- 1INRES Molecular Phytomedicine, University of Bonn, Bonn, Germany
- 2Institute of Bioorganic Chemistry, Heinrich Heine University Düsseldorf, Forschungszentrum Jülich, Stetternicher Forst, Jülich, Germany
- 3IBG-1: Bioorganic Chemistry, Forschungszentrum Jülich GmbH, Jülich, Germany
Bacterial metabolites represent an invaluable source of bioactive molecules which can be used as such or serve as chemical frameworks for developing new antimicrobial compounds for various applications including crop protection against pathogens. Prodiginines are tripyrrolic, red-colored compounds produced by many bacterial species. Recently, due to the use of chemical-, bio-, or mutasynthesis, a novel group of prodiginines was generated. In our study, we perform different assays to evaluate the effects of prodigiosin and five derivatives on nematodes and plant pathogenic fungi as well as on plant development. Our results showed that prodigiosin and the derivatives were active against the bacterial feeding nematode Caenorhabditis elegans in a concentration- and derivative-dependent manner while a direct effect on infective juveniles of the plant parasitic nematode Heterodera schachtii was observed for prodigiosin only. All compounds were found to be active against the plant pathogenic fungi Phoma lingam and Sclerotinia sclerotiorum. Efficacy varied depending on compound concentration and chemical structure. We observed that prodigiosin (1), the 12 ring- 9, and hexenol 10 derivatives are neutral or even positive for growth of Arabidopsis thaliana depending on the applied compound concentration, whereas other derivatives appear to be suppressive. Our infection assays revealed that the total number of developed H. schachtii individuals on A. thaliana was decreased to 50% in the presence of compounds 1 or 9. Furthermore, female nematodes and their associated syncytia were smaller in size. Prodiginines seem to indirectly inhibit H. schachtii parasitism of the plant. Further research is needed to elucidate their mode of action. Our results indicate that prodiginines are promising metabolites that have the potential to be developed into novel antinematodal and antifungal agents.
Introduction
Plant pests (including insects, mites, plant parasitic nematodes), pathogens (e.g., viruses, bacteria, fungi), and weeds are major problems in crop production causing a share of the total global yield losses of up to 18%, 16%, and 34%, respectively (Oerke, 2006). In order to minimize crop losses, chemical, biological, and cultural means side by side with the use of resistant plants are management strategies in use (Bridge, 1996; Heydari and Pessarakli, 2010; Habash and Al-Banna, 2011; Timper, 2014). Each of these control means has its own challenges, but so far, pesticides are the fastest and the most effective combat means utilized. However, due to environmental hazards and toxicity to humans many pesticides are being banned. Therefore, effective and sustainable alternatives are needed.
Microbial metabolites represent a valuable source of compounds that can be used for development of new drugs and plant protection agents. The bacterial prodiginines are nice examples with a wide range of bioactivities and intensively used in drug discovery research (Darshan and Manonmani, 2015). Prodiginines are tripyrrolic dark red compounds produced as secondary metabolites by many bacterial species within Serratia (Williams et al., 1956), Streptomyces (Gerber, 1975; Kawasaki et al., 2008), Hahella (Kim et al., 2007), and Vibrio (Starič et al., 2010), for instance. The family of prodiginines comprises diverse members (as shown in Figure 1), some having linear alkyl side chains such as prodigiosin (1) and undecylprodigiosin (2) while others have cyclic moieties such as cycloprodigiosin (3), metacycloprodigiosin (4), or streptorubin B (5) (Williamson et al., 2006; Stankovic et al., 2014; Hu et al., 2016). In pharmaceutical studies, prodiginines were often used for their antimalarial and antitumor activities (Pérez-Tomás et al., 2003; Papireddy et al., 2011). They also exhibit inhibitory activities on various microbes. For example, Escherichia coli exhibited a leaky outer membrane and cells had severely decreased respiration activity upon exposure to prodigiosin (1) (Danevčič et al., 2016b). Likewise, treating Pseudomonas aeruginosa with prodigiosin (1), isolated from Serratia marcescens, altered the cell surface hydrophobicity, and biofilm integrity significantly. The treatment also caused nucleic acid degradation of P. aeruginosa (Kimyon et al., 2016). Recent research has shown potential applications of prodiginines in food industry as coloring agents, antioxidants, and antimicrobial additives to increase product shelf life (Arivizhivendhan et al., 2018).
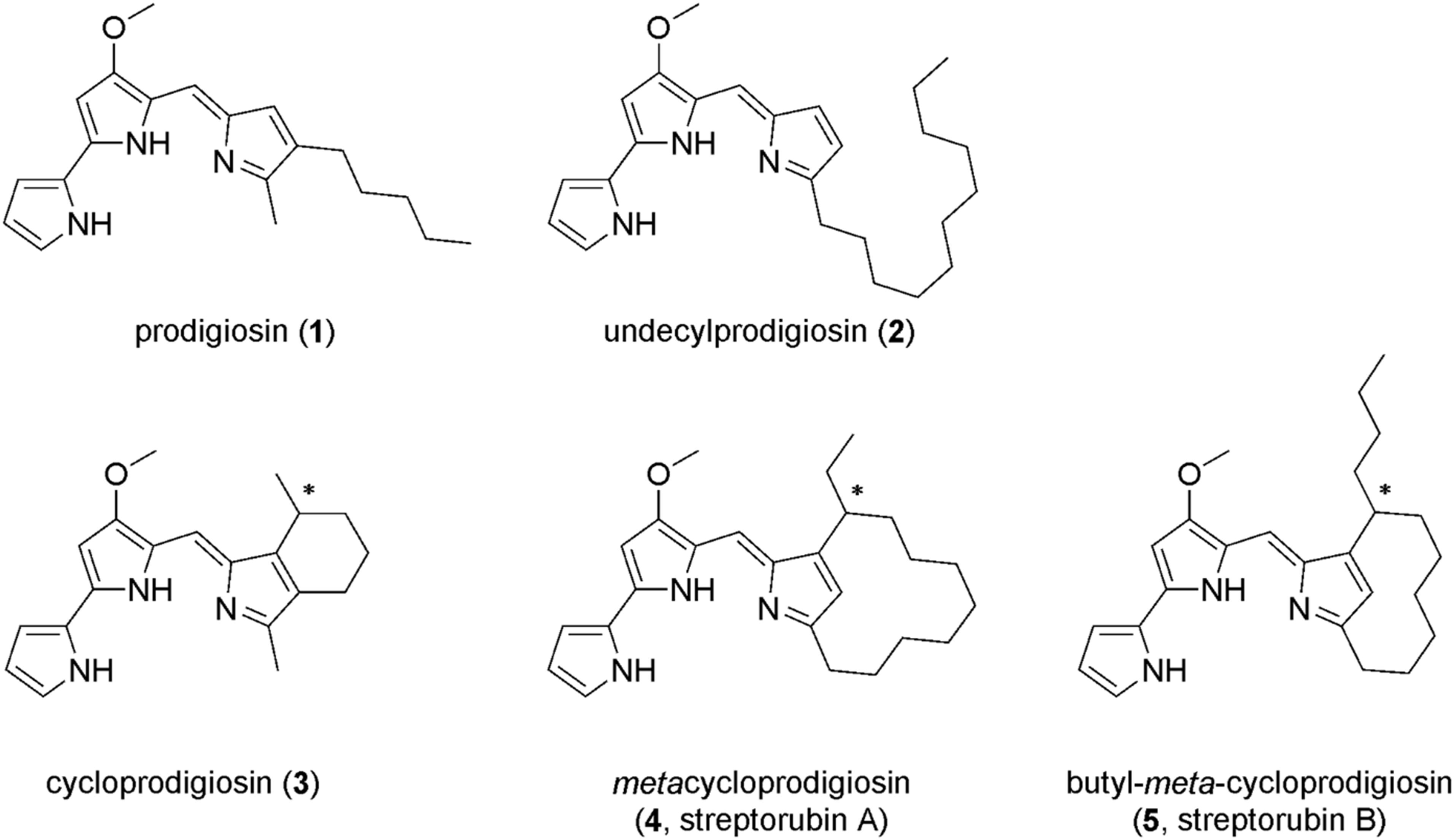
Figure 1. Examples of natural prodiginines: prodigiosin (1), undecylprodigiosin (2), cycloprodigiosin (3), metacycloprodigiosin (4), and butyl-meta-cycloprodigiosin (5).
In agricultural research, activities of prodigiosin (1) against several plant pathogens are described. It was shown that prodigiosin (1) totally inhibited spore germination of Botrytis cinerea, the causal agent of gray mold (Someya et al., 2001). The purified red pigment of Serratia marcescens was found to be effective against juvenile stages of the plant parasitic nematodes Radopholus similis and Meloidogyne javanica at low concentrations of 83 and 79 μg/mL, respectively, and inhibited nematode egg-hatching ability. After deep analysis of the bioactive red supernatant, prodigiosin (1) was detected in the supernatant (Rahul et al., 2014). This evidence supports the potential of the red pigment prodigiosin (1) to be applied in several fields.
