- 1Center of Plant, Soil Interaction and Natural Resources Biotechnology, Scientific and Technological Bioresource Nucleus (BIOREN), Universidad de La Frontera, Temuco, Chile
- 2Laboratorio de Biología Vegetal, Instituto de Ciencias Ambientales y Evolutivas, Facultad de Ciencias, Universidad Austral de Chile, Valdivia, Chile
- 3Centro de Investigación en Suelos Volcánicos (CISVo), Valdivia, Chile
- 4Departamento de Ciencias Químicas y Recursos Naturales, Facultad de Ingeniería y Ciencias, Universidad de La Frontera, Temuco, Chile
- 5Laboratorio de Epigenética Vegetal, Departamento de Silvicultura, Facultad de Ciencias Forestales, Universidad de Concepción, Concepción, Chile
- 6Facultad de Ciencias Agrarias, Instituto de Ingeniería Agraria y Suelos, Universidad Austral de Chile, Valdivia, Chile
- 7School of Biological Sciences, The University of Western Australia, Perth, WA, Australia
Southern South American Proteaceae thrive on young volcanic substrates, which are extremely low in plant-available phosphorus (P). Most Proteaceae exhibit a nutrient-acquisition strategy based on the release of carboxylates from specialized roots, named cluster roots (CR). Some Proteaceae colonize young volcanic substrates which has been related to CR functioning. However, physiological functioning of other Proteaceae on recent volcanic substrates is unknown. We conducted an experiment with seedlings of five Proteaceae (Gevuina avellana, Embothrium coccineum, Lomatia hirsuta, L. ferruginea, and L. dentata) grown in three volcanic materials. Two of them are substrates with very low nutrient concentrations, collected from the most recent deposits of the volcanoes Choshuenco and Calbuco (Chile). The other volcanic material corresponds to a developed soil that exhibits a high nutrient availability. We assessed morphological responses (i.e., height, biomass, and CR formation), seed and leaf macronutrient and micronutrient concentrations and carboxylates exuded by roots. The results show that G. avellana was less affected by nutrient availability of the volcanic substrate, probably because it had a greater nutrient content in its seeds and produced large CR exuding carboxylates that supported their initial growth. Embothrium coccineum exhibited greater total plant height and leaf P concentration than Lomatia species. In general, in all species leaf macronutrient concentrations were reduced on nutrient-poor volcanic substrates, while leaf micronutrient concentrations were highly variable depending on species and volcanic material. We conclude that Proteaceae from temperate rainforests differ in their capacity to grow and acquire nutrients from young and nutrient-poor volcanic substrates. The greater seed nutrient content, low nutrient requirements (only for G. avellana) and ability to mobilize nutrients help explain why G. avellana and E. coccineum are better colonizers of recent volcanic substrates than Lomatia species.
Introduction
Temperate forest ecosystems of southern South America are a unique biome that is biogeographically isolated with a highly endemic flora (Quintero et al., 2014). These ecosystems are frequently affected by catastrophic disturbances such as volcanic eruptions and earthquakes that usually trigger orogenic uplift and landslides (Veblen and Ashton, 1978; Veblen et al., 1992, 1996), which lead to soil rejuvenation, leaving bare areas where the process of primary succession begins again. Following these catastrophic events, volcanic eruptions leave large parts of the landscape covered with rocks formed from lava flow, stones or sandy substrates, which are generally nutrient-poor and with almost no organic matter. Likewise, it is common to find volcanic soils in southern Chile containing large amounts of active aluminum (Al3+), oxides and hydroxides of iron (Fe) and Al, humus-Al/Fe complexes and amorphous and poorly crystallized minerals (e.g., allophane), which strongly sorb phosphorus (P) (Borie and Rubio, 2003; Matus et al., 2006; Borie et al., 2019). Therefore, volcanic soils may contain large amounts of total P, but with a very low availability for plants.
The colonization of nutrient-impoverished environments involves species with specialized root structures (e.g., cluster roots) or symbiotic associations (e.g., mycorrhizas, nitrogen (N)-fixing structures, or/and P-solubilizing bacteria) (Lambers et al., 2008). Among them, some species of the Proteaceae family frequently colonize young volcanic substrates (Delgado et al., 2018). Species belonging to this family have been extensively studied, because many of them inhabit extremely nutrient-poor soils in southwestern Australia and South Africa, and are highly efficient at both acquiring and utilizing nutrients, especially P (Lambers et al., 2015a). The main nutrient-acquisition strategy of Proteaceae involves the formation of cluster roots (CR), which are clusters of dense hairy rootlets growing in longitudinal rows along lateral roots (Purnell, 1960) that efficiently mobilize nutrients from the soil by actively releasing exudates (Lamont, 2003; Shane and Lambers, 2005a; Lambers et al., 2006). Carboxylates are the main root exudates and are involved in several key processes in the rhizosphere including nutrient acquisition and metal mobilization/detoxification (Jones, 1998; Ryan et al., 2001; Chen and Liao, 2016). Carboxylates have negative charges, allowing the complexation of metal cations and the displacement of anions such as phosphate, from the soil matrix. Additionally, carboxylates increase the availability of some micronutrients through their solubilizing and reducing capacity, for example, Mn4+ to Mn2+ and Fe3+ to Fe2+, which are the forms that are taken up by roots that use Strategy I (i.e., roots of dicots and non-graminaceous monocots that release reducing/chelating substances and increase the plasma membrane-bound reductase activity) (Marschner et al., 1986; Dinkelaker et al., 1995). These micronutrients, like others transition metal cations such as zinc (Zn2+), can also be mobilized by carboxylates at the root surface, where they are taken up by plasma membrane transporters with a low specificity (Lambers et al., 2015b). Thus, carboxylate exudation by CR (which occurs in large quantities compared with that of non-CR) is an adaptive trait allowing species to thrive in nutrient-poor soils.
Southern South America is inhabited by six Proteaceae species: Embothrium coccineum J. R. Forst. & G. Forst., Lomatia hirsuta Lam., L. ferruginea Cav. R. Br., L. dentata Ruiz et Pavon R. Br., Orites myrtoidea Poepp. & Endl., and Gevuina avellana Mol. (Donoso, 2006). These species may co-occur along their geographical range between 36 and 44°S. However, O. myrtoidea, has the narrowest distribution (35–38°S) and occurs only in the Andes Mountains (Hechenleitner et al., 2005). Additionally, L. dentata and L. hirsuta may occur a little further north (32°S) and L. ferruginea and E. coccineum, may occur much further south (50° and 56°S, respectively) (Steubing et al., 1983). In their natural habitat, these Proteaceae grow in a wide range of soil conditions (Souto et al., 2009; Delgado et al., 2018, 2019) and some of them, such as E. coccineum, O. myrtoidea, and G. avellana, can thrive in young volcanic substrates with very low nutrient availability (Donoso, 2006; Zúñiga-Feest et al., 2018; Ávila-Valdés et al., 2019; Zúñiga-Feest et al., 2020). Conversely, Lomatia species perform better in relatively more fertile soils. In fact, Lomatia hirsuta is a successful pioneer on landslides or after forest clearing, while L. ferruginea and L. dentata grow better under the shade of other trees growing on more developed soils (Donoso, 2006; Zúñiga-Feest et al., 2020).
The South American Proteaceae are considered outliers from the main centers of Proteaceae diversity, which are found in South western Australia and South Africa (Prance and Plana, 1998), both ancient landscapes with severely P-impoverished soils (Lambers et al., 2010). The South American Proteaceae differ from the Proteaceae of Southwest Australia and South Africa, because, in general, they have lower seed P concentrations (Groom and Lamont, 2010; Delgado et al., 2015b). This suggests that seedling establishment of southern South American Proteaceae depends more heavily on nutrients in the substrate than on nutrient reserves in their seeds. In fact, except for the larger-seeded G. avellana (354 mg dry weight seed–1), the southern South American Proteaceae produce small seeds (≤17 mg seed–1 dry weight) (Delgado et al., 2014), and form CR, even before they shed their cotyledons (e.g., ∼1 month-old E. coccineum seedlings; Delgado et al., 2015b). We propose that CR formation is a key strategy for nutrient uptake at very early life stages. However, the ability of these species to establish and thrive in soils with a low P availability such as recent volcanic substrates, is not fully understood.
Recently, Zúñiga-Feest et al. (2020) reported that G. avellana grows faster than L. dentata in nutrient-poor volcanic sand, but this trend is the opposite when these plants are grown on the same volcanic substrate, supplemented with a complete nutrient solution. This finding suggests that these species differ in their ability to grow in soils/substrates with different nutrient availability, possibly due to their different nutritional demand or nutrient-acquisition strategy. Although all Proteaceae from southern south America can grow on nutrient-poor volcanic soils, it is unknown how they adjust their growth, biomass allocation to CR, and carboxylate exudation when they grow on volcanic soil/substrate with different nutrient availability. In order to address this question, we conducted an experiment where seedlings of five Proteaceae (E. coccineum, L. hirsuta, L. ferruginea, L. dentata, and G. avellana) were grown in three volcanic materials with different nutrient availability. We hypothesized that the colonizing ability strongly depends on nutrient demand of the species and their ability to sustain growth under low-nutrient conditions. Specifically, we expected that the seedling performance of the only larger-seeded species, G. avellana, will be less affected by nutrient availability of the soil/substrate, because the higher nutrient content in its seeds will support their initial growth. Additionally, E. coccineum, the species reported as colonizer of volcanic substrates, will perform well in both nutrient-rich volcanic soil and young nutrient-poor substrates due to its greater nutrient-uptake capacity and faster rates of root carboxylate exudation. In contrast, species belonging to the genus Lomatia, will perform better in nutrient-rich soils, because they have greater nutritional demands. Therefore, the aim of this study was to assess the differences in seedling performance of five temperate rainforest Proteaceae and the morpho-physiological traits involved in soils/substrates with different nutrient availability, aiming to understand their differential colonizing ability under field conditions. Understanding how these species perform in different soil conditions will be key to support restoration activities with these native species and the information generated could be useful to extrapolate to other species that present similar morpho-physiological traits.
Materials and Methods
Sampling Sites
Three volcanic materials were collected from the localities of Ensenada (41° 10′52.48′′S – 72° 27′16.74′′W), Choshuenco (39° 33′ 12′′S – 72° 8′ 43.44′′W) and Experimental Station “Agropecuaria Austral” (ESAA) (39° 45′30′ – 73° 14′55′′W), Chile. At one extreme, substrates from the localities of Choshuenco and Ensenada correspond to recent volcanic deposits. Thus, from the Ensenada site, we collected deposits of volcanic sand from the last eruption of “Calbuco” volcano (on 23–24 April, 2015), and from the Choshuenco site, deposits of volcanic sand were collected at the foot of the “Mocho-Choshuenco” volcano, of which the last eruption was recorded in 1864 (Rawson et al., 2015). At the other extreme, soil collected at the ESAA, belonging to the Universidad Austral de Chile, corresponds to a developed soil that originated from volcanic ashes (Duric Hapludand; CIREN, 2003) that is locally named Trumao (Valdivia soil Serie). The three volcanic materials were taken to the greenhouse of the Universidad Austral, and sieved through a 5-mm sieve to remove organic material (e.g., roots, leaves, etc.) and other larger debris. These volcanic materials were analyzed chemically in the Soil Laboratory of the Faculty of Agricultural Sciences at the Universidad Austral de Chile, using the methods described in Delgado et al. (2018), showing differences in their nutrient concentrations and other chemical parameters. For example, soil from ESAA presented, on average, a two and six times greater N and P availability, respectively, than the Choshuenco and Ensenada substrates. Likewise, soil from ESAA contained nine and 20 times more total P than Choshuenco and Ensenada substrates, respectively. The youngest volcanic substrate, collected at Ensenada, showed the lowest values of exchangeable cations (Ca2+, K+, Na+, and Mg2+) and the highest percentage of Al saturation (Table 1). Additionally, water-retention curves and pore-size distribution were measured for disturbed volcanic materials used in this experiment. For the determination of water-retention curves, saturated samples of each material (230 cm3) were drained at decreasing water potential values (0, −6, −15, −33, and −1,500 kPa). The distribution of soil pores was obtained from the water-retention curve as described by Dörner et al. (2010) and, according to pore size classified by Ingram et al. (2015), the lowest amount of plant-available water was found in the youngest volcanic substrate, collected at Ensenada site (Table 1, Supplementary Figure 1).
