- 1Functional and Tropical Plant Ecology, Bayreuth Centre of Ecology and Environmental Research (BayCEER), University of Bayreuth, Bayreuth, Germany
- 2Smithsonian Tropical Research Institute, Ancón, Panama
- 3Department of Agriculture and Natural Resources Sciences, Namibia University of Science and Technology (NUST), Windhoek, Namibia
- 4Plant Ecology Group, Institute of Evolution and Ecology, University of Tübingen, Tübingen, Germany
The plant economics spectrum hypothesizes a correlation among resource-use related traits along one single axis, which determines species’ growth rates and their ecological filtering along resource gradients. This concept has been mostly investigated and shown in perennial species, but has rarely been tested in annual species. Annuals evade unfavorable seasons as seeds and thus may underlie different constraints, with consequences for interspecific trait-trait, trait-growth, and trait-environment relations. To test the hypotheses of the plant economics spectrum in annual species, we measured twelve resource-use related leaf and root traits in 30 winter annuals from Israel under controlled conditions. Traits and their coordinations were related to species’ growth rates (for 19 species) and their distribution along a steep rainfall gradient. Contrary to the hypotheses of the plant economics spectrum, in the investigated annuals traits were correlated along two independent axes, one of structural traits and one of carbon gain traits. Consequently, species’ growth rates were related to carbon gain traits, but independent from structural traits. Species’ distribution along the rainfall gradient was unexpectedly neither associated with species’ scores along the axes of carbon gain or structural traits nor with growth rate. Nevertheless, root traits were related with species’ distribution, indicating that they are relevant for species’ filtering along rainfall gradients in winter annuals. Overall, our results showed that the functional constraints hypothesized by the plant economics spectrum do not apply to winter annuals, leading to unexpected trait-growth and trait-rainfall relations. Our study thus cautions to generalize trait-based concepts and findings between life-history strategies. To predict responses to global change, trait-based concepts should be explicitly tested for different species groups.
Introduction
Trait-based schemes, such as the plant economics spectrum, characterize general combinations and trade-offs among functional traits and their relations to environmental conditions (e.g., Grime, 1977; Westoby et al., 2002; Wright et al., 2004; Reich, 2014). They are widely used for assessing and predicting community assembly and ecosystem functioning under current and future conditions (e.g., Westoby et al., 2002; Wright et al., 2004; Reich, 2014). However, the plant economics spectrum has been mainly developed and tested for woody and perennial herbaceous species (reviewed in Reich, 2014), whereas investigations of this concept in annual species are virtually missing (but see Brouillette et al., 2014). The universality of the plant economics spectrum to reflect functional constraints and interspecific trait-environment relations across life-history strategies (annuals vs. perennials) therefore remains unclear.
Annual species substantially contribute to species diversity, primary production, and ecosystem services in many dryland ecosystems worldwide (Noy-Meir, 1973; Tielbörger et al., 2014; Ruppert et al., 2015). These ecosystems have been characterized as particularly vulnerable to global change (Sala et al., 2000; Schröter et al., 2005), underscoring the relevance of assessing and predicting species’ responses to climate and land-use change. Environmental changes may affect annual plant communities even faster than perennial ones due to their short life cycle. Understanding interspecific trait-trait relations and the ecological filtering of annuals is therefore timely and of practical relevance.
The plant economics spectrum hypothesizes an interspecific trade-off between trait attributes conferring rapid resource acquisition (i.e., acquisitive or fast attributes, e.g., high assimilation rate and nutrient concentrations, low tissue density, Table 1) and those conserving resources (i.e., conservative or slow attributes, e.g., low assimilation rate and nutrient concentrations, and high tissue density) at the whole plant level, i.e., among and within leaves, stems, and roots (Reich, 2014). Due to functional constraints to avoid resource shortage or excess, interspecific variation of resource-use related leaf, stem, and root traits should thus be coordinated along one single axis of variation (Freschet et al., 2010; Reich, 2014).
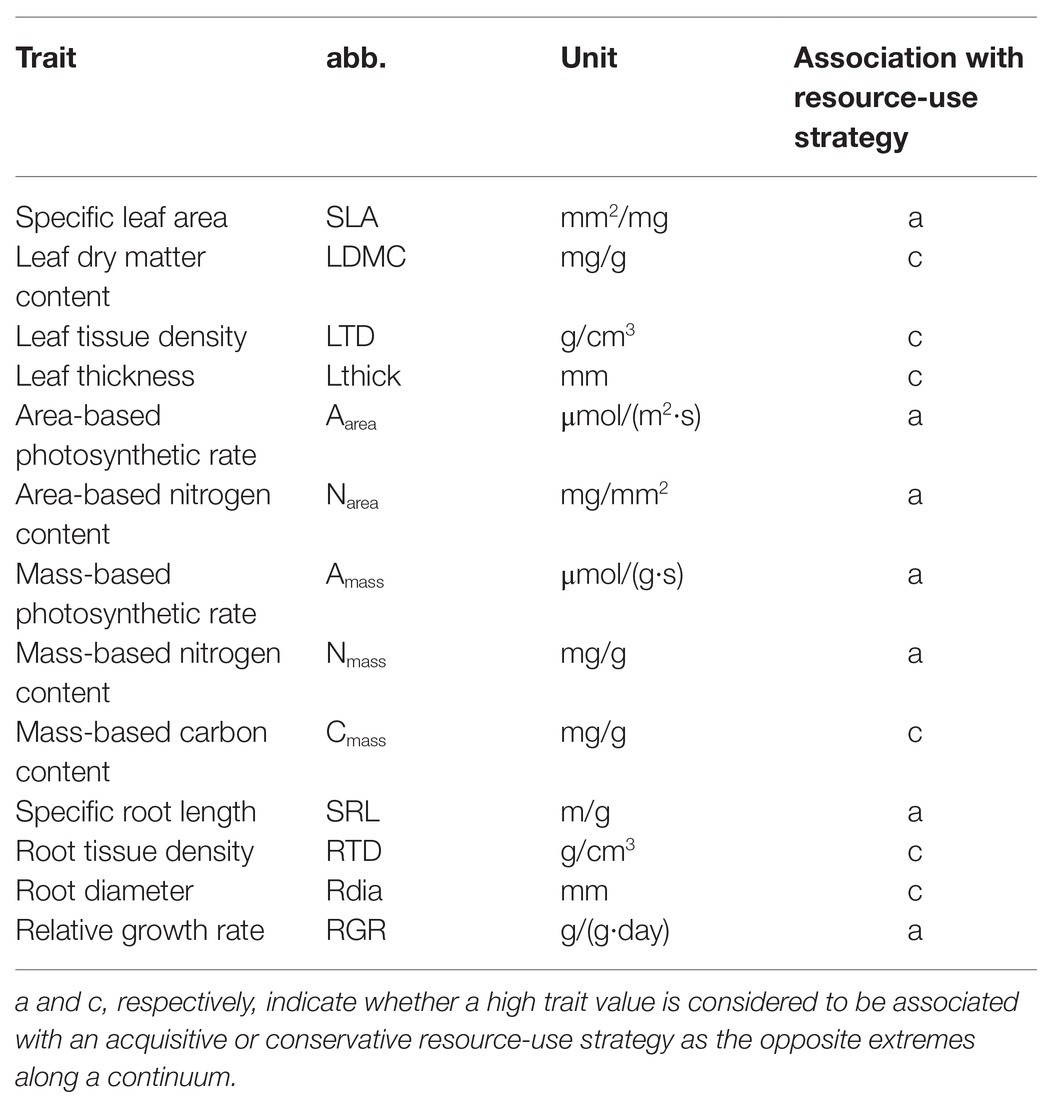
Table 1. Studied resource-use related traits, and relative growth rate with their abbreviation (abb.), unit, and hypothesized association with the resource-use strategy according to the plant economics spectrum.
According to the plant economics spectrum, the trade-off among resource-use related traits should influence species’ growth rates and their ecological filtering along resource gradients (Reich, 2014). Species with acquisitive trait attributes should exhibit high growth rates under high resource availability, but exhibit low performance under resource-poor conditions because of their higher resource demand (Reich, 2014). In contrast, species with conservative trait attributes minimize performance losses under resource-poor conditions (i.e., they exhibit high stress resistance) but at the cost of lower growth rates (Reich, 2014). The resulting interspecific growth-stress resistance trade-off should lead to ecological filtering of species along resource gradients, with acquisitive/fast species predominating under high resource availability, and conservative/slow species under low resource availability (Grime and Hunt, 1975; Reich, 2014).
