- 1Instituto de Biología Molecular y Celular de Rosario (IBR-CONICET) Ocampo y Esmeralda PREDIO CCT-Facultad de Ciencias Bioquímicas y Farmacéuticas (UNR), Rosario, Argentina
- 2Max Planck Institute of Molecular Plant Physiology, Potsdam, Germany
Plants, as sessile organisms, are continuously threatened by multiple factors and therefore their profitable production depends on how they can defend themselves. We have previously reported on the characterization of fitness mutants which are more tolerant to environmental stresses due to the activation of defense mechanisms. Here, we demonstrate that in fitness mutants, which accumulate moderate levels of salicylic acid (SA) and have SA signaling activated, pathogen infection is restricted. Also, we demonstrate that NPR1 is essential in fitness mutants for SA storage and defense activation but not for SA synthesis after Pseudomonas syringae (Pst) infection. Additionally, these mutants do not appear to be metabolically impared, resulting in a higher seed set even after pathogen attack. The FITNESS transcriptional network includes defense-related transcription factors (TFs) such as ANAC072, ORA59, and ERF1 as well as jasmonic acid (JA) related genes including LIPOXYGENASE2 (LOX2), CORONATINE INSENSITIVE1 (COI1), JASMONATE ZIM-domain3 (JAZ3) and JAZ10. Induction of FITNESS expression leads to COI1 downregulation, and to JAZ3 and JAZ10 upregulation. As COI1 is an essential component of the bioactive JA perception apparatus and is required for most JA-signaling processes, elevated FITNESS expression leads to modulated JA-related responses. Taken together, FITNESS plays a crucial role during pathogen attack and allows a cost-efficient way to prevent undesirable developmental effects.
Introduction
Annual crop yield losses due to pathogen attack are a major concern for producers. Although chemical control has been increased, the use of synthetic chemicals represents a threat to global food security and agricultural sustainability (Nelson et al., 2017), and many farmers have started to look into more natural ways for food production. Enhancing plant resistance is an alternative way to avoid the use of chemicals. However, genes that play roles in disease resistance might also affect other important traits such as the response to abiotic factors or yield.
Some plant transcription factors (TFs) have been described as master regulators of essential aspects – such as development and stress response – using different approaches. We recently described the function of FITNESS in Arabidopsis thaliana, which leads to the deregulation of reactive oxygen species (ROS) levels in planta when FITNESS is overexpressed using the CaMV 35S promoter (Osella et al., 2018). FITNESS protein possesses a single CCT (CONSTANS, CONSTANS-like, and TOC1) domain, first described in the protein CONSTANS (Robson et al., 2001). It is included in a family of uncharacterized genes named CCT motif family genes (Cockram et al., 2012). Genes encoding CCT domain proteins have been implicated in processes such as photoperiodic flowering (Putterill et al., 1995), regulation of the circadian rhythm (Strayer et al., 2000), and plant architecture (Ordoñez-Herrera et al., 2018). There are also some examples of CCT members involved in biotic stresses, like ZmCCT10, which is responsive to Gibberella stalk rot resistance in maize (Zea mays; Wang et al., 2017), and OsCOL9, identified as an early response gene in rice (Oryza sativa) after Magnaporthe oryzae infection (Liu et al., 2016).
Under normal growth conditions, transgenic Arabidopsis plants constitutively overexpressing FITNESS (FITNESSox lines) accumulate high ROS levels and show reduced growth and seed set. On the contrary, plant performance was increased in the mutant fitness-1 and fitness-2, compared to Col-0 wild type (WT), resulting in a higher seed set. To better understand the observed phenotypes’ basis, we previously analyzed the global transcriptome and metabolome of the lines with altered FITNESS expression. Transcript abundance of genes related to the biosynthesis and signaling of the plant hormone salicylic acid (SA) was increased in fitness mutants. In line with this, a moderate increase in the levels of free SA was measured (Osella et al., 2018).
Salicylic acid plays an essential role in plant defense against biotrophic and hemibiotrophic pathogens (Fu and Dong, 2013). In Arabidopsis, the bacterium Pseudomonas syringae causes extensive chlorosis and necrotic spots in leaves (Whalen et al., 1991), and basal resistance is predominantly dependent on SA (Wildermouth et al., 2001). After the pathway is activated at the infection site, a similar response is triggered in distal parts of the plant, inducing a broad-spectrum resistance called systemic acquired resistance or SAR (Conrad et al., 2015). The regulatory protein NON-EXPRESSOR OF PATHOGENESIS-RELATED GENES 1 (NPR1), which acts downstream of SA, plays an important role in SA-dependent defense signaling (Backer et al., 2019). Mutations in this gene impair the transcriptional reprograming exerted by SA and the establishment of SAR. NPR1 activation depends on the redox state of the cell. SA accumulation leads to a reduced cellular environment, which triggers the reduction of an oligomeric cytosolic NPR1 complex and its translocation into the nucleus. Once there, it interacts with basic leucine-zipper TFs, such as the TGACG-binding TFs (TGAs), and activates the expression of defense-related genes called PATHOGENESIS-RELATED (PR) genes. PR1 is one of the best-characterized genes of the PR family, and experimentally it is used as a robust marker for SA-responsive gene expression. Expression of both NPR1 and PR1, among others, is elevated in fitness mutants, indicating a constitutive activation of defense responses in the absence of pathogen stress. Constitutive activation of defense responses has frequently been associated with plant growth and productivity penalties. The general assumption is that resources are channeled toward defense responses, thereby compromising plant growth (Zhu et al., 2013). For example, the Arabidopsis mutant suppressor of npr1, constitutive1 (snc1), which accumulates SA and shows NPR1-independent pathogen resistance, is dwarf due to a constitutive defense response (Zhang et al., 2003). Also, the overexpression of Arabidopsis NPR1 (AtNPR1) in rice leads to broad-spectrum resistance associated with the development of a lesion mimic/cell death phenotype and decreased seed production (Fitzgerald et al., 2004; Quilis et al., 2008).
How defense responses repress plant growth at the molecular level is not well understood. Hormone crosstalk has emerged as a major player in regulating tradeoffs needed to balance growth and defense (Hout et al., 2014). Ample evidence exists that SA- and jasmonic acid (JA)-dependent signal transduction pathways cross-communicate during plant defense and enable plants to mount responses specifically tailored to the inducing attacker, improving defense responses. The interplay between SA- and JA-responses boosts the immune response against single attackers (Spoel and Dong, 2008). By analyzing plants with altered FITNESS expression levels, we aimed to determine whether SA pathway activation exerted in fitness mutants triggers an effective defense against bacterial attack and whether this response is associated with a decline in seed productivity.
The present study demonstrates a strongly enhanced resistance to Pseudomonas syringae pv. tomato DC3000 (Pst) in fitness mutants. Given that the exact mechanism by which SA activates NPR1 is not completely understood yet (Backer et al., 2019), fitness mutants provide an important genetic model for investigating the basis for enhanced plant resistance induction. Additionally, a higher yield relative to WT was measured in fitness mutants after pathogen attack. Our results suggest that low levels of FITNESS lead to the optimization of the stress responses gene network to integrate dynamic environmental inputs. This reconfiguration acts synergistically to maximize the plant’s reproductive success.
Materials and Methods
General
Chemicals and reagents were obtained from Sigma-Aldrich (St Louis, MO, United States) or Merck (Buenos Aires, Argentina). Standard molecular techniques were performed as described (Sambrook and Russell, 2001). DNA sequencing was performed by the University of Maine DNA sequencing facility (United States, Orono, ME). For sequence analyses, the tools provided by the National Center for Biotechnology Information1, the Arabidopsis Information Resource (TAIR)2, WolF Sort3, and GPS-SUMO 2.04 were used. Restriction enzymes for cloning and reagents for quantitative real-time PCR (qPCR) were provided by Promega and Invitrogen Life Technologies (Buenos Aires, Argentina).
Constructs and Plants
Arabidopsis thaliana accession Col-0 was employed as wild type in all experiments. FITNESS loss-of-function mutants (fitness-1 and fitness-2), FITNESS overexpressing line1 (FITNESSox1) and a mutant line rescued by constitutive overexpression of the full-length FITNESS cDNA transcriptionally fused to the cauliflower mosaic virus 35S promoter (fitness-1/35S-FITNESS) used in this work were previously described (Osella et al., 2018). The double knockout mutant deficient in both NPR1 and FITNESS was generated by crossing the two single knockout mutants derived from Col-0 and self-pollination of the F1 generation. For selection of the fitness-1/npr1 knockout line, genomic DNA leaf extracts of the F2 generation were prepared from 25-day-old plants according to Rezadoost et al. (2016). The mutation of the FITNESS allele was determined by PCR amplification using primers FITNESS_For, FITNESS_Rev, specific for the WT allele amplification and the pair promFITNESS_For and LB for the T-DNA insertion. The primer pair PP2a_For and PP2a__Rev was used as control of amplification. npr1-1 single mutation was confirmed by sequencing using primers npr1_For and npr1_Rev. (Supplementary Table 1 and Supplementary Figure 1). Relative transcript levels of FITNESS in these lines are shown in Supplementary Figure 2.
