- State Key Laboratory of Crop Genetics and Germplasm Enhancement, Key Laboratory of Landscaping, Ministry of Agriculture and Rural Affairs, College of Horticulture, Nanjing Agricultural University, Nanjing, China
Heat stress limits the growth and development of chrysanthemum seedlings. Although melatonin (MT) has been linked to the heat stress response in plants, research on the underlying molecular mechanisms is scarce. In this study, the regulatory networks of MT on heat stress in chrysanthemum seedlings were explored. Physiological measurements suggested that MT not only reduced malondialdehyde accumulation, hydrogen peroxide content, and superoxide anion free radical generation rate, but also significantly promoted osmotic regulation substance synthesis (proline and soluble protein), antioxidant accumulation (GSH and AsA), and the antioxidant enzyme activities (SOD, POD, CAT, and APX) in chrysanthemum leaves under heat stress. Furthermore, MT increased the fresh weight, dry weight, chlorophyll content, photosynthesis rate, and gas exchange indexes. Further, RNA-seq results revealed 33,497 and 36,740 differentially expressed genes in the S/Con and SMT/ConMT comparisons, respectively. The differences in the comparisons revealed that MT regulated heat shock transcription factors (HSFs) and heat shock proteins (HSPs), and the genes involved in Ca2+ signal transduction (CNGCs and CAM/CMLs), starch and sucrose metabolism (EDGL, BGLU, SuS, and SPS), hormone (PP2Cs, AUX/IAAs, EBFs, and MYC2), chlorophyll metabolism (HEMA and PORA), flavonoid biosynthesis (CHS, DFR, and FNS), and carotenoid biosynthesis (DXPS, GGDP, and PSY). MT effectively improved chrysanthemum seedling heat-resistance. Our study, thus, provides novel evidence of a gene network regulated by MT under heat stress.
Introduction
High temperature stress restricts plant growth and development, thereby, severely reducing crop yields (Wilson et al., 2014; Lesk et al., 2016). Heat damage includes leaf curling and yellowing, whole leaf wilting, and leaf edge scorching (Sharma et al., 2016). Moreover, reactive oxygen species (ROS) are produced in excess under heat stress, which in turn causes a series of complex metabolic alterations, including, changes in enzyme activity, in proteins and nucleic acids, and in cell membrane and cytoskeleton stability (Ahammed et al., 2016). Plants have their own antioxidant systems that can effectively scavenge ROS (Baxter et al., 2014). However, excessive accumulation of ROS causes severe disruption of ROS homeostasis, resulting in the oxidation of lipids, DNA, and proteins (Vanderauwera et al., 2011; Baxter et al., 2014). High ambient temperatures enhance transpiration, causing tissue dehydration and even plant death (Locato et al., 2009). Urgent measures are required for the amelioration of heat-induced plant damage; a research area that has received widespread attention (Baninasab and Ghobadi, 2010), particularly, because the plant heat-response system can be overwhelmed by conditions of chronic and severe heat stress.
Acting as an antioxidant and growth regulator (Fleta-Soriano et al., 2017), melatonin was first discovered in the pineal gland of bovines (Arnao and Hernandez-Ruiz, 2014). It plays a vital role in the growth and development of plants, and their resistance to stress (Arnao and Hernandez-Ruiz, 2015), assisting plants to survive and thrive (Reiter et al., 2015). Endogenous regulation and exogenous spraying of melatonin can improve plant resistance to biotic and abiotic stress (Hasan et al., 2015; Shi et al., 2015; Xu et al., 2016). A recent study in Lolium perenne revealed that exogenous spraying of melatonin reduced abscisic acid content under heat stress, as well as increased the concentration of endogenous melatonin and cytokinin (Zhang et al., 2017). Heat stress causes misfolding of proteins in plant cells; in tomato, melatonin reduced the ratio of insoluble protein to total protein, thereby protecting plant proteins against heat-induced denaturation (Xu et al., 2016). The application of melatonin increased superoxide dismutase (SOD) (Zhang et al., 2017), and ascorbic acid (AsA)-GSH cycle-related enzyme activities, such as that of ascorbate peroxidase (APX). Melatonin can modulate Ca2+ influx through a non-selective Ca2+ permeable cation channel (Celik and Nazıroğlu, 2012), and stimulate Ca2+ transport across the cellular membranes (Santofimia-Castaño et al., 2014). In kiwifruit seedlings, melatonin can effectively modulate carbon fixation and improve photosynthesis under heat stress by regulating the transcription of triosephosphate isomerase (TIM), ribose 5-phosphate isomerase A (RPI), and phosphoenolpyruvate carboxykinase (PCK) genes (Liang et al., 2019). Melatonin also increase the biosynthesis of polyphenols such as total phenols, flavonoids, and anthocyanins in grape berries (Meng et al., 2019). In addition, melatonin was shown to induce the transcription of heat shock proteins (HSPs) and to promote the degradation of denatured proteins in response to abiotic stress (Shi et al., 2015; Wang et al., 2015; Xu et al., 2016). In plants, melatonin affects root architecture (Pelagio-Flores et al., 2012), organ development (Arnao and Hernandez-Ruiz, 2014), photosynthesis (Arnao and Hernandez-Ruiz, 2015), defense (Weeda et al., 2014), senescence (Byeon et al., 2012; Wang et al., 2013), and stress responses (Kostopoulou et al., 2015; Zhang et al., 2015). Specifically, melatonin effectively maintains photosynthesis in tomato plants growing under heat stress (Ahammed et al., 2018). In maize seedlings, melatonin enhances thermotolerance by modulating antioxidant defense, methylglyoxal detoxification, and osmoregulation systems (Li et al., 2019). In wheat seedlings, melatonin suppressed the heat stress-induced damage by modulating the antioxidant machinery (Buttar et al., 2020). However, whether MT could enhance the thermotolerance of chrysanthemum and the underlying mechanisms is not known.
Chrysanthemum is one of the most widely cultivated cut flowers in the world, thus having high ornamental and economic values. In summer, under heat stress conditions, chrysanthemum seedlings grow slowly, and the leaves curl, turn yellow, and wither. In severe cases, chrysanthemum seedlings can die. If chrysanthemum encounters extreme heat stress during the reproductive growth period, its flowers will die prior to propagation, which will seriously restrict the development of the chrysanthemum industry and the value of ornamental chrysanthemum. Therefore, it is important to identify methods for improving the resistance of chrysanthemum to heat stress conditions. In this study, we explored the melatonin-mediated enhancement of chrysanthemum seedling-stress resistance through its regulation of the physiological and molecular responses involved. Physiologically, our main analyses focused on osmotic regulation substances, peroxides, antioxidant contents, and antioxidant enzymes. Moreover, we highlighted the genes involved in the ROS, the heat shock transcription factor (HSF)-HSP, Ca2+ signal transduction, carbon fixation, the starch and sucrose metabolism pathways, hormone signal transduction, and the chlorophyll, flavonoid, and carotenoid metabolic pathways. We elucidated the gene regulatory networks involving melatonin under heat stress; furthermore, our study provides a sound theoretical basis for research on melatonin to improve heat tolerance in plants.
Materials and Methods
Plant Materials and Growing Conditions
The chrysanthemum cultivar “Jinba” was obtained from the Chrysanthemum Germplasm Resource Preserving Center (Nanjing Agricultural University, China). Rooted seedlings were transplanted into pots filled with a 1:1 soil/vermiculite mixture and placed in a greenhouse under a 16:8 h light:dark regime; a 25/15°C day/night temperature regime, and 70% relative humidity, until they had formed approximately ten fully expanded functional leaves, excluding young leaves at the same developmental phase. To screen for a suitable melatonin concentration, five concentrations (0, 50, 100, 200, and 400 μM) were tested in a preliminary experiment. The results of preliminary experiments indicated that 200 μM solution was selected as the treatment concentration, owing to it had a stronger effect on the physiological indexes, including growth indicators (i.e., fresh and dry weights), osmotic regulators [i.e., malondialdehyde (MDA), and proline content], and antioxidant enzyme activities [SOD and peroxidase (POD)]. Then chrysanthemum seedlings were uniformly sprayed with 200 μM melatonin every other day for 6 days until the leaves and stems were fully moistened, without the occurrence of dripping. The total volume of melatonin solution sprayed per plant per day was approximately 10 mL with spraying prevention of soils. Control plants (Con) were sprayed with distilled H2O (no melatonin). Melatonin was first dissolved in a small amount of alcohol and then formulated to 200 μM melatonin in distilled water. Each treatment was applied to 30 chrysanthemum seedlings and the experiment was performed in triplicates. Subsequently, the chrysanthemum seedlings and controls pre-treated with melatonin or water were subjected to the following treatments: Con, 25°C/15°C with water; stress (S) plants, 40°C/30°C with water; stress with melatonin (SMT), 40°C/30°C with MT, and control with melatonin (ConMT), 25°C/15°C with MT. At 0, 6, 12, 24, and 48 h, leaf samples were collected from the four treatments and stored at −80°C to determine physiological indicators. After 6 days of heat stress, we observed the phenotype of chrysanthemum seedlings pre-treated with melatonin or water, and measured their fresh weight, dry weight, chlorophyll content, photosynthesis rate, and gas exchange indexes.
Physiological Measurements
Malondialdehyde content was measured by the thiobarbituric acid method after Schmedes and Hølmer (1989). The proline content (Pro) was established by the acid ninhydrin method (Li, 2000), and the Coomassie Brilliant Blue G-250 method was used to determine the content of soluble protein (Bradford, 1976). The soluble sugar content was identified by anthrone colorimetry (Li, 2000), and the hydrogen peroxide (H2O2) level was determined according to Willekens et al. (1997). The presence of superoxide anion free radicals (O2•–) was detected using nitroblue tetrazolium staining (Kamrul et al., 2015). Reduced glutathione (GSH) and reduced AsA levels were measured using the method of Ma and Cheng (2003). The activity of SOD was determined based on the method of Giannopolittis and Ries (1997), peroxidase (POD) by the method of Scebba et al. (2001), and catalase (CAT) was determined using the improved method of Kato and Shimizu (1987). APX activity was determined as described by Nakano and Asada (1981). The above measurements were repeated three times, and the average value was used as the representative value for each treatment.
Measurement of Growth, Photosynthesis Rate and Gas Exchange
Three chrysanthemum seedlings were randomly selected 0 and 6 days after treatment. The seedlings were subsequently cut, rinsed with water, their surface wiped clean with filter paper, and weighed (g), after which, they were oven dried at 105°C for 10 min and then at 80°C to constant weight prior to measurement of dry-mass weight (g). Additionally, three chrysanthemum seedlings were randomly selected, and 0.2 g of fresh-leaf samples were obtained, wiped clean of surface dirt, and then the midrib was removed; the foliar blades were mixed and placed in a test tube, added 10 mL of 95% ethanol and incubated for 12 h in the dark. Absorbance was measured at 665 and 649 nm, and used to calculate chlorophyll a and chlorophyll b, respectively, and total chlorophyll. Rubisco enzyme activity was determined according to the method described by Xia et al. (2009). A portable LI-6800 IRGA (Li-COR, Lincoln, NE, United States) was used to determine net photosynthetic rate (Pn), stomatal conductance (gs), intercellular CO2 concentration (Ci), and transpiration rate (Tr). Three plants were randomly selected for each treatment and mature leaves at plant mid height were selected for the determination of photosynthesis and gas exchange. The open air path and red and blue light sources were selected; quantum flux density was set to 800 μmol⋅m–2⋅s–1, and the airflow rate in the sample chamber was set to 500 μmol⋅s–1; CO2 concentration, relative humidity, and temperature were set to 390–410 μmol⋅mol–1, 30–40%, and 25°C, respectively.
RNA Sequencing and Bioinformatics Analysis
According to the measured physiological indicators, we found that the difference between SMT and S was most significant at 24 h after treatment; therefore, we chose 24 h samples for transcriptomic analysis. Total RNA was extracted from Con-24 h, ConMT-24 h, S-24 h, and SMT-24 h using an RNA isolation kit (Waryong, Beijing, China). The leaves collected from five potted plants were considered as one biological replicate, and samples of three such biological replicates were subjected to the DNBSEQ platform for RNA sequencing. Adaptor-polluted, low-quality, and high-content unknown base (N) reads were removed from the raw data (Grabherr et al., 2011). Trinity software (Pertea et al., 2003) was used for de novo assembly of clean reads (removed PCR duplication to improve assembly efficiency), and the obtained unigenes were assigned a presumptive function according to homolog deposits in the NR, NT, Swiss-Prot, KEGG, COG, and Pfam databases. The transcriptome datasets are available in the NCBI repository1, Accession No. for library PRJNA732569. We identified differentially expressed genes (DEGs) using a false discovery rate (FDR) ≤ 0.05, and | log2FoldChange| ≥ 1.0. The heat map of the DEGs was generated using the MeV software.
Quantitative Real-Time PCR Assay
Eight genes were randomly chosen for quantitative verification to confirm the accuracy of the transcriptome data. Primers used for qRT-PCR were designed using the Primer5 software (Supplementary Table 1). The reference gene EF-1α (GenBank: KF305681) was selected as an expression control (Wang et al., 2015). Each sample was represented by three biological replicates and three technical replicates. The specific steps of qRT-PCR were as described previously (Ren et al., 2014). The relative transcriptional expression of DEGs was calculated using the 2–Δ Δ CT method (Livak and Schmittgen, 2001).
Statistical Analysis
Using Duncan’s test for data analysis, differences among Con, ConMT, S, and SMT groups were identified as significant at P < 0.05. SPSS v17.0 software (SPSS Inc., Chicago, IL, United States) was used for statistical analyses.
