- 1Beijing Key Laboratory of New Technology in Agricultural Application, National Demonstration Center for Experimental Plant Production Education, Plant Science and Technology College, Beijing University of Agriculture, Beijing, China
- 2National Engineering Research Center for Vegetables, Institute of Vegetable Science, Beijing Academy of Agriculture and Forestry Sciences, Beijing, China
Lettuce (Lactuca sativa L.) is a leafy vegetable whose edible organs usually are leaf or stems, and thus high-temperature induced bolting followed by flower initiation is an undesirable trait in lettuce production. However, the molecular mechanism that controls lettuce bolting and flowering upon thermal treatments is largely unknown. Here, we identified a Lettuce auxin response factor 3 (LsARF3), the expression of which was enhanced by heat and auxin treatments. Interestingly, LsARF3 is preferentially expressed in stem apex, suggesting it might be associated with lettuce bolting. Transgenic lettuce overexpressing LsARF3 displayed early bolting and flowering, whereas knockout of LsARF3 dramatically delayed bolting and flowering in lettuce under normal or high temperature conditions. Furthermore, Exogenous application of IAA failed to rescue the late-bolting and -flowering phenotype of lsarf3 mutants. Several floral integrator genes including LsCO, LsFT, and LsLFY were co-expressed with LsARF3 in the overexpression and knockout lettuce plants. Yeast one-hybrid (Y1H) experiments suggested that LsARF3 could physically interact with the LsCO promoter, which was further confirmed by a dual luciferase assay in tobacco leaves. The results indicated that LsARF3 might directly modulate the expression of LsCO in lettuce. Therefore, these results demonstrate that LsARF3 could promote lettuce bolting in response to the high temperature by directly or indirectly activating the expression of floral genes such as LsCO, which provides new insights into lettuce bolting in the context of ARFs signaling and heat response.
Introduction
Lettuce (Lactuca sativa L.) is a popular leafy vegetable which is harvested and consumed during its vegetative growth. Thus, early bolting can result in a significant reduction in crop yield and culinary quality, and consequently is an undesirable trait in lettuce cultivation and breeding (Simonne et al., 2002; Han et al., 2021). Bolting is characterized as the elongation of the floral stem, accompanied with the initiation of the flowering (Pouteau and Albertini, 2009). It has been proposed that bolting and flowering are regulated by an elaborate network of genetic pathways that incorporate endogenous and environmental cues, such as vernalization, photoperiod, circadian clock, gibberellin, nutrient supply, and ambient temperature (Andrés and Coupland, 2012; Kinoshita and Richter, 2020; Yan et al., 2021). The molecular basis of photoperiodic induction of floral transition has been studied intensively in the model plant Arabidopsis (Blümel et al., 2015). It has been demonstrated that the bolting and flowering signals from internal or external factors usually converge on a few integrator genes which serve as flowering inducers or repressors during floral transition. Floral inducers including FLOWERING LOCUS T (FT), FD, SUPPRESSOR OF CONSTANS 1 (SOC1), and LFEAY (LFY) activate the transcription of the majority of genes floral genes, promoting Arabidopsis bolting and flowering (Johansson and Staiger, 2015). Besides, nuclear proteins CONSTANS (CO) and GIGANTEA (GI) also promote flowering through photoperiod and circadian pathways, making them master regulators affecting floral transition (Valverde, 2011; Brandoli et al., 2020). On the contrary, transcription factor FLOWERING LOCUS C (FLC) is the major flowering repressor, which antagonizes gibberellin and photoperiod pathways and delays bolting and flowering (Sheldon et al., 2000). Arabidopsis flc mutant exhibits accelerated flowering independent of vernalization, whereas some accessions with functional FLC genes do need vernalization for summer flowering (Shen et al., 2022). Although progresses have been achieved in the photoperiod and vernalization, our knowledge of the effects of ambient temperature on flowering and bolting remains intangible.
It is well-known that ambient temperature has pronounced, albeit complex, effects on bolting and flowering time (Mcclung et al., 2016). In Arabidopsis, bolting and flowering time are strongly influenced by high temperatures (Andrés and Coupland, 2012; Takeno, 2016). Previous studies suggested that, when exposed to high growth temperature, Arabidopsis can accelerate its floral transition and seed production to survive the adverse environment. Similarly, most lettuce cultivars prefer to grow in cool conditions (16°C–18°C), while heat temperature (>24°C, may be variable depending on the genetic background) promotes lettuce bolting and flowering (Katz and Weaver, 2003; Yamori et al., 2022). Some lettuce cultivars such as GB-30 are also extremely sensitive to the thermal treatments and can initiate their floral stem elongation, as well as flowering, within one-week growth at a greenhouse with the temperature above 33°C (Hao et al., 2021), thus leading to severe economic loss to lettuce growers, especially under frequently occurred heat waves in summers. Thus, investigations on heat-induced flowering are of great interest to biologists as well as breeders.
In Arabidopsis, at least two bHLH transcription factors PHYTROCHROME INTERACTING FACTOR4 (PIF4) and PIF5 promote flowering through the induction of FT and its paralog TWIN SISTER OF FT (TSF) during thermally induce flowering (Kunihiro et al., 2011; Kumar et al., 2012; Mcclung et al., 2016). Furthermore, PIF4 can physically interact with CO and other components to form a complex, thereby contributing to the upregulation of FT and TSF (Kumar et al., 2012). These results indicate that “heat signals” are relayed to FT and/or its homologs, upregulation of which contributes to the acceleration of bolting and flowering in response to elevated temperature. Studies in lettuce suggested that heat shock transcription factors (HSFs) might be involved in the floral induction pathway, and its binding motifs were identified in the promoter regions of LsSOC1 and LsMADS55, putative lettuce orthologues to Arabidopsis SOC1 and APETALA1(Chen et al., 2018; Ning et al., 2019). Knockdown or RNA inference lines of LsSOC1 are insensitive to high temperature, and overexpression of LsMADS55 resulted in early flowering in transgenic Arabidopsis lines, suggesting that their important roles in heat-promoted bolting and flowering (Chen et al., 2018; Ning et al., 2019). Thus, it has been postulated that heat-accelerated floral transition in lettuce might be regulated by a number of HSF transcription factors (Chen et al., 2018; Ning et al., 2019). Proteomic analysis revealed that HEAT SHOCK PROTEIN 70 (HSP70) is differentially expressed between heat-sensitive and heat-tolerate lettuce cultivars, further supporting the possibility of the HSP-modulated flowering pathway in lettuce (Li et al., 2017). Another study compared the transcriptome of heat-treated (bolted) and control (non-bolted) lettuce and found that C2H2 zinc finger, AP2/EREBP, and WRKY transcription factors might be associated with heat-accelerated bolting (Liu et al., 2018). Although there is no experimental evidence that PIFs were involved in thermally induced floral transition in lettuce, current findings suggested that the floral transition in lettuce might be modulated under different regulatory pathways from that of Arabidopsis.
Auxin is a key phytohormone that regulates many physiological and developmental processes in plants, such as embryogenesis, organogenesis, directional growth, and organ structure (Enders and Strader, 2015). Auxin response factors (ARFs) represent the core component of the auxin signal cascade, as they are responsible for the transcriptional regulation of a defined set of genes in the auxin pathway (Roosjen et al., 2018). Most ARF protein contains a conserved amino-terminal DNA-binding domain (DBD) responsible for the binding to TGTCTC motif, a variable middle region that confers activator or repressor activity, and a C-terminal PB1 (formally called IAA/Aux domain) involved in homo- and hetero-dimerization (Liu et al., 2014a; Li et al., 2016; Roosjen et al., 2018). However, Arabidopsis ARF3/ETTIN (ETT) encodes a truncated ARF proteins which lack PB1 domains (Nemhauser et al., 2000; Guilfoyle and Hagen, 2007), and thus ARF3 could be insensitive to auxin. Nevertheless, ARF3 functions in some auxin-involved pathways, including polarity specification, gynoecium patterning, floral meristem maintenance and termination, and early flower development, in Arabidopsis (Nemhauser et al., 2000; Liu et al., 2014b; Simonini et al., 2017; Kuhn et al., 2020). For example, ARF3 controls floral meristem determinacy by directly repressing the expression of ISOPENTENYLTRANSFERASE (IPT) family genes (Cheng et al., 2013). ARF3 is a direct target of APETALA2 (AP2), which is associated with its role in floral meristem determinacy (Liu et al., 2014b). Transcription of ARF3 itself is repressed by TAS3-derived trans-activating small interfering RNAs (tasiRNAs), which ensures leaf polarity in Arabidopsis (Hunter et al., 2006). Noteworthy, a recent advance showed that a number of drought stress-related genes were upregulated in ARF3 overexpressing Arabidopsis, indicating that ARF3 is important for those genes’ expression under dehydration stress (Zheng et al., 2018). Therefore, it can be concluded that ARF3 is emerging as a key regulator of abiotic stress and development in Arabidopsis. However, how ARF3 participates in the accelerated floral transition under thermal treatments remains to be elucidated.
Our previous study showed that the majority of LsARFs were positively respond to heat stress and lettuce bolting (Qi, 2019; Wang et al., 2022b). Of these heat-inducible LsARF genes, LsARF3 expression was stimulated by elevated temperatures and maintained higher expression level with the progression of heat stress, indicating LsARFs might be implicated in the heat-accelerated floral transition (Qi, 2019). However, the molecular mechanism by which ARF3 regulates thermally induced bolting and flowering is still unclear. In this study, we demonstrate that the expression level of LsARF3 was increased upon the floral transition and thermal treatments. Transgenic lettuce overexpressing LsARF3 exhibited early bolting and flowering phenotypes. By contrast, the floral transition of lettuce lsarf3 mutants was delayed under thermal treatment, and exogenous application of auxin failed to rescue the late bolting of lsarf3 mutants. We also showed that several floral genes such as LsCO, LsFT, and LsLFY, might be directly regulated by LsARF3 through co-expression and Yeast one-hybridization analysis. Those findings not only identify a new player in thermally accelerated bolting but also contribute to a better understanding of ARFs signaling and heat response in lettuce.
