- 1State Key Laboratory for Agrobiotechnology, Key Laboratory of Crop Heterosis and Utilization (MOE), Key Laboratory of Crop Genetic Improvement, China Agricultural University, Beijing, China
- 2Institute of Evolution, University of Haifa, Haifa, Israel
- 3College of Agronomy Hebei Agricultural University, Hebei Agricultural University, Baoding, China
Wheat leaf rust, caused by the fungus Puccinia triticina Eriks. (Pt), is a destructive disease found throughout common wheat production areas worldwide. At its adult stage, wheat cultivar Liaochun10 is resistant to leaf rust and the gene for that resistance has been mapped on chromosome 2BS. It was designated LrLC10 and is the same gene as cataloged gene Lr13 by pedigree analysis and allelism test. We fine-mapped it using recessive class analysis (RCA) of the homozygous susceptible F2 plants derived from crosses using Liaochun10 as the resistant, male parent. Taking advantage of the re-sequencing data of Liaochun10 and its counterpart susceptible parent, we converted nucleotide polymorphisms in the LrLC10 interval between the resistant and susceptible parents into molecular markers to saturate the LrLC10 genetic linkage map. Four indel markers were added in the 1.65 cM map of LrLC10 flanked by markers CAUT163 and Lseq22. Thirty-two recombinants were identified by those two markers from the 984 F2 homozygous susceptible plants and were further genotyped with additional ten markers. LrLC10 was finally placed in a 314.3 kb region on the Chinese Spring reference sequence (RefSeq v1.0) that contains three high confidence genes: TraesCS2B01G182800, TraesCS2B01G182900, and TraesCS2B01G183000. Sequence analysis showed several variations in TraesCS2B01G182800 and TraesCS2B01G183000 between resistant and susceptible parents. One KASP marker and an indel marker were designed based on the differences in those two genes, respectively, and were validated to be diagnostic co-segregating markers for LrLC10. Our results both improve marker-assisted selection and help with the map-based cloning of LrLC10.
Introduction
Globally, common wheat (Triticum aestivum) is one of the most commonly cultivated crops, comprising 20% of human caloric intake and 15% of cultivated area in the world (FAOSTAT, 2015; WAP, 2017). Wheat leaf rust, caused by Puccinia triticina Eriks. (Pt) is one of the most damaging diseases of wheat, especially in coastal regions or areas with high temperatures and humidity during the wheat maturing seasons (Kolmer et al., 2018). In China, past widespread wheat leaf rust epidemics have caused severe yield losses (Dong, 2001; Zhou et al., 2013). In the future, due to impending climate change, leaf rust is expected to damage wheat production even more (Jiang et al., 2018). Utilization of wheat resistant cultivars considered a most effective, economical and environmentally-friendly strategy for controlling this disease (Bariana et al., 2007; Singh et al., 2013).
Currently, about 80 leaf rust resistant genes have been reported and formally named in common wheat or its relatives (McIntosh et al., 2017; Qureshi et al., 2018), and by using different types of molecular markers, most of these genes have been mapped on the wheat chromosomes1. Development of robust molecular markers linked to resistance genes is essential in wheat disease resistance breeding, especially for resistance gene pyramiding. Nevertheless, among these designated leaf rust resistance genes, only a few have tightly linked molecular markers for marker-assisted selection2.
Because of limited wheat genomic sequence data, developing molecular markers for wheat genes has been difficult. But now, by combining the T. aestivum ‘Chinese Spring’ (CS) IWGSC RefSeq v1.0 genome3 with annotations of high-quality gene models, these difficulties have been reduced, especially for gene location and markers development (Clavijo et al., 2017). Discovery of the highly abundant, locus-specific wheat nucleotide variations that can be used to identify the relevant genes are now within grasp because of affordable next-generation sequencing (Varshney et al., 2014; Xu et al., 2017). Compared with other kinds of markers, kompetitive allele-specific PCR (KASP) assays accelerate the conversion of DNA variations into available gene-linked markers. Taking advantage of such whole genomic sequences, Wu et al. (2018b) mapped wheat yellow rust resistance gene Yr26 on a 0.003-cM interval on chromosome 1B near the centromere, Narang et al. (2019) defined wheat leaf rust resistance gene LrP and yellow rust resistance gene YrP on a 15.71 Mb region on 5DS in the CS RefSeq v1.0 genome assembly, and Wu et al. (2019) localized the Pm52 locus within a 5.6 Mb interval on the long arm of chromosome 2B (2BL).
Bulked segregant analysis (BSA) can rapidly identify markers linked to target genes (Michelmore et al., 1991), and it has been improved by bulking homozygous recessive plants and using recessive class analysis (RCA) to map specific genes (Zhang et al., 1994). RCA is highly efficient, with a lower probability of misclassification and more reliability than using a random F2 population. Furthermore, this approach avoids creating F2:3 families and screening the entire F2 population, thus saving time in fine mapping and map-based cloning. RCA has been proven to map genes efficiently and reliably (Zhang et al., 1994; Yao et al., 1997; Mei et al., 1999; Chen et al., 2006; Kiswara et al., 2014). In wheat, rapid gene mapping using RCA has been used to map a sterile female gene (Dou et al., 2009) and a stripe rust resistance gene, YrLM168a (Feng et al., 2015), and to successfully map and clone the powdery mildew resistance gene Pm60 (Zou et al., 2018).
In wheat, Lr13, first identified in the Canadian cultivar “Manitou” in 1966, is an important adult-plant leaf rust resistance gene (McIntosh et al., 1995) that is widely found in wheat cultivars (e.g., ‘Frontana,’ ‘Frondoso,’ and ‘Fronteria’) and used in many breeding programs throughout the world (Roelfs, 1988; Pathan and Park, 2006). In China, Lr13 is one of the main resistance genes and confers effective resistance to leaf rust (Singh et al., 1999; Yuan et al., 2007; Yuan and Chen, 2011; Ren et al., 2015; Zhang et al., 2019). Previous studies indicated that Lr13 was located on chromosome 2BS (McIntosh et al., 1995), and Bansal et al. (2008) reported Lr13 was delimited to a 13.8 cM interval flanked by markers Xksm58 and Xstm773b. Recently, using a segregating population of Lr13 near-isogenic lines with simple sequence repeat and KASP markers, Zhang et al. (2016) mapped Lr13 to a small interval of 10.7 cM, and the closest marker was kwh37 (4.9 cM). A morphological marker, hybrid necrosis gene Ne2m, was found linked to Lr13 by Singh and Gupta (1991), but it cannot be used to accurately detect Lr13 (Anand et al., 1991). Therefore, a co-segregated and diagnostic marker for Lr13 in molecular breeding is yet unavailable.
In this study, we confirmed that the leaf rust resistance gene LrLC10 in Liaochun10 is Lr13 by pedigree analysis, and finely mapped it to a close interval with recessive class analysis (RCA) through the markers developed according to the re-sequencing data of the parental lines. We also developed molecular markers that were closely linked to LrLC10 and that can be used to facilitate marker-assisted selection of LrLC10 in wheat resistance breeding.
Materials and Methods
Plant Materials
The spring wheat cultivar Liaochun10, is highly resistant to leaf rust, was crossed with two susceptible wheat lines Han 87-1 (87-1) and 7D49 (a wild emmer wheat introgression line created by the crossing IW123/Zheng98//87-1∗2), to construct two F2 segregating populations of 3,908 plants. Wild emmer wheat IW123 was donated by T. Fahima and E. Nevo, University of Haifa, Israel and Lr13-carrier line RL4031 was provided by Zaifeng Li, College of Plant Protection, Heibei Agricultural University, China. The 1,395 F2 plants derived from the Liaochun10 × RL4031 cross were used to test allelism. A total of 35 cultivars with known presence/absence of Lr13 were chosen to validate the co-segregating markers (Table 1). A panel of 524 Chinese wheat accessions/landraces was used to test the Lr13 frequencies (Supplementary Table S1). Through all the experiments, a susceptible, common wheat line, Xuezao, was used to check for successful inoculation.
