- 1Department of Biology, Northeastern University, Boston, MA, United States
- 2Department of Chemical Engineering, Northeastern University, Boston, MA, United States
- 3Department of Bioengineering, Northeastern University, Boston, MA, United States
- 4Department of Chemistry and Chemical Biology, Northeastern University, Boston, MA, United States
The Catharanthus roseus plant is the exclusive source of the valuable anticancer terpenoid indole alkaloids, vinblastine (VB) and vincristine (VC). The recent availability of transcriptome and genome resources for C. roseus necessitates a fast and reliable method for studying gene function. In this study, we developed an Agrobacterium-mediated transient expression method to enable the functional study of genes rapidly in planta, conserving the compartmentalization observed in the VB and VC pathway. We focused on (1) improving the transformation method (syringe versus vacuum agroinfiltration) and cultivation conditions (seedling age, Agrobacterium density, and time point of maximum transgene expression), (2) improving transformation efficiency through the constitutive expression of the virulence genes and suppressing RNA silencing mechanisms, and (3) improving the vector design by incorporating introns, quantitative and qualitative reporter genes (luciferase and GUS genes), and accounting for transformation heterogeneity across the tissue using an internal control. Of all the parameters tested, vacuum infiltration of young seedlings (10-day-old, harvested 3 days post-infection) resulted in the strongest increase in transgene expression, at 18 – 57 fold higher than either vacuum or syringe infiltration of other seedling ages. Endowing the A. tumefaciens strain with the mutated VirGN54D or silencing suppressors within the same plasmid as the reporter gene further increased expression by 2 – 10 fold. For accurate measurement of promoter transactivation or activity, we included an internal control to normalize the differences in plant mass and transformation efficiency. Including the normalization gene (Renilla luciferase) on the same plasmid as the reporter gene (firefly luciferase) consistently yielded a high signal and a high correlation between RLUC and FLUC. As proof of principle, we applied this approach to investigate the regulation of the CroSTR1 promoter with the well-known activator ORCA3 and repressor ZCT1. Our method demonstrated the quantitative assessment of both the activation and repression of promoter activity in C. roseus. Our efficient Agrobacterium-mediated seedling infiltration (EASI) protocol allows highly efficient, reproducible, and homogenous transformation of C. roseus cotyledons and provides a timely tool for the community to rapidly assess the function of genes in planta, particularly for investigating how transcription factors regulate terpenoid indole alkaloid biosynthesis.
Introduction
Plants produce a vast array of specialized metabolites that enable adaptation to environmental challenges. For instance, plants produce compounds that can attract pollinators or deter pathogens and herbivores (Weng et al., 2012). For humans, these specialized metabolites are an important source of useful chemicals, particularly medicines (Balunas and Kinghorn, 2005; Wurtzel and Kutchan, 2016).
During the past five years, the availability of transcriptome and genome resources for medicinal plants has grown substantially; examples include the Medicinal Plant Genomics Resource1, PhytoMetaSyn2, and 1000 Plants Initiative3. In addition, advances in metabolomic technologies now enable single-cell and spatial metabolite profiling (Wurtzel and Kutchan, 2016). These approaches are accelerating the identification of candidate genes important for the biosynthesis of these medicinal compounds and for the regulation of their biosynthetic genes. There is a timely need for the functional study of this growing list of genes. For this reason, we developed a fast and reliable method to study gene function in the medicinal plant, Catharanthus roseus (L.) G. Don (van der Heijden et al., 2004; Facchini and De Luca, 2008).
Catharanthus roseus produces two potent anticancer terpenoid indole alkaloids (TIA), vinblastine and vincristine, which were serendipitously discovered in the 1950s while investigating the plant’s reputation for the treatment of diabetes (Noble, 1990). Since the anticancer TIAs are only found in trace amounts in C. roseus leaves (Andersson et al., 1996), they are the target of production by biotechnological means (van der Heijden et al., 2004). The first comprehensive transcriptome resource for C. roseus was released in 2012 (Góngora-Castillo et al., 2012) and the first partial genome in 2015 (Kellner et al., 2015). The availability of these and other resources enabled the pathway for catharanthine and vindoline biosynthesis, the two precursors to the anticancer compounds vinblastine and vincristine, to be fully elucidated (Qu et al., 2015, 2018; Caputi et al., 2018). The pathway involves 28 known enzymes distributed across several cell types in the leaf including internal phloem-associated parenchyma cells, epidermis, laticer/idioblasts, and different subcellular compartments (reviewed in Pan et al., 2016). Multiple transcription factors and transporter genes involved in trafficking of the pathway intermediates across compartments have also been identified (Menke et al., 1999; Pasquali et al., 1999; van der Fits and Memelink, 2000; Chatel et al., 2003; Pauw et al., 2004; Suttipanta et al., 2011; Zhang et al., 2011; Yu and De Luca, 2013; Van Moerkercke et al., 2015, 2016; Paul et al., 2017; Payne et al., 2017; Patra et al., 2018). Previous attempts to increase alkaloid production by expressing single pathway genes or altering the expression of transcription factors have resulted in limited success, suggesting the importance of investigating the complex regulation underlying their biosynthesis (Rizvi et al., 2016; Schweizer et al., 2018; Sun et al., 2018; reviewed in Pan et al., 2016). Tools are needed to rapidly assess the regulation of catharanthine and vindoline biosynthesis in appropriate tissue type, toward engineering increased production in the future.
Methods to evaluate gene function are limited in C. roseus compared to Arabidopsis thaliana, tobacco, and other model plants. As in other plants, development of transgenic plants via regeneration from tissue culture is time-consuming, expensive, and genotype-dependent (Zhao et al., 2017). Few reports exist regarding the development of transgenic plants in C. roseus (Pan et al., 2012; Wang et al., 2012). In C. roseus, the development of transgenic hairy roots is commonly pursued because an efficient and reliable method exists (Choi et al., 2004; Rizvi et al., 2015). However, the disadvantage of using hairy roots is that many genes of the TIA pathway are regulated differently in roots than leaves (reviewed in Pan et al., 2016), and hairy roots require 4–6 months for development. Therefore, the generation of hairy root lines is unsuitable for quick screening of leaf-specific candidate genes.
For evaluating gene function more rapidly, model plants like tobacco have been used for promoter transactivation studies of C. roseus genes (Van Moerkercke et al., 2015, 2016; Paul et al., 2017; Patra et al., 2018; Schweizer et al., 2018). However, the vindoline pathway is exclusively found in C. roseus. Therefore, the regulation of the vindoline pathway, including monitoring expression and metabolite levels, needs to be studied in C. roseus tissue. Initial attempts to transiently transform C. roseus were successfully made in calli, cell suspensions, and protoplasts using methods such as biolistic transformation, Agrobacterium, or electroporation (van der Fits and Memelink, 1997; Guirimand et al., 2009; Suttipanta et al., 2011). However, these systems lack the specialized cells or subcellular compartmentalization observed in cotyledons and leaves where vindoline biosynthesis predominantly occurs (Aerts et al., 1994; Pan et al., 2016). Other drawbacks of biolistic or protoplast transformations include low transformation rates, the requirement of specialized equipment, and extensive optimization. Subsequent transient transformation attempts in C. roseus have focused on Agrobacterium-mediated transient transformation of detached leaves (Di Fiore et al., 2004), petals (Van Moerkercke et al., 2016, adapted from Verweij et al., 2008), and seedlings (Makhzoum et al., 2011; Weaver et al., 2014). However, we observed low transformation efficiency in mature tissue (unpublished data). Additionally, the petal transient transformation method requires flowers harvested from plants grown under special photoperiods to induce flowering year-round in a greenhouse. Of these tissues, seedlings are ideal because they have intact plant tissue structure, are obtained in a matter of days, and can be cultivated in tissue culture boxes in the laboratory.
In this study, we developed a transient expression method in C. roseus seedlings to enable functional study of genes rapidly in planta, conserving the compartmentalization observed in the vindoline pathway. We completely revised our Agrobacterium-mediated transient expression method (Weaver et al., 2014) by focusing on three main aspects: (1) improving throughput (syringe versus vacuum infiltration) and cultivation conditions (seedling age, Agrobacterium density, and time point of maximum transgene expression), (2) improving transformation efficiency through the constitutive expression of the virulence genes (via the constitutively active VirG) and suppressing RNA silencing mechanisms (via viral silencing suppressors), and (3) improving the vector design by incorporating introns, quantitative and qualitative reporter genes (luciferase and GUS genes), and accounting for transformation heterogeneity across the tissue using an internal control.
We developed this quantitative transient expression assay for studying the regulation underlying TIA biosynthesis. For this application, the firefly and Renilla luciferase reporter genes were selected due to their sensitivity, dynamic range, and time-resolution for the accurate measurement of transcription factor and promoter activity. As proof of principle, we applied our transient expression assay to demonstrate the transactivation of the Strictosidine synthase (STR1) promoter by the Octadecanoid-Responsive Catharanthus AP2 (ORCA3) transcriptional activator (van der Fits and Memelink, 2001) and by a repressor from the Zinc finger protein family of Catharanthus (ZCT1) (Pauw et al., 2004). Overall, we developed a reproducible, robust, and highly efficient transient expression method, known as efficient Agrobacterium-mediated seedling infiltration (EASI) protocol, for studying gene function in C. roseus seedlings. This low-cost method requires no specialized equipment and offers high transformation rates, high throughput, and rapid generation of results.
Materials and Methods
Preparation of C. roseus Seedlings
Catharanthus roseus seeds were surface-sterilized by submerging 0.8 g of seeds (Little Bright Eye, NEseeds) in 20 ml of 5% PPM (Plant Preservative Mixture, Caisson Laboratory) for 16 h at 25°C in the dark (day 0). After discarding the 5% PPM, the seeds were spread evenly on top of full-strength Gamborg’s media (3.1 g/L of Gamborg’s basal salts, 1 ml/L of Gamborg’s vitamins (1000×), 6% micropropagation agar type 1, Phytotechnology Laboratory), inside a sterile MagentaTM box (Sigma) (day 1). Seeds were kept in the dark at 25°C for 7 days when most seeds germinated and were at the hook stage (i.e., seedlings had the cotyledons pointing downward and hypocotyl was around 1 cm long). On day 8, the seedlings in the Magenta boxes were then transferred to 16 h of light (Erligpowht 45W LED Red Blue Lights) and 8 h of dark at room temperature for 2 days (except in section “Optimum Transgene Expression Occurs With Vacuum Infiltration of Young Seedlings” with the optimization of seedling age). On day 10, seedlings were transferred to complete darkness for 16 h before Agrobacterium-infiltration to increase the competency for transient transformation (Lee and Yang, 2006). We counted seedlings age from the day they were spread on Gamborg’s media to start germination.
Agroinfiltration of C. roseus Seedlings
The transient expression assay utilized the non-tumorigenic Agrobacterium tumefaciens GV3101 (pMP90) strain (Koncz et al., 1992). Agrobacteria were transformed with expression plasmids by electroporation and plated on LB agar media with gentamicin (10 μg/ml) and appropriate antibiotics for selection of expression vectors. After verification by colony PCR, a single colony was cultivated in antibiotic-containing LB media overnight at 26°C and 250 RPM. Glycerol stocks were prepared from freshly growing liquid cultures and stored at −80°C for long-term storage.
Four days before agroinfiltration, Agrobacteria from the glycerol stocks were freshly streaked onto LB agar plates with gentamicin (10 μg/ml) and appropriate antibiotics and incubated 2–3 days at 26°C. A fresh single colony from the plate was used to inoculate 10 ml of LB liquid medium containing appropriate antibiotics and incubated overnight at 26°C at 250 RPM. To induce the virulence genes, Agrobacteria were centrifuged (7000×g for 6 min), resuspended in 10 ml of Agrobacterium minimal media with appropriate antibiotics and 100 μM acetosyringone (AS), and incubated for 3 h at 26°C and 250 RPM. The Agrobacterium minimal media consists of 5 g/L glucose, 1 g/L NH4Cl, 0.3 g/L MgSO4, 0.150 g/L KCl, 0.010 g/L CaCl2, 0.0025 g/L FeSO4, 3 g/L K2HPO4, 1 g/L NaH2PO4, and 0.195 g/L MES, buffered at pH = 5.5 (Agrobacterium Minimal Medium, bioWORLD).
In preparation for agroinfiltration, Agrobacteria cells were centrifuged (7000×g for 6 min) and resuspended to the desired OD at 600 nm in infiltration medium (Lee and Yang, 2006; 10 mM MgSO4, 10 mM MES, pH 5.6) freshly supplemented with 200 μM AS. Agroinfiltration was carried out with 10, 14, and 21-day old seedlings using either a needless syringe or under vacuum. For vacuum infiltration, 0.01% Silwet® L-77 was added to the infiltration media.
Syringe Infiltration
A needleless 5 ml syringe was used to infiltrate Agrobacterium into the abaxial side of individual cotyledons in place. This method could not be used with 10-day-old seedlings as the cotyledons were smaller than the syringe. After syringe infiltration, seedlings were kept in Magenta boxes containing Gamborg’s media and maintained in the dark for 48 h and then transferred back to a 16 h/8 h photoperiod for 24 h.
Vacuum Infiltration
Seedlings (25–30) were gently transferred from the Magenta box to a 100 ml glass bottle containing 50 ml of infiltration media. Uncapped bottles were placed inside a vacuum desiccator, and vacuum was applied twice for 4 min, with an interval of no vacuum for 4 min. After vacuum infiltration, seedlings were transferred to Magenta boxes containing fresh Gamborg’s media, gently planting the roots into the media to help seedlings stand up. Seedlings were maintained in the dark for 48 h and then transferred to a 16 h/8 h photoperiod for 24 h (typically) or the desired time.