The bio- and chemical-based derivatization of prodiginines introduces new compounds. These compounds could contribute to an improved microbe resistance management or having stronger bioactivities. Recently, nature inspired prodiginines were produced by combining organic syntheses with a mutasynthesis approach using the HV1-certified bacterium Pseudomonas putida KT2440 as host strain (Weist and Süssmuth, 2005; Kennedy, 2008; Kirschning and Hahn, 2012; Klein et al., 2017, 2018; Classen and Pietruszka, 2018). The previously constructed mutasynthesis strain harbors the prodigiosin (1) gene cluster from S. marcescens, but the bifurcated biosynthetic pathway is blocked upon gene deletion, thus, only one out of two prodiginine building blocks, namely 4-methoxy-2,2’-bipyrrole-5-carbaldehyde (MBC), is produced biosynthetically (Domröse et al., 2015; Klein et al., 2017). The other precursor and especially derivatives thereof are chemically synthesized and fed during cultivation, enabling the production of new and non-natural prodiginines (Klein et al., 2017, 2018). The obtained compounds possessed antibacterial activities against several species with minimum inhibitory concentrations (MIC) ranging from 0.1 to 12 μMM. In the same studies, they demonstrated that the produced prodiginines exhibit modulating activities of autophagy in human breast cancer cells. The novel compounds with such bioactivities could be good candidates to be used against plant pathogens. Their attraction is increased by the fact that prodiginines act on microorganisms in a mode different compared to conventional pesticides.
In our study we present the bioactivities of prodigiosin (1), several cyclic derivatives 6–9 and a hydroxylated prodiginine 10 against different organisms, particularly focusing on the plant parasitic nematode Heterodera schachtii. First, we examined the bioactivities of prodiginines on two nematode species belonging to bacterial feeding and plant parasitic nematodes. We further investigated the effect of the compounds on plant growth using the model plant A. thaliana and subsequently evaluated the efficacy of selected compounds in combating nematode parasitism in the pathosystem A. thaliana–H. schachtii. Finally, we tested the bioactivities of prodiginines on other pathogens by using two species of plant pathogenic fungi.
Materials and Methods
Prodiginine Production
Production of Prodiginines 1, 6–9 via Biosynthesis or Mutasynthesis
Prodigiosin (1) was produced by using the strain P. putida pig-r2 as previously described (Domröse et al., 2015). The prodiginine derivatives 6–9 (Figure 2) were produced via mutasynthesis as previously described (Klein et al., 2017, 2018). For details, see Supplementary Material.
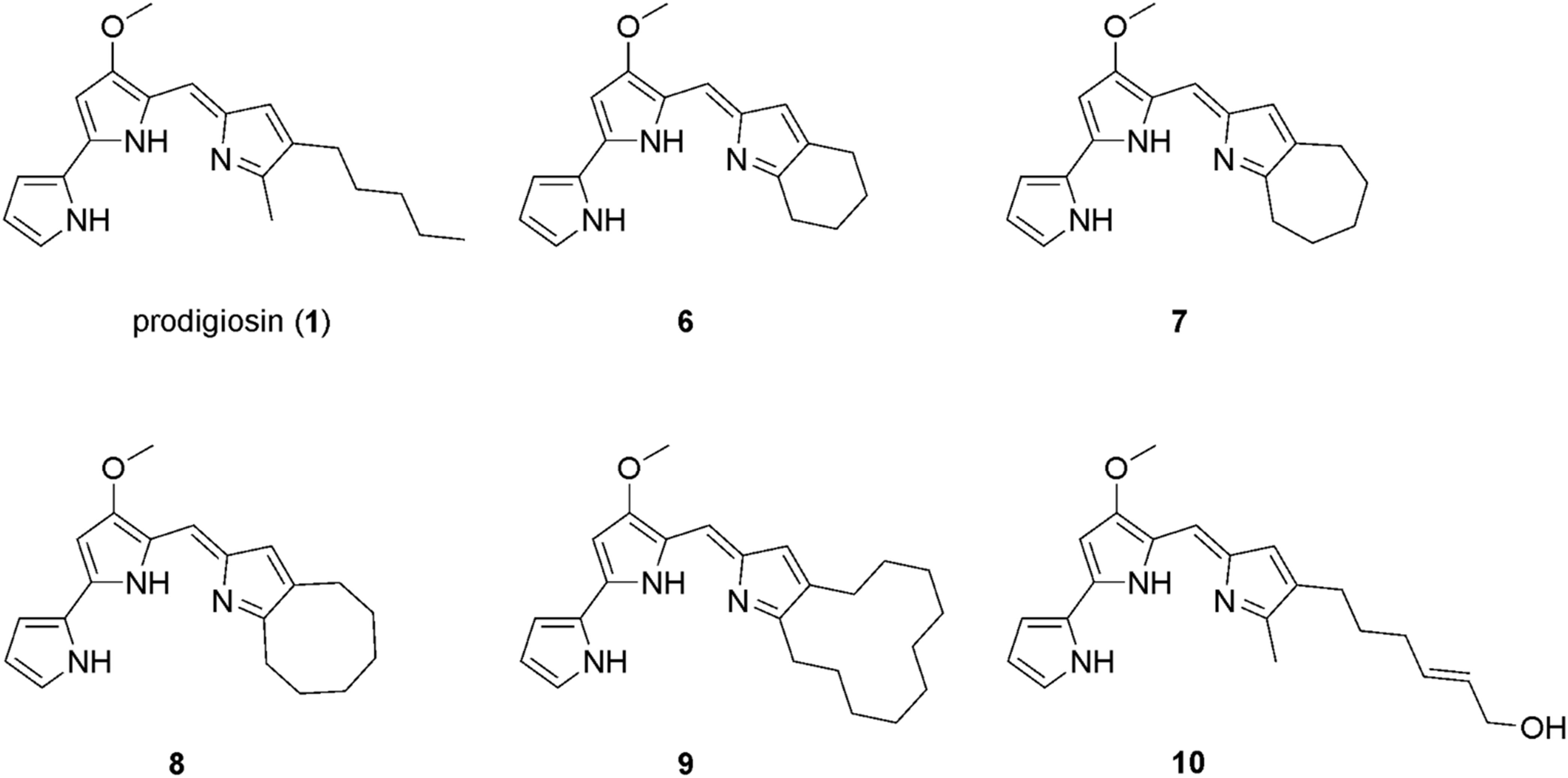
Figure 2. Chemical structure of prodigiosin (1) and the derivatives 6–10 that were investigated in this study.
Chemical Synthesis of Prodiginines
Synthesis of 10 is described in detail in Supplementary Material.
Nematode Culture
The wild type of the bacterial feeding nematode C. elegans, used in this work, was maintained on nematode growth medium (NGM) and was fed with E. coli strain OP50. In the toxicity assay the synchronized first stage nematodes were used (Al Banna et al., 2018). The plant parasitic nematode H. schachtii Schmidt used in the experiments was reared on white mustard (Sinapis alba L. cv. Albatros) plants which were grown aseptically on 0.2% Knop agar medium. Mature cysts were collected in funnels and hatched in 3 mM ZnCl2 (Sijmons et al., 1991). The hatched pre-parasitic J2s were collected and used in the experiments.
Effect of Prodigiosin Derivatives on Nematodes
To investigate the effect of prodigiosin derivatives on nematode motility, two nematode species were used. Both nematodes, C. elegans and H. schachtii, were challenged with the selected compounds 1, 6–10. The activity experiment was conducted in 96-well plates (Greiner Bio-One) under aseptic conditions. Each well contained 90–100 nematodes incubated in 60 μL of the compound-containing test solution. The used dilutions were 100, 50, 25, 12.5, and 6.25 μM dissolved in 0.5% (v/v) dimethyl sulfoxide (DMSO) diluted in ddH2O. Nematodes soaked in 0.5% (v/v) DMSO served as control. Plates were incubated for two days at 24°C in the dark. Numbers of active (moving) and inactive (not moving) nematodes were evaluated using a dissecting microscope. Nematode movement was provoked by adding 2 μL of 1 M sodium hydroxide (NaOH). Those nematodes that remained immobile after challenging with NaOH were regarded as dead. The percentages of nematode motility were calculated. The experiment was set up in three biological replications (each contains three wells per concentration). To decide whether H. schachtii J2s were really killed or just paralyzed by prodigiosin (1), J2s were washed free of the compound after a 48 h incubation period and subsequently left in water for another 48 h. This was necessary as some H. schachtii J2s incubated in 1 showed a slight movement of the tail region after adding NaOH what made a reliable decision difficult. Data were collected and statistically analyzed by one-way analysis of variance (ANOVA) followed by Dunnett’s post-hoc test to determine significant differences to the control (SigmaPlot 12.5).
Plant Growth Test
In order to investigate the effect of prodiginines on plants, A. thaliana ecotype Col-0 was grown aseptically on agar medium supplemented with modified 0.2% Knop solutions at 16 h light and 8 h dark at 25°C as described previously (Sijmons et al., 1991). Five-days-old plants were transferred to 6-well plates containing 2 mL liquid Murashige and Skoog medium (MS) supplemented with the prodiginines 1, 6–10 at different concentrations (50, 25, 12.5 μM), MS medium supplemented with 1 μM bacterial flagellin (flg22) or with 0.5% (v/v) DMSO were used as controls. Fresh weight of the roots and the shoots were measured after 15 days of incubation. The experiments were performed in five technical replicates and independently repeated three times. Data were statistically analyzed using one-way ANOVA followed by Dunnett’s post-hoc test.
