- 1Anhui Province Key Laboratory of Wetland Ecosystem Protection and Restoration, School of Resources and Environmental Engineering, Anhui University, Hefei, China
- 2Department of Biological Sciences, Tennessee State University, Nashville, TN, United States
Plant species may acquire different forms of nitrogen (N) to reduce competition for the same resource, but how plants respond to neighbors with different densities in their N uptake is still poorly understood. We investigated the effects of competition regime on the uptake of different N forms by two hygrophytes, Carex thunbergii and Polygonum criopolitanum, by conducting a hydroponic test of excised roots and an in situ experiment in a subtropical wetland ecosystem. The two species were grown either in monocultures or mixtures with various neighbor densities. Root functional traits and N uptake rates of different N forms were measured. Our results showed that N uptake was mainly determined by N form, rather than species identity. Both species were able to use organic N sources, but they took up relatively more N supplied as than as or glycine, irrespective of competition treatments. Both species preferred when grown in monoculture, but in the presence of competitors, the preference of fast-growing C. thunbergii persisted while P. criopolitanum acquired more and glycine, with stronger responses being observed at the highest neighbor density. The hydroponic test suggested that these divergences in N acquisition between two species might be partially explained by different root functional traits. To be specific, N uptake rates were significantly positively correlated with root N concentration and specific root length, but negatively correlated with root dry matter content. Our results implicated that C. thunbergii has a competitive advantage with relatively more stable N acquisition strategy despite a lower N recovery than P. criopolitanum, whereas P. criopolitanum could avoid competition with C. thunbergii via a better access to organic N sources, partly mediated by competition regimes.
Introduction
Nitrogen (N) is an essential element for plant growth, and its availability limits the productivity of most terrestrial ecosystems (Lebauer and Treseder, 2008). N limitation is common, mainly because the inorganic N ( and ) contents in the soil are low and a large fraction of soil N exists in organic form which is not readily accessible to plants (Jiang et al., 2017). In the past 30 years, an increasing number of studies have indicated that it might be a common phenomenon for plants to take up low molecular-weight organic N directly from soil solution (Näsholm et al., 2009). Plants may use distinct nutrient acquisition strategies in response to changing environment, which is closely correlated with species co-existence (McKane et al., 2002) and ecosystem functioning (Boudsocq et al., 2012). Such strategies include that of nutrient uptake, transportation, utilization, and resorption. Due to the differences in mobility, energy consumption in assimilation, characteristics of various N transport genes of different N forms, and tolerance to ion toxicity among species (Zhang et al., 2018), plant species have different capacities in taking up , , or organic N as their primary N source. Some species have a preference for specific N forms (McKane et al., 2002), while other species showed flexibility in N uptake (Houlton et al., 2007). Whether a plant can change its N acquisition pattern will largely determine its ability to adapt to environmental changes (Gao et al., 2020). Studies on the acquisition of organic N by plants are mainly focused on alpine and arctic ecosystems with slow N mineralization rate (e.g., McKane et al., 2002; Xu et al., 2006). Recently, studies have confirmed that tropical forest species can also take up organic N (Andersen et al., 2017; Liu et al., 2018). Given that the N acquisition pattern may be ecosystem specific (Zhou et al., 2019), it is not clear whether the differences in N acquisition strategies among coexisting plants apply across different ecosystems (Yang et al., 2018).
The complexity of N acquisition strategies largely stems from the interplay between plant species and their interactions with various abiotic variables (Moreau et al., 2019). On the one hand, all species in the community are facing resource competition from other coexisting species, the uptake capacity of different N sources is affected by interspecific competition (e.g., Miller et al., 2007; Simon et al., 2014). Plants have evolved different nutrient acquisition strategies, and the most important one is niche differentiation, which shows that there are preference differences among species for different forms of N to avoid competition (Simon et al., 2017; Huangfu et al., 2019). For example, Huangfu et al. (2019) found that exotic plant Flaveria bidentis and coexisting species Amaranthus retroflexus preferred to take up N in the form of in monoculture, while the former tended to increase the acquisition of , while the latter had more uptakes of when they were grown together in competition. So the flexibility of N acquisition is considered to be an important strategy for plants to reduce niche overlap and mitigate N competition (niche partitioning) to achieve coexistence (McKane et al., 2002; Ashton et al., 2010). However, researches testing for niche separation achieved mixed results (Kuster et al., 2016, references therein), as 15N uptake by plants under interspecific competition can be either increased or decreased (Hong et al., 2017).
On the other hand, environmental conditions can cause this inconsistency in plant N acquisition strategy. Plant preference for N is closely related to relative availability of different N forms in soil solution (Zhang et al., 2018). For example, Schimel and Bennett (2004) reported that, with the change of available N supply (such as from boreal forest to tropical forest), the N form preference of plant community switches from organic N to and to . In inorganic N deficient soils, plants tend to use organic N, while in high fertility soils, they use inorganic ones (Scott and Rothstein, 2011). Water content is also related N transformation processes within the soil as it affects the aeration. In aerobic and arid soils, is usually the predominant N form available to plants (Xu et al., 2011), whereas is the main available N source in flooded and anaerobic soils (such as paddy fields, Houlton et al., 2007). Due to the different transport capacity of different forms of N, water shortage can reduce the N transport to the root surface, thus affecting the uptake process. On the contrary, higher soil water content is conducive to the transformation of organic matter to produce low molecular-weight organic N (e.g., glycine) for plant acquisition (Dijkstra et al., 2015). Consequently, plant uptake of organic or/and inorganic N differs in various ecosystems (Inselsbacher and Näsholm, 2012).
In response to the environmental variation, plants have evolved various root functional traits in term of the N uptake capacity (Hong et al., 2018). Among these, both fine root traits (Legay et al., 2020) and their associated mycorrhizal fungi (Liese et al., 2018) play crucial roles in soil N acquisition, facilitating species coexistence via promoting diversity of resource acquisition strategies (Lambers et al., 2008; Zemunik et al., 2015). In the littoral zone of Shengjin Lake, approximately 17% of the total area consists of Carex meadow (Cao and Fox, 2009) which was historically dominated by Polygonum criopolitanum one decade ago. As a result, these species coexist in various plant densities. The difference of N acquisition strategy may be affected by the competition regime among species, severing as a potential mechanism driving the community composition. However, we are lacking evidence of whether niche complementarities of inorganic and organic N acquisition exist, and how N acquisition respond to plant competition, as well as the roles of these root functional traits and neighbor density on the outcome of this competition in this wetland ecosystems. In this study, we aimed to test how plant competition influenced the acquisition of different forms of N by two species with different growth strategies, and the possible density dependence involved. We conducted two experiments consisting of a hydroponic test of excised roots of two dominant species (Carex thunbergii and P. criopolitanum), and an in situ experiment in the littoral zone of Shengjin Lake. We proposed the following three hypotheses: (1) the two species had different physiological preferences for N forms in the absence of interspecific competition; (2) root functional traits would contribute to the plant nutrient acquisition with species having more exploitative traits (e.g., high specific root length and root N content) being more efficient to take up N, and vice versa; (3) N uptake capacity of focal species was affected by plant competition in a density-dependent and species-specific way.
Materials and Methods
Study Site
The Shengjin Lake National Nature Reserve (30° 15′ N-30°30′ N, 116°55′ E-117°15′ E) is located in the southern Anhui Province, China. It is the main habitat for overwintering waterfowls (Meng et al., 2020). The climate in this area belongs to subtropical monsoon. The mean annual precipitation is ca. 1600 mm, most falling between May and August, and the mean annual air temperature is 16.4°C. Soils belong to Inceptisols according to the American soil taxonomy (Shi et al., 2004). Multiple shallow ephemeral wetlands were formed due to summer monsoonal flooding and drawdown in water levels during the autumn through spring of the coming year. The littoral zone plants communities mainly consisted of C. thunbergii, P. criopolitanum, Echinochloa caudata, Miscanthus floridulus, Paspalum distichum, Artemisia annua, Alternanthera philoxeroides, and Rumex dentatus (L.) (Meng et al., 2020). We selected two co-existing plant species that varied in their abundances: C. thunbergii and P. criopolitanum. C. thunbergii is generally more abundant at higher elevation, and a deep-rooted rhizomatous clonal sedge hygrophyte (Cyberaeae, accounting for more than 70% of total coverage across the littoral zone), and the forb P. criopolitanum’s abundance increases with decreasing elevation (accounting for about 5% of total coverage). As such, the vegetation is also known as “Carex meadow.” P. criopolitanum (Polygonaceae) is mycorrhizal (Wang and Qiu, 2006), while Cyberaeae is primarily non-mycorrhizal (Smith and Read, 2008). In this ecosystem, these two species have a common phenology characterized by two growing periods: the first growing season begins following flood recession in autumn; they spend the winter dormant, then resuming active growth from spring to summer (Yuan et al., 2017). Initial soil physical and chemical properties were listed in Table 1.
Laboratory Hydroponic Experiment
The usage of excised root segments in hydroponic experiment allowed to characterize the physiological uptake for different N sources in the absence of interspecific competition and to test our hypothesis 1 (Legay et al., 2020). The experiment consisted of culture of excised fine roots of two focal species in vials in a completely randomized factorial design considering two species and four N isotope labeled solutions. We used a total of 40 experimental units with five replicates per treatment (2 species × 4 solutions × 5 replicates) = 40 vials. The four solutions contained three forms of N, , NH4 +, and glycine in combination (1:1:1) with same total N concentration (500 μM), but only one form of the three N sources was 15N labeled in the first three solutions, while the fourth had no 15N labeled as a control treatment to account for the natural abundance of 15N. This concentration supplied enough N for the test period and allowed us to test for physiological N uptake. Though many organic N sources are shown to be directly absorbed by plants, glycine was repeatedly reported to be abundant amino acid in soils and represent a model organic N source, and recent study has found that glycine is among the organic N with the fastest uptake rate, mainly due to its high mobility (Tian et al., 2020).
