- Sugarbeet and Potato Research Unit, Edward T. Schafer Agricultural Research Center, Agricultural Research Service, United States Department of Agriculture, Fargo, ND, United States
Seed germination is a critical first stage of plant development but can be arrested by factors including dormancy and environmental conditions. Strategies to enhance germination are of interest to plant breeders to ensure the ability to utilize the genetic potential residing inside a dormant seed. In this study, seed germination in two sugarbeet (Beta vulgaris ssp. vulgaris L.) lines F1004 and F1015 through incubating seeds in hydrogen peroxide (H2O2) solution was improved over 70% relative to germinating seeds through water incubation. It was further found that low germination from water incubation was caused by physical dormancy in F1015 seeds with initial seed imbibition blocked by the seed pericarp, and physiological dormancy in F1004 seeds with germination compromised due to the physiological condition of the embryo. To identify genes that are differentially expressed in response to cellular activities promoted by H2O2 during overcoming different type of dormancies, an RNA-Seq study was carried out and found H2O2 treatment during germination accelerated the degradation of seed stored mRNAs that were synthesized before or during seed storage to provide protections and maintain the dormant state. Comparison of transcripts in H2O2-treated seeds between the two sugarbeet lines identified differentially expressed genes (DEGs) that were higher in F1004 for alleviating physiological dormancy were known to relative to gene expression regulation. The research established that H2O2 overcomes both physical and physiological dormancies by hastening the transition of seeds from dormancy into germination. More DEGs related to gene expression regulation were involved in relieving physiological dormancy which provides new knowledge about the role of exogenous H2O2 as a signaling molecule for regulating gene activities during germination. Moreover, the protocol using H2O2 to promote germination will be useful for rescuing plant germplasms with poor germination.
Introduction
Seed germination is the initial developmental stage responsible for producing plants of the next generation to maintain the germplasm and multiply individuals of the species. From initial water uptake to the development of the embryonic axis, the germination process involves numerous events including protein hydration, subcellular structural changes, respiration rate increases, macromolecular syntheses, cell elongation and division, and many other metabolic activities (Bewley and Black, 1994). Seed germination, therefore, is a very complex process and can be influenced by factors of extrinsic (water, temperature, light, and oxygen) and intrinsic (mainly the dormancy due to physiological condition within seed) natures (Raven et al., 2005). Adjusting extrinsic factors to provide more favorable conditions may not always lead to successful germination if seeds are in a dormant state (Baskin and Baskin, 2004). Consequently, breaking seed dormancy by stimulating key processes related to germination often becomes vital for seedling establishment and proliferation in the new generation.
Seed dormancy is a mechanism that evolved to slow down or inhibit germination under unsuitable conditions to help plant species survive under adverse conditions. In general, seed dormancy includes exogenous physical dormancy due to physical restrictions caused by the seed coat as well as endogenous physiological dormancy caused by physiological conditions within the embryo itself (Baskin and Baskin, 2004). Physical dormancy can be broken naturally during seed imbibition or artificially through scarification, dry heat, fire, or application of hot water, acid, or other chemicals to open seed coats and allow seeds to take up the water and oxygen needed for germination (Emery, 1988). In contrast, overcoming physiological dormancy, which arises from the presence of germination inhibitors or other unknown physiological factors, may require a biochemical trigger to initiate the germination process (Keeley, 1991).
Hydrogen peroxide (H2O2) has been shown to stimulate germination (Fontaine et al., 1994; Katzman et al., 2001; Zeinalabedini et al., 2009), but the mechanism by which it acts is largely unknown. H2O2 and other reactive oxygen species (ROS) have been detected in seeds during water imbibition and the early stages of germination (Schopfer et al., 2001; Bailly, 2004; El-Maarouf-Bouteau and Bailly, 2008; Kranner et al., 2010; Zhang et al., 2014; Wojtyla et al., 2016). H2O2 accumulation has also been shown to be a signaling cue for regulating seed dormancy and germination (Bailly et al., 2008; Bailly, 2019). According to the “oxidative window” hypothesis proposed by Bailly et al. (2008), only a critical range of ROS concentrations alleviates dormancy while levels below or above the critical range impair germination. Verma et al. (2015) postulated that stored proteins were oxidatively modified by H2O2 and ROS, and the modified proteins were then recognized by storage organs as signals to mobilize nutrients for the rapid growth that is needed for germination. Oxidation induced alterations in enzymatic and binding properties of proteins have been observed by Davies (2005), and protein oxidations were also observed during germination of pea (Barba-Espín et al., 2010) and Arabidopsis (Job et al., 2005). Therefore, selective oxidation of the seed proteome and transcriptome remodeling by H2O2 and ROS may play a role in releasing dormancy to start germination (Diaz-Vivancos et al., 2013).
Another possible mechanism by which H2O2 promotes germination is via crosstalk with phytohormones such as abscisic acid (ABA) and gibberellic acid (GA) which have known roles in maintaining and releasing dormancy, respectively (Finch-Savage and Leubner-Metzger, 2006; Finkelstein et al., 2008; Nambara et al., 2010; Weitbrecht et al., 2011; Graeber et al., 2012; Rajjou et al., 2012; Diaz-Vivancos et al., 2013; Golldack et al., 2013; Rodríguez et al., 2015; Shu et al., 2016; Wojtyla et al., 2016; Ishibashi et al., 2017; Carrera-Castaño et al., 2020). For example, ROS and H2O2 concentrations increased with addition of GA in germinating seeds of radish (Schopfer et al., 2001) and Arabidopsis (Liu et al., 2010; Lariguet et al., 2013), thereby changing oxidative conditions and enhancing germination. Bahin et al. (2011) proposed that H2O2 affects GA signaling by modulating phytohormone balance and initiating germination in barley, which was later supported by Lariguet et al. (2013) as they observed that oxidative levels were enhanced by GA treatment but declined with ABA treatment in Arabidopsis seeds. Other research has indicated that H2O2 may release the inhibitory effect of ABA to initiate germination in Arabidopsis (Müller et al., 2006, 2007) and lettuce (Zhang et al., 2014).
H2O2 and ROS have also been shown to be signal molecules that regulate expression of germination-related genes. Lariguet et al. (2013) suggested that H2O2 may regulate the expression of genes encoding enzymes that hydrolyze the testa and endosperm of Arabidopsis seeds. Bray and West (2005) and Weitbrecht et al. (2011) proposed that ROS induce the expression of DNA ligase for DNA repair during Arabidopsis seed imbibition since the excess ROS can induce DNA damage and affect the accumulation of transcripts. Also, H2O2 and ROS have been shown to alter seed physiology during germination, thus affecting gene transcription (Weitbrecht et al., 2011) and the degradation of stored mRNAs in seeds (El-Maarouf-Bouteau et al., 2015). Overall, information currently available regarding the effect on gene expression and other cell activities when breaking seed dormancy through H2O2 and ROS is extremely limited.
Sugarbeet (Beta vulgaris ssp. vulgaris L.) is a relatively new crop with economic importance worldwide. Sugarbeet seeds are woody fruits commonly called seedballs. Inside each seedball, one (monogerm) or up to five (multigerm) kidney-shaped seeds are tightly wrapped in a woody pericarp that is composed of impervious sclerenchyma cells (Ignatz et al., 2019). The inner dense layer of pericarp restricts water and oxygen uptake by the enclosed seeds (Coumans et al., 1976; Richard et al., 1989; Tohidloo et al., 2015; Chomontowski and Podlaski, 2020), but the basal pore at the bottom of the pericarp is comprised of loose cells that provide a site of entry for water and oxygen. Hermann et al. (2007) analyzed the germination of sugarbeet fruit and isolated individual seed using selected plant hormone treatments and found that ethylene or the ethylene precursor, 1-aminocyclopropane-1-carboxylic acid (ACC), promoted radicle emergence of both fruits and the isolated seeds, while ABA inhibited radicle emergence from isolated seeds but not fruits. They thus proposed that the pericarp may block entry of exogenous ABA and restrict leaching of endogenous ACC. In other research, McGrath et al. (2000) used H2O2 solutions to discriminate vigor of seeds from various sugarbeet lines or seed lots since H2O2 stimulated germination and thus provided the optimal germinating conditions. These studies confirmed that ABA inhibits and ethylene and H2O2 promote sugarbeet germination. However, gene expression regulation during the release of seed dormancy toward germination remains unknown.
While germinating seeds of sugarbeet germplasms that were cold stored for many years, we encountered many lines with poor germination when seeds were incubated in water. Our preliminary tests indicated that incubating these seeds in a H2O2 solution improved germination. Research, therefore, was conducted to develop an efficient protocol of using H2O2 to enhance sugarbeet germination. Additionally, gene expression was compared between sugarbeet lines and between H2O2- and water-treated seeds to understand the mechanisms by which H2O2 promotes germination in seeds whose germination is restricted by physical or physiological dormancy.
Materials and Methods
Plant Materials
Seedballs from two sugarbeet lines F1004 (PI 590763) and F1015 (PI 605413) were used in this research. F1004 was released in 1984 and developed from mass selection for resistance to storage rot (Campbell and Bugbee, 1984). F1015 was released in 1996 as the first publicly available sugarbeet germplasm line with sugarbeet root maggot (Tetanops myopaeformis von Röder) resistance (Campbell et al., 2000). Both lines produce multigerm seedballs. Seedballs of F1004 and F1015 were stored in the USDA-ARS sugarbeet genetics laboratory in Fargo, ND, United States at 4°C and 40% relative humidity for 24 and 7 years, respectively. Seedballs of F1004 were polished by manually rubbing seedballs to remove the out loose layer of the pericarp, while seedballs of F1015 were raw fruits without any treatment. Preliminary tests indicated that germination of both lines was very low when seeds were germinated by incubating in distilled water or wetted soil. In addition, a set of 50 additional sugarbeet lines that was cold stored in the same lab for 10–37 years was used for validating the protocol of using H2O2 to enhance germination.
Germination Experiments
Germination experiments were designed to determine the optimum length of time to incubate seeds in a H2O2 solution and evaluate the effects of environmental conditions such as seed hydration, temperature, and light on H2O2-stimulated germination. Also, germination of seeds that were manually isolated from seedballs was performed to identify the nature of seed dormancy for the two sugarbeet lines.
For determining the optimum length of time for incubating seeds in a H2O2 solution, five H2O2 treatments along with a water control were used. For each treatment, seedballs were incubated in a 1% H2O2 solution for 1, 2, 3, 4, or 7 days, then incubated in distilled water for the remainder of the 8-days period. Germination was evaluated daily. After 8 days, ungerminated seeds from each treatment were incubated for an additional 2 days in distilled water, and seeds were considered as having no germinability if no germination occurred at this time. Each treatment included three replications for each line with each replication comprised of 100 seedballs in a 9-cm diameter petri plate. Therefore, a total of 300 seedballs from each line was tested in each treatment. All petri plates were incubated at room temperature under fluorescent lights with light intensity around 11.9 μmol m–2 s–1 for approximately 9 h per day. On the first day, seedballs in each plate were incubated in 15 mL 1% H2O2 or distilled water, depending on the treatment. On each of the following days, incubating solution was decanted off and seedballs were washed three times with distilled water. To maintain treatment conditions but leave seedballs partially uncovered by liquid, 5 mL fresh 1% H2O2 or distilled water was added to each plate according to the treatment. Seedballs were considered germinated when radicles emerged and percentages of germinated seedballs were recorded and compared among treatments.
