- 1Institut de Biologie Physico-Chimique, UMR 8226, CNRS, Sorbonne Université, Paris, France
- 2Université Paris-Saclay, Saint-Aubin, France
Chlamydomonas reinhardtii is a model unicellular organism for basic or biotechnological research, such as the production of high-value molecules or biofuels thanks to its photosynthetic ability. To enable rapid construction and optimization of multiple designs and strains, our team and collaborators have developed a versatile Chlamydomonas Modular Cloning toolkit comprising 119 biobricks. Having the ability to use a wide range of selectable markers is an important benefit for forward and reverse genetics in Chlamydomonas. We report here the development of a new selectable marker based on the resistance to the antibiotic blasticidin S, using the Bacillus cereus blasticidin S deaminase (BSR) gene. The optimal concentration of blasticidin S for effective selection was determined in both liquid and solid media and tested for multiple laboratory strains. In addition, we have shown that our new selectable marker does not interfere with other common antibiotic resistances: zeocin, hygromycin, kanamycin, paromomycin, and spectinomycin. The blasticidin resistance biobrick has been added to the Chlamydomonas Modular Cloning toolkit and is now available to the entire scientific community.
Introduction
Chlamydomonas reinhardtii is a model microalga widely used for basic and biotechnological research such as photosynthesis, cilia/flagella, production of biofuels, or other molecules of interest (Georgianna and Mayfield, 2012; Barahimipour et al., 2016; Salomé and Merchant, 2019). In the last decades Chlamydomonas has been shown to be amenable to powerful genetic approaches including CRISPR-Cas9 gene editing (Jiang et al., 2014; Greiner et al., 2017; Kao and Ng, 2017). The creation of the Chlamydomonas Library Project (CLiP), which contains more than 62,000 mutants covering roughly 83% of Chlamydomonas genes (Li et al., 2019), has also greatly contributed to the development of Chlamydomonas reverse genetics. A wide range of molecular tools for engineering of the nuclear genome are also available in Chlamydomonas, most of which have been grouped in a Modular Cloning toolkit (Chlamy MoClo toolkit). This collection contains 119 biobricks (promoters, terminators, reporter genes, selectable markers, targeting peptides, antibiotic resistance genes, riboswitch, miRNA backbone, etc.), which can be easily assembled through Golden Gate cloning (Crozet et al., 2018). Most applications related to the manipulation of nuclear gene expression require transformation of Chlamydomonas cells followed by selection of transformants on plates using a selectable marker, which is in most cases an antibiotic resistance gene enabling selection on a medium containing the appropriate antibiotic. In the case of multiple consecutive transformations several selectable markers are required. Therefore, it is essential for advanced genetic engineering to have as many selectable markers as possible. At the moment, six antibiotic resistances are commonly used in Chlamydomonas as selectable markers: zeocin (Stevens et al., 1996), hygromycin (Berthold et al., 2002), kanamycin (Barahimipour et al., 2016), paromomycin (Sizova et al., 2001), sulfadiazine (Tabatabaei et al., 2019), and spectinomycin (Meslet-Cladière and Vallon, 2011).
Blasticidin S (hereafter referred to as “blasticidin”) is an antibiotic that inhibits cytosolic protein synthesis by blocking ribosomal translation termination (Svidritskiy et al., 2013). Blasticidin S deaminase (BSR) detoxifies blasticidin by catalyzing its deamination (Seto et al., 1966; Endo et al., 1987). The BSR gene has been successfully used as a selectable marker in mammals (Izumi et al., 1991), plants (Kamakura et al., 1990), yeasts (Kimura et al., 1994; Fukuda and Kizaki, 1999), and algae, including the diatom Phaeodactylum tricornutum (Buck et al., 2018) and the Chlamydomonas-related volvocine alga Volvox carteri (Ortega-Escalante et al., 2018).
We report here that blasticidin can be used as an antibiotic in Chlamydomonas, for all six laboratory strains tested. We show that BSR is functional in Chlamydomonas and can be used as a selectable marker, without conferring resistance to the other commonly used antibiotics. We also show that BSR can be used in combination with all other antibiotic resistance genes available in Chlamydomonas.
Materials and Methods
Strains, Media, and Growth Conditions
If not otherwise specified, the reference strain used in the present study is CC-4533 (CMJ030), the CLiP library recipient strain used to generate the insertional mutants (Li et al., 2019). We also used other common laboratory strains CC-4051 (4A+) (Kim et al., 2005), CC-400 (cw15) (Roy Davies, 1972), CC-4425 (D66) (Schnell and Lefebvre, 1993), UVM4 (Neupert et al., 2009), and CC-124 (137c) (Pröschold et al., 2005). Chlamydomonas cells were grown on agar plates or liquid medium, using Tris-acetate-phosphate (TAP) medium (Gorman and Levine, 1965) at 25°C, under continuous light (40–60 μmol photon⋅m–2⋅s–1) and shaking (130 rpm). Growth analysis were performed in the Algem® labscale double photobioreactor systems (Algenuity, Stewartby, United Kingdom) under continuous light (200 μmol photons⋅m–2⋅s–1) and 120 rpm agitation in TAP or high salt medium (HSM) (Sueoka, 1960), with bubbling air. The absorbance at 740 nm was recorded every 10 min using the built-in sensor. The maximal growth rate was determined as the maximal slope of the growth curve (Δabs/Δtime). Linearity of the growth curve was estimated through linear regression every 200 min over a period of 13 h for TAP or 300 min over a period of 40 h for HSM condition. The slopes of these linear regressions were selected only for regressions displaying an R2 < 99.5%. Finally, the maximal slope was chosen as the maximal growth rate (Supplementary Figure S2B). Antibiotics used were blasticidin S (Sigma-Aldrich: SBR00022), zeocin (Invitrogen: R25005), hygromycin B (Sigma-Aldrich: H9773), kanamycin (Sigma-Aldrich: K1377), paromomycin (Sigma-Aldrich: P8692), or spectinomycin (Sigma-Aldrich: S4014). Chlamydomonas multi-well plates and Petri dishes were scanned using a Perfection V800 scanner (Epson).
