- State Key Laboratory of Tea Plant Biology and Utilization, International Joint Laboratory on Tea Chemistry and Health Effects, Anhui Agricultural University, Hefei, China
C-repeat (CRT)/dehydration responsive element (DRE)-binding factor CBFs, a small family of genes encoding transcriptional activators, play important roles in plant cold tolerance. In this study, a comprehensive genome-wide analysis was carried out to identify and characterize the functional dynamics of CsCBFs in tea plant (Camellia sinensis). A total of 6 CBF genes were obtained from the tea plant genome and named CBF1-6. All of the CsCBFs had an AP2/ERF DNA-binding domain and nuclear localization signal (NLS) sequence. CsCBF-eGFP fusion and DAPI staining analysis confirmed the nuclear localization of the CsCBFs. Transactivation assays showed that the CsCBFs, except CsCBF1, had transcriptional activity. CsCBF expression was differentially induced by cold, heat, PEG, salinity, ABA, GA, MeJA, and SA stresses. In particular, the CsCBF genes were significantly induced by cold treatments. To further characterize the functions of CsCBF genes, we overexpressed the CsCBF3 gene in Arabidopsis thaliana plants. The resulting transgenic plants showed increased cold tolerance compared with the wild-type Arabidopsis plant. The enhanced cold tolerance of the transgenic plants was potentially achieved through an ABA-independent pathway. This study will help to increase our understanding of CsCBF genes and their contributions to stress tolerance in tea plants.
Introduction
Abiotic stresses in the natural environment, including low temperature, drought, and high salinity, seriously affect the growth, development, distribution, and productivity of plants (Kulik et al., 2011). Plants have evolved a complex set of adaptive mechanisms to survive these adverse environmental conditions, and these mechanisms involve a number of biochemical and physiological changes (Ramanjulu and Bartels, 2002). Different studies have suggested that transcription factors can activate the expression of related genes to protect plants from adversity (Agarwal and Jha, 2010). CBFs/DREBs (C-repeat binding factors/dehydration responsive element-binding factors) belong to the AP2/ERF (APETALA2/ethylene-responsive element-binding factor) transcription factor family (Riechmann and Meyerowitz, 1998) and have been reported to play pivotal roles in freezing tolerance and cold acclimation (Stockinger et al., 1997). The AP2/ERF family contains a highly conserved AP2/ERF domain that harbors a DNA-binding motif of ~60 amino acids (Sakuma et al., 2002). When plants suffer from low temperatures, related regulatory proteins are modified to regulate the expression of the CBF gene. For example, the inducers of CBF expression 1 (ICE1), calmodulin-binding transcription activator 3 (CAMTA3) and brassinazole-resistant 1 (BZR1), positively regulate CBF expression, whereas MYB15, ethylene-insensitive 3 (EIN3), and 14-3-3 negatively regulate CBF expression (Liu et al., 2017). Subsequently, CBF/DREB1 proteins bind to the cold- and dehydration-responsive DNA regulatory element designated the CRT (C-repeat)/DRE (dehydration response element), which is present in the promoters of COR (cold-regulated) genes and contains the core motif of G/ACCGAC (Yamaguchi-Shinozaki and Shinozaki, 1994; Huang et al., 2012), and to the promoters of other cold responsive genes, such as COR15A, RD29A, and COR78 (Liu et al., 1998; Lucas et al., 2011; Akhtar et al., 2012), and stimulates their transcription (Baker et al., 1994; Jaglo et al., 2001; Zhao T. et al., 2012). Previous studies have identified a number of CBF/DREB1 genes and verified their functions in Arabidopsis thaliana (Novillo et al., 2007) and other plant species, such as cotton (Gossypium hirsutum) (Shan et al., 2007), wheat (Triticum aestivum) (Shen et al., 2003), rice (Oryza sativa) (Wang et al., 2008), maize (Zea mays) (Qin et al., 2004), soybean (Glycine max) (Kidokoro et al., 2015), and tomato (Lycopersicon esculentum) (Zhang et al., 2004). These findings suggested the conserved roles of CBF/DREB1 genes in the regulation of freezing tolerance across diverse plant species. Nevertheless, CBFs play different roles in responses to stress in different plant species (Ebrahimi et al., 2015). In addition to the cold response, CBFs could also respond to other abiotic stresses and hormones, such as heat, drought, salt, and abscisic acid (ABA) (Dubouzet et al., 2003; Xiao et al., 2006; Nada and Abogadallah, 2015).
Tea plants are the world's most important nonalcoholic beverage crop with a wealth of health benefits (Mukhopadhyay et al., 2016). The growth of tea plants is seriously affected by abiotic stresses (Wang et al., 2014), particularly extreme temperature and drought (Das et al., 2016; Liu et al., 2016; Hou et al., 2018; Zhou et al., 2018). Although several recent studies have demonstrated that CsCBF1 enables cold stress in tea plants (Wang et al., 2012), overexpression of CsDREB increases salt and drought tolerance in transgenic Arabidopsis thaliana (Wang et al., 2017). However, given that the CBF transcription factor is a polygenic family, it is still unclear whether there are other new CBF genes in tea plants that respond to low temperature. In the present study, we thoroughly investigated the CBF genes in tea plant using tea plant genome and transcriptome datasets, with the aim of providing novel insights into the functional dynamics of CBF genes in tea plant. The overall obtained results provide a foundation for additional studies of the biological functions of CsCBFs under abiotic stresses and a new perspective for resistance breeding in tea plants.
Materials and Methods
Plant Materials and Stress Treatments
One-year-old tea cutting seedlings of the Shuchazao cultivar were planted in a pot and grown with a natural photoperiod in a greenhouse (12 h light and 12 h dark photoperiod, 25°C temperature and 70% relative humidity) (Li et al., 2016) at the State Key Laboratory of Tea Plant Biology and Utilization, Anhui Agricultural University (Hefei, China). For low and high temperature stresses, tea plants were grown at 4 and 38°C in a plant growth chamber. For the GA, ABA, SA, and MeJA treatments, a working solution of 100 μM was foliar sprayed on plants (Pan et al., 2017). For drought and salinity, the plants were transferred to a 20% PEG6000 and 200 mM NaCl solution (Li et al., 2010; Yue et al., 2014). The second or third mature leaves were harvested for gene analysis 0, 4, 12, and 24 h (Agarwal M. et al., 2006) after treatments. After collection, the samples were immediately frozen in liquid nitrogen and stored at −80°C for RNA extraction. Three biological replicates were conducted.
Identification of CsCBF Genes and Phylogenetic Construction
The protein sequences of AtCBFs were downloaded from the NCBI (https://www.ncbi.nlm.nih.gov/) database and used as queries to search homologous sequences against the published genomes of tea plant (Wei et al., 2018; Xia E. et al., 2019) with the BlastP program at an e-value of 10−3 (Pan et al., 2017). The biophysical properties of the CsCBFs were computed using the online ProtParam tool (https://web.expasy.org/protparam/). The protein sequences of Camellia sinensis and Arabidopsis thaliana were aligned by DNAMAN6.0. Multiple sequence alignments of Camellia sinensis and Arabidopsis thaliana were analyzed using MEGA 6.0 (http://www.megasoftware.net/). The phylogenetic tree was constructed using the neighbor-joining algorithm with 1,000 replicates.
Characterization of CsCBF Genes and Proteins
The promoter sequences ~2 kb upstream of the transcription start site of each CsCBF gene were identified, and the cis-elements were analyzed by the online tool Plant CARE (http://bioinformatics.psb.ugent.be/webtools/plantcare/html/). The full-length amino acid sequences of CsCBF were entered into the MEME (http://meme-suite.org/tools/meme) analysis tool to find their conserved motifs. Parameters of MEME are following: number of different motifs: 10, Minimum/Maximum motif width: 6/100.
Subcellular Localization of CsCBFs
The full-length ORFs of CsCBF sequences were cloned into the pK7WGF2.0 vector containing the enhanced green fluorescent protein (eGFP) reporter gene by the gateway method. The isolated DNA was transformed into Agrobacterium strain GV3101. Six 35S::eGFP-CsCBF constructs and the control plasmids without CsCBF coding sequences were separately infiltrated into tobacco (Nicotiana tabacum) leaves by the Agrobacterium-mediated genetic transformation method. DAPI (4',6-diamidino-2-phenylindole dihydrochloride) was used to identify nuclei. The cells of transformed tobacco leaves were observed by an Olympus IX81 fluorescence microscope (Olympus, Japan).
