- 1Laboratory of Phytopathology, Wageningen University & Research, Wageningen, Netherlands
- 2Department of Bioscience, Wageningen University & Research, Wageningen, Netherlands
- 3Laboratory of Molecular Biology, Wageningen University & Research, Wageningen, Netherlands
- 4Department of Plant Breeding, Wageningen University & Research, Wageningen, Netherlands
Fire blight represents a widespread disease in Lilium spp. and is caused by the necrotrophic Ascomycete Botrytis elliptica. There are >100 Lilium species that fall into distinct phylogenetic groups and these have been used to generate the contemporary commercial genotypes. It is known among lily breeders and growers that different groups of lilies differ in susceptibility to fire blight, but the genetic basis and mechanisms of susceptibility to fire blight are unresolved. The aim of this study was to quantify differences in fire blight susceptibility between plant genotypes and differences in virulence between fungal isolates. To this end we inoculated, in four biological replicates over 2 years, a set of 12 B. elliptica isolates on a panel of 18 lily genotypes representing seven Lilium hybrid groups. A wide spectrum of variation in symptom severity was observed in different isolate-genotype combinations. There was a good correlation between the lesion diameters on leaves and flowers of the Lilium genotypes, although the flowers generally showed faster expanding lesions. It was earlier postulated that B. elliptica pathogenicity on lily is conferred by secreted proteins that induce programmed cell death in lily cells. We selected two aggressive isolates and one mild isolate and collected culture filtrate (CF) samples to compare the cell death inducing activity of their secreted compounds in lily. After leaf infiltration of the CFs, variation was observed in cell death responses between the diverse lilies. The severity of cell death responses upon infiltration of the fungal CF observed among the diverse Lilium hybrid groups correlated well to their fire blight susceptibility. These results support the hypothesis that susceptibility to fire blight in lily is mediated by their sensitivity to B. elliptica effector proteins in a quantitative manner. Cell death-inducing proteins may provide an attractive tool to predict fire blight susceptibility in lily breeding programs.
Introduction
Since the dawn of time, lily (Lilium spp., Liliaceae, L.) represents one of the most prestigious ornamental flowering plants in the Occidental culture as it was considered the symbol of divine purity. Either as bulb, potted plant or cut flower, lily is one of the main flower bulb crops sold worldwide (Kamenetsky, 2014). The genus Lilium consists of approximately 120 described species (Angiosperm Phylogeny Group (APG), 2020). Based on their geographical distribution and morphological traits (De Jong, 1974), ribosomal DNA sequences (Nishikawa et al., 1999; Nishikawa, 2007) and the chloroplast genome (Du et al., 2017; Kim et al., 2019), the genus Lilium is phylogenetically divided in seven taxonomic sections (Pelkonen and Pirttilä, 2012; Angiosperm Phylogeny Group (APG), 2020). The assortment of commercial lily genotypes includes thousands of hybrids that were generated via intrasectional crosses between different Lilium species and these have been divided into four hybrid groups: Asiatic (A), Longiflorum (L), Trumpet (T), and Oriental (O). To combine ornamental traits and obtain more vigorous plants, a variety of intersectional hybrids were subsequently generated using artificial pollination, embryo rescue and polyploidization techniques (van Tuyl et al., 1991; Lim et al., 2008; van Tuyl and Arens, 2011), resulting in the interspecific hybrid groups LA (Longiflorum-Asiatic), LO (Longiflorum-Oriental), OA (Oriental-Asiatic) and OT (Oriental-Trumpet). Despite its flourishing breeding and cultivation history, the lily industry is threatened by pests and diseases. Breeding efforts have thus far predominantly focused on ornamental traits and to lesser extent on the resistance to pests and pathogens. Given the current challenges more focus on disease resistance is needed.
Among several pests and diseases the most destructive is fire blight, a fungal disease caused by the filamentous Ascomycetes Botrytis cinerea and Botrytis elliptica (Doss et al., 1986; Fang Hsieh et al., 2001; Furukawa et al., 2005; Chastagner et al., 2017). Both fungi initially cause small brownish necrotic lesions on leaves and flowers. Outgrowth of necrotic lesions leads to rapid death of the entire plant. While the generalist B. cinerea was reported only to occur on damaged plant tissue and especially on cut lilies, the specialist B. elliptica inflicts serious economic losses since it is able to cause disease on undamaged, vigorous plants (Hou and Chen, 2003; Staats et al., 2007; Chastagner et al., 2017). The fungus can infect both leaves and flowers. Leaf infection can be devastating (hence the name “lily fire blight”), however this is usually controlled by intensive chemical control. Flower infection is a postharvest problem and is therefore economically more damaging, as it affects the quality at the retailer or leads to dissatisfied consumers. Both B. cinerea and B. elliptica belong to the genus Botrytis (Sclerotinaceae, Micheli ex Pers.) which includes around 35 described species (Valero-Jiménez et al., 2019). According to their necrotrophic lifestyle, Botrytis species kill the host cells to gain nutrients from the dead plant tissue for growth and reproduction (van Kan, 2006). The fungus is able to trigger the host plant cells to commit suicide via Programmed Cell Death (PCD) (van Kan, 2006; Veloso and van Kan, 2018). In the lily–B. elliptica interaction, PCD induction is mediated by secreted proteins, and the generalist B. cinerea and the tulip specialist B. tulipae acquired the ability to cause necrotic lesions when inoculated on lily leaves, previously infiltrated with B. elliptica secreted proteins (van Baarlen et al., 2004).
There are anecdotic reports among lily growers and breeders that certain lilies are less susceptible to B. elliptica than others. Published studies by Doss et al. (1986) reported a large variation in disease incidence (25–98%) and lesion size among 35 cultivars, of which the hybrid type was in most cases undescribed. A more recent study by Jang et al. (2018) also reported large variation in susceptibility among cultivars from four hybrid types and seven Korean genotypes from distinct indigenous species, with Asiatic hybrid genotypes generally falling in the highly resistant group, and the Korean genotypes generally falling in the susceptible group. A study by Gao et al. (2018), however, classified Oriental × Trumpet and Oriental hybrid cultivars as resistant, while Asiatic and Trumpet hybrids were classified as susceptible. Crosses between resistant and susceptible cultivars provided evidence for very complex inheritance of susceptibility to B. elliptica in hybrid progenies, with hybrid offspring displaying a large spectrum of susceptibility (Beers et al., 2005; Hu et al., 2017).
These previous studies, however, show several limitations that hamper obtaining a clear overview of the variation in susceptibility in lily and the variation in virulence in the B. elliptica population. Several studies included a single fungal isolate (Beers et al., 2005; Hu et al., 2017; Gao et al., 2018; Jang et al., 2018) and distinct inoculation methods. Sometimes agar plugs were used as inoculum (Hu et al., 2017; Gao et al., 2018), while in another case, leaf tips were inoculated with fungal conidia (Beers et al., 2005). Some studies were performed with cultivars of undescribed hybrid groups (Doss et al., 1986; Balode, 2009; Daughtrey and Bridgen, 2013) or performed in the field under unknown disease pressure and uncontrolled conditions (Balode, 2009; Daughtrey and Bridgen, 2013). Only a single study (Daughtrey and Bridgen, 2013) comprised repetitions over multiple years, and the method for disease scoring differed between all studies. Furthermore the variation in susceptibility of lily flowers was not addressed in any of the above mentioned studies.
We aimed to analyze and quantify the disease development under controlled conditions in a panel of commercial lily genotypes from seven hybrid groups upon inoculation with a collection of B. elliptica isolates. Disease assays were principally performed on leaves, and a subset of genotypes was also inoculated on flowers. We selected three fungal isolates that differed in virulence and evaluated the capacity of their culture filtrates (CFs) to cause cell death in leaves of different lily genotypes. These experiments enabled us to investigate a correlation between the susceptibility of lily genotypes to fire blight and their sensitivity to cell death induction in response to fungal secreted compounds.
Materials and Methods
Plant Material and Growth Conditions
Eighteen Lilium genotypes (Table 1) were used in this study representing the hybrid groups Asiatic (A), Longiflorum (L), Oriental (O) or intersectional hybrid groups Longiflorum × Asiatic (LA), Longiflorum × Oriental (LO), Oriental × Asiatic (OA), Oriental × Trumpet (OT), respectively. Material was provided by breeding companies as fresh bulbs and stored in potting soil at −1°C in darkness until planting. For commercial reasons, the names of cultivars have been anonymized. Lilies used in the disease assays in early spring (April–May 2019 and 2020) were planted as bulbs in the second week of February 2019 and 2020, whereas lily bulbs used in the disease assays in late spring (May–June 2019 and 2020) were planted in the third week of March 2019 and 2020. For repetitions of the assay, each of the 18 lily genotypes was planted in batches of 10 bulbs in plastic crates containing potting soil and grown in a greenhouse under natural day/night light regime at a minimum night temperature between 12 and 15°C and a maximum day temperature between 24 and 26°C. Temporal differences in shoot emergence from soil, vegetative growth and transition into flowering were observed. Disease assays in the early spring were conducted with mature leaves (as defined by Bar and Ori, 2014) of 6–7 weeks old plants in their vegetative stage, whereas disease assays in the late spring were conducted with mature leaves of 4–5 weeks old plants in their vegetative stage.
Disease assays on flowers were conducted in June 2020. Lilies used for disease assays on flowers were planted as bulbs in the second week of May 2020 and grown in a greenhouse under natural day/night light regime. Depending on the genotype, flower buds started to develop after 4–6 weeks. Lilies carrying flower buds at initiation of bud color were harvested as cut flowers and transported to the laboratory to be inoculated. Fungal inoculations on lily flowers were conducted within 2 days after bud opening. From the same lilies used for inoculation on flowers, leaves were detached to be inoculated with fungal conidia.
Fungal Material and Growth Conditions
Botrytis elliptica isolates used in this research (Table 2) were stored as conidia suspensions in 20% glycerol at −80°C. To obtain conidia for inoculation, fungi were grown on Malt Extract Agar (50 g/L, Oxoid) at 20°C and sporulation was induced by illumination with UV-A lamps. After harvesting, the conidia were collected and washed in demineralized water, counted with the Bürker-Türk counting chamber, adjusted to a concentration of 106 conidia/mL and stored until use in darkness at 4°C. Disease assays with B. cinerea were conducted similarly using isolate B05.10 (van Kan et al., 2017).
Disease Assays on Lily Leaves
Four rounds of disease assays were conducted in 2019 and 2020, both in the early (April–May) and late spring (May–June) season. In each round of assays all 18 lily genotypes were tested with 12 B. elliptica isolates. Nine lily genotypes were tested in two consecutive weeks, each week with six B. elliptica isolates, for a total of 4 weeks per round. Each disease assay was carried out as follows. From each genotype, 30 mature leaves (as defined by Bar and Ori, 2014) were detached from 10 plants and transported in clean plastic bags to the laboratory for inoculation. Per genotype, three random detached leaves were placed in plastic boxes on plastic grids placed on a layer of wet filter paper, with a total of three lily genotypes per box. The leaves were inoculated with each B. elliptica isolate at a concentration of 105 conidia/mL in Potato Dextrose Broth (12 g/L, PDB, Oxoid). Leaves were abaxially inoculated with four 2 μL droplets of conidial suspension on well separated spots avoiding leaf edges and leaf veins. In total, 12 inoculations were performed per genotype and per isolate in each round of disease assay. Mock inoculation was carried out in separate boxes on three detached leaves with 2 μL droplets of PDB. Plastic boxes were closed with a transparent plastic lid and sealed with tape to maintain high humidity. After 4 days of incubation at constant temperature (19–21°C) and under regular day/night light regime, inoculated leaves were photographed and lesion diameters measured with a caliper. Given the ellipsoidal shape of necrotic spots, the lesion diameter was measured as a conjugate diameter1 (accessed January 2021). Specifically, we measured the length of the chord that connects the middle of the top-left quadrant with the middle of the bottom-right quadrant of the ellipse (illustrated with white dotted lines in Supplementary Figure 1A).
Disease Assays on Lily Flowers
To compare the susceptibility to B. elliptica of flowers and leaves and to test the pathogenicity of B. cinerea on lily, combined disease assays on flower and leaf tissues were conducted in June 2020. In these experiments, leaves and flowers of 12 lily genotypes were used, representing all but one of the hybrid groups (Table 1). Conidia of three B. elliptica isolates that showed variation in aggressiveness in leaf assays (Table 2) and of B. cinerea isolate B05.10 were inoculated on flowers and leaves with 2 μL droplets of conidial suspension at a concentration of 5 × 104 conidia/mL PDB (6 g/L). Disease assays on flowers were carried out with two just opened flowers that were harvested from the inflorescence of lilies and fixed into wet oasis foams, which were placed into plastic boxes containing tap water. Of the six tepals of each flower, five were inoculated with one droplet of conidial suspension and one was marked with paper tape and mock inoculated. Simultaneously to the flowers, three leaves per genotype were inoculated with the same fungal isolates. After 3 days of incubation at constant temperature (19–21°C) and under regular day/night light regime, the inoculated plant material was photographed and lesion diameters were measured.
