- 1Henan Key Laboratory of Ion-Beam Bioengineering, College of Agricultural, Zhengzhou University, Zhengzhou, China
- 2School of Physics, Key Laboratory of Materials Modification by Laser, Ion and Electron Beams, Ministry of Education, Dalian University of Technology, Dalian, China
Non-thermal plasma holds great potentials as an efficient, economical, and eco-friendly seed pretreatment method for improving the seed germination and seedling growth, but the mechanisms are still unclear. Therefore, a plant model organism Arabidopsis thaliana was used to investigate the physio-biochemical responses of seeds to non-thermal plasma at different treatment times by measuring the plant growth parameters, redox-related parameters, calcium (Ca2+) level and physicochemical modification of seed surface. The results showed that short-time plasma treatment (0.5, 1, and 3 min) promoted seed germination and seedling growth, whereas long-time plasma treatment (5 and 10 min) exhibited inhibitory effects. The level of superoxide anion (O2•−) and nitric oxide (NO) and the intensity of infrared absorption of the hydroxyl group were significantly higher in short-time plasma treated Arabidopsis seeds, and the level of hydrogen peroxide (H2O2) was remarkably increased in long-time plasma treated seeds, indicating that O2•−, ·OH, and NO induced by plasma may contribute to breaking seed dormancy and advancing seed germination in Arabidopsis, while plasma-induced H2O2 may inhibit the seed germination. The intensity of hydroxyl group and the contents of H2O2, malondialdehyde, and Ca2+ in Arabidopsis seedlings were obviously increased with the plasma treatment time. Catalase, superoxide dismutase, and peroxidase activities as well as proline level in short-time treated seedlings were apparently higher than in control. The etching effects of plasma on seed surface were dose-dependent, spanning from slight shrinkages to detached epidermis, which also significantly increased the oxidation degree of seed surface. Therefore, the improved activities of antioxidant systems, moderate ·OH, H2O2, and Ca2+ accumulation and seed surface modification induced by plasma all contribute to the enhanced seedling growth of Arabidopsis after short-time plasma treatment.
Introduction
In the past few decades, atmospheric non-thermal plasma has shown promising applications in the biomedical field, thus leading to the emergence of “plasma medicine” (Fridman et al., 2010). Nowadays, the agricultural applications of non-thermal plasma for decontaminating seeds, improving seed quality and crop yields as well as curing plant disease have attracted much attention due to the fact that food shortage is becoming one of the most serious global problems in this century because of the continuously growing population and decreasing arable land (Koga et al., 2015). Numerous works have shown that non-thermal plasma is a fast, uniform, economic, effective, and eco-friendly approach for stimulating seed germination and seedling growth compared with the conventional seed pretreatment methods, e.g. ultraviolet and gamma radiation, scarification, hot water soaking, and chemical reagent treatment (Li et al., 2014; Randeniya and De Groot, 2015; Mildaziene et al., 2018; Štěpánová et al., 2018). In the last years many authors studied the stimulation of the seed germination and the plant growth by using plasma generator (Živković et al., 2004; Šerá et al., 2010; Dobrin et al., 2015; Bafoil et al., 2018). Some studies have found that plasma treatment can increase seed activity including earlier germination, higher germination rate, faster growth, and other growth parameters (Šerá et al., 2010; Jiang et al., 2014), enzyme activity (Henselová et al., 2012; Surowsky et al., 2013), and the yield of plants (Yin et al., 2005). However, the detailed mechanisms underlying the stimulatory effects of plasma on seeds are still not fully understood.
Among the various constituents in plasma, reactive oxygen species (ROS) [e.g., hydroxyl radical (OH), superoxide anion (O2•−), hydrogen peroxide (H2O2), and singlet oxygen (1O2)] and reactive nitrogen species (RNS) [e.g., nitric oxide (NO), nitrite (NO2¯), nitrite (NO3¯) and peroxynitrite (OONO¯)] are considered as the major agents for plasma-induced biological effects (Iseni et al., 2016). The ROS and RNS in plants can play dual roles, beneficial or harmful, depending on the amount (Panngom et al., 2018), which activates a variety of physiological and metabolic behaviors (such as breaking dormancy, accelerating germination, and enhancing antioxidant capacity) at low doses, while causes oxidative stress in seeds at high doses (Romero-Puertas et al., 2019). Plants are well equipped with an intrinsic antioxidant defense system comprising enzymatic antioxidants, such as superoxide dismutase (SOD), catalase (CAT), and peroxidase (POD), as well as non-enzymatic antioxidants, for instance, ascorbate, glutathione, and proline to resist the oxidative stress (Liu et al., 2018). These antioxidants are essential for stimulating physiological and developmental processes and resisting stresses (Liu et al., 2007). On the other hand, plasma-generated reactive species could also directly etch the seed husk, increasing the seed coat permeability for oxygen, water, and other nutrition species, consequently improving the seed growth characteristics (Zhang and Kirkham, 1994). Besides ROS and RNS, intracellular Ca2+ is also recognized as an important signaling molecule for many physiological processes in plants, such as hormone secretion, seed germination, cell division, cell expansion, pollen tube growth, and fertilization (Demidchik et al., 2018). Moreover, as an essential nutrient for plants, calcium deficiency or excessive calcium concentration can both lead to disorders in plants and humans (White and Broadley, 2003). However, there is still little available information about the effects of plasma on intracellular Ca2+ during seed germination and seedling development.
Considering the above factors, the intracellular redox state, Ca2+ level, and physicochemical modification of seed surface were all involved in this study to comprehensively unravel the physio-biochemical mechanism of plasma-regulated seed germination and early seedling growth using a plant model organism Arabidopsis thaliana.
Materials and Methods
Plant Material and Growth Conditions
The wild-type Arabidopsis thaliana seeds (Columbia-0, col) with different air-dry storage times at room temperature after harvest were used for plasma treatment. The seeds after 10-month storage were considered as the dormant seeds, while the seeds after 1-month storage were considered as the non-dormant seeds, which were only used for germination test. The seeds are collected from various plants when they are mature at the same time. Then the seeds were randomly divided into three parts for repeated testing (30–50 samples per group). Arabidopsis seeds were treated with sterilized water (75% ethanol: 30% hydrogen peroxide = 4:1) for 30 s and dried off naturally. Then the seeds were grown in petri dishes containing 1/2 Murashige and Skoog mineral salts (MS) medium (Sigma) including 1% (w/v) agar, 1% (w/v) sucrose (pH 6.2–6.4) and required vernalization in the dark at 4°C during 48 h. The incubating conditions were at 23°C under fluorescent light 140 ± 20 µmolm−2s−1 with 16/8 h light–dark cycle (Chen et al., 2015; Li et al., 2015; Qi et al., 2015).
Non-Thermal Plasma System and Treatment Conditions
Figure 1A shows the schematic diagram of the experimental setup. A dielectric barrier surface discharge device was used to generate the atmospheric non-thermal plasma, consisting of a copper plane electrode, a fiberglass dielectric layer, and a copper hexagonal mesh electrode. The mesh electrode was grounded and the plane electrode was connected to a 7.95 kHz high-voltage alternating-current power supply. The surface plasmas were generated in the hexagon mesh elements with a good mesh-to-mesh homogeneity (Figure 1B). The waveforms of discharge voltage and current were recorded by an oscilloscope. The plasma device was placed in an acrylic chamber to offer a stable gas environment for discharging. The temperature and humidity of the chamber during experiment were detected by a temperature-humidity sensor. More details of the plasma device can be found in reference (Gao et al., 2017). Air in the chamber was used as the working gas. As shown in Figure 1C, the applied discharge voltage had a peak-to-peak value of 8.47 kV, corresponding to a discharge power of 2.5 W. The humidity was kept at about 65% RH, and the temperature was slightly increased from 33.08 to 33.27°C during 20-min plasma discharge (Figure 1D).
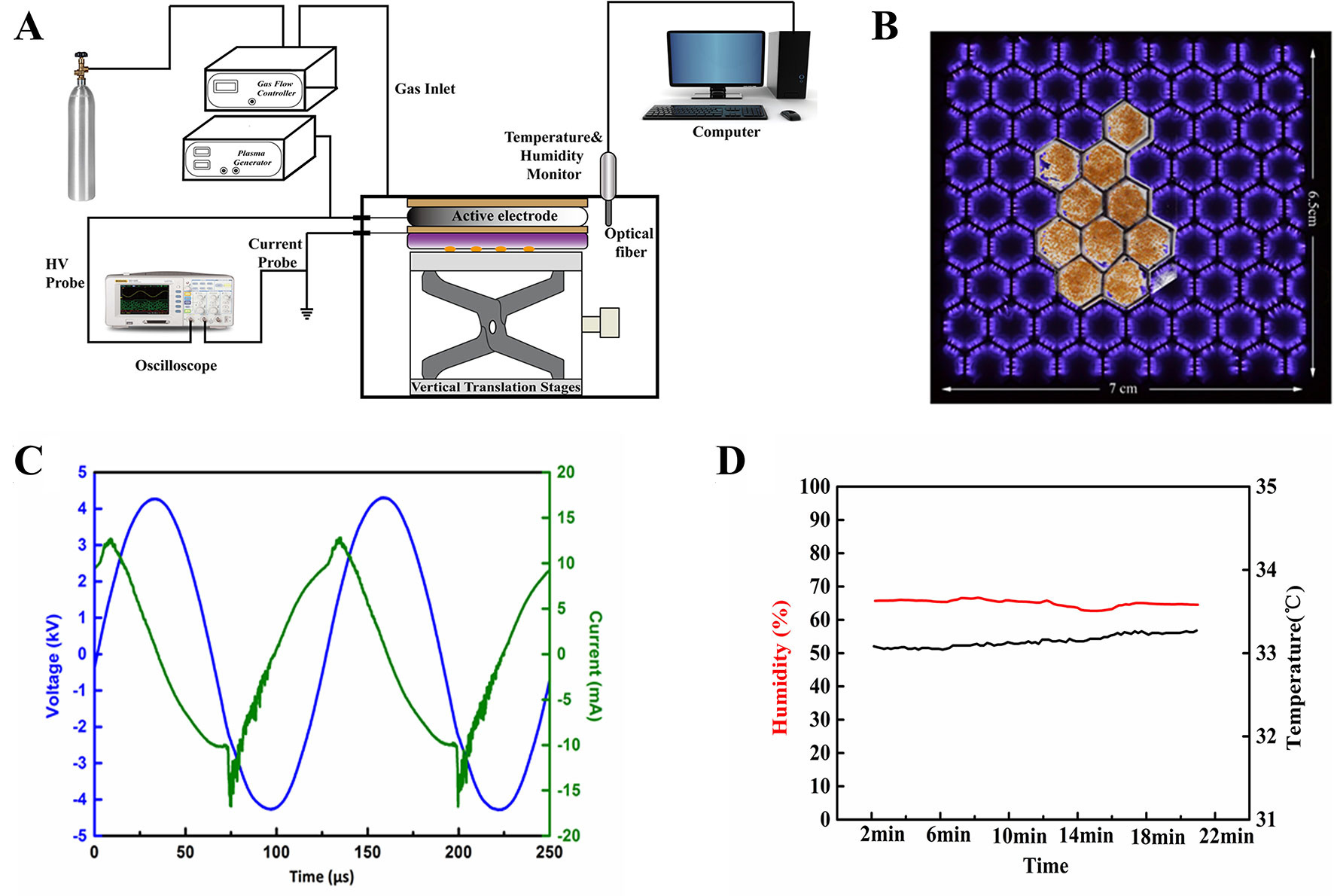
Figure 1 (A) Schematic diagram of the experimental setup used in this study. (B) Photo of air surface discharge treatment of Arabidopsis seeds. (C) Waveforms of the applied voltage and the discharge current. (D) Profiles of temperature and humidity in the atmospheric pressure discharge reactor.
