- 1Université de la Réunion, UMR PVBMT, Saint Pierre, Réunion
- 2CIRAD, UMR PVBMT, Saint Pierre, Réunion
- 3Instituto de Ciencias Agrarias, Consejo Superior de Investigaciones Científicas, ICA-CSIC, Madrid, Spain
- 4CIRAD, UMR PVBMT, Antananarivo, Madagascar
Introduction: Huanglonbing (HLB) is the most serious disease of citrus in the world, associated with three non-cultivable phloem-restricted bacteria Candidatus Liberibacter asiaticus (CLas), Ca L. africanus (CLaf) and Ca L. americanus (CLam). CLas is transmitted by the Asian citrus psyllid Diaphorina citri, and has spread to several countries. The African psyllid Trioza erytreae, the vector of CLaf occurs in Africa and neighbouring islands. Only two major citrus-growing regions - Australia/New Zealand and the Mediterranean Basin - are still HLB-free in the world. However, T. erytreae has recently been introduced into continental Europe (Portugal and Spain) and has become a potential threat to citrus production. The transmission of CLas by T. erytreae had been postulated but never tested. To evaluate the risk of T. erytreae transmitting CLas, comparative transmissions of CLas by T. erytreae and D. citri were assessed.
Methods: Transmission tests were performed on excised leaves and seedlings of Citrus volkameriana with different inoculation access periods (in series) for both insect species. Quantifications of bacterial titers were made in excised leaves, seedlings three and six months after inoculation and on individual insects.
Results: Our results showed that T. erytreae was able to efficiently acquire CLas. Furthermore, T. erytreae carried significantly higher bacterial titers than D. citri, and was able to efficiently transmit the bacteria to seedlings at a similar rate that D. citri highlighting the high risk of spread of the most aggressive variant of HLB (CLas) by T. erytreae in Europe.
Discussion: Thus, extreme precautions to prevent any entry of CLas into Europe should be adopted.
Introduction
All Citrus trees belong to the family Rutaceae, subfamily Aurantioideae and are native to the tropical and subtropical regions of Southeast Asia, northeast India, Yunnan Province in southwest China, northern Myanmar, the Indochinese peninsula and the Malaysian archipelago (Zhong and Nicolosi, 2020). It is the world’s largest fruit crop, cultivated in more than 140 countries in the world [143.755, 6 million tons in 2020, (FAO, 2021)]. The main threat of this tree crop is a disease called Huanglongbing (HLB) or citrus greening, which has provoked the decline of many citrus industries that have faced the disease (Gottwald, 2010). The disease was first reported in China in 1919 but the causal agents were only discovered in 1970 by electron microscopy (EM) (Bové, 2006). It is caused by three main species of uncultivable, phloem-restricted, Gram-negative bacteria of the genus Liberibacter. Three different bacteria species pathogenic to Citrus inducing HLB had been described so far: the Asian species named Candidatus Liberibacter asiaticus (CLas), the African species Ca. Liberibacter africanus (CLaf) and the American species Ca. Liberibacter americanus (CLam) (Bové, 2006). The former species is described as the most aggressive species among all three causing more severe symptoms of HLB, and being able to grow in a wide range of temperatures, even above 30°C (Bové, 2006). Symptoms affect the entire citrus plant, from roots to leaves, even altering the chemical characteristics and sensory attributes of the fruit, and eventually killing the plant (Bové, 2006; Baldwin et al., 2010; Dala-Paula et al., 2019).
HLB disease is transmitted by grafting and mainly by two species of phloem-sap feeding psyllids (Bové, 2006). One species originating from Asia, the Asian citrus psyllid (ACP), Diaphorina citri Kuwayama, 1908 (Hemiptera: Psyllidae) is the vector of CLas (Martinez and Wallace, 1967) and CLam (Teixeira et al., 2005a; Texeira et al., 2005b). The second species originating from Africa, the African citrus psyllid (AfCP), Trioza erytreae (Del Guercio, 1918) (Hemiptera: Triozidae) (McClean and Oberholzer, 1965) is the vector of CLaf (Bové, 2006). Both psyllid species are very sensitive to changes in temperature and relative humidity. The AfCP, develops under cool and moist climate, the optimum temperature for its preimaginal development is between 17°C and 25°C, and its juvenile stages can be completed in 17 and 43 days at 25 and 14°C, respectively (Catling, 1973). The ACP has a higher heat tolerance and its development from egg to adult can be completed in 17 days with optimal population growth potential at 25-28°C (Hall, 2008).
Huanglongbing disease affects several countries in Asia, sub-Saharan Africa, the islands of the Indian Ocean and the Americas (except for Bolivia, Chile, Perú, and Uruguay), but the Mediterranean Basin and Australia are still free from it (Ferrarezi et al., 2020). However, T. erytreae has recently been described in southern Europe in Portugal and Spain and is extending its range in both countries eastwards reaching the Basque region just at the border of southern France and southwestwards, reaching the Algarve region in southern of Portugal (Cocuzza et al., 2017; EPPO, 2021; Benhadi-Marín et al., 2022). Most studies on CLas transmission have been conducted with D. citri, the species widely present in Asia and America, while those on CLaf have exclusively used T. erytreae as a vector as the pathogen and the vector were previously restricted to the African continent. However, in recent years CLas has been introduced into Africa where both psyllid vector species are present and the epidemiological implications of such introduction are unknown (Ajene et al., 2020b). As one of CLas’s potential vectors is already present in southern Europe, the high heat tolerance, strong symptoms induction leading to the death of trees, high aggressiveness, and wide distribution of CLas, make this disease the main threat to this major citrus-growing area. However, the transmission ability of CLas by T. erytreae has never been fully proven, as i) when the experiment was carried out (in the 1970s), it was not possible to discriminate HLB species, and ii) it was tested on very few plants (Massonie et al., 1976). Recently, CLas was reported from field-collected adults of T. erytreae feeding on HLB infected citrus trees in Ethiopia and Uganda (Ajene et al., 2020b), but no transmission assay was conducted to prove its transmission capacity. Thus, there is no information on the risk of spread of CLas in a geographical area where T. erytreae is present. To clarify the transmission capacity of CLas by T. erytreae, we compared the transmission capacities of the two psyllids D. citri and T. erytreae for CLas in laboratory experiments. These experiments were conducted in La Réunion, an island in the southwest part of the Indian Ocean where the two-psyllid species are present, and the two HLB species CLas and CLaf have been reported (Catling, 1972; Garnier et al., 1996; Lu et al., 2021; Wang et al., 2021).
Material and methods
Insect material
Laboratory colonies of D. citri and T. erytreae were started from adult field collected individuals in La Réunion in 2019 and 2020, respectively. These adults were transferred in separate cages and growth chambers to start laboratory colonies for all further experiments in insectarium facilities of the Plant Protection Platform, La Réunion.
Diaphorina citri adults were collected on orange jasmine [Murraya paniculata (L.) Jack] at 400 m asl in Le Tampon locality in La Réunion. The colony was maintained on the same plant with fluctuating temperatures (26°C day/20°C night (+/- 2°C); LD 12:12h photoperiod; 80+/-20% relative humidity).
Trioza erytreae adults were initially collected on Tangor Ortanic Mandarin at 700 m asl in Salazie locality in La Réunion after an intensive survey, as this species was considered almost extinct in the 90s (Aubert, 1987; Aubert et al., 1996). The colony was maintained on Volkamer lemon (C. limonia Osbeck ‘Volkameriana’) with fluctuating temperatures (23°C day/17°C night (+/- 2°C); LD 12:12h photoperiod; 80 +/-20% relative humidity) that should be in the optimal range for development of this species (Cocuzza et al., 2017).
Individuals from the two colonies were qPCR-assayed for CLas and CLaf absence. The two colonies were regularly checked to ensure their bacteria-free status.
Plant material and HLB inoculum
In all further laboratory transmission experiments, leaves or seedlings of Volkameriana were used. Those plants were grown in the laboratory growth chamber facilities to ensure their HLB-free status.
