- 1College of Plant Sciences, Tarim University, Xinjiang, China
- 2State Key Laboratory of Cotton Biology, Key Laboratory of Cotton Genetic Improvement, Ministry of Agriculture, Institute of Cotton Research of Chinese Academy of Agricultural Sciences, Anyang, China
- 3Western Agriculture Research Centre, Chinese Academy of Agricultural Sciences, Changji, China
Cotton is one of the most economically important crops worldwide. Seed size is a vital trait for plants connected with yield and germination. GW2 encodes a RING_Ubox E3 ubiquitin ligase that controls seed development by affecting cell growth. Here, are few reports on GW2-like genes in cotton, and the function of GW2 in cotton is poorly understood. In the present study, a genome-wide analysis identified 6 and 3 GW2-like genes in each of the two cultivated tetraploids (Gossypium hirsutum and G. barbadense) and each of their diploid ancestral species (G. arboreum, G. raimondii), respectively. GhGW2-2D has the same functional domain and high sequence similarity with AtDA2 in Arabidopsis. Overexpression of GhGW2-2D in Arabidopsis significantly reduced seed and seedling size, suggesting GhGW2-2D is a potential target for regulating cotton seed size. These results provided information on the genetic and molecular basis of GW2-like genes in cotton, thus establishing a foundation for functional studies of cotton seeds.
Introduction
Cotton is one of the most valuable commercial crops worldwide. Cotton fiber is important for textile manufacturing, and cottonseed is a major source of nutrients in human and livestock feed (Bellaloui et al., 2015; Bellaloui et al., 2021). Despite cottonseeds being of high economic value, they have not received sufficient attention in the past years (Li et al., 2009). Understanding the characteristics of cottonseeds is important for optimizing cotton plant growth, for instance, large seeds usually have higher germination and seedling vigor indices (Bewley, 1997; Pahlavani et al., 2008; Zhao et al., 2019). Seed size is an important trait that is limited by both genetic and environmental (Hu et al., 2021). Numerous studies conducted in rice (Oryza sativa) and Arabidopsis thaliana have provided an insight into the molecular mechanisms controlling seed size (Xing and Zhang, 2010; Valluru et al., 2014; Li et al., 2019). These mechanisms include several signaling pathways that control seed size, such as G-protein signaling, the ubiquitin-proteasome pathway, mitogen-activated protein kinase (MAPK) signaling, the HAIKU (IKU) pathway, phytohormones, and some transcriptional regulators (Li and Li, 2016; Li et al., 2019). The ubiquitin proteasome pathway plays an important role in controlling seed size (Li and Li, 2016; Li et al., 2019; Hao et al., 2021). Several RING-type E3 ubiquitin ligases have been reported to control seed size. For example, Grain Width 2 (GW2), encoding RING_Ubox type E3 ubiquitin ligase, was found to affect grain size and improve grain yield potential (Song et al., 2007). GW2 directly interacts with EXPANSIN-like1 (EXPLA1), a cell wall-loosening protein that increases cell growth, and inactivates it through ubiquitination, thereby reducing seed growth (Choi et al., 2018). The function of GW2-like genes in plants has been reported in many types of crops. In spikelet hulls, a loss-of-function mutation in GW2 has been reported to increase cell proliferation, leading to wide and heavy grains with a high yield of rice (Yamaguchi et al., 2020). Seeds of GW2-knockout mutants have a high protein content and essential dietary minerals in the endosperm (Achary and Reddy, 2021). Reducing the abundance of GW2 transcript with RNA interference in the durum wheat cultivar Svevo increased the grain starch content, width, and surface area (Sestili et al., 2019). In addition, GW2 homologs were also reported to control grain size in maize and wheat (Li et al., 2010; Su et al., 2011). The gene AtDA2 shares significant homology with OsGW2 (Xia et al., 2013), which encodes a protein that can monoubiquitinate DA1 to activate its peptidase activity, thereby causing the cleavage of DA1 substrates (Dong et al., 2017). In Arabidopsis, AtDA2 also interacts with AtDA1 and da2-1 has larger seeds and organs than wild type, suggesting a conserved function between OsGW2 and AtDA2 in seed size control (Xia et al., 2013).
Nonetheless, the function of GW2 in cotton is still poorly understood. In recent years, complete sequencing of the cotton genome has facilitated the comprehensive identification and analysis of the cotton genes (Wang K. et al., 2012; Li et al., 2014, 2015; Zhu et al., 2017). In this study, we identified several GW2-like genes in Gossypium hirsutum (6), G. barbadense (6), G. arboreum (3), and G. raimondii (3). The sequence characteristics, chromosomal distribution, evolutionary relationship, and the expression patterns of GW2-like genes in cotton were analyzed. As the homologous gene of AtDA2 and OsGW2, GhGW2-2D was also found to encode a RING_Ubox domain in cotton, which may negatively regulate seed size. Then we overexpressed GhGW2-2D into Arabidopsis thaliana ecotype Col-0 to further investigate the function of GhGW2-2D. The results of this study lay the foundation for future studies on GW2-like genes in the improvement of cotton seed size.
Materials and Methods
Sequence Retrieval and Identification of GW2-Like Genes in Cotton
The four completed genome assemblies of G. arboreum (A2, CRI_V1.0) (Du et al., 2018), G. raimondii (D5, JGI v2_a2.1) (Paterson et al., 2012), hirsutum acc. TM-1 (AD1, ZJU) (Hu et al., 2019), and G. barbadense acc.H7124 (AD2, ZJU) (Hu et al., 2019) were downloaded from the CottonGen database1 (Yu et al., 2014). The protein sequences of AtDA2 and OsGW2 were acquired from the Arabidopsis Information Resource2 and the Rice Genome Annotation Project3, respectively. The protein sequences of AtDA2 and OsGW2 were used as queries to identify GW2-like genes against four genome databases of Gossypium with e-values of 1e––10. The candidate sequences were submitted to the NCBI batch CDD program4 and SMART database5 (Letunic et al., 2015) for further confirmation.
Chromosomal Location
The chromosomal distribution and the loci of all GW2-like genes were determined based on the results of identification genome annotation data. Molecular markers of reported QTLs were download from CottonQTLdb6 (Said et al., 2013, 2015a,b). Subsequently, the location images of GW2-like genes were drawn using the MapChart software (Voorrips, 2002).
Phylogenetic Analysis
The phylogenetic tree of GW2-like genes was analyzed and generated using multiple sequence alignments of four cultivated cotton species using ClusterW7 (Larkin et al., 2007). A phylogenetic tree was constructed using MEGA 7.08 (Kumar et al., 2016) with pairwise distance and the neighbor-joining (NJ) method.
Gene Duplication and Synteny Analysis
Gene duplication events and genomic synteny were analyzed using MCScanX and visualized using the TBtools software (Wang Y. et al., 2012; Cheng et al., 2020). The synonymous (Ks) and non-synonymous (Ka) substitution ratios were calculated using TBtools software.