Infection Assay
In order to investigate the impact of prodigiosin derivatives on nematode parasitism, prodigiosin (1) and prodiginine 9 were selected to be tested. The compounds and the used concentration were chosen based on the previous results of the plant development assay. Compounds were added to the 0.2% Knop agar medium to yield a final concentration of 14 μM. A. thaliana Col-0 plants were grown aseptically on the medium with 16 h light and 8 h dark at 25°C as described previously (Sijmons et al., 1991). The infection assay with A. thaliana plants was performed as described previously (Habash et al., 2017). Briefly, roots of 10 days old seedlings were inoculated with 60–70 H. schachtii J2s per plant. Twelve days after inoculation (DAI), numbers of adult males and females were counted per plant. Furthermore, sizes of females and associated syncytia were measured after 13 DAI using Leica M165C Binocular (Leica Microsystems, Wetzlar, Germany) and Leica Application Suite software. A. thaliana plants grown on 0.2% Knop agar medium supplemented with 0.5% (v/v) DMSO were used as control. Experiments were repeated three times and statistically analyzed using one-way ANOVA followed by Dunn’s post-hoc test. Each experiment consisted of 12 plants per treatment.
Effect of Prodigiosin Derivatives on Plant Pathogenic Fungi
The plant pathogenic fungi Phoma lingam and Sclerotinia sclerotiorum were used in this study to evaluate the bioactivity of the compounds on hyphal growth. Both isolates were obtained from the Leibniz-Institut DSMZ (Deutsche Sammlung von Mikroorganismen und Zellkulturen GmbH, Germany) and subcultured on potato dextrose agar (PDA) at 24°C. To test prodiginine bioactivities, PDA was supplemented with the chemicals to several final dilutions. The used concentrations were 50, 25 and 12.5 μM. Fungal discs were cut from the culture media and placed in the middle of PDA plates containing the chemicals. PDA plates with 0.5% (v/v) DMSO alone were used as control. All plates were incubated for 7 days at 24°C. Subsequently, the diameter of the fungal colony was measured, and inhibition percentage was calculated. Experiments were repeated four times, each experiment consisted of 3 technical replicates. Differences between the treatments were statistically analyzed by using one-way ANOVA followed by Dunnett’s post-hoc.
Results
Activity of Prodiginines Against Nematodes Is Dependent on Compound Structure and Concentration as Well as Nematode Species
The bioactivity of prodigiosin (1) and the derivatives 6–10 was determined using two representative soil nematodes with different feeding behavior, the bacterial feeding model nematode C. elegans and the plant parasitic nematode H. schachtii. The two tested nematode species responded differently to exposure to prodiginines. Results revealed that the impact of the tested compounds on C. elegans depends on prodiginine structure and concentration (Figure 3). Prodigiosin (1) showed the highest nematicidal activity against C. elegans juveniles with 0.127 μM being the effective concentration that killed half of the treated nematodes (EC50). This was followed by 7 (35.9 μM), 8 (79.1 μM), and then the other three derivatives. For compounds 10, 6, and 9 a reliable EC50 could not be determined as only 26, 27, and 33% effectivity, respectively, could be observed at the highest test concentration.
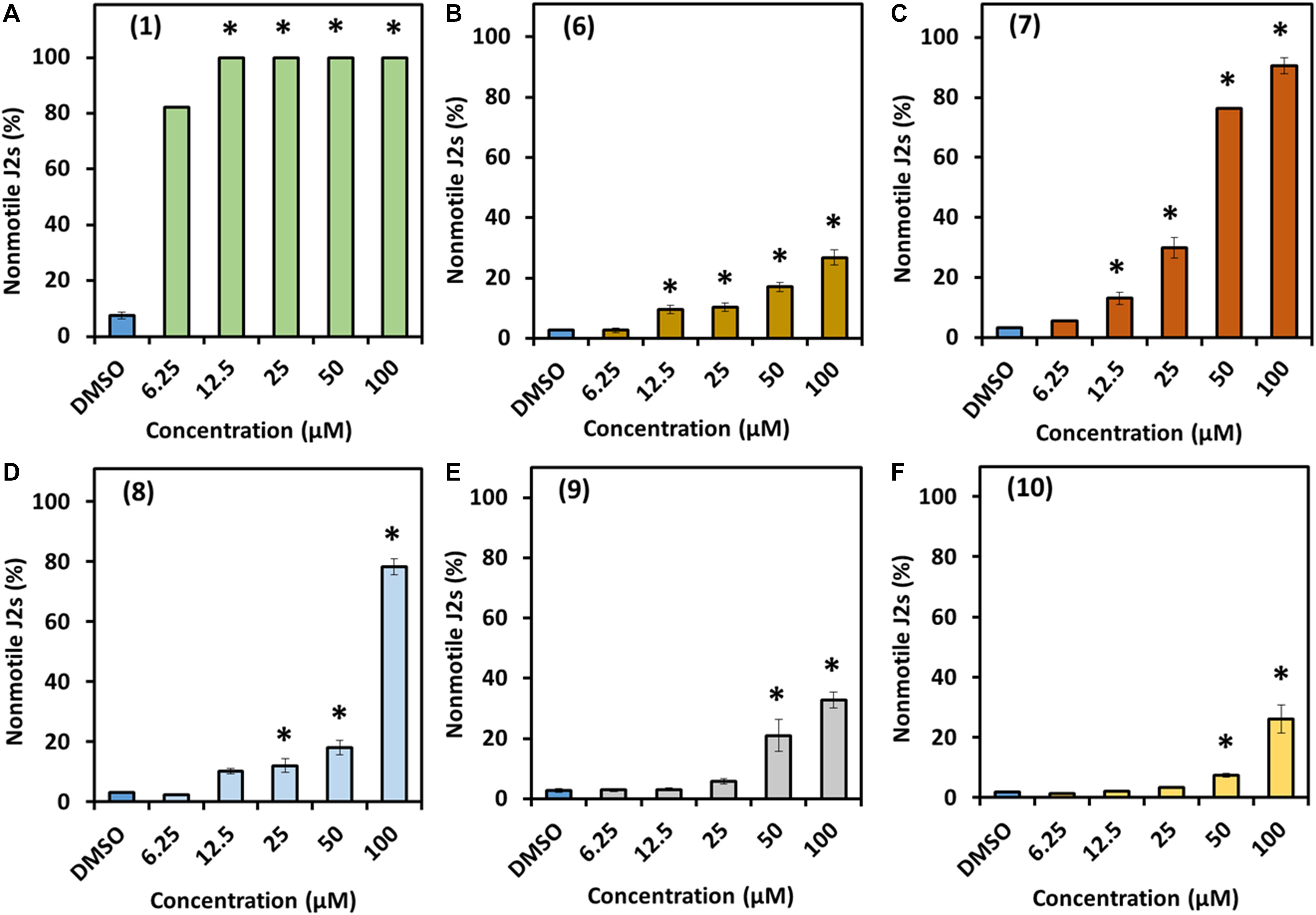
Figure 3. Direct effect of prodigiosin (1) and derivatives 6–10 on C. elegans first-stage juveniles. (A) Compound 1, (B) compound 6, (C) compound 7, (D) compound 8, (E) compound 9, and (F) compound 10. Values are means ± standard error of three biological replicates (n = 9). Asterisks indicate significant differences to the control based on one-way ANOVA followed by Dunnett’s post-hoc test (P < 0.05).
The compounds were much less effective against H. schachtii J2s and rather had a static effect. In fact, prodigiosin (1) was the only derivative able to cause considerable paralysis of the second stage juveniles. The calculated 48 h EC50 value was 13.3 μM and the J2s were able to recover even from the 100 μM treatment as only 27% of the J2s were observed to be dead after a 48 h recovery phase. The impact of a 48 h exposure of the J2s to any other derivative only had a very low neglectable effect with a maximum of about 10% immobile nematodes (100 μM) thus not enabling us to calculate the EC50 (Table 1 and Figure 4).
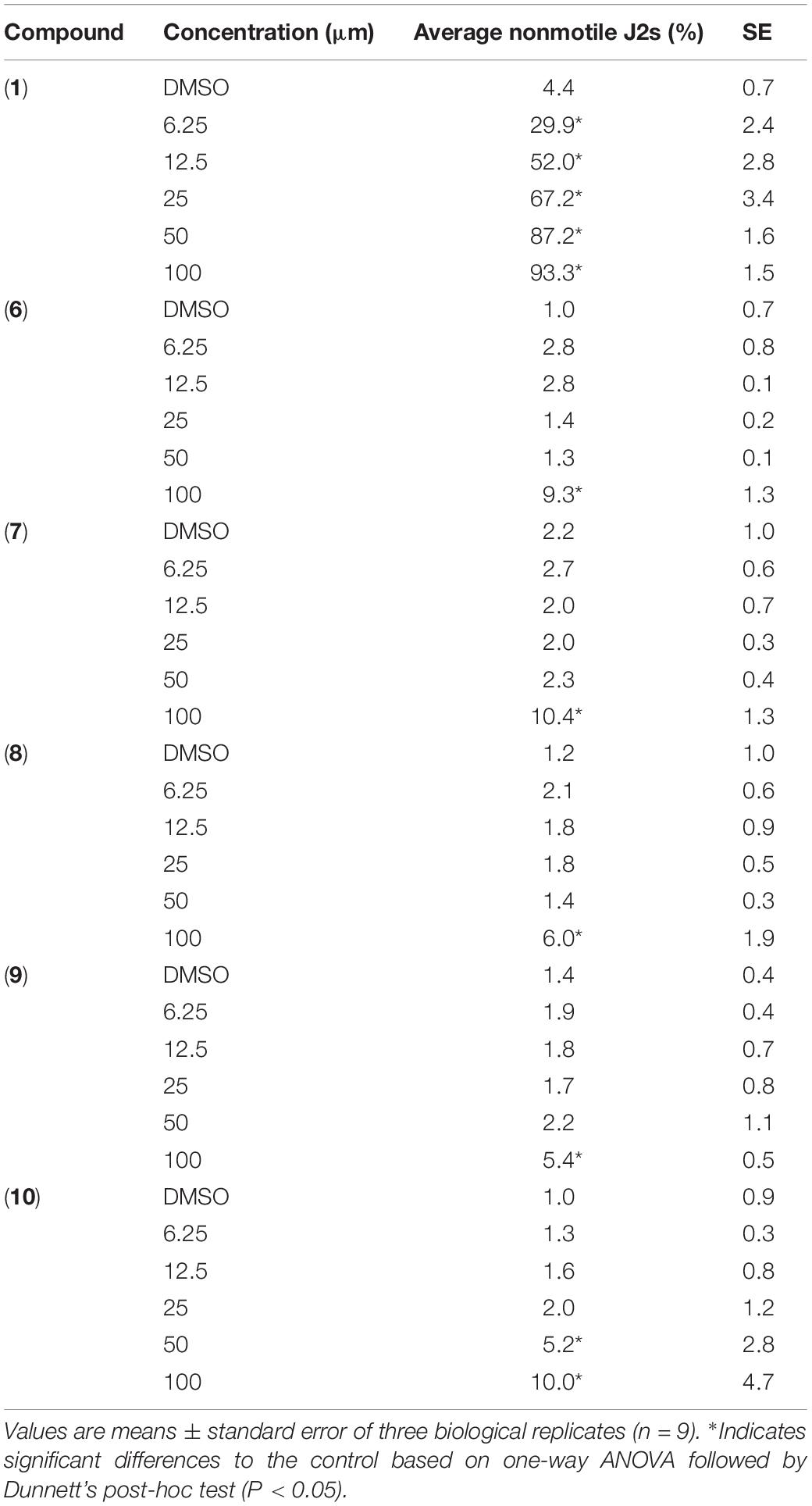
Table 1. Direct effect of prodigiosin (1) and derivatives 6–10 on H. schachtii second-stage juveniles.
