- 1Dipartimento di Scienze Agrarie e Ambientali, Università degli Studi di Milano, Milan, Italy
- 2Dipartimento di Scienze e Tecnologie Biologiche ed Ambientali, Università del Salento – Centro Ecotekne, Lecce, Italy
- 3Faculty of Viticulture and Winemaking, Caucasus International University, Tbilisi, Georgia
- 4National Wine Agency of Georgia, Tbilisi, Georgia
Grapevine (Vitis vinifera) is one of the most widely cultivated plant species of agricultural interest, and is extensively appreciated for its fruits and the wines made from its fruits. Considering the high socio-economic impact of the wine sector all over the world, in recent years, there has been an increase in work aiming to investigate the biodiversity of grapevine germplasm available for breeding programs. Various studies have shed light on the genetic diversity characterizing the germplasm from the cradle of V. vinifera domestication in Georgia (South Caucasus). Georgian germplasm is placed in a distinct cluster from the European one and possesses a rich diversity for many different traits, including eno-carpological and phenological traits; resistance to pathogens, such as oomycetes and phytoplasmas; resistance to abiotic stresses, such as sunburn. The aim of this review is to assess the potential of Georgian cultivars as a source of useful traits for breeding programs. The unique genetic and phenotypic aspects of Georgian germplasm were unraveled, to better understand the diversity and quality of the genetic resources available to viticulturists, as valuable resources for the coming climate change scenario.
Grapevine: A High Socio-Economic Impact Crop Strongly Threatened by Climate Change
The genus Vitis is present in 10 distribution areas, all in the northern hemisphere: five in North America, where 29 species have been described; four in Asia, with at least 11 species; and only one, Vitis vinifera, in a wide range that includes the Mediterranean, sub-Mediterranean, and Caucasian floristic regions with a spread toward the Pontic, Caspian, and Central Asiatic areas (Mullins et al., 1992). V. vinifera is one of the most widely cultivated plant species of agricultural interest and the only species extensively used in the global wine industry, covering approximately 7.4 Mha in 2018, and producing more than 77.8 mt of grapes (wine, table and dried grapes) and a world wine trade worth around EUR 32 billion1. Regions of its cultivation are located roughly between the 35th and 55th northern parallels and between the 25th and 35th southern parallels, in areas with average annual temperatures between 10 and 20°C. These environments are characterized by the alternation of a favorable growing season and an unfavorable cold one. However, the cold winters are not too intense (minimum temperatures range between −10 and 15°C) and the favorable season (average temperature higher than 10°C) is long enough (>200 days) for grapes to ripen (Gladstones, 1992).
Viticulture depends on environmental resources (i.e., climate and soil conditions) in terms of yields and quality (van Leeuwen and Darriet, 2016). The current climatic phase, characterized by the increase of average global temperature, has led to changes in the environmental conditions of agricultural areas that need to be tackled with suitable tools, in a context of adaptation and mitigation. Due to the socio-economic impact of the wine sector in Europe and around the world, over recent years, there has been an increase in work aiming to study the impact of climate change on viticulture (Hannah et al., 2013; Morales-Castilla et al., 2020).
Santos et al. (2020) proposed a list of measures to be adopted in viticulture to face with the climate change. The list divided the measures in two categories: the short-term adaptation strategies and the long-term adaptation strategies. The short-term strategies include crop cultural practices and techniques to delay ripening time, plant protection against extreme heat, irrigation, pest and disease control and soil management. Among the long-term strategies, there are: change in training systems, varietal/clonal and rootstock selection and vineyard relocation. Breeding programs for new varieties which will be better able to perform in the environmental conditions expected in the future could be one of the most promising solutions, although this strategy is included in the long-term ones. An appropriate cultivar selection reduces the inputs required for plant management, increasing the sustainability of production. Great sources of biodiversity in the V. vinifera species have been recently found in its domestication cradle, located in Georgia (South Caucasus) (Imazio et al., 2013) (Figure 1).
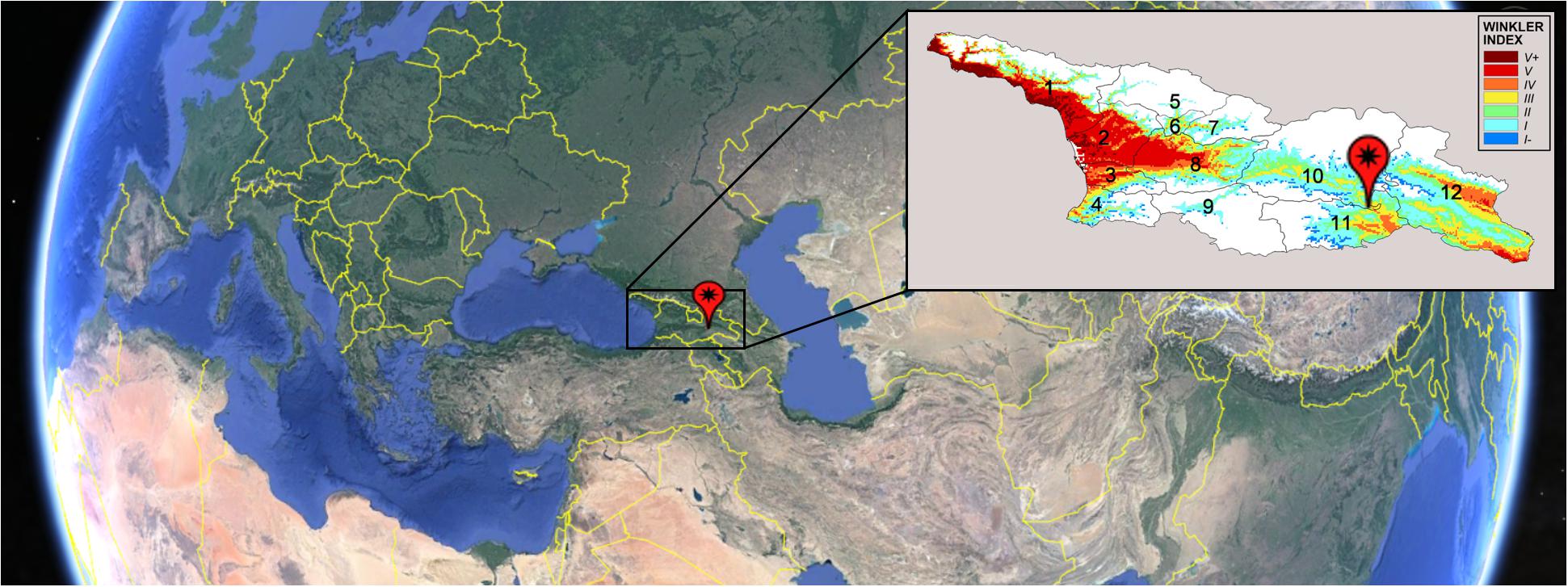
Figure 1. Map of Georgia and location of 12 Georgian wine-growing regions: 1 – Abkhazeti; 2 – Samegrelo; 3 – Guria; 4 – Adjara; 5 – Svaneti; 6 – Lechkhumi; 7 – Racha; 8 – Imereti; 9 – South Kartli; 10 – Inner Kartli; 11 – Lower Kartli; 12 – Kakheti. Image is obtained by Google Earth. Pin indicates Tbilisi position. Map on the right reports the Winkler classification based on yearly average Winkler index calculated for the period 1994–2013 in Georgia (Caucasus). The analysis is limited to the areas below 1250 m above sea level. Description of Winkler indices: (I–) GDD (Growth Degree Days) < 850, viticultural climate is very cool, vinicultural aptitude is very early ripening grapes for fresh and fruity wines or sparkling wine bases. (I) GDD 850–1400, viticultural climate is cool, vinicultural aptitude is early ripening grapes for fresh and fruity wines or sparkling wine bases. (II) GDD 1400–1650, viticultural climate is temperate cool, vinicultural aptitude is early ripening grapes for wines to be aged. Medium ripening grapes for white or red wines ready to drink. (III) GDD 1650–1950, viticultural climate is temperate, vinicultural aptitude is medium ripening grapes for white or red wines ready to be aged. (IV) GDD 1950–2200, viticultural climate is temperate warm, vinicultural aptitude is late ripening grapes for white or red wines ready to be aged. (V) GDD 2200–2700, viticultural climate is hot, vinicultural aptitude is late ripening grapes for bodied red wines to be aged. (V+) GDD > 2700, viticultural climate is very hot; vinicultural aptitude is very late ripening grapes for bodied red wines to be aged.
Georgia counts 48,000 hectares of vineyards and a production of wine and table grapes of 159,000 and 8,000 tons, respectively (see text footnote 1). In 2015, about 100 M liters of wine were produced, 80% of them obtained by white and 20% from red berry grapes. More than 90% of the 2015 production was supported by Kakheti region, producing mainly white and red wines from Rkatsiteli and Saperavi grapes, in the ratio 7:3.
The aim of this review is to assess the potential of Georgian cultivars as sources of useful traits for new breeding programs, aiming to face the future challenges that await viticulture worldwide. To do this, we reviewed the particular genetic and phenotypic aspects (such as berry traits and resistance to pathogens) of Georgian germplasm, in the hope of better understanding the diversity and quality of the genetic resources available to viticulturists, coming directly from the origin of domestication.
South Caucasus, the First Grapevine Domestication Center
Vitis vinifera is indigenous to Eurasia and it is suggested that the ancestors of the first Vitis genus appeared about 65 million years ago (Olmo et al., 1995). Nowadays, V. vinifera species includes both cultivated (V. vinifera subsp. sativa) and wild (V. vinifera subsp. silvestris) subspecies, the latter considered the progenitor of subspecies sativa (This et al., 2006). Its domestication process seems to be strongly linked to the alcoholic and gustative superiority of its fermented juice (the wine) in comparison to that of other fleshy fruits (fruit wines), although it is not well known which process predated the other (Terral et al., 2010). The main changes driving grapevine domestication were identified in the flower morphology (appearance of hermaphrodite flowers), larger berry size, higher berry sugar content, a wide range of berry color and aromatic content, characters which ensure yield, quality and a greater sugar content for a better fermentation (This et al., 2006). The major questions about grapevine domestication process are related to the number of events occurred, single event versus multiple events, and the geographical location where these events took place. For a vine domestication center to be born, different conditions need to occur. Among these, there is a strong awareness in practicing and developing viticulture by entire peasant villages. To bring out such a situation, many factors have to converge: territories with a (relatively) high population density, with stable settlements and in positions at crossroads of trade flows and cultural trends (Forni, 2012). It would be reasonable to expect that such situation could have occurred in several areas, differing in chronology and level of development. The most accredited hypothesis suggests that V. vinifera was domesticated from its wild form in the South Caucasus, between the Caspian and Black Seas, around 6,000–5,800 BC, and then spread throughout Europe and Mediterranean areas thanks to the spread of civilizations (McGovern et al., 2017). Recently, Zhou et al. (2019), proposing a four-state domestication process for grapevine, date the beginning of this process around 20,000 years ago, when South Caucasian human populations started to manage and harvest the local wild populations (Stage 1). In the same region around 8,000 years ago, the humans started with the conscious or unconscious selection of desirable phenotypical traits (Stage 2), although this transition is not well documented yet. Another force driving the transition from Stage 1 to Stage 2 is the bottleneck. Nevertheless, genetic evidences showed that grapevine did not experience a severe bottleneck (Myles et al., 2011; Zhou et al., 2017), making the conscious or unconscious selection as a unique force shaping the genetic diversity of grapevine during the domestication process. Stage 3 consists on spreading of newborn crop in new locations and the consequence of local domestication or introgression events. Reviewing the most comprehensive studies on grapevine genetic population, it turned out that an East-to-West grapevine gene flow after the first domestication process occurred, with some evidence of putative secondary domestication centers along the main migration routes due to genetic relationships between wild and cultivated accessions, especially in the Mediterranean Basin and Central Asia (Grassi et al., 2003; Arroyo-García et al., 2006; Myles et al., 2011; Zhou et al., 2017; Riaz et al., 2018). The coexistence of wild populations together with domesticated ones is often and the bidirectional gene flow (wild-to-cultivated and cultivated-to-wild) has been well documented (De Andrés et al., 2012; Ekhvaia et al., 2014; Riaz et al., 2018; D’Onofrio, 2020; Maraš et al., 2020), supporting the occurrence of secondary domestication events from local wild populations or introgression events. These events, the geographical origin and human usage were found to strongly shape the genetic structure of grapevine germplasm (Bacilieri et al., 2013). Another aspect, although less investigated, is the role of wild Vitis species in the sativa domestication process. It seems that wild Vitis species have contribute to the current structure of grapevine germplasm (Zhou et al., 2019). The last stage proposed by Zhou et al. (2017) (Stage 4) takes into account the modern breeding programs, a relative recent event occurred over the last few hundred years and led to the birth of so-called anthropic crossings, with the aim of satisfying specific requirements.
Georgian Territory, Climate, and Grapevine Production
Georgia is a large basin of the mid latitudes, bordered by the Greater Caucasus in the North and the Lesser Caucasus in the South, and opening toward the Black Sea in the West and toward the Caspian depression in the East (Figure 1). Those geographical features strongly characterize the climate of its 12 wine-growing regions that, following the Köppen – Geiger classification (Köppen and Geiger, 1936), are characterized by profoundly different climatic conditions, ranging from hot summer continental climates to warm summer continental or hemiboreal climates, that translate into different classes of the Winkler index (Figure 1).
In relation to the climatic conditions of each wine-growing region, the Georgian varietal assortment is strongly differentiated as well, being adapted to a very wide range of cold and summer stresses (Table 1). Worldwide wine-growing regions experiencing the same climatic conditions of Georgia may provide benefit by this so differentiated varietal spectrum.
It is interesting to highlight that, in 1994 Georgia faced an abrupt rise in temperatures, similarly to what happened in Western Europe in the late 1980s (Reid et al., 2016), with 1987 as the most likely year of change (Mariani et al., 2012). This delay could be explained by the progressive dilution of the Atlantic circulation signal as it moves into the European continent (Cola et al., 2017). The increase of temperature determined an advance in grapevine phenology, which was more significant at the higher altitudes, where more favorable thermal conditions were established. On the other hand, at lower altitudes the phenological advance was partially depleted by the increase of super-optimal thermal conditions (increasing the occurrence of stress conditions during ripening). For instance, in the case of the widely diffused cultivar Rkatsiteli, the average advance of veraison was less than 6 days for the 250–500 m asl elevation belt and around 18 days for the 750–1000 m one (Cola et al., 2017).
