- 1State Key Laboratory Breeding Base of Dao-di Herbs, National Resource Center for Chinese Materia Medica, China Academy of Chinese Medical Sciences, Beijng, China
- 2College of Pharmacy, Nanjing University of Chinese Medicine, Nanjing, China
- 3National Agricultural Technology Extension and Service Center, Beijing, China
- 4Key Laboratory of Biology and Cultivation of Herb Medicine, Ministry of Agriculture and Rural Affairs, Beijing, China
Root rot disease caused by Fusarium oxysporum is a devastating disease of Salvia miltiorrhiza and dramatically affected the production and quality of Sa. miltiorrhiza. Besides the agricultural and chemical control, biocontrol agents can be utilized as an additional solution. In the present study, an actinomycete that highly inhibited F. oxysporum was isolated from rhizosphere soil and identified as based on morphological and molecular characteristics. Greenhouse assay proved that the strain had significant biological control effect against Sa. miltiorrhiza root rot disease and growth-promoting properties on Sa. miltiorrhiza seedlings. To elucidate the biocontrol and plant growth-promoting properties of St-220, we employed an analysis combining genome mining and metabolites detection. Our analyses based on genome sequence and bioassays revealed that the inhibitory activity of St-220 against F. oxysporum was associated with the production of enzymes targeting fungal cell wall and metabolites with antifungal activities. Strain St-220 possesses phosphate solubilization activity, nitrogen fixation activity, siderophore and indole-3-acetic acid production activity in vitro, which may promote the growth of Sa. miltiorrhiza seedlings. These results suggest that St. albidoflavus St-220 is a promising biocontrol agent and also a biofertilizer that could be used in the production of Sa. miltiorrhiza.
Introduction
Salvia miltiorrhiza is a well-important traditional Chinese medicinal plant with terrific economic, social, and medicinal benefits (Su et al., 2015). Its dried root, called Danshen for its medicinal use, has been used for hundreds of years (Jiang et al., 2019), primarily for the treatment of various cardiovascular and cerebrovascular diseases in China and other Asia countries. In addition, Sa. miltiorrhiza is also used as a health-promotion food (Shi et al., 2019). To fit the large demand of Danshen, the planting areas of Sa. miltiorrhiza has reached to 100 thousand hectares in China by the year of 2020. However, the production of Sa. miltiorrhiza was severely limited by root rot disease caused by Fusarium oxysporum. The average incidence of Sa. miltiorrhiza root rot disease in China is 10% ~ 30%. Moreover, in some plots where the disease severely happened, the incidence could reach to 80%, causing irreversible losses to farmers (Wang et al., 2018a).
Currently, the root rot disease on Sa. miltiorrhiza cannot be effectively controlled by using physical and chemical methods (Ye et al., 2003). Additionally, the long-term overuse of fungicides has caused many adverse effects on environment, animal and human health, soil quality, and pathogen controlling (Wang et al., 2014, 2018a; Raza et al., 2017). Consequently, it is important and urgent to develop alternative methods and agents that are less toxic and more effective in controlling root rot. Utilization of functional microbes that not only antagonistic to phytopathogens but also friendly to environment is considered an economical and effective method to control root rot disease and improve plant health. The use of functional microorganisms and their biological products can provide growers an option to not only avoid the problem of chemical residues on plants and soil, but also to reduce pathogen resistance (Handelsman and Stabb, 1996; Abbas et al., 2020; Sun et al., 2020). Strains of Streptomyces are considered as biocontrol agents due to their production of various active compounds with agricultural applications. In addition, they are able to survive in harsh environments and colonize the root of plants belonging to multiple species including Sa. miltiorrhiza (Suárez-Moreno et al., 2019; Jose et al., 2021; Wu et al., 2021). Moreover, Streptomyces strains have multiple strategies to suppress fungal pathogens such like nutrients competition, cell wall degradation, virulence factors degradation and plant immunity induction (Chen et al., 2018). Certain Streptomyces can also improve nutrient absorption and in turn boost plant development by producing auxins, solubilizing inorganic phosphate, fixing nitrogen and other methods (Goudjal et al., 2013; Vijayabharathi et al., 2015; Liu et al., 2016; Raaijmakers and Mazzola, 2016; Jones and Elliot, 2017). Streptomyces SCA2-4 T, isolated from the rhizosphere soil of prickly pear (Opuntia stricta), exhibited a strong antagonistic activity against F. oxysporum f. sp. cubense tropical race 4 causing banana Fusarium wilt (Qi et al., 2021). Streptomyces NEAU-S7GS2 isolated from the root of soybean does not only prevent Sclerotinia stem rot of soybean, but also promotes the soybean growth (Liu et al., 2019). Therefore, Streptomyces species offers abundant resources of biofungicides or biofertilizers for agricultural usage (Liu et al., 2019).
In the present study, St. albidoflavus strain St-220 was isolated from the rhizosphere soil of Sa. miltiorrhiza, and was identified based on its morphological and molecular characteristics. Additionally, the plant growth-promoting activity and antifungal activity of St-220 was also evaluated in vitro and in greenhouse conditions. To demonstrate the antifungal and growth-promoting mechanisms, we carried out an analysis combining genome mining and metabolites detection based on the genome sequence of St-220.The pathways for synthesis of secondary metabolites including antibiotics and plant growth-promoting compounds were investigated, and genes encoding the antifungal enzymes were also predicted. These results provided essential and deep insights into the biocontrol properties of St. albidoflavus St-220.
Materials and methods
Actinomyces and Fusarium strains
Salvia miltiorrhiza along with the rhizosphere soil were collected from Sa. miltiorrhiza plantation in Laiwu City, Shandong Province, China (36°18′N 117°50′E). The rhizosphere soil of Sa. miltiorrhiza were obtained from the root surface. The isolation of actinomycetes was performed according to the methods described previously with modifications (Wang et al., 2021). Briefly, 10 ml of soil suspension containing 1 g rhizosphere soil and 10 ml sterile water was incubated in a shaker at 100 rpm for 30 min, then diluted into 10−3 g/ml, 10−4 g/ml, and 10−5 g/ml. Two hundred microliters of the diluted suspension were added to Gause’s agar medium (containing 2% soluble starch, 0.051% K2HPO4, 0.025% MgSO4, 0.001% FeSO4, and 2% Agar B, pH 7.2–7.4) amended with 20 μg mL−1 nalidixic acid, respectively, and cultured at 28°C. For purification, single colonies grown on the plates were separately transferred to another plates and then stored at −80°C in 20% glycerol. The phytopathogenic fungi F. oxysporum was isolated from plant tissues of Sa. miltiorrhiza with root rot disease collected from a field in Yuzhou, Henan, in August 2019.
Antagonistic effects of Streptomyces strains on Fusarium oxysporum
The inhibition ability of Streptomyces against F. oxysporum was determined using the conventional improved scribe inoculation method (Chen et al., 2018). A mycelium plug of F. oxysporum in the center of potato dextrose agar (PDA) plates. Streptomyces strains were inoculated by streaking symmetrically at the two sides of the plug, 25 mm to the plate center. Petri dishes not inoculated with Streptomyces were used as controls, and three times each experiment was performed. After incubation for 5 ~ 7 days at 28°C, the colony diameters were measured, and the growth inhibition (GI) was calculated according to the following formula (Qi et al., 2019):
where D and d represented the diameters of fungal colonies on the control and treated plates, respectively.
Control effect of St-220 on Salvia miltiorrhiza root rot disease in greenhouse condition
Before planting, Sa. miltiorrhiza seeds were soaked in 75% ethanol for 5 min, and then soaked in 5% bleach for 10 min for surface disinfection. After rinsed with sterile water for three times, the seeds were placed in a culture bottle with a sterile mixture of soil and vermiculite (2:1). To make inoculum, a mycelium plug of F. oxysporum was inoculated in PDA liquid culture and incubated in a dark shaker at 28°C 180 rpm for 10 days, then the culture was cloth-filtered and the flow-through was saved as spore suspension, which was then adjusted to 1 × 107 cfu/ml for use. To make cell suspensions of strain St-220, 500 μl of glycerol suspension was inoculated in 500 ml Gause’s liquid medium and incubated at 28°C 160 rpm for 10 days. The two-leaf Sa. miltiorrhiza seedlings were inoculated by drenching with 10 ml inoculum of F. oxysporum (Fo), 10 ml St-220 cell suspension mixed with 10 ml inoculum of F. oxysporum (Fo + St), and 10 ml of sterile water (CK), respectively. The inoculated seedlings were grown in a growth chamber with temperature of 30°C/26°C, photoperiod of 12/12 h and 50% humidity. At 30 days after inoculation (DAI), disease symptoms were observed and evaluated using a severity scale: 0 for no symptoms; 1 was suffered disease symptoms less than 20% (only 1 leaf yellowing or wilting); 2 and 3 were plants suffering from disease symptoms in the range of 20%–40% (more than 2 but less than half of the leaves turn yellow or wither) and 40%–80%, respectively; 4 was Sa. miltiorrhiza showing severe disease symptoms with only the top 1 to 2 leaves being healthy; level 5 was plants that have died (Li et al., 2022). The disease index (DI) was calculated based on the formula where A is the disease scale (0, 1, 2, 3, 4, and 5), B is the number of seedlings at each level of the scale, and C is the total number of seedlings for each treatment. Disease incidence and control efficiency were calculated according to the following formulas:
Plant traits including fresh and dry weight of the root and shoot of the seedlings, and the diameter and length of the roots were measured at 30 DAI. Ten seedlings in five culture bottles were inoculated for each treatment, and the experiment was repeated for three times.
In vitro assessment of plant growth promotion traits
To evaluate the growth-promoting properties, the Phosphate solubilization, biological nitrogen fixation, siderophore and indoleacetic acid (IAA) production of the St-220 strain was determined. For this purpose, St-220 was cultured in 100 ml Gause’s liquid medium for 5 days at 28°C in an orbital shaker (150 rpm.), and each assay was performed with three biological replicates for each strain.
Phosphate solubilization
An improved Pikovskaya (PVK) solid medium was used to evaluate the ability of strain St-220 on insoluble organic phosphate solubilization. The plate was inoculated with strain St-220 and kept at 28°C for 7 days. Positive phosphate solubilization was evident by a clear halo around strain St-220 (Gupta et al., 1994). Plates inoculated with sterile water were as control. The experiment was repeated three times.
Biological nitrogen fixation
Assay for nitrogen-fixing activity of the strains was performed according to a modified procedure described previously (Roy, 1958): strain St-220 colony was inoculated on nitrogen-free agar medium (Ashby’s Nitrogen-free medium) and then incubated at 28°C 7 days for 3 times. That the strain grew after three consecutive transfers indicated nitrogen fixation activity.