Results
Physiological Changes of Heat Treatment
To investigate the effects of MT on the resistance of chrysanthemum seedlings to heat stress, the physiological alterations were measured. First, the MDA content was detected, and showed a significant increase in S of 137.5, 144, 178.26, and 152.08% at 6, 12, 24, and 48 h, respectively, and a significant decrease in S + MT of 35.79, 35.25, 25.78, and 17.36% over the same time periods, respectively (Figure 1A). Second, the content of osmotic adjustment substances in chrysanthemum seedlings under heat stress was measured, and compared with control, the proline content of S significantly increased by 154.58, 252.4, 313.31, and 552.32% at 6, 12, 24, and 48 h, respectively. Additionally, the proline content of S + MT increased significantly compared to S by 56.16, 49.88, 65.61, and 10.74% over the same time frames, respectively (Figure 1B). The soluble protein content of S increased by 50.23 and 58.74% compared with control at 24 and 48 h, respectively, whereas that of S + MT began to accumulate at 12 h, increasing by 51.98, 18.17, and 30.1% compared with S at 12, 24, and 48 h, respectively (Figure 1C). The soluble sugar content of S exceeded that of control by 52.22, 87.99, and 42.71% at 12, 24, and 48 h, respectively, while S + MT remained at a higher level than S at 48 h, increasing 29.19% (Figure 1D).
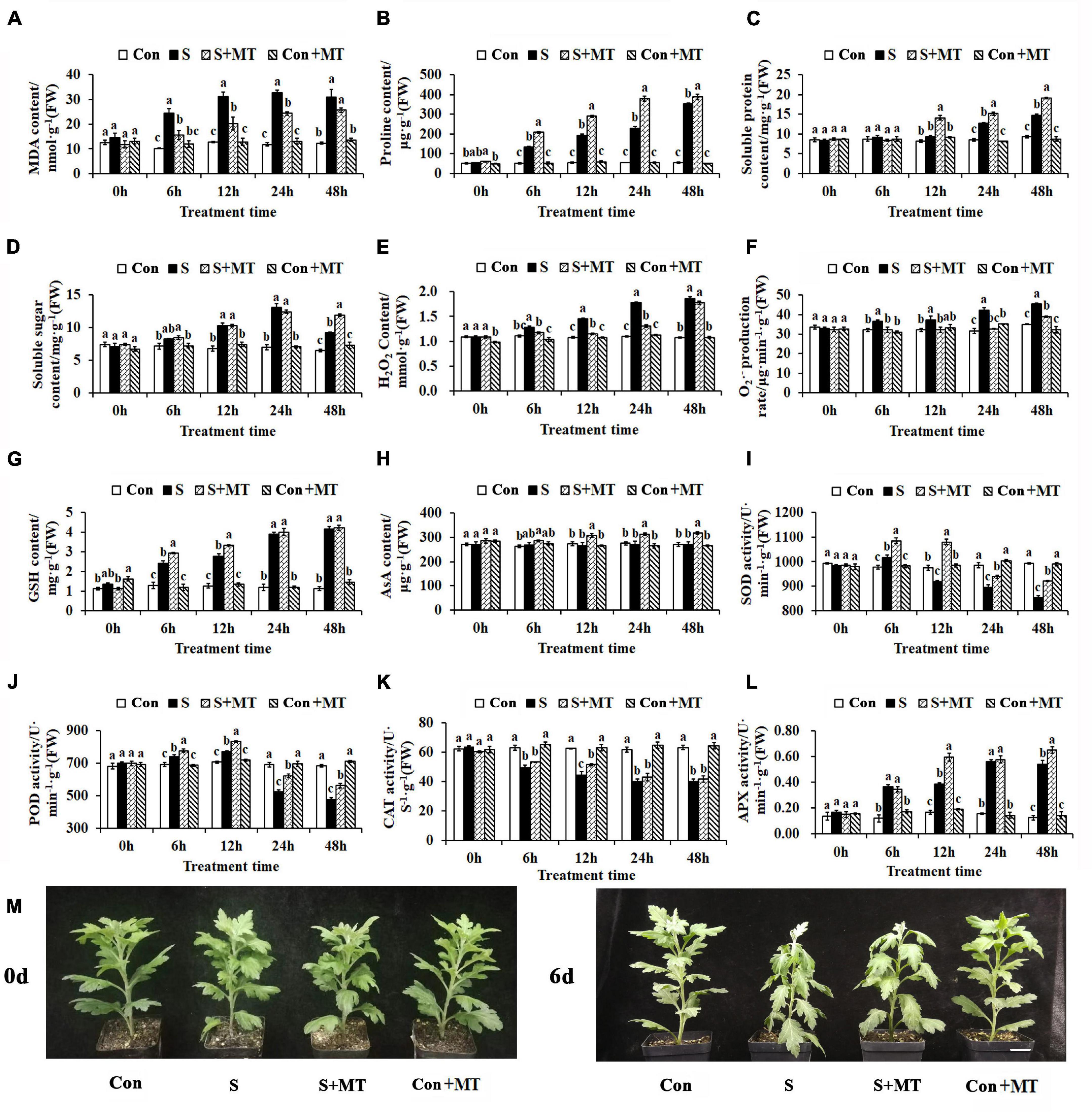
Figure 1. Physiological responses of chrysanthemum seedlings under heat stress. (A–E) The content of MDA (A), proline (B), soluble protein (C), soluble sugar (D), and hydrogen peroxide (E). (F) The generation rate of superoxide anion free radicals. (G) The content of reduced glutathione. (H) The ascorbic acid content. (I–L) The activities of SOD (I), POD (J), CAT (K), and APX (L). (M) Phenotypes after 6 days of heat stress treatment. Con, control; S, heat stress; S + MT, heat stress with exogenous melatonin treatment; Con + MT, control with exogenous melatonin treatment. Error bars indicate SE (n = 3). Duncan’s multiple range test was used to analyze significant differences. Different letters indicate significant differences at P < 0.05.
Third, the influence of melatonin treatment on peroxide content in chrysanthemum seedlings under heat stress was investigated. The H2O2 content of S increased by 14.99, 34.62, 62.35, and 74.16% compared with control at 6, 12, 24, and 48 h, respectively, whereas that of S + MT decreased when compared to S by 8.30, 20.89, 26.60, and 4.57% at the respective time periods (Figure 1E). The generation rate of superoxide anion free radicals of S was significantly increased by 13.55% (6 h), 16.08% (12 h), 33.52% (24 h), and 29.73% (48 h) compared with control, while the generation rate of S + MT was significantly lower than that of S at all times measured and maintained at the control level at 6–24 h (Figure 1F). The antioxidant content of chrysanthemum seedlings under heat stress was measured, and compared with control, the reduced glutathione (GSH) content of S was 87.91, 120.22, 226.19, and 270.89% higher than the control at 6, 12, 24, and 48 h, respectively. Compared with S, the GSH content of S + MT increased by 20.47 and 19.39% at 6 and 12 h, respectively (Figure 1G). The AsA content of S + MT increased when compared to S by 14.46% (12 h), 15.43% (24 h), and 16.91% (48 h) (Figure 1H). Antioxidant enzyme activities were also detected. The SOD activity of S + MT was significantly higher than that of S, which increased by 6.73, 17.41, 4.74, and 7.94% at 6, 12, 24, and 48 h, respectively (Figure 1I). Similarly, POD of S + MT showed greater activity than that of S at all times measured, with increases of 5.06, 7.76, 19.18, and 17.7% compared with S at the four respective times (Figure 1J). The CAT activity of S + MT was increased by 6.65% (6 h), 15.15% (12 h), 7.14% (24 h), and 3.37% (48 h), respectively, compared with S, but there was a significant difference only at 12 h (Figure 1K). Compared with control, the APX activity of S increased by 205.00, 132.14, 261.54, and 333.33% at 6, 12, 24, and 48 h, respectively. At 12 and 48 h, APX was significantly more active in S + MT than S by 53.85 and 19.78%, respectively (Figure 1L). The chrysanthemum seedlings were subjected to heat stress for 6 day; those of Con + MT and CK grew vigorously, followed by S + MT, whereas the S seedlings were the most wilted (Figure 1M). Collectively, these results show that melatonin can effectively alleviate the damage aroused by ROS in chrysanthemum seedlings under heat stress, and is beneficial to the synthesis of osmotic regulation substances and antioxidant contents. Meanwhile, melatonin treatment can effectively reduce the content of MDA and peroxide, and improve the resistance of chrysanthemum seedlings to heat stress.
Heat-Induced Changes in Growth and Photosynthetic Parameters
Melatonin treatment alleviated the damage caused by heat stress in chrysanthemum seedlings, whose fresh and dry weights were reduced by 42.27 and 28.77%, respectively, compared with control, after 6 days in the S treatment, while those corresponding to the seedlings under the S + MT treatment were significantly increased by 30.97 and 22.12%, respectively (Figures 2A,B). Similarly, chlorophyll a, chlorophyll b, and total chlorophyll content decreased by 44.12, 38.08, and 42.44%, respectively, in S, compared with the control, while the three increased by 36.79, 40.88, and 38.01%, respectively, under the S + MT treatment, compared to the corresponding values measured under the S treatment (Figures 2C–E). Rubisco is the rate-limiting enzyme in photosynthesis. In this study, Rubisco activity was significantly reduced by 45.28% relative to control after 6 days of heat stress. However, melatonin significantly increased Rubisco activity by 48.28% under S + MT, compared to S (Figure 2F). Additionally, photosynthesis rate (Pn) and gas exchange parameters including, Pn, gs, Ci, and Tr, all decreased by 42.32, 78.28, 54.79, and 71.45%, respectively, compared with control, while they increased by 29.11, 114.08, 25.0, and 60.32%, respectively, under the S + MT treatment compared with the S treatment (Supplementary Table 2).
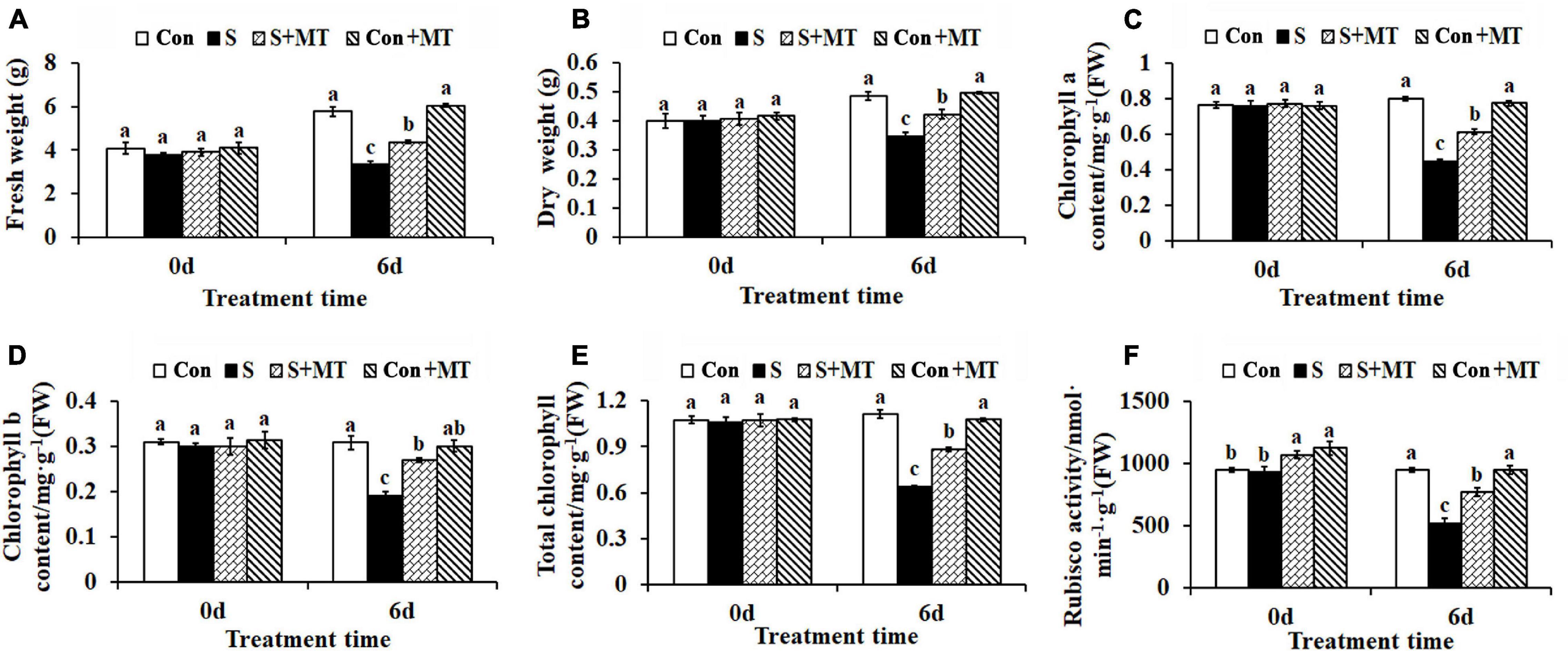
Figure 2. Effects of melatonin on chrysanthemum seedling growth, chlorophyll content and Rubisco enzyme activity under heat stress. (A) Fresh weight. (B) Dry weight. (C) Chlorophyll a. (D) Chlorophyll b. (E) Total chlorophyll. (F) Rubisco activity. Con, control; S, heat stress; S + MT, heat stress with exogenous melatonin treatment; Con + MT, control with exogenous melatonin treatment. Error bars indicate SE (n = 3). Duncan’s multiple range test was used to analyze significant differences. Different letters indicate significant differences at P < 0.05.
