- 1Centre for Integrative Conservation, Xishuangbanna Tropical Botanical Garden, Chinese Academy of Sciences, Beijing, China
- 2University of Chinese Academy of Sciences, Beijing, China
- 3School of Biological and Chemical Sciences, Pu’er University, Pu’er, China
Bacteria communities associated with plants have been given increasing consideration because they are arguably beneficial to their host plants. To understand the ecological and evolutionary impact of these mutualistic associations, it is important to explore the vast unknown territory of bacterial genomic diversity and their functional contributions associated with the major branches of the tree-of-life. Arguably, this aim can be achieved by profiling bacterial communities by applying high throughput sequencing approaches, besides establishing model plant organisms to test key predictions. This study utilized the Illumina Miseq reads of bacterial 16S rRNA sequences to determine the bacterial diversity associated with the endosphere of the leaves of the highly specialized rock spleenwort Asplenium delavayi (Aspleniaceae). By documenting the bacterial communities associated with ferns collected in natural occurrence and cultivation, this study discovered the most species-rich bacterial communities associated with terrestrial ferns reported until now. Despite the substantial variations of species diversity and composition among accessions, a set of 28 bacterial OTUs was found to be shared among all accessions. Functional analyses recovered evidence to support the predictions that changes in bacterial community compositions correspond to functional differentiation. Given the ease of cultivating this species, Asplenium delavayi is introduced here as a model organism to explore the ecological and evolutionary benefits created by mutualistic associations between bacteria and ferns.
Introduction
Advancements in DNA sequencing technologies enable not only the study of the plant genomes (Marks et al., 2021) including the exploration of plant pan-genomes (Bayer et al., 2020), but also to unravel the cryptic contribution of arguably ubiquitous endophytic phytomicrobiomes—also called the plant endosphere or the holobiome of plants—to the adaption of land plants to their environment (Vorholt, 2012; Smith et al., 2017; Lyu et al., 2021; Cernava and Berg, 2022). By affecting traits of their host plants positively, some endophytic microorganisms, e.g., bacteria and fungi, support the functionality of ecological networks and the evolutionary success of land plants (Vandenkoornhuyse et al., 2015; Chen et al., 2020; Compant et al., 2020; Harrison and Griffin, 2020; Lyu et al., 2021; Tan et al., 2021). Furthermore, endophytic microbiomes have been increasingly considered to be treasure chests to discover bioactive metabolites (Gouda et al., 2016; Ek-Ramos et al., 2019; Wu et al., 2021; Cernava and Berg, 2022), to promote sustainable agriculture (Chen Q. L. et al., 2021; French et al., 2021), and to counteract the impact of the global climate change (Suryanarayanan and Shaanker, 2021; Perreault and Laforest-Lapointe, 2022). Unfortunately, the holobiome of the vast majority of plants is still awaiting to be discovered, which applies particularly to the bacterial diversity in the endo-phyllosphere—meaning the niche spaces inside the leaves of land plants (Vorholt, 2012; Khare et al., 2018; Cordovez et al., 2019; Harrison and Griffin, 2020; Chaudhry et al., 2021; Hawkes et al., 2021; Chialva et al., 2022). Many studies considered bacterial taxa enhancing plant defense (Matsumoto et al., 2021; Cernava and Berg, 2022; Shalev et al., 2022) besides other plant growth-promoting functions (Glick, 2012; Backer et al., 2018; Leontidou et al., 2020), but current studies expanded their focus on a wide range of ecological aspects such as the contribution of bacterial communities to the rapid adaption to local environments enforced by bacterial communities (Li et al., 2021) that coincide with diversification patterns (Abedlfattah et al., 2021) as argued in the SYMPHY proposal (Tripp et al., 2017) and the Plant Holobiont Theory (Lyu et al., 2021). Notable aspects studied include the enhanced resistance of plants to environmental stresses supported by endophytic bacteria, e.g., salt tolerance (Vaishnav et al., 2019) and heavy metal tolerance (Zhu et al., 2014; Xu et al., 2016; Gu et al., 2018; Banach et al., 2020; Yang et al., 2020; Antenozio et al., 2021) or even support the adaptation to extreme environments, e.g., deserts (Zhang et al., 2019) and karst habitats (Li F. et al., 2019). Endophytic bacteria have been also considered as promoters of horizontal gene transfer (Tiwari and Bae, 2020; Zilber-Rosenberg and Rosenberg, 2021). Finally, some endophytic bacteria associated with land plants may even play an essential role in mitigation of climate active gases such as isoprene and chloromethane to the atmosphere by forming communities degrading these gases within the phyllosphere (Crmbie et al., 2018; Jaeger et al., 2018; Kroeber et al., 2021).
Considering the rationale that mutualistic interactions between bacteria and plants provide unknown benefits, the study was designed to explore this untapped source by evaluating the prediction that the endo-phyllosphere of ferns hosts mostly unknown but highly diverse endophytic bacterial communities. These associations are expected to provide undiscovered benefits supporting the evolutionary success of this ancient plant lineage. This rationale considers recent findings supporting the hypothesis that bacteria associations of plants are constrained by genotypic and environmental factors (Morella et al., 2020), including plant neighborhood (Meyer et al., 2022) but also phylogenetic relationships (Harrison and Griffin, 2020; Lajoie and Kembel, 2021). An extensive review of published studies confirmed the lack of small number of surveys preventing careful analyses of ubiquity and trends in the microbial communities associated with the phyllosphere of ferns (see Supplementary Table 1). Arguably, the best-studied microbiomes of ferns are associated with the water fern Azolla filiculloides (Dijkhuzien et al., 2017; Banach et al., 2020), the arsenic hyperaccumulator Pteris vittata (Zhu et al., 2014; Xu et al., 2016; Gu et al., 2018; Yang et al., 2020; Antenozio et al., 2021), and the xeric fern Pellaea calomelanos (Mahlangu and Serepa-Dlamini, 2018a,b; Tshishonga and Serepa-Dlamini, 2019, 2020; Diale et al., 2021). The later studies explicitly focused on the genomes of cultivated endophytic bacteria, whereas other studies explored specifically antibacterial properties (Das et al., 2017a,b,c, 2018), growth-promoting abilities (Mukherjee et al., 2017; Rakshit and Sukul, 2021a,b), and ecological aspects in general (Jackson et al., 2006; de Araújo-Barros et al., 2010; Li F. et al., 2019). Enhancing our understanding of the endophytic bacterial communities are expected to specify the evolutionary history of the origin of the bacterial proteins such as Tma12 recovered in some fern genomes ferns arguably as a consequence of horizontal gene transfer (Li F. W. et al., 2019; Yadav et al., 2019). In summary, understanding the microbial communities associated with ferns will supply crucial information to understand the interactions between ferns and their environment, and significantly contributes to studies aiming to trace the origin of bacterial protein blueprints occurring in fern genomes.
To tackle this pivotal gap in our understanding of fern biology, we intend to establish a fern model system to explore the ecological and evolutionary aspects of endophyllospheric bacterial communities. To achieve this, we employed a metabarcoding approach applying high throughput sequencing of 16S ribosomal RNA as now widely utilized in metagenomic studies of microbial communities (Langille et al., 2013; Xia et al., 2020) to identify the bacterial diversity associated with the leaf endosphere of the poorly known spleenwort Asplenium delavayi (Franch.) Copel. This morphologically distinct but rare species forms dense stands on mossy rock and ledges in canyons (Figure 1A). The distribution range includes southwestern China and adjacent regions in Bhutan, Northern India, Laos, Myanmar, and Thailand. The small-sized ferns produce several densely clustered leaves with a simple, orbicular to reniform shaped lamina that has a thick herbaceous texture (Figure 1A). Vegetative reproduction via proliferous roots facilitates not only the establishment of dense clusters in its natural habitats but also enable easy proliferation of individuals in culture conditions (Figure 1B). Relatively little is known about the ecology of this fern, but our own observations suggest a rapid turn-over of leaves in response to water stress during the dry season. Given the arguably stressful environment, a rich but highly variable community of endophytic bacteria is expected to occur in natural populations with the prominent bacterial representatives shared.
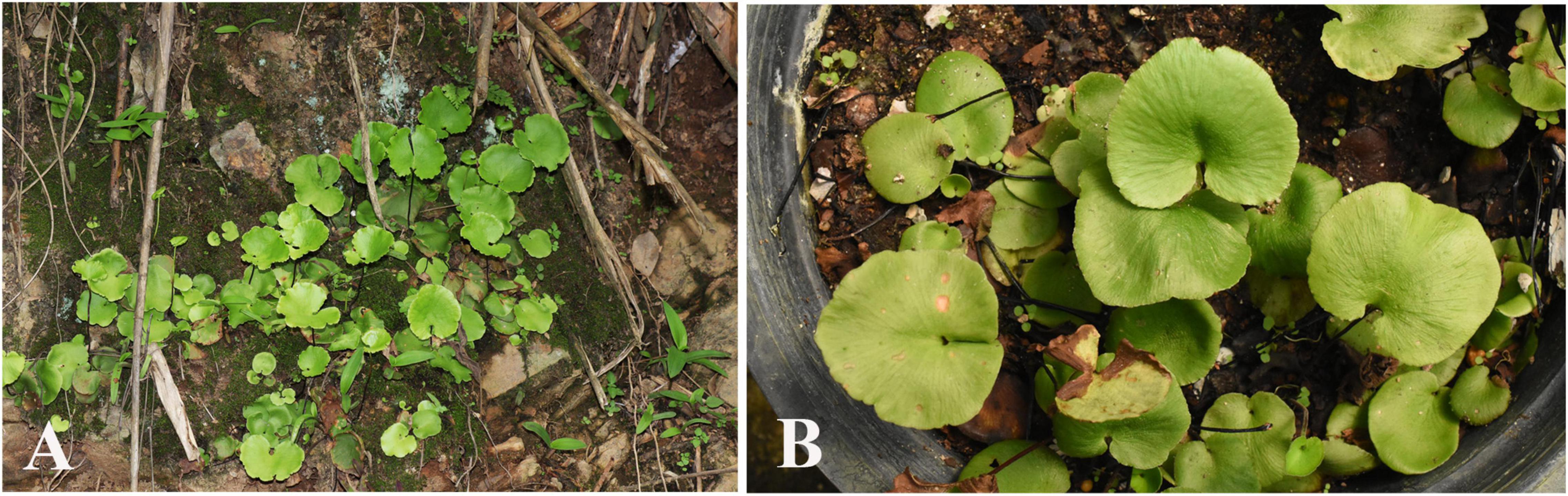
Figure 1. Sporophytes of the introduced model fern organism Asplenium delavayi (A) natural occurrences in Yunnan, (B) cultivated individuals at XTBG.