Cell Death Quantification
Dead cells were detected using Evans blue (Sigma-Aldrich: E2129) at a final concentration of 0.2% w/v. Dead cells appear in blue whereas living cells that are impermeable to Evans blue remain green (Gaff and Okong’o-Ogola, 1971). Cells were observed with a microscope (Olympus BX43, Tokyo, Japan). For each sample multiple microscopic fields were analyzed and Evans blue-positive cells scored. For each value the percentage of dead cells was calculated on a minimum of 100 individuals.
Plasmid Construction
Protein and nucleic acid designs were performed in silico on Serial Cloner 2.6.1 software. Bacillus cereus BSR protein sequence (NCBI accession number: WP_076871832.1) was reverse translated using Chlamydomonas nuclear genome codon usage table1. The resulting BSR coding sequence was domesticated by removing BbsI restriction sites, designed for the position B3–B5 of the common Plant MoClo syntax (Patron et al., 2015), synthetized (Twist Bioscience), and cloned by Golden Gate reaction with BsaI-HFv2 (New England Biolabs) in pICH41308 (Weber et al., 2011) yielding plasmid pCM0-120, numbered according to the Chlamydomonas MoClo toolkit nomenclature (Crozet et al., 2018). Two other parts from the toolkit were used to build the BSR module (pCM1-029), the promoter PA/R coupled to the 5′UTR of RBCS2 (pCM0-020) and the 3′UTR of RBCS2 coupled to terminator TRBCS2 (pCM0-115) (Schroda et al., 2002; Crozet et al., 2018). The other antibiotic resistance genes were built using these same regulatory sequences and the coding sequence (CDS) from pCM0-077 (zeocin), pCM0-073 (hygromycin), pCM0-074 (paromomycin), pCM0-075 (kanamycin) and pCM0-076 (spectinomycin) generating the plasmids pCM1-030 (zeocin), pCM1-031 (hygromycin), pCM1-032 (kanamycin), pCM1-027 (paromomycin), and pCM1-033 (spectinomycin), respectively. All plasmid sequences are available in Supplementary Files S1–S7.
Chlamydomonas Transformation
Transformations were performed as previously described (Crozet et al., 2018). Briefly, Chlamydomonas cells were grown in TAP to early exponential phase (1–2 × 106 cells/mL), concentrated 100 times in TAP + 60 mM sucrose. After incubation of 250 μL of cells with DNA (55 fmol of purified resistance module excised with BbsI-HF; New England Biolabs) at 4°C for 20 min, they were electroporated (2000 V/cm, 25 μF, no shunt resistance) and incubated for 16–20 h in 10 mL of TAP + 60 mM sucrose prior to be plated on TAP-agar complemented with appropriate antibiotic(s). Transformants were selected on TAP-agar medium containing blasticidin S (50 mg/L, unless otherwise specified), zeocin (15 mg/L), hygromycin B (20 mg/L), kanamycin (50 mg/L), paromomycin (20 mg/L), and/or spectinomycin (100 mg/L). Plates and transformants were analyzed after 5–7 days of growth in continuous light (50 μmol photon m–2 s–1) at 25°C.
Insert Detection
Cells were pelleted (5 min, 2500 × g, room temperature) and lysed in 400 μL of extraction buffer (200 mM Tris-HCl pH 7.5; 200 mM NaCl; 25 mM EDTA; 0.5% SDS) for 10 min at 37°C under agitation (1400 rpm). After centrifugation (3 min, 17,000 × g, room temperature), the supernatant was harvested and the genomic DNA was precipitated with one volume of isopropanol for 10 min at room temperature, washed with 70% ethanol, dried and resuspended in water. PCR was performed using the Quick-Load® Taq 2× Master Mix (New England Biolabs) according to the manufacturer recommendations with the primers BSR.5 (5′-GCTGTACGAGGACAACAAGC-3′), TRBCS2.3 (5′-ACGGAGGATCGTTACAACC-3′), CBLP.5 (5′-GACGTCATCCACTGCCTGTG-3′), and CBLP.3 (5′-CGACGCATCCTCAACACACC-3′).
Results
Chlamydomonas Is Sensitive to Blasticidin
To test the sensitivity of Chlamydomonas to blasticidin, the cells were grown in the presence of increasing blasticidin concentrations (25, 50, or 75 mg/L) in both solid and liquid cultures. Blasticidin was very effective to kill Chlamydomonas cells and the minimum efficient concentration was 50 mg/L in both conditions (Figure 1A). Many reference strains are used within the community and they present an important genetic diversity and substantial phenotypic differences (Gallaher et al., 2015). Compared to the reference strain of this study (CC-4533), some strain specific phenotypes, such as the absence of the cell wall, could alter blasticidin resistance. To assess whether this genetic diversity among common reference strains affects their sensitivity to blasticidin, CC-4051 (4A+), CC-400 (cw15), CC-4425 (D66+), UVM4, and CC-124 (137c) were cultivated on a solid medium supplemented or not with blasticidin (50 mg/L). In all cases, none of the strains survived in the presence of blasticidin, regardless of the initial number of cells tested (Figure 1B).
