- 1Beijing Key Laboratory of Ornamental Plants Germplasm Innovation and Molecular Breeding, National Engineering Research Center for Floriculture and College of Landscape Architecture, Beijing Forestry University, Beijing, China
- 2Ningxia State Farm, Yinchuan, China
- 3Zhejiang Provincial Key Laboratory of Germplasm Innovation and Utilization for Garden Plants, School of Landscape Architecture, Zhejiang Agriculture and Forestry University, Hangzhou, China
Tree peony (Paeonia suffruticosa) is a well-known Chinese ornamental plant with showy flower color. However, the color fading problem during vase time seriously blocks its development in the cut flower market. In this study, we found that exogenous glucose supply improved the color quality of P. suffruticosa ‘Tai Yang’ cut flowers with increased total soluble sugar and anthocyanin contents of petals. Besides, the promotion effect of glucose was better than the osmotic control of 3-O-methylglucose (3OMG) treatment and the glucose analog mannose treatment. The structural genes, including PsF3H, PsF3′H, PsDFR, PsAOMT, and PsUF5GT, were remarkably upregulated under glucose treatment. Meanwhile, the regulatory genes, including PsbHLH1, PsbHLH3, PsMYB2, PsWD40-1, and PsWD40-2, also showed a strong response to glucose treatment. Among these five regulatory genes, PsMYB2 showed less response to 3OMG treatment but was highly expressed under glucose and mannose treatments, indicating that PsMYB2 may have an important role in the glucose signal pathway. Ectopic overexpression of PsMYB2 in Nicotiana tabacum resulted in a strong pigmentation in petals and stamens of tobacco flowers accompanied with multiple anthocyanin biosynthetic genes upregulated. More importantly, the overexpression of PsMYB2 enhanced the ability of glucose-induced anthocyanin accumulation in Arabidopsis thaliana seedlings since PsMYB2-overexpressing Arabidopsis showed higher expression levels of AtPAL1, AtCHS, AtF3H, AtF3′H, AtDFR, and AtLDOX than those of wild type under glucose treatment. In summary, we suggested that glucose supply promoted petal coloration of P. suffruticosa ‘Tai Yang’ cut flower through the signal pathway, and PsMYB2 was a key component in this process. Our research made a further understanding of the mechanism that glucose-induced anthocyanin biosynthesis of P. suffruticosa cut flowers during postharvest development, laying a foundation for color retention technology development of cut flowers.
Introduction
In China, due to the various flower colors and long cultivation history, tree peony possesses the reputation of “the King of Flowers” and becomes a competitive candidate for “Chinese National Flower” (Suo et al., 2008). However, a fast decrease in the ornamental quality along with color fading that always appears in the cut flowers during vase time greatly impairs its commercial value as cut flower. Therefore, color quality declining of tree peony during postharvest development is urgently needed to be solved.
As a class of secondary metabolites of flavonoids, anthocyanins are the primary flower pigments in higher plants whose accumulations are tightly linked with flower development, making flowers show a series of colors, mainly ranging from red to purple and blue (Mol et al., 2008; Tanaka et al., 2008). Anthocyanin biosynthesis and accumulation are controlled by a complex environmental and developmental regulations (Weiss, 2000), such as UV light, nitrogen source, osmotic stress, and sugars. Among them, sugars are often used as the energy sources to improve the quality of cut flowers, such as lisianthus (Eustoma grandiflorum) (Jamal Uddin et al., 2001; Shimizu and Ichimura, 2005), oriental lily (Lilium brownii) (Han, 2003), and phlox (Phlox paniculata) (Sankhla et al., 2005), thereby indirectly improving the coloration of petals. However, the recent studies found that sugars not only serve as energy and structural materials in anthocyanin biosynthesis but also important signal molecules regulating its production (Rolland et al., 2002; Li et al., 2019). In Arabidopsis, higher anthocyanin accumulation was detected in the seedlings cultivated on sugar-containing plates, whereas the addition of sugar analogs had no such effect (Solfanelli et al., 2006). Similarly, exogenous sugars including glucose, fructose, or sucrose induced a large amount of anthocyanin production in detached hypocotyls of radish (Raphanus sativus) seedlings, but the osmotic control of 3OMG hardly caused anthocyanin accumulation (Hara et al., 2003). In addition, the cases that sugar enhanced anthocyanin accumulation through signal pathways were also found in radish hypocotyl (Hara et al., 2003), apple (Malus domestica) calli (Hu et al., 2016), petunia (Petunia hybrida) corolla (Moalem-Beno et al., 1997), and grape (Vitis vinifera) cells (Vitrac et al., 2000).
At present, the detailed information of sugars serving as signal molecules to regulate anthocyanin biosynthesis largely has not been revealed. A few studies suggested that this process was related to the regulation of gene expression levels involved in anthocyanin biosynthesis (Weiss, 2000; Rolland et al., 2002; Rook and Bevan, 2003; Mol et al., 2008; Naing et al., 2021). For example, in radish hypocotyl, the anthocyanin accumulation caused by sucrose treatment was related to higher transcripts of CHS and ANS (Hara et al., 2003). CHS-A from petunia was also a sugar-dependent expression gene in transgenic Arabidopsis (Tsukaya et al., 1991). In grape berry, research showed that sugar-induced anthocyanin accumulation was closely associated with incubation time and the RNA levels of F3H under different sugars (Zheng et al., 2009). Further transcriptome analysis suggested that sugar-induced anthocyanin production was not only related to the changed expression levels of structural genes but also the participation of regulatory genes, gathered with a massive modification in signaling pathways (Dai et al., 2013). Actually, acting upstream of structural genes involved in anthocyanin biosynthetic pathways (Dooner et al., 1991), transcription factors (TFs) are considered to be the important responders and performers in sugar-induced anthocyanin accumulation (Teng et al., 2005; Ai et al., 2016; Hu et al., 2016). In the recent years, more and more TFs that participate in sugar-induced anthocyanin production have been found and identified, including members of DELLA, WRKY, bHLH, MYB, and GARP families, and the MYB TFs are the most discovered and identified among them (Smeekens, 2000; Teng et al., 2005; Li et al., 2014; Hu et al., 2016; Chen et al., 2019; Zhao et al., 2021).
The R2R3-MYB transcription factor is the largest group of plant MYB factors that contain an R2R3 MYB domain at the N-terminal and an [DE]Lx2[RK]x3Lx6Lx3R motif in the R3 repeat for interacting with bHLH proteins, playing essential roles in the regulation of secondary metabolism including anthocyanin biosynthesis (Yan et al., 2021). To date, more and more R2R3-MYB TFs participating in the regulation of anthocyanin biosynthesis are discovered and identified in various fruit and flowers, such as cherry (Prunus avium) (Shen et al., 2014), apple (Jiang et al., 2014), lily (Yamagishi et al., 2010), and petunia (Quattrocchio et al., 1999). During flower color development, R2R3-MYBs are not only in charge of enzyme gene regulation but also responsible for the initiation of anthocyanin biosynthesis in response to a variety of developmental and environmental changes (Dooner et al., 1991). For instance, ABA-induced anthocyanin enhancement in sweet cherry was mediated by PacMYBA regulation (Shen et al., 2014). During the storage of kiwifruit (Actinidia chinensis), AcMYBA1-1 and AcMYB5-1 responded to low temperatures and induced the expression of several structural genes involved in anthocyanin biosynthesis (Li et al., 2017). More importantly, R2R3-MYB often appears in sugar response and plays a vital role in this process. For example, in Arabidopsis, AtMYB75, a key transcription factor for DFR activation, activated the anthocyanin biosynthesis when seedlings were exposed to high light or sucrose treatment (Teng et al., 2005; Li et al., 2016). AtMyb56 regulated the anthocyanin accumulation by controlling the transcript level of AtGPT2 in response to sucrose treatment (Jeong et al., 2018). Besides, AtMYBL2 acted downstream of the transcription factor AtGLK1 and participated in Arabidopsis sucrose-induced anthocyanin biosynthesis (Zhao et al., 2021).
For the research on the anthocyanin biosynthesis in tree peony, many studies confirmed that it was mainly under the control of transcription factors including R2R3-MYB, bHLH, and WD40 repeats (Gu et al., 2019; Zhang et al., 2019; Qi et al., 2020). For example, PsMYB12 interacted with bHLH and WD40 protein to activate PsCHS expression, which was specific to the petal blotches (Gu et al., 2019). In flower color research of tree peony, people paid more attention to studies the such as double-color formation (Zhang et al., 2018), blotch formation (Zhang Y. et al., 2015; Gu et al., 2019), and petal pigmentation (Shi et al., 2015). However, the research on the effects of external factors such as light, temperature, and sugar on anthocyanin biosynthesis and their regulatory mechanism is insufficient. Previous study found that glucose treatment had a better color retention effect on P. suffruticosa ‘Luoyang Hong’ cut flower, and it promoted the anthocyanin accumulation of petals through signal pathways (Zhang C. et al., 2015). Research showed that the molecular mechanisms of petal coloration, as well as gene expression patterns involved in anthocyanin biosynthesis, varied among different anthocyanin compositions (Qian et al., 2021). As we know, P. suffruticosa ‘Luoyang Hong’ is a purple-red flower type cultivar with cyanidin (Cy)-dominated biosynthetic pathway in anthocyanin synthesis (Wang et al., 2001). Thus, whether glucose has the same pigmentation effect on pelargonidin (Pg)-based red pigment tree peony cut flowers remains to be determined.
In this study, P. suffruticosa ‘Tai Yang,’ belonging to pelargonidin (Pg)-based red pigment type, was selected as the material. The glucose and glucose analogs were applied to P. suffruticosa ‘Tai Yang’ cut flowers during vase time. The total anthocyanin (TA) content and individual anthocyanin were quantified through high-performance liquid chromatography (HPLC). The gene expression levels including nine structural genes and seven anthocyanin-related regulatory genes that maigt play positive role in glucose-induced anthocyanin production were detected to explore the possible regulation mechanism. Based on the comparison of gene expression between treatments, we found that the R2R3-MYB transcription factor PsMYB2 showed a strong behavior to glucose treatment through the signal pathway in P. suffruticosa ‘Tai Yang’ cut flowers. The positive regulation effect of PsMYB2 on anthocyanin biosynthesis was identified through transgenic tobacco. Additionally, the role of PsMYB2 under glucose treatment was further studied in its transgenic Arabidopsis seedlings. These findings provided valuable data for elucidating the mechanism of glucose-induced anthocyanin biosynthesis and developing color retention technology for cut flowers.
Materials and Methods
Materials and Treatments
Tree peony (P. suffruticosa ‘Tai Yang’) used in this study was cultivated in peony planting base in Heze, Shandong Province, China. Referring to our previous study (Guo et al., 2004), flowers that developed to stage 1 (S1, soft bud stage) were harvested, with flower branches about 30 cm in length and upper compound leaves retained. After harvesting, flowers were transported to the laboratory within 12 h. Before further treatment, all flower branches were retrimmed to 25 cm length with leaves removed, and then, the flower’s stems were placed into distilled water for 1 h for water recovery. After that, the flowers at S1 were randomly divided into four groups and inserted into glass bottles added with 100 ml of different holding solutions. The holding solution with distilled water only was the control (CK, in vase flowers), and the distilled water containing glucose (Glu), 3-O-methylglucose (3OMG, as osmotic control), and mannose (Man, for HXK-depend signaling pathway detecting), respectively, were the other three treatments. According to previous research that 333 mM glucose had a good color retention effect on tree peony cut flowers (Zhang C. et al., 2015), the concentration of glucose, 3OMG, and mannose in this study was set to 333 mM. Besides, all solutions contained 0.05% (v/v) NaClO to prevent the growth of bacteria. There were a total of thirty cut flowers per treatment. The vase condition was maintained at room temperature at 20–23°C and relative humidity of 50–60%, under 40 μmol m–2s–1 light intensity with 12-h photoperiod. The solutions or distilled water were replaced every day at fixed times. Referring to the opening stages given in Figure 1A (stage 2, S2, pre-opening stage; stage 3, S3, initial opening stage; stage 4, S4, half opening stage; and stage 5, S5, full opening stage), the middle petals of flowers at four developmental stages under different treatments were sampled and quickly frozen in liquid nitrogen. All samples were stored at –80°C for anthocyanin determination and gene expression analysis. In addition, the flowers that grew naturally in the field condition and opened to S5 were sampled for anthocyanin compositions analysis of P. suffruticosa ‘Tai Yang’ flowers.
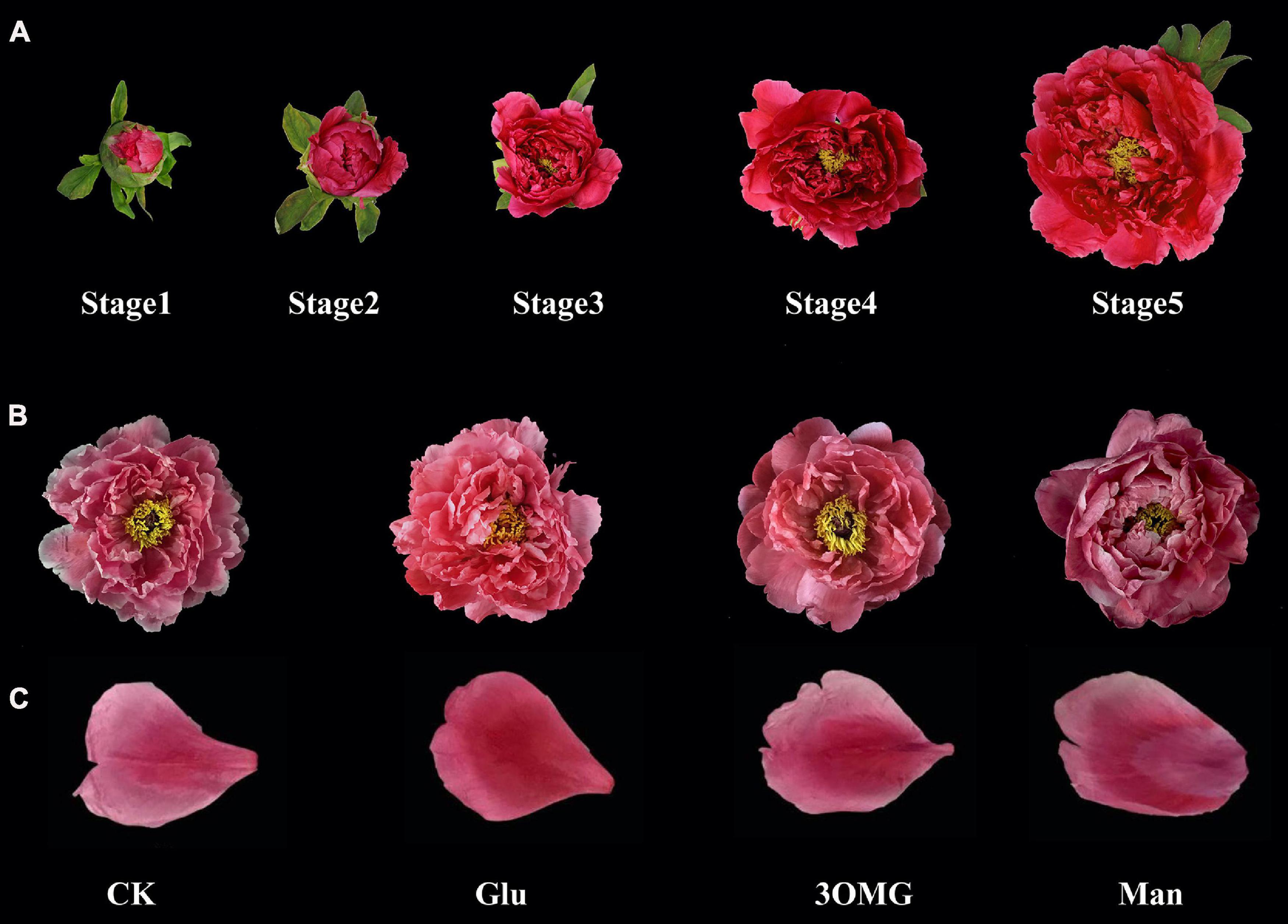
Figure 1. P. suffruticosa ‘Tai Yang’ (A) On-tree flowers of P. suffruticosa ‘Tai Yang’ at five developmental stages. Stage 1, soft bud stage; stage 2, pre-opening stage; stage 3, initial opening stage; stage 4, half opening stage; stage 5, full opening stage. (B) Flowers at stage 5 under four treatments. (C) Petals of flowers under four treatments. There were four treatments including CK, Glu, 3OMG, and Man, respectively. CK was the control group that cut flowers treated with distilled water. Glu, 3OMG, and Man were the cut flowers treated with glucose, 3-O-methylglucose, and mannose at 333 mM, respectively.
