- 1Key Laboratory of Plant Nutrition and Fertilizer, Ministry of Agriculture and Rural Affairs/Institute of Agricultural Resources and Regional Planning, Chinese Academy of Agricultural Sciences, Beijing, China
- 2Institute of Geographic Sciences and Natural Resources Research, Chinese Academy of Sciences, Beijing, China
- 3Institute of Plant Nutrition and Resources, Beijing Academy of Agriculture and Forestry Sciences, Beijing, China
Organic manure has been proposed to substitute part of the chemical fertilizers. However, past research was usually conducted in regimes with excessive nitrogen (N) fertilization, which was not conducive to the current national goal of green and sustainable development. Therefore, exploring the potential of organic fertilizer substitution for mineral N fertilizer under regimes with reduced N inputs is important to further utilize organic fertilizer resources and establish sustainable nutrient management recommendations in the winter wheat (Triticum aestivum L.) – summer maize (Zea mays L.) rotation system in North-central China. In this study, a 4-year field experiment was conducted to investigate the effects of different chicken manure substitution ratios on crop yield, N recovery efficiency (REN), soil N and soil organic matter contents, to clarify the optimal organic substitution ratio of N fertilizer under reduced N application (from 540 kg N ha−1 year−1 to 400 kg N ha−1 year−1). Six substitution ratios were assessed: 0%, 20%, 40%, 60%, 80% and 100% under 200 kg N ha−1 per crop season, respectively, plus a control with no N application from chemical fertilizer or chicken manure. Results showed that the highest yield was achieved under the 20% substitution ratio treatment, with 1.1% and 2.3% higher yield than chemical N alone in wheat season and maize seasons, respectively. At the chicken manure substitution ratios of 20% in wheat season and 20%-40% in maize season, the highest REN reached to 31.2% and 26.1%, respectively. Chicken manure application reduced soil residual inorganic N with increasing substitution ratio. All organic substitution treatments increased soil organic matter and total N content. Implementing 20% organic substitution in wheat season and 20%-40% in maize season under the reduced N application regime in the North-central China is therefore recommended in order to achieve high crop yields and REN, improve soil fertility and enhance livestock manure resource utilization.
1 Introduction
As a large agricultural producer, China feeds 22% of the world’s population with 9% of its arable land (FAO, 2020). As a by-product of animal husbandry, a large amount of livestock and poultry manure has been generated. At present, China’s agriculture produces 5.7 billion tons of organic resources annually, of which livestock and poultry manure accounts for 66.7% (Niu and Ju, 2017). These manure resources are high-quality nutritional resources for agricultural production, but the utilization rate is less than 40% as reported by the Ministry of Agriculture and Rural Affairs of the People’s Republic of China (2017). Surveys showed that less than 10% of the arable land in China was applied with organic fertilizers (Chen et al., 2018). Therefore, improving the utilization rate of agricultural wastes and organic materials is a necessary way to achieve the development of green agriculture in the future.
Organic fertilizers are rich in nutrients and contain a variety of organic acids and peptides that help to improve soil fertility and crop yield (Valdez Velarca, 2016). For example, addition of exogenous carbon with organic fertilizers can promote the formation of soil aggregates and provide a favorable environment for microbial activities (Tripathi et al., 2014). Application of organic fertilizers can enrich mineralized N source and increase the soluble N content of the soil (Farrell et al., 2011; Wang et al., 2013), and the free amino acids produced by soil microbial decomposition microorganisms can be directly absorbed by crops (Näsholm et al., 2000). Compared with chemical fertilizers that quickly release nutrients into the soil, organic fertilizers can continuously supply nutrients, improve soil structure, promote nutrient cycling and transformation, and eventually enhance soil and crop productivity.
However, some disadvantages of organic fertilizers, such as a high volume for transportation and low nutrient contents resulting in slow crop response, lead farmers to focus more on chemical fertilization. To achieve high yields, chemical fertilizer application rate in China has increased 4.1 times over the past 40 years, with a 48% increase in N fertilizer (National Bureau of Statistics of China, 2020). This results in a series of problems, such as soil quality degradation, low nutrient use efficiency and serious environmental pollution (Galloway et al., 2008). One-third of the global total N pollution was caused by China (West et al., 2014). Using organic fertilizers to substitute part of the chemical fertilizers (hereafter called organic fertilizer substitution) has been suggested as an important measure to reduce chemical fertilizer inputs and mitigate their environmental consequences. Several previous studies have confirmed that long-term organic fertilizer substitution can improve soil fertility, and achieve high and stable crop yields compared to chemical fertilizer application only (Mi et al., 2016; Xie et al., 2016; Ding et al., 2018). In addition, organic fertilizer substitution was found to reduce soil inorganic N leaching (Elbl et al., 2020).
Improving fertilization techniques and reducing chemical N fertilizer application through combined organic-inorganic fertilization has become a pressing research topic in soil science and plant nutrition, as well as a trend for efficient use of agricultural resources in China’s agricultural development. The role of combined organic-inorganic fertilization in reducing chemical fertilizer application and increasing yields has been recognized (Zhang et al., 2018). However, many studies have focused on organic fertilizer substitution under high fertilizer application rates (Zhao et al., 2016; Xu et al., 2021), with limited research on reducing total nutrient input.
As an important grain production region in China, the North-central China has 18 million hectares of farmland, and its wheat and maize production is crucial to ensure national food security. Nevertheless, the N fertilizer application rate in this region is the highest among grain crops in China (Ju et al., 2009). The region is also a major livestock and poultry production region, for example with Handan as the second biggest city of chicken industry in China. Optimal fertilization is now receiving great attention as an essential measure to address the overuse of chemical fertilizers. In this context, chicken manure is a good alternative nutrient source for crop production, however, how to apply chicken fertilizer under reduced or optimized fertilization conditions needs further exploration. In this study, therefore, we investigated the effects of different ratios of chicken manure substitution under a regime of reduced N input in a winter wheat-summer maize rotation system in the North-central China. The objectives were to (1) assess the substitution effects on crop yield and N use efficiency, and on soil organic matter and soil N changes, and (2) identify the optimal ratio of chicken manure substitution.
2 Materials and methods
2.1 Study site and experimental design
A field experiment of winter wheat-summer maize rotation was conducted from June 2016 to June 2020 in Handan County, Hebei Province, North-central China (36°45’ 25’’ N, 115°19’ 21’’ E). The region has a temperate climate, and air temperature and precipitation were measured at an automatic meteorological station near the study site (Supplementary Figure 1). The soil type is fluvo-aquic soil, and the initial soil chemical properties at the 0-20 cm depth were: organic matter 1.83%, soil total N 0.12%, pH 8.56 (soil:water 1:2.5), Olsen-P 21.9 mg kg−1, and ammonium acetate (NH4OAc)-K 175 mg kg−1.
A fertilizer application survey with local farmers showed that N fertilizer application rate was up to 540 kg ha−1 year−1. In this study, the N application was reduced by 25% than farmers’ conventional rate, i.e., at 200 kg N ha−1 per crop season (400 kg ha−1 year−1). The experiment was set up with six different ratios of chicken manure substitution for chemical N fertilizer: 0%, 20%, 40%, 60%, 80% and 100%, which were noted as M0%, M20%, M40%, M60%, M80% and M100%, respectively. Moreover, a treatment with no N application (N0) was used as control (Supplementary Table 1). The field experiment employed a randomized block design with three replications, and each plot had an area of 30 m2. The amounts of P and K fertilizers applied were 33 kg P ha−1 and 75 kg K ha−1, respectively. The organic fertilizer used was chicken manure that had a pH 8.01 and contained 32.8% organic matter, 1.94% total N, 2.44% total P, and 1.45% total K on a dry matter basis. All fertilizers were applied as basal fertilizer in the maize season, and in two separate applications of 50%:50% during the basal and jointing stages in wheat season. Chicken manure was spread evenly on the soil surface and turned into the soil before planting each season. Other management measures for controlling weeds, pests, and diseases, were taken by spraying of herbicides (Tribenuron-methyl and 2.4-D for wheat; Atrazine and Propisochlor for maize) and insecticides (Carbendazim, Acephate, Omethoate, and Tradimefon for wheat; Carbofuran for maize) in accordance with the farmers’ conventional practices.
