- 1School of Life Sciences, Yunnan University, Kunming, Yunnan, China
- 2School of Agriculture and Life Sciences, Kunming University, Yunnan, China
The gaseous molecule carbon monoxide (CO) can freely pass through the cell membrane and participate in signal transduction in the cell to regulate physiological activities in plants. Here, we report that CO has a positive regulatory role in stomatal development. Exogenous CO donor CORM-2 [Tricarbonyldichlororuthenium (II) dimer] treatment resulted in an increase of stomatal index (SI) on the abaxial epidermis of cotyledons in wild-type, which can be reversed by the addition of the CO biosynthesis inhibitor ZnPPIX [Protoporphyrin IX zinc (II)]. Consistent with this result, mutation of the CO biosynthesis gene HY1 resulted in a decrease of SI in hy1-100 plants, while overexpression of HY1 led to an increase of SI. Further investigation revealed that CO acts upstream of SPCH and YDA in the stomatal development pathway, since the loss of function mutants spch-1 and yda-2 were insensitive to CORM-2. The expression of EPF2 was inhibited by CORM-2 treatment in wild type and is lower in hy1 than in wild-type plants. In contrast, the expression of STOMAGEN was promoted by CORM-2 treatment and is higher in HY1-overexpression lines. Loss of function mutants of both epf2 and stomagen are insensitive to CORM-2 treatment. These results indicated that CO positively regulates stomatal initiation and distribution by modulating the expression of EPF2 and STOMAGEN.
Introduction
Stomata are small pores in plants that mediate gas exchange on the epidermis of above ground organs such as the cotyledon, leaf, and stem. Stoma can respond quickly to multiple stimuli and its movement (opening and closing) affect the efficiency of both photosynthesis and respiration. In the dicotyledonous plant Arabidopsis thaliana, the formation of a stoma requires at least one asymmetric cell division (ACD) and one symmetric cell division (SCD), which is accompanied by a successive transition of cell fate (Pillitteri and Torii, 2012). Early in the development of young leaves, specific cells (meristemoid mother cells, MMCs) enter into the stomatal lineage and acquire the capability to divide asymmetrically, which produces two daughter cells, a small meristemoid (M) and a large SLGC (stomatal lineage ground cell). Meristemoids can undergo more ACDs (usually 1 to 3 times) to renew itself before the transition into a guard mother cell (GMC) (Nadeau and Sack, 2002). Finally, the GMC divide equally and produce two kidney-like guard cells (GCs) (Bergmann and Sack, 2007). Transcription factors, especially the basic helix-loop-helix (bHLH) family members, play core roles in stomatal development. SPEECHLESS (SPCH), MUTE, and FAMA (bHLH097) function in the successive divisions of stomatal lineage precursors, by forming heterodimers with ICE1/SCREAM or SCRM2 (Kanaoka et al., 2008). The SPCH/SCRMs regulates the ACD of MMCs and Ms and maintains their self-renewing capability (MacAlister et al., 2007). The MUTE/SCRMs module controls the transition of M to GMC and the exit of precursor from a stem cell identity (Pillitteri et al., 2007). The FAMA/SCRMs module regulates the terminal division of GMC and GC fate (Ohashi-Ito and Bergmann, 2006).
Distribution of stomata on the epidermis obeys the rule of “one-cell-spacing”,which is ensured by a complex cell-cell signaling pathway (Nadeau and Sack, 2002). Signaling peptides from EPFs (EPIDERMAL PATTERNING FACTOR) and CLVs (CLAVATAS/ESR-RELATED) families are secreted and perceived by receptors from ER (ERECTA) family and co-receptors TMM (TOO MANY MOUTHS) (Hara et al., 2007; Hara et al., 2009; Sugano et al., 2010; Lee et al., 2015; Vatén et al., 2018), which initiates a MAPK (MITOGEN-ACTIVATED PROTEIN KINASE) signaling cascade by phosphorylation. The MAPK cascade consists of the MAPKKK protein YDA(YODA), MAPKK protein MKK4/5/7/9, and the MAPK proteins MPK3 and MPK6. Activated MPK3/6 can phosphorylate SPCH and decrease its stability (Bergmann et al., 2004; Wang et al., 2007; Lampard et al., 2009). Members from the EPF family play vital roles in stomatal development. EPF1 and EPF2 secreted from epidermal cells act as a negative regulator, while STOMAGEN/EPFL9 from mesophyll acts as a positive regulator of stomatal density (Hara et al., 2009; Hunt and Gray, 2009; Sugano et al., 2010). EPF2 binds with ER-TMM and activates MAPK, which restricts the initiation of stomatal development by phosphorylating SPCH and decreasing its abundance (Bergmann et al., 2004; Lee et al., 2015). STOMAGEN competitively binds to ER-TMM to inhibit the activation of the MAPK cascade triggered by EPF2 (Lee et al., 2012; Lee et al., 2015). Thus, peptides from the same family fine-tune the density and patterning of stomata on the epidermis.
Carbon monoxide (CO), as a signaling molecule, functions in the regulation of multiple plant development processes and stress responses, such as lateral root formation (Guo et al., 2008; Chen and Kao, 2012; Samma et al., 2014), seed germination (Liu et al., 2007; Liu et al., 2010; Jia et al., 2018), cadmium tolerance (Han et al., 2008; Han et al., 2014), salt acclimation (Xie et al., 2011), stomatal movement (Cao et al., 2007; Song et al., 2008; Xie et al., 2016), and boron and iron homeostasis (Wei et al., 2011; Li et al., 2013; Yang et al., 2016; Lv et al., 2017). CO interacts with hormones and other signaling molecules to regulate the above processes. For example, CO promotes the accumulation of IAA during lateral root formation and dependent on the function of NO (Nitric Oxide) in tomato (Guo et al., 2008) and rice (Chen and Kao, 2012). CO can mediate ABA-induced closure of stomata in Vicia faba through interaction with NO and ROS (Cao et al., 2007; Song et al., 2008; Xie et al., 2016). In addition, crosstalk with NO, H2O2, and other small signaling molecules also play vital roles in CO-mediated stress responses to salt, drought, and heavy metals (Wang and Liao, 2016).
In plant cells, CO is mainly produced by heme oxidation, which is catalyzed by four HOs (heme oxygenase enzymes) in Arabidopsis (Muramoto et al., 2002; Emborg et al., 2006; Gisk et al., 2009). The four HOs are HY1(HO1), HO2, HO3, and HO4. HO1, encoded by HY1, can decompose heme and produce CO, as well as ions (Fe2+) and biliverdin IXa (BV) (Emborg et al., 2006; Gisk et al., 2009). BV is a precursor of the phytochrome chromophore, which participates in plant photomorphogenesis (Emborg et al., 2006).
Although broadly involved in multiple developmental processes, whether CO participates in the regulation of stomatal development remains unknown. Here we demonstrate that CO acts upstream of YDA to promote stomatal development in Arabidopsis cotyledons. Promotion of CO biosynthesis both endogenously and exogenously results in the increase of SI, while blocking of CO production chemically or genetically inhibits stomatal development. CO acts upstream of YDA to promote the stomatal index, as yda-2 is insensitive to CORM-2 treatment. We also found that CO can modulate the expression of EPF2 and STOMAGEN, and through their action regulate stomatal development.
Results
CO promotes stomatal development
To determine whether CO is involved in the regulation of stomatal density and distribution, Arabidopsis wild-type Columbia-0 (Col-0) seedlings were treated with CO donor CORM-2 at concentrations of 0, 10, 20, and 40 µM for 14 days (Figure 1A). We found that the stomatal index (SI) was significantly increased in treated seedlings compared with the control plants. The promotion effect of CORM-2 is concentration-dependent (Figures 1A, B). An increased SI (26.11±2.55)was found at a concentration of 10 μM compared with the control (24.09±1.90). The strongest promotion of SI was observed with 40 µM CORM-2 (33.91±1.90) (Figure 1B). Next, wild-type seedlings (Col-0) were treated with the HY1 inhibitor ZnPPIX to suppress CO production, which caused a significant decrease of SI in the wild type (19.87±1.37) (Figures 1A, B). Furthermore, ZnPPIX treatment also reversed the CORM-2-induced increase in SI (23.90±1.92) (Figures 1A, B). These results suggested that the CO donor treatment facilitated stomatal development.
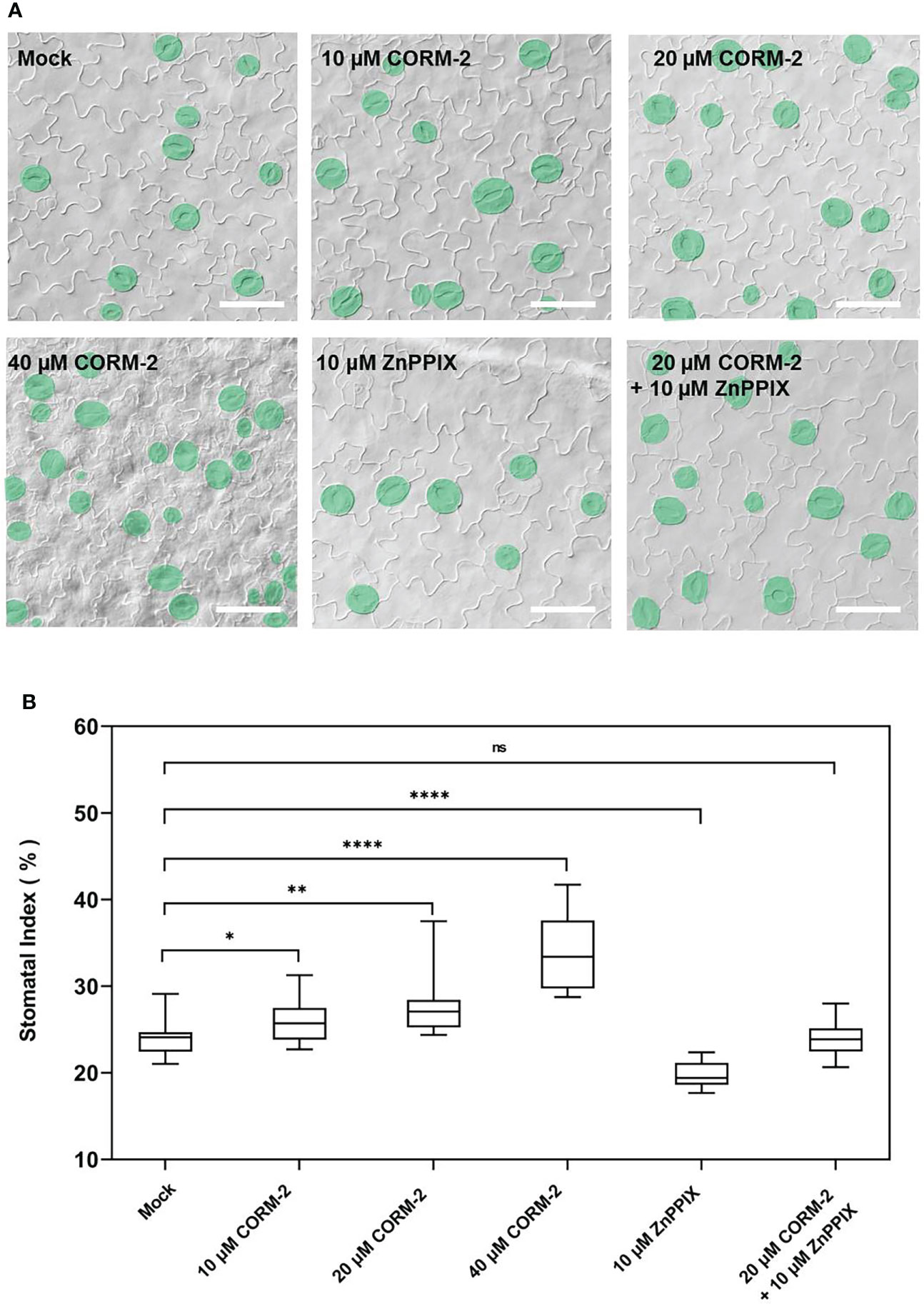
Figure 1 Exogenous CORM-2 treatment promotes stomatal development. (A) Differential interference contrast (DIC) images of the cotyledon epidermis of 14d old Wild-type (Col-0) plants with CORM-2 and ZnPPIX treatments. Bars = 50 μm. (B) Stomatal index (SI) of Wild-type (Col-0) with or without treatments. Error bars show standard deviation (SD) (n = 16). Statistical significance of different results was analyzed using Student’s t-test: ns Difference is not significant (p-value > 0.05). * Difference is significant (p-value < 0.05). ** Difference is highly significant (p-value < 0.01). **** Difference is highly significant (p-value < 0.0001).
