- 1School of Biological and Food Engineering, Anyang Institute of Technology, Anyang, China
- 2Taihang Mountain Forest Pests Observation and Research Station of Henan Province, Linzhou, China
- 3International Joint Research Laboratory for Global Change Ecology, School of Life Sciences, Henan University, Kaifeng, China
Perennial cropping has been an alternative land use type due to its widely accepted role in increasing soil carbon sequestration. However, how soil organic carbon (SOC) changes and its underlying mechanisms under different cropping years are still elusive. A chronosequence (0-, 3-, 6-, 20-year) of perennial mugwort cropping was chosen to explore the SOC dynamics and the underlying mechanisms in agricultural soils of Northern China Plain. The results revealed that SOC first increased and then decreased along the 20-year chronosequence. The similar patterns were also found in soil properties (including soil ammonium nitrogen, total nitrogen and phosphorus) and two C-degrading hydrolytic enzyme activities (i.e., α-glucosidase and β-glucosidase). The path analysis demonstrated that soil ammonium nitrogen, total nitrogen, and plant biomass affected SOC primarily through the indirect impacts on soil pH, total phosphorus availability, and C-degrading hydrolytic enzyme activities. In addition, the contributions of soil properties are greater than those of biotic factors (plant biomass) to changes in SOC across the four mugwort cropping years. Nevertheless, the biotic factors may play more important roles in regulating SOC than abiotic factors in the long run. Moreover, SOC reached its maximum and was equaled to that under the conventional rotation when cropping mugwort for 7.44 and 14.88 years, respectively, which has critical implications for sustainable C sequestration of agricultural soils in Northern China Plain. Our observations suggest that short-term but not long-term perennial mugwort cropping is an alternative practice benefiting soil C sequestration and achieving the Carbon Neutrality goal in China.
Introduction
Soils store approximately 1550 Gt organic carbon (C), dominating the global soil C pool (2500 Gt), which is 3.3 times than the atmospheric C pool (Lal, 2004). Soil organic carbon (SOC) is vital for maintaining key functions of agricultural soils, such as water and nutrient retention, microbial activity, as well as the maintenance of a physicochemical balance (Trost et al., 2013; Miller et al., 2015; Wang et al., 2019; Wiesmeier et al., 2019). Increasing SOC in agricultural soils by optimized management is a priority for achieving food security and mitigating climate change (Lal, 2004; Ledo et al., 2020; Jia et al., 2021; Beillouin et al., 2022). Previous studies have revealed that agricultural managements/land use types have substantial impacts on SOC stocks (Ledo et al., 2019; Ledo et al., 2020; Zhu et al., 2021; Kan et al., 2022; Mondal and Chakraborty, 2022). For example, proper agricultural management (such as, perennial cropping) can elevate SOC sequestration in subtropical upland soils (Zhu et al., 2021). By contrast, land use conversion from natural to agricultural ecosystems (annual or perennial crops) could lead to SOC loss (Don et al., 2011; Deng et al., 2016; Ledo et al., 2020). In fact, intensified land use changes have been observed in recent decades (Song et al., 2018; Winkler et al., 2021), however, little efforts have been made to explore the effects and underlying mechanisms of the conversion from annual to perennial crops on SOC at spatial-temporal scale.
In addition to provide food, energy feedstock, fiber, and medicinal component, perennial cropping have been also demonstrated to be an effective land use type for increasing SOC (Glover et al., 2010; Ledo et al., 2020; Zhu et al., 2021). It has been shown that conversion from natural ecosystems to perennial crops could have diverse impacts on SOC stocks. For example, land use changes from natural ecosystem to perennial crops could lead to positive (Post and Kwon, 2000; Robertson et al., 2017), negative (Don et al., 2011; Crowther et al., 2016; Deng et al., 2016), or neutral effects on SOC (Fialho and Zinn, 2012; Qin et al., 2016). The divergent impacts of perennial cropping on SOC may be attributed to different perennial crop species and soil properties. In addition, perennial cropping could result in shifts of soil physicochemical properties, such as soil C and N nutrients (Zan et al., 2001; Glover et al., 2010). These changes may have the potential to affect the growth of crops and the sustainability of soil-crop systems (Bezemer et al., 2006; Hawkers et al., 2013). Nevertheless, with the increasing of perennial cropping years, soil C sequestration may decrease due to the reduced capacity of self-sustainability (Rowe et al., 2016) or continuous cropping obstacles (Chen et al., 2020; Wang et al., 2020b; Tan et al., 2021), which pose great challenges for sustainable soil-crop systems.
In general, perennial crops have greater biomass than annual crops, providing high organic matter input into soils with crop residues, root exudates, and fine root turnover (Glover et al., 2010; Anderson-Teixeira et al., 2013; Zhu et al., 2021). Plant roots could affect SOC dynamics by root litter and rhizodeposition (Dijkstra et al., 2021). For example, SOC may gain when plant roots increase SOC stabilization through forming soil aggregates, which are less accessible to decomposition (Schimdt et al., 2011; Slessarev et al., 2020). By contrast, SOC may also lose when plant roots stimulate SOC destabilization by aggregate destruction with tillage (Hartley et al., 2012; Cheng et al., 2014; Dijkstra et al., 2021). In addition, recent evidence reveals that preference for root nitrogen uptake as ammonium nitrogen over nitrate nitrogen could lead to rhizosphere acidification, and thus inhibit the rhizosphere priming effect, with consequently promote SOC sequestration (Wang et al., 2016; Wang and Tang, 2018). Moreover, SOC could also be regulated by soil extracellular enzyme activity (Chen et al., 2018; Chen et al., 2020; Chen and Sinsabaugh, 2021). Extracellular enzymes secreted by soil microbial communities degrade complex polymers into substrates assimilating and respiring CO2 during the decomposition of soil organic matter (Burns et al., 2013; Bödeker et al., 2014), during which the extracellular enzymes play a critical role during the decomposition of soil organic matter (Sinsabaugh et al., 2008; Cenini et al., 2016). Due to less soil disturbance associated with lower tillage, conversion from annual to perennial cropping may have the potential to increase soil microbial enzyme activities (Haney et al., 2010; Dou et al., 2013). In addition, soil chemical properties under less soil disturbance can provide steady nutrient supply for extracellular enzymes (Cattaneo et al., 2014; Zhang et al., 2021a). Thus, conversion from annual to perennial cropping may have substantial impacts on SOC through affecting enzyme activities. Nevertheless, the relative contributions of diverse soil and plant properties to SOC under the conversion from annual to perennial cropping remain unclear.
Considering that perennial crops dominate 30% of global croplands (Ledo et al., 2018) and usually have greater biomass than conventional crops, perennial cropping has the substantial potential to sequester more C into agricultural soils and thus mitigate climate change (Ledo et al., 2019; Ledo et al., 2020). As a perennial crop, mugwort (Artemisia argyi Lévl. et Van) has been widely cultivated in recent years due to its medicinal value in Northern China Plain (Jiang et al., 2019). In this study, a chronosequence (0, 3, 6, and 20 years) of perennial mugwort cropping was identified and chosen in Northern China Plain. Soil and climate conditions were similar among the four sampling sites, which provided us the opportunity for using the space-for-time substitution method to explore the scientific questions: 1) what are SOC dynamics along the chronosequences of mugwort cropping? 2) which factors drive the changes in SOC under different cropping years of mugwort?
Materials and methods
Site description and sample collection
This study was conducted in Tangyin County (35°45′-36°01′ N, 114°13′-114°42′ E), Anyang, Henan, where is one of the origins of mugwort, a genuine herb. This region has a warm temperate continental monsoon climate, with mean annual temperature of 13.4°C and precipitation of 582.0 mm, respectively. The soil is classified as cinnamon soil according to the Chinese soil classification system. The surface soil contained organic matter of 16.7 mg/g and total nitrogen content of 1.07 mg/g. With the development of the traditional Chinese medicine industry, parts of croplands were gradually converted from maize-wheat rotation to perennial mugwort cropping in Tangyin County in recent years, which provides the opportunity to assess the effects of the chronosequence of perennial mugwort cropping on soil organic carbon.
We identified and selected a chronosequence (continuous maize-wheat rotation (Control-Y0), perennial mugwort cropping for 3 (Y3), 6(Y6), and 20 years(Y20)) of perennial mugwort cropping in this study region. Given the distances among the four chronosequence periods were close (less than 5 km), we considered that soil types among the four chronosequence periods were not heterogeneous. In each chronosequence period, three blocks were randomly established. The aboveground plant parts of each plot in each block were harvested and put into tagged mash bags in late September of 2020. Then, three 20 cm depth (0-10 cm, 10-20 cm) cylindrical holes were excavated using a soil auger (5 cm in diameter) in the plots. Soil samples were passed through a 0.25 mm sieve and roots were collected then oven-dried at 105°C for 48 hours. Then, soil samples were divided into two subsamples. One subsample was stored at 4°C for measuring soil physicochemical properties. Another subsample was stored at -20°C for measuring soil extracellular enzymatic activities (α- (AG) and β-glucosidase (BG)).
Soil chemical properties and extracellular enzyme activities
Soil organic carbon and total N content were measured by an elemental analyzer with a dry combustion method (Vario MAX CN, Elementar Co., Germany). The total phosphorus (P) content was determined by H2SO4-HClO4 digestion and then P molybdenum blue colorimetric analysis (Murphy and Riley, 1962). The concentrations of ammonium (NH4+) and nitrate-nitrogen (NO3-) were extracted with 2M KCl solution and measured by a flow injection analyzer (SAN-System, Netherlands). Soil pH was measured with a combination glass-electrode [soil:water = 1:2.5 (W/V)] (Tian et al., 2022).
