- 1Department of Biology, Centre of Molecular and Environmental Biology, University of Minho – Campus de Gualtar, Braga, Portugal
- 2Centre for the Research and Technology of Agro-Environmental and Biological Sciences, University of Trás-os-Montes and Alto Douro, Vila Real, Portugal
- 3EA 2106 Biomolécules et Biotechnologie Végétales, UFR des Sciences Pharmaceutiques, Université de Tours, Tours, France
- 4Department of Biological Engineering, Centre of Biological Engineering (CEB), University of Minho – Campus de Gualtar, Braga, Portugal
Vineyard calcium (Ca) sprays have been increasingly used by grape growers to improve fruit firmness and thus maintain quality, particularly in periods of heavy rains and hail. The observation that Ca visibly modified berry size, texture, and color in the most prominent white cultivar of the DOC region ‘Vinhos Verdes’, cultivar (cv.) Loureiro, led us to hypothesize that Ca induced metabolic rearrangements that resulted in a substantial delay in fruit maturation. Targeted metabolomics by ultra-performance liquid chromatography coupled to mass spectrometry and directed transcriptomics were thus combined to characterize the metabolic and transcriptional profiles of cv. Loureiro berries that, together with firmness, °Brix, and fruit weight measurements, allowed to obtain an integrated picture of the biochemical and structural effects of Ca in this cultivar. Results showed that exogenous Ca decreased amino acid levels in ripe berries while upregulating PAL1 expression, and stimulated the accumulation of caftaric, coutaric, and fertaric acids. An increase in the levels of specific stilbenoids, namely E-piceid and E-ω-viniferin, was observed, which correlated with the upregulation of STS expression. Trace amounts of anthocyanins were detected in berries of this white cultivar, but Ca treatment further inhibited their accumulation. The increased berry flavonol content upon Ca treatment confirmed that Ca delays the maturation process, which was further supported by an increase in fruit firmness and decrease in weight and °Brix at harvest. This newly reported effect may be specific to white cultivars, a topic that deserves further investigation.
Introduction
Calcium (Ca) supplements have been increasingly used in fresh fruits and vegetables toward improved fitness, sanitation, nutritional enrichment, and decay prevention (Martín-Diana et al., 2007). Diverse supplementation strategies have been optimized, from routinely spraying the fruits throughout their development in the tree, applying a single treatment at pre-harvest, or supplying Ca only at postharvest, through spraying, dipping, or even impregnation techniques (Saftner et al., 1997; Martín-Diana et al., 2005, 2007; Manganaris et al., 2007; Wang et al., 2014; Correia et al., 2019). The refinement of these methodologies showed that the beneficial effects of Ca can be achieved at both pre-harvest and postharvest stages, supporting its potential to protect the grape berries against abiotic and biotic stresses (Alcaraz-López et al., 2005; Romanazzi et al., 2012; Ciccarese et al., 2013; González-Fontes et al., 2017; Aldon et al., 2018). Accordingly, increased fruit resistance to infection by Botrytis cinerea during storage was achieved by Ca dips after harvest (Fu et al., 2020), an effect that was also reported upon vineyard Ca sprays between fruit set and veraison stages (Amiri et al., 2009; Ciccarese et al., 2013). In line with these observations, Ca sprays during berry development reduce the incidence of microcracks on the fruit surface and the lodging of filamentous fungi in these structures, reducing fruit decay at postharvest (Martins et al., 2020b, 2021a).
The effects of Ca on fruit texture are connected to its structural function in the cell wall and membranes, mediating cross-links between pectin molecules, and inhibiting the activity of polygalacturonases responsible for fruit softening (Hocking et al., 2016; Martins et al., 2020b), but a myriad of developmental and stress–response processes mediated by Ca may occur because it is a pivotal secondary messenger (González-Fontes et al., 2017; Aldon et al., 2018). Recent studies reported increased bulk anthocyanin content in berries cultivar (cv.) Manicure Finger sprayed with Ca around veraison stage (Yu et al., 2020). In contrast, a general repression in the synthesis of anthocyanins in the grape berry was shown through a targeted metabolomics approach, following Ca treatments throughout the entire fruiting season in vineyards cv. Vinhão (Martins et al., 2020a). This effect was also reported in grape cell cultures cv. Gamay Fréaux var. Teinturier, which became significantly less pigmented upon Ca treatment, due to a general repression of the entire flavonoid pathway (Martins et al., 2018, 2020a). These effects were underlaid by the Ca-driven regulation of core enzymes of secondary metabolism, at gene expression and protein activity levels, besides vacuolar transporters mediating anthocyanin accumulation (Martins et al., 2018). Transcriptomics studies in berries cv. Manicure Finger also showed the involvement of Ca-activated transcription factors in the regulation of anthocyanin levels (Yu et al., 2020). In cv. Vinhão berries, the inhibition of anthocyanin synthesis was accompanied by a general accumulation of stilbenoids, including E-resveratrol, E-ε-viniferin, E-piceid, and pallidol, demonstrating the powerful ability of Ca in diverting polyphenolic biosynthetic routes (Martins et al., 2020a).
The studies reported above provided a good overview of the effects of Ca over the metabolism of red wine grape cultivars (Martins et al., 2018, 2020a; Yu et al., 2020); however, how the phenolics metabolism of berries from white cultivars is affected by Ca is still puzzling. Previous studies indicated a qualitative improvement in the general color of grape berries of the white cv. Asgari (Amiri et al., 2009), but information on the metabolic mechanisms underlying these effects is lacking. The present study aimed at filling a gap in the literature, following the observation that Ca visibly modified berry size, texture, and color in the white cv. Loureiro, in two consecutive seasons. Our main hypothesis is that Ca induces specific metabolic rearrangements that result in a substantial delay in fruit maturation. Integrated metabolomics and directed transcriptomics were combined to study the effect of Ca over key genes involved in polyphenol biosynthesis and in grape berry metabolic profile at harvest time. The determination of technical and biochemical parameters such as firmness, °Brix, and fruit weight further enlightened the mechanisms of Ca-driven modulation of fruit structure and metabolism in cv. Loureiro, possibly posing as a model for other white cultivars.
Materials and Methods
Vineyard Treatments and Sample Collection
Field trials were performed in grapevines cv. Loureiro, the most prominent white cultivar of the Portuguese DOC region of ‘Vinhos Verdes’ (edaphoclimatic conditions specified in Supplementary Figure 1), cultivated in a commercial vineyard with coordinates: N41°28′28″ latitude, W8°34′59″ longitude, 165 m altitude. The plants were oriented in southwest to northeast, spaced at 2.2 m between rows, 1.0 m along the row and trained on a vertical shoot position trellis system, uniformly pruned on a unilateral Royat cordon. Grapevine aerial parts were evenly sprayed with a solution of 2% (w/v) CaCl2 and 0.1% (v/v) Silwet L-77 used as a surfactant, as previously optimized (Saftner et al., 1997; Martins et al., 2020a, b, 2021a). Treatments were performed in the early morning, and 3 L of the solution was used for every 10 plants. Three applications were performed throughout the fruiting season, every 30 days, the first performed at the pea size (E-L 31; Coombe, 1995), the second performed at veraison stage (E-L 35), and the last performed 1 week before harvest (E-L 38). Control plants were sprayed with a solution containing the surfactant agent only. Both control and Ca-treated grapevines were healthy, cultivated under the same microclimate, and subjected to the same routine phytosanitary treatments with Topaze and Ridomil Gold R WG, according to the instructions of the suppliers. Rows of control and Ca-treated vines were intercalated with vines with no treatment At the harvest time, berries were randomly collected from 10 Ca-treated and control grapevines. Six independent sets (n = 6) of approximately 30 berries each were frozen immediately in liquid nitrogen and stored at −80°C for further characterization of metabolite profile. The determination of technical/biochemical parameters and gene expression analysis were performed in three pools of two independent sets (n = 3).
Determination of Berry Weight, Ca Content, °Brix, and Firmness
Grape berry fresh weight was assessed with an analytical scale Mettler Toledo AG245 (Martins et al., 2020a). Ca content was determined in profusely washed berries, using a previously optimized adaptation of the technique described by Spare (1964). Briefly, berries were ground in liquid nitrogen and 200 mg of fresh weight were used for extraction of soluble contents in 1.5 mL milli-Q H2O. After centrifuging the extracts at 12,000 × g for 3 min, 300 μL of 0.008% (w/v) murexide reagent was added to 500 μl of the supernatant. Following incubation for 15 min at RT, the OD490nm was recorded and Ca concentration was determined through a calibration curve of CaCl2 solution at 2–150 μM (Martins et al., 2020b). The °Brix was determined in aliquots of grape juice using a digital wine refractometer Hanna HI 96813, as described previously (Martins et al., 2020c). Fruit firmness was assessed in 24 intact fruits containing the pedicel, by determining the tension necessary to perforate the fruit skin. Tests were performed on a Shimadzu (model AG-IS) equipped with a 50-N load cell and a 1-mm diameter needle. Force–stroke plots were assembled in Trapezium 2.0 Software and results were expressed in MPa, as described previously (Martins et al., 2020b).