Transcriptome Sequencing and Analysis
To explore the crucial genes and regulatory network at play in chrysanthemum seedlings in response to heat stress and melatonin, we performed transcriptome sequencing on chrysanthemum leaves. Twelve cDNA libraries were sequenced on the DNBSEQ platform, and the total raw reads, total clean reads, and total clean bases obtained were a minimum of 40.48 Mb, 37.58 Mb, and 5.64 Gb (Q20 values > 95.53%, Q30 values > 89.86%, and clean reads > 91.73%), respectively (Supplementary Table 3). After assembly and de-redundancy, 145,639 unigenes were obtained. The total length, average length, N50, N70, N90, and GC content were 174,088,211 bp, 1195 bp, 1667 bp, 1193 bp, 616 bp, and 39.58%, respectively (Supplementary Table 4).
The results showed 17,262 downregulated and 16,235 upregulated DEGs in the stress/control (S/Con) comparison; in contrast, in the stress with melatonin/control with melatonin (SMT/ConMT) comparison, downregulated and upregulated DEGs were 18,785 and 17,955, respectively (Figure 3A and Supplementary Table 5). Furthermore, 4378 and 830 DEGs were generated in the comparisons of ConMT/Con and SMT/S, respectively, suggesting that melatonin may control more genes to cope with heat stress. Among the different comparisons, overlapping DEGs were further analyzed. The results showed 329 overlapping DEGs between ConMT/Con and SMT/S, and 25,226 were found between S/Con and SMT/ConMT (Figure 3B). To verify the veracity of our transcriptome data, we randomly selected eight genes for qRT-PCR, and the results proved that RNA-seq was reliable (Figure 4).
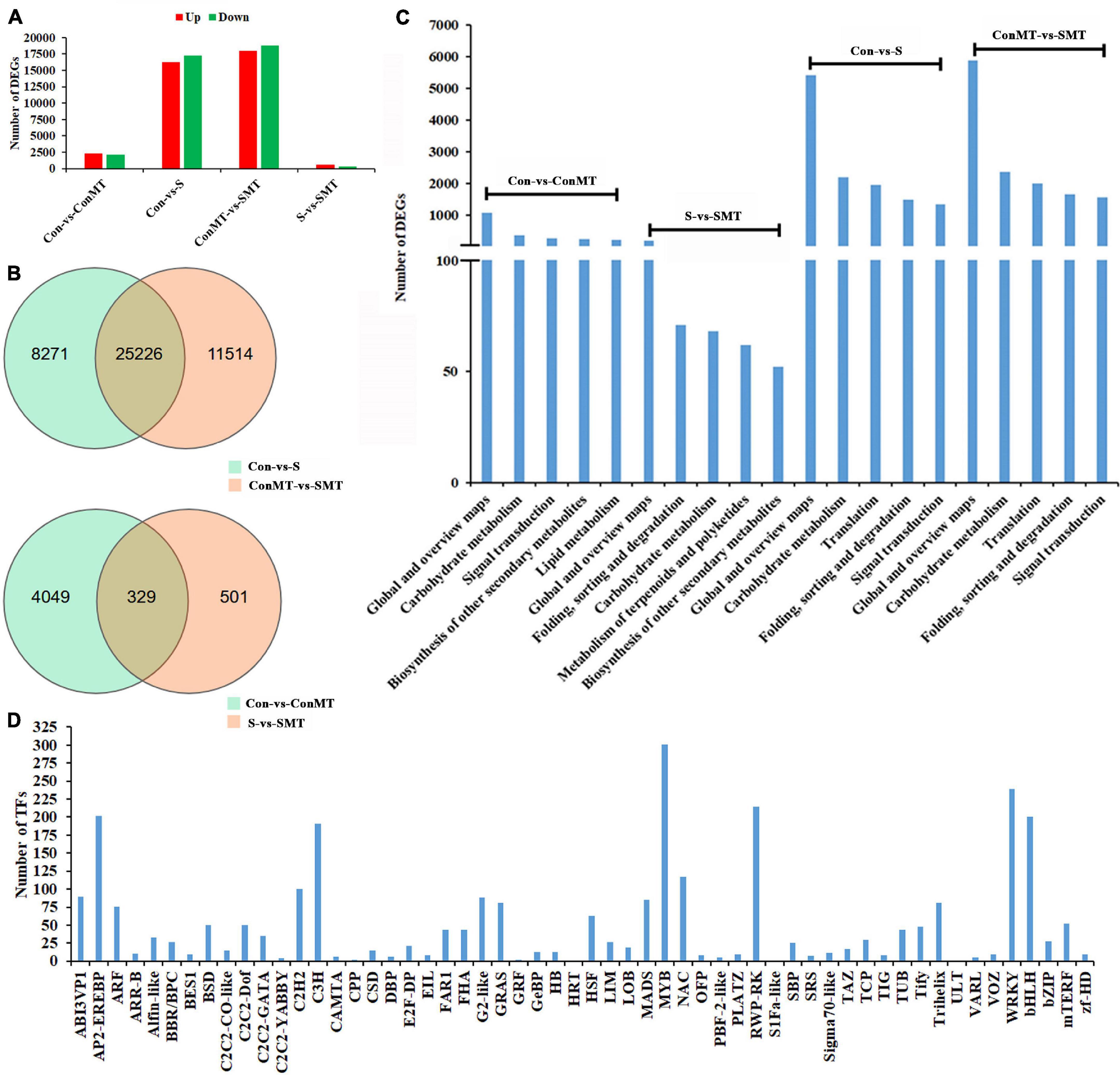
Figure 3. Effects of exogenous melatonin and heat stress on gene expression. (A) Number of differentially expressed genes in different comparisons. (B) Overlapped differentially expressed genes in different comparisons. (C) KEGG analysis in different comparisons (top 5). (D) Number of TFs families. Con, control; S, heat stress; SMT, heat stress with exogenous melatonin treatment; ConMT, control with exogenous melatonin treatment.
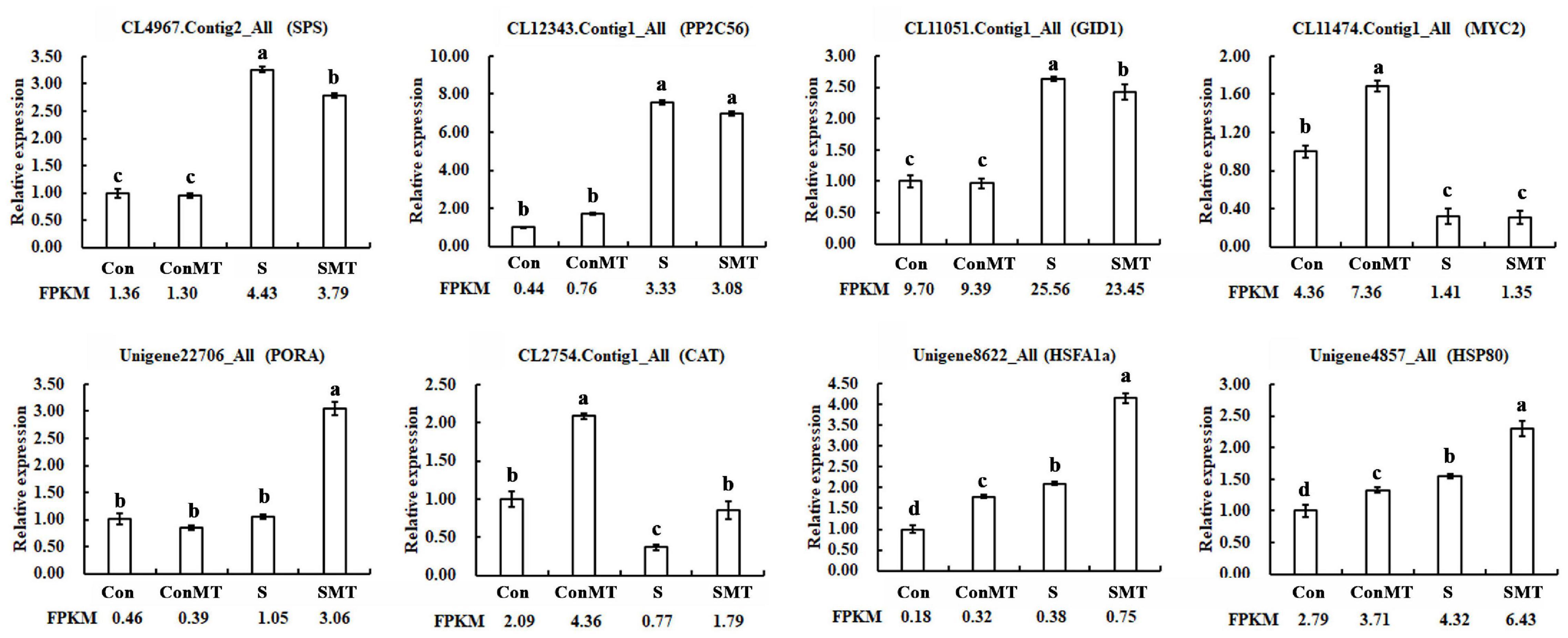
Figure 4. Use of qRT-PCR to verify the accuracy of RNA-Seq. Con, control; S, heat stress; SMT, heat stress with exogenous melatonin treatment; ConMT, control with exogenous melatonin treatment. Error bars represent SE (n = 3). Duncan’s multiple range test was used for significant difference analysis, and different letters indicate a significant difference at P < 0.05.
In addition, KEGG enrichment analysis was conducted to explore the potential functions of DEGs in response to melatonin and heat stress (Supplementary Table 6). The KEGG pathway in Figure 3C indicates that the DEGs in S/Con and SMT/ConMT were enriched in global and overview maps, carbohydrate metabolism, translation, and signal transduction. In addition, DEGs involved in carbohydrate metabolism and biosynthesis of other secondary metabolites were observed in the ConMT/Con and SMT/S comparisons. Annotation results suggested that highly representative pathways might be indispensable for chrysanthemum survival rate and melatonin regulation under heat stress.
Figure 3D and Supplementary Table 7 list 56 families of TFs. Among them, the number of differentially expressed transcription factors of HSF, MADS, MYB, NAC, TCP, WRKY, and bHLH were 63, 85, 301, 117, 30, 239, and 200, respectively. These results revealed that exogenous melatonin regulated the differential expression of many transcription factors, thus confirming that melatonin plays a vital role in heat stress.
Genes Involved in the Metabolism of ROS-Scavengers, Heat Shock Transcription Factors and Heat Shock Proteins
Based on the FPKM value, we analyzed the transcriptome data to further clarify the molecular mechanism of exogenous melatonin treatment of chrysanthemum leaves under heat stress conditions, and found 12 enzymes, 3 HSFs, and 4 HSPs related genes (Figure 5 and Supplementary Table 8). The expression of glutamate cysteine ligase (GCL) and GDP-D-mannose 3′, 5′-epimerase (GME) were significantly more active in SMT than in S (Figure 5, #1 to #2). The DEGs encoding antioxidant enzymes 1 glutathione reductase (GR), 1 SOD, 3 POD (peroxidase), 3 CAT (catalase), and 2 APX (Figure 5, #3 to #12) respectively, were significantly upregulated in SMT than in S. The expression of the heat-response-related genes HSFB3, HSFA1a, HSFA2b, HSP23, HSP70, HSP80, and HSP90 was the greatest in the SMT treatment, followed by S (Figure 5, #13 to #19). This shows that spraying chrysanthemum leaves with melatonin under intense heat conditions can effectively improve the stress resistance of the plants.
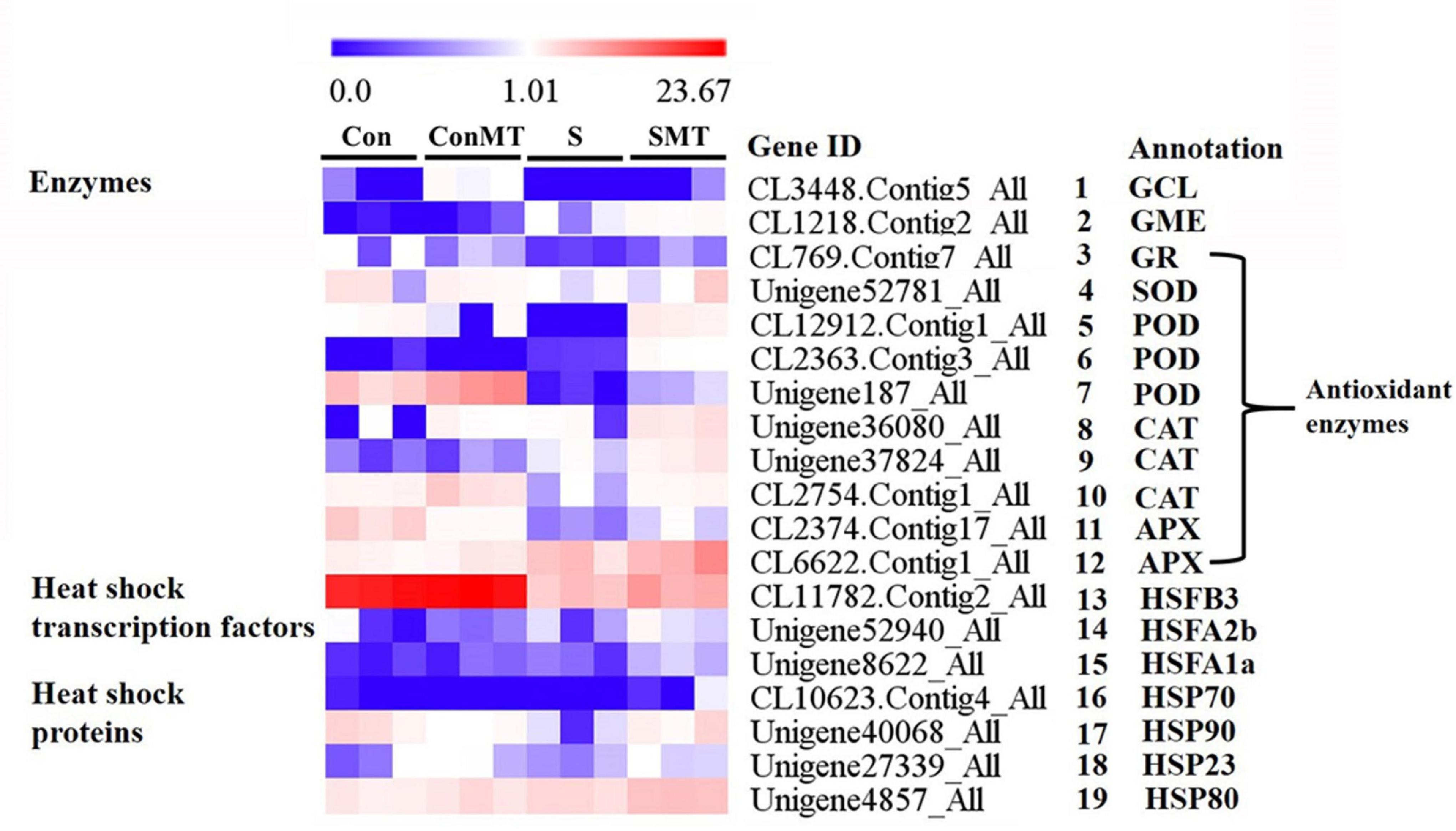
Figure 5. Differentially expressed genes related to enzymes, heat shock transcription factors and heat shock proteins under heat stress in four comparisons. The bar indicates the expression (FPKM) of each gene in Con, ConMT, S, SMT as indicated by blue, white, and red squares. Blue and red represent low and high expression, respectively. Con, control; S, heat stress; SMT, heat stress with exogenous melatonin treatment; ConMT, control with exogenous melatonin treatment. More detailed information is shown in Supplementary Table 8.