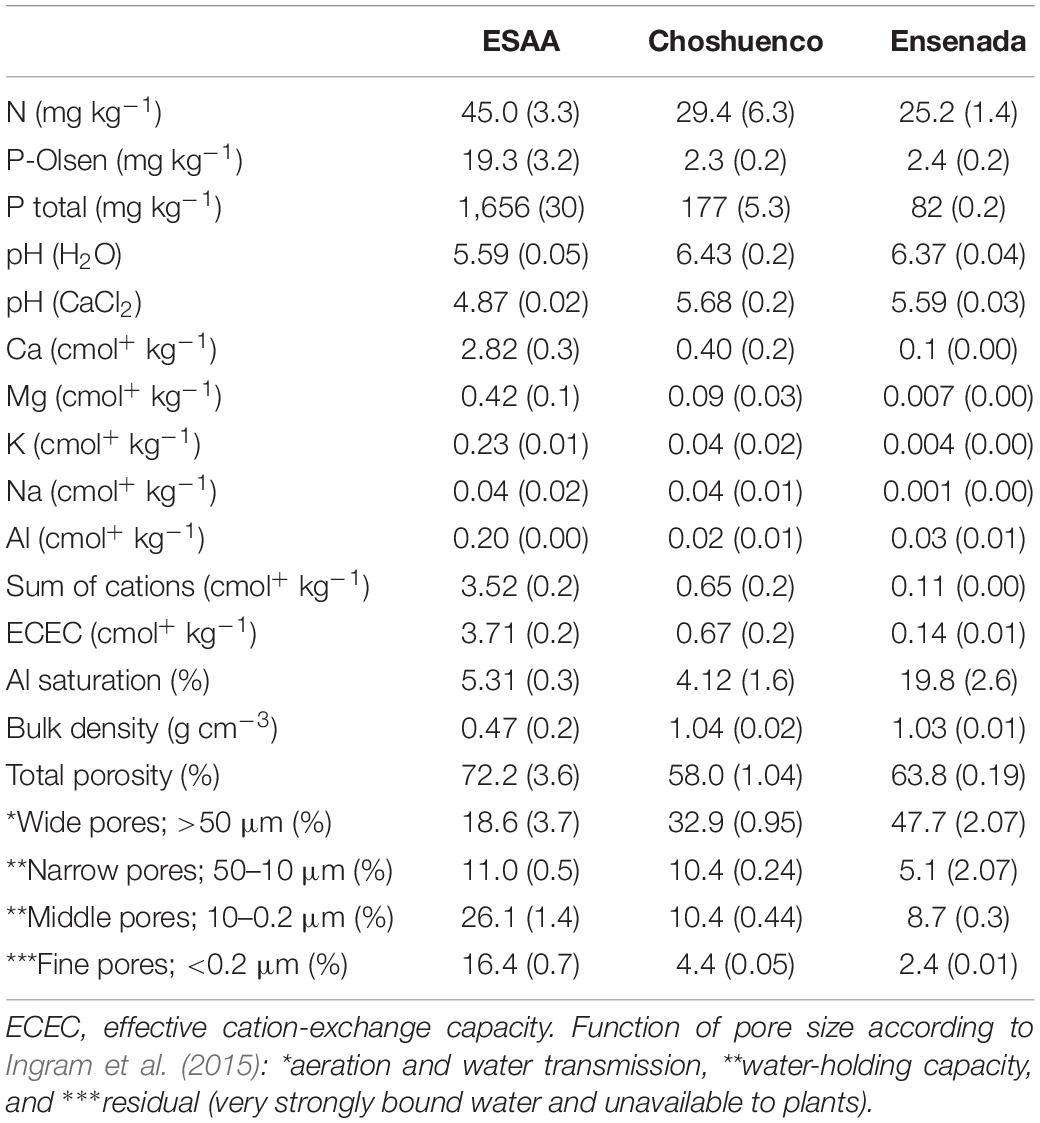
Table 1. Chemical and physical analysis of the substrates used in the experiment. Each value corresponds to the average of three soil samples ± standard error (SE).
Plant Material
In March 2016, seeds of G. avellana, E. coccineum, L. hirsuta, L. ferruginea, and L. dentata were collected from the Botanical Garden of the Universidad Austral de Chile, Valdivia. The seeds were taken to the laboratory (Universidad Austral de Chile, Valdivia) and stored at 4°C for 3 months to perform stratification requirements (Donoso and Escobar, 1986). Then, in order to stimulate germination, seeds were treated with 250 mg L–1 of gibberellic acid and placed in a temperature-controlled chamber at 20°C. After 1 month, the germinated seeds were planted in the different volcanic materials described above.
Experimental Design
Forty-five plants of each species were randomly separated into three groups of 15 seedlings each. Individual plants of each group were planted in 1-L pots of one of the collected materials from each site. The plants were maintained in the greenhouse for 9 months, from July 2016 to April 2017. The average temperature during the experiment was 19.2°C, with maximum and minimum temperatures of 34.8 and 7.2, respectively. The average light intensity was 261 μmol photons m°2 s°1 between 10:30 and 11:30 AM, with maximum and minimum values of 618 and 72 μmol photons m°2 s°1, respectively. The plants were irrigated regularly to field capacity with tap water.
Height and Biomass Determination
At the end of the experiment, the height of the stem was recorded for all seedlings. In addition, seedlings were harvested and separated into leaves, stems, non-cluster roots and CR, and dried in an oven at 60°C for 48 h. Subsequently, the different plant organs were weighed on an analytical balance (AS220-C2 Radwag, Randon, Poland) to determine total biomass and biomass distribution. The number of mature (living) and senesced CR were also determined. For this, the color was used to distinguish between mature (white) and senesced (dark-brown) CR, as described in Delgado et al. (2015a). Additionally, to better understand the nutrient limitation on growth, we used reaction norm approach described by Sadras and Richards (2014), where values of total biomass and total height of each species were expressed in relative terms with respect to total biomass and total height of seedlings grown in the nutrient-poorest substrate.
Collection and Determination of Root Exudates
Exudates were collected from the total root system of each plant following the methodology described in Delgado et al. (2014). Briefly, the roots were washed with tap water, incubated in CaSO4 (0.2 mM) and shaken for 2 h. Subsequently, the solution was filtered, to avoid the presence of microorganisms, with a sterile syringe containing a filter of 0.22 μm. The liquid samples containing the exudates were frozen at −20°C and then lyophilized using a freeze-dryer (Model FD8508, Bondiro, Ilshin Lab, Co. Ltd., Korea). Finally, lyophilized samples were re-suspended in water for high-performance liquid chromatography (HPLC) and quantified using HPLC equipment (JASCO, LC-Net II/ADC, Tokyo, Japan) following the protocol described by Delgado et al. (2013). Citrate, malate, oxalate, and succinate were used as standards. These determinations were carried out at the Institute of Agroindustries of the Universidad de La Frontera, Temuco. The values were expressed as a rate of carboxylates exuded per gram of fresh weight (FW) per hour (μmol g–1 FW h–1). The exudates from six seedlings per species grown in the different substrates were analyzed.
Foliar and Seed Mineral Concentrations
Leaves were dried at 60°C in a forced-air oven for 48 h and pulverized to analyze P, N, Mn, Fe, Cu, Zn, and Al concentration. Nitrogen was determined through acid digestion, Kjeldahl distillation and titration (Baker and Thompson, 1992). To determine the other elements, samples were ashed at 500°C for 8 h and then treated with 2 M hydrochloric acid. Phosphorus was determined by colorimetry using the vanadate phosphomolybdate method. Manganese, Fe, Cu, Zn, and Al concentrations were quantified using a simultaneous multielement atomic absorption spectrophotometer (Model 969, Unicam, Cambridge, United Kingdom) using the methodology described by Sadzawka et al. (2004). Additionally, in order to evaluate the influence of seed nutrient content on plant performance, macro- and micronutrients were determined in the seeds of all species. For this, 0.5 g of seeds were milled and the nutrients were determined using the same methodologies described for leaf nutrient concentration. We used the dry weight of the seeds previously reported by Delgado et al. (2014) to determine the nutrient content of the seeds.
Statistical Analyses
To determine if there were significant differences in the responses of the species and the different volcanic materials, as well as possible interactions between the factors studied, the data were evaluated using a two-way ANOVA with a Tukey’s a posteriori test (P ≤ 0.05). To determine significant differences in seeds nutrient concentrations and content, we used one-way ANOVA with Tukey’s a posteriori test (P ≤ 0.05). Additionally, relationships between total plant biomass and nutrient content in seeds were tested by linear regression. ANOVAs and regression analyses were performed using the Sigma Plot v.12 and Graphpad prims v.8, respectively. Finally, a principal component analysis (PCA) was performed to associate chemical variables of the three volcanic materials with the plant traits, using the R Studio program.
Results
Height and Biomass Determination
All species showed the best performance, i.e., total height and total dry biomass, when grown in ESAA soil (Figures 1A,B). For seedlings grown in ESAA soil, the total height and total dry biomass were about six to eight times and three to 19 times greater than those of the seedlings grown on Choshuenco and Ensenada substrates, respectively (Supplementary Figure 3). The growth response varied significantly (P ≤ 0.05) among species, even on the same substrate. Thus, in ESAA soil, E. coccineum and L. dentata seedlings showed the greatest growth in total height, being significantly greater than those in G. avellana, L. hirsuta, and L. ferruginea seedlings (Figure 1A). However, the greatest total dry biomass was found in G. avellana which was significantly (P ≤ 0.05) greater than that in the other species (Figure 1B). On the poorest substrates, Choshuenco and Ensenada, G. avellana was also the species showing the greatest total dry biomass, followed by E. coccineum and the Lomatia species. In general, we observed no significant (P ≤ 0.05) differences in total height and total dry biomass of the species grown on the Ensenada and Chohuenco substrates.