Further experiments showed that endogenous CO has the same effects on the development of stomata. We examined the stomatal phenotypes of hy1-100 (a CO-deficient mutant) and the HY1-OE line, which overexpressed HY1 to generate higher endogenous CO (Xie et al., 2011; Yang et al., 2016). hy1-100 is a loss of function mutant (CS236) which has an A to G substitution in the first intron (Muramoto et al., 1999), and no HY1 protein can be detected in this mutant (Xie et al., 2011). As previously reported, the mutation led to a longer hypocotyl (Figure 2A) in hy1-100 homozygous plants than in wild type seedlings (Xie et al., 2011; Yang et al., 2016). We also found that the SI of hy1-100 (18.85±1.42) was lower than that of wild type (24.09±1.90) (Figures 2B, D). In contrast, the SI was higher in HY1-OE transgenic plants (30.44±2.24) than in wild type (24.09±1.90) (Figures 2B, D). Most importantly, CORM-2 treatment effectively increased the SI in hy1-100 to a level higher than the wild type, which can be effectively reversed by the addition of ZnPPIX (Figures 2C, E). ZnPPIX alone also led to a small decrease of SI in hy1-100 (Figures 2C, E), which might be due to the presence of the other HOs in this mutant. In addition, treatment with hemin led to an increased SI in cotyledons of wild type, while it failed to induce the increase of SI in hy1-100 seedlings (Figures 3A, B). These findings indicated that HY1-mediated hemin oxidation and CO biosynthesis positively affect stomatal development.
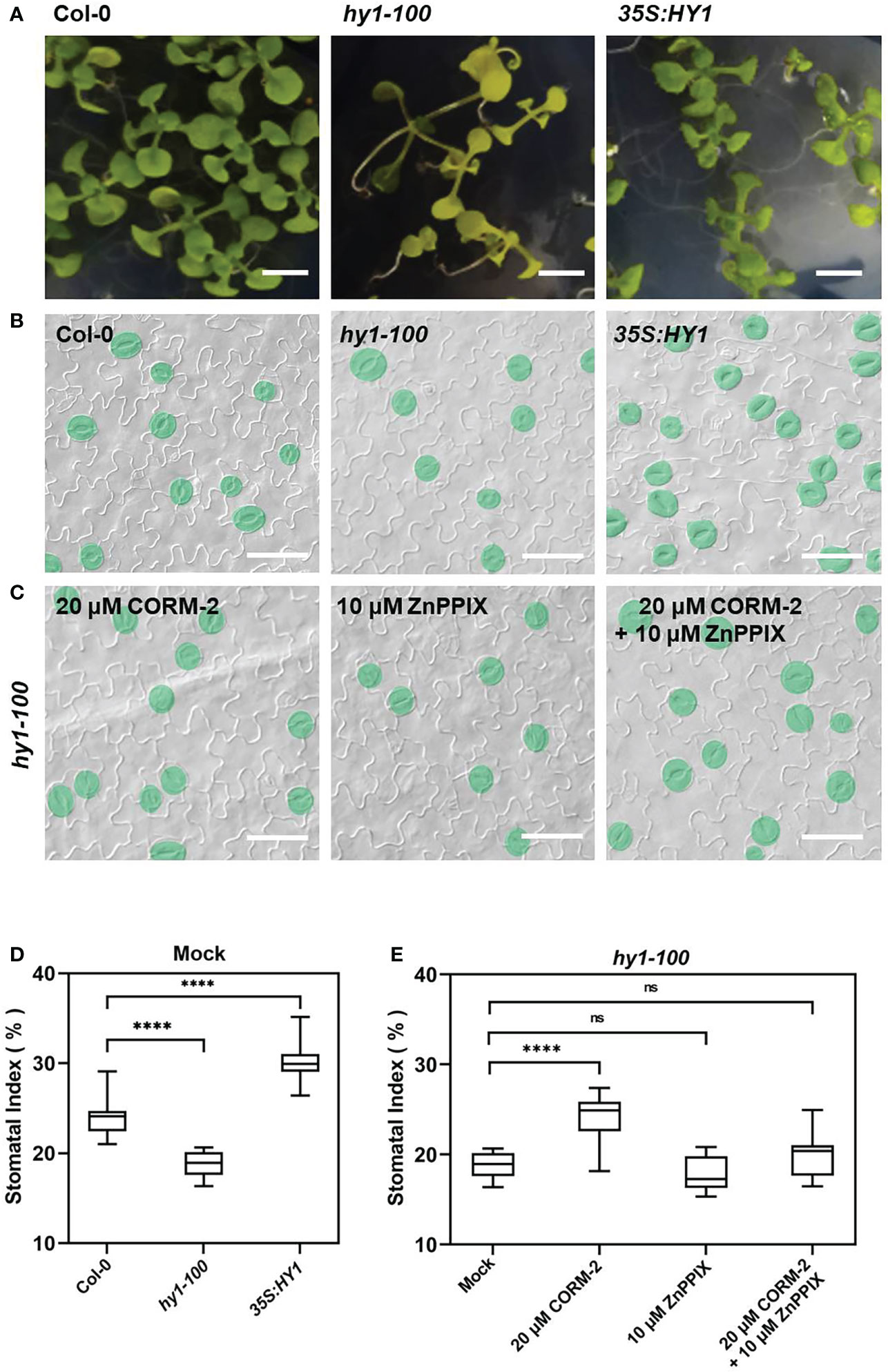
Figure 2 Stomatal development phenotype affected by loss-of (hy1-100) or overexpressed (35S:HY1) function of HY1. (A) Seedlings of 21d old of Wild-type (Col-0), hy1-100, and 35S:HY1 plants. Bars = 2 mm. (B) DIC images of the cotyledon epidermis of 14d old Wild-type (Col-0), hy1-100 and 35S:HY1 plants. Bars = 50 μm. (C) DIC images of the cotyledon epidermis of 14d old hy1-100 with CORM-2 (20 μM) and ZnPPIX (10 μM) treatment. Bars = 50 μm. (D) SIs of the Wild-type (Col-0), hy1-100, and and 35S:HY1 plants. Error bars show standard deviation (SD) (n = 16) (E) SIs of the hy1-100 mutant treated with CORM-2 (20 μM), ZnPPIX (10 μM) and their combination treatments (20 μM CORM-2 and 10 μM ZnPPIX). Error bars show standard deviation (SD) (n = 16). Statistical significance of different results was analyzed using Student’s t-test: ns Difference is not significant (p-value > 0.05). **** Difference is highly significant (p-value < 0.0001).
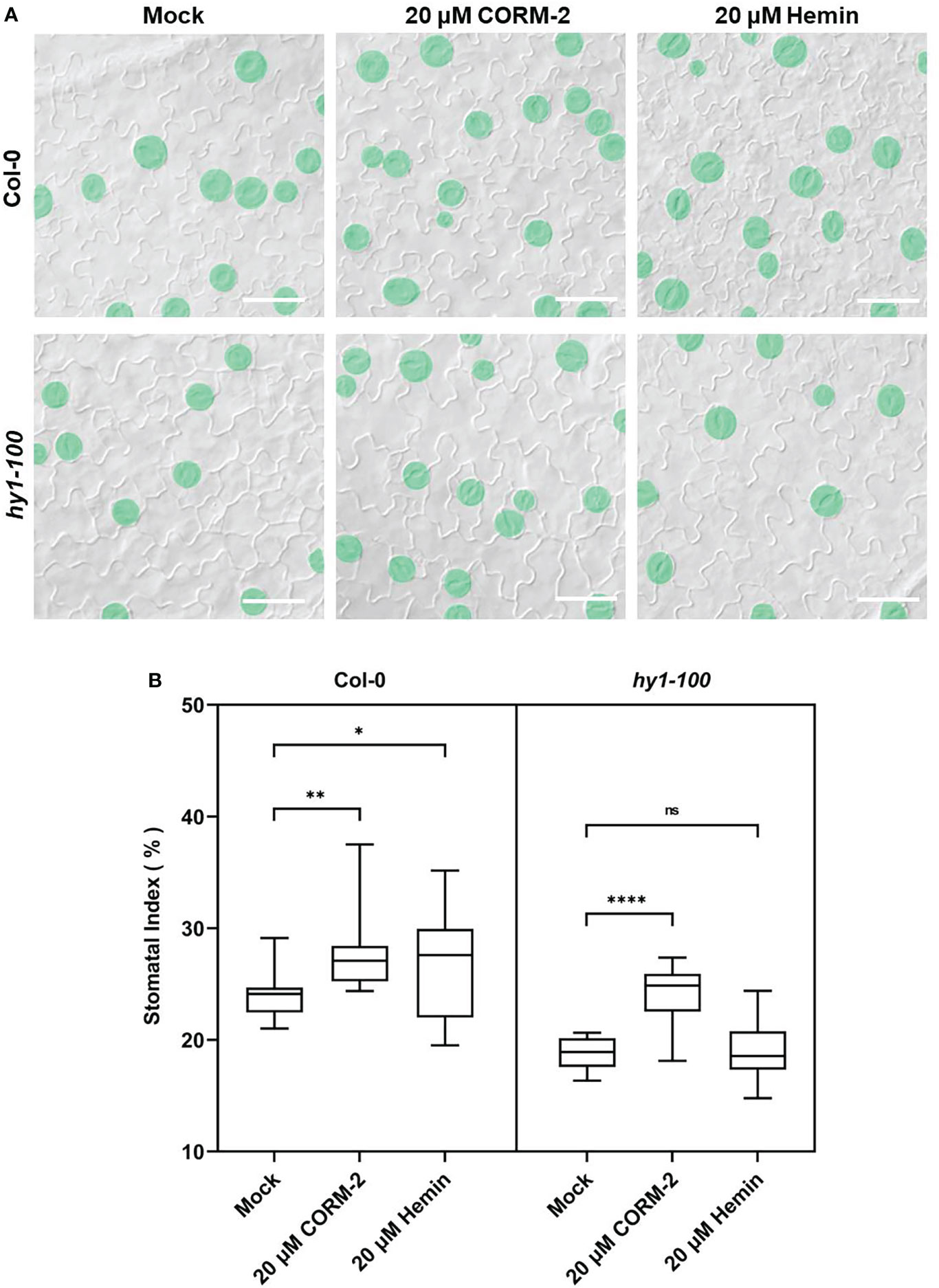
Figure 3 CORM-2 but not Hemin can restore the stomatal phenotype of hy1-100. (A) DIC images of the cotyledon epidermis of 14d old Wild-type (Col-0) and hy1-100 plants with CORM-2 and hemin treatment. Bars = 50 μm. (B) SIs of Wild-type (Col-0) and hy1-100 with or without CORM-2 (20 μM) and Hemin (20 μM) treatment. Error bars show standard deviation (SD) (n = 16). Statistical significance of different results was analyzed using Student’s t-test: ns Difference is not significant (p-value > 0.05). * Difference is significant (p-value < 0.05). ** Difference is highly significant (p-value < 0.01). **** Difference is highly significant (p-value < 0.0001).