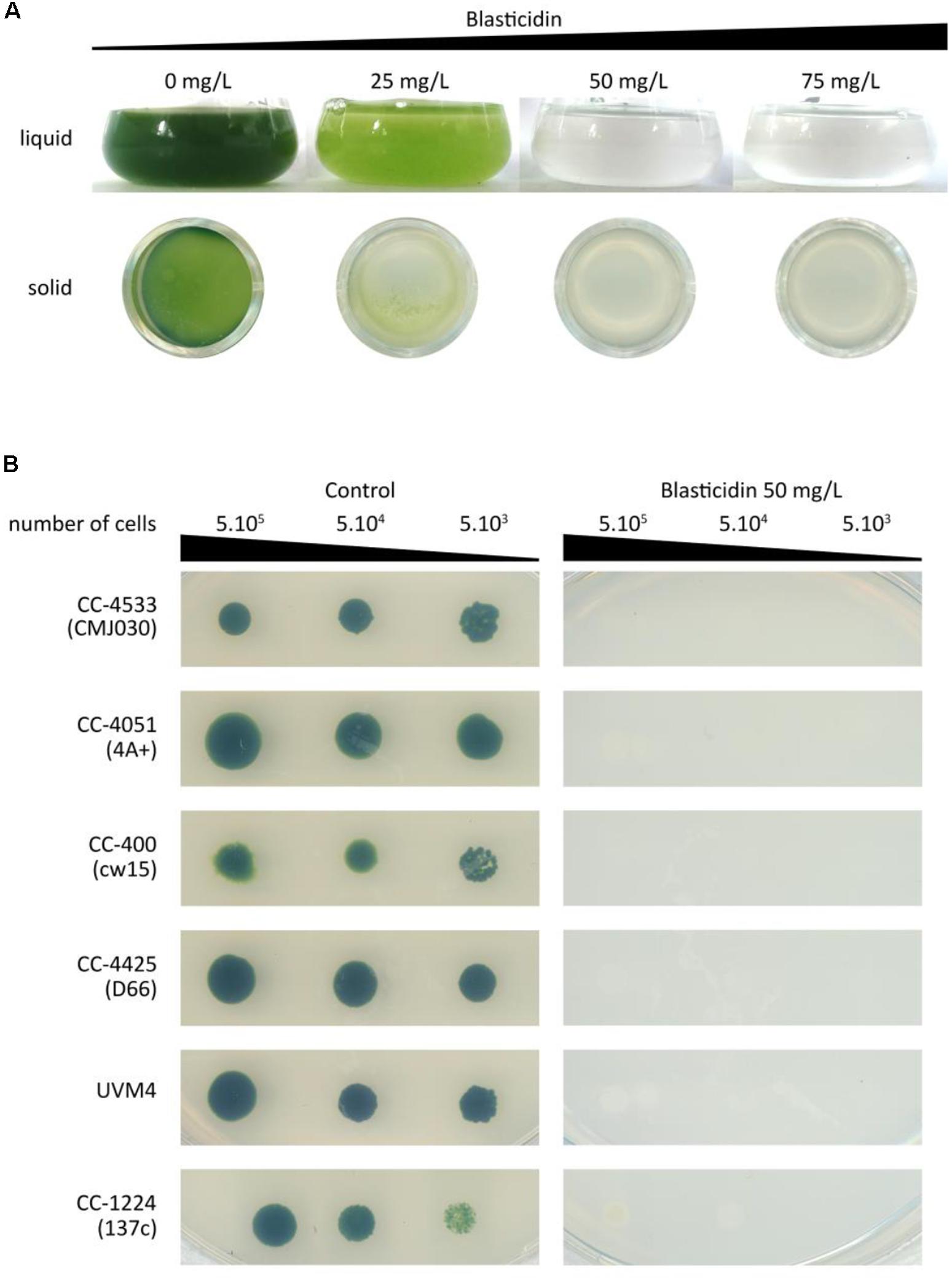
Figure 1. Sensitivity of Chlamydomonas to blasticidin. (A) Chlamydomonas CC-4533 (CMJ030) was grown with increasing concentrations of blasticidin: 25, 50, or 75 mg/L, in both liquid and 24-well agar plates. For both liquid and solid media, cultures were photographed after 3 days of growth. (B) Spot tests were performed for six standard laboratory strains with or without blasticidin (50 mg/L). The results shown are representative of three biological replicates.
Blasticidin S Deaminase Can Be Used as a Selectable Marker in Chlamydomonas
To test whether the BSR module could be used as a selectable marker in Chlamydomonas, we engineered B. cereus BSR coding sequence to fit the optimal codon bias of Chlamydomonas nuclear genome. This has been already shown to improve transgene expression efficiency, including selection markers (Barahimipour et al., 2015, 2016). The engineered BSR coding sequence was then domesticated by removing BbsI and BsaI recognition motif and adding appropriate fusion sites of the Plant MoClo syntax (Patron et al., 2015). It was finally assembled with the parts PA/R (the hybrid promoter of HSP70A coupled to the 5′UTR of RBCS2) and TRBCS2 (coupling the 3′UTR and terminator of RBCS2) to form a functional module. PA/R is a chimeric constitutive promoter made up of HSP70A and RBCS2 promoters that was proven to be very efficient in Chlamydomonas by significantly reducing gene silencing (Schroda et al., 2002). We also chose this promoter/terminator combination because it allows successful expression of the same BSR gene in Volvox (Ortega-Escalante et al., 2018). The resulting construct pCM1-029 (pCM stands for plasmid Chlamydomonas MoClo) is represented in Figure 2A using the MoClo nomenclature (Crozet et al., 2018). pCM0-120 and pCM1-029 plasmid sequences are available in Supplementary Files S1, S2, respectively. Cells transformed with pCM1-029 or an empty vector were incubated on plates containing blasticidin. Transformants appeared only when the cells were transformed with pCM1-029 (Blasticidin resistant cells are hereafter referred to as “BlastR”), as shown in Figure 2B, indicating that BSR can be used as a selectable marker in Chlamydomonas. The insertion of the BSR module was confirmed by PCR in four independent BlastR strains (Supplementary Figure S1).
