- 1Plant Genetic Engineering Laboratory, Department of Biotechnology, Bharathiar University, Coimbatore, India
- 2Functional Cell Reprogramming and Organism Plasticity (FunCROP), University of Évora, Évora, Portugal
- 3Cell and Molecular Biology of Plants (BPOCEMP)/Industrial Biotechnology and Bioproducts, Department of Sciences of the Vidaydela Agriculture, University of the Armed Forces-ESPE, Milagro, Ecuador
- 4Faculty of Engineering, State University of Milagro (UNEMI), Milagro, Ecuador
- 5Functional Genomics and Bioinformatics Group, Department of Biochemistry and Molecular Biology, Federal University of Ceará, Fortaleza, Brazil
- 6Council for Agricultural Research and Economics (CREA), Research Centre for Animal Production and Aquaculture, Lodi, Italy
- 7Wageningen Plant Research, Wageningen University & Research, Wageningen, Netherlands
- 8National Institute of Plant Genome Research, New Delhi, India
- 9CERNAS-Research Center for Natural Resources, Environment and Society, Department of Environment, Escola Superior Agrária de Coimbra, Coimbra, Portugal
Somatic embryogenesis (SE) is the most striking and prominent example of plant plasticity upon severe stress. Inducing immature carrot seeds perform SE as substitute to germination by auxin treatment can be seen as switch between stress levels associated to morphophysiological plasticity. This experimental system is highly powerful to explore stress response factors that mediate the metabolic switch between cell and tissue identities. Developmental plasticity per se is an emerging trait for in vitro systems and crop improvement. It is supposed to underlie multi-stress tolerance. High plasticity can protect plants throughout life cycles against variable abiotic and biotic conditions. We provide proof of concepts for the existing hypothesis that alternative oxidase (AOX) can be relevant for developmental plasticity and be associated to yield stability. Our perspective on AOX as relevant coordinator of cell reprogramming is supported by real-time polymerase chain reaction (PCR) analyses and gross metabolism data from calorespirometry complemented by SHAM-inhibitor studies on primed, elevated partial pressure of oxygen (EPPO)–stressed, and endophyte-treated seeds. In silico studies on public experimental data from diverse species strengthen generality of our insights. Finally, we highlight ready-to-use concepts for plant selection and optimizing in vivo and in vitro propagation that do not require further details on molecular physiology and metabolism. This is demonstrated by applying our research & technology concepts to pea genotypes with differential yield performance in multilocation fields and chickpea types known for differential robustness in the field. By using these concepts and tools appropriately, also other marker candidates than AOX and complex genomics data can be efficiently validated for prebreeding and seed vigor prediction.
Background
Environmental changes challenge plant plasticity at both individual and evolutionary levels. Moreover, environment can blur borders between hormone-related individual physiological characteristics and species-relevant, genetic traits by acting on meristems/stem cells (Cutri et al., 2013). Environmental changes are transmitted to plants via complex, diverse signaling. External signals are translated via secondary messengers to adaptive mild or severe molecular-physiological responses. Adaptive growth and developmental regulation associate to complex concentration- and spatiotemporal-dependent balances of networking hormones. Transcription and epigenetic factors are often rated as master regulators. They play critical roles during adaptation and link to genome-wide structural changes, such as copy number variability (CNV) and chromatin remodeling (Arnholdt-Schmitt, 2004; Gabur et al., 2019). This complex scenario connects to wide concerted gene networks, where DNA sequences form the structural primary basis for metabolism (Arnholdt-Schmitt, 2005a). Reactive oxygen species (ROS), redox-related pathways, and nitric oxide are known to integrate environment signaling via DNA break and repair mechanisms, hormone actions, and communication from cell to nucleus (retrograde communication) to adjust metabolism and physiology for individual genotype adaptation (anterograde communication). ROS induced ROS release’ (RIRR) is a process in which cellular compartments or organelles release ROS, which triggers production of ROS at other sites (Zandalinas and Mittler, 2018). RIRR is explored as crucial pathway to confront abiotic or biotic challenges. Crosstalk between ROS and autophagy linked to hormone signaling is associated to stress tolerance.
Mitochondria play a major role in managing stress response and cell network integration (Galluzzi et al., 2012). They adjust their structures, mobility, and activities upon external and internal stimuli to optimize growth and development via mitochondrial retrograde response signaling. Expression of transcription factor (TF) ANAC017 in complex hierarchy of 12 downstream TFs can lead to higher expression of genes related to mitochondrial stress and cell death/autophagy (Meng et al., 2019). Somatic embryogenesis (SE) is stress-inducible (Zavattieri et al., 2010). The balance between survival, embryo development, and programmed cell death (PCD) together with suspensor elimination seems critical for SE efficiency in angiosperms and gymnosperms (Smertenko and Bozhkov, 2014). Mitochondria play crucial role in manifesting two waves of PCDs during SE in conifers (in Arnholdt-Schmitt et al., 2016). They perceive signals from environment through adaptive membrane fluidity and transfer signals via respiration mediated by ROS for adaptive metabolic adjustment at cell, tissue, organ, and organism level. Metabolic profiling in young maize roots is promising for predicting heterosis in field (de Abreu E Lima et al., 2017). Calorespirometry is the only technology that enables linking temperature-dependent, metabolic adjustment based on respiration traits to growth performance (Hansen et al., 2005; Hansen et al., 2009; Scafaro et al., 2017). In a simple, rapid way, it allows exploring almost simultaneously rates of both heat emission and CO2 production (Hansen et al., 2005; Arnholdt-Schmitt, 2017). Calorespirometry data from Prosopis cineraria correlated closely with embryogenic tissue response. Therefore, calorespirometry was recommended as an early predictive tool for reprogramming events (Kim et al., 2006). In carrot, alternative oxidase 1 AOX1 and AOX2a expression peaks during de novo growth induction, which coincided with a critical time point that can be used for biomass prediction using calorespirometry (Campos et al., 2016). In maize, salicylic acid–induced earlier seed germination could be sensed by increased heat rates (Moravcová et al., 2018). Calorespirometry enables studying in unicellular systems the effect of isolated AOX genes on growth performance linked to gross metabolism and cell density (Arnholdt-Schmitt and Patil, 2017). Recently, calorespirometry is being developed as a general tool for conventional and molecular plant/holobiont selection associated to plant robustness and germination efficiency (Arnholdt-Schmitt et al., 2015; Arnholdt-Schmitt et al., 2018).
Carrot Seeds Provide a Powerful Experimental System to Study Cell Fate and Developmental Plasticity
Seed germination and induction of SE are adaptive responses to environmental changes. Germination of dry seeds occurs upon water imbibition in an oxygen-containing environment. This process, visible by radicle emergence, can be seen as environment-induced stress. Seeds recover from the dry and oxygen-low status and acclimate to water- and oxygen-enriching environment. On the other hand, SE is known as adaptive response to higher stress levels (Zavattieri et al., 2010; Fehér, 2014; Winkelmann, 2016). In vitro induction of SE is the most striking example of stress-inducible cell reprogramming due to totipotency acquisition (Frederico et al., 2009a). This response emerges when survival mechanisms are activated by extreme conditions. It was proposed that SE can be used as an experimental system to identify differential genotype behavior linked to stress tolerance (Frederico et al., 2009a; Afuape et al., 2013).
In early steps of zygotic and SE, auxin distribution plays a predominant role for polarity and pattern formation (Winkelmann, 2016; Robert et al., 2018; Figueiredo and Köhler, 2018). The effect of exogenous and endogenous auxin on growth and development depends on complex feedback networks associated to spatiotemporal biogenesis, transport, and gradients of endogenous auxin at the tissue and cell levels. Mitochondrial disturbance was unambiguously shown to be harmful for auxin signaling (Kerchev et al., 2014). Abscisic acid (ABA) and gibberellins are primary hormones that regulate seed dormancy and germination in a concentration-dependent manner (Miransari and Smith, 2014; Shu et al., 2016). However, ABA-mediated seed dormancy seems to be inevitably controlled through auxin-responsive factors (ARFs) besides micro RNAs (miRNAs) (Liu et al., 2013). Also, accumulation of endogenous auxins was found to be concomitant with germination initiation (Bialek et al., 1992). Germination can be promoted or inhibited through supplying auxin depending on its concentration, which is species-dependent (Garrard, 1954). In carrot seeds, zygotic embryos are still immature after desiccation (Homrichhausen et al., 2003). When carrot seeds are treated with high auxin from imbibition, germination is suppressed, and at the same time, embryogenic calli are induced that can later give rise to multiple propagules (Frederico et al., 2009a). Thus, the combined experimental system including germination and SE induction provides excellent opportunity to study changes between different levels of stress severity. This makes it especially useful to reveal relative importance of potentially relevant factors that interacts during stress adaptation.
AOX—A Mere Stress Indicator/Alleviator or Rather an Efficient Coordinator of Adaptive Morphophysiological Plasticity?