By generating a library of bacterial sequences of a variable region of the 16S rRNA gene, we scrutinized evidence to support or reject the following research predictions. (1) The leaves of this fern host a diverse community composed mainly of bacterial lineages previously reported to be associated with the holobiome of plants. (2) Despite distinct differences in the composition of the bacterial communities, a set of bacteria occurs in all accessions. (3) Differences in the microbiome coincides with functional differentiation. Finally, we aim to establish a protocol to explore the secrets of the leaf endosphere by using a cost-effective high throughput sequencing approach in combination with existing DNA databases and interpretation tools introduced to study microbial communities in general (Langille et al., 2013; Louca et al., 2016).
Materials and Methods
Sample Collection Protocol
The endosphere microbiota associated with the leaves of Asplenium delavayi were assessed using the high-throughput sequencing approach employing the MiSeq Illumina platform (Illumina, United States). Leaf samples were collected from two natural occurrences, accession AD0615 located in Yuanjiang County, Yuxi Prefecture, Yunnan, China (23°50′38.7″, 102°7′49.6″″, alt. 1,250 m) and accession AD0618 from Mojiang County, Pu’er Prefecture, Yunnan, China (23°33′12″, 101°33′8″, alt. 1,410 m). For comparison, we sampled leaves from plants cultivated at Xishuangbanna Tropical Botanical Garden, Chinese Academy of Sciences (accession number AD0620). No specific permissions were required for sampling in any location, nor were any endangered or protected species involved. Only a few leaves were sampled for each accession to avoid destroying the local populations, with one individual removed per location as a voucher deposited at the herbarium of XTBG (HITBC). For accessions AD0615 and AD0618, four subsamples were obtained by randomly collecting four individuals (1 to 4) per each forementioned locations. For each subsample, three leaves were aseptically cut by an ethanol sterilized scalpel, quickly put in sterile Ziploc plastic bags, and transferred on ice to our laboratory for further processing. For the cultivated accession AD0620, three leaves were sampled using the same procedure. To remove epiphylls, surface sterilization was carried out before microbial DNA extraction. Samples were sequentially washed with sterile Millipore water (30 s), followed by immersion in 70% (v/v) ethanol (2 min), 2.5% sodium hypochlorite solution (5 min) supplemented with 0.1% Tween 80, 70% (v/v) ethanol (30 s), and finally rinsing the samples five times with sterile Millipore water. We spread the last rinse water onto solidified LB media and incubated for 48 hours at 30°C to check if the sterilization was effective. If contamination was found, the surface sterilization procedure was repeated with some modifications of sterilant concentration. Finally, approximately 2.0 g of surface-sterilized leaves per sample were homogenized by portioning the samples into small fragments using a sterile scalpel and macerating them in sterile 10 mM phosphate saline (PBS) buffer (130 mM NaCl, 7 mM Na2HPO4, 3 mM NaH2PO4, pH 7.4).
Library Preparation
Total genomic DNA was extracted from the surface-sterilized samples using the E.Z.N.A.™ Mag-Bind Soil DNA Kit (Omega, United States), following the manufacturer’s instructions. Each extraction’s DNA concentration and integrity were determined by using Qubit v.3.0 (Invitrogen, United States) and 1% agarose gel electrophoresis, respectively. To avoid amplification of chloroplast 16S sequences, we took advantage of the modified primer pair 799F/1492R specifically designed to mistarget the host plant organelle 16S RNA (Chelius and Triplett, 2001). PCR amplifications were carried out with the described mixtures—a final volume of 30 μL included 20 ng of gDNA mixed with 1μL of each primer at 10 μM and 12.5 μL of the 2 × Hieff® Robust PCR Master Mix (Yeasen, Shanghai, China) and filled with 9 μL of PCR grade water—and the program as DNA denaturing at 94°C for 3 min, followed by 5 cycles of denaturing at 95°C for 30 s, annealing at 45°C for 30 s, elongation at 72°C for 30 s, then 20 cycles of denaturing at 95°C for 30 s, annealing at 55°C for 30 s, elongation at 72°C for 30 s and a final extension at 72°C for 5 min. PCR products were prepared for 16S sequencing following the Illumina Miseq system library preparation recommended protocol1. Second-round PCR was performed using the barcoded V5-V7 primers with Illumina adapters. The PCR products were purified using the AMPure XP magnetic beads and then pair-end sequenced using the MiSeq Illumina platform (Illumina, United States) at Sangon Biotech Co., Ltd. (Shanghai, China), according to the manufacturer’s instructions.
Data Analyses
Raw Illumina reads were trimmed and filtered, applying a sliding window approach with a window size of 10 and a minimum quality of 20 as implemented in Trimmomatic v0.38 (Bolger et al., 2014). These reads were further processed by removing adapter sequences, and sequence pairs with one or both reads shorter than 200 bp using Cutadapt v 1.18 (Martin, 2011), followed by sequence assembling by employing Pear v0.9.8 (Zhang et al., 2014), and finally processed with Mothur v.1.43.0 (Schloss et al., 2009; Kozich et al., 2013). PCR and sequencing errors were reduced by removing ambiguous bases and homopolymers of more than eight nucleotides. The assembled sequences were aligned against the Silva SSU database release 138.1 (Quast et al., 2013) and de-noised by subjecting to a 2% pre-clustering allowing for up to 4 differences between sequences to reduce the contribution of possible sequencing errors. UCHIME (Edgar, 2013) was employed to remove potential chimeric sequences with > 3% errors before the remaining sequences were clustered into operational taxonomic units (OTUs) using a 97% identity threshold implemented in UC LUST (Wang et al., 2020). Raw sequencing data were deposited in NCBI’s Sequence Read Archive (SRA) in BioProject ID PRJNA794952. All recognized OTUs were classified into phylum, class, order, family, genus, and species levels using the Ribosomal Database Project Release 11.5 (Wang et al., 2007). We further analyzed the data with the packages phyloseq (McMurdie and Holmes, 2013) and vegan v. 2.5-7 (Oksanen et al., 2020) in R v3.6.0 (Core Development Team R, 2020). To examine the microbial communities associated with A. delavayi leaf samples, filtered reads were pooled into OTUs to identify the bacterial representatives. Rarefaction analyses using rarefy in vegan v. 2.5.-7 (Oksanen et al., 2020) were carried out to assess the richness and uniformity of the species composition. Phylogenetic relationships were estimated for all samples by conducting maximum likelihood analyses using RAxML v.8.2 (Stamatakis, 2014). The observed microbial diversity was explored by calculating five indices commonly used to assess microbial diversity by employing the mothur pipeline (Schloss et al., 2009; Kozich et al., 2013). The utilized indices were: ACE, Chao 1, Good’s Coverage, Shannon Diversity Index, and Simpson Diversity Index. The taxonomic distribution of microbial communities was visualized using the “ggplot2” v. 3.3.5 (Wickham et al., 2021), whereas Venn diagrams were generated to visualize the numbers of shared and unique OTUs among the three accessions. To contrast the species composition and functional differentiation among the nine libraries, the metagenomic functional content of the microbial communities was observed using the FAPROTAX 1.2.4 (Louca et al., 2016) and PICRUSt package (Langille et al., 2013) utilizing the KEGG database (Kanehisa et al., 2016, 2021) and COG databases (Tatusov et al., 2000). Principal coordinate analyses were carried out to detect differentiation between samples based on species composition or functional trait composition. The observed lineages of endophytic bacteria were taken into context of previously reported association of bacteria and ferns (Supplementary Table 1), including their plant association status as documented in PLaBAse v.1.01 (Patz et al., 2021).
Results
The total number of 16S bacterial sequences per sample ranged from 33,821 (AD0618-1) to 55,387 for AD0618-2 (Table 1), accumulating to a total number of sequences of 408,903. These sequences represented 831 OTUs, of which 28 were shared among the nine studied samples (Table 2). The proportion of these 28 shared OTUs varied between 51.4% (AD0620-1) to 99.2% (AD0615-1). Sequencing depth was sufficient to characterize the bacterial communities of all samples, given the graphs leveled off for both OTU rarefaction (Figure 2A) and Shannon-Index rarefaction (Figure 2B). The Good’s coverage of bacterial OTUs was consistently 1.0 for all samples studied (Table 1). Ignoring chloroplast sequences and unclassified sequences, the OTUs were assigned to 19 phyla, 34 classes, 74 orders, and 153 families (Table 3). A total of 30.63% of the OTUs and 3.72% of the sequences were not classified up to the family level (Table 4). Out of the 19 phyla, only four namely Actinobacteria, Bacteroidetes, Firmicutes, and Proteobacteria, were found across all accessions, and each included more than 10% of the OTUs recovered (Table 3 and Supplementary Tables 1, 2). These four phyla contributed 90% or more of the total number of sequences obtained (Tables 1, 3).