Materials and methods
Plant materials and treatment
Lettuce (Lactuca sativa L.) of GB-30, a variety, which bolts easily and comes from seeding plant protection Co., LTD in Inner Mongolia Bameng Fidelity, were conserved in our laboratory. Seeds were sown in a mixture of sand, soil, and peat (volume ratio of 1:1:1), and grown in the growth chamber under normal conditions (20°C/13°C (day/night), 14 light and 10 h darkness, and 60% relative humidity). Standard pest control and water management practices were used. The seedlings were transplanted into 6 cm × 8 cm pots at the trefoil stage. When the plants developed the sixth true leaf, they were divided into two groups. The control group continued growing under the normal growth conditions as described above. The other group was moved to another growth chamber and treated with high temperatures of 33°C and 25°C during the day and night, respectively. The other environmental factors were unchanged. After high-temperature treatment for 0, 2, 4, 6, 8, 10, 12, 24 h and 0, 8, 16, 24 days, shoot apex of lettuce were harvested for RNA extractions. Root, stem (stalk), stem apex, leaf, flower, fruit, and seed tissue samples from lettuce grown under the normal temperature conditions were also collected. All samples were immediately frozen in liquid nitrogen and stored at −80 ° C for further measurements on gene expression analysis. Every five plants were used as one replicate for three replicates.
RNA extraction, synthesis of cDNA, LsARF3 cloning, and bioinformatics analysis
Total RNA was extracted from specific tissues using the Quick RNA isolation Kit (Huayueyang Biotech, Beijing, China), following the user manual from the manufacturer. NanoDrop 2000 Spectrophotometer (Thermo Fisher Scientific, Wilmington, DE, United States) and 1% agarose gel electrophoresis were used to determine RNA quality and concentration. The extracted RNA was digested with EasyScript® One-Step gDNA Removal and cDNA Synthesis SuperMix (Transgen, Beijing, China) was used to synthesize cDNA.
The open reading frame of the cDNA was amplified with gene-specific primers of LsARF3-F/R (Supplementary Table1) based on LsARF3 (AccessionXM_023880987.2) available at NCBI. The whole cDNA of LsARF3 was then amplified with mixed super fidelity polymerase (Vazyme Biotech Co., Ltd.), under thermal cycling conditions of 95°C denaturation for 3 min, followed by 35 cycles of 95°C for 15 s, 60°C for 15 s, 72°C for 2 min, and a final extension of 72°C for 5 min. A single band of expected size 1794 bp was purified from gel and sub-cloned into pEASY vector (TransGen Biotech, Beijing, China).
A homology search of sequences was carried out through BLAST in NCBI, and multiple alignments were analyzed using DNAMAN 9.0. A phylogenetic tree was constructed using the neighbor-joining method (NJ) with MEGA 7.0 software.
Quantitative real-time PCR
The total RNA of fresh lettuce samples was extracted by quick RNA Isolation Kit (Huayueyang, China), and the cDNA was obtained by transcript one step gDNA removal and cDNA synthesis Supermix reagent (Transgen, China). Then RR430b TB green fast qPCR mix (Takara, Japan) preparation system, and the fluorescence data were obtained by Bole bio rad CFX96 real-time fluorescence quantitative PCR instrument. 18 s rRNA (HM047292.1) was selected as the internal reference gene, and the internal reference gene and samples were biologically repeated three times and technically repeated three times (Supplementary Table1). The relative gene expression is based on the 2−Δ ΔCt method.
Subcellular localization
pRI101 Carrying GFP label after transformation was selected, and pRI101-LsARF3 vector was constructed through homologous recombination. The 35S promoter was used for directing the expression of the fusion gene. Subcellular localization of LsARF3 by fusion with a green fluorescent protein (GFP) in the C-terminal region. It was injected into tobacco with five leaves by Agrobacterium infection. After dark culture for 2 days, it was observed and photographed under a laser confocal microscope.
Overexpression and CRISPR/Cas9 plasmid construction
Using LsARF3 clone plasmid as a template, primer LsARF3-OE-F/R (Supplementary Table1), amplify the target band and add a homologous arm. NdeI restriction endonuclease single enzyme digested the expression vector pRI101-GFP to linearize the plasmid. Clonexpress II one-step cloning kit homologous recombinant PCR product and linearized plasmid were transferred to DH5α competent cell. After colony PCR, the positive clones were sent to sequencing, and the correct sequenced plasmid was transferred into Agrobacterium GV3101. The sequencing primer was pRI101-F/R (Supplementary Table1).
According to the principle of adjacent motif recognition (PAM) of the original spacer sequence of the CRISPR/Cas9 system and the design principle of guide RNAs (gRNAs) of gene knockout target site, CRISPR gRNA is used to design the website1 and NCBI-Blast potential knockout site detection, and select the two most suitable gRNAs. Four primers were designed according to two gRNA sequences. CRISPR/Cas9 plasmid pKSE401-DT1T2 carries two tandem repeat gRNA expression boxes and Cas9 enzyme. Using pCBC-DT1T2 plasmid as a template, four primers DT1-BsF + DT1-F0/DT1-BsR + DT1-R0 (Supplementary Table1) were amplified to obtain a tandem repeat expression box fragment carrying gRNA1 and gRNA2 with homologous arm. Bsai-HFv2 restriction enzyme linearized pKSE401 vector, ClonExpress II One Step Cloning Kit homologous recombinant PCR product and linearized plasmid, and transferred to DH5α competent cell. After colony PCR, the positive clones were sent for sequencing. The plasmids in which both two gRNAs were successfully constructed were transferred into Agrobacterium GV3101. The colony PCR and sequencing primers were U626-IDF/U629-IDR (Supplementary Table1).
Genetic transformation of lettuce
To generate the LsARF3 transgenic lettuce, Agrobacterium-mediated leaf disk transformation of lettuce. Cotyledons of 4-day-old seedlings were pre-cultured in an MS medium supplemented with 0.5 mg/l 6-BA, and 0.05 mg/l NAA for 1 day. The cotyledons were then immersed for 5 min in an Agrobacterium culture (OD600 = 0.1–0.3) with the liquid MS medium. The cotyledons were dried and then co-cultured for 30 h in an MS medium containing 0.5 mg/l Kinetin, and 0.05 mg/l NAA under dark conditions. After co-cultivation, the cotyledons were transformed into a regeneration and selection MS medium (0.5 mg/l 6-BA, 0.05 mg/l NAA, 300 mg/l Timentin, 100 mg/l Kanamycin). After every week, the medium was refreshed until the shooting. Two-to-three-centimeter newly developed shoots were then transformed into a rooting MS medium (0.05 mg/l NAA and 300 mg/l Timentin) until true roots developed. Once the regenerated seedlings had enough and strong roots, they were transplanted to nutrient soil and continued to grow in the greenhouse.
The identification method of overexpression positive seedlings is as follows: T0 takes the leaves of transgenic seedlings to extract DNA, takes DNA as template, pRI101-F/R as identification primer, amplifies bands, and those with clear bands are positive seedlings. After the seeds of T0 generation positive seedlings were harvested and sown, the leaves of T1 generation transgenic seedlings were taken to extract RNA and reverse transcribed to synthesize cDNA. The cDNA was used as the template and GFP-F/R (Supplementary Table1) was used as the identification primer to amplify the bands. If the expression increased significantly, it was a positive seedling. Through the above methods, we have obtained three T1 lines of overexpressed LsARF3. The identification method of gene editing positive seedlings is as follows: take the leaf DNA of transgenic seedlings as the template, U626-IDF/U629-IDR and Cas9-F/R as the primers, and those with two bright bands in amplification are transgenic positive seedlings. On the genomic DNA sequence of leaf lettuce, the primers CRISPR-DNA-F/R (Supplementary Table1) were designed at about 200 bp before and after the two gRNAs to amplify the target sequence of positive seedlings, and the PCR products were sent to sequencing to identify the editing type. Unedited and homozygous editing types can be directly determined by the sequencing results of PCR products. If the sequencing results of PCR products are bimodal, it is necessary to connect the PCR products to the Blunt-Zero cloning vector, and send at least 10 monoclonal antibodies to each sample for sequencing. The results were consistent and could be regarded as positive seedlings. Through the above methods, three homozygous T1 lines of LsARF3 gene editing were obtained.
The phenotypic changes of LsARF3-OE and WT plants were observed and recorded at normal temperature (NT), the stem length changes were measured every day. The lsarf3 mutants and WT plants were treated with high temperature (HT) when they had six leaves. After treatment, the changes in phenotype and stem length were observed and recorded every day. In both LsARF3-OE and lsarf3 mutants, when the stem length was significantly different compared to WT plants, flower bud differentiation of stem apexs were observed regularly by paraffin section method (Zhang et al., 2016) to determine bolting time.
Exogenous IAA application
When the seedlings of lsarf3 mutants and WT plants grow to six leaves, they are sprayed every other week at a concentration of 40 mg/Kg for four consecutive weeks. The plant phenotype was observed and photographed every day, the stem length was measured every 8 days, the progress of flower bud differentiation of stem apex was determined by the paraffin section method (Zhang et al., 2016), and the transcript level of LsARF3 was detected by qRT-PCR at 24 days after exogenous IAA application.
Yeast one-hybrid assay
For the Yeast one-hybrid (Y1H) screening assay, the promoter of LsCO was amplified with specific primers (Supplementary Table 1). LsCO promoter was cloned into the pHIS2 vector, and the full-length coding region of LsARF3 was ligated into the pGADT7 vector, respectively. The combined pHIS2 vector was linearized and transformed to Y187 yeast strain together with an empty AD vector or pGADT7-LsARF3. The transformed yeast cells were screened on SD/−Leu-Trp-media and SD/−Leu-Trp-His + 60 mM 3AT-medium.
Dual-luciferase assay
Dual-luciferase assay was performed according to the previous study (Ma et al., 2019). The LsCO promoter (2,000 bp) was cloned into the pGreenII 0800-LUC vector at the PstI and BamHI restriction enzyme sites. The LsARF3 coding sequence was cloned into the pGreenII 62-SK vector. For the co-expression analysis, leaves from 5- to 6-week-old tobacco plants were transformed with the recombinant plasmids; the no-effector construct was used as a negative control. The Dual-Luciferase® Reporter Assay System (Promega, Bei-jing, China) was used to analyze firefly luciferase (LUC) and Renilla luciferase (REN) activities. The LUC:REN ratio was used to determine activation or repression.