Since CO biosynthesis catalyzed by HY1 of hemin oxidation is accompanied by the production of Fe2+ and BV. Fe-EDTA and BV were used to treat wild type seedlings to detect whether they have any effect on stomatal development. There was not a significant difference between the Fe-EDTA, BV treatment and the control plants (Figure S1). These results precluded the possibility that the effect of HY1 on stomatal development is due to Fe2+ and BV, further proving that CO could promote stomatal development.
CO regulates the expression of master regulators of stomatal development
To analyze how CO was involved in stomatal development, plants expressing a meristemoids specific reporter gene pTMM:TMM-GFP (Nadeau and Sack, 2002) was used for treatment. TMM-GFP-positive cells increased in the 20 µM CORM-2-treated seedlings (526.60±20.55 per mm2) while decreased in the 10 µM ZnPPIX-treated plants (121.60±17.30 per mm2) compared with the control (278.90±26.80 per mm2) (Figures 4A, B). Another reporter gene, pSPCH:SPCH-GFP, which expresses in the stomatal lineage precursor cells (MacAlister et al., 2007), was also treated with CORM-2 and ZnPPIX. Compared with the control (in which the number of cells expressing pSPCH:SPCH-GFP was 124.20±30.22 per mm2), more cotyledon epidermal cells (319.80±33.92 per mm2) expressed the marker pSPCH:SPCH-GFP in response to 20 µM CORM-2 treatment, while less cells (32.20±14.04 per mm2) in response to 10 µM ZnPPIX (Figures 4A, B). A third reporter, pFAMA:FAMA-GFP, which expresses in GMC and young GCs (Ohashi-Ito and Bergmann, 2006) was also used for treatment. We found that FAMA-GFP-positive cells also increased in CORM-2-treated cotyledons (234.0±25.18 per mm2) and decrease in ZnPPIX-treated cotyledons (116.20±17.80per mm2) than in controls (177.60±17.80 per mm2). These results show that exogenous CORM-2 can lead to the increase of the number of stomatal lineage cells on the cotyledon epidermis, while ZnPPIX has the opposite effect.
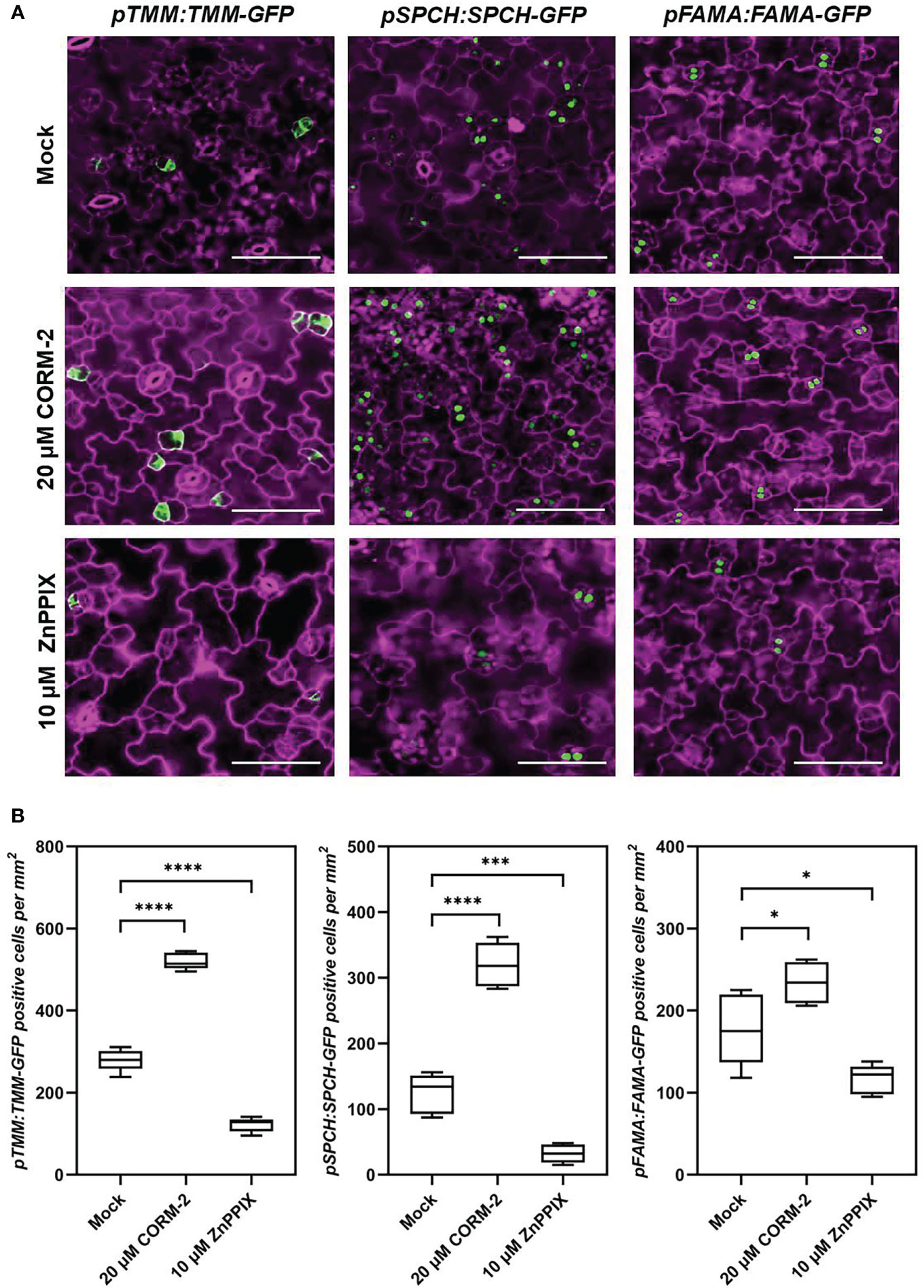
Figure 4 CO regulates the expression of key regulators of stomatal development. (A) Confocal images of the cotyledon epidermis of 4d old Wild–type (Col-0) plants expressing pTMM:TMM-GFP (pTMM:TMM-GFP in Col-0; first lane), pSPCH:SPCH-GFP (pSPCH:SPCH-GFP in Col-0; second lane), and pFAMAp:FAMA-GFP (pFAMA:FAMA-GFP in Col-0; third lane) with or without CORM-2 (20 μM) and ZnPPIX (10 μM) treatment. Bars = 50 μm. (B) Number of GFP-positive cells on the cotyledon epidermis of 4d old Wild–type (Col-0) plants expressing pTMM:TMM-GFP, pSPCH:SPCH-GFP, and pFAMAp:FAMA-GFP with or without CORM-2 (20 μM) and ZnPPIX (10 μM) treatment. Error bars show standard deviation (SD) (n = 5). Statistical significance of different results was analyzed using Student’s t-test: * Difference is significant (p-value < 0.05). *** Difference is highly significant (p-value < 0.001). **** Difference is highly significant (p-value < 0.0001).
CO acts upstream of the SPCH and YDA cascade to promote stomatal development
The bHLH transcription factor SPCH is needed for the first entry division of stomatal lineage precursors. Without SPCH, as in the loss of function mutant spch-1, no cell can enter into the stomatal lineage leading to the formation of an epidermis with only pavement cells (MacAlister et al., 2007). The promotion of stomatal initiation by CO also needs the function of SPCH, as both CORM-2 and ZnPPIX treatment cannot rescue stomata formation in the spch-1 mutant background (Figure 5A), which also suggested that CO functions upstream of SPCH to promote the initiation of stomatal divisions.