CLas-infected leaves of plants to be used as CLas sources for positive controls and CLas acquisition by insects in transmission tests were collected from infected citrus plants grown in a specific orchard at 160 m asl in Saint-Pierre. Two different CLas-infected cultivars were used as infected sources for the transmission tests: ForEl 41 (V1), a hybrid between the mandarin Fortune and the Tangor Ellendale and Pet Yala Mandarin (V8) a Citrus suhuiensis (Koizumi et al., 1993). The CLas-infected status of both infected-source plants was checked prior to the start of the experiments. Both cultivars were chosen because they had different initial bacterial titers (Cq).
DNA extraction and quantitative CLas molecular detection
Each adult psyllid was individually extracted using the Animal Blood and Tissue kit (Qiagen©, Europe) following the manufacturer’s instructions. The DNA was extracted for all psyllids that were used in the experiments, even the collected dead ones that died before the last inoculation access period (IAP).
Each leaf used for the IAP and the acquisition access period (AAP) was individually extracted for its DNA content. To do this, the midrib was separated from each leaf, using a sterile scissor (sterilized after each midrib sampled) directly after sample collection, then stored at -80°C until further use. Then, 0.2 g of each frozen midrib was subjected to a first grinding step in 1mL of Tris-EDTA-SDS buffer (Cellier et al., 2020) using ceramic beads in a FastPrep®96 homogenizer (MP Biomedicals, France). This shredded material was then extracted with the DNeasy Plant Mini kit (Qiagen©, Europe) following the manufacturer’s instructions. All samples were stored at −80°C.
To determine the CLas titer for each extracted individual psyllid or leaf we used a Real time PCR StepOne PLUS (Applied Biosystem©, Life Tech) with the following primer sets amplifying a region of the CLas 16s rDNA: HLBas TCGAGCGCGTATGCAATACG and HLBr GCGTTATCCCGTAGAAAAAGGTAG and Taqman probe HLBp FAM-AGACGGGTGAGTAACGCG (Li et al., 2006) following a modified protocol of Cellier et al. (2020). Each reaction was made in a final volume of 13 µL comprising: 6.50 μl of GoTaq® Probe Master Mix (Promega ©, Europe), 0.25 µM of primers, 0.13 μM of HLBp probe, 3.67μl of water and 2 μl of DNA. The PCR parameters were: 95°C for 10 min, 45 cycles (95°C for 15 sec, 58°C for 1 min). Each sample was run in duplicate and the average Cq value of the two runs was used for assessing CLas status. In each qPCR reaction plate, at least 4 negative controls were added (i.e., for plants, DNA samples came from healthy non-CLas positive plants, for psyllids it came from DNA samples extracted from non-CLas positive insects of each species). The positivity threshold was chosen as the lowest Cq of the controls.
HLB transmission assays
To evaluate the ability of acquisition, retention and transmission of CLas by T. erytreae and D. citri, we carried out serial inoculation tests.
The whole experiment consisted in four steps (Figure 1). The first step was the AAP where approximately 40 nymphs at the 3rd to 4th instar stage of each species were introduced into tubes containing CLas-infected detached leaves from a young field-collected plant (either V1 or V8 mandarin cultivars). In addition, a laboratory-grown detached young leaf of Volkameriana free of CLas was used as a negative control. Each petiole was inserted into an Eppendorf tube filled with Murashige and Skoog (MS) medium, itself inserted in a glass tube closed by a fine mesh. The nymphs were left to feed and develop on the infected leaves inside the tubes for an AAP of about 14 days until the adults emerged. As nymphs acquire CLas in a much more efficient way than adults, the nymphal stage was chosen for an optimal acquisition (Ammar et al., 2016). After the 14-day AAP, leaves were directly collected and midrib sampled as described above, and frozen at -80°C for further tests.
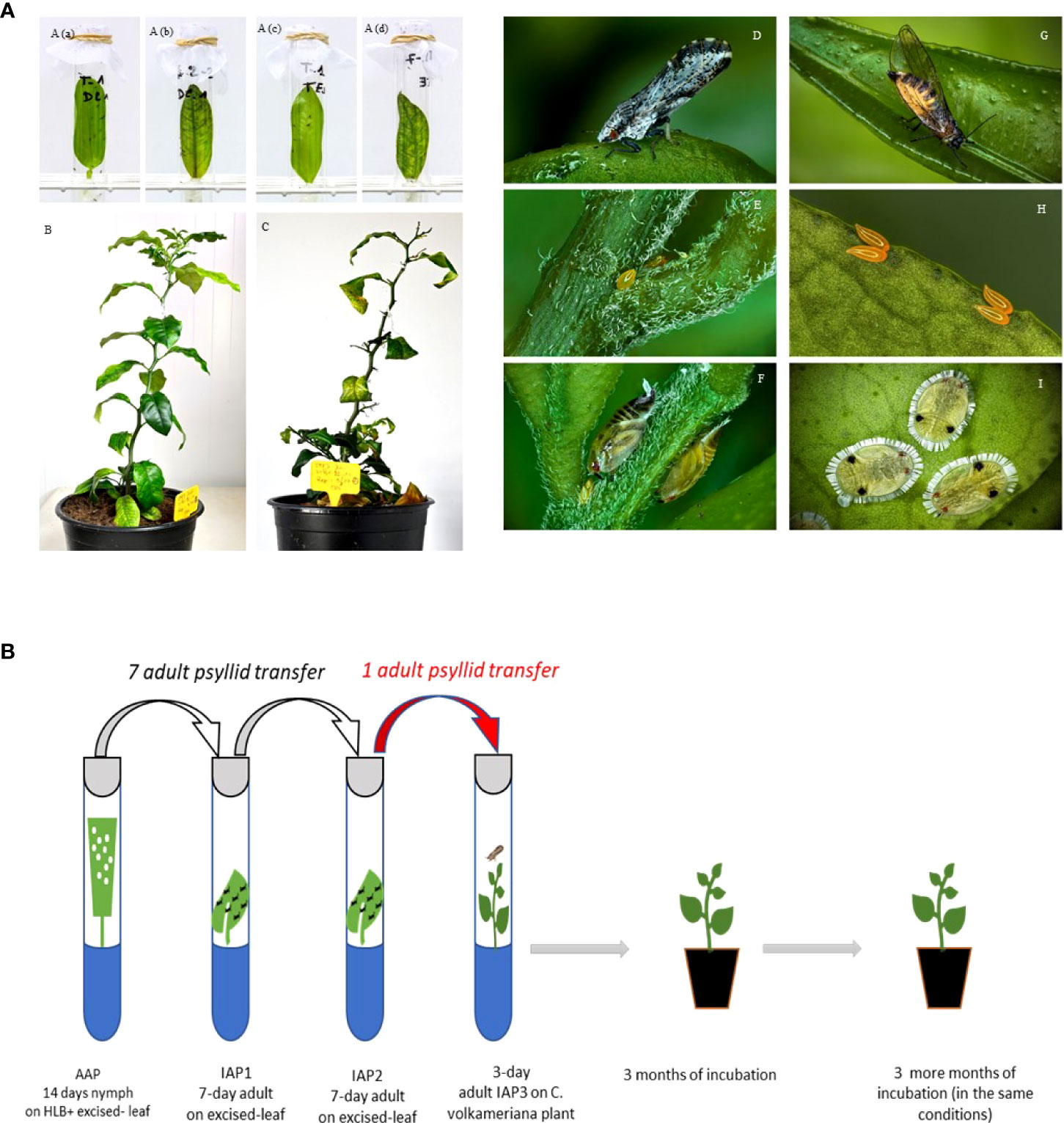
Figure 1 Part (A) Different plant material (excised citrus leaves and plants) and insect stages of both psyllid species that were used in the CLas transmission experiments. Acquisition access period (AAP) of Diaphorina citri (Aa, Ab) and Trioza erytreae (Ac, Ad) on HLB+ (Ab, Ad) or HLB- (Aa, Ac) excised leaves; CLas + 3-month (B) and 6-month (C) old plant in IAP 3; Diaphorina citri adult (D), eggs (E) and nymphs (F); Trioza erytreae adult (G), eggs (H) and nymphs (I). All pictures were taken by Antoine Franck (CIRAD, UMR PVBMT). Part (B) Experimental set up.