The seeds were fixed on a quartz plate (5 cm × 5 cm) in a shape of the hexagonal mesh electrode with double-sided tape (Figure 1B), and closely placed under the mesh electrode. Arabidopsis thaliana seeds were treated by surface air plasma for 0, 0.5, 1, 3, 5, and 10 min, respectively. The seeds without plasma treatment were served as a control check (ck).
Optical Emission Spectroscopy
Optical emission spectroscopy (OES) in the 200–800 nm range was employed to analyze the major excited reactive species in non-thermal plasma by AvaSpec-2048-8 Fiber Optic Spectrometer (Avantes, USA) (Ji et al., 2015; Yu et al., 2015).
Measurement of Seed Germination and Seedling Growth
A seed was considered as germinated when the radicle elongated to 2 mm. The germination was counted every 12 h for 3 days. On the fifth day of germination, the primary roots of control and non-thermal plasma-treated seeds vertically cultured in petri dish were photographed with a digital camera (Nikon D5100). The length of primary roots was measured using the Image J software with the Segmentation Line tool after setting scales. The whole seedlings of 7-day-old were collected for fresh weight measurement (Zhang et al., 2016).
Measurement of Reactive Nitrogen Species and Reactive Oxygen Species in Arabidopsis thaliana
The contents of RNS (NO, NO2−, NO3−, ONOO−) and ROS (O2•−, H2O2, .OH) in Arabidopsis seeds immediately after plasma treatment (day-0) and 7-day-old Arabidopsis seedlings germinated from the plasma-treated seeds (day-7) were detected. To test the effect of plasma treatment on NO, NO2−, NO3−, ONOO−, O2•−, H2O2, and .OH content in Arabidopsis seeds and seedlings, three different tissue extracts were carried out under conditions of different test purposes.
NO2− Contents Were Measured Using the Method of Griess Assay, Which Involves a Diazo-Coupling Procedure (Griess, 1879). Samples (0.5 G) Were Ground in 5 Ml of 50 Mm Ice-Cold Phosphate Buffer Solution (Ph 7.8); These Samples Were Centrifuged At 12,000 Rpm/Min for 10 Min At 4°C. The Supernatant Was Used for Determining the Content of NO, NO2−, and NO3−. NO2− reacts with sulfartilic acid under acidic conditions to form a diazoniurn cation, which subsequently couples to the aromatic amine 1-naphthylamine to produce a red-violet colored (540 nm), water-soluble azo dye. in this process, the total NO content can be represented indirectly by adding nitrate reductase (Beyotime, S0024), and NO3− can be directly measured by UV absorbance detection at 210–220 nm (Wang et al., 2017). a standard curve was plotted with known concentrations of NO, NO2̄, and NO3¯ t o measure their concentration in the experimental samples.
ONOO ̄ was determined according to the method of Huang et al. (2007). Folic acid was prepared by dissolving appropriate amount of folic acid in 0.001 mol/L NaOH and kept frozen, and the working barbital buffer solution was prepared by dissolving 4.125 g barbital sodium in 500 ml distilled water and add 0.7 ml 1.0 mol/L HCl. 0.2 g samples were collected and ground in 2 ml alcohol, centrifuged at 12,000 rpm/min for 10 min at 4°C; the supernatant was used for determining the content of ONOŌ. An aqueous solution of 1 ml folic acid was rapidly mixed with 3 ml working barbital buffer solution and then 300 µl seeds or seedling extracts were immediately added for 5 min at room temperature. High fluorescence product was generated by the introduction of peroxynitrite into the solution of folic acid, resulting in dramatic increase in spectra characteristics with excitation maximum at 380 nm and fluorescence emission maximum at 460 nm.
The H2O2 content was measured by the method of Wang et al. (2017a). A total of 0.2 g samples (seeds or 0.2 g of fresh weight (FW) from 7-d-old seedlings) were extracted in 5 ml of pre-cooled acetone at 4°C ice bath, centrifuged at 10,000 rpm/min for 15 min at 4°C. The supernatant was removed and reacted with 0.1 ml mixture solution of titanium tetrachloride (TiCl4) in 10% hydrochloric acid (HCl) (v/v). Reaction for 5 min at room temperature (25°C), the mixture was centrifuged at 8,000 rpm/min for 10 min at 4°C. The precipitate was washed 3–5 times with cold acetone and dissolved in 3 ml 1 M H2SO4. Absorption at 410 nm was recorded (UV mini-1240, SHIMADZU, Japan), and the H2O2 concentration was calculated from a standard curve prepared in the same manner.
The most frequently used approach to detect O2•− is the nitroblue tetrazolium (NBT) colorimetric (Jambunathan, 2010). Nitroblue tetrazolium chloride (0.1% [w/v] NBT was prepared in 10 mM sodium azide, 50 mM potassium phosphate. 0.5 g samples were ground in 5 ml of the above solution, centrifuged at 12,000 rpm/min for 10 min at 4°C; the supernatant absorbance of the samples was read at 580 nm.
The spectral characteristics of hydroxyl groups were detected using attenuated total reflectance fourier transform infrared measurements (ATR-FTIR) in the spectral range of 4,000–400 cm−1 (NICOLET IS10, Thermo Fisher Scientific, USA) (Sharma and Uttam, 2019). The absorption band 3,700–3,100 cm−1 ascribed with -OH stretching confirmed the presence of chemical compounds with hydroxyl groups (Mishra and Mohanty, 2019). 0.2 g samples were collected and ground in 2 ml alcohol, centrifuged at 12,000 rpm/min for 10 min at 4°C, and the supernatant was used for determining the intensity of hydroxyl groups. Supplemental Figure 1 indicates that the intensity of the absorption band 3700–3100 cm−1 ascribed with hydroxyl group can characterize the level of hydroxyl radicals in seeds, which was detected by terephthalic acid (TA) method (Barreto et al., 1994).
Measurement of Lipid Peroxidation and Antioxidants in Arabidopsis thaliana
The contents of malondialdehyde (MDA) and antioxidants (proline, CAT, SOD and POD) in Arabidopsis seeds immediately after plasma treatment (day-0) and 7-day-old Arabidopsis seedlings germinated from the plasma-treated seeds (day-7) were detected.
MDA is the end product of lipid peroxidation and reflects the degree of damage due to adverse conditions. The levels of lipid peroxidation in Arabidopsis were determined by the trichloroacetic acid (TCA) reaction (Shalata and Neumann, 2001). Samples of seeds or seedlings (0.2 g) were collected and ground sufficiently with 5 ml 10% TCA and a little of SiO2. After centrifugation at 5,000 rpm/min for 10 min, the supernatant (2 ml) was combined with 0.6% (w/v) thiobarbituric acid (2 ml) and incubated in boiling water for 15 min. The reactions were stopped by placing the tubes in an ice bath. The mixture was centrifuged at 5,000 rpm/min for 15 min and the supernatants were read at 532 and 450 nm.
The proline content was measured using colorimetric methods according to (Bates et al., 1973). The irradiated samples 0.2 g seeds and 0.2 g FW of 7-day-old whole seedlings germinated from irradiated seeds were homogenized in liquid nitrogen. Tissues were suspended in 5 ml of 3% sulfosalicylic acid and incubated in boiling water for 10 min. After centrifugation, 2 ml supernatant was mixed with 4 ml acid ninhydrin, 2 ml acetic acid and 2 ml distilled water to a final volume of 10 ml. All the mixtures were incubated in boiling water for 1 h. The reaction mixtures were then extracted with 4 ml toluene, and the upper phases were collected. The absorbance of samples was read at 520 nm, and a standard curve was plotted with known concentrations of proline to measure the proline concentration in experimental samples.