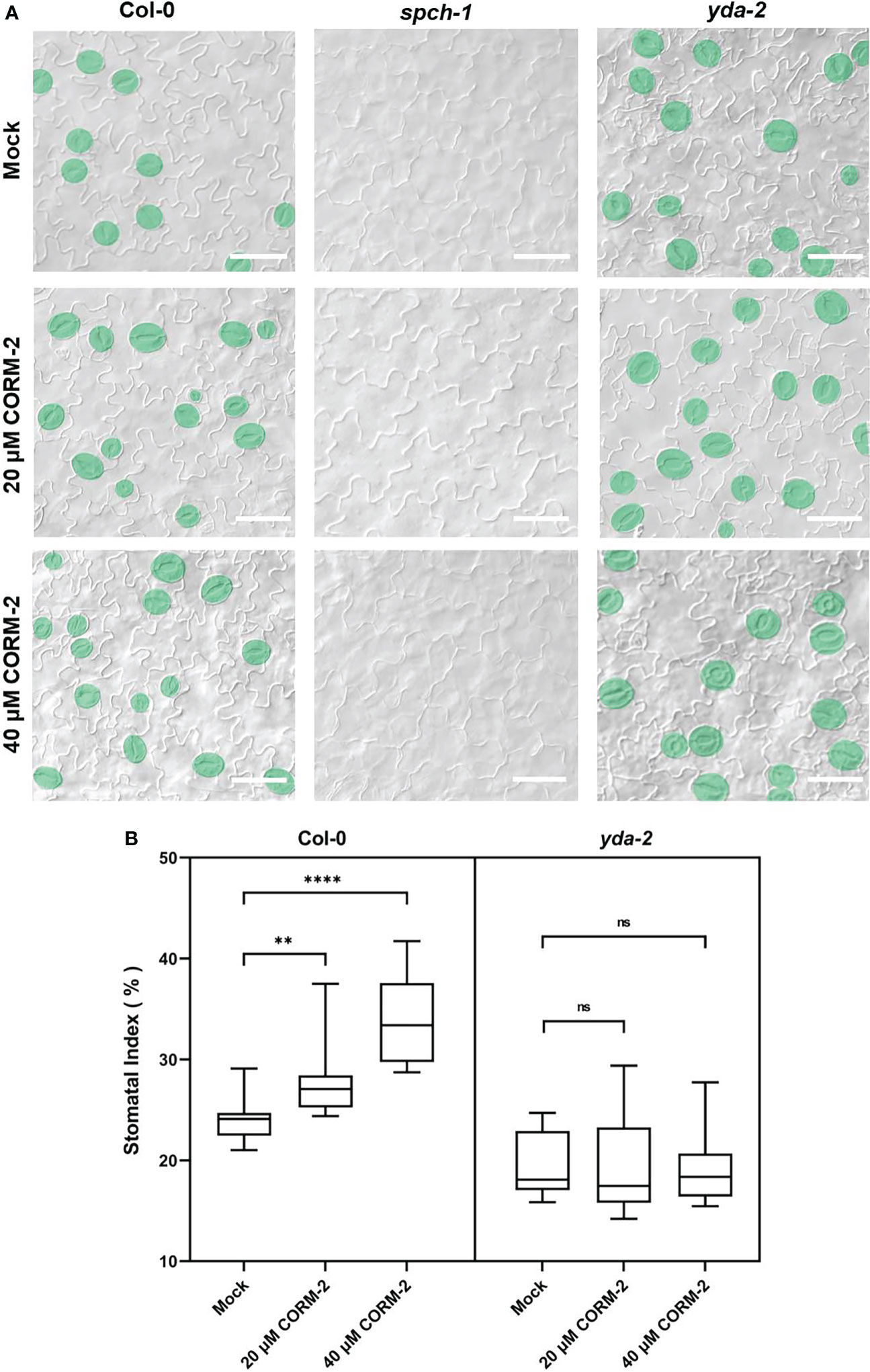
Figure 5 CO acts upstream of SPCH and YDA during stomatal development. (A) DIC images of the abaxial cotyledon epidermis of 14d old Wild–type (Col-0), spch-1, and yda-2 plants with or without CORM-2 (20μM or 40μM) treatment. Bars = 50 μm. (B) SIs of Wild–type (Col-0), spch-1, and yda-2 plants with or without CORM-2 (20μM or 40μM) treatment. Error bars show standard deviation (SD) (n = 16). Statistical significance of different results was analyzed using Student’s t-test: ns Difference is not significant (p-value > 0.05). ** Difference is highly significant (p-value < 0.01). **** Difference is highly significant (p-value < 0.0001).
YDA is an upstream regulator of SPCH. It encodes for a MAPKKK protein in the MAPK cascade in stomatal development. YDA can integrate environmental factors with stomatal initiation by phosphorylation of SPCH through its downstream targets MPK3 and MPK6 (Gudesblat et al., 2012; Kim et al., 2012). To detect whether the promotion of stomatal development by CO is dependent on the function of YDA-initiated MAPK signaling, a loss of function mutant of yda-2 was treated with CORM-2 and ZnPPIX. The results showed that yda-2 was insensitive to CORM-2 and ZnPPIX. As shown in Figure 5B, no significant difference of SI was found in yda-2 cotyledons in the presence of CORM-2 and ZnPPIX. These results indicated that CO regulated stomatal development in a manner dependent on the YDA-initiated pathway and functions upstream of the YDA cascade.
TMM-ERs complexes are membrane receptor kinases which bind different ligands from outside and transduce the signaling into the cell though activating the YDA cascade (Nadeau and Sack, 2002; Lee et al., 2015). To detect whether TMM and ERs were required for CO to regulate stomatal development, loss of function mutants of tmm-1, er-105, erl1, and erl2 were treated with CORM-2. CORM-2 treatment induced a slight, but significant increase of SI in tmm1 at a concentration of 20 µM, which indicated that tmm-1 was less sensitive to CORM-2 treatment compared with wild type (Figures 6A, B). The er-105, erl1, and erl2 mutants were also less sensitive to CORM-2 treatment because their SIs were less affected by treatment than wild type (Figures 6A, B). The changed sensitivity of the above mutants to CORM-2 indicated that regulation of stomatal development by CO may depend on the function of TMM and ERs.
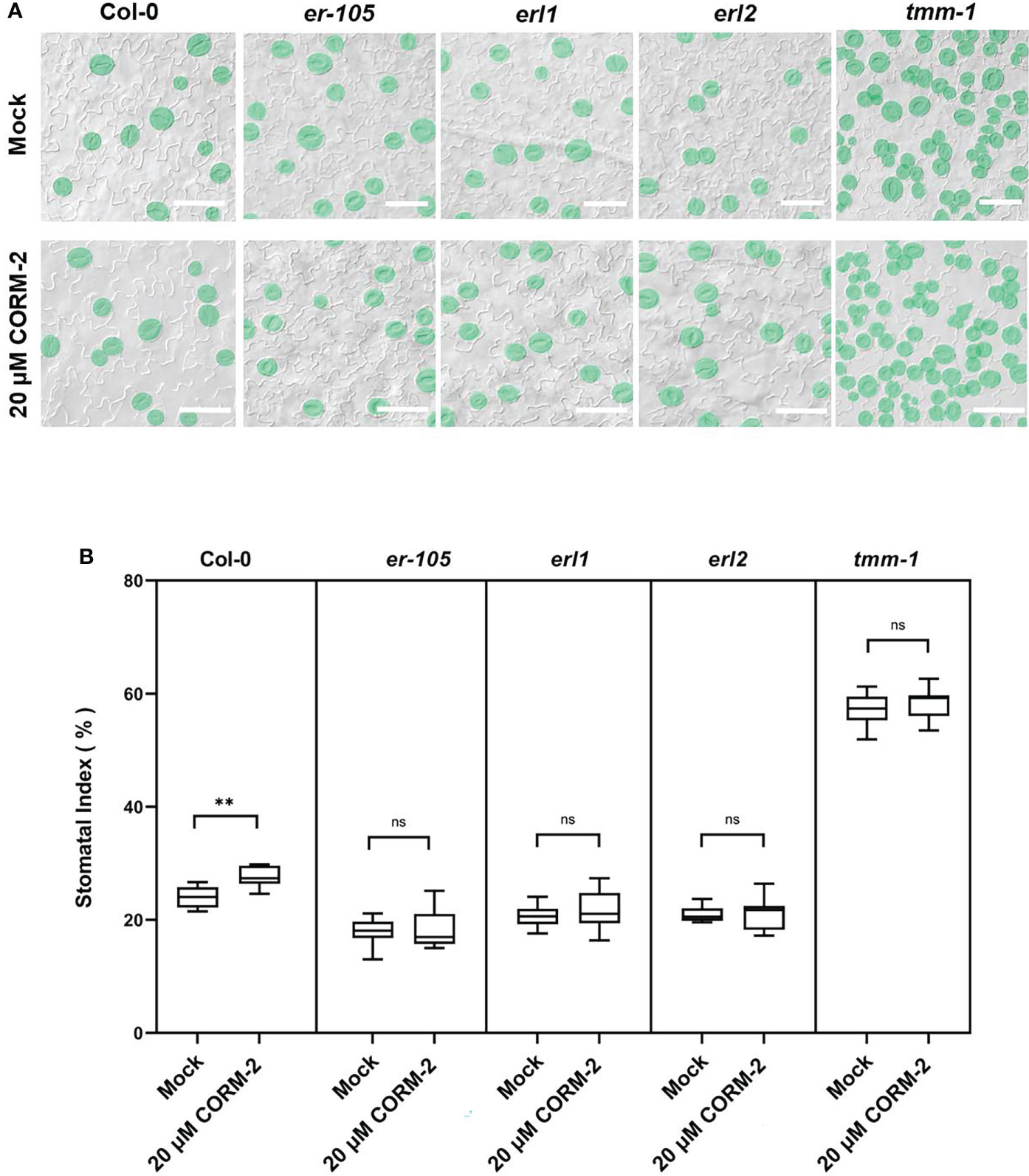
Figure 6 Response of Col, tmm-1, er-105, erl1, and er2 seedlings to CORM-2 treatment. (A) DIC images of the abaxial cotyledon epidermis of 14d old Wild–type (Col-0), tmm-1, er-105, erl1, and erl2 plants with or without CORM-2 (20 20) treatment. Bars = 50 μm. (B) SIs of Wild–type (Col-0), tmm-1, er-105, erl1, and erl2 plants with or without CORM-2 (20 μM) treatment. Error bars show standard deviation (SD) (n = 8). Statistical significance of different results was analyzed using Student’s t-test: ns Difference is not significant (p-value > 0.05). ** Difference is highly significant (p-value < 0.01).
CO regulates the expression of EPF2 and STOMAGEN
ER and ERL1 form a complex with TMM and specifically bind the peptides from the EPFs family to modify the density and distribution of stomata (Lee et al., 2015). Since TMM and ER/ERL1 may function in CO-regulated stomatal development, we asked whether the expression of EPFs was affected by CO. We used the promoters of EPFs to express a GFP marker (pEPF1:GFP, pEPF2:GFP and pSTOMAGEN:nucGFP) to detect the effect of CORM-2 and ZnPPIX on their expression. The expression of pEPF2:GFP was inhibited by CORM-2 and promoted by ZnPPIX treatment (Figures 7A, B). In contrast, there was no difference in pEPF1:GFP expression in the presence of CORM-2 and ZnPPIX (Figures S2A, S2B). Moreover, the expression of pSTOMAGEN:nucGFP increased after CORM-2 treatment and decreased after ZnPPIX treatment (Figures 7A, C).
