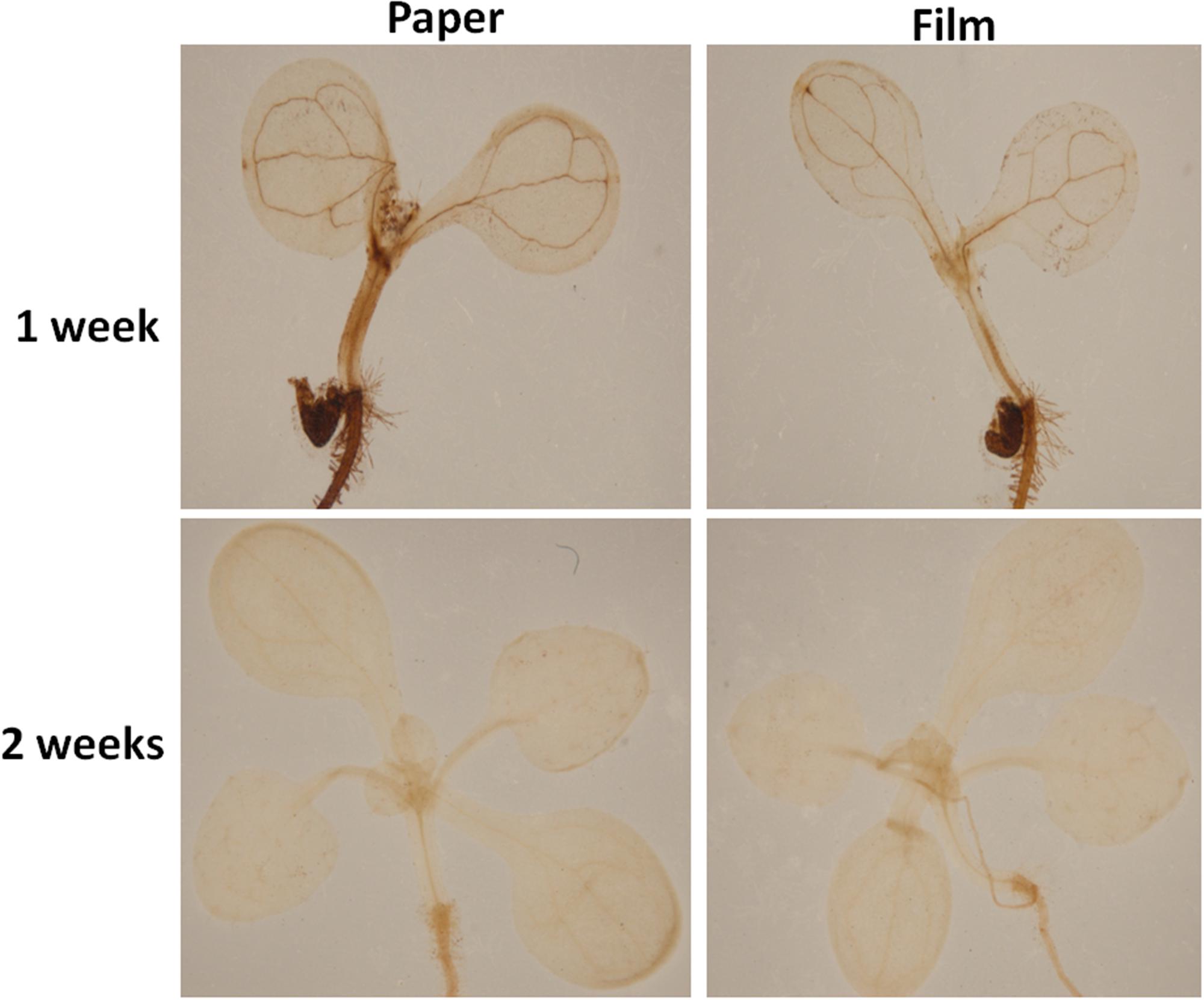
Figure 3. H2O2 accumulation in Col seedlings after 1 and 2 weeks of growth measured using DAB solution staining. DAB is oxidized by hydrogen peroxide in a reaction typically catalyzed by horseradish peroxidase (HRP). The oxidized DAB forms a brown precipitate, at the location of the HRP, which can be visualized using light microscopy. All seedlings were grown on standard MS media with sucrose shown in section “Materials and Methods”. One (of six) typical seedlings is shown for every treatment. The experiment was repeated twice with similar results.
Reactive oxygen species have also emerged as important regulators of plant development in which they play roles in processes as diverse as hormonal signal transduction and the modulation of cell wall polymer structure and tip growth (Potikha and Levine, 1999; Schopfer, 2001; Joo et al., 2005; Kerstin et al., 2009).
Different Sealing Methods Influence Transcriptional Networks
To identify the transcriptional networks controlled during the growth process using the different sealing methods, we compared the transcriptomic profiles of whole seedlings of different age (1-, 2-, and 3-week-old) using genome-wide RNA-sequencing (RNA-seq) (Figure 1). RNA was isolated from a pooled seedlings sample of three biological replicates. We sequenced the libraries on an Illumina HiSeq 2000. The reads were aligned onto the Arabidopsis reference genome assembly (TAIR10), and the raw reads can be found in Supplementary Table S1. 725, 490, and 10 differentially expressed genes (DEG) were found in paper tape-sealed seedlings after 7, 14, and 21 days of growth, respectively, relative to parafilm-sealed plants. These genes were divided into two groups using a hierarchical clustering algorithm (Figure 4). Genes differentially expressed in seedlings with the two sealing methods grown for 7 days were categorized and annotated based on biological processes, molecular functions, and cellular components (Figure 5). The most impressive changes happened in defense, heat, and water response as well as oxidative stress response. Differentially expressed genes in seedlings grown for 14 days and 21 days with two sealing method categorized according to biological processes, molecular functions, and cellular components can be found in Supplementary Figures S1 and S2, respectively.