Siderophore production
Chrome azurol blue agar was used to assess siderophore production, and the pH was adjusted to 7.2 with KOH as suggested previously (Schwyn and Neilands, 1987). The presence of a yellow halo indicates the production of siderophores.
Indoleacetic acid production
The IAA production activity of St-220 was determined by the method of Salkowski colorimetry (Tang and Bonner, 1948). The activated St-220 was inoculated to 0.5 g/L Gause’s agar liquid medium containing tryptophan, and then cultured at 28°C, 150 r/min in a shaker for 7 days to obtain the fermentation broth. One milliliter of the broth was centrifuged at 12,000 rpm for 5 min, then the supernatant was mixed with 2 ml Salkowski reagent containing 15 ml concentrated H2SO4, 25 ml distilled water and 0.75 ml of 0.5 M FeCl3.6H2O (de Oliveira-Longatti et al., 2014).
After incubation in darkness at room temperature for 30 min, the mixture turned pink when IAA was generated. Serial dilutions of a standard IAA solution (0, 5, 10, 15, 20, 25, 30, 35, and 40 μg/ml) were used to construct the calibration plot (Abbasi et al., 2019).
Plant growth-promotion experiments
Seeds of Sa. miltiorrhiza were disinfected as described previously, and then planted in pots (7 cm × 7 cm × 10 cm) containing 100 g of sterilized soil substrate (nutrient soil: vermiculite = 1:1) for germination. When grew two leaves, seedlings that are similar in height were selected for use. To make inoculum, strain St-220 was grown on Gause’s liquid medium and incubated at 28°C 160 rpm for 10 days, then the harvested cell suspension was adjusted to 1 × 107 cfu/ml. For inoculation, 20 ml of St-220 inoculum were applied to each pot, and 20 ml of sterile water was separately applied as negative control. The seedlings were inoculated every 10 days until the plant traits were investigated. Five replicates for each treatment, and the experiment was repeated three times. All the pots were placed in growth chamber at 30/26°C and 12/12 h, 50% humidity. Plant traits including root and shoot fresh weight, total dry weight, length and diameter of the root was measured at 40 DAI.
Sequencing, assembly, annotation, and bioinformatics analysis of the genome of St-220
DNA extraction
To obtain the genomic DNA, a single colony of St-220 was transferred to Gause’s liquid medium and then incubated at 28°C at 160 rpm for 5 days. The obtained cell suspension was then centrifuged and the supernatant was yield for DNA extraction by the SDS method (Lim et al., 2016). The DNA purity and quantity were examined by using Qubit® 2.0 Fluorometer (Thermo Scientific). The 16 s rDNA of St-220 was sequenced and compared to existing databases for identification.
Sequencing, assembly, and annotation
The obtained genomic DNA of St-220 was used for the whole genome sequencing by using the Illumina NovaSeq PE150 sequencing platform at Novogene Technology Co., Ltd. (Beijing, China). A series of de novo assemblies were carried out with different software (SOAP, SPAdes; Li et al., 2008; Simpson et al., 2009; Bankevich et al., 2012). The protein coding genes (CDSs), rRNA and tRNA were predicted by Glimmer version 3.02, RNAmmer 1.2 and tRNA-scan-SE version 2.0, respectively, (Lowe and Eddy, 1997; Delcher et al., 2007; Lagesen et al., 2007). For gene annotation, BLAST searches was carried out in several databases including NCBI Non-redundant (NR), Clusters of Orthologous Groups (COG; Jensen et al., 2007), Pfam (Finn et al., 2014), Swiss-Prot (Zhou et al., 2021), Carbohydrate-Active enZYmes (CAZy; Zhang et al., 2018), Kyoto Encyclopedia of Genes and Genomes (KEGG; Kanehisa and Goto, 2006) and Gene Ontology (GO; Ashburner et al., 2000). The online software antiSMASH version 6.0 was employed to definite antibiotic and secondary metabolite gene clusters (Blin et al., 2021).
Identification and characterizations of strain St-220
Phylogenetic analyses
For identification of St-220, a phylogenetic tree was constructed based on the 16S rDNA and five housekeeping genes (atpD, gyrB, recA, rpoB, and trpB) concatenated sequences. Multiple alignment of the sequences and construction of phylogenetic tree using maximum likelihood were generated by using Clustal X (Larkin et al., 2007) and PhyloSuitev1.2.2 (Zhang et al., 2020), respectively. Calculation of orthoANI values (orthologous average nucleotide identity) was performed by JSpeciesWS (Richter et al., 2016) and an online tool ANI-Blast (ANIb) Calculator. The ANIb values were used for assessing two strains are same species. A Genome-to-Genome Distance Calculator (GGDC) web server version 3.0 (Rigden and Fernández, 2022) was used to determine DNA–DNA hybridization (DDH) values in silico.
Cultural and morphological characterizations
The morphological characteristics of the St-220 strain were observed under scanning electron microscopy (SEM; model S-3400 N, Hitachi, Ltd., Tokyo, Japan) when grown on PDA medium for 14 days. The mycelium and substrate mycelium characteristics of St-220 were investigated after incubation at 28°C for 14 days on PDA and Gause’s agar medium, respectively.
Statistical analysis
Statistical analyses including Student’s t-tests and ANOVA with Dunnett’s test were performed with R scripts. Difference was considered significant when the p value was <0.05.
Results
In vitro antagonistic effects of streptomyces strains against Fusarium oxysporum
A total of 163 strains of actinomycetes were isolated from the rhizosphere soil of Sa. miltiorrhiza in the plantation, and 11 strains showed an inhibitory effect on F. oxysporum, of which strain St-220 showed the most obvious inhibitory effect on mycelia growth of F. oxysporum (Supplementary Table S1). After 7 days incubation, the F. oxysporum incubated with St-220 showed narrow and oval colonies compared to the negative control (Figure 1A). To calculate the inhibition rate, the mean diameters of mycelia colonies were estimated by measuring the perpendicular length of each colony. The mean diameter of F. oxysporum mycelia colonies reached to 8.50 cm, while that of F. oxysporum grown with St-220 reached to 3.46 cm, with an inhibitory rate of 53.40% (Figure 1B).
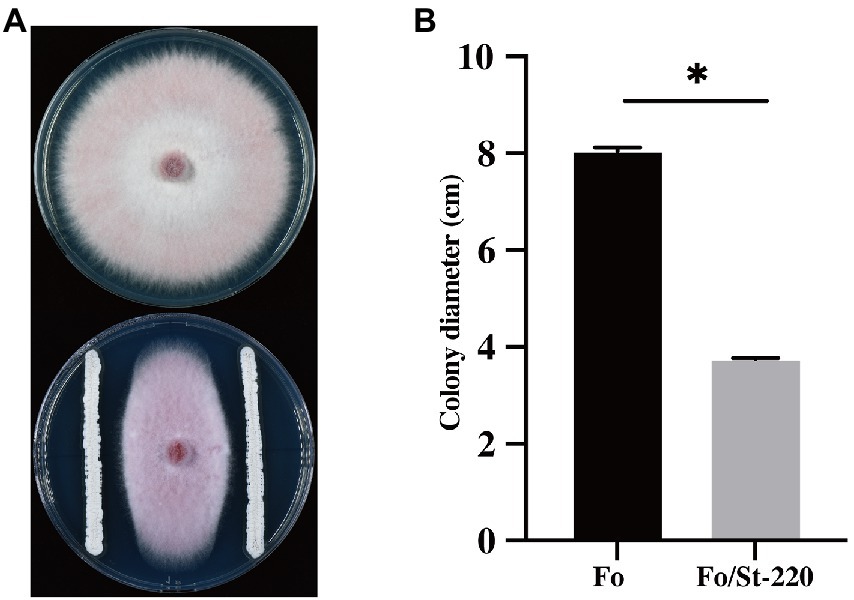
Figure 1. The antifungal activity of strain St-220 against Fusarium oxysporum. (A) F. oxysporum colony grew on PDA plate alone (upper) and with St-220 (lower) at 7 days after inoculation. (B) Colony diameter of F. oxysporum in each treatment. Bars with ∗ above are statistically different (p < 0.05).
Control effect of St-220 on root rot disease of Salvia miltiorrhiza in greenhouse condition
After treated with cell suspension of strain St-220 for 30 days, Sa. miltiorrhiza seedlings in the pathogen treatment group displayed morphological indications of disease, with leaves turning yellow and wilting and roots rotting (Figure 2A). The disease incidence and disease index of the treatment group inoculated with F. oxysporum (Fo) were 86.67% and 68.00%, respectively, while the disease incidence and disease index of the treatment group inoculated with F. oxysporum and strain St-220 (Fo + St) were 20% and 22.66%, respectively. Strain St-220 significantly (p < 0.05) reduced disease incidence by 76.92% and disease index by 66.67% (Supplementary Table S2). Compared with the treatment Fo, the total fresh weight (Figure 2C), dry weight (Figure 2D), shoot height and root length (Figures 2B,E) of the Fo + St treatment significantly increased by 138.45%, 39.73%, 137.43%, and 72.12%, respectively. Meanwhile, root fresh weight, root dry weight, and root diameter were also increased (Figures 2C–F). Therefore, St-220 has the biological control impact on Sa. miltiorrhiza root rot in greenhouse condition.