Transactivation Assay Analysis
Each CsCBF gene was amplified and cloned into the pGBKT7 vector. Six pGBKT7-CsCBF vectors, the pGBKT7-AtCBF2 vector (positive control) and the pGBKT7 vector (negative control) were separately transformed into the Y2HGold yeast strain. The transformed yeast cells were incubated on SD/-Trp, SD/-Trp/-His-Ade, and SD/-Trp-His-Ade-x-gal plates at 30°C for 3 d.
RNA Isolation and Quantitative RT-PCR
Total RNA was extracted using the RNAprep Pure Plant Kit (Tiangen, Beijing, China). A Nanodrop 2000 spectrophotometer (Thermo Scientific, Wilmington, USA) was used to measure the concentration of isolated RNA, and the quality was assessed using 1.2% formaldehyde–agarose gel electrophoresis. cDNA was synthesized for qRT-PCR by the PrimeScript™ RT Reagent Kit with gDNA Eraser (Takara, Tokyo, Japan) and diluted 10-fold for PCR amplification.
The specific primers for qRT-PCR were designed by Primer Premier 5 software and synthesized by Sangon Biotech Co. (Shanghai, China). The qRT-PCR reaction program was performed under the following conditions: 95°C for 30 s, followed by 40 cycles at 95°C for 5 s, and 60°C for 30 s. The reaction volume was 25 μL, which contained 4 μL of diluted cDNA, 6.5 μL of deionized water, 12.5 μL of SYBR® Premix Ex Taq™ II (Tli RNaseH Plus; TaKaRa), 1 μL of forward primer, and 1 μL of reverse primer. CsGAPDH was used as the reference gene (Pan et al., 2017). The relative gene expression levels were calculated using the comparative 2−ΔΔCt method (Livak and Schmittgen, 2001). Regarding the heatmaps, the 2−ΔΔCt values of the transcripts of the CsCBF genes were normalized as the log2-fold change. In the stress-treated plant samples, the values were normalized to plant samples of the 0 h treatment and expressed as a log2-fold change. qRT-PCR experiments were conducted with three independent total RNA samples.
Functional Analysis of CsCBF3-Overexpressing Transgenic Arabidopsis thaliana
Expression of CsCBF3 shows significant changes during cold treatment and we selected it for overexpression. The ORF of CsCBF3 was cloned into the pBI121 vector. The construct was transformed into Agrobacterium strain GV3101 by electroporation, and the Arabidopsis plants were transformed using the floral dip method. Arabidopsis ecotype Columbia-0 (col-0) was used as the wild type in this study. Transformed plants were selected on the basis of their resistance to kanamycin, and 4-weeks-old homozygous T3 plants were used for further experiments. Two transgenic lines were treated at −4 and −8°C for 12 h and then grown under 25°C conditions for analysis of survival rate.
Results
Identification and Characterization of CBF Genes in Tea Plant
We initially identified 10 CBF genes from genome and transcriptome databases of tea plant. Six of them were successfully cloned and deposited into the NCBI GenBank database under the accession numbers CsCBF1 (EU563238.1), CsCBF2 (KC702795.1), CsCBF3 (MH017428.1), CsCBF4 (KF988866.1), CsCBF5 (MH165878.1), and CsCBF6 (MN544638.1). Alignment of the sequences obtained from cDNA and genomic DNA indicated that the CsCBF genes were intronless. The CDS length of the CsCBFs ranged from 720 to 879 bp, and the genes encoded proteins with lengths varying from 239 to 292 amino acids. The molecular weights were between 26.43 and 32.86 kDa, and the pI-values ranged from 4.84 to 8.09. Most of the CsCBF proteins presented grand average hydropathicity (GRAVY) values of <0, implying their hydrophilic nature (Table 1).
Sequence Alignment and Phylogenetic Analysis
We investigated the amino acid characteristics of CBF proteins of C. sinensis and A. thaliana. Alignment of the amino acid sequences of the CsCBFs and AtCBFs revealed that the CsCBFs contained one highly conserved DNA-binding domain (AP2 domain) consisting of 59 amino acids. A putative nuclear localization signal (NLS) sequence (PKKRAGRKKFK) was detected in the N-terminal region. In addition, the C-terminal regions of the CsCBF proteins were highly diverged, particularly CsCBF6 (Figure 1).
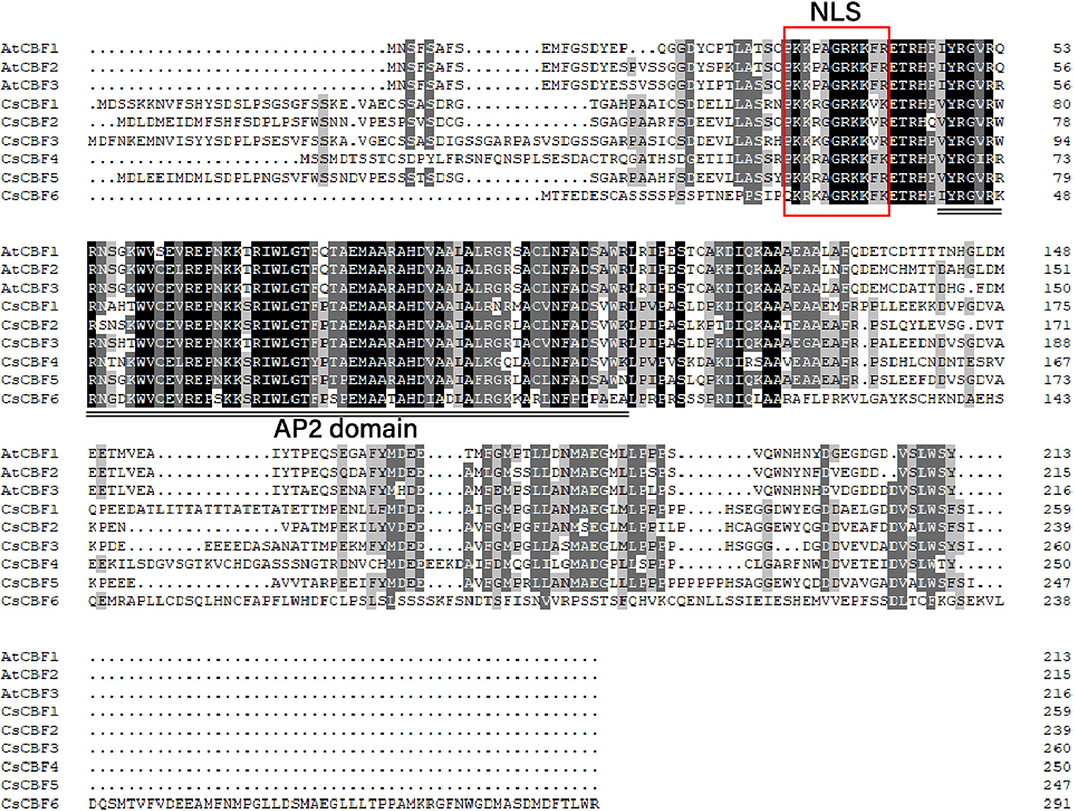
Figure 1. Sequence alignment of the amino acid sequence of CBFs in C. sinensis and Arabidopsis. Identical and conserved amino acids are shaded in black and gray, respectively. The conserved nuclear localization signal (NLS) and AP2 DNA-binding domain are shown. The NLS sequence is indicated by a red rectangle, and the conserved AP2 domain is underlined (double line).
A phylogenetic tree was constructed using the neighbor-joining method with the MEGA program to explore the evolutionary relationships among CBF homologs in C. sinensis and A. thaliana (Figure 2). The 60 collected DREBs from C. sinensis and A. thaliana were clustered into six groups. All 10 CsCBF proteins were clustered together with AtDDF1-2 and AtCBF1-4 in Group A1. The result showed that CsCBFs belongs to DREB-A1 subfamily.