α-glucosidase (AG) and β-glucosidase (BG) are two key C-degrading hydrolytic enzymes. The activities of the two enzymes were determined by a colorimetric method described previously using fluorescently-labeled substrates (German et al., 2011; Allison et al., 2018). Fresh soil sample (0.2 g dry weight) was homogenized in 100 mL 25 mM maleate buffer (pH = 6.0). 125 µL of fluorometric substrate solution in 25 mM maleate buffer was mixed with 125 µL soil homogenate in each well of a 96-well microplate. Then, we incubated the 96-well microplate for 4 hours for analyzing the two hydrolytic enzymes (AG and BG). Fluorescent signals for the two enzymes were acquired at 365 nm excitation and 450 nm emission (BioTek Synergy H1 microplate reader, Winooski, VT, USA). The enzyme activity was expressed as nmol h-1 g-1 dry soil based on the method described by German et al. (2011).
Statistical analyses
All data are presented as mean values ± standard deviation (SD) for the three plots in each cropping type. Two-way ANOVAs were used to explore the effects of soil depth and cropping year on all the variables included in the study. In addition, changes in soil chemical properties among the four cropping types were assessed using one-way ANOVA with Duncan multiple comparisons. After that, regression analyses were conducted between SOC and cropping years to fit the SOC dynamic. The correlations among variables were explored by the Pearson correlation method. Significant differences were evaluated at the 0.05 probability level.
Random Forest (RF) models were used to partition independent influences of NH4+, NO3-, NH4+/NO3-, TN, TP, pH, AG, BG, root biomass (RB), and aboveground biomass (AGB) on SOC. Then, path analysis was employed to explore a mechanistic understanding of the direct and indirect effects of soil properties and enzyme activities, as well as RB and AGB on SOC along the chronosequence of perennial mugwort cropping. We first examined whether there were any collinear relationships between the factors by calculating the variance inflation factor (VIF). The factors were excluded if VIF was more than 5. Due to there were no significant relationships of SOC with NO3- and RB/AGB, or weak relationships between SOC and NH4+/NO3- in linear regression analyses, NO3-, RB/AGB, and NH4+/NO3- were not included in the path analysis. The path analysis was achieved based on the maximum likelihood method, Chi-square (χ2), degree of freedom (df), root-mean-squared error (RMSE), AIC value, and goodness of fit index (GFI). The path analysis was performed using AMOS 20.0 (AMOS Development Corporation, Chicago, IL, USA) and other statistical analyses were performed using SAS 8.0 (SAS Institute Inc., Cary, NC, USA) and R v.4.1.1 (R Development Core Team). Excel 2019 (Microsoft Crop., Redmond, WA, USA) and GraphPad Prism 9.0 software (GraphPad Inc., San Diego, California, USA) were used to plot the graphs.
Results
Soil properties
Soil ammonium nitrogen (NH4+), nitrate-nitrogen (NO3-), NH4+/NO3-, total N and phosphorus (P), pH, α-glucosidase (AG) and β-glucosidase (BG), as well as SOC showed significant variations among different mugwort cropping years across the two depths (Figures 1, 2, all P < 0.001, Table 1). NH4+, TN, TP, AG, BG, and SOC showed a trend of first increase and then decrease along the chronosequence (Y0-Y20, Figures 1A, D, E, G, H, 2A, B). TN and TP, BG, as well as SOC were lowest in 20-year mugwort cropping soils (Figures 1D, E, H). In addition, the highest of NH4+/NO3-, TN, AG, BG, and SOC were found in the 3-year mugwort cropping soils (Figures 1C, D, G, H). When simulating the SOC against with year (R2 = 0.77, P < 0.001, Figure 2B), we found that SOC reached its maximum (20.82 g/kg) in the year of 7.44 and was equal to that under the conventional rotation when cropping mugwort for 14.88 years (Figure S1).
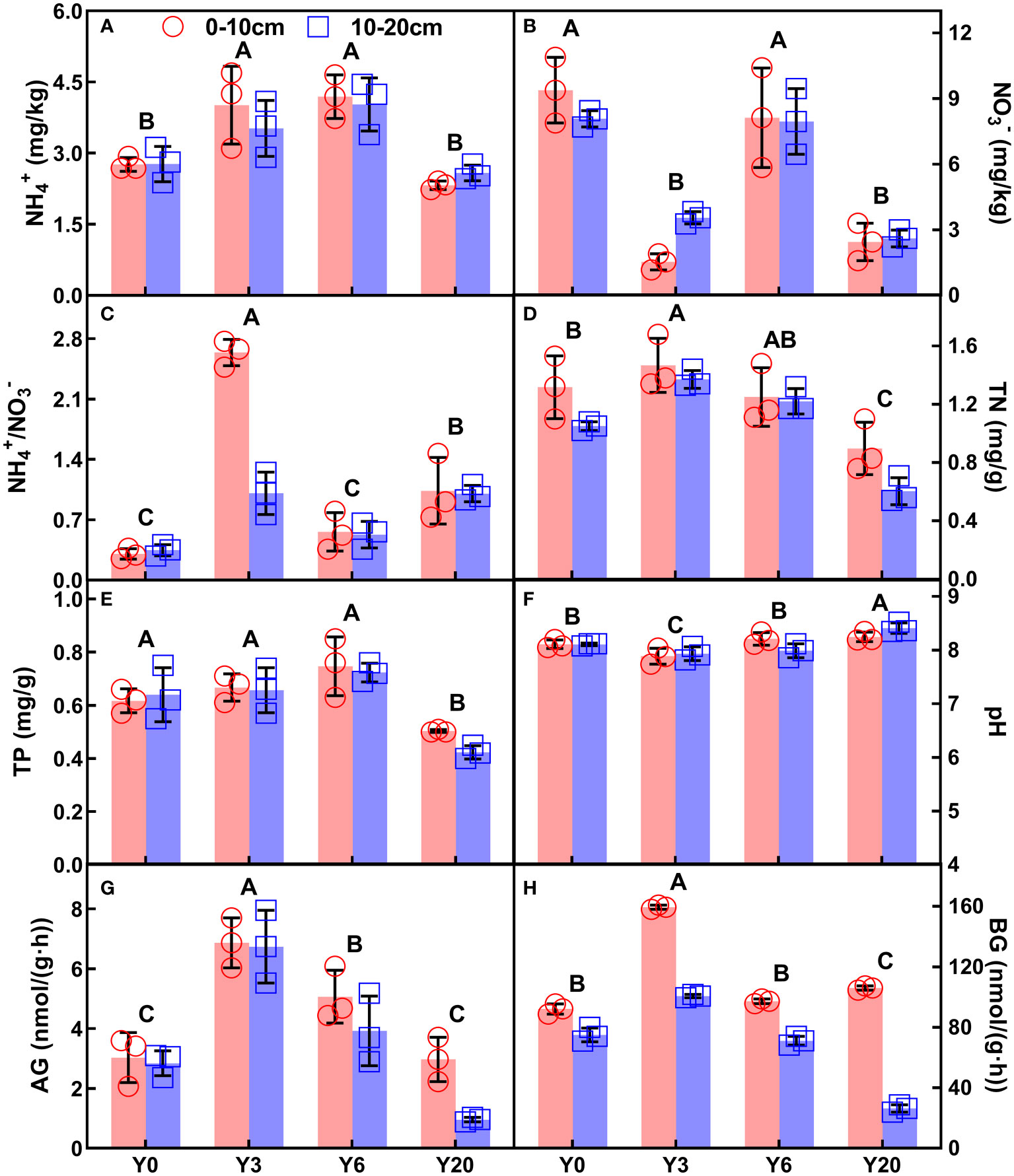
Figure 1 NH4+ (A), NO3- (B), the ration of NH4+ to NO3- (NH4+/NO3-, C), TN (D), TP (E), pH (F), AG (G), and BG (H) (Mean ±SD) of different soil depths (0-10 cm and 10-20 cm) along the chronosequence of mugwort cropping. Y0: continuous maize-wheat rotation; Y3: mugwort cropping for 3 years (since 2017); Y6: mugwort cropping for 6 years (since 2014); Y20: mugwort cropping for 20 years. Different letters indicate significant differences among the four cropping years at P < 0.05. See Table 1 for abbreviations.