The interspecific trait-trait, trait-growth, and trait-environment relations expected by the plant economics spectrum have been demonstrated in woody and perennial herbaceous species from various ecosystems (reviewed in Reich, 2014). Only a few studies showed trait-trait or trait-environment relations deviating from the hypotheses of this concept (Baraloto et al., 2010; Fortunel et al., 2012; Kramer-Walter et al., 2016). However, these few studies are significant, because they indicate that the plant economics spectrum may not be universally applicable across different ecosystems, life forms, and/or life-history strategies.
In contrast to perennial species, annuals are characterized by early reproduction at small vegetative size, a short lifespan, high reproductive allocation, and especially annuals from drylands by a pronounced between-year seed dormancy, acting as bet-hedging mechanism against unpredictable reproductive failure (Grime, 1977; Philippi and Seger, 1989; Kooyers, 2015). Additionally, annuals have been assumed and shown to exhibit pronounced acquisitive trait attributes and high growth rates (Grime, 1977; Garnier and Laurent, 1994; Roumet et al., 2006; Kooyers, 2015). This trait combination enables them to evade unfavorable conditions in time, i.e., annuals exhibit an escape strategy (sensu Levitt, 1980; Kooyers, 2015). Although all annuals show this strategy, they differ in their trait attributes and occur in a wide range of environmental conditions (Bilton et al., 2016; Li and Shipley, 2017; Blumenthal et al., 2020). The consequences of annual’s life-history on interspecific trait-trait, and trait-growth relations, and on their ecological filtering along resource gradients, however, remain almost unexplored.
To our knowledge, interspecific studies testing the plant economics spectrum in annuals are missing. Intraspecifically, one study has addressed differences of resource-use related leaf traits along a rainfall and nutrient gradient in an annual desert forb (Brouillette et al., 2014). Populations showed the expected trait-trait correlations, but contrary to the assumptions of the plant economics spectrum, acquisitive trait attributes were associated with low resource availability (dry and nutrient-poor habitats) and conservative attributes with high resource availability (wetter, nutrient-rich habitats; Brouillette et al., 2014). However, to rigorously test the applicability of the plant economics spectrum in annuals, we need multi-species studies that directly link comparative trait assessments across species with their growth rates and their distribution along resource gradients.
In the present study, we addressed this gap and tested interspecific trait-trait, trait-growth, and trait-environment relations in winter annuals from rangelands in Israel. Israel is characterized by a high diversity of annual species (Tielbörger et al., 2014) and by steep rainfall gradients, ranging from arid conditions (short growing season, low, unpredictable rainfall) to mesic-Mediterranean conditions (longer growing season, high, predictable rainfall). The region thus provides an ideal study system to investigate the variation of traits and growth rates across annual species, and to relate them to their distribution along rainfall gradients to assess species’ ecological filtering.
Winter annuals grow and reproduce in the mild, rainy season (winter), and survive the dry, hot season (summer) as seeds, i.e., they escape the dry season. Accordingly in winter annuals, arid (i.e., resource poor) conditions should favor species with pronounced escape traits, which are considered to be associated with acquisitive trait attributes and high growth rates (see above, Grime, 1977; Kooyers, 2015). This trait combination should enable them to grow and reproduce within the short rainfall season. Toward the opposite, moist side of rainfall gradients, resource availability and competition intensity increase, but rainfall season is still interrupted by occasional dry spells (Noy-Meir, 1973; Schiffers and Tielbörger, 2006; Ziv et al., 2014). Under these conditions, annuals with sufficient drought resistance to withstand dry spells in the vegetative and reproductive phase should be favored, since they can utilize the whole length of the growing season to attain larger heights for an increased competitive effect. Higher stress (drought) resistance is associated with conservative trait attributes and slow growth according to the hypotheses of the plant economics spectrum (Reich, 2014). Ecological filtering in winter annuals should favor species with acquisitive (instead of conservative) traits in arid conditions and species with conservative (instead of acquisitive) traits in more mesic Mediterranean conditions. The expected interspecific trait changes along rainfall gradients in winter annuals are thus opposite to the predictions of the plant economics spectrum and the patterns in perennials (Reich, 2014), but consistent with the findings on intraspecific trait variation in an annual forb (Brouillette et al., 2014).
In the present study, we measured twelve traits that are considered relevant for resource-use by the plant economics spectrum (Table 1) in 30 winter annual species from Israel under common, controlled conditions. We analyzed trait-trait relations, as well as the relations of traits to species’ growth rates (for 19 species) and to their distribution across a steep regional rainfall gradient. Specifically, we addressed the following hypotheses:
Resource-use related leaf and root traits are correlated along one main axis of variation, reflecting a trade-off between acquisitive and conservative trait attributes.
Species’ growth rates are influenced by their trait combinations, i.e., their positions along the main trait axis. Species with acquisitive traits exhibit high growth rates, while species with conservative traits exhibit low growth rates.
Species’ distributions along a rainfall gradient are related with their trait combinations and growth rates. Fast-growing annuals with acquisitive traits are associated with arid conditions, whereas slow-growing species with conservative traits are associated with wetter conditions.
Materials and Methods
Study System
Israel in the Eastern Mediterranean Basin comprises steep regional rainfall gradients from both East-West (across 50 km) and North-South (across 350 km) with high and more predictable mean annual rainfall (MAR) in mesic-Mediterranean areas in the north and west (up to 800 mm/year ± 18%, mean ± CV) and less, very unpredictable rainfall in the desert in the south (20 mm/year ± 55%) and toward the Dead Sea (east). The length of the rainfall season, which corresponds to the main growing season, as well as primary productivity and competition intensity decrease toward arid conditions, while average temperature hardly changes (Schiffers and Tielbörger, 2006; Tielbörger et al., 2014). The region is characterized by semi-arid shrublands with mostly winter annual species dominating the inter-shrub matrix. They account for up to 90% of species diversity, and between 55% and 99% of net primary production (Tielbörger et al., 2014).
Study Species and Plant Material
The study focused on 30 winter annual species comprising 22 forbs (including six legumes) and eight grasses (Supplementary Table S1). Species selection considered the following criteria: (1) high abundance in the region, (2) inclusion of several plant families, (3) wide differences in their distribution along the rainfall gradients (based on BioGIS, 2018), and (4) seed availability. The 30 species belonged to 27 genera and seven families, and all had C3 photosynthesis. Seeds were collected in the mid-range of the regional rainfall gradient from natural habitats in two sites that are about 40 km apart from each other (Lahav, N 31°23' E 34°54', 300 mm MAR and Matta, N 31°42' E 35°3', 540 mm MAR; for details see Tielbörger et al., 2014) in April 2012. The sampling comprised at least 50 plants per species distributed in an area of 1.0–1.5 km2.
The field-collected seeds were germinated and grown under common conditions with natural light and ample water supply in a greenhouse in Tübingen (Germany) during winter 2013/2014 to produce F1 seeds (inbred lines) with homogenized parental effects. F1 seeds were over-summered for two months (mid-June to mid-August) in a greenhouse in Bayreuth (Germany) to break summer dormancy before the start of the experiments (see Tielbörger et al., 2012). The plants for trait measurements were grown from F1 seeds under common conditions in a greenhouse in Bayreuth (Germany) during winter 2017/2018 (except for growth rate, grown in 2018/2019 under similar conditions). The comparative approach focused on trait differences across species, the level considered in the plant economics spectrum, and allowed to exclude intraspecific trait variation introduced by phenotypic plasticity or ecotypic differentiation.
Plant Cultivation in the Greenhouse
Plants were germinated and grown in cylindrical pots (1 L volume, diameter 6.5 cm, depth 36 cm, Deepot Cells, Stuewe & Sons, Oregon, United States) with a 1:1 mixture of sand and compost supplemented with 5 g of amorphous silicon (Aerosil 300, Evonik Industries AG, Essen, Germany). Temperature was set to 20–23°C in winter and 20–26°C in spring. Natural light was supplemented by artificial lights, and day length was adapted to natural variation in Israel for unbiased phenology. All plants received water in ample supply and were fertilized several times with Wuxal Super (NPK fertilizer 8–8-6, Wilhelm Haug GmbH & Co) to preclude nutrient limitation. The pots with the different species were randomly distributed in the greenhouse and rearranged every two weeks. Plants were grown until the end of their life cycle (31–34 weeks after sowing) indicated by leaf senescence in most of the species.
Trait Measurements
Twelve resource-use related traits, including leaf and root traits as well as structural and carbon gain related traits, were measured on 5–14 individuals per species (Supplementary Table S2). Leaf traits were assessed on one randomly chosen, healthy, mature leaf per plant 8–12 weeks after sowing. Root traits were measured 14–20 weeks after sowing.