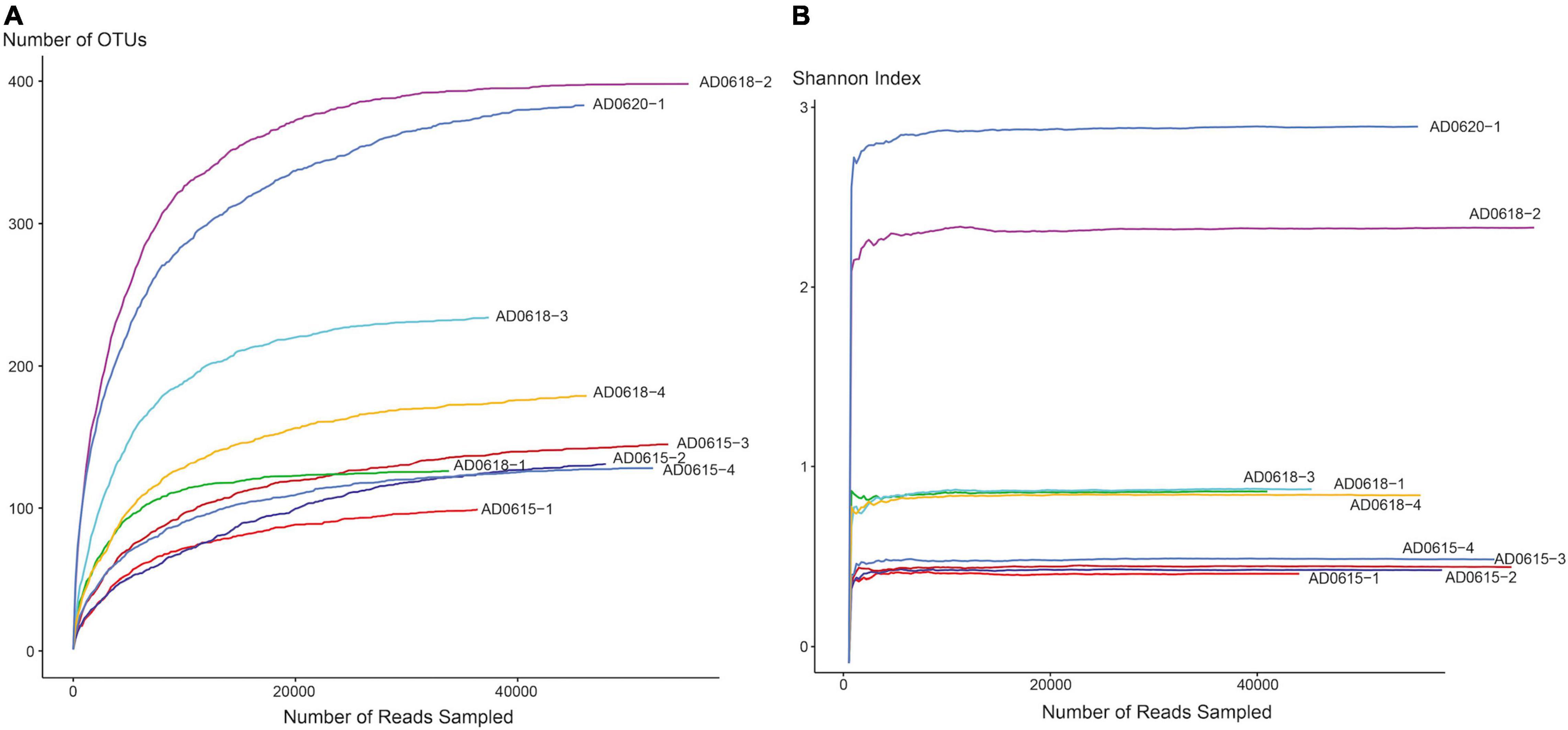
Figure 2. Rarefaction curves for OTU (A) and Shannon-Index (B) of leaf-associated bacterial communities in nine accessions of Asplenium delavayi. The X-axis shows the number of reads, whereas Y-axis shows the accumulation of the number of OTUs, and the increase of Shannon Index, respectively, for each of the nine accessions studied. Endpoints of graphs correspond to the number of reads per sample.
The four subsamples of accession AD0615 showed highly similar bacterial diversity as documented by the average values and standard errors of four biodiversity indices, namely Shannon-Index = 0.52 ± 0.02, ACE-Index = 138.49 ± 12.38, Chao-Index 136.92 ± 0 = 13.08, and Simpson-Diversity Index = 0.85 ± 0.003. These samples show a notable dominance by Firmicutes with an average contribution of 92.91% ± 0.12 (Table 1). In contrast, subsamples of AD0618 showed increased variation in the bacterial diversity as documented by average values of the four biodiversity indices, namely Shannon-Index = 1.28 ± 0.36, ACE-Index = 2377.44 ± 58.39, Chao-Index 2,377.44 ± 58.01, and Simpson-Diversity0Index = 0.65 ± 0.10. These values coincided with a highly reduced contribution of Firmicutes with an average contribution of 82.40% ± 5.13. The cultivated accession AD0620 strongly diverged from the two wild collections (Table 1), especially by the strong decline of Firmicutes with 49.80% (Table 1).
In total, 28 OTUs were shared among all samples obtained, although their frequency varied substantially (Table 1). These 28 OTUs represented four phyla, seven classes, 15 orders, 20 families, and 21 genera (Figure 3 and Supplementary Table 2). Accessions with a Shannon Index > 1.00, namely AD0618-2 and AD0620, also had the lowest frequency of the shared OTUs. The frequency of unique OTUs was negatively correlated with the 28 shared OTUs with r2 = 0.701 and p = 0.004 (Table 1 and Figures 4, 5). These trends altered the composition of the phyla, classes, order, and family, respectively (Figure 4). The class formed by unclassified sequences was most predominant in AD0618-2 and AD0620-1. The number of shared OTUs suggests conservation of the endophytic community in this taxon, and only two accessions showed evidence for substantial expansion of bacterial diversity.
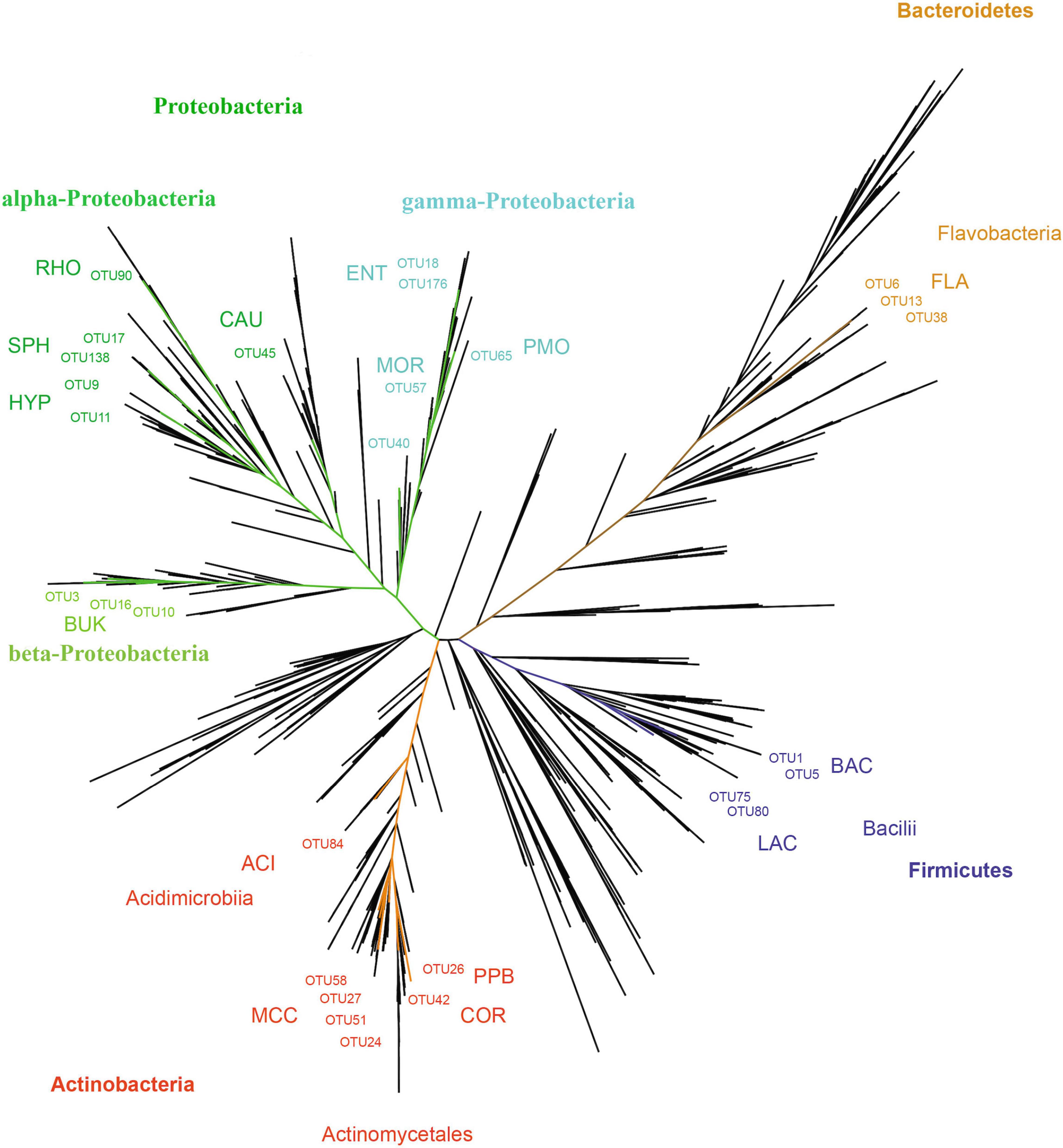
Figure 3. Unrooted phylogram showing the relationships of OTUs. The 28 OTUs shared among all samples are marked and classified. The main lineages (phylum) detached are marked using a color code: Actinobacteria (red), Bacteroidetes (brown), Firmicutes (blue), Protobacteria (brown). Besides phyla, the placenta of classes and orders are marked with the later abbreviated: ACI = Acidimicrobiales, BAC = Bacillales, BUK = Burkholderiales, CAU = Caulobacterales, COR = Corynebacteriales, ENT = Enterobacteriales, FLA = Flavobacteriales, HYP = Hyphomicrobiales, LAC = Lactobacillales, MCC = Micrococcales, MOR = Moraxellales, PMO = Pseudomonadales, PRO = Propionibacteriales. RHO = Rhodobacterales, SPH = Sphingomonadales.
