- 1Instituto de Recursos Naturales y Agrobiología, Spanish National Research Council (CSIC), Seville, Spain
- 2Andalusian Institute of Agricultural and Fisheries Research and Training (IFAPA) Centro Las Torres, Seville, Spain
Resistance to the defoliating pathotype of Verticillium dahliae has been evaluated in a pool of 68 wild genotypes of olive belonging to the SILVOLIVE collection. Resistance was evaluated by assessing symptom severity using a 0–4 rating scale, estimating the relative area under the disease progress curve (RAUDPC), determining the percentage of dead plants (PDP), and measuring the evolution of morphological parameters in inoculated plants over time. In addition, the density levels of V. dahliae in the stem of root-inoculated genotypes have been quantified by means of quantitative real-time PCR at 35 and 120 days after inoculation (dai). Fifteen genotypes (22%) were cataloged as resistant to V. dahliae (i.e., disease parameters did not significantly differ from those of the resistant cultivar Frantoio, or were even lower). Resistant genotypes are characterized by presenting fewer symptoms and a lower amount of V. dahliae DNA at 120 dai than at 35 dai, indicating their ability to control the disease and reduce the density of the pathogen. The rest of the evaluated genotypes showed variable levels of susceptibility. Overall analysis of all genotypes showed high correlation between symptomatology and the amount of V. dahliae DNA in the stem of inoculated genotypes at 120 dai, rather than at 35 dai. However, correlation at 120 dai was not observed in the set of resistant genotypes, suggesting that resistance to defoliating V. dahliae in olive is based on the occurrence of different mechanisms such as avoidance or tolerance. These mechanisms are valuable for designing breeding programs and for the identification of target genes and resistant rootstocks to better control Verticillium wilt in the olive grove.
Introduction
Olive (Olea europaea L) is one of the most cultivated woody crops in the world, having high socio-economic importance in all olive-producing areas (Blázquez-Martínez, 1996; Civantos, 2004). Verticillium wilt is considered the most important soilborne disease affecting olive (Bubici and Cirulli, 2011; López-Escudero and Mercado-Blanco, 2011; Tsror, 2011), causing severe losses to growers, nurseries and olive industries (Jiménez-Díaz et al., 2012). The causal agent, Verticillium dahliae Kleb., is a soilborne fungus that penetrates the roots of the host plant and colonizes its vascular system. The combined action of the fungal colonization and the defense response of the infected plant, e.g., formation of gels, gums, and tyloses (Fradin and Thomma, 2006; Yadeta and Tomma, 2013) provokes the obstruction of xylem vessels, reducing the transport of water and nutrients and producing wilt symptoms, defoliation, dieback of shoots, and the eventual death of the whole plant (Gharbi et al., 2016). In addition, V. dahliae produces mycotoxins that can seriously damage the metabolism of the plant and contribute to plant wilting (Fradin and Thomma, 2006; Luo et al., 2014). Resistance has been evaluated on the basis of external symptoms, vascular browning, and isolation of the fungus from plant tissues (López-Escudero et al., 2004; Martos-Moreno et al., 2006; Colella et al., 2008; Markakis et al., 2009; Bubici and Cirulli, 2012; García-Ruiz et al., 2014) even in naturally infected soils (Trapero et al., 2013). In addition, the qPCR quantification of V. dahliae in inoculated plants has been used to determine the resistance of different olive genotypes (Mercado-Blanco et al., 2003; Markakis et al., 2009; Jiménez-Fernández et al., 2016).
Verticillium dahliae isolates from olive can be classified into defoliating (D) and non-defoliating (ND) pathotypes, depending on the virulence and the symptoms caused in the infected plant (Schnathorst and Sibbett, 1971; Bejarano-Alcázar et al., 1996). The D pathotype is highly virulent and is able to cause the drop of leaves and the complete death of the tree (Sánchez-Hernández et al., 1998). The ND pathotype is mildly virulent and does not cause defoliation of the tree. Although ND isolates can eventually cause wilting and death of susceptible cultivars, remission of symptoms with time has been observed (López-Escudero and Mercado-Blanco, 2011). The D pathotype has progressively spread and displaced the ND pathotype in most olive cropping areas in southern Spain, severely affecting olive production yields (López-Escudero et al., 2010; Jiménez-Díaz et al., 2011, 2012; Areal and Riesgo, 2014).
Besides having a wide range of host species, including many common crops such as sunflower and cotton (Hiemstra and Harris, 1998; Pegg and Brady, 2002), V. dahliae can also asymptomatically colonize other plant species and persist in organic plant residues (Bhat and Subbarao, 1999; Pegg and Brady, 2002). In addition, the fungus is well adapted to long-term survival in soil because it can form resistant structures named microsclerotia (Wilhelm, 1955). All these properties increase the inoculum density in the soil and the dispersion of the pathogen, making the use of crop rotation an unwise strategy to control Verticillium wilt. In recent years, changes in cropping practices including drip irrigation and high-density plantings have also influenced the worsening of the phytosanitary status of olive crops (López-Escudero and Blanco-López, 2005; Villalobos et al., 2006; Pérez-Rodríguez et al., 2015). All these factors, joined to the inefficacy of chemical control, make eradication of V. dahliae a very difficult task in olive plantations (López-Escudero et al., 2004; Hegazi et al., 2012; Trapero et al., 2013; García-Ruiz et al., 2014). Because single control measures are generally ineffective, integrated approaches are frequently implemented (López-Escudero and Mercado-Blanco, 2011; Montes-Osuna and Mercado-Blanco, 2020). Cultural methods e.g., control of irrigation, avoiding rotation with susceptible crops, and pruning of branches before defoliation, can contribute to the decrease of the density of inoculum in the soil. Soil solarization, the application of organic amendments to the soil (Varo-Suárez et al., 2018), and the use of biological control agents are sustainable and promising control strategies (Areal and Riesgo, 2014; Gómez-Lama Cabanas et al., 2018; Azabou et al., 2020; Boutaj et al., 2020; Castro et al., 2020; Mulero-Aparicio et al., 2020). Preventive or curative chemical fungicides can only partially control the disease (Tjamos, 1993; Fradin and Thomma, 2006; Tsror, 2011; Gómez-Gálvez et al., 2020). Biotechnological approaches such as the preventive detection of V. dahliae by using specific and highly sensitive molecular methods such as quantitative real-time PCR (qPCR), allows the identification of both healthy plant material and free-pathogen pre-planting soils.
The use of resistant cultivars is probably the most effective and sustainable strategy for the control of Verticillium wilt disease. However, most of the commercial cultivars used in Spain (including the most used cultivars Picual, Hojiblanca, Cornicabra, and Arbequina) are susceptible or highly susceptible to V. dahliae (López-Escudero et al., 2004; Hegazi et al., 2012; Trapero et al., 2013, 2015; García-Ruiz et al., 2014). The few available resistant cultivars such as Frantoio are not used in olive production because they have lower agronomic quality. However, their use as rootstock has demonstrated to confer resistance to V. dahliae to the grafted variety (Porras-Soriano et al., 2003; Bubici and Cirulli, 2012). However, this resistance is slightly broken in soils with moderate and high inoculum density of V. dahliae (Trapero et al., 2013) or is not durable in the long term in highly infected soils (Valverde et al., 2021). Therefore, new genotypes with long-lasting resistance to V. dahliae are needed as cultivars or rootstocks for adequate control of Verticillium wilt in olive plantations. Breeding programs have been developed with the aim of identifying resistant cultivars (Arias-Calderón et al., 2015a,b,c; Serrano et al., 2021) although satisfactory planting material with appropriate agronomic traits and long-term resistance are not available yet. Several breeding cycles could be necessary to obtain new cultivars with improved agronomic performance. Finally, the use of resistant cultivars (Porras-Soriano et al., 2003; Bubici and Cirulli, 2012) or wild-olive genotypes (Arias-Calderón et al., 2015c; Jiménez-Fernández et al., 2016) as rootstocks has emerged as a feasible alternative for getting V. dahliae resistance, although a limited number of genotypes have been evaluated.
The SILVOLIVE collection comprises an extensive number of wild genotypes belonging to all the known subspecies of Olea europaea, providing a natural source of genetic variability with high potential to be used for breeding and as rootstocks of commercial varieties (Díaz-Rueda et al., 2020). When used as rootstocks, different genotypes have demonstrated to regulate morphological parameters of the grafted scion and could potentially provide the grafted cultivar with resistance to V. dahliae. Taking advantage of the genetic and phenotypic variability of the SILVOLIVE collection, our objectives were (i) to evaluate the resistance to V. dahliae of 68 artificially inoculated wild olive genotypes and three control reference cultivars, the highly susceptible Picual, the moderately susceptible Arbequina and the resistant Frantoio; (ii) to determine by qPCR technology if quantification of fungal DNA in the basal stem of inoculated plants is a reliable tool to predict resistance to V. dahliae and what is the optimal infection time to assess the degree of susceptibility according to DNA quantification (35 or 120 dai); and (iii) to go in depth into the mechanisms of resistance/tolerance based in the quantification of both fungal density and the development of symptoms at different times after inoculation.
Materials and Methods
Plant Material
Plant material consisted in 6 months-old, 20–30 cm high olive plantlets belonging to the SILVOLIVE collection, which comprises genotypes from all known subspecies of Olea europaea (europaea, laperrinei, cuspidata, cerasiformis, guanchica, and maroccana) (Díaz-Rueda et al., 2020). A total of 71 genotypes were evaluated including 68 wild-olive genotypes, and three commercial varieties (Frantoio, Arbequina, and Picual) used as highly resistant, moderately susceptible, and extremely susceptible reference controls, respectively (Table 1). Wild-olive genotypes were micropropagated from the in-vitro SILVOLIVE germplasm collection (Díaz-Rueda et al., 2020). Seedlings were cut into uninodal segments and incubated in Rugini medium (Rugini, 1984) supplemented with 1 mg/L zeatin in a growth chamber with 16 h light photoperiod (34 μM intensity with 70% red: 30% blue light-emitting diodes, LEDs) at 25 ± 2°C. For whole plant regeneration, grown shoots were transferred to rooting medium (50% strength Rugini medium) supplemented with α-naphthalacetic acid (0.8 mg/L). Rooted seedlings were acclimatized ex-vitro for 3 weeks, transplanted into 1-L pots and then grown under common greenhouse conditions (Díaz-Rueda et al., 2020).
