- 1Shandong Academy of Grape, Shandong Academy of Agricultural Science, Jinan, China
- 2College of Horticulture and Landscape Architecture, Henan Institute of Science and Technology, Xinxiang, China
- 3College of Horticulture, Henan Agricultural University, Zhengzhou, China
In grapevines, the MYB transcription factors play an important regulatory role in the phenylpropanoid pathway including proanthocyanidin, anthocyanin, and flavonoid biosynthesis. However, the role of MYB in abiotic stresses is not clear. In this study, an R2R3-MYB transcription factor, VyMYB24, was isolated from a high drought-tolerant Chinese wild Vitis species V. yanshanesis. Our findings demonstrated that it was involved in plant development and drought tolerance. VyMYB24 is a nuclear protein and is significantly induced by drought stress. When over-expressed in tobacco, VyMYB24 caused plant dwarfing including plant height, leaf area, flower size, and seed weight. The GA1+3 content in transgenic plants was reduced significantly, and spraying exogenous gibberellin could recover the dwarf phenotype of VyMYB24 transgenic plants, suggesting that VyMYB24 might inhibit plant development by the regulation of gibberellin (GA) metabolism. Under drought stress, the VyMYB24 transgenic plants improved their tolerance to drought with a lower wilting rate, lower relative electrical conductivity, and stronger roots. Compared to wild-type tobacco plants, VyMYB24 transgenic plants accumulated less reactive oxygen, accompanied by increased antioxidant enzyme activity and upregulated gene expression levels of superoxide dismutase (SOD), peroxidase (POD), and catalase (CAT) genes. In addition, transgenic plants accumulated more proline, and their related synthetic genes NtP5CR and NtP5CS genes were significantly upregulated when exposed to drought. Besides, abiotic stress-responsive genes, NtDREB, NtERD10C, NtERD10D, and NtLEA5, were upregulated significantly in VyMYB24 transgenic plants. These results indicate that VyMYB24 plays a positive regulatory role in response to drought stress and also regulates plant development, which provides new evidence to further explore the molecular mechanism of drought stress of the MYB gene family.
Introduction
In their lifespan, plants are often subjected to various biotic or abiotic stresses such as drought, salinization, cold, disease, insect pests, etc. Of them, drought stress is the most common. At present, more than one-third of the land area worldwide is located in arid or semi-arid regions (Khresat et al., 2004). Water deficit triggers wilt, restricts plant growth, and inhibits photosynthesis, resulting in significant financial losses. The plant's response to drought is a complex gene expression network. The water deficit signal is transferred to the cell membrane or nucleus, which directly triggers a second messenger system (Ca2+/IP3/CDPK phosphorylation and dephosphorylation) and activates the expression of a transcription factor or drought-responsive genes that function in transcriptional regulation and synthesis of protective enzymes and metabolites. This finally causes changes in cell physiological and biochemical processes to adapt to drought stress (Zhu, 2002).
The MYB family is one of the largest transcription factor families in higher plants. There are 190 MYB members in Arabidopsis thaliana (Stracke et al., 2001), 157 in maize (Du et al., 2012), 183 in rice (Chen et al., 2006), and 192 in Populus (Wilkins et al., 2009). The MYB transcription factor contains a highly conserved DNA binding domain (MYB domain) at the N terminal. According to the number of adjacent MYB domains, MYB transcription factors can be divided into four subfamilies: MYB-related, R2R3-MYB, 3R-MYB, and 4R-MYB (Dubos et al., 2010). Among them, the R2R3-MYB transcription factor has more members and various functions including secondary metabolism (Zhou et al., 2019; Ke et al., 2021), cell differentiation (Lu et al., 2021), and organ morphogenesis (Lau et al., 2015). Increasingly, recent reports show that R2R3-MYB proteins have been found to play an important regulatory role in response to abiotic stresses such as drought, cold, and salinity stresses. In Arabidopsis, AtMYB60 promoted root elongation to increase water uptake in the early stage of drought, and ABA was involved in drought resistance of AtMYB60 by regulating stomatal closure (Oh et al., 2011). The AtMYB15 participated in the response to cold stress by forming the complex inducer of CBF expression 1 (ICE1) and specifically combined it with the MYB element of CBF gene promoter (Agarwal et al., 2006a). In Oryza sativa, OsMYB30 interacted with the Jasmonate ZIM-domain gene (OsJAZ9) to regulate the expression of β-amylase, which degraded starch to maltose and enhanced the tolerance to cold stress as a soluble substance (Lv et al., 2017; Zeng et al., 2019). Abscisic acid (ABA) was involved in the drought tolerance of OsMYB48-1 by upregulating the expression of the ABA biosynthesis genes OsNCED4 and OsNCED5, as well as the stress-responsive genes RAB21, OsLEA1, RAB16C, and RAB16D (Xiong et al., 2014). In wheat, the heterologous expression of TaMyb1D in tobacco suppressed the expression of the phenylalanine metabolism-related genes and reduced the accumulation of flavonoids and lignin, thus enhancing the tolerance to drought and oxidative stress (Wei et al., 2017).
In grapevines, 108 members of the R2R3 MYB subfamily were identified (Matus et al., 2008). Previous studies on grapevine MYB genes revealed an unexpected wealth of genes. For example, grapevine MYB14 and MYB15 genes regulated the stilbene accumulation (Höll et al., 2013; Duan et al., 2016); VIMYBA1 genes, VvMYBC2-L1, VvMYB4, and VvMYB5a, modulated anthocyanin and proanthocyanidin biosynthesis (Deluc et al., 2008; Cutanda-Perez et al., 2009; Huang et al., 2014; Perez-Diaz et al., 2016); VvMYBPA1 and VvMYB5b genes induced phenylpropanoid pathway (Deluc et al., 2006; Bogs et al., 2007); and VvMYBF1 controlled flavonoid synthesis (Czemmel et al., 2009). However, the possible role of grapevine R2R3-MYB in drought stress is not clear.
Vitis yeshanensis J. X. Chen. is an important germplasm resource native to North China with high resistance to drought (Cui et al., 2020). In our previous study, a drought stress-induced differential expression gene VIT_14s0066g01090, VyMYB24, was screened from the transcriptome of V. yanshensis, which was upregulated in response to drought treatment. Based on its specific expression under drought stress, the present study mainly focuses on the potential role of the VyMYB24 gene in responding to drought tolerance and elaborates on its function in development regulation. Our study aimed to provide valuable information for exploring the more complex functions of grapevine MYB genes and contribute to future grapevine improvement with high drought resistance.
Materials and methods
Plant material and stress treatments
Vitis yeshanensis accession Yanshan-1 was grown in the grape repository of Shandong Institute of Pomology (Tai'an, Shandong, China). Two-year cutting plants in pots were cultivated in a greenhouse under a 16/8 h photoperiod (2000 lx) and the relative humidity of approximately 70–80% at 25–28°C. The drought treatment was conducted when the soil water content reached 70% in pots, and the leaves were sampled at 0, 1, 2, 3, 4, 5, 7, and 9 days. For salt treatment, the plants were watered with a 0.1 mol/L NaCl solution till flowing from the bottom of the pot. For temperature treatment, the plants were placed in the illumination incubator at 40°C. The leaves were collected at 0, 3, 6, 9, 12, 24, 36, and 48 h after salt treatment and high-temperature treatment, respectively. The leaf samples were frozen with liquid nitrogen and stored at −80°C. Each treatment included six pots of grape plants with a uniform growth trend. The normally managed plants were considered as control (CK).
The tobacco plants (Nicotiana benthamiana L.) were sowed in vitro on an MS medium under a 16/8 h photoperiod at 25°C. Ten-day-old seedlings were transferred into the soil with pots of 7 cm length, 7 cm width, and 10 cm height. Phenotypic indexes of transgenic plants, such as plant height, leaves, flowers, and fruit, were observed and recorded during different plant development periods. Seed weight was expressed as the mean value of three seeds. For GA treatment, three-week tobacco plants were sprayed using 10−5 M GA3 two times at intervals of five days, and the plant height was measured after three weeks.
Four-week-old tobacco plants were chosen to perform the drought treatment without watering under a 16/8 h photoperiod at 28°C. The control plants were watered normally. After 10 days of treatment, the physiological indexes were calculated. On the 12th day after drought stress, the wilting rate was recorded, and the fresh weight of the root was measured. The wilting rate was calculated based on the number of plants with more than 50% leaves with wilting phenotype to the total plants. Each treatment included 90 plants in three biological repeats.
VyMYB24 cloning
Total RNA was extracted from Yanshan-1 leaves using E.Z.N.A. total RNA Kit I (OMEGA). The first cDNA was amplified by the SMART MMLV Reverse Transcriptase (TaKaRa). Specific primers were designed according to the DEG VIT_14s0066g01090 MYB gene with the forward primer: 5′-atggataaaaaaccctgcaattctcag-3′ and the reverse primer: 5′-ttaatctccattaagtagctgcatag-3′. The VyMYB24 gene was cloned using PrimeSTAR® HS DNA Polymerase (Takara, Beijing, China). PCR was performed in 30 cycles of 98°C for 10 s and 60°C for 10 s, followed by 72°C for 10 min. The obtained product was cloned into pGEM-T Easy Vector System (Promega, USA) at 4°C overnight, transformed into Escherichia coli strain TOP10 (Tiangen, Beijing, China), screened on the LB plate, and then sequenced by Sangon Biotech Co., Ltd (Shanghai, China).
Quantitative real-time PCR analysis
Total RNA extraction and cDNA amplification were performed according to the above method. The Quantitative real-time PCR (qRT-PCR) was run on a CFX96 real-time PCR cycler (Bio-Rad Laboratories, Hercules, CA, USA) using SYBR Premix Ex TaqTM II Kit (Takara, Beijing, China), with the following cycling conditions: 30 s at 95°C and 40 cycles of 5 s at 95°C and 30 s at 60°C. The Actin gene was used as an internal reference. Relative mRNA ratios were calculated using the 2−ΔΔCT (Livak and Schmittgen, 2001). The specific primers used for qPCR are listed in Supplementary Table 1.
Subcellular localization of VyMYB24
The VyMYB24 coding region was fused to GFP in the pBI221-GFP plasmid to construct the VvMYB24-GFP vector. For subcellular localization assays, Arabidopsis protoplasts were isolated according to the previous protocol (Yoo et al., 2007). The vector was transferred to the protoplasts using the PEG6000 method. The transfected protoplasts were incubated for 12–16 h at 28°C in the dark. Fluorescence was detected using a confocal laser scanning microscope (LSM510; Carl Zeiss Thornwood, New York, NY, USA).