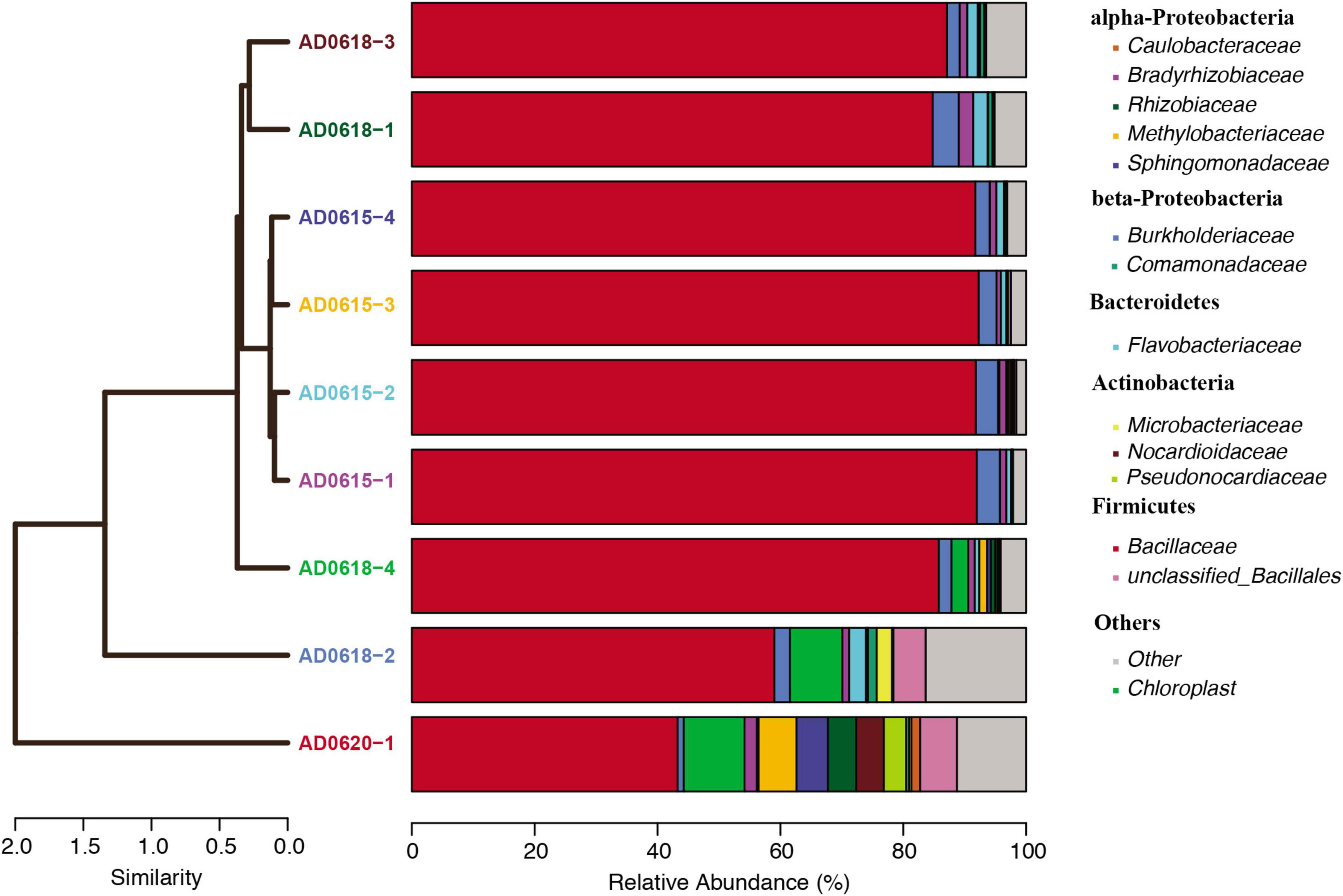
Figure 4. Relative abundance of bacterial lineages plotted for each accession. The cluster analyses illustrated the similarity among lineages visualized as a tree, whereas the relative abundance blocks visualized the proportion of each major bacterial lineage recovered. Color code showed on the right side.
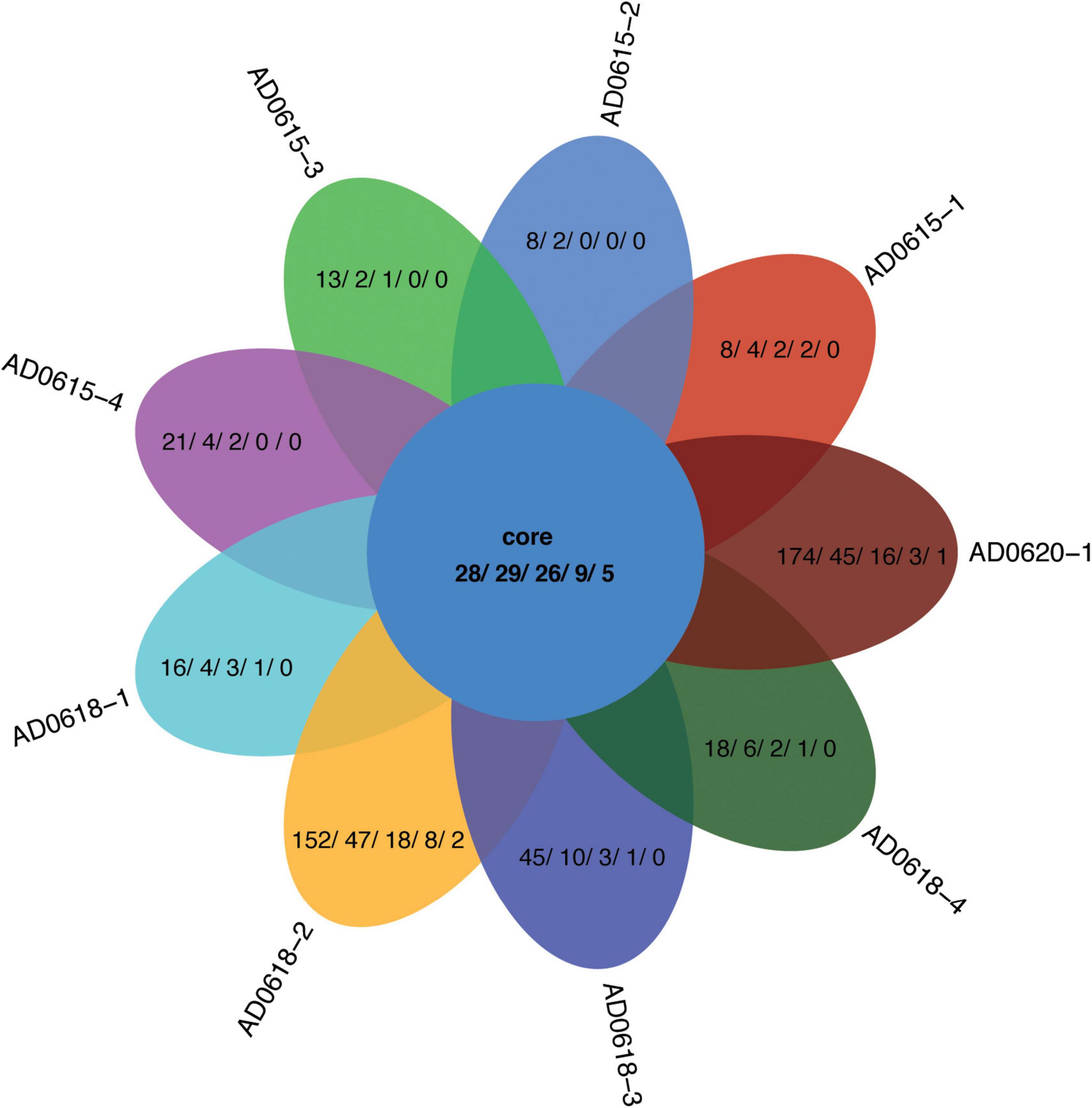
Figure 5. Venn diagram showing the distribution contrast of the OTUs shared among all accessions and the unique OTUs per accession not shared with all other accessions. Numbers given represent the OTU/Phylum/Class/Order/Family, respectively.
Contrasting functional differentiation with diversity richness patterns recovered functional differentiation among the individuals collected from the same location. In both analyses, AD0620-1 and AD0618-2 were highly isolated, consistent with the strongly differentiated community composition (Figures 6, 7). In diversity analysis, AD0618-1 and AD0618-3 were highly similar and separated from AD0618-4 that clustered together with the four subsamples of accession AD0615 (Figure 5). Functional analyses utilizing FAPROTAX or PICRUST recovered AD0618-4 separated from other samples of AD0618 by showing similar functional trends as AD0615-1 and AD0615-2, AD0615-3, and AD0615-4. However, in contrast to diversity patterns, AD0615-1 was highly similar to AD0618-3 in the functional analyses (Figure 6). Functional analysis via FAPROTAX recovered a large portion of undefined functional hits (Table 5) but predicted functions shared among accessions belonging to pathways involving chemoheterotrophy, fermentation, methylotrophy and nitrogen household (Table 5). Consistent with the expanded diversity, AD0618-2 and AD0620 showed distinct trends such as reduction of the proportion of the functional group “other” and the highest proportion of hits for “Chemoheterotrophy” (Table 5), besides hits to functional groups such as “cellulolysis”, “manganese oxidation”, and “thiosulfate respiration” only found in these accessions (Table 5).