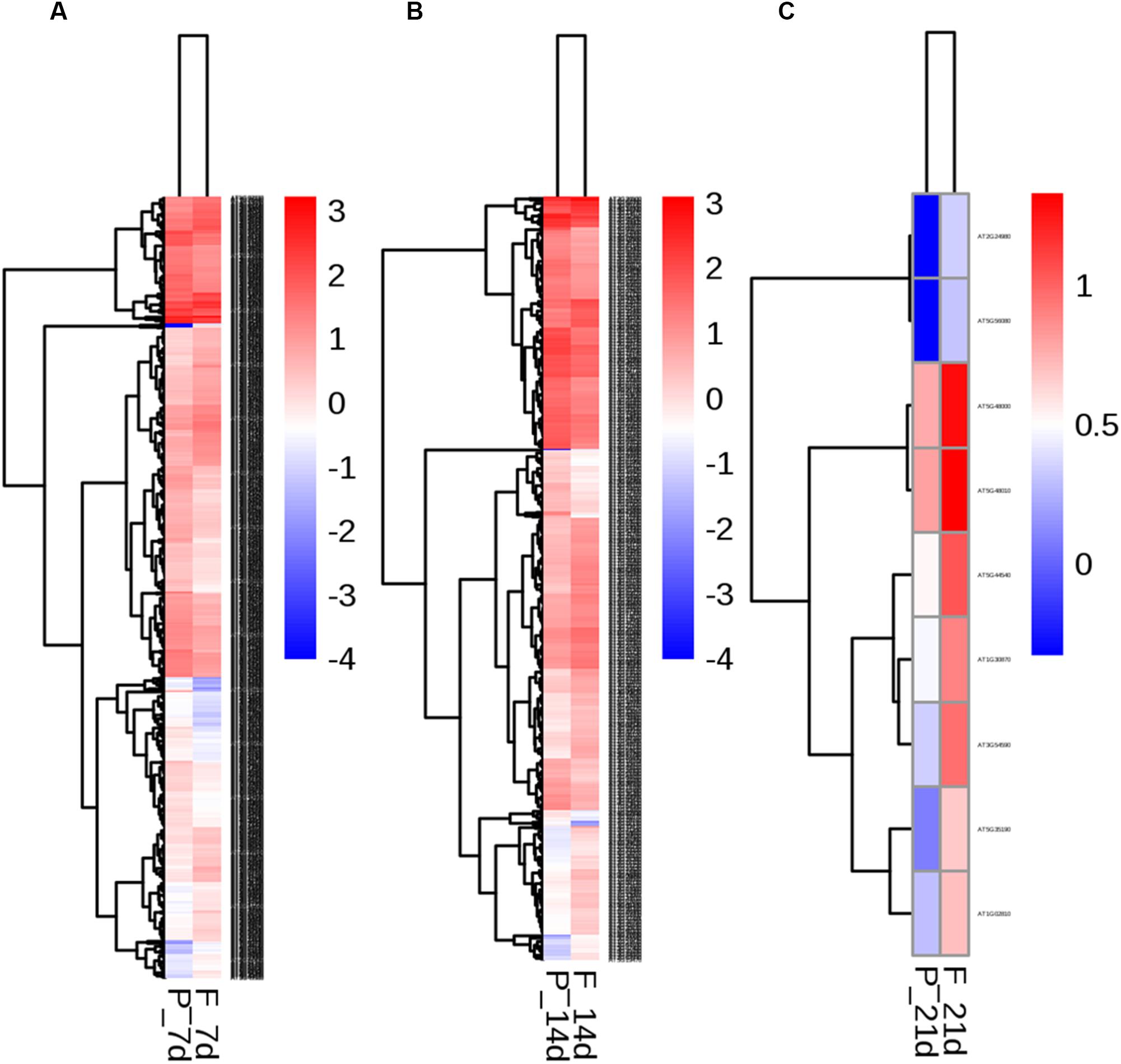
Figure 4. Two-way hierarchical clustering heat map of genes differentially expressed in seedlings in parafilm and paper tape-sealed petri dishes for 7 (A), 14 (B), and 21 days (C). Differentially expressed transcripts were clustered. The color key in the right-hand corner is for the colors in the heat map. Detail information of these DEGs can be found in Tables 3–6.
