- IRTA, Postharvest Programme, Edifici Fruitcentre, Parc Científic i Tecnològic Agroalimentari de Lleida, Parc de Gardeny, Lleida, Spain
The development of brown rot caused by the necrotrophic fungi Monilinia spp. in stone fruit under field and postharvest conditions depends, among others, on environmental factors. The effect of temperature and humidity are well studied but there is little information on the role of light in disease development. Herein, we studied the effect of two lighting treatments and a control condition (darkness) on: (i) several growth parameters of two Monilinia spp. (M. laxa and M. fructicola) grown in vitro and (ii) the light effect in their capacity to rot the fruit (nectarines) when exposed to the different lighting treatments. We also assessed the effect of such abiotic factors in the development of the disease on inoculated nectarines during postharvest storage. Evaluations also included testing the effect of fruit bagging on disease development as well as on ethylene production. Under in vitro conditions, lighting treatments altered colony morphology and conidiation of M. laxa but this effect was less acute in M. fructicola. Such light-induced changes under in vitro development also altered the capacity of M. laxa and M. fructicola to infect nectarines, with M. laxa becoming less virulent. The performance of Monilinia spp. exposed to treatments was also determined in vivo by inoculating four bagged or unbagged nectarine cultivars, indicating an impaired disease progression. Incidence and lesion diameter of fruit exposed to the different lighting treatments during postharvest showed that the effect of the light was intrinsic to the nectarine cultivar but also Monilinia spp. dependent. While lighting treatments reduced M. laxa incidence, they enhanced M. fructicola development. Preharvest conditions such as fruit bagging also impaired the ethylene production of inoculated fruit, which was mainly altered by M. laxa and M. fructicola, while the bag and light effects were meaningless. Thus, we provide several indications of how lighting treatments significantly alter Monilinia spp. behavior both in vitro and during the interaction with stone fruit. This study highlights the importance of modulating the lighting environment as a potential strategy to minimize brown rot development on stone fruit and to extent the shelf-life period of fruit in postharvest, market, and consumer’s house.
Introduction
Species of Monilinia are responsible of brown rot disease on stone fruit both in the field and during postharvest. In particular, Monilinia laxa is found worldwide (Obi et al., 2018) and is the main causal agent of brown rot in Europe (Rungjindamai et al., 2014), while Monilinia fructicola is more virulent (Kreidl et al., 2015) and its presence has been increasing in Spanish orchards since 2006 (De Cal et al., 2009; Villarino et al., 2013). These pathogens are necrotrophic since they can colonize fruit tissues causing cellular death (Garcia-Benitez et al., 2016), rotting most parts of the tree, from buds to fruit (Villarino et al., 2010). Sources of inoculum can be primary [e.g., from mummified fruit (Gell et al., 2008)] or secondary [e.g., from infected fruit (Villarino et al., 2012)], resulting in a polycyclic disease (reviewed in Oliveira Lino et al., 2016).
Environmental conditions are critical for brown rot development. Temperature and wetness period are the most studied factors and are demonstrated to influence penetration and spread of both M. laxa (Gell et al., 2008) and M. fructicola (Luo and Michailides, 2001). Solar radiation, wind speed and rainfall factors also play an important role in the spread of M. laxa and M. fructicola (Gell et al., 2009) but detailed information is scarce. During plant-pathogen interactions, light quantity and quality (Idnurm and Crosson, 2009) and photoperiod (Tisch and Schmoll, 2010) not only influence the behavior of the pathogen, but also the interaction with its hosts (Carvalho and Castillo, 2018).
Fungi are able to adapt their metabolic pathways when perceiving light (Tisch and Schmoll, 2010; Corrochano, 2019) through a complex of photoreceptors and so regulate their behavior and development (Bahn et al., 2007), such as the development of sexual or vegetative reproductive structures and tropism of unicellular structure (Corrochano, 2019). The light alters gene expression patterns of Monilinia spp. (De Miccolis Angelini et al., 2018) and, in fact, some photoreceptors and related regulatory proteins (e.g., velvet regulatory family) have recently been described in M. laxa (Rodríguez-Pires et al., 2021). However, how the fungus perceives and modulates light responses needs further investigation. For instance, Botrytis cinerea, a species of the same family of Monilinia spp., produces sclerotia in constant darkness and conidia under the light, which is enhanced when growing under light-dark cycles compared to constant light (Schumacher, 2017). Hence, the presence of light but also its intensity, quality and photoperiod can alter fungal development both under in vitro conditions and on fruit. Thus said, little is known regarding how light can affect the infection process of phytopathogenic fungi, and only one study incubating M. laxa-inoculated stone fruit under different white light conditions and photoperiods have been conducted (Rodríguez-Pires et al., 2020).
Likewise, light regulates plant growth and development (Folta and Carvalho, 2015), including responses to biotic stresses (Roeber et al., 2020). Perception of light can control the establishment of the systemic acquired resistance, which would lead to an enhancement of disease resistance in several plant-pathogen interactions (Métraux, 2002; Roberts and Paul, 2006). When both the pathogen-host interaction and light conditions take place, plant circadian rhythm controls the pathogen host, leading to a daytime-dependent response (Griebel and Zeier, 2008). After an interaction, the host induces a hormone signaling cascade, which in turn, triggers defense mechanisms (Pandey et al., 2016). Ethylene is one of the multiple hormones which mediates the host response against necrotrophic pathogens (McDowell and Dangl, 2000), although it also modulates the response to numerous abiotic stresses (reviewed in Müller and Munné-Bosch, 2015). In fact, recent studies have demonstrated the link between the jasmonate/ethylene pathway and the photoreceptor-mediated light response, and its importance on the resistance to the pathogen B. cinerea (Xiang et al., 2020).
The solar radiation that fruit receives in the field varies along the year, being low at the beginning and higher at the end of the stone fruit season. Nowadays, growers are implementing some alternative practices to control pests and diseases during preharvest (Usall et al., 2015) in substitution to those based on chemicals. Among them, fruit bagging (Allran, 2017) has been proved to be effective in controlling brown rot incidence in peach and plum (Keske et al., 2011, 2014). However, these alternatives, together with the use of colored shade nets (Ilić and Fallik, 2017), have also an effect on the incidence of solar radiation that fruit receives during its development, altering many fruit physicochemical properties (Sharma et al., 2014; Ilić and Fallik, 2017; Zhou et al., 2019), which ultimately could impair the fruit response to pathogens.
Therefore, the understanding of the light effect on the pathogens but also on the capacity of fruit to respond properly to infections is critical to establish an optimal practice in the field but also along the postharvest in packinghouses and through the distribution chain. Thus said, this study aimed to understand the effect of the darkness (control) and two lighting treatments on the behavior of M. laxa and M. fructicola in vitro and during the interaction with nectarine fruit. In particular, we assessed the (i) effect of the three treatments on the ecophysiology of Monilinia spp. in vitro in two different culture media; (ii) effect of the three treatments in the capacity of the two species after being exposed to the lighting treatments to infect fruit; (iii) effect of fruit bagging on fruit susceptibility to brown rot at postharvest; (iv) effect of the three treatments in the ethylene production and the development of the disease of the inoculated fruit exposed to different lighting treatments.
Materials and Methods
Fungal Material and Incubation Treatments
The species of Monilinia used in this study were single-conidia strains of M. laxa (ML8L) and M. fructicola (CPMC6), deposited in the Spanish Culture Type Collection (CECT 21100 and CECT 21105, respectively). Fungal cultures and conidial suspensions were maintained and prepared as described by Baró-Montel et al. (2019c). Fungal suspensions were prepared at 105 conidia mL–1 and used to inoculate plates or fruit depending on the experiment.
Both in vitro and in vivo experiments were conducted in a growth chamber with the following incubation and lighting treatments: (1) “Control”, at 20°C, 45–55 % RH and complete darkness; (2) “Treatment 1,” consisting of 4 fluorescents of low light intensity and incandescent white TL-D 36 W/827 (Ta = 2700 K, 3350 lm, 350 – 740 nm, 630 nm max) (Philips), and photoperiod of 12 h light (22 ± 1°C, 50 ± 10 % RH) / 12 h dark (20°C, 90 % RH); (3) “Treatment 2,” consisting of 4 fluorescents of high light intensity and cool white TL-D 58W/840 (Ta = 4000 K, 5000 lm, 300 – 740 nm, 550 nm max) (Philips), and photoperiod of 16 h light (21 ± 1°C, 50 ± 10 % RH) / 8 h dark (20°C, 90 % RH).
In vitro Ecophysiology
To evaluate the light effect on the two strains of Monilinia spp., Potato Dextrose Agar (PDA; Biokar Diagnostics, 39 g L–1) and/or PDA plates supplemented with 25 % tomato pulp (PDA-T) were inoculated with one drop of 10 μL of the conidial suspension (105 conidia mL–1) of each species on the center of Petri dishes. Plates were incubated under the three incubations treatments mentioned above. During and after 7 days under each treatment, ecophysiological parameters for both species were evaluated: growth parameters (including colony morphology, conidiation, conidia morphology, and growth rate), conidial viability and germination. All experiments consisted of three replicates per treatment, culture media and Monilinia spp. and each experiment was repeated twice.
Growth Parameters
Four growth parameters were investigated for each Monilinia spp. grown in PDA and PDA-T media. The colony growth rate, the total conidiation, a visual inspection of colony features according to EPPO standard PM 7/18 (3) (Bulletin OEPP/EPPO, 2020) and the conidia morphology of cultures were assessed. The colony growth rate (cm day–1) was determined as the slope of the lineal equation obtained from the individual measurements of the mean of the colony diameter in two perpendicular directions by plotting growth diameter (cm) vs. time (days). Conidiation was calculated by rubbing the conidia from the surface of the PDA-T plates with a known volume of sterile water containing 0.01 % Tween-80 (w/v), filtering through two layers of sterile cheesecloth and then titrating the conidia using a haemocytometer. The concentration of conidiation (conidia mL–1) was calculated and expressed as total conidiation in relation to control. Comparison of conidia morphology from plates subjected to different treatments was assessed by rubbing the PDA and PDA-T plates with sterile water containing 0.01 % Tween-80 (w/v) and filtering through two layers of sterile cheesecloth. Images at 40x magnification were taken in an optical microscope (Leica DM5000B, Leica Microsystems CMS GmbH, Germany). The images were acquired using a Leica color digital camera (Leica DFC 420).
Conidial Viability
To test the conidial viability (i.e., the ability of conidia to form new colonies) after exposing the Monilinia spp. grown in PDA-T media for 7 days under the different light regimes, colony-forming units (CFUs) were measured by performing serial ten-fold dilutions on PDA medium. Plates were incubated for 3 to 4 days at 20°C under darkness.
Germination of Conidia
Percentage of germinated conidia (%) was studied under optical microscopy, as described by Casals et al. (2010) with some modifications. Droplets (10 μL) of the conidial suspension (105 conidia mL–1) were placed around PDA plates, and immediately incubated under each treatment. Samplings were carried out each 30 min or 1 h until 6 h. To stop germination at each incubation time, 1 mL of 25 % ammonia was applied onto a filter paper placed on the cover of the Petri dish. Conidia were considered germinated when cell wall deformation forming a germ tube was observed.
Light Effect on the Ability of Monilinia spp. to Infect Fruit
To evaluate whether the capacity of Monilinia spp. to infect fruit was altered by treatments, an inoculation of nectarines with the two species previously exposed to the three treatments was conducted. Experiments were performed with two organically grown cultivars of nectarines [P. persica var. nucipersica (Borkh.) Schneider]. “Fantasia” and “Venus” cultivars were obtained from an orchard located in Alfarràs and Ivars de Noguera (Lleida, Catalonia, Spain), respectively. Fruit for analysis was further homogenized by using a portable DA-Meter (TR-Turoni, Forli, Italy), based on the single index of absorbance difference.
Fruit Inoculations
Cultures of Monilinia spp. exposed to each treatment were used to artificially inoculate nectarines. One drop (10 μL) of conidial suspensions (105 conidia mL–1) of M. laxa or M. fructicola was placed on PDA-T plates and cultures were maintained under each afore-mentioned treatment (section “Fungal material and incubation treatments”) for 7 days. Conidial suspensions of both species were prepared as described above (section “Fungal material and incubation treatments”). Non-wounded fruit was inoculated with one drop (10 μL) of conidial suspension (105 conidia mL–1). A total of 20 fruits per cultivar, species, and treatment were used. Fruit were stored in a growth chamber, inside plastic boxes with wet filter paper (distilled water), under darkness and controlled incubation conditions (20 ± 1°C, 97 ± 3 % RH).