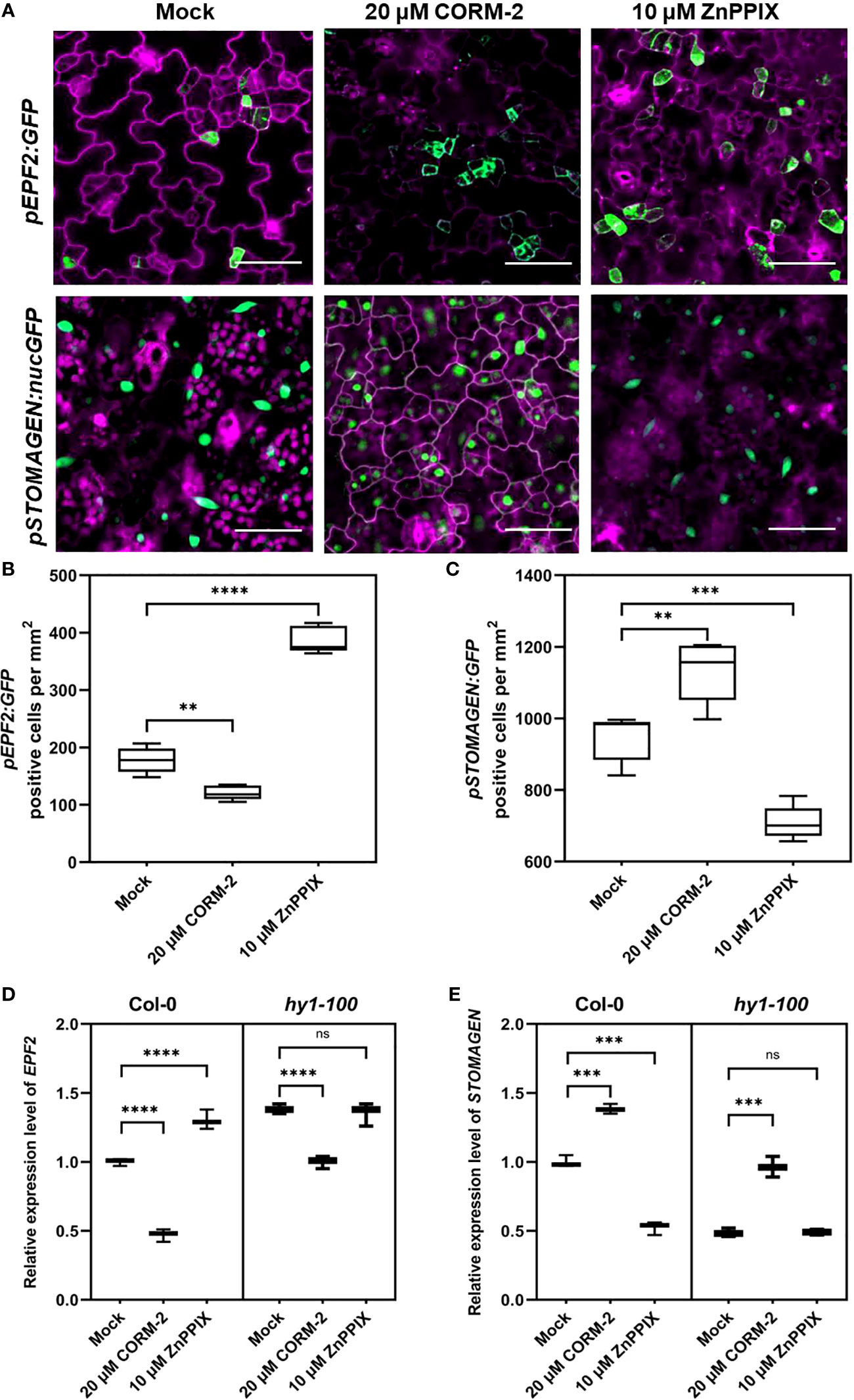
Figure 7 CO regulates the expression of EPF2 and STOMAGEN. (A) Confocal images of the abaxial cotyledon epidermis of 4d old Wild–type (Col-0) harboring pEPF2:GFP and pSTOMANGEN:nucGFP with or without CORM-2 (20 μM) treatments and ZnPPIX (10 μM) treatment. Bars = 50 μm. (B, C) Number of GFP-positive cells on the cotyledon epidermis of 4d old Wild–type (Col-0) plants expressing pEPF2:GFP (B) and pSTOMAGEN:nucGFP (C). Error bars show standard deviation (SD) (n = 5). (D, E) Relative mRNA level of EPF2 (D) and STOMAGEN (E) in Wild–type (Col-0) and hy1-100 seedlings (4dap) with or without CORM-2 (20 μM) treatments and ZnPPIX (10 μM) treatment. Error bars show standard deviation (SD) (n = 3). Statistical significance of different results was analyzed using Student’s t-test: ns Difference is not significant (p-value > 0.05). ** Difference is highly significant (p-value < 0.01). *** Difference is highly significant (p-value < 0.001). **** Difference is highly significant (p-value < 0.0001).
Real-time PCR experiments confirmed the function of CO on the EPFs genes expression. A significant increase (by 38.33 %) of STOMAGEN and a decrease (by 53.00 %) of EPF2 were found after CORM-2 treatment in wild type (Figures 7D, E). In contrast, no difference in the expression level of EPF1 was seen with CORM-2 and ZPPIX treatment (Figure S2B). In accordance with these results, a lower mRNA level of STOMAGEN and a higher expression of EPF2 were found in hy1-100 than in wild type (Figures 7D, E). In addition, exogenous CORM-2 treatment can restore the expression of STOMAGEN and EPF2 in hy1-100 to wild type levels (Figures 7D, E). These results showed that CO can promote the expression of STOMAGEN and inhibit the expression of EPF2.
CO promotes the stomatal development in a way dependent on EPF2 and STOMAGEN
Since CO can regulate the expression of EPF2 and STOMAGEN, we then asked whether the promotion of stomatal development by CO was dependent on the function of EPF2 and STOMAGEN. First, a CRISPR/Cas9 system was employed to edit the STOMAGEN gene and produce a loss-of-function mutant. The homozygous stomagen-cr mutant contained a 1-bp insertion between 798 and 799bp of the coding region (Figure 8A), which caused a decrease of the mRNA level (by 84.34 %) (Figure 8B) and SI (by 7.58 %) on the cotyledon epidermis (Figure 8C), a phenotype similar to previously reported RNAi lines (Sugano et al., 2010). We then treated this mutant with 40 μM CORM-2, and found that it was insensitive to the chemical as no significant change of SI was found after treatment (Figures 8C, D). As well, an epf2 loss of function mutant was also treated with 40 μM CORM-2 and the SI did not significantly change (Figures 8C, D). Taken together, these results verified that CO regulates stomatal development in a way dependent on the function of EPF2 and STOMAGEN.
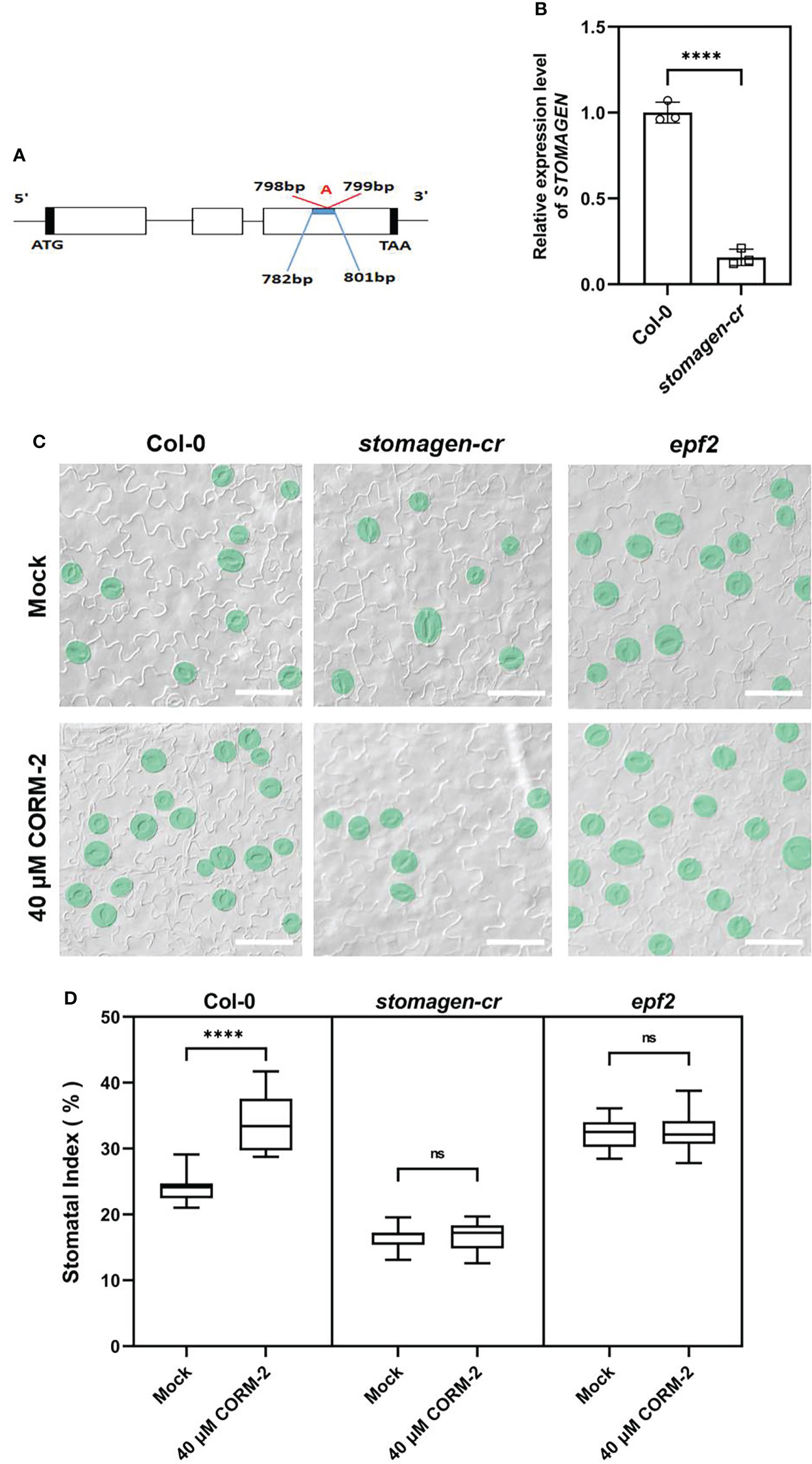
Figure 8 Regulation of stomatal development by CO is dependent on the function of EPF2 and STOMAGEN. (A) Insertion site of CRSIPR-Cas9 line of the stomagen mutant. (B) Expression level of STOMAGEN in Wild–type (Col-0) and stomagen-cr mutant produced by CRISPR-Cas9. Error bars show standard deviation (SD) (n = 3). (C) DIC images of the abaxial cotyledon epidermis of 14d old Wild–type (Col-0), epf2, and stomagen-cr plants with or without CORM-2 (40 μM) treatment. Bars = 50 μm. (D) SIs of Wild–type (Col-0), epf2, and stomagen-cr plants with or without CORM-2 (40 μM) treatments. Error bars show standard deviation (SD) (n = 16). Statistical significance of different results was analyzed using Student’s t-test: ns Difference is not significant (p-value > 0.05); **** Difference is highly significant (p-value < 0.0001).
