- 1Shenzhen Key Laboratory of Marine Bioresource and Eco-Environmental Science, Shenzhen Engineering Laboratory for Marine Algal Biotechnology, Guangdong Provincial Key Laboratory for Plant Epigenetics, College of Life Sciences and Oceanography, Shenzhen University, Shenzhen, China
- 2Marine Resources Big Data Center of South China Sea, Southern Marine Science and Engineering Guangdong Laboratory, Zhanjiang, China
The histone acetyltransferases (HATs), together with histone deacetylases, regulate the gene transcription related to various biological processes, including stress responses in eukaryotes. This study found a member of HATs (HpGCN5) from a transcriptome of the economically important microalgae Haematococcus pluvialis. Its expression pattern responding to multiple abiotic stresses and its correlation with transcription factors and genes involved in triacylglycerols and astaxanthin biosynthesis under stress conditions were evaluated, aiming to discover its potential biological function. The isolated HpGCN5 was 1,712 bp in length encoding 415 amino acids. The signature domains of Acetyltransf_1 and BROMO were presented, as the GCN5 gene from Arabidopsis and Saccharomyces cerevisiae, confirming that HpGCN5 belongs to the GCN5 subfamily of the GNAT superfamily. The phylogenetic analysis revealed that HpGCN5 is grouped with GNAT genes from algae and is closer to that from higher plants, compared with yeast, animal, fungus, and bacteria. It was predicted that HpGCN5 is composed of 10 exons and contains multiple stress-related cis-elements in the promoter region, revealing its potential role in stress regulation. Real-time quantitative PCR revealed that HpGCN5 responds to high light and high salt stresses in similar behavior, evidenced by their down-regulation exposing to stresses. Differently, HpGCN5 expression was significantly induced by SA and Nitrogen-depletion stresses at the early stage but was dropped back after then. The correlation network analysis suggested that HpGCN5 has a strong correlation with major genes and a transcription factor involved in astaxanthin biosynthesis. Besides, the correlation was only found between HpGCN5 and a few genes involved in triacylglycerols biosynthesis. Therefore, this study proposed that HpGCN5 might play a role in the regulation of astaxanthin biosynthesis. This study firstly examined the role of HATs in stress regulation and results will enrich our understanding of the role of HATs in microalgae.
Introduction
Eukaryotes have a complex gene expression regulation mechanism to adapt to developmental and environmental changes, by activation or repression of target genes through chromatin remodeling which is generally regulated by histone modification, DNA methylation, and nucleosome remodeling (Kadonaga, 1998; Loidl, 2004; Pfluger and Wagner, 2007). Acetylation is considered as the most important type of histone modification, which is accomplished by histone acetyltransferases (HATs) and histone deacetylases (HDACs), compared with methylation, phosphorylation, etc. (Kuo and Allis, 1998). It has been recorded that HATs can neutralize the positive charges on lysine residues through acetylation to loosen the chromatin, which facilitates the binding of transcriptional regulatory proteins to DNA and thereby promotes gene transcription (Roth et al., 2001). Besides, HATs also can acetylate other non-histone components, such as transcription factors, transcription co-regulators, DNA-binding proteins, and non-nuclear proteins, to regulate the gene expression (Panagopoulos et al., 2001).
According to the amino acid sequence characterization, HATs can be classified into four groups, namely, the CBP family (p300/CREB-binding protein), GNAT (GCN5-related N-terminal acetyltransferases)/MYST superfamily, TAFII250 family, and the mammals-unique HAT family (Pandey et al., 2002). Compared with other three families, the GNAT/MYST superfamily has been widely studied. It has been reported that the difference between GNAT and MYST is that there are four motifs in the HAT domain in GNAT members, while only one in MYST members (Sterner and Berger, 2000). Previous studies divided GNAT members into four subfamilies, namely, GCN5, ELP3, HAT1, and HAT2 (Roth et al., 2001). In Arabidopsis, the homolog of each subfamily was found in the genome, except for the HAT2 subfamily (Pandey et al., 2002). Later, experiments confirmed that the members of GNAT play an important role in plant growth, development, and stress responses (Stockinger et al., 2001; Vlachonasios et al., 2003; Chinnusamy and Zhu, 2009; Fang et al., 2014; Hu, 2015; Ueda and Seki, 2020).
In the microalgae, histone modification has been reported in Ostreococcus tauri, Ostreococcus lucimarinus, Bathycoccus prasinos, and Micromonas sp., in the manner of methylation (Kim et al., 2015). However, HATs have been rarely reported in microalgae. To date, only a gene CrGNAT encoding the acetyltransferase in Chlamydomonas reinhardtii has been reported to regulate the responses to heavy metals (Wang et al., 2015). As an economically important microalga, Haematococcus pluvialis is popular for its superior biosynthesis of astaxanthin, which is a super antioxidant (Shah et al., 2016). In general, the production of astaxanthin is commonly induced by stress conditions in H. pluvialis (Shah et al., 2016). Even though the fact of histone modification playing an important role in the stress response has been widely demonstrated in plants (Ueda and Seki, 2020), the fundamental research on histone modification in stress responses in H. pluvialis is still not carried out. In this study, the candidates of histone acetyltransferases were isolated and characterized in H. pluvialis, aiming to have a first view of the role of HATs in abiotic stress responses and its potential role in astaxanthin and triacylglycerols biosynthesis. The results will potentially extend the understanding of the mechanism of stress-induced astaxanthin biosynthesis.
Materials and Methods
Algae Culture and Growth Conditions
Haematococcus pluvialis strain 192.80 used in this study was from Experimental Phycology and Culture Collection of Algae (EPSAG), Goettingen University (Goettingen, Germany). Algal cells were cultured in a 250 ml flask with 100 ml ESP basal medium with peptone as recommended by EPSAG (https://www.uni-goettingen.de/en/186449.html), in a growth chamber under continuous illumination (25 μmol photon m−2s−1) at 22°C, with gently shaking by hand daily. At the middle logarithmic growth stage (about 1.5 × 105 cell/ml), algal cells were subjected to four independent abiotic stresses, including, high salt (HS), salicylic acid (SA), high light (HL), and Nitrogen-depletion (N-).
For the HS treatment and SA treatment, filter-sterilized sodium acetate or salicylic acid solution was added into the cultures at the final concentration of 45 mM and 0.18 M, respectively. For the HL treatment, algal cells were exposed to continuous irradiance at 500 μmol photon m−2s−1. For the N- treatment, algal cells were harvested by mild centrifugation at 1,000 rpm for 10 min and resuspended in the BG11 medium (Allen, 1968) without nitrate components. For the HL and HS stresses, algal cells were treated for 24 h and samples were collected at 0, 1.5, 3, 6, 12, and 24 h. For the SA and N- stresses, algal cells were treated for 5 days and samples were collected daily. For each treatment, a total of 18 cultures with 100 ml in 250 ml flasks were prepared and three cultures were randomly harvested at each collection time as three biological replicates.