To determine specific leaf area (SLA = LA/DW) and leaf dry matter content (LDMC = DW/FW, LDMC is the inverse of leaf water content, LWC in mg/g, LWC = 1,000-LDMC), we measured saturated fresh weight (FW) of the leaf after hydrating plants overnight (approx. 15 h), and dry weight (DW) after oven-drying. Leaf area (LA) was quantified with an Area-meter (Model LI 3100, Li-Cor Bioscience, Lincoln, NE, United States). Leaf thickness (Lthick) was measured with a micrometer (Mitutoyo M110-25, graduation 0.01 mm) at three points in the center of the leaf blade, avoiding the midrib and primary veins, and averaged. Leaf tissue density (LTD) was calculated as ratio of dry weight to leaf volume (leaf volume = LA·Lthick).
Maximum photosynthetic rate per leaf area (Aarea) was measured with an infra-red gas analyzer Li-Cor 6400 (Li-Cor, Lincoln, NE, United States) between 8.30 and 11.00 h at a light intensity of 2000 μmol·photons/(m2·s1) (based on light response curves for a species subset), 25°C, and 400 ppm CO2. If the leaf did not fill the measurement chamber, photosynthetic rate was re-calculated based on leaf area measurements with an Area Meter (see above). Usually, one leaf per individual was measured, but in species with very small or thin leaves (e.g., a few grasses, Filago, and Helianthemum) several leaves were jointly arranged in the measurement chamber. The leaves used for photosynthesis measurements (few exceptions in Psilurus incurvus, Rostraria cristata) were oven-dried and ground to analyze their mass-based nitrogen content (Nmass) and carbon content (Cmass) with an EA-IRMS coupling (Elemental Analyzer NA 1108, CE Instruments, Milan, Italy; Interface ConFlo III, Finnigan MAT, Bremen, Germany; Isotope ratio mass spectrometer: delta S, Finnigan MAT, Bremen, Germany). Photosynthetic rate and nitrogen content were converted to their area- or mass-based equivalent (Amass, Narea) via SLA based on species’ average values.
Morphological root traits were determined on three subsamples of fine roots (diameter < 2 mm, stored in 35% ethanol before the measurements) from the upper, middle, and lower part of the root (except whole roots in Filago and Helianthemum salicifolium). The root samples were stained with toluidine blue (0.2 g/l) and scanned (Scanner Epson Perfection V800/V850 photo scanner, 600 dpi). Images were analyzed with WinRHIZO © Reg 2017 (Regent Instruments Inc., Quebec, Canada) to determine mean diameter (Rdia), volume, and length of the scanned root sample. Samples were oven-dried to measure dry weight, and to calculate specific root length (SRL = root length/root dry weight) and root tissue density (RTD = root mass/root volume). Calculations of SRL and RTD considered diameter heterogeneity by using summed root length and volume from 40 diameter classes (see Rose, 2017), respectively.
Species’ average relative growth rate (RGR) was assessed in 19 species (see Supplementary Table S1) in a separate plant set that was grown under similar conditions (see Plant cultivation) in winter 2018/19. RGR was calculated based on species-specific averages of aboveground biomass (AB) in week 16 (t1) and week 22–23 (t2) after sowing as: RGR = (AB2–AB1)/[AB1 · (t2-t1)].
Species’ Distribution Along Rainfall Gradients
Species’ distribution along the regional rainfall gradients in Israel was characterized based on their occurrences (presence/absence data) in independent biological records (BioGIS, 2018). The BioGIS database provides the mean annual rainfall niche of each species, which is modeled as the average of local mean annual rainfall across all occurrence sites of a respective species. Our study species covered mean annual rainfall niches between 120 mm/year (association with arid conditions) and 580 mm/year (association with Mediterranean conditions, Supplementary Table S1).
Statistical Analyses
Trait differences across species were tested with F-tests on linear models (LM) with species’ identity as explanatory factor. Traits (except Rdia and Cmass) were natural log-transformed to improve normality and homoscedasticity. Pairwise trait correlations were calculated with Spearman rank correlation coefficients based on species’ average values.
The main axes of correlations among the resource-use related traits were assessed with a principal component analysis (PCA) at the plant level (i.e., combining leaf and root traits) based on species’ average trait values. Since mass- but not area-based traits are explicitly included in the leaf economics spectrum (Wright et al., 2004), we equivalently considered only Amass, Nmass, and Cmass in the PCA. The main trait correlations though were similar between a PCA with these mass-based traits and a PCA considering instead Aarea, Narea, and Carea (Pearson correlation coefficients among species’ scores along the trait axes |0.48| ≤ r ≤ |0.87|). We also calculated PCAs separately for leaf and for root traits. The trait coordinations in leaf and root traits were similar to those observed at the whole plant level (Supplementary Table S3). Species’ scores along the principal components (PC, in the following referred to as trait axes) calculated for the whole plant level were therefore used to characterize species’ trait combinations.
We tested for differences among life forms (i.e., grasses, non-legume forbs, legumes) in species’ scores along the main trait axes (PC 1, PC 2) with F-tests on LMs separately calculated for each trait axis and Tukey post-hoc tests. Additionally, the main axes of trait correlations were separately assessed for forbs (including legumes) and grasses with PCAs as described above for all species. Trait correlations within life forms were similar to the ones among all species (Pearson correlation coefficients among species’ scores along the trait axes |0.60| ≤ r ≤ |0.98|). The relations between species’ scores along the trait axes or single traits, species’ growth rate and distribution were therefore calculated for the whole species set.
The relations between species’ scores along the main trait axes (PC 1, PC 2, i.e., species’ trait combinations) and their relative growth rates were tested with F-tests on LMs separately calculated for each trait axis. The relations between the species’ scores along the trait axes and relative growth rates did not qualitatively differ depending on whether the species’ scores were derived from a PCA with the full species set (30 species, Figure 1) or from a PCA with the species set with growth rate measurements (19 species, Supplementary Figure S1). For consistency among all analyses, we therefore presented the findings with the PCA based on the full species set. Relations between single traits and relative growth rates were also tested with F-tests on LMs separately calculated for each trait. Relative growth rates were natural log-transformed in the LM with Cmass to improve normality and homoscedasticity.
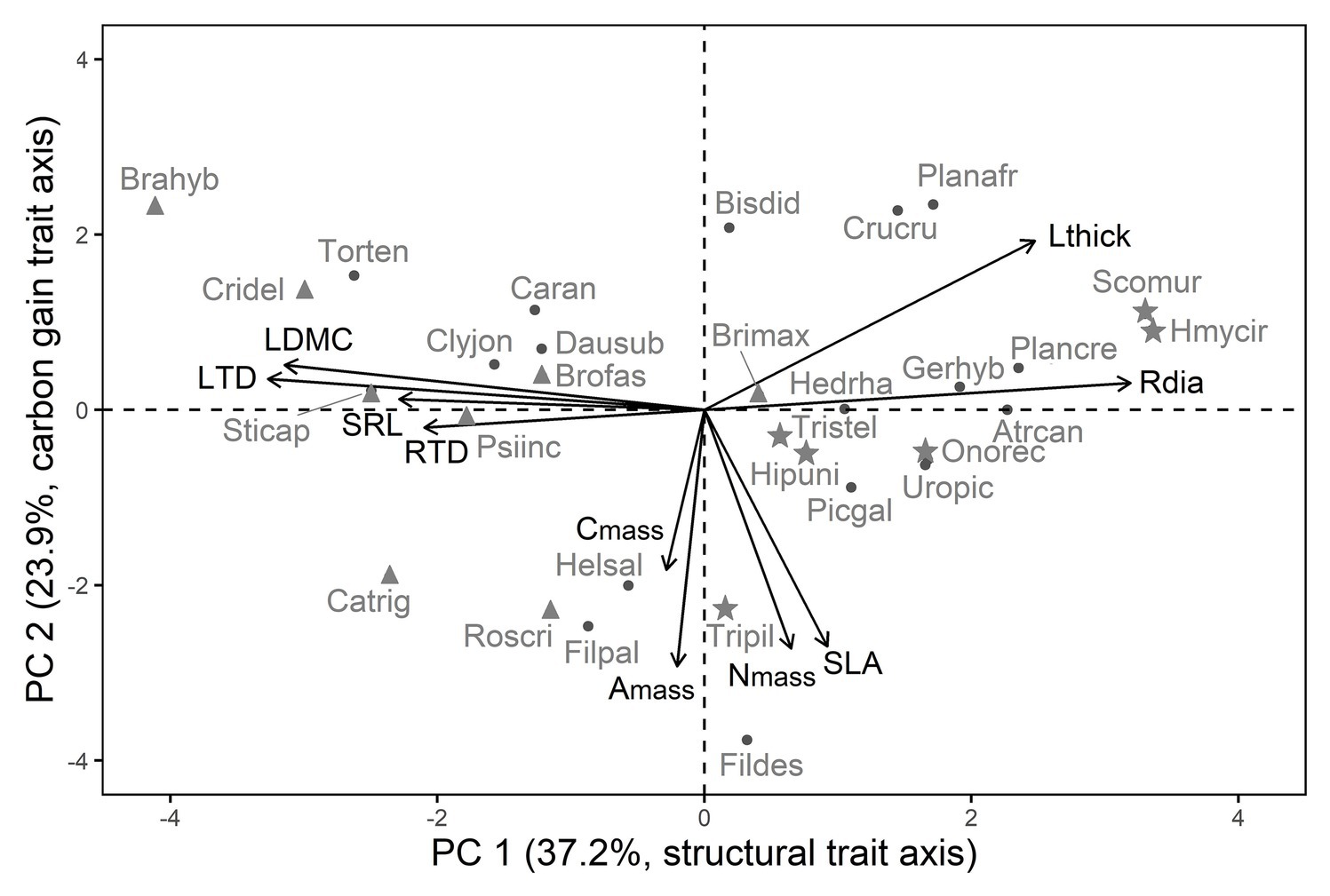
Figure 1. Resource-use related traits are correlated along two axes in 30 winter annual species, the first axis corresponds to structural traits (PC 1), and the second to carbon gain traits (PC 2). Trait abbreviations and trait loadings are given in Tables 1, 2, and species abbreviations are given in Supplementary Table S1, respectively. Symbols indicate life form: triangles for grasses, points for non-legume forbs, asterisks for legumes. Grasses differed from non-legume forbs and legumes in their species’ scores along PC 1, but not along PC 2.