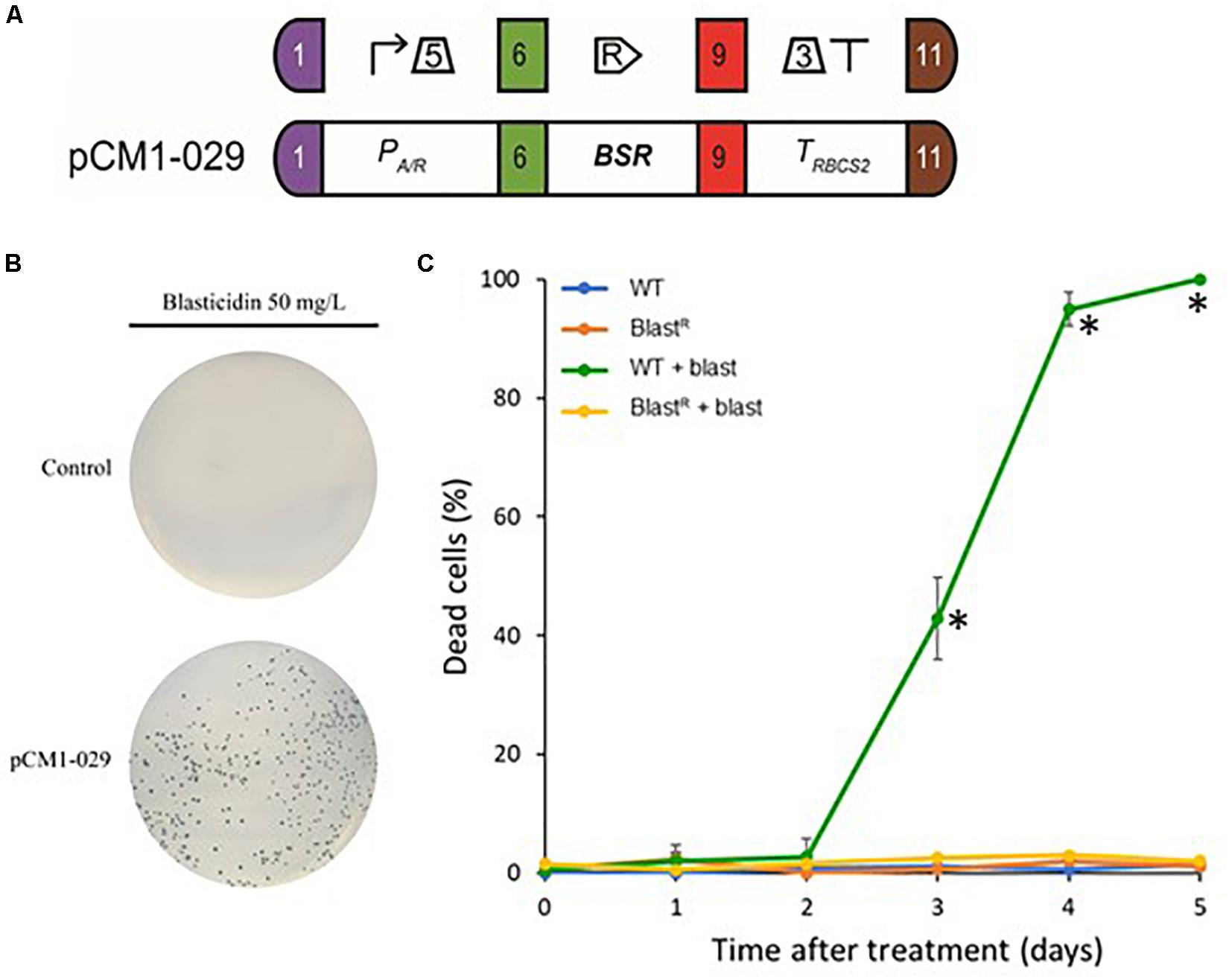
Figure 2. Blasticidin S deaminase expression in Chlamydomonas. (A) The blasticidin resistance module pCM1-029 is built from a constitutive promoter (PA/R + 5′UTR of RBCS2), the BSR coding sequence and a terminator (3′UTR of RBCS2 + TRBCS2) and was assembled using Golden Gate cloning. The numbers 1, 6, 9, and 11 stand for the standard fusion sites used for cloning (Crozet et al., 2018). SBOL2.0 visual syntax is shown above the module (Roehner et al., 2016). (B) CC-4533 cells were transformed with pCM1-029 or an empty vector (pICH47742) (Weber et al., 2011) and plated on TAP-agar supplemented with blasticidin (50 mg/L). Results are representative of three biological replicates. (C) The percentage of dead cells was evaluated using Evans blue in wild-type and BlastR cultures with or without blasticidin, several days after treatment. Values represent the means and standard deviations of three independent experiments, symbol (∗) shows the samples found to be significantly different doing an ANOVA analysis for each time points (p < 0.001).
To precisely evaluate the effect of blasticidin on the mortality of wild-type and BlastR cells, we used Evans blue as a death marker (Gaff and Okong’o-Ogola, 1971). To avoid the heterogeneity that comes from the position of the transgene insertion locus, dozens of BlastR colonies were collected from a Petri dish and then mixed in liquid culture (BlastR culture). Wild-type and BlastR cultures were grown in a 24-well plate with or without blasticidin, and the percentage of dead cells was evaluated several days after treatment. In the wild-type strain treated with blasticidin, the percentage of dead cells increased after 3 days and all the cells died after 5 days, while no significant levels of death was detected for BlastR and untreated cells (Figure 2C). To assess a potential detrimental effect of BSR on Chlamydomonas, wild-type and BlastR growth were quantified in a photobioreactor, in both mixotrophic (TAP) and autotrophic (HSM) conditions. No differences in the growth rate were observed suggesting that BSR does not affect growth or photosynthesis (Supplementary Figure S2).
Blasticidin Can Be Used in Combination With Other Antibiotics
To test the possible interactions of blasticidin with the other most commonly used selectable markers in Chlamydomonas, we first generated strains resistant to these antibiotics by transformation of wild-type cells with plasmids containing modules conferring resistance to zeocin (pCM1-030), hygromycin (pCM1-031), kanamycin (pCM1-032), paromomycin (pCM1-027), and spectinomycin (pCM1-033) under the control of the same regulatory sequences used for BSR in pCM1-029. Transformants were selected on plates containing the appropriate antibiotic and pooled to take into account position effect, as for BlastR culture. These cultures were called ZeoR, HygroR, KanaR, ParoR, and SpecR. The wild-type strain and the six antibiotic resistant cultures were cultivated in 96-well plates until exponential phase (5 × 106 cells/mL) prior to treatment with the different antibiotics. Five days after the addition of antibiotics, only the cells carrying the corresponding resistance gene survived the treatment, and importantly, only the BlastR culture had survived upon blasticidin treatment (Figure 3A). It is to be noticed that KanaR strains are also resistant to paromomycin (Figure 3A), as previously reported (Barahimipour et al., 2016).