Production of B. elliptica Culture Filtrate and Leaf Infiltration
Botrytis elliptica CF were obtained by growing each isolate (Be9401, Be0006, and Be9732) in a 250 mL flask containing 50 mL of liquid medium with 3g/L Gamborg B5 salts (Duchefa, Haarlem, Netherlands), 10 mM potassium phosphate pH = 6 (LabChem, Tiel, Netherlands), 1% sucrose (Duchefa) and 30% lily leaf extract in demineralized water. The leaf extract was obtained by grinding with a blender 30 g of fresh harvested leaf material of genotypes A 3, L 2 and OT 3 (10 g each) in 250 mL demineralized water. The homogenate was centrifuged (3500 rpm, 20 min) and the supernatant was concentrated by freeze drying. The obtained concentrate was filter-sterilized (0.45 μm pore size, Millipore) and added to the liquid medium. The liquid culture was started at a spore concentration of 104 conidia/mL medium. Flasks were closed with a cotton plug and fungal cultures were grown in a shaking incubator for 7 days (20°C, 150 rpm). When the liquid cultures were harvested, pH of the CF was adjusted to 6.0 with 1M KOH, and the CF was passed through a layer of Miracloth (Calbiochem, San Diego, CA, United States), filter-sterilized (0.45 μm pore size, Millipore, Amsterdam, Netherlands) and ice-chilled. A mock liquid medium was prepared and processed in the same way without fungal conidia added. For leaf infiltration with the CF samples and mock solution, three detached leaves were used of non-flowering plants from 12 different lily genotypes, representing all seven lily hybrid groups (Table 1). The leaves were placed in moist plastic boxes and 0.1 mL CF sample from each fungal culture was abaxially infiltrated in four different spots per leaf with the aid of a 1 mL sterile plastic syringe. After 3 days incubation at 19–21°C under ambient daylength, leaves were photographed and the response evaluated.
Statistical Analysis
The statistical analysis was performed using R (Version 4.0.2). Lesion diameters measured in disease assays on lily leaves and flowers were plotted on a box-plot diagram using ggplot2 (Version 3.2.2). To determine the contributions of plant and pathogen genotype, and the seasonal effect on observed variation, the following linear mixed model was used:
whereby: ls, lesion diameter measured; be, B. elliptica isolate; group, lily hybrid group; season, late or early spring; year, 2019 or 2020, as random variable.
As the data were not balanced, a REML model was built using lmer() from the lme4 package (Version 1.1-22; Bates et al., 2015). The year was added to the model as a random effect to correct for year effect. To quantify the impact of the year on the variation observed, a variance component analysis was performed with lme4. Estimated marginal means (EMMs) of lesion diameters per fungal isolate, per hybrid group, were obtained using emmeans (Version 1.5.0). Differences in EMMs were calculated using the pairwise comparison function of emmeans, and p-values for differences in EMMS were adjusted by Tukey’s method. Pairwise differences for the average lesion diameters within isolate, for each hybrid group were plotted on a dot plot using ggplot().
Lesion diameters measured in lily flowers and in leaves of cut lilies were analyzed with the following linear model.
whereby: ls, lesion diameter measured; be, B. elliptica isolate; group, lily hybrid group.
This simpler, linear model was chosen because the flower infection assays were performed only in one season (early summer 2020).
EMMs were obtained using emmeans (). Differences in EMMs were calculated and plotted similarly to the EMMs for lesion diameter on leaves.
Results
To assess the susceptibility of lily genotypes to B. elliptica and the virulence of B. elliptica isolates in a quantitative manner, we inoculated 12 fungal isolates (Table 2) on leaves of 18 lily genotypes (Table 1) representing three hybrid groups (Asiatic, Longiflorum and Oriental) and four intersectional hybrid groups (LA, LO, OA, OT). Lesion development was quantified over the course of 3–4 days post inoculation (dpi) (raw data available in Supplementary Material 1). The experiments were conducted in four replicates over 2 years and each experiment contained 12 inoculation spots per genotype-isolate combination.
Disease Assays in Leaves Distinguish Sections and Interspecific Hybrids
Upon inoculation with conidial suspensions, disease symptoms initially appeared as small necrotic spots of brownish, collapsed abaxial epidermal tissue which were surrounded by a water soaked translucent area (Supplementary Figures 1, 2). In the most susceptible genotypes the lesions became visible from 2 dpi onward (Supplementary Figure 2). In the following days, the necrotic areas expanded, a larger portion of the abaxial leaf epidermis collapsed and the mesophyll tissue started to shrink inwards until the necrotic tissue could be observed also on the adaxial side of the leaf. With disease progression, the typical fire blight ellipsoidal necrotic lesions developed and especially for the most virulent isolates, growth of fungal mycelium was observed on the dead leaf tissue. Moreover, a dark discoloration was observed in the leaf vasculature and leaf yellowing was observed at a distance from the necrotic lesion. Mock inoculated leaves remained symptomless for the entire incubation period (Supplementary Figure 2). At 4 dpi, symptoms were quantified by measuring the lesion diameter. Despite the variation in lesion diameters among the specific genotype-isolate combinations, a general trend was noticed. Upon inoculation with all B. elliptica isolates, lily genotypes belonging to the groups A, LA, and OA developed larger lesions when compared to genotypes belonging to the groups L, O, LO, and OT (Figures 1, 2). As illustrated in Supplementary Figure 3, experiments in late spring (May–June 2019 and 2020) developed slightly larger lesions as compared to the experiments conducted in early spring (April–May 2019 and 2020). The largest variation in lesion diameter was observed among the groups A, LA, OA, and OT where lesion diameters ranged from 0 to 30 mm in the A genotypes and from 0 to 20 mm in the LA, OA and OT groups (Figure 1). By contrast, lily genotypes belonging to the groups L, O, and LO showed lesion diameters that ranged from 0 to 12 mm (Figure 1). This trend was consistently observed in both years and seasons (Supplementary Figure 3).
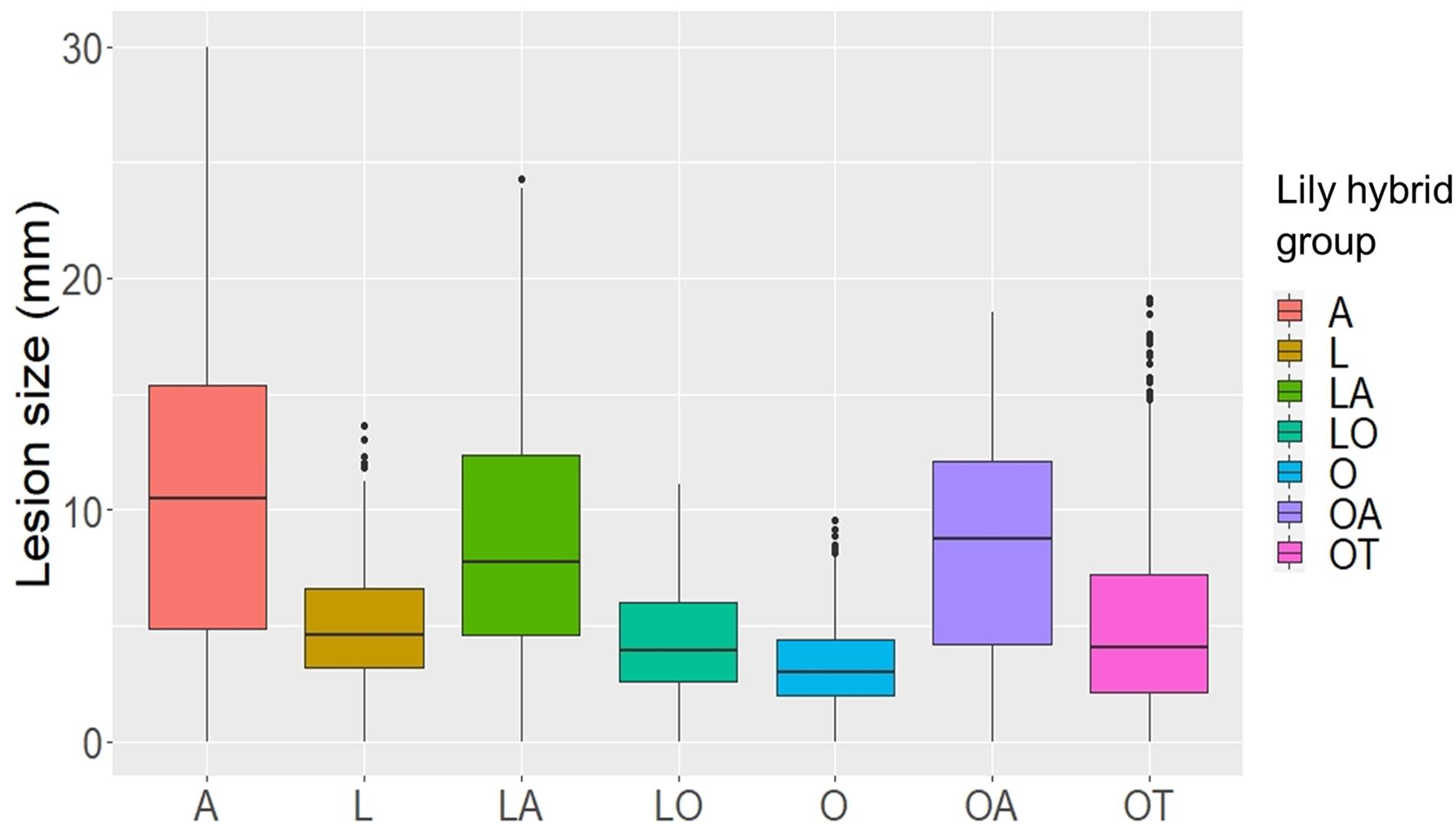
Figure 1. Lesion diameters at 4 dpi upon inoculation of 12 different B. elliptica isolates on leaves of seven different lily hybrid groups. Each box represents all compiled lesion diameters (in mm) for a given lily hybrid group in all four repetitions of the disease assays.
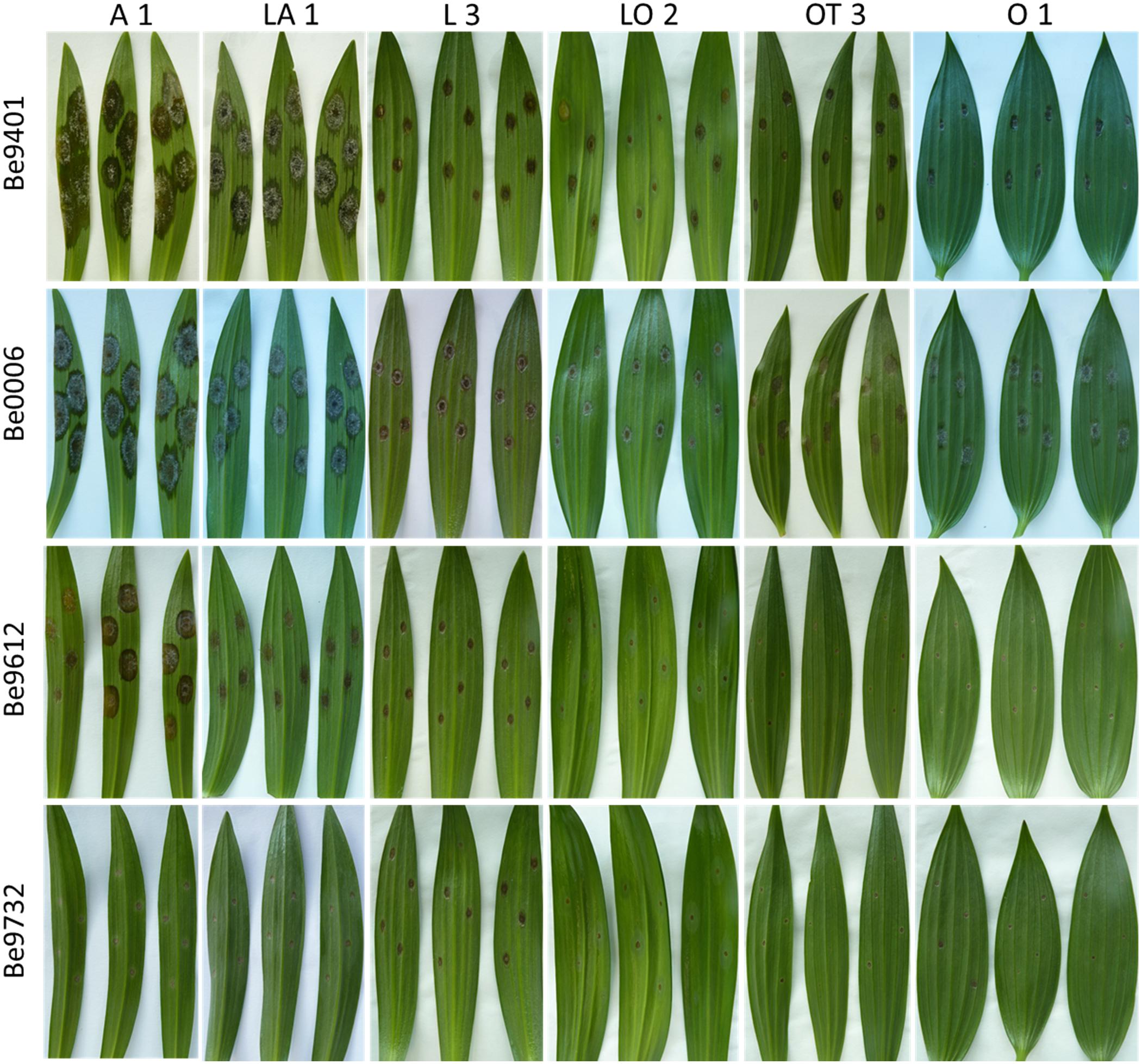
Figure 2. Necrotic lesions at 4 dpi upon inoculation with four distinct B. elliptica isolates (specified in left margin) on leaves of six lily genotypes (specified at the top). For each repetition of the disease assay, three representative leaves are shown.