Transcriptomic Identification and Experimental Cloning of HpGCN5
An Iso-seq transcriptome database was previously obtained using RNA extracted from H. pluvialis grown under the high light stress condition. To identify the potential histone acetyltransferase, a query database was constructed by using histone acetyltransferases recorded in NCBI. TBLASTN program was then performed locally using the query database against the Iso-seq database. The candidates of histone acetyltransferases were screened out using a threshold e-value of 1e-5. Aiming to dissociate the GNAT members from potential histone acetyltransferase, the maximum likelihood phylogenetic tree was conducted by MEGA7 software using their putative proteins and GNAT genes from Arabidopsis (GenBank accession: AAK31318.1), Saccharomyces cerevisiae (Genbank accession: NP_011768.1), and Chlamydomonas reinhardtii (Genbank accession: XP_001693868.1). Finally, the candidates of GNAT in H. pluvialis were manually checked by SMART and Pfam analyses to avoid errors normally generated by the large-scale bioinformatic analysis. Hence, the candidate of GCN5 belonging to the GNAT family would be identified based on the domain analysis.
Thereby, the candidate of the GCN5 gene (HpGCN5) was experimentally isolated from H. pluvialis by RT-PCR and T-A cloning strategy. For the RT-PCR, the cDNA template was obtained from the RNA used for the Iso-seq database construction, with the help of Perfect Real-Time PrimeScript™ RT Reagent Kit with gDNA Eraser (TaKaRa, China). Gene-specific primers used to isolate the target gene were designed based on its nucleotides from the Iso-seq database (GF1: 5′-GACACTAGGAGGACATCAGGACAAAT-3′; GR1: 5′-GTGTACCAACAAGCGACTGCGACT-3′). The RT-PCR reaction consisted of 1 μl cDNA, 1.0 μl each forward and reverse primer (10 μM), 10 μl 2 × Platinum™ SuperFi II Green PCR Master Mix (Invitrogen Life technologies, United States), and 7.2 μl ddH2O. The amplification was performed on the Bio-Rad T100 thermal cycler (Bio-Rad, United States) and the condition was as follows: initial denaturation at 98°C for 2 min; 35 cycles of 98°C for 10 s, 60°C for 10 s, 72°C for 1.5 min; and a final extension at 72°C for 5 min. Agarose gel electrophoresis, PCR products purification, T-A cloning including vector construction, E. coli transformation, colony PCR identification, and sequencing were performed as previously reported (Huang et al., 2019).
Bioinformatics Analysis
The ORF and deduced amino acids of isolated HpGCN5 were carried out by EditSeq (DNASTAR software, Lasergene, United States). To determine the sequence identity and divergence, multiple sequence alignment was performed by MegAlign (DNASTAR software, Lasergene, United States). The domain of deduced amino acid was predicted by SMART online at http://smart.embl-heidelberg.de/. To carry out the potential gene structure of HpGCN5, the cDNA sequence was aligned with the genome sequence of H. pluvialis (BIG Data Center GSA Database accession no. PRJCA000614) (Luo et al., 2019). The schematic diagram of gene structure was displayed by Gene Structure Display Server 2.0 (http://gsds.cbi.pku.edu.cn/). Based on the genome sequence, the cis-elements within 2,000 bp upstream of the transcription start site of HpGCN5 were searched in the PlantCARE database (http://bioinformatics.psb.ugent.be/webtools/plantcare/html/) (Lescot et al., 2002). The phylogenetic relationship was analyzed by MEGA7 software with the Minimum Evolution method (Kumar et al., 2016).
To evaluate if HpGCN5 is involved in the stress response, triacylglycerols biosynthesis, and astaxanthin biosynthesis, the correlations between HpGCN5 and associated genes were analyzed using transcriptome data driven from H. pluvialis treated with SAHL (salicylic acid combined with high light) and SAHS (salicylic acid combined with high salt) that were previously reported by our research group (Hu et al., 2021). First, a gene cluster was constructed, including HpGCN5 and 310 differentially expressed transcripts (49 were annotated as transcription factors, 169 were associated with carotenoids biosynthesis, and 92 were associated with triacylglycerols biosynthesis). The expression data of the gene cluster were retrieved from the SAHL and SAHS transcriptome data (NCBI SRA database accession no. PRJNA675306), respectively. Finally, the Pearson statistical analysis was performed at a significance of 0.05, and the correlation network was constructed using genes with a correlation >0.8.
Expression Pattern Analyses Responding to Various Abiotic Stresses
The quantitative real-time PCR (qRT-PCR) was performed to evaluate the expression pattern of HpGCN5 responding to various abiotic stresses in this study. For each sample collected from each time point of each stress treatment, total RNA was exacted by RNA fast 200 Kit (Fastagen, China) and the first strand of cDNA was synthesized by PrimeScript™ RT reagent Kit with gDNA Eraser (Perfect Real-Time) (TaKaRa, China), according to the corresponding user instruction. The qRT-PCR reaction consisted of 6 μl TB Green™ Premix Ex Taq ™II (Tli RNaseH Plus) (TaKaRa, China), 1 μl cDNA, 0.5 μl each forward and reverse primer (10 μM), and 4 μl ddH2O. The amplification was as follows: initial denaturation at 95°C for 30 s, 40 cycles of 95°C for 5 s, and 60°C for 30 s. The amplification was monitored on an ABI QuantStudio™ 6 Flex System (Applied Biosystems, United States). To quantify the expression level of HpGCN5 in each sample, HpActin was employed as an internal reference (Wen et al., 2015). Primers targeting HpGCN5 were qGF (5′-ATCATGCACGGCCTCTCCCACAGC-3′) and qGR (5′-GACTTCAGCCGACCCCTCCTTCAG-3′), and targeting HpActin were qAF (5′-AGCGGGAGATAGTGCGGGACA-3′) and qAR (5′-ATGCCCACCGCCTCCATGC-3′), respectively. The expression level of HpGCN5 in each sample was calculated by the 2−ΔΔCt method (Mackay et al., 2002). All reactions were run in triplicate.
Statistical Analysis
Expression levels of HpGCN5 between each sample collected from the same abiotic stress were compared by Pearson's t-test. The significance was determined at the level of 0.05, 0.01, and 0.001.