Characterization of the Grape Berry Metabolic Profile
Grape berries were ground in liquid nitrogen and converted into a fine powder. Metabolites were extracted from freeze-dried samples, using a proportion of 1 mL of 80% (v/v) methanol per 25 mg of dry weight. Samples were sonicated for 30 min and macerated overnight at 4°C in the dark, centrifuged at 18,000g for 10 min, and the supernatants were recovered. Ultra-performance liquid chromatography coupled to mass spectrometry (UPLC–MS)-targeted metabolomic analysis was performed as optimized previously (Billet et al., 2018a,b,c; Martins et al., 2020a), using an ACQUITY UPLC system coupled to a photo diode array detector and a Xevo TQD mass spectrometer (Waters, Milford, MA, United States) equipped with an electrospray ionization source controlled by Masslynx 4.1 software (Waters, Milford, MA, United States). Analyte separation was achieved by using a Waters Acquity HSS T3 C18 column (150 × 2.1 mm, 1.8 μm) with a flow rate of 0.4 mL/min at 55°C. Chromatographic separation and identification of analytes were achieved as optimized previously, using the same standards specified by Martins et al. (2020a, 2021b). UPLC–MS analyses were achieved using the selected ion monitoring (SIM) mode of the targeted molecular ions. SIM chromatograms were integrated using the subroutine QuanLynx 4.1 for data mining. Peak integration was performed using the ApexTrack algorithm with a mass window of 0.1 Da and relative retention time window of 1 min followed by Savitzky–Golay smoothing (iteration = 1 and width = 1). To evaluate the robustness of measurements and analytical variability, a pool of all samples was prepared to obtain a quality control sample and the samples were randomly injected. Relative quantification was determined for L-proline (m1), L-leucine (m2), L-isoleucine (m3), L-phenylalanine (m4), L-tyrosine (m5), L-tryptophan (m6), cyanidin-3-O-glucoside (m7), peonidin-3-O-glucoside (m8), delphinidin-3-O-glucoside (m9), cyanidin-3-O-(6-O-acetyl)-glucoside (m10), malvidin-3-O-glucoside (m11), malvidin-3-O-(6-O-acetyl)-glucoside (m12), petunidin-3-O-(6-p-coumaroyl)-glucoside (m13), malvidin-3-O-(6-p-coumaroyl)-glucoside (m14), malvidin-3,5-O-diglucoside (m15), gallic acid (m16), citric acid (m17), E-resveratrol (m18), E-piceatannol (m19), catechin (m20), epicatechin (m21), coutaric acid (m22), caftaric acid (m23), fertaric acid (m24), E-piceid (m25), kaempferol-3-O-glucoside (m26), pallidol (m27), E-ε-viniferin (m28), E-ω-viniferin (m29), E-δ-viniferin (m30), quercetin-3-O-glucoside (m31), quercetin-3-O-glucuronide (m32), myricetin-hexoside 1 (m33), myricetin-hexoside 2 (m34), quercetin derivative (m35), procyanidin B1 (m36), procyanidin B2 (m37), procyanidin B3 (m38), procyanidin B4 (m39), kaempferol-3-O-rutinoside (40), procyanidin gallate (41), procyanidin trimer 1 (42), and procyanidin trimer 2 (43).
RNA Extraction and Quantitative Real-Time PCR Analysis
Total RNA was extracted from 0.3 g of freshly ground samples according to the method of Reid et al. (2006), as previously optimized (Martins et al., 2020a, b). RNA was purified with the GRS Total RNA kit – Plant (Grisp Research Solutions, Porto, Portugal), and the cDNA was obtained from 1 μg of mRNA by reverse transcription with an Xpert cDNA Synthesis Kit and oligo (dT) primers (Grisp Research Solutions). Quantitative real-time PCR (qRT-PCR) reactions were performed in triplicate, as previously described (Martins et al., 2020a, b). The sequences of the gene-specific primers used are detailed in Supplementary Table 1. Genes encoding core enzymes of secondary metabolism were selected, namely, PAL1 (phenylalanine ammonia lyase), STS (stilbene synthase), CHS3 (chalcone synthase), CHI1 (chalcone isomerase), F3′5′H (flavonoid 3′,5′-hydroxylase), F3H1 (flavanone 3-hydroxylase), FLS1 (flavonol synthase), DFR (dihydroflavonol 4-reductase), LAR1 (leucoanthocyanidin reductase), ANS (anthocyanidin synthase), BAN and ANR (anthocyanidin reductases), and UDP–glucose:flavonoid-3-O-glucosyltransferase (UFGT) (Jeong et al., 2004; Bogs et al., 2005; Castellarin et al., 2007; Tavares et al., 2013; Martins et al., 2018). The expression of LAC encoding a laccase involved in the oxidation of E-resveratrol was also studied, together with key genes involved in cell wall and cuticle structure, namely, PME1 (pectin methylesterase), PG1 (polygalacturonase), EXP6 (expansin), CesA3 (cellulose synthase), CER9 (E3 ubiquitin ligase), and CYP15 (cytochrome P450 monooxygenase/hydroxylase) (Martins et al., 2018, 2020b). Dissociation curves allowed confirmation of the specificity of the PCR reactions. Expression of target genes was normalized to that of the reference genes glyceraldehyde 3-phosphate dehydrogenase (GAPDH) and actin (ACT1) (Martins et al., 2020a) using the ΔΔCq method in CFX Manager Software 3.1 (Bio-Rad Laboratories, Inc., Hercules, CA, United States).
General Statistical Analysis
Results were statistically analyzed through the Student’s t-test in Prism6 (GraphPad Software, Inc.). The significance level of differences between the control and the Ca-treated samples is marked in graphs with asterisks: ∗P ≤ 0.05; ∗∗P ≤ 0.01; ∗∗∗P ≤ 0.001; ****P ≤ 0.0001. A multivariate statistical data analysis (MVA) of the samples was performed with SIMCA P+ version 15 (Umetrics AB, Umeå, Sweden), after mean-centering all variables and scaling unit-variance. Metabolic variables affected by Ca treatment were revealed through the principal component analysis (PCA) applied as the unsupervised MVA method.
Results
Results showed that Ca treatment throughout the fruiting season visibly modified the size (decreased), texture, and color of the berries at harvest time, and these effects were consistent in two consecutive seasons (Figure 1A). Accordingly, the fresh weight of berries from Ca-treated plants was 35% lower than that of the control fruits. In parallel, Ca sprays increased fruit Ca content by 30% and decreased the °Brix from 18.4 to 16.7 °B, in line with the immature appearance of the fruits (Figure 1B). In addition, the force necessary to perforate the skin of berries from Ca-treated plants, expressed as tension, was significantly higher than that of the control fruits (Figure 2A). This change was associated to a 56-fold increase in the expression of cell wall PME1 (Figure 2B). Likewise, transcript levels of EXP6 also increased by 5.6-fold. In contrast, the expression of PG1 decreased by 66% upon Ca treatment. The same effect was observed for CYP15 involved in the cuticle structure, that was downregulated by 82%. Other genes involved in the cell wall and cuticle structures, namely CesA3 and CER9 were not significantly affected by the Ca treatment.