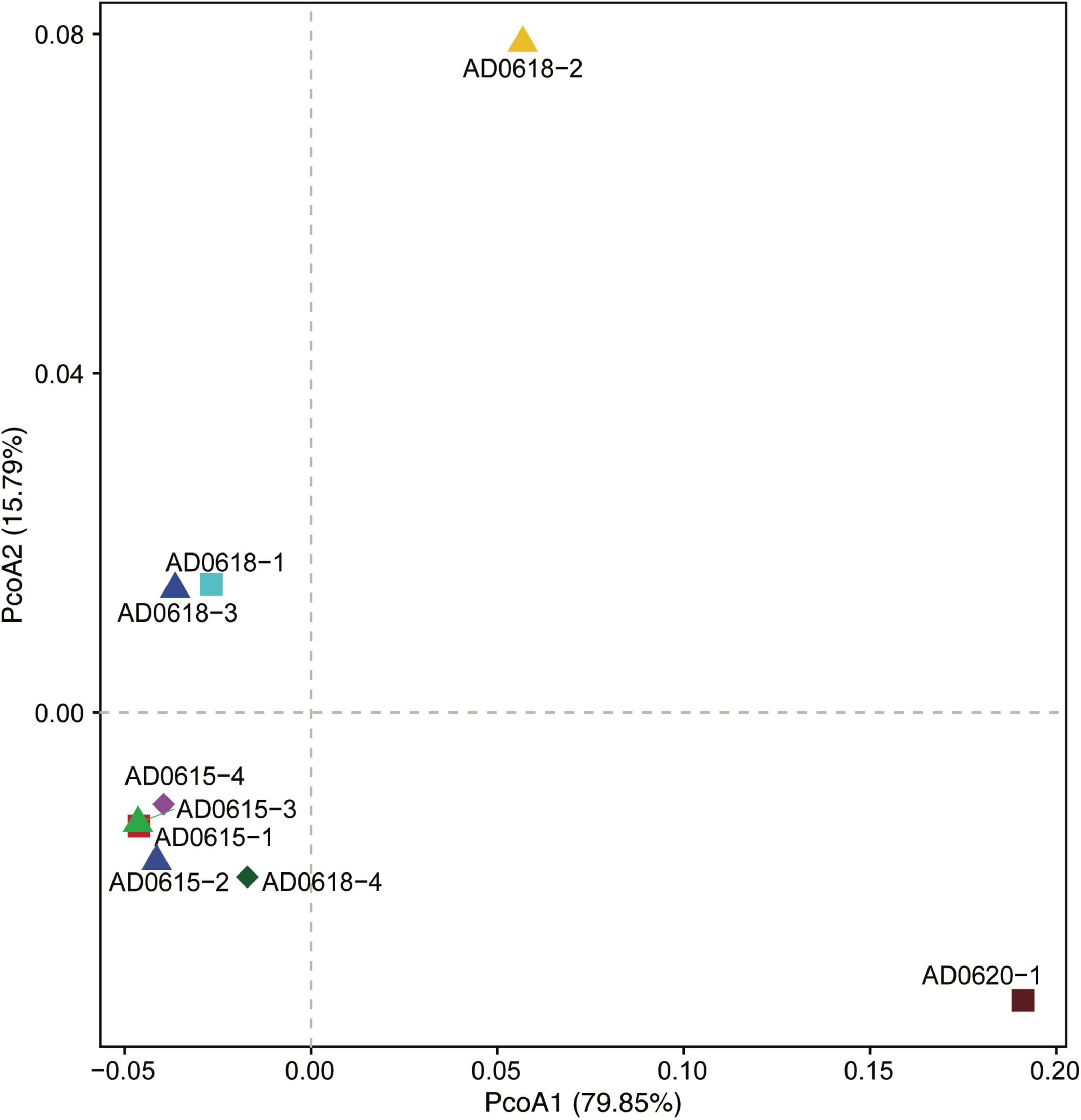
Figure 6. Principal Coordinate Analyses illustrating the similarities and differences of the bacterial communities’ diversity among the nine accessions studied. Axis1 explains 79.85%, and Axis2 explains 15.79% of the total variation. All accessions of ADO615 show high similarity, whereas AD0618 show a stronger differentiation among its four accessions, mainly along PCoA2.
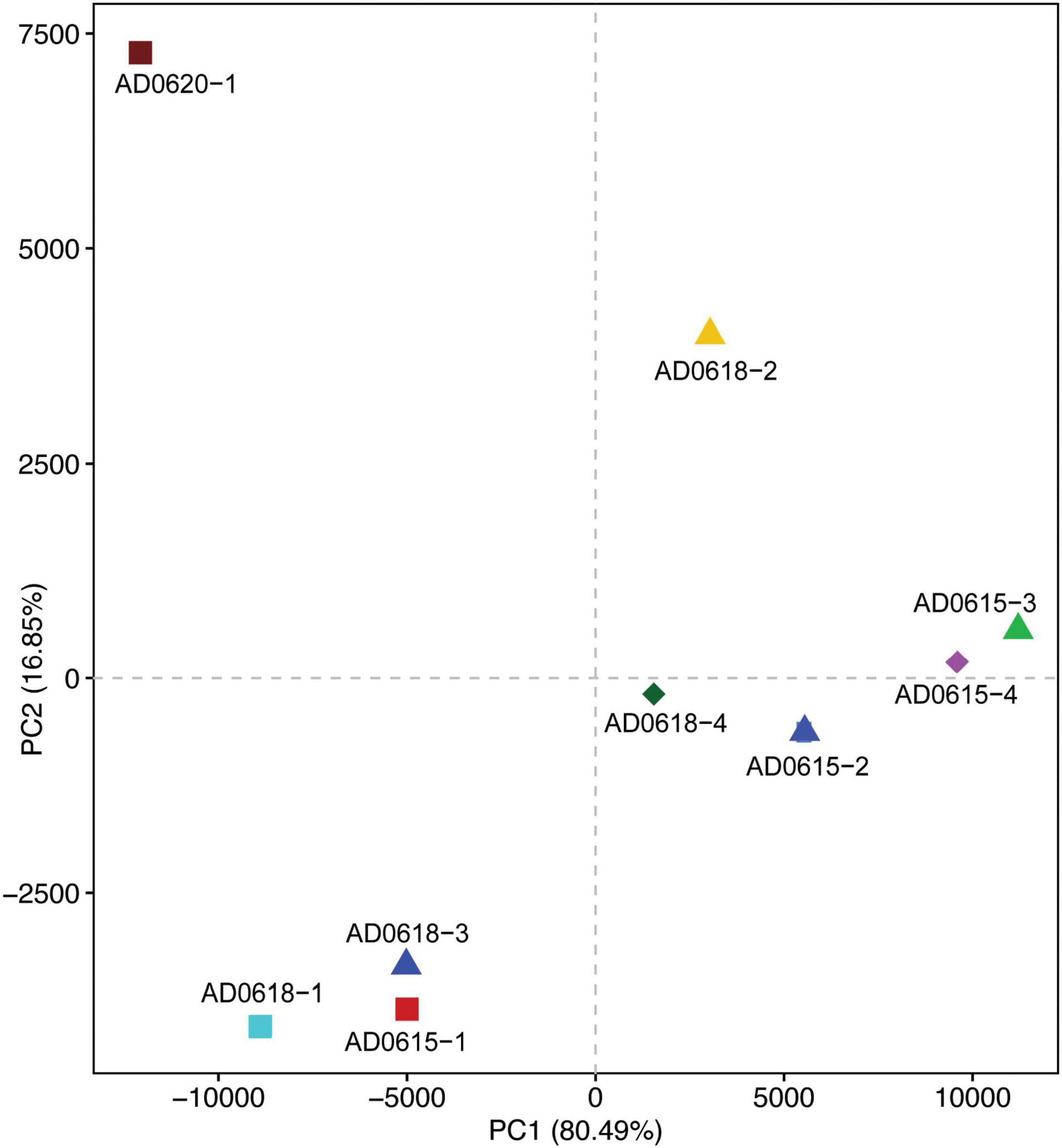
Figure 7. Principal Component Analyses illustrating the similarities and distinction of the functional attributes of the bacterial communities among the nine accessions studied. The shown scatter plot was based on FAPROTAX. Axis1 explained 80.49% and Axis2 explained 16.85% of the variation. Chemoheterotrophy was the main contributor to axis1 besides methanol/methyl and nitrogen processing pathways, which were also main contributors to axis 2 besides fermentation. Scatter plots based on PICRUSt cog and ko analyses showed highly similar results (results not shown).
Discussion
The leaves of the spleenwort Asplenium delavayi host a rich endophytic bacterial community exceeding any previous record of fern bacterial association considering the number of phyla, classes, orders, families-, and genera documented (see Supplementary Table 1). This result may be partly explained by approaches employed and the research foci selected in previous studies. None of the previous studies explicitly targeted the documentation of the bacteria communities colonizing the leaf endosphere using a high throughput sequencing approach. Instead, several studies employed the more traditional approach to cultivate bacteria to discover strains with antimicrobial activities (Das et al., 2017a,b,c, 2018), or to document the variation used by growth regimes (de Araújo-Barros et al., 2010). 16S rRNA sequences were utilized in several studies, but these studies were designed to address particular research foci such as the discovery of chloromethane degraders (Jaeger et al., 2018; Kroeber et al., 2021) or heavy-metal resistant strains (Zhu et al., 2014; Xu et al., 2016; Gu et al., 2018; Antenozio et al., 2021). Two studies are arguably most similar to our study in their research focus and strategy. These studies aimed to document the bacterial communities associated with the leaves of resurrection fern Pleopeltis polypodioides (Jackson et al., 2006) and the xeric fern Pellaea calomelanos (Mahlangu and Serepa-Dlamini, 2018a), respectively. However, only the study of Pleopeltis polypodioides (Jackson et al., 2006) recovered also a notable diverse bacterial community, including 7 phyla, 11 classes, 15 orders, and 20 families (Supplementary Table 1). Similar to our study, 16S rRNA sequences were targeted, but the sequencing protocols available in 2016 were arguably less potent than the approaches available in 2021. Furthermore, we have to point out the minimal number of fern species studied, namely 17 species (Supplementary Table 1) and the lack of evidence for several orders of ferns. Nevertheless, discovering a rich endophytic bacterial community associated with Asplenium delavayi provides a unique opportunity to explore the ecological and evolutionary aspects of these associations by establishing this easily cultivated fern species as a model system.
In this context, it is crucial to note that our study recovered a set of OTUs found in all samples studied. These 28 OTUs not only dominated the communities of all samples except for AD0618-2 and AD0620-2, but also contributed about half of the sequences in those accessions (Table 2). The vast majority of these OTUs belong to families repeatedly reported to be associated with plants, including the phyllosphere, and are considered to comprise “plant growth-promoting bacteria” (Glick, 2012; Backer et al., 2018; Patz et al., 2021). In recent years, increasing consideration has been given to the utilization of bacteria (Sessitsch et al., 2019), for example to improve crop production on nutrient-poor soils instead of using artificial fertilizers (Chen L. et al., 2021). To improve our capability to use these interactions, studies are not only needed to focus on economically important plants, but assessments of the diversity and evolutionary significance of these associations are also essential. Major challenges observed in this study are the large amount of sequence data lacking a classification due to the limited exploration of the 16S rRNA diversity of bacteria as well as the limitations of functional trait databases such as FAPROTAX (Louca et al., 2016). Nevertheless, the discovered bacterial communities associated with Asplenium delavayi showed potentially involvement in nitrogen household and methane degrading (Table 5). Both issues were highlighted as major topics in studies on “plant growth-promoting bacteria” (Li et al., 2021), management of environments in a globally warming world (Jaeger et al., 2018; Kroeber et al., 2021), and set up of new strategies for disease prevention (Cernava and Berg, 2022). However, not all the bacteria associated with fern leaves may provide benefits and some may even represent pathogens (Kloepper et al., 2013). To unravel the complexities and dynamics of these mutualistic interactions, we herein propose a need to carry out carefully designed experiments targeting Asplenium delavayi as a model system. Despite the limited numbers of samples, this study showed variation in richness and composition likely associated with differences in the environment, e.g., natural habitat versus cultivated conditions.