Plants were collected from the Shengjin Lake Experimental Station in the fall of 2019. We collected plant-soil monoliths (10 cm width × 10 cm length × 10 cm depth) of target species grew in monoculture in the field using a spade. The monoliths were immediately transported to the laboratory and immersed in water until the soil had been loosely dispersed in the water, and the roots could be easily separated. The intact roots of each species were then washed carefully with tap water. In this study, we sieved and collected only fine roots (<2 mm in diameter), and transplanted to the test vials. In addition, subsamples were stored in refrigerated containers at 4°C for root functional traits analysis (see below). Excised root segments (around 6 cm in length each) of each species were randomly assigned to vials. To minimize the potential decline in N uptake ability, root N uptake of C. thunbergii and P. criopolitanum started within 30 min after excision. Samples were incubated in one of four solutions with a 15N excess of 99% atom. Solution volumes (50 ml) and fresh weights of excised roots (ca. 1.5 g in fresh) were selected to maintain a general constant concentration of N over experimental period. We added 0.5 mM CaCl2 to maintain membrane integrity. After 2 h, roots were repeatedly washed for 180 s with a 1 mM CaCl2 to remove any 15N label absorbed on the root surface, followed by rinsing using running demineralized water. Root samples were then oven-dried in paper bags at 65°C for 72 h, ground (MM2, Retsch, Haan, Germany) to a fine powder and aliquots of dried sample (ca. 2 mg) was analyzed by IRMS for 15N atom% and N concentrations (Huangfu et al., 2019).
In situ 15N Labeling Experiment
To determine whether competition regime modified plant N-form preference (hypothesis 3), the in situ 15N labeling experiment was conducted at Yang’etou of Shengjin Lake Experimental Station in November 2019, where two species also co-occurred at various abundances in the intermediate elevation in a mosaic pattern, allowing the effect of plant interspecific competition at different densities on N uptake to be tested.
To test whether neighbor density affected 15, 15, and 15N-glycine acquisition by plants, we established three blocks of 60 labeling plots (10 cm × 10 cm) in a randomized 4 × 5 factorial design within 0.2 ha area (200 m × 10 m) in the littoral zone. Blocks (10 m × 10 m each) were relatively flat in topography and homogeneous in vegetation composition, and at least 10 m away from the nearest blocks. 15N-, 15N-, and 15N-glycine and control treatments were randomly assigned to plots within each block. To reflect field dominance patterns, the plant composition consisted of five intra- and interspecific treatments with various densities of two species, from monodominant to co-dominant communities, each with four individual plants: (C. thunbergii: P. criopolitanum at 4:0, 3:1, 2:2, 1:3, 0:4). At the time of labeling, the microclimatic conditions and growth stage were similar. We carefully selected target species of similar size and marked them to clarify which treatment was applied. The plant individuals within each plot grew as near as possible to ensure that they could compete for soil resources from each other, while non-target plants were eliminated using scissors (Miller et al., 2007). Surrounding vegetation was clipped to the ground within a 10 cm radius of focal species to limit their interactions in term of N acquisition (Ashton et al., 2010). This was conducted one month prior of N labeling, with repeated clipping to control the regrowth. Tracer solutions were prepared as above, except that each labeling solution consisted of equal concentrations (2 mg N plot–1 for each N form) of , , and glycine. The amount of N added to the plot was small and minimized the possible N fertilization effect on plant growth as compared to background total N content (6 mg N plot−1≈2.7 mg N kg–1 soil ≪ 150 mg N kg–1 soil), while allowed the detection of 15N within plant biomass. A solution equivalent to 6 mg 15N was injected into one plot at four equal squares to a depth of 10 cm using a glass syringe, with each square receiving 10 mL of labeled solution, thus adding a total of 40 mL per plot. Higher solution volume was used to increase its diffusion to uniformly label the soil profile. Each injection point was equally spaced from each other within a 2 cm radius of the nearest target plant. Before each injection, we drilled a hole at 10 cm soil depths with a screw-driver to avoid clogging of the needle. Injections were applied on November 7, 2019, when maximal growth was assumed with the mean daily temperature was around 23°C. This date of the experiment was carefully chosen as no rainfall occurred within 5 days before the N treatment to minimize hydrologic transfers of labeled solution within soil column. Considering potential diurnal effects in N uptake, in situ labeling was always conducted between 09:00 and 11:00, coinciding with peak photosynthetic activity.
Plant and Soil Sampling and Analyses
Two hours after 15N labeling, leaves and stems were individually harvested using scissors for both species. Thereafter, we collected plant-soil monoliths measuring 10 cm × 10 cm × 15 cm in each plot, and collected the belowground parts (root, rhizome) by washing with deionized water. The sampled plant materials were placed in a portable icebox before transportation to the laboratory within 2 h. In the laboratory, the roots were immediately rinsed with a 1 mM CaCl2 to remove any 15N label absorbed on the root surface, followed by rinsing with running demineralized water, and finally all plant materials were oven-dried at 60°C for 72 h, ground and analyzed for total N and 15N/14N ratios as mentioned above.
Fresh fine root subsamples in hydroponic experiment were placed in a transparent tray and covered with deionized water. The tray was scanned using an Epson Expression 10,000 XL photo scanner at 600 dpi (Seiko Epson Corporation, Japan) and analyzed for each species using WinRhizo software (Winrhizo, Regent Instruments, Inc., Québec, QC, Canada) to obtain root length (cm). This information was used to calculate specific root length (SRL; Hong et al., 2018). We also measured the fresh and dry weights of each root sample. Altogether, we obtained root functional traits, including root dry matter content (RDMC, mg/g), SRL (m/g), root 15N natural abundance (%) and N content (RNC, mg/g). Among these, RDMC was calculated by dividing dry mass by fresh mass, and SRL was calculated by dividing the length of the roots by their dry mass. We also set aside subsample (ca. 0.05 g) of fine root from each species to determined root colonization by AM fungi as we previously described (Huangfu et al., 2019), and root AM fungi colonization (%) was calculated.
We collected bulk soil samples to a depth of 15 cm with five replications before labeling, sieved (2 mm), and stored at 4°C for measurement of soil properties. The characteristics of soils including moisture content (40.92 ± 2.71%) and temperature (28 ± 1.24°C) were measured in situ when the labeling was done. Soil total N was analyzed according to the Kjeldahl digestion procedure (Bremner and Tabatabai, 1972). and was extracted with 2 M KCl and determined using an auto-analyzer (AA3, Bran-Luebbe, Germany). The soil glycine content was also determined in the extracts (Näsholm et al., 1987) using an HPLC (Waters 515, Waters Inc., United States).
Calculations and Statistical Analysis
The 15N atom percent excess (APE) was calculated as the difference of the atom% 15N in plants between the 15N labeled and control treatments:
The net uptake rates of N for each plant species (NUR, μg N g–1 d.w. root h–1) were calculated following McKane et al. (2002) and Miller and Bowman (2003):
The 15N uptake (μg 15N plant–1), defined as the amount of 15N recovered from N pool (whole plant), was calculated by multiplying APE (%) with the moles of N in the plants as follows:
The percentage of 15N recovered in plants (15Nrecovery) was calculated using the following equation:
where 15Nuptake (μg 15N plant–1) refers to the 15N mass uptake by plants, and 15Nadded (μg 15N plant–1) refers to the total 15N mass added to the soil per plot. The contributions of each N form were calculated by dividing the N uptake rate by the sum of uptake rates of the three N forms.
The normal distribution of the data and the homogeneity of variances were tested using the Kolmogorov–Smirnov and Levene’s test, respectively. For the hydroponic experiment, we used the two-way analysis of variance (ANOVA) to estimate the effects of species, N form (the main factors) and their interactions on the N uptake rates. A significant interaction between species and N form would indicate that plant took up different N sources in a species-specific way. To test hypothesis 1, differences between N forms were established according to the Tukey’s HSD post hoc test. Data of the two species were analyzed separately. To test hypothesis 2, we also investigated the relationships between root functional traits and total N uptake rate as well as uptake rates of different forms of N using Pearson correlation analysis. The differences in root traits and AM fungi colonization between species were determined using paired t-test. For the in situ experiment, three-way ANOVA was used to test plant composition, species, N form (the main factors) and their interactions on N uptake rates and 15N recovery separately, followed by the Tukey post hoc test. An interaction between plant composition and N form would indicate different N acquisition strategies depending on competition regimes. To test hypothesis 3, the difference in contribution of each N form (calculated by dividing the N uptake rate by the sum of uptake rates of the three forms) among different plant combinations were determined by one-way ANOVA for each species. Differences in total biomass and the root to shoot ratios for the two species were analyzed by two-way ANOVA, testing the main and interactive effects of species and competition regime. We performed all statistics using the SPSS software 17.0 (IBM Inc., Chicago, IL, United States). Figures were designed using OriginPro 9.1. Differences were tested for significance at P = 0.05.
Results
The Root Functional Traits
All root functional traits except 15N natural abundance (measured in subsection of “Plant and Soil Sampling and Analyses”) were significantly different between the two plant species. C. thunbergii had a significantly higher mean RDMC (210 vs 40 mg/g), lower SRL (32.10 vs 51.75 m/g), lower N concentration (6.43 vs 16.45 mg/g), and AM fungi colonization than P. criopolitanum (4.39 vs 73.5%, Table 2).