Generation of VyMYB24 transgenic tobacco plants
The open reading frame sequence of VyMYB24 was amplified with the forward primer 5′-ggggtaccATGGATAAAAAACCCTGCAATTC-3′ and reverse primer 5′-gggtcgacATCTCCATTAAGTAGCTGCAT-3′. The resulting fragments were digested by restriction endonucleases KpnI and SalI and inserted into the pCAMBIA2300 vector to produce the pCAMBIA2300:: VyMYB24 construct. These constructs were transformed into N. benthamiana via Agrobacterium GV3101 using the leaf disc method according to the protocol described by Horsch et al. (1985). Transgenic tobacco plants were placed on the MS medium with added kanamycin (50 mg·L−1). The T2 transgenic tobacco plants were grown in a greenhouse under a 16/8 h photoperiod at 25°C.
Evaluation of chlorophyll content, relative electrical conductivity, and malondialdehyde (MDA) content
Total chlorophyll concentration was measured according to the method described by Costa et al. (2008). About 0.1 g of leaves were extracted using 80% acetone and analyzed using a UV-Visible Spectrophotometer (UV2600, Shimadzu, Kyoto, Japan). The absorbance was measured at 645 and 663 nm, respectively. For relative electrical conductivity, 0.3 g of fresh leaves were cut into pieces and placed in a tube with 10 ml sterile deionized water for 3 h; the electrical conductivity (R1) was measured with a Crison conductivity meter (Basic 30, Crison Instruments, SA). Then, the samples were bathed in boiling water at 100°C for 20 min and cooled to room temperature. The electrical conductivity (R2) was measured. The relative electrical conductivity was calculated with R1/R2×100%. The MDA content was measured by the thiobarbituric acid (TBA) method described by Zhao et al. (2009) and expressed as U/mg protein.
Histochemical ROS staining and antioxidant enzyme activity analysis
For 3, 3′-diaminobenzidine (DAB) and tetranitroblue tetrazolium chloride (NBT) staining, the leaves were infiltrated in the DAB solution (0.1%, in 50 nM Tris-HCl, pH 3.8) or the NBT solution (0.5 mg/ml, in 0.25 mM phosphate buffer, pH 7.6). After overnight in dark, the leaf samples were put in 95% alcohol and bathed in boiling water at 100°C for 10 min. After cooling, the leaves were kept in a bleaching solution (lactic acid: glycerin: H2O = 1:1:4) and photographed. The H2O2 and contents were measured by the ammonium molybdate method or hydroxylamine oxidation method described by Kong et al. (2011). For drought tolerance analysis, the enzyme activity of the antioxidant enzyme, including superoxidase dismutase (SOD), peroxidase (POD), and catalase (CAT) in tobacco leaves, were evaluated using A001-1, A084-3, and A007-1-1 kits (Nanjing Jiancheng Co. Ltd, China), respectively.
Measurement of proline and gibberellin (GA) content
The osmosis substance proline was measured by the ninhydrin method described by Kong et al. (2011), and GA contents were measured by the enzyme-linked immunosorbent assay (ELISA) method as described previously (Shan et al., 2007; Wang et al., 2012). Briefly, 0.5–1.0 g fresh samples were ground in 80% methanol with butylated hydroxytoluene (1 mmol L−1) under an ice bath condition and transferred into a 10 ml centrifuge tube for 4 h at 4°C. After centrifugation, the supernatants were passed through a C18 Sep-Pak cartridge, washed with methanol or diethyl ether, and then dried in nitrogen. The residues were dissolved in phosphate-buffered saline solution (PBS) and transferred into 96-well microtitration plates and then coated with synthetic GA1-ovalbumin for 30 min at 37°C. After washing the plate with PBS four times, the horseradish peroxidase-labeled goat anti-rabbit immunoglobulins were added and incubated for 30 min at 37°C. After washing the plate with PBS, ortho phenylenediamine and H2O2 were added for coloration in the dark for 15 min at 37°C, and the reaction was stopped by adding 2 mol L−1 H2SO4. The absorbance was measured using the ELISA analyzer (Thermo Multiskan MK3) at 490 nm. Three biological replicates of each assay were performed.
Results
Isolation and sequence analysis of VyMYB24
Using the PCR method, the full length of VyMYB24 cDNA was cloned, and its open reading frame was 570 bp encoding 190 amino acids and 21.569 kD of the predicted protein molecular weight. Based on the genome of Vitis vinifera (https://www.genoscope.cns.fr/externe/GenomeBrowser/Vitis), the VyMYB24 gene was located at 16764247–16767889 on Chromosome14, and the gDNA of 3643 bp contained three exons (136, 130, and 304 bp, respectively) and two introns (93 and 2980 bp) (Figure 1A). The protein sequence analysis showed that VyMYB24 contained a conservative R2R3 domain, which had a high similarity of 78.65, 70.24, and 68.84% with NtMYB305, AmMYB305, and AmMYB340, respectively, from Nicotiana tabacum and Antirrhinum majus (Figure 1B). The phylogenetic analysis of VyMYB24 and 15 MYB proteins from Arabidopsis showed that VyMYB24 belonged to the same cluster as AtMYB24, AtMYB21, and AtMYB57, which were involved in the regulation of anther development (Cheng et al., 2009) (Figure 1C).
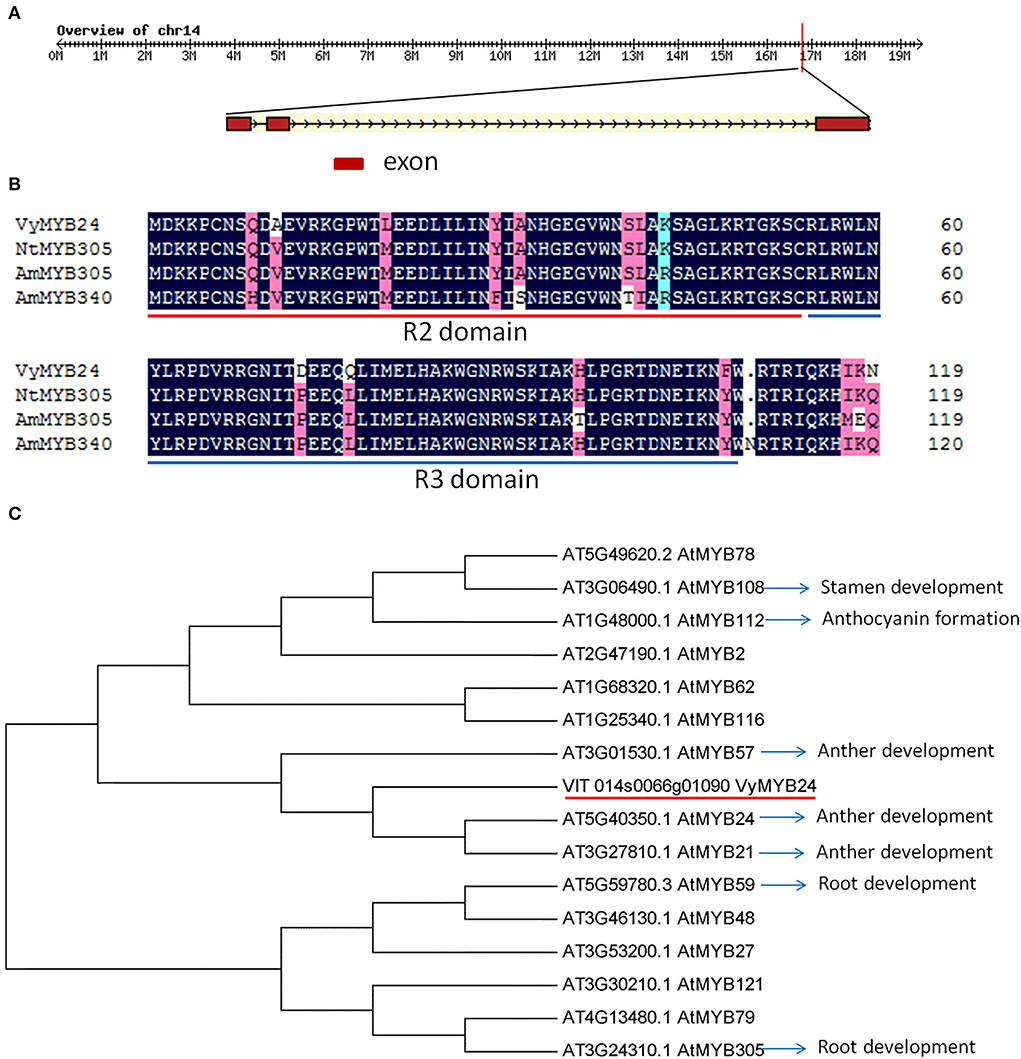
Figure 1. Features of VyMYB24 protein. (A) The genomic sequence of VyMYB24. (B) Alignment of VyMYB24 protein with other R2R3-MYB proteins from N. tabacum and A. majus. R2 and R3 are the two repeats of the MYB DNA-binding domain. GeneBank accession numbers are as follows: NtMYB305 (XP_016506729), AmMYB305 (P81391), and AmMYB340 (P81396). (C) Phylogenetic analysis of VyMYB24 protein with other 15 R2R3-MYB proteins from Arabidopsis. The phylogenetic tree was generated using the neighbor-joining method by MEGA5.0. Part of the R2R3-MYB genes was involved in plant development.
VyMYB24 gene was localized in the nucleus
To confirm whether VyMYB24 was localized in the cell nucleus, the plasmid pBI221-VvMYB24-GFP was transformed into Arabidopsis mesophyll protoplasts. The results showed that the VvMYB6-GFP fusion protein was located solely in the nucleus, whereas the control GFP protein was distributed throughout the cell, including the nucleus, the cytoplasm, and the cell wall (Figure 2), suggesting VyMYB24 as a nuclear protein.
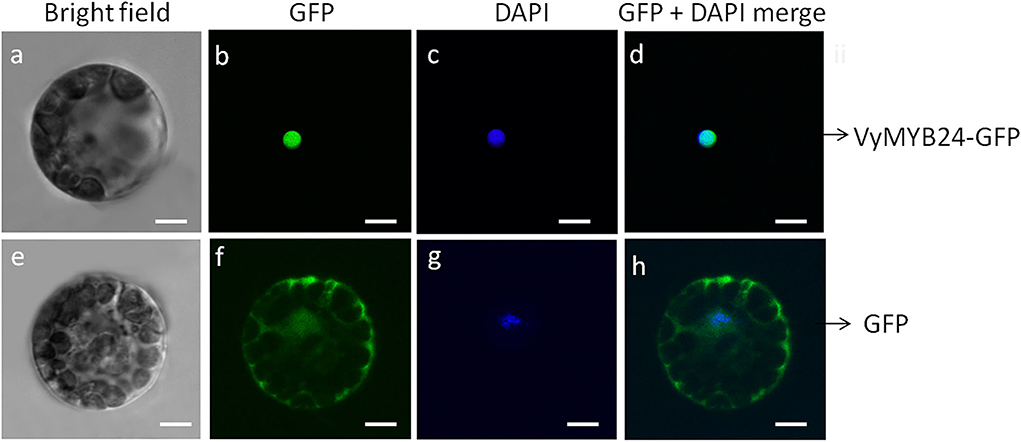
Figure 2. Subcellular localization of the VyMYB24 protein in Arabidopsis leaf protoplasts. The fluorescent signal was detected by confocal laser-scanning microscopy. (a,e) Bright field; (b,f) GFP; (c,g) DAPI; (d,h) GFP + DAPI merge. Bars correspond to 10 μm.