Plants of Nicotiana benthamiana and Nicotiana tabacum used in the experiment were cultivated for about 4–8 weeks at a 22°C chamber with 14-h light/10-h dark condition and 60–70% relative humidity. Then, they were selected for the transient expression analysis and stable transformation materials by Agrobacterium tumefaciens, respectively.
Arabidopsis for genetic transformation were the Columbia ecotype (Col-0). The seedlings were grown under a 16-h/8-h (light/dark) cycle at 23/21°C until required.
Petal Color Measurement
Flower surface color was assessed using the Spectrophotometer NF333 (Nippon Denshoku Industries Co., Ltd., Japan). The color reading was taken five times from the upper epidermis region of middle petals (the 4th–6th layer petals) and then averaged as one replicate. A total of three replicates were required for each flower color evaluation. The color measurements at every developmental stage (S2–S5) for each treatment were generated from five single cut flowers. The flower color values of L* (lightness), a* (from green to red), and b* (from blue to yellow) were generated under daylight conditions with the instrument. In addition, the C* (chroma) value was calculated with the formula of C* = (a*2 + b*2)0.5 (Voss, 1992).
Anthocyanin Content Measurement
For anthocyanin composition analysis of P. suffruticosa flowers, the petals of each sample with about 0.3 g fresh weight were extracted with 5 ml extraction solvent (CH3OH: HCl: H2O = 70: 0.1: 29.9; v/v/v) at 4°C in the dark for 24 h, shaking the mixture with a vortex per 8 h during this period. The contents and changes of anthocyanin composition in the tree peony petals under four treatments were qualified with the method of HPLC according to a protocol reported by Zhang et al. (2018) with a few modifications. First, the supernatants were filtered with a 0.22-μm syringe filter and analyzed on an Agilent 1100 High Performance Liquid Chromatograph (Agilent, United States). The mobile phase (A) was 1% formic acid, and the mobile phase (B) was 15% methanol acetonitrile. The program parameter was set as follows: 0 min, 5% B; 42 min, 8% B; and 45 min, 8% B. The injection volume was 10 μl, and the flow rate was 1.0 ml min–1. The C18 column of ODS-80Ts QA (4.6 and 150 mm) (Tosoh, Japan) was used for anthocyanin separating with a column temperature of 35°C at 515 nm.
The TA content analysis of tobacco flowers and Arabidopsis seedlings were measured with the spectrophotometric method. Samples of tobacco flowers and Arabidopsis seedlings with approximately 100 and 20 mg, respectively, were incubated in 1% HCl/methanol solution in the darkness at 4°C for 24 h. The absorbance of the supernatant was monitored at 530 and 657 nm, and the TA contents were calculated with the formula of (A530−0.25 × A657) × M–1. M was the fresh weight (g) of the samples. There were three biological replicates for each sample.
Soluble Sugar Content Measurement
The frozen petals of P. suffruticosa flower under four treatments with approximately 0.2 g for each sample were ground in liquid nitrogen and extracted with 80% ethanol (v/v) in 80°C water bath for 30 min. The mixture was centrifuged at 10,000 × g for 5 min at room temperature, and the supernatant was collected into the volumetric flask. This extraction step was repeated two times. The soluble sugar content was measured with anthrone-sulfuric acid at a wavelength of 620 nm (Hedge et al., 1962).
Total RNA Extraction, cDNA Synthesis, and Gene Expression Analysis
Total RNAs were respectively extracted from tissues of P. suffruticosa, tobacco, and Arabidopsis using the EASY Spin Plant RNA Rapid Extraction Kit (Aidlab, China) according to the manufacturer’s instructions. The concentration and quality were evaluated with a NanoDrop 2000C spectrophotometer (NanoDrop, United States). Subsequently, RNase-free DNase I (Takara, Japan) was used to remove the potential DNA contamination in RNA. About 1 μg of total RNA was used to synthesize the cDNA using M-MLV (Takara, Japan) according to the manufacturer’s protocol.
Real-time PCRs for P. suffruticosa, tobacco, and Arabidopsis were carried out on a CFX96 real-time PCR machine (Bio-Rad, United States) in a total reaction mixture of 20 μl in each containing 10 μl of SYBR Green Premix Ex Taq TM (TaKaRa, Japan), 1 μl of each primer (10 mM), 2 μl of diluted cDNA, and 6 μl of ddH2O. The qPCR amplification protocol parameter was as follows: 30 s at 95°C, followed by 40 cycles of 5 s at 95°C, 30 s at 62°C, and 30 s at 72°C, followed by 65 to 95°C melting curve detection. Primers for the individual genes are listed in Supplementary Table 1. The internal control gene of qPCR assay in P. suffruticosa, tobacco, and Arabidopsis were Psubiquitin, NtTub1, and AtActin2, respectively. Relative gene expression levels were obtained via the 2–ΔΔCt method. Each measurement was taken with three biological replicates.
Cloning, Sequence, and Subcellular Localization Analysis of PsMYB2
In our previous study, an Illumina/Solexa library of P. suffruticosa ‘Luoyang Hong’ flower petal was constructed and sequenced. A gene (GenBank accession number: KJ466975) encoding R2R3-MYB transcription factor PsMYB2 was obtained with reverse transcriptase-polymerase chain reaction (RT-PCR), and the open reading frame (ORF) was identified in our previous study (Zhang et al., 2014). The complete coding region of PsMYB2 was amplified from the cDNA templates of P. suffruticosa ‘Tai Yang’ using PrimeSTAR HS DNA Polymerase (Takara, Japan). Primer sequences used for PsMYB2 cloning are shown in Supplementary Table 1. Multiple sequence alignment was generated by the DNAMAN software version 6. The phylogenetic tree was constructed with MYB TFs from other species using the neighbor-joining method of MEGA11 software, and the tree nodes were evaluated with 1,000 bootstrap replicates.
For subcellular localization analysis, the coding region of PsMYB2 was cloned to a pBI121-GFP vector, forming a pBI121-GFP-PsMYB2 construct. Primer sequences used for vector construction are listed in Supplementary Table 1. The plasmids of pBI121-GFP-PsMYB2 and empty pBI121-GFP were transformed into the GV3101 strain and then were transiently expressed in leaves of 4 to 6-week-old tobacco plants via agrobacterium injection. The green fluorescent protein (GFP) signals were detected with a fluorescence microscope (TCS SP8, Leica, Germany) after 48–72 h of infiltration.
Tobacco and Arabidopsis Stable Transformation
To detect the function of PsMYB2, the coding region of PsMYB2 was cloned to the pBI121 vector driving by the CaMV35s promoter, constructing a pBI121-PsMYB2 overexpression vector. Primer sequences used for vector construction are shown in Supplementary Table 1. Then, the pBI121-PsMYB2 plasmid was stably transferred into tobacco and Arabidopsis by leaf disk method (Horsch et al., 1985) and feather dip method (Clough and Bent, 1998), respectively. Transformed tobacco plants and Arabidopsis seedlings were selected using kanamycin (100 mg ml–1) as a selective marker, and the transgenic lines hosting PsMYB2 were further identified by PCR amplification assay (Supplementary Figures 1A,B). A total of three independent T2 progeny PsMYB2-overexpressing tobaccos and two independent homozygous T3 Arabidopsis seeds with higher PsMYB2 gene expression levels were selected for further analysis.
Arabidopsis Treatment With Glucose
Homozygous T3 seeds of transgenic PsMYB2 Arabidopsis were plated on the one-half-strength MS medium with 0.7% plant agar, pH 5.8, including vitamins. The culture medium added with 100 mM glucose was the treatment group, and no sugar added was the control group (CK). In addition, the culture medium added with 100 mM 3OMG was used as the osmotic control group (3OMG). About 100 seeds were sowed for each treatment. The seeds were cultured on plates at 4°C in the dark for 4 days. Then, the seeds were cultured at 22°C under continuous fluorescent light. After 5 days of growth, seedlings were harvested and soon frozen in the liquid nitrogen. Then, all samples were stored at –80°C for TA content measurement and gene expression analysis.
Statistical Analysis
Statistical data were calculated with software SPSS version 20.0 (IBM, NY, United States). All values were shown as the mean ± standard errors at least three replicates. Data differences in flower color, soluble sugar content, anthocyanin content, and gene expression levels of P. suffruticosa ‘Tai Yang’ cut flowers were calculated through Duncan’s multiple range test at 0.05 probability.
Results
The Flower Color Changes of Four Treatments at Different Developmental Stages in P. suffruticosa ‘Tai Yang’
As shown in Figures 1B,C, the flower color under the glucose treatment was more flamboyant with the naked eye, and the coloration of the middle petal under glucose treatment was much uniform than other treatments. The petal color changes at four developmental stages under different treatments are shown in Figure 2. The petal color of cut flowers was presented as lightness (L*), redness (a*), yellowness (b*), and chroma (C*). Compared with the CK, the L* value of the cut flowers was decreased by the glucose, 3OMG, and mannose treatments during the whole flower opening process, except for no difference of 3OMG treatment at S3. At the full opening stage (S5), L* values were reduced by 10, 8, and 5% under glucose, 3OMG, and mannose treatments, respectively, compared with the CK treatment (Figure 2A).
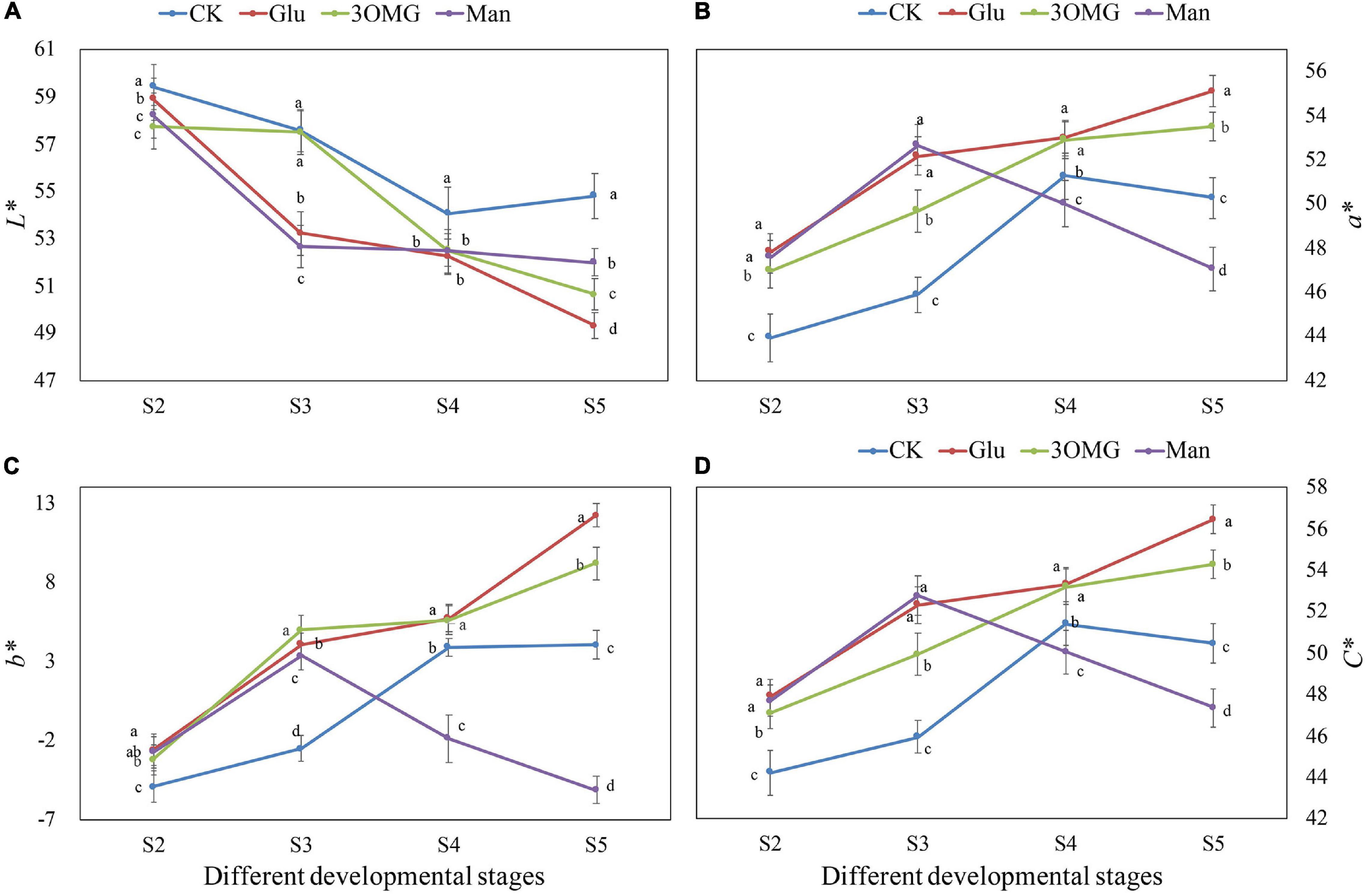
Figure 2. The flower color values of P. suffruticosa ‘Tai Yang’ cut flowers under four treatments. (A) Lightness (L*). (B) Redness (a*). (C) Yellowness (b*). (D) Chroma (C*). There were four treatments including CK, Glu, 3OMG, and Man, respectively. CK was the control group that cut flowers treated with distilled water. Glu, 3OMG, and Man were the cut flowers treated with glucose, 3-O-methylglucose, and mannose at 333 mM, respectively. S2, pre-opening stage; S3, initial opening stage; S4, half opening stage; S5, full opening stage. Each value was expressed as the mean ± standard errors (SE) of triplicate replications. Different small letters indicated significant differences among treatments at the same developmental stage according to Duncan’s multiple range test at p < 0.05.