Field Evaluation of Leaf Rust Symptoms at the Adult Stage
Wheat leaf rust isolate PHT (provided by the Institute of Plant Protection, Chinese Academy of Agricultural Sciences, Beijing, China) was used as the inoculum. PHT isolate was avirulent on Liaochun10 and RL4031.
The populations were sown at the experiment farm of China Agriculture University, Beijing, China. At the late tillering stage (Feekes stage 5) at least one tiller of each plant was inoculated by injecting urediniospore suspended in 0.1% Tween 20 into the leaf bundle with a 10 mL syringe. The urediniospore was propagated in the greenhouse on the susceptible control, Xuezao.
The infection type of the flag leaf and the top second leaf of each individual was evaluated about 1–2 month post-inoculation when the susceptible control was fully infected, based on an infection type scale of 0–4, where 0 indicated no visible symptoms, 0; indicated hypersensitive flecks, and 1–4, indicated small uredinia with necrosis, small- to medium-sized uredinia with green islands and surrounded by necrosis or chlorosis, medium- to large-sized uredinia with chlorosis, and large uredinia without chlorosis, respectively. Values 0–2 were categorized as resistant and 3–4 were classified as susceptible (Roelfs et al., 1992). A second assessment was conducted for each plant 4 days after the first examination.
Allelism Tests
We used an F2 population derived from Liaochun10 × RL4031 to determine the allelic relationships between genes Lr13 and LrLC10. The responses of each F2 plant to Pt race PHT was determined by the rust response method described above.
Development of Molecular Markers
The sequences of all the markers anchored in the LrLC10 (Lr13) genetic linkage map were used as queries to search against the Chinese Spring reference genome sequence (RefSeq v1.0) to define the genome interval of the resistance gene on chromosome arm 2BS. Near the LrLC10 locus, single-nucleotide polymorphisms (SNPs) or insertion/deletion (indel) polymorphisms were found based on re-sequencing result of the two parents (the concrete method refer to Chai et al., 2018) and the 300 bp flanking sequence of those indel sites which were ≥5 bp that were obtained from the Chinese Spring reference genome sequence4, Primer3 (v.0.4.0)5 was used to design the indel markers. The SNPs or indels (<5 bp) were converted into kompetitive allele-specific PCR (KASP) markers, which were designed using PolyMarker6. The markers used in this study were listed in Table 2.
Marker Genotyping Assays
PCR amplification was conducted in a 10 μL reaction volume consisting of 5 μL 2 × Tag PCR StarMix with loading dye, 50–100 ng/μL DNA 1.5 μL, 1.5 μL primer (mixture of forward and reverse primer, 2 μM), and 2 μL H2O. PCR proceeded with initial denaturation at 94°C for 5 min, then 35 cycles at 94°C for 30 s, 30 s at 50–60°C for primer annealing (depending on the specific primers), 72°C for 30 s of extension; and the final extension at 72°C for 5 min. The PCR product was separated in either 8% or 10% non-denaturing polyacrylamide gels (acrylamide:bisacrylamide = 39:1) that were silver stained and photographed. KASP assays were performed following the protocol described in Wu et al. (2018a).
Construction of the Genetic Linkage Map
We performed chi-square analysis of the leaf rust test data from the segregating F2 populations to confirm the goodness-of-fit of the observed ratios to theoretical expectations. The recombination frequencies of the resistance gene and the markers were calculated according to Chen et al. (2006). Using the Kosambi mapping function, we converted the recombination frequencies to centimorgans (Kosambi, 1943) and drew the genetic map using Mapdraw v2.1 (Liu and Meng, 2003).
Results
Genetic Analysis of Wheat Leaf Rust Resistance Gene LrLC10 in Two Segregating Populations
The parental lines 87-1 and 7D49 were highly susceptible to Pt race PHT, [infection type (IT) = 3], whereas Liaochun10 was highly resistant (IT = 0, Figure 1). We examined the two segregating F2 populations that grew from crossing the susceptible lines with Liaochun10. The 87-1 crossed with Liaochun10 produced the F2 population including 3,057 plants, of which 2,300 were resistant and 757 were susceptible to Pt isolate PHT (χ23:1 = 0.092, P > 0.05). In the F2 population derived from 7D49 crossed with Liaochun10, 624 plants were resistant and 227 were susceptible to Pt race PHT (χ23:1 = 1.268, P > 0.05). These results indicated that leaf rust resistance in Liaochun10 is controlled by a single dominant gene.
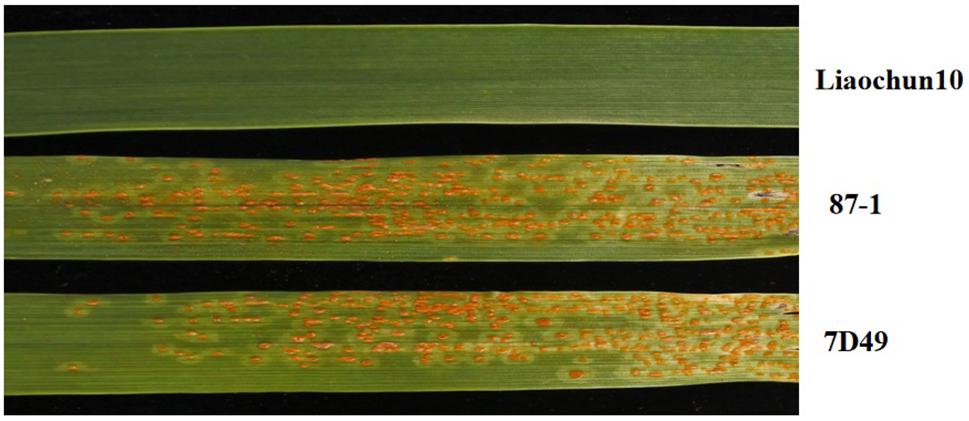
Figure 1. Phenotype of resistant parent Liaochun10, susceptible parent 87-1, and 7D49 30 days after inoculation with Pt race PHT.
Allelism Test of LrLC10 and Lr13
Liaochun10, RL4031, and the 1,395 F2 plants from the cross of Liaochun10 × RL4031 were evaluated against Pt race PHT. We found no susceptible plants, thus confirming that LrLC10 in Liaochun10 was on the same locus as Lr13. Since there was Lr13 donor parents Frontanan and UP301 in the Liaochun10 pedigree (Singh and Gupta, 1991; He et al., 2001; Pathan and Park, 2006), we concluded that leaf rust resistance gene LrLC10 in Liaochun10 is Lr13.
Molecular Mapping of Leaf Rust Resistance Gene LrLC10 (Lr13)
We chose 92 extremely susceptible individuals from the 7D49 × Liaochun10 F2 population to be re-genotyped using markers linked to LrLC10 that were established by Lv et al. (2017) (Figures 2A,B). To define the LrLC10 physical interval, we searched the sequences of all markers anchored in the genetic map against the Chinese Spring reference genomic sequence (RefSeq v1.0) and found that the relative physical positions of those markers were generally consistent with the genetic map (Figures 2B,C). Two flanking markers, CAUT163 and Xbarc18, spanned an approximately 100 Mb region (153,676,602–255,348,323) in the reference genome, and here we detected numerous sequence variations between the parents when we analyzed the re-sequencing data. Twenty indel primer pairs were designed based on those insertion/deletion polymorphisms we found between parental lines in the 11 Mb (159,000,000–170,000,000) section that was 6 Mb from marker CAUT163 going toward LrLC10. Among these, 4 markers (Lseq22, Lseq29, Lseq31, and Lseq35) were successfully added to the genetic map and the LrLC10 gene was delimited within a 1.65 cM area between markers CAUT163 and Lseq22, an interval corresponding to a 5.7 Mb (153,676,602–159,302,377) region in the CS reference genome (Figure 2C).