C. roseus Developmental Stages
We evaluated the Agrobacterium-mediated transformation of C. roseus seedlings at three early developmental stages to minimize the time required to conduct the transient expression protocol. The developmental stages consisted of the following: (1) 10-day-old seedlings (7 days in the dark followed by 3 days in 16 h/8 h photoperiod) with small, open, and green cotyledons, (2) 14-day-old seedlings (7 days in the dark followed by 7 days in 16 h/8 h photoperiod) with bigger cotyledons but no visible true leaves, and (3) 21-day-old seedlings (7 days in the dark followed by 14 days in 16 h/8 h photoperiod) with the first set of true leaves at approximately 4 mm in length.
Transient Transformation Evaluation
We evaluated the Agrobacterium-mediated transformation of C. roseus seedlings both qualitatively using the GUS gene and quantitatively using the luciferase gene. For qualitative evaluation, seedlings were transiently transformed with a plasmid encoding an intron-containing β-glucuronidase gene (GUS) under the control of the cauliflower mosaic virus 2X 35S promoter (CaMV2X35S, Supplementary Figure S1 and Figure 6) and visualized with GUS staining.
For quantitative evaluation, we measured the bioluminescence of seedlings transformed with the intron-containing firefly luciferase gene (FLUC-I) under the control of different promoters and the intron-containing Renilla luciferase gene (RLUC-I) under the control of the Agrobacterium tumefaciens promoter (AtuMAS, Figure 1). The FLUC signal was either normalized to the RLUC signal or protein content as quantified by the Bradford protein assay (Bio-Rad). For each experiment, one condition served as reference and its average normalized FLUC activity was set to 100%. To calculate the relative % activity, the normalized FLUC activity of each sample was divided by the average normalized FLUC activity of the reference. Calculating the relative % activity facilitated the statistical analysis of pooled data from three independent assays. For each condition of the assay, 10 biological repeats were conducted (if not otherwise specified); each biological repeat consisted of two 10-day-old seedlings or a single 17- and 24-day old seedlings. Statistical analysis of experimental data was carried out using JMP®, Version 13 (SAS Institute Inc., Cary, NC, United States, 1989–2007).
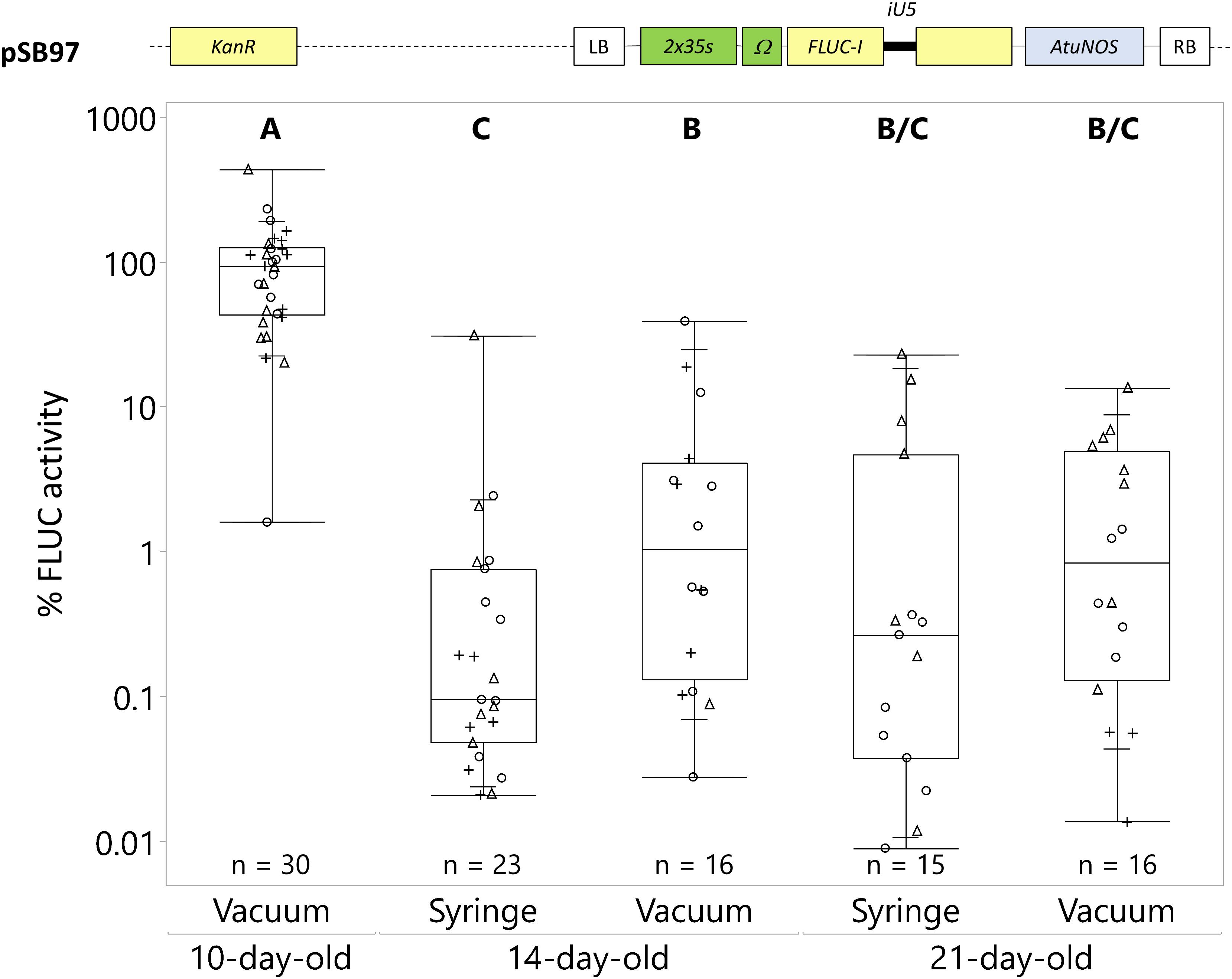
Figure 1. Catharanthus roseus seedling age and infection method significantly affect transient transformation efficiency. C. roseus seedlings at 10, 14, and 21-day-old were infiltrated with A. tumefaciens GV3101 (pMP90) containing the FLUC-I reporter (plasmid pSB97) at OD600 = 0.2, using either syringe or vacuum infiltration. Samples were taken at 3 dpi. FLUC activity was normalized to protein content. The average FLUC activity to protein content of 10-day-old vacuum infiltrated seedlings was used as reference (set to 100%) to determine the relative FLUC activity of each sample. The experiment was carried out in three independent assays (represented by the +, ○, Δ symbols). The box plot horizontal line shows the median, ends of the boxes show interquartile range, small marks show the 10th and 90th quantile and whiskers show lowest/highest data point. Different letters indicate values that are significantly different (Tukey-Kramer HSD test on log-transformed data, p < 0.05).
GUS Staining and Imaging
Plant tissue was stained with 5-bromo-4-chloro-3-indolyl glucuronide (X-Gluc) by incubating tissue in GUS staining solution (50 mM Na3PO4 buffer at pH 7.2, 1.0 mM EDTA, 0.1 mM K3Fe(CN)6, 0.1 mM K4Fe(CN)6, 20% (v/v) methanol, 700 μg/ml X-Gluc) at 25°C for 24 h. Plant tissue was then washed in 70% EtOH until the chlorophyll was completely removed. Images were acquired with a Nikon SMZ800 microscope (Nikon, Tokyo, Japan) and SPOT Insight CCD 2.0 Mp Color camera (Diagnostic Instruments, Inc., Sterling Heights, MI, United States).
Protein Extraction
Transformed seedlings were harvested, flash-frozen in liquid nitrogen, and kept at −80°C until protein extraction. Frozen tissue was pulverized using 3 mm beads in a bead beater at 4 m/s for 20 s (MP Biomedicals), transferred to ice, and 130 μl of extraction buffer was added (50 mM Na3PO4 at pH7.2, 1.0 mM EDTA) with 10 mM β-mercaptoethanol and 0.1% Triton-X 100 added fresh. After vortexing and centrifugation (21,000×g for 2 min), approximately 110 μl of supernatant was transferred to a 96-well plate and maintained on ice.
Firefly and Renilla Luciferase Assay
FLUC activity was analyzed using the Luc-PairTM Firefly Luciferase HT Assay while both FLUC and RLUC activity was analyzed using the Luc-PairTM Duo-Luciferase HT Assay Kit (Genecopeia). The plant protein extract (20 μl) was mixed with 20 μl of the appropriate reaction solution from the corresponding kit at room temperature. Samples were dispensed in every other well of a 96-well white plates (CorningTM 3992) to avoid cross-talk between wells. Luminescence was read with a plate reader for 2 s to acquire relative light units (RLU).
Protein Quantification
The Bradford assay (BioRad) was used to quantify protein content. A protein standard curve from 62.5 to 3000 ng/μl of bovine serum albumin was prepared and a second order polynomial equation was used to fit the data. Samples were diluted 1:2 to ensure the values were within range of the calibration curve.
Vector Construction
During vector construction, all PCR reactions required using Phusion High-Fidelity DNA Polymerase (New England BioLabs) to avoid introducing errors during PCR, and all primers used in this study are listed in Supplementary Table S1.
Vector Construction With Golden Gate Based Modular Cloning (MoClo)
All final vectors were constructed using Golden Gate based modular cloning (MoClo, Weber et al., 2011). MoClo uses standardized genetic elements (parts in L0 vectors) to assemble these into transcriptional units (L1 vectors). Multiple transcriptional units from L1 vectors can then be assembled in the final L2 vectors. Backbone vectors and Level 0 parts were taken from the Golden Gate MoClo Plant Parts Kit and Golden Gate MoClo Plant Toolkit (Weber et al., 2011; Engler et al., 2014) if not otherwise specified. Higher level vectors were assembled as described in Weber et al. (2011) to obtain the final vectors needed in this study.
The construction of novel L0 parts is described in Supplementary Materials (Methods). All new promoters and coding sequences were cloned into the L0 acceptor vectors as described in Weber et al. (2011). All newly constructed L0 MoClo vectors and final L2 vectors are deposited at Addgene (Addgene IDs 123180-123200).
The HcPro sequence (GB0067) was a gift from Dr. Diego Orzaez (Addgene plasmid # 68196, Sarrion-Perdigones et al., 2013). The vector is MoClo compatible.
Construction of a VirGN54D Containing L2 Acceptor Vector
pAGM4723 (Weber et al., 2011) was modified by inserting the VirGN54D gene using NEBuilder (New England BioLabs). An 1153 bp fragment containing the VirGN54D gene was amplified from pAD1289 (Hansen et al., 1994) with primers 1 and 2. The linearized pAGM4723 vector was obtained by amplification with primers 3 and 4. The VirGN54D fragment and linearized vector were assembled as described in the NEBuilder manual. The constructed vector, containing VirGN54D in the backbone, was named pSB90 – pAGM4723_VirGN54D and was deposited at Addgene (Addgene ID 123187).
Results
Optimum Transgene Expression Occurs With Vacuum Infiltration of Young Seedlings
In preliminary experiments, we assessed the amenability of C. roseus leaves to Agrobacterium-mediated transformation and observed high transformation with seedlings but decreased transformation with increasing leaf age (data not shown). Since seedlings exhibited the highest reporter gene expression and could be produced in a short period of time, we tested both syringe and vacuum infiltration on young seedlings (10, 14, and 21-day-old). Cotyledons of 10-day-old seedlings were too small to carry out syringe infiltration; therefore only the effect of vacuum infiltration was evaluated at this seedling age.
The highest transformation efficiency occurred in 10-day-old seedlings using vacuum infiltration, resulting in an 18–57 fold higher FLUC activity compared to any other condition (Figure 1). Both transformation methods produced significantly lower and less efficient transformations in 14 and 21-day-old seedlings. The transformation efficiency was not statistically different between vacuum infiltration and syringe infiltration in 14 and 21-day-old seedlings. Overall, vacuum infiltration of 10-day-old seedlings consistently resulted in high transformation efficiency with minimal tissue damage, unlike syringe infiltration. Also, vacuum infiltration required less tissue handling. Therefore, vacuum infiltration of 10-day-old seedlings was used for the optimization of other factors involved in the transient transformation process.
High Transgene Expression Occurs at Agrobacterium Density of OD600 = 0.2 and 0.4
Agrobacterium-mediated transient transformation protocols in diverse plant species use bacterial density (OD600) in the infection medium from 0.01 to 2 (Wroblewski et al., 2005; Cazzonelli and Velten, 2006; Marion et al., 2008; Hosein et al., 2012). While low Agrobacterium densities yield low transgene expression, high densities (>OD600 = 1.0) can result in lower efficiency (Hosein et al., 2012), tissue yellowing, or wilting (Wroblewski et al., 2005). An optimum bacterial density yields higher transgene expression (Wroblewski et al., 2005; Marion et al., 2008) without tissue damage. Therefore, we evaluated the transient transformation efficiency of 10-day-old C. roseus seedlings using OD600 = 0.02, 0.2, and 0.4 during vacuum infiltration.
We found that the bacterial density had a significant effect on C. roseus transformation efficiency (Figure 2). Transformation efficiency increased directly with bacterial density. The average transformation efficiency at OD600 = 0.02, 0.2 (reference), and 0.4 was 24, 100, and 177%, respectively (Figure 2). Even though OD600 = 0.4 yielded the highest transformation efficiency with little tissue damage, we used OD600 = 0.2 in subsequent vacuum infiltrations to accommodate the combination of two A. tumefaciens strains carrying effector and reporter plasmids (see section “Dual-Luciferase Reporter System Is the Preferred Normalization Strategy for Reducing Inter-Experimental Variability” and “Transactivation of the C. roseus STR1 Promoter With ORCA3 and ZCT1”), which in total resulted in the highest bacterial concentration tested, OD600 = 0.4.