Then, 5 to 7 newly emerged adults were transferred to a tube containing a healthy Volkameriana detached receptor leaf for the first 7-day of IAP1. Then, the adults (including those developed on non-infected leaves that were used as control) were transferred to another tube containing a healthy Volkameriana detached receptor leaf for the last 7-day of IAP (IAP2). After IAP1 and 2, the excised leaves were left in the tubes for an extra incubation step (in the same thermal conditions) for 7 extra days to allow bacterial multiplication according to Ammar et al. (Ammar et al., 2013). Then, leaves were collected and midrib sampled as described above, and frozen at -80°C for further tests.
To assess the effect of temperature on CLas bacterial titer in the leaves and insects during the different AAP and IAP1 and IAP2 tests, we carried out experiments at two different temperature regimes: 23°C day/17°C night or 26°C day/20°C night.
Finally, a further and final IAP (IAP3) was carried out using a single adult from the serial IAP tests which was individually transferred to healthy 2-leaf stage Volkameriana receptor seedlings (young seedlings of 3 to 4 leaves were transferred in tubes on Ms medium) for a 3-day of IAP period. Adults of both species that developed on uninfected leaves were also transferred individually to healthy Volkameriana seedlings under the same conditions as described above and used as controls. After the 3-day of IAP3, all adults were carefully collected and stored individually in absolute ethanol at -80°C for further tests. After IAP3, the seedlings were transferred to 1 L pots in a growth chamber at 26°C+/-2°C (LD 12:12h photoperiod, 80+/- 10% relative humidity) for an incubation period of 3 months. After this incubation period, the 4th upper leaf (from the top) was collected and midrib sampled as described above, and frozen at -80°C for further tests. Plants were then kept for an extra period of 3 months and were checked further for new positives after this period (Figure 1).
Statistical analysis
Analyses were performed using R software version 4.2.0 (R Development Core Team, 2015). To evaluate the effect of temperature, psyllid species, initial CLas-infected source cultivars and their interactions, we built linear mixed models on Cq values for AAP, IAP1, IAP2 leaves, IAP3 seedlings and psyllid adults, and generalized linear mixed models on the proportion of positive psyllid adults or IAP3 seedlings (Bates et al., 2015), with the trials as a random effect. Deviance tests were calculated to test the fixed effects. For pairwise comparisons in case of significant fixed effects, we calculated estimated marginal means (Lenth, 2022) and used the Benjamini-Hochberg method for correction of the p-values. Confidence intervals (CI) at 95% were calculated using the functions MeanCI (DescTools package) and/or t.test (R stats package) with conf.level=0.95.
Results
All field collected leaves of both mandarin cultivars, V1 and V8, were CLas positive. All plants or excised leaves where insects from the two species tested CLas negative were always found CLas negative (negative controls).
For AAP leaves, the positivity threshold was 29.27. A high significant difference on Cqs between the two CLas-infected cultivars was found (Deviance test, P = 1.41 -08) with significantly lower predicted averaged Cq for V1 than V8. There was no significant effect of temperature (Deviance test, P = 0.60) nor between the two psyllid species tested, T. erytreae and D. citri (Test of deviance, P = 0.22) (Figure 2; Table 1) on the Cq values.
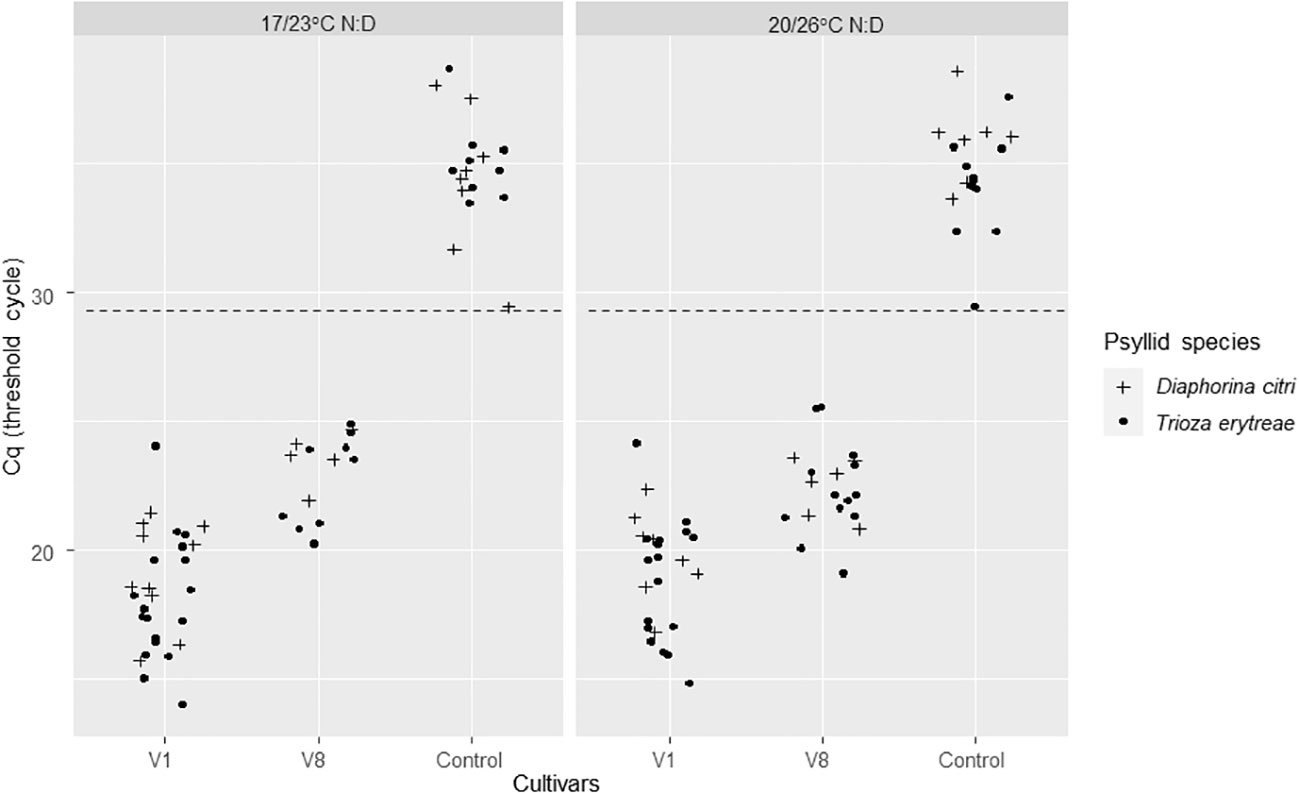
Figure 2 CLas Cq values of excised ForEl 41 (V1) and Pet Yala (V8) mandarin cultivars where Diaphorina citri and Trioza erytreae where in 7-day acquisition access periods (AAP) as nymphs. The control leaves came from a CLas – plant of Volkameriana citrus. The positivity threshold (dash line) chosen as the lowest Cq of the controls was of 29.27. AAPs were made at two different sets of temperatures: 17/23°C; night/day or 20/26°C; night/day.