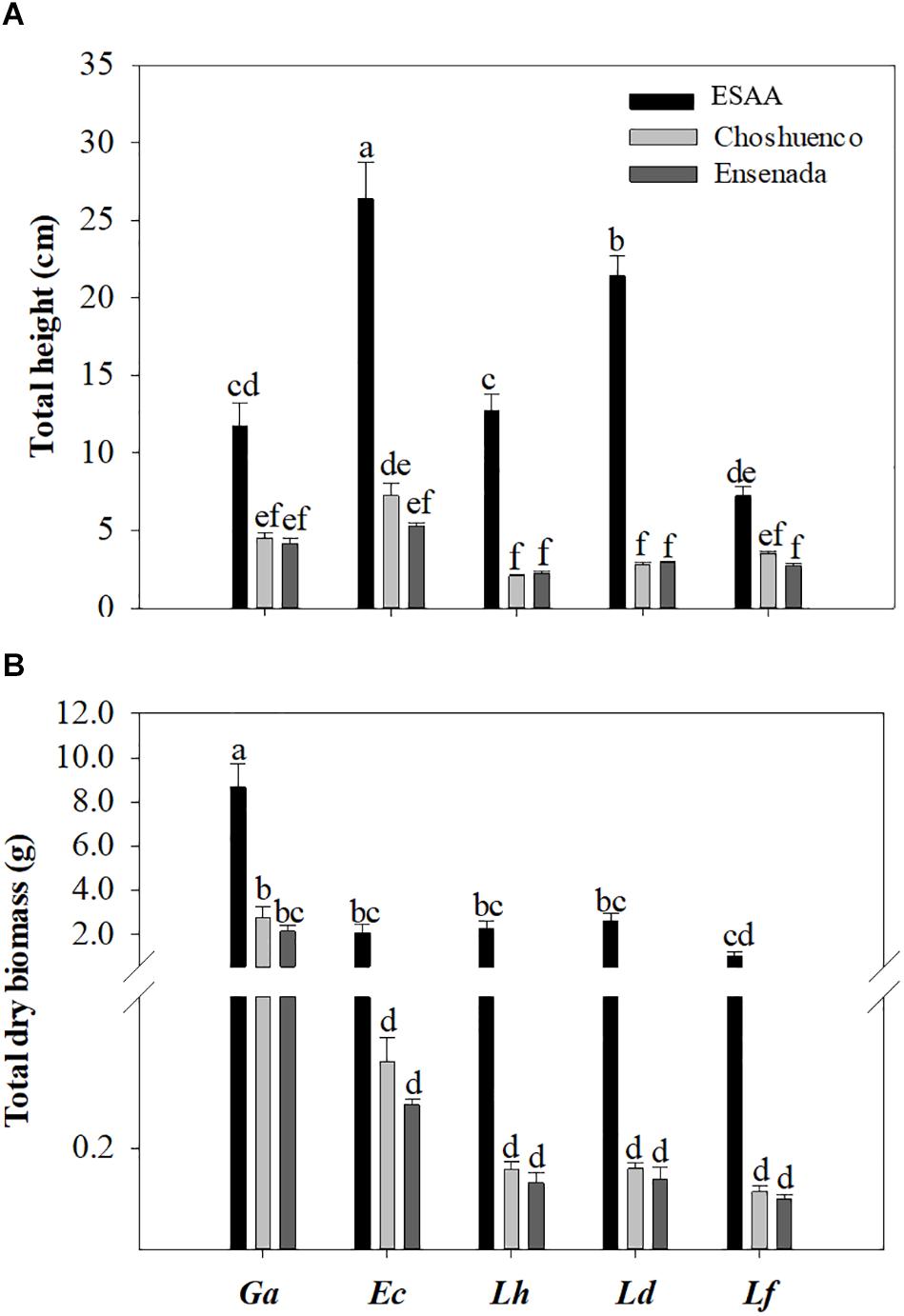
Figure 1. Mean total height (A) and total dry biomass (B) per plant of Gevuina avellana (Ga), Embothrium coccineum (Ec), Lomatia hirsuta (Lh), L. dentata (Ld), and L. ferruginea (Lf) grown in three volcanic materials: ESAA, Choshuenco and Ensenada. Each bar corresponds to the average per plant (n = 15) ± standard error (SE). Different letters indicate significant differences among species and volcanic materials (P ≤ 0.05).
Except for L. ferruginea, shoot/root ratio was significantly (P ≤ 0.05) affected by volcanic material. Thus, the highest shoot/root ratio values were found in the plants grown in soil from ESAA compared with those grown on substrates from Ensenada and Chohuenco (Figure 2A). Similarly, the CR/Total plant dry biomass ratio was also significantly affected by substrate, with higher values on the poorest substrates, Choshuenco and Ensenada (Figure 2B). Interestingly, G. avellana showed a significantly (P ≤ 0.05) higher CR/Total plant dry biomass ratio on Choshuenco substrate than on the other two substrates (Figure 2B).
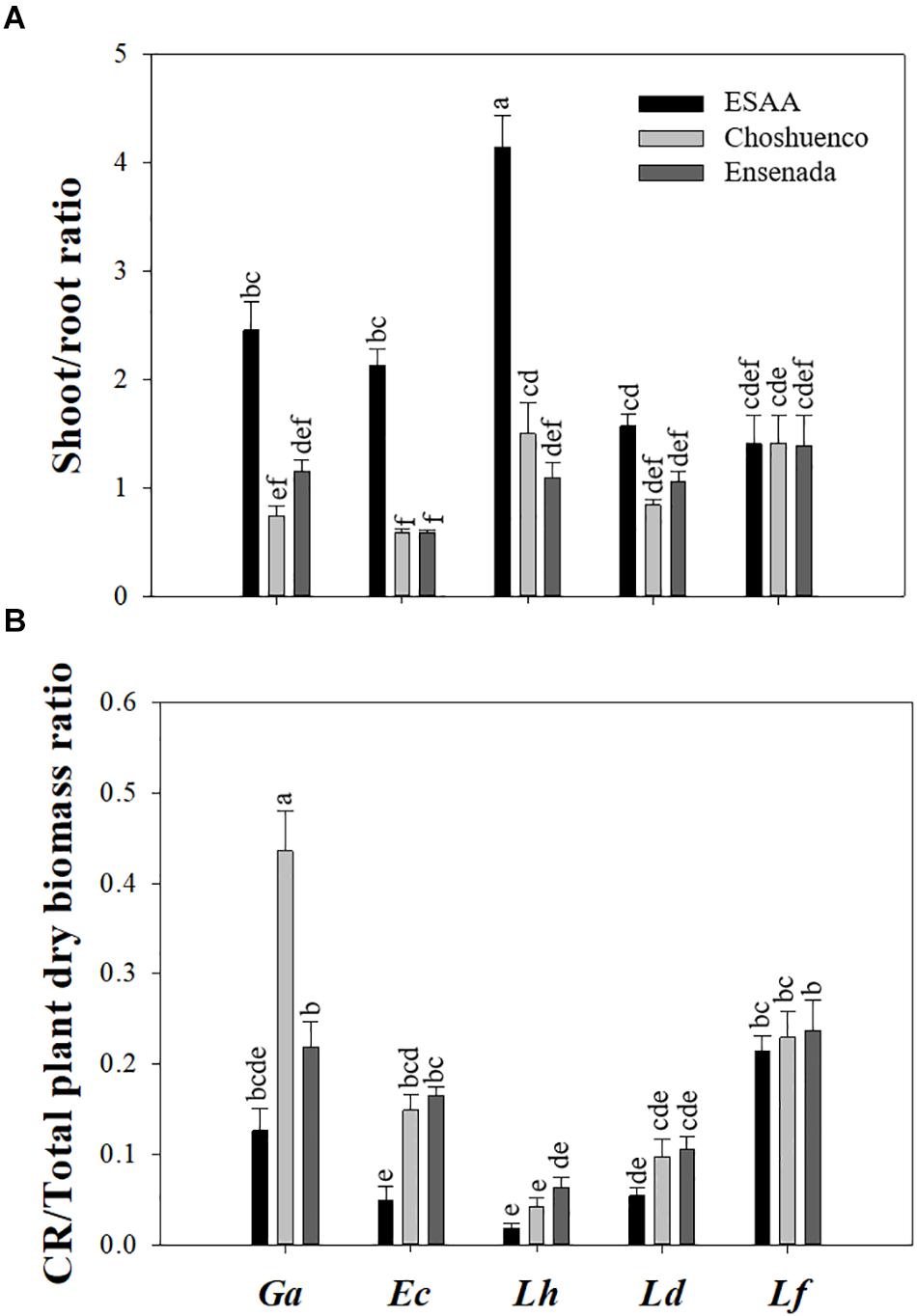
Figure 2. Shoot/root ratio (A) and cluster roots (CR)/total plant dry biomass ratio (B) of Gevuina avellana (Ga), Embothrium coccineum (Ec), Lomatia hirsuta (Lh), L. dentata (Ld), and L. ferruginea (Lf) grown in three volcanic materials: ESAA, Choshuenco and Ensenada. Each bar corresponds to the average per plant (n = 15) ± standard error (SE). Different letters indicate significant differences among species and volcanic materials (P ≤ 0.05).
Number and Biomass of Cluster Roots (CR) and Carboxylate Exudation Rate From Whole Root Systems
All seedlings produced CR, even those grown in the soil richest in nutrients (ESAA). In fact, a greater average number and biomass of CR was observed when the seedlings were grown in the richest soil than in the nutrient-poor substrates, Choshuenco and Ensenada (Figure 3A). In ESAA soil, L. dentata and L. ferruginea were the species that presented the largest number of CR. However, their biomass was similar or less than that of the other species, especially in the nutrient-poor substrates. Conversely, G. avellana was the species with the greatest CR biomass in all volcanic materials, and it also exhibited the fastest carboxylate-exudation rate, especially on the nutrient-poor substrates (Figure 3B). The main carboxylate exuded by roots of G. avellana was succinate, whereas Lomatia species exuded only oxalate. Embothrium coccineum had similar or more CR biomass than Lomatia species, but exuded carboxylates at a slower rate than these species (Figure 3A).
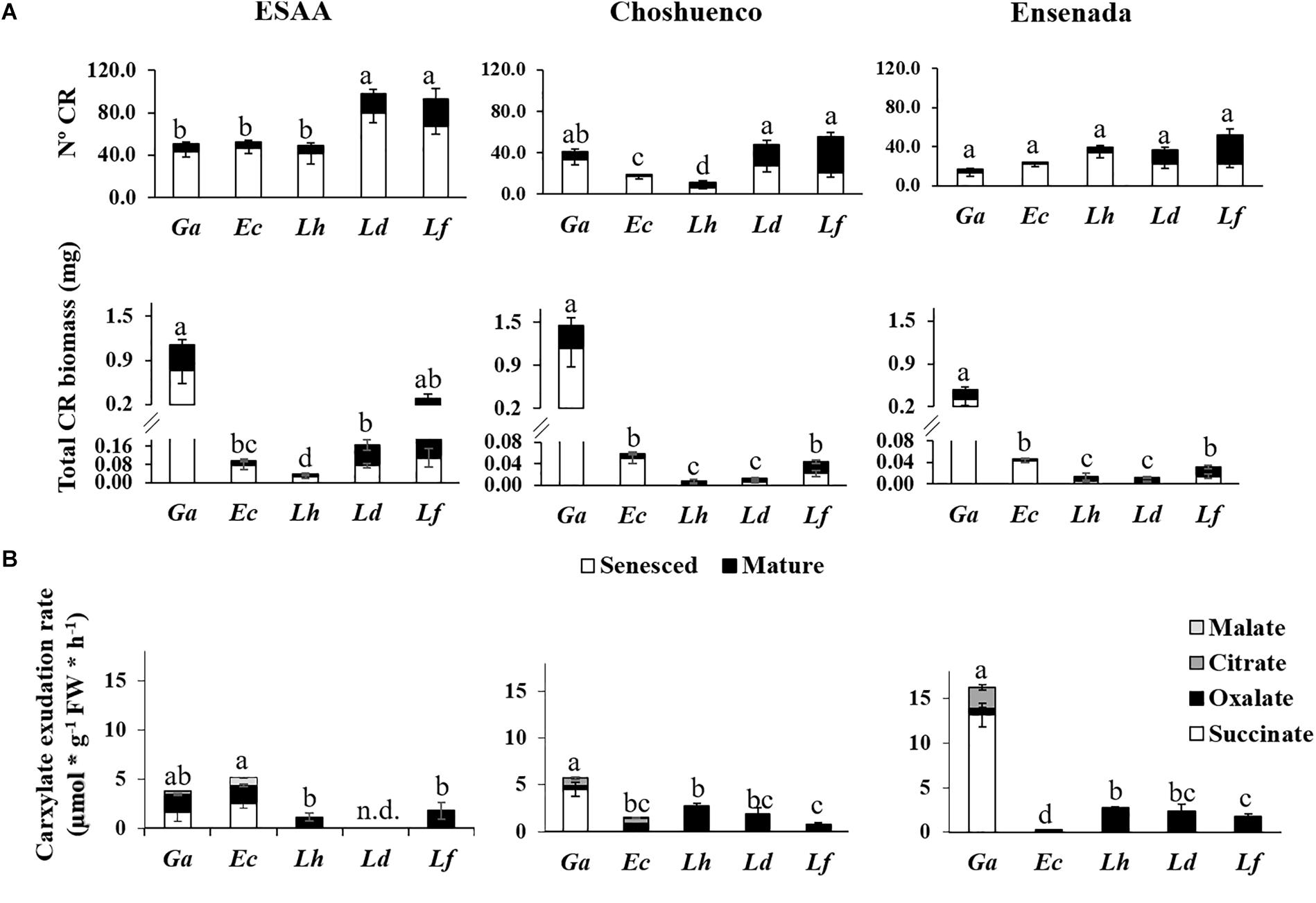
Figure 3. (A) Mean number and biomass of senesced and mature cluster roots (CR) per plant (n = 15) and (B) carboxylate-exudation rates from entire root systems (n = 6) of Gevuina avellana (Ga), Embothrium coccineum (Ec), Lomatia hirsuta (Lh), L. dentata (Ld), and L. ferruginea (Lf) grown in three volcanic materials: ESAA, Choshuenco and Ensenada. Different letters in (A) indicate significant differences in total (sum of mature and senesced) number and biomass of CR among species on the same volcanic material (P ≤ 0.05). Different letters in (B) indicate significant differences in total (sum of carboxylates) carboxylate exudation rate among species on the same volcanic material (P ≤ 0.05).