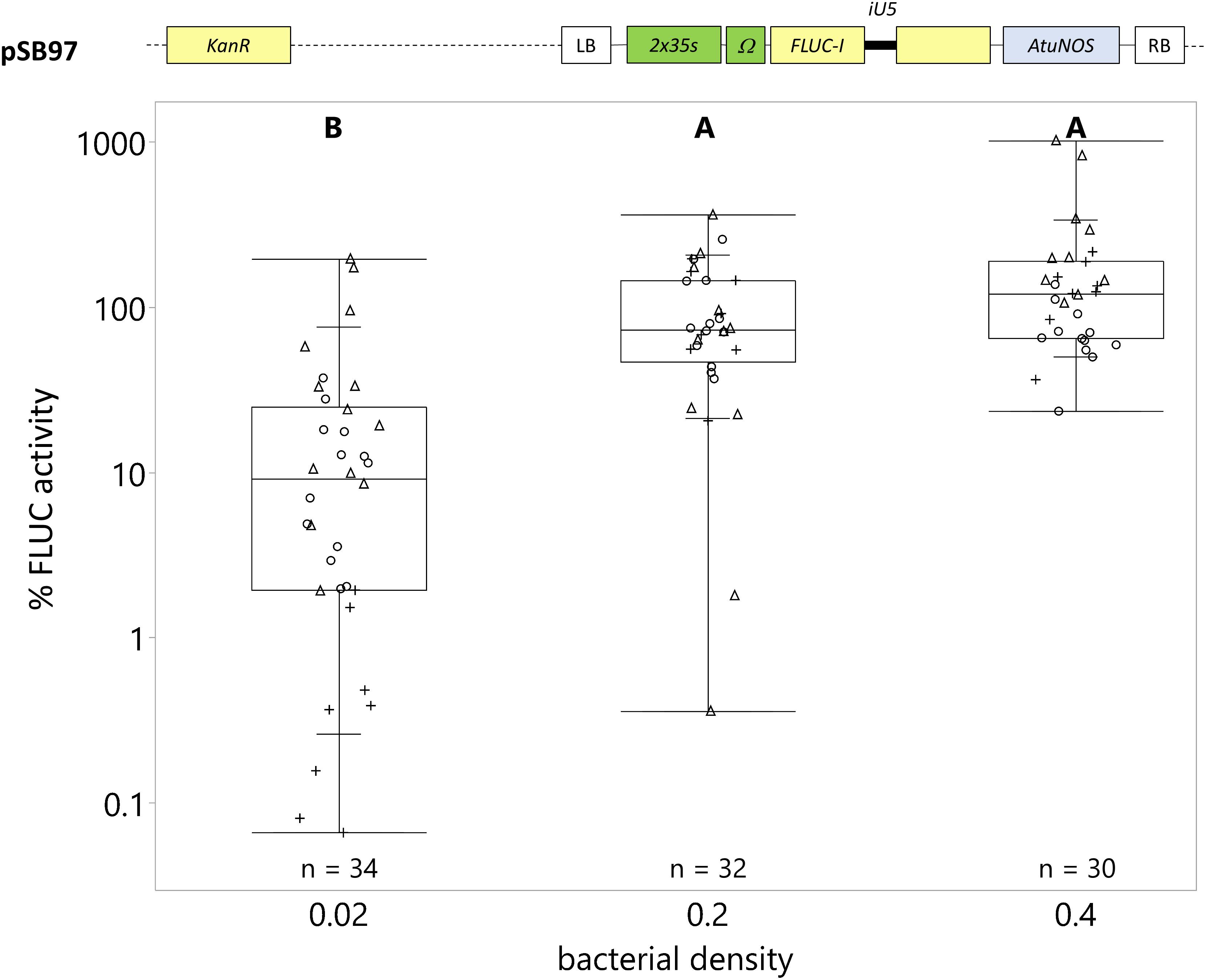
Figure 2. Transgene expression is directly correlated with bacterial density during vacuum infiltration. C. roseus seedlings (10-day-old) were vacuum infiltrated with A. tumefaciens containing the FLUC-I reporter (plasmid pSB97) at OD600 = 0.02, 0.2, and 0.4. Samples were taken at 3 dpi. FLUC activity was normalized to protein content. The average FLUC activity to protein content of seedlings infected at OD600 = 0.2 was used as reference (set to 100%) to determine the relative FLUC activity of each sample. The experiment was carried out in three independent assays (represented by the +, ○, Δ symbols). The box plot horizontal line shows the median, ends of the boxes show interquartile range, small marks show the 10th and 90th quantile and whiskers show lowest/highest data point. Different letters indicate values that are significantly different (Tukey-Kramer HSD test on log-transformed data, p < 0.05).
Optimum Transgene Expression Occurs 3 Days Post-infection
Transgene expression is commonly evaluated 2–4 days post-infection (dpi) with Agrobacterium (Sparkes et al., 2006; Marion et al., 2008; Wu et al., 2014; Lu et al., 2017). Longer cultivation periods after infection may favor the accumulation of proteins or metabolites of interest. Therefore, we evaluated FLUC activity in seedlings 1, 2, 3, 6, and 9 dpi to obtain a time course of expression.
The strongest FLUC activity was detected 3 dpi (Figure 3). At 1 dpi, FLUC activity was detectable at low levels, at 0.35% of the average FLUC activity at 3 dpi (reference, 100%). The average FLUC activity increased at 2 and 3 dpi (65 and 100%, respectively). At 6 and 9 dpi, the FLUC activity decreased to 40 and 21%, respectively. Therefore, we evaluated all further experiments at 3 dpi, unless otherwise noted.
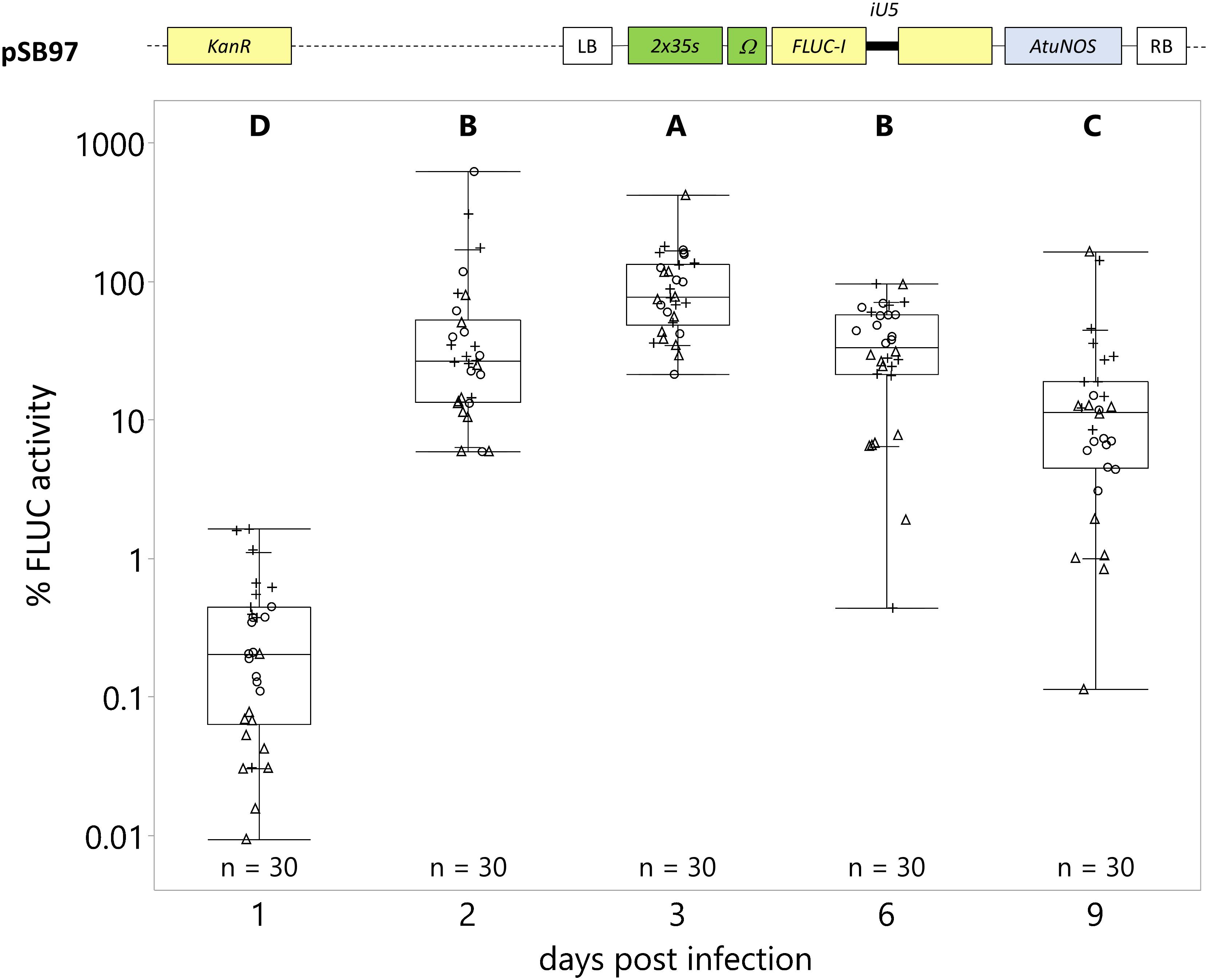
Figure 3. Strongest transgene expression is detected in seedlings 3 days post-infection (dpi). C. roseus seedlings (10-days-old) were vacuum infiltrated with A. tumefaciens containing the FLUC-I reporter (plasmid pSB97) at OD600 = 0.2. Samples were taken 1, 2, 3, 6, and 9 dpi. FLUC activity was normalized to protein content. The average FLUC activity to protein content of samples collected at 3 dpi was used as reference (set to 100%) to determine the relative FLUC activity of each sample. The experiment was carried out in three independent assays (represented by the +, ○, Δ symbols). The box plot horizontal line shows the median, ends of the boxes show interquartile range, small marks show the 10th and 90th quantile and whiskers show lowest/highest data point. Different letters indicate values that are significantly different (Tukey-Kramer HSD test on log-transformed data, p < 0.05).
Constitutive Expression of Virulence Genes Enhances Transgene Expression
In Agrobacterium, the virulence (Vir) region of the Ti-plasmid encodes genes essential for DNA transfer to the plant. Transcription of the Vir genes is regulated by VirA and VirG in response to phenolic inducer(s) produced by plants such as acetosyringone (AS). Specifically, phosphorylation of VirG by VirA results in the transcription of Vir genes. In the mutated VirGN54D, an asparagine is substituted to aspartic acid at position 54, resulting in the inducer-independent activity of VirG (Pazour et al., 1992). Generally, AS is included during the pre-induction and infection steps to drive the expression of the Vir genes. In this paper, the A. tumefaciens strain used (GV3101) contains the pMP90 plasmid, which encodes all the native virulence genes. In this experiment, we evaluated whether the presence of VirGN54D could either replace AS or work synergistically with AS to improve transient transformation efficiency. The VirGN54D was either encoded on the same plasmid (pSB96) or on a different plasmid as the reporter gene (pSB97 + pAD1289) and electroporated into the GV3101 (pMP90) strain.
Both VirGN54D and AS significantly increased C. roseus transformation efficiency (Figure 4). The addition of AS increased transformation efficiency when VirGN54D was either absent or on separate plasmids (absent and −AS = transformation efficiency of 37%; absent and +AS = 100% (reference); separate and −AS = 42%; separate and +AS = 141%; Figure 4). When encoded on the same plasmid as the reporter gene, VirGN54D with AS achieved the highest transformation efficiency (same and +AS = 220%; Figure 4). Therefore, we used VirGN54D in the backbone of the same plasmid as the reporter gene combined with AS addition in subsequent optimization experiments.