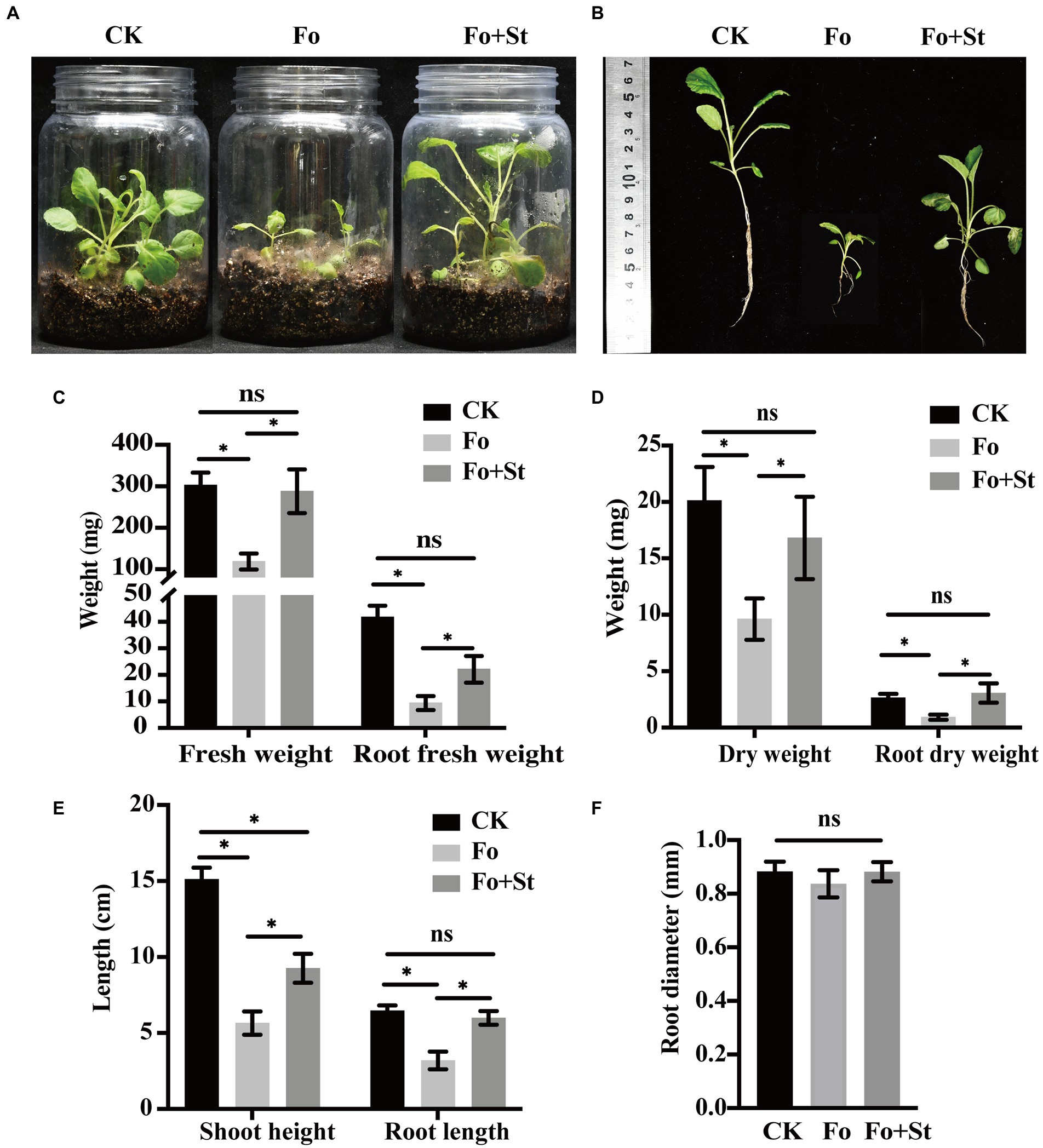
Figure 2. Control effect of St-220 on root rot disease of Salvia miltiorrhiza seedlings. (A) Symptoms of root rot developed on seedlings inoculated with Fusarium oxysporum (Fo) and mixture of F. oxysporum and St-220 (Fo + St) at 30 DAI, while no symptoms were observed on seedlings inoculated with sterile water (CK). (B) The entire plant of the seedlings in CK, Fo, and Fo + St treatment. Measurement of the fresh weight (C), dry weight (D), shoot height, root length (E), and root diameter (F) of seedlings inoculated. Data are mean ± SE (n = 10). Means were compared with ANOVA analysis in combination with Tukey post-test. Means were considered statistically different when p < 0.05, Bars with ∗ above are statistically different, ns above are not statistically different.
Biological characteristics involved in plant growth-promoting activity of St-220
To explore the potential mechanism of St-220 on plant growth-promoting activity, four biological characteristics of strain St-220 were tested. In phosphate solubilizing activity assay, a distinct circle around the colony was generated after 7 days of strain St-220 growing on PVK medium (Figure 3A), demonstrating that strain St-220 possessed phosphate solubilizing activity. Strain St-220 was able to grow on Ashby’s nitrogen-free medium after 3 successive transfers suggesting nitrogen-fixing activity (Figure 3B). The siderophore generating carrier activity of strain St-220 was indicated by the creation of a prominent yellow halo surrounding the colony after 7 days of growth in Chrome Azurol Blue agar (Figure 3C). The IAA production activity of strain St-220 was also determined (Figure 3D). Strain St-220 produced maximum 30.40 μg/ml of IAA at 7 DAI, according to a standard curve based on series dilution [y = 0.0094x + 0.0430 (R2 = 0.9735, where y is the absorbance value at wavelength of 530 nm, x is the concentration of IAA)] (Supplementary Figure S1).
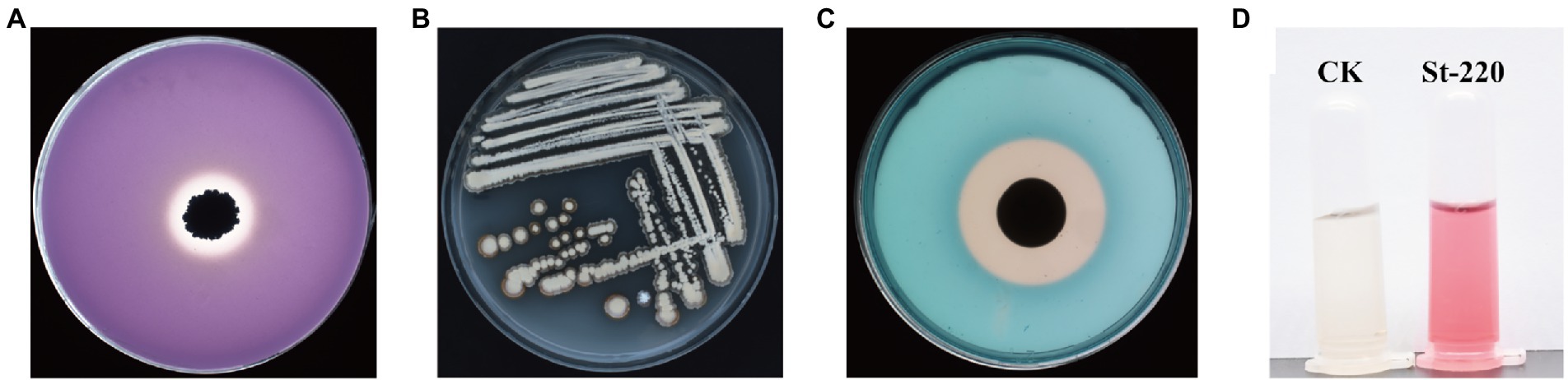
Figure 3. Evaluation of strain St-220 for key traits related to direct plant growth-promotion. (A) Qualitative phosphate solubilization assay. (B) Biological nitrogen fixation activity assay. (C) Siderophores qualitative production assay. (D) Production of indole acetic acid activity assay.
Plant growth-promotion activity of St-220 on Salvia miltiorrhiza
To investigate the growth-promoting impact of strain St-220 on Sa. miltiorrhiza, a greenhouse experiment was performed and the plant traits was assessed at 40 DAI. The results suggested that strain St-220 was able to stimulate Sa. miltiorrhiza growth in contrast to non-inoculated plants, since it exhibited increases in shoot height and fresh weight in roots and plants. St-220 significantly increased the root fresh weight, total fresh weight, total dry weight and root dry weight of Sa. miltiorrhiza seedlings by 85.22%, 105.50%, 60.88%, and 36.72%, respectively (Figure 4A). Shoot length and root length also showed an increase (Figures 4B-D).
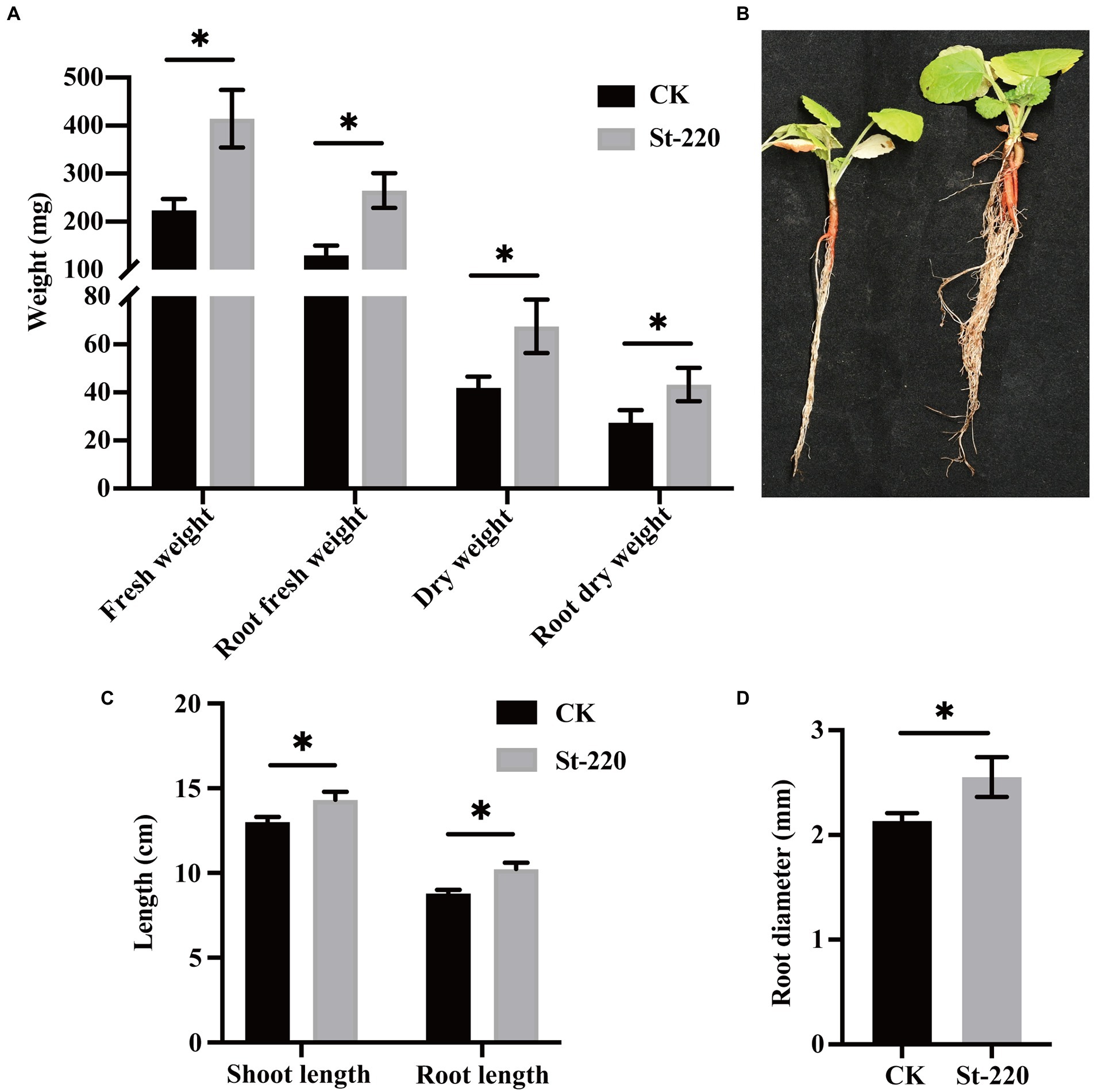
Figure 4. The growth-promoting effect of St-220 on Salvia miltiorrhiza seedlings. The growth-promoting activity of strains St-220 was measured under greenhouse conditions, and the data were recorded at 40 days after inoculation. (A) the biomass of Sa. miltiorrhiza seedlings. (B) overall development of Sa. miltiorrhiza seedlings inoculated with sterile water (left) and St-220 (right). The shoot height, root length (C) and root diameter (D) of Sa. miltiorrhiza seedlings inoculated with sterile water and cell suspension of St-220. Data are mean ± SE (n = 10). Means were considered statistically different when p < 0.05, Bars with ∗ above are statistically different, ns above are not statistically different.