VyMYB24 caused dwarf phenotype in the transgenic tobacco plants
To further explore the potential function of the VyMYB24 gene, transgenic tobacco plants were obtained. Compared with the wild-type plants, the transgenic VyMYB24 tobacco lines (OE1 and OE3) all exhibited the dwarf phenotype at 2, 6, 8, and 10 weeks (Figures 3A–D). The height of transgenic plant lines OE1 and OE3 were 54.07 and 48.15% of wild-type tobacco plants at two weeks; it comparatively reduced to 49.01 and 35.83% at six weeks, increased to 64.44 and 58.73% at eight weeks, and reduced again to 59.91 and 56.78% of the wild-type plants at 10 weeks (Figure 3E). In addition, we also found that leaf areas were smaller in transgenic tobacco plants (Figures 3F,H); the leaf areas of the transgenic plant lines OE1 and OE3 were 86.56 and 56.76% of the wild-type plants at six weeks. The transgenic tobacco plants also accumulated more chlorophyll (Figures 3F,G), reaching 119.10% (OE1) and 138.22% (OE3) of the wild type. Meanwhile, shorter flowers and smaller seeds were observed in the transgenic VyMYB24 lines (Figures 3I–L); the flower length of OE1 and OE3 were 82.15 and 81.50% of the wild-type plants and their seed weight were only 49.16 and 40.74% of wild type. In summary, the VyMYB24 gene significantly modulated plant growth and development.
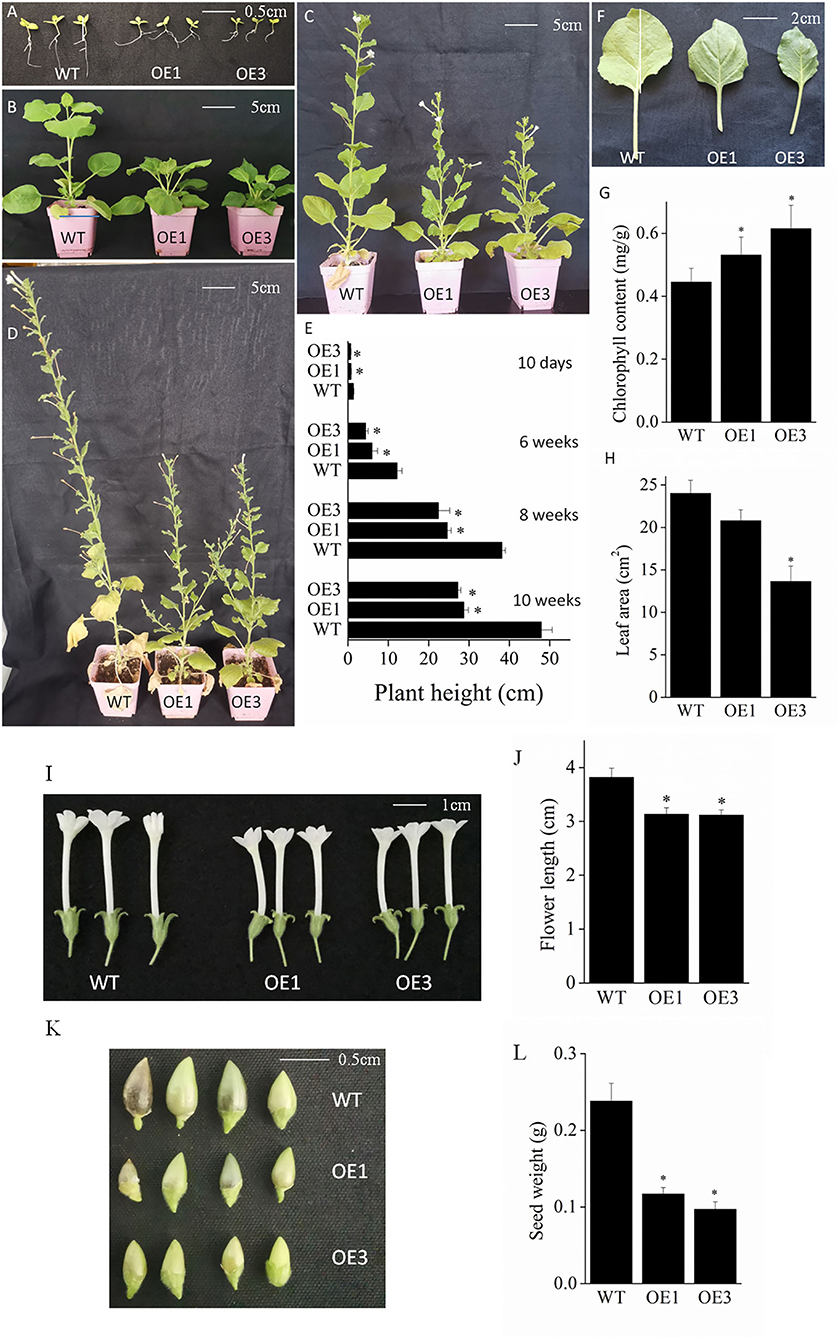
Figure 3. Phenotypic analysis of VyMYB24 transgenic tobacco plants. The phenotypes of 10-day seedlings (A), six-week plants (B), eight-week plants (C), and ten-week plants (D) were observed, and the plant height was measured (E). Leaves from six-week plants (F) were chosen to measure the leaf area (G) and the chlorophyll content (H). The flowers (I) and seeds (K) were photographed and the flower length (J) and seed weight (L) were evaluated. Bars represent mean ± SD. A significant difference from the WT was confirmed by a one-way ANOVA test, *p < 0.05.
VyMYB24 gene regulated GAs biosynthesis in transgenic plants
As an endogenous hormone, GA plays a key role in regulating plant height development. To examine the reason for the dwarf phenotype of a transgenic plant, the GA content was measured using the ELISA method. As shown in Figure 4A, GA1+3 content was significantly lower in the transgenic VyMYB24 lines compared with the wild type. The GA1+3 content of transgenic plant lines OE1 and OE3 were 47.72 and 46.17% of that of wild type, respectively. Meanwhile, the GA metabolism-related genes were checked using the qRT-PCR method. As shown in Figure 4B, the genes related to GA synthesis (GA20ox1, GA20ox2, GA20ox3, GA3ox2, and GA3ox3) were down-regulated significantly in the transgenic lines except for GA3ox1, while the genes related to GA degradation genes (GA2ox2, GA2ox4, GA2ox5, and GA2ox6) were positively regulated significantly (Figure 4B). To further verify the above results, the phenotype recovery test was conducted. After exogenous spraying of GA for three weeks, the transgenic and wild-type tobacco plants all grew significantly taller (Figure 4C). However, the height difference between transgenic and wild-type plants were 15.1–16.7% under GA spraying conditions, which was markedly lesser than without GA spraying (46.9–59.4%) (Figure 4D), indicating that exogenous GA could recover the dwarfing phenotype of transgenic plants.
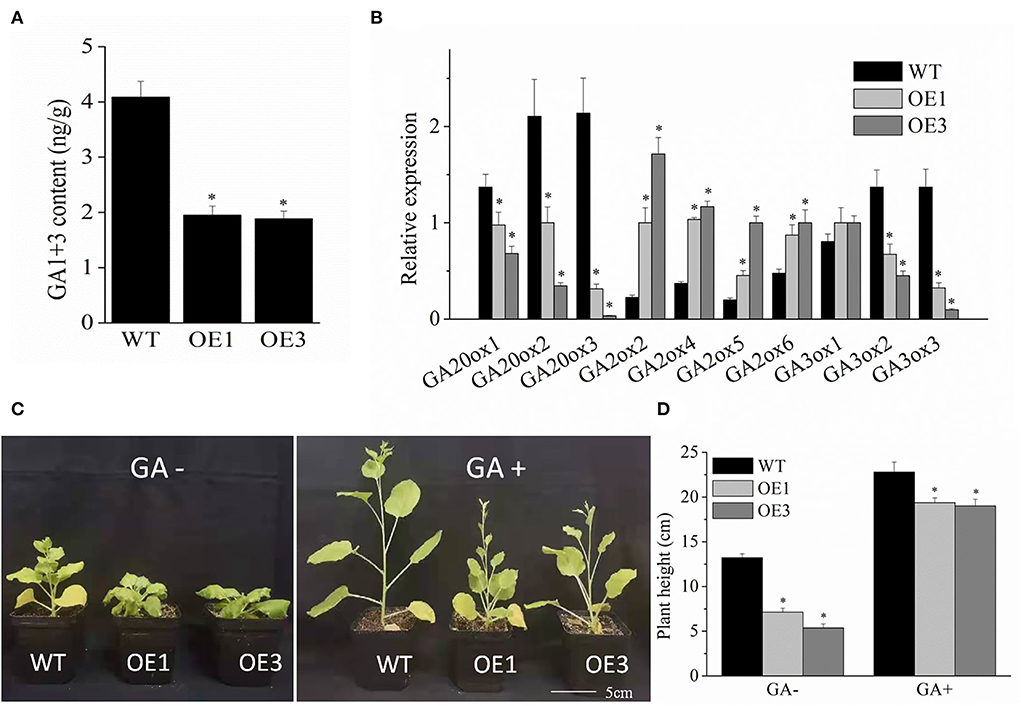
Figure 4. The VyMYB24 gene regulated GA biosynthesis in the transgenic lines. (A) The content of GA1+3 was measured by the ELISA method. (B) Expression profiles of the GA biosynthesis-related genes. (C) Phenotypic characteristics of the transgenic lines and wild-type plants were sprayed with 10−5 M GA after three weeks, and the plant height was measured (D). Bars represent mean ± SD. A significant difference from the WT was confirmed by a one-way ANOVA test, *p < 0.05.
Expression profiles of VyMYB24 gene under stresses
To determine the response of the VyMYB24 gene to abiotic stresses, the gene expression was evaluated in grapevine leaves under drought, high temperature, and salt stresses using the qRT-PCR method. We found that the VyMYB24 gene expression was induced by three abiotic stresses (Figure 2). The transcript abundance of VyMYB24 reached its peak at 36 h, which was 3.18 times higher than at 0 h under high temperature, and 2.72 times higher at 48 h than at 0 h under salt stress. It was noted that the VyMYB24 gene responded remarkably to drought stress (Figure 5) with 25.96 higher folds at 9 d than at 0 d, suggesting that the VyMYB24 gene was greatly involved in the drought stress.