Variation Between Genotypes Within Sections and Interspecific Hybrids
Differences in lesion diameters were not only detected between the different lily hybrid groups but also between individual genotypes within the same hybrid group (Figure 3). The most evident differences were observed when comparing lily genotypes of the groups A (Figure 3A), LA (Figure 3B), and OT (Figure 3C). In genotypes A1 and A2 the lesion diameters ranged from 15 to 25 mm, whereas in genotype A3 only the more aggressive fungal isolates caused lesions larger than 10 mm (Figure 3A). In the genotypes LA1 and LA2 lesion diameters ranged from 15 to 20 mm upon inoculation with the more aggressive isolates whereas in genotype LA3 the lesion diameters ranged from 10 to 15 mm (Figure 3B). In genotype OT1 lesion diameters ranged from 12 to 18 mm whereas in genotypes OT2 and OT3 lesion diameters ranged from 4 to 12 mm (Figure 3C).
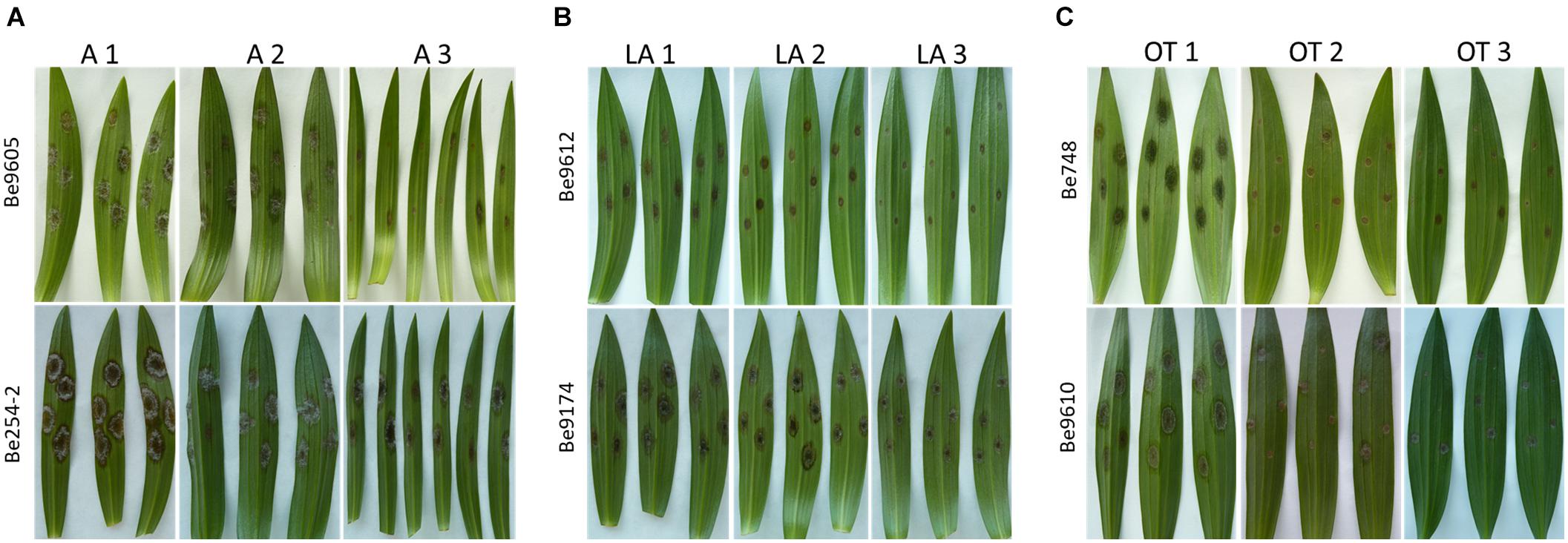
Figure 3. Necrotic lesions at 4 dpi on leaves of three different lily genotypes belonging to the hybrid group Asiatic inoculated with Be9605 and Be254-2 (A), hybrid group LA inoculated with Be9612 and Be9174 (B), and hybrid group OT inoculated with Be748 and Be9610 (C).
Variation in Virulence Between Fungal Isolates
The variation in lesion diameter and symptom development was also related to differences in virulence of the tested B. elliptica isolates (Figures 2, 4). All 12 B. elliptica isolates were able to cause disease symptoms on leaves of all lily genotypes. Inoculation with Be9401 and Be0006 resulted in lesions ranging from 10 to 30 mm on leaves of susceptible hybrid groups A, LA, and OA, and ranging from 8 to 20 mm on OT genotypes. These two isolates were classified as aggressive. By contrast, inoculation with isolates Be9612, Be9714, and Be9732 resulted in lesions ranging from 3 to 12 mm in the susceptible hybrid groups A and LA, and even smaller lesions on less susceptible lily groups (Figure 4), classifying these three isolates as mild pathogens. The lesions diameters of the other seven isolates classified these as intermediate aggressive. All isolates, including the aggressive ones, developed lesions < 10 mm when inoculated on leaves of the groups O and LO and lesions < 15 mm on leaves of groups L and OT (Figure 4) confirming the relatively resistant nature of these lily hybrid groups.
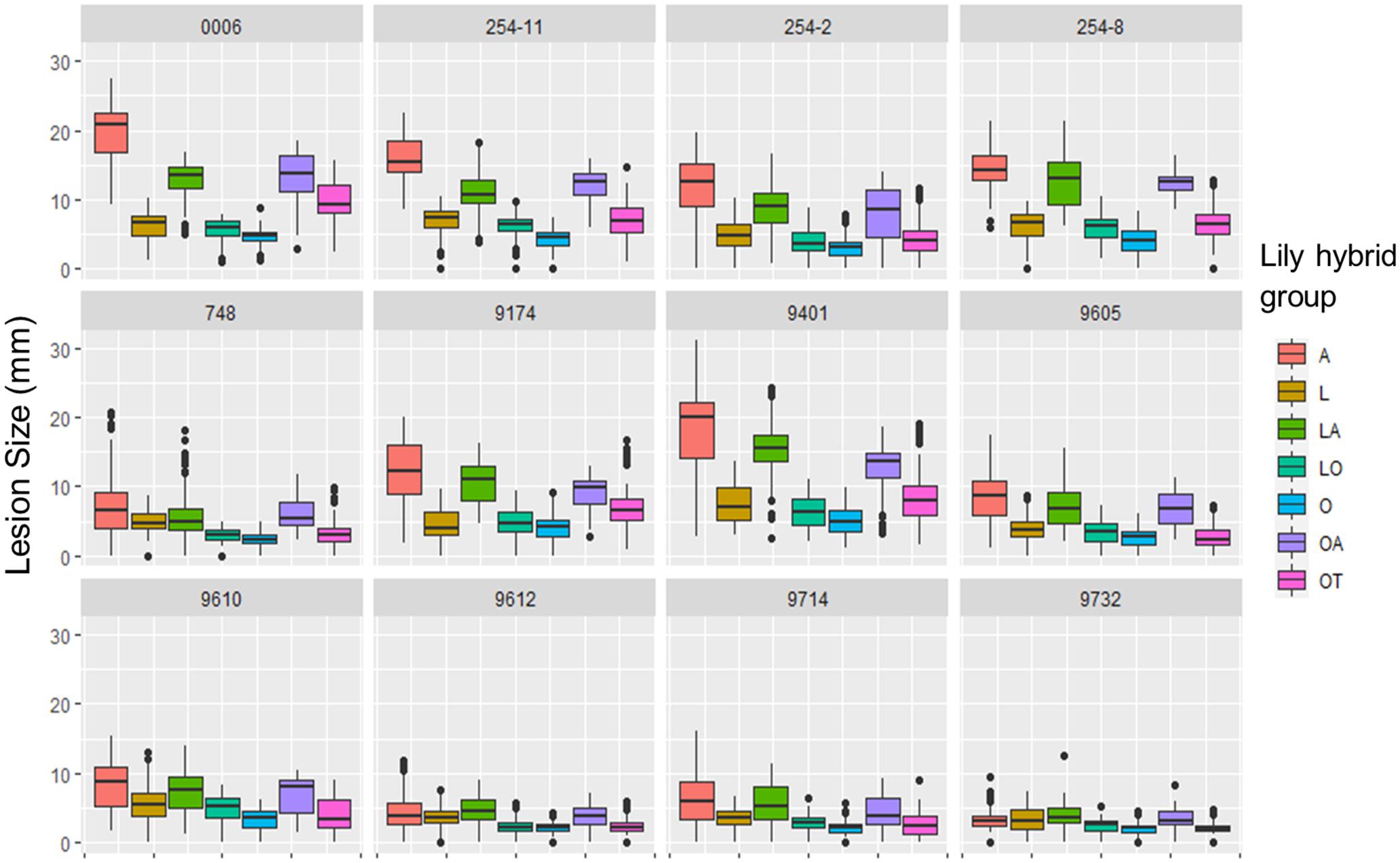
Figure 4. Lesion diameters at 4 dpi upon inoculation of 12 different B. elliptica isolates on leaves of 18 different lily genotypes. Lesion diameters measured in mm are plotted for each single isolate in 12 separate panels. Each of the colored boxes represents all lesion diameters measured for a given lily hybrid group in all four repetitions of the disease assays.
Statistical Analysis of the Variation in Lesion Diameter in the Disease Assays on Lily Leaves
The linear mixed model of lesion diameter on leaves shows that both the fungal diversity and lily group diversity, as well as the interaction between fungal isolate and lily group were the main contributors to the variation in lesion diameter (Table 3). The model shows that also the season and the interaction terms which included the season had a significant contribution on the variation in lesion diameter, while the year contributed to the variance only to a minimal extent (Table 3). Figure 5 and Supplementary Figure 4 show the differences in EMMs calculated for each lily hybrid group in the early and late spring, respectively (see also Supplementary Material 2). Each colored dot represents the estimated difference of the lesion diameter in one particular B. elliptica isolate – lily hybrid group interaction in comparison to the lesion diameter for the same B. elliptica isolate during the interaction with a different lily hybrid group. Three main patterns can be recognized:
(1) For all isolates in both seasons, dots representing the groups A (red), LA (green), and OA (violet) show a positive value in differences of EMMs. This indicates that in general, all isolates caused bigger lesions when inoculated on genotypes of these groups in comparison to genotypes of the groups L, O, LO, and OT. On the other hand, dots representing the groups O (light blue), LO (blue-green), and OT (pink) show negative values in differences of EMMs indicating that in general all isolates caused smaller lesions when inoculated on genotypes of these lily hybrids in comparison to genotypes of the groups A, LA, and OA. Finally, EMMs calculated for the interaction of each B. elliptica isolate with genotypes of group L (brown dots) cluster around the base line and in most of the cases they remain within the confidence intervals.
(2) For the more aggressive isolates (Be0006 and Be9401), larger differences in EMMs are observed when comparing the interaction of these isolates with the different hybrid groups. This indicates that the plant genotype significantly influenced the outcome of the isolate–plant interaction.
(3) The differences in EMMs for the isolates Be9612, Be9714, and Be9732 showed little variation, both within lily groups and between groups.