Identification of St-220 strain
After 2-week incubation on PDA, the colony morphology of St-220 revealed a firm surface with white aerial mycelia and faintly whitish-yellow spores (Figure 5A), which is consist with typical morphological characteristics of the Streptomyces genus. Both substrate and aerial mycelia were grown well without fragmentation. The flexuous spore chains formed by cylindrical spores were observed under our scanning electron microscope observation (Figure 5B).
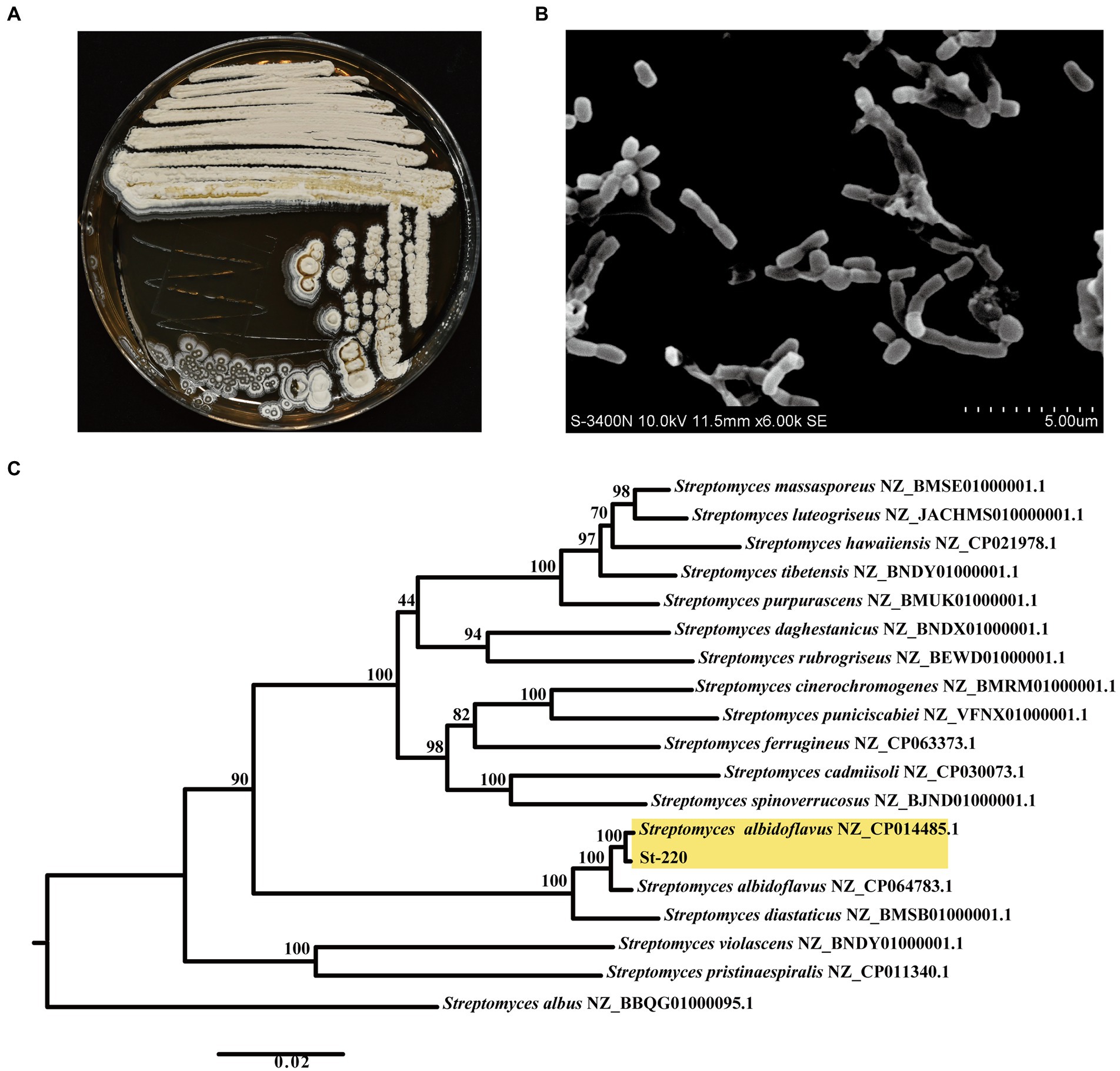
Figure 5. Morphological and molecular identification of strain St-220. (A) Colony morphology of the strain St-220 on PDA medium after 14 days of incubation at 28°C. (B) Spores of St-220 observed under scanning electron microscope after incubated on PDA medium for 14 days at 28°C. (C) A phylogenetic tree using the maximum likelihood method based on the sequences of 16S rDNA and 5 housekeeping genes with 1,000 bootstraps.
The 16S rDNA sequence of St-220 was amplified by PCR and sequenced, and in turn searched in the EzTaxon database, and the strains with high similarity were screened. The sequences of the 16S rDNA and 5 housekeeping genes (atpD, gyrB, recA, rpoB, and trpB) were concatenated and used to construct a phylogenetic tree using the Maximum-Likelihood method with 1,000 bootstraps. The results suggested that strain St-220 and St. albidoflavus clustered into a same clade (Figure 5C). To further confirm our result, the Average Nucleotide Identity (ANI) and DNA–DNA hybridization (DDH) values between St-220 and other 13 Streptomyces strains were calculated. The genome of St. albidoflavus showed the highest ANI and DDH value of 98.87% and 93.90, among the test strains, respectively, (Supplementary Table S3), which was greater than the threshold value of 95% ~ 96% and 70 for species delineation (Richter and Rosselló-Móra, 2009). Altogether, strain St-220 is recognized as a new member of the St. albidoflavus species.
Genome features of St-220
To have a deep insight in the molecular mechanisms of inhibitory effect and plant growth-promoting, the whole genome of St-220 was sequenced and analyzed. After adapter trimming, the reads were de novo assembled into 175 contigs. The genome size of St-220 is 7,310,412 bp with G + C content of 73.41%. The whole genome sequence for St-220 have been deposited in the GenBank database with accession number of JAMFMD000000000. Genomic analysis revealed that the genome of St-220 contained 6,327 CDSs accounting for ~85.43% of the genome (Table 1).
Functional analysis revealed that 5,148, 4,152, 4,798 out of the 6,244 identified CDSs were assigned to COG, GO, and KEGG categories, respectively. In COG categories, the highest ratio the metabolism process was assigned gene numbers with ratio of 36.77%, followed by the category of information storage and processing (17.89%), and the category of cellular processes and signaling (28.61%; Figure 6A). Gene ontology analysis revealed that the category of biological process contained the most GO terms and genes (8,166), followed by molecular function (5,437) and cellular component (2,872; Figure 6B). KEGG pathway analysis showed that the metabolism pathway had the most genes involved, followed by the pathway of environmental information processing (Figure 6C). Additionally, 274 genes were identified in CAZy database and classified into six families. A total of 111 proteins were predicted as belonging to the Glycoside Hydrolase family, of which 88 to Carbohydrate-Binding Modules, 47 to Glycosyl Transferases, 20 to Carbohydrate Esterases, 7 to Auxiliary Activities, and 1 to the Polysaccharide Lyases family (Figure 6D).
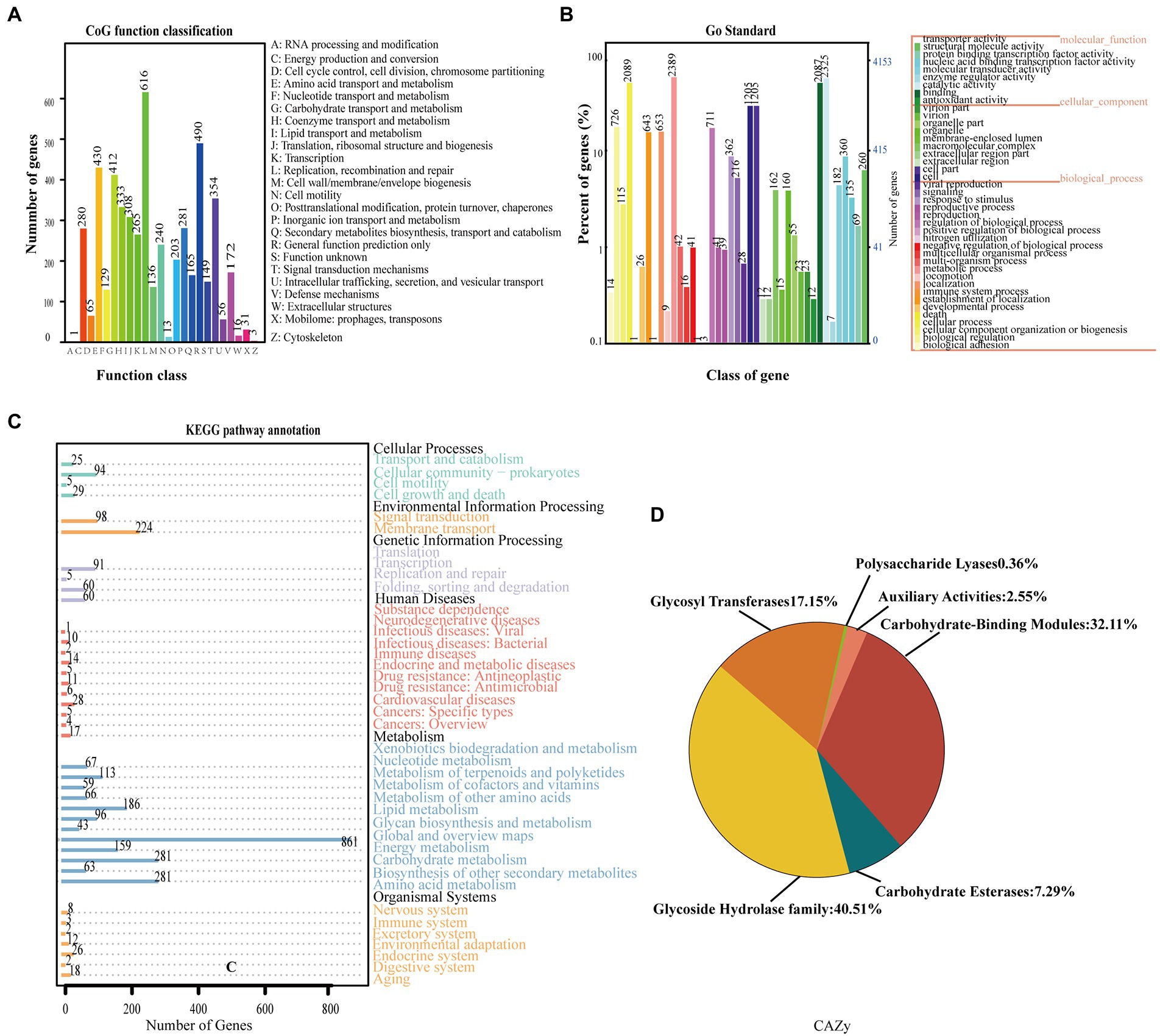
Figure 6. Analysis of genome structure and metabolic pathway of strain Streptomyces albidoflavus St-220. (A) COG annotation of strain St. albidoflavus St-220. genome. (B) GO functional categories of St. albidoflavus St-220. (C) Pathway annotation of strain St. albidoflavus St-220 genome according to the KEGG database. The vertical axis represented the level two classification of KEGG pathway. The horizontal axis represented the gene number annotated in this classification. Different colors of the columns represented different classifications of KEGG pathway. (D) Gene count distributions of carbohydrate-active enzyme (CAZy) families.