Alternative oxidase is key enzyme in mitochondrial alternative respiration (AR), an additional cyanide-insensitive pathway for driving electrons to oxygen, thereby decreasing ROS production. This alleviates various stress conditions, including drought, low oxygen, and temperature. AOX gene family is nucleus encoded, composed of one to six members distributed in two subfamilies (AOX1 and AOX2) (Costa et al., 2014; Costa et al., 2017). Vanlerberghe et al. (2009) postulated a “master role” for AOX through degree of homeostasis signaling. It can improve cell survival rates and seems to determine threshold for inducing PCD. This was shown in various organisms across kingdoms (Rogov et al., 2014; Fernandez Del-Saz et al., 2018). The role of AtAOX1a in maintaining cellular redox homeostasis, protecting cells against oxidative damage, improving the chance of survival and sustaining growth under oxidative stress in Saccharomyces cerevisiae was reported (Vishwakarma et al., 2016). Ivanova et al. (2014) highlighted reciprocal interaction of mitochondrial stress and auxin signaling for AtAOX1a regulation. It has been confirmed by many recent studies that AOX acts as a positive indicator for plant performance and the role of AOX in complex networks (Scheibe, 2018; Selinski et al., 2018; Wang et al., 2018). Positive effects are mediated mainly through AOX down-regulation with the help of transcriptional suppression and protein activity regulation that confers regulatory and metabolic flexibility (Fernandez Del-Saz et al., 2018; Selinski et al., 2018). AOX1 and AOX2 have differences in their conserved regions flanking CysI (conserved cysteine residue) (Costa et al., 2009a; Costa et al., 2014). They are differentially regulated by tricarboxylic acid cycle metabolites related to different structures and functionalities (Albury et al., 2009; Elliott et al., 2014; Selinski et al., 2017). However, Anthony Moore stressed that AOX activity conferred by all gene family members together is important (personal communication), since the mechanism of enzymatic activity is the same, irrelevant of AOX gene and organism (Elliott et al., 2014). Coregulation versus differential regulation of DcAOX1 and DcAOX2a in carrot was connected to earlier or later stages in embryo development (Frederico et al., 2009a). Co- upregulated transcription of both genes was observed during de novo growth induction from quiescent root phloem tissue (Campos et al., 2016).
In 2006, the hypothesis was raised that AOX can serve as a functional marker for efficient cell reprogramming under stress (Arnholdt-Schmitt et al., 2006; Clifton et al., 2006; Arnholdt-Schmitt, 2015), and a symposium and special issue (Physiol Plantarum, 2009) were exclusively dedicated to its role in yield stability. The role of AOX for target cell or tissue reprogramming and plant plasticity was studied in diverse in vitro and in vivo systems, such as in olive rooting (Santos Macedo et al., 2009; Hedayati et al., 2015; Velada et al., 2018), in carrot SE (Frederico et al., 2009a; Arnholdt-Schmitt et al., 2016), carrot primary cultures (Campos et al., 2009; Campos et al., 2016), and germination of Hypericum perforatum (Velada et al., 2016). AOX gene diversity is characterized extensively (Costa et al., 2009b; Polidoros et al., 2009; Cardoso et al., 2015; Nobre et al., 2016; Nogales et al., 2016a), and the importance of ecotyping (Costa and Svensson, 2015), epigenetics (Noceda et al., 2015; Costa and Arnholdt-Schmitt, 2017), and new software based on artificial intelligence (Quaresma et al., 2015) for functional marker development was highlighted. Considering the plant–endophyte interaction for functional genomics was stressed in general (Arnholdt-Schmitt et al., 2014; Nogales et al., 2016b), and involvement of AOX from both partners in symbiotic systems is indicated (Campos et al., 2015; Mercy et al., 2017).
During soybean germination, Yentur and Leopold (1976) observed transition from alternative to normal respiration at 4 to 8 hours after seed imbibition (HAI). AR was linked to germination initiation, seedling growth, and chlorophyll synthesis. Similar results were reported in other species (Esashi et al., 1979). In cocklebur seeds, AR increased by 5 to 7 HAI and contributed heavily to germination at its earliest stage. By applying AOX-inhibitor salicylhydroxamic acid (SHAM) to seeds, Esashi et al. (1981) showed that the most sensitive phase to SHAM ranged from 6 to 12 HAI. These authors also observed that seeds with higher germination potential showed higher capacities of AR. In Orobanche, a parasitic plant, “clear-cut effect” germination through AOX inhibition corresponds to changes in oxygen consumption and had functional implication for controlling germination and pathogenicity (Nun et al., 2003). In early stages, germination was sensitive to lower O2-tension, indicating relevance of AR, since AOX has lower affinity to O2 than cytochrome oxidase (Bonner, 1973, cited in Yentur and Leopold, 1976). Avena fatua showed maximal germination efficiency when seeds were treated by cyanide or azide during 4 to 16 and 4 to 12 HAI respectively. While stimulation of respiration by azide appeared to be SHAM sensitive, once induced, both azide- and cyanide-stimulated respirations were insensitive to SHAM (Tilsner and Upadhyaya, 1987). In dry and mature seeds of Arabidopsis thaliana, and during early germination, Saisho et al. (2001) and Clifton et al. (2006) found high expression of AOX2, with a rapid decrease of transcripts after 12 HAI. However, AR capacity seemed dependent on both family members, AOX2 and AOX1a (Saisho et al., 2001). A critical role of ROS for germination was indicated by positive effects of H2O2 and adverse response to AOX inhibition; the importance of exogenous H2O2 in germination was also confirmed in other plant species (Barba-Espín et al., 2012). Auxins can induce oxidative stress and substitute H2O2 treatment. In olive, auxin-dependent de novo root induction was linked to H2O2 (Santos Macedo et al., 2009, and references herein). An inhibitory effect of SHAM on olive rooting was observed in micro-shoots and semi-hardwood cuttings (Santos Macedo et al., 2012; Porfirio et al., 2016). SHAM inhibition appeared to be specific to rooting and did not interfere with preceding callus formation (Santos Macedo et al., 2012). Promising association between rooting ability and OeAOX2 gene polymorphism in olive cuttings was first suggested by Santos Macedo et al. (2009) and later confirmed in genetic studies by Hedayati et al. (2015). Recently, Velada et al. (2018) associated OeAOX1a and OeAOX1d transcript accumulation to induction, initiation, and expression phase of rooting. Both genes were coregulated and dramatically increased in early stages without visible root induction with a maximum peak of transcript accumulation at 8 HAI and rapid decrease from 1 DAIs (days after imbibition). At 4 DAIs, a second smaller peak was observed corresponding to initiation of first meristemoids and morphogenetic zones. A third increment occurred for both genes around 10 to 14 DAIs, which corresponds to callus formation from 12 to 14 DAIs before root emergence starts at around 22 DAIs. At the earliest increments, OeAOX1a was more pronounced than AOX1d, and higher transcript levels were observed at 14 DAIs when cell division activity increased. It remains to be seen whether differences in transcript accumulation might be related to distinct auxin responsive elements observed within both promoter regions.
In SE, less information is available on AR, but a role of AOX was proposed by Arnholdt-Schmitt et al. (2006; 2016). In Abies alba, AOX was found to act during SE induction via ROS capturing as an anti-apoptotic factor (Petrussa et al., 2009). Frederico et al. (2009a) reported early up-regulation of AOX transcripts during initiation of carrot somatic embryo development upon auxin depletion. Transcript level increase was consistent with a 2.5-fold up-regulated AOX1 and 1.5-fold down-regulated AOX2a. During late embryo development, differential regulation was observed, and it was reversibly suppressed by SHAM supply and depletion. This research was aimed to identify promising marker candidates for assisting SE in recalcitrant species such as conifers, finally promising functional marker candidates could be identified from DcAOX2b (Frederico et al., 2009b; Frederico, 2017).
In Figure 1, the advanced rationale of our perspective on the coordinative role of AOX in stress management and its practical impact for plant holobiont breeding and applied functional hologenomics (Nogales et al., 2016b) are explained. It starts from original research (A–C) until proof-of-concept validation (D) (methods are provided in Supplementary file). In conclusion, we found that (a) the level of early AOX transcript stress signaling can be relevant and associated to subsequent changes in metabolism and morpho-physiology; (b) when strong morphological reprogramming is induced (by adding highly concentrated, stressful auxin that induces SE) versus expression of already induced morpho-physiology (seed imbibition in auxin-free medium that initiates germination), an early higher AOX transcript peak appears (Supplementary Figure S1); (c) higher early AOX stress signaling is linked to slower AOX stress signaling recovery associated to slower global metabolic adjustment and increase in carbon use efficiency (CUE); (d) very first hours of seed germination are relevant for both, individual development (germination efficiency, seed vigor) and identification of differences in seed vigor due to genetics or seed treatment; (e) differences in seed vigor and plant field performance can be predicted from early hours after seed imbibition by SHAM-inhibition trials and global metabolism data based on respiration traits (oxycaloric equivalent); (f) arbuscular mycorrhizal fungi (AMF) inoculation interfered with germination efficiency affecting root length growth rather than by initiating germination; (g) AMF inoculation interacts with SHAM treatment on root growth and palliates effects of the inhibitor on germination efficiency (Supplementary Figure S2); (h) AMF inoculation effects can be modified by endophytes available in seeds.