The a* value changing trend of cut flowers under four treatments varied, a* value under CK and mannose treatments increased first, reaching the highest level at S4 and S3, respectively, and then declined (Figure 2B). In contrast, it remained to increase from S2 to S5 under glucose and 3OMG treatments. During the entire opening stages, the a* value of glucose and 3OMG treatment continued to be higher than CK. The a* value of mannose treatment was higher than that of the CK at the early stages (S2–S3) and then became lower than CK due to the continuous decline in the later stages (S4-S5). At the full opening stage (S5), the a* value of glucose treatment was 55.12, which was the highest among the four treatments, followed by 3OMG, CK, and mannose treatments with the values of 53.50, 50.28, and 47.07, respectively (Figure 2B). Besides, the b* and C* value changing trends were the same as a* values in four treatments (Figures 2C,D). At the full opening stage, the glucose treatment owned the highest value of b* among four treatments, followed by 3OMG, CK, and mannose (Figure 2C). Similarly, C* value of glucose treatment at S5 was the highest with the value of 56.47, which was 4, 12, and 19% higher than 3OMG, CK, and mannose, respectively (Figure 2D).
Total Soluble Sugar and Anthocyanin Contents of Flowers at Different Developmental Stages
The soluble sugar content in four treatments showed an upward trend with the opening of cut flowers (Figure 3A). In the CK flowers, soluble sugar content increased with the flowers opening, reaching a maximum value of 55.16 ug g–1 at S4, and then no longer increased. However, compared with the CK, the soluble sugar content of cut flowers under the glucose, 3OMG, and mannose treatments continued to increase from S2 to S5, showing greater increases than that of the CK at all corresponding stages (Figure 3A). At the early opening stages (S2–S3), the soluble sugar contents under the glucose and mannose treatments were about 1.80 times that of the CK treatment, which was higher than that of the 3OMG treatment. During the late opening stages (S4–S5), mannose’s enhancement on sugar content became weaker with no difference with 3OMG treatment. In contrast, sugar content under glucose treatment was still remained at the highest level among four treatments. At the full opening stage (S5), the soluble sugar contents under glucose, 3OMG, mannose, and CK were 113.46 > 90.21 > 88.99 > 54.84 ug g–1, respectively.
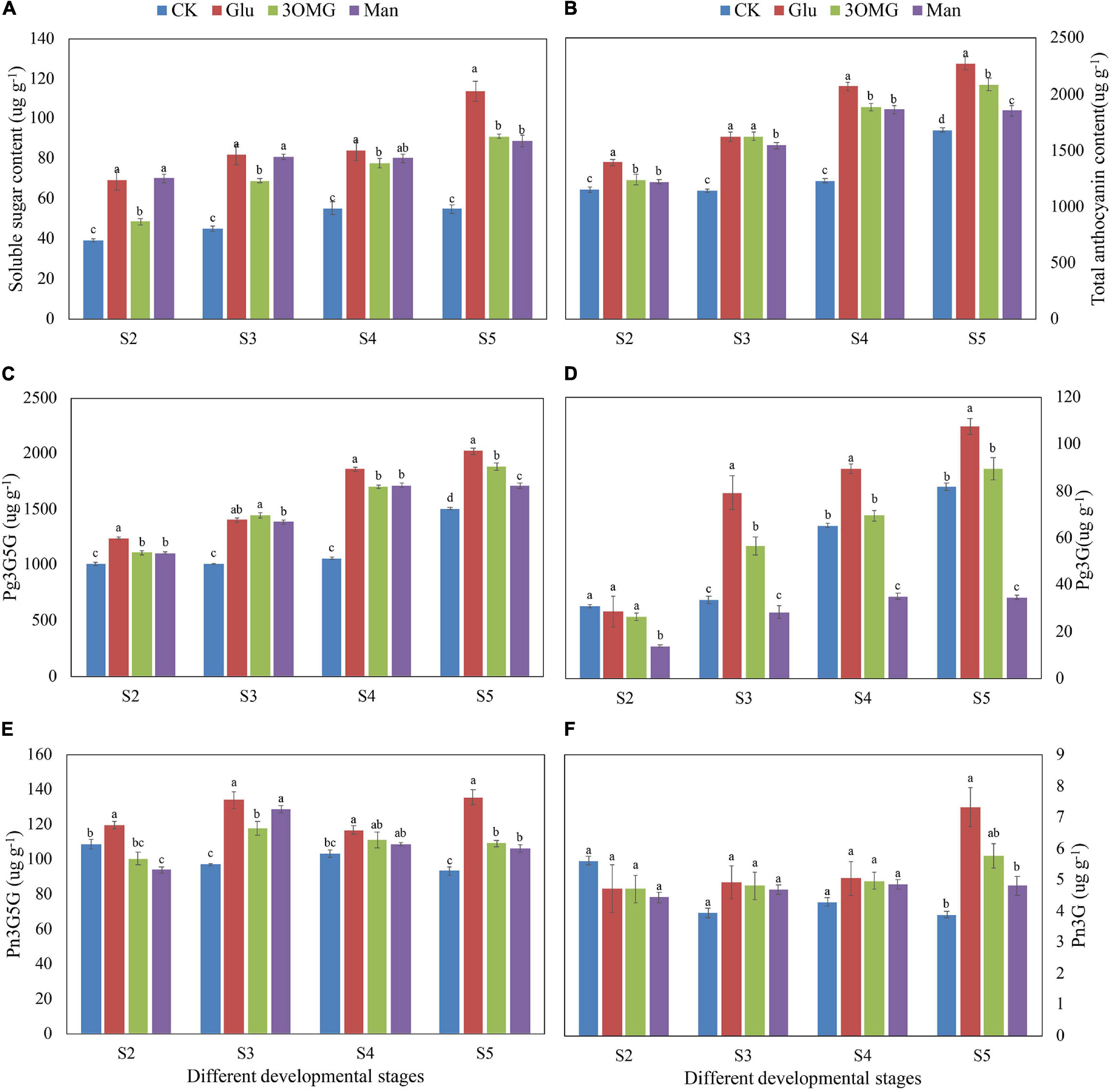
Figure 3. The soluble sugar contents in petals of P. suffruticosa ‘Tai Yang’ cut flowers under four treatments (A). The total anthocyanin contents in petals of P. suffruticosa ‘Tai Yang’ cut flowers under four treatments (B). The contents of four anthocyanin compositions in the petals of P. suffruticosa ‘Tai Yang’ cut flowers under four treatments (C–F). (C) Pelargonidin-3,5-di-O-glucoside (Pg3G5G); (D) pelargonidin 3-O-glucoside (PG3G); (E) peonidin-3,5-di-O-glucoside (Pn3G5G); (F) peonidin-3-O-glucoside (Pn3G). There were four treatments including CK, Glu, 3OMG, and Man, respectively. CK was the control group that cut flowers treated with distilled water. Glu, 3OMG and Man were the cut flowers treated with glucose, 3-O-methylglucose and mannose at 333 mM, respectively. S2, pre-opening stage; S3, initial opening stage; S4, half opening stage; S5, full opening stage. Each value was expressed as the mean ± standard errors (SE) of triplicate replications. Different small letters indicated significant differences among treatments at the same developmental stage according to Duncan’s multiple range test at p < 0.05.
The changes in TA content in petals under four treatments were similar to that of soluble sugar content, with a gradually increasing trend overall during the flowers opening (Figure 3B). Compared with the CK treatment, the TA content in petals showed greater increases under glucose, 3OMG, and mannose treatments at corresponding stages. Among four treatments, glucose treatment made the highest TA content, higher than 3OMG, mannose, and CK, except for S3 with no significance with 3OMG. In addition, TA content under 3OMG treatment was higher than that of mannose, but it did not reach significance in S2 and S4 stages. When the cut flowers opened to the best viewing period (S5), the TA content order of petals was glucose > 3OMG > Man > CK, with the content of 2,276.47, 2,087.29, 1,855.62, and 1,684.42 ug g–1, respectively.
Qualitative and Quantitative Analysis of Anthocyanin Changes at Different Developmental Stages in P. suffruticosa ‘Tai Yang’ Under Four Treatments
First, the anthocyanin compositions of P. suffruticosa ‘Tai Yang’ flowers were detected. According to HPLC data analysis, there were four anthocyanin compositions in the petals of the flowers at S5 that grew naturally in the field condition, including pelargonidin-3-O glucoside (Pg3G), pelargonidin-3,5-di-O-glucoside (Pg3G5G), peonidin-3-O-glucoside (Pn3G), and peonidin-3,5-di-O-glucoside (Pn3G5G). Among them, Pg3G5G had the highest proportion of 69%, followed by Pg3G, Pn3G5G, and Pn3G with the ratios of 25, 5, and 1%. The results are shown in Supplementary Table 2. During postharvest development, four anthocyanin compositions’ changes in cut flower petals under different treatments were as follows:
As the main anthocyanin composition of P. suffruticosa ‘Tai Yang’ flowers, in the CK flowers, with the opening of cut flowers, Pg3G5G content increased and reached the highest level at the full opening stage (S5) (Figure 3C). Compared with the CK treatment, glucose, 3OMG, and mannose treatments showed greater increases. But Pg3G5G content under the glucose treatment was much greater than other treatments at the corresponding stages, except for S3. At the full opening stage, Pg3G5G content was 2,025.72 ug g–1 in glucose, which was 1.35, 1.08, and 1.18 times that of the CK, 3OMG, and mannose treatments, respectively (Figure 3C).
In the CK flowers, Pg3G content showed a linear upward trend from S2 to S5, reaching the maximum at S5 with the value of 81.66 ug g–1, which was 50.97 ug g–1greater than that of the pre-opening stage (S2) (Figure 3D). Compared with the CK flowers, Pg3G content in glucose was greatly enhanced during the later developmental stages (S3–S5) (Figure 3D). When flowers fully opened (S5), Pg3G content reached the highest level with the value of 107.58 ug g–1, which was 1.32 times that of the control at S5 (Figure 3D). In the 3OMG treatment, Pg3G content from S3 to S5 was also higher than CK, but the difference was only significant at S3 (Figure 3D). Unlike glucose and 3OMG treatments, mannose inhibited the increase in Pg3G content, and Pg3G content was much lower than that of CK from S2 to S5, except for no difference at S3 (Figure 3D).
With the opening of cut flowers, Pn3G5G content was unstable in CK flowers, showing a descending trend overall from S2 to S5. At full opening stage (S5), Pn3G5G content of CK was 93.46 ug g–1, which was 15.45 ug g–1 less than that of the pre-opening stage (S2) (Figure 3E). Compared with the CK flowers, Pn3G5G content was increased by the glucose treatment, which was higher than CK from S2 to S5. Specifically, at S5, Pn3G5G content (135.85 ug g–1) under glucose treatment was 1.45 times that of the CK flowers (Figure 3E). In addition, 3OMG and mannose treatments also enhanced the accumulation of Pn3G5G but were both lower than that of the glucose treatment (Figure 3E).
The content of Pn3G was the least in P. suffruticosa ‘Tai Yang’ flowers. With the opening of flowers, Pn3G content in the CK decreased from S2 to S3 and then remained stable from S3 to S5 (Figure 3F). As Figure 3F shows, from S3 to S4, although the Pn3G contents of glucose, 3OMG, and mannose treatments were higher than that of the CK, they did not reach the statistical significance. However, at the full opening stage (S5), Pn3G content under the glucose treatment was the highest with the value of 7.32 ug g–1, which was higher than that of the mannose and CK treatments (Figure 3F).
Gene Expression Changes of Nine Structural Genes at Different Developmental Stages in P. suffruticosa ‘Tai Yang’ Under Four Treatments
The abundance of structural gene expression will directly affect the amount of anthocyanin biosynthesis and composition. Gene expression levels of nine structural genes at different developmental stages under four treatments are shown in Figure 4. Compared with the CK treatment, at S2 (Figure 4A), more than half of the genes, including PsCHS, PsF3H, PsDFR, PsAOMT, and PsUF3GT, were upregulated by glucose. However, 3OMG only showed an upregulation effect on PsDFR and PsAOMT. For mannose treatment, it showed a promotion effect on PsF3′H and PsDFR. Moreover, the PsF3′H expression level of mannose was higher than that of other treatments. With the development of flowers, at the initial opening stage (S3) (Figure 4B), glucose continued to upregulate the expression of PsF3H and PsDFR genes, and the expression of PsF3′H and PsUF5GT also began to be promoted by glucose. At this stage, the genes upregulated by 3OMG treatment were the same as glucose treatment, and the PsDFR expression level under 3OMG treatment was higher than glucose. Compared with the CK, although the mannose treatment showed inhibition on most genes at S3, it continued to favor the induction on the PsF3′H gene expression, which was higher than glucose and 3OMG, with about 0.82 and 0.60 times higher than theirs, respectively. The genes upregulated by glucose and 3OMG treatment were still the same at S4 (Figure 4C), including PsF3H, PsDFR, PsAOMT, and PsUF5GT. The PsUF5GT expression level under glucose treatment was higher than that of 3OMG, whereas PsDFR was the opposite. At the same stage, except for PsF3′H, the expression levels of the other eight structural genes were all inhibited by mannose treatment (Figure 4C). The full opening stage (S5) was the best viewing period for tree peony cut flowers, where the cut flowers were kept for the longest time. During this period, genes including PsF3H, PsF3′H, PsAOMT, and PsUF5GT showed high expression levels under glucose treatment (Figure 4D). Although several genes were promoted by 3OMG treatment at this stage, its regulation effect was not as good as glucose treatment overall. For example, the expression of PsF3′H and PsUF5GT under glucose treatment was about 1.52 and 1.48 times of 3OMG treatment, respectively (Figure 4D). For mannose treatment, the genes inhibited by mannose treatment reduced at S5. Furthermore, the expression levels of PsF3H, PsF3′H, and PsUF3GT were promoted by mannose (Figure 4D).