In parallel, it is worth noticing the high variability in the plant phenology among Georgian cultivars, both in the sprouting date and in the ripening period. A delayed budburst period could represent an avoidance mechanism against spring frosts. Considering Georgian cultivars, bud swelling of ‘Partala’ vines was recorded at the end of March, and, thus, a higher susceptibility to spring frost is expected when compared to the other cultivars that sprouted in April (Maghradze et al., 2014). Global warming generally resulted in the increase of cases of temperature above the optimal range (24–26°C) during summer and in particular during grape ripening (Cola et al., 2020). A delay in the maturation process, obtained through the selection of late-ripening cultivars, could ensure thermal conditions during ripening more suitable for berry metabolism. Maghradze et al. (2012) studied the phenology of Georgian cultivars in northern Italy in comparison to Chardonnay and Cabernet Sauvignon grown in the same area, and they found a relatively late ripening with respect to the reference varieties: nevertheless, a very wide range of variability was observed. Similar results were found in other comparative evaluation carried out in Georgian ampelographic collections and are reported by Maghradze et al. (2014) and Rustioni et al. (2014). Some extreme cases are: early ripening cultivars – Kartuli Saadreo, Meskhuri Mtsvane, Buza, Budeshuri Tsiteli and Daisi; late ripening cultivars – Ojaleshi, Akomshtali, Kamuri, Shavi, Tavkara, Khushia Shavi, Satsuravi, Maghlari Tvrina, Mtevandidi, Argvetula, Dziganidzis Shavi, Adanasuri, Mamukas Vazi, Otskhanuri Sapere, Gorula, Saperavi Meskhuri, Ghrubela and Shavtita. The same results were obtained when comparing the phenological timing of Georgian varieties internationally grown. The phenological model developed by Mariani et al. (2013) for Cabernet Sauvignon and Chardonnay and adapted to the Georgian varieties Saperavi, Rkatsiteli, Mtsvane Kakhuri (Cola et al., 2017) was applied to a long time series of daily temperature (Perugia–Italy. 1990–2019). Figure 2 shows the late phenological timing of Georgian varieties (average values are shown).
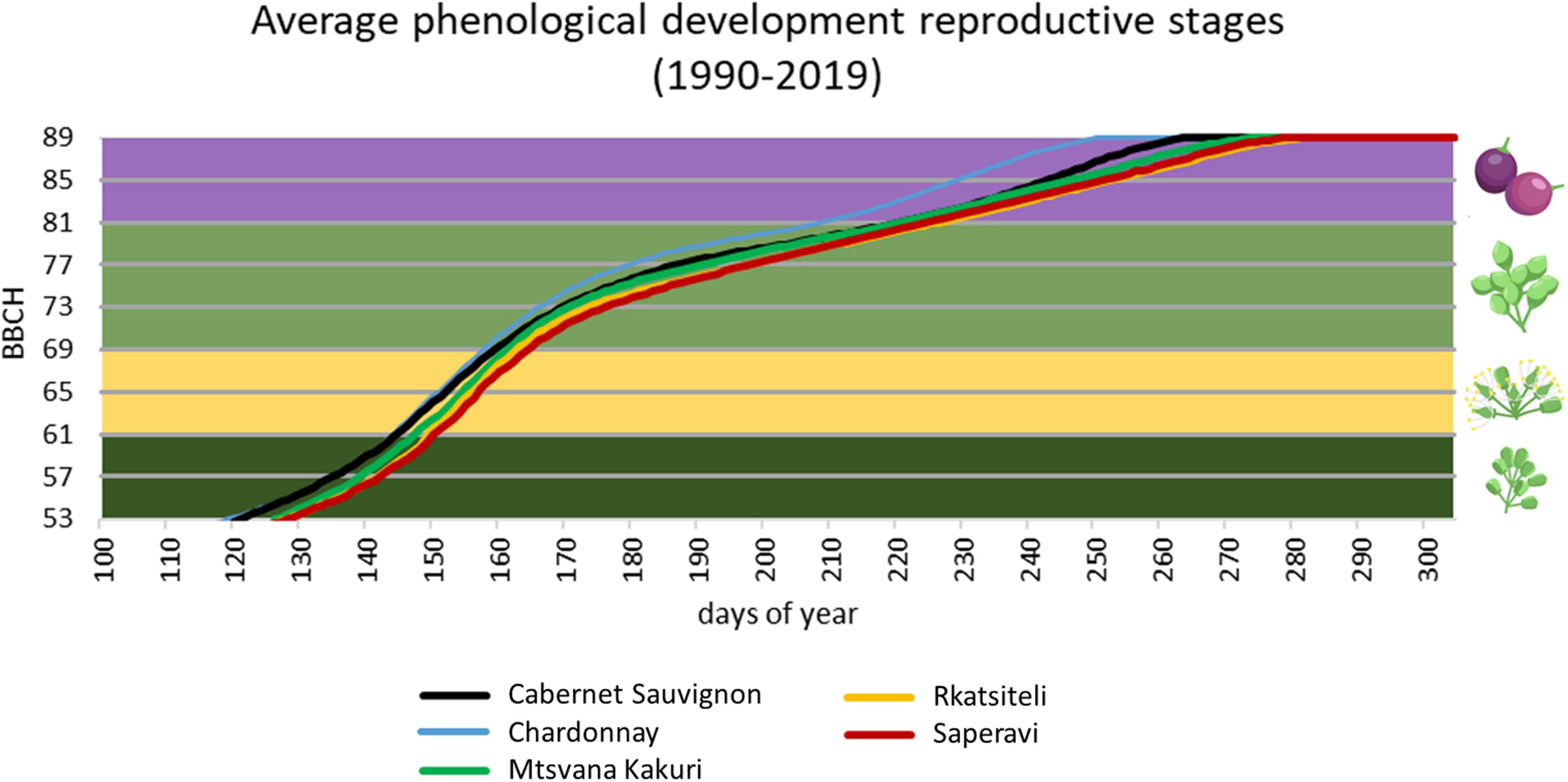
Figure 2. Phenological timing simulation for three relevant Georgian cultivars, compared with Chardonnay and Cabernet sauvignon, using meteorological data of Perugia (Italy) (years 1990–2019). Phenology is represented following the reference BBCH scale: (i) 53–59 development of flowers; (ii) 60–69 flowering; (iii) 70–79 development of fruits; (iv) 80–89 ripening.
The Ampelographic Collections of Georgian Germplasm
To date, fifty grapevine varieties are recommended for cultivation in Georgia. Most of them (37) are wine grape cultivars, while the others (13) are generally used to produce grapes for fresh consumption. Predominantly, the Georgian vineyards are cultivated with autochthonous varieties: among the recommended wine grapes, 31 are local varieties and seven are international cultivars, while, considering the table grapes, nine of them have a local origin: four are traditional, autochthonous, Georgian cultivars, five are from local breeding outputs, and five are allochthonous varieties (Chkhartishvili and Maghradze, 2012). Beside the recommended autochthonous varieties, other cultivars enlarge the intraspecific biodiversity preserved in Georgia: Tsertsvadze (2012) described 48 grapevine native cultivars in the ‘Caucasus and Northern Black Sea Region Ampelography’ and further studies are in progress to continuously increase the number of recognized Georgian cultivars preserving this important source of biodiversity. Based on information available, more than 700 Georgian accessions can be counted (Supplementary Table 1), most of them are germplasm accessions and the rest are classified as major (20) and minor (8) cultivars. These accessions are available in nine Georgian collections (Table 2) and other collections hold by foreign Institutions, such as Italy (443 accessions), Ukraine (309 accessions), Russia (191 accessions), Moldova (122 accessions), Uzbekistan (32 accessions), France (20 accessions), and Slovakia (7 accessions). Although the number of accessions is high, only a limited number of them were genotyped and phenotyped (see sections “Georgian Germplasm as a Source of Genetic Variability” and “The Phenotypical Characterization of Georgian Germplasm Collections”). This limited number of information makes a not so easy determination of the exact number of autochthonous Georgian varieties. Further efforts are needed to better understand the genetic diversity of this valuable germplasm and to identify synonyms, homonyms and misidentifications.
Georgian Germplasm as a Source of Genetic Variability
Historical information coupled with archeological and palaeobotanical findings pointed to Georgia as a cradle for grapevine domestication (Zohary and Hopf, 2000; McGovern, 2003; McGovern et al., 2017). Molecular analysis produced the same evidence. Genetic diversity of Georgian germplasm was investigated, by both nuclear SSR (simple sequence repeat) (Laucou et al., 2011; Imazio et al., 2013; Ekhvaia et al., 2014) and SNPs (single nucleotide polymorphisms) (De Lorenzis et al., 2015; Laucou et al., 2018) molecular markers, although a number of autochthonous cultivars, collected in local ampelographic collections, still remain to be studied (Supplementary Table 1). Thanks to two European research programs, GrapeGen06 (2007–2010) (Laucou et al., 2011), first, and then COST Action FA1003 (2011–2014) (Failla, 2015), a strong and still active network of scientific collaborations has been developed between European and Georgian researchers, to genetically characterize and preserve the Georgian genetic resources of vines.
All the outcomes about the genetic characterization of Georgian germplasm reported the uniqueness and originality of this germplasm when compared to the European and Central Asian germplasm (Myles et al., 2011; Bacilieri et al., 2013; Imazio et al., 2013; Riaz et al., 2018; De Lorenzis et al., 2019). The Georgian cultivars showed the distinctive features of a domestication center, such as high levels of genetic diversity and heterozygosity, the presence of alleles absent or poorly represented in other countries, and differentiation from the European varieties, clustering in a well-separated branch (as reported in the Figure 3, where SSR and SNP genetic profiles of varieties from France, Georgia, Italy and Spain were re-elaborated to perform a discriminant analysis of principal component, using data published in De Lorenzis et al., 2015, 2019, Laucou et al., 2018, and Riaz et al., 2018). A differentiation inside the germplasm, based on the geographical origin of cultivars, was identified as well: the varieties putatively originated in Kartli and Kakheti (Eastern regions) differ from the ones originating in Abkhazeti, Samegrelo, Guria, Adjara, Imereti, Racha, and Lechkhumi (Western regions). The origin of this subdivision lies in the geographical subdivision of Georgia into two major parts, due to the Likhi Mountains running in a North-to-South direction across Georgia (Imazio et al., 2013; De Lorenzis et al., 2015), confirming that, despite long-standing cultivation, the Georgian cultivars maintain their originality.
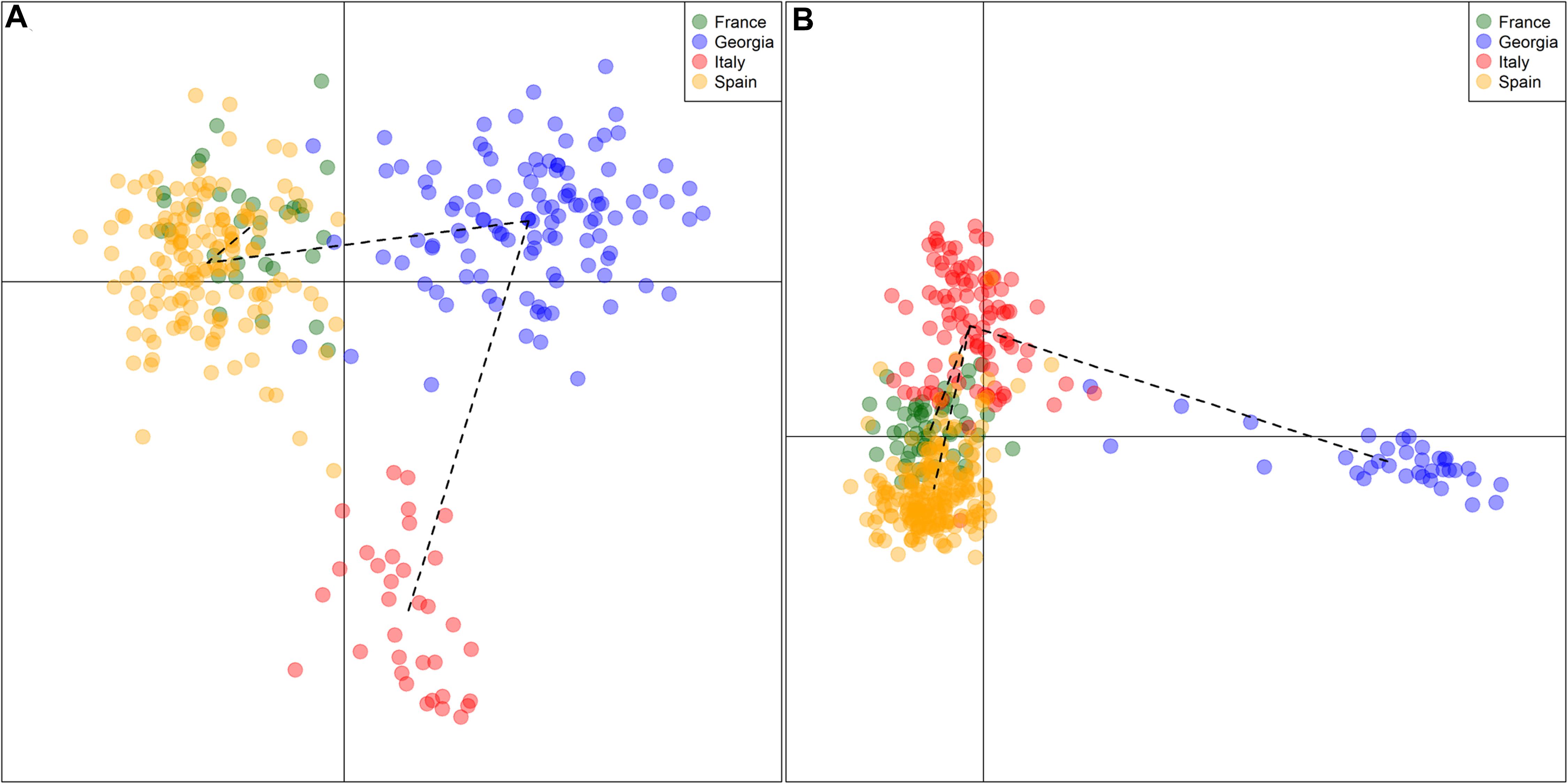
Figure 3. Two-dimension DAPC (discriminant analysis of principal component) scatter plot. Reworking of genetic profiles of grapevine cultivars coming from France, Georgia, Italy, and Spain, genotyped by 20 SSRs (A) and 18k SNPs (B), using data reported in De Lorenzis et al. (2015), Laucou et al. (2018), Riaz et al. (2018), and De Lorenzis et al. (2019). DAPC was performed to identify genetic clusters using the package adegenet of R software. Black dotted lines represent a minimum-spanning tree.
