- 1Department of Agronomy, College of Agriculture, University of Sargodha, Sargodha, Pakistan
- 2College of Life Sciences, Yan’an University, Yan’an, China
- 3Future Regions Research Centre, Federation University Australia, Mount Helen, VIC, Australia
- 4Institute of Molecular Biology and Biotechnology, The University of Lahore, Lahore, Pakistan
- 5Department of Agronomy, University of Agriculture Faisalabad, Faisalabad, Pakistan
- 6State Key Laboratory of Grassland Agroecosystems, School of Life Sciences, Institute of Arid Agroecology, Lanzhou University, Lanzhou, China
- 7Department of Plant Breeding and Genetics, The University of Haripur, Haripur, Pakistan
It is known that the impact of elevated CO2 (eCO2) will cause differential photosynthetic responses in plants, resulting in varying magnitudes of growth and productivity of competing species. Because of the aggressive invasive nature of Acacia longifolia ssp. longifolia, this study is designed to investigate the effect of eCO2 on gas exchange parameters, water use efficiency, photosystem II (PSII) activities, and growth of this species. Plants of A. longifolia ssp. longifolia were grown at 400 ppm (ambient) and 700 ppm (elevated) CO2 under 100 and 60% field capacity. Leaf gas exchange parameters, water use efficiency, intrinsic water use efficiency, instantaneous carboxylation efficiency, and PSII activity were measured for 10 days at 2-day intervals. eCO2 mitigated the adverse effects of drought conditions on the aforementioned parameters compared to that grown under ambient CO2 (aCO2) conditions. A. longifolia, grown under drought conditions and re-watered at day 8, indicated a partial recovery in most of the parameters measured, suggesting that the recovery of this species under eCO2 will be higher than that with aCO2 concentration. This gave an increase in water use efficiency, which is one of the reasons for the observed enhanced growth of A. longifolia under drought stress. Thus, eCO2 will allow to adopt this species in the new environment, even under severe climatic conditions, and foreshadow its likelihood of invasion into new areas.
Introduction
A substantial increase in atmospheric CO2 level has already occurred since the Industrial Revolution, and it is predicted that it will approach 700 ppm by the year 2050 (IPCC, 2018). This increase in atmospheric CO2 will cause global warming (Boucher et al., 2009) that leads to water scarcity, a key constraint of crop productivity. Despite that, elevated CO2 (eCO2) is believed to increase the photosynthetic rate and suppress their photorespiration (Li et al., 2019). eCO2 increased the photosynthesis and stimulated biomass and yield parameters (Kirschbaum, 2011) along with decreased water use per unit area of vegetation by partial stomatal closure (Pastore et al., 2020). Studies have also shown that eCO2 causes a 20–30% increase in net assimilation rate, together with an increase in dry matter and yield of crops (Cruz et al., 2018; Pan et al., 2018; Xie et al., 2018). Increasing atmospheric CO2 has significant effects on physiological processes related to plant growth, even though responses will be modified under drought stress (Qaderi et al., 2006, 2013). It is well documented that enhanced biomass production and alteration in photosynthetic activity and water relation under eCO2 are dependent on the availability of other limiting resources (Robredo et al., 2007). According to Elgawad et al. (2015), eCO2 has been shown to mitigate the stress impact of drought, and they quote examples of how the reduction of drought stress affects barley and alfalfa crops. Despite these beneficial effects on crops, ongoing increases in atmospheric CO2 may have important consequences for weed-crop competition, leading to subsequent yield losses (Ziska et al., 2019).
It has been noted that climate change further increases the risk of plant invasiveness by facilitating a change in the ecosystem through the increased competitiveness of the weed under eCO2 conditions (Bradley et al., 2010; Le et al., 2019). Previous studies have shown that invasive plants exhibit greater climatic change tolerance in invaded habitats compared to native species due to evolutionary plasticity (Clements and Ditommaso, 2011; Davidson et al., 2011). In this respect, eCO2 and water stress, sowing to climate change, can directly affect plant physiology and morphology (Lobell and Gourdji, 2012; Salazar-Parra et al., 2012). It is postulated that because invasive species benefit from eCO2, this will enable them to acclimatize to their new environments in the future (Dukes et al., 2011; Pastore et al., 2020). It is predicted that rising atmospheric CO2 may cause global warming, which could be the basis of change in precipitation patterns and drought occurrence, and could possibly affect those regions not currently subjected to drought (Robredo et al., 2007; Dai, 2013; Dai et al., 2018). It is also postulated that different plant species showed different responses with rising atmospheric CO2 concentration under drought conditions (Pastore et al., 2020). Researchers have observed that plants are grown under eCO2 and dry more slowly as water is withheld due to lower stomatal conductance and transpiration rate (Dikšaitytė et al., 2019; Pastore et al., 2020).
Acacia species, which are native to Australia, have been deliberately exported for some years to a number of temperate countries in order to stabilize degraded soil (especially sand dunes) for ornamental purposes and fuelwood (Kull et al., 2011). However, the aggressive growth properties of most of the Acacia species have made them particularly invasive (Corlett, 2010), with the result that these species are currently included in the Global Invasive Species Database. It is well established that invasive alien species affected the growth and wellbeing of native species by altering the ecosystem and introducing direct competition for resources (Werner et al., 2008; Alba et al., 2019). According to Blossey and Notzold (1995) hypothesis, competitive abilities can be maximized by increasing vegetative growth or reproductive growth for success in a particularly new environment. This alteration in the mix of biota presents environmental managers with an urgent challenge to stem the infiltration of invasive species in order to maintain indigenous biodiversity (Qaderi et al., 2013). In many situations, it is evident that these invasive plant species are considered to be the major cause of biodiversity loss and are also thought to contribute to global environmental change (Bellard et al., 2014).
Acacia longifolia ssp. longifolia, which is the focus of this article, belongs to the Fabaceae family and is commonly known as either Sydney golden wattle, long-leaved wattle, or shallow wattle (Marchante et al., 2010). It is indigenous to South Eastern Australia (Costermans, 1981), but currently it is widely distributed throughout Australia (Marchante et al., 2015). Whilst sandy coastal areas are the preferred habitat of A. longifolia, it also grows successfully in woodlands, grasslands, and along roadsides (Marchante et al., 2010). Its ability to fix nitrogen enables this species to colonize successfully in nutrient-poor soil (Sampaio, 2019). Due to its aggressive growth habit, this species is currently recognized as a problematic invasive plant in adjoining native areas in Australia, as well as in introduced environments in Portugal, Southern Africa, and Spain (Souza-Alonso et al., 2018). Many reports have indicated that this species can outcompete native plants by rapid growth even under disturbed conditions (Osunkoya et al., 2005; Marchante et al., 2015). This invasive character of A. longifolia ssp. longifolia can be attributed to its prolific annual seed production and physical seed dormancy due to its hard seed coat, which facilitates its soil seed bank persistence and its consequent emergence in times of stress (Welgama et al., 2019).
The interest of this article is that many species of Acacia respond to eCO2 concentrations and record increased growth as a result of enhanced carbon assimilation (Evans et al., 2000; Le et al., 2019). In this respect, Evans et al. (2000) studied the effect of eCO2 on photosynthetic activity of 10 Acacia species (i.e., Acacia aneura, Acacia colei, Acacia coriacea, Acacia tetragonophylla, Acacia irrorata, Acacia dealbata, Acacia mearnsii, Acacia implexa, Acacia melanoxylon, and Acacia saligna) under normal irrigation conditions. Except for A. aneura, results revealed that growth and gas exchange measurements of all species were enhanced under 700 ppm CO2 compared to that under 350 ppm, with the degree of enhancement being species-specific. A. longifolia ssp. longifolia was not included in the previous study, but other studies have revealed a wealth of information on its ecology and biology. Nonetheless, more information about physiological processes and biomass production regarding A. longifolia ssp. longifolia will increase the understanding of the potential future impacts of climate change on this species. As a consequence, this study was designed to investigate the effects of drought on A. longifolia ssp. longifolia under two atmospheric CO2 concentrations (400 and 700 ppm), noting changes in physiological processes, water use efficiency, and growth.
Materials and Methods
Experimental Site and Sowing Conditions
Experiments were conducted at Federation University, Mt Helen, Australia (37°37.39°S, 143°53.27°E) in two CO2 chambers (2.1 m length, 2.1 m width, and 2.0 m height) (Steridium Pty Ltd., Brendale, QLD, Australia). One CO2 chamber was set at 400 ppm CO2 concentration [ambient CO2 (aCO2)], whilst the other was set at 700 ppm CO2 concentration (eCO2). The average chamber temperature was maintained at 22°C day/18°C night alternating temperature with 60% humidity. Mature seeds of A. longifolia ssp. longifolia were collected from the Grampians National Park Victoria (37°12.16°S, 142°23.35°E). Two moisture levels (well-watered and drought) were also maintained in each CO2 chamber. Twenty plastic pots (13 cm wide and 14 cm height) were filled each with 800 g of 2:1 mixture of garden soil and commercially available potting mixture. The seeds of A. longifolia ssp. longifolia were surface-scarified with sandpaper No. 1000 to remove the physical dormancy, imposed by the seed coat. Five scarified seeds were sown in each pot.
Effects on Photosynthetic Response by Drought Conditions and Elevated CO2 Levels
Out of 20 pots, 10 pots were placed in the chamber with aCO2 concentration, and the remaining half were placed in the chamber with eCO2 concentration. The pots were placed in large plastic trays, and water was added to these trays to reduce the disturbance of the potting mixture until emergence occurred. At the four-leaf stage, the seedlings were thinned to two per pot. After 30 days from sowing, the first measurements of gas exchange parameters were recorded, and these measurements were termed “zero-day.” Subsequently, moisture treatments of well-watered and drought were commenced. The well-watered treatments were maintained at 100% field capacity and drought treatments at 60% field capacity. These water regimes were selected as different plant species showed variable responses with eCO2 concentration under drought conditions. The water holding capacity of the soil used in the pots was calculated according to the method suggested by Bajwa et al. (2017). Of the 10 pots in each chamber, half were subjected to well-watered, and the remaining half were subjected to drought. The pots were weighed in order to maintain the field capacity levels, and the weight of the growing plant was much smaller than that of the soil in pot. There were five replications (one pot for each replication) for each treatment, and each replication consisted of two plants. In the drought treatments, water was withheld until day 8 in both CO2 chambers, after which drought treatment pots were re-watered to investigate the recovery response of the A. longifolia ssp. longifolia plants. The amount of water added was calculated based on pot weight, and 60% field capacity was maintained till day 10. In the well-watered treatments, water was added on alternate days. To evaluate the effect of CO2 and drought, physiological parameters were measured using the LI-COR portable infrared CO2 gas analyzer (LI-6400 XT portable photosynthesis system, LI-COR, Biosciences, Lincoln, Nebraska, United States). Measurements were recorded with the following adjustments of LI-COR: block temperature was set at 20°C, photosynthetic photon flux density (PPFD) was 1,000 μmol m–2 s–1, leaf cuvette area was set at 2 cm2, and the flow rate was adjusted at 500 μmol m–2 s–1. The CO2 concentration in the chamber was noted before each measurement. Net photosynthetic rate, stomatal conductance, transpiration rate, and intercellular CO2 concentration were measured on alternate days. Water use efficiency was calculated by dividing the net photosynthetic rate with transpiration rate, intrinsic water use efficiency was calculated by dividing the net photosynthetic rate with stomatal conductance, and instantaneous carboxylation efficiency was calculated by dividing the net photosynthetic rate by the intercellular CO2 concentration. Photosystem II (PSII) activity, such as minimum fluorescence, maximum fluorescence, quantum yield of PSII, photochemical efficiency of PSII, photochemical quenching, non-photochemical quenching, and photosynthetic electron transport rate, was calculated according to the methods described by Maxwell and Johnson (2000).