Gene Structure Analysis and Identified Motifs
The structural information of GFF3 files of four cotton species were download from CottonGen database (see text footnote 1). The protein length, molecular weight, and isoelectric point of the encoded proteins were predicted using the ProtParam tool9 (Gasteiger et al., 2003). To clarify the conserved motifs and protein domains, the online program MEME10 (Bailey et al., 2009) and NCBI database were used, respectively. The structure of GW2-like genes was predicted using TBtools software (Cheng et al., 2020).
Plant Material, RNA Extraction, and qRT-PCR Analysis
Wild-type cotton (TM-1) and Arabidopsis (Col-0) were planted in the experimental field and greenhouse of the Institute of Cotton Research of Chinese Academy of Agricultural Sciences under conventional field management conditions in Anyang, China. To detect the relative expression of GW2-like genes, different samples were collected from WT cotton at different stages of ovule development. All ovule samples were self-pollinated. Total RNA was isolated from samples using Fast Pure Plant Total RNA Isolation Kit (Vazyme, Nanjing, China). The qRT-PCR analysis primers were listed in Supplementary Table 1. Gh_D03G0370 (GhActin3) (Li et al., 2005) and AT3G18780 (AtActin2) (Wang et al., 2016) were used as internal controls for the qPCR experiments.
Constructs and Transformation
The 1,272 bp complete coding sequence (CDS) of GhGW2-2D was amplified using the primers 35S:GhGW2-2D-F and 35S:GhGW2-2D-R (Supplementary Table 1). The 35S:GhGW2-2D construct were cloned into a pBI121 vector digested with BamHI and SacI using a PCR-based homologous recombination system. The 35S:GhGW2-2D plasmid transferred into Col-0 plants using Agrobacterium GV3101 and the transformants were selected on medium supplemented with kanamycin (50 mg/L). Three homozygous T3 generation lines were used for further analysis. The seed and cotyledon were conducted by scanning to generate a digital image and then measured by ImageJ software.
Results
Identification of GW2-Like Genes in Gossypium
In order to identify all GW2-like proteins in tetraploid cotton (G. hirsutum and G. barbadense) and their diploid ancestors (G. arboreum and G. raimondii), the protein sequences of AtDA2 and OsGW2 in rice and Arabidopsis were used as queries. We identified three, three, six, and six GW2-like genes in G. arboreum, G. raimondii, G. barbadense, and G. hirsutum, respectively. The length of these cotton GW2-like gene sequences ranged from 1,260 to 1,290 bp, encoding polypeptides from 419 to 429 amino acids long. The predicted isoelectric point of proteins varied from 4.70 to 4.93. According to the results of evolutionary analysis, all members were divided into three subgroups. More details of the gene names, locus IDs, and other features are shown in Table 1.
Chromosomal Location of GW2-Like Genes
All GW2-like genes were unevenly mapped onto chromosomes based on the genome annotation data of the four species (Supplementary Figure 1 and Table 1). In the G. arboreum genome, the three identified GaGW2s were three located on chromosomes A01, A05, and A13. In G. raimondii, the three GrGW2 genes were distributed on three chromosomes: chromosome 02 (D01), chromosome 09 (D05), and chromosome 13 (D13). In G. hirsutum, six GhGW2 genes were mapped on three At subgenome chromosomes (A01, A05, and A13) and three Dt subgenome chromosomes (D01, D05, and D13). The chromosomal distribution of GW2-like genes in the G. barbadense genome was resemble to that of GhGW2s. Therefore, it could infer that GW2-like genes were highly conserved in the evolution from diploid to tetraploid in cotton.
Gene Structure and Protein Domain Analysis of GW2-Like Genes
An efficient way to predict gene function is to analyze its gene structure and the encoded protein domains. In this study, the GW2-like gene structures of four cotton species were visualized to further understand their differences and potential functional diversity.
A phylogenetic tree of GW2-like genes in G. hirsutum and G. barbadense and their ancestral diploids was constructed using the NJ method (Figure 1A). All GW2-like proteins were divided into three subgroups. Each group contained six members, one from G. arboreum, one from G. raimondii, two from G. hirsutum, and two from G. barbadense. All GW2-like genes have eight exons that were highly conserved in the four species of cotton (Figure 1B).
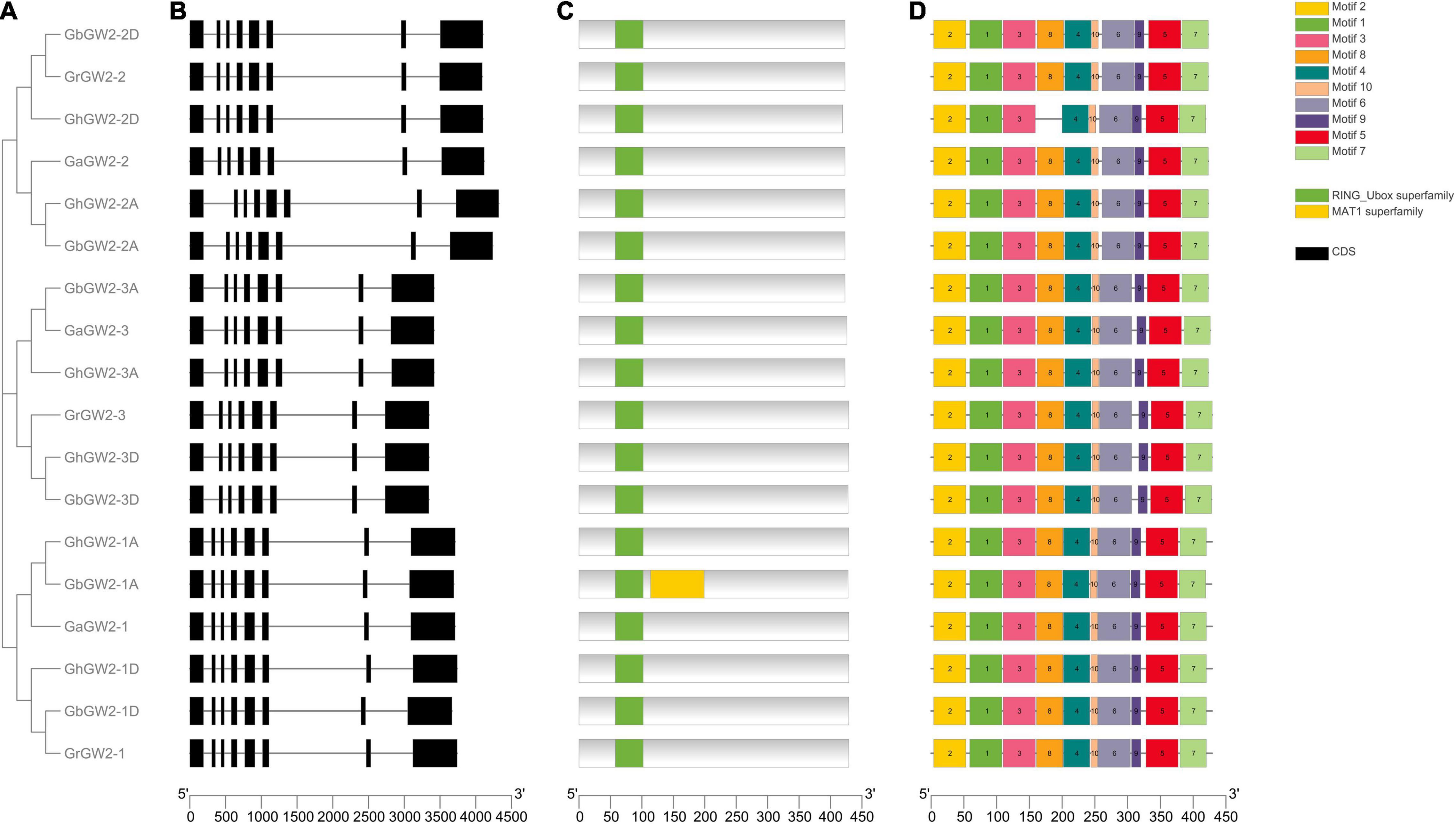
Figure 1. Phylogenetic analysis, gene structure, and protein domain analyses of GW2-like genes in Gossypium. (A) Phylogenetic tree of GW2-like genes in Gossypium. Phylogenetic tree was constructed using MEGA 7.0 by Neighbor-Joining method with 1,000 bootstrap replicates. (B) The exon-intron structure of GW2-like genes. (C) GW2-like protein domain prediction. (D) The motifs prediction of GW2-like protein.