Genetic variation provides the foundation for any breeding programs, and natural genetic diversity represented historically the major source of variability for crop improvement and adaptation to changing environmental conditions. Given the uniqueness of Georgian germplasm, its strong link with the regions of origin coupled with the evidence of this country being the center of domestication makes this germplasm very attractive for investigation from the perspectives of phenology, grape phenotype and resistance to biotic and abiotic stresses, as sources of new variability for future breeding programs.
The Phenotypical Characterization of Georgian Germplasm Collections
The collaboration among European and Asian researchers makes feasible the comparisons among ampelographic collections using common protocols. Among them, phenotyping was considered in the framework of the COST Action FA1003 (Rustioni et al., 2014), allowing the description of numerous autochthonous cultivars (Abashidze et al., 2015; Cornea and Savin, 2015; Goryslavets et al., 2015; Maghradze et al., 2015; Ujmajuridze and Mamasakhlisashvili, 2015). This work, finally, produced a general overview of the V. vinifera variability concerning eno-carpological traits (Rustioni et al., 2019). Table 3 reports the distribution of the Georgian records with respect to the variability described for the V. vinifera species (data obtained by the reworking of the results published in Rustioni et al., 2014, 2019, and Abashidze et al., 2015). To emphasize some results, showing the differences among the two groups of data, Figure 4 shows the frequency distribution of the Georgian records in comparison with the data collected for the entire V. vinifera species, concerning some specific traits (titratable acidity, percentage of skin, skin phenolic content). Briefly, the main differences highlighted in Table 3 in terms of oenological applications are that Georgian grapes have, with respect to the V. vinifera species population, higher concentrations in both sugars and acids and thicker skins, ensuring acceptable amounts of phenolics despite the lower accumulation per unit of tissue. Details concerning the results of this comparison are discussed in Sections “Fruit Morphology and Technological Quality of Georgian Cultivars” and “Abiotic Stress Adaptations and Secondary Metabolisms.” It is worth noting that the phenotypic variability reported is due to the genotype, to the environmental growing conditions, and to their interactions. Thus, further studies will be necessary to discriminate these effects, highlighting the role of genotypes.
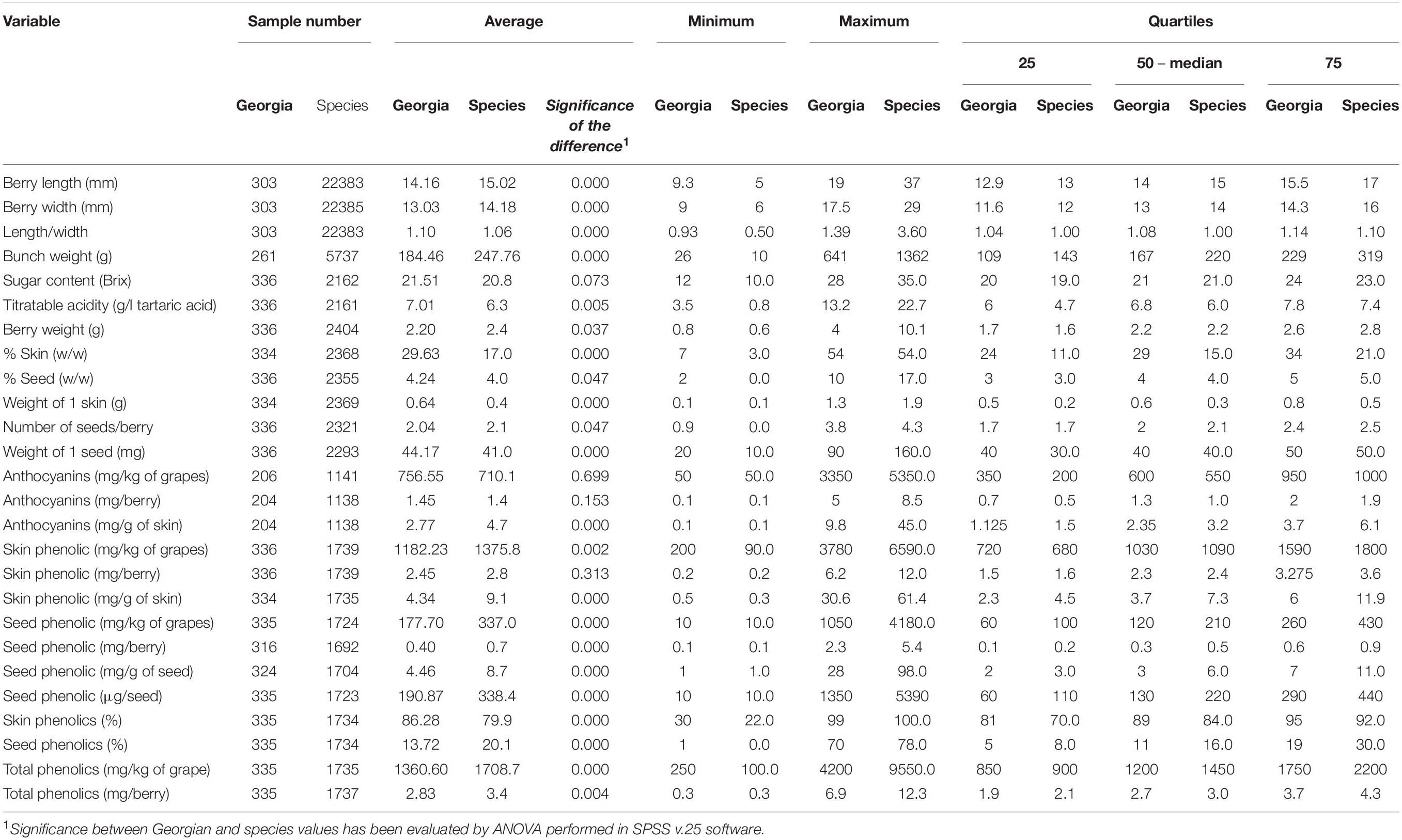
Table 3. Physical dimensions and chemical components distribution of Georgian grapevine germplasm with respect to the variability described for the entire V. vinifera species (reworking of the results published in Rustioni et al., 2014, 2019 and Abashidze et al., 2015).
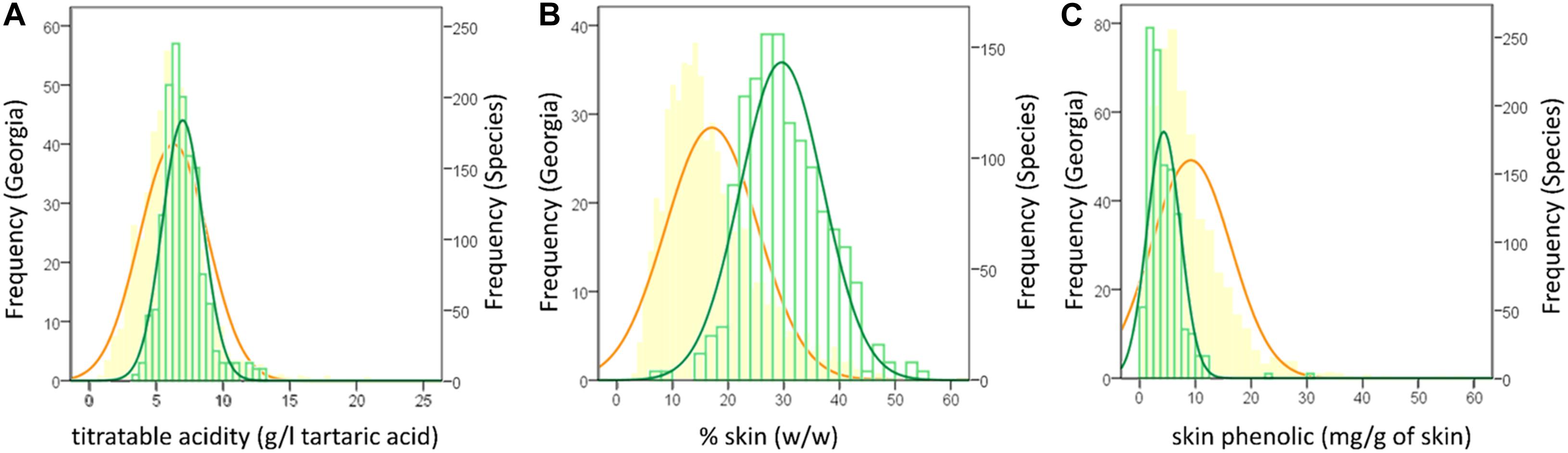
Figure 4. Frequency distribution of the entire V. vinifera species (orange) in comparison with the Georgian (green) records concerning the traits: titratable acidity (A); % of skin (B) and skin phenolics (C) (reworking of the results published in Rustioni et al., 2014, 2019 and Abashidze et al., 2015).
Fruit Morphology and Technological Quality of Georgian Cultivars
Despite the wide variability for berry shapes within the species, Georgian grapes generally have round or slightly elongated small berries (Table 3) (OIV, descriptor 223). This is probably due to the ancient traditions that, during millennia of winemaking activities (Chkhartishvili and Maghradze, 2012; McGovern et al., 2017), favored the selection of wine grapes over table grapes.
Considering technological maturity, Georgian records generally show higher concentrations in both sugars and acids than foreign varieties (Table 3). In the perspective of climate change, the high sugar content could represent a problem, due to the risk of further increases related to the higher temperatures during the anticipated ripening periods (Keller, 2010; Mira de Orduña, 2010; van Leeuwen and Destrac-Irvine, 2017). The increased sugar concentrations expected in hot ripening conditions may cause growth inhibition or lysis in the yeasts responsible for wine fermentation (Mira de Orduña, 2010). Furthermore, high sugar stress could modify the yeast metabolisms, increasing the accumulation of by-products (such as glycerol and acetic acid) that, together with the increased alcoholic content, affects the wine perceptions of consumers and, thus, it could modify the expected characteristics of traditional wines (Mira de Orduña, 2010). However, the deviation of Georgian data with respect to the species variability concerning the sugar content is rather limited (significance of the difference = 0.073), neither covering the maximum records of the one obtained when analyzing wider grapevine genetic pools (Table 3) (Rustioni et al., 2019). Furthermore, the expected sugar content increase is usually ascribed to the earlier ripening anticipated in climate changed conditions (Palliotti et al., 2014; Martínez-Moreno et al., 2019), and it is important to remind the prevalence of late ripening cultivars among the Georgian grapevines (Maghradze et al., 2012). Finally, the sugar content seems to be well counterbalanced by the acidity (Table 3). The distribution of Georgian grapes concerning the titratable acidity, showed a right shift (Figure 4A), demonstrating the ability of these cultivars to keep a high acidic concentration despite the sweetness of the berries. This is a crucial point for viticulture adaptation to climate change, because high temperatures usually cause a decrease in acids, especially due to malic acid degradation (Keller, 2010; Mira de Orduña, 2010; van Leeuwen and Destrac-Irvine, 2017).
In the perspective of climate change adaptation, it is very interesting to note a particular feature of Georgian grapes concerning the proportions among skin, seeds and pulp, at the expense of the latter (Table 3). The Georgian records shown in Figure 4B, demonstrate an important shift toward thicker skins with respect to the general V. vinifera species. This is due to the lighter berries and heavier skins (Table 3). Considering the effect of climate change on the berries, a thicker skin could represent a more resistant barrier against the stressful environment. In fact, it has been shown that a possible adaptation to climate change could be related to berry skin thickening (van Leeuwen and Destrac-Irvine, 2017).
Abiotic Stress Adaptations and Secondary Metabolisms
Grape epicuticular waxes also have important protective roles against dehydration (Pangavhane et al., 1999; Di Matteo et al., 2000; Doymaz, 2006; Muganu et al., 2011) and pathogen infections (Marois et al., 1986; Rosenquist and Morrison, 1988; Percival et al., 1993). Furthermore, a study conducted on Georgian cultivars, suggested a possible eco-physiological role of epicuticular waxes in reducing heating stresses by an interaction with infrared radiation (Rustioni et al., 2012). However, a comparison among Georgian cultivars and grape varieties cultivated in other regions is not available and, thus, we should suppose that this mechanism is not exclusive for Georgian cultivars.
Excesses of photosynthetically active radiation (PAR) could causeproblems in grapes due to chlorophyll overexcitation (Rustioni et al., 2015; Rustioni, 2017). Rkatsiteli response to photo-oxidativesunburn was tested by Rustioni et al. (2015). It was considered among the “tolerant cultivars,” as it showed relatively low susceptibility to sunburn (recorded asbrowning appearance) at all the phenological periods studied. Inparticular, the correlation between chlorophyll contents and browningsymptoms had a high R2 (0.989), but the slopecoefficient (60.2) together with the average Browning Intensity Index(27.5) indicated a light symptom appearance in Rkatsiteli grapes. Inphoto-oxidative sunburn, browning symptoms appear due to the reactiveoxygen species (ROS) scavenging activity of phenolics through theiroxidation and consequent polymerization that produce brown pigments(Felicetti and Schrader, 2008; Rustioni et al., 2015). Often, plants face stresses through secondary metabolites, and the crucial role of phenolics against photodamage is well known (Close and McArthur, 2002; Graham et al., 2004; Rustioni, 2017). However, if the substrate for these oxidative polymerizations (phenolics) are in low concentrations, the sunburn browning symptoms could appear less intense: this is likely in the case of Rkatsiteli. Abashidze et al. (2015) reported 404.7 ± 58.3 mg/kg of grapes as average skin phenolics for this cultivar, which falls in the first 10th percentile of the V. vinifera variability concerning this trait (Rustioni et al., 2019) (Table 3). Considering total phenolic compounds, Georgian cultivars appeared to accumulate low amounts of these molecules in skins (Figure 4C and Table 3), but the difference is still exacerbated by seed phenolics (Table 3). In fact, the average percentage of phenolics arising from seeds is much lower in data coming from Georgia (13.7% in Georgian records in comparison with 20.1% of species characteristics). Of course, considering the eco-physiological role of phenolics, this trait could be considered as a downside of Georgian cultivars. However, in a production perspective, it could be an important advantage.