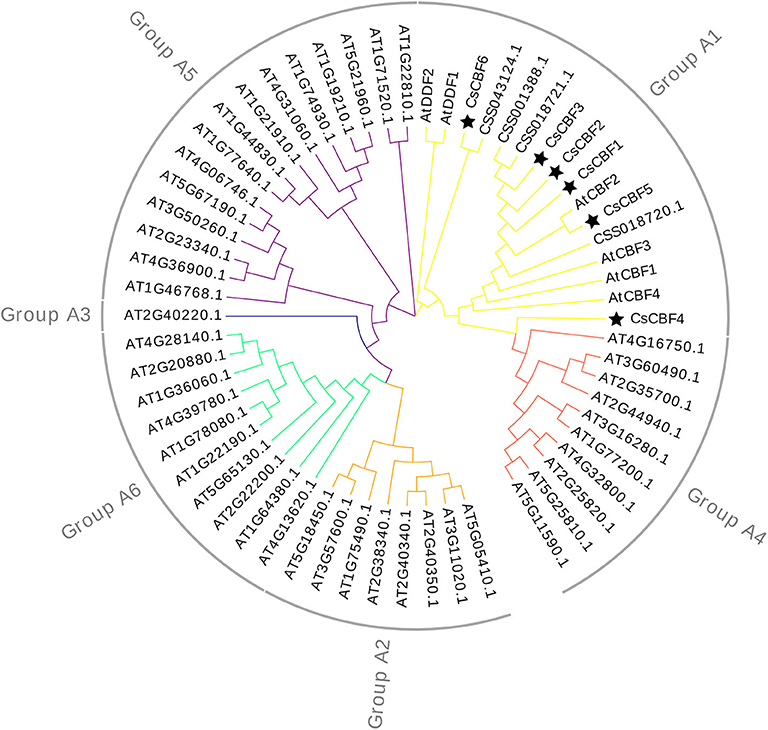
Figure 2. Phylogenetic analysis of CBF proteins from C. sinensis and Arabidopsis. All protein sequences are listed in Table S1.
Motif Analysis
To explore the diversification of CBF genes in tea plants, we examined the conserved motifs within the CsCBFs proteins using the MEME program (Figure 3). Ten motifs were identified. Multilevel consensus sequences and the E-value of each motif are shown. The results revealed conserved motif distribution among CsCBF proteins. Motif 1 and motif 2 were found in all CBFs. Motifs 3–6 could be found in CsCBF1, 2, 3, and 5. Motif 7 was mainly identified in the C-terminal regions of CBF1, 2, and 3. Motif 8 was unique to CsCBF6 and motif 9 was unique to CsCBF4. Motif 10 was distributed in CBF1, 2, and 3. Motif analysis supports the results of the phylogenetic analysis.
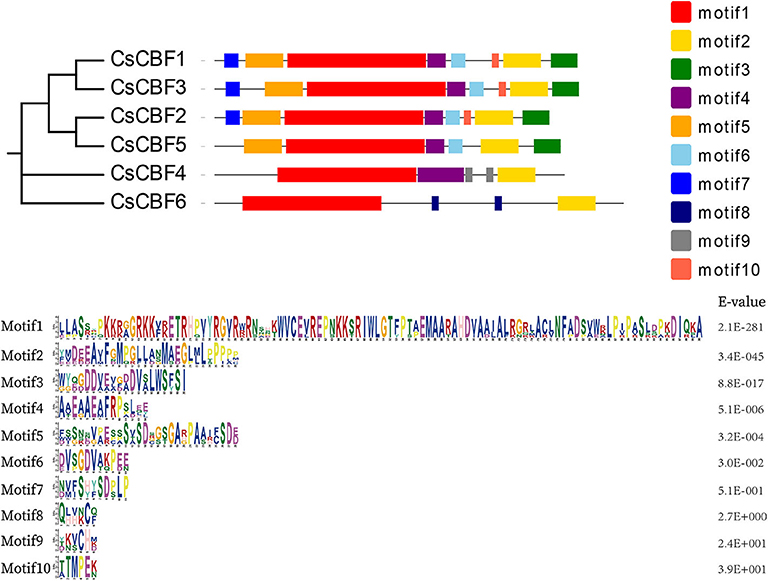
Figure 3. Motif analysis of CsCBF proteins. Different motifs are represented by different colored boxes.
Subcellular Localization of CsCBF Proteins
To investigate the subcellular localization of CsCBF proteins, six 35S::eGFP-CsCBF constructs, one for each CsCBF, and a 35S::eGFP (positive control) construct were generated and transiently expressed in tobacco leaves. As shown in Figure 4, eGFP alone resulted in a diffuse distribution of green fluorescence throughout the entire cell. In contrast, CsCBF-eGFP proteins localized predominantly to the nucleus, which was further confirmed by 4′,6-diamidino-2-phenylindole (DAPI) staining. The results indicated that CsCBFs are nuclear localized proteins.
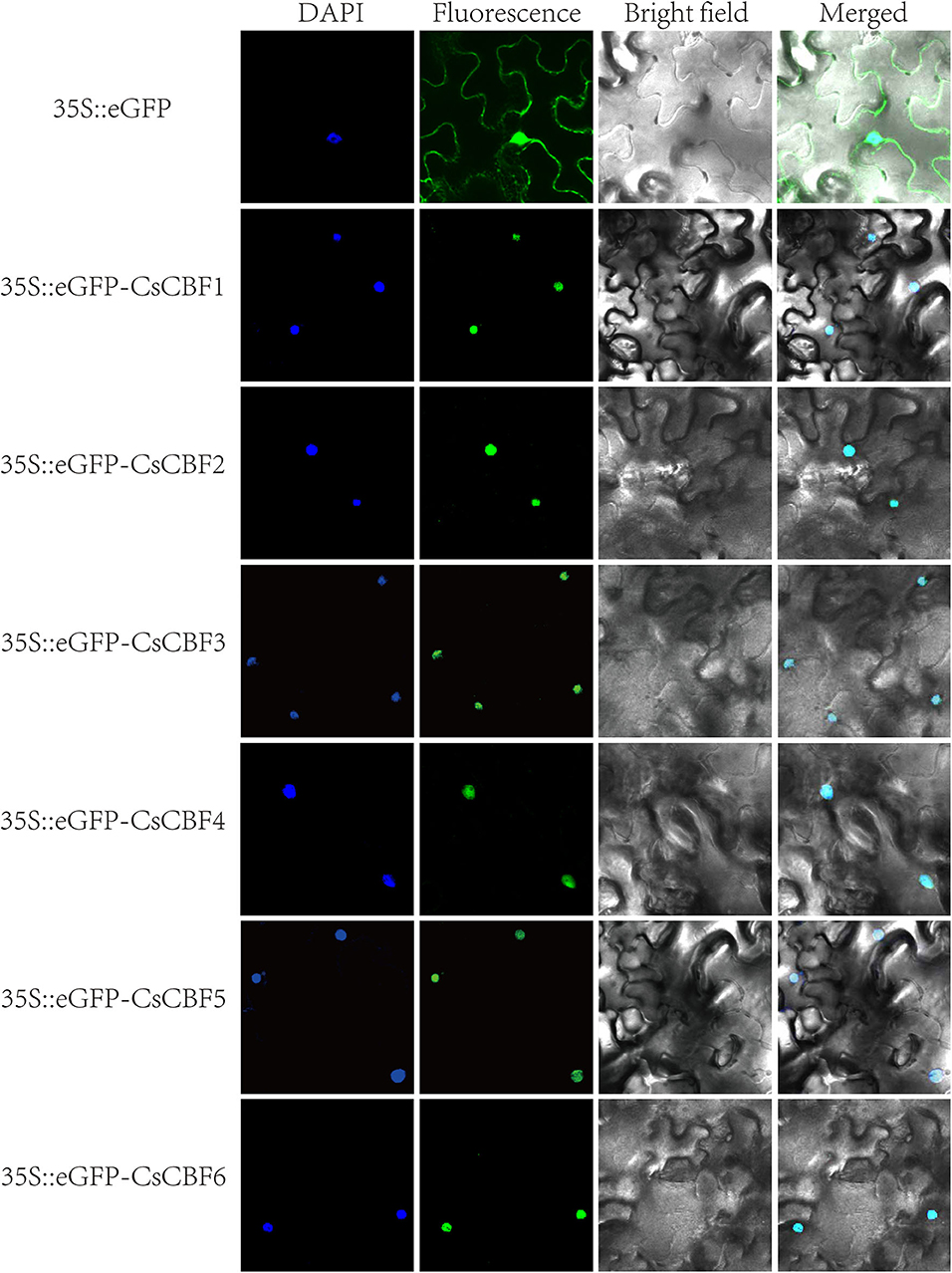
Figure 4. Subcellular localization of CsCBF proteins in tobacco epidermal cells. The 35S::GFP vector was used as a positive control. Images show 4',6-diamino-phenylindole (DAPI) staining fluorescence, GFP fluorescence, and bright light individually and in combination to demonstrate the morphology of the cells.