For the establishment of an inducible overexpression (IOE) construct, the FITNESS CDS was amplified by PCR with primers that contain a PacI or SpeI site (Supplementary Table 1). PCR products were ligated into pGEM-T Easy vector (Promega, Mannheim, Germany). After sequence confirmation, the vector was digested with PacI and SpeI, and the fragment containing the FITNESS CDS was ligated into the pER8 vector (Zuo et al., 2000). Constructs were transformed into Arabidopsis Col-0 wild type using Agrobacterium tumefaciens (strain GV3101)-mediated transformation employing a floral dip method. Arabidopsis IOE lines were selected on MS medium containing hygromycin. Subsequently, stable transgenic T3 lines showing an increased expression level by qPCR after estradiol induction were used for detailed analysis. For expression experiments, total RNA was isolated from 2-week-old FITNESS-IOE plants after treatment with 10 μM estradiol in 0.01% Silwet 77 for 4 and 6 h; plants treated as before but without estradiol were used as controls.
Plant Growth Conditions
Individual Arabidopsis plants were grown in controlled growth chambers in 6-cm pots at approximately 70% relative humidity with a 16 h light/8 h dark period for long day (LD) conditions (23°C, 120 μmol m–2 s–1). To perform all experiments, all plants were grown alongside each other under carefully controlled conditions. All experiments were repeated at least three times.
For root growth analysis, surface-sterilized seeds were germinated on 0.5× MS plates, supplemented with 0.8% agar. After 4 days, seedlings were transferred to new plates containing 0, 10, or 50 μM Methyl Jasmonate (Sigma-Aldrich) and placed vertically for 12 days in a controlled growth chamber under the same conditions as stated before.
Bacterial Strain, Plant Inoculation Conditions, and Bacterial Proliferation Assay
The virulent hemibiotrophic bacterial pathogen Pseudomonas syringae pv. tomato DC3000 (Pst) was used for infections as described in Liu et al. (2015). Briefly, bacteria were suspended in sterile 10 mM MgCl2 at approximately 107 c.f.u. ml–1. The suspension was infiltrated into fully expanded Arabidopsis leaves through the abaxial surface. Three leaves of six biological replicates per genotype were inoculated. Leaf discs were taken from the inoculated leaves 30 h post-inoculation. To assess bacterial growth each sample was plated at least three times. After incubation at 28°C for 48 h, bacterial colonies were counted.
RNA Extraction and qPCR
Total RNA was extrated using TRIzol (Invitrogen Life Technologies) following the manufacturer’s procedure. Three biological replicates were prepared for each genotype. RNA quality and quantity, as well as RNA reverse transcription and qPCR, were performed as previously described (Osella et al., 2018). For qPCR, primer sequences are given in Supplementary Table 1. PCR reactions were carried out in a Mastercycler ep Realplex thermocycler (Eppendorf, Westbury, NY, United States) using a SYBR Green fluorescence-based assay. The relative expression ratio for each gene was calculated as previously described (Pfaffl, 2001). The PCR efficiency for each reaction was calculated based on the profile of the emitted fluorescence in the exponential phase (Rutledge and Stewart, 2008). Transcript levels were normalized to the transcript level of PROTEIN PHOSPHATASE 2A (PP2A, At1g13320) gene (Czechowski et al., 2005). Reagents for qPCR were provided by Promega and Invitrogen Life Technologies (Buenos Aires, Argentina).
Quantification of Damaged Leaf Area
The relative damage area was measured using ImageJ software at 6 days after infection with a bacterial suspension of Pst. Measurements are relative to the total leaf area. At least nine leaves per genotype were used for this analysis.
Determination of SA Levels
Free and conjugated SAs were extracted from leaves (200 mg fresh weight) of 3-week-plants using the procedure described by Aboul-Soud et al. (2004). Three biological replicates were prepared for each genotype. Gas chromatography-mass spectrometry (GC-MS) was used to measure free and conjugated SA, and their absolute concentrations (nmol g FW–1) were determined by comparison with calibration curve response ratios of various concentrations of standard solutions, including the internal standard ribitol (Roessner-Tunali et al., 2003; Zanor et al., 2009).
Statistical Analyses
Statistical analysis were performed using Student’s t-test embedded in the Microsoft Excel software. Only a return of P < 0.05 was designated statistically significant. For multiple comparisons, one-way analysis of variance (ANOVA) followed by Fisher’s least significant difference multiple comparison test was used. Statistically significant differences (P < 0.05) are indicated by different letters. The multiple comparison tests as well as the correlation coefficient and significances between phytohormone and transcript levels were calculated using the InfoStat software (Di Rienzo et al., 2011).
Results
The Knock-Down of FITNESS Reveals Its Role in Pathogen Resistance Against Pseudomonas syringae pv. tomato DC3000
In a previous study, we presented evidence that the Arabidopsis fitness mutants exhibit increased tolerance to oxidative stress associated with an increase in plant productivity (Osella et al., 2018). The mutants also accumulated moderate SA levels accompanied by an induction of several SA-signaling marker genes, such as NPR1, ENHANCED DISEASE SUSCEPTIBILITY1 (EDS1), and PHYTOALEXIN DEFICIENT4 (PAD4). The induction of SA responses led us to test whether this activation of defenses is protective to the plant when challenged with pathogens. As mentioned before, SA plays an essential role in plant defense against biotrophic and hemibiotrophic pathogens. We, therefore, analyzed the expression of FITNESS upon Pst infection. Leaf material was used to test FITNESS transcripts levels after inoculation of Arabidopsis WT plants with the bacterial pathogen. After 24 h of infection, FITNESS transcript levels were significantly reduced (Figure 1A). To elucidate the possible involvement of FITNESS in plant defense against bacterial pathogens we tested Arabidopsis plants with altered FITNESS expression levels for their response to Pst infection, including the fitness-1 and -2 mutants (a T-DNA insertion mutant and a CRISPR/Cas9 mutant, respectively), and a transgenic line overexpressing FITNESS under the control of the 35S CaMV promoter (hereafter, FITNESSox1), as previously described (Osella et al., 2018). Pressure-infiltration with Pst (1 × 107 c.f.u. ml–1) was used to inoculate WT plants along with the mentioned lines. Compared with WT, fitness-1 and -2 exhibited enhanced disease resistance while the FITNESSox1 line showed increased susceptibility toward Pst. Extended chlorosis was observed in all lines tested except the fitness mutants. Finally, the infection resulted in accelerated cell death in the inoculated leaves (Figure 1B). We also monitored the ability of Pst to multiply endophytically in the inoculated tissue and observed greater bacterial proliferation in FITNESSox1 than WT plants (at 30 h post-inoculation; hpi). The opposite was evident in fitness-1 and -2 plants that had a lower bacterial titer than WT plants suggesting that the absence of FITNESS is associated with bacterial growth restriction (Figure 1C). To further explore these observations, we analyzed the fitness-1/35S-FITNESS line after pathogen attack. We observed a higher bacterial load in this line than in the fitness-1 mutant, similar to that observed in the WT (Figure 1C). Taken together, our data suggest that FITNESS acts as a negative regulator of immunity to a bacterial pathogen.