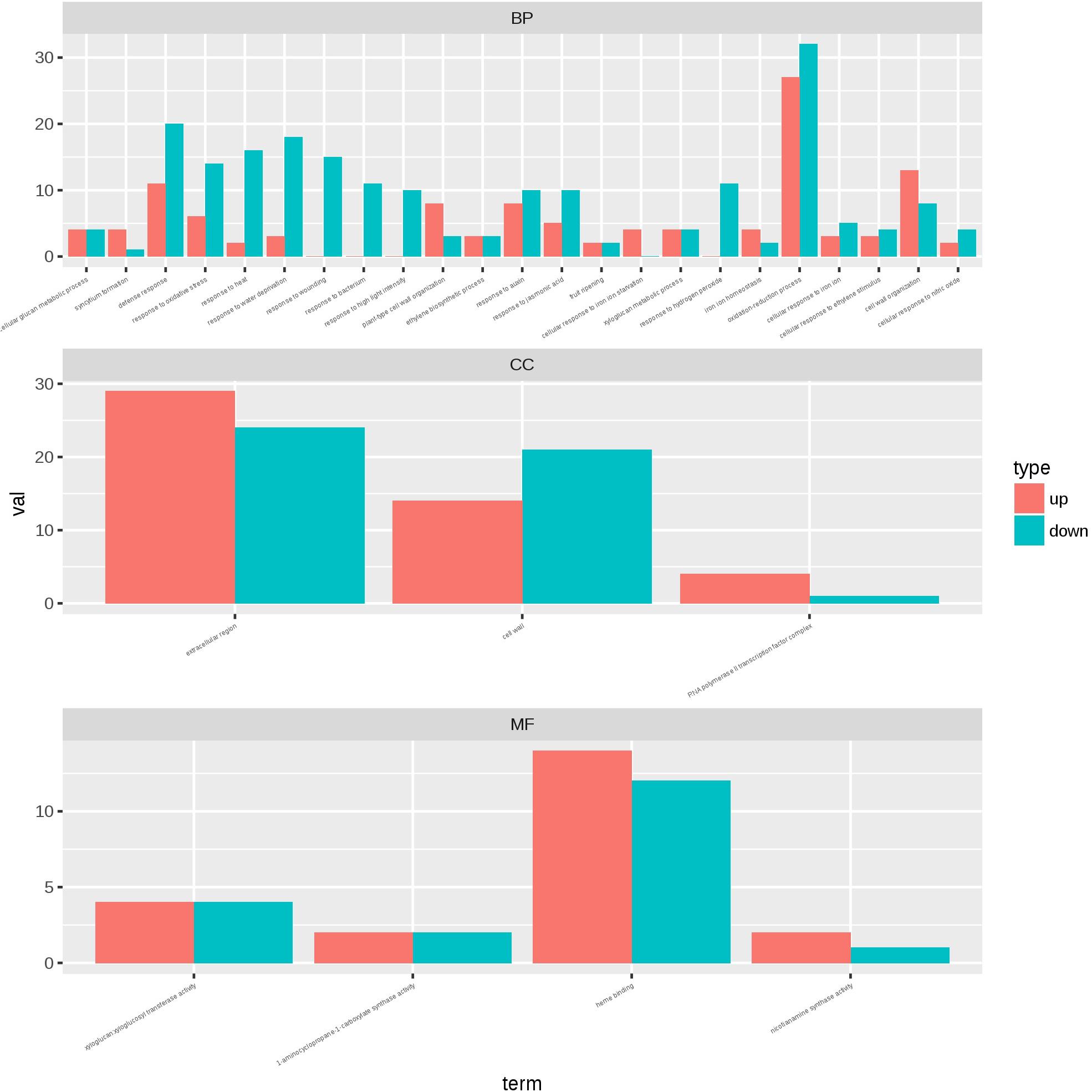
Figure 5. Gene ontology plant term enrichment. Genes differentially expressed in seedlings with the two sealing methods grown for 7 days were categorized and annotated based on biological processes, molecular functions, and cellular components. Three biological repeats were prepared for RNA-seq analysis and the data was generated in the same experiment.
We listed all the DEGs in 7- and 14-day-old young seedlings (fold change > 2 or < -2), while little difference was found between 21-day-old plants using the different sealing methods. For paper-sealed 7-day-old seedlings, seven of cold-inducible genes, four of pathogenesis-related genes, three of heat shock genes, and several of stress-responsive genes (late embryogenesis genes and trichomes) and nutrition, phytohormone-responsive, lipid metabolism and development-related genes were downregulated compared to those in parafilm-sealed plants. In our previous study of the freezing tolerance of Arabidopsis sealed with parafilm in a Petri dish, we found it is very hard to obtain stable results using young seedlings. Therefore, we could not find the suitable treatment method to differentiate between wild-type plants and mutants, and we finished all the phenotypic experiments with adult plants in soil. From the current results, we may obtain some clue to explain why the phenotyping was so difficult, and the difficulty may result from the stress indicated by induce of stress responsive genes caused by the close culture system (Table 3). Similarly, after growth for 14 days, seven genes in the DREB family (involved in cold and drought tolerance), two pathogenesis-related genes, and several phytohormone-related genes (ET, JA, and cytokinin) were less expressed in paper tape-sealed plants compared to those in parafilm-sealed seedlings (Table 4). In contrast, in 7-day-old paper-sealed seedlings most upregulated genes were related to nutrient acquisition processes (iron deficiency responsive genes, phosphate responsive genes), defense-related genes, phytohormone-responsive genes and root development-related genes. The upregulation of BR increased expression of 1, GA synthesis (R2R3-MYB, also found in 14-day-old seedlings) and GA methyltransferase 2 (GAMT2, also found in 14-day-old seedlings) of paper tape-sealed plants and that might be a reason for better growth of seedlings (Tables 5, 6). Peroxidase 33, which is responsible for generating H2O2, was also much more elevated in paper-sealed than in parafilm-sealed plants and it may explain the increase in pink signal (Figure 3).