Both seeds (day-0) and seedlings (day-7) were homogenized in a mortar with 2 ml of 50 mM ice-cold phosphate buffer (pH 7.8) containing 1 mM EDTA. The homogenate was collected and centrifuged at 12,000 rpm/min for 15 min at 4°C. The supernatant was used for determining the activities of CAT, SOD and POD based on the Refs (Zhang and Kirkham, 1994; Jiang et al., 2014; Qi et al., 2015), respectively. All procedures were performed at 4°C.
The activity of CAT was determined using the method that involves measuring the initial rate of disappearance of H2O2, as described (Qi et al., 2015). The enzyme extract (0.1 ml) which was added to the reaction solution (3 ml) consisted of 50 mM phosphate buffer (pH7.0), 20 mM H2O2. A decrease in H2O2 was monitored at 240 nm for at least 3 min.
The SOD activity was determined using the nitro-blue tetrazolium (NBT) method (Zhang and Kirkham, 1994). 3 ml reaction mixture contained 50 mM phosphate buffer (pH7.8), 0.1 mM EDTA, 130 mM methionine, 0.75 mM NBT, 0.02 mM riboflavin, and 0.1 ml enzyme extract. Riboflavin was added as the last component, and the reaction mixtures were illuminated for 15 min at a light intensity of 5,000 lx. Non-illuminated and illuminated reactions without the supernatant served as calibration standards. One unit of SOD activity was defined as the amount of enzyme required to cause 50% inhibition of the reduction of NBT monitored at 560 nm.
The guaiacol method was used to measure the POD activity (Jiang et al., 2014). The enzyme extract (0.02 ml) was added to the reaction mixture containing 0.02 ml guaiacol solution and 0.01 ml H2O2 solution in 3 ml of phosphate buffer solution (pH 7.0). The addition of the enzyme extract started the reaction, and the increase in absorbance was recorded at 470 nm for 5 min.
Assessment of Intracellular Ca2+
The Ca2+ content was tested using the probe fluorescein Fluo-2-acetoxymethyl ester (Fura-2 AM) (S1052, Beyotime). The 4-day-old seedlings were stained with 0.5 µM Fura-2 AM for 45 min at room temperature (25°C), and washed with fresh liquid media three times before observation. All the experiments were captured for six different fields. The images were taken by fluorescence microscope (Leica DM4000B, Germany).
Scanning Electron Microscopy-Energy Dispersive X-ray Spectroscopy
The seeds were dried completely under the ethanol dehydration (20%, 40%, 60%, 80%, 100%; 15 min each time), and then the surface was sputter-coated with gold and observed by scanning electron microscopy (SEM) (JSM-6700F/INCA-ENERGY, JEOL, Japan) (Chen et al., 2012). The elements on seed surface were analyzed by energy dispersive X-ray (EDX) spectroscopy with a X-MaxN (51-XMX1146) (Gómez-Ramírez et al., 2017).
Statistical Analysis
Experiments were performed using a completely randomized designs with three independent replicate experiments (n = 3). Differences were analyzed with One-way analysis of variance (ANOVA) and least significant difference (LSD) test. Analyses were performed using SPSS statistical software package (SPSS Inc., version 17.0, USA). Differences at p < 0.05 were considered significant.
Results and Discussions
The Major Excited Species in Air Plasma by Optical Emission Spectroscopy
OES was used to investigate the excited active species generated in the surface air plasma ranging from 200 to 800 nm. As observed in Figure 2, the spectra was dominated by N2 second positive system (C3∏u→B3∏g) emissions at 297.6, 313.6, 315.9, 333.8, 337.1, 353.7, 357.7, 371.0, 375.5, 380.5, 385.8, 394.3, 399.8, 405.9, 420.1, 427.0, and 434.3 nm as well as by N2+ first negative system (B2∑u→X2∑g) emissions at 391.0 and 400–450 nm as a result of the direct or step-wise electron impact excitations and the pooling reaction of the nitrogen metastable state (Yu et al., 2015). The NOγ-system (A2∑+→X2∏) emission bands from 200 to 280 nm and atomic oxygen emission at 777.2 nm were also observed. The OES results indicated that abundant excited nitrogen molecules and ion, atomic oxygen and NO existed in the air plasma, which can react with each other to form various kinds of RNS and ROS (hydroxyl [OH], hydrogen peroxide [H2O2], nitrite [NO2−], nitrate [NO3−], ozone [O3], etc.) due to their high activity (Liang et al., 2012). These reactive species could not only react with the seed coat of Arabidopsis, but also regulate its growth and development as secondary messengers.
Effect of Plasma on Seed Germination and Seedling Growth
Figure 3A shows the germination rate of dormant Arabidopsis seeds exposed to non-thermal plasma at different times during 3-day cultivation. On day 3, about 20% seeds still can’t germinate in the control group, while the seeds after short-time plasma treatment (0.5, 1, and 3 min) almost completely germinated (98.7%, 96.0%, 96.7%), indicating that plasma could break the seed dormancy in Arabidopsis. Similar results were also reported by Šerá et al. (2009), who found that plasma can break seed dormancy and accelerate seed germination in Lamb’s Quarters seeds. It is generally accepted that the elevated ROS and RNS production in the embryos is necessary for seed transition from the dormant to the non-dormant state, and to accomplish the germination process. Thus, the plasma-generated ROS and RNS may mainly contribute to the seed dormancy alleviation in Arabidopsis seeds. For long-time plasma treatment, 5-min plasma exposure initially promoted the seed germination, then slightly inhibited after 48 h. 10-min plasma exposure significantly inhibited the seed germination, suggesting that the plasma either reduces the seed viability or deepens their dormancy. And previous studies have suggested that a prolonged plasma treatment or a treatment with more reactive and energetic plasma active species would have negative effects on germination (Bafoil et al., 2019). For the non-dormant Arabidopsis seeds (Figure 3B), the final germination of untreated seeds rose to almost 100% after 36-h cultivation, while 0.5 and 1-min plasma exposure resulted in the same final germination rate and higher germination speed compared with that of control, indicating that besides seed dormancy release, the germination-promoting effects of non-thermal plasma may be also attributed to other factors such as the modification of seed coat (Volin et al., 2000), improvement of wettability and water imbibition of seeds (Jiang et al., 2014; Bormashenko et al., 2015), as well as the increase of soluble sugar and soluble protein concentrations in seeds (Henselová et al., 2012).
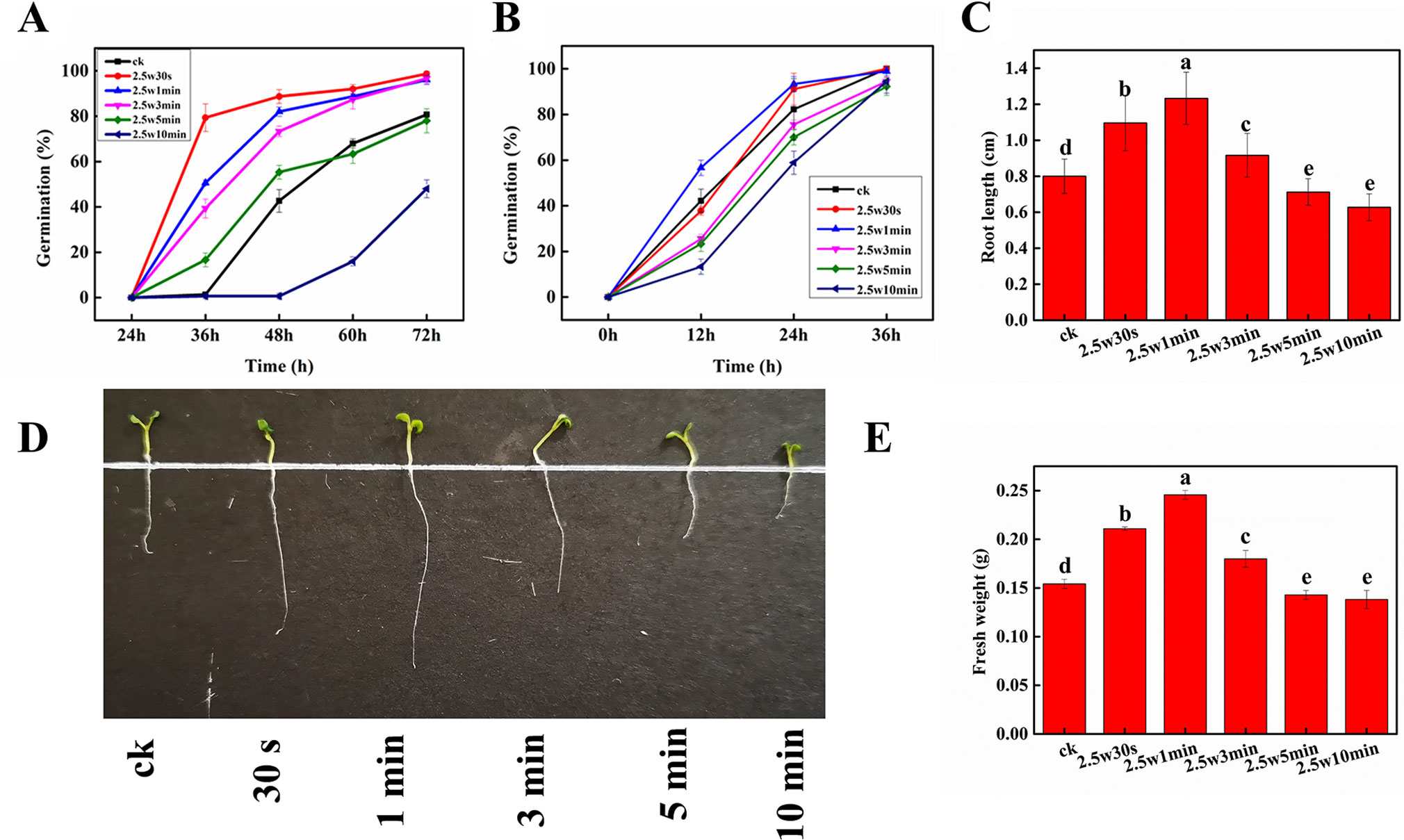
Figure 3 Effect of plasma on germination of dormant (A) and non-dormant (B) seeds, radical length (C, D) and fresh weight (E) of Arabidopsis seeds. Different letters (a–e) indicate significant differences among treatments according to LSD test (p 0.05).