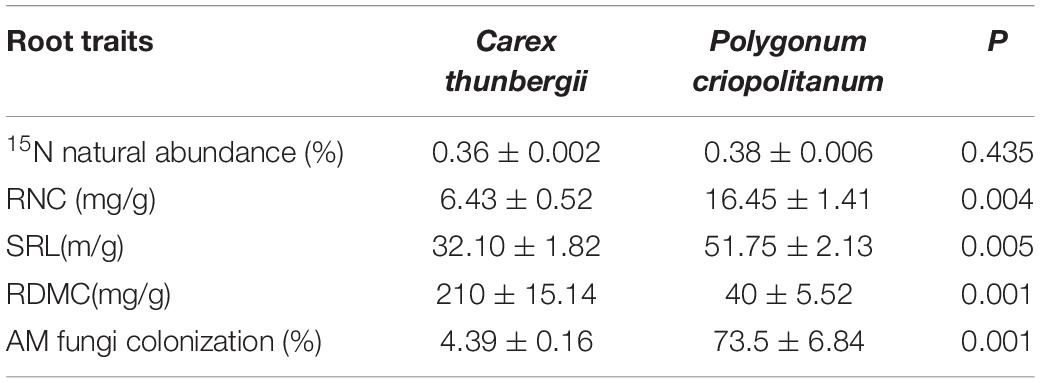
Table 2. Variation of root functional traits of two plant species (Mean ± SE) tested by paired t-test. RNC, SRL, and RDMC indicated root nitrogen content, specific root length, root dry matter content, and AM fungi colonization, respectively.
The Plant Uptake Rates for Different N Forms: Hydroponic Experiment
The net N uptake rates (including , , and glycine-N) differed among two plant species (F = 135.13, P < 0.001) and N forms (F = 84.42, P < 0.001). The sum of net uptake rates of three N forms was almost four times higher for P. criopolitanum than for C. thunbergii (60.12 vs 12.30 μg N g–1 d.w. root h–1, Figure 1). Further, there were significant interactions of plant species × N form (F = 16.63, P < 0.001). To be specific, both species took up N in the form of at the highest rate (P < 0.05). While and glycine-N contributed over one third to net N uptake for P. criopolitanum, they only contributed around 9% to net N uptake for C. thunbergii.
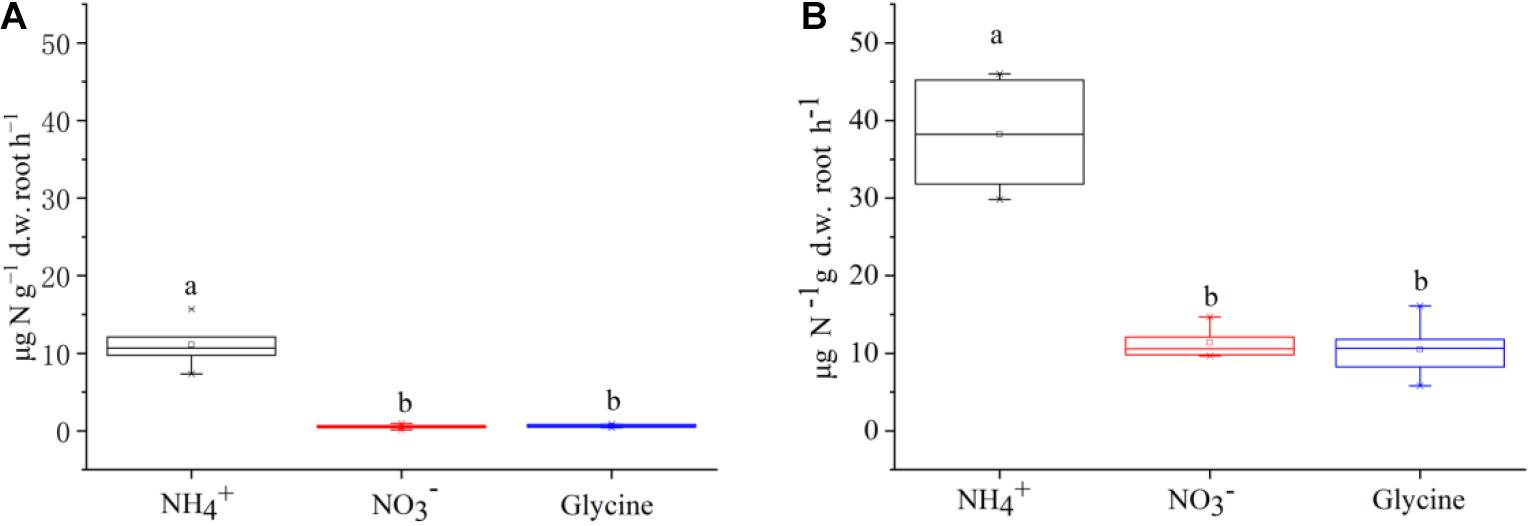
Figure 1. Uptake rates of glycine, , and by Carex thunbergii (A) and Polygonum criopolitanum (B) using excised roots under different treatments. Values are presented as means ± SE of five replicates. Different lowercase letters indicate significant differences in uptake rates among N forms for each species (one-way ANOVA, P < 0.05).
The Effect of Plant Competition on Plant Growth and Uptake Rates of Different N Forms: in situ 15N Labeling Experiment
There were no significant difference among plant combinations in total biomass for either species (F = 1.57, P = 0.205). However, interspecific competition altered their biomass allocation patterns. Specially, P. criopolitanum allocated more biomass into belowground part with increasing density of competitor, leading to higher root to shoot ratio (Supplementary Figure 1, P < 0.05). Net uptake rates of different N forms were significantly affected by species, plant composition, N form, and their interactions (Table 3, all P < 0.05 except for species × plant composition). With the exception of combinations dominated by either species, net uptake rates of inorganic N were comparable for C. thunbergii and P. criopolitanum, and higher for than for . The comparisons of uptake rates for glycine were largely species- or plant composition-dependent (Figures 2, 3).
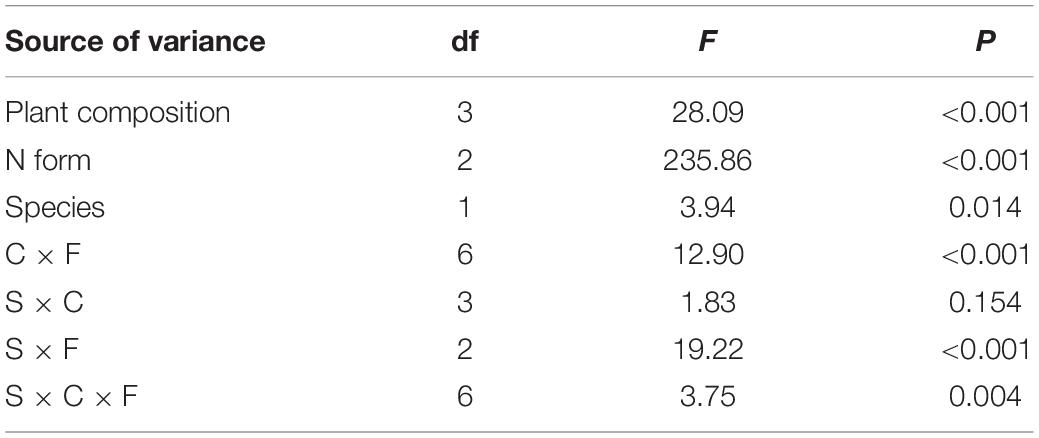
Table 3. The three-way analysis of variance (ANOVA) results for the nitrogen (N) uptake rates of Carex thunbergii and Polygonum criopolitanum with plant composition (C), species (S), and N form (F) as the main factors.
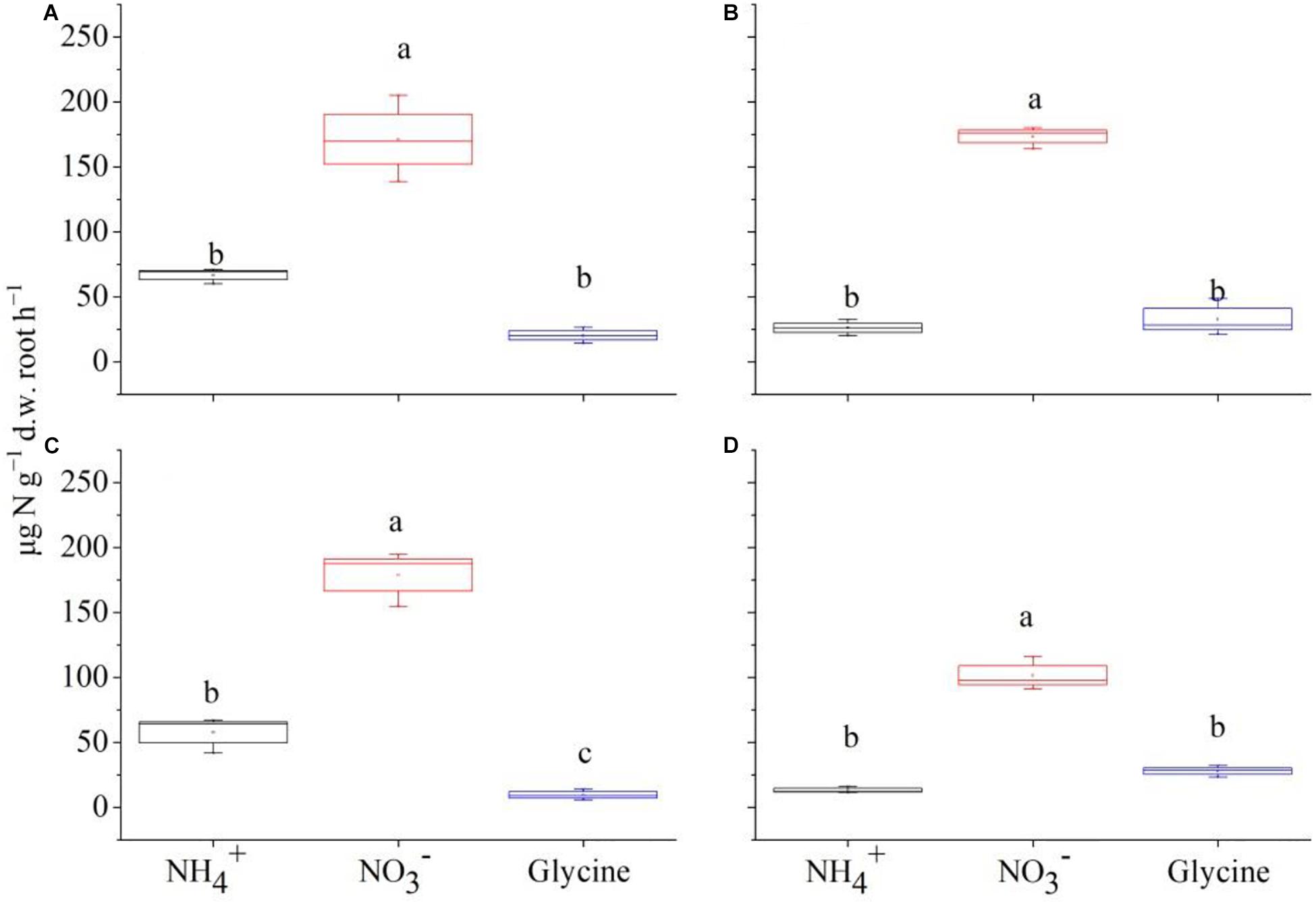
Figure 2. The mass-specific net rate of nitrogen (μg N g–1 d.w. root h–1) in the form of , , and glycine-N by Carex thunbergii under competition regime of 4:0 (C. thunbergii: Polygonum criopolitanum, A), 3:1 (B), 2:2 (C), and 1:3 (D), respectively. The values are means ± SE of three replicates. Different letters above each symbol indicate significant differences of mass-specific net rate of N uptake among three different N forms within a specific competition regime treatment at P < 0.05.