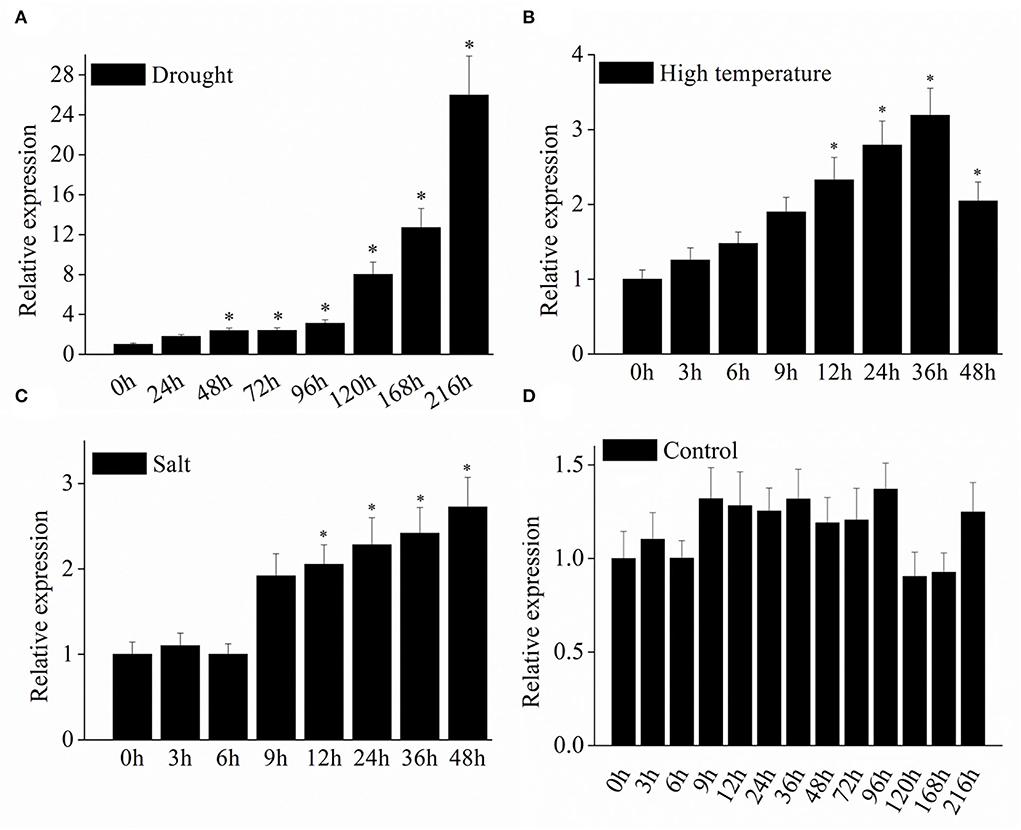
Figure 5. The expression profiles of the VyMYB24 gene in V. yanshanesis accession Yanshan-1 leaves under abiotic stresses. The two-year-old Yanshan-1 seedlings were treated with drought (A), high temperature of 40°C (B), and high salt of 0.1 M NaCl (C) with normal as CK (D). The data represents the means ± SD of three replicates and the statistical analyses were performed with a one-way ANOVA test, *p < 0.05.
VyMYB24 gene enhanced the drought tolerance
As seen in Figures 6A,B, at 12 d after drought stress, most of the wild-type tobacco plants displayed a serious wilting phenotype, while fewer transgenic plants exhibited wilting phenotype. The wilting rate of transgenic plants was 26.7–30%, which was significantly lower than that of wild-type plants (80%) (Figure 6C). Moreover, the transgenic plants had stronger roots (Figure 6D). Under normal watering, the root fresh weight of transgenic plant lines OE1 and OE3 was 0.60 and 0.44 g, but only 0.28 g for the wild-type plants. Drought stress hinders root growth in plants. However, our results demonstrated that the roots were stronger in the transgenic VyMYB24 plants than in wild-type ones, and the root fresh weight of transgenic plant lines OE1 and OE3 were more by 2.85 and 2.77- folds in wild-type plants (Figure 6E). Collectively, the VyMYB24 gene improved the drought tolerance in transgenic lines due to a lower wilting rate and stronger roots.
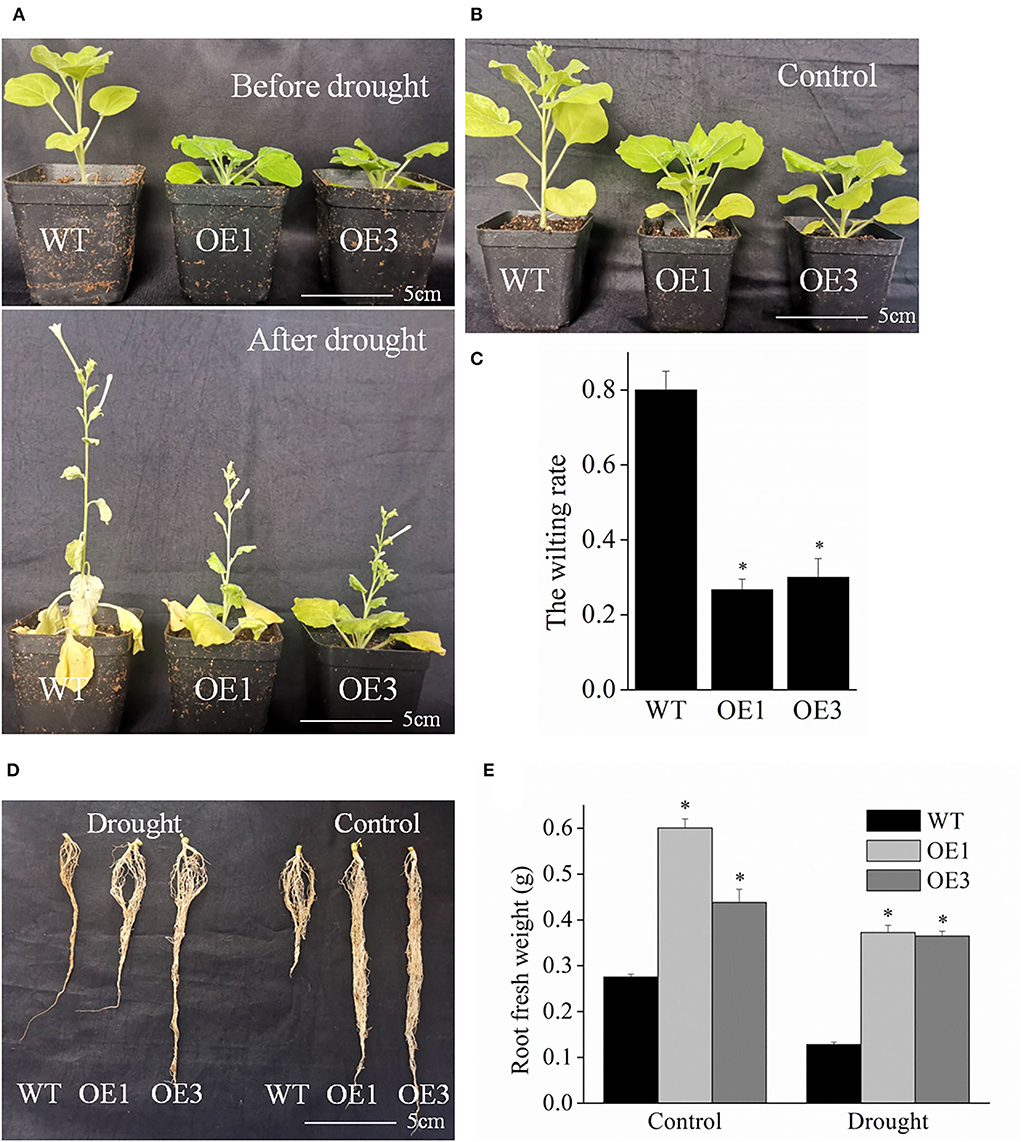
Figure 6. The VyMYB24 gene improved the drought resistance in the tobacco transgenic lines. (A) Four-week-old transgenic and wild-type plants were treated with drought stress for 12 days with the normal as CK (B). (C) The wilting rate of plants was measured, and the wilting rate was calculated based on the numbers of the plants with more than 50% leaves with wilting phenotype to the total plants. (D) The root phenotypes were observed before or after drought treatment, and the root fresh weight of plants was measured (E). Bars represent mean ± SD. A significant difference from the WT was confirmed by a one-way ANOVA test, *p < 0.05.
ROS accumulation by histochemical staining
MDA is an important physiological index to evaluate membrane lipid peroxidation. Under drought stress, the VyMYB24 transgenic plant lines accumulated less MDA, about 54.07 and 48.15% lesser than wild-type plants (Figure 7A), indicating that the integrity of cell membrane was less destroyed in transgenic plants. Furthermore, the relative electrical conductivity was 0.38–0.42 in the transgenic plants, significantly lower than that of the wild-type plants, which was 0.73 under drought stress (Figure 7B).