Root length and fresh weight were measured to estimate the effect of non-thermal plasma on Arabidopsis plant growth. As shown in Figures 3C–E, during 10-min plasma treatment, the values of root length and fresh weight all peaked at 1 min, and then decreased with longer plasma treatment time. Compared with untreated seedlings, 0.5, 1, and 3-min plasma treatment led to a distinct increase of 36.9, 53.9, and 14.5% in root length as well as 36.7, 59.2, and 16.7% in fresh weight (p < 0.05), respectively. While a decrease of 11.0 and 21.6% in root length as well as 7.3 and 10.4% in fresh weight (p < 0.05) was observed in 5 and 10-min treated seedlings, respectively. These results indicated that short-time plasma exposure (≤3 min) could enhance the seed germination and seedling growth of Arabidopsis, but long-time plasma treatment (≥5 min) exhibited inhibitory effects. This phenomenon is so-called dose-dependent effect induced by non-thermal plasma, which has also been observed in various plants (Guo et al., 2017).
Effect of Plasma on Reactive Oxygen Species and Reactive Nitrogen Species in Arabidopsis
Given that ROS and RNS as the signal molecules play important roles in seed dormancy alleviation, seed germination and seedling growth (Singh et al., 2016), seven RNS and ROS (NO, NO2 ̄, NO3 ̄, ONOO ̄, O2•−, H2O2, and OH) were measured in Arabidopsis seeds immediately after plasma treatment (day-0) and 7-day-old Arabidopsis seedlings germinated from the plasma-treated seeds (day-7) to investigate the effect of non-thermal plasma on the level of ROS and RNS during seed germination and seedling growth in Arabidopsis. As shown in Figure 4, except for NO2 ̄, the other ROS and RNS contents in Arabidopsis seeds were all increased compared with untreated samples, indicating that the reactive species produced by plasma could permeate into the interior of seeds through the seed coat. The levels of NO and H2O2 in Arabidopsis seeds were increased with plasma treatment time (Figures 4A, E), while the level of O2•− and the intensity of infrared absorption of hydroxyl group were all increased firstly and then decreased with prolonged plasma treatment time (Figures 4F, G), indicating that the short-lived ROS (O2•− and OH) may convert into the long-lived ROS (H2O2) for long-time plasma treatment, consequently leading to a markedly higher H2O2 content in 5 and 10-min treated seeds. For example, O2•− can convert into H2O2 and O2 by SOD, and the excessive OH can form H2O2via the reaction (OH + OH → H2O2) (Zhang et al., 2018). For NO2− contents, there was no significant difference among 0, 0.5, and 1-min treated seeds, while 3, 5, and 10-min plasma treatment significantly decreased NO2− contents(Figure 4B). The NO3 ̄ contents ONOO − contents had similar change trend, which was increased in the first 5 min and significantly reduced at 10 min (Figures 4C, D). Combined with the results of germination, it is concluded that O2•−, OH, and NO induced by plasma can break seed dormancy and accordingly improve the seed germination in short-time plasma treated seeds, while high H2O2 concentration can cause oxidative damages in long-time plasma treated seeds, consequently inhibiting the seed germination. Similar results were also reported by Whitaker et al. (2010), who found that reagents that generate OH and O2•− can break seed dormancy in Bidens pilosa L. seeds, while H2O2 tended to inhibit rather than promote total germination, indicating that it is OH and O2•− rather than H2O2 implicated in the release of seed dormancy in this species. For Arabidopsis seedlings, the level of H2O2 and the intensity of infrared absorption of hydroxyl group had similar change pattern, which were all significantly increased with the plasma treatment time except for 0.5-min treated seedlings (Figures 4E, H). These results suggested that moderate H2O2 and OH have positive effects on seedling growth of Arabidopsis, while excessive H2O2 and OH could inhibit the seedling growth. The O2•− contents in Arabidopsis seedlings were very low compared with that of seeds (Figure 4F), indicating that O2•− almost has no effects on the seedling growth. Compared with untreated seedlings, the NO concentrations in 1 and 3-min treated seedlings were significantly increased, while 5 and 10-min plasma treatment significantly decreased the NO concentration, indicating that NO also plays an important role in improving the seedling growth after short-time plasma treatment.
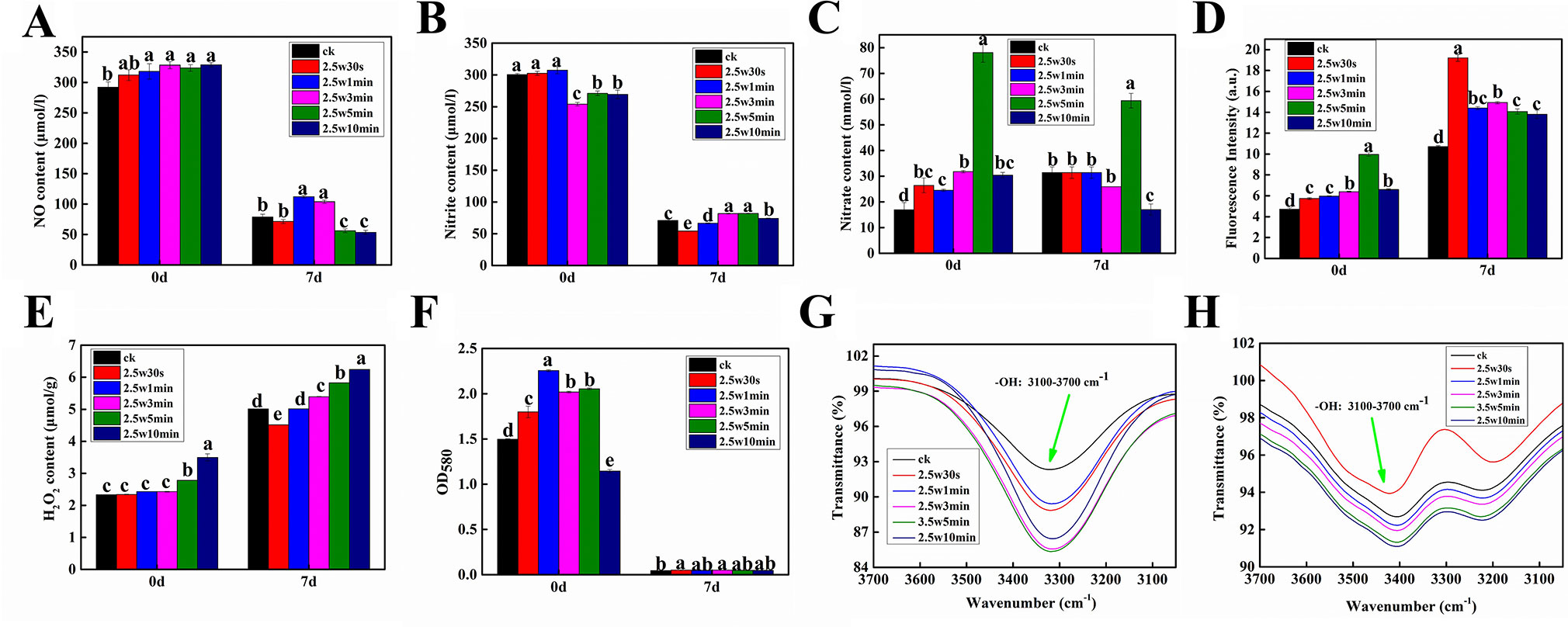
Figure 4 Effect of plasma on the level of NO (A), nitrite (B), nitrate (C), peroxynitrite anion (D), H2O2 (E), superoxide anion (F) as well as the intensity of infrared absorption of hydroxyl group (G, H) in Arabidopsis seeds immediately after plasma treatment (day-0) and 7-day-old Arabidopsis seedlings germinated from the plasma-treated seeds (day-7).
Effect of Plasma on Lipid Peroxidation and Antioxidants in Arabidopsis
Due to the fact that the intracellular redox state is determined by ROS and antioxidants and the cell would suffer oxidative stress when the antioxidants cannot scavenge excessive ROS (Kumar and Prasad, 2018), four typical antioxidants (proline, CAT, SOD, and POD) and a commonly used oxidative stress indicator (MDA) were measured in Arabidopsis seeds immediately after plasma treatment (day-0) and 7-day-old Arabidopsis seedlings germinated from the plasma-treated seeds (day-7) to evaluate the effects of non-thermal plasma on the cellular redox homeostasis of Arabidopsis. SOD, POD, and CAT together form the first and most important line of antioxidant enzyme defense systems against ROS. SOD catalyzes the dismutation of O2•− to H2O2 and O2, then both CAT and POD decompose H2O2 into H2O and O2 (Sun et al., 2014). Proline can scavenge OH as a non-enzymatic antioxidant (Soares et al., 2016). As shown in Figure 5A, the MDA level was significantly increased in plasma-treated seeds compared with the untreated seeds. The proline level and SOD and POD activity in Arabidopsis seeds were all increased firstly and then decreased with the plasma treatment time (Figures 5B, D, E). The CAT activity in plasma-treated seeds was significantly decreased compared with the untreated seeds (Figure 5C). However, we didn’t obtain a clear change law of these parameters following the change of plasma treatment time, which may due to the fact that these parameters didn’t have enough time to respond adequately to the ROS and RNS in seeds when they were immediately measured after plasma treatment.