Genome analysis of secondary metabolite clusters
In our genome mining analysis, the strain St-220 was predicted on produce a plenty of secondary metabolites. By using the antiSMASH, 21 gene clusters for secondary metabolites biosynthesis were predicted and found located in the chromosome of St-220 (Supplementary Table S4), of which 10 gene clusters were involved in the biosynthesis of metabolites antimicrobial activities including ectoine, desferrioxamine B, surugamide A, antimycin, geosmin, indigoidine, isorenieratene, and candicidin (Figure 7). Furthermore, the Region 12.1 was predicted as involved in the desferrioxamine B and E biosynthesis (Supplementary Table S4), which participated the removal of excess iron in the environment.
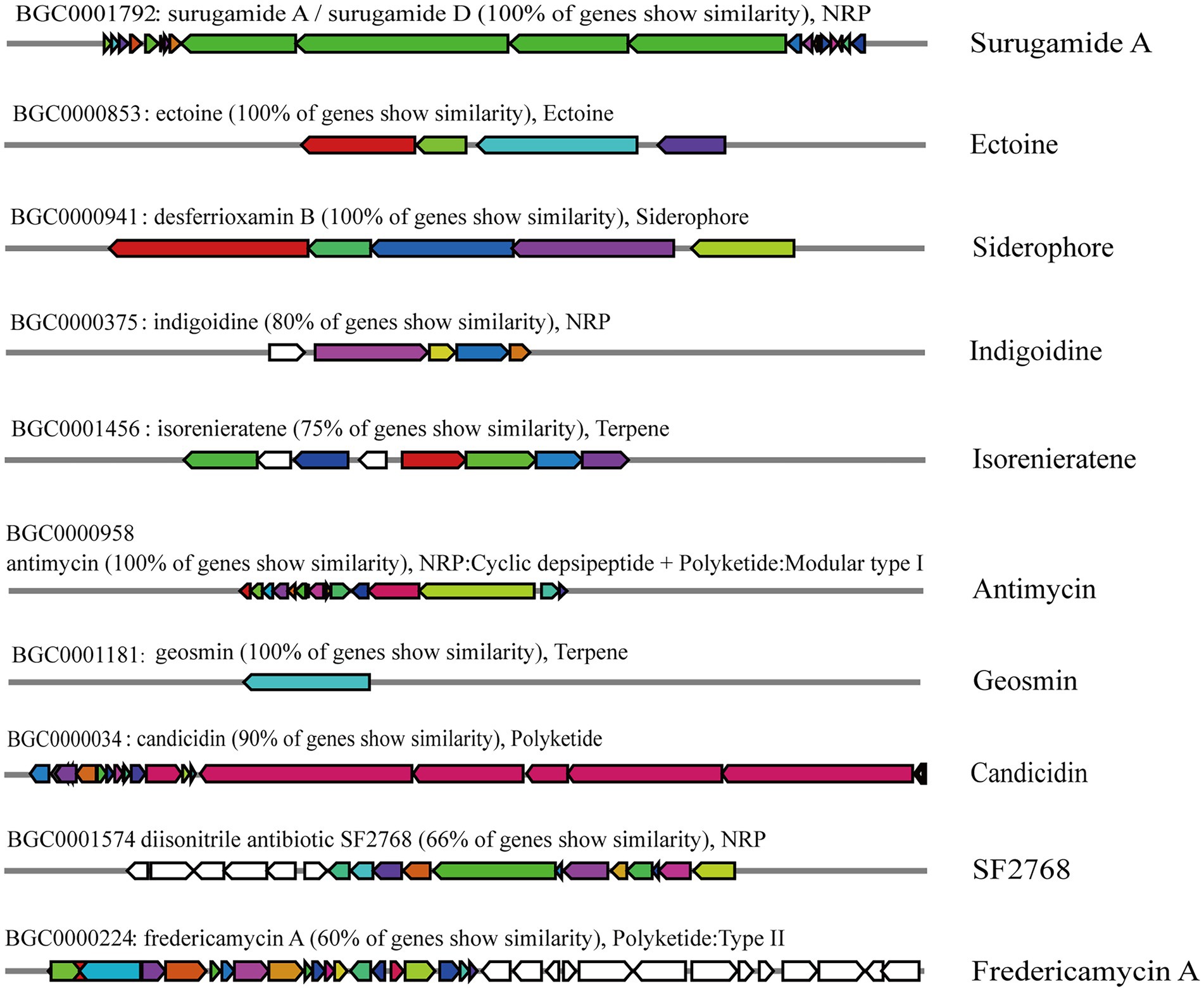
Figure 7. Genome-wide analysis of gene clusters related to the biosynthesis of secondary metabolites using the online antiSMASH v6.0 software.
Genes associated with fungal cell wall degrading enzymes
The genome of strain St-220 harbors 15 genes encoding enzymes involved in chitin degrading, including six β-N-acetyl hexosaminidase, eight chitinases, and one chitosanase. In addition, St-220 has four chitin-binding proteins belonging to the AA10 family, which enhance the binding abilities of enzymes to insoluble substrates. Four genes in the genome of St-220 were further found to encode endo-1, 3-β-glucanase for degradation of glucan (Supplementary Table S5). Moreover, St-220 contains various genes encoding enzymes that play roles in the degradation of cellulose, protein, and lipids (Supplementary Table S5).
Genes associated with plant growth-promotion
Our genomic analysis identified several genes related to the plant growth-promoting activities of St-220. These genes participated in 3 trp-dependent biosynthesis pathways of indole-3-acetic acid, including the indole acetamide (IAM), the tryptamine (TAM) and the indole-acetonitrile (IAN) pathways. In the IAM pathway, tryptophan is converted to IAM by tryptophan monooxygenase enzyme, and then amidase enzyme converts IAM to IAA. Nine encoding genes associated with the IAM pathway were found in the St-220 chromosome, of which six encoding tryptophan 2-monooxygenase and three encoding amidas (Supplementary Table S6). In the TAM pathway, tryptophan is firstly converted to TAM, then amine oxidase converts TAM to indole-3-acetaldehyde (IAAld), and finally IAAd is converted to IAA by aldehyde dehydrogenase. Two genes encoding monoamine oxidase and four genes encoding aldehyde dehydrogenase were found to be present in the genome of St-220. The fact that St-220 harbors two separate pathways for IAA biosynthesis suggested that the IAA production plays a role in life maintenance and plant growth-promoting activity. Moreover, St-220 also contains a gene encoding putative1-aminocyclopropane-1-carboxylic acid (ACC) deaminase involved in the decomposition of ACC (Supplementary Table S6) and we made a case that the St-220 could improve the ability of plants to survive under stress conditions by inhibiting ethylene synthesis.
The genome of strain St-220 contains multiple genes involved in the degradation of inorganic polyphosphates and the dissolution of organic phosphates, including a ppx gene encoding exopoly phosphatase, a ppa gene encoding inorganic pyrophosphatase, and three phoD genes encoding alkaline phosphatase. Furthermore, a pstABCS cluster involved in the transport and degradation of phosphonates is found in the chromosome of St-220 (Supplementary Table S7).
The genome of Strain St-220 contains one nitrogen fixation protein NifU, and an ammonium transporter protein that was involved in the ability of nitrogen fixation. The strain St-220 genome also contains nine nitrate reductase genes (Supplementary Table S8).
The St-220 genome harbors plenty of genetic elements involved in siderophore biosynthesis and iron complex transport (Supplementary Table S9). Moreover, one cluster involved in siderophore biosynthesis is also present in the chromosome sequence of St-220.
Discussion
Root rot disease caused by F. oxysporum is one of the most severe soil-borne disease worldwide, and also the main constraint of Sa. miltiorrhiza production in China. In the present study, an actinomycete strain St-220 with biocontrol activity was isolated from roots of Sa. miltiorrhiza and identified as Streptomyces albidoflavus. The strain showed inhibition rate of 53.40% against F. oxysporum in the dual culture assay and control effect of 77.33% on root rot disease incidence in greenhouse condition. In addition, St. albidoflavus St-220 strain also promoted the growth of Sa. miltiorrhiza by increasing biomass including total fresh weight, root fresh weight, total dry weight and root dry weight, as well as shoot and root length. These results indicate that St. albidoflavus St-220 is a promising biocontrol agent for the control of root rot disease and biofertilizer for Sa. miltiorrhiza.
Streptomyces albidoflavus St-220 have both biological control activity and plant growth-promoting activity
Some Streptomyces strains could significantly improve the biocontrol of Fusarium root rot disease and promote the growth of plant seedlings (El-Tarabily et al., 2009; Goudjal et al., 2016; Tamreihao et al., 2016; Chen et al., 2021). They were generally identified by three properties: IAA production, the abilities to solubilize phosphate and fix nitrogen, and siderophores production (Vurukonda et al., 2018). IAA is a phytohormone that regulates the growth of plant roots by stimulating the development of root (Lwin et al., 2012), and is also an important trait of plant growth-promoting microorganism. Tomato seedlings significantly increased in fresh and dry weight after treated with IAA producing strain S. fradiae (Myo et al., 2019). Phosphorus as a macronutrient is dispensable for plants (Ågren and Weih, 2020). Most of the phosphorus, however, present in the form of insoluble in the soil, and cannot be directly utilized by plants (Rawat et al., 2021). The Streptomyces strains with growth-promoting activity can dissolve the insoluble phosphate for plant growth. Inoculation of Streptomyces sp. strain 7.1 with inorganic phosphate solubilizing activity significantly increased the fresh weight of roots and stems of rice (Suárez-Moreno et al., 2019). Nitrogen is critical to whole life cycle of plants. The atmospheric nitrogen was transformed into ammonia that could be utilized by plants through nitrogen fixation (Dobbelaere et al., 2003). The siderophores secreted by biocontrol agents could suppress the pathogen and protect plants from pathogen infection by iron-competition and restructuring rhizosphere microbiome (Amano et al., 2011; Yu et al., 2013; Gu et al., 2020). For example, the endophytic Streptomyces strains SNL1 and SNL2 producing siderophores have antagonistic activities against F. oxysporum f. sp. cubenese causing Fusarium wilt of banana (Cao et al., 2005). Streptomyces can further promote plant mineral nutrient supply by synthesizing siderophores. Streptomyces sp. GMKU 3100 producing siderophore was able to promote the growth of rice and mung bean, whereas its siderophore-deficient mutant did not differ from the uninoculated control (Rungin et al., 2012).
