- Institute of Pomology and Olericulture, College of Horticulture, Sichuan Agricultural University, Chengdu, China
Fruit aromas are composed of a complex mixture of volatile organic compounds, which are essential attributes associated with the overall flavor and consumer preference. Muscadine grape (MG; Vitis rotundifolia Michx.) is an aroma-dense fruit crop. However, there is very scarce information on its volatile profiles. In this study, the volatile constituents of five newly introduced MG cultivars, including Alachua, Carlos, Fry, Granny Val, and Noble, were profiled using headspace solid-phase microextraction-gas chromatography-mass spectrometry (HS-SPME-GC/MS) combined with multivariate statistical analysis. A total of 44 compounds, including esters, aldehydes, alcohols, fatty acids, terpenes, ketones, and furan, were identified and relatively quantified. Principal component analysis (PCA) and partial least-squares discriminant analysis (PLS-DA) evidently discriminated against the five MG cultivars based on their volatile profiles. The specific volatiles that contributed the most to this discrimination were highlighted. Geraniol and cinnamyl alcohol were demonstrated to be essential for characterizing the Alachua MG cultivar, whereas ethyl trans-2-butenoate and propyl acetate were shown to be important compounds to characterize the Noble MG cultivar. The results further showed that 2-Ethyl-1-hexanol, (Z)-3-hexenal, and (E)-2-hexenol were closely related to Carlos, Fry, and Granny Val cultivars, respectively. This investigation is the first in-depth exploration of the volatile profiles of the aroma-dense muscadine grape, which is essential for future genetic or biotechnological improvements to attain a cultivar with the desired flavor.
Introduction
Grapes (Vitis vinifera L.) are among the most economically important and the earliest domesticated fruit crops throughout the world (Reisch et al., 2012; Ramos-Madrigal et al., 2019). Similar to fresh fruit, grapes are an essential nutrient-dense food source, being part of the human diet, while as processed products, i.e., dried raisins, fruit preserves, primarily wine, and spirits, they have added economic values and represent a globally traded commodity with significant economic value (Reisch et al., 2012; Ramos-Madrigal et al., 2019). Most of the grapes cultivated for fruit production are either of the species V. vinifera, hybrids of V. vinifera, belonging to the genus Vitis L., or, to a lower extent, to the closely related subgenus Muscadinia (Reisch et al., 2012; Lin et al., 2019).
Taxonomically, the muscadine grape (MG) (Muscadinia rotundifolia Michx., syn. V. rotundifolia Michx.) is closely related to Vitis species (Olien, 1990; Liu et al., 2016; Wen et al., 2018). Genetically, the basic chromosome of MG (x = 20) is interestingly one chromosome greater than the 19 basic chromosomes of the V. vinifera L. (Olien, 1990). Indigenous to the warm and humid climate of the southeastern United States, MG was the first American grape species to be cultivated (Olien, 1990) and has been cultivated for more than 400 years (Stanley, 1997; Conner, 2009). It can be found growing naturally from Delaware to Central Florida state, i.e., south-north distribution, and from Texas and Oklahoma to the eastern coastal plain, i.e., east-west span, in the United States, along the Mississippi River to Missouri and near the Appalachian Mountains from the east and west (Olien, 1990).
Muscadine grape has relatively high intrinsic resistance to disease, including downy and powdery mildews, gray mold, and anthracnose, especially Pierce's disease (Xylella fasti diosa) (Olien, 1990). Over the past few decades, MG has drawn increasing attention from consumers, growers, and breeders because it constitutes an important source of dietary fibers, amino acids, minerals, vitamins, antioxidant phytochemicals, such as polyphenolic compounds, and other health-promoting compounds (Sandhu and Gu, 2010; Wang et al., 2010). Since the resurgence of the interest in MG in the United States to date, more than 100 MG cultivars have been released (Hoffmann et al., 2020), and MG has also been extended to California, Chile, and China (Hoffmann et al., 2020). For example, five MG cultivars, including Alachua, Carlos, Fry, Granny Val, and Noble, have been successfully introduced and cultivated in southern China (Wei et al., 2017; Ren et al., 2020).
In addition to having significantly more phytonutrients than the average table grape cultivars (Marshall et al., 2012), MG has a distinct musky aroma and unique flavor (Kambiranda et al., 2016), which is essential for its current wine, juice, and table grape markets. Volatile organic compounds (VOCs), such as esters, ketones, terpenes, aldehydes, alcohols, C13 norisoprenoids, and benzenoids, are significant determinants of the grape aroma and perceived flavor (Gürbüz et al., 2014; Lin et al., 2019). The VOCs of fruits are complex and vary significantly depending on the analytical methods used and a plethora of factors, such as the cultivar, sample type, ripening stage, environmental conditions, abiotic and biotic stress (Sánchez-Palomo et al., 2005; Kalua and Boss, 2009; Wu et al., 2020). VOCs are the main contributors to the fresh and fruity note of wines (Perez-Coello and Diaz-Maroto, 2009) and are important nutritional constituents, shaping the sensory properties of foods and influencing the perception of consumers (Berger, 2009). Each fruit species has a specific combination of various VOCs with different concentration and perception thresholds (El Hadi et al., 2013). The differences of VOCs between the table and wine grapes have been well investigated, whereas only a few studies have reported the VOC compositions of MG (Lee et al., 2016).
Headspace solid-phase microextraction (HS-SPME) is a passive sampling approach that collects compounds present in the vapor phase above samples, which does not interfere with the samples and omits the compounds in the vapor phase above the matrix during heating (Lubes and Goodarzi, 2017). Compared with solvent extraction, HS-SPME is rapid, simple, and reproducible, and yields more realistic VOC discrimination results (Lubes and Goodarzi, 2017). HS-SPME coupled with gas chromatography-mass spectrometry (GC-MS) has been extensively employed to study the volatile profiles of many fruit varieties, vegetables, and beverages.
Muscadine grape is an aroma-dense fruit. However, relatively few publications have documented its aromatic VOC compositions. Therefore, a systematic, qualitative, and quantitative investigation of the MG VOCs is highly required and of great importance. In this study, HS-SPME-GC-MS was employed to compare, both qualitatively and quantitatively, the volatile constituents of five MG cultivars that were newly introduced in China. Multivariate statistical analysis methods, including principal component analysis (PCA), and partial least-squares discriminate analysis (PLS-DA), were utilized to highlight the differences among cultivars and identify the chemical biomarkers discriminating the five MG cultivars. Determining the VOCs of MG and exploring the differences in VOCs among cultivars is essential for future genetic or biotechnological improvements targeting aroma-dense grape cultivars.
Materials and Methods
Fruit Materials
This study was conducted using 5-year-old (in 2020) newly introduced MG cultivars (Alachua, Carlos, Fry, Granny Val, and Noble). Trees were grown in the fields of the experimental station of the College of Horticulture, Sichuan Agricultural University (30°42′N, 103°51′E), China. In this area, all vines were subjected to identical and standard viticultural practices for table grape cultivars throughout the experiment, including winter pruning, pest and pathogen control, basal fertilizer, and irrigation.