Aggressiveness Parameters
Disease symptoms were examined to calculate incidence (percentage of fruit with brown rot symptoms) and severity (lesion diameter length in cm of rotted fruit) along 7 days after inoculation. The incubation period (number of days to the observation of the onset of brown rot symptoms) and the latency period (number of days to the observation of conidiation) were also recorded. In fruit inoculated with M. fructicola, the conidiation was determined on the fruit surface after 7 days post-inoculation (dpi) for each treatment. For that, peels of the infected area of 3–4 inoculated fruits were obtained, immersed in a sterile filter bag with 40 mL sterile water containing 0.01 % Tween-80 (w/v) and homogenized in a Stomacher (Seward, London, United Kingdom) set at 12 strokes s–1 for 120 s. The filtered volume was recovered and the conidia was counted using a haemocytometer. The concentration of conidia (conidia g fresh peel–1) was calculated as the mean of each group of 3–4 fruit.
Light Effect on the Monilinia spp.-Fruit Interaction
To evaluate the light effect on the interaction of Monilinia spp. with nectarine, inoculated fruit with both M. laxa and M. fructicola were incubated under each afore-mentioned treatment. For that, experiments were conducted with organically grown cultivars of nectarines; two early-mid (“Fantasia” and “Venus”) and two late (“Nectatinto” and “Albared”) cultivars, obtained from an orchard located in Alfarràs, Ivars de Noguera, Gimenells and Alfarràs (Lleida, Catalonia, Spain), respectively. The light effect was assessed on unbagged and bagged fruit, which was bagged with white paper bags at least one month before harvest. Bagged and unbagged fruit were harvested in the same sun-side of trees due to the influence of fruit canopy position to all fruit’s characteristics (Minas et al., 2018). Bags were removed just before conducting assays. Fruit for analysis was further homogenized by using a portable DA-Meter (TR-Turoni, Forli, Italy), based on the single index of absorbance difference.
Fruit Inoculations and Conidia Establishment
Inoculation was carried out by placing one drop (50 μL) of the conidial suspension (105 conidia mL–1) on the colored side of non-wounded fruit. A mock inoculation (mock) was performed by inoculating sterile water containing 0.01 % Tween-80 (w/v). Inoculated fruit was first incubated at high humidity conditions for 24 h for the establishment of conidia on the fruit surface. For that, fruit was placed on boxes covered with a wet paper and a plastic bag, and then stored in a growth chamber, at controlled conditions (20°C, 90 ± 3 % RH). After that, fruit were immediately placed under each lighting treatment.
Aggressiveness Parameters and Ethylene Measurements
Fruit were daily examined to calculate brown rot incidence, severity and incubation period along 7 days, as described above (section “Aggressiveness parameters”). Experiments were conducted with 4 replicates of 5 fruits each per cultivar, bagging condition, treatment and Monilinia spp. Ethylene production of both mock-inoculated fruit and Monilinia spp. inoculated fruit was determined as described by Giné-Bordonaba et al. (2017). Measurements were conducted at four time points along the infection time course until 7 dpi. At each sampling point, fruit were placed in 3.8 L sealed flasks and left to incubate for 2 h. After ethylene measurements, fruit were placed back under each lighting treatment. Experiments were conducted with four replicates of three fruits each.
Statistical Analysis
Data were statistically analyzed with JMP software version 14.2.0 (SAS Institute Inc., Cary, NC, United States). Prior to the analysis, all data were checked for the assumptions of parametric statistics and transformed when needed. Data of in vitro assays (growth rate, total conidiation and conidial viability), conidiation on fruit surface and severity were used as original data. Incubation and latency period (dpi) were subjected to square root transformation. Data of ethylene production (μL kg–1 h–1) were subjected to Log transformation. All these data were subjected to analysis of variance (ANOVA). Conidia germination (%) was analyzed using the generalized linear model (GLM) based on a Poisson distribution and Log-link function. Brown rot incidence (%) was analyzed using the GLM based on a binomial distribution and logit-link function. When the analysis was statistically significant, orthogonal contrasts (P ≤ 0.05) were performed for means separation among treatments. When comparisons were conducted between two means (bagged vs. unbagged), Student’s T-test (P ≤ 0.05) was used. For means comparison of inoculated fruit (mock, M. laxa and M. fructicola), Tukey’s HSD test (P ≤ 0.05) was conducted.
Results
Light Differentially Alters the Phenotype of M. laxa and M. fructicola
To evaluate the light effect on the in vitro behavior of Monilinia spp., we assessed several ecophysiological parameters after exposing M. laxa and M. fructicola to two lighting treatments and control condition (constant darkness) for 7 days (Figure 1). Under both treatments, colony features were very different from those grown under control condition, for each Monilinia spp. in both culture media (Figures 1A,B). The colonies of M. laxa in both culture media subjected to both lighting treatments showed more hazel colors if compared to those white and gray colors observed in the control condition. Monilinia laxa significantly grew faster under both lights than under control condition in both media. Monilinia fructicola grown on PDA-T and subjected to both lighting treatments presented lobed culture’s margin, while when growing under control condition, colonies presented entire margins. Only treatment 2 was able to significantly reduce its growth rate when growing on PDA but not in PDA-T medium. Conidia morphology examination showed that, except for M. laxa on PDA where few conidia were detected, both treatments altered conidia shapes of both M. laxa and M. fructicola (Figures 1A,B). While conidia from control condition cultures presented the typical ovoid and limoniform morphologies, lighting treatments induced an increase of irregular morphologies such as globose, cylindrical, or ellipsoidal (Figures 1A,B).
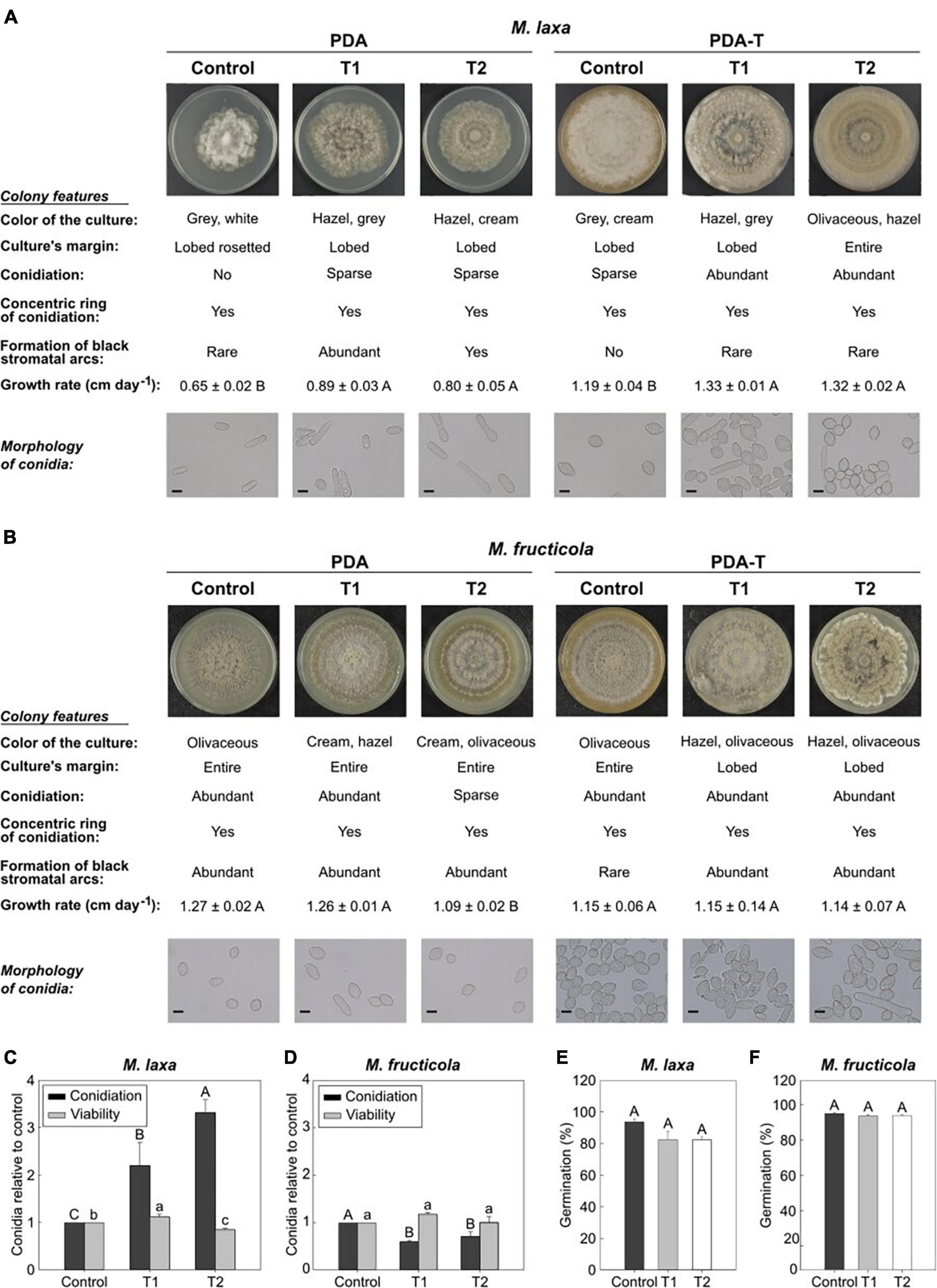
Figure 1. In vitro ecophysiology of Monilinia spp. after exposure to treatments 1 and 2 and control condition (constant darkness). Images of Monilinia cultures, description of colony features, growth rate (cm day–1) and microscopy images (40×) of M. laxa (A) and M. fructicola (B) grown on PDA and PDA-T and incubated under each light condition. Data for growth rate represent the mean of replicates (n = at least 4) ± standard error of the means. Different letters indicate significant differences (P ≤ 0.05) among incubation conditions according to orthogonal contrasts. Scale bar for microscopy images is indicated (10 μm). Conidiation and conidial viability of M. laxa (C) and M. fructicola (D) grown on PDA-T incubated under each light condition. Data is represented relative to the control condition (control = 1). Different uppercase and lowercase letters indicate significant differences (P ≤ 0.05) of conidiation and conidial viability, respectively, among incubation conditions according to orthogonal contrasts. Germination (%) after 6 h of M. laxa (E) and M. fructicola (F) on PDA medium. Different letters indicate significant differences (P ≤ 0.05) among incubation conditions according to orthogonal contrasts. For panels (C–F), bars represent the mean of replicates (n = at least 4) and error bars represent the standard error of the means.
The visual inspection of Monilinia cultures demonstrated that M. laxa produced more conidia in PDA-T plates exposed to both treatments 1 and 2 (2.19 and 3.31-fold significantly higher, respectively) if compared to control condition (constant darkness) (Figure 1C). However, we were not able to observe M. laxa conidiation on the PDA medium incubated under control condition (Figure 1A). In fact, almost no conidia were visualized in microscopic inspections in PDA plates (Figure 1A) as exposed above. In contrast, conidiation of M. fructicola was significantly reduced in PDA-T plates exposed to both treatments 1 and 2 (0.59 and 0.71-fold, respectively) if compared to control condition (Figure 1D). Conidiation in PDA plates was like that on PDA-T plates, where both treatments 1 and 2 significantly reduced (0.43 and 0.29-fold, respectively) the number of conidia in illuminated plates compared to control condition. Regarding the conidial viability, results showed that treatment 2 significantly reduced the number of CFUs of M. laxa, although on treatment 1 it was slightly higher (1.12-fold) than on control condition (Figure 1C). In contrast, we did not observe any effect of lighting treatment on the conidial viability of M. fructicola (Figure 1D). Finally, exposition to light affected the germination’s capability of neither M. laxa (Figure 1E) nor M. fructicola (Figure 1F).
Contrary to M. fructicola, M. laxa Becomes Less Virulent Once Exposed to Lighting Treatment
To test how changes observed under in vitro ecophysiological parameters affected the capacity of both Monilinia spp. to infect fruit, we assessed the development of the disease on nectarines inoculated with M. laxa or M. fructicola which were previously grown under each lighting treatment. In ‘Fantasia’ nectarines inoculated with M. laxa, both treatments 1 and 2 significantly reduced incidence (55 and 61 %, respectively) and severity (2.4 and 2.0 cm, respectively) since the first time point compared to control condition (constant darkness) (90 % of incidence and 3.9 cm of severity) (Figure 2A). No differences in the incubation period were observed among treatments (Figure 2B). Only 10% of fruit inoculated with M. laxa which was grown under treatment 1 and control condition showed conidiation on the fruit surface. In line with these results, fruit inoculated with M. laxa grown under treatment 1 revealed a higher latency period (1.17-fold) than those inoculated with the pathogen held under control condition (Figure 2B). Besides, under the treatment 2, the fungal development did not even show any conidiation (Figure 2B). Thus, although both treatments improved the behavior of M. laxa in vitro, they made the pathogen impair and delay its capacity to infect and in consequence, made it less virulent.