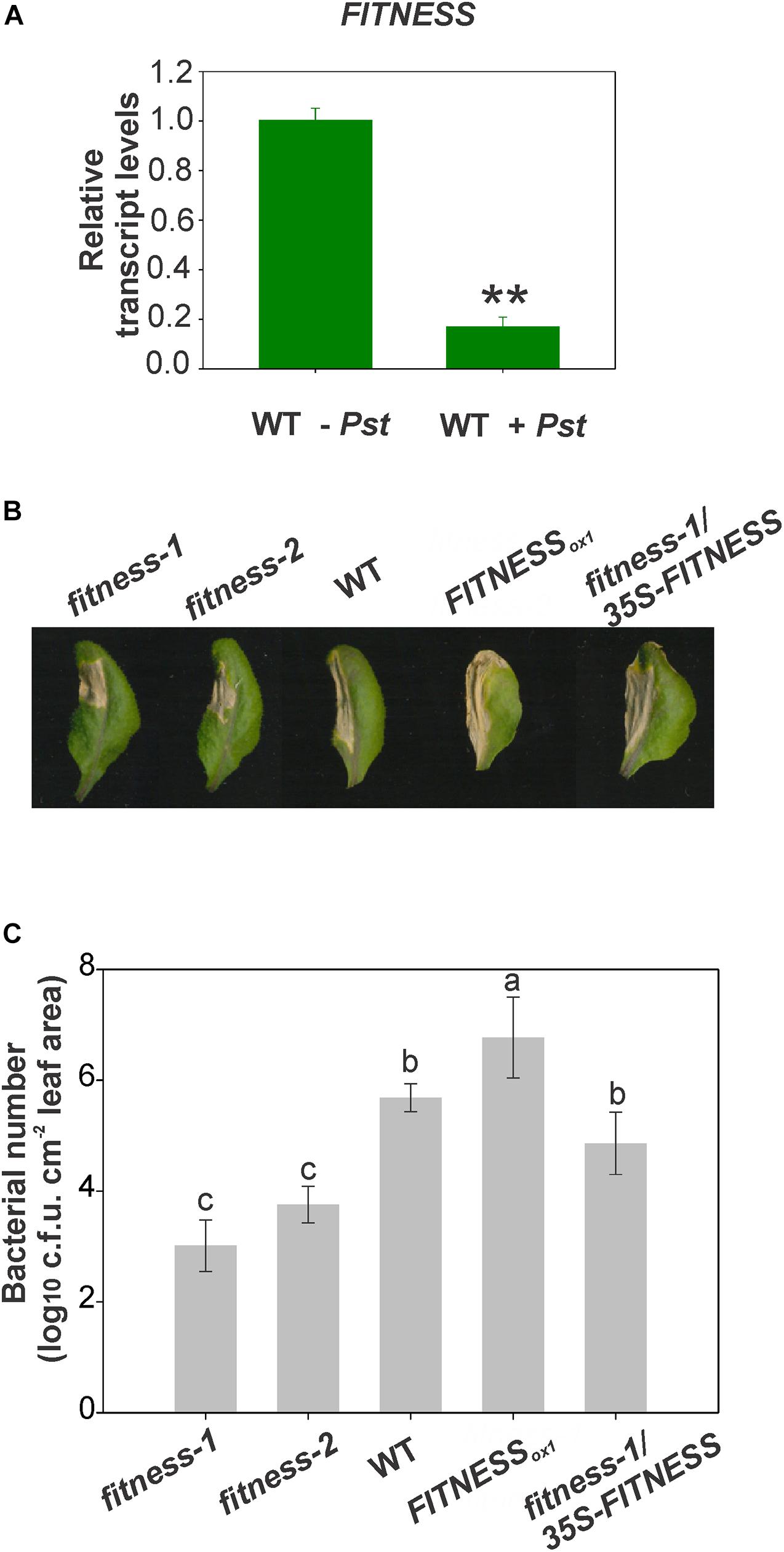
Figure 1. FITNESS knock down leads to Pst resistance. (A) FITNESS expression is reduced upon pathogen attack. RNA from 25-day-old wild-type Arabidopsis thaliana plants mock-infiltrated (WT-Pst) or after 24 h of Pseudomonas syringae pv tomato DC3000 (WT + Pst) treatment was used. Means of three biological replicates are shown; error bars represent SE. Statistical analyses were performed using Student’s t-test embedded in the Microsoft Excel software, statistically significant differences (P < 0.01) are indicated by **. (B) FITNESS is involved in disease resistance to Pst in Arabidopsis. Disease symptoms from representative leaves of 4-week-old plants expressing different levels of FITNESS are shown. Photographs were taken 4 days post-infection. (C) Bacterial titers, expressed as log10 (c.f.u. cm–2 leaf area), in the leaves of 4-week-old plants infiltrated with Pst. The experiments were repeated three times with similar results. Values were averaged from at least six biological replicates per genotype, and error bars represent SE. Bars with the same letter indicate no significant difference between the samples (analysis of variance + Fisher least significant difference, P < 0.05).
Salicylic Acid-Triggered Defense in Fitness Mutants Is NPR1-Dependent
The role of NPR1 as a central positive regulator of SAR transducing the SA signal to activate downstream PR gene expression has been well demonstrated, and mutations in NPR1 lead to compromised disease resistance and loss of PR gene expression (Li et al., 2006). Several genes involved in the SA response are up-regulated in fitness mutants (Osella et al., 2018). To assess whether SA-triggered defense in fitness mutants is dependent on NPR1, we generated fitness-1/npr1 double mutants by crossing the single mutants and screening for double homozygous mutants by a polymerase chain reaction in the F2 generation (Figure 2A and Supplementary Figure 1). We then tested the lines’ responses to Pst and measured PR1 transcript levels as a proxy of SA response activation. As expected, WT plants showed a ∼20-fold elevation of PR1 transcript abundance after Pst infection. Noteworthy, this level of PR1 expression is similar to that observed in the fitness-1 mutant before bacterial infection. Moreover, after infection, a further increase in PR1 transcript level was observed in the fitness-1 mutant, suggesting that this mutant can increase the defense responses beyond the defense levels observed in WT plants after infection. Results shown in Figure 2B indicate that a low level of PR1 transcripts is present in the fitness-1/npr1 double mutant, similar to that observed in npr1. However, after Pst infection, the major induction of PR1 transcripts observed in the fitness-1 mutant was absent in both the npr1 and fitness-1/npr1 mutants (Figure 2C). Altogether, these results suggest that the activation of the SA-signaling pathway in the fitness mutants requires active NPR1. Moreover, fitness-1/npr1 mutants behave similarly to npr1 mutants leading to high Pst counts after infection and to an increased size of the damaged area compared to those measured in WT plants and the fitness-1 mutant reinforcing the fact that disease control in the fitness mutants is exerted through NPR1 signaling (Figures 2D,E).
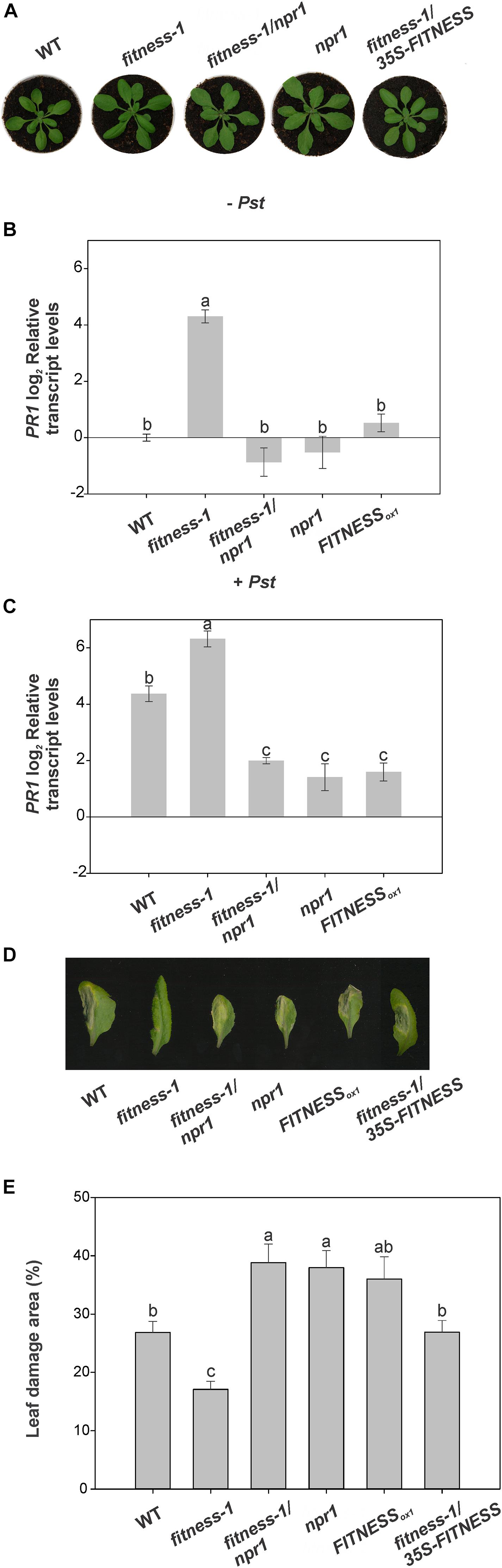
Figure 2. Pst resistance in fitness mutants is NPR1-dependent. (A) FITNESS requires NPR1 to induce Pst resistance in Arabidopsis. Representative photos of 25-day-old wild-type (WT), fitness-1, fitness-1/npr1, npr1, and fitness-1/35S-FITNESS plants. (B,C) Relative transcript levels of PR1 in 25-day-old plants after 24 h mock treatment (B) or treatment with Pst (C). The fitness-1/npr1 mutant fails to induce PR1. Means of three biological replicates are shown. Error bars represent ± SE. (D) Disease symptoms of representative leaves of 4-week-old plants after Pst treatment. (E) Relative damage area of Pst-treated leaves. Samples were taken 6 days after infection. Values were averaged from at least nine biological replicates per genotype, and error bars represent SE. In panels (B,C,E), bars with the same letter indicate no significant difference between samples (analysis of variance + Fisher least significant difference, P < 0.05).