To determine the effect of seed hydration on H2O2 enhancement of germination, five treatments were used with seedballs incubated in distilled water for 0, 1, 2, 3, or 4 days prior to treatment with a 1% H2O2 solution. The effect of light on germination was tested using seedballs incubated either in water or H2O2 solution for 8 days at room temperature in the dark or under the natural light obtained through a window, with an approximate light intensity of 26.8 μmol m–2 s–1 for about 12 h per day. To test the influence of temperature on germination in H2O2 solution, two treatments were used. For the first treatment, seedballs were incubated in a 1% H2O2 solution at 4°C for 3 days and then incubated in H2O2 for an additional 4 days at room temperature; for the second treatment seedballs were incubated in 1% H2O2 at room temperature throughout the experiment. All experiments utilized three replicate petri plates per treatment for each of the two lines with each replicate comprised of 100 seedballs. Seedballs were rinsed with water each day and fresh 1% H2O2 solution or distilled water were added as described above. For validating the H2O2 germination protocol, two plates each containing 100 seedballs, were used for H2O2 treatment and water control, respectively, for each of 50 sugarbeet lines described above. Seeds were rinsed daily as described for germination experiments, and germination percentage was recorded on the 8th day. Analysis of variance (ANOVA) was conducted using the aov function in R1 to test the significance of each factor affecting germination, and the LSD.test function in R2 was used to calculate LSDs (least significant differences) at P < 0.05 for each treatment in each line to compare the significance of treatments on germination. Analyses of correlation, t-tests, and standard deviation calculations were conducted using functions of Microsoft Excel.
To determine if endogenous physiological or exogenous physical dormancy was responsible for the low germination of the two lines when seedballs were germinated in water, individual seeds were carefully isolated from seedballs. A hammer was used to slightly crack the pericarp, and seeds were carefully excised from broken seedballs. The isolated seeds were visually inspected and any seeds with visible damage were excluded. A total of 50 isolated seeds from each line were placed in a 9-cm diameter petri plate and incubated in 5 mL of distilled water. Seeds were washed daily by decanting the old rinse solution and washing seeds with water. Germination was checked daily.
RNA Extraction, Library Construction and Sequencing
RNA-sequencing (RNA-seq) was used to analyze gene transcripts during seed imbibition and up to germination. From germination tests using H2O2 solution it was determined that seeds very likely had lost viability if they showed no germination after 8 days in H2O2 solution. Samples for RNA extraction thus were prepared using an 8-days period with seedballs treated with 1% H2O2 solution or distilled water using two replications for each line. A total of 64 plates (two lines × two treatments × two replications × 8 days) were used with each plate comprised of 100 seedballs. Eight plates (two lines × two treatments × two replications) were sampled each day. Since the purpose of this experiment was to detect genes expressed during seed imbibition and metabolic reawakening under H2O2 or water treatment, germinated seedballs with roots that extended out of the seed coat were excluded to minimize mRNAs that were transcribed in the root tissue that were irrelevant to germination. Therefore, seedballs with emerged radicles were counted each day and removed from each plate before collecting samples for RNA extraction. Collected samples were immediately frozen in liquid nitrogen and stored at –80°C until processing.
Total RNA was extracted and purified using a Qiagen-RNeasy Micro Kit according to the manufacturer’s instructions. Based on a preliminary test that almost no RNA was extracted from samples using seed pericarp only (data not shown), the whole seedballs thus were used for total RNA extraction. An Agilent 2100 Bioanalyzer (Agilent RNA 6000 Nano Kit) was used to determine RNA concentration, RIN value, the 28S/18S ratio, and fragment length distribution, and a NanoDrop 2000 spectrophotometer (Thermo Scientific, Inc., Waltham, MA, United States) was used to assess the purity of the RNA samples. To prepare sequencing libraries, mRNAs were isolated using poly-T oligo-conjugated magnetic beads, and cDNA was synthesized using reverse transcriptase Super Script II (Invitrogen, Waltham, MA, United States) at 42°C and random primers after RNA fragmentation. Second strand cDNA was synthesized using DNA Polymerase I and RNase H. The cDNA fragments were modified by the addition of A-tails and ligation of sequencing adapters and purified and enriched through PCR amplification. After a quality check, samples were pooled, and single strand DNA circles (ssDNA circles) were made. DNA nanoballs (DNBs) were generated by rolling circle replication (RCR) of ssDNA circles and the DNBs were loaded into patterned nanoarrays. Sequence data comprised of 100-bp pair-end reads were collected on a DNBseq platform using the combinatorial probe-anchor synthesis (cPAS) method (Fehlmann et al., 2016).
Transcriptome and Gene Expression Level Analysis
Raw reads were filtered by removing adapters and trimming low quality bases (Phred score < 20) at the end of reads using the program SOAPnuke3. Filtered reads were aligned to the sugarbeet genome sequence, RefBeet v1.2.2 (Dohm et al., 2014; available from NCBI4) using the tool HISAT2 (Hierarchical Indexing for Spliced Alignment of Transcripts) (Kim et al., 2015). Transcript assemblies were generated using the computer package StringTie (Martin and Wang, 2011; Pertea et al., 2015) followed by use of Cuffcompare (Trapnell et al., 2012) to compare reconstructed transcripts to the reference annotation. The computer package CPC (Kong et al., 2007) was used to predict novel transcripts and merge novel transcripts with the reference genome to get a complete transcript reference. Transcript abundance was normalized to FPKM (fragment per kilobase of transcript per million reads mapped) values which were calculated using the computer package RSEM (Li and Dewey, 2011). Differentially expressed genes (DEGs) were detected using PoissonDis that is based on the Poisson distribution (Audic and Claverie, 1997) and limited to DEGS with a fold change > 2 and a false detection rate (FDR) at P < 0.001. Comparisons of gene expression were performed between treatments (H2O2 treatment vs. water control) to identify transcriptional changes in seed due to H2O2 and between the two sugarbeet lines (F1004 vs. F1015) to reveal transcriptional differences corresponding to different types of seed dormancy.
Gene Functional Assignment and Expression Level Comparison
Gene ontology (GO) analysis was done according to Young et al. (2010) and GO functional enrichment determined using phyper, a function of R5. Gene functional annotation used the gene IDs and annotations in RefBeet (Dohm et al., 2014). For novel genes that did not align with any annotated genes in the sugarbeet genome, DNA sequences were compared with those stored in the NCBI GenBank database6 to infer gene function based on sequence similarity with a critical expectation value (E value) threshold set at 1.0 × 10–5 (Altschul et al., 1997). Pathway analysis of DEGs was conducted through KEGG (Aoki-Kinoshita and Kanehisa, 2007). Online protein databases (such as UniProt, SwissProt, and TrEMBL) were also searched using amino acid sequences or protein names as queries to identify possible biological processes or pathways of the proteins encoded by DEGs.
Results
Effect of H2O2 Treatment Time on Germination
In the water control, none of F1015 but 7.3% of F1004 seedballs germinated (Table 1). The 1-day treatment in 1% H2O2 solution had almost no effect on enhancing germination when compared with the water control (Table 1). This suggests that during the first day of incubating seedballs in H2O2 solution or water, only the pericarp rather than the enclosed seeds, were taking up water, and thus seed imbibition was unlikely to start on the first day. As the days of H2O2-treatment increased, germination of both lines increased by about 10% in the 2-day H2O2 treatment and by another 30% in each of the 3- and 4-days H2O2 treatments (Table 1). The highest germination percentage occurred in the 7-days H2O2 treatment in both lines with germination reaching 80.0% in F1004 and 86.7% in F1015 (Table 1), an increase of about 10% in F1004 and 20% in F1015 compared to the percentage observed in seedballs of the 4-days H2O2 treatment. This suggests that few seedballs require five or more days incubating in H2O2 solution to promote germination. The ungerminated seeds from the 7-days H2O2 treatment were likely unviable since they were mostly unable to germinate even after extending the H2O2 treatment time. Therefore, incubating seeds in H2O2 solution enhanced sugarbeet germination with the highest germination percentage achieved using a 7-days treatment.
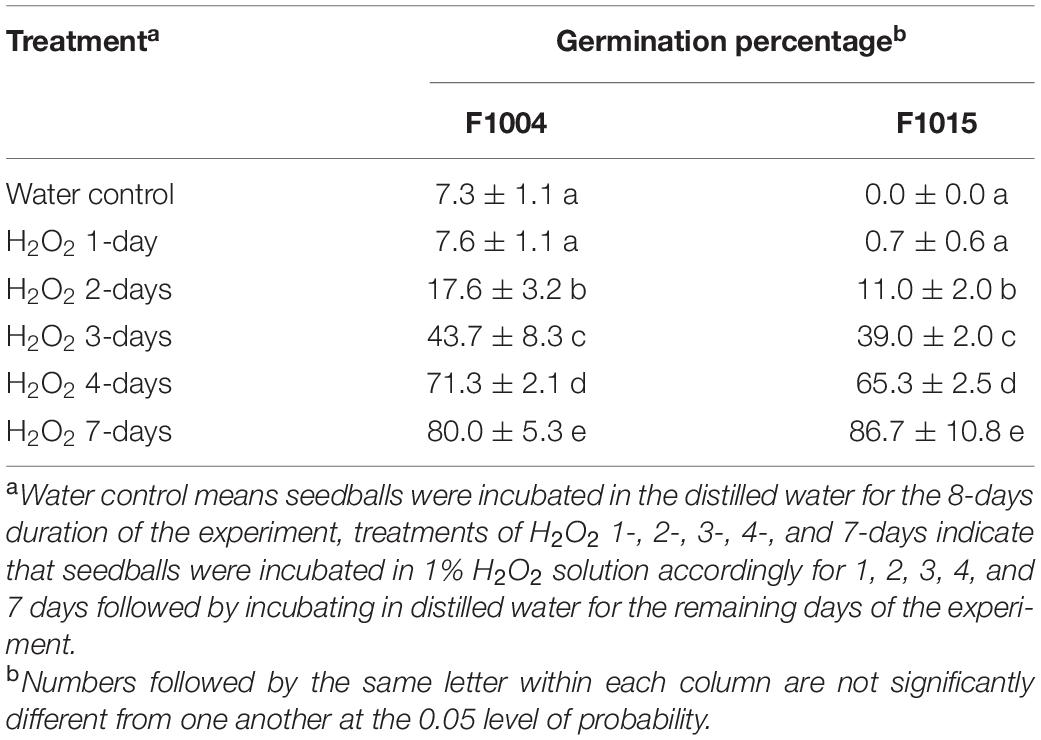
Table 1. Germination induced by incubating seedballs of sugarbeet lines F1004 and F1015 in 1% H2O2 solution for varying durations under fluorescent lights at room temperature.
Effects of Environmental Factors on Germination Induced by H2O2
For evaluating the effect of seed hydration on germination in H2O2 solution, seedballs were preincubated in water for 1–4 days to achieve different levels of hydration. Overall, all water-preincubated seedballs germinated more poorly in both lines, with the reduction in germination more severe as seedballs were incubated for longer durations in water (Table 2). Germination of seedballs preincubated in water for 1 day followed by H2O2 treatment was reduced by approximately 30% compared to seedballs that receive no water pretreatment. A further 20–30% reduction in germination was observed with 2 and 3 days of water preincubation. Seedballs preincubated in water for 4 days followed by H2O2 treatment showed a similar germination percentage to the water control (Table 2), indicating that H2O2 treatment did not enhance germination if seeds were preincubated in water for 4 or more days. Therefore, increasing hydration in seeds did not improve germination under H2O2 treatment.