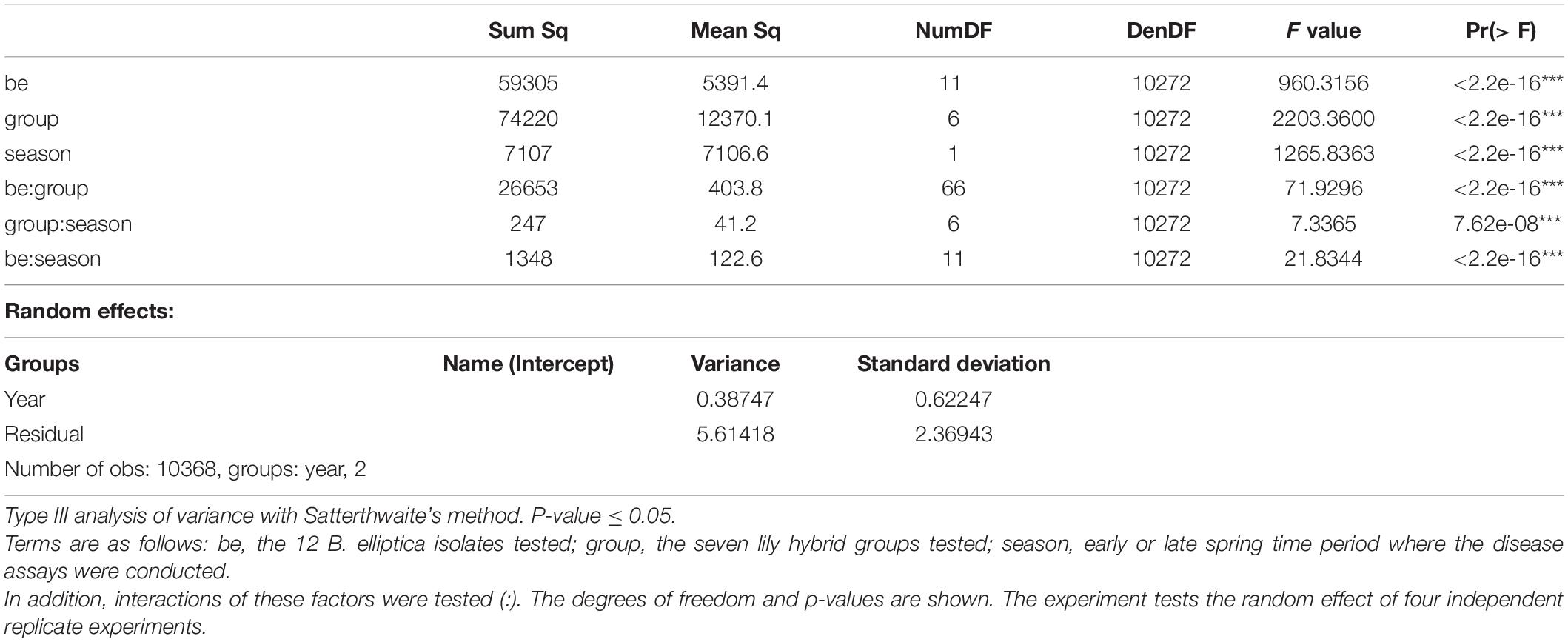
Table 3. ANOVA results of the interaction in leaves between 18 Lilium genotypes and 12 B. elliptica isolates measured as lesion diameter.
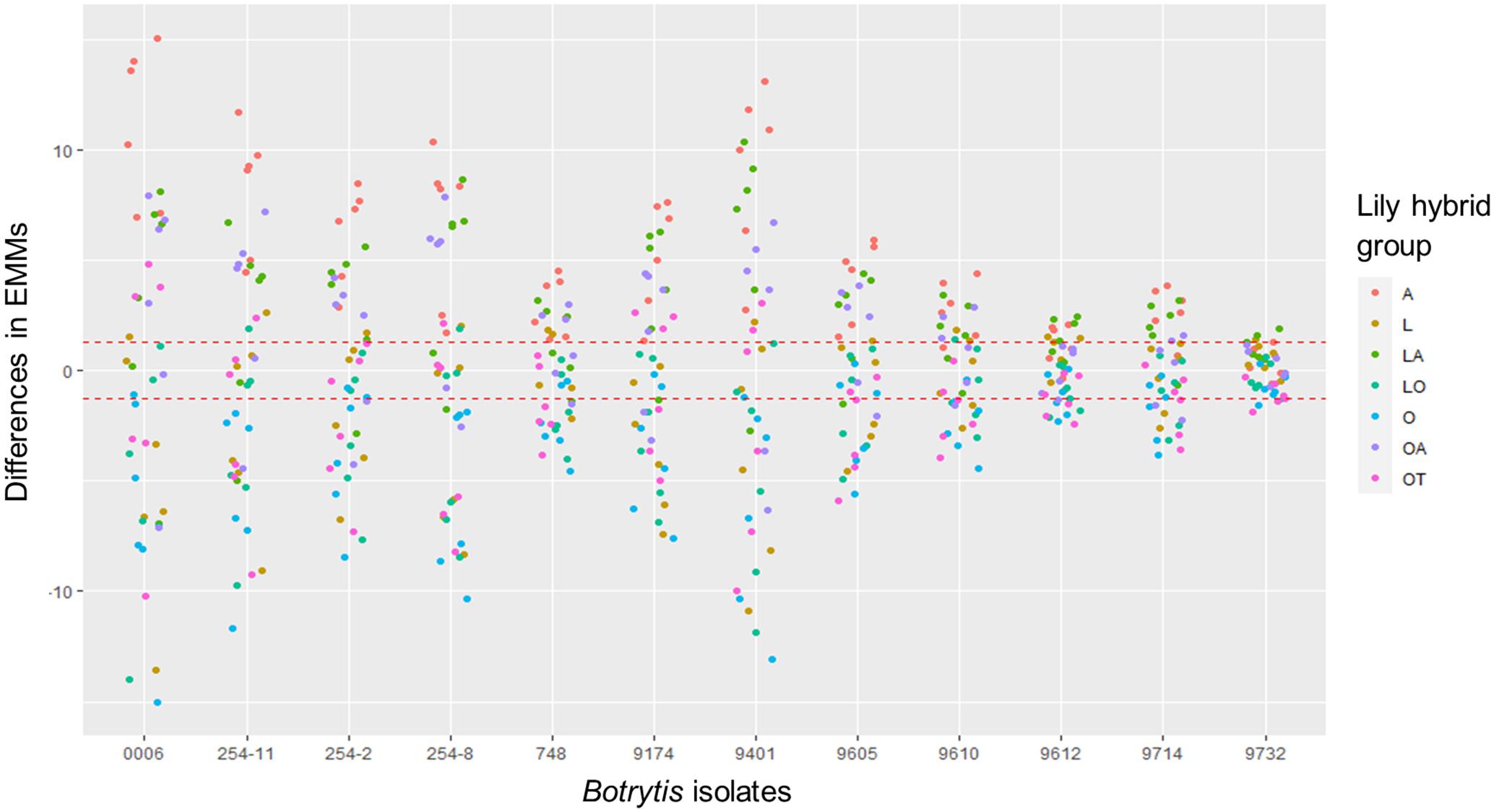
Figure 5. Dot plot showing differences in EMMs of lesion diameters on lily leaves in early spring for a given B. elliptica isolate, calculated using lily hybrid group as predicted factor. Red dotted line indicates the average p-value cut off for estimated differences which are significantly different from 0 (p > 0.05). P-values were adjusted using Tukey’s comparison for a family of 84 estimates.
Disease Assays in Lily Flowers
The most appreciated organ in lily is the flower and fire blight is known to affect also the reproductive organs, especially at the cut flower stage (Munafo and Gianfagna, 2011). We examined the susceptibility of flowers among representatives of the seven lily hybrid groups. A pilot experiment revealed that the conidial density for flower inoculation needed to be reduced to prevent excessively rapid lesion outgrowth. The optimal density for discriminative infection assays on flowers was 5 × 104/mL conidia, while for leaves we used a density of 105/mL. For logistic reasons, the experiment could not be conducted with all 18 lily genotypes and 12 isolates. A panel of 12 lily genotypes (Table 1) was inoculated with three B. elliptica isolates (two aggressive isolates and one mild isolate; Table 2) and also one B. cinerea isolate and disease progression was monitored over time. B. cinerea was included because it was reported to cause necrotic lesions on lily flowers in the postharvest stage.
Because cutting of the inflorescence caused natural perigone abscission upon prolonged incubation, even in mock-treated samples and symptoms on lily flowers developed faster than on leaves and the size of necrotic lesions on tepals was therefore measured at 3 dpi (Table 4). All three B. elliptica isolates and B. cinerea were able to cause disease symptoms on lily flowers. As depicted in Figures 6, 7, flowers of lily genotypes belonging to the groups A and LA showed the most severe symptoms, irrespective of the isolate used. By contrast, lily genotypes of the groups O, LO, and OT showed smaller lesions upon inoculation with all four fungi (Figure 6, fifth and sixth column from the left). Inoculated flowers of L developed lesions with diameters that were in-between those observed on the groups A, O, LA, LO, and OT. Differences in lesion diameters were also observed among the fungal isolates when tested on flowers. B. elliptica isolates Be9401 and Be0006 caused larger lesions on flowers of all lily genotypes tested, as compared to Be9732 and B. cinerea (Figure 7 and Table 4).
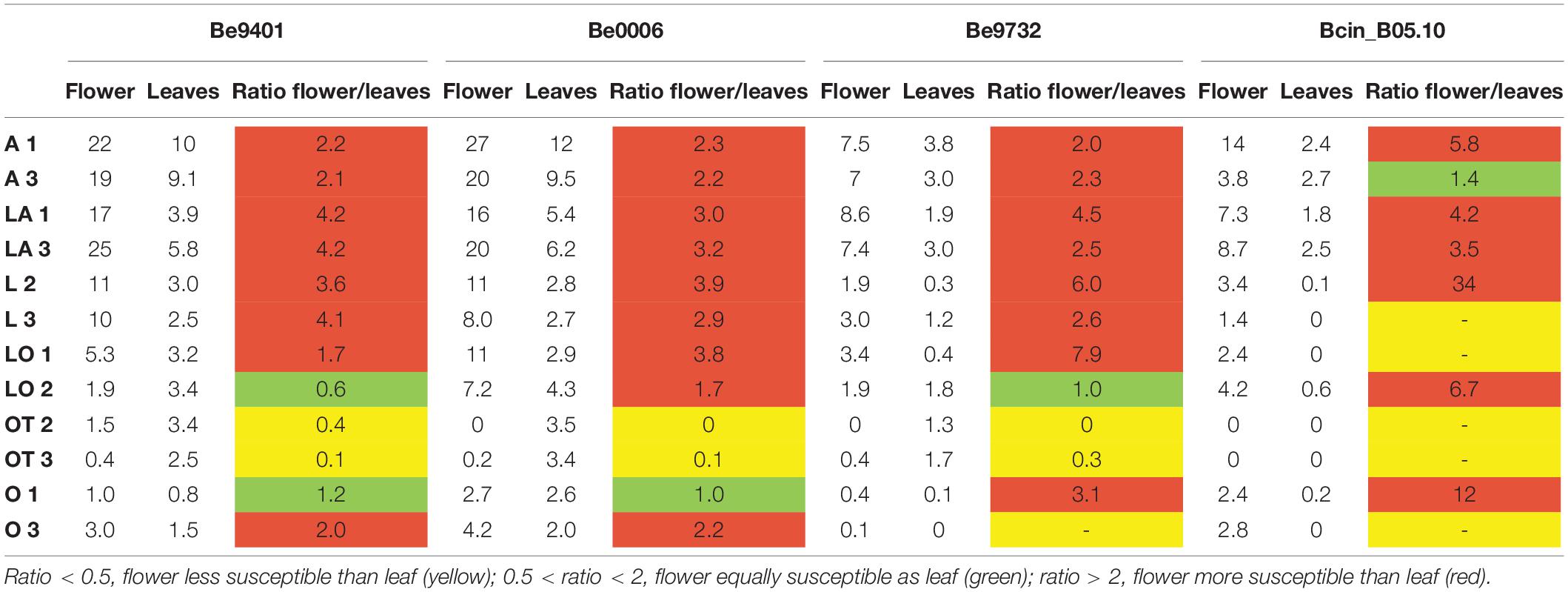
Table 4. Ratios between average lesion diameters (given in mm) on lily flowers and leaves inoculated with three different B. elliptica isolates and one B. cinerea isolate at 3 dpi.