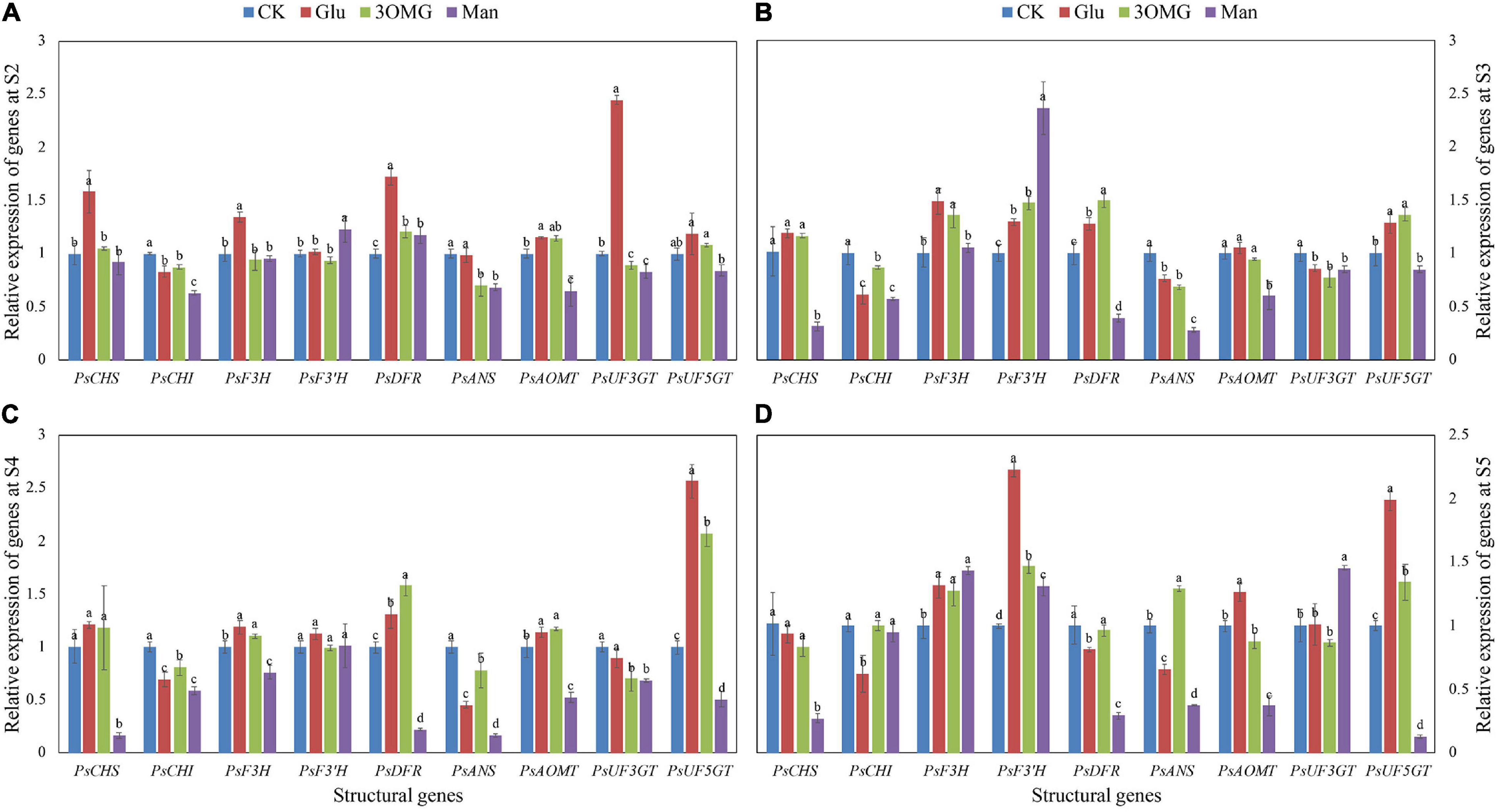
Figure 4. Relative expression levels of nine anthocyanin biosynthetic structural genes in petals of P. suffruticosa ‘Tai Yang’ cut flowers under four treatments. (A) S2, pre-opening stage; (B) S3, initial opening stage; (C) S4, half opening stage; (D) S5, full opening stage. There were four treatments including CK, Glu, 3OMG, and Man, respectively. CK was the control group that cut flowers treated with distilled water. Glu, 3OMG, and Man were the cut flowers treated with glucose, 3-O-methylglucose, and mannose at 333 mM, respectively. Each value was expressed as the mean ± standard errors (SE) of triplicate replications. Different small letters indicated significant differences among treatments at the same developmental stage according to Duncan’s multiple range test at p < 0.05.
Gene Expression Changes of Seven Regulatory Genes at Different Developmental Stages in P. suffruticosa ‘Tai Yang’ Under Four Treatments
The expression of structural genes during anthocyanin biosynthesis is directly controlled by regulatory genes. In this study, seven regulatory genes, including three MYB TFs, two bHLH TFs, and two WD40 TFs, were monitored during postharvest development of flowers. Compared with CK treatment, except for PsMYB57 and PsMYB114L, all genes, including PsMYB2, PsbHLH1, PsbHLH3, PsWD40-1, and PsWD40-2, continuously showed high expression levels under glucose treatment from S2 to S5 (Figure 5). Specifically, in PsMYB2, PsbHLH1, and PsbHLH3, their expression levels at the corresponding stages were 1.30–2.40 times that of the CK. Among these five genes continuously induced by glucose, PsWD40-1 and PsWD40-2 showed a sustained high expression state under 3OMG treatment (Figure 5). Additionally, the PsWD40-2 expression level under 3OMG was greater than glucose at late opening stages (S4–S5) (Figures 5C,D). Although the gene expression levels of PsbHLH1 at S3 and PsbHLH3 at S5 under 3OMG treatment had no difference with CK treatment, they were higher than that of CK treatment at the other three stages (Figure 5). The expression level of PsbHLH1 at S2 was especial, which was 2.18 times that of the CK treatment and higher than that of glucose treatment (Figure 5A). Besides, the expression level of PsbHLH3 gene was lower than that of glucose in all stages (Figure 5). The expression levels of these five regulatory genes under mannose were lower than those of glucose and 3OMG treatments overall. Still, the genes including PsMYB2, PsbHLH1, and PsWD40-2 were upregulated by mannose treatment in the most stages (at least 3 stages), compared to CK treatment (Figure 5). For example, except for no difference with the CK treatment at the pre-opening stage (S2), PsMYB2 continued to be highly expressed from S3 to S5 under mannose treatment (Figures 5B–D), and especially at the S5, its expression level was higher than that of glucose and 3OMG treatments with 0.75 times higher than that of the CK (Figure 5D). It seemed that glucose treatment had a weaker promotion effect on PsMYB57 and PsMYB114L. Compared with the CK, PsMYB57 was only highly expressed at the S4 to S5 stages under glucose treatment, and the expression level of PsMYB114L was inhibited by glucose treatment at S3 to S4 stages (Figures 5B,C). Although 3OMG treatment also inhibited PsMYB114L expression level at late opening stages (S3–S5), PsMYB57 expression was enhanced by 3OMG treatment, which was 2.01, 2.19, and 1.45 times that of the CK at S3, S4, and S5, respectively. In addition, although the expression level of PsMYB114L was continuously downregulated by mannose treatment throughout four opening stages (S2–S5) (Figure 5), PsMYB57 was highly expressed under mannose treatment during the middle opening stages (S3–S4) (Figures 5B,C).
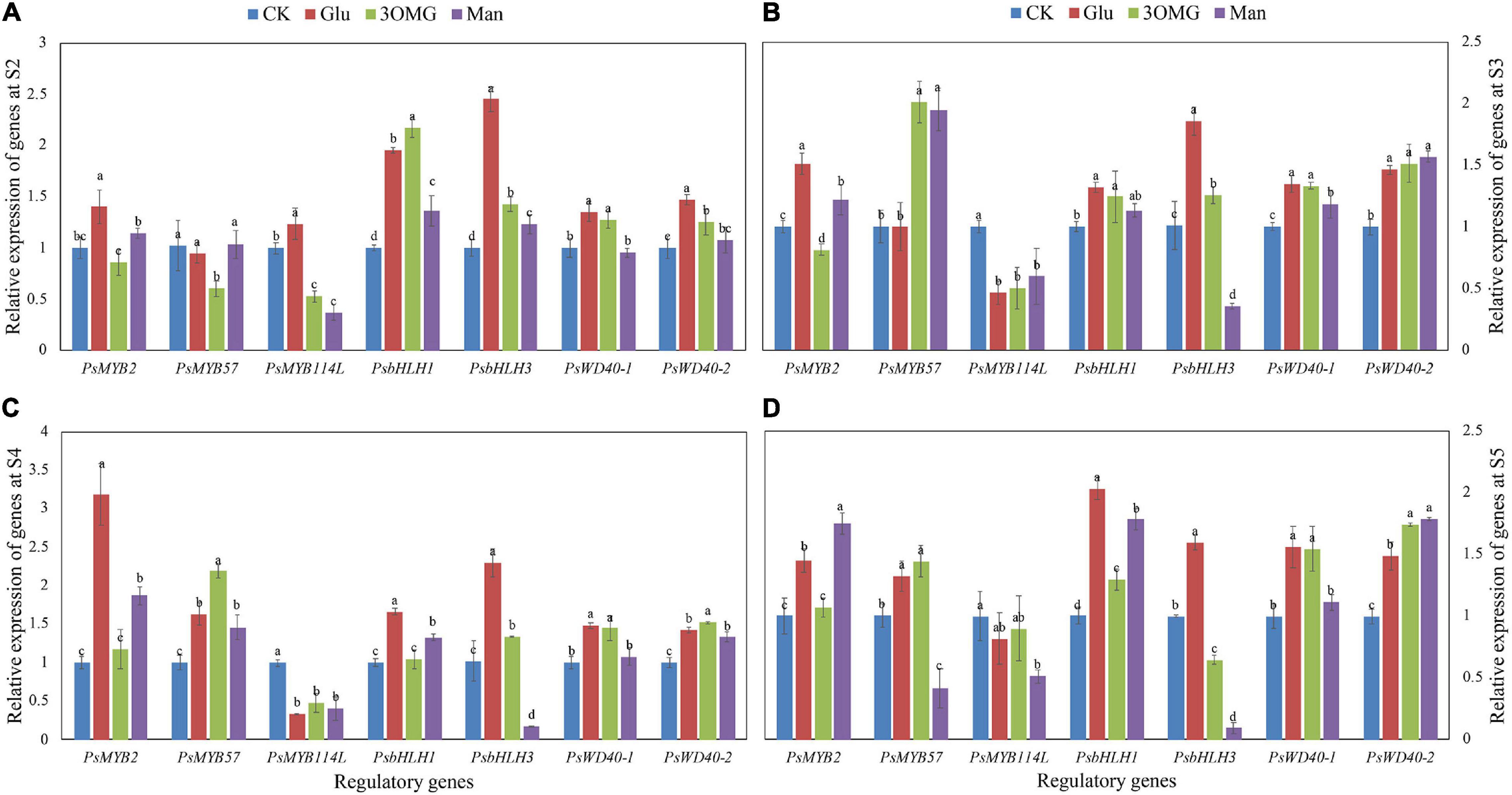
Figure 5. Relative expression levels of seven anthocyanin biosynthetic regulatory genes in petals of P. suffruticosa ‘Tai Yang’ cut flowers under four treatments. (A) S2, pre-opening stage; (B) S3, initial opening stage; (C) S4, half opening stage; (D) S5, full opening stage. There were four treatments including CK, Glu, 3OMG, and Man, respectively. CK was the control group that cut flowers treated with distilled water. Glu, 3OMG, and Man were the cut flowers treated with glucose, 3-O-methylglucose, and mannose at 333 mM, respectively. Each value was expressed as the mean ± standard errors (SE) of triplicate replications. Different small letters indicated significant differences among treatments at the same developmental stage according to Duncan’s multiple range test at p < 0.05.
Through the expression analysis of regulatory genes under four treatments, both mannose and glucose showed the promotion effects on PsMYB2, PsbHLH1, and PsWD40-2. Among these three genes, PsbHLH1 and PsWD40-2 were also upregulated by 3OMG treatment, whereas only PsMYB2 was hardly induced by 3OMG treatment (Figure 5). Thus, we speculated that PsMYB2 might be an essential transcription factor in glucose inducing anthocyanin biosynthesis of P. suffruticosa ‘Tai Yang’ cut flowers through the HXK-dependent signaling pathway. Therefore, we conducted further research on PsMYB2.
Sequence and Subcellular Localization Analysis of PsMYB2
First, the full-length cDNA sequence of PsMYB2, encoding an R2R3-MYB protein, was obtained by PCR from a tree peony cDNA library of P. suffruticosa ‘Tai Yang.’ The sequencing result indicated that it had a 918 bp ORF encoding a protein of 305 amino acids. Multiple sequence alignment of amino acids showed that it contained a conserved R2R3 DNA-binding domain at the N-terminus and a bHLH interaction motif ([D/E]Lx2[R/K]x3Lx6Lx3R) in the R3 domain (Figure 6A). In addition, two specific motifs were found in the C-terminal variable region of PsMYB2: Motif 2 and Motif 3 (Figure 6A). Motif 2 was called C1 (Lx3GIDPxTHKPL), found in the proteins belonging to the MYB subfamily 4, and initially characterized by Kranz et al. (1998). Another one was Motif 3 and temporarily named C3 (DDxF[S/P] SFL[N/D]SLIN[E/D]). This conserved motif was only found in a few MYB protein sequences gathered to the VvMYB5a/b cluster. Besides, Gly and DNEI (Asp–Asn–Glu1–Ile), in the R2 and R3, respectively, were found in PsMYB2 protein, which was related to the biosynthesis of anthocyanin and proanthocyanidin (Heppel et al., 2013). Finally, no C2 motif was found in the PsMYB2 protein (Jin et al., 2000).