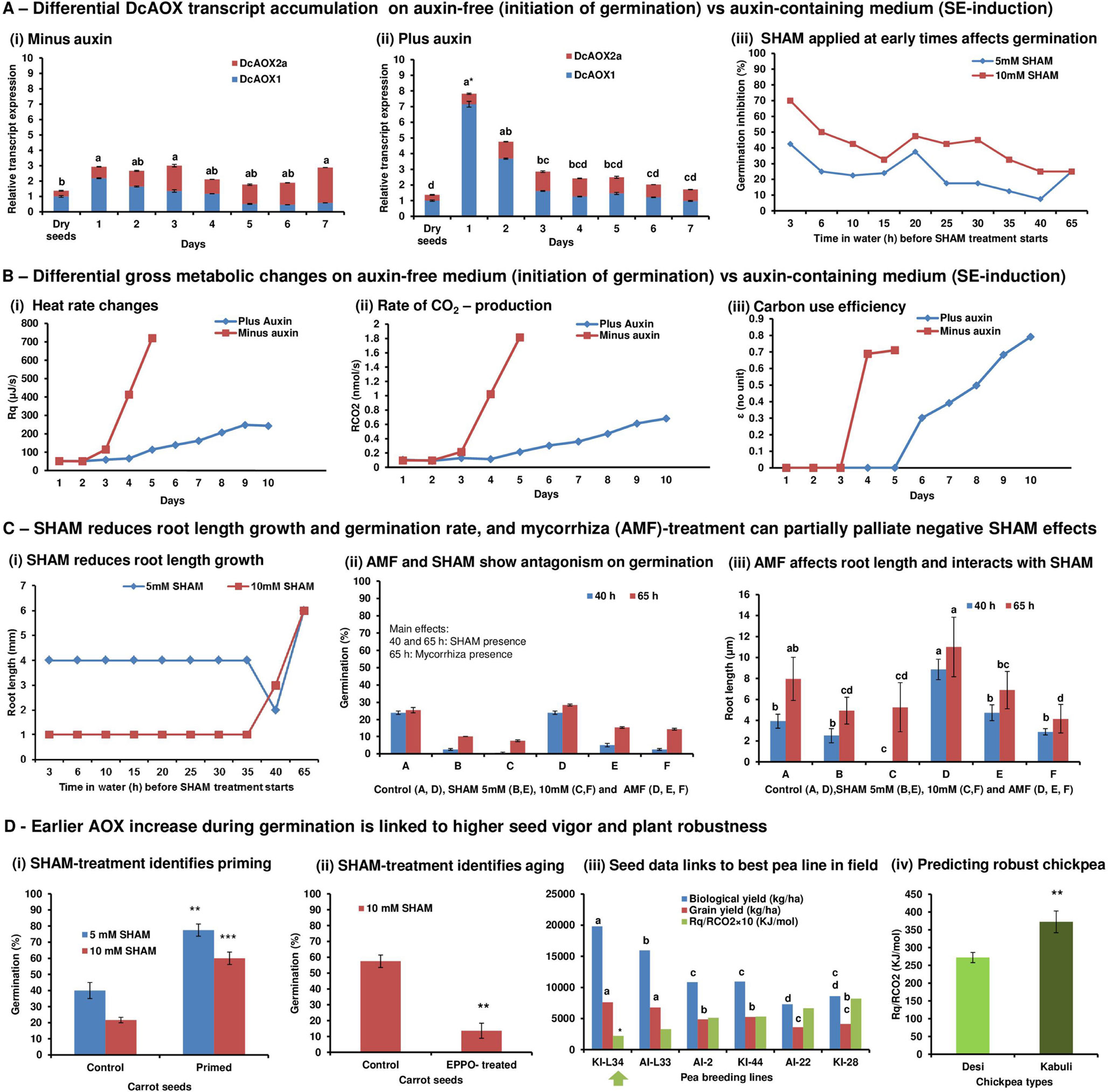
Figure 1 (A) Differential DcAOX transcript accumulation on auxin-free (initiation of germination) versus auxin-containing medium (SE induction). At day 1 of carrot seed cultivation with and without auxin, a significant increase in AOX transcript accumulation was observed (Ai, ii). This was mainly due to AOX1 (Figure S1) and confirms its role as stress indicator as shown in many other systems (see references in text). However, when SE was induced, this peak was strikingly higher, which indicates higher stress. In the following days, the level of AOX transcripts remained stable in the absence of auxin, since decreasing levels of AOX1 were compensated by slightly variable, but increasing levels of AOX2a. In this equilibrated situation, growth of seed embryos were initiated, which was visible by root emergence. AOX2 transcript levels increased to significantly higher values at day 7 when seedling growth was established. On the contrary, when SE was induced, slower stress recovery was indicated; the high peak of AOX transcripts was rapidly going down due to significantly decreasing amounts of AOX1 transcripts. However, AOX2a transcripts remained at stable level. From day 4, the overall transcripts was stabilized at a low level. In auxin-containing medium that mediated SE induction, no increase in AOX2a could be observed during the experimental time period (Aii). Sequential SHAM inhibition during the initiation of germination confirmed that most critical events for germination happen from 3 to 15 HAI (Aiii). (B) Differential gross metabolic changes on auxin-free medium (initiation of germination) versus auxin-containing medium (SE-induction). From day 2 of carrot seed imbibition, i.e. 1 day after AOX stress signaling was observed, metabolic heat emission rate (Bi) and rate of CO2 production (Bii) increased. At that time, we started to observe emergence of radicles. From day 3, carbon was efficiently translated into growth indicated by an increased value for carbon use efficiency (CUE) (Biii). When SE was induced by auxin at imbibition, metabolic heat emission rate was transiently suppressed, and a slighter increase in heat rate and RCO2 started only after day 4. Nevertheless, this increase did not reach the same level as observed already at day 4 during germination and remained still low until end of experiment at day 10 after imbibition. While during germination CUE increased rapidly to 0.7, a value that indicates cell division growth (Hansen et al., 2005), during SE this value was reached only from day 9, which can thus be supposed to indicate initiation of embryonic callus formation. (C) SHAM reduces root length growth and germination rate and mycorrhiza (AMF) treatment can partially palliate negative SHAM effects. Sequential SHAM treatment during the first 35 HAI changed the mean length of emerging roots in comparison to control seeds (only in water until 65 HAI) in a concentration-dependent manner when observed at 65 HAI (Ci). However, no differential effects were observed when treatment start varied from 3 to 35 HAI. When SHAM was supplied only at 40 HAI, root length could no longer be differentially affected by inhibitor concentration. Influence of SHAM and AMF on the germination rate and root length was represented in graphs (Cii, iii). AMF-inoculated seeds increased germination efficiency by affecting root length growth rather than by affecting early initiation of germination. AMF interacted with SHAM treatment on root growth and could partly compensate SHAM-reduced germination rate. Additionally, endophytes available in the seeds blocked the positive effect of added AMF on root growth (visible already at 40 HAI) and affected % of germination only when observed at 65 HAI (shown in Figure S2). Differences in root length between treatments for each time are stated with different letters (α = 0.05). (D) Earlier AOX increase during germination is linked to higher seed vigor and plant robustness. As proof-of-concept trials, control and primed, coated commercial F1 carrot seeds (cv. Nerac 2) (Di), control, and elevated partial pressure of oxygen (EPPO) stress–treated carrot seeds (cv. Nantaise 2/Milan) (Dii), Pea seeds from breeding lines with top-ranking, mid-ranking and bottom ranking biological and grain yielding ability over three test environments (Annicchiarico et al., 2019) were compared for 18 each of two RIL populations (Diii) and two chickpea types known for differential yield performance and multistress tolerance in field were compared (Div). (Di) Primed, coated seeds show higher germination efficiency and have improved seedling vigor in field (seeds and information provided by BejoSamen). These seeds show increased earlier metabolic heat emission rate and CO2 production (data not shown). When treated with SHAM from 10 HAI, germination efficiency in primed, coated seeds could not be reduced as strongly as the control. This is congruent with our expectation that AOX signaling is critically relevant for germination efficiency. From the described results (Panels A–C), primed seeds could be expected to demonstrate an earlier stress-related AOX peak than control seeds and a more efficient stress recovery indicated by rapidly lowered AOX1 transcript levels. Thus, primed seeds could supposedly be less sensitive to early SHAM inhibition at 10 HAI during germination. In fact, this could be shown homogeneously across all three repetitions by using 3× bulked samples of 40 seeds. Further, heat rate increase of primed seeds could completely be suppressed when SHAM was applied at 2 HAI, while this did not happen in control seeds where heat rate increase was only postponed (data not shown). (Dii) EPPO-stressed seeds induce aging and showed already 2 weeks after having treated the dry seeds, a significantly reduced speed of germination at T50 (data not shown). In agreement with the expectation that higher vigor control seeds are at the start of SHAM treatment at 10 HAI, which are already less sensitive to the AOX inhibitor, EPPO-stressed seeds display lower germination rates homogeneously in all three repetitions. (Diii) Pea breeding lines that were grown by a breeder in three locations demonstrated significant differences in yield performance. Only the best breeding line KI-L34 was selected by the breeder for cultivar registration based on complex field data. By applying calorespirometry at 10 HAI at a constant temperature (25°C) and using oxycaloric equivalent (Rq/RCO2) values, the breeding lines could be ranked a posteriori with an inverse relationship to yield data. The breeder-selected line for registration was in fact the only one, significantly different from all others. Thus, applying our method would provide a highly innovative, predictive biomarker for early plant selection on yield ability. (Div) Early chickpea plant vigor is critical for plant productivity under terminal drought conditions (Sivasakthi et al., 2017). From the two principle chickpea types, Desi and Kabuli, it is known from vast field experience that Desi is clearly superior in terms of multistress tolerance and yield performance (Purushothaman et al., 2014). By applying our approach, we can discriminate both types and predict a posteriori the known better yield stability of Desi by a lower oxycaloric equivalent (Rq/RCO2) value due to differential carbon use at 10 HAI.