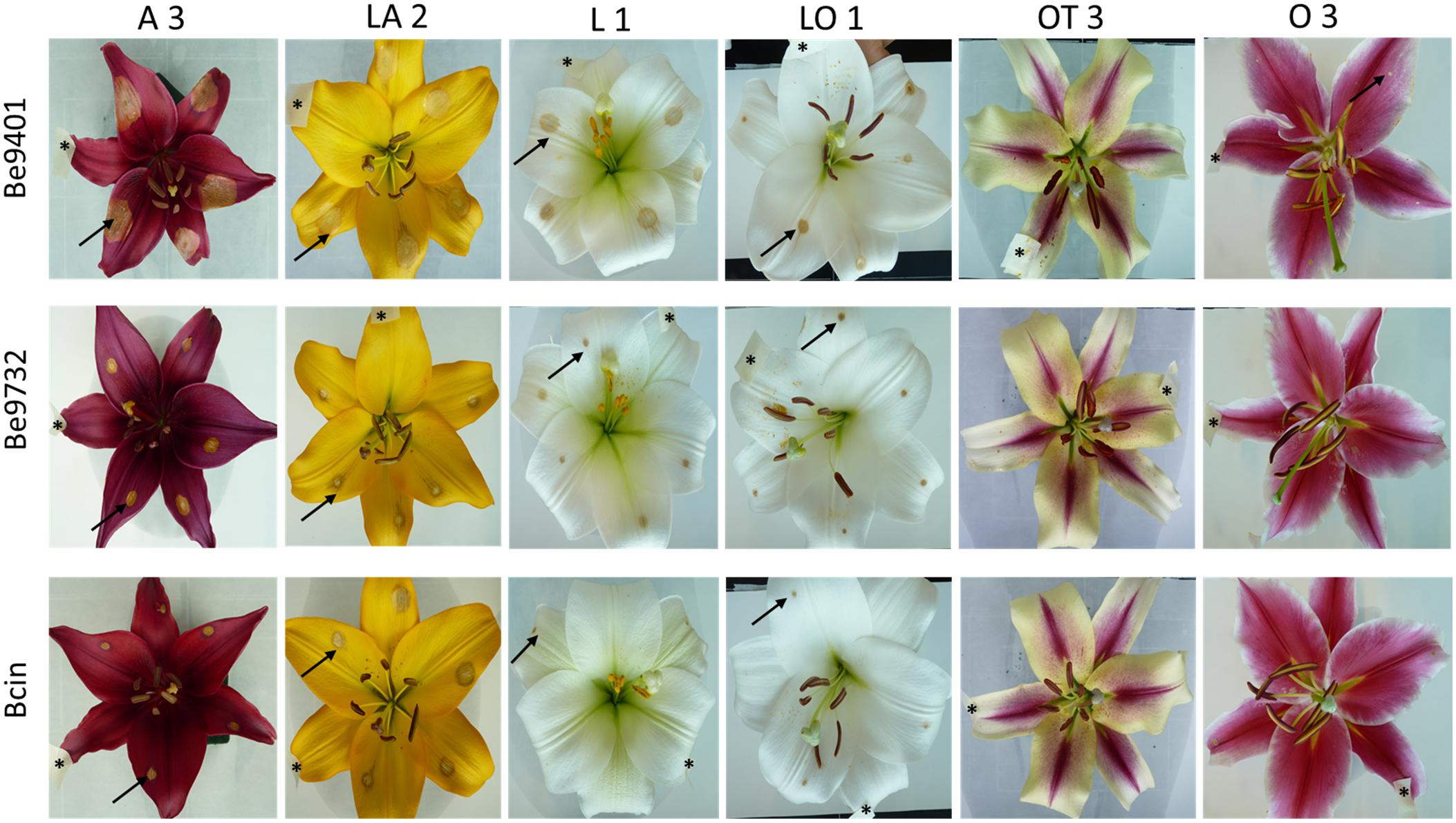
Figure 6. Necrotic lesions at 3 dpi upon inoculation of B. elliptica isolates Be9401, Be9732 and one B. cinerea isolate on flowers of lily genotype A 3, LA 2, L 1, LO 1, OT 3, and O 3. For each cultivar, one representative flower is shown that was inoculated on five different tepals. Black arrows indicate necrotic lesions observed on the tepals. Mock inoculation was labeled with a piece of paper tape on the tepal and is marked with a black asterisk (*).
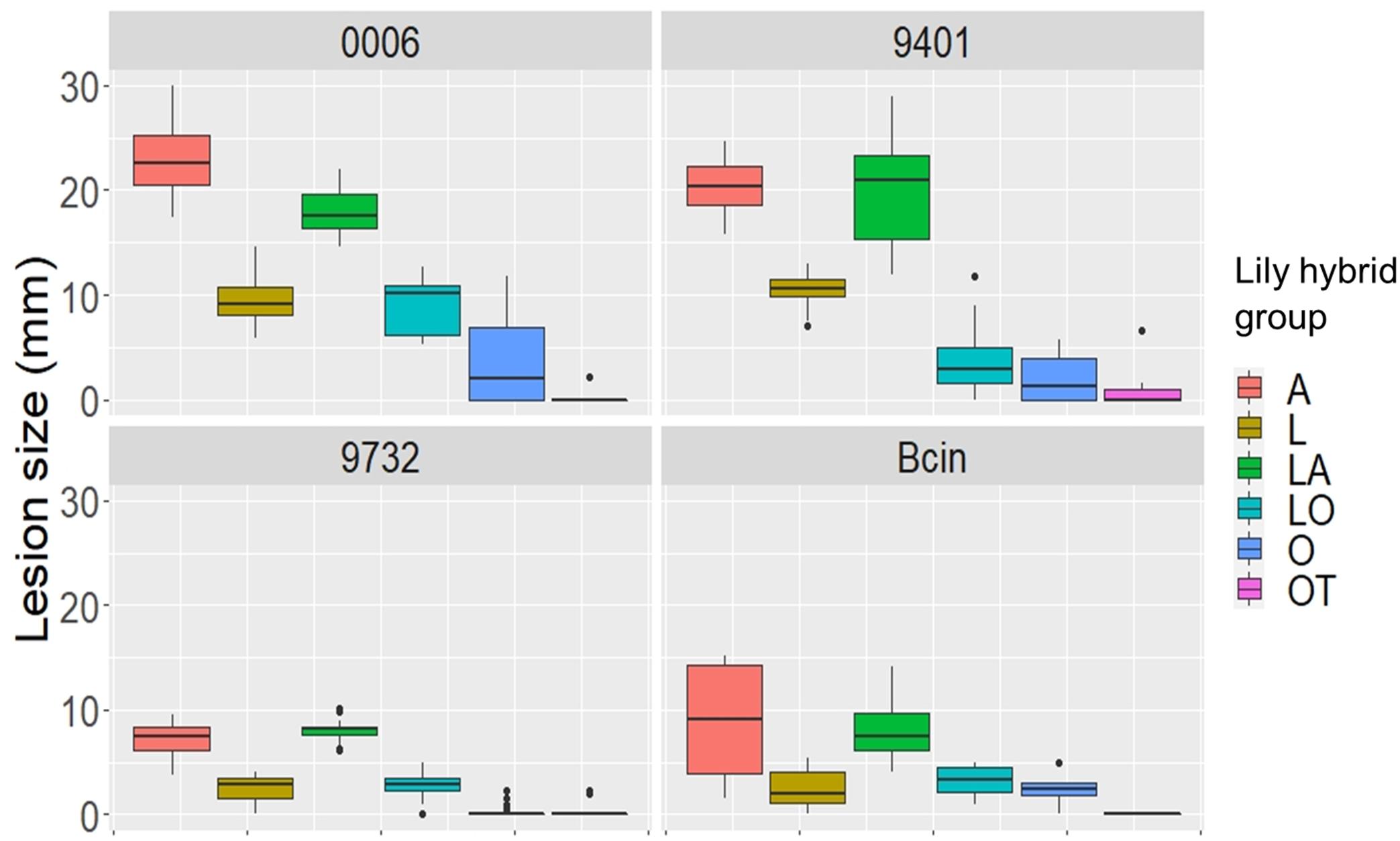
Figure 7. Lesion diameters at 3 dpi upon inoculation of B. elliptica isolates Be9401, Be9732, and Be0006 and one B. cinerea isolate on flowers of 12 lily genotypes. Lesion diameters measured in mm of each single fungus are plotted separately in the four panels. Each of the colored boxes represents all lesion diameters measured for a given lily hybrid group.
To compare Botrytis susceptibility of flowers and leaves as consistently as possible, we inoculated at the same spore density (5 × 104/mL) conidia of the same three B. elliptica isolates and B. cinerea on leaves which were detached from the same lilies used to perform the flower assays. Overall, it was observed that at 3 dpi, inoculated leaves of lilies belonging to the groups A, L, LA, and LO developed smaller leaf lesions, as compared to flowers (Table 4). The trends in relative susceptibility between the hybrid groups and trends in differences in virulence among the isolates (Supplementary Figures 5, 6) were consistent with trends observed in the leaf inoculations on the entire set of lily genotypes with all fungal isolates (Figures 1, 2, 4). We calculated the ratios of lesion diameters on flowers and leaves from the same plants at 3 dpi and observed that for genotypes from the groups A, L, and LA, those ratios exceeded 1.5 for all three B. elliptica isolates, indicating that flowers were substantially more susceptible than leaves. For genotypes belonging to the hybrid group OT, the ratios were <0.5, indicating that leaves were more susceptible than flowers. For genotypes belonging to the hybrid groups O and LO, the ratios differed depending on the genotype-isolate combination (Table 4). Inoculation with B. cinerea conidia resulted in development of lesions of highly variable sizes on flowers of all hybrid groups, except OT (no lesions). By contrast, B. cinerea caused only small necrotic lesions on leaves of genotypes belonging to the groups A and LA, but rarely caused any lesions on genotypes of the other groups (Table 4 and Supplementary Figure 6).
The statistical analysis of the data for flower and leaf inoculations of cut lilies showed that the genetic diversity of fungal and plant genotype, and the interaction between fungal isolate–plant genotype significantly contributed to the variation observed in lesion diameter (Supplementary Tables 1, 2). When the differences in EMMs were calculated for the lesion diameters observed on flowers and on leaves of cut lilies (Supplementary Figures 7, 8), it was found that for all Botrytis isolates tested, dots representing the hybrid groups A (red dots) and LA (green dots) show a significant positive difference in EMMs. On the other hand, dots representing the groups O (light blue dots), LO (blue-green dots), and OT (pink dots) show negative differences in EMMs. The differences in EMMs calculated for the interaction of each B. elliptica isolate with L show a reduced spread and in several cases they remain within the confidence intervals. Moreover, it can be noticed that the differences in EMMs were larger when the interactions of the most aggressive isolates (Be0006 and Be9401) with a given lily genotype were compared, while smaller differences in EMMs were observed for the milder isolates (Be9732 and B. cinerea).
Leaf Infiltration Assays With CF Samples
Crude CF samples were collected from the same three B. elliptica isolates that were used in flower and leaf inoculations to test whether disease susceptibility and virulence correlate to effector sensitivity (of the lily genotype) and effector production (by the fungal isolate). CF samples were infiltrated in leaves of 12 lily genotypes (Table 1). At 3 days post infiltration, the leaf responses were observed (Figure 8 and Supplementary Figure 2). Lily genotypes displayed cell death responses of the infiltrated leaf area to different extents. A severe cell death response (tissue collapse, drying and slight browning) was observed for genotypes from the groups A, L, LA (Figure 8) and OA (Supplementary Figure 2) when infiltrated with CF samples from the aggressive Be9401 and Be0006. By contrast, a mild response (translucence, discoloration and softening of infiltrated tissue) was observed for genotypes representing the groups O, LO and OT when infiltrated with CF samples from these same isolates. The infiltration of genotype L3 with the CF from Be9401 resulted in symptoms very distinct from other combinations, typified by a dark discoloration, yet with a transparent appearance indicative of severe tissue degradation (Figure 8). The response of genotype L3 to CF samples from Be0006 and Be9732 was similar to that of many other genotypes tested. Moreover, CF from Be9401 did not cause similarly dark, macerated tissues in other lily genotypes. There were no visible responses in any lily genotype to infiltration with the CF sample obtained from Be9732 (Figure 8, third row). As expected, leaf infiltration with culture medium in which no fungi were grown did not induce any visible symptoms (Supplementary Figure 2).