Statistical analysis
Three independent biological replicates sampled from different plants were used for each determination. Data were presented as means ± standard errors (SEs) of three replicates. Statistical analysis of the bioassays were performed with SPSS statistical software (version 19.0, SPSS Inc., Chicago, IL, United States). Student’s t-test was used to test for significance at levels of p < 0.05 (∗) or p < 0.01 (∗∗).
Results
Identification and expression analysis of LsARF3 gene in lettuce
To explore the roles of the ARF3 transcription factor on lettuce bolting, LsARF3 was identified through a BLAST search against lettuce genome using Arabidopsps ARF3 (AtARF3) as query sequence. Based on phylogenetic relationships, we found that Lsat_1_v5_gn_4_51680 shared high sequence homology with AtARF3 proteins as well as other ARF3 reported in 11 plant species such as artichoke, populus, asparagus, and etc. Phylogenetic analysis of all these ARF3 proteins showed that they could be divided into two groups (Figure 1A). Among these ARF3s, members from lettuce, Arabidopsis, and artichoke were grouped into the first subclade, suggesting their close relationship; ARFs from poplars, cucumbers, and other plant species consist the second group. Analysis of the conserved domains of LsARF3 protein suggested that the LsARF3 had two conserved domains: B3, and Auxin_resp (Figure 1B). The B3 domain is the motif the is responsible for the binding to target DNA regions, while Auxin_resp motif is the conserved domain specific to the ARFs. Combined with the sequence alignments and phylogenetic analysis, it can be inferred that LsARF3/Lsat_1_v5_gn_4_51680 is the lettuce orthologs of the AtARF3, which might encode the ARF3 transcription factors.
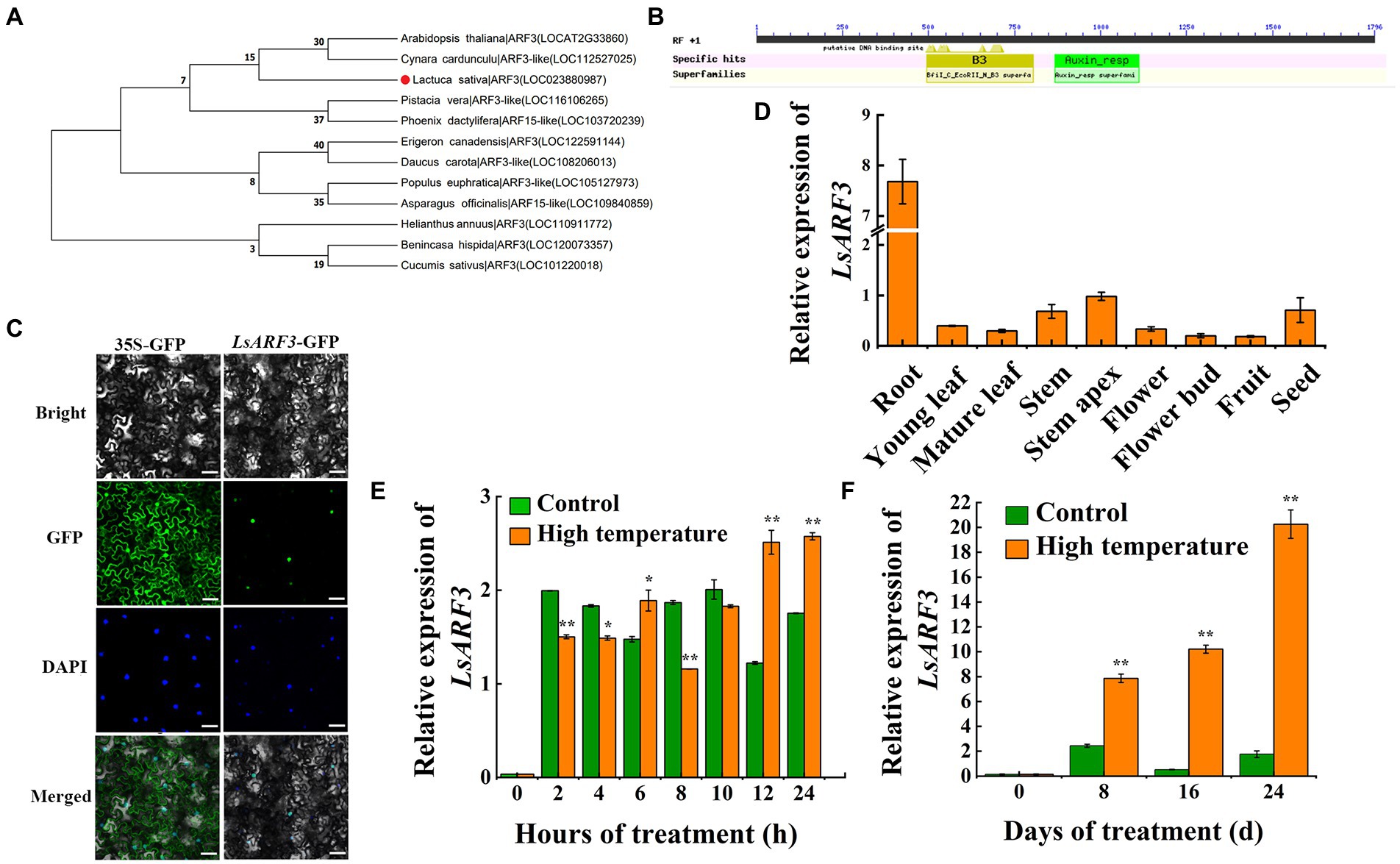
Figure 1. Identification and expression analysis of LsARF3 in lettuce. (A) Phylogenetic tree of LsARF3 protein. (B) Analysis of conserved domains of LsARF3 protein in lettuce. (C) Subcellular localization of LsARF3 fusion protein in lettuce cells. Plasmid with green fluorescent protein (GFP) alone served as the control. Bar = 50 μm. (D) Expression level of LsARF3 in different organs of lettuce. Gene encoding 18 s rRNA was selected as the internal control. (E) Expression level of LsARF3 in stem apex within 24 h of high temperature stress. (F) Expression level of LsARF3 in stem apex within 24 days of high temperature stress. Significant differences were tested by Student’s t-test (*indicates p < 0.05 and **indicates p < 0.01).
The subcellular localization results suggest that LsARF3 is a nuclear protein (Figure 1C), which is consistent with its roles as transcription factor. In the analysis of tissue/organ-specific expression, LsARF3 had a relatively higher level of expression at lettuce root, stem apex, and seed (Figure 1D), indicating that its functions might be associated with the meristem of the stem apex and root apex. After high-temperature treatment, the expression level of LsARF3 gene decreased in the early stage and increased in late stage with significant difference within 24 h (Figure 1E). Furthermore, within 24 days of high-temperature treatment, the LsARF3 gene in the stem apex was significantly higher, compared to the control group, and the increasing trend was more significant with the extended treatment time (Figure 1F), further suggesting that LsARF3 expression was induced by high temperature. Since our previous study showed that high temperature promotes the bolting of lettuce (Hao et al., 2018), It is reasonable that LsARF3 might be involved in the regulation of accelerated bolting under high temperature.
CRISPR/Cas9-mediated knockout of LsARF3 delays bolting of lettuce
To further verify the LsARF3 function, the CRISPR/Cas9 system was used to knock out LsARF3 in lettuce. Two single-guide RNAs were designed for knockout of the LsARF3 genes, and they were precisely targeted to the first or the second exons of LsARF3. Twelve T0 transgenic lines were identified by PCR using Cas9 gene-specific primers. Sequencing of LsARF3 gene of T1 plants identified three lines of homozygous mutants, lsarf3-4, lsarf3-6, lsarf3-9, for phenotypic analysis. As shown in the Figure 2A, the lsarf3-4 carries a C to G mutation at the first exon, the lsarf3-6 harbors a 82-bp deletion at the first exon, and lsarf3-9 brings insertions and deletions in the second exon. All those changes in the amino acid sequence could result in the frameshift mutations in the LsARF3 proteins. As shown in the Figure 2A, three lines of lsarf3 mutants delayed bolting upon high temperatures. After grown in normal conditions, WT lettuce started anthesis at 161 days post germination (DPG), while lsarf3 mutants did not finished the floral transition yet (Figure 2A), suggesting that LsARF3 might play vital role in lettuce bolting and flowering. In previous studies, we have demonstrated that the GB-30 lettuce initiates stem elongation after 8-day heat treatments (Hao et al., 2018). In this study, the stem lengths of lsarf3 mutants were significant lower than that of WT from 16 days after high-temperature treatment (Figure 2B). Microscopic observation suggested that lsarf3 mutants entered the initial stage of flower bud differentiation after high-temperature treatment for 32 days (Figure 2C), suggesting that lsarf3 mutants had started bolting approximately after 32-day heat treatments. Measurements of bolting time, budding time, and anthesis time of the first flower indicated that lsarf3 mutants were about 20, 32, and 48 days later than WT plants, respectively (Figures 2D–F). At the same time, we observed the budding time of mutant plants at normal temperature, and found that it began to bud about 161 days, about 20 days later than WT (Figure 2A). Together, the results indicated that absence of functional LsARF3 postponed floral transition in the knockout lines, resulting in delayed bolting and flowering under normal or heat conditions.