Foliar Nutrient Concentrations
Foliar nutrient concentrations varied significantly (P ≤ 0.05) among species and volcanic material they were grown in (Figure 4). Due to the fact that the mineral N concentration in the Choshuenco and Ensenada substrates is about half that in the ESAA soil (Table 1), we found that all species grown in nutrient-poor substrates showed significantly (P ≤ 0.05) lower leaf N concentrations than when grown in ESAA soil (Figure 4). In addition, leaf P concentrations were also significantly (P ≤ 0.05) higher in plants grown in ESAA soil (Figure 4). Interestingly, the only exception was G. avellana, which had similar foliar P concentrations in the three volcanic materials, independent of the basal P concentration in them. Embothrium coccineum was the species showing the significantly (P ≤ 0.05) highest leaf P concentration when grown in the ESSA soil. Similarly, this species along with G. avellana, presented significantly (P ≤ 0.05) higher leaf P concentrations than the Lomatia species when grown in Choshuenco and Ensenada substrates. In these nutrient-poor volcanic substrates, L. hirsuta and L. dentata showed similar foliar P concentrations, but significantly (P ≤ 0.05) higher values than those found in L. ferruginea (Figure 4). Interestingly, leaf N:P ratios in Lomatia species was greater than 16 when grown on the poorest substrate, in contrast to G. avellana and E. coccineum, in which it was around 10 (Figure 5).
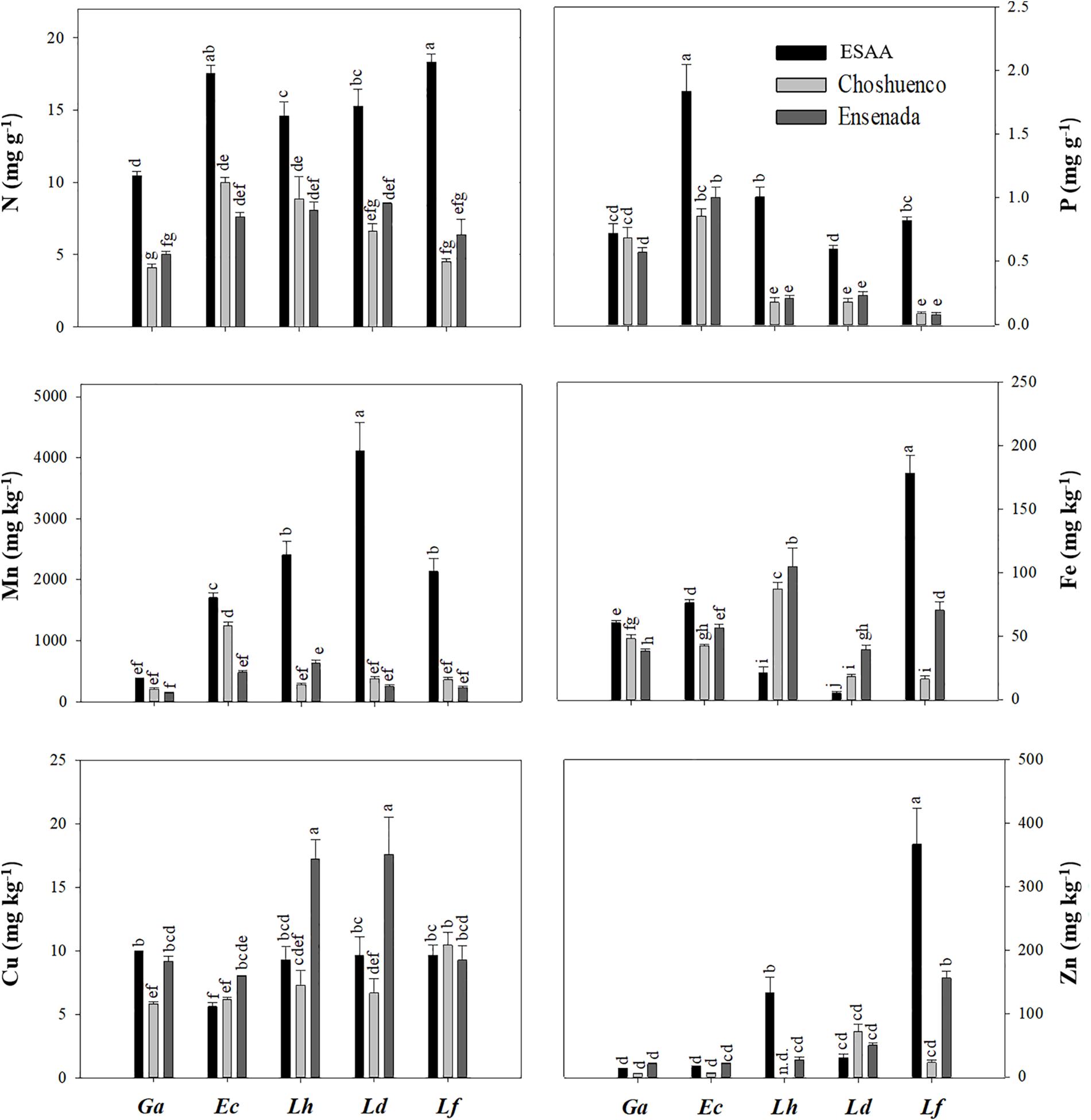
Figure 4. Foliar nitrogen (N), phosphorus (P), manganese (Mn), iron (Fe), copper (Cu), and zinc (Zn) concentrations in Gevuina avellana (Ga), Embothrium coccineum (Ec), Lomatia hirsuta (Lh), L. dentata (Ld), and L. ferruginea (Lf) grown in three volcanic materials: ESAA, Choshuenco, and Ensenada. Each bar corresponds to the average per plant (n = 6) ± standard error (SE). Different letters indicate significant differences among species and volcanic materials (P ≤ 0.05).
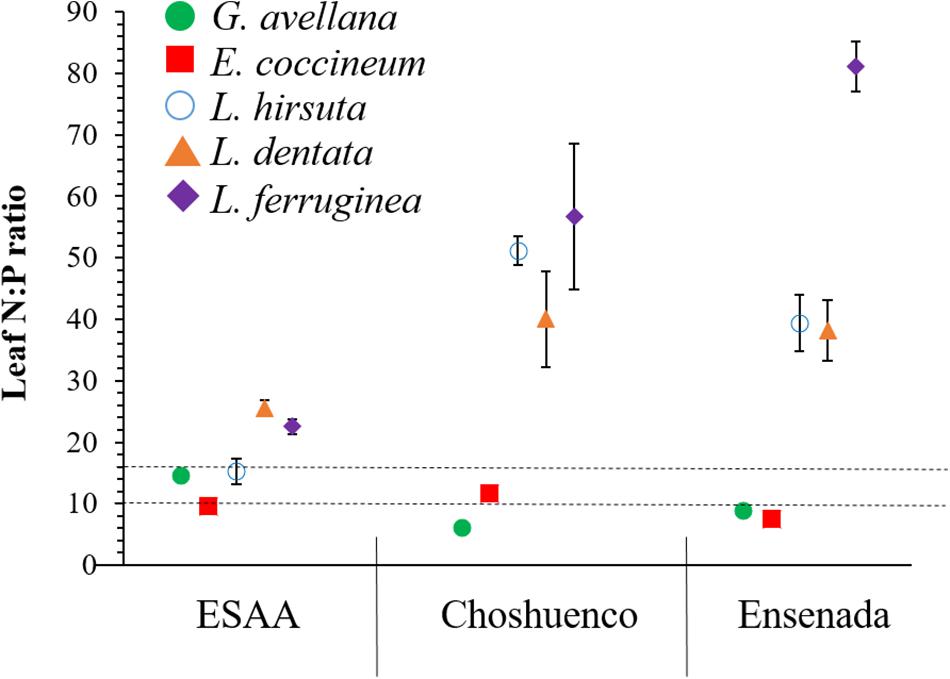
Figure 5. Leaf nitrogen:phosphorus (N:P) ratio (n = 6) in Gevuina avellana, Embothrium coccineum, Lomatia hirsuta, L. dentata, and L. ferruginea grown in three volcanic materials: ESAA, Choshuenco, and Ensenada. Horizontal lines indicate N limitation (values < 10), P limitation (values > 16) or both N and P limitation (values 10–16) according to Koerselman and Meuleman (1996).
In general, the plants that grew on the ESAA soil presented higher values of leaf Mn concentration than those on the Choshuenco and Ensenada substrates. Lomatia dentata was the species that had the highest Mn concentration in its leaves, while G. avellana was the species that had the lowest leaf Mn concentration on all substrates (Figure 4).
Except for L. ferruginea, leaf Cu concentration varied significantly (P ≤ 0.05) among the volcanic materials. In general, plants grown on Ensenada substrate showed the highest values of leaf Cu concentration, especially in L. hirsuta and L. dentata (Figure 4). Lomatia ferruginea was the species with the highest leaf concentrations of Fe and Zn, especially on ESAA soil and Ensenada substrate. In the other species, the leaf Fe and Zn concentration was variable and depending on the volcanic material. For example, the leaf Fe concentrations in G. avellana and E. coccineum were higher in ESAA soil, while in L. hirsuta and L. dentata the concentrations were higher in the nutrient-poor substrates, Choshuenco and Ensenada. With respect to leaf Zn concentration, the general tendency was to find lower values in plants grown in the Choshueco substrate, except for L. dentata, where a higher leaf Zn concentration was found than in plants grown in ESAA soil and Ensenada substrate.