FITNESS Controls the Accumulation of Free and Conjugated SA
As reported before, fitness mutants accumulate moderate levels of free SA relative to WT in control conditions (Osella et al., 2018). Thus, we quantitatively measured free and conjugated SA in plants with altered FITNESS levels in Pst-treated leaves after 24 h of infection and in mock-treated controls. In mock-infiltrated plants, besides the accumulation of free SA observed in fitness mutants, FITNESSox1 plants had levels similar to WT plants (Figures 3A,B, left panels). Moreover, the fitness-1/35S-FITNESS line had lower free SA levels than the fitness mutants. An interesting feature was observed after Pst infection. All lines tested showed an increase in free SA compared to the control mock condition, indicating a further activation of SA synthesis (Figures 3A,B, right panels). Interestingly, fitness-1/npr1 mutants showed SA levels similar to those in fitness mutants indicating that the failure in the double mutant’s defense responses is related to downstream signaling events. Even FITNESSox1 plants showed an increase in free SA after Pst infection. However, this increase was less prominent than the one in WT plants. To explain all these observations, we measured the transcript levels of ICS1, which encodes for the enzyme ISOCHORISMATE SYNTHASE1 and is mainly responsible for the stress-induced accumulation of SA (Wildermouth et al., 2001). After mock infection, ICS1 transcript levels in fitness-1/npr1 mutants were similar to those measured in npr1 mutants and lower than those measured in fitness mutants, reinforcing the idea that FITNESS modulates SA synthesis (Figure 3C). After Pst infection, ICS1 transcript levels were additionally induced in all lines mirroring the measured SA levels (Figure 3C).
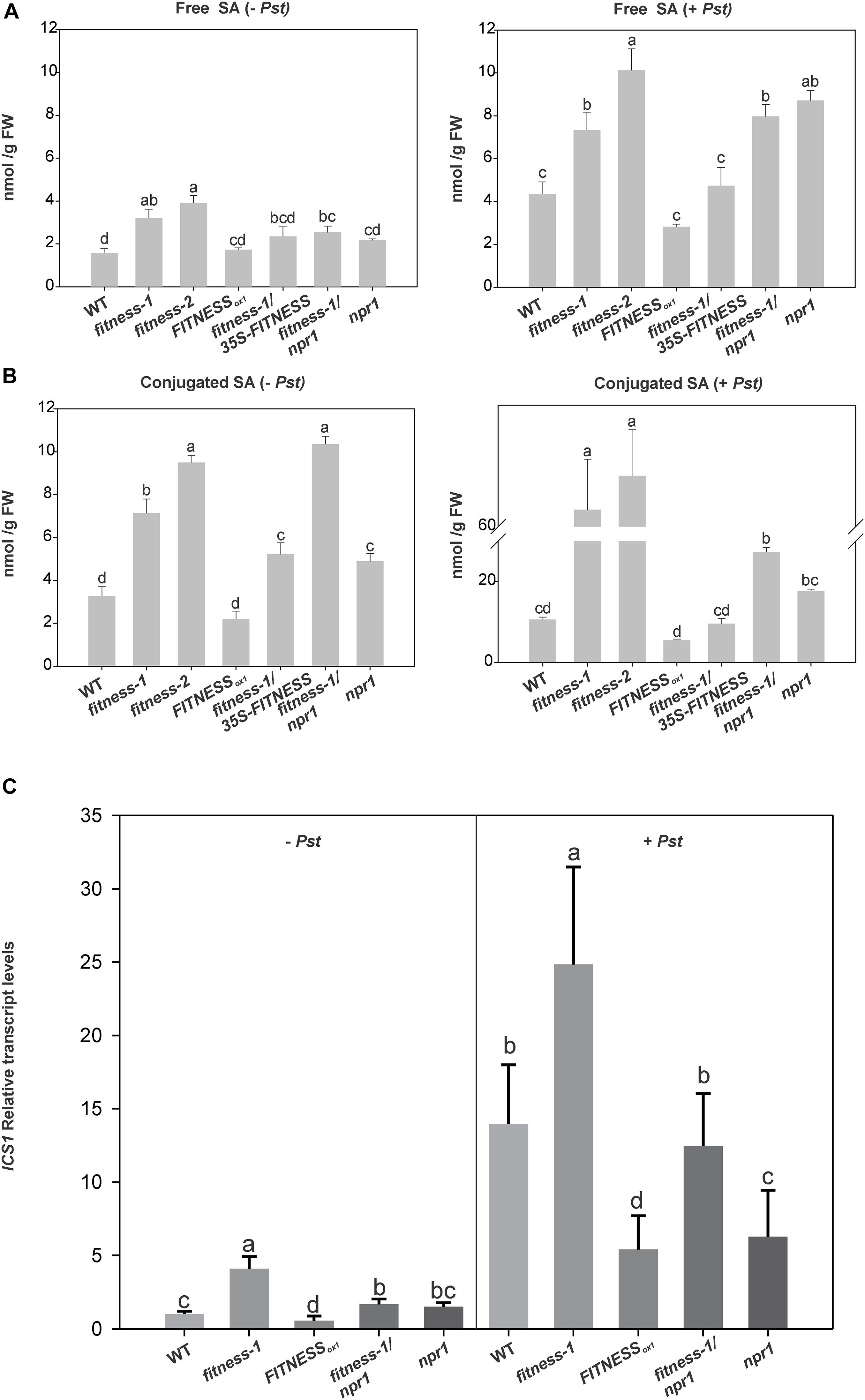
Figure 3. FITNESS controls the accumulation of free and conjugated SA. (A,B) Free and conjugated salicylic acid (SA) levels in plants with altered levels of FITNESS. Plants were mock treated (left panels) or Pst treated (right panels). Means of three biological replicates per genotype are shown. Error bars represent ± SE Bars with the same letter indicate no significant difference between samples (analysis of variance + Fisher least significant difference, P < 0.05). (C) Relative transcripts levels of ICS1 in 25-day-old plants with altered level of FITNESS expression, mock-(left) or Pst-(right) infiltrated. Means of three biological replicates per genotype are shown. Error bars represent ± SE Bars with the same letter are not significantly different from one another (analysis of variance + Fisher least significant difference, P < 0.05).
The levels of conjugated and free SAs showed similar trends, except for the fitness-1/npr1 double mutant, which accumulated less conjugated SA than the fitness mutants after Pst infection (Figure 3B, right panel). Most of the SA is conjugated by SA-glucosyltransferases and transported to vacuoles for storage. Two genes in Arabidopsis encode active SA-glucosyltransferases (Thompson et al., 2017), UDP-GLYCOSYLTRANSFERASE 74F1 (UGT74F1) and UGT74F2. We observed a significant increase in the transcript levels of UGT74F2 in fitness-1 mutant compared to WT (Osella et al., 2018).
Altogether, these results suggest that FITNESS acts as a negative regulator of SA biosynthesis. Enzymes, which influence flux and metabolite concentrations, are highly regulated. Knowing which genes affect certain metabolite levels open an opportunity to detect transcriptionally regulated pathways and novel genes controlling, or affecting, metabolic pathways (Redestig and Costa, 2011). The most commonly applied method to find metabolite-transcript co-responses is to calculate the Pearson correlation coefficient between the variables. To test the hypothesis that FITNESS and SA levels are linked, we calculated the Pearson correlation coefficient. We found a significant negative correlation between total SA content and FITNESS transcript levels (−0.65, P = 0.023), reinforcing the model that FITNESS is a new regulator of SA-mediated responses in Arabidopsis.
Transcript Levels of Genes Involved in SA- and JA-Related Processes Are Altered in FITNESS Lines
As mentioned before, a genome-wide transcriptome study was previously done to identify genes present in the FITNESS transcriptomic network and one of the GO terms found significantly enriched was “response to SA” (SA, GO:0009751). Several genes related to SA-signaling like ACCELERATED CELL DEATH 6 (ACD6), LATE UPREGULATED IN RESPONSE TO HYALOPERONOSPORA PARASITICA (LURP1), and ICS1 showed increased expression in fitness mutants and a decreased expression in FITNESSox1 plants. Also, as earlier stated, the transcripts of both NPR1, a key component of the SA signaling cascade, and PR1, a marker gene for SA signaling, were induced in fitness mutants (Osella et al., 2018). NPR1 is important for SA-induced SAR since NPR1 loss-of-function plants are almost unable to activate PR genes’ expression. NPR1 was proposed to regulate PR1 expression through its interaction with TGA TFs (Fan and Dong, 2002). In Arabidopsis, there are ten TGA TFs reported, and several interact constitutively with NPR1 (Zhou et al., 2000). However, two of them, namely TGA1 and TGA4, only interact with NPR1 upon SA induction (Després et al., 2003). Interestingly, only these two TFs showed increased transcript levels in fitness mutants (Osella et al., 2018); of note, TGA1 is one of the proteins that interact with FITNESS in a yeast two-hybrid assay (Trigg et al., 2017).
To further characterize the FITNESS regulatory network, we used estradiol (EST) – inducible overexpressing (FITNESS-IOE) plants. We first tested EST-dependent FITNESS expression 2, 4, and 6 h after EST treatment, using 15-day-old FITNESS-IOE plants. As controls, we used 0.01% Silwet 77-treated FITNESS-IOE lines. FITNESS expression increased by more than 10-fold after 4 h EST treatment, reaching its maximum. Six hours after the treatment, FITNESS transcript levels declined again (Supplementary Figure 3). To find possible FITNESS target genes, we used 15-day-old FITNESS-IOE seedlings harvested 4 h after either treatment with 0.01% Silwet 77 (control) or 10 μM EST and performed a targeted transcript profiling. We selected 42 genes and checked their expression by qPCR. The list included 31 non-TF- and 11 TF-encoding genes that were either significantly altered in fitness mutants or involved in specific hormone or stress signaling pathways (Figure 4). Among them, four genes (LOX2, ANAC072, JAZ3, and JAZ10) were highly induced, and eight were significantly repressed (ICS1, COI1, WAK1, PCC1, ACD6, EDS1, ORA59, ERF1) 4 h after the induction of FITNESS expression (Figure 4).