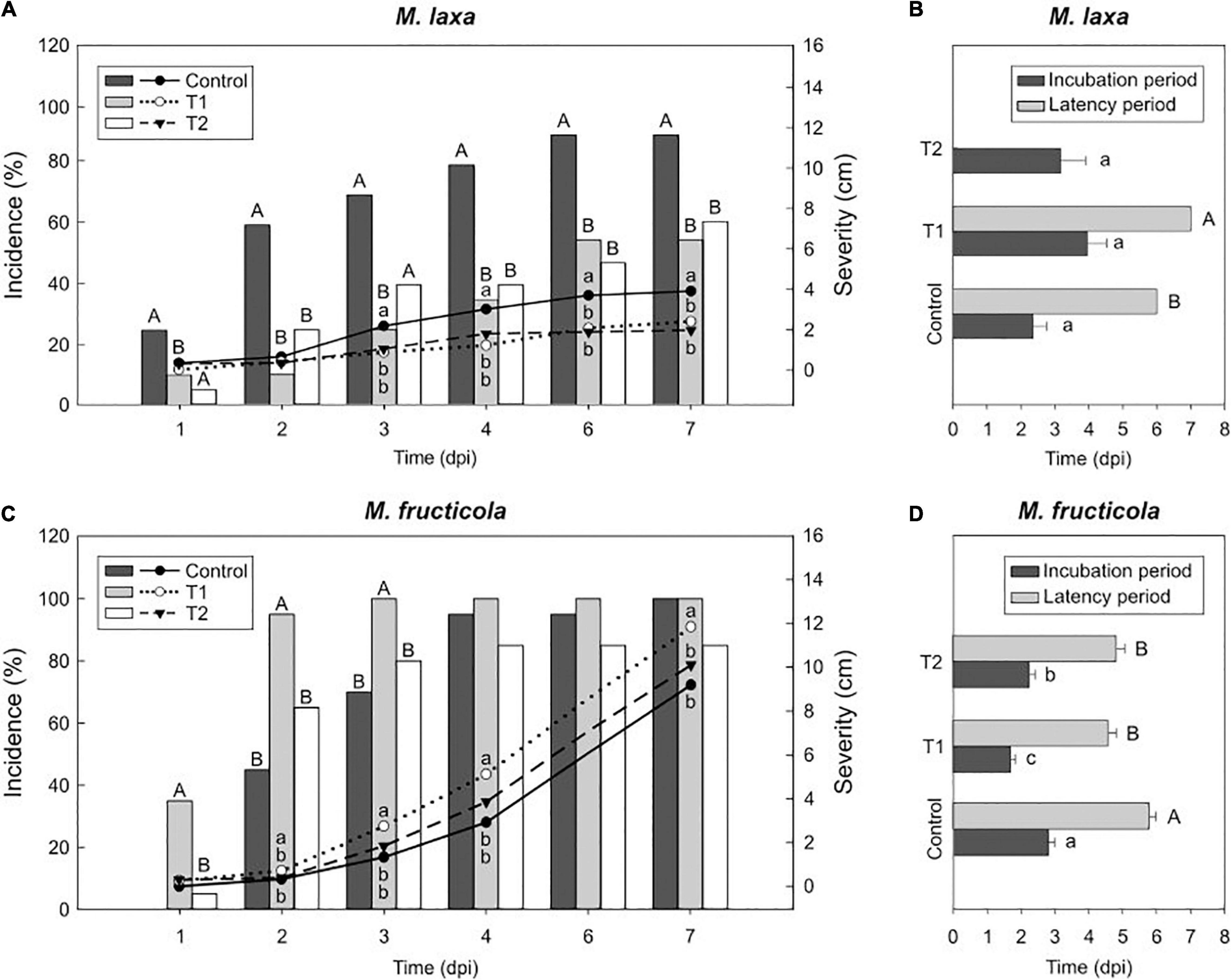
Figure 2. Light effect on the capacity of Monilinia spp. to infect fruit in “Fantasia” cultivar. Incidence (% of brown rot, bars) and severity (lesion diameter length in cm of rotted fruit, lines) of M. laxa (A) and M. fructicola (C) in “Fantasia” nectarines along the infection time course (dpi, days post-inoculation) after growing the fungi for 7 days under treatments 1 and 2 and control condition (constant darkness). Bars represent the mean of incidence on fruit (n = 20). Lines represent the mean of diameter length of rotted fruit. Different uppercase and lowercase letters indicate significant differences (P ≤ 0.05) of incidence and severity, respectively, among incubation conditions according to orthogonal contrasts at each time point. No letters indicate no significant differences. Incubation and latency periods (dpi) of M. laxa (B) and M. fructicola (D) in “Fantasia” nectarines after growing the fungi for 7 days under treatments 1 and 2 and control condition. Bars represent the mean of fruits with symptoms (n = 2 to 20) and error bars represent the standard error of the means. Different lowercase and uppercase letters indicate significant differences (P ≤ 0.05) of incubation and latency periods, respectively, among incubation conditions according to orthogonal contrasts.
Regarding M. fructicola, the incidence of fruit inoculated with M. fructicola grown under treatment 1 significantly peaked at early time points (up to 100 %), although such differences completely subsided through time (Figure 2C). Interestingly, only M. fructicola subjected to that treatment 1 was able to cause significantly higher lesion diameter on fruit (up to 11.8 cm) than that in the two other conditions (9.2 cm under control condition and 10.1 cm under treatment 2) (Figure 2C). In addition, both treatments accelerated the onset of disease symptoms. The incubation periods of fruit inoculated with M. fructicola exposed to treatment 2 and treatment 1 were significantly lower (1.25 and 1.65-fold, respectively) than when the pathogen was grown under control condition (constant darkness) (Figure 2D). Between 94 and 100% of inoculated fruit, irrespective of treatment in which the fungus was grown, presented conidiation on the fruit surface. However, the latency of M. fructicola under either lighting treatment significantly accelerated the onset of conidiation symptoms (between 4.6 and 4.8 days of average) compared to control condition (an average of 5.8 days) (Figure 2D). Finally, regarding the concentration of conidia in the fruit surface, treatment 1 induced M. fructicola to produce significantly more conidia on fruit (1.92-fold) compared to control condition, whereas treatment 2 was like control condition (Supplementary Figure 1). Hence, while lighting treatments seemed to make M. laxa lose virulence, it accelerated the onset of disease symptoms and conidiation of M. fructicola. All these experiments were also conducted in another nectarine cultivar (“Venus”) and results showed similar tendencies of fruit susceptibility to brown rot (Supplementary Figure 2).
Fruit Bagging Can Alter Its Susceptibility to Monilinia spp. in a Cultivar-Dependent Manner
To test the effect of fruit bagging on fruit susceptibility to brown rot, we conducted a disease evaluation of four different nectarine cultivars inoculated with M. laxa and M. fructicola and incubated under control condition (constant darkness). In inoculated fruit with either M. laxa or M. fructicola, results showed two tendencies of fruit susceptibility (Supplementary Table 1). Unbagged “Fantasia” nectarines were more susceptible to both Monilinia spp. than fruit that was bagged during preharvest (“bagged fruit”). However, the other cultivars (“Venus,” “Nectatinto” and “Albared”) showed that unbagged fruit was slightly more resistant to both Monilinia spp. than bagged fruit. Hence, results pointed out that the effect of fruit bagging in fruit susceptibility to brown rot could be cultivar-dependent.
Light Reduces M. laxa Disease in Nectarines but Enhance M. fructicola Development
To further investigate the light effect in brown rot progress at postharvest, we assessed some aggressiveness features after incubating the inoculated fruit under the lighting treatments. Results demonstrated that the effect of light on the host-pathogen interaction was cultivar-dependent. While we observed significant differences in incidence and severity of early-mid cultivars such as “Fantasia” (Figures 3A,C, 4A,C), we detected almost no differences in late cultivars such as “Nectatinto” and “Albared” (data not shown). The disease behavior on the later cultivars was similar among all lighting treatments. In addition, the incubation period was slightly higher in the early cultivars than in the late ones (data not shown).
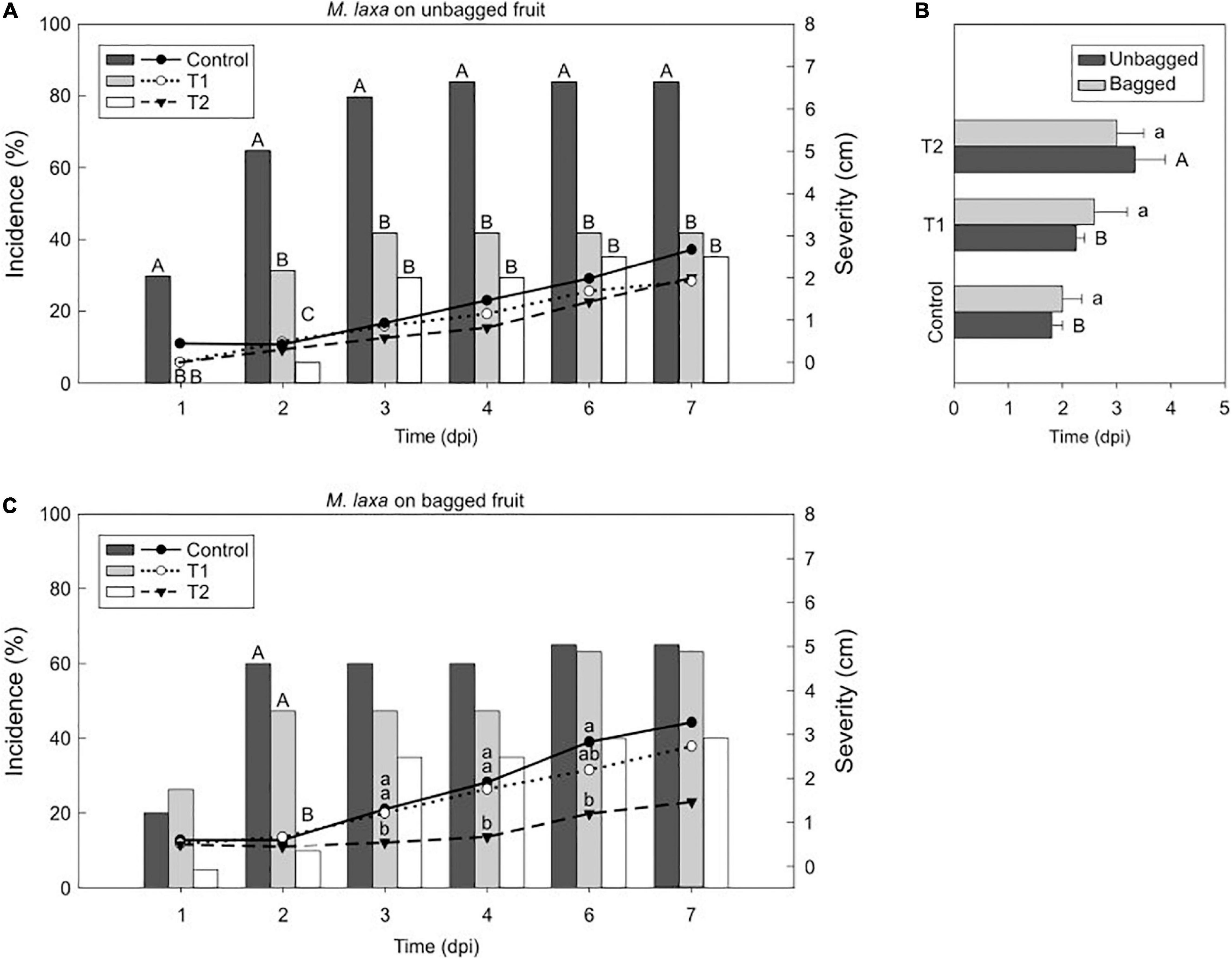
Figure 3. Light effect on the M. laxa-nectarine interaction. Incidence (% of brown rot, bars) and severity (lesion diameter length in cm of rotted fruit, lines) of M. laxa in unbagged (A) and bagged (C) “Fantasia” nectarines along the infection time course (dpi, days post-inoculation) incubated for 7 days under treatments 1 and 2 and control condition (constant darkness). Bars represent the mean of incidence on fruits (n = 20). Lines represent the mean of diameter length of rotted fruit. Different uppercase and lowercase letters indicate significant differences (P ≤ 0.05) of incidence and severity, respectively, among incubation conditions according to orthogonal contrasts at each time point. No letters indicate no significant differences. The incubation period (dpi) of M. laxa in bagged and unbagged “Fantasia” nectarines (B) after 7 days of incubation under treatments 1 and 2 and control condition. Bars represent the mean of fruits with symptoms (n = 2 to 20) and error bars represent the standard error of the means. Different uppercase and lowercase letters indicate significant differences (P ≤ 0.05) among incubation conditions in unbagged and bagged fruit, respectively, according to orthogonal contrasts.