Genes Involved in Ca2+ Signal Transduction, Carbon Fixation, and Starch and Sucrose Metabolism
We explored different genes related to calcium signal transduction, photosynthetic biological carbon sequestration, and starch and sucrose metabolism (Figure 6). In S/Con and SMT/ConMT comparisons, heat stress induced WRKYs and decreased the expressions of cyclic nucleotide gated channel (CNGCs) and mitogen-activated protein kinase kinase kinases (MAPKKKs). In S/Con, the expression of respiratory burst oxidase (RBOH) was increased, whereas, in SMT/S, it was reduced (Supplementary Table 9). In the SMT/S comparisons, six DEGs involved in the Ca2+ signaling pathway were upregulated (Figure 6A). There were four genes in the calmodulin/calmodulin-like protein (CaM/CML) gene family, including two CML, one CAM2, and one CAM4, while two genes (CNGC4 and CNGC20) belonged to the CNGC gene family, and the remaining gene was in the RBOH group.
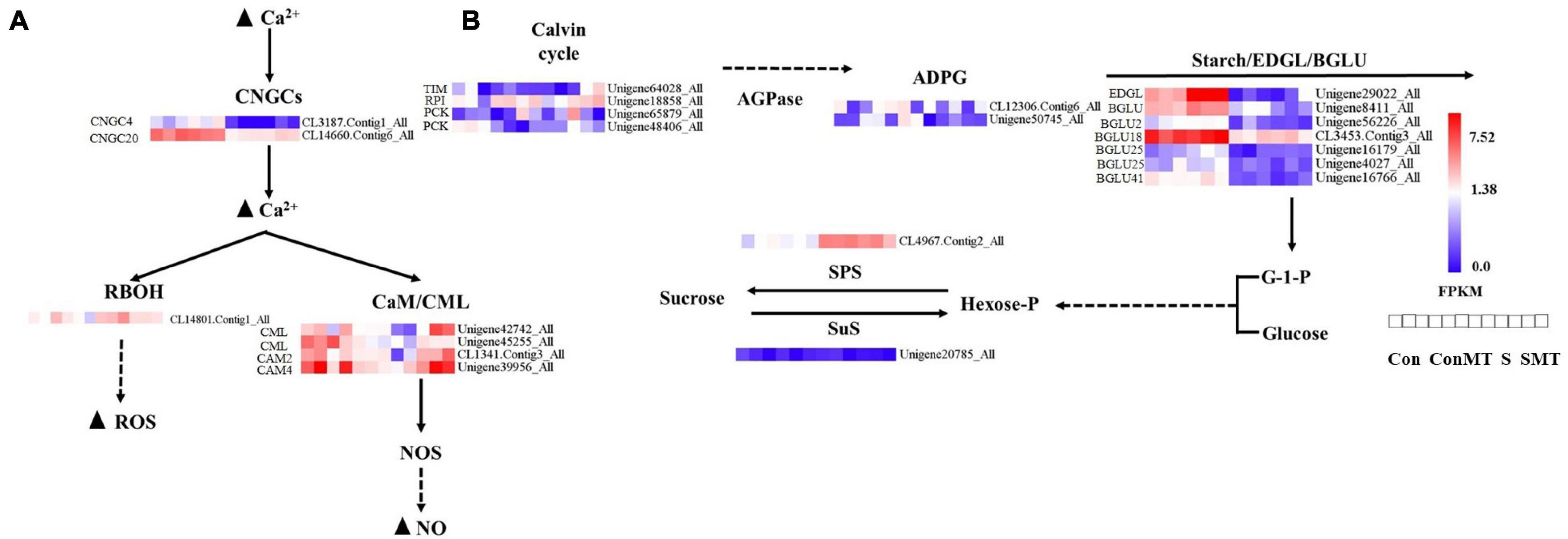
Figure 6. Differentially expressed genes caused by heat stress. (A) Calcium signal transduction. (B) The metabolism of starch and sugars. The bar represents the expression (FPKM) of each gene in Con, ConMT, S, SMT as indicated by blue, white, and red squares. Blue and red represent low and high expression, respectively. Con, control; S, heat stress; SMT, heat stress with exogenous melatonin treatment; ConMT, control with exogenous melatonin treatment. More detailed information is shown in Supplementary Table 12.
The majority of the genes in the carbon fixation pathway had inhibited expression in relation to heat stress. We emphasized the DEGs in SMT/S. Specifically, the transcription of TIM, PCK, and RPI were upregulated by melatonin (Figure 6B and Supplementary Table 10). Our results (Supplementary Table 11) showed that in ConMT/Con, five genes were induced, including one β-D-xylosidase (BXL), one endoglucanase (EDGL), one trehalose-phosphate phosphatase (TPP), and two pectinesterase genes. One sucrose synthase (SuS) encoding gene was reduced, while polygalacturonase, TPP, and pectinesterase were significantly increased in SMT/S. The expression of DEGs encoding ADP-glucose pyrophosphorylase (AGPase), β-glucosidase (BGLU), EDGL, and endoglucane-1, 3-β-glucosidase (EGLC) was reduced under heat stress; conversely, the expression of genes encoding sucrose phosphate synthase (SPS), TPP, α-amylase, and β-amylase were induced both in S/Con and in SMT/ConMT comparisons (Supplementary Tables 11, 12).
Genes Involved in Plant Hormone Signal Transduction
Aiming to clarify the regulatory networks involving melatonin in the plant responses to heat stress, we analyzed the differential expression of genes related to plant-hormone signal transduction (Figure 7 and Supplementary Table 13).
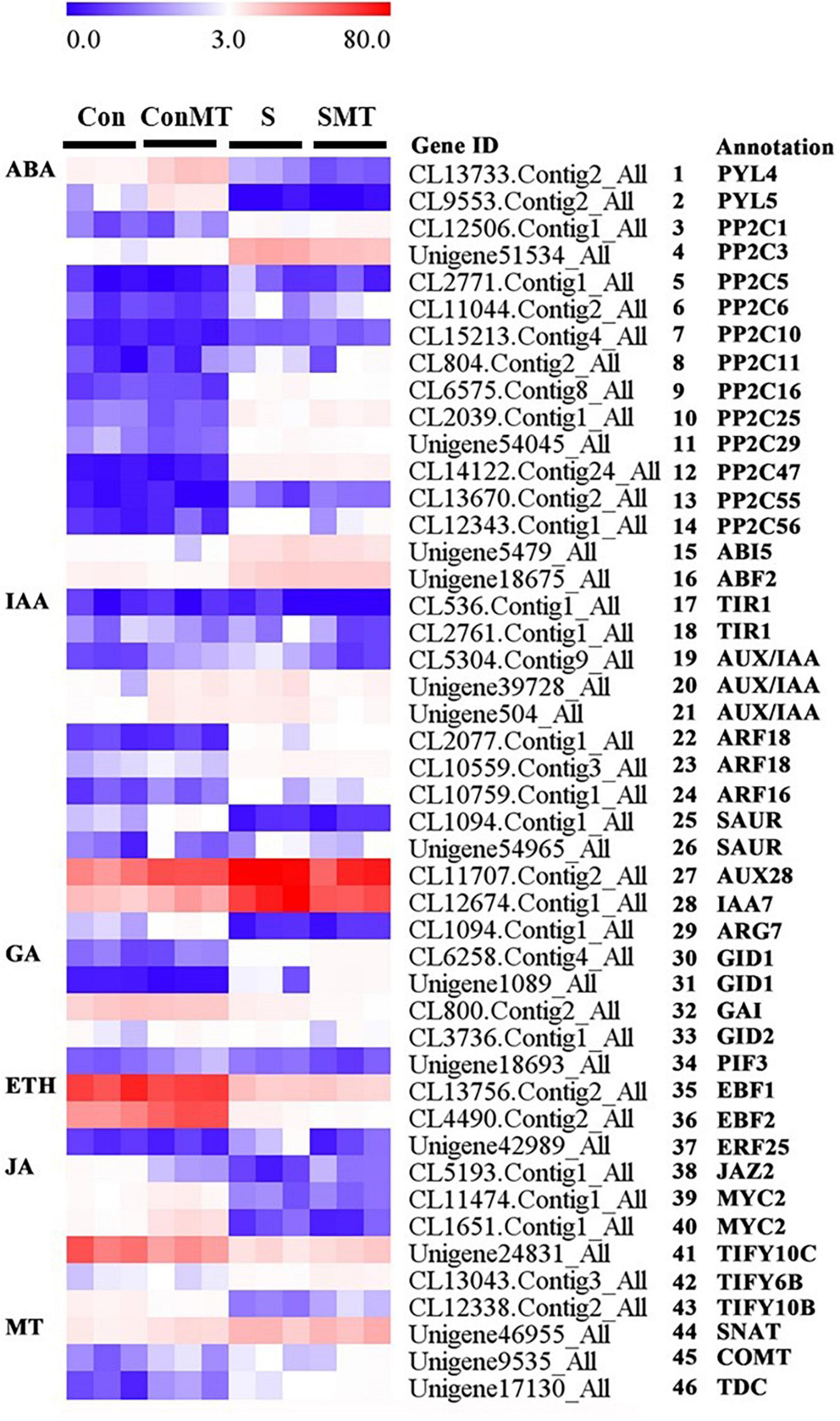
Figure 7. Differentially expressed genes related to plant hormone under heat stress. The bar represents the expression (FPKM) of each gene in Con, ConMT, S, SMT, as indicated by blue, white, and red squares. Blue and red represent low and high expression, respectively. Con, control; S, heat stress; SMT, heat stress with exogenous melatonin treatment; ConMT, control with exogenous melatonin treatment. More detailed information is provided in Supplementary Table 13.
In S/Con and SMT/ConMT, ABA signaling related genes protein phosphatase 2C (PP2C1, PP2C3, PP2C5, PP2C6, PP2C10, PP2C11, PP2C16, PP2C25, PP2C29, PP2C47, PP2C55, and PP2C56) (Figure 7, #3 to #14), the ABA-insensitive (ABI5) (Figure 7, #15) gene and ABF2 (Figure 7, #16) were induced under heat stress, whereas the expression of PYL4 and PYL5 (Figure 7, #1 to #2) were inhibited.
Five DEGs including, one IAA-induced protein (ARG7) (Figure 7, #29), one AUX28 (Figure 7, #27), one IAA7 (Figure 7, #28), and two small auxin-up RNA (SAUR) (Figure 7, #25 to #26), were detected in ConMT/Con as induced and related to auxin signal transduction. Heat stress-induced auxin response factors (ARFs) and the expression trends of genes encoding SAURS were inconsistent. In SMT/S, the expression of the auxin receptor transport inhibitor response 1 (TIR1) (Figure 7, #17 to #18) decreased significantly, whereas in S/Con, there was no change in the expression of TIR1. Therefore, auxin/indole acetic acid protein (AUX/IAAs) (Figure 7, #19 to #21), ARFs (ARF16 and ARF18) (Figure 7, #22 to #24), SAUR, and the auxin-responsive proteins IAA7 and ARG7 were expressed as hubs in S/Con, whereas in the SMT/ConMT comparison, TIR1, AUX/IAAs, ARF18, SAUR, and ARG7 were pivotal genes.
In terms of GA signaling, heat stress induced the GA receptor (GID1) (Figure 7, #30 to #31), whereas the DELLA proteins (GAI) (Figure 7, #32) were reduced in S/Con and SMT/ConMT, and the expression levels of GID2 (F-box proteins) (Figure 7, #33) and photosensitive interaction factor 3 (PIF3) (Figure 7, #34) were repressed in the latter.