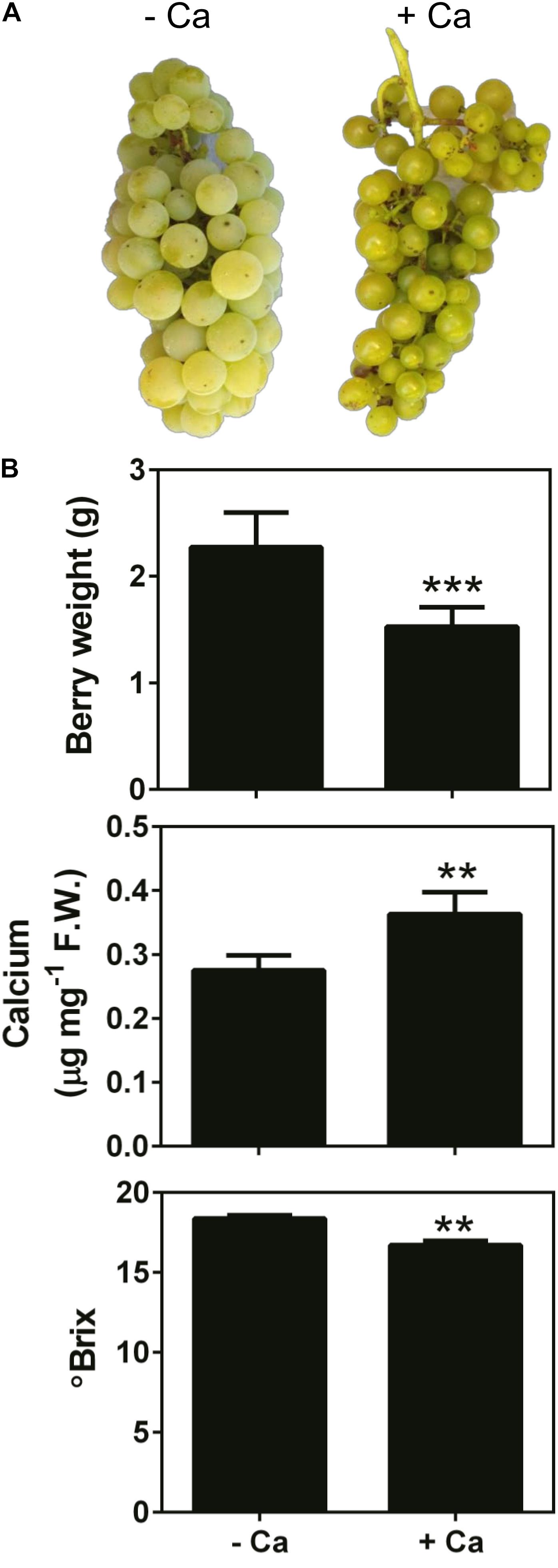
Figure 1. Appearance (A), weight, Ca content, and °Brix (B) of mature berries from vines cv. Loureiro treated with Ca (+ Ca) or without treatment (– Ca). Images in panel (A) are representative of two consecutive seasons. Results are expressed as mean ± SD and asterisks denote statistical significance as compared to control (– Ca): **P ≤ 0.01; ***P ≤ 0.001; n = 3.
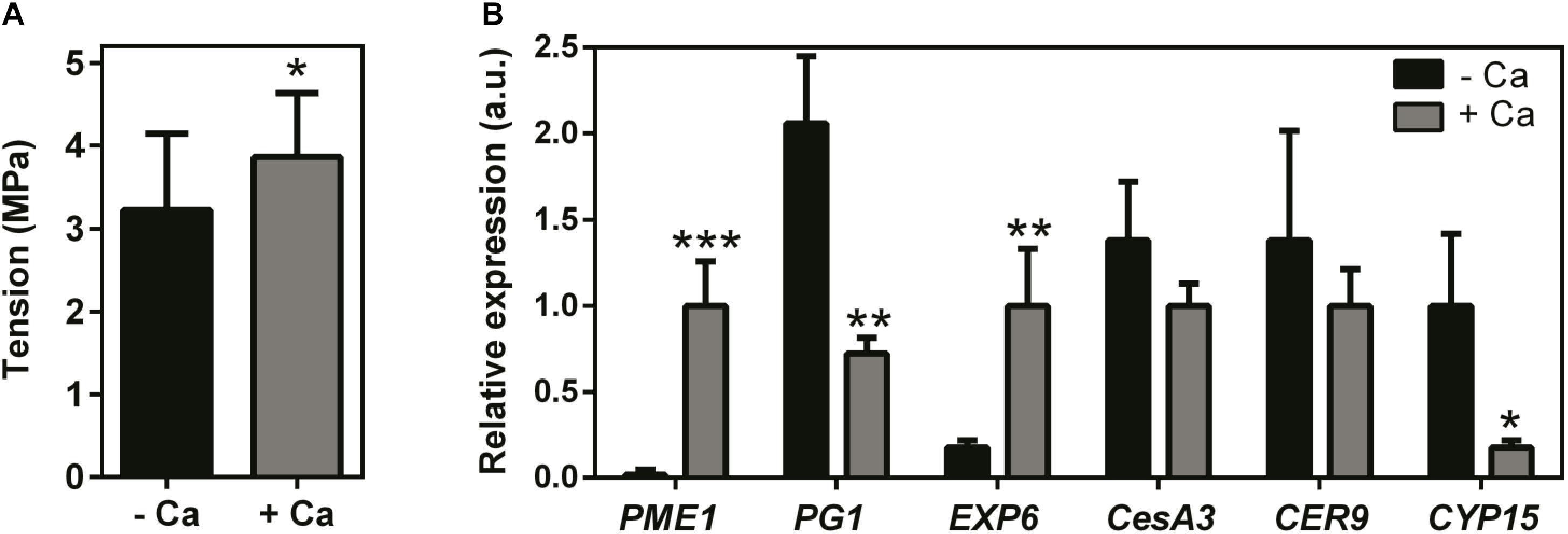
Figure 2. Firmness (A) and transcript levels of genes involved in cell wall and cuticle structures (B), in berries from vines cv. Loureiro treated with Ca (+ Ca) or without treatment (– Ca). Firmness is expressed as the tension necessary to perforate the fruit skin; n = 24. Transcript levels are shown for pectin methylesterase (PME1), polygalacturonase (PG1), expansin (EXP6), cellulose synthase (CesA3), E3 ubiquitin ligase (CER9), and cytochrome P450 monooxygenase/hydroxylase (CYP15). Results are expressed as mean ± SD and asterisks denote statistical significance as compared to control (– Ca): *P ≤ 0.05; **P ≤ 0.01; ***P ≤ 0.001; n = 3.
Targeted metabolomics analysis by UPLC–MS allowed the detection of 44 metabolites, including 5 phenolic and organic acids, 6 amino acids, 9 flavan-3-ols, 7 flavonols, 9 anthocyanins, and 7 stilbenoids (Supplementary Table 2). Unsupervised PCA score plot of the first two components explained 52.9% of the variance and readily discriminated the metabolic profiles of berries from control and Ca-treated vines (Figure 3A). In general, amino acids and anthocyanins di-OH mostly accumulated in control berries, while most phenolic acids were more abundant in berries from Ca-treated vines (Figure 3B). A detailed analysis of berry metabolic profiles showed that all amino acids detected, including L-phenylalanine, were significantly reduced in berries from Ca-treated vines, decreasing by up to 40% in comparison to the control fruits (Figure 4). In contrast, phenolic acids increased by up to 1.9-fold, among which coutaric, caftaric, and fertaric acids. The levels of citric and gallic acids were not affected by Ca treatment. Regarding stilbenoids, a significant increase of 1.8-fold was observed in E-piceid levels upon Ca treatment, together with a 6.5-fold increase in E-ω-viniferin content. For the remaining stilbenoids, which included E-resveratrol, E-ε-viniferins, and E-δ-viniferins, a tendential but not significant decrease was observed. Regarding flavan-3-ols, only the content in epicatechin was significantly affected by Ca treatment, for which a reduction of 20% was observed. Thus, the apparent increase in procyanidin levels observed in the loading plot was not statistically significant (Figure 3B). The accumulation of specific flavonols, namely kaempferol-3-O-rutinoside, quercetin-3-O-glucuronide, and myricetin-hexoside 2, was favored by the Ca treatment and their content increased by up to 2.8-fold in comparison to the control fruits. The corresponding glucosides were not significantly affected by the Ca treatment, nor myricetin-hexoside 1 (Figure 4). Anthocyanins detected in berries of cv. Loureiro included cyanidin, peonidin, petunidin, delphinidin, and malvidin conjugates, the latter being the most diverse. The Ca treatment specifically reduced cyanidin-3-O-glucoside levels by 40% and malvidin-3-O-(6-p-coumaroyl)-glucoside content by 60%. The effect of Ca on the remaining anthocyanins was not statistically significant.