Climate changes often produce disequilibria in the berry ripening processes, increasing the quantity of phenolic compounds (Keller, 2010; van Leeuwen and Destrac-Irvine, 2017) that, often, do not reach an optimal ripening quality. Unripe phenolics could strongly compromise the wine quality, being involved in the perception of bitterness and astringency (Kontoudakis et al., 2011). Seed phenolics, due to their intrinsic characteristics, are often considered as an undesirable source of defects, so it is true that technologies have been developed to separate seeds to prevent phenolic extractions in wines (Canals et al., 2008) or to artificially ripen them under controlled conditions (Rustioni et al., 2018; VanderWeide et al., 2020). In this perspective, and considering that climate change is expected to make it harder to reach an equilibrated phenolic ripening in grapes, the lower phenolic concentration of Georgian cultivars (especially in the seeds) could be considered as a positive trait to deal with future difficult ripening conditions.
Another important class of phenolic molecules is represented byanthocyanin pigments. Among the 48 native Georgian grapevinevarieties described by Tsertsvadze (2012), 21 of them are white grapecultivars, while the other 27 have pigmented berries (22 black, 2 red, 1 gray, and 2 pink). Among the native Georgian grapevarieties described by Ketskhoveliet al. (1960), 245 of them are not pigmented (241 white and 4 yellow) grape cultivars, while the other 278 have pigmented berries (221 black, 27 red, 5 gray, and 25 pink). The reflectance spectra of 51 Georgian cultivars, together with other 69 accessions originated from other countries, were studied by Rustioni et al. (2013). Based on this first screening, some of these cultivars were selected to highlight dysfunctions in anthocyanin accumulation: Ubakluri, Ghrubela Kartlis, Rkatsiteli Vardisperi (and Marguli Sapere among the reference cultivars). Ubakluri shows a very light color, due to a very low pigment accumulation. Ghrubela Kartlis, due to the prevalence of peonidin-3-O-glucosides among the anthocyanins, has a gray appearance. Rkatsiteli Vardisperi, with the salmon pink color due to the high proportion of cyanidin-3-O-glucosides, is considered a berry color mutant resulting from a retro-transposon-induced mutation of the Rkatsiteli white-skinned cultivar (Rustioni et al., 2016; De Lorenzis et al., 2020). These color peculiarities could be interesting for future selections, especially considering the importance of appearance for table grape markets.
The environmental conditions (e.g., light and temperature) can affect the pigment accumulation in skins and the modulation of the anthocyanin biosynthetic pathway in berries could be considered as a grapevine eco-physiological adaptation mechanism (Keller, 2010; Rustioni et al., 2011; De Lorenzis et al., 2016). Considering anthocyanins (Table 3), Georgian data generally show slightly higher contents of pigments with respect to the V. vinifera species average when expressed as mg/kg of grapes or mg/berry. However, this appears mainly due to the thick Georgian berry skins, and, thus, it is not due to a higher accumulation in this tissue, but to the higher quantity of pigmented tissue itself. In fact, when considering the anthocyanin accumulation in skins, the average Georgian record is 2.77 mg/g of skin, while the species average is nearly twice higher (4.7 mg/g of skin).
Resistance to Grapevine Fungal Diseases
The grapevine varieties cultivated worldwide belong to the Eurasian grapevine, V. vinifera, and are susceptible, at different levels, to several pathogens (fungi, bacteria, and viruses), while non-vinifera species, from North American and Asian, are resistant to fungi and tolerant to viruses and some bacteria (Oliver and Fuchs, 2011; Armijo et al., 2016). Amongst the various diseases which directly affect grapevines, powdery mildew (caused by the ascomycete Erysiphe necator) and downy mildew (caused by the oomycete Plasmopara viticola) are two of the most important (Bois et al., 2017). Disease management became an unavoidable task for European viticulture in the second half of the nineteenth century, when the two pathogens were introduced into Europe and the European grapevine growers were faced with their destructive effects (Töpfer et al., 2011). The P. viticola introduction was a probable consequence of the massive importation of American grapevine species to be used as rootstock for V. vinifera and contrast the destructive effects of phylloxera, caused by Daktulosphaira vitifoliae, on the Eurasian grapevine species (Granett et al., 2001; Gessler et al., 2011). The search for suitable tools for disease management rapidly became a priority for the viticulturists. The discovery of the efficacy of sulfur and copper in controlling the diseases was a key point, but great attention was also paid to the development of resistant cultivars. The American Vitaceae soon proved to be the best sources of resistance, due to co-evolution with the pathogens, and extensive breeding programs, based on interspecific crosses between American Vitis species (e.g., Vitis riparia, Vitis rupestris, Vitis berlandieri and Vitis labrusca) and V. vinifera, were undertaken at the beginning of the XX century (Gessler et al., 2011). Nevertheless, the interest in searching for resistant plants decreased over time, probably due to the discovery of new fungicides (Russell, 2005), that were widely employed for disease control, and the inheritance of the specific foxy off-flavors from the non-vinifera parent species.
Recently, public concern about sustainability in agriculture and newregulations on plant protection products have renewed the interest ofgrowers in the cultivation of resistant varieties (Merdinoglu et al., 2018). In fact, although viticulture in the whole of the EU only occupies alow percentage of arable land, the industry is responsible for a highuse of fungicides to fight downy mildew infections (Eurostat2). Furthermore, studies on the effects of CO2 and temperature on downy and powdery mildews showed that the disease incidence of downy mildew increases with rises in gas and temperature, while an increase in CO2 did not influence powdery mildew incidence (Pugliese et al., 2011). In view of the coming climate change, that will potentially favor the pathogens’ development, it is also important to search for new resistance genes, focusing on alternative species, such as V. vinifera, to the non-vinifera ones.
V. vinifera Resistant Cultivars Against P. viticola
The identification of P. viticola dates back to 1838, when Schweinitz, one of the founders of American mycology, collected the first samples from wild Vitis species in South Carolina (Gessler et al., 2011). In Europe, downy mildew was first reported during 1878 in Bordeaux and then it spread all over the old continent and beyond, reaching Australia and New Zealand between 1919 and 1926 (Emmett et al., 1992). All traditional European grapevine cultivars showed high susceptibility to the pathogen, leading to severe pandemics across Europe (Boso and Kassemeyer, 2008; Gessler et al., 2011). Today, the pathogen is found in warm and humid climates worldwide.
Symptoms of downy mildew (Figure 5) are observable on infected organs as yellowish oily lesions (sometimes red, in black cultivars) on the upper surface of the leaves (Figures 5A,B) followed by sporulation on the underside of the leaf (Figure 5C); malformations and necrosis on herbaceous shoots and inflorescences (Figures 5D,E); change of color to violet and withering on berries (Figure 5F), that detach from the rachis leaving a dry stem scar (Gessler et al., 2011). The disease negatively impacts grape production at both qualitative and quantitative levels: the loss of photosynthetic tissues limits the sugar amount in berries, that produce low quality wines; the shoot and bunch damage leads to poor yields. Severe infections, in the absence of disease control, can result in total loss of leaves and in some cases, total yield loss (Töpfer et al., 2011; Toffolatti et al., 2018).
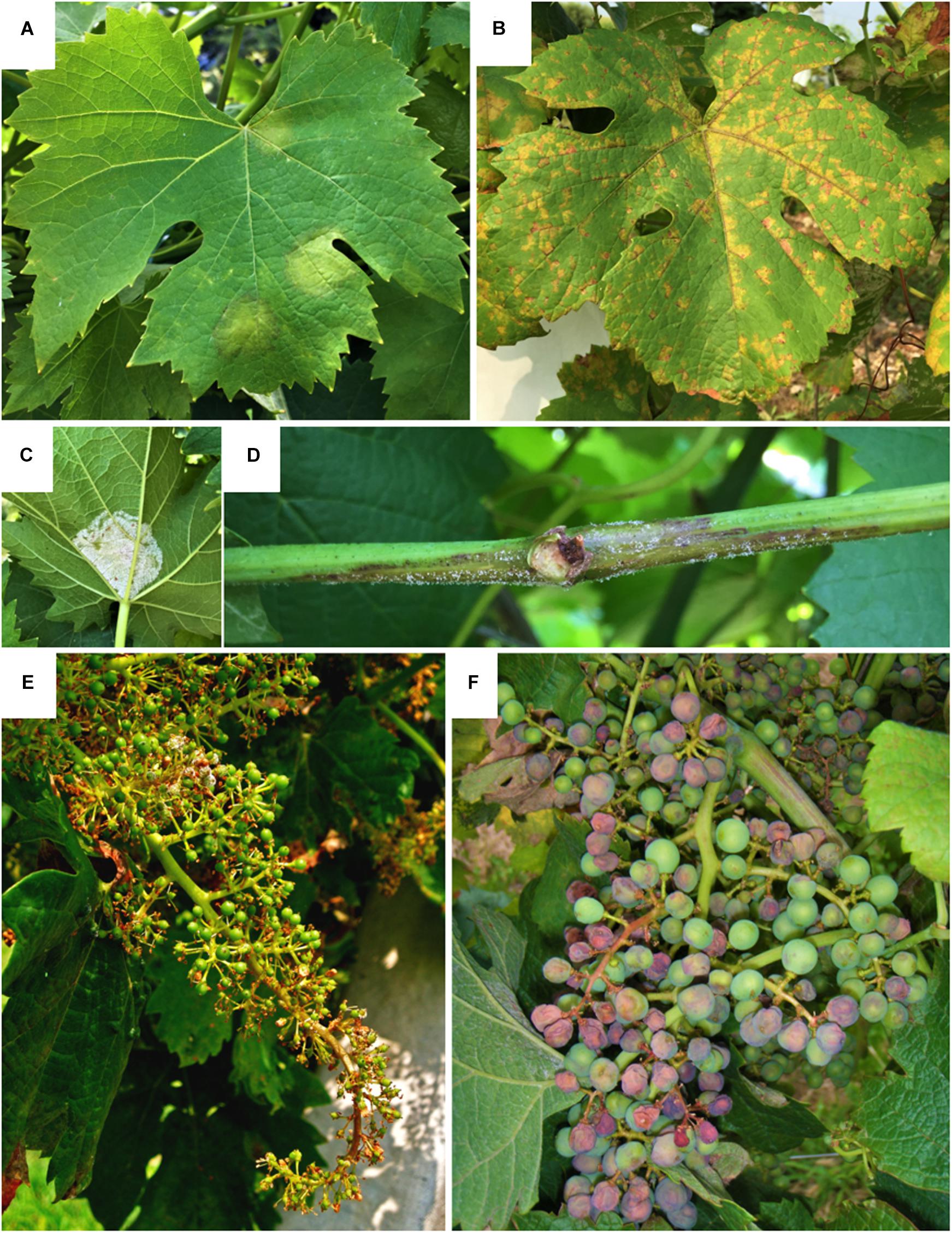
Figure 5. Symptoms of grapevine downy mildew on leaves (A–C), shoot (D) and bunches (E,F). (A) Oilspot (yellow circular spots with an oily appearance) on the upper side of the leaf; (B) mosaic symptom (yellow spot restricted by veins to form yellow-to-brown small, angular spots in a mosaic pattern) on the upper side of the leaf; (C) sporulation (sporangiophores and sporangia appearing as a bright white, fluffy growth) on the undersides of leaves; (D) shoot covered by sporulation turning brown; (E) distorted bunch (U-shaped) turning necrotic; (F) shrinking berries turning violet.
Most of the Vitis taxa native to North America are to some extent resistant to P. viticola (Unger et al., 2007). The resistance response to P. viticola results in rapid plant cell death after pathogen recognition and local necrosis induction. This mechanism, known as the hypersensitive response (HR), is an actively triggered procedure initiated by fungal elicitors or other elicitors (Balint-Kurti, 2019) that leads to bursts of production of ROS and nitric oxide (NO). Consequently, the host cells collapse and shrink, hampering the fungal infection (Toffolatti et al., 2016). Cell death is visible to the naked eye as small necrotic spots on plant tissues.
The Georgian grapevine germplasm is characterized by very high genetic diversity, with cultivars differing from major European ones (Imazio et al., 2013). Considering that this high variability could also be a source of resistance to important pathogens, studies have been undertaken to assess the resistance levels of Georgian accessions to P. viticola. The first one, carried out by Bitsadze et al. (2015), showed that 20 accessions were characterized by medium to high levels of resistance to downy mildew in a collection of 61 native Georgian varieties. Given the promising results, it appeared worthwhile to keep screening Georgian germplasm. In Toffolatti et al. (2016), a total of 93 accessions were studied over a period of 3 years in field surveys and in the laboratory. A small group of varieties, including Kamuri Shavi, Mgaloblishvili and Ubakluri, showed low disease severity values, but only Mgaloblishvili showed a strong and constant phenotypical resistance against the pathogen. In Supplementary Table 1, a list of Georgian resistant varieties is reported. Indeed, recent studies on the transcriptome of Mgaloblishvili showed that the cultivar possesses a unique response to P. viticola that is based on the overexpression of genes that are not modulated or downregulated in susceptible (Pinot Noir, a V. vinifera cv) and resistant (Bianca, interspecific hybrid) cultivars (Toffolatti et al., 2018). The resistance mechanism of Mgaloblishvili is based on the overexpression of genes encoding: (i) receptors for pathogen recognition (PAMP – Pathogen Associated Microbial Patterns-receptors) and for damage at the cell wall (DAMP – Damage Associated Microbial Patterns); (ii) an NB–LRR receptor of fungal effectors (named Lr10); (iii) ethylene signaling; (iv) synthesis of terpenes, such as valencene, and flavonoids; and (v) strengthening of cell walls. Besides genes involved in resistance, susceptibility genes were identified as well. Susceptibility genes are essential for plant-pathogen interaction and their disruption leads to resistance, as with mlo gene, whose knockdown is involved in resistance to E. necator (Pessina et al., 2016). The candidate gene related to susceptibility to P. viticola in V. vinifera encodes an LOB domain-containing (LBD) protein (Toffolatti et al., 2020) that has been previously found in the interaction between Arabidopsis thaliana and Fusarium oxysporum (Thatcher et al., 2012). The new genome editing tools, providing several protocols to introduce knockout on target sequences, makes the understanding of plant pathogen-resistance mechanism mediated by susceptibility genes a very attractive alternative for the development of durable disease-resistant varieties (Zaidi et al., 2018).