Besides the commonly associated lineages such as Actinobacteria, Bacteroidetes, Firmicutes, and Proteobacteria, some accessions comprised a small portion of sequences identified as belonging to the phylum Chloroflexi. This poorly known lineage of bacteria has been proposed to be the origin of the Tma12 insecticidal protein found in ferns (Li F. W. et al., 2019). The discovery of Chloroflexi occurring in the endophyllosphere of ferns provides additional support to the hypothesis of a horizontal gene transfer of this gene from bacteria to ferns. In general, endophytic bacteria have been considered to support HGT events in plants (Tiwari and Bae, 2020; Zilber-Rosenberg and Rosenberg, 2021), but relatively few reports can be directly associated to support this hypothesis. Thus, we suggest giving enhanced attention to the occurrence of bacterial sequences in sequencing reads obtained in the whole genome sequencing project. Instead of deleting as a result of contaminations, they may be best considered highly informative evidence to study the bacterial communities associated with ferns.
By reviewing the published evidence of endophytic bacterial communities associated with ferns, we clearly showed an insufficient grasp on the potential of the crucial ecological and evolutionary contribution of these associations. Together with our new records, assessments are available for only 17 species belonging to 15 genera, 11 families, and six orders. Furthermore, previous studies have rarely aimed to identify all the bacteria associated with ferns. In turn, our study showed the potential of a high throughput sequencing approaches enabled by the advancements of genome sequencing to document the bacterial diversity associated with ferns including the capacity to carry out well designed experiments to test variation of bacterial communities associated with fern leaves under different environmental conditions.
Conclusion
Asplenium delavayi is an outstanding candidate to be utilized as a model system to carry out studies with the aim to improve our understanding of the ecological and evolutionary significance of endophyllospheric bacterial associations of ferns and plants in general. For the first time, a highly diverse bacterial community was recorded for a terrestrial fern, whereas previous studies exploring fern-bacterial communities focused mainly on the water fern Azolla (Dijkhuzien et al., 2017; Li F. W. et al., 2019; Banach et al., 2020). The latter has been considered a highly specialized and unique example for symbiotic interactions between ferns and bacteria, including coinciding diversification of bacteria and these remarkable water ferns (Tripp et al., 2017). Still, our new findings suggest a more widespread and potential ubiquitous occurrence of mutualistic interactions between ferns and bacteria as considered in the concepts of the plant holobiont theory (Lyu et al., 2021), and symbiome phylogenetics (Tripp et al., 2017). Furthermore, the study supported the expectation that high throughput sequencing approaches enable the rapid assessment of bacterial communities associated with plants, including differentiation among accessions collected from different localities and cultivation conditions. Also, in as much as the work made a substantial descriptive profiling of the present organisms and their prospective functions, it did not give a comprehensive insight of whether these organisms are active members of the microbiome. Therefore, we suggest the extension of our current findings by integrating additional meta-transcriptomics with microbiome data as to have a deeper understanding of the microbes’ functions.
Data Availability Statement
The datasets presented in this study can be found in online repositories. The names of the repository/repositories and accession number(s) can be found below: https://www.ncbi.nlm.nih.gov/, PRJNA794952.
Author Contributions
HL and HS designed the project. VM designed and carried out the DNA library preparation and analyses. PZ, KW, AZ, and SS collected the populations occurring in Yunnan. HS and HL wrote the manuscript. All authors approved the final manuscript before submission and listed have made a substantial, direct, and intellectual contribution to the study.
Funding
This study was supported by the One Thousand Talent Program (grant no. WQ2017530454), Key Project of Yunnan Provincial Science and Technology Department (grant no. 202101AS070012), and Yunnan Postdoctoral Funding (grant no. Y8BSH11B09).
Conflict of Interest
The authors declare that the research was conducted in the absence of any commercial or financial relationships that could be construed as a potential conflict of interest.
Publisher’s Note
All claims expressed in this article are solely those of the authors and do not necessarily represent those of their affiliated organizations, or those of the publisher, the editors and the reviewers. Any product that may be evaluated in this article, or claim that may be made by its manufacturer, is not guaranteed or endorsed by the publisher.
Acknowledgments
We thank the gardeners of the XTBG Horticulture and Landscape Center for their support by cultivating this species for many years and the curators of herbaria for providing information about the occurrences of this fern.
Supplementary Material
The Supplementary Material for this article can be found online at: https://www.frontiersin.org/articles/10.3389/fpls.2022.891155/full#supplementary-material
Footnotes
References
Abedlfattah, A., Tack, A. J. M., Wasserman, B., Liu, J., Berg, G., and Norelli, J. (2021). Evidence for host-microbiome co-evolution in apple. New Phytol. 234, 2088–2100. doi: 10.1111/nph.17820
Antenozio, L. M., Gianenlli, G., Marabottini, R., Brunetti, P., Allevato, E., and Marzi, D. (2021). Phytoextraction efficiency of Pteris vittata grown on a naturally As-rich soil and characterization of As-resistant rhizosphere bacteria. Sci. Rep. 11:6794. doi: 10.1038/s41598-021-86076-7
Backer, R., Rokem, J. S., Ilangumaran, G., Lamont, J., Praslickova, D., and Ricci, E. (2018). Plant growth-promoting Rhizobacteria: context, mechanisms of action, and roadmap to commercialization of biostimulants for sustainable agriculture. Front. Plant Sci. 9:1473. doi: 10.3389/fpls.2018.01473
Banach, A. M., Kuzniar, A., Grzadziel, J., and Wolinska, A. (2020). Azolla filiculloides L. as a source of metal-tolerant microorganisms. PLoS One 15:e0232699. doi: 10.1371/journal.pone.0232699
Bayer, P. E., Golicz, A. A., Scheben, A., Batley, J., and Edwards, D. (2020). Plant pan-genomes are the new reference. Nat. Plants 6, 914–920. doi: 10.1038/s41477-020-0733-0
Bolger, A. M., Lohse, M., and Usadel, B. (2014). Trimmomatic: a flexible trimmer for Illumina sequence data. Bioinformatics 30, 2114–2120. doi: 10.1093/bioinformatics/btu170
Cernava, T., and Berg, G. (2022). The emergence of disease-preventing bacteria within the plant microbiota. Environ. Microbiol. [Epub ahead of print]. doi: 10.1111/1462-2920-15896
Chaudhry, V., Runge, P., Sengupta, P., Doehlemann, G., Parker, J. E., and Kemen, E. (2021). Shaping the leaf microbiota: plant-microbe interactions. J. Exp. Bot. 71, 36–56. doi: 10.1093/jxb/eraa417
Chelius, M. K., and Triplett, E. W. (2001). The diversity of Archaea and Bacteria in association with the roots of Zea mays L. Micr. Ecol. 41, 252–263. doi: 10.1007/S002480000087
Chen, L., Li, K., Shang, J. Y., Wu, Y., Chen, T., and Wanyan, Y. Q. (2021). Plant growth-promoting bacteria improve maize growth through reshaping the rhizobacterial community in low-nitrogen and low-phosphorous soil. Biol. Fertil. Soils 57, 1075–1088. doi: 10.1007/s00374-021-01598-6
Chen, Q. L., Hu, H. W., He, Z. Y., Qui, L., Zhu, Y. G., and He, J. Z. (2021). Potential of indigenous crop microbiomes for sustainable agriculture. Nat. Food 2, 233–240. doi: 10.1038/s43016-21-00253-5
Chen, T., Nomura, K., Wang, X. L., Sohrabi, R., Xu, J., and Yao, L. Y. (2020). A plant genetic network for preventing dysbiosis in the phyllosphere. Nature 580, 653–657. doi: 10.1038/s41586-020-2185-0
Chialva, M., Lanfranco, L., and Bonfante, P. (2022). The plant microbiota: composition, functions, and engineering. Curr. Opin. Biotech. 73, 135–142. doi: 10.1016/j.copbio.2021.07.003
Compant, S., Cambon, M. C., Vacher, C., Mitter, B., Samad, A., and Sessitsch, A. (2020). The plant endosphere world-bacterial life within plants. Environ. Microbiol. 23, 1812–1829. doi: 10.1111/1462-2920.15240
Cordovez, V., Dini-Andreote, F., Carrion, V., and Raaijmakers, J. M. (2019). Ecology and evolution of plant microbiomes. Ann. Rev. 73, 69–88. doi: 10.1146/annurev-micro-090817-062524
Core Development Team R (2020). A Language and Environment for Statistical Computing. R Foundation Statistical Computation v. 3.6.0. Available online at: http:/www.r-project.org. (accessed on Dec 20, 2021).