Transactivation Assay of CsCBFs
To examine the transcriptional activities of CsCBFs, full length sequences of six CsCBFs and AtCBF2 (positive control) were fused to the vector pGBKT7 containing the GAL4 DNA-binding domain and subsequently transformed into yeast. The yeast cells harboring pGBKT7-CsCBF2, pGBKT7-CsCBF3, pGBKT7-CsCBF4, pGBKT7-CsCBF5, pGBKT7-CsCBF6, and pGBKT7-AtCBF2 grew well on the selection media without Trp, His or adenine (SD/-Trp-His-Ade) and were positive for α-galactosidase activity. The yeast cells with the empty vector pGBKT7 (negative control) and pGBKT7-CsCBF1, which is missing the transcriptional activation domain GAL4 AD, were unable to grow on the same medium. The results indicated that CsCBF2, CsCBF3, CsCBF4, CsCBF5, and CsCBF6 have transcriptional activity, while CsCBF1 has no transcriptional activity (Figure 5).
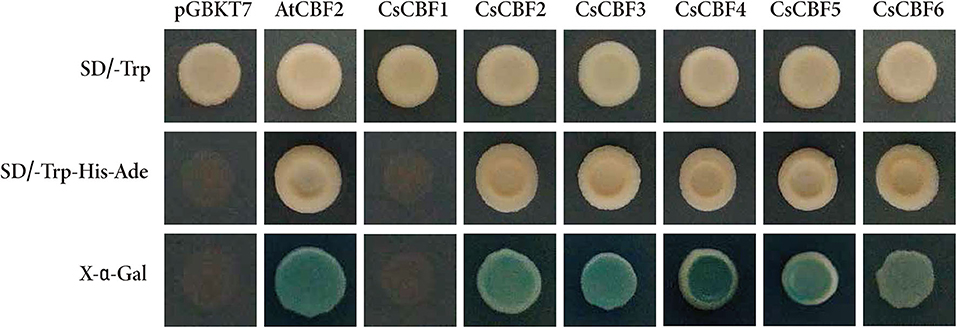
Figure 5. Transcriptional activity analysis of CsCBFs. The entire ORFs of CsCBF sequences were fused to the vector pGBKT7 containing the GAL4 DNA-binding domain. The images in the top row and the middle row show the transformed yeast cells growing on SD medium without Trp and SD medium without Trp, His, or Ade. The bottom row exhibits the α-galactosidase activity of the transformed yeast cells.
Cis-Element Analysis of the Promoter Regions of CsCBF Genes
To further investigate the regulatory mechanism of CsCBFs, sequences 2,000 bp upstream of the start codon of the CsCBF genes were extracted from the tea plant genome database (Xia E. et al., 2019). As shown in Table 2, all CsCBF proteins contained the MeJA-responsive element (CGTCA), stress responsiveness element (MYB), and water stress and dehydration responsiveness element (MYC). The abscisic acid-responsive element (ABRE) was found in CsCBF1-pro, CsCBF3-pro, CsCBF4-pro, CsCBF5-pro, and CsCBF6-pro. Ethylene-responsive elements (EREs) were distributed in CsCBF1-pro, CsCBF2-pro, CsCBF3-pro, and CsCBF5-pro. The gibberellin-responsive element (GARE) was unique to CsCBF4-pro. The light-responsive element (G-box) was found in CsCBF1-pro, CsCBF3-pro, CsCBF4-pro, CsCBF5-pro, and CsCBF6-pro. The low-temperature-responsive element (LTR) was shown in CsCBF2-pro, CsCBF4-pro, CsCBF5-pro, and CsCBF6-pro. The Myb-binding site (MBS) was distributed in CsCBF2-pro, CsCBF3-pro, and CsCBF6-pro. Salicylic acid-responsive elements (TCA-element) were unique to CsCBF4-pro and CsCBF5-pro. Defense and stress-responsive elements (TC-rich repeat) were found in CsCBF1-pro, CsCBF5-pro, and CsCBF6-pro. Auxin-responsive elements (TGA elements) were found in CsCBF2-pro and CsCBF4-pro (Table 2).
Expression Analysis of CsCBFs Under Various Abiotic Stresses
We investigated the expression profile of CsCBF genes in response to eight stress treatments (cold, heat, PEG, salinity, ABA, GA, MeJA, and SA) by qRT-PCR (Figure 6). Under cold treatment, all CsCBFs showed high expression levels during 24 h. Notably, the expression of CsCBF1,2,3,4, and 5 was much higher than that of CsCBF6. The expression of CsCBF1, 2, and 3 increased a 100-fold or even 1000-fold throughout the entire incubation time, while the expression of CsCBF4, 5, and 6 increased with processing time but reached a maximum at 12 h. Under heat stress, CsCBF1, 2, and 5 presented an expression trend of rising first and then falling, while the expression of CsCBF4 and 6 declined during processing, and the expression of CsCBF3 fell to 12 h and then returned to normal. Under PEG stress, CsCBF2 had a strong increasing response. CsCBF3 and 5 showed no response to the treatment. The expression of CsCBF4 and 6 was downregulated and reached a minimum at 12 h, and then the expression level returned to the control level. CsCBF1 showed a decrease over time. Under salt stress, CsCBF1 and 4 showed a trend of increasing expression over time. The expression of CsCBF2, 3, and 5 first dropped and reached the lowest level at 4 h, then began to rise, and finally was overall positively expressed. CsCBF6 expression decreased. The results of the treatment of four plant hormones were as follows: under ABA treatment, the expression of CsCBF1 and 2 increased and peaked at 4 h. CsCBF3 and 5 had almost no response. CsCBF4 was downregulated and reached its minimum at 12 h, then it returned to the control level. CsCBF6 slowly increased with the treatment process. Under GA treatment, CsCBF1 and 6 both decreased and reached a minimum at 4 and 12 h, respectively, and finally returned to the control level. CsCBF2 and 4 both increased and reached a maximum at 4 and 12 h, respectively. CsCBF3 showed no response to GA. CsCBF5 showed a downward adjustment in the later stages of processing. Under MeJA treatment, the expression of CsCBF1, 2, 3, 4, and 5 was downregulated. CsCBF6 rose in 4 h and then decreased with time. Under SA treatment, CsCBF1, 3, and 5 were downregulated and reached a minimum at 12 h. CsCBF2 was positive in the first 4 h and then decreased to negative over time. CsCBF4 was downregulated and reached its minimum at 12 h, then it returned to the control level. CsCBF6 was negatively regulated and then positively regulated.
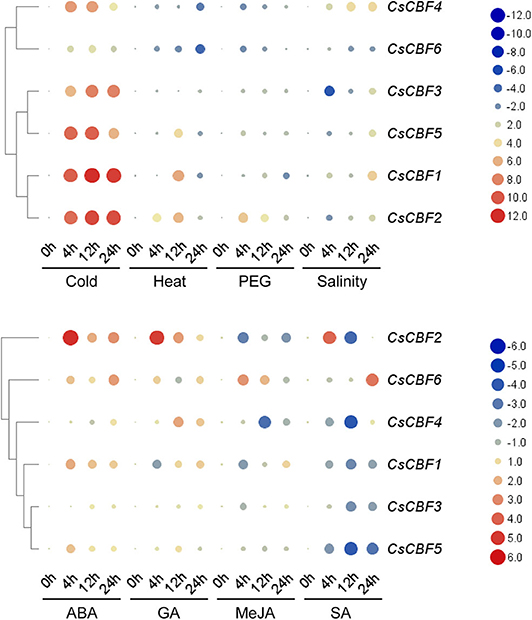
Figure 6. Expression patterns of CsCBF genes in response to cold, heat, PEG, salinity, ABA, GA, MeJA, and SA stresses in tea plant cultivars. The relative gene expression levels were calculated using the 2−ΔΔCt method and expressed as the fold change relative to expression of the 0-h treatment. GAPDH was used as a housekeeping gene. The mean expression values were again normalized using logarithm with the base of two. The color bar in all heat maps represents the expression values: red represents upregulation, black represents no significant difference in expression, and green denotes downregulation.
In general, the results illustrated that cold, ABA and GA could induce high expression of most of the CsCBF genes.
Overexpression of CsCBF3 in Arabidopsis thaliana Improves Tolerance to Cold Stress
To confirm the in vivo functions of the CsCBF3 gene during low-temperature stress in plants, we transferred CsCBF3 into A. thaliana. The expression of CsCBF3 was detected using real-time PCR assay in overexpressed (OE) plants but not in wild-type (WT) plants (Figure 7A). Two overexpressed lines (OE-9 and OE-14) were treated in low temperature conditions. Thirty seeds of wild-type and overexpressed plants were selected for treatment. Under normal growth conditions (25°C), there were no obvious differences in survival rate between the WT and transgenic plants. A 3-days recovery after low-temperature treatment at −4 and −8°C for 12 h, the survival rates of WT plants significantly decreased compared to those of transgenic plants (Figure 7B). Recovery for 3 d after −4°C treatment, ~31% of wild-type plants survived, while 73% of OE-9 survived. Recovery for 3 d after −8°C treatment, the survival rates of wild-type plants and OE-14 were 11 and 58%, respectively (Figure 7C).