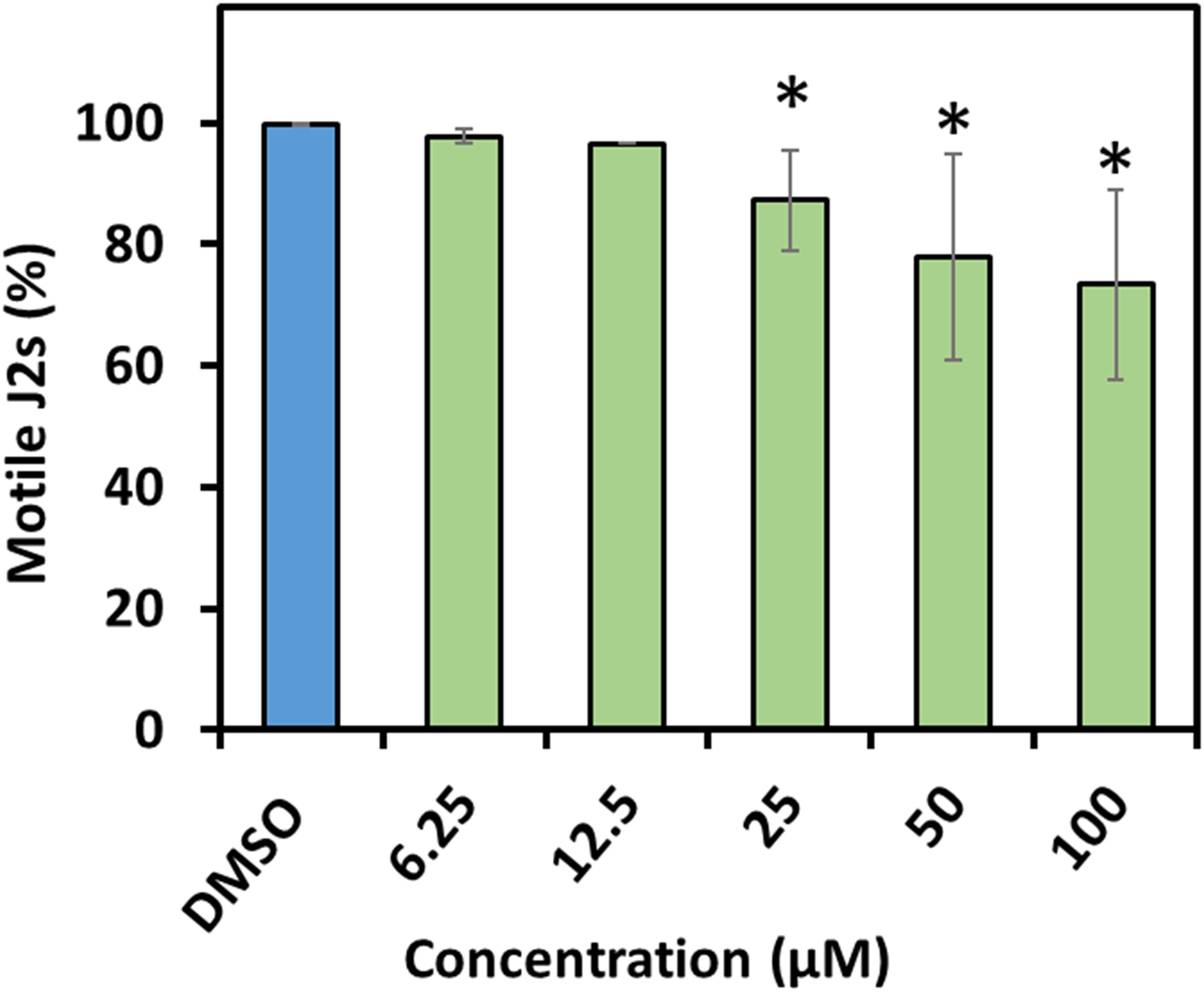
Figure 4. Recovery of second stage juveniles of H. schachtii after exposure to prodigiosin (1). Percentage of recovered nematodes after 48 h incubation in prodigiosin (1) followed by washing and incubation for 48 h in water. Values are means ± standard error of three biological replicates (n = 9). Asterisks indicate significant differences to the control based on one-way ANOVA followed by Dunnett’s post-hoc test (P < 0.05).
Effect of Prodigiosin (1) and Derivatives 6–10 on Plant Growth
The effect of prodiginines on plant growth was tested in order to obtain a suitable concentration for the subsequent infection assay and to get an indication which compound could be best suited for a possible use as plant protection agent in agricultural applications. Therefore, shoot weight and root weight were determined. The applied concentrations between 12.5 and 50 μM of the derivatives 6 and 8 inhibited plant growth as shoot weight was decreased by more than 70% compared to the DMSO control. Compound 7 was also detrimental for the plant but less severe. Cultivating the plants in 25 and 50 μM significantly decreased plant shoot weight, whereas the impact of 12.5 μM was not significant compared to the DMSO control. On the other hand, incubating plants in prodigiosin (1, 12.5 μM) and derivative 10 (12.5 and 25 μM) increased shoot weight by up to 70%. Higher concentrations of both derivatives had no significant effect. All concentrations of 9 had no significant effect on shoot weight compared with the control (Figure 5).
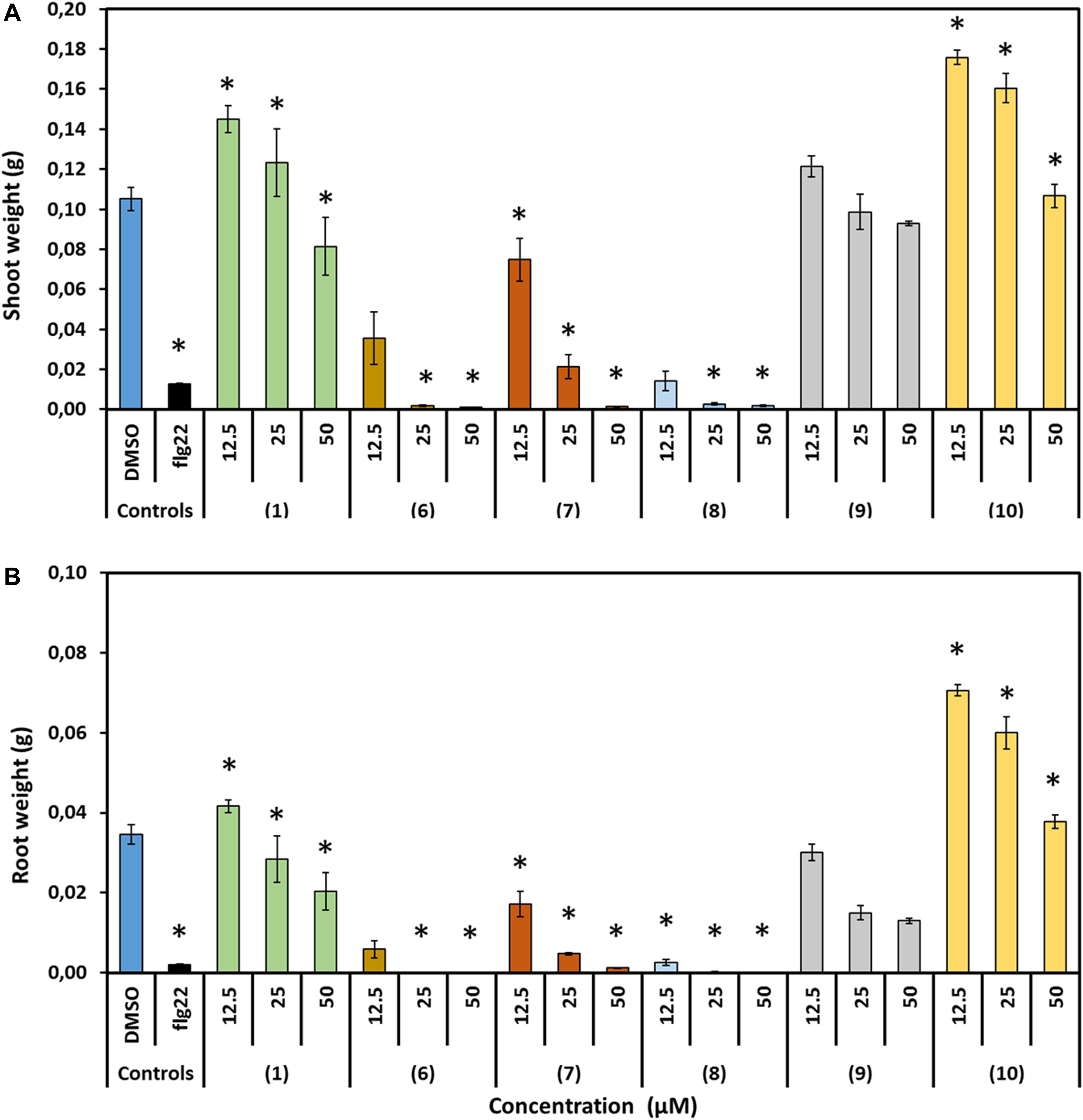
Figure 5. Effect of prodigiosin (1) and derivatives 6–10 on A. thaliana growth. Figures show growth parameters representing development of A. thaliana cultivated on media mixed with prodigiosin (1) or different derivatives (6–10) in three concentrations compared with those growing on medium mixed with DMSO as control. Flg22 (1 μM) treated plants served as controls for successful growth inhibition. (A) Average fresh shoot weight (g). (B) Average fresh root weight (g). Data are based on three independent experiments. Each bar represents the mean ± standard error of n = 15. Asterisks indicate significant differences to the control based on one-way ANOVA followed by Dunnett’s post-hoc test (P < 0.05).