Results
Transcriptome-Wide Identification of GCN5 Candidates
Based on the local TBLASTN using NCBI-recorded histone acetyltransferase from various species, 52 transcripts were hit. Since some transcripts shared the same amino acids, their putative amino acids were used for further analysis, instead of nucleotides. It was found that 52 transcripts were associated with 26 genes, which were subjected to the phylogenetic analysis. Results suggested that two genes, namely, Gene005060 and Gene010338, were clustered with AtGCN5 and ScGCN5 from Arabidopsis and S. cerevisiae, respectively, while Gene002197 was clustered with CrGNAT from C. reinhardtii (Supplementary Figure S1). Subsequently, these three candidates were subjected to further SMART analysis to figure out the possible protein domains. Results suggested that all three candidates contained the signature Acetyltransf_1 domain (Pfam accession: PF.00583), the same as AtGCN5, ScGCN5, and CrGNAT did, indicating that these three candidates should be the members of the GNAT family (Supplementary Figure S2). Furthermore, two of them (Gene005060 and Gene010338) contained the additional BROMO domain (Pfam accession: PF00439), as AtGCN5 and ScGCN5 did. Hence, Gene005060 and Gene010338 were selected as the candidates for HpGCN5 genes.
Experimental Cloning and Sequence Characterization of HpGCN5
Based on previous bioinformatics analysis, Gene005060 and Gene010338 were considered the HpGCN5 candidate genes. However, only Gene010338 was successfully experimental cloned, which was renamed HpGCN5. The isolated cDNA sequence of HpGCN5 was 1,712 bp in length and the open reading frame (ORF) was predicted to be 1,245 bp in length encoding 415 amino acids. By BLASTN against the genome sequence of Haematococcus pluvialis (BIG Data Center GSA Database accession no. PRJCA000614) using the HpGCN5 cDNA sequence, a scaffold was carried out. Based on the alignment, the HpGCN5 cDNA sequence covered 5,214 bp in the scaffold and was divided into 10 exons (Figure 1, Supplementary Data 1). The putative amino acid was analyzed by SMART and the results turned out two specific domains, Acetyltransf_1 (PF00583) and BROMO (PF00439) were presented indicating that HpGCN5 should be a member of proteins encoding acetyltransferase. The alignment using putative amino acids suggested high conservation in Acetyltransf_1 and BROMO domain between HpGCN5 and GNAT genes from other algae species, including Chlamydomonas sp., Tetrabaena socialis, Gonium pectoral, Scenedesmus sp., Coccomyxa subellipsoidea, Trebouxia sp., and Scenedesmus sp. (Figure 2). Meanwhile, cis-acting regulatory elements, were predicted in the 2,000 bp upstream of the coding region of HpGCN5. Twelve cis-elements were carried out, including five involved in light responsiveness (ACE, G-Box, GTGGC-motif, LAMP-element, and Sp1), five involved in small molecular hormone responsiveness (ABRE, CGTCA-motif, TGACG-motif, P-box, and TCA-element), one involved in anaerobic induction (ARE), and one involved in drought inducibility (MBS) (Table 1).

Figure 1. The schematic diagram of HpGCN5 gene structure, which was predicted by aligning with the corresponding genome sequence.
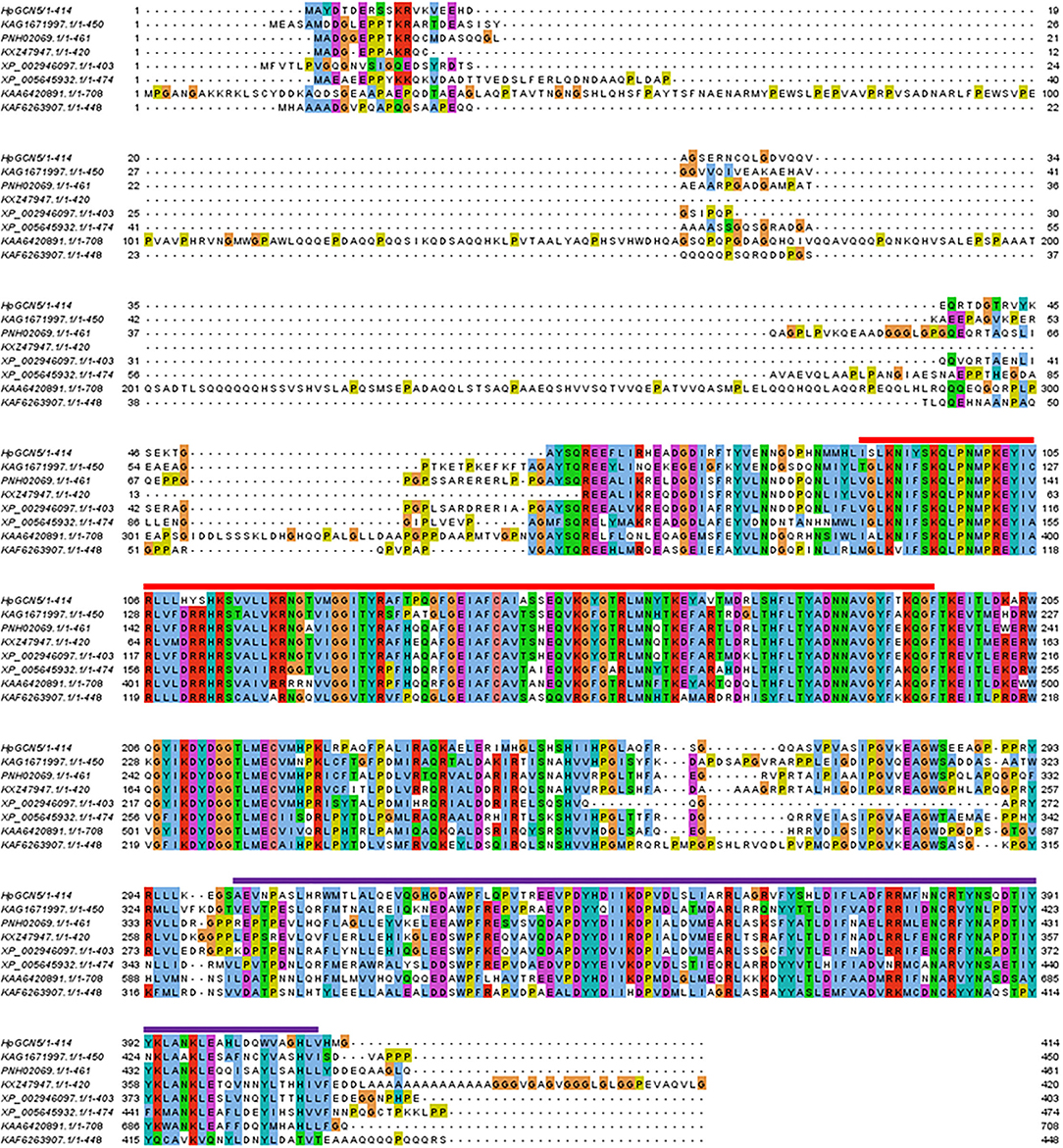
Figure 2. The alignment of amino acids of GCN5 gene from Haematococcus pluvialis and its homologous from other species including KAG1671997.1 from Chlamydomonas sp., PNH02069.1 from Tetrabaena socialis, KXZ47947.1 from Gonium pectoral, XP002946097.1 from Volvox carteri, XP 005645932.1 from Coccomyxa subellipsoidea, KAA6420891.1 from Trebouxia sp., and KAF6263907.1 from Scenedesmus sp. The Acetyltransf_1 domain (Pfam accession: PF00583) was shown by the red line and the BROMO domain (Pfam accession: PF00439) was shown by the purple line.