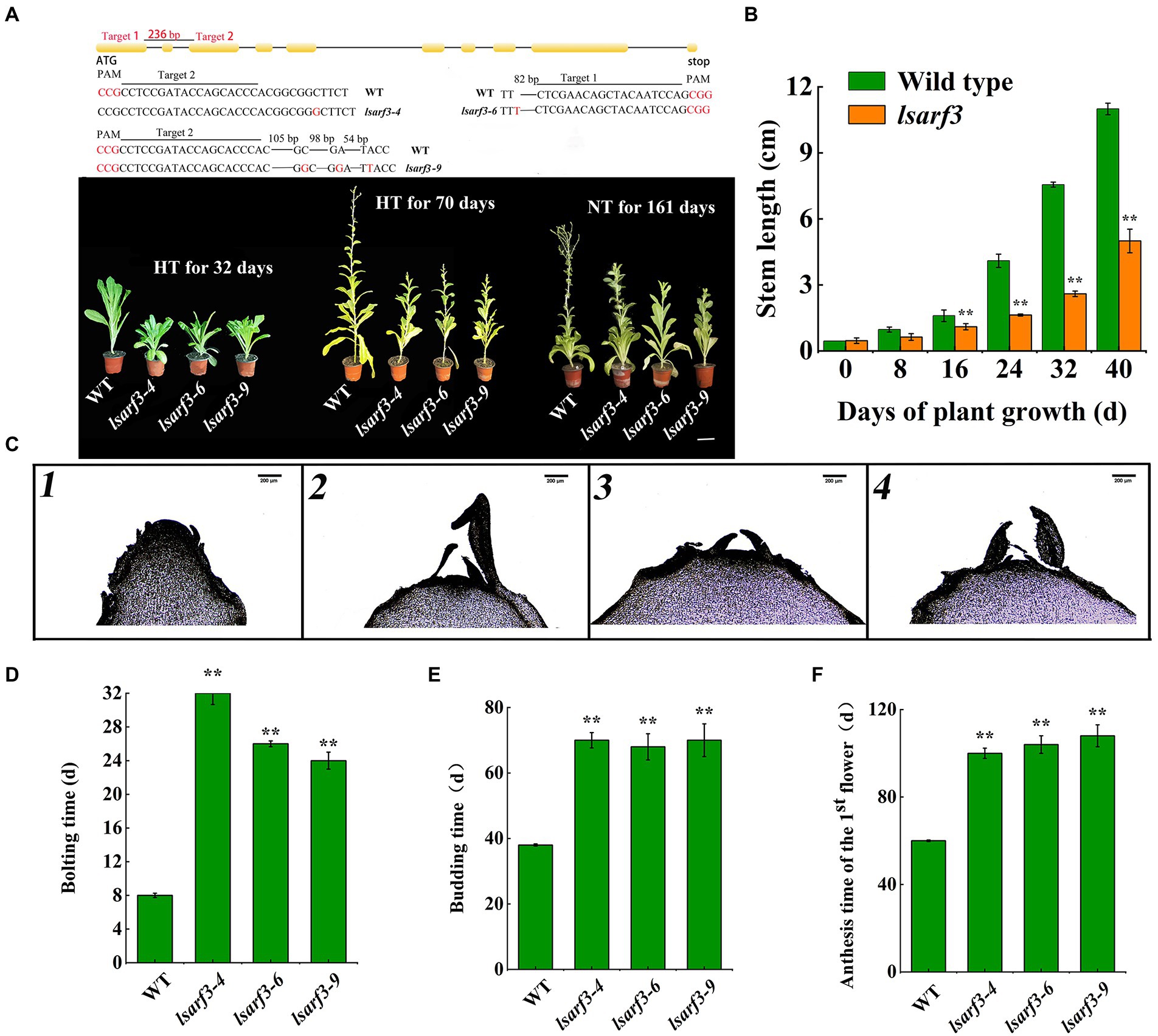
Figure 2. Knockout of LsARF3 in lettuce via CRISPR-Cas9. (A) Phenotypes of WT and lsarf3 mutant lines growing in high temperature condition for 32 and 70 days, and in normal temperature condition for 161 days, Bar = 6 cm. HT represents high temperature condition. (B) Stem length change of WT and lsarf3 mutant lines. (C) Flower bud differentiation of stem apex in high temperature condition for 32 days. 1.WT; 2. lsarf3-4; lsarf3-6; 3. lsarf3-9. Bar =200 μm. (D) Bolting time of WT and lsarf3 mutants. (E) Budding time of WT and lsarf3 mutants. (F) Anthesis time of the 1st flower in WT and lsarf3 mutants. Significant differences were tested by Student’s t-test (**indicates p < 0.01).
Overexpression of LsARF3 gene accelerates bolting of lettuce
To further investigate that the LsARF3 roles in lettuce bolting, we generated the LsARF3-overexpressing (OE) transgenic lettuce plants. The LsARF3-OE plants displayed accelerated bolting and flowering (Figure 3A). The three OE lines (OE-1, OE-7, OE-6) revealed that the expression levels of the LsARF3 gene were nearly 4 times, 8 times, and 16 times higher than that of wild-type plants (WT), respectively (Figure 3B). Microscopic analysis showed that LsARF3-OE lines began the initiation stage of flower bud differentiation at 60 days, whereas the growth cone of WT control was prominent and wrapped in the middle of the leaf primordium that showed a semicircular shape, indicating they were still in the vegetative growth stages (Figure 3C). In addition, the stem length of LsARF3-OE lines was higher than that of WT with a significant or extremely significant difference from growing 40 days (Figure 3D). From phenotype and microscopic observation, it also supported the observations that the LsARF3-OE lines had entered the bolting stage after growing for 60 days. Similarly, the bolting time, budding time, and first flower anthesis time of LsARF3-OE lines were 48, 33, and 40 days earlier than that of WT, respectively (Figures 3E–G), reflecting that the LsARF3 overexpression could accelerate bolting and flowering in lettuce.
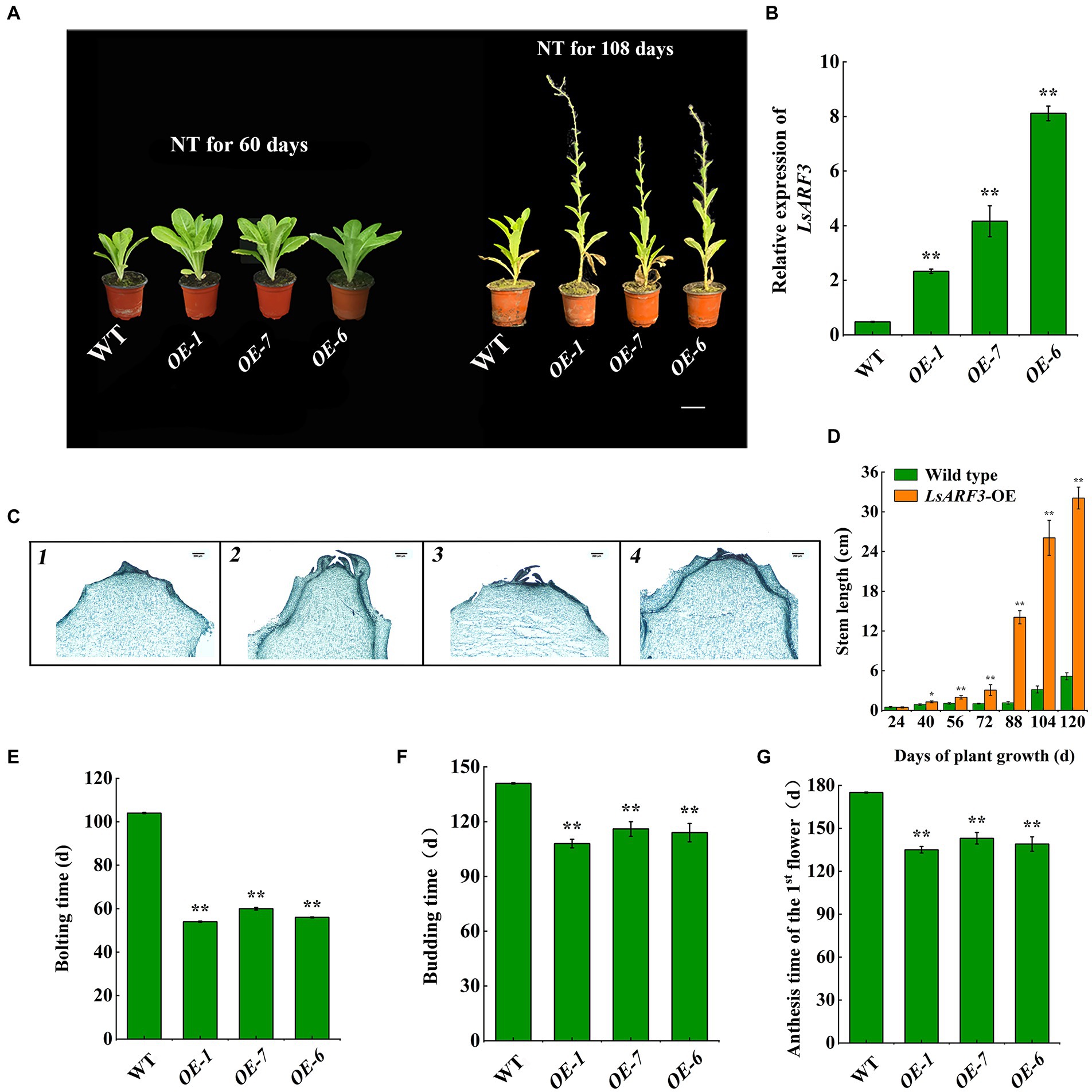
Figure 3. Overexpression of LsARF3 in lettuce. (A) Phenotypes of WT and LsARF3-OE lines. Bar = 6 cm. NT represents normal temperature condition. (B) Expression level of LsARF3 in WT and LsARF3-OE lines. (C) Flower bud differentiation of stem apex at 60 days during growing. 1.WT; 2. OE-1; OE-7; 3. OE-6. Bar =200 μm. (D) Stem length change of WT and LsARF3-OE lines. (E) Bolting time of WT and LsARF3-OE lines. (F) The budding time of WT and LsARF3-OE lines. (G) Anthesis time of the 1st flower of WT and LsARF3-OE lines. Significant differences were tested by Student’s t-test (*indicates p < 0.05 and **indicates p < 0.01).