Comparable effects of the compounds on root weight could be observed. Compound 10 was highly promoting root growth at concentrations of 25 or 12.5 μM. Prodigiosin (1) treatment was neutral except for the 50 μM concentration were root weight was significantly reduced. Root weight was decreased significantly when plants were incubated in 50 μM of all other derivatives. The effect was less severe when the concentration was decreased to 25 or 12.5 μM but still root weight was significantly decreased compared to the control with the exception of 9, where this inhibitory effect was demolished when plants were incubated in 12.5 μM.
Prodiginines Inhibit Nematode Parasitism
Due to the observed neutral to positive effect of prodiginines on plant growth and compound availability, 1 and 9 were selected to investigate their effect on H. schachtii parasitism of A. thaliana. A final concentration of 14 μM of each molecule was incorporated into the plant growth medium. Medium mixed with DMSO in a final concentration of 0.5% was used as control. Results showed that the number of developed females and males was significantly decreased on the plant roots in presence of compound 1 or 9 compared with the control (Figure 6). The number of females was decreased by up to 60%, while the males were up to 42% less in the treatments compared to the control. In total, a reduction of up to 50% in total infection was observed when plant roots are exposed to prodigiosin (1) or the derivative 9.
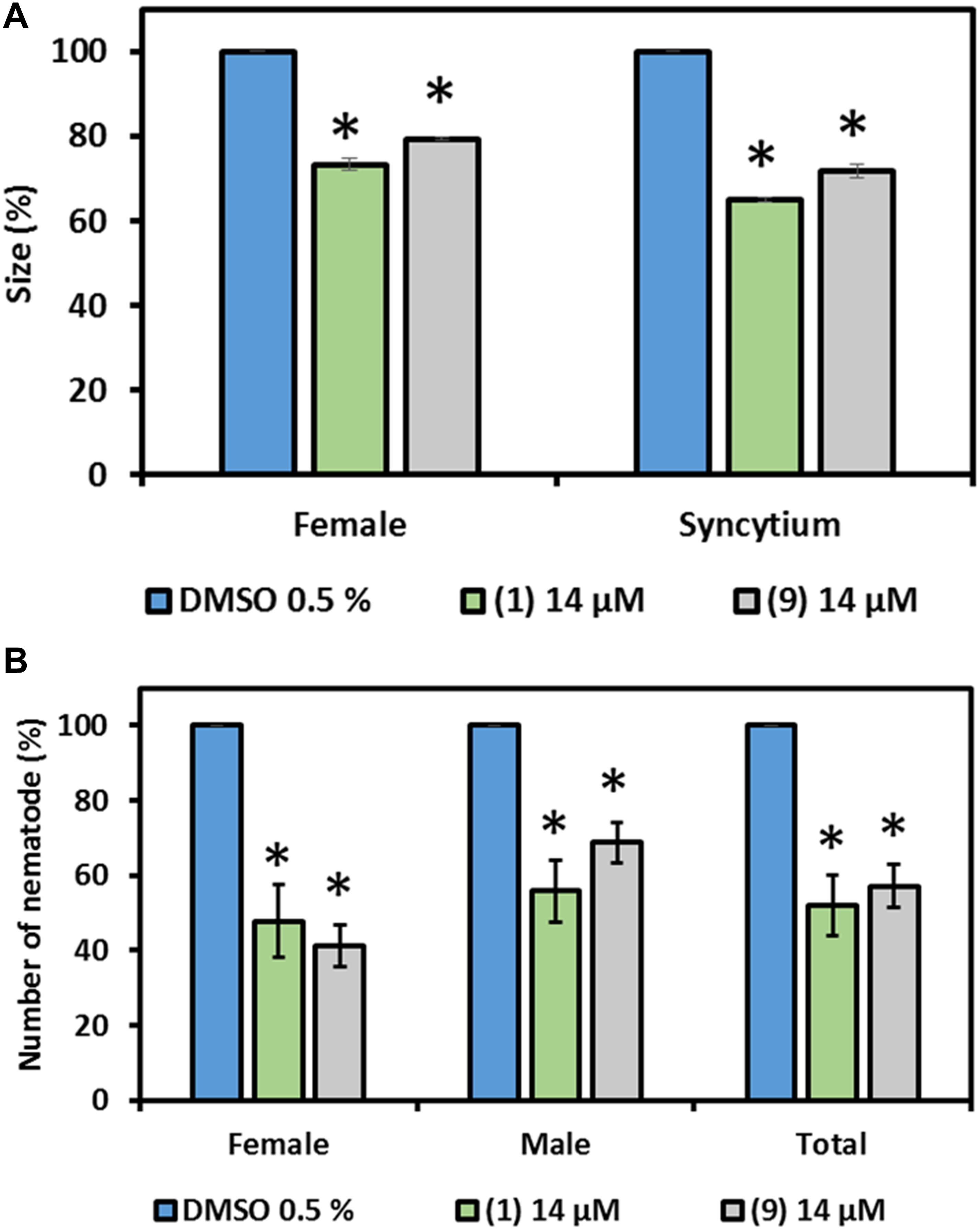
Figure 6. Effect of prodigiosin (1) and derivative 9 on H. schachtii parasitism of A. thaliana. Plants were grown on Knoṕs agar mixed with prodigiosin (1) and derivative 9 and were infected with J2s of H. schachtii. Susceptibility parameters were (A) average percentage of females, males and total nematodes per plant compared with control and (B) average percentage of sizes of females and syncytia at 13 DAI. Plants grown on medium mixed with DMSO (0.5% final concentration) were used as control. Data are based on three independent experiments. Each bar represents the mean ± standard error of n > 35. Asterisks indicate significant differences to the control based on one-way ANOVA followed by Dunnett’s post-hoc test (P < 0.05).
Furthermore, the size of developed females and their associated syncytia were measured in order to investigate nematode development. Both, developed females and their associated syncytia were smaller in size compared with the control in the presence of compound 1 or 9 in the medium. The female size reached 77 and 80% of that of the females of the control in presence of prodigiosin (1) and 9, respectively. The inhibitory effect on syncytium development was higher as the average size determined was 60 and 75% of the size in the control in presence of prodigiosin (1) and 9, respectively (Figure 6).
Bioactivities Against Plant Pathogenic Fungi
We further investigated the bioactivity of prodiginines against the two plant pathogenic fungi P. lingam and S. sclerotiorum. Both fungi share host plants with H. schachtii. Besides observing a possible antifungal activity, we aimed at getting an indication whether the changes in bioactivity documented for the structurally different prodiginines against nematodes follow a similar pattern in their activity against fungi. The two fungi responded differently to direct compound exposure and the degree of inhibition of hyphal growth varied with the prodiginine and concentration used (Figure 7). All tested derivatives except 10 significantly inhibited the hyphal growth of P. lingam at 50 μm. When the fungus was cultured on medium containing 25 μM of the compounds, prodigiosin (1), 6 and 8 showed significant inhibition. While none of the used compound showed inhibitory activity on hyphal growth at 12.5 μM.