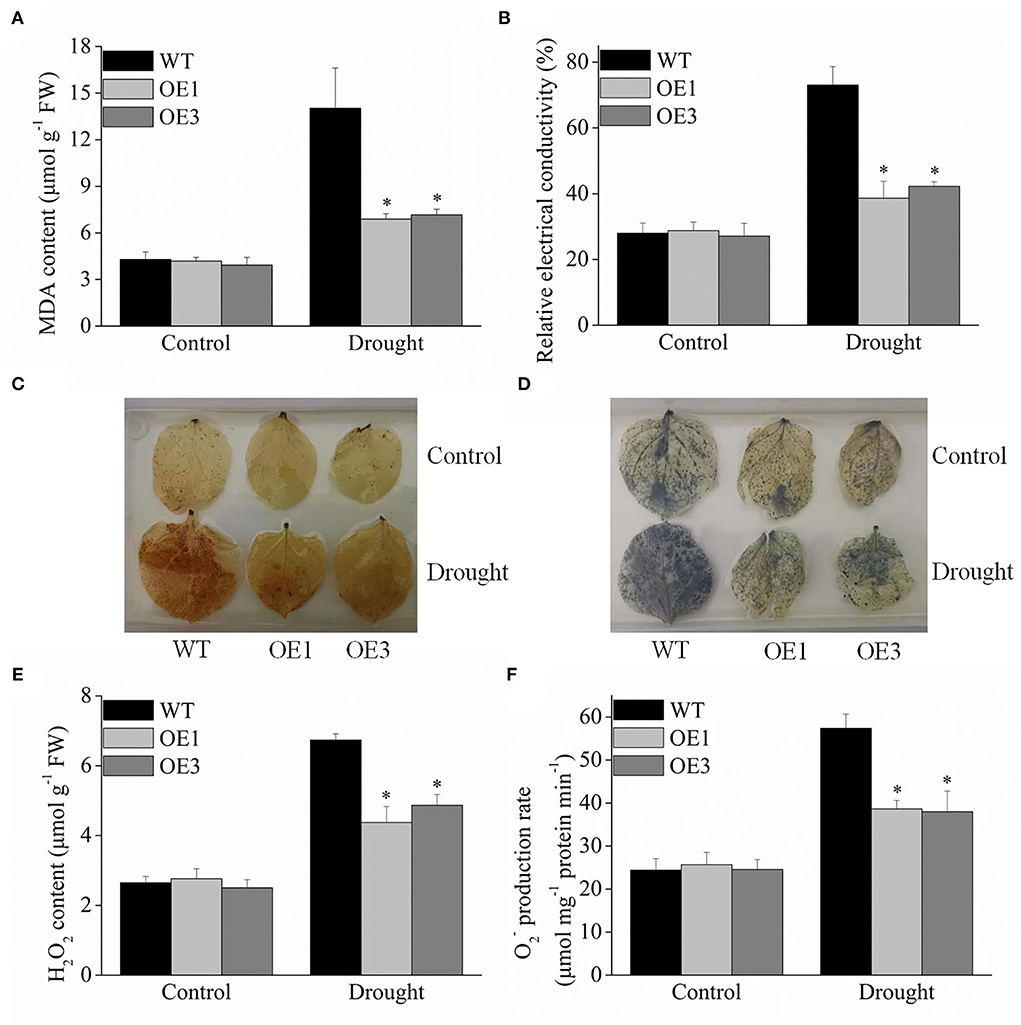
Figure 7. Oxidation conditions in VyMYB24 transgenic and wild-type plants exposed to drought stress. Four-week-old tobacco plants were treated with drought stress for 10 days, and the MDA content (A) and relative electrical conductivity (B) were checked. (C), (D) Detection of H2O2 and by DAB staining and NBT staining in transgenic and wild-type plants. H2O2 content (E) and production rate (F) were evaluated. Bars represent mean ± SD. A significant difference from the WT was confirmed by a one-way ANOVA test, *p < 0.05.
DAB and NBT staining are commonly used to locate the H2O2 and in plant tissues. Under normal conditions, the tobacco leaves of the control appeared lighter in color with no significant difference between the transgenic and wild plants. Under drought treatment for 10 d, more yellowish-brown plaques were distributed on wild-type tobacco leaves compared to the transgenic plants using DAB staining (Figure 7C). When stained with NBT, the wild-type leaves appeared darker blue, while slight blue color was observed in the transgenic tobacco leaves (Figure 7D). Meanwhile, the H2O2 and contents were also evaluated. Under normal conditions, H2O2 and no significant differences were observed between the transgenic and wild-type plants. Under drought stress, the wild-type tobacco plants significantly accumulated more H2O2 and , and the H2O2 content in the transgenic plant lines OE1 and OE3 was 64.93 and 72.57% of wild type (Figure 7E), and was 67.38 and 66.20% of the wild-type ones (Figure 7F).
Analysis of antioxidant enzyme activity
Reactive oxygen-scavenging enzymes play an important role in plant response to environmental stresses. Under normal conditions, the enzyme activity of SOD, POD, and CAT were not significantly different between the transgenic and wild-type plants, However, the transgenic VyMYB24 tobacco plants had a significantly higher enzyme activity compared to the wild-type ones under drought stress (Figure 8). The SOD activity in OE1 and OE3 was 191 and 170% of wild type ones, the POD activity was 140 and 132% of wild type, and the CAT activity was 184 and 168% of wild type. Meanwhile, proline was significantly accumulated in the VyMYB24 transgenic plants under drought stress (Figure 8), especially in OE3 – two-folds higher than the wild-type ones.
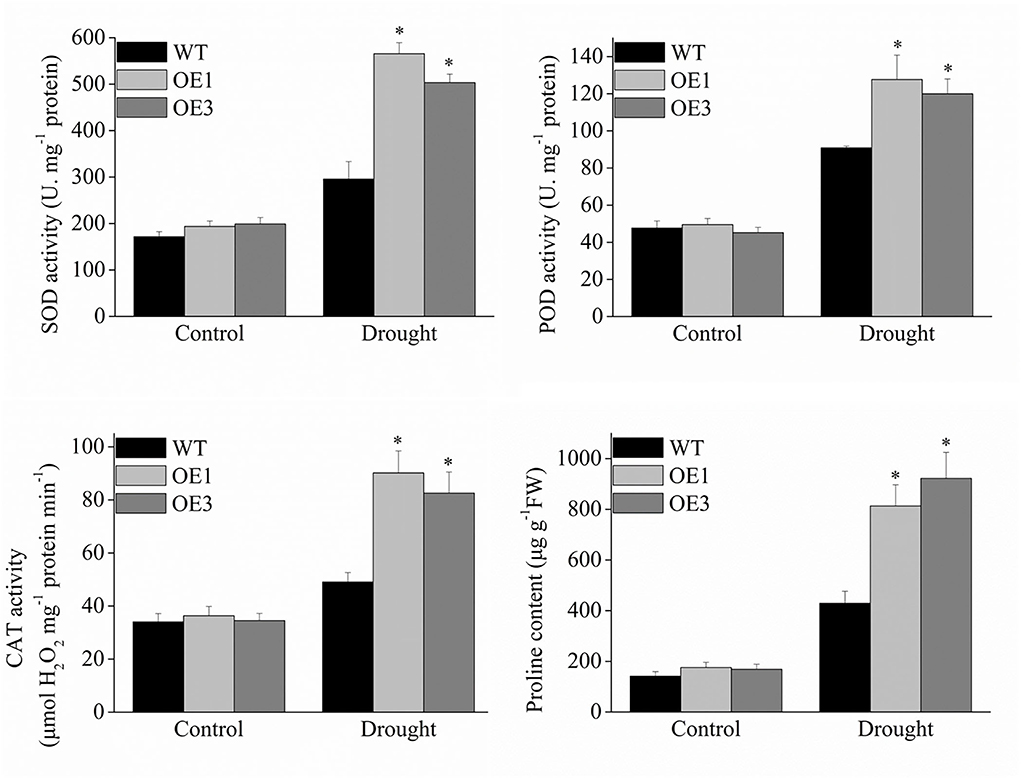
Figure 8. The total activities of the antioxidant enzymes and proline content in transgenic and wild-type plants under drought stress. Four-week-old tobacco plants were treated with drought stress for 10 days, and the activities of SOD, POD, CAT, and proline content were measured. Bars represent mean ± SD. A significant difference from the WT was confirmed by a one-way ANOVA test, *p < 0.05.
Expression profiles of the stress-related genes
To further explore the molecular mechanism underlying the enhanced drought resistance in transgenic lines, nine stress-related genes including NtSOD, NtPOD, NtCAT, NtLEA5, NtP5CS NtP5CR, NtDREB, NtERD10C, and NtERD10D were investigated using the qRT-PCR method. The results showed that all nine stress-related genes were all up-regulated by drought stress in transgenic lines compared to the wild type (Figure 9), especially, NtERD10C, NtERD10D, and NtLEA5.
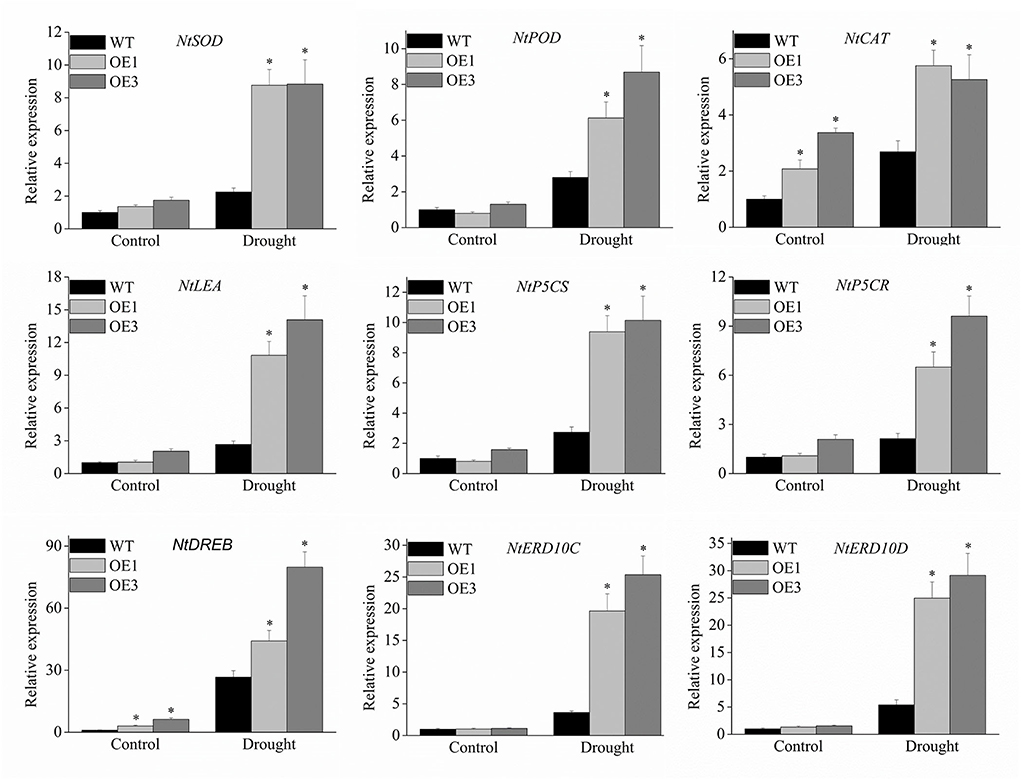
Figure 9. Expression profiles of the stress-responsive genes in transgenic plants under drought stress. The expression data were normalized against the expression of NtActin. Bars represent mean ± SD. A significant difference from the WT was confirmed by a one-way ANOVA test, *p < 0.05.
Discussion
Grapevine (Vitis vinifera L.), as a commercial horticultural crop, is cultivated worldwide. However, drought, as the major environmental stressor, seriously impacts the growth and development of the plant but more particularly affects berry and wine quality and grapevine yield (Costa et al., 2007; Chaves et al., 2010). Several reports demonstrate that MYB transcription factors regulate diverse plant developmental processes, affecting biotic and abiotic stresses (Liu et al., 2011; Lv et al., 2017; Zeng et al., 2019; Zhou et al., 2019; Lu et al., 2021). However, very little is known about how MYB transcription factors mediate the response to drought stress in grapevines. In the present study, the VyMYB24 gene was obtained based on the transcriptome analysis of V. yeshanensis accession Yanshan-1 under drought stress. Sequence analysis showed that the VyMYB24 protein contained a conservative R2R3 domain and is localized in the nucleus of the Arabidopsis leaf protoplasts, which was in accordance with the typical characteristics of transcription factors (Stracke et al., 2001).