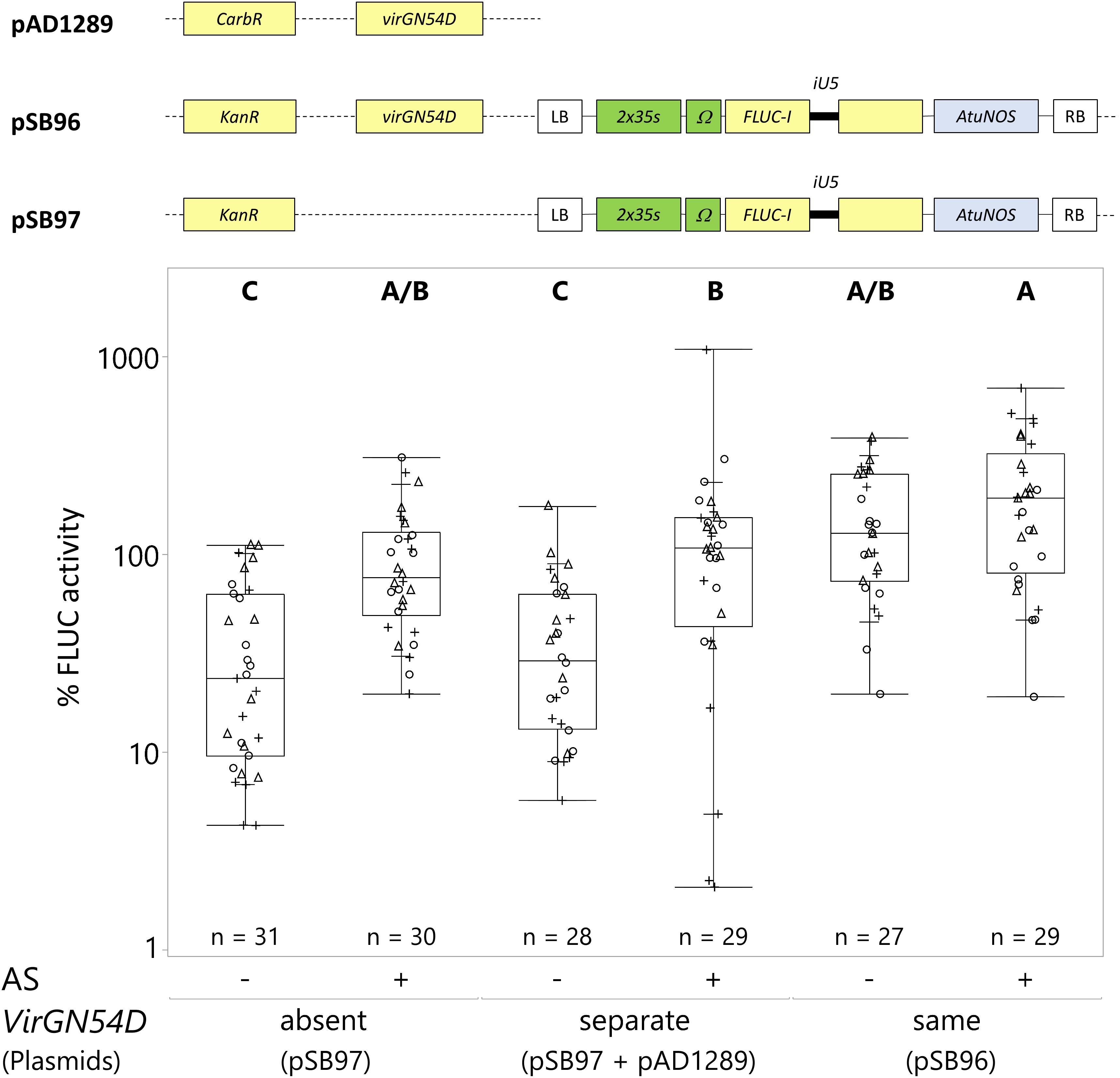
Figure 4. VirGN54D and acetosyringone synergistically improve C. roseus transient transformation. C. roseus seedlings (10-days-old) were vacuum infiltrated with one strain of A. tumefaciens at OD600 = 0.2, without (–) or with (+) AS. The A. tumefaciens strain contained either the reporter gene (pSB97) with no VirGN54D gene or with VirGN54D gene on a separate plasmid (pSB97+pAD1289), or with VirGN54D on the same plasmid as the FLUC-I reporter (pSB96). Samples were taken at 3 dpi. FLUC activity was normalized to protein content. The average FLUC activity to protein content of seedlings treated with AS but in the absence of VirGN54D was used as reference (set to 100%) to determine the relative FLUC activity of each sample. The experiment was carried out in three independent assays (represented by the +, ○, Δ symbols). The box plot horizontal line shows the median, ends of the boxes show interquartile range, small marks show the 10th and 90th quantile and whiskers show lowest/highest data point. Different letters indicate values that are significantly different (Tukey-Kramer HSD test on log-transformed data, p < 0.05).
Silencing Suppressors Enhance
Transgene Expression With Prolonged
Cultivation Post-infection
Silencing suppressors can disrupt the plant’s innate RNA silencing mechanisms and can therefore increase transgene expression levels over time in transient transformations (Pumplin and Voinnet, 2013). We evaluated the effect of the following three silencing suppressors on C. roseus transient transformation efficiency. The Tobacco etch potyvirus (TEV) helper-component proteinase (HcPro) was one of the first silencing suppressors discovered in plants (Anandalakshmi et al., 1998; Brigneti et al., 1998; Kasschau and Carrington, 1998). HcPro interferes with transgene-induced or virus-induced RNA silencing at the posttranscriptional level via modes that are not completely understood (reviewed in Voinnet, 2001). The Tomato bushy stunt virus (TBSV) suppressor of gene silencing, P19 (Silhavy et al., 2002), binds to small 21-nt RNAs, preventing their activity in AGO proteins and reducing the AGO1 protein accumulation through induction of miR168 (Várallyay et al., 2010). The V2 from Tomato yellow leaf curl virus (TYLCV) prevents new double-strand RNA synthesis by binding the RNA-DEPENDENT RNA POLYMERASE 6 (RDR6) cofactor SUPPRESSOR OF GENE SILENCING 3 (SGS3) (Zrachya et al., 2007; Glick et al., 2008).
In this study, we compared the effect of these silencing suppressors on transgene expression under Agrobacterium-mediated transient transformation after 3 dpi (optimum from section “Optimum Transgene Expression Occurs 3 Days Post-infection”) and 6 dpi, when the plant’s RNA silencing process is activated. Expression of the FLUC-I reporter gene in the presence of silencing suppressors (V2, HcPro, and P19) was compared against a no silencing control (tGFP-PLUS). At 3 dpi, only HcPro significantly increased FLUC activity (212%) relative to tGFP-PLUS (100%, Figure 5). At 6 dpi, transgene expression decreased in the absence of the silencing suppressors. For instance, the FLUC activity in the tGFP-PLUS control at 6 dpi was 38% of its expression at 3 dpi (consistent with our results in section “Optimum Transgene Expression Occurs 3 Days Post-infection”). HcPro and P19 strongly and significantly increased FLUC activity at 6 dpi. For instance, the FLUC activity was 8–11 fold higher compared to the tGFP-PLUS control at 6 dpi (HcPro = 310%; P19 = 446%). The silencing suppressor V2 did not significantly increase transgene expression at 3 or 6 dpi in our experiments and seems less suitable in the C. roseus system. Therefore, we recommend including HcPro and P19 silencing suppressor for applications requiring prolonged cultivation (6 dpi) post Agrobacterium infiltration.
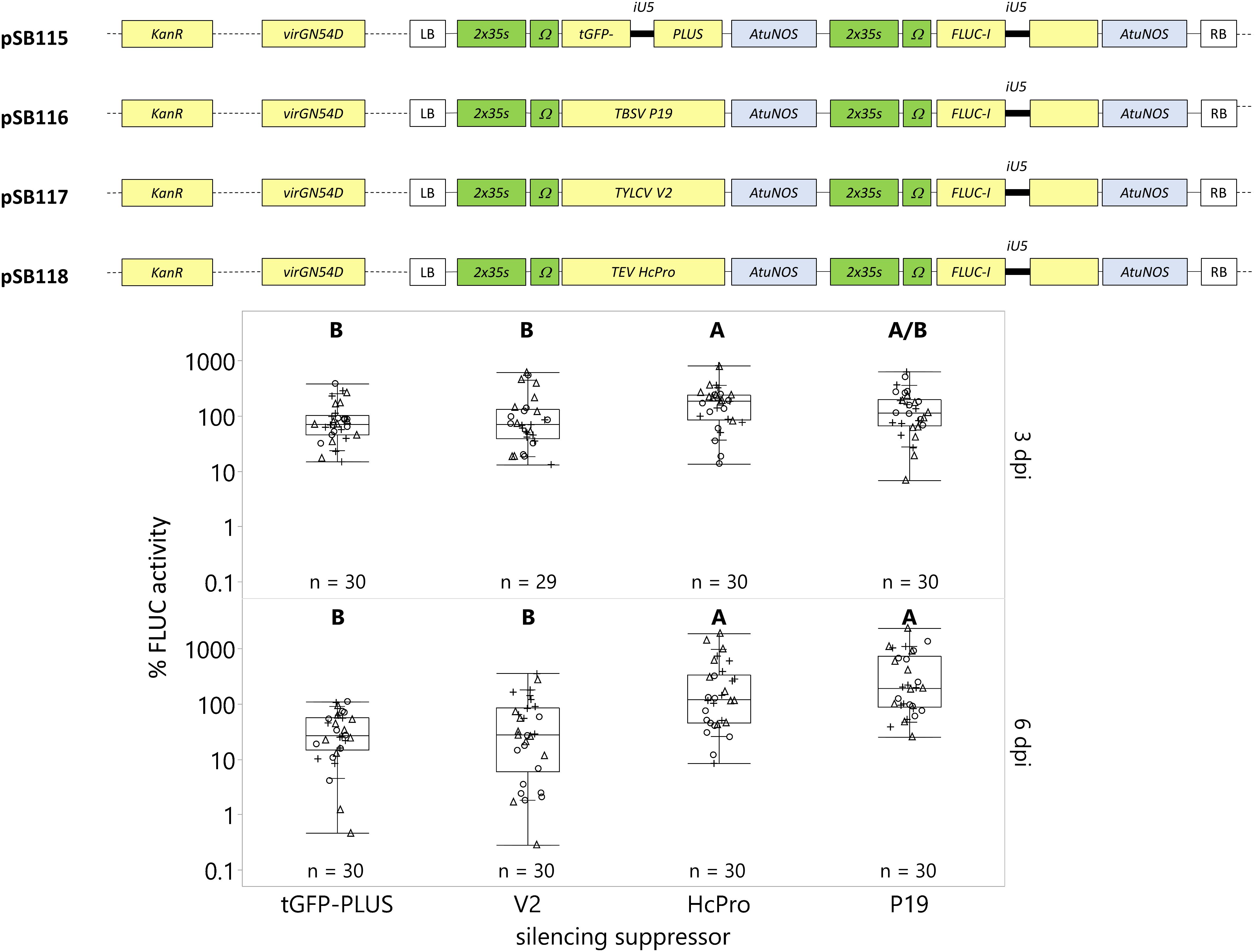
Figure 5. The silencing suppressors HcPro and P19 enhance transgene expression with prolonged cultivation post-infection. C. roseus seedlings (10-days-old) were vacuum infiltrated with A. tumefaciens containing the FLUC-I reporter and silencing suppressor within the same plasmid (TYLCV V2 – pSB117, TEV HcPro – pSB118, TBSV P19 – pSB116) or control (tGFP-PLUS – pSB115) at OD600 = 0.2. Samples were taken at 3 and 6 dpi. FLUC activity was normalized to protein content. The average FLUC activity to protein content of control samples (tGFP-PLUS) collected 3 dpi was used as reference (set to 100%) to determine the relative FLUC activity of each sample. The experiment was carried out in three independent assays (represented by the +, ○, Δ symbols). The box plot horizontal line shows the median, ends of the boxes show interquartile range, small marks show the 10th and 90th quantile, and whiskers show lowest/highest data point. Different letters indicate significantly different values within the 3 or 6 dpi groups (Tukey-Kramer HSD test on each group, on log-transformed data, p < 0.05).
Transformation of C. roseus Seedlings Leads to an Even Histochemical GUS Staining of the Cotyledons With Minimal Tissue Damage
From our studies using luciferase reporters, we obtained the highest transformation efficiency using the following conditions: (1) 10-day-old seedlings transformed with A. tumefaciens GV3101 (pMP90) strain at OD600 = 0.2 using vacuum infiltration and harvested 3 dpi, and (2) the A. tumefaciens strain transformed with the intron-containing reporter gene and the VirGN54D within the same plasmid synergistically activated with AS.
To determine whether EASI results in even transformation efficiency across seedling leaves, we transformed seedlings with the reporter β-glucuronidase (GUS), which enzymatically cleaves X-Gluc to produce a blue precipitate. This protocol resulted in minimal tissue damage at the harvest time point (3 dpi, Figure 6A). Endogenous β-glucuronidase-like (GUS-like) activity can be detected in specific tissues of various plant species (Hu et al., 1990); however, we detected no GUS-like activity in C. roseus seedlings after transformation with A. tumefaciens lacking a GUS reporter (Figure 6B). Transformation of seedlings with A. tumefaciens containing the CaMV2x35s driven GUS reporter at OD600 = 0.2 led to an even transformation of the cotyledons (Figure 6C). Hence, our EASI protocol promotes homogenous and high expression of the transgene in the cotyledons of C. roseus seedlings.