The relations of species’ scores along the main trait axes (PC 1, PC 2, i.e., species’ trait combination) or single traits with species’ distribution along the rainfall gradient (i.e., mean annual rainfall niche) were tested with F-tests on LMs separately calculated for each axis or trait. Relations were also calculated with minimum and maximum rainfall niche based on BioGIS (2018), but qualitative results hardly changed (results not shown).
Adjusted significance level according to Holm-Bonferroni sequential correction (Holm, 1979; Gaetano, 2013) was applied to the multiple tests for species’ differences in the traits, and for trait (axes) relations to species’ growth rates and distribution. Since we tested only pre-planned hypotheses (see Table 1), we interpreted the results based on unadjusted significance level (see Armstrong, 2014).
All analyses were conducted with R 3.6.1 (R Core Team, 2019).
Results
The twelve resource-use related traits differed significantly across the 30 winter annuals, with almost 2–5 fold variation, and in relative growth rate with about 26 fold variation, respectively (F-values between 2.4 and 93.2, all p < 0.001, Supplementary Table S2). The attribute range of several leaf traits in the studied annuals almost corresponded to 60–80% of the globally documented trait ranges in plant species from different ecosystems and life forms worldwide (Kattge et al., 2020, see details in Supplementary Table S2). In all traits, the values of the investigated annuals fall within the acquisitive end of the global trait range (e.g., high SLA, Nmass, Supplementary Table S2).
Traits were correlated along two PCs, instead of one as we had expected (Figure 1; Table 2). PC 1 corresponded in positive direction with Rdia and Lthick, and in negative direction with LDMC, SRL, RTD, and LTD (Figure 1; Table 2). PC 1 thus summarized structural leaf and root traits (in the following referred to as structural trait axis). PC 2 was highly negatively correlated with Amass, Nmass, Cmass, and SLA, four traits associated with carbon gain (in the following referred to as carbon gain trait axis, Figure 1; Table 2). The independence between structural and carbon gain traits was also reflected in the pairwise trait correlations (Supplementary Table S4).
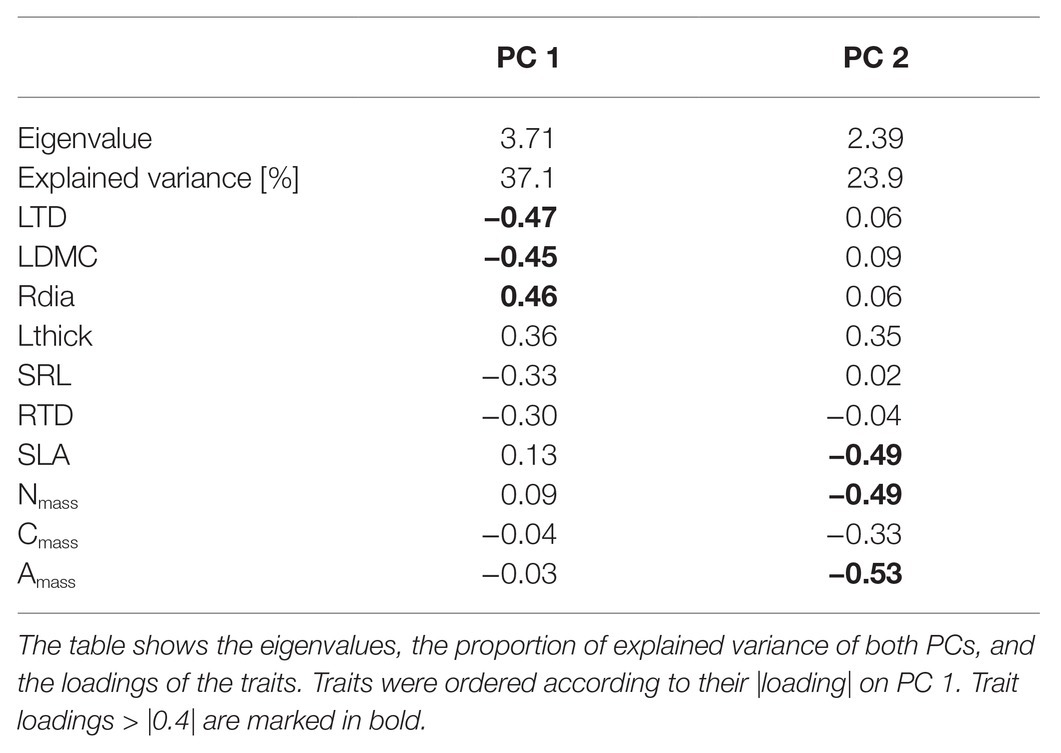
Table 2. Trait loadings on the first two principal components (PC) of a principal component analysis (PCA) with ten resource-use related traits in 30 winter annual species (see Figure 1).
Grasses differed from non-legume forbs and legumes in their scores along the structural trait axis (PC 1, LM statistics F2,27 = 11.23, p < 0.001, R2 = 0.41) with higher LTD and LDMC in grasses, but not along the carbon gain axis (PC 2, F2,27 = 0.12, p = 0.89, Figure 1). Non-legume forbs and legumes exhibited similar scores along both trait axes (Figure 1). The trait correlations along PC 1 and PC 2 within each life form (i.e., forbs vs. grasses) were similar to the ones among all species (Supplementary Figure S2).
Species’ scores along the carbon gain trait axis (PC 2) were related to relative growth rate, i.e., species with higher Amass, Nmass, Cmass, and SLA grew faster, but scores on the structural trait axis were unrelated to relative growth rate (Figure 2; Supplementary Table S5). Among single traits, only Amass and Aarea were positively related with relative growth rate (Supplementary Table S5).
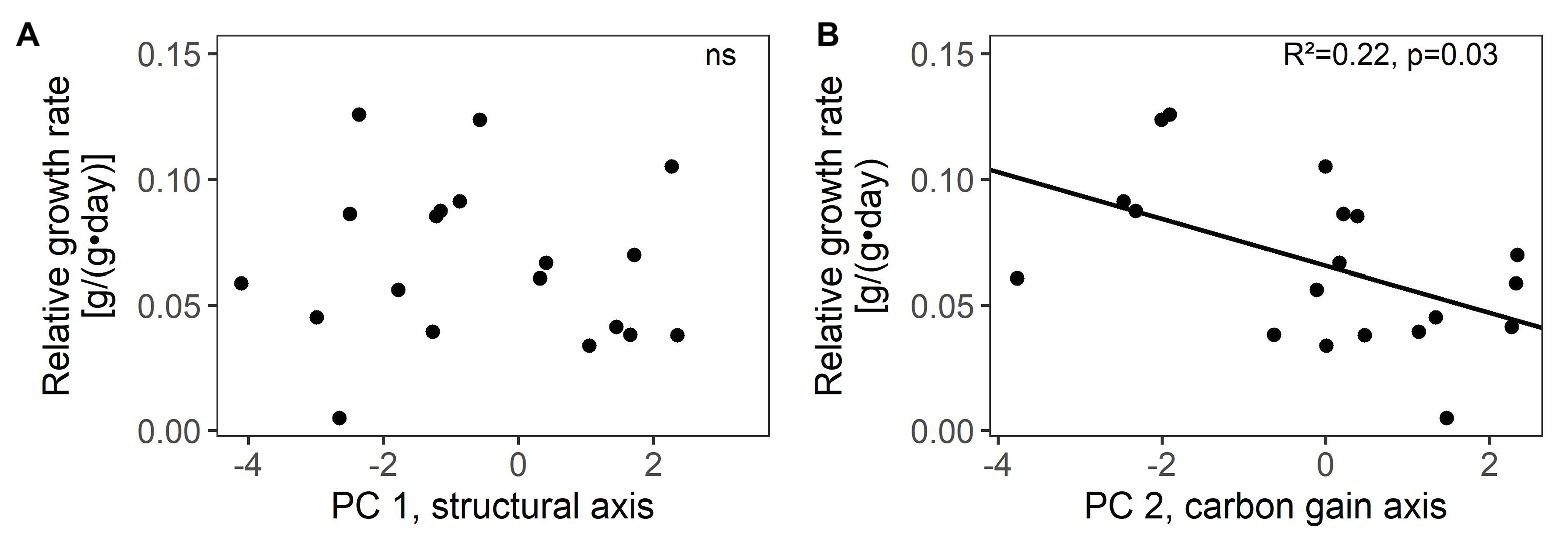
Figure 2. Relations between relative growth rates and species’ scores along the two main trait axes of resource-use related traits: (A) structural trait axis (PC 1) and (B) carbon gain trait axis (PC 2) in 19 winter annual species. R2 values and significance are given (ns not significant, for details see Supplementary Table S5). Species’ scores were based on the PCA in Figure 1 with 30 species.