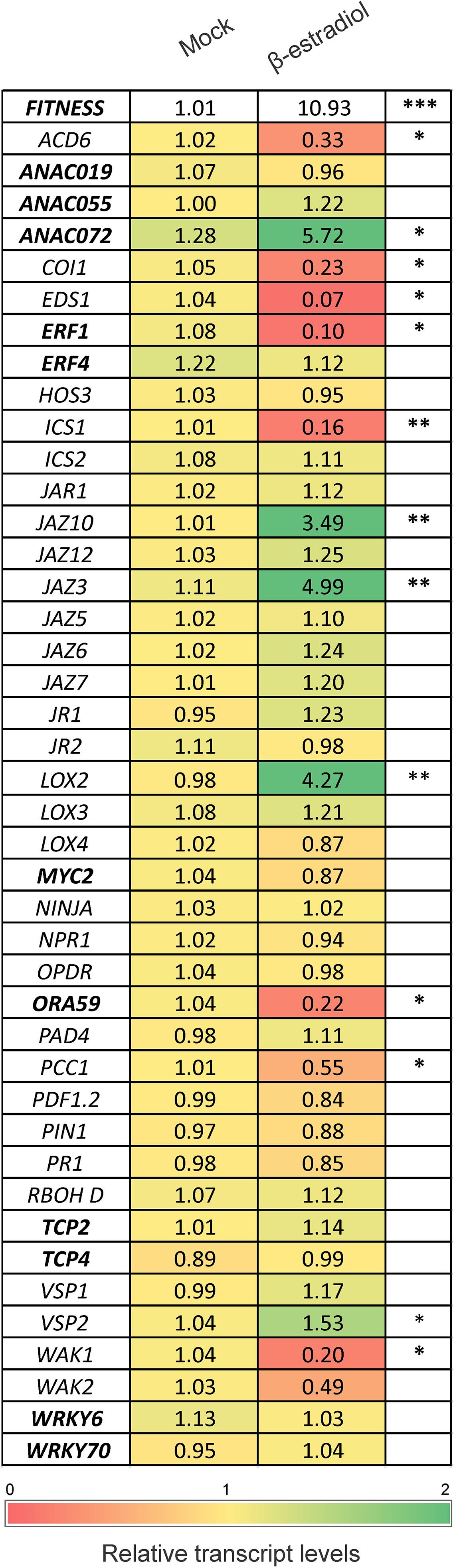
Figure 4. Relative transcript levels of defense-/stress-related genes possibly involved in the FITNESS regulatory network. Expression of selected genes possibly involved in the FITNESS regulatory network. Heat map showing differential expression of genes in the FITNESS-IOE line after 4 h of treatment with 10 μM estradiol vs. mock treatment. Data are expressed as relative transcript levels accompanied with Student’s t-test (*P < 0.05; ** P < 0.01; *** P < 0.001). Values were averaged from at least three biological replicates per treatment. Green and red colors denote up- and down-regulated genes, respectively, compared to mock treatment. Genes encoding for TFs are highlighted in bold.
Noteworthy, many of these putative target genes are involved in JA synthesis or signaling. LOX2 (LIPOXYGENASE2) encodes a key enzyme in the octadecanoid pathway leading to JA biosynthesis (Bell et al., 1995). ANAC072, together with the homologs ANAC019 and ANAC055, act in the JA-mediated inhibition of the SA pathway by suppressing ICS1 expression. Accordingly, the transcripts levels of ICS1 were significantly repressed in FITNESS-IOE lines, which have higher transcript levels of ANAC072 (Figure 4). Also, JAZ3 and JAZ10, which act by inhibiting JA-responsive genes, were induced in FITNESS-IOE lines. However, COI1 (CORONATINE INSENSITIVE 1) transcript levels were significantly reduced. COI1 encodes an F-box protein of the Skp/Cullin/F-box complex (SCFCOI1). It is an essential component of the bioactive JA perception apparatus, and is required for most JA-signaling processes (Katsir et al., 2008). It has been reported that Methyl Jasmonate (MeJA) inhibits root growth in Arabidopsis (Staswick et al., 1992). Therefore, we examined whether primary root growth in Arabidopsis plants with altered expression levels of FITNESS was sensitive to MeJA. FITNESSox1 line roots were significantly less inhibited than WT roots (43 vs. 52%) in the presence of 50 μM MeJA when compared to control conditions. On the other side, fitness mutants showed the highest growth inhibition (62 and 66% for fitness-1 and -2, respectively). Similar trends were observed when the roots were grown in the presence of 10 μM MeJA (Supplementary Figure 4). These results suggest that FITNESS modulates JA-related responses.
We also analyzed the JA signaling branches downstream of JAZ repressors, namely, the MYC TF branch, which is related to wound responses (with VEGETATIVE STORAGE PROTEIN2 [VSP2] as a marker gene), and the ERF TF branch, which links JA to necrotrophic pathogen resistance (with PLANT DEFENSIN1.2 [PDF1.2] as a marker gene) in FITNESS-IOE lines at 6h after estradiol induction. VSP1 and 2 transcript levels showed a significant induction (Supplementary Figure 5). On the other side, expression of PDF1.2, which is a marker gene for JA-ethylene (ET)-mediated responses, was significantly decreased 6h after FITNESS induction. This is in agreement with the fact that PDF1.2 is a direct target of the ERF TFs ORA59 and ERF1, and expression of the genes encoding them was strongly reduced in FITNESS-IOE lines.
Reproductive Output of Fitness Mutants After Pst Treatment
Based on the concept that an active immune system is associated with production costs, we aimed to measure the reproductive output of fitness mutants after Pst treatment. To this end, the different lines and mutants were sprayed with a Pst suspension at approximately 108 c.f.u. ml–1, and then were allowed to grow until seed set. The quantification showed that the total seed yield per plant was higher in the fitness-1 mutant than all other lines (Figure 5). The seed yield of the fitness-1/npr1 double mutant was similar to that of WT plants leading to the model that in fitness mutants, the higher yield was related to their elevated SA content (see above). This observation opens up new possibilities for the generation of plants combining resistance traits without compromising yield.
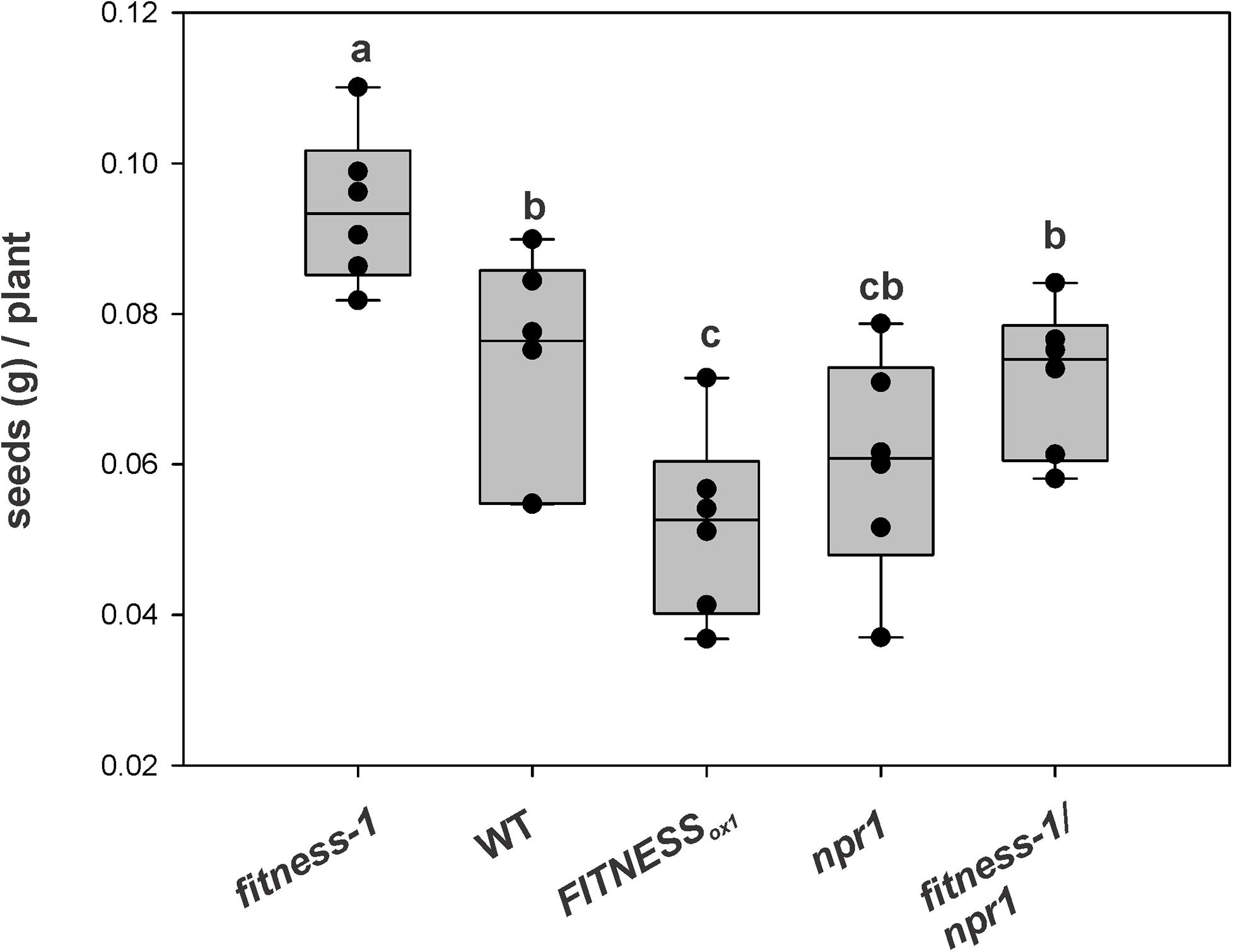
Figure 5. Seed productivity of different FITNESS lines after pathogen attack. At least six plants per line were sprayed with a Pst suspension at approximately 108 c.f.u. ml–1. Bars with the same letter indicate no significant difference between samples (analysis of variance + Fisher least significant difference, P < 0.05). The experiment was repeated twice with similar results.