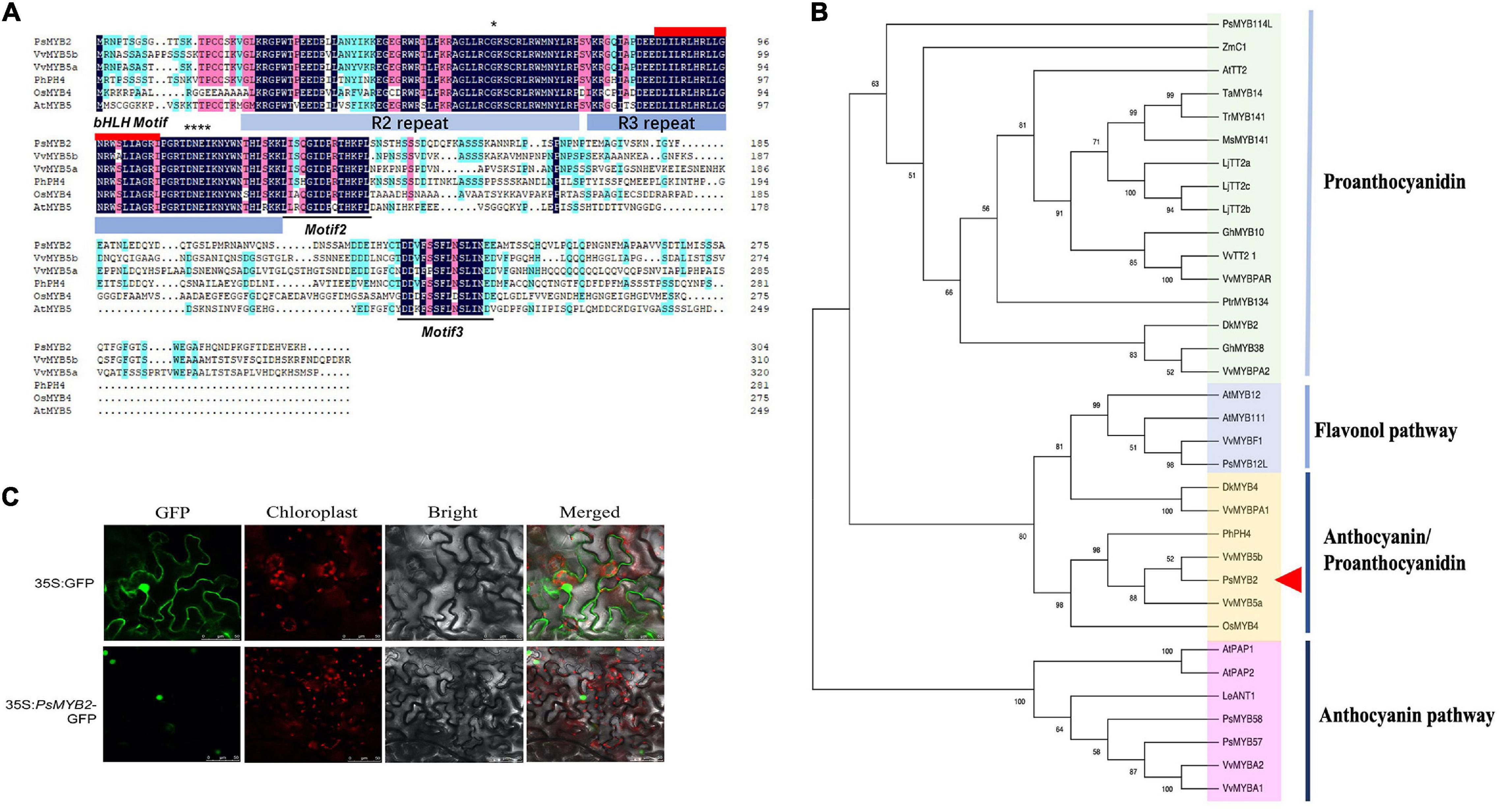
Figure 6. Sequence analyses of PsMYB2. (A) Protein sequence alignment analysis of the PsMYB2. Sequence alignment was performed using the DNAMAN software of version 6. GenBank accession numbers of these MYB proteins were as follows: VvMYB5b, Vitis vinifera, AAX51291.1; VvMYB5a, Vitis vinifera, AAS68190.1; PhPH4, Petunia hybrida AAY51377.1; OsMYB4, Oryza sativa, BAA23340.1; AtMYB5, Arabidopsis thaliana, NP_187963.1. (B) Phylogenetic analysis of PsMYB2 with MYB transcription factors from other species. The phylogenetic tree was constructed with MEGA11 software through neighbor-joining method and the tree nodes were evaluated with 1000 bootstrap replicates. GenBank accession numbers of the MYB proteins were as follows: ZmC1, Zea mays, AAA33482.1; AtTT2, Arabidopsis thaliana, Q9FJA2.1; LjTT2c, Lotus japonicus, BAG12895.1; LjTT2b, Lotus japonicus, BAG12894.2; LjTT2a, Lotus japonicus, BAG12893.1; TaMYB14, Trifolium arvense, AFJ53053.1; TrMYB141, Trifolium repens, AFJ53048.1; MsMYB141, Medicago sativa, AFJ53055.1; GhMYB10, Gossypium hirsutum, NP_001314513.1; PtrMYB134, Populus trichocarpa, XP_002308528.1; VvTT2_1, Vitis vinifera, RVW44110.1; VvMYBPAR, Vitis vinifera, BAP39802.1; DkMYB2, Diospyros kaki, BAI49719.1; GhMYB38, Gossypium hirsutum, AAK19618.1; VvMYBPA2, Vitis vinifera, ACK56131.1; AtMYB12, Arabidopsis thaliana, NP_182268.1; AtMYB111, Arabidopsis thaliana, NP_199744.1; VvMYBF1, Vitis vinifera, ACT88298.1; PsMYB12L, Paeonia suffruticosa, QBK15080.1; DkMYB4, Diospyros kaki, BAI49721.1; VvMYBPA1, Vitis vinifera, CAJ90831.1; PhPH4, Petunia hybrida, AAY51377.1; VvMYB5b, Vitis vinifera, AAX51291.1; VvMYB5a, Vitis vinifera, AAS68190.1; OsMYB4, Oryza sativa, BAA23340.1; PsMYB58, Paeonia suffruticosa, QZJ84669.1; AtPAP1, Arabidopsis thaliana, AAG42001.1; AtPAP2, Arabidopsis thaliana, NP_176813.1; SlANT1, Solanum lycopersicum, AAQ55181.1; PsMYB57, Paeonia suffruticosa, QIG55740.1; VvMYBA2, Vitis vinifera, BAD18978.1; VvMYBA1, Vitis vinifera, BAD18977.1. (C) The subcellular localization of PsMYB2. 35S: GFP, control group; 35S: PsMYB2-GFP, PBI121-GFP-PsMYB2.
To better understand the feature of PsMYB2, R2R3-MYBs associated with different functions were selected for phylogenetic analysis with the neighbor-joining method. The result showed that the R2R3-MYBs were robustly separated into 4 sub-branches with more than 60% bootstrap percentages (Figure 6B). Among 34 R2R3-MYBs, PsMYB2 was gathered into VvMYB5a/b cluster with 98% bootstrap percentages of this cluster, indicating that the VvMYB5a/b sub-branch was very stable. Based on the different roles in the flavonoid biosynthetic pathway, they were named as flavonol, anthocyanin, anthocyanin/proanthocyanidin, and proanthocyanidin, respectively (Figure 6B). PsMYB2 was grouped into the clade of anthocyanin/proanthocyanidin and closed to the VvMYB5a and VvMYB5b, which were positively associated with anthocyanin and proanthocyanidin production (Deluc et al., 2006, 2008). Sequence similarity showed that the PsMYB2 protein sequence displayed 55 and 50% identical to VvMYB5b and VvMYB5a, respectively.
Furthermore, the subcellular localization of PsMYB2 was also detected. Both recombinant vector PBI121-GFP-PsMYB2 and the control vector (PBI121-GFP) were transiently expressed into the Nicotiana benthamiana leaves. The results showed that GFP fluorescence was observed in the nucleus and cytomembrane in the control group, whereas the group with the PBI121-GFP-PsMYB2 vector only showed fluorescence signals in the nucleus (Figure 6C). Thus, we speculated that the PsMYB2 transcription factor was localized and functioned in the nucleus.
Overexpression of PsMYB2 in Tobacco Promotes the Anthocyanin Accumulation of Tobacco Flowers
To verify its function on anthocyanin biosynthesis, we constructed a PsMYB2 overexpression vector and stably transformed it into tobacco. As shown in Figure 7A, the darker flower petals were visually observed in tobacco line 5 and 6 overexpressing PsMYB2 with the naked eye. Moreover, intense pigmentation was observed on the stamens of all transgenic tobacco flowers (lines 3, 5, and 6). The anthocyanin accumulation in the petal and stamen of lines 5 and 6 was much greater than that of the wild type. Although there was no difference in the anthocyanin content in the petals between line 3 transgenic tobacco and the wild type, the anthocyanin content in the stamen of the line 3 transgenic tobacco was significantly higher than that of the wild type, which was about 5 times that of the wild type.
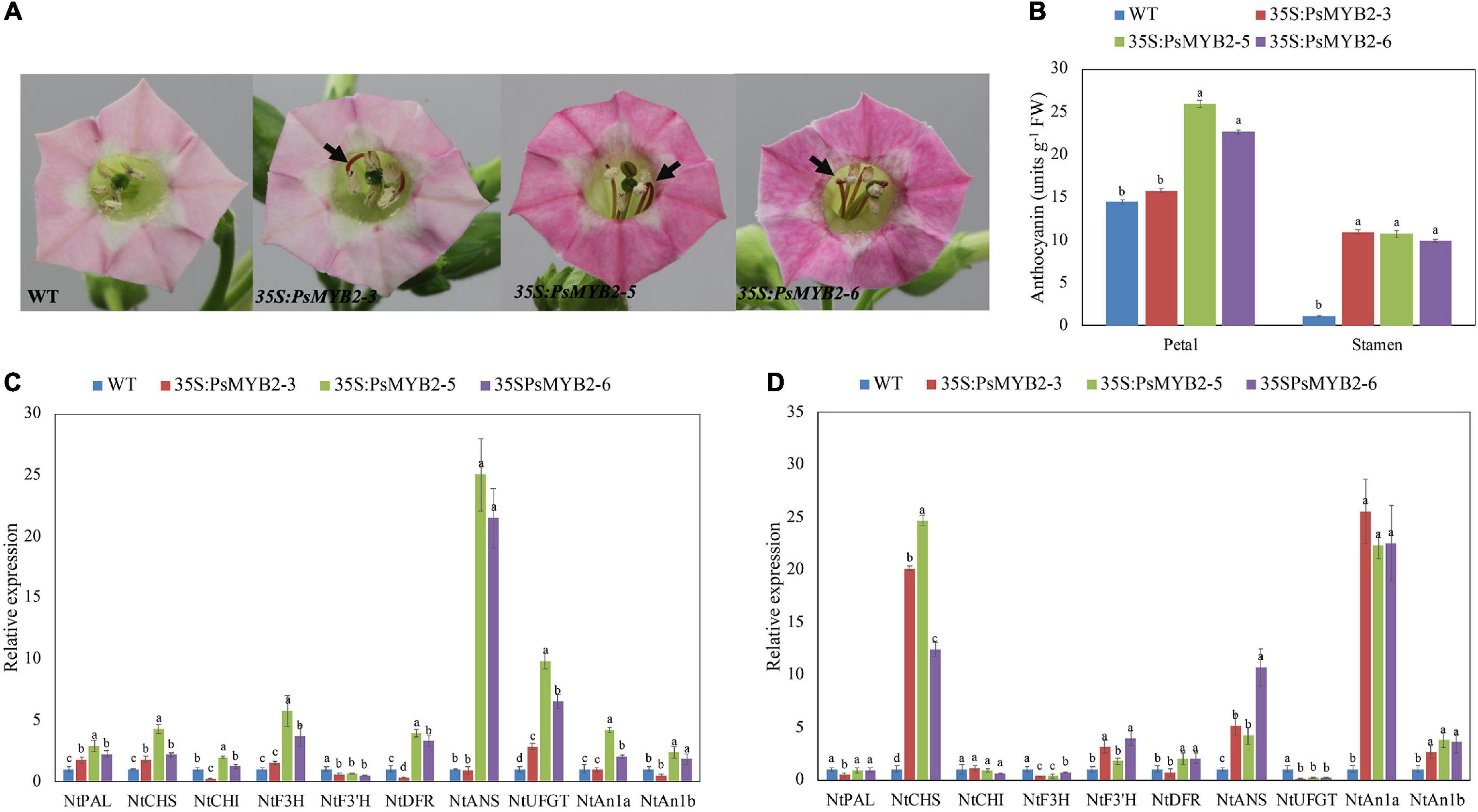
Figure 7. Overexpression of PsMYB2 in tobacco. (A) Overexpression of PsMYB2 increased the anthocyanin accumulation in tobacco flowers. Flowers of transgenic tobacco showed increased pigmentation in petal and stamen, compared to wild-type flowers. (B) The TA content in the petal and stamen of the transgenic flowers was higher than that of the wild type. (C,D) Were the gene expression levels related to anthocyanin biosynthesis in the petal and stamen of tobacco flowers, respectively. Each value was expressed as the mean ± standard errors (SE) of triplicate replications. Different small letters indicated significant differences among PsMYB2 transgenic lines and wild-type plants according to Duncan’s multiple range test at p < 0.05. WT, wild-type plant; 35S:PsMYB2 (3, 5, and 6), plant overexpressing PsMYB2.
As higher pigmentation in the petal and stamen of transgenic tobacco flowers, we extracted RNA of the transgenic and wild-type plants for further gene expression analysis. As shown in Figure 7C, except for line 3, the transcription levels of multiple anthocyanin biosynthetic genes were upregulated in flower petals. The expression levels of NtCHS, NtF3H, NtANS, and NtUFGT in line 5 were 3.30 times higher than those of the wild type. In stamens (Figure 7D), NtCHS and NtANS were upregulated in all transgenic tobacco lines. Particularly, NtCHS gene expression level in lines 3, 5, and 6 were 19.65, 24.08, and 12.10 times that of wild type, respectively. Furthermore, except for the petal of line 3, the two bHLH regulatory genes, NtAn1a and NtAn1b, involved in the regulation of tobacco anthocyanin production were all greatly upregulated in the PsMYB2 transgenic petal and stamen (Figures 7C,D). Gene expression level of NtAn1a in stamens of lines 3, 5, and 6 was 23.83, 20.69, and 21.91 times higher than that of the wild type, respectively (Figure 7D).
PsMYB2 Induced Anthocyanin Accumulation of Transgenic Arabidopsis Seedlings in Response to Glucose Treatment
A total of two independent T3 homozygous of PsMYB2 transgenic Arabidopsis were used to study the role of PsMYB2 under glucose treatment in transgenic Arabidopsis seedlings. In the experiment, 3OMG was introduced as an osmotic control, and no glucose added was the control treatment (CK). As shown in Figure 8B, there were no differences in anthocyanin content between 35S:PsMYB2 lines and wild-type seedlings in CK treatment. The anthocyanin accumulations were increased by 3OMG and glucose treatments, but the TA content under glucose treatment increased the most (Figure 8B). Under the osmotic control of 3OMG, the anthocyanin content of the 35S:PsMYB2 lines 3 and 10 was 5.97 and 6.09 units g–1, respectively, which had no difference with the wild-type plants. Compared to the wild type, the TA content of 35S:PsMYB2 transgenic lines 3 and 10 under glucose treatment was 9.66 and 8.71 units g–1, respectively, which were higher than that of wild-type seedlings (Figure 8B), and the anthocyanin extraction solutions of 35S:PsMYB2 lines were much darker than the wild type (Figure 8A).
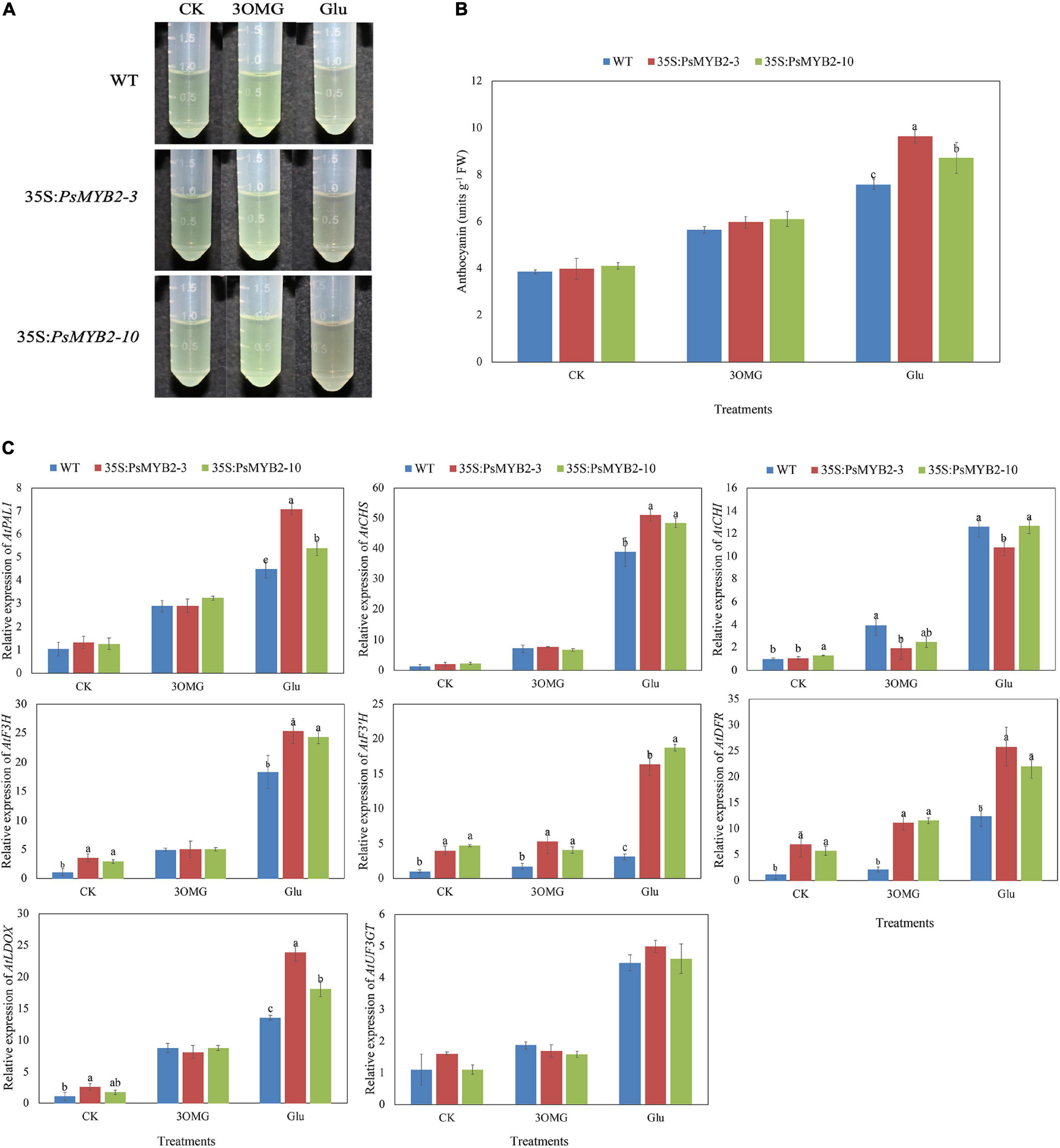
Figure 8. Overexpression of PsMYB2 increased the ability of glucose-induced anthocyanin accumulation in Arabidopsis. (A) Anthocyanin extraction solutions for Arabidopsis seedlings under three treatments. (B) Total anthocyanin content in Arabidopsis seedlings under three treatments. (C) The relative expression levels of anthocyanin biosynthetic pathway genes in Arabidopsis under three treatments. Different small letters indicated significant differences among PsMYB2 transgenic lines and wild-type plants according to Duncan’s multiple range test at p < 0.05. WT, wild-type plant; 35S:PsMYB2 (3 and 10), plant overexpressing PsMYB2.