For each cultivar, fruit samples (Figure 1) were randomly collected from three clusters of four different plants in September (the maturation stage), which is considered ideal for commercialization in Sichuan based on the observations during the previous years. Specifically, the solid soluble content of Alachua, Carlos, Fry, Granny Val, and Noble samples ranged from 16.28 to 17.7°Brix, from 14.2 to 16.18°Brix, from 14.62 to 16.3°Brix, from 14.9 to 18.03°Brix, and from 14.7 to 15.38°Brix, respectively. Each cluster was clipped off at the end of the peduncle. Samples were stored in a cold chamber and transported to the laboratory within 2 h. Three berries from the top, central, and bottom parts of each cluster were pooled together. A total of 36 berries were picked and randomly divided into three biological replicates. A 0.5 cm thickness slice was obtained from the equatorial region of each fruit, immediately frozen in liquid nitrogen, and stored at −80°C until the further determination of VOCs.
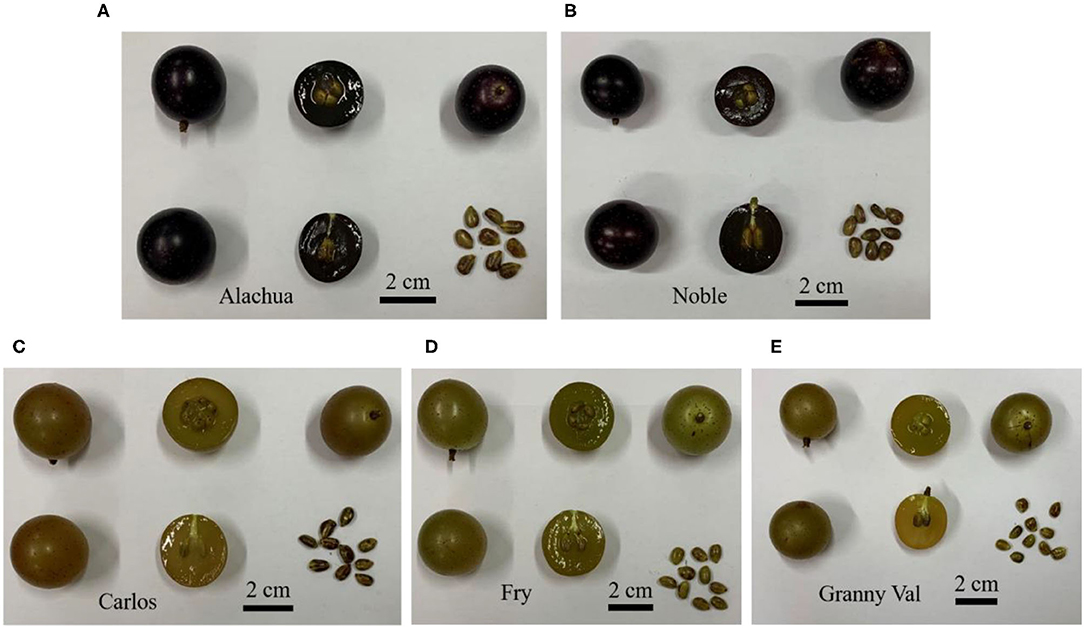
Figure 1. Fruit phenotypes of the Alachua (A), Noble (B), Carlos (C), Fry (D), and Granny Val (E) muscadine grape cultivars. Scale bars denote 2 cm.
Chemicals and Solvents
The study used n-Alkane (C7-C30) standards and the available authentic standards, including ethyl acetate (≥99.5%), propyl acetate (≥99.5%), butyl acetate (99.7%), ethyl trans-2-butenoate, ethyl hexanoate (≥99%), ethyl heptanoate (≥99%), ethyl octanoate (≥99%), ethyl 3-hydroxybutyrate (≥ 98%), hexyl hexanoate (≥ 98%), hexanal (≥95%), (Z)-3-hexenal (50% in triacetin), (E)-2-hexenal (≥97%), nonanal (≥99.5%), benzaldehyde (≥99.5%), (E)-2-decenal (≥95%), citral (≥95%), 1-butanol (≥99.4%), 1-hexanol (≥99.9%), (E)-2-hexenol (96%), 1-octen-3-ol (≥98%), 1-heptanol (≥99.5%), 2-ethylhexanol (≥99%), 1-octanol (≥99%), 2-octen-1-ol (97%), (Z)-5-octen-1-ol (≥97%), (E)-5-decen-1-ol (≥97%), phenylethyl alcohol (≥99%), cinnamyl alcohol (≥96%), acetic acid (≥99.7%), hexanoic acid (≥98%), heptanoic acid (≥99%), octanoic acid (99%), nonanoic acid (≥99.5%), limonene (mixture of D- and L-form at ratio of 1:1, ≥95%), linalool (≥99%), citronellol (≥95%), nerol (≥97%), geraniol (≥98.5%), 2-octanone (≥99.5%), acetophenone (≥99.5%), 2-pentylfuran (≥97%), 2-octanol (≥97%), and sodium chloride (NaCl, ≥99%) which were all purchased from Sigma-Aldrich (St. Louis, MO, USA). Geranic acid (sum of isomers, 98%) was purchased from Alfa Aesar Corporation (Tianjin, China). Ultrapure water was prepared using a Milli-Q water purification system (Millipore Corporation, Bedford, MA, USA) with a 0.22 μm filter.
Fruit Sample Preparation for HS-SPME
The VOCs from the whole fruit were extracted using HS-SPME. Fruit samples, including the seeds, flesh, and skins, were pooled together and fully ground into a fine powder in liquid nitrogen. For each extraction sample, 100 mg of powder, spiked with 10 μl of 2-octanol, was accurately weighed and transferred to a 20-ml glass sample container (Thermo Scientific, Bellefonte, PA, USA). Samples were overlaid with a 5 ml saturated sodium chloride (NaCl) solution to inhibit enzyme degradation. A tiny stirring bar was added to facilitate VOC release before the glass vial was capped. The homogenized samples were incubated for 15 min in a 60°C water bath with continuous agitation (125 rpm). Thereafter, the VOCs were collected using a 2 cm DVB/CAR/PMDS SPME fiber (50/30 μm, Supelco Inc., Bellefonte, PA, USA) by exposing the fiber to the headspace for another 30 min under the same conditions. The fibers were activated before sampling according to the instructions of the manufacturer. After this incubation step, the SPME fiber was inserted directly into the injection port of the GC system for thermal desorption (4 min at 250°C) in a splitless mode.
GC-MS Operating Conditions
Volatile organic compounds were analyzed using an Agilent 7890 gas chromatography system equipped with a 5977B mass spectrometer (Agilent Technologies Inc., Santa Clara, CA, USA). Helium (99.999% purity) was used as the carrier gas with a front inlet purge flow rate of 3 ml min−1 and a constant gas flow rate through the column of 1 ml min−1. VOC separation was carried out using an Agilent DB-Wax (30m × 250 μm ×0.25 μm, Agilent Technologies Inc., Santa Clara, CA, USA) column. The oven temperature program was initiated at 40°C for 4 min, then ramped up to 245°C at a rate of 5 °C min−1, and held for 5 min. The transfer line, ion source, and quadrupole mass detector temperature values were set to 250, 230, and 150°C, respectively. Mass spectra in electron impact ionization (ME-EI) mode were recorded at ionization energy of 70 eV. Data acquisition was performed using the mass spectrometer scanning mode from m/z 20 to 500. The solvent delay time was 0 min.
VOC Identification and Quantification
The row peak obtained from GC-MS was first processed using Chroma TOF 4.3X software (LECO Corporation, St Joseph, MI, USA). The parameters used for raw peak extraction, data baseline filtering and calibration of the baseline, peak alignment, deconvolution analysis, peak identification, integration, and spectrum match of the peak area were the same for all samples. VOCs were identified by matching the retention time in conjunction with the MS fragments with the data of previous studies using a similar chromatographic column and the built-in commercial MS databases, such as the NIST reference library (National Institute of Standards and Technology, Gaithersburg, MA, USA). The VOCs were checked against authentic standards when they were accessible. The VOC concentrations were obtained via peak normalization and semi-quantified to the internal reference standard in the same GC-MS run, which was added at the beginning of the VOC extraction step, as mentioned above. Correspondingly, the peak area of each VOC was converted into a relative concentration value for the following statistical analyses.
Statistical Analyses
The normalized data were exported to R free software v. 3.2.3 (R Core Team, 2020) for statistical analysis. The means and SEs were determined for all the detected variables. Significant variances were validated using one-way ANOVA and Tukey's honestly significant difference (HSD) test (P < 0.05). The data were then imported into SIMCA software version 14.1 (Umetrics, Umea, Sweden) for multivariate statistical analyses. PCA was first conducted to visualize the main correlations in the whole data matrix, followed by PLS-DA to discriminate the MG varieties further. A permutation test (100 times) was applied to validate the PLS-DA results and to avoid overfitting (Saccenti, 2012). Afterward, the variable importance in projection (VIP) was used to define which VOCs significantly contribute to discriminate the five MG cultivars (Chong and Jun, 2005).
Results and Discussion
Characterization of the Fruit VOCs of the Five MG Cultivars
Headspace-SPME combined with GC-MS analysis and the comparison of mass spectra with the NIST17 library and the available authentic standards resulted in the identification of 44 metabolites in the five MG cultivars, whose chromatographic profiles are shown in Supplementary Figure 1. These metabolites include eight esters, ten aldehydes, twelve alcohols, six fatty acids, five terpenes, two ketones, and one furan based on their chemical nature (Table 1). We found that this chemical classification agreed with the major constituents for grape volatiles that have been reported so far (Lee et al., 2016; Mencarelli and Bellincontro, 2018; Lin et al., 2019; Wu et al., 2020; Golombek et al., 2021; Ju et al., 2021).
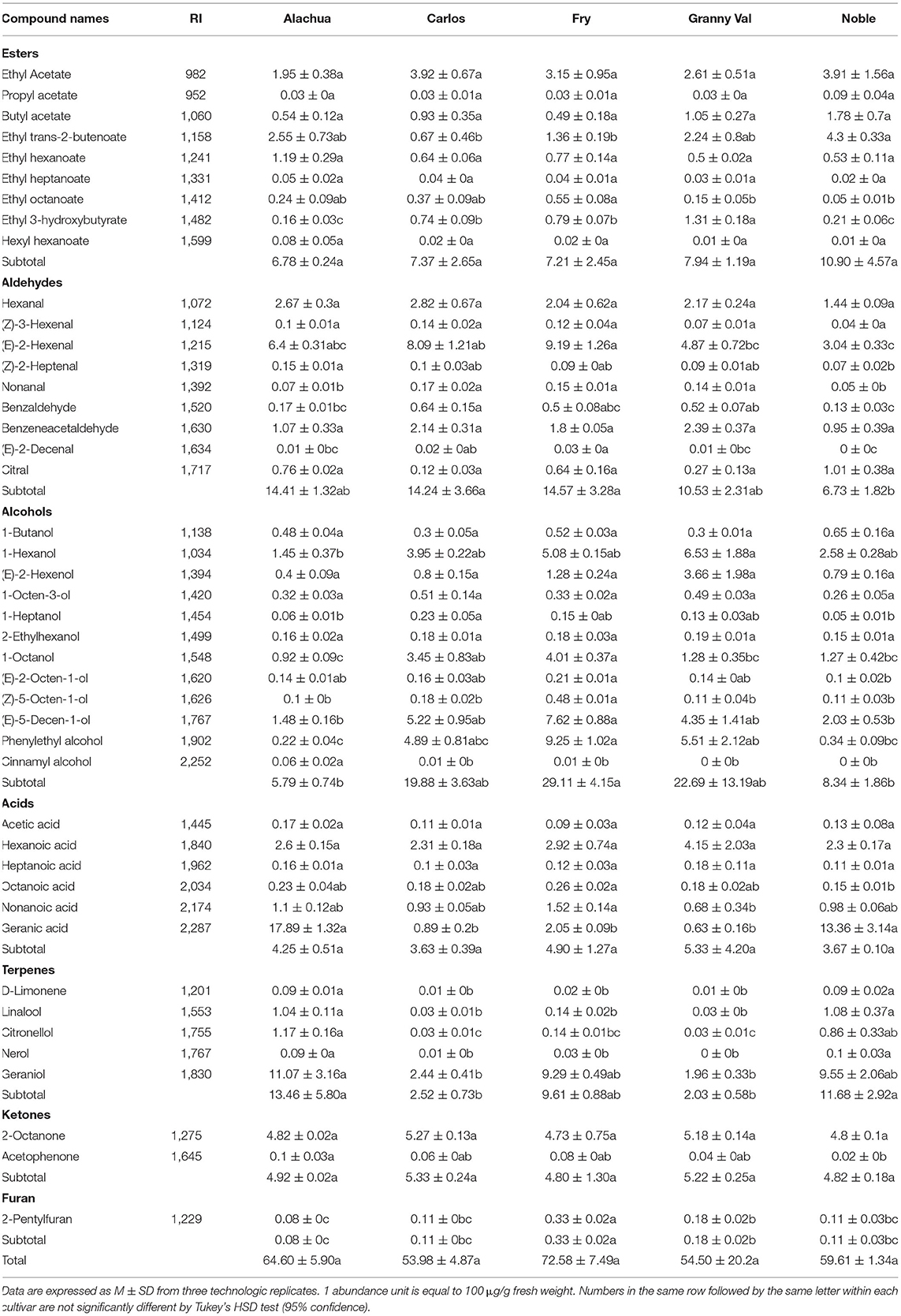
Table 1. The chemical compositions and relative concentrations of fruit volatiles of five muscadine grape cultivars.
The level of each VOC was evaluated using its relative area toward the internal standard. Geranic acid, geraniol, 2-octanone, and (E)-2-hexenal components were found to be with the greatest concentration values in the Alachua and Noble MG cultivars. The main components quantified in the Carlos, Fry, and Granny Val MG cultivars were (E)-5-decen-1-ol, phenylethyl alcohol, 1-hexanol, 2-octanone, and (E)-2-hexenal (Table 1). A log transformation was performed to allow the in-depth analysis of all the VOCs detected (Figure 2). One-way ANOVA combined with Tukey's HSD test (P < 0.05) was used to estimate the significant differences in VOC content among the five MG cultivars, resulting in 29 significantly different (P < 0.05) metabolites (Figure 2).
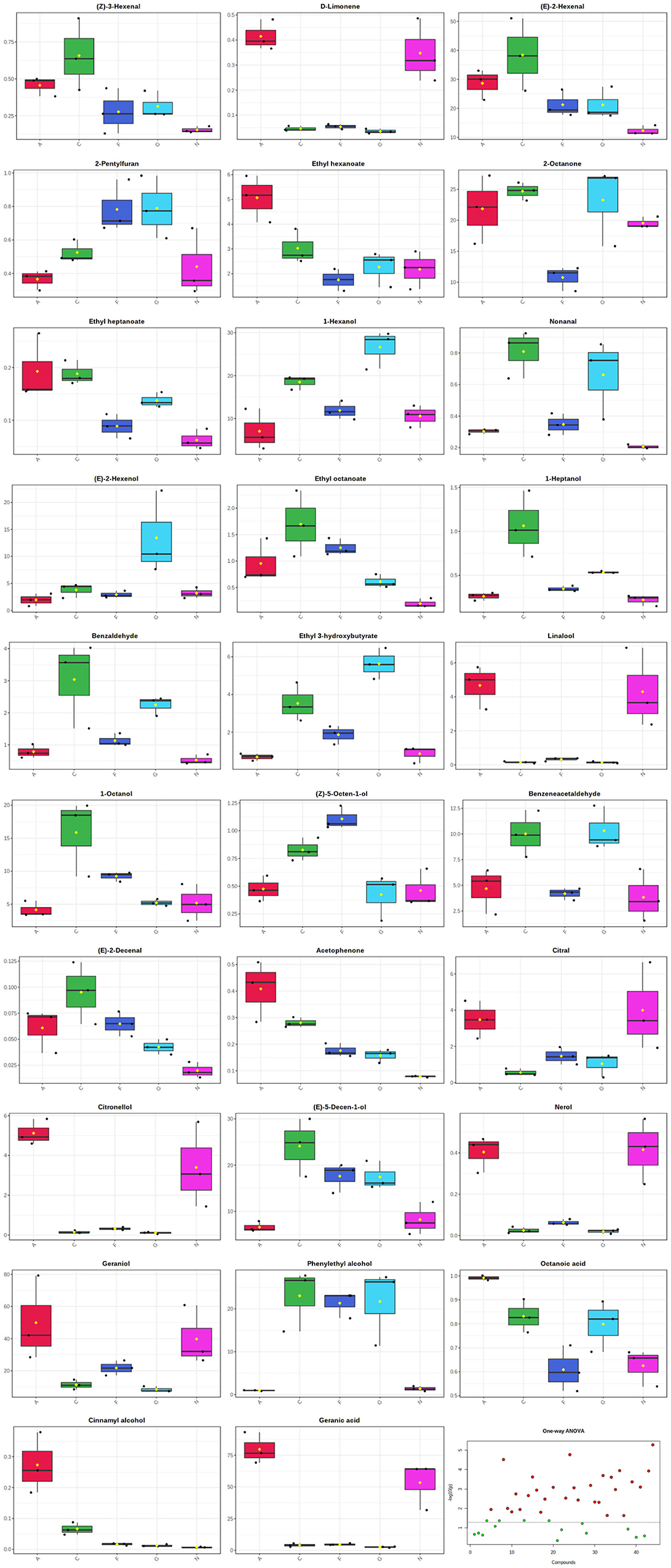
Figure 2. Box and whisker plots showing the significantly different volatiles identified in the five muscadine grape cultivars. Vertical bars represent the normalized relative concentration of volatiles, and the x-axis depicts the different muscadine grape cultivars. A, Alachua; C, Carlos; F, Fry; G, Granny Val; N, Noble.
The cultivar (Ju et al., 2021), cultural practices (Golombek et al., 2021), and postharvest biological control (Mencarelli and Bellincontro, 2018) were the most influential factors affecting the volatile compositions and the production of grapes. In the present study, all vines were grown under the same conditions using the same horticultural practices. In addition, strictly identical extraction conditions and analytical parameters were used for all samples. The influence of the environmental factors and technical parameters on the composition and production of volatiles was negligible. Therefore, by bringing together these extensive factors, we demonstrated in our study that the main source of variance is closely related to the cultivar. The predominant compounds contributing to the MG volatile profiles agree with those found previously, among which the hexenal, (E)-2-hexenal, and 1-hexanol were reported as the major constituents of six clones of spine grape berries (Ju et al., 2021), and geraniol and ethyl acetate were reported as the main constituents of Cowart MG berries (Lee et al., 2016). The (E)-2-hexenal and 1-hexanol identified here have been reported as the dominant C6 volatile compounds in V. vinifera cultivars. However, there was a wide variation in the concentration and percentage of the C6 volatiles contributing to the total volatiles (Yang et al., 2009). This finding indicated that the composition and concentration of VOCs in MG cultivars could vary with the genetic background.
Multivariate PCA and PLS-DA Analyses of the HS-SPME-GC-MS Data
Multivariate analysis of the dataset using PCA was primarily performed to visualize the overall differentiation and intrinsic variation of VOCs among the five MG cultivars (Figure 3A). PCA is an unsupervised chemometric method that reduces dimensionality and visualizes the main correlations and variability of a complex dataset (Vidal et al., 2016). In the PCA score scatter plot, the cultivars Carlos, Fry, and Granny Val were located at the right side of the score plot towards the center, while the distribution of samples from the center to the left side of the score plot comprised the cultivars Alachua and Noble. A clear differentiation among the five MG cultivars can be observed, in particular, the bronze-colored cultivars are distributed far away from the purple-black colored cultivars, as depicted in Figure 3A. All the investigated samples were located within the 95% confidence interval, which indicated that no outliers existed in the whole dataset. The accumulated variance contribution rate R2X reached 0.667, with the first and second principal components (PCs) carrying data variance of 36.7 and 18.9%, respectively. The Q2 value of the PCA model was 0.254 (Figure 3A).
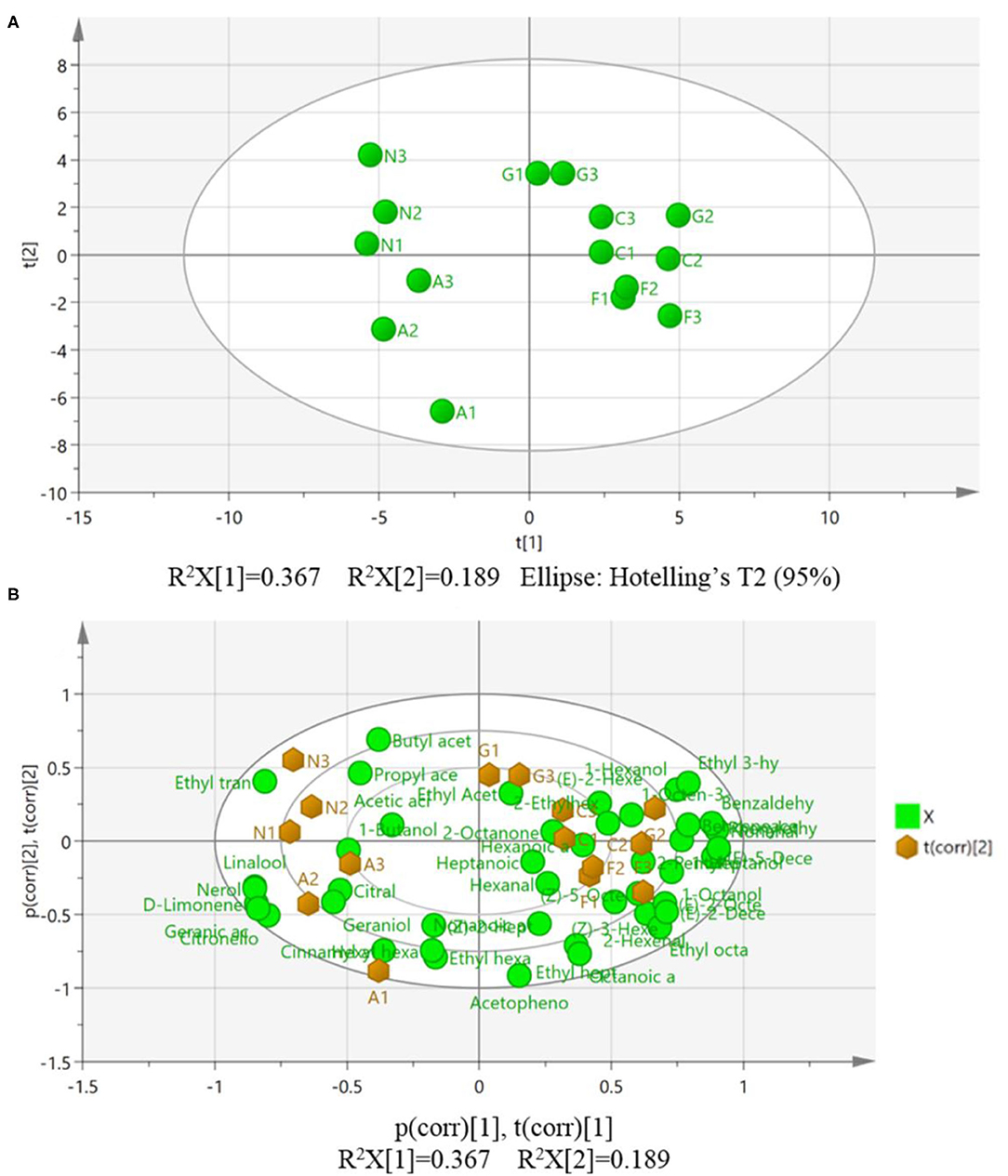
Figure 3. Principal component analysis (PCA) showing the main differences in the fruit volatiles of the five muscadine grape cultivars. (A) PCA score plot of all the investigated samples; (B) PCA biplot of the variables associated with 15 investigated samples. A, Alachua; C, Carlos; F, Fry; G, Granny Val; N, Noble; t[1], the first principal component score; t[2], the second principal component score.
Since in a PCA model, the directions in the score plot correspond to the directions in the loading plot, a comparison of these two plots can be used to identify which variables (loadings) have the greatest influence on the class separation of the different samples (scores) (Lubes and Goodarzi, 2017). Based on these criteria, the individual volatiles attributed to the variation in the five MG cultivars were graphically illustrated in a biplot (Figure 3B).
Partial least-squares discriminant analysis is a supervised multivariate statistical analysis and a variant of the PLS regression approach, which is widely used to construct a multidimensional model to predict features, discriminating between different samples, and further potential biomarkers explorations (Kalivodová et al., 2015). Therefore, PLS-DA was subsequently applied to perform an even better separation of the MG cultivars. Similarly, the score scatters plot of PLS-DA showed that the clusters of bronze-colored cultivars were located far from the purple black-color cultivars. Compared with the PCA model (Figure 3A), a noticeable improvement in the distinction of the five MG cultivars was observed (Figure 4A). All data points were within the 95% confidence interval (Figure 4A) and consistent with the results of the PCA model.
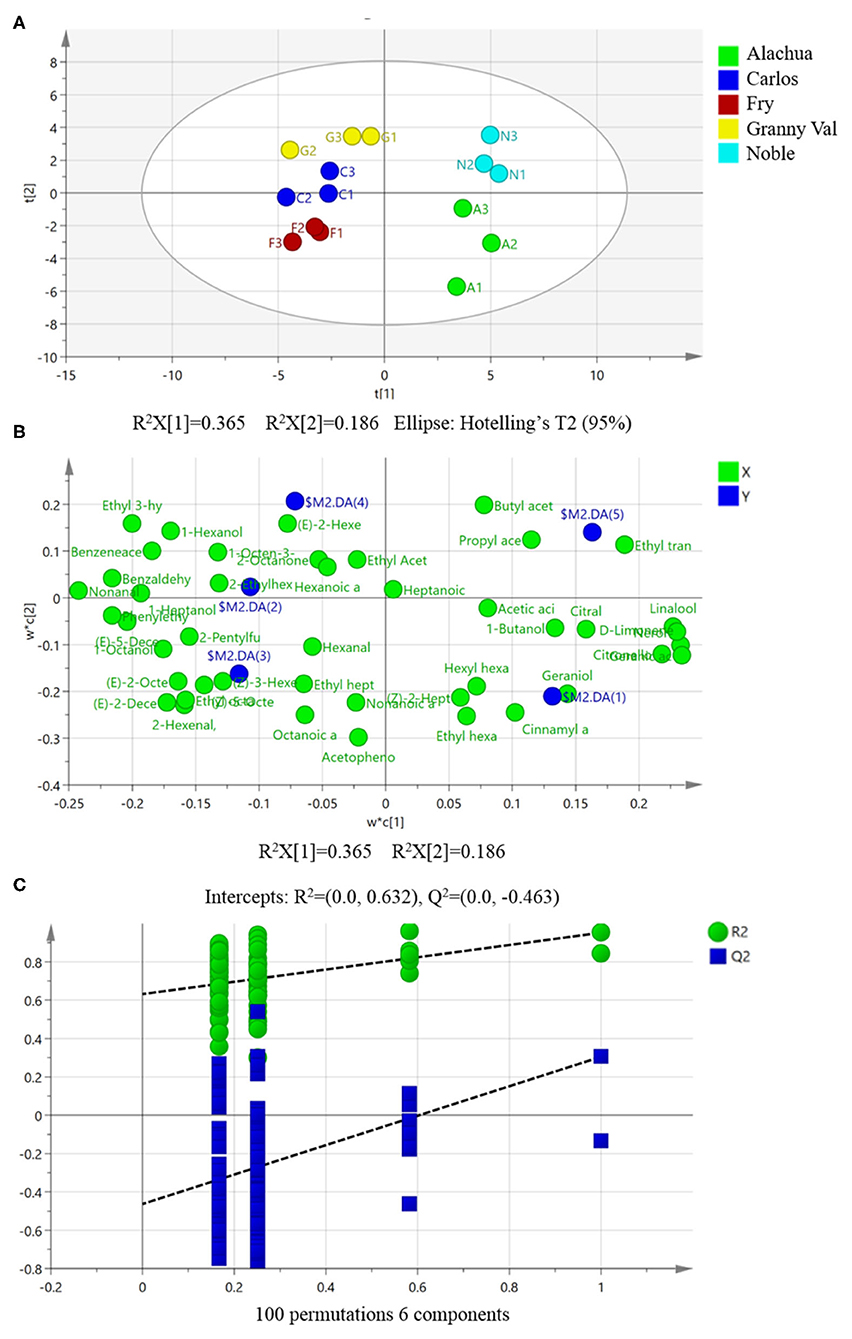
Figure 4. Partial least-squares discriminate analysis (PLS-DA) showing a better separation among the five muscadine grape cultivars based on the fruit volatiles. (A) PLS-DA score plot of all the investigated samples; (B) loadings plot of variables associated with different muscadine grape cultivars; (C) permutation test for PLS-DA model validation. A, Alachua; C, Carlos; F, Fry; G, Granny Val; N, Noble; t[1], the first principal component score; t[2], the second principal component score.
The PLS-DA model revealed that the corresponding values of R2X(cum), R2Y(cum), and Q2(cum) were 0.823, 0.927, and 0.503, respectively (Figure 4A). In the PLS-DA model, the R2X and R2Y values were utilized to describe the total explained variation in X and Y, respectively, and were represented by the PCs. At the same time, the Q2 parameter was used to assess the robustness of the model (Kalivodová et al., 2015). The R2Y and Q2 parameters of PLS-DA were significantly elevated (>0.5) (Figure 4A), indicating a valid and robust model (Kalivodová et al., 2015).
In this study, the PLS-DA model was shown to be a tool that could be used to investigate whether the five MG cultivars can be effectively discriminated (Figure 4A) and enable the visualization of the volatiles that contributed the most to the corresponding discrimination of the five MG cultivars (Figure 4B). The loadings of the variables on the two PLS-DA components are graphically illustrated in Figure 4B. More specifically, geraniol and cinnamyl alcohol were demonstrated to be essential for characterizing the Alachua MG cultivar in the loading plot of the PLS-DA. At the same time, ethyl trans-2-butenoate and propyl acetate were essential compounds to characterize the Noble MG cultivar. Furthermore, 2-Ethyl-1-hexanol, (Z)-3-hexenal, and (E)-2-hexenol were closely related to the Carlos, Fry, and Granny Val cultivars, respectively (Figure 4B).
The study further preformed 100 permutation tests to validate the PLS-DA model and are summarized in Figure 4C. The permutated models resulted in all R2 (representing the explained variance) and Q2 (representing the predictive capability) values (Y-axis data) on the left being lower than the original points on the right, indicating that the original model is statistically viable (Figure 4C).
Specific Volatile Markers of the Five MG Cultivars
The potential markers to distinguish different samples can be selected using the PLS-DA model (Lubes and Goodarzi, 2017). The VIP scores in the PLS-DA model estimate the importance of each x variable for each x variate in the prediction model and summarize the contribution that a variable makes to the model (Chong and Jun, 2005). Generally, a VIP score greater than 1 is considered a criterion for variable selection. A VIP score lower than 0.5 indicates that the variable is unimportant for the model classification and discrimination (Chong and Jun, 2005). In this study, by setting a threshold value of 1 for the VIP score in the PLS-DA model, 24 volatile metabolites were identified as crucial differential volatiles (Table 2). Combining the VIP scores in Table 2 and the ANOVA results in Figure 2, five volatile markers that discriminate the five MG cultivars, namely 2-pentylfuran, 1-heptanol, ethyl hexanoate, (Z)-3-hexenal, and phenylethyl alcohol, were obtained.
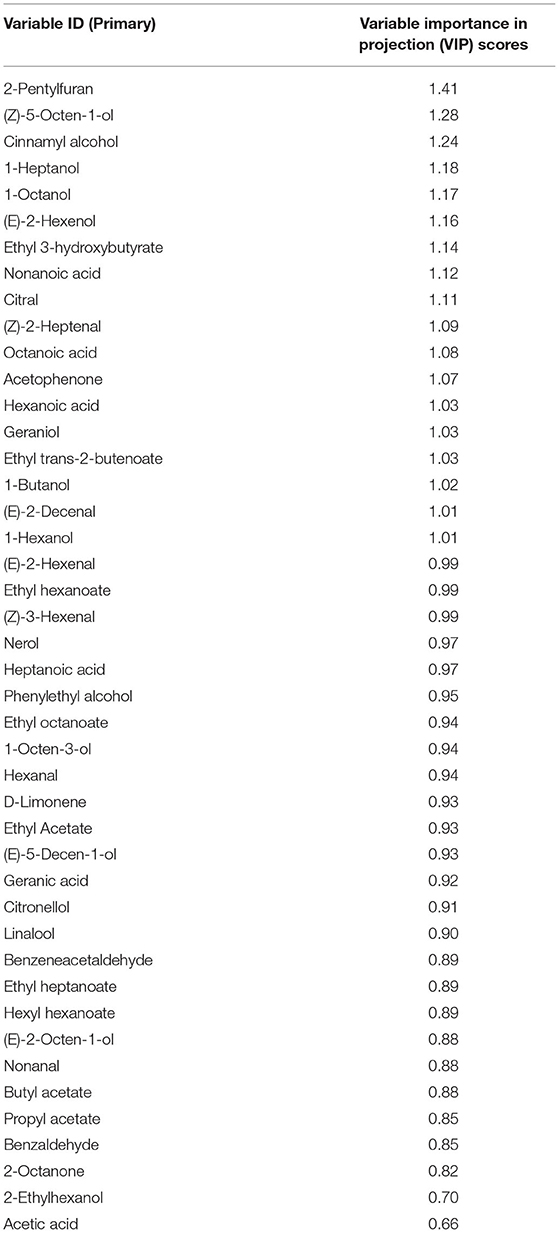
Table 2. The variable importance in projection (VIP) scores within the partial least-squares discriminate analysis (PLS-DA) model.
Subsequently, a hierarchical cluster analysis (HCA) dendrogram was constructed to visualize the content differences of the potentially characterized VOCs (Figure 5). The HCA results revealed that the key volatiles were grouped into two classes. The first class comprised nine volatiles more abundant volatiles in the Carlos, Fry, and Granny Val MG cultivars. The second contained 15 volatiles correlated with the Alachua and Noble MG cultivars. This result was in accordance with the above multivariate analysis results.
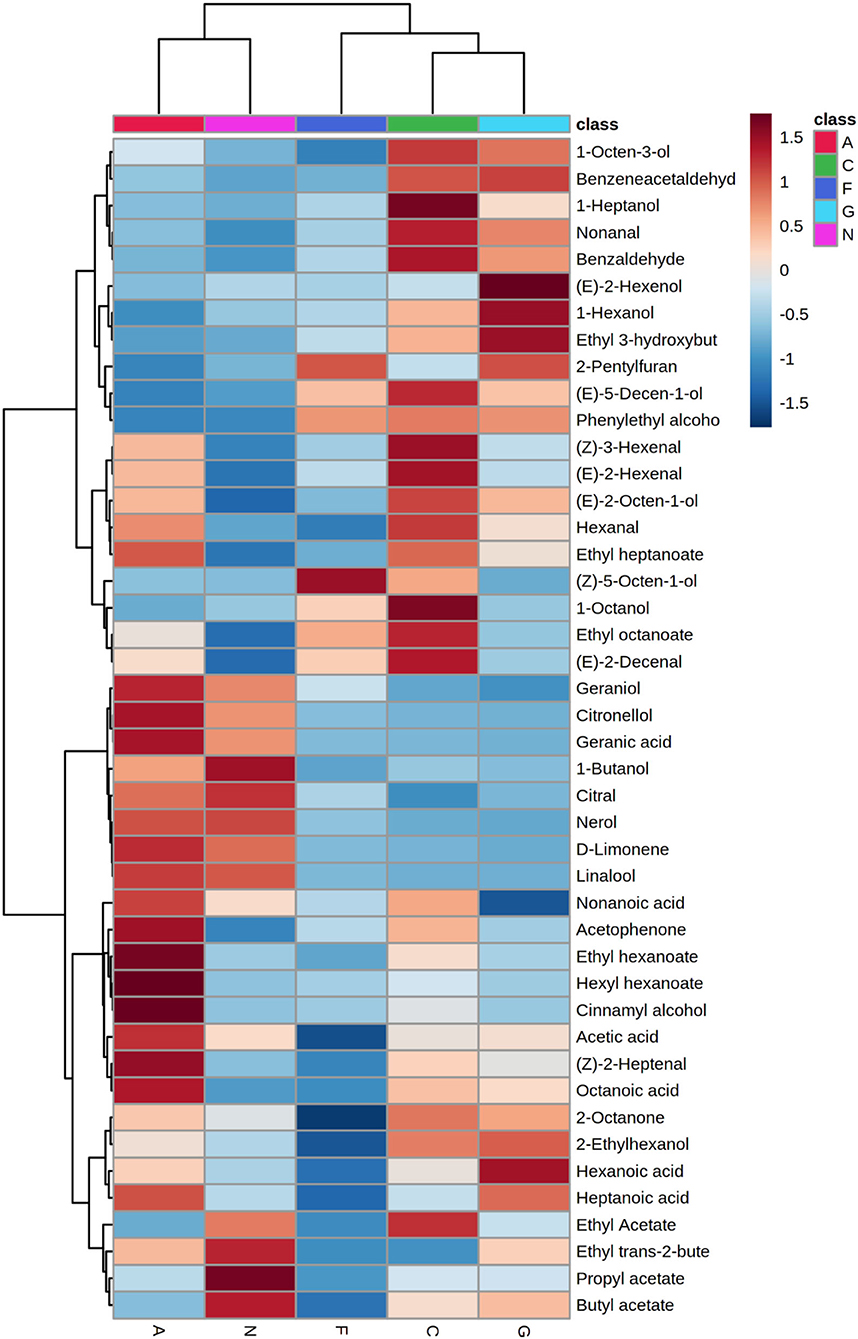
Figure 5. Hierarchical clustering heatmap of the different volatiles in the five muscadine grape cultivars. Each colored cell on the map corresponds to a relative concentration of a volatile, with the samples in the rows and compounds in the columns. A, Alachua; C, Carlos; F, Fry; G, Granny Val; N, Noble.
Fruit aroma is highly influential on the overall flavor and consumer preference (Lin et al., 2019). As an aroma-dense fruit, the MG grape is a promising cultivar for future breeding efforts to attain the desired volatile aroma of table grapes and resulting wine. The characterization of the volatile profiles of the aroma-dense MG cultivars is the first step in elucidating the possible molecular mechanisms underlying volatile synthesis and the future genetic improvement of grape aroma. In this study, the volatile profiles of five newly introduced MG cultivars were comprehensively investigated and evaluated. The results opened the avenues to attain a cultivar with the desired flavor in the future.
Conclusions
Muscadine grape is an aroma-dense fruit and has been increasingly appreciated by growers, breeders, and consumers worldwide. Recently, five MG cultivars, namely Alachua, Carlos, Fry, Granny Val, and Noble, have been successfully introduced and cultivated in southern China. In the current study, the volatile constituents of the berries of the five MG cultivars were isolated using HS-SPME and analyzed using GC-MS. The results identified 44 different compounds in the studied cultivars by comparing the mass spectra and retention index with authentic standards, NIST libraries, and literature data. These volatiles were divided into esters, aldehydes, alcohols, fatty acids, terpenes, ketones, and furan based on their chemical nature. ANOVA, combined with Tukey's HSD test, revealed that the significant differences among the five cultivars are due to the quantitative differences of the 29 volatiles. Multivariate PCA and PLS-DA analyses showed a clear differentiation among the five cultivars, particularly the bronze-colored cultivars and the purple-black colored cultivars. The volatiles that contribute the most to the corresponding discrimination of five cultivars were highlighted. The implications of these findings were also discussed. This investigation is the first in-depth exploration of the volatile profiles of the aroma-dense MG.
Data Availability Statement
The original contributions presented in the study are included in the article/Supplementary Material, further inquiries can be directed to the corresponding author/s.
Author Contributions
HD, QD, and HX conceived and designed the research and checked and revised the manuscript. HD, RH, ML, YL, and YZ performed the experiments. HD, LL, DL, XZ, ML, and XL analyzed the data. HD prepared and wrote the manuscript. All authors contributed to this article and approved the submitted version.
Funding
This study was financially supported by the Sichuan Science and Technology Programs (2020YFH0144 and 2020JDKP0039) and Sichuan Science Technology Resource Sharing Service Platform (Grape Resources).
Conflict of Interest
The authors declare that the research was conducted in the absence of any commercial or financial relationships that could be construed as a potential conflict of interest.
Publisher's Note
All claims expressed in this article are solely those of the authors and do not necessarily represent those of their affiliated organizations, or those of the publisher, the editors and the reviewers. Any product that may be evaluated in this article, or claim that may be made by its manufacturer, is not guaranteed or endorsed by the publisher.
Acknowledgments
We thank all the members of HD's laboratory for so much help and so many great moments. Professor Jiang Lu is gratefully acknowledged for providing the five Muscadine grape cultivars. HD expresses her great and deep appreciation to her supervisor, Dr. Changyong Zhou, who gave her the greatest supporting in the work and life when she was a visiting scholar in Southwest University.
Supplementary Material
The Supplementary Material for this article can be found online at: https://www.frontiersin.org/articles/10.3389/fpls.2021.728891/full#supplementary-material
References
Berger, R. G. (2009). Biotechnology of flavours-the next generation. Biotechnol. Lett. 31, 1651–1659. doi: 10.1007/s10529-009-0083-5
Chong, I. G., and Jun, C. H. (2005). Performance of some variable selection methods when multicollinearity is present. Chemom. Intell. Lab. Syst. 78, 103–112. doi: 10.1016/j.chemolab.2004.12.011
Conner, P. J. (2009). Performance of Muscadine grape cultivars in Southern Georgia. J. Am. Pomol. Soc. 63, 101–107. Available online at: https://muscadines.caes.uga.edu/content/dam/caes-subsite/muscadine-grape-breeding/docs/Grape-Cultivar-Final.pdf (accessed June 21, 2021).
El Hadi, M. A. M., Zhang, F. J., Wu, F. F., Zhou, C. H., and Tao, J. (2013). Advances in fruit aroma volatile research. Molecules 18, 8200–8229. doi: 10.3390/molecules18078200
Golombek, P., Wacker, M., Buck, N., and Durner, D. (2021). Impact of UV-C treatment and thermal pasteurization of grape must on sensory characteristics and volatiles of must and resulting wines. Food Chem. 338, 128003. doi: 10.1016/j.foodchem.2020.128003
Gürbüz, O., Rouseff, J., and Rouseff, R. L. (2014). Comparison of Muscadine ester volatiles profiles of wines produced using pectinase pre-treatment and traditional methods. In: Flavour Science Proceedings from XIII Weurman Favour Research Symposium, eds. V. Ferreira and R. Lopez (London: Elsevier Inc.) pp. 429–433. doi: 10.1016/B978-0-12-398549-1.00080-5
Hoffmann, M., Conner Professor, P., Breeding Program, M., Brannen, P., Burrack, H., Mitchem, W., et al. (2020). Muscadine grape production guide for the Southeast, 2020. https://smallfruits.org/files/2020/07/muscadine-grape-production-guide-southeast.pdf. (accessed June 22, 2021)
Ju, Y., lun Yue, X., feng Cao, X., ying Wei, X., feng Fang, Y., and lin (2021). First study on the fatty acids and their derived volatile profiles from six Chinese wild spine grape clones (Vitis davidii Foex). Sci. Hortic. (Amsterdam). 275, 109709. doi: 10.1016/j.scienta.2020.109709
Kalivodová, A., Hron, K., Filzmoser, P., Najdekr, L., Janečková, H., and Adam, T. (2015). PLS-DA for compositional data with application to metabolomics. J. Chemom. 29, 21–28. doi: 10.1002/cem.2657
Kalua, C. M., and Boss, P. K. (2009). Evolution of volatile compounds during the development of cabernet sauvignon grapes (Vitis vinifera l.). J. Agric. Food Chem. 57, 3818–3830. doi: 10.1021/jf803471n
Kambiranda, D., Basha, S. M., Singh, R. K., He, H., Calvin, K., and Mercer, R. (2016). In Depth Proteome Analysis of Ripening Muscadine Grape Berry cv. Carlos reveals proteins associated with flavor and aroma compounds. J. Proteome Res. 15, 2910–2923. doi: 10.1021/acs.jproteome.5b01064
Lee, B., Lin, P., ching Cha, H., soo Luo, J., and Chen, F. (2016). Characterization of volatile compounds in Cowart muscadine grape (Vitis rotundifolia) during ripening stages using GC-MS combined with principal component analysis. Food Sci. Biotechnol. 25, 1319–1326. doi: 10.1007/s10068-016-0207-3
Lin, J., Massonnet, M., and Cantu, D. (2019). The genetic basis of grape and wine aroma. Hortic. Res. 6, 81. doi: 10.1038/s41438-019-0163-1
Liu, X. Q., Ickert-Bond, S. M., Nie, Z. L., Zhou, Z., Chen, L. Q., and Wen, J. (2016). Phylogeny of the Ampelocissus-Vitis clade in Vitaceae supports the New World origin of the grape genus. Mol. Phylogenet. Evol. 95, 217–228. doi: 10.1016/j.ympev.2015.10.013
Lubes, G., and Goodarzi, M. (2017). Analysis of volatile compounds by advanced analytical techniques and multivariate chemometrics. Chem. Rev., 117, 6399–6422. doi: 10.1021/acs.chemrev.6b00698
Marshall, D. A., Stringer, S. J., and Spiers, J. D. (2012). Stilbene, ellagic acid, flavonol, and phenolic content of Muscadine grape (Vitis rotundifolia Michx.) cultivars. Pharmac. Crops 3, 69–77. doi: 10.2174/2210290601203010069
Mencarelli, F., and Bellincontro, A. (2018). Recent advances in postharvest technology of the wine grape to improve the wine aroma. J. Sci. Food Agric. 28, 303–325. doi: 10.1002/jsfa.8910
Olien, W. C. (1990). The Muscadine grape: botany, viticulture, history, and current industry. HortScience 25, 732–739. doi: 10.21273/HORTSCI.25.7.732
Perez-Coello, M. S., and Diaz-Maroto, M. S. (2009). Volatile and aroma compounds. In: Wine Chemistry and Biochemistry, eds. V. Moreno-Arribas and M. C. Polo (New York: Springer) pp. 249–436.
R Core Team (2020). R: A Language and Environment for Statistical Computing. R Foundation for Statistical Computing. Available online at: http://www.r-project.org/index.html (accessed June 21, 2021).
Ramos-Madrigal, J., Runge, A. K. W., Bouby, L., Lacombe, T., Samaniego Castruita, J. A., Adam-Blondon, A. F., et al. (2019). Palaeogenomic insights into the origins of French grapevine diversity. Nat. Plants 5, 595–603. doi: 10.1038/s41477-019-0437-5
Reisch, B. I., Owens, C. L., and Cousins, P. S. (2012). Grape. In: Fruit Breeding, eds. M. L. Badenes and D. H. Byrne (Boston, MA: Springer Science+Business Media, LLC) pp. 225–262. doi: 10.1007/978-1-4419-0763-9_7
Ren, J., Huang, J., Shen, Y., Liang, D., and Xia, H. (2020). Comparative analysis of fruit quality and nutritional components of four muscadine grape cultivars. Food Ferment. Ind. 46, 158–163. doi: 10.13995/j.cnki.11-1802/ts.021453
Saccenti, E. (2012). Double-check: Validation of diagnostic statistics for PLS-DA models in metabolomics studies. Metabolomics 8, S3–S16. doi: 10.1007/s11306-011-0330-3
Sánchez-Palomo, E., Díaz-Maroto, M. C., and Pérez-Coello, M. S. (2005). Rapid determination of volatile compounds in grapes by HS-SPME coupled with GC-MS. Talanta 66, 1152–1157. doi: 10.1016/j.talanta.2005.01.015
Sandhu, A. K., and Gu, L. (2010). Antioxidant capacity, phenolic content, and profiling of phenolic compounds in the seeds, skin, and pulp of Vitis rotundifolia (Muscadine Grapes) as determined by HPLC-DAD-ESI-MSn. J. Agric. Food Chem. 58, 4681–4692. doi: 10.1021/jf904211q
Vidal, R., Ma, Y., and Sastry, S. S. (2016). Principal component analysis. In: Generalized Principal Component Analysis, Interdisciplinary Applied Mathematics 40, eds. R. Vidal, Y. Ma, and S. Sastry (New York: Springer-Verlag) 25–62. doi: 10.1007/978-0-387-87811-9
Wang, X., Tong, H., Chen, F., and Gangemi, J. D. (2010). Chemical characterization and antioxidant evaluation of muscadine grape pomace extract. Food Chem. 123, 1156–1162. doi: 10.1016/j.foodchem.2010.05.080
Wei, Z., Luo, J., Huang, Y., Guo, W., Zhang, Y., Guan, H., et al. (2017). Profile of polyphenol compounds of five muscadine grapes cultivated in the United States and in newly adapted locations in China. Int. J. Mol. Sci. 18, 1–18. doi: 10.3390/ijms18030631
Wen, J., Lu, L. M., Nie, Z. L., Liu, X. Q., Zhang, N., Ickert-Bond, S., et al. (2018). A new phylogenetic tribal classification of the grape family (Vitaceae). J. Syst. Evol. 56, 262–272. doi: 10.1111/jse.12427
Wu, Y., Zhang, W., Song, S., Xu, W., Zhang, C., Ma, C., et al. (2020). Evolution of volatile compounds during the development of Muscat grape ‘Shine Muscat' (Vitis labrusca × V. vinifera). Food Chem. 309, 125778. doi: 10.1016/j.foodchem.2019.125778
Keywords: volatile organic compounds, headspace solid-phase microextraction, gas chromatography-mass spectrometry, principal component analysis, partial least-squares discriminate analysis
Citation: Deng H, He R, Long M, Li Y, Zheng Y, Lin L, Liang D, Zhang X, Liao M, Lv X, Deng Q and Xia H (2021) Comparison of the Fruit Volatile Profiles of Five Muscadine Grape Cultivars (Vitis rotundifolia Michx.) Using HS-SPME-GC/MS Combined With Multivariate Statistical Analysis. Front. Plant Sci. 12:728891. doi: 10.3389/fpls.2021.728891
Received: 22 June 2021; Accepted: 22 September 2021;
Published: 25 October 2021.
Edited by:
Jinhe Bai, Horticultural Research Laboratory (USDA-ARS), United StatesReviewed by:
Liping Du, Tianjin University of Science and Technology, ChinaBo Zhang, Zhejiang University, China
Copyright © 2021 Deng, He, Long, Li, Zheng, Lin, Liang, Zhang, Liao, Lv, Deng and Xia. This is an open-access article distributed under the terms of the Creative Commons Attribution License (CC BY). The use, distribution or reproduction in other forums is permitted, provided the original author(s) and the copyright owner(s) are credited and that the original publication in this journal is cited, in accordance with accepted academic practice. No use, distribution or reproduction is permitted which does not comply with these terms.
*Correspondence: Qunxian Deng, MTMyNDg1NjI5OUBxcS5jb20=; Hui Xia, c3VzYW54aWFfMjAwMUAxNjMuY29t