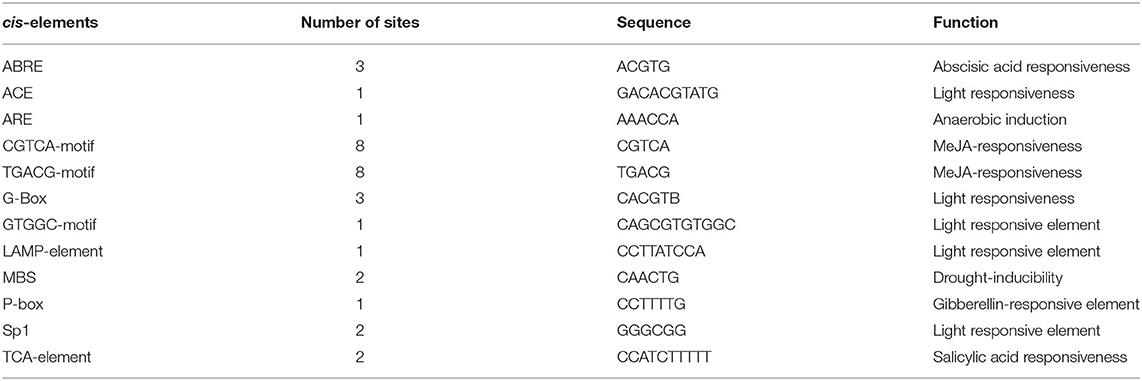
Table 1. Stress-related cis-elements in the promoter region of HpGCN5 identified from the PlantCARE database.
Molecular Evolution of HpGCN5
To determine the evolutionary pattern of histone acetyltransferase genes, an evolutionary relationship tree was constructed using amino acids obtained in this study and retrieved from GenBank by the Minimum Evolution method. The amino acids used in the tree were from multiple organisms, including algae, higher plants, yeast, animal, fungus, and bacteria (Figure 3). Results found that HpGCN5 was grouped with most histone acetyltransferase genes from algae, which was distinguished from other species, including higher plants, yeast, animal, fungus, and bacteria. Compared with yeast, animal, fungus, and bacteria, GNAT from algae was closer to higher plants, except XP_001693617.1 and XP_001706134.1 from algae Chlamydomonas reinhardtii. Within the algae group, HpGCN5 found in H. pluvialis was dissociated from the other seven algae species.
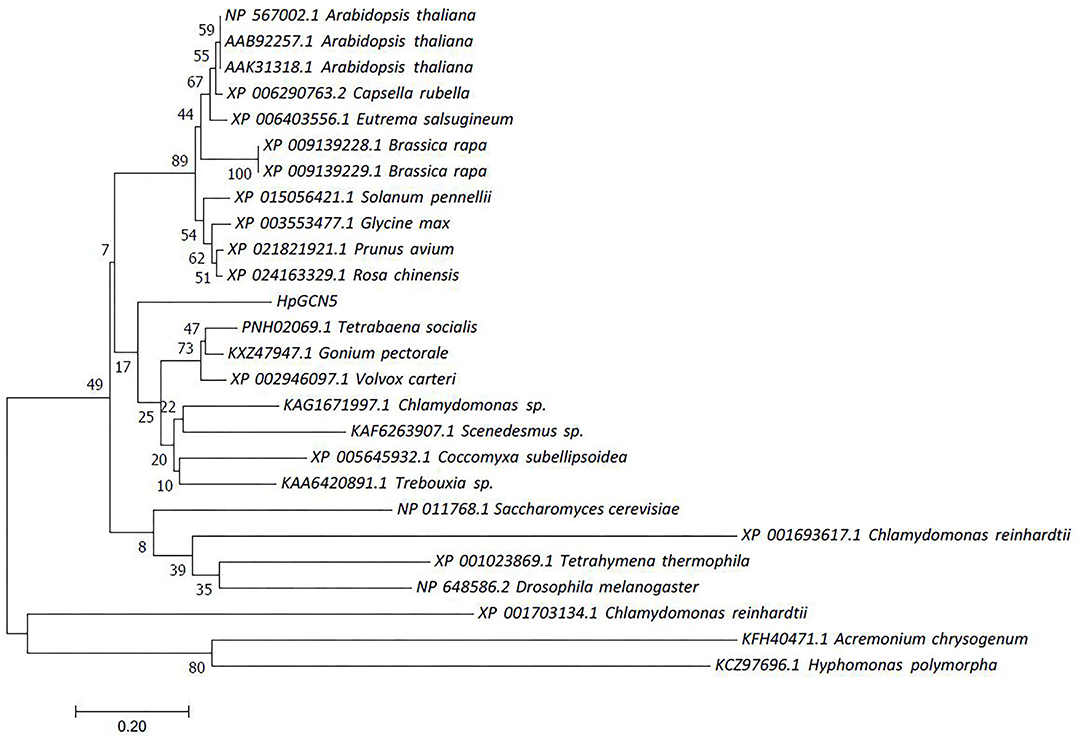
Figure 3. Phylogenetic relationships of the deduced amino acids of HpGCN5 with its homologous from other species.
Time-Course Expression Profile of HpGCN5 Under Multiple Abiotic Stresses
To further characterize the biological function of HpGCN5, qRT-PCR was carried out to evaluate its expression pattern under multiple stresses in a time-course manner, since stress-related cis-elements were present in the promoter region. Expression level changes were observed suggesting that HpGCN5 should be involved in the stress responses (Figure 4). It was shown that the responses of HpGCN5 to high light (HL) and high salt (HS) stresses were similar, which was different from that of salicylic acids (SA) and Nitrogen-depletion (N-) stresses. A significant decrease in the transcriptional level of HpGCN5 was found at the initial stage of HL and HS stresses and the down-regulation was continued in all examined samples. Conversely, a significant increase in the transcriptional level of HpGCN5 was displayed on Day 1 under SA and N- stresses, respectively. However, the transcriptional level was dramatically dropped back on Day 2 and continued to decrease until Day 3, then started to increase on Day 4 till Day 5. The highest transcriptional level was found on Day 1 under N- stress, which is about 4.5-fold as the control (Day 0).