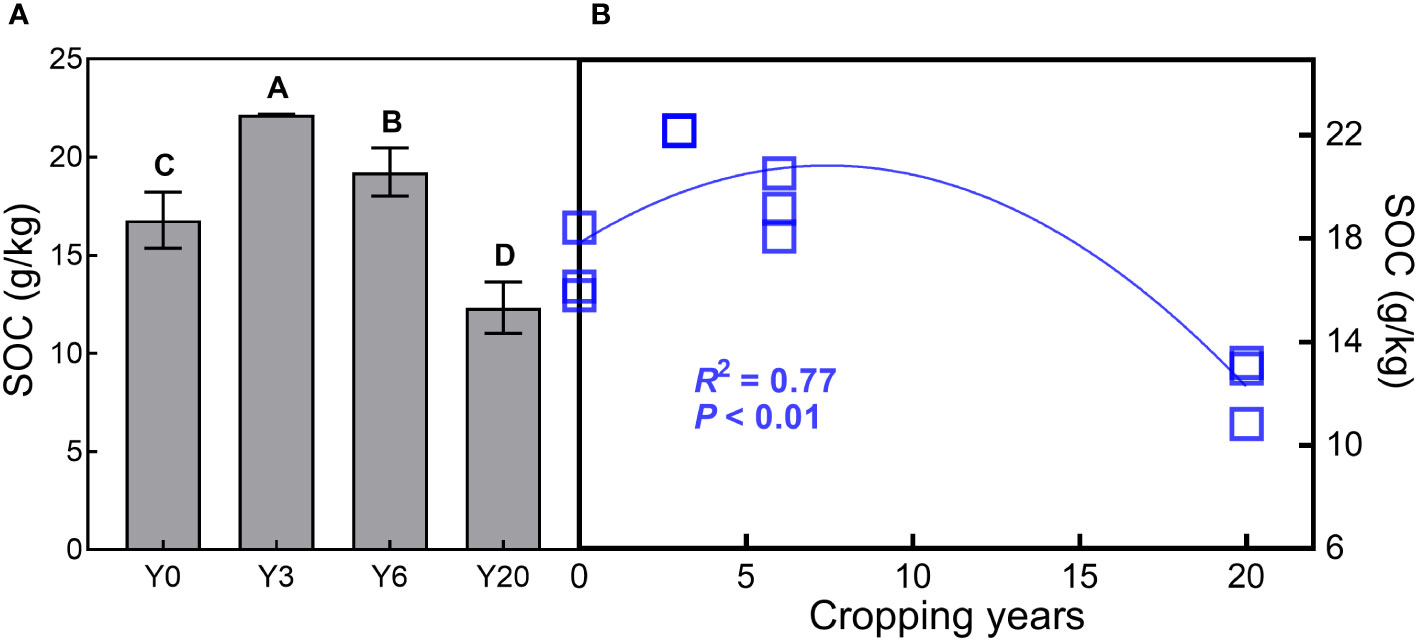
Figure 2 Soil organic carbon content across the two soil depths (SOC, g/kg, n=3, Mean ± SD, A), and the relationship of SOC with cropping years (B). Different letters indicate significant difference among the four cropping years at P < 0.05. See Figure 1 for abbreviations.
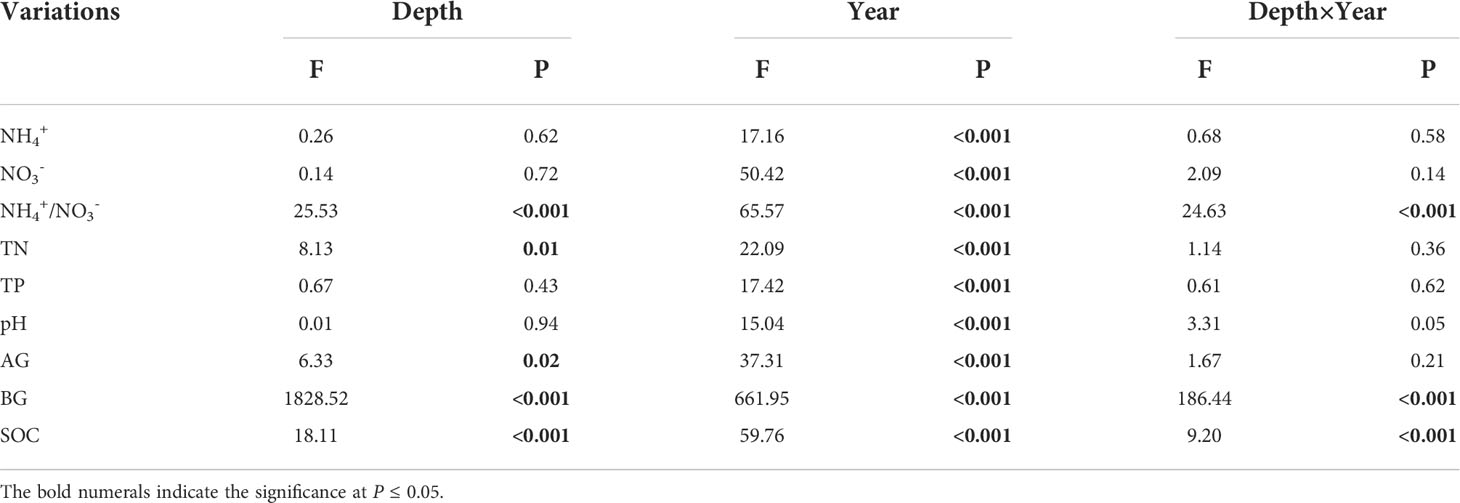
Table 1 Effects of soil depth (Depth) and cropping year (Year) on soil ammonium-nitrogen content (NH4+), nitrate-nitrogen content (NO3-), the ratio of NH4+ to NO3- (NH4+/NO3-), total nitrogen content (TN), total phosphorus content (TP), pH, two C-acquiring enzymes: α- (AG) and β-glucosidase (BG), and soil organic carbon content (SOC).
Plant properties
The lowest root biomass was observed in 0-year mugwort cropping (Table 2). There was no difference in root biomass among the 3-year, 6-year, or 20-year mugwort cropping. Aboveground biomass showed a decreasing trend along the chronosequence of mugwort cropping (Table 2). The ratio of RB to AGB (RB/AGB) in 0-year mugwort cropping soils was lower than that in the other three chronosequence cropping periods. No differences in RB/AGB were detected among the 3-year, 6-year, or 20-year mugwort cropping (Table 2).

Table 2 Mean values (Mean ± 1SE) of root biomass (RB), aboveground biomass (AGB), and the ratio of RB to AGB (RB/AGB) across the plots along the chronosequence of mugwort cropping.
Relationships among soil and plant properties
Soil organic carbon (SOC) enhanced with increasing NH4+, NH4+/NO3-, TN, TP, as well as AG and BG (Figures 3A, C–E, G, H), whereas decreased with the augment of pH (Figures 3F, G). In addition, the quadratic relationships of SOC with root and aboveground biomass were observed (Figures 4A, B). There was no relationship of SOC with RB/AGB (Figure 4C). The RF models showed that AG and BG accounted for 17.59% and 10.93% of SOC variance, respectively (Figure 5). Path diagrams showed interdependence relationships of TN with NH4+ (r = 0.52, P = 0.03) and pH (r = -0.53, P = 0.01), as well as of RB with AG (r = 0.44, P < 0.01) and AGB (r = -0.81, P < 0.01), respectively (Figure 6).
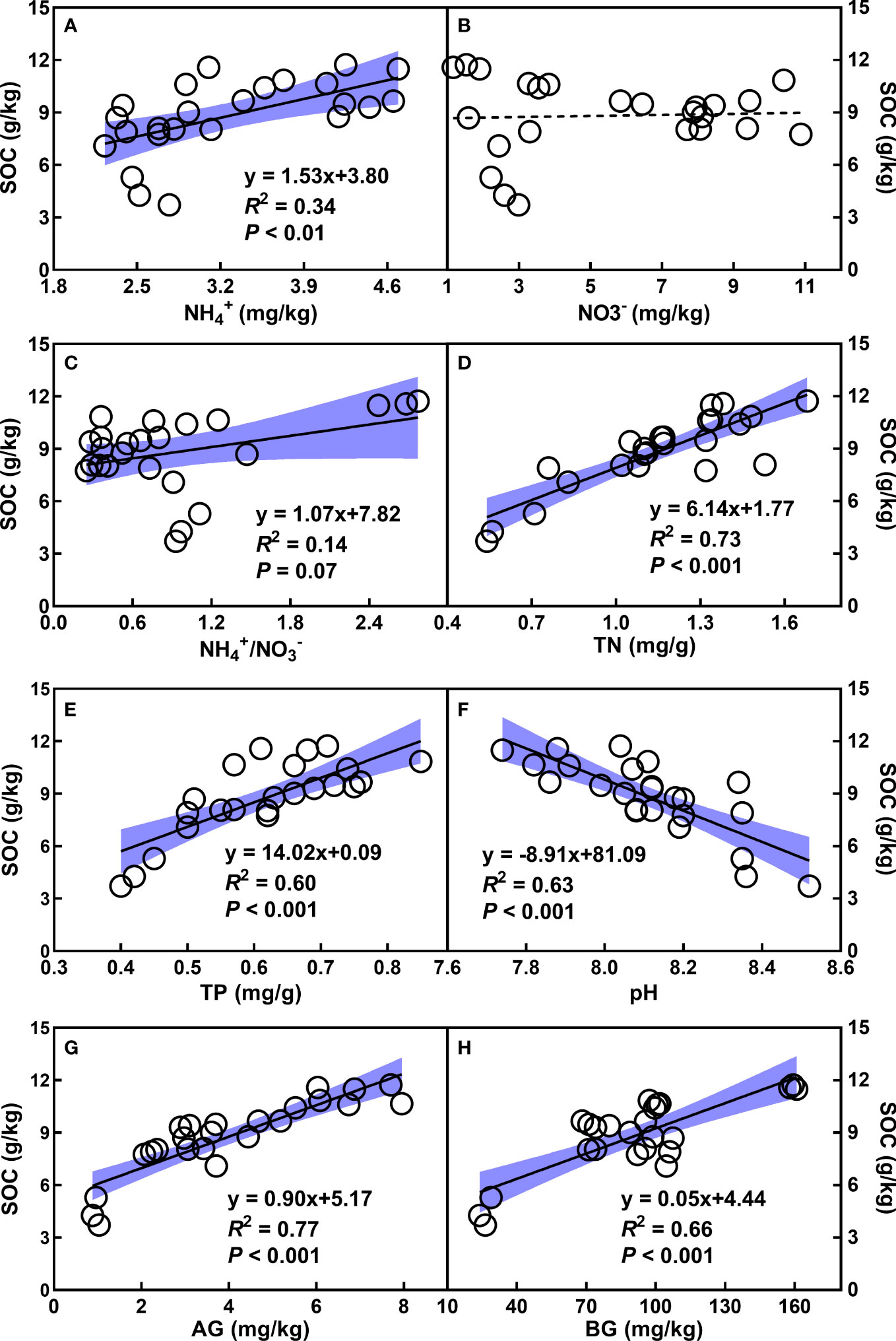
Figure 3 Relationships of SOC (g/kg) with NH4+ (A), NO3- (B), the ratio of NH4+ to NO3- (C), TN (D), TP (E), pH (F), AG (G), and BG (H), respectively. The shadow areas represent 95% confidence intervals. See Table 1 for abbreviations.