The center gene ethylene-responsive transcription factor 25 (ERF25) plays an important role in the heat stress response. In SMT/ConMT and S/Con, EIN3-Binding F-Box protein (EBF1 and EBF2) (Figure 7, #35 to #36) were inhibited. In the SMT/S comparison, ERF25 was downregulated (Figure 7, #37).
In terms of jasmonic acid signaling, in both S/Con and SMT/ConMT comparisons, heat stress repressed the expression of TIFY10C (Figure 7, #41) and MYC2 (Figure 7, #39 to #40) but there was a similar upregulation of TIFY6B (Figure 7, #42) in the latter comparison, whereas TIFY10B (Figure 7, #43) and TIFY10C were induced in SMT/S.
To reveal how melatonin biosynthesis-related genes were altered in response to heat stress or exogenous melatonin, we analyzed the expression of SNAT, COMT, and TDC genes. In all, ConMT/Con, S/Con, and SMT/S comparisons, SNAT (Figure 7, #44), COMT (Figure 7, #45), and TDC (Figure 7, #46) genes were all upregulated.
Genes Involved in Chlorophyll, Flavonoid, Carotenoid Metabolism
The differential expression of genes involved in chlorophyll, flavonoid, and carotenoid metabolism (Figure 8 and Supplementary Table 14) was identified, which is essential for determining the potential regulatory network of melatonin under heat stress. There were nine differential transcriptions associated with chlorophyll, five genes involved in chlorophyll biosynthesis (Figure 8, #1 to #5), two in the chlorophyll cycle (Figure 8, #6 to #7), and two genes involved in chlorophyll degradation (Figure 8, #8 to #9). The chlorophyll biosynthesis [HEMA, HEMF, CHLH, and protochlorophyllide oxidoreductase (PORA)] and chlorophyll cycle (NYC1/NOL and HCAR) genes had increased abundance in the SMT/S comparison, whereas they remained unchanged in the ConMT/Con comparison. Two transcripts associated with chlorophyll degradation [CLH and pheophytinase (PPH)] were down-regulated in SMT/S comparison, whereas they were upregulated in the S/Con comparison and remained unchanged in the ConMT/Con comparison. The transcripts associated with flavonoid biosynthesis were (Figure 8, #10 to #14) chalcone isomerase (CHI), chalcone synthase (CHS), dihydroflavonol 4-reductase (DFR), flavanone 3-hydroxylase (F3H), and flavone synthase (FNS). In SMT/S comparison, these genes were all significantly upregulated, but there was no consistent trend in the other comparisons. In addition, under heat stress conditions, genes related to carotenoid synthesis were affected. In this study, we found nine (Figure 8, #15 to #23) key genes for carotenoid synthesis, two 1-deoxy-D-xylulose-5-phosphate synthase (DXPS) (Figure 8, #15 to #16), two geranylgeranyl diphosphate synthase (GGDP) (Figure 8, #17 to #18), one phytoene synthase (PSY) (Figure 8, #19), one violaxanthin de-epoxidase (VDE) (Figure 8, #20), one zeaxanthin epoxidase (ZEP) (Figure 8, #21), and two 1-deoxy-D-xylulose-5-phosphate reductoisomerase (DXR) (Figure 8, #22 to #23), all of which were significantly upregulated in SMT/S comparisons. In contrast, the expression of DXPS, PSY, VDE, and ZEP were significantly downregulated in S/Con comparisons, but there was no significant difference between GGDP and DXR.
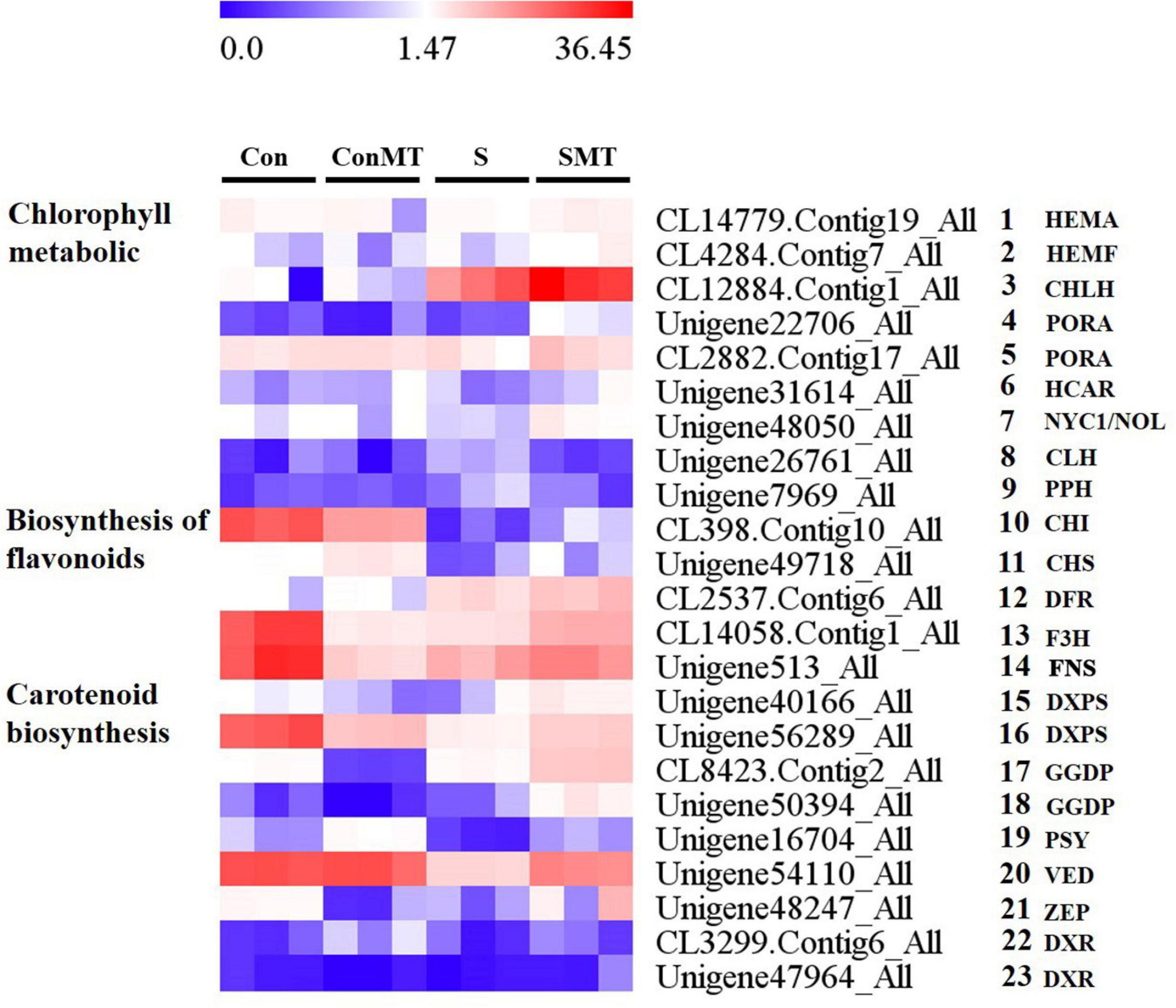
Figure 8. Heat stress modified the expression profiles of chlorophyll, flavonoids, and carotenoid metabolic related genes in four comparisons. The bar represents the expression (FPKM) of each gene in Con, ConMT, S, SMT as indicated by blue, white, and red squares. Blue and red represent low and high expression, respectively. Con, control; S, heat stress; SMT, heat stress with exogenous melatonin treatment; ConMT, control with exogenous melatonin treatment. More detailed information was shown in Supplementary Table 14.
Discussion
Effects of Exogenous Melatonin on Osmotic Regulation Substances and Reactive Oxygen Species in Chrysanthemum Seedlings Under Heat Stress
To avoid injury due to heat stress, plants have developed a series of strategies (Chen and Yang, 2019). Thus, for example, H2O2 plays a critical role in heat stress (Konigshofer et al., 2008; Banti et al., 2010). AtRbohB and AtRbohD are the main synthetases of O2•– production by heat stress (Chen and Yang, 2019). In our study, the expression of RBOH was significantly upregulated under S, compared to Con treatment; furthermore, we detected that H2O2 content and O2•–⋅production rate increased significantly in chrysanthemum seedlings under heat stress. Melatonin can directly remove ROS to stabilize cell membranes and avoid lipid peroxidation under stress (Zhang et al., 2015). Here, the expression of RBOH, and H2O2 and O2•–⋅levels were reduced in SMT/S. The SOD, POD, CAT, and APX activities of chrysanthemum seedlings enhanced the ability of chrysanthemum seedlings to remove ROS, which is consistent with the results of a related study on lupine (Garnczarska and Bednarski, 2004) and other previous studies (Qi et al., 2018). This could relate to the fact that MT in chrysanthemum heightens antioxidant enzyme activity by increasing the expression of the related genes and reducing the degradation of biological macromolecules, thereby enhancing the ability to remove ROS. When plants are subjected to oxidative stress, GSH is one of the effective scavengers produced by intracellular metabolism and the peroxide processes, which makes plants more resistant to environmental stress, therefore, when plants encounter adversity, the contents of AsA and GSH will change (Yin et al., 2008). Compared with SMT, AsA and GSH contents were lower under S, suggesting that MT enhanced GR activity to accelerate the process of regeneration, and AsA and glutathione synthesized these compounds to relatively high concentrations in chrysanthemum seedlings subjected to heat stress; thereby, improving plant resistance under these conditions.
Effects of Exogenous Melatonin on Heat Shock Transcription Factor-Heat Shock Protein in Chrysanthemum Seedlings Under Heat Stress
Heat stress increases endogenous melatonin production in Arabidopsis leaves; furthermore, exogenous melatonin enhances the heat resistance of this genus (Shi et al., 2015). Exogenous melatonin and heat stress remarkably induced the expression of A1 heat-shock factors (HSFA1s) in Arabidopsis thaliana, which are major regulators of the heat stress response (Shi et al., 2015). Studies have shown that exogenous melatonin-enhanced heat resistance was notably attenuated in quadruple knockout HSFA1 mutants, while HSFA1-activated the thermal response gene-transcripts (HSFA2, HSP90, and HSP101), implying they might participate in melatonin-mediated thermotolerance (Shi et al., 2015). HSPs are closely related to heat stress, and Hsp70 gene overexpression can enhance plant tolerance to this condition (Wang et al., 2004). Consistent with previous reports, the transcription of heat response-related genes (HSFB3, HSFA1a, HSFA2b, HSP23, HSP70, HSP80, and HSP90) under SMT was significantly greater than that of S, suggesting that heat-responsive genes played a vital part in melatonin-mediated heat resistance of chrysanthemum leaves.
Effects of Exogenous Melatonin on Ca2+ Signal Transduction in Chrysanthemum Seedlings Under Heat Stress
In plants, calcium signal transduction is the basic mechanism responsible for sensing and responding to environmental stimuli (Duszyn et al., 2019). In signal transduction, CNGCs are involved in the absorption of Ca2+ ions as ligand-gated protein channels. A stress-induced Ca2+ increase can activate CNGC, leading to a CaM/CML response (Gao et al., 2020). When plants are subjected to heat stress, the specific Ca2+ channel on the cell membrane opens, allowing Ca2+ to flow along the concentration gradient and activate multiple calcium/calmodulin-binding protein kinases and Ca2+-dependent protein kinases, which initiates the expression of downstream genes related to heat stress. In Ganoderma lucidum, plasma membrane-mediated extracellular calcium influx, intracellular calcium store release, and other calcium ions from different sources are involved in regulating the increase in intracellular calcium content under heat stress (Zhang et al., 2016), thereby improving the heat tolerance of plants. In our study, in the SMT/S comparisons, CNGCs and CAM/CMLs were upregulated. Therefore, MT enhanced the stress resistance of plants by activating the expression of CNGCs and CAM/CMLs.
Effects of Exogenous Melatonin on Carbon Fixation, and Starch and Sucrose Metabolism in Chrysanthemum Seedlings Under Heat Stress
Heat stress can cause changes in photosynthesis of plants, thereby shortening their life cycle and reducing their productivity (Barnabas et al., 2008). Increasing temperatures inhibit the activities of various enzymes in the Calvin cycle during photosynthesis (Morales et al., 2003). However, by regulating the transcription of TIM, RPI, and PCK genes, MT can effectively modulate carbon fixation and improve photosynthesis under heat stress (Liang et al., 2019). In SMT/S comparison, MT induced the expression of genes encoding TIM, RPI, and PCK.
The high utilization rate of carbohydrates under high-temperature stress is closely related to heat resistance (Roitsch and González, 2004). As sugars act as signaling molecules in the stress response pathway, sucrose and its metabolites can modulate the developmental processes of plants and their response to heat stress through changes in the distribution of carbon, and sugars signaling (Yang X. et al., 2019). Stress induction accelerates the conversion of starch to sugars, thereby, playing a protective role as it contributes to osmotic regulation and a quick energy supply (Dong and Beckles, 2019). When the endosperm develops at high temperatures, starch accumulation decreases (Zhao et al., 2008). SPS plays a vital role in the synthesis of sucrose (Winter and Huber, 2000), our results reveal that the decrease in starch content and induction of α-amylase, β-amylase, and SPS genes under heat stress may promote starch degradability. Exogenous melatonin induced the accumulation of starch and balanced the content of sugars. In line with this hypothesis, exogenous melatonin reportedly improves photosynthesis in plants, which in turn promotes starch accumulation (Sharma et al., 2020). Interestingly, SuS was downregulated in the SMT/S comparisons, and EDGL was downregulated in S/Con and SMT/ConMT comparisons. Moreover, BGLU (BGLU2, BGLU12, and BGLU18) genes were specifically downregulated in the S/Con and SMT/ConMT comparison. Studies have shown that the deficiency of BGLU18 delays the accumulation of dehydration-induced ABA, indicating that melatonin can stabilize ABA content and abiotic stress responses by regulating BGLU18-mediated ABA-glucose ester hydrolysis (Han et al., 2020). In conclusion, heat stress activated sucrose metabolism and starch degradation, while melatonin enhanced resistance to heat stress by positively regulating the accumulation of carbohydrates and the ratio of starch to sucrose.