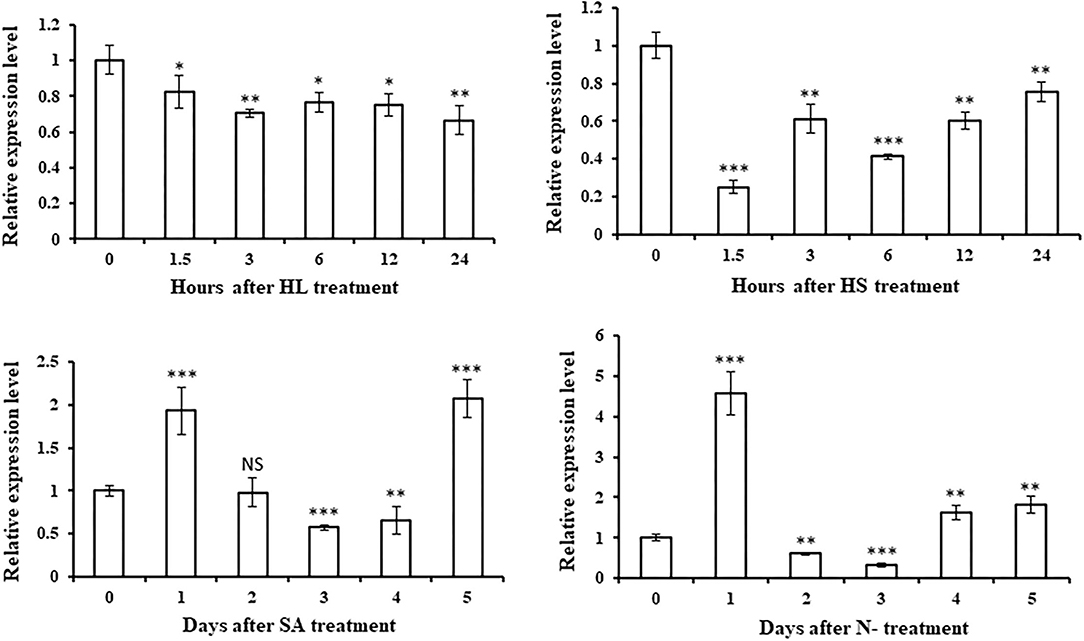
Figure 4. The relative expression levels of HpGCN5 under multiple abiotic stresses quantified by qRT-PCR assay. HL indicates the high light stress, HS indicates the high salt stress, SA indicates the salicylic acid stress, and N- indicates the Nitrogen-depletion stress. *, **, *** indicates the statistical significance between the treatment and control at the level of 0.05, 0.01, and 0.001, respectively. NS indicates no statistical significance.
Correlation Network of HpGCN5
To estimate if HpGCN5 is associated with other genes under stress conditions, the correlation network of HpGCN5 was constructed. The analyzed targets included 310 differentially expressed transcripts associated with transcription factors, carotenoids, and triacylglycerols biosynthesis identified in H. pluvialis grown under SAHL and SAHS conditions. It was found that seven transcripts correlated with HpGCN5 according to their expression pattern under the SAHL stress condition, whereas 24 transcripts had a correlation with HpGCN5 under the SAHS stress condition, implying that HpGCN5 might be more sensitive to SAHS stress than to SAHL stress (Figure 5, Table 2). In detailed, the seven transcripts in SAHL transcriptome included four transcripts associated with carotenoids biosynthesis, two transcripts associated with triacylglycerols biosynthesis, and one transcript involved in transcriptional regulation. The highest correlation factor was −0.957231, which was between HpGCN5 and MSTRG.12069.1 (a transcript involved in triacylglycerols biosynthesis). The 24 transcripts in SAHS transcriptome included 15, 4, and 5 associated with carotenoids, triacylglycerols, and transcriptional regulation, respectively. The highest factor was −0.994516, which was between HpGCN5 and Ch_GLEAN_10006000 (a transcript involved in carotenoids biosynthesis). It is interesting that transcripts involved in triacylglycerols biosynthesis were negatively correlated with HpGCN5 under SAHL stress, but positively correlated with HpGCN5 under SAHS stress. It indicated that either HpGCN5 or triacylglycerols biosynthesis genes respond to SAHL and SAHS stress in a different way.
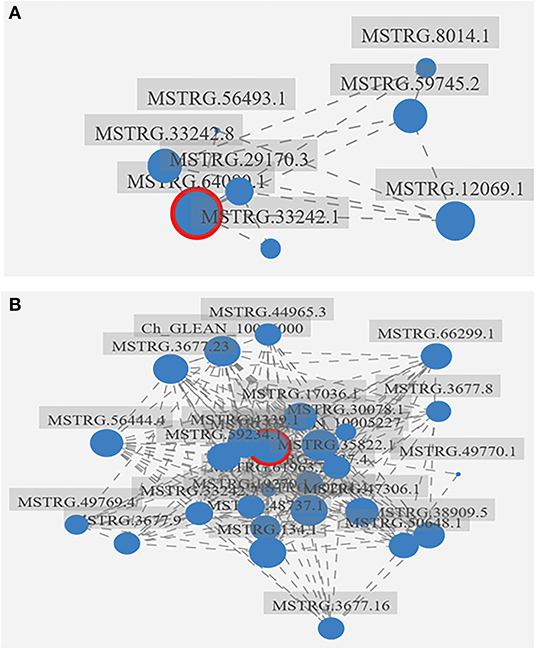
Figure 5. The correlation network of HpGCN5 and genes involved in carotenoids biosynthesis, triacylglycerols biosynthesis, and transcriptional regulation. (A) The correlation network constructed by using SAHL transcriptome data; (B) the correlation network constructed by using SAHS transcriptome data. The HpGCN5 was red cycled. The big blue dots indicate the nod in the network, and the bigger the nod, the more correlated transcripts.
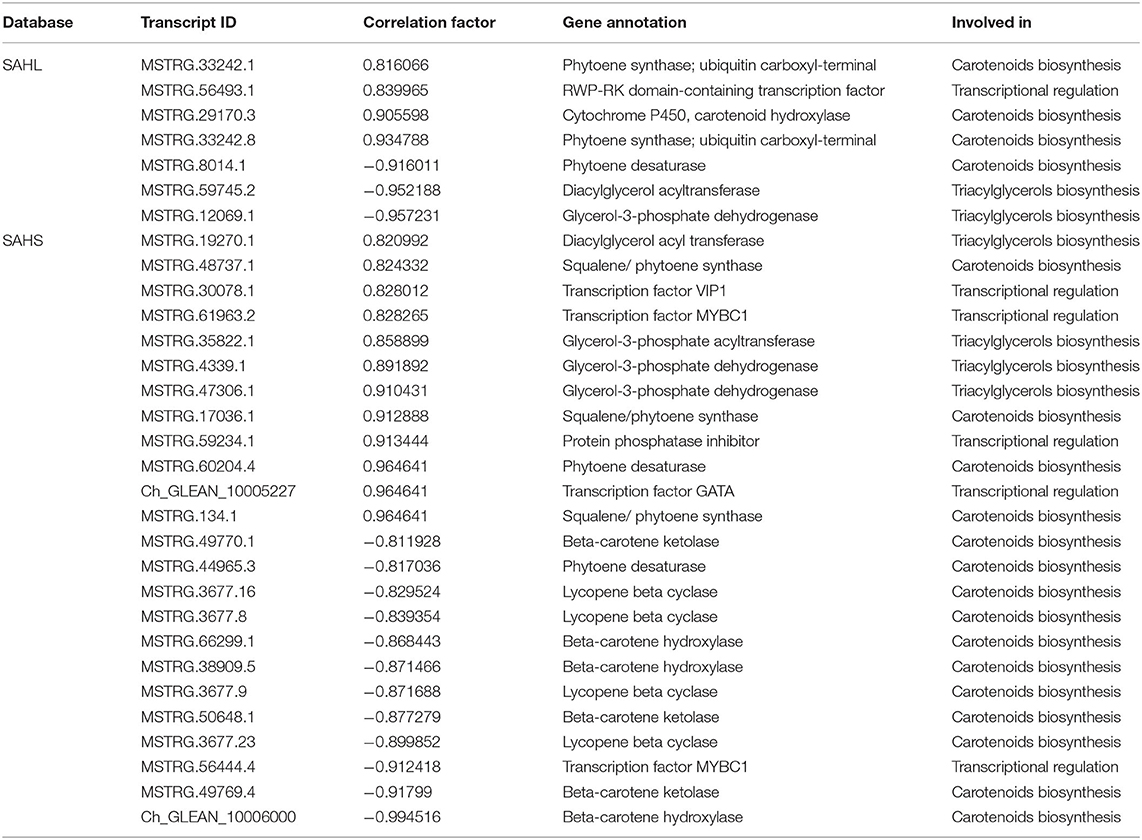
Table 2. The annotation of transcripts presented in the correlation network constructed using transcriptome data of SAHL and SAHS, respectively.