VyMYB24 Inhibited the Development of Transgenic Plants
Members of MYB from other species were involved in plant development, including organ morphogenesis, flower development, and plant growth (Cheng et al., 2009; Seo et al., 2009; Lau et al., 2015). Homology analysis of protein sequences showed that VyMYB24 has a high similarity to NtMYB305 from N. tabacum and AmMYB330 from Antirrhinum (Figure 1B), which could inhibit plant development in transgenic plants that displayed dwarf phenotype (Tamagnone et al., 1998; Liu, 2010). Our results further demonstrated that overexpressing VyMYB24 caused plant dwarfing, resulting in growth parameters smaller than wild plants, including plant height, leaf area, flower length, and seed weight (Figure 3). A similar phenotype was also found in Arabidopsis, overexpressing the MYB96 plants also exhibited dwarf growth, altered leaf morphology, and reduced lateral roots (Seo et al., 2009). In addition, the phylogenetic analysis showed that VyMYB24 fell into the same cluster as AtMYB24, AtMYB21, and AtMYB57 from Arabidopsis, which were involved in anther development (Cheng et al., 2009), but VyMYB24 did not affect the development of anthers in transgenic plants, and no male sterile phenotype was found (Figures 3I,K). Therefore, it was inferred that VyMYB24 was involved in just regulating plant height.
VyMYB24 enhanced the tolerance to drought
The expression analysis under different stresses showed that VyMYB24 was induced significantly by drought stress compared to high temperature and salt stresses (Figure 5). This prompted us to explore the possible role of the VyMYB24 gene under drought stress. Treated with drought treatment, transgenic plants displayed a lower wilting rate and lower relative electrical conductivity compared to the wild-type plants (Figure 6), suggesting that VyMYB24 could improve the plant's tolerance to drought. Moreover, VyMYB24 transgenic plants had more strong roots compared to wild-type plants. This result was similar to MdSIMYB1 from Malus Domestica, where the heterologous expression of MdSIMYB1 in tobacco enhanced the tolerance to drought and exhibited robust root growth (Wang et al., 2014). Similarly, apple gene MdMYB88 transgenic plants had more roots and higher hydraulic conductivity under drought stress (Geng et al., 2018). The plant root is a critical organ that absorbs water and mineral nutrients from the soil. A developed root can absorb more water from the soil which contributes to drought resistance (Gullo et al., 1998; Hoecker et al., 2006). The above results suggested that strong roots might play a role in the drought resistance of VyMYB24 transgenic plants.
Reactive oxygen can regulate plant development as a signal molecule, and the production and the elimination of reactive oxygen keep a balance under normal conditions. When exposed to stress, the plant will accumulate excess reactive oxygen, which causes membrane lipid peroxidation, resulting in membrane system damage and, even worse, the death of the plant (Gechev et al., 2006). Plants exhibit an active oxygen scavenging system. Through a series of enzymatic reactions, the SOD enzyme can convert into molecular oxygen, and POD enzyme and CAT enzyme can convert H2O2 into H2O and O2. Previous studies showed that many MYB genes such as from Arabidopsis, OsMYB6 from rice (Tang et al., 2019), GaMYB85 from cotton (Butt et al., 2017), TaMYB33 from wheat (Qin et al., 2012), and MdSIMYB1 from apple (Wang et al., 2014) could regulate the production of reactive oxygen by the antioxidant enzyme system. In the present study, VyMYB24 transgenic plants produced less reactive oxygen compared with the wild-type plants under drought stress. Meanwhile, VyMYB24 transgenic had increased SOD, POD, and CAT activity than wild-type plants, and NtSOD, NtPOD, and NtCAT genes were significantly upregulated. The above result suggested that VyMYB24 might reduce the production of reactive oxygen by upregulation of antioxidant enzyme activity.
Under stress treatment, stress-related genes, such as P5CR, P5CS, DREB, ERD, and LEA, were significantly induced, which promoted the synthesis of protective enzymes or active downstream genes. In this study, NtP5CR and NtP5CS genes were upregulated significantly compared to wild-type plants, and more specifically, proline was accumulated in VyMYB24 transgenic when exposed to drought. Proline plays an important role in osmotic regulation, which can hold water and prevent dehydration under drought stress (Hong et al., 2000). As key regulatory enzymes, P5CR and P5CS are involved in the biosynthesis of proline (Delauney and Verma, 1993). Many drought tolerance MYB genes also promote the accumulation of proline under drought stress, such as GmMYBJ1 from soybean (Su et al., 2014), FtMYB9 from Tartary buckwheat (Gao et al., 2017), MdMYB10 from apple (Gao et al., 2011), and SoMYB18 from sugarcane (Shingote et al., 2015). The DREB transcription factors belong to the AP2/EREBP family, which can combine with dehydration-responsive elements to activate downstream stress-related genes (Agarwal et al., 2006b). In Arabidopsis, overexpressing DREB1A in tobacco improved drought and cold stress tolerance and significantly activated the stress-related genes NtERD10C and NtEED10D, which are late embryogenesis abundant proteins (Kasuga et al., 2004). In this study, NtDREB, NtERD10C, NtERD10D, and NtLEA5 genes were upregulated significantly in VyMYB24 when exposed to drought stress. These results indicated that stress-related genes participated in the drought tolerance of VyMYB24.
The role of GA in development and drought resistance
As an important hormone, GA plays a key role in regulating plant development and the reduction of GA levels and has been shown to contribute to plant growth restriction (Yamaguchi, 2008). In this study, the GA1+3 content was reduced significantly in transgenic plants (Figure 4A); meanwhile, exogenous GA spraying could recover the dwarf phenotype of VyMYB24 transgenic plants (Figures 4C,D). Similar to the DREB transcription factor transgenic GhDREB1 from cotton, GhDREB1 transgenic plants displayed a GA-deficient phenotype and could be rescued by exogenous GA3 application (Huang et al., 2009). During GA metabolism, regulation of the key genes of the GA biosynthetic or degradable pathway, such as GA20ox, GA3ox, and GA2ox, often results in different levels of bioactivity, followed by accelerated or retarded plant development. GA12 is converted to GA4 through oxidations on C-20 and C-3 by GA 20-oxidase (GA20ox) and GA3-oxidase (GA3ox), respectively, and GAs are metabolically deactivated by a class of GA 2-oxidase (GA2ox) (Yamaguchi, 2008). In VyMYB24 transgenic plants, the GA synthesis-related genes were downregulated and the GA degradation-related genes were upregulated compared with wild-type (Figure 4B). The results were obtained in Arabidopsis, where the NAC transcription factor, JUB1, affects growth by negatively regulating genes encoding key enzymes of gibberellin GA3ox1 (Shahnejat-Bushehri et al., 2016). Given the above results, VyMYB24 might inhibit plant development by the regulation of GA metabolism.
Gibberellin plays a key role in response to environmental stress. When exposed to abiotic stress, such as drought, salt, or cold, there is a reduction of endogenous bioactive GAs (Achard et al., 2006; Magome et al., 2008). Inhibition of the biosynthesis of gibberellins (GAs) and the application of GAs to retardant-treated plants and GA-deficient mutants reverse their enhanced stress tolerance as well as their dwarf growth condition (Gilley and Fletcher, 1998; Vettakkorumakankav et al., 1999; Rademacher, 2000). In Arabidopsis, upregulation of the biosynthetic gene GA20ox or exogenous application of GA reduced the tolerance to drought, while downregulation of the biosynthetic gene GA20ox or GA3ox could enhance the tolerance to drought (Colebrook et al., 2014). Arabidopsis NAC transcription factor JUB1, which negatively regulated the biosynthesis of GA, increased the tolerance to drought, salt, and heat stress (Thirumalaikumar et al., 2018). So, we speculated that VyMYB24 might enhance drought tolerance by negatively regulating GA biosynthetic pathway. Moreover, there is evidence that GA signaling may integrate multiple hormone signaling pathways in response to stress, such as the stress hormone jasmonic acid (Colebrook et al., 2014). Whether there are other hormones involved in drought tolerance of VyMYB24 needs further study.
Conclusion
In conclusion, grapevine VyMYB24, belonging to the R2R3-MYB gene, was first identified from V. yanshanesis with high drought tolerance. Specifically, the present study found that VyMYB24 enhanced the tolerance to drought stress by stimulating strong roots and by regulating the antioxidant enzyme system, proline accumulation, and stress-related gene expression. Moreover, VyMYB24 could inhibit plant development by negatively regulating the biosynthesis of GA. Therefore, the current study expanded the potential function of the grapevine MYB gene and provided new evidence to further explore the molecular mechanism of drought stress in the MYB gene family.
Data availability statement
The original contributions presented in the study are included in the article/Supplementary material, further inquiries can be directed to the corresponding authors.
Author contributions
ZZ, BL, and JS conceived and designed the experiment. ZZ and RQ conducted the experiment and data analysis. WX, GC, GY, XL, ZH, and GL contributed to the data analysis. ZZ and JS wrote the manuscript. WX, GC, GY, XL, ZH, GL, and BL drafted the discussion and revised the manuscript. All authors contributed to the article and approved the final version.
Funding
This work was supported by the Major Science and Technology Innovation Major Project of Shandong Province (Grant No. 2019JZZY010727), the Shandong Province Natural Science Foundation of China (Grant No. ZR2020MC137), the Agricultural Improved Variety Project of Shandong Province (Grant No. 2020LZGC008), and the Key Science and Technology Project of Henan Province (Grant No. 222102110199).
Conflict of interest
The authors declare that the research was conducted in the absence of any commercial or financial relationships that could be construed as a potential conflict of interest.
Publisher's note
All claims expressed in this article are solely those of the authors and do not necessarily represent those of their affiliated organizations, or those of the publisher, the editors and the reviewers. Any product that may be evaluated in this article, or claim that may be made by its manufacturer, is not guaranteed or endorsed by the publisher.