Comparison of 35S:PsMYB2 lines with wild-type plants showed that the overexpression of PsMYB2 enhanced the anthocyanin accumulation of seedlings treated with glucose. We further extracted RNA of samples under different treatments and detected the gene expression changes in 35S:PsMYB2 lines and wild-type seedlings (Figure 8C). In the CK treatment with no sugar added, genes including AtF3H, AtF3′H, and AtDFR were highly expressed in the 35S:PsMYB2 lines compared with the wild type. For 3OMG treatment, the expression levels of most structural genes in both transgenic and wild-type Arabidopsis were upregulated. However, the increase in expression levels of several genes in PsMYB2 transgenic Arabidopsis was lower than that of wild type (Figure 8C). Under 3OMG treatment, only gene expression levels of AtF3′H and AtDFR in 35S:PsMYB2 lines were higher than that of the wild type. Different with 3OMG treatment, although the expression levels of all structural genes in both wild type and 35S:PsMYB2 lines were increased under glucose treatment, the genes including AtPAL1, AtCHS, AtF3H, AtF3′H, AtDFR, and AtLDOX in PsMYB2 transgenic Arabidopsis were higher than those of the wild type. Particularly, the gene expression level of AtF3′H in lines 3 and 10 under glucose treatment was 5.25 and 6.02 times that of wild-type Arabidopsis, respectively (Figure 8C).
Discussion
Glucose Supply Promote Anthocyanin Accumulation in P. suffruticosa ‘Tai Yang’ Cut Flower
Cases of exogenous sugar supply enhancing the coloration of detached tissues or organs, including flowers, fruits, and roots, have been found in many plants, such as oriental lily (Han, 2003), phlox (Sankhla et al., 2005), strawberry (Fragaria ananassa) (Li et al., 2019), and radish (Hara et al., 2003). Similarly, in this study, glucose supply greatly enhanced the pigmentation of P. suffruticosa ‘Tai Yang’ cut flowers during postharvest development. At the best viewing period (S5), the flowers treated with glucose were more flamboyant, with uniform coloration of the middle petals (Figures 1B,C), matching with the color values of higher redness (a*), yellowness (b*), chroma (C*), and lower lightness (L*) (Figure 2). With the opening of cut flowers, the accumulations of anthocyanin and soluble sugar content in flower petals all increased (Figures 3A,B). Anthocyanin production occurring simultaneously with the accumulation of sugars was also found in radish hypocotyls (Hara et al., 2003), Begonia semperflorens (Zhang et al., 2013), apricot (Prunus armeniaca) (Huang et al., 2019), and grape berries (Yu et al., 2020). Sugars that have a special promotion effect on specific anthocyanin compositions are found in many studies. For example, during the storage, sucrose treatment specifically promoted pelargonidin derivatives synthesis in strawberry fruit (Li et al., 2019). In pigmented cells of Vitis vinifera suspension cultures, peonidin 3-glucoside was enhanced by higher sucrose levels (Bao Do and Cormier, 1991). Different from the previous studies, glucose had a wide range of promotion effects on anthocyanin compositions including Pg3G5G, Pg3G, and Pn3G5G in this study (Figures 3C–E).
Sugars not only serve as energy and structural materials in anthocyanin biosynthesis but also promote its accumulation through signal pathways (Rolland et al., 2002; Li et al., 2019), and glucose as a signal molecule to regulate anthocyanin production has been studied in many plants (Hara et al., 2003; Solfanelli et al., 2006; Zheng et al., 2009). The research showed that the phosphorylation process of HXK was considered an important sensing and transduction process of sugar regulation pathways involved in glucose signal (Jang et al., 1997). Thus, the glucose analog 3OMG, which can be transported inside the cell but not substrates for HXK (Colombo et al., 1978), is often introduced to distinguish glucose signal regulation. In this study, 3OMG treatment had a continuous promotion effect on anthocyanin accumulation from S2 to S5, including the compositions of Pg3G5G and Pn3G5G (Figures 3C,E), which was different from previous studies that 3OMG treatment hardly enhanced anthocyanin accumulation (Hara et al., 2003; Solfanelli et al., 2006; Zhang C. et al., 2015). The sugars in vase solution (metabolized sugar and non-metabolized sugar) could improve the water absorption of cut flowers and increase the osmotic concentration of petals, therefore maintaining the cut flowers at a good water balance condition (Ho and Nichols, 1977; Pun and Ichimura, 2003). 3OMG in this study might work as osmotic pressure and increase the water absorption of flower branches, so that the anthocyanin accumulations were improved. According to the research of Moalem-Beno et al. (1997), 3OMG also slightly promoted the anthocyanin content in the petunia corolla. Although 3OMG treatment had anthocyanin promotion effect on P. suffruticosa ‘Tai Yang’ cut flower, the color and anthocyanin content of flower petals under 3OMG treatment were lower than those of glucose (Figures 1B,C, 3), which suggested that glucose promoted anthocyanin production was not due to osmotic regulation.
Mannose is a kind of poorly metabolized glucose analog but can be phosphorylated by hexokinases to mannose-6P, modulating some sugar-regulatory processes (Loreti, 2001). Like the previous study of Zhang C. et al. (2015), the middle part of the petals showed an abnormal coloration under mannose treatment (Figure 1C), which might be related to the toxic effect of mannose or mannose-6P (Loreti, 2001). However, the TA content overall was increased by mannose treatment with higher accumulations of Pg3G5G and Pn3G5G (Figures 3B,C,E), which was different from the result that mannose hardly influenced the accumulation of anthocyanin in radish hypocotyls (Hara et al., 2003). In grape berry cells (Vitrac et al., 2000) and petunia flowers (Neta-Sharir et al., 2000), mannose could function like glucose and sucrose to regulate some sugar-regulatory processes at low concentrations and induce anthocyanin biosynthesis.
Several Structural Genes Involved in Anthocyanin Biosynthesis Were Induced by Glucose Treatment in P. suffruticosa ‘Tai Yang’ Cut Flower During Postharvest Development
Studies showed that sugar-induced anthocyanin accumulation was through the upregulation of genes related to the anthocyanin biosynthetic pathway (Rolland et al., 2002; Van den Ende and El-Esawe, 2014; Abdullah et al., 2018). During the postharvest storage of strawberries, sucrose promoted anthocyanin production accompanied by higher CHS activity in fruit (Li et al., 2019). In broccoli sprouts, the gene expression levels of CHI, F3H, DFR, LDOX, and GST were promoted by sucrose treatment with the anthocyanin content increased (Guo et al., 2011). Similarly, several structural genes, including PsF3H, PsF3′H, PsDFR, PsAOMT, and PsUF5GT, were upregulated under glucose treatments (Figure 4), which was in accordance with the anthocyanin enhancement. In grape berry, the expression level of F3H was transiently induced from 2 to 4 h by different sugars, including glucose 100 mM, fructose 100 mM, and sucrose 150 mM (Zheng et al., 2009). During the treatment, PsF3H showed a continuous responding behavior to glucose treatment from S2 to S5 (Figure 4). It might be due to the stronger promotion effect of glucose in P. suffruticosa, like the previous study in Zhang C. et al. (2015). It is known that PsF3′H is a crucial gene for cyanidin-derived anthocyanin biosynthesis (Shi et al., 2015), and PsAOMT is responsible for converting cyanidin into peonidin (Du et al., 2015). Thus, these two genes are the nodes for the production of Pn3G5G and Pn3G in P. suffruticosa ‘Tai Yang.’ Under glucose treatment, PsAOMT kept higher expression levels from S3 to S5 and PsF3′H showed a much higher expression level at the S5 (Figure 4). We believed that the increased expression levels of PsAOMT and PsF3′H were directly related to the higher contents of Pn3G5G and Pn3G at the full opening stage in glucose-treated flowers (Figures 3E,F). Similarly, the previous study in P. suffruticosa ‘Luoyang Hong,’ a cyanidin-based purple-red color flower, found that the expression level of PsF3′H was increased by glucose treatment, showing a positive correlation with TA production (Zhang C. et al., 2015). PsUF5GT is a gene functioning in the biosynthesis of 3G5G-type anthocyanin in tree peony (Shu et al., 2008). We also observed the accordance between the expression level of PsUF5GT and the accumulations of Pg3G5G and Pn3G5G in glucose-treated flowers from S3 to S5 (Figures 3C,E, 4), suggesting that PsUF5GT could be a key gene in the glucose-induced anthocyanin accumulation in P. suffruticosa ‘Tai Yang.’ UFGT that played an essential role in the sugar-induced anthocyanin accumulation was also found in grape berries through genome-wide transcriptome analysis (Dai et al., 2013).
According to the previous study in petunia, 3OMG applied with GA3 slightly promoting CHS expression was considered to partly benefit from the modifications in the osmotic potential of the cells (Moalem-Beno et al., 1997). In this research, among the five structural genes upregulated by glucose treatments, we found that only PsDFR showed greater sensitivity to osmotic pressure (Figure 4). In contrast, the gene expression levels of PsF3H, PsF3′H, PsAOMT, and PsUF5GT under glucose treatment were much better than that of osmotic treatment overall (Figure 4), indicating that these genes were mainly regulated by glucose signal rather than osmotic control. PsF3′H was the only gene continuously promoted by mannose treatment from S2 to S5 (except for S4 with no significance) (Figure 4). Mannose, simulating sugars, enhanced the anthocyanin accumulation through the hexokinase-dependent (HXK-dependent) pathway with the upregulation of F3H expression in grape (Zheng et al., 2009). In this study, anthocyanin enhancement of Pn3G5G might be related to the upregulation of PsF3′H by mannose via the HXK-dependent pathway.
Glucose Treatment Showed a Stronger Promotion Effect on Regulatory Genes and PsMYB2 Might Be a Crucial Regulatory Gene in Response to Glucose Signal
Anthocyanin regulatory genes are the important mediators that lead to the initiation of anthocyanin biosynthesis in response to various developmental and environmental regulations (Dooner et al., 1991), as well as an important role in sugar-induced anthocyanin production (Teng et al., 2005; Dai et al., 2013; Ai et al., 2016). In Arabidopsis, sucrose specifically induced the anthocyanin biosynthesis, and the expression level of AtMYB75 was upregulated by several 100-folds (Solfanelli et al., 2006). However, the Arabidopsis, whose AtMYB75 protein function was almost lost due to mutation, hardly responded to sucrose with less anthocyanin accumulation in seedlings, suggesting an indispensable role of AtMYB75 in sucrose-induced anthocyanin accumulation (Solfanelli et al., 2006). High glucose medium enhanced the anthocyanin level in grape berry, and genome-wide transcriptome analysis suggested that the regulatory genes played a curial role in this process (Dai et al., 2013). In our study, we believed that TFs including PsMYB2, PsbHLH1, PsbHLH3, PsWD40-1, and PsWD40-2 were the important components in glucose-induced anthocyanin biosynthesis of P. suffruticosa ‘Tai Yang’ cut flowers since they kept higher expression levels throughout the flower opening process (S2–S5) (Figure 5). In addition, not all regulatory genes responded to glucose treatment. PsMYB57, which had been identified to be a positive regulator of anthocyanin biosynthesis in tree peony (Zhang et al., 2020), was highly expressed under 3OMG treatment rather than glucose treatment (Figure 5), indicating that PsMYB57 might play important role in osmotic regulation. Besides, we suggested that PsMYB114L might play a weaker role in postharvest anthocyanin biosynthesis, since it was not highly expressed under glucose, 3OMG, or mannose treatments (Figure 5).
Among five TFs promoted by glucose, we found that only PsMYB2 was highly expressed under mannose but less responsive to 3OMG treatment (Figure 5), which indicated that PsMYB2 was likely to be an important transcription factor for glucose-induced anthocyanin biosynthesis through signal pathway. In the previous study of Zhang C. et al. (2015), the regulatory gene PsMYB2 was also upregulated by glucose and mannose treatments but hardly promoted by 3OMG during the whole flower opening process. Therefore, we conducted further research on PsMYB2.
PsMYB2 Is a Positive Transcription Factor in Anthocyanin Biosynthesis
Flower color is an important economic trait for P. suffruticosa, and high color quality during the vase period tents to attract more people to purchase it. Studies show that R2R3-MYBs TFs play the essential roles in regulating anthocyanin biosynthesis (Koes et al., 2005). According to the gene expression analysis among four treatments, we suspected that PsMYB2 might be a vital transcription factor that promoted the biosynthesis of anthocyanin in response to glucose signal. Since the function of PsMYB2 had not been studied by others, we first examined its role in anthocyanin biosynthesis. Phylogenetic analyses indicated that the PsMYB2 protein was different from MYB TFs identified in tree peony recently, which participated in the regulation of anthocyanin biosynthesis, such as PsMYB57 (Zhang et al., 2020), PsMYB58 (Zhang et al., 2021), and PsMYB114L (Zhang et al., 2019). It belonged to a small cluster including VvMYB5a/b-like TFs (Figure 6B). Furthermore, sequence alignment found that PsMYB2 not only had R2R3 MYB domain, bHLH interaction motif, and C1 motif (Figure 6A) but also contained a C3 motif that was specifically identified in the members of the VvMYB5a/b cluster (Yan et al., 2021), indicating a similar function that PsMYB2 may have.