Effects on Biomass Productivity by Drought Conditions and Elevated CO2 Levels
Growth parameters, including plant height, number of leaves, number of branches, stem diameter, leaf thickness, leaf area, leaf fresh weight, dry weight, and root-shoot fresh and dry weights, were measured at the conclusion of the experiment on day 10. Plants were removed from pots, and the stem was cut from the roots. Root fresh weight was measured after careful washing and air drying. Stem, leaves, and roots were placed in a separate paper bag and dried in an oven at 70°C for 72 h.
Statistical Analysis
Data for gas exchange parameters were presented in graphs along with the SE of each mean, and the graphs were prepared using the SigmaPlot version 11 software. To investigate the effect of time of observation (time), water conditions (water), and CO2 levels (CO2), physiological parameters were analyzed with Statistix version 8.1 using three-way ANOVA. All the main effects and two- and three-way interactions were examined using the Tukey’s honestly significant difference (HSD) test at a 5% probability level. Data of growth parameters were subjected to two-way ANOVA to assess the effect of CO2 concentrations and water regimes on the growth of A. longifolia ssp. longifolia. Five replications (two plants per pot) were used for each combination of treatments. The significance among the treatment means was separated by using the Tukey’s HSD at p ≤ 0.05.
Results
Effects on Photosynthetic Response by Drought Conditions and Elevated CO2 Levels
Photosynthetic activities were measured for A. longifolia ssp. longifolia when grown under two moisture regimes (i.e., well-watered and drought) at 400 ppm CO2 concentration (aCO2 concentration) and 700 ppm CO2 concentration (eCO2 concentration). Figure 1A shows that eCO2 concentration increased the net photosynthetic rate by 2–4 μmol CO2 m–2 s–1 when compared to that with aCO2 concentration under well-watered conditions. However, a variable response was noted with both CO2 concentrations when A. longifolia ssp. longifolia was grown under drought conditions. At aCO2 concentration, the net photosynthetic rate decreased to 0.07 μmol CO2 m–2 s–1 at day 8, whilst plants under eCO2 concentration recorded a net photosynthetic rate of 6.17 μmol CO2 m–2 s–1 on the same day. After all these measurements, water was added to the drought treatments on day 8 to investigate the recovery response of the plants in terms of the net photosynthetic rate. Photosynthesis was recovered at a high rate with eCO2 and increased from 6.17 to 9.8 μmol CO2 m–2 s–1, whilst under aCO2 concentration, it increased from 0.17 to 5.1 μmol CO2 m–2 s–1 (Figure 1A). The ANOVA results showed that time (p = 0.001), water (p < 0.001), CO2 (p < 0.001), time × water (p = 0.001), and time × CO2 (p = 0.001) were significant for photosynthetic rate of A. longifolia, whereas the interaction of water × CO2 and time × water × CO2 was non-significant (Table 1).
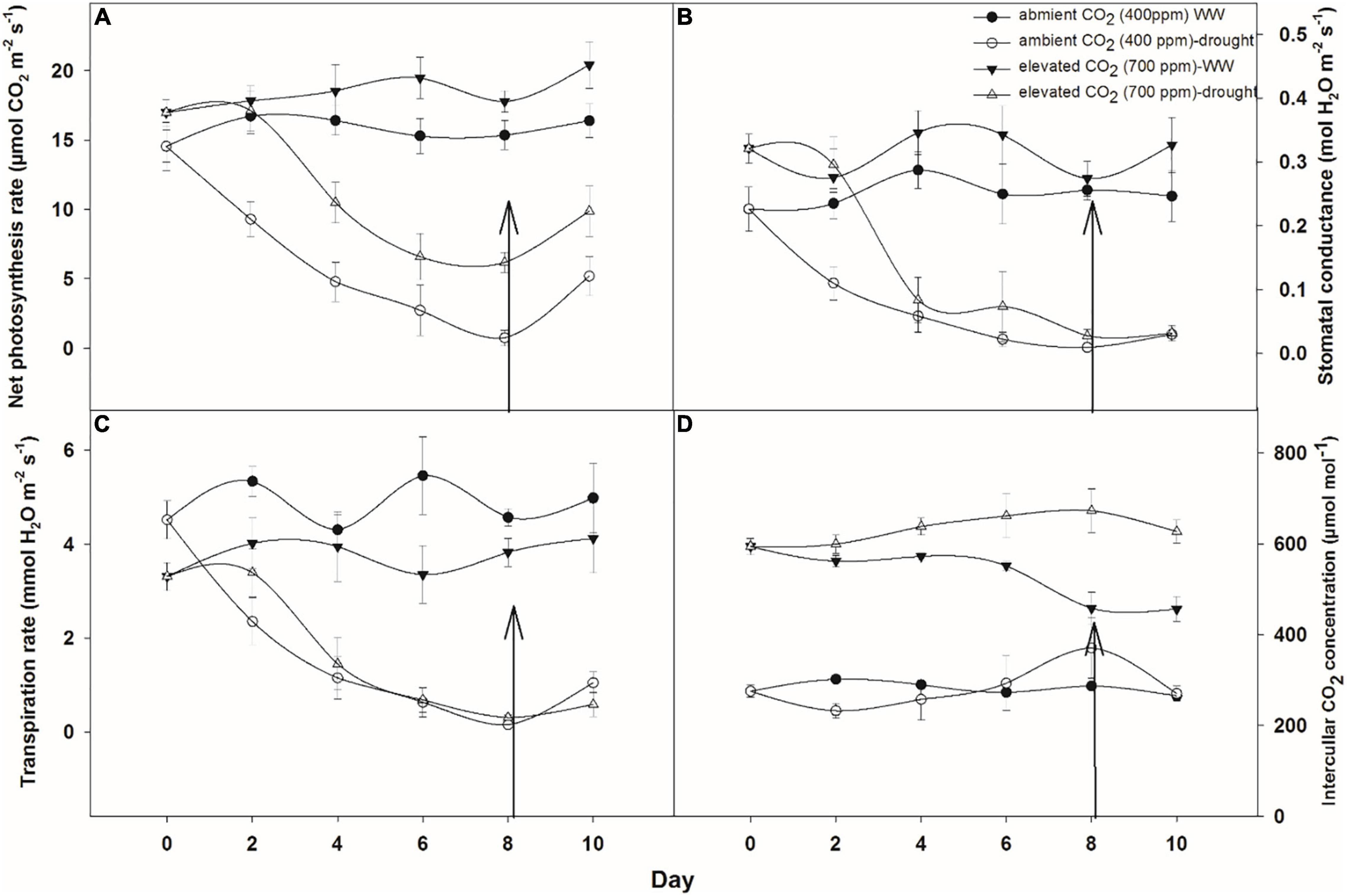
Figure 1. Effect of CO2 concentrations and moisture conditions on photosynthetic rate (A), stomatal conductance (B), transpiration rate (C), and intercellular CO2 concentration (D) of A. longifolia ssp. longifolia. Nail on the data point represents the SE of the mean, and vertical arrow line after day 8 represents the addition of water in the drought treatments. WW, well-watered.
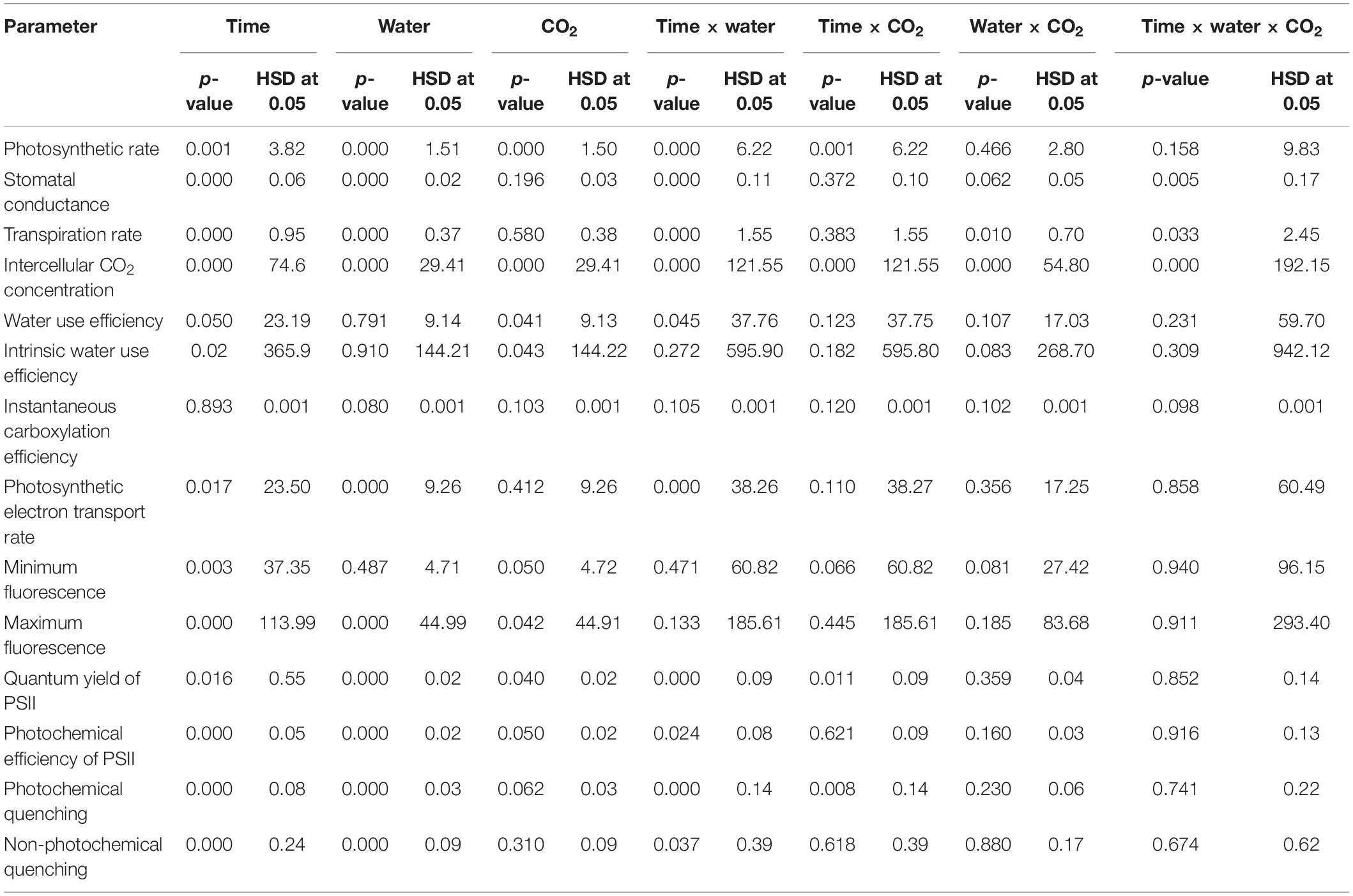
Table 1. Statistical significances for physiological parameters of A. longifolia ssp. longifolia in response to time of observation, water conditions, and CO2 levels.