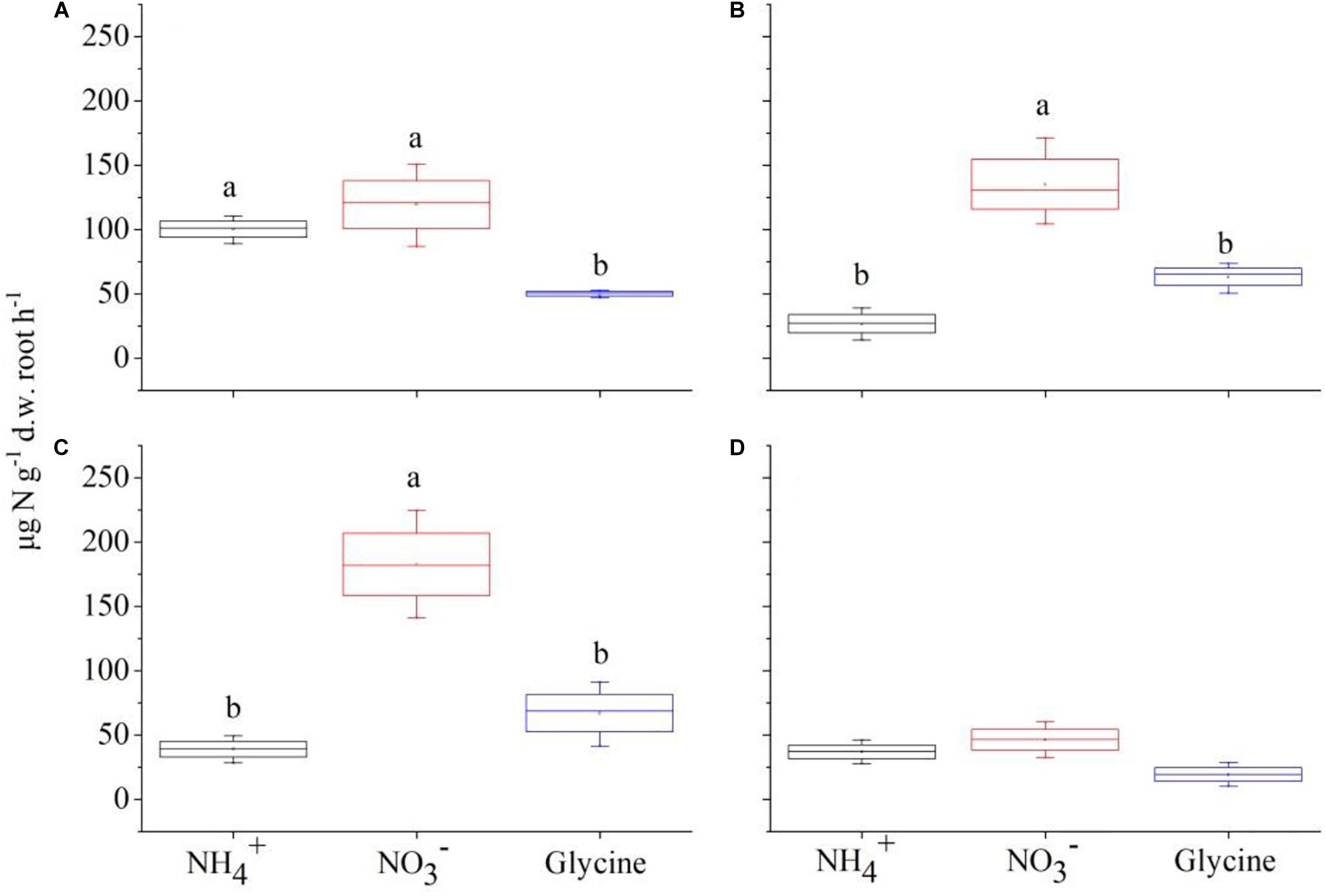
Figure 3. The mass-specific net rate of nitrogen (μg N g–1 d.w. root h–1) in the form of , , and glycine-N by Polygonum criopolitanum under competition regime of 4:0 (Polygonum criopolitanum: Carex thunbergii, A), 3:1 (B), 2:2 (C), and 1:3 (D), respectively. The values are means ± SE of three replicates. Different letters above each symbol indicate significant differences of mass-specific net rate of N uptake among three different N forms within a specific competition regime treatment at P < 0.05.
For C. thunbergii, in contrast to monoculture, interspecific competition significantly decreased uptake rates at plant combination of 3:1 and 2:2 (C. thunbergii: P. criopolitanum), while the uptake rates of and glycine were not affected (Figures 2B,C). However, when the community was dominated by P. criopolitanum (at 1:3), the uptake rates of both and were greatly reduced (P < 0.05, Figure 2D). Relatively, P. criopolitanum in monoculture acquired N in the form of and at comparable rates. Similarly, interspecific competition from C. thunbergii significantly decreased uptake rates at plant composition of 3:1 and 2:2 (P < 0.05, Figures 3B,C). Finally, interspecific competition significantly decreased N uptake rates in the form of by P. criopolitanum when two species growing at 3:1, as a result, three forms of N contributed equally to the total N uptake (P > 0.05, Figure 3D).
Recovery of 15N by Plants
Two-way ANOVA showed that species (F = 402.58, P < 0.001) and plant composition (F = 104.98, P < 0.001), as well as their interaction (F = 89.09, P < 0.001) had significant effects on total N recovery (%). Specifically, P. criopolitanum generally recovered more 15N than C. thunbergii. In the monoculture treatment, for example, total 15N recovery in P. criopolitanum (89.53 ± 3.27%) was four times higher than in C. thunbergii (19.12 ± 0.56%) 2 h after labeling (Figures 4, 5, P < 0.05). Overall, plant composition did not affect total 15N recovery by C. thunbergii with the exception when growing at 1:3 (13.73 ± 0.54%, F = 6.81, P = 0.014, Figure 4D). On the contrary, the total 15N recovery in P. criopolitanum decreased with the increase of competitor density (40.34 ± 3.39%, 27.17 ± 2.06%, 30.55 ± 2.18% for 1:3, 2:2, 3:1, respectively, F = 107.52, P < 0.001, Figure 5).
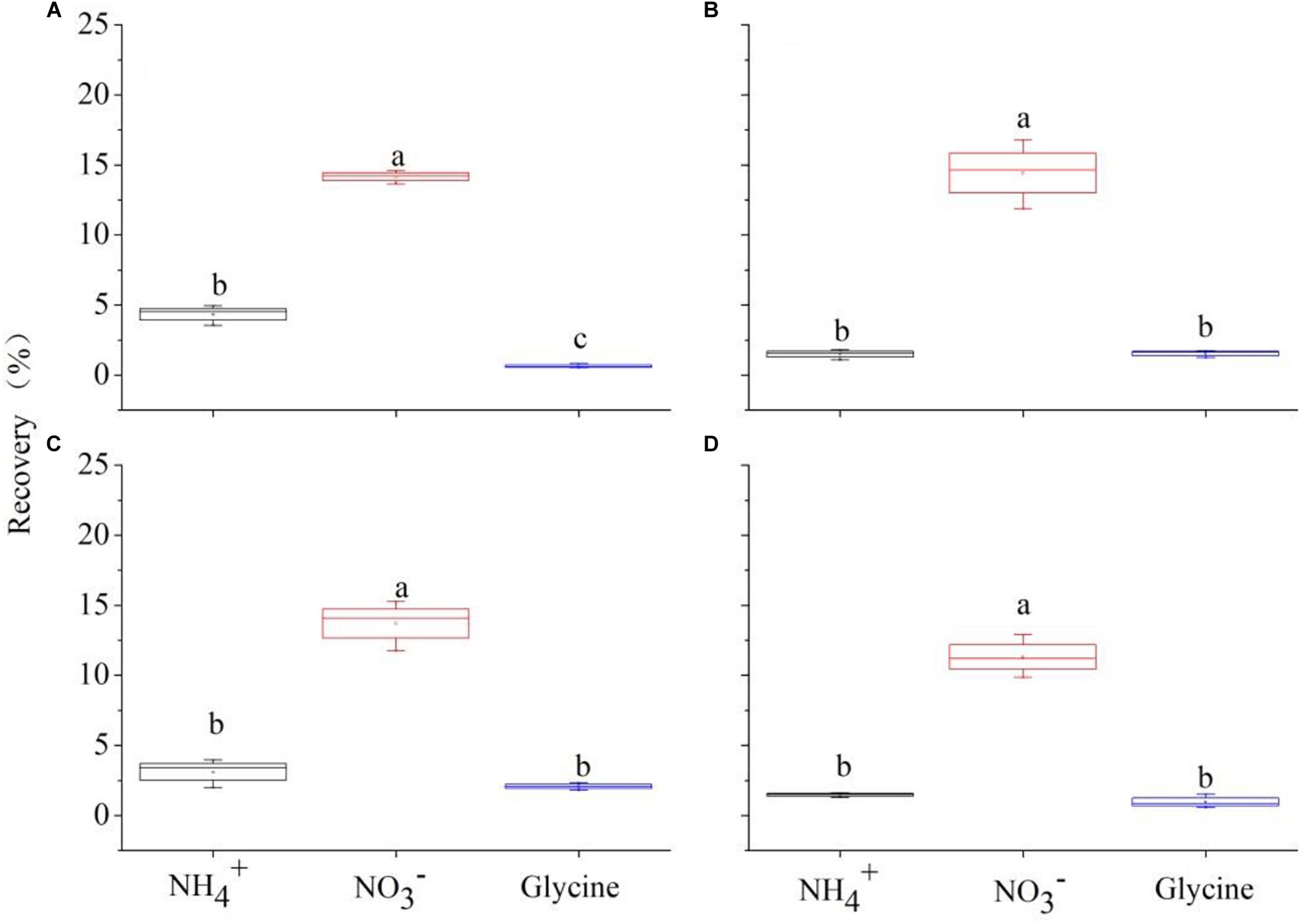
Figure 4. 15N recovered by Carex thunbergii (% of added 15N) from , , and glycine-N 2 h after 15N injection under competition regime of 4:0 (Carex thunbergii: Polygonum criopolitanum, A), 3:1 (B), 2:2 (C), and 1:3 (D), respectively. The bars and error bars show means ± SE (n = 3).