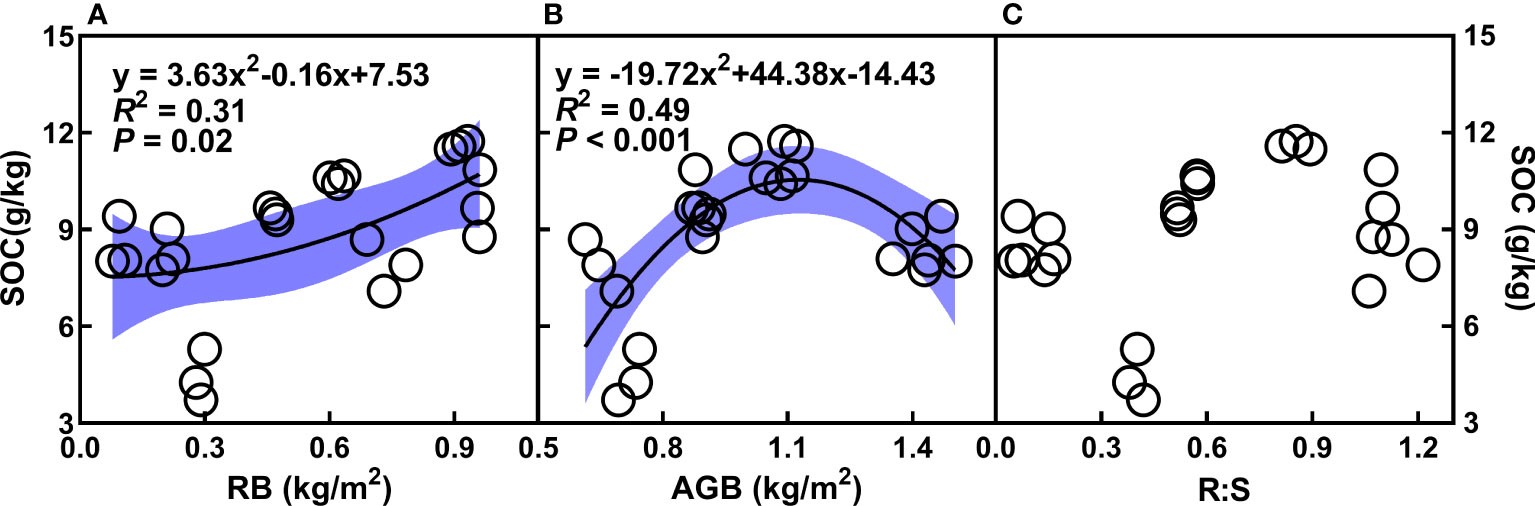
Figure 4 Relationships of SOC (g/kg) with root (RB, kg/m2, A) and aboveground biomass (AGB, g/m2, B), as well as the ratio of root biomass to aboveground biomass (RB/AGB, C) under the four cropping durations. The shadow areas represent 95% confidence intervals.
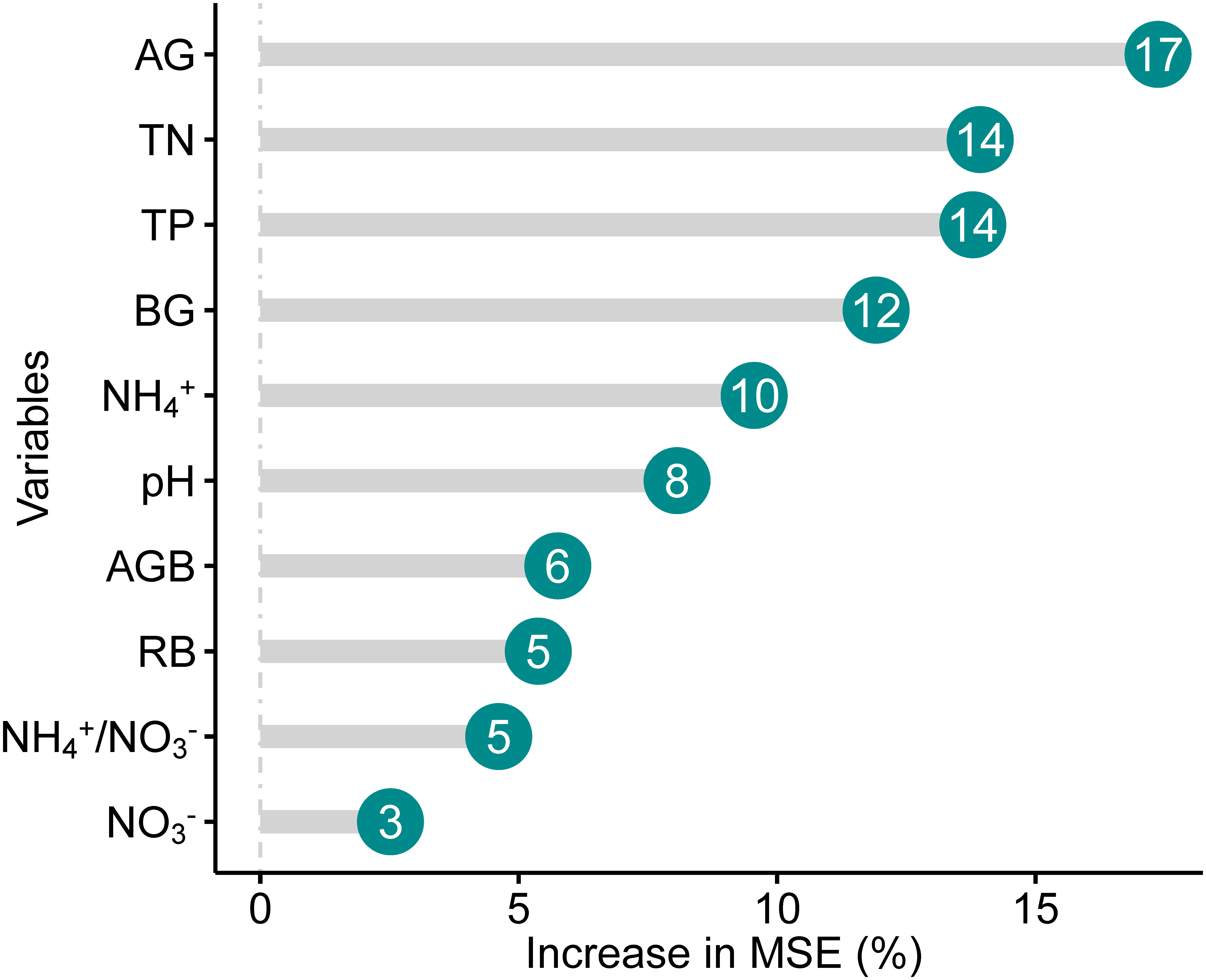
Figure 5 Relative contributions (Increase in MSE, %) of different factors to SOC. See Table 1 for abbreviations.