A. longifolia ssp. longifolia exhibited a significant difference in stomatal conductance under eCO2 and aCO2 concentrations (Figure 1B). eCO2 inhibited the stomatal conductance compared to that with aCO2 under well-watered conditions. Drought stress resulted in a progressive decline in stomatal conductance up to day 8 with aCO2 (0.009 mol H2O m–2 s–1). However, this decline was less with eCO2, since 0.027 mol H2O m–2 s–1 stomatal conductance was recorded on day 8. After the addition of water to drought treatments on day 8, stomatal conductance recovered slightly, but this recovery was larger with eCO2 than that with aCO2. The results of ANOVA in Table 1 indicate that the stomatal conductance of A. longifolia spp. longifolia in response to time, water, CO2, and time × water was significant (p < 0.04).
The eCO2 concentration inhibited the transpiration rate under well-watered conditions compared to that with aCO2 (Figure 1C). The transpiration rate reduced progressively with an increase in the duration of drought under both CO2 regimes. During the initial days of drought stress, transpiration did not reduce significantly with eCO2, whilst aCO2 reduced the transpiration rate linearly, and it was measured at 0.6 mmol H2O m–2 s–1 on the 8th day of observation. The addition of water to drought treatments after the 8th day of observation resulted in higher recovery (from 0.6 to 1.0 4 mmol H2O m–2 s–1) in the transpiration rate of A. longifolia ssp. longifolia with aCO2, compared to that when grown under eCO2. Response of transpiration rate of A. longifolia spp. longifolia to time, water, time × water, and water × CO2 was significant (Table 1).
When A. longifolia ssp. longifolia was grown under well-watered conditions, eCO2 caused an increase in intracellular CO2 concentration, being recorded between 450 and 590 μmol mol–1 compared with aCO2 where intercellular CO2 concentration was between 270 and 300 μmol mol–1 (Figure 1D). Drought stress exerted significant effects on intercellular CO2 concentration, and it increased with an increase in drought duration under both CO2 concentrations, attaining maximum value on the 8th day of measurement. An increase in intracellular CO2 concentration was higher with eCO2 compared to that with aCO2. The addition of water to drought treatments on the 8th day slightly reduced the value of intercellular CO2 concentration, whilst it was significantly reduced under aCO2 with the addition of water. The ANOVA results showed that the main effects (time, water, and CO2) and two-way or three-way interactions for intracellular CO2 concentration were significant (p < 0.02).
Under well-watered conditions, eCO2 increased the water use efficiency by 0.76 mmol CO2 mol–1 H2O in comparison with that with aCO2, whereas the effects of drought on water use efficiency of A. longifolia ssp. longifolia were different under both CO2 concentrations. eCO2 linearly increased the water use efficiency and recorded a maximum on the 8th day of measurement (21.6 mmol CO2 mol–1 H2O). Whilst drought conditions reduced the water use efficiency of A. longifolia ssp. longifolia when grown under aCO2, the recorded minimum water use efficiency (0.038 mmol CO2 mol–1 H2O) was measured on the 8th day. The addition of water to drought treatments when grown under aCO2 recovered the water use efficiency by 4.09 mmol CO2 mol–1 H2O till the 10th day of measurement. In contrast to aCO2, the recovery in water use efficiency did not occur with eCO2, and it was reduced with the addition of water to drought treatments when grown under eCO2 concentration (Figure 2A). The ANOVA results showed that the effect of time, CO2, and time × water on water use efficiency of A. longifolia spp. longifolia was significant (Table 1).
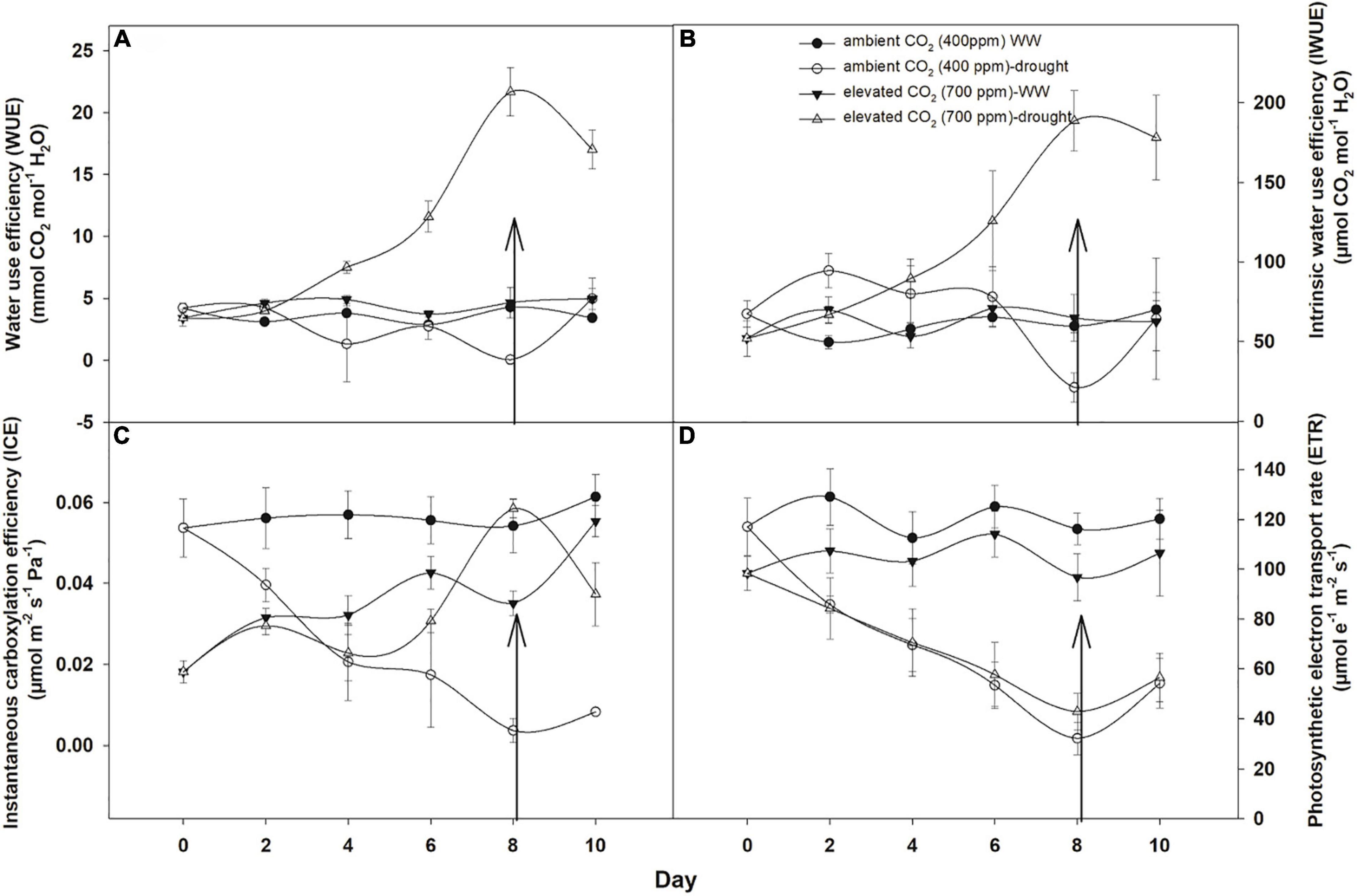
Figure 2. Effect of CO2 concentrations and moisture conditions on water use efficiency (A), intrinsic water use efficiency (B), instantaneous carboxylation efficiency (C), and photosynthetic electron transport rate (D) of A. longifolia ssp. longifolia. Nail on the data point represents the SE of the mean, and vertical arrow line after day 8 represents the addition of water in the drought treatments. WW, well-watered.
Intrinsic water use efficiency of A. longifolia ssp. longifolia was the same under both CO2 concentrations when grown under well-watered conditions (Figure 2B). However, the effect of drought was different for intrinsic water use efficiency under both CO2 concentrations. eCO2 in drought conditions increased the intrinsic water use efficiency linearly up to the 8th day of measurement. Whilst aCO2 sustained the intrinsic water use efficiency under drought conditions till the 6th day of measurement, after this time, the intrinsic water use efficiency decreased to a minimum value (21.1 μmol CO2 mol–1 H2O). The addition of water to the drought treatments had no effects in terms of recovery of intrinsic water use efficiency of A. longifolia ssp. longifolia when grown under eCO2. In contrast, significant recovery in intrinsic water use efficiency was noted for aCO2 with the addition of water on the 8th day of measurement (Figure 2B). The ANOVA showed that the effect of time (p = 0.02) and CO2 (p = 0.04) on intrinsic water use efficiency was significant.
Instantaneous carboxylation efficiency of A. longifolia ssp. longifolia was higher with aCO2 than that with eCO2 when grown under well-watered conditions (Figure 2C). Drought stress had variable effects on instantaneous carboxylation efficiency under both CO2 concentrations. eCO2 under drought conditions had no effects on instantaneous carboxylation efficiency up to the 4th day of observation. Subsequently, it increased the instantaneous carboxylation efficiency and recorded the maximum value (0.05 μmol m–2 s–1 Pa–1) on the 8th day of measurement. This contrasts with aCO2 under drought conditions, which progressively decreased the instantaneous carboxylation efficiency with an increase in drought duration. The addition of water to drought treatments when grown under eCO2 decreased the instantaneous carboxylation efficiency, while the addition of water to aCO2 treatment did not affect instantaneous carboxylation efficiency (Figure 2C). According to the results of ANOVA, the effect of time, water, and CO2 on instantaneous carboxylation efficiency was non-significant.
Under well-watered conditions, the photosynthetic electron transport rate was higher with aCO2 concentration than that with eCO2 concentration (Figure 2D). Drought significantly affected the photosynthetic electron transport efficiency with every increment in the drought days, and the photosynthetic electron transport rate reached a minimum value (32 μmol e–1 m–2 s–1 for aCO2 and 42 μmol e–1 m–2 s–1 for eCO2) on the 8th day of measurement under both CO2 concentrations. The addition of water to drought treatments recovered the photosynthetic electron transport rate under both CO2 concentrations. The photosynthetic electron transport rate of A. longifolia spp. longifolia was significant in response to time and CO2; however, all the interactions were non-significant (Table 1).
The minimum and maximum fluorescence values of A. longifolia ssp. longifolia when grown under well-watered conditions were higher with eCO2 compared to those with aCO2 (Figures 3A,B). The effect of drought was more pronounced on minimum fluorescence under eCO2 as fluorescence decreased linearly till the 8th day of observation with an increase in drought duration under eCO2. In comparison, aCO2 under drought conditions had no effects on minimum fluorescence till the 6th day of observation, and after that, it began to reduce on day 8 (Figure 3A). The addition of water to drought treatments recovered the minimum fluorescence for both CO2 concentrations. Maximum fluorescence under drought conditions was higher with eCO2 when compared to that with aCO2, and a decline in maximum fluorescence occurred in both CO2 concentrations with an increase in drought duration till the 8th day of measurement. The addition of water to drought treatments slightly recovered the maximum fluorescence in both CO2 concentrations (Figure 2D). The effect of time (p = 0.003) and CO2 (p = 0.05) on minimum fluorescence was significant, whereas the effect of time (p < 0.001), water (p < 0.001), and CO2 (p = 0.042) on maximum fluorescence was significant (Table 1).