Discussion
CO is a positive regulator of stomatal development
Mounting evidence indicates that CO can participate in a variety of developmental processes and stress responses in plants (Wang and Liao, 2016). For instance, CO positively affects seed germination (Liu et al., 2007; Liu et al., 2010; Jia et al., 2018) and lateral root formation (Guo et al., 2008; Chen and Kao, 2012; Samma et al., 2014), and protects plant against heavy metals (Han et al., 2008; Wei et al., 2011; Zheng et al., 2011; Yang et al., 2016), osmotic stress (Liu et al., 2010; Xie et al., 2011) and oxidative stress (Wu et al., 2010). Stomata are small pores through which plants exchange water and gases with the atmosphere, and both environmental factors and gaseous signaling molecules can modulate their development (Wu et al., 2010; Fu et al., 2016; Han et al., 2018; Deng et al., 2020). CO has been extensively reported to affects stomatal movement (She and Song, 2008; Song et al., 2008). However no reports focus on the effect of CO on stomatal development. In this study, we report that CO can promote stomatal initiation to increase stomatal index. Treatment with CORM-2 facilitates a dose-dependent increase of stomatal index (Figures 1A-C). CORM-2 treatment also promotes the expression of marker genes, including the MMCs- and Ms-expressed pTMM:TMM-GFP and pSPCH:SPCH-GFP, and GMC- and GC-expressed pFAMA:FAMA-GFP) (Figure 4). In contrast, treatment with the CO biosynthesis inhibitor ZnPPIX and a mutation of HY1 that disrupted CO generation dampened stomatal development in Arabidopsis cotyledons (Figures 1, 2B). In addition, the restoration of the capacity for CO generation through CORM-2 restored a wild type stomatal index in the hy1-100 mutant plants (Figures 2C, E).
Possible regulation mechanism of CO in stomatal development
In Arabidopsis, peptides from the EPFs family are perceived by membrane-located receptors to fine-tune the initiation and distribution of stomata (Lee et al., 2012; Jewaria et al., 2013; Lee et al., 2015). Environmental factors and hormonal signaling molecules regulate stomatal initiation by mediating the expression of EPFs (Engineer et al., 2014; Zhang et al., 2014; Hepworth et al., 2015; Caine et al., 2016; Wang et al., 2021). EPF2 is perceived by ER and its co-receptor TMM. Binding of EPF2 with the ER-TMM complex triggers rapid phosphorylation of the downstream of MAPK cascade (Bergmann et al., 2004; Lee et al., 2012; Lee et al., 2015). STOMAGEN can competitively bind to ER-TMM to replace EPF2, and diminish the downstream activation of MAPK elicited by EPF2 binding (Lee et al., 2015). In this study, we found that CO regulates the expression of STOMAGEN and EPF2. First, CORM-2 treatment downregulated EPF2 expression and upregulated STOMAGEN expression in the wild type (Figures 7B, C). Secondly, the expression level of EPF2 was higher in hy1-100 than in the wild type (Figure 7D). A lower expression level of STOMAGEN in hy1-100 compared to the wild type were also detected (Figure 7E). Third, the higher expression of EPF2 and lower expression of STOMAGEN in hy1-100, were rescued by treatment with CORM-2 (Figures 7D, E). Fourth, loss of function mutants of epf2 and stomagen were insensitive to CORM-2 treatment (Figures 8C, D), which confirmed that CO needs the functions of EPF2 and STOMAGEN to promote stomatal development. In conclusion, our data suggest that CO plays a positive role in the regulation of stomatal initiation in Arabidopsis cotyledons via modulating the expression of EPF2 and STOMAGEN.
HY1 is involved in the regulation of two pathways for stomatal development in Arabidopsis
HY1 plays a crucial role in the endogenous production of CO, as HY1, encoding a heme oxygenase, degrades hemin producing Fe2+, BV, and CO. In this report, we precluded the possibility that the effect of HY1 on stomatal development is due to the byproducts Fe2+ and BV (Figure S1). Moreover, a previous study reported that the hy1-100 mutant has a higher JA level than wild type (Zhai et al., 2007), and JAs negatively regulates stomatal initiation in Arabidopsis cotyledons (Han et al., 2018). Therefore, based on our results and these reports, we propose the possible regulation mechanism of HY1 catalyzed hemin oxidation in stomatal development as shown in Figure 9. On one hand, as a product of heme oxidation catalyzed by HY1 in the chloroplast, CO promotes the expression of STOMAGEN in mesophyll cells and also diffuses to epidermal cells where it inhibits the expression of EPF2. Less EPF2 from epidermal cells and more STOMAGEN synergistically release downstream inhibition of SPCH through the MAPK pathway. On the other hand, HY1 inhibits the accumulation of JAs and removes the inhibition of JAs in stomatal initiation. In addition, JAs inhibits the expression of HY1 conversely (Zhai et al., 2007), which leads to the formation of a feedback loop between the production of JAs and HY1 activity. In the wild type, the formation of the feedback loop fine-tunes the expression level of SPCH and thus the stomatal production. In the hy1 mutant, the block of this feedback resulted in low levels of CO and overaccumulation of JAs which synergistically inhibit the formation of stomata on the cotyledon epidermis. In our previous research, another gaseous signaling molecule, H2S, also regulates stomata through JA signaling (Deng et al., 2020). Therefore, JA signaling is important to regulate stomata development through gaseous signaling molecules. Nevertheless, the mechanism underlying the crosstalk of JA and CO/H2S remains largely unknown.
Moreover, CO is also involved in the regulation of darkness-induced NO synthesis (She and Song, 2008), and the crosstalk between CO and NO has been shown in seed germination regulation (Liu et al., 2010). As a positive regulator, NO can promote the expression of SPCH, MUTE, and SCRM2 and inhibit the expression of MPK6 (Fu et al., 2016). Furthermore, CO is also involved in IAA-regulated adventitious root development (Xuan et al., 2008). A previous study reported that the auxin-related transcription factor ARF5/MONOPTEROS (MP) can repress the expression of STOMAGEN by directly binding to its promoter (Zhang et al., 2014). While little is known about the cross talk between CO and auxin in stomatal development, it is reasonable to deduce that CO may regulate the expression of STOMAGEN through the ARF5-mediated auxin pathway, a hypothesis which requires further experimental verification. As well, further investigation is required to establish the relationship between CO and auxin through the EPF family, which will provide insights into the complicated regulatory mechanism governing the initiation of stomata.
Materials and methods
Materials and plant growth conditions
Arabidopsis thaliana plants were grown on half-strength Murashige and Skoog (MS) medium at 22°C under a 16-h light/8-h dark photoperiod. Chemicals were purchased from the Shanghai Sangon Biotechnology Co. Ltd. (Shanghai, China). COMR-2 and ZnPPIX were obtained from Sigma (St. Louis, Missouri, USA), and Taq DNA polymerases were from Takara Biotechnology (Dalian, China). The mutants used in this study are: tmm-1 (Yang and Sack, 1995), spch-1 (MacAlister et al., 2007), yda-2 (CS6393, Lukowitz et al., 2004), epf2 (Hara et al., 2007), and 35S:HY1 transgenic plants (Xie et al., 2011). Transgenic plants with stomatal lineage-specific markers (pTMM:TMM-GFP, pSPCH:SPCH-GFP, pFAMA:FAMA-GFP, pEPF1:GFP, and pEPF2:GFP) were obtained from Fred Sack (University of British Columbia). The mutants hy1-100 (CS236), er-105 (CS6588), erl1 (SALK_081669C), and erl2 (SALK_144166C) were from the Nottingham Arabidopsis Stock Centre (NASC).
Chemical treatment
Stock solution (at 10 mM) of COMR-2 and ZnPPIX (Sigma) were dissolved in DMSO (dimethylsulphoxide). Final use concentration solutions were made by diluting stocks with sterilized medium. In this study, 10, 20, and 40 μM were used as final concentrations for CORM-2, and 10 μM for ZnPPIX. An equivalent concentration of DMSO was added in the plates as control. Seeds were sterilized and germinated on half-MS medium supplemented with or without chemicals. All the treatments have at least three replicates.
Microscopy
An OLYMPUS BX51 microscope was used to take DIC images from samples which were stored in Hoyer’s solution. The 14 dag (day after germination) cotyledons were used for observation. The clearing of samples was as follows: first seedlings were immersed in 70% ethanol. After being cleared overnight at room temperature, samples were transferred into the Hoyer’s solution for storage. To observe the expression of marker genes, a ZEISS LSM800 laser confocal scanning microscope was used for shoot GFP fluorescence images and PI (propidium iodide) (Sigma-Aldrich) staining. Confocol images were cleared using magenta for the PI channel.
Stomatal index measurement
An OLYMPUS BX51 microscope was used to visual the samples placed in water. Stomatal index (SI) is defined as the ratio of stomata and stomatal lineage precursors (MMC and M) in total epidermal cells per unit area. For stomatal index, number of stomata, stomatal lineage precursors (MMC and M) and all epidermal cells of 0.37 mm2 were counted to acquire a SI value for different cotyledons from different plants. Cotyledons from at least eight different plants were selected from the used genotypes.
RNA extraction and qRT-PCR
Total RNA extraction and qRT-PCR were performed as described previously (Han et al., 2018; Deng et al., 2020). Trizol reagent (Invitrogen) was used to extract total RNA. For qRT-PCR, 1 μg DNase-treated RNA in 20 μl reaction volumes were used to synthesis the first-strand using M-MuLV reverse transcriptase (Monad, China) with oligo (dT)18 primer. 2×TB Green Premix Ex Taq II (Tli RNaseH) was used to perform PCR on an Applied Biosystems QuantStudioTM 6 flex system real-time PCR machine, according to the manufacturer’s instructions. Three replicates for each sample were used for qRT-PCR analysis. The Actin gene was used as an internal control. Gene-specific primers used to detect transcripts are listed in Supplemental Table S1.
Construction of plasmids
For the construction of stomagen-cr lines, target sites were designed by the use of CRISPR-GE to avoid off-targets (http://skl.scau.edu.cn/; Ma et al., 2015). The editing vectors were constructed as described in a previous report (Zhang et al., 2019). The sgRNA containing a single target site driven by the AtU3b promoter was cloned into the pMH-SA vector by the restriction enzyme sites AscI. Homozygous mutant lines were identified by sequencing.
For the construction of pSTOMAGEN:nucGFP, the 2,000 bp promoter sequence of AT4G12970 (STOMAGEN) was amplified by PCR and cloned in front of nucGFP in the Poca30 binary vector. The primers and restriction enzyme sites used to amplify sequences and generate vectors are listed in Supplemental Table S1.
Accession numbers
Arabidopsis Genome Initiative numbers for the genes used in this article are as follows: HY1 (AT2G26670); TMM (AT1G80080); ER (AT2G26330); ERL1 (AT5G62230); ERL2 (AT5G07180); YODA (AT1G63700); SPCH (AT5G53210); FAMA (AT3G24140); EPF1 (AT2G20875); EPF2 (AT1G34245); STOMAGEN (AT4G12970) and ACTIN (AT3G18780).