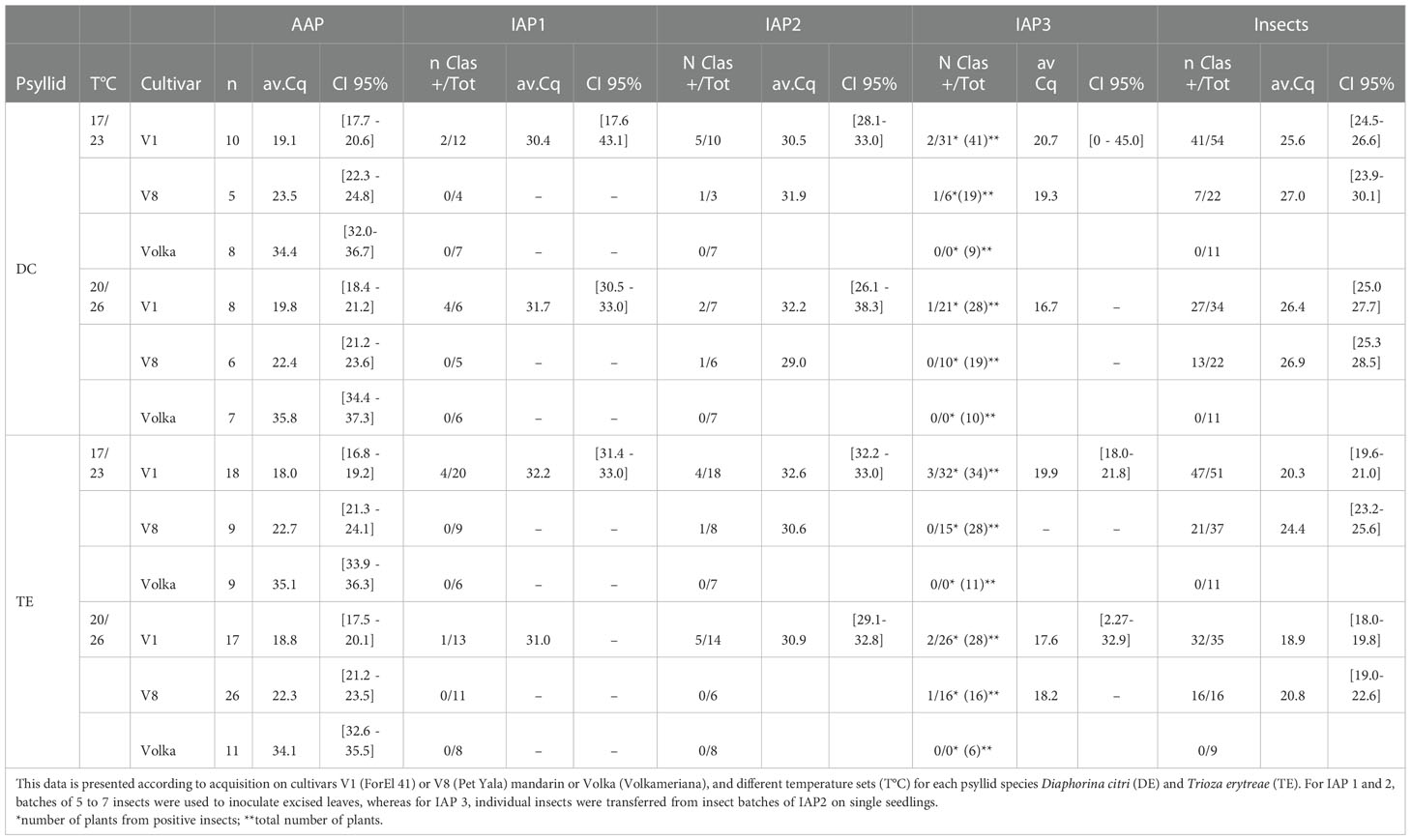
Table 1 Average CLas Cq (av. Cq) and numbers of repetitions (n) for each AAP, IAPs, and insects with 95% confidence intervals (CI 95%).
For IAP1 excised leaves, the positivity threshold was 32.86. A significant difference between psyllid species (Deviance test, P = 0.02) and between cultivars on Cq values was found (Deviance test, P = 0.03) (Figure 3A; Table 1). In IAP1, 22.22% (n=27) of the excised leaves were tested CLas positive by D. citri, and 9.43% (n=53) by T. erytreae after the 7-day IAP. When looking at the initial CLas-infected sources used for acquisition (AAP) (V1 and V8 cultivars), all IAP1 positive excised leaves (for both psyllid species) were derived from the V1 cultivar (Figure 3A; Table 1).
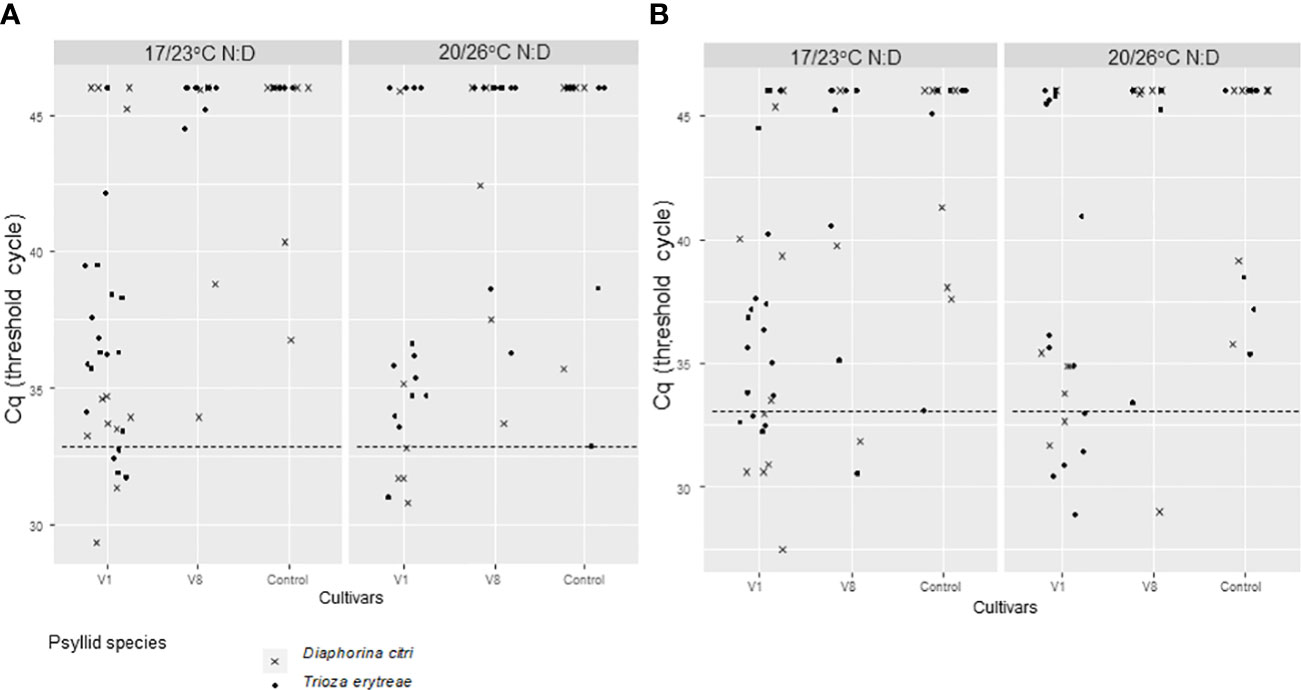
Figure 3 CLas Cq of excised Volkameriana citrus leaves where Diaphorina citri and Trioza erytreae where in 7-day inoculation access periods IAP1 (A) and IAP2 (B) as adults. The 7-insect batches that were previously on AAP on CLas + V1 (ForEl 41) or V8 (Pet Yala) mandarin cultivars and CLas- Volkameriana (Figure 2) where transferred on new Volkameriana CLas – excised leaved for two 7-day IAPs and tested by qPCR for CLas detection. The positivity threshold (dash line) chosen as the lowest Cq of the controls was of 32.9 for IAP1 and 33.1 for IAP2. IAPs were made at two different sets of temperatures: 17/23°C; night/day or 20/26°C; night/day.
For IAP2 excised leaves, the Cq threshold was 33.12. No significant effect of temperature, initial CLas-infected source cultivar, or psyllid species was observed on the number of positive psyllids (Deviance test, P > 0.09). We observed 34.62% (n=26) of the excised leaves that were CLas positive when inoculated by D. citri, and 33.33% (n=45) when inoculated by T. erytreae after the last 7-day IAP on observed data (Figure 3B; Table 1). When predicted values were calculated by emmeans (D. citri predicted values= 23% [1-89%, 95% CI]; T. erytreae predicted values=4% [0-51%, 95% CI]), strong discrepancies were observed with the observed values, most probably due to too small samples. When looking at the initial CLas-infected source plants leaves used for acquisition (AAP) (both V1, V8), 66.67% (n=9) and 90% (n=10) IAP2 positive excised leaves were derived from V1 cultivar for D. citri and T. erytreae, respectively (Figure 3B; Table 1).
For IAP3 seedlings, the positivity threshold was 33.28. No significant effect of temperature, initial CLas-infected source cultivar, or psyllid species was observed (Deviance tests, P > 0.51). In total, the predicted transmission rates on 3-month old plants, using one insect by plant, were 7% [1-26%, 95% CI] for D. citri and 9% [3-29%, 95% CI] for T. erytreae (Figure 4; Table 1) with averaged Cq from 17 to 22 (Table 1). No more positive plants were observed after three more months (i.e., 6-month old plants).