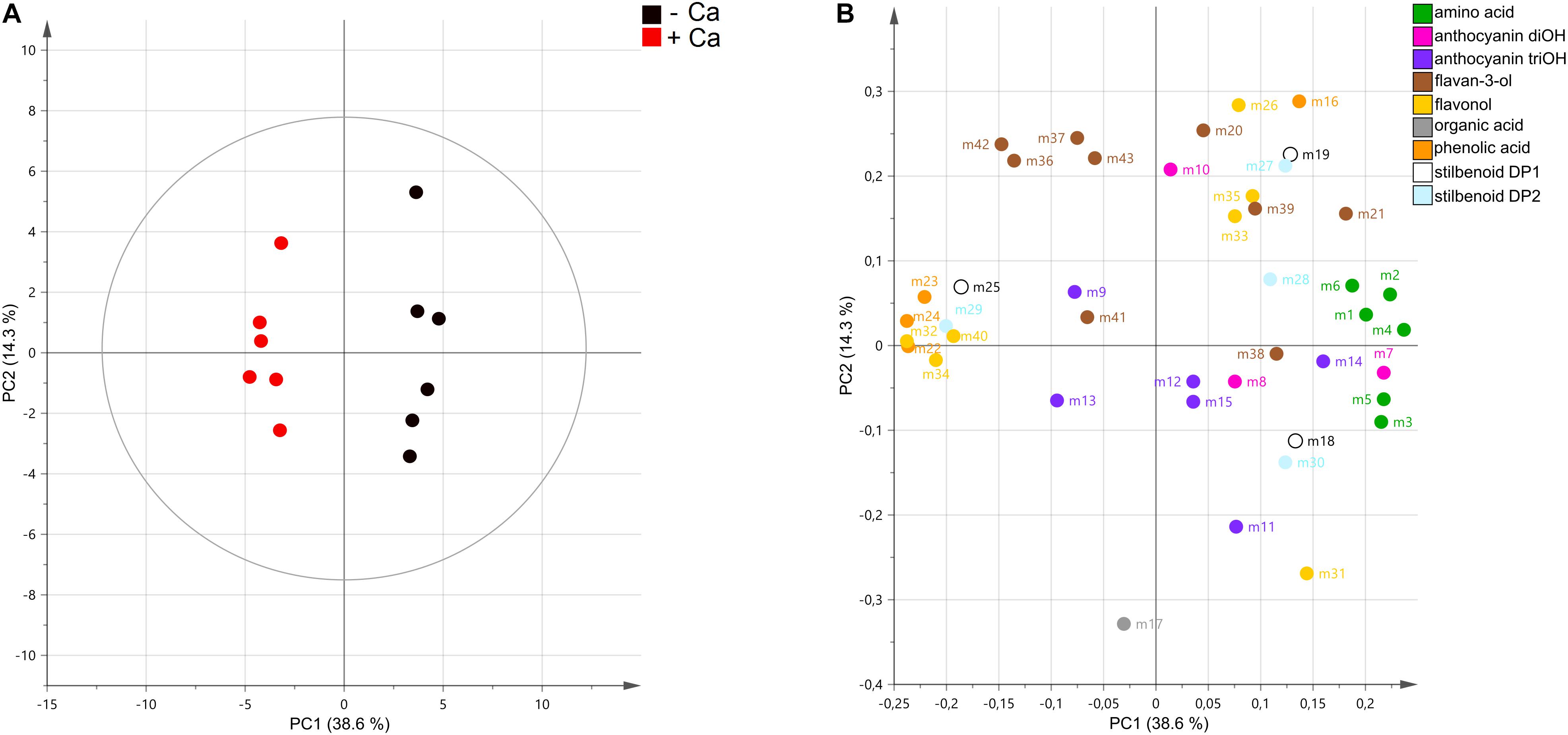
Figure 3. Unsupervised classification using the principal component analysis on metabolomic data from grape berries from vines cv. Loureiro treated with Ca (+ Ca) or without treatment (– Ca) (n = 6). Variables in the score plot (A) were colored according to the treatment, and variables in loading plot (B) were colored according to the metabolic class. Numbers indicate the ID of metabolites, as follows: L-proline (m1), L-leucine (m2), L-isoleucine (m3), L-phenylalanine (m4), L-tyrosine (m5), L-tryptophan (m6), cyanidin-3-O-glucoside (m7), peonidin-3-O-glucoside (m8), delphinidin-3-O-glucoside (m9), cyanidin-3-O-(6-O-acetyl)-glucoside (m10), malvidin-3-O-glucoside (m11), malvidin-3-O-(6-O-acetyl)-glucoside (m12), petunidin-3-O-(6-p-coumaroyl)-glucoside (m13), malvidin-3-O-(6-p-coumaroyl)-glucoside (m14), malvidin-3,5-O-diglucoside (m15), gallic acid (m16), citric acid (m17), E-resveratrol (m18), E-piceatannol (m19), catechin (m20), epicatechin (m21), coutaric acid (m22), caftaric acid (m23), fertaric acid (m24), E-piceid (m25), kaempferol-3-O-glucoside (m26), pallidol (m27), E-ε-viniferin (m28), E-ω-viniferin (m29), E-δ-viniferin (m30), quercetin-3-O-glucoside (m31), quercetin-3-O-glucuronide (m32), myricetin-hexoside 1 (m33), myricetin-hexoside 2 (m34), quercetin derivative (m35), procyanidin B1 (m36), procyanidin B2 (m37), procyanidin B3 (m38), procyanidin B4 (m39), kaempferol-3-O-rutinoside (40), procyanidin gallate (41), procyanidin trimer 1 (42), and procyanidin trimer 2 (43).
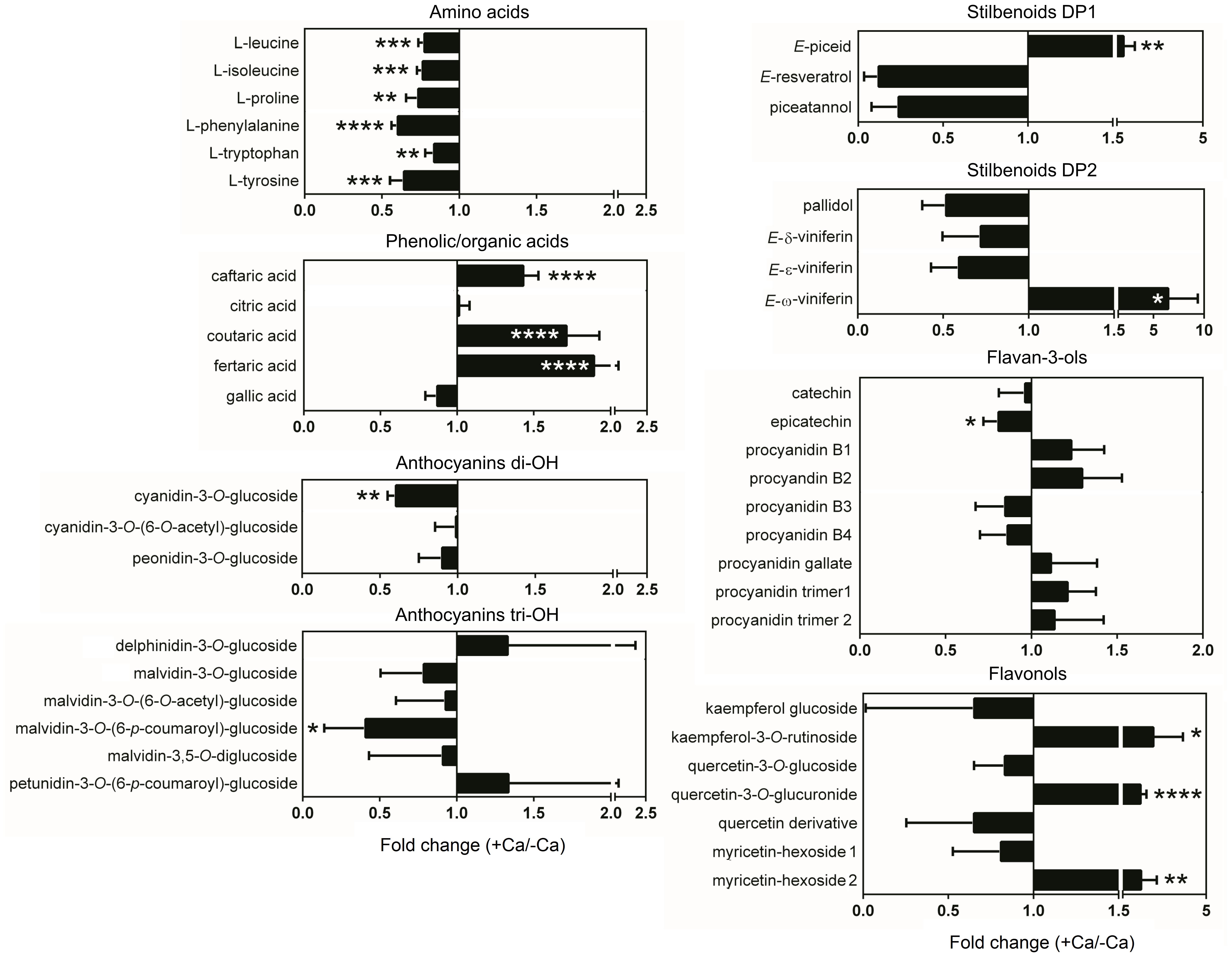
Figure 4. Effect of Ca on the metabolite profile of grape berries cv. Loureiro. Results are expressed as fold changes of the mean ± SD values obtained for Ca treatment (+ Ca) relative to the control (– Ca), and asterisks indicate statistical significance of + Ca vs. – Ca for each metabolite: *P ≤ 0.05; **P ≤ 0.01; ***P ≤ 0.001; ****P ≤ 0.0001; n = 6.