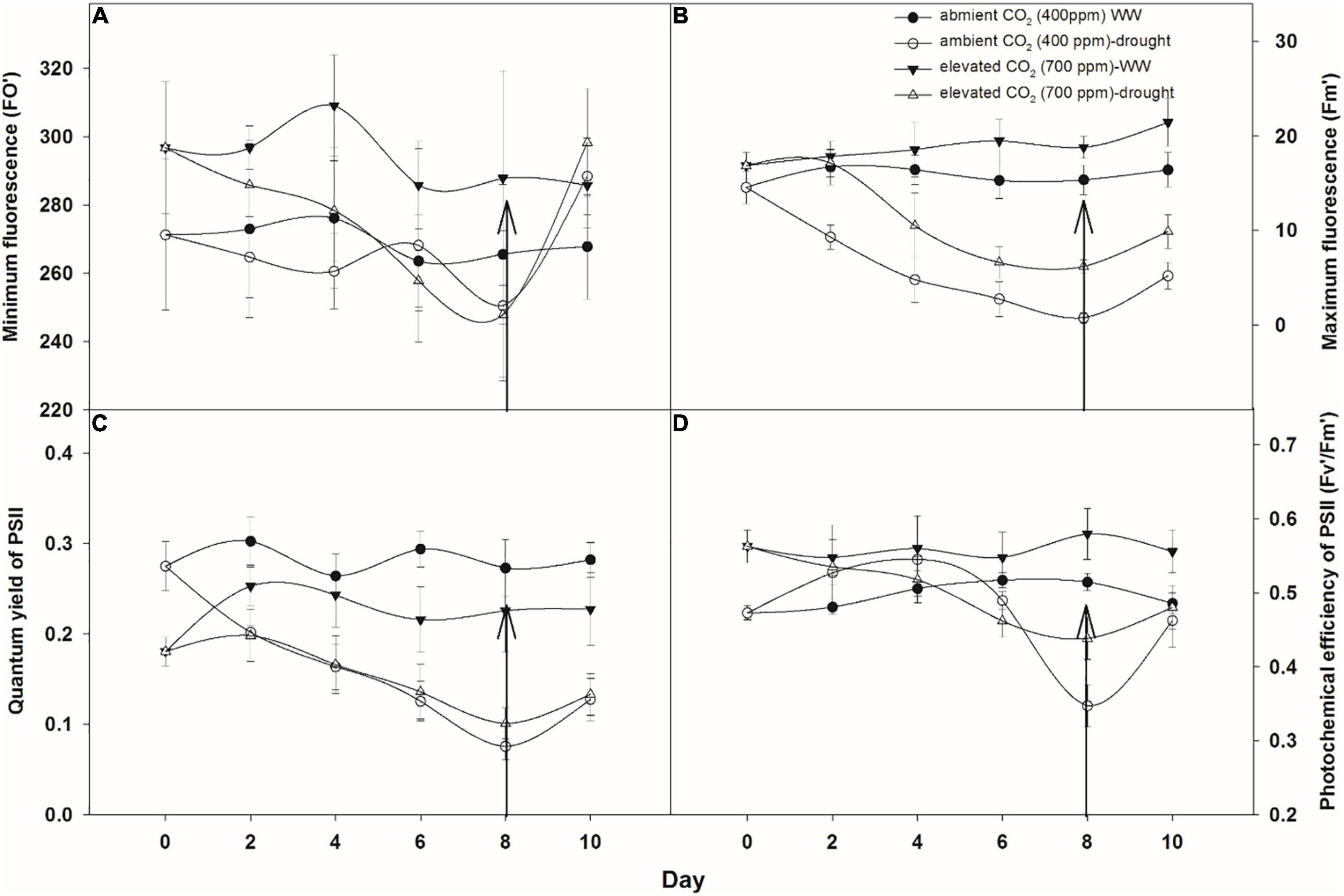
Figure 3. Effect of CO2 concentrations and moisture conditions on minimum fluorescence (A), maximum fluorescence (B), quantum yield of photosystem II (PSII) (C), and photochemical efficiency of PSII (D) of A. longifolia ssp. longifolia. Nail on the data point represents the SE of the mean, and vertical arrow line after day 8 represents the addition of water in the drought treatments. WW, well-watered.
The quantum yield of PSII was relatively higher when A. longifolia ssp. longifolia was grown under aCO2 in well-watered conditions (Figure 3C). Drought stress decreased the yield progressively in both CO2 concentrations. eCO2 slightly mitigated the adverse effects of drought on the quantum yield of PSII as the yield was 0.1 on the 8th day of measurement compared to that with aCO2 where it was 0.07 on the same day (Figure 3C). The addition of water to drought treatments recovered the yield in the same way for both CO2 concentrations. The ANOVA table showed that time (p = 0.016), water (p < 0.001), CO2 (p = 0.040), time × CO2 (p < 0.001), and time × water (p = 0.011) have significant effects on quantum yield of PSII (Table 1). Under well-watered conditions, the photochemical efficiency of PSII was significantly higher with eCO2 compared to that with aCO2 throughout the observation period (days 0–10) (Figure 3D). Data showed that eCO2 mitigated the adverse effects of drought on the photochemical efficiency of PSII as no decline in photochemical efficiency of PSII occurred until day 4, and after that, the photochemical efficiency of PSII slightly reduced till the 8th day of observation. The drought had drastic effects on the photochemical efficiency of PSII under aCO2 which decreased to 0.34. However, the addition of water to drought treatments when grown under aCO2 recovered the photochemical efficiency of PSII significantly compared to that in eCO2 (Figure 3D). The ANOVA table showed that time (p < 0.001), water (p < 0.001), CO2 (p = 0.050), and time × CO2 (p = 0.024) have significant effects on the photochemical efficiency of PSII (Table 1).
Photochemical and non-photochemical quenching was higher with aCO2 when A. longifolia ssp. longifolia was grown under well-watered conditions (Figures 4A,B). eCO2 mitigated the adverse effects of drought on photochemical quenching, and a decline in this parameter was less with an increase in drought duration as compared to that with aCO2. eCO2 had no effects on non-photochemical quenching under drought conditions. The addition of water to drought treatments recovered the photochemical and non-photochemical quenching under both CO2 concentrations (Figures 4A,B). The results of ANOVA indicated that the effect of time, water, and time × water was significant on photochemical and non-photochemical quenching of A. longifolia (Table 1).
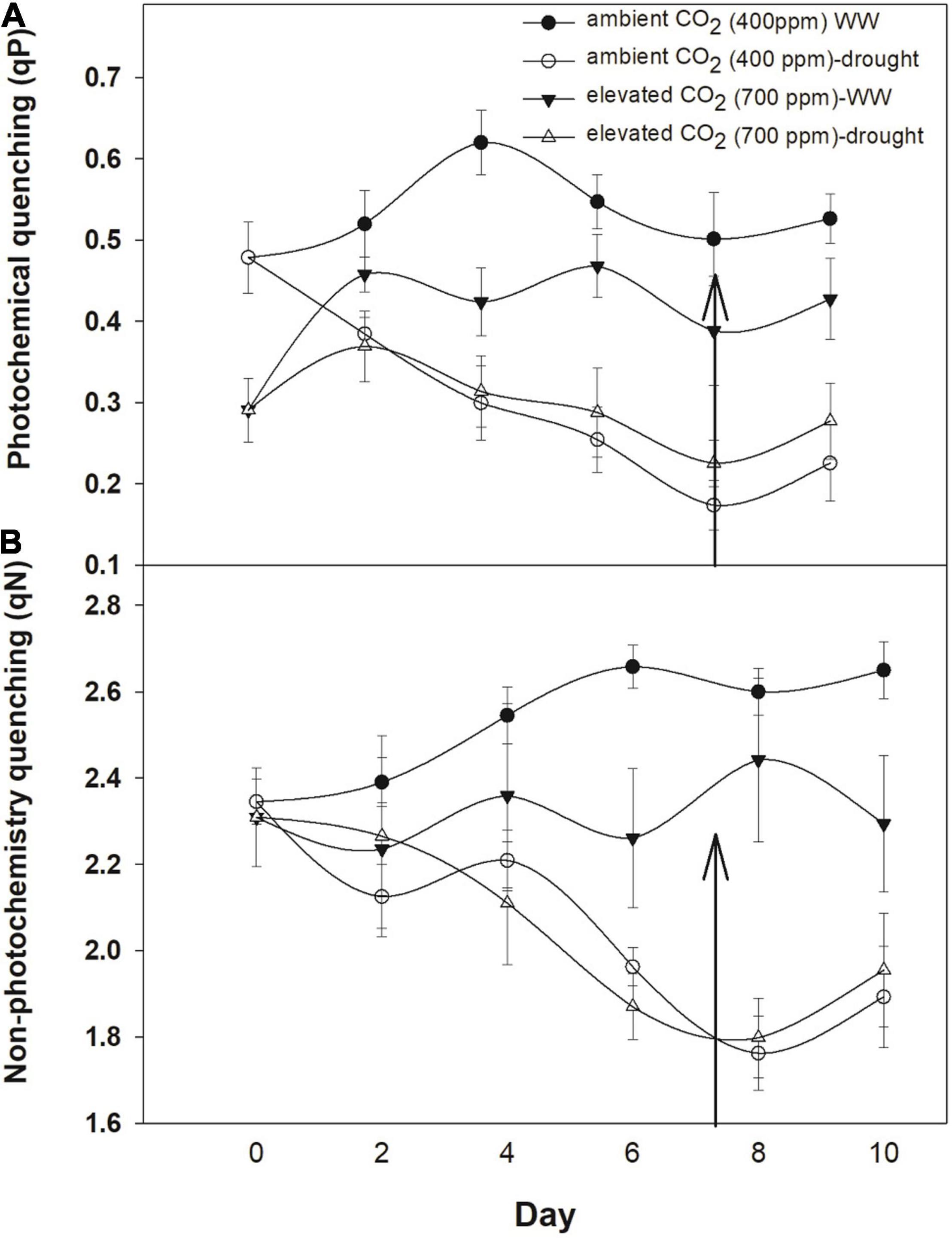
Figure 4. Effect of CO2 concentrations and moisture conditions on photochemical quenching (A) and non-photochemical quenching (B) of A. longifolia ssp. longifolia. Nail on the data point represents the SE of the mean, and vertical arrow line after day 8 represents the addition of water in the drought treatments. WW, well-watered.