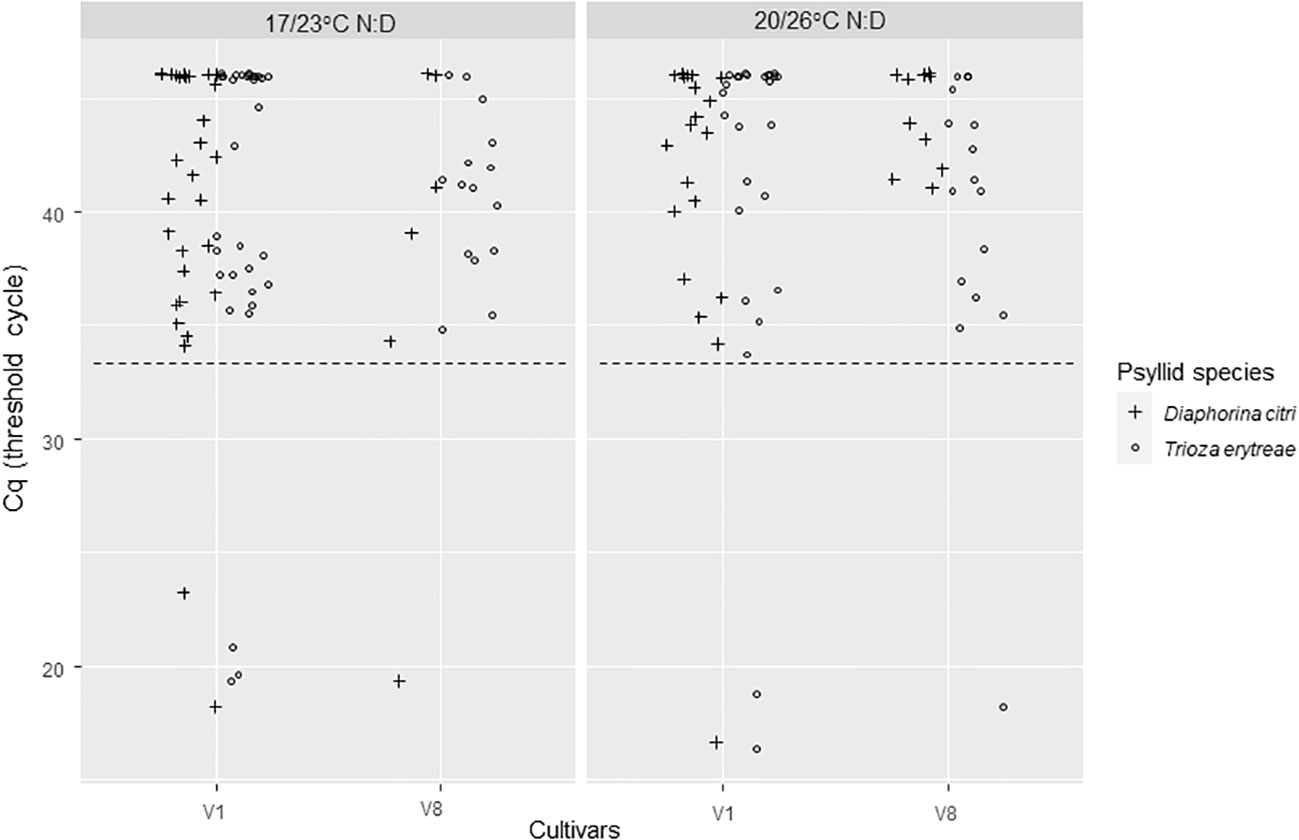
Figure 4 CLas Cq of 3-month Volkameriana citrus plants where Diaphorina citri and Trioza erytreae where in 3-day inoculation access periods at the IAP3. One adult insect that were previously on IAP2 (Figure 3) was transferred on a Volkameriana CLas – seedlings for a 3-day IAP. Plants where further left to grow in growth chambers in the lab for 3-month, then tested by qPCR for CLas detection. The positivity threshold (dash line) chosen as the lowest Cq of the controls was of 33.28. IAPs were made at two different sets of temperatures: 17/23°C; night/day or 20/26°C; night/day.
The CLas infection status and bacterial titer for all adult psyllids that were used in the experiment (except the ones that were lost) were assessed after the IAP 3 (Figure 5). The positivity threshold for this experiment was 31.66. Significant differences were found in the proportion of CLas positive psyllid adults between the two species (Deviance test, P = 0.003) with 78% [63-88%, 95% CI] of D. citri adults and 92% [80-97%, 95% CI] of T. erytreae adults that were CLas positive. There was an effect of the initial CLas-infected source cultivars used for acquisition (Deviance test, P = 1.28e-5). No temperature effect was noticed in the proportion of positive psyllid adults. However, when taking into account Cq values, significant interactions were found between the initial cultivars AAP cultivars and psyllid species (Test of deviance, P= 0.025) as well as for temperature ranges and psyllid species (Test of deviance; P=0.04) (Figure 5; Supplementary Data Figure 1). These significant interactions were mostly due to T. erytreae samples that had much higher Cq values when cultivar V8 was used as a CLas-infected source for the AAP at the lowest temperature range (Supplementary Data Figure 1). Higher differences were found for T. erytreae, with lower averaged Cq values for V1 (20.18 [19.07-21.30, 95% CI] and of 18.57 [17.32-19.83, 95% CI] at 23°C and 26°C, respectively) than for V8 (24.24 [22.79-25.68, 95% CI] and of 20.89 [19.23-22.55, 95% CI] at 23°C and 26°C, respectively). For D. citri, higher Cq values were observed on average compared to T. erytreae, but with fewer differences between V1 (25.37 [24.23-26.51, 95% CI] and 26.14 [24.87-27.42, 95% CI] at 23°C and 26°C, respectively) and V8 (27.15 [24.88-29.41, 95% CI] and 26.44 [24.73-28.15, 95% CI] at 23°C and 26°C, respectively) were observed (Supplementary Data Figure 1).
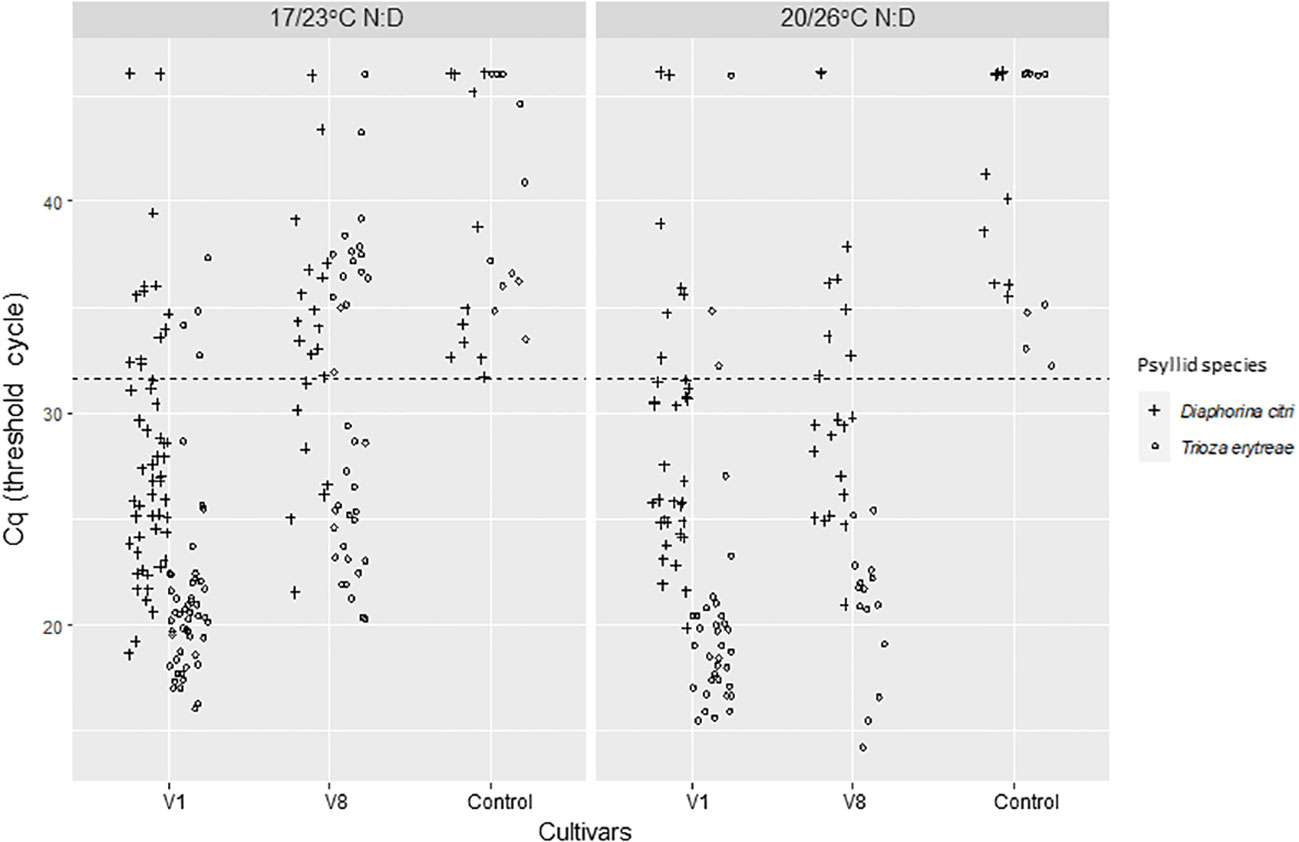
Figure 5 CLas Cq of Diaphorina citri and Trioza erytreae that where used in the whole experiment and collected after the last 3-day IAP3. The positivity threshold (dash line) chosen as the lowest Cq of the controls was of 31.66. IAPs were made at two different sets of temperatures: 17/23°C; night/day or 20/26°C; night/day.