The NCBI and SMART databases were used to predict the domains of the GW2-like proteins. All GW2-like proteins had a typical E3 ubiquitin ligase RING_Ubox domain (Figure 1C), which can bind to a specific E2 ubiquitin-binding enzyme and determines the specificity of the ubiquitinated substrate. The MEME online program was used to predict the motifs of the GW2-like family genes. The results showed that most members in the same subgroup had resemble gene structures and functional domains (Figure 1D).
Adaptive Evolution Analysis of the GW2-Like Genes
Gene replication and subsequent functionalization are important driving forces for genome and species evolution. In addition, gene duplication events are important for amplification of gene families. To clarify the amplification mechanism and evolution of GW2-like genes in the four cotton species, we conducted interspecific collinearity analysis and gene repeat event analysis (Figure 2 and Table 2).
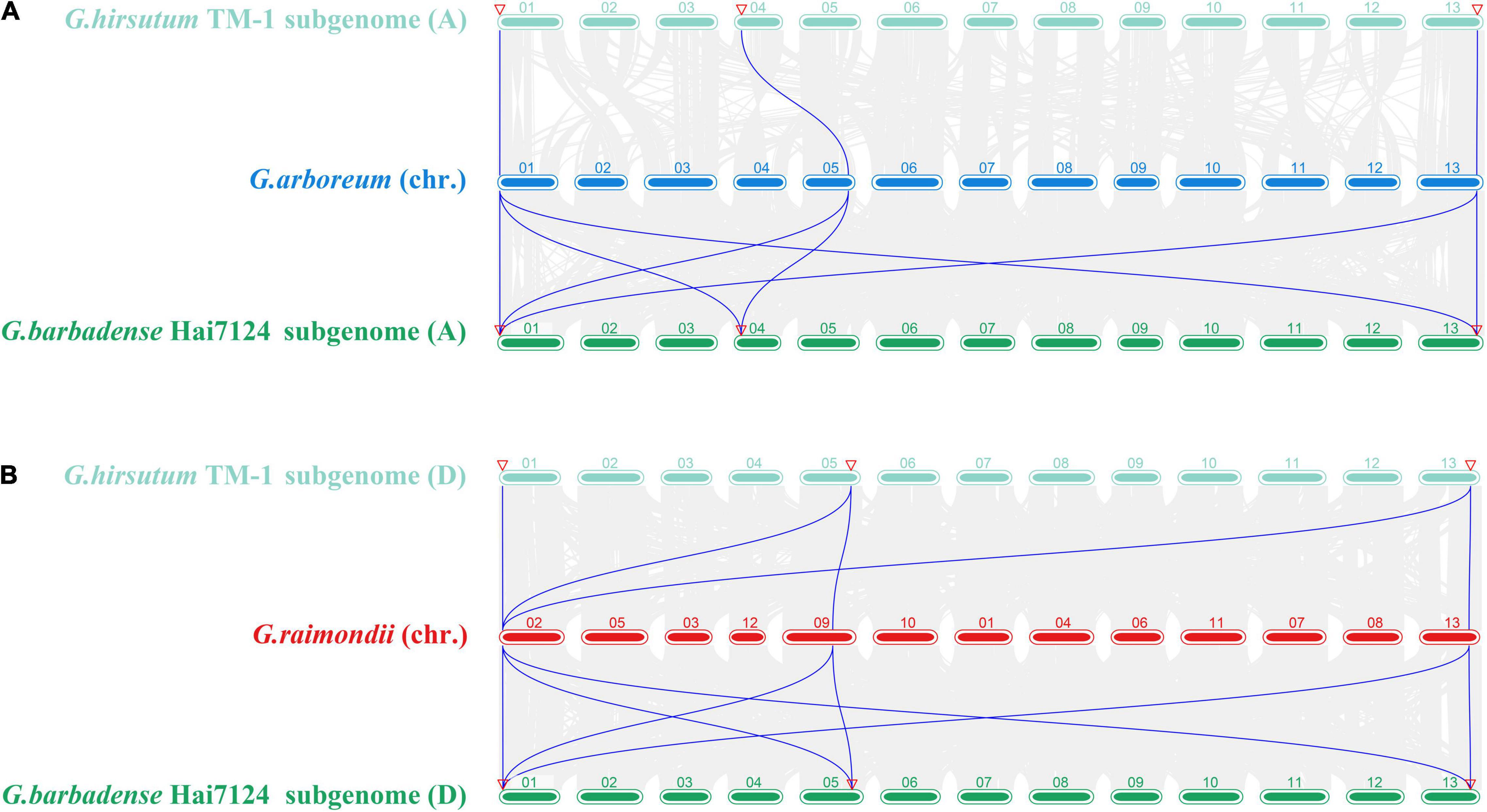
Figure 2. Analysis of synteny among multiple Gossypium genomes about GW2-like. (A) Synteny analysis among G. arboreum, G. hirsutum (At subgenome), and G. barbadense (At subgenome). (B) Synteny analysis among G. raimondii, G. hirsutum (Dt subgenome), and G. barbadense (Dt subgenome).