To validate generality of our insights, we searched for in silico data. We reviewed public transcriptome data on germination of Arabidopsis and soybean and on auxin-induced SE from Arabidopsis wild type (Col-0) and nonembryogenic mutant clf/swn (Table 1). Taken all findings together, higher initial expression of AOX1a appears to be essential for efficient root emergence, while higher expression of AOX2 (a/b/c type) is required for further development. Related to SE, in wild type (Col-0), AtAOX1 transcript increase was important to overcome epigenetic SE barriers. It was potentiated through injury, while AOX2 expression remained stable. In clf/swn, AOX1 did not increase, and AOX2 was barely detected. Appropriate in silico studies on plant–endophyte interaction were not available from germination trials and SE. However, endophytes modulate AOX transcripts in a species-, stress-, and development-dependent manner (Supplementary Table S1). It is confirmed that endophytes interact specifically with stress-related AOX gene family members. In Arabidopsis, salt stress increased AOX1a expression, while Enterobacter species reduced its expression. However, when salt stress was applied together with endophytes, mRNA levels of AOX1a were maintained low (de Zélicourt et al., 2018) (Table S1).
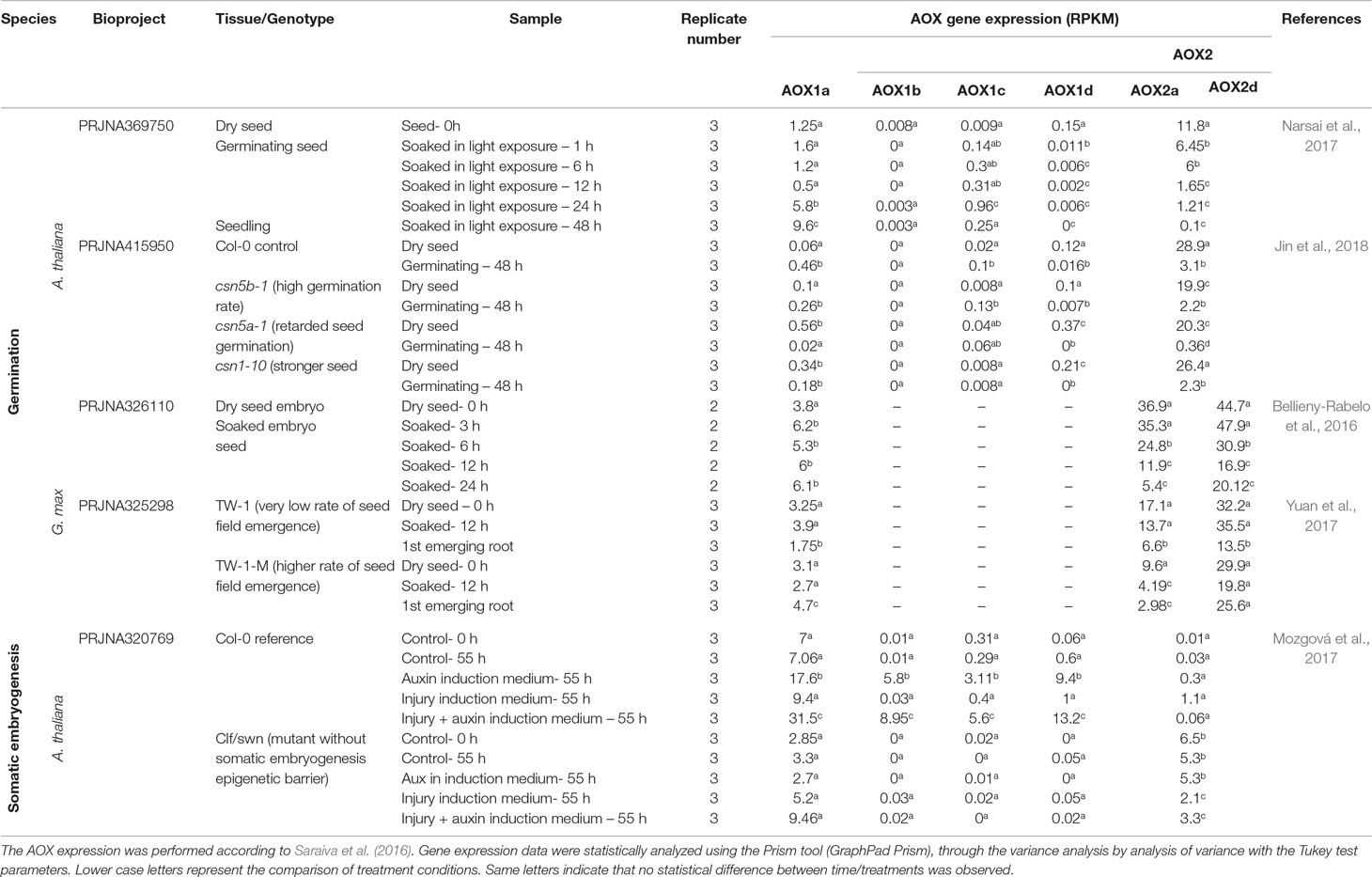
Table 1 Expression of AOX genes during germination (Arabidopsis and soybean) and somatic embryogenesis (Arabidopsis) using RNA-seq data.
Outlook
Biologists aim to understand biological and ecological complexity. However, for economy, it is important that biological systems work properly as expected. This contrasting view from application is the reason why top-down approaches are required for crop improvement rather than bottom-up strategies starting with complexity (Arnholdt-Schmitt, 2005a). First, species-specific target tissues/cells need to be identified that associate to outlined traits, such as yield stability; for example, adaptive growth and development responses depend on stem cells, and molecular characterization needs to start at that cell level. This insight is widely agreed within the community working with AOX (Arnholdt-Schmitt, 2005b; Nogales et al., 2015; Ragonezi and Arnholdt-Schmitt, 2017, Fernandez Del-Saz et al., 2018; Selinski et al., 2018). For breeding purposes, early selection at seed level is highly advantageous. Individual genetic information is physically concentrated due to high percentages of stem cells. Thus, it is easily accessible for characterization without requiring necessarily tissue or cell isolation. Moreover, during seed imbibition, the embryo is still at an early point of epigenetic programming and provoked for the first time to show its basic genetic capacity for stress management. These might be the reasons why successful prediction of later plant responses can already be done from germinating seeds.
Biological systems are unique and have their individual internal complexity that drives adaptive responses. Nevertheless, breeding and agricultural management can improve the probability that biological systems will function as expected. This strategy was successful over centuries. However, biological, ecological, and socio-economical systems underlie permanent, mutually dependent changes and require continuous adaptation. Plants with high general plasticity or robustness provide a better chance to sustain changing complex conditions and to enable the highest economic output.
Until the 1970s, it was believed that individual enzymes might play a key role in crop improvement for stable yields. However, this view changed (see in Arnholdt-Schmitt, 2005a) along with growing knowledge on biological complexity, the crucial role of quantitative genetics, and the importance of (not only social, but also) biological and genome-wide networks (systems biology). This is also the reason why a master role of AOX has been recently questioned (Scheibe, 2018; Wang et al., 2018). It is not easily accepted that one enzyme or pathway might play a critical, unique role for determining general fitness and productivity of plants. This drastic view is related to believing more in networks instead of individual components. However, the presented new fundamental insights applied to seeds with differential vigor strengthen our perspective that the small enzyme “clan” of AOX can play a key coordinative role for predicting seed and plant performance. Nevertheless, this view does not exclude that other types of marker candidates may also be successful. SHAM inhibits AOX, but it can affect also other enzymes and pathways. In contrary to other AOX inhibitors, such as propyl gallate, SHAM distinguishes AOX gene variants (Berthold, 1998), which might make this inhibitor especially interesting for genotype discrimination. Therefore, studying complex effects of SHAM on initiating germination versus SE in the proposed experimental carrot system might help identifying further molecular or metabolic candidates for genotyping. However, our extended in silico search (not shown) for candidates from auxin metabolism (Porfirio et al., 2016), urease, and others strengthened the master role of AOX. Besides, we got hints for a crucial role of growth- and development-related, genome-wide CNV for the most important repetitive elements in plants. Thus, it remains to be explored whether AOX functionality connects also to adaptive growth determination via global genome regulation as indicated by Arnholdt-Schmitt (1993, 1995, and 2004).