2.2 Sampling and chemical analysis
At harvest of maize, two rows of plants in the middle of each plot were collected to determine maize yield and converted to 15.5% of the standard moisture content for the final maize grain yield. Another five plants were collected from each plot and separated into straw and grain to determine the harvest index and straw weight. While for wheat, three representative 1 m × 1 m subplots in each plot were sampled to determine grain yield and converted to 13.5% of standard moisture content for the final wheat grain yield. Another sample of wheat plants in a 50 cm long row was collected randomly from each plot, and separated into straw and grain to determine the harvest index and straw weight.
The harvested straw and grain from subsamples were dried to constant weight at 60°C for 72 h to determine dry matter weight, and then ground and digested with H2SO4-H2O2 using a Kjeldahl method to determine N concentration for straw and grain. The soils from the 0-100cm depth at 20 cm per layer were sampled before sowing and after harvest in each plot to determine soil inorganic N concentrations (NO3-N+NH4-N) using flow injection analyzer (model AA3 -A001-02E, Brambleau, Germany). Soil water content was measured by oven drying the samples at 105°C. Soil total N and organic carbon were determined by the Kjedahl method and the dichromate oxidation method, respectively (Chinese Society of Soil Science, 2000).
2.3 Calculation and statistical analysis
The accumulated recovery efficiency (REN) of N fertilizer application (include chemical and organic N) was used to assess N use efficiency as follows:
where i is the season (i = 1,2,…), with maize or wheat counted as one season separately in a rotation; UF and UCK represent the total N uptake (kg ha−1) in the aboveground biomass in the treatments with N application and control, respectively; and FN is the N fertilizer application rate (kg ha−1).
Soil organic carbon (SOC) storage (Mg ha−1) in the topsoil layer (0-20 cm depth) was calculated as:
where T is the SOC storage (Mg ha−1); BD is the soil bulk density (g cm−3); C is SOC content (g kg−1); and h (cm) is the soil thickness of top layer.
The amount of SOC sequestration (Mg ha−1) and the SOC sequestration rate (Mg ha−1 year−1) were calculated as follows:
where SOCf and SOCi are the SOC stocks in June 2020 and June 2016, respectively.
A one-way ANOVA analysis was conducted to evaluate the effects of chicken manure substitution ratio on maize and wheat yield, REN, soil total N and soil organic matter. Mean values of variables in each treatment were compared using Duncan’s multiple comparisons to identify significant differences among treatments at a significant level of P<0.05 using IBM SPSS Statistics 19 software (IBM Corp., Armonk, NY, USA). Graphs were plotted using the SigmaPlot software (SigmaPlot 14.0).
3 Results
3.1 Grain yield
All treatments with chicken manure substitution significantly increased wheat yield compared to N0 treatment (P<0.001). The M20% treatment obtained the highest average yield across all seasons with an average increase of 1.1% compared to the treatment with only chemical fertilizer (M0%). However, there were significant difference in wheat yields among the treatments with different substitution ratios. With increasing substitution ratios, wheat yields decreased by 1.5%, 2.6%, 8.9%, and 16.3% for M40%, M60%, M80%, and M100% treatments, respectively, compared to M0%. This indicated that high N fertilizer substitution ratios did not promote wheat yield under the reduced N input regime (Table 1).
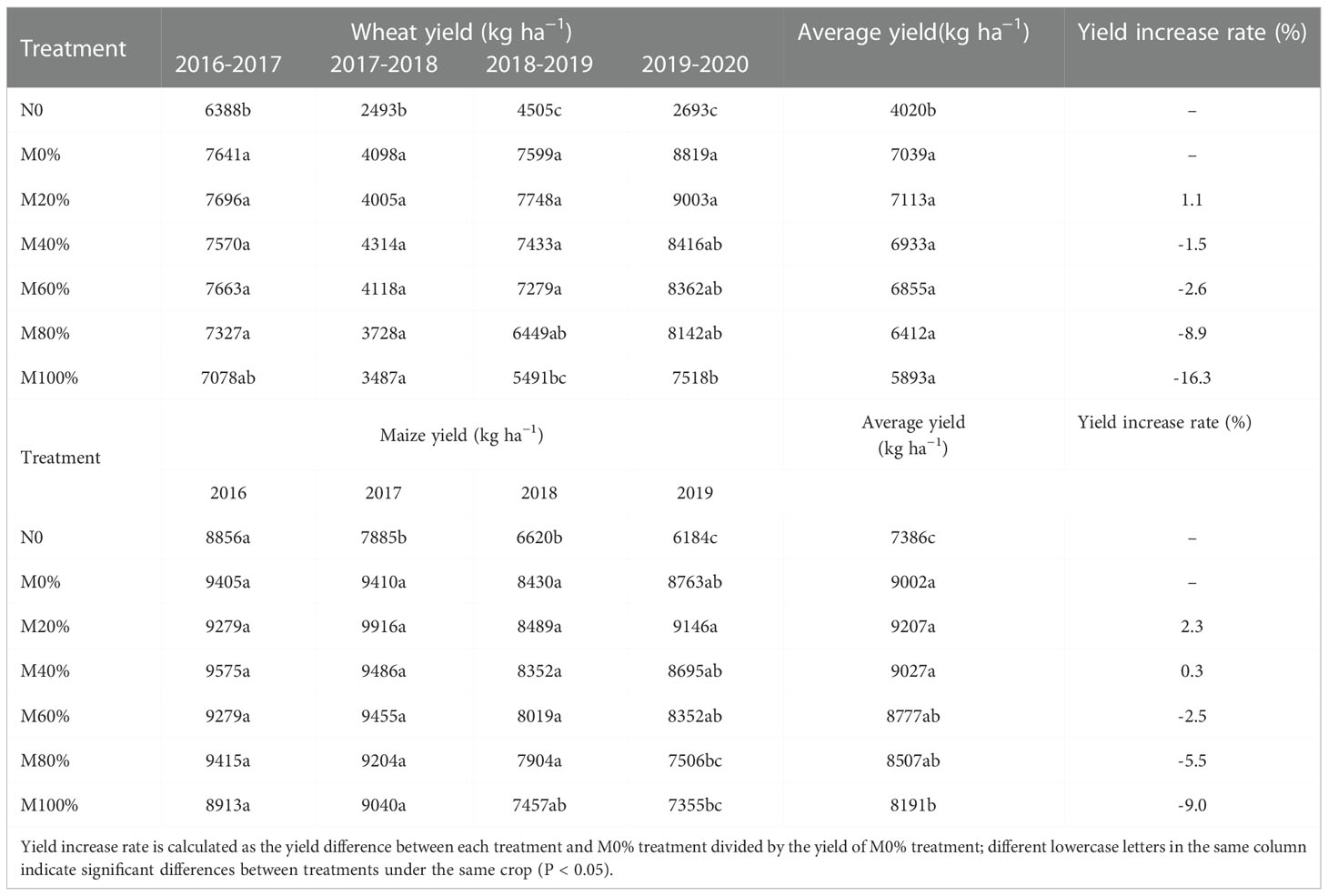
Table 1 Yield of wheat and maize under treatments with different ratios of organic substitution for mineral N fertilizer.
For the maize season, although each treatment with chicken manure substitution increased maize yield compared to N0 treatment, maize yield remained high level without N application, reaching a 4-year average of 7386 kg ha−1 (Table 1). In terms of average maize yield across all seasons, the highest value was also observed in the M20% treatment, with a 2.3% increase compared to M0%. However, unlike wheat, the M40% and M0% treatments had similar yields. Although in 2016 and 2017 maize yields under the M40% treatment increased by 1.8% and 0.8%, respectively, compared to M0% treatment, the average maize yield increased by 0.3% across all seasons. In contrast, maize yields under the other chicken manure alternative treatments were lower than the M0% treatment, with reductions of 2.5%, 5.5% and 9.0% under M60%, M80% and M100% treatments, respectively.