Crmbie, A. T., Larke-Mejia, N. L., Emery, H., Dawson, R., Pratscher, J., and Murphy, G. P. (2018). Poplar phyllosphere harbors disparate isoprene-degrading bacteria. Proc. Natl. Acad. Sci. U. S. A. 115, 13081–13086. doi: 10.1073/pnas.1812668115
Das, G., Park, S., Choi, J., and Baek, K. H. (2018). Anticandidal potential of endophytic bacteria isolated from Dryopteris uniformis (Makino). Jundishapur J. Microbiol. 12:e69878. doi: 10.5812/jjm.69878
Das, G., Park, S. J., and Baek, K. H. (2017a). Diversity of endophytic bacteria in a fern species Dryopteris uniformis (Makino) Makino and evaluation of their antibacterial potential against five food borne pathogenic bacteria. Foodborne Pathog. Dis. 14, 260–268. doi: 10.1089/fdp.2016.2243
Das, G., Patra, J. K., and Baek, K. H. (2017b). Antibacterial properties of endophytic bacteria isolated from a fern species Equisetum arvense L. against food-borne pathogenic bacteria Staphylococcus aureus and Escherichia coli O157:H7. Foodborne Pathog. Dis. 14, 50–58. doi: 10.1089/fdp.2016.2192
Das, G., Patra, J. K., Choi, J., and Baek, K. H. (2017c). Anticandidal effect of endophytic bacteria isolated from Equisetum arvense L. Against Candida albicans and Candida glabrata. Brazil. Arch. Biol. Technol. 6:e17160433. doi: 10.1590/1678-4324-2017160433
de Araújo-Barros, I., Araujo, W. L., and Azevedo, J. L. (2010). The effect of different growth regimes on the endophytic bacterial communities of the fern, Dicksonia sellowiana Hook. (Dicksoniaceae). Brazil. J. Microbiol. 41, 965–965. doi: 10.1590/S1517-838220100004000014
Diale, M. O., Kayitesi, E., and Serepa-Dlamini, M. H. (2021). Genome in silico and in vitro analysis of the probiotic properties of a bacterial endophyte, Bacillus paranthracis strain MGSD3. Front. Genet. 12:672149. doi: 10.3389/fgene.2021.672149
Dijkhuzien, L. W., Brouwer, P., Bolhuis, H., Reichart, G. J., Koppers, N., and Huettel, B. (2017). Is there foul play in the leaf pocket? the metagenome of floating fern Azolla reveals endophytes that do not fix N2 but may denitrify. New Phytol. 217, 453–466. doi: 10.1111/nph.14843
Edgar, R. C. (2013). PUARSE: highly accurate OTU sequences from microbial amplicon reads. Nat. Methods 10, 996–998. doi: 10.1038/nmeth.2604
Ek-Ramos, M. J., Gomez-Flores, R., Orozco-Flores, A. A., Rodriguez-Padilla, C., Gonzalez-Ochoa, G., and Tame-Guerra, P. (2019). Bioactive products from plant-endophytic gram-positive bacteria. Front. Microbiol. 10:463. doi: 10.3389/fmicb.2019.00463
French, E., Kaplan, I., Iyer-Pascuzzi, A., Nakatsu, C. H., and Enders, L. (2021). Emerging strategies for precision microbiome management in diverse agroecosystems. Nat. Plants 7, 256–267. doi: 10.1038/s41477-020-00830-9
Glick, B. R. (2012). Plant growth-promoting bacteria: mechanisms and applications. Sci. Rep. 2012:963401. doi: 10.6064/2012/963401
Gouda, S., Das, G., Sen, S. K., Shin, H. S., and Patra, J. K. (2016). Endophytes: a treasure house of bioactive compounds of medicinal importance. Front. Microbiol. 7:1538. doi: 10.3389/fmicb.2016.01538
Gu, Y. F., Wang, Y. Y., Sun, Y. H., Zhao, K., Xiang, Q. J., Yu, X. M., et al. (2018). Genetic diversity and characterization of arsenic-resistant endophytic bacteria isolated from Pteris vittata, an arsenic hyperaccumulator. BMC Microbiol. 18:42. doi: 10.1186/s12866-018-1184-x
Harrison, J. G., and Griffin, E. A. (2020). The diversity and distribution of endophytes across biomes, plant phylogeny and host tissues: how far have we come and where do we go here? Environ. Microbiol. 22, 2107–2123. doi: 10.1111/1462-2929.14968
Hawkes, C. V., Kjoeller, R., Raaijmakers, J. M., Riber, L., Christensen, S., and Rasmussen, S. (2021). Extension of plant phenotype by the foliar microbiome. Ann. Rev. Plant Biol. 72, 823–846. doi: 10.1146/annurev-arplant-080620-114342
Jackson, E. F., Echlin, H. L., and Jackson, C. R. (2006). Changes in the phyllosphere community of the resurrection ferns, Polypodium polypodioides, associated with rainfall and wetting. FEMS Microbiol. Ecol. 58, 236–246. doi: 10.1111/j.1574-6941.2006.00152.x
Jaeger, N., Besaury, L., Rohling, A. N., Koch, F., Delort, A. M., Gasc, C., et al. (2018). Chloromethane formation and degradation in the fern phyllosphere. Sci. Total Environ. 634, 1278–1287. doi: 10.1016/j.scitotenv.2018.03.316
Kanehisa, M., Sato, Y., and Kawashima, M. (2021). KEGG mapping tools for uncovering hidden features in biological data. Protein Sci. 31, 47–53. doi: 10.1002/pro.4172
Kanehisa, M., Sato, Y., Kawashima, M., Furumichi, M., and Tanabe, M. (2016). KEGG as a reference resource for gene and protein annotation. Nucleic Acids Res. 44, D457–D462. doi: 10.1093/nar/gkv1070
Khare, E., Mishra, J., and Arora, N. K. (2018). Multifaceted interactions between endophytes and plant: developments and prospects. Front. Microbiol. 9:2732. doi: 10.3389/fmicb.2018.02732
Kloepper, J. S., McInroy, J. A., and Hu, C. H. (2013). Symptoms of fern distortion syndrome resulting from inoculation with opportunistic endophytic fluorescent Pseudomonas spp. PLoS One 8:e58531. doi: 10.1371/journal.pone.0058531
Kozich, J. J., Westcott, S. L., Baxter, N. T., Highlander, S. K., and Schloss, P. D. (2013). Development of a dual-index sequencing Strategy and curation pipeline for analyzing amplicon sequence data on the MiSeq Illumina sequencing platform. Appl. Environ. Microbiol. 79, 5112–5120. doi: 10.1128/AEM.01043-13
Kroeber, E., Wende, S., Kanukollu, S., Buchen-Tschiskale, C., Besaury, L., Keppler, F., et al. (2021). 13C-chloromethane incubations provide evidence for novel bacterial chloromethane degraders in a living tree fern. Environ. Microbiol. 23, 4450–4465. doi: 10.1111/1562-2920.15638
Lajoie, G., and Kembel, S. W. (2021). Plant-bacteria associations are phylogenetically structured in the phyllosphere. Mol. Ecol. 30, 5572–5587. doi: 10.1111/mec.16131
Langille, M. G. I., Zaneveld, J., Caporaso, J. G., McDonald, D., Knights, D., Reyes, J. A., et al. (2013). Predictive functional profiling of microbial communities using 16S rRNA marker gene sequences. Nat. Biotech. 31, 814–821. doi: 10.1038/nbt.2676
Leontidou, K., Genitsaris, S., Papadopoulou, A., Kamou, N., Bosmali, I., Matsi, T., et al. (2020). Plant growth promoting rhizobacteria isolated from halophytes and drought-tolerant plants: genomic characterisation and exploration of phyto-beneficial traits. Sci. Rep. 10:14857. doi: 10.1038/s41598-020-71652-0
Li, E. Q., de Jonge, R., Liu, C., Jiang, H. N., Friman, V.-P., Bakker, P. A. H. M., et al. (2021). Rapid evolution of bacterial mutualism in the plant rhizosphere. Nat. Commun. 12:3829. doi: 10.1038/s41467-012-24005-y
Li, F., He, X. H., Sun, Y. Y., Zhang, X. M., Tang, X. X., Li, Y. K., et al. (2019). Distinct endophytes are used by diverse plants for adaptation to karst regions. Sci. Rep. 9:5246. doi: 10.1038/s41598-019-41802-0
Li, F. W., Brouwer, P., Careetero-Paulet, L., Chen, S. F., de Vries, J., and Delaux, P. M. (2019). Fern genomes elucidate land plant evolution and cyanobacterial symbioses. Nat. Plants 4, 460–472. doi: 10.1038/s41477-018-0188-8
Louca, S., Parfrey, L. W., and Doebeli, M. (2016). Decoupling function and taxonomy in the global ocean microbiome. Science 353, 1272–1277. doi: 10.1126/science.aaf4507
Lyu, D. M., Zajonc, J., Page, A., Tanney, C. A. S., Shah, A., and Monjezi, N. (2021). Plant holobiont Theory: the phytomicrobiome plays a central role in evolution and success. Microorganism 9:675. doi: 10.3390/microorganisms9040675
Mahlangu, S. G., and Serepa-Dlamini, M. H. (2018a). First report of bacterial endophytes from the leaves of Pellaea calomemanos in South Africa. South Afr. J. Sci. 114, 55–63.
Mahlangu, S. G., and Serepa-Dlamini, M. H. (2018b). Draft genome sequence of Pantoaea ananatis strain MHSD5 isolated from surface sterilized leaves of medicinal plant, Pellaea calomemanos obtained in South Africa. Data Brief 19, 1727–1732. doi: 10.1016/j.dib.2018.06.039
Marks, R. A., Hotaling, S., Frandsen, P. B., and VanBuren, R. (2021). Representation and participation across 20 years of plant genome sequencing. Nat. Plants 7, 1571–1578. doi: 10.1038/s-41477-021-01031-8
Martin, M. (2011). Cutadapt remotes adapter sequences from high-throughput sequencing reads. EMBnet J. 17, 10–12.
Matsumoto, H., Fa, X. Y., Wang, Y., Kusstatscher, P., Duan, J., and Wu, S. L. (2021). Bacterial seed endophytes shapes disease resistance in rice. Nat. Plants 7, 60–72. doi: 10.1038/s-41477-020-0082605
McMurdie, P. J., and Holmes, S. (2013). phyloseq: an R package for reproducible interactive analysis and graphics of microbial census data. PLoS One 8:e61217. doi: 10.371/journal.pone.0061217
Meyer, K. M., Porch, R., Muscettola, I. E., Vasconcelos, A. L. S., Sherman, J. K., Metcalf, J. E., et al. (2022). Plant neighborhood shapes diversity and reduces interspecific variation of the phyllosphere microbiome. ISME J. 16, 1376–1387. doi: 10.1038/S41396-021-01184-6
Morella, N. M., Weng, F. C. H., Jourbert, P. M., Metcalf, C. J. E., Linwow, S., and Koskella, B. (2020). Successive passaging of a plant-associated microbiome reveals robust habitat and host genotype-dependent selection. Proc. Natl. Acad. Sci. U. S. A. 117, 1148–1159. doi: 10.1073/pnas.1908600116
Mukherjee, A., Bhattacharjee, P., Das, R., Pal, A., and Paul, A. K. (2017). Endophytic bacteria with plant growth promoting abilities from Ophioglossum reticulatum L. AIMS Microbiol. 3, 596–612. doi: 10.3934/microbiol.2017.3.596
Oksanen, J., Blanchet, F. G., Friendly, M., Kindt, R., Legendre, P., McGlinn, D., et al. (2020). Vegan: Community Ecology Package v 2.6.2. Available online at: https://rdrr.io/cran/vegan/ (accessed on Dec 20, 2021).