Discussion
Our results demonstrated the ability of T. erytreae to acquire and transmit CLas equally well as D. citri, with no effect of the initial bacterial inoculum or temperature ranges tested.
When testing the bacterial titer of different mandarin cultivars as CLas-infected source plants for the initial inoculum (AAP) we were able to choose two cultivars (V1 and V8) that were found to have significantly different bacterial titers, both showing strong symptoms in the field. The highest bacterial titer was observed for V1, a cultivar known to be HLB susceptible (Koizumi et al., 1993). These differences in bacterial titers between cultivars had already been shown in the field and could reflect the capacity of the V8 cultivar to diminish bacterial multiplication (Alves et al., 2021). Another hypothesis to explain those observed variations could be that a different variant or strain of CLas had infected V1 and V8 cultivars, even in trees next to each other in a same orchard. Genetic studies of the CLas species revealed that even within a given region, it might comprise several different variants (Bastianel et al., 2005; Teixeira et al., 2008).
To our knowledge, this is the first time that plant-to-plant transmission experiments using CLas-infected plants and T. erytreae as the vector species have been performed. Both excised leaves and seedlings were used for both acquisition and inoculation tests. Our results clearly show the efficacy of T. erytreae to acquire and transmit efficiently the bacteria (i.e., in a similar way as D. citri its primary vector) highlighting the threat of having this vector invading the commercial citrus growing areas of southern Europe (Cocuzza et al., 2017; Benhadi-Marín et al., 2022). More importantly, our results highlight that the most aggressive and destructive C. Liberibacter species (CLas) can be spread efficiently by T. erytreae in regions where D. citri has not yet been established. Our findings have important epidemiological implications as CLas in addition to CLaf can be efficiently spread in regions where T. erytreae is present. This stresses the importance of putting in place extreme precautions to prevent the introduction of CLas-infected material in countries where T. erytreae is already established such as Spain or Portugal that have a very important citrus industry.
The initial AAP inoculum bacterial titer was shown to be important since most of the effective transmissions to the final IAP by the two-psyllid species on seedlings came from the V1 cultivar with the highest initial bacterial titer. A 14-day AAP using nymphs should have ensured a better acquisition rate for insects (Inoue et al., 2009; Ammar et al., 2016), which was verified here with CLas-positive rates obtained for D. citri and T. erytreae of 67 and 83%, respectively. Nonetheless, despite such a positive number of CLas-positive insects, only 7% and 9% of transmissions were recorded on IAP3 seedlings for single insect transmission of T. erytreae and D. citri, respectively. Similar results were observed with single insect transmission of D. citri (with transmission rates of 6.7% after 31 days post AAP) showing intermittent and random transmission over series of IAP (Canale et al., 2017). To be noticed, recent results obtained for D. citri with CLas on citrus seedlings with young shoots gave much higher transmission rates (over 56% of transmission), however this could be possibly explained by the experimental design, slightly different (regarding to AAP and IAP times) and the citrus cultivar used as the recipient of CLas (Lopes and Cifuentes-Arenas, 2021). Indeed, Volkameriana cultivar was considered as a moderately tolerant cultivar to CLas compared to sensitive sweet oranges, but still with comparable high bacterial titers (Folimonova et al., 2009). Furthermore, different bacterial strains or psyllid populations between both studies could also play a role in these discrepancies, as in La Réunion the CLas strain is slightly different from others (Lu et al., 2021) such as the ACP psyllid populations which were found genetically different from the new invasive populations in Kenya (Wang et al., 2021) as being present on the island for over 50 years (Bové, 2006).
T. erytreae had significantly higher bacterial titers than D. citri (Supplementary Data Figure 1), but with no higher transmission rates on seedlings in IAP3, or on excised leaves in IAP1 and IAP2, compared to D. citri. Trioza erytreae had been shown to acquire (1 hour AAP was enough) and efficiently transmit CLaf within less than 7 days post AAP, quicker than D. citri with CLas (van Vuuren and van der Merwe, 1992; Ammar et al., 2013; Ammar et al., 2016). So, these lesser transmission rates observed for T. erytreae with CLas (compared to CLaf) might reflect a lesser adaptation of T. erytreae to CLas, as CLas never co-evolved due to their non-overlapping geographical distribution until recently (case of Ethiopia and Kenya where both CLas and T. erytreae are together (Ajene et al., 2020a; Ajene et al., 2020b)). This geographical isolation also contributes to explaining why all transmission tests with CLas have been conducted exclusively with D. citri, and all the CLaf transmission tests have been conducted with T. erytreae. Our results showing the almost equal transmission ability of CLas by T. erytreae need however to be further refined and further experiments should be conducted to better determine the latency period, the persistence or the intermittence of transmission over the life span of T. erytreae.
According to our results T. erytreae has the capacity of acquisition of CLas bacteria at its larval stage and ability to transmit it during its feeding at the adult stage to a CLas-free plant. This implies that CLas, known to be transmitted in a propagative-circulative manner by D. citri (Inoue et al., 2009; Ammar et al., 2016; Canale et al., 2017; Ammar et al., 2018), might also have the same capacity to multiply and circulate up to the salivary glands of T. erytreae, a psyllid of a different area of origin and from another family. Nonetheless, further experiments are needed to determine the pathways of passage and multiplication of the bacteria in the insect body and to be able to compare them with the system of D. citri and CLas.
No significant effect was observed between both temperature regimes tested (17-23°C/20-26°C) on transmission rates of both psyllid species on excised leaves or seedlings. Similar results were observed in the USA on CLas-infected plants incubated at 20°C, 27°C, and 32°C where similar bacterial titers and symptom induction were obtained (Folimonova et al., 2009). Those tested temperature ranges are also compatible with the ones where T. erytreae is already present in Southern Europe (Cocuzza et al., 2017; Benhadi-Marín et al., 2022). This area is then at high risk if the bacteria is introduced, consequences would be tremendously important knowing that this region, still HLB-free, is the 5th worldwide citrus producer, after China, the USA, Brazil, and India (FAO, 2021).Furthermore, a recent study has proven also the risk of the potential introduction of this vector in high citrus growing areas of Mexico that would be most suitable for this vector than D. citri with CLas already present (Espinosa-Zaragoza et al., 2021). Preventive measures and an action plans were proposed in previous studies to reduce the risk of introduction and/or establishment of CLas and CLaf in the Mediterranean area (Durán-Vila et al., 2014; Aragón et al., 2022). Thus, in the light of the results of our study and the recent increase in the range of T. erytreae, these proposed measures should be taken into account even more. In conclusion, this study clearly demonstrated the ability of T. erytreae to acquire and transmit CLas. The presence of T. erytreae in the southern Europe is of great concern given its ability to disseminate the most aggressive variant of HLB (CLas). Thus, extreme precautions to prevent any entry of CLas into Europe should be adopted.