The molecular nature of the metabolic shifts triggered by Ca in berries of cv. Loureiro vines was investigated through the analysis of transcript levels of genes encoding key enzymes of secondary metabolism. The expression of PAL1, encoding PAL, was upregulated by 63% upon Ca treatment (Figure 5). Likewise, STS encoding stilbene synthases was upregulated by 62%. Coincidentally, the expression of CHS3 encoding chalcone synthase was 62% lower in berries from Ca-treated vines than in the control berries. The same effect was observed for F3H1 encoding flavanone 3-hydroxylase, whose transcript levels decreased by 50% upon Ca treatment. Further in the flavonoid pathway, DFR encoding dihydroflavonol reductase was upregulated by 30% upon Ca treatment, whereas ANS encoding anthocyanidin synthase was downregulated by 49%. Seven other genes of the flavonoid pathway including UFGT were not significantly affected by the Ca treatment, nor did LAC (laccase) was involved in the E-resveratrol oxidation. An overview of the effects of Ca in cv. Loureiro berries is shown in Figure 6.
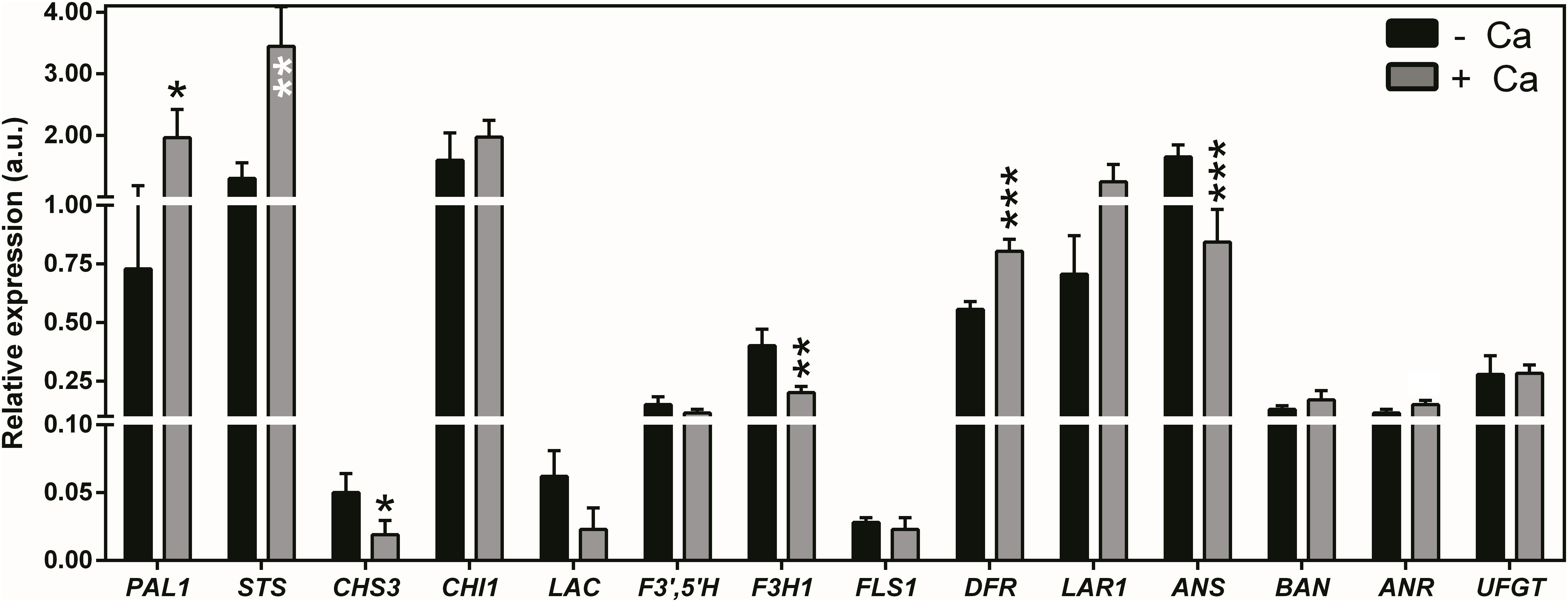
Figure 5. Transcript levels of genes encoding enzymes of secondary metabolism core branches in berries from vines cv. Loureiro treated with Ca (+ Ca) or without treatment (– Ca). Transcript levels are shown for phenylalanine ammonia lyase (PAL1), stilbene synthase (STS), polyphenol oxidase/laccase (LAC), chalcone synthase (CHS3), chalcone isomerase (CHI1), flavonoid 3′,5′-hydroxylase (F3′,5′H), flavanone 3-hydroxylase (F3H1), flavonol synthase (FLS1), dihydroflavonol 4-reductase (DFR), leucoanthocyanidin reductase (LAR1), anthocyanidin synthase (ANS), anthocyanidin reductases (BAN and ANR), and anthocyanidin 3-O-glucosyltransferase (UDP–glucose:flavonoid-3-O-glucosyltransferase, UFGT). Expression levels were normalized to the transcript levels of GAPDH and (ACT1) (housekeeping genes). Results are expressed as mean ± SD and asterisks denote statistical significance as compared to control (– Ca): *P ≤ 0.05; **P ≤ 0.01; and ***P ≤ 0.001; n = 3.
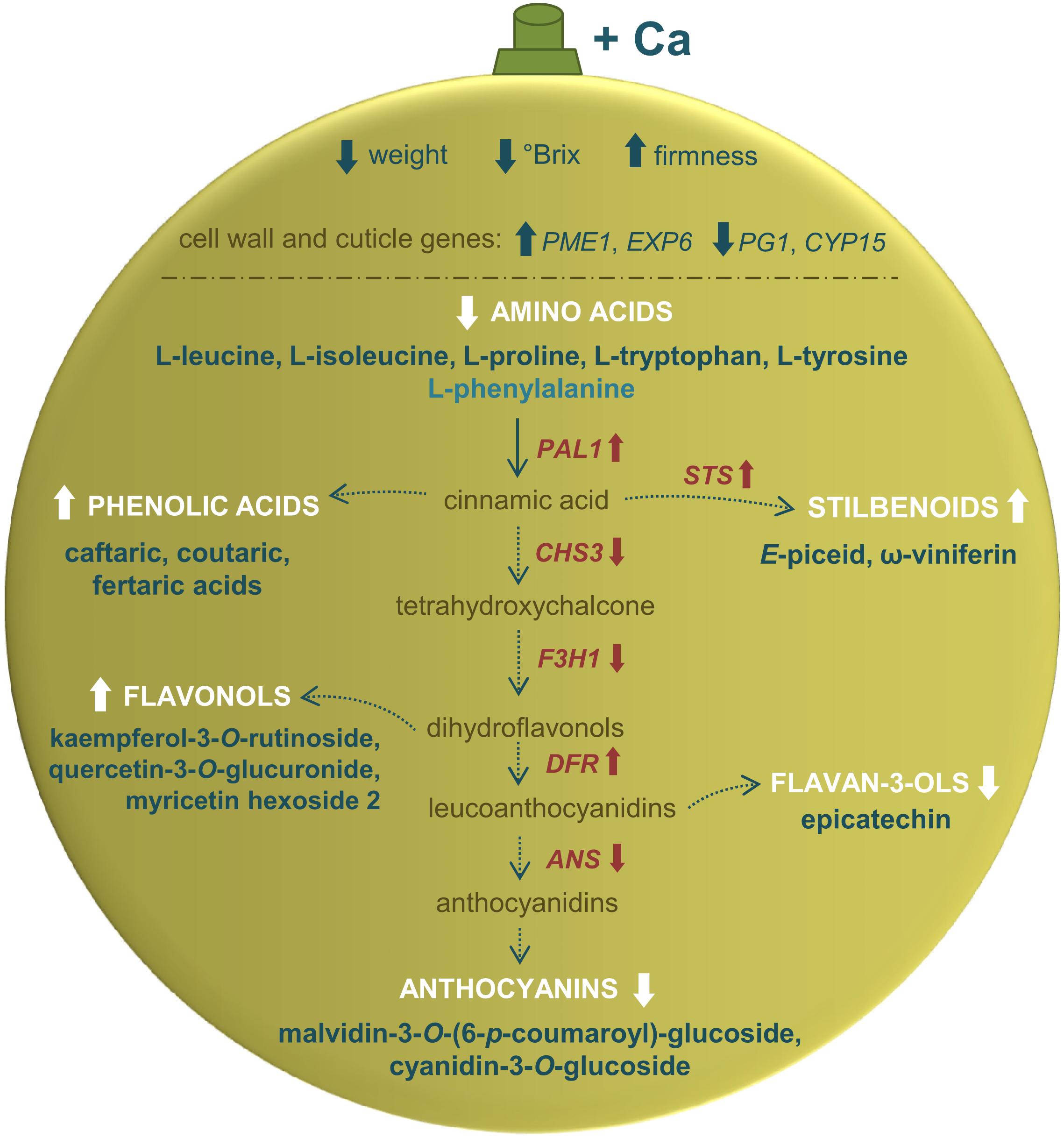
Figure 6. Physiological, metabolic, and transcriptional changes driven by exogenous Ca in grape berries from vines cv. Loureiro. Arrows pointing upward indicate increase in comparison to control berries, while arrows pointing downward indicate decrease. The pathway was based on the information from Kyoto Encyclopedia of Genes and Genomes – KEGG database.