Species’ scores along the carbon gain and structural trait axes (PC 1, PC 2) as well as relative growth rates were independent from their distribution along the rainfall gradient (mean annual rainfall niche; Figures 3A–C; Supplementary Table S6). However, among single traits, root traits were related with species’ distribution. Species with higher RTD and smaller Rdia were associated with arid conditions (Figures 3D,E; Supplementary Table S6).
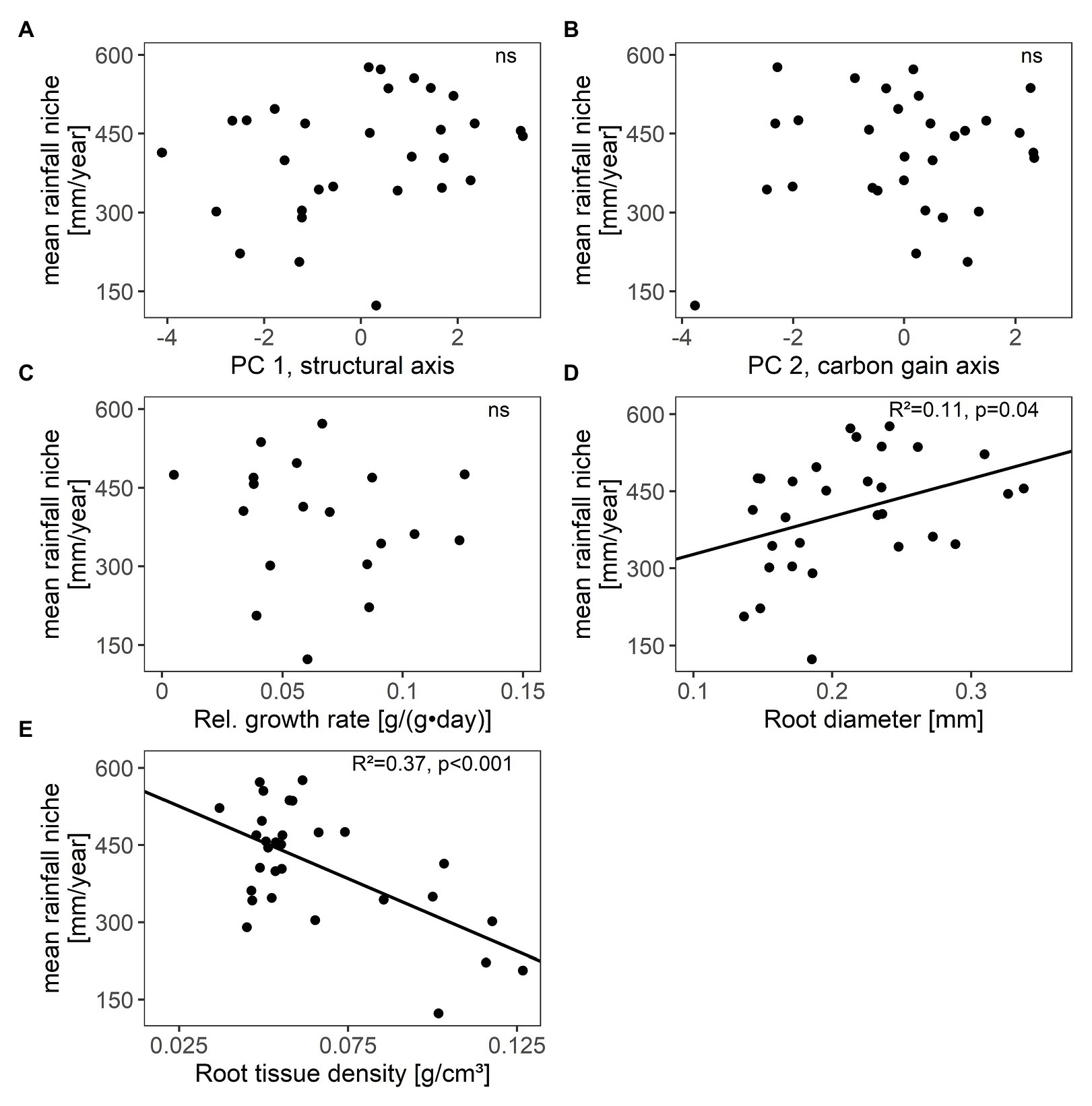
Figure 3. Relations between species’ mean annual rainfall niche (i.e., species’ distribution along the rainfall gradient) and (A,B) their scores along the two main axes of resource-use related traits (PC 1, PC 2), (C) relative growth rate, (D) root diameter, and (E) root tissue density in 30 winter annual species (relative growth rate was only assessed in 19 species). R2 values and significance were given (ns not significant, for details see Supplementary Table S6). Further single traits were unrelated to species’ mean annual rainfall niche (see Supplementary Table S6).
Discussion
The main premise of the plant economics spectrum is that resource-use related traits are correlated along a single axis, comprising both structural and carbon gain traits, as well as traits of different plant organs (Reich, 2014). In contrast, in the investigated winter annuals structural and carbon gain traits were decoupled, and correlated along two independent axes. The unexpected decoupling contradicts the predictions of the plant economics spectrum and had pervasive consequences on trait relations to growth rates and species’ distributions along the rainfall gradient that were also inconsistent with the hypotheses of the plant economics spectrum.
Trait-Trait Relations: Structural and Carbon Gain Traits Are Independent
The decoupling between structural and carbon gain traits we found in the investigated winter annuals has, to our knowledge, not been observed in perennial herbaceous or woody species. Instead, perennial herbaceous or woody species predominantly show the expected correlation of resource-use related traits along a single axis (e.g., Freschet et al., 2010; Liu et al., 2010; de la Riva et al., 2016a). In perennials, variation of leaf structure, especially of leaf tissue density and thickness, is mainly due to differences in carbon content (Roderick et al., 1999; de la Riva et al., 2016b). Higher carbon contents are associated with a higher proportion of sclerenchyma, thicker mesophyll layers and/or thicker mesophyll cell walls (de la Riva et al., 2016b; Onoda et al., 2017). This leaf structure leads to lower mass-based photosynthetic rate due to higher diffusion resistance to carbon dioxide, shading of chloroplasts, or lower proportion of mass-based nitrogen content (Niinemets, 1999; Shipley et al., 2005; Onoda et al., 2017). In the studied winter annuals, however, structural trait variation (i.e., the structural trait axis) was independent from carbon content. The unexpected decoupling between structural and carbon gain traits implies that structural trait variation is due to components that do not constrain photosynthetic rate.
Our study species exhibited high water contents, leaf hairiness, and silicon accumulation. These traits are assumed to increase species’ ability to cope with the environmental conditions in drylands by maximizing water storage, reflecting sunlight, decreasing transpiration, and deterring grazing herbivores (Ehleringer and Mooney, 1978; Woodman and Fernandes, 1991; Sack et al., 2003; Katz, 2019). They have additionally been proposed to dilute the relations between mass-based nitrogen content and structural leaf traits expected by the leaf economics spectrum (Grubb, 2016). Consistently, they influenced leaf tissue density and thickness in our study species. Leaf tissue density was strongly influenced by silicon instead of carbon content (Supplementary Table S4; Supplementary Method 1); and in forbs, high tissue density was additionally associated with long and/or dense leaf hairs (pers. obs.). Similarly, high leaf thickness mainly resulted from high water content and was negatively associated with carbon content (Supplementary Table S4). Despite their influence on leaf structure, silicon content, leaf water content, and leaf hairiness did not affect mass-based photosynthetic rate, since they did not constrain the proportion of mass-based nitrogen content (Supplementary Table S4), or the diffusion resistance to carbon dioxide. Decoupling between structural and carbon gain traits, which is in contrast to the hypothesis of the plant economics spectrum, thus might not be restricted to winter annuals, but should also emerge in other species groups, in which traits other than carbon content lead to leaf structure variation, e.g., in perennial grasses with high silicon accumulation or succulent perennials (compare to Grubb, 2016).