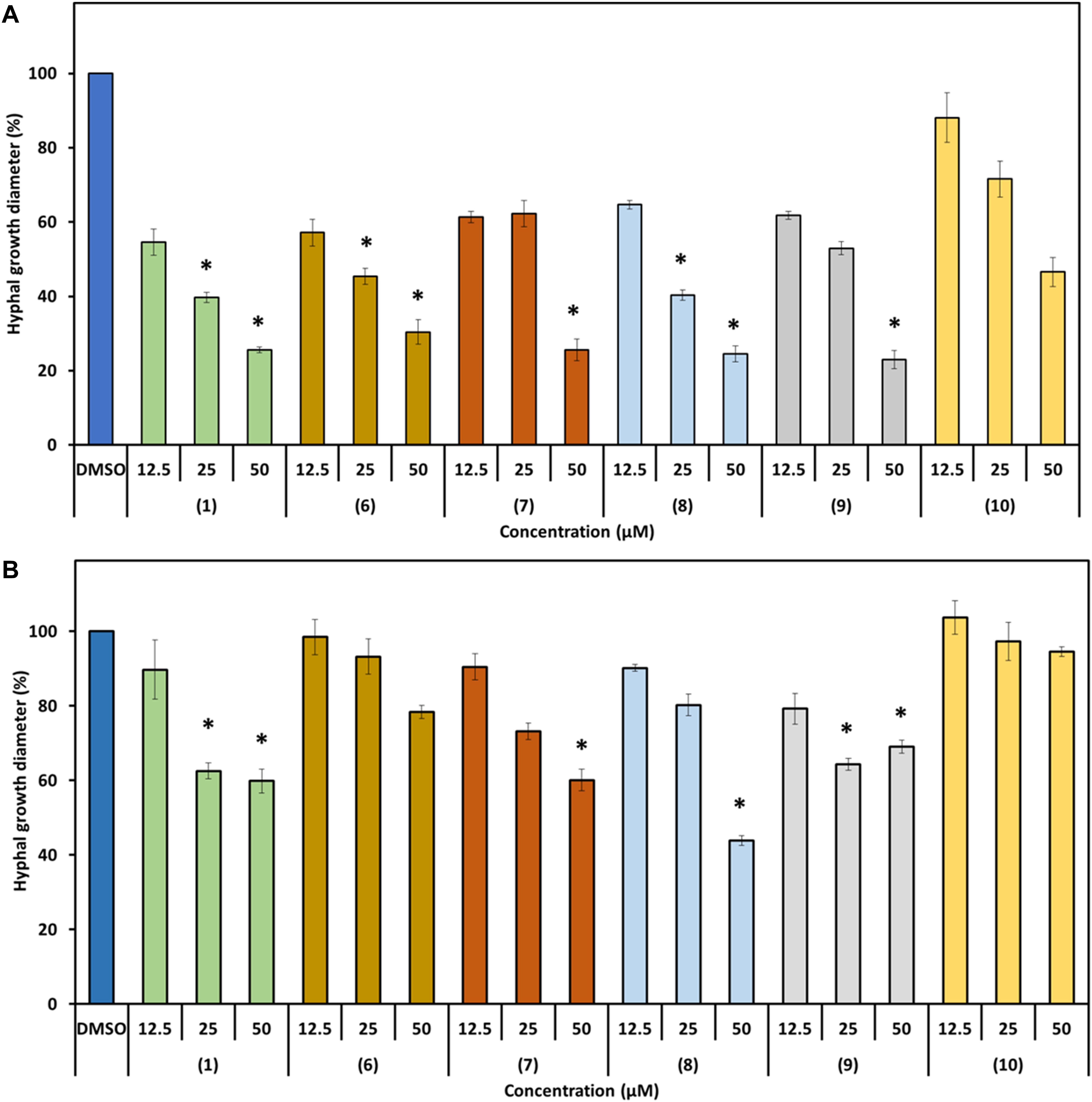
Figure 7. Effect of prodigiosin (1) and derivatives 6–10 on fungal hyphal growth. Figures show percentage of hyphal growth of the plant pathogenic fungi cultivated on media mixed with prodigiosin (1) or the derivatives 6–10 in three concentrations compared with those cultivated on medium mixed with DMSO as control. (A) P. lingam. (B) S. sclerotiorum. Values are means ± standard error of four biological replicates (n = 12). Asterisks indicate significant differences to the control based on one-way ANOVA followed by Dunnett’s post-hoc test (P < 0.05).
S. sclerotiorum was less sensitive toward the used prodiginines. All compounds inhibited hyphal growth except derivatives 6 and 10, even when 50 μM was used (Figure 7B). Fungal growth varied depending on the tripyrrol applied and ranged from 44% (8) to 100% (10) compared to the control (Figure 6B).
Discussion
Management of plant pathogens in agricultural crop production is indispensable, no matter whether organic, integrated or conventional farming practices are applied. In particular, control of plant parasitic nematodes is extremely challenging. Rising public health concerns about pesticides harmful for the environment led to several effective compounds being banned from the market. This in return, limited the pathogen management options of the farmer during production processes. These reasons urge to find environmentally safe and sustainable alternatives to control pathogens and ensure yield and food quality.
Naturally occurring bacterial metabolites represent a valuable source of active compounds that can be used in different applications including pathogen control. In the last decade, several studies investigated these potential compounds (Schrader et al., 2010; Pathma et al., 2011; Zhai et al., 2018). Prodigiosin (1) which is a tripyrrolic red-colored pigment produced by many bacterial species got attention due to its bioactivities and was intensively studied (Darshan and Manonmani, 2015; Yip et al., 2019). Derivatization of compounds is one of the important tools to increase the availability as well as the activity of compounds. Various structure activity studies have already proven that variation of the prodiginine structure leads to different biological activities (D’Alessio et al., 2000; Papireddy et al., 2011; Marchal et al., 2014a, b; Kancharla et al., 2015). Synthetic biology is one of the approaches that helps this process and provides the tools necessary for applying mutasynthesis or biotransformation concepts for a controlled production of prodiginines in a safe heterologous bacterial host (Klein et al., 2017, 2018). Also biocatalysis approaches employing only the final enzyme of the prodigiosin (1) gene cluster and chemically synthesized precursors can lead to new prodiginine structures (Brass et al., 2019).
In our current study, we introduce several lines of results showing the potential of prodigiosin (1) and several derivatives including a new prodiginine with a terminal hydroxy group adjacent to an allylic double bond and their bioactivities toward several organisms including nematodes, fungi and plant. We first tested all compounds against the model nematode C. elegans and the plant parasitic nematode H. schachtii in direct exposure assays. The nematode C. elegans is frequently used in studies involved in investigating compounds’ bioactivities against multicellular organisms (Sun et al., 2017; Al Banna et al., 2018; Liao, 2018). The nematode H. schachtii is one of the devastating pathogens of several economically valuable crops (Steele, 1965; Muller, 1999). We observed that all derivatives are nematicidal for C. elegans while basically only the lead structure prodigiosin (1) is static for H. schachtii J2s. The nematicidal activity of the compounds toward C. elegans is clearly dependent on the molecule structure and the concentration with compounds 6, 9, and 10 having the lowest efficacy. The difference in compound activity toward the two nematode species could be due to differences in feeding habit. C. elegance has a wider mouth part which is an open canal that allows to uptake compounds from the environment while in H. schachtii the uptake through the mouth is controlled by the spear-like stylet and feeding exclusively occurs at the host plant during the sedentary phase. Thus, the mobile infective juvenile stage of H. schachtii used here is not feeding at all consequently limiting uptake of compounds from the environment to passive processes. Same results were demonstrated when both, C. elegans and Meloidogyne incognita, were exposed to the nanoparticles of silicon carbide. C. elegans was much more sensitive and exhibited high mortality as well as accumulation of the nanoparticles in the body after the exposure, while very low mortality and particle accumulation were observed in case of M. incognita (Al Banna et al., 2018). Furthermore, the two related nematodes, H. schachtii and Globodera rostochiensis, behaved differently upon exposure to exogenous application of several amino acids. In these studies, G. rostochiensis was shown to be sensitive to the application of methionine while no effect was observed in case of H. schachtii (Evans and Trudgill, 1971; Blümel et al., 2018). Both evidences support the hypothesis that different nematode anatomy and biology affect the nematodes’ sensitivity toward compounds. The nematicidal activities of prodigiosin (1) itself was also observed previously. It was shown that the survival of juvenile stages of Radopholus similis and Meloidogyne javanica was affected after treatment with the red pigment extracted from S. marcescens with LC50 (lethal concentration) value of 83 and 79 μg/mL, for R. similis and M. javanica, respectively. After deep analysis of the pigment using several liquid chromatography approaches and spectroscopic analysis, they confirmed the presence of prodigiosin as a bioactive metabolite (Rahul et al., 2014). All these findings support our results and show that different nematode species could behave differently due to exposer to compounds.
In order to introduce such compounds as agrochemicals, the effect on the plant has to be tested. No previous work was done studying the effect of prodiginines on plant development. Here, we report the effect of prodigiosin (1) and derivatives on plant growth of the model plant A. thaliana for the first time. Arabidopsis growth was different between treatments and was depending on concentration and prodiginine structure. We observed that prodigiosin (1) and the 12-ring derivative 9 are neutral for plant growth when low concentrations are applied. In case of the prodiginine 10, plant growth was even highly promoted at low concentrations.
Based on the plant growth experiment results and compound availability, prodigiosin (1) and the 12-ring derivative (9) were chosen and a proper concentration was defined to be used to test the effect of the molecules on nematode parasitism on A. thaliana. The presence of 14 μM of both compounds in the plant growth medium affected H. schachtii parasitism and decreased the total number of adult nematodes per plant up to 50%. Furthermore, the compounds affected nematode development which is reflected by the smaller developed females and associated syncytia. Interestingly, although prodigiosin (1) paralyzes the J2s in liquid medium, we could not observe an impact of prodigiosin-supplemented agar on J2 motility. Therefore, we suggest that the observed effect of the two compounds on H. schachtii parasitism could be explained by compound-triggered plant defense responses. Similar observations were reported when no direct effect was detected after incubating J2s of H. schachtii in amino acids but still decreased parasitism when amino acids were integrated in the growth medium (Blümel et al., 2018). The logical explanation of such an outcome is that the presence of the compounds in the growth medium is activating plant defense responses against the nematode. These tests should be complemented by soil-based experiments in the future to confirm the observed control potential under field conditions. Particularly because in natural environments, factors like compound leaching, soil properties and compound stability affect efficacy.