Data availability statement
The original contributions presented in the study are included in the article/Supplementary Material. Further inquiries can be directed to the corresponding author.
Author contributions
Conceptualization, BR, HD; Data collection, BR, MG, PT, FM, SR; Data analysis and interpretation, PT, FC, BR, HD; Writing—original draft, HD; Writing, review and editing, BR, HD, AF, FC; Funding acquisition, BR, HD, AF. All authors contributed to the article and approved the submitted version.
Funding
This work was supported, in whole or in part, by the European Union grant, program H2020 entitled: PRE-HLB: Preventing HLB epidemics for ensuring citrus survival in Europe. H2020-SFS-2018–2 Topic SFS-05–2018-2019–2020—new and emerging risks to plant health (Project n° 817526) and the “Conseil Régional de La Réunion” and the European Agricultural Fund for Rural Development (EAFRD).
Acknowledgments
We acknowledge the advice received from Olivier Pruvost, Gilles Cellier and Isabelle Robène, and the technical help received from Karine Boyer. We greatly thank Antoine Franck (CIRAD, UMR PVBMT) for taking and providing all pictures presented in Figure 1 of this manuscript. We thank Frédéric Labbé for the proofreading of our manuscript. The authors acknowledge the Plant Protection Platform (3P, IBiSA), where all experiments were conducted.
Conflict of interest
The authors declare that the research was conducted in the absence of any commercial or financial relationships that could be construed as a potential conflict of interest.
Publisher’s note
All claims expressed in this article are solely those of the authors and do not necessarily represent those of their affiliated organizations, or those of the publisher, the editors and the reviewers. Any product that may be evaluated in this article, or claim that may be made by its manufacturer, is not guaranteed or endorsed by the publisher.
Supplementary material
The Supplementary Material for this article can be found online at: https://www.frontiersin.org/articles/10.3389/fpls.2022.1089762/full#supplementary-material
References
Ajene, I. J., Khamis, F., Ballo, S., Pietersen, G., van Asch, B., Seid, N., et al. (2020a). Detection of Asian citrus psyllid (Hemiptera: Psyllidae) in Ethiopia: a new haplotype and its implication to the proliferation of huanglongbing. J. Economic Entomol. 113 (4), 1640–1647. doi: 10.1093/jee/toaa113
Ajene, I. J., Khamis, F. M., van Asch, B., Pietersen, G., Seid, N., Rwomushana, I., et al. (2020b). Distribution of Candidatus liberibacter species in Eastern Africa, and the first report of Candidatus liberibacter asiaticus in Kenya. Sci. Rep. 10, 1–10. doi: 10.1038/s41598-020-60712-0
Alves, M. N., Lopes, S. A., Raiol-Junior, L. L., Wulff, N. A., Girardi, E. A., Ollitrault, P., et al. (2021). Resistance to ‘Candidatus liberibacter asiaticus,’the huanglongbing associated bacterium, in sexually and/or graft-compatible citrus relatives. Front. Plant Sci. 11. doi: 10.3389/fpls.2020.617664
Ammar, E.-D., Hall, D. G., Hosseinzadeh, S., Heck, M. (2018). The quest for a non-vector psyllid: natural variation in acquisition and transmission of the huanglongbing pathogen ‘Candidatus liberibacter asiaticus’ by Asian citrus psyllid isofemale lines. PLoS One 13 (4), e0195804. doi: 10.1371/journal.pone.0195804
Ammar, E.-D., Ramos, J. E., Hall, D. G., Dawson, W. O., Shatters, R. G., Jr (2016). Acquisition, replication and inoculation of Candidatus liberibacter asiaticus following various acquisition periods on huanglongbing-infected citrus by nymphs and adults of the Asian citrus psyllid. PLoS One 11 (7), e0159594. doi: 10.1371/journal.pone.0159594
Ammar, E.-D., Walter, A. J., Hall, D. G. (2013). New excised-leaf assay method to test inoculativity of Asian citrus psyllid (Hemiptera: Psyllidae) with Candidatus liberibacter asiaticus associated with citrus huanglongbing disease. J. Economic Entomol. 106 (1), 25–35. doi: 10.1603/EC12245
Aragón, C., Dalmau, V., Escrivà, C., Ferrer, A., Forner-Giner, M.Ă., Galvañ, A., et al. (2022). Being prepared for huanglongbing disease of citrus: A simulation exercise workshop for contingency planning held in Valencia, Spain. EPPO Bulletin, 1–8. doi: 10.1111/epp.12892
Aubert, B. (1987). Trioza erytreae del guercio and Diaphorina citri kuwayama (Homoptera: Psylloidea), the two vectors of citrus greening disease: biological aspects and possible control strategies. Fruits 42 (3), 149–162.
Aubert, B., Grisoni, M., Villemin, M., Rossolin, G. (1996). “A case study of huanglongbing (greening) control in reunion,” in Proc. 13th Conference of the International Organization of Citrus Virologists (IOCV), University of California, Riverside. 276–278.
Baldwin, E., Plotto, A., Manthey, J., McCollum, G., Bai, J., Irey, M., et al. (2010). Effect of liberibacter infection (Huanglongbing disease) of citrus on orange fruit physiology and fruit/fruit juice quality: chemical and physical analyses. J. Agric. Food Chem. 58 (2), 1247–1262. doi: 10.1021/jf9031958
Bastianel, C., Garnier-Semancik, M., Renaudin, J., Bové, J., Eveillard, S. (2005). Diversity of “Candidatus liberibacter asiaticus,” based on the omp gene sequence. Appl. Environ. Microbiol. 71 (11), 6473–6478. doi: 10.1128/AEM.71.11.6473-6478.2005
Bates, D., Maechler, M., Bolker, B., Walker, S. (2015). Fitting linear mixed-effects models using lme4. J. Stat. Software 67, 1–48. doi: 10.48550/arXiv.1406.5823
Benhadi-Marín, J., Fereres, A., Pereira, J. A. (2022). Potential areas of spread of Trioza erytreae over mainland Portugal and Spain. J. Pest Sci. 95 (1), 67–78. doi: 10.1007/s10340-021-01440-w
Bové, J. M. (2006). Huanglongbing: a destructive, newly-emerging, century-old disease of citrus. J. Plant Pathol. 88 (1), 7–37.
Canale, M. C., Tomaseto, A. F., Haddad, M., Della Coletta-Filho, H., Lopes, J. R. S. (2017). Latency and persistence of ‘Candidatus liberibacter asiaticus’ in its psyllid vector, Diaphorina citri (Hemiptera: Liviidae). Phytopathology 107 (3), 264–272. doi: 10.1094/PHYTO-02-16-0088-R
Catling, H. (1972). The distribution of psyllid vectors of citrus greening disease in reunion. Rapport de mission, document IRFA Réunion.
Catling, H. (1973). Notes on the biology of the south African citrus psylla, Trioza erytreae (Del guercio) (Homoptera: Psyllidae). J. Entomological Soc. South Afr. 36, 299–306.
Cellier, G., Redondo, C., Cubero, J., Roselló, M., de Andrade, E., Cruz, L., et al. (2020). Comparison of the performance of the main real-time and conventional PCR detection tests for ‘Candidatus liberibacter’spp., plant pathogenic bacteria causing the huanglongbing disease in citrus spp. Eur. J. Plant Pathol. 157 (4), 919–941. doi: 10.1007/s10658-020-02052-3
Cocuzza, G. E. M., Alberto, U., Hernández-Suárez, E., Siverio, F., Di Silvestro, S., Tena, A., et al. (2017). A review on Trioza erytreae (African citrus psyllid), now in mainland Europe, and its potential risk as vector of huanglongbing (HLB) in citrus. J. Pest Sci. 90 (1), 1–17. doi: 10.1007/s10340-016-0804-1
Dala-Paula, B. M., Plotto, A., Bai, J., Manthey, J. A., Baldwin, E. A., Ferrarezi, R. S., et al. (2019). Effect of huanglongbing or greening disease on orange juice quality, a review. Front. Plant Sci. 9. doi: 10.3389/fpls.2018.01976
Del Guercio, G. (1918). Notes and Observations on Agricultural Entomology. Preliminary Notes. Instituto Agricolo Coloniale Italiano. 282 p.