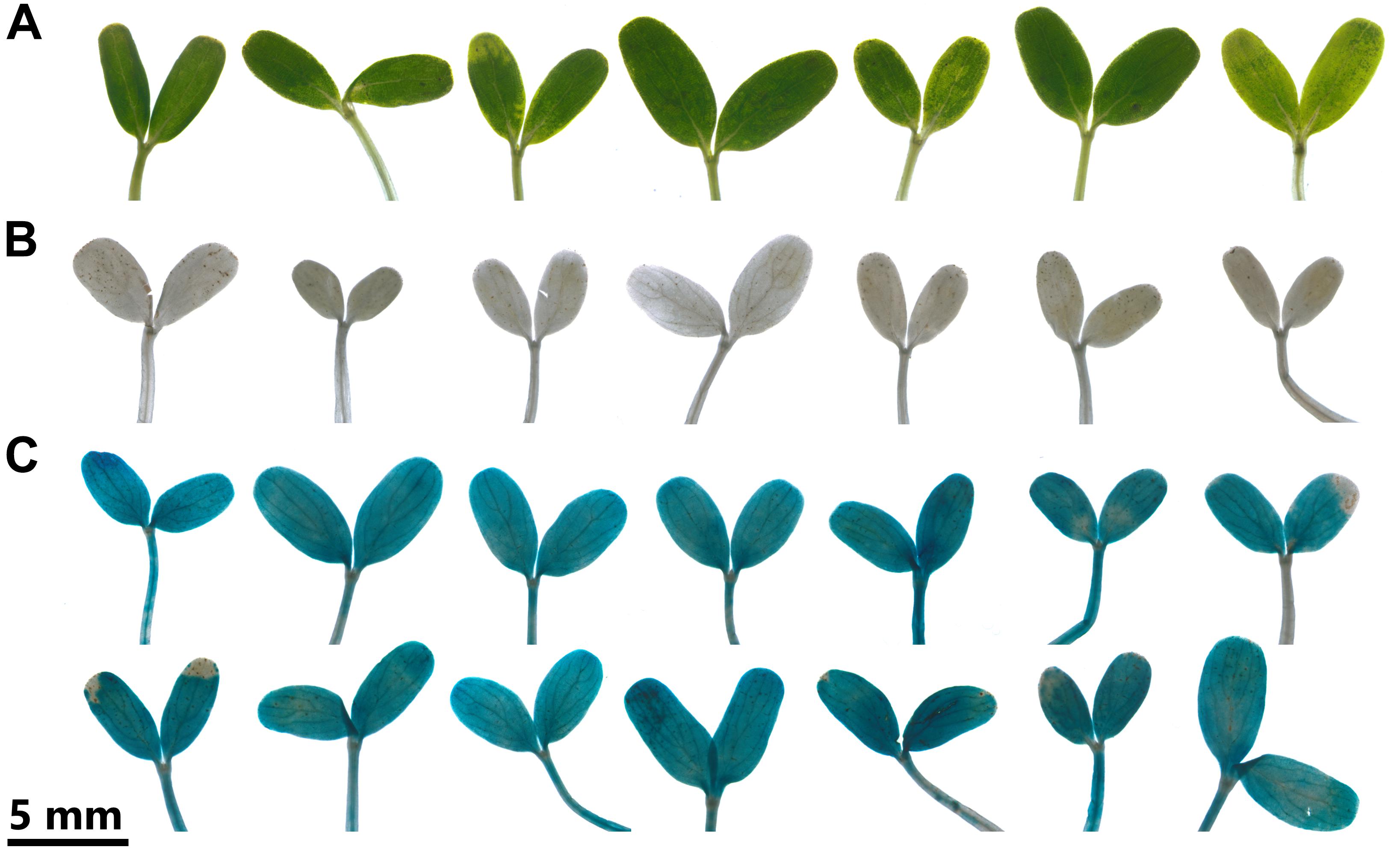
Figure 6. Catharanthus roseus seedlings after transformation with A. tumefaciens. C. roseus seedlings (10-day-old) were vacuum infiltrated with A. tumefaciens lacking the vector encoding the GUS reporter at OD600 = 0.4 (A,B) or containing the vector encoding the GUS reporter at OD600 = 0.2 (pSB161, C). Samples were taken at 3 dpi. Seedlings (B,C) underwent histochemical GUS assay and then were destained in 70% ethanol.
Dual-Luciferase Reporter System Is the Preferred Normalization Strategy for Reducing Inter-Experimental Variability
For accurate measurement of expression in promoter transactivation or promoter activity studies, a sensitive reporter gene with a wide dynamic range and rapid readout is desired. Luciferase assays provide high sensitivity and time-resolution for measuring promoter activity. While the GUS reporter is a valuable reporter for visualizing the evenness of expression across the tissue (Ruijter et al., 2003; Koo et al., 2007; Velten et al., 2008), GUS has a longer mRNA and protein half-life, which can bias the interpretation of promoter activity (Ruijter et al., 2003). Therefore, two luciferases (firefly luciferase and Renilla luciferase) were selected for quantifying promoter activity and normalizing transformation efficiency.
Normalization of FLUC signal to protein content has been used to account for variations in the amount of tissue (Ow et al., 1986). To account for variations in transformation efficiency across seedlings within an experiment, the activity of Renilla luciferase (RLUC) is generally used to normalize the FLUC reporter signal. Previous Agrobacterium-mediated transient assays normalized the transformation signal in the following ways: the reporter and normalization signal either (1) on the same plasmid (Liu et al., 2014; Moyle et al., 2017), (2) on different plasmids transformed into the same Agrobacterium strain (Cazzonelli and Velten, 2006), or (3) on different plasmids in different Agrobacterium strains and co-infiltrated into the seedlings (Van Moerkercke et al., 2011). To determine the optimal method for normalizing transformation efficiencies, we expressed RLUC-I and FLUC-I under the control of constitutive promoters (Figure 7) using these three approaches and determined the correlation between the two signals.
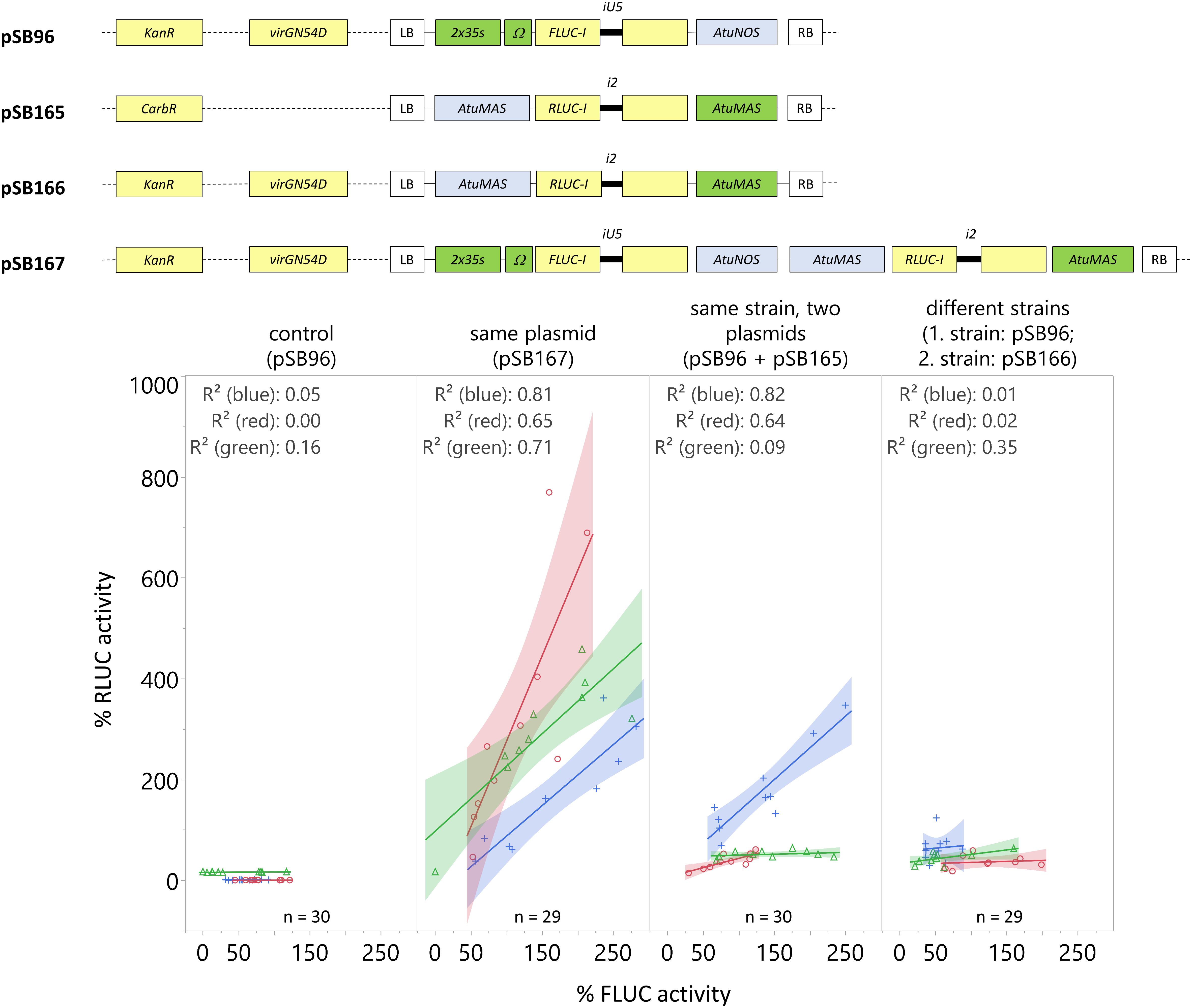
Figure 7. Including the normalization reporter RLUC-I on the same plasmid as the reporter FLUC-I provides consistently high RLUC signal, and is highly correlated with FLUC signal. C. roseus seedlings (10-day-old) were vacuum infiltrated with A. tumefaciens containing: (I) the FLUC-I reporter (Negative control – pSB96), (II) the FLUC-I reporter and the RLUC-I reporter within the same plasmid (Same plasmid – pSB167), (III) the FLUC-I reporter and the RLUC-I reporter in different plasmids but same strain of A. tumefaciens (Same strain, Two plasmids – pSB96, pSB165), (IV) the FLUC-I reporter and the RLUC-I reporter in different plasmids and different strains of A. tumefaciens, co-infiltrated into seedlings (Different strains – pSB96, pSB166) at OD600 = 0.2 for each strain. Samples were taken 3 dpi. The experiment was carried out in three independent assays (represented by blue, red, and green lines). For each independent assay, the FLUC activity of each sample was normalized to the average FLUC signal, and the RLUC activity of each sample was normalized to the average RLUC signal. Linear fit and corresponding R-squared values for the correlation between FLUC and RLUC signal are shown for each condition, in each experiment. The shaded region represents the 95% confidence interval of the linear fit.
In Figure 7 (from left to right), the negative control, containing FLUC-I only (pSB96), showed no RLUC signal, confirming that the FLUC signal was properly quenched. Including the normalization gene (RLUC-I) on the same plasmid as the reporter gene (FLUC-I) consistently yielded a high signal and a high correlation between RLUC and FLUC for all replicate experiments (R2 from 0.65 to 0.81, Figure 7). Infiltrating seedlings with A. tumefaciens carrying the normalization and reporter genes on separate plasmids showed variable results. Co-infiltrating seedlings with two strains of A. tumefaciens carrying FLUC-I and RLUC-I separately resulted in minimal correlation between FLUC and RLUC signal.
Therefore, we recommend including the normalization gene (RLUC-I) on the same plasmid as the reporter gene (FLUC-I) to account for variations in transformation efficiency across seedlings within an experiment.
Transactivation of the C. roseus STR1 Promoter With ORCA3 and ZCT1
Next, we applied EASI to study the transactivation of the CroSTR1 promoter by a known activator (ORCA3, van der Fits and Memelink, 2000, 2001) and repressor (ZCT1, Pauw et al., 2004) in C. roseus seedlings. Seedlings were infiltrated with two A. tumefaciens strains (OD600 = 0.2 each or final OD600 = 0.4), one containing the reporter construct and the other containing the effector construct. The reporter construct consisted of the CroSTR1 promoter driving FLUC-I and the AtuNOS promoter driving RLUC-I for normalization. The CroSTR1 and AtuNOS promoters were placed in opposite orientations on the T-DNA, maximizing the distance between the promoters to avoid crosstalk between the promoters. The effector construct consisted of the CaMV2x35s promoter driving GUS (negative control), ORCA3, or ZCT1. An effector construct expressing GUS was used as the reference and GUS staining was used to evaluate transformation success.
Overexpression of ORCA3 led to a 9-fold increase in expression compared to the overexpression of the GUS control (Figure 8). However, overexpression of ZCT1 did not repress the CroSTR1 promoter activity (Figure 9). Therefore, the ratio of the strains was tested to optimize reporter signal and effector activity (1 part reporter strain to 3, 6, or 12 parts of effector strain while maintaining a final OD600 = 0.4). Supplementary Figure S2 shows a decrease in the activity of the normalization gene (RLUC-I) as the ratio of effector to reporter increases, as expected. Transactivation with ratios of 1:3, 1:6, and 1:12 led to a decrease of the CroSTR1 promoter activity, but the activity of the RLUC was too low and unreliable at the 1:12 ratio and will be excluded in future studies (Supplementary Figure S2). The ratio that showed high reliable signal and significance was the 1:6 ratio. At the 1:6 ratio, the overexpression of ZCT1 repressed the expression of the CroSTR1 promoter by 38%. Therefore, EASI is an effective method for assessing both activation and repression of promoter activity in C. roseus.