Effects on Biomass Productivity by Drought Conditions and Elevated CO2 Levels
Plant height was significantly affected by water regimes and CO2 concentrations (Figure 5A). eCO2 produced 6.4 cm taller plants (25.1 cm) of A. longifolia ssp. longifolia under well-watered conditions compared with aCO2 (18.7 cm). Under drought conditions, eCO2 mitigated the adverse effects of drought on the height of A. longifolia ssp. longifolia and recorded 21.1 cm taller plants. Plants subjected to drought when grown under aCO2 attained a height of only 13.6 cm. Under well-watered conditions, the number of leaves per plant was statistically the same with both CO2 concentrations. Stem diameter was not improved with eCO2 under well-watered conditions in comparison with aCO2, whilst eCO2 enhanced the stem diameter by 0.3 mm when grown under drought conditions (Figure 5B). Drought significantly affected the number of leaves per plant under both CO2 concentrations, but eCO2 slightly improved the number of leaves by mitigating the drought effects, hence, producing four more leaves than that with aCO2 (Figure 5C). The number of branches was the same for both CO2 concentrations under well-watered conditions (Figure 5D). However, eCO2 mitigated the adverse effects of drought and produced a higher number of branches per plant (4.2) compared to that with aCO2 where branches (3.2) were produced under drought conditions. Drought stress affected the leaf thickness and leaf area per plant under both CO2 concentrations, and this effect was more when plants were grown under aCO2 (Figures 6A,B). The effect of eCO2 was significant for leaf thickness and non-significant for leaf area per plant when A. longifolia ssp. longifolia was grown under well-watered conditions. eCO2 increased the plant height, stem diameter, number of leaves, and number of branches by 34, 12, 13, and 6%, respectively, over aCO2 under well-watered conditions. In contrast, under drought conditions, an increase of 55, 10, 31, and 31% in plant height, stem diameter, number of leaves, and number of branches, respectively, was observed with eCO2 over aCO2 (data not shown).
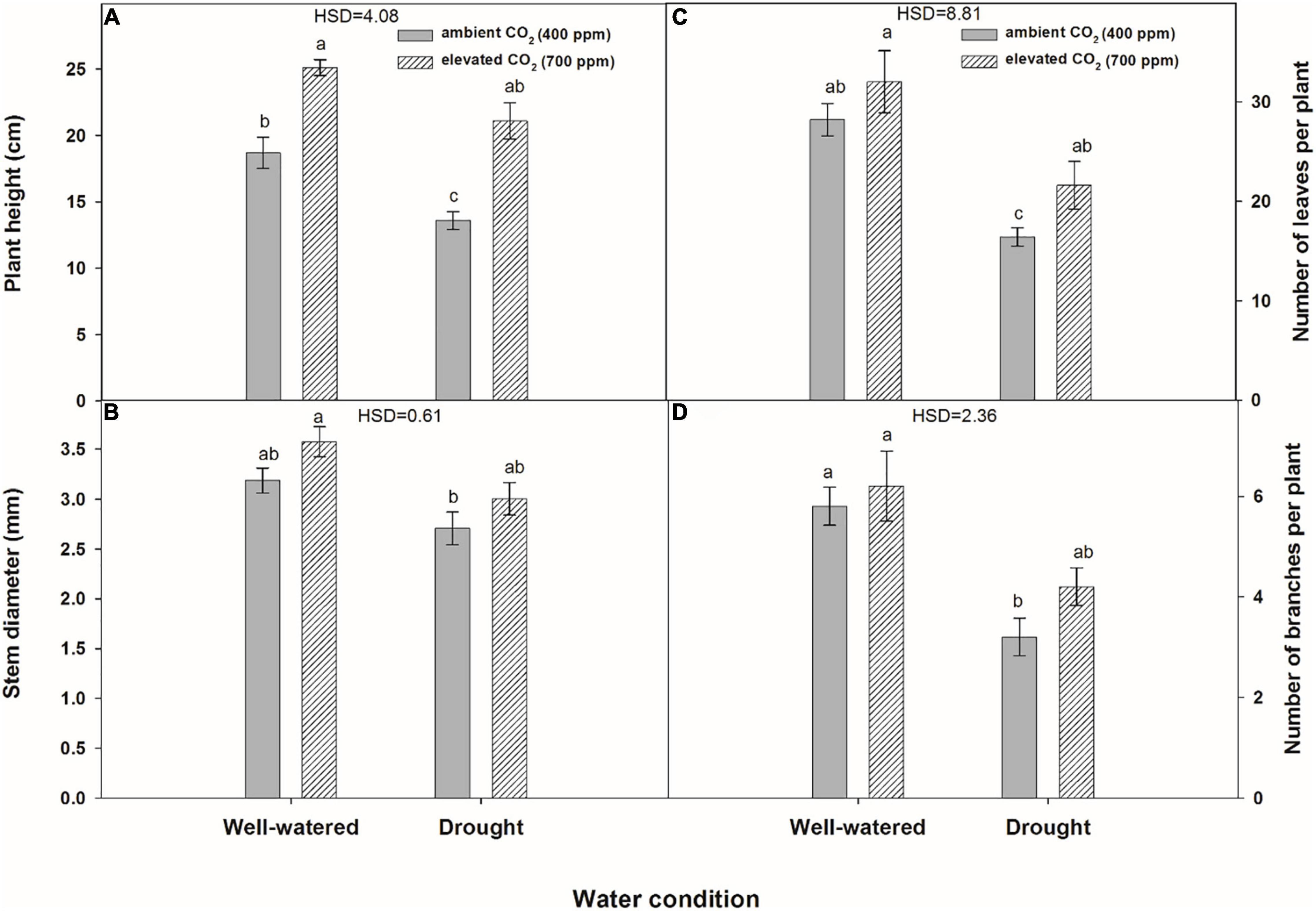
Figure 5. Effect of CO2 concentrations and moisture conditions on plant height (A), stem diameter (B), number of leaves per plant (C), and number of branches per plant (D) of A. longifolia ssp. longifolia. Nail on the bar represents the SE of the mean.
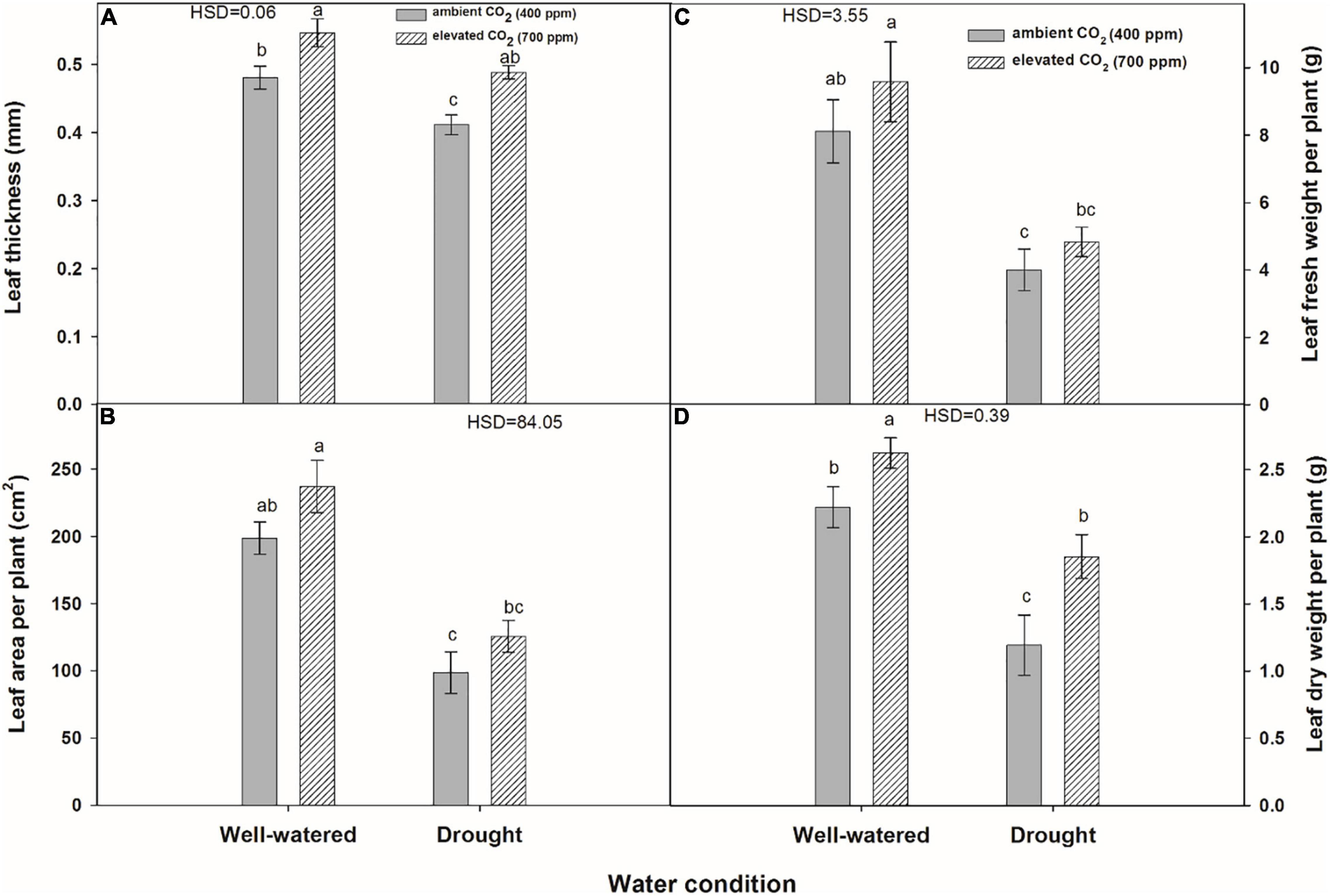
Figure 6. Effect of CO2 concentrations and moisture conditions on leaf thickness (A), leaf area per plant (B), leaf fresh weight per plant (C), and leaf dry weight per plant (D) of A. longifolia ssp. longifolia. Nail on the bar represents the SE of the mean.
Leaf fresh weight was significantly affected by water regimes, and drought caused the lowest fresh weight of leaves per plant (4.8 g) under aCO2 (Figure 6C). Leaf dry weight was significantly affected by water regimes and CO2 concentrations. eCO2 produced higher leaf dry weight under both water conditions when compared to that with aCO2 (Figure 6D). The effect of water regimes and CO2 concentrations was significant for root fresh weight per plant of A. longifolia ssp. longifolia (Figure 7A). eCO2 recorded maximum fresh weight of root when grown under well-watered conditions. However, eCO2 did not enhance the root fresh weight under drought conditions in comparison with that of aCO2. Root dry weight was also enhanced by eCO2 when A. longifolia ssp. longifolia was grown under well-watered conditions (Figure 7B). The effect of water conditions and CO2 concentrations was non-significant for the shoot fresh and shoot dry weights of A. longifolia ssp. longifolia (Figures 7C,D). eCO2 increased the leaf fresh weight, leaf dry weight, root fresh weight, and root dry weight by 18, 19, 77, and 25% under well-watered conditions and 8, 57, 39, and 38% under drought conditions, respectively, over aCO2 (data not shown).