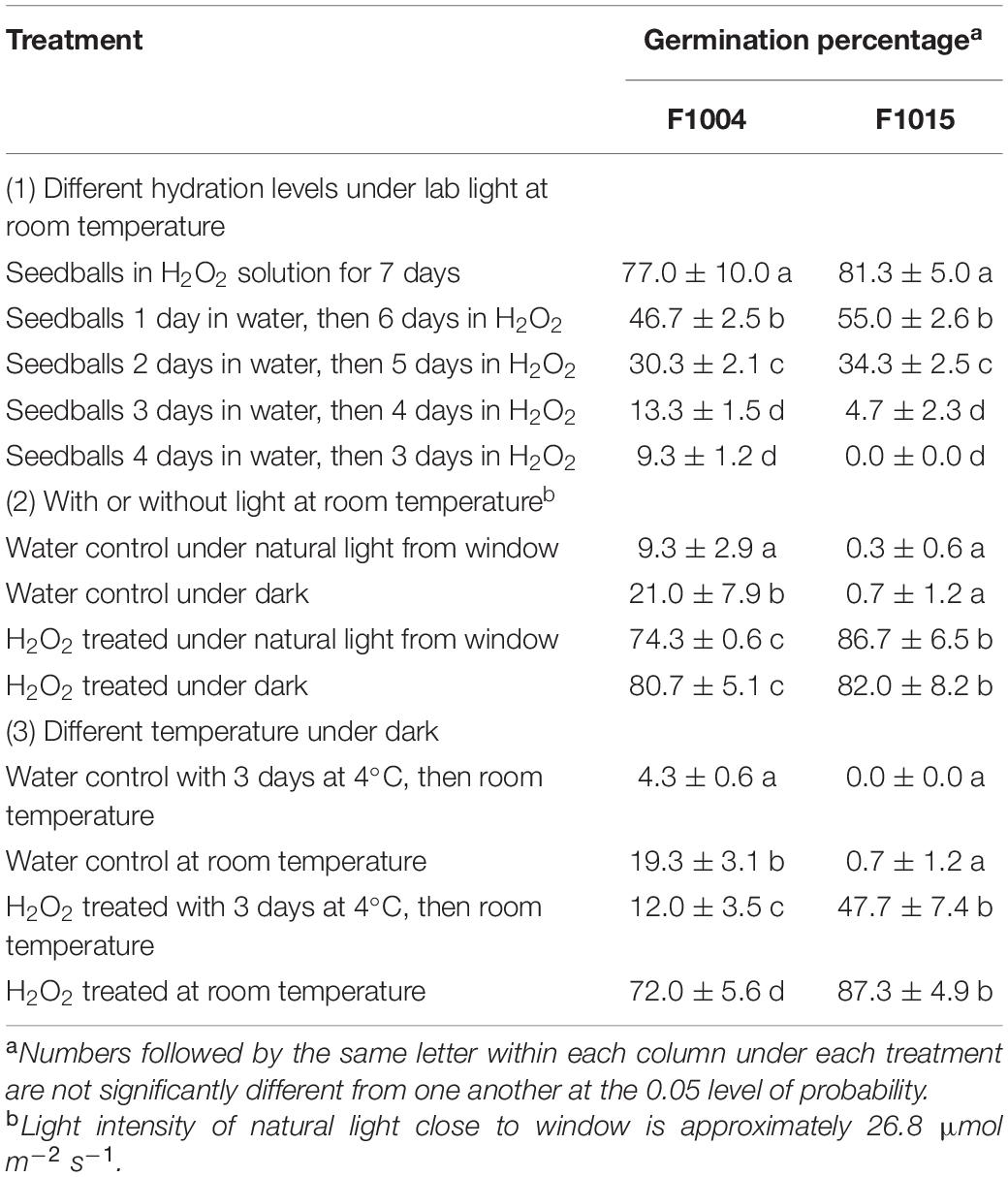
Table 2. Seedball germination to determine effects of hydration, light, and temperature on germination induced by H2O2 solution.
Dark conditions increased germination by about 10% in water-incubated F1004 seedballs but had no effect on water-incubated F1015 seedballs. When using H2O2 treatment to induce germination, dark conditions increased germination of F1004 seedballs by 6.4% but had no effect on germination of F1015 seedballs (Table 2). Therefore, dark conditions are limitedly effective in increasing germination when utilizing a H2O2 treatment, with its effectiveness dependent on genotype.
Three days of cold treatment greatly decreased germination of both lines when germinated in H2O2 solution or water (Table 2). Only 4.3% of cold-treated F1004 seedballs germinated in the water control, which was significantly reduced from the 19.3% germination observed in the water control at room temperature. With the H2O2 treatment, germination of seedballs that received the 3-days cold treatment was 12.0% in F1004 and 47.7% in F1015. These germination percentages were much lower than those (80.7 and 82.0% in F1004 and F1015, respectively) obtained with seedballs that were not cold treated (Table 2). Therefore, no cold treatment was needed when using H2O2 to induce germination. Overall, these results suggest that using H2O2 solution to continuously treat seeds for 7 days in the dark at room temperature provided the highest germination percentage.
Validation of the H2O2 Protocol to Promote Germination
The ability of H2O2 to promote germination was tested using seeds from 50 randomly selected sugarbeet lines that had been cold stored for 10–37 years. Average germination percentage of these seeds using the H2O2 treatment reached 73.7% with a range of 38.0–99.0%. This was 49.7% greater than germination observed in water controls (average at 24.0% with a range of 1.0–70.0%). Analysis by t-tests indicated that mean percentages of the two treatment were significantly different at probability level of P < 0.01 (Table 3), which proved that the H2O2 protocol significantly enhanced germination of sugarbeet. Correlation analysis found that germination percentages of the 50 lines under the two treatments were highly correlated (r = 0.715, P < 0.01, Table 3), which means the H2O2 protocol will have a better chance to improve germination if seeds were able to germinate in water incubation. Particularly, the protocol showed a promising ability of rescuing germplasms such as F1011, F1012 and F1013 that had very low germination percentages using a water incubation (Table 3).
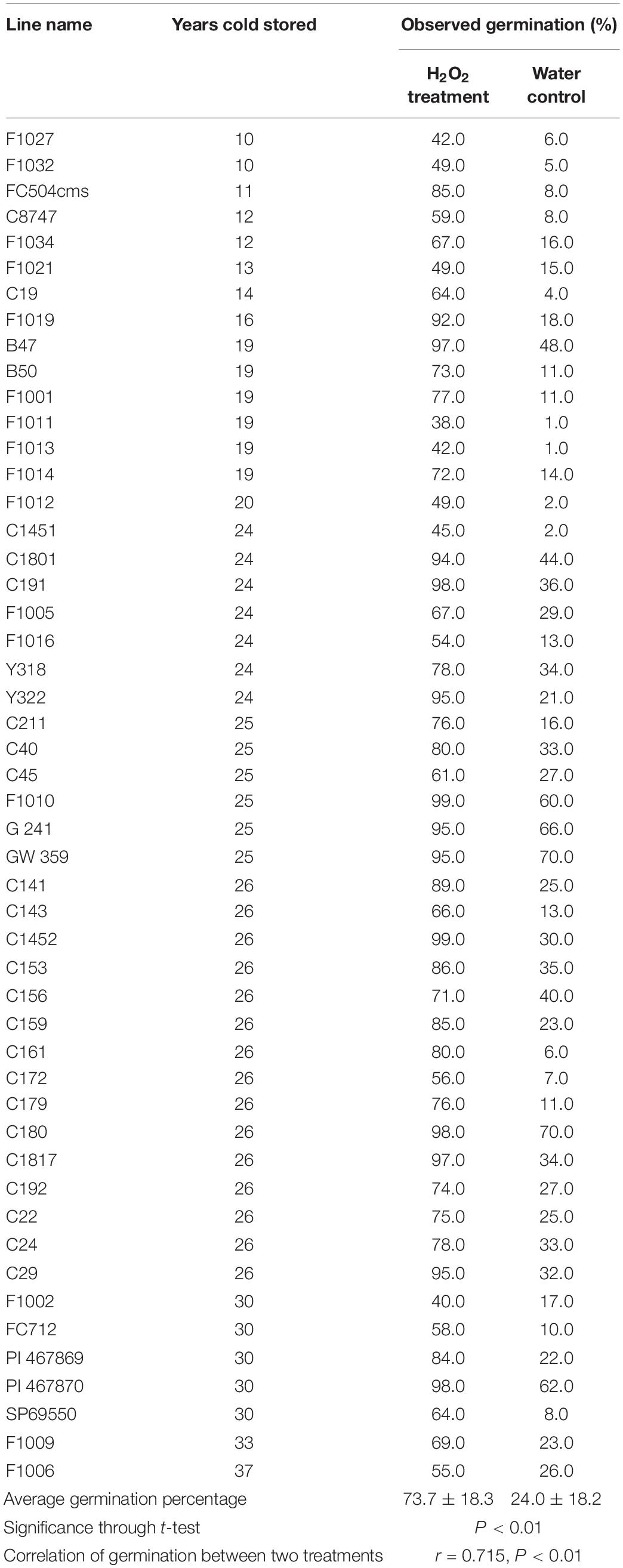
Table 3. Germination percentage of seeds of 50 sugarbeet lines that were cold stored for 10–37 years after incubation in 1% H2O2 or water for 8 days.
Germination of Individual Isolated Seeds
When germinating seeds isolated from seedballs, only 4% of F1004 seeds germinated in water incubation for 4 days (Table 4), a germination percentage that was similar to that achieved when incubating F1004 seedballs in water. However, 60% of isolated seeds of F1015 germinated when incubated in water (Table 4), which was much higher than the 0% germination of seedballs incubated in water but close to the germination percentage achieved under H2O2 treatment. Therefore, difficulties in germinating F1015 seedballs when incubated in water were mainly caused by the physical barrier of the seed coat. In contrast, the consistently low germination rate in F1004 seedballs and isolated seeds indicated that endogenous physiological dormancy was likely to be responsible for inhibiting germination.
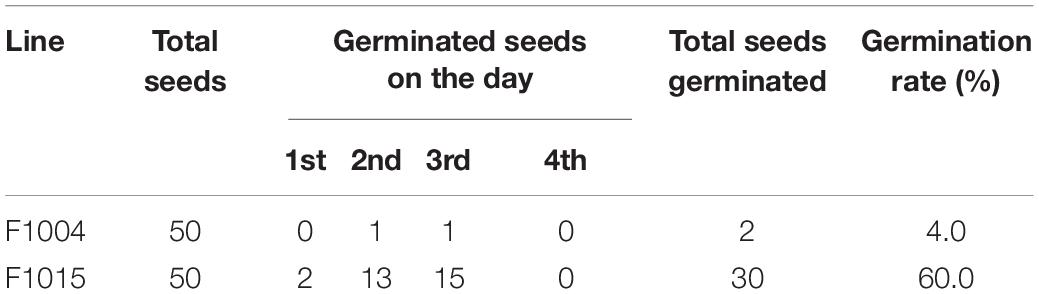
Table 4. Germination of seeds isolated from the seedballs of F1004 and F1015 in distilled water at room temperature under natural light.