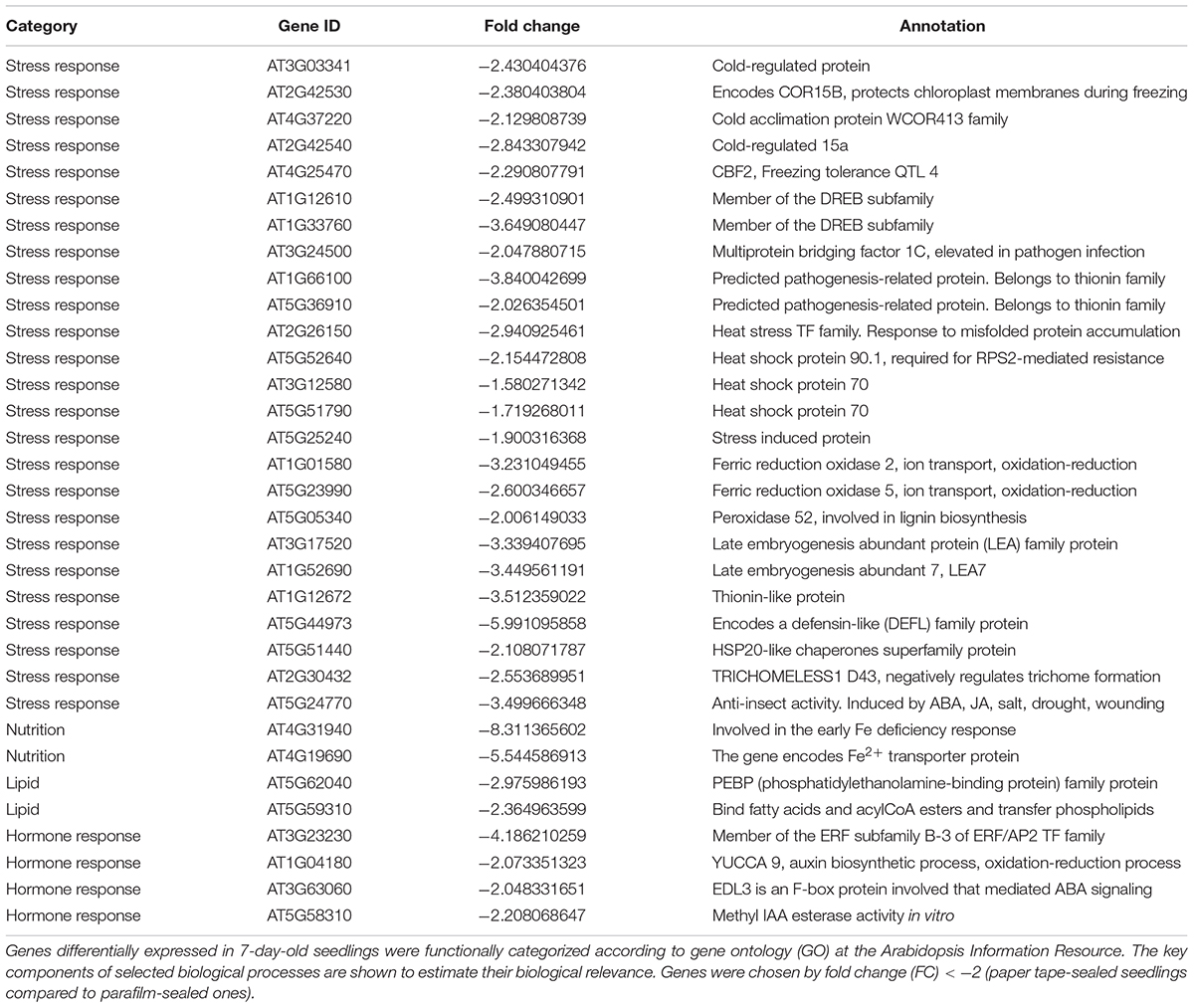
Table 3. Transcript profiles of key genes involved in selected biological processes (P_7d_vs_F_7d.DEG_down).
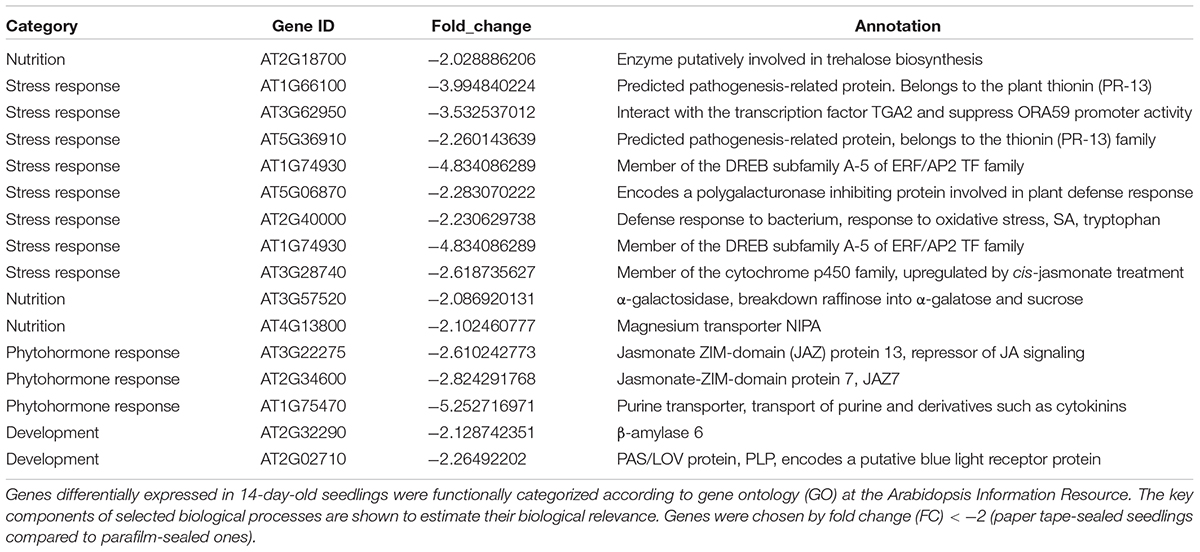
Table 4. Transcript profiles of key genes involved in selected biological processes (P_14d_vs_F_14d.DEG_down).