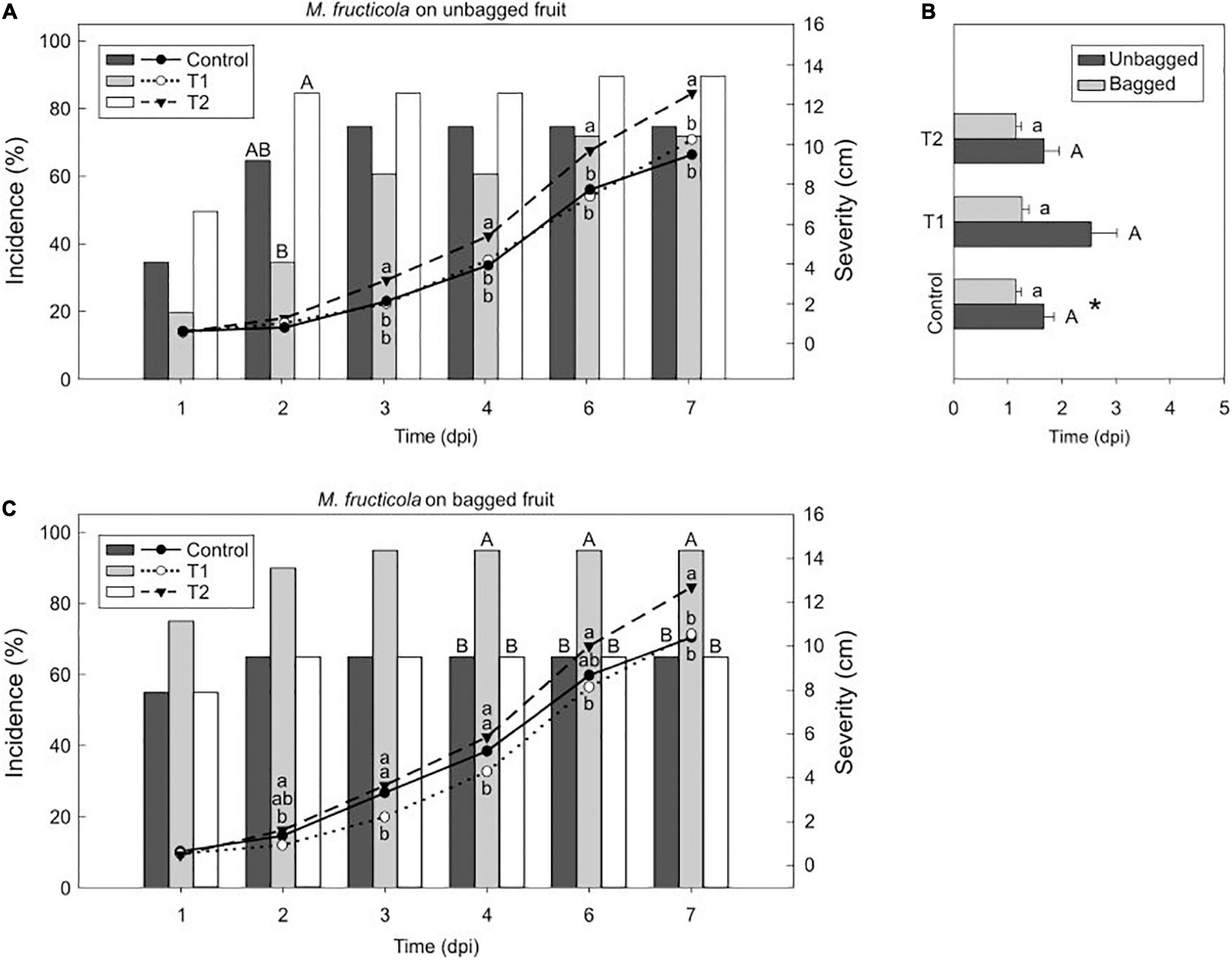
Figure 4. Light effect on the M. fructicola-nectarine interaction. Incidence (% of brown rot, bars) and severity (lesion diameter length in cm of rotted fruit, lines) of M. fructicola in unbagged (A) and bagged (C) “Fantasia” nectarines along the infection time course (dpi, days post-inoculation) incubated for 7 days under treatments 1 and 2 and control condition (constant darkness). Bars represent the mean of incidence on fruits (n = 20). Lines represent the mean of diameter length of rotted fruit. Different uppercase and lowercase letters indicate significant differences (P ≤ 0.05) of incidence and severity, respectively, among incubation conditions according to orthogonal contrasts at each time point. No letters indicate no significant differences. The incubation period (dpi) of M. fructicola in bagged and unbagged “Fantasia” nectarines (B) after 7 days of incubation under treatments 1 and 2 and control condition. Bars represent the mean of fruits with symptoms (n = 2 to 20) and error bars represent the standard error of the means. Different uppercase and lowercase letters indicate significant differences (P ≤ 0.05) among incubation conditions in unbagged and bagged fruit, respectively, according to orthogonal contrasts. Symbol (*) indicates significant differences between bagging conditions under control condition according to Student’s T-test (P ≤ 0.05).
Afterward, we selected the “Fantasia” cultivar for further analysis. Monilinia laxa incidence on unbagged nectarines maintained under control condition (constant darkness) was significantly higher (84 %) than that under treatment 1 and 2 (42 % and 35 %, respectively) (Figure 3A). The lesion diameter revealed the same tendency as incidence, although with no significant differences along time (Figure 3A). In the same line, the incubation period was significantly higher in inoculated unbagged fruit exposed to the treatment 2 than treatment 1 and control condition (1.5- and 1.8-fold, respectively) (Figure 3B). Regarding nectarines that were bagged during preharvest, there was no difference in neither incidence (ranging from 40 to 65 %) (Figure 3C) nor incubation period (between 2 and 3 days) among treatments (Figure 3B). However, the severity of M. laxa-inoculated fruit subjected to both control condition and treatment 1 was significantly higher than that under treatment 2 at 3 and 4 dpi (Figure 3C), although such differences subsided along the infection time course.
In ‘Fantasia’ unbagged nectarines inoculated with M. fructicola, the incidence at 2 dpi under treatment 2 (85 %) was significantly higher than those incidences under control condition and treatment 1 (65 % and 35 %, respectively). Treatment 2 also significantly increased severity (up to 12.6 cm) in unbagged fruit along time compared to that under control condition and treatment 1 (9.5 and 10.2 cm, respectively) (Figure 4A). However, the incubation period was similar among all treatments (Figure 4B). In bagged fruit, treatment 1 rose disease incidence (95 %) and was significant from 4 dpi onward, compared to the other treatments tested (65 % both). Contrary, treatment 2 significantly increased severity (12.7 cm) compared to that under control condition and treatment 1 (10.4 and 10.5 cm, respectively) (Figure 4C). No differences were observed among treatments when analyzing the incubation period of bagged or unbagged fruit (Figure 4B). Interestingly, the M. fructicola-incubation period of bagged fruit incubated under control condition was significantly lower (1.4- fold) than unbagged fruit at the same condition (Figure 4B). Overall, light seemed to negatively affect the disease incidence and severity of M. laxa whereas it caused the opposite effect for M. fructicola.
Ethylene Production in M. laxa-Fruit Interaction Is Bag and Lighting Treatment-Dependent
In addition, to assess the development of the disease in Monilinia spp.-inoculated nectarines, we also evaluated the ethylene production of the pathosystem under the different experimental treatments (Figure 5). Firstly, we determined the bagging effect on ethylene production in Monilinia-inoculated fruit incubated under control condition (constant darkness) (Figures 5A–C). Results denoted that while unbagged and bagged mock-inoculated fruit produced similar ethylene levels (Figure 5A), on M. laxa inoculated nectarines, the levels of ethylene produced by the unbagged fruit were significantly higher than those in the bagged fruit (Figure 5B). Contrary to M. laxa, M. fructicola induced a peak of ethylene at 6 dpi in both unbagged and bagged fruit and results only showed significant differences between bagging conditions at 2 dpi (Figure 5C).
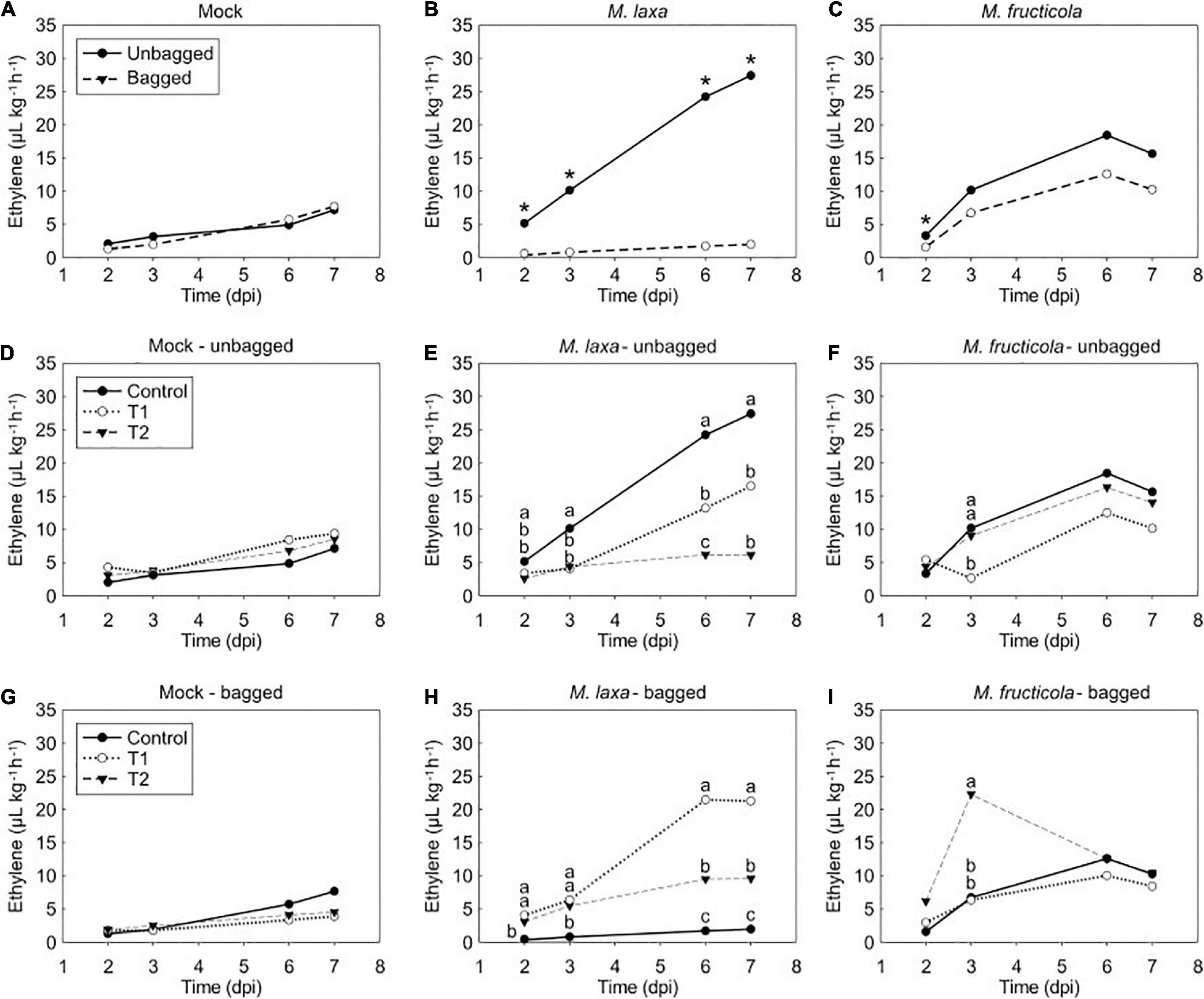
Figure 5. Ethylene production of mock fruit, M. laxa- and M. fructicola-fruit interaction among bag and lighting treatments through time in “Fantasia” nectarines. Ethylene measurements of mock (A), M. laxa-fruit (B) and M. fructicola-fruit (C) along the infection time course (dpi, days post-inoculation) under control condition (constant darkness). Symbols (*) indicate significant differences between bagging conditions at each time point according to Student’s T-test (P ≤ 0.05). Ethylene measurements in mock fruit (D,G), M. laxa-fruit (E,H) and M. fructicola-fruit (F,I) in unbagged (D–F) and bagged conditions (G–I) along the infection time course (dpi) under each lighting treatment. Different letters indicate significant differences (P ≤ 0.05) among light conditions according to orthogonal contrasts at each time point. No letters indicate no significant differences. In all graphics, values represent the mean of ethylene measurements of each replicate (n = 4).