Germinating Seeds for RNA-seq Analysis
Of the seedballs used for RNA-seq analysis, germination after 8 days in a H2O2 solution reached 82.0% in F1015 and 66.4% in F1004, which far exceeded the 0% germination in F1015 and the 7.6% germination in F1004 from water-incubated controls (Figure 1). Germination of seedballs in H2O2 treatment in both lines occurred predominantly on the 3rd, 4th, 5th, and 6th days, with maximum germination on the 4th day (Figure 1). This agreed with observations made in previous germination tests that seed imbibition started on the second day, germination peaked at the 3rd and 4th days and finished on the 7th and 8th days of the treatment. Therefore, seed samples on the first day of H2O2 and water treatments were collected as controls for gene expression before germination started, and samples collected on the second day of the treatment represented gene expression after imbibition began in a few seeds under each treatment. Seed samples from the 3rd and 4th days of the treatments, when germination was greatest, were pooled and presumed to have the greatest expression of genes related to germination. Pooled samples from the 5th and 6th days, and the 7th and 8th days of the treatments were collected to assist in identifying genes that diminish in expression during germination.
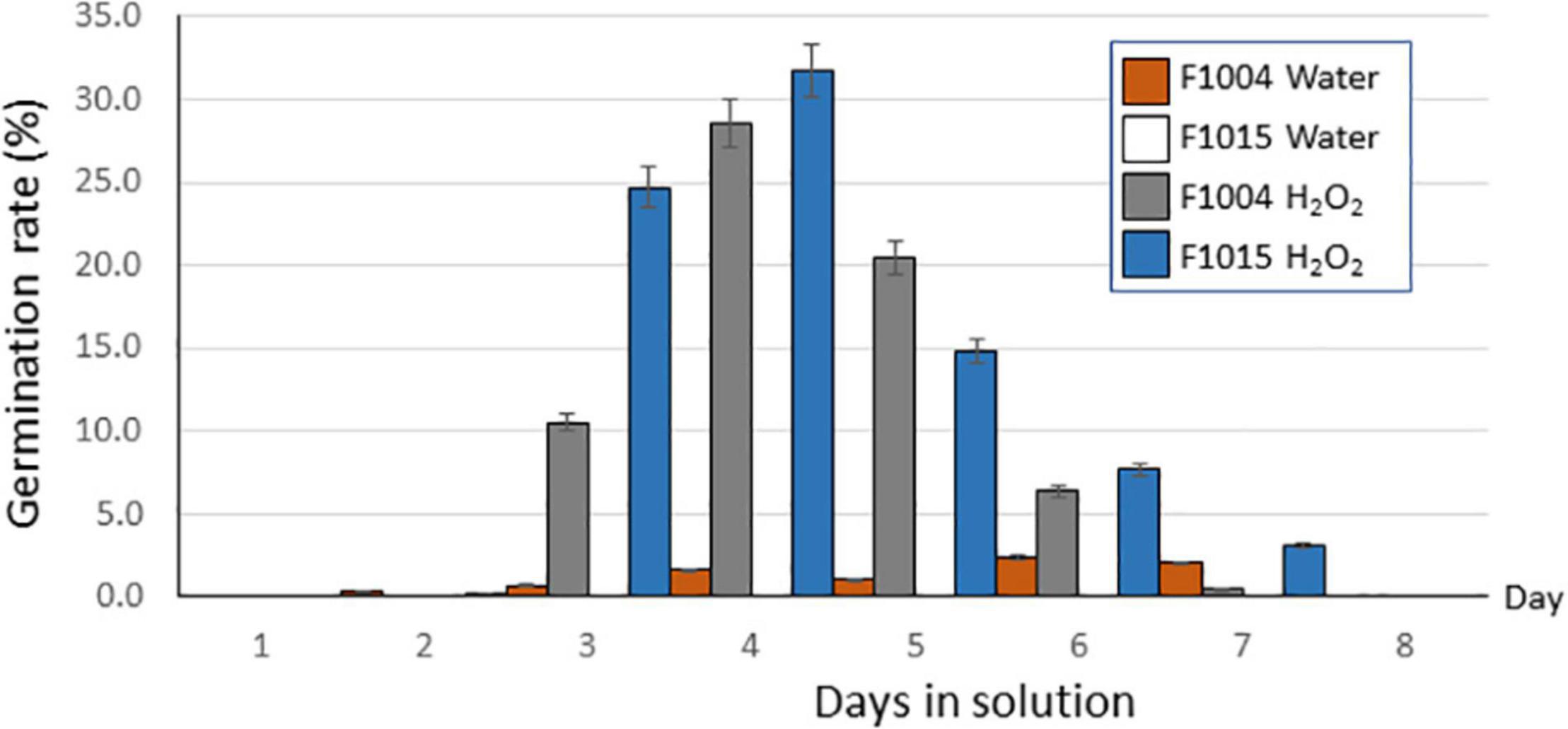
Figure 1. Percent of seeds germinated as a function of time in solution for seedballs of sugarbeet lines F1004 and F1015 that were germinated in a 1% H2O2 solution or distilled water for 8 days. No seeds of F1015 were germinated under water control.
RNA-seq Sequencing Summary
RNA extracted from the F1004 seed sample on the second day in the water control was of low quality and was removed from RNA-seq analysis. RNA extracted from the remaining 19 samples that were collected from two sugarbeet lines (F1004 and F1015) under two treatments (H2O2 treatment vs. water control) on different days (1st, 2nd, pools of 3rd and 4th, 5th and 6th, and 7th and 8th), however, were used to produce RNA sequencing libraries. A total of 86.8 Gb bases of RNA sequence was generated with an average of 4.6 Gb bases per sample. After quality trimming and filtering, 868 million clean reads remained, yielding an average of 45.7 million reads per sample. About 76.7% of the sequences obtained mapped to the sugarbeet RefBeet reference genome with 59.0% of them mapped uniquely to single location (Supplementary Table 1). Overall, a total of 26,668 genes were identified with 22,287 annotated and 4,401 that were novel genes with unknown functions.
Comparison of Gene Expression Between H2O2 Treatment and Water Control
Comparisons of transcriptome profiles from seed samples collected on different days all showed significant gene expressions difference between H2O2 treatment and water control in both F1004 and F1015 (Supplementary Figure 1). Combining all expression differences in different samples and genotypes identified a common set of 173 DEGs, of which 90 genes were expressed at higher levels in seeds treated by H2O2 and 83 genes were expressed at higher levels in seed samples from the water control (Table 5 and Supplementary Table 2). The 90 upregulated DEGs from the H2O2 treatment mostly were expressed at low levels in samples collected on the first day of the treatments but increased in samples collected on the second day and the pooled samples from the 3rd and 4th days, then declined in pooled samples for the 5th and 6th, and 7th and 8th days of the treatment (Figure 2), which mirrored germination during the 8 days of the experiment (Figure 1). An analysis of gene functions found 62 (68.9%) genes were related to growth, with 23 and 11 genes involved in the cell cycle and DNA replication, respectively, suggesting that H2O2 treatment promoted gene activities related to cell cycle and growth. Of the remaining H2O2-promoted DEGs, five were of unknown function, and 12 (13.3%) and 11 (12.2%) were related to the regulation of gene expression and stress responses, respectively. Of the DEGs related to gene expression regulation, nine were transcription regulators, two were involved in signal transduction, and one was involved in a phytohormone-signaling pathway. From the 11 DEGs related to stress responses, seven were involved in responses to oxidative stress, two were involved in defense responses, and two were involved in response to cold and osmotic stress (Table 5 and Supplementary Table 2).
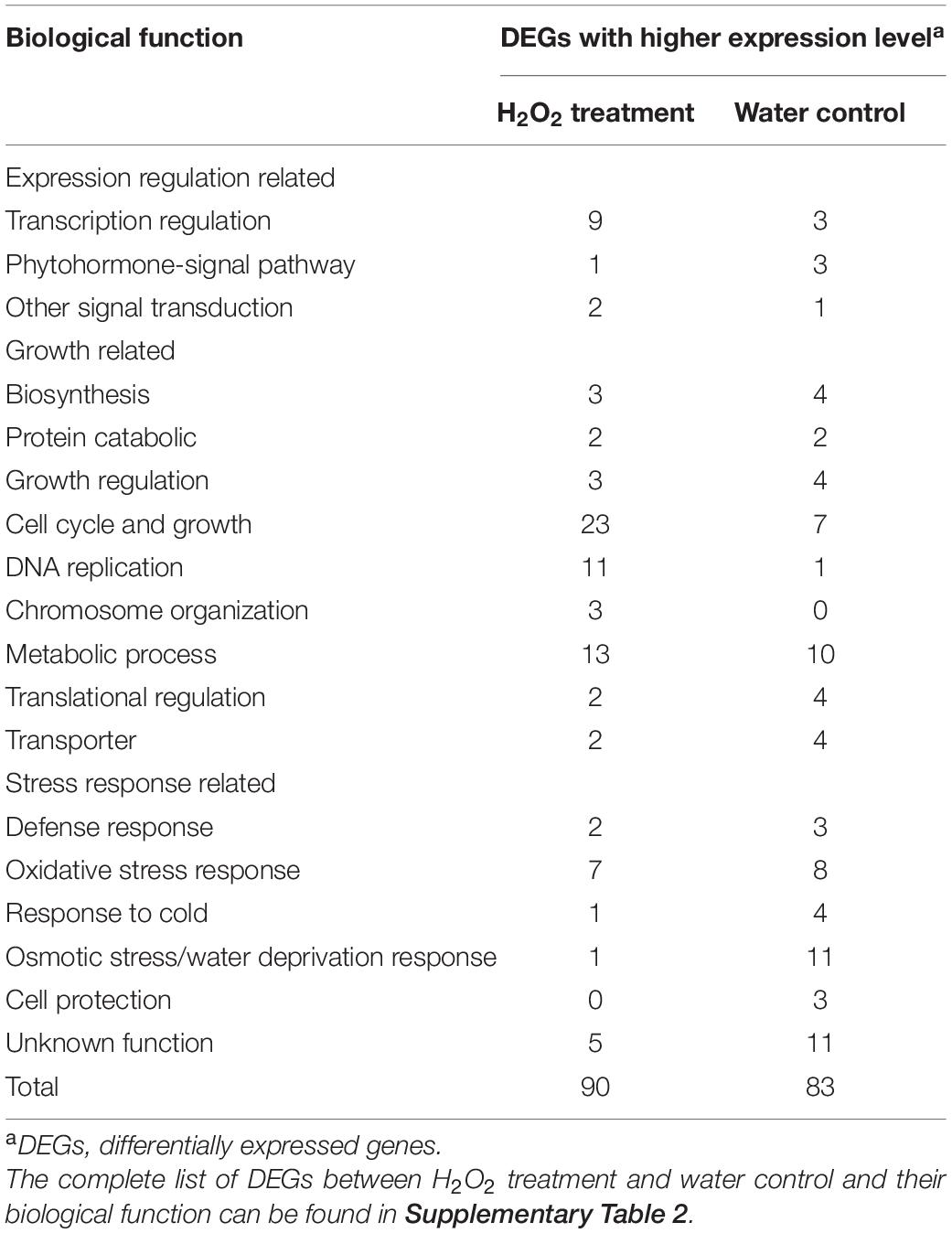
Table 5. Comparison of the biological functions of genes that were differentially expressed in seedballs germinated in 1% H2O2 solution (H2O2 treatment) or distilled water (water control).