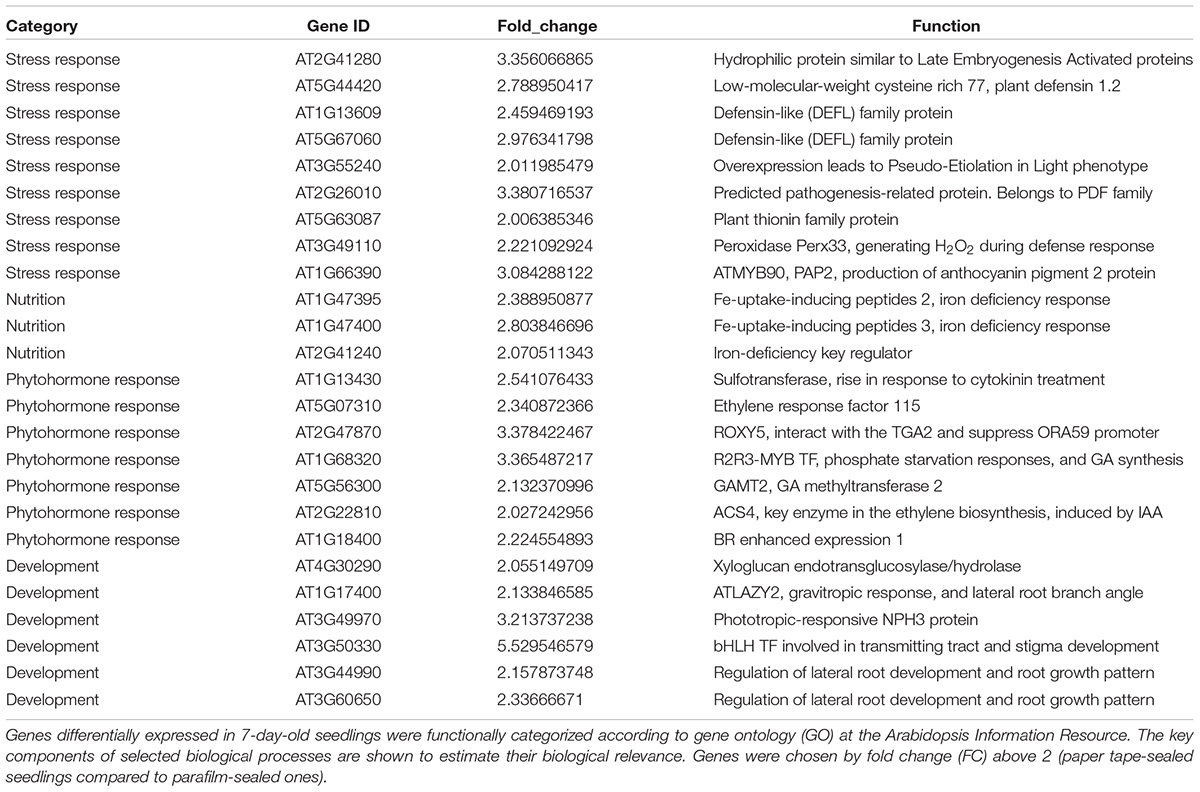
Table 5. Transcript profiles of key genes involved in selected biological processes (P_7d_vs_F_7d.DEG_up).
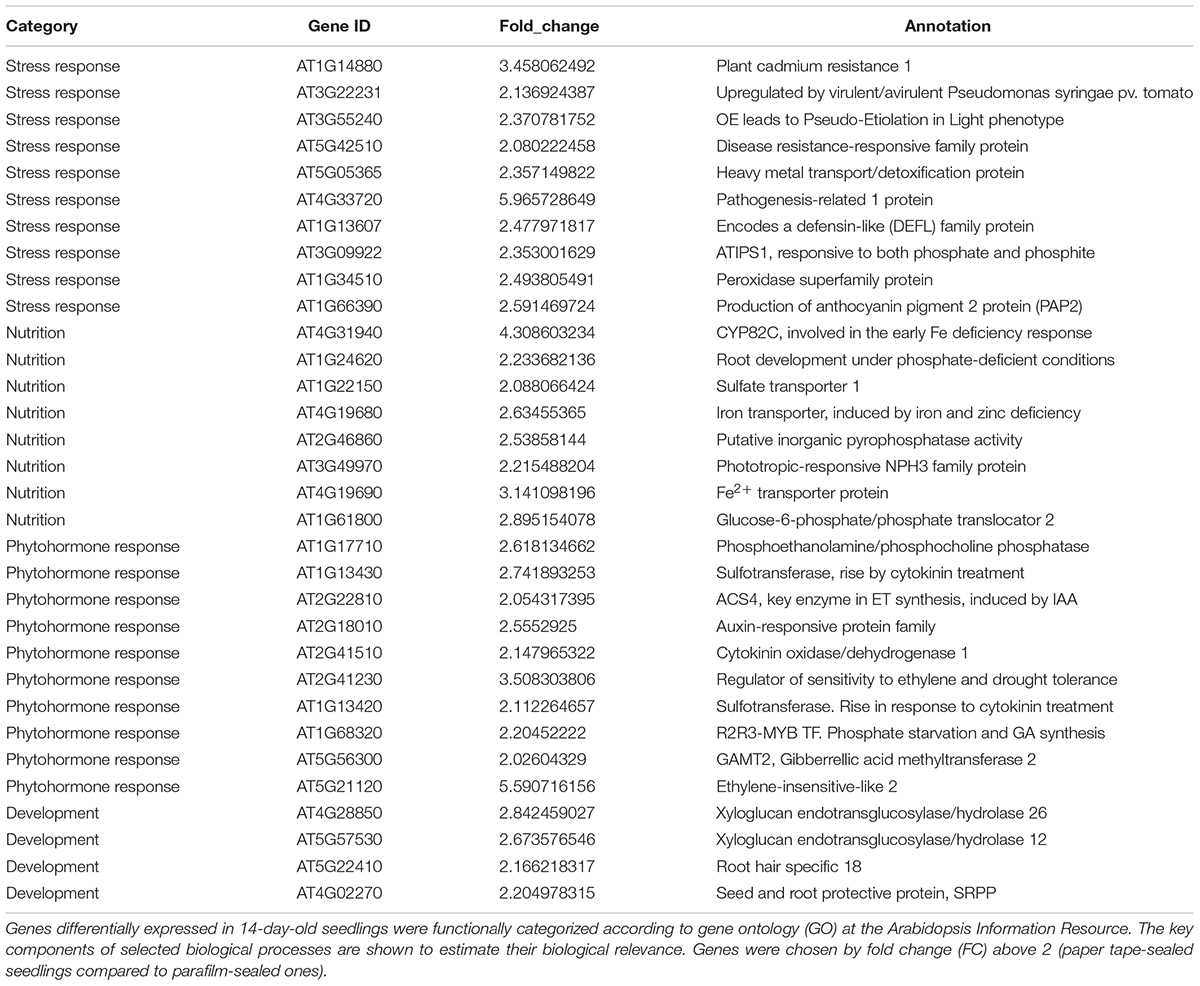
Table 6. Transcript profiles of key genes involved in selected biological processes (P_14d_vs_F_14d.DEG_up).