The average annual yield of the wheat-maize rotation system showed a trend of increasing and then decreasing as the manure substitution ratio increased. This indicates that the higher percentage of chicken manure substitution for chemical fertilizer is not more effective in increasing crop yield. The results of this study showed that in the wheat-maize system, the M20% treatment had the highest average annual yield of 16,320 kg ha−1 with 25% less N application compared to farmers’ practices and the yield was 1.7% higher than that of M0%. In contrast, the lowest yield was observed in the M100% treatment with only 14,085 kg ha−1, which was 12.2% lower than that of M0%.
3.2 Nitrogen use efficiency
During the wheat season, REN tended to increase and then decrease with increasing chicken manure substitution ratio (Figure 1A). The REN reached the maximum at M40%, M20%, M40%, and M20% in the four wheat seasons, respectively. The highest REN of 31.2% was found both in the M20% and M40% treatments, which was 0.6% higher than in M0%, but the difference was not significant. The REN showed a decreasing trend when the chicken manure substitution ratio was greater than 60% and reached a significant reduction level when it was greater than 80%. Therefore, increasing the chicken manure substitution ratio of chemical N fertilizer under optimal N application rate did not result in a significant increase in REN, but an optimal substitution ratio exists to achieve highest yield.
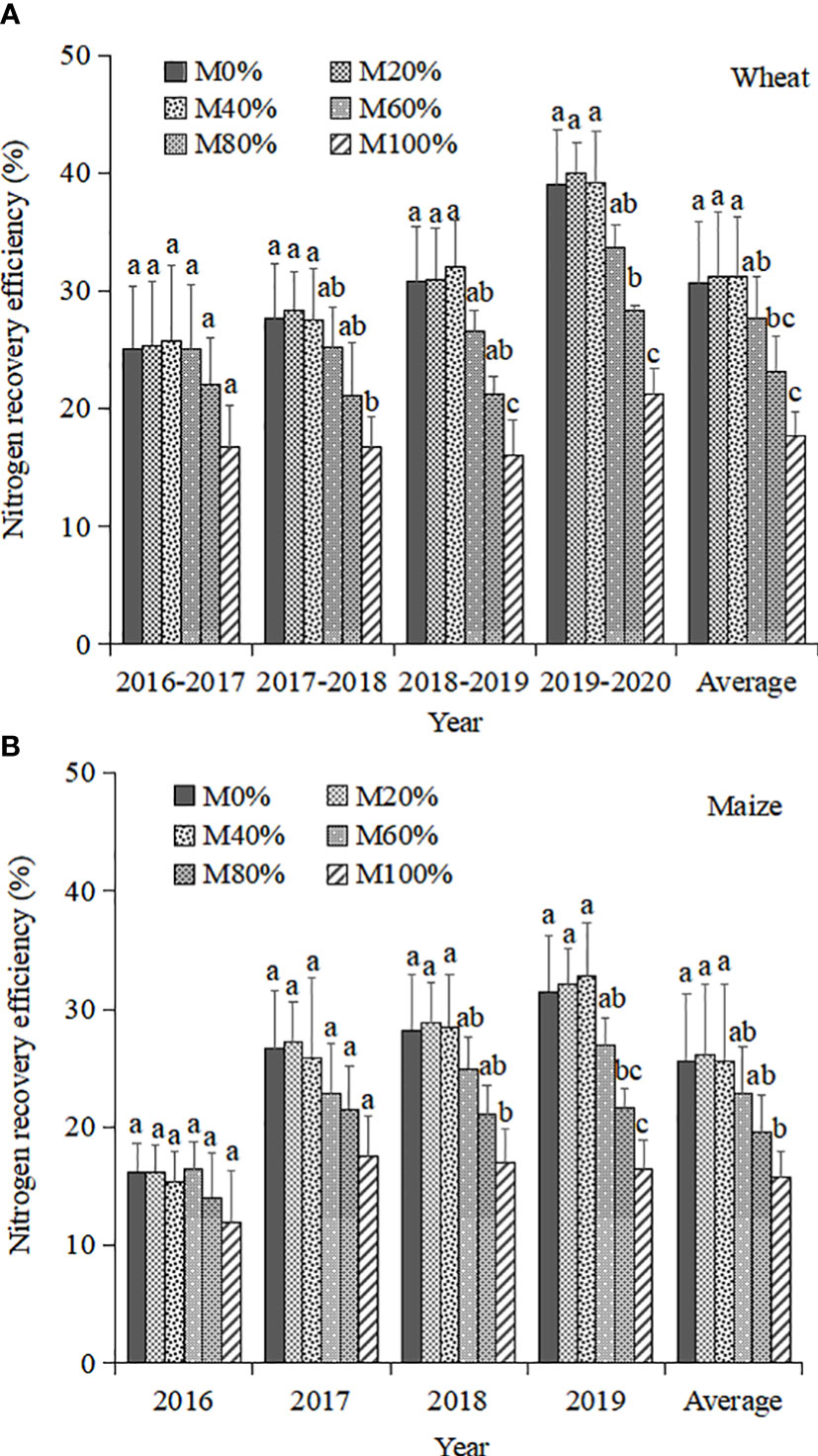
Figure 1 Nitrogen recovery efficiency of wheat (A) and maize (B) under treatments with different ratios of chicken manure substitution for mineral nitrogen fertilizer.
The REN in the maize season followed the same trend as in the wheat season (Figure 1B). The highest REN was achieved at M60%, M20%, M20%, and M40% in the 4-year maize seasons, respectively. Across all maize seasons, on average, the highest REN was 26.1% and was achieved in the M20% treatment, which was 0.5% higher than the M0% treatment, but with no significant difference. A significant decrease in REN occurred when the chicken manure substitution ratio was greater than 40%. The reason for the lower REN in maize season compared to wheat season was that the experiment started in maize season, and thus the high initial soil N content resulted in a significantly lower REN in the first maize season (2016) than in other years.
The higher REN was attributed to the increase in N accumulation. Compared to M0%, higher N uptake could be maintained under M20% and M40% treatments, with the highest total N uptake across four-year under M20% treatment (Supplementary Figure 2). On the contrary, the crop N uptake gradually decreased when the substitution ratio was greater than 40%. Compared with the M0% treatment, the cumulative N uptake under the M100% treatment was 12.9% and 9.9% lower in the wheat and maize seasons, respectively (Supplementary Figure 2). This indicates that chemical N fertilizer is necessary to maintain soil N supply and meet crop nutrient requirements.
3.3 Soil nutrients and organic matter
Soil residual mineral N at harvest showed a decreasing trend with increasing chicken manure substitution ratio (Figure 2). All substitution ratios reduced soil residual mineral N at harvest compared to M0% treatment, with the smallest reduction of 61.3% under M100% treatment. The mineral N content of the different soil layers also presented a tendency to decrease with increasing chicken manure substitution percentage, especially in the 0−60 cm depth. The treatments significantly reduced the mineral N content in the 20−40 cm soil layer compared to the initial soil, by 20.3%, 32.8%, 41.7%, 50.2%, 62.8%, and 78.0% under the M0%, M20%, M40%, M60%, M80%, and M100% treatments, respectively. This indicates that a reasonable chicken manure substitution ratio can reduce soil mineral N residues and prevent N leaching.