New Resistant Loci Associated With Resistance to P. viticola in V. vinifera
The investigation of the genetic basis of P. viticola resistance through QTL (Quantitative Trait Loci) analysis on a range of North American and Asian Vitis species has led to the identification of 28 resistance (R) loci (Figure 6). These R loci (designated Rpv for Resistance to P. viticola) confer different degrees of resistance to disease, ranging from partial to total resistance (Dry et al., 2019). The major loci on this list are: (i) Rpv1, identified in Muscadinia rotundifolia, that confers a not total resistance to P. viticola infection and is associated with a gene encoding a TIR-NB-LRR protein (MrRPV1) (Merdinoglu et al., 2003; Feechan et al., 2013); (ii) Rpv2, identified in M. rotundifolia, that confers total resistance to downy mildew and is associated to a cluster of TIR-NB-LRR genes (Dry et al., 2019); (iii) Rpv3, identified in V. labrusca, Vitis lincecumii, V. riparia and V. rupestris, that confers partial resistance to downy mildew (Bellin et al., 2009; Gaspero et al., 2011; Welter et al., 2017); (iv) Rpv8 and Rpv12, identified in V. amurensis, that confer a high resistance to P. viticola infection and are associated with the cluster of genes encoding NB-LRR proteins (Blasi et al., 2011; Venuti et al., 2013); (v) Rpv15, identified in Vitis piasezkii, that confers strong resistance to P. viticola infection (Dry et al., 2019). The other R loci are considered minor loci due to their ability to confer low degrees of resistance and they are only useful when combined with major R loci.
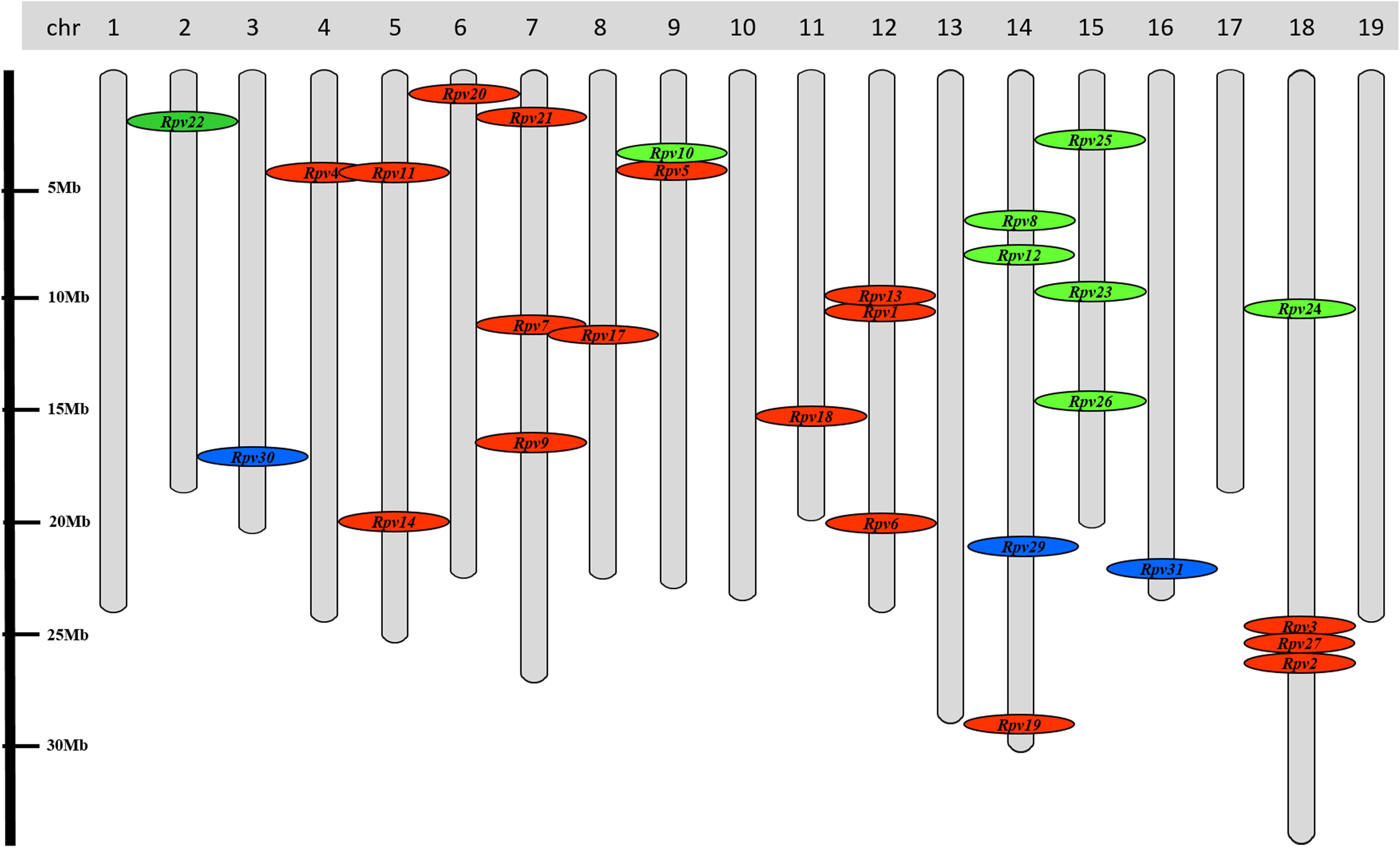
Figure 6. Distribution of resistance loci to P. viticola (Rpv) overall the 19 grapevine chromosomes. In red: loci identified in Northern American Vitis species. In green: loci identified in Asian Vitis species. In blue: loci identified in V. vinifera genetic background (Georgian germplasm). No information is now available for Rpv15 and Rpv16 detected in Vitis piasezkii Maxim (Pap et al., unpublished).
Very recently, new promising downy mildew R loci (Rpv29, Rpv30, and Rpv31) have been identified, through a GWAS (Genome Wide Association Study), in the genetic background of the Georgian V. vinifera germplasm (Figure 6) (Sargolzaei et al., 2020). These new R loci, mapping on chromosome 14, 3 and 16 for Rpv29, 30 and 31, respectively, and conferring from high to very high resistance to downy mildew, seem to be associated with receptors of pathogen effectors, signaling mediated by protein ubiquitination and a cluster of Lr10-like (NB-LRR) effector receptors.
Low Susceptibility of Georgian Grapevine Cultivars to Phytoplasma-Associated Diseases
Flavescence dorée (FD) and Bois noir (BN) are the more important diseases of the grapevine yellows (GY) complex, responsible for severe yield losses in vineyards worldwide (Belli et al., 2010). FD and BN are associated with phytoplasmas, phloem-limited bacteria transmitted by insect vectors (Weintraub and Beanland, 2006). Even if their symptoms were indistinguishable (desiccation of inflorescences, berry shrivel, leaf discolorations, reduction of growth, and irregular ripening of wood), FD and BN are associated with phytoplasmas distinct at both genetic and ecological level (Belli et al., 2010). The FD phytoplasma is efficiently transmitted from grapevine to grapevine by the insect Scaphoideus titanus, which sustains its whole life cycle on Vitis spp. (Oliveira et al., 2019). Consequently, geographic areas hosting large vector populations and FD phytoplasma can be damaged by strong FD epidemics. Due to this aspect, FD phytoplasma is a quarantine pathogen, to be controlled through mandatory measures (Oliveira et al., 2019). On the other hand, BN phytoplasma (‘Candidatus Phytoplasma solani’) (Quaglino et al., 2013) is occasionally transmitted to grapevine by the insect Hyalesthes obsoletus, a polyphagous vector living preferentially on Urtica dioica (nettle), Convolvulus arvensis (bindweed), and Vitex agnus-castus (chaste tree) (Langer and Maixner, 2004; Kosovac et al., 2016). The epidemiological cycle associated with BN is extremely complex and was recently discovered to include other highly polyphagous insect vectors and a very broad range of secondary wild hosts (Mori et al., 2015; Quaglino et al., 2019). Moreover, the typical management strategies for phytoplasma diseases, based on the control of the vector(s) with insecticides and the removal of infected plants, are not effective against BN. Thus, it is difficult to organize effective prevention and containment measures. An ambitious strategy is based on the selection of plant varieties as the source of resistance-genes for plant breeding programs (Bianco et al., 2019). Unfortunately, none of the Vitis species and V. vinifera varieties studied have been found to be resistant or tolerant to the GY phytoplasmas (Laimer et al., 2009).
Surveys conducted in vineyards of Khaketi and Shida Kartli regions in eastern Georgia highlighted a wide diffusion of BN, while FD was not reported (Quaglino et al., 2014). Moreover, most autochthonous Georgian grapevine cultivars were found to be only mildly symptomatic, maintaining complete berry production, while internationally known cultivars exhibited severe symptoms (Quaglino et al., 2016) (Figure 7). As largely reported for phytoplasma-associated diseases of stone fruits, symptom intensity observed in infected plants can be influenced both by the virulence of the pathogen and the susceptibility level of the plant host (Kison and Seemuller, 2001; Seemüller and Schneider, 2007). Molecular characterization, supported by phylogenetic analyses, revealed that BN phytoplasma strains identified in Georgia constitute a bindweed-related population which is genetically distinct from the one found in central-western Europe. Interestingly, the presence of the same phytoplasma strain in grapevine cultivars showing a range of symptom intensity suggested a low susceptibility of Georgian local cultivars to BN (Quaglino et al., 2016) (Supplementary Table 1). Studies in progress are focusing on (i) identifying genetic traits associated with this low susceptibility to BN in the perspective of improving breeding programs to produce novel tolerant and/or resistant grapevine cultivars; (ii) investigating the susceptibility of Georgian grapevine cultivars to FD.
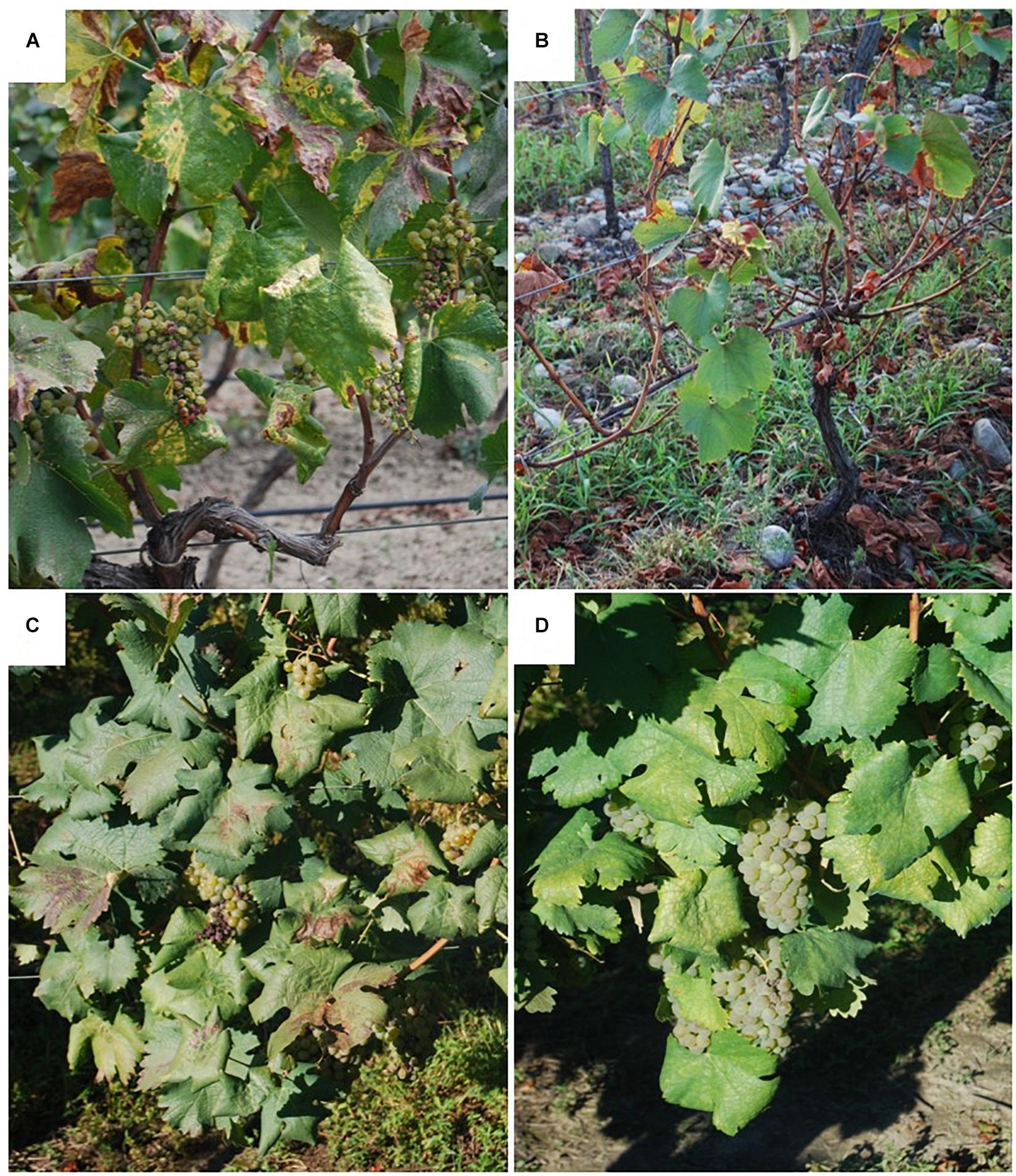
Figure 7. Symptoms observed on ‘Candidatus Phytoplasma solani’ infected grapevine cultivars in Georgia. Severe symptoms on international cultivar Chardonnay (A) and Georgian cultivar Kisi (B); moderate symptoms on Georgian cultivar Goruli Mtsvane (C); mild symptoms on Georgian cultivar Tsitska (D).