Effects of Exogenous Melatonin on Hormone Signal Transduction in Chrysanthemum Seedlings Under Heat Stress
Studies have shown that heat stress induces plant hormones, such as ABA, AUX, GA, and ETH, which are considered to play a vital role in plant heat tolerance (Kotak et al., 2007). In this study, the differential expression of hormone-related genes was involved in different treatments, further, these genes were related to the increase in heat resistance of chrysanthemum seedlings upon spray treatment with exogenous melatonin under heat stress.
PYR/PYL are ABA receptors that interact with PP2C to reduce the inhibitory effect on SnRK2, thereby regulating the downstream gene ABF (Yang X. et al., 2019; Yang Y. et al., 2019). In the present study, heat stress induced the expression of PP2Cs, ABI5, and ABF2. Studies have shown that overexpression of ABA receptors PYR/PYL might promote ABA signal transduction, thereby increasing plant resistance to abiotic and biotic stress conditions (Liu et al., 2020). Melatonin plays a vital part upstream of the ABA signal (Arnao and Hernandez-Ruiz, 2018), and in this study, melatonin induced the expression level of PYL4 genes, while the effects of melatonin on the expression of PP2Cs did not involve DEGs; therefore, we hypothesized that the MT-mediated expression of PYL4 enhanced heat stress resistance.
Auxin plays a negative regulatory role in the process of plant resistance to stress, and Aux/IAAs and TIR1/AFB receptors activate a complex regulatory network to regulate the expression of ARF genes (Kazan, 2013; Jung et al., 2015). In accordance with our results, melatonin significantly reduced the transcription of TIR1 and Aux/IAA under heat stress. Studies have found that the IAA content increases under heat stress conditions (Franklin et al., 2011), and in our study, IAA7 was significantly upregulated by heat stress treatment. In addition, melatonin can regulate the interaction between Aux/IAA and TIR1 to resist heat stress (Naser and Shani, 2016), which was consistent with our study results, suggesting that Aux/IAA multimers significantly inhibited auxin-signal transduction and might improve resistance to heat stress by affecting ROS metabolism. With an increase in GA content, GA interacts with DELLA protein (GAI) after binding to its receptor GID1, thereby causing it to be ubiquitinated and degraded, ultimately activating the GA response (Wang et al., 2021). Under salt stress, melatonin promoted the expression of GA biosynthesis genes GA20ox and GA3ox in cucumber seedlings, causing the upregulation of GA3 and GA4 (Zhang et al., 2014, 2016). Heat stress decreased GA levels by repressing the expression of GA biosynthetic genes, such as GA20ox1, GA20ox2, GA20ox3, GA3ox1, and GA3ox2 (Toh et al., 2008). Although GA is normally identified as possessing an antagonistic effect on ABA, there is a strong interaction between DELLA proteins and ABF2 (Wang et al., 2020). In accordance with our results, GAI, GID1, GID2, and phytochrome interacting factor 3 were the core components of the GA signal pathway. In studies of tomatoes (Inaba and Chachin, 1988) and apples (Lurie and Klein, 2006), it was found that heat stress (above 38°C) reduced the rate of fruit ripening and the production of ethylene and enhanced respiration. The production of ethylene can improve the resistance of plants to heat stress. Exogenous melatonin slightly increased ethylene generation by inducing the expression of 1-aminocyclopropane-1-carboxylic acid synthase. In contrast, melatonin in etiolated lupine seedlings significantly inhibited ethylene synthesis (Arnao and Hernández-Ruiz, 2007). In the present study, the hub genes EBF2 and ERF25 were downregulated by MT under heat stress. SNAT, COMT, and TDC are the melatonin biosynthetic genes (Arnao and Hernandez-Ruiz, 2014). Exogenous melatonin application induced the accumulation of endogenous MT and upregulated the expression of SNAT and COMT genes in loquat seedlings during a stress period (Wang et al., 2021). In Agaricus bisporus, exogenous melatonin application promoted endogenous MT accumulation by increasing the expression levels of TDC, T5H, SNAT, and ASMT, which was helpful in protecting membrane integrity (Shekari et al., 2021). Consistent with the previous studies, here, an exogenous melatonin spray promoted the expression of melatonin biosynthesis-related genes SNAT, COMT, and TDC under heat stress.
Effects of Exogenous Melatonin on Chlorophyll, Flavonoid, and Carotenoid Metabolism in Chrysanthemum Seedlings Under Heat Stress
Heat stress negatively affects various physiological processes of plants, including photosynthesis, and flavonoid and carotenoid metabolism. Heat stress can lead to the degradation of plant chlorophyll, the decrease in photosynthetic rate, the hindrance of photosynthetic electron transfer, and the decrease of enzyme activity related to carbon assimilation (Zhou et al., 2016). Melatonin can improve plant photosynthesis under adversity and improve its resistance to stressors (Biswojit et al., 2018). Consistent with previous results, chlorophyll a, chlorophyll b, and total chlorophyll contents were significantly higher under the SMT than those under the S treatment. The chlorophyll biosynthesis (HEMA, HEMF, CHLH, and PORA) and chlorophyll cycle (NYC1/NOL) genes were upregulated, and chlorophyll degradation (CLH and PPH) genes were down-regulated in SMT/S comparison.
Flavonoids are important secondary metabolites in plants. Flavonoids can eliminate various types of ROS, thus resulting in a strong antioxidant capacity (Hernandez et al., 2009). When plants encounter heat stress and other adversity stresses, and a large amount of ROS accumulates in their bodies, flavonoids can degrade this excess ROS and maintain the ROS metabolism balance in plants. Studies have found that melatonin up-regulates the biosynthesis of polyphenols such as total phenols, flavonoids, and anthocyanins in grape berries (Meng et al., 2019). Similar results were found in cabbage, tomato (Sun et al., 2015), and other plants. Melatonin can enhance the activity of phenylalanine ammonia lyase, cinnamic acid-4-hydroxylase, CHS, F3H, leucoanthocyanin reductase, and anthocyanin reductase, and enhance the transcriptional abundance of the corresponding genes, thereby promoting the production of flavonoids, such as anthocyanins in the leaves of kiwifruit, and delaying senescence (Liang et al., 2018). In our study, CHI, CHS, DFR, F3H, and FNS were all significantly upregulated in the SMT/S comparison, suggesting that exogenous melatonin can improve the stress resistance of chrysanthemum under heat stress conditions.
In addition, studies have found that lutein and some other terpenoids can stabilize and protect the thylakoid membrane from abiotic stress (Camejo et al., 2006). Meanwhile, after overexpressing the chyB gene in Arabidopsis, the resistance to heat stress was higher, which indicates that zeaxanthin can prevent oxidative damage of the membrane (Meiri et al., 2010). The survival rate of Pinctada fucata decreased with an increase in temperature from 26 to 34°C and with decreasing total carotenoid content. Conversely, a higher total carotenoid content was accompanied by a higher survival rate. This compound, along with and total antioxidant capacity reduced evidently at 30°C with increasing stress (Meng et al., 2016). In our study, the carotenoid synthesis-related genes, such as DXPS, GGDP, PSY, VDE, ZEP, and DXR were significantly upregulated in SMT/S comparisons, and the expression of DXPS, PSY, VDE, and ZEP were significantly downregulated in S/Con comparisons, but there was no significant difference between GGDP and DXR. The results showed that melatonin effectively alleviated the degradation of carotenoids under heat stress conditions and improved the stress resistance of chrysanthemums.
Overall, exogenous spraying of melatonin improves the resistance of chrysanthemum leaves under heat stress conditions, including physiological and transcription analyses. The physiological aspects mainly include MDA, osmotic regulation substances, peroxides, non-enzymatic antioxidant, and antioxidant enzymes. RNA-seq involves the ROS, HSF-HSP, calcium ion-calmodulin, carbon fixation, starch and sucrose metabolism, hormone, and chlorophyll, flavonoid, and carotenoid pathway-related genes. Based on the above results, we propose the model of the MT-regulated adaptive response to high temperature stress in chrysanthemum leaves (Figure 9).
Data Availability Statement
The original contributions presented in the study are included in the article/Supplementary Material, further inquiries can be directed to the corresponding author/s.
Author Contributions
WF, JfJ, and FC conceived and designed the project. XX and YD collected the materials. YD and JyJ carried out the lab work and measured the morphological traits. XX and AS performed the analysis. XX wrote the manuscript with the help from JfJ. FC and SC supervised the experiment. All authors read and approved the final version of the manuscript.
Funding
This work was supported by the National Key Research and Development Program of China (2019YFD1001500), the Fundamental Research Funds for the Central Universities (KJQN202126), the China Postdoctoral Science Foundation (2019M661871), the National Natural Science Foundation of China (32002075), the Earmarked Fund for Jiangsu Agricultural Industry Technology System [JATS (2020)406], as well as by a Project Funded by the Priority Academic Program Development of Jiangsu Higher Education Institutions.
Conflict of Interest
The authors declare that the research was conducted in the absence of any commercial or financial relationships that could be construed as a potential conflict of interest.
Publisher’s Note
All claims expressed in this article are solely those of the authors and do not necessarily represent those of their affiliated organizations, or those of the publisher, the editors and the reviewers. Any product that may be evaluated in this article, or claim that may be made by its manufacturer, is not guaranteed or endorsed by the publisher.
Supplementary Material
The Supplementary Material for this article can be found online at: https://www.frontiersin.org/articles/10.3389/fpls.2021.673236/full#supplementary-material
Supplementary Table 1 | Primer sequences of transcriptome qRT-PCR.
Supplementary Table 2 | Effects of melatonin treatment on photosynthesis and gas exchange parameters in chrysanthemum seedlings.
Supplementary Table 3 | Summary of sequencing reads after filtering in chrysanthemum leaves were treated with exogenous melatonin.
Supplementary Table 4 | Quality metrics of unigenes in chrysanthemum leaves were treated with exogenous melatonin.
Supplementary Table 5 | Differentially expressed genes in Con-vs.-ConMT, Con-vs.-S, ConMT-vs.-SMT, and S-vs.-SMT comparisons.
Supplementary Table 6 | KEGG analysis (top 5) in Con-vs.-ConMT, Con-vs.-S, ConMT-vs.-SMT, and S-vs.-SMT comparisons.
Supplementary Table 7 | Number of TFs families.
Supplementary Table 8 | Detailed information of the changes in the expression of enzymes, heat shock transcription factors, and heat shock proteins related genes in Con, ConMT, S, and SMT comparisons.
Supplementary Table 9 | Detailed information of the changes in the expression of WRKY, Ca2+ signal transduction, and MAPKKKs in Con, ConMT, S, and SMT comparisons.
Supplementary Table 10 | Detailed information of the changes in the expression of calvin cycle in Con, ConMT, S, and SMT comparisons.
Supplementary Table 11 | Detailed information of the changes in the expression of starch and sucrose in Con, ConMT, S, and SMT comparisons.
Supplementary Table 12 | Detailed information of the changes in the expression of the vital genes in the Ca2+ signal transduction, starch and sugars metabolism in Con, ConMT, S, and SMT comparisons.
Supplementary Table 13 | Detailed information of the changes in the expression of hormone related genes in Con, ConMT, S, and SMT comparisons.
Supplementary Table 14 | Detailed information of the changes in the expression of chlorophyll, flavonoids, and carotenoid metabolic related genes in Con, ConMT, S, and SMT comparisons.
Abbreviations
ABI5, ABA-insensitive; AGPase, ADP-glucose pyrophosphorylase; ARF, auxin response factor; ARG7, IAA-induced protein; APX, ascorbate peroxidase; AsA, ascorbic acid; AUX/IAAs, auxin/indole acetic acid protein; BGLU, β-glucosidase; BXL, β-D-xylosidase; CAT, catalase; CAM/CMLs, calmodulin/calmodulin-like protein; CHI, chalcone isomerase; CHLH, magnesium-chelatase subunit ChlH; CHS, chalcone synthase 2; Con, Control; ConMT, control with melatonin; CLH, hydroxymethyl chlorophyll a reductase; CNGCs, cyclic nucleotide gated channel; DEGs, differentially expressed genes; DFR, dihydroflavonol 4-reductase; DXPS, 1-deoxy-D-xylulose-5-phosphate synthase; DXR, 1-deoxy-D-xylulose-5-phosphate reductoisomerase; EBFs, EIN3-Binding F-Box protein; EDGL, endoglucanase; EGLC, endoglucane-1, 3- β-glucosidase; ERF25, ethylene-responsive transcription factor 25; FDR, false discovery rate; FNS, flavone synthase; F3H, flavanone 3-hydroxylase; GCL, glutamate cysteine ligase; GGDP, geranylgeranyl diphosphate synthase; GME, GDP-D-mannose 3′, 5′-epimerase; GR, glutathione reductase; GSH, glutathione; HEMA, glutamyl-tRNA reductase; HEMF, coproporphyrinogen III oxidase; H2O2, hydrogen peroxide; HSFs, heat shock transcription factors; HSPs, heat shock proteins; MAPKKKs, mitogen-activated protein kinase kinase kinases; MDA, malondialdehyde; MT, melatonin; NYC1/NOL, chlorophyllide a oxygenase; O2•–, superoxide anion free radicals; PCK, phosphoenolpyruvate carboxykinase; PIF3, photosensitive interaction factor 3; POD, peroxidase; PORA, protochlorophyllide oxidoreductase; PP2Cs, protein phosphatase 2C; PPH, pheophytinase; Pro, proline; PSY, phytoene synthase; RBOH, respiratory burst oxidase; ROS, reactive oxygen species; RPI, ribose 5-phosphate isomerase A; S, stress; SAUR, small auxin-up RNA; SOD, superoxide dismutase; S/Con, stress/control; SMT, stress with melatonin; SMT/ConMT, stress with melatonin/control with melatonin; SPS, sucrose phosphate synthase; SuS, sucrose synthase; TIM, triosephosphate isomerase; TIR1, transport inhibitor response 1; TPP, trehalose-phosphate phosphatase; VDE, violaxanthin de-epoxidase; ZEP, zeaxanthin epoxidase.