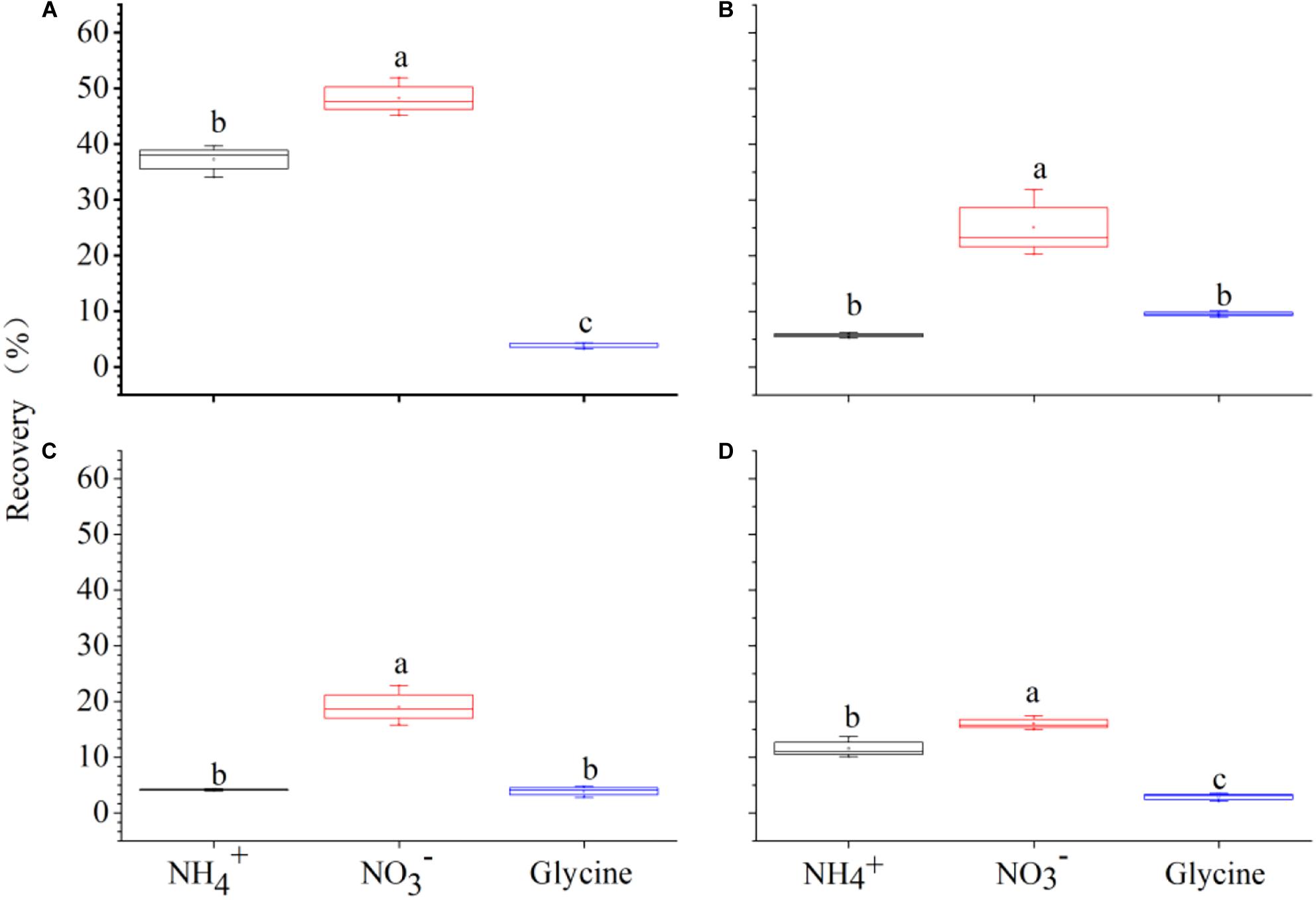
Figure 5. 15N recovered by Polygonum criopolitanum (% of added 15N) from , , and glycine-N 2 h after 15N injection under competition regime of 4:0 (Polygonum criopolitanum: Carex thunbergii, A), 3:1 (B), 2:2 (C), and 1:3 (D), respectively. The bars and error bars show means ± SE (n = 3).
Relatively, N form had a more important effect on 15N recovery (F = 482.62) than species (F = 463.47) and plant composition (F = 120.86), and 15N recovery was also influenced by its interactions with species and plant composition (all P < 0.001, Table 4). For C. thunbergii, its N recovery under competition was context-dependent. When growing with low and medium competition from P. criopolitanum (at 3:1 and 2:2 ratios), C. thunbergii increased N recovery in the form of and glycine while recovery of was generally stable. When the competition continued to increase (i.e., at 1:3 ratio), both and were reduced (Figure 4), leading to an overall decrease in total N recovery. In contrast, interspecific competition had a remarkable effect on 15N recovery in any form by P. criopolitanum (P < 0.05, Figure 5). Consequently, 15N recoveries of and by P. criopolitanum were greatly reduced under competition compared to the monoculture. The recovery of -15N was reduced by 48.06% (at ratio of 1:3) to 66.74% (at ratio of 3:1, all P < 0.05). Collectively, the uptake of glycine by P. criopolitanum was significantly enhanced in the presence of C. thunbergii (Figure 5B, P < 0.05). Similar results were observed for at ratio of 3:1, leading to overall similar total N recovery among three competition treatments (Figure 5, P > 0.05).
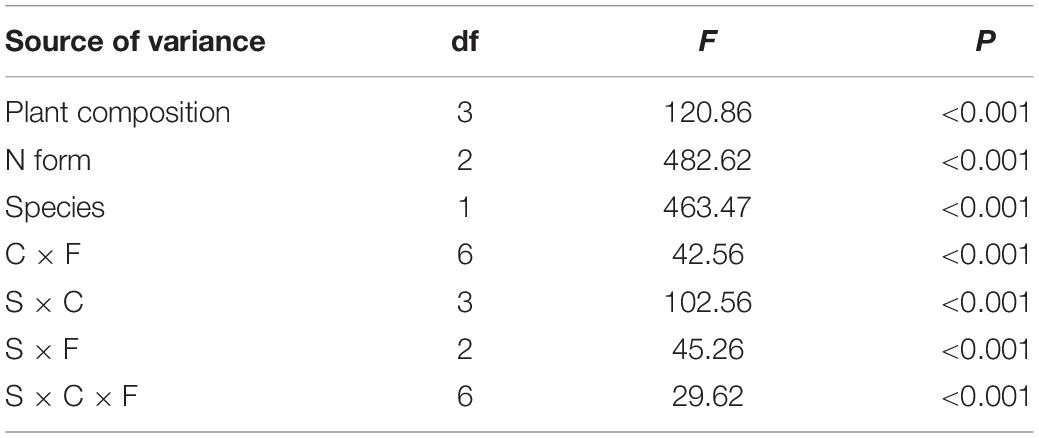
Table 4. The analysis of variance (ANOVA) results for the 15N recovery of Carex thunbergii and Polygonum criopolitanum with plant composition (C), species (S), and N form (F) as the main factors.
Although competition did not change the order of uptake preference for the three N forms among two focal species, they did change the contributions of three N forms (Figure 6). For all treatments, uptake of accounted for more than 50% of total N uptake (sum of the uptake of the three N forms), with the highest value for C. thunbergii (77.91% on average). The difference of two species in N acquisition observed was mainly associated with the contributions of and glycine. Though competition increased the uptake of glycine, its contribution to N recovery by P. criopolitanum seemed to be more important (Figure 6, P < 0.05). The contribution of glycine to P. criopolitanum was increased by 19.28, 9.92, and 5.06% of the total N uptake at ratios of 3:1, 2:2, 1:3, respectively, and was significantly higher than that to C. thunbergii (5.47, 7.65, and 3.72%, Figure 6, P < 0.05). While two plant species decreased uptake under competition, the contribution of to P. criopolitanum was nearly 38% of the total N uptake, which was comparable to the control with no competition (41%, Figure 6, P > 0.05).