Discussion
In this study, the robust resistance of fitness mutants, and the enhanced sensitivity upon FITNESS overexpression, demonstrate the importance of FITNESS as a negative regulator of plant immunity in response to infection by the hemibiotrophic pathogen Pst. The induction or repression of defense genes is orchestrated by signaling networks directed by plant hormones, of which SA and JA are the major players (Caarls et al., 2015). Moreover, NPR1 acts as a master key in plant defense signaling networks mediating cross-talk between SA and JA/ET responses (Backer et al., 2019). Global gene expression studies revealed that FITNESS functions to modulate SA-responsive gene expression. Also, the levels of free and conjugated SA were negatively linked to FITNESS expression. FITNESS loss of function leads to the accumulation of both SA forms. On the contrary, FITNESS overexpression reduces the phytohormone synthesis and accumulation. We further confirmed that the fitness-1/35S-FITNESS line accumulates lower SA levels than the fitness mutants supporting the model that FITNESS negatively influences SA synthesis. We also showed that the basal SA content in fitness mutants provides the benefit of limiting bacterial pathogen infection. However, SA accumulation after Pst infection in fitness mutants does not depend on the presence of active NPR1. This conclusion can be drawn from the fact that both the fitness-1/npr1 double mutant and the fitness-1 single-gene mutant accumulate similar SA levels after Pst infection. This agrees with previous reports that show NPR1 is not necessary for SA synthesis (Marek et al., 2010). However, the level of conjugated SA, which accounts for its storage form, is decreased in the fitness-1/npr1 double mutant. Plants unable to synthesize or accumulate SA are more susceptible to infection by certain pathogens (van Wees and Glazebrook, 2003). This is consistent with the increased susceptibility to Pst infection in the fitness-1/npr1 compared to the fitness-1 mutant. As mentioned before, SA-glucosyltransferases convert SA to its conjugated form. We found UGT74F2 to be transcriptionally induced in the fitness-1 mutant, in agreement with high conjugated SA levels after Pst infection. By searching in publicly available transcriptome data, we also observed that UGT74F1 and UGT74F2 are transcriptionally down-regulated in the npr1 mutant compared to WT after SA treatment5 (GSE51626; Singh et al., 2015). Taking all this into account, we conclude that NPR1 is essential in fitness mutants for SA storage and defense activation but not for SA synthesis after Pst infection.
In Arabidopsis, simultaneous activation of SA and JA mediated resistance is restricted by the strong negative effect of SA on the JA/ET responses (Spoel and Dong, 2008; Pieterse et al., 2009). Notably, altered levels of FITNESS led to alteration of the network of TFs, which control defense responses. After FITNESS inducible expression, ANAC072 transcript levels were significantly induced, while ORA59 and ERF1 transcript levels were significantly repressed. Recent studies showed that ANAC072 and its homologs, ANAC019 and ANAC055, are involved in plants’ response to bacterial pathogens, JA-mediated defense, and thermotolerance (Bu et al., 2008; Zheng et al., 2012; Guan et al., 2014). Also, ANAC072 acts by inhibiting ICS1 expression (Zheng et al., 2012) and accordingly, ICS1 transcript levels were downregulated in FITNESS-IOE plants.
ORA59 and ERF1, which integrate JA and ET signals to promote antimicrobial compounds’ expression, were strongly downregulated in FITNESS-IOE lines. Also, the expression of COI1 was strongly downregulated in FITNESS-IOE lines. Considering that COI1 loss of function abolishes JA-dependent responses and that ORA59 functions downstream of COI1, we propose that elevated FITNESS expression leads to an inhibition of JA responses. We also found that transcripts of two JAZ proteins, namely JAZ3 and JAZ10, which act as repressors of JA signaling, are highly upregulated after FITNESS induction. Arabidopsis possesses thirteen JAZ genes, and the specific role of each one is still unclear. Proteasomal degradation of JAZ proteins results in JA responses’ derepression and activation of JA-responsive genes (Memelink, 2009). The aforementioned results suggest that JAZ degradation upon the perception of biologically active JAs, and JA perception itself, are affected due to COI1 repression.
Summarizing, our work demonstrates that in fitness mutants, changes induced in the transcriptional network lead to an efficient defense response without a compromise in plant productivity after bacterial pathogen attack. Although this is in conflict with the proposed incompatibility between growth and defense, growth inhibition due to activation of defense responses is not a default program. For example, Arabidopsis accession C24 has no yield penalties, although it has an elevated pathogen resistance due to constitutive high levels of disease resistance (Bechtold et al., 2010). Additionally, by analyzing the reported seed yield of several mutants known for their differential SA accumulation, no predictive pattern can be envisaged between yield and defenses activation. cpr6-1 and lsd1 mutants both accumulate SA and show increased defense responses but low seed yields (Dietrich et al., 1997; Clarke et al., 1998; Mateo et al., 2004; Bechtold et al., 2010; Bernacki et al., 2019) and acd5 mutants which also accumulate SA, have decreased seed yield and decreased tolerance to pathogens (Greenberg et al., 2000). On the contrary, higher seed yields were reported in SA accumulation affected lines (van Wees and Glazebrook, 2003; Abreu and Munné-Bosch, 2009; Lu et al., 2009). The impact of FITNESS on reproductive performance was previously reported for plants grown under non-stress conditions (Osella et al., 2018). Here, we go beyond our initial observation and show that a lack of FITNESS leads to reproductive success even after a bacterial pathogen attack. Plant diseases are a constant threat to agricultural production. Yield losses due to pathogen attacks include direct and indirect consequences. On one side, the yield might be compromised, but also crop pests are critical concerning food security. A significant proportion of global crop production is annually lost due to pests and diseases (Savary et al., 2012). While pesticides help reduce these losses, there is growing concern about pesticide resistance and their impacts on health and the environment (Schwarzenbacher et al., 2014). Therefore, the identification and downregulation of FITNESS orthologs opens new avenues for future research in improving crop species toward reducing the impact of pests and diseases on crop yield to support global food production. In conclusion, we presented evidence that FITNESS is a new component of the plant defense response network and an integration node for balancing the reproductive output during stress responses.
Data Availability Statement
The original contributions presented in the study are included in the article/Supplementary Material, further inquiries can be directed to the corresponding author.
Author Contributions
DM performed most of the experiments. LRT isolated and characterized the fitness-1/npr1 double mutant. DM and SB designed and constructed the FITNESS inducible overexpressing line. MIZ conceived the project and wrote the manuscript with contributions by all authors.
Funding
Research funding was provided to MIZ by the Agencia Nacional de Promoción Científica y Tecnológica (ANPCyT) from Argentina (Préstamo BID PICT-2012-2709 and PICT-2017-1301) and to SB by the Max Planck Institute of Molecular Plant Physiology.
Conflict of Interest
The authors declare that the research was conducted in the absence of any commercial or financial relationships that could be construed as a potential conflict of interest.
Acknowledgments
DM was a fellowship recipient of Consejo Nacional de Investigaciones Científicas y Técnicas (CONICET) and was awarded a Bayer Jeff Schell short-term fellowship to perform research in SB’s lab at the Max Planck Institute of Molecular Plant Physiology, Potsdam-Golm, Germany. MIZ is a career member of CONICET. We thank Dr. Betiana Garavaglia for the kind gift of the bacterial strain used in this work and Dr. Carolina Grandellis for providing the npr1-1 seeds.
Supplementary Material
The Supplementary Material for this article can be found online at: https://www.frontiersin.org/articles/10.3389/fpls.2021.606791/full#supplementary-material
Footnotes
- ^ http://www.ncbi.nlm.nih.gov/
- ^ http://www.arabidopsis.org/
- ^ http://www.genscript.com/wolf-psort.html
- ^ http://sumosp.biocuckoo.org/online.php
- ^ https://www.ncbi.nlm.nih.gov/gds
References
Aboul-Soud, M. A. M., Cook, K., and Loake, G. J. (2004). Measurement of salicylic acid by a high-performance liquid chromatography procedure based on ion-exchange. Chromatographia 59, 129–133.