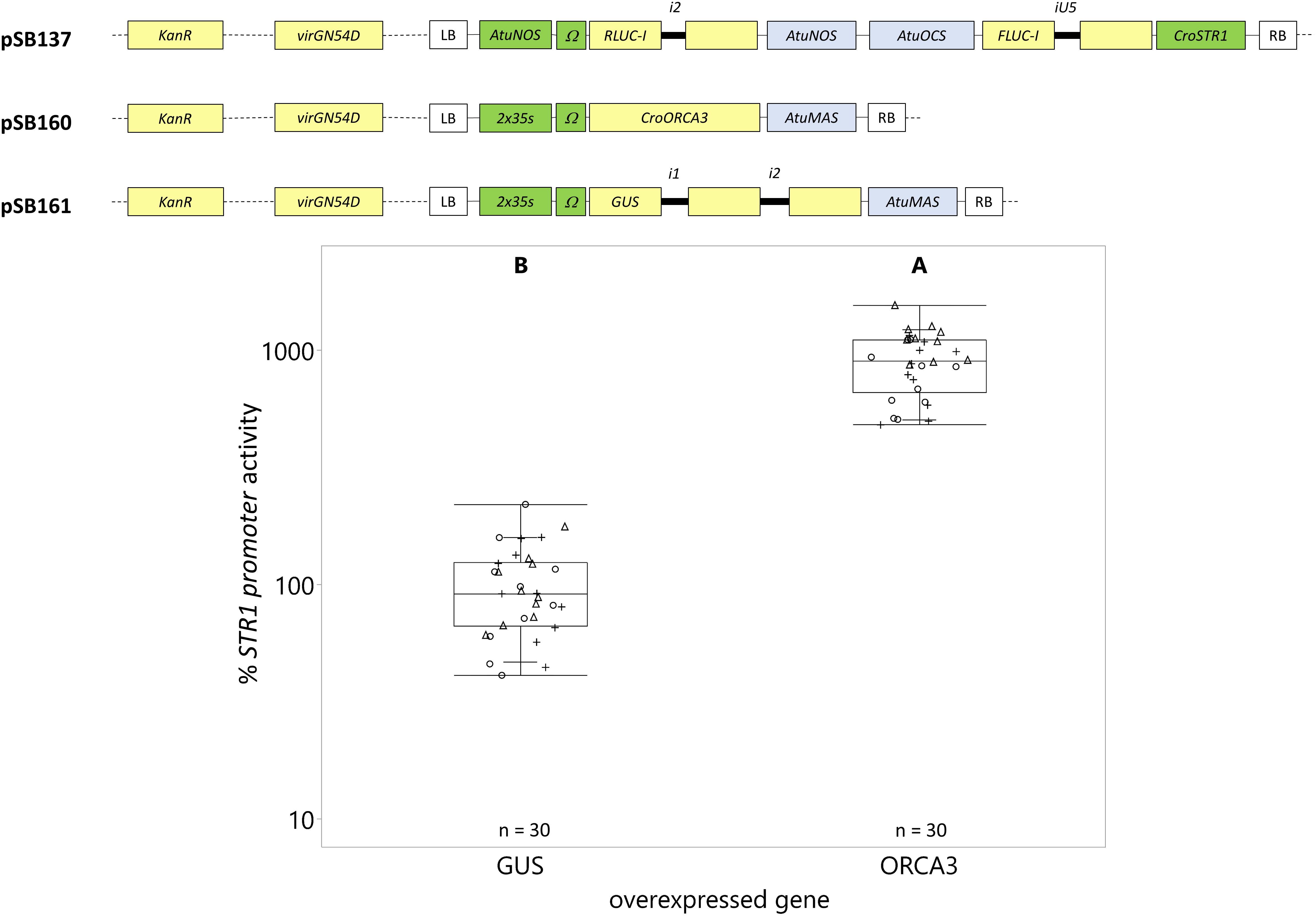
Figure 8. Transactivation of the CroSTR1 promoter with ORCA3. C. roseus seedlings (10-days-old) were vacuum infiltrated with a combination of two strains of A. tumefaciens (1:1 volume ratio): (I) strain containing the CroSTR1 promoter driven FLUC-I reporter and the AtuNOS promoter driven RLUC-I normalization reporter (plasmid pSB137) at OD600 = 0.2, and (II) strain containing a CaMV2x35s driven gene for transactivation (GUS as control – pSB161 or ORCA3 – pSB160) at OD600 = 0.2. Samples were taken 3 dpi. The CroSTR1 promoter activity is the FLUC to RLUC activity ratio for each sample normalized to the FLUC to RLUC activity ratio of the GUS control (set to 100%). The experiment was carried out in three independent assays (represented by the +, ○, Δ symbols). The box plot horizontal line shows the median, ends of the boxes show interquartile range, small marks show the 10th and 90th quantile, and whiskers show lowest/highest data point. Different letters indicate significantly different values. ORCA3 overexpression significantly increased the expression of the STR1 promoter (Student’s t-test on log-transformed data, p < 0.0001).
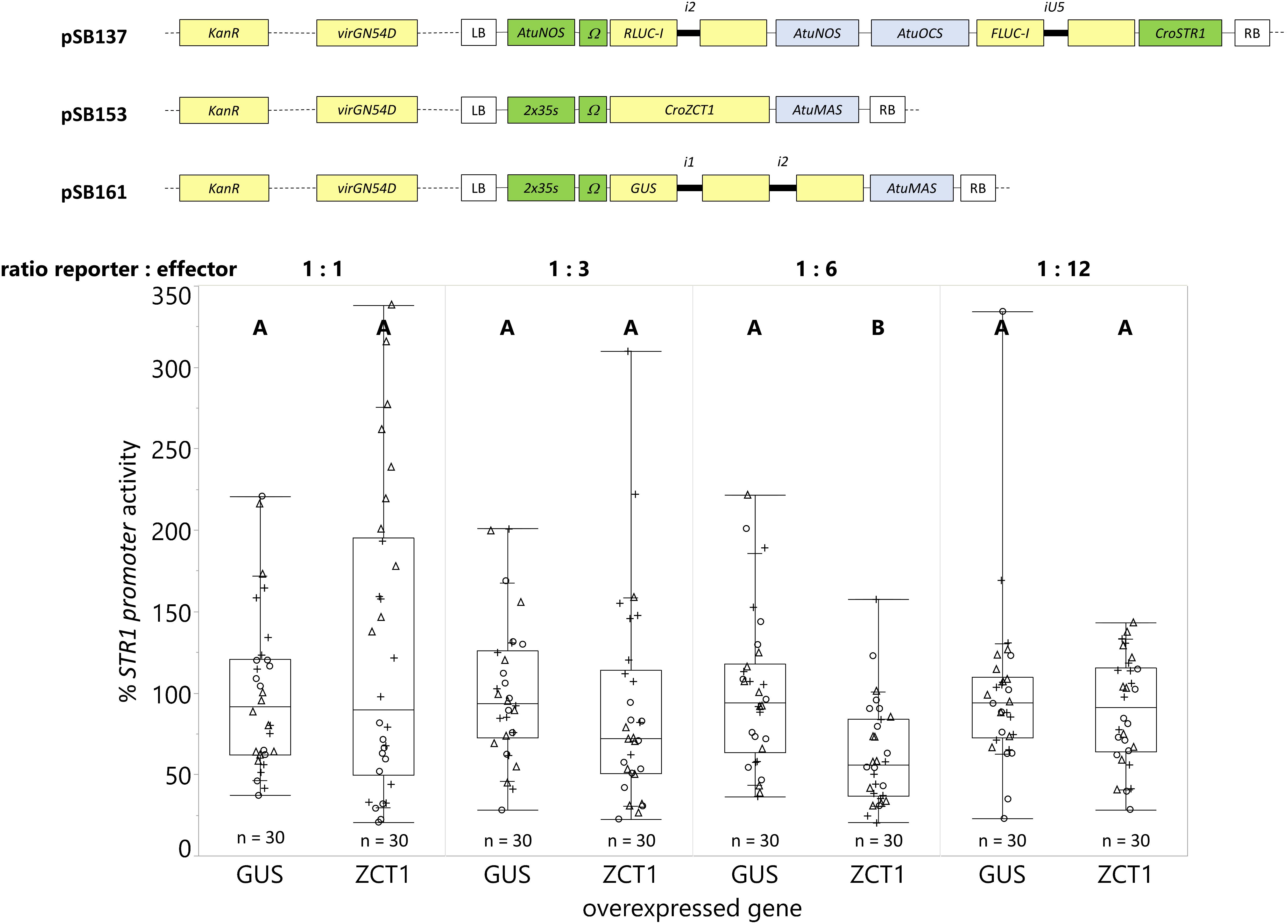
Figure 9. A ratio of 1-part reporter strain and 6-parts effector strain provides the highest sensitivity in transactivation assays. C. roseus seedlings (10-days-old) were vacuum infiltrated with a combination of two strains of A. tumefaciens (total OD600 = 0.4): (I) strain containing the CroSTR1 promoter driven FLUC-I reporter and the AtuNOS promoter driven RLUC-I normalization reporter (plasmid pSB137), and (II) strain containing a CaMV2x35s driven effector for transactivation (GUS as control – pSB161 or ZCT1 – pSB153). Samples were taken 3 dpi. For each reporter to effector ratio, the CroSTR1 promoter activity is the FLUC to RLUC activity ratio for each sample normalized to the FLUC to RLUC activity ratio of the GUS control (set to 100%). The experiment was carried out in three independent assays (represented by the +, ○, Δ symbols). The box plot horizontal line shows the median, ends of the boxes show interquartile range, small marks show the 10th and 90th quantile, and whiskers show lowest/highest data point. Different letters indicate significantly different values. ZCT1 overexpression significantly decreased the expression of the STR1 promoter in the 1:6 ratio, but not in the other ratios (Student’s t-test comparing each condition to its GUS control on log-transformed data, p < 0.05).
Discussion
In this paper, we present the development of an efficient Agrobacterium-mediated seedling infiltration (EASI) method for transformation of C. roseus seedlings, and demonstrate its applicability for the study of transcriptional regulation of TIA biosynthetic genes. This protocol can now be applied for the rapid assessment of the function of genes in planta, particularly for overexpressing transcription factor candidates and monitoring the expression of the genes involved in TIA biosynthesis. Our EASI method is complementary to viral induced gene silencing, which is used to silence the target gene (Liscombe and O’Connor, 2011). Previously, we developed an Agrobacterium co-cultivation method for transforming C. roseus leaf tissue (Weaver et al., 2014). Here, we have completely revised and markedly improved our previous transient expression method and increased transformation efficiency by (1) using vacuum infiltration, (2) introducing a constitutively active VirG gene, and (3) improving construct design, as detailed below.
Significant Increases in Transformation Efficiency With Vacuum Infiltration of Young Seedlings
Of all the parameters tested, vacuum infiltration of young seedlings (10-day-old) resulted in the strongest increase in transgene expression, at 18–57 times higher than either vacuum or syringe infiltration of other seedling ages. Other parameters, including Agrobacterium density and time point of maximum transgene expression, increased transgene expression to a lesser extent, up to 100% increase (Figures 2, 3). Vacuum infiltration offers other advantages over syringe infiltration, including ease of scale-up, minimum handling, and minimal tissue damage compared to syringe infiltration of individual seedlings (Figure 1). Minimizing tissue damage is important because tissue damage could activate expression of stress responsive transcription factors and confound transactivation results.
Increased Transformation Efficiency With Constitutively Active VirG and Silencing Suppressors
Incorporating the constitutively active VirG (VirGN54D, Pazour et al., 1992) also resulted in increased expression, particularly when the mutated VirG gene was encoded on the same plasmid as the reporter gene. It is possible including VirGN54D on the same plasmid as the reporter gene requires lower energy consumption or is less stressful for Agrobacterium to maintain (i.e., one plasmid with one antibiotic resistance gene) than on two separate plasmids (i.e., with two different antibiotic resistances). This construct (reporter and VirGN54D on the same plasmid) in combination with AS addition led to the highest transgene expression with a 120% increase above that of the reference condition (VirGN54D absent, + AS).
Commonly, transient transgene expression is high at 2–3 dpi but decreases with prolonged cultivation periods, as tissue dies due to the Agrobacterium infection or silencing of the transgene occurs. For example, Zrachya et al. (2007) transformed Nicotiana benthamiana leaves by agroinfiltration and observed high mRNA levels of the transgene (GFP) at 2 dpi but undetectable transgene expression at 7 dpi. The silencing suppressors V2, P19, and HcPro prolonged transgene expression in Nicotiana benthamiana (Zrachya et al., 2007). Similarly, we demonstrate that HcPro significantly increased transgene activity by 212% compared to the control at 3 dpi and up to 10-fold compared to the control at 6 dpi (Figure 5), while V2 is a less potent silencing suppressor in C. roseus.
Construct Design Facilitates Both High Expression Level and Appropriate Normalization of Transformation Efficiency
When performing transient expression assays, the reporter gene activity needs to be normalized to account for potential differences in transformation efficiency and in amount of analyzed tissue. Normalization of reporter gene activity by protein content can account for variations in the amount of tissue, but not in transformation efficiency. Transforming two different A. tumefaciens strains with the respective reporter genes (e.g., firefly luciferase and Renilla luciferase) is the most convenient approach; no complex vector needs to be constructed and the plasmids can have the same origin of replication and antibiotic selection marker. Unfortunately, we found that both the overall signal and the correlation between the two reporter gene activities in this configuration were low (Figure 7). Another convenient approach is to transform one A. tumefaciens strain with two different vectors; the vectors should have different origins of replications with different incompatibly groups, and different antibiotic resistances to allow selection of colonies containing both vectors. Although good reporter gene expression and correlation was observed in the first experiment (Figure 7; R2 = 0.823), this trend was not consistent in subsequent replicate experiments (R2 = 0.644 and 0.090). Incorporating two plasmids within the same strain adds additional stress to the Agrobacterium, and may introduce variations in plasmid copy number or in T-DNA transfer rates.
In contrast, designing the construct with the reporter gene (FLUC-I) and the normalization gene (RLUC-I) on the same plasmid yielded a high signal and a high correlation between RLUC and FLUC (Figure 7). We therefore recommend the construction of one vector with both reporter genes, which has not always been a common practice. While cloning is more extensive, the construction of one vector with both reporter genes avoids the risk of plasmid instability associated with two vectors within the same strain. Furthermore, Gibson assembly (Gibson et al., 2009) or Golden Gate-based methods like MoClo (Weber et al., 2011) now provide easy to use and fast methods for the construction of complex vectors.
Application of EASI for the Transactivation of the C. roseus STR1 Promoter With ORCA3 and ZCT1
We chose the well-studied transactivation and repression of the CroSTR1 promoter by the activator ORCA3 and repressor ZCT1 to demonstrate the applicability of the EASI method. ORCA3 expression induced a 9-fold increase in the CroSTR1 promoter activity (Figure 8). This is comparable to the previously reported transactivation of the CroSTR1 promoter with ORCA3, which led to an ∼10- to 25-fold increase in tobacco protoplasts (Van Moerkercke et al., 2015; Paul et al., 2017) and a ∼2.5-fold increase in N. benthamiana leaves (Van Moerkercke et al., 2015). The CroSTR1 promoter can be repressed by ZCT1 in C. roseus cells (Pauw et al., 2004). We did not observe any significant repression in C. roseus seedlings using a 1 to 1 ratio of the Agrobacterium strain containing the CroSTR1 promoter driving reporter to the Agrobacterium strain containing the ZCT1 effector (Figure 9). A ratio of 1:6 provided maximum effect (38% decrease) from the effector strain while maintaining relatively high and reliable reporter gene expression. These results demonstrate the application of the EASI method to study novel transcriptional regulation of TIA biosynthesis in C. roseus.