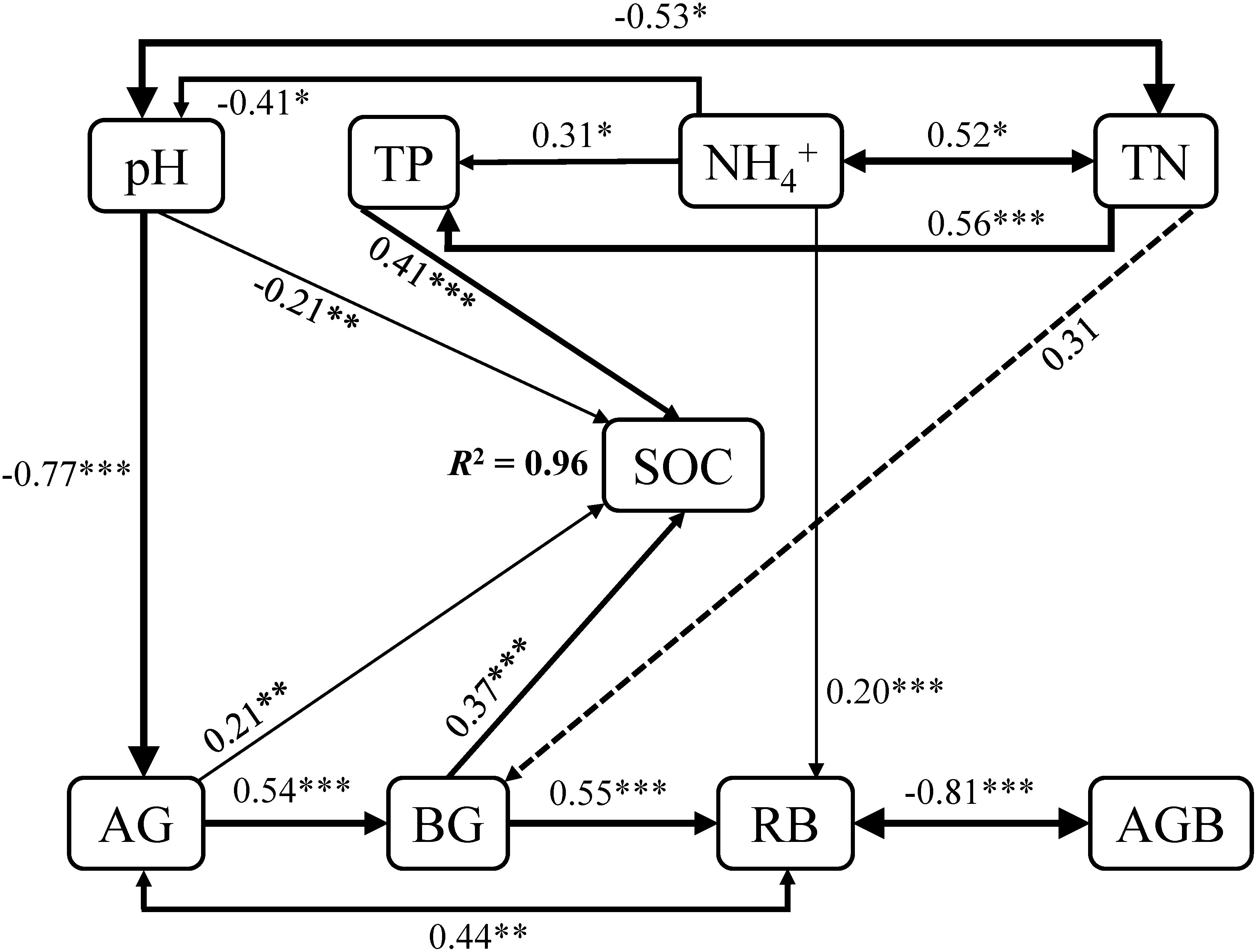
Figure 6 Path analysis showing the effects of pH, TP, NH4+, TN, AG, BG, as well as RB and AGB on SOC. The final path analysis adequately fitted the data χ2 = 28.55, df = 20, P = 0.10, GFI = 0.83, AIC = 78.55, RSME = 0.001. Solid and dashed arrows suggest significant and non-significant paths, respectively. The width of the solid arrows indicates the strength of the relationships. R2 shows the proportion of SOC explained by variables. Significant level: * P < 0.05; ** P < 0.01; *** P < 0.001.
In addition, neither AGB nor RB had effects on SOC directly, whereas affect SOC by altering AG and BG indirectly. Changes in RB had positive impacts on AG (r = 0.44, P < 0.01), with consequently alter the SOC (r = 0.21, P < 0.01). Moreover, changes in NH4+ have impacts on SOC mainly through affecting pH (r = -0.41, P = 0.03; r = -0.21, P < 0.01) and TP (r = 0.31, P = 0.05; r = 0.41, P < 0.001). RB increased with increments of NH4+, indicating that NH4+ could also mediate SOC through altering RB (r = 0.20, P < 0.001), and thus AG (Figure 6). Furthermore, the interdependence relationship of TN with pH, as well as the positive dependence of TP on TN, suggesting that changes in TN had noticeable influences on SOC by altering TP (r = 0.56, P < 0.001; r = 0.41, P < 0.001) and pH (r = -0.53, P = 0.01; r = -0.21, P < 0.01). Given that the positive relationship of BG with AG (r = 0.54, P < 0.001), variables that affect AG could thus alter BG, and consequently change SOC (Figure 6).
Discussion
Changes in SOC along the chronosequence of perennial mugwort cropping
In this study, SOC under 3- and 6-year cropping of perennial mugwort is greater than that under conventional rotation, which indicates that short-term perennial mugwort could be an effective practice for improving soil C sequestration. This finding is consistent with those reported by previous studies, which have revealed that perennial cropping can have fundamental positive impacts on soil C sequestration (Ledo et al., 2019; Ledo et al., 2020; Zhu et al., 2021). However, mugwort cropping begins to decrease SOC after 7.44 years (especially after 14.88 years: SOC is lower than that under the conventional rotation), suggesting that long-term perennial mugwort cropping could not benefit for soil C sequestration, which may lead to positive feedbacks to climate change (Lal, 2004; Ledo et al., 2020; Jia et al., 2021).
When analyzed by different soil depths, SOC under mugwort cropping at the 20-year chronosequence was equivalent to that under maize cropping (Y0) at the depth of 10 cm (Figure S1). Therefore, the observations of reduced SOC across the two depths can be largely attributed to decreased SOC at the depth of 20 cm (Figure S1), indicating that subsoil SOC may decrease more quickly than topsoil SOC under mugwort cropping at the long-term scale. The findings that decreased patterns of SOC with soil depth observed in this study are consistent with those reported in previous studies (Rentschler et al., 2019; Zhu et al., 2021). The above observations contribute to the decreased SOC across the two depths over the chronosequence of mugwort cropping. The trends reported in this study make it reasonably clear that short-term mugwort cropping is beneficial to SOC sequestration, whereas long-term (more than 14.88 years) mugwort cropping has the reverse impacts on SOC sequestration, indicating that mugwort should be rotated with other crops to prevent the decrease of SOC compared with that under conventional crops. However, recent evidence reveals that SOC storage of deep soil may show change compared with that of surface soil under perennial cropping (Ledo et al., 2020). For example, conversion from annual to perennial crops can result in an average 20% enhancement in SOC at 0-30 cm and 10% increase across the 0-100 cm soil profile (Ledo et al., 2020). Therefore, accurate evaluation on the dynamics of SOC under perennial cropping needs to consider deeper profiles (such as, up to 60 cm or 100 cm depth) in future studies (Ledo et al., 2020; Kan et al., 2022; Chen et al., 2022). Nevertheless, lack of cropping chronosequence between 6 and 20 years, during which there may be uncertainties in SOC shifts. Therefore, more cropping years are needed to be considered in future field studies.
Changes in aboveground and root biomass and their impacts on SOC
Aboveground and root biomass play important roles in regulating SOC through providing C input (Anderson-Teixeira et al., 2013; Waring et al., 2014; Deng et al., 2019; Zhou et al., 2020). In this study, the aboveground biomass of mugwort was lower than that of maize, whereas root biomass of mugwort was greater than that of maize (Table 2). Under the short-term mugwort cropping, higher root biomass could stimulate root turnover and C exudation, and thus increase SOC (Beare et al., 2014). Under the long-term (more than 14 years) mugwort cropping, although root biomass of mugwort is still greater compared with that of maize, the turnover of mugwort root may be lower than that of maize root due to continuous cropping obstacle, which is demonstrated in other crops (Chen et al., 2020; Wang et al., 2020b; Tan et al., 2021), leading to reduced root exudation (Leifeld et al., 2015; McNally et al., 2017), and consequently result in lower SOC. In addition, decreased aboveground biomass under perennial mugwort cropping, leading to a reduction of crop C input into soil, which may further depress SOC at a long-term scale (Cai et al., 2022; Krauss et al., 2022).
Changes in soil properties and their impacts on SOC
The finding of short-term (Y3 and Y6) consecutive mugwort cropping increased NH4+, total N and P of surface soil (Figures 1A, D, E, 2A) are consistent with that reported in a previous study (Zhu et al., 2021). In the current study, although short-term mugwort cropping decreased aboveground biomass, increased root biomass combining the enhanced soil NH4+, total N and P nutrient could stimulate the turnover rate of roots (Sun et al., 2017), which is beneficial to sequestrate C into the soil. The observations of positive and quadratic relationships of SOC with root biomass and aboveground biomass, respectively (Figures 4A, B) support the above discussion. In addition, less tillage associated with conversion from annual crops (Y0) to perennial mugwort could decrease the fragmentation of soil aggregates and thus decomposition of soil organic matters (Liu et al., 2021b; Kan et al., 2022; Lessmann et al., 2022; Mondal and Chakraborty, 2022), which can increase the storage of SOC. Moreover, it has been documented that enzyme activity plays a vital role in regulating organic carbon in agricultural soils and shows a positive relationship with SOC (Cattaneo et al., 2014; Zhang et al., 2021a). The observations of positive dependences of SOC with AG and BG in this study are consistent with those found in previous studies (Cattaneo et al., 2014; Zhang et al., 2021a). In this study, increased root biomass could stimulate root turnover and associated substrate accumulation (e.g., dead root, root exudation, etc.). The extent of increased substrate accumulation may exceed that decomposed by enzymes, and thus resulting in the positive relationships between SOC and enzyme activities, with consequently increase SOC. Furthermore, decreased pH may inhibit soil respiration and thus the C mineralization process (Kemmitt et al., 2006; Lazicki et al., 2022), with consequently contribute to the increased SOC under the short-term mugwort cropping. Less soil disturbance under consecutive mugwort cropping with lower tillage may also protect the aggregates from fragmentation and thus the loss of SOC (Zhu et al., 2014; Lal, 2015; Liu et al., 2021b; Kan et al., 2022; Mondal and Chakraborty, 2022). As a consequence, consecutive perennial mugwort cropping could increase SOC sequestration at the short-term scale in the temperate regions of Northern China Plain.