We further evaluated the light effect on both unbagged and bagged fruit inoculated with each species. Results demonstrated no significant differences in the ethylene production of mock-inoculated fruit among treatments regardless of the bagging condition in which come from Figures 5D,G. Ethylene levels of unbagged fruit inoculated with M. laxa were significantly higher at 7 dpi when incubated under control condition than when exposed to treatments 1 and 2 (4.5 and 2.7-fold, respectively) (Figure 5E). In contrast, bagged fruit inoculated with M. laxa and incubated under control condition displayed an opposite ethylene pattern (Figure 5H). Under lighting treatments, fruit inoculated with M. laxa slowly increased ethylene production of the pathosystem along time and was significantly higher than under control condition, resulting in a 10.9- and 4.9-fold increase under treatment 1 and 2, respectively. Regarding unbagged fruit inoculated with M. fructicola, all incubation treatments showed similar ethylene patterns, which peaked at 6 dpi. Only at 3 dpi, fruit incubated under treatment 1 significantly produced lower ethylene levels than the other treatments (Figure 5F). Bagged fruit inoculated with M. fructicola revealed a similar pattern to unbagged fruit. In that case, only ethylene levels of the M. fructicola-fruit interaction exposed to treatment 2 significantly peaked at 3 dpi (Figure 5I).
When comparing the ethylene emission pattern among mock-inoculated fruit and Monilinia spp. inoculated fruit on unbagged nectarines (Supplementary Figure 3), results clearly demonstrated that the ethylene pattern emitted by both Monilinia spp.-inoculated fruit incubated under control treatment was significantly higher than the one produced by mock fruit. The ethylene production of M. laxa-fruit interaction increased progressively along time, producing a similar pattern to mock fruit, although to a different extent, depending on the incubation treatment. In fact, M. laxa-inoculated fruit maintained under control treatment produced significantly higher levels (3.8-fold) than those of the mock fruit (Supplementary Figure 3A), while a slight difference of 1.8-fold between mock and M. laxa-inoculated fruit was observed under the treatment 1 at 7 dpi (not statistically different) (Supplementary Figure 3B). Conversely, the presence of M. fructicola stimulated an ethylene peak at 6 dpi that was 3.8, 1.5, and 1.6-fold higher under control treatment, treatment 1 and 2, respectively, if compared to mock-inoculated fruit (Supplementary Figure 3). Overall, both Monilinia spp. induced the ethylene levels of the pathosystem but in a lighting treatment-dependent manner.
Discussion
Light is essential both in the preharvest period (i.e., solar radiation) and postharvest chain (i.e., artificial lighting) of fruit. The combination of light quality, intensity, and photoperiod constitute a source of information for the fruit but also to pathogens, and in turn, can influence the onset of symptoms of the development of the disease on the fruit surface. Scarce information regarding in vitro development of Monilinia spp. or brown rot infection on stone fruit under the effect of light is available. Some studies have been conducted with discrete sections of the spectrum such as long-wave UV (De Cal and Melgarejo, 1999), in other Monilinia spp. such as M. fructigena (Marquenie et al., 2003) and the effect of visible white light in M. laxa isolates (Rodríguez-Pires et al., 2020, 2021). However, a study aiming to decipher the effect of lighting treatments on the two main Monilinia spp. of stone fruit has never been conducted. Accordingly, we characterized for the first time, the effect of different lighting treatments on both the in vitro fungal development of M. fructicola and M. laxa, and during the interaction of Monilinia spp. – nectarine fruit, using similar artificial lighting treatment previously applied to M. laxa-stone fruit studies (Rodríguez-Pires et al., 2020).
Altered Conidia Morphology Impairs the Conidial Viability in a Monilinia spp.-Dependent Manner
Monilinia laxa demonstrated a broader photomorphogenesis response to light than M. fructicola under in vitro conditions. In this study, cultures grown on either PDA or PDA-T media and incubated under control condition (constant darkness) were similar to other M. laxa or M. fructicola isolates grown on similar conditions (Tran et al., 2020; Rodríguez-Pires et al., 2021). After exposure to both lighting treatments, but especially under treatment 2, M. laxa mycelia turned mainly hazel whereas the colony color of M. fructicola was not altered at any condition (Figures 1A,B). To regulate fungal biology, fungi sense light through photoreceptors and use it as an input of information (Tisch and Schmoll, 2010). One of the most common and long-term effects of light responses is the induction of pigment expression, such as carotenoid biosynthesis in many microorganisms (Fuller et al., 2015; Corrochano, 2019), and, in fact, the carotenoid production in the closely related organism B. cinerea has been suggested (Schumacher et al., 2014). In turn, carotenoids are highly implicated in protecting cells from reactive oxygen species (ROS) (Avalos and Limón, 2015). Light also induces the biosynthesis of other pigments such as melanin and mycosporines in several fungi (Fuller et al., 2015). The role of melanin in M. fructicola has been described on not only the protection against environmental stresses such as desiccation, UV irradiation, and temperature (Rehnstrom and Free, 1996), but also on the conidia turgor adjustment and full virulence to infect stone fruit (Yu et al., 2020). Visible light can cause oxidative stress in B. cinerea cells, which could be, in part, due to an alteration in the homeostasis of cellular ROS levels (Canessa et al., 2013). In fact, our results revealed how both treatments altered the morphology of conidia after 7 days of incubation under each light condition (Figures 1A,B) if compared to typically limoniform (or also cylindrical in the case of M. laxa) conidia shapes (Yin et al., 2015) observed under control condition. Therefore, these findings suggest that under these lighting treatments, conidia were submitted to stress that ultimately affected cell turgor. However, the impaired morphology could also rely on the result of the phototropism generated in response to light, which has been described in conidia, apothecia and conidial germ tubes of B. cinerea (Jarvis, 1972). Regarding conidial viability, studies on how light alters the ability to form new colonies of Monilinia spp. are nonexistence. We demonstrated that M. laxa, but not M. fructicola, increased its conidial viability under treatment 1 but reduced it under treatment 2 in relation to control condition (Figures 1C,D). In fact, Lafuente et al. (2018) already demonstrated that continuous blue light and complete darkness increased Penicillium digitatum cell viability in vitro compared to non-continuous light. These results are in line with what we observed for M. laxa, since the spectrum of lights used in this study do emit small wavelengths around blue. Alternatively, the altered conidia morphology could explain the reduction of M. laxa cell viability under treatment 2. Thus, the relation between turgor and the ability to form new colonies is a point of interest. Although some studies point out the role of light in controlling the conidial germination (Corrochano, 2019; Yu and Fischer, 2019), herein we did not observe an effect either on M. laxa or M. fructicola (Figures 1E,F).
Monilinia laxa Coped With Light Stress and Its in vitro Development Was Favored
Light altered the in vitro fungal expansion, especially in M. laxa. Under standard conditions (growing on PDA medium at 22–25°C and darkness), M. fructicola grows faster and produces more conidia than M. laxa (Villarino et al., 2016; Tran et al., 2020), like observed in the present study (Figure 1). However, lighting treatments made M. laxa to grow and produce more conidia (compared to control condition) than M. fructicola on PDA-T medium. Another reported light effect, widely described in B. cinerea (Schumacher, 2017), is that light can regulate biological responses, such as vegetative mycelial growth and the transition from sexual to asexual reproduction (conidiation) (Corrochano, 2019). In fact, the endogenous circadian clock also controls conidiation (Hevia et al., 2015). In this line, Canessa et al. (2013) reported that a photoperiod of cool white light and control condition reduced the growth rate and increased conidiation of a strain of B. cinerea. Our results suggest that M. laxa and M. fructicola behaved similarly to B. cinerea in terms of conidiation and growth rate, respectively. Botrytis cinerea perceives and reacts to the entire visible spectrum and beyond, and several fungal biological responses have been described for each monochromatic section of the spectrum (Schumacher, 2017; Veloso and van Kan, 2018). Green light (around 540 nm) represses mycelial growth (Zhu et al., 2013), whereas blue (around 450 nm) and red (around 650 nm) light restrain conidiation (Tan, 1975). Both treatment 1 and 2 tested herein emit three wavelength peaks around 440, 550, and 630 nm. Remarkably, the orange/red wavelength of treatment 1 is higher than the treatment 2 one. Hence, although M. laxa is able to sense and express green light photoreceptors (Rodríguez-Pires et al., 2021), its growth was increased rather than repressed. Zhu et al. (2013) found that under green light, B. cinerea cells showed deformed mitochondria and enlarged central vacuoles, probably as a result of the vacuoles’ action to eliminate cell structures damaged due to the light stress (Shoji et al., 2010), and in consequence, the growth rates of B. cinerea were retarded. However, under such light stress, M. laxa could be coping with it through autophagy of damaged organelles structures to support mycelial growth, as has been demonstrated when nutrient availability is limited (Shoji et al., 2010). A contrary effect was observed for M. fructicola which suggests the different ability of both species to sense and respond to light. The mechanisms underlying such differences are encouraged. An example pathway of interest related to light is the light-responsive transcription factor (LTF1), which controls development but also is required for maintenance of the redox homeostasis in mitochondria and full virulence in B. cinerea (Schumacher et al., 2014). Overall results showed that growth rate was in line with conidial viability and the reviewed results evidence the different ability of both Monilinia spp. to cope with lighting treatments.
Blue and red light have been described to repress conidiation in B. cinerea (Tan, 1975). Thus, although M. laxa is able to sense and express blue and red-light photoreceptors (Rodríguez-Pires et al., 2021), it increased its conidiation, whereas the conidia production of M. fructicola seemed to be affected by these sections of the spectrum (Figures 1C,D). Recent studies have shown that red light drastically increases conidiation of M. laxa compared to control condition (constant darkness) while does not affect or alter M. fructicola conidiation when compared to control condition (Verde-Yáñez et al., unpublished). Conidiation is regulated by light-responsive transcription factors, such as FL (fluffy) for undifferentiated mycelia, and it is induced by blue light through the blue-light photoreceptor WHITE COLLAR COMPLEX in the fungal model Neurospora crassa (Olmedo et al., 2010). However, our results revealed a fluffy phenotype of M. laxa when growing on PDA-T medium and incubated under control condition. Hence, other transcription factors should be responsible for the increased conidiation in M. laxa and in-depth studies should be conducted. Blue light has also been shown to act as an antimicrobial agent (Kahramanoğlu et al., 2020), which could, in part, explain the reduced conidiation observed in M. fructicola, highlighting again the different ability of both species to respond to light.
The Light-Induced Impaired Fungal Development Ultimately Alters Their Capacity to Infect Fruit
Light also affects the ability of pathogens to infect and rot fruit, such as described in several pathosystems (Islam et al., 1998; Lafuente et al., 2018). Among the aspects of fungal behavior and development that light can govern, light can regulate secondary metabolism, also related to the balance between sexual development toward conidia (Tisch and Schmoll, 2010; Schumacher, 2017). Our results demonstrated that after incubating M. laxa and M. fructicola under each lighting treatment for 7 days prior to fruit inoculation, both treatments reduced the ability of M. laxa to infect fruit, whereas only treatment 1 seemed to increase the virulence of M. fructicola (Figure 2). The colored phenotype and/or the altered conidia morphology observed in M. laxa grown on PDA-T medium maintained under lighting treatments could in part, explain its reduced capacity to infect. Similar results and hypotheses have been described for the P. digitatum-orange pathosystem. In line with spore viability, continuous blue light (450 nm) and complete darkness exposition of P. digitatum cultures lead to increased capability to infect oranges if compared to cultures submitted to non-continuous light (Lafuente et al., 2018). The authors suggested that the anomalous morphology of spores was more responsible for the lower capacity to infect fruit rather than the other parameters evaluated (metabolic activity and ethylene production). Alternatively, mutants of B. cinerea producing conidia in either light or darkness are associated with reduced virulence in primary leaves of French bean (Schumacher et al., 2012). However, how these altered features ultimately impair viability and capacity to infect fruit needs further investigation. Interestingly, fruit inoculated with M. laxa, previously incubated under treatment 1, showed conidia on fruit surface only after 7 dpi, slightly later than under control conditions (constant darkness) (6 dpi) and no conidiation was observed under treatment 2 (Figure 2B). In the line with what observed in P. digitatum, opposite incubation conditions (continuous light vs. complete darkness) can induce similar fungal phenotypes and responses, such as those observed herein regarding M. laxa. Contrary to M. laxa, in M. fructicola, the effect of light was mainly observed at the beginning of the infection course, showing the highest diameter length, accelerating the appearance of the onset of brown rot symptoms, and inducing more conidia on the fruit surface (Figures 2C,D and Supplementary Figure 1). Overall, results suggest that altered conidia morphology and reduced in vitro conidiation could positively impair its virulence on the fruit surface. Studies regarding the effect of light in photoreceptors related to conidiation (blue and red) and their signaling cascade would be interesting to be evaluated prior to and after fruit infection.