Auxin cannot recovery the delayed bolting phenotype of lsarf3 mutant
Our previous studies have shown that exogenous IAA promoted bolting, and it occurred begin 8 days after exogenous application (Wang et al., 2022b). To explore whether LsARF3 was involved in auxin-induced bolting of lettuce, the exogenous IAA was applicated to WT and lsarf3 mutants. After the application of exogenous IAA, the plants showed obvious differences (Figure 4A). It was found that the expression levels of LsARF3 in lsarf3 mutants was extremely significant lower than that of the WT(Figure 4B). Miscroscopy experiments demonstrated that, after exogenous IAA application for 24 days, WT plants entered the stage of flower bud differentiation, but knockout mutants were insensitive to auxin applications (Figure 4C). The stem of WT lettuce elongated rapidly after IAA application, while it was not observed in the lsarf3 mutants. After 24 days after IAA application, the stem lengths of lsarf3 mutants were shorter by 58.3% to that of the WT (Figure 4D). Thus, it could be concluded that WT plants started bolting on 24th Day after IAA application, but at that time, lsarf3 mutants were still in the vegetative stage. Afterward, the stems become visible and elongated above the rosette leaves in WT plants; in contrast, the stem of lsarf3 mutants is still embedded in the rosette leaves of transgenic lettuces. It took 90 days to entered budding stage for WT lettuces, whereas lsarf3 lines kept at vegetative stages (Figure 4A). In other words, the defects in bolting time of lsarf3 mutants could not fixed by exogenous IAA applications (Figures 2A,E, 4A). Together, the results suggested that exogenous auxin accelerated bolting in WT, but it cannot recover the delayed bolting phenotype of lsarf3 mutants.
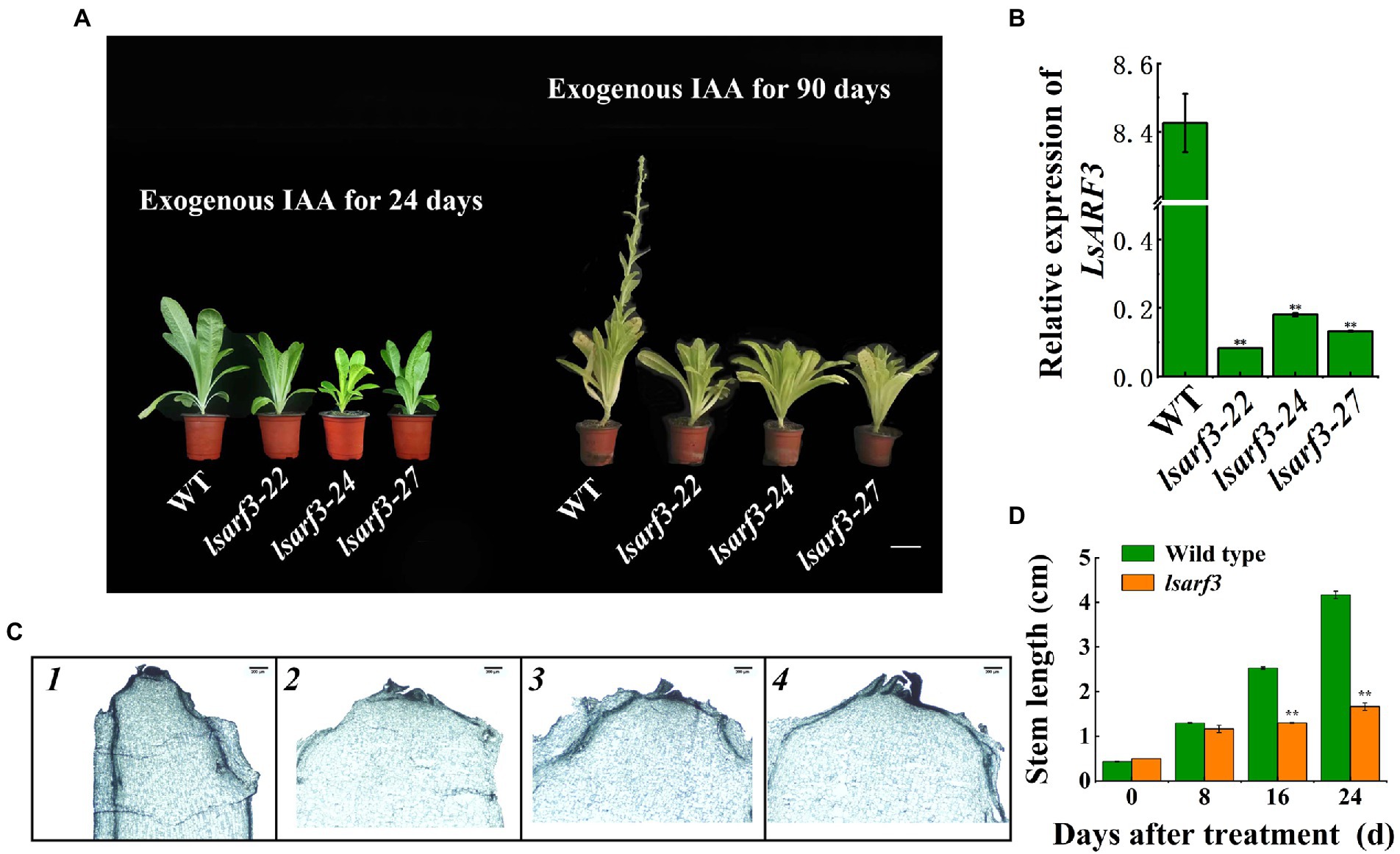
Figure 4. Effects of exogenous IAA on bolting and LsARF3 expression in lsarf3 mutants. (A) Plant morphological character after exogenous IAA application. (B) LsARF3 gene expression after exogenous IAA application for 24 days. (C) Flower bud differentiation of stem apex after exogenous IAA application for 24 days. (D) Stem length change after exogenous IAA application. Significant differences were tested by Student’s t-test (**indicates p < 0.01).
The LsARF3 positively regulated the expression of flowering-related genes
To explore the molecular mechanism underlying LsARF3-mediated bolting upon thermal treatments, the expression profiles of selected flowering-related genes in LsARF3-OE and mutant lines were analyzed in the shoot apex samples from three lsarf3 mutant lines and wild type plants. The results showed that the expression levels of LsCO, LsFT, LsLFY, LsGI, and LsSOC1 were significantly upregulated in these three LsARF3-OE lines, while their expression levels of LsFLC and LsFLM were much lower compared to that of WT lettuce plants (Figure 5A). Conversely, the expression of LsCO, LsFT, LsLFY, LsGI, and LsSOC1 were attenuated in lsarf3 mutants, and accordingly, the transcript levels of LsFLC and LsFLM were significantly increased compared to WT plants (Figure 5B). The results suggested that LsCO, LsFT, LsLFY, LsGI, and LsSOC1 exhibited similar expression pattern in the LsARF3-OE and knockout lines. Therefore, it is possible that those flowering-related genes could be the potential downstream genes of LsARF3, thereby controlling the floral transition and the bolting of lettuce.
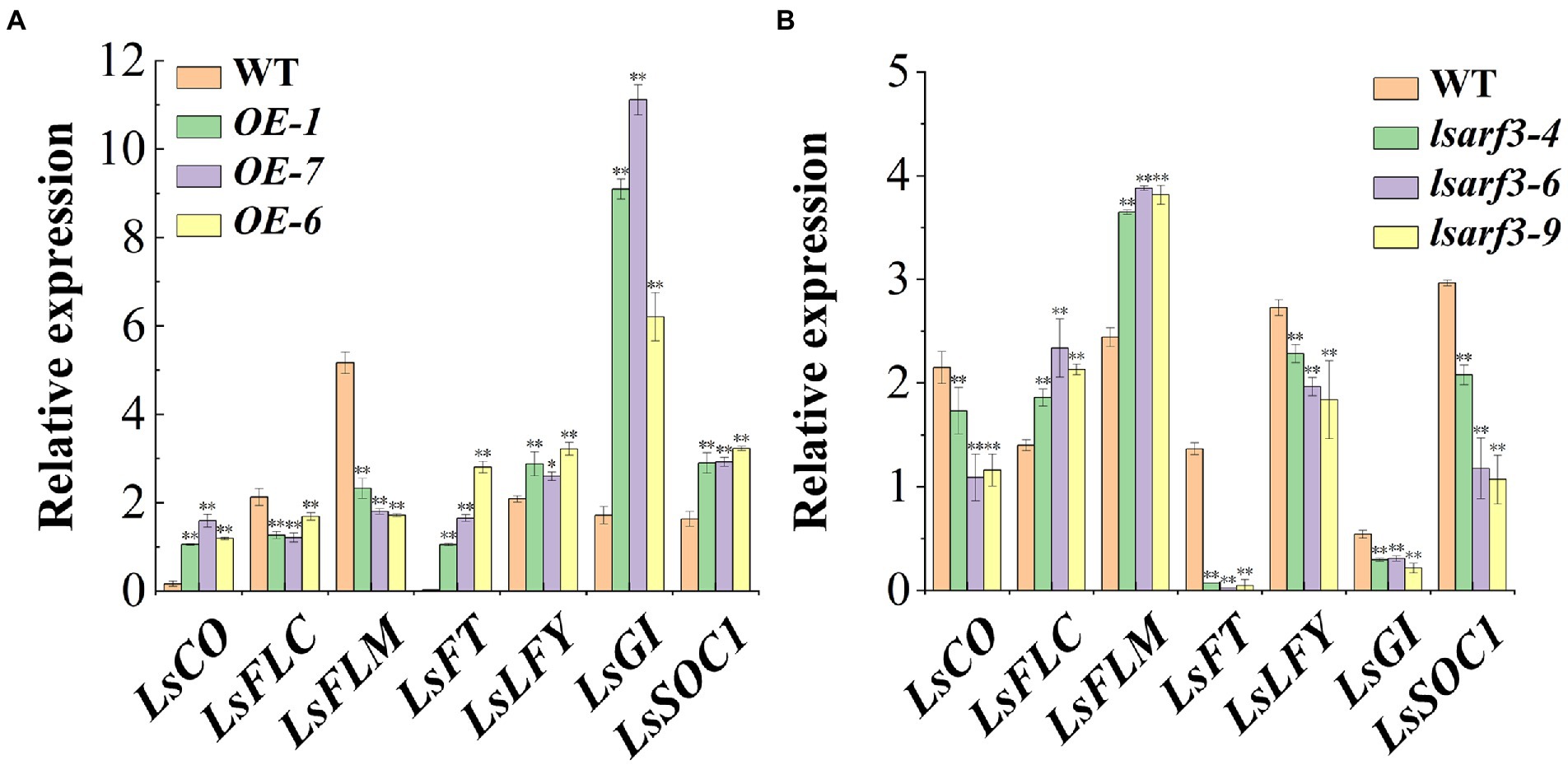
Figure 5. Transcript levels of flowing related genes in WT, LsARF3-OE and lsarf3 mutants. (A) Expression levels of flowering related genes in WT and LsARF3-OE lines. (B) Expression levels of flowering related genes in the WT and lsarf3 mutants. Significant differences were tested by Student’s t-test (*indicates p < 0.05 and **indicates p < 0.01).