Despite the decoupling of structural and carbon gain traits, the structural traits of roots and leaves were correlated in the studied winter annuals (SRL, RTD, Rdia, and LTD, LDMC, Lthick, respectively), consistent with findings in perennials (Freschet et al., 2010; Reich, 2014; de la Riva et al., 2016a). The structural analogy between leaves and roots thus is not a consequence of the correlation of resource-use related traits along one axis, but might result from tissues pervading the entire plant, such as xylem and phloem vessels (Wahl and Ryser, 2000; Hummel et al., 2007).
Trait-Growth Relations: Growth Rate Is Independent From Structural Traits
The plant economics spectrum proposes that species’ positions along the single axis of resource-use related traits determine their growth rates (Reich, 2014). Consistent with this hypothesis, winter annuals with higher carbon gain trait attributes exhibited higher relative growth rates. However, structural traits were unrelated to relative growth rate, reflecting their uncoupling from carbon gain. Winter annuals with a wide variation of structural traits thus exhibited similar relative growth rates in contrast to the findings in perennials and the hypothesis of the plant economics spectrum (Lambers and Poorter, 1992; Reich, 2014).
The structural traits should, however, influence species’ stress resistance. In our study species, the structural trait axis was associated with silicon content and leaf hairiness (see above), traits assumed to deter grazing herbivores (Woodman and Fernandes, 1991; Katz, 2019), as well as with turgor loss point, i.e., the water potential at which leaves lose turgor (Supplementary Figure S3; Supplementary Method 1). Turgor loss point is considered as a major physiological determinant of species’ drought response (Bartlett et al., 2012; Sun et al., 2020). A relation between structural traits and species’ drought and grazing resistance has also been indicated across life forms in semi-arid ecosystems (Blumenthal et al., 2020).
Independence between growth rate and structural traits thus implies that the assumptions of the growth-stress resistance trade-off (see Grime and Hunt, 1975; Reich, 2014) do not apply to the studied winter annuals. Growth rates indeed turned out to be independent of species’ stress resistance (to grazing and drought) in our study species (Kurze et al., in review). Similar findings emerged in a few studies of perennial herbaceous species (Fernández and Reynolds, 2000; Jung et al., 2020).
Trait-Environment Relations: Species’ Filtering Along the Rainfall Gradient Is Only Reflected in Root Traits
We expected that arid conditions favor winter annuals with acquisitive trait attributes and high growth rates due to their ability to reproduce within a short period and thus to escape drought (see Levitt, 1980; Kooyers, 2015). However, in the investigated annuals, species’ distribution along the rainfall gradient was unrelated to their scores along the structural and carbon gain trait axis and to growth rate. It is improbable that this unanticipated result is due to our focus on trait variation across species, which did not consider ecotypic variation. Ecotypic trait variation is usually considerably smaller than interspecific variation (Garnier et al., 2001; Kazakou et al., 2014; Siefert et al., 2015). This has been also shown in some of our study species (Bergholz et al., 2017; Kurze et al., 2017; Álvarez-Cansino et al., unpublished data). Additionally, at the intraspecific level, resource-use related leaf traits did not show directional changes along the rainfall gradient in our study system (Bergholz et al., 2017; Kurze et al., 2017).
Rather, this unexpected finding likely emerged from the independence of growth rate and stress resistance, which facilitates similar ecological success of species with alternative trait combinations and supports the co-occurrence of annuals with a wide range of structural and carbon gain traits and growth rates along the rainfall gradient. Theoretical models (Marks and Lechowicz, 2006) and studies on interspecific variation of leaf traits in woody and perennial herbaceous species support that different trait combinations can be successful in the same environment (Wright et al., 2004; Cernusak et al., 2011; Forrestel et al., 2017; Muir et al., 2017). In our study species, the structural trait axis was associated with traits related to both drought and grazing resistance (e.g., turgor loss point, leaf silicon content, leaf hairiness, see above), which should be differentially filtered along the rainfall gradient and may offset each other (Carmona et al., 2012; Rota et al., 2017). Similarly, fast and slow growth rates may confer species a high competitive effect and high competitive response under wetter conditions (Goldberg and Landa, 1991; Liancourt et al., 2009). Consequently, contrary to the expectations of the plant economics spectrum, neither species’ trait combinations along the structural or carbon gain trait axis nor growth rates led to ecological filtering along the rainfall gradient in winter annuals.
Nevertheless, fine root traits were associated with species’ distribution along the rainfall gradient in the studied annuals. Fine root traits should be more directly related to species’ drought resistance than the combination of structural traits (reflected in the structural trait axis), which comprised traits of both drought and grazing resistance. Root traits have indeed previously been shown to be more strongly related to the drought survival of annual species than leaf traits (Harrison and LaForgia, 2019). In our study species, root diameter decreased and root tissue density increased with increasing species’ association to arid conditions. Species with these trait attributes are assumed to exhibit higher drought resistance, since thin and dense roots decrease the resistance to radial water inflow and are less prone to cavitation due to smaller xylem vessels (Wahl and Ryser, 2000; Comas et al., 2013). Woody species with these root trait attributes have been shown to be favored under low water availability (Nicotra et al., 2002; de la Riva et al., 2018). The root trait changes along the rainfall gradient observed in our study species thus imply that the low water availability during the growing season imposes an ecological filter under arid conditions. Arid conditions thus favor winter annuals with high drought resistance in the vegetative phase conferred by root traits but not with acquisitive traits or high growth rate to escape drought, as we initially expected.
Conclusion
Our findings showed that the functional constraints hypothesized by the plant economics spectrum do not apply to winter annuals. Winter annuals can thus not be ranked along a single axis of resource-use related traits from acquisitive (or fast) to conservative (or slow), as proposed by the plant economics spectrum (Reich, 2014). The limited applicability of the plant economics spectrum to winter annuals cautions to generalize functional constraints, trait-growth, or trait-environment relations among life-history groups. Explicitly testing and establishing these relations for species groups that may be subjected to different constraints is a precondition for using trait-based approaches to understand and predict species’ performance, community composition, or ecosystem functioning.
Data Availability Statement
The raw data supporting the conclusions of this article will be made available by the authors, without undue reservation.
Author Contributions
SK conceived the ideas for this manuscript, and LÁ-C, BE, SK, MB, and KT designed the study. SK coordinated and conducted the trait measurements, analyzed the data, and wrote the manuscript with contributions by LÁ-C and BE. All co-authors commented on subsequent drafts of the manuscripts and gave final approval for publication.
Funding
This work was supported by the German Research Foundation (grant numbers AL 1952/1-1, TI 338/12-1). Open Access Publication was funded by the German Research Foundation and the University of Bayreuth in the funding program Open Access Publishing.
Conflict of Interest
The authors declare that the research was conducted in the absence of any commercial or financial relationships that could be construed as a potential conflict of interest.
Acknowledgments
We thank Fabian Nützel for assistance in raising the plants, Burkhard Stumpf for support in setting up the experiment and in several trait measurements, Christine Peterek for analyses of the morphological root traits, Jörg Schaller for advice on the measurement of silicon content, many students whose help was invaluable in the greenhouse experiments, and Jens Kattge for providing data from the TRY database. The BayCEER Laboratory of Isotope Biogeochemistry at the University of Bayreuth carried out the analyses of the leaf carbon and nitrogen contents, and the BayCEER Keylab for Experimental Biogeochemistry carried out the analyses of leaf silicon contents.
Supplementary Material
The Supplementary Material for this article can be found online at: https://www.frontiersin.org/articles/10.3389/fpls.2021.640862/full#supplementary-material
References
Armstrong, R. A. (2014). When to use the Bonferroni correction. Ophthalmic Physiol. Opt. 34, 502–508. doi: 10.1111/opo.12131
Baraloto, C., Paine, C. E. T., Poorter, L., Beauchene, J., Bonal, D., Domenach, A.-M., et al. (2010). Decoupled leaf and stem economics in rain forest trees. Ecol. Lett. 13, 1338–1347. doi: 10.1111/j.1461-0248.2010.01517.x
Bartlett, M. K., Scoffoni, C., and Sack, L. (2012). The determinants of leaf turgor loss point and prediction of drought tolerance of species and biomes: a global meta-analysis. Ecol. Lett. 15, 393–405. doi: 10.1111/j.1461-0248.2012.01751.x
Bergholz, K., May, F., Ristow, M., Giladi, I., Ziv, Y., and Jeltsch, F. (2017). Two Mediterranean annuals feature high within-population trait variability and respond differently to a precipitation gradient. Basic Appl. Ecol. 25, 48–58. doi: 10.1016/j.baae.2017.11.001
Bilton, M. C., Metz, J., and Tielbörger, K. (2016). Climatic niche groups: a novel application of a common assumption predicting plant community response to climate change. Perspect. Plant Ecol. Evol. Syst. 19, 61–69. doi: 10.1016/j.ppees.2016.02.006
BioGIS (2018). Israel biodiversity information system. Available at: http://www.biogis.huji.ac.il (Accessed October 30, 2018).