We tested the bioactivities of the prodiginines against two plant pathogenic fungi. Both fungi can infect the same host plants as H. schachtii, thus a double impact of the compounds would increase their value. Our results showed that the direct effect of the prodiginines against the fungi P. lingam and S. sclerotiorum was even stronger compared to nematodes. The hyphal growth of both tested fungi was affected by the presence of prodigiosin (1) and derivatives 6–10 in the growth medium. Earlier, it was shown that prodigiosin (1) is active against several fungi and oomycetes. The purified prodigiosin (1) from S. marcescens F-1-1 inhibited the germination of cystospores and the growth of hyphae of Phytophthora capsici. It also appeared to be active against the plant pathogenic fungi Cochliobolus miyabeanus, Pythium spinosum and P. ultimum (Okamoto et al., 1998). According to our results, hyphal growth of P. lingam was affected more than that of S. sclerotiorum. Such a difference is dependent on fungal species and also demonstrated by previous studies. Chen et al. (2014) presented that the novel fungicide 3-[5-(4-chlorophenyl)-2,3-dimethyl-3-isoxazolidinyl] pyridine (SYP-Z048) affected several pathogenic fungi and the effect was variable between the tested fungi.
Comparing the results of the assays, we show that prodigiosin (1) is the most effective and derivative 10 is the least effective obviously reflecting the variability in compound sensitivity between different organisms. Particularly, P. lingam appears to be very sensitive to all compounds whereas H. schachtii only responded to prodigiosin (1). Interestingly, the compounds’ impact on plant development does not completely follow the same trend as seen for nematodes and fungi. Although plant growth promotion is highest for the prodiginine with the overall lowest activity against nematodes and fungi (compound 10), prodigiosin (1) is neutral for plant growth depending on the concentration applied.
Compound 9 is a very good example that structural modification of a bioactive lead structure can guide compound activity toward desired properties. The lead structure 1 and derivative 9 have a comparable impact on plant development (neutral) and on parasitism of H. schachtii on A. thaliana (inhibition). Intriguingly, 1 is highly toxic for C. elegans, which is a representative of non-target organisms, whereas 9 does not affect this nematode’s vitality at concentrations suitable to control H. schachtii and P. lingam, which are two important pathogens on sugar beet.
Further detailed investigations are needed to unravel the mode of action against nematodes. Although studies with nematodes are missing so far, mechanistic insights of the effect of prodigiosin in other organisms were reported. For example, it was shown that the motility of the bacteria Bacillus cereus, Staphylococcus aureus, Pseudomonas aeruginosa, and Escherichia coli was inhibited upon exposure to prodigiosin. The inhibition was combined with activation of several features of programmed cell death (Darshan and Manonmani, 2015). In another study, the exposure of B. subtilis to prodigiosin was associated with growth inhibition and cell membrane leakage. The authors suggested that prodigiosin affects the bacterial cell by inducing cell lysis activities (Danevčič et al., 2016a). Similarly, E. coli exhibited a leaky outer membrane and cells had severely decreased respiration activity upon exposure to prodigiosin (Danevčič et al., 2016b).
Our current results demonstrate the bioactivities of prodigiosin (1) and its derivatives (6–10) against plant pathogenic fungi and parasitic nematodes and thereby introduce new natural compounds that have the potential to be developed into novel plant protection agents.
Data Availability Statement
All datasets presented in this study are included in the article/Supplementary Material.
Author Contributions
AS, FG, JP, TC, and SH conceived the research concept and designed the experiments. SH performed the assays on plants, nematodes, and fungi. HB, AK, DK, and TW produced and synthesized the used prodiginines. SH drafted the manuscript with input from all authors. All authors reviewed and approved the final manuscript.
Funding
This research and the scientific activities of the Bioeconomy Science Center were financially supported by the Ministry of Culture and Science within the framework of the NRW Strategieprojekt BioSC (No. 313/323-400-00213). The funding body had no role in the design of the study and collection, analysis, and interpretation of data or in writing the manuscript.
Conflict of Interest
The study was the basis for a patent application. Patent applicant: Forschungszentrum Juelich GmbH; inventors: SH, HB, AK, DK, TW, TC, JP, FG, and AS application number: 10 2020 116 516; status: examination phase.
Acknowledgments
The authors gratefully acknowledge the Bioeconomy Science Center and the Ministry of Culture and Science of the German State of North Rhine-Westphalia (MKW) for funding the work.
Supplementary Material
The Supplementary Material for this article can be found online at: https://www.frontiersin.org/articles/10.3389/fpls.2020.579807/full#supplementary-material
References
Al Banna, L., Salem, N., Ghrair, A. M., and Habash, S. S. (2018). Impact of silicon carbide nanoparticles on hatching and survival of soil nematodes Caenorhabditis elegans and Meloidogyne incognita. Appl. Ecol. Environ. Res. 16, 2651–2662. doi: 10.15666/aeer/1603_26512662
Arivizhivendhan, K. V., Mahesh, M., Boopathy, R., Swarnalatha, S., Regina Mary, R., and Sekaran, G. (2018). Antioxidant and antimicrobial activity of bioactive prodigiosin produces from Serratia marcescens using agricultural waste as a substrate. J. Food Sci. Technol. 55, 2672–2681. doi: 10.1007/s13197-018-3188-9
Blümel, R. C., Fischer, D. F., and Grundler, F. M. W. (2018). Effects of exogenous amino acid applications on the plant-parasitic nematode Heterodera schachtii. Nematology 20, 713–727. doi: 10.1163/15685411-00003169
Brass, H. U. C., Klein, A. S., Nyholt, S., Classen, T., and Pietruszka, J. (2019). Condensing enzymes from pseudoalteromonadaceae for prodiginine synthesis. Adv. Synth. Catal. 361:adsc.201900183. doi: 10.1002/adsc.201900183
Bridge, J. (1996). Nematode management in sustainable and subsistence agriculture. Annu. Rev. Phytopathol. 34, 201–225. doi: 10.1146/annurev.phyto.34.1.201
Chen, F., Han, P., Liu, P., Si, N., Liu, J., and Liu, X. (2014). Activity of the novel fungicide SYP-Z048 against plant pathogens. Sci. Rep. 4:6473. doi: 10.1038/srep06473
Classen, T., and Pietruszka, J. (2018). Complex molecules, clever solutions – Enzymatic approaches towards natural product and active agent syntheses. Bioorg. Med. Chem. 26, 1285-1303. doi: 10.1016/j.bmc.2017.06.045
D’Alessio, R., Bargiotti, A., Carlini, O., Colotta, F., Ferrari, M., Gnocchi, P., et al. (2000). Synthesis and immunosuppressive activity of novel prodigiosin derivatives. J. Med. Chem. 43, 2557–2565. doi: 10.1021/jm001003p
Danevčič, T., Vezjak, M. B., Tabor, M., Zorec, M., and Stopar, D. (2016a). Prodigiosin induces autolysins in actively grown Bacillus subtilis cells. Front. Microbiol. 7:27. doi: 10.3389/fmicb.2016.00027
Danevčič, T., Vezjak, M. B., Zorec, M., and Stopar, D. (2016b). Prodigiosin - A multifaceted Escherichia coli antimicrobial agent. PLoS One 11:e0162412. doi: 10.1371/journal.pone.0162412
Darshan, N., and Manonmani, H. K. (2015). Prodigiosin and its potential applications. J. Food Sci. Technol. 52, 5393–5407. doi: 10.1007/s13197-015-1740-4
Domröse, A., Klein, A. S., Hage-Hülsmann, J., Thies, S., Svensson, V., Classen, T., et al. (2015). Efficient recombinant production of prodigiosin in Pseudomonas putida. Front. Microbiol 6:972. doi: 10.3389/fmicb.2015.00972
Evans, K., and Trudgill, D. L. (1971). Effects of amino acids on the reproduction of Heterodera rostochiensis. Nematologica 17, 495–500. doi: 10.1163/187529271X00215
Gerber, N. N. (1975). A new prodiginne (prodigiosin-like) pigment from Streptomyces. Antimalarial activity of several prodiginnes. J. Antibiot. 28, 194–199. doi: 10.7164/antibiotics.28.194
Habash, S., and Al-Banna, L. (2011). Phosphonate fertilizers suppressed root knot nematodes Meloidogyne javanica and M. incognita. J. Nematol. 43, 95–100.