By contrast, at the long-term scale (20-year mugwort cropping), changes in soil properties (soil NH4+, total N and P, as well as AG and BG) were reversed compared with those at the short-term scale (Figure 1). The decreased above soil properties (soil NH4+, total N and P) may be ascribed to two reasons. First, long-term monoculture could lead to intensified intraspecific competition and continuous cropping obstacles (Chen et al., 2020; Wang et al., 2020b; Liu et al., 2021a; Tan et al., 2021), and thus result in lower nutrient use efficiency, which depress the aboveground biomass (Table 2), further decrease the transfer of C from plant to the soil (Wang et al., 2020b), with consequently inhibit the enzyme activities (i.e., AG and BG, Figures 1G, H) and soil nutrient availability. Second, decreased soil total N and P associated with reduced nutrient use efficiency may result from the leaching effects, and exacerbate the positive feedbacks of aboveground biomass reduction. Decreased soil total N could lead to lower soil C/N, which further reduce the stability and resistance to the decomposition of soil organic matters, resulting in decreased SOC (McNally et al., 2017; Wang and Tang, 2018; Ni et al., 2022). Regression and path analyses showed that there were indirect impacts of aboveground biomass on SOC through affecting root biomass and thus AG and BG activities (Figures 4, 6). In addition, given the important role of pH in regulating C mineralization (Kemmitt et al., 2006; Zhang et al., 2021b), increased pH associated with decreased soil total N could stimulate soil respiration (C mineralization process) and consequently reduce SOC under long-term mugwort cropping (Figures 1, 3, 6), which is consistent with that found in a recent study (Lu et al., 2022). Irrespective of the temporal impacts of mugwort cropping on SOC, the contributions of C-degrading hydrolytic enzyme activities (i.e., AG and BG) and soil nutrients (e.g., TN and TP, Figure 5) were greater than those of biotic factors (aboveground and root biomass) on SOC along the chronosequence cropping. These observations indicate that soil properties play more important roles than biotic factors in regulating SOC at the spatial scale in agricultural soils, which is also supported by the path analysis (Figure 6). It has been demonstrated that long-term conservation tillage (such as, no-tillage) could increase the abundances of plant pathogens, resulting in root rot and thus reduction of plant biomass (Wang et al., 2020a), with consequently further decrease the substrate supply for accumulation of SOC in the long run. As a consequence, the importance of biotic factors may exceed than that of abiotic factors under the long-term mugwort cropping. Overall, our study has critical implications for sustainable mugwort cropping and mitigation of climate change. The findings of first increased and then decreased SOC along the chronosequence of mugwort cropping suggest that short-term (less than 7.44 years) mugwort cropping could be beneficial for maximized C sequestration, whereas long-term mugwort cropping has a negative impact on SOC. These observations indicate that long-term mugwort cropping (more than 14 years) should be avoided to access the goals of peak C emission and neutrality (Wang et al., 2021).
Conclusions
Using a data set of SOC along a chronosequence of perennial mugwort cropping in Northern China Plain, we demonstrated that SOC increased under the short-term cropping and reached the maximum in the year of 7.44, whereas decreased under the long-term cropping (more than 14.88 years). Soil properties and C-degrading hydrolytic enzyme activities contributed more than biotic factors (aboveground and root biomass) in regulating SOC across the chronosequence. Nevertheless, the role of biotic factors may exceed that of abiotic factors in mediating SOC under long-term mugwort cropping. Given its economic benefit and critical role in regulating SOC sequestration, short-term (less than 7 years) perennial mugwort cropping is an alternative practice to maximumly sequestrate more C into the soil in Northern China Plain. Our findings that contrast impacts of short-term and long-term perennial mugwort cropping on SOC suggest that long-term perennial mugwort cropping may not beneficial for maintaining soil C storage and achieving the goals of C neutrality of China.
Data availability statement
The original contributions presented in the study are included in the article/Supplementary Material. Further inquiries can be directed to the corresponding authors.
Author contributions
ZZ originally formulated the idea, ZZ, FT, and XZ developed methodology, ZZ and FT conducted fieldwork, ZZ and FT generated data analyses, ZZ, KZ, and SH wrote the manuscript. All authors contributed to the article and approved the submitted version.
Funding
This work was financially supported by the Postdoctoral Innovation and Practice Base of Anyang Institute of Technology (BSJ2020021, BHJ2021007). This work was financially supported by the Postdoctoral Innovation and Practice Base of Anyang Institute of Technology (BSJ2020021, BHJ2021007) and the National Natural Science Foundation of China (32201366).
Acknowledgments
The authors thank Qingfeng Li and Mingdong Chen for their help in the field sampling, Prof. Guoyong Li for his suggestions for improving this manuscript.
Conflict of interest
The authors declare that the research was conducted in the absence of any commercial or financial relationships that could be construed as a potential conflict of interest.
Publisher’s note
All claims expressed in this article are solely those of the authors and do not necessarily represent those of their affiliated organizations, or those of the publisher, the editors and the reviewers. Any product that may be evaluated in this article, or claim that may be made by its manufacturer, is not guaranteed or endorsed by the publisher.
Supplementary material
The Supplementary Material for this article can be found online at: https://www.frontiersin.org/articles/10.3389/fpls.2022.975169/full#supplementary-material
References
Allison, S. D., Romero-Olivares, A. L., Lu, Y., Taylor, J. W., Treseder, K. K. (2018). Temperature sensitivities of extracellular enzyme Vmax and Km across thermal environments. Global Change Biol. 24, 2884–2897. doi: 10.1111/gcb.14045
Anderson-Teixeira, K. J., Masters, M. D., Black, C. K., Zeri, M., Hussain, M. Z., Bernacchi, C. J., et al. (2013). Altered belowground carbon cycling following land-use change to perennial bioenergy crops. Ecosystems 16, 508–520. doi: 10.1007/s10021-012-9628-x
Beare, M., McNeill, S., Curtin, D., Parfitt, R., Jones, H., Dodd, M., et al. (2014). Estimating the organic carbon stabilisation capacity and saturation deficit of soils: A new Zealand case study. Biogeochemistry 120, 71–87. doi: 10.1007/s10533-014-9982-1
Beillouin, D., Cardinael, R., Berre, D., Boyer, A., Corbeels, M., Fallot, A., et al. (2022). A global overview of studies about land management, land-use change, and climate change effects on soil organic carbon. Global Change Biol. 28, 1690–1702. doi: 10.1111/gcb.15998
Bezemer, T. M., Lawson, C. S., Hedlund, K., Edwards, A. R., Brook, A. J., Igual, J. M., et al. (2006). Plant species and functional group effects on abiotic and microbial soil properties and plant-soil feedback responses in two grasslands. J. Ecol. 94, 893–904. doi: 10.1111/j.1365-2745.2006.01158.x
Bödeker, I. T. M., Karina, E. C., Wietse de, B., Francis, M., Olson, Å., Lindahl, B. D. (2014). Ectomycorrhizal Cortinarius species participate in enzymatic oxidation of humus in northern forest ecosystems. New Phytol. 203, 245–256. doi: 10.1111/nph.12791
Burns, R. G., DeForest, J. L., Marxsen, J., Sinsabaugh, R. L., Stromberger, M. E., Wallenstein, M. D., et al. (2013). Soil enzymes in a changing environment: Current knowledge and future directions. Soil Biol. Biochem. 58, 216–234. doi: 10.1016/j.soilbio.2012.11.009
Cai, A. D., Han, T. F., Ren, T. J., Sanderman, J., Rui, Y. C., Wang, B., et al. (2022). Declines in soil carbon storage under no tillage can be alleviated in the long run. Geoderma 425, 116028. doi: 10.1016/j.geoderma.2022.116028
Cattaneo, F., Di Gennaro, P., Barbanti, L., Giovannini, C., Labra, M., Moreno, B., et al. (2014). Perennial energy cropping systems affect soil enzyme activities and bacterial community structure in a south European agricultural area. Appl. Soil Ecol. 84, 213–222. doi: 10.1016/j.apsoil.2014.08.003