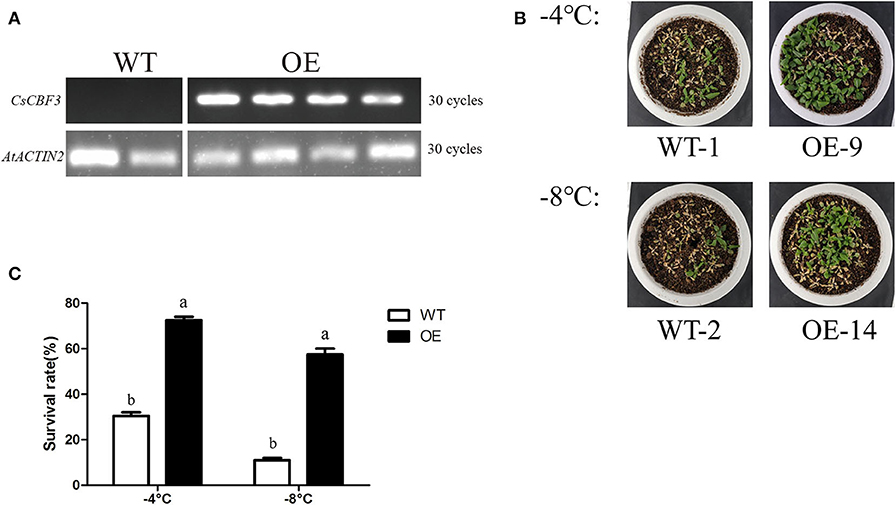
Figure 7. Low-temperature tolerance of transgenic and wild-type A. thaliana. (A) Expression level of CsCBF3 in wild-type and transgenic plants. (B) A 3-days recovery growing situation of WT and OE plants after −4 and −8°C low temperature treatment. (C) Survival rates of wild-type and transgenic plants (P < 0.05).
In addition, we detected the expression of downstream target ABA-dependent stress-induced genes (AtRD29B, AtRAB18, AtABI1, and AtABI2) and ABA-independent stress-induced genes (AtCOR15a and AtRD29A) to explore the potential CsCBF3-associated regulatory pathway. Under unstressed conditions, the expression of AtCOR15a and AtRD29A in transgenic plants was significantly higher than that in wild-type plants. The expression of AtRD29B, AtRAB18, AtABI1, and AtABI2 showed slight differences in WT and OE plants (Figure 8). These results indicated that CsCBF3 may affect the expression of ABA-independent stress-induced genes to increase plant tolerance to cold stress.
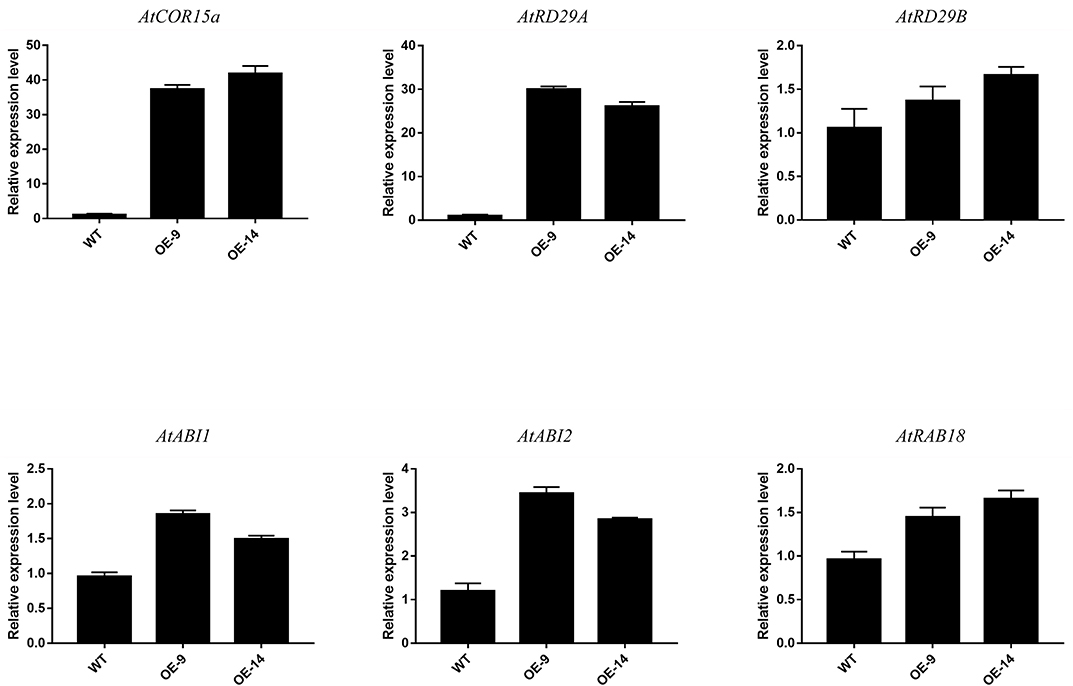
Figure 8. Relative expression level of the downstream target gene of CsCBF3 in wild-type and transgenic A. thaliana plants under cold stress.
Discussion
Low temperature is a major abiotic factor that limits crop productivity. When plants suffer from cold stress, CBF/DREB transcription factors are triggered and regulate ~12% of the cold responsive transcriptome, showing important roles in cold tolerance (Sun et al., 2014). To date, two CsCBF genes' biological function have been reported in tea plant. Wang et al. (2012) and Ban et al. (2017) found that CsCBF1 was not expressed at normal temperature (20°C) but was significantly induced at low temperature (4°C). In addition, Wang et al. (2012) used a DNA-binding assay to demonstrate that CsCBF1 can specifically bind to the CRT/DRE cis-element, suggesting that CsCBF1 can regulate downstream genes containing CRT elements such as COR15 and RD29A. Wang et al. (2017) showed that overexpression of a CsDREB gene in Arabidopsis thaliana plants could increase the salt and drought tolerance of transgenic plants. Wang et al. (2019) identified five CsCBFs from tea plant genome sequences (Wei et al., 2018), namely CsCBF1-5. However, we failed to clone CsCBF2 (TEA010423), which might be caused by draft nature of the current genome assembly with low assembly quality. The CsCBF1 identified by Wang et al. (2012) was consistent with that identified in this study but totally different from that identified by Wang et al. (2019) (Data Sheet S3). Similarly, we were not only unable to find homologous gene of the CsCBF3 identified by Yin et al. (2016) in the current tea plant genome assembly, but also failed to clone it in this study. We here successfully cloned a total of 6 CsCBFs (CsCBF1-6) based on the latest version of tea plant genome annotation (Xia E. et al., 2019). Of them, CsCBF1-4 were in agreement with those identified by Wang et al. (2019), and CsCBF5 and 6 were newly discovered (Data Sheet S3). Sequence analysis showed that CsCBFs contain an AP2 DNA-binding domain and two signature motifs of the CBF family (Figure 1). These results showed that CsCBF1~6 typically belong to the CBF family. The C-terminus of CsCBF6 is longer than those of the other CsCBFs, and it may cause functional divergence in plants under cold temperatures. Expression of CsCBF6 is much less than other CsCBFs verified this (Figure 6).
It is widely accepted that transcription factors must be present in the nucleus to perform their functions (Wang et al., 2008; Yang et al., 2011). Bioinformatics analysis showed the presence of nuclear localization signal (NLS) sequences in CsCBFs. In our vivo targeting experiment using a CsCBFs-fused GFP as a florescent marker demonstrated that the fusion protein was localized to the nucleus of tobacco leaf, suggesting that CsCBFs are nuclear proteins and functions as transcription factors. The results are consistent with the findings in cotton (Shan et al., 2007) and eggplant (Zhou et al., 2018). We designed a yeast single hybrid experiment to verify the transcriptional activities of CsCBFs. The results showed that CsCBF2-6 had transcriptional activity in yeast Y2HGold cells, but not CsCBF1. This finding is similar to the findings of Sakuma and Zhao (Sakuma et al., 2006; Zhao X. et al., 2012). A plausible explanation is probably because the secondary structure of the CsCBF1 protein itself is abnormal or CsCBF1 is required to be activated by a posttranslational modification.