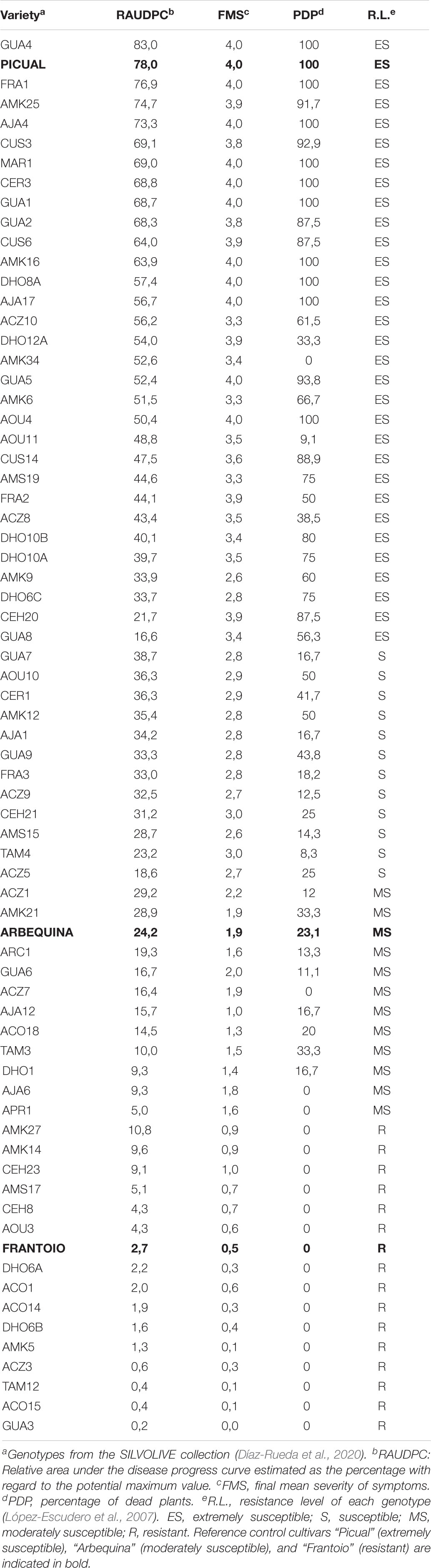
Table 1. Mean disease parameters assessed in the wild olive genotypes inoculated with the defoliating isolate VD117 of Verticillium dahliae.
Fungal Isolate and Inoculum Production
The defoliating V. dahliae pathotype VD-117, obtained from the culture collection of IFAPA research center, Córdoba (Spain) (Bejarano-Alcázar et al., 1996), was used in the inoculation tests for resistance assessment. Conidial suspensions for resistance tests were prepared by transferring five 8 mm-Potato Dextrose Agar (PDA) discs of actively growing mycelium of VD-117 isolate to flasks containing 100 mL potato dextrose broth (PDB) and incubated at 150 rpm in an orbital shaker at 24°C in the dark for 7 days. The conidial suspension was filtered through four layers of sterile cheesecloth and adjusted to 1 × 107 conidia/ml with sterile distilled water using a haemocytometer.
Inoculation of Olive Plants
For assessing resistance to V. dahliae in the 68 wild-olive genotypes (Supplementary Figure 2), four experiments were carried out over four different years: 2016, 2017, 2018, and 2019. The reference cultivars were repeated in the four trials, and a number of wild genotypes have been repeated in, at least, two different assays. Thus, ACZ1 and ACO15 have been repeated in three different assays while APR, ACZ7, ACZ9, ARC, GUA8, GUA9, CUS6, GUA5, AMK16, AMK5, AMK6, and DHO6A have been repeated in two different assays. Repetitions of both reference cultivars and wild-type genotypes in different trials and years have shown consistent results. Twenty-four plants were inoculated for each genotype. Plant roots were washed under abundant tap water to remove the substrate. Secondary roots were cut in 4–5 positions and the whole bare root system was dipped in the V. dahliae conidial suspension for 15 min. Control plants were treated by immersion of roots in PDB:sterile distilled water (1:1, v:v). Each plant was individually transplanted into 1 L pots containing sterilized 2:1 silt:peat moss (v:v). A completely randomized blocks design with four blocks and six plants (repetitions) per block for each genotype was used. Plants were maintained at 24/18°C and 60/40% relative humidity (day/night) in a greenhouse with a daily 14-h photoperiod supplemented with fluorescent illumination (360 mmol/m2). Plants were watered as needed, and fertilized weekly with Hoagland’s nutrient solution (Hoagland and Arnon, 1950). The most resistant genotypes from each pathogenicity test were re-evaluated in the following experiment, so that susceptible and highly susceptible genotypes were evaluated once, whereas moderately susceptible and resistant genotypes were evaluated twice.
Symptoms Assessment
Symptoms of aerial organs were evaluated on each plant every 2 weeks, starting 35 days after inoculation. Symptoms were registered following a 0–4 rating scale according to the percentage of Maximum Intensity Symptoms (MIS): chlorosis, leaf curl, stunting, leaf and shoot necrosis or defoliation: 0 = 0% MIS or no symptoms; 1 = 25% MIS; 2 = 50% MIS; 3 = 75% MIS; 4 = 100% MIS or dead plants.
At the end of the experiment, the following disease parameters were estimated from these scale values: (i) the relative area under the disease progress curve (RAUDPC), calculated for each cultivar considering its percentage with regard to the maximum possible value that could be reached in the period of assessment, based on the calculation formula according to (Campbell and Madden, 1990):
where Si = mean severity of the experimental unit in the observation i; △t = the number of days between observations; Smax = maximum disease rating (=4); T = experimental period in days (=120); n = number of observations; (ii) the final mean severity of symptoms (FMS), calculated according to López-Escudero et al. (2007); (iii) the percentage of dead plants (PDP) from the total of inoculated plants. Using all these parameters, the resistance level (RL) of each genotype was determined according to (López-Escudero et al., 2007): ES = extremely susceptible; S = susceptible; MS = moderately susceptible; R = resistant. In assay 3, morphological parameters such as the number of nodes, the accumulated length of branches, and the plant height of each plant were also measured over time at 0, 35, 50, 70, 85, 100, and 120 dai. The relative growth rate (RGR) was calculated as the number of new nodes per day.
Quantification of Verticillium dahliae in Plant Tissues by Real-Time PCR
Quantification of V. dahliae levels in the inoculated plants was carried out by qPCR at two times, 35 and 120 dai, using half of the inoculated plants for each time of analysis (three inoculated plants/block and four blocks, in a total of 12 plants per analyzed time). Plants were removed from the pots and two thirds of the stem, corresponding to the basal portion, was deprived of leaves, and immediately frozen at −80°C until analysis. Stems of plants from the same experimental block were grouped in a composite sample, giving rise to four biological replicates per treatment and harvesting time. Samples were ground in a mortar in presence of liquid nitrogen until getting a fine powder. Total DNA was extracted using the Isolate II Plant DNA Kit (Bioline, London, United Kingdom) following the manufacturers’ instructions. DNA concentration was accurately determined in triplicate measurements by using a fluorescent spectrophotometer (ModulusTM II Microplate Multimode reader, Turner Biosystems, United States). PCR reactions amplifying an intergenic spacer of V. dahliae previously characterized (Bilodeau et al., 2012) were performed in a 20 mL final volume, in 96-well plates in a CFX Connect thermocycler (Bio-Rad). The reaction cocktail contained 1x SensiMix (SensiMixTM Probe Kit, Bioline), 1 mM each forward and reverse primers (Bilodeau et al., 2012), 5 mM TaqMan probe labeled with 6′FAM fluorescein (Bilodeau et al., 2012), 0.1 mg/mL BSA, and 3 μL of extracted DNA adjusted to a concentration of 15 ng/mL. Amplifications were carried out at 95°C for 10 min, and 55 cycles of 15 s at 95°C and 30 s at 62°C. For normalization of DNA loading, a conserved region of the plant housekeeping cytochrome oxidase gene (cox) was amplified in a parallel qPCR reaction with the same conditions as those used for V. dahliae amplification (Garrido et al., 2009). The standard curve for V. dahliae quantification was obtained through serial dilutions of genomic DNA from V. dahliae VD-117 (10 ng to 1 fg) in a fixed background of plant DNA (20 ng/ml) obtained from healthy olive stems. V. dahliae DNA was extracted from 0.1 g VD-117 mycelium grown for 10-days in PDA medium, using the Isolate II Plant DNA Kit, as described above. The standard curve for cox quantification was obtained through serial dilutions of DNA from non-treated olive plants (10 ng to 0.1 pg). The relative amounts of V. dahliae and cox DNA in the inoculated olive plants were obtained by extrapolation of cycle threshold value from the respective standard curves through the CFX Manager software (Bio-Rad). The threshold position of the DNA standard curves generated from different plates was manually fixed at the same position for all treatments and experiments for better comparison (Vaerman et al., 2004). The efficiencies of the reactions were calculated from the slope of the standard curves according to the following formula: E = 10 (–1/slope). Four biological replicates (one DNA extractions × each block × each time) and three technical replicates (PCR reaction) of standards, samples and DNA template-free controls were used. Results were expressed as means ± standard errors of the V. dahliae:cox ratios (ng V. dahliae DNA ⋅ ng plant DNA–1), previously defined as mean normalized quantity (MNQ) in Garrido et al. (2009). Quantifications were also calculated as ng of V. dahliae DNA per ng of plant DNA, and similar quantitative results were obtained than when using cox normalization (data not shown).
Statistical Analysis and Normalization
Statistical analyses were performed with Statistix 9.0 (Analytical Software, Tallahassee, FL, United States). Data from varieties that were repeated twice were subjected to analysis of variance (ANOVA) using the assays as a factor. Where significant differences were not detected, data were pooled for the calculation of the means. For those varieties repeated with differences in their results, the chosen data was that with the highest severity. Means of FMS, RAUDPC and DNA content at 120 dai were compared between each genotype and the means of Frantoio, Arbequina, and Picual reference controls, respectively, by the LSD test at P = 0.05. Percentage data were previously transformed by arcsine (Y/100)1/2. Linear regression analyses were performed for estimating relationships between biometric parameters and FMS or RAUDPC in inoculated plants at 120 dai, respectively.
Results
Development of Symptoms in Inoculated Wild Olive Genotypes
In preliminary assays, not reported here, quantification of Verticillium DNA content and symptoms was carried out at times shorter than 120 dai. We noticed that, in order to obtain clear results on the resistance/tolerance mechanisms displayed by olive wild genotypes, both Verticillium DNA content and symptoms had to be recorder in 120 dai trials. The inoculated cultivars used as control references developed symptoms of Verticillium wilt according to their previously established RLs (Table 1, Figure 1, and Supplementary Figure 1). Thus, Picual plants presented severe wilting symptoms, showing early defoliation around 21 dai, chlorosis, leaf curl, leaf and shoot necrosis, stunting, and death. Picual plants exhibited a RAUDPC of 78.0% and reached a final mean severity (FMS) index of 4 on the 0–4 symptoms scale. Arbequina, considered as moderately susceptible, showed mild symptoms starting around 50 dai, consisting on chlorosis, moderate defoliation, leaf, and shoot necrosis. RAUDPC and FMS values in Arbequina plants were 24.2%, and 1.9, respectively. Frantoio plants behaved as resistant and displayed very slight symptoms showing occasional defoliation or leaf curl, although most of the inoculated plants did not show symptoms. Frantoio plants achieved a RAUDPC value of 2.7%, and an FMS value of 0.5. At the end of the experiment, the PDP was 100% for Picual, 23.1% for Arbequina and 0% for Frantoio varieties. RAUDPC, FMS, and PDP values were significantly different (P < 0.05) between each of the Picual, Arbequina, and Frantoio cultivars (Figure 2).