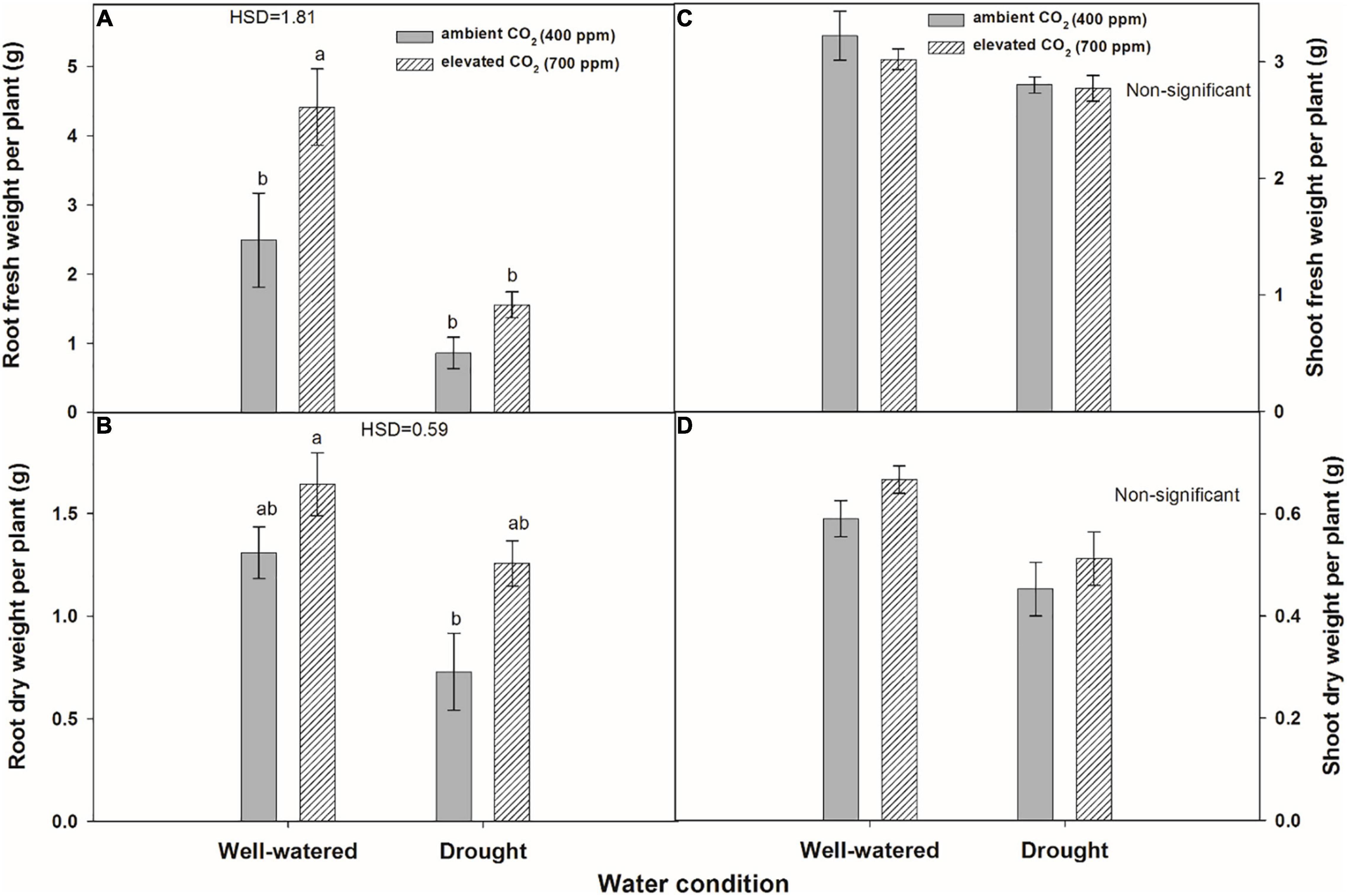
Figure 7. Effect of CO2 concentrations and moisture conditions on root fresh weight per plant (A), root dry weight per plant (B), shoot fresh weight per plant (C), and shoot dry weight per plant (D) of A. longifolia ssp. longifolia. Nail on the bar represents the SE of the mean.
Discussion
eCO2 and drought stress are the main components of climate change that directly affect the physiology and morphology of plants (Lobell and Gourdji, 2012; Salazar-Parra et al., 2012; Chadha et al., 2020; Dawood et al., 2021). This study exhibits that CO2 concentrations (ambient at 400 ppm and elevated at 700 ppm) had variable effects on gas exchange, water use efficiency, PSII activities, and growth of A. longifolia ssp. longifolia when grown under well-watered and drought conditions. Drought conditions had negative effects on photosynthetic activity, water use efficiency, and growth of A. longifolia ssp. longifolia, but eCO2 mitigated the adverse effects of drought on the physiological and morphological parameters of this plant. Prior study showed that drought stress causes various responses of photosynthetic mechanisms depending upon species (Prieto et al., 2009; Weller et al., 2020). Furthermore, leaf photosynthesis is generally believed to increase with eCO2 even under stressful environments (Meibaum et al., 2012). In our study, the net photosynthetic rate of A. longifolia ssp. longifolia leaves increased by 12% with eCO2. According to Evans et al.’s (2000) study on growth and gas exchange, measurements of nine Acacia species were recorded to be enhanced under 700 μl L–1 CO2 concentration. A study by Ibrahim et al. (2020) reported that Acacia mangium was well adapted to higher temperatures and eCO2 compared to native health forest species, including Buchanania arborescens Blume, Dillenia suffruticosa Martelli, Calophyllum inophyllum L., and Ploiarium alternifolium Melch. eCO2 induced a low transpiration rate under well-watered and drought conditions. The decline in transpiration rate might have been due to the stomatal closure as a result of the effect of eCO2 and drought. It is postulated that eCO2 increases cytosolic-free calcium within guard cells, which may act as a messenger in the signal transduction pathway for maintaining the turgor pressure of the guard cells (Kim et al., 2010). Low transpiration rate and stomatal conductance of A. longifolia ssp. longifolia under eCO2 conserve soil moisture (Habermann et al., 2019), and this increases the net photosynthetic rate and growth under eCO2. Soil moisture conservation in other species exposed to eCO2 has been observed (Wullschleger et al., 2002; Qaderi et al., 2013; Miranda-Apodaca et al., 2018; Dikšaitytė et al., 2019). According to Yu et al. (2012), plants grown under eCO2 in drought conditions resulted in greater stomatal closure than that of the plants grown under aCO2. A study by Weller et al. (2021) showed that stomatal conductance of Amaranthus retroflexus L. (redroot pigweed) was reduced under eCO2. Furthermore, eCO2 reduced the drought-induced damage, and plant recovery was notable upon re-watering the plants grown under water stress. However, in previous studies, it was noted that the degree of recovery depends upon species and duration of drought, as barley plants were not able to recover stomatal closure when plants were re-watered after 16 days of drought stress under eCO2 (Robredo et al., 2007). In our study, stomatal conductance of A. longifolia ssp. longifolia was recovered with the addition of water to drought-treated plants. In contrast, higher transpiration rate and stomatal conductance of A. longifolia ssp. longifolia under aCO2 with both moisture conditions caused a faster decrease in soil moisture contents which affected the photosynthesis activity and growth of the plant. These findings share some similarities with tall fescue, in which a lower stomatal conductance was observed under drought conditions in the aCO2 treatment (Yu et al., 2012). Furthermore, our findings are inconsistent with those of Robredo et al. (2007) who concluded that under eCO2, water stress was developed more slowly due to a slower rate of moisture depletion. Intercellular CO2 concentration was increased with an increase in drought duration, and this showed higher increase with eCO2, which could have been due to the combined effect of eCO2 and drought, thereby causing stomatal closure. Conversely, our findings are in line with those of Robredo et al. (2007) and Wang et al. (2015) who reported that eCO2 caused stomatal closure with high intercellular CO2 concentration at 700 ppm, which led to a higher rate of carbon assimilation.
Under well-watered conditions, water use efficiency of A. longifolia ssp. longifolia increased by 0.75 mmol CO2 mol–1 H2O under eCO2 in comparison with that of aCO2. Water use efficiency was seen to be further enhanced when plants were grown under drought conditions. This might have been the result of decreased transpiration rate under eCO2. Previous studies have shown that eCO2 increased water use efficiency by lowering the transpiration rate and moisture depletion at eCO2 (Schmid et al., 2016; Tom-Dery et al., 2019; Hebbar et al., 2020; Ma et al., 2020). For example, photosynthetic activities and water use efficiency of invasive A. mangium were reported to be stimulated by eCO2 when compared to that with native B. arborescens and D. suffruticosa (Ibrahim et al., 2020). Therefore, regarding the increase in water use efficiency of A. longifolia ssp. longifolia under eCO2, our results are supported by Saxe et al. (1998) and Gilbert et al. (2011) who reported that intrinsic water use efficiency increased by 25–30% as a result of reduced stomatal conductance and enhanced photosynthetic rate under eCO2. Soil moisture conservation due to eCO2 concentration has been observed in other species (Wullschleger et al., 2002; Madhu and Hatfield, 2013; Kimball, 2016), and this may reduce the impact of limited water conditions on growth (Reddy et al., 2010). Our results are in accordance with the findings of Pérez-López et al. (2013) and Wang et al. (2015) who reported that instantaneous carboxylation efficiency declined with eCO2.
Drought significantly affected the photosynthetic electron transport rate under both CO2 concentrations, but this decline was less marked with eCO2. A higher rate of photosynthetic electron transport occurring in a drought stress environment coupled with eCO2 was also reported by Kitao et al. (2007). Drought stress decreased the quantum yield of PSII, whilst eCO2 mitigated the adverse effects of drought on the quantum yield of PSII in our study. Similarly, the photochemical efficiency of PSII was also increased with eCO2, which led to the conclusion that a CO2-enriched environment reduces the risk of damage by drought stress. These findings are also supported by Perveen et al. (2010), Zhao et al. (2010), and Wang et al. (2018) who showed that eCO2 decreased the quantum yield of PSII and increased the photochemical efficiency of PSII. Drought stress caused a decline in photochemical quenching; however, eCO2 reduced the rate of decline even with an increase in drought duration. This suggested that eCO2 reduced the risk of photoinhibition (Arena et al., 2011).
Water stress generally has negative effects on plant growth, but under eCO2, growth appears to have a positive effect (Serret et al., 2018). The interactive effect of drought and eCO2 is important to consider when predicting the effects of climate change on plant species (Martínez-Carrasco et al., 2005; Battaglia and Bruce, 2017). Our results showed that eCO2 significantly increased plant height, number of leaves, stem diameter, leaf thickness, leaf area, leaf dry weight, and root dry weight of A. longifolia ssp. longifolia when compared to aCO2 under both moisture conditions. This enhanced growth with eCO2 might have been due to increased photosynthesis and water use efficiency, and reduced stomatal conductance resulting in decreased transpiration rate. Ziska et al. (2019) predicted that plant biomass would increase as a result of increased photosynthetic rate and water use efficiency. The reports of Qaderi et al. (2006) showed that eCO2 increased the availability of building blocks for the production of proteins and structural molecules, which increased the leaf area and specific leaf mass. This was supported by the observation that plants grown under aCO2 and water stress had the lowest biomass productions. Previous studies have shown that eCO2 alleviated the drought impacts by reinforcement in CO2 uptake, decreasing the oxidative pressure in the chloroplast electron transport chain, changing the biomass accumulation, and altering the partitioning to favor water acquisition. All these changes enable the plants to cope more effectively with water shortages (Avila et al., 2020). Another study demonstrated that drought-imposed limitations on photosynthesis were based on a significant decrease in stomatal conductance and intercellular concentration (Salmon et al., 2020). Given that the increased photosynthesis under eCO2 resulted in more ATP use for ribulose 1,5-bishosphate (RuBP) regeneration (López-Calcagno et al., 2020). eCO2 levels also improve the allometric adjustment linked to drought tolerance (Avila et al., 2020). The aggressive nature of A. longifolia ssp. longifolia for growth under eCO2 will affect the growth and wellbeing of native species by altering the ecosystem and introducing direct competition for resources in a new environment.