The overexpression of PsMYB2 in tobacco showed similar phenotypic changes to the studies of VvMYB5a/b-like genes overexpressing in tobaccos. Anthocyanin accumulation in petal and stamen was enhanced in PsMYB2 transgenic tobacco accompanied with higher anthocyanin content (Figures 7A,B), just like tobaccos overexpressing with VvMYB5a (Deluc et al., 2006), VvMYB5b (Deluc et al., 2006), EsMYB9 (Huang et al., 2013), or FhMYB5 (Li et al., 2018). Further gene expression analysis explained the increased anthocyanin accumulation in PsMYB2 transgenic tobacco. In petals, most of the anthocyanin biosynthetic genes were upregulated (Figure 7C). Although genes upregulated in stamens were less, the expression levels of NtCHS and NtANS genes were strongly upregulated (Figure 7D). Similarly, almost all anthocyanin biosynthetic genes, including NtCHS, NtCHI, NtF3H, NtDFR, and NtANS, were remarkably upregulated in tobaccos overexpressing with VvMYB5a or VvMYB5b (Deluc et al., 2006, 2008). Besides, we found that the two bHLH regulatory genes, NtAn1a and NtAn1b that regulated the anthocyanin biosynthesis of tobacco, were also upregulated in the PsMYB2 transgenic petals and stamens (Figures 7C,D). Similarly, the enhancement of NtAn1a and NtAn1b was also found in tobaccos overexpressing with VvMYB5a/b-like TFs including EsMYB9, McMYB12, and LcMYB5 (Huang et al., 2017; Tian et al., 2017; Lai et al., 2019). Combined with the bHLH motif found in the PsMYB2 protein domain (Figure 6A) and the research that MYB usually interacted with a bHLH protein forming a complex to regulate anthocyanin structural genes (Yan et al., 2021), we thought that PsMYB2 might be able to interact with NtAn1a or NtAn1b, thus leading to the upregulation of NtAn1a and NtAn1b in tobacco. In addition, we noticed that the tobacco petals of transgenic line 3 did not appear darkening color, which may be related to the low expression levels of NtAn1a and NtAn1b in the petals of this line.
In conclusion, we suggested that PsMYB2 was a positive transcription factor in anthocyanin biosynthesis, as well as a new member of the VvMYB5b cluster.
Overexpression of PsMYB2 Enhanced the Ability of Glucose-Induced Anthocyanin Accumulation in Arabidopsis
In Arabidopsis, gene changes leading to the altered responding ability to sugar also showed changed ability of sugar-induced anthocyanin production (Mita et al., 1997a,b; Baier et al., 2004). For example, TTG1, a gene encoding WD40 protein, was a key component for sucrose-induced anthocyanin production, and sucrose hardly induced anthocyanin accumulation in the ttgl mutant (Shirley et al., 1995). Sucrose-induced anthocyanin biosynthesis depended on the function of the AtMYB75 protein, and the loss of AtMYB75 activity caused a reduced response to sucrose-induced anthocyanin accumulation in Arabidopsis (Teng et al., 2005). Oppositely, in this study, overexpressing PsMYB2 in Arabidopsis increased the sensitivity of seedlings to glucose treatment. Under glucose treatment, the anthocyanin content of PsMYB2 transgenic Arabidopsis seedlings was higher than that of wild-type seedlings (Figure 8B), in accordance with much darker anthocyanin extraction solutions (Figure 8A). This result was similar to the previous research in petunia that transgenic lines overexpressing R2R3-MYB (RsMYB1) isolated from Raphanus sativus showed greater anthocyanin accumulation in response to sucrose treatment compared to wild-type plants (Ai et al., 2016). Further gene expression analysis showed that the expression levels of anthocyanin biosynthetic genes were upregulated under glucose treatment both in wild type and PsMYB2 transgenic Arabidopsis. But the transcript of multiple structural genes including AtPAL1, AtCHS, AtF3H, AtF3′H, AtDFR, and AtLDOX in PsMYB2 transgenic Arabidopsis was much higher than that of wild-type seedlings (Figure 8C). Similarly, the transcript levels of the anthocyanin biosynthetic genes, including PAL, CHS, CHI, F3H, DFR, and ANS, were elevated in RsMYB1 transgenic plants under sucrose treatment (Ai et al., 2016). Besides, there were no differences in anthocyanin content between the PsMYB2 transgenic and wild-type plants under the 3OMG treatment (osmotic control), illustrating that glucose-induced anthocyanin accumulation was not due to osmotic pressure. Overall, we suggested that the overexpression of PsMYB2 increased the sensitivity of seedlings to glucose treatment leading to the higher anthocyanin accumulation in Arabidopsis seedlings.
Conclusion
Exogenous glucose supply had a good color retention effect on P. suffruticosa ‘Tai Yang’ cut flower during postharvest development, and it was mainly through glucose signal pathway rather the osmotic pressure. Multiple anthocyanin biosynthetic genes were upregulated by glucose treatment, in accordance with anthocyanin accumulations of Pg3G, Pg3G5G, and Pn3G5G. PsMYB2 was a new positive transcription factor for anthocyanin biosynthesis in tree peony, as well as a new member of the VvMYB5b cluster. More importantly, it could be a key component in glucose-induced anthocyanin accumulation through signal pathway in P. suffruticosa ‘Tai Yang’ cut flower.
Data Availability Statement
The original contributions presented in this study are included in the article/Supplementary Material, further inquiries can be directed to the corresponding author.
Author Contributions
LD and CZ designed and supervised this study. LZ and LY performed the experiments and analyzed the data. XK and YZ assisted with doing the experiments. LZ wrote the article. All authors read and approved the manuscript.
Funding
This work was supported by the National Key Research and Development Project (No. 2018YFD1000407) and the National Natural Science Foundation of China (No. 31572164).
Conflict of Interest
The authors declare that the research was conducted in the absence of any commercial or financial relationships that could be construed as a potential conflict of interest.
Publisher’s Note
All claims expressed in this article are solely those of the authors and do not necessarily represent those of their affiliated organizations, or those of the publisher, the editors and the reviewers. Any product that may be evaluated in this article, or claim that may be made by its manufacturer, is not guaranteed or endorsed by the publisher.
Supplementary Material
The Supplementary Material for this article can be found online at: https://www.frontiersin.org/articles/10.3389/fpls.2022.874526/full#supplementary-material
References
Abdullah, M., Cheng, X., Shakoor, A., Cao, Y. P., Lin, Y., Cai, Y. P., et al. (2018). New opinion of sugar and light crosstalk in the induction of anthocyanins biosynthesis in fruits. Internat. J. Agricult. Biol. 20, 2465–2474. doi: 10.17957/Ijab/15.0790
Ai, T. N., Naing, A. H., Arun, M., Lim, S. H., and Kim, C. K. (2016). Sucrose-induced anthocyanin accumulation in vegetative tissue of Petunia plants requires anthocyanin regulatory transcription factors. Plant Sci. 252, 144–150. doi: 10.1016/j.plantsci.2016.06.021
Baier, M., Hemmann, G., Holman, R., Corke, F., Card, R., Smith, C., et al. (2004). Characterization of mutants in Arabidopsis showing increased sugar-specific gene expression, growth, and developmental responses. Plant Physiol. 134, 81–91. doi: 10.1104/pp.103.031674
Bao Do, C., and Cormier, F. (1991). Effects of low nitrate and high sugar concentrations on anthocyanin content and composition of grape (Vitis vinifera L.) cell suspension. Plant Cell Rep. 9, 500–504. doi: 10.1007/BF00232105
Chen, Q., Xu, X., Xu, D., Zhang, H., Zhang, C., and Li, G. (2019). WRKY18 and WRKY53 coordinate with HISTONE ACETYLTRANSFERASE1 to regulate rapid responses to sugar. Plant Physiol. 180, 2212–2226. doi: 10.1104/pp.19.00511
Clough, S. J., and Bent, A. F. (1998). Floral dip: a simplified method for Agrobacterium-mediated transformation of Arabidopsis thaliana. Plant J. 16, 735–743. doi: 10.1046/j.1365-313x.1998.00343.x
Colombo, R., De Michelis, M. I., and Lado, P. (1978). 3-O-Methyl glucose uptake stimulation by auxin and by fusicoccin in plant materials and its relationships with proton extrusion. Planta 138, 249–256. doi: 10.1007/BF00386819
Dai, Z. W., Meddar, M., Renaud, C., Merlin, I., Hilbert, G., Delrot, S., et al. (2013). Long-term in vitro culture of grape berries and its application to assess the effects of sugar supply on anthocyanin accumulation. J. Exp. Bot. 65, 4665–4677. doi: 10.1093/jxb/ert489
Deluc, L., Barrieu, F., Marchive, C., Lauvergeat, V., Decendit, A., Richard, T., et al. (2006). Characterization of a grapevine R2R3-MYB transcription factor that regulates the phenylpropanoid pathway. Plant Physiol. 140, 499–511. doi: 10.1104/pp.105.067231
Deluc, L., Bogs, J., Walker, A. R., Ferrier, T., Decendit, A., Merillon, J. M., et al. (2008). The transcription factor VvMYB5b contributes to the regulation of anthocyanin and proanthocyanidin biosynthesis in developing grape berries. Plant Physiol. 147, 2041–2053. doi: 10.1104/pp.108.118919
Dooner, H. K., Robbins, T. P., and Jorgensen, R. A. (1991). Genetic and developmental control of anthocyanin biosynthesis. Annu Rev. Genet. 25, 173–199. doi: 10.1146/annurev.ge.25.120191.001133
Du, H., Wu, J., Ji, K. X., Zeng, Q. Y., Bhuiya, M. W., Su, S., et al. (2015). Methylation mediated by an anthocyanin, O-methyltransferase, is involved in purple flower coloration in Paeonia. J. Exp. Bot. 66, 6563–6577. doi: 10.1093/jxb/erv365
Gu, Z., Zhu, J., Hao, Q., Yuan, Y. W., Duan, Y. W., Men, S., et al. (2019). A novel R2R3-MYB transcription factor contributes to petal blotch formation by regulating organ-specific expression of PsCHS in tree peony (Paeonia suffruticosa). Plant Cell Physiol. 60, 599–611. doi: 10.1093/pcp/pcy232
Guo, R., Yuan, G., and Wang, Q. (2011). Sucrose enhances the accumulation of anthocyanins and glucosinolates in broccoli sprouts. Food Chem. 129, 1080–1087. doi: 10.1016/j.foodchem.2011.05.078
Guo, W. W., Dong, L., Wang, L. Y., Chen, R. X., and Liu, A. Q. (2004). The postharvest characteristics and water balance of some cultivars of tree-peony cut flowers. Scientia Silvae Sinicae 40, 89–93. doi: 10.1016/j.jce.2003.10.003
Han, S. S. (2003). Role of sugar in the vase solution on postharvest flower and leaf quality of oriental lily ‘Stargazer’. HortScience 38, 412–416. doi: 10.21273/hortsci.38.3.412
Hara, M., Oki, K., Hoshino, K., and Kuboi, T. (2003). Enhancement of anthocyanin biosynthesis by sugar in radish (Raphanus sativus) hypocotyl. Plant Sci. 164, 259–265. doi: 10.1016/S0168-9452(02)00408-9
Hedge, J. E., Hofreiter, B. T., Whistler, R. L., and Miller, J. (1962). Methods in carbohydrate chemistry. Acad. Press 17:1. doi: 10.1016/B978-0-12-746206-6.50001-1
Heppel, S. C., Jaffé, F. W., Takos, A. M., Schellmann, S., Rausch, T., Walker, A. R., et al. (2013). Identification of key amino acids for the evolution of promoter target specificity of anthocyanin and proanthocyanidin regulating MYB factors. Plant Mole. Biol. 82, 457–471. doi: 10.1007/s11103-013-0074-8
Ho, L. C., and Nichols, R. (1977). Translocation of 14C-sucrose in relation to changes in carbohydrate content in rose corollas cut at different stages of development. Ann. Bot. 41, 227–242. doi: 10.1093/oxfordjournals.aob.a085272
Horsch, R. B., Fry, J. E., Hoffmann, N. L., Wallroth, M., Eichholtz, D., Rogers, S. G., et al. (1985). A aimple and general method for transferring genes into plants. Science 227, 1229–1231. doi: 10.1126/science.227.4691.1229
Hu, D. G., Sun, C. H., Zhang, Q. Y., An, J. P., You, C. X., and Hao, Y. J. (2016). Glucose sensor MdHXK1 phosphorylates and stabilizes MdbHLH3 to promote anthocyanin biosynthesis in apple. PLoS Genet. 12:e1006273. doi: 10.1371/journal.pgen.1006273
Huang, W., Lv, H., and Wang, Y. (2017). Functional characterization of a novel R2R3-MYB transcription factor modulating the flavonoid biosynthetic pathway from Epimedium sagittatum. Front. Plant Sci. 8:1274. doi: 10.3389/fpls.2017.01274
Huang, W., Sun, W., Lv, H., Luo, M., Zeng, S., Pattanaik, S., et al. (2013). A R2R3-MYB transcription factor from Epimedium sagittatum regulates the flavonoid biosynthetic pathway. PLoS One 8:e70778. doi: 10.1371/journal.pone.0070778
Huang, Z., Wang, Q., Xia, L., Hui, J., Li, J., Feng, Y., et al. (2019). Preliminarily exploring of the association between sugars and anthocyanin accumulation in apricot fruit during ripening. Scientia Horticulturae 248, 112–117. doi: 10.1016/j.scienta.2019.01.012
Jamal Uddin, A. F. M., Hashimoto, F., Kaketani, M., Shimizu, K., and Sakata, Y. (2001). Analysis of light and sucrose potencies on petal coloration and pigmentation of lisianthus cultivars (in vitro). Scientia Horticulturae 89, 75–84. doi: 10.1016/s0304-4238(01)00224-2
Jang, J. C., Leon, P., Zhou, L., and Sheen, J. (1997). Hexokinase as a sugar sensor in higher plants. Plant Cell 9, 5–19. doi: 10.1105/tpc.9.1.5
Jeong, C. Y., Kim, J. H., Lee, W. J., Jin, J. Y., Kim, J., Hong, S. W., et al. (2018). AtMyb56 regulates anthocyanin levels via the modulation of AtGPT2 expression in response to sucrose in Arabidopsis. Mol. Cells 41, 351–361. doi: 10.14348/molcells.2018.2195
Jiang, R., Tian, J., Song, T., Zhang, J., and Yao, Y. (2014). The Malus crabapple transcription factor McMYB10 regulates anthocyanin biosynthesis during petal coloration. Scientia Horticulturae 166, 42–49. doi: 10.1016/j.scienta.2013.12.002