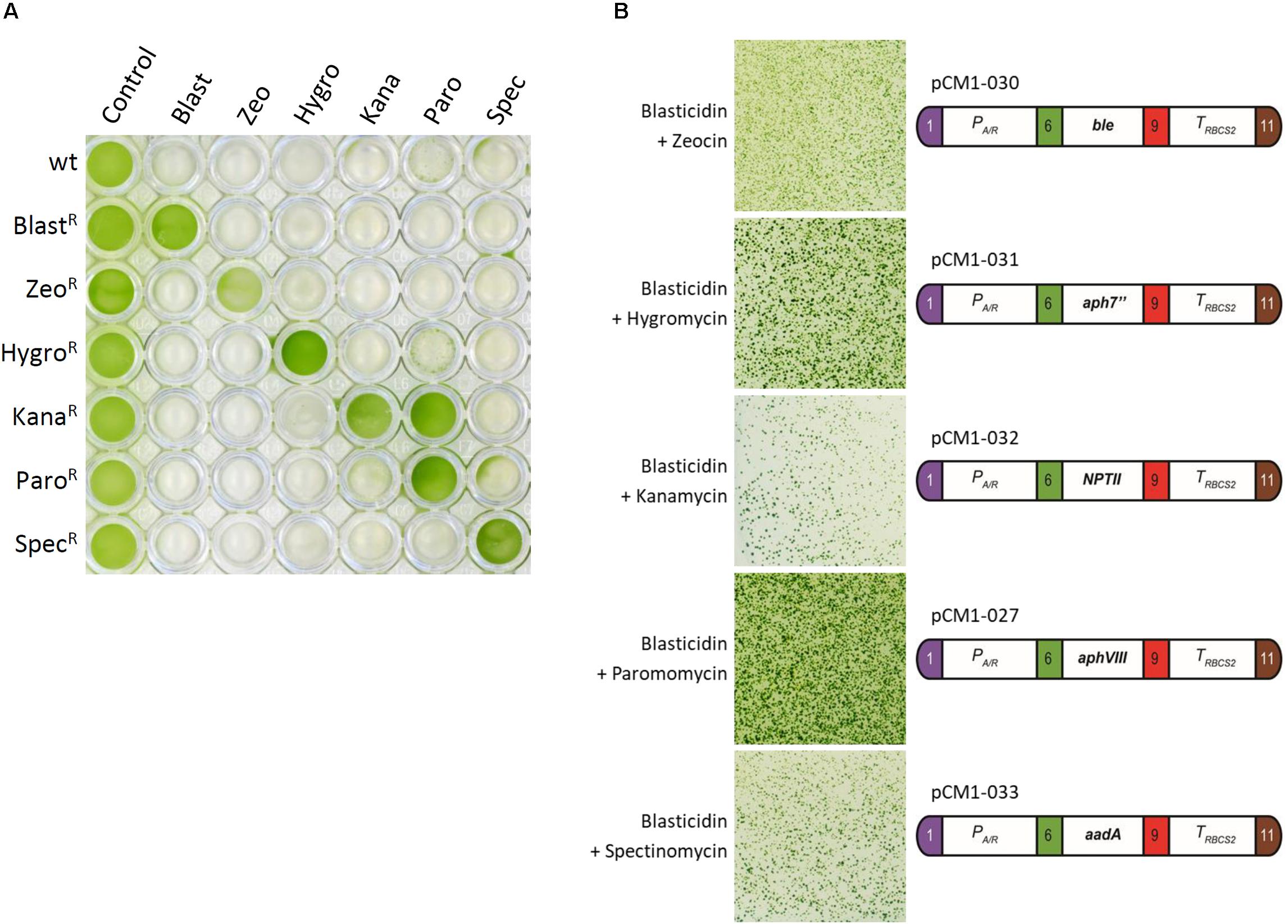
Figure 3. Cross reactivity test. (A) Liquid cultures in exponential phase of wild-type strain and ZeoR, HygroR, KanaR, ParoR, and SpecR cultures were placed in a 96-well plate and treated with blasticidin (Blast, 50 mg/L), zeocin (Zeo, 15 mg/L), hygromycin B (Hygro, 20 mg/L), kanamycin (Kana, 50 mg/L), paromomycin (Paro, 20 mg/L), and spectinomycin (Spec, 100 mg/L) for 5 days. (B) A blasticidin resistant strain carrying pCM1-029 was independently transformed with the resistance modules to zeocin (pCM1-030), hygromycin (pCM1-031), kanamycin (pCM1-032), paromomycin (pCM1-027), and spectinomycin (pCM1-033). The numbers 1, 6, 9, and 11 stand for the standard fusion sites used for cloning (Crozet et al., 2018). Transformants were selected on plate containing both blasticidin and the appropriate antibiotic. The results showed are representative of three biological replicates. The full experiment with the different controls is available in Supplementary Figure S3.
If the resistance modules tested display no cross reactivity to one another, they can be combined to allow double selection. To use the BSR module and blasticidin in combination with another selectable marker, there must then be no interference between the different antibiotics and resistance modules. To verify possible interference, a single BlastR strain was transformed with either pCM1-030 (zeocin resistance), pCM1-031 (hygromycin resistance), pCM1-032 (kanamycin resistance), pCM1-027 (paromomycin resistance), or pCM1-033 (spectinomycin resistance) (Figure 3B). Transformants were selected on plates containing both blasticidin and the appropriate antibiotic. For each combination tested, transformants resistant to both antibiotics could be obtained (Figure 3B), indicating that no interference exists between the selectable markers tested.
Discussion
Here we report the development of a new selectable marker for C. reinhardtii. Our data show that using blasticidin at a concentration of 50 mg/L ensures proper selection for all common laboratory strains of Chlamydomonas (Figure 2C). This concentration is slightly higher than the efficient concentration reported for V. carteri (Ortega-Escalante et al., 2018) or diatoms (Buck et al., 2018), but remains comparable with other antibiotics used in Chlamydomonas (Crozet et al., 2018).