Discussion
Results in the present study demonstrated the beneficial effect of Ca sprays over the firmness of berries cv. Loureiro, complementing the few studies conducted in other white cultivars, namely Thompson Seedless, Asgari, and Italia (Amiri et al., 2009; Bonomelli and Ruiz, 2010; Ciccarese et al., 2013), and are in agreement with some previous reports on red cultivars such as Vinhão and Crimson (Alcaraz-López et al., 2005; Martins et al., 2020b). Electron microscopy studies on cv. Vinhão berries showed that increased firmness was accompanied by a reduction in the incidence of microcracks on the fruit surface which became smoother than that of the control fruits (Martins et al., 2020b). The tight regulation of genes involved in the cell wall and cuticle structures by Ca in berries cv. Loureiro likely explained the improved fruit firmness. The observed inhibition of PG1 and CYP15 expression was in accordance to previous results in cv. Vinhão berries (Martins et al., 2020b), thus it seems that both white and red varieties share the same targets related to the prevention of fruit softening in response to Ca. PGs degrade pectin molecules in the cell wall and a particularly close correlation between PG1 levels and grape berry softening has been reported (Deytieux-Belleau et al., 2008). In turn, cuticular CYPs such as CYP15 are involved in the synthesis of wax triterpenoids, which also determine the fruit quality (Fukushima et al., 2011; Lara et al., 2014). Contrary to previous studies in cv. Vinhão berries (Martins et al., 2020b), PME1 and EXP6 were upregulated upon Ca treatment in cv. Loureiro. PME and EXP are involved in various physiological processes underlying both reproductive and vegetative plant development, including seed germination, root tip elongation, and soft fruit ripening (Sampedro and Cosgrove, 2005; Pelloux et al., 2007). PME effects on the latter process arise from its contribution in the degree of demethylated polygalacturonans that are prone to degradation by PGs and the availability of homogalacturonan carboxylic groups for Ca2+ binding (Deytieux-Belleau et al., 2008). Accordingly, the induction of PME mRNAs has been associated to the decrease in the degree of methyl-esterification of insoluble pectins during grape berry development (Barnavon et al., 2001).
Results in the present study showed a reduction in the weight and °Brix of mature berries from vines sprayed with Ca, suggesting a delay in fruit maturation, which could be anticipated from the immature appearance of the fruits. This result was not observed in vines of the red cultivar Vinhão subjected to the same Ca application protocol (Martins et al., 2020b). However, decreased °Brix following Ca treatment was reported previously for another white grape cv. Asgari (Amiri et al., 2009). This effect was accompanied by a change in fruit skin color, berries remaining greener, and not attaining the characteristic golden color of ripe fruits (Amiri et al., 2009), much like the observations in the present study with the cv. Loureiro.
In this study, trace amounts of anthocyanins were detected by UPLC–MS in cv. Loureiro berries, as reported in other white cultivars such as Chardonnay, Sauvignon Blanc, Riesling, Pinot Blanc, and Muscat Blanc, also by chromatographic methods (Arapitsas et al., 2015; Niu et al., 2017). In contrast, earlier studies (Boss et al., 1996) reported the absence of these pigments in white cultivars; however, the quantification methods were much less sensitive. Results in the present study and in previous reports suggested that anthocyanin diversity is similar in both white and red grape varieties (Arapitsas et al., 2015; Niu et al., 2017; Martins et al., 2020a). The inhibitory effect of Ca over the anthocyanins malvidin-3-O-(6-p-coumaroyl)-glucoside and cyanidin-3-O-glucoside observed in the present study for fruits cv. Loureiro is in line with previous studies in cv. Vinhão, and is consistent with the downregulation of ANS and UFGT (Martins et al., 2020a).
The consistent decrease in fruit amino acid levels upon Ca treatment observed in the present study might bring about changes during wine fermentation, as many of these metabolites constitute the yeast assimilable nitrogen fraction of the must (Vilanova et al., 2007). In particular, the decrease in L-phenylalanine levels was tightly linked to the upregulation of PAL1 encoding the enzyme responsible for its conversion to cinnamic acid, the first catalytic step of plant secondary metabolism (Teixeira et al., 2013). This effect correlated with the increase in phenolic acids, produced in downstream routes initially fed by this substrate. Caftaric acid is known to account for the color of white wines, as it can be hydrolyzed to caffeic acid during the wine-making process, the oxidation of the latter contributing to wine browning (Cilliers and Singleton, 1990). Caftaric acid and other hydroxycinnamates including coutaric acid, also detected in the present study, were shown to be effective markers of wine differentiation, together with resveratrol, piceid, and epicatechin (Andrés-Lacueva et al., 2002; Lampíř, 2013). In the present study, Ca treatment induced STS expression and consequently, stilbenoid synthesis, in analogy to previous reports in berries of cv. Vinhão vines located in the same vineyard (Martins et al., 2020a). Although in cv. Vinhão a general increase in most stilbenoids including E-resveratrol and E-ε-viniferin was reported, in cv. Loureiro a targeted accumulation of E-piceid and E-ω-viniferin was observed, suggesting a specific action of Ca effect depending on the cultivar. The interaction of Ca with other metabolites differentially present in each cultivar may underlie these effects; accordingly, previous studies showed that the combination of Ca and plant hormones such as jasmonic or abscisic acid greatly determines the redirecting of secondary metabolism toward the synthesis of specific compounds such as different types of viniferins (Martins et al., 2018, 2021b). The targeted action of Ca over specific polyphenols was evident in other metabolic classes, including anthocyanins (discussed above), flavonols, and flavan-3-ols. The large increase of the flavonols kaempferol-3-O-rutinoside, quercetin-3-O-glucuronide, and myricetin-hexoside 2 observed in cv. Loureiro was not reported previously in cv. Vinhão (Martins et al., 2020a). Flavonols are exclusively found in the grape berry skin and seeds, peaking at veraison stage of fruit development (Teixeira et al., 2013). Thus, the increase in their levels upon Ca treatment supports the delay in fruit maturation in cv. Loureiro, as discussed previously. In parallel, only epicatechin was affected in this cultivar, suggesting a minor influence of Ca over flavan-3-ols contrary to that observed in cv. Vinhão and cv. Gamay Fréaux var. Teinturier cell cultures where a general repression of the flavonoid pathway was reported (Martins et al., 2018, 2020a).
Conclusion
Results in the present study confirmed the postulated hypothesis, showing that vineyard Ca sprays induce precise metabolic rearrangements in cv. Loureiro berries that result in a substantial delay in fruit maturation. A specific integrated effect of Ca over biochemical and structural properties of cv. Loureiro berries is thus suggested: by inhibiting the action of polygalacturonases responsible for degradation of cell wall pectin and fruit softening, Ca prevents fruit growth and other processes associated with fruit maturation, leading to increased flavonol content and firmness, at the expense of fruit size and °Brix. This effect may be specific for white cultivars, a topic that deserves further investigation. The results may pave the way for the optimization of protocols of Ca treatments in the field aimed to prevent early fruit ripening in specific cultivars from wine regions most affected by climate change, possibly consisting of a good alternative to crop forcing. Additional benefits on the resistance to biotic and abiotic stresses and on shelf-life could also be expected, in accordance to previous studies (Romanazzi et al., 2012; Martins et al., 2021a).
Data Availability Statement
The original contributions presented in the study are included in the article/Supplementary Material, further inquiries can be directed to the corresponding author.