Seed Nutrient Concentrations and Contents
Gevuina avellana had the lowest macro- and micronutrient concentrations in its seeds compared with the other Proteaceae (Supplementary Table 1). However, due to the larger size of its seeds, the total nutrient content was significantly (P ≤ 0.05) greater (Table 2). Thus, the values of N and P content were, on average, nine times greater in G. avellana than in E. coccineum, while, values of N and P content were on average 26 and 17 times greater in G. avellana than in Lomatia species, respectively. The micronutrient contents varied among species, but in general the trend was that G. avellana had the highest micronutrient content, followed by E. coccineum and then Lomatia species. The regression analyses revealed a relationship between total plant biomass and nutrient content in seeds (Supplementary Figure 4).
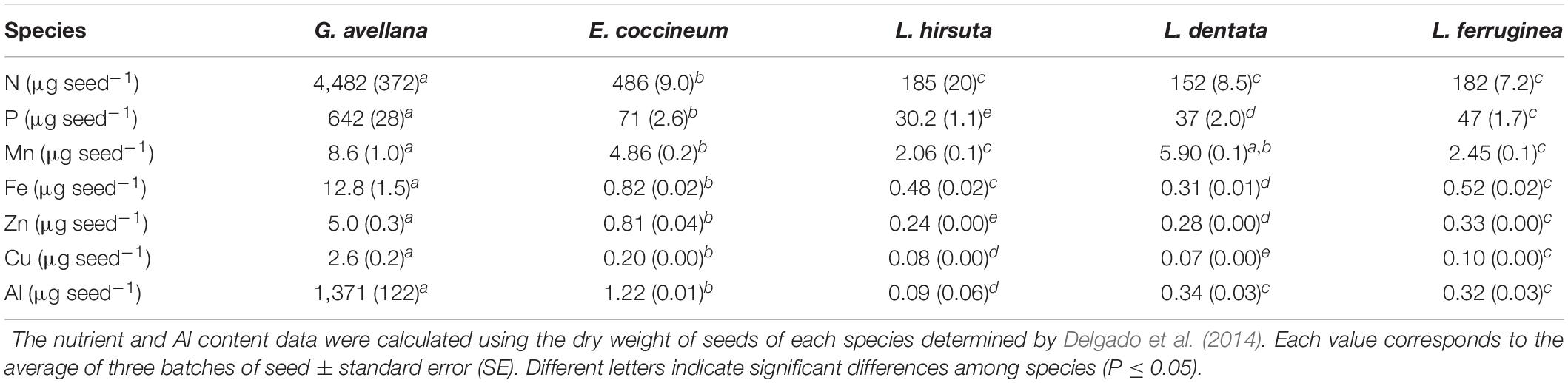
Table 2. Nitrogen (N), phosphorus (P), manganese (Mn), iron (Fe), copper (Cu), zinc (Zn), and aluminum (Al) content in seeds of Gevuina avellana, Embothrium coccineum, Lomatia hirsuta, L. dentata, and L. ferruginea.
Volcanic Materials and Plant Traits
From the PCA performed on volcanic materials and plant traits, we found clear separations across the horizontal axe between groups of plants that were grown on the nutrient-rich volcanic soil (EEAA) versus those grown on young and nutrient-poor volcanic substrates (Choshuenco and Ensenada) (Figure 6). Likely, species grown on EEAA were joined because they presented, in general, higher leaf macronutrient (N and P) concentrations, total height and shoot:root ratio than those grown on Choshuenco and Ensenada substrates. In addition, among species grown on young and nutrient-poor volcanic substrates, E. coccineum was separated from Lomatia species, mainly explained by their differences in the foliar N:P ratio. Finally, the PCA also revealed that G. avellana plants were separated from the rest of the species (lower quadrant), probably because it presented the highest N and P content in the seeds, leaf Al concentration, total biomass and CR:total plant biomass ratio (Figure 6).
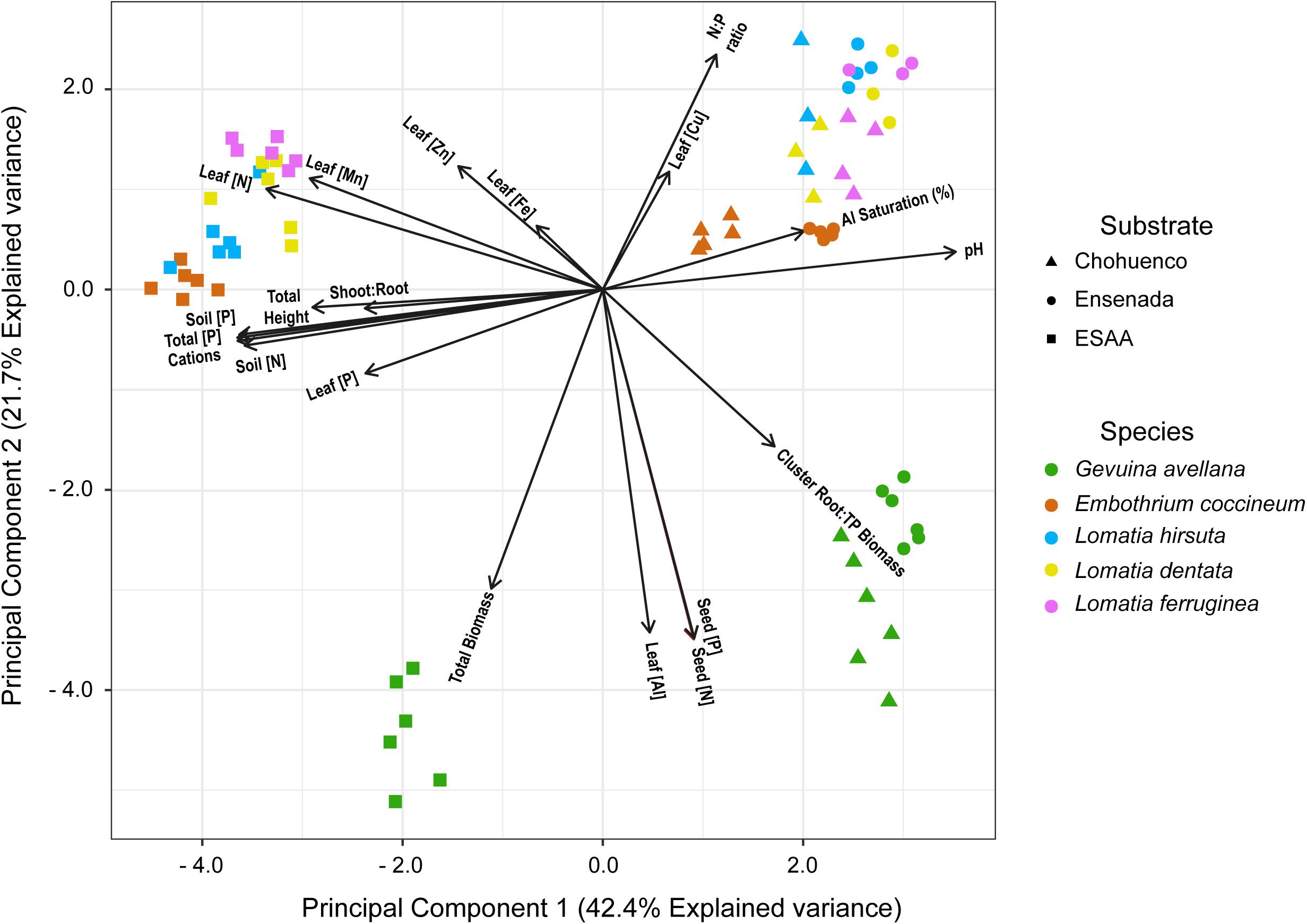
Figure 6. Principal component analysis representing different plant traits of five southern South American Proteaceae [e.g., leaf nitrogen (N), phosphorus (P), manganese (Mn), iron (Fe), zinc (Zn), copper (Cu), and aluminum (Al) concentrations (mg g– 1), leaf N:P ratio, seed nitrogen (N) and phosphorus (P) concentration, total height, total biomass, shoot:root ratio, cluster root:total plant biomass ratio (cluster root: TP biomass)] and soil variables [e.g., soil mineral N concentration (soil (N), mg kg– 1), available P soil (soil P, mg kg– 1), soil pH (pH), sum of cations (cations), Al saturation (%) and total P (mg kg– 1)] of three volcanic materials (ESAA, Choshuenco, and Ensenada) where these species were grown.
Discussion
In general, G. avellana showed greater total dry biomass and E. coccineum presented greater height in all substrates compared with the other species (Figures 1A,B). Most likely, the reason why G. avellana, in its early stages, produced more biomass than the other Proteaceae species is its larger seeds (Delgado et al., 2014) and higher nutrient content compared with those of the other species (Table 2), favoring its initial growth (Supplementary Figure 4). This trend was maintained in E. coccineum, which is the species that has the second-largest seed nutrient content (Table 2). Even though there is clear evidence that seed size and nutrient content play an important role in early stablisment of species on nutrient-poor soils (Milberg and Lamont, 1997; Denton et al., 2007), the greater growth of G. avellana and E. coccineum in poor soils cannot be explained by the nutrient content in their seeds alone, since in the case of G. avellana, almost all the plants (88%) had shed their cotyledons 2 months after starting the experiment (data not shown). Thus, these plants must have additional strategies to sustain growth and development under nutrient deprivation. For example, plants tend to allocate a greater proportion to root biomass and thus increase the chance to acquire nutrients (Bloom et al., 1985; Chapin et al., 1987; Gedroc et al., 1996). Our study supports this idea, because shoot/root ratios of all species (with the exception of L. ferruginea) were significantly lower in plants grown on the poorest substrates than on EEAA soil (Figure 2A). Likewise, the CR/total plant dry biomass ratio was higher in plants grown on recent volcanic substrates than in those grown in the nutrient-rich soil (Figure 2B), showing that, as reported in Hakea prostrata R. Br. (Proteaceae) (Shane et al., 2003), the biomass allocation to CR is greater when plants grow under nutrient deprivation. Interestingly, L. ferruginea was the only species that did not adjust its relative biomass distribution (e.g., shoot/root ratio, CR/total plant dry biomass ratio) in response to nutrient availability, suggesting a constitutive biomass-distribution pattern in this species.
In combination with root morphological traits as a strategy to explore the soil for nutrients, species bearing CR modify their metabolism and enhance the biosynthesis and release of carboxylates (Neumann et al., 1999; Shane et al., 2004, 2013), which play an important role in nutrient mobilization from the soil. Carboxylate composition of root exudates depends on the soil/substrate and species (Lambers et al., 2002; Veneklaas et al., 2003; Shi et al., 2019). In our study, a mixture of carboxylates (succinate, malate, citrate and malate) was found in root exudates of G. avellana and E. coccineum, while in Lomatia species only oxalate was detected (Figure 3B). Succinate was the main carboxylate exuded by the roots of G. avellana, which is the species with the highest total CR biomass (Figures 3A,B). This carboxylate is unusual in other species bearing CR, where citrate or malate are usually the major carboxylates exuded (Roelofs et al., 2001; Shane et al., 2004; Delgado et al., 2014; Ávila-Valdés et al., 2019), although it has been detected in the root exudates of Medicago sativa L. seedlings (Lipton et al., 1987) and two Phaseolus vulgaris L. genotypes (Atemkeng et al., 2011) when plants were subjected to low-P stress. Interestingly, Lomatia species only exuded oxalate. Zúñiga-Feest et al. (2020) reported similar results in root exudates of L. dentata. These authors suggest that oxalate could play an important role in P mobilization, because this organic anion has effects similar to citrate in P mobilization (Gerke et al., 2000). On the other hand, E. coccineum had more CR biomass than Lomatia species, but exuded carboxylates at a slower rate than Lomatia species, probably because at the time of the plant harvest (autumn), most CR had senesced in E. coccineum, as reported by Donoso-Ñanculao et al. (2010). These authors observed that there is a seasonal variation in CR formation in E. coccineum, with a greater proportion of mature CR relative to total root biomass in spring than in autumn. Seasonal variation of CR formation in Lomatia species has not been assessed, but apparently these species can maintain or produce mature CR for longer than the other species, especially L. dentata and L. ferruginea (Figure 3A). Although in the present study E. coccineum did not exude large amounts of carboxylates, Delgado et al. (2014) reported that CR of E. coccineum can exude large amounts of citrate and malate, even more than CR of H. prostrata (Shane et al., 2004), a species that occurs in extremely nutrient-poor soils, when grown in similar hydroponic conditions. Therefore, the composition and quantity of exudates depend on the time exudates are collected and on experimental conditions.