Discussion
Haematococcus pluvialis is a green microalga that naturally accumulates astaxanthin, which is a superior antioxidant with excellent commercial value (Shah et al., 2016). It has been documented that the biosynthesis and accumulation of astaxanthin is an internal stress response to remove free radicals produced when grown under unfavorable conditions (Shah et al., 2016). However, the molecular mechanism of stress responses in H. pluvialis is unclear. In higher plants, it has been reported that the histone modification, including histone acetylation, plays an important role in the stress response (Sterner and Berger, 2000; Stockinger et al., 2001; Pfluger and Wagner, 2007; Chinnusamy and Zhu, 2009; Fang et al., 2014; Hu, 2015; Ueda and Seki, 2020). Unfortunately, the discovery and functional analysis of histone modification in microalgae are rare.
In this study, a gene encoding histone acetyltransferase was identified from the Iso-seq transcriptome database. Its responses to multiple abiotic stresses were evaluated and its correlation with transcription factors and genes involved in triacylglycerols and astaxanthin biosynthesis was predicted, aiming to discover its potential role in stress regulation. Based on the bioinformatics analysis, three candidates were found to have the signature Acetyltransf_1 domain in GNAT genes while two of them contained the BROMO domain in GCN5 genes replying there might have two candidates of HpGCN5. A previous study found that various organisms, including Arabidopsis, S. cerevisiae, S. pombe, D. melanogaster, C. elegans, and even human beings, only have a single representative of GCN5 (Pandey et al., 2002). The experimental isolation was only success in Gene010338 (HpGCN5) but failed in Gene005060. A BLASTN has been performed using Gene005060 against the genome sequence of H. pluvialis. Results suggested the existence of an intron with 3,287 bp (data not shown), which might challenge the reality of Gene005060. It is well known that the technique of Iso-seq using single-module long reads reduced the accuracy of nucleotides for long transcripts (Gonzalez-Garay, 2016), leading to the possibility that Gene005060 might be a false prediction during transcriptome data processing when using the genome of H. pluvialis as the reference to correct the nucleotides. Therefore, we might conclude that H. pluvialis also only have a single representative of GCN5, as other species do.
According to the sequence analysis, HpGCN5 contained 10 exons encoding 415 amino acids (Figure 1). The domain analysis revealed that HpGCN5 contained a single Acetyltransf_1 and a single BROMO domain (Figure 2) that further assigned HpGCN5 into the subgroup of GCN5 belonging to the GNAT/MYST superfamily of HATs. In Arabidopsis, a single BROMO domain was found in BRD, CHR, HAF, GTE, and HAG1 proteins, leading to the prediction that plants do not have multi-bromodomain proteins like fungi and animals (Pandey et al., 2002). Similarly, a single BROMO domain was also found in HpGCN5. Therefore, HpGCN5 might evolutionarily be close to higher plants than fungi and animals and this was further confirmed by the phylogenetic relationship analysis (Figure 3). Within the microalgae clade in the phylogenetic tree, it showed that HpGCN5 was dissociated from the other seven algae species. Even though both Acetyltransf_1 and BROMO domain were presented in the other seven algae species and shared high amino acids similarity among species, large variation was found in the non-domain region (Figure 2), leading to the prediction that H. pluvialis might have a different ancestor of HpGCN5 from other algae species.
To explore the biological function of HpGCN5, the cis-element in the promoter and expression patterns under stress conditions were investigated. It was found that there were 12 cis-elements and 10 of them are associated with stress responsiveness, including light and hormones (Table 1). Therefore, the transcriptional expression levels of HpGCN5 under high light and salicylic acid stress were evaluated. Results turned out high light inhibited while salicylic acid-induced the expression of HpGCN5 (Figure 4). In H. pluvialis, researchers indicated that except high light and hormones, other stress conditions, such as salinity, nitrogen starvation, high temperature, and additional iron supplement, could also accelerate the accumulation of astaxanthin (Raman and Ravi, 2011; Gao et al., 2012a,b, 2015; Hong et al., 2015; He et al., 2018; Zhao et al., 2020; Hu et al., 2021). Therefore, the responses of HpGCN5 to high salt and Nitrogen-depletion were also evaluated. It is interesting that the high salt stress also inhibited the expression of HpGCN5 as high light did, whereas, the Nitrogen-depletion treatment generated similar expression changes of HpGCN5 as salicylic acid did (Figure 4). It is possible that the histone acetylation process controlled by HpGCN5 should be similar when induced by high light and high salt and it is different from that by hormones and Nitrogen-depletion. It is further revealed that the mechanism of high light and high salt response should be different from hormones and Nitrogen-depletion at the histone modification level.