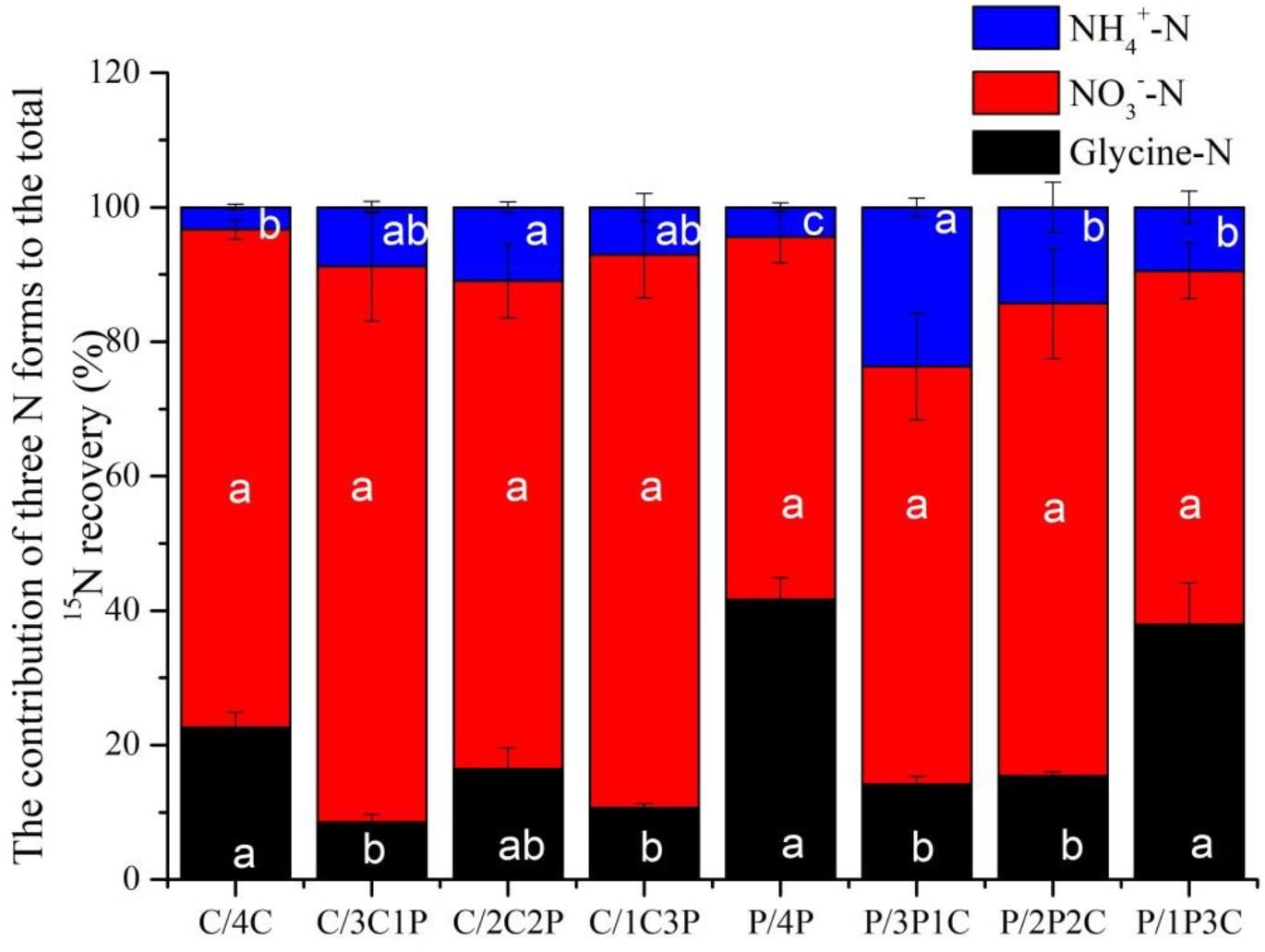
Figure 6. Contributions of N recovery in three forms for Carex thunbergii and Polygonum criopolitanum in the field experiment. Treatment codes: C and P signify C. thunbergii, and P. criopolitanum individuals, respectively, followed by the specific combination tested, in which the composition of each component species is indicated by numerals. Values indicate the mean ± SE (n = 3). For each species, bars with different lowercase letters denote a significant difference between combinations within same N form at P < 0.05.
Correlations Between Root Traits and N Uptake Rates (Hypothesis 2)
Correlation analysis revealed that both RNC and SRL were significantly positively correlated with the total N uptake rate and its components (all r > 0.75, P < 0.01), and RDMC was significantly negatively correlated with these variables (r < −0.85, P < 0.01). There was no significant relationship between the 15N natural abundance value and N uptake rates (P > 0.50, Supplementary Table 1).
Discussion
Our results provided evidence of niche complementarity for the resource use of species regulated by competition regimes. Two species differed in their N acquisition strategies in response to competition: both preferred when grown in monoculture, while in the presence of competitors, the preference of fast-growing C. thunbergii persisted but P. criopolitanum acquired more and glycine-N, especially with increasing neighbor densities.
N Uptake Variations Dominated by N Form
Previous researchers revealed that N uptake is related to N form, N availability in soils, and species identity (Britto and Kronzucker, 2013). In our in situ study, N form acted as the dominant factor driving N uptake, and plant species showed various influences. Though it was often found that plants obtain N from soil proportionally to its availability, contrary to our hypothesis one, both species in this study preferred N in the form of , followed by , and lastly glycine, regardless of N availability in the soil. Similar result has been reported with Fagus grandifolia, which takes up approximately five times more than , despite the fact that content is lower than in soils (Templer and Dawson, 2004). Limited niche partitioning of N uptake among the coexisting species has been reported in subtropical ecosystems (Yang et al., 2018), which might be largely explained by species-specific differences (Miller and Bowman, 2003) rather than the relative dominance among N forms in soils (Schimel and Bennett, 2004). Relatively, is easily diffused to the root surface through water flow due to the difference in the mobility of different N forms (Hodge et al., 2000), whereas is preferably immobilized by microorganisms in the field (Romero et al., 2015). This process is particularly of importance in wetland ecosystems where soil moisture is often higher than that of most terrestrial ecosystems. In this context, sufficient water can selectively enhance the delivery of to the root surface, and consequently affect their uptake by plants (Boudsocq et al., 2012).
Another consideration for domination is associated with pH of growth media. Plant growth preference for N forms can shift from at pH of 5.5 to at pH of 6.4 (Teyker and Hobbs, 1992). Therefore, in contrast to , seemed less important, at least for C. thunbergii in this system. Compared to extreme ecosystems such as alpine or arctic region, the general less contribution of glycine in the wetland ecosystems might reflect the lower availability of glycine in the soil, largely conforming with habitat filtering selection patterns (Schimann et al., 2008). Our judicious estimates of contribution of glycine to the total N uptakes ranged between 3 and 25% by wetland species was lower than that reported by the dominant species in alpine meadows (13–35%, Xu et al., 2006) and alpine wetlands (20–40%) (Gao et al., 2014).
Species-Specific N Uptake in Response to Plant Competition
In line with our hypothesis 3, interactions between two species indicated asymmetric competition in term of N uptake pattern: the presence of competitor caused less plasticity in dominant species (C. thunbergii) than subdominant species (P. criopolitanum), supporting niche preemption hypothesis which predicated that the species with low abundance switches to a different N sources while species with high abundance takes up N mostly from (Ashton et al., 2010). Functional traits have been recognized to be related to plant N acquisition strategies (Hong et al., 2018). In this study, the lack of niche partitioning of N acquisition between two species might be explained by their root N niche separation in space instead of chemical form of N (von Felten et al., 2012). C. thunbergii, a rhizomatous clonal sedge characterized by high deep rooting and branching frequency (Gao et al., 2012), is capable of exploiting more soil volumes and thus reducing the diffusion path of N to root surfaces (Fitter, 1991). Critically, rhizomes of C. thunbergii might be beneficial in assisting N translocation among different clone parts rooting under different nutrient patches (Stuefer, 1998), showing a much stable N acquisition strategy. P. criopolitanum is a shallow-rooted forb, which might take up N concentratedly in the upper soil and must shift its N niche when growing with competitors by acquisition of a greater variety of N forms (Mommer et al., 2011), showing a relatively flexible N acquisition strategy. Future work is needed to develop occupancy models for species root systems in natural communities with more root functional traits included.
In spite of this, thinner roots of P. criopolitanum (as shown by high SRL) allow it to maximize N capture, leading to more efficient N acquisition (Hong et al., 2018), which was evident in the hydroponic experiment when equal root mass used. In supporting of our hypothesis 2, therefore, root functional traits such as high SRL can be similarly functional or more effective in nutrient foraging than placing roots selectively in nutrient hotspots (Mommer et al., 2011). Considering larger size of C. thunbergii than that of P. criopolitanum, this seeming counter-intuitive pattern might be ascribed to the luxury N utilization of P. criopolitanum, whereby excess N resources were taken up relative to the immediate growth rate (e.g., Liu et al., 2020). The increasing root to shoot ratios of P. criopolitanum, along with its higher N content than C. thunbergii (Table 2; Meng et al., 2020), provided additional evidence that this forb may have more efficient N uptake ability due to relative exploitative traits.
N niche partitioning could also be mediated by mycorrhizal symbiosis with plant species (Gerz et al., 2018). Previous studies have shown that symbiosis with arbuscular mycorrhizal (AM) fungi which are highly effective at acquiring N, especially in the form of (Jin et al., 2005). As such, more recovery of by P. criopolitanum was likely due to the possibility that the effects of interspecific competition were mitigated by its symbiotic AM fungi in N acquisition and reduced dependency on external N supply. Moreover, increasing glycine uptake by P. criopolitanum may be due to the fact that it was subordinate in the soil N pool, as a compensation, help host plant buffer competition from the competitors under N-limited conditions (McKane et al., 2002; Silvertown, 2004; Miller et al., 2007).