LsARF3 was bind to the putative promoter region of LsCO
Given that LsARF3 could co-expressed with a number of flowering-related genes such as LsCO, LsFT, and LsGI, we expect that the transcription of these genes might be directly regulated by LsARF3. Based on the analysis of the 2 kb promoter sequences of LsCO, LsFT, and LsGI, an AuxRE cis-elements in the LsCO promoter was identified, suggesting LsCO was a possible LsARF3 direct target (Figure 6A). To test the hypothesis, a yeast one-hybrid (Y1H) assay was conducted to determine whether LsARF3 can bind directly to the LsCO promoter. As shown in Figure 6B, the Y1H results showed that the yeast cells containing the bait vector combined with the promoter region of LsCO could grow on the SD/−Leu-Trp-His medium supplemented with 60 mM 3AT, when co-transformed with pGADT7-LsARF3 construct. However, the yeast cells transformed with the empty pGADT7 vector did not grow on the same selective medium. Hence, the results suggested that LsARF3 could in vivo bind to the promoter of LsCO and activate the reporter gene in yeast. This finding was further confirmed by a dual luciferase assay in tobacco leaves with LsCO promoter activity being monitored with a ProLsCO::LUC reporter construct. Overexpression of LsARF3 significantly increased the activity of LsCO promoter (Figure 6C), further indicating that LsARF3 could promote LsCO expression via directly binding to its promoter.
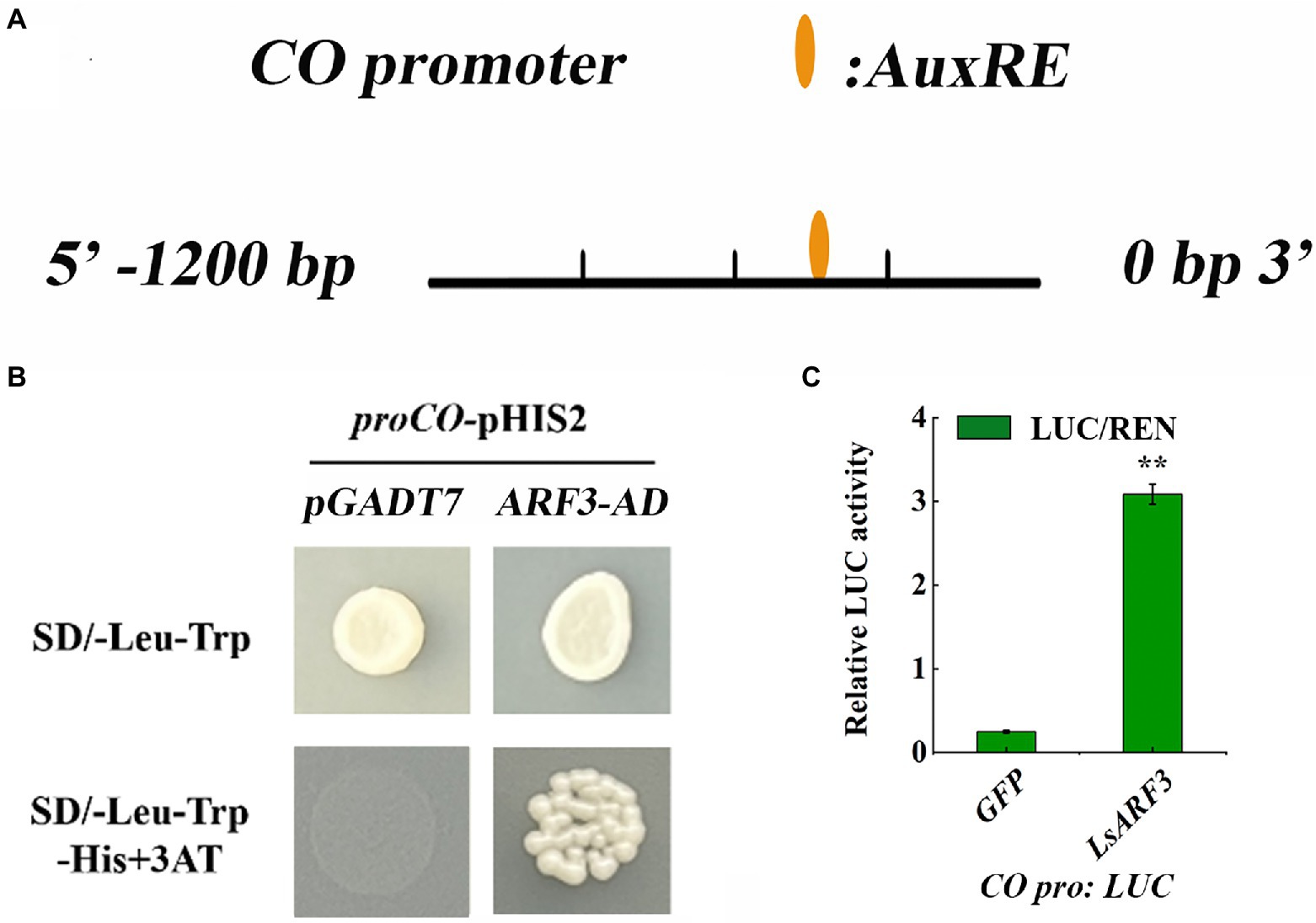
Figure 6. LsARF3 binds to the promoter of LsCO by Yeast one-Hybrid (Y1H) assay. (A) The AuxRE element in the promoter of LsCO gene. (B) Yeast one-hybrid (Y1H) experiment showing the binding of LsARF3 -AD to the promoter of LsCO. (C) Dual luciferase reporter assay. The GFP effector was used as a negative control, and the LUC/REN ratios of GFP were set as 1. Significant differences were tested by Student’s t-test (*indicates p < 0.05).
Discussion
In addition to endogenous cues, environmental variables substantially affect the floral transition among a wide range of plant species (Mcclung et al., 2016; Nagalla et al., 2021). Earlier studies have shown that high temperatures could induce premature bolting and early flowering in lettuce (Han et al., 2021). Our experiments demonstrated that exogenous applications of auxin could promote plant bolting and expressions of some LsARFs were associated with bolting time, implying the important contributions of auxin and ARFs to the lettuce bolting, as well as flowering. It has been reported that ARF3 is characterized by its roles in organ polarity, gynoecium patterning, self-incompatibility, and meristem activity in Arabidopsis. However, whether or how ARF3 mediates plant bolting remains unknown. Here, we reveal that LsARF3 controls the lettuce bolting by regulating the expression of floral transition-related genes. Our results illustrate a novel regulator model in thermally accelerated bolting and flowering pathway, which expands our understanding of how plants control developmental events in response to high temperature.
Auxin was implicated in the heat-induced bolting in lettuce
The transition from vegetative to reproductive growth is critical to plant development because the proper timing of flowering ensures seed production required for the survival of species (Takeno, 2016). Given that auxin regulates diverse developmental processes from phototropism, root/shoot tropisms, to apical dominance in plants (Enders and Strader, 2015), it is perhaps not surprising that auxin is involved in the transition to bolting and flowering in lettuce. It was demonstrated that, under normal growth conditions, the IAA content in lettuce stems was significantly increased upon bolting, and exogenous application of IAA could promote bolting of some lettuce cultivars after 4-day treatments (Wang et al., 2022b). Recently, another study also showed that overexpression of LsRGL1, encoding a functional DELLA repressor, resulted in the reduction of IAA biosynthesis, while auxin feeding restored the bolting time of LsRGL1 overexpression lines (Wang et al., 2022a). Their observations suggested that auxin was closely involved in the lettuce bolting, but whether auxin is involved in the accelerated bolting under thermal stresses remains to be examined. In our analysis, we found that IAA treatments indeed promoted the elongation of inflorescence stems under heat stresses. Accordingly, we observed that the IAA content in lettuce stems kept increasing with the progression of heat treatments (Hao et al., 2018). Therefore, our results demonstrated that auxin, at least in part, participated the thermal stress-induced bolting in lettuce, linking abiotic auxin signaling pathways with inflorescence development under abiotic stresses.
LsARF3 might function as transcription activators in auxin signaling cascade
ARF3 and its orthologues are found in various angiosperms, including monocots and dicots, but so far, its roles in plant development were mainly obtained from the model plant Arabidopsis (Hobecker et al., 2017; Brackmann et al., 2018; Zhang et al., 2018). To our knowledge, the functions of ARF3 orthologues in monocots such as lettuce remain largely intangible. Previous extensive evidence demonstrated that ARF3/ETTIN was involved in the regulation of organ polarity determination and pattering, gynoecium morphogenesis, self-incompatibility, flower development, and other auxin-responsive behaviors in Arabidopsis (Nemhauser et al., 2000; Liu et al., 2014b; Zhang et al., 2018). For example, ettin mutants exhibited severe defects in leaf polarity specification and gynoecium patterning (Nemhauser et al., 2000). Zhang and his collogues also reported that ARF3 regulates floral meristem determinacy by directly inhibiting the expression of ISOPENTENYLTRANSFERASE responsible for the rate-limiting step of cytokinin biosynthesis (Zhang et al., 2018). A recent study suggested that Medicago truncatula plants overexpressing MtARF3 exhibited curling leaf margins with more serrations which resemble the phenotypes of the palm1 mutant to a large extent; further experiments confirmed that MtARF3 restricted spatiotemporal expression of PALMATE-LIKE PENTAFOLIATA1 (PALM1) through selective interaction with the promoter of PALM1 (Peng et al., 2017). Expression of OsARF3 construct with altered tasi-RNA targeting sites resulted in dwarf and tufty phenotypes, irregular phyllotaxis, with rolled-up or thread-like leaves in transgenic rice lines, indicating its function and regulation are conserved in higher plants (Song et al., 2012). Taken at face value, most studies supported that ARF3s serves as transcriptional repressors in the auxin-regulated pathways. Nevertheless, our results indicated that overexpression of LsARF3 promoted plant growth and early bolting and flowering under normal or heat-stress conditions. Surprisingly, no developmental defects in leaf or flower organs were observed in the LsARF3 overexpression or knockout lines, despite the slightly reduced flower fertility, which was not reported, to our knowledge, in other ARF3 transgenic plants yet. Furthermore, the expressions of several floral genes including LsCO, LsFT, LsSOC1, and LsLFY were positively correlated with that of LsARF3 in the overexpression or loss-of-function lines, implying their transcription could be induced by LsARF3 during the lettuce bolting process. Thus, it is likely that LsARF3 was able to activate the expression of those floral integrator genes at high temperatures. Importantly, it is also worth noting that LsARF3 might function as a transcriptional activator in lettuce, whereas other ARF3 homologs reported in Arabidopsis, legume, or rice, act as transcriptional repressors in the auxin signaling pathways. The discrepancy between LsARF3 and its orthologues in other plant species implies that it might participate in some auxin signaling pathways in ways different from its orthologues in other plant species.