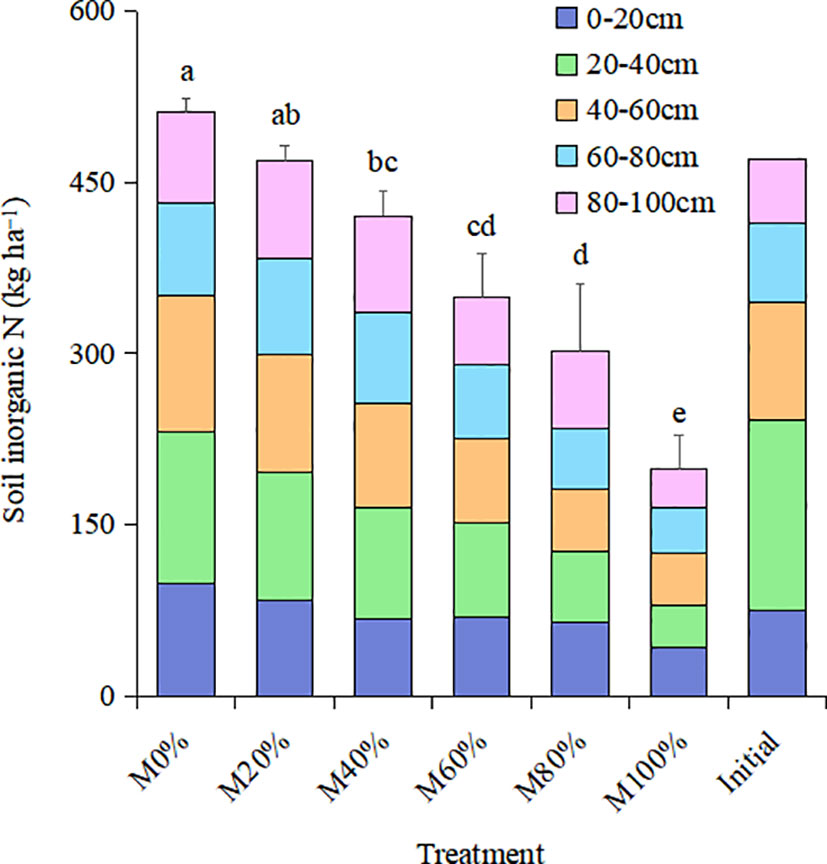
Figure 2 Soil inorganic nitrogen at harvest in 2020 at different soil layers with different organic substitution ratios of nitrogen fertilizer.
Chicken manure substitution for chemical N fertilizer reduced the soil mineral N content but increased soil total N content (Table 2). The soil total N content at each chicken manure substitution ratio ranged from 0.125% (M0%) to 0.163% (M100%), and was significantly higher than that of M0% treatment except for M20%. The soil total N content increased by 5.6%, 15.2%, 20.0%, 21.6%, and 30.4% in the treatment ranging from low to high chicken manure substitution ratios compared to M0% treatment.
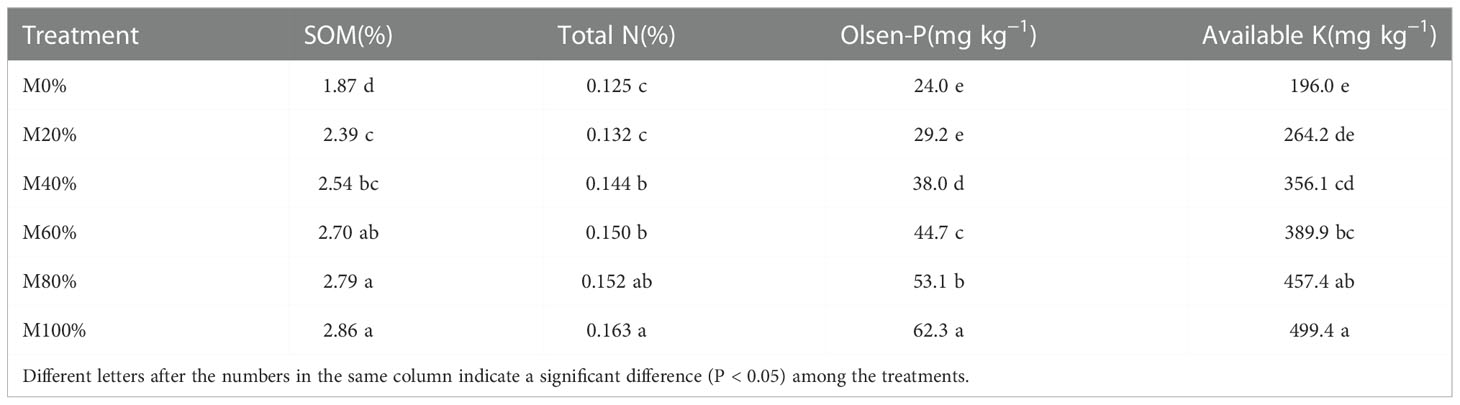
Table 2 Soil organic matter (SOM), total N, Olsen-P and available K at harvest in 2020 for different organic substitution ratios of N fertilizer.
In addition, chicken manure substitution also increased the content of Olsen-P and available K in each treatment (Table 2), with similar trends to soil total N. Except for M20%, the soil Olsen-P and available K contents were significantly higher under each chicken manure substitution treatment compared to the chemical fertilizer treatment alone. As compared to M0%, the soil Olsen-P content increased by 21.6%, 58.3%, 86.3%, 121.3%, and 159.6%, and the soil available K content increased by 34.8%, 81.7%, 98.9%, 133.4%, and 154.8%, respectively, with an increase in chicken manure substitution ratio.
Application of chicken manure significantly increased the soil organic matter content for each substitution ratio compared with M0% treatment, and the soil organic matter content increased with the increase in chicken manure substitution percentage (Table 2). The highest soil organic matter content of 2.86% was observed in the M100% treatment. All treatments with fertilizers increased soil organic matter content than the N0 treatment (1.77%), with the increase ranging from 5.4% in M0% to 61.2% in M100%. Compared with M0% (1.87%), the soil organic matter increased by 27.8%, 35.8%, 44.4%, 49.2%, and 52.9%, respectively, as the chicken manure substitution ratio ranged from 20% to 100%. Among the treatments, organic matter content was not significant between M20% (2.39%) and M40% (2.54%) treatments, and among M60% (2.70%), M80% (2.79%) and M100% (2.86%) treatments. The increase in soil organic matter was due to C inputs, and a significantly positive relationship was found between soil organic carbon (SOC) and C inputs (Figure 3). SOC sequestration rate per year increased with the increase of chicken manure substitution ratio in this study, from 0.23 Mg ha−1 year−1 in the M20% treatment to 0.42 Mg ha−1 year−1 in the M100% treatment.
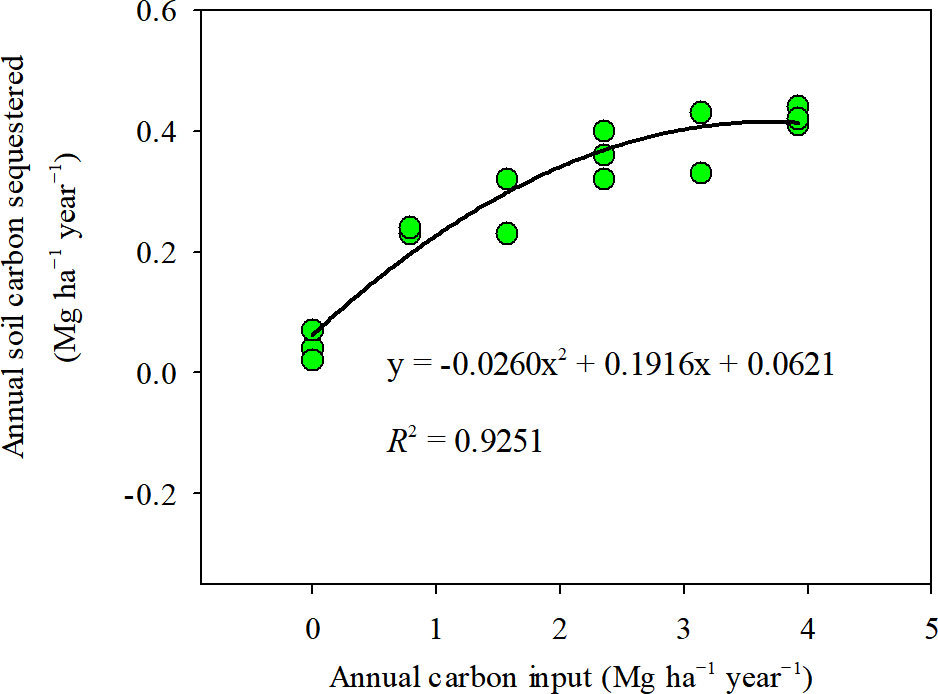
Figure 3 Relationship between annual soil organic carbon sequestration rate and annual carbon input in the wheat-maize rotation system.