In order to avoid misinterpretation of the roots exudates collected in a single moment, some authors have suggested using other techniques as a proxy of the cumulative effect of carboxylates. Thus, leaf Mn concentration can be used as a proxy for carboxylate exudation or P-acquisition potential (Shane and Lambers, 2005b; Hayes et al., 2014; Lambers et al., 2015b, 2021; Pang et al., 2018; Shi et al., 2019). This is because carboxylates exuded by roots simultaneously mobilize P and other nutrients from the rhizosphere, especially Mn. The measurement of leaf Mn concentration is a valuable indicator of the cumulative effect of carboxylate exudation (Shane and Lambers, 2005b; Pang et al., 2018). Our results show that Lomatia species grown on the ESAA soil, had higher leaf Mn concentrations than G. avellana and E. coccineum (Figure 4), indicating that Lomatia roots released more carboxylates when grown in a nutrient-rich soil. These results are consistent with the fact that L. dentata and L. ferruginea produced a greater number and more biomass of CR (Figure 3A) when plants were grown on ESAA soil. Therefore, our findings suggest that the non-colonizing species, L. dentata and L. ferruginea, when grown on nutrient-richer soils responded rapidly forming CR and, presumably, maintained rapid rates of carboxylate exudation over time, as evidenced by their high leaf Mn concentration. Among Proteaceae that grew in the young and nutrient-poor substrates, E. coccineum was the species that presented the highest leaf Mn concentration. This species is commonly found colonizing volcanic substrates/soils (Donoso, 2006) which might be explained by its high capacity to exude carboxylates (in this study supported by its high leaf Mn concentration). In contrast, Gevuina avellana is a species that also can colonize and thrive on recent volcanic substrates. However, the relatively low Mn concentration in its leaves (Figure 4) suggests a relatively low carboxylate-exudation capacity. Recently, Delgado et al. (2019) reported that G. avellana hyperaccumulates aluminum (Al) in its leaves. That study was carried out at various sites along the natural geographical distribution of Proteaceae species (37.23°–51.22°S), and showed that leaf Mn concentration of G. avellana was much lower than that of non-Al hyperaccumulator species of the Proteaceae family from the same region. A similar trend was found in the present study, where G. avellana plants hyperaccumulated Al in its leaves (Supplementary Figure 2), but had the lowest leaf Mn concentrations compared with the other species (Figure 4). In this context, several studies have shown negative correlations between Mn and Al concentrations in leaves (Foy et al., 1973; Blair and Taylor, 1997; Fernando et al., 2009). However, the antagonistic uptake of these two metal ions remains unclear, because the two metals are taken up by different transport systems (Nevo and Nelson, 2006; Xia et al., 2010).
Interestingly, the Proteaceae species we studied differed in their leaf nutrient concentrations. For example, G. avellana was the species with the lowest leaf N concentration, especially in ESAA soil. This might be related to its nutrient-conservation strategy, since species with long leaf lifespan, such as G. avellana (4.3–5.4 years; Lusk and Corcuera (2011), tend to produce thicker leaves (Wright et al., 2004) with low N concentration on a weight basis (Reich et al., 1991; Reich et al., 1998), which enhances their robustness and decreases their palatability. Another trait revealing its nutrient-conservation strategy is the similar P concentrations found in leaves of G. avellana seedlings grown on the three volcanic materials evaluated (Figure 4). These results suggest that this species has a low P requirement and tightly down-regulates its P-uptake capacity when grow in soil with higher P availability (ESAA). Similar results have been found in other species bearing CR (e.g., Viminaria juncea (Schrad.) Hoffmanns (Fabaceae) (De Campos et al., 2013b), Euplassa cantareirae Sleumer (Proteaceae) (De Britto Costa et al., 2015), and this trait might be related to the ability of those species to avoid toxicity caused by excess soil P (Shane and Lambers, 2005c; De Campos et al., 2013a). We suggest that this ability allows G. avellana to develop well in a wide range of soil conditions, from deep soils with high fertility to volcanic substrates such as lava and slag (Donoso, 2006; Delgado et al., 2018). Some of the other studied Proteaceae can also grow in a wide range of soil conditions, but they probably use the P to grow faster when they occur in more fertile soils. Thus, G. avellana was the species that presented minor changes (four-fold) in plant biomass when grown in recent volcanic substrates versus nutrient-rich soil. Conversely, E. coccineum, L. ferruginea, L. hirsuta, and L. dentata produced, on average, up to 7, 10, 16, and 18 times more biomass, respectively, in fertile soil than in recent volcanic substrates (Figure 1B, Supplementary Figure 3). Zúñiga-Feest et al. (2020) also found that G. avellana has a more conservative relative growth rate when it grows in sand watered with different nutrient concentrations (full nutrient solution, without P, without N, water), while L. dentata grows faster when watered with complete nutrient solution. These results support the idea that Lomatia species maximize their growth under nutrient-rich soils, probably to compete in more fertile and diverse plant communities.
On the poorest substrates, Choshuenco and Ensenada, the leaf N:P ratio in Lomatia species was greater than 16, whereas in G. avellana and E. coccineum the N:P ratio was less than or close to 10 (Figure 5). According to the N:P ratios for vegetation representing the nature of nutrient limitation (Koerselman and Meuleman, 1996), our finding indicate P limitation in the leaves of Lomatia species and N limitation in the leaves of G. avellana and E. coccineum. These results contrast with those previously reported by Delgado et al. (2018), who determined the N:P ratios in the same species of this study, finding that adult plants growing in a wide variety of climatic and edaphic conditions are mainly limited by P. We postulate that newly emerged seedlings of Lomatia species have higher P requirements for triggering CR formation than G. avellana and E. coccineum. These results were more evident for L. dentata and L. ferruginea, which were limited by P even in the nutrient-richest soil (Figure 5), where they also produced more total CR biomass (Figure 3A). Alternatively, these results suggest that CR of E. coccineum and G. avellana could be more effective at acquiring P than the CR of Lomatia species which is evidenced by the fact that leaves of G. avellana and E. coccineum showed the highest leaf P concentration, even on the poorest substrates.
With respect to micronutrient concentrations, these were highly variable depending on species and volcanic material, especially in Lomatia species, which presented greater differences in their foliar copper (L. hirsuta and L. dentata) and zinc (L. ferruginea) concentrations than G. avellana and E. coccineum. Additionally, L. ferruginea showed the widest range of foliar iron (Fe) concentrations, reaching the highest Fe concentrations when grown on ESAA soil. This is consistent with Delgado et al. (2019), who found a wide variation in leaf Fe concentration under natural conditions. According to Delgado et al. (2019), this high variation in leaf Fe concentration is not correlated with soil Fe availability, and, therefore, it would be interesting to study the factors that influence the different Fe-uptake rates in these species, which apparently show the same trend as those of zinc uptake (Figure 4).
In summary, our study reveals that, although Proteaceae is a family widely known to produce carboxylate-releasing CR (Lambers et al., 2021), there are great differences among species in relation to their ability to thrive on soil/substrates with different nutrient availability (Figure 6). Probably, species that are better adapted to grow on relatively more fertile soils (e.g., Lomatia species), have decreased and/or lost the functionality of their CR when they grow in extremely nutrient-poor soils, perhaps because they are not able to recover the costs associated with the formation and functioning of these root structures. In fact, it has recently been reported that Xylomelum occidentale, a Proteaceae growing on soil that is moderately less P-impoverished than those in representative Proteaceae habitats in south-western Australia, do not produce functional CR (Zhong et al., 2021). In this context, it is necessary to mention that the better performance of these species under different soil nutrient conditions cannot be fully explained by a single trait. In fact, the plant adaptations to certain environmental conditions involve a complex network of physiological, biochemical and molecular responses, which, until now, are far from being fully understood in native plants. In our study, we contributed to the understanding of the autoecology of southern South American Proteaceae through the identification of some traits, such as seed nutrient content, nutrient requirements and ability to mobilize nutrients, that help us to explain – at least in part – the differential colonization capacities and performance under field conditions of these species.
Conclusion
We conclude that Proteaceae species vary widely in their ability to grow and acquire nutrients in young and nutrient-poor volcanic substrates. Gevuina avellana and E. coccineum performed better on young nutrient-poor volcanic substrates than Lomatia species. On the one hand, the seedling growth of larger-seeded G. avellana was less affected by nutrient availability of the soil/substrate, probably because it had a greater nutrient content in its seeds and produced CR exuding a large amount of carboxylates that supported their initial growth. On the other hand, E. coccineum exhibited greater total plant height and leaf P concentration than Lomatia species, presumably due to greater carboxylate exudation over time, as evidenced by their higher leaf Mn concentration (used as a proxy for carboxylate exudation) in one of the nutrient-poor volcanic substrates. Understanding the ecophysiology and functioning of these species in nutrient-poor soils can provide valuable tools to be used in restoration with these native species or other species with similar traits.
Data Availability Statement
The original contributions presented in the study are included in the article/Supplementary Material, further inquiries can be directed to the corresponding author/s.
Author Contributions
MD contributed to conceptualization, design of methodology, organization (i.e., graphs and tables), and interpretation of the data, along with writing the original draft. AZ-F contributed in the conception of this study and contributed with important intellectual content at all stages. MR-D contributed to perform chemical analysis of leaf samples as well as carboxylates determination. PB contributed to writing the original draft. SR contributed in the setup, supervision and harvest of the experiment. AB-B contributed to soil/substrates collection in the field as well as in the setup, supervision and harvest of the experiment. SV contributed to chemical analysis of soil and seed samples as well as statistical analysis. MP contributed to soil physical analysis, design of figures, and important intellectual content at the final stage. HL contributed to writing and editing of the manuscript. All authors revised critically the manuscript and approved the final version.
Funding
This study was financed by the Agencia Nacional de Investigación y Desarrollo (ANID, ex CONICYT) of Chilean government through FONDECYT initiation project No. 11170368 (MD) and No. 11200377 (PB), Postdoctoral projects No. 3150187 (MD) and No. 3160551 (AB-B), and FONDECYT Regular projects No. 1180699 (AZ-F) and No. 1171286 (MR-D).
Conflict of Interest
The authors declare that the research was conducted in the absence of any commercial or financial relationships that could be construed as a potential conflict of interest.
Acknowledgments
We thank Andrea Ávila and Cristian Muñoz for their enthusiastic support in the collection of volcanic substrates. Besides, we thank Ruth Espinoza for her valuable support in chemical analysis. Finally, we thank Fernanda Winkler and Cecilia Reyes for their help in harvesting the experiment and Felipe Ramirez for seeds collection and setup the experiment.