Conclusion
The described method achieves uniform and high transformation of the cotyledons and has the potential for many different applications in C. roseus, such as studying transcriptional regulation and protein localization. Therefore, EASI provides a timely tool for the community to rapidly assess the function of genes discovered through the availability of C. roseus transcriptome and genome resources. In addition, the vectors constructed in this study have been made available through Addgene to other researchers and can be applied for optimizing transient transformations and transactivation assays in other plant systems. These constructs include intron-containing reporter genes (for Agrobacterium-mediated transformation), the constitutively expressed virulence G gene, and the silencing suppressors, which together can result in highly efficient transient transformation.
Author Contributions
SM, DB-F, EC, and CL-P conceived and designed the research. SM, DB-F, LC, and SS performed the experiments. SM, DB-F, LC, SS, EC, and CL-P analyzed the data. SM, DB-F, LC, EC, and CL-P wrote the manuscript.
Funding
This work was supported by the National Science Foundation (NSF) MCB Award #1516371 and BBBE Award #1033889 to CL-P and EC.
Conflict of Interest Statement
The authors declare that the research was conducted in the absence of any commercial or financial relationships that could be construed as a potential conflict of interest.
Acknowledgments
We thank Prof. Anath Das (Department of Biochemistry, Molecular Biology and Biophysics, University of Minnesota) for providing the mutated VirGN54D construct (pAD1289).
Supplementary Material
The Supplementary Material for this article can be found online at: https://www.frontiersin.org/articles/10.3389/fpls.2019.00755/full#supplementary-material
FIGURE S1 | Binary vectors used for C. roseus transformations. The plasmid pAD1289 was kindly provided by Prof. A. Das. All pSB vectors were constructed using MoClo (Weber et al., 2011; Engler et al., 2014). Level 1 vector parts for pSB96, pSB115-118, pSB137, pSB153, pSB160-161, and pSB166-167 were assembled in the newly constructed L2 acceptor vector pSB90 (see section “Materials and Methods” for detail). pSB97 and pSB153 were assembled in the pAGM4723 vector (Weber et al., 2011). Promoters including 5′UTRs are shown in green, coding sequences in yellow, and terminators in blue. Introns are indicated with a black bar within the coding sequence. Vectors and vector files of all pSB plasmids are deposited at Addgene (Addgene ID 123180–123200).
FIGURE S2 | ZCT1 does not repress the AtuNOS promoter and a 1:12 ratio does not provide sufficient RLUC signal for normalization. C. roseus seedlings (10-days old) were vacuum infiltrated with a combination of two strains of A. tumefaciens (total OD600 = 0.4): (I) strain containing the CroSTR1 promoter driven FLUC-I reporter and the AtuNOS promoter driven RLUC-I normalization reporter (plasmid pSB137), and (II) strain containing a CaMV2x35s driven effector for transactivation (GUS as control – pSB161 or ZCT1 – pSB153). Samples were taken 3 dpi. The experiment was carried out in three independent assays (represented by the +, ○, Δ symbols). The box plot horizontal line shows the median, ends of the boxes show interquartile range, small marks show the 10th and 90th quantile, and whiskers show lowest/highest data point. ZCT1 overexpression did not significantly decrease the expression of the AtuNOS promoter (student’s t-test comparing each condition to its GUS control on log-transformed data, p < 0.05).
TABLE S1 | Primers used for the construction of new vectors.
TABLE S2 | Construction of level 0 vector parts.
Footnotes
- ^ http://medicinalplantgenomics.msu.edu
- ^ https://bioinformatics.tugraz.at/phytometasyn/
- ^ https://sites.google.com/a/ualberta.ca/onekp/
References
Aerts, R. J., Gisi, D., De Carolis, D., De Luca, V., and Baumann, T. W. (1994). Methyl jasmonate vapor increases the developmentally controlled synthesis of alkaloids in Catharanthus and Cinchona seedlings. Plant J. 5, 635–643. doi: 10.1111/j.1365-313x.1994.00635.x
Anandalakshmi, R., Pruss, G. J., Ge, X., Marathe, R., Mallory, A. C., Smith, T. H., et al. (1998). A viral suppressor of gene silencing in plants. Proc. Natl. Acad. Sci. U.S.A. 95, 13079–13084. doi: 10.1073/pnas.95.22.13079
Andersson, C.-M., Hallberg, A., and Högberg, T. (1996). Advances in the development of pharmaceutical antioxidants. Adv. Drug Res. 28, 65–180. doi: 10.1016/S0065-2490(96)80004-9
Balunas, M. J., and Kinghorn, A. D. (2005). Drug discovery from medicinal plants. Life Sci. 78, 431–441. doi: 10.1016/j.lfs.2005.09.012
Brigneti, G., Voinnet, O., Li, W., and Ji, L. (1998). Viral pathogenicity determinants are suppressors of transgene silencing in Nicotiana benthamiana. EMBO 17, 6739–6746. doi: 10.1093/emboj/17.22.6739
Caputi, L., Franke, J., Farrow, S. C., Chung, K., Payne, R. M. E., Nguyen, T.-D., et al. (2018). Missing enzymes in the biosynthesis of the anticancer drug vinblastine in Madagascar periwinkle. Science 360, 1235–1239. doi: 10.1126/SCIENCE.AAT4100
Cazzonelli, C. I., and Velten, J. (2006). An in vivo, luciferase-based, Agrobacterium-infiltration assay system: implications for post-transcriptional gene silencing. Planta 224, 582–597. doi: 10.1007/s00425-006-0250-z
Chatel, G., Montiel, G., Pré, M., Memelink, J., Thiersault, M., Saint-Pierre, B., et al. (2003). CrMYC1, a Catharanthus roseus elicitor- and jasmonate-responsive bHLH transcription factor that binds the G-box element of the strictosidine synthase gene promoter. J. Exp. Bot. 54, 2587–2588. doi: 10.1093/jxb/erg275
Choi, P. S., Kim, Y. D., Choi, K. M., Chung, H. J., Choi, D. W., and Liu, J. R. (2004). Plant regeneration from hairy-root cultures transformed by infection with Agrobacterium rhizogenes in Catharanthus roseus. Plant Cell Rep. 22, 828–831. doi: 10.1007/s00299-004-0765-3
Di Fiore, S., Hoppmann, V., Fischer, R., and Schillberg, S. (2004). Transient gene expression of recombinant terpenoid indole alkaloid enzymes in Catharanthus roseus leaves. Plant Mol. Biol. Rep. 22, 15–22. doi: 10.1093/mp/sst058
Engler, C., Youles, M., Gruetzner, R., Ehnert, T. M., Werner, S., Jones, J. D., et al. (2014). A golden gate modular cloning toolbox for plants. ACS Synth. Biol. 3, 839–843. doi: 10.1021/sb4001504
Facchini, P. J., and De Luca, V. (2008). Opium poppy and Madagascar periwinkle: model non-model systems to investigate alkaloid biosynthesis in plants. Plant J. 54, 763–784. doi: 10.1111/j.1365-313X.2008.03438.x
Gibson, D. G., Young, L., Chuang, R. Y., Venter, J. C., Hutchison, C. A., and Smith, H. O. (2009). Enzymatic assembly of DNA molecules up to several hundred kilobases. Nat. Methods 6, 343–345. doi: 10.1038/nmeth.1318
Glick, E., Zrachya, A., Levy, Y., Mett, A., Gidoni, D., Belausov, E., et al. (2008). Interaction with host SGS3 is required for suppression of RNA silencing by tomato yellow leaf curl virus V2 protein. Proc. Natl. Acad. Sci. U.S.A. 105, 157–161. doi: 10.1073/pnas.0709036105
Góngora-Castillo, E., Childs, K. L., Fedewa, G., Hamilton, J. P., Liscombe, D. K., Magallanes-Lundback, M., et al. (2012). Development of transcriptomic resources for interrogating the biosynthesis of monoterpene indole alkaloids in medicinal plant species. PLoS One 7:e52506. doi: 10.1371/journal.pone.0052506
Guirimand, G., Burlat, V., Oudin, A., Lanoue, A., St-Pierre, B., and Courdavault, V. (2009). Optimization of the transient transformation of Catharanthus roseus cells by particle bombardment and its application to the subcellular localization of hydroxymethylbutenyl 4-diphosphate synthase and geraniol 10-hydroxylase. Plant Cell Rep. 28, 1215–1234. doi: 10.1007/s00299-009-0722-2
Hansen, G., Das, A., and Chilton, M. D. (1994). Constitutive expression of the virulence genes improves the efficiency of plant transformation by Agrobacterium. Proc. Natl. Acad. Sci. U.S.A. 91, 7603–7607. doi: 10.1073/pnas.91.16.7603
Hosein, F. N., Lennon, A. M., and Umaharan, P. (2012). Optimization of an Agrobacterium-mediated transient assay for gene expression studies in Anthurium andraeanum. J. Am. Soc. Hortic. Sci. 137, 263–272. doi: 10.21273/jashs.137.4.263
Hu, C. Y., Chee, P. P., Chesney, R. H., Zhou, J. H., Miller, P. D., and O’Brien, W. T. (1990). Intrinsic GUS-like activities in seed plants. Plant Cell Rep. 9, 1–5. doi: 10.1007/BF00232123
Kasschau, K. D., and Carrington, J. C. (1998). A counterdefensive strategy of plant viruses. Cell 95, 461–470. doi: 10.1016/S0092-8674(00)81614-1
Kellner, F., Kim, J., Clavijo, B. J., Hamilton, J. P., Childs, K. L., Vaillancourt, B., et al. (2015). Genome-guided investigation of plant natural product biosynthesis. Plant J. 82, 680–692. doi: 10.1111/tpj.12827
Koncz, C., Schell, J., and Rédei, G. P. (1992). “T-DNA transformation and insertion mutagenesis,” in Methods in Arabidopsis Research, eds C. Koncz, N. H. Chua, and J. Schell (Singapore: World Scientific), 224–273. doi: 10.1142/9789814439701_0009
Koo, J., Kim, Y., Kim, J., Yeom, M., Lee, I. C., and Nam, H. G. (2007). A GUS/luciferase fusion reporter for plant gene trapping and for assay of promoter activity with luciferin-dependent control of the reporter protein stability. Plant Cell Physiol. 48, 1121–1131. doi: 10.1093/pcp/pcm081
Lee, M. W., and Yang, Y. (2006). “Transient Expression Assay by Agroinfiltration of Leaves,”,” in Arabidopsis Protocols, eds J. Salinas and J. J. Sanchez-Serrano (New Jersey, NJ: Humana Press), 225–230. doi: 10.1385/1-59745-003-0:225
Liscombe, D. K., and O’Connor, S. E. (2011). A virus-induced gene silencing approach to understanding alkaloid metabolism in Catharanthus roseus. Phytochemistry 72, 1969–1977. doi: 10.1016/j.phytochem.2011.07.001
Liu, Q., Wang, F., and Axtell, M. J. (2014). Analysis of complementarity requirements for plant MicroRNA targeting using a Nicotiana benthamiana quantitative transient assay. Plant Cell 26, 741–753. doi: 10.1105/tpc.113.120972
Lu, J., Bai, M., Ren, H., Liu, J., and Wang, C. (2017). An efficient transient expression system for gene function analysis in rose. Plant Methods 13:116. doi: 10.1186/s13007-017-0268-1
Makhzoum, A., Petit-Paly, G., St. Pierre, B., and Bernards, M. A. (2011). Functional analysis of the DAT gene promoter using transient Catharanthus roseus and stable Nicotiana tabacum transformation systems. Plant Cell Rep. 30, 1173–1182. doi: 10.1007/s00299-011-1025-y
Marion, J., Bach, L., Bellec, Y., Meyer, C., Gissot, L., and Faure, J.-D. (2008). Systematic analysis of protein subcellular localization and interaction using high-throughput transient transformation of Arabidopsis seedlings. Plant J. 56, 169–179. doi: 10.1111/j.1365-313X.2008.03596.x
Menke, F. L., Champion, A., Kijne, J. W., and Memelink, J. (1999). A novel jasmonate-and elicitor-responsive element in the periwinkle secondary metabolite biosynthetic gene Str interacts with a jasmonate-and elicitor-inducible AP2-domain transcription factor, ORCA2. EMBO J. 18, 4455–4463. doi: 10.1093/emboj/18.16.4455
Moyle, R. L., Carvalhais, L. C., Pretorius, L.-S., Nowak, E., Subramaniam, G., Dalton-Morgan, J., et al. (2017). An optimized transient dual luciferase assay for quantifying MicroRNA directed repression of targeted sequences. Front. Plant Sci. 8:1631. doi: 10.3389/fpls.2017.01631
Noble, R. L. (1990). The discovery of the vinca alkaloids–chemotherapeutic agents against cancer. Biochem. Cell Biol. 68, 1344–1351. doi: 10.1139/o90-197