Conclusion
This study reveals that eCO2 improved the photosynthetic activity, water use efficiency, and general growth parameters of A. longifolia ssp. longifolia under both well-watered and drought conditions. Therefore, this species is able to compensate for a lack of soil moisture with greater growth of its root and shoot, and it takes an advantage of eCO2 to increase overall biomass. This ability to grow under limited water availability with eCO2 indicated that this species will be able to adapt to new environments even under severe variation in climatic conditions, and foreshadows its likelihood of invasion into new areas. This species has a potential threat to the ecosystem in future due to changing climatic conditions.
Data Availability Statement
The raw data supporting the conclusions of this article will be made available by the authors, without undue reservation.
Author Contributions
SKF conceived and designed the study. MMJ conducted the experiments. All authors were involved in writing, reviewing the manuscript.
Funding
This publication was supported by the Natural Science Basic Research Program of Shaanxi Province (Grant No. 2018JQ5218) and the National Natural Science Foundation of China (Grant No. 51809224) of the Top Young Talents of Shaanxi Special Support Program.
Conflict of Interest
The authors declare that the research was conducted in the absence of any commercial or financial relationships that could be construed as a potential conflict of interest.
Publisher’s Note
All claims expressed in this article are solely those of the authors and do not necessarily represent those of their affiliated organizations, or those of the publisher, the editors and the reviewers. Any product that may be evaluated in this article, or claim that may be made by its manufacturer, is not guaranteed or endorsed by the publisher.
Supplementary Material
The Supplementary Material for this article can be found online at: https://www.frontiersin.org/articles/10.3389/fpls.2022.817730/full#supplementary-material
Abbreviations
aCO2, ambient CO2; eCO2, elevated CO2; PPFD, photosynthetic photon flux density; PSII, photosystem II; RuBP, ribulose 1,5-bishosphate.
References
Alba, C., Fahey, C., and Flory, S. L. (2019). Global change stressors alter resources and shift plant interactions from facilitation to competition over time. Ecology 100:e02859. doi: 10.1002/ecy.2859
Arena, C., Vitale, L., and De Santo, A. V. (2011). Influence of irradiance on photosynthesis and PSII photochemical efficiency in maize during short-term exposure at high CO2 concentration. Photosynthetica 49, 267–274. doi: 10.1007/s11099-011-0034-y
Avila, R. T., de Almeida, W. L., Costa, L. C., Machado, K. L., Barbosa, M. L., de Souza, R. P., et al. (2020). Elevated air [CO2] improves photosynthetic performance and alters biomass accumulation and partitioning in drought-stressed coffee plants. Environ. Exper. Bot. 177:104137. doi: 10.1016/j.envexpbot.2020.104137
Bajwa, A. A., Chauhan, B. S., and Adkins, S. (2017). Morphological, physiological and biochemical responses of two Australian biotypes of Parthenium hysterophorus to different soil moisture regimes. Environ. Sci. Poll. Res. 24, 16186–16194. doi: 10.1007/s11356-017-9176-1
Battaglia, M., and Bruce, J. (2017). Direct climate change impacts on growth and drought risk in blue gum (Eucalyptus globulus) plantations in Australia. Aus. For. 80, 216–227. doi: 10.1080/00049158.2017.1365403
Bellard, C., Leclerc, C., Leroy, B., Bakkenes, M., Veloz, S., Thuiller, W., et al. (2014). Vulnerability of biodiversity hotspots to global change. Glob. Ecol. Biogeogr. 23, 1376–1386.
Blossey, B., and Notzold, R. (1995). Evolution of increased competitive ability in invasive nonindigenous plants: a hypothesis. J. Ecol. 83, 887–889. doi: 10.1007/s004420050899
Boucher, O., Friedlingstein, P., Collins, B., and Shine, K. P. (2009). The indirect global warming potential and global temperature change potential due to methane oxidation. Environ. Res. Lett. 4:044007. doi: 10.1039/c2cs35095e
Bradley, B. A., Blumenthal, D. M., Wilcove, D. S., and Ziska, L. H. (2010). Predicting plant invasions in an era of global change. Trends Ecol. Evol. 25, 310–318. doi: 10.1016/j.tree.2009.12.003
Chadha, A., Florentine, S., Javaid, M., Welgama, A., and Turville, C. (2020). Influence of elements of climate change on the growth and fecundity of Datura stramonium. Environ. Sci. Poll. Res. 27, 35859–35869. doi: 10.1007/s11356-020-10251-y
Clements, D., and Ditommaso, A. (2011). Climate change and weed adaptation: can evolution of invasive plants lead to greater range expansion than forecasted? Weed Res. 51, 227–240. doi: 10.1111/j.1365-3180.2011.00850.x
Corlett, R. T. (2010). Invasive aliens on tropical East Asian islands. Biodivers. Conserv. 19, 411–423.
Costermans, L. F. (1981). Native trees and shrubs of south-eastern Australia. Native trees and shrubs of south-eastern Australia. Australia: New Holland Publishers
Cruz, J. L., LeCain, D. R., Alves, A. A., Coelho Filho, M. A., and Coelho, E. F. (2018). Elevated CO2 reduces whole transpiration and substantially improves root production of cassava grown under water deficit. Arch. Agro. Soil Sci. 64, 1623–1634. doi: 10.1080/03650340.2018.1446523
Dai, A. (2013). Increasing drought under global warming in observations and models. Nat. Clim. Change 3, 52–58.
Dai, A., Zhao, T., and Chen, J. (2018). Climate change and drought: A precipitation and evaporation perspective. Curr. Clim. Change Rep. 4, 301–312. doi: 10.1007/s40641-018-0101-6
Davidson, A. M., Jennions, M., and Nicotra, A. B. (2011). Do invasive species show higher phenotypic plasticity than native species and, if so, is it adaptive? Meta-Anal. Ecol. Lett. 14, 419–431. doi: 10.1111/j.1461-0248.2011.01596.x
Dawood, M. F., Zaid, A., and Latef, A. A. H. A. (2021). Salicylic acid spraying-induced resilience strategies against the damaging impacts of drought and/or salinity stress in two varieties of Vicia faba L. Seedlings. J. Plant Grow. Regul. 41, 1–24.
Dikšaitytė, A., Viršilė, A., Žaltauskaitė, J., Januškaitienė, I., and Juozapaitienė, G. (2019). Growth and photosynthetic responses in Brassica napus differ during stress and recovery periods when exposed to combined heat, drought and elevated CO2. Plant Physiol. Biochem. 142, 59–72. doi: 10.1016/j.plaphy.2019.06.026
Dukes, J. S., Chiariello, N. R., Loarie, S. R., and Field, C. B. (2011). Strong response of an invasive plant species (Centaurea solstitialis L.) to global environmental changes. Ecol. Appl. 21, 1887–1894. doi: 10.1890/11-0111.1
Elgawad, H., Farfan-Vignolo, E. R., de Vos, D., and Asard, H. (2015). Elevated CO2 mitigates drought and temperature-induced oxidative stress differently in grasses and legumes. Plant Sci. 231, 1–10. doi: 10.1016/j.plantsci.2014.11.001
Evans, J. R., Schortemeyer, M., McFarlane, N., and Atkin, O. K. (2000). Photosynthetic characteristics of 10 Acacia species grown under ambient and elevated atmospheric CO2. Funct. Plant Biol. 27, 13–25.
Gilbert, M. E., Zwieniecki, M. A., and Holbrook, N. M. (2011). Independent variation in photosynthetic capacity and stomatal conductance leads to differences in intrinsic water use efficiency in 11 soybean genotypes before and during mild drought. J.Exper. Bot. 62, 2875–2887. doi: 10.1093/jxb/erq461
Habermann, E., Dias, de Oliveira, E. A., Contin, D. R., San Martin, J. A., Curtarelli, L., et al. (2019). Stomatal development and conductance of a tropical forage legume are regulated by elevated [CO2] under moderate warming. Front. Plant Sci. 10:609. doi: 10.3389/fpls.2019.00609
Hebbar, K., Apshara, E., Chandran, K., and Prasad, P. V. (2020). Effect of elevated CO2, high temperature, and water deficit on growth, photosynthesis, and whole plant water use efficiency of cocoa (Theobroma cacao L.). Int. J. Biometeorol. 64, 47–57. doi: 10.1007/s00484-019-01792-0
Ibrahim, M. H., Sukri, R. S., Tennakoon, K. U., Le, Q.-V., and Metali, F. (2020). Photosynthetic Responses of Invasive Acacia mangium and Co-Existing Native Heath Forest Species to Elevated Temperature and CO2 Concentrations. J.Sustain. Forestry 40, 1–21. doi: 10.1080/10549811.2020.1792317
IPCC (2018). IPCC Special Report on Global Warming of 1.5°C. United Nations Intergovernmental Panel on Climate Change. Switzerland: IPCC
Kim, T.-H., Böhmer, M., Hu, H., Nishimura, N., and Schroeder, J. I. (2010). Guard cell signal transduction network: advances in understanding abscisic acid, CO2, and Ca2+ signaling. Annu. Rev. Plant Biol. 61, 561–591. doi: 10.1146/annurev-arplant-042809-112226
Kimball, B. A. (2016). Crop responses to elevated CO2 and interactions with H2O, N, and temperature. Curr. Opin. Plant Biol. 31, 36–43.
Kirschbaum, M. U. (2011). Does enhanced photosynthesis enhance growth? Lessons learned from CO2 enrichment studies. Plant Physiol. 155, 117–124. doi: 10.1104/pp.110.166819
Kitao, M., Lei, T. T., Koike, T., Kayama, M., Tobita, H., and Maruyama, Y. (2007). Interaction of drought and elevated CO2 concentration on photosynthetic down-regulation and susceptibility to photoinhibition in Japanese white birch seedlings grown with limited N availability. Tree Physiol. 27, 727–735. doi: 10.1093/treephys/27.5.727
Kull, C. A., Shackleton, C. M., Cunningham, P. J., Ducatillon, C., Dufour-Dror, J. M., Esler, K. J., et al. (2011). Adoption, use and perception of Australian acacias around the world. Divers. Distrib. 17, 822–836.
Le, Q.-V., Tennakoon, K. U., Metali, F., and Sukri, R. S. (2019). Photosynthesis in co-occurring invasive Acacia spp. and native Bornean heath forest trees at the post-establishment invasion stage. J. Sustain. For. 38, 230–243.
Li, D., Zhang, X., Li, L., Aghdam, M. S., Wei, X., Liu, J., et al. (2019). Elevated CO2 delayed the chlorophyll degradation and anthocyanin accumulation in postharvest strawberry fruit. Food Chem. 285, 163–170. doi: 10.1016/j.foodchem.2019.01.150
Lobell, D. B., and Gourdji, S. M. (2012). The influence of climate change on global crop productivity. Plant Physiol. 160, 1686–1697. doi: 10.1104/pp.112.208298
López-Calcagno, P. E., Brown, K. L., Simkin, A. J., Fisk, S. J., Vialet-Chabrand, S., Lawson, T., et al. (2020). Stimulating photosynthetic processes increases productivity and water-use efficiency in the field. Nat. Plants 6, 1054–1063. doi: 10.1038/s41477-020-0740-1
Ma, Y., Wu, Y., and Song, X. (2020). Seasonal responses of maize growth and water use to elevated CO2 based on a coupled device with climate chamber and weighing lysimeters. Sci. Total Environ. 140344. doi: 10.1016/j.scitotenv.2020.140344
Madhu, M., and Hatfield, J. (2013). Dynamics of plant root growth under increased atmospheric carbon dioxide. Agro. J. 105, 657–669.