Collinearity analysis was performed for G. arboreum and G. hirsutum (A subgenome), G. arboreum and G. barbadense (A subgenome), G. raimondii and G. hirsutum (D subgenome), G. raimondii and G. barbadense (D subgenome), respectively (Figure 2). A total of three, six, five, and seven pairs of collinear genes were found, respectively. The GbGW2-like genes in G. barbadense had more collinear gene pairs with G. arboreum (A2) and G. raimondii (D5), while the GhGW2-like genes in G. hirsutum had fewer collinear gene pairs with G. arboreum (A2) and G. raimondii (D5), indicating that GW2-like genes in G. barbadense were more related to two possible ancestral species of G. arboreum and G. raimondii than GW2-like genes in G. hirsutum.
In order to elucidating the evolutionary dynamics and selection pressure of protein coding, the non-synonymous (Ka) and synonymous (Ks) replacement rates were calculated using full-length sequences between each pair of linear homologous genes. Generally, a Ka/Ks ratio>1, = 1 and <1 indicates diversified selection, neutral selection, and purification selection, respectively. The Ka/Ks ratio of all gene pairs was less than 1, suggesting that these genes had undergone purification selection (Table 2). Above results indicated that the GW2-like genes in G. hirsutum and G. barbadense were relatively conserved.
Expression Profiles of GhGW2-Like Genes in Cotton
To investigate the tissue-specific expression profiles of GW2-like genes, published TM-1 expression data, including root, stem, leaf, torus, petal, stamen, pistil, calycle, fiber and ovule at various developmental stages were used. Most GhGW2 genes were extensively expressed in many tissues (Supplementary Figure 2). GhGW2-1A and GhGW2-1D were relatively highly expressed in pollen, petal, and pistil. GhGW2-2A was highly expressed in 20 DPA fiber and roots. GhGW2-2D was highly expressed in the stem, root and early stages of ovule. GhGW2-3A and GhGW2-3D had similar expression trends with GhGW2-1A that highly expressing in flowers and pistils (Supplementary Figure 2A). The expression patterns of GbGW2-like genes in G. barbadense and G. hirsutum shared alike (Supplementary Figure 2B), indicating that these genes may have similar functions. qRT-PCR of diffident ovule development stages showed that GhGW2-1A, GhGW2-2A and GhGW2-1D had a similar tendency with highly expressed in early stages and later stages, and less expressed in 10–20 DPA ovules (Figures 3A–C). GhGW2-2D expression was highest in 10 DPA ovule and 30 DPA ovule, suggesting it may involve in cotton ovules development (Figure 3D). GhGW2-3A revealed an extensive expression in all ovule development stages (Figure 3E). GhGW2-3D was expressed at very low levels in all ovule development stages except in 30 DPA ovule (Figure 3F).
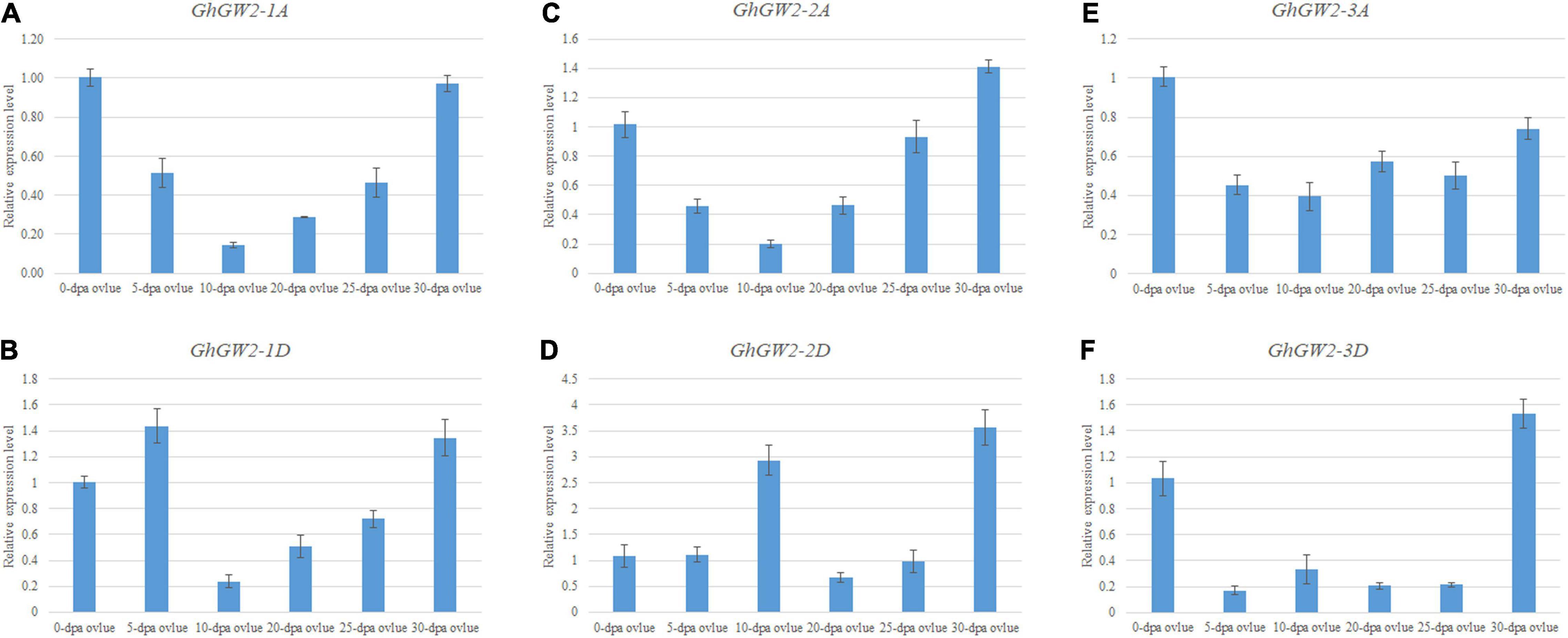
Figure 3. qRT-PCR of GhGW2s in ovules. Relative expression level of GhGW2-1A (A), GhGW2-1D (B), GhGW2-2A (C), GhGW2-2D (D), GhGW2-3A (E), GhGW2-3D (F). GhActin3 was selected as internal and the 1 Ct value of 0-DPA-ovules was set as the control. The data presented are the means ± SD of three replicates.
GhGW2-like genes had distinct expression patterns in different tissues of TM-1, suggesting that GhGW2-like genes may have different functions in cotton growth.
Generation of GhGW2-2D-Overexpressing Arabidopsis Lines
Based on the sequence alignment and homology analysis, GhGW2-2D was selected for further study because of its similarity to OsGW2 and AtDA2 (Figure 4). Moreover, the expression of GhGW2-2D was relatively high in the stage of ovule development. Plants overexpressing GhGW2-2D were generated and identified using semi-quantitative PCR (Figure 5A), and a total of three transgenic lines with high expression of GhGW2-2D were identified. qRT-PCR was used to further assess the relative expression levels of the three diverse transgenic lines (Figure 5B).