The Development of the Disease Relays on the Pathogen’s Light Effect Rather Than on the Fruit Itself
Plants are continuously exposed to a variety of abiotic stresses, which could drive to a modulation of the plant phenotype. Light is one of the major and influential inputs for their physiology and is perceived through plant photoreceptors (Folta and Carvalho, 2015). For that reason, in response to light, the mechanisms to face biotic stresses can also be altered. When Monilinia spp.-inoculated unbagged fruit were incubated under each treatment (Figures 3A,B, 4A,B), results revealed a comparable fungal development than the one observed when the pathogens were previously incubated under each lighting treatment prior to fruit inoculation. Both lighting treatments reduced M. laxa incidence, whereas control condition (constant darkness) reduced M. fructicola in unbagged fruit, elucidating that the fruit responses were Monilinia spp. dependent rather than dependent on light conditions. In Arabidopsis thaliana plants inoculated with B. cinerea, constant light and a photoperiod of light/dark considerably reduced the lesion areas compared to constant darkness (Canessa et al., 2013), in concordance with what we observed in M. laxa. Similar to that described in fungi, plant photoreceptors also perceive narrow-bandwidth wavelengths, which in turn activate specific internal responses (Folta and Carvalho, 2015). For instance, Zhu et al. (2013) demonstrated that white and green light decreased lesion diameter in B. cinerea-inoculated grapes and only green light reduced diameter in B. cinerea-inoculated tomatoes. Herein, we demonstrated that light had a major effect on Monilinia spp. rather than on fruit integrity, suggesting that pathogens are differentially modulating fruit responses.
Preharvest Fruit Conditions Influence the Disease Plant Response
Preharvest conditions are also crucial for fruit integrity and in turn, in its capacity to face any stress. Fruit bagging is an emerging agricultural practice mainly down to reduce the amount of fungicide on fruit surface. Bagging the fruit alters the solar radiation that irradiates fruit, and hence, influencing internal quality parameters (Sharma et al., 2014) skin color (Zhou et al., 2019) and marketable yield at harvest (Allran, 2017). Therefore, fruit bagging may result in changed defense response against pathogens. Herein, while bagged “Fantasia” cultivar was less susceptible to brown rot, bagged fruit of the other cultivars were more susceptible to both Monilinia spp. under control condition (Supplementary Table 1). Hence, findings point out that different solar radiation received by the unbagged and bagged fruit can differentially affect the fruit defense mechanisms in front of brown rot in a cultivar-dependent manner. Several studies conducted to test the bagging effect have also shown contradictory results when comparing cultivars, and fruit- and cultivar-specific responses have been suggested as one of the main causes (Sharma et al., 2014). In fact, fruit have different intrinsic characteristics depending on the stone fruit cultivar that leads to a different brown rot susceptibility (Baró-Montel et al., 2019a; Obi et al., 2019). Out of the responses of the host to counteract the pathogen’s intrusion, fruit activates stress responses through activating the antioxidant metabolism such as glutathione and redox-related amino acids (Balsells-Llauradó et al., 2020). Hence, analyzing intrinsic properties differing among cultivars such as quality parameters and fruit antioxidant metabolism, could ultimately be correlated with brown rot development, and thus, could shed light on the incidence differences among cultivars. In addition to that, fruit bagging can also affect to microclimate around the fruit, increasing temperature and humidity and in turn, affecting to transpiration, respiration and cuticle in peel cells (Ali et al., 2021).
The development of Monilinia spp. in bagged fruit, incubated under each incubation treatment (Figures 3C, 4C), was slightly different from the one observed in unbagged fruit. Hence, preventing the fruit from solar radiation may have caused not only an impact on the fruit’s intrinsic characteristics but also on the response to face the pathogens. Therefore, results highlight not only the importance of the light effect in preharvest (solar radiation), but also its effect in postharvest (artificial lighting). Solar light comprises a broad range of electromagnetic waves. The red light fraction of the spectra is of interest since not only was suggested to alter the behavior of Monilinia spp. (Section “Altered conidia morphology impair the conidial viability in a Monilinia spp.-dependent manner” and “Monilinia laxa coped with light stress and its in vitro development was favored”), but it can also have a positive effect on fruits in front of M. laxa, but not in front of M. fructicola. For instance, the previous incubation of strawberry leaves under red light significantly increased its resistance to B. cinerea (Meng et al., 2019). Further from the visible light, UV-C irradiation can induce resistance in several fruit and vegetables (reviewed in Romanazzi et al., 2016). Light quality can strongly modulate phenolic compounds, flavonoids, carotenoids, and anthocyanins (reviewed in Ilić and Fallik, 2017), being chlorophyll and carotenoids directly activated by photons. In particular, the activation of phenylpropanoids biosynthesis is enhanced by light in Xanthomonas oryza-treated rice leaves (Guo et al., 1993) and by the combination of red and blue light in lettuce (Heo et al., 2012). In addition, the expression of the zeaxanthin epoxidase, a flavoprotein from the carotenoid biosynthesis, that is active under light (Latowski et al., 2000), is upregulated in inoculated-fruit with M. laxa compared to healthy fruit along time (Balsells-Llauradó et al., 2020). Accordingly, future studies aiming to unravel the different fruit properties such as secondary metabolites in response to light would contribute to a better understanding of the fruit’s capability to face the pathogens.
Ethylene Production in the Host-Pathogen Interaction Is Mainly Influenced by Monilinia spp. Rather Than the Bag and Light Effect
Ethylene has been implicated in modulating the plant response not only to abiotic stresses but also to necrotrophic pathogens (McDowell and Dangl, 2000; Müller and Munné-Bosch, 2015). Hence, ethylene modulations induced by fruit bagging, lighting treatments and Monilinia spp. were assessed on the nectarine-Monilinia spp. interaction. Several studies have described that light affects ethylene levels and other hormones (e.g., cytokinins) and suggest a crosstalk among light and both hormones (reviewed in Zdarska et al., 2015), influencing plant development. However, our results showed that the ethylene produced by mock-inoculated fruit was affected by neither the bag nor the lighting treatments analyzed (Figures 5A,D,G). Specifically, ethylene emission increased along time, following the production pattern of a climacteric fruit until ripening (Oetiker and Yang, 1995). In other crops, such as grapes, lighting treatment does neither induce ethylene compared to dark (Zhu et al., 2012). Only Gong et al. (2015) found that blue light can induce changes in ethylene production to accelerate postharvest ripening in peaches, although the lighting treatments tested herein only emit a short intensity of blue light wavelength. Hence, ‘Fantasia’ cultivar was not affected by these abiotic conditions in terms of ethylene production.
Some fungi can also produce ethylene although its function in fungal development or as a virulence factor is inconclusive (Chague, 2010). Recently, white and blue lights have been shown to significantly increase the ethylene production rate of several fungi (such as B. cinerea) under in vitro conditions compared to dark, and that even B. cinerea could be the ethylene producer in an interaction with A. thaliana seedlings (Guo et al., 2020). However, the ethylene production by Monilinia spp. has not been deciphered to date. Herein, overall changes in the ethylene pattern of the pathogen-fruit pathosystem were due to the interaction with the pathogen and to bagging and lighting treatments. Among all the host responses that plant ethylene mediates (McDowell and Dangl, 2000), this hormone is also implicated in ripening and senescence processes, which can be conducive to disease susceptibility (Liu et al., 2015; Pandey et al., 2016). In fact, a different ethylene pattern was observed for both pathogens in interaction with fruit (Figure 5 and Supplementary Figure 3), pointing out to either a different response of the host to cope with the two Monilinia spp. or a different Monilinia species-dependent modulation to avoid the ethylene-mediated defense response. Other studies also reported a different modulation of ethylene production by both Monilinia spp.-fruit interaction in artificially inoculated peaches (Baró-Montel et al., 2019b) and peach petals (Vall-llaura et al., 2020). Although several hypotheses have been suggested, its role in promoting defense or susceptibility is still controversial (van Loon et al., 2006). In addition, results highlighted that the incubation under lighting treatments and the presence of the bag did alter the ethylene production, especially in M. laxa-inoculated fruit. These results could in turn explain the altered fruit’s capability to respond to these species (Figures 5B,E,H and Supplementary Figures 3A,B). Accordingly, unbagged fruit incubated under control condition demonstrated an increased M. laxa incidence and a lower incubation period (Figure 3), revealing that this species took advantage of the increased ethylene production. However, in M. laxa-inoculated bagged fruit both lighting treatments significantly induced ethylene production, such as the ethylene-induced in the B. cinerea-grapes pathosystem (Zhu et al., 2012). In addition to the plant ethylene role in biotic interactions, Xiang et al. (2020) suggested that the main downstream regulators of phytochromes (the phytochrome-interacting factors, PIFs) acted upstream of the ethylene response factor 1 (ERF1) to negatively regulate the resistance to B. cinerea in A. thaliana. With that, those authors suggested that the PIF-mediated defense against the pathogen is closely related to the jasmonate/ethylene signaling pathway. Thus, molecular studies related to the signaling downstream phytochromes need further investigation to understand the dual ethylene responses occurring during the nectarine-Monilinia spp. interaction under light conditions.
Concluding Remarks
To avoid or delay the appearance of brown rot symptoms and conidiation on the fruit surface and hence, reduce economic losses driven from contamination through conidia spreading along the postharvest chain, environmental light conditions should be considered. Our study highlights the different behavior of M. laxa and M. fructicola in both in vitro and in vivo development and further studies aiming to investigate the differences that underlie the impaired photomorphogenesis due to lighting treatments, such genes related to conidiation, of both species is encouraged. White lighting treatment has not only impaired the fungal development but also the host response to the pathogen attack. Light received for the fruit during preharvest modifies its intrinsic properties that ultimately would influence its capability to prevent or overcome the infection caused by Monilinia spp. During postharvest, light incidence also affected the nectarine-Monilinia spp. interaction since fungal development was altered in a species-dependent manner. Thus, deciphering the light-dependent modulation of the fruit properties that will give rise to improved defense response, but also the light-effect that triggers fungal development, will allow contributing to the development of new strategies to control brown rot at both preharvest and postharvest.
Data Availability Statement
The original contributions presented in the study are included in the article/Supplementary Material, further inquiries can be directed to the corresponding author.
Author Contributions
JU, RT, and NV conceived and designed the experiments. MB-L, NT, and CC carried out fruit inoculations, in vitro studies, pathological studies, and ethylene measurements. MB-L, NV, and RT wrote the article. CC, NT, and JU contributed to improving the final version of the manuscript. All authors contributed to the article and approved the submitted version.
Funding
This work was supported by national project AGL2017-84389-C2-1-R from the Spanish Government (MINECO), by a Ph.D. grant CPD2016-0159 (MB-L) from INIA and by funding received from the CERCA Programme/Generalitat de Catalunya.
Conflict of Interest
The authors declare that the research was conducted in the absence of any commercial or financial relationships that could be construed as a potential conflict of interest.
Publisher’s Note
All claims expressed in this article are solely those of the authors and do not necessarily represent those of their affiliated organizations, or those of the publisher, the editors and the reviewers. Any product that may be evaluated in this article, or claim that may be made by its manufacturer, is not guaranteed or endorsed by the publisher.
Acknowledgments
We want to acknowledge Sandra Serrano-Prieto and Lucía Verde-Yáñez for the technical support and Dr. Jordi Giné-Bordonaba for ethylene experimental design advice.
Supplementary Material
The Supplementary Material for this article can be found online at: https://www.frontiersin.org/articles/10.3389/fpls.2021.666985/full#supplementary-material
Supplementary Figure 1 | Conidiation of M. fructicola on “Fantasia” cultivar surface. The concentration of conidia is represented relative to control condition (dark). Different letters indicate statistically differences among treatments according to orthogonal contrasts (P < 0.05).