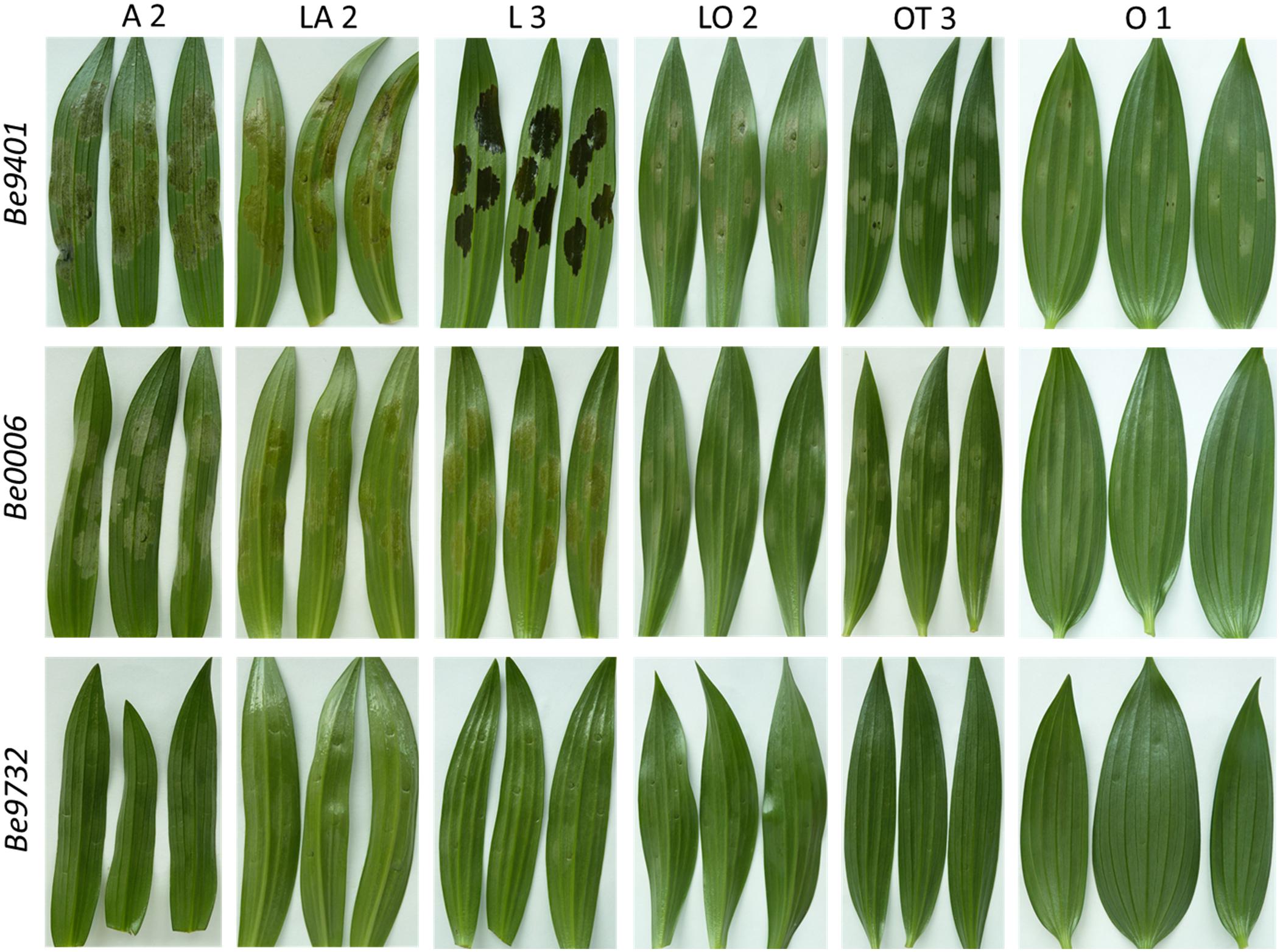
Figure 8. Cell death symptoms caused by infiltration of CF samples obtained from liquid cultures of three B. elliptica isolates (specified in left margin) on leaves of six lily genotypes (specified at the top).
Discussion
Variation in Fire Blight Susceptibility in Lilium
Our data unveil significant phenotypic variation in fire blight susceptibility within the phylogenetically complex genus Lilium. Genotypes belonging to the hybrid groups A, LA, and OA showed significantly more severe symptoms than genotypes of hybrid groups L, O, LO, and OT upon inoculation with all B. elliptica isolates tested (Figures 1, 2, 4, 5). This trend was consistently observed in all repetitions of the disease assays, both in leaves and flowers. These observations are in line with other studies, however our research provides more comprehensive data, as it included 18 lily genotypes, representing seven of the eight existing hybrid groups, as well as a set of 12 B. elliptica isolates collected over multiple years.
Asiatic (A) hybrids are obtained from lily species which belong to the taxonomic section Sinomartagon that is closely related to L. longiflorum (L). On the other hand, Trumpet (T) hybrids are generated from species which are closely related to species of the section Archelirion from which Oriental (O) hybrids derive (Nishikawa et al., 1999; Arzate-Fernández et al., 2005; Shahin et al., 2014; Kim et al., 2019). Intersectional hybrids LA, OA and OT were developed after chromosome doubling in the F1 progeny and backcrossing to A or O hybrids, respectively, in order to obtain a more vigorous, triploid genotype (van Tuyl and Arens, 2011). Breeding efforts have thus far predominantly focused on ornamental traits and to lesser extent on the resistance to pests and pathogens. Genetic loci associated with resistance to Fusarium oxysporum f. sp. lilii and lily mottle virus were mapped via QTL analysis (Arens et al., 2014). However, traits and loci related to Botrytis susceptibility remain unknown. This may be due to the fact that resistance to necrotrophic pathogens is often inherited as a recessive and quantitative trait (Wolpert and Lorang, 2016; Kariyawasam et al., 2016; Liu et al., 2017; Koladia et al., 2017; Faris et al., 2020) and may have not been considered during the breeding selection.
Although the A, LA, and OA genotypes tested were in general the more susceptible groups, there was substantial variation in lesion diameters and symptom severity between individual genotypes within these groups (Figures 3A,B). Lilium species of the section Sinomartagon (from which the Asiatic hybrids derive) are characterized by large genetic diversity because of their polyphyletic origin (Huang et al., 2018; Kim and Kim, 2018; Kim et al., 2019). Our results reveal that traits for reduced fire blight susceptibility (i.e., quantitative resistance) may be present in the germplasm of the Asiatic hybrids. Depending on the genotype(s) of the parents and of the line to which the F1 hybrid was backcrossed, traits conferring recessive resistance to fire blight may have been retained to different extents in the genomes of the new hybrid genotypes. It remains important to investigate a wider assortment of Asiatic hybrids for fire blight susceptibility. On the other hand, different genotypes of the hybrid group Longiflorum, showed little variation in lesion diameter upon inoculation with all B. elliptica isolates (Figures 1, 4). The genetic diversity of Longiflorum genotypes is narrower as compared to other sections, because either a single or very few species have been involved in the generation of these genotypes (van Tuyl, 1985).
Flower Pathology in Botrytis–Lilium Interaction
Botrytis spp. are sometimes considered opportunistic pathogens which exploit wounded and senescing plant tissues to promote host infection. This assumption is supported by our results in the disease assays on flowers. Inoculated lily tepals developed larger necrotic lesions when compared to leaves of the same plant. Fungi of the genus Botrytis require PCD induction to infect plant tissue (van Kan, 2006; Veloso and van Kan, 2018) and during natural senescence of flower tissue, tepal cells begin to die before flower wilting becomes visible (van Doorn et al., 2003; van Doorn and Woltering, 2008; Rogers, 2013; Shibuya et al., 2016). In several monocot taxa (Alstroemeria, Hemerocallis, Iris, and Lilium) flower senescence shows typical hallmarks of PCD such as closure of plasmodesmata, starvation of tepal cells due to ATP depletion, formation of small vesicles, vacuolar swelling and tonoplast rupture. The progression of the PCD process involves the action of caspases and other intracellular proteases (Battelli et al., 2011), causing loss of cell integrity and breakdown of the cytoplasmic content including plastids and mitochondria, as well as the release of hydrolytic enzymes that degrade cell wall polysaccharides (van Doorn and Woltering, 2008; Rogers, 2013; Shibuya et al., 2016). Chromatin condensation and DNA fragmentation are considered as hallmark diagnostic features for PCD (van Baarlen et al., 2004; Yamada et al., 2006). Even though the PCD regulatory mechanisms at subcellular and molecular level are not fully understood, the micro-environment that is created during flower senescence (water-, sugar-, and amino acid-rich apoplast) represents an attractive growth substrate for necrotrophic pathogens. Therefore, the autonomous initiation and progression of PCD in cut flowers may benefit both B. elliptica and B. cinerea in their infection process and thereby cause more severe symptoms in lily flowers than in leaves.
The disease symptoms on lily flowers upon inoculation with B. cinerea, which is not specific to lily (van Baarlen et al., 2004), were comparable to those caused by the mild B. elliptica isolate Be9732. These observations suggest that these two fungi lack virulence factors that specifically promote infection in lily, however, they may benefit from the endogenous flower senescence process.
The relative ranking of flower susceptibility of lily hybrid types to fire blight showed a similar pattern to that in leaves. A and LA genotypes showed significantly larger lesions on flowers when compared to O, OT, and LO, with L genotypes showing intermediate lesion diameters. The ratio of lesion diameters in flowers versus leaves of the exact same plants (Table 4) showed a pattern that yet remains to be understood. The flowers of genotypes from the groups A, L, and LA were more susceptible than their leaves. By contrast, the leaves of genotypes from the OT group were more susceptible than their flowers, while genotypes from the groups O and LO showed a mixture of ratios, depending on the genotype-isolate combination. Whether B. elliptica uses different virulence factors for the infection of lily flowers and leaves will be subject of further studies.
Fire Blight Susceptibility and Fungal Virulence Correlate to Effector Sensitivity and Effector Production
Botrytis elliptica secreted proteins can induce PCD when infiltrated in lily leaves (van Baarlen et al., 2004). We hypothesize that disease development in the B. elliptica-lily interaction is determined in a quantitative manner by the production by B. elliptica isolates of effector proteins that can induce PCD in the host, as well as by the sensitivity of lily genotypes to these effectors. Crude CF samples from cultures of B. elliptica were able to cause PCD in lily leaves to different extents. Genotypes belonging to the hybrid groups A, L, LA, and OA showed a more severe necrotic response upon CF infiltration, as compared to genotypes belonging to the groups O, LO, and OT (Figure 7 and Supplementary Figure 2F). These observations support the hypothesis that lily genotypes that developed the most severe disease symptoms in leaves and flowers were the same genotypes that showed a more pronounced necrotic response upon CF infiltration. Conversely, CF samples that caused the strongest necrotic response were obtained from B. elliptica isolates that caused the largest lesions on lily leaves and flowers in the disease assays, while CF from a less aggressive isolate caused little visible symptoms in any lily genotype.
The response observed in Longiflorum leaves upon infiltration with the CF sample from Be9401 was an outlier, despite the similar disease symptoms that this fungus caused upon inoculation. These leaves showed a much more severe response than all other infiltrated lily leaves. The infiltrated leaf segment had a dark brown color and yet appeared transparent, as if the tissue architecture was completely destroyed and the cell content dissolved. The dark pigmentation may have been generated by the combined activity of fungal and plant (poly)phenol oxidases which caused the oxidation of phenolic compounds released from dying lily cells during PCD (Schouten et al., 2002; Boeckx et al., 2015). At the same time, the leaf tissue collapse likely resulted from the action of fungal cell wall degrading enzymes in the CF sample, which cause maceration leading to loss of cell integrity and tissue architecture (van Kan, 2006). It is also important to consider that, differently from the situation in the disease assays, where necrotic lesions expand gradually over time upon fungal colonization, leaf infiltration with CF samples leads to the immediate and homogeneous exposure of all leaf cells to the PCD inducing compounds. Thereby, the activity of such compounds might become more effective and cause a stronger and faster response in the infiltrated leaf tissue. The physiological processes underlying the distinct response of the Longiflorum genotype could be unraveled by comparing the protein composition of CF samples from Be9401 with those of Be0006 and Be9732, the latter two causing a milder response in Longiflorum leaves (Figure 8, second row).
The broad spectrum of susceptibility (in the lily genotypes) and virulence (in the fungal isolates) suggests that there might be multiple effectors, each of them contributing quantitatively to PCD induction. In the Parastagonospora nodorum-wheat interaction, seven distinct cell death inducing effectors have been reported, each requiring a specific plant susceptibility gene (Friesen et al., 2008; Friesen and Faris, 2010; Zhang et al., 2011; Friesen et al., 2012; Gao et al., 2015; Shi et al., 2015). Three of these susceptibility genes, Snn1 (Shi et al., 2016) and Tsn1 (Faris et al., 2010) and Snn3 (Zhang et al., 2021), have been cloned from wheat and appeared to encode proteins from distinct families of plant receptor genes that also contain many resistance genes. Recent work (Downie et al., 2018) has identified several additional QTLs in wheat germplasm that might also be involved in effector recognition to mediate susceptibility.
We hypothesize that the lily-B. elliptica interaction relies on similar effector-receptor interactions. At this point it is unknown how many effector proteins and their cognate receptors are at play in this plant–fungus interaction. The difference in susceptibility of the lily genotypes may be related to variation in the numbers and types of functional receptor proteins. In view of the huge genome size of lily, and the hybrid (diploid or triploid) nature of most genotypes, unraveling the molecular nature of such receptor genes will require a major effort. The B. elliptica effector proteins may be useful tools for mapping sensitivity genes and identify molecular markers for breeding purposes.
The difference in virulence between fungal isolates may be related to the repertoire of active PCD-inducing effector proteins that they secrete. Whether the most virulent isolates produce a different spectrum of effector proteins or possess more active allelic variants, or they produce larger quantities of effector proteins, remains to be analyzed once such effector genes are identified. Our next step will be to use mass spectrometry and identify proteins in the crude CFs that were generated in this study. The annotated genomes of two B. elliptica isolates (Valero-Jiménez et al., 2019, 2020) are instrumental in the identification of effector genes.
Perspectives for Lily Breeding
We propose that the sensitivity of lily genotypes to B. elliptica effector proteins that induce PCD is predictive of the susceptibility of that individual plant to a fungal isolate that produces such effector(s). Given the logistic and experimental challenges of performing disease assays with living pathogens in a commercial breeding setting, PCD inducing proteins might provide an attractive alternative as prediction tool for fire blight susceptibility. Testing breeding material by a simple infiltration (of pure proteins or mixtures) and monitoring cell death responses presents a fast, low-tech solution to identify the most sensitive genotypes and eliminate them from the breeding program in early stages. This offers opportunities to pursue a breeding program only with a subset of breeding lines that will display increased fire blight resistance.