Data Availability
All datasets generated for this study are included in the manuscript and the Supplementary Files.
Author Contributions
GM performed AOX transcript analyses, calorespirometry, and SHAM studies demonstrated in Figures 1A, B and Ci as part of her PhD studies. RB measured endophyte effects (Figures 1Cii + iii). BA-S analyzed primed seeds (Figure 1Di), and EM elevated partial pressure of oxygen (EPPO)–treated seeds (Figure 1Dii). EPPO treatment was performed by SG and JK. SS together with EM analyzed pea genotypes; pea lines had been bred and produced in multi-location field trials by PA; JC and KL performed all in silico studies (Table 1 and S1). CN performed statistics analyses on all carrot trials and together with JC was strongly involved in interpreting all data; RS supervised together with SRK the laboratory work of GM and RB. AK and JGK proposed and integrated chickpea studies, and AK performed together with EM calorespirometry analyses. All were involved in manuscript writing and data interpretation. SRK managed final organization of the manuscript for submission; all coauthors approved the final manuscript. BA-S designed and coordinated the overall project.
Conflict of Interest Statement
The authors declare that the research was conducted in the absence of any commercial or financial relationships that could be construed as a potential conflict of interest.
Acknowledgments
GM is grateful to UGC, India, for doctoral grant from BSR fellowship. GM, RS, and BA-S acknowledge support for academic cooperation and researchers mobility by the India-Portugal Bilateral Cooperation Programme (2013-2015), funded by “Fundação para a Ciência e Tecnologia” (FCT), Portugal, and the Department of Science and Technology (DST), India. GM also thanks Drs. Isabel Velada and Helia Cardoso for transferring skills on real-time PCR analyses during her stay at the University of Évora. RB thanks RS for the opportunity to accept him for a short-term collaboration in his laboratory and is grateful to SRK for integration and his overall supervision. RS is thankful to Dr. P. Muthukumar, Associate Professor, Department of Botany, Bharathiar University, for providing AMF. PA, SG, JK, EM, and BA-S acknowledge support through the European Horizon2020 project LIVESEED GRANT NO. 727230. BA-S thanks the company Bejo samen GmbH for providing control and primed, coated seeds of cv. Nerac 2. AK, JGK, EM, and BA-S acknowledge financial support for researchers mobility through FCT (Portugal) and DST (India) supported by the bilateral cooperation program India-Portugal 2017-2019. JC is grateful to CNPq for the Researcher fellowship. KL acknowledges doctoral grant from CNPq. CN acknowledges support by UNEMI [Universidad Estatal de Milagro (State University of Milagro), Ecuador] through financing in 2016 the project “Involvement of oxidative stress-regulator genes in oliveadventitious rooting.” so as to the international scientific network BIOALI-CYTED, which both contributed to present insights and to establish FunCROP contacts. BA-S is grateful for the continued availability of Thomas Nothnagel, Julius Kühn-Institut, Quedlinburg, Germany, since 2005 to help with his expertise in breeding and providing freely appropriate carrot seed material that helped her to establish the basic methodologies for research translation. BA-S also appreciates in this sense support through the companies Sativa (Switzerland) and Vitalis (The Netherlands) as LIVESEED partners. BA-S and EM want to express special thanks to Véronique Chable (LIVESEED partner) for support and her spirit to advocate “innovation” to the benefit of society. BA-S is grateful to the Portuguese company NORLEQ for support and excellent technical service to apply calorespirometry’. Finally, we would like to recognize the reviewer’s competent and strong efforts to help us improving our ms: we enjoyed the open-minded discussion.
Supplementary Material
The Supplementary Material for this article can be found online at: https://www.frontiersin.org/articles/10.3389/fpls.2019.01134/full#supplementary-material
References
Afuape, S. O., Sayre, R., Tawanda, Z., Kahya, S. S. (2013). Transgenic cassava lines carrying heterologous alternative oxidase (AtAOX1a) showed impaired quantitative and qualitative response to embryogenesis. Afr. J. Biotechnol. 12 (27), 4303–4309. doi: 10.5897/AJB12.2923
Albury, M. S., Elliott, C., Moore, A. L. (2009). Towards a structural elucidation of the alternative oxidase in plants. Physiol. Plant 137 (4), 316–327. doi: 10.1111/j.1399-3054.2009.01270.x
Annicchiarico, P., Nazzicari, N., Pecetti, L., Romani, M., Russi, L. (2019). Pea genomic selection for Italian environments. BMC Genomics 20 (1), 603. doi: 10.1186/s12864-019-5920-x
Arnholdt-Schmitt, B. (1993). Rapid changes in amplification and methylation pattern of genomic DNA in cultured carrot root explants (Daucus carota L.). Theor. Appl. Genet. 85 (6-7), 793–800. doi: 10.1007/BF00225021
Arnholdt-Schmitt, B. (1995). Physiological aspects of genome variability in tissue culture. II. Growth phase-dependent quantitative variability of repetitive BstNI fragments of primary cultures of Daucus carota L. Theor. Appl. Genet. 91 (5), 816–823. doi: 10.1007/BF00220965
Arnholdt-Schmitt, B. (2004). Stress-induced cell reprogramming. A role for global genome regulation? Plant Physiol. 136 (1), 2579–2586. doi: 10.1104/pp.104.042531
Arnholdt-Schmitt, B. (2005a). Efficient cell reprogramming as a target for functional-marker strategies? Towards new perspectives in applied plant-nutrition research. J. Plant Nutr. Soil Sci. 168 (4), 617-624. doi: 10.1002/jpln.200420493
Arnholdt-Schmitt, B. (2005b). Functional markers and a ‘systemic strategy’: convergency between plant breeding, plant nutrition and molecular biology. Plant Physiol. Biochem. 43 (9), 817–820. doi: 10.1016/j.plaphy.2005.08.011
Arnholdt-Schmitt, B. (2015). “From AOX gene diversity to functional marker development,” in Alternative respiratory pathways in higher plants. Eds. Gupta, K. J., MuR, L. A. J., Neelwarn, E. B. (Oxford: John Wiley & Sons, Inc.), 235–239.
Arnholdt-Schmitt, B. (2017). “Respiration traits as novel markers for plant robustness under the threat of climate change: a protocol for validation,” in Plant respiration and internal oxygen. Ed. Gupta, K. J. (New York, NY: Humana Press), 183–191. doi: 10.1007/978-1-4939-7292-0_15
Arnholdt-Schmitt, B., Patil, V. K., (2017). “Studying individual plant AOX gene functionality in early growth regulation: a new approach,” in Plant respiration and internal oxygen: methods and protocol biology. Eds. Walker, John M., Gupta, K. J. (New York, NY: Humana Press), 235–244. doi: 10.1007/978-1-4939-7292-0_20
Arnholdt-Schmitt, B., Costa, J. H., de Melo, D. F. (2006). AOX—a functional marker for efficient cell reprogramming under stress? Trends Plant Sci. 11 (6), 281–287. doi: 10.1016/j.tplants.2006.05.001
Arnholdt-Schmitt, B., Hansen, L. D., Nogales, A. (2015). Calorespirometry, oxygen isotope analysis and functional-marker–assisted selection (‘CalOxy-FMAS’) for genotype screening: a novel concept and tool kit for predicting stable plant growth performance and functional marker identification. Brief. Funct. Genomics. 15 (1), 10–15. doi: 10.1093/bfgp/elv008
Arnholdt-Schmitt, B., Mohanapriya, G., Ramalingam, S., Santos Macedo, E., Coasta, J.H., (2018). “Predicting biomass production from plant robustness and germination efficiency by calorespirometry,” in Biofuels: greenhouse gas mitigation and global warming, next generation biofuels and role of biotechnology, vol. 81. Eds. Kumar, Ashwani, Ogita, Shinjiro, Yau, Yuan-Yeu (India: Springer Nature), ISBN: 978-81-322-3761-7. doi: 10.1007/978-81-322-3763-1_5
Arnholdt-Schmitt, B., Ragonezi, C., Cardoso, H., (2016). “A central role of mitochondria for stress-induced somatic embryogenesis,” in In vitro embryogenesis in higher plants. Eds. M. A. Garmana and Lambard (New York, NY: Humana Press), 87–100. doi: 10.1007/978-1-4939-3061-6_4
Arnholdt-Schmitt, B., Valadas, V., Döring, M. (2014). Functional marker development is challenged by the ubiquity of endophytes—a practical perspective. Brief. Funct. Genomics 15 (1), 16–21. doi: 10.1093/bfgp/elu049
Barba-Espín, G., Hernández, J. A., Diaz-Vivancos, P. (2012). Role of H2O2 in pea seed germination. Plant Signal Behav. 7 (2), 193–195. doi: 10.4161/psb.18881
Bellieny-Rabelo, D., De Oliveira, E. A., Ribeiro, E. S., Costa, E. P., Oliveira, A. E., Venancio, T.M., et al. (2016). Transcriptome analysis uncovers key regulatory and metabolic aspects of soybean embryonic axes during germination. Sci. Rep. 6, 36009. doi: 10.1038/srep36009
Berthold, D. A. (1998). Isolation of mutants of the Arabidopsis thaliana alternative oxidase (ubiquinol: oxygen oxidoreductase) resistant to salicylhydroxamic acid. Biochim. Biophys. Acta 1364 (1), 73–83. doi: 10.1016/S0005-2728(98)00015-2
Bialek, K., Michalczuk, L., Cohen, J. D. (1992). Auxin biosynthesis during seed germination in Phaseolus vulgaris. Plant Physiol. 100 (1), 509–517. doi: 10.1104/pp.100.1.509
Bonner, W. D. (1973). Mitochondria and plant respiration. Miller, Lawrence Peter. Phytochemistry 3, 221–261.