Footnotes
References
Ahammed, G. J., Li, X., Zhou, J., Zhou, Y. H., and Yu, J. Q. (2016). “Role of hormones in plant adaptation to heat stress,” in Plant Hormones Under Challenging Environmental Factors, eds G. Ahammed and J. Q. Yu (Dordrecht: Springer), 1–21. doi: 10.1007/978-94-017-7758-2_1
Ahammed, G. J., Xu, W., Liu, A., and Chen, S. (2018). COMT1 silencing aggravates heat stress-induced reduction in photosynthesis by decreasing chlorophyll content, photosystem II activity, and electron transport efficiency in tomato. Front. Plant Sci. 9:998. doi: 10.3389/fpls.2018.00998
Arnao, M. B., and Hernández-Ruiz, J. (2007). “Inhibition of ACC oxidase activity by melatonin and indole-3-acetic acid in etiolated lupin hypocotyls,” in Advances in Plant Ethylene Research, eds A. Ramina, C. Chang, J. Giovannoni, H. Klee, P. Perata, and E. Woltering (Dordrecht: Springer), 101–103. doi: 10.1007/978-1-4020-6014-4_21
Arnao, M. B., and Hernandez-Ruiz, J. (2014). Melatonin: plant growth regulator and/or biostimulator during stress? Trends Plant Sci. 19, 789–797. doi: 10.1016/j.tplants.2014.07.006
Arnao, M. B., and Hernandez-Ruiz, J. (2015). Functions of melatonin in plants: a review. J. Pineal Res. 59, 133–150. doi: 10.1111/jpi.12253
Arnao, M. B., and Hernandez-Ruiz, J. (2018). Melatonin and its relationship to plant hormones. Ann. Bot. 121, 195–207. doi: 10.1093/aob/mcx114
Baninasab, B., and Ghobadi, C. (2010). Influence of paclobutrazol and application methods on high-temperature stress injury in cucumber seedlings. J. Plant Growth Regul. 30, 213–219. doi: 10.1007/s00344-010-9188-2
Banti, V., Mafessoni, F., Loreti, E., Alpi, A., and Perata, P. (2010). The heat-inducible transcription factor HsfA2 enhances anoxia tolerance in Arabidopsis. Plant Physiol. 152, 1471–1483. doi: 10.1104/pp.109.149815
Barnabas, B., Jager, K., and Feher, A. (2008). The effect of drought and heat stress on reproductive processes in cereals. Plant Cell Environ. 31, 11–38. doi: 10.1111/j.1365-3040.2007.01727.x
Baxter, A., Mittler, R., and Suzuki, N. (2014). ROS as key players in plant stress signalling. J. Exp. Bot. 65, 1229–1240. doi: 10.1093/jxb/ert375
Biswojit, D., Mubasher, H., Muhammad, I., Sangeeta, M., Min, L., Shuang, L., et al. (2018). Exogenous melatonin mitigates acid rain stress to tomato plants through modulation of leaf ultrastructure, photosynthesis and antioxidant potential. Molecules 23, 388–395. doi: 10.3390/molecules23020388
Bradford, M. M. (1976). A rapid and sensitive method for the quantitation of microgram quantities of protein utilizing the principle of protein-dye binding. Anal. Biochem. 72, 248–254. doi: 10.1016/0003-2697(76)90527-3
Buttar, Z. A., Wu, S. N., Arnao, M. B., Wang, C., Ullah, I., and Wang, C. (2020). Melatonin suppressed the heat stress-induced damage in wheat seedlings by modulating the antioxidant machinery. Plants 9:809. doi: 10.3390/plants9070809
Byeon, Y., Park, S., Kim, Y. S., Park, D. H., Lee, S., and Back, K. (2012). Light-regulated melatonin biosynthesis in rice during the senescence process in detached leaves. J. Pineal Res. 53, 107–111. doi: 10.1111/j.1600-079X.2012.00976.x
Camejo, D., Jimenez, A., Alarcon, J. J., Torres, W., Gomez, J. M., and Sevilla, F. (2006). Changes in photosynthetic parameters and antioxidant activities following heat-shock treatment in tomato plants. Funct. Plant Biol. 33, 177–187. doi: 10.1071/FP05067
Celik, O., and Nazıroğlu, M. (2012). Melatonin modulates apoptosis and TRPM2 channels in transfected cells activated by oxidative stress. Physiol. Behav. 107, 458–465. doi: 10.1016/j.physbeh.2012.09.013
Chen, Q., and Yang, G. (2019). Signal function studies of ROS, especially RBOH-dependent ROS, in plant growth, development and environmental stress. J. Plant Growth Regul. 39, 157–171. doi: 10.1007/s00344-019-09971-4
Dong, S., and Beckles, D. M. (2019). Dynamic changes in the starch-sugar interconversion within plant source and sink tissues promote a better abiotic stress response. J. Plant Physiol. 234-235, 80–93. doi: 10.1016/j.jplph.2019.01.007
Duszyn, M., Wieawska, B., Szmidt-Jaworska, A., and Jaworski, K. (2019). Cyclic nucleotide gated channels (CNGCs) in plant signalling—Current knowledge and perspectives. J. Plant Physiol. 241:153035. doi: 10.1016/j.jplph.2019.153035
Fleta-Soriano, E., Díaz, L., Bonet, E., and Munné-Bosch, S. (2017). Melatonin may exert a protective role against drought stress in maize. J. Agron. Crop Sci. 203, 286–294. doi: 10.1111/jac.12201
Franklin, K. A., Lee, S. H., Patel, D., Kumar, S. V., Spartz, A. K., Gu, C., et al. (2011). Phytochrome-interacting factor 4 (PIF4) regulates auxin biosynthesis at high temperature. Proc. Natl. Acad. Sci. U.S.A. 108, 20231–20235. doi: 10.1073/pnas.1110682108
Gao, T., Zhang, Z., Liu, X., Wu, Q., Chen, Q., Liu, Q., et al. (2020). Physiological and transcriptome analyses of the effects of exogenous dopamine on drought tolerance in apple. Plant Physiol. Biochem. 148, 260–272. doi: 10.1016/j.plaphy.2020.01.022
Garnczarska, M., and Bednarski, W. (2004). Effect of a short-term hypoxic treatment followed by re-aeration on free radicals level and antioxidative enzymes in lupine roots. Plant Physiol. Biochem. 42, 233–240. doi: 10.1016/j.plaphy.2004.01.005
Giannopolittis, C., and Ries, S. (1997). Superoxide dismutase (II) purification and quantitative relationship with water-soluble protein in seedlings. Plant Physiol. 59, 315–318. doi: 10.1104/pp.59.2.315
Grabherr, M. G., Haas, B. J., Yassour, M., Levin, J. Z., Thompson, D. A., Amit, I., et al. (2011). Full-length transcriptome assembly from RNA-Seq data without a reference genome. Nat. Biotechnol. 29, 644–652. doi: 10.1038/nbt.1883
Han, Y., Watanabe, S., Shimada, H., and Sakamoto, A. (2020). Dynamics of the leaf endoplasmic reticulum modulate beta-glucosidase-mediated stress-activated ABA production from its glucosyl ester. J. Exp. Bot. 71, 2058–2071. doi: 10.1093/jxb/erz528
Hasan, M. K., Ahammed, G. J., Yin, L., Shi, K., Xia, X., Zhou, Y., et al. (2015). Melatonin mitigates cadmium phytotoxicity through modulation of phytochelatins biosynthesis, vacuolar sequestration, and antioxidant potential in Solanum lycopersicum L. Front. Plant Sci. 6:601. doi: 10.3389/fpls.2015.00601
Hernandez, I., Alegre, L., Van Breusegem, F., and Munne-Bosch, S. (2009). How relevant are flavonoids as antioxidants in plants? Trends Plant Sci. 14, 125–132. doi: 10.1016/j.tplants.2008.12.003
Inaba, M., and Chachin, K. (1988). Influence of and recovery from high-temperature stress on harvested mature green tomatoes. Hortic. Sci. 23, 190–192.
Jung, H., Lee, D. K., Choi, Y. D., and Kim, J. K. (2015). OsIAA6, a member of the rice Aux/IAA gene family, is involved in drought tolerance and tiller outgrowth. Plant Sci. Int. J. Exp. Plant Biol. 236, 304–312. doi: 10.1016/j.plantsci.2015.04.018
Kamrul, H. M., Jalal, A. G., Yin, L. L., Shi, K., Xia, X. J., Zhou, Y. H., et al. (2015). Melatonin mitigates cadmium phytotoxicity through modulation of phytochelatins biosynthesis, vacuolar sequestration, and antioxidant potential in Solanum lycopersicum L. Front. Plant Sci. 6:601. doi: 10.3389/fpls.2015.00601
Kato, M., and Shimizu, S. (1987). Chlorophyll metabolism in higher plants. VII. Chlorophyll degradation in senescing tobacco leaves: phenolic-dependent peroxidative degradation. Can. J. Bot. 65, 729–735. doi: 10.1139/b87-097
Kazan, K. (2013). Auxin and the integration of environmental signals into plant root development. Ann. Bot. 112, 1655–1665. doi: 10.1093/aob/mct229
Konigshofer, H., Tromballa, H. W., and Loppert, H. G. (2008). Early events in signalling high-temperature stress in tobacco BY2 cells involve alterations in membrane fluidity and enhanced hydrogen peroxide production. Plant Cell Environ. 31, 1771–1780. doi: 10.1111/j.1365-3040.2008.01880.x
Kostopoulou, Z., Therios, I., Roumeliotis, E., Kanellis, A. K., and Molassiotis, A. (2015). Melatonin combined with ascorbic acid provides salt adaptation in Citrus aurantium L. seedlings. Plant Physiol. Biochem. 86, 155–165. doi: 10.1016/j.plaphy.2014.11.021
Kotak, S., Larkindale, J., Lee, U., von Koskull-Doring, P., Vierling, E., and Scharf, K. D. (2007). Complexity of the heat stress response in plants. Curr. Opin. Plant Biol. 10, 310–316. doi: 10.1016/j.pbi.2007.04.011
Lesk, C., Rowhani, P., and Ramankutty, N. (2016). Influence of extreme weather disasters on global crop production. Nature 529, 84–87. doi: 10.1038/nature16467
Li, H. S. (2000). Principles and Techniques of Plant Physiology and Biochemistry Experiments. Beijing: Higher Education Press, 164–165.
Li, Z. G., Xu, Y., Bai, L. K., Zhang, S. Y., and Wang, Y. (2019). Melatonin enhances thermotolerance of maize seedlings (Zea mays L.) by modulating antioxidant defense, methylglyoxal detoxification, and osmoregulation systems. Protoplasma 256, 471–490. doi: 10.1007/s00709-018-1311-4
Liang, D., Ni, Z., Xia, H., Xie, Y., Lv, X., Wang, J., et al. (2019). Exogenous melatonin promotes biomass accumulation and photosynthesis of kiwifruit seedlings under drought stress. Sci. Hortic. 246, 34–43. doi: 10.1016/j.scienta.2018.10.058
Liang, D., Shen, Y., Ni, Z., Wang, Q., Lei, Z., Xu, N., et al. (2018). Exogenous melatonin application delays senescence of kiwifruit leaves by regulating the antioxidant capacity and biosynthesis of flavonoids. Front. Plant Sci. 9:426. doi: 10.3389/fpls.2018.00426
Liu, L., Liu, C. Y., Wang, H., Yu, S. Y., Guan, T. S., Huang, Y. F., et al. (2020). The abscisic acid receptor gene VvPYL4 positively regulates grapevine resistance to Plasmopara viticola. Plant Cell Tissue Organ Cult. (PCTOC) 142, 483–492. doi: 10.1007/s11240-020-01872-9
Livak, K. J., and Schmittgen, T. D. (2001). Analysis of relative gene expression data using real-time quantitative PCR and the 2−ΔΔCT method. Methods 25, 402–408. doi: 10.1006/meth.2001.1262
Locato, V., de Pinto, M. C., and De Gara, L. (2009). Different involvement of the mitochondrial, plastidial and cytosolic ascorbate-glutathione redox enzymes in heat shock responses. Physiol. Plant. 135, 296–306. doi: 10.1111/j.1399-3054.2008.01195.x
Lurie, S., and Klein, J. D. (2006). Heat treatment of ripening apples: differential effects on physiology and biochemistry. Physiol. Plant. 78, 181–186. doi: 10.1111/j.1399-3054.1990.tb02078.x
Ma, F., and Cheng, L. (2003). The sun-exposed peel of apple fruit has higher xanthophyll cycle-dependent thermal dissipation and antioxidants of the ascorbate-glutathione pathway than the shaded peel. Plant Sci. 165, 819–827. doi: 10.1016/S0168-9452(03)00277-2
Meiri, D., Tazat, K., Cohen-Peer, R., Farchi-Pisanty, O., Aviezer-Hagai, K., Avni, A., et al. (2010). Involvement of Arabidopsis ROF2 (FKBP65) in thermotolerance. Plant Mol. Biol. 72, 191–203. doi: 10.1007/s11103-009-9561-3
Meng, J. F., Yu, Y., Shi, T. C., Fu, Y. S., Zhao, T., Zhang, Z. W., et al. (2019). Melatonin treatment of pre-veraison grape berries modifies phenolic components and antioxidant activity of grapes and wine. Food Sci. Technol. 39, 35–42. doi: 10.1590/1678-457x.24517
Meng, Z., Zhang, B., Liu, B., Li, H., and Yu, D. (2016). High carotenoids content can enhance resistance of selected Pinctada fucata families to high temperature stress. Fish Shellfish Immunol. 61, 211–218. doi: 10.1016/j.fsi.2016.12.032
Morales, D., Rodriguez, P., Dell’Amico, J., Nicolas, E., Torrecillas, A., and Sanchez-Blanco, M. J. (2003). High-temperature preconditioning and thermal shock imposition affects water relations, gas exchange and root hydraulic conductivity in tomato. Biol. Plant. 46, 203–208. doi: 10.1023/B:BIOP.0000022252.70836.fc
Nakano, Y., and Asada, K. (1981). Hydrogen peroxide is scavenged by ascorbate-specific peroxidase in spinach chloroplasts. Plant Cell Physiol. 22, 867–880.