Such an “effectoromics” assay has multiple advantages. First of all, screening a population of young lily plants by protein infiltration represents a non-destructive assay since the infiltrated plant survives, and there is no (natural or artificial) infection of the fungus in the precious breeding population. Effector-sensitive genotypes can be discarded in a pre-breeding stage thereby saving time and costs. Multiple repeats on different organs of the same plant can be performed to obtain a more consistent scoring for effector sensitivity. Several thousand individual plants can readily be tested in 1 day. Furthermore, the good correlation that was observed between leaf and flower susceptibility is an encouraging incentive to presume that leaf infiltration of effector proteins is also predictive for fire blight susceptibility in flower. After a cross it usually takes 2 years before new offspring plants will be able to flower and testing leaves as a proxy for flower susceptibility gains precious time and saves greenhouse space and labor enabling to extend breeding efforts.
In other pathosystems, purified effectors have been implemented as tools to screen plant germplasm to predict disease resistance traits (Wolpert et al., 2002; Faris et al., 2010; Tan et al., 2012) and make recommendations to breeders. The development of a similar procedure to identify and eliminate the fire blight susceptible genotypes in a lily breeding population will support breeders in their efforts to develop new genotypes that may thrive with lower input of fungicide applications.
Data Availability Statement
The original contributions presented in the study are included in the article/Supplementary Material, further inquiries can be directed to the corresponding author/s.
Author Contributions
JK, PA, and MM designed the study. MM and TQ conducted the experiments and statistical analyses. MM wrote the draft manuscript. JK, PA, and RI edited the final manuscript. JK, PA, and RI supervised the study. All authors contributed to the article and approved the submitted version.
Funding
This research is funded by NWO-Science domain (NWO-ENW), project GSGT.GSGT.2018.008.
Conflict of Interest
Plant material was provided by lily breeding companies. For reasons of competitiveness and publicity, the companies have requested that the names of cultivars would be anonymized in the manuscript. Other than this request, companies have neither had any influence on the research design nor on the interpretation of results, nor have they been involved in writing the manuscript.
Acknowledgments
The plant material used was kindly provided by De Jong Lelies, Hobaho, Mak Breeding, Royal van Zanten, Vletter-den Haan, and World Breeding. The authors are grateful to Rinie Verwoert, Rohan van Genderen, and Wilfred Krijnen for their essential support in the cultivation of the lilies; to Sikke van der Weg for his contribution in optimizing the disease assays in lily flowers; to Dr. Sabine Schnabel (Biometris, Wageningen University and Research) for her contribution in the statistical analysis.
Supplementary Material
The Supplementary Material for this article can be found online at: https://www.frontiersin.org/articles/10.3389/fpls.2021.660337/full#supplementary-material
Supplementary Figure 1 | (A) Fire blight symptoms observed upon and conidia inoculation of B. elliptica isolates Be9605 on leaf of lily cultivar OT-1 at 3 dpi. White dotted lines represent the chord of the ellipsoidal necrotic spots used to measure the lesion diameter. (B) Close-up of two representative necrotic lesions highlighted in the black quadrant in (A). Yellow arrows indicate area showing translucence, maceration softening and water soaking. Red arrows indicate necrotic collapsed tissue.
Supplementary Figure 2 | Symptoms observed upon mock (left) and conidia inoculation of B. elliptica isolates Be9401 and Be9732 at 2 (A), 3 (B), and 4 dpi (C) on leaves of lily cultivar OA. Red arrows indicate translucence and maceration around the necrotic spot. Black arrows indicate dark brown coloration in the leaf vasculature. Cell death response observed at 3 days post infiltration of mock liquid medium (D), Be9732 CF sample (E) and Be9401 CF sample (F) in leaves of lily genotype OA.
Supplementary Figure 3 | Lesion diameters at 4 dpi upon inoculation of 12 different B. elliptica isolates on leaves of 18 different lily genotypes in early spring 2019 and 2020 (A) and in late spring 2019 and 2020 (B). Each box represents all compiled lesion diameters measured in mm for a given lily hybrid group in the two seasonal repetitions of the disease assays.
Supplementary Figure 4 | Dot plot showing differences in EMMs of lesion diameters on lily leaves in late spring for a given B. elliptica isolate, calculated using lily hybrid group as predicted factor. Red dotted line indicates the average p-value cut off for estimated differences which are significantly different from 0 (p > 0.05). P-values were adjusted using Tukey’s comparison for a family of 84 estimates.
Supplementary Figure 5 | Lesion diameters at 3 dpi upon inoculation of B. elliptica isolates Be9401, Be9732, and Be0006 and B. cinerea isolate B05.10 on detached leaves of 12 cut lily genotypes. Lesion diameters measured in mm of each single fungus are plotted separately in the four panels. Each of the colored boxes represents all lesion diameters measured for a given lily hybrid group.
Supplementary Figure 6 | Necrotic lesions developing at 3 dpi upon inoculation of Be9401, Be9732 and Bcin on leaves of cut lily genotypes A 3, LA 2, L 1, LO 1, OT 3, and O 3 from which also the flowers were tested. For each cultivar, 12 inoculations were performed on two or three leaves detached from fresh harvested lilies.
Supplementary Figure 7 | Dot plot showing differences in EMMs of lesion diameters on lily flowers for a given Botrytis isolate calculated using lily hybrid group as predicted factor. Red dotted line indicates the average p-value cut off for estimated differences which are significantly different from 0 (p > 0.05). P-values were adjusted using Tukey’s comparison for a family of 24 estimated.
Supplementary Figure 8 | Dot plot showing differences in EMMs of lesion diameters measured on leaves of lilies for a given Botrytis isolate calculated using lily hybrid group as predicted factor. Red dotted line indicates the average p-value cut off for estimated differences which are significantly different from 0 (p > 0.05). P-values were adjusted using Tukey’s comparison for a family of 24 estimated.
Supplementary Table 1 | ANOVA results of the interaction in flowers between 12 Lilium genotypes and three B. elliptica and one B. cinerea isolates measured as lesion diameter. Analysis of Variance with Satterthwaite’s method. P-value ≤ 0.05. The experiment tests the random effect of one experiment. Terms are as follow: be, the three B. elliptica and one B. cinerea isolates tested; group, the six lily hybrid groups tested. In addition, interactions of these factors were tested (:). The degrees of freedom and p-value are shown.
Supplementary Table 2 | ANOVA results of the interaction in leaves of cut lilies between 12 Lilium genotypes and three B. elliptica and one B. cinerea isolates measured as lesion diameter. Analysis of Variance with Satterthwaite’s method. P-value ≤ 0.05. The experiment tests the random effect of one experiment. Terms are as follow: be, the three B. elliptica and one B. cinerea isolates tested; group, the six lily hybrid groups tested. In addition, interactions of these factors were tested (:). The degrees of freedom and p-value are shown.
Supplementary Material 1 | Lesion diameters measured at 4 dpi on 18 leaves of different lily genotype inoculated with 12 different B. elliptica isolates scored in the disease assays conducted in early (April–May) and late (May–June) spring 2019 and 2020. Lesion diameters measured at 3 dpi on flowers and leaves of 12 cut lilies genotypes inoculated with three B. elliptica isolates (Be9401, Be0006, and Be9732) and one B. cinerea isolate Bcin_B05.10.
Supplementary Material 2 | Results of Multiple Comparison Analysis and EEMs conducted on lesion diameters scored in the four repetition of the main disease assays in lily leaves and on lesion diameters scored in the disease assays on flowers and leaves of cut lilies.
Footnotes
References
Angiosperm Phylogeny Group (APG) (2020). KAVITHA. Available online at: http://www.mobot.org/MOBOT/research/APweb/orders/lilialesweb.htm#Liliaceae (accessed September 19, 2020).
Arens, P., Shahin, A., and van Tuyl, J. M. (2014). (Molecular) Breeding of Lilium. Acta Hortic. 1027, 113–127. doi: 10.17660/actahortic.2014.1027.12
Arzate-Fernández, A. M., Miwa, M., Shimada, T., Yonekura, T., and Ogawa, K. (2005). Genetic diversity of Miyamasukashi-yuri (Lilium maculatum Thunb. var. bukosanense), an endemic and endangered species at Mount Buko, Saitama, Japan. Plant Spec. Biol. 20, 57–65.
Balode, A. (2009). Breeding for resistance against Botrytis in lily. Acta Hortic. 836, 143–148. doi: 10.17660/actahortic.2009.836.20
Bar, M., and Ori, N. (2014). Leaf development and morphogenesis. Development. 141, 4219–4230. doi: 10.1242/dev.106195
Bates, D., Kliegl, R., Vasishth, S., and Baayen, H. (2015). Parsimonious mixed models. arXiv [Preprint] ∗∗arXiv:1506.04967,Google Scholar
Battelli, R., Lombardi, L., Rogers, H. J., Picciarelli, P., Lorenzi, R., and Ceccarelli, N. (2011). Changes in ultrastructure, protease and caspase-like activities during flower senescence in Lilium longiflorum. Plant Sci. 180, 716–725. doi: 10.1016/j.plantsci.2011.01.024
Beers, C. M., Barba-Gonzalez, R., van Silfhout, A. A., Ramanna, M. S., and van Tuyl, J. M. (2005). Mitotic and meiotic polyploidization in lily hybrids for transferring Botrytis resistance. Acta Hortic. 673, 449–452. doi: 10.17660/actahortic.2005.673.57
Boeckx, T., Winters, A. L., Webb, K. J., and Kingston-Smith, A. H. (2015). Polyphenol oxidase in leaves: is there any significance to the chloroplastic localization? J. Exp. Bot. 66, 3571–3579. doi: 10.1093/jxb/erv141
Chastagner, G. A., van Tuyl, J. M., Verbeek, M., Miller, W. B., and Westerdahl, B. B. (2017). “Diseases of Lily,” in the Handbook of Florists’ Crops Diseases and Plant Disease Management, eds R. J. McGovern and W. H. Elmer (Cham: Springer International Publishing AG).
Daughtrey, M. L., and Bridgen, M. P. (2013). Evaluating resistance responses to Botrytis elliptica in field grown lilies. Acta Hortic. 1002, 313–318. doi: 10.17660/actahortic.2013.1002.41
Doss, R. P., Chastagner, G. A., and Riley, K. L. (1986). Screening ornamental lilies for resistance to Botrytis elliptica. Sci. Hortic. 30, 237–246. doi: 10.1016/0304-4238(86)90102-0
Downie, R. C., Bouvet, L., Furuki, E., Gosman, N., Gardner, K. A., Mackay, placeI. J., et al. (2018). Assessing European wheat sensitivities to Parastagonospora nodorum necrotrophic effectors and fine-mapping the Snn3-B1 locus conferring sensitivity to the effector SnTox3. Front. Plant Sci. 9:881.
Du, Y. P., Bi, Y., Yang, F. P., Zhang, M. F., Chen, X. Q., Xue, J., et al. (2017). Complete chloroplast genome sequences of Lilium: insights into evolutionary dynamics and phylogenetic analyses. Sci. Rep. 7, 1–10.
Fang Hsieh, T., Wen, H. J., and Hsiang, T. (2001). Light and scanning electron microscopy studies on the infection of oriental lily leaves by Botrytis elliptica. Eur. J. Plant Pathol. 107, 571–581.
Faris, J. D., Overlander, M. E., Kariyawasam, G. K., Carter, A., Xu, S. S., and Liu, Z. (2020). Identification of a major dominant gene for race-nonspecific tan spot resistance in wild emmer wheat. Theor. Appl. Genet. 133, 829–841. doi: 10.1007/s00122-019-03509-8
Faris, J. D., Zhang, Z., Lu, H., Lu, S., Reddy, L., Cloutier, S., et al. (2010). A unique wheat disease resistance-like gene governs effector-triggered susceptibility to necrotrophic pathogens. Proc.Natl. Acad. Sci. USA 107, 13544–13549. doi: 10.1073/pnas.1004090107
Friesen, T. L., placeChu, C. G., Xu, S. S., and Faris, J. D. (2012). SnTox5-Snn5: a novel Stagonospora nodorum effector-wheat gene interaction and its relationship with the SnToxA-Tsn1 and SnTox3-Snn3-B1 interactions. Mol. Plant Pathol. 13, 1101–1109. doi: 10.1111/j.1364-3703.2012.00819.x
Friesen, T. L., and Faris, J. D. (2010). Characterization of the wheat-Stagonospora nodorum disease system: what is the molecular basis of this quantitative necrotrophic disease interaction? country-regionplaceCan. J. Plant Pathol. 32, 20–28. doi: 10.1080/07060661003620896
Friesen, T. L., Zhang, Z., Solomon, P. S., Oliver, R. P., and Faris, J. D. (2008). Characterization of the interaction of a novel Stagonospora nodorum host-selective toxin with a wheat susceptibility gene. Plant Physiol. 146, 682–693.
Furukawa, T., Ushiyama, K., and Kishi, K. (2005). Botrytis blight of Taiwanese toad lily caused by Botrytis elliptica (placeCityBerkeley) Cooke. J. Gen. Plant Pathol. 71, 95–97. doi: 10.1007/s10327-004-0167-y
Gao, X., Cui, Q., Cao, Q. Z., Zhao, Y. Q., Liu, Q., He, H. B., et al. (2018). Evaluation of resistance to Botrytis elliptica in Lilium hybrid cultivars. Plant. Physiol. Bioch. 123, 392–399. doi: 10.1016/j.plaphy.2017.12.025
Gao, Y., Faris, J. D., Liu, Z., Kim, Y. M., Syme, R. A., Oliver, R. P., et al. (2015). Identification and characterization of the SnTox6-Snn6 interaction in the Parastagonospora nodorum-wheat pathosystem. Mol. Plant Microbe Interact. 28, 615–625. doi: 10.1094/mpmi-12-14-0396-r
Hou, P. F., and Chen, C. Y. (2003). Early stages of infection of lily leaves by Botrytis elliptica and B. cinerea. Plant Pathol. 12, 103–108.
Hu, F., Liu, G., Hu, Y., Guo, R., Zhu, L., Luo, F., et al. (2017). Authenticity identification and leaf blight resistance evaluation of the F1 hybrids from two Lilium cultivars ‘Sorbonne’and ‘Francia’. Physiol. Mol. Plant Pathol. 100, 194–200. doi: 10.1016/j.pmpp.2017.10.005
Huang, J., Yang, L. Q., Yu, Y., Liu, Y. M., Xie, D. F., Li, J., et al. (2018). Molecular phylogenetics and historical biogeography of the tribe Lilieae (Liliaceae): bi-directional dispersal between biodiversity hotspots in placeEurasia. Ann. Bot. 122, 1245–1262.
Jang, J. Y., Subburaj, S., Lee, G. J., and Kim, H. S. (2018). In vitro screening for Botrytis leaf blight resistance in Lilium species. Sci. Hortic. 239, 133–140. doi: 10.1016/j.scienta.2018.05.009
Kamenetsky, R. (2014). Flower biology in Lilium: achievements and research challenges. Acta Hortic. 1027, 65–74. doi: 10.17660/actahortic.2014.1027.6
Kariyawasam, G. K., Carter, A. H., Rasmussen, J. B., Faris, J. D., Xu, S. S., Mergoum, M., et al. (2016). Genetic relationships between race-nonspecific and race-specific interactions in the wheat–Pyrenophora tritici-repentis pathosystem. Theor. Appl. Genet. 129, 897–908. doi: 10.1007/s00122-016-2670-x
Kim, H. T., Lim, K. B., and Kim, J. S. (2019). New insights on Lilium phylogeny based on a comparative phylogenomic study using complete plastome sequences. Plants. 8, 547. doi: 10.3390/plants8120547
Kim, J. S., and Kim, J. H. (2018). Updated molecular phylogenetic analysis, dating and biogeographical history of the lily family (Liliaceae: Liliales). Bot. J. Linn. 187, 579–593. doi: 10.1093/botlinnean/boy031
Koladia, V. M., Faris, J. D., Richards, J. K., Brueggeman, R. S., Chao, S., and Friesen, T. L. (2017). Genetic analysis of net form net blotch resistance in barley lines CIho 5791 and Tifang against a global collection of P. teres f. teres isolates. Theor. Appl. Genet. 130, 163–173. doi: 10.1007/s00122-016-2801-4
Lim, K. B., Barba-Gonzalez, R., Zhou, S., Ramanna, M. S., and Van Tuyl, J. M. (2008). “Interspecific hybridization in lily (Lilium): taxonomic and commercial aspects of using species hybrids in breeding,” in 1st Floriculture, ornamental and plant biotechnology: advances and topical issues, Vol. 5, ed. J. A. Teixeira de Silva (Isleworth: Global Science Books), 146–151.
Liu, Z., Zurn, J. D., Kariyawasam, G., Faris, J., Shi, G., Jansen, J., et al. (2017). Inverse gene-for-gene interactions contribute additively to tan spot susceptibility in wheat. Theor. Appl. Genet. 130, 1267–1276. doi: 10.1007/s00122-017-2886-4
Munafo, J. P. Jr., and Gianfagna, T. J. (2011). Antifungal activity and fungal metabolism of steroidal glycosides of Easter lily (Lilium longiflorum Thunb.) by the plant pathogenic fungus, Botrytis cinerea. J. Agr. Food Chem. 59, 5945–5954. doi: 10.1021/jf200093q
Nishikawa, T. (2007). Molecular phylogeny of the genus Lilium and its methodical application to other taxon. Ph.D. Thesis. CityTokyo: placePlaceNameTokyo PlaceTypeUniversity of Agriculture and Technology.
Nishikawa, T., CityplaceOkazaki, K., Uchino, T., Arakawa, K., and Nagamine, T. (1999). A molecular phylogeny of Lilium in the internal transcribed spacer region of nuclear ribosomal DNA. J. Mol. Evol. 49, 238–249. doi: 10.1007/pl00006546
Pelkonen, V. P., and Pirttilä, A. M. (2012). Taxonomy and phylogeny of the genus Lilium. Floriculture Orn. Biotech. 6, 1–8.
Rogers, H. J. (2013). From models to ornamentals: how is flower senescence regulated? Plant Mol. Biol. 82, 563–574. doi: 10.1007/s11103-012-9968-0
Schouten, A., Wagemakers, L., Stefanato, F. L., Kaaij, R. M., and van Kan, J. A. L. (2002). Resveratrol acts as a natural profungicide and induces self-intoxication by a specific laccase. Mol. Microbiol. 43, 883–894. doi: 10.1046/j.1365-2958.2002.02801.x
Shahin, A., Smulders, M. J., van Tuyl, J. M., Arens, P., and Bakker, F. T. (2014). Using multi-locus allelic sequence data to estimate genetic divergence among four Lilium (Liliaceae) cultivars. Frontiers in plant science. 5:567.
Shi, G., Friesen, T. L., Saini, J., Xu, S. S., Rasmussen, J. B., and Faris, J. D. (2015). The wheat Snn7 gene confers susceptibility on recognition of the Parastagonospora nodorum necrotrophic effector SnTox7. Plant Genome 8, doi: 10.3835/plantgenome2015.02.0007 ∗page,
Shi, G., Zhang, Z., Friesen, T. L., Raats, D., Fahima, T., Brueggeman, R. S., et al. (2016). The hijacking of a receptor kinase–driven pathway by a wheat fungal pathogen leads to disease. Science Advances 2, e1600822. doi: 10.1126/sciadv.1600822
Shibuya, K., Yamada, T., and Ichimura, K. (2016). Morphological changes in senescing petal cells and the regulatory mechanism of petal senescence. J. Exp. Bot. 67, 5909–5918. doi: 10.1093/jxb/erw337
Staats, M., Van Baarlen, P., and van Kan, J. A. L. (2007). AFLP analysis of genetic diversity in populations of Botrytis elliptica and Botrytis tulipae from the country-regionplaceNetherlands. Eur. J. Plant Pathol. 117, 219–235. doi: 10.1007/s10658-006-9085-5
Tan, K. C., Ferguson-Hunt, M., Rybak, K., Waters, O. D. C., Stanley, A. W., Bond, S. C., et al. (2012). Quantitative variation in effector activity of ToxA isoforms from Stagonospora nodorum and Pyrenophora tritici-repentis. Mol. Plant-Microbe Interaction. 25, 515–522. doi: 10.1094/mpmi-10-11-0273
Valero-Jiménez, C. A., Veloso, J., Staats, M., and van Kan, J. A. L. (2019). Comparative genomics of plant pathogenic Botrytis species with distinct host specificity. BMC Genomics. 20:203.
Valero-Jiménez, C., Steentjes, M., Slot, J., Shi-Kunne, X., Scholten, O., and van Kan, J. (2020). Dynamics in secondary metabolite gene clusters in otherwise highly syntenic and stable genomes in the fungal genus Botrytis. Genome Biol. Evol. 12, 2491–2507.
van Baarlen, P., Staats, M., and van Kan, J. A. L. (2004). Induction of programmed cell death in lily by the fungal pathogen Botrytis elliptica. Mol. Plant Pathol. 5, 559–574. doi: 10.1111/j.1364-3703.2004.00253.x
van Doorn, W. G., Balk, P. A., van Houwelingen, A. M., Hoeberichts, F. A., Hall, R. D., CityplaceVorst, O., et al. (2003). Gene expression during anthesis and senescence in Iris flowers. Plant Mol. Biol. 53, 845–863. doi: 10.1023/b:plan.0000023670.61059.1d
van Doorn, W. G., and Woltering, E. J. (2008). Physiology and molecular biology of petal senescence. J. Exp. Bot. 59, 453–480. doi: 10.1093/jxb/erm356
van Kan, J. A. L. (2006). Licensed to kill: the lifestyle of a necrotrophic plant pathogen. Trends Plant Sci. 11, 247–253. doi: 10.1016/j.tplants.2006.03.005
van Kan, J. A. L., Stassen, J. H., Mosbach, A., van der Lee, T. A., Faino, L., Farmer, A. D., et al. (2017). A gapless genome sequence of the fungus Botrytis cinerea. Mol. Plant Pathol. 18, 75–89. doi: 10.1111/mpp.12384
van Tuyl, J. M. (1985). Effect of temperature on bulb growth capacity and sensitivity to summer sprouting in Lilium longiflorum Thunb. Sci. Hortic. 25, 177–187. doi: 10.1016/0304-4238(85)90088-3
van Tuyl, J. M., and Arens, P. (2011). Lilium: Breeding history of the modern cultivar assortment. Acta Hort. 900, 223–230. doi: 10.17660/actahortic.2011.900.27
van Tuyl, J. M., van Diën, M. P., van Creij, M. G. M., van Kleinwee, T. C. M., Franken, J., and Bino, R. J. (1991). Application of in vitro pollination, ovary culture, ovule culture and embryo rescue for overcoming incongruity barriers in interspecific Lilium crosses. Plant Science. 74, 115–126. doi: 10.1016/0168-9452(91)90262-7
Veloso, J., and van Kan, J. A. L. (2018). Many shades of grey in Botrytis-host plant interactions. Trends Plant Sci. 23, 613–622. doi: 10.1016/j.tplants.2018.03.016
Wolpert, T. J., Dunkle, L. D., and Ciuffetti, L. M. (2002). Host-selective toxins and avirulence determinants: what’s in a name? Annu. Rev. Phytopathol. 40, 251–285.
Wolpert, T. J., and Lorang, J. M. (2016). Victoria Blight, defense turned upside down. Physiol. Mol. Plant Pathol. 95, 8–13. doi: 10.1016/j.pmpp.2016.03.006
Yamada, T., Ichimura, K., and van Doorn, W. G. (2006). DNA degradation and nuclear degeneration during programmed cell death in petals of Antirrhinum, Argyranthemum, and Petunia. J. Exp. Bot. 57, 3543–3552. doi: 10.1093/jxb/erl100
Zhang, Z., Friesen, T. L., Xu, S. S., Shi, G., Liu, Z., Rasmussen, J. B., et al. (2011). Two putatively homoeologous wheat genes mediate recognition of SnTox3 to confer effector-triggered susceptibility to Stagonospora nodorum. The Plant Journal. 65, 27–38. doi: 10.1111/j.1365-313x.2010.04407.x
Keywords: Botrytis fire blight, Lilium, effector proteins, necrotroph, programmed cell death
Citation: Malvestiti MC, Immink RGH, Arens P, Quiroz Monnens T and van Kan JAL (2021) Fire Blight Susceptibility in Lilium spp. Correlates to Sensitivity to Botrytis elliptica Secreted Cell Death Inducing Compounds. Front. Plant Sci. 12:660337. doi: 10.3389/fpls.2021.660337
Received: 29 January 2021; Accepted: 01 June 2021;
Published: 28 June 2021.
Edited by:
Paloma Melgarejo, Ministerio de Agricultura, Alimentación y Medio Ambiente, SpainReviewed by:
Zhaohui Liu, North Dakota State University, United StatesCeledonio González, University of La Laguna, Spain
Copyright © 2021 Malvestiti, Immink, Arens, Quiroz Monnens and van Kan. This is an open-access article distributed under the terms of the Creative Commons Attribution License (CC BY). The use, distribution or reproduction in other forums is permitted, provided the original author(s) and the copyright owner(s) are credited and that the original publication in this journal is cited, in accordance with accepted academic practice. No use, distribution or reproduction is permitted which does not comply with these terms.
*Correspondence: Jan A. L. van Kan, amFuLnZhbmthbkB3dXIubmw=