CBF encodes a member of the DREB subfamily A-1 of ERF/AP2 transcription factor family. CBF/DREB genes from different plant species may have inconsistent expression profiles in response to various stresses (Zhou et al., 2016). There are six members in this subfamily, including CBF1, CBF2, CBF3, and CBF4 in Arabidopsis thaliana. AtCBF1-3 gene is involved in response to low temperature and abscisic acid. AtCBF4 gene is involved in response to drought stress and abscisic acid treatment, but not to low temperature (Novillo et al., 2004). EgCBF3 and FeDREB1 can be upregulated not only by cold but also by osmotic and high-salt stresses (Ebrahimi et al., 2015; Fang et al., 2015). CaDREBLP1 is not upregulated by low temperature but by dehydration and salt (Hong and Kim, 2005). We examined the expression patterns of CsCBF genes in relation to various environmental stresses. At normal growth temperatures, CsCBF genes is not transcribed, or is transcribed at lower levels, while CsCBF genes except CsCBF4,6 are rapidly, transiently and strongly induced by cold stress. CsCBF genes were induced to varying degrees by other abiotic stress treatment including exposure to high temperature, drought, exogenous hormones, or salinity. CsCBF4 had a strong response to salinity stress, which was similar to the study by Wang et al. (2017) in CsDREB. AtCBF2 gene is involved in a negative regulatory or feedback circuit of the CBF pathway (CBF2/DREB1C is a negative regulator of CBF1/DREB1B and CBF3/DREB1A expression and plays a central role in stress tolerance in Arabidopsis). Whether there is redundancy or feedback circuit function among CsCBFs genes needed to be further research.
Numerous studies have demonstrated that the expression of CBFs is regulated by GA, JA, ABA, ETH, and brassinosteroids (BRs) (Shan et al., 2007; Hu et al., 2013; Eremina et al., 2015; Barrero-Gil and Salinas, 2017). Different numbers plant hormone-responsive cis-elements were detected in the CsCBF promoters including abscisic acid, MeJA, ethylene, gibberellin, salicylic acid, auxin-responsive element. Results consistent with Wang et al. (2019). The molecular regulatory mechanisms of CsCBFs at the crossroads of plant hormone signaling in cold stress response need to be further elucidation. Light responsiveness motifs were also found in CsCBF promoters. Previous studies have showed that light is required for many cold-responsive genes, and there is a complex cross-talk between light and cold (Catalá et al., 2011). Large numbers of stress responsiveness cis-elements were found in CsCBF promoters, giving the reason tea plant can defend the cold stress. Sequence analysis of the CsCBF promoters revealed the existence of different numbers of MYB and MYC binding site, suggesting that induction of the CsCBF genes in response to low temperature is involved in the regulation of transcription factors, such as ICE1 and MYB15 in Arabidopsis (Chinnusamy et al., 2003; Agarwal M. et al., 2006).
To confirm in vivo functions of the CsCBF3 gene during low-temperature stress in plants, we ectopically expressed CsCBF3 into Arabidopsis. The results showed that in the case of CsCBF3 overexpression, transgenic plants showed enhanced resistance to cold damage. We also observed overexpression of CsCBF3 resulting in delayed flowering and dwarfism. Under a cold environment, 4-weeks-old overexpression plants had a much higher survival rate than wild-type plants. Similar function was observed in AtCBF1-3, GhDREB1, SmCBF, LpCBF3 (Xiong and Fei, 2006; Novillo et al., 2007; Shan et al., 2007; Zhou et al., 2018). To clarify how CsCBF3-overexpressing transgenic plants cope with low temperature stress, we examined the relative expression level of the downstream target gene of CBF identified in Arabidopsis plants. The results showed that the genes belonging to the ABA-independent pathway had a higher expression than the ABA-dependent genes when transgenic Arabidopsis plants were exposed to cold stress. Zhang et al. (2004) demonstrated the LeCBF1 in a heterologous system could activate the Arabidopsis cold related (COR) genes involved in increasing freezing tolerance, but that LeCBF1 in tomato plants did not up-regulate equivalent genes (Zhang et al., 2004). The function of CsCBF3 overexpression in tea plant can't be studied because of genetic transformation system has not been established. We speculated that CsCBF3 overexpression in transgenic plants improved cold tolerance mainly through the ABA-independent pathway, which was consistent with CBFs belonging to the ABA-independent pathway.
Conclusion
CBF/DREB transcription factors were identified in tea plants. CsCBF proteins were localized to the nucleus. CsCBFs had transcriptional activity except CsCBF1. CsCBF gene expression could be affected by abiotic stress and plant hormones. Ectopic expression of CsCBF3 in Arabidopsis induced cold tolerance, and the mechanism of CsCBF3 regulation of downstream target genes was mainly the ABA-independent pathway.
Data Availability Statement
The datasets generated for this study can be found in the NCBI, CsCBF1 (EU563238.1), CsCBF2 (KC702795.1), CsCBF3 (MH017428.1), CsCBF4 (KF988866.1), CsCBF5 (MH165878.1), CsCBF6 (MN544638.1).
Author Contributions
YL and EX designed the study. ZH, QB, JH, XZ, YC, JM, and ML conducted the experiments and analyzed the data. ZH and QB prepared the manuscript. All authors consent to the manuscript.
Funding
This research work was supported by Key R&D Program of China (Project No. 2018YFD1000601), Natural Science Foundation of China (Project No. 31270729), Special Project for Central Guiding Science and Technology Innovation of Region in Anhui Province (Project No. 2016080503B024), and Changjiang Scholars and Innovative Research Team in University (Project No. IRT1101).
Conflict of Interest
The authors declare that the research was conducted in the absence of any commercial or financial relationships that could be construed as a potential conflict of interest.
Acknowledgments
The authors are thankful to Prof. Zhaoliang Zhang and for providing plant expression vector PK7WGF2.0 and PGBKT7.
Supplementary Material
The Supplementary Material for this article can be found online at: https://www.frontiersin.org/articles/10.3389/fpls.2020.00921/full#supplementary-material
Table S1. Primers used in this study.
Table S2. Name of genes in this study.
Data Sheet S1. The amino acid sequences of CBF genes used in phylogenetic tree construction.
Data Sheet S2. Expression level of CsCBFs under abiotic stresses.
Data Sheet S3. Expression level of downstream target genes of CsCBF3 in Arabidopsis.