Jin, H., Cominelli, E., Bailey, P., Parr, A., Mehrtens, F., Jones, J., et al. (2000). Transcriptional repression by AtMYB4 controls production of UV-protecting sunscreens in Arabidopsis. EMBO J. 19, 6150–6161. doi: 10.1093/emboj/19.22.6150
Koes, R., Verweij, W., and Quattrocchio, F. (2005). Flavonoids: a colorful model for the regulation and evolution of biochemical pathways. Trends Plant Sci. 10, 236–242. doi: 10.1016/j.tplants.2005.03.002
Kranz, H. D., Denekamp, M., Greco, R., Jin, H., Leyva, A., Meissner, R. C., et al. (1998). Towards functional characterisation of the members of the R2R3-MYB gene family from Arabidopsis thaliana. Plant J. 16, 263–276. doi: 10.1046/j.1365-313x.1998.00278.x
Lai, B., Du, L. N., Hu, B., Wang, D., Huang, X. M., Zhao, J. T., et al. (2019). Characterization of a novel litchi R2R3-MYB transcription factor that involves in anthocyanin biosynthesis and tissue acidification. BMC Plant Biol. 19:62. doi: 10.1186/s12870-019-1658-5
Li, B., Xia, Y., Wang, Y., Qin, G., and Tian, S. (2017). Characterization of genes encoding key enzymes involved in anthocyanin metabolism of kiwifruit during storage period. Front. Plant Sci. 8:341. doi: 10.3389/fpls.2017.00341
Li, D., Zhang, X., Xu, Y., Li, L., Aghdam, M. S., and Luo, Z. (2019). Effect of exogenous sucrose on anthocyanin synthesis in postharvest strawberry fruit. Food Chem. 289, 112–120. doi: 10.1016/j.foodchem.2019.03.042
Li, S., Wang, W., Gao, J., Yin, K., Wang, R., Wang, C., et al. (2016). MYB75 phosphorylation by MPK4 is required for light-induced anthocyanin accumulation in Arabidopsis. Plant Cell 28, 2866–2883. doi: 10.1105/tpc.16.00130
Li, Y., Shan, X., Zhou, L., Gao, R., Yang, S., Wang, S., et al. (2018). The R2R3-MYB factor FhMYB5 from Freesia hybrida contributes to the regulation of anthocyanin and proanthocyanidin biosynthesis. Front. Plant Sci. 9:1935. doi: 10.3389/fpls.2018.01935
Li, Y., Van den Ende, W., and Rolland, F. (2014). Sucrose induction of anthocyanin biosynthesis is mediated by DELLA. Mol. Plant 7, 570–572. doi: 10.1093/mp/sst161
Loreti, E. (2001). Why and how do plant cells sense sugars? Ann. Bot. 88, 803–812. doi: 10.1006/anbo.2001.1526
Mita, S., Hirano, H., and Nakamura, K. (1997a). Negative regulation in the expression of a sugar-inducible gene in Arabidopsis thaliana. A recessive mutation causing enhanced expression of a gene for beta-amylase. Plant Physiol. 114, 575–582. doi: 10.1104/pp.114.2.575
Mita, S., Murano, N., Akaike, M., and Nakamura, K. (1997b). Mutants of Arabidopsis thaliana with pleiotropic effects on the expression of the gene for beta-amylase and on the accumulation of anthocyanin that are inducible by sugars. Plant J. 11, 841–851. doi: 10.1046/j.1365-313x.1997.11040841.x
Moalem-Beno, D., Tamari, G., Leitner-Dagan, Y., Borochov, A., and Weiss, D. (1997). Sugar-dependent gibberellin-induced chalcone synthase gene expression in petunia corollas. Plant Physiol. 113, 419–424. doi: 10.1104/pp.113.2.419
Mol, J., Jenkins, G., Schäfer, E., Weiss, D., and Walbot, V. (2008). Signal perception, transduction, and gene expression involved in anthocyanin biosynthesis. Crit. Rev. Plant Sci. 15, 525–557. doi: 10.1080/07352689609382369
Naing, A. H., Xu, J., Park, K. I., Chung, M. Y., and Kim, C. K. (2021). Sucrose enhances anthocyanin accumulation in Torenia by promoting expression of anthocyanin biosynthesis genes. Horticulturae 7:8. doi: 10.3390/horticulturae7080219
Neta-Sharir, I., Shoseyov, O., and Weiss, D. (2000). Sugars enhance the expression of gibberellin-induced genes in developing petunia flowers. Physiol. Plant. 109, 196–202. doi: 10.1034/j.1399-3054.2000.100212.x
Pun, U. K., and Ichimura, K. (2003). Role of sugars in senescence and biosynthesis of ethylene in cut flowers. Japan Agricult. Res. Q. JARQ 37, 219–224. doi: 10.6090/jarq.37.219
Qi, Y., Zhou, L., Han, L., Zou, H., Miao, K., and Wang, Y. (2020). PsbHLH1, a novel transcription factor involved in regulating anthocyanin biosynthesis in tree peony (Paeonia suffruticosa). Plant Physiol. Biochem. 154, 396–408. doi: 10.1016/j.plaphy.2020.06.015
Qian, J., Lai, W., Jiang, L., Zhan, H., Zhai, M., Fu, J., et al. (2021). Association between differential gene expression and anthocyanin biosynthesis underlying the diverse array of petal colors in Zinnia elegans. Scientia Horticulturae 277:109809. doi: 10.1016/j.scienta.2020.109809
Quattrocchio, F., Wing, J., van der Woude, K., Souer, E., de Vetten, N., Mol, J., et al. (1999). Molecular analysis of the anthocyanin2 gene of petunia and its role in the evolution of flower color. Plant Cell 11, 1433–1444. doi: 10.1105/tpc.11.8.1433
Rolland, F., Moore, B., and Sheen, J. (2002). Sugar sensing and signaling in plants. Plant Cell 14(Suppl. 1), S185–S205. doi: 10.1105/tpc.010455
Rook, F., and Bevan, M. W. (2003). Genetic approaches to understanding sugar-response pathways. J. Exp. Bot. 54, 495–501. doi: 10.1093/jxb/erg054
Sankhla, N., Mackay, W. A., and Davis, T. D. (2005). Corolla abscission and petal color in cut phlox flower heads: effects of sucrose and thidiazuron. Acta Horticult. 389–394. doi: 10.17660/ActaHortic.2005.669.51
Shen, X., Zhao, K., Liu, L., Zhang, K., Yuan, H., Liao, X., et al. (2014). A role for PacMYBA in ABA-regulated anthocyanin biosynthesis in red-colored sweet cherry cv. Hong Deng (Prunus avium L.). Plant Cell Physiol. 55, 862–880. doi: 10.1093/pcp/pcu013
Shi, Q., Zhou, L., Wang, Y., Li, K., Zheng, B., and Miao, K. (2015). Transcriptomic analysis of Paeonia delavayi wild population flowers to identify differentially expressed genes involved in purple-red and yellow petal pigmentation. PLoS One 10:e0135038. doi: 10.1371/journal.pone.0135038
Shimizu, H., and Ichimura, K. (2005). Effects of silver thiosulfate complex (STS), sucrose and their combination on the quality and vase life of cut eustoma flowers. J. Japan. Soc. Horticult. Sci. 74, 381–385. doi: 10.2503/jjshs.74.381
Shirley, B. W., Kubasek, W. L., Storz, G., Bruggemann, E., Koornneef, M., Ausubel, F. M., et al. (1995). Analysis of Arabidopsis mutants deficient in flavonoid biosynthesis. Plant J. 8, 659–671. doi: 10.1046/j.1365-313x.1995.08050659.x
Shu, Q. Y., Zhu, J., Men, S. Q., Hao, Q., and Wang, L. S. (2008). Establishing virus induced gene silencing (VIGS) system in tree peony using PsUFGT genes. Acta Horticult. Sinica 45, 168–176. doi: 10.16420/j.issn.0513-353x.2017-0522
Smeekens, S. (2000). Sugar-induced signal transduction in plants. Annu Rev. Plant Physiol. Plant Mol. Biol. 51, 49–81. doi: 10.1146/annurev.arplant.51.1.49
Solfanelli, C., Poggi, A., Loreti, E., Alpi, A., and Perata, P. (2006). Sucrose-specific induction of the anthocyanin biosynthetic pathway in Arabidopsis. Plant Physiol. 140, 637–646. doi: 10.1104/pp.105.072579
Suo, Z. L., Zhao, X. Q., Zhao, J. P., Zhao, X. C., and Chen, F. F. (2008). ‘Sisters on Spring Outing’ (Paeonia suffruticosa ‘Zi Mei You Chun’) (Paeoniaceae): a unique Chinese tree peony cultivar possessing side flowers and bicolored floral discs. HortScience 43, 532–534. doi: 10.21273/hortsci.43.2.532
Tanaka, Y., Sasaki, N., and Ohmiya, A. (2008). Biosynthesis of plant pigments: anthocyanins, betalains and carotenoids. Plant J. 54, 733–749. doi: 10.1111/j.1365-313X.2008.03447.x
Teng, S., Keurentjes, J., Bentsink, L., Koornneef, M., and Smeekens, S. (2005). Sucrose-specific induction of anthocyanin biosynthesis in Arabidopsis requires the MYB75/PAP1 gene. Plant Physiol. 139, 1840–1852. doi: 10.1104/pp.105.066688
Tian, J., Zhang, J., Han, Z. Y., Song, T. T., Li, J. Y., Wang, Y. R., et al. (2017). McMYB12 transcription factors co-regulate proanthocyanidin and anthocyanin biosynthesis in Malus crabapple. Sci. Rep. 7:43715. doi: 10.1038/srep43715
Tsukaya, H., Ohshima, T., Naito, S., Chino, M., and Komeda, Y. (1991). Sugar-dependent expression of the CHS-A gene for chalcone synthase from petunia in transgenic Arabidopsis. Plant Physiol. 97, 1414–1421. doi: 10.1104/pp.97.4.1414
Van den Ende, W., and El-Esawe, S. K. (2014). Sucrose signaling pathways leading to fructan and anthocyanin accumulation: a dual function in abiotic and biotic stress responses? Environ. Exp. Bot. 108, 4–13. doi: 10.1016/j.envexpbot.2013.09.017
Vitrac, X., Larronde, F., Krisa, S., Decendit, A., Deffieux, G., and Merillon, J. M. (2000). Sugar sensing and Ca2+-calmodulin requirement in Vitis vinifera cells producing anthocyanins. Phytochemistry 53, 659–665. doi: 10.1016/s0031-9422(99)00620-2
Voss, D. H. (1992). Relating colorimeter measurement of plant color to the royal horticultural society colour chart. HortScience 27, 1256–1260. doi: 10.21273/hortsci.27.12.1256
Wang, L. S., Shiraishi, A., Hashimoto, F., Aoki, N., Shimizu, K., and Sakata, Y. (2001). Analysis of petal anthocyanins to investigate flower coloration of zhongyuan (Chinese) and daikon island (Japanese) tree peony cultivars. J. Plant Res. 114, 33–43. doi: 10.1007/pl00013966
Weiss, D. (2000). Regulation of flower pigmentation and growth: multiple signaling pathways control anthocyanin synthesis in expanding petals. Physiol. Plant. 110, 152–157. doi: 10.1034/j.1399-3054.2000.110202.x
Yamagishi, M., Shimoyamada, Y., Nakatsuka, T., and Masuda, K. (2010). Two R2R3-MYB genes, homologs of petunia AN2, regulate anthocyanin biosyntheses in flower tepals, tepal spots and leaves of asiatic hybrid lily. Plant Cell Physiol. 51, 463–474. doi: 10.1093/pcp/pcq011
Yan, H., Pei, X., Zhang, H., Li, X., Zhang, X., Zhao, M., et al. (2021). MYB-mediated regulation of anthocyanin biosynthesis. Int. J. Mol. Sci. 22:6. doi: 10.3390/ijms22063103
Yu, J., Zhu, M., Wang, M., Xu, Y., Chen, W., and Yang, G. (2020). Transcriptome analysis of calcium-induced accumulation of anthocyanins in grape skin. Scient. Horticult. 260:108871. doi: 10.1016/j.scienta.2019.108871
Zhang, C., Fu, J., Wang, Y., Gao, S., Du, D., Wu, F., et al. (2015). Glucose supply improves petal coloration and anthocyanin biosynthesis in Paeonia suffruticosa ‘Luoyang Hong’ cut flowers. Postharv. Biol. Technol. 101, 73–81. doi: 10.1016/j.postharvbio.2014.11.009
Zhang, C., Wang, Y., Fu, J., Dong, L., Gao, S., and Du, D. (2014). Transcriptomic analysis of cut tree peony with glucose supply using the RNA-Seq technique. Plant Cell Rep. 33, 111–129. doi: 10.1007/s00299-013-1516-0
Zhang, K. M., Li, Z., Li, Y., Li, Y. H., Kong, D. Z., and Wu, R. H. (2013). Carbohydrate accumulation may be the proximate trigger of anthocyanin biosynthesis under autumn conditions in Begonia semperflorens. Plant Biol. 15, 991–1000. doi: 10.1111/j.1438-8677.2012.00721.x
Zhang, X., Xu, Z., Yu, X., Zhao, L., Zhao, M., Han, X., et al. (2019). Identification of two novel R2R3-MYB transcription factors, PsMYB114L and PsMYB12L, related to anthocyanin biosynthesis in Paeonia suffruticosa. Internat. J. Mole. Sci. 20:5. doi: 10.3390/ijms20051055
Zhang, X., Zhao, L., Xu, Z., and Yu, X. (2018). Transcriptome sequencing of Paeonia suffruticosa ‘Shima Nishiki’ to identify differentially expressed genes mediating double-color formation. Plant Physiol. Biochem. 123, 114–124. doi: 10.1016/j.plaphy.2017.12.009
Zhang, Y., Cheng, Y., Ya, H., Xu, S., and Han, J. (2015). Transcriptome sequencing of purple petal spot region in tree peony reveals differentially expressed anthocyanin structural genes. Front. Plant Sci. 6:964. doi: 10.3389/fpls.2015.00964
Zhang, Y., Xu, S., Cheng, Y., Wang, J., Wang, X., Liu, R., et al. (2020). Functional identification of PsMYB57 involved in anthocyanin regulation of tree peony. BMC Genet. 21:124. doi: 10.1186/s12863-020-00930-7
Zhang, Y., Xu, S., Ma, H., Duan, X., Gao, S., Zhou, X., et al. (2021). The R2R3-MYB gene PsMYB58 positively regulates anthocyanin biosynthesis in tree peony flowers. Plant Physiol. Biochem. 164, 279–288. doi: 10.1016/j.plaphy.2021.04.034
Zhao, D., Zheng, Y., Yang, L., Yao, Z., Cheng, J., Zhang, F., et al. (2021). The transcription factor AtGLK1 acts upstream of MYBL2 to genetically regulate sucrose-induced anthocyanin biosynthesis in Arabidopsis. BMC Plant Biol. 21:242. doi: 10.1186/s12870-021-03033-2
Keywords: cut flower, anthocyanin biosynthesis, tree peony, PsMYB2, glucose signal
Citation: Zhang L, Yan L, Zhang C, Kong X, Zheng Y and Dong L (2022) Glucose Supply Induces PsMYB2-Mediated Anthocyanin Accumulation in Paeonia suffruticosa ‘Tai Yang’ Cut Flower. Front. Plant Sci. 13:874526. doi: 10.3389/fpls.2022.874526
Received: 12 February 2022; Accepted: 16 May 2022;
Published: 14 June 2022.
Edited by:
Maria F. Drincovich, Centro de Estudios Fotosintéticos y Bioquímicos (CEFOBI), ArgentinaReviewed by:
Lu Wang, Tea Research Institute (CAAS), ChinaYanzhao Zhang, Luoyang Normal University, China
Dong Liu, Jiangxi Agricultural University, China
Copyright © 2022 Zhang, Yan, Zhang, Kong, Zheng and Dong. This is an open-access article distributed under the terms of the Creative Commons Attribution License (CC BY). The use, distribution or reproduction in other forums is permitted, provided the original author(s) and the copyright owner(s) are credited and that the original publication in this journal is cited, in accordance with accepted academic practice. No use, distribution or reproduction is permitted which does not comply with these terms.
*Correspondence: Li Dong, ZG9uZ2xpQGJqZnUuZWR1LmNu