For the upregulated genes in 14-day-old paper-sealed seedlings compared to those in parafilm-sealed plants, most were involved in nutrient acquisition (iron transporter and phosphate-responsive genes), heavy metal transport genes (cadmium), and phytohormone-responsive genes (Table 5). After 3 weeks of growth, few differently expressed genes were found between plants of the two sealing methods, even though significant effects on growth were observed by naked eyes.
In summary, most abiotic stress-related genes were much more highly expressed in parafilm-sealed plants, while most nutrient responsive and growth promoting phytohormone genes were downregulated compared to those in paper-sealed seedlings. The expression patterns of these genes are consistent with the phenotype results.
Discussion
Growth, development and quality of in vitro cultured plants are strongly influenced by their environment (Kristiansen et al., 1999; Mroginski et al., 1999). The chemical composition of the culture medium is generally accepted as of the utmost importance (Morard and Henry, 1998; Goleniowski and Trippi, 1999). External conditions, such as light, temperature and humidity, also have significant effects on plant growth.
In conventional tissue culture, the sealing film is usually used to seal the culture vessel, mainly to prevent pollution and excessive water evaporation, but a stressful environment is formed. Loose sealing can reduce the vitrification of carnations (Hakkaart and Versluijs, 1983; Dillen and Buysens, 1989) and promote the growth of seedlings. Lavender buds cultured in the “open culture system” with the appliance’s stomatal sealing have high vitality and regeneration rate (Tsuro et al., 2000), and our results demonstrated that parafilm might not be the optimal sealing method, because the seal is airtight and our RNA-seq results also demonstrated that fewer stress-related genes were highly expressed and that nutrition and phytohormone-responsive genes were upregulated in paper tape-sealed seedlings compared to parafilm-sealed plants after 1 and 2 weeks of growth.
More O2 content were detected in paper tape-sealed Petri dishes at early growth stage (7 days) and distinct CO2 content in parafilm-sealed and paper tape-sealed Petri dishes were measured. Since plants photosynthesize and need CO2 to generate glucose and store starch, and chemical reactions are pushed toward their end product, one would expect that more CO2 would be better, for increased growth and survival rates. However, it is not always the case, and it was shown that doubling the atmospheric CO2 concentration from 340–410 ppm to 610 ppm might have negative effect on plant growth and survival, and excess CO2 reduces the rate of transpiration of some plants (Mansfield and Majernik, 1970), and the water flow from the soil/medium to the leaves also drops, causing a runoff of water, and this in turn stalls nutrient uptake. In our case, the measured CO2 concentration in the parafilm-sealed Petri dishes reached 620 ppm level, so the above scenario may be plausible. It should be also noted that CO2 tolerances are species dependent. For example, CO2 tolerance in cotton plants is low, and starch buildup has been observed in the entire plant, but especially in the root systems and the stem (Hendrix et al., 1994). Future experiments and optimal and limiting CO2/O2 ratios for Arabidopsis plants, and link them with the changes in plant phenotype and gene expression changes reported in this work.
In addition, in order to observe root growth, the Petri dishes are oriented in a vertical position. Water easily accumulates at the bottom of a Petri dish and causes bacteria to multiply and serious stress on the root after long time culture. However, sealing with ventilated paper tape will keep the proper humidity of the medium, although the tape may fail for volatile substances in the medium, such as alcohol, and in antibiotic screening tests. However, we measured the moisture content of the medium sealed with two method, and we find the water content ranged from 96 to 97.2% and no difference was observed between different sealing method.
Inevitably, cultures enclosed in parafilm-sealed containers need a compensating pathway for diffusive gas exchange is contrived or replaced by some system of convective flow that carries gasses to and from the tissue. Obvious ways to achieve leakage include loosening the closure or inserting a gas-permeable membrane (e.g., polypropylene).