Variations of N Uptake Ability Regulated by the Neighbor Density
One way to test plant species partitioning in resource use is manipulating the neighbor density and evaluate if this manipulation changes the use patterns of interspecific competitor at community level (Silvertown, 2004). In support of our hypothesis 3, the neighbor density significantly influenced N uptake pattern of plant species (thereby N preference), in particular for P. criopolitanum. Such a change in N acquisition as a result of the shifts in competition regime has been invoked, but has rarely been tested (Miller et al., 2007). In a recent study, Huangfu and Li (2019) revealed that the density of an invasive species in a community was crucial in determining not only its direct ecological impacts (e.g., growth performance) but also the strength of trophic cascades (e.g., N uptake pattern) of neighboring native species. The density-dependent model predicated that plant growth, as well as associated ecological functions (e.g., nutrient uptake) would decreases as density increases when resource competition is the dominating factor in plant interactions (Barto and Cipollini, 2009). This is the case for the relationship between C. thunbergii and P. criopolitanum in our study, likely due to their N niche overlap (Huangfu and Li, 2019). Therefore, the finding of lower total N recovery by C. thunbergii in competition when P. criopolitanum dominated a community compared to monoculture signified a mediation of plant competition. Particularly, slightly higher total N uptake by P. criopolitanum at ratio of 3:1 than that at 2:2 could be found when interspecific competition from both species was lowered, assumedly due to the niche shift under competition (Miller et al., 2007). In this case, for instance, the increased planting density of C. thunbergii reduced the soil volume for root of P. criopolitanum, thereby the selection for separations of N in chemical forms should become strong. Overall, our results indicated there was stronger competition between species for than other N forms with increasing neighbor densities, and subdominant species could shift its preference for to meet their N demand by flexible uptake for alternative N forms. Recognition of the density-dependency in the provision of essential ecosystem services leads to a new conceptual insight into how competition regime change key ecosystem processes. Furthermore, given that was in high demand by focal species, especially C. thunbergii, not much of would lose via denitrification or leaching at sites where they dominated.
We recognize that only two plant species were included in this study. Consequently, the lack of replication at the species level limited the scope of inference of the results and interpretation of N foraging differences between species. Further research incorporating a large number of wetland species from different functional groups is needed to better understand the relationship between plant functional traits and associated resources acquisition strategies.
Conclusion and Implications
Two plant species shared common preferences for N sources when grew in monoculture, but they showed divergent responses and species-specific patterns in N acquisition strategies under different competition regimes. Collectively, the higher and glycine but lower acquisition by P. criopolitanum compared to C. thunbergii in competition suggested that the subdominant species could have a relative advantage over dominant species via better exploitation of less accessible soil source (Xi et al., 2017). These divergences in N acquisition between two species might be partially explained by different root functional traits and the degree of N uptake plasticity. Therefore, the species coexistence is likely realized under the field conditions via niche partitioning in N uptake (Kahmen et al., 2006).
Data Availability Statement
The original contributions presented in the study are included in the article/Supplementary Material, further inquiries can be directed to the corresponding author.
Author Contributions
XJ: methodology, software, validation, formal analysis, investigation, data curation, and writing–original draft. CH: conceptualization, methodology, validation, formal analysis, investigation, data curation, writing, review and editing, and supervision funding acquisition. DH: methodology, validation, and review and editing. All authors contributed to the article and approved the submitted version.
Conflict of Interest
The authors declare that the research was conducted in the absence of any commercial or financial relationships that could be construed as a potential conflict of interest.
Acknowledgments
This work was supported, in part, by the High-level Talent Introduction Starting Funding of Anhui University. DH was supported by the United States National Science Foundation projects.
Supplementary Material
The Supplementary Material for this article can be found online at: https://www.frontiersin.org/articles/10.3389/fpls.2020.584370/full#supplementary-material
References
Andersen, K. M., Mayor, J. R., and Turner, B. L. (2017). Plasticity in nitrogen uptake among plant species with contrasting nutrient acquisition strategies in a tropical forest. Ecology 98, 1388–1398. doi: 10.1002/ecy.1793
Ashton, I. W., Miller, A. E., Bowman, W. D., and Suding, K. N. (2010). Niche complementarity due to plasticity in resource use: plant partitioning of chemical N forms. Ecology 91, 3252–3260. doi: 10.1890/09-1849.1
Barto, E. K., and Cipollini, D. (2009). Density-dependent phytotoxicity of Impatiens pallida plants exposed to extracts of Alliaria petiolata. J. Chem. Ecol. 35, 495–504. doi: 10.1007/s10886-009-9629-1
Boudsocq, S., Niboyet, A., Lata, J. C., Raynaud, X., Loeuille, N., Mathieu, J., et al. (2012). Plant preference for ammonium versus nitrate: a neglected determinant of ecosystem functioning? Am. Nat. 180, 60–69. doi: 10.1086/665997
Bremner, J. M., and Tabatabai, M. A. (1972). Use of an ammonia electrode for determination of ammonia in Kjedahl analysis of soils. Commun. Soil Sci. Plant Anal. 3, 159–165. doi: 10.1080/00103627209366361
Britto, D. T., and Kronzucker, H. J. (2013). Ecological significance and complexity of N-source preference in plants. Ann. Bot. 112, 957–963. doi: 10.1093/aob/mct157
Cao, L., and Fox, A. D. (2009). Birds and people both depend on China’s wetlands. Nature 460:173. doi: 10.1038/460173b
Dijkstra, F. A., He, M. Z., Johan, M. P., Harrison, J. J., and Keitel, C. (2015). Plant and microbial uptake of nitrogen and phosphorus affected by drought using 15N and 32P tracers. Soil Biol. Biochem. 82, 135–142. doi: 10.1016/j.soilbio.2014.12.021
Fitter, A. H. (1991). “The ecological significance of root system architecture: an economic approach,” in Plant Root Growth: An Ecological Perspective, ed. D. Atkinson (Oxford: Blackwell Scientific Publications), 229–243.
Gao, J. Q., Yi, M., Xu, X. L., Zhang, X. W., and Yu, F. H. (2014). Spatiotemporal variations affect uptake of inorganic and organic nitrogen by dominant plant species in an alpine wetland. Plant Soil 381, 271–278. doi: 10.1007/s11104-014-2130-9
Gao, L., Cui, X., Hill, P. W., and Guo, Y. (2020). Uptake of various nitrogen forms by co-existing plant species in temperate and cold-temperate forests in northeast China. Appl. Soil Ecol. 147:103398. doi: 10.1016/j.apsoil.2019.103398
Gao, Y., Xing, F., Jin, Y., Nie, D., and Wang, Y. (2012). Foraging responses of clonal plants to multi-patch environmental heterogeneity: spatial preference and temporal reversibility. Plant Soil 359, 137–147. doi: 10.1007/s11104-012-1148-0
Gerz, M., Guillermo, B. C., Ozinga, W. A., Zobel, M., and Moora, M. (2018). Niche differentiation and expansion of plant species are associated with mycorrhizal symbiosis. Ecology 106, 254–264. doi: 10.1111/1365-2745.12873
Hodge, A., Robinson, D., and Fitter, A. (2000). Are microorganisms more effective than plants at competing for nitrogen? Trends Plant Sci. 5, 304–308. doi: 10.1016/S1360-1385(00)01656-3
Hong, J., Ma, X., Yan, Y., Zhang, X., and Wang, X. (2018). Which root traits determine nitrogen uptake by alpine plant species on the Tibetan Plateau? Plant Soil 424, 63–72. doi: 10.1007/s11104-017-3434-3
Hong, J., Ma, X., Zhang, X., and Wang, X. (2017). Nitrogen uptake pattern of herbaceous plants: coping strategies in altered neighbor species. Biol. Fertil. Soils 53, 729–735. doi: 10.1007/s00374-017-1230-0
Houlton, B. Z., Sigman, D. M., Schuur, E. A. G., and Hedin, L. O. (2007). A climate-driven switch in plant nitrogen acquisition within tropical forest communities. Proc. Natl. Acad. Sci. U.S.A. 104, 8902–8906. doi: 10.1073/pnas.0609935104
Huangfu, C. H., and Li, K. L. (2019). Growing density interacts with competitor identity to modulate nitrogen form preference of an invasive plant. Ecol. Indic. 107:105641. doi: 10.1016/j.ecolind.2019.105641
Huangfu, C. H., Li, K. L., and Hui, D. F. (2019). Influences of plant interspecific competition and arbuscular mycorrhizal fungi on nitrogen form preference of an invasive plant. Biogeochemistry 145, 295–313. doi: 10.1007/s10533-019-00607-z
Inselsbacher, E., and Näsholm, T. (2012). The below-ground perspective of forest plants: soil provides mainly organic nitrogen for plants and mycorrhizal fungi. New Phytol. 195, 329–334. doi: 10.1111/j.1469-8137.2012.04169.x
Jiang, L., Wang, S., Zhe, P., Wang, C., Kardol, P., Zhong, L., et al. (2017). Effects of grazing on the acquisition of nitrogen by plants and microorganisms in an alpine grassland on the Tibetan Plateau. Plant Soil 416, 297–308. doi: 10.1007/s11104-017-3205-1