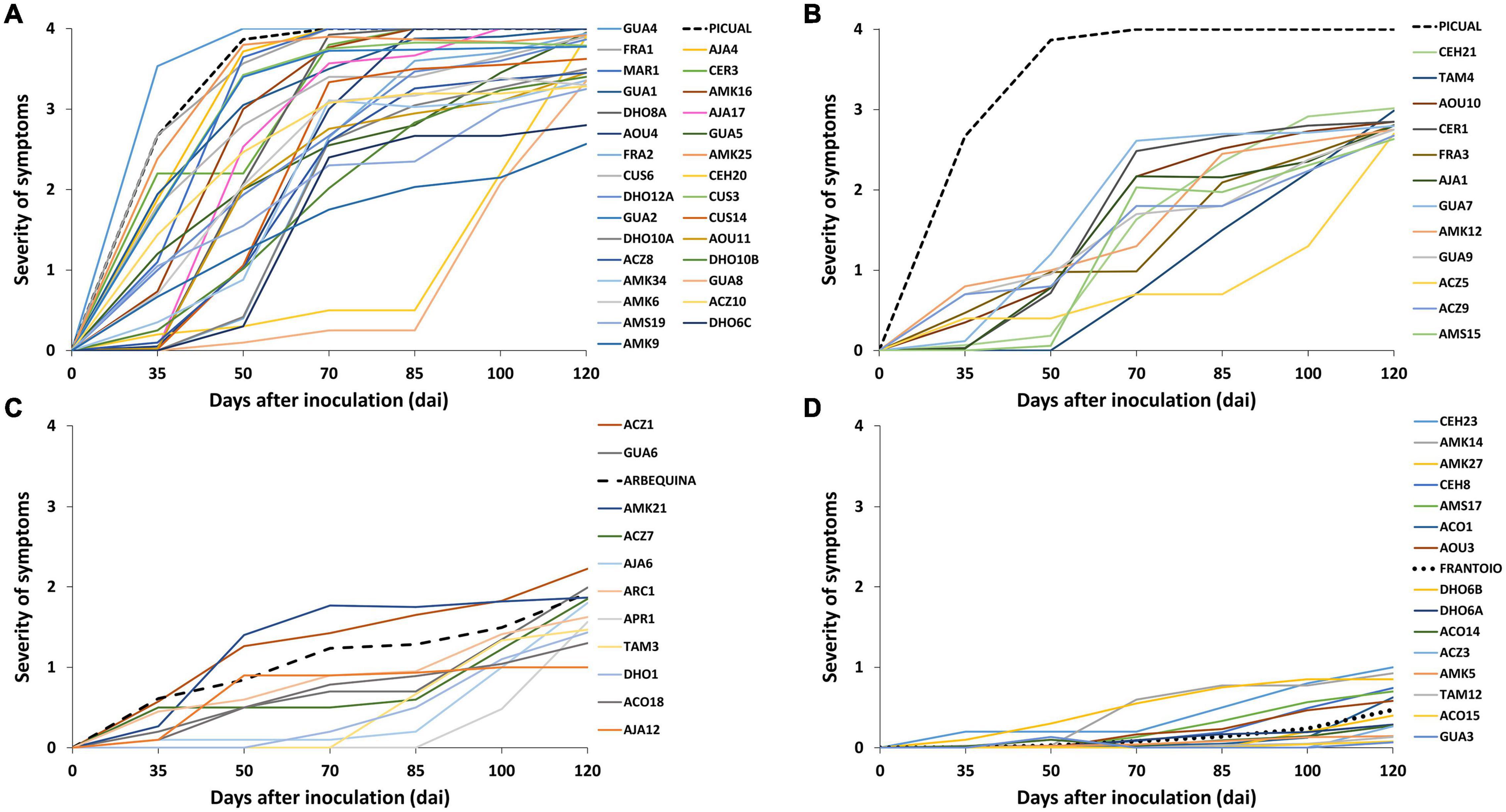
Figure 1. Progress of the severity of symptoms recorded in wild olive genotypes inoculated with the defoliating isolate VD117 of Verticillium dahliae (assays 1–4). Values are the means of 24 plants per assay. Severity of symptoms was assessed each time on a 0–4 rating scale according to the percentage of Maximum Intensity Symptoms (MIS): chlorosis, leaf and shoot necrosis or defoliation: 0 = 0% MIS or no symptoms; 1 = 25% MIS; 2 = 50% MIS; 3 = 75% MIS; 4 = 100% MIS or dead plants. (A) extremely susceptible, (B) susceptible, (C) moderately susceptible, and (D) resistant genotypes. The extremely susceptible cultivar Picual was maintained in panel 1B as a reference. Reference control cultivars Picual, Arbequina, and Frantoio are indicated in dashed lines.
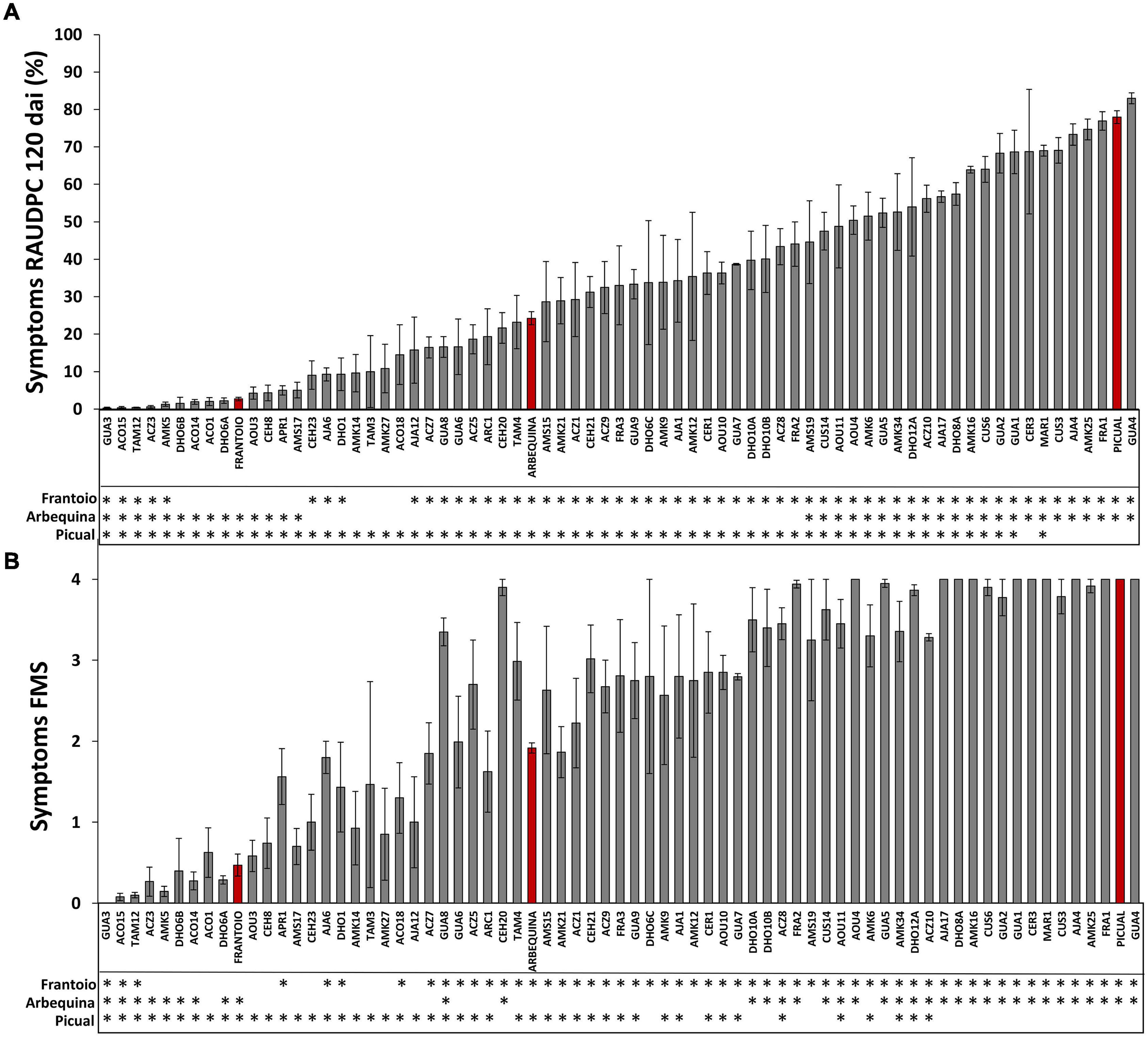
Figure 2. Symptoms of infection of olive genotypes inoculated with the Verticillium dahliae defoliating isolate VD117. The Relative Area Under the Disease Progress Curve (RAUDPC) (A) and the Final Mean Severity (FMS) (B) of all wild olive genotypes was evaluated at 120 days after inoculation (dai). Red bars correspond to the reference cultivars: the resistant Frantoio (RAUDPC < 10, FMS < 1.5 and PDP = 0); the moderately susceptible Arbequina (RAUDPC 31–50, FMS 1.5–2.5 and PDP = 0–30); and the extremely susceptible Picual (RAUDPC > 71, FMS > 3.0 and PDP > 51), according to the levels of resistance described by López-Escudero et al. (2007). Asterisks indicate significant differences with cvs. Frantoio, Arbequina, and Picual, respectively. Results are the mean of 24 plants and error bars correspond to the standard error. Data were subjected to analysis of variance (ANOVA) and multiple comparisons of means were analyzed by LSD (Least Significant Difference) at P = 0.05. Multiple range test was performed by Statistix 9.0 (Analytical Software, Tallahase, FL, United States).
A wide variability of the disease progress was observed in wild olive genotypes (Supplementary Figure 1). Wild olive genotypes and reference olive cultivars were classified into four groups of resistance (Table 1) according to the criteria defined by López-Escudero et al. (2007): extremely susceptible (Figure 1A); susceptible (Figure 1B); moderately susceptible (Figure 1C); and resistant (Figure 1D) genotypes. Thirty out of 68 wild olive genotypes assayed (44%) were extremely susceptible to the defoliating isolate VD117 of V. dahliae. With the exception of DHO12A, AMK34, AOU11, and ACZ8, all extremely susceptible genotypes showed a mortality rate of at least 50% at 120 dai (Table 1). A group of 12 genotypes (18%) exhibited RAUDPC values ranging from 18.6 to 38.7%, FMS between 2.6 and 3.0, and PDP from 8.3 to 50%, and were considered as susceptible (Table 1 and Figure 1B). Eleven genotypes (16%) showed disease parameters similar to those of Arbequina, i.e., RAUDPC values from 5.0 to 29.2%, FMS between 1.0 and 2.2, and PDP from 0 to 33.3%, and were classified as moderately susceptible (Table 1 and Figure 1C).
A group of 15 wild olive genotypes (22%) were defined as resistant and presented low RAUDPC values (0.2–10.8%), moderate or no symptoms (FMS from 0.0 to 1.0), and no dead plants (PDP 0%) (Table 1 and Figure 1D). Within this group, genotypes GUA3, ACO15, TAM12, ACZ3, and AMK5 exhibited lower symptoms than Frantoio, with significantly lower values in RAUDPC and/or FMS parameters, pointing to greater resistance to Verticillium wilt than the resistant cultivar of reference (Figure 2). The resistant genotypes DHO6B, ACO14, ACO1, DHO6A, AOU3, CEH8, AMS17, AMK14, and AMK27 showed non-significant differences with the resistant cultivar Frantoio in RAUDPC and FMS values (Figure 2).