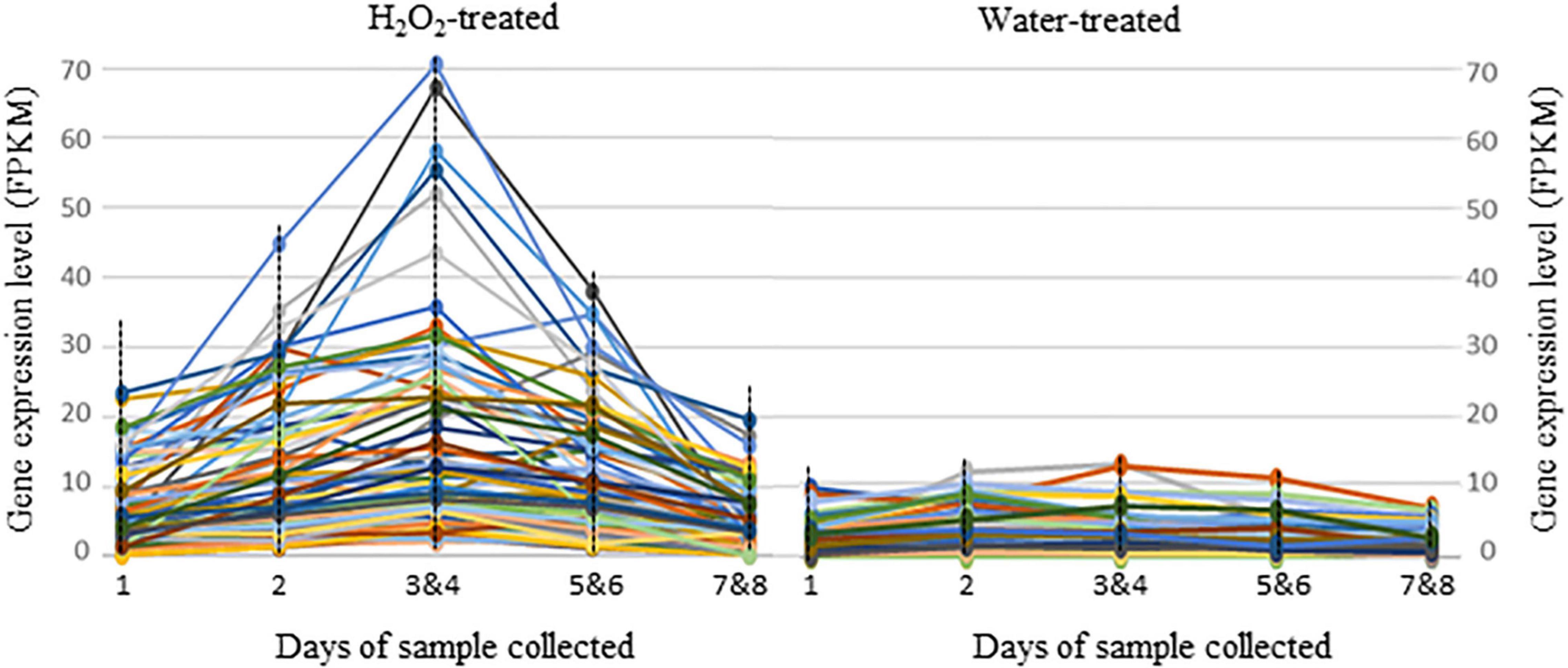
Figure 2. Expression level changes for the 90 DEGs (differentially expressed genes) that were more highly expressed in seeds of sugarbeet lines F1004 and F1015 under H2O2 treatment. The samples labeled as 1, 2, 3&4, 5&6, and 7&8 correspond to seedball samples collected on the 1st, 2nd, 3rd and 4th, 5th and 6th, and 7th and 8th days of the treatment. Lines with different colors represent different genes. Expression levels and function of genes were shown in Supplementary Table 2.
Of the 83 DEGs with greater expression in the water controls, 36 (43.4%) were related to growth, 29 (34.9%) were involved in stress responses, and seven (8.4%) were regulators of gene expression. Expression of these DEGs decreased as seeds were incubated for longer durations in either H2O2 solution or distilled water, but expression declined more rapidly in samples treated with H2O2 (Figure 3). Of the DEGs with biological functions related to stress responses, eight were involved in response to oxidative stress, four were related to response to cold, 11 were involved in response to osmotic or water deprivation stress, and three corresponded to cell protection. All of these functions suggest that transcripts that were more highly expressed in water-incubated seeds were unlikely to be genes expressed during imbibition since seedballs were not subjected to cold or water deprivation during this time. Rather, these DEGs were presumably stored mRNAs that were produced while seeds were stored under dry and cold conditions or were transcribed when water content declined during seed maturation. Expression of these DEGs progressively decreased during the duration of the experiment in seedballs under both treatments, which is also consistent with a degradation of stored mRNAs during imbibition. Therefore, the faster decline in mRNAs that were likely to be present in stored seedballs under H2O2 treatment suggests that H2O2 accelerated the transition of seeds from dormancy to germination.
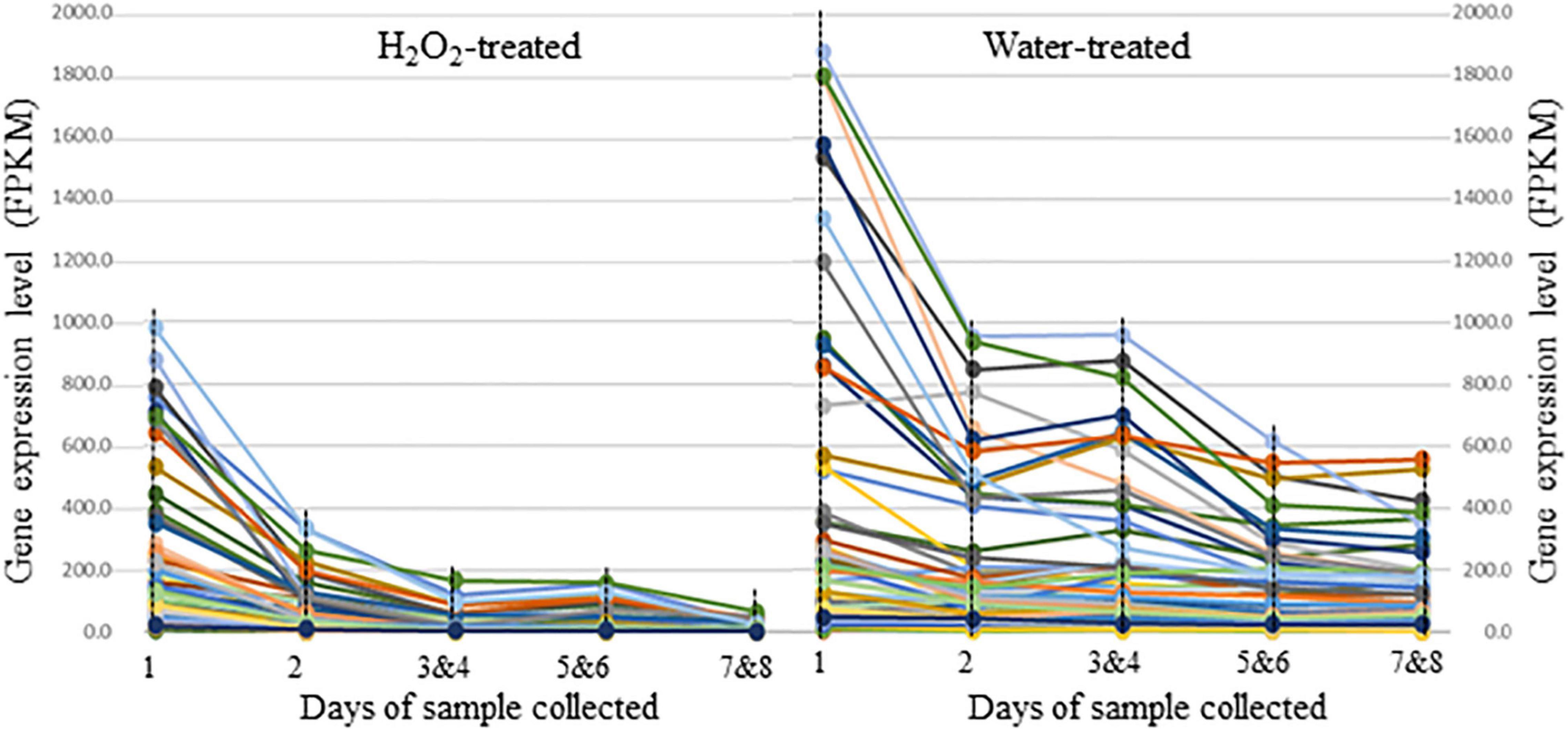
Figure 3. Expression level changes for the 83 DEGs (differentially expressed genes) that were more highly expressed in seeds of sugarbeet lines F1004 and F1015 germinated in water relative to seeds germinated in H2O2. Samples labeled as 1, 2, 3&4, 5&6, and 7&8 correspond to seedball samples that were collected on the 1st, 2nd, 3rd and 4th, 5th and 6th, and 7th and 8th days. Lines with different colors represented different genes. Expression levels and function of genes were shown in Supplementary Table 2.
Comparison of Gene Expression Between F1004 and F1015 Under H2O2 Treatment
Germination tests using isolated seeds indicated that germination was restricted by physiological dormancy in F1004 and physical dormancy in F1015. H2O2 treatment increased germination for both lines, presumably by improving water and oxygen penetration through the pericarp in F1015 and by triggering gene expression changes to overcome physiological dormancy in F1004. Therefore, comparison of gene expression between these two lines when their germination was promoted by H2O2 could reveal changes in gene expression that are related to the alleviation of physiological dormancy.
Since previous experiments found that incubating seeds in H2O2 solution for 1 day had no effect on germination and seeds that remained ungerminated after 7 days of H2O2 treatment were likely to be unviable, the transcription data from seedballs treated with H2O2 for 2–6 days in the two lines were used to identify DEGs between F1004 and F1015. In total, 370 DEGs were detected between the two lines with 101 and 269 DEGs more highly expressed in F1015 and F1004, respectively (Table 6 and Supplementary Table 3). The significantly higher number of DEGs in F1004 suggests that overcoming physiological dormancy required alteration of a greater number of gene activities than were needed to overcome the physical dormancy of F1015.