Patz, S., Gautam, A., Becker, M., Ruppel, S., Rodriguez-Palenzuela, P., and Huson, D. H. (2021). PLaBAse: a comprehensive web resource for analyzing the plant promoting potential of plant associated bacteria. BioRxiv [Preprint]. doi: 10.1101/2021.12.13.472471
Perreault, R., and Laforest-Lapointe, I. (2022). Plant-microbe interactions i the phylalosphere: facing challenges of the anthraxocene. ISME J. 16, 339–345. doi: 10.1038/s41396-021-01109-3
Quast, C., Presse, E., Yilmaz, P., Gerken, J., Schweer, T., Yarza, O., et al. (2013). The SILVA ribosomal RNA gene database project: improved data processing and web-based tools. Nucleic Acids Res. 41, D590–D596. doi: 10.1093/nar/gks1219
Rakshit, A., and Sukul, S. (2021a). Report of indole acetic acid (IAA) producing endophytic bacteria from the edible fern Ampelopteris prolifera (Retz.) Copel. Int. J. Bot. St. 6, 314–321.
Rakshit, A., and Sukul, S. (2021b). Studies on two indole acetic acid (IAA) producing endophytic bacteria from Thelypteris interruptus (Willd.) K.Iwats. and their effect on seed germination. Res. J. Agric. Sci. 12, 2037–2043.
Schloss, P. D., Westcott, S. L., Ryabin, T., Hall, J. R., Hartmann, M., and Hollister, E. B. (2009). Introducing mother: open-source, platform-independent, community supported software for describing and comparing microbial communities. Appl. Environ. Microbol. 75, 7537–7541. doi: 10.1128/AEM.01541-09
Sessitsch, A., Pfaffenbichler, N., and Mitter, B. (2019). Microbiome applications from lab to field: facing complexity. Trends Plant Sci. 24, 194–198. doi: 10.1916/j.tplants.2018.12/004
Shalev, O., Karasov, T. L., Lundberg, D. S., Ashkenazy, H., Ayutthaya, P. P. A., and Weigel, D. (2022). Commensal Pseudomonas strains facilitate protective response against pathogens in the host plant. Nat. Ecol. Evol. 6, 383–396. doi: 10.1038/s41559-022-01673-7
Smith, D. L., Gravel, V., and Yergeau, E. (2017). Editorial: singling in the phytomicrobiome. Front. Plant Sci. 8:611. doi: 10.3389/flps.2017.00611
Stamatakis, A. (2014). RAxML 8: a tool for phylogenetic analyses and post-analyses of large phylogenies. Bioinformatics 30, 1312–1313. doi: 10.1093/bioinformatics/btu033
Suryanarayanan, T. S., and Shaanker, R. U. (2021). Can endophytes fast-track plant adaptations to climate change? Fungal Ecol. 50:101039.
Tan, J. Q., Kerstetter, J. E., and Turcotte, M. M. (2021). Eco-evolutionary interaction between microbiome presence and rapid biofilm evolution determines plant host fitness. Nat. Ecol. Evol. 5, 670–676. doi: 10.1038/s41559-021-01406-2
Tatusov, R. L., Galperin, M. Y., Natale, D. A., and Koonin, E. V. (2000). The COG database: a tool for genome-scale analysis of protein functions and evolution. Nucleic Acid Res. 28, 33–36. doi: 10.1093/nar.28.1.33
Tiwari, P., and Bae, H. H. (2020). Horizontal gene transfer and endophytes; an implication for the acquisition of novel traits. Plants 9:305. doi: 10.3390/plants9030305
Tripp, E. A., Zhang, N., Schneider, H., Huang, Y., Mueller, G. M., Hu, Z. H., et al. (2017). Reshaping Darwin’s tree” impact of the symbiome. Trends Ecol. Evol. 32, 552–555. doi: 10.1016/j.tree.2017.05.002
Tshishonga, K., and Serepa-Dlamini, M. H. (2019). Draft genome sequence of Enterobacter hormaechei strain MHSD6, a plant endophyte isolated from medicinal plant Pellaea calomelanos. Microbiol. Resour. Announ. 8, e1251–19. doi: 10.1128/MRA.01251-19
Tshishonga, K., and Serepa-Dlamini, M. H. (2020). Draft genome sequence of Pseudoarthrobacer phenanthrenivorans Strain MGSD1, a bacterial endophyte isolated from the medicinal plant Pellaea calomelanos. Evol. Bioinform. Online 16:1176934320913257. doi: 10.1177/1176934320913257
Vaishnav, A., Shukla, A. K., Sharma, A., Kumar, R., and Choudhary, D. K. (2019). Endophytic bacteria in plant salt stress tolerance: current and future prospects. J. Plant Growth Regul. 38, 650–668. doi: 10.1007/s00344-018-9880-1
Vandenkoornhuyse, P., Quaiser, A., Dihamel, M., Le Van, A., and Durfesne, A. (2015). The importance of the microbiome of plant holobiont. New Phytol. 206, 1196–1206. doi: 10.1111/nph.13312
Vorholt, J. A. (2012). Microbial life in the phyllosphere. Nat. Rev. Microbiol. 10, 828–840. doi: 10.1038/nrmicro2910
Wang, Q., Garrity, G. M., Tiedje, J. M., and Cole, J. R. (2007). Native Bayesian classifier for rapid assignment of rRNA sequences into the new bacterial taxonomy. Appl. Environ. Microbiol. 73, 5261–5267. doi: 10.1128/AEM.00062-07
Wang, Y. R., Chai, W. C., Wang, W. P., Shu, N., Zhang, Z. D., Hou, Q. C., et al. (2020). Analysis of microbial diversity and functional differences in different types of high-temperature Daqu. Food Sci. Nutri. 17, 1003–1016. doi: 10.1002/fsn3.2068
Wickham, H., Chang, W., Henry, L., Pedersen, T. L., Takahashi, K., Wilke, C., et al. (2021). ggplot2: create Elegant Data Visualisations Using the Grammar of Graphics. Available online at: https://cran.r-project.org/web/packages/ggplot2/index.html. (accessed on Dec 20, 2021).
Wu, W., Chen, W. H., Liu, S. Y., Wu, J. J., Zhu, Y. T., Qin, L. P., et al. (2021). Beneficial relationships between endophytic bacteria and medicinal plants. Front. Plant Sci. 12:646146. doi: 10.3389/flps.2021.646146
Xia, A. N., Liu, J., Kang, D. C., Zhang, H. G., Zhang, R. H., and Liu, Y. G. (2020). Assessment of endophytic bacterial diversity in rose by high-throughput sequencing analysis. PLoS One 15:e0230924. doi: 10.1371/journal.pone.0230924
Xu, J. Y., Han, Y. H., Chen, Y. S., Zhu, L. J., and Ma, L. Q. (2016). Arsenic transformation and plant growth promotion characteristics of As-resistant endophytic bacteria from As-hyperaccumulator Pteris vittata. Chemosphere 144, 1233–1240. doi: 10.1016/j.chemosphere.2015.09.102
Yadav, S. K., Archana Singh, R., Singh, P. K., and Vasudev, P. G. (2019). Insecticidal fern protein Tma12 is possibly a lytic polysaccharide monooxygenase. Plants 249, 1987–1996. doi: 10.1007/s00425-019-03135-0
Yang, C. Y., Ho, Y. N., Makita, R., Inoue, C., and Chien, M. G. (2020). A multifunctional rhizobacterial strain with wide application in different ferns facilitates arsenic phytoremediation. Sci. Total Environ. 7212:134504. doi: 10.1016/j.scitotenv.2019.134504
Zhang, J., Kobert, K., Flouri, T., and Stamatakis, A. (2014). PEAR: a fast and accurate Illumina paired-end read merger. Bioinformatics 30, 614–620.
Zhang, Q., Acuna, J. J., Inostroza, N. G., Mora, M. L., Radic, S., Sadowsky, M. J., et al. (2019). Endophytic bacterial communities associated with roots and leaves of plants growing in Chilean extremes environments. Sci. Rep. 9:4950. doi: 10.1038/s41598-019-41160-x
Zhu, L. J., Guan, D. X., Luo, J., Rathinasabapathi, B., and Ma, L. Q. (2014). Characterization of arsenic-resistant endophytic bacteria from hyperaccumulators Pteris vittata and Pteris multifida. Chemosphere 113, 9–16. doi: 10.1016/j.chemosphere/2014.03.081
Keywords: ferns, high-throughput sequencing, model organisms, phyllosphere, plant genomics, plant-growth promoting bacteria, symbiome phylogenetics, endophytic bacterial communities
Citation: Masocha VF, Liu H, Zhan P, Wang K, Zeng A, Shen S and Schneider H (2022) Bacterial Microbiome in the Phyllo-Endosphere of Highly Specialized Rock Spleenwort. Front. Plant Sci. 13:891155. doi: 10.3389/fpls.2022.891155
Received: 07 March 2022; Accepted: 30 May 2022;
Published: 07 July 2022.
Edited by:
Camille Eichelberger Granada, Universidade do Vale do Taquari – Univates, BrazilReviewed by:
Anton Hartmann, Ludwig Maximilian University of Munich, GermanyAlessandra Turrini, University of Pisa, Italy
Copyright © 2022 Masocha, Liu, Zhan, Wang, Zeng, Shen and Schneider. This is an open-access article distributed under the terms of the Creative Commons Attribution License (CC BY). The use, distribution or reproduction in other forums is permitted, provided the original author(s) and the copyright owner(s) are credited and that the original publication in this journal is cited, in accordance with accepted academic practice. No use, distribution or reproduction is permitted which does not comply with these terms.
*Correspondence: Harald Schneider, aGFyYWxkQHh0YmcuYWMuY24=
†These authors have contributed equally to this work