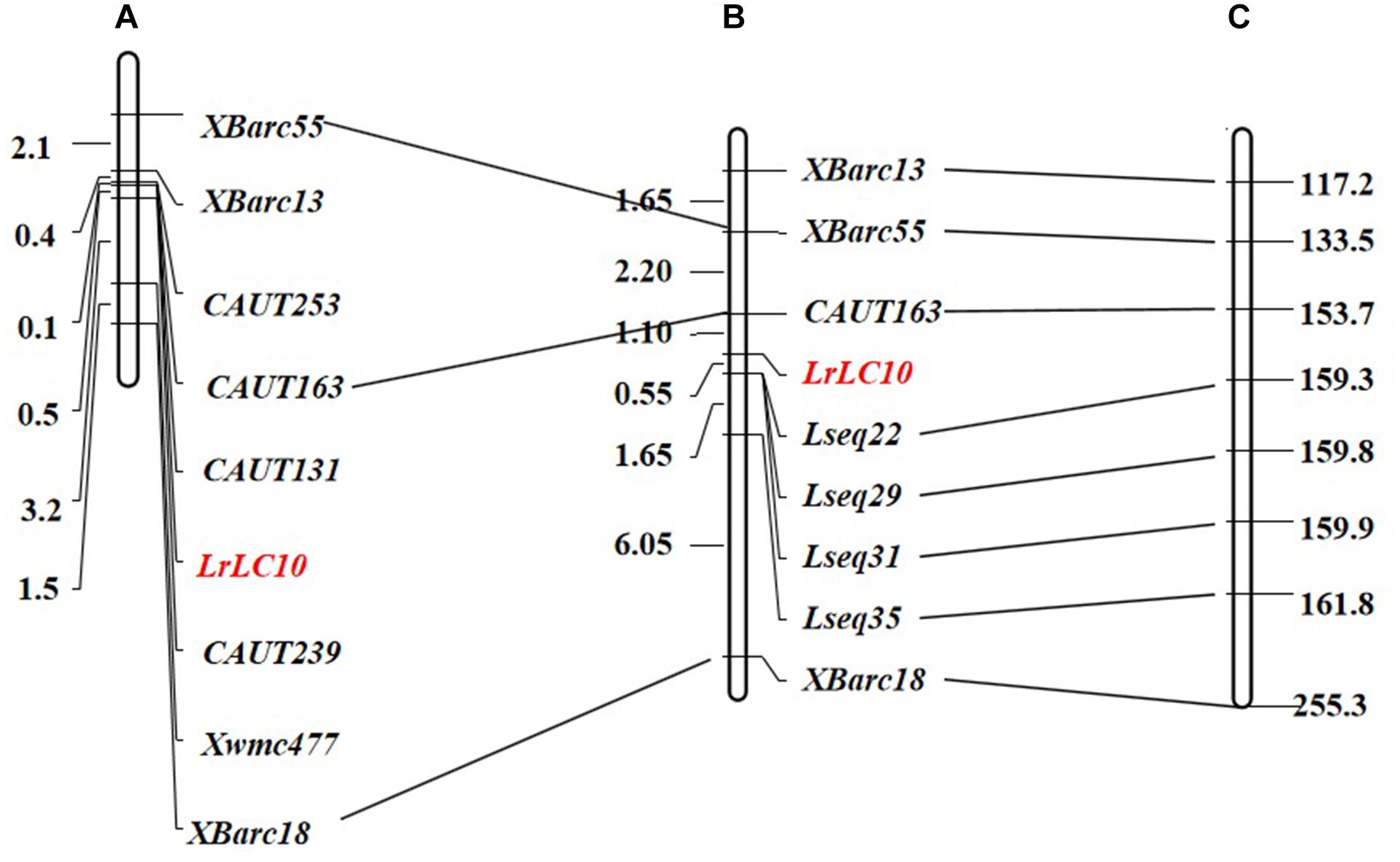
Figure 2. Genetic linkage map of leaf rust resistance gene LrLC10 and corresponding physical position on the Chinese Spring RefSeq v1.0. (A) Lv et al. (2017) genetic map. (B) The genetic linkage map of LrLC10, genetic distances are shown to the left in cM. (C) The corresponding physical location of the polymorphic linkage markers of LrLC10 on the chromosome 2BS of Chinese Spring RefSeq v1.0, physical distances are shown to the right in Mb.
Development of Tightly Linked Markers to LrLC10 (Lr13)
We used those co-dominant flanking markers, CAUT163 and Lseq22, to identify recombinants in the 984 homozygous, susceptible F2 plants. Thirty-two recombinant plants were identified and then used for fine mapping of LrLC10.
Based on the parents’ re-sequencing data that corresponded to the 5.7 Mb interval of the CS RefSeq v1.0, we designed 80 indel primers and a KASP marker. They were tested on 3 parental lines and 10 markers were polymorphic between the parents and used to finely map LrLC10 (Figure 3).
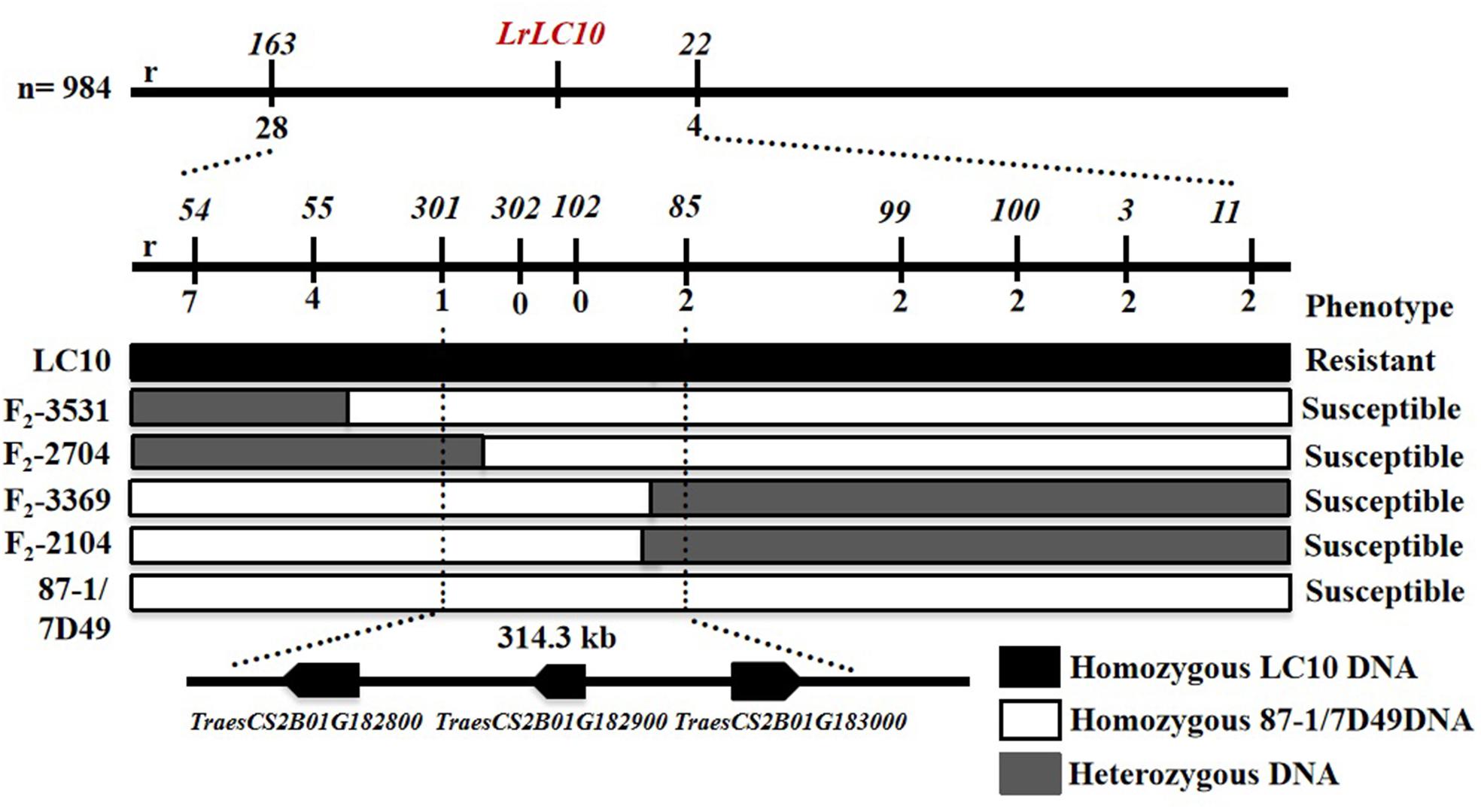
Figure 3. Fine mapping of LrLC10 and three annotated genes localize in the mapping interval on Chinese Spring reference genome. Phenotypes and genotypes of four F2 recombinants including (F2-3531, F2-2704, F2-3369, F2-2104) are showed. The name and phenotype of F2 individuals were labeled in the left and right, respectively. Black, white and gray blocks present genomic region of LC10, 87-1/7D49 and heterozygous, respectively. r indicated the number of recombinants.