Campos, C., Cardoso, H., Nogales, A., Svensson, J., Lopez-Ráez, J. A., Pozo, M. J., et al. (2015). Intra and inter-spore variability in Rhizophagus irregularis AOX gene. PLoS One 10 (11), e0142339. doi: 10.1371/journal.pone.0142339
Campos, M. D., Nogales, A., Cardoso, H. G., Kumar, S. R., Nobre, T., Sathishkumar, R., et al. (2016). Stress-induced accumulation of DcAOX1 and DcAOX2a transcripts coincides with critical time point for structural biomass prediction in carrot primary cultures (Daucus carota L). Front. Genet. 7, 1. doi: 10.3389/fgene.2016.00001
Campos, M. D., Cardoso, H. G., Linke, B., Costa, J. H., De Melo, D. F., Justo, L., et al. (2009). Differential expression and co-regulation of carrot AOX genes (Daucus carota). Physiol. Plant 137 (4), 578–591. doi: 10.1111/j.1399-3054.2009.01282.x
Cardoso, H. G., Nogales, A., Frederico, A. M., Svensson, J. T., Macedo, E. S., Valadas, V., et al. (2015). “Natural AOX gene diversity,” in Alternative respiratory pathways in higher plants. Eds. Gupta, K. J., MuR, L. A. J., Neelwarn, E. B. (Oxford: John Wiley and Sons, Inc.), 241–254.
Clifton, R., Millar, A. H., Whelan, J. (2006). Alternative oxidases in Arabidopsis: a comparative analysis of differential expression in the gene family provides new insights into function of non-phosphorylating bypasses. Biochim. Biophys. Acta 1757 (7), 730–741. doi: 10.1016/j.bbabio.2006.03.009
Costa, J. H., Svensson, J. T., (2015). “AOX gene diversity in Arabidopsis ecotypes,” in Alternative respiratory pathway in higher plants. Eds. Gupta, K. J., MuR, L. A. J., Neelwarn, E. B. (Oxford: John Wiley & Sons, Inc.), 255–260.
Costa, J. H., Cardoso, H. G., Campos, M. D., Zavattieri, A., Frederico, A. M., de Melo, D. F., et al. (2009a). Daucus carota L.—an old model for cell reprogramming gains new importance through a novel expansion pattern of alternative oxidase (AOX) genes. Plant Physiol. Biochem. 47 (8), 753–759. doi: 10.1016/j.plaphy.2014.11.013
Costa, J. H., Cardoso, H. G., Campos, M. D., Zavattieri, A., Frederico, A. M., de Melo, D. F., et al. (2014). Corrigendum to “Daucus carota L.—an old model for cell reprogramming gains new importance through a novel expansion pattern of alternative oxidase (AOX) genes.” Plant Physiol. Biochem. 47, 753–759. doi: 10.1016/j.plaphy.2014.11.013
Costa, J. H., De Melo, D. F., Gouveia, Z., Cardoso, H. G., Peixe, A., Arnholdt-Schmitt, B. (2009b). The alternative oxidase family of Vitis vinifera reveals an attractive model to study the importance of genomic design. Physiol. Plant 137 (4), 553–565. doi: 10.1111/j.1399-3054.2009.01267.x
Costa, J. H., dos Santos, C. P., da Cruz Saraiva, K. D., Arnholdt-Schmitt, B. (2017). “A step-by-step protocol for classifying AOX proteins in flowering plants,” in Plant respiration and internal oxygen: methods and protocol. Eds. Walker, J. M., Gupta, K. J., Dr (New York, NY: Humana Press), 225–234. doi: 10.1007/978-1-4939-7292-0_19
Costa, J. H., McDonald, A. E., Arnholdt-Schmitt, B., de Melo, D. F. (2014). A classification scheme for alternative oxidases reveals the taxonomic distribution and evolutionary history of the enzyme in angiosperms. Mitochondrion 19, 172–183. doi: 10.1016/j.mito.2014.04.007
Costa, J. H., Arnholdt-Schmitt, B. (2017). “A driving bioinformatics approach to explore co-regulation of AOX gene family members during growth and development,” in Methods in molecular biology. Eds. John, M., Gupta, K. J. (New York, NY: Humana Press). doi: 10.1007/978-1-4939-7292-0_18
Cutri, L., Nave, N., Ami, M. B., Chayut, N., Samach, A., Dornelas, M. C. (2013). Evolutionary, genetic, environmental and hormonal-induced plasticity in the fate of organs arising from axillary meristems in Passiflora spp. Mech. Dev. 130 (1), 61–69. doi: 10.1016/j.mod.2012.05.006
de Abreu, E, Lima, F., Westhues, M., Cuadros-Inostroza, Á., Willmitzer, L., Melchinger, A. E., Nickoloski, Z. (2017). Metabolic robustness in young roots underpins a predictive model of maize hybrid performance in the field. Plant J. 90 (2), 319–329. doi: 10.1111/tpj.13495
de Zélicourt, A., Synek, L., Saad, M. M., Alzubaidym, H., Jalal, R., Xie, Y., et al. (2018). Ethylene induced plant stress tolerance by Enterobacter sp. SA187 is mediated by 2-keto-4-methylthiobutyric acid production. PLoS One 14, e1007273. doi: 10.1371/journal.pgen.1007273
Elliott, C., Young, L., May, B., Shearman, J., Albury, M. S., Kido, Y., et al. (2014). Purification and characterization of recombinant DNA encoding the alternative oxidase from Sauromatum guttatum. Mitochondrion 19, 261–268. doi: 10.1016/j.mito.2014.03.002
Esashi, Y., Kusuyama, K., Tazaki, S., Ishihara, N. (1981). Necessity of a balance between CN-sensitive and CN-resistant respirations for germination of cocklebur seeds. Plant Cell Physiol. 22 (1), 65–71.
Esashi, Y., Ohhara, Y., Okazaki, M., Hishinuma, K. (1979). Control of cocklebur seed germination by nitrogenous compounds: nitrite, nitrate, hydroxylamine, thiourea, azide and cyanide. Plant Cell Physiol. 20 (2), 349–361. doi: 10.1093/oxfordjournals.pcp.a075819
Fehér, A. (2014). Somatic embryogenesis—stress-induced remodeling of plant cell fate. Biochim. Biophys. Acta 1849 (4), 385–402. doi: 10.1016/j.bbagrm.2014.07.005
Fernandez Del-Saz, N. F., Ribas-Carbo, M., McDonald, A. E., Lambers, H., Fernie, A. R., Florez-Sarasa, I. (2018). An in vivo perspective of the role (s) of the alternative oxidase pathway. Trends Plant Sci. 23 (3), 206–219. doi: 10.1016/j.tplants.2017.11.006
Figueiredo, D. D., Köhler, C. (2018). Auxin: a molecular trigger of seed development. Genes Dev. 32(7–8), 479–490. doi: 10.1101/gad.312546.118
Frederico, A. M., Campos, M. D., Cardoso, H. G., Imani, J., Arnholdt-Schmitt, B. (2009a). Alternative oxidase involvement in Daucus carota somatic embryogenesis. Physiol. Plant 137 (4), 498–508. doi: 10.1111/j.1399-3054.2009.01278.x
Frederico, A. M., Zavattieri, M. A., Campos, M. D., Cardoso, H. G., McDonald, A. E., Arnholdt-Schmitt, B. (2009b). The gymnosperm Pinus pinea contains both AOX gene subfamilies, AOX1 and AOX2. Physiol. Plant 137 (4), 566–577. doi: 10.1111/j.1399-3054.2009.01279.x
Frederico, A. M. (2017). “Exploring alternative oxidase (AOX) as a functional marker candidate for efficient somatic embryogenesis,” in Daucus carota L. PhD thesis (Portugal: University of Evora).