Cenini, V. L., Fornara, D. A., McMullan, G., Ternan, N., Carolan, R., Crawley, M. J., et al. (2016). Linkages between extracellular enzyme activities and the carbon and nitrogen content of grassland soils. Soil Biol. Biochem. 96, 198–206. doi: 10.1016/j.soilbio.2016.02.015
Chen, J., Elsgaard, L., van Groenigen, K. J., Olesen, J. E., Liang, Z., Jiang, Y., et al. (2020). Soil carbon loss with warming: New evidence from carbon-degrading enzymes. Global Change Biol. 26, 1944–1952. doi: 10.1111/gcb.14986
Cheng, W., Parton, W. J., Gonzalez-Meler, M. A., Phillips, R., Asao, S., McNickle, G. G., et al. (2014). Synthesis and modeling perspectives of rhizosphere priming. New Phytol. 201, 31–44. doi: 10.1111/nph.12440
Chen, J., Lærke, P. E., Jørgensen, U. (2022). Land conversion from annual to perennial crops: A win-win strategy for biomass yield and soil organic carbon and total nitrogen sequestration. Agricult. Ecosystem Environ. 330, 107907. doi: 10.1016/j.agee.2022.107907
Chen, J., Luo, Y. Q., van Groenigen, K. J., Hungate, B. A., Cao, J. J., Zhou, X. H., et al. (2018). A keystone microbial enzyme for nitrogen control of soil carbon storage. Sci. Adv. 4, eaaq1689. doi: 10.1126/sciadv.aaq1689
Chen, J., Sinsabaugh, R. L. (2021). Linking microbial functional gene abundance and soil extracellular enzyme activity: Implications for soil carbon dynamics. Global Change Biol. 27, 1322–1325. doi: 10.1111/gcb.15506
Chen, P., Wang, Y. Z., Liu, Q. Z., Zhang, Y. T., Li, X. Y., Li, H. Q., et al. (2020). Phase changes of continuous cropping obstacles in strawberry (Fragaria × ananassa dush.) production. Appl. Soil Ecol. 155, 103626. doi: 10.1016/j.apsoil.2020.103626
Crowther, T. W., Todd-Brown, K. E. O., Rowe, C. W., Wieder, W. R., Carey, J. C., Machmuller, M. B., et al. (2016). Quantifying global soil carbon losses in response to warming. Nature 540, 104–108. doi: 10.1038/nature20150
Deng, L., Peng, C., Huang, C., Wang, K., Liu, Q., Liu, Y., et al. (2019). Drivers of soil microbial metabolic limitation changes along a vegetation restoration gradient on the loess plateau, China. Geoderma 353, 188–200. doi: 10.1016/j.geoderma.2019.06.037
Deng, L., Zhu, G., Tang, Z., Shangguan, Z. (2016). Global patterns of the effects of land-use changes on soil carbon stocks. Global Ecol. Conserv. 5, 127–138. doi: 10.1016/j.gecco.2015.12.004
Dijkstra, F. A., Zhu, B., Cheng, W. X. (2021). Root effects on soil organic carbon: A double-edged sword. New Phytol. 230, 60–65. doi: 10.1111/nph.17082
Don, A., Schumacher, J., Freibauer, A. (2011). Impact of tropical land-use change on soil organic carbon stocks–a meta-analysis. Global Change Biol. 17, 1658–1670. doi: 10.1111/j.1365-2486.2010.02336.x
Dou, F. G., Hons, F. M., Ocumpaugh, W. R., Read, J. C., Hussey, M. A., Muir, J. P. (2013). Soil organic carbon pools under switchgrass grown as a bioenergy crop compared to other conventional crops. Pedosphere 23, 409–416. doi: 10.1016/S1002-0160(13)60033-8
Fialho, R., Zinn, Y. (2012). Changes in soil organic carbon under eucalyptus plantations in Brazil: A comparative analysis. Land Degradation Dev. 25, 428–437. doi: 10.1002/ldr.2158
German, D. P., Weintraub, M. N., Grandy, A. S., Lauber, C. L., Rinkes, Z. L., Allison, S. D. (2011). Optimization of hydrolytic and oxidative enzyme methods for ecosystem studies. Soil Biol. Biochem. 43, 1387–1397. doi: 10.1016/j.soilbio.2011.03.017
Glover, J. D., Reganold, J. P., Bell, L. W., Borevitz, J., Brummer, E. C., Buckler, E. S., et al. (2010). Increased food and ecosystem security via perennial grains. Science 328, 1638–1639. doi: 10.1126/science.1188761
Haney, R. L., Kiniry, J. R., Johnson, M. V. (2010). Soil microbial activity under different grass species: Underground impacts of biofuel cropping. Agric. Ecosyst. Environ. 139, 754–758. doi: 10.1016/j.agee.2010.10.003
Hartley, I. P., Garnett, M. H., Sommerkorn, M., Hopkins, D. W., Fletcher, B. J., Sloan, V. L., et al. (2012). A potential loss of carbon associated with greater plant growth in the European Arctic. Nat. Climate Change 2, 875–879. doi: 10.1038/nclimate1575
Hawkers, C. V., Kivlin, S. N., Du, J., Eviner, V. T. (2013). The temporal development and additivity of plant-soil feedback in perennial grasses. Plant Soil 369, 141–150. doi: 10.1007/s11104-012-1557-0
Jiang, Z. H., Gao, X., Zhang, K. P., Sekaran, G., Cao, B. R., Zhao, Q. Q., et al. (2019). The essential oils and eucalyptol from Artemisia vulgaris l. prevent acetaminophen-induced liver injury by activating Nrf2-Keap1 and enhancing APAP clearance through non-toxic metabolic pathway. Front. Pharmacol. 10. doi: 10.3389/fphar.2019.00782
Jia, H. X., Wang, X., Xiao, J. J., Jang, S. L., Zhao, Y. F., Ye, W. L. (2021). Simulated soil organic carbon stocks in northern china’s cropland under different climate change scenarios. Soil Tillage Res. 213, 105088. doi: 10.1016/j.still.2021.105088
Kan, Z. R., Liu, W. X., Liu, W. S., Lal, R., Dang, Y. P., Zhao, X., et al. (2022). Mechanisms of soil organic carbon stability and its response to no-till: A global synthesis and perspective. Global Change Biol. 28, 693–710. doi: 10.1111/gcb.15968
Kemmitt, S. J., Wright, D., Goulding, K. W. T., Jones, D. L. (2006). pH regulation of carbon and nitrogen dynamics in two agricultural soils. Soil Biol. Biochem. 38, 898–911. doi: 10.1016/j.soilbio.2005.08.006
Krauss, M., Wiesmeier, M., Don, A., Cuperus, F., Gattinger, A., Gruber, S., et al. (2022). Reduced tillage in organic farming affects soil organic carbon stocks in temperate Europe. Soil Tillage Res. 216, 105262. doi: 10.1016/j.still.2021.105262
Lal, R. (2004). Soil carbon sequestration impacts on global climate change and food security. Science 304, 1623–1627. doi: 10.1126/science.1097396
Lal, P. (2015). Sequestering carbon and increasing productivity by conservation agriculture. J. Soil Water Conserv. 70, 55–62. doi: 10.2489/jswc.70.3.55A
Lazicki, P., Rodrigues, J. L. M., Geisseler, D. (2022). Acid stress and compost addition decouple carbon and nitrogen cycling in an agricultural soil: An incubation study. Appl. Soil Ecol. 169, 104219. doi: 10.1016/j.apsoil.2021.104219
Ledo, A., Heathcote, R., Hastings, A., Smith, P., Hillier, J. (2018). Perennial-GHG: A new generic model to estimate biomass accumulation and greenhouse gas emissions in perennial food and bioenergy crops. Environ. Model. Software 102, 292–305. doi: 10.1016/j.envsoft.2017.12.005
Ledo, A., Hillier, J., Smith, P., Aguilera, E., Blagodatskiy, S., Brearley, F. Q., et al. (2019). A global, empirical, harmonized dataset of soil organic carbon changes under perennial crops. Sci. Data 6, 57. doi: 10.1038/s41597-019-0062-1
Ledo, A., Smith, P., Zerihun, A., Whitaker, J., Vicente-Vicente, J. L., Qin, Z. C., et al. (2020). Changes in soil organic carbon under perennial crops. Global Change Biol. 26, 4158–4168. doi: 10.1111/gcb.15120
Leifeld, J., Meyer, S., Budge, K., Sebastia, M. T., Zimmermann, M., Fuhrer, J. (2015). Turnover of grassland roots in mountain ecosystems revealed by their radiocarbon signature: Role of temperature and management. PloS One 10, e0119184. doi: 10.1371/journal.pone.0119184
Lessmann, M., Ros, G. H., Young, M. D., de Vries, W. (2022). Global variation in soil carbon sequestration potential through improved cropland management. Global Change Biol. 28, 1162–1177. doi: 10.1111/gcb.15954
Liu, S., Wang, Z. Y., Niu, J. F., Dang, K. K., Zhang, S. K., Wang, S. Q., et al. (2021a). Changes in physicochemical properties, enzymatic activities, and the microbial community of soil significantly influence the continuous cropping of Panax quinquefolius l. (American ginseng). Plant Soil 463, 427–446. doi: 10.1007/s11104-021-04911-2
Liu, X. T., Wu, X. P., Liang, G. P., Zheng, F. J., Zhang, M. N., Li, S. P. (2021b). A global meta-analysis of the impacts of no-tillage on soil aggregation and aggregate-associated organic carbon. Land Degradation Dev. 32, 5292–5305. doi: 10.1002/ldr.4109
Lu, X. F., Gilliam, F. S., Guo, J. Y., Hou, E. Q., Kuang, Y. W. (2022). Decrease in soil pH has greater effects than increase above-ground carbon inputs on soil organic carbon in terrestrial ecosystems of China under nitrogen enrichment. J. Appl. Ecol. 59, 768–778. doi: 10.1111/1365-2664.14091
McNally, S. R., Laughlin, D. C., Rutledge, S., Dodd, M. B., Six, J., Schipper, L. A. (2017). Herbicide application during pasture renewal initially increases root turnover and carbon input to soil in perennial ryegrass and white clover pasture. Plant Soil 412, 133–142. doi: 10.1007/s11104-016-3050-7