Among the 32 recombinant plants, 28 showed recombination between marker CAUT163 and LrLC10, while 4 recombination events were detected between marker Lseq22 and LrLC10. We used the 10 polymorphic markers located between the flanking markers to examine these recombinants and found that the closest flanking markers were Lseq301 (with 1 recombination event) and Lseq85 (with 2 recombination events) and markers Lseq302 and Lseq102 co-segregated with LrLC10 (Figure 3). These results suggest that LrLC10 locus is located in a 314.3-kb region between markers Lseq85 and Lseq301 (157,688,415–158,002,717) in the CS RefSeq v1.0 (Figure 3). Markers Lseq85 and Lseq301 were designed based on the 5 bp and 6 bp deletions, respectively, in Liaochun10 as compared to 7D49. The KASP marker Lseq302 was based on the SNP (A/T) detected in exon 2 of TraesCS2B01G182800 between Liaochun10 and 7D49 (Figure 4A and Table 3), while Lseq102 was developed based on a 9-bp deletion in the TraesCS2B01G183000 coding region between Liaochun10 and 7D49 (Figure 4B and Table 3).
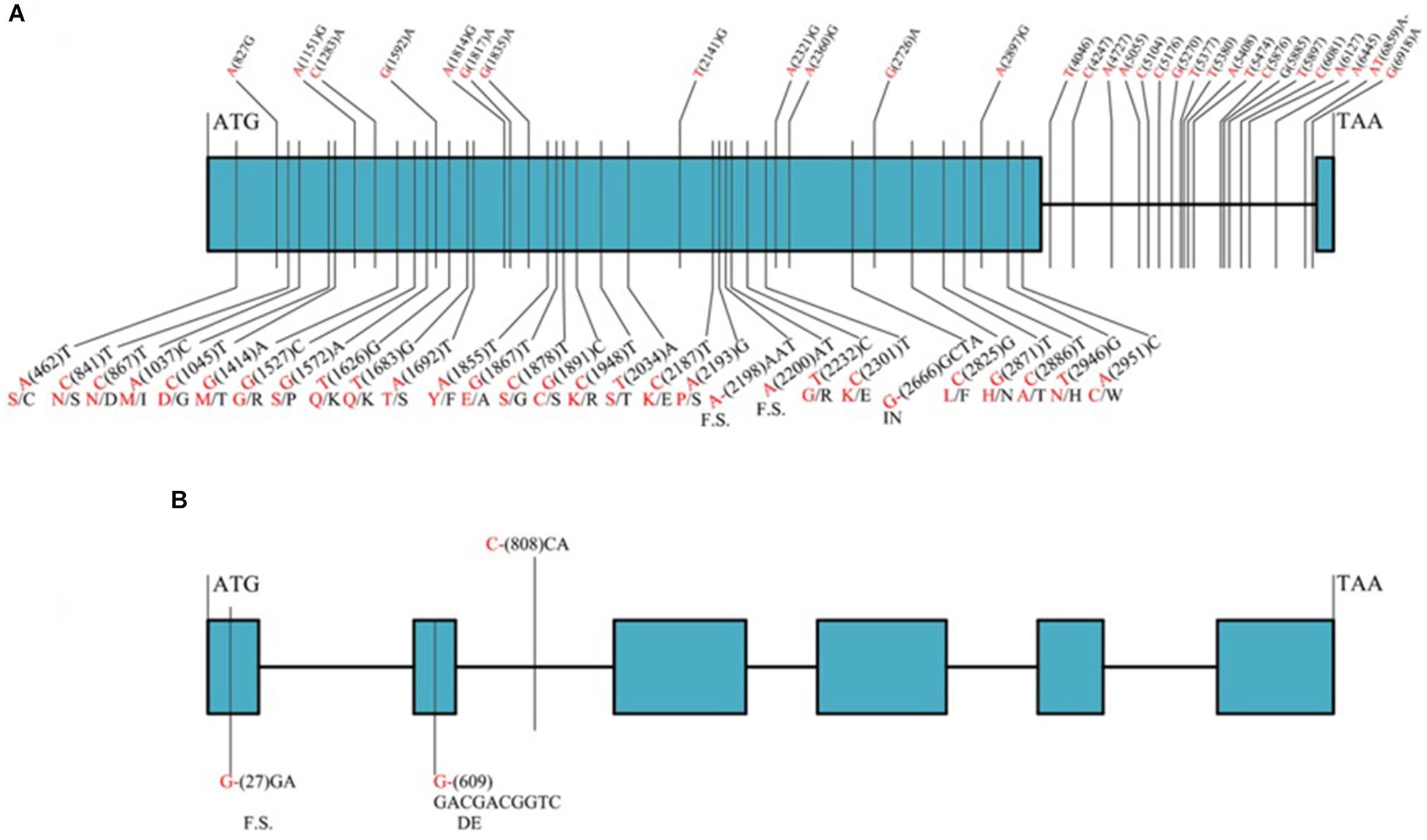
Figure 4. Structure of annotated genes [TraesCS2B01G182800 and TraesCS2B01G183000 (A,B)] showing the nucleotide and amino acid sequences polymorphism between resistant and susceptible parents. Introns, exons are shown in lines, blue boxes. Red and black fonts represent resistant and susceptible parents, respectively. The numbers in bracket represent the positions of nucleotide sequences relative to ATG. F.S. represents frame shift, - indicates nucleotide deletion, IN represents amino acid insertion, DE represents amino acid deletion.
Validation of LrLC10-Co-segregating Markers for Marker-Assisted Selection
We wanted to test if these co-segregating markers (Lseq302 and Lseq102) could be used for marker-assisted selection of LrLC10 in different backgrounds. We tested 25 wheat leaf rust resistant accessions and 10 susceptible cultivars with those 2 markers to evaluate their utility. Twenty-three of the resistant accessions had the same marker genotypes as Liaochun10 and all the susceptible cultivars’ genotypes were identical to 87-1 and 7D49 (Figures 5A,B and Table 1). Moreover, we found that the F1 plants of the crosses of those 14 of the 23 resistant accessions with Xuezao, which was proved to carry the hybrid necrosis gene Ne1 (unpublished results), showed progressive necrosis (Table 1). According to Zhang et al. (2016), Lr13 and Ne2m are the same gene; so by inference, the F1 plants’ phenotypes indicate that those 14 cultivars have leaf rust resistance gene Lr13. These results suggest that markers Lseq302 and Lseq102 can be used to identify Lr13.