A Brief Interlude on the Status of Georgian Wild Compartment
The V. vinifera subsp. silvestris is considered the progenitor of cultivated species. In the last two decades, an increase interest in preserving wild genetic resources has led to surveys on Georgian land aimed to localize and gather wild grapevine material. The plant material collected in these surveys is summarized in the Supplementary Table 2. These accessions are now partially (more than 100) available in Georgian collections (Saguramo, Skra and other collections) and some other in USDA National Clonal Germplasm Repository of Davis (CA, United States) and in the collection of Milan University. This subspecies is seriously worldwide endangered by human activities, such as urbanization, forest cleaning and setting fires (Arnold et al., 2005). The Georgian one is not an exception. Indeed, very small wild populations have been identified overall the Georgian land (Ocete Rubio et al., 2012). Populations with both male and female individuals were detected, but in Zhinvali and Sabue populations no female individuals were identified. Generally, in the Georgian populations the number of male individuals is higher than the female ones (Supplementary Table 2). Most of the wild Georgian populations showed severe downy and powdery mildew symptoms, although three individuals showed high resistance to P. viticola infection (Supplementary Table 2) (Ocete Rubio et al., 2012; Bitsadze et al., 2015). Nevertheless, remarkable is the absence of symptoms caused by phylloxera in the populations sampled by Ocete Rubio et al. (2012). In the same populations, symptoms caused by two mites, Colomerus vitis and Calepitrimerus vitis, have been observed, although the damages were not serious and appeared to do not affect the viability of the plants.
From the genetic point of view, some of these accessions weregenotyped by SSR and SNP molecular markers(Supplementary Table 2) (Imazio et al., 2013; Ekhvaia et al., 2014; De Lorenzis et al., 2015; Riaz et al., 2018). Results reported in Imazio et al. (2013) and De Lorenzis et al. (2015) clearly discriminatedthe wild individuals from the cultivated ones, two subspecies that diverged at least 22,000 years ago (Zhou et al., 2017; Liang et al., 2019). The Georgian accessions were differentiated by European wild accessions and cultivated accessions (Riaz et al., 2018). Interesting, Ekhvaia et al. (2014) identified absence of genetic isolation among some of the analyzed wild populations due to gene flow among them.
At the phenotypical level, few information is available and further studies need to deeply investigate the enological potential of this compartment. Nevertheless, preliminary results showed that musts obtained by Georgian wild grapes could be added to the must of traditional cultivars to improve the wine color (Maghradze et al., 2020).
What’s Next?
Climate change will impact many aspects of human life, the environment, agriculture and food. Regarding viticulture, data available on climate change have already demonstrated impacts on wine growing areas, resulting in changes in grape chemical composition as well as grape phenology. Because of their isolated geographical origin and huge genetic variability, the Georgian grapevine germplasm is of great interest as a worthwhile resource for breeding programs. The Georgian germplasm has distinguished itself by including cultivars characterized by late ripening, which could potentially reduce issues related to excessive temperatures in summertime, distinctive eno-carpological traits, which affect the grape and wine quality, specific response to abiotic stresses, such as sunburn, and resistance traits related to biotic stresses, such as P. viticola and phytoplasmas.
Given the reasons stated in this review, the screening and assessment of Georgian germplasm should be promoted at the phenotypical, agronomical, physiological and genetic level. A number of gaps has still to be filled, such as their attitude to abiotic (drought, salinity, iron chlorosis) and biotic stresses, as well as the whole genome analysis of the most performing Georgian cultivars, in order to identify the genetic regions related to such valuable traits. A step toward this direction has been performed by Tabidze et al. (2017), sequencing the whole genome of four major Georgian varieties (Chkhaveri, Saperavi, Meskhetian green, and Rkatsiteli) and releasing information useful to understand the complexity of grape genome and for further comparative analysis. Aside from traditional breeding programs, these invaluable resources could be exploited in breeding programs based on the use of New Breeding Technologies (NBTs), by means of genome editing applied to both resistance and, with even more practical advantages, susceptibility candidate genes to abiotic and biotic stresses. In this way, it will be possible to exploit the valuable traits carried by this unique source of genetic variability for new varieties able to meet the challenges awaiting viticulture in the era of climate change.
Author Contributions
GDL and SLT conceived the work. MS, VR, and GDLwrote the introduction and genetic variability section. GC wrote theclimate section. LR wrote the section on phenotype. SLT wrote the section on resistance to fungal pathogens. FQ wrote the section on susceptibility to phytoplasma diseases. DM, OF, and PAB critically revised the manuscript. All the authors read and approved the final version of the manuscript.
Funding
This research was supported by University of Milan, DiSAA, Research[cpsenter]Support Plan 2018, Linea 2 A, Project “Dal phenotyping al genome[cpsenter]editing: strategie per limitare i danni da peronospora e legno nero[cpsenter]in vite (ResVite)” and by the National Wine Agency of Georgia within[cpsenter]the ‘Research Project for the Study of the Georgían Grapes and Wine[cpsenter]Culture.’ University of Milan supported the article processing charges.
Conflict of Interest
The authors declare that the research was conducted in the absence of any commercial or financial relationships that could be construed as a potential conflict of interest.
Acknowledgments
We would like to thank Dr. Lesley Currah for supporting us on English language editing.
Supplementary Material
The Supplementary Material for this article can be found online at: https://www.frontiersin.org/articles/10.3389/fpls.2021.630122/full#supplementary-material
Supplementary Table 1 | List of Georgianautochthonous and breeding grapevine accessions based on Ketskhoveliet al. (1960) and VIVC. Table includes information related to berry color, origin, usage, spreading, genotyping and resistance to plant disease. Synonyms, homonyms and misidentifications are not verified. SSR, simple sequence repeat; SNP, single nucleotide polymorphism; VIVC, Vitis International Variety Catalogue (https://www.vivc.de/).
Supplementary Table 2 | List of Georgian wild grapevine accessions. Table includes information related to flower sex, origin, genotyping and resistance to plant disease. SSR, simple sequence repeat; SNP, single nucleotide polymorphism; VIVC, Vitis International Variety Catalogue (https://www.vivc.de/). F, female; H, hermaphrodite; M, male; UNIMI, University of Milan.
Footnotes
References
Abashidze, E., Mdinaradze, I., Chipashvili, R., Vashakidze, L., Maghradze, D., Rustioni, L., et al. (2015). Evaluation of eno-carpological traits in Georgian grapevine varieties from Skra germplasm repository. Vitis 54, 151–154.
Armijo, G., Schlechter, R., Agurto, M., Muñoz, D., Nuñez, C., and Arce-Johnson, P. (2016). Grapevine pathogenic microorganisms: understanding infection strategies and host response scenarios. Front. Plant Sci. 7:382. doi: 10.3389/fpls.2016.00382
Arnold, C., Schnitzler, A., Douard, A., Peter, R., and Gillet, F. (2005). Is there a future for wild grapevine (Vitis vinifera subsp. silvestris) in the Rhine Valley? Biodivers. Conserv. 14, 1507–1523. doi: 10.1007/s10531-004-9789-9
Arroyo-García, R., Ruiz-García, L., Bolling, L., Ocete, R., López, M. A., Arnold, C., et al. (2006). Multiple origins of cultivated grapevine (Vitis vinifera L. ssp. sativa) based on chloroplast DNA polymorphisms. Mol. Ecol. 15, 3707–3714. doi: 10.1111/j.1365-294x.2006.03049.x
Bacilieri, R., Lacombe, T., Le Cunff, L., Di Vecchi-Staraz, M., Laucou, V., Genna, B., et al. (2013). Genetic structure in cultivated grapevines is linked to geography and human selection. BMC Plant Biol. 13:25. doi: 10.1186/1471-2229-13-25
Balint-Kurti, P. (2019). The plant hypersensitive response: concepts, control and consequences. Mol. Plant Pathol. 20, 1163–1178. doi: 10.1111/mpp.12821
Belli, G., Bianco, P. A., and Quaglino, F. (2010). Grapevine yellows in Italy: past, present and future. J. Plant Pathol. 92, 303–326.
Bellin, D., Peressotti, E., Merdinoglu, D., Wiedemann-Merdinoglu, S., Adam-Blondon, A. F., Cipriani, G., et al. (2009). Resistance to plasmopara viticola in grapevine ‘Bianca’ is controlled by a major dominant gene causing localised necrosis at the infection site. Theor. Appl. Genet. 120, 163–176. doi: 10.1007/s00122-009-1167-2
Bianco, P. A., Romanazzi, G., Mori, N., Myrie, W., and Bertaccini, A. (2019). “Integrated management of phytoplasma diseases,” in Phytoplasmas: Plant Pathogenic Bacteria–II, eds A. Bertaccini, P. G. Weintraub, G. P. Rao, and N. Mori (Singapore: Springer Singapore).
Bitsadze, N., Aznarashvili, M., Vercesi, A., Chipashvili, R., Failla, O., and Maghradze, D. (2015). Screening of Georgian grapevine germplasm for susceptibility to downy mildew (Plasmopara viticola). Vitis J. Grapevine Res. 54, 193–196. doi: 10.17660/ActaHortic.2014.1032.25
Blasi, P., Blanc, S., Prado, E., Rühl, E. H., Mestre, P., and Merdinoglu, D. (2011). Construction of a reference linkage map of Vitis amurensis and genetic mapping of Rpv8, a locus conferring resistance to grapevine downy mildew. Theor. Appl. Genet. 123, 43–53. doi: 10.1007/s00122-011-1565-0
Bois, B., Zito, S., Calonnec, A., and Ollat, N. (2017). Climate vs grapevine pests and diseases worldwide: the first results of a global survey. J. Int. des Sci. la Vigne du Vin 51, 133–139. doi: 10.20870/oeno-one.2016.0.0.1780
Boso, S., and Kassemeyer, H. H. (2008). Different susceptibility of European grapevine cultivars for downy mildew. Vitis J. Grapevine Res. 47, 39–49.
Canals, R., del Carmen, Llaudy, M., Canals, J. M., and Zamora, F. (2008). Influence of the elimination and addition of seeds on the colour, phenolic composition and astringency of red wine. Eur. Food Res. Technol. 226, 1183–1190. doi: 10.1007/s00217-007-0650-8
Chkhartishvili, N., and Maghradze, D. (2012). Viticulture and winemaking in Georgia. Am. J. Enol. Vitic. 51, 169–239.
Close, D. C., and McArthur, C. (2002). Rethinking the role of many plant phenolics – protection from photodamage not herbivores? Oikos 99, 166–172. doi: 10.1034/j.1600-0706.2002.990117.x
Cola, G., Failla, O., Maghradze, D., Megrelidze, L., and Mariani, L. (2017). Grapevine phenology and climate change in Georgia. Int. J. Biometeorol. 61, 761–773. doi: 10.1007/s00484-016-1241-9
Cola, G., Mariani, L., Maghradze, D., and Failla, O. (2020). Changes in thermal resources and limitations for Georgian viticulture. Aust. J. Grape Wine Res. 26, 29–40. doi: 10.1111/ajgw.12412
Cornea, V., and Savin, G. (2015). Exploration and revaluation of old autochthonous varieties in the Republic of Moldova. Vitis Geilweilerhof- 54, 115–119.
De Andrés, M. T., Benito, A., Pérez-Rivera, G., Ocete, R., Lopez, M., Gaforio, L., et al. (2012). Genetic diversity of wild grapevine populations in Spain and their genetic relationships with cultivated grapevines. Mol. Ecol. 21, 800–816. doi: 10.1111/j.1365-294x.2011.05395.x
De Lorenzis, G., Chipashvili, R., Failla, O., and Maghradze, D. (2015). Study of genetic variability in Vitis vinifera L. germplasm by high-throughput Vitis18kSNP array: the case of Georgian genetic resources. BMC Plant Biol. 15:154. doi: 10.1186/s12870-015-0510-9
De Lorenzis, G., Mercati, F., Bergamini, C., Cardone, M. F., Lupini, A., Mauceri, A., et al. (2019). SNP genotyping elucidates the genetic diversity of Magna Graecia grapevine germplasm and its historical origin and dissemination. BMC Plant Biol. 19:7. doi: 10.1186/s12870-018-1576-y
De Lorenzis, G., Rustioni, L., Parisi, S. G., Zoli, F., and Brancadoro, L. (2016). Anthocyanin biosynthesis during berry development in corvina grape. Sci. Hortic. 212, 74–80. doi: 10.1016/j.scienta.2016.09.039
De Lorenzis, G., Rustioni, L., Pozzi, C., and Failla, O. (2020). Disfunctions in the anthocyanin accumulation of Vitis vinifera L. varieties studied by a targeted resequencing approach. J. Berry Res. 10, 1–19. doi: 10.3233/jbr-190478
Di Matteo, M., Cinquanta, L., Galiero, G., and Crescitelli, S. (2000). Effect of a novel physical pretreatment process on the drying kinetics of seedless grapes. J. Food Eng. 46, 83–89. doi: 10.1016/S0260-8774(00)00071-6
D’Onofrio, C. (2020). Introgression among cultivated and wild grapevine in Tuscany. Front. Plant Sci. 11:202. doi: 10.3389/fpls.2020.00202
Doymaz, Í (2006). Drying kinetics of black grapes treated with different solutions. J. Food Eng. 76, 212–217. doi: 10.1016/j.jfoodeng.2005.05.009
Dry, I., Riaz, S., Fuchs, M., Sosnowski, M., and Thomas, M. (2019). “Scion breeding for resistance to biotic stresses,” in The Grape Genome, eds D. Cantu and A. M. Walker (Berlin: Springer), 319–347. doi: 10.1007/978-3-030-18601-2_15
Ekhvaia, J., Gurushidze, M., Blattner, F. R., and Akhalkatsi, M. (2014). Genetic diversity of Vitis vinifera in Georgia: relationships between local cultivars and wild grapevine, V. vinifera L. subsp. sylvestris. Genet. Resour. Crop Evol. 61, 1507–1521. doi: 10.1007/s10722-014-0125-2
Emmett, R. W., Wicks, T. J., and Magarey, R. (1992). “Downy mildew of grapes,” in Plant Diseases of International Importance, eds J. Kumar, H. S. Chaube, U. S. Singh, and A. N. Mukhopadhyay (Prentice Hall, NJ: Englewood Cliffs), 90–128.