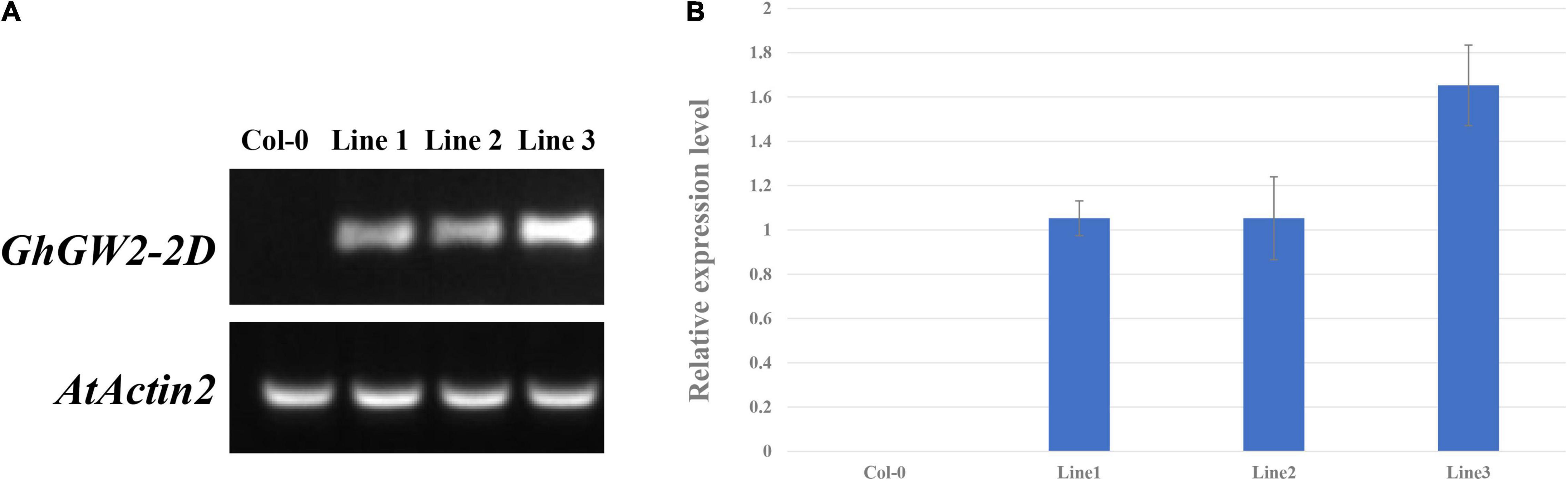
Figure 5. (A) Identification of GhGW2-2D transgenic plants using semi-quantitative PCR. (B) Relative expression level of GhGW2-2D in three transgenic Arabidopsis lines. The Ct value of GhGW2-2D in transgenic line 1 was set as the control. The data presented are the means ± SD of three replicates.
Overexpression of GhGW2-2D Decreases Seed Size
To evaluate the applicability of transgenic breeding to alter seed size, different developmental stages of transgenic Arabidopsis were characterised. Overexpression of GhGW2-2D reduced seed size (Figures 6A–D). The seed areas of lines 1, 2, and 3 decreased by 25, 24, and 26%, respectively, compared with that of the wild type Col-0 (Figure 6I). The growth of nine-day-old seedlings was measured after germinating. The transgenic lines had smaller cotyledons than Col-0 (Figures 6E–H). Compared with that of wild type Col-0, the cotyledon area of lines 1, 2, and 3 decreased by 31, 33, and 42%, respectively (Figure 6J). These results further support the role of GhGW2-2D in limiting seed and organ growth.
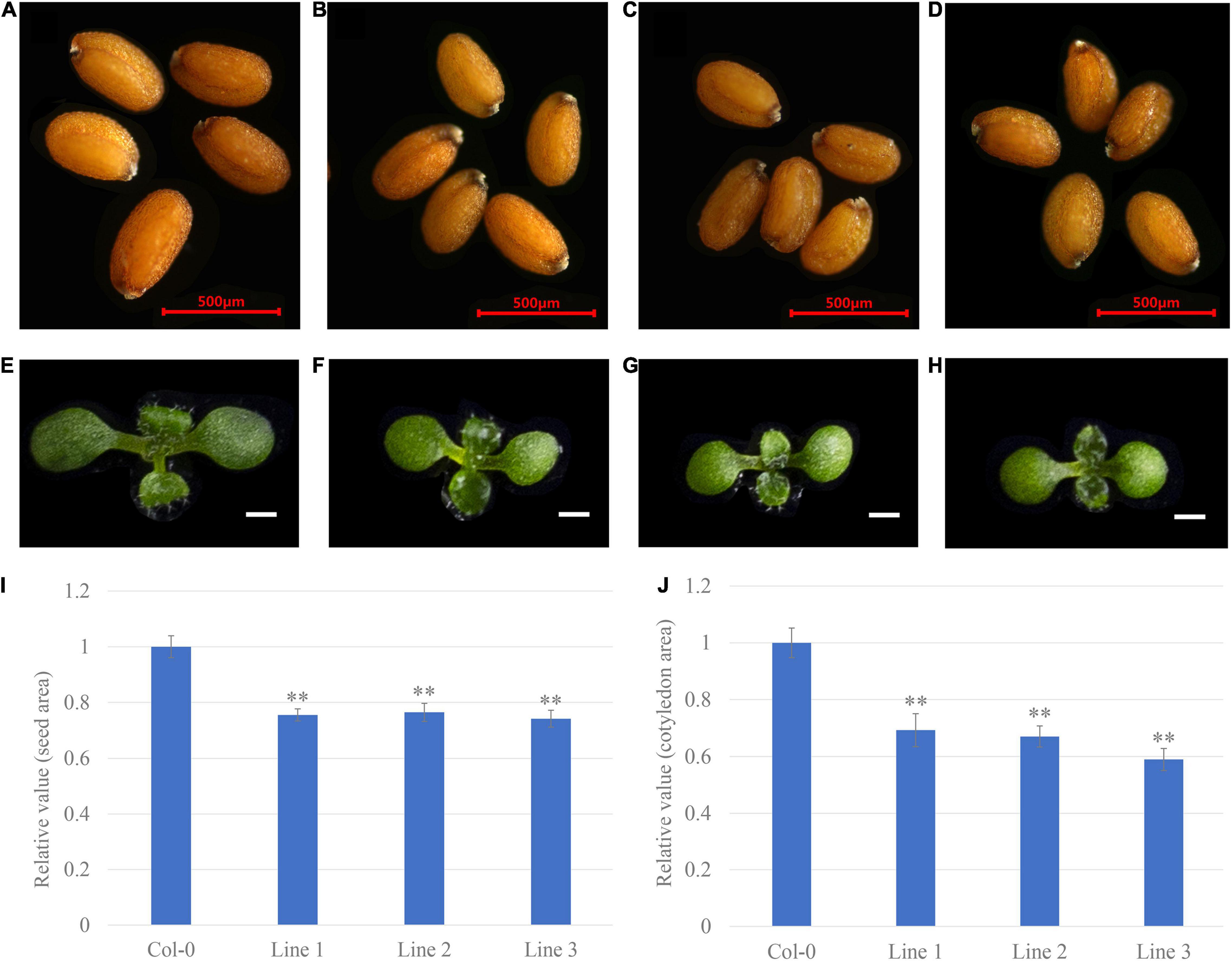
Figure 6. The overexpression of GhGW2-2D decreases the seed size in Arabidopsis. (A–D) Seed phenotype of Col-0 (A) and transgenic lines 1 (B), 2 (C), and 3 (D) (n = 30). Bar = 500 μm. (E–H) Seedling phenotype of Col-0 (E) and transgenic lines 1 (F), 2 (G), and 3 (H) (n = 20). Bar = 1 mm. The relative seed (I) and cotyledon (J) area of seeds from transgenic lines compared to Col-0. Values in (I) and (J) are given as mean ± SE relative to the respective Col-0 values, set at 1. ** represents significant differences at the P < 0.01 level.