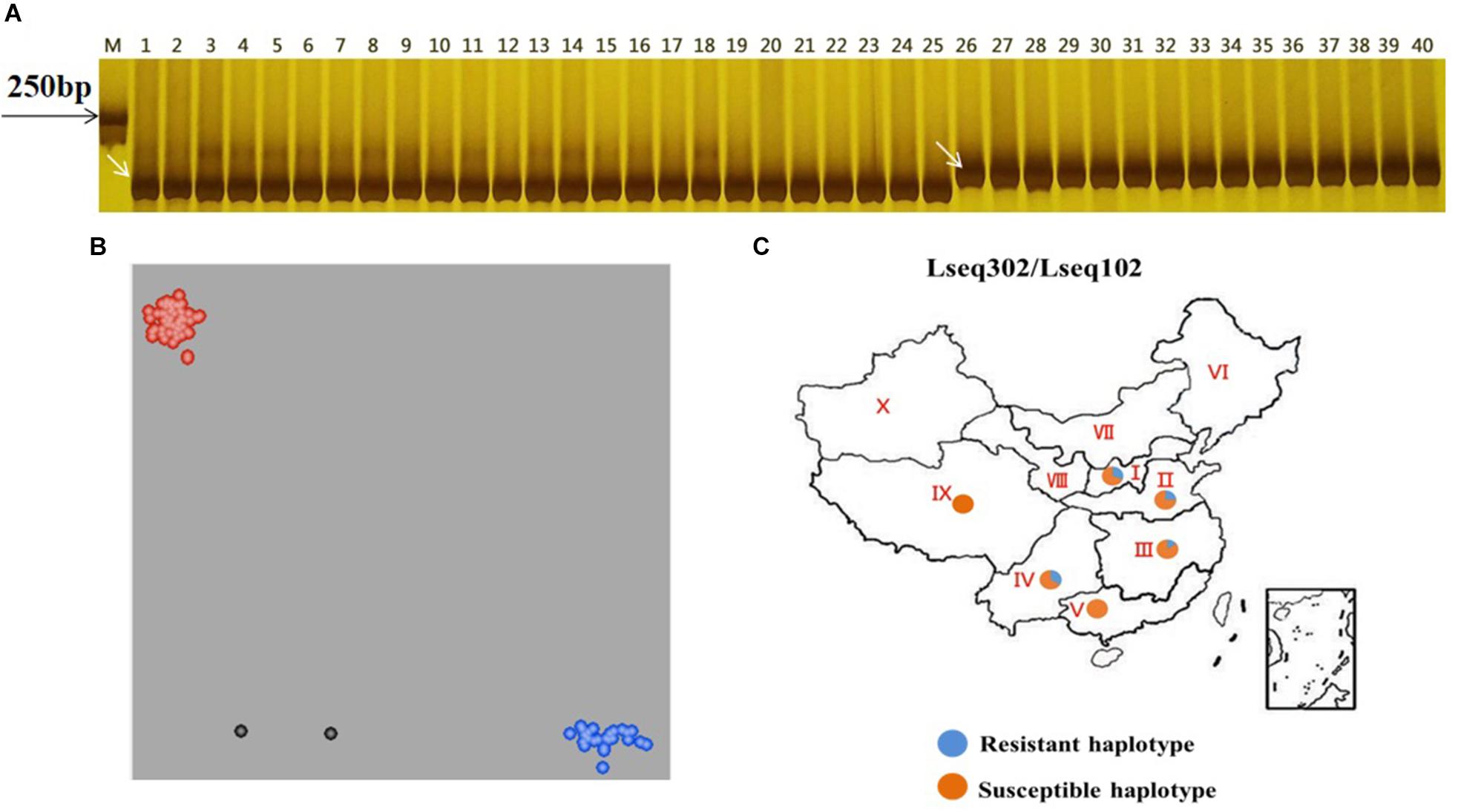
Figure 5. Validation of Lr13 diagnostic markers (A,B) and haplotype distribution of Lseq302 and Lseq102 in 524 common wheat cultivars/landraces from China (C). A co-segregated marker Lseq102 genotyped several Lr13 carriers and susceptible accessions in PAGE gel. The number 1 and 24 present Liaochun10 and 87-1, respectively, 2–23, 25–31 indicate Lr13 carriers and susceptible lines, respectively. M: Marker; B plots of KASP marker Lseq302 tested 23 Lr13 carriers (blue dots), 10 susceptible lines (red dots) and other cultivars with unknown leaf rust resistance. Black dots are water controls; C I, north China winter wheat region; II, Yellow and Huai River valley winter wheat region; III, middle and lower Yangtze River valley winter wheat region; IV, south-western winter wheat region; V, south China winter wheat region; VI, north-eastern spring wheat region; VII, northern spring wheat region; VIII, north-western spring wheat region; IX, Qinghai–Tibet spring–winter wheat region; X, Xinjiang winter–spring wheat region.
To evaluate the distribution of Lseq302-L and Lseq102-L (the marker alleles of Lseq302/Lseq102 in Liaochun10) in China, a panel of 524 common wheat accessions/landraces from China was tested with these markers. Lseq302-L and Lseq102-L always co-existed in all the cultivars, forming a specific haplotype block. The haplotype of Lseq302-L/Lseq102-L was present in various frequencies in 4 of 10 agro-ecological production zones: I, North China winter wheat region (30.76%); II, Yellow and Huai River valleys winter wheat region (24.56%); III, middle and lower Yangtze River valley winter wheat region (14.81%); and V, South China winter wheat region (33.33%) (Figure 5C and Table 4).
Discussion
Seven leaf rust resistance genes (Lr13, Lr16, Lr23, Lr35, Lr48, Lr73, and LrZH22) had been mapped on wheat chromosome 2BS (Seyfarth et al., 1999; Park et al., 2014; Nsabiyera et al., 2016; Wang et al., 2016; Zhang et al., 2016; Chhetri et al., 2017; Kassa et al., 2017). We found that LrLC10 is located in the region 157,688,415–158,002,717 bp (Figure 3) (2BS1-0.53-0.75) on the reference sequence of Chinese Spring (RefSeq v1.0). Of those 7 Lr genes, only Lr13 and LrZH22 were reported to be located in the same region as LrLC10 (Wang et al., 2016; Zhang et al., 2016). LrZH22 confers resistance to leaf rust at both the seedling and adult stages (Wang et al., 2016), whereas resistance gained from LrLC10 is effective only after the four-leaf stage. Up to now, the relationship between LrLC10 and Lr13 was unknown, but we confirmed that LrLC10 is Lr13. The pedigree of Liaochun10 is 1048 (Ke71F4370-10/Mexipak66//UP301) × Liao70181-2 (Liaochun6/Jinghong1) and the parents of Liaochun6 are Frontana and Liaochun1 (He et al., 2001). Since the leaf rust resistance gene in Frontana and UP301 is Lr13 (Singh and Gupta, 1991; Pathan and Park, 2006), the leaf rust resistance gene in Liaochun10 may be derived from the Frontana or UP301 parent.
In the process of fine mapping LrLC10, enough polymorphic markers were found to narrow down the genetic interval covering the targeted gene. We accomplished this by re-sequencing the parental lines and developing indel and SNP markers based on sequence information in the targeted region. Four indel markers revealed polymorphisms and localized LrLC10 gene in a 1.65 cM genetic interval, which corresponded to a 5.7 Mb interval on the Chinese Spring reference genomic sequence (Figures 2B,C). Based on sequence diversities between the parental lines on the candidate interval, we designed 9 indel markers and a KASP marker and used these to test the 32 recombinants that we identified using LrLC10-flanking markers CAUT163 and Lseq22 derived from 984 homozygous, susceptible F2 individuals. LrLC10 was finally delimited into a 314.3-kb genomic interval on the Chinese Spring reference sequence v1.0 by markers Lseq301 and Lseq85 (Figure 3). This mapping of LrLC10 demonstrated our methods efficiently developed molecular markers based from the re-sequencing data of the parents.
Lr13 is one of the most widely distributed leaf rust resistance genes in wheat (McIntosh et al., 1995), but it has become ineffective in some regions, such as Mexico and South America (Singh and Rajaram, 1992). However, it is effective in combination with other resistance genes, such as Lr3ka, Lr34, and Lr16 (Kolmer, 1992). Therefore, we need diagnostic molecular markers for Lr13 to facilitate selection or stacking it with other Lr genes. In our study, KASP marker Lseq302 and indel marker Lseq102 co-segregated with Lr13 and were effective in diverse wheat backgrounds (Figures 5A,B, Table 1, and Supplementary Table S1). Therefore, these diagnostic markers may be used for efficient marker-assisted selection of Lr13, thus enabling researchers to either pyramid it with other adult plant resistant genes to achieve durable leaf rust resistance or stack it with stripe rust, stem rust, and powdery mildew resistance genes (e.g., Yr27, Sr40, and pm42) on chromosome 2BS, to create multi-resistance accessions (McDonald et al., 2004; Hua et al., 2009; Wu et al., 2009).