Unique roles of LsARF3 in heat-induced bolting in lettuce
In the last decade, several genes or quantitative trait loci (QTLs) that affect the timing of floral transition have been reported to be associated with heat-induced bolting and flowering (Hartman et al., 2013; Han et al., 2021). In Arabidopsis, FT, SOC1, and CO are major floral integrators because diverse floral induction pathways are mainly converged upon these developmental regulators (Yoo et al., 2005; Valverde, 2011; Pin and Nilsson, 2012). Accordingly, their lettuce orthologues of these floral genes were functionally characterized to unveil their potential roles in floral transition, in particular under heat stresses (Chen et al., 2017; Liu et al., 2018). Overexpression of LsFT and LsSOC1 can promote flowering, whereas their knockdown by RNA interference in lettuce resulted in significantly delayed bolting and insensitivity to high temperatures (Fukuda et al., 2011; Chen et al., 2017). Although lettuce COs have not been characterized, at least one CO-like gene was found to be located within flowering QTL, qFLT5.3, which implies its possible contributions to implicated in bolting initiation in lettuce (Seki et al., 2020). In the present study, AuxRE element was identified in the promoter region of LsCO gene, suggesting that CO expression might be regulated by ARF transcription factors. Indeed, LsCO was constitutively activated in transgenic lettuce overexpressing the LsARF3; by contrast, deletion of LsARF3 dramatically attenuated the expression level in lettuce, implying that LsCO might be the target of LsARF3. Consequently, upregulation of LsARF3 promoted lettuce bolting, while its knockout lines failed to accelerate bolting despite auxin feeding plus thermal treatments. Furthermore, yeast one-hybridization analysis verified that LsARF3 could physically interact with AuxRE cis-elements of the LsCO promoter. Therefore, our findings reinforced the idea that LsARF3 probably directly operated the transcription of LsCO under flowering-inducible conditions. However, the question of whether LsARF3 directly activates the expression of LsFT and LsSOC1 to facilitate bolting will be examined in future experiments.
Based on our experiments, one possible scenario for interpreting lettuce early bolting upon heat stress is to postulate an auxin-ARF3 mediated signaling pathway: thermal treatment could activate the auxin biosynthesis and/or transport in inflorescence stem, resulting in the increase of local auxin levels and subsequent LsARF3 transcription. Subsequently, LsARF3 might directly activate the transcription of LsCO and indirectly induce the expression of LsFT and LsSOC1 in an auxin-dependent manner, eventually leading to the accelerated bolting and flowering in lettuce. Overall, our study provides new insights into lettuce bolting in the context of ARFs signaling and heat response. However, we cannot exclude the possibility that other ARFs might be also involved in lettuce floral transition, and further experiments are still needed to investigate the functions of ARF genes in this important vegetable.
Data availability statement
The original contributions presented in the study are included in the article/Supplementary material, further inquiries can be directed to the corresponding authors.
Author contributions
JH and YH conceived and supervised the project. YL performed the experiments and analyzed the data. NL and JH wrote the manuscript. JZ, YF, ZL, ZR, NL, and CL gave advises and edited the manuscript. All authors contributed to the article and approved the submitted version.
Funding
This work was financially supported by the National Natural Science Foundation of China (grant no. 32072560) and the Support Plan for Spark Action on Scientific and Technological Innovation of Beijing University of Agriculture (BUA-HHXD2022003).
Conflict of interest
The authors declare that the research was conducted in the absence of any commercial or financial relationships that could be construed as a potential conflict of interest.
Publisher’s note
All claims expressed in this article are solely those of the authors and do not necessarily represent those of their affiliated organizations, or those of the publisher, the editors and the reviewers. Any product that may be evaluated in this article, or claim that may be made by its manufacturer, is not guaranteed or endorsed by thepublisher.
Supplementary material
The Supplementary material for this article can be found online at: https://www.frontiersin.org/articles/10.3389/fpls.2022.958833/full#supplementary-material
Footnotes
References
Andrés, F., and Coupland, G. (2012). The genetic basis of flowering responses to seasonal cues. Nat. Rev. Genet. 13, 627–639. doi: 10.1038/nrg3291
Blümel, M., Dally, N., and Jung, C. (2015). Flowering time regulation in crops—what did we learn from Arabidopsis? Curr. Opin. Biotechnol. 32, 121–129. doi: 10.1016/j.copbio.2014.11.023
Brackmann, K., Qi, J., Gebert, M., Jouannet, V., Schlamp, T., Grunwald, K., et al. (2018). Spatial specificity of auxin responses coordinates wood formation. Nat. Commun. 9:875. doi: 10.1038/s41467-018-03256-2
Brandoli, C., Petri, C., Egea-Cortines, M., and Weiss, J. (2020). Gigantea: uncovering new functions in flower development. Genes 11:11. doi: 10.3390/genes11101142
Chen, Z., Han, Y., Ning, K., Ding, Y., Zhao, W., Yan, S., et al. (2017). Inflorescence development and the role of LsFT in regulating bolting in lettuce (Lactuca sativa L.). front. Plant Sci. 8:2248. doi: 10.3389/fpls.2017.02248
Chen, Z., Zhao, W., Ge, D., Han, Y., Ning, K., Luo, C., et al. (2018). LCM-seq reveals the crucial role of LsSOC1 in heat-promoted bolting of lettuce (Lactuca sativa L.). Plant J. 95, 516–528. doi: 10.1111/tpj.13968
Cheng, Z. J., Wang, L., Sun, W., Zhang, Y., Zhou, C., Su, Y. H., et al. (2013). Pattern of auxin and cytokinin responses for shoot meristem induction results from the regulation of cytokinin biosynthesis by AUXIN RESPONSE FACTOR3. Plant Physiol. 161, 240–251. doi: 10.1104/pp.112.203166
Enders, T. A., and Strader, L. C. (2015). Auxin activity: past, present, and future. Am. J. Bot. 102, 180–196. doi: 10.3732/ajb.1400285
Fukuda, M., Matsuo, S., Kikuchi, K., Kawazu, Y., Fujiyama, R., and Honda, I. (2011). Isolation and functional characterization of the FLOWERING LOCUS T homolog, the LsFT gene, in lettuce. J. Plant Physiol. 168, 1602–1607. doi: 10.1016/j.jplph.2011.02.004
Guilfoyle, T. J., and Hagen, G. (2007). Auxin response factors. Curr. Opin. Plant Biol. 10, 453–460. doi: 10.1016/j.pbi.2007.08.014
Han, R., Truco, M. J., Lavelle, D. O., and Michelmore, R. W. (2021). A composite analysis of flowering time regulation in lettuce. Front. Plant Sci. 12:632708. doi: 10.3389/fpls.2021.632708
Hao, J. H., Zhang, L. L., Li, P.-P., Sun, Y. C., Li, J. K., Qin, X. X., et al. (2018). Quantitative proteomics analysis of lettuce (Lactuca sativa L.) reveals molecular basis-associated auxin and photosynthesis with bolting induced by high temperature. Int. J. Mol. Sci. 19:2967.