We successfully engineered BSR coding sequence to adapt it to Chlamydomonas and no addition of intron was necessary for efficient expression, contrary to what was reported for V. carteri (Ortega-Escalante et al., 2018). Now that we have developed the blasticidin resistance module, seven antibiotic-based selectable markers are available for Chlamydomonas. This new tool can become important for advanced synthetic biology strategies requiring successive transformations of the same strain in combination with new engineering tools including the CLiP library (Li et al., 2019), the CRISPR/Cas technology (Jiang et al., 2014; Greiner et al., 2017; Kao and Ng, 2017) and the MoClo toolkit (Crozet et al., 2018). It is also important to increase the number of selectable markers available because the use of certain antibiotics should be taken with care. For instance zeocin is not always recommended since it can potentially cause DNA damages (Chankova et al., 2007; Čížková et al., 2019) and subsequent unwanted mutations. The kanamycin selectable marker NPTII is also conferring resistance to paromomycin (Barahimipour et al., 2015; Figure 3A) which makes it impossible to use in CLiP strains that are paromomycin resistant (Li et al., 2019). We show here that it is possible to use BSR and blasticidin in combination with all the other commonly used selectable markers in Chlamydomonas. BSR gene has been integrated as a new biobrick into the Chlamydomonas MoClo toolkit, and is now available to the entire community through the Chlamydomonas Resource Center2.
Data Availability Statement
All datasets generated for this study are included in the article/Supplementary Material.
Author Contributions
FC, JL, PC, SL, and AD designed the study and analyzed the data. FC, JL, NB, PC, SL, and AD wrote the manuscript. FC, JL, NB, and AD performed the experiments.
Funding
This work was supported in part by the CNRS, Sorbonne Université, and Université Paris-Saclay, by Agence Nationale de la Recherche Grant 17-CE05-0001 CalvinDesign and by LABEX DYNAMO ANR-LABX-011.
Conflict of Interest
The authors declare that the research was conducted in the absence of any commercial or financial relationships that could be construed as a potential conflict of interest.
Acknowledgments
We thank Sandrine Bujaldon for providing the Chlamydomonas reinhardtii strains CC-4051, CC-400, and CC-124; Kevin Sarkissian for his help in the cloning; and Cyrielle Durand, Théo Le Moigne, Dr. Christophe Marchand, and Dr. Julien Henri for stimulating discussions and suggestions.
Supplementary Material
The Supplementary Material for this article can be found online at: https://www.frontiersin.org/articles/10.3389/fpls.2020.00242/full#supplementary-material
FIGURE S1 | Detection of the BSR module. pCM1-029 plasmid and the genomic DNA of the wild type (CC-4533) and four independent transformants BlastR were used to amplify by PCR a fragment of the BSR resistance module. A product at the expected size (546 bp) was amplified only for pCM1-029 (positive control) and the BlastR transformants. Amplification of CBLP (Cre06.g278222) was used as a positive control for the experiment.
FIGURE S2 | Growth of wild-type (CC-4533) and BlastR strains in a controlled photobioreactor. (A) Measurement of growth as detected by absorbance at 740 nm every 10 min, in TAP (mixotrophy) and HSM medium (autotrophy), light was set to 200 μmol photons⋅m–2⋅s–1 with temperature and shaking set at 25°C and 120 rpm, respectively. (B) Quantification of the average growth rate of wild-type and BlastR cells, corresponding to the growth curves shown in Graph (A). Error bars represent the standard error to the mean of two biological replicates.
FIGURE S3 | Transformation of a blasticidin resistant strain with zeocin, hygromycin, kanamycin, paromomycin and spectinomycin resistance plasmids. A blasticidin resistant strain (BlastR) carrying pCM1-029 was independently transformed with the resistance modules to zeocin (pCM1-030), hygromycin (pCM1-031), kanamycin (pCM1-032), paromomycin (pCM1-027), and spectinomycin (pCM1-033) or an empty vector (pICH50881). The numbers 1, 6, 9, and 11 stand for the standard fusion sites used for cloning (Crozet et al., 2018). BlastR transformed cells were selected on plate containing both blasticidin and the appropriate antibiotic. CC-4533 was also transformed with the same five resistance modules or the empty vector and selected on the corresponding antibiotic with or without addition of blasticidin. The results showed are representative of three biological replicates.
FILE S1 | Annotated sequence of pCM0-120, the level 0 plasmid containing BSR coding sequence engineered for Chlamydomonas nuclear genome and designed for the position B3–B5 of the Chlamydomonas MoClo toolkit (Crozet et al., 2018).
FILE S2 | Annotated sequence of pCM1-029, the level 1 plasmid made up of the promoter AR (PA/R + 5′UTR of RBCS2), BSR coding sequence and the terminator of RBCS2 (3′UTR of RBCS2 + TRBCS2) conferring blasticidin resistance in Chlamydomonas (Crozet et al., 2018).
FILE S3 | Annotated sequence of pCM1-027, the level 1 plasmid made up of the promoter AR (PA/R + 5′UTR of RBCS2), aphVIII coding sequence and the terminator of RBCS2 (3′UTR of RBCS2 + TRBCS2) conferring paromomycin resistance in Chlamydomonas (Sizova et al., 2001; Crozet et al., 2018).
FILE S4 | Annotated sequence of pCM1-030, the level 1 plasmid made up of the promoter AR (PA/R + 5′UTR of RBCS2), Ble coding sequence and the terminator RBCS2 (3′UTR of RBCS2 + TRBCS2) conferring zeocin resistance in Chlamydomonas (Stevens et al., 1996; Crozet et al., 2018).
FILE S5 | Annotated sequence of pCM1-031, the level 1 plasmid made up of the promoter AR (PA/R + 5′UTR of RBCS2), aph7″ coding sequence and the terminator RBCS2 (3′UTR of RBCS2 + TRBCS2) conferring hygromycin resistance in Chlamydomonas (Berthold et al., 2002; Crozet et al., 2018).
FILE S6 | Annotated sequence of pCM1-032, the level 1 plasmid made up of the promoter AR (PA/R + 5′UTR of RBCS2), NPTII coding sequence and the terminator RBCS2 (3′UTR of RBCS2 + TRBCS2) conferring kanamycin resistance in Chlamydomonas (Barahimipour et al., 2016; Crozet et al., 2018).