To date, several leaf rust resistance genes have been isolated in wheat: Lr1, Lr10, Lr21, Lr34, Lr67, and Lr22a (Feuillet et al., 2003; Huang et al., 2003; Cloutier et al., 2007; Krattinger et al., 2009; Moore et al., 2015; Thind et al., 2017). Among those genes, Lr34 encodes an ATP-binding cassette transporter that carries resistance-related metabolites that affect the growth of pathogenic bacteria (Krattinger et al., 2009). Lr67 encodes a hexose transporter, LR67res, that through heterodimerization with the Lr67- susceptible functional transporter LR67sus, exerted a dominant-negative effect that restricted the growth of multiple biotrophic pathogens by reducing their glucose uptake (Moore et al., 2015). Lr34 and Lr67 provide wheat with resistance to multiple fungal pathogens. The others are typical resistance genes with nucleotide-binding site leucine-rich repeat (NBS-LRR) domains (Feuillet et al., 2003; Huang et al., 2003; Cloutier et al., 2007; Thind et al., 2017). However, LrLC10 (Lr13) was shown to be a race-specific resistance gene (Dyck et al., 1966), thus it most likely has the R-gene structure of NLR.
In this study, we delimited LrLC10 to a 314.3 kb region on short arm of chromosome 2B in Chinese Spring reference genome sequence (RefSeq v1.0). Three high confidence genes (TraesCS2B01G182800, TraesCS2B01G182900, and TraesCS2B01G183000) have different functions based on the annotation of Chinese Spring reference genome7, which encode a typical NBS-LRR protein, Ribonuclease, and an F-box domain containing Leucine-rich repeats protein were located in this region. DNA sequence comparison showed that the parents did not differ in TraesCS2B01G182900. Compared to 7D49, Liaochun10 had a 9 bp deletion in its TraesCS2B01G183000 coding region, resulting in a deletion of three amino acids, and another 1-bp deletion that led to a translational frame shift (Figure 4B and Table 3). In TraesCS2B01G182800, we found many sequence variations, including 56 SNPs and 4 indels, between Liaochun10 and its rust-susceptible parent. Among them, we found 18 SNPs and one indel in the intron region, and 38 SNPs and three indels in exon 2. Among those 38 SNPs, 26 caused amino acid substitutions, and two of the indels led to a translational frame shift. A 3-bp insertion in 7D49 resulted in an amino acid insertion that was not found in Liaochun10 (Figure 4A and Table 3). Based on the TraesCS2B01G182800 and TraesCS2B01G183000 sequence polymorphisms between the parental lines, we developed the markers Lseq102 and Lseq302 and found that they co-segregated with LrLC10 (Figure 3). In total, our results suggest that LrLC10 (Lr13) might be one of those two annotated genes. However, there is still a chance that the sequence corresponding to LrLC10 is absent in the CS genomic sequence. Therefore, our analysis of re-sequencing data based on the wheat reference genome sequence is not enough to be absolutely certain of its identity. Because of this, a library of the resistant parent must be constructed so that a physical map would enable the cloning of LrLC10. Recently, some alternative methods (e.g., MutRenSeq, TACCA, and MutChromSeq) have been used to clone wheat disease resistance genes (Steuernagel et al., 2016; Sánchez-Martín et al., 2016; Thind et al., 2017) and may possibly be used to clone LrLC10. After all, the fine genetic map and co-segregating markers developed in our present study may aid the map-based cloning and the marker-assisted selection of LrLC10 (Lr13).
Data Availability Statement
The datasets generated for this study can be found in the European Variation Archive (EVA) using accession number PRJEB37197.
Author Contributions
LQ and CX conceived the project. LQ, HW, WW, YuL, and JMu performed the experiments. MG and YiL assisted in revising the manuscript. WG analyzed the re-sequencing data. JMa, ZH, and QS provided materials. LQ wrote the manuscript. CX revised the manuscript.
Funding
This work was financially supported by the National Key Research and Development Project of China 2016YFD0101802) and National Natural Science Foundation of China (31671676 and 31872865).
Conflict of Interest
The authors declare that the research was conducted in the absence of any commercial or financial relationships that could be construed as a potential conflict of interest.
Acknowledgments
We are grateful to Dr. Zhen Wang for assistance in revising the manuscript; Dr. Xiaobo Wang for providing the DNA of 524 Chinese wheat accessions/landraces.
Supplementary Material
The Supplementary Material for this article can be found online at: https://www.frontiersin.org/articles/10.3389/fpls.2020.00470/full#supplementary-material
Footnotes
- ^ https://shigen.nig.ac.jp/wheat/komugi/
- ^ https://maswheat.ucdavis.edu/protocols/index.htm
- ^ https://www.wheatgenome.org/
- ^ https://www.wheatgenome.org/
- ^ http://bioinfo.ut.ee/primer3-0.4.0
- ^ http://www.polymarker.info/
- ^ https://urgi.versailles.inra.fr/download/iwgsc/IWGSC_RefSeq_Annotations/v1.0/
References
Anand, D., Saini, R. G., and Gupta, A. K. (1991). Linkage distance between the wheat leaf rust resistance gene Lr13 and a gene for hybrid necrosis Ne2m. J. Genet. Breed. 45, 245–246.
Bansal, U. K., Hayden, M. J., Venkata, B. P., Khanna, R., Saini, R. G., Bariana, H. S., et al. (2008). Genetic mapping of adult plant leaf rust resistance genes Lr48 and Lr49 in common wheat. Theor. Appl. Genet. 117, 307–312. doi: 10.1007/s00122-008-0775-6
Bariana, H. S., Brown, G. N., Bansal, U. K., Miah, H., Standen, G. E., and Lu, M. (2007). Breeding triple rust resistant wheat cultivars for Australia using conventional and marker-assisted selection technologies. Aust. J. Agric. Res. 58, 576–587. doi: 10.1071/AR07124
Chai, L., Chen, Z., Bian, R., Zhai, H., Cheng, X., Peng, H., et al. (2018). Dissection of two quantitative trait loci with pleiotropic effects on plant height and spike length linked in coupling phase on the short arm of chromosome 2D of common wheat (Triticum aestivum L.). Theor. Appl. Genet. 131, 2621–2637. doi: 10.1007/s00122-018-3177-4
Chen, J. W., Wang, L., Pang, X. F., and Pan, Q. H. (2006). Genetic analysis and fine mapping of a rice brown planthopper (Nilaparvata lugens Stål) resistance gene bph19(t). Mol. Genet. Genomics 275, 321–329. doi: 10.1007/s00438-005-0088-2
Chhetri, M., Bariana, H., Wong, D., Sohail, Y., Hayden, M., and Bansal, U. (2017). Development of robust molecular markers for marker-assisted selection of leaf rust resistance gene Lr23 in common and durum wheat breeding programs. Mol. Breed. 37:21. doi: 10.1007/s11032-017-0628-6
Clavijo, B. J., Venturini, L., Schudoma, C., Accinelli, G. G., Kaithakottil, G., Wright, J., et al. (2017). An improved assembly and annotation of the allohexaploid wheat genome identifies complete families of agronomic genes and provides genomic evidence for chromosomal translocations. Genome Res. 27, 885–896. doi: 10.1101/gr.217117.116
Cloutier, S., McCallum, B. D., Loutre, C., Banks, T. W., Wicker, T., Feuillet, C., et al. (2007). Leaf rust resistance gene Lr1, isolated from bread wheat (Triticum aestivum L.) is a member of the large psr567 gene family. Plant Mol. Biol. 65, 93–106. doi: 10.1007/s11103-007-9201-8
Dou, B., Hou, B., Xu, H., Lou, X., Chi, X., Yang, J., et al. (2009). Efficient mapping of a female sterile gene in wheat (Triticum aestivum L.). Genet. Res. 91, 337–343. doi: 10.1017/S0016672309990218
Dyck, P. L., Samborski, D. J., and Anderson, R. G. (1966). Inheritance of adult-plant leaf rust resistance derived from the common wheat varieties Exchange and Frontana. Can. J. Genet. Cytol. 8, 665–671. doi: 10.1139/g66-082
FAOSTAT (2015). FAO statistical Pocketbook 2015: World Food and Agriculture. Rome: Food and Agriculture Organization of the United Nations.