Supplementary material
The Supplementary Material for this article can be found online at: https://www.frontiersin.org/articles/10.3389/fpls.2022.966641/full#supplementary-material
References
Achard, P., Cheng, H., De Grauwe, L., Decat, J., Schoutteten, H., Moritz, T., et al. (2006). Integration of plant responses to environmentally activated phytohormonal signals. Science 311, 91–94. doi: 10.1126/science.1118642
Agarwal, M., Hao, Y., Kapoor, A., Dong, C. H., Fujii, H., Zheng, X., et al. (2006a). A R2R3 type MYB transcription factor is involved in the cold regulation of CBF genes and in acquired freezing tolerance. J. Biol. Chem. 281, 37636–37645. doi: 10.1074/jbc.M605895200
Agarwal, P. K., Agarwal, P. A., Reddy, M. K., and Sopory, S. K. (2006b). Role of DREB transcription factors in abiotic and biotic stress tolerance in plants. Plant Cell Rep. 25, 1263–1274. doi: 10.1007/s00299-006-0204-8
Bogs, J., Jaffe, F. W., Takos, A. M., Walker, A. R., and Robinson, S. P. (2007). The grapevine transcription factor VvMYBPA1 regulates proanthocyanidin synthesis during fruit development. Plant Physiol. 143, 1347–1361. doi: 10.1104/pp.106.093203
Butt, H. I., Yang, Z., Gong, Q., Chen, E., Wang, X., Zhao, G., et al. (2017). GaMYB85, an R2R3 MYB gene, in transgenic Arabidopsis plays an important role in drought tolerance. BMC Plant Biol. 17:142. doi: 10.1186/s12870-017-1078-3
Chaves, M. M., Zarrouk, O., Francisco, R., Costa, J. M., Santos, T., Regalado, A. P., et al. (2010). Grapevine under deficit irrigation: hints from physiological and molecular data. Ann. Bot. 105, 661–676. doi: 10.1093/aob/mcq030
Chen, Y., Yang, X., Kun, H., Liu, M., Li, J., Gao, Z., et al. (2006). The MYB transcription factor superfamily of Arabidopsis: expression analysis and phylogenetic comparison with the rice MYB family. Plant Mol. Biol. 60, 107–124. doi: 10.1007/s11103-005-2910-y
Cheng, H., Song, S., Xiao, L., Soo, H., Cheng, Z., Xie, D., et al. (2009). Gibberellin acts throught jasmonate to control the expression of MYB21, MYB24, and MYB57 to promote stamen filament growth in Arabidopsis. PLoS Genet. 5:e1000440. doi: 10.1371/journal.pgen.1000440
Colebrook, E. H., Thomas, S. G., Phillips, A. P., and Hedden, P. (2014). The role of gibberellins signaling in plant responses to abiotic stress. J. Exp. Biol. 217, 67–75. doi: 10.1242/jeb.089938
Costa, J. M., Ortuño, M. F., and Chaves, M. M. (2007). Deficit irrigation as strategy to save water: physiology and potential application to horticulture. J. Integr. Plant Biol. 49, 1421–1434. doi: 10.1111/j.1672-9072.2007.00556.x
Costa, M. D., Reis, P. A., Valente, M. A., Irsigler, A. S., Carvalho, C. M., Loureiro, M. E., et al. (2008). A new branch of endoplasmic reticulum stress signaling and the osmotic signal converge on plant-specific asparagine-rich proteins to promote cell death. J. Biol. Chem. 283, 20209–20219. doi: 10.1074/jbc.M802654200
Cui, X., Xue, J., Zhang, B., Chen, C., Tang, Y., Zhang, P., et al. (2020). Physiological change and screening of differentially expressed genes of wild Chinese Vitis yeshanesis and American Vitis riparia in response to drought stress. Sci. Hortic. 266:109140. doi: 10.1016/j.scienta.2019.109140
Cutanda-Perez, M. C., Ageorges, A., Gomez, C., Vialet, S., Terrier, N., Romieu, C., et al. (2009). Ectopic expression of VlmybA1 in grapevine activates a narrow set of genes involved in anthocyanin synthesis and transport. Plant Mol. Biol. 69, 633–648. doi: 10.1007/s11103-008-9446-x
Czemmel, S., Stracke, R., Weisshaar, B., Cordon, N., Harris, N. N., Walker, A. R., et al. (2009). The grapevine R2R3-MYB transcription factor VvMYBF1 regulates flavonol synthesis in developing grape berries. Plant Physiol. 151, 1513–1530. doi: 10.1104/pp.109.142059
Delauney, A. J., and Verma, D. P. S. (1993). Proline biosynthesis and osmoregulation in plant. Plant J. 4, 251–223. doi: 10.1046/j.1365-313X.1993.04020215.x
Deluc, L., Barrieu, F., Marchive, C., Lauvergeat, V., Decendit, A., Richard, T., et al. (2006). Characterization of a grapevine R2R3-MYB transcription factor that regulates the phenylpropanoid pathway. Plant Physiol. 140, 499–511. doi: 10.1104/pp.105.067231
Deluc, L., Bogs, J., Walker, A., Ferrier, T., Decendit, A., Merillon, J. M., et al. (2008). The transcription factor VvMYB5b contributes to the regulation of anthocyanin and proanthocyanidin biosynthesis in developing grape berries. Plant Physiol. 147, 2041–2053. doi: 10.1104/pp.108.118919
Du, H., Feng, B. R., Yang, S. S., Huang, Y. B., and Tang, Y. X. (2012). The R2R3- MYB transcription factor gene family in maize. PLoS ONE 7:e37463. doi: 10.1371/journal.pone.0037463
Duan, D., Fischer, S., Merz, P., Bogs, J., Riemann, M., and Nick, P. (2016). An ancestral allele of grapevine transcription factor MYB14 promotes plant defence. J. Exp. Bot. 67, 1795–1804. doi: 10.1093/jxb/erv569
Dubos, C., Stracke, R., Grotewold, E., Weisshaar, B., Martin, C., and Lepiniec, L. (2010). MYB transcription factors in Arabidopsis. Trends Plant Sci. 15, 573–581. doi: 10.1016/j.tplants.2010.06.005
Gao, F., Zhou, J., Deng, R., Zhao, H., Li, C., Chen, H., et al. (2017). Overexpression of a tartary buckwheat R2R3-MYB transcription factor gene, FtMYB9, enhances tolerance to drought and salt stresses in transgenic Arabidopsis. J. Plant Physiol. 214, 81–90. doi: 10.1016/j.jplph.2017.04.007
Gao, J., Zhang, Z., Peng, R., Xiong, A., Xu, J., Zhu, B., et al. (2011). Forced expression of Mdmyb10, a myb transcription factor gene from apple, enhances tolerance to osmotic stress in transgenic Arabidopsis. Mol. Biol. Rep. 38, 205–211. doi: 10.1007/s11033-010-0096-0
Gechev, T. S., Breusegem, F. V., Stone, J. M., Denev, I., and Laloi, C. (2006). Reactive oxygen species as signals that modulate plant stress responses and programmed cell death. BioEssays 28, 1091–1101. doi: 10.1002/bies.20493
Geng, D., Chen, P., Shen, X., Zhang, Y., Li, X., Jiang, L., et al. (2018). MdMYB88 and MdMYB124 enhance drought tolerance by modulating root vessels and cell wall in apple. Plant Physiol. 178, 1296–1309. doi: 10.1104/pp.18.00502
Gilley, A., and Fletcher, R. A. (1998). Gibberellin antagonizes paclobutrazol-induced stress protection in wheat seedlings. J. Plant Physiol. 153, 200–207. doi: 10.1016/S0176-1617(98)80066-7
Gullo, M. L., Nardini, A., and Salleo, S. (1998). Changes in root hydraulic conductance (KR) of Olea oleaster seedlings following drought stress and irrigation. New Phytol. 140, 25–31. doi: 10.1046/j.1469-8137.1998.00258.x
Hoecker, N., Keller, B., Piepho, H. P., and Hochholdinger, F. (2006). Manifestation of heterosis during early maize (Zea mays L.) root development. Theor. Appl. Genet. 112, 421–429. doi: 10.1007/s00122-005-0139-4
Höll, J., Vannozzi, A., Czemmel, S., D'Onofrio, C., Walker, A. R., Rausch, T., et al. (2013). The R2R3-MYB transcription factors MYB14 and MYB15 regulate stilbene biosynthesis in Vitis vinifera. Plant Cell 25, 4135–4149. doi: 10.1105/tpc.113.117127
Hong, Z., Lakkineni, K., Zhang, Z., and Verma, D. P. S. (2000). Removal of feedback inhibition of Δ1-pyrroline-5-carboxylate synthetase results in creased proline accumulation and protection of plants from osmotic stress. Plant Physiol. 122, 1129–1136. doi: 10.1104/pp.122.4.1129
Horsch, R. B., Fry, J. E., Hoffmann, N. L., Eichholtz, D., Rogers, S. G., and Farley, R. T. (1985). A simple and general method for transferring genes into plants. Science 227, 1229–1231. doi: 10.1126/science.227.4691.1229
Huang, J., Yang, M., Liu, P., Yang, G., Wu, C., Zheng, C. (2009). GhDREB1 enhances abiotic stress tolerance, delays GA-mediated development and represses cytokinin signaling in transgenic Arabidopsis. Plant Cell Environment. 32, 1132–1145. doi: 10.1111/j.1365-3040.2009.01995.x
Huang, Y. F., Vialet, S., Guiraud, J. L., Torregrosa, L., Bertrand, Y., Cheynier, V., et al. (2014). A negative MYB regulator of proanthocyanidin accumulation, identified through expression quantitative locus mapping in the grape berry. New Phytol. 201, 795–809. doi: 10.1111/nph.12557
Kasuga, M., Miura, S., Shinozaki, K., and Yamaguchi-Shinozaki, K. (2004). A combination of the Arabidopsis DREB1A gene and stress-inducible rd29A promoter improved drought- and low-temperature stress tolerance in tobacco by gene transfer. Plant Cell Physiol. 45, 346–350. doi: 10.1093/pcp/pch037
Ke, Y., Abbas, F., Zhou, Y., Yu, R., and Fan, Y. (2021). Auxin-responsive R2R3-MYB transcription factors HcMYB1 and HcMYB2 activate volatile coronarium flowers. Front. Plant Sci. 12:710826. doi: 10.3389/fpls.2021.710826
Khresat, S. A., Rawajfih, Z., Buck, B., and Monger, H. C. (2004). Geomorphic features and soil formation of arid lands in northeastern Jordan. Arch. Agron. Soil Sci. 50, 607–615. doi: 10.1080/03650340400005572
Kong, X. P., Sun, L. P., Zhou, Y., Zhang, M. Y., Liu, Y., Pan, J., et al. (2011). ZmMKK4 regulates osmotic stress through reactive oxygen species scavenging in transgenic tobacco. Plant Cell Rep. 30, 2097–2104. doi: 10.1007/s00299-011-1116-9
Lau, S. E., Schwarzacher, T., Othman, R. Y., and Harikrishna, J. A. (2015). dsRNA silencing of an R2R3-MYB transcription factor affects flower cell shape in a Dendrobium hybrid. BMC Plant Biol. 15:194. doi: 10.1186/s12870-015-0577-3
Liu, G. (2010). Functional Study of Transcription Factor MYB305 in Tobacco Flower Development. Graduate Theses and Dissertations. Iowa State University.