FILE S7 | Annotated sequence of pCM1-029, the level 1 plasmid made up of the promoter AR (PA/R + 5′UTR of RBCS2), aadA coding sequence and the terminator RBCS2 (3′UTR of RBCS2 + TRBCS2) conferring spectinomycin resistance in Chlamydomonas (Meslet-Cladière and Vallon, 2011; Crozet et al., 2018).
Footnotes
References
Barahimipour, R., Neupert, J., and Bock, R. (2016). Efficient expression of nuclear transgenes in the green alga Chlamydomonas: synthesis of an HIV antigen and development of a new selectable marker. Plant Mol. Biol. 90, 403–418. doi: 10.1007/s11103-015-0425-8
Barahimipour, R., Strenkert, D., Neupert, J., Schroda, M., Merchant, S. S., and Bock, R. (2015). Dissecting the contributions of GC content and codon usage to gene expression in the model alga Chlamydomonas reinhardtii. Plant J. 84, 704–717. doi: 10.1111/tpj.13033
Berthold, P., Schmitt, R., and Mages, W. (2002). An engineered Streptomyces hygroscopicus aph 7 Gene Mediates dominant resistance against hygromycin B in Chlamydomonas reinhardtii. Protist 153, 401–412. doi: 10.1078/14344610260450136
Buck, J. M., Río Bártulos, C., Gruber, A., and Kroth, P. G. (2018). Blasticidin-S deaminase, a new selection marker for genetic transformation of the diatom Phaeodactylum tricornutum. PeerJ 6:e5884. doi: 10.7717/peerj.5884
Chankova, S. G., Dimova, E., Dimitrova, M., and Bryant, P. E. (2007). Induction of DNA double-strand breaks by zeocin in Chlamydomonas reinhardtii and the role of increased DNA double-strand breaks rejoining in the formation of an adaptive response. Radiat. Environ. Biophys. 46, 409–416. doi: 10.1007/s00411-007-0123-2
Čížková, M., Slavková, M., Vítová, M., Zachleder, V., and Bišová, K. (2019). Response of the green alga Chlamydomonas reinhardtii to the DNA damaging agent zeocin. Cells 8:E735. doi: 10.3390/cells8070735
Crozet, P., Navarro, F. J., Willmund, F., Mehrshahi, P., Bakowski, K., Lauersen, K. J., et al. (2018). Birth of a photosynthetic chassis: a MoClo toolkit enabling synthetic biology in the microalga Chlamydomonas reinhardtii. ACS Synth. Biol. 7, 2074–2086. doi: 10.1021/acssynbio.8b00251
Endo, T., Furuta, K., Kaneko, A., Katsuki, T., Kobayashi, K., Azuma, A., et al. (1987). Inactivation of blasticidin S by Bacillus cereus. I. inactivation mechanism. J. Antibiot. 40, 1791–1793. doi: 10.7164/antibiotics.40.1791
Fukuda, H., and Kizaki, Y. (1999). A new transformation system of Saccharomyces cerevisiae with blasticidin S deaminase gene. Biotechnol. Lett. 21, 969–971. doi: 10.1023/A:1005613206138
Gaff, D. F., and Okong’o-Ogola, O. (1971). The Use of non-permeating pigments for testing the survival of cells. J. Exp. Bot. 22, 756–758. doi: 10.1093/jxb/22.3.756
Gallaher, S. D., Fitz-Gibbon, S. T., Glaesener, A. G., Pellegrini, M., and Merchant, S. S. (2015). Chlamydomonas genome resource for laboratory strains reveals a mosaic of sequence variation, identifies true strain histories, and enables strain-specific studies. Plant Cell 27, 2335–2352. doi: 10.1105/tpc.15.00508
Georgianna, D. R., and Mayfield, S. P. (2012). Exploiting diversity and synthetic biology for the production of algal biofuels. Nature 488, 329–335. doi: 10.1038/nature11479
Gorman, D. S., and Levine, R. P. (1965). Cytochrome f and plastocyanin: their sequence in the photosynthetic electron transport chain of Chlamydomonas reinhardi. Proc. Natl. Acad. Sci. U.S.A. 54, 1665–1669. doi: 10.1073/pnas.54.6.1665
Greiner, A., Kelterborn, S., Evers, H., Kreimer, G., Sizova, I., and Hegemann, P. (2017). Targeting of photoreceptor genes in Chlamydomonas reinhardtii via zinc-finger nucleases and CRISPR/Cas9. Plant Cell 29, 2498–2518. doi: 10.1105/tpc.17.00659
Izumi, M., Miyazawa, H., Kamakura, T., Yamaguchi, I., Endo, T., and Hanaoka, F. (1991). Blasticidin S-resistance gene (bsr): a novel selectable marker for mammalian cells. Exp. Cell Res. 197, 229–233. doi: 10.1016/0014-4827(91)90427-V
Jiang, W., Brueggeman, A. J., Horken, K. M., Plucinak, T. M., and Weeks, D. P. (2014). Successful transient expression of Cas9 and Single Guide RNA Genes in Chlamydomonas reinhardtii. Eukaryot Cell 13, 1465–1469. doi: 10.1128/EC.00213-14
Kamakura, T., Yoneyama, K., and Yamaguchi, I. (1990). Expression of the blasticidin S deaminase gene (bsr) in tobacco: fungicide tolerance and a new selective marker for transgenic plants. Mol. Gen. Genet. 223, 332–334. doi: 10.1007/bf00265072
Kao, P.-H., and Ng, I.-S. (2017). CRISPRi mediated phosphoenolpyruvate carboxylase regulation to enhance the production of lipid in Chlamydomonas reinhardtii. Bioresour. Technol. 245, 1527–1537. doi: 10.1016/j.biortech.2017.04.111
Kim, K.-S., Feild, E., King, N., Yaoi, T., Kustu, S., and Inwood, W. (2005). Spontaneous mutations in the ammonium transport gene AMT4 of Chlamydomonas reinhardtii. Genetics 170, 631–644. doi: 10.1534/genetics.105.041574