Abreu, M. F., and Munné-Bosch, S. (2009). Salicylic acid deficiency in NahG transgenic lines and sid2 mutants increases seed yield in the annual plant Arabidopsis thaliana. J. Exp Bot. 60, 1261–1271. doi: 10.1093/jxb/ern363
Backer, R., Naidoo, S., and van den Berg, N. (2019). The nonexpressor of pathogenesis-related genes 1 (NPR1) and related family: mechanistic insights in plant resistance. Front. Plant Sci. 10:102.
Bechtold, U., Lawson, T., Mejia-Carranza, J., Meyer, R. C., Brown, I. R., Altmann, T., et al. (2010). Constitutive salicylic acid defences do not compromise seed yield, drought tolerance and water productivity in the Arabidopsis accession C24. Plant Cell Environ. 33, 1959–1973. doi: 10.1111/j.1365-3040.2010.02198.x
Bell, E., Creelman, R. A., and Mullet, J. E. (1995). A chloroplast lipoxygenase is required for wound-induced jasmonic acid accumulation in Arabidopsis. Proc. Natl. Acad. Sci. USA 92, 8675–8679. doi: 10.1073/pnas.92.19.8675
Bernacki, M. J., Czarnocka, W., Rusaczonek, A., Witoń, D., Kęska, S., Czyż, J., et al. (2019). LSD1-, EDS1- and PAD4-dependent conditional correlation among salicylic acid, hydrogen peroxide, water use efficiency and seed yield in Arabidopsis thaliana. Physiol. Plant 165, 369–382. doi: 10.1111/ppl.12863
Bu, Q., Jiang, H., Li, C. B., Zhai, Q., Zhang, J., Wu, X., et al. (2008). Role of the Arabidopsis thaliana NAC transcription factors ANAC019 and ANAC055 in regulating jasmonic acid-signaled defense responses. Cell Res. 18, 756–767. doi: 10.1038/cr.2008.53
Caarls, L., Pieterse, C. M. J., and Van Wees, S. C. M. (2015). How salicylic acid takes transcriptional control over jasmonic acid signaling. Front. Plant Sci. 6:170.
Clarke, J. D., Liu, Y., Klessig, D. F., and Dong, X. (1998). Uncoupling PR gene expression from NPR1 and bacterial resistance: characterization of the dominant Arabidopsis cpr6-1 Mutant. Plant Cell 10, 557–569. doi: 10.2307/3870732
Cockram, J., Thiel, T., Steuernagel, B., Stein, N., Taudien, S., Bailey, P. C., et al. (2012). Genome dynamics explain the evolution of flowering time CCT domain gene families in the Poaceae. PLoS One 7:e45307. doi: 10.1371/journal.pone.0045307
Conrad, U., Beckers, G. J. M., Langebach, C. J. G., and Jaskiewicz, M. R. (2015). Priming for enhanced defense. Annu. Rev. Phytopathol. 53, 97–119. doi: 10.1146/annurev-phyto-080614-120132
Czechowski, T., Stitt, M., Altmann, T., Udvardi, M. K., and Scheible, W.-R. (2005). Genome-wide identification and testing of superior reference genes for transcript normalization in Arabidopsis. Plant Physiol. 139, 5–17. doi: 10.1104/pp.105.063743
Després, C., Chubak, C., Rochon, A., Clark, R., Bethune, T., Desveaux, D., et al. (2003). The Arabidopsis NPR1 disease resistance protein is a novel cofactor that confers redox regulation of DNA binding activity to the basic domain/leucine zipper transcription factor TGA1. Plant Cell 15, 2181–2191. doi: 10.1105/tpc.012849
Di Rienzo, J., Casanoves, F., Balzarini, M., Gonzalez, L., Tablada, M., and Robledo, C. (2011). Grupo InfoStat Versión 2011. Vol. 8. Argentina: Grupo InfoStat, FCA, Universidad Nacional de Córdoba, 195–199.
Dietrich, R. A., Richberg, M. H., Schmidt, R., Dean, C., and Dangl, J. L. (1997). A novel zinc finger protein is encoded by the Arabidopsis LSD1 gene and functions as a negative regulator of plant cell death. Cell 88, 685–694. doi: 10.1016/s0092-8674(00)81911-x
Fan, W., and Dong, X. (2002). In vivo interaction between NPR1 and transcription factor TGA2 leads to salicylic acid-mediated gene activation in Arabidopsis. Plant Cell 14, 1377–1389. doi: 10.1105/tpc.001628
Fitzgerald, H. A., Chern, M.-S., Navarre, R., and Ronald, P. C. (2004). Overexpression of (At)NPR1 in rice leads to a BTH-and environment-induced lesion-mimic/cell death phenotype. Mol. Plant Microbe Interact. 17, 140–151. doi: 10.1094/mpmi.2004.17.2.140
Fu, Z. Q., and Dong, X. (2013). Systemic acquired resistance: turning local infection into global defense. Annu. Rev. Plant Biol. 64, 839–863. doi: 10.1146/annurev-arplant-042811-105606
Greenberg, J. T., Silverman, F. P., and Liang, H. (2000). Uncoupling salicylic acid-dependent cell death and defense-related responses from disease resistance in the Arabidopsis Mutant acd5. Genetics 156, 341–350.
Guan, Q., Yue, X., Zeng, H., and Zhu, J. (2014). The protein phosphatase RCF2 and its interacting partner NAC019 are critical for heat stress–responsive gene regulation and thermotolerance in Arabidopsis. Plant Cell 26, 438–453. doi: 10.1105/tpc.113.118927
Hout, B., Yao, J., Montgomery, B. L., and He, S. Y. (2014). Growth–defense tradeoffs in plants: a balancing act to optimize fitness. Mol. Plant 7, 1267–1287. doi: 10.1093/mp/ssu049
Katsir, L., Schilmiller, A. L., Staswick, P. E., He, S. H., and Howe, G. A. (2008). COI1 is a critical component of a receptor for jasmonate and the bacterial virulence factor coronatine. Proc. Natl. Acad. Sci. 105, 7100–7105. doi: 10.1073/pnas.0802332105
Li, J., Brader, G., Kariola, T., and Palva, E. T. (2006). WRKY70 modulates the selection of signaling pathways in plant defense. Plant J. 46, 477–491. doi: 10.1111/j.1365-313x.2006.02712.x
Liu, H., Dong, S., Sun, D., Liu, W., Gu, F., Liu, Y., et al. (2016). CONSTANS-Like 9 (OsCOL9) interacts with receptor for activated C-kinase 1(OsRACK1) to regulate blast resistance through salicylic acid and ethylene signaling pathways. PLoS One 11:e0166249. doi: 10.1371/journal.pone.0166249
Liu, X., Sun, Y., Kørner, C. J., Du, X., Vollmer, M. E., and Pajerowska-Mukhtar, K. M. (2015). Bacterial leaf infiltration assay for fine characterization of plant defense responses using the Arabidopsis thaliana-Pseudomonas syringae pathosystem. J. Vis. Exp. 104:e53364.
Lu, H., Salimian, S., Gamelin, E., Wang, G., Fedorowski, J., LaCourse, W., et al. (2009). Genetic analysis of acd6-1 reveals complex defense networks and leads to identification of novel defense genes in Arabidopsis. Plant J. 58, 401–412. doi: 10.1111/j.1365-313x.2009.03791.x
Marek, G., Carver, R., Ding, Y., Sathyanarayan, D., Zhang, X., and Mou, Z. (2010). A high-throughput method for isolation of salicylic acid metabolic mutants. Plant Methods 6:21. doi: 10.1186/1746-4811-6-21
Mateo, A., Mühlenbock, P., Rustérucci, C., Chang, C. C.-C., Miszalski, Z., Karpinska, B., et al. (2004). Lesion simulating disease 1 is required for acclimation to conditions that promote excess excitation energy. Plant Phys. 136, 2818–2830. doi: 10.1104/pp.104.043646
Memelink, J. (2009). Regulation of gene expression by jasmonate hormones. Phytochemistry 70, 1560–1570. doi: 10.1016/j.phytochem.2009.09.004
Nelson, R., Wiesner-Hanks, T., Wisser, R., and Balint-Kurti, P. (2017). Navigating complexity to breed disease-resistant crops. Nat. Rev. Genet. 19, 21–23. doi: 10.1038/nrg.2017.82
Ordoñez-Herrera, N., Trimborn, L., Menje, M., Henschel, M., Robers, L., Kaufholdt, D., et al. (2018). The transcription factor COL12 Is a substrate of the COP1/SPA E3 ligase and regulates flowering time and plant architecture. Plant Phys. 176, 1327–1340. doi: 10.1104/pp.17.01207
Osella, A. V., Mengarelli, D. A., Mateos, J., Dong, S., Yanovsky, M. J., Balazadeh, S., et al. (2018). FITNESS, a CCT domain-containing protein, deregulates reactive oxygen species levels and leads to fine-tuning trade-offs between reproductive success and defence responses in Arabidopsis. Plant Cell Environ. 41, 2328–2341. doi: 10.1111/pce.13354
Pfaffl, M. W. (2001). A new mathematical model for relative quantification in real-time RT-PCR. Nucleic Acids Res. 29:e45.