Eurostat (2007). Available online at: http://ec.europa.eu/eurostat/de
Failla, O. (2015). East-West collaboration for grapevine diversity exploration and mobilization of adaptive traits for breeding: a four years story. Vitis Geilweilerhof- 54, 1–4.
Feechan, A., Anderson, C., Torregrosa, L., Jermakow, A., Mestre, P., Wiedemann-Merdinoglu, S., et al. (2013). Genetic dissection of a TIR-NB-LRR locus from the wild North American grapevine species Muscadinia rotundifolia identifies paralogous genes conferring resistance to major fungal and oomycete pathogens in cultivated grapevine. Plant J. 76, 661–674. doi: 10.1111/tpj.12327
Felicetti, D., and Schrader, L. (2008). Photooxidative sunburn of apples: characterization of a third type of apple sunburn. Int. J. Fruit Sci. 8, 160–172. doi: 10.1080/15538360802526472
Forni, G. (2012). “The origin of ‘old world’ viticulture,” in Caucasus and Northern Black Sea Region, eds D. Maghradze, L. Rustioni, A. Scienza, J. Turok, and O. Failla (Dresden: Julius Kuhn-Institut), 27–38.
Gaspero, G., Copetti, D., Coleman, C., Castellarin, S. D., Eibach, R., Kozma, P., et al. (2011). Selective sweep at the Rpv3 locus during grapevine breeding for downy mildew resistance. Theor. Appl. Genet. 124, 277–286. doi: 10.1007/s00122-011-1703-8
Gessler, C., Pertot, I., and Perazzolli, M. (2011). Plasmopara viticola : a review of knowledge on downy mildew of grapevine and effective disease management. Phytopathol. Mediterr. 50, 3–44.
Gladstones, J. (1992). Viticulture and Environment : A Study of the Effects of Environment on Grapegrowing and Wine Qualities, With Emphasis on Present and Future Areas for Growing Winegrapes in Australia. Broadview, SA: Winetitles.
Goryslavets, S., Bacilieri, R., Risovannaya, V., Memetova, E., and Laucou, V. (2015). Genetic diversity of ancient grape cultivars of the Crimea region. Vitis J. Grapevine Res. 54, 37–41.
Graham, L. E., Kodner, R. B., Fisher, M. M., Graham, J. M., Wilcox, L. W., Hackney, J. M., et al. (2004). “9 – Early landplant adaptations to terrestrial stress: A focus on phenolics,” The Evolution of Plant Physiology, eds R. Alan, R. Hemsley, and I. Poole (Academic Press), 155–169. doi: 10.1016/B978-012339552-8/50010-X
Granett, J., Walker, M. A., Kocsis, L., and Omer, A. D. (2001). Biology and management of Grape Phylloxera. Annu. Rev. Entomol. 46, 387–412.
Grassi, F., Labra, M., Imazio, S., Spada, A., Sgorbati, S., Scienza, A., et al. (2003). Evidence of a secondary grapevine domestication centre detected by SSR analysis. Theor. Appl. Genet. 107, 1315–1320. doi: 10.1007/s00122-003-1321-1
Hannah, L., Roehrdanz, P. R., Ikegami, M., Shepard, A. V., Shaw, M. R., Tabor, G., et al. (2013). Climate change, wine, and conservation. Proc. Natl. Acad. Sci. U.S.A. 110, 6907–6912. doi: 10.1073/pnas.1210127110
Imazio, S., Maghradze, D., Lorenzis, G., Bacilieri, R., Laucou, V., This, P., et al. (2013). From the cradle of grapevine domestication: molecular overview and description of Georgian grapevine (Vitis vinifera L.) germplasm. Tree Genet. Genomes 9, 641–658. doi: 10.1007/s11295-013-0597-9
Keller, M. (2010). Managing grapevines to optimize fruit development in a challenging environment: a climate change primer for viticulturists. Environ. Sustain. Vitic. Pract. Pract. 16, 259–292. doi: 10.1201/b18226
Ketskhoveli, N., Ramishvili, M., and Tabidze, D. (1960). “Ampelography of Georgia,” in Georgian and Russian (Tbilisi: Georgian Academy of Science), 20–439.
Kison, H., and Seemuller, E. (2001). Differences in strain virulence of the european stone fruit yellows phytoplasma and susceptibility of stone fruit trees on various rootstocks to this pathogen. J. Phytopathol. 149, 533–541. doi: 10.1046/j.1439-0434.2001.00671.x
Kontoudakis, N., Esteruelas, M., Fort, F., Canals, J. M., De Freitas, V., and Zamora, F. (2011). Influence of the heterogeneity of grape phenolic maturity on wine composition and quality. Food Chem. 124, 767–774. doi: 10.1016/j.foodchem.2010.06.093
Kosovac, A., Radonjić, S., Hrnc̃ić, S., Krstić, O., Toševski, I., and Jović, J. (2016). Molecular tracing of the transmission routes of bois noir in Mediterranean vineyards of Montenegro and experimental evidence for the epidemiological role of Vitex agnus-castus (Lamiaceae) and associated Hyalesthes obsoletus (Cixiidae). Plant Pathol. 65, 285–298. doi: 10.1111/ppa.12409
Laimer, M., Lemaire, O., Herrbach Herrbach, E., Goldschmidt, V., Minafra, A., Bianco, P. A., et al. (2009). Resistance to viruses, phytoplasmas and their vectors in the grapevine in Europe: a review. J. Plant Pathol. 91, 7–23.
Langer, M., and Maixner, M. (2004). Molecular characterisation of grapevine yellows associated phytoplasmas of the stolbur-group based on RFLP-analysis of non-ribosomal DNA. Vitis J. Grapevine Res. 43, 191–199.
Laucou, V., Lacombe, T., Dechesne, F., Siret, R., Bruno, J.-P., Dessup, M., et al. (2011). High throughput analysis of grape genetic diversity as a tool for germplasm collection management. Theor. Appl. Genet. 122, 1233–1245. doi: 10.1007/s00122-010-1527-y
Laucou, V., Launay, A., Bacilieri, R., Lacombe, T., Adam-Blondon, A.-F., Bérard, A., et al. (2018). Extended diversity analysis of cultivated grapevine Vitis vinifera with 10K genome-wide SNPs. PLoS One 13:e0192540. doi: 10.1371/journal.pone.0192540
Liang, Z., Duan, S., Sheng, J., Zhu, S., Ni, X., Shao, J., et al. (2019). Whole-genome resequencing of 472 Vitis accessions for grapevine diversity and demographic history analyses. Nat. Commun. 10, 1–12.
Maghradze, D., Melyan, G., Salimov, V., Chipashvili, R., Iñiguez, M., Puras, P., et al. (2020). Wild grapevine (Vitis sylvestris C.C.Gmel.) wines from the Southern Caucasus region. OENO One 54, 809–822. doi: 10.20870/oeno-one.2020.54.4.3720
Maghradze, D., Rustioni, L., Scienza, A., FaiLLa, and Os. (2012). Phenological diversity of georgian grapevine cultivars in Northern Italy. J. Am. Pomol. Soc. 66, 56–67.
Maghradze, D., Salimov, V., Melyan, G., Musayev, M., Ocete, C. A., Chipashvili, R., et al. (2015). Sanitary status of the Eurasian wild grapevine in the South Caucasian region. Vitis J. Grapevine Res. 54, 203–205.
Maghradze, D., Vashakidze, L., Abashidze, E., Chipashvili, R., Mdinaradze, I., Failla, O., et al. (2014). Multidisciplinary study of traditional grape cultivars from Kartli province of Georgia (the Caucasus region) and activities for their preservation. Acta Hortic. 1032, 235–241. doi: 10.17660/actahortic.2014.1032.33
Maraš, V., Tello, J., Gazivoda, A., Mugoša, M., Perišić, M., Raic̃ević, J., et al. (2020). Population genetic analysis in old Montenegrin vineyards reveals ancient ways currently active to generate diversity in Vitis vinifera. Sci. Rep. 10:15000.
Mariani, L., Alilla, R., Cola, G., Monte, G. D., Epifani, C., Puppi, G., et al. (2013). IPHEN—a real-time network for phenological monitoring and modelling in Italy. Int. J. Biometeorol. 57, 881–893. doi: 10.1007/s00484-012-0615-x
Mariani, L., Parisi, S. G., Cola, G., and Failla, O. (2012). Climate change in Europe and effects on thermal resources for crops. Int. J. Biometeorol. 56, 1123–1134. doi: 10.1007/s00484-012-0528-8
Marois, J. J., Nelson, J. K., Morrison, J. C., Lile, L. S., and Bledsoe, A. M. (1986). The influence of berry contact within grape clusters on the development of botrytis cinerea and epicuticular wax. Am. J. Enol. Vitic. 37, 293–296.
Martínez-Moreno, A., Sanz, F., Yeves, A., Gil-Muñoz, R., Martínez, V., Intrigliolo, D. S., et al. (2019). Forcing bud growth by double-pruning as a technique to improve grape composition of Vitis vinifera L. cv. Tempranillo in a semi-arid Mediterranean climate. Sci. Hortic. 256:108614. doi: 10.1016/j.scienta.2019.108614
McGovern, P., Jalabadze, M., Batiuk, S., Callahan, M. P., Smith, K. E., Hall, G. R., et al. (2017). Early Neolithic wine of Georgia in the South Caucasus. Proc. Natl. Acad. Sci. U.S.A. 114, 10309–10318. doi: 10.1073/pnas.1714728114
Merdinoglu, D., Schneider, C., Prado, E., Wiedemann-Merdinoglu, S., and Mestre, P. (2018). Breeding for durable resistance to downy and powdery mildew in grapevine. OENO One 52, 203–209. doi: 10.20870/oeno-one.2018.52.3.2116
Merdinoglu, D., Wiedeman-Merdinoglu, S., Coste, P., Dumas, V., Haetty, S., Butterlin, G., et al. (2003). Genetic analysis of downy mildew resistance derived from Muscadinia rotundifolia. Acta Hortic. 603, 451–456. doi: 10.17660/actahortic.2003.603.57
Mira de Orduña, R. (2010). Climate change associated effects on grape and wine quality and production. Food Res. Int. 43, 1844–1855. doi: 10.1016/j.foodres.2010.05.001
Morales-Castilla, I., de Cortázar-Atauri, I. G., Cook, B. I., Lacombe, T., Parker, A., van Leeuwen, C., et al. (2020). Diversity buffers winegrowing regions from climate change losses. Proc. Natl. Acad. Sci. U.S.A. 117, 2864–2869. doi: 10.1073/pnas.1906731117
Mori, N., Quaglino, F., Tessari, F., Pozzebon, A., Bulgari, D., Casati, P., et al. (2015). Investigation on ‘bois noir’ epidemiology in north-eastern Italian vineyards through a multidisciplinary approach. Ann. Appl. Biol. 166, 75–89. doi: 10.1111/aab.12165
Muganu, M., Bellincontro, A., Barnaba, Paolocci, M., Bignami, C., and Scossa, et al. (2011). Influence of bunch position in the canopy on berry epicuticular wax during ripening and on weight loss in postharvest dehydration process. Am. J. Enol. Vitic. 62, 91–98. doi: 10.5344/ajev.2010.10012
Mullins, M. G., Bouquet, A., and Williams, L. E. (1992). Biology of the Grapevine. Cambridge: Cambridge University Press.
Myles, S., Boyko, A. R., Owens, C. L., Brown, P. J., Grassi, F., Aradhya, M. K., et al. (2011). Genetic structure and domestication history of the grape. Proc. Natl. Acad. Sci. U.S.A. 108, 3530–3535. doi: 10.1073/pnas.1009363108
Ocete Rubio, R., Ocete Rubio, E., Ocete Pérez, C., Ángeles Pérez, Izquierdo, M., Rustioni, L., et al. (2012). Ecological and sanitary characteristics of the Eurasian wild grapevine (Vitis vinifera L. ssp. sylvestris (Gmelin) Hegi) in Georgia (Caucasian region). Plant Genet. Resour. Character. Util. 10, 155–162. doi: 10.1017/S1479262112000160
Oliveira, M. J. R. A., Roriz, M., Vasconcelos, M. W., Bertaccini, A., and Carvalho, S. M. P. (2019). Conventional and novel approaches for managing “flavescence dorée” in grapevine: knowledge gaps and future prospects. Plant Pathol. 68, 3–17. doi: 10.1111/ppa.12938
Oliver, J. E., and Fuchs, M. (2011). Tolerance and resistance to viruses and their vectors in Vitis sp.: a virologist’s perspective of the literature. Am. J. Enol. Vitic. 62, 438–451. doi: 10.5344/ajev.2011.11036
Olmo, H. P., McGovern, P. E., Fleming, S. J., and Katz, S. H. (1995). The Origins and Ancient History of Wine, Vol. 31. Abingdon: Routledge, 43.