Previous studies have revealed that Streptomyces strains with above properties showed plant growth-promoting activity. S. violaceusniger AC12AB was found to have properties of IAA production, siderophores production, nitrogen fixation and phosphates solubilization. It significantly promoted the potato crop up to 26.8% in field trial (Sarwar et al., 2019). Barley plants inoculated with S. roseocinereus MS1B15, a strain with IAA-producing, phosphate solubilizing, and nitrogen-fixing activity, significantly increased shoot and spike length (Chouyia et al., 2020). In this study, application of the St-220 resulted in a significant increase in the biomass of Sa. miltiorrhiza seedlings. To elucidate the way that the St-220 promotes the growth, the activities of IAA production, phosphorus solubilization, nitrogen fixation and siderophores production was determined and the synthesis pathway was found in further genomic analysis.
Genomic analysis revealed the potential antifungal and root growth-promoting mechanism of St-220
The strains of Streptomyces genus employ their secondary metabolites as weapons to inhibit phytopathogenic fungi (Amin et al., 2021; Hotta, 2021; Mahasneh et al., 2021; Terra et al., 2021). In this study, genome sequencing revealed that the chromosome of the St. albidoflavus St-220 contained 21 conserved biosynthesis gene clusters (BGCs), of which 10 showed high similarities in structure with known BGCs encoding terpenes, non-ribosomal peptides, polyketides, siderophores, and ectoines, which had been proven to participate in the regulation of antimicrobial activities of Streptomyces strains (van Bergeijk et al., 2020). Among these compounds, the surugamide A, indigoidine Antimycin and Candicidin SF2768 were found to have antifungal activities (Xu et al., 2017; Santos-Beneit et al., 2022), indicating the potential mechanism of the inhibitory effect of St-220 against F. oxysporum.
Chitin, the most important component of fungal cell wall, is the preliminary target that biocontrol agents aim at. Streptomyces strains produce chitinases to break through the fungal cell wall. For instance, S. griseus secret ChiIS, which belongs to glycosyl hydrolase family 19, to inhibit the growth of Aspergillus nidulans, F. culmorum, and S. sclerotiorum (Hoster et al., 2005). Chitinase produced by Streptomyces sp. TK-VL_333 showed antifungal activity against F. oxysporum (Kavitha and Vijayalakshmi, 2011). The purified and crude chitinase from S. luridiscabiei U05 inhibited the growth of F. oxysporum and Alternaria alternata (Swiontek Brzezinska et al., 2019). In this study, multiple genes (chitinases, β-N-acetyl hexosaminidase, chitosanase) encoding enzymes involved in chitin degradation were found in the genome of St. albidoflavus St-220, indicating that the St-220 deployed several weapons targeting the fungal cell wall for its biocontrol effect.
The genome mining has also confirmed the potential mechanism of St. albidoflavus St-220 on promoting root growth of Sa. miltiorrhiza. In our greenhouse assay, St. albidoflavus St-220 promoted the growth of Sa. miltiorrhiza seedlings by increasing the plant biomass, especially the length, diameter, fresh and dry weight of the plant roots (Figure 4). To have a deep perspective on the root promoting mechanism, we tested and found that St. albidoflavus St-220 has the biological characteristics involved in plant promoting activity including phosphate solubilization, nitrogen fixation, IAA production and siderophore production. The actinobacterial strains, such as St. alfalfae strain XN-04, Streptomyces sp. NEAU-S7GS2, and St. chartreusis strain WZS021, have root growth-promoting activities on cotton, soyabean and sugarcane, respectively, and genes related to IAA, siderophores, phosphate solubilization were identified in their genomes (Wang et al., 2018b, Liu et al., 2019, Chen et al., 2021). In various studies, IAA has been shown to increase plant root size and distribution, as well as root hairs, resulting in higher nutrient uptake from the soil (Datta and Basu, 2000; Gumiere et al., 2014; Liao et al., 2017; Ulrich et al., 2021). A number of encoding genes directly involved in the synthesis of indoleacetic acid were found in the genome of St-220, including two genes encoding monoamine oxidase and four genes encoding aldehyde dehydrogenase. Many plant-associated actinomycetes are able to solubilize phosphorus into a form that can be used by plants by secreting phosphatases and phytases (Suárez-Moreno et al., 2019). In our present study, the genomic sequences of strain St-220 were found to encode acid and alkaline phosphatases, as well as phytases, suggesting a potential root stimulation of St-220. Additionally, 12 genes related to nitrogen fixation were also found in the genome of strain St-220. The nitrogen fixation plays a key role in the promoting activity of biocontrol agents on plant root growth and development (Dobbelaere et al., 2003). Our genome mining confirmed that the St. albidoflavus St-220 harbors predicted genes involved in pathways regarding IAA and siderophores production, phosphate solubilization and nitrogen fixation, which may play roles in simulating growth and development of plant roots. Therefore, we speculated that St. albidoflavus St-220 promotes plant growth in greenhouse condition through employing genes involved in a variety of metabolites synthesis pathways that may related to growth-promoting effects. Our results revealed the antifungal and growth-promoting activities of the St. albidoflavus St-220, and suggested the St-220 could be developed as a promising biological fertilizer.
Conclusion
Strain St-220 has inhibitory activity against F. oxysporum causing root rot disease of Sa. miltiorrhiza, and also promotes the growth of Sa. miltiorrhiza seedlings. The strain was identified as St. albidoflavus by its morphological and molecular characteristics. Our genome sequencing identified many pathways involved in synthesis of secondary metabolites with antifungal and growth-promoting activities, indicating the versatility of St-220 for being developed as a BCA against Fusarium wilt of Sa. miltiorrhiza.
Data availability statement
The original contributions presented in the study are publicly available. This data can be found at: https://www.ncbi.nlm.nih.gov/, JAMFMD010000000.
Author contributions
TW, LG, and LH conceived and designed the experiments. YD and TW performed the experiments and analyzed the data. YD, TW, JJ, YW, CL, KS, JS, BY and CK contributed reagents, materials, and analysis tools. All authors contributed to the article and approved the submitted version.
Funding
This research was funded by National Natural Science Foundation of China (82104341), the Key Project at Central Government Level: The Ability Establishment of Sustainable Use for Valuable Chinese Medicine Resources (2060302), and Scientific and technological innovation project of China Academy of Chinese Medical Sciences (CI2021A03905).
Conflict of interest
The authors declare that the research was conducted in the absence of any commercial or financial relationships that could be construed as a potential conflict of interest.
Publisher’s note
All claims expressed in this article are solely those of the authors and do not necessarily represent those of their affiliated organizations, or those of the publisher, the editors and the reviewers. Any product that may be evaluated in this article, or claim that may be made by its manufacturer, is not guaranteed or endorsed by the publisher.