Blumenthal, D. M., Mueller, K. E., Kray, J. A., Ocheltree, T. W., Augustine, D. J., and Wilcox, K. R. (2020). Traits link drought resistance with herbivore defence and plant economics in semi-arid grasslands: the central roles of phenology and leaf dry matter content. J. Ecol. 108, 2336–2351. doi: 10.1111/1365-2745.13454
Brouillette, L. C., Mason, C. M., Shirk, R. Y., and Donovan, L. A. (2014). Adaptive differentiation of traits related to resource use in a desert annual along a resource gradient. New Phytol. 201, 1316–1327. doi: 10.1111/nph.12628
Carmona, C. P., Azcárate, F. M., de Bello, F., Ollero, H. S., Lepš, J., and Peco, B. (2012). Taxonomical and functional diversity turnover in Mediterranean grasslands: interactions between grazing, habitat type and rainfall. J. Appl. Ecol. 49, 1084–1093. doi: 10.1111/j.1365-2664.2012.02193.x
Cernusak, L. A., Hutley, L. B., Beringer, J., Holtum, J. A. M., and Turner, B. J. (2011). Photosynthetic physiology of eucalypts along a sub-continental rainfall gradient in northern Australia. Agric. For. Meteorol. 151, 1462–1470. doi: 10.1016/j.agrformet.2011.01.006
Comas, L. H., Becker, S. R., Cruz, V. V., Byrne, P. F., and Dierig, D. A. (2013). Root traits contributing to plant productivity under drought. Front. Plant Sci. 4:442. doi: 10.3389/fpls.2013.00442
de la Riva, E. G., Olmo, M., Poorter, H., Ubera, J. L., and Villar, R. (2016b). Leaf mass per area (LMA) and its relationship with leaf structure and anatomy in 34 Mediterranean woody species along a water availability gradient. PLoS One 11:e0148788. doi: 10.1371/journal.pone.0148788
de la Riva, E. G., Tosto, A., Pérez-Ramos, I. M., Navarro-Fernández, C. M., Olmo, M., Anten, N. P., et al. (2016a). A plant economics spectrum in Mediterranean forests along environmental gradients: is there coordination among leaf, stem and root traits? J. Veg. Sci. 27, 187–199. doi: 10.1111/jvs.12341
de la Riva, E. G., Violle, C., Pérez-Ramos, I. M., Marañón, T., Navarro-Fernández, C. M., Olmo, M., et al. (2018). A multidimensional functional trait approach reveals the imprint of environmental stress in Mediterranean woody communities. Ecosystems 21, 248–262. doi: 10.1007/s10021-017-0147-7
Ehleringer, J. R., and Mooney, H. A. (1978). Leaf hairs: effects on physiological activity and adaptive value to a desert shrub. Oecologia 37, 183–200. doi: 10.1007/BF00344990
Fernández, R. J., and Reynolds, J. F. (2000). Potential growth and drought tolerance of eight desert grasses: lack of a trade-off? Oecologia 123, 90–98. doi: 10.1007/s004420050993
Forrestel, E. J., Donoghue, M. J., Edwards, E. J., Jetz, W., du Toite, J. C. O., and Smith, M. D. (2017). Different clades and traits yield similar grassland functional responses. Proc. Natl. Acad. Sci. U. S. A. 114, 705–710. doi: 10.1073/pnas.1612909114
Fortunel, C., Fine, P. V. A., and Baraloto, C. (2012). Leaf, stem and root tissue strategies across 758 Neotropical tree species. Funct. Ecol. 26, 1153–1161. doi: 10.1111/j.1365-2435.2012.02020.x
Freschet, G. T., Cornelissen, J. H. C., van Logtestijn, R. S. P., and Aerts, R. (2010). Evidence of the ‘plant economics spectrum’ in a subarctic flora. J. Ecol. 98, 362–373. doi: 10.1111/j.1365-2745.2009.01615.x
Gaetano, J. (2013). Holm-Bonferroni sequential correction: An EXCEL calculator. Version 1.2. Available at: https://doi.org/10.13140/RG.2.1.4466.9927 (Accessed October 1, 2015).
Garnier, E., and Laurent, G. (1994). Leaf anatomy, specific mass and water content in congeneric annual and perennial grass species. New Phytol. 128, 725–736. doi: 10.1111/j.1469-8137.1994.tb04036.x
Garnier, E., Laurent, G., Bellmann, A., Debain, S., Berthelier, P., Ducout, B., et al. (2001). Consistency of species ranking based on functional leaf traits. New Phytol. 152, 69–83. doi: 10.1046/j.0028-646x.2001.00239.x
Goldberg, D. E., and Landa, K. (1991). Competitive effect and response: hierarchies and correlated traits in the early stages of competition. J. Ecol. 79, 1013–1030. doi: 10.2307/2261095
Grime, J. P. (1977). Evidence for the existence of three primary strategies in plants and its relevance to ecological and evolutionary theory. Am. Nat. 111, 1169–1194. doi: 10.1086/283244
Grime, J. P., and Hunt, R. (1975). Relative growth-rate: its range and adaptive significance in a local flora. J. Ecol. 63, 393–422. doi: 10.2307/2258728
Grubb, P. J. (2016). Trade-offs in interspecific comparisons in plant ecology and how plants overcome proposed constraints. Plant Ecol. Divers. 9, 3–33. doi: 10.1080/17550874.2015.1048761
Harrison, S., and LaForgia, M. (2019). Seedling traits predict drought-induced mortality linked to diversity loss. Proc. Natl. Acad. Sci. U. S. A. 116, 5576–5581. doi: 10.1073/pnas.1818543116
Hummel, I., Vile, D., Violle, C., Devaux, J., Ricci, B., Blanchard, A., et al. (2007). Relating root structure and anatomy to whole-plant functioning in 14 herbaceous Mediterranean species. New Phytol. 173, 313–321. doi: 10.1111/j.1469-8137.2006.01912.x
Jung, E., Gaviria, J., Sun, S., and Engelbrecht, B. M. J. (2020). Comparative drought resistance of temperate grassland species: evaluation of performance trade-offs and the relation to moisture association. Oecologia 192, 1023–1036. doi: 10.1007/s00442-020-04625-9
Kattge, J., Díaz, S., Tautenhahn, S., Werner, G. D. A., Aakala, T., Abedi, M., et al. (2020). TRY plant trait database–enhanced coverage and open access. Glob. Chang. Biol. 26, 119–188. doi: 10.1111/gcb.14904
Katz, O. (2019). Silicon content is a plant functional trait: implications in a changing world. Flora 254, 88–94. doi: 10.1016/j.flora.2018.08.007
Kazakou, E., Violle, C., Roumet, C., Navas, M.-L., Vile, D., Kattge, J., et al. (2014). Are trait-based species rankings consistent across data sets and spatial scales? J. Veg. Sci. 25, 235–247. doi: 10.1111/jvs.12066
Kooyers, N. J. (2015). The evolution of drought escape and avoidance in natural herbaceous populations. Plant Sci. 234, 155–162. doi: 10.1016/j.plantsci.2015.02.012
Kramer-Walter, K. R., Bellingham, P. J., Millar, T. R., Smissen, R. D., Richardson, S. J., and Laughlin, D. C. (2016). Root traits are multidimensional: specific root length is independent from root tissue density and the plant economic spectrum. J. Ecol. 104, 1299–1310. doi: 10.1111/1365-2745.12562
Kurze, S., Bareither, N., and Metz, J. (2017). Phenology, roots and reproductive allocation, but not the LHS scheme, shape ecotypes along an aridity gradient. Perspect. Plant Ecol. Evol. Syst. 29, 20–29. doi: 10.1016/j.ppees.2017.09.004
Lambers, H., and Poorter, H. (1992). Inherent variation in growth rate between higher plants: a search for physiological causes and ecological consequences. Adv. Ecol. Res. 34, 187–261. doi: 10.1016/S0065-2504(03)34004-8
Levitt, J. (1980). Responses of plants to environmental stresses: Water, radiation, salt, and other stresses. Vol. 2. New York: Academic Press.