While parafilm-sealed Petri dishes are convenient and offer much advantages, they also possess a stressful environment and cause significant perturbation to plant gene expression profile. This alters plant metabolism and adaptive responses. Similar problems exist with the currently used Petri dish system with light inducing stress in roots. Simple darkenning of the root part is providing remedy. Qu et al. (2017) reported that an improved plant-growing method (IPG) based on a dark growth chamber found that the light intensity was dramatically reduced deeper in the dark chamber. The flavone, flavonol, and flavonoid biosynthesis pathways were significantly different between IPG and traditional method. Besides different gene expression patterns, compared to traditional plant-growing method (TPG), IPG produced plants with less total root length, lateral root length and root hair density, while their primary roots were longer. Moreover, root gravitropism, PIN2 (an auxin efflux carrier) abundance, H+ efflux or Ca2+ influx in root apices, were weaker in IPG-grown roots than those in TPG-grown roots (Xu et al., 2013). Yokawa et al. (2011, 2014) also reported that illumination of roots which naturally grow in darkness, even for a few seconds, induces an immediate and strong burst of ROS. In conclusion, in transparent Petri dishes, regular light exposure affects root morphology and behavior. An improved plant culture method using a shaded/darkened environment for roots that mimics normal underground light conditions was recommended (Yokawa et al., 2013). Besides, Buer et al. (2000) also reported that the gaseous environment within the Petri dish could be an important reason which causes the wave-like growth of Arabidopsis thaliana roots on semi-solid medium. Wrapping plates with surgical tape (loosely sealed) increased the wave characteristics and produced the slanting root growth wherein the wave response decreased in Nescofilm (airtight) sealed Petri dishes. Root response on tightly sealed plates could differ from responses on loosely wrapped plates. Plate wrapping materials, and other growth conditions occasionally, are taken for granted, while wide-ranging phenotypes require careful attention when results obtained from such systems are then extrapolated to glasshouse- or field-grown plants.
Author Contributions
LX, SL, and WZ conceived and designed the research. LX and TJ conducted the experiments. WZ provided the reagents and items for the experiments. SL analyzed the data. LX wrote the manuscript. SS and WZ revised the manuscript. All authors read and approved the manuscript.
Conflict of Interest Statement
The authors declare that the research was conducted in the absence of any commercial or financial relationships that could be construed as a potential conflict of interest.
Acknowledgments
We thank Dr. Libing Yuan for experiment design and Dr. Jihong Hu for RNA-seq analysis.
Supplementary Material
The Supplementary Material for this article can be found online at: https://www.frontiersin.org/articles/10.3389/fpls.2019.00637/full#supplementary-material
FIGURE S1 | Gene ontology plant term enrichment. Genes differentially expressed in seedlings with two sealing methods grown for 14 days were categorized and annotated based on biological processes, molecular functions, and cellular components.
FIGURE S2 | Gene ontology plant term enrichment. Genes differentially expressed in seedlings with two sealing methods grown for 21 days were categorized and annotated based on biological processes, molecular functions, and cellular components.
TABLE S1 | RNA-seq reads.
Footnotes
References
Baker, C. J., and Orlandi, E. W. (1995). Active oxygen in plant pathogenesis. Annu. Rev. Phytopathol. 33, 299–321. doi: 10.1146/annurev.phyto.33.1.299
Buer, C. S., Masle, J., and Wasteneys, G. O. (2000). Growth conditions modulate root-wave phenotypes in Arabidopsis. Plant Cell Physiol. 41, 1164–1170. doi: 10.1093/pcp/pcd042
Chen, Q. F., Xu, L., Tan, W. J., Chen, L., Qi, H., Xie, L. J., et al. (2015). Disruption of the Arabidopsis defense regulator genes SAG101, EDS1, and PAD4 confers enhanced freezing tolerance. Mol. Plant. 8, 1536–1549. doi: 10.1016/j.molp.2015.06.009
Dillen, W., and Buysens, S. (1989). A simple technique to overcome vitrification in Gypsophila paniculata L. Plant Cell Tissue Organ Cult. 19, 181–188. doi: 10.1007/bf00043345
Eckardt, N. A. (2001). Arabidopsis genome conference 2000. How a small weed changed the world. Plant Cell 13, 5–10. doi: 10.2307/3871149
Goleniowski, M., and Trippi, V. S. (1999). Effect of growth medium composition on psilostachyinolides and altamisine production. Plant Cell Tissue Organ Cult. 56, 215–218. doi: 10.1023/a:1006273927530
Hakkaart, F. A., and Versluijs, J. M. A. (1983). Some factors affecting glassiness in carnation meristem tip cultures. Netherlands J. Plant Pathol. 89, 47–53. doi: 10.1007/BF01974443
Hendrix, D., Mauney, J., Kimball, B., Lewin, K., Nagy, J., and Hendrey, G. (1994). Influence of elevated CO2 and mild water stress on nonstructural carbohydrates in field-grown cotton tissues. Agric. For. Meteorol. 70, 153–162. doi: 10.1016/0168-1923(94)90054-X
Jackson, M. B., Belcher, A. R., and Brain, P. (1994). “Measuring shortcomings in tissue culture aeration and their consequences for explant development,” in Physiology, Growth and Development of Plants in Culture, eds P. J. Lumsden, J. R. Nicholas, and Davies (Berlin: Springer), 191–203. doi: 10.1007/978-94-011-0790-7-18
Joo, J. H., Yoo, H. J., Hwang, I., Lee, J. S., Nam, K. H., and Yun, S. B. (2005). Auxin-induced reactive oxygen species production requires the activation of phosphatidylinositol 3-kinase. FEBS Lett. 579, 1243–1248. doi: 10.1016/j.febslet.2005.01.018
Kerstin, M., Linkies, A., Vreeburg, R. A. M., Fry, S. C., and Leubner-Metzger, K. L. (2009). In vivo cell wall loosening by hydroxyl radicals during cress seed germination and elongation growth. Plant Physiol. 150, 1855–1865. doi: 10.1104/pp.109.139204
Klerk, G. J. D., Hanecakova, J., and Jan, J. (2008). Effect of medium-pH and MES on adventitious root formation from stem disks of apple. Plant Cell Tissue Organ Cult. 95, 285–292. doi: 10.1007/s11240-008-9442-5
Kristiansen, K., Rnstrup, H., and Brandt, K. (1999). In vitro PPFD and media composition affect both in and ex vitro performance of alstroemeria butterfly-hybrids. Plant Cell Tissue Organ Cult. 56, 145–153. doi: 10.1023/a:1006208119297
Lager, I., Andreasson, O., Dunbar, T. L., Andreasson, E., Escobar, M. A., Rasmusson, A. G., et al. (2010). Changes in external pH rapidly alter plant gene expression and modulate auxin and elicitor responses. Plant Cell Environ. 33, 1513–1528. doi: 10.1111/j.1365-3040.2010.02161.x
Leifert, C., Pryce, S., Lumsden, P. J., and Waites, W. M. (1992). Effect of medium acidity on growth and rooting of different plant species growing in vitro. Plant Cell Tissue Organ Cult. 30, 171–179. doi: 10.1007/bf00040019
Low, P. S., and Merida, J. R. (2010). The oxidative burst in plant defense: function and signal transduction. Physiol. Plant. 96, 533–542. doi: 10.1111/j.1399-3054.1996.tb00469.x
Mansfield, T. A., and Majernik, O. (1970). Can stomata play a part in protecting plants against air pollutants? Environ. Pollut. 1, 149–154. doi: 10.1016/0013-9327(70)90015-7
Mehdy, M. C. (1994). Active oxygen species in plant defense against pathogens. Plant Physiol. 105, 467–472.