Supplementary material
The Supplementary material for this article can be found online at: https://www.frontiersin.org/articles/10.3389/fpls.2022.976813/full#supplementary-material
References
Abbas, E., Osman, A., and Sitohy, M. (2020). Biochemical control of Alternaria tenuissima infecting post-harvest fig fruit by chickpea vicilin. J. Sci. Food Agric. 100, 2889–2897. doi: 10.1002/jsfa.10314
Abbasi, S., Safaie, N., Sadeghi, A., and Shamsbakhsh, M. (2019). Streptomyces strains induce resistance to Fusarium oxysporum f. sp. lycopersici race 3 in tomato through different molecular mechanisms. Front. Microbiol. 10:1505. doi: 10.3389/fmicb.2019.01505
Ågren, G. I., and Weih, M. (2020). Multi-dimensional plant element stoichiometry-looking beyond carbon, nitrogen, and phosphorus. Front. Plant Sci. 11:23. doi: 10.3389/fpls.2020.00023
Amano, S., Sakurai, T., Endo, K., Takano, H., Beppu, T., Furihata, K., et al. (2011). A cryptic antibiotic triggered by monensin. J. Antibiot. 64:703. doi: 10.1038/ja.2011.69
Amin, D. H., Sayed, H. A. E., Elissawy, A. M., EL-Ghwas, D. E., and Singab, A. N. B. (2021). Antimicrobial profile of actinomycin d analogs secreted by egyptian desert Streptomyces sp. DH7. Antibiotics 10:1264. doi: 10.3390/antibiotics10101264
Ashburner, M., Ball, C. A., Blake, J. A., Botstein, D., Butler, H., Cherry, J. M., et al. (2000). Gene ontology: tool for the unification of biology. Nat. Genet. 25, 25–29. doi: 10.1038/75556
Bankevich, A., Nurk, S., Antipov, D., Gurevich, A. A., Dvorkin, M., Kulikov, A. S., et al. (2012). SPAdes: a new genome assembly algorithm and its applications to single-cell sequencing. J. Comput. Biol. 19, 455–477. doi: 10.1089/cmb.2012.0021
Blin, K., Shaw, S., Kloosterman, A. M., Charlop-Powers, Z., Van Wezel, G. P., Medema, M. H., et al. (2021). antiSMASH 6.0: improving cluster detection and comparison capabilities. Nucleic Acids Res. 49, W29–W35. doi: 10.1093/nar/gkab335
Cao, L., Qiu, Z., You, J., Tan, H., and Zhou, S. (2005). Isolation and characterization of endophytic streptomycete antagonists of Fusarium wilt pathogen from surface-sterilized banana roots. FEMS Microbiol. Lett. 247, 147–152. doi: 10.1016/j.femsle.2005.05.006
Chen, J., Hu, L., Chen, N., Jia, R., Ma, Q., and Wang, Y. (2021). The biocontrol and plant growth-promoting properties of Streptomyces alfalfae XN-04 revealed by functional and genomic analysis. Front. Microbiol. 12:745766. doi: 10.3389/fmicb.2021.745766
Chen, Y., Wang, J., Yang, N., Wen, Z., Sun, X., Chai, Y., et al. (2018). Wheat microbiome bacteria can reduce virulence of a plant pathogenic fungus by altering histone acetylation. Nat. Commun. 9, 3429–3414. doi: 10.1038/s41467-018-05683-7
Chouyia, F. E., Romano, I., Fechtali, T., Fagnano, M., Fiorentino, N., Visconti, D., et al. (2020). P-solubilizing Streptomyces roseocinereus MS1b15 with multiple plant growth-promoting traits enhance barley development and regulate rhizosphere microbial population. Front. Plant Sci. 11:1137. doi: 10.3389/fpls.2020.01137
Datta, C., and Basu, P. S. (2000). Indole acetic acid production by a rhizobium species from root nodules of a leguminous shrub, Cajanus cajan. Microbiol. Res. 155, 123–127. doi: 10.1016/s0944-5013(00)80047-6
de Oliveira-Longatti, S. M., Marra, L. M., Lima Soares, B., Bomfeti, C. A., Da Silva, K., Avelar Ferreira, P. A., et al. (2014). Bacteria isolated from soils of the western amazon and from rehabilitated bauxite-mining areas have potential as plant growth promoters. World J. Microbiol. Biotechnol. 30, 1239–1250. doi: 10.1007/s11274-013-1547-2
Delcher, A. L., Bratke, K. A., Powers, E. C., and Salzberg, S. L. (2007). Identifying bacterial genes and endosymbiont DNA with glimmer. Bioinformatics 23, 673–679. doi: 10.1093/bioinformatics/btm009
Dobbelaere, S., Vanderleyden, J., and Okon, Y. (2003). Plant growth-promoting effects of diazotrophs in the rhizosphere. CRC Crit. Rev. Plant Sci. 22, 107–149. doi: 10.1080/713610853
El-Tarabily, K. A., Nassar, A. H., Hardy, G., and Sivasithamparam, K. (2009). Plant growth promotion and biological control of Pythium aphanidermatum, a pathogen of cucumber, by endophytic actinomycetes. J. Appl. Microbiol. 106, 13–26. doi: 10.1111/j.1365-2672.2008.03926.x
Finn, R. D., Bateman, A., Clements, J., Coggill, P., Eberhardt, R. Y., Eddy, S. R., et al. (2014). Pfam: the protein families database. Nucleic Acids Res. 42, D222–D230. doi: 10.1093/nar/gkt1223
Goudjal, Y., Toumatia, O., Sabaou, N., Barakate, M., Mathieu, F., and Zitouni, A. (2013). Endophytic actinomycetes from spontaneous plants of Algerian Sahara: indole-3-acetic acid production and tomato plants growth promoting activity. World J. Microbiol. 29, 1821–1829. doi: 10.1007/s11274-013-1344-y
Goudjal, Y., Zamoum, M., Sabaou, N., Mathieu, F., and Zitouni, A. (2016). Potential of endophytic Streptomyces spp. for biocontrol of Fusarium root rot disease and growth promotion of tomato seedlings. Biocontrol Sci. Tech. 26, 1691–1705. doi: 10.1080/09583157.2016.1234584
Gu, S. H., Wei, Z., Shao, Z. Y., Friman, V., Cao, K., Yang, T., et al. (2020). Competition for iron drives phytopathogen control by natural rhizosphere microbiomes. Nat. Microbiol. 5, 1002–1010. doi: 10.1038/s41564-020-0719-8
Gumiere, T., Ribeiro, C. M., Vasconcellos, R. L., and Cardoso, E. J. (2014). Indole-3-acetic acid producing root-associated bacteria on growth of Brazil pine (Araucaria angustifolia) and slash pine (Pinus elliottii). Antonie Van Leeuwenhoek 105, 663–669. doi: 10.1007/s10482-014-0120-9
Gupta, R., Singal, R., Shankar, A., Kuhad, R. C., and Saxena, R. K. (1994). A modified plate assay for screening phosphate solubilizing microorganisms. J. Appl. Microbiol. 40, 255–260. doi: 10.2323/JGAM.40.255
Handelsman, J., and Stabb, E. V. (1996). Biocontrol of soilborne plant pathogens. Plant Cell 8:1855. doi: 10.1105/tpc.8.10.1855
Hoster, F., Schmitz, J. E., and Daniel, R. (2005). Enrichment of chitinolytic microorganisms: isolation and characterization of a chitinase exhibiting antifungal activity against phytopathogenic fungi from a novel Streptomyces strain. Appl. Microbiol. Biotechnol. 66, 434–442. doi: 10.1007/s00253-004-1664-9
Hotta, K. (2021). Basic and applied research on multiple aminoglycoside antibiotic resistance of actinomycetes: an old-timer's recollection. J. Ind. Microbiol. Biotechnol. 48, 9–10. doi: 10.1093/jimb/kuab059
Jensen, L. J., Julien, P., Kuhn, M., Mering, C., Muller, J., Doerks, T., et al. (2007). eggNOG: automated construction and annotation of orthologous groups of genes. Nucleic Acids Res. 36, D250–D254. doi: 10.1093/nar/gkm796
Jiang, Z., Gao, W., and Huang, L. (2019). Tanshinones, critical pharmacological components in salvia miltiorrhiza. Front. Pharmacol. 10:202. doi: 10.3389/fphar.2019.00202
Jones, S. E., and Elliot, M. A. (2017). Streptomyces exploration: competition, volatile communication and new bacterial behaviours. Trends Microbiol. 25, 522–531. doi: 10.1016/j.tim.2017.02.001
Jose, P. A., Maharshi, A., and Jha, B. (2021). Actinobacteria in natural products research: progress and prospects. Microbiol. Res. 246:126708. doi: 10.1016/j.micres.2021.126708
Kanehisa, M., and Goto, S. (2006). KEGG: Kyoto encyclopedia of genes and genomes. Nucleic Acids Res. 34, D354–D357. doi: 10.1093/nar/gkj102
Kavitha, A., and Vijayalakshmi, M. (2011). Partial purification and antifungal profile of chitinase produced by Streptomyces tendae TK-VL_333. Ann. Microbiol. 61, 597–603. doi: 10.1007/s13213-010-0178-1
Lagesen, K., Hallin, P., Rødland, E. A., Stærfeldt, H.-H., Rognes, T., and Ussery, D. W. (2007). RNAmmer: consistent and rapid annotation of ribosomal RNA genes. Nucleic Acids Res. 35, 3100–3108. doi: 10.1093/nar/gkm160
Larkin, M. A., Blackshields, G., Brown, N. P., Chenna, R., McGettigan, P. A., McWilliam, H., et al. (2007). Clustal W and Clustal X version 2.0. Bioinformatics 23, 2947–2948. doi: 10.1093/bioinformatics/btm404
Li, R., Li, Y., Kristiansen, K., and Wang, J. (2008). SOAP: short oligonucleotide alignment program. Bioinformatics 24, 713–714. doi: 10.1093/bioinformatics/btn025
Li, Y., Jiang, S., Jiang, J., Gao, C., Qi, X., Zhang, L., et al. (2022). Synchronized efficacy and mechanism of alkaline fertilizer and biocontrol fungi for Fusarium oxysporum f. sp. cubense tropical race 4. J. Fungi 8:261. doi: 10.3390/jof8030261
Liao, X., Lovett, B., Fang, W., and St Leger, R. J. (2017). Metarhizium robertsii produces indole-3-acetic acid, which promotes root growth in Arabidopsis and enhances virulence to insects. Microbiology 163, 980–991. doi: 10.1099/mic.0.000494
Lim, H., Lee, E. H., Yoon, Y., Chua, B., and Son, A. (2016). Portable lysis apparatus for rapid single-step DNA extraction of Bacillus subtilis. J. Appl. Microbiol. 120, 379–387. doi: 10.1111/jam.13011
Liu, X., Dou, G., and Ma, Y. (2016). Potential of endophytes from medicinal plants for biocontrol and plant growth promotion. J. Gen. Plant Pathol. 82, 165–173. doi: 10.1007/s10327-016-0648-9
Liu, D., Yan, R., Fu, Y., Wang, X., Zhang, J., and Xiang, W. (2019). Antifungal, plant growth-promoting, and genomic properties of an endophytic actinobacterium Streptomyces sp. NEAU-S7GS2. Front. Microbiol. 10:2077. doi: 10.3389/fmicb.2019.02077
Lowe, T. M., and Eddy, S. R. (1997). tRNAscan-SE: A program for improved detection of transfer RNA genes in genomic sequence. Nucleic Acids Res. 25, 955–964. doi: 10.1093/nar/25.5.955
Lwin, K. M., Myint, M. M., Tar, T., and Aung, W. Z. M. (2012). Isolation of plant hormone (indole-3-acetic acid-IAA) producing rhizobacteria and study on their effects on maize seedling. Eng. J. 16, 137–144. doi: 10.4186/ej.2012.16.5.137
Mahasneh, A. A., Odat, J. D., Al-Joubori, B. M., and Saadoun, I. (2021). Phenotypic and molecular analysis of dominant occurring antibiotic active-producing Streptomyces soil flora in northern Jordan. Saudi J. Biol. Sci. 28, 4500–4510. doi: 10.1016/j.sjbs.2021.04.048
Myo, E. M., Ge, B., Ma, J., Cui, H., Liu, B., Shi, L., et al. (2019). Indole-3-acetic acid production by Streptomyces fradiae NKZ-259 and its formulation to enhance plant growth. BMC Microbiol. 19:155. doi: 10.1186/s12866-019-1528-1