Supplementary Material
The Supplementary Material for this article can be found online at: https://www.frontiersin.org/articles/10.3389/fpls.2021.636056/full#supplementary-material
References
Atemkeng, M. F., Remans, R., Michiels, J., Tagne, A., and Ngonkeu, E. L. M. (2011). Inoculation with Rhizobium etli enhances organic acid exudation in common bean (Phaseolus vulgaris L.) subjected to phosphorus deficiency. Afr. J. Agric. Res. 6, 2235–2242. doi: 10.5897/AJAR10.024
Ávila-Valdés, A., Piper, F. I., and Zúñiga-Feest, A. (2019). Cluster root formation and function vary in two species with contrasting geographic ranges. Plant Soil 440, 25–38. doi: 10.1007/s11104-019-04056-3
Baker, W., and Thompson, T. (1992). “Determination of total nitrogen in plant samples by Kjeldahl,” in Plant Analysis Reference Procedures for the Southern Region of the United States. Southern Cooperative Series Bulletin, ed. C. O. Plank (Athens, Georgia: The University of Georgia), 13–16.
Blair, L. M., and Taylor, G. J. (1997). The nature of interaction between aluminum and manganese on growth and metal accumulation in Triticum aestivum. Environ. Exp. Bot. 37, 25–37. doi: 10.1016/S0098-8472(96)01036-2
Bloom, A. J., Chapin Iii, F. S., and Mooney, H. A. (1985). Resource limitation in plants-an economic analogy. Annu. Rev. Ecol. Syst. 16, 363–392.
Borie, F., Aguilera, P., Castillo, C., Valentine, A., Seguel, A., Barea, J. M., et al. (2019). Revisiting the nature of phosphorus pools in Chilean volcanic Soils as a basis for Arbuscular Mycorrhizal management in plant P acquisition. J. Soil Sci. Plant Nutr. 145, 1–12. doi: 10.1007/s42729-019-00041-y
Borie, F., and Rubio, R. (2003). Total and organic phosphorus in chilean volcanic soils. Gayana Bot. 60, 69–78. doi: 10.4067/S0717-66432003000100011
Chapin, F. S., Bloom, A. J., Field, C. B., and Waring, R. H. (1987). Plant responses to multiple environmental factors. Bioscience 37, 49–57. doi: 10.2307/1310177
Chen, Z. C., and Liao, H. (2016). Organic acid anions: an effective defensive weapon for plants against aluminum toxicity and phosphorus deficiency in acidic soils. J. Genet. Genomics 43, 631–638. doi: 10.1016/j.jgg.2016.11.003
CIREN (2003). Estudio Agrologico X Región. Descripciones de Suelos. Materiales y Simbolos. Santiago: CIREN Publication.
De Britto Costa, P., Abrahão, A., Viani, R. A. G., Brancalion, P. H. S., Lambers, H., Sawaya, A. C. H. F., et al. (2015). Cluster-root formation and carboxylate release in Euplassa cantareirae (Proteaceae) from a neotropical biodiversity hotspot. Plant Soil 403, 267–275. doi: 10.1007/s11104-015-2630-2
De Campos, M. C., Pearse, S. J., Oliveira, R. S., and Lambers, H. (2013a). Downregulation of net phosphorus-uptake capacity is inversely related to leaf phosphorus-resorption proficiency in four species from a phosphorus-impoverished environment. Ann. Bot. 111, 445–454. doi: 10.1093/aob/mcs299
De Campos, M. C., Pearse, S. J., Oliveira, R. S., and Lambers, H. (2013b). Viminaria juncea does not vary its shoot phosphorus concentration and only marginally decreases its mycorrhizal colonization and cluster-root dry weight under a wide range of phosphorus supplies. Ann. Bot. 111, 801–809. doi: 10.1093/aob/mct035
Delgado, M., Zúñiga-Feest, A., Alvear, M., and Borie, F. (2013). The effect of phosphorus on cluster-root formation and functioning of Embothrium coccineum (R. et J. Forst.). Plant Soil 373, 765–773. doi: 10.1007/s11104-013-1829-3
Delgado, M., Suriyagoda, L., Zúñiga-Feest, A., Borie, F., and Lambers, H. (2014). Divergent functioning of Proteaceae species: the South American Embothrium coccineum displays a combination of adaptive traits to survive in high-phosphorus soils. Funct. Ecol. 28, 1356–1366. doi: 10.1111/1365-2435.12303
Delgado, M., Valle, S., Barra, P. J., Reyes-Díaz, M., and Zúñiga-Feest, A. (2019). New aluminum hyperaccumulator species of the Proteaceae family from southern South America. Plant Soil 444, 475–487. doi: 10.1007/s11104-019-04289-2
Delgado, M., Zúñiga-Feest, A., Almonacid, L., Lambers, H., and Borie, F. (2015a). Cluster roots of Embothrium coccineum (Proteaceae) affect enzyme activities and phosphorus lability in rhizosphere soil. Plant Soil 395, 189–200. doi: 10.1007/s11104-015-2547-9
Delgado, M., Zúñiga-Feest, A., and Borie, F. (2015b). Ecophysiological role of Embothrium coccineum, a Proteaceae species bearing cluster roots, at increasing phosphorus availability in its rhizosphere. J. Soil Sci. Plant Nutr. 15, 307–320. doi: 10.4067/S0718-95162015005000028
Delgado, M. F., Valle, S., Reyes-Díaz, M., Barra, P. J., and Zúñiga-Feest, A. (2018). Nutrient use efficiency of southern South America Proteaceae species. Are there general patterns in the Proteaceae family? Front. Plant Sci. 9:883. doi: 10.3389/fpls.2018.00883
Denton, M. D., Veneklaas, E. J., and Lambers, H. (2007). Does phenotypic plasticity in carboxylate exudation differ among rare and widespread Banksia species (Proteaceae)? New Phytol. 173, 592–599. doi: 10.1111/j.1469-8137.2006.01956.x
Dinkelaker, B., Hengeler, C., and Marschner, H. (1995). Distribution and function of proteoid roots and other root clusters. Bot. Acta 108, 193–200. doi: 10.1111/j.1438-8677.1995.tb00850.x
Donoso, C. (2006). Las Especies Arbóreas de los Bosques Templados de Chile y Argentina Autoecología. Valdivia: Marisa Cúneo Ediciones.
Donoso, C., and Escobar, B. (1986). Germinación de las Proteáceas arbóreas chilenas. Bosque (Chile) 7, 85–94. doi: 10.4206/bosque.1986.v7n2-04
Donoso-Ñanculao, G., Castro, M., Navarrete, D., Bravo, L. A., and Corcuera, L. J. (2010). Seasonal induction of cluster roots in Embothrium coccineum JR Forst. & G. Forst. in the field: factors that regulate their development. Chil. J. Agric. Res. 4, 559–566. doi: 10.4067/S0718-58392010000400005
Dörner, J., Sandoval, P., and Dec, D. (2010). The role of soil structure on the pore functionality of an Ultisol. J. Soil Sci. Plant Nutr. 10, 495–508. doi: 10.4067/S0718-95162010000200009
Fernando, D., Guymer, G., Reeves, R., Woodrow, I., Baker, A., and Batianoff, G. (2009). Foliar Mn accumulation in eastern Australian herbarium specimens: prospecting for new Mn hyperaccumulators and its potential application in taxonomy. Ann. Bot. 103, 931–939. doi: 10.1093/aob/mcp013
Foy, C., Fleming, A., and Schwartz, J. (1973). Opposite alluminum and manganese tolerances of two wheat varieties. Agron. J. 65, 123–126. doi: 10.2134/agronj1973.00021962006500010037x
Gedroc, J., Mcconnaughay, K., and Coleman, J. (1996). Plasticity in root/shoot partitioning: optimal, ontogenetic, or both? Funct. Ecol. 10, 44–50. doi: 10.2307/2390260
Gerke, J., Beißner, L., and Römer, W. (2000). The quantitative effect of chemical phosphate mobilization by carboxylate anions on P uptake by a single root. I. The basic concept and determination of soil parameters. J. Plant Nutr. Soil Sci. 163, 207–212. doi: 10.1002/(SICI)1522-2624(200004)163:2<207::AID-JPLN207<3.0.CO;2-P
Groom, P. K., and Lamont, B. B. (2010). Phosphorus accumulation in Proteaceae seeds: a synthesis. Plant Soil 334, 61–72. doi: 10.1007/s11104-009-0135-6
Hayes, P., Turner, B. L., Lambers, H., and Laliberté, E. (2014). Foliar nutrient concentrations and resorption efficiency in plants of contrasting nutrient-acquisition strategies along a 2-million-year dune chronosequence. J. Ecol. 102, 396–410. doi: 10.1111/1365-2745.12196
Hechenleitner, P., Gardner, M., Thomas, P., Echeverría, C., Escobar, B., Brownless, P., et al. (2005). Plantas Amenazadas del Centro-Sur de Chile. Chile: Universidad Austral de Chile y Real Jardín Botánico de Edimburgo.
Ingram, D. S., Vince-Prue, D., and Gregory, P. J. (2015). Science and the Garden: The Scientific Basis of Horticultural Practice. New York, NY: John Wiley & Sons.
Jones, D. L. (1998). Organic acids in the rhizosphere–a critical review. Plant Soil 205, 25–44. doi: 10.1023/A:1004356007312
Koerselman, W., and Meuleman, A. F. (1996). The vegetation N:P ratio: a new tool to detect the nature of nutrient limitation. J. Appl. Ecol. 33, 1441–1450. doi: 10.2307/2404783
Lambers, H., Brundrett, M. C., Raven, J. A., and Hopper, S. D. (2010). Plant mineral nutrition in ancient landscapes: high plant species diversity on infertile soils is linked to functional diversity for nutritional strategies. Plant Soil 334, 11–31. doi: 10.1007/s11104-010-0444-9
Lambers, H., Finnegan, P. M., Jost, R., Plaxton, W. C., Shane, M. W., and Stitt, M. (2015a). Phosphorus nutrition in Proteaceae and beyond. Nat. Plants 1:15109. doi: 10.1038/nplants.2015.109
Lambers, H., Hayes, P. E., Laliberté, E., Oliveira, R. S., and Turner, B. L. (2015b). Leaf manganese accumulation and phosphorus-acquisition efficiency. Trends Plant Sci. 20, 83–90. doi: 10.1016/j.tplants.2014.10.007
Lambers, H., Juniper, D., Cawthray, G. R., Veneklaas, E. J., and Martínez-Ferri, E. (2002). The pattern of carboxylate exudation in Banksia grandis (Proteaceae) is affected by the form of phosphate added to the soil. Plant Soil 238, 111–122. doi: 10.1023/A:1014289121672
Lambers, H., Raven, J. A., Shaver, G. R., and Smith, S. E. (2008). Plant nutrient-acquisition strategies change with soil age. Trends Ecol. Evol. 23, 95–103. doi: 10.1016/j.tree.2007.10.008
Lambers, H., Shane, M. W., Cramer, M. D., Pearse, S. J., and Veneklaas, E. J. (2006). Root structure and functioning for efficient acquisition of phosphorus: matching morphological and physiological traits. Ann. Bot. 98, 693–713. doi: 10.1093/aob/mcl114
Lambers, H., Wright, I. J., Pereira, C. G., Bellingham, P. J., Bentley, L. P., Boonman, A., et al. (2021). Leaf manganese concentrations as a tool to assess belowground plant functioning in phosphorus-impoverished environments. Plant Soil 1–19. doi: 10.1007/s11104-020-04690-2
Lamont, B. B. (2003). Structure, ecology and physiology of root clusters – a review. Plant Soil 248, 1–19. doi: 10.1023/A:1022314613217
Lipton, D. S., Blanchar, R. W., and Blevins, D. G. (1987). Citrate, malate, and succinate concentration in exudates from P-sufficient and P-stressed Medicago sativa L. seedlings. Plant Physiol. 85, 315–317. doi: 10.1104/pp.85.2.315
Lusk, C. H., and Corcuera, L. J. (2011). Effects of light availability and growth rate on leaf lifespan of four temperate rainforest Proteaceae. Rev. Chil. Hist. Nat. 84, 269–277. doi: 10.4067/S0716-078X2011000200011
Marschner, H., Römheld, V., and Kissel, M. (1986). Different strategies in higher plants in mobilization and uptake of iron. J. Plant Nutr. 9, 695–713. doi: 10.1080/01904168609363475
Matus, F., Amigo, X., and Kristiansen, S. M. (2006). Aluminium stabilization controls organic carbon levels in Chilean volcanic soils. Geoderma 132, 158–168. doi: 10.1016/j.geoderma.2005.05.005
Milberg, P., and Lamont, B. B. (1997). Seed/cotyledon size and nutrient content play a major role in early performance of species on nutrient-poor soils. New Phytol. 137, 665–672.