Evolution of morphological parameters after treatment was measured in all genotypes analyzed in assay 3 (Figure 3 and Supplementary Figure 2). After inoculation, the relative growth rate was strongly inhibited in susceptible, but not in resistant genotypes (Figure 3A). Resistant genotypes inoculated with the fungus showed little or no growth inhibition when compared to their non-inoculated controls (Figure 3B). In contrast, a remarkable growth inhibition was detected in the inoculated Arbequina (Figure 3A), and full growth inhibition was observed in the inoculated Picual susceptible cultivar (Figure 3B). Production of new nodes was similar in inoculated and non-inoculated resistant genotypes compared to the scarce or null production of new nodes observed in susceptible genotypes (Supplementary Figures 2A,B, respectively). Accumulated secondary shoot length was more inhibited in susceptible than in resistant genotypes (Supplementary Figures 2C,D). This is, at least in part, a result of the reduction of the stem internodal elongation in inoculated plants (Supplementary Figures 2E,F). At the end of the experiment, average growth inhibition by Verticillium wilt (relative to non-inoculated plants), measured as production of new nodes, accumulated secondary shoot length, and plant height, was significantly lower in the group of resistant genotypes than in the moderately or extremely susceptible ones (Figure 4A). Significant negative correlations were observed between FMS and the production of new nodes (R2 = −0.8646); the accumulated secondary shoot length (R2 = −0.698); and the plant height (R2 = −0.5723), respectively (Figure 4B).
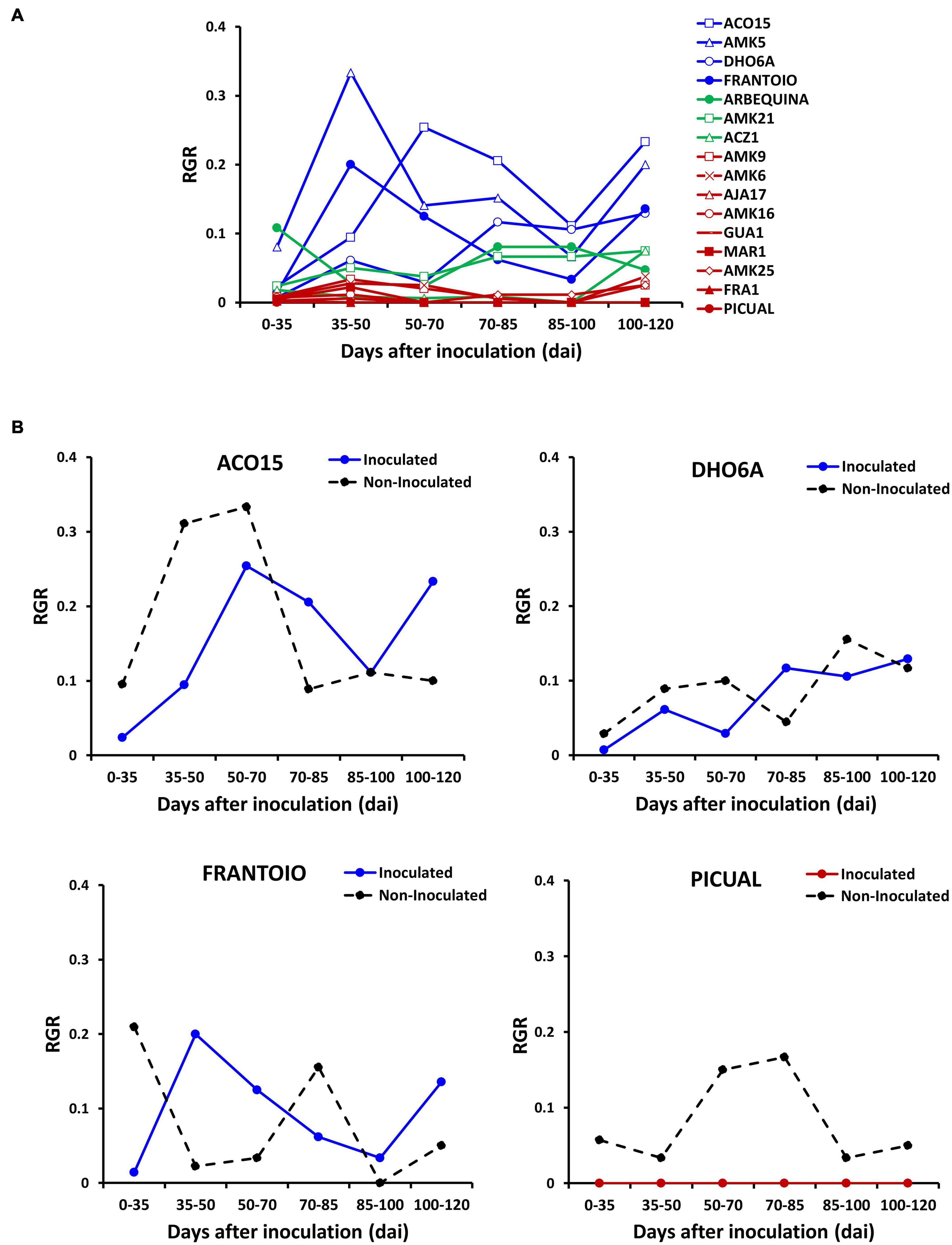
Figure 3. Relative growth rate (RGR) quantified as the production of new nodes per day in wild genotypes and cultivars of assay 3. (A) RGR values of plants inoculated with the defoliating isolate VD117 of Verticillium dahliae were compared between resistant (blue), moderately susceptible (green) and susceptible or extremely susceptible (red) genotypes. (B) Comparison of inoculated (plain lines) vs. non-inoculated (dashed black lines) RGR in resistant wild genotypes and in representative cultivars: resistant Frantoio and extremely susceptible Picual.
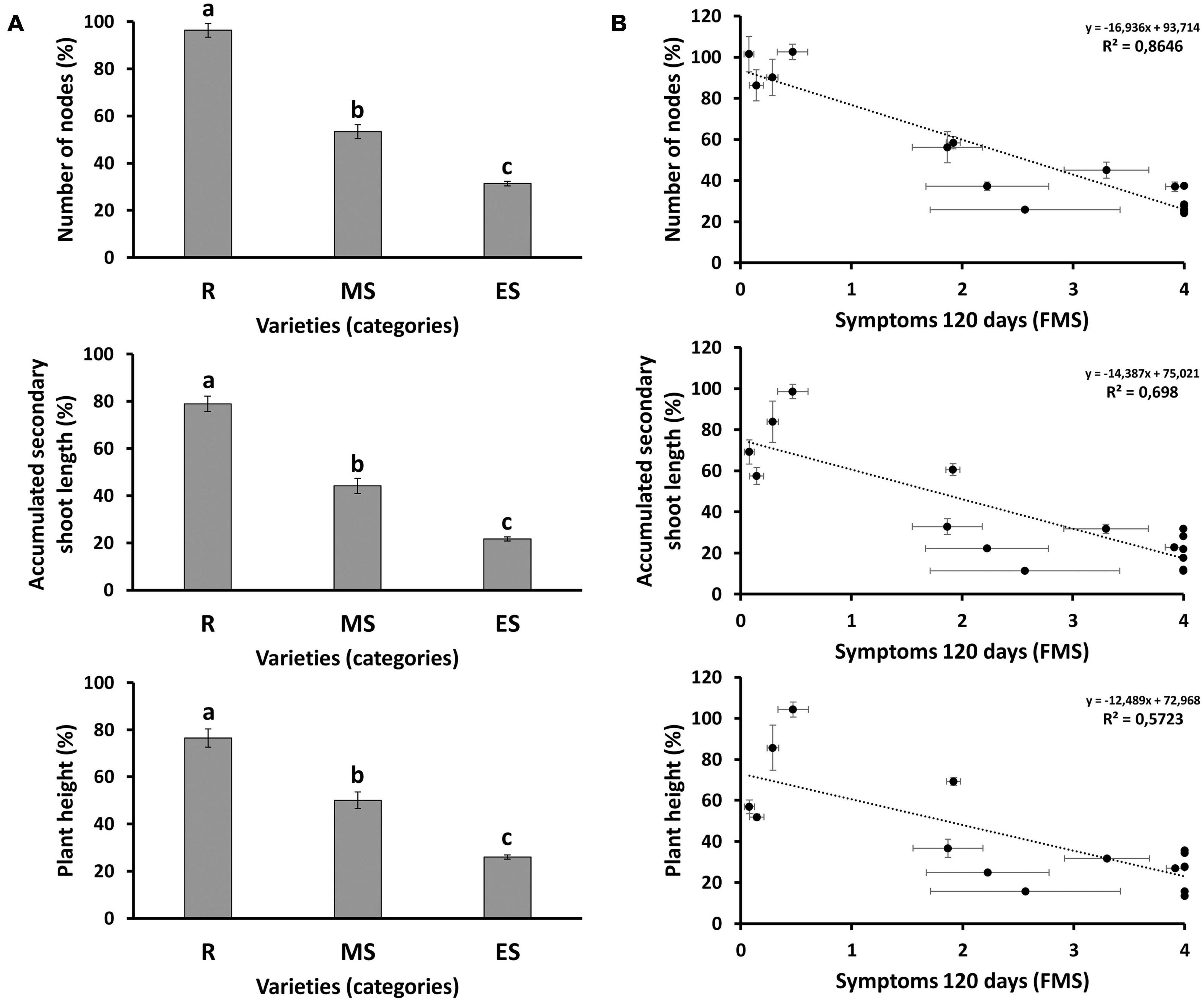
Figure 4. (A) Morphological parameters in different groups of susceptibility at 120 days after inoculation (dai). Results are given as the average value of the different genotypes included in each group of susceptibility: R, resistant genotypes; MS, moderately susceptible genotypes; ES, extremely susceptible genotypes. For each genotype, the morphological value was normalized with the average value of the respective non-inoculated plants. Letters above bars represent significant differences at P < 0.05. (B) Correlations between morphological parameters and symptoms, measured as final mean severity of symptoms (FMS) at 120 dai. Scatter plots including the regression line, the regression equation and the coefficient of determination (R2) are given for each morphological parameter: number of nodes; accumulated secondary shoot length; and plant height. Bars and dots represent the average value of 12 plants normalized with the average value of the respective non-inoculated plants for each genotype. Error bars correspond to standard errors of the mean.
Quantification of Verticillium dahliae in Inoculated Wild Olive Genotypes
The limit of detection of V. dahliae in the basal stems of inoculated plants using the TaqMan-based qPCR protocol was 15 fg of fungal DNA. No differences between the efficiency and Ct values of the standard curves were found between experiments, so that the data from experiments 1–4 could be compared. Average values of V. dahliae DNA content (MNQ) in the basal stem of the reference cultivars at 120 dai were: 3,130.93 × 10–3 in the susceptible Picual; 1,594.41 × 10–3 in the moderately susceptible Arbequina; and 0.41 × 10–3 in the resistant Frantoio (Supplementary Table 1 and Figure 5A).