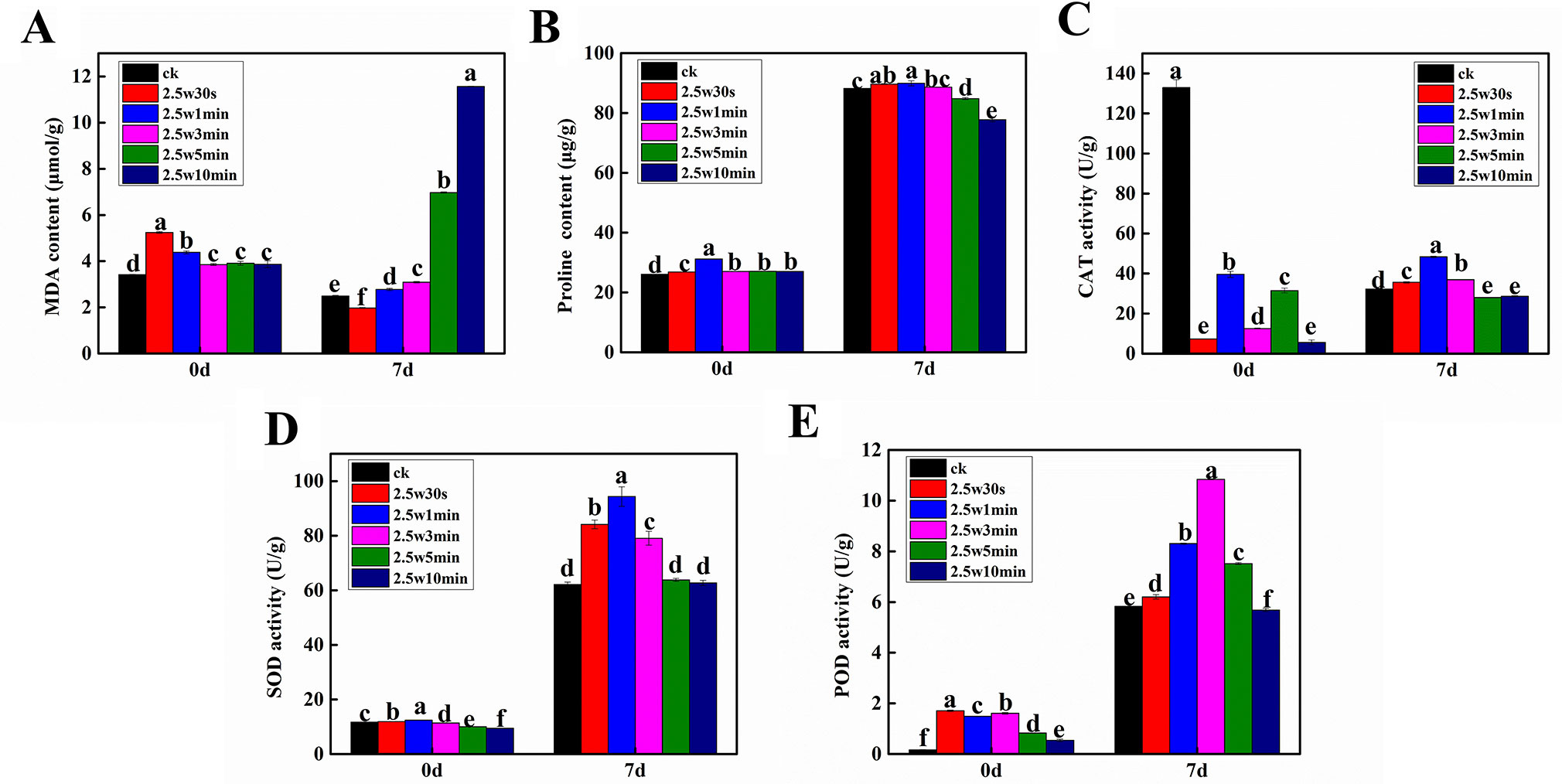
Figure 5 Effect of plasma on the level of MDA (A) and proline (B) as well as the activity of CAT (C), SOD (D) and POD (E) in Arabidopsis seeds immediately after plasma treatment (day-0) and 7-day-old Arabidopsis seedlings germinated from the plasma-treated seeds (day-7).
For Arabidopsis seedlings, the concentration of MDA was increased with the plasma treatment time. Except for 0.5-min treated seedlings, the MDA level was higher than in untreated samples after plasma exposure (Figure 5A). Especially, 5 and 10-min treatments caused a dramatic increase of 180.0% and 364.3% in MDA content. These results revealed that short-time plasma treatment (≤3 min) only slightly elevated the oxidative level in Arabidopsis seedlings, while the long-time treated seedlings (≥5 min) were subjected to severe oxidative stress. In Figures 5B–E all significantly enhanced relative to untreated samples, which is due to the elevated oxidative stress caused by plasma. Similar results have also been reported by other groups in various plants. Puač et al. (2014) showed that plasma induced higher SOD and CAT activity in meristematic cells of Daucus carota both immediately and 2 weeks after plasma treatment. Hosseini et al. (2018) found that nitrogen plasma increased POD and CAT activity in artichoke seeds. Zahoranová et al. demonstrated that SOD, CAT, and guaiacol peroxidase (POX) activity of maize seeds were enhanced by plasma (Zahoranová et al., 2016). Besides 5-min treated seedlings having a higher POD activity compared with control, the long-time plasma treatment (≥5 min) either caused a significant decrease in the proline content and activity of CAT and POD or had no effects on the SOD activity. These results reveal that the alteration of redox state may play important roles in plasma-regulated seedling growth of Arabidopsis. It is hypothesized that the antioxidant systems with enhanced activities can effectively scavenge the slightly increased ROS in Arabidopsis seeds after short-time plasma exposure (≤3 min), consequently leading to growth promotion. Conversely, the long-time plasma treated seedlings (≥5 min) didn’t have enough antioxidants to combat with the severe oxidative stress, accordingly displaying inhibited seedling growth.
Effect of Plasma on Intracellular Ca2+
Given that Ca2+ is not only a necessary nutrient for plants, but also an important signal molecule for many physiological processes, the intracellular Ca2+ level of Arabidopsis seedlings was measured with calcium-specific fluorescent dye (Fluo-2 AM) via fluorescence microscope. Several studies have shown that excessive tissue calcium concentration will result in cellular toxicity, in overly rigid cell walls and in developmental abnormalities (Conn et al., 2011; Cybulska et al., 2011). As presented in Figure 6, the green fluorescence intensity was significantly increased with the plasma treatment time, indicating that plasma led to the Ca2+ accumulation in Arabidopsis seedlings. Similar to ROS, Ca2+ also has dose-dependent effects on plants. Low level of Ca2+ promotes plant growth, while excessive Ca2+ causes cytotoxicity to plants via affecting the ion homeostasis and osmotic pressure (Volk et al., 1997). High calcium concentration in plant tissue can result in cellular toxicity or developmental abnormalities (Hocking et al., 2016). Considering the plant growth results, it is concluded that intracellular Ca2+ may also participate in the plasma-regulated seedling growth of Arabidopsis.
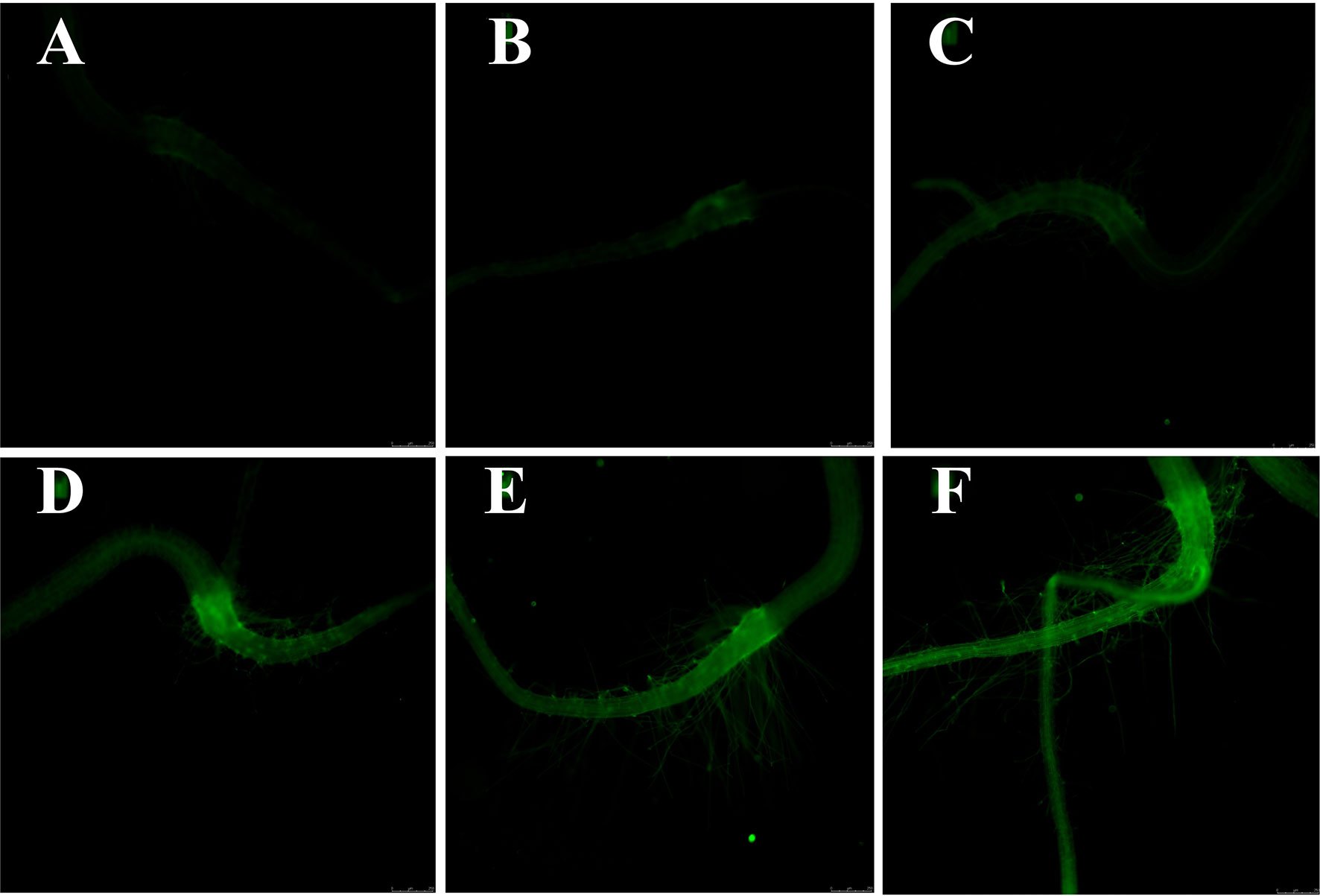
Figure 6 The cytosolic Ca2+ fluorescence microscope images (10×) of (A) 0, (B) 0.5, (C) 1, (D) 3, (E) 5, and (F) 10-min plasma treated Arabidopsis seedlings. The magnification for all images was 5×.