Supplementary Figure 2 | Light effect on the capacity of Monilinia spp. to infect fruit in “Venus” cultivar. Incidence (% of brown rot, bars) and severity (lesion diameter length in cm of rotted fruit, lines) of M. laxa (A) and M. fructicola (C) in “Venus” nectarines along the infection time course (dpi, days post inoculation) after growing the fungi during 7 days under control and treatments 1 and 2. Bars represent the mean of incidence on fruit (n = 20). Lines represent the mean of diameter length of rotted fruit. Different uppercase and lowercase letters indicate significant differences (P ≤ 0.05) of incidence and severity, respectively, among treatments according to orthogonal contrasts at each time point. No letters indicate no significant differences. Incubation and latency periods (days) of M. laxa (B) and M. fructicola (D) in “Venus” nectarines after growing the fungi during 7 days under control and treatments 1 and 2. Bars represent the mean of fruits with symptoms (n = 1 to 20) and error bars represent the standard error of the means. Different lowercase and uppercase letters indicate significant differences (P ≤ 0.05) of incubation and latency periods, respectively, among treatments according to orthogonal contrasts. Conidiation of M. fructicola on fruit surface (E). The concentration of conidia is represented relative to control (dark). Different letters indicate statistically differences among treatments according to orthogonal contrasts (P < 0.05).
Supplementary Figure 3 | Ethylene production of mock-inoculated fruit, M. laxa and M. fructicola-inoculated fruit on unbagged nectarines. Ethylene measurements of mock, M. laxa, M. fructicola-inoculated fruit incubated during 7 days under control condition (A) and treatments 1 (B) and 2 (C). Different letters indicate significant differences among inoculums at each time point according to Tukey’s HSD test (P ≤ 0.05). No letters indicate no significant differences. In all graphics, values represent the mean of ethylene measurements of each replicate (n = 4).
Supplementary Table 1 | Monilinia spp. incidence (%) on unbagged and bagged fruit from different cultivars after 7 days of incubation under control condition (dark). No significant differences between bagging conditions were found according to generalized linear model (GLM).
References
Ali, M. M., Anwar, R., Yousef, A. F., Li, B., Luvisi, A., De Bellis, L., et al. (2021). Influence of bagging on the development and quality of fruits. Plants 10, 1–17. doi: 10.3390/plants10020358
Allran, J. (2017). Investigation Of Peach Fruit Bagging To Produce High Quality Fruit And To Manage Pests And Diseases. Available online at: https://tigerprints.clemson.edu/all_theses/2695/ (accessed October 10, 2019)
Avalos, J., and Limón, M. C. (2015). Biological roles of fungal carotenoids. Curr. Genet. 61, 309–324. doi: 10.1007/s00294-014-0454-x
Bahn, Y. S., Xue, C., Idnurm, A., Rutherford, J. C., Heitman, J., and Cardenas, M. E. (2007). Sensing the environment: lessons from fungi. Nat. Rev. Microbiol. 5, 57–69. doi: 10.1038/nrmicro1578
Balsells-Llauradó, M., Silva, C. J., Usall, J., Vall-llaura, N., Serrano-Prieto, S., Teixidó, N., et al. (2020). Depicting the battle between nectarine and Monilinia laxa: the fruit developmental stage dictates the effectiveness of the host defenses and the pathogen’s infection strategies. Horticult. Res. 7:167. doi: 10.1038/s41438-020-00387-w
Baró-Montel, N., Torres, R., Casals, C., Teixidó, N., Segarra, J., and Usall, J. (2019a). Developing a methodology for identifying brown rot resistance in stone fruit. Eur. J. Plant Pathol. 154, 287–303. doi: 10.1007/s10658-018-01655-1
Baró-Montel, N., Vall-llaura, N., Giné-Bordonaba, J., Usall, J., Serrano-Prieto, S., Teixidó, N., et al. (2019b). Double-sided battle: the role of ethylene during Monilinia spp. infection in peach at different phenological stages. Plant Physiol. Biochem. 144, 324–333. doi: 10.1016/j.plaphy.2019.09.048
Baró-Montel, N., Vall-llaura, N., Usall, J., Teixidó, N., Naranjo-Ortíz, M. A., Gabaldón, T., et al. (2019c). Pectin methyl esterases and rhamnogalacturonan hydrolases: weapons for successful Monilinia laxa infection in stone fruit? Plant Pathol. 68, 1381–1393. doi: 10.1111/ppa.13039
Bulletin OEPP/EPPO (2020). PM 7/18 (3) Monilinia fructicola. EPPO Bull. 50, 5–18. doi: 10.1111/epp.12609
Canessa, P., Schumacher, J., Hevia, M. A., Tudzynski, P., and Larrondo, L. F. (2013). Assessing the effects of light on differentiation and virulence of the plant pathogen Botrytis cinerea: characterization of the white collar complex. PLoS One 8:e84223. doi: 10.1371/journal.pone.0084223
Carvalho, S. D., and Castillo, J. A. (2018). Influence of light on plant–phyllosphere interaction. Front. Plant Sci. 9:1482. doi: 10.3389/fpls.2018.01482
Casals, C., Vinas, I., Torres, R., Griera, C., and Usall, J. (2010). Effect of temperature and water activity on in vitro germination of Monilinia spp. J. Appl. Microbiol. 108, 47–54.
Chague, V. (2010). “Ethylene production by fungi: biological questions and future developments towards a sustainable polymers industry,” in Handbook of Hydrocarbon and Lipid Microbiology, ed. K. N. Timmis (Berlin: Springer-Verlag), 3011–3020. doi: 10.1007/978-3-540-77587-4_224
Corrochano, L. M. (2019). Light in the fungal world: from photoreception to gene transcription and beyond. Annu. Rev. Genet. 53, 149–170. doi: 10.1146/annurev-genet-120417-031415
De Cal, A., and Melgarejo, P. (1999). Effects of long-wave UV light on Monilinia growth and identification of species. Plant Dis. 83, 62–65. doi: 10.1094/pdis.1999.83.1.62
De Cal, A., Gell, I., Usall, J., Viñas, I., and Melgarejo, P. (2009). First report of brown rot caused by Monilinia fructicola in peach orchards in Ebro Valley Spain. Plant Dis. 93:763. doi: 10.1094/PDIS-93-7-0763A
De Miccolis Angelini, R. M., Abate, D., Rotolo, C., Gerin, D., Pollastro, S., and Faretra, F. (2018). De novo assembly and comparative transcriptome analysis of Monilinia fructicola, Monilinia laxa and Monilinia fructigena, the causal agents of brown rot on stone fruits. BMC Genomics 19:436. doi: 10.1186/s12864-018-4817-4
Folta, K. M., and Carvalho, S. D. (2015). Photoreceptors and control of horticultural plant traits. HortScience 50, 1274–1280. doi: 10.21273/hortsci.50.9.1274
Fuller, K. K., Loros, J. J., and Dunlap, J. C. (2015). Fungal photobiology: visible light as a signal for stress, space and time. Curr. Genet. 61, 275–288. doi: 10.1007/s00294-014-0451-0
Garcia-Benitez, C., Melgarejo, P., De Cal, A., and Fontaniella, B. (2016). Microscopic analyses of latent and visible Monilinia fructicola infections in nectarines. PLoS One 11:e0160675. doi: 10.1371/journal.pone.0160675
Gell, I., De Cal, A., Torres, R., Usall, J., and Melgarejo, P. (2008). Relationship between the incidence of latent infections caused by Monilinia spp. and the incidence of brown rot of peach fruit: Factors affecting latent infection. Eur. J. Plant Pathol. 121, 487–498. doi: 10.1007/s10658-008-9268-3
Gell, I., De Cal, A., Torres, R., Usall, J., and Melgarejo, P. (2009). Conidial density of Monilinia spp. on peach fruit surfaces in relation to the incidences of latent infections and brown rot. Eur. J. Plant Pathol. 2009, 415–424.
Giné-Bordonaba, J., Echeverria, G., Ubach, D., Aguiló-Aguayo, I., López, M. L., and Larrigaudière, C. (2017). Biochemical and physiological changes during fruit development and ripening of two sweet cherry varieties with different levels of cracking tolerance. Plant Physiol. Biochem. 111, 216–225. doi: 10.1016/j.plaphy.2016.12.002
Gong, D., Cao, S., Sheng, T., Shao, J., Song, C., Wo, F., et al. (2015). Effect of blue light on ethylene biosynthesis, signalling and fruit ripening in postharvest peaches. Sci. Hortic. (Amsterdam). 197, 657–664. doi: 10.1016/j.scienta.2015.10.034
Griebel, T., and Zeier, J. (2008). Light regulation and daytime dependency of inducible plant defenses in Arabidopsis: phytochrome signaling controls systemic acquired resistance rather than local defense. Plant Physiol. 147, 790–801. doi: 10.1104/pp.108.119503
Guo, A., Reimers, J., and Leach, J. E. (1993). Effect of light on incompatible interactions between Xanthomonas oryzae pv oryzae and rice. Physiol. Mol. Plant Pathol. 42, 413–425.
Guo, H., Liu, A., Wang, Y., Wang, T., Zhang, W., Zhu, P., et al. (2020). Measuring light-induced fungal ethylene production enables non-destructive diagnosis of disease occurrence in harvested fruits. Food Chem. 310:125827. doi: 10.1016/j.foodchem.2019.125827
Heo, J.-W., Kang, D.-H., Bang, H.-S., Hong, S.-G., Chun, C.-H., and Kang, K.-K. (2012). Early growth, pigmentation, protein content, and phenylalanine ammonia-lyase activity of red curled lettuces grown under different lighting conditions. Korean J. Hortic. Sci. Technol. 30, 6–12. doi: 10.7235/hort.2012.11118
Hevia, M. A., Canessa, P., Müller-Esparza, H., and Larrondo, L. F. (2015). A circadian oscillator in the fungus Botrytis cinerea regulates virulence when infecting Arabidopsis thaliana. Proc. Natl. Acad. Sci. U.S.A. 112, 8744–8749. doi: 10.1073/pnas.1508432112
Idnurm, A., and Crosson, S. (2009). The photobiology of microbial pathogenesis. PLoS Pathog. 5:11–13. doi: 10.1371/journal.ppat.1000470
Ilić, Z. S., and Fallik, E. (2017). Light quality manipulation improves vegetable quality at harvest and postharvest: a review. Environ. Exp. Bot. 139, 79–90. doi: 10.1016/j.envexpbot.2017.04.006
Islam, S. Z., Honda, Y., and Sonhaji, M. (1998). Phototropism of conidial germ tubes of Botrytis cinerea and its implication in plant infection processes. Plant Dis. 82, 850–856. doi: 10.1094/PDIS.1998.82.8.850
Jarvis, W. R. (1972). Phototropism in Botrytis cinerea. Trans. Br. Mycol. Soc. 58, 526–587. doi: 10.1016/s0007-1536(72)80108-6
Kahramanoğlu, I., Nisar, M. F., Chen, C., Usanmaz, S., Chen, J., and Wan, C. (2020). Light: an alternative method for physical control of postharvest rotting caused by fungi of citrus fruit. J. Food Qual. 2020, 8821346. doi: 10.1155/2020/8821346
Keske, C., Amorim, L., and Mio, L. (2011). Peach brown rot incidence related to pathogen infection at different stages of fruit development in an organic peach production system. Crop Prot. 30, 802–806. doi: 10.1016/j.cropro.2011.03.005
Keske, C., Treutter, D., and Neumüller, M. (2014). “Effect of bagging on brown rot incidence in European Plum. Ecofruit,” in Proceedings of the 16th International Conference Organization Growth Proceedings, 17-19 February 2014, Hohenheim, 228–231.
Kreidl, S., Edwards, J., and Villalta, O. N. (2015). Assessment of pathogenicity and infection requirements of Monilinia species causing brown rot of stone fruit in Australian orchards. Australas. Plant Pathol. 44, 419–430. doi: 10.1007/s13313-015-0362-7
Lafuente, M. T., Alférez, F., and González-Candelas, L. (2018). Light-emitting diode blue light alters the ability of Penicillium digitatum to infect citrus fruits. Photochem. Photobiol. 94, 1003–1009. doi: 10.1111/php.12929
Latowski, D., Burda, K., and Strzałka, K. (2000). A mathematical model describing kinetics of conversion of violaxanthin to zeaxanthin via intermediate antheraxanthin by the xanthophyll cycle enzyme violaxanthin de-epoxidase. J. Theor. Biol. 206, 507–514. doi: 10.1006/jtbi.2000.2141
Liu, M., Pirrello, J., Chervin, C., Roustan, J. P., and Bouzayen, M. (2015). Ethylene control of fruit ripening: revisiting the complex network of transcriptional regulation. Plant Physiol. 169, 2380–2390. doi: 10.1104/pp.15.01361
Luo, Y., and Michailides, T. J. (2001). Factors affecting latent infection of prune fruit by Monilinia fructicola. Phytopathology 91, 864–872.