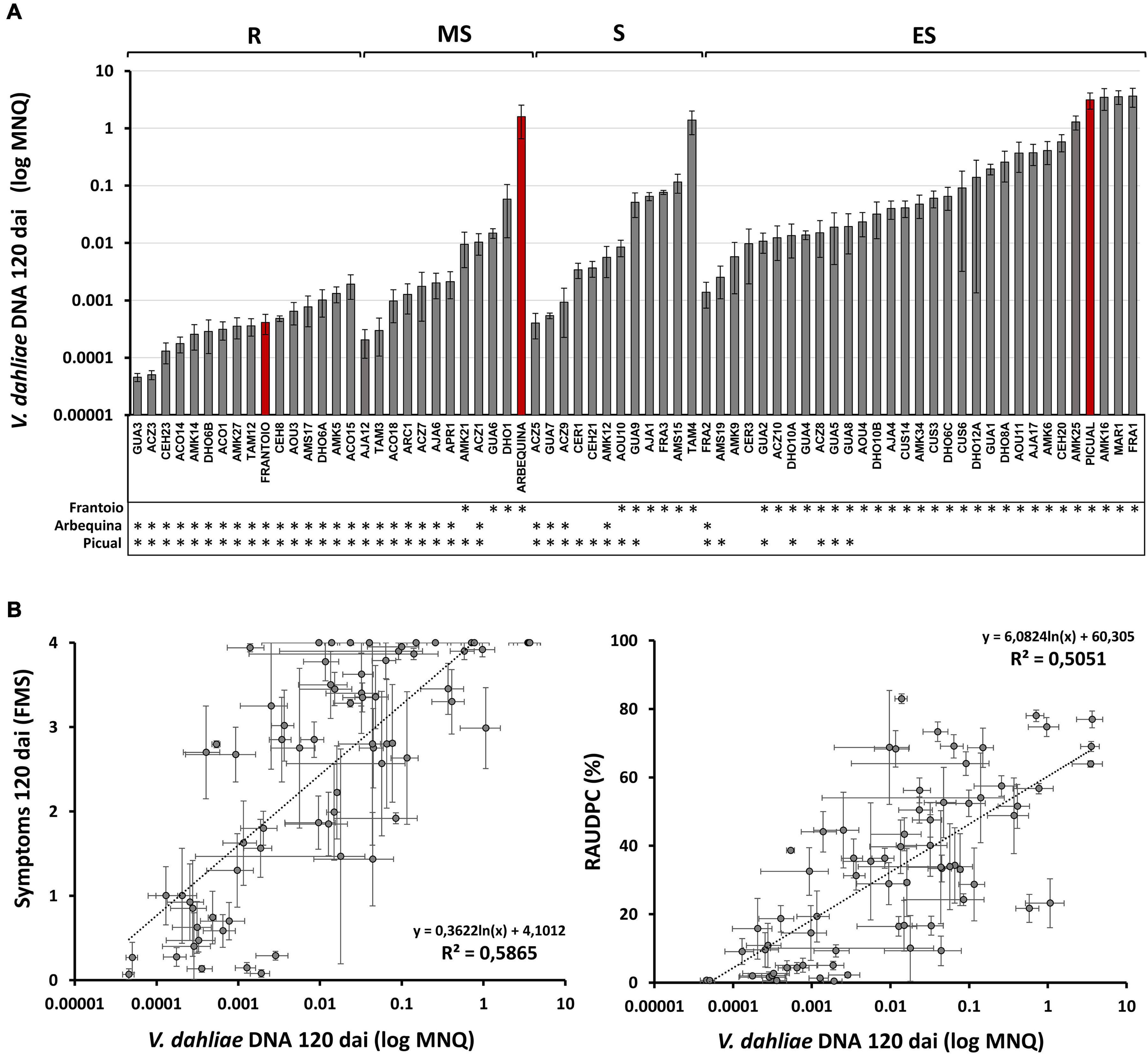
Figure 5. (A) Mean Normalized Quantity (MNQ) of Verticillium dahliae DNA of wild olive genotypes evaluated at 120 days after inoculation (dai) with the V. dahliae defoliating isolate VD117. Red bars represent cvs. Frantoio, Arbequina, and Picual as reference cultivars of resistant, moderately susceptible, and extremely susceptible genotypes, respectively, according to the resistance level described by López-Escudero et al. (2007). Asterisks indicate significant differences with cvs. Frantoio, Arbequina, and Picual, respectively. (B) Scatter plots including regression line, regression equation and coefficient of determination (R2) for the relationships between the Mean Normalized Quantity (MNQ) of V. dahliae DNA and the Final Mean Severity (FMS) (left) or the RAUDPC (right) at 120 dai. Results are the mean of 12 plants per assay, or 24 plants for those genotypes repeated in two different assays. Error bars in two dimensions indicate the standard error of the mean.
According to the classification of RLs of genotypes shown in Table 1, average MNQ values of V. dahliae DNA at 120 dai ranged as follows (Supplementary Table 1): from 3,658.79 × 10–3 (FRA1) to 1.39 × 10–3 (FRA2) in extremely susceptible genotypes; from 1,392.34 × 10–3 (TAM4) to 0.40 × 10–3 (ACZ5) in susceptible genotypes; from 58.25 × 10–3 (DHO1) to 0.2 × 10–3 (AJA12) in moderately susceptible genotypes; and from 1.90 × 10–3 (ACO15) to 0.04 × 10–3 (GUA3) in resistant genotypes (Supplementary Table 1). Therefore, the highest amounts of V. dahliae DNA were detected in extremely susceptible genotypes, whereas the lowest DNA amounts of V. dahliae DNA were detected in resistant genotypes. In the group of resistant genotypes, GUA3, ACZ3, CEH23, ACO14, AMK14, DHO6B, ACO1, AMK27, and TAM12 showed lower content of V. dahliae DNA than the resistant cultivar Frantoio at 120 dai, although differences were not statistically significant. The lowest content of V. dahliae DNA was detected in ACZ3 and GUA3 genotypes, about ten times lower than that of the resistant Frantoio.
Correlation Patterns Between Verticillium dahliae DNA Content and Plant Symptoms
In agreement with the results previously shown, a statistically significant positive linear correlation was established between the amount V. dahliae in plant shoot tissues and the plant symptoms at 120 dai, measured as FMS (R2 = 0.5865) or RAUDPC (R2 = 0.5051) (Figure 5B). This positive correlation was observed not only when the genotypes of the different assays were analyzed together (Figure 5B), but also when they were analyzed as separated assays (Supplementary Figure 3). However, in the subset of resistant genotypes, no correlation could be observed between the V. dahliae DNA content and symptoms at 120 dai calculated as RAUDPC (R2 = 0.1987) or FMS (R2 = 0.0202) (Supplementary Figure 4A). DNA of V. dahliae was also quantified at 35 dai (in assays 1, 2, and 3), but a poor correlation between the amount of V. dahliae DNA and symptoms was observed at this early period of infection (FMS R2 = 0.21; Supplementary Figure 4B). And no correlation at all was observed between V. dahliae DNA content at 35 dai and symptoms at 120 dai (FMS R2 = 0.0782; Supplementary Figure 4B), suggesting that diagnosis of Verticillium wilt based upon DNA quantification cannot be performed during an early stage of infection.
We took advantage of having available DNA content values at early and late infection periods in assays 1, 2, and 3, to study the occurrence of different evolution patterns of infection over time, and to compare it with the degree of susceptibility to the disease in the different genotypes (Figure 6A). We observed that the lack of correlation between V. dahliae DNA content and symptoms in the group of resistant genotypes was even more pronounced at 35 than at 120 dai (Supplementary Figure 5). Thus, genotypes with very low or no symptoms such as ACO15 and DHO6A, showed significantly higher V. dahliae DNA content at 35 dai than genotypes such as Frantoio or CEH23, with lower V. dahliae DNA and higher symptoms (Supplementary Figure 5 and Figure 2). The data suggest the occurrence of genotypes such as ACO15 and DHO6A that tolerate the presence of relatively high amounts of the fungus. Therefore, different response patterns in the evolution of the fungus in plant tissues after infection can be distinguished (Figure 6B). The first one (pattern 1) consisted of a significant decrease in the amount of V. dahliae DNA between 35 and 120 dai. All the resistant genotypes, and the moderately susceptible AMK21 genotype, followed this pattern (Figure 6B). Pattern 2 showed no significant variation in the quantity of V. dahliae DNA between 35 and 120 dai. This pattern included 43% of moderately susceptible, 33% of susceptible, and 15% of extremely susceptible genotypes to V. dahliae, but none of the resistant genotypes (Figure 6B). Finally, in pattern 3, a significant increase in V. dahliae DNA at 120 dai respect to 35 dai was observed, including most of extremely susceptible (85%), 67% susceptible, 43% moderately susceptible genotypes, and none of the resistant genotypes (Figure 6B). The results point to the occurrence of different mechanisms of resistance to Verticillium wilt in wild olive genotypes.
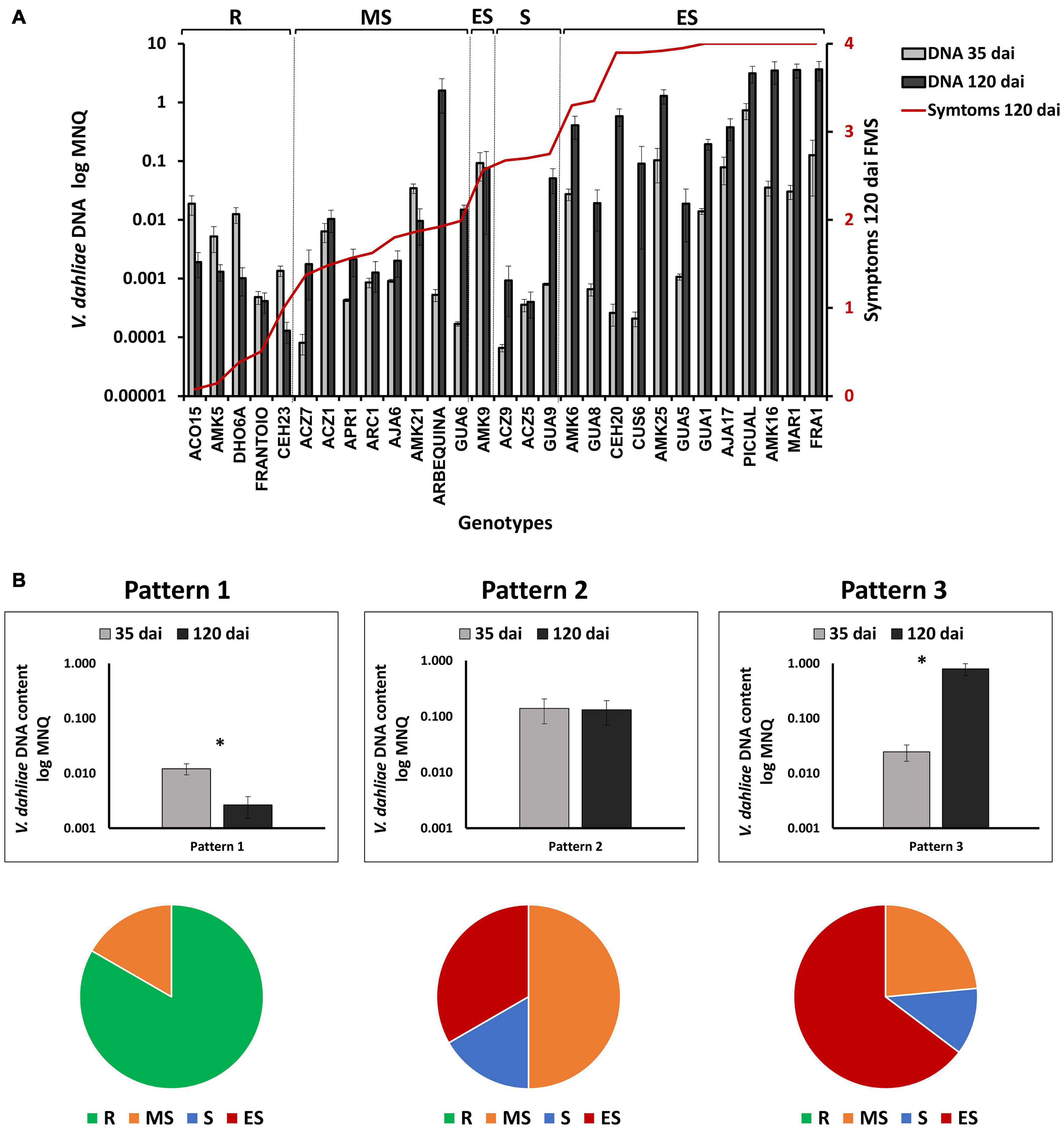
Figure 6. (A) Evolution of Verticillium dahliae content after inoculation in genotypes tested in assays 1, 2, and 3 (A) Quantification of V. dahliae DNA (Mean Normalized Quantity, MNQ) in wild olive genotypes inoculated with the defoliating isolate VD117 of V. dahliae at 35 and 120 days after inoculation (dai). Bars represent the mean of 12 plants per assay, or 24 plants for those genotypes repeated in two different assays. The red line represents the average final mean severity (FMS) at 120 dai for each genotype. (B) Patterns of variation of V. dahliae DNA quantity between 35 and 120 dai (upper diagram), and relative content of genotypes according to the resistance level described by López-Escudero et al., 2007; lower diagram) for each pattern. Asterisks indicate significant differences at P < 0.05. The data were subjected to analysis of variance (ANOVA) and multiple comparisons of means were analyzed by Tukey’s HSD (Honestly significant difference). Error bars represent the standard error of the mean. R, resistant; MS, moderately susceptible; S, susceptible; ES, extremely susceptible.