Feng, J., Chen, G., Wei, Y., Liu, Y., Jiang, Q., Li, W., et al. (2015). Identification and mapping stripe rust resistance gene YrLM168a using extreme individuals and recessive phenotype class in a complicate genetic background. Mol. Genet. Genomics 290, 2271–2278. doi: 10.1007/s00438-015-1077-8
Feuillet, C., Travella, S., Stein, N., Albar, L., Nublat, A., and Keller, B. (2003). Map-based isolation of the leaf rust disease resistance gene Lr10 from the hexaploid wheat (Triticum aestivum L.) genome. Proc. Natl. Acad. Sci. U.S.A. 100, 15253–15258. doi: 10.1073/pnas.2435133100
He, Z. H., Rajaram, S., and Huang, G. Z. (2001). A History of Wheat Breeding in China. Mexico City: CIMMYT.
Hua, W., Liu, Z., Zhu, J., Xie, C., Yang, T., Zhou, Y., et al. (2009). Identification and genetic mapping of pm42, a new recessive wheat powdery mildew resistance gene derived from wild emmer (Triticum turgidum var. dicoccoides). Theor. Appl. Genet. 119, 223–230. doi: 10.1007/s00122-009-1031-4
Huang, L., Brooks, S. A., Li, W., Fellers, J. P., Trick, H. N., and Gill, B. S. (2003). Map-Based cloning of leaf rust resistance gene Lr21 from the large and polyploid genome of bread wheat. Genetics 164, 655–664.
Jiang, B., Liu, T., Li, H., Han, H., Li, L., Zhang, J., et al. (2018). Physical mapping of a novel locus conferring leaf rust resistance on the long arm of Agropyron cristatum Chromosome 2P. Front. Plant Sci. 9:817. doi: 10.3389/fpls.2018.00817
Kassa, M. T., You, F. M., Hiebert, C. W., Pozniak, C. J., Fobert, P. R., Sharpe, A. G., et al. (2017). Highly predictive SNP markers for efficient selection of the wheat leaf rust resistance gene Lr16. BMC Plant Biol. 17:45. doi: 10.1186/s12870-017-0993-7
Kiswara, G., Lee, J. H., Hur, Y. J., Cho, J. H., Lee, J. Y., Kim, S. Y., et al. (2014). Genetic analysis and molecular mapping of low amylose gene du12(t) in rice (Oryza sativa L.). Theor. Appl. Genet. 127, 51–57. doi: 10.1007/s00122-013-2200-z
Kolmer, J. A. (1992). Enhanced leaf rust resistance in wheat conditioned by resistance gene pairs with Lr13. Euphytica 61, 123–130. doi: 10.1007/BF00026802
Kolmer, J. A., Su, Z., Bernardo, A., Bai, G., and Chao, S. (2018). Mapping and characterization of the new adult plant leaf rust resistance gene Lr77 derived from Santa Fe winter wheat. Theor. Appl. Genet. 131, 1553–1560. doi: 10.1007/s00122-018-3097-3
Kosambi, D. D. (1943). The estimation of map distance from recombination values. Ann. Eugen. 12, 172–175. doi: 10.1111/j.1469-1809.1943.tb02321.x
Krattinger, S. G., Lagudah, E. S., Spielmeyer, W., Singh, R. P., Huerta-Espino, J., McFadden, H., et al. (2009). A putative ABC transporter confers durable resistance to multiple fungal pathogens in wheat. Science 323, 1360–1363. doi: 10.1126/science.1166453
Liu, R., and Meng, J. (2003). MapDraw: a microsoft excel macro for drawing genetic linkage maps based on given genetic linkage data. Hereditas 25, 317–321. doi: 10.16288/j.yczz.2003.03.017
Lv, X., Tang, H., Geng, M., Mi, Y., Li, Y., Li, F., et al. (2017). Comparative genomics analysis of leaf rust resistance gene LrLC10 in common wheat cultivar Liaochun10. J. China Agric. Univ. 22, 01–09.
McDonald, D. B., McIntosh, R. A., Wellings, C. R., Singh, R. P., and Nelson, J. C. (2004). Cytogenetical studies in wheat XIX. location and linkage studies on gene Yr27 for resistance to stripe (yellow) rust. Euphytica 136, 239–248. doi: 10.1023/B:EUPH.0000032709.59324.45
McIntosh, R. A., Dubcovsky, J., Rogers, W. J., Morris, C., and Xia, X. (2017). Catalogue of Gene Symbols for Wheat: 2017 Supplement. Avaliable at: https://shigen.nig.ac.jp/wheat/komugi/genes/macgene/supplement2017.pdf. (accessed September 20, 2017).
McIntosh, R. A., Wellings, C. R., and Park, R. F. (1995). Wheat Rusts: An Atlas of Resistance Genes. Melbourne: Csiro Publishing.
Mei, M. H., Dai, X. K., Xu, C. G., and Zhang, Q. (1999). Mapping and genetic analysis of the genes for photoperiod-sensitive genic male sterility in rice using the original mutant Nongken 58S. Crop Sci. 39, 1711–1715. doi: 10.2135/cropsci1999.3961711x
Michelmore, R. W., Paran, I., and Kesseli, R. V. (1991). Identification of markers linked to disease-resistance genes by bulked segregant analysis: a rapid method to detect markers in specific genomic regions by using segregating populations. Proc. Natl. Acad. Sci. U.S.A. 88, 9828–9832. doi: 10.1073/pnas.88.21.9828
Moore, J. W., Herrera-Foessel, S., Lan, C., Schnippenkoetter, W., Ayliffe, M., Huerta-Espino, J., et al. (2015). A recently evolved hexose transporter variant confers resistance to multiple pathogens in wheat. Nat. Genet. 47, 1494–1498. doi: 10.1038/ng.3439
Narang, D., Kaur, S., Steuernagel, B., Ghosh, S., Dhillon, R., Bansal, M., et al. (2019). Fine mapping of Aegilops peregrina co-segregating leaf and stripe rust resistance genes to distal-most end of 5DS. Theor. Appl. Genet. 132, 1473–1485. doi: 10.1007/s00122-019-03293-5
Nsabiyera, V., Qureshi, N., Bariana, H. S., Wong, D., Forrest, K. L., Hayden, M. J., et al. (2016). Molecular markers for adult plant leaf rust resistance gene Lr48 in wheat. Mol. Breed. 36:65. doi: 10.1007/s11032-016-0488-5
Park, R. F., Mohler, V., Nazari, K., and Singh, D. (2014). Characterisation and mapping of gene Lr73 conferring seedling resistance to Puccinia triticina in common wheat. Theor. Appl. Genet. 127, 2041–2049. doi: 10.1007/s00122-014-2359-y
Pathan, A. K., and Park, R. F. (2006). Evaluation of seedling and adult plant resistance to leaf rust in European wheat cultivars. Euphytica 149, 327–342. doi: 10.1007/s10681-005-9081-4
Qureshi, N., Bariana, H., Kumran, V. V., Muruga, S., Forrest, K. L., Hayden, M. J., et al. (2018). A new leaf rust resistance gene Lr79 mapped in chromosome 3BL from the durum wheat landrace Aus26582. Theor. Appl. Genet. 131, 1091–1098. doi: 10.1007/s00122-018-3060-3
Ren, X., Liu, T., Liu, B., Gao, L., and Chen, W. Q. (2015). Postulation of seedling leaf rust resistance genes in 84 Chinese winter wheat cultivars. J. Integrat. Agric. 14, 1992–2001. doi: 10.1016/S2095-3119(14)61002-9
Roelfs, A. P. (1988). Resistance to Leaf and Stem Rusts in Wheat. Breeding Strategies for Resistance to the Rusts of Wheat. Mexico: CIMMYT.
Roelfs, A. P., Singh, R. P., and Saari, E. E. (1992). Rust Diseases of Wheat: Concepts and Methods of Disease Management. Mexico: CIMMYT.