Marquenie, D., Geeraerd, A. H., Lammertyn, J., Soontjens, C., Van Impe, J. F., Michiels, C. W., et al. (2003). Combinations of pulsed white light and UV-C or mild heat treatment to inactivate conidia of Botrytis cinerea and Monilia fructigena. Int. J. Food Microbiol. 85, 185–196.
McDowell, J. M., and Dangl, J. L. (2000). Signal transduction in the plant immune response. Trends Biochem. Sci. 25, 79–82. doi: 10.1016/S0968-0004(99)01532-7
Meng, L., Höfte, M., and Van Labeke, M. C. (2019). Leaf age and light quality influence the basal resistance against Botrytis cinerea in strawberry leaves. Environ. Exp. Bot. 157, 35–45. doi: 10.1016/j.envexpbot.2018.09.025
Métraux, J. P. (2002). Systemic acquired resistance. Euphytica 124, 237–243. doi: 10.1016/B978-0-12-374984-0.01509-6
Minas, I. S., Tanou, G., and Molassiotis, A. (2018). Environmental and orchard bases of peach fruit quality. Sci. Hortic. (Amsterdam). 235, 307–322. doi: 10.1016/j.scienta.2018.01.028
Müller, M., and Munné-Bosch, S. (2015). Ethylene response factors: a key regulatory hub in hormone and stress signaling. Plant Physiol. 169, 32–41. doi: 10.1104/pp.15.00677
Obi, V. I., Barriuso, J. J., and Gogorcena, Y. (2018). Peach brown rot: still in search of an ideal management option. Agriculture 8, 1–34. doi: 10.3390/agriculture8080125
Obi, V. I., Barriuso, J. J., Usall, J., and Gogorcena, Y. (2019). Breeding strategies for identifying superior peach genotypes resistant to brown rot. Sci. Hortic. (Amsterdam). 246, 1028–1036. doi: 10.1016/j.scienta.2018.10.027
Oetiker, J. H., and Yang, S. F. (1995). The role of ethylene in fruit ripening. Acta Hortic. 398, 167–178. doi: 10.17660/actahortic.1995.398.17
Oliveira Lino, L., Pacheco, I., Mercier, V., Faoro, F., Bassi, D., Bornard, I., et al. (2016). Brown rot strikes Prunus fruit: An ancient fight almost always lost. J. Agric. Food Chem. 64, 4029–4047. doi: 10.1021/acs.jafc.6b00104
Olmedo, M., Ruger-Herreros, C., and Corrochano, L. M. (2010). Regulation by blue light of the fluffy gene encoding a major regulator of conidiation in Neurospora crassa. Genetics 184, 651–658. doi: 10.1534/genetics.109.109975
Pandey, D., Rajendran, S. R. C. K., Gaur, M., Sajeesh, P. K., and Kumar, A. (2016). Plant defense signaling and responses against necrotrophic fungal pathogens. J. Plant Growth Regul. 35, 1159–1174. doi: 10.1007/s00344-016-9600-7
Rehnstrom, A. L., and Free, S. J. (1996). The isolation and characterization of melanin-deficient mutants of Monilinia fructicola. Physiol. Mol. Plant Pathol. 49, 321–330. doi: 10.1006/pmpp.1996.0057
Roberts, M. R., and Paul, N. D. (2006). Seduced by the dark side: integrating molecular and ecological perspectives onfence against pests and pathogens. New Phytol. 170, 677–699. doi: 10.1111/j.1469-8137.2006.01707.x
Rodríguez-Pires, S., Garcia-Companys, M., Espeso, E. A., Melgarejo, P., and De Cal, A. (2020). Influence of light on the Monilinia laxa stone fruit interaction. Plant Pathol. 70, 326–335. doi: 10.1111/ppa.13294
Rodríguez-Pires, S., De Cal, A., Espeso, E. A., Rasiukeviciut, N., and Melgarejo, P. (2021). Light-photoreceptors and proteins related to Monilinia laxa. J. Fungi 7, 1–17 doi: 10.3390/jof7010032
Roeber, V. M., Bajaj, I., Rohde, M., Schmülling, T., and Cortleven, A. (2020). Light acts as a stressor and influences abiotic and biotic stress responses in plants. Plant Cell Environ. 44, 645–664. doi: 10.1111/pce.13948
Romanazzi, G., Sanzani, S. M., Bi, Y., Tian, S., Gutiérrez Martínez, P., and Alkan, N. (2016). Induced resistance to control postharvest decay of fruit and vegetables. Postharvest Biol. Technol. 122, 82–94. doi: 10.1016/j.postharvbio.2016.08.003
Rungjindamai, N., Jeffries, P., and Xu, X.-M. (2014). Epidemiology and management of brown rot on stone fruit caused by Monilinia laxa. Eur. J. Plant Pathol. 140, 1–17. doi: 10.1007/s10658-014-0452-3
Schumacher, J. (2017). How light affects the life of Botrytis. Fungal Genet. Biol. 106, 26–41. doi: 10.1016/j.fgb.2017.06.002
Schumacher, J., Pradier, J. M., Simon, A., Traeger, S., Moraga, J., Collado, I. G., et al. (2012). Natural variation in the VELVET gene bcvel1 affects virulence and light-dependent differentiation in Botrytis cinerea. PLoS One 7:e47840. doi: 10.1371/journal.pone.0047840
Schumacher, J., Simon, A., Cohrs, K. C., Viaud, M., and Tudzynski, P. (2014). The transcription factor BcLTF1 regulates virulence and light responses in the necrotrophic plant pathogen Botrytis cinerea. PLoS Genet. 10:e1004040. doi: 10.1371/journal.pgen.1004040
Sharma, R. R., Reddy, S. V. R., and Jhalegar, M. J. (2014). Pre-harvest fruit bagging: a useful approach for plant protection and improved post-harvest fruit quality – a review. J. Hortic. Sci. Biotechnol. 89, 101–113. doi: 10.1080/14620316.2014.11513055
Shoji, J. Y., Kikuma, T., Arioka, M., and Kitamoto, K. (2010). Macroautophagy-mediated degradation of whole nuclei in the filamentous fungus Aspergillus oryzae. PLoS One 5:e15650. doi: 10.1371/journal.pone.0015650
Tan, K. K. (1975). Interaction of near-ultraviolet, blue, red, and far-red light in sporulation of Botrytis cinerea. Trans. Br. Mycol. Soc. 64, 215–222. doi: 10.1016/s0007-1536(75)80105-7
Tisch, D., and Schmoll, M. (2010). Light regulation of metabolic pathways in fungi. Appl. Microbiol. Biotechnol. 85, 1259–1277. doi: 10.1007/s00253-009-2320-1
Tran, T. T., Li, H., Nguyen, D. Q., Sivasithamparam, K., Jones, M. G. K., and Wylie, S. J. (2020). Comparisons between genetic diversity, virulence and colony morphology of Monilinia fructicola and Monilinia laxa isolates. J. Plant Pathol. 102, 743–751. doi: 10.1007/s42161-020-00498-2
Usall, J., Casals, C., Sisquella, M., Palou, L., and De Cal, A. (2015). Alternative technologies to control postharvest diseases of stone fruits. Stewart Postharvest Rev. 11, 1–6. doi: 10.2212/spr.2015.4.2
Vall-llaura, N., Giné-Bordonaba, J., Usall, J., Larrigaudière, C., Teixidó, N., and Torres, R. (2020). Ethylene biosynthesis and response factors are differentially modulated during the interaction of peach petals with Monilinia laxa or Monilinia fructicola. Plant Sci. 299:110599. doi: 10.1016/j.plantsci.2020.110599
van Loon, L. C., Rep, M., and Pieterse, C. M. J. (2006). Significance of inducible defense-related proteins in infected plants. Annu. Rev. Phytopathol. 44, 135–162. doi: 10.1146/annurev.phyto.44.070505.143425
Veloso, J., and van Kan, J. A. L. (2018). Many shades of grey in Botrytis–Host plant interactions. Trends Plant Sci. 23, 613–622. doi: 10.1016/j.tplants.2018.03.016
Villarino, M., Eguen, B., Lamarca, N., Segarra, J., Usall, J., Melgarejo, P., et al. (2013). Occurrence of Monilinia laxa and M. fructigena after introduction of M. fructicola in peach orchards in Spain. Eur. J. Plant Pathol. 137, 835–845. doi: 10.1007/s10658-013-0292-6
Villarino, M., Melgarejo, P., and De Cal, A. (2016). Growth and aggressiveness factors affecting Monilinia spp. survival peaches. Int. J. Food Microbiol. 227, 6–12. doi: 10.1016/j.ijfoodmicro.2016.01.023
Villarino, M., Melgarejo, P., Usall, J., Segarra, J., and De Cal, A. (2010). Primary inoculum sources of Monilinia spp. in Spanish peach orchards and their relative importance in brown rot. Plant Dis. 94, 1048–1054. doi: 10.1094/pdis-94-8-1048
Villarino, M., Melgarejo, P., Usall, J., Segarra, J., Lamarca, N., and De Cal, A. (2012). Secondary inoculum dynamics of Monilinia spp. and relationship to the incidence of postharvest brown rot in peaches and the weather conditions during the growing season. Eur. J. Plant Pathol. 133, 585–598. doi: 10.1007/s10658-011-9931-y
Xiang, S., Wu, S., Zhang, H., Mou, M., Chen, Y., Li, D., et al. (2020). The PIFs redundantly control plant defense response against Botrytis cinerea in Arabidopsis. Plants 9, 1–13. doi: 10.3390/plants9091246
Yin, L. F., Chen, S. N., Chen, G. K., Schnabel, G., Du, S. F., Chen, C., et al. (2015). Identification and characterization of three Monilinia species from plum in China. Plant Dis. 99, 1775–1783. doi: 10.1094/PDIS-12-14-1308-RE
Yu, F.-Y., Chiu, C.-M., Lee, Y.-Z., Lee, S.-J., Chou, C.-M., You, B.-J., et al. (2020). Polyketide synthase gene expression in relation to chloromonilicin and melanin production in Monilinia fructicola. Phytopatholog 110, 1465–1475. doi: 10.1094/phyto-02-20-0059-r
Yu, Z., and Fischer, R. (2019). Light sensing and responses in fungi. Nat. Rev. Microbiol. 17, 25–36. doi: 10.1038/s41579-018-0109-x
Zdarska, M., Dobisová, T., Gelová, Z., Pernisová, M., Dabravolski, S., and Hejátko, J. (2015). Illuminating light, cytokinin, and ethylene signalling crosstalk in plant development. J. Exp. Bot. 66, 4913–4931. doi: 10.1093/jxb/erv261
Zhou, H., Yu, Z., and Ye, Z. (2019). Effect of bagging duration on peach fruit peel color and key protein changes based on iTRAQ quantitation. Sci. Hortic. (Amsterdam). 246, 217–226. doi: 10.1016/j.scienta.2018.10.072
Zhu, P., Xu, L., Zhang, C., Toyoda, H., and Gan, S. S. (2012). Ethylene produced by Botrytis cinerea can affect early fungal development and can be used as a marker for infection during storage of grapes. Postharvest Biol. Technol. 66, 23–29. doi: 10.1016/j.postharvbio.2011.11.007
Keywords: necrotroph, brown rot, nectarine, photomorphogenesis, preharvest, postharvest, bagging, ethylene
Citation: Balsells-Llauradó M, Torres R, Vall-llaura N, Casals C, Teixidó N and Usall J (2021) Light Intensity Alters the Behavior of Monilinia spp. in vitro and the Disease Development on Stone Fruit-Pathogen Interaction. Front. Plant Sci. 12:666985. doi: 10.3389/fpls.2021.666985
Received: 11 February 2021; Accepted: 09 August 2021;
Published: 08 September 2021.
Edited by:
Maria Del Mar Jimenez-Gasco, The Pennsylvania State University (PSU), United StatesReviewed by:
Graham Robert David McGrann, Science and Advice for Scottish Agriculture (SASA), United KingdomGianfranco Romanazzi, Marche Polytechnic University, Italy
Copyright © 2021 Balsells-Llauradó, Torres, Vall-llaura, Casals, Teixidó and Usall. This is an open-access article distributed under the terms of the Creative Commons Attribution License (CC BY). The use, distribution or reproduction in other forums is permitted, provided the original author(s) and the copyright owner(s) are credited and that the original publication in this journal is cited, in accordance with accepted academic practice. No use, distribution or reproduction is permitted which does not comply with these terms.
*Correspondence: Rosario Torres, cm9zYXJpby50b3JyZXNAaXJ0YS5jYXQ=