Marchante, H., Freitas, H., and Hoffmann, J. H. (2010). Seed ecology of an invasive alien species, Acacia longifolia (Fabaceae), in Portuguese dune ecosystems. Am. J. Bot. 97, 1780–1790. doi: 10.3732/ajb.1000091
Marchante, H., Marchante, E., Freitas, H., and Hoffmann, J. H. (2015). Temporal changes in the impacts on plant communities of an invasive alien tree. Acacia longifolia. Plant Ecol. 216, 1481–1498.
Martínez-Carrasco, R., Pérez, P., and Morcuende, R. (2005). Interactive effects of elevated CO2, temperature and nitrogen on photosynthesis of wheat grown under temperature gradient tunnels. Environ. Exper. Bot. 54, 49–59. doi: 10.1016/j.envexpbot.2004.05.004
Maxwell, K., and Johnson, G. N. (2000). Chlorophyll fluorescence: a practical guide. J. Exper. Bot. 51, 659–668. doi: 10.1093/jxb/51.345.659
Meibaum, B., Riede, S., Schröder, B., Manderscheid, R., Weigel, H.-J., and Breves, G. (2012). Elevated CO2 and drought stress effects on the chemical composition of maize plants, their ruminal fermentation and microbial diversityin vitro. Arch. Anim. Nutr. 66, 473–489. doi: 10.1080/1745039x.2012.735080
Miranda-Apodaca, J., Pérez-López, U., Lacuesta, M., Mena-Petite, A., and Muñoz-Rueda, A. (2018). The interaction between drought and elevated CO2 in water relations in two grassland species is species-specific. J. Plant Physiol. 220, 193–202. doi: 10.1016/j.jplph.2017.11.006
Osunkoya, O. O., Othman, F. E., and Kahar, R. S. (2005). Growth and competition between seedlings of an invasive plantation tree, Acacia mangium, and those of a native Borneo heath-forest species, Melastoma beccarianum. Ecol. Res. 20, 205–214.
Pan, C., Ahammed, G. J., Li, X., and Shi, K. (2018). Elevated CO2 improves photosynthesis under high temperature by attenuating the functional limitations to energy fluxes, electron transport and redox homeostasis in tomato leaves. Front. Plant Sci. 9:1739. doi: 10.3389/fpls.2018.01739
Pastore, M. A., Lee, T. D., Hobbie, S. E., and Reich, P. B. (2020). Interactive effects of elevated CO2, warming, reduced rainfall, and nitrogen on leaf gas exchange in five perennial grassland species. Plant Cell Environ. 43:1862–1878 doi: 10.1111/pce.13783
Pérez-López, U., Miranda-Apodaca, J., Muñoz-Rueda, A., and Mena-Petite, A. (2013). Lettuce production and antioxidant capacity are differentially modified by salt stress and light intensity under ambient and elevated CO2. J. Plant Physiol. 170, 1517–1525. doi: 10.1016/j.jplph.2013.06.004
Perveen, S., Shahbaz, M., and Ashraf, M. (2010). Regulation in gas exchange and quantum yield of photosystem II (PSII) in salt-stressed and non-stressed wheat plants raised from seed treated with triacontanol. Pak. J. Bot. 42, 3073–3081.
Prieto, P., Penuelas, J., Llusia, J., Asensio, D., and Estiarte, M. (2009). Effects of long-term experimental night-time warming and drought on photosynthesis, Fv/Fm and stomatal conductance in the dominant species of a Mediterranean shrubland. Acta Physiol. Plantarum 31, 729–739.
Qaderi, M. M., Kurepin, L. V., and Reid, D. M. (2006). Growth and physiological responses of canola (Brassica napus) to three components of global climate change: temperature, carbon dioxide and drought. Physiol. Plantarum 128, 710–721.
Qaderi, M. M., Lynch, A. L., Godin, V. J., and Reid, D. M. (2013). Single and interactive effects of temperature, carbon dioxide, and watering regime on the invasive weed black knapweed (Centaurea nigra). Ecoscience 20, 328–338.
Reddy, A. R., Rasineni, G. K., and Raghavendra, A. S. (2010). The impact of global elevated CO2 concentration on photosynthesis and plant productivity. Curr. Sci. 46–57.
Robredo, A., Pérez-López, U., de la Maza, H. S., González-Moro, B., Lacuesta, M., Mena-Petite, A., et al. (2007). Elevated CO2 alleviates the impact of drought on barley improving water status by lowering stomatal conductance and delaying its effects on photosynthesis. Environ. Exper. Bot. 59, 252–263.
Salazar-Parra, C., Aguirreolea, J., Sánchez-Díaz, M., Irigoyen, J. J., and Morales, F. (2012). Photosynthetic response of Tempranillo grapevine to climate change scenarios. Ann. Appl. Biol. 161, 277–292.
Salmon, Y., Lintunen, A., Dayet, A., Chan, T., Dewar, R., Vesala, T., et al. (2020). Leaf carbon and water status control stomatal and nonstomatal limitations of photosynthesis in trees. New Phytol. 226, 690–703. doi: 10.1111/nph.16436
Sampaio, C. V. R. D. S. (2019). Influence of Abiotic Factors on Growth and Nodulation of Acacia longifolia. Ph.D Dissertation, Departamento De Biologia Animal, Faculdade De Ciencias, Universidade De Lisboa, Portugal.
Saxe, H., Ellsworth, D. S., and Heath, J. (1998). Tree and forest functioning in an enriched CO2 atmosphere. New Phytol. 139, 395–436.
Schmid, I., Franzaring, J., Müller, M., Brohon, N., Calvo, O., Högy, P., et al. (2016). Effects of CO2 enrichment and drought on photosynthesis, growth and yield of an old and a modern barley cultivar. J. Agro. Crop Sci. 202, 81–95.
Serret, M. D., Yousfi, S., Vicente, R., Piñero, M. C., Otálora-Alcón, G., Del Amor, F. M., et al. (2018). Interactive effects of CO2 concentration and water regime on stable isotope signatures, nitrogen assimilation and growth in sweet pepper. Front. Plant Sci. 8:2180. doi: 10.3389/fpls.2017.02180
Souza-Alonso, P., González, L., López-Nogueira, A., Cavaleiro, C., and Pedrol, N. (2018). Volatile organic compounds of Acacia longifolia and their effects on germination and early growth of species from invaded habitats. Chem. Ecol. 34, 126–145.
Tom-Dery, D., Eller, F., Fromm, J., Jensen, K., and Reisdorff, C. (2019). Elevated CO2 does not offset effects of competition and drought on growth of shea (Vitellaria paradoxa CF Gaertn.) seedlings. Agrofor. Syst. 93, 1807–1819.
Wang, A., Lam, S. K., Hao, X., Li, F. Y., Zong, Y., Wang, H., et al. (2018). Elevated CO2 reduces the adverse effects of drought stress on a high-yielding soybean (Glycine max (L.) Merr.) cultivar by increasing water use efficiency. Plant Physiol. Biochem. 132, 660–665. doi: 10.1016/j.plaphy.2018.10.016
Wang, M., Xie, B., Fu, Y., Dong, C., Hui, L., Guanghui, L., et al. (2015). Effects of different elevated CO2 concentrations on chlorophyll contents, gas exchange, water use efficiency, and PSII activity on C3 and C4 cereal crops in a closed artificial ecosystem. Photosynth. Res. 126, 351–362.
Welgama, A., Florentine, S., Marchante, H., Javaid, M. M., and Turville, C. (2019). The germination success of Acacia longifolia subsp. longifolia (Fabaceae): a comparison between its native and exotic ranges. Aus. J. Bot. 67, 414–424.
Weller, S., Florentine, S., Javaid, M. M., Welgama, A., Chadha, A., Chauhan, B. S., et al. (2021). Amaranthus retroflexus L.(Redroot Pigweed): Effects of elevated CO2 and soil moisture on growth and biomass and the effect of radiant heat on seed germination. Agronomy, 11:728.
Weller, S. L., Javaid, M. M., and Florentine, S. K. (2020). Evaluation of the growth response of arid zone invasive species Salvia verbenaca cultivars to atmospheric carbon dioxide and soil moisture. Rangeland J. 42, 45–53.
Werner, C., Peperkorn, R., Máguas, C., and Beyschlag, W. (2008). Competitive balance between the alien invasive Acacia longifolia and native Mediterranean species. Plant invasions: human perception, ecological impacts and management. Leiden: Backhuys Publishers, 261–275.
Wullschleger, S., Tschaplinski, T., and Norby, R. (2002). Plant water relations at elevated CO2, implications for water-limited environments. Plant Cell Environ. 25, 319–331. doi: 10.1073/pnas.1605036113
Xie, X., Li, R., Zhang, Y., Shen, S., and Bao, Y. (2018). Effect of elevated [CO2] on assimilation, allocation of nitrogen and phosphorus by maize (Zea Mays L.). Commun. Soil Sci. Plant Anal. 49, 1032–1044.
Yu, J., Chen, L., Xu, M., and Huang, B. (2012). Effects of elevated CO2 on physiological responses of tall fescue to elevated temperature, drought stress, and the combined stresses. Crop Sci. 52, 1848–1858.
Zhao, X., Mao, Z., and Xu, J. (2010). Gas exchange, chlorophyll and growth responses of Betula Platyphylla seedlings to elevated CO2 and nitrogen. Int. J. Biol. 2, 143.
Keywords: elevated CO2, photosynthetic processes, water use efficiency, photosystem II, drought, Acacia longifolia ssp. longifolia
Citation: Javaid MM, Wang X, Florentine SK, Ashraf M, Mahmood A, Li F-M and Fiaz S (2022) Effects on Photosynthetic Response and Biomass Productivity of Acacia longifolia ssp. longifolia Under Elevated CO2 and Water-Limited Regimes. Front. Plant Sci. 13:817730. doi: 10.3389/fpls.2022.817730
Received: 18 November 2021; Accepted: 15 February 2022;
Published: 31 March 2022.
Edited by:
Shabir Hussain Wani, Sher-e-Kashmir University of Agricultural Sciences and Technology, IndiaReviewed by:
Awais Shakoor, Universitat de Lleida, SpainAbbu Zaid, Govt. Degree College Doda, India
Copyright © 2022 Javaid, Wang, Florentine, Ashraf, Mahmood, Li and Fiaz. This is an open-access article distributed under the terms of the Creative Commons Attribution License (CC BY). The use, distribution or reproduction in other forums is permitted, provided the original author(s) and the copyright owner(s) are credited and that the original publication in this journal is cited, in accordance with accepted academic practice. No use, distribution or reproduction is permitted which does not comply with these terms.
*Correspondence: Muhammad Mansoor Javaid, mmansoorjavaid@gmail.com; Xiukang Wang, wangxiukang@yau.edu.cn