Durán-Vila, N., Janse, J., Foissac, X., Melgarejo, P., Bove, J.-M. (2014). Addressing the threat of huanglongbing in the Mediterranean region: a challenge to save the citrus industry. J. Plant Pathol. 96 (4SUP), 4–3-S4. 8. doi: 10.4454/jpp.v96i2SUP.3297
EPPO (2021) Update on the situation of trioza erytreae in Portugal. Available at: https://gd.eppo.int/reporting/article-7215.
Espinosa-Zaragoza, S., Perez-De la O, N. B., Aguirre-Medina, J. F., Lopez-Martinez, V. (2021). Does the African citrus psyllid, Trioza erytreae (Del Guercio)(Hemiptera: Triozidae), represent a phytosanitary threat to the citrus industry in Mexico? Insects 12 (5), 450. doi: 10.3390/insects12050450
Ferrarezi, R. S., Vincent, C. I., Urbaneja, A., Machado, M. A. (2020). Unravelling citrus huanglongbing disease. Front. Plant Sci. 11. doi: 10.3389/fpls.2020.609655
Folimonova, S. Y., Robertson, C. J., Garnsey, S. M., Gowda, S., Dawson, W. O. (2009). Examination of the responses of different genotypes of citrus to huanglongbing (citrus greening) under different conditions. Phytopathology 99 (12), 1346–1354. doi: 10.1094/PHYTO-99-12-1346
Garnier, M., Jagoueix, S., Toorawa, P., Grisoni, M., Mallessard, R., Dookun, A., et al. (1996). “Both huanglongbing (greening) liberobacter species are present in Mauritius and Réunion,” in International Organization of Citrus Virologists Conference Proceedings (1957-2010).
Gottwald, T. R. (2010). Current epidemiological understanding of citrus huanglongbing. Annu. Rev. Phytopathol. 48, 119–39. doi: 10.1146/annurev-phyto-073009–114418
Hall, D. G. (2008). “Biology, history and world status of Diaphorina citri.,” in Proceedings of the International Workshop on Huanglongbing and Asian Citrus Psyllid, Mexico, Vol. 8. 1–11.
Inoue, H., Ohnishi, J., Ito, T., Tomimura, K., Miyata, S., Iwanami, T., et al. (2009). Enhanced proliferation and efficient transmission of Candidatus liberibacter asiaticus by adult diaphorina citri after acquisition feeding in the nymphal stage. Ann. Appl. Biol. 155 (1), 29–36. doi: 10.1111/j.1744-7348.2009.00317.x
Koizumi, M., Prommintara, M., Linwattana, G., Kaisuwan, T. (1993). “Field evaluation of citrus cultivars for greening disease resistance in Thailand,” in Twelfth IOCV conference Proceedings (1975-2010). 12 (12), (California: U.o.C. International Organization of Citrus Virologists, Riverside), Eds. Moreno, P., Da Graca, J. V., Timmer, L. W.. 12 (12).
Li, W., Hartung, J. S., Levy, L. (2006). Quantitative real-time PCR for detection and identification of candidatus liberibacter species associated with citrus huanglongbing. J. microbiological Methods 66 (1), 104–115. doi: 10.1016/j.mimet.2005.10.018
Lopes, S. A., Cifuentes-Arenas, J. C. (2021). Protocol for successful transmission of ‘Candidatus liberibacter asiaticus’ from citrus to citrus using Diaphorina citri. Phytopathology® 111 (12), 2367–2374. doi: 10.1094/PHYTO-02-21-0076-R
Lu, J., Delatte, H., Reynaud, B., Beattie, G. A., Holford, P., Cen, Y., et al. (2021). Genome sequence resource of ‘Candidatus liberibacter asiaticus’ from Diaphorina citri kuwayama (Hemiptera: Liviidae) from la Réunion. Plant Dis. 105 (4), 1171–1173. doi: 10.1094/PDIS-09-20-1998-A
Martinez, A., Wallace, J. (1967). Citrus leaf mottle-yellows disease in the Philippines and transmission of the causal virus by a psyllid, Diaphorina citri. Plant Dis. Rep. 51 (8), 692–695.
Massonie, G., Garnier, M., Bové, J. (1976). “Transmission of Indian citrus decline by Trioza erytreae (Del guercio), the vector of south African greening,” in International Organization of Citrus Virologists Conference Proceedings (1957-2010).
McClean, A., Oberholzer, P. (1965). Citrus psylla, a vector of the greening disease of sweet orange-research note. South Afr. J. Agric. Sci. 8 (1), 297–298.
R Development Core Team (2015). R : A language and environment for statistical computing (Vienna, Austria: R Foundation for Statistical Computing).
Teixeira, D. C., Saillard, C., Couture, C., Martins, E. C., Wulff, N. A., Eveillard-Jagoueix, S., et al. (2008). Distribution and quantification of candidatus liberibacter americanus, agent of huanglongbing disease of citrus in são paulo state, Brasil, in leaves of an affected sweet orange tree as determined by PCR. Mol. Cell. Probes 22 (3), 139–150. doi: 10.1016/j.mcp.2007.12.006
Teixeira, D. C., Saillard, C., Eveillard, S., Danet, J. L., da Costa, P. I., Ayres, A. J., et al. (2005a). 'Candidatus liberibacter americanus', associated with citrus huanglongbing (greening disease) in são paulo state, Brazil. Int. J. Systematic Evol. Microbiol. 55 (Pt 5), 1857–1862. doi: 10.1099/ijs.0.63677-0
Texeira, D., Ayres, J., Kitajima, E., Danet, L., Jagoueix-Eveillard, S., Saillard, C., et al. (2005b). First report of a huanglongbing-like disease of citrus in são paulo state, Brazil and association of a new liberibacter species,”Candidatus liberibacter americanus”, with the disease. Plant Dis. 89 (1), 107–107. doi: 10.1094/PD-89-0107A
van Vuuren, S., van der Merwe, M. (1992). Efficacy of citrus psylla. Trioza erytreae. as a vector of citrus greening disease. Phytophylactica 14 (3), 285–288.
Wang, Y., Halbert, S., Mohamed, S., Delatte, H., Reynaud, B., Beattie, G., et al. (2021). Mitochondrial genomes reveal diverse lineages of Diaphorina citri kuwayama (Hemiptera: Sternorrhyncha: Psyllidae) in Kenya and la Réunion. Biol. Invasions 23 (10), 3109–3117. doi: 10.1007/s10530-021-02560-1
Keywords: Huanglonbing, Diaphorina citri, transmission efficiency, HLB (citrus greening), CLAS
Citation: Reynaud B, Turpin P, Molinari FM, Grondin M, Roque S, Chiroleu F, Fereres A and Delatte H (2022) The African citrus psyllid Trioza erytreae: An efficient vector of Candidatus Liberibacter asiaticus. Front. Plant Sci. 13:1089762. doi: 10.3389/fpls.2022.1089762
Received: 04 November 2022; Accepted: 01 December 2022;
Published: 22 December 2022.
Edited by:
Ivo Tosevski, Institute for Plant Protection and Environment (IZBIS), SerbiaReviewed by:
Xavier Foissac, Institut National de recherche pour l’agriculture, l’alimentation et l’environnement (INRAE), FranceSubhas Hajeri, Citrus Pest Detection Program, United States
Copyright © 2022 Reynaud, Turpin, Molinari, Grondin, Roque, Chiroleu, Fereres and Delatte. This is an open-access article distributed under the terms of the Creative Commons Attribution License (CC BY). The use, distribution or reproduction in other forums is permitted, provided the original author(s) and the copyright owner(s) are credited and that the original publication in this journal is cited, in accordance with accepted academic practice. No use, distribution or reproduction is permitted which does not comply with these terms.
*Correspondence: Delatte Hélène, aGVsZW5lLmRlbGF0dGVAY2lyYWQuZnI=
†ORCID: Hélène Delatte, orcid.org/0000-0001-5216-5542