Gabur, I., Chawla, H, S., Snowdon, R. J., Parkin, I. A. P. (2019). Connecting genome structural variation with complex traits in crop plants. Theor. Appl. Genet. 132, 733–750. doi: 10.1007/s00122-018-3233-0
Galluzzi, L., Kepp, O., Trojel-Hansen, C., Kroemer, G. (2012). Mitochondrial control of cellular life, stress, and death. Circ. Res. 111 (9), 1198–1207. doi: 10.1161/CIRCRESAHA.112.268946
Garrard, A. (1954). The effects of β-indolylacetic acid on the germination and root growth of certain members of the cruciferae. New Phytol. 53 (2), 165–176. doi: 10.1111/j.1469-8137.1954.tb05234.x
Hansen, L. D., Criddle, R. S., Smith, B. N., (2005). “Calorespirometry in plant biology,” in Plant respiration: advances in photosynthesis and respiration, vol. 18. Eds. Lambers, H., Ribas-Carbo, M. (Dordrecht: Springer), 17–30. doi: 10.1007/1-4020-3589-6_2
Hansen, L. D., Thomas, N. R., Arnholdt-Schmitt, B. (2009). Temperature responses of substrate carbon conversion efficiencies and growth rates of plant tissues. Physiol. Plant 137 (4), 446–458. doi: 10.1111/j.1399-3054.2009.01287.x
Hedayati, V., Mousavi, A., Razavi, K., Cultrera, N., Alagna, F., Mariotti, R., et al. (2015). Polymorphisms in the AOX2 gene are associated with the rooting ability of olive cuttings. Plant Cell Rep. 34 (7), 1151–1164. doi: 10.1007/s00299-015-1774-0
Homrichhausen, T. M., Hewitt, J. R., Nonogaki, H. (2003). Endo-β-mannanase activity is associated with the completion of embryogenesis in imbibed carrot (Daucus carota L.) seeds. Seed Sci. Res. 13 (3), 219–227. doi: 10.1079/SSR2003139
Ivanova, A., Law, S. R., Narsai, R., Duncan, O., Lee, J. H., Zhang, B., et al. (2014). A functional antagonistic relationship between auxin and mitochondrial retrograde signaling regulates alternative oxidase1a expression in Arabidopsis. Plant Physiol. 165 (3), 1233–1254. doi: 10.1104/pp.114.237495
Jin, D., Wu, M., Li, B., Bücker, B., Keil, P., Zhang, S., et al. (2018). The COP9 signalosome regulates seed germination by facilitating protein degradation of RGL2 and ABI5. PLoS Genet. 14 (2), e1007237. doi: 10.1371/journal.pgen.1007237
Kerchev, P. I., De Clercq, I., Denecker, J., Mühlenbock, P., Kumpf, R., Nguyen, L., et al. (2014). Mitochondrial perturbation negatively affects auxin signaling. Mol. Plant 7 (7), 1138–1150. doi: 10.1093/mp/ssu071
Kim, G. H., Burger, D. W., Lieth, H., Choi, N. H., Jeong, B. R. (2006). Metabolic heat and CO2 evolution rates measured by calorimetry during different in vitro induction processes of cineraria. Thermochim. Acta 447 (1), 52–56. doi: 10.1016/j.tca.2006.04.015
Liu, X., Zhang, H., Zhao, Y., Feng, Z., Li, Q., Yang, H. Q., et al. (2013). Auxin controls seed dormancy through stimulation of abscisic acid signaling by inducing ARF-mediated ABI3 activation in Arabidopsis. Proc. Natl. Acad. Sci. U.S.A. 110 (38), 15485–15490. doi: 10.1073/pnas.1304651110
Meng, X., Li, L., De Clercq, I., Narsai, R., Xu, Y., Hartmann, A., et al. (2019). ANAC017 coordinates organellar functions and stress responses by reprogramming retrograde signaling. Plant Physiol. 180, 634–653. doi: 10.1104/pp.18.01603
Mercy, L., Lucic-Mercy, E., Nogales, A., Poghosyan, A., Schneider, C., Arnholdt-Schmitt, B. (2017). A functional approach towards understanding the role of the mitochondrial respiratory chain in an endomycorrhizal symbiosis. Front Plant Sci 8, 417. doi: 10.3389/fpls.2017.00417
Miransari, M., Smith, D. L. (2014). Plant hormones and seed germination. Environ. Exper. Bot. 99, 110–121. doi: 10.1016/j.envexpbot.2013.11.005
Moravcová, Š., Tůma, J., Dučaiová, Z. K., Waligórski, P., Kula, M., Saja, D., et al. (2018). Influence of salicylic acid pretreatment on seeds germination and some defence mechanisms of Zea mays plants under copper stress. Plant Physiol. Biochem. 122, 19–30. doi: 10.1016/j.plaphy.2017.11.007
Mozgová, I., Muñoz-Viana, R., Hennig, L. (2017). PRC2 represses hormone-induced somatic embryogenesis in vegetative tissue of Arabidopsis thaliana. PLoS Genet. 13 (1), e1006562. doi: 10.1371/journal.pgen.1006562
Narsai, R., Gouil, Q., Secco, D., Srivastava, A., Karpievitch, Y. V., Liew, L. C., et al. (2017). Extensive transcriptomic and epigenomic remodelling occurs during Arabidopsis thaliana germination. Genome Biol. 18 (1), 172. doi: 10.1186/s13059-017-1302-3
Nobre, T., Oliveira, M., Arnholdt-Schmitt, B. (2016). Wild carrot differentiation in Europe and selection at DcAOX1 gene? PLoS One 11 (10), e0164872. doi: 10.1371/journal.pone.0164872
Noceda, C., Svensson, J. T., Nogales, A., Arnholdt-Schmitt, B., (2015). “AOX gene diversity can affect DNA methylation and genome organization relevant for functional marker development,” in Alternative respiratory pathway in higher plants. Eds. Gupta, K. J., MuR, L. A. J., Neelwarn, E. B. (Oxford: John Wiley & Sons, Inc.), 281–286. doi: 10.1002/9781118789971.ch17
Nogales, A., Nobre, T., Cardoso, H. G., Muñoz-Sanhueza, L., Valadas, V., Campos, M. D., et al. (2016a). Allelic variation on DcAOX1 gene in carrot (Daucus carota L.): an interesting simple sequence repeat in a highly variable intron. Plant Gene 5, 49–55. doi: 10.1016/j.plgene.2015.11.001
Nogales, A., Nobre, T., Valadas, V., Ragonezi, C., Döring, M., Polidoros, A., et al. (2016b). Can functional hologenomics aid tackling current challenges in plant breeding? Brief. Funct. Genomics. 15 (4), 288–297. doi: 10.1093/bfgp/elv030
Nogales, A., Muñoz-Sanhueza, L., Hansen, L. D., Arnholdt-Schmitt, B. (2015). Phenotyping carrot (Daucus carota L.) for yield-determining temperature response by calorespirometry. Planta 241 (2), 525–538. doi: 10.1007/s00425-014-2195-y
Nun, N. B., Plakhine, D., Joel, D. M., Mayer, A. M. (2003). Changes in the activity of the alternative oxidase in Orobanche seeds during conditioning and their possible physiological function. Phytochemistry 64 (1), 235–241. doi: 10.1016/S0031-9422(03)00165-1
Petrussa, E., Bertolini, A., Casolo, V., Krajňáková, J., Macrì, F., Vianello, A. (2009). Mitochondrial bioenergetics linked to the manifestation of programmed cell death during somatic embryogenesis of Abies alba. Planta 231 (1), 93–107. doi: 10.1007/s00425-009-1028-x
Physiol Plantarum. (2009). AOX special issue coordinated by Birgit Arnholdt-Schmitt. http://onlinelibrary.wiley.com/doi/10.1111/ppl.2009.137.issue-4/issuetoc.