Kimura, M., Kamakura, T., Zhou Tao, Q., Kaneko, I., and Yamaguchi, I. (1994). Cloning of the blasticidin S deaminase gene (BSD) from Aspergillus terreus and its use as a selectable marker for Schizosaccharomyces pombe and Pyricularia oryzae. Mol. Gen. Genet. 242, 121–129. doi: 10.1007/BF00391004
Li, X., Patena, W., Fauser, F., Jinkerson, R. E., Saroussi, S., Meyer, M. T., et al. (2019). A genome-wide algal mutant library and functional screen identifies genes required for eukaryotic photosynthesis. Nat. Genet. 51, 627–635. doi: 10.1038/s41588-019-0370-376
Meslet-Cladière, L., and Vallon, O. (2011). Novel shuttle markers for nuclear transformation of the green alga Chlamydomonas reinhardtii. Eukaryotic Cell 10, 1670–1678. doi: 10.1128/EC.05043-11
Neupert, J., Karcher, D., and Bock, R. (2009). Generation of Chlamydomonas strains that efficiently express nuclear transgenes. Plant J. 57, 1140–1150. doi: 10.1111/j.1365-313X.2008.03746.x
Ortega-Escalante, J. A., Kwok, O., and Miller, S. M. (2018). New Selectable Markers for Volvox carteri Transformation. Protist 170, 52–63. doi: 10.1016/j.protis.2018.11.002
Patron, N. J., Orzaez, D., Marillonnet, S., Warzecha, H., Matthewman, C., Youles, M., et al. (2015). Standards for plant synthetic biology: a common syntax for exchange of DNA parts. New Phytol. 208, 13–19. doi: 10.1111/nph.13532
Pröschold, T., Harris, E. H., and Coleman, A. W. (2005). Portrait of a species: Chlamydomonas reinhardtii. Genetics 170, 1601–1610. doi: 10.1534/genetics.105.044503
Roehner, N., Beal, J., Clancy, K., Bartley, B., Misirli, G., Grünberg, R., et al. (2016). Sharing structure and function in biological design with SBOL 2.0. ACS Synth. Biol. 5, 498–506. doi: 10.1021/acssynbio.5b00215
Roy Davies, D. (1972). Cell wall organisation in Chlamydomonas reinhardi. Mol. Gen. Genet. 115, 334–348. doi: 10.1007/BF00333172
Salomé, P. A., and Merchant, S. S. (2019). A series of fortunate events: introducing Chlamydomonas as a reference organism. Plant Cell 31, 1682–1707. doi: 10.1105/tpc.18.00952
Schnell, R. A., and Lefebvre, P. A. (1993). Isolation of the Chlamydomonas regulatory gene NIT2 by transposon tagging. Genetics 134, 737–747.
Schroda, M., Beck, C. F., and Vallon, O. (2002). Sequence elements within an HSP70 promoter counteract transcriptional transgene silencing in Chlamydomonas. Plant J. 31, 445–455. doi: 10.1046/j.1365-313X.2002.01371.x
Seto, H., Ôtake, N., and Yonehara, H. (1966). Biological transformation of Blasticidin S by Aspergillus fumigatus sp. Agric. Biol. Chem. 30, 877–886. doi: 10.1080/00021369.1966.10858693
Sizova, I., Fuhrmann, M., and Hegemann, P. (2001). A Streptomyces rimosus aphVIII gene coding for a new type phosphotransferase provides stable antibiotic resistance to Chlamydomonas reinhardtii. Gene 277, 221–229. doi: 10.1016/s0378-1119(01)00616-3
Stevens, D. R., Purton, S., and Rochaix, J.-D. (1996). The bacterial phleomycin resistance gene ble as a dominant selectable marker in Chlamydomonas. Mol. Gen. Genet. 251, 23–30. doi: 10.1007/BF02174340
Sueoka, N. (1960). Mitotic replication of deoxyribonucleic acid in Chlamydomonas Reinhardi. Proc. Natl. Acad. Sci. U.S.A. 46, 83–91. doi: 10.1073/pnas.46.1.83
Svidritskiy, E., Ling, C., Ermolenko, D. N., and Korostelev, A. A. (2013). Blasticidin S inhibits translation by trapping deformed tRNA on the ribosome. Proc. Natl. Acad. Sci. U.S.A. 110, 12283–12288. doi: 10.1073/pnas.1304922110
Tabatabaei, I., Dal Bosco, C., Bednarska, M., Ruf, S., Meurer, J., and Bock, R. (2019). A highly efficient sulfadiazine selection system for the generation of transgenic plants and algae. Plant Biotechnol. J. 17, 638–649. doi: 10.1111/pbi.13004
Keywords: blasticidin, Chlamydomonas reinhardtii, antibiotic resistance, selectable marker, synthetic biology, modular cloning, algal biotechnology
Citation: de Carpentier F, Le Peillet J, Boisset ND, Crozet P, Lemaire SD and Danon A (2020) Blasticidin S Deaminase: A New Efficient Selectable Marker for Chlamydomonas reinhardtii. Front. Plant Sci. 11:242. doi: 10.3389/fpls.2020.00242
Received: 13 November 2019; Accepted: 17 February 2020;
Published: 05 March 2020.
Edited by:
Dimitris Petroutsos, UMR 5168 Laboratoire de Physiologie Cellulaire Vegetale (LPCV), FranceReviewed by:
Xenie Johnson, Commissariat à l’Energie Atomique et aux Energies Alternatives (CEA), FranceVéronique Larosa, University of Liège, Belgium
Ryutaro Tokutsu, National Institute for Basic Biology, Japan
Copyright © 2020 de Carpentier, Le Peillet, Boisset, Crozet, Lemaire and Danon. This is an open-access article distributed under the terms of the Creative Commons Attribution License (CC BY). The use, distribution or reproduction in other forums is permitted, provided the original author(s) and the copyright owner(s) are credited and that the original publication in this journal is cited, in accordance with accepted academic practice. No use, distribution or reproduction is permitted which does not comply with these terms.
*Correspondence: Antoine Danon, YW50b2luZS5kYW5vbkBpYnBjLmZy
†These authors have contributed equally to this work