Effect of Plasma on the Physicochemical Modification of Seed Surface
The structural and chemical changes of seed coat after plasma treatment were observed by scanning electron microscopy-energy dispersive X-ray spectroscopy (SEM-EDX). SEM images show that the seed husk of Arabidopsis undergoes physical distortion to varying degrees with the increasing plasma treatment time (Figures 7A–L). There were no visible morphological alterations after 0.5-min plasma treatment. However, the epidermis was obviously deformed and shrinked in 1 and 3-min treated seeds. After 5-min plasma treatment, the epidermis was detached from the seed. For 10-min plasma treated seeds, the seed surface was even smoothed without the protection of epidermis. Plasma-induced etching effects on seed surface are closely related to the seed type and surface morphology (Butscher et al., 2016). Arabidopsis seed epidermis with a highly compact surface texture is more fragile and easier to be detached from the seed surface after plasma treatment, which is conducive to absorbing water and nutrients in the surrounding environment for seeds, consequently enhancing the seed germination (Stolárik et al., 2015; Wang et al., 2017b). Similar results were reported by Bormashenko et al. and Khamsen et. al., who also demonstrated that the seed surface modification caused by plasma is beneficial for seed germination (Bormashenko et al., 2015; Khamsen et al., 2016).
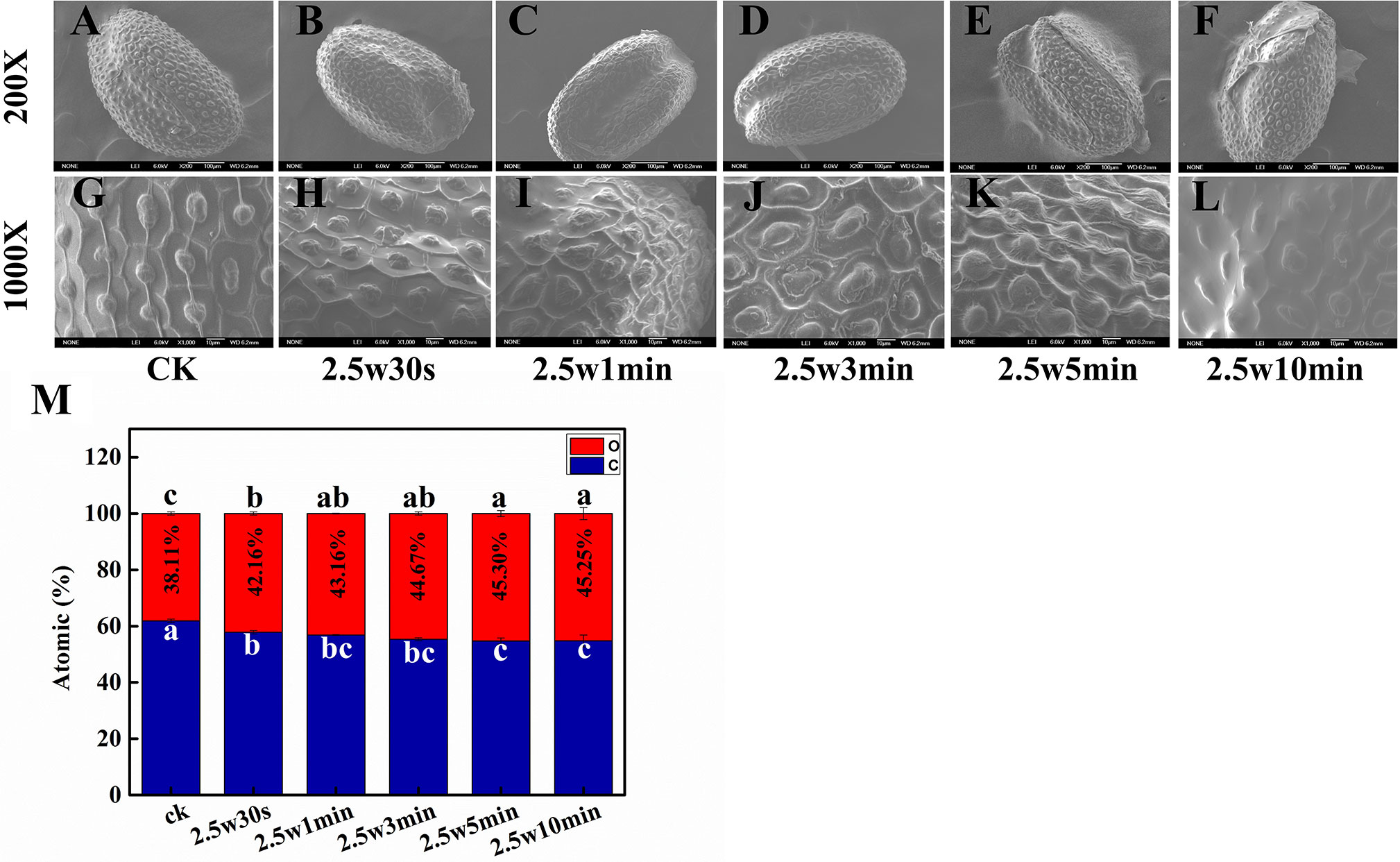
Figure 7 SEM images of (A, G) 0, (B, H) 0.5, (C, I) 1, (D, J) 3, (E, K) 5 and (F, L) 10-min plasma treated Arabidopsis seed surface. The magnification for all images was 200× (top) and 1000× (bottom). (M) Effect of plasma on atomic ratio of C and O in Arabidopsis seed surface.
The etching effects of plasma on seed surface were most probably attributed to the oxidation of RONS in plasma. To verify this hypothesis, the chemical composition of the seed surface after plasma treatment has also been analyzed by EDX. C and O are the main elements of the seed surface of Arabidopsis. As shown in Figure 7M, relative to untreated samples with an O ratio of 38.11%, the O ratio of seed surface was significantly increased from 42.16 to 45.25% with plasma treatment time from 0.5 to 10 min, indicating that plasma could indeed increase the oxidation degree of Arabidopsis seed surface. The SEM-EDX results indicated that the moderate surface deformation of Arabidopsis seed induced by short-time plasma treatment (≤3 min) via oxidative etching is a predominant mechanism for improved seed germination and seedling growth.
Conclusion
In conclusion, plasma exhibited a dose-effect on seed germination and early seedling growth of Arabidopsis, ranging from stimulation (≤3 min) to inhibition (≥5 min) depended on the plasma treatment time. For Arabidopsis seeds, O2•−, ·OH, and NO play important roles in the improvement of seed germination, while H2O2 could inhibit the seed germination. For Arabidopsis seedlings, the positive effects of short-time plasma treatment are ascribed to the enhanced activities of antioxidant system, moderate intracellular ·OH, H2O2, NO, and Ca2+ accumulation and mild seed surface modification. Whereas, severe oxidative stress caused by high level of ·OH and H2O2, excessive intracellular Ca2+, and severely damaged seed surface induced by long-time plasma treatment can result in the inhibitory effects. This work extends the available knowledge of physio-biochemical mechanism underlying plasma-regulated seed germination and seeding growth in Arabidopsis, which would be beneficial to promote the application of non-thermal plasma as a promising pre-sowing seed approach in agriculture.
Data Availability Statement
All datasets generated for this study are included in the article/Supplementary Material.
Author Contributions
DC and RM conceived the original screening and research plans. ZJ supervised the experiments. YY, ZW, HD, and ZJ provided technical assistance to DC. DC, YY, JW, and RM designed the experiments. DC, YY, and JW performed most of the experiments. DC, YY, JW, and ZW made great contribution on data collecting and analyze. DC, RM, and ZJ contributed on interpretation of data for the work. DC conceived the project and wrote the article with contributions of all the authors. ZW, HD, and RM revised it critically for important intellectual content. ZJ supervised and complemented the writing. RM, and ZJ agrees to serve as the author responsible for contact and ensures communication.
Funding
This work was funded by the National Natural Science Fund of China (11605159 and 11704343), Chinese Postdoctoral Science Foundation (2017M612412), the Foundation of Key Technology Research Project of Henan Province (182102110090).
Conflict of Interest
The authors declare that the research was conducted in the absence of any commercial or financial relationships that could be construed as a potential conflict of interest.
Acknowledgement
We are very grateful for Mr. Pengtao Yuan (School of Pharmacy, East China University of Science and Technology, Shanghai, China, 200237) and Hangbo Xu (Henan Key Laboratory of Ion-beam Bioengineering, College of Agricultural, Zhengzhou University, Zhengzhou, China, 450052) for their technical assistance and helpful discussions.