Discussion
Seed size is a vital characteristic of flowering plants (Orsi and Tanksley, 2009). Cottonseed kernels are regarded as the best source of vegetable protein and oil and are associated with seed size (Poehlman, 1994; Pahlavani et al., 2009). Advances have revealed that plants with large seeds accumulate more nutrients, which may affect seed germination, plant growth, and development (Wang, 2008). Thus, understanding the mechanisms controlling seed size is essential for agricultural production and germplasm improvement.
Recent studies have shown that GW2-like genes have a far-reaching impact on seed size and weight. GW2, which encodes an E3 ubiquitin ligase involved in the regulation of cell division, was found to affect grain size and improve grain yield potential (Song et al., 2007). The seeds of the offspring of mutant da2-1 plants were bigger than those of the control, and the 1,000 grain weight was increased (Xia et al., 2013). Moreover, the loss of the function of this gene has been reported to increase the number of cells and the grain size of rice (Yamaguchi et al., 2020). Altogether, these studies highlight that the GW2 gene has an important value in breeding and plays an important regulatory role in controlling seed development.
We identified 3, 3, 6, and 6 GW2-like genes in G. raimondii, G. arboreum, G. barbadense, and G. hirsutum, respectively. The chromosomal distribution, evolutionary relationship, and expression patterns of GW2-like genes in cotton were analyzed. It has been found that all GW2-like genes have a typical RING_U-box domain, which can interact with a specific E2 ubiquitin binding enzyme and ubiquitinate specific substrates (Song et al., 2007). Gene structure analysis revealed that all GW2-like genes have eight exons, which indicates that the gene may be functionally conserved during evolution. According to the selection pressure analysis, the Ka/Ks ratio of GW2-like was less than 1, further supporting the evolutionary conservation of these genes. The GhGW2-2D was co-localized with quantitative trait loci (QTL) for seed size (boll weight, BW), suggesting that its sequence variations may be genetically associated with the natural variation in seed size (Supplementary Figure 3). Processing and analysis of published RNA-Seq data, we found that GhGW2-2D was highly expressed in roots, stems, and during early ovule development. Moreover, GhGW2-2D is most similar to AtDA2 and OsGW2 and has the same protein conserved functional domain. Therefore, this gene was selected for the subsequent analysis.
To date, only few studies have investigated GW2-like genes in cotton. In the present study, a comprehensive analysis of GW2-like genes in the four sequenced cotton species was performed, which provided more details of the cotton GW2-like genes. Overexpression of GhGW2-2D in Arabidopsis decreased seed size, indicating that the product encoded by this gene may play a negative regulatory role in seed development. DA1 interacts with the E3 ubiquitin ligase DA2 to regulate seed and organ size in Arabidopsis, Triticum aestivum, and G. hirsutum (Xia et al., 2013; Liu et al., 2020; Yang et al., 2021). It may be interesting to further explore the molecular network controlling seed size in cotton. These findings could provide an important theoretical and experimental basis for growth and regulating the development of seeds and organs in cotton, which has great significance in creating excellent germplasm.
Data Availability Statement
The original contributions presented in the study are included in the article/Supplementary Material, further inquiries can be directed to the corresponding authors.
Author Contributions
LH and SY planned the experiments and wrote the manuscript. LWu and YX participated in the study. JS, MW, WP, and JY provided advice for experiments and manuscript writing. XM and SH conceived and designed the research and manuscript revision. All authors read and approved the final manuscript.
Funding
This present study was supported by the Natural Science Foundation of Xinjiang Uygur Autonomous Region of China (Grant Nos. 2021D01B113 and 2020D01A135), the National Natural Science Foundation of China (Grant Nos. 32060449 and 31660410), and Agricultural Science and Technology Innovation Program of Chinese Academy of Agricultural Sciences.
Conflict of Interest
The authors declare that the research was conducted in the absence of any commercial or financial relationships that could be construed as a potential conflict of interest.
Publisher’s Note
All claims expressed in this article are solely those of the authors and do not necessarily represent those of their affiliated organizations, or those of the publisher, the editors and the reviewers. Any product that may be evaluated in this article, or claim that may be made by its manufacturer, is not guaranteed or endorsed by the publisher.
Supplementary Material
The Supplementary Material for this article can be found online at: https://www.frontiersin.org/articles/10.3389/fpls.2022.860922/full#supplementary-material
Supplementary Figure 1 | Chromosomal localization of GW2-like genes in Gossypium. All GW2-like genes were mapped onto chromosomes of G. arboreum (A), G. raimondii (B), G. hirsutum (C), and G. barbadense (D). The scale represents megabases (Mb).
Supplementary Figure 2 | Expression profiles of GW2-like genes in G. hirsutum TM1 and G. barbadense Hai7124. (A) Expression patterns of GhGW2-like genes. (B) Expression patterns of GbGW2-like genes.
Supplementary Figure 3 | A co-localization analysis of GhGW2-like genes with seed size quantitative trait loci (QTLs). GhGW2-like genes and co-localized QTL are shown in red.