With the advantage of previously constructed transcriptome data using H. pluvialis grown under SAHL and SAHS stress conditions for different periods, this study evaluated the possibility of HpGCN5 involved in the astaxanthin and triacylglycerol formation, as well as in the transcriptional regulation responding to stress conditions. The formation of astaxanthin and triacylglycerol is a common phenotypical response when grown under stress conditions in H. pluvialis (Shah et al., 2016). Meanwhile, the transcription factor has commonly played an important role in gene expression regulation (Hobert, 2008). Hence, the genes involved in carotenoids and triacylglycerols formation and transcriptional regulation were evaluated for their correlation with HpGCN5. It turns out major genes in the astaxanthin biosynthesis pathway, including phytoene desaturase (PDS), lycopene beta cyclase (LCY), beta-carotene hydroxylase (CrtR), and beta-carotene ketolase (BKT), were negatively correlated with HpGCN5 with factors over 0.8 (Figure 5, Table 2). It is surprising that only a few genes involved in triacylglycerols biosynthesis showed a correlation with HpGCN5 (Figure 5, Table 2), even though the triacylglycerols biosynthesis is also a responsive phenotype for H. pluvialis grown under stress conditions (Shah et al., 2016). Furthermore, MYB transcription factors in H. pluvialis should play an important role in astaxanthin accumulation by regulating astaxanthin synthesis-related genes (Gao et al., 2015; Wang et al., 2021). This study observed a strong correlation between HpGCN5 and MYBC1 (Table 2). Therefore, it might predict that HpGCN5 indirectly regulated the synthesis of astaxanthin by directly regulating MYB transcription factors. More research can be conducted to further clarify the mechanism of astaxanthin accumulation regulated by HpGCN5.
Data Availability Statement
The datasets presented in this study can be found in online repositories. The names of the repository/repositories and accession number(s) can be found in the article/Supplementary Material.
Author Contributions
DH, HL, and CW contributed to the conception, design of the study, and edited the manuscript. DH and WL performed experiments. DH and QH analyzed the data. DH wrote the draft of the manuscript. All authors contributed to the article and approved the submitted version.
Funding
This work was funded by the Natural Science Foundation of Guangdong Province (2019A1515011701) and the Shenzhen Scientific Project (JCYJ20210324093604011).
Conflict of Interest
The authors declare that the research was conducted in the absence of any commercial or financial relationships that could be construed as a potential conflict of interest.
Publisher's Note
All claims expressed in this article are solely those of the authors and do not necessarily represent those of their affiliated organizations, or those of the publisher, the editors and the reviewers. Any product that may be evaluated in this article, or claim that may be made by its manufacturer, is not guaranteed or endorsed by the publisher.
Acknowledgments
We thank Dr. Zhangli Hu for his support to accomplish experiments in this study.
Supplementary Material
The Supplementary Material for this article can be found online at: https://www.frontiersin.org/articles/10.3389/fpls.2022.903764/full#supplementary-material
Supplementary Figure S1. Maximum Likelihood analysis of GCN5 candidates from Haematococcus pluvialis and reported genes from Arabidopsis (AAK31318.1), Saccharomyces cerevisiae (NP 011768.1), and Chlamydomonas reinhardtii (XP_001693868.1).
Supplementary Figure S2. The domain of GCN5 candidates from Haematococcus pluvialis and reported genes from Arabidopsis (AAK31318.1), Saccharomyces cerevisiae (NP 011768.1), and Chlamydomonas reinhardtii (XP_001693868.1) according to SMART analysis.
Supplementary Data 1. The corresponding genome sequence of HpGCN5 downloaded from the BIG Data Center GSA Database accession no. PRJCA000614.
References
Allen, M. M. (1968). Simple conditions for growth of unicellular blue-green algae on plates. J. Phycol. 4, 1–4. doi: 10.1111/j.1529-8817.1968.tb04667.x
Chinnusamy, V., and Zhu, J. (2009). Epigenetic regulation of stress responses in plants. Curr. Opin. Plant Biol. 12, 133–139. doi: 10.1016/j.pbi.2008.12.006
Fang, H., Liu, X., Thorn, G., Duan, J., and Tian, L. (2014). Expression analysis of histone acetyltransferases in rice under drought stress. Biochem. Bioph. Res. Co. 443, 400–405. doi: 10.1016/j.bbrc.2013.11.102
Gao, Z. Q., Li, Y., Wu, G. X., Li, G. Q., Sun, H. F., Deng, S. Z., et al. (2015). Transcriptome analysis in Haematococcus pluvialis: astaxanthin induction by salicylic Acid (SA) and jasmonic Acid (JA). PLoS ONE. 10, 1–14. doi: 10.1371/journal.pone.0140609
Gao, Z. Q., Meng, C. X., Zhang, X. W., Xu, D., Miao, X. X., Wang, Y. T., et al. (2012a). Induction of salicylic acid (SA) on transcriptional expression of eight carotenoid genes and astaxanthin accumulation in Haematococcus pluvialis. Enzyme Micro. Technol. 51, 225–230. doi: 10.1016/j.enzmictec.2012.07.001
Gao, Z. Q., Meng, C. X., Zhang, X. W., Xu, D., Zhao, Y. F., Wang, Y. T., et al. (2012b). Differential expression of carotenogenic genes, associated changes on astaxanthin production and photosynthesis features induced by JA in H. pluvialis. PLoS ONE. 7, 1–9. doi: 10.1371/journal.pone.0042243
Gonzalez-Garay, M. L. (2016). Introduction to isoform sequencing using pacific biosciences technology (Iso-Seq). In: Wu, J,. (eds) Transcriptomics and Gene Regulation. Translational Bioinformatics, vol 9, pp. 141–160, Springer, Dordrecht. doi: 10.1007/978-94-017-7450-5_6
He, B. G., Hou, L. L., Dong, M. M., Shi, J. W., Huang, X. Y., Ding, Y. T., et al. (2018). Transcriptome analysis in Haematococcus pluvialis: astaxanthin induction by high light with acetate and Fe2+. Int. J. Mol. Sci. 19, 1–18. doi: 10.3390/ijms19010175
Hobert, O. (2008). Gene regulation by transcription factors and microRNAs. Science 319, 1785–1786. doi: 10.1126/science.1151651
Hong, M., Hwang, S. K., Chang, W. S., Kim, B. W., Lee, J., and Sim, S. J. (2015). Enhanced autotrophic astaxanthin production from Haematococcus pluvialis under high temperature via heat stress-driven Haber-Weiss reaction. Appl. Microbiol. Biotechnol. 99, 5203–5215. doi: 10.1007/s00253-015-6440-5