Author Contributions
VM and HG conceptualized the work. VM performed field trials, sample processing, firmness measurements, quantification of biochemical parameters, and gene expression analyses. AL and MU performed metabolomic analysis and data treatment. AT performed statistical analysis. HG and AL contributed with resources and funding acquisition. VM, HG, and AL wrote the manuscript. All authors edited and reviewed the manuscript, contributed to the article, and approved the submitted version.
Funding
This work was supported by the “Contrato-Programa” UIDB/04050/2020 funded by Portuguese national funds through the FCT IP. This work was also supported by FCT, CCDR-N (Norte Portugal Regional Coordination and Development Commission), European Funds (FEDER/POCI/COMPETE2020) through the project AgriFood XXI (NORTE-01-0145-FEDER-000041) and the research projects BerryPlastid (PTDC/BIA-FBT/28165/2017 and POCI-01-0145-FEDER-028165), and MitiVineDrought (PTDC/BIA-FBT/30341/2017 and POCI-01-0145-FEDER-030341). AT was supported by a postdoctoral researcher contract/position within the project “BerryPlastid”. The Région-Centre Val de Loire (France) supported this work under the grant agreement to Projects CEPATLAS and VINODRONE to AL. This work also benefited from the networking activities within the European COST Action CA 17111 INTEGRAPE, the CoLAB VINES & WINES, and the CoLAB 4FOOD – Collaborative Laboratory for Innovation in the Food Industry.
Conflict of Interest
The authors declare that the research was conducted in the absence of any commercial or financial relationships that could be construed as a potential conflict of interest.
Publisher’s Note
All claims expressed in this article are solely those of the authors and do not necessarily represent those of their affiliated organizations, or those of the publisher, the editors and the reviewers. Any product that may be evaluated in this article, or claim that may be made by its manufacturer, is not guaranteed or endorsed by the publisher.
Acknowledgments
The authors thank Ana Garcia (CIIMAR, Portugal) for providing access to the experimental fields used in this study. The authors also acknowledge Senentxu Lanceros-Méndez and Pedro Costa (Department of Physics, University of Minho, Portugal) for providing access to the Shimadzu Autograph used in fruit firmness measurements.
Supplementary Material
The Supplementary Material for this article can be found online at: https://www.frontiersin.org/articles/10.3389/fpls.2021.742887/full#supplementary-material
Supplementary Figure 1 | Edaphoclimatic conditions of the vineyard located in the DOC region ‘Vinhos Verdes’ where field trials were conducted.
Supplementary Table 1 | Accession numbers and specific primers forward (F) and reverse (R) of sequences used in quantitative real-time PCR studies.
Supplementary Table 2 | Polyphenolic compounds quantified in mature grape berries from cv. Loureiro vines treated with Ca (+ Ca) or without treatment (− Ca).
References
Alcaraz-López, C., Botía, M., Alcaraz, C. F., and Riquelme, F. (2005). Induction of fruit calcium assimilation and its influence on the quality of table grapes. Span. J. Agric. Res. 3, 335–343.
Aldon, D., Mbengue, M., Mazars, C., and Galaud, J. P. (2018). Calcium signalling in plant biotic interactions. Int. J. Mol. Sci. 19:665. doi: 10.3390/ijms19030665
Amiri, E. M., Fallahi, E., and Safari, G. (2009). Effects of preharvest calcium sprays on yield, quality and mineral nutrient concentrations of ‘Asgari’ table grape. Int. J. Fruit Sci. 9, 294–304. doi: 10.1080/15538360903241377
Andrés-Lacueva, C., Ibern-Gomez, M., Lamuela-Raventos, R. M., Buxaderas, S., and de la Torre-Boronat, M. C. (2002). Cinnamates and resveratrol content for sparkling wine characterization. Am. J. Enol. Vitic. 53, 147–150.
Arapitsas, P., Oliveira, J., and Mattivi, F. (2015). Do white grapes really exist? Food Res. Int. 69, 21–25. doi: 10.1016/j.foodres.2014.12.002
Barnavon, L., Doco, T., Terrier, N., Ageorges, A., Romieu, C., and Pellerin, P. (2001). Involvement of pectin methyl-esterase during the ripening of grape berries: partial cDNA isolation, transcript expression and changes in the degree of methyl-esterification of cell wall pectins. Phytochemistry 58, 693–701. doi: 10.1016/S0031-9422(01)00274-6
Billet, K., Delanoue, G., Arnault, I., Besseau, S., Oudin, A., Courdavault, V., et al. (2018a). Vineyard evaluation of stilbenoid-rich grape cane extracts against downy mildew: a large-scale study. Pest Manag. Sci. 75, 1252–1257. doi: 10.1002/ps.5237
Billet, K., Houillé, B., Besseau, S., Mélin, C., Oudin, A., Papon, N., et al. (2018b). Mechanical stress rapidly induces E-resveratrol and E-piceatannol biosynthesis in grape canes stored as a freshly-pruned byproduct. Food Chem. 240, 1022–1027. doi: 10.1016/j.foodchem.2017.07.105
Billet, K., Houillé, B., de Bernonville, T. D., Besseau, S., Oudin, A., Courdavault, V., et al. (2018c). Field-based metabolomics of Vitis vinifera L. stems provides new insights for genotype discrimination and polyphenol metabolism structuring. Front. Plant Sci. 9:798. doi: 10.3389/fpls.2018.00798
Bogs, J., Downey, M. O., Harvey, J. S., Ashton, A. R., Tanner, G. J., and Robinson, S. P. (2005). Proanthocyanidin synthesis and expression of genes encoding leucoanthocyanidin reductase and anthocyanidin reductase in developing grape berries and grapevine leaves. Plant Physiol. 139, 652–663. doi: 10.1104/pp.105.064238
Bonomelli, C., and Ruiz, R. (2010). Effects of foliar and soil calcium application on yield and quality of table grape cv. ‘Thompson Seedless’. J. Plant Nutr. 33, 299–314. doi: 10.1080/01904160903470364
Boss, P. K., Davies, C., and Robinson, S. P. (1996). Expression of anthocyanin biosynthesis pathway genes in red and white grapes. Plant Mol. Biol. 32, 565–569. doi: 10.1007/BF00019111
Castellarin, S. D., Pfeiffer, A., Sivilotti, P., Degan, M., Peterlunger, E., and Di Gaspero, G. (2007). Transcriptional regulation of anthocyanin biosynthesis in ripening fruits of grapevine under seasonal water deficit. Plant Cell Environ. 30, 1381–1399. doi: 10.1111/j.1365-3040.2007.01716.x
Ciccarese, A., Stellacci, A. M., Gentilesco, G., and Rubino, P. (2013). Effectiveness of pre-and post-veraison calcium applications to control decay and maintain table grape fruit quality during storage. Postharvest Biol. Tech. 75, 135–141. doi: 10.1016/j.postharvbio.2012.08.010
Cilliers, J. J., and Singleton, V. L. (1990). Nonenzymic autoxidative reactions of caffeic acid in wine. Am. J. Enol. Vitic. 41, 84–86.
Coombe, B. G. (1995). Growth stages of the grapevine: adoption of a system for identifying grapevine growth stages. Aust. J. Grape Wine Res. 1, 104–110. doi: 10.1111/j.1755-0238.1995.tb00086.x
Correia, S., Queirós, F., Ribeiro, C., Vilela, A., Aires, A., Barros, A. I., et al. (2019). Effects of calcium and growth regulators on sweet cherry (Prunus avium L.) quality and sensory attributes at harvest. Sci. Hortic. 248, 231–240. doi: 10.1016/j.scienta.2019.01.024
Deytieux-Belleau, C., Vallet, A., Donèche, B., and Geny, L. (2008). Pectin methylesterase and polygalacturonase in the developing grape skin. Plant Physiol. Biochem. 46, 638–646. doi: 10.1016/j.plaphy.2008.04.008
Fu, W., Zhang, M., Zhang, P., Liu, Z., Dong, T., Zhang, S., et al. (2020). Transcriptional and metabolite analysis reveal a shift in fruit quality in response to calcium chloride treatment on “Kyoho” grapevine. J. Food Sci. Technol. 58, 2246–2257. doi: 10.1007/s13197-020-04735-5
Fukushima, E. O., Seki, H., Ohyama, K., Ono, E., Umemoto, N., Mizutani, M., et al. (2011). CYP716A subfamily members are multifunctional oxidases in triterpenoid biosynthesis. Plant Cell Physiol. 52, 2050–2061. doi: 10.1093/pcp/pcr146
González-Fontes, A., Navarro-Gochicoa, M. T., Ceacero, C. J., Herrera-Rodríguez, M. B., Camacho-Cristóbal, J. J., and Rexach, J. (2017). “Understanding calcium transport and signaling, and its use efficiency in vascular plants,” in Plant Macronutrient Use Efficiency–Molecular and Genomic Perspectives in Crop Plants, eds M. A. Hossain, T. Kamiya, D. J. Burritt, L.-S. P. Tran, and T. Fujiwara (Cambridge, MA: Academic Press), 165–180.