Naser, V., and Shani, E. (2016). Auxin response under osmotic stress. Plant Mol. Biol. 91, 661–672. doi: 10.1007/s11103-016-0476-5
Pelagio-Flores, R., Muñoz-Parra, E., Ortiz-Castro, R., and López-Bucio, J. (2012). Melatonin regulates Arabidopsis root system architecture likely acting independently of auxin signaling. J. Pineal Res. 53, 279–288. doi: 10.1111/j.1600-079X.2012.00996.x
Pertea, G., Huang, X., Liang, F., Antonescu, V., Sultana, R., Karamycheva, S., et al. (2003). TIGR Gene Indices clustering tools (TGICL): a software system for fast clustering of large EST datasets. Bioinformatics 19, 651–652. doi: 10.1093/bioinformatics/btg034
Qi, Z. Y., Wang, K. X., Yan, M. Y., Kanwar, M. K., Li, D. Y., Wijaya, L., et al. (2018). Melatonin alleviates high temperature-induced pollen abortion in Solanum lycopersicum. Molecules 23:386. doi: 10.3390/molecules23020386
Reiter, R. J., Tan, D. X., Zhou, Z., Cruz, M. H., Fuentes-Broto, L., and Galano, A. (2015). Phytomelatonin: assisting plants to survive and thrive. Molecules 20, 7396–7437. doi: 10.3390/molecules20047396
Ren, L. P., Sun, J., Chen, S. M., Gao, J. J., Dong, B., Liu, Y. N., et al. (2014). A transcriptomic analysis of Chrysanthemum nankingense provides insights into the basis of low temperature tolerance. BMC Genomics 15:844. doi: 10.1186/1471-2164-15-844
Roitsch, T., and González, M. C. (2004). Function and regulation of plant invertases: sweet sensations. Trends Plant Sci. 9, 606–613. doi: 10.1016/j.tplants.2004.10.009
Santofimia-Castaño, P., Ruy, D. C., Fernandez-Bermejo, M., Salido, G. M., and Gonzalez, A. (2014). Pharmacological dose of melatonin reduces cytosolic calcium load in response to cholecystokinin in mouse pancreatic acinar cells. Mol. Cell. Biochem. 397, 75–86. doi: 10.1007/s11010-014-2174-4
Scebba, F., Sebastiani, L., and Vitagliano, C. (2001). Activities of antioxidant enzymes during senescence of Prunus armeniaca leaves. Biol. Plant. 44, 41–46. doi: 10.1023/A:1017962102950
Schmedes, A., and Hølmer, G. (1989). A new thiobarbituric acid (TBA) method for determining free malondialdehyde (MDA) and hydropeoxides selectively as a measure of lipid peroxidation. J. Am. Oil Chem. Soc. 66, 813–817. doi: 10.1007/BF02653674
Sharma, A., Wang, J., Xu, D., Tao, S., Chong, S., Yan, D., et al. (2020). Melatonin regulates the functional components of photosynthesis, antioxidant system, gene expression, and metabolic pathways to induce drought resistance in grafted Carya cathayensis plants. Sci. Total Environ. 713, 136675.1–136675.13. doi: 10.1016/j.scitotenv.2020.136675
Sharma, L., Priya, M., Bindumadhava, H., Nair, R. M., and Nayyar, H. (2016). Influence of high temperature stress on growth, phenology and yield performance of mungbean [Vigna radiata (L.) Wilczek] under managed growth conditions. Sci. Hortic. 213, 379–391. doi: 10.1016/j.scienta.2016.10.033
Shekari, A., Hassani, R. N., Aghdam, M. S., Rezaee, M., and Jannatizadeh, A. (2021). The effects of melatonin treatment on cap browning and biochemical attributes of Agaricus bisporus during low temperature storage. Food Chem. 348:129074. doi: 10.1016/j.foodchem.2021.129074
Shi, H. T., Tan, D. X., Reiter, R. J., Ye, T. T., Yang, F., and Chan, Z. L. (2015). Melatonin induces class A1 heat-shock factors (HSFA1s) and their possible involvement of thermotolerance in Arabidopsis. J. Pineal Res. 58, 335–342. doi: 10.1111/jpi.12219
Sun, Q., Zhang, N., Wang, J., Zhang, H., Li, D., Shi, J., et al. (2015). Melatonin promotes ripening and improves quality of tomato fruit during postharvest life. J. Exp. Bot. 66, 657–668. doi: 10.1093/jxb/eru332
Toh, S., Imamura, A., Watanabe, A., Nakabayashi, K., Okamoto, M., Jikumaru, Y., et al. (2008). High temperature-induced abscisic acid biosynthesis and its role in the inhibition of gibberellin action in Arabidopsis seeds. Plant Physiol. 146, 1368–1385. doi: 10.1104/pp.107.113738
Vanderauwera, S., Suzuki, N., Miller, G., van de Cotte, B., Morsa, S., and Ravanat, J. L. (2011). Extranuclear protection of chromosomal DNA from oxidative stress. Proc. Natl. Acad. Sci. U.S.A. 108, 1711–1716. doi: 10.1073/pnas.1018359108
Wang, D., Chen, Q., Chen, W., Guo, Q., Xia, Y., Wang, S., et al. (2021). Physiological and transcription analyses reveal the regulatory mechanism of melatonin in inducing drought resistance in loquat (Eriobotrya japonica Lindl.) seedlings. Environ. Exp. Bot. 181:104291. doi: 10.1016/j.envexpbot.2020.104291
Wang, P., Sun, X., Chang, C., Feng, F., Liang, D., Cheng, L., et al. (2013). Delay in leaf senescence of Malus hupehensis by long-term melatonin application is associated with its regulation of metabolic status. J. Pineal Res. 55, 424–434. doi: 10.1111/jpi.12091
Wang, P., Sun, X., Wang, N., Tan, D. X., and Ma, F. (2015). Melatonin enhances the occurrence of autophagy induced by oxidative stress in Arabidopsis seedlings. J. Pineal Res. 58, 479–489. doi: 10.1111/jpi.12233
Wang, W., Vinocur, B., Shoseyov, O., and Altman, A. (2004). Role of plant heat-shock proteins and molecular chaperons in the abiotic stress response. Trends Plant Sci. 9, 244–252. doi: 10.1016/j.tplants.2004.03.006
Wang, Z., Liu, L., Cheng, C., Ren, Z., Xu, S., and Li, X. (2020). GAI functions in the plant response to dehydration stress in Arabidopsis thaliana. Int. J. Mol. Sci. 21:819. doi: 10.3390/ijms21030819
Weeda, S., Zhang, N., Zhao, X., Ndip, G., Guo, Y., and Buck, G. A. (2014). Arabidopsis transcriptome analysis reveals key roles of melatonin in plant defense systems. PLoS One 9:e93462. doi: 10.1371/journal.pone.0093462
Willekens, H., Chamnongpol, S., Davey, M., Schraudner, M., Langebartels, C., Montagu, M. V., et al. (1997). Catalase is a sink for H2O2 and is indispensable for stress defence in C3 plants. EMBO J. 16, 4806–4816. doi: 10.1093/emboj/16.16.4806
Wilson, R. A., Sangha, M. K., Banga, S. S., Atwal, A. K., and Gupta, S. (2014). Heat stress tolerance in relation to oxidative stress and antioxidants in Brassica juncea. J. Environ. Biol. 35, 383–387.
Winter, H., and Huber, S. C. (2000). Regulation of sucrose metabolism in higher plants: localization and regulation of activity of key enzymes. Crit. Rev. Biochem. Mol. Biol. 35, 253–289. doi: 10.1080/10409230008984165
Xia, X. J., Huang, L. F., Zhou, Y. H., Mao, W. H., Shi, K., Wu, J. X., et al. (2009). Brassinosteroids promote photosynthesis and growth by enhancing activation of Rubisco and expression of photosynthetic genes in Cucumis sativus. Planta 230, 1185–1196. doi: 10.1007/s00425-009-1016-1
Xu, W., Cai, S. Y., Zhang, Y., Wang, Y., Ahammed, G. J., Xia, X. J., et al. (2016). Melatonin enhances thermotolerance by promoting cellular protein protection in tomato plants. J. Pineal Res. 61, 457–469. doi: 10.1111/jpi.12359
Yang, X., Zhao, T., Rao, P., Gao, K., Yang, X., Chen, Z., et al. (2019). Transcriptome profiling of Populus tomentosa under cold stress. Ind. Crops Prod. 135, 283–293. doi: 10.1016/j.indcrop.2019.04.056
Yang, Y., Gao, S., Su, Y., Lin, Z., Guo, J., Li, M., et al. (2019). Transcripts and low nitrogen tolerance: regulatory and metabolic pathways in sugarcane under low nitrogen stress. Environ. Exp. Bot. 163, 97–111. doi: 10.1016/j.envexpbot.2019.04.010
Yin, H., Chen, Q., and Yi, M. (2008). Effects of short-term heat stress on oxidative damage and responses of antioxidant system in Lilium longiflorum. Plant Growth Regul. 54, 45–54. doi: 10.1007/s10725-007-9227-6
Zhang, J., Shi, Y., Zhang, X., Du, H., Xu, B., and Huang, B. (2017). Melatonin suppression of heat-induced leaf senescence involves changes in abscisic acid and cytokinin biosynthesis and signaling pathways in perennial ryegrass (Lolium perenne L.). Environ. Exp. Bot. 138, 36–45. doi: 10.1016/j.envexpbot.2017.02.012
Zhang, N., Sun, Q., Zhang, H., Cao, Y., Weeda, S., Ren, S., et al. (2015). Roles of melatonin in abiotic stress resistance in plants. J. Exp. Bot. 66, 647–656. doi: 10.1093/jxb/eru336
Zhang, N., Zhang, H., Zhao, B., Sun, Q., Cao, Y., Li, R., et al. (2014). The RNA-seq approach to discriminate gene expression profiles in response to melatonin on cucumber lateral root formation. J. Pineal Res. 56, 39–50. doi: 10.1111/jpi.12095
Zhang, X., Ren, A., Li, M. J., Cao, P. F., Chen, T. X., Zhang, G., et al. (2016). Heat stress modulates mycelium growth, heat shock protein expression, ganoderic acid biosynthesis, and hyphal branching of Ganoderma lucidum via cytosolic Ca2+. Appl. Environ. Microbiol. 82, 4112–4125. doi: 10.1128/AEM.01036-16
Zhao, H., Dai, T., Jiang, D., and Cao, W. (2008). Effects of high temperature on key enzymes involved in starch and protein formation in grains of two wheat cultivars. J. Agron. Crop Sci. 194, 47–54. doi: 10.1111/j.1439-037X.2007.00283.x
Keywords: chrysanthemum, melatonin, high temperature, physiology, RNA-seq
Citation: Xing X, Ding Y, Jin J, Song A, Chen S, Chen F, Fang W and Jiang J (2021) Physiological and Transcripts Analyses Reveal the Mechanism by Which Melatonin Alleviates Heat Stress in Chrysanthemum Seedlings. Front. Plant Sci. 12:673236. doi: 10.3389/fpls.2021.673236
Received: 27 February 2021; Accepted: 24 August 2021;
Published: 22 September 2021.
Edited by:
Frank Bedon, La Trobe University, AustraliaReviewed by:
Juan de Dios Alché, Estación Experimental de Zaidín, Consejo Superior de Investigaciones Científicas (CSIC), SpainWei Shan, South China Agricultural University, China
Qi Chen, Kunming University of Science and Technology, China
Copyright © 2021 Xing, Ding, Jin, Song, Chen, Chen, Fang and Jiang. This is an open-access article distributed under the terms of the Creative Commons Attribution License (CC BY). The use, distribution or reproduction in other forums is permitted, provided the original author(s) and the copyright owner(s) are credited and that the original publication in this journal is cited, in accordance with accepted academic practice. No use, distribution or reproduction is permitted which does not comply with these terms.
*Correspondence: Weimin Fang, ZmFuZ3dtQG5qYXUuZWR1LmNu; Jiafu Jiang, amlhbmdqaWFmdUBuamF1LmVkdS5jbg==