Data availability statement
The original contributions presented in the study are included in the article/Supplementary Material. Further inquiries can be directed to the corresponding authors.
Author contributions
LJZ and XLC designed experiments. XJW, LYZ, SSY, YL, YYR and ZJZ performed experiments. XJW, LJZ, SSY and ZRH analyzed data. XLC, LYZ, and LJZ wrote the manuscript. All authors contributed to the article and approved the submitted version.
Funding
This work was funded by the Natural Science Foundation of China (31260283 and 31560078) and the Natural Science Foundation of Yunnan Province (NO.202001BB050011) to XLC.
Acknowledgments
We appreciate Fred Sack (University of British Columbia) for sharing tmm-1, spch-1, epf2, yda-2, pTMM:TMM-GFP, pSPCH:SPCH-GFP, pFAMA:FAMA-GFP, pEPF1:GFP, and pEPF2:GFP, the Nottingham Arabidopsis Stock Centre (NASC) for providing hy1-100 (CS236), er-105 (SALK_061943), erl1 (SALK_081669), and erl2 (SALK_007643), and Gang Liang (Xishuangbanna Tropical Botanical Garden, Chinese Academy of Sciences) for 35S:HY1.
Conflict of interest
The authors declare that the research was conducted in the absence of any commercial or financial relationships that could be construed as a potential conflict of interest.
Publisher’s note
All claims expressed in this article are solely those of the authors and do not necessarily represent those of their affiliated organizations, or those of the publisher, the editors and the reviewers. Any product that may be evaluated in this article, or claim that may be made by its manufacturer, is not guaranteed or endorsed by the publisher.
Supplementary material
The Supplementary Material for this article can be found online at: https://www.frontiersin.org/articles/10.3389/fpls.2022.1029703/full#supplementary-material
Supplementary Figure 1 | Fe-EDTA and BV have no effect on the stomatal development of wild type. (A) DIC images of the abaxial cotyledon epidermis of 14d old Wild–type (Col-0) plants with or without Fe-EDTA (40 μM), BV (20 μM) and CORM-2 (40 μM). Bars = 50 μm. (B) SIs of Wild–type (Col-0) plants with or without Fe-EDTA (40 μM), BV (20 μM) and CORM-2 (40 μM) treatments. Error bars show standard deviation (SD) (n = 8). Statistical significance of different results was analyzed using Student’s t-test: ns Difference is not significant (p-value > 0.05). **** Difference is highly significant (p-value < 0.0001).
Supplementary Figure 2 | Expression of EPF1 is not affected by CO. (A) Confocal images of the abaxial cotyledon epidermis of 4 d old Wild–type (Col-0) harboring pEPF1:GFP with or without CORM-2 (20 μM) and ZnPPIX (10 μM) treatment. Bars = 50 μm. (B) Number of GFP-positive cells on the cotyledon epidermis of 4d old Wild–type (Col-0) plants expressing pEPF1:GFP with or without CORM-2 (20 μM) and ZnPPIX (10 μM) treatment. Error bars show standard deviation (SD) (n = 5). (C) Expression of EPF1 in Wild–type (Col-0) and hy1-100 with or without CORM-2 (20 μM) and ZnPPIX (10 μM) treatment. Error bars show standard deviation (SD) (n = 3). Statistical significance of different results was analyzed using Student’s t-test: ns Difference is not significant (p-value > 0.05).
Supplementary Table 1 | Primers used in this study.
Abbreviations
bHLH, basic Helix-Loop-Helix; BV, Biliverdin IXa; CO, Carbon monoxide; CORM-2, Tricarbonyldichlororuthenium (II) dimer; EPFs, Epidermal Patterning Factors; ER, ERECTA; GC, Guard Cell; GMC, Guard Mother Cell; HO, Heme Oxygenase; MAPK, Mitogen-Activated Protein Kinase; MMC, Meristemoid Mother Cell; SI, Stomatal Index; SLGC, Stomatal Lineage Ground Cell; SPCH, SPEECHLESS; TMM, TOO MANY MOUTHS; YDA, YODA; ZnPPIX, Protoporphyrin IX zinc (II).
References
Bergmann, D. C., Lukowitz, W., Somerville, C. R. (2004). Stomatal development and pattern controlled by a MAPKK kinase. Science 304, 1494–1497. doi: 10.1126/science.1096014
Bergmann, D. C., Sack, F. D. (2007). Stomatal development. Annu. Rev. Plant Biol. 58, 163–181. doi: 10.1146/annurev.arplant.58.032806.104023
Caine, R. S., Chater, C. C., Kamisugi, Y., Cuming, A. C., Beerling, D. J., Gray, J. E., et al. (2016). An ancestral stomatal patterning module revealed in the non-vascular land plant physcomitrella patens. Development 143, 3306–3314. doi: 10.1242/dev.135038
Cao, Z. Y., Huang, B. K., Wang, Q. Y., Xuan, W., Ling, T. F., Zhang, B., et al. (2007). Involvement of carbon monoxide produced by heme oxygenase in ABA-induced stomatal closure in vicia faba and its proposed signal transduction pathway. Chin. Sci. Bull. 52, 2365–2373. doi: 10.1007/s11434-007-0358-y
Chen, Y. H., Kao, C. H. (2012). Heme oxygenase is involved in nitric oxide- and auxin-induced lateral root formation in rice. Plant Cell Rep. 31, 1085–1091. doi: 10.1007/s00299-012-1228-x
Deng, G. B., Zhou, L. J., Wang, Y. Y., Zhang, G. S., Chen, X. L. (2020). Hydrogen sulfide acts downstream of jasmonic acid to inhibit stomatal development in arabidopsis. Planta 251, 42. doi: 10.1007/s00425-019-03334-9
Emborg, T. J., Walker, J. M., Noh, B., Vierstra, R. D. (2006). Multiple heme oxygenase family members contribute to the biosynthesis of the phytochrome chromophore in arabidopsis. Plant Physiol. 140, 856–868. doi: 10.1104/pp.105.074211
Engineer, C. B., Ghassemian, M., Anderson, J. C., Peck, S. C., Hu, H., Schroeder, J. I. (2014). Carbonic anhydrases, EPF2 and a novel protease mediate CO2 control of stomatal development. Nature 513, 246–250. doi: 10.1038/nature13452
Fu, Z. W., Wang, Y. L., Lu, Y. T., Yuan, T. T. (2016). Nitric oxide is involved in stomatal development by modulating the expression of stomatal regulator genes in arabidopsis. Plant Sci. 252, 282–289. doi: 10.1016/j.plantsci.2016.08.005
Gisk, B., Yasui, Y., Kohchi, T., Frankenberg-Dinkel, N. (2009). Characterization of the haem oxygenase protein family in arabidopsis thaliana reveals a diversity of functions. Biochem. J. 425, 425–434. doi: 10.1042/BJ20090775
Gudesblat, G. E., Schneider-Pizoń, J., Betti, C., Mayerhofer, J., Vanhoutte, I., Dongen, V. W., et al. (2012). SPEECHLESS integrates brassinosteroid and stomata signaling pathways. Nat. Cell Biol. 14, 548–554. doi: 10.1038/ncb2471
Guo, K., Xiad, K., Yang, Z. (2008). Regulation of tomato lateral root development by carbon monoxide and involvement in auxin and nitric oxde. J. Exp. Bot. 59, 3443–3452. doi: 10.1093/jxb/ern194
Han, X., Hu, Y. R., Zhang, G. S., Jiang, Y. J., Chen, X. L., Yu, D. Q. (2018). Jasmonate negatively regulates stomatal development in arabidopsis cotyledons. Plant Physiol. 176, 2871–2885. doi: 10.1104/pp.17.00444
Han, B., Yang, Z., Xie, Y., Nie, L., Cui, J., Shen, W. B. (2014). Arabidopsis HY1 confers cadmium tolerance by decreasing nitric oxide production and improving iron homeostasis. Mol. Plant 7, 388–403. doi: 10.1093/mp/sst122
Han, Y., Zhang, J., Chen, X., Gao, Z., Xuan, W., Xu, S., et al. (2008). Carbon monoxide alleviates cadmium-induced oxidative damage by modulating glutathione metabolism in the roots of medicago sativa. New Phytol. 177, 155–166. doi: 10.1111/j.1469-8137.2007.02251.x
Hara, K., Kajita, R., Torii, K. U., Bergmann, D. C., Kakimoto, T. (2007). The secretory peptide gene EPF1 enforces the stomatal one-cell-spacing rule. Genes Dev. 21, 1720–1725. doi: 10.1101/gad.1550707
Hara, K., Yokoo, T., Kajita, R., Onishi, T., Yahata, S., Peterson, K. M. (2009). Epidermal cell density is autoregulated via a secretory peptide, EPIDERMAL PATTERNING FACTOR 2 in arabidopsis leaves. Plant Cell Physiol. 50, 1019–1031. doi: 10.1093/pcp/pcp068
Hepworth, C., Doheny-Adams, T., Hunt, L., Cameron, D. D., Gray, J. E. (2015). Manipulating stomatal density enhances drought tolerance without deleterious effect on nutrient uptake. New Phytol. 208, 336–341. doi: 10.1111/nph.13598
Hunt, L., Gray, J. E. (2009). The signaling peptide EPF2 controls asymmetric cell divisions during stomatal development. Curr. Biol. 19, 864–869. doi: 10.1016/j.cub.2009.03.069
Jewaria, P. K., Hara, T., Tanaka, H., Kondo, T., Betsuyaku, S., Sawa, S., et al. (2013). Differential effects of the peptides stomagen, EPF1 and EPF2 on activation of MAP kinase MPK6 and the SPCH protein level. Plant Cell Physiol. 54, 1253–1262. doi: 10.1093/pcp/pct076
Jia, Y. J., Li, R. J., Yang, W. J., Chen, Z., Hu, X. Y. (2018). Carbon monoxide signal regulates light-initiated seed germination by suppressing SOM expression. Plant Sci. 272, 88–98. doi: 10.1016/j.plantsci.2018.04.009
Kanaoka, M. M., Pillitteri, L. J., Fujii, H., Yoshida, Y., Bogenschutz, N. L., Takabayashi, J., et al. (2008). SCREAM/ICE1 and SCREAM2 specify three cell-state transitional steps leading to arabidopsis stomatal differentiation. Plant Cell 20, 1775–1785. doi: 10.1105/tpc.108.060848
Kim, T. W., Michniewicz, M., Bergmann, D. C., Wang, Z. Y. (2012). Brassinosteroid regulates stomatal development by GSK3-mediated inhibition of a MAPK pathway. Nature 482, 419. doi: 10.1038/nature10794