Hu, Q., Huang, D., Li, A., Hu, Z., Gao, Z., Yang, Y., et al. (2021). Transcriptome-based analysis of the effects of salicylic acid and high light on lipid and astaxanthin accumulation in Haematococcus pluvialis. Biotechnol. Biofuel. 14, 82. doi: 10.1186/s13068-021-01933-x
Hu, Z. (2015). Histone acetyltransferase GCN5 is essential for heat stress-responsive gene activation and thermotolerance in Arabidopsis. Plant J. 84, 1178–1191. doi: 10.1111/tpj.13076
Huang, D., Liu, W., Li, A., Wang, C., and Hu, Z. (2019). Discovery of geranylgeranyl pyrophosphate synthase (GGPPS) paralogs from Haematococcus pluvialis based on Iso-Seq analysis and their function on astaxanthin biosynthesis. Mar. Drugs 17, 696. doi: 10.3390/md17120696
Kadonaga, J. T. (1998). Eukaryotic transcription: an interlaced network of transcription factors and chromatin-modifying machines. Cell 92, 307–313. doi: 10.1016/S0092-8674(00)80924-1
Kim, E. J., Ma, X., and Cerutti, H. (2015). Gene silencing in microalgae: mechanisms and biological roles. Bioresour. Technol. 184, 23–32. doi: 10.1016/j.biortech.2014.10.119
Kumar, S., Stecher, G., and Tamura, K. (2016). MEGA7: Molecular evolutionary genetics analysis version 7.0 for bigger datasets. Mol. Biol. Evol. 33, 1870–1874. doi: 10.1093/molbev/msw054
Kuo, M. H., and Allis, C. D. (1998). Roles of histone acetyltransferases and deacetylases in gene regulation. Bioassays 20, 615–626. doi: 10.1002/(SICI)1521-1878(199808)20:8<615::AID-BIES4>3.0.CO;2-H
Lescot, M., Déhais, P., Moreau, Y., De Moor, B., Rouz,é, P., and Rombauts, S. (2002). PlantCARE: a database of plant cis-acting regulatory elements and a portal to tools for in silico analysis of promoter sequences. Nucleic Acids Res. Datab. 30, 325–327. doi: 10.1093/nar/30.1.325
Loidl, P. (2004). A plant dialect of the histone language. Trends Plant Sci. 9, 84–90. doi: 10.1016/j.tplants.2003.12.007
Luo, Q., Bian, C., Tao, M., Huang, Y., Zheng, Y., Lv, Y., et al. (2019). Genome and transcriptome sequencing of the astaxanthin-producing green microalga, Haematococcus pluvialis. Genome Biol. Evol. 11, 166–173. doi: 10.1093/gbe/evy263
Mackay, I. M., Arden, K. E., and Nitsche, A. (2002). Real-time PCR in virology. Nucleic Acids Res. 30, 1292–1305. doi: 10.1093/nar/30.6.1292
Panagopoulos, I., Fioretos, T., Isaksson, M., Samuelsson, U., Billström, R., Strömbeck, B., et al. (2001). Fusion of the MORF and CBP genes in acute myeloid leukemia with the t(10,16) (q22,p13). Hum. Mol. Genet. 10, 395–404. doi: 10.1093/hmg/10.4.395
Pandey, R., MuÈller, A., Napoli, C. A., Selinger, D. A., Pikaard, C. S., Richards, E. J., et al. (2002). Analysis of histone acetyltransferase and histone deacetylase families of Arabidopsis thaliana suggests functional diversification of chromatin modification among multicellular eukaryotes. Nucleic Acids Res. 30, 5036–5055. doi: 10.1093/nar/gkf660
Pfluger, J., and Wagner, D. (2007). Histone modifications and dynamic regulation of genome accessibility in plants, Curr. Opin. Plant Biol. 10, 645. doi: 10.1016/j.pbi.2007.07.013
Raman, V., and Ravi, S. (2011). Effect of salicylic acid and methyl jasmonate on antioxidant systems of Haematococcus pluvialis. Acta Physiol. Plant 33, 1043–1049. doi: 10.1007/s11738-010-0623-6
Roth, S. Y., Denu, J. M., and Allis, C. D. (2001). Histone acetyltransferases. Annu. Rev. Biochem. 70, 81–120. doi: 10.1146/annurev.biochem.70.1.81
Shah, M. R., Yuanmei, L., Cheng, J. J., and Maurycy, D. (2016). Astaxanthin-producing green microalga Haematococcus pluvialis: from single cell to high value commercial products. Front. Plant Sci. 7, 531. doi: 10.3389/fpls.2016.00531
Sterner, D. E., and Berger, S. L. (2000). Acetylation of histones and transcription-related factors. Microbiol. Mol. Biol. Rev. 64, 435–459. doi: 10.1128/MMBR.64.2.435-459.2000
Stockinger, E. J., Mao, Y., Regier, M. K., Triezenberg, S. J., and Thomashow, M. F. (2001). Transcriptional adaptor and histone acetyltransferase proteins in Arabidopsis and their interactions with CBF1, a transcriptional activator involved in cold regulated gene expression. Nucleic Acids Res. 29, 1524–1533. doi: 10.1093/nar/29.7.1524
Ueda, M., and Seki, M. (2020). Histone modifications form epigenetic regulatory networks to regulate abiotic stress response. Plant Physiol. 182, 15–26. doi: 10.1104/pp.19.00988
Vlachonasios, K. E., Thomashow, M. F., and Triezenberg, S. J. (2003). Disruption mutations of ADA2b and GCN5 transcriptional adaptor genes dramatically affect Arabidopsis growth, development, and gene expression. Plant Cell 15, 626–638. doi: 10.1105/tpc.007922
Wang, C., Wang, K., Ning, J., Luo, Q., Yang, Y., Huang, D., et al. (2021). Transcription factors from Haematococcus pluvialis involved in the regulation of astaxanthin biosynthesis under high light-sodium acetate stress. Front. Bioeng. Biotechnol. 9, 650178. doi: 10.3389/fbioe.2021.650178
Wang, Y., Cheng, Z. Z., Chen, X., Zheng, Q., and Yang, Z. M. (2015). CrGNAT gene regulates excess copper accumulation and tolerance in Chlamydomonas reinhardtii. Plant Sci. 240, 120–129. doi: 10.1016/j.plantsci.2015.09.004
Wen, Z., Liu, Z., Hou, Y., Liu, C., Gao, F., Zheng, Y., et al. (2015). Ethanol induced astaxanthin accumulation and transcriptional expression of carotenogenic genes in Haematococcus pluvialis. Enzym. Microb. Technol. 78, 10–17. doi: 10.1016/j.enzmictec.2015.06.010
Keywords: Haematococcus pluvialis, HpGCN5, abiotic stresses, correlation network, astaxanthin
Citation: Huang D, Liu W, Hu Q, Li H and Wang C (2022) The Histone Acetyltransferase HpGCN5 Involved in the Regulation of Abiotic Stress Responses and Astaxanthin Accumulation in Haematococcus pluvialis. Front. Plant Sci. 13:903764. doi: 10.3389/fpls.2022.903764
Received: 24 March 2022; Accepted: 19 April 2022;
Published: 20 May 2022.
Edited by:
Zhengquan Gao, Shandong University of Technology, ChinaReviewed by:
Zhao Xiao Hu, Huazhong Agricultural University, ChinaNianjun Xu, Ningbo University, China
Copyright © 2022 Huang, Liu, Hu, Li and Wang. This is an open-access article distributed under the terms of the Creative Commons Attribution License (CC BY). The use, distribution or reproduction in other forums is permitted, provided the original author(s) and the copyright owner(s) are credited and that the original publication in this journal is cited, in accordance with accepted academic practice. No use, distribution or reproduction is permitted which does not comply with these terms.
*Correspondence: Chaogang Wang, charlesw@szu.edu.cn