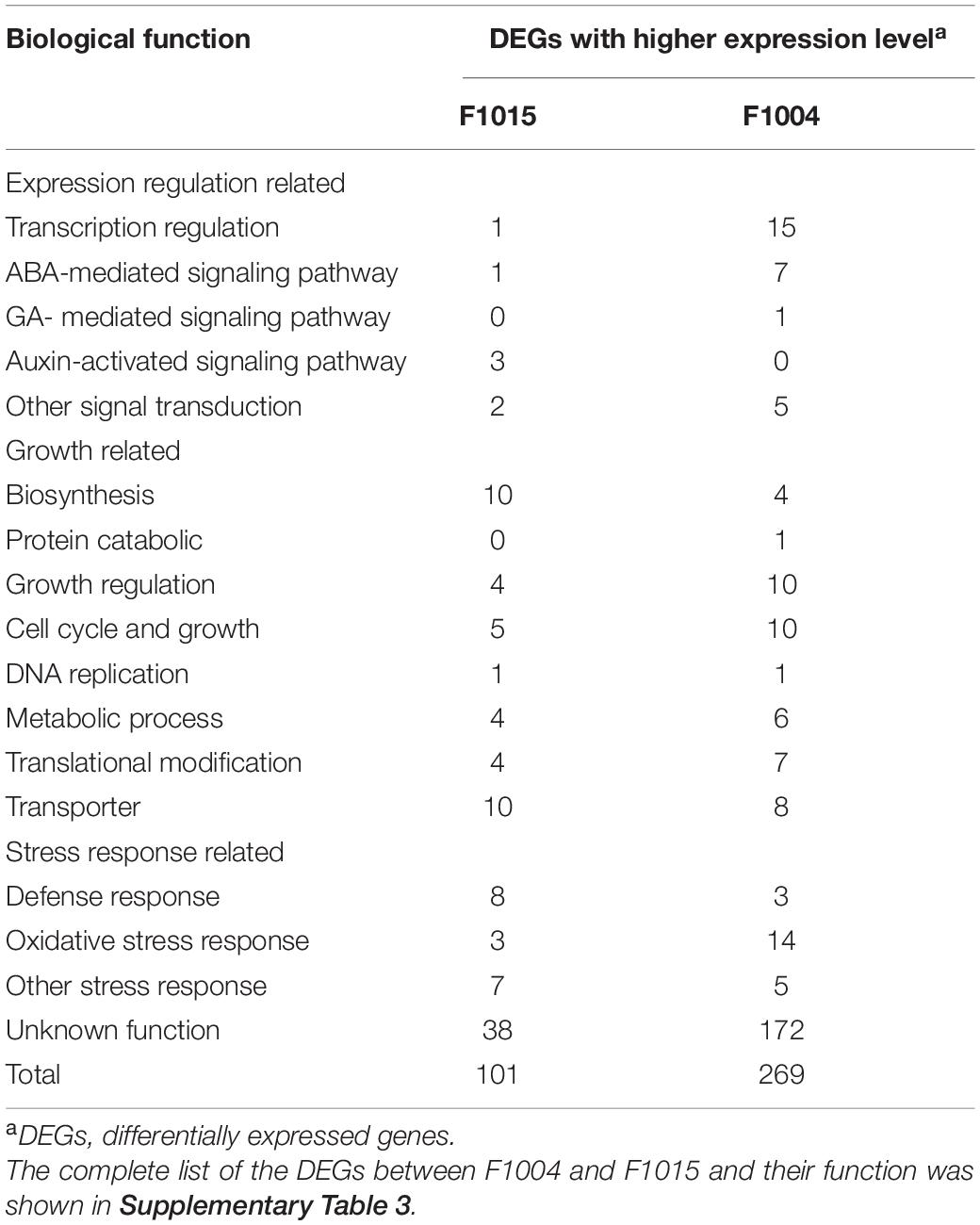
Table 6. Comparison of the biological functions of genes that were differentially expressed in seedballs of sugarbeet lines F1004 and F1015 during germination induced using a 1% H2O2 solution.
Analysis of DEGs by biological function indicated a large difference between the two lines in genes involved in gene expression regulation. For example, F1004 had 28 upregulated DEGs involved in expression regulation of which 15 were transcription regulators, seven were involved in the ABA-mediated signaling pathway, one was involved in the GA-mediated signaling pathway, and five were involved in other signal transduction processes. In contrast, only seven DEGs that were involved in gene expression regulation were expressed at a higher level in F1015, including one transcription regulator, one involved in ABA-mediated signaling, three that were involved in auxin-activated signaling pathways, and two involved in other signal transduction processes (Table 6 and Supplementary Table 3). Overall, this suggests that ABA-mediated signaling pathways, along with a significant number of transcription regulators, played important roles in cell regulation toward overcoming physiological dormancy.
Another significant difference in gene expression between the two lines were the number of DEGs involved in responses to oxidative stress with 14 expressed at higher levels in F1004 but only three that were more highly expressed in F1015. The H2O2 treatment created an oxidative environment for both lines, but the greater number of genes in line F1004 involved in response to oxidative stress indicates that some oxidative stress response genes may participate in regulating germination rather than merely providing cell protection against oxidative stress. In addition, 38 and 172 DEGs were non-annotated with unknown functions in F1015 and F1004, respectively (Table 6 and Supplementary Table 3). The larger number of unknown DEGs in F1004 provides further evidence that release of physiological dormancy requires more complex changes in gene transcription than release of physical dormancy.
Discussion
Germination is a key developmental stage in a plant’s life cycle. This research demonstrated that incubating sugarbeet seeds in a 1% H2O2 solution for 7 days or more at room temperature in the dark maximizes germination percentage. Research also established that H2O2 treatment can break both physical and physiological dormancies. By using this protocol, germination percentage in 50 sugarbeet lines were significantly increased (Table 3). Acceptable germination was also achieved in germinating an additional 2,000 sugarbeet germplasms through this method (data not shown). Additionally, H2O2 treatment greatly improved germination of some wheat and sunflower lines that germinated poorly in water, although post-germination root growth of wheat and sunflower was inhibited by H2O2 (data not shown). Lower H2O2 concentrations and shorter treatment times reduced the harmful effects of H2O2 in these species and indicate that H2O2 concentration and treatment time need to be optimized for each plant species to avoid damage to young seedlings by H2O2. Fortunately, no harmful effects of H2O2 were observed when germinating sugarbeet seeds, although seedlings of a few sugarbeet genotypes showed signs of damage if germinated seeds remained in H2O2 solution beyond 7 days. Thus, timely transplanting the established seedlings into soil is necessary to minimize the toxic effects of H2O2.
Cold treatment is normally considered helpful for breaking seed dormancy and Yamauchi et al. (2004) found that GA biosynthesis in Arabidopsis thaliana seeds was likely stimulated through upregulating the cold-inducible GA biosynthesis genes GA3ox2 and GA3ox2 under a low temperature. However, this research indicated that low temperature was not helpful for breaking dormancy of sugarbeet seeds and greatly reduced the effect of H2O2 in promoting germination. This finding may be due to lower catalase activity at low temperature that reduces H2O2 catabolism as reported in MacRae and Ferguson (1985). Soaking seeds in water before planting has been recommended by Habib (2010) for enhancing sugarbeet germination in fields, suggesting that an increase in seed hydration may be helpful for germination. However, in our studies, incubation in water for 1–3 days prior to H2O2 treatment impaired the ability of H2O2 to promote germination. As the seeds used in this research germinated poorly in water due to their strong dormancy, viability of some seeds may be lost during water pre-incubation due to the extension of the incubation period, which thus reduces germination by delaying H2O2 treatment. Light is normally considered as a factor that influences germination in many plant species (Shinomura, 1997), and this research also found that darkness increased germination of F1004 seeds in water incubation (Table 2). But for all seeds treated with H2O2 incubation, no significant effect of darkness in enhancing germination was observed. It is possible that H2O2 has the overwhelm effect of promoting germination and makes effect of darkness for germination unobservable. Overall, using the H2O2 protocol from this research for germinating seeds, there was no need to pretreat seeds with low temperature or a water incubation, but darkness is recommended. An additional benefit of using H2O2 to promote germination is its antimicrobial properties (McDonnell, 2014) that protect germinating seeds from pathogens. Similar protective effects of H2O2 against pathogens were also made by Schopfer et al. (2001) and Morkunas et al. (2004). Therefore, the protocol using H2O2 to enhance germination developed here will be useful for rescuing germplasms of sugarbeet or other plant species that have been exposed to pathogens.
Normally, dry quiescent seeds resume metabolic activity during imbibition. However, the seedballs of the two sugarbeet lines used in this research had very low germination percentage when incubated in distilled water. Preincubating seedballs in distilled water greatly reduced germination even when immediately followed by H2O2 incubation. In contrast, seedballs consistently incubated in a H2O2 solution had much improved germination, establishing that H2O2 promotes imbibition and alters gene expression to encourage germination. Comparison of gene transcription profiles of seedballs treated with H2O2 or water also found that the majority of DEGs that were more highly expressed under H2O2 treatment were related to growth activities with many involved in cell proliferation, DNA replication and metabolism. In addition, twelve DEGs that were upregulated in H2O2 treated seedballs participated in gene expression regulation with nine genes encoding transcription regulators and two genes involved in signal transduction processes (Table 5 and Supplementary Table 2). This suggests that exogenous H2O2 promotes the regulation of gene expression toward germination. However, it is unknown whether endogenous H2O2 contributes to the expression of genes related to germination under H2O2 treatment. Further studies, therefore, are needed to separate the external and internal effects of H2O2 on sugarbeet seed germination to characterize the mechanism by which H2O2 regulates gene expression and initiates germination.
In contrast, of the 83 DEGs that were more highly expressed in water-treated seedballs, 29 genes were involved in stress responses, with 24 DEGs contributing to responses to cold, osmotic stress, oxidative stress, and water deprivation and four involved in cell protection (Table 5 and Supplementary Table 2). Since no cold, osmotic or water deprivation stress occurred during germination in this study, the DEGs that were more highly expressed in water-treated samples were likely mRNAs synthesized prior to or during cold storage of the seeds. The continuous reduction of these DEGs during H2O2 or water treatment provides further indication that they were mRNAs stored in seeds. A much sharper reduction of these stored mRNAs in H2O2 treated seedballs suggests that the faster degradation of these mRNAs improved germination. Therefore, seed dormancy and germination are not only affected by transcriptional regulation during germination, but also by the degradation of mRNAs stored in seeds as has been previously observed in sunflower by El-Maarouf-Bouteau et al. (2015).
The faster degradation of mRNAs in H2O2 treated seedballs may be largely due to oxidative damage. Under the oxidative conditions created by incubating in a H2O2 solution, oxidative damage to mRNA can occur due to mRNA cellular localization, its single-stranded structure, and a lack of mRNA repair mechanisms in cells (Kong and Lin, 2010). Oxidative damage to stored mRNA can inhibit protein synthesis and protein degradation, thereby interrupting the processes that maintain dormancy as well as acting as a positive signal to initiate processes related to germination (El-Maarouf-Bouteau et al., 2013; Chmielowska-Bak et al., 2015). However, we found the level of stored mRNAs was greatly reduced in seeds on the first day of H2O2 treatment relative to the corresponding sample in the water control (Figure 3), even though germination was not improved for seeds incubated in H2O2 for only 1 day. This indicates that the degradation of mRNA alone is not sufficient to overcome seed dormancy.
Germination tests using individual sugarbeet seeds isolated from the woody seedballs found that poor germination under water treatment in F1015 was primarily due to the physical dormancy imposed by the dense inner layer of pericarp, whereas the low germination of F1004 was mostly caused by physiological dormancy of the embryo that inhibited germination. This research established that H2O2 breaks both physical and physiological dormancies. Overcoming physical dormancy with H2O2 and ROS has been demonstrated in lettuce since exogenous ROS increase the percentage of endosperm caps that ruptured during germination (Zhang et al., 2014). Oxidative reactions involving proteins and other intercellular substances are believed to loosen cell walls (Wojtyla et al., 2016) and promote water and oxygen penetration through the mechanic barriers of the seed. Mechanisms by which H2O2 treatment breaks physiological dormancy, however, are largely unknown.
A comparison of transcripts in H2O2-treated seed samples of F1004 and F1015 provided insight into genes that may be involved in overcoming physiological dormancy. F1004 had more upregulated DEGs than F1015 which suggests that alleviating physiological dormancy involves more gene activities and biological processes than alleviating physical dormancy (Table 6 and Supplementary Table 3). Of all DEGs between the two lines, F1004 had 28 DEGs involved in transcription regulation, ABA-mediated signaling pathways, GA-mediated signaling pathways and other signal transduction processes, whereas F1015 only had seven upregulated DEGs related to gene expression regulation. More DEGs involved in ABA or GA-mediated signaling pathways in F1004 indicates that the well-known action between ABA and GA plays a role in releasing physiological dormancy and initiating germination. The greater prevalence of DEGs related to transcription regulators in F1004 also indicate that H2O2 was involved in gene expression regulatory changes that assisted in breaking physiological dormancy. Several studies have found that H2O2 directly acts as a signaling molecule and participates in crosstalk with phytohormones to regulate germination (Barba-Espín et al., 2010; El-Maarouf-Bouteau et al., 2015). Therefore, H2O2 interactions with phytohormones likely have a role in regulating gene expression to promote the transition of F1004 seeds from dormancy to germination.