3.4 Nitrogen reduction potential under reduced fertilizer application
From the average annual yield of the crop rotation system, crop yield decreased with the increase of the chicken manure substitution ratio, and only the M20% treatment showed a certain advantage in yield compared with conventional chemical fertilizer (M0%) by 1.7% increase (not significant). This indicated that the high chicken manure substitution ratio for chemical fertilizers played a negative effect on yield. Therefore, it is important to give a reasonable range of substitution under the reduced N input regime. The analysis of the relationship between the substitution ratio and the average annual yield, REN and soil organic matter content at the end of the experiment in the rotational system showed a significant quadratic curve (Figure 4). Using the highest yield obtained as a benchmark, the chicken manure substitution ratio in this study was 17.2% under the 200 kg N ha−1 per crop season, which was estimated to result in a yield of 16192 kg ha−1 year−1, accumulated REN of 39.9%, and an increase in the soil organic matter by 19.8%. However, the results obtained at this point were still lower than the performance of the M20% treatment, which was mainly limited by the number of treatments with low substitution ratios (below 20%). Therefore, in this study, considering the yield and REN, it is possible to replace 20% of chemical N fertilizer under the reduced N input regime. Of course, a reasonable chicken manure substitution ratio will be further increased with the continuous mineralization of the previous organic N inputs, and the current 20% substitution ratio is also the result of a 4-year field experiment.
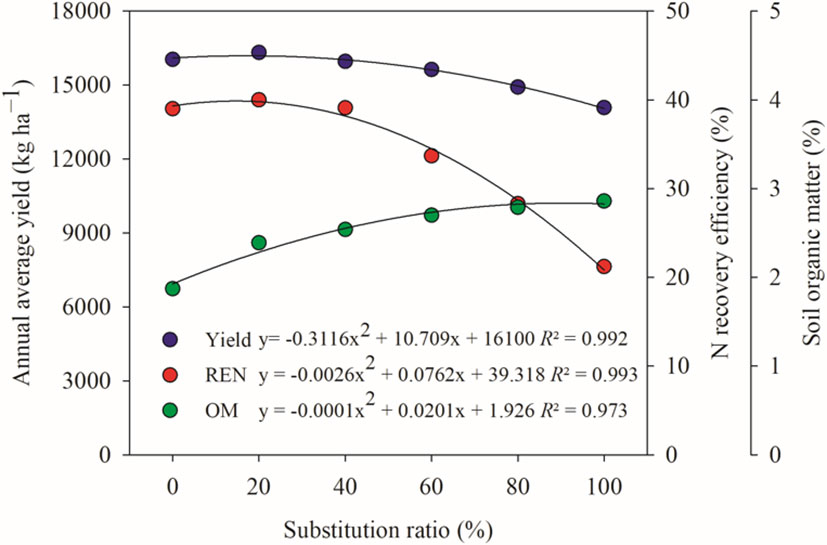
Figure 4 Relationship between different N fertilizer substitution ratios and average annual yield, N recovery efficiency (REN) and soil organic matter (OM) content in the wheat-maize rotation system.
4 Discussion
In the current nutrient management, N fertilizer still plays the greatest role in increasing yields in wheat and maize rotation systems. However, excessive N fertilizer application has caused negative impacts on soil and the environment. Organic fertilizer substitution, as one of the important measures for reducing N fertilizer, has played a positive role in chemical N fertilizer reduction and crop yield improvement (Yan et al., 2015). Organic fertilizer application not only enriches the soil with a large amount of nutrients, but also brings exogenous microorganisms into the soil (McGill et al., 1986), promotes the formation and decomposition of soil organic matter (Guo et al., 2016), accelerates nutrient cycling and contributes to crop yield increase (Han et al., 2021). Meanwhile, some small molecules from organic fertilizer application can serve to delay organ senescence in wheat and maize, prolong filling time, increase ear grain weight, and provide balanced nutrients for crops during later nutritional and reproductive growth, thus achieving yield increase (Redding et al., 2016; Sarkar et al., 2021).
Numerous studies have shown that organic fertilizers have great potential to reduce chemical N fertilizer and increase crop yields under current high N application. For example, organic fertilizer substitution of chemical N up to 50%-70% resulting in yield increase of 40.5% for maize (Xu et al., 2021), 23.3% for wheat (Li et al., 2022), 8.3% for early rice and 9.6% for late rice (Dai et al., 2021). However, most of these studies were conducted on organic fertilizer inputs under the premise of higher chemical N application, while with the implementation of various policies, limited standards and application of advanced technologies, N fertilizer use in China is on a decreasing trend (FAO, 2021)and will eventually return to reasonable levels. The study of organic substitution under reduced application is important for the sustainability of chemical fertilizer reduction. This study also showed that chemical N fertilizer can be replaced by 20% in wheat season and by 20%-40% in maize season with organic fertilizer under optimized N fertilizer application rate, meanwhile ensuring crop yield, which is consistent with some previous studies (Zhang et al., 2010; Duan et al., 2014). However, it has also been shown that organic fertilizers application with chemical fertilizers did not increase the yield of wheat and maize (Xin et al., 2017), and the main reason for this difference was that the N in organic fertilizer was not mineralized during the experimental period (Zhao et al., 2010). Compared to chemical fertilizers that have a fast-acting nature, a high ratio of organic fertilizer inputs would reduce crop nutrient uptake because of its slow nutrient release rate, resulting in inadequate supply of N in the early stage (Pang and Letey, 2000; Berry et al., 2002). In addition to chemical N input, the major part of soil available N source is from mineralization (Nyawade et al., 2020). As chicken manure is readily mineralized to an available inorganic N form, a combination of organic and inorganic applications provides a balanced and continuous supply of nutrients for the crop life cycle. Therefore, a certain amount of available nutrients at crop early growth period is necessary to maintain crop yield and support nutrient uptake (Paul et al., 2016).
The percentage of organic fertilizer substitution for chemical N fertilizer should be significantly lower under the regime of reduced fertilizer application compared to the regime of high chemical fertilizer input, otherwise it will negatively affect the crop yield. In this study, the yield of wheat−maize rotation showed a decreasing trend with increasing percentage of chicken manure substitution. However, the chicken manure substitution ratio in maize season can reach 40%, which is twice that of wheat season. The difference was mainly due to the increased mineralization of organic N by the simultaneous rain and heat in maize season.
It is indisputable that organic fertilizer application can increase soil organic matter (Kaur and Singh, 2014; Tripathi et al., 2014), and long-term application can serve to improve soil fertility. It is worth to note that a higher annual C addition with chicken manure produced a significant increase of sequestrated C by 0.19 Mg ha−1 year−1 in M20% to by 0.38 Mg ha−1 year−1 in M100% than M0% in this study. These findings indicated that the tested soils had great potential to sequestrate considerable amounts of C and build up SOC pool. The increase in SOC could be explained by the external C addition to the soil by manure application (Xu et al., 2023), because there was a positive relationship between SOC and C input (Figure 3), while the similar SOC content was observed between M0% and initial value. In addition, application of organic fertilizers exerts advantages in reducing soil bulk density (Bronick and Lal, 2005) and increasing soil porosity (Guo et al., 2016), and positively affects soil aggregation, macro porosity and water holding capacity, thus maintaining a high level of soil fertility (Petersen and Hoyle, 2016). Guo et al. (2016) showed that soil organic matter can be increased by 28-87% after 5 years of organic substitution under a wheat-maize rotation system. In addition, various nutrients in organic fertilizers complement the soil nutrient pool (Valdez Velarca, 2016; Guo et al., 2016), such as soil Olsen-P and available K contents (Table 2), and a good soil environment in turn promotes accumulation and hydrolysis of organic matter (Duan et al., 2017; Zhang et al., 2019; Liu et al., 2022). Various small molecule compounds in organic fertilizers also play a positive role in crop growth.