References
Agarwal, M., Hao, Y., Kapoor, A., Dong, C. H., Fujii, H., Zheng, X., et al. (2006). A R2R3 type MYB transcription factor is involved in the cold regulation of CBF genes and in acquired freezing tolerance. J. Biol. Chem. 281, 37636–37645. doi: 10.1074/jbc.M605895200
Agarwal, P. K., and Jha, B. (2010). Transcription factors in plants and ABA dependent and independent abiotic stress signaling. Biol. Plant. 54, 201–212. doi: 10.1007/s10535-010-0038-7
Akhtar, M., Jaiswal, A., Taj, G., Jaiswal, J. P., Qureshi, M. I., and Singh, N. K. (2012). DREB1/CBF transcription factors: their structure, function and role in abiotic stress tolerance in plants. J. Genet. 91, 385–395. doi: 10.1007/s12041-012-0201-3
Baker, S. S., Wilhelm, K. S., and Thomashow, M. F. (1994). The 5′-region of Arabidopsis thaliana COR15a has cis-acting elements that confer cold-, drought- and ABA-regulated gene expression. Plant. Mol. Biol. 24, 701–713. doi: 10.1007/BF00029852
Ban, Q., Wang, X., Pan, C., Wang, Y., Kong, L., Jiang, H., et al. (2017). Comparative analysis of the response and gene regulation in cold resistant and susceptible tea plants. PLoS ONE 12:e0188514. doi: 10.1371/journal.pone.0188514
Barrero-Gil, J., and Salinas, J. (2017). CBFs at the crossroads of plant hormone signaling in cold stress response. Mol. Plant. 10, 542–544. doi: 10.1016/j.molp.2017.03.004
Catalá, R., Medina, J., and Salinas, J. (2011). Integration of low temperature and light signaling during cold acclimation response in Arabidopsis. Proc. Natl. Acad. Sci. U. S. A. 108, 16475–16480. doi: 10.1073/pnas.1107161108
Chinnusamy, V., Ohta, M., Kanrar, S., Lee, B. H., Hong, X., Agarwal, M., et al. (2003). ICE1: a regulator of cold-induced transcriptome and freezing tolerance in Arabidopsis. Genes Dev. 17, 1043–1054. doi: 10.1101/gad.1077503
Das, A., Mukhopadhyay, M., and Mondal, T. K. (2016). Generation and characterization of expressed sequence tags in young roots of tea (Camellia assamica). Biol. Plant. 60, 48–54. doi: 10.1007/s10535-015-0565-3
Dubouzet, J. G., Sakuma, Y., Ito, Y., Kasuga, M., Dubouzet, E. G., Miura, S., et al. (2003). OsDREB genes in rice, Oryza sativa L., encode transcription activators that function in drought-, high-salt- and cold-responsive gene expression. Plant. J. 33, 751–763. doi: 10.1046/j.1365-313X.2003.01661.x
Ebrahimi, M., Abdullah, S. N. A., Aziz, M. A., and Namasivayam, P. (2015). A novel CBF that regulates abiotic stress response and the ripening process in oil palm (Elaeis guineensis) fruits. Tree Genet. Genomes 11:56. doi: 10.1007/s11295-015-0874-x
Eremina, M., Rozhon, W., and Poppenberger, B. (2015). Hormonal control of cold stress responses in plants. Cell. Mol. Life Sci. 73, 797–810. doi: 10.1007/s00018-015-2089-6
Fang, Z., Zhang, X., Gao, J., Wang, P., Xu, X., Liu, Z., et al. (2015). A buckwheat (Fagopyrum esculentum) DRE-binding transcription factor gene, FeDREB1, enhances freezing and drought tolerance of transgenic Arabidopsis. Plant. Mol. Biol. Rep. 33, 1510–1525. doi: 10.1007/s11105-015-0851-4
Hong, J., and Kim, W. T. (2005). Isolation and functional characterization of the Ca-DREBLP1 gene encoding a dehydration-responsive element binding-factor-like protein 1 in hot pepper (Capsicum annuum L. cv. Pukang). Planta 220, 875–888. doi: 10.1007/s00425-004-1412-5
Hou, Y., Wu, A., He, Y., Li, F., and Wei, C. (2018). Genome-wide characterization of the basic leucine zipper transcription factors inCamellia sinensis. Tree Genet. Genomes. 14:27. doi: 10.1007/s11295-018-1242-4
Hu, Y., Jiang, L., Wang, F., and Yu, D. (2013). Jasmonate regulates the inducer of CBF expression–C-repeat binding factor/DRE binding factor1 cascade and freezing tolerance in Arabidopsis. Plant. Cell 25, 2907–2924. doi: 10.1105/tpc.113.112631
Huang, G. T., Ma, S. L., Bai, L. P., Zhang, L., Ma, H., Jia, P., et al. (2012). Signal transduction during cold, salt, and drought stresses in plants. Mol. Biol. Rep. 39, 969–987. doi: 10.1007/s11033-011-0823-1
Jaglo, K. R., Kleff, S., Amundsen, K. L., Zhang, X., Haake, V., Zhang, J. Z., et al. (2001). Components of the Arabidopsis C-repeat/dehydration-responsive element binding factor cold-response pathway are conserved in Brassica napus and other plant species. Plant. Physiol. 127, 910–917. doi: 10.1104/pp.010548
Kidokoro, S., Watanabe, K., Ohori, T., Moriwaki, T., Maruyama, K., Mizoi, J., et al. (2015). Soybean DREB1/CBF-type transcription factors function in heat and drought as well as cold stress-responsive gene expression. Plant. J. 81, 505–518. doi: 10.1111/tpj.12746
Kulik, A., Wawer, I., Krzywinska, E., Bucholc, M., and Dobrowolska, G. (2011). SnRK2 protein kinases—key regulators of plant response to abiotic stresses. OMICS 15, 859–872. doi: 10.1089/omi.2011.0091
Li, H., Huang, W., Wang, G. L., Wu, Z. J., and Zhuang, J. (2016). Expression profile analysis of ascorbic acid-related genes in response to temperature stress in the tea plant, Camellia sinensis (L.) O. Kuntze. Genet. Mol. Res. 15:gmr.15048756. doi: 10.4238/gmr.15048756
Li, X. W., Feng, Z. G., Yang, H. M., Zhu, X. P., Liu, J., and Yuan, H. Y. (2010). A novel cold-regulated gene from Camellia sinensis, CsCOR1, enhances salt- and dehydration-tolerance in tobacco. Biochem. Biophys. Res. Commun. 394, 354–359. doi: 10.1016/j.bbrc.2010.03.011
Liu, Q., Kasuga, M., Sakuma, Y., Abe, H., Miura, S., Yamaguchi-Shinozaki, K., et al. (1998). Two transcription factors, DREB1 and DREB2, with an EREBP/AP2 DNA binding domain separate two cellular signal transduction pathways in drought- and low-temperature-responsive gene expression, respectively, in Arabidopsis. Plant. Cell 10, 1391–1406. doi: 10.1105/tpc.10.8.1391
Liu, Z., Jia, Y., Ding, Y., Shi, Y., Li, Z., Guo, Y., et al. (2017). Plasma membrane CRPK1-mediated phosphorylation of 14-3-3 proteins induces their nuclear import to fine-tune CBF signaling during cold response. Mol. Cell 66, 117–128.e5. doi: 10.1016/j.molcel.2017.02.016
Liu, Z., Wu, Z., Li, X., Huang, Y., Li, H., Wang, Y., et al. (2016). Identification, classification, and expression profiles of heat shock transcription factors in tea plant (Camellia sinensis) under temperature stress. Gene 576, 52–59. doi: 10.1016/j.gene.2015.09.076
Livak, K. J., and Schmittgen, T. D. (2001). Analysis of relative gene expression data using real-time quantitative PCR and the 2(-Delta Delta C(T)) Method. Methods 25, 402–408. doi: 10.1006/meth.2001.1262
Lucas, S., Durmaz, E., Akpinar, B. A., and Budak, H. (2011). The drought response displayed by a DRE-binding protein from Triticum dicoccoides. Plant. Physiol. Biochem. 49, 346–351. doi: 10.1016/j.plaphy.2011.01.016
Mukhopadhyay, M., Mondal, T. K., and Chand, P. K. (2016). Biotechnological advances in tea (Camellia sinensis [L.] O. Kuntze): a review. Plant. Cell Rep. 35, 255–287. doi: 10.1007/s00299-015-1884-8
Nada, R. M., and Abogadallah, G. M. (2015). Developmental acquisition of salt tolerance in the halophyte Atriplex halimus L. is related to differential regulation of salt inducible genes. Plant. Growth Reg. 75, 165–178. doi: 10.1007/s10725-014-9941-9
Novillo, F., Alonso, J. M., Ecker, J. R., and Salinas, J. (2004). CBF2/DREB1C is a negative regulator of CBF1/DREB1B and CBF3/DREB1A expression and plays a central role in, stress tolerance in Arabidopsis. Proc. Natl. Acad. Sci. U. S. A. 101, 3985–3990. doi: 10.1073/pnas.0303029101
Novillo, F., Medina, J., and Salinas, J. (2007). Arabidopsis CBF1 and CBF3 have a different function than CBF2 in cold acclimation and define different gene classes in the CBF regulon. Proc. Natl. Acad. Sci. U. S. A 104, 21002–21007. doi: 10.1073/pnas.0705639105
Pan, C., Tian, K., Ban, Q., Wang, L., Sun, Q., He, Y., et al. (2017). Genome-wide analysis of the biosynthesis and deactivation of gibberellin-dioxygenases gene family in Camellia sinensis (L.) O. Kuntze. Genes 8:235. doi: 10.3390/genes8090235
Qin, F., Sakuma, Y., Li, J., Liu, Q., Li, Y. Q., Shinozaki, K., et al. (2004). Cloning and functional analysis of a novel DREB1/CBF transcription factor involved in cold-responsive gene expression in zea mays L. Plant Cell Physiol. 45, 1042–1052. doi: 10.1093/pcp/pch118
Ramanjulu, S., and Bartels, D. (2002). Drought- and desiccation-induced modulation of gene expression in plants. Plant Cell Environ. 25, 141–151. doi: 10.1046/j.0016-8025.2001.00764.x
Riechmann, J. L., and Meyerowitz, E. M. (1998). The AP2/EREBP family of plant transcription factors. Biol. Chem. 379, 633–646.