Another notable difference between F1004 and F1015 was the number of DEGs involved in oxidative stress responses. Of these DEGs, 14 were highly expressed in F1004 and only three were more highly expressed in F1015. Since H2O2 treatment released physical dormancy of F1015 seeds, gene expression in H2O2-treated F1015 seeds was expected to be similar to gene activities that occurred in seeds without dormancy during germination. The three upregulated DEGs in F1015 that were involved in responding to oxidative stress, therefore, were likely to contribute to cell protection against the oxidative conditions of H2O2 treatment. In contrast, the significantly greater number of DEGs for oxidative responses in F1004 may contribute to regulating oxidation levels in seeds to allow them to maintain the “oxidative window” that is critical for germination as proposed by Bailly et al. (2008) in addition to their role in cell protection responses. In addition, there were 172 unannotated DEGs that were more highly expressed in F1004. Annotation of these genes would be potentially helpful to further understanding mechanisms by which sugarbeet seeds overcome physiological dormancy.
Conclusion
This research developed an effective protocol to enhance sugarbeet germination by continuously incubating seedballs in a 1% H2O2 solution in the dark for 7 days. This method provides a useful method for rescuing plant germplasms with poor germination. Gene expression analysis indicated that H2O2 treatment not only promoted gene activities related to cell proliferation and growth, but also led to faster degradation of mRNAs stored in seeds to promote the transition from seed dormancy to germination. Comparison of transcripts during germination in seeds of F1004 and F1015 with physiological and physical dormancies, respectively, found a greater number of DEGs were involved in overcoming physiological dormancy of F1004, with many DEGs related to regulating gene expression and responses to oxidative stress. This finding suggests that regulating gene expression along with adjusting the oxidative level in seeds played key roles in breaking the physiological dormancy of this sugarbeet line.
Data Availability Statement
The datasets presented in this study can be found in online repositories. The names of the repository/repositories and accession number(s) can be found below: National Center for Biotechnology Information (NCBI) BioProject database under accession number PRJNA787080 (https://www.ncbi.nlm.nih.gov/bioproject/PRJNA787080/).
Author Contributions
CC designed the project, conducted bench work and data analysis, and prepared the manuscript. RP conducted bench work. MB involved project design and reviewed the manuscript. KF helped on data analysis and revised the manuscript. All authors contributed to the article and approved the submitted version.
Funding
This research was supported by the Beet Sugar Development Foundation (BSDF), and the USDA-ARS CRIS project No. 3060-21000-044-000-D. Mention of trade names or commercial products in this article is solely for the purpose of providing specific information and does not imply recommendation or endorsement by the US Department of Agriculture. The US Department of Agriculture is an equal opportunity provider and employer.
Conflict of Interest
The authors declare that the research was conducted in the absence of any commercial or financial relationships that could be construed as a potential conflict of interest.
Publisher’s Note
All claims expressed in this article are solely those of the authors and do not necessarily represent those of their affiliated organizations, or those of the publisher, the editors and the reviewers. Any product that may be evaluated in this article, or claim that may be made by its manufacturer, is not guaranteed or endorsed by the publisher.
Supplementary Material
The Supplementary Material for this article can be found online at: https://www.frontiersin.org/articles/10.3389/fpls.2022.888519/full#supplementary-material
Supplementary Figure 1 | Plots of transcriptome comparisons through Poisson Distribution method between the paired treatments of incubating seedballs of F1004 and F1015 in H2O2 solution and water. For each of plots, X-axis represents the value of log2 transformed mean expression level, Y-axis represents value of log2 transformed expression level fold change calculated from dividing level of H2O2 treatment with that in water control. Red dots represent up-regulated DEGs in H2O2 treatment, blue dots represent down-regulated ones, and gray points represent non-DEGs.
Footnotes
- ^ https://www.rdocumentation.org/packages/stats/versions/3.6.2/topics/aov
- ^ https://www.rdocumentation.org/packages/agricolae/versions/1.3-5/topics/LSD.test
- ^ https://github.com/BGI-flexlab/SOAPnuke
- ^ https://www.ncbi.nlm.nih.gov/genome/?term=Beta%20Vulgaris
- ^ https://stat.ethz.ch/R-manual/R-devel/library/stats/html/Hypergeometric.html
- ^ https://www.ncbi.nlm.nih.gov/
References
Altschul, S. F., Madden, T. L., Schäffer, A. A., Zhang, J., Zhang, Z., Miller, W., et al. (1997). Gapped BLAST and PSI-BLAST: a new generation of protein database search programs. Nucleic Acids Res. 25, 3389–3402. doi: 10.1093/nar/25.17.3389
Aoki-Kinoshita, K. F., and Kanehisa, M. (2007). Gene annotation and pathway mapping in KEGG. Methods Mol. Biol. 396, 71–91. doi: 10.1007/978-1-59745-515-2_6
Audic, S., and Claverie, J. M. (1997). The significance of digital gene expression profiles. Genome Res. 7, 986–995. doi: 10.1101/gr.7.10.986
Bahin, E., Bailly, C., Sotta, B., Kranner, I., Corbineau, F., and Leymarie, J. (2011). Crosstalk between reactive oxygen species and hormonal signaling pathways regulates grain dormancy in barley. Plant Cell Environ. 34, 980–993. doi: 10.1111/j.1365-3040.2011.02298.x
Bailly, C. (2004). Active oxygen species and antioxidants in seed biology. Seed Sci. Res. 14, 93–107. doi: 10.1079/SSR2004159
Bailly, C. (2019). The signaling role of ROS in the regulation of seed germination and dormancy. Biochem. J. 476, 3019–3032. doi: 10.1042/BCJ20190159
Bailly, C., El-Maarouf-Bouteau, H., and Corbineau, F. (2008). From intracellular signaling networks to cell death: the dual role of reactive oxygen species in seed physiology. C. R. Biol. 331, 806–814. doi: 10.1016/j.crvi.2008.07.022
Barba-Espín, G., Diaz-Vivancos, P., Clemente-Moreno, M. J., Albacete, A., Faize, L., Faize, M., et al. (2010). Interaction between hydrogen peroxide and plant hormones during germination and the early growth of pea seedlings. Plant Cell Environ. 33, 981–994. doi: 10.1111/j.1365-3040.2010.02120.x
Baskin, J. M., and Baskin, C. C. (2004). A classification system for seed dormancy. Seed Sci. Res. 14, 1–16. doi: 10.1079/SSR2003150
Bewley, J. D., and Black, M. (1994). Seeds. Physiology of Development and Germination. New York, NY: Plenum Press.
Bray, C. M., and West, C. E. (2005). DNA repair mechanisms in plants: crucial sensors and effectors for the maintenance of genome integrity. New Phytol. 168, 511–528. doi: 10.1111/j.1469-8137.2005.01548.x
Campbell, L. G., and Bugbee, W. M. (1984). Development of sugarbeet germplasm lines resistant to storage rot. Farm Res. 41, 18–19.
Campbell, L. G., Anderson, A. W., and Dregseth, R. J. (2000). Registration of F1015 and F1016 sugarbeet germplasm with resistance to the sugarbeet root maggot. Crop Sci. 40, 867–868. doi: 10.2135/cropsci2000.403863x
Carrera-Castaño, G., Calleja-Cabrera, J., Pernas, M., Gómez, L., and Oñate-Sánchez, L. (2020). An updated overview on the regulation of seed germination. Plants (Basel) 9:703. doi: 10.3390/plants9060703
Chmielowska-Bak, J., Izbianska, K., and Deckert, J. (2015). Products of lipid, protein and mRNA oxidation as signals and regulators of gene expression. Front. Plant Sci. 6:405. doi: 10.3389/fpls.2015.00405
Chomontowski, C., and Podlaski, S. (2020). Impact of sugar beet seed priming using the SMP method on the properties of the pericarp. BMC Plant Biol. 20:32. doi: 10.1186/s12870-020-2246-4
Coumans, M., Côme, D., and Gaspar, T. (1976). Stabilized dormancy in sugarbeet fruits. I. Seed coats as a physicochemical barrier to oxygen. Bot. Gazette 137, 274–278. doi: 10.1086/336870
Davies, M. J. (2005). The oxidative environment and protein damage. Biochim. Biophys. Acta 1703, 93–109. doi: 10.1016/j.bbapap.2004.08.007
Diaz-Vivancos, P., Barba-Espín, G., and Hernández, J. A. (2013). Elucidating hormonal/ROS networks during seed germination: insights and perspectives. Plant Cell Rep. 32, 1491–1502. doi: 10.1007/s00299-013-1473-7
Dohm, J. C., Minoche, A. E., Holtgräwe, D., Capella-Gutiérrez, S., Zakrzewski, F., Tafer, H., et al. (2014). The genome of the recently domesticated crop plant sugar beet (Beta vulgaris). Nature 505, 546–549. doi: 10.1038/nature12817
El-Maarouf-Bouteau, H., and Bailly, C. (2008). Oxidative signaling in seed germination and dormancy. Plant Signal. Bechav. 3, 175–182. doi: 10.4161/psb.3.3.5539
El-Maarouf-Bouteau, H., Meimoun, P., Job, C., Job, D., and Bailly, C. (2013). Role of protein and mRNA oxidation in seed dormancy and germination. Front. Plant Sci. 4:77. doi: 10.3389/fpls.2013.00077
El-Maarouf-Bouteau, H., Sajjad, Y., Bazin, J., Langlade, N., Cristescu, S. M., Balzergue, S., et al. (2015). Reactive oxygen species, abscisic acid and ethylene interact to regulate sunflower seed germination. Plant Cell Environ. 38, 364–374. doi: 10.1111/pce.12371
Emery, D. E. (1988). Seed Propagation of Native California Plants. Santa Barbara, CA: Santa Barbara Botanic Garden.
Fehlmann, T., Reinheimer, S., Geng, C., Su, X., Drmanac, S., Alexeev, A., et al. (2016). cPAS-based sequencing on the BGISEQ-500 to explore small non-coding RNAs. Clin. Epigenet. 8:123. doi: 10.1186/s13148-016-0287-1
Finch-Savage, W. E., and Leubner-Metzger, G. (2006). Seed dormancy and the control of germination. New Phytol. 171, 501–523. doi: 10.1111/j.1469-8137.2006.01787.x
Finkelstein, R., Reeves, W., Ariizumi, T., and Steber, C. (2008). Molecular aspects of seed dormancy. Annu. Rev. Plant Biol. 59, 387–415. doi: 10.1146/annurev.arplant.59.032607.092740
Fontaine, O., Huault, C., Pavis, N., and Billard, J. P. (1994). Dormancy breakage of Hordeum vulgare seeds: effect of hydrogen peroxide and stratification on glutathione level and glutathione reductase activity. Plant Physiol. Biochem. 32, 677–683.