Footnotes
- ^ https://www.cottongen.org
- ^ http://www.arabidopsis.org
- ^ http://rice.uga.edu/expression.shtml
- ^ https://www.ncbi.nlm.nih.gov/Structure/bwrpsb/bwrpsb.cgi
- ^ http://smart.embl-heidelberg.de/
- ^ http://www2.cottonqtldb.org:8081/
- ^ http://www.ebi.ac.uk/Tools/msa/clustalw2
- ^ http://www.megasoftware.net
- ^ https://web.expasy.org/
- ^ http://meme-suite.org/
References
Achary, V. M. M., and Reddy, M. K. (2021). CRISPR-Cas9 mediated mutation in GRAIN WIDTH and WEIGHT2 (GW2) locus improves aleurone layer and grain nutritional quality in rice. Sci. Rep. 11:21941. doi: 10.1038/s41598-021-00828-z
Bailey, T. L., Boden, M., Buske, F. A., Frith, M., Grant, C. E., Clementi, L., et al. (2009). MEME SUITE: tools for motif discovery and searching. Nucleic Acids Res. 37, W202–W208. doi: 10.1093/nar/gkp335
Bellaloui, N., Stetina, S. R., and Turley, R. B. (2015). Cottonseed protein, oil, and mineral status in near-isogenic Gossypium hirsutum cotton lines expressing fuzzy/linted and fuzzless/linted seed phenotypes under field conditions. Front. Plant Sci. 6:137. doi: 10.3389/fpls.2015.00137
Bellaloui, N., Turley, R. B., and Stetina, S. R. (2021). Cottonseed protein, oil, and minerals in cotton (Gossypium hirsutum L.) lines differing in curly leaf morphology. Plants 10:525. doi: 10.3390/plants10030525
Bewley, J. D. (1997). Seed germination and dormancy. Plant Cell 9, 1055–1066. doi: 10.1105/tpc.9.7.1055
Cheng, J., Chen, Y., Hu, Y., Zhou, Z., Hu, F., Dong, J., et al. (2020). Fine mapping of restorer-of-fertility gene based on high-density genetic mapping and collinearity analysis in pepper (Capsicum annuum L.). Theor. Appl. Genet. 133, 889–902. doi: 10.1007/s00122-019-03513-y
Choi, B. S., Kim, Y. J., Markkandan, K., Koo, Y. J., Song, J. T., and Seo, H. S. (2018). GW2 functions as an E3 ubiquitin ligase for rice expansin-like 1. Int. J. Mol. Sci. 19:1904. doi: 10.3390/ijms19071904
Dong, H., Dumenil, J., Lu, F. H., Na, L., Vanhaeren, H., Naumann, C., et al. (2017). Ubiquitylation activates a peptidase that promotes cleavage and destabilization of its activating E3 ligases and diverse growth regulatory proteins to limit cell proliferation in Arabidopsis. Genes Dev. 31, 197–208. doi: 10.1101/gad.292235.116
Du, X., Huang, G., He, S., Yang, Z., Sun, G., Ma, X., et al. (2018). Resequencing of 243 diploid cotton accessions based on an updated A genome identifies the genetic basis of key agronomic traits. Nat. Genet. 50, 796–802. doi: 10.1038/s41588-018-0116-x
Gasteiger, E., Gattiker, A., Hoogland, C., Ivanyi, I., Appel, R. D., and Bairoch, A. (2003). ExPASy: the proteomics server for in-depth protein knowledge and analysis. Nucleic Acids Res. 31, 3784–3788. doi: 10.1093/nar/gkg563
Hao, J., Wang, D., Wu, Y., Huang, K., Duan, P., Li, N., et al. (2021). The GW2-WG1-OsbZIP47 pathway controls grain size and weight in rice. Mol. Plant 14, 1266–1280. doi: 10.1016/j.molp.2021.04.011
Hu, S., Yang, H., Gao, H., Yan, J., and Xie, D. (2021). Control of seed size by jasmonate. Sci. China Life Sci. 64, 1215–1226. doi: 10.1007/s11427-020-1899-8
Hu, Y., Chen, J., Fang, L., Zhang, Z., Ma, W., Niu, Y., et al. (2019). Gossypium barbadense and Gossypium hirsutum genomes provide insights into the origin and evolution of allotetraploid cotton. Nat. Genet. 51, 739–748. doi: 10.1038/s41588-019-0371-5
Kumar, S., Stecher, G., and Tamura, K. (2016). MEGA7: molecular evolutionary genetics analysis version 7.0 for bigger datasets. Mol. Biol. Evol. 33, 1870–1874. doi: 10.1093/molbev/msw054
Larkin, M. A., Blackshields, G., Brown, N. P., Chenna, R., McGettigan, P. A., McWilliam, H., et al. (2007). Clustal W and clustal X version 2.0. Bioinformatics 23, 2947–2948. doi: 10.1093/bioinformatics/btm404
Letunic, I., Doerks, T., and Bork, P. (2015). SMART: recent updates, new developments and status in 2015. Nucleic Acids Res. 43, D257–D260. doi: 10.1093/nar/gku949
Li, F., Fan, G., Lu, C., Xiao, G., Zou, C., Kohel, R. J., et al. (2015). Genome sequence of cultivated upland cotton (Gossypium hirsutum TM-1) provides insights into genome evolution. Nat. Biotechnol. 33, 524–530. doi: 10.1038/nbt.3208
Li, F., Fan, G., Wang, K., Sun, F., Yuan, Y., Song, G., et al. (2014). Genome sequence of the cultivated cotton Gossypium arboreum. Nat. Genet. 46, 567–572. doi: 10.1038/ng.2987
Li, N., and Li, Y. (2016). Signaling pathways of seed size control in plants. Curr. Opin. Plant Biol. 33, 23–32. doi: 10.1016/j.pbi.2016.05.008
Li, N., Xu, R., and Li, Y. (2019). Molecular networks of seed size control in plants. Annu. Rev. Plant Biol. 70, 435–463. doi: 10.1146/annurev-arplant-050718-095851
Li, Q., Li, L., Yang, X., Warburton, M. L., Bai, G., Dai, J., et al. (2010). Relationship, evolutionary fate and function of two maize co-orthologs of rice GW2 associated with kernel size and weight. BMC Plant Biol. 10:143. doi: 10.1186/1471-2229-10-143
Li, W., Zhou, Z., Meng, Y., Xu, N., and Fok, M. (2009). Modeling boll maturation period, seed growth, protein, and oil content of cotton (Gossypium hirsutum L.) in China. Field Crops Res. 112, 131–140. doi: 10.1016/j.fcr.2009.02.009
Li, X. B., Fan, X. P., Wang, X. L., Cai, L., and Yang, W. C. (2005). The cotton ACTIN1 gene is functionally expressed in fibers and participates in fiber elongation. Plant Cell 17, 859–875. doi: 10.1105/tpc.104.029629
Liu, H., Li, H., Hao, C., Wang, K., Wang, Y., Qin, L., et al. (2020). TaDA1, a conserved negative regulator of kernel size, has an additive effect with TaGW2 in common wheat (Triticum aestivum L.). Plant Biotechnol. J. 18, 1330–1342. doi: 10.1111/pbi.13298
Orsi, C. H., and Tanksley, S. D. (2009). Natural variation in an ABC transporter gene associated with seed size evolution in tomato species. PLoS Genet. 5:e1000347. doi: 10.1371/journal.pgen.1000347
Pahlavani, M., Miri, A., and Kazemi, G. (2009). Response of oil and protein content to seed size in cotton(Gossypium hirsutum L., cv. Sahel). Plant Breed. Seed Sci. 59, 53–64. doi: 10.2478/v10129-009-0004-8
Pahlavani, M. H., Miri, A. A., and Kazemi, G. (2008). Response of oil and protein content to seed size in cotton. Int. J. Agric. Biol. 10, 643–647.