Hao, J. H., Su, H. N., Zhang, L. L., Liu, C. J., Han, Y. Y., Qin, X. X., et al. (2021). Quantitative proteomic analyses reveal that energy metabolism and protein biosynthesis reinitiation are responsible for the initiation of bolting induced by high temperature in lettuce (Lactuca sativa L.). BMC Genomics 22:427. doi: 10.1186/s12864-021-07664-5
Hartman, Y., Uwimana, B., Hooftman, D. A., Schranz, M. E., Van De Wiel, C. C., Smulders, M. J., et al. (2013). Genomic and environmental selection patterns in two distinct lettuce crop-wild hybrid crosses. Evol. Appl. 6, 569–584. doi: 10.1111/eva.12043
Hobecker, K. V., Reynoso, M. A., Bustos-Sanmamed, P., Wen, J., Mysore, K. S., Crespi, M., et al. (2017). The MicroRNA390/TAS3 pathway mediates symbiotic nodulation and lateral root growth. Plant Physiol. 174, 2469–2486. doi: 10.1104/pp.17.00464
Hunter, C., Willmann, M. R., Wu, G., Yoshikawa, M., De La Luz Gutierrez-Nava, M., and Poethig, S. R. (2006). Trans-acting siRNA-mediated repression of ETTIN and ARF4 regulates heteroblasty in Arabidopsis. Development 133, 2973–2981. doi: 10.1242/dev.02491
Johansson, M., and Staiger, D. (2015). Time to flower: interplay between photoperiod and the circadian clock. J. Exp. Bot. 66, 719–730. doi: 10.1093/jxb/eru441
Kinoshita, A., and Richter, R. (2020). Genetic and molecular basis of floral induction in Arabidopsis thaliana. J. Exp. Bot. 71, 2490–2504. doi: 10.1093/jxb/eraa057
Kuhn, A., Ramans Harborough, S., Mclaughlin, H. M., Natarajan, B., Verstraeten, I., Friml, J., et al. (2020). Direct ETTIN-auxin interaction controls chromatin states in gynoecium development. Elife 9:e51787. doi: 10.7554/eLife.51787
Kumar, S. V., Lucyshyn, D., Jaeger, K. E., Alós, E., Alvey, E., Harberd, N. P., et al. (2012). Transcription factor PIF4 controls the thermosensory activation of flowering. Nature 484, 242–245. doi: 10.1038/nature10928
Kunihiro, A., Yamashino, T., Nakamichi, N., Niwa, Y., Nakanishi, H., and Mizuno, T. (2011). Phytochrome-interacting factor 4 and 5 (PIF4 and PIF5) activate the homeobox ATHB2 and auxin-inducible IAA29 genes in the coincidence mechanism underlying photoperiodic control of plant growth of Arabidopsis thaliana. Plant Cell Physiol. 52, 1315–1329. doi: 10.1093/pcp/pcr076
Li, Y., Li, T., Han, Y., and Fan, S. (2017). Cloning and function analysis of heat-shock-protein LsHsp70-2711 gene under high temperature stress in leaf lettuce (Lactuca sativa L.). Sci. Agric. Sin. 50, 1486–1494. doi: 10.3864/j.issn.0578-1752.2017.08.012
Li, S. B., Xie, Z. Z., Hu, C. G., and Zhang, J. Z. (2016). A review of Auxin response factors (ARFs) in plants. Front. Plant Sci. 7:47. doi: 10.3389/fpls.2016.00047
Liu, X., Dinh, T. T., Li, D., Shi, B., Li, Y., Cao, X., et al. (2014b). AUXIN RESPONSE FACTOR 3 integrates the functions of AGAMOUS and APETALA2 in floral meristem determinacy. Plant J. 80, 629–641. doi: 10.1111/tpj.12658
Liu, X., Lv, S., Liu, R., Fan, S., Liu, C., Liu, R., et al. (2018). Transcriptomic analysis reveals the roles of gibberellin-regulated genes and transcription factors in regulating bolting in lettuce (Lactuca sativa L.). PLoS One 13:e0191518. doi: 10.1371/journal.pone.0191518
Liu, N., Wu, S., Van Houten, J., Wang, Y., Ding, B., Fei, Z., et al. (2014a). Down-regulation of AUXIN RESPONSE FACTORS 6 and 8 by microRNA 167 leads to floral development defects and female sterility in tomato. J. Exp. Bot. 65, 2507–2520. doi: 10.1093/jxb/eru141
Ma, X. S., Li, C. Q., Huang, X. M., Wang, H. C., Wu, H., Zhao, M. L., et al. (2019). Involvement of HD-ZIP I transcription factors LcHB2 and LcHB3 in fruitlet abscission by promoting transcription of genes related to the biosynthesis of ethylene and ABA in litchi. Tree Physiol. 39, 1600–1613. doi: 10.1093/treephys/tpz097
Mcclung, C. R., Lou, P., Hermand, V., and Kim, J. A. (2016). The importance of ambient temperature to growth and the induction of flowering. Front. Plant Sci. 7:1266. doi: 10.3389/fpls.2016.01266
Nagalla, A. D., Nishide, N., Hibara, K. I., and Izawa, T. (2021). High ambient temperatures inhibit Ghd7-mediated flowering repression in Rice. Plant Cell Physiol. 62, 1745–1759. doi: 10.1093/pcp/pcab129
Nemhauser, J. L., Feldman, L. J., and Zambryski, P. C. (2000). Auxin and ETTIN in Arabidopsis gynoecium morphogenesis. Development 127, 3877–3888. doi: 10.1242/dev.127.18.3877
Ning, K., Han, Y., Chen, Z., Luo, C., Wang, S., Zhang, W., et al. (2019). Genome-wide analysis of MADS-box family genes during flower development in lettuce. Plant Cell Environ. 42, 1868–1881. doi: 10.1111/pce.13523
Peng, J., Berbel, A., Madueño, F., and Chen, R. (2017). AUXIN RESPONSE FACTOR3 regulates compound leaf patterning by directly repressing PALMATE-LIKE PENTAFOLIATA1 expression in Medicago truncatula. Front. Plant Sci. 8:1630. doi: 10.3389/fpls.2017.01630
Pin, P. A., and Nilsson, O. (2012). The multifaceted roles of FLOWERING LOCUS T in plant development. Plant Cell Environ. 35, 1742–1755. doi: 10.1111/j.1365-3040.2012.02558.x
Pouteau, S., and Albertini, C. (2009). The significance of bolting and floral transitions as indicators of reproductive phase change in Arabidopsis. J. Exp. Bot. 60, 3367–3377. doi: 10.1093/jxb/erp173
Qi, Z.-Y. (2019). Expression of ARFs (Auxin Response Factors) Gene Family and Functional Analysis of LsARF8 in Leaf Lettuce (Lactuca sativa L.) during Bolting Induced by High Temperature. Ms. Master. Beijing University of Agriculture.
Roosjen, M., Paque, S., and Weijers, D. (2018). Auxin response factors: output control in auxin biology. J. Exp. Bot. 69, 179–188. doi: 10.1093/jxb/erx237
Seki, K., Komatsu, K., Tanaka, K., Hiraga, M., Kajiya-Kanegae, H., Matsumura, H., et al. (2020). A CIN-like TCP transcription factor (LsTCP4) having retrotransposon insertion associates with a shift from Salinas type to empire type in crisphead lettuce (Lactuca sativa L.). Hortic. Res. 7:15. doi: 10.1038/s41438-020-0241-4
Sheldon, C. C., Rouse, D. T., Finnegan, E. J., Peacock, W. J., and Dennis, E. S. (2000). The molecular basis of vernalization: the central role of FLOWERING LOCUS C (FLC). Proc. Natl. Acad. Sci. U. S. A. 97, 3753–3758. doi: 10.1073/pnas.97.7.3753
Shen, L., Zhang, Y., and Sawettalake, N. (2022). A molecular switch for FLOWERING LOCUS C activation determines flowering time in Arabidopsis. Plant Cell 34, 818–833. doi: 10.1093/plcell/koab286
Simonini, S., Bencivenga, S., Trick, M., and Ostergaard, L. (2017). Auxin-induced modulation of ETTIN activity orchestrates gene expression in Arabidopsis. Plant Cell 29, 1864–1882. doi: 10.1105/tpc.17.00389
Simonne, A. H., Simonne, E. H., Eitenmiller, R. R., and Coker, C. E. H. (2002). Bitterness and composition of lettuce varieties grown in the southeastern United States. HortTechnology 12, 721–726. doi: 10.21273/HORTTECH.12.4.721
Song, X., Wang, D., Ma, L., Chen, Z., Li, P., Cui, X., et al. (2012). Rice RNA-dependent RNA polymerase 6 acts in small RNA biogenesis and spikelet development. Plant J. 71, 378–389. doi: 10.1111/j.1365-313X.2012.05001.x
Takeno, K. (2016). Stress-induced flowering: the third category of flowering response. J. Exp. Bot. 67, 4925–4934. doi: 10.1093/jxb/erw272
Valverde, F. (2011). CONSTANS and the evolutionary origin of photoperiodic timing of flowering. J. Exp. Bot. 62, 2453–2463. doi: 10.1093/jxb/erq449
Wang, Y., Li, B., Li, Y., Du, W., Zhang, Y., Han, Y., et al. (2022b). Application of exogenous auxin and gibberellin regulates the bolting of lettuce (Lactuca sativa L.). Open Life Sci. 17, 438–446. doi: 10.1515/biol-2022-0043
Wang, S., Luo, C., Sun, L., Ning, K., Chen, Z., Yang, J., et al. (2022a). LsRGL1 controls the bolting and flowering times of lettuce by modulating the gibberellin pathway. Plant Sci. 316:111175. doi: 10.1016/j.plantsci.2021.111175
Yamori, N., Levine, C. P., Mattson, N. S., and Yamori, W. (2022). Optimum root zone temperature of photosynthesis and plant growth depends on air temperature in lettuce plants. Plant Mol. Biol. doi: 10.1007/s11103-022-01249-w
Yan, W., Wang, B., Chan, E., and Mitchell-Olds, T. (2021). Genetic architecture and adaptation of flowering time among environments. New Phytol. 230, 1214–1227. doi: 10.1111/nph.17229
Yoo, S. K., Chung, K. S., Kim, J., Lee, J. H., Hong, S. M., Yoo, S. J., et al. (2005). CONSTANS activates SUPPRESSOR OF OVEREXPRESSION OF CONSTANS 1 through FLOWERING LOCUS T to promote flowering in Arabidopsis. Plant Physiol. 139, 770–778. doi: 10.1104/pp.105.066928
Zhang, Y. M., Ma, H. L., Calderón-Urrea, A., Tian, C. X., Bai, X. M., and Wei, J. M. (2016). Anatomical changes to protect organelle integrity account for tolerance to alkali and salt stresses in Melilotus officinalis. Plant and Soil 406, 327–340. doi: 10.1007/s11104-016-2875-4
Zhang, K., Wang, R., Zi, H., Li, Y., Cao, X., Li, D., et al. (2018). AUXIN RESPONSE FACTOR3 regulates floral meristem determinacy by repressing cytokinin biosynthesis and signaling. Plant Cell 30, 324–346. doi: 10.1105/tpc.17.00705
Keywords: lettuce, bolting, Auxin response factor, LsARF3, LsCO, overexpression, knockout
Citation: Li Y, Zhu J, Feng Y, Li Z, Ren Z, Liu N, Liu C, Hao J and Han Y (2022) LsARF3 mediates thermally induced bolting through promoting the expression of LsCO in lettuce (Lactuca sativa L.). Front. Plant Sci. 13:958833. doi: 10.3389/fpls.2022.958833
Edited by:
Judy Brusslan, California State University, Long Beach, United StatesReviewed by:
Miaoyun Xu, Biotechnology Research Institute (CAAS), ChinaAlfred (Heqiang) Huo, University of Florida, United States
Copyright © 2022 Li, Zhu, Feng, Li, Ren, Liu, Liu, Hao and Han. This is an open-access article distributed under the terms of the Creative Commons Attribution License (CC BY). The use, distribution or reproduction in other forums is permitted, provided the original author(s) and the copyright owner(s) are credited and that the original publication in this journal is cited, in accordance with accepted academic practice. No use, distribution or reproduction is permitted which does not comply with these terms.
*Correspondence: Jinghong Hao, aGFvamluZ2hvbmcyMDEzQDEyNi5jb20=; Yingyan Han, aHl5YmFjQDEyNi5jb20=