However, organic fertilizer is a double-edged sword for soil N. On the one hand, changes in the soil microenvironment can facilitate N cycling and transformation (He et al., 2015). On the other hand, N mineralization from organic fertilizer requires a time process that can negatively affect crop growth in the absence of available N (Choi et al., 2004; Guo et al., 2016). The greater C content in soil increases both nitrate immobilization and N demand (Liu et al., 2020). In this study, as the chicken manure substitution ratio increased, the soil total N content increased significantly by 1.4% in M20% to 7.6% in M100% compared to the chemical fertilizer application alone, but the soil mineral N content decreased significantly, which led to a significant decrease in crop yield. The study has shown that soil organic N content can be increased by up to 90% after organic fertilizer application (Rezig et al., 2013). The addition of organic fertilizer enhances immobilization by slowly releasing mineral N from the decomposition processes, while facilitating the interaction between soil C and N cycles, thus significantly reducing N losses to the environment. Under the same N application rate in this study, the marked contrasting effects observed between total N and mineral N content suggested that manure input was favorable for N retention as complex organic forms (Tang et al., 2022). Therefore, replacing part of chemical fertilizer with manure is an effective method to reduce inorganic N surplus observed in soil of conventionally excessive chemical N fertilization. However, the organic N will be continuously mineralized along with the prolonged period of manure application, so the substitution ratio should also be increased. Further research and practice are still required to identify the optimal substitution ratio by considering long-term effects of manure applications.
The application of organic-inorganic fertilizers can decrease chemical N fertilizer inputs while reducing the instantaneous accumulation of inorganic N in the soil. Meanwhile, slow-release N from organic fertilizers reduces soil N losses while meeting nutrient requirements for later crop growth (Luo et al., 2011; Zhao et al., 2013). Conventional production patterns that rely on high amounts of chemical N fertilizer inputs not only lead to soil N accumulation and thereby increasing the risk of N leaching, but also increase the negative impacts on the ecosystem through ammonia volatilization and greenhouse gas emissions (Yao et al., 2013; Lassaletta et al., 2014; Wuepper et al., 2020). The recycling of organic resources such as livestock manure to farmland (Lassaletta et al., 2014; Bonaudo et al., 2014), i.e., partially replace chemical fertilizers, is one of the key strategies to mitigate soil nutrient losses (Elbl et al., 2020).
In recent years, studies on the application of organic materials in maintaining soil nutrient balance and reducing nutrient losses have been widely reported (Steiner et al., 2008; Beesley et al., 2011; Zhang et al., 2018). Tang et al. (2022) found that a 50% substitution with pig manure increased the diversity of bacteria, the relative abundance of specific microbiota involved in N cycling, the amount of denitrifiers, and the proportion of N2O-reducers, which resulted in a 44% reduction in agricultural inputs and less environmental impacts per unit of product. However, the reduction of fertilizer inputs and N losses should not be at the expense of crop yield, and organic fertilizer inputs should be kept at a reasonable range. This study showed that a 25% N fertilizer was reduced comparing with farmers’ conventional fertilizer application, and a further 20% chemical N fertilizer in wheat season and 20%-40% chemical N fertilizer in maize season can be replaced by chicken manure compared with the treatment of chemical fertilizer application alone (200 kg N ha−1 per season). This fertilization method would not only maintain crop yield but also reduce soil residual N while increasing N uptake. Liu et al. (2022) also showed that 30% organic substitution improved soil quality and increased maize yields. In the case of rice, application of 25%-50% organic fertilizer substitution could simultaneously increase yield and achieve agricultural sustainability (Song et al., 2022). These studies supported the results of our study. Therefore, adopting an appropriate organic substitution ratio under optimal fertilizer application conditions can effectively balance yield and environmental protection.
5 Conclusions
The results of a 4-year field experiment in the wheat-maize rotation of North-central China showed that partial substitution of chemical N fertilizer with chicken manure improved crop yield and N recovery efficiency, reduced soil mineral N residues, and significantly increased soil organic matter and total N content. Under the current chemical N application rate in this study (200 kg N ha−1 per season), organic substitutions by 20% in wheat season and 20%-40% in maize season were found to be suitable for the study area in terms of achieving high yield, N use efficiency and soil fertility. The study showed that manure fertilizer application increased soil Olsen-P and available K contents. However, the synergistic role of the manure application in optimizing the three macronutrients application should be explored in the future.
Data availability statement
The original contributions presented in the study are included in the article/Supplementary Material. Further inquiries can be directed to the corresponding authors.
Author contributions
LN analyzed the data and wrote the manuscript. XX designed the research and performed methodology. YZ and GZ participated in experimental research. SZ, SQ, and WD participated in data collation, investigation and validation. XX and PH commented on data interpretation and modified the manuscript. All authors contributed to the article and approved the submitted version.
Funding
This research was supported by the China Agriculture Research System of MOF and MARA, National Key Research and Development Program of China (2016YFD0200101), and the Agricultural Science and Technology Innovation Program of Chinese Academy of Agricultural Sciences (GY2022-11-2).
Acknowledgments
We are grateful to farmers in our study for their patience and support.
Conflict of interest
The authors declare that the research was conducted in the absence of any commercial or financial relationships that could be construed as a potential conflict of interest.
Publisher’s note
All claims expressed in this article are solely those of the authors and do not necessarily represent those of their affiliated organizations, or those of the publisher, the editors and the reviewers. Any product that may be evaluated in this article, or claim that may be made by its manufacturer, is not guaranteed or endorsed by the publisher.
Supplementary material
The Supplementary Material for this article can be found online at: https://www.frontiersin.org/articles/10.3389/fpls.2022.1050179/full#supplementary-material
References
Beesley, L., Moreno-Jiménez, E., Gomez-Eyles, J. L., Harris, E., Robinson, B., Sizmur, T. (2011). A review of biochars’ potential role in the remediation, revegetation and restoration of contaminated soils. Environ. pollut. 159, 3269–3282. doi: 10.1016/j.envpol.2011.07.023
Berry, P. M., Sylvester-Bradley, R., Philipps, L., Hatch, D. J., Cuttle, S. P., Rayns, F. W., et al. (2002). Is the productivity of organic farms restricted by the supply of available nitrogen? Soil Use Manage. 18, 248–255. doi: 10.1111/j.1475-2743.2002.tb00266.x
Bonaudo, T., Bendahan, A. B., Sabatier, R., Ryschawy, J., Bellon, S., Leger, F., et al. (2014). Agroecological principles for the redesign of integrated crop–livestock systems. Eur. J. Agron. 57, 43–51. doi: 10.1016/j.eja.2013.09.010
Bronick, C. J., Lal, R. (2005). Soil structure and management: A review. Geoderma 124, 3–22. doi: 10.1016/j.geoderma.2004.03.005
Chen, X. H., Ma, L., Ma, W. Q., Wu, Z. G., Cui, Z. L., Hou, Y., et al. (2018). What has caused the use of fertilizers to skyrocket in China? Nutr. Cycl. Agroecosyst. 110, 241–255. doi: 10.1007/s10705-017-9895-1
Chinese Society of Soil Science (2000). Methods of soil and plant analysis (Beijing: China Agriculture Scientech Press).