Lampard, G. R., Lukowitz, W., Ellis, B. E., Bergmann, D. C. (2009). Novel and expanded roles for MAPK signaling in arabidopsis stomatal cell fate revealed by cell type-specific manipulations. Plant Cell 21, 3506–3517. doi: 10.1105/tpc.109.070110
Lee, J. S., Hnilova, M., Maes, M., Lin, Y. C., Putarjunan, A., Han, S. K., et al. (2015). Competitive binding of antagonistic peptides fine-tunes stomatal patterning. Nature 522, 439–443. doi: 10.1038/nature14561
Lee, J. S., Kuroha, T., Hnilova, M., Khatayevich, D., Kanaoka, M. M., McAbee, J. M., et al. (2012). Direct interaction of ligand-receptor pairs specifying stomatal patterning. Genes Dev. 26, 126–136. doi: 10.1101/gad.179895.111
Li, H., Song, J. B., Zhao, W. T., Yang, Z. M. (2013). AtHO1 is involved in iron homeostasis in an NO-dependent manner. Plant Cell Physiol. 54, 1105–1117. doi: 10.1093/pcp/pct063
Liu, Y. H., Xu, S., Ling, T. F., Xu, L. L., Shen, W. B. (2010). Heme oxygenase/carbon monoxide system participates in regulating wheat seed germination under osmotic stress involving the nitric oxide pathway. J. Plant Physiol. 167, 1371–1379. doi: 10.1016/j.jplph.2010.05.021
Liu, K., Xu, S., Xuan, W., Ling, T., Cao, Z., Huang, B., et al. (2007). Carbon monoxide counteracts the inhibition of seed germination and alleviates oxidative damage caused by salt stress in oryza sativa. Plant Sci. 172, 544–555. doi: 10.1016/j.plantsci.2006.11.007
Lukowitz, W., Roeder, A., Parmenter, D., Somerville, C. (2004). A MAPKK kinase gene regulates extra-embryonic cell fate in arabidopsis. Cell 116, 0–119. doi: 10.1016/S0092-8674(03)01067-5
Lv, Q., Wang, L., Wang, J. Z., Li, P., Chen, Y. L., Du, J., et al. (2017). SHB1/ HY1 alleviates excess boron stress by increasing BOR4 expression level and maintaining boron homeostasis in arabidopsis roots. Front. Plant Sci. 8. doi: 10.3389/fpls.2017.00790
MacAlister, C. A., Ohashi-Ito, K., Bergmann, D. C. (2007). Transcription factor control of asymmetric cell divisions that establish the stomatal lineage. Nature 445, 537–540. doi: 10.1038/nature05491
Ma, X., Zhang, Q., Zhu, Q., Liu, W., Chen, Y., Qiu, R., et al. (2015). A robust CRISPR/Cas9 system for convenient, high-efficiency multiplex genome editing in monocot and dicot plants. Mol. Plant 8, 1274–1284. doi: 10.1016/j.molp.2015.04.007
Muramoto, T., Kohchi, T., Yokota, A., Hwang, I., Goodman, H. M. (1999). The arabidopsis photomorphogenic mutant hy1 is deficient in phytochrome chromophore biosynthesis as a result of a mutation in a plastid heme oxygenase. Plant Cell 11, 335–348. doi: 10.1105/tpc.11.3.335
Muramoto, T., Tsurui, N., Terry, M. J., Yokota, A., Kohchi, T. (2002). Expression and biochemical properties of a ferredoxin-dependent heme oxygenase required for phytochrome chromophore synthesis. Plant Physiol. 130, 1958–1966. doi: 10.1104/pp.008128
Nadeau, J. A., Sack, F. D. (2002). Control of stomatal distribution on the Arabidopsis leaf surface. Science 296, 1697–1700. doi: 10.1126/science.1069596
Ohashi-Ito, K., Bergmann, D. C. (2006). Arabidopsis FAMA controls the final proliferation/differentiation switch during stomatal development. Plant Cell 18, 2493–2505. doi: 10.1105/tpc.106.046136
Pillitteri, L. J., Sloan, D. B., Bogenschutz, N. L., Torii, K. U. (2007). Termination of asymmetric cell division and differentiation of stomata. Nature 445, 501–505. doi: 10.1038/nature05467
Pillitteri, L. J., Torii, K. U. (2012). Mechanisms of stomatal development. Annu. Revi. Plant Biol. 63, 591–614. doi: 10.1186/2041-9139-3-11
Samma, M. K., Shen, W., Wang, J., et al. (2014). Interaction between HY1 and H2O2 in auxin-induced lateral root formation in arabidopsis. Plant Mol. Biol. 85, 49–61. doi: 10.1007/s11103-013-0168-3
She, X. P., Song, X. G. (2008). Carbon monoxide-induced stomatal closure involves generation of hydrogen peroxide in vicia faba guard cells. J. Integr. Plant Biol. 50, 1539–1548. doi: 10.1111/j.1744-7909.2008.00716.x
Song, X. G., She, X. P., Zhang, B. (2008). Carbon monoxide-induced stomatal closure in Vicia faba is dependent on nitric oxide synthesis. Physiol. Plant. 132, 514–525. doi: 10.1111/j.1399-3054.2007.01026.x
Sugano, S. S., Kajita, R., Miyazaki, A., Okawa, K., Tamai, A., Mori, M., et al. (2010). Stomagen positively regulates stomatal density in arabidopsis. Nature 463, 241–244. doi: 10.1038/nature08682
Vatén, A., Soyars, C. L., Tarr, P. T., Nimchuk, Z. L., Bergmann, D. C. (2018). Modulation of asymmetric division diversity through cytokinin and SPEECHLESS regulatory interactions in the stomatal lineage. Dev. Cell 47, 53–66. doi: 10.1016/j.devcel.2018.08.007
Wang, M., Liao, W. (2016). Carbon monoxide as a signaling molecule in plants. Front. Plant Sci. 7. doi: 10.3389/fpls.2016.00572
Wang, H., Ngwenyama, N., Liu, Y., Walker, J. C., Zhang, S. (2007). Stomatal development and patterning are regulated by environmentally responsive mitogen-activated protein kinases in arabidopsis. Plant Cell 19, 63–73. doi: 10.1105/tpc.106.048298
Wang, S., Zhou, Z., Rahiman, R., Lee, G. S. Y., Yeo, Y. K., Yang, X., et al. (2021). Light regulates stomatal development by modulating paracrine signaling from inner tissues. Nat. Commun. 12, 3403. doi: 10.1038/s41467-021-23728-2
Wei, Y. Y., Zheng, Q., Liu, Z. P., Yang, Z. M. (2011). Regulation of tolerance of chlamydomonas reinhardtii to heavy metal toxicity by heme oxygenase-1 and carbon monoxide. Plant Cell Physiol. 52, 1665–1675. doi: 10.1093/pcp/pcr102
Wu, M. Z., Huang, J. J., Xu, S., Ling, T. F., Xie, Y. J., Shen, W. B. (2010). Haem oxygenase delays programmed cell death in wheat aleurone layers by modulation of hydrogen peroxide metabolism. J. Exp. Bot. 62, 235–248. doi: 10.1093/jxb/erq261
Xie, Y. J., Mao, Y., Duan, X. L., Zhou, H., Lai, D. W., Zhang, Y. H., et al. (2016). Arabidopsis HY1-modulated stomatal movement: An integrative hub is functionally associated with ABI4 in dehydration-induced ABA responsiveness. Plant Physiol. 170, 1699–1713. doi: 10.1104/pp.15.01550
Xie, Y., Xu, S., Han, B., Wu, M. Z., Shen, W. B. (2011). Evidence of arabidopsis salt acclimation induced by up-regulation of HY1 and the regulatory role of RbohD-derived reactive oxygen species synthesis. Plant J. 66, 280–292. doi: 10.1111/j.1365-313X.2011.04488.x
Xuan, W., Zhu, F. Y., Xu, S., Huang, B. K., Ling, T. F., Qi, J. Y., et al. (2008). The heme oxygenase/carbon monoxide systemis involved in the auxin-induced cucumber adventition rooting process. Plant Physiol. 148, 881–893. doi: 10.1104/pp.108.125567
Yang, L., Ji, J., Wang, H., Harris-Shultz, K. R., Abd Allah, E. F., Luo, Y., et al. (2016). Carbon monoxide interacts with auxin and nitric oxide to cope with iron deficiency in arabidopsis. Front. Plant Sci. 7. doi: 10.3389/fpls.2016.00112
Yang, M., Sack, F. D. (1995). The too many mouths and four lips mutations affect stomatal production in arabidopsis. Plant Cell 7, 2227–2239. doi: 10.1105/tpc.7.12.2227
Zhai, Q. Z., Li, C. B., Zheng, W. G., Wu, X., Zhao, J., Zhou, G., et al. (2007). Phytochrome chromophore deficiency leads to overproduction of jasmonic acid and elevated expression of jasmonate-responsive genes in arabidopsis. Plant Cell Physiol. 48, 1061–1071. doi: 10.1093/pcp/pcm076
Zhang, J. Y., He, S. B., Yang, H. Q. (2014). Auxin inhibits stomatal development through MONOPTEROS repression of a mobile peptide gene STOMAGEN in mesophyll. Proc. Natl. Acad. Sci. U.S.A. 111, E3015–E3023. doi: 10.1073/pnas.1400542111
Zhang, H. M., Li, Y., Pu, M. N., Xu, P., Liang, G., Yu, D. Q. (2019). Positive regulation of IRON DEFICIENCY RESPONSE2 (OsPRI2) and OsPRI3 are involved in the maintenance of fe homeostasis. Plant Cell Environ. 43, 261–274. doi: 10.1111/pce.13655
Keywords: Arabidopsis thaliana, stomatal development, carbon monoxide, EPF2, STOMAGEN
Citation: Weng X, Zhu L, Yu S, Liu Y, Ru Y, Zhang Z, He Z, Zhou L and Chen X (2022) Carbon monoxide promotes stomatal initiation by regulating the expression of two EPF genes in Arabidopsis cotyledons. Front. Plant Sci. 13:1029703. doi: 10.3389/fpls.2022.1029703
Received: 27 August 2022; Accepted: 21 October 2022;
Published: 11 November 2022.
Edited by:
Luciana Renna, University of Florence, ItalyReviewed by:
Marie Hronkova, Biology Centre of the Czech Academy of Sciences and South Bohemia University, CzechiaNoelia Pastor-Cantizano, University of Valencia, Spain
Copyright © 2022 Weng, Zhu, Yu, Liu, Ru, Zhang, He, Zhou and Chen. This is an open-access article distributed under the terms of the Creative Commons Attribution License (CC BY). The use, distribution or reproduction in other forums is permitted, provided the original author(s) and the copyright owner(s) are credited and that the original publication in this journal is cited, in accordance with accepted academic practice. No use, distribution or reproduction is permitted which does not comply with these terms.
*Correspondence: Xiaolan Chen, Y2hlbnhpYW9sYW5AeW51LmVkdS5jbg==; Lijuan Zhou, emhvdWxpanVhbjEyMDFAMTYzLmNvbQ==
†These authors have contributed equally to this work