Golldack, D., Li, C., Mohan, H., and Probst, N. (2013). Gibberellins and abscisic acid signal crosstalk: living and developing under unfavorable conditions. Plant Cell Rep. 32, 1007–1016. doi: 10.1007/s00299-013-1409-2
Graeber, K., Nakabayashi, K., Miatton, E., Leubner-Metzgber, G., and Soppe, W. J. J. (2012). Molecular mechanisms of seed dormancy. Plant Cell Environ. 35, 1769–1786. doi: 10.1111/j.1365-3040.2012.02542.x
Habib, M. (2010). Sugarbeet (Beta vulgaris L.) seed pre-treatment with water and HCl to improve germination. Afr. J. Biotechnol. 9, 1338–1342.
Hermann, K., Meinhard, J., Dobrev, P., Linkies, A., Pesek, B., Heß, B., et al. (2007). 1-Aminocyclopropane-1-carboxylic acid and abscisic acid during the germination of sugar beet (Beta vulgaris L.): a comparative study of fruits and seeds. J. Exp. Bot. 58, 3047–3060. doi: 10.1093/jxb/erm162
Ignatz, M., Hourston, J. E., Turečková, V., Strnad, M., Meinhard, J., Fischer, U., et al. (2019). The biochemistry underpinning industrial seed technology and mechanical processing of sugar beet. Planta 250, 1717–1729. doi: 10.1007/s00425-019-03257-5
Ishibashi, Y., Aoki, N., Kasa, S., Sakamoto, M., Kai, K., Tomokiyo, R., et al. (2017). The interrelationship between abscisic acid and reactive oxygen species plays a key role in barley seed dormancy and germination. Front. Plant Sci. 8:275. doi: 10.3389/fpls.2017.00275
Job, C., Rajjou, L., Lovigny, Y., Belghazi, M., and Job, D. (2005). Patterns of protein oxidation in Arabidopsis seeds and during germination. Plant Physiol. 138, 790–802. doi: 10.1104/pp.105.062778
Katzman, L. S., Taylor, A. G., and Langhans, R. W. (2001). Seed enhancements to improve spinach germination. Hort Sci. 36, 979–981. doi: 10.21273/hortsci.36.5.979
Keeley, J. E. (1991). Seed germination and life history syndromes in the California chaparral. Bot. Rev. 57, 91–116.
Kim, D., Langmead, B., and Salzberg, S. L. (2015). HISAT: a fast spliced aligner with low memory requirements. Nat. Methods 12, 357–360. doi: 10.1038/nmeth.3317
Kong, L., Zhang, Y., Ye, Z., Liu, X., Zhao, S., Wei, L., et al. (2007). CPC: assess the protein-coding potential of transcripts using sequence features and support vector machine. Nucleic Acids Res. 35, W345–W349. doi: 10.1093/nar/gkm391
Kong, Q. M., and Lin, C. G. (2010). Oxidative damage to RNA: mechanisms, consequences and diseases. Cell. Mol. Life Sci. 67, 1817–1829. doi: 10.1007/s00018-010-0277-y
Kranner, I., Roach, T., Beckett, R. P., Whitaker, C., and Minibayeva, F. V. (2010). Extracellular production of reactive oxygen species during seed germination and early seedling growth in Pisum sativum. J. Plant Physiol. 167, 805–811. doi: 10.1016/j.jplph.2010.01.019
Lariguet, P., Ranocha, P., De Meyer, M., Barbier, O., Penel, C., and Dunand, C. (2013). Identification of a hydrogen peroxide signalling pathway in the control of light-dependent germination in Arabidopsis. Planta 238, 381–395. doi: 10.1007/s00425-013-1901-5
Li, B., and Dewey, C. N. (2011). RSEM: accurate transcript quantification from RNA-Seq data with or without a reference genome. BMC Bioinformatics 12:323. doi: 10.1186/1471-2105-12-323
Liu, Y., Ye, N., Liu, R., Chen, M., and Zhang, J. (2010). H2O2 mediates the regulation of ABA catabolism and GA biosynthesis in Arabidopsis seed dormancy and germination. J. Exp. Bot. 61, 2979–2990. doi: 10.1093/jxb/erq125
MacRae, E., and Ferguson, I. (1985). Changes in catalase activity and hydrogen peroxide concentration in plants in response to low temperature. Physiol. Plant. 65, 51–56. doi: 10.1111/j.1399-3054.1985.tb02358.x
Martin, J., and Wang, Z. (2011). Next-generation transcriptome assembly. Nat. Rev. Genet. 12, 671–682. doi: 10.1038/nrg3068
McDonnell, G. (2014). The Use of Hydrogen Peroxide for Disinfection and Sterilization Applications. PATAI’S Chemistry of Functional Groups. Hoboken, NJ: John Wiley & Sons, Ltd, 1–34. doi: 10.1002/9780470682531.pat0885
McGrath, J., Derrico, C. A., Morales, M., Copeland, L. O., and Christenson, D. R. (2000). Germination of sugar beet (Beta vulgaris L.) seed submerged in hydrogen peroxide and water as a means to discriminate cultivar and seedlot vigor. Seed Sci. Technol. 28, 607–620.
Morkunas, I., Bednarski, W., and Kozłowska, M. (2004). Response of embryo axes of germinating seeds of yellow lupine to Fusarium oxysporum. Plant Physiol. Biochem. 42, 493–499. doi: 10.1016/j.plaphy.2004.05.007
Müller, K., Hess, B., and Leubner-Metzger, G. (2007). “A role for reactive oxygen species in endosperm weakening,” in Seeds: Biology, Development and Ecology, eds S. Adkins, S. Ashmore, and S. Navie (Wallingford: CAB International), 287–295. doi: 10.1079/9781845931971.0287
Müller, K., Tintelnot, S., and Leubner-Metzger, G. (2006). Endosperm-limited Brassicaceae seed germination: abscisic acid inhibits embryo-induced endosperm weakening of Lepidium sativum (cress) and endosperm rupture of cress and Arabidopsis thaliana. Plant Cell Physiol. 47, 864–877. doi: 10.1093/pcp/pcj059
Nambara, E., Okamoto, M., Tatematsu, K., Yano, R., Seo, M., and Kamiya, Y. (2010). Abscisic acid and the control of seed dormancy and germination. Seed Sci. Res. 20, 55–67. doi: 10.1017/S0960258510000012
Pertea, M., Pertea, G. M., Antonescu, C. M., Chang, T., Mendell, J. T., and Salzberg, S. L. (2015). StringTie enables improved reconstruction of a transcriptome from RNA-seq reads. Nat. Biotechnol. 33, 290–295. doi: 10.1038/nbt.3122
Rajjou, L., Duval, M., Gallardo, K., Catusse, J., Bally, J., Job, C., et al. (2012). Seed germination and vigor. Ann. Rev. Plant Biol. 63, 507–533. doi: 10.1146/annurev-arplant-042811-105550
Raven, P. H., Evert, R. F., and Eichhorn, S. E. (2005). Physiology of Seed Plants: Plant Nutrition and Soils. Biology of Plants, 7th Edn. New York, NY: WH Freeman and Company.
Richard, G., Raymond, P., Corbineau, F., and Pradet, A. (1989). Effect of the pericarp on sugar beet (Beta vulgaris L.) seed germination: study of the energy metabolism. Seed Sci. and Technol. 17, 485–498.
Rodríguez, M. V., Barrero, J. M., Corbineau, F., Gubler, F., and Benech-Arnold, R. L. (2015). Dormancy in cereals (not too much, not so little): about the mechanisms behind this trait. Seed Sci. Res. 25, 99–119. doi: 10.1017/S0960258515000021
Schopfer, P., Plachy, C., and Frahry, G. (2001). Release of reactive oxygen intermediates (superoxide radicals, hydrogen peroxide, and hydroxyl radicals) and peroxidase in germinating radish seeds controlled by light, gibberellin, and abscisic acid. Plant Physiol. 125, 1591–1602. doi: 10.1104/pp.125.4.1591
Shinomura, T. (1997). Phytochrome regulation of seed germination. J Plant Res. 110, 151–161. doi: 10.1007/bf02506854
Shu, K., Liu, X. D., Xie, Q., and He, Z. H. (2016). Two faces of one seed: hormonal regulation of dormancy and germination. Mol. Plant 9, 34–45. doi: 10.1016/j.molp.2015.08.010
Tohidloo, G., Taleghani, D. F., Chegini, S., Chegini, M. A., Farzad, P., Khodaie, A. H., et al. (2015). Effect of polishing and washing on germination quality and viability of sugar beet seed. Int. J. Biosci. 6, 209–215. doi: 10.12692/ijb/6.2.209-215
Trapnell, C., Roberts, A., Goff, L., Pertea, G., Kim, D., Kelley, D. R., et al. (2012). Differential gene and transcript expression analysis of RNA-seq experiments with TopHat and Cufflinks. Nat. Protoc. 7, 562–578. doi: 10.1038/nprot.2012.016
Verma, G., Mishra, S., Sangwan, N., and Sharma, S. (2015). Reactive oxygen species mediate axis-cotyledon signaling to induce reserve mobilization during germination and seedling establishment in Vigna radiata. J. Plant Physiol. 184, 79–88. doi: 10.1016/j.jplph.2015.07.001
Weitbrecht, K., Müller, K., and Leubner-Metzger, G. (2011). First on the mark: early seed germination. J. Exp. Bot. 62, 3289–3309. doi: 10.1093/jxb/err030
Wojtyla, Ł, Lechowska, K., Kubala, S., and Garnczarska, M. (2016). Different modes of hydrogen peroxide action during seed germination. Front. Plant Sci. 7:66. doi: 10.3389/fpls.2016.00066
Yamauchi, Y., Ogawa, M., Kuwahara, A., Hanada, A., Kamiya, Y., and Yamaguchi, S. (2004). Activation of gibberellin biosynthesis and response pathways by low temperature during imbibition of Arabidopsis thaliana seeds. Plant Cell 166, 367–378. doi: 10.1105/tpc.018143
Young, M. D., Wakefield, M. J., Smyth, G. K., and Oshlack, A. (2010). Gene ontology analysis for RNA-seq: accounting for selection bias. Genome Biol. 11:R14. doi: 10.1186/gb-2010-11-2-r14
Zeinalabedini, M., Majourhat, K., Hernández, J. A., and Martínez-Gómez, P. (2009). Breaking seed dormancy in long-term stored seeds from Iranian wild almond species. Seed Sci. Technol. 37, 267–275. doi: 10.15258/sst.2009.37.2.01
Keywords: sugar beet, seed dormancy, RNA-Seq, gene expression, differentially expressed gene, transcript analysis
Citation: Chu C, Poore RC, Bolton MD and Fugate KK (2022) Mechanism of Sugarbeet Seed Germination Enhanced by Hydrogen Peroxide. Front. Plant Sci. 13:888519. doi: 10.3389/fpls.2022.888519
Received: 02 March 2022; Accepted: 28 March 2022;
Published: 25 April 2022.
Edited by:
Petr Smýkal, Palacký University Olomouc, CzechiaReviewed by:
Martin Cerny, Mendel University in Brno, CzechiaJana Balarynová, Palacký University Olomouc, Czechia
Juan Pablo Renzi, Instituto Nacional de Tecnología Agropecuaria, Argentina
Copyright © 2022 Chu, Poore, Bolton and Fugate. This is an open-access article distributed under the terms of the Creative Commons Attribution License (CC BY). The use, distribution or reproduction in other forums is permitted, provided the original author(s) and the copyright owner(s) are credited and that the original publication in this journal is cited, in accordance with accepted academic practice. No use, distribution or reproduction is permitted which does not comply with these terms.
*Correspondence: Chenggen Chu, Y2hlbmdnZW4uY2h1QHVzZGEuZ292