Liu, H., Zhou, X., Dong, N., and Liu, X. (2011). Expression of a wheat MYB gene in transgenic tobacco enhances resistance to Ralstonia solanacearum, and to drought and salt stresses. Funct. Integr. Genom. 11, 431–443. doi: 10.1007/s10142-011-0228-1
Livak, K. J., and Schmittgen, T. D. (2001). Analysis of relative gene expression data using real-time quantitative PCR and the 2(-Delta Delta C(T)) Method. Methods 25, 402–408. doi: 10.1006/meth.2001.1262
Lu, H. C., Lam, S. H., Zhang, D., Hsiao, Y., Li, B., Niu, S., et al. (2021). R2R3-MYB genes coordinate conical cell development and cuticular wax biosynthesis in Phalaenopsis Aphrodite. Plant Physiol. 188, 318–331. doi: 10.1093/plphys/kiab422
Lv, Y., Yang, M., Hu, D., Yang, Z., Ma, S., Li, X., et al. (2017). The OsMYB30 transcription factor suppresses cold tolerance by interacting with a JAZ protein and suppressing beta-Amylase expression. Plant Physiol. 173, 1475–1491. doi: 10.1104/pp.16.01725
Magome, H., Yamaguchi, S., Hanada, A., Kamiya, Y., and Oda, K. (2008). The DDF1 transcriptional activator upregulates expression of a gibberellin-deactivating gene, GA2ox7, under high-salinity stress in Arabidopsis. Plant J. 56, 613–626. doi: 10.1111/j.1365-313X.2008.03627.x
Matus, J. T., Aquea, F., and Arce-Johnson, P. (2008). Analysis of the grape MYB R2R3 subfamily reveals expanded wine quality-related clades and conserved gene structure organization across Vitis and Arabidopsis genomes, BMC Plant Biol. 8:83. doi: 10.1186/1471-2229-8-83
Oh, J. E., Kwon, Y., Kim, J. H., Noh, H., Hong, S. W., and Lee, H. (2011). A dual role for MYB60 in stomatal regulation and root growth of Arabidopsis thaliana under drought stress. Plant Mol. Biol. 77, 91–103. doi: 10.1007/s11103-011-9796-7
Perez-Diaz, J. R., Perez-Diaz, J., Madrid-Espinoza, J., Gonzalez-Villanueva, E., Moreno, Y., and Ruiz-Lara, S. (2016). New member of the R2R3-MYB transcription factors family in grapevine suppresses the anthocyanin accumulation in the flowers of transgenic tobacco. Plant. Mol. Biol. 90, 63–76. doi: 10.1007/s11103-015-0394-y
Qin, Y., Wang, M., Tian, Y., He, W., Han, L., and Xia, G. (2012). Over-expression of TaMYB33 encoding a novel wheat MYB transcription factor increases salt and drought tolerance in Arabidopsis. Mol. Biol. Rep. 39, 7183–7192. doi: 10.1007/s11033-012-1550-y
Rademacher, W. (2000). Growth retardants: effects on gibberellin biosynthesis and other metabolic pathways. Annu. Rev. Plant Physiol. Plant Mol. Biol. 51, 501–531. doi: 10.1146/annurev.arplant.51.1.501
Seo, P. J., Xiang, F., Qiao, M., Park, J. Y., Lee, Y. N., Kim, S. G., et al. (2009). The MYB96 transcription factor mediates abscisic acid signaling during drought stress response in Arabidopsis. Plant Physiol. 151, 275–289. doi: 10.1104/pp.109.144220
Shahnejat-Bushehri, S., Tarkowska, D., Sakuraba, Y., and Balazadeh, S. (2016). Arabidopsis NAC transcription factor JUB1 regulates GA/BR metabolism and signalling. Nat. Plants 2:16013. doi: 10.1038/nplants.2016.13
Shan, D., Huang, J., Yang, Y., Guo, Y., Wu, C., Yang, G., et al. (2007). Cotton GhDREB1 increases plant tolerance to low temperature and is negatively regulated by gibberellic acid. New Phytol. 176, 70–81. doi: 10.1111/j.1469-8137.2007.02160.x
Shingote, P. R., Kawar, P. G., Pagariya, M. C., Kuhikar, R. S., Thorat, A. S., and Babu, K. H. (2015). SoMYB18, a sugarcane MYB transcription factor improves salt and dehydration tolerance in tobacco. Acta Physiol. Plant. 37:217. doi: 10.1007/s11738-015-1961-1
Stracke, R., Werber, M., and Weisshaar, B. (2001). The R2R3–MYB gene family in Arabidopsis thaliana. Curr. Opin. Plant Biol. 4, 447–456. doi: 10.1016/S1369-5266(00)00199-0
Su, L., Li, J., Liu, D., Zhai, Y., Zhang, H., Li, X., et al. (2014). A novel MYB transcription factor, GmMYBJ1, from soybean confers drought and cold tolerance in Arabidopsis thaliana. Gene 538, 46–55. doi: 10.1016/j.gene.2014.01.024
Tamagnone, L., Merida, A., Parr, A., Mackay, S., Culianez-Macia, F. A., Roberts, K., et al. (1998). The AmMYB308 and AmMYB330 transcription factors from Antirrhinum regulate phenylpropanoid and lignin biosynthesis in transgenic tobacco. Plant Cell 10, 135–154. doi: 10.1105/tpc.10.2.135
Tang, Y., Bao, X., Zhi, Y., Wu, Q., Guo, Y., Yin, X., et al. (2019). Overexpression of a MYB Family Gene, OsMYB6, increases drought and salinity stress tolerance in transgenic rice. Front. Plant Sci. 10:168. doi: 10.3389/fpls.2019.00168
Thirumalaikumar, V. P., Devkar, V., Mehterov, N., Ali, S., Ozgur, R., Turkan, I., et al. (2018). NAC transcription factor JUNGBRUNNEN1 enhances drought tolerance in tomato. Plant Biotechnol. J. 16, 354–366. doi: 10.1111/pbi.12776
Vettakkorumakankav, N. N., Falk, D., Saxena, P., and Fletcher, R. A. (1999). A crucial role for gibberellins in stress protection of plants. Plant Cell Physiol. 40, 542–548. doi: 10.1093/oxfordjournals.pcp.a029575
Wang, G., Zhang, M., Gong, J., Guo, Q., Feng, Y., and Wang, W. (2012). Increased gibberellins contents contribute to accelerated growth and development of transgenic tobacco overexpressing a wheat ubiquitin gene. Plant Cell Rep. 31, 2215–2227. doi: 10.1007/s00299-012-1331-z
Wang, R., Cao, Z., and Hao, Y. (2014). Overexpression of a R2R3 MYB gene MdSIMYB1 increases tolerance to multiple stresses in transgenic tobacco and apples. Physiol Plant. 150, 76–87. doi: 10.1111/ppl.12069
Wei, Q., Zhang, F., Sun, F., Luo, Q., Wang, R., Hu, R., et al. (2017). A wheat MYB transcriptional repressor TaMyb1D regulates phenylpropanoid metabolism and enhances tolerance to drought and oxidative stresses in transgenic tobacco plants. Plant Sci. 265, 112–123. doi: 10.1016/j.plantsci.2017.09.020
Wilkins, O., Nahal, H., Foong, J., Provart, N. J., and Campbell, M. M. (2009). Expansion and diversification of the Populus R2R3–MYB family of transcription factors. Plant Physiol. 149, 981–993. doi: 10.1104/pp.108.132795
Xiong, H., Li, J., Liu, P., Duan, J., Zhao, Y., Guo, X., et al. (2014). Overexpression of OsMYB48-1, a novel MYB-related transcription factor, enhances drought and salinity tolerance in rice. PloS ONE:e92913. doi: 10.1371/journal.pone.0092913
Yamaguchi, S. (2008). Gibberellin metabolism and its regulation. Annu. Rev. Plant Bio. 59, 225–251. doi: 10.1146/annurev.arplant.59.032607.092804
Yoo, S. D., Cho, Y. H., and Sheen, J. (2007). Arabidopsis mesophyll protoplasts: a versatile cell system for transient gene expression analysis. Nat. Protoc. 2, 1565–1572. doi: 10.1038/nprot.2007.199
Zeng, Y., Wen, J., Zhao, W., Wang, Q., and Huang, W. (2019). Rational improvement of rice yield and cold tolerance by editing the three genes OsPIN5b, GS3, and OsMYB30 With the CRISPR-Cas9 system. Front. Plant Sci. 10:1663. doi: 10.3389/fpls.2019.01663
Zhao, L., Liu, F., Xu, W., Di, C., Zhou, S., Xue, Y., et al. (2009). Increased expression of OsSPX1 enhances cold/subfreezing tolerance in tobacco and Arabidopsis thaliana. Plant Biotechnol. J. 7, 550–561. doi: 10.1111/j.1467-7652.2009.00423.x
Zhou, H., Wang-Lin, K., Wang, F., Espley, R. V., Ren, F., Zhao, J., et al. (2019). Activator-type R2R3-MYB genes induce a repressor-type R2R3-MYB gene to balance anthocyanin and proanthocyanidin accumulation. New Phytol. 221, 1919–1934. doi: 10.1111/nph.15486
Keywords: MYB genes, V. yanshanesis, plant development, drought tolerance, gibberellin
Citation: Zhu Z, Quan R, Chen G, Yu G, Li X, Han Z, Xu W, Li G, Shi J and Li B (2022) An R2R3-MYB transcription factor VyMYB24, isolated from wild grape Vitis yanshanesis J. X. Chen., regulates the plant development and confers the tolerance to drought. Front. Plant Sci. 13:966641. doi: 10.3389/fpls.2022.966641
Received: 11 June 2022; Accepted: 14 July 2022;
Published: 08 September 2022.
Edited by:
Ping Wang, Iowa State University, United StatesReviewed by:
Hui Xia, Sichuan Agricultural University, ChinaLibo Xing, Northwest A&F University, China
Jianfu Jiang, Zhengzhou Fruit Research Institute (CAAS), China
Copyright © 2022 Zhu, Quan, Chen, Yu, Li, Han, Xu, Li, Shi and Li. This is an open-access article distributed under the terms of the Creative Commons Attribution License (CC BY). The use, distribution or reproduction in other forums is permitted, provided the original author(s) and the copyright owner(s) are credited and that the original publication in this journal is cited, in accordance with accepted academic practice. No use, distribution or reproduction is permitted which does not comply with these terms.
*Correspondence: Bo Li, c2R0YWxpYm9AMTYzLmNvbQ==; Jiangli Shi, c2psaTMwQGhlbmF1LmVkdS5jbg==