Ow, D. W., De Wet, J. R., Helinski, D. R., Howell, S. H., Wood, K. V., and DeLuca, M. (1986). Transient and stable expression of the firefly luciferase gene in plant cells and transgenic plants. Science 234, 856–859. doi: 10.1126/science.234.4778.856
Pan, Q., Rianika, N., and Verpoorte, R. (2016). Monoterpenoid indole alkaloids biosynthesis and its regulation in Catharanthus roseus: a literature review from genes to metabolites. Phytochem. Rev. 15, 221–250. doi: 10.1007/s11101-015-9406-4
Pan, Q., Wang, Q., Yuan, F., Xing, S., Zhao, J., Choi, Y. H., et al. (2012). Overexpression of ORCA3 and G10H in Catharanthus roseus plants regulated alkaloid biosynthesis and metabolism revealed by NMR-metabolomics. PLoS One 7:e4038. doi: 10.1371/journal.pone.0043038
Pasquali, G., Erven, A. S., Ouwerkerk, P. B., Menke, F. L., and Memelink, J. (1999). The promoter of the strictosidine synthase gene from periwinkle confers elicitor-inducible expression in transgenic tobacco and binds nuclear factors GT-1 and GBF. Plant Mol. Biol. 39, 1299–1310. doi: 10.1023/A:1006177414456
Patra, B., Pattanaik, S., Schluttenhofer, C., and Yuan, L. (2018). A network of jasmonate-responsive bHLH factors modulate monoterpenoid indole alkaloid biosynthesis in Catharanthus roseus. New Phytol. 217, 1566–1581. doi: 10.1111/nph.14910
Paul, P., Singh, S. K., Patra, B., Sui, X., Pattanaik, S., and Yuan, L. (2017). A differentially regulated AP2/ERF transcription factor gene cluster acts downstream of a MAP kinase cascade to modulate terpenoid indole alkaloid biosynthesis in Catharanthus roseus. New Phytol. 213, 1107–1123. doi: 10.1111/nph.14252
Pauw, B., Hilliou, F. A., Martin, V. S., Chatel, G., De Wolf, C. J., Champion, A., et al. (2004). Zinc finger proteins act as transcriptional repressors of alkaloid biosynthesis genes in Catharanthus roseus. J. Biol. Chem. 279, 52940–52948. doi: 10.1074/jbc.M404391200
Payne, R. M. E., Xu, D., Foureau, E., Teto Carqueijeiro, M. I. S., Oudin, A., de Bernonville, T. D., et al. (2017). An NPF transporter exports a central monoterpene indole alkaloid intermediate from the vacuole. Nat. Plants 3:16208. doi: 10.1038/nplants.2016.208
Pazour, G. J., Ta, C. N., and Das, A. (1992). Constitutive mutations of Agrobacterium tumefaciens transcriptional activator virG. J. Bacteriol. 174, 4169–4174. doi: 10.1128/jb.174.12.4169-4174.1992
Pumplin, N., and Voinnet, O. (2013). RNA silencing suppression by plant pathogens: defence, counter-defence and counter-counter-defence. Nat. Rev. Microbiol. 11, 745–760. doi: 10.1038/nrmicro3120
Qu, Y., Easson, M. L., Froese, J., Simionescu, R., Hudlicky, T., and De Luca, V. (2015). Completion of the seven-step pathway from tabersonine to the anticancer drug precursor vindoline and its assembly in yeast. Proc. Natl. Acad. Sci. U.S.A. 112, 6224–6229. doi: 10.1073/pnas.1501821112
Qu, Y., Safonova, O., and De Luca, V. (2018). Completion of the canonical pathway for assembly of anticancer drugs vincristine/vinblastine in Catharanthus roseus. Plant J. 97, 257–266. doi: 10.1111/tpj.14111
Rizvi, N. F., Cornejo, M., Stein, K., Weaver, J., Cram, E. J., and Lee-Parsons, C. W. T. (2015). An efficient transformation method for estrogen-inducible transgene expression in Catharanthus roseus hairy roots. Plant Cell Tissue Organ. Cult. 120, 475–487. doi: 10.1007/s11240-014-0614-1
Rizvi, N. F., Weaver, J. D., Cram, E. J., and Lee-Parsons, C. W. T. (2016). Silencing the transcriptional repressor, ZCT1, illustrates the tight regulation of terpenoid indole alkaloid biosynthesis in Catharanthus roseus hairy roots. PLoS One 11:e0159712. doi: 10.1371/journal.pone.0159712
Ruijter, N. C. A., De Verhees, J., Van Leeuwen, W., and Van Der Krol, A. R. (2003). Evaluation and comparison of the GUS, LUC and GFP reporter system for gene expression studies in plants. Plant Biol. 5, 103–115. doi: 10.1055/s-2003-40722
Sarrion-Perdigones, A., Vazquez-Vilar, M., Palaci, J., Castelijns, B., Forment, J., Ziarsolo, P., et al. (2013). GoldenBraid 2.0: a comprehensive DNA assembly framework for plant synthetic biology. Plant Physiol. 162, 1618–1631. doi: 10.1104/pp.113.217661
Schweizer, F., Colinas, M., Pollier, J., Van Moerkercke, A., Vanden Bossche, R., de Clercq, R., et al. (2018). An engineered combinatorial module of transcription factors boosts production of monoterpenoid indole alkaloids in Catharanthus roseus. Metab. Eng. 48, 150–162. doi: 10.1016/j.ymben.2018.05.016
Silhavy, D., Molnár, A., Lucioli, A., Szittya, G., Hornyik, C., Tavazza, M., et al. (2002). A viral protein suppresses RNA silencing and binds silencing-generated, 21-to 25-nucleotide double-stranded RNAs. EMBO J. 21, 3070–3080. doi: 10.1093/emboj/cdf312
Sparkes, I. A., Runions, J., Kearns, A., and Hawes, C. (2006). Rapid, transient expression of fluorescent fusion proteins in tobacco plants and generation of stably transformed plants. Nat. Protoc. 1, 2019–2025. doi: 10.1038/nprot.2006.286
Sun, J., Zhao, L., Shao, Z., Shanks, J., and Peebles, C. A. M. (2018). Expression of tabersonine 16-hydroxylase and 16-hydroxytabersonine-O-methyltransferase in Catharanthus roseus hairy roots. Biotechnol. Bioeng. 115, 673–683. doi: 10.1002/bit.26487
Suttipanta, N., Pattanaik, S., Kulshrestha, M., Patra, B., Singh, S. K., and Yuan, L. (2011). The transcription factor CrWRKY1 positively regulates the terpenoid indole alkaloid biosynthesis in Catharanthus roseus. Plant Physiol. 157, 2081–2093. doi: 10.1104/pp.lll.181834
van der Fits, L., and Memelink, J. (1997). Comparison of the activities of CaMV 35S and FMV 34S promoter derivatives in Catharanthus roseus cells transiently and stably transformed by particle bombardment. Plant Mol. Biol. 33, 943–946. doi: 10.1023/A:1005763605355
van der Fits, L., and Memelink, J. (2000). ORCA3, a jasmonate-responsive transcriptional regulator of plant primary and secondary metabolism. Science 289, 295–297. doi: 10.1126/science.289.5477.295
van der Fits, L., and Memelink, J. (2001). The jasmonate-inducible AP2/ERF-domain transcription factor ORCA3 activates gene expression via interaction with a jasmonate-responsive promoter element. Plant J. 25, 43–53. doi: 10.1046/j.1365-313x.2001.00932.x
van der Heijden, R., Jacobs, D. I., Snoeijer, W., Hallard, D., and Verpoorte, R. (2004). The Catharanthus alkaloids: pharmacognosy and biotechnology. Curr. Med. Chem. 11, 607–628.
Van Moerkercke, A., Haring, M. A., and Schuurink, R. C. (2011). The transcription factor emission of benzenoids II activates the MYB ODORANT1 promoter at a MYB binding site specific for fragrant petunias. Plant J. 67, 917–928. doi: 10.1111/j.1365-313X.2011.04644.x
Van Moerkercke, A., Steensma, P., Gariboldi, I., Espoz, J., Purnama, P. C., Schweizer, F., et al. (2016). The basic helix-loop-helix transcription factor BIS2 is essential for monoterpenoid indole alkaloid production in the medicinal plant Catharanthus roseus. Plant J. 88, 3–12. doi: 10.1111/tpj.13230
Van Moerkercke, A., Steensma, P., Schweizer, F., Pollier, J., Gariboldi, I., Payne, R., et al. (2015). The bHLH transcription factor BIS1 controls the iridoid branch of the monoterpenoid indole alkaloid pathway in Catharanthus roseus. Proc. Natl. Acad. Sci. U.S.A. 112, 8130–8135. doi: 10.1073/pnas.1504951112
Várallyay, É., Válóczi, A., Ágyi, Á., Burgyán, J., and Havelda, Z. (2010). Plant virus-mediated induction of miR168 is associated with repression of ARGONAUTE1 accumulation. EMBO J. 29, 3507–3519. doi: 10.1038/emboj.2010.215
Velten, J., Pogson, B., and Cazzonelli, C. I. (2008). Luciferase as a reporter of gene activity in plants. Transgenic Plant J. 2, 1–13.
Verweij, W., Spelt, C., Di Sansebastiano, G. P., Vermeer, J., Reale, L., Ferranti, F., et al. (2008). An H+ P-ATPase on the tonoplast determines vacuolar pH and flower colour. Nat. Cell Biol. 10, 1456–1462. doi: 10.1038/ncb1805
Voinnet, O. (2001). RNA silencing as a plant immune system against viruses. Trends Genet. 17, 449–459. doi: 10.1016/S0168-9525(01)02367-8
Wang, Q., Xing, S., Pan, Q., Yuan, F., Zhao, J., Tian, Y., et al. (2012). Development of efficient Catharanthus roseus regeneration and transformation system using Agrobacterium tumefaciens and hypocotyls as explants. BMC Biotechnol. 12:34. doi: 10.1186/1472-6750-12-34
Weaver, J., Goklany, S., Rizvi, N., Cram, E. J., and Lee-Parsons, C. W. T. (2014). Optimizing the transient fast agro-mediated seedling transformation (FAST) method in Catharanthus roseus seedlings. Plant Cell Rep. 33, 89–97. doi: 10.1007/s00299-013-1514-2
Weber, E., Engler, C., Gruetzner, R., Werner, S., and Marillonnet, S. (2011). A modular cloning system for standardized assembly of multigene constructs. PLoS One 6:e16765. doi: 10.1371/journal.pone.0016765
Weng, J.-K., Philippe, R. N., and Noel, J. P. (2012). The rise of chemodiversity in plants. Science 336, 1667–1670. doi: 10.1126/science.1217411
Wroblewski, T., Tomczak, A., and Michelmore, R. (2005). Optimization of Agrobacterium-mediated transient assays of gene expression in lettuce, tomato and Arabidopsis. Plant Biotechnol. J. 3, 259–273. doi: 10.1111/j.1467-7652.2005.00123.x
Wu, H.-Y., Liu, K.-H., Wang, Y.-C., Wu, J.-F., Chiu, W.-L., Chen, C.-Y., et al. (2014). AGROBEST: an efficient Agrobacterium-mediated transient expression method for versatile gene function analyses in Arabidopsis seedlings. Plant Methods 10, 1–16. doi: 10.1186/1746-4811-10-19
Wurtzel, E. T., and Kutchan, T. M. (2016). Plant metabolism, the diverse chemistry set of the future. Science 353, 1232–1236. doi: 10.1126/science.aad2062
Yu, F., and De Luca, V. (2013). ATP-binding cassette transporter controls leaf surface secretion of anticancer drug components in Catharanthus roseus. Proc. Natl. Acad. Sci. U.S.A. 110, 15830–15835. doi: 10.1073/pnas.1307504110
Zhang, H., Hedhili, S., Montiel, G., Zhang, Y., Chatel, G., Pré, M., et al. (2011). The basic helix-loop-helix transcription factor CrMYC2 controls the jasmonate-responsive expression of the ORCA genes that regulate alkaloid biosynthesis in Catharanthus roseus. Plant J. 67, 61–71. doi: 10.1111/j.1365-313X.2011.04575.x
Zhao, X., Meng, Z., Wang, Y., Chen, W., Sun, C., Cui, B., et al. (2017). Pollen magnetofection for genetic modification with magnetic nanoparticles as gene carriers. Nat. Plants 3, 956–964. doi: 10.1038/s41477-017-0063-z
Keywords: Catharanthus roseus, agroinfiltration, transient transformation, transactivation, ORCA3, ZCT1, mutated VirG, viral silencing suppressors
Citation: Mortensen S, Bernal-Franco D, Cole LF, Sathitloetsakun S, Cram EJ and Lee-Parsons CWT (2019) EASI Transformation: An Efficient Transient Expression Method for Analyzing Gene Function in Catharanthus roseus Seedlings. Front. Plant Sci. 10:755. doi: 10.3389/fpls.2019.00755
Received: 31 January 2019; Accepted: 24 May 2019;
Published: 11 June 2019.
Edited by:
Roger Deal, Emory University, United StatesReviewed by:
Ling Yuan, University of Kentucky, United StatesBenoit Lacroix, Stony Brook University, United States
Kexuan Tang, Shanghai Jiao Tong University, China
Copyright © 2019 Mortensen, Bernal-Franco, Cole, Sathitloetsakun, Cram and Lee-Parsons. This is an open-access article distributed under the terms of the Creative Commons Attribution License (CC BY). The use, distribution or reproduction in other forums is permitted, provided the original author(s) and the copyright owner(s) are credited and that the original publication in this journal is cited, in accordance with accepted academic practice. No use, distribution or reproduction is permitted which does not comply with these terms.
*Correspondence: Carolyn W. T. Lee-Parsons, Y2EubGVlQG5vcnRoZWFzdGVybi5lZHU=
†These authors have contributed equally to this work