Discussion
Control of Verticillium wilt in olive is nowadays a challenge that must be addressed under an integrated management strategy (López-Escudero and Mercado-Blanco, 2011; Montes-Osuna and Mercado-Blanco, 2020), in which the search for sources of resistance should be of highest priority. Most of the commercial olive varieties domesticated for higher fruit and olive oil yields and quality are susceptible to V. dahliae. First steps in the search for resistant genotypes based on germplasm collections composed of commercial varieties, wild genotypes or breeding crosses have been addressed (Caballero and Del Río, 2008; Arias-Calderón et al., 2015c; Jiménez-Fernández et al., 2016), but still with no practical transference of results to olive production. In this work, the resistance to a defoliating isolate of V. dahliae has been evaluated for a high number of wild olive genotypes belonging to the SILVOLIVE collection. This collection is composed of individuals representative of all known subspecies of Olea europaea (europaea, cuspidata, laperrinei, cerasiformis, guanchica, and maroccana), which confers it a high diversity and genetic variability (Díaz-Rueda et al., 2020). Our results provide keys to better understand the resistance mechanisms to Verticillium wilt and a source of genotypes to be used in breeding programs or as rootstocks to improve the control of the disease in the olive grove.
Wild Olive Genotypes: A Valuable Source of Resistance to Verticillium Wilt
A wide spectrum of RLs was found among the SILVOLIVE genotypes tested (Table 1 and Figures 1, 2). This is in accordance with the variability of RLs previously described in olive cultivars, wild olive genotypes, and the offspring of breeding crosses (Colella et al., 2008; Arias-Calderón et al., 2015b; Jiménez-Fernández et al., 2016). The percentage of resistant genotypes found in our work was 22% (15 out of 68), similar to another screening of comparable dimensions that reported 23% (13 out of 55) resistant genotypes (Arias-Calderón et al., 2015c). The resistant genotypes displayed similar or even better behavior than the resistant cultivar Frantoio, i.e., delay of the disease progress, scarce wilt symptoms, lower reduction of growth parameters, and no incidence of dead plants (Table 1 and Figures 1–3). The mean content of V. dahliae DNA quantified in the stem of root-inoculated resistant genotypes was 1,647 (ACO15) to 68,043 (GUA3) times lower than that of the susceptible cultivar Picual. Other genotypes previously reported as resistant showed DNA contents that were 249–1,537 times lower than Picual (Jiménez-Fernández et al., 2016). The content of V. dahliae DNA was 837 (ACO15) to 34,565 (GUA3) times lower than that of the moderately susceptible Arbequina, which is the most widely used cultivar in super-intensive olive orchards. Four of the six subspecies of O. europaea are represented in the resistant pool of the SILVOLIVE collection (europaea, cuspidata, laperrine, and guanchica) with no relationship between resistance and olive subspecies. We have not found resistant genotypes among the subspecies maroccana and cerasiformis, probably due to the low number of genotypes assayed (MAR1 from subsp. maroccana and CER1 and CER3 from subsp. cerasiformis). These genotypes were classified as susceptible (CER3) or extremely susceptible (MAR1, CER1) to V. dahliae despite being polyploid genotypes, a characteristic previously related to resistance to abiotic and biotic stress in plants (Sattler et al., 2016; Ruiz et al., 2020; Russo et al., 2020).
The presence of genotypes that showed significant higher RLs than Frantoio is remarkable (Figure 2). The resistant genotype GUA3 belongs to the subspecies guanchica (Canary Islands, Spain). Progenies of this subspecies have been previously reported as resistant to the D pathotype of V. dahliae (Arias-Calderón et al., 2015b). However, most of the guanchica genotypes assayed in our study were classified as susceptible (GUA7 and GUA9) or extremely susceptible (GUA1, GUA2, GUA4, GUA5, GUA7, and GUA8), indicating that guanchica subspecies include genotypes with different levels of resistance to the fungus.
Verticillium dahliae inoculation did not significantly inhibit the relative growth rate of resistant genotypes (Figure 3). Most susceptible genotypes showed severe reduction of the internodal length (Supplementary Figure 2E), probably as a result of impaired cell elongation. This phenomenon may be due to the loss of hydraulic conductivity in infected plants as a consequence of vascular occlusion by accumulation of defense metabolites such as tyloses and gels (Yadeta et al., 2013; Gharbi et al., 2017) or by cavitation of xylem vessels (Pouzoulet et al., 2014; Trapero et al., 2018).
Strategies for the Control of Verticillium Wilt in Resistant Genotypes
Different patterns of V. dahliae DNA at 35 and 120 dai vs. different degree of symptoms developed by wild genotypes, point to the occurrence of different mechanisms of resistance to Verticillium wilt. On the one hand, we propose that genotypes with relatively high content of V. dahliae at 35 dai and low level at 120 dai (e.g., ACO15, AMK5, and DHO6A) tolerate moderate infection levels at the short term, and control the infection at the medium- and long-term, reducing the amount of fungus in the plant tissues and exhibiting minimal symptoms (Figures 2, 3). On the other hand, genotypes like Frantoio and CEH23 maintained low V. dahliae DNA levels at 35 and 120 dai, suggesting a more effective disease avoidance ability, since they prevent the fungus to proliferate in the shorter and longer terms. The occurrence of the two resistance mechanisms, tolerance and avoidance, may be the reason explaining the lack of correlation between the content of V. dahliae DNA and symptoms in the pool of resistant genotypes (Supplementary Figure 5). Anyway, it is clearly stated here the fact that resistant genotypes limit the spread of V. dahliae. Thus, a significant decrease in the amount of fungus DNA at 120 days compared to 35 days is observed in most resistant plants, a phenomenon that did not occur in non-resistant genotypes.
Different physiological, cellular and molecular mechanisms of resistance have been proposed in V. dahliae resistant genotypes (Gómez-Lama Cabanas et al., 2015; Trapero et al., 2018): the reinforcement of the cell wall by deposition of lignin and suberin at the site of infection (Gharbi et al., 2017); the production of reactive oxygen species such as H2O2 (Gharbi et al., 2017); and the early activation of plant defense mechanisms (Gharbi et al., 2016), such as the induction of genes coding for chitinases and β-1,3-glucanase to degrade the pathogen cell wall. Elucidating what type of molecular mechanisms determines the tolerance vs. the avoidance response to Verticillium wilt is of prime interest.
Potential Use of Genotypes as Resistant Rootstocks
Therefore, grafting susceptible cultivars of economic relevance, such as Picual and Arbequina, onto resistant genotypes is a necessary approach to identify the most convenient strategy of resistance. From the resistance mechanisms previously proposed, tolerance to V. dahliae would be optimal to be implemented in cultivars through breeding programs, while the disease avoidance would be optimal for rootstocks. Thus, although exhibiting minimal symptoms, the tolerance strategy of ACO15, AMK5, and DHO6A may have the disadvantage of allowing the fungus to proliferate and reach the grafted scion during the early infection period. From this perspective, the strategy of minimizing the proliferation of the fungus in the rootstock, represented by the resistant Frantoio and CEH23 genotypes, could be more appropriate. We are currently conducting these assays with grafted plants to clarify these points.
These and other resistant genotypes can be used as rootstocks to improve Verticillium wilt resistance in the grafted plant as previously shown (Bubici and Cirulli, 2012; Porras-Soriano et al., 2003). Furthermore, other traits previously characterized in these genotypes make them of special interest for their potential use as rootstocks. Thus, ACO15, AMK5, and DHO6A (europaea subspecies, Marrakech, Morocco), ACZ3 (europaea subspecies, Cádiz Mountains, Spain) and TAM12 (europaea subspecies, Tamri, Morocco) were classified as very low to intermediate vigor and high branching genotypes. Vigor reduction is a desirable trait in genotypes to be used as rootstocks for high and super high-density hedgerow orchards, a trait that can be transmitted to the grafted scion (Díaz-Rueda et al., 2020). It may be also the case of high branching, which means increased canopy density and high number of potential fruiting sites.
DNA Quantification of Verticillium dahliae as a Tool for Diagnosis of Verticillium Wilt
In planta quantification of V. dahliae DNA through TaqMan qPCR technology allowed specific detection and accurate quantification of the pathogen in this work, as previously shown (Mercado-Blanco et al., 2003; Gramaje et al., 2013; Jiménez-Fernández et al., 2016). DNA of V. dahliae was detected in all genotypes assayed, including the highly resistant ones, demonstrating that the fungus penetrated the root and colonized the stem of the plant. The lack of correlation between V. dahliae DNA at 35 dai and plant symptoms indicates that a screening of resistant genotypes cannot be performed at early infection times (e.g., 35 dai). For instance, some genotypes behaved as resistant up to at least 85 dai, after which they suffered an abrupt increase in symptoms and V. dahliae DNA content, which determined them to be finally classified as extremely susceptible (Figure 1A). This indicates that the plants can prevent proliferation of the fungus for a time, after which the barriers of resistance are overcome, and the disease eventually develops. This is in line with results showing that disease symptoms can appear long after planting olive cultivars in naturally infected soils (Trapero et al., 2013; Valverde et al., 2021). To postulate resistant genotypes as useful for the control of Verticillium wilt, it is necessary to assess the resistance of susceptible cultivars grafted on the resistant wild genotypes identified in this work. In this regard, we are currently evaluating the resistance of commercial cultivars grafted on different wild olive genotypes that have demonstrated resistance to the disease. Field evaluation in naturally infected soils is also needed to test the long-term persistence of the resistance trait.