Qi, D., Zou, L., Zhou, D., Chen, Y., Gao, Z., Feng, R., et al. (2019). Taxonomy and broad-spectrum antifungal activity of Streptomyces sp. SCA3-4 isolated from rhizosphere soil of Opuntia stricta. Front. Microbiol. 10:1390. doi: 10.3389/fmicb.2019.01390
Qi, D. F., Zou, L., Zhou, D., Zhang, M., Wei, Y., Zhang, L., et al. (2021). Identification and antifungal mechanism of a novel actinobacterium Streptomyces huiliensis sp. nov. against Fusarium oxysporum f. sp. cubense tropical race 4 of banana. Front. Microbiol. 12:3399. doi: 10.3389/fmicb.2021.722661
Raaijmakers, J. M., and Mazzola, M. (2016). Soil immune responses soil microbiomes may be harnessed for plant health. Science 352, 1392–1393. doi: 10.1126/science.aaf3252
Rawat, P., Das, S., Shankhdhar, D., and Shankhdhar, S. C. (2021). Phosphate-solubilizing microorganisms: mechanism and their role in phosphate solubilization and uptake. J. Soil Sci. Plant Nutr. 21, 49–68. doi: 10.1007/s42729-020-00342-7
Raza, W., Ling, N., Zhang, R., Huang, Q., Xu, Y., and Shen, Q. (2017). Success evaluation of the biological control of Fusarium wilts of cucumber, banana, and tomato since 2000 and future research strategies. Crit. Rev. Biotechnol. 37, 202–212. doi: 10.3109/07388551.2015.1130683
Richter, M., and Rosselló-Móra, R. (2009). Shifting the genomic gold standard for the prokaryotic species definition. Proc. Natl. Acad. Sci. U. S. A. 106, 19126–19131. doi: 10.1073/pnas.0906412106
Richter, M., Rosselló-Móra, R., Oliver Glöckner, F., and Peplies, J. (2016). JSpeciesWS: a web server for prokaryotic species circumscription based on pairwise genome comparison. Bioinformatics 32, 929–931. doi: 10.1093/bioinformatics/btv681
Rigden, D. J., and Fernández, X. M. (2022). The 2022 nucleic acids research database issue and the online molecular biology database collection. Nucleic Acids Res. 50, D1–D10. doi: 10.1093/nar/gkab1195
Roy, A. B. (1958). A new species of Azotobacter producing heavy slime and acid. Nature 182, 120–121. doi: 10.1038/182120a0
Rungin, S., Indananda, C., Suttiviriya, P., Kruasuwan, W., Jaemsaeng, R., and Thamchaipenet, A. (2012). Plant growth enhancing effects by a siderophore-producing endophytic streptomycete isolated from a Thai jasmine rice plant (Oryza sativa L. cv. KDML105). Antonie Van Leeuwenhoek 102, 463–472. doi: 10.1007/s10482-012-9778-z
Santos-Beneit, F., Ceniceros, A., Nikolaou, A., Salas, J. A., and Gutierrez-Merino, J. (2022). Identification of antimicrobial compounds in two Streptomyces sp. strains isolated from beehives. Front. Microbiol. 13:742168. doi: 10.3389/fmicb.2022.742168
Sarwar, A., Latif, Z., Zhang, S., Hao, J., and Bechthold, A. (2019). A potential biocontrol agent Streptomyces violaceusniger AC12AB for managing potato common scab. Front. Microbiol. 10:202. doi: 10.3389/fmicb.2019.00202
Schwyn, B., and Neilands, J. (1987). Universal chemical assay for the detection and determination of siderophores. Anal. Biochem. 160, 47–56. doi: 10.1016/0003-2697(87)90612-9
Shi, M., Huang, F., Deng, C., Wang, Y., and Kai, G. (2019). Bioactivities, biosynthesis and biotechnological production of phenolic acids in salvia miltiorrhiza. Crit. Rev. Food Sci. Nutr. 59, 953–964. doi: 10.1080/10408398.2018.1474170
Simpson, J. T., Wong, K., Jackman, S. D., Schein, J. E., Jones, S. J., and Birol, I. (2009). ABySS: a parallel assembler for short read sequence data. Genome Res. 19, 1117–1123. doi: 10.1101/gr.089532.108
Su, C. Y., Ming, Q. L., Qian, L. M., Rahman, K., Han, T., and Qin, L. P. (2015). Salvia miltiorrhiza: traditional medicinal uses, chemistry, and pharmacology. Chin. J. Nat. Med. 13, 163–182. doi: 10.1016/S1875-5364(15)30002-9
Suárez-Moreno, Z. R., Vinchira-Villarraga, D. M., Vergara-Morales, D. I., Castellanos, L., Ramos, F. A., Guarnaccia, C., et al. (2019). Plant-growth promotion and biocontrol properties of three Streptomyces spp. isolates to control bacterial rice pathogens. Front. Microbiol. 10:290. doi: 10.3389/fmicb.2019.00290
Sun, Z. B., Li, S. D., Ren, Q., Xu, J. L., Lu, X., and Sun, M. H. (2020). Biology and applications of Clonostachys rosea. J. Appl. Microbiol. 129, 486–495. doi: 10.1111/jam.14625
Swiontek Brzezinska, M., Jankiewicz, U., Kalwasińska, A., Świątczak, J., and Żero, K. (2019). Characterization of chitinase from Streptomyces luridiscabiei U05 and its antagonist potential against fungal plant pathogens. J. Phytopathol. 167, 404–412. doi: 10.1111/jph.12809
Tamreihao, K., Ningthoujam, D. S., Nimaichand, S., Singh, E. S., Reena, P., Singh, S. H., et al. (2016). Biocontrol and plant growth promoting activities of a Streptomyces corchorusii strain UCR3-16 and preparation of powder formulation for application as biofertilizer agents for rice plant. Microbiol. Res. 192, 260–270. doi: 10.1016/j.micres.2016.08.005
Tang, Y., and Bonner, J. (1948). The enzymatic inactivation of indole acetic acid; the physiology of the enzyme. Am. J. Bot. 35, 570–578. doi: 10.2307/2438053
Terra, L., Ratcliffe, N., Castro, H. C., Vicente, A. C., and Dyson, P. (2021). Biotechnological potential of Streptomyces siderophores as new antibiotics. Curr. Med. Chem. 28, 1407–1421. doi: 10.2174/0929867327666200510235512
Ulrich, K., Kube, M., Becker, R., Schneck, V., and Ulrich, A. (2021). Genomic analysis of the endophytic Stenotrophomonas strain 169 reveals features related to plant-growth promotion and stress tolerance. Front. Microbiol. 12:687463. doi: 10.3389/fmicb.2021.687463
van Bergeijk, D. A., Terlouw, B. R., Medema, M. H., and van Wezel, G. P. (2020). Ecology and genomics of Actinobacteria: new concepts for natural product discovery. Nat. Rev. Microbiol. 18, 546–558. doi: 10.1038/s41579-020-0379-y
Vijayabharathi, R., Sathya, A., and Gopalakrishnan, S. (2015). “Plant growth-promoting microbes from herbal vermicompost,” in Plant Growth-Promoting Rhizobacteria (PGPR) and Medicinal Plants. eds. D. Egamberdieva, S. Shrivastava, and A. Varma (Switzerland: Springer), 71–88.
Vurukonda, S. S. K. P., Giovanardi, D., and Stefani, E. (2018). Plant growth promoting and biocontrol activity of Streptomyces spp. as endophytes. Int. J. Mol. Sci. 19:952. doi: 10.3390/ijms19040952
Wang, T. L., Guan, W., Sun, K., Wang, S., Chi, X. L., and Guo, L. P. (2018a). Progress in researches on pathogens, epidemiology and integrated control of diseases on Salvia miltiorrhiza in China. Chin. J. Chin. Med. 43, 2402–2406. doi: 10.19540/j.cnki.cjcmm.20180329.001
Wang, Z., Solanki, M. K., Yu, Z. X., Anas, M., Dong, D., Xing, Y., et al. (2021). Genome characteristics reveal the biocontrol potential of actinobacteria isolated from sugarcane rhizosphere. Front. Microbiol. 12:797889. doi: 10.3389/fmicb.2021.797889
Wu, Y. R., Li, C. B., Wu, Y. H., Li, L., Li, B., Li, W. B., et al. (2021). Diversity and function of culturable actinobacteria in the root-associated of salvia miltiorrhiza Bunge. PeerJ 9:e11749. doi: 10.7717/peerj.11749
Wang, X., Chen, M. L., Yang, G., Li, X. M., Li, P. Y., and Chen, M. (2014). Effect of glomus versiforme and trichodema harzianum on growth and quality of salvia miltiorrhiza. J. Tradit. Chin. Med. 39, 1574–1578. doi: 10.4268/cjcmm20140906
Wang, Z., Solanki, M. K., Yu, Z. X., Yang, L. T., An, Q. L., Dong, D. F., et al. (2018b). Draft genome analysis offers insights into the mechanism by which Streptomyces chartreusis WZS021 increases drought tolerance in sugarcane. Front. Microbiol. 9:3262. doi: 10.3389/fmicb.2018.03262
Xu, F., Nazari, B., Moon, K., Bushin, L. B., and Seyedsayamdost, M. R. (2017). Discovery of a cryptic antifungal compound from Streptomyces albus J1074 using high-throughput elicitor screens. J. Am. Chem. Soc. 139, 9203–9212. doi: 10.1021/jacs.7b02716
Ye, P. S., Zeng, H. L., Jiang, H. Z., and Li, Q. F. (2003). Study on root rot disease of radix Salviae Miltiorrhizae and its control by microorganisms. Modern. TCM. 5, 63–65. doi: 10.3969/j.issn.1674-3849.2003.02.016
Yu, D., Xu, F., Valiente, J., Wang, S., and Zhan, J. (2013). An indigoidine biosynthetic gene cluster from Streptomyces chromofuscus ATCC 49982 contains an unusual IndB homologue. J. Ind. Microbiol. Biotechnol. 40, 159–168. doi: 10.1007/s10295-012-1207-9
Zhang, H., Yohe, T., Huang, L., Entwistle, S., Wu, P., Yang, Z., et al. (2018). dbCAN2: a meta server for automated carbohydrate-active enzyme annotation. Nucleic Acids Res. 46, W95–W101. doi: 10.1093/nar/gky418
Zhang, D., Gao, F., Jakovlić, I., Zou, H., Zhang, J., Li, W. X., et al. (2020). PhyloSuite: An integrated and scalable desktop platform for streamlined molecular sequence data management and evolutionary phylogenetics studies. Mol. Ecol. Resour. 20, 348–355. doi: 10.1111/1755-0998.13096
Keywords: biocontrol agents, plant growth-promotion, Streptomyces albidoflavus, Salvia miltiorrhiza, root rot disease
Citation: Du Y, Wang T, Jiang J, Wang Y, Lv C, Sun K, Sun J, Yan B, Kang C, Guo L and Huang L (2022) Biological control and plant growth promotion properties of Streptomyces albidoflavus St-220 isolated from Salvia miltiorrhiza rhizosphere. Front. Plant Sci. 13:976813. doi: 10.3389/fpls.2022.976813
Edited by:
Minmin Li, Institute of Food Science and Technology (CAAS), ChinaReviewed by:
Ran Wang, Beijing Academy of Agriculture and Forestry Sciences, ChinaJie-Yin Chen, Institute of Plant Protection (CAAS), China
Copyright © 2022 Du, Wang, Jiang, Wang, Lv, Sun, Sun, Yan, Kang, Guo and Huang. This is an open-access article distributed under the terms of the Creative Commons Attribution License (CC BY). The use, distribution or reproduction in other forums is permitted, provided the original author(s) and the copyright owner(s) are credited and that the original publication in this journal is cited, in accordance with accepted academic practice. No use, distribution or reproduction is permitted which does not comply with these terms.
*Correspondence: Tielin Wang, d3RsODJAMTYzLmNvbQ==; Lanping Guo, Z2xwMDFAMTI2LmNvbQ==; Luqi Huang, aHVhbmdsdXFpMDFAMTI2LmNvbQ==
†These authors have contributed equally to this work and share first authorship