Sánchez-Martín, J., Steuernagel, B., Ghosh, S., Herren, G., Hurni, S., Adamski, N., et al. (2016). Rapid gene isolation in barley and wheat by mutant chromosome sequencing. Genome Biol. 17:221. doi: 10.1186/s13059-016-1082-1
Seyfarth, R., Feuillet, C., Schachermayr, G., Winzeler, M., and Keller, B. (1999). Development of a molecular marker for the adult plant leaf rust resistance gene Lr35 in wheat. Theor. Appl. Genet. 99, 554–560. doi: 10.1007/s001220051268
Singh, A., Knox, R. E., DePauw, R. M., Singh, A. K., Cuthbert, R. D., Campbell, H. L., et al. (2013). Identification and mapping in spring wheat of genetic factors controlling stem rust resistance and the study of their epistatic interactions across multiple environments. Theor. Appl. Genet. 126, 1951–1964. doi: 10.1007/s00122-013-2109-6
Singh, R. P., Chen, W. Q., and He, Z. H. (1999). Leaf rust resistance of spring, facultative, and winter wheat cultivars from china. Plant Dis. 83, 644–651. doi: 10.1094/PDIS.1999.83.7.644
Singh, R. P., and Gupta, A. K. (1991). Genes for leaf rust resistance in Indian and Pakistani wheats tested with Mexican pathotypes of Puccinia recondita f. sp. tritici. Euphytica 57, 27–36. doi: 10.1007/BF00040475
Singh, R. P., and Rajaram, S. (1992). Genetics of adult-plant resistance of leaf rust in ‘Frontana’ and three CIMMYT wheats. Genome 35, 24–31. doi: 10.1139/g92-004
Steuernagel, B., Periyannan, S. K., Hernández-Pinzón, I., Witek, K., Rouse, M. N., Yu, G., et al. (2016). Rapid cloning of disease-resistance genes in plants using mutagenesis and sequence capture. Nat. Biotechnol. 34, 652–655. doi: 10.1038/nbt.3543
Thind, A. K., Wicker, T., Šimková, H., Fossati, D., Moullet, O., Brabant, C., et al. (2017). Rapid cloning of genes in hexaploid wheat using cultivar-specific long-range chromosome assembly. Nat. Biotechnol. 35, 793–796. doi: 10.1038/nbt.3877
Varshney, R. K., Terauchi, R., and McCouch, S. R. (2014). Harvesting the promising fruits of genomics: applying genome sequencing technologies to crop breeding. PLoS Biol. 12:e1001883. doi: 10.1371/journal.pbio.1001883
Wang, C., Yin, G., Xia, X., He, Z., Zhang, P., Yao, Z., et al. (2016). Molecular mapping of a new temperature-sensitive gene LrZH22 for leaf rust resistance in Chinese wheat cultivar Zhoumai 22. Mol. Breed. 36:18. doi: 10.1007/s11032-016-0437-3
WAP (2017). “World agricultural production,” in Circular Series, WAP 01–17, Washington, DC: United States Department of Agriculture-Foreign Agricultural Service.
Wu, J., Liu, S., Wang, Q., Zeng, Q., Mu, J., Huang, S., et al. (2018a). Rapid identification of an adult plant stripe rust resistance gene in hexaploid wheat by high-throughput SNP array genotyping of pooled extremes. Theor. Appl. Genet. 131, 43–58. doi: 10.1007/s00122-017-2984-3
Wu, J., Zeng, Q., Wang, Q., Liu, S., Yu, S., Mu, J., et al. (2018b). SNP-based pool genotyping and haplotype analysis accelerate fine-mapping of the wheat genomic region containing stripe rust resistance gene Yr26. Theor. Appl. Genet. 131, 1481–1496. doi: 10.1007/s00122-018-3092-8
Wu, P., Hu, J., Zou, J., Qiu, D., Qu, Y., Li, Y., et al. (2019). Fine mapping of the wheat powdery mildew resistance gene Pm52 using comparative genomics analysis and the Chinese Spring reference genomic sequence. Theor. Appl. Genet. 132, 1451–1461. doi: 10.1007/s00122-019-03291-7
Wu, S., Pumphrey, M., and Bai, G. (2009). Molecular mapping of stem-rust-resistance gene in wheat. Crop Sci. 49:1681. doi: 10.2135/cropsci2008.11.0666
Xu, Y., Li, P., Zou, C., Lu, Y., Xie, C., Zhang, X., et al. (2017). Enhancing genetic gain in the era of molecular breeding. J. Exp. Bot. 68, 2641–2666. doi: 10.1093/jxb/erx135
Yao, F., Xu, C., Yu, S., Li, J., Gao, Y., Li, X., et al. (1997). Mapping and genetic analysis of two fertility restorer loci in the wild-abortive cytoplasmic male sterility system of rice (Oryza sativa L.). Euphytica 98, 183–187. doi: 10.1023/A:1003165116059
Yuan, J., and Chen, W. (2011). Estimate on the effectiveness of main resistant genes for leaf rust in Chinese Wheat. J. Triticeae Crops 31, 994–999.
Yuan, J., Liu, T., and Chen, W. (2007). Postulation of leaf rust resistance genes in 47 new wheat cultivars (lines) at seedling stage cultivars (lines) at seedling stage. Sci. Agric. Sinica 40, 1925–1935.
Zhang, P., Gebrewahid, T. W., Zhou, Y., Li, Q., Li, Z., and Liu, D. (2019). Seedling and adult plant resistance to leaf rust in 46 Chinese bread wheat landraces and 39 wheat lines with known Lr genes. J. Integrat. Agric. 18, 1014–1023. doi: 10.1016/S2095-3119(19)62575-X
Zhang, P., Hiebert, C. W., McIntosh, R. A., McCallum, B. D., Thomas, J. B., Hoxha, S., et al. (2016). The relationship of leaf rust resistance gene Lr13 and hybrid necrosis gene Ne2m on wheat chromosome 2BS. Theor. Appl. Genet. 129, 485–493. doi: 10.1007/s00122-015-2642-6
Zhang, Q., Shen, B. Z., Dai, X. K., Mei, M. H., Maroof, M. A. S., and Li, Z. (1994). Using bulked extremes and recessive class to map genes for photoperiod-sensitive genic male sterility in rice. Proc. Natl. Acad. Sci. U.S.A 91, 8675–8679. doi: 10.1073/pnas.91.18.8675
Zhou, H., Xia, X., He, Z., Li, X., Wang, C., Li, Z., et al. (2013). Molecular mapping of leaf rust resistance gene LrNJ97 in Chinese wheat line Neijiang 977671. Theor. Appl. Genet. 126, 2141–2147. doi: 10.1007/s00122-013-2124-7
Keywords: wheat, LrLC10 (Lr13), leaf rust resistance, fine mapping, marker-assisted selection (MAS)
Citation: Qiu L, Wang H, Li Y, Wang W, Liu Y, Mu J, Geng M, Guo W, Hu Z, Ma J, Sun Q and Xie C (2020) Fine Mapping of the Wheat Leaf Rust Resistance Gene LrLC10 (Lr13) and Validation of Its Co-segregation Markers. Front. Plant Sci. 11:470. doi: 10.3389/fpls.2020.00470
Received: 26 November 2019; Accepted: 30 March 2020;
Published: 06 May 2020.
Edited by:
Luigi Cattivelli, Research Centre for Genomics and Bioinformatics, Council for Agricultural and Economics Research, ItalyReviewed by:
Urmil Bansal, University of Sydney, AustraliaPablo Daniel Olivera, University of Minnesota Twin Cities, United States
Copyright © 2020 Qiu, Wang, Li, Wang, Liu, Mu, Geng, Guo, Hu, Ma, Sun and Xie. This is an open-access article distributed under the terms of the Creative Commons Attribution License (CC BY). The use, distribution or reproduction in other forums is permitted, provided the original author(s) and the copyright owner(s) are credited and that the original publication in this journal is cited, in accordance with accepted academic practice. No use, distribution or reproduction is permitted which does not comply with these terms.
*Correspondence: Chaojie Xie, eGllY2oxMjdAMTI2LmNvbQ==