In conclusion, we have identified 15 wild genotypes displaying similar or better resistance to Verticillium wilt than the resistant cultivar Frantoio. Measurement of Verticillium DNA content at early and late stages of infection, together with correlations with plant symptoms, made it possible to identify specific patterns of response in wild olive genotypes, pointing to the occurrence of different strategies of resistance to Verticillium wilt, such as avoidance and tolerance mechanisms. Therefore, this work represents a valuable source of resistant genotypes to be used as rootstocks and in breeding programs. Our findings contribute to the improvement of an integrated, effective, and sustainable strategy for optimal control of Verticillium wilt in the olive grove.
Data Availability Statement
The raw data supporting the conclusions of this article will be made available by the authors when required, without undue reservation.
Author Contributions
PD-R participated in all experimental tasks, particularly in plant material production, DNA quantification by qPCR and data analysis, as well as in writing the manuscript. AA contributed in the inoculation of the plants and participated in data analysis. LR-C contributed in the inoculation of the plants and DNA quantification by qPCR. NC designed the experiments, participated in inoculation of the plants, and wrote the manuscript. JC-F conceived the project, obtained the funds for its financing, and supervised the experiments and the manuscript. All authors contributed to the article and approved the submitted version.
Funding
This work has been funded through the CSIC/EU-FEDER RECUPERA-2020 project (Ref. 20134R089), the IFAPA contract CAICEM 15-02 and the Spanish National Research Council “Proyectos Intramurales” CSIC-201640E069, CSIC-201740E041, CSIC-201940E077, and CSIC-202040E057.
Conflict of Interest
The authors declare that the research was conducted in the absence of any commercial or financial relationships that could be construed as a potential conflict of interest.
Acknowledgments
We would like to thank Viveros Sevilla S. L. for supplying plant material and for the use of their greenhouse facilities. We would also like to thank the technical assistance of Francisco Durán, Pilar Alcántara, Miriam Pérez, Procopio Peinado, Álvaro F. García, Carlos Rivero, and Antonella Pisanu.
Supplementary Material
The Supplementary Material for this article can be found online at: https://www.frontiersin.org/articles/10.3389/fpls.2021.662060/full#supplementary-material
References
Areal, F., and Riesgo, L. (2014). Farmers’ views on the future of olive farming in Andalusia, Spain. Land Use Policy 36, 543–553. doi: 10.1016/j.landusepol.2013.10.005
Arias-Calderón, R., Leon, L., Bejarano-Alcazar, J., Belaj, A., de la Rosa, R., and Rodriguez-Jurado, D. (2015a). Resistance to Verticillium wilt in olive progenies from open-pollination. Sci. Hortic. 185, 34–42. doi: 10.1016/j.scienta.2015.01.015
Arias-Calderón, R., Rodriguez-Jurado, D., Bejarano-Alcazar, J., Belaj, A., de la Rosa, R., and Leon, L. (2015b). Evaluation of Verticillium wilt resistance in selections from olive breeding crosses. Euphytica 206, 619–629. doi: 10.1007/s10681-015-1463-7
Arias-Calderón, R., Rodriguez-Jurado, D., Leon, L., Bejarano-Alcazar, J., de la Rosa, R., and Belaj, A. (2015c). Pre-breeding for resistance to Verticillium wilt in olive: fishing in the wild relative gene pool. Crop Prot. 75, 25–33. doi: 10.1016/j.cropro.2015.05.006
Azabou, M. C., Gharbi, Y., Medhioub, I., Ennouri, K., Barham, H., Tounsi, S., et al. (2020). The endophytic strain Bacillus velezensis OEE1: an efficient biocontrol agent against Verticillium wilt of olive and a potential plant growth promoting bacteria. Biol. Control 142:104168. doi: 10.1016/j.biocontrol.2019.104168
Bejarano-Alcázar, J., Blanco-Lopez, M. A., Melero-Vara, J. M., and Jiménez-Díaz, R. M. (1996). Etiology, importance, and distribution of Verticillium wilt of cotton in southern Spain. Plant Dis. 80, 1233–1238. doi: 10.1094/pd-80-1233
Bhat, R. G., and Subbarao, K. V. (1999). Host range specificity in Verticillium dahliae. Phytopathology 89, 1218–1225. doi: 10.1094/phyto.1999.89.12.1218
Bilodeau, G., Koike, S., Uribe, P., and Martin, F. (2012). Development of an assay for rapid detection and quantification of Verticillium dahliae in soil. Phytopathology 102, 331–343. doi: 10.1094/PHYTO-05-11-0130
Blázquez-Martínez, J. (1996). Origine e Diffusione Della Coltivazione. Enciclopedia Mondiale dell’olivo. Madrid: COI.
Boutaj, H., Chakhchar, A., Meddich, A., Wahbi, S., El Alaoui-Talibi, Z., Douira, A., et al. (2020). Bioprotection of olive tree from Verticillium wilt by autochthonous endomycorrhizal fungi. J. Plant Dis. Prot. 127, 349–357. doi: 10.1007/s41348-020-00323-z
Bubici, G., and Cirulli, M. (2011). “Verticillium wilt of olives,” in Olive Diseases and Disorders, eds L. Schena, G. E. Agosteo, and S. O. Cacciola (Kerala: Research Signpost).
Bubici, G., and Cirulli, M. (2012). Control of Verticillium wilt of olive by resistant rootstocks. Plant Soil 352, 363–376. doi: 10.1007/s11104-011-1002-9
Caballero, J. M., and Del Río, C. (2008). “The olive world germplasm bank of Spain,” in Proceedings of the 15th International Symposium on Olive Growing, Lovaina, 31–38. doi: 10.17660/actahortic.2008.791.1
Campbell, C., and Madden, L. (1990). Introduction to Plant Disease Epidemiology. New York, NY: John Wiley & Sons.
Castro, D., Torres, M., Sampedro, I., Martinez-Checa, F., Torres, B., and Bejar, V. (2020). Biological control of Verticillium Wilt on olive trees by the salt-tolerant strain bacillus velezensis XT1. Microorganisms 8:1080. doi: 10.3390/microorganisms8071080
Civantos, L. (2004). “La olivicultura en el mundo y en España,” in El Cultivo del Olivo, eds D. Barranco, R. Fernández Escobar, and L. Rallo (Madrid: Ediciones Mundi-Prensa), 19–35.
Colella, C., Miacola, C., Amenduni, M., D’Amico, M., Bubici, G., and Cirulli, M. (2008). Sources of Verticillium wilt resistance in wild olive germplasm from the Mediterranean region. Plant Pathol. 57, 533–539. doi: 10.1111/j.1365-3059.2007.01785.x
Díaz-Rueda, P., Franco-Navarro, J., Messora, R., Espartero, J., Rivero-Núñez, C., Aleza, P., et al. (2020). SILVOLIVE, a germplasm collection of wild subspecies with high genetic variability as a source of rootstocks and resistance genes for olive breeding. Front. Plant Sci. 11:629. doi: 10.3389/fpls.2020.00629
Fradin, E. F., and Thomma, B. (2006). Physiology and molecular aspects of Verticillium wilt diseases caused by V-dahliae and V-albo-atrum. Mol. Plant Pathol. 7, 71–86. doi: 10.1111/j.1364-3703.2006.00323.x
García-Ruiz, G. M., Trapero, C., Del Rio, C., and Lopez-Escudero, F. J. (2014). Evaluation of resistance of Spanish olive cultivars to Verticillium dahliae in inoculations conducted in greenhouse. Phytoparasitica 42, 205–212. doi: 10.1007/s12600-013-0353-6
Garrido, C., Carbu, M., Fern ndez-Acero, F. J., Boonham, N., Colyer, A., Cantoral, J. M., and Budge, G. (2009). Development of protocols for detection of Colletotrichum acutatum and monitoring of strawberry anthracnose using real-time PCR. Plant Pathol. 58, 43–51. doi: 10.1111/j.1365-3059.2008.01933.x
Gharbi, Y., Barkallah, M., Bouazizi, E., Cheffi, M., Gdoura, R., and Triki, M. A. (2016). Differential fungal colonization and physiological defense responses of new olive cultivars infected by the necrotrophic fungus Verticillium dahliae. Acta Physiol. Plant 38:242.
Gharbi, Y., Barkallah, M., Bouazizi, E., Gdoura, R., and Triki, M. A. (2017). Differential biochemical and physiological responses of two olive cultivars differing by their susceptibility to the hemibiotrophic pathogen Verticillium dahliae. Physiol. Mol. Plant Pathol. 97, 30–39. doi: 10.1016/j.pmpp.2016.12.001
Gómez-Gálvez, F. J., Vega-Macias, V., Hidalgo-Moya, J. C., Hidalgo-Moya, J. J., and Rodriguez-Jurado, D. (2020). Application to soil of disinfectants through irrigation reduces Verticillium dahliae in the soil and Verticillium wilt of olive. Plant Pathol. 69, 272–283. doi: 10.1111/ppa.13114
Gómez-Lama Cabanas, C., Ruano-Rosa, D., Legarda, G., Pizarro-Tobias, P., Valverde-Corredor, A., Carlos Trivino, J., et al. (2018). Bacillales members from the olive rhizosphere are effective biological control agents against the defoliating pathotype of Verticillium dahliae. Agriculture 8:90. doi: 10.3390/agriculture8070090
Gómez-Lama Cabanas, C., Schiliro, E., Valverde-Corredor, A., and Mercado-Blanco, J. (2015). Systemic responses in a tolerant olive (Olea europaea L.) cultivar upon root colonization by the vascular pathogen Verticillium dahliae. Front. Microbiol. 6:928. doi: 10.3389/fmicb.2015.00928
Gramaje, D., Perez-Serrano, V., Montes-Borrego, M., Navas-Cortes, J. A., Jiménez-Díaz, R. M., and Landa, B. B. (2013). A Comparison of real-time pcr protocols for the quantitative monitoring of asymptomatic olive infections by Verticillium dahliae pathotypes. Phytopathology 103, 1058–1068. doi: 10.1094/phyto-11-12-0312-r
Hegazi, E., Hegazi, A., and Allatif, M. (2012). Resistence of olive cultivars to Verticillium wilt. J. Appl. Sci. Res. 8, 2758–2765.
Hiemstra, J., and Harris, D. (1998). A Compendium of Verticillium Wilt in Tree Species. Wageningen: Ponsen & Looije.
Hoagland, D., and Arnon, D. (1950). The Water Culture Method for Growing Plants Without Soils. Berkeley, CA: Agricultura Expermental Station.