Pieterse, C. M., Leon-Reyes, A., Van der Ent, S., and Van Wees, S. C. (2009). Networking by small-molecule hormones in plant immunity. Nat. Chem. Biol. 5, 308–316. doi: 10.1038/nchembio.164
Putterill, J., Robson, F., Lee, K., Simon, R., and Coupland, G. (1995). The CONSTANS gene of Arabidopsis promotes flowering and encodes a protein showing similarities to zinc finger transcription factors. Cell 80, 847–857. doi: 10.1016/0092-8674(95)90288-0
Quilis, J., Peńas, G., Messeguer, J., Brugidou, C., and San Segundo, B. (2008). The Arabidopsis AtNPR1 inversely modulates defense responses against fungal bacterial, or viral pathogens while conferring hypersensitivity to abiotic stresses in transgenic rice. Mol. Plant Microbe Interact. 21, 1215–1231. doi: 10.1094/mpmi-21-9-1215
Redestig, H., and Costa, I. G. (2011). Detection and interpretation of metabolite-transcript coresponses using combined profiling data. Bioinformatics 27, i357–i365. doi: 10.1093/bioinformatics/btr231
Rezadoost, M. H., Kordrostami, M., and Kumleh, H. H. (2016). An efficient protocol for isolation of inhibitor-free nucleic acids even from recalcitrant plants. 3 Biotech. 6:61.
Robson, F., Costa, M. M., Hepworth, S. R., Vizir, I., Piñeiro, M., Reeves, P. H., et al. (2001). Functional importance of conserved domains in the flowering-time gene CONSTANS demonstrated by analysis of mutant alleles and transgenic plants. Plant J. 28, 619–631. doi: 10.1046/j.1365-313x.2001.01163.x
Roessner-Tunali, U., Hegemann, B., Lytovchenko, A., Carrari, F., Bruedigam, C., Granot, D., et al. (2003). Metabolic profiling of transgenic tomato plants overexpressing hexokinase reveals that the influence of hexose phosphorylation diminishes during fruit development. Plant Physiol. 133, 84–99. doi: 10.1104/pp.103.023572
Rutledge, R. G., and Stewart, D. (2008). Critical evaluation of methods used to determine amplification efficiency refutes the exponential character of real-time PCR. BMC Mol. Biol. 9:96. doi: 10.1186/1471-2199-9-96
Sambrook, J. F., and Russell, D. W. (2001). Molecular Cloning: a Laboratory Manual. Cold Spring: Harbor Laboratory Press.
Savary, S., Ficke, A., Aubertot, J.-N., and Hollier, C. (2012). Crop losses due to diseases and their implications for global food production losses and food security. Food Secur. 4, 519–537. doi: 10.1007/s12571-012-0200-5
Schwarzenbacher, R. E., Luna, E., and Ton, J. (2014). The discovery of the BABA receptor: scientific implications and application potential. Front. Plant Sci. 25:304.
Singh, M., Bag, S. K., Bhardwaj, A., Ranjan, A., Mantri, S., Nigam, D., et al. (2015). Global nucleosome positioning regulates salicylic acid mediated transcription in Arabidopsis thaliana. BMC Plant Biol. 15:13. doi: 10.1186/s12870-014-0404-2
Spoel, S. H., and Dong, X. (2008). Making sense of hormone crosstalk during plant immune responses. Cell Host Microbe. 3,, 348–351. doi: 10.1016/j.chom.2008.05.009
Staswick, P. E., Su, W., and Howell, S. H. (1992). Methyl jasmonate inhibition of root growth and induction of a leaf protein are decreased in an Arabidopsis thaliana mutant. Proc. Natl. Acad. Sci. USA 89, 6837–6840. doi: 10.1073/pnas.89.15.6837
Strayer, C., Oyama, T., Schultz, T. F., Raman, R., Somers, D. E., Mas, P., et al. (2000). Cloning of the Arabidopsis clock gene TOC1, an autoregulatory response regulator homolog. Science 289, 768–771. doi: 10.1126/science.289.5480.768
Thompson, A. M. G., Iancu, C. V., Neet, K. E., Dean, J. V., and Choe, J.-Y. (2017). Differences in salicylic acid glucose conjugations by UGT74F1 and UGT74F2 from Arabidopsis thaliana. Sci. Rep. 7:46629.
Trigg, S. A., Garza, R. M., MacWilliams, A., Nery, J. R., Bartlett, A., Castanon, R., et al. (2017). CrY2H-seq: a massively multiplexed assay for deep-coverage interactome mapping. Nat. Methods 14, 819–825. doi: 10.1038/nmeth.4343
van Wees, S. C., and Glazebrook, J. (2003). Loss of non-host resistance of Arabidopsis NahG to Pseudomonas syringae pv. phaseolicola is due to degradation products of salicylic acid. Plant J. 33, 733–742. doi: 10.1046/j.1365-313x.2003.01665.x
Wang, C., Yang, Q., Wang, W., Li, Y., Guo, Y., Zhang, D., et al. (2017). A transposon-directed epigenetic change in ZmCCT underlies quantitative resistance to Gibberella stalk rot in maize. New Phytol. 215, 1503–1515. doi: 10.1111/nph.14688
Whalen, M. C., Innes, R. W., Bent, A. F., and Staskawicz, B. J. (1991). Identification of Pseudomonas syringae pathogens of Arabidopsis and a bacterial locus determining avirulence on both Arabidopsis and soybean. Plant Cell 3, 49–59. doi: 10.2307/3869199
Wildermouth, M. C., Dewdney, J., Wu, G., and Ausubel, F. M. (2001). Isochorismate synthase is required to synthesize salicylic acid for plant defence. Nature 29, 562–565. doi: 10.1038/35107108
Zanor, M. I., Osorio, S., Nunes-Nesi, A., Carrari, F., Lohse, M., Usadel, B., et al. (2009). RNA interference of LIN5 in Solanum lycoperisum confirms its role in controlling Brix content, uncovers the influence of sugars on the levels of fruit hormones and demonstrates the importance of sucrose cleavage for normal fruit development and fertility. Plant Physiol. 150, 1204–1218. doi: 10.1104/pp.109.136598
Zhang, Y., Goritschnig, Y., Dong, X., and Li, X. (2003). A gain-of-function mutation in a plant disease resistance gene leads to constitutive activation of downstream signal transduction pathways in suppressor of npr1-1, constitutive 1. Plant Cell 15, 2636–2646. doi: 10.1105/tpc.015842
Zheng, X., Spivey, N., Zeng, W., Liu, P., Fu, Z. Q., Klessig, D. F., et al. (2012). Coronatine promotes Pseudomonas syringae virulence in plants by activating a signaling cascade that inhibits salicylic acid accumulation. Cell Host Microbe 14, 587–596. doi: 10.1016/j.chom.2012.04.014
Zhou, J. M., Trifa, Y., Silva, H., Pontier, D., Lam, E., Shah, J., et al. (2000). NPR1 differentially interacts with members of the TGA/OBF family of transcription factors that bind an element of the PR-1 gene required for induction by salicylic acid. Mol. Plant Microbe Interact. 13, 191–202. doi: 10.1094/mpmi.2000.13.2.191
Zhu, Y., Du, B., Qian, J., Zou, B., and Hua, J. (2013). Disease resistance gene-induced growth inhibition is enhanced by rcd1 independent of defense activation in Arabidopsis. Plant Physiol. 161, 2005–2013. doi: 10.1104/pp.112.213363
Keywords: pathogen, Arabidopsis, salicylic acid, NPR1, defense
Citation: Mengarelli DA, Roldán Tewes L, Balazadeh S and Zanor MI (2021) FITNESS Acts as a Negative Regulator of Immunity and Influences the Plant Reproductive Output After Pseudomonas syringae Infection. Front. Plant Sci. 12:606791. doi: 10.3389/fpls.2021.606791
Received: 15 September 2020; Accepted: 11 January 2021;
Published: 04 February 2021.
Edited by:
Aardra Kachroo, University of Kentucky, United StatesReviewed by:
Ramesh Raina, Syracuse University, United StatesArchana Singh, University of Delhi, India
Copyright © 2021 Mengarelli, Roldán Tewes, Balazadeh and Zanor. This is an open-access article distributed under the terms of the Creative Commons Attribution License (CC BY). The use, distribution or reproduction in other forums is permitted, provided the original author(s) and the copyright owner(s) are credited and that the original publication in this journal is cited, in accordance with accepted academic practice. No use, distribution or reproduction is permitted which does not comply with these terms.
*Correspondence: María Inés Zanor, zanor@ibr-conicet.gov.ar
†ORCID: Salma Balazadeh, orcid.org/0000-0002-5789-4071; María Inés Zanor, orcid.org/0000-0002-8903-0673