Li, Y., and Shipley, B. (2017). An experimental test of CSR theory using a globally calibrated ordination method. PLoS One 12:e0175404. doi: 10.1371/journal.pone.0175404
Liancourt, P., Tielbörger, K., Bangerter, S., and Prasse, R. (2009). Components of ‘competitive ability’ in the LHS model: implication on coexistence for twelve co-occurring Mediterranean grasses. Basic Appl. Ecol. 10, 707–714. doi: 10.1016/j.baae.2009.05.003
Liu, G., Freschet, G. T., Pan, X., Cornelissen, J. H. C., Li, Y., and Dong, M. (2010). Coordinated variation in leaf and root traits across multiple spatial scales in Chinese semi-arid and arid ecosystems. New Phytol. 188, 543–553. doi: 10.1111/j.1469-8137.2010.03388.x
Marks, C. O., and Lechowicz, M. J. (2006). Alternative designs and the evolution of functional diversity. Am. Nat. 167, 55–66. doi: 10.1086/498276
Muir, C. D., Conesa, M. A., Roldán, E. J., Molins, A., and Galmés, J. (2017). Weak coordination between leaf structure and function among closely related tomato species. New Phytol. 213, 1642–1653. doi: 10.1111/nph.14285
Nicotra, A., Babicka, N., and Westoby, M. (2002). Seedling root anatomy and morphology: an examination of ecological differentiation with rainfall using phylogenetically independent contrasts. Oecologia 130, 136–145. doi: 10.1007/s004420100788
Niinemets, Ü. (1999). Components of leaf dry mass per area - thickness and density - alter leaf photosynthetic capacity in reverse directions in woody plants. New Phytol. 144, 35–47. doi: 10.1046/j.1469-8137.1999.00466.x
Noy-Meir, I. (1973). Desert ecosystems: environment and producers. Annu. Rev. Ecol. Syst. 4, 25–51. doi: 10.1146/annurev.es.04.110173.000325
Onoda, Y., Wright, I. J., Evans, J. R., Hikosaka, K., Kitajima, K., Niinemets, U., et al. (2017). Physiological and structural trade-offs underlying the leaf economics spectrum. New Phytol. 214, 1447–1463. doi: 10.1111/nph.14496
Philippi, T., and Seger, J. (1989). Hedging one’s evolutionary bets, revisited. Trends Ecol. Evol. 4, 41–44. doi: 10.1016/0169-5347(89)90138-9
R Core Team (2019). R: A language and environment for statistical computing. R foundation for statistical computing, Vienna, Austria. Available at: https://www.R-project.org/ (Accessed March 15, 2020).
Reich, P. B. (2014). The world-wide ‘fast–slow’ plant economics spectrum: a traits manifesto. J. Ecol. 102, 275–301. doi: 10.1111/1365-2745.12211
Roderick, M. L., Berry, S. L., Saunders, A. R., and Noble, I. R. (1999). On the relationship between the composition, morphology and function of leaves. Funct. Ecol. 13, 696–710. doi: 10.1046/j.1365-2435.1999.00369.x
Rose, L. (2017). Pitfalls in root trait calculations: how ignoring diameter heterogeneity can lead to overestimation of functional traits. Front. Plant Sci. 8:898. doi: 10.3389/fpls.2017.00898
Rota, C., Manzano, P., Carmona, C. P., Malo, J. E., and Peco, B. (2017). Plant community assembly in Mediterranean grasslands: understanding the interplay between grazing and spatio-temporal water availability. J. Veg. Sci. 28, 149–159. doi: 10.1111/jvs.12470
Roumet, C., Urcelay, C., and Díaz, S. (2006). Suites of root traits differ between annual and perennial species growing in the field. New Phytol. 170, 357–368. doi: 10.1111/j.1469-8137.2006.01667.x
Ruppert, J. C., Harmoney, K., Henkin, Z., Snyman, H. A., Sternberg, M., Willms, W., et al. (2015). Quantifying drylands’ drought resistance and recovery: the importance of drought intensity, dominant life history and grazing regime. Glob. Chang. Biol. 21, 1258–1270. doi: 10.1111/gcb.12777
Sack, L., Cowan, P. D., Jaikumar, N., and Holbrook, N. M. (2003). The ‘hydrology’ of leaves: co-ordination of structure and function in temperate woody species. Plant Cell Environ. 26, 1343–1356. doi: 10.1046/j.0016-8025.2003.01058.x
Sala, O. E., Chapin, F. S., Armesto, J. J., Berlow, E., Bloomfield, J., Dirzo, R., et al. (2000). Global biodiversity scenarios for the year 2100. Science 287, 1770–1774. doi: 10.1126/science.287.5459.1770
Schiffers, K., and Tielbörger, K. (2006). Ontogenetic shifts in interactions among annual plants. J. Ecol. 94, 336–341. doi: 10.1111/j.1365-2745.2006.01097.x
Schröter, D., Cramer, W., Leemans, R., Prentice, I. C., Araújo, M. B., Arnell, N. W., et al. (2005). Ecosystem service supply and vulnerability to global change in Europe. Science 310, 1333–1337. doi: 10.1126/science.1115233
Shipley, B., Vile, D., Garnier, E., Wright, I. J., and Poorter, H. (2005). Functional linkages between leaf traits and net photosynthetic rate: reconciling empirical and mechanistic models. Funct. Ecol. 19, 602–615. doi: 10.1111/j.1365-2435.2005.01008.x
Siefert, A., Violle, C., Chalmandrier, L., Albert, C. H., Taudiere, A., Fajardo, A., et al. (2015). A global meta-analysis of the relative extent of intraspecific trait variation in plant communities. Ecol. Lett. 18, 1406–1419. doi: 10.1111/ele.12508
Sun, S., Jung, E., Gaviria, J., and Engelbrecht, B. M. J. (2020). Drought survival is positively associated to high turgor loss points in temperate perennial grassland species. Funct. Ecol. 34, 788–798. doi: 10.1111/1365-2435.13522
Tielbörger, K., Bilton, M. C., Metz, J., Kigel, J., Holzapfel, C., Lebrija-Trejos, E., et al. (2014). Middle-eastern plant communities tolerate 9 years of drought in a multi-site climate manipulation experiment. Nat. Commun. 5:5102. doi: 10.1038/ncomms6102
Tielbörger, K., Petrů, M., and Lampei, C. (2012). Bet-hedging germination in annual plants: a sound empirical test of the theoretical foundations. Oikos 121, 1860–1868. doi: 10.1111/j.1600-0706.2011.20236.x
Wahl, S., and Ryser, P. (2000). Root tissue structure is linked to ecological strategies of grasses. New Phytol. 148, 459–471. doi: 10.1046/j.1469-8137.2000.00775.x
Westoby, M., Falster, D. S., Moles, A. T., Vesk, P. A., and Wright, I. J. (2002). Plant ecological strategies: some leading dimensions of variation between species. Annu. Rev. Ecol. Evol. Syst. 33, 125–159. doi: 10.1146/annurev.ecolsys.33.010802.150452
Woodman, R. L., and Fernandes, G. W. (1991). Differential mechanical defense: herbivory, evapotranspiration, and leaf-hairs. Oikos 60, 11–19. doi: 10.2307/3544986
Wright, I. J., Reich, P. B., Westoby, M., Ackerly, D. D., Baruch, Z., Bongers, F., et al. (2004). The worldwide leaf economics spectrum. Nature 428, 821–827. doi: 10.1038/nature02403
Keywords: annual species, drylands, growth rate, life-history strategy, leaf structure, rainfall gradient, resource-use strategy, root morphology
Citation: Kurze S, Engelbrecht BMJ, Bilton MC, Tielbörger K and Álvarez-Cansino L (2021) Rethinking the Plant Economics Spectrum for Annuals: A Multi-Species Study. Front. Plant Sci. 12:640862. doi: 10.3389/fpls.2021.640862
Edited by:
Iván Prieto, Spanish National Research Council, SpainReviewed by:
Keith John Bloomfield, Imperial College London, United KingdomLuciana Gonzalez Paleo, Museo Paleontologico Egidio Feruglio - CONICET, Argentina
Copyright © 2021 Kurze, Engelbrecht, Bilton, Tielbörger and Álvarez-Cansino. This is an open-access article distributed under the terms of the Creative Commons Attribution License (CC BY). The use, distribution or reproduction in other forums is permitted, provided the original author(s) and the copyright owner(s) are credited and that the original publication in this journal is cited, in accordance with accepted academic practice. No use, distribution or reproduction is permitted which does not comply with these terms.
*Correspondence: Susanne Kurze, c3VzYW5uZS5rdXJ6ZUB1bmktYmF5cmV1dGguZGU=