Meinke, D. W., Cherry, J. M., Dean, C., Rounsley, S. D., and Koornneef, M. (1998). Arabidopsis thaliana: a model plant for genome analysis. Science 282, 662–682. doi: 10.1126/science.282.5389.662
Morard, P., and Henry, M. (1998). Optimization of the mineral composition of in vitro culture media. J. Plant Nutr. 21, 1565–1576. doi: 10.1080/01904169809365504
Mroginski, L. A., Rouvier, S. M., Fabisik, J. C., Levit, M., Marassi, M. A., Sansberro, P. A., et al. (1999). Effect of medium composition and light supply on in vitro shoot proliferation in Ilex paraguariensis (Aquifoloaceae). J. Plant Nutr. 22, 359–368. doi: 10.1080/01904169909365633
Msogoya, T. J., Maerere, A. P., Nzogela, Y., and Kusolwa, P. M. (2008). Changes in acidity of plant growth media during heat sterilization. J. Appl. Biosci. 10, 488–490.
Owen, H. R., Wengerd, D., and Miller, A. R. (1991). Culture medium pH is influenced by basal medium, carbohydrate source, gelling agent, activated charcoal, and medium storage method. Plant Cell Rep. 10, 583–586.
Potikha, T. S., and Levine, A. (1999). The involvement of hydrogen peroxide in the differentiation of secondary walls in cotton fibers. Plant Physiol. 119, 849–858. doi: 10.1104/pp.119.3.849
Qu, Y., Liu, S., Bao, W., Xue, X., Ma, Z., Yokawa, K., et al. (2017). Expression of root genes in Arabidopsis seedlings grown by standard and improved growing methods. Int. J. Mol. Sci. 18:951. doi: 10.3390/ijms18050951
Ramage, C. M., and Williams, R. R. (2002). Mineral nutrition and plant morphogenesis. Vitro Cell Dev. Biol. Plant 38, 116–124. doi: 10.1079/IVP2002269
Schopfer, P. (2001). Hydroxyl radical-induced cell-wall loosening in vitro and in vivo: implications for the control of elongation growth. Plant J. 28, 679–688. doi: 10.1046/j.1365-313x.2001.01187.x
Tsuro, M., Koda, M., and Inoue, M. (2000). Efficient plant regeneration from multiple shoots formed in the leaf-derived callus of Lavandula vera, using the “open culture system”. Sci. Hortic. 86, 81–88. doi: 10.1016/s0304-4238(00)00140-0
Xin, Z., and Zhen, S. (2007). EasyGO: gene ontology-based annotation and functional enrichment analysis tool for agronomical species. BMC Genomics 8:246. doi: 10.1186/1471-2164-8-246
Xu, W., Ding, G., Yokawa, K., Baluška, F., Li, Q. F., Liu, Y., et al. (2013). An improved agar-plate method for studying root growth and response of Arabidopsis thaliana. Sci. Rep. 3:1273. doi: 10.1038/srep01273
Yokawa, K., Fasano, R., Kagenishi, T., and Baluška, F. (2014). Light as stress factor to plant roots - case of root halotropism. Front. Plant Sci. 5:718. doi: 10.3389/fpls.2014.00718
Yokawa, K., Kagenishi, T., and Baluška, F. (2013). Root photomorphogenesis in laboratory-maintained Arabidopsis seedlings. Trends Plant Sci. 18, 117–119. doi: 10.1016/j.tplants.2013.01.002
Keywords: Arabidopsis, transcriptome analysis, gas exchange, abiotic stress, sterile culture
Citation: Xu L, Li S, Shabala S, Jian T and Zhang W (2019) Plants Grown in Parafilm-Wrapped Petri Dishes Are Stressed and Possess Altered Gene Expression Profile. Front. Plant Sci. 10:637. doi: 10.3389/fpls.2019.00637
Received: 29 December 2018; Accepted: 29 April 2019;
Published: 15 May 2019.
Edited by:
Patrick H. Masson, University of Wisconsin-Madison, United StatesReviewed by:
Frantisek Baluska, University of Bonn, GermanyJohn Sedbrook, Illinois State University, United States
Copyright © 2019 Xu, Li, Shabala, Jian and Zhang. This is an open-access article distributed under the terms of the Creative Commons Attribution License (CC BY). The use, distribution or reproduction in other forums is permitted, provided the original author(s) and the copyright owner(s) are credited and that the original publication in this journal is cited, in accordance with accepted academic practice. No use, distribution or reproduction is permitted which does not comply with these terms.
*Correspondence: Wenying Zhang, d3l6aGFuZ0B5YW5ndHpldS5lZHUuY24=
†These authors have contributed equally to this work