Habash, S. S., Sobczak, M., Siddique, S., Voigt, B., Elashry, A., and Grundler, F. M. W. (2017). Identification and characterization of a putative protein disulfide isomerase (HsPDI) as an alleged effector of Heterodera schachtii. Sci. Rep 7:13536. doi: 10.1038/s41598-017-13418-9
Heydari, A., and Pessarakli, M. (2010). A review on biological control of fungal plant pathogens using microbial antagonists. J. Biol. Sci. 10, 273–290. doi: 10.3923/jbs.2010.273.290
Hu, D. X., Withall, D. M., Challis, G. L., and Thomson, R. J. (2016). Structure, chemical synthesis, and biosynthesis of prodiginine natural products. Chem. Rev. 116, 7818–7853. doi: 10.1021/acs.chemrev.6b00024
Kancharla, P., Kelly, J. X., and Reynolds, K. A. (2015). Synthesis and structure-activity relationships of tambjamines and b-ring functionalized prodiginines as potent antimalarials. J. Med. Chem. 58, 7286–7309. doi: 10.1021/acs.jmedchem.5b00560
Kawasaki, T., Sakurai, F., and Hayakawa, Y. (2008). A Prodigiosin from the Roseophilin Producer Streptomyces griseoviridis. J. Nat. Prod. 71, 1265–1267. doi: 10.1021/np7007494
Kennedy, J. (2008). Mutasynthesis, chemobiosynthesis, and back to semi-synthesis: Combining synthetic chemistry and biosynthetic engineering for diversifying natural products. Nat. Prod. Rep. 25, 25-34. doi: 10.1039/b707678a
Kim, D., Lee, J. S., Park, Y. K., Kim, J. F., Jeong, H., Oh, T. K., et al. (2007). Biosynthesis of antibiotic prodiginines in the marine bacterium Hahella chejuensis KCTC 2396. J. Appl. Microbiol. 102, 937–944. doi: 10.1111/j.1365-2672.2006.03172.x
Kimyon, Ö, Das, T., Ibugo, A. I., Kutty, S. K., Ho, K. K., Tebben, J., et al. (2016). Serratia secondary metabolite prodigiosin inhibits Pseudomonas aeruginosa biofilm development by producing reactive oxygen species that damage biological molecules. Front. Microbiol 7:972. doi: 10.3389/fmicb.2016.00972
Kirschning, A., and Hahn, F. (2012). Merging chemical synthesis and biosynthesis: A new chapter in the total synthesis of natural products and natural product libraries. Angew. Chem. Int. Ed. 51, 4012-4022. doi: 10.1002/anie.201107386
Klein, A. S., Brass, H. U. C., Klebl, D. P., Classen, T., Loeschcke, A., Drepper, T., et al. (2018). Preparation of cyclic prodiginines by mutasynthesis in Pseudomonas putida kt2440. Chembiochem 19, 1545–1552. doi: 10.1002/cbic.201800154
Klein, A. S., Domröse, A., Bongen, P., Brass, H. U. C., Classen, T., Loeschcke, A., et al. (2017). New prodigiosin derivatives obtained by mutasynthesis in Pseudomonas putida. ACS Synth. Biol. 6, 1757–1765. doi: 10.1021/acssynbio.7b00099
Liao, V. H. C. (2018). Use of Caenorhabditis elegans to study the potential bioactivity of natural compounds. J. Agric. Food Chem. 66, 1737–1742. doi: 10.1021/acs.jafc.7b05700
Marchal, E., Rastogi, S., Thompson, A., and Davis, J. T. (2014a). Influence of B-ring modifications on proton affinity, transmembrane anion transport and anti-cancer properties of synthetic prodigiosenes. Org. Biomol. Chem. 12, 7515–7522. doi: 10.1039/c4ob01399a
Marchal, E., Smithen, D. A., Uddin, M. I., Robertson, A. W., Jakeman, D. L., Mollard, V., et al. (2014b). Synthesis and antimalarial activity of prodigiosenes. Org. Biomol. Chem. 12, 4132–4142. doi: 10.1039/c3ob42548g
Muller, J. (1999). The economic importance of Heterodera schachtii in Europe. Helminthologia 36, 205–213.
Oerke, E. C. (2006). Crop losses to pests. J. Agric. Sci. 144, 31–43. doi: 10.1017/S0021859605005708
Okamoto, H., Sato, Z., Sato, M., Koiso, Y., Iwasaki, S., and Isaka, M. (1998). Identification of antibiotic red pigments of Serratia marcescens F-1-1, a biocontrol agent of damping-off of cucumber, and antimicrobial activity against other plant pathogens. Jap. J. Phytopathol. 64, 294–298. doi: 10.3186/jjphytopath.64.294
Papireddy, K., Smilkstein, M., Kelly, J. X., Shweta, S., Salem, S. M., Alhamadsheh, M., et al. (2011). Antimalarial activity of natural and synthetic prodiginines. J. Med. Chem. 54, 5296–5306. doi: 10.1021/jm200543y
Pathma, J., Rahul, G. R., Kamaraj, K. R., Subashri, R., and Sakthivel, N. (2011). Secondary metabolite production by bacterial antagonists. J. Biol. Control. 25, 165–181. doi: 10.18641/JBC/25/3/39985
Pérez-Tomás, R., Montaner, B., Llagostera, E., and Soto-Cerrato, V. (2003). The prodigiosins, proapoptotic drugs with anticancer properties. Biochem. Pharmacol. 66, 1447–1452. doi: 10.1016/S0006-2952(03)00496-9
Rahul, S., Chandrashekhar, P., Hemant, B., Chandrakant, N., Laxmikant, S., and Satish, P. (2014). Nematicidal activity of microbial pigment from Serratia marcescens. Nat. Prod. Res. 28, 1399–1404. doi: 10.1080/14786419.2014.904310
Schrader, K. K., Andolfi, A., Cantrell, C. L., Cimmino, A., Duke, S. O., Osbrink, W., et al. (2010). A survey of phytotoxic microbial and plant metabolites as potential natural products for pest management. Chem. Biodivers. 7, 2261–2280. doi: 10.1002/cbdv.201000041
Sijmons, P. C., Grundler, F. M. W., von Mende, N., Burrows, P. R., and Wyss, U. (1991). Arabidopsis thaliana as a new model host for plant-parasitic nematodes. Plant J. 1, 245–254. doi: 10.1111/j.1365-313X.1991.00245.x
Someya, N., Nakajima, M., Hirayae, K., Hibi, T., and Akutsu, K. (2001). Synergistic antifungal activity of chitinolytic enzymes and prodigiosin produced by biocontrol bacterium, Serratia marcescens strain B2 against gray mold pathogen, Botrytis cinerea. J. Gen. Plant Pathol. 67, 312–317. doi: 10.1007/PL00013038
Stankovic, N., Senerovic, L., Ilic-Tomic, T., Vasiljevic, B., and Nikodinovic-Runic, J. (2014). Properties and applications of undecylprodigiosin and other bacterial prodigiosins. Appl. Microbiol. Biotechnol. 98, 3841–3858. doi: 10.1007/s00253-014-5590-1
Starič, N., Danevčič, T., and Stopar, D. (2010). Vibrio sp. DSM 14379 pigment production-A competitive advantage in the environment. Microb. Ecol. 60, 592–598. doi: 10.1007/s00248-010-9671-0
Steele, A. E. (1965). The host range of the sugar beet nematode, Heterodera schachtii Schmidt. J. Sugarb. Res. 13, 574–603.
Sun, C. L., Zhang, H., Liu, M., Wang, W., and Crowder, C. M. (2017). A screen for protective drugs against delayed hypoxic injury. PLoS One 12:e0176061. doi: 10.1371/journal.pone.0176061
Weist, S., and Süssmuth, R. D. (2005). Mutational biosynthesis - A tool for the generation of structural diversity in the biosynthesis of antibiotics. Appl. Microbiol. Biotechnol 68, 141–150. doi: 10.1007/s00253-005-1891-8
Williams, R. P., Green, J. A., and Rappo-Port, D. A. (1956). Studies on pigmentation of Serratia marcescens, I. Spectral and paper chromatographic properties of prodigiosin. J. Bacteriol. 71, 115–120.
Williamson, N. R., Fineran, P. C., Leeper, F. J., and Salmond, G. P. C. (2006). The biosynthesis and regulation of bacterial prodiginines. Nat. Rev. Microbiol. 4, 887–899. doi: 10.1038/nrmicro1531
Yip, C. H., Yarkoni, O., Ajioka, J., Wan, K. L., and Nathan, S. (2019). Recent advancements in high-level synthesis of the promising clinical drug, prodigiosin. Appl. Microbiol. Biotechnol. 103, 1667–1680. doi: 10.1007/s00253-018-09611-z
Keywords: prodiginines, natural product, plant pathogens, plant protection, nematode
Citation: Habash SS, Brass HUC, Klein AS, Klebl DP, Weber TM, Classen T, Pietruszka J, Grundler FMW and Schleker ASS (2020) Novel Prodiginine Derivatives Demonstrate Bioactivities on Plants, Nematodes, and Fungi. Front. Plant Sci. 11:579807. doi: 10.3389/fpls.2020.579807
Received: 03 July 2020; Accepted: 18 September 2020;
Published: 16 October 2020.
Edited by:
Juan Emilio Palomares-Rius, Institute for Sustainable Agriculture (IAS) (CSIC), SpainReviewed by:
Paola Leonetti, National Research Council (CNR), ItalyCatherine Lilley, University of Leeds, United Kingdom
Copyright © 2020 Habash, Brass, Klein, Klebl, Weber, Classen, Pietruszka, Grundler and Schleker. This is an open-access article distributed under the terms of the Creative Commons Attribution License (CC BY). The use, distribution or reproduction in other forums is permitted, provided the original author(s) and the copyright owner(s) are credited and that the original publication in this journal is cited, in accordance with accepted academic practice. No use, distribution or reproduction is permitted which does not comply with these terms.
*Correspondence: Samer S. Habash, c2FtZXJAdW5pLWJvbm4uZGU=; orcid.org/0000-0002-4493-1451; A. Sylvia S. Schleker, c3lsdmlhLnNjaGxla2VyQHVuaS1ib25uLmRl