Polidoros, A. N., Mylona, P. V., Arnholdt-Schmitt, B. (2009). AOX gene structure, transcript variation and expression in plants. Physiol. Plant 137, 342–353. doi: 10.1111/j.1399-3054.2009.01284.x
Porfirio, S., Calado, M. L., Noceda, C., Cabrita, M. J., da Silva, M. G., Azadi, P., et al. (2016). Tracking biochemical changes during adventitious root formation in olive (Olea europaea L.). Sci. Hort. 204, 41–53. doi: 10.1016/j.scienta.2016.03.029
Purushothaman, R., Upadhyaya, H. D., Gaur, P. M., Gowda, C. L. L., Krishnamurthy, L. (2014). Kabuli and desi chickpeas differ in their requirement for reproductive duration. Field Crops Res. 163, 24–31. doi: 10.1016/j.fcr.2014.04.006
Quaresma, P., Gonçalves, T., Abreu, S., Costa, J. H., Mashayekhi, K., Arnholdt-Schmitt, B., et al., (2015). “Artificial intelligence for the detection of AOX functional markers,” in Alternative respiratory pathway in higher plants. Eds. Gupta, K. J., MuR, L. A. J., Neelwarn, E. B. (Oxford: John Wiley & Sons, Inc.), 261–266. doi: 10.1002/9781118789971.ch14
Ragonezi, C., Arnholdt-Schmitt, B. (2017). Laser capture microdissection for amplification of alternative oxidase (AOX) genes in target tissues in Daucus carota L. Methods Mol. Biol. 1670, 245–252. doi: 10.1007/978-1-4939-7292-0_21
Robert, H. S., Park, C., Gutièrrez, C. L., Wójcikowska, B., Pěnčík, A., Novák, O., et al. (2018). Maternal auxin supply contributes to early embryo patterning in Arabidopsis. Nat. Plants. 4 (8), 548–553. doi: 10.1038/s41477-018-0204-z
Rogov, A. G., Sukhanova, E. I., Uralskaya, L. A., Aliverdieva, D. A., Zvyagilskaya, R. A. (2014). Alternative oxidase: distribution, induction, properties, structure, regulation, and functions. Biochemistry (Moscow) 79 (13), 1615–1634. doi: 10.1134/S0006297914130112
Saisho, D., Nakazono, M., Lee, K. H., Tsutsumi, N., Akita, S., Hirai, A. (2001). The gene for alternative oxidase-2 (AOX2) from Arabidopsis thaliana consists of five exons unlike other AOX genes and is transcribed at an early stage during germination. Genes Genet. Syst. 76 (2), 89–97. doi: 10.1266/ggs.76.89
Santos Macedo, E., Sircar, D., Cardoso, H. G., Peixe, A., Arnholdt-Schmitt, B. (2012). Involvement of alternative oxidase (AOX) in adventitious rooting of Olea europaea L. microshoots is linked to adaptive phenylpropanoid and lignin metabolism. Plant Cell Rep. 31 (9), 1581–1590. doi: 10.1007/s00299-012-1272-6
Santos Macedo, E., Cardoso, H. G., Hernández, A., Peixe, A. A., Polidoros, A., Ferreira, A., et al, (2009). Physiologic responses and gene diversity indicate olive alternative oxidase as a potential source for markers involved in efficient adventitious root induction. Physiol. Plant 137 (4), 532–552. doi: 10.1111/j.1399-3054.2009.01302.x
Saraiva, K. D., Oliveira, A. E., Santos, C. P., Lima, K. T., Sousa, J. M., Melo, D. F., et al. (2016). Phylogenetic analysis and differential expression of EF1α genes in soybean during development, stress and phytohormone treatments. Mol. Genet. Genomics. 291, 1505–1522. doi: 10.1007/s00438-016-1198-8
Scafaro, A. P., Negrini, A. C. A., O’Leary, B., Rashid, F. A. A., Hayes, L., Fan, Y., et al. (2017). The combination of gas-phase fluorophore technology and automation to enable high-throughput analysis of plant respiration. Plant Methods. 13 (1), 16. doi: 10.1186/s13007-017-0169-3
Scheibe, R. (2018). Maintaining homeostasis by controlled alternatives for energy distribution in plant cells under changing conditions of supply and demand. Photosynth. Res. 139 (1-3), 81–91. doi: 10.1007/s11120-018-0583-z
Selinski, J., Hartmann, A., Kordes, A., Deckers-Hebestreit, G., Whelan, J., Scheibe, R. (2017). Analysis of posttranslational activation of alternative oxidase isoforms. Plant Physiol. 174 (4), 2113–2127. doi: 10.1104/pp.17.00681
Selinski, J., Scheibe, R., Day, D. A., Whelan, J. (2018). Alternative oxidase is positive for plant performance. Trends Plant Sci. 23 (7), 588–597. doi: 10.1016/j.tplants.2018.03.012
Shu, K., Liu, X. D., Xie, Q., He, Z. H. (2016). Two faces of one seed: hormonal regulation of dormancy and germination. Mol. Plant 9 (1), 34–45. doi: 10.1016/j.molp.2015.08.010
Sivasakthi, K., Tharanya, M., Kholová, J., Wangari Muriuki, R., Thirunalasundari, T., Vadez, V. (2017). Chickpea genotypes contrasting for vigor and canopy conductance also differ in their dependence on different water transport pathways. Front Plant Sci. 8, 1663. doi: 10.3389/fpls.2017.01663
Smertenko, A., Bozhkov, P. V. (2014). Somatic embryogenesis: life and death processes during apical–basal patterning. J. Exp. Bot. 65 (5), 1343–1360. doi: 10.1093/jxb/eru005
Tilsner, H. R., Upadhyaya, M. K. (1987). Action of respiratory inhibitors on seed germination and oxygen uptake in Avena fatua L. Ann. Bot. 59 (5), 477–482. doi: 10.1093/oxfordjournals.aob.a087339
Vanlerberghe, G. C., Cvetkovska, M., Wang, J. (2009). Is the maintenance of homeostatic mitochondrial signaling during stress a physiological role for alternative oxidase? Physiol. Plant 137 (4), 392–406. doi: 10.1111/j.1399-3054.2009.01254.x
Velada, I., Cardoso, H. G., Ragonezi, C., Nogales, A., Ferreira, A., Valadas, V., et al. (2016). Alternative oxidase gene family in Hypericum perforatum L.: characterization and expression at the post-germinative phase. Front. Plant Sci. 7, 1043. doi: 10.3389/fpls.2016.01043
Velada, I., Grzebelus, D., Lousa, D., Soares, C., Santos Macedo, E., Peixe, A., et al. (2018). AOX1-subfamily gene members in Olea europaea cv. “Galega vulgar”—gene characterization and expression of transcripts during IBA-induced in vitro adventitious rooting. Int. J. Mol. Sci. 19 (2), 597. doi: 10.3390/ijms19020597
Vishwakarma, A., Dalal, A., Tetali, S. D., Kirti, P. B., Padmasree, K. (2016). Genetic engineering of AtAOX1a in Saccharomyces cerevisiae prevents oxidative damage and maintains redox homeostasis. FEBS Open Bio. 6 (2), 135–146. doi: 10.1002/2211-5463.12028
Wang, Y., Berkowitz, O., Selinski, J., Xu, Y., Hartmann, A., Whelan, J. (2018). Stress responsive mitochondrial proteins in Arabidopsis thaliana. Free Radic. Biol. Med. 122, 28–39. doi: 10.1016/j.freeradbiomed.2018.03.031
Winkelmann, T. (2016). “Somatic versus zygotic embryogenesis: learning from seeds,” in In vitro embryogenesis in higher plants: methods in molecular biology, vol. 1359. Eds. Germana, M., Lambardi (New York, NY: Humana Press), 25–46. ISSN: 978-1-4939-3060-9. doi: 10.1007/978-1-4939-3061-6_2
Yentur, S., Leopold, A. C. (1976). Respiratory transition during seed germination. Plant Physiol. 57 (2), 274–276. doi: 10.1104/pp.57.2.274
Yuan, F., Yu, X., Dong, D., Yang, Q., Fu, X., Zhu, S., et al. (2017). Whole genome-wide transcript profiling to identify differentially expressed genes associated with seed field emergence in two soybean low phytate mutants. BMC Plant Biol. 17 (1), 16. doi: 10.1186/s12870-016-0953-7
Zavattieri, M. A., Frederico, A. M., Lima, M., Sabino, R., Arnholdt-Schmitt, B. (2010). Induction of somatic embryogenesis as an example of stress-related plant reactions. Electron. J. Biotechnol. 13 (1), 12–13. doi: 10.2225/vol13-issue1-fulltext-4
Keywords: environmental stress, developmental plasticity, metabolic biomarker, endophytes, seed technology, plant performance prediction
Citation: Mohanapriya G, Bharadwaj R, Noceda C, Costa JH, Kumar SR, Sathishkumar R, Thiers KLL, Santos Macedo E, Silva S, Annicchiarico P, Groot SPC, Kodde J, Kumari A, Gupta KJ and Arnholdt-Schmitt B (2019) Alternative Oxidase (AOX) Senses Stress Levels to Coordinate Auxin-Induced Reprogramming From Seed Germination to Somatic Embryogenesis—A Role Relevant for Seed Vigor Prediction and Plant Robustness. Front. Plant Sci. 10:1134. doi: 10.3389/fpls.2019.01134
Received: 06 April 2019; Accepted: 16 August 2019;
Published: 20 September 2019.
Edited by:
Sandra Isabel Correia, University of Coimbra, PortugalReviewed by:
Elena Corredoira, Instituto de Investigaciones Agrobiológicas de Galicia (IIAG), SpainTaras P. Pasternak, University of Freiburg, Germany
Copyright © 2019 Mohanapriya, Bharadwaj, Noceda, Costa, Kumar, Sathishkumar, Thiers, Santos Macedo, Silva, Annicchiarico, Groot, Kodde, Kumari, Gupta and Arnholdt-Schmitt. This is an open-access article distributed under the terms of the Creative Commons Attribution License (CC BY). The use, distribution or reproduction in other forums is permitted, provided the original author(s) and the copyright owner(s) are credited and that the original publication in this journal is cited, in accordance with accepted academic practice. No use, distribution or reproduction is permitted which does not comply with these terms.
*Correspondence: Birgit Arnholdt-Schmitt, ZXVfY2hhaXJAdWV2b3JhLnB0; Ramalingam Sathishkumar, cnNhdGhpc2hAYnVjLmVkdS5pbg==