Choi, W. J., Ro, H. M., Chang, S. X. (2004). Recovery of fertilizer-derived inorganic-15N in a vegetable field soil as affected by application of an organic amendment. Plant Soil. 263, 191–201. doi: 10.1023/B:PLSO.0000047726.09394.d3
Dai, X. L., Song, D. L., Zhou, W., Liu, G. R., Liang, G. Q., He, P., et al. (2021). Partial substitution of chemical nitrogen with organic nitrogen improves rice yield, soil biochemical indictors and microbial composition in a double rice cropping system in south China. Soil Tillage Res. 205, 104753. doi: 10.1016/j.still.2020.104753
Ding, W. C., Xu, X. P., He, P., Ullah, S., Zhang, J. J., Cui, Z. L., et al. (2018). Improving yield and nitrogen use efficiency through alternative fertilization options for rice in China: A meta-analysis. Field Crops Res. 227, 11–18. doi: 10.1016/j.fcr.2018.08.001
Duan, Y. H., Xu, M. G., Gao, S. D., Yang, X. Y., Huang, S. M., Liu, H. B., et al. (2014). Nitrogen use efficiency in a wheat–corn cropping system from 15 years of manure and fertilizer applications. Field Crops Res. 157, 47–56. doi: 10.1016/j.fcr.2013.12.012
Duan, P. P., Zhang, Y. L., Cong, Y. H., Xu, W. J., Yu, N., Zhang, Y. L. (2017). The dynamics of soil-soluble nitrogen and soil-retained nitrogen in greenhouse soil. Acta Agr. Scand. B-S P. 67, 51–61. doi: 10.1080/09064710.2016.1218923
Elbl, J., Šimečková, J., Škarpa, P., Kintl, A., Brtnický, M., Vaverková, M. D. (2020). Comparison of the agricultural use of products from organic waste processing with conventional mineral fertilizer: potential effects on mineral nitrogen leaching and soil quality. Agronomy 10, 226. doi: 10.3390/agronomy10020226
Farrell, M., Hill, P. W., Farrar, J., Bardgett, R. D., Jones, D. L. (2011). Seasonal variation in soluble soil carbon and nitrogen across a grassland productivity gradient. Soil Biol. Biochem. 43, 835–844. doi: 10.1016/j.soilbio.2010.12.022
Food and Agriculture Organization (2020). World food and agriculture - Statistical yearbook 2020. Rome. https://doi.org/10.4060/cb1329en [Accessed August 5, 2022].
Food and Agriculture Organization (2021). https://www.fao.org/faostat/zh/#data [Accessed August 22, 2022].
Galloway, J. N., Townsend, A. R., Erisman, J. W., Bekunda, M., Cai, Z. C., Freney, J. R., et al. (2008). Transformation of the nitrogen cycle: recent trends, questions, and potential solutions. Science 320, 889–892. doi: 10.1126/science.1136674
Guo, L. Y., Wu, G. L., Li, Y., Li, C. H., Liu, W. J., Meng, J., et al. (2016). Effects of cattle manure compost combined with chemical fertilizer on topsoil organic matter, bulk density and earthworm activity in a wheat–maize rotation system in Eastern China. Soil Tillage Res. 156, 140–147. doi: 10.1016/j.still.2015.10.010
Han, S., Delgado-Baquerizo, M., Luo, X. S., Liu, Y. R., Van Nostrand, J. D., Chen, W. L., et al. (2021). Soil aggregate size-dependent relationships between microbial functional diversity and multifunctionality. Soil Biol. Biochem. 154, 108143. doi: 10.1016/j.soilbio.2021.108143
He, Y. T., Zhang, W. J., Xu, M. G., Tong, X. G., Sun, F. X., Wang, J. Z., et al. (2015). Long-term combined chemical and manure fertilizations increase soil organic carbon and total nitrogen in aggregate fractions at three typical cropland soils in China. Sci. Total Environ. 532, 635–644. doi: 10.1016/j.scitotenv.2015.06.011
Ju, X. T., Xing, G. X., Chen, X. P., Zhang, S. L., Zhang, L. J., Liu, X. J., et al. (2009). Reducing environmental risk by improving n management in intensive Chinese agricultural systems. Proc. Natl. Acad. Sci. 106, 3041–3046. doi: 10.1073/pnas.0813417106
Kaur, J., Singh, J. P. (2014). Long-term effects of continuous cropping and different nutrient management practices on the distribution of organic nitrogen in soil under rice-wheat system. Plant Soil Environ. 60, 63–68. doi: 10.17221/440/2013-PSE
Lassaletta, L., Billen, G., Grizzetti, B., Anglade, J., Garnier, J. (2014). 50-year trends in nitrogen use efficiency of world cropping systems: The relationship between yield and nitrogen input to cropland. Environ. Res. Lett. 9, 105011. doi: 10.1088/1748-9326/9/10/105011
Li, X. Y., Li, B., Chen, L., Liang, J. Y., Huang, R., Tang, X. Y., et al. (2022). Partial substitution of chemical fertilizer with organic fertilizer over seven years increases yields and restores soil bacterial community diversity in wheat–rice rotation. Eur. J. Agron. 133, 126445. doi: 10.1016/j.eja.2021.126445
Liu, H. W., Du, X. F., Li, Y. B., Han, X., Li, B., Zhang, X. K., et al. (2022). Organic substitutions improve soil quality and maize yield through increasing soil microbial diversity. J. Clean. Prod. 347, 131323. doi: 10.1016/j.jclepro.2022.131323
Liu, S. B., Wang, J. Y., Pu, S. Y., Blagodatskaya, E., Kuzyakov, Y., Razavi, B. S. (2020). Impact of manure on soil biochemical properties: a global synthesis. Sci. Total Environ. 745, 141003. doi: 10.1016/j.scitotenv.2020.141003
Luo, L. G., Itoh, S., Zhang, Q. W., Yang, S. Q., Zhang, Q. Z., Yang, Z. L. (2011). Leaching behavior of nitrogen in a long-term experiment on rice under different n management systems. Environ. Monit. Assess. 177, 141–150. doi: 10.1007/s10661-010-1624-z
McGill, W. B., Cannon, K. R., Robertson, J. A., Cook, F. D. (1986). Dynamics of soil microbial biomass and water-soluble organic c in Breton l after 50 years of cropping to two rotations. Can. J. Soil Sci. 66, 1–19. doi: 10.4141/cjss86-001
Ministry of Agriculture and Rural Affairs of the People's Republic of China (2017) Ministry of Agriculture on the issuance of "resource utilization of livestock and poultry manure Action Program (2017-2020). Available at: http://www.moa.gov.cn/nybgb/2017/dbq/201801/t20180103_6134011.htm [Accessed August 14, 2022].
Mi, W., Wu, L., Brookes, P. C., Liu, Y., Zhang, X., Yang, X. (2016). Changes in soil organic carbon fractions under integrated management systems in a low-productivity paddy soil given different organic amendments and chemical fertilizers. Soil Tillage Res. 163, 64–70. doi: 10.1016/j.still.2016.05.009
National Bureau of Statistics of China. National data (2020). https://data.stats.gov.cn/ [Accessed August 14, 2022].
Näsholm, T., Huss-Danell, K., Högberg, P. (2000). Uptake of organic nitrogen in the field by four agriculturally important plant species. Ecology 81, 1155–1161. doi: 10.1890/0012-9658(2000)081[1155:UOONIT]2.0.CO;2
Niu, X. S., Ju, X. T. (2017). Organic fertilizer resources and utilization in China. J. Plant Nutr. Fert. 23, 1462–1479. doi: CNKI:SUN:ZWYF.0.2017-06-008
Nyawade, S. O., Karanja, N. N., Gachene, C. K., Gitari, H. I., Schulte-Geldermann, E., Parker, M. (2020). Optimizing soil nitrogen balance in a potato cropping system through legume intercropping. Nutr. Cycl. Agroecosyst. 117 (1), 43–59. doi: 10.1007/s10705-020-10054-0
Pang, X. P., Letey, J. (2000). Organic farming challenge of timing nitrogen availability to crop nitrogen requirements. Soil Sci. Soc Am. J. 64, 247–253. doi: 10.2136/sssaj2000.641247x
Paul, J., Choudhary, A. K., Sharma, S., Bohra, M., Dixit, A. K., Kumar, P. (2016). Potato production through bio-resources: Long-term effects on tuber productivity, quality, carbon sequestration and soil health in temperate Himalayas. Sci. Hortic. 213, 152–163. doi: 10.1016/j.scienta.2016.10.022
Petersen, E. H., Hoyle, F. C. (2016). Estimating the economic value of soil organic carbon for grains cropping systems in Western Australia. Soil Res. 54, 383–396. doi: 10.1071/SR15101
Redding, M. R., Lewis, R., Kearton, T., Smith, O. (2016). Manure and sorbent fertilisers increase on-going nutrient availability relative to conventional fertilisers. Sci. Total Environ. 569, 927–936. doi: 10.1016/j.scitotenv.2016.05.068
Rezig, F. A. M., Mubarak, A. R., Ehadi, E. A. (2013). Impact of organic residues and mineral fertilizer application on soil–crop system: II soil attributes. Arch. Agron. Soil Sci. 59, 1245–1261. doi: 10.1080/03650340.2012.709623
Sarkar, S., Mukherjee, I. (2021). IEffect of organic amendment on mobility behavior of flupyradifurone in two different Indian soils. Bull. Environ. Contam. Toxicol. 107 (1), 160–166. doi: 10.1007/s00128-021-03209-4
Song, W. F., Shu, A. P., Liu, J. A., Shi, W. C., Li, M. C., Zhang, W. X., et al. (2022). Effects of long-term fertilization with different substitution ratios of organic fertilizer on paddy soil. Pedosphere 32, 637–648. doi: 10.1016/S1002-0160(21)60047-4
Steiner, C., Glaser, B., Geraldes Teixeira, W., Lehmann, J., Blum, W. E. H., Zech, W. (2008). Nitrogen retention and plant uptake on a highly weathered central Amazonian ferralsol amended with compost and charcoal. J. Plant Nutr. Soil Sci. 171, 893–899. doi: 10.1002/jpln.200625199
Tang, Q., Cotton, A., Wei, Z. J., Xia, Y. Q., Daniell, T., Yan, X. Y. (2022). How does partial substitution of chemical fertiliser with organic forms increase sustainability of agricultural production? Sci. Total Environ. 803, 149933. doi: 10.1016/j.scitotenv.2021.149933
Tripathi, R., Nayak, A. K., Bhattacharyya, P., Shukla, A. K., Shahid, M., Raja, R., et al. (2014). Soil aggregation and distribution of carbon and nitrogen in different fractions after 41 years long-term fertilizer experiment in tropical rice–rice system. Geoderma 213, 280–286. doi: 10.1016/j.geoderma.2013.08.031
Valdez Velarca, M. (2016). Effects of nitrogen fertilization on organic rice production (Texas: Texas A&M University).