Jin, H., Pfeffer, P. E., Douds, D. D., Piotrowski, E., Lammers, P. J., and Shachar-Hill, Y. (2005). The uptake, metabolism, transport and transfer of nitrogen in an arbuscular mycorrhizal symbiosis. New Phytol. 168, 687–696. doi: 10.1111/j.1469-8137.2005.01536.x
Kahmen, A., Renker, C., Unsicker, S. B., and Buchmann, N. (2006). Niche complementarity for nitrogen: an explanation for the biodiversity and ecosystem functioning relationship? Ecology 87, 1244–1255. doi: 10.1890/0012-9658(2006)87[1244:NCFNAE]2.0.CO;2
Kuster, T. M., Wilkinson, A., Hill, P. W., Jones, D. L., and Bardgett, R. D. (2016). Warming alters competition for organic and inorganic nitrogen between co-existing grassland plant species. Plant Soil 406, 117–129. doi: 10.1007/s11104-016-2856-7
Lambers, H., Raven, J. A., Shaver, G. R., and Smith, S. E. (2008). Plant nutrient acquisition strategies change with soil age. Trends Ecol. Evol. 23, 95–103. doi: 10.1016/j.tree.2007.10.008
Lebauer, D. S., and Treseder, K. K. (2008). Nitrogen limitation of net primary productivity in terrestrial ecosystems is globally distributed. Ecology 89, 371–379. doi: 10.1890/06-2057.1
Legay, N., Grassein, F., Arnoldi, C., Segura, R., Laîné, P., Lavorel, S., et al. (2020). Studies of and uptake ability of subalpine plants and resource-use strategy identified by their functional traits. Oikos 129, 830–841. doi: 10.1111/oik.07282
Liese, R., Lübbe, T., Albers, N. W., and Meier, I. C. (2018). The mycorrhizal type governs root exudation and nitrogen uptake of temperate tree species. Tree Physiol. 38, 83–95. doi: 10.1093/treephys/tpx131
Liu, M., Li, H., Song, J., Song, M., Qiao, N., Tian, Y., et al. (2020). Interactions between intercropped Avena sativa and Agropyron cristatum for nitrogen uptake. Plant Soil 447, 611–621. doi: 10.1007/s11104-019-04389-z
Liu, S. L., Hou, X. Y., Yang, M., Cheng, F. Y., Coxixo, A., Wu, X., et al. (2018). Factors driving the relationships between vegetation and soil properties in the Yellow River Delta, China. Catena 165, 279–285. doi: 10.1016/j.catena.2018.02.004
McKane, R. B., Johnson, L. C., Shaver, G. R., Nadelhoffer, K. J., Rastetter, E. B., Fry, B., et al. (2002). Resource-based niches provide a basis for plant species diversity and dominance in arctic tundra. Nature 415, 68–71. doi: 10.1038/415068a
Meng, Y., Hui, D., and Huangfu, C. (2020). Site conditions interact with litter quality to affect home-field advantage and rhizosphere effect of litter decomposition in a subtropical wetland ecosystem. Sci. Total Environ. 737:141442. doi: 10.1016/j.scitotenv.2020.141442
Miller, A. E., and Bowman, W. D. (2003). Alpine plants show species-level differences in the uptake of organic and inorganic nitrogen. Plant Soil 250, 283–292. doi: 10.1023/A:1022867103109
Miller, A. E., Bowman, W. D., and Suding, K. N. (2007). Plant uptake of inorganic and organic nitrogen: neighbor identity matters. Ecology 88, 1832–1840. doi: 10.1890/06-0946.1
Mommer, L., Visser, E. J. W., van Ruijven, J., de Caluwe, H., Pierik, R., and de Kroon, H. (2011). Contrasting root behaviour in two grass species: a test of functionality in dynamic heterogeneous conditions. Plant Soil 344, 347–360. doi: 10.1007/s11104-011-0752-8
Moreau, D., Bardgett, R. D., Finlay, R. D., Jones, D. L., and Philippot, L. (2019). A plant perspective on nitrogen cycling in the rhizosphere. Funct. Ecol. 33, 540–552. doi: 10.1111/1365-2435.13303
Näsholm, T., Kielland, K., and Ganeteg, U. (2009). Uptake of organic nitrogen by plants. New Phytol. 182, 31–48. doi: 10.1111/j.1469-8137.2008.02751.x
Näsholm, T., Sandberg, G., and Ericsson, A. (1987). Quantitative analysis of amino acids in conifer tissues by high-performance liquid chromatography and fluorescence detection of their 9-fluorenylmethyl chloroformate derivatives. J. Chromatogr. A 396, 225–236. doi: 10.1016/S0021-9673(01)94060-9
Romero, C. M., Engel, R., Chen, C., and Wallander, R. (2015). Microbial immobilization of nitrogen-15 labelled ammonium and nitrate in an agricultural soil. Soil Sci. Soc. Am. J. 79, 595–602. doi: 10.2136/sssaj2014.08.0332
Schimann, H., Ponton, S., Hättenschwiler, S., Ferry, B., Lensi, R., Domenach, A. M., et al. (2008). Differing nitrogen use strategies of two tropical rainforest late successional tree species in French Guiana: evidence from 15N natural abundance and microbial activities. Soil Biol. Biochem. 40, 487–494. doi: 10.1016/j.soilbio.2007.09.011
Schimel, J. P., and Bennett, J. (2004). Nitrogen mineralization: challenges of a changing paradigm. Ecology 85, 591–602. doi: 10.1890/03-8002
Scott, E. E., and Rothstein, D. E. (2011). Amino acid uptake by temperate tree species characteristic of low- and high-fertility habitats. Oecologia 167, 547–557. doi: 10.1007/s00442-011-2009-x
Shi, X. Z., Yu, D. S., Sun, W. X., Wang, H. J., Zhao, Q. G., and Gong, Z. T. (2004). Reference benchmarks relating to great groups of genetic soil classification of China with soil taxonomy. Chin. Sci. Bull. 49, 1507–1511. doi: 10.1360/03wd0476
Silvertown, J. (2004). Plant coexistence and the niche. Trends Ecol. Evol. 19, 605–611. doi: 10.1016/j.tree.2004.09.003
Simon, J., Dannenmann, M., Pena, R., Gessler, A., and Rennenberg, H. (2017). Nitrogen nutrition of beech forests in a changing climate: importance of plant-soil-microbe water, carbon, and nitrogen interactions. Plant Soil 418, 89–114. doi: 10.1007/s11104-017-3293-y
Simon, J., Li, X., and Rennenberg, H. (2014). Competition for nitrogen between European beech and sycamore maple shifts in favour of beech with decreasing light availability. Tree Physiol. 34, 49–60. doi: 10.1093/treephys/tpt112
Smith, S. E., and Read, D. (2008). Mycorrhizal Symbiosis, 3rd Edn. Amsterdam: Elsevier Academic Press.
Stuefer, J. F. (1998). Two types of division of labour in clonal plants: benefits, costs and constraints. Perspect. Plant Ecol. Evol. Syst. 1, 47–60. doi: 10.1078/1433-8319-00051
Templer, P. H., and Dawson, T. E. (2004). Nitrogen uptake by four tree species of the Catskill Mountains, New York: implications for forest N dynamics. Plant Soil 262, 251–261. doi: 10.1023/B:PLSO.0000037047.16616.98
Teyker, R. H., and Hobbs, D. C. (1992). Growth and root morphology of corn as influenced by nitrogen. Agron. J. 84, 694–700. doi: 10.2134/agronj1992.00021962008400040030x
Tian, Y., Yu, M., Xu, F., Ouyang, S., Xu, X., Gao, Q., et al. (2020). Uptake of amino acids and inorganic nitrogen by two dominant temperate grasses. Rhizosphere 14:100199. doi: 10.1016/j.rhisph.2020.100199
von Felten, S., Niklaus, P. A., Scherer-Lorenzen, M., Hector, A., and Buchmann, N. (2012). Do grassland plant communities profit from N partitioning by soil depth? Ecology 93, 2386–2396. doi: 10.1890/11-1439.1
Wang, B., and Qiu, Y. L. (2006). Phylogenetic distribution and evolution of mycorrhizas in land plants. Mycorrhiza 16, 299–363. doi: 10.1007/s00572-005-0033-6
Xi, N., Zhu, B., and Zhang, D. (2017). Contrasting grass nitrogen strategies reflect interspecific trade-offs between nitrogen acquisition and use in a semi-arid temperate grassland. Plant Soil 418, 267–276. doi: 10.1007/s11104-017-3296-8
Xu, X., Ouyang, H., Kuzyakov, Y., Richter, A., and Wanek, W. (2006). Significance of organic nitrogen acquisition for dominant plant species in an alpine meadow on the Tibet Plateau, China. Plant Soil 285, 221–231. doi: 10.1007/s11104-006-9007-5
Xu, X. L., Ouyang, H., Cao, G., Richter, A., Wanek, W., and Kuzyakov, Y. (2011). Dominant plant species shift their nitrogen uptake patterns in response to nutrient enrichment caused by a fungal fairy in an alpine meadow. Plant Soil 341, 495–504. doi: 10.1007/s11104-010-0662-1
Yang, L., He, T., Yu, Y., Li, Z., and Li, D. (2018). Community-wide consistence in plant N acquisition during post-agricultural succession in a karst area, southwest China. New For. 49, 197–214. doi: 10.1007/s11056-017-9613-5
Yuan, S., Yang, Z., Liu, X., and Wang, H. (2017). Key parameters of water level fluctuations determining the distribution of Carex in shallow lakes. Wetlands 37, 1005–1014. doi: 10.1007/s13157-017-0934-0
Zemunik, G., Turner, B. L., Lambers, H., and Laliberté, E. (2015). Diversity of plant nutrient-acquisition strategies increases during long-term ecosystem development. Nat. Plants 1:15050. doi: 10.1038/nplants.2015.50
Zhang, Z., Li, N., Xiao, J., Zhao, C., Zou, T., Li, D., et al. (2018). Changes in plant nitrogen acquisition strategies during the restoration of spruce plantations on the eastern Tibetan Plateau, China. Soil Biol. Biochem. 119, 50–58. doi: 10.1016/j.soilbio.2018.01.002
Keywords: nitrogen uptake, organic nitrogen, plant competition, neighbor density, functional traits
Citation: Jia X, Huangfu C and Hui D (2020) Nitrogen Uptake by Two Plants in Response to Plant Competition as Regulated by Neighbor Density. Front. Plant Sci. 11:584370. doi: 10.3389/fpls.2020.584370
Received: 20 July 2020; Accepted: 20 November 2020;
Published: 10 December 2020.
Edited by:
Boris Rewald, University of Natural Resources and Life Sciences, Vienna, AustriaReviewed by:
Florian Fort, UMR 5175 Centre d’Ecologie Fonctionnelle et Evolutive (CEFE), FranceLina Fusaro, Institute for Bioeconomy, CNR, Italy
Copyright © 2020 Jia, Huangfu and Hui. This is an open-access article distributed under the terms of the Creative Commons Attribution License (CC BY). The use, distribution or reproduction in other forums is permitted, provided the original author(s) and the copyright owner(s) are credited and that the original publication in this journal is cited, in accordance with accepted academic practice. No use, distribution or reproduction is permitted which does not comply with these terms.
*Correspondence: Chaohe Huangfu, huangfuch@ahu.edu.cn