Supplementary Material
The Supplementary Material for this article can be found online at: https://www.frontiersin.org/articles/10.3389/fpls.2019.01322/full#supplementary-material
References
Bafoil, M., Jemmat, A., Martinez, Y., Merbahi, N., Eichwald, O., Dunand, C., et al. (2018). Effects of low temperature plasmas and plasma activated waters on Arabidopsis thaliana germination and growth. PloS One 13, e0195512. doi: 10.1371/journal.pone.0195512
Bafoil, M., Le Ru, A., Merbahi, N., Eichwald, O., Dunand, C., Yousfi, M. (2019). New insights of low-temperature plasma effects on germination of three genotypes of Arabidopsis thaliana seeds under osmotic and saline stresses. Sci. Rep. 9, 1–10. doi: 10.1038/s41598-019-44927-4
Bates, L. S., Waldren, R. P., Teare, I. D. (1973). Rapid determination of free proline for water-stress studies. Plant Soil. 39, 205–207. doi: 10.1007/BF00018060
Barreto, J. C., Smith, G. S., Strobel, N. H. P., McQuillin, P. A., Miller, T. A. (1994). Terephthalic acid: A dosimeter for the detection of hydroxyl radicals in vitro. Life Sci. 56, PL89–PL96. doi: 10.1016/0024-3205(94)00925-2
Bormashenko, E., Shapira, Y., Grynyov, R., Whyman, G., Bormashenko, Y., Drori, E. (2015). Interaction of cold radiofrequency plasma with seeds of beans (Phaseolus vulgaris). J. Exp. Bot. 66, 4013–4021. doi: 10.1093/jxb/erv206
Butscher, D., Van Loon, H., Waskow, A., Von Rohr, P. R., Schuppler, M. (2016). Plasma inactivation of microorganisms on sprout seeds in a dielectric barrier discharge. Int. J. Food Microbiol. 238, 222–232. doi: 10.1016/j.ijfoodmicro.2016.09.006
Chen, G. Q., Ren, L., Zhang, J., Reed, B. M., Zhang, D., Shen, X. H. (2015). Cryopreservation affects ROS-induced oxidative stress and antioxidant response in Arabidopsis seedlings. Cryobiology 70, 38–47. doi: 10.1016/j.cryobiol.2014.11.004
Chen, H. H., Chen, Y. K., Chang, H. C. (2012). Evaluation of physicochemical properties of plasma treated brown rice. Food Chem. 135, 74–79. doi: 10.1016/j.foodchem.2012.04.092
Conn, S. J., Gilliham, M., Athman, A., Schreiber, A. W., Baumann, U., Moller, I., et al. (2011). Cell-specific vacuolar calcium storage mediated by cax1 regulates apoplastic calcium concentration, gas exchange, and plant productivity in arabidopsis. Plant Cell 23, 240–257. doi: 10.1105/tpc.109.072769
Cybulska, J., Zdunek, A., Konstankiewicz, K. (2011). Calcium effect on mechanical properties of model cell walls and apple tissue. J. Food Eng. 102, 217–223. doi: 10.1016/j.jfoodeng.2010.08.019
Demidchik, V., Shabala, S., Isayenkov, S., Cuin, T. A., Pottosin, I. (2018). Calcium transport across plant membranes: mechanisms and functions. New Phytol. 220, 49–69. doi: 10.1111/nph.15266
Dobrin, D., Magureanu, M., Mandache, N. B., Ionita, M.-D. (2015). The influence of non-thermal plasma treatment on wheat germination. Innov. Food Sci. Emerg. Technol. 29, 255–260. doi: 10.1016/j.ifset.2015.02.006
Fridman, G., Friedman, G., Gutsol, A., Shekhter, A. B., Vasilets, V. N., Fridman, (2010). A. Applied plasma medicine. Plasma Processes Polym. 5, 503–533. doi: 10.1002/ppap.200700154
Gao, L., Feng, C. L., Wang, Z. W., Ding, H. (2017). High sensitive and high temporal and spatial resolved image of reactive species in atmospheric pressure surface discharge reactor by laser induced fluorescence. Rev. Sci. Instrum. 88, 053107. doi: 10.1063/1.4983060
Gómez-Ramírez, A., López-Santos, C., Cantos, M., García, J. L., González-Elipe, A. R., et al. (2017). Surface chemistry and germination improvement of Quinoa seeds subjected to plasma activation. Sci. Rep. 7, 5924. doi: 10.1038/s41598-017-06164-5
Griess., P. (1879). bemerkungen zu der abhandlung der hh. weselsky und benediktueber einige azoverbindungen. Ber. Dtsch. Chem. Ges. 12, 426–428. doi: 10.1002/cber.187901201117
Guo, Q., Meng, Y. R., Qu, G. Z., Wang, T. C., Yang, F. N., Liang, D. L., et al. (2017). Improvement of wheat seed vitality by dielectric barrier discharge plasma treatment. Bioelectromagnetics 39, 120–131. doi: 10.1002/bem.22088
Henselová, M., Martinka, M., Zahoranová, A. (2012). Growth, anatomy and enzyme activity changes in maize roots induced by treatment of seeds with low-temperature plasma. Biologia 67, 490–497. doi: 10.2478/s11756-012-0046-5
Surowsky, B., Fischer, A., Schlueter, O., Knorr, D. (2013). Cold plasma effects on enzyme activity in a model food system. Innov. Food Sci. Emerg. Technol. 19, 146–152. doi: 10.1016/j.ifset.2013.04.002
Hocking, B., Tyerman, S. D., Burton, R. A., Gilliham, M. (2016). Fruit calcium: transport and physiology. Front. Plant Sci. 7, 1–17. doi: 10.3389/fpls.2016.00569
Hosseini, S. I., Mohsenimehr, S., Hadian, J., Ghorbanpour, M., Shokri, B. (2018). Physico-chemical induced modification of seed germination and early development in artichoke (Cynara scolymus L.) using low energy plasma technology. Phys. Plasmas. 25, 013525. doi: 10.1063/1.5016037
Huang, J. C., Li, D. J., Diao, J. C., Hou, J., Yuan, J. L., Zou, G. L. (2007). A novel fluorescent method for determination of peroxynitrite using folic acid as a probe. Talanta 72, 1283–1287. doi: 10.1016/j.talanta.2007.01.033
Iseni, S., Bruggeman, P. J. P., Weltmann, K., Reuter, S. (2016). Nitrogen metastable (N2 (A3Σu+)) in a cold argon atmospheric pressure plasma jet: Shielding and gas composition, Appl. Phys. Lett. 108, 184101. doi: 10.1063/1.4948535
Jambunathan, N. (2010). Determination and detection of reactive oxygen species (ros), lipid peroxidation, and electrolyte leakage in plants. Plant Stress Tolerance. 639, 291–297 doi: 10.1007/978-1-60761-702-018
Ji, S. H., Kim, T., Panngom, K., Hong, Y. J., Pengkit, A., Park, D. H., et al. (2015). Assessment of the effects of nitrogen plasma and plasma-generated nitric oxide on early development of Coriandum sativum. Plasma Process. Polym. 12, 1164–1173. doi: 10.1002/ppap.201500021
Jiang, J. F., Lu, Y. F., Li, J. G., Li, L., He, X., Shao, H., et al. (2014). Effect of seed treatment by cold plasma on the resistance of tomato to Ralstonia solanacearum (Bacterial Wilt). PloS One 9, e 97753. doi: 10.1371/journal.pone.0097753
Khamsen, N., Onwimol, D., Teerakawanich, N., Dechanupaprittha, S., Kanokbannakorn, W., Hongesombut, K., et al. (2016). Rice (Oryza sativa L.) seed sterilization and germination enhancement via atmospheric hybrid nonthermal discharge plasma. ACS Appl. Mater. Inter. 8, 19268–19275. doi: 10.1021/acsami.6b04555
Koga, K., Thapanut, S., Amano, T., Seo, H., Itagaki, N., Hayashi, N. (2015). Simple method of improving harvest by nonthermal air plasma irradiation of seeds of Arabidopsis thaliana (l.). Appl. Phys. Express 9, 016201. doi: 10.7567/APEX.9.016201
Kumar, A., Prasad, M. N. V. (2018). Plant-lead interactions: transport, toxicity, tolerance, and detoxification mechanisms. Ecotox. Environ. Safe 166, 401–418. doi: 10.1016/j.ecoenv.2018.09.113
Li, L., Jiang, J. F., Li, J. G., Shen, M. C., He, X., Shao, H. L., et al. (2014). Effects of cold plasma treatment on seed germination and seedling growth of soybean. Sci. Rep. 4, 5859. doi: 10.1038/srep05859
Liang, Y. D., Wu, Y., Sun, K., Chen, Q., Shen, F. X., Zhang, J., et al. (2012). Rapid inactivation of biological species in the air using atmospheric pressure nonthermal plasma. Environ. Sci. Technol. 46, 3360–3368. doi: 10.1021/es203770q
Li, L., Li, J. G., Shen, M. C., Zhang, C. l., Dong, Y. H. (2015). Cold plasma treatment enhances oilseed rape seed germination under drought stress. Sci. Rep. 5, 13033. doi: 10.1038/srep13033
Liu, H. Y., Yu, X., Cui, D. Y., Sun, M. H., Sun, W. N., Tang, Z. C., et al. (2007). The role of water channel proteins and nitric oxide signaling in rice seed germination. Cell Res. 17, 638–649. doi: 10.1038/cr.2007.34
Liu, S. L., Yang, R. J., Tripathi, D. K., Li, X., He, W., Wu, M. X., et al. (2018). The interplay between reactive oxygen and nitrogen species contributes in the regulatory mechanism of the nitro-oxidative stress induced by cadmium in Arabidopsis. J. Hazard. Mater. 344, 1007–1024. doi: 10.1016/j.jhazmat.2017.12.004
Mildaziene, V., Pauzaite, G., Naucienė, Z., Malakauskiene, A., Zukiene, R., Januskaitiene, I., et al. (2018). Pre-sowing seed treatment with cold plasma and electromagnetic field increases secondary metabolite content in purple coneflower (echinacea purpurea) leaves. Plasma Processes Polym. 15, e1700059. doi: 10.1002/ppap.201700059