Paterson, A. H., Wendel, J. F., Gundlach, H., Guo, H., Jenkins, J., Jin, D., et al. (2012). Repeated polyploidization of Gossypium genomes and the evolution of spinnable cotton fibres. Nature 492, 423–427. doi: 10.1038/nature11798
Said, J. I., Knapka, J. A., Song, M., and Zhang, J. (2015a). Cotton QTLdb: a cotton QTL database for QTL analysis, visualization, and comparison between Gossypium hirsutum and G. hirsutum × G. barbadense populations. Mol. Genet. Genomics 290, 1615–1625. doi: 10.1007/s00438-015-1021-y
Said, J. I., Song, M., Wang, H., Lin, Z., Zhang, X., Fang, D. D., et al. (2015b). A comparative meta-analysis of QTL between intraspecific Gossypium hirsutum and interspecific G. hirsutum × G. barbadense populations. Mol. Genet. Genomics 290, 1003–1025. doi: 10.1007/s00438-014-0963-9
Said, J. I., Lin, Z., Zhang, X., Song, M., and Zhang, J. (2013). A comprehensive meta QTL analysis for fiber quality, yield, yield related and morphological traits, drought tolerance, and disease resistance in tetraploid cotton. BMC Genomics 14:776. doi: 10.1186/1471-2164-14-776
Sestili, F., Pagliarello, R., Zega, A., Saletti, R., Pucci, A., Botticella, E., et al. (2019). Enhancing grain size in durum wheat using RNAi to knockdown GW2 genes. Theor. Appl. Genet. 132, 419–429. doi: 10.1007/s00122-018-3229-9
Song, X. J., Huang, W., Shi, M., Zhu, M. Z., and Lin, H. X. (2007). A QTL for rice grain width and weight encodes a previously unknown RING-type E3 ubiquitin ligase. Nat. Genet. 39, 623–630. doi: 10.1038/ng2014
Su, Z., Hao, C., Wang, L., Dong, Y., and Zhang, X. (2011). Identification and development of a functional marker of TaGW2 associated with grain weight in bread wheat (Triticum aestivum L.). Theor. Appl. Genet. 122, 211–223. doi: 10.1007/s00122-010-1437-z
Valluru, R., Reynolds, M. P., and Salse, J. (2014). Genetic and molecular bases of yield-associated traits: a translational biology approach between rice and wheat. Theor. Appl. Genet. 127, 1463–1489. doi: 10.1007/s00122-014-2332-9
Voorrips, R. E. (2002). MapChart: software for the graphical presentation of linkage maps and QTLs. J. Hered. 93, 77–78. doi: 10.1093/jhered/93.1.77
Wang, K., Wang, Z., Li, F., Ye, W., Wang, J., Song, G., et al. (2012). The draft genome of a diploid cotton Gossypium raimondii. Nat. Genet. 44, 1098–1103. doi: 10.1038/ng.2371
Wang, X. Y. (2008). Effect of seed size on the plant growth at seedling stage in cotton. J. Anhui Agric. Sci. 36, 9939–9940.
Wang, Y., Tang, H., Debarry, J. D., Tan, X., Li, J., Wang, X., et al. (2012). MCScanX: a toolkit for detection and evolutionary analysis of gene synteny and collinearity. Nucleic Acids Res. 40:e49. doi: 10.1093/nar/gkr1293
Wang, Z., Li, N., Jiang, S., Gonzalez, N., Huang, X., Wang, Y., et al. (2016). SCF(SAP) controls organ size by targeting PPD proteins for degradation in Arabidopsis thaliana. Nat. Commun. 7:11192. doi: 10.1038/ncomms11192
Xia, T., Li, N., Dumenil, J., Li, J., Kamenski, A., Bevan, M. W., et al. (2013). The ubiquitin receptor DA1 interacts with the E3 ubiquitin ligase DA2 to regulate seed and organ size in Arabidopsis. Plant Cell 25, 3347–3359. doi: 10.1105/tpc.113.115063
Xing, Y., and Zhang, Q. (2010). Genetic and molecular bases of rice yield. Annu. Rev. Plant Biol. 61, 421–442. doi: 10.1146/annurev-arplant-042809-112209
Yamaguchi, K., Yamamoto, T., Segami, S., Horikawa, M., Chaya, G., Kitano, H., et al. (2020). gw2 mutation increases grain width and culm thickness in rice (Oryza sativa L.). Breed. Sci. 70, 456–461. doi: 10.1270/jsbbs.20018
Yang, S., Huang, L., Song, J., Liu, L., Bian, Y., Jia, B., et al. (2021). Genome-wide analysis of DA1-like genes in Gossypium and functional characterization of GhDA1-1A controlling seed size. Front. Plant Sci. 12:647091. doi: 10.3389/fpls.2021.647091
Yu, J., Jung, S., Cheng, C. H., Ficklin, S. P., Lee, T., Zheng, P., et al. (2014). CottonGen: a genomics, genetics and breeding database for cotton research. Nucleic Acids Res. 42, D1229–D1236. doi: 10.1093/nar/gkt1064
Zhao, W., Yan, Q., Yang, H., Yang, X., Wang, L., Chen, B., et al. (2019). Effects of mepiquat chloride on yield and main properties of cottonseed under different plant densities. J. Cotton Res. 2:10. doi: 10.1186/s42397-019-0026-1
Keywords: cotton, GW2-like, expression pattern, seed size, GhGW2-2D
Citation: Huang L, Yang S, Wu L, Xin Y, Song J, Wang L, Pei W, Wu M, Yu J, Ma X and Hu S (2022) Genome-Wide Analysis of the GW2-Like Genes in Gossypium and Functional Characterization of the Seed Size Effect of GhGW2-2D. Front. Plant Sci. 13:860922. doi: 10.3389/fpls.2022.860922
Received: 24 January 2022; Accepted: 15 February 2022;
Published: 07 March 2022.
Edited by:
Baohua Wang, Nantong University, ChinaReviewed by:
Jinfa Zhang, New Mexico State University, United StatesGuanghui Xiao, Shaanxi Normal University, China
Copyright © 2022 Huang, Yang, Wu, Xin, Song, Wang, Pei, Wu, Yu, Ma and Hu. This is an open-access article distributed under the terms of the Creative Commons Attribution License (CC BY). The use, distribution or reproduction in other forums is permitted, provided the original author(s) and the copyright owner(s) are credited and that the original publication in this journal is cited, in accordance with accepted academic practice. No use, distribution or reproduction is permitted which does not comply with these terms.
*Correspondence: Xiaoyan Ma, bWF4eV9jYWFzQDEyNi5jb20=; Shoulin Hu, aHVzaG91bGluZ2h1QDE2My5jb20=
†These authors have contributed equally to this work