Miller, B. A., Koszinski, S., Wehrhan, M., Sommer, M. (2015). Comparison of spatial association approaches for landscape mapping of soil organic carbon stocks. Soil 1, 217–233. doi: 10.5194/soil-1-217-2015
Mondal, S., Chakraborty, D. (2022). Global meta-analysis suggests that no-tillage favourably changes soil structure and porosity. Geoderma 405, 115443. doi: 10.1016/j.geoderma.2021.115443
Murphy, J., Riley, J. P. (1962). A modified single solution method for the determination of phosphate in natural waters. Analytica Chimica Acta 27, 31–36. doi: 10.1016/S0003-2670(00)88444-5
Ni, H. W., Liu, C. Y., Sun, B., Liang, Y. T. (2022). Response of global farmland soil organic carbon to nitrogen application over time depends on soil type. Geoderma 406, 115542. doi: 10.1016/j.geoderma.2021.115542
Post, W. M., Kwon, K. C. (2000). Soil carbon sequestration and land-use change: Processes and potential. Global Change Biol. 6, 317–327. doi: 10.3334/CDIAC/TCM.009
Qin, Z., Dunn, J. B., Kwon, H., Mueller, S., Wander, M. M. (2016). Soil carbon sequestration and land use change associated with biofuel production: Empirical evidence. Global Change Biol. Bioenergy 8, 66–80. doi: 10.1111/gcbb.12237
Rentschler, T., Gries, P., Bruelheide, H., Kühn, P., Seitz, S., Shi, X., et al. (2019). Comparison of catchment scale 3D and 2.5D modelling of soil organic carbon stocks in Jiangxi province, PR China. PloS One 14, e0220881. doi: 10.1371/journal.pone.0220881
Robertson, A. D., Whitaker, J., Morrison, R., Davies, C. A., Smith, P., McNamara, N. P. (2017). A Miscanthus plantation can be carbon neutral without increasing soil carbon stocks. Global Change Biol. Bioenergy 9, 645–661. doi: 10.1111/gcbb.12397
Rowe, R. L., Keith, A. M., Elias, D., Dondaini, M., Smith, P., Oxley, J., et al. (2016). Initial soil c and land-use history determine soil c sequestration under perennial bioenergy crops. Global Change Biol. Bioenergy 8, 1046–1060. doi: 10.1111/gcbb.12311
Schimdt, M. W. I., Torn, M. S., Abiven, S., Dittmar, T., Guggenberger, G., Janssens, I. A., et al. (2011). Persistence of soil organic matter as an ecosystem property. Nature 478, 49–56. doi: 10.1038/nature10386
Sinsabaugh, R. L., Lauber, C. L., Weinstraub, M. N., Ahmed, B., Allison, S. D., Crenshaw, C., et al. (2008). Stoichiometry of soil enzyme activity at global scale. Ecol. Lett. 11, 1252–1264. doi: 10.1111/j.1461-0248.2008.01245.x
Slessarev, E. W., Nuccio, E. E., McFarlane, K. J., Ramon, C. E., Saha, M., Firestone, M. K., et al. (2020). Quantifying the effects of switchgrass (Panicum virgatum) on deep organic c stocks using natural abundance 14C in three marginal soils. Global Change Biol. Bioenergy 12, 834–847. doi: 10.1111/gcbb.12729
Song, X.-P., Hansen, M. C., Stehman, S. V., Potapov, P. V., Tyukavina, A., Vermote, E. F., et al. (2018). Global land change from 1982 to 2016. Nature 560, 639–643. doi: 10.1038/s41586-018-0411-9
Sun, Y. F., Wan, H. W., Zhao, Y. J., Chen, S. P., Bai, Y. F. (2017). Spatial patterns and drivers of root turnover in grassland ecosystems in China. Chin. J. Plant Ecol. 42, 337–348. doi: 10.17521/cjpe.2017.0241
Tan, G., Liu, Y. J., Peng, S. G., Yin, H. Q., Meng, D. L., Tao, J. M., et al. (2021). Soil potentials to resist continuous cropping obstacle: Three field cases. Environ. Res. 200, 111319. doi: 10.1016/j.envres.2021.111319
Tian, F. R., Zhou, Z. X., Wang, X. F., Zhang, K. P., Han, S. J. (2022). Changes in soil microbial community along a chronosequence of perennial mugwort cropping in northern China plain. Agronomy 12, 1568. doi: 10.3390/agronomy12071568
Trost, B., Prochnow, A., Drastig, K., Meyer-Aurich, A., Ellmer, F., Baumecker, M. (2013). Irrigation, soil organic carbon and N2O emissions. a review. Agron. Sustain. Dev. 33, 733–749. doi: 10.1007/s13593-013-0134-0
Wang, S., Fan, J., Zhong, H., Li, Y., Zhu, H., Qiao, Y., et al. (2019). A multi-factor weighted regression approach for estimating the spatial distribution of soil organic carbon in grasslands. Catena 174, 248–258. doi: 10.1016/j.catena.2018.10.050
Wang, F., Harindintwali, J. D., Yuan, Z. Z., Wang, M., Wang, F. M., Li, S., et al. (2021). Technologies and perspectives for achieving carbon neutrality. Innovation 2, 100180. doi: 10.1016/j.xinn.2021.100180
Wang, H., Li, X., Li, X., Wang, J., Li, X., Guo, Q., et al. (2020a). Long-term no-tillage and different residue amounts alter soil microbial community composition and increase the risk of maize root rot in northeast China. Soil Tillage Res. 196, 104452. doi: 10.1016/j.still.2019.104452
Wang, X., Tang, X. (2018). The role of rhizosphere pH in regulating the rhizosphere priming effect and implications for the availability of soil-derived nitrogen to plants. Ann. Bot. 121, 143–151. doi: 10.1093/aob/mcx138
Wang, X., Tang, C., Severi, J., Butterly, C. R., Baldock, J. A. (2016). Rhizosphere priming effect on soil organic carbon decomposition under plant species differing in soil acidification and root exudation. New Phytol. 211, 864–873. doi: 10.1111/nph.13966
Wang, Y., Zhang, Y., Li, Z. Z., Zhao, Q., Huang, X. Y., Huang, K. F. (2020b). Effect of continuous cropping on the rhizosphere soil and growth of common buckwheat. Plant Production Sci. 23, 81–90. doi: 10.1080/1343943X.2019.1685895
Waring, B. G., Weintraub, S. R., Sinsabaugh, R. L. (2014). Ecoenzymatic stoichiometry of microbial nutrient acquisition in tropical soils. Biogeochemistry 117, 101–113. doi: 10.1007/s10533-013-9849-x
Wiesmeier, M., Urbanski, L., Hobley, E., Lang, B., von Lützow, M., Marin-Spiotta, E., et al. (2019). Soil organic carbon storage as a key function of soils-a review of drivers and indicators at various scales. Geoderma 333, 149–162. doi: 10.1016/j.geoderma.2018.07.026
Winkler, K., Fuchs, R., Rounsevell, M., Herold, M. (2021). Global land use changes are four times greater than previously estimated. Nat. Commun. 12, 2501. doi: 10.1038/s41467-021-22702-2
Zan, C. S., Fyles, J. W., Girouard, P., Samson, R. A. (2001). Carbon sequestration in perennial bioenergy, annual corn and uncultivated systems in southern Quebec. Agric. Ecosyst. Environ. 86, 135–144. doi: 10.1016/S0167-8809(00)00273-5
Zhang, B. G., Cai, Y. J., Hu, S. J., Chang, S. X. (2021a). Plant mixture effects on carbon-degrading enzymes promote soil organic carbon accumulation. Soil Biol. Biochem. 163, 108457. doi: 10.1016/j.soilbio.2021.108457
Zhang, J. W., Wu, X. F., Shi, Y. J., Jin, C. J., Yang, Y. H., Wei, X. W., et al. (2021b). A slight increase in soil pH benefits soil organic carbon and nitrogen storage in a semi-arid grassland. Ecol. Indic. 130, 108037. doi: 10.1016/j.ecolind.2021.108037
Zhou, L. H., Liu, S. S., Shen, H. H., Zhao, M. Y., Xu, L. C., Xing, A. J., et al. (2020). Soil extracellular enzyme activity and stoichiometry in china’s forests. Funct. Ecol. 34, 1461–1471. doi: 10.1111/1365-2435.13555
Zhu, H. H., Wang, S., Zhu, Q. H., Huang, D. Y. (2021). Perennial ramie cropping sustainably increases c sequestration of subtropical upland soils. Geoderma 381, 114688. doi: 10.1016/j.geoderma.2020.114688
Keywords: biomass, cropping years, enzyme activity, perennial cropping, soil organic carbon
Citation: Zhou Z, Tian F, Zhao X, Zhang K and Han S (2022) Short-term but not long-term perennial mugwort cropping increases soil organic carbon in Northern China Plain. Front. Plant Sci. 13:975169. doi: 10.3389/fpls.2022.975169
Received: 21 June 2022; Accepted: 23 September 2022;
Published: 10 October 2022.
Edited by:
Abraham J. Escobar-Gutiérrez, Institut National de recherche pour l’agriculture, l’alimentation et l’environnement (INRAE), FranceReviewed by:
Narendra Kumar Lenka, Indian Institute of Soil Science (ICAR), IndiaPengshuai Shao, Binzhou University, China
Copyright © 2022 Zhou, Tian, Zhao, Zhang and Han. This is an open-access article distributed under the terms of the Creative Commons Attribution License (CC BY). The use, distribution or reproduction in other forums is permitted, provided the original author(s) and the copyright owner(s) are credited and that the original publication in this journal is cited, in accordance with accepted academic practice. No use, distribution or reproduction is permitted which does not comply with these terms.
*Correspondence: Zhenxing Zhou, enpob3VoZW51QDE2My5jb20=; Kunpeng Zhang, emhhbmdrdW5wZW5nYWdAMTYzLmNvbQ==
†These authors have contributed equally to this work and share first authorship