Wang, X. L., Ye, J., Perez, P. G., Tang, D. M., Huang, D. F. (2013). The impact of organic farming on the soluble organic nitrogen pool in horticultural soil under open field and greenhouse conditions: a case study. Soil Sci. Plant Nutr. 59, 237–248. doi: 10.1080/00380768.2013.770722
West, P. C., Gerber, J. S., Engstrom, P. M., Mueller, N. D., Brauman, K. A., Carlson, K. M., et al. (2014). Leverage points for improving global food security and the environment. Science 345, 325–328. doi: 10.1126/science.1246067
Wuepper, D., Le Clech, S., Zilberman, D., Mueller, N., Finger, R. (2020). Countries influence the trade-off between crop yields and nitrogen pollution. Nat. Food. 1, 713–719. doi: 10.1038/s43016-020-00185-6
Xie, Z. J., Tu, S. X., Shah, F., Xu, C. X., Chen, J. R., Han, D., et al. (2016). Substitution of fertilizer-n by green manure improves the sustainability of yield in double-rice cropping system in south China. Field Crops Res. 188, 142–149. doi: 10.1016/j.fcr.2016.01.006
Xin, X. L., Qin, S. W., Zhang, J. B., Zhu, A. N., Yang, W. L., Zhang, X. F. (2017). Yield, phosphorus use efficiency and balance response to substituting long-term chemical fertilizer use with organic manure in a wheat-maize system. Field Crops Res. 208, 27–33. doi: 10.1016/j.fcr.2017.03.011
Xu, F., Liu, Y. L., Du, W. C., Li, C. L., Xu, M. L., Xie, T. C., et al. (2021). Response of soil bacterial communities, antibiotic residuals, and crop yields to organic fertilizer substitution in north China under wheat–maize rotation. Sci. Total Environ. 785, 147248. doi: 10.1016/j.scitotenv.2021.147248
Xu, W. X., Liu, W. J., Tang, S. R., Yang, Q., Meng, L., Wu, Y. Z., et al. (2023). Long-term partial substitution of chemical nitrogen fertilizer with organic fertilizers increased SOC stability by mediating soil c mineralization and enzyme activities in a rubber plantation of hainan island, China. Appl. Soil Ecol. 182, 104691. doi: 10.1016/j.apsoil.2022.104691
Yan, G. X., Yao, Z. S., Zheng, X. H., Liu, C. Y. (2015). Characteristics of annual nitrous and nitric oxide emissions from major cereal crops in the north China plain under alternative fertilizer management. Agric. Ecosyst. Environ. 207, 67–78. doi: 10.1016/j.agee.2015.03.030
Yao, Z. S., Zheng, X. H., Wang, R., Xie, B. H., Butterbach-Bahl, K., Zhu, J. G. (2013). Nitrous oxide and methane fluxes from a rice–wheat crop rotation under wheat residue incorporation and no-tillage practices. Atmospheric Environ. 79, 641–649. doi: 10.1016/j.atmosenv.2013.07.006
Zhang, X., Wang, H., Hui, X. L., Wang, Z. H., Liu, J. S. (2019). Effects of different fertilization and fallowing practices on soil carbon and nitrogen mineralization in a dryland soil with low organic matter. J. Soil Sci. Plant Nutr. 19, 108–116. doi: 10.1007/s42729-019-0016-x
Zhang, H. M., Xu, M. G., Shi, X. J., Li, Z. Z., Huang, Q. H., Wang, X. J. (2010). Rice yield, potassium uptake and apparent balance under long-term fertilization in rice-based cropping systems in southern China. Nutr. Cycl. Agroecosyst. 88, 341–349. doi: 10.1007/s10705-010-9359-3
Zhang, M., Yao, Y. L., Tian, Y. H., Ceng, K., Zhao, M., Zhao, M., et al. (2018). Increasing yield and n use efficiency with organic fertilizer in Chinese intensive rice cropping systems. Field Crops Res. 227, 102–109. doi: 10.1016/j.fcr.2018.08.010
Zhao, B. Z., Chen, J., Zhang, J. B., Xin, X. L., Hao, X. Y. (2013). How different long-term fertilization strategies influence crop yield and soil properties in a maize field in the north China plain. J. Plant Nutr. Soil Sci. 176, 99–109. doi: 10.1002/jpln.201200076
Zhao, B. Q., Li, X. Y., Li, X. P., Shi, X. J., Huang, S. M., Wang, B. R., et al. (2010). Long-term fertilizer experiment network in China: crop yields and soil nutrient trends. Agron. J. 102, 216–230. doi: 10.2134/agronj2009.0182
Zhao, J., Ni, T., Li, J., Lu, Q., Fang, Z. Y., Huang, Q. W., et al. (2016). Effects of organic-inorganic compound fertilizer with reduced chemical fertilizer application on crop yields, soil biological activity and bacterial community structure in a rice-wheat cropping system. Appl. Soil Ecol. 99, 1–12. doi: 10.1016/j.apsoil.2015.11.006
Keywords: crop production, nutrient management, N recovery efficiency, soil C sequestration, soil organic matter
Citation: Ning L, Xu X, Zhang Y, Zhao S, Qiu S, Ding W, Zou G and He P (2022) Effects of chicken manure substitution for mineral nitrogen fertilizer on crop yield and soil fertility in a reduced nitrogen input regime of North-Central China. Front. Plant Sci. 13:1050179. doi: 10.3389/fpls.2022.1050179
Received: 21 September 2022; Accepted: 02 December 2022;
Published: 15 December 2022.
Edited by:
Anoop Kumar Srivastava, Central Citrus Research Institute (ICAR), IndiaReviewed by:
Surani Chathurika, University of Sri Jayewardenepura, Sri LankaMazhar Rafique, The University of Haripur, Pakistan
Copyright © 2022 Ning, Xu, Zhang, Zhao, Qiu, Ding, Zou and He. This is an open-access article distributed under the terms of the Creative Commons Attribution License (CC BY). The use, distribution or reproduction in other forums is permitted, provided the original author(s) and the copyright owner(s) are credited and that the original publication in this journal is cited, in accordance with accepted academic practice. No use, distribution or reproduction is permitted which does not comply with these terms.
*Correspondence: Xinpeng Xu, eHV4aW5wZW5nQGNhYXMuY24=; Ping He, aGVwaW5nMDJAY2Fhcy5jbg==