Jiménez-Díaz, R. M., Cirulli, M., Bubici, G., del Mar Jimenez-Gasco, M., Antoniou, P. P., and Tjamos, E. C. (2012). Verticillium Wilt, a major threat to olive production: current status and future prospects for its management. Plant Dis. 96, 304–329. doi: 10.1094/pdis-06-11-0496
Jiménez-Díaz, R. M., Olivares-Garcia, C., Landa, B. B., del Mar Jiménez-Gasco, M., and Navas-Cortes, J. A. (2011). Region-wide analysis of genetic diversity in Verticillium dahliae populations infecting olive in southern Spain and agricultural factors influencing the distribution and prevalence of vegetative compatibility groups and pathotypes. Phytopathology 101, 304–315. doi: 10.1094/phyto-07-10-0176
Jiménez-Fernández, D., Trapero-Casas, J. L., Landa, B. B., Navas-Cortes, J. A., Bubici, G., Cirulli, M., et al. (2016). Characterization of resistance against the olive-defoliating Verticillium dahliae pathotype in selected clones of wild olive. Plant Pathol. 65, 1279–1291.
López-Escudero, F., and Mercado-Blanco, J. (2011). Verticillium wilt of olive: a case study to implement an integrated strategy to control a soil-borne pathogen. Plant Soil 344, 1–50. doi: 10.1007/s11104-010-0629-2
López-Escudero, F., Mercado-Blanco, J., Manuel Roca, J., Valverde-Corredor, A., and Angel Blanco-Lopez, M. (2010). Verticillium wilt of olive in the Guadalquivir Valley (southern Spain): relations with some agronomical factors and spread of Verticillium dahliae. Phytopathol. Mediterr. 49, 370–380.
López-Escudero, F. J., and Blanco-López, M. A. (2005). Recovery of young olive trees from Verticillium dahliae. Eur. J. Plant Pathol. 113, 367–375. doi: 10.1007/s10658-005-3145-0
López-Escudero, F. J., del Rio, C., Caballero, J. M., and Blanco-López, M. A. (2004). Evaluation of olive cultivars for resistance to Verticillium dahliae. Eur. J. Plant Pathol. 110, 79–85. doi: 10.1023/b:ejpp.0000010150.08098.2d
López-Escudero, F. J., Mwanza, C., and Blanco-Lopez, M. A. (2007). Reduction of Verticillium dahliae microsclerotia viability in soil by dried plant residues. Crop Prot. 26, 127–133. doi: 10.1016/j.cropro.2006.04.011
Luo, X., Xie, C., Dong, J., Yang, X., and Sui, A. (2014). Interactions between Verticillium dahliae and its host: vegetative growth, pathogenicity, plant immunity. Appl. Microbiol. Biotechnol. 98, 6921–6932. doi: 10.1007/s00253-014-5863-8
Markakis, E. A., Tjamos, S. E., Antoniou, P. P., Paplomatas, E. J., and Tjamos, E. C. (2009). Symptom development, pathogen isolation and real-time QPCR quantification as factors for evaluating the resistance of olive cultivars to Verticillium pathotypes. Eur. J. of Plant Pathol. 124, 603–611. doi: 10.1007/s10658-009-9447-x
Martos-Moreno, C., Lopez-Escudero, F. J., and Blanco-Lopez, M. A. (2006). Resistance of olive cultivars to the defoliating pathotype of Verticillium dahliae. Hortscience 41, 1313–1316. doi: 10.21273/hortsci.41.5.1313
Mercado-Blanco, J., Collado-Romero, M., Parrilla-Araujo, S., Rodriguez-Jurado, D., and Jiménez-Díaz, R. M. (2003). Quantitative monitoring of colonization of olive genotypes by Verticillium dahliae pathotypes with real-time polymerase chain reaction. Physiol. Mol. Plant Pathol. 63, 91–105. doi: 10.1016/j.pmpp.2003.10.001
Montes-Osuna, N., and Mercado-Blanco, J. (2020). Verticillium wilt of olive and its control: what did we learn during the last decade? Plants 9:735. doi: 10.3390/plants9060735
Mulero-Aparicio, A., Varo, A., Agusti-Brisach, C., Lopez-Escudero, F. J., and Trapero, A. (2020). Biological control of Verticillium wilt of olive in the field. Crop Prot. 128:104993. doi: 10.1016/j.cropro.2019.104993
Pérez-Rodríguez, M., Alcantara, E., Amaro, M., Serrano, N., Lorite, I. J., Arquero, O., et al. (2015). The influence of irrigation frequency on the onset and development of Verticillium wilt of olive. Plant Dis. 99, 488–495. doi: 10.1094/pdis-06-14-0599-re
Porras-Soriano, A., Soriano Martin, M. L., and Porras Piedra, A. (2003). Grafting olive cv. Cornicabra on rootstocks tolerant to Verticillium dahliae reduces their susceptibility. Crop Protection 22, 369–374. doi: 10.1016/S0261-2194(02)00180-1
Pouzoulet, J., Pivovaroff, A., Santiago, L., and Rolshausen, P. (2014). Can vessel dimension explain tolerance toward fungal vascular wilt diseases in woody plants? Lessons from Dutch elm disease and esca disease in grapevine. Front. Plant Sci. 5:253. doi: 10.3389/fpls.2014.00253
Rugini, E. (1984). In vitro-propagation of some olive (Olea europaea sativa L.) cultivars with different root-ability, and medium development using analytical data from developing shoots and embryos. Sci. Hortic. 24, 123–134. doi: 10.1016/0304-4238(84)90143-2
Ruiz, M., Oustric, J., Santini, J., and Morillon, R. (2020). Synthetic Polyploidy in Grafted Crops. Front. Plant Sci. 11:540894. doi: 10.3389/fpls.2020.540894
Russo, R., Caruso, M., Arlotta, C., Lo Piero, A. R., Nicolosi, E., and Di Silvestro, S. (2020). Identification of field tolerance and resistance to mal secco disease in a citrus germplasm collection in sicily. Agronomy 10:1806. doi: 10.3390/agronomy10111806
Sánchez-Hernández, M., Ruiz-Dávila, A., Pérez de Algaba, A., Blanco-López, M., and Trapero-Casas, A. (1998). Occurrence and etiology of death of young olive trees in southern Spain. Eur. J. Plant Pathol. 104, 347–357.
Sattler, M. C., Carvalho, C. R., and Clarindo, W. R. (2016). The polyploidy and its key role in plant breeding. Planta 243, 281–296. doi: 10.1007/s00425-015-2450-x
Schnathorst, W., and Sibbett, G. (1971). Relation of strains of Verticillium albo-atrum to severity of Verticillium wilt in Gossypium hirsutum and Olea europaea in California. Plant Dis. Rep. 55:780.
Serrano, A., Rodriguez Jurado, M. D., Roman, B., Bejarano-Alcazar, J., de la Rosa, R., and Leon, L. (2021). Verticillium wilt evaluation of olive breeding selections under semi-controlled conditions. Plant Dis. (in press). doi: 10.1094/PDIS-08-20-1829-RE
Tjamos, E. (1993). Prospects and strategies in controlling Verticillium wilt of olive. EPPO Bull. 23, 505–512. doi: 10.1111/j.1365-2338.1993.tb01361.x
Trapero, C., Alcantara, E., Jimenez, J., Amaro-Ventura, M. C., Romero, J., Koopmann, B., et al. (2018). Starch hydrolysis and vessel occlusion related to wilt symptoms in olive stems of susceptible cultivars infected by Verticillium dahliae. Front. Plant Sci. 9:72. doi: 10.3389/fpls.2018.00072
Trapero, C., Rallo, L., Lopez-Escudero, F. J., Barranco, D., and Diez, C. M. (2015). Variability and selection of Verticillium wilt resistant genotypes in cultivated olive and in the Olea genus. Plant Pathol. 64, 890–900. doi: 10.1111/ppa.12330
Trapero, C., Serrano, N., Arquero, O., Del Rio, C., Trapero, A., and Lopez-Escudero, F. J. (2013). Field resistance to Verticillium Wilt in selected olive cultivars grown in two naturally infested soils. Plant Dis. 97, 668–674. doi: 10.1094/pdis-07-12-0654-re
Tsror, L. (2011). Epidemiology and control of Verticillium wilt on olive. Isr. J. Plant Sci. 59, 59–69. doi: 10.1560/ijps.59.1.59
Vaerman, J. L., Saussoy, P., and Ingargiola, I. (2004). Evaluation of real-time PCR data. J.Biol. Regul. Homeost. Agents 18, 212–214.
Valverde, P., Trapero, C., Arquero, O., Serrano, N., Barranco, D., Munoz Diez, C., et al. (2021). Highly infested soils undermine the use of resistant olive rootstocks as a control method of Verticillium wilt. Plant Pathol. 70, 144–153. doi: 10.1111/ppa.13264
Varo-Suárez, A., Raya-Ortega, M. C., Agusti-Brisach, C., Garcia-Ortiz-Civantos, C., Fernandez-Hernandez, A., Mulero-Aparicio, A., et al. (2018). Evaluation of organic amendments from agro-industry waste for the control of Verticillium wilt of olive. Plant Pathol. 67, 860–870. doi: 10.1111/ppa.12798
Villalobos, F., Testi, L., Hidalgo, J., Pastor, M., and Orgaz, F. (2006). Modelling potential growth and yield of olive (Olea europaea L.) canopies. Eur. J. Agron. 24, 296–303. doi: 10.1016/j.eja.2005.10.008
Wilhelm, S. (1955). Longevity of the Verticillium wilt fungus in the laboratory and field. Phytopathology 45, 180–181.
Yadeta, K., and Tomma, B. (2013). The xylem as battle-ground for plant hosts and vascular wilt pathogens. Front. Plant Sci. 4:97. doi: 10.3389/fpls.2013.00097
Keywords: SILVOLIVE, Verticillium wilt, resistance, Olea europaea subspecies, tolerance, olive crop, qPCR
Citation: Díaz-Rueda P, Aguado A, Romero-Cuadrado L, Capote N and Colmenero-Flores JM (2021) Wild Olive Genotypes as a Valuable Source of Resistance to Defoliating Verticillium dahliae. Front. Plant Sci. 12:662060. doi: 10.3389/fpls.2021.662060
Received: 31 January 2021; Accepted: 31 May 2021;
Published: 01 July 2021.
Edited by:
Sabrina Sarrocco, University of Pisa, ItalyReviewed by:
David Ruano-Rosa, Instituto Tecnológico Agrario de Castilla y León, SpainOfere Francis Emeriewen, Julius Kühn Institute (JKI), Germany
Copyright © 2021 Díaz-Rueda, Aguado, Romero-Cuadrado, Capote and Colmenero-Flores. This is an open-access article distributed under the terms of the Creative Commons Attribution License (CC BY). The use, distribution or reproduction in other forums is permitted, provided the original author(s) and the copyright owner(s) are credited and that the original publication in this journal is cited, in accordance with accepted academic practice. No use, distribution or reproduction is permitted which does not comply with these terms.
*Correspondence: Nieves Capote, bWFyaWFuLmNhcG90ZUBqdW50YWRlYW5kYWx1Y2lhLmVz; José M. Colmenero-Flores, Y2hlbWFjZkBpcm5hc2UuY3NpYy5lcw==