Neumann, G., Massonneau, A., Martinoia, E., and Römheld, V. (1999). Physiological adaptations to phosphorus deficiency during proteoid root development in white lupin. Planta 208, 373–382. doi: 10.1007/s004250050572
Nevo, Y., and Nelson, N. (2006). The NRAMP family of metal-ion transporters. Biochim. Biophys. Acta Mol. Cell Res. 1763, 609–620. doi: 10.1016/j.bbamcr.2006.05.007
Pang, J., Bansal, R., Zhao, H., Bohuon, E., Lambers, H., Ryan, M. H., et al. (2018). The carboxylate−releasing phosphorus−mobilizing strategy can be proxied by foliar manganese concentration in a large set of chickpea germplasm under low phosphorus supply. New Phytol. 219, 518–529. doi: 10.1111/nph.15200
Prance, G., and Plana, V. (1998). The American proteaceae. Aust. Syst. Bot. 11, 287–299. doi: 10.1071/SB97023
Purnell, H. M. (1960). Studies of the family Proteaceae. I. Anatomy and morphology of the roots of some Victorian species. Aust. J. Bot. 8, 38–50. doi: 10.1071/BT9600038
Quintero, C., Garibaldi, L. A., Grez, A., Polidori, C., and Nieves-Aldrey, J. L. (2014). “Galls of the temperate forest of southern South America: Argentina and Chile,” in Neotropical Insect Galls, eds G. Fernandes and J. Santos (Dordrecht: Springer), 429–463.
Rawson, H., Naranjo, J. A., Smith, V. C., Fontijn, K., Pyle, D. M., Mather, T. A., et al. (2015). The frequency and magnitude of post-glacial explosive eruptions at Volcán Mocho-Choshuenco, southern Chile. J. Volcanol. Geotherm. Res. 299, 103–129. doi: 10.1016/j.jvolgeores.2015.04.003
Reich, P., Uhl, C., Walters, M., and Ellsworth, D. (1991). Leaf lifespan as a determinant of leaf structure and function among 23 Amazonian tree species. Oecologia 86, 16–24. doi: 10.1007/BF00317383
Reich, P. B., Walters, M. B., Ellsworth, D. S., Vose, J. M., Volin, J. C., Gresham, C., et al. (1998). Relationships of leaf dark respiration to leaf nitrogen, specific leaf area and leaf life-span: a test across biomes and functional groups. Oecologia 114, 471–482.
Roelofs, R. F. R., Rengel, Z., Cawthray, G. R., Dixon, K. W., and Lambers, H. (2001). Exudation of carboxylates in Australian Proteaceae: chemical composition. Plant Cell Environ. 24, 891–903. doi: 10.1046/j.1365-3040.2001.00741.x
Ryan, P. R., Delhaize, E., and Jones, D. L. (2001). Function and mechanism of organic anion exudation from plant roots. Annu. Rev. Plant Physiol. Plant Mol. Biol. 52, 527–560. doi: 10.1146/annurev.arplant.52.1.527
Sadras, V., and Richards, R. (2014). Improvement of crop yield in dry environments: benchmarks, levels of organisation and the role of nitrogen. J. Exp. Bot. 65, 1981–1995. doi: 10.1093/jxb/eru061
Sadzawka, A., Grez, R., Carrasco, M., and Mora, M. (2004). Métodos de Análisis de Tejidos Vegetales. Chile: Comisión de normalización y acreditación sociedad chilena de la ciencia del suelo.
Shane, M. W., Cramer, M. D., Funayama-Noguchi, S., Cawthray, G. R., Millar, A. H., Day, D. A., et al. (2004). Developmental physiology of cluster-root carboxylate synthesis and exudation in harsh hakea. Expression of phosphoenolpyruvate carboxylase and the alternative oxidase. Plant Physiol. 135, 549–560. doi: 10.1104/pp.103.035659
Shane, M. W., De Vos, M., De Roock, S., Cawthray, G. R., and Lambers, H. (2003). Effects of external phosphorus supply on internal phosphorus concentration and the initiation, growth and exudation of cluster roots in Hakea prostrata R.Br. Plant Soil 248, 209–219. doi: 10.1023/a:1022320416038
Shane, M. W., Fedosejevs, E. T., and Plaxton, W. C. (2013). Reciprocal control of anaplerotic phosphoenolpyruvate carboxylase by in vivo monoubiquitination and phosphorylation in developing proteoid roots of phosphate-deficient harsh hakea. Plant Physiol. 161, 1634–1644. doi: 10.1104/pp.112.213496
Shane, M. W., and Lambers, H. (2005a). Cluster roots: a curiosity in context. Plant Soil 274, 101–125. doi: 10.007/s11104-004-2725-7
Shane, M. W., and Lambers, H. (2005b). Manganese accumulation in leaves of Hakea prostrata (Proteaceae) and the significance of cluster roots for micronutrient uptake as dependent on phosphorus supply. Physiol. Plant. 124, 441–450. doi: 10.1111/j.1399-3054.2005.00527.x
Shane, M. W., and Lambers, H. (2005c). Systemic suppression of cluster-root formation and net P-uptake rates in Grevillea crithmifolia at elevated P supply: a proteacean with resistance for developing symptoms of ‘P toxicity’. J. Exp. Bot. 57, 413–423. doi: 10.1093/jxb/erj004
Shi, J., Strack, D., Albornoz, F. E., Han, Z., and Lambers, H. (2019). Differences in investment and functioning of cluster roots account for different distributions of Banksia attenuata and B. sessilis, with contrasting life history. Plant Soil 447, 85–98. doi: 10.1007/s11104-019-03982-6
Souto, C. P., Premoli, A. C., and Reich, P. B. (2009). Complex bioclimatic and soil gradients shape leaf trait variation in Embothrium coccineum (Proteaceae) among austral forests in Patagonia. Rev. Chil. Hist. Nat. 82, 209–222. doi: 10.4067/S0716-078X2009000200004
Steubing, L., Alberdi, M., and Wenzel, H. (1983). Seasonal changes of cold resistance of Proteaceae of the South Chilean laurel forest. Plant Ecol. 52, 35–44. doi: 10.1007/BF00040015
Veblen, T., Donoso, C., Kitzberger, T., and Rebertus, A. (1996). “Ecology of southern Chilean and Argentinean Nothofagus forests,” in The Ecology and Biogeography of Nothofagus Forests, eds T. T. Veblen, R. Hill, and J. Read (New Haven, CT: Yale University Press), 293–353.
Veblen, T. T., and Ashton, D. H. (1978). Catastrophic influences on the vegetation of the Valdivian Andes. Chile. Vegetatio 36, 149–167. doi: 10.1007/BF02342598
Veblen, T. T., Kitzberger, T., and Lara, A. (1992). Disturbance and forest dynamics along a transect from Andean rain forest to Patagonian shrubland. J. Veg. Sci. 3, 507–520. doi: 10.2307/3235807
Veneklaas, E. J., Stevens, J., Cawthray, G. R., Turner, S., Grigg, A. M., and Lambers, H. (2003). Chickpea and white lupin rhizosphere carboxylates vary with soil properties and enhance phosphorus uptake. Plant Soil 248, 187–197. doi: 10.1023/a:1022367312851
Wright, I. J., Reich, P. B., Westoby, M., Ackerly, D. D., Baruch, Z., Bongers, F., et al. (2004). The worldwide leaf economics spectrum. Nature 428, 821–827. doi: 10.1038/nature02403
Xia, J., Yamaji, N., Kasai, T., and Ma, J. F. (2010). Plasma membrane-localized transporter for aluminum in rice. Proc. Natl. Acad. Sci. U.S.A. 107, 18381–18385. doi: 10.1073/pnas.1004949107
Zhong, H., Zhou, J., Azmi, A., Arruda, A. J., Doolette, A. L., Smernik, R. J., et al. (2021). Xylomelum occidentale (Proteaceae) accesses relatively mobile soil organic phosphorus without releasing carboxylates. J. Ecol. 109, 246–259. doi: 10.1111/1365-2745.13468
Zúñiga-Feest, A., Muñoz, G., Bustos-Salazar, A., Ramírez, F., Delgado, M., Valle, S., et al. (2018). The nitrogen fixing specie Sophora cassioides (Fabaceae), is nutritionally favored and their rhizosphere bacteria modified when is co-cultivated with the cluster root forming Embothrium coccineum (Proteaceae). J. Soil Sci. Plant Nutr. 18, 597–616. doi: 10.4067/S0718-95162018005001801
Zúñiga-Feest, A., Sepúlveda, F., Delgado, M., Valle, S., Muñoz, G., Pereira, M., et al. (2020). Gevuina avellana and Lomatia dentata, two Proteaceae species from evergreen temperate forests of South America exhibit contrasting physiological responses under nutrient deprivation. Plant Soil 1–16. doi: 10.1007/s11104-020-04640-y
Keywords: carboxylates, cluster roots, colonization, nutrients, Proteaceae, volcanic substrate-soils
Citation: Delgado M, Zúñiga-Feest A, Reyes-Díaz M, Barra PJ, Ruiz S, Bertin-Benavides A, Valle S, Pereira M and Lambers H (2021) Ecophysiological Performance of Proteaceae Species From Southern South America Growing on Substrates Derived From Young Volcanic Materials. Front. Plant Sci. 12:636056. doi: 10.3389/fpls.2021.636056
Received: 30 November 2020; Accepted: 01 February 2021;
Published: 19 February 2021.
Edited by:
Iván Prieto, Center for Edaphology and Applied Biology of Segura, Spanish National Research Council, SpainReviewed by:
Yunpeng Nie, Chinese Academy of Sciences (CAS), ChinaLina Fusaro, Institute for Bioeconomy, Italian National Research Council, Italy
Copyright © 2021 Delgado, Zúñiga-Feest, Reyes-Díaz, Barra, Ruiz, Bertin-Benavides, Valle, Pereira and Lambers. This is an open-access article distributed under the terms of the Creative Commons Attribution License (CC BY). The use, distribution or reproduction in other forums is permitted, provided the original author(s) and the copyright owner(s) are credited and that the original publication in this journal is cited, in accordance with accepted academic practice. No use, distribution or reproduction is permitted which does not comply with these terms.
*Correspondence: M. Delgado, bWFiZWwuZGVsZ2Fkb0B1ZnJvbnRlcmEuY2w=; A. Zúñiga-Feest, YWxlamFuZHJhenVuaWdhZmVlc3RAZ21haWwuY29t