Mishra, R. K., Mohanty, K. (2019). Pyrolysis of three waste biomass: effect of biomass bed thickness and distance between successive beds on pyrolytic products yield and properties. Renew. Energ. 14, 549–558. doi: 10.1016/j.renene.2019.04.044
Panngom, K., Chuesaard, T., Tamchan, N., Jiwchan, T., Srikongsritong, K., Park, G. (2018). Comparative assessment for the effects of reactive species on seed germination, growth and metabolisms of vegetables. Sci. Hortic-amsterdam. 227, 889–891. doi: 10.1016/j.scienta.2017.09.026
Puač, N., Živković, S., Selaković, N., Milutinović, M., Boljević, J., Malović, G., et al. (2014). Long and short term effects of plasma treatment on meristematic plant cells. Appl. Phys. Lett. 104, 214106. doi: 10.1063/1.4880360
Qi, W. C., Zhang, L., Wang, L., Xu, H. B., Jin, Q. S., Jiao, Z. (2015). Pretreatment with low-dose gamma irradiation enhances tolerance to the stress of cadmium and lead in Arabidopsis thaliana seedlings. Ecotox. Environ. Safet. 115, 243–249. doi: 10.1016/j.ecoenv.2015.02.026
Randeniya, L. K., De Groot, G. J. J. B. (2015). Non-thermal plasma treatment of agricultural seeds for stimulation of germination, removal of surface contamination and other benefits: a review. Plasma Processes Polym. 12, 608–623. doi: 10.1002/ppap.201500042
Romero-Puertas, M. C., Terrón-Camero, L. C., Peláez-Vico, M., Olmedilla, A., Sandalio, L. M. (2019). Reactive oxygen and nitrogen species as key indicators of plant responses to Cd stress. Environ. Expe. Bot. 161, 107–119. doi: 10.1016/j.envexpbot.2018.10.012
Šerá, B., Šerý, M., Štrañák, V., Špatenka, P., Tichý, M. (2009). Does cold plasma affect breaking dormancy and seed germination? a study on seeds of lamb’s quarters (Chenopodium album agg.). Plasma Sci. Technol. 11, 750–754. doi: 10.1088/1009-0630/11/6/22
Šerá, B., Špatenka, P., Šerý, M., Vrchotová, N., Hrušková, I. (2010). Influence of plasma treatment on wheat and oat germination and early growth. IEEE Trans. Plasma Sci. 38, 2963–2968. doi: 10.1109/tps.2010.2060728
Singh, R., Singh, S., Parihar, P., Mishra, R. K., Tripathi, D. K., Singh, V. P., et al. (2016). Reactive oxygen species (ROS): beneficial companions of plants’ developmental processes. Front. Plant Sci. 7, 1299. doi: 10.3389/fpls.2016.01299
Shalata, A., Neumann, P. M. (2001). Exogenous ascorbic acid (vitamin c) increases resistance to salt stress and reduces lipid peroxidation. J. Exp. Bot. 52, 2207–2211. doi: 10.1093/jexbot/52.364.2207
Sharma, S., Uttam, K. N. (2019). Non-invasive monitoring of biochemical response of wheat seedlings toward titanium dioxide nanoparticles treatment using attenuated total reflectance fourier transform infrared and laser induced fluorescence spectroscopy. Anal. Lett., 52, 1629–1652. doi: 10.1080/00032719.2018.1563940
Soares, A., Pinto, A., Azenha, M., Teixeira, J., Azevedo, R. A., Fidalgo, F. (2016). Effect of 24-epibrassinolide on ROS content, antioxidant system, lipid peroxidation and Ni uptake in Solanum nigrum L. under Ni stress. Environ. Expe. Bot. 122, 115–125. doi: 10.1016/j.envexpbot.2015.09.010
Štěpánová, V., Slavíček, P., Kelar, J., Prášil, J., Smékal, M., Stupavská1, M., et al. (2018). Atmospheric pressure plasma treatment of agricultural seeds of cucumber (cucumis sativus l.) and pepper (capsicum annuum l.) with effect on reduction of diseases and germination improvement. Plasma Processes Polym. 15, e1700076. doi: 10.1002/ppap.201700076
Stolárik, T., Henselová, M., Martinka, M., Novák, O., Zahoranová, A., Černák, M. (2015). Effect of low-temperature plasma on the structure of seeds, growth and metabolism of endogenous phytohormones in pea (Pisum sativum L.). Plasma Chem. Plasma P. 35, 659–676. doi: 10.1007/s11090-015-9627-8
Sun, Z. C., Liu, Y. G., Huang, Y. Q., Zeng, G. M., Wang, Y. Q., Hu, X. J., et al. (2014). Effects of indole-3-acetic, kinetin and spermidine assisted with edds on metal accumulation and tolerance mechanisms in ramie (Boehmeria nivea (l.) gaud.). Eco. Eng. 71, 108–112. doi: 10.1016/j.ecoleng.2014.07.054
Volk, T., Hensel, M., Kox, W. (1997). Transient Ca2+ changes in endothelial cells induced by low doses of reactive oxygen species: Role of hydrogen peroxide. Mol. Cell. Biochem. 171, 11–21. doi: 10.1023/A:1006886215193
Volin, J. C., Denes, F. S., Young, R. A., Park, S. M. T. (2000). Modification of seed germination performance through cold plasma chemistry technology. Crop Sci. 40, 1706–1718. doi: 10.2135/cropsci2000.4061706x
Whitaker, C., Beckett, R. P., Minibayeva, F. V., Kranner, I. (2010). Alleviation of dormancy by reactive oxygen species in Bidens pilosa L. seeds. South Afr. J. Bot. 76, 601–605. doi: 10.1016/j.sajb.2010.04.014
Wang, Q. H., Yu, L. J., Liu, Y., Lin, L., Lu, R. G., Zhu, J. P., et al. (2017). Methods for the detection and determination of nitrite and nitrate: a review. Talanta 165, 709–720. doi: 10.1016/j.talanta.2016.12.044
Wang, X. J., Ma, R. N., Cui, D. J., Cao, Q., Shan, Z., Jiao, Z. (2017a). Physio-biochemical and molecular mechanism underlying the enhanced heavy metal tolerance in highland barley seedlings pre-treated with low-dose gamma irradiation. Sci. Rep. 7, 14233. doi: 10.1038/s41598-017-14601-8
Wang, X. Q., Zhou, R. W., Groot, G. D., Bazaka, K., Murphy, A. B., Ostrikov, K. (2017b). Spectral characteristics of cotton seeds treated by a dielectric barrier discharge plasma. Sci. Rep. 7, 5601. doi: 10.1038/s41598-017-04963-4
White, P. J., Broadley, M. R. (2003). Calcium in plants. Ann. Bot. 92, 487–511. doi: 10.1093/aob/mcg164
Yin, M. Q., Huang, M. J., Ma, B. Z., Ma, T. C. (2005). Stimulating effects of seed treatment by magnetized plasma on tomato growth and yield. Plasma Sci. Technol. 7, 3142–3147. doi: 10.1088/1009-0630/7/6/017
Yu, S., Chen, Q., Liu, J., Wang, K., Jiang, Z., Sun, Z., et al. (2015). Dielectric barrier structure with hollow electrodes and its recoil effect. Appl. Phys. Lett. 106, 244101. doi: 10.1063/1.4922395
Zahoranová, A., Henselová, M., Hudecová, D., Kaliňáková, B., Kováčik, D., Medvecká, V., et al. (2016). Effect of cold atmospheric pressure plasma on the wheat seedlings vigor and on the inactivation of microorganisms on the seeds surface. Plasma Chem. Plasma P. 36, 397. doi: 10.1007/s11090-015-9684-z
Zhang, L., Qi, W. C., Xu, H. B., Wang, L., Jiao, Z. (2016). Effects of low-energy N+-beam implantation on root growth in Arabidopsis seedlings. Ecotox. Environ. Safe 124, 111–119. doi: 10.1016/j.ecoenv.2015.10.003
Zhang, J. X., Kirkham, M. B. (1994). Drought-stress-induced changes in activities of superoxide dismutase, catalase, and peroxidase in wheat species. Plant Cell Physiol. 35, 785–791.doi: 10.1093/oxfordjournals.pcp.a078658
Zhang, X. H., Zhou, R. W., Bazaka, K., Liu, Y., Zhou, R. S., Chen, G. L., et al. (2018). Quantification of plasma produced OH radical density for water sterilization. Plasma Process. Polym. 15, 1–12. doi: 10.1002/ppap.201700241
Keywords: non-thermal plasma, Arabidopsis thaliana, reactive oxygen/nitrogen species, seed germination, seedling growth, physio-biochemical mechanism
Citation: Cui D, Yin Y, Wang J, Wang Z, Ding H, Ma R and Jiao Z (2019) Research on the Physio-Biochemical Mechanism of Non-Thermal Plasma-Regulated Seed Germination and Early Seedling Development in Arabidopsis. Front. Plant Sci. 10:1322. doi: 10.3389/fpls.2019.01322
Received: 21 May 2019; Accepted: 23 September 2019;
Published: 08 November 2019.
Edited by:
Christophe Dunand, Université Toulouse III Paul Sabatier, FranceReviewed by:
Thomas Roach, University of Innsbruck, AustriaFrancisco Javier Medina, Superior Council of Scientific Investigations, Spain
Copyright © 2019 Cui, Yin, Wang, Wang, Ding, Ma and Jiao. This is an open-access article distributed under the terms of the Creative Commons Attribution License (CC BY). The use, distribution or reproduction in other forums is permitted, provided the original author(s) and the copyright owner(s) are credited and that the original publication in this journal is cited, in accordance with accepted academic practice. No use, distribution or reproduction is permitted which does not comply with these terms.
*Correspondence: Ruonan Ma, bWFydW9uYW5Aenp1LmVkdS5jbg==; Zhen Jiao, amlhb3poZW5Aenp1LmVkdS5jbg==