Palliotti, A., Tombesi, S., Silvestroni, O., Lanari, V., Gatti, M., and Poni, S. (2014). Changes in vineyard establishment and canopy management urged by earlier climate-related grape ripening: a review. Sci. Hortic. 178, 43–54. doi: 10.1016/j.scienta.2014.07.039
Pangavhane, D. R., Sawhney, R. L., and Sarsavadia, P. N. (1999). Effect of various dipping pretreatment on drying kinetics of Thompson seedless grapes. J. Food Eng. 39, 211–216. doi: 10.1016/s0260-8774(98)00168-x
Percival, D., Sullivan, J., and Fisher, K. (1993). Effect of cluster exposure, berry contact and cultivar on cuticular membrane formation and occurrence of bunch rot (BotrytiscinereaPERS.: FR.) with 3 Vitis vinifera L. cultivars. Vitis 32, 87–97.
Pessina, S., Lenzi, L., Perazzolli, M., Campa, M., Dalla Costa, L., Urso, S., et al. (2016). Knockdown of MLO genes reduces susceptibility to powdery mildew in grapevine. Hortic. Res. 3:16016. doi: 10.1038/hortres.2016.16
Pugliese, M., Gullino, M. L., and Garibaldi, A. (2011). Effect of climate change on infection of grapevine by downy and powdery mildew under controlled environment. Commun. Agric. Appl. Biol. Sci. 76, 579–582.
Quaglino, F., Maghradze, D., Casati, P., Chkhaidze, N., Lobjanidze, M., Ravasio, A., et al. (2016). Identification and characterization of new ‘ Candidatus Phytoplasma solani’ strains associated with bois noir disease in Vitis vinifera L. cultivars showing a range of symptom severity in Georgia, the Caucasus Region. Plant Dis. 100, 904–915. doi: 10.1094/pdis-09-15-0978-re
Quaglino, F., Maghradze, D., Chkhaidze, N., Casati, P., Failla, O., and Bianco, P. A. (2014). First report of ‘ Candidatus Phytoplasma solani’ and ‘ Ca. P. convolvuli’ associated with grapevine bois noir and bindweed yellows, respectively, in Georgia. Plant Dis. 98, 1151–1151. doi: 10.1094/pdis-01-14-0026-pdn
Quaglino, F., Sanna, F., Moussa, A., Faccincani, M., Passera, A., Casati, P., et al. (2019). Identification and ecology of alternative insect vectors of ‘Candidatus Phytoplasma solani’ to grapevine. Sci. Rep. 9:19522.
Quaglino, F., Zhao, Y., Casati, P., Bulgari, D., Bianco, P. A., Wei, W., et al. (2013). ‘ Candidatus Phytoplasma solani’, a novel taxon associated with stolbur- and bois noir-related diseases of plants. Int. J. Syst. Evol. Microbiol. 63, 2879–2894. doi: 10.1099/ijs.0.044750-0
Reid, P. C., Hari, R. E., Beaugrand, G., Livingstone, D. M., Marty, C., Straile, D., et al. (2016). Global impacts of the 1980s regime shift. Glob. Chang. Biol. 22, 682–703.
Riaz, S., De Lorenzis, G., Velasco, D., Koehmstedt, A., Maghradze, D., Bobokashvili, Z., et al. (2018). Genetic diversity analysis of cultivated and wild grapevine (Vitis vinifera L.) accessions around the Mediterranean basin and Central Asia. BMC Plant Biol. 18:137. doi: 10.1186/s12870-018-1351-0
Rosenquist, J. K., and Morrison, J. C. (1988). The development of the cuticle and epicuticular wax of the grape berry. Vitis 27, 63–70.
Russell, P. (2005). A century of fungicide evolution. J. Agric. Sci. 143, 11–25. doi: 10.1017/s0021859605004971
Rustioni, L. (2017). Oxidized polymeric phenolics: could they be considered photoprotectors? J. Agric. Food Chem. 65, 7843–7846. doi: 10.1021/acs.jafc.7b03704
Rustioni, L., Basilico, R., Fiori, S., and Leoni, A. (2013). Grapecolour phenotyping : development of a method based on the reflectance spectrum. Phytochem. Anal. 24, 453–459. doi: 10.1002/pca.2434
Rustioni, L., Cola, G., Fiori, S., Failla, O., Bacilieri, R., Maul, E., et al. (2014). Application of standard methods for the grapevine (Vitis vinifera L.) phenotypic diversity exploration: phenological traits. Acta Hortic. 1032, 253–260. doi: 10.17660/ActaHortic.2014.1032.35
Rustioni, L., Cola, G., Maghradze, D., Abashidze, E., Argiriou, A., Aroutiounian, R., et al. (2019). Description of the Vitis vinifera L. phenotypic variability in eno-carpological traits by a Euro-Asiatic collaborative network among ampelographic collections. Vitis 58, 37–46.
Rustioni, L., Cola, G., VanderWeide, J., Murad, P., Failla, O., and Sabbatini, P. (2018). Utilization of a freeze-thaw treatment to enhance phenolic ripening and tannin oxidation of grape seeds in red (Vitis vinifera L.) cultivars. Food Chem. 259, 139–146. doi: 10.1016/j.foodchem.2018.03.120
Rustioni, L., Lorenzis, G., and De, and Monica, H. (2016). Plant Physiology and Biochemistry Pink berry grape (Vitis vinifera L.) characterization : reflectance spectroscopy, HPLC and molecular markers. Plant Physiol. Biochem. 98, 138–145. doi: 10.1016/j.plaphy.2015.11.018
Rustioni, L., Maghradze, D., and Failla, O. (2012). Optical properties of berry epicuticular waxes in four Georgian Grape Cultivars (Vitis vinifera L.). South Afric. J. Enol. Vitic. 33, 138–143.
Rustioni, L., Milani, C., Parisi, S., and Failla, O. (2015). Chlorophyll role in berry sunburn symptoms studied in different grape (Vitis vinifera L.) cultivars. Sci. Hortic. 185, 145–150. doi: 10.1016/j.scienta.2015.01.029
Rustioni, L., Rossoni, M., Cola, G., Mariani, L., and Failla, O. (2011). Bunch exposure to direct solar radiation increases ortho-diphenol anthocyanins in Northern Italy climatic condition. J. Int. DES Sci. 45, 85–99. doi: 10.20870/oeno-one.2011.45.2.1489
Santos, J. A., Fraga, H., Malheiro, A. C., Moutinho-Pereira, J., Dinis, L.-T., Correia, C., et al. (2020). A review of the potential climate change impacts and adaptation options for European viticulture. Appl. Sci. 10:3092. doi: 10.3390/app10093092
Sargolzaei, M., Maddalena, G., Bitsadze, N., Maghradze, D., Bianco, P. A., Failla, O., et al. (2020). Rpv29, Rpv30 and Rpv31: three novel genomic loci associated with resistance to Plasmopara viticola in Vitis vinifera. Front. Plant Sci. 11:562432. doi: 10.3389/fpls.2020.562432
Seemüller, E., and Schneider, B. (2007). Differences in virulence and genomic features of strains of ‘ Candidatus Phytoplasma mali’, the apple proliferation agent. Phytopathology 97, 964–970. doi: 10.1094/PHYTO-97-8-0964
Tabidze, V., Pipia, I., Gogniashvili, M., Kunelauri, N., Ujmajuridze, L., Pirtskhalava, M., et al. (2017). Whole genome comparative analysis of four Georgian grape cultivars. Mol. Genet. Genomics 292, 1377–1389. doi: 10.1007/s00438-017-1353-x
Terral, J. F., Tabard, E., Bouby, L., Ivorra, S., Pastor, T., Figueiral, I., et al. (2010). Evolution and history of grapevine (Vitis vinifera) under domestication: new morphometric perspectives to understand seed domestication syndrome and reveal origins of ancient European cultivars. Ann. Bot. 105, 443–455. doi: 10.1093/aob/mcp298
Thatcher, L. F., Powell, J. J., Aitken, E. A. B., Kazan, K., and Manners, J. M. (2012). The lateral organ boundaries domain transcription factor LBD20 functions in Fusarium wilt susceptibility and jasmonate signaling in Arabidopsis. Plant Physiol. 160, 407–418. doi: 10.1104/pp.112.199067
This, P., Lacombe, T., and Thomas, M. R. (2006). Historical origins and genetic diversity of wine grapes. Trends Genet. 22, 511–519. doi: 10.1016/j.tig.2006.07.008
Toffolatti, S. L., De Lorenzis, G., Brilli, M., Moser, M., Shariati, V., Tavakol, E., et al. (2020). Novel aspects on the interaction between grapevine and Plasmopara viticola: dual-RNA-Seq analysis highlights gene expression dynamics in the pathogen and the plant during the battle for infection. Genes 11:261. doi: 10.3390/genes11030261
Toffolatti, S. L., De Lorenzis, G., Costa, A., Maddalena, G., Passera, A., Bonza, M. C., et al. (2018). Unique resistance traits against downy mildew from the center of origin of grapevine (Vitis vinifera). Sci. Rep. 8:12523. doi: 10.1038/s41598-018-30413-w
Toffolatti, S. L., Maddalena, G., Salomoni, D., Maghradze, D., Bianco, P. A., and Failla, O. (2016). Evidence of resistance to the downy mildew agent Plasmopara viticola in the Georgian Vitis vinifera germplasm. Vitis J. Grapevine Res. 55, 121–128. doi: 10.5073/vitis.2016.55.121-128
Töpfer, R., Hausmann, L., and Eibach, R. (2011). “Molecular breeding,” in Genetics, Genomics and Breeding of Grapes, eds A. F. Adam-Blondon, M. M. Zapater, and C. Kole (Enfield, NJ: Science Publishers), 160–185.
Tsertsvadze, N. (2012). “Georgia: native varieties of grapevines,” in Caucasus and Northern Black Sea Region Ampelography, (Maghradze, Rustioni, Turok, Scienza, Failla eds) (JuliusKühn-Institut: Siebeldingen), 177–239.
Ujmajuridze, L., and Mamasakhlisashvili, L. (2015). Agricultural and biological characteristics of Georgian grapevine varieties. VITIS J. Grapevine Res. 54, 163–164.
Unger, S., Büche, C., Boso, S., and Kassemeyer, H.-H. (2007). The course of colonization of two different vitis genotypes by Plasmopara viticola indicates compatible and incompatible host-pathogen interactions. Phytopathology 97, 780–786. doi: 10.1094/phyto-97-7-0780
van Leeuwen, C., and Darriet, P. (2016). The impact of climate change on viticulture and wine quality. J. Wine Econ. 11, 150–167. doi: 10.1017/jwe.2015.21
van Leeuwen, C., and Destrac-Irvine, A. (2017). Modified grape composition under climate change conditions requires adaptations in the vineyard. OENO One 51, 147–154. doi: 10.20870/oeno-one.2017.51.2.1647
VanderWeide, J., Forte, A., Peterlunger, E., Sivilotti, P., Medina-Meza, I. G., Falchi, R., et al. (2020). Increase in seed tannin extractability and oxidation using a freeze-thaw treatment in cool-climate grown red (Vitis vinifera L.) cultivars. Food Chem. 308:125571. doi: 10.1016/j.foodchem.2019.125571
Venuti, S., Copetti, D., Foria, S., Falginella, L., Hoffmann, S., Bellin, D., et al. (2013). Historical introgression of the Downy Mildew Resistance Gene Rpv12 from the Asian Species Vitis amurensis into Grapevine Varieties. PLoS One 8:e61228. doi: 10.1371/journal.pone.0061228
Weintraub, P. G., and Beanland, L. (2006). Insect vectors of phytoplasmas. Annu. Rev. Entomol. 51, 91–111. doi: 10.1146/annurev.ento.51.110104.151039
Welter, L. J., Tisch, C., Kortekamp, A., Topfer, R., and Zyprian, E. (2017). Powdery mildew responsive genes of resistant grapevine cultivar “regent.”. Vitis J. Grapevine Res. 56, 181–188. doi: 10.5073/vitis.2017.56.181-8
Zaidi, S. S.-A., Mukhtar, M. S., and Mansoor, S. (2018). Genome editing: targeting susceptibility genes for plant disease resistance. Trends Biotechnol. 36, 898–906. doi: 10.1016/j.tibtech.2018.04.005
Zhou, Y., Massonnet, M., Sanjak, J. S., Cantu, D., and Gaut, B. S. (2017). Evolutionary genomics of grape (Vitis vinifera ssp. vinifera) domestication. Proc. Natl. Acad. Sci. U.S.A. 114, 11715–11720. doi: 10.1073/pnas.1709257114
Zhou, Y., Muyle, A., and Gaut, B. S. (2019). “Evolutionary genomics and the domestication of grapes,” in The Grape Genome, eds D. Cantu and M. A. Walker (Cham: Springer Nature Switzerland), 39–55. doi: 10.1007/978-3-030-18601-2_3
Keywords: Vitis vinifera L., genetic diversity, phenotypical characterization, resistance to diseases, climate change
Citation: Sargolzaei M, Rustioni L, Cola G, Ricciardi V, Bianco PA, Maghradze D, Failla O, Quaglino F, Toffolatti SL and De Lorenzis G (2021) Georgian Grapevine Cultivars: Ancient Biodiversity for Future Viticulture. Front. Plant Sci. 12:630122. doi: 10.3389/fpls.2021.630122
Received: 16 November 2020; Accepted: 13 January 2021;
Published: 05 February 2021.
Edited by:
Chiara Pastore, University of Bologna, ItalyReviewed by:
Goran Zdunic, Institute for Adriatic Crops and Karst Reclamation, CroatiaAnnarita Marrano, University of California, Davis, United States
Javier Tello, Institute of Vine and Wine Sciences (ICVV), Spain
Copyright © 2021 Sargolzaei, Rustioni, Cola, Ricciardi, Bianco, Maghradze, Failla, Quaglino, Toffolatti and De Lorenzis. This is an open-access article distributed under the terms of the Creative Commons Attribution License (CC BY). The use, distribution or reproduction in other forums is permitted, provided the original author(s) and the copyright owner(s) are credited and that the original publication in this journal is cited, in accordance with accepted academic practice. No use, distribution or reproduction is permitted which does not comply with these terms.
*Correspondence: Silvia L. Toffolatti, c2lsdmlhLnRvZmZvbGF0dGlAdW5pbWkuaXQ=; Gabriella De Lorenzis, Z2FicmllbGxhLmRlbG9yZW56aXNAdW5pbWkuaXQ=