Sakuma, Y., Liu, Q., Dubouzet, J. G., Abe, H., Shinozaki, K., and Yamaguchi-Shinozaki, K. (2002). DNA-binding specificity of the ERF/AP2 domain of Arabidopsis DREBs, transcription factors involved in dehydration- and cold-inducible gene expression. Biochem. Biophys. Res. Commun. 290, 998–1009. doi: 10.1006/bbrc.2001.6299
Sakuma, Y., Maruyama, K., Osakabe, Y., Qin, F., Seki, M., Shinozaki, K., et al. (2006). Functional analysis of an Arabidopsis transcription factor, DREB2A, involved in drought-responsive gene expression. Plant. Cell 18, 1292–1309. doi: 10.1105/tpc.105.035881
Shan, D. P., Huang, J. G., Yang, Y. T., Guo, Y. H., Wu, C. A., Yang, G. D., et al. (2007). Cotton GhDREB1 increases plant tolerance to low temperature and is negatively regulated by gibberellic acid. New Phytol. 176, 70–81. doi: 10.1111/j.1469-8137.2007.02160.x
Shen, Y. G., Zhang, W. K., He, S. J., Zhang, J. S., Liu, Q., and Chen, S. Y. (2003). An EREBP/AP2-type protein in Triticum aestivum was a DRE-binding transcription factor induced by cold, dehydration and ABA stress. Theor. Appl. Genet. 106, 923–930. doi: 10.1007/s00122-002-1131-x
Stockinger, E. J., Gilmour, S. J., and Thomashow, M. F. (1997). Arabidopsis thaliana CBF1 encodes an AP2 domain-containing transcriptional activator that binds to the C-repeat/DRE, a cis-acting DNA regulatory element that stimulates transcription in response to low temperature and water deficit. Proc. Natl. Acad. Sci. U. S. A. 94, 1035–1040. doi: 10.1073/pnas.94.3.1035
Sun, J., Peng, X., Fan, W., Tang, M., Liu, J., and Shen, S. (2014). Functional analysis of BpDREB2 gene involved in salt and drought response from a woody plant Broussonetia papyrifera. Gene 535, 140–149. doi: 10.1016/j.gene.2013.11.047
Wang, M., Zhuang, J., Zou, Z., Li, Q., Xin, H., and Li, X. (2017). Overexpression of a Camellia sinensis DREB transcription factor gene (CsDREB) increases salt and drought tolerance in transgenic Arabidopsis thaliana. J. Plant Biol. 60, 452–461. doi: 10.1007/s12374-016-0547-9
Wang, P., Chen, X., Guo, Y., et al. (2019). Identification of CBF transcription factors in tea plants and a survey of potential CBF target genes under low temperature. Int. J. Mol. Sci. 20:5137. doi: 10.3390/ijms20205137
Wang, Q., Guan, Y., Wu, Y., Chen, H., Chen, F., and Chu, C. (2008). Overexpression of a rice OsDREB1F gene increases salt, drought, and low temperature tolerance in both Arabidopsis and rice. Plant Mol. Biol. 67, 589–602. doi: 10.1007/s11103-008-9340-6
Wang, W., Wang, Y., Du, Y., Zhao, Z., Zhu, X., Jiang, X., et al. (2014). Overexpression of Camellia sinensis H1 histone gene confers abiotic stress tolerance in transgenic tobacco. Plant Cell Rep. 33, 1829–1841. doi: 10.1007/s00299-014-1660-1
Wang, Y., Jiang, C. J., Li, Y. Y., Wei, C. L., and Deng, W. W. (2012). CsICE1 and CsCBF1: two transcription factors involved in cold responses in Camellia sinensis. Plant. Cell Rep. 31, 27–34. doi: 10.1007/s00299-011-1136-5
Wei, C., Yang, H., Wang, S., Zhao, J., Liu, C., Gao, L., et al. (2018). Draft genome sequence of Camellia sinensis var sinensis provides insights into the evolution of the tea genome and tea quality. Proc. Natl. Acad. Sci. U. S. A. 115, E4151–E4158. doi: 10.1073/pnas.1719622115
Xia, E., Li, F., Tong, W., Yang, H., Wang, S., Zhao, J., et al. (2019). The tea plant reference genome and improved gene annotation using long-read and paired-end sequencing data. Sci. Data 6, 122–122. doi: 10.1038/s41597-019-0127-1
Xia, E. -H., Li, F. D., Tong, W., Li, P. H., Wu, Q., Zhao, H. J., et al. (2019). Tea Plant Information archive: a comprehensive genomics and bioinformatics platform for tea plant. Plant Biotechnol. J. 17, 1938–1953. doi: 10.1111/pbi.13111
Xiao, H., Siddiqua, M., Braybrook, S., and Nassuth, A. (2006). Three grape CBF/DREB1 genes respond to low temperature, drought and abscisic acid. Plant. Cell Environ. 29, 1410–1421. doi: 10.1111/j.1365-3040.2006.01524.x
Xiong, Y., and Fei, S. Z. (2006). Functional and phylogenetic analysis of a DREB/CBF-like gene in perennial ryegrass (Lolium perenne L.). Planta 224, 878–888. doi: 10.1007/s00425-006-0273-5
Yamaguchi-Shinozaki, K., and Shinozaki, K. (1994). A novel cis-acting element in an Arabidopsis gene is involved in responsiveness to drought, low-temperature, or high-salt stress. Plant Cell Online 6, 251–264. doi: 10.1105/tpc.6.2.251
Yang, W., Liu, X. D., Chi, X. J., Wu, C. A., Li, Y. Z., Song, L. L., et al. (2011). Dwarf apple MbDREB1 enhances plant tolerance to low temperature, drought, and salt stress via both ABA-dependent and ABA-independent pathways. Planta 233, 219–229. doi: 10.1007/s00425-010-1279-6
Yin, Y., Ma, Q., Zhu, Z., Cui, Q. Y., Shen, C. S., Chen, X., et al. (2016). Functional analysis of CsCBF3 transcription factor in tea plant (Camellia sinensis) under cold stress. Plant Growth Reg. 80, 335–343. doi: 10.1007/s10725-016-0172-0
Yue, C., Cao, H., Wang, L., Zhou, Y., Hao, X., Zeng, J., et al. (2014). Molecular cloning and expression analysis of tea plant aquaporin (AQP) gene family. Plant. Physiol. Biochem. 83, 65–76. doi: 10.1016/j.plaphy.2014.07.011
Zhang, X., Fowler, S. G., Cheng, H., Lou, Y., Rhee, S. Y., Stockinger, E. J., et al. (2004). Freezing-sensitive tomato has a functional CBF cold response pathway, but a CBF regulon that differs from that of freezing-tolerant Arabidopsis. Plant. J. 39, 905–919. doi: 10.1111/j.1365-313X.2004.02176.x
Zhao, T., Liang, D., Wang, P., Liu, J., and Ma, F. (2012). Genome-wide analysis and expression profiling of the DREB transcription factor gene family in Malus under abiotic stress. Mol. Genet. Genomics 287, 423–436. doi: 10.1007/s00438-012-0687-7
Zhao, X., Lei, H., Zhao, K., Yuan, H., and Li, T. (2012). Isolation and characterization of a dehydration responsive element binding factor MsDREBA5 in Malus sieversii Roem. Sci. Hortic. 142, 212–220. doi: 10.1016/j.scienta.2012.05.020
Zhou, L., Li, J., He, Y., Liu, Y., and Chen, H. (2018). Functional characterization of SmCBF genes involved in abiotic stress response in eggplant (Solanum melongena). Sci. Horticulturae 233, 14–21. doi: 10.1016/j.scienta.2018.01.043
Keywords: Camellia sinensis, CBF gene family, cold stress, functional genomics, transgenic Arabidopsis thaliana
Citation: Hu Z, Ban Q, Hao J, Zhu X, Cheng Y, Mao J, Lin M, Xia E and Li Y (2020) Genome-Wide Characterization of the C-repeat Binding Factor (CBF) Gene Family Involved in the Response to Abiotic Stresses in Tea Plant (Camellia sinensis). Front. Plant Sci. 11:921. doi: 10.3389/fpls.2020.00921
Received: 29 January 2020; Accepted: 05 June 2020;
Published: 23 July 2020.
Edited by:
Eric Ruelland, UMR7618 Institut d'écologie et des sciences de l'environnement de Paris (IEES), FranceReviewed by:
Parviz Heidari, Shahrood University of Technology, IranJavier Barrero-Gil, Instituto Nacional de Investigación y Tecnología Agraria y Alimentaria, Spain
Copyright © 2020 Hu, Ban, Hao, Zhu, Cheng, Mao, Lin, Xia and Li. This is an open-access article distributed under the terms of the Creative Commons Attribution License (CC BY). The use, distribution or reproduction in other forums is permitted, provided the original author(s) and the copyright owner(s) are credited and that the original publication in this journal is cited, in accordance with accepted academic practice. No use, distribution or reproduction is permitted which does not comply with these terms.
*Correspondence: Enhua Xia, eGlhZW5odWFAZ21haWwuY29t; Yeyun Li, bHl5QGFoYXUuY29t
†These authors have contributed equally to this work