Hocking, B., Tyerman, S. D., Burton, R. A., and Gilliham, M. (2016). Fruit calcium: transport and physiology. Front. Plant Sci. 7:569. doi: 10.3389/fpls.2016.00569
Jeong, S. T., Goto-Yamamoto, N., Kobayashi, S., and Esaka, M. J. P. S. (2004). Effects of plant hormones and shading on the accumulation of anthocyanins and the expression of anthocyanin biosynthetic genes in grape berry skins. Plant Sci. 167, 247–252. doi: 10.1016/j.plantsci.2004.03.021
Lampíř, L. (2013). Varietal differentiation of white wines on the basis of phenolic compounds profile. Czech J. Food Sci. 31, 172–179.
Lara, I., Belge, B., and Goulao, L. F. (2014). The fruit cuticle as a modulator of postharvest quality. Postharvest Biol. Technol. 87, 103–112. doi: 10.1016/j.postharvbio.2013.08.012
Manganaris, G. A., Vasilakakis, M., Diamantidis, G., and Mignani, I. (2007). The effect of postharvest calcium application on tissue calcium concentration, quality attributes, incidence of flesh browning and cell wall physicochemical aspects of peach fruits. Food Chem. 100, 1385–1392. doi: 10.1016/j.foodchem.2005.11.036
Martín-Diana, A. B., Rico, D., Barry-Ryan, C., Frías, J. M., Mulcahy, J., and Henehan, G. T. (2005). Comparison of calcium lactate with chlorine as a washing treatment for fresh-cut lettuce and carrots: quality and nutritional parameters. J. Sci. Food Agric. 85, 2260–2268. doi: 10.1002/jsfa.2254
Martín-Diana, A. B., Rico, D., Frias, J. M., Barat, J. M., Henehan, G. T. M., and Barry-Ryan, C. (2007). Calcium for extending the shelf life of fresh whole and minimally processed fruits and vegetables: a review. Trends Food Sci. Technol. 18, 210–218. doi: 10.1016/j.tifs.2006.11.027
Martins, V., Billet, K., Garcia, A., Lanoue, A., and Gerós, H. (2020a). Exogenous calcium deflects grape berry metabolism towards the production of more stilbenoids and less anthocyanins. Food Chem. 313:126123. doi: 10.1016/j.foodchem.2019.126123
Martins, V., Garcia, A., Alhinho, A. T., Costa, P., Lanceros-Méndez, S., Costa, M. M. R., et al. (2020b). Vineyard calcium sprays induce changes in grape berry skin, firmness, cell wall composition and expression of cell wall-related genes. Plant Physiol. Biochem. 150, 49–55. doi: 10.1016/j.plaphy.2020.02.033
Martins, V., Garcia, A., Costa, C., Sottomayor, M., and Gerós, H. (2018). Calcium-and hormone-driven regulation of secondary metabolism and cell wall enzymes in grape berry cells. J. Plant Physiol. 231, 57–67. doi: 10.1016/j.jplph.2018.08.011
Martins, V., López, R., Garcia, A., Teixeira, A., and Gerós, H. (2020c). Vineyard calcium sprays shift the volatile profile of young red wine produced by induced and spontaneous fermentation. Food Res. Int. 131:108983. doi: 10.1016/j.foodres.2020.108983
Martins, V., Soares, C., Spormann, S., Fidalgo, F., and Gerós, H. (2021a). Vineyard calcium sprays reduce the damage of postharvest grape berries by stimulating enzymatic antioxidant activity and pathogen defense genes, despite inhibiting phenolic synthesis. Plant Physiol. Biochem. 162, 48–55. doi: 10.1016/j.plaphy.2021.02.025
Martins, V., Unlubayir, M., Teixeira, A., Gerós, H., and Lanoue, A. (2021b). Calcium and methyl jasmonate cross-talk in the secondary metabolism of grape cells. Plant Physiol. Biochem. 165, 228–238. doi: 10.1016/j.plaphy.2021.05.034
Niu, S., Hao, F., Mo, H., Jiang, J., Wang, H., Liu, C., et al. (2017). Phenol profiles and antioxidant properties of white skinned grapes and their coloured genotypes during growth. Biotechnol. Biotechnol. Equip. 31, 58–67. doi: 10.1080/13102818.2016.1258329
Pelloux, J., Rusterucci, C., and Mellerowicz, E. J. (2007). New insights into pectin methylesterase structure and function. Trends Plant Sci. 12, 267–277. doi: 10.1016/j.tplants.2007.04.001
Reid, K. E., Olsson, N., Schlosser, J., Peng, F., and Lund, S. T. (2006). An optimized grapevine RNA isolation procedure and statistical determination of reference genes for real-time RT-PCR during berry development. BMC Plant Biol. 6:27. doi: 10.1186/1471-2229-6-27
Romanazzi, G., Lichter, A., Gabler, F. M., and Smilanick, J. L. (2012). Recent advances on the use of natural and safe alternatives to conventional methods to control postharvest gray mold of table grapes. Postharvest Biol. Technol. 63, 141–147. doi: 10.1016/j.postharvbio.2011.06.013
Saftner, R. A., Buta, J. G., Conway, W. S., and Sams, C. E. (1997). Effect of surfactants on pressure infiltration of calcium chloride solutions into Golden Delicious’ apples. J. Am. Soc. Hortic. Sci. 122, 386–391. doi: 10.21273/JASHS.122.3.386
Sampedro, J., and Cosgrove, D. J. (2005). The expansin superfamily. Genome Biol. 6:242. doi: 10.1186/gb-2005-6-12-242
Spare, P. D. (1964). A stable murexide reagent for the estimation of calcium in micro quantities of serum. Clin. Chem. 10, 726–729. doi: 10.1093/clinchem/10.8.726
Tavares, S., Vesentini, D., Fernandes, J. C., Ferreira, R. B., Laureano, O., Ricardo-Da-Silva, J. M., et al. (2013). Vitis vinifera secondary metabolism as affected by sulfate depletion: diagnosis through phenylpropanoid pathway genes and metabolites. Plant Physiol. Biochem. 66, 118–126. doi: 10.1016/j.plaphy.2013.01.022
Teixeira, A., Eiras-Dias, J., Castellarin, S. D., and Gerós, H. (2013). Berry phenolics of grapevine under challenging environments. Int. J. Mol. Sci. 14, 18711–18739. doi: 10.3390/ijms140918711
Vilanova, M., Ugliano, M., Varela, C., Siebert, T., Pretorius, I. S., and Henschke, P. A. (2007). Assimilable nitrogen utilisation and production of volatile and non-volatile compounds in chemically defined medium by Saccharomyces cerevisiae wine yeasts. Appl. Microbiol. Biotechnol. 77, 145–157. doi: 10.1007/s00253-007-1145-z
Wang, Y., Xie, X., and Long, L. E. (2014). The effect of postharvest calcium application in hydro-cooling water on tissue calcium content, biochemical changes, and quality attributes of sweet cherry fruit. Food Chem. 160, 22–30. doi: 10.1016/j.foodchem.2014.03.073
Keywords: anthocyanins, amino acids, calcium, flavonols, fruit firmness, grape berry ripening, polyphenolic metabolism, white grape cultivars
Citation: Martins V, Unlubayir M, Teixeira A, Lanoue A and Gerós H (2021) Exogenous Calcium Delays Grape Berry Maturation in the White cv. Loureiro While Increasing Fruit Firmness and Flavonol Content. Front. Plant Sci. 12:742887. doi: 10.3389/fpls.2021.742887
Received: 16 July 2021; Accepted: 09 August 2021;
Published: 27 August 2021.
Edited by:
M. Teresa Sanchez-Ballesta, Instituto de Ciencia y Tecnología de Alimentos y Nutrición (ICTAN), SpainReviewed by:
Michaela Griesser, University of Natural Resources and Life Sciences Vienna, AustriaNazareth Torres, University of California, Davis, United States
Copyright © 2021 Martins, Unlubayir, Teixeira, Lanoue and Gerós. This is an open-access article distributed under the terms of the Creative Commons Attribution License (CC BY). The use, distribution or reproduction in other forums is permitted, provided the original author(s) and the copyright owner(s) are credited and that the original publication in this journal is cited, in accordance with accepted academic practice. No use, distribution or reproduction is permitted which does not comply with these terms.
*Correspondence: Viviana Martins